- 1ISMAR-CNR Bologna, Bologna, Italy
- 2ISP-CNR Bologna, Bologna, Italy
- 3Lamont-Doherty Earth Observatory, Columbia University, New York, NY, United States
- 4Biology Department, Woods Hole Oceanographic Institution, Woods Hole, MA, United States
- 5Stazione Zoologica Anton Dohrn, Naples, Italy
Over 25 mounds have been identified in the Corsica Channel (Mediterranean Sea) through multibeam bathymetric mapping at depth of 400–430 m, with dimensions ranging from 70 to 330 m, achieving maximum heights of 25 m. Two mounds have been explored in detail using a remotely operated vehicle, revealing thick coral growth with a predominance of the branching scleractinian Madrepora oculata as main frame builder and subordinate Desmophyllum pertusum. The solitary scleractinians Desmophyllum dianthus and Javania cailleti add to the biodiversity here, which accounts for at least 50 macro- and megabenthic species. In consideration of the remarkable surface (ca. 5.3 km2) covered by living corals, their density and healthy appearance, and discontinuity with other major cold-water coral (CWC) occurrences in the Mediterranean Sea, we propose that this area represents a distinct CWC province in a sector already known for the presence of pre-modern CWC mounds. Noticeably, well-developed contourite drift systems occur in the Corsica Channel, lending support to their strict spatial link with coral establishment at depth. The ecosystemic value of the new CWC province calls for proper conservation measures to ensure their present Good Environmental Status.
Introduction
The concept of “Cold-Water Coral Province” is not rigorously codified in literature thus far, vaguely referring by convention to geographically discrete areas with important deep-sea scleractinian presence (e.g., Taviani et al., 2011; Hebbeln et al., 2014; Mohn et al., 2014; Wienberg et al., 2018). It is anyhow of practical use because (i) it identifies those situations where the area covered by cold (or deep)-water corals (CWCs) is often many tens square kilometers, far exceeding occasional to sparse CWC occurrences; and (ii) it helps to narrow the areal of seabed meritorious of special attention for management and protection purposes. To date, seven CWC provinces, structured by the colonial scleractinians Madrepora oculata and, subordinately, Desmophyllum pertusum (Lophelia pertusa; Addamo et al., 2016), have been identified in the Mediterranean Sea (Taviani et al., 2017, 2019; Chimienti et al., 2019). From east to west, the CWC provinces are Bari Canyon (e.g., Freiwald et al., 2009; Angeletti et al., 2014, 2020) and Santa Maria di Leuca (Taviani et al., 2005; Vertino et al., 2010) in the Adriatic and Ionian seas, South Malta (Schembri et al., 2007; Freiwald et al., 2009; Knittewis et al., 2019), South Sardinia Nora Canyon (Taviani et al., 2017) in the Tyrrhenian Sea, Cassidaigne (Fabri et al., 2014, 2019) and Cap de Creus canyons (Orejas et al., 2009) in the Gulf of Lion, and Alboran Sea (Lo Iacono et al., 2019, with references therein).
There is a consolidated consensus that CWCs establish under a bottom current regime of high intensity, with the Levantine Intermediate Water (LIW) mass as the main driver controlling their distribution in the Mediterranean Basin (Freiwald et al., 2009; Taviani et al., 2016, 2017; Orejas and Jiménez, 2019). Along the LIW path, the recent visual surveys have, indeed, led to the discovery of healthy CWC grounds in the Nora and Dorhn canyons in the Tyrrhenian Sea (Taviani et al., 2017, 2019).
Bathing the Tyrrhenian Italian margin, the LIW reaches the Ligurian Sea by flowing through the Corsica Channel. Besides some anecdotal evidence reporting live Madrepora oculata in this region (F. Serena, M. Borghi, M. Vacchi, personal communication), to date, the presence of CWCs in the Corsica Channel concerns Late Pleistocene buried coral mounds (Remia and Taviani, 2005; McCulloch et al., 2010). Substantial evidence of live CWC populations between Tyrrhenian and Ligurian sites (Tunesi et al., 2001; Fanelli et al., 2017) is, however, still missing.
Exploration of the southern Ligurian and northern Tyrrhenian seas in the frame of the Italian Marine Strategy Framework Directive in 2016 and 2017 documented in the present study proves prolonged CWC regional presence, although not necessarily continuous.
Areal extent and CWC density and richness warrant the identification of the new “Corsica Channel Cold-Water Coral Province” as described below.
The discovery of these healthy and lush coral mounds site bears to the distribution, organization, and connectivity of the Mediterranean CWC communities.
Setting
The area is located in the Corsica Channel between the Corsica Island to the west and the Tuscan Archipelago to the east. The Corsica Channel or (Trough) is a narrow (10–35 km wide), shallow (maximum depth of ca. 900 m in the south), and north-south oriented feature, separating the Corsica from the Tuscan shelves, and connecting the Tyrrhenian and Ligurian basins in the Western Mediterranean Sea (Figure 1). The narrowest and shallowest zone is off Capraia Island, where the Tuscan and Corsica shelves (at 200 m) are separated by the Corsica Sill that is 9.5 km wide. Modified Atlantic Water [(MAW) from 0 to 200 m], northward flowing, is the main water mass circulating in the shallow (i.e., shelf) Corsica Channel (e.g., Astraldi and Gasparini, 1992; Millot, 1999; Millot and Taupier-Letage, 2005). Secluded in the eastern side, the LIW flows northward at depths between ∼250 and 600–700 m (e.g., Artale and Gasparini, 1990; Vetrano et al., 2010; Toucanne et al., 2012). Alongslope water mass movements are responsible for long-lasting well-developed contourite drifts from the south (off Montecristo Island) to the north near the Gorgona Island, which extend almost continuously for more than 100 km (e.g., Cattaneo et al., 2017; Rebesco and Taviani, 2019, with references therein). These contourite depositional processes started in the middle Pliocene under the anticlockwise influence of the Coriolis force and as a response to the topographic setting (Stanley et al., 1980; Marani et al., 1993; Roveri, 2002). Morphology and size (maximum width of 10 km: Miramontes et al., 2016) of the contourite drifts change remarkably due to the differences in the basin morphology and depth (Roveri et al., 2002; Miramontes et al., 2016). Turbiditic systems characterize, on the contrary, the western side of the Corsica Trough (Bellaiche et al., 1994; Gervais et al., 2004; Calvès et al., 2013), among which the Golo Turbidite System is active at least since the Late Pleistocene (e.g., Deptuck et al., 2008; Sømme et al., 2011). Finally, the strong hydrodynamics marking this area is also responsible for sediment starvation and hardground formation along the slope (Remia et al., 2004).
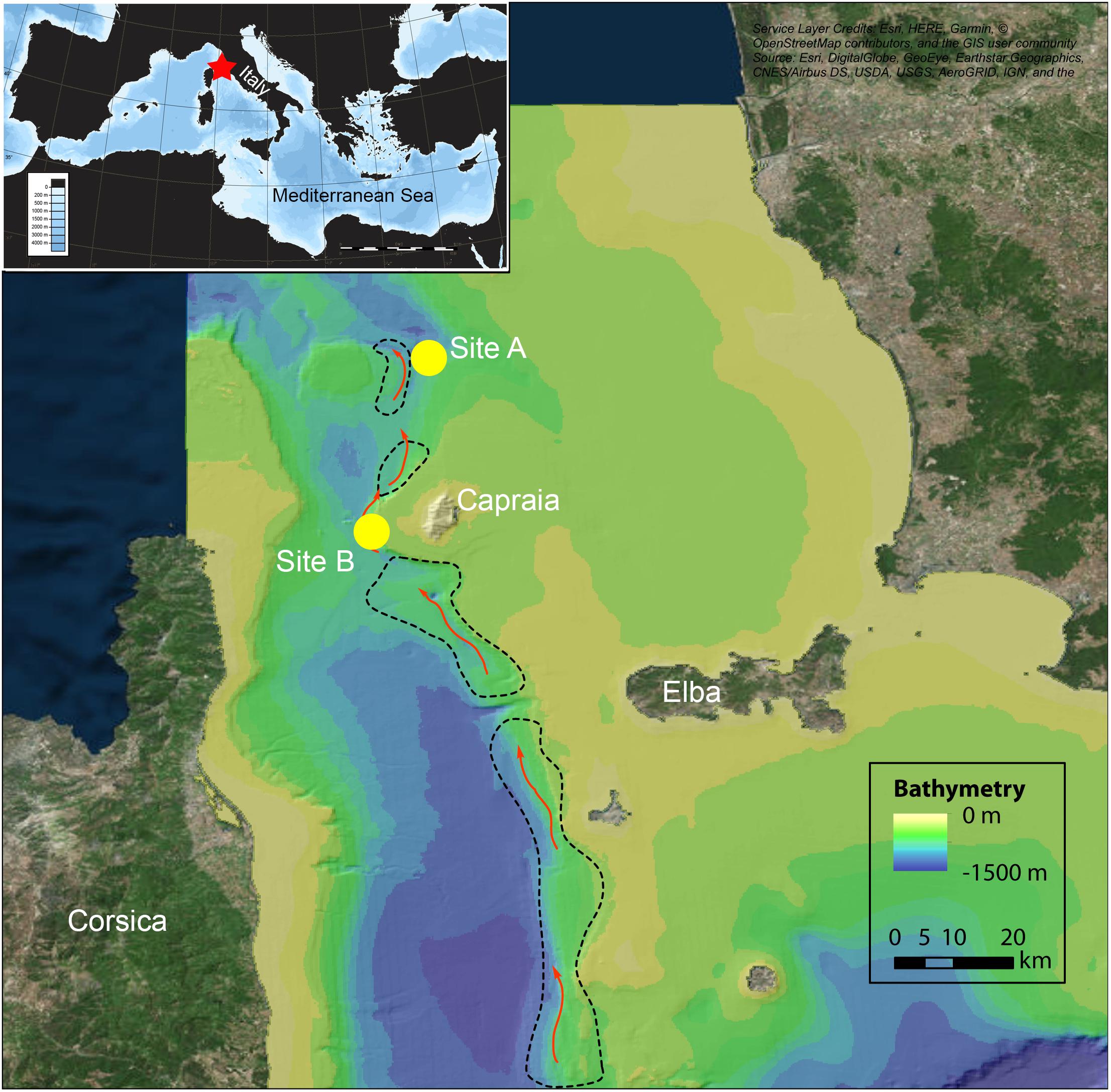
Figure 1. Location map of the Corsica Channel (red star in the inset Basemap data: ESRI ArcGIS) showing the narrowest area of the channel between Corsica Island and the Tuscan Archipelago. Bathymetry from http://www.emodnet-bathymetry.eu/. Dashed line indicates the main contouritic deposits (modified from Miramontes et al., 2016), and red arrows show the main path of the Levantine Intermediate Water. Yellow circles indicate the two explored sites.
Materials and Methods
The study area has been surveyed in the last 20 years by different technologies during cruises LM99 (December 1999–January 2000) and CORTI (December 2003–January 2004) onboard R/V Urania and during cruises Strategia Marina-Ligure/Tirreno (July–August 2016) and MSFD-IT-1-2017 (July 2017) onboard R/V Minerva Uno.
Swath bathymetry and backscatter data considered in this study have been acquired using a hull-mounted Reson Seabat 7160 Multibeam Echosounder with a nominal frequency of 44 kHz, swath coverage of ca. 4 × greater than water depth and 512 beams per second acquired with Reson PDS2000 software. All data were plotted in the Transverse Mercator–UTM 33N-WGS84 Coordinate System. Morphobathymetric map, with a cell size of 5 × 5 m2, was obtained using CARIS SIS and HIPS software (Figure 2). Seabottom sampling was performed with a large volume (60 l) modified Van Veen grab, epibenthic and heavy-chained dredges. Samples were stored at the ISMAR-CNR Repository in Bologna.
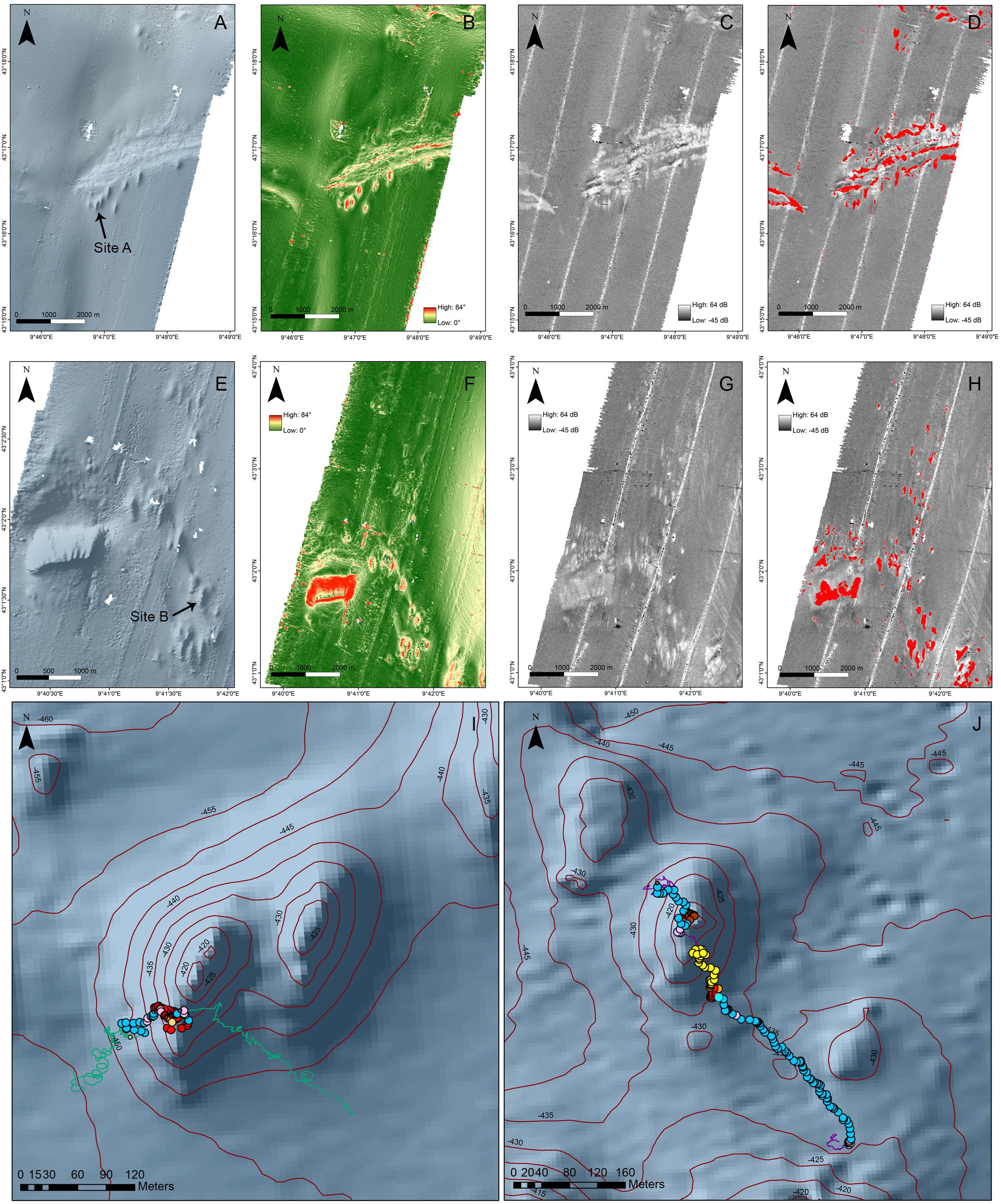
Figure 2. Site A (A–D) and Site B (E–H) morphobathymetrical and backscatter data. Panels (A,E) represent the morphobathymetric map of the two coral mound areas; (B,F) slope map of the study areas, calculated from the digital terrain model (DTM), clearly showing the difference between the side slope of the coral mounds reaching a maximum value of 39° and the regional surrounding slope about 1°–8°; (C,G) backscatter photomosaic of the surveyed area and (D,H) automatic selection of all features with backscatter intensity >15 dB. (I,J) Habitat mapping performed on Site A remotely operated vehicle (ROV) survey and Site B, respectively; blue dots represent muddy sediments, red dots show living Madrepora oculata, yellow dots indicate the presence of coral rubble, and pink dots refer to cold-water coral (CWC) occurrences (e.g., living M. oculata and Desmophyllum pertusum).
Seawater physical attributes of the studied area were obtained from 20 depth profiles (Figure 3) sourced from the World Ocean Atlas 2018 (WOA2018) database and were visualized using Ocean Data View software, version 5.3.0 (Schlitzer, 2020). Metadata on bottom sampling and oceanographic data are reported in Table 1.
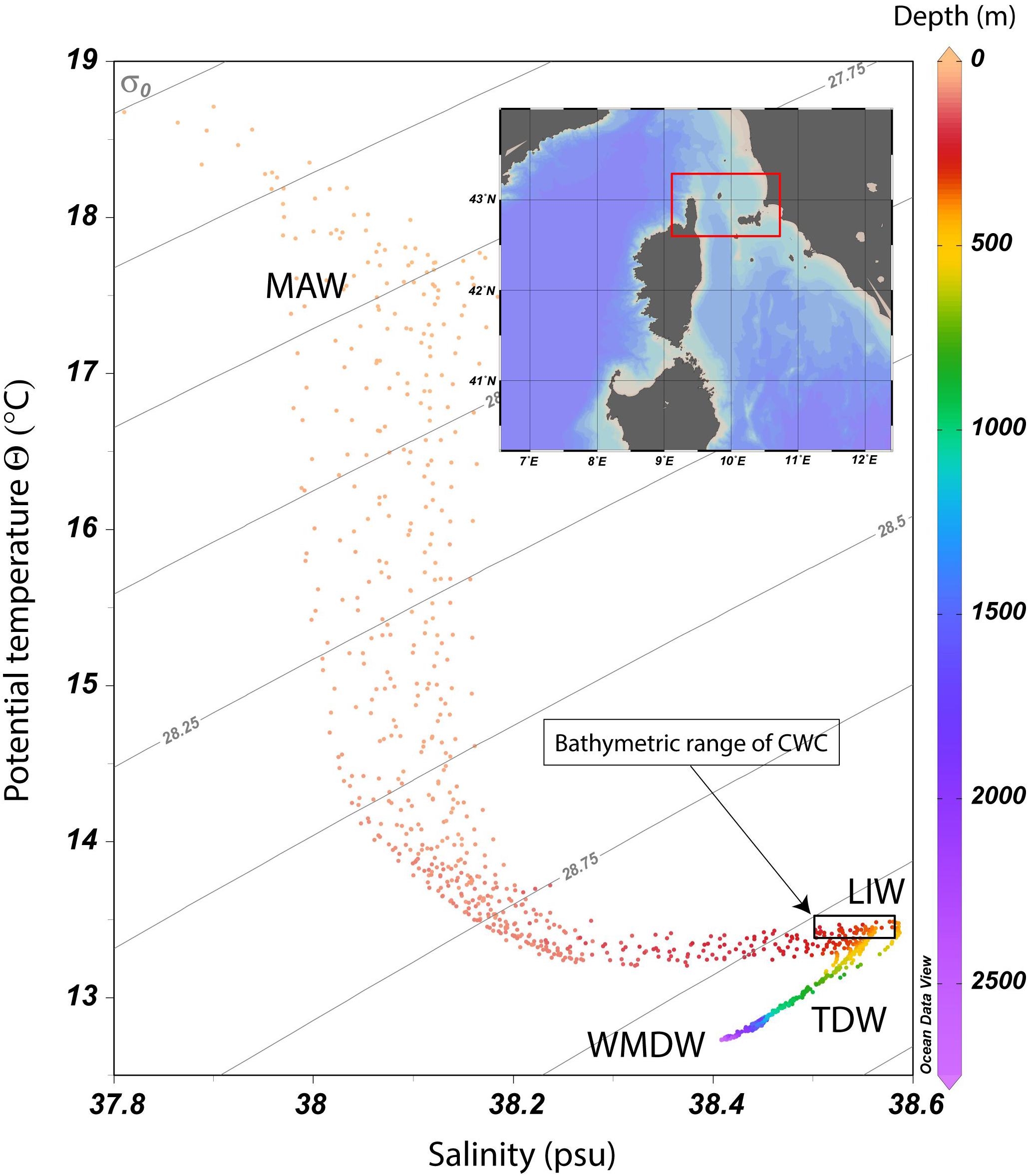
Figure 3. T-S plot obtained from 20 depth profiles sourced from the World Ocean Atlas 2018 (WOA18) database in the area of the Corsica Channel (red square: 43.375°N-9.125°E; 43.375°N-10.625°E; 42.625°N-9.125°E; 42.625°N-10.625°E). Isopycnals are calculated with the reference pressure at 0 m (σ0). MAW, Modified Atlantic Water; LIW, Levantine Intermediate Water; TDW, Tyrrhenian Deep Water; WMDW, Western Mediterranean Deep Water. The plot was created using Ocean Data View (Schlitzer, R., Ocean Data View, http://odw.awi.de, 2020).
Two remotely operated vehicle (ROV) dives (Stations MS17-I-54 and MS17-I-135) were conducted using a Pollux III (Global Electric Italiana) equipped with a low-resolution CCD video camera for navigation and a high-resolution (2,304 × 1,296 pixels) video camera in order to visually groundtruth the mounds identified by swath bathymetry. Three laser beams at 20 cm of each other provided the scale bar on the videos. The ROV was equipped with an underwater acoustic tracking system that gives position and depth every 1 s. Still-photo footage, one frame every 10 s, was georeferenced using Adelie, and ESRI ArcGIS software, provided taxonomic information. Macro- (>2 cm) and mega-benthic organisms were identified to the lowest possible taxonomic rank. Taxa unidentifiable at the species level from images alone (e.g., most sponges) were categorized only as morpho-species or morphological categories (e.g., Bell and Barnes, 2001; Bo et al., 2012; Cau et al., 2017; Foglini et al., 2019). Taxonomic identifications follow the World Register of Marine Species database (WoRMS Editorial Board, 20201).
Results
Hydrography
The local intermediate water mass close to the CWC occurrences in the Corsica Channel has a mean potential temperature of 13.4°C, salinity of 38.56 psu, and potential density of 29.07 kg/m3 (Figure 3), which are typical values of the LIW in this area of the Mediterranean Sea (Vetrano et al., 2010). The LIW flows through the Mediterranean Sea westward, entering the Tyrrhenian Sea from the Strait of Sicily and separates in two branches: one crossing northward the Corsica Channel and one flowing toward the eastern Sardinia (Millot and Taupier-Letage, 2005; Vetrano et al., 2010; Ozer et al., 2017).
Seafloor Bathymetry
Morphobathymetry and backscatter data have been combined with high-resolution Chirp profiles to reconstruct the origin of the elevated and elongated seafloor features observed. We identified 28 mounded features in a 5.3-km2 area at a depth of 400–430 m, ranging in length from 70 to 330 m and up to 25 m high. All these structures are north-south oriented, suggesting a preferential growth strongly influenced by currents. Two main patches of mounded features occur (Figures 2A,E), one ca. 20 km south of Gorgona including eight mounds (Site A) and another ca. 12 km east of Capraia clustering 20 mounds (Site B). Slope map, calculated from the digital terrain model (DTM), clearly shows the difference between the side slope of the mounds and the slope of the surrounding region (Figures 2B,F). In fact, the first ranges from 12° to 35° reaching a maximum of 39°, while the latter is ca. 1°–8°.
Backscatter analysis reveals that two different intensity classes characterize the mounds (Figures 2C,G). High intensity (>15 dB) typifies the major mounds, characterized by hard and firm substrates, while low intensity (<15 dB) characterizes the surrounding areas dominated by mobile sediments (i.e., muds). To better identify the mounds, we applied a selection algorithm, developed in ArcGIS 10.5, integrating features elevation, represented by the Bathymetric Position Index (BPI), with the seabed reflectivity value. The BPI was computed from the high-resolution bathymetry using the Benthic Terrain Modeler tool (BTM, V. 3.0) in the ArcGIS software, selecting an inner radius of 5 pixels and an outer of 20 pixels, corresponding to 25 and 100 m, respectively. The algorithm considers and selects all positive BPI as potential mounds (features elevating from the seafloor). This selection was then filtered by extracting a reflectivity intensity value in correspondence of georeferenced living coral occurrences, obtaining a 15-dB value as a threshold for cnidarian-dominated mounds (Figures 2D,H); Focal Statistic tool of ArcGIS (radius of 5 pixels) was used to exclude false positive.
Additionally, smooth rounded features, ranging from 30 to 250 m in length and from 20 to 80 m in width and achieving a maximum height of ca. 5 m covering an area of 1.94 km2, could represent buried mounds.
Biological and Habitat Characteristics Based Upon Video Surveys
The ROV dive MS17-I-54 explored Site A whereas ROV MS17-I-135 surveyed Site B between 430–415 m and 450–425 m water depths, respectively, for a total coverage of 978 m (Figures 2I,J and Table 1). ROV surveys revealed the existence of lush and healthy coral mounds dominated by the colonial scleractinian Madrepora oculata (Figure 4–7).
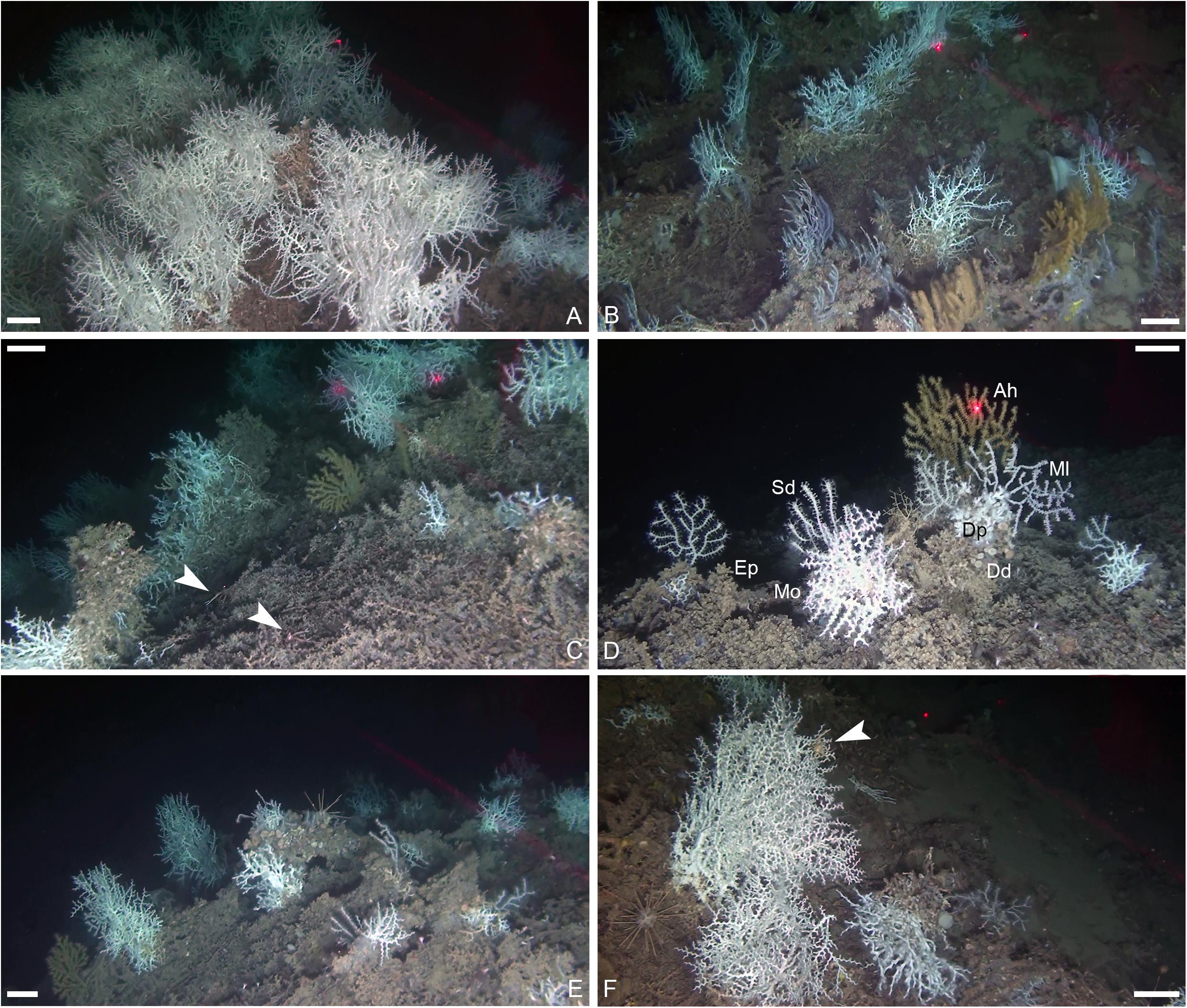
Figure 4. Corsica Channel coral mounds: (A) spatially closed live Madrepora oculata bushes on mound crest (Site A), bar = 20 cm; (B) cnidarian aggregation at mound crest (Site B) showing preferred growth orientation, bar = 10 cm; (C) dense M. oculata growth on dead coral frames at the mound flank, white arrows indicate specimens of Munida tenuimana sheltering on frames, bar = 10 cm; (D) cnidarian aggregation characterized by M. oculata (Mo), Desmophyllum pertusum (Dp), cluster of Desmophyllum dianthus (Dd), Swiftia dubia (Sd), Acanthogorgia hirsuta (Ah), Muriceides lepida (MI) and Epizoanthus sp. (Ep), bar = 20 cm; (E) bush-like M. oculata and D. pertusum colonies growing on dead coral frames, bar = 20 cm; (F) M. oculata and D. pertusum colonies on dead frames, white arrow indicate the decapod Anamathia rissoana, bar = 10 cm. Panels (C to F) are all from Site B.
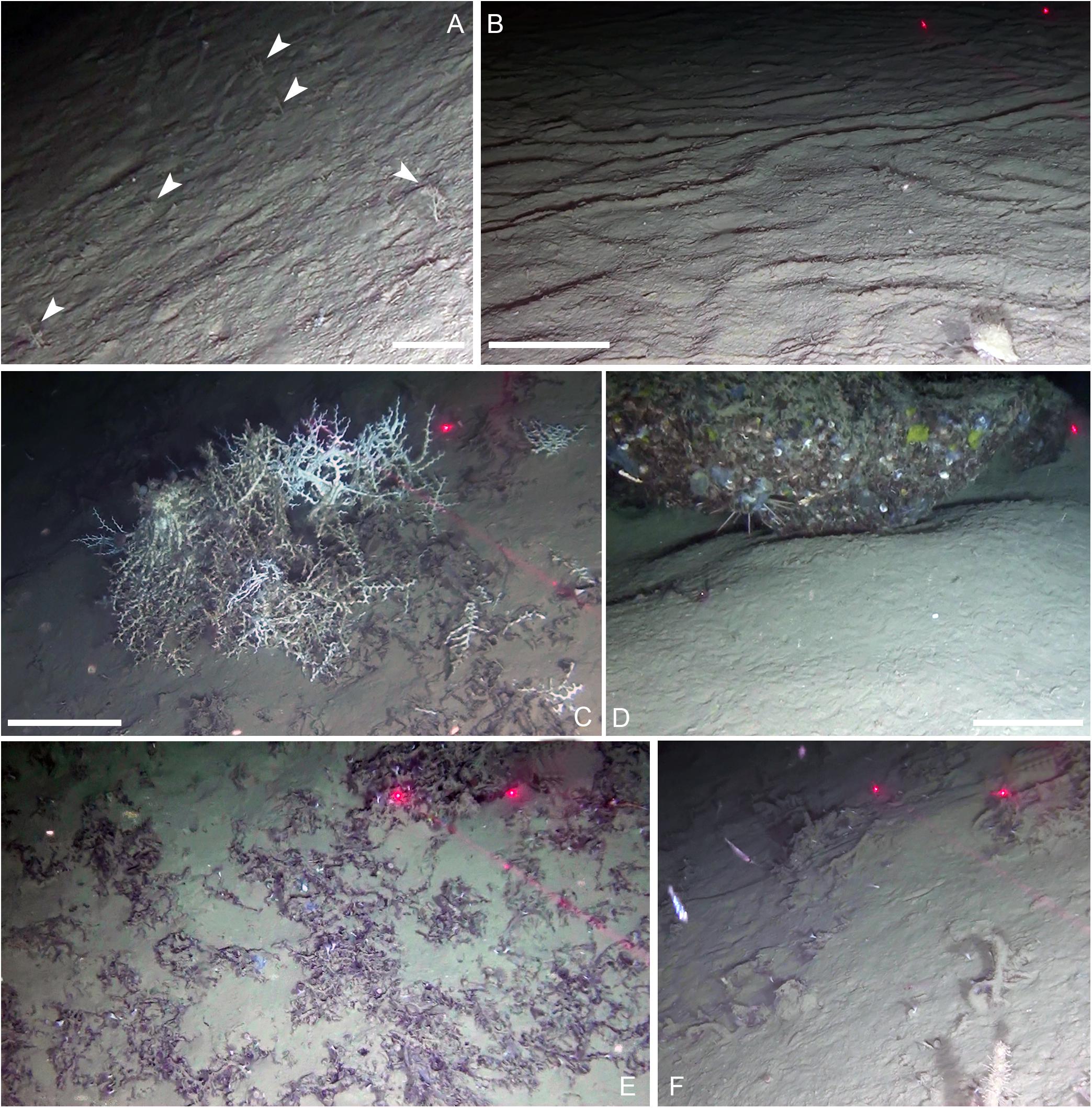
Figure 5. Muddy bottoms (A) characterized by dense occurrence of the macroforaminifer Pelosina sp. at Site A, bar = 2 cm, while dense bioturbation (B) prevents colonization at site B, bar = 20 cm; (C) isolated Madrepora oculata bush on a dead coral frame (Site A), bar = 20 cm; (D) hardground substrate characterizes flanks of coral mound at Site B, note the presence of the vagrant echinoderm Cidaris cidaris, bar = 20 cm; (E) muddy coral rubble dominates mounds’ flanks at Site A, bar = 20 cm; (F) dead Dendrophyllia cornigera frame (Site A) sparse on muddy sediment, bar = 20 cm.
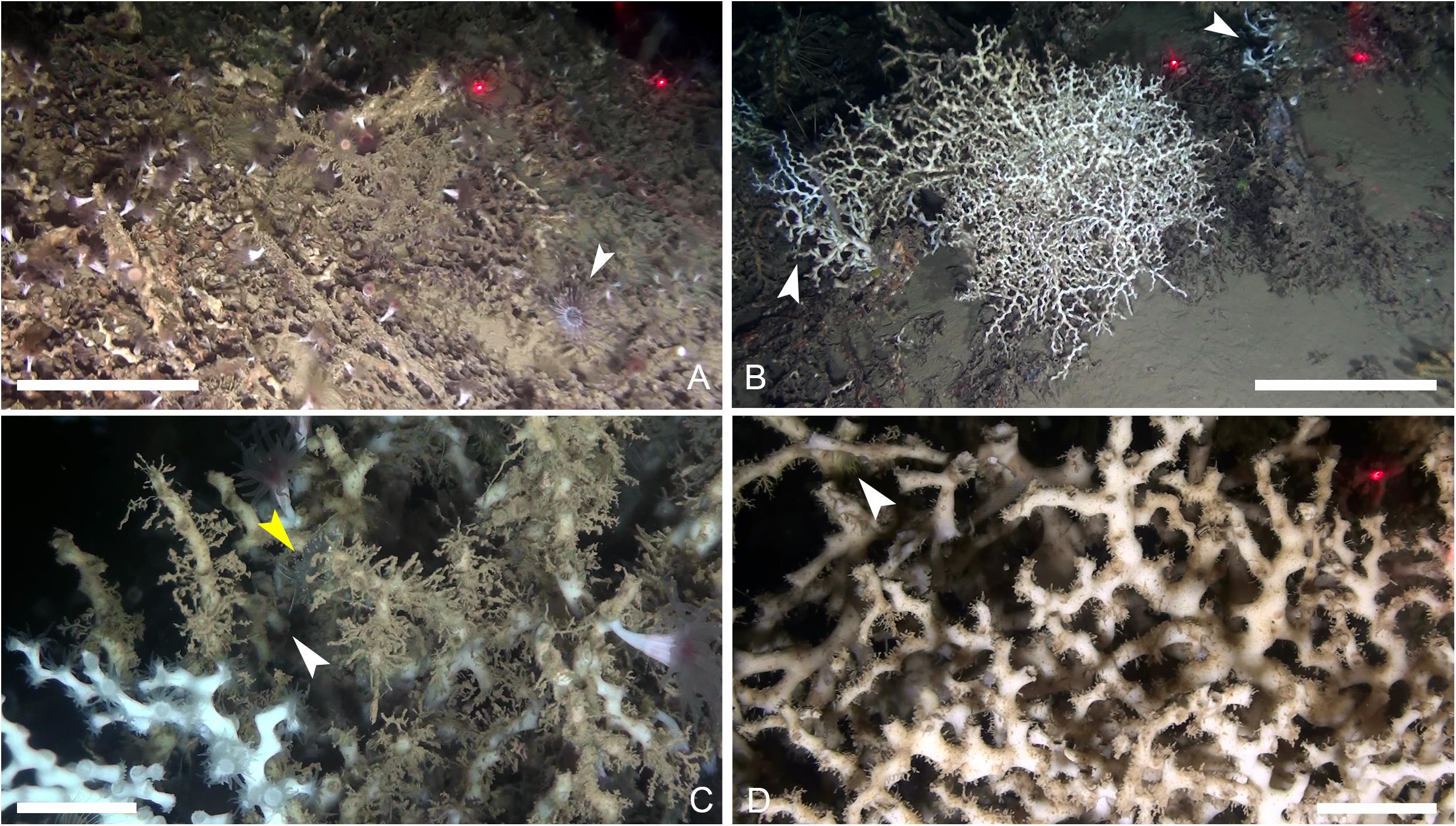
Figure 6. Associated biodiversity (A) dense solitary coral growth Desmophyllum dianthus and Javania cailleti on dead coral frames at Site A, arrow pointed at cf. Pachycerianthus, bar = 20 cm; (B) isolated large Madrepora oculata colony with juvenile Desmophyllym pertusum (right side) colony (Site B), bar = 20 cm; (C) close-up on M. oculata frame (Site A), white arrow indicates the polychaete Eunice norvegica, an undetermined nudibranch is pointed at by a yellow arrow, bar = 5 cm; (D) close-up on a dead coral frame (Site B), white arrow indicates a grazing undetermined nudibranch, bar = 5 cm.
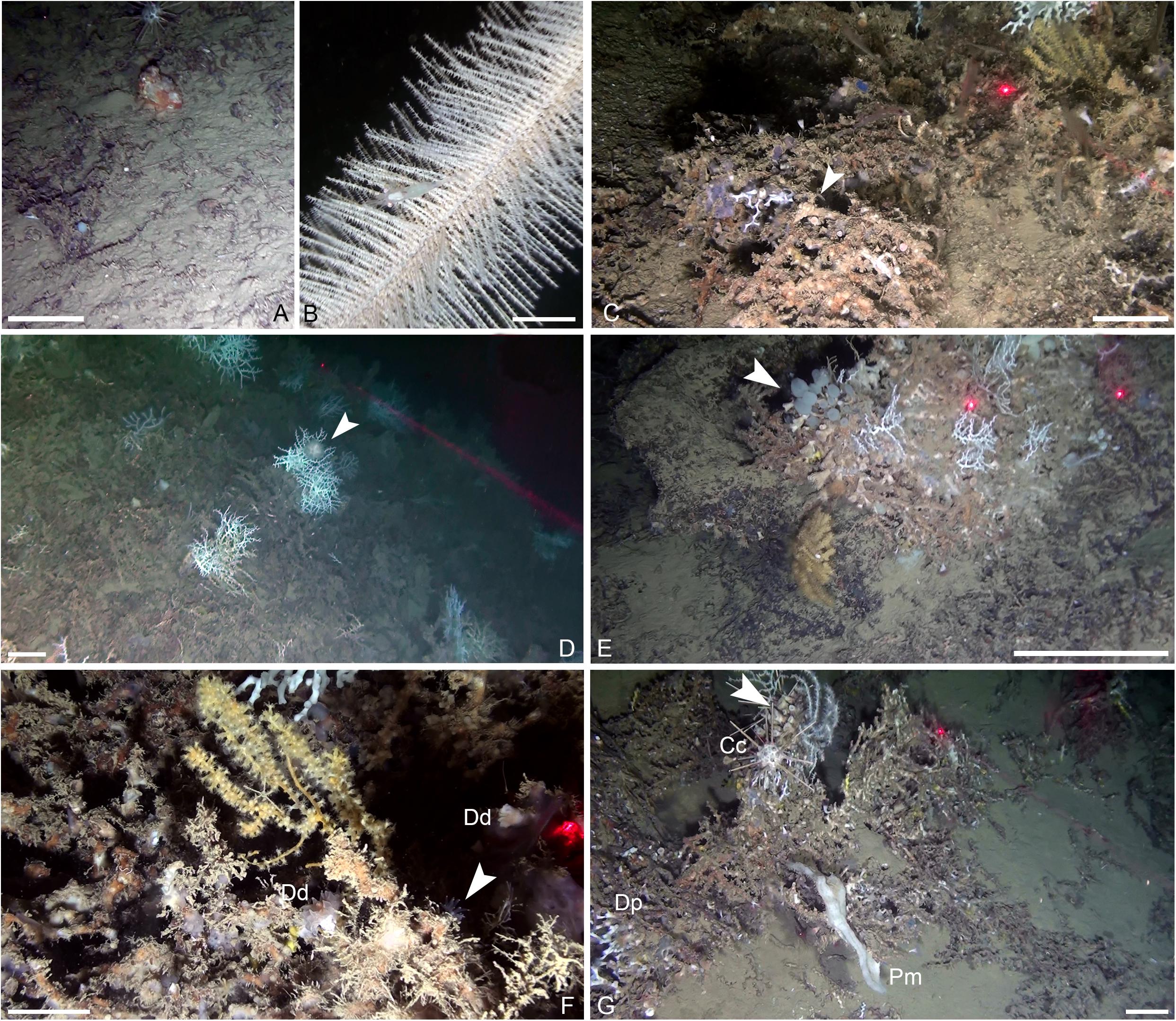
Figure 7. The vagrant Octopus salutii on muddy coral rubble at Site B (A), bar = 10 cm; (B) detail of Stylocheiron sp. on Parantipathes larix (Site A), bar = 3 cm; (C) arrow indicates the carnivorous coralliophilinid Hirtomurex squamosus feeding on a dead coral frame, bar = 5 cm; (D) isolated Madrepora oculata bushes on dead frames, arrow pointed at Echinus cf. melo grazing on M. oculata, bar = 20 cm; (E) the rare lollipop sponge Sympagella delauzei (arrow) associated with Desmophyllum dianthus and Acanthogorgia hirsuta, bar = 20 cm; (F) close-up on cnidarian assemblage with juvenile D. dianthus (Dd) and A. hirsuta with grazing undetermined nudibranch (arrow), bar = 5 cm; (G) dead coral frames colonized by the large fan-shaped sponge Pachastrella monilifera (Pm), juvenile Desmophyllum pertusum (Dp, on the left) and a cluster of the solitary Desmophyllum dianthus (arrow), the echinoderm Cidaris cidaris (Cc) grazing on a coral frame, bar = 10 cm. Panels (C–G) all from Site B.
(1) ROV MS17-I-54. The ROV landed at −450 m on the muddy bottom (Figure 5A) in the western side of a north-south-oriented mound, whose crest topped at −420 m. Along the entire transect, the biodiversity is relatively high, counting more than 20 megabenthic taxa (Table 2). At 435 m, the substrate changes, and black-coated Madrepora oculata rubble (Figures 5C,E) emerges providing suitable substrate for caryophylliid and antipatharians such as Parantipathes larix (Figure 7B). Abundant live M. oculata colonies (>50 cm) characterize the mound crest at 425 m (Figure 4A), together with common solitary scleractinians Desmophyllum dianthus (up to 29 ind m–2) and Javania cailleti (Figure 6A). M. oculata colonies host the polychaetes Eunice norvegica (Figure 6C), whose encalcification contributes to strengthening coral frames and Serpula vermicularis (e.g., Mueller et al., 2013; Sanfilippo et al., 2013; Taviani et al., 2017). The actinian Amphianthus dohrnii settles on living M. oculata, while the sea-pen Kophobelemnon stelliferum and the alcyonacea Acanthogorgia hirsuta colonize muddy bottoms and coral rubble, respectively. Dead Dendrophyllia cornigera frames have been rarely ascertained on coral rubble (Figure 5F). Surprisingly, no living colonies of this noticeable scleractinian (Castellan et al., 2019) have yet been detected in this area. A specimen of an undetermined nudibranch was observed grazing on living M. oculata (Figure 6C). The brachiopods Gryphus vitreus and Terebratulina retusa were recognized on coral rubble while Munida cf. tenuimana and Anamathia rissoana represent the commonest crustaceans on coral colonies. No echinoderms have been identified along this transect. Finally, the tiny species Gadiculus argenteus and the scorpaenid Helicolenus dactylopterus characterize the demersal fish fauna.
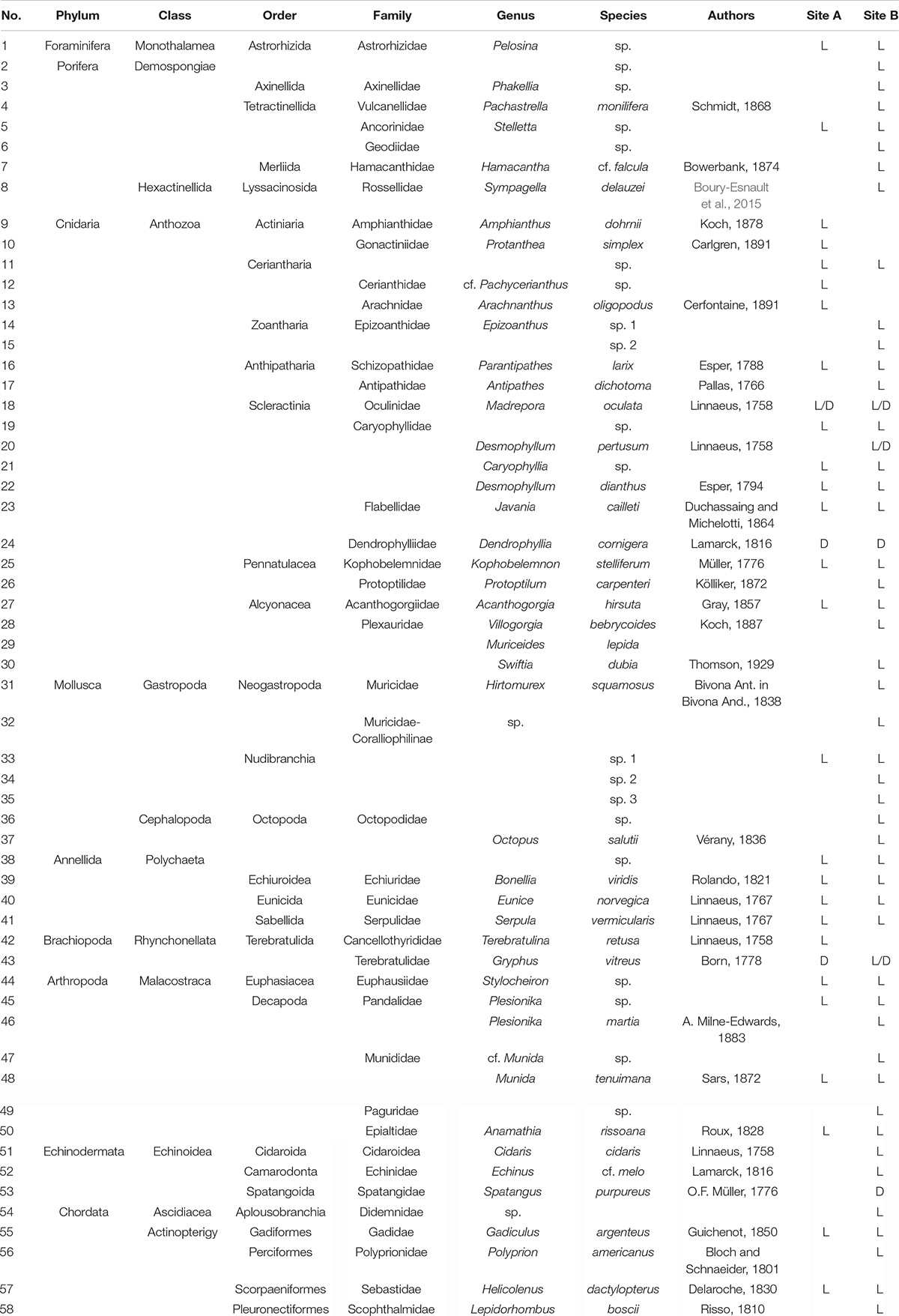
Table 2. List of macroorganisms observed in the new “Corsica Channel CWC Province.” L, living observed organisms; D, dead only; L/D, specimens observed both living and dead.
(2) ROV MS17-I-135. The substrate typology rapidly evolves from the muddy bottom at the mound toe (430 m) to a loosely packed coral and coral rubble at its crest (412 m), which is exploited by live coral growth (Figures 4B,D–F). Living colonies of M. oculata (>50 cm in size) dominate the steep mound’s flank up to the crest at 412 m (104 colonies in 128 m of linear exploration) (Figure 4C). Subordinately, tiny colonies of Desmophyllum pertusum (nine colonies in 128 m of linear exploration) and specimens of D. dianthus (>19 ind m–2) and J. cailleti contribute to the coral biodiversity in this area (Figure 6B). Antipatharians (Antipathes dichotoma) and alcyonaceans (Acanthogorgia hirsuta, Villogorgia bebrycoides, and Swiftia dubia) are quite common (Figures 4D,E, 7F). Dead coral frameworks offer substrate for antipatharians such as P. larix and other solitary scleractinians (e.g., caryophylliids). They also provide habitat for many other taxa such as Epizoanthus sp. and the sponges Phakellia sp., Pachastrella monilifera (Figure 7G), Stelletta sp., Hamacantha cf. falcula, and the recently described Sympagella delauzei (Boury-Esnault et al., 2015; Figure 7E). The carnivorous coralliophilinid gastropod Hirtomurex squamosus, often associated with CWC (Taviani and Colantoni, 1979; Taviani et al., 2009; Angeletti and Taviani, 2011), at least three undetermined nudibranchs and the rare cephalopod Octopus salutii (Figure 7A) inhabit coral frames (Figures 6D, 7C,E,F). Serpula vermicularis is the most abundant polychaete on hard substrates (cf. Sanfilippo et al., 2013), while Bonellia viridis is relatively common on muddy bottoms. The crustaceans Munida tenuimana and Anamathia rissoana and the echinoderms Cidaris cidaris (Figure 5D) and Echinus melo (Figure 7D) represent the principal vagrant benthic component, as often may find associated with Mediterranean CWC (Rueda et al., 2019).
A sparse benthic fauna inhabits muddy sediments, including single occurrences of the cnidarian Kophobelemnon stelliferum and of the rare pennatulacea Protoptilum carpenteri (e.g., Prampolini et al., 2020). The macro foraminifera Pelosina sp. is abundant (up to 25 ind. 0.3 m–2) at Site A (Figure 5A), while it occurs rarely on the highly bioturbated muddy bottoms of Site B (Figure 5B). Ceriantids (cf. Pachycerianthus and Arachnanthus oligopodus) and sparse presence of Bonellia viridis characterize the sessile fauna inhabiting muddy sediments on both sites. Rare decapods (Plesionika martia) and the demersal fish Lepidorhombus boscii represent vagrant fauna. The echinoid Spatangus purpureus represents the commonest vagile organism at Site B, further testified by the abundance of its skeletal remains; pagurids and other crustaceans are also common presences.
The Corsica Channel hosts at least 20 cnidarian species (Table 2), all known from the Mediterranean Basin (e.g., Chimienti et al., 2019; Rueda et al., 2019, with references therein) including the scleractinians M. oculata, D. pertusum, D. dianthus, and J. cailleti; alcyonarians A. hirsuta, V. bebrycoides, M. lepida, and S. dubia; antipatharians P. larix and A. dichotoma; and pennatulaceans K. stelliferum and P. carpenteri.
Discussion
Mound Morphology and Genesis
Coral mounds of the Corsica Channel are roughly parallel to each other, displaying oval to elongated shapes, mean length of ca. 200 m, width of 110 m, heights of up to 25 m, and very steep flanks up to 35°. Mounds develop at present, adjacent spatially to contouritic deposits, similar to other Mediterranean localities (e.g., off Pantelleria: Martorelli et al., 2011; east and west Melilla: Corbera et al., 2019; Hebbeln et al., 2019; Rebesco and Taviani, 2019, with references therein). Active mounds of the sites A and B seem to develop where the bottom currents decelerate but are still sufficiently intense to sustain coral growth, provide nutrients, and prevent excess silting. Between sites A and B, seismic and bathymetric records show the occurrence of north-south-aligned morphological features with elongated shapes and dimensions comparable to the active mounds under scrutiny. These structures cover an area of ca. 2 km2 and might represent buried CWC mounds. This is also consistent with the documented existence of Madrepora-dominated buried mounds previously described in this region (Remia and Taviani, 2005). In response to climatic changes, the past regime might have been somehow different in direction and strength from today, as for turbidity and nutrient supply. This might account for the demise of such Pleistocene CWC mounds in the study area as recorded by U/Th dated coral mounds (McCulloch et al., 2010), and the newly observed buried features here discussed.
Anyhow, morphological similarities and comparable alignments of active (modern) and buried (past) mounds in the Corsica Channel suggest a similar environmental control on coral mound development, at least since the latest Pleistocene, with the LIW acting as the main driver.
Cnidarian Assemblage and Associate Fauna
The new “Corsica Channel CWC Province” presents biological characteristics similar to Atlantic and Mediterranean counterparts. Differently from their Atlantic equivalent, where the main frame builder is Desmophyllum pertusum, here, Madrepora oculata shows the highest abundance, contributing primarily to form CWC mounds (e.g., Buhl-Mortensen et al., 2017; Corbera et al., 2019). In terms of biodiversity richness, faunal data compare well with other sites in the Tyrrhenian Sea such as the Nora Canyon (78 species, see Taviani et al., 2017), northeastern Sardinian margin (58 species, see Moccia et al., 2019), and the Dohrn Canyon (64 species, see Taviani et al., 2019). Overall, more than 50 mega- and macro-benthic taxa have been identified by the ROV surveys plus four different demersal species (Table 2). Sessile megafauna observed in the explored mounds shows a preferential spatial distribution, appearing more dense at the crest of the mounds, in particular M. oculata and the alcyonarians A. hirsuta, V. bebrycoides, M. lepida, and S. dubia. However, when dense aggregations of M. oculata cover the crest of the mounds, the associated species occur in areas with more exposed dead framework. Moreover, increasing of cnidarian density and colonies’ size have been observed on mound crests. Similar situations have been reported in other Mediterranean sites (Orejas and Jiménez, 2019, and references therein) where the presence of healthy and lush CWC is linked to the flowing of the LIW.
The new “Corsica Channel CWC Province” fits in the path of the LIW, confirming this water mass as the main controlling factor of the distribution of CWC provinces in the Mediterranean Sea (e.g., Taviani et al., 2017, 2019). Our findings are biogeographically relevant also in terms of connectivity and connectance with other coral sites and provinces in the Mediterranean Basin (Figure 8).
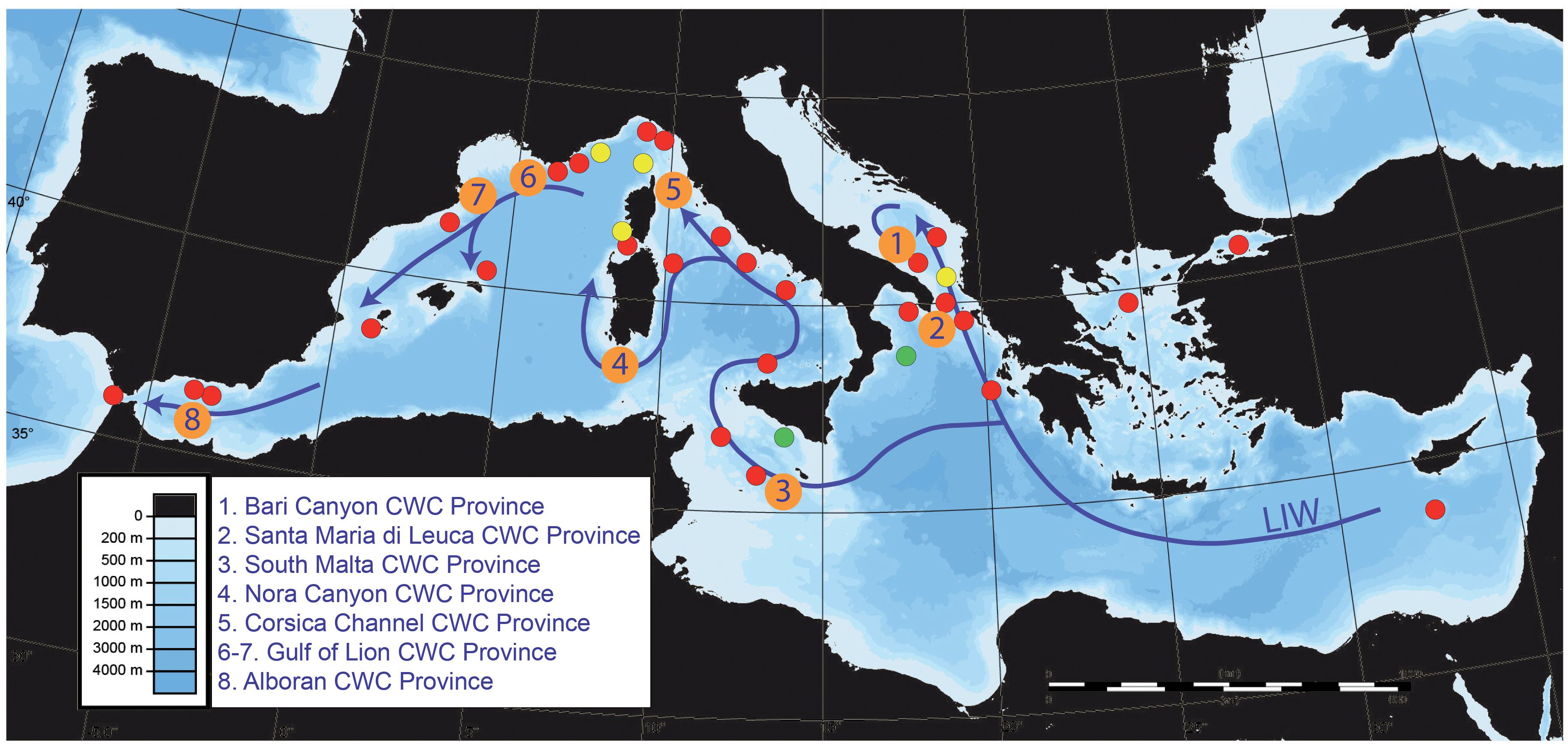
Figure 8. Map of the Mediterranean Sea showing the eight coral provinces (numbered orange dots) and main coral occurrences (red dots) with respect to the main path of the Levantine Intermediate Water (LIW). Yellow dots indicate coral occurrence on anthropic structures (e.g., shipwreck), while green dots on derelict fishing gears. CWC, cold-water coral.
Anthropic Impact
Insubstantial or null anthropic impact on the CWC grounds in the Corsica Channel was evidenced by ROV images. Differently from other Mediterranean CWC sites (e.g., D’Onghia et al., 2017; Taviani et al., 2017, 2019; Giusti et al., 2019; Enrichetti et al., 2020, with references therein), the limited visual information available shows that the coral grounds here seem less impacted by anthropic activities in terms of macrolitter and derelict fishing gears. The paucity of obvious anthropic macroimpact could imply a rather pristine status of the CWC habitat there. We cannot exclude, however, the occurrence of other forms of direct and indirect impact, including the pervasive microplastics (e.g., Danovaro et al., 2020).
Governance and Protection
International organizations have been taking several actions and recommendations to preserve CWC ecosystems. However, the application and eventual implementation of such conservation measures and policies in the Mediterranean Sea still remain limited (see Rees et al., 2018; Otero and Marin, 2019, with references therein).
Due to its ecosystemic importance in the Mediterranean panorama, pristine condition, presence of several threatened and target species of Vulnerable Marine Ecosystem (VME), and the potential exposure to anthropic impact, the “Corsica Channel CWC Province” surely deserves earning proper governance and protection, in line with current views (e.g., Armstrong et al., 2009, 2014; Fabri et al., 2014; Taviani et al., 2017, 2019; Danovaro et al., 2020). Although fisheries do not seem to represent a threat to date, and littering appears scant here as documented by video images, a principle of caution should be enforced in the future for ensuring the Good Environmental Status for the area. In fact, the “Corsica Channel CWC Province” hosts a number of species listed in protection protocols, such as RAC/SPA/BD, IUCN Red List of Threatened Species and EU-Habitats Directive. The designation of such a new CWC province in a protection program is highly desirable, also in light of its role within the Mediterranean CWC province network. As a first propositive step, we suggest two suitable areas to be considered for new transnational high-seas marine protected areas, with an extension of 1.47 km2 and 3.86 km2 for sites A and B, respectively, and including most of the best CWC “reefs” identified thus far (Figure 9 and Table 3).
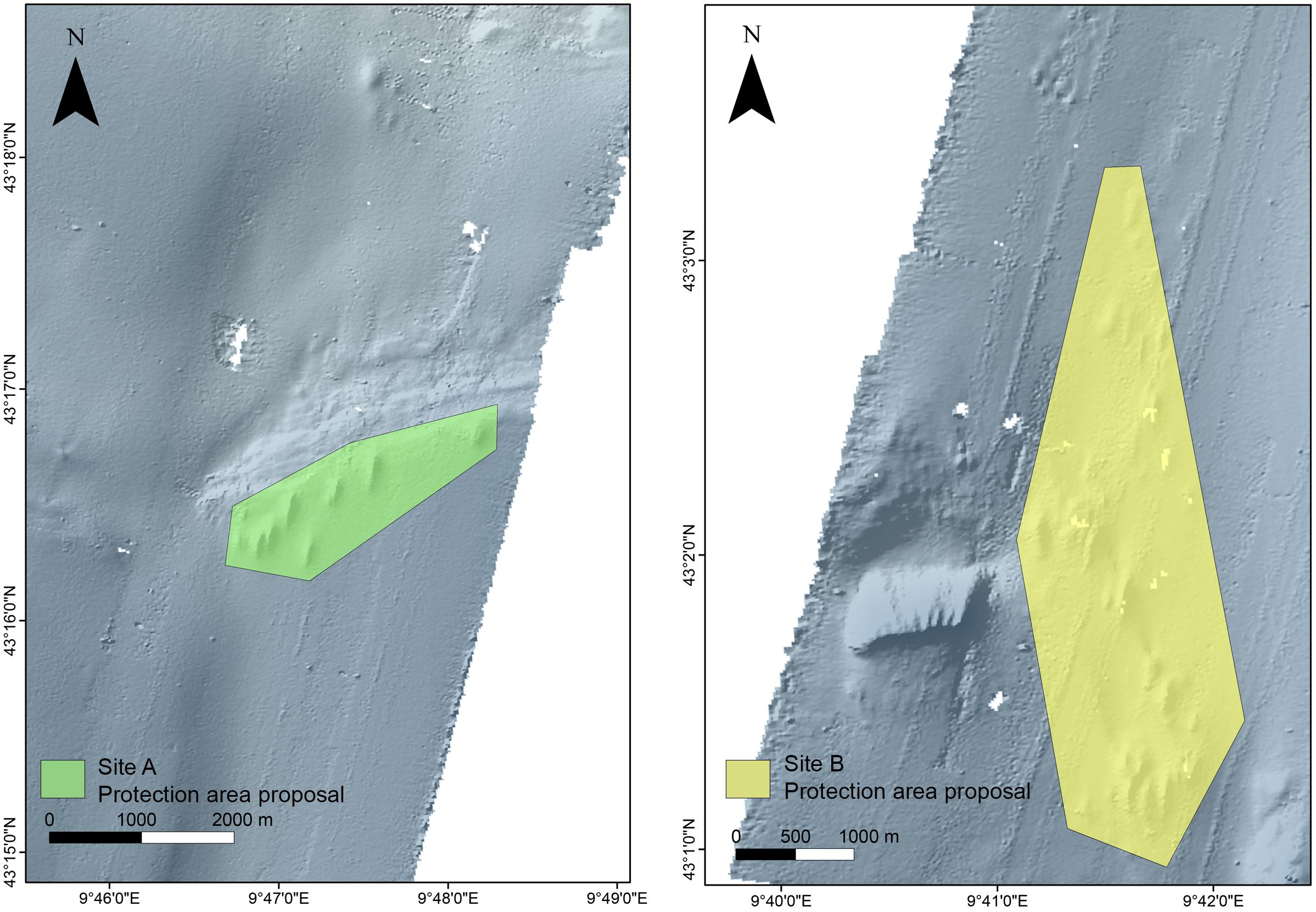
Figure 9. Map showing the areas proposed for protection of cold-water coral (CWC) mounds. Protection area for Site A (left) has an extension of 1.47 km2, while Site B area (right) extends for 3.86 km2.
Conclusion
(1) In consideration of the remarkable surface covered by living corals (ca. 5.3 km2), their density, healthy appearance, and discontinuity with other major CWC occurrences in the Mediterranean Sea, this area in the Corsica Channel is erected as a distinct and new CWC province for this basin. The high levels of biodiversity documented advise for the consideration of proper management and protection of these CWC grounds.
(2) This new “Corsica Channel Cold-Water Coral Province” is located off the Tuscan Archipelago in the Corsica Channel, in a sector previously known for the presence of pre-modern CWC mounds and presence of well-developed contourite drift systems.
(3) In line with the main Mediterranean CWC sites, the new province is aligned along the main path of the LIW and represents a potential connection with other coral sites and provinces throughout the Mediterranean Basin.
Data Availability Statement
All datasets generated for this study are included in the article/Supplementary Material.
Author Contributions
LA, AR, GC, and MT analyzed the data and wrote the manuscript together with PM. All authors contributed to the discussion and preparation of the manuscript.
Funding
This work was partly supported by the “Convenzione MATTM-CNR per i Programmi di Monitoraggio per la Direttiva sulla Strategia Marina (MSFD, Art. 11, Dir. 2008/56/CE)” and is part of the DG Environment programme IDEM (grant agreement no. 11.0661/2017/750680/SUB/EN V.C2) and the MIUR-PRIN GLIDE.
Conflict of Interest
The authors declare that the research was conducted in the absence of any commercial or financial relationships that could be construed as a potential conflict of interest.
Acknowledgments
This paper is part of the keynote presentation by MT at the 7th International Symposium on Deep-Sea Corals held in Cartagena, Colombia, July 29–August 2, 2019. We are grateful to the organizers Juan Armando Sánchez, Santiago Herrera, and Luisa Dueñas for their kind invitation to contribute an article to the Symposium’s special issue. We thank captain, crew, and scientific staff of RR/VV Urania and Minerva Uno cruises for their skillful and efficient cooperation during operations at sea. Manuscript finalized and revised at the time of the COVID-19 pandemic. This is ISMAR-Bologna scientific contribution no. 1998.
Supplementary Material
The Supplementary Material for this article can be found online at: https://www.frontiersin.org/articles/10.3389/fmars.2020.00661/full#supplementary-material
Footnotes
References
Addamo, A. M., Vertino, A., Stolarski, J., García-Jiménez, R., Taviani, M., and Machordom, A. (2016). Merging scleractinian genera: the overwhelming genetic similarity between solitary Desmophyllum and colonial Lophelia. BMC Evol. Biol. 16:108. doi: 10.1186/s12862-016-0654-8
Angeletti, L., Prampolini, M., Foglini, F., Grande, V., and Taviani, M. (2020). “Cold-water coral habitat in the Bari Canyon System, Southern Adriatic Sea (Mediterranean Sea),” in Seafloor Geomorphology as Benthic Habitat, eds P. T. Harris and E. Baker (Amsterdam: Elsevier), 811–824. doi: 10.1016/b978-0-12-814960-7.00049-x
Angeletti, L., and Taviani, M. (2011). Entrapment, preservation and incipient fossilization of benthic predatory molluscs within deep-water coral frames in the Mediterranean Sea. Geobios 44, 543–548. doi: 10.1016/j.geobios.2011.02.004
Angeletti, L., Taviani, M., Canese, S., Foglini, F., Mastrototaro, F., Argnani, A., et al. (2014). New deep-water cnidarian sites in the southern Adriatic Sea. Mediterranean Mar. Sci. 15, 263–273.
Armstrong, C. W., Foley, N. S., Kahui, V., and Grehan, A. (2014). Cold water coral reef management from an ecosystem service perspective. Mar. Policy 50, 126–134. doi: 10.1016/j.marpol.2014.05.016
Armstrong, C. W., Grehan, A., Kahui, V., Mikkelsen, E., Reithe, S., and Van Den Hove, S. (2009). Bioeconomic modeling and the management of cold-water coral resources. Oceanography 22, 86–91. doi: 10.5670/oceanog.2009.10
Artale, V., and Gasparini, G. P. (1990). Simultaneous temperature and velocity measurements of the internal wave field in the Corsican Channel (eastern Ligurian Sea). J. Geophys. Res. Oceans 95, 1635–1645. doi: 10.1029/JC095iC02p01635
Astraldi, M., and Gasparini, G. P. (1992). The seasonal characteristics of the circulation in the north Mediterranean basin and their relationship with the atmospheric-climatic conditions. J. Geophys. Res.Oceans 97, 9531–9540. doi: 10.1029/92JC00114
Bell, J. J., and Barnes, D. K. A. (2001). Sponge morphological diversity: a qualitative predictor of species diversity? Aquat. Conserv. Mar. Freshw. Ecosyst. 11, 109–121. doi: 10.1002/aqc.436
Bellaiche, G., Droz, L., Gaullier, V., and Pautot, G. (1994). Small submarine fans on the eastern margin of Corsica: sedimentary significance and tectonic implications. Mar. Geol. 117, 177–185. doi: 10.1016/0025-3227(94)90013-2
Bo, M., Bertolino, M., Bavestrello, G., Canese, S., Giusti, M., Angiolillo, M., et al. (2012). Role of deep sponge grounds in the Mediterranean Sea: a case study in southern Italy. Hydrobiologia 687, 163–177. doi: 10.1007/s10750-011-0964-1
Boury-Esnault, N., Vacelet, J., Reiswig, H. M., Fourt, M., Aguilar, R., and Chevaldonné, P. (2015). Mediterranean hexactinellid sponges, with the description of a new Sympagella species (Porifera, Hexactinellida). J. Mar. Biol. Assoc. U.K. 95, 1353–1364. doi: 10.1017/S0025315414001891
Buhl-Mortensen, L., Serigstad, B., Buhl-Mortensen, P., Olsen, M., Ostrowski, M., Blazewicz-Paszkowycz, M., et al. (2017). First observations of the structure and megafaunal community of a large Lophelia reef on the Ghanaian shelf (the Gulf of Guinea). Deep Sea Res. Part II Top. Stud. Oceanogr. 137, 148–156. doi: 10.1016/j.dsr2.2016.06.007
Calvès, G., Toucanne, S., Jouet, G., Charrier, S., Thereau, E., Etoubleau, J., et al. (2013). Inferring denudation variations from the sediment record; an example of the last glacial cycle record of the Golo Basin and watershed, East Corsica, western Mediterranean Sea. Basin Res. 25, 197–218. doi: 10.1111/j.1365-2117.2012.00556.x
Castellan, G., Angeletti, L., Taviani, M., and Montagna, P. (2019). The yellow coral Dendrophyllia cornigera in a warming ocean. Front. Mar. Sci. 6:692. doi: 10.3389/fmars.2019.00692
Cattaneo, A., Miramontes, E., Samalens, K., Garreau, P., Caillaud, M., Marsset, B., et al. (2017). Contourite identification along Italian margins: the case of the Portofino drift (Ligurian Sea). Mar. Petroleum Geol. 87, 137–147. doi: 10.1016/j.marpetgeo.2017.03.026
Cau, A., Follesa, M. C., Moccia, D., Bellodi, A., Mulas, A., Marzia, B., et al. (2017). Leiopathes glaberrima millennial forest from SW Sardinia as nursery ground for the small spotted catshark Scyliorhinus canicula. Aquat. Conserv. Mar. Freshw. Ecosyst. 27, 731–735. doi: 10.1002/aqc.2717
Chimienti, G., Bo, M., Taviani, M., and Mastrototaro, F. (2019). “Occurrence and biogeography of Mediterranean cold-water corals,” in Mediterranean Cold-Water Corals: Past, Present and Future, eds C. Orejas and C. Jiménez (Cham: Springer International Publishing), 295–333. doi: 10.1007/978-3-319-91608-8_19
Corbera, G., Lo Iacono, C., Gràcia, E., Grinyó, J., Pierdomenico, M., Huvenne, V. A. I., et al. (2019). Ecological characterisation of a Mediterranean cold-water coral reef: cabliers Coral Mound Province (Alboran Sea, western Mediterranean). Prog. Oceanogr. 175, 245–262. doi: 10.1016/j.pocean.2019.04.010
Danovaro, R., Fanelli, E., Canals, M., Ciuffardi, T., Fabri, M.-C., Taviani, M., et al. (2020). Towards a Marine strategy for the deep Mediterranean sea: analysis of current ecological status. Mar. Policy 112:103781. doi: 10.1016/j.marpol.2019.103781
Deptuck, M. E., Piper, D. J. W., Savoye, B., and Gervais, A. (2008). Dimensions and architecture of late Pleistocene submarine lobes off the northern margin of East Corsica. Sedimentology 55, 869–898. doi: 10.1111/j.1365-3091.2007.00926.x
D’Onghia, G., Calculli, F., Capezzuto, F., Carlucci, R., Carluccio, A., Grehan, A., et al. (2017). Anthropogenic impact in the Santa Maria di Leuca cold-water coral province (Mediterranean Sea): observations and conservations traits. Deep Sea Res. Part II Top. Stud. Oceanogr. 145, 87–101. doi: 10.1016/j.dsr2.2016.02.012
Enrichetti, F., Dominguez-Carrió, C., Toma, M., Bavestrello, G., Canese, S., and Bo, M. (2020). Assessment and distribution of seafloor litter on the deep Ligurian continental shelf and shelf break (NW Mediterranean Sea). Mar. Pollut. Bull. 151:110872. doi: 10.1016/j.marpolbul.2019.110872
Fabri, M.-C., Pedel, L., Beuck, L., Galgani, F., Hebbeln, D., and Freiwald, A. (2014). Megafauna of vulnerable marine ecosystems in French mediterranean submarine canyons: spatial distribution and anthropogenic impacts. Deep Sea Res. Part II Top. Stud. Oceanogr. 104, 184–207. doi: 10.1016/j.dsr2.2013.06.016
Fabri, M.-C., Vinha, B., Allais, A.-G., Bouhier, M.-E., Dugornay, O., Gaillot, A., et al. (2019). Evaluating the ecological status of cold-water coral habitats using non-invasive methods: an example from Cassidaigne Canyon, northwestern Mediterranean Sea. Prog. Oceanogr. 178:102172. doi: 10.1016/j.pocean.2019.102172
Fanelli, E., Delbono, I., Ivaldi, R., Pratellesi, M., Cocito, S., and Peirano, A. (2017). Cold-water coral Madrepora oculata in the eastern Ligurian Sea (NW Mediterranean): historical and recent findings. Aquat. Conserv. Mar. Freshw. Ecosyst. 27, 965–975. doi: 10.1002/aqc.2751
Foglini, F., Grande, V., Marchese, F., Bracchi, V. A., Prampolini, M., Angeletti, L., et al. (2019). Application of hyperspectral imaging to underwater habitat mapping, Southern Adriatic Sea. Sensors 19:2261. doi: 10.3390/s19102261
Freiwald, A., Beuck, L., Rüggeberg, A., Taviani, M., Hebbeln, D. and R/V Meteor Cruise M70-1 Participants (2009). The white coral community in the central Mediterranean Sea revealed by ROV surveys. Oceanography 22, 58–74. doi: 10.5670/oceanog.2009.06
Gervais, A., Savoye, B., Piper, D. J. W., Mulder, T., Cremer, M., and Pichevin, L. (2004). Present morphology and depositional architecture of a sandy confined submarine system: the Golo turbidite system (eastern margin of Corsica). Geol. Soc. Lond. Spec. Public. 222, 59–89. doi: 10.1144/GSL.SP.2004.222.01.05
Giusti, M., Canese, S., Fourt, M., Bo, M., Innocenti, C., Goujard, A., et al. (2019). Coral forests and derelict fishing gears in submarine canyon systems of the Ligurian Sea. Prog. Oceanogr. 178:102186. doi: 10.1016/j.pocean.2019.102186
Hebbeln, D., Bender, M., Gaide, S., Titschack, J., Vandorpe, T., Van Rooij, D., et al. (2019). Thousands of cold-water coral mounds along the Moroccan Atlantic continental margin: distribution and morphometry. Mar. Geol. 411, 51–61. doi: 10.1016/j.margeo.2019.02.001
Hebbeln, D., Wienberg, C., Wintersteller, P., Freiwald, A., Becker, M., Beuck, L., et al. (2014). Environmental forcing of the Campeche cold-water coral province, southern Gulf of Mexico. Biogeosciences 11, 1799–1815. doi: 10.5194/bg-11-1799-2014
Knittewis, L., Evans, J., Aguilar, R., Álvarez, H., Borg, J. A., García, S., et al. (2019). “Recent discoveries of extensive cold-water coral assemblages in maltese waters,” in Mediterranean Cold-Water Corals: Past, Present and Future, eds C. Orejas and C. Jiménez (Cham: Springer), 253–256. doi: 10.1007/978-3-319-91608-8_22
Lo Iacono, C., Savini, A., Huvenne, V. A. I., and Gràcia, E. (2019). “Habitat mapping of cold water corals in the Mediterranean Sea,” in Mediterranean Cold-Water Corals: Past, Present and Future, eds C. Orejas and C. Jiménez (Cham: Springer International Publishing), 157–172. doi: 10.1007/978-3-319-91608-8_15
Marani, M., Argnani, A., Roveri, M., and Trincardi, F. (1993). Sediment drifts and erosional surfaces in the central Mediterranean: seismic evidence of bottom-current activity. Sediment. Geol. 82, 207–220. doi: 10.1016/0037-0738(93)90122-L
Martorelli, E., Petroni, G., Chiocci, F. L. and The Pantelleria Scientific Party (2011). Contourites offshore Pantelleria Island (Sicily Channel, Mediterranean Sea): depositional, erosional and biogenic elements. Geo Mar. Lett. 31, 481–493. doi: 10.1007/s00367-011-0244-0
McCulloch, M., Taviani, M., Montagna, P., López Correa, M., Remia, A., and Mortimer, G. (2010). Proliferation and demise of deep-sea corals in the Mediterranean during the Younger Dryas. Earth Planet. Sci. Lett. 298, 143–152. doi: 10.1016/j.epsl.2010.07.036
Millot, C. (1999). Circulation in the Western Mediterranean Sea. J. Mar. Syst. 20, 423–442. doi: 10.1016/S0924-7963(98)00078-5
Millot, C., and Taupier-Letage, I. (2005). “Circulation in the Mediterranean Sea,” in The Mediterranean Sea Handbook of Environmental Chemistry, ed. A. Saliot (Berlin: Springer), 29–66. doi: 10.1007/b107143
Miramontes, E., Cattaneo, A., Jouet, G., Théreau, E., Thomas, Y., Rovere, M., et al. (2016). The Pianosa contourite depositional system (Northern Tyrrhenian Sea): drift morphology and Plio-Quaternary stratigraphic evolution. Mar. Geol. 378, 20–42. doi: 10.1016/j.margeo.2015.11.004
Moccia, D., Cau, A., Alvito, A., Canese, S., Cannas, R., Bo, M., et al. (2019). New sites expanding the “Sardinian cold-water coral province” extension: a new potential cold-water coral network? Aquat. Conservat. Mar. Freshw. Ecosyst. 29, 153–160. doi: 10.1002/aqc.2975
Mohn, C., Rengstorf, A., White, M., Duineveld, G., Mienis, F., Soetaert, K., et al. (2014). Linking benthic hydrodynamics and cold-water coral occurrences: a high-resolution model study at three cold-water coral provinces in the NE Atlantic. Prog. Oceanogr. 122, 92–104. doi: 10.1016/j.pocean.2013.12.003
Mueller, C. E., Lundälv, T., Middelburg, J. J., and van Oevelen, D. (2013). The symbiosis between Lophelia pertusa and Eunice norvegica stimulates coral calcification and worm assimilation. PLoS One 8:e58660. doi: 10.1371/journal.pone.0058660
Orejas, C., Gori, A., Lo Iacono, C., Puig, P., Gili, J.-M., and Dale, M. R. (2009). Cold-water corals in the Cap de Creus canyon, northwestern Mediterranean: spatial distribution, density and anthropogenic impact. Mar. Ecol. Prog. Ser. 397, 37–51. doi: 10.3354/meps08314
Orejas, C., and Jiménez, C. (eds) (2019). Mediterranean Cold-Water Corals: Past, Present and Future. Cham: Springer International Publishing.
Otero, M. M., and Marin, P. (2019). “Conservation of cold-water corals in the Mediterranean: current status and future prospects for improvement,” in Mediterranean Cold-Water Corals: Past, Present and Future, eds C. Orejas and C. Jiménez (Cham, CH: Springer International Publishing), 535–545. doi: 10.1007/978-3-319-91608-8_46
Ozer, T., Gertman, I., Kress, N., Silverman, J., and Herut, B. (2017). Interannual thermohaline (1979-2014) and nutrient (2002-2014) dynamics in the levantine surface and intermediate water masses, SE Mediterranean Sea. Glob. Planet. Chang. 151, 60–67. doi: 10.1016/j.gloplacha.2016.04.001
Prampolini, M., Angeletti, L., Grande, V., Taviani, M., and Foglini, F. (2020). “Tricase Submarine Canyon: cold-water coral habitats in the southwesternmost Adriatic Sea (Mediterranean Sea),” in Seafloor Geomorphology as Benthic Habitat, eds P. T. Harris and E. Baker (Amsterdam: Elsevier), 793–810. doi: 10.1016/B978-0-12-814960-7.00048-8
Rebesco, M., and Taviani, M. (2019). “A Turbulent Story: Mediterranean Contourites and Cold-Water Corals,” in Mediterranean Cold-Water Corals: Past, Present and Future, eds C. Orejas and C. Jiménez (Cham: Springer International Publishing), 35–46. doi: 10.1007/978-3-319-91608-8_4
Rees, S. E., Foster, N. L., Langmead, O., Pittman, S., and Johnson, D. E. (2018). Defining the qualitative elements of Aichi Biodiversity Target 11 with regard to the marine and coastal environment in order to strengthen global efforts for marine biodiversity conservation outlined in the United Nations sustainable development goal 14. Mar. Policy 93, 241–250. doi: 10.1016/j.marpol.2017.05.016
Remia, A., Montagna, P., and Taviani, M. (2004). Submarine diagenetic products on the sediment-starved Gorgona slope, Tuscan Archipelago (Tyrrhenian Sea). Chem. Ecol. 20, 131–153. doi: 10.1080/02757540310001642823
Remia, A., and Taviani, M. (2005). Shallow-buried Pleistocene Madrepora-dominated coral mounds on a muddy continental slope, Tuscan Archipelago, NE Tyrrhenian Sea. Facies 50, 419–425. doi: 10.1007/s10347-004-0029-2
Roveri, M. (2002). Sediment drifts of the Corsica Channel, northern Tyrrhenian Sea. Geol. Soc. Lond. Mem. 22, 191–208. doi: 10.1144/GSL.MEM.2002.022.01.14
Rueda, J. L., Urra, J., Aguilar, R., Angeletti, L., Bo, M., García-Ruiz, C., et al. (2019). “Cold-water coral associated fauna in the Mediterranean Sea and Adjacent Areas,” in Mediterranean Cold-Water Corals: Past, Present and Future, eds C. Orejas and C. Jiménez (Cham: Springer International Publishing), 295–333. doi: 10.1007/978-3-319-91608-8_29
Sanfilippo, R., Vertino, A., Rosso, A., Beuck, L., Freiwald, A., and Taviani, M. (2013). Serpula aggregates and their role in deep-sea coral communities in the southern Adriatic Sea. Facies 59, 663–677. doi: 10.1007/s10347-012-0356-7
Schembri, P. J., Dimech, M., Camilleri, M., and Page, R. (2007). Living deep-water Lophelia and Madrepora corals in Maltese waters (Strait of Sicily, Mediterranean Sea). Cahiers Biol. Mar. 48, 77–83.
Schlitzer, R. (2020). Ocean Data View. Available online at: http://odv.awi.de (accessed July 2, 2020).
Sømme, T. O., Piper, D. J. W., Deptuck, M. E., and Helland-Hansen, W. (2011). Linking onshore-offshore sediment dispersal in the Golo source-to-sink system (Corsica, France) during the Late Quaternary. J. Sediment. Res. 81, 118–137. doi: 10.2110/jsr.2011.11
Stanley, D. J., Rehault, J.-P., and Stuckenrath, R. (1980). Turbid-layer bypassing model: The Corsican Trough, northwestern Mediterranean. Mar. Geol. 37, 19–40. doi: 10.1016/0025-3227(80)90010-9
Taviani, M., Angeletti, L., Antolini, B., Ceregato, A., Froglia, C., López Correa, M., et al. (2011). Geo-biology of Mediterranean deep-water coral ecosystems. Mar. Res. CNR 6, 705–719.
Taviani, M., Angeletti, L., Beuck, L., Campiani, E., Canese, S., Foglini, F., et al. (2016). Reprint of “On and off the beaten track: Megafaunal sessile life and Adriatic cascading processes”. Mar. Geol. 375, 146–160. doi: 10.1016/j.margeo.2015.10.003
Taviani, M., Angeletti, L., Canese, S., Cannas, R., Cardone, F., Cau, A., et al. (2017). The “Sardinian cold-water coral province” in the context of the Mediterranean coral ecosystems. Deep Sea Res. Part II Top. Stud. Oceanogr. 145, 61–78. doi: 10.1016/j.dsr2.2015.12.008
Taviani, M., Angeletti, L., Cardone, F., Montagna, P., and Danovaro, R. (2019). A unique and threatened deep water coral-bivalve biotope new to the Mediterranean Sea offshore the Naples megalopolis. Sci. Rep. 9, 1–12. doi: 10.1038/s41598-019-39655-8
Taviani, M., Angeletti, L., Dimech, M., Mifsud, C., Freiwald, A., Harasewych, M. G., et al. (2009). Coralliophilinae (Gastropoda: Muricidae) associated with deep-water coral banks in the Mediterranean. Nautilus 123, 106–112.
Taviani, M., and Colantoni, P. (1979). Thanatocoenoses würmiennes associées aux coraux blancs. Rapp. Comm. Int. Mer. Médit. 25–26, 141–142.
Taviani, M., Freiwald, A., and Zibrowius, H. (2005). “Deep coral growth in the Mediterranean Sea: an overview,” in Cold-Water Corals and Ecosystems, eds A. Freiwald and J. M. Roberts (Berlin: Springer-Verlag), 137–156. doi: 10.1007/3-540-27673-4_7
Toucanne, S., Jouet, G., Ducassou, E., Bassetti, M.-A., Dennielou, B., Angue Minto’o, C. M., et al. (2012). A 130,000-year record of Levantine Intermediate Water flow variability in the Corsica Trough, western Mediterranean Sea. Q. Sci. Rev. 33, 55–73. doi: 10.1016/j.quascirev.2011.11.020
Tunesi, L., Diviacco, G., and Mo, G. (2001). “Observation by submersible on the biocoenosis of the deep-sea corals off Portofino Promontory (Northwestern Mediterranean Sea),” in Proceedings of the First International Symposium on Deep-Sea Corals, eds J. H. M. Willison, J. Hall, S. F. Gass, E. L. R. Kenchington, M. Butler, and P. Doherty (Halifax: Ecology Action Centre Nova Scotia Museum), 76–85.
Vertino, A., Savini, A., Rosso, A., Di Geronimo, I., Mastrototaro, F., Sanfilippo, R., et al. (2010). Benthic habitat characterization and distribution from two representative sites of the deep-water SML Coral Province (Mediterranean). Deep Sea Res. Part II Top. Stud. Oceanogr. 57, 380–396. doi: 10.1016/j.dsr2.2009.08.023
Vetrano, A., Napolitano, E., Iacono, R., Schroeder, K., and Gasparini, G. P. (2010). Tyrrhenian Sea circulation and water mass fluxes in spring 2004: observations and model results. J. Geophys. Res. 115:C06023. doi: 10.1029/2009JC005680
Wienberg, C., Titschack, J., Freiwald, A., Frank, N., Lundälv, T., Taviani, M., et al. (2018). The giant Mauritanian cold-water coral mound province: oxygen control on coral mound formation. Q. Sci. Rev. 185, 135–152. doi: 10.1016/j.quascirev.2018.02.012
WoRMS Editorial Board (2020). World Register of Marine Species. Available online at: http://www.marinespecies.org (accessed July 2, 2020).
Keywords: cold-water corals, Mediterranean Sea, hydrology, biodiversity, contourites, protection
Citation: Angeletti L, Castellan G, Montagna P, Remia A and Taviani M (2020) The “Corsica Channel Cold-Water Coral Province” (Mediterranean Sea). Front. Mar. Sci. 7:661. doi: 10.3389/fmars.2020.00661
Received: 27 December 2019; Accepted: 21 July 2020;
Published: 20 August 2020.
Edited by:
Juan Armando Sanchez, University of Los Andes, Colombia, ColombiaReviewed by:
Nadia Santodomingo, Natural History Museum, United KingdomElena Quintanilla, University of Giessen, Germany
Copyright © 2020 Angeletti, Castellan, Montagna, Remia and Taviani. This is an open-access article distributed under the terms of the Creative Commons Attribution License (CC BY). The use, distribution or reproduction in other forums is permitted, provided the original author(s) and the copyright owner(s) are credited and that the original publication in this journal is cited, in accordance with accepted academic practice. No use, distribution or reproduction is permitted which does not comply with these terms.
*Correspondence: Lorenzo Angeletti, bG9yZW56by5hbmdlbGV0dGlAYm8uaXNtYXIuY25yLml0