- 1Department of Natural Resources and Environmental Management, University of Haifa, Haifa, Israel
- 2Morris Kahn Marine Research Station, Department of Marine Biology, Leon H. Charney School of Marine Sciences, University of Haifa, Haifa, Israel
- 3Department of Marine Biology, Leon H. Charney School of Marine Sciences, University of Haifa, Haifa, Israel
- 4GEOMAR Helmholtz Centre for Ocean Research Kiel, Kiel, Germany
- 5Israel Oceanographic and Limnological Research, Haifa, Israel
The aim of this brief research report was to define the consequential shifts in biomass and trophic structure of an ecosystem surrounding an offshore monoculture fish farm in Israel. It attempts to clarify the impact of the industry expansion and input of artificial fish pellets on functional group biomasses. We account for the direct addition of artificial food pellets, the metabolic wastes from the caged fish in a mass-balance food web model (Ecopath), as well as the temporal expansion of the farm’s production capacity to 21,000 t over a 30-year period (Ecosim). In the static mass-balance model of the food web, the addition of the fish cages at its current production size of 1000 t does not adversely affect the system, and trophic energy transfer is still dependent on primary production versus the detrital pathway. The model suggests a semi-stable ecosystem with low trophic interactions. With time, the increase in fish farming at the site is characterized by an increase of all functional group biomasses at the site over the 30-year period. The accumulation in detritus most notably correlates to greater biomass for all benthic functional niches and their secondary consumers. It is, therefore, apt to develop an indicator species list to indicate negative site disturbance. In summary, the sediment column condition will be the main indicator for ecosystem stability, as well as the increase in apex predators that are attracted to the site from the accumulation of discards at the cage bottom.
Introduction
Aquaculture is a growing industry throughout many areas of the world (Duarte et al., 2009), with a reported 54.1 million tonnes (t) of finfish farmed in marine and coastal waters in 2016 (FAO, 2018). The benefits of providing added food security through fish proteins also assists to reduce the pressure of over-harvesting wild fisheries (Tidwell and Allan, 2001), however, these correspond with increasing fish aggregations in the local vicinity from the artificial structure (Dempster et al., 2002) and artificial pellets wastage from unconsumed food (Sanchez-Jerez et al., 2011). In Israel, 100 km2 of marine space has been allocated for potential offshore development (Ayalon et al., 2015). Currently, one open-sea farm (74 m depth) is operating since 2017, farming approx. 1,000 t of gilthead sea bream (Sparus aurata), and the farm is projected to produce 21,000 t over 14 km2 of space in the next two decades.
The eastern Mediterranean Sea is a region afflicted by increasing sea temperature and the greatest influx of invasive marine species in the world, termed Lessepsian migrants (Por, 1978; Edelist et al., 2013). The system is unusual in that the deep waters exhibit a high nitrate to phosphate (N:P) ratio (28:1) and are phosphorus-limited year-round (Krom et al., 1991). There have been innumerable studies investigating the effects of fish farming on the surrounding environment, but few which have used mass-balance modeling to elucidate the effect. In a heavily impacted marine region such as the Levantine Basin, ecosystem-scale models are beneficial when combined with niche micro-research objectives, and Ecopath with Ecosim (hereafter abbreviated to EwE, Christensen et al., 2005) models provide a common infrastructure to accomplish this and apply it to sustainable management and policy.
Ecopath with Ecosim is a common tool for analyzing trophic flows within a given ecosystem (White and San Diego-McGlone, 1999). The simple user friendly interface and other prominent features are well documented (Christensen, 1995) as providing a bridge from science to policy and be informative for both marine managers and ecologists. Previously, Corrales et al. (2017a, b) produced an EwE model of the Israeli EEZ of 40 functional groups, with only phytoplankton and detritus groups considered at the lower trophic levels (TL) and group parameters adjusted from previous studies. A separate publication of an Ecosim model from the same area modeled the ecosystem under the impacts of increasing sea surface temperature (SST), influx of invasive species, and with respect to recent changes (2018) in Israeli fisheries policies (Corrales et al., 2018). It determined that the empty functional niches brought about from increased SST would be filled by invasive fish species, and a decline in biomass would characterize the new system under the pressure from climate change, which would influence fisheries catch. The system’s decline in biomass was most affected by the SST increase, regardless of policy shifts in fisheries. Both models focused on the impact of alien species and/or climate change on the Levantine Basin, and did not look at the specific pressures from the mariculture industry. Similar models exist in the Mediterranean Sea (López et al., 2008; Bayle-Sempere et al., 2013) but are nearer to shore and are culturing fish in more productive settings.
This study focuses on the present-day trophic flow and community structure of an ecosystem surrounding an offshore fish farm. It is the first attempt to model the impacts from fish culture in an ultra-oligotrophic marine setting using EwE, inclusive of the microbial loop and interactions, and models the impact from increasing production 21-fold (i.e., loading of artificial pellets). The impacts to the organisms, which directly consume dissolved and particulate effluent, are specifically emphasized (i.e., primary producers, benthos and pelagic nekton). We addressed identifying in trophic structure, defined which impacts were greatest to the marine system, and how these sit within a management context for Israel, considering results from previous local studies. The trophic interrelationships and energy shifts caused by expanding the fish farm and inputting more nutrients were clarified, in order to visualize their impact on the system’s TL in terms of biomass.
Methods
Ecopath Model of Ashdod
The energy fluxes and trophic structure were modeled as a “snapshot” using EwE software, a static mass-balance model for a chosen period. The algorithm assumes that the ecosystem is balanced (i.e., production is equal to consumption; Polovina, 1984) through the equation:
where for an i group, Pi is production, Bi is biomass (t km2) in tonnes wet weight, EE is ecotrophic efficiency, Qi is the predator consumption, BAi is the biomass accumulation rate for I, and Ei is the net migration rate of the group. The diets of functional groups were incorporated through the predator-prey matrix as a fractional form of the predator’s average diet composition (Supplementary Table A). Data on fisheries landing was also included in the model (t km–2; Supplementary Table B).
Input Parameters for Ecopath Model
The model is composed of 34 functional groups (FGs; guild of organisms occupying a functional niche) and includes 2 primary producers, 10 groups of invertebrates, squids, and plankton consumers, 15 groups of fishes (teleostei and demersal elasmobranchii), 1 group of reared fish (S. aurata), 1 group of sea turtles, 1 group of bacteria, 1 group of dolphins, and 3 groups of detritus (detritus, pellets, and discards).
Detailed FG names and all data sources may be found in the Supplementary Table C. Reared gilthead seabream biomass was calculated from the harvested biomass from the first year of operations (1000 t; GiliOcean Ltd., personal communication) and fit to the whole model area (50 km2) since the study concerns impact to the wild fisheries outside the cage system. The model area was chosen because an initial study from 2008 to 2011 of the fish farms showed no impact one km from the cage site (IOLR report H47/2007), and the maximum size of the fish cages will not exceed 14 km2. The P/B and Q/B estimates for farmed sea bream were taken from Bayle-Sempere et al. (2013). For the additional detritus groups, artificial food pellet input was estimated assuming 1000 t harvested biomass within 1 year (fish were fed 1–2% of stocked biomass per day, dependent on season).
As the artificial food pellets do not consume living biomass (Bayle-Sempere et al., 2013), it was automatically assigned TL 1 in the Ecopath model and considered detritus import as it is not generated within the system. The Bacteria functional group was included as bacterial abundance is higher than normal for the basin (i.e., >0.5 t km–2 in EwE); in some cases, the microbial loop “replaces” the insufficient biomass of primary producers in atypical, oligotrophic systems (Robarts et al., 1996; Plagányi, 2007). Reared fish and the artificial food pellets were restricted to 33% of the entire habitat area. The sharks around cages and large pelagic fish were assumed to have similar immigration/emigration rates (t km–2). Pellet wastage (1% lost to the water column, GiliOcean Ltd., pers. comm.) was assumed to be entirely consumed on the way down, as visually estimated and confirmed through local studies (Pitta et al., 2009; Kroeger et al., 2019). Fecal loading from the caged fish biomass was automatically configured into the model’s algorithm, so it was unnecessary to include this detritus group. Landings and discards information was taken from Corrales et al. (2018).
Ecopath Model Balancing and Analysis
The model was balanced primarily following Heymans et al. (2016). The preliminary criteria for balancing the model was determined using EE < 1, with a slight modification in the diet composition for Detritus groups, Turtles and Cages (fish) (± 3% maximum, Supplementary Table D; Coll et al., 2006). Since all values for non-fish functional groups were derived from Corrales et al. (2017a) or estimated via EE, the estimates of P/B, Q/B, and P/Q are higher than the Microbenthos. All respiration to assimilation, and production to respiration ratios were <1, while the respiration/biomass (R/B) ratio was higher for motile species than sessile.
The trophic flow diagram and a series of flow indices were estimated to determine the adequacy of the model’s inputs, and trophic representation of the marine ecosystem. The connectance schematic is a classic snapshot of the TL and their flows respective to each other. Flows, ecological indicators and calculated statistical estimates were considered to determine the impact of artificial food pellets and analyzed in comparison to other regional Ecopath models concerning aquaculture in the Mediterranean (assuming similar hydrographic conditions, functional niche species, and marine ecosystem characteristics; Odum, 1971; Coll et al., 2006; Bayle-Sempere et al., 2013; Forrestal et al., 2012; Piroddi et al., 2017).
Ecosim Model Parameterization
Ecosim is a series of differential equations that estimate shifts in functional group biomass over time (Christensen and Walters, 2004). Ecosim uses the Ecopath model as its initial conditions and then projects the system forward (with the potential to modify fisheries, environmental forcing etc., as desired). In this case, the Ecosim model was run over a 30-year period from 2017 to 2047, with the artificial Pellets group increasing, step-wise, up to 21× the number of pellets through the import of a time series for the caged biomass (assuming an increase from 1000 t stocking biomass to 21,000 t maximum allowable production for year 2047). The stepped increase was set to 2,000 t every 2 years from the baseline 1,000 t production; this increase was chosen to mimic the allotment set by the Ministry of Environmental Protection (2015). The base proportion of free nutrients was set at the lowest possible setting (0.3) to trigger low nutrient effects in the model, which increases competition among producer groups. In the Ecosim parameters, a nutrient forcing function was added to force nutrients to increase alongside the increase in Pellets and Cages biomass; the nutrient concentration affects producer groups through the assumption of uptake as dictated by Michaelis–Menten (Christensen et al., 2008). The vulnerability parameter was reduced for primary producer groups and artificial pellets, as their predation increases with the increase in feeding and nutrient effluent. The vulnerabilities were increased for turtles and large pelagics, due to commercial fisheries and bycatch, which are also assumed to increase with greater farm production. The results of the dynamic temporal increase in cage biomass through Ecosim as indicated in Table 2 to see the impact of artificial feeding and metabolic waste on the ecosystem against other regional model outputs.
Results
Trophic Structure and Network Analysis
The flow diagram was typical of a 5-tier trophic outline, with primary producers and detritus groups populating TL 1, being succeeded by Demersal and Benthopelagic FGs (TL 2), and with mesopelagic and pelagic apex consumers (TLs 3–5; Figure 1). There are three primary energy pathways: (1) originating from phytoplankton to Micro/Mesoplankton species (2) originating from detrital loading to TL 2 benthic and demersal-related species (3) originating from pellets to higher TL FGs (medium to large pelagic fish, who eat the artificial pellets “wasted” and not consumed in the cages.
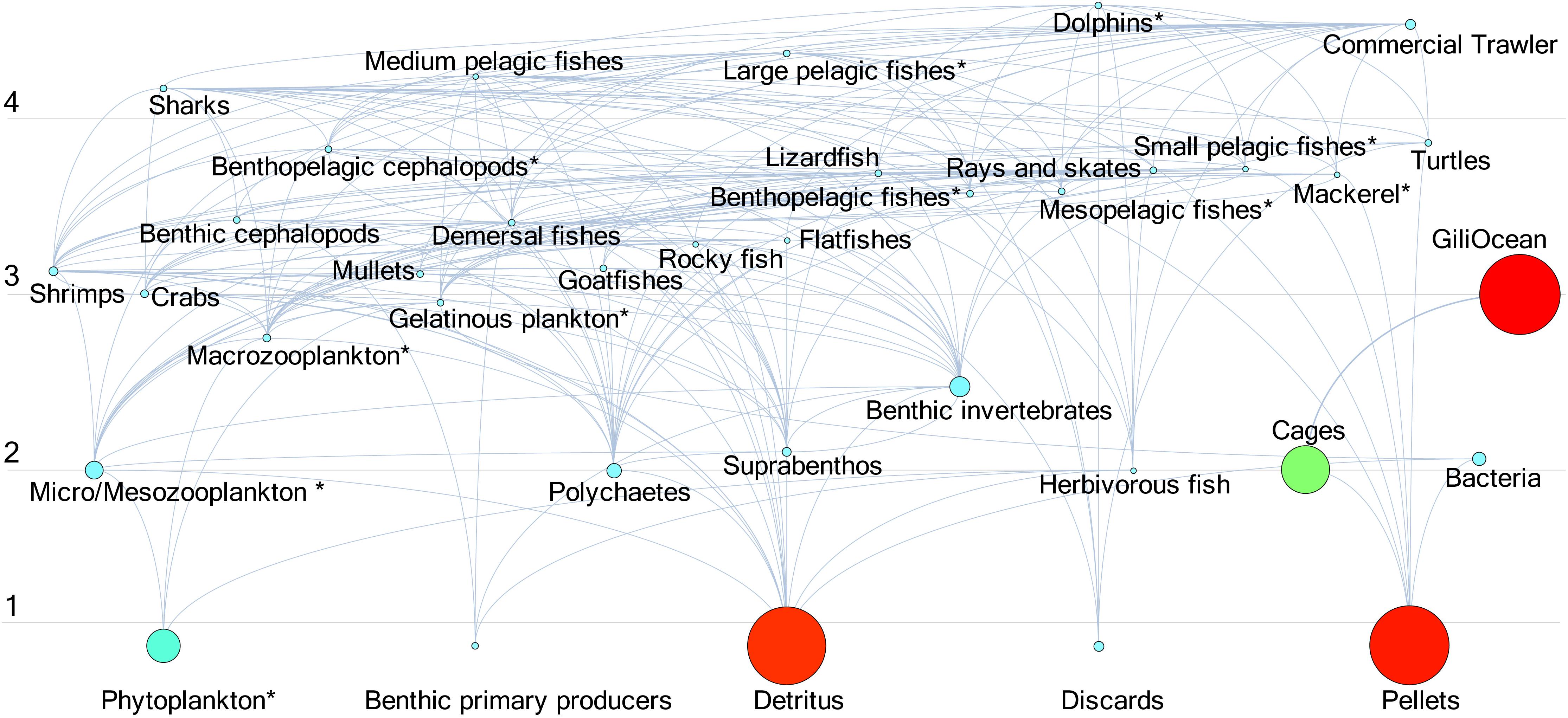
Figure 1. Flow diagram of 34 functional group biomasses and fisheries fleets (GiliOcean, Commercial Trawler).
The detrital groups (Detritus, Discards, and Pellets) had much lower reported EE values (0.334, 0.102, and 0.319, respectively). The Phytoplankton, Benthic primary producers, and Bacteria had EE’s of 0.306, 0.462, and 0.075, respectively. Mid-range EE values for Sharks, Turtles, Dolphins were typical of a higher TL status. FGs “cages” had a very low EE, as they fed almost exclusively on artificial pellets or discards, respectively. Production/Consumption (P/Q; Table 1) ranged from 0.01 (Dolphins) to 0.36 (Cages), with P/Q decreasing with higher TL. Respiration on assimilation (R/A) ranged from 0.587 (Micro/Mesozooplankton) to 0.99 (Dolphins), with the highest values correlating with TLs 3 and 4. It should be noted the farmed fish have additional protection by netting.
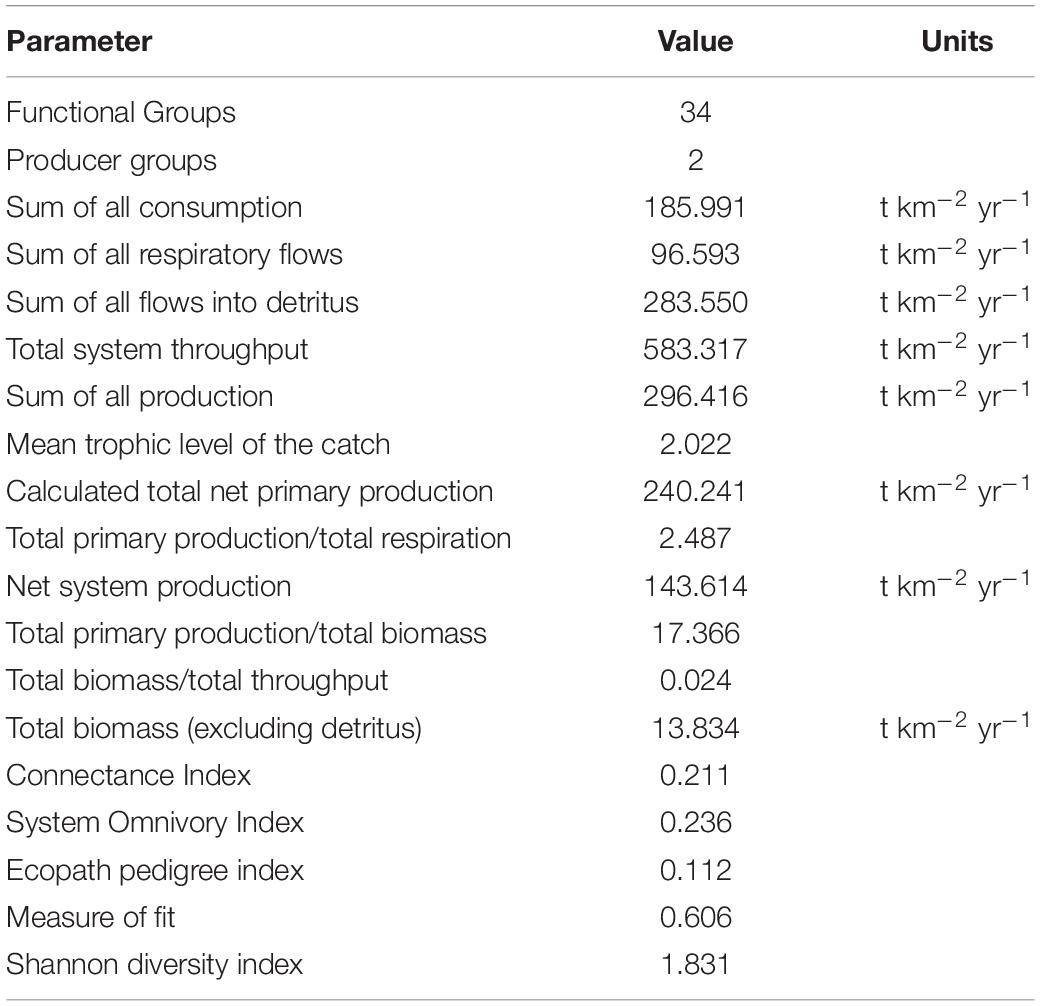
Table 1. Statistics, ecological indicators (Odum, 1971) and flows of the Ecopath model.
Transfer efficiency (TE) from producer groups to TL 4 was the highest, likely due to the direct consumption of artificial pellets by pelagic FGs located outside the cages in the upper TLs and increased abundance of prey for apex predators who aren’t detritivores. The mean TE from producers to TL 2–5 was 18.7%, and from detritus to these FG was 18.8% (overall 21.7%). This is slightly higher when compared to the mean TE of 19% in the Israeli EwE of Corrales et al. (2017a) and suggests the cages and pellets addition to the system does not adversely impact the system. The contribution of energy flows by top predators was low, indicating a slower transfer and distribution, and that much of the energy is maintained in the bottom TLs and detritus groups.
The ratios of total consumption and respiration to total system throughput (TST) are indicative of lower energy usage around the fish farm (Table 1). However, total production to TST was much higher, indicating greater efficiency of the farming operations itself. Total primary production (TPP) to total respiration is much higher in comparison to other regional studies, and when aligned with the total biomass to TST ratio of 0.022, reflects the lower accumulation of heterotrophic biomass in this study and the Levantine Basin. The TPP/total biomass is a respectable 17.366, indicative of a stable system as per Odum’s principles (Odum’s 1969).
The keystone species of the ecosystem are: Sharks > Benthic invertebrates > Benthic cephalopods > Phytoplankton > Micro/Mesoplankton. These sentinel species’ biomass are crucial to ecosystem functionality. In addition, all fish FG TLs were in agreement (within 0.5 measure) of TL estimates that are reported in FishBase.org (Froese and Pauly, 2012). Both the flow diagram and statistical output indicates that the upper and TL groups dictate the ecosystem structure, with a clear trophic cascade dynamic.
Most planktonic groups negatively impacted on their conspecifics, indicating a competitive landscape. Negative impacts are also displayed for predators on their known prey groups (Figure 2). Other notable impacts and evidence for competition are apparent when reviewing (1) Suprabenthos on Polychaetes, (2) Demersal fishes in competition with Flatfishes and Mullets, (3) Commercial trawlers on Sharks, and Dolphins, (4) and direct fishing of Large pelagics and Rocky fish, Goatfishes, and Benthopelagic cephalopods from commercial trawlers as expected. There was no observed impact of cages or pellets on the other groups. Large pelagic fish impacted/competed with Medium pelagic fish, whilst Detritus was mainly beneficial to all predator groups, especially Suprabenthos and Polychaetes.
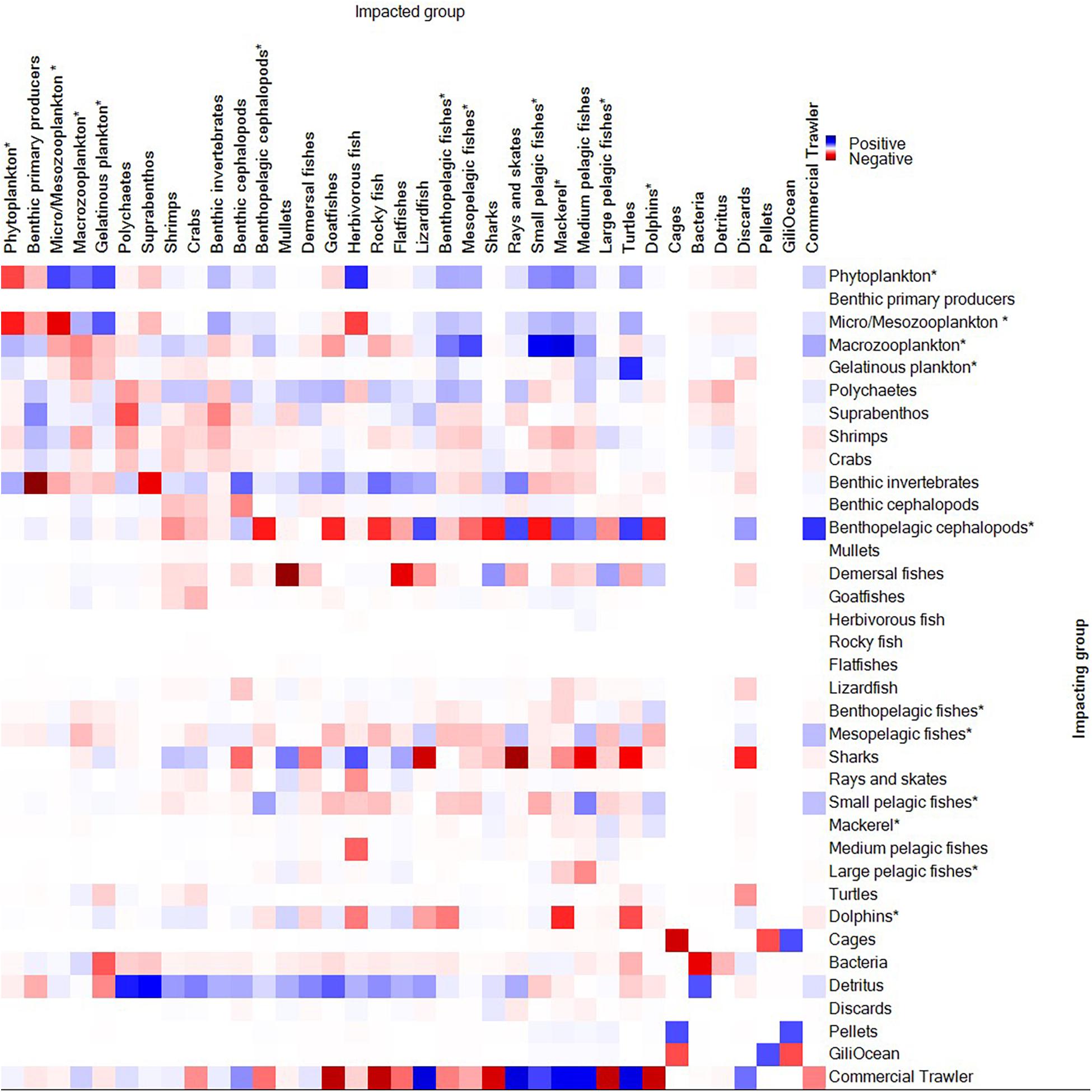
Figure 2. Output of Mixed Trophic Impact analysis of selected functional groups (impacting vs. impacted). Positive impacts are indicated as blue, and negative impacts are indicated by red shades.
Ecosim – Temporal Expansion of Ashdod Fish Farm
The linear increase to 21,000 t (over 50 km2 space and over 30 years) resulted in an increase in biomass of benthic invertebrates and some herbivorous/demersal fish groups; overall, the biomass of the system increases 1–10 times the baseline biomass of the system (Table 2). This table does not include FG Cages or Pellets, as the caged biomass is nearly entirely exported from the system at harvest, and Discards are treated as detritus; the Pellets are imported into the system and not a part of the natural ecosystem. The apex predators’ (Dolphins, Sharks, Turtles) populations double but remain within a normal range compared to central and western Mediterranean region. The lower TL groups (except Bacteria) remain stable throughout the 30 year period and no crash in biomass is exhibited. It appears that Mullets, Goatfishes, Lizardfish, and benthic groups are most affected by the increase of farming activity (due to their diet), with Discards, Detritus and Bacteria also increasing 7–9 times their baseline biomass input.
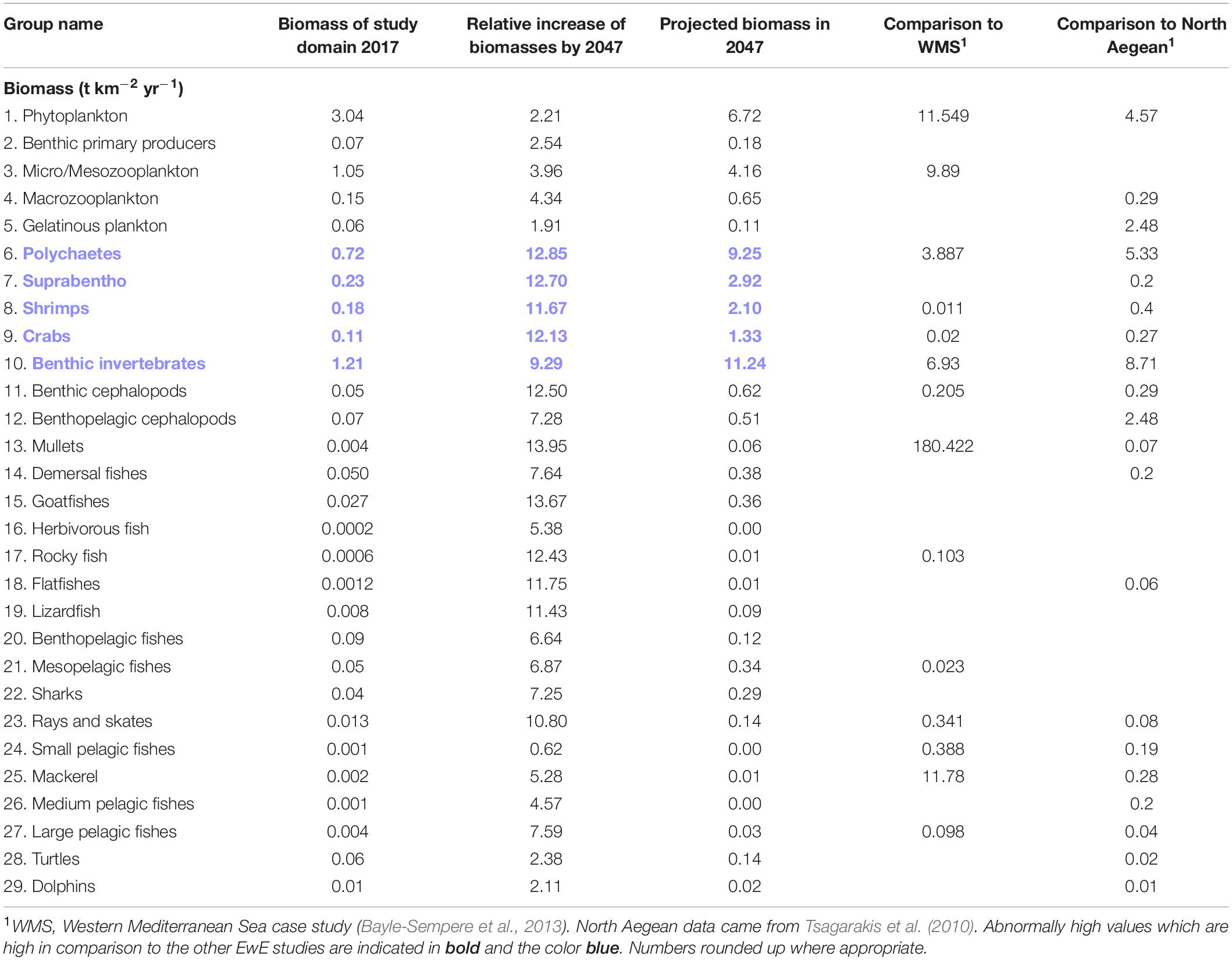
Table 2. Relative biomass increases of all functional groups over 30-year period (from 1000 t farm production to 21,000 t).
Discussion
The model addressed effects of increasing artificial fish pellet feeding into the system, over time, on the wild biomass surrounding the fish farm. The input parameters and resulting model’s fitness was acceptable according to measure of fit (0.611). However, the model did not address dispersion (i.e., spatial considerations) of the waste products from the farmed fish, nor was the model suitable to describe the biogeochemical implications of such a farm. It is a simple, initial description of the system structure currently, and over time. The model’s confidence was met through the PREBAL “rules of thumb” routine, abridging the system information efficiently and thoroughly, and describing the trophic structure and its temporal shift with increased fish production. This was the first EwE of a mariculture system in an ultraoligotrophic setting, and to include the microbial loop. This study also separated the microbial loop from the detritus/primary production pathways, as bacterial biomass is notably greater than in other marine regions and it is common to include this group where production is insufficient for consumption.
The inclusion of the microbial loop into the model was essential for clarifying the primary productivity and detrital pathways. The higher bacterial biomass, EE and position in the food chain indicates that it may be compensating for the very low biomass of primary producers in the basin. The input of fish pellets, although mostly consumed by the farmed fish, provides a food source/attractant for the wild populations of apex predators. Benthic groups are also impacted by the build-up of organic matter on the seawater-sediment interface (indicated by lower EE estimates). As the Ecopath model area currently has such a low biomass, this increase in organic matter may be consumed by the greater biomass of benthos. The total primary productivity in relation to total biomass was higher, indicating that the artificial pellets are not overwhelming the system as a primary producer at current levels.
The flow diagram visually complemented other Mediterranean trophic flows (the Levantine model; Piroddi et al., 2017), the TL estimates from Froese and Pauly (2012), and the typical guidelines of a food web (Odum, 1969). The addition of an artificial pellets group was considered a standalone energy source and crucial to defining specific impacts to the surrounding system. The low EE of Phytoplankton and Benthic primary producer’s biomass is contradictory to the EwE “norm”: typically, EE for Primary producers is nearly one (1) for oligotrophic systems in open seas. While this should be the case, the Eastern Mediterranean Sea system (EMS) system also displays strong seasonal changes in productivity and is abnormal in that the summer is nitrogen and phosphorus co-limited offshore, and switches to P-limitation during winter months (Krom et al., 1991). The low EE indicates a higher bacterial loading compared to other models in the Mediterranean, however, the producer groups typically exhibit lower to moderate ecotrophic efficiencies. Likewise, all the non-living groups (Detritus, Discards, Pellets) had lower EE, indicating moderate accumulation and a system less equipped to recycle nutrients.
Odum (1971) demonstrates that a TPP over Total Respiration (TPP/TR) value greater than 1 indicates a mature ecosystem. However, in conjunction with the model’s lower reported values of system omnivory and connectance indices (and higher-than-usual TPP/TR and TPP/Total Biomass) indicates a simpler, semi-stable yet mature ecosystem, with the caged, reared fish occupying TL 2. This is further supported by the connectance index (0.211) which was slightly higher than the fish farm study by Bayle-Sempere et al. (2013) but had a similar omnivory index (0.236). All three are indicators of ecosystem maturation.
The greater ratio of TPP to TB means the pellets do not replace or assume the role than primary production in the system. Most EwE models do not clarify the detrital TL 1 input so definitively. However, it was necessary to elaborate on this input to clarify a bottom-up disturbance in the trophic structure. The biomass and flows to detritus were mostly from TL 2, and the greater exports of this TL were related to the addition of fish cages. The mean TE of the Ecopath model (21.7%) was similar to the Ecopath model in Israel (TE 19%, Corrales et al. (2017a), and the high similarity in producer/detritus TE indicated that the addition of pellets as detritus did not overtake the role of the primary producers in the system. In addition, the Shannon Diversity index of 1.8 is favorable in comparison to the recommendation by Karakassis and Sanchez-Jerez (2012).
Other models in the Mediterranean Sea region are within 3 km of their respective shoreline, and thus experience greater nutrient availability and productivity ranges (Tsagarakis et al., 2010; Bayle-Sempere et al., 2013). Thus, these Ecopath models studying impacts from aquaculture were chosen for comparison to our model’s projected biomasses. A coastal fish farm in south-eastern Spain (Bayle-Sempere et al., 2013) had a far higher input of artificial pellets (32,000 t km–2 compared to our 63 t km–2), yet, the position further offshore of the Ashdod farm has a much lesser effect to the marine benthos, due to its far greater maximal depth. Indeed, the fact that the model’s indices are higher indicates the Israeli fish farm (at current production level) may not be significantly impacting the surroundings, and the empty functional niches in the Levant may be partly fulfilled by the import of nutrients to the system. However, as most of the capacity for growth in the model was expressed as overhead (but only by a small margin), this may indicate low to moderate flexibility in assimilating novel sources of production (i.e., stimuli) from the fish farm (Samson and Knopf, 2013).
The key findings from the Ecopath model are that the dominance of detritus over grazing pathways in the system, and that the detrital and lower TL have significant positive impacts on other groups in the system, suggesting “bottom-up” control of the food web. Odum (1969) suggested that as systems mature, they become more dependent on detritivores than herbivores. The relatively high system ascendency and overhead for the model suggests that this system has a fairly high level of development, and has strength in reserve (resilience).
When the increase in cage production is expressed over time, the Ecosim output suggests that the detritus groups act to attract and support greater abundance in the ecosystem around the fish farm (mostly benthic and demersal species). The highest increases in biomass were in Mullets and Goatfishes (14-fold), Polychaetes and Suprabenthos (approx. 13-fold) and other benthic and herbivorous group consumers (8–12-fold). Aligning with a Greek mesocosm focusing on the effects of nutrient waste from fish cages (Pitta et al., 2016), the model depicts rapid transfer of energy from lower to higher TL, with some effect on biomass of apex predators (a modeled increase equivalent of two adult sharks per km2). This rapid consumption of primary producers is typical of an oligotrophic environment. Thus, these cages aren’t a risk but an “oasis” of bioavailable nutrient as the production (and thus soluble and particulate effluent) expands. It appears the system flips from bottom up to top down control, as the ecosystem structure and food web linkages are forced and dependent on the artificial source of energy from the artificial food pellets input, and the increases in benthos.
For nearly all groups, the increased input of artificial pellets and resulting input of nutrients to the ultra-oligotrophic marine system only serve to bring their biomasses to within a normal range reported by other regional EwE studies not focused on fish farming (i.e., “baseline conditions”). It is noted that the only other regional EwE model for comparison was situated in lesser exposed sites, closer to shore. The pellets’ forced increase by 21 times resulted in 64 times biomass relative to the initial 63 t km–2 put in 2017. This, in conjunction with the low EE of the Ecopath model, suggests that there will be moderate to high accumulation/surplus of pellets accumulating over time. The groups which exceed the other studies are all constrained to the benthos niche (Polychaetes, Suprabenthos, Shrimps, Crabs, and Invertebrates), as a reflection of the increased particulate detritus to the farming site. The increase of discard biomass is moderate, alongside an increase in sharks near the fish farm cages. As fish production increases, the consequential effect on biomass for all pelagic groups is smaller, while benthic groups steadily increase.
This is the first attempt to clarify impacts of mariculture in a far offshore, ultraoligotrophic setting. The model demonstrated the effects from the current production levels and projected the increases to all TL biomasses over a 30 year period as a result of increased farming. The increase of soluble nutrient loading, expressed through a forcing function, increased primary production at the site, but the detrital and artificial pellets loading suggests that the surplus of accumulation may have negative implications on biodiversity as farm activity increases.
More research on the microbial loop is needed – the EMS experiences greater secondary production from of bacteria than the western Mediterranean (Turley et al., 2000). Their high affinity for nutrients, high surface area/biovolume ratio, and lower N:P ratios means that bacteria can sequester particulate N and P in competition with primary producers. As the EMS is impoverished with P, high grazing rates from both groups might mean the transfer of energy bypasses the producers to the upper TL (Pitta et al., 2016).
Most importantly, there was no observed crash in upper and lower TL biomasses (apex predators and primary producer groups). The primary and apex producers increase moderately, with no extreme biomass fluctuations. The latter groups’ increment indicates a need for greater R&D on automated discards and dead fish removal system at the base of the case, in order to limit apex predator attraction. This study’s temporal model could also be expanded to include realistic scenarios of SST increase, acidification, salinity, invasive species, and present fisheries (i.e., trawling).
Data Availability Statement
The datasets generated for this study are available on request to the corresponding author.
Author Contributions
LL, DT, and MG conceived of the presented idea. LL and MG developed the theory. LL performed the computations. MG verified the analytical methods. DT and OA supervised the findings of this work. All authors discussed the results and contributed to the final manuscript.
Funding
This research was supported by the Kahn Foundation, the Viktor Petri Foundation, and the Mediterranean Sea off the Coast of Israel (MERCI) funded this project in regards to partial assistance during the analysis of results, and presentation at conferences.
Conflict of Interest
The authors declare that the research was conducted in the absence of any commercial or financial relationships that could be construed as a potential conflict of interest.
Supplementary Material
The Supplementary Material for this article can be found online at: https://www.frontiersin.org/articles/10.3389/fmars.2020.00556/full#supplementary-material
References
Ayalon, O., Eshet, T., Trop, T., Liebes, I., Zerbib, M., Kerem, E., et al. (2015). Sustainable Mariculture in Israel. S. Neaman Publ. 142 pp. (Hebrew). Available online at: http://www.neaman.org.il/Neaman2011/Templates/showpage.asp?DBID=1&LNGID=1&TMID=580&FID=964&IID=1402 (accessed June 5, 2015).
Bayle-Sempere, J. T., Arreguín-Sánchez, F., Sanchez-Jerez, P., Salcido-Guevara, L. A., Fernandez-Jover, D., and Zetina-Rejón, M. J. (2013). Trophic structure and energy fluxes around a Mediterranean fish farm. Ecol. Model. 248, 135–147. doi: 10.1016/j.ecolmodel.2012.08.028
Christensen, V. (1995). Ecosystem maturity – towards quantification. Ecol. Model. 77, 3–32. doi: 10.1016/0304-3800(93)e0073-c
Christensen, V., Walters, C., Pauly, D., and Forrest, R. (2008). Ecopath with Ecosim Version 6 User Guide. Vancouver: University of British Columbia.
Christensen, V., and Walters, C. J. (2004). Ecopath with Ecosim: methods, capabilities and limitations. Ecol. Model. 172, 109–139. doi: 10.1016/j.ecolmodel.2003.09.003
Christensen, V., Walters, C. J., and Pauly, D. (2005). Ecopath with Ecosim: A User’s Guide. Vancouver: University of British Columbia.
Coll, M., Palomera, I., Tudela, S., and Sarda, F. (2006). Trophic flows, ecosystem structure and fishing impacts in the South Catalan Sea, Northwestern Mediterranean. J. Mar. Syst. 59, 63–96. doi: 10.1016/j.jmarsys.2005.09.001
Corrales, X., Coll, M., Ofir, E., Heymans, J. J., Steenbeek, J., Goren, M., et al. (2018). Future scenarios of marine resources and ecosystem conditions in the Eastern Mediterranean under the impacts of fishing, alien species and sea warming. Sci. Rep. 8:14284.
Corrales, X., Coll, M., Ofir, E., Piroddi, C., Goren, M., Edelist, D., et al. (2017a). Hindcasting the dynamics of an Eastern Mediterranean marine ecosystem under the impacts of multiple stressors. Mar. Ecol. Prog. Ser. 580, 17–36. doi: 10.3354/meps12271
Corrales, X., Ofir, E., Coll, M., Goren, M., Edelist, D., Heymans, J. J., et al. (2017b). Modeling the role and impact of alien species and fisheries on the Israeli marine continental shelf ecosystem. J. Mar. Syst. 170, 88–102. doi: 10.1016/j.jmarsys.2017.02.004
Dempster, T., Sanchez-Jerez, P., Bayle-Sempere, J. T., Giménez-Casalduero, F., and Valle, C. (2002). Attraction of wild fish to sea-cage fish farms in the south-western Mediterranean Sea: spatial and short-term temporal variability. Mar. Ecol. Prog. Ser. 242, 237–252. doi: 10.3354/meps242237
Duarte, C. M., Holmer, M., Olsen, Y., Soto, D., and Marbà, N. (2009). Will the oceans help feed humanity? Bioscience 59, 967–976. doi: 10.1525/bio.2009.59.11.8
Edelist, D., Rilov, G., Golani, D., Carlton, J. T., and Spanier, E. (2013). Restructuring the Sea: profound shifts in the world’s most invaded marine ecosystem. Divers. Distrib. 19, 69–77. doi: 10.1111/ddi.12002
Forrestal, F., Coll, M., Die, D., and Christensen, V. (2012). Ecosystem effects of bluefin tuna Thunnus thynnus thynnus aquaculture in the NW Mediterranean Sea. Mar. Ecol. Prog. Ser. 456, 215–231. doi: 10.3354/meps09700
Froese, R., and Pauly, D. (eds) (2012). FishBase. World Wide Web Electronic Publication. Available online at: www.fishbase.org (accessed September, 2018).
Heymans, J. J., Coll, M., Link, J. S., Mackinson, S., Steenbeek, J., Walters, C., et al. (2016). Best practice in Ecopath with Ecosim food-web models for ecosystem-based management. Ecol. Model. 331, 173–184. doi: 10.1016/j.ecolmodel.2015.12.007
Karakassis, I., and Sanchez-Jerez, P. S. (2012). “COMMITTEE ON AQUACULTURE (CAQ) environmental quality standards for mediterranean marine finfish farming based on the response of experts to a Delphi questionnaire (draft version 1),” in Proceedings of the Fifth Coordinating Meeting of the Working Groups (CMWG), Rome. Available online at: http://gfcmsitestorage.blob.core.windows.net/documents/web/CAQ/CMWG/5/GFCM_CAQ_2012_CMWG-5_Inf.10.pdf
Kroeger, L. A., Tsemel, A., Tchernov, D., and Ayalon, O. (2019). A theoretical approach concerning nutrient emissions to inform monitoring and management of mariculture farms. Mar. Sci. 2, 125–133. doi: 10.29199/arms.201031
Krom, M. D., Kress, N., Brenner, S., and Gordon, L. I. (1991). Phosphorus limitation of primary productivity in the eastern Mediterranean Sea. Limnol. Oceanogr. 36, 424–432. doi: 10.4319/lo.1991.36.3.0424
López, B. D., Bunke, M., and Shirai, J. A. B. (2008). Marine aquaculture off Sardinia Island (Italy): ecosystem effects evaluated through a trophic mass-balance model. Ecol. Model. 212, 292–303. doi: 10.1016/j.ecolmodel.2007.10.028
Ministry of Environmental Protection (2015). Monitoring the Impact Of The Fish Farms In The Port Of Ashdod On The Marine Environment. New Delhi: Ministry of Environmental Protection.
Odum, E. P. (1969). The strategy of ecosystem development. Science 164, 262–270. doi: 10.1126/science.164.3877.262
Piroddi, C., Coll, M., Liquete, C., Macias, D., Greer, K., Buszowski, J., et al. (2017). Historical changes of the Mediterranean Sea ecosystem: modelling the role and impact of primary productivity and fisheries changes over time. Sci. Rep. 7:44491.
Pitta, P., Nejstgaard, J. C., Tsagaraki, T. M., Zervoudaki, S., Egge, J. K., Frangoulis, C., et al. (2016). Confirming the “Rapid phosphorus transfer from microorganisms to mesozooplankton in the Eastern Mediterranean Sea” scenario through a mesocosm experiment. J. Plank Res. 38, 502–521. doi: 10.1093/plankt/fbw010
Pitta, P., Tsapakis, M., Apostolaki, E. T., Tsagaraki, T., and Holmer, M. (2009). Ghost nutrients’ from fish farms are transferred up the food web by phytoplankton grazers. Mar. Ecol. Prog. Ser. 374, 1–6. doi: 10.3354/meps07763
Plagányi, ÉE. (2007). Models For An Ecosystem Approach to Fisheries. FAO Fisheries Technical Paper No. 477. Rome: FAO.
Polovina, J. J. (1984). Model of a coral reef ecosystem I. The ECOPATH model and its application to French Frigate Shoals. Coral Reefs 3, 1–11. doi: 10.1007/bf00306135
Por, F. D. (1978). Lessepsian Migrations: The Influx Of Red Sea Biota Into The Mediterranean By Way Of The Suez Canal. Berlin: Springer Verlag.
Robarts, R. D., Zohary, T., Waiser, M. J., and Yacobi, Y. Z. (1996). Bacterial abundance, biomass, and production in relation to phytoplankton biomass in the Levantine Basin of the southeastern Mediterranean Sea. Mar. Ecol. Prog. Ser. 137, 273–281. doi: 10.3354/meps137273
Sanchez-Jerez, P., Fernandez, J. D., Uglem, I., Arechavala, L. P., Dempster, T., et al. (2011). Coastal Fish Farms as Fish Aggregation Devices (FADs). Boca Raton, FL: CRC Press.
Tidwell, J. H., and Allan, G. L. (2001). Fish As Food: Aquaculture’s Contribution. Heidelberg: EMBO.
Tsagarakis, K., Coll, M., Giannoulaki, M., Somarakis, S., and Papaconstantinou, C. (2010). Food-web traits of the North Aegean Sea ecosystem (Eastern Mediterranean) and comparison with other Mediterranean ecosystems. Estuar. Coast. Shelf Sci. 88, 233–248. doi: 10.1016/j.ecss.2010.04.007
Turley, C. M., Bianchi, M., Christaki, U., Conan, P., Harris, J. R. W., Psarra, S., et al. (2000). Relationship between primary producers and bacteria in an oligotrophic sea–the Mediterranean and biogeochemical implications. Mar. Ecol. Prog. Ser. 193, 11–18. doi: 10.3354/meps193011
Keywords: Ecopath mass-balance, oligotrophic, Mediterranean Sea – eastern, fish farm, Ecosim, biomass, modeling
Citation: Livne L, Grossowicz M, Tchernov D and Ayalon O (2020) Predicting Impacts of Offshore Monoculture Farm Expansion in Ultra-Oligotrophic Waters of the Levantine Basin. Front. Mar. Sci. 7:556. doi: 10.3389/fmars.2020.00556
Received: 30 January 2020; Accepted: 16 June 2020;
Published: 17 July 2020.
Edited by:
Heliana Teixeira, University of Aveiro, PortugalReviewed by:
Elizabeth A. Fulton, Commonwealth Scientific and Industrial Research Organisation (CSIRO), AustraliaJean-Claude Dauvin, Université de Caen Normandie, France
Copyright © 2020 Livne, Grossowicz, Tchernov and Ayalon. This is an open-access article distributed under the terms of the Creative Commons Attribution License (CC BY). The use, distribution or reproduction in other forums is permitted, provided the original author(s) and the copyright owner(s) are credited and that the original publication in this journal is cited, in accordance with accepted academic practice. No use, distribution or reproduction is permitted which does not comply with these terms.
*Correspondence: Leigh Livne, leigh.livne@gmail.com