- 1Marine and Freshwater Research Centre, Department of Natural Science, School of Science and Computing, Galway-Mayo Institute of Technology, Galway, Ireland
- 2Sorbonne Université, Faculté des Sciences et Ingénierie, Paris, France
- 3Institute of Zoology, Zoological Society of London, London, United Kingdom
- 4Age Dynamics, Lyngby, Denmark
- 5Environment and Sustainability Institute, University of Exeter, Penryn, United Kingdom
- 6Marine Environmental Monitoring, Penwalk, Cardigan, United Kingdom
- 7Scottish Marine Animal Stranding Scheme, SRUC Veterinary Services, Inverness, United Kingdom
Harbor porpoises exhibit early maturation, relatively short gestation/lactation periods and a faster rate of reproduction as compared to other cetacean species. Intrinsic and extrinsic factors can influence both population vital rates and population structure, which ultimately cause changes in dynamics within and between populations. Here, we undertook a retrospective analysis of mortality data collected over a 24-year period for assessing life history traits of the North-east Atlantic harbor porpoise population. We use time-period specific models for key life history relationships that considered cause of death of individuals (as a proxy for health status), sex and management unit (MU). Sexual variation in asymptotic length, asymptotic age, average length at 50% maturity (L50) and average age at 50% maturity (A50) were observed, with females attaining a larger asymptotic length, larger L50, and delaying attainment of both sexual and physical maturity, compared to males. While females are constrained in their minimum body size due to giving birth to proportionally larger offspring, males exhibited more plasticity in size at sexual maturity, enabling re-allocation of available energy resources toward reproduction. Data were then used to compare biological parameters among two porpoise MUs in United Kingdom waters, both of which in the current study exhibited reduced reproductive rates compared to other geographic regions. In both MUs, females significantly increased their A50 and males significantly declined in their L50. An increase in the age at asymptotic length was also observed in both sexes, along with a significant decline in the Gompertz growth rate parameter that was more apparent in the female data. While availability of suitable prey resources may be a limiting factor, a combination of other factors cannot be ruled out. Porpoises in the Celtic and Irish Seas MU were significantly larger in their maximum length, asymptotic length and L50 compared to porpoises in the North Sea MU throughout the study period, suggesting limited gene flow between these two MUs. These results justify the maintenance of these harbor porpoise MUs or assessment units, as two separate units, within the range of the North-east Atlantic population, and for indicator assessments under the EU’s Marine Strategy Framework Directive.
Introduction
Among cetaceans, a fast-slow continuum exists in life history strategies, with some species leading a fast life, i.e., early maturation, relatively short gestation and lactation periods, annual reproduction and dying younger, as observed in the harbor porpoise (Phocoena phocoena) (Read and Hohn, 1995). Other species such the killer whale (Orcinus orca) and short-finned pilot whale (Globicephala macrorhynchus) (Foote, 2008) lead a slow life, i.e., late maturation, longer gestation and lactation periods, extended calving intervals and lifespans. Body size has been recognized as one of the parameters that correlate with life history traits in mammals, which may explain the fast-slow continuum with, for example, larger animals exhibiting traits of a slower life (Calder, 1984; Harvey et al., 1989; Promislow and Harvey, 1990; Harvey and Nee, 1991; Kraus et al., 2005; Dobson and Oli, 2007). However, phylogeny has to be taken into account given that large baleen whales can also exhibit fast lane characteristics, such as early maturation (Lockyer, 1984). After controlling for the effects of body size, the fast-slow continuum can be explained by population mortality rates, including those of specific age-sex classes that have been correlated with life history traits (Harvey et al., 1989; Harvey and Nee, 1991; Kraus et al., 2005). In cetaceans, for example, short-finned pilot whales and resident killer whales have selected for an extension of the female post-reproductive life-span, in contrast to the accelerated mortality rate observed in the long-finned pilot whale (Globicephala melas) that leads to less females surviving to the post-reproductive stage (Foote, 2008) – though all three species typically exhibit other characteristics of a ‘slow’ life. Additionally, ecological conditions need to be borne in mind when considering both species and population-level variations in life history adaptations (Ferguson and Higdon, 2006), as well as changes in local population density (Stoffel et al., 2018).
Population dynamics, such as population growth rates, are affected by both intrinsic (e.g., density dependence) and extrinsic (e.g., pollution, anthropogenic mortality, environmental change, and interspecific competition) mechanisms through altering vital rates and population structure (Wade, 2009; Koons et al., 2016). The harbor porpoise, which exhibits reverse sexual dimorphism (Read and Tolley, 1997), is the smallest cetacean species in the North-east Atlantic. Harbor porpoises live on an energetic knife-edge and are known as the aquatic shrew. They forage during the day and night in order to meet their high metabolic demands due to, among other things, their small size, cold water habitat and feeding on small-sized prey (Wisniewska et al., 2016). Evidence of density-dependent compensatory responses has been observed in porpoise populations. Due to either high bycatch rates in sink gillnet fisheries and/or changes in the availability of herring and their energy content, female porpoises in the North-west Atlantic population attained sexual maturity at a significant younger age and shorter body length during the period 1985–88, compared to 1969–1973 (Read and Gaskin, 1990; Read, 2001). In waters off Greenland, a measured effect of climate change was observed. Harbor porpoises increased their consumption of Atlantic cod (Gadus morhua) during warmer water temperature periods as it became more readily available and this resulted in an improved body condition of porpoises, i.e., larger blubber fat deposits, compared to colder water periods (Heide-Jørgensen et al., 2011). Work by Murphy et al. (2015) reported a lower pregnancy rate and higher age at sexual maturity in porpoises inhabiting United Kingdom waters compared to other geographic regions. Findings may be attributed to exposure to pollutants possibly impacting, among other things, fetal (and newborn) survival rates, though other extrinsic and/or intrinsic factors may also be at play (Murphy et al., 2010, 2015).
One harbor porpoise population exists in the North-east Atlantic extending from waters off France to Norway, which is characterized by significant genetic isolation by distance, i.e., increasing differentiation with geographic distance (Fontaine et al., 2007). For conservation management purposes, harbor porpoises within the North-east Atlantic have been delineated into five Management Units (MUs), or Assessment Units (AUs) under the European Marine Strategy Framework Directive; (1) Kattegat and Belt Seas, (2) North Sea, (3) western Scotland and Northern Ireland, (4) Celtic and Irish Seas (including French Atlantic waters), and the (5) Iberian Peninsula (ICES WGMME, 2013, 2014; IAMMWG, 2015). Boundaries were based partially on genetic analysis as well as measurements of time-integrated ecological tracers and morphological differences, ICES areas/divisions boundaries, and the spatial extent of human activities (ICES WGMME, 2013, 2014; IAMMWG, 2015; OSPAR, 2017). Subsequent genetic and morphometric analyses of stranded and bycaught porpoises in United Kingdom waters, using samples and data obtained between 1990 and 2002, revealed that porpoises off the southwest United Kingdom were genetically distinct, and of a larger body size, compared to other regions (Fontaine et al., 2017). Although the more recent analysis by Fontaine et al. (2017) showed clear evidence for separate Celtic and Irish Seas, and North Sea MUs, it did not provide a strong justification for a western Scotland and Northern Ireland MU based on genetic structure. Based on new findings, NAMMCO and IMR (2019) re-delineated the boundaries of the three MUs in United Kingdom waters – while still retaining the three units.
Porpoises in both the North Sea MU and Celtic and Irish Seas MU are subject to major anthropogenic threats such incidental capture in fishing gear (Tregenza et al., 1997; ICES WGBYC, 2015, 2018; OSPAR, 2017; NAMMCO and IMR, 2019), pollution (Pierce et al., 2008; Law et al., 2010; Murphy et al., 2010, 2015; Jepson et al., 2016; NAMMCO and IMR, 2019), and disturbance associated with underwater noise (Brandt et al., 2011; Thompson et al., 2013; Pirotta et al., 2014; NAMMCO and IMR, 2019). Thus, estimates of biological parameters are required for each MU where such data are essential for effective implementation of conservation management strategies including a management framework for determining bycatch triggers and limits (Winship, 2009; Murphy et al., 2019b), and also estimating the population consequences of disturbance, e.g., noise (King et al., 2015). From the 1940s onwards, harbor porpoises became scarce in the southern North Sea, English Channel and off the Atlantic coast of north-west France (Smeenk, 1987; Evans, 1992; Reijnders, 1992; Addink and Smeenk, 1999; Camphuysen, 2004; Evans et al., 2008; Jung et al., 2009). Since the mid-to-late 1990s, there has been an observed southern movement/re-distribution of animals in the North-east Atlantic population, including in the North Sea (Camphuysen, 2004; Jung et al., 2009; Hammond et al., 2013) that may have altered life history strategies and traits within each MU. Further, the current re-population of the English Channel, particularly the central Channel (Hammond et al., 2017; Laran et al., 2017) where the division between both MUs occurs (see Figure 1) could be from animals migrating from either MU. Recent analysis has suggested that while the abundance of harbor porpoise in the North Sea has been relatively stable since around 2005, the abundance of porpoises in the Celtic and Irish Seas area may be declining slowly since 2009 – though further work is required to substantiate these results (NAMMCO and IMR, 2019).
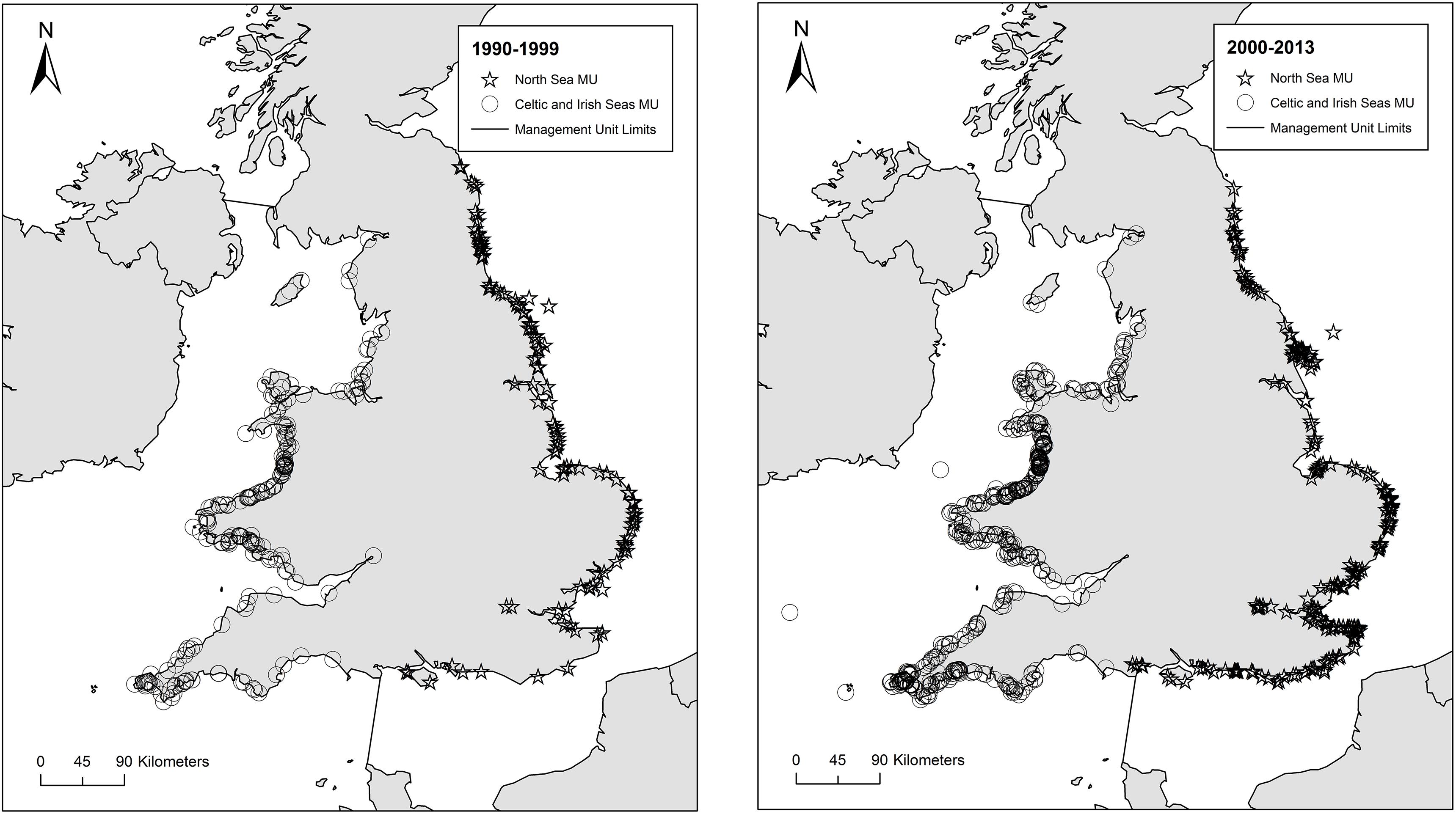
Figure 1. Locations of stranded and bycaught harbor porpoises from the North Sea MU (stars) and Celtic and Irish Seas MU (circles) between (a) 1990 and 1999 and (b) 2000 and 2013. See associated text for n values. Maps were created with ArcGIS version 10.3.1©2015 ESRI.
The current study aims to assess demographic characteristics and determine key biological parameters in male and female harbor porpoises within the North Sea MU and Celtic and Irish Seas MU, and any significant variations among them. In cetaceans, estimations of life history parameters are primarily undertaken using retrospective analysis of mortality samples and data, particularly for more pelagic species, or non-resident species. This study avails of samples and data collected by the UK Cetacean Strandings Investigation Programme (CSIP) over a 24-year period to determine growth parameters, the average age and size attained at sexual maturity, and pregnancy rates of stranded and bycaught harbor porpoises. The dataset was further divided into two time periods to assess temporal variation in said parameters – time periods reflected available samples and were based on data/samples collected before and after the main period of re-distribution in the North-east Atlantic population. Using time-period specific models for key life history relationships, considering causes of death of individuals, sex and management unit, the study aims to identify what changes may have occurred within that 24-year period. Further, we discuss the justification for maintaining these separate MUs, even considering the large-scale movements of animals within the range of the North-east Atlantic population.
Materials and Methods
Samples
Harbor porpoises assessed within the current project were collected along English and Welsh coastlines, or from fisheries, and necropsied by the UK Cetacean Strandings Investigation Programme following European Cetacean Society guidelines on gross necropsy and tissue sampling protocols (Kuiken and Garcia Hartmann, 1991). Analyses utilized data and samples collected from 1226 individuals sampled between 1990 and 2013, comprising 188 females and 236 males from the North Sea MU and 400 females and 402 males from the Celtic and Irish Seas MU (see Figure 1). To assess temporal changes in biological parameters the dataset was divided into two time periods 1990–1999 and 2000–2013, as described previously.
Data collected on each individual included date and location of the stranding/bycatch event, decomposition code, sex, and body length. Causes of death were determined by diagnostic criteria (Jepson et al., 2005; Deaville and Jepson, 2011). Females were identified as sexually mature if one or more corpora (lutea or albicanta) were present on their ovaries (Murphy et al., 2015), and sexual maturity in males was assessed based on histological analysis of gonadal tissue (after Murphy et al., 2005). Age was determined by counting dentinal growth layers groups present in the teeth of the animal, outlined in Murphy et al. (2014). As teeth were processed and aged by a number of different researchers throughout the study period, cross-calibration exercises were carried out to ensure consistency in age readings. Age was determined for 645 individuals. Period 1 (1990–1999) represented a 10-year period with a large age sample size (n = 372) and sufficient data per year. Period 2 (2000–2012) represents a 13-year period with a smaller age sample size (n = 273), particularly for the years 2006 to 2012.
Growth Model
Non-linear least squares were used to fit Gompertz growth models (Gompertz, 1825) with a period effect on the parameters of the model. The Gompertz growth model was fit assuming first that for each animal i:
Where Li is the body length of animal i, indices denote: MU j, time period k and sex s; a is the mean asymptotic body length; b is the displacement parameter; and c is the growth rate parameter; ti is the age (years) of animal i. Note that Equation (1) is the full model where all parameters can vary by management unit, time period and sex. All combinations of sub-models, where the parameters are constrained by grouping variable, were fit and finally the best fitting model for inference chosen via corrected Akaike Information Criterion AICc. All growth models were fit using the nls procedure in R (R Core Team, 2019), assuming normally distributed errors. Residual diagnostics were checked for departures from normality and independence. For seven of eight sexes, management units and time periods, Shapiro-Wilk’s tests failed to reject a normal distribution assumption. For males in the Celtic and Irish Sea in the period 1990–1999 there were more large-sized one-year old males than the model predicted. Given that only one age class of this group of individuals were not normally distributed, we retain the overall normal distributional assumption across all groups. Durbin-Watson tests for autocorrelation indicated no autocorrelation in the residuals for all groups, except for females in the Celtic and Irish Seas for the period 1990–1999 (DW = 1.65, p-value = 0.034). As a plot of the autocorrelation for this group indicated no significant correlation at lag 1, we therefore concluded that there was weak evidence for autocorrelation in this group and retain an independent assumption.
Average Age or Length Attained at Sexual Maturity
Age at sexual maturity, or age at 50% maturity (A50), was determined using a binomial logistic regression fitted to age and sexual maturity status data. The binomial distribution with a logit link is commonly used for modeling binary data (Mccullagh and Nelder, 1989), and is thus employed within the current study. A Generalized Linear Model (GLM) was used with covariate effects on the probability of being sexually mature. GLMs were fitted to the binary maturity observations with age, management unit, time period, sex and cause of death as explanatory variables. All two-way interactions were allowed in the first models and compared. All valid sub-models were fit, and the best fitting model used for inference chosen by the lowest AICc. Age at 50% maturity was estimated from the best fitting model by the negative of the slope over the intercept of the estimated logit (Venables and Ripley, 2002).
Length at sexual maturity, or length at 50% maturity (L50), was determined using the same methodology described for A50, but including length as the predictor variable. Following Murphy et al. (2015), causes of death were categorized in three groups: infectious disease, trauma (bycatch, boat/ship strike, bottlenose dolphin attacks, and dystocia) and others (live stranding, starvation, neoplasia, and not established).
The average age at attainment of sexual maturity (ASM) and its variance was also determined using the sum-of-fraction immature algorithm (SOFI) (Hohn, 1989) for each cause of death group including data from both management units and time periods.
Where, if Ii ≠ Ni, pi = Ii/Ni, and qi = (Mi)/Ni; if Ii = Ni,, pi = (Ii − ½)/Ni, and qi = (Mi + ½)/Ni, and if Mi = Ni, pi = (Ii + ½)/Ni, and qi = (Mi−½)/Ni.
Confidence interval (at p = 0.05) = ASM ± 1.96 √s2
j = the first indeterminate age class
k = the last indeterminate age class
pi = fraction of immature specimens in age class i
qi = fraction of mature specimens in age class i (pi + qi = 1)
Ii = number of immature specimens in age class i
Mi = number of mature specimens in age class i
Ni = number of specimens in age class i (Ni = Ii + Mi)
In order to calculate the average length attained at sexual maturity (LSM), the SOFI method was modified to use constant length intervals (5 cm) instead of ages, after Danil and Chivers (2007).
j = the lower limit of the length class with the smallest mature animal
imin = length class with the shortest mature animal
imax = length class the longest mature animal
pi = proportion of immature animals in length class i
xi = proportion of length classes combined in length class i
ni = total number of animals in the ith length class
xi = interval width of length class i
ni = total number of animals in the ith length class
Pregnancy Rates
The pregnancy rate (PR) was estimated by calculating the proportion of pregnant females in the sexually mature sample. Females that were sampled during the mating/conception period for the species in United Kingdom waters (May-September; Learmonth et al., 2014) were not included in the analysis, due to the increased possibility that embryos or small fetuses were not detected during early stages of gestation (Murphy et al., 2015).
Results
Sample Statistics
Within the whole sample of 1226 individuals, 578 individuals (47.15%) died as a result of trauma, 257 individuals (20.96%) died from infectious diseases, and 391 individuals (31.89%) were classed as ‘other’ causes of death such as live stranding, starvation, neoplasia, or was not established.
Harbor porpoises in United Kingdom waters are a relatively young population, with an observed increase in the mean age of individuals during the study period that was more evident in North Sea porpoises. Although a maximum age of 22 years was reported, approximately 80% of necropsied porpoises were ≤5 years old, and only 5% were aged 12 years or older (n = 645). Maximum age reported in North Sea porpoises declined during the whole study period from 22 to 16 years, however, mean age increased in both sexes, which was statistically significant for males (p = 0.012, see Table 1). Within the Celtic and Irish Seas MU, an increase in maximum age was observed in females (15 to 21 years) but declined in males (18 to 15 years). Although mean age increased in both sexes, this was not statistically significant (see Table 1).
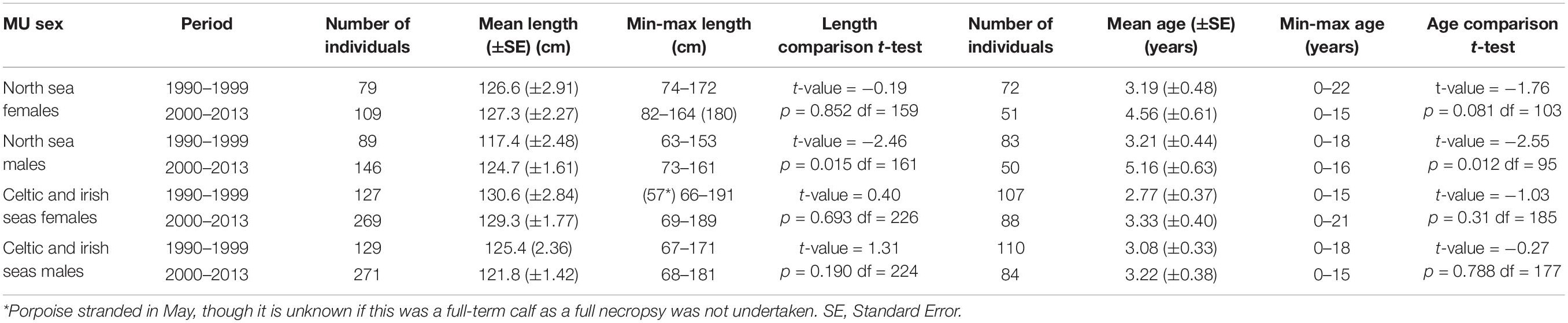
Table 1. Results of t-tests comparing temporal variations in mean lengths and mean ages for female and male harbor porpoises in the North Sea MU and Celtic and Irish Seas MU for the two time periods, 1990–1999 and 2000–2013.
Age-frequency distributions of harbor porpoise MUs shows the overall dominance of young individuals but with some changes between time periods (Figure 2). The number of aged individuals <1-year-old was almost double during the first period in both MUs (42 vs. 20 in the North Sea; 72 vs. 40 in the Celtic and Irish Seas). In the North Sea MU, more young harbor porpoises (≤5 years) were necropsied during the first period (1990–1999; 80%) compared to the second (2000–2012; 62%). Whereas, in the Celtic and Irish Seas MU, the number of individuals aged 5 years or less between the two time periods did not change (79 vs. 79%).
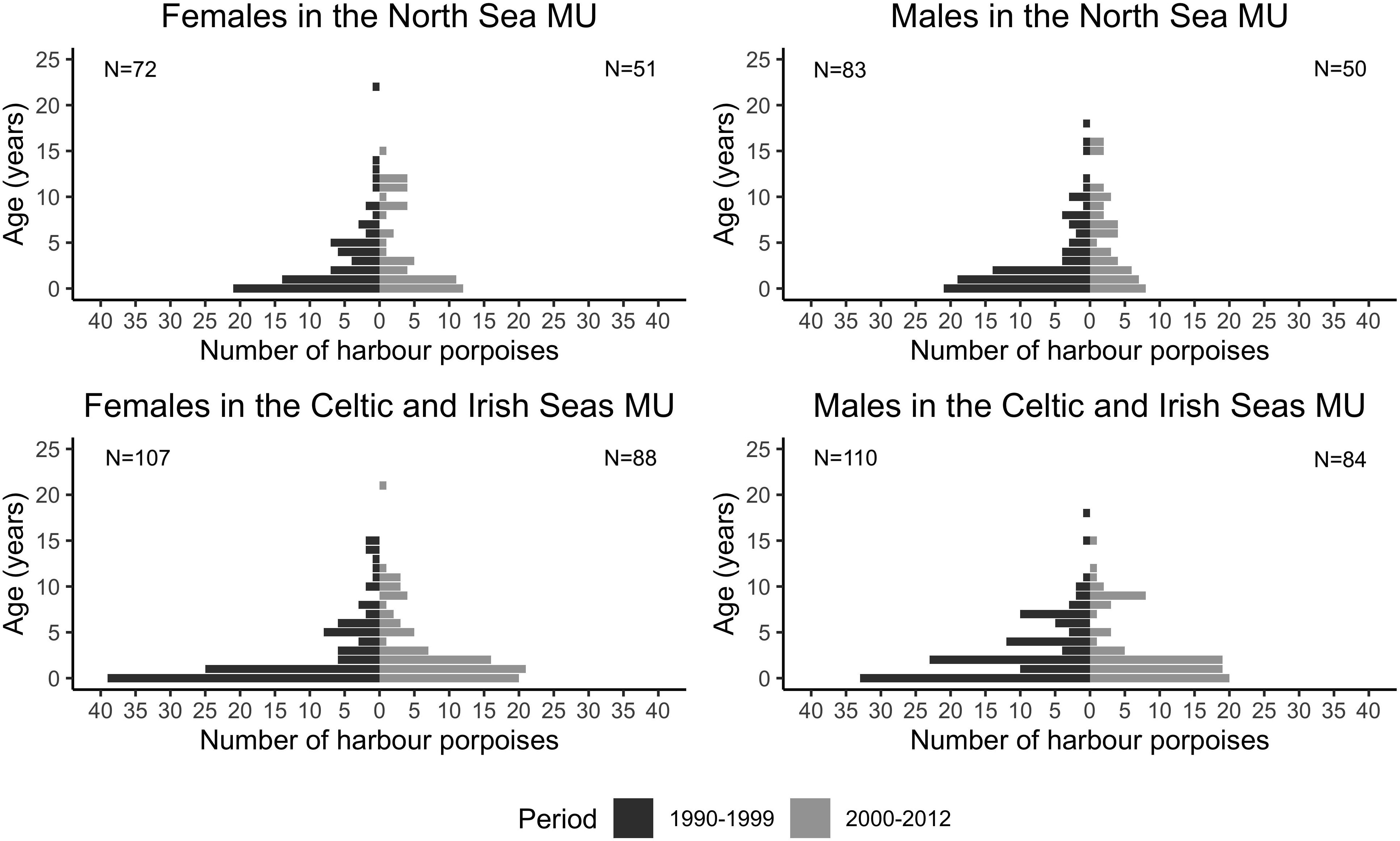
Figure 2. Age-frequency distribution and sample sizes of female and male harbor porpoises sampled within the North Sea MU and Celtic and Irish seas MU during the two time periods, 1990–1999 and 2000–2012.
Growth
Age and length data from 638 harbor porpoises were available for estimations of growth parameters and assessing temporal changes in said parameters within the study period. Reverse sexual size dimorphism was evident in the species and was observed in both MUs (comparing best fitting model with sex-generic asymptote test: F = 22.41, df1 = 9, df2 = 11, p < 0.001; see Table 2 and Figure 3). Female porpoises attained an asymptotic size 10.2% larger than males in the North Sea MU and 11.2% larger than males in the Celtic and Irish Seas MU. Sexual size dimorphism (SSD) ratios (male asymptotic length/female asymptotic length) of 0.91 and 0.90 were obtained for the North Sea, and Celtic and Irish Seas MUs, respectively.
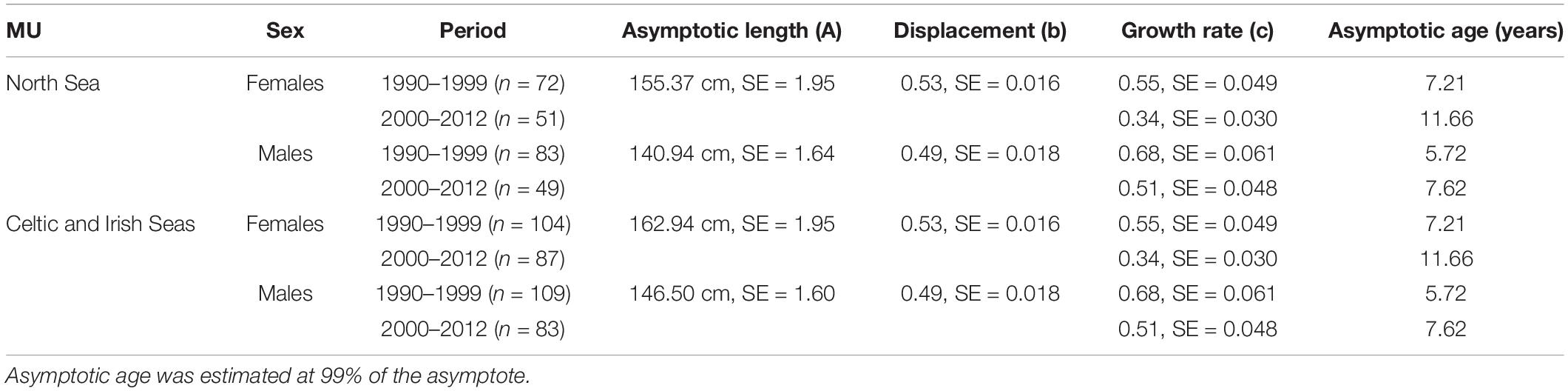
Table 2. Asymptotic length (A), displacement (b), and growth rate (c) and their respective standard errors (SE) estimated using the Gompertz growth model for female and male harbor porpoises in the North Sea MU and Celtic and Irish Seas MU for the two time periods (and a year-based approach), 1990–1999 and 2000–2012.
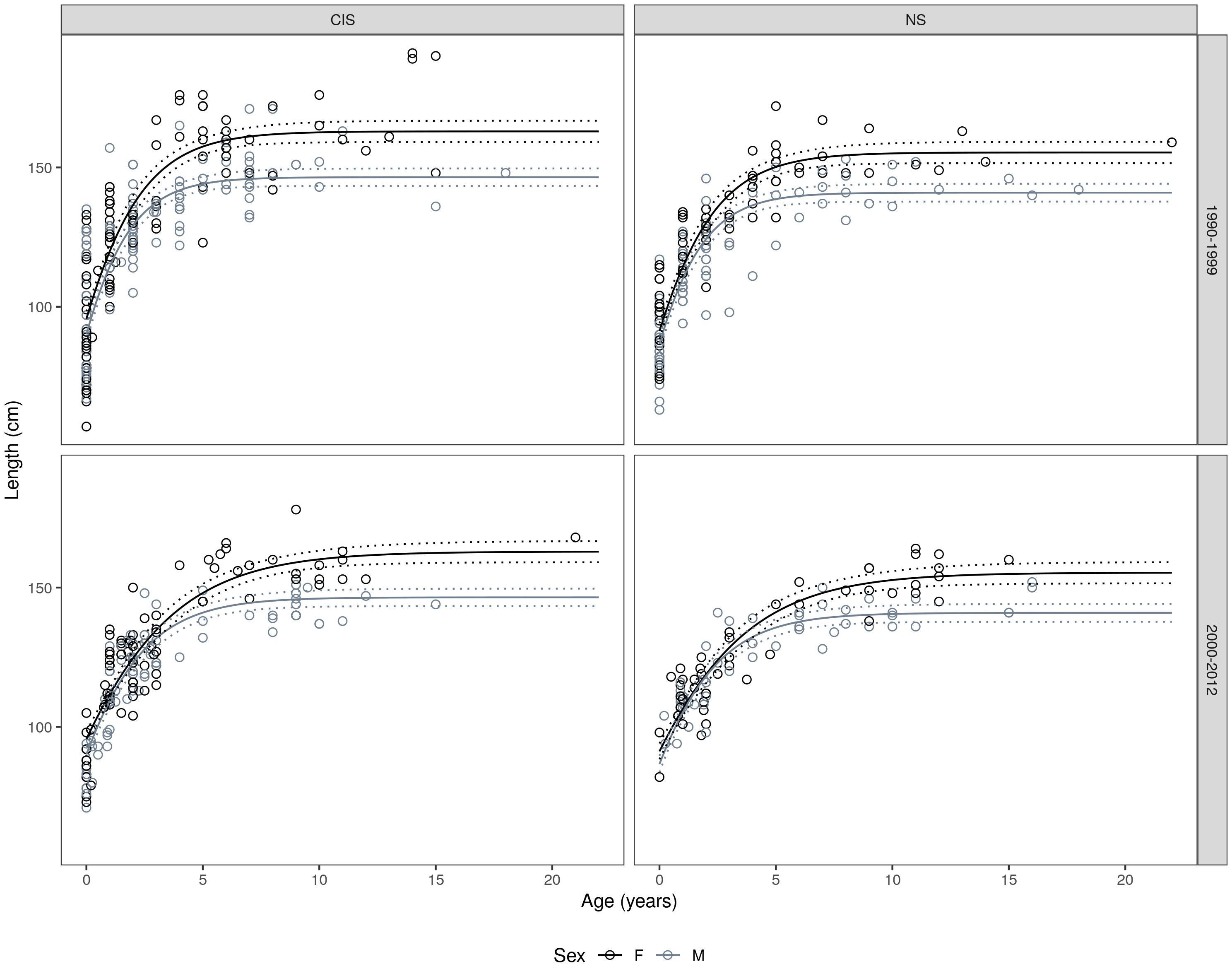
Figure 3. Gompertz growth models fitted to the length-at-age data; fitted by management unit, time period, and sex (see Table 2 for n values and growth parameters). Stippled lines represent the 95% confidence intervals for the growth curves.
A large scatter in the length of neonates was observed in Period 1 (see Figure 3). This was attributed to individuals less than 1 year old being assigned an age of zero, whereas during period 2 individuals less than 1 year old were assigned ages of 0, 0.25, 0.5, and 0.75, depending on the deposition of the first growth layer group in the dentine tissue. Resulting from this, single growth curves were used to describe growth in the species – though earlier work on harbor porpoises in the Sea of Azov and the Black Sea recommended the application of a two-stage growth model for the species in some cases, with the intersection point ranging between 1 to 2 years, depending on the area and sex (Gol’din, 2004).
For both sexes, significant geographic variation in the asymptotic lengths were observed between MU’s (F = 15.325, df0 = 9, df1 = 11, p < 0.001). Females attained a larger asymptotic size in the Celtic and Irish Seas (CIS) MU, compared to the North Sea (NS) MU (162.9 vs. 155.4 cm), and a similar picture was observed in males (CIS: 146.5 vs. NS: 140.9 cm) (Figure 4). Best fitting growth model included MU and sex differences on the mean asymptotic length; sex-specific displacement parameter effects; and sex-specific and time period differences in the growth rate (Table 2).
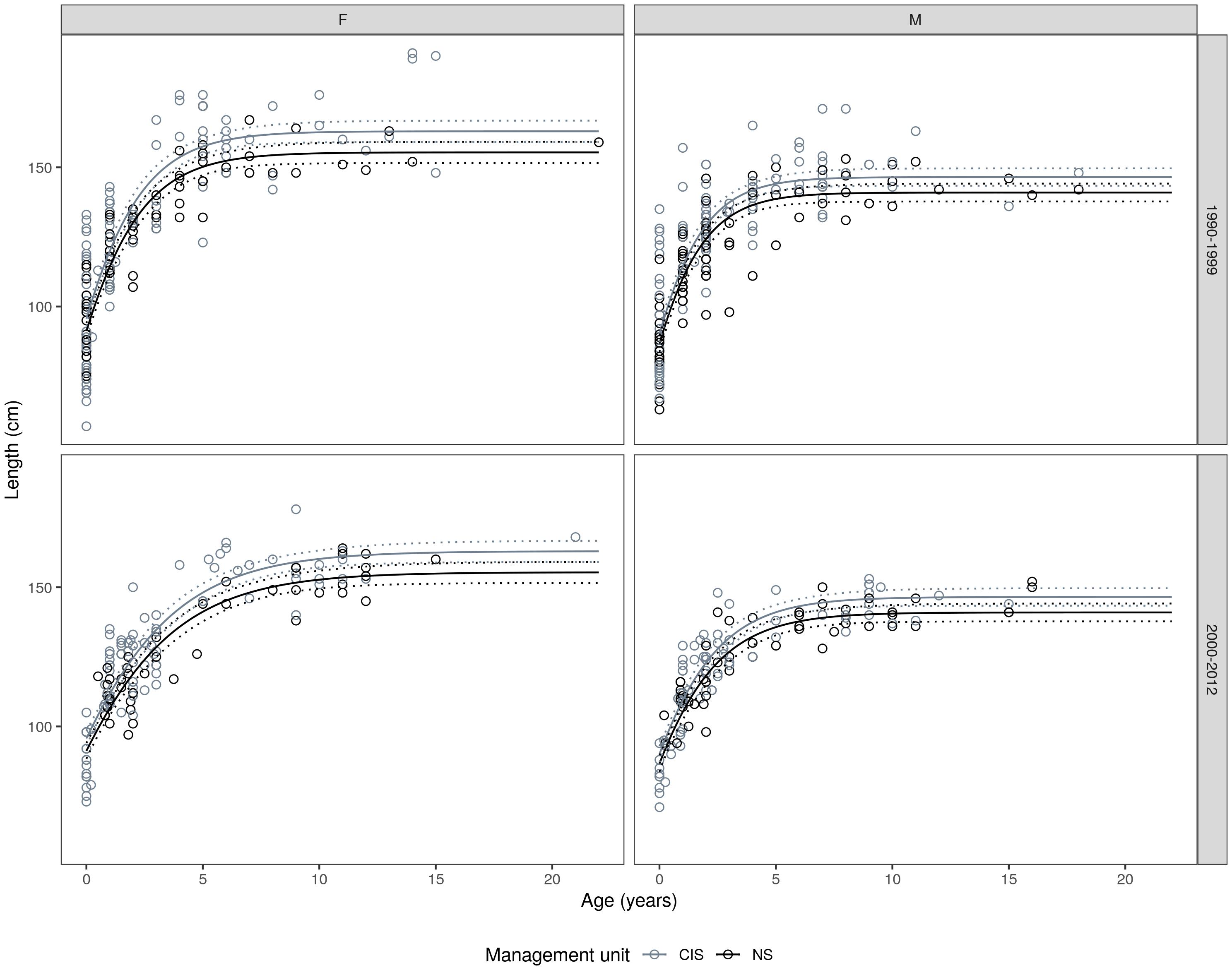
Figure 4. Gompertz growth models fitted to the length-at-age data; fitted by sex, time period, and management unit (see Table 2 for n values and growth parameters). Stippled lines represent the 95% confidence intervals for the growth curves.
In both MUs, and for both sexes, a decline in growth rates (parameter c) was observed which was more apparent in the female data (Table 2). Although no significant temporal variation in the asymptotic length was observed in either sex when comparing between time-periods within each MU (F = 1.140, df0 = 11, df1 = 15, p = 0.337), what was apparent is at a given age, porpoises from period 1 (1990–1999) were of a larger size compared to porpoises in period 2 (2000–2012) (see Figure 5). This was more pronounced in female and male porpoises from the Celtic and Irish Seas MU, and female porpoises in the North Sea MU.
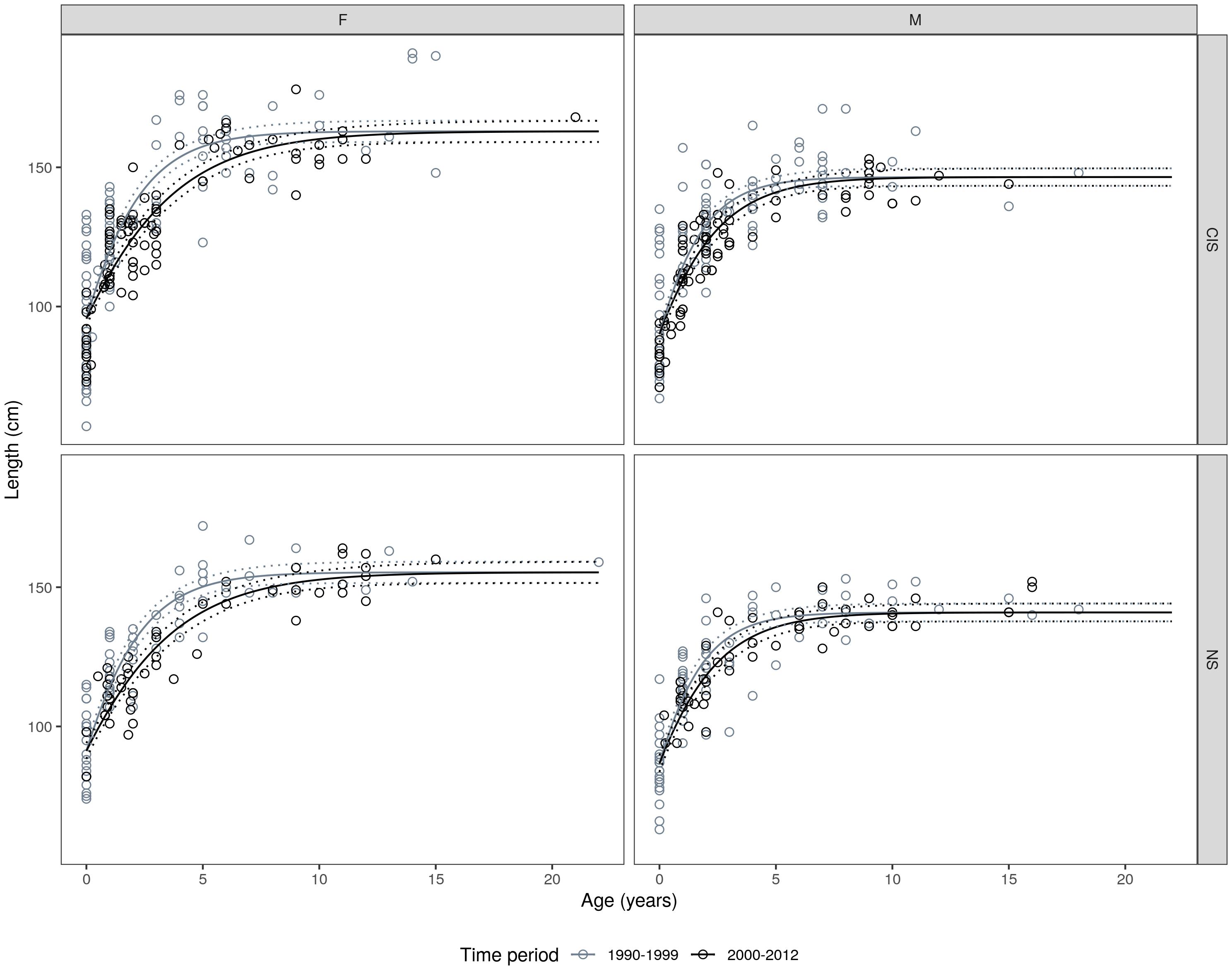
Figure 5. Gompertz growth models fitted to the length-at-age data; fitted by sex, management unit, and time period (see Table 2 for n values and growth parameters). Stippled lines represent the 95% confidence intervals for the growth curves.
Asymptotic age, defined as the age at 99% of the asymptote, was estimated and females attained asymptotic length at an older age compared to males (varying between 2 and 4 years) in both MUs and time-periods (see Table 2). Similar asymptotic ages were estimated for both sexes between MUs, and both MUs showed an increase in asymptotic age over time.
Age or Length at Sexual Maturity
Age and sexual maturity status data were available for 556 harbor porpoises collected between 1990 and 2012. The best fitting model for A50 maturity had a sex and time period specific intercept and a common age effect (slope). There was no difference between management units in the A50 for either sex with MU retained only in the fourth best fitting model with a difference of 2.03 AICc units from the best fitting model. The effect of sex was significant when tested against a null model with time period-specific intercepts (omitting the sex effect) (D = 8.175, df = 2, p = 0.017). Additionally, the effect of time period was significant when tested against a null model with sex-specific intercepts (omitting the period effect) (D = 6.89, df = 2, p = 0.032). Female harbor porpoises attained sexual maturity at an older age than males, which was more evident in period 2 (Table 3 and Figure 6). In males, ages at 50% maturity were similar between time periods, 3.56 years in period 1 and 3.62 years in period 2. Whereas females attained an A50 a year later in period 2 in both management units; 3.8 years in period 1 and 4.8 years in period 2 (Figure 7, Table 3, and Supplementary Material S1).
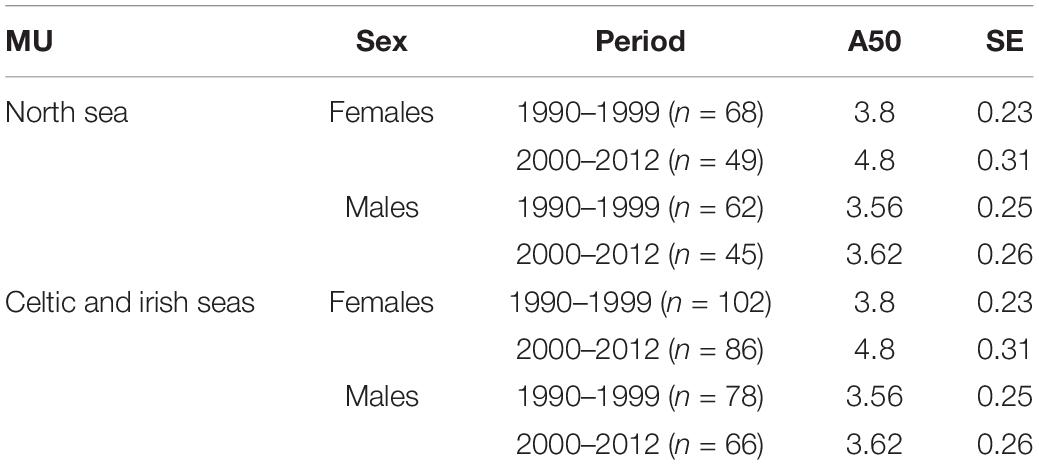
Table 3. Age at 50% maturity (A50) for female and male harbor porpoises within the North Sea MU and Celtic and Irish Seas MU for two time periods, 1990–1999 and 2000–2012.
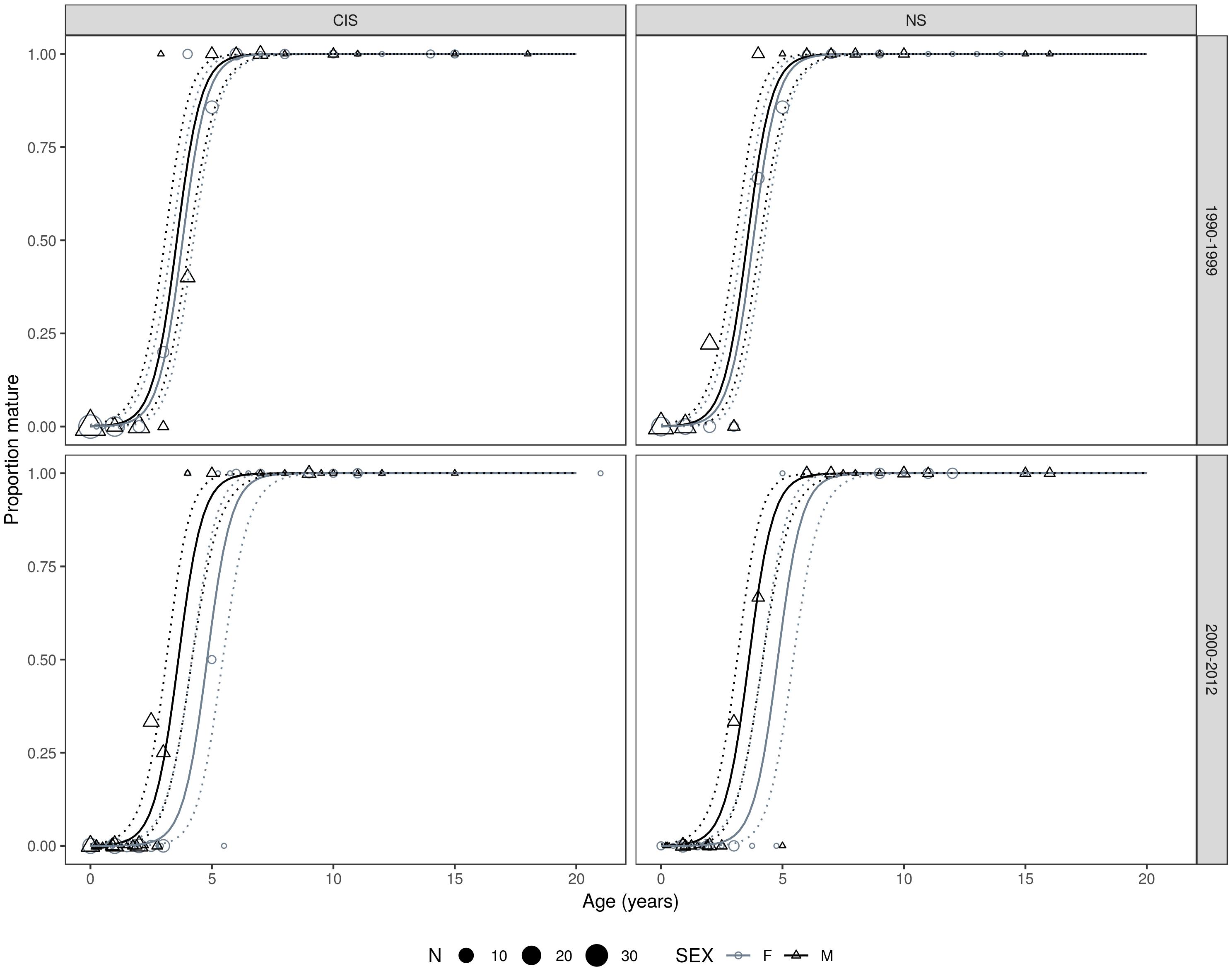
Figure 6. Best fitting age (years) at maturity model fits by management unit, time period, and sex. Points are scaled proportional to the number of observations for a given proportion. Solid and dashed curves represent the mean and 95% confidence intervals on the estimated proportion mature, respectively.
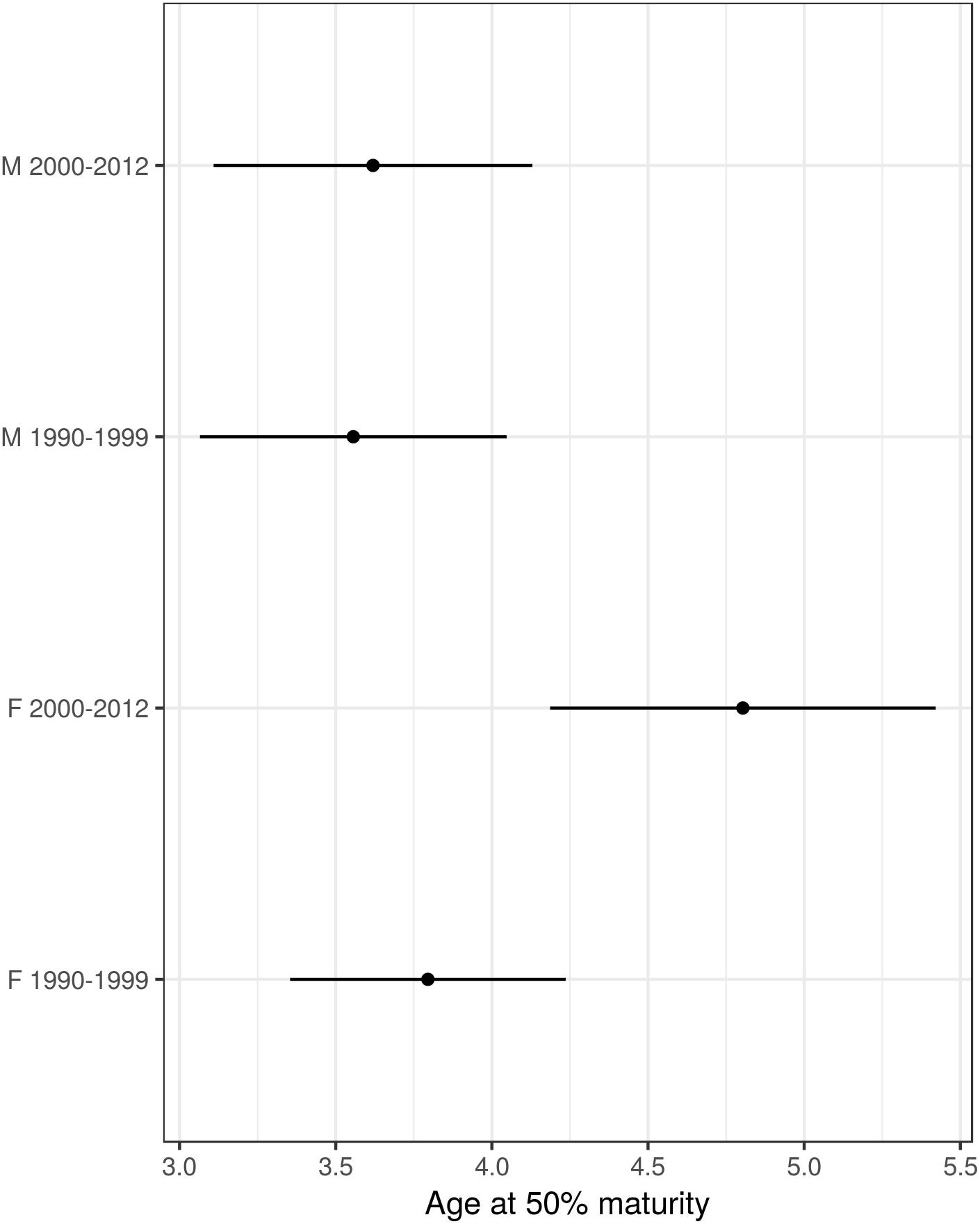
Figure 7. Age at 50% maturity (years) estimates from the best fitting maturity model for age (years) (common age effect and separate intercepts by sex and time period). Points represent the mean value with horizontal lines denoting the 95% confidence intervals.
Although an individuals’ overall health status may affect when they attain sexual maturity (i.e., delayed attainment of sexual maturity in individuals with poor nutritional and/or health status), cause of death did not appear in the top ten best fitting models (with a difference in AICc from the best fitting model of 3.22). To assess further, the average age at attainment of sexual maturity (ASM) and its variance was determined using the sum-of-fraction immature algorithm (Hohn, 1989) for each cause of death group including data from both management units and time periods. For females, a comparable ASM was determined for animals that died from trauma (4.39 years, SE = 0.07) and infectious disease (4.67 years, SE = 0.19). An ASM could not be determined using the SOFI method for the ‘other’ cause of death group, as insufficient data were obtained for the indeterminant age classes. In males, a younger ASM was determined for animals that died from trauma (3.5 years, SE = 0.05). Whereas, an older ASM was estimated for both the infectious disease (4.41 years, SE = 0.13) and ‘other’ cause of death groups (4.17 years, SE = 0.27), though this was based on a very small sample sizes of individuals within the indeterminate age classes (n = 15 and 5, respectively) (see Supplementary Material S2).
Length and sexual maturity status data were available for approximately 74% (n = 902) of the harbor porpoise sample, and the temporal variations in L50 were assessed using data collected between 1990 and 2013. The best fitting model for L50 had main effects of time period, sex and MU and an interaction between time period and sex on the intercept and stock-specific slopes over length. Including cause of death as a covariate resulted in a model that was ranked 14th among all tested models with a difference of 2.07 AICc units from the best fitting model.
Female harbor porpoises attained sexual maturity at a larger size than males, which was more apparent in period 2 particularly within the Celtic and Irish Seas MU (Table 4 and Figure 8). Highly significant effects of MU were found when comparing the best fitting model against a null model that omitted MU (D = 95.13, df = 4, p < 0.001). Harbor porpoises in the Celtic and Irish Seas attained a L50 at a larger body size than individuals in the North Sea (Figure 9). Female porpoises in the Celtic and Irish Seas obtained a L50 at 146.9 cm in period 2 compared to 139.2 cm in the North Sea. Whereas male porpoises in the Celtic and Irish Seas obtained a L50 at 133.5 cm in period 2 compared to 129.5 cm in the North Sea. For males the effect of time period was significant when tested against a null model omitting time period for the male only data (D = 6.72, df = 1, p = 0.01). In both MUs, males declined in their L50 during the study period – attaining a smaller L50 in period 2 (2000–2013) compared to period 1 (1990–1999) (Table 4, Figure 10, and Supplementary Material S3). Whereas for the female-only data, no significant differences were found between the time periods (D = 0.176, df = 1, p = 0.675) (Figure 10 and Supplementary Material S3).
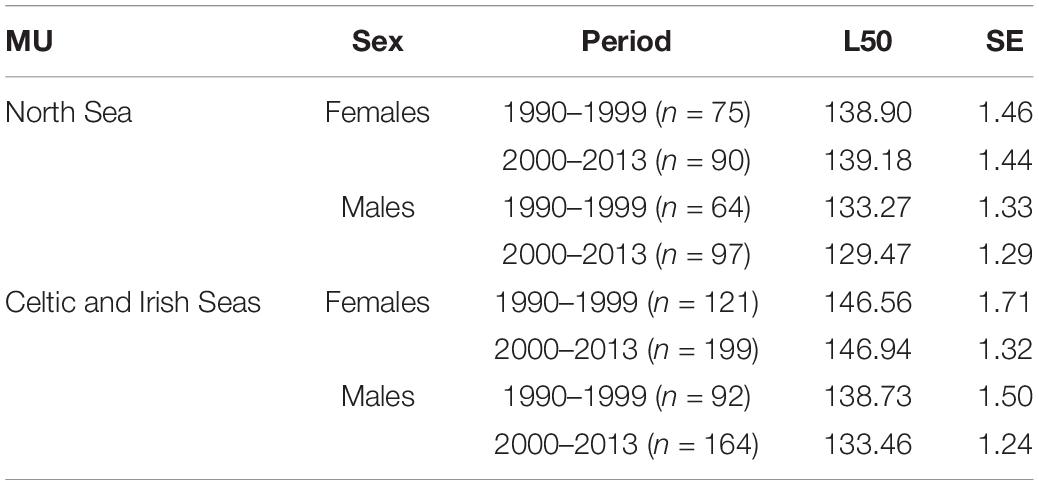
Table 4. Length at 50% maturity (L50) for female and male harbor porpoises within the North Sea MU and Celtic and Irish Seas MU for two time periods, 1990–1999 and 2000–2013.
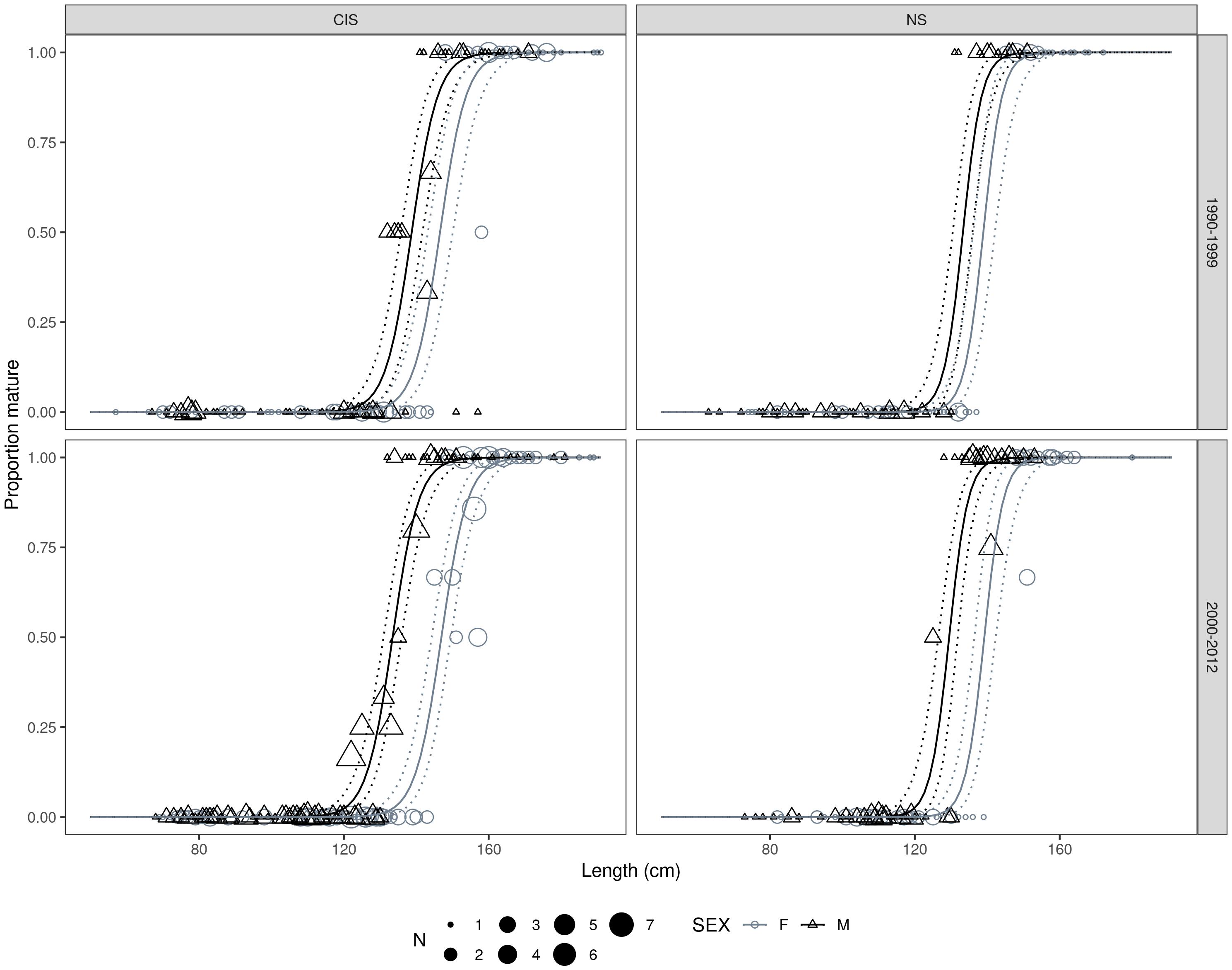
Figure 8. Best fitting length (cm) at maturity model fits by management unit, time period, and sex. Points are scaled proportional to the number of observations for a given proportion. Solid and dashed curves represent the mean and 95% confidence intervals on the estimated proportion mature, respectively.
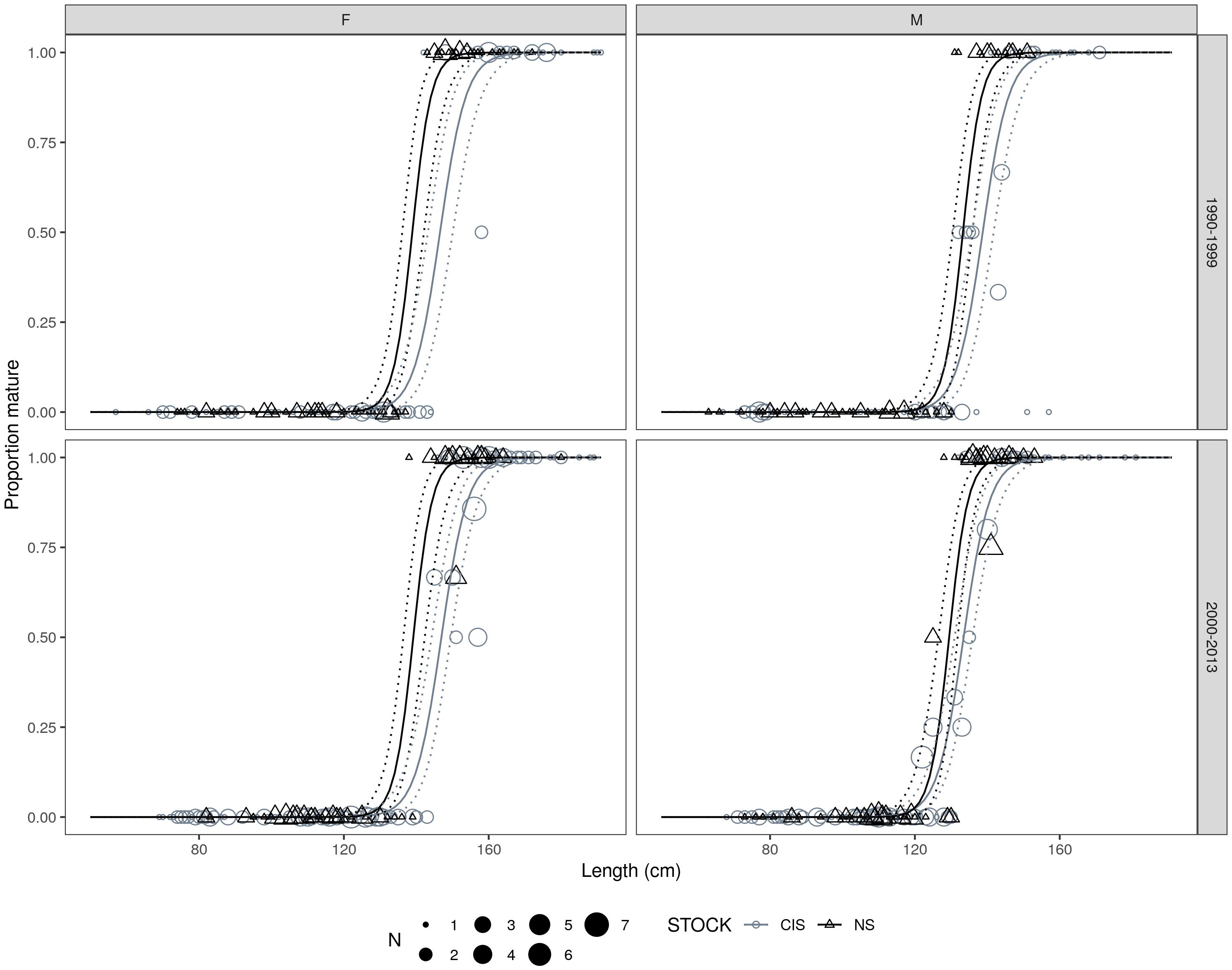
Figure 9. Best fitting length (cm) at maturity model fits by sex, time period, and management unit. Points are scaled proportional to the number of observations for a given proportion. Solid and dashed curves represent the mean and 95% confidence intervals on the estimated proportion mature, respectively.
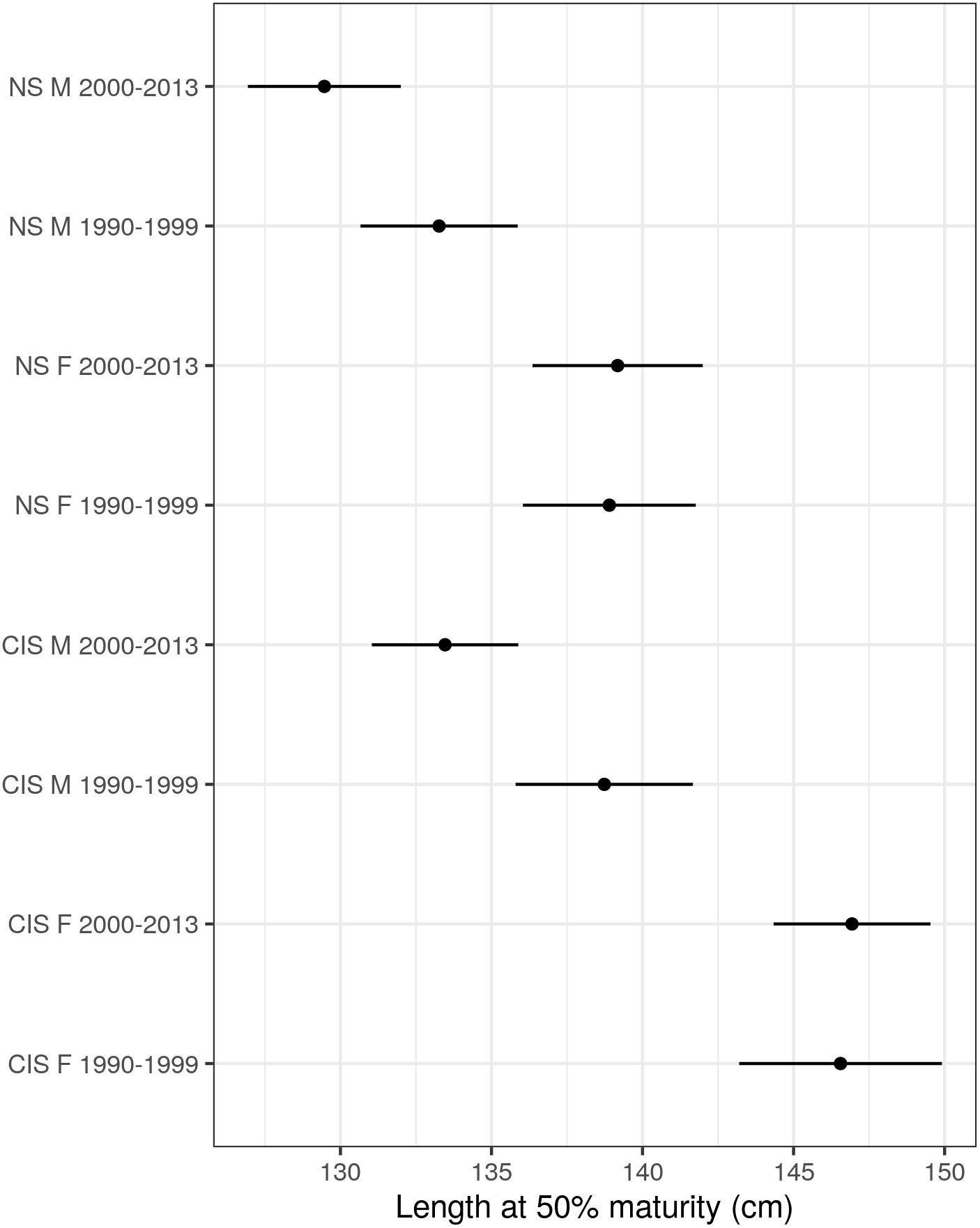
Figure 10. Length at 50% maturity (cm) estimates from the best fitting maturity model for length (common length effect and separate intercepts by sex, time period, and management unit). Points represent the mean value with horizontal lines denoting the 95% confidence intervals.
Pregnancy Rates
Using data from all English and Welsh waters that were obtained outside the conception period, a pregnancy rate of 47% was estimated. There was a significant difference in the proportion of pregnant females in the Celtic and Irish Seas sample compared to the North Sea sample; with an overall estimate of 60% for the Celtic and Irish Seas compared to just 29% for the North Sea (χ2 = 9.765, df = 1, p = 0.002, n = 102; Table 5). Differing results between MUs were attributed to the sample composition as 78% of the North Sea sample for estimation of the pregnancy rate died either from infectious disease or ‘other’ causes. Whereas the Celtic and Irish Sea sample was largely composed of animals that died as a result of trauma (60%). A decrease (though not significant) was observed in the proportion of pregnant females in Celtic and Irish Seas sample, declining from 68% in period 1 to 54% in period 2 (χ2 = 1.143, df = 1, p = 0.285, n = 60), and a slight non-significant increase was observed in the proportion of pregnant females in the North Sea sample between both periods, increasing from 26% in period 1 to 30% in period 2 (χ2 = 0.083, df = 1, p = 0.769, n = 42; see Table 5).
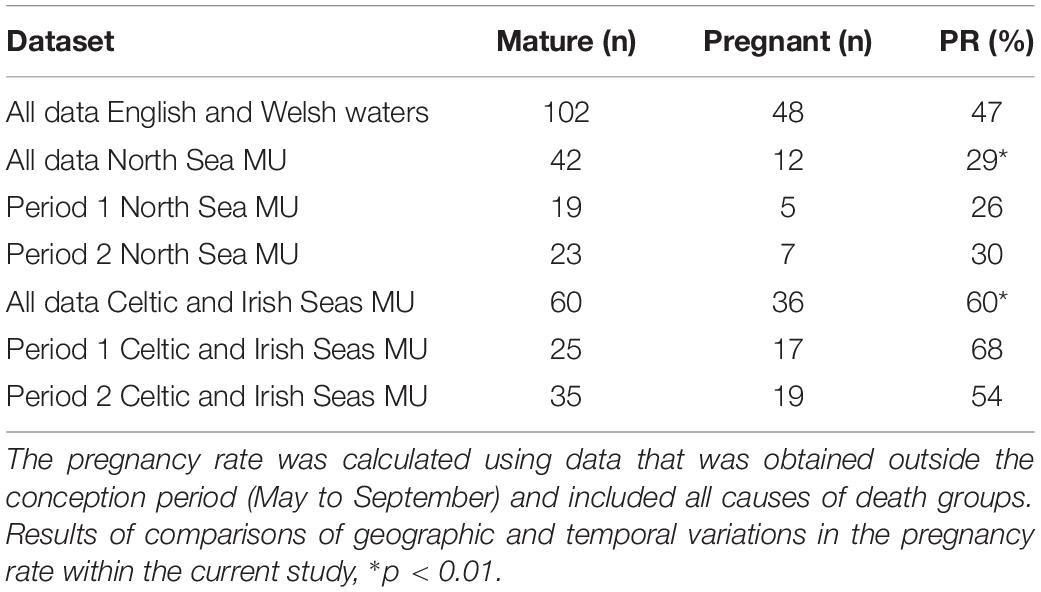
Table 5. Estimated pregnancy rates (PR: percentage pregnant) using all data, and separately for both MUs and time periods.
Discussion
Life History Traits of Harbor Porpoises
Harbor porpoises exhibit reverse sexual size dimorphism with females attaining a larger asymptotic size (Read and Hohn, 1995; Read and Tolley, 1997; Galatius, 2005), between 10.2 and 11.2% larger in the current study, due to an extended period of growth compared to males. Females also attained asymptotic size at an older age than males – between 2-to-4 years older in the current study, depending on the MU and time period. In both MUs and for both time periods, males exhibited faster growth rate parameters than females (Table 2). This was notable during the early phase of development, particularly during period 2 (2000–2012), following which, growth rates slowed down in males (compared to females) just prior to the onset/attainment of sexual maturation (see Supplementary Material S4). This resulted in males attaining their asymptotic length not only at a smaller body size, but also a younger age than females (Figure 3). Female-bias in sexual size dimorphism is not unique in cetaceans. In the 13 baleen whale species, females are approximately 5% longer than males in their asymptotic length (Ralls and Mesnick, 2009). The larger-size may enable female mysticetes to store more energy resources during extended non-feeding periods, which is essential for maintenance of pregnancy and lactation at that time (Ralls and Mesnick, 2009).
Using the Gompertz growth model, female harbor porpoises were longer in body length at a given age, including at birth, which was more pronounced in physically immature individuals during the first period (1990–1999) in the current study (Figure 5). Paedomorphosis (retaining juvenile skeletal proportions and features) has been reported to occur in porpoises, with males exhibiting a greater degree of paedomorphosis relative to females (Barnes, 1985; Galatius and Kinze, 2003; Galatius, 2005). While it has been suggested that porpoises have selected for a smaller body size through paedomorphosis (Barnes, 1985), there may be limits on the minimum body size that female harbor porpoises can attain due to giving birth to proportionally larger sized offspring (Read and Tolley, 1997) – up to 83 cm in length in the current study for a full-term fetus whose mother died as a result of dystocia and stillbirth, though normally maximum neonatal length is 75 cm for the species in this region (Lockyer, 2003). It has been hypothesized that the proportionally larger-size reduces the surface-area-to-volume ratio in newborns, and thus limits both body heat and energy loss (Stuart and Morejohn, 1980; Read and Tolley, 1997; Lockyer, 2003; Galatius, 2005). The thoracic and lumbar regions in adult female harbor porpoises exhibit less paedomorphic body proportions (Galatius, 2005) and also a faster growth rate (in the thoracic region) compared to males (Read and Tolley, 1997), which enables females to carry a large fetus (Galatius, 2005).
Females, on average, attained sexual maturity at a larger body size (5.6 to 13.5 cm larger depending on the MU and time-period) and at an older age (0.2 to 1.2 years older, increasing between period 1 and period 2) than male porpoises in the current study. Similar results were observed in other geographic areas in the North Atlantic (Lockyer, 2003; Read, 2016), apart from waters off Scotland where males attained sexual maturity at an older age than females; 4.35 years in females, 5.00 years in males (Learmonth et al., 2014). As a large proportion of stranded animals assessed in the Scottish study were in a poor health status (28%), it was suggested that this may have biased estimates (upward) of reproductive parameters (Learmonth et al., 2014). While there may be an evolutionary consequence to female porpoises reducing their adult body size at both sexual and physical maturity, males appear to have more plasticity, and even significantly reduced their length at 50% sexual maturity during the sampling period of the current study – while no significant difference was observed in females in this parameter (see Table 4).
Male harbor porpoises compete, more than likely, via sperm competition (Fontaine and Barrette, 1997; Macleod, 2010), and male sexual approaches have been described as ‘rapid,’ ‘high energy,’ and ‘precision timing,’ and include aerial display behaviors (Keener et al., 2018). Thus, selective pressures toward a smaller body size for ease of maneuverability may be a key factor where contest competition is not at play. Further, attaining sexual maturity at a young age can increase a males’ reproductive output, while attaining a smaller length at sexual maturity may enable available energy resources to be re-allocated toward maximizing their number of offspring. Harbor porpoises have been reported to have ‘megatestes’ (Fontaine and Barrette, 1997), with a percentage testes mass of 4% reported in the species (Harrison, 1969; Read, 1990; Fontaine and Barrette, 1997). Thus, the male strategy in harbor porpoises is to grow fast, attain sexual maturation at an early age and minimum body size, which enables individuals to re-allocate their available energy resources to reproduction; due to their higher reproductive demands in maintaining such large testicular mass during the breeding period.
For a cetacean species, harbor porpoises have been reported to live life in the fast lane. Not only do porpoises mature early, they also have relatively short gestation and lactation periods (Read and Hohn, 1995; Learmonth et al., 2014) and in other geographic areas, annual reproduction. For example, pregnancy rates of 93 and 98% were reported in female porpoises in the Gulf of Maine and Bay of Fundy in the North-west Atlantic (1989–1993) (Read and Hohn, 1995) and porpoises off Iceland (1991–1997) (Ólafsdóttir et al., 2003), respectively. Substantially lower pregnancy rates have been reported in other geographic regions, including the current study, where many females are not on a 1-year calving cycle (Hohn and Brownell, 1990; Murphy et al., 2015; Read, 2016). A pregnancy rate of 47% was determined for English and Welsh waters within the current study, which equates roughly to an overall 2-year calving interval for the dataset. An earlier study including samples from English, Welsh and Scottish waters obtained a similar pregnancy rate of 50% for harbor porpoises where only animals that died as a result of trauma were assessed – thus, accounting for potential biases when using stranded (diseased) animals (n = 29 stranded and bycaught porpoises; 1990–2012) (Murphy et al., 2015). While some mature female porpoises may be on a 1 or 2-year calving cycle in United Kingdom waters, many individuals experienced reproductive dysfunction and also reproductive failure through either fetal or newborn mortality (Murphy et al., 2015). Although the underlying mechanism for reproductive failure was not established, it was proposed that exposure to anthropogenic pollutants, such as polychlorinated biphenyls, may have been a contributing factor through causing immunosuppression and/or due to their endocrine disrupting properties (Murphy et al., 2015).
Life history patterns in species and populations are defined not only by phylogeny and body size, but also the amount of energy in the environment and how variable it is (Ferguson and Higdon, 2006), as well as (fluctuating) density dependent selection (Stoffel et al., 2018). Increased resource provision has been attributed to the higher reproductive rates observed in the North-west Atlantic, including a significant decline in age and length at sexual maturity, and a significant increase in calf length (Read and Gaskin, 1990; Read and Hohn, 1995; Read, 2001). In contrast, female harbor porpoises significantly increased their average age at 50% maturity (A50) within the sampling period of the current study in both MUs – while no significant difference was observed in males. This resulted in sexual maturity being attained in females at a considerably older age in period 2 (4.8 years; 2000–2012) than what was reported previously for porpoises in the North-west Atlantic and off Iceland using somewhat comparable methodologies (approx. 3.2 years; Read and Hohn, 1995; Ólafsdóttir et al., 2003). Though recently, a similar A50 was estimated for harbor porpoises inhabiting German waters of the North and Baltic Seas (4.95 ± 0.6 years in both regions; Kesselring et al., 2017). In all United Kingdom waters, the proportion of necropsied harbor porpoise displaying evidence of starvation has been on the increase since the early 2000s (Deaville and Jepson, 2011; Deaville, 2016; Murphy et al., 2019a), and emaciation has been reported as a common cause of death in porpoises found stranded along the Dutch North Sea coast, which all suggests a decline in prey resource availability and/or quality (Leopold, 2015). Analysis of stomach contents of porpoises necropsied in the Netherlands highlighted that periods of decreased quality or quantity of prey can be detrimental to the species, due to its large surface-to-volume ratio (Leopold, 2015).
Another pre-requisite for living life in the fast lane is a shorter lifespan, though this may be strongly coupled with available energy resources (Ferguson and Higdon, 2006). Relatively ‘young’ porpoise populations inhabit the North-east Atlantic and other geographic regions, where few porpoises live to an old age (Read and Hohn, 1995; Lockyer, 2003). The maximum lifespan of porpoises in the North-east Atlantic is 24 years, including a female porpoise in captivity in Denmark at the time of writing, but most individuals in the wild do not live beyond 12-years of age (Lockyer, 2003; Lockyer and Kinze, 2003; Learmonth et al., 2014; this study). Further, between 62 and 80% of porpoises sampled in the current study, depending on the MU and time period, were less than or equal to 5-years of age. Although it cannot be ruled out that sampling biases toward particular age-sex classes as a result of their cause of death may have occurred, what is noteworthy is that the number of aged individuals less than or equal to 1-year of age was approximately double during the earlier period (1990–1999) of the study. Within both MUs, the maximum age declined in both sexes (22 to 16 years), apart from female porpoises in the Celtic and Irish Seas where an increase was observed (15 to 21 years) – though only one female was aged older than 12 years in the second period. In contrast, mean age was reported to increase in both MUs and for both sexes. However, this was only statistically significant for males in the North Sea (3.21 vs. 5.16 years) which may have been due to more young individuals (≤5 years) being necropsied during the first period. This was also reflected in the length profiles of necropsied individuals, where a larger proportion of larger-sized male porpoises from the North Sea were necropsied during the second period (see Supplementary Material S5), resulting in mean body lengths of males increasing significantly from 117.4 to 124.7 cm between periods 1 and 2, respectively (see Table 1).
Status of the Celtic and Irish Seas and North Sea Harbor Porpoise MUs
A comparison of life history parameters between both MUs, Celtic and Irish Seas (including western English Channel and French Atlantic waters) (CIS) and North Sea (including eastern English Channel) (NS), suggests differing life history traits that were apparent throughout the entire sampling period in the current study, even after the observed large-scale distributional movements in the species in the North-east Atlantic. Male and female harbor porpoises inhabiting the CIS were significantly larger in their maximum length, asymptotic length and length at sexual maturity compared to porpoises in the NS (see Tables 1–4). These results showing a divergent life-history pattern among MUs, confirmed those of an earlier genetics study of porpoises in United Kingdom waters that analyzed samples collected between 1990 and 2002, and where the body size of porpoises off the south-west coast of the United Kingdom (as well as some porpoises in the Irish Sea) was significantly larger than other regions, including waters off Scotland and the eastern English Channel (Fontaine et al., 2017). Fontaine et al. (2017) suggested a genetic basis to the size-related trait in porpoises, with observed morphological differences correlated with observed genetic differences in the species. As sampling was extended to 2013 in the current study, results further suggest that gene flow between the CIS and NS MUs is still somewhat limited or that unidirectional gene flow may be maintaining the apparent genetic and phenotypic differences.
Within the North-east Atlantic, the harbor porpoise is typically distributed in continental shelf waters (coastal waters <200 m depth) from the Barents Sea to North Africa. Recent changes in distribution have been observed within the region with a largescale re-distribution of individuals from northern to southern North Sea waters occurring between 1994 and 2005 (Camphuysen, 2004; Thomsen et al., 2006; Hammond et al., 2013, 2017), along with increased sightings in the Celtic Sea (Hammond et al., 2013), and a re-population of the English Channel and French Atlantic waters (Jung et al., 2009; Pikesley et al., 2012; Hammond et al., 2013, 2017; Laran et al., 2017; OSPAR, 2017). This general southward shift in distribution was suggested to be related to changes (declines/shifts) in local prey distribution and/or availability (Camphuysen, 2004; Hammond et al., 2013). Analysis of microsatellite markers revealed the existence of one harbor porpoise population ranging from French waters in the southern Bay of Biscay to arctic waters off Norway and Iceland, including the North Sea (Fontaine et al., 2007). Separate (sub-)populations have been proposed in the Belt Sea and Baltic Sea proper (Wiemann et al., 2010; Galatius et al., 2012; Sveegaard et al., 2015; Lah et al., 2016), whereas, a separate sub-species Phocoena phocoena meridionalis, of a larger-sized morphotype (Donovan and Bjorge, 1995), has been described in Iberian and Mauritian waters (Fontaine et al., 2014). Although the North-east Atlantic population was reported as ‘continuous’ and extending over thousands of kilometers (based on both genetic analyses using microsatellite markers and distributional data), as noted earlier, significant isolation by distance was detected that was more apparent in the southern extent of their range (Fontaine et al., 2007).
Further work by Alfonsi et al. (2012) discussed the return of harbor porpoises to French Atlantic waters during the previous 11-year period and assessed the genetic profile of ‘French’ porpoises using both mtDNA and microsatellite markers. Results revealed two genetically distinct groups and suggested that French Atlantic porpoises were an admixture of individuals from waters further north (northern ecotype), as well as the Iberian-Mauritian sub-species (southern ecotype). Porpoises not only re-distributed southwards within range of the North-Atlantic population, individuals within P. p. meridionalis migrated northwards crossing the putative environmental barriers described in the region for the sub-species, namely the Capbreton canyon (Fontaine et al., 2007, 2010; Alfonsi et al., 2012). Harbor porpoises off the south-west coast of the United Kingdom also exhibit an admixed ancestry of the northern and southern ecotypes, which extends the contact zone for admixed individuals from the northern Bay of Biscay to the Celtic Sea and English Channel (Fontaine et al., 2017). Within all United Kingdom waters, significant isolation by distance was observed in juvenile, but not adult porpoises which suggests reduced dispersal of mature (female) porpoises from natal areas during the breeding period (Fontaine et al., 2017). Thus, explaining the larger-sized morphotype described within the CIS MU in the current study. Why individuals from the southern ecotype are larger in size is unknown. Though, as observed in other species, these individuals may be adapted to dealing with resource shortages (food availability and prey quality) and density independent mortalities, which favors selection of a larger body size (Perrin, 1989; Ferguson and Larivière, 2008; Ferguson et al., 2018), or due to mechanisms for heat conservation (Ferguson et al., 2018). Interestingly, porpoises within the CIS also display reduced genetic diversity resulting from unidirectional gene flow from the southern ecotype, with low genetic diversity, to the northern ecotype (Fontaine et al., 2017). While the abundance of the P. p. meridionalis has been relatively stable over a 12-year period in Iberian waters, abundance is relatively lowl (2,900, CV = 0.32 in 2016 and 2,880, CV = 0.72 in 2005) compared to that of the North-east Atlantic P. phocoena population (Hammond et al., 2017).
Although temporal variation in asymptotic size was not observed for either sex (in either MU), what was apparent was, at a given age (female) porpoises were of a larger size in the 1990s compared to the 2000s and 2010s (see Figure 5). Further, a significant decline in the Gompertz growth rate parameter was observed in both MUs that was more evident in the female data. This is congruent with the decline of a visually discernible ‘larger-sized morphotype’ upon necropsy from the CIS MU during the study period (UK CSIP observation). As the dataset was divided by time period based on year, this may have masked changes that occurred in asymptotic size within the study period, as individuals born during the 1990s, could have died during the 2000s. Thus, to assess further the dataset was divided by cohort, whereby animals were classified depending on the year they were born, based on their ages and year of death. The two time-periods were retained (1990–1999 and 2000–2012), and analysis by cohort resulted in the transfer of 111 individuals from the previous year-based 2000–2012 period to the cohort-based 1990–1999 period. The best fitting model had separate asymptotes by MU and sex, similar to the year-based approach. Period differences were observed in the displacement parameter (‘b’ parameter) (see Supplementary Material S6). As the cohort-based approach significantly reduced the number of individuals available for the analysis within the second period, further work is required to increase the number of aged individuals in the study, in order to improve the resolution of the effects by cohort-based period.
Female porpoises in the CIS MU had a significantly higher pregnancy rate (60%) compared to animals inhabiting the NS MU (29%). However, this may be reflective of sampling biases and the larger proportion of individuals that died from infectious disease or other causes such as starvation, live stranding, neoplasia or not established in the latter MU. Thus, cause of death, which was used a proxy for health status, had significant implications on estimations of the pregnancy rate. Contrastingly, cause of death did not appear in the top ten best fitting models for estimations of either length or age at 50% sexual maturity. Limited work has been undertaken on estimations of reproductive parameters in either MU. Sørensen and Kinze (1994) estimated a higher pregnancy rate of 73% for all Danish waters using samples collected between 1985 and 1991, though the sampling range included waters of the ‘Vulnerable’ western Baltic sub-population (Helcom, 2013). Whereas Winship (2009), estimated a pregnancy rate of 60% for a limited number of ‘healthy’ North Sea porpoises, i.e., died as a result of trauma, that were sampled between 1987 and 2005 by Danish and English fisheries (n = 17 mature females). While this pregnancy rate estimate is comparable to that estimated for the CIS MU in the current study, an updated estimate is required using contemporary data from a larger effective sample size of porpoises sampled throughout the North Sea MU area. In contrast to the observed geographic variation in length-based traits within the current study, there was no significant geographic variation in the A50 among MUs for either sex. Though as noted earlier, a significant temporal increase in the A50 was observed in females in both MUs, increasing by 1 year during the study period (Table 3). Further, an increase in the asymptotic age was also observed in both sexes over the study period – increasing between 2 and 4 years.
While the abundance of harbor porpoises in the NS [355,408 porpoises (CV = 0.225) in 2005; 345,306 porpoises (CV = 0.180) in 2016] and West Scotland/Ireland [44,976 porpoises (CV = 0.317) in 2005; 42,920 (CV = 0.151) porpoises in 2016 (Hammond et al., 2013, 2017; NAMMCO and IMR, 2019)] MUs have remained relatively stable over a 10 year period even in light of the various pressures on individuals in the region (Hammond et al., 2017; NAMMCO and IMR, 2019), the abundance of porpoises in the CIS declined from 88,696 (CV = 0.339) to 35,252 (CV = 0.192) individuals between 2005 and 2016 – though there was insufficient power in the estimates to detect a trend (Hammond et al., 2013, 2017; NAMMCO and IMR, 2019). If the decline in abundance in the CIS MU area is real, it may reflect further movements of porpoises within the range of the North-east Atlantic population, increased mortality and morbidity due to anthropogenic activities, and/or a decline in female fecundity in the region (Murphy et al., 2019a).
Although no significant temporal variation was observed in the pregnancy rate in the CIS MU, the pregnancy rate declined from 68 to 54%. Taken in conjunction with a significant increase in the A50 suggests a decline in the reproductive output in females during the study period. If a real decline in abundance occurred possibly due to anthropogenic activities such as incidental capture in fishing gear, density dependent compensatory responses in life history parameters resulting from increased mortalities would have been expected, which would have increased a female’s reproductive output. Other factors may indeed be at play, including impacts of exposure to anthropogenic toxins, nutritional stress, and/or low genetic diversity, on porpoise reproductive health and fitness. Further genetic analysis of contemporary samples from porpoises in United Kingdom and adjacent waters is required to ascertain if the change in abundance in the CIS MU is due to further movements within the distributional range of the population (Murphy et al., 2019a). Though analysis of demographic parameters in the current study using samples and data collected up to 2013 suggests reduced movements between the CIS and NS management units.
Conclusion
Harbor porpoises are cetaceans that live life in the fast lane, with their reproductive strategy and energetic investment focused on reproducing at a relatively young age and small body size compared to other odontocetes. This was apparent particularly in males, who also exhibited plasticity in their length at sexual maturity. While females, who attain sexual and physical maturity at an older age (due to an extended period of growth) than males and a larger body size, approx. 10–11% larger in asymptotic size in the current study, are constrained in their minimum body size as they need to give birth to proportionally larger-size offspring. Harbor porpoises have relatively short lifespans, and although the species can reproduce annually, geographic variation in demographic parameters is apparent. Substantially lower pregnancy rates and attainment of sexual maturity at a considerably older age was observed in the North-east Atlantic population compared to some other geographic regions. Causes for the reduced reproductive output at a population level could be many. For example, a lack of availability of suitable prey due to either ecosystem-wide changes or the population approaching carrying capacity, and/or exposure to environmental pollutants, that may have endocrine disrupting properties or cause immunodeficiency and increased disease risk (Murphy et al., 2015).
For wide-ranging pelagic species such as the harbor porpoise, undertaking a retrospective analysis of mortality data is often the only source of information on demographic parameters. Although this may incur biases, results of the current study suggested that estimations of the age and length at sexual maturity were not impacted by the overall health status of individuals, though an individual’s health status did impact estimations of the pregnancy rate. Data were used to compare life history traits among the CIS and NS MUs in the North-east Atlantic, with the former MU area composed of an admixture of individuals of a northern ecotype of P. phocoena and a southern ecotype of P. p. meridionalis (Fontaine et al., 2017). Male and female harbor porpoises in the CIS were significantly larger in their maximum length, asymptotic length and length at sexual maturity compared to porpoises in the NS throughout the study period (1990–2013), which suggests limited gene flow and supports the justification for maintaining these two areas as separate management units or assessment units, within the range of the North-east Atlantic population, for indicator assessments under the EU’s Marine Strategy Framework Directive.
Interestingly, in both MUs during the study period, females significantly increased their average age attained at sexual maturity and males significantly declined in their average length attained at sexual maturity. An increase in the age at asymptotic length was also observed. Both sexes appeared to decline in length at a given age, with a significant decline in the Gompertz growth rate parameter reported – that was more apparent in the female data. While availability of suitable prey resources may be the limiting factor, a combination of other factors was not ruled out. Including a decline in the unidirectional gene flow in the North-east Atlantic from the larger-form southern ecotype (with a relatively small population size) to the northern ecotype and requires further investigation.
Data Availability Statement
The datasets generated for this study are available on request to the corresponding author.
Ethics Statement
Ethical review and approval was not required for the animal study because samples used in this study were obtained from the UK Cetacean Strandings Investigation Programme. No research was undertaken on live animals.
Author Contributions
SM, CM, and MACP contributed to reconstruction and synthesis, designed the statistical analysis, performed the analysis, and contributed to the manuscript preparation and writing. PJ, RD, SM, CL, JB, MP, RP, and ND contributed to reagents, materials, and analysis tools. PJ, RD, SM, CL, JB, MP, RP, ND, and MACP performed the laboratory experiments. PJ, RD, CL, JB, MP, RP, and ND provided expertise and editing. All authors contributed to the article and approved the submitted version.
Funding
While the samples and laboratory analysis were funded by previous projects, only data arising from that work were supplied to the current study. No funding was received to undertake the analysis within the current paper, or to publish the results of this work.
Conflict of Interest
RP was employed by company Marine Environmental Monitoring and CL was employed by company Age Dynamics.
The remaining authors declare that the research was conducted in the absence of any commercial or financial relationships that could be construed as a potential conflict of interest.
Acknowledgments
We thank Simon Northridge, Bob Reid, Tony Patterson, Harry Ross, Jason Barley, John Baker, Thijs Kuiken, Vic Simpson, Bob Monies, Adrian Colloff, and Sue Quinney and the staff of the AHVLA at Polwhele (Truro) as well as the volunteers of the Cornwall Wildlife Trust Marine Strandings Network for their contributions to data collection. Analysis of reproductive and teeth samples was part-funded by the Marie Curie International Outgoing Fellowship within the Seventh European Community Framework Programme (Project Cetacean-stressors, PIOF-GA-2010-276145 to SM and PJ), the Agreement on the Conservation of Small Cetaceans of the Baltic, North East Atlantic, Irish and North Seas (ASCOBANS) (Grants SSFA/2008 and SSFA/ASCOBANS/2010/5 to SM) and under contract to the United Kingdom Department of the Environment, Food and Rural Affairs (Project MF0736 to the Sea Mammal Research Unit). Samples examined in this research were collected under the collaborative Cetacean Strandings Investigation Programme (http://ukstrandings.org/), which is funded by United Kingdom Defra and the UK’s Devolved Administration in Wales (http://sciencesearch.defra.gov.uk/Default.aspx?Menu=Menu&Module=More&Location=None&Completed=0&ProjectID=15331) (grants to PJ and RD). We also thank the reviewers for their time and insightful comments on the manuscript.
Supplementary Material
The Supplementary Material for this article can be found online at: https://www.frontiersin.org/articles/10.3389/fmars.2020.502352/full#supplementary-material
References
Addink, M. J., and Smeenk, C. (1999). The harbour porpoise Phocoena phocoena in Dutch coastal waters: analysis of stranding records for the period 1920-1994. Lutra 41, 55–80.
Alfonsi, E., Hassani, S., Carpentier, F.-G., Dabin, W., Van Canneyt, O., Fontaine, M. C., et al. (2012). A European Melting Pot of Harbour Porpoise in the French Atlantic Coasts Inferred from Mitochondrial and Nuclear Data. PLoS One 7:e44425. doi: 10.1371/journal.pone.0044425
Barnes, L. G. (1985). Evolution, taxonomy and antitropical distributions of the porpoises (Phocoenidae, mammalia). Mar. Mamm. Sci. 1, 149–165. doi: 10.1111/j.1748-7692.1985.tb00003.x
Brandt, M. J., Diederichs, A., Betke, K., and Nehls, G. (2011). Responses of harbour porpoises to pile driving at the Horns Rev II offshore wind farm in the Danish North Sea. Mar. Ecol. Prog. Series 421, 205–216. doi: 10.3354/meps08888
Camphuysen, K. (2004). The return of the harbour porpoise (Phocoena phocoena) in Dutch coastal waters. Lutra 47, 135–144.
Danil, K., and Chivers, S. J. (2007). Growth and reproduction of female short-beaked common dolphins, Delphinus delphis, in the eastern tropical Pacific. Can. J. Zool. 85, 108–121. doi: 10.1139/z06-188
Deaville, R. (2016). Annual Report for the period 1st January – 31st December 2015. (Contract number MB0111). Available online at: http://ukstrandings.org/csip-reports/ (accessed August 30, 2019).
Deaville, R., and Jepson, P. D. (2011). CSIP Final Report for the period 1st January 2005-31st December 2010. UK Cetacean Strandings Investigation Programme: Report to the UK Department for Food and Rural Affairs and the Devolved Administrations. Available onlie at: http://randd.defra.gov.uk/Document.aspx?Document=FinalCSIPReport2005-2010_finalversion061211released[1].pdf (accessed August 30, 2019).
Dobson, F. S., and Oli, M. K. (2007). Fast and slow life histories of mammals. Ecoscience 14, 292–297. doi: 10.2980/1195-6860(2007)14[292:faslho]2.0.co;2
Donovan, G., and Bjorge, A. (1995). Harbour Porpoises in the North Atlantic: Edited Extract from the Report of the IWC Scientific Committee, Dubin 1995. (Cambridge: International whaling commission), 1–25.
Evans, P. G. H. (1992). Status of Cetaceans in British and Irish Waters Report to UK Department of. Environment. Oxford: Sea Watch Foundation.
Evans, P. G. H., Lockyer, C. H., Smeenk, C. S., Addink, M., and Read, A. J. (2008). “Harbour porpoise Phocoena phocoena,” in Mammals of the British Isles. Handbook, 4th Edn, eds S. Harris, and D. W. Yalden, (Southampton: The Mammal Society), 50–55.
Ferguson, S. H., and Higdon, J. W. (2006). How seals divide up the world: environment, life history, and conservation. Oecologia 150, 318–329. doi: 10.1007/s00442-006-0489-x
Ferguson, S. H., and Larivière, S. (2008). How social behaviour links environment and body size in mammalian carnivores. Open Ecol. J. 1, 1–7. doi: 10.2174/1874213000801010001
Ferguson, S. H., Zhu, X., Young, B. G., Yurkowski, D. J., Thiemann, G. W., Fisk, A. T., et al. (2018). Geographic variation in ringed seal (Pusa hispida) growth rate and body size. Can. J. Zool. 96, 649–659. doi: 10.1139/cjz-2017-0213
Fontaine, M. C., Baird, S. J. E., Piry, S., Ray, N., Tolley, K. A., Duke, S., et al. (2007). Rise of oceanographic barriers in continuous populations of a cetacean: the genetic structure of harbour porpoises in Old World waters. BMC Biol. 5:30. doi: 10.1186/1741-7007-5-30
Fontaine, M. C., Roland, K., Calves, I., Austerlitz, F., Palstra, F. P., Tolley, K. A., et al. (2014). Postglacial climate changes and rise of three ecotypes of harbour porpoises, Phocoena phocoena, in western Palearctic waters. Mol. Ecol. 23, 3306–3321. doi: 10.1111/mec.12817
Fontaine, M. C., Thatcher, O., Ray, N., Piry, S., Brownlow, A., Davison, N. J., et al. (2017). Mixing of porpoise ecotypes in southwestern UK waters revealed by genetic profiling. R. Soc. Open Sci. 4:160992. doi: 10.1098/rsos.160992
Fontaine, M. C., Tolley, K. A., Michaux, J. R., Birkun, A., Ferreira, M., Jauniaux, T., et al. (2010). Genetic and historic evidence for climate-driven population fragmentation in a top cetacean predator: the harbour porpoises in European water. Proc. R. Soc. B Biol. Sci. 277, 2829–2837. doi: 10.1098/rspb.2010.0412
Fontaine, P. M., and Barrette, C. (1997). Megatestes: anatomical evidence for sperm competition in the harbor porpoise. Mammalia 61, 65–71.
Foote, A. D. (2008). Mortality rate acceleration and post-reproductive lifespan in matrilineal whale species. Biol. Lett. 4, 189–191. doi: 10.1098/rsbl.2008.0006
Galatius, A. (2005). Sexually dimorphic proportions of the harbour porpoise (Phocoena phocoena) skeleton. J. Anat. 206, 141–154. doi: 10.1111/j.1469-7580.2005.00381.x
Galatius, A., and Kinze, C. C. (2003). Ankylosis patterns in the postcranial skeleton and hyoid bones of the harbour porpoise (Phocoena phocoena) in the Baltic and North Sea. Can. J. Zool. 81, 1851–1861. doi: 10.1139/z03-181
Galatius, A., Kinze, C. C., and Teilmann, J. (2012). Population structure of harbour porpoises in the Baltic region: evidence of separation based on geometric morphometric comparisons. J. Mar. Biol. Assoc. U.K. 92, 1669–1676. doi: 10.1017/s0025315412000513
Gol’din, P. E. (2004). Growth and body size of the harbour porpoise, Phocoena phocoena (Cetacea, Phocoenidae), in the Sea of Azov and the Black Sea. Vestn. Zool. 38, 59–73.
Gompertz, S. (1825). On the nature of the function expressive of the law of human mortality, and on a new mode of determining the value of life contingencies. Philos. Trans. R. Soc. 115, 513–585. doi: 10.1098/rstl.1825.0026
Hammond, P. S., Lacey, C., Gilles, A., Viquerat, S., Börjesson, P., Herr, H., et al. (2017). Estimates of Cetacean Abundance in European Atlantic Waters in Summer 2016 from the SCANS-III Aerial and Shipboard Surveys. SCANS-III Project Report 1. (St Andrews: Sea Mammal Research Unit), 39.
Hammond, P. S., Macleod, K., Berggren, P., Borchers, D. L., Burt, M. L., Cañadas, A., et al. (2013). Cetacean abundance and distribution in European Atlantic shelf waters to inform conservation and management. Biol. Conserv. 164, 107–122. doi: 10.1016/j.biocon.2013.04.010
Harrison, R. J. (1969). “Reproduction and reproductive organs,” in Biology of Marine Mammals, ed. H. T. Andersen, (New York, NY: Academic Press), 253–247.
Harvey, P. H., and Nee, S. (1991). How to live like a mammal. Nature 350, 23–24. doi: 10.1038/350023a0
Harvey, P. H., Read, A. F., and Promislow, D. E. L. (1989). Life history variation in placental mammals: unifying data with theory. Oxf. Surv. Evol. Biol. 6, 13–31.
Heide-Jørgensen, M. P., Iversen, M., Nielsen, N. H., Lockyer, C., Stern, H., and Ribergaard, M. H. (2011). Harbour porpoises respond to climate change. Ecol. Evol. 1, 579–585. doi: 10.1002/ece3.51
Helcom, (2013). Red List Marine Mammal Expert Group. Available online at: www.helcom.fi (accessed August 30, 2019).
Hohn, A. A. (1989). Variation in Life-History Traits: The Influence of Introduced Variation. Ph.D. thesis, University of California, Berkeley, CA.
Hohn, A. A., and Brownell, R. L. Jr. (1990). Harbour Porpoise in Central Californian Waters: Life History and Incidental Catches. SC/42/SM47. Report to the International Whaling Commission. Cambridge: International Whaling Commission.
IAMMWG, (2015). Management Units for cetaceans in UK waters (January 2015). JNCC Report No. 547. Peterborough: JNCC.
ICES WGBYC, (2015). Report of the Working Group on Bycatch of Protected Species (WGBYC), 2-6 February 2015. (Copenhagen: ICES), 82.
ICES WGBYC, (2018). Report from the Working Group on Bycatch of Protected Species (WGBYC), 1–4 May 2018. Reykjavik: ICES.
ICES WGMME, (2013). Report of the Working Group on Marine Mammal Ecology (WGMME), 4-7 February 2013. Paris: ICES.
ICES WGMME, (2014). Report of the Working Group on Marine Mammal Ecology (WGMME), 10-13 March 2014. (Woods Hole, MA: ICES), 234.
Jepson, P. D., Bennett, P. M., Deaville, R., Allchin, C. R., Baker, J. R., and Law, R. J. (2005). Relationships between polychlorinated biphenyls and health status in harbour porpoises (Phocoena phocoena) stranded in the United Kingdom. Environ. Toxicol. Chem. 24, 238–248. doi: 10.1897/03-663.1
Jepson, P. D., Deaville, R., Barber, J. L., Aguilar, À, Borrell, A., Murphy, S., et al. (2016). PCB pollution continues to impact populations of orcas and other dolphins in European waters. Sci. Rep. 6:18573.
Jung, J. L., Stephan, E., Louis, M., Alfonsi, E., Liret, C., Carpentier, F. G., et al. (2009). Harbour porpoises (Phocoena phocoena) in north-western France: aerial survey, opportunistic sightings and strandings monitoring. J. Mar. Biol. Assoc. U.K. 89, 1045–1050. doi: 10.1017/s0025315409000307
Keener, W., Webber, M. A., Szczepaniak, I. D., Markowitz, T. M., and Orbach, D. N. (2018). The sex life of harbor porpoises (Phocoena phocoena): lateralized and aerial behavior. Aquat. Mammala 44, 620–632. doi: 10.1578/am.44.6.2018.620
Kesselring, T., Viquerat, S., Brehm, R., and Siebert, U. (2017). Coming of age: - Do female harbour porpoises (Phocoena phocoena) from the North Sea and Baltic Sea have sufficient time to reproduce in a human influenced environment? PLoS One 12:e0186951. doi: 10.1371/journal.pone.0186951
King, S. L., Schick, R. S., Donovan, C., Booth, C. G., Burgman, M., Thomas, L., et al. (2015). An interim framework for assessing the population consequences of disturbance. Methods Ecol. Evol. 6, 1150–1158. doi: 10.1111/2041-210x.12411
Koons, D. N., Iles, D. T., Schaub, M., and Caswell, H. (2016). A life-history perspective on the demographic drivers of structured population dynamics in changing environments. Ecol. Lett. 19, 1023–1031. doi: 10.1111/ele.12628
Kraus, C., Thompson, D. L., Kunkele, J., and Trillmich, F. (2005). Living slow and dying young? Life-history strategy and age-specific survival rates in a precocial small mammal. J. Anim. Ecol. 74, 171–180. doi: 10.1111/j.1365-2656.2004.00910.x
Kuiken, T., and Garcia Hartmann, M. (1991). “Cetacean pathology: dissection techniques and tissue sampling,” in Proceedings of the First ECS Workshop, Leiden, 13–14.
Lah, L., Trense, D., Benke, H., Berggren, P., Gunnlaugsson, O., Lockyer, C., et al. (2016). Spatially Explicit Analysis of Genome-Wide SNPs Detects Subtle Population Structure in a Mobile Marine Mammal, the Harbor Porpoise. PLoS One 11:e0162792. doi: 10.1371/journal.pone.0162792
Laran, S., Authier, M., Blanck, A., Doremus, G., Falchetto, H., Monestiez, P., et al. (2017). Seasonal distribution and abundance of cetaceans within French waters: Part II: the Bay of Biscay and the English Channel. Deep Sea Res. II 141, 31–40. doi: 10.1016/j.dsr2.2016.12.012
Law, R. J., Bersuder, P., Barry, J., Deaville, R., Reid, R. J., and Jepson, P. D. (2010). Chlorobiphenyls in the blubber of harbour porpoises (Phocoena phocoena) from the UK: levels and trends 1991-2005. Mar. Pollut. Bull. 60, 470–473. doi: 10.1016/j.marpolbul.2009.12.003
Learmonth, J. A., Murphy, S., Luque, P. L., Reid, R. J., Patterson, I. A. P., Brownlow, A., et al. (2014). Life history of harbor porpoises (Phocoena phocoena) in Scottish (UK) waters. Mar. Mamm. Sci. 30, 1427–1455. doi: 10.1111/mms.12130
Leopold, M. F. (2015). Eat or be Eaten: Porpoise Diet Studies. Ph.D. thesis, Wageningen University, Wageningen, NL.
Lockyer, C. (1984). “Review of baleen whale (Mysticeti) reproduction and implications for management,” in Reproduction in Whales, Dolphins and Porpoises, eds W. F. Perrin, R. L. Brownell, Jr., and D. P. Demaster, (Cambridge: International Whaling Commission), 27–50.
Lockyer, C. (2003). “Harbour porpoises in the North Atlantic: biological parameters,” in Harbour Porpoises in the North Atlantic. Nammco Scientific Publications, Vol. 5, eds T. Haug, G. Desportes, G. A. Vikingsson, and L. Witting, (Tromso: The North Atlantic Marine Mammal Commission), 71–91. doi: 10.7557/3.2740
Lockyer, C., and Kinze, C. (2003). “Status, ecology and life history of the harbour porpoise (Phocoena phocoena) in Danish waters,” in Harbour porpoises in the North Atlantic. Nammco Scientific Publications, Vol. 5, eds T. Haug, G. Desportes, G. A. Vikingsson, and L. Witting, (Tromso: The North Atlantic Marine Mammal Commission).
Macleod, C. D. (2010). The relationship between body mass and relative investment in testes mass in cetaceans: implications for inferring interspecific variations in the extent of sperm competition. Mar. Mamm. Sci. 26, 370–380. doi: 10.1111/j.1748-7692.2009.00348.x
Mccullagh, P., and Nelder, J. A. (1989). Generalized Linear Models, Vol. 37. Boca Raton, FL: CRC Press.
Murphy, S., Barber, J. L., Learmonth, J. A., Read, F. L., Deaville, R., Perkins, M. W., et al. (2015). Reproductive failure in UK harbour porpoises Phocoena phocoena: legacy of pollutant exposure? PLoS One 10:e0131085. doi: 10.1371/journal.pone.0131085
Murphy, S., Caurant, F., Evans, P. G. H., Tougaard, J., and Hammond, P. S. (2019a). Area Status Report West Scotland/Ireland & Celtic and Irish Seas. Annex 7. Report of Joint IMR/NAMMCO International Workshop on the Status of Harbour Porpoises in the North Atlantic. Tromsø: North Atlantic Marine Mammal Commission.
Murphy, S., Collet, A., and Rogan, E. (2005). Mating strategy in the male common dolphin Delphinus delphis: what gonadal analysis tells us. J. Mammal. 86, 1247–1258. doi: 10.1644/1545-1542(2005)86[1247:msitmc]2.0.co;2
Murphy, S., Evans, P. G. H., Pinn, E., and Pierce, G. J. (2019b). Conservation management of common dolphins: Lessons learned from the North-East Atlantic. Aquat. Conserv. 1–30. doi: 10.1002/aqc.3212
Murphy, S., Perrott, M., Mcvee, J., Read, F. L., and Stockin, K. A. (2014). Deposition of Growth Layer Groups in Dentine Tissue of Captive Common Dolphins Delphinus delphis. NAMMCO Scientific Publication Volume 10: Age Estimation of Marine Mammals with a Focus on Monodontids. Available online at: http://dx.doi.org/10.7557/3.3017 (accessed August 30, 2019).
Murphy, S., Pierce, G. J., Law, R. J., Bersuder, P., Jepson, P. D., Learmonth, J. A., et al. (2010). Assessing the effect of persistent organic pollutants on reproductive activity in common dolphins and harbour porpoises. NAFO/ICES/NAMMCO symposium “The Role of Marine Mammals in the Ecosystem in the 21st Century”. J. Northwest Atlantic Fish. Sci. 42, 153–173. doi: 10.2960/j.v42.m658
NAMMCO, and IMR. (2019). North Atlantic Marine Mammal Commission and the Norwegian Institute of Marine Research. Report of Joint IMR/NAMMCO International Workshop on the Status of Harbour Porpoises in the North Atlantic. Bergen: Norwegian Institute of Marine Research.
Ólafsdóttir, D., Víkingsson, G. A., Halldórsson, S. D., and Sigurjónsson, J. (2003). “Growth and reproduction in harbour porpoises (Phocoena phocoena) in Icelandic waters,” in Harbour porpoises in the North Atlantic. Nammco Scientific Publications, Vol. 5, eds T. Haug, G. Desportes, G. A. Vikingsson, and L. Witting, (Tromso: The North Atlantic Marine Mammal Commission), 195–210. doi: 10.7557/3.2747
OSPAR, (2017). The Intermediate Asssessment 2017. Assessment of the Marine Environment in OSPAR’s Waters. Available online at: https://oap.ospar.org/en/ospar-assessments/intermediate-assessment-2017/ (accessed August 30, 2019).
Perrin, M. R. (1989). “Alternative life-history styles of small mammals,” in Alternative Life-History Styles of Animals. Perspectives in vertebrate science, Vol. 6, ed. M. N. Bruton, (Dordrecht: Springer).
Pierce, G. J., Santos, M. B., Murphy, S., Learmonth, J. A., Zuur, A. F., Rogan, E., et al. (2008). Bioaccumulation of persistent organic pollutants in female common dolphins (Delphinus delphis) and harbour porpoises (Phocoena phocoena) from western European seas: Geographical trends, causal factors and effects on reproduction and mortality. Environ. Pollut. 153, 401–415. doi: 10.1016/j.envpol.2007.08.019
Pikesley, S. K., Witt, M. J., Hardy, T., Loveridge, J., Loveridge, J., Williams, R., et al. (2012). Cetacean sightings and strandings: evidence for spatial and temporal trends. J. Mar. Biol. Assoc. U.K. 92, 1809–1820. doi: 10.1017/S0025315411000464
Pirotta, E., Brookes, K. L., Graham, I. M., and Thompson, P. M. (2014). Variation in harbour porpoise activity in response to seismic survey noise. Biol. Lett. 10:20131090. doi: 10.1098/rsbl.2013.1090
Promislow, D. E. L., and Harvey, P. H. (1990). Living fast and dying young: a comparative analysis of life-history variation among mammals. J. Zool. 220, 417–437. doi: 10.1111/j.1469-7998.1990.tb04316.x
R Core Team, (2019). R: A Language and Environment for Statistical Computing. Vienna: R Foundation for Statistical Computing.
Ralls, K., and Mesnick, S. L. (2009). “Sexual dimorphism,” in Encyclopedia of Marine Mammals, eds W. F. Perrin, B. Wursig, and J. G. M. Thewissen, (London: Academic press), 1005–1011.
Read, A. J. (1990). Reproductive Seasonality in Harbor Porpoises, Phocoena- Phocoena, from the Bay of Fundy. Can. J. Zool. 68, 284–288. doi: 10.1139/z90-042
Read, A. J. (2001). Trends in the maternal investment of harbour porpoises are uncoupled from the dynamics of their primary prey. Proc. R. Soc. Lond. Ser. B Biol. Sci. 268, 573–577. doi: 10.1098/rspb.2000.1419
Read, A. J., and Gaskin, D. E. (1990). Changes in growth and reproduction of harbour porpoises, Phocoena phocoena, from the Bay of Fundy. Can. J. Fish. Aquat. Sci. 47, 2158–2163. doi: 10.1139/f90-240
Read, A. J., and Hohn, A. A. (1995). Life in the fast lane: the life history of harbour porpoises from the Gulf of Maine. Mar. Mamm. Sci. 11, 423–440. doi: 10.1111/j.1748-7692.1995.tb00667.x
Read, A. J., and Tolley, K. A. (1997). Postnatal growth and allometry of harbour porpoises from the Bay of Fundy. Can. J. Zool. 75, 122–130. doi: 10.1139/z97-016
Read, F. L. (2016). Understanding Cetacean and Fisheries Interactions in the North-West Iberian Peninsula. Ph.D. thesis, Universidade de Vigo, Pontevedra, 309.
Reijnders, P. J. H. (1992). Harbour porpoises Phocoena phocoena in the North Sea: numerical responses to changes in environmental conditions. Neth. J. Aquat. Ecol. 26, 75–85. doi: 10.1007/bf02298029
Smeenk, C. (1987). The Harbour Porpoise Phocoena phocoena (L., 1758) in The Netherlands: stranding records and decline. Lutra 30, 77–90.
Sørensen, T. B., and Kinze, C. C. (1994). Reproduction and reproductive seasonality in Danish harbour porpoises, Phocoena phocoena. Ophelia 39, 159–176. doi: 10.1080/00785326.1994.10429541
Stoffel, M. A., Humble, E., Paijmans, A. J., Acevedo-Whitehouse, K., Chilvers, B. L., Dickerson, B., et al. (2018). Demographic histories and genetic diversity across pinnipeds are shaped by human exploitation, ecology and life-history. Nat. Commun. 9:4836.
Stuart, L. J., and Morejohn, G. V. (1980). Developmental patterns in osteology and external morphology in Phocoena phocoena. Rep. Int. Whal. Comm. (special issue 3)133–142.
Sveegaard, S., Galatius, A., Dietz, R., Kyhn, L., Koblitz, J. C., Amundin, M., et al. (2015). Defining management units for cetaceans by combining genetics, morphology, acoustics and satellite tracking. Glob. Ecol. Conserv. 3, 839–850. doi: 10.1016/j.gecco.2015.04.002
Thompson, P. M., Brookes, K. L., Graham, I. M., Barton, T. R., Needham, K., Bradbury, G., et al. (2013). Short-term disturbance by a commercial two-dimensional seismic survey does not lead to long-term displacement of harbour porpoises. Proc. R. Soc. B Biol. Sci. 280:20132001. doi: 10.1098/rspb.2013.2001
Thomsen, F., Laczny, M., and Piper, W. (2006). A recovery of harbour porpoises (Phocoena phocoena) in the southern North Sea? A case study off Eastern Frisia, Germany. Helgol. Mar. Res. 60, 189–195. doi: 10.1007/s10152-006-0021-z
Tregenza, N. J. C., Berrow, S. D., Hammond, P. S., and Leaper, R. (1997). Harbour porpoise (Phocoena phocoena L.) by-catch in set gillnets in the Celtic Sea. ICES J. Mar. Sci. 54, 896–904. doi: 10.1006/jmsc.1996.0212
Venables, W. N., and Ripley, B. D. (2002). Modern Applied Statistics with S. New York, NY: Springer.
Wade, P. R. (2009). “Population Dynamics,” in Encyclopedia of Marine Mammals, eds W. F. Perrin, B. Würsig, and J. G. M. Thewissena, (London: Academic Press), 913–918.
Wiemann, A., Andersen, L. W., Berggren, P., Siebert, U., Benke, H., Teilmann, J., et al. (2010). Mitochondrial Control Region and microsatellite analyses on harbour porpoise (Phocoena phocoena) unravel population differentiation in the Baltic Sea and adjacent waters. Conserv. Genet. 11, 195–211. doi: 10.1007/s10592-009-0023-x
Winship, A. J. (2009). Estimating the Impact of Bycatch and Calculating Bycatch Limits to Achieve Conservation Objectives as Applied to Harbour Porpoise in the North Sea. Ph.D. thesis, University of St Andrews, St Andrews.
Keywords: harbor porpoise, cetacean, life history, age, growth, strandings, bycatch, monitoring
Citation: Murphy S, Petitguyot MAC, Jepson PD, Deaville R, Lockyer C, Barnett J, Perkins M, Penrose R, Davison NJ and Minto C (2020) Spatio-Temporal Variability of Harbor Porpoise Life History Parameters in the North-East Atlantic. Front. Mar. Sci. 7:502352. doi: 10.3389/fmars.2020.502352
Received: 02 October 2019; Accepted: 30 September 2020;
Published: 26 November 2020.
Edited by:
Jeremy Kiszka, Florida International University, United StatesReviewed by:
Daniel Paul Costa, University of California, Santa Cruz, United StatesPavel Gol’Din, National Academy of Sciences of Ukraine (NAN Ukraine), Ukraine
Copyright © 2020 Murphy, Petitguyot, Jepson, Deaville, Lockyer, Barnett, Perkins, Penrose, Davison and Minto. This is an open-access article distributed under the terms of the Creative Commons Attribution License (CC BY). The use, distribution or reproduction in other forums is permitted, provided the original author(s) and the copyright owner(s) are credited and that the original publication in this journal is cited, in accordance with accepted academic practice. No use, distribution or reproduction is permitted which does not comply with these terms.
*Correspondence: Sinéad Murphy, sinead.murphy@gmit.ie; sinead.noirin.murphy@gmail.com; Cóilín Minto, coilin.minto@gmit.ie
†These authors share first authorship
‡Present address: Marie A. C. Petitguyot, Instituto de Investigaciones Marinas, Centro Superior de Investigaciones Científicas (IIM-CSIC), Vigo, Spain