The Role of Manipulating Photoperiod and Temperature in Oocyte Development of the Polychaete Hediste diversicolor (O.F. Müller, 1976)
- 1Department of Biology, Norwegian University of Science and Technology, Trondheim, Norway
- 2SINTEF Ocean, Trondheim, Norway
- 3College of Marine Sciences, Hainan University, Haikou, China
Three different experiments were conducted to examine the oocyte development of the polychaete Hediste diversicolor in response to changing photoperiod and temperature at three different periods of oogenesis. In experiment I, worms collected during summer were reared under constant or decreasing photoperiod and temperatures to test the combined effects of the summer–autumn photoperiod and temperature transition on oogenesis. The result showed females collected during summer showed the highest oocyte growth when exposed to constant temperatures combined with decreasing photoperiod and decreasing temperature combined with constant photoperiod. In experiment II, worms collected in late autumn were under mimicked or shortened seasonal changes in photoperiod to evaluate the effect of accelerated change on oocyte growth. The result showed worms had 1.5 times faster oocyte growth rates when exposed to accelerated rate of change in photoperiod (2.5 times faster). In experiment III, worms collected in spring were exposed to different temperature regimes to examine the effect of raising temperature on the synchronization of oocyte growth and maturation. The results showed worms collected in spring showed increased temperatures will increase oocyte maturation synchronicity. It was concluded temperature and photoperiod transition can increase the oocyte development.
Introduction
The world’s aquaculture production has shown an annual growth of 8% over the last decades, and the sector is expected to continue to grow in the future (Food and Agriculture Organization [FAO], 2017). This sustainable growth demands alternative feeds and feed ingredients. Cultivation of polychaetes can help the aquaculture industry produce new feed resources as well as recycle particulate aquaculture waste into new marine biomass. Hediste diversicolor (Müller, 1776) (Nereididae, Polychaeta) is widely distributed from North Sea and Baltic Sea to Mediterranean. They can obtain good growth rates and nutritional composition when fed cheap feed sources such as fish waste (Bischoff et al., 2009; Wang et al., 2019). If industrialized polychaete cultivation using particulate organic wastes from land-based aquaculture is to be realized, the stocking material for future grow-out systems must be supplied from dedicated, centralized broodstock and hatchery facilities. To produce polychaete larvae year-round, closing and controlling the life cycle of the target species is paramount (Olive, 1999).
The biggest challenge of polychaete aquaculture is mass production and the supply of offspring (Mandario, 2018). Semelparous Nereid species spend 1–3 years before reaching maturity in their natural habitat; however, they can be manipulated to mature earlier if cultured under appropriate photoperiod and temperature conditions (Chu and Levin, 1989; Bentley and Pacey, 1992; Olive et al., 1997). Moreover, by controlling environmental factors such as photoperiod and temperature, the onset of reproduction in polychaetes can be either triggered or suppressed (Chu and Levin, 1989; Olive et al., 1997).
Response to photoperiod change has been studied in some polychaete species, and seasonal photoperiod changes are reported to affect time of breeding and lifespan in polychaetes (Olive, 1995; Olive et al., 1998). Short photoperiod (<8 h of light) and decreasing temperature induce rapid oocyte growth in Nereis virens (Olive et al., 1998), whereas oocyte growth increased when polychaete Kefersteinia cirrata is exposed to long photoperiod (>16 h light) and increasing temperature (Olive and Pillai, 1983). Garwood and Olive (1982) suggested that long photoperiod (>11 h light) coupled with an endogenous timer synchronizes spawning of the first cohort of oocytes in Harmothoe imbricate L. The authors also mentioned that in H. imbricate vitellogenesis was favored by low temperature and increasing photoperiod.
Environmental cues such as long photoperiod and optimal temperature were reported to be essential for polychaete growth, timing of maturation, and rate of oocyte development (Bentley and Pacey, 1992; Nesto et al., 2012). Photoperiod controls gametogenesis via imposing seasonality on life cycle, while temperature is more related to the metabolism of the polychaetes, which would initiate the onset from somatic growth to gametes development, and therefore was closely related to age-at-maturity (Olive et al., 1997). Temperature may also act as a circannual clock, as polychaetes reared at constant high temperatures seemed to spawn asynchronously (Batista et al., 2003), while decreasing temperature in winter and increasing temperature from winter to early spring supports the synchronization of gamete maturation and spawning (Bartels-Hardege and Zeeck, 1990; Lawrence and Soame, 2004). There may be a synergic effect between temperature and photoperiod that may affect brood growth and oogenesis. Chu and Levin (1989) found increasing temperature with increasing or fixed long photoperiods led to more rapid brood growth rate than decreasing temperature and temperature under winter–spring transition.
In Nereids, oogenesis is mainly regulated by brain hormones until the oocytes reach diameters of approximately 70 μm, whereas somatic growth is influenced by external factors such as photoperiod (Pfannenstiel, 1978). Clark and Ruston (1963) have also shown that the oocyte growth of H. diversicolor increased rapidly at a size of 70–150 μm, and gradually slowed down after reaching a diameter of around 180 μm. To examine oocyte growth under different external factors, photoperiod and temperature, only worms with oocytes larger than 80 μm were used in this study.
Batista et al. (2003) indicated that Nereis diversicolor cultured at 13.8–16.6°C with an initial wet weight of 7.8 mg⋅ind–1 was found to precociously spawn within 3 months of cultivation. However, if there is no cue to synchronize the timing of maturation, spawning will be spontaneous (Nesto et al., 2012). Asynchronized spawning of females and males will result in fertilization failure, especially when a small number of worms are cultured. So to find factors to control the timing of maturation is important to polychaete farming.
This study investigated oocyte development under different photoperiod and temperature. The aim of this study was to assess the potential of improving the oogenesis process in H. diversicolor by inducing with different environmental abiotic stimuli, in order to develop cultivation protocols for the industrial production of H. diversicolor as a novel marine protein and lipid source for the aquafeed market.
Materials and Methods
Sample Collection
Polychaetes H. diversicolor were collected in from the intertidal zone of Leangen Bay, Trondheim, Norway (63.4390549 N, 10.4705787 E) during low tide at sediment depths of 0–20 cm. They were collected gently by hand and immediately transported to the lab in plastic containers filled with wet newspapers and seaweed. Worms collected about twice a month between the end of October and April were used to characterize natural oocyte maturation (sampling date shown in Figure 1).
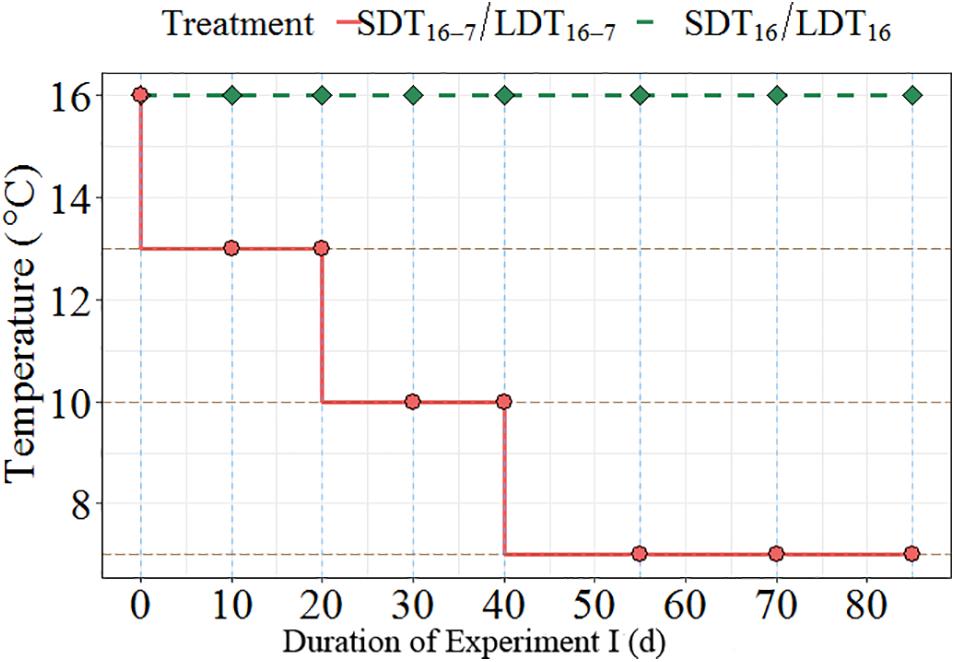
Figure 1. Temperature regimes used for Experiment I showed temperature decline over the experimental period of 82 days. The solid dots represent the days when samplings were conducted.
Combined Effects of Temperature and Photoperiod on Oogenesis (Experiment I)
Worms were collected in July (0.13 ± 0.05 g wet weight) and placed in a flow-through system (XHAB XR3 stand alone, AQUATIC HABITATS, Pentair Aquatic Eco-Systems Inc., United States) for somatic growth at 16°C and constant light (16 h light) for 82 days before the experiment. To examine the effect of photoperiod and temperature on oogenesis, worms were exposed to two different light regimes [long days (LD), 16 h light, vs. short days (SD), 8 h light] and two different temperature regimes [constant temperature (T16), 16°C, vs. gradually decreasing temperature (T16–7), from 16 to 7°C]. The experiment was set as a factorial design. This resulted in four experimental treatments (Table 1). The changes in temperature are shown in Figure 1.
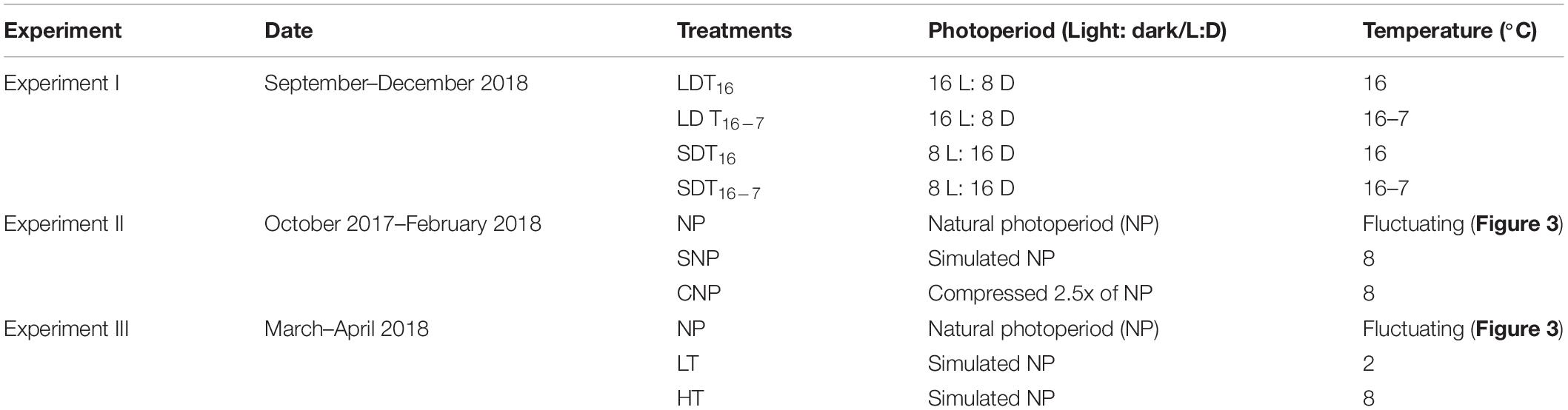
Table 1. Schematic view of all experimental conditions used in this study for H. diversicolor oogenesis.
Six randomly selected female worms per treatment were placed separately in cups (200 mL). Each cup contained one worm, and two cups were placed in one container (5 L). The cups were filled with 5 cm chamotte (ceramic clay with grain size 0.5–2 mm, Alt for Keramikk AS, Norway) with 5 cm deep water level above. The water was changed every other day before feeding with fish feed (Gemma Diamond 1.0, Skretting). The oocytes were sampled at the beginning of the experiment and seven times thereafter over the course of the experiment (Figure 1).
Before oocyte samplings, the worms were anesthetized with 7% ethanol for nearly 2 min. Coelomic samples were taken using 1 mL syringe (SABRE, needle diameter: 0.5 mm) assisted with forceps wrapped in rubber to avoid damage (Olive et al., 1998). The needle was inserted into the worms’ body cavity laterally, and little coelomic fluid and eggs was extracted. In order to release the small amount of coelomic fluid and eggs from the syringe, some seawater was sucked together. During the entire experiment, this procedure was repeated eight times per worm. The oocytes were then transferred into a sample glass (30 mL), adding seawater till the 20 mL calibration mark and stirred gently. Subsamples (50 μL, n = 10) were taken using a micropipette and the number of oocytes in each droplet was counted using a microscope (Eclipse TS100). The diameter of the oocytes was measured using a camera (Nikon Digital Sight, DS-U3) attached to the microscope and analyzed with imaging software (NIS-Elements BR version 4.30). The average oocyte diameter was calculated for 50 oocytes per worm. After sampling, the worms were returned to their cups. Full recovery of the worms after sampling was confirmed by visually observing them construct new burrows in 3–5 min.
Effect of Compressed Photoperiod on Oocyte Growth (Experiment II)
Worms collected in late autumn were kept in a climate chamber (Fitoclima S600 PHCI, Aralab) at 8°C with LED lighting adjusted to equal irradiances for all available wavelengths within 400–735 nm (Heliospectra, 5–10 μmol⋅m–2⋅s–1). The worms were kept for 124 days under simulated natural photoperiod reflecting the light conditions at the location where the worms were sampled (hereafter SNP) vs. a compressed light regime sped up 2.5 times compared to the rate of the SNP (124 days compressed to 48 days, hereafter CNP). The photoperiods for this experiment were based on sunrise–sunset tables for Trondheim, Norway. The photoperiod and sampling interval is shown in Figure 2. The worms were placed in 1 L containers (12.4 × 8.5 × 11 cm, W × D × H) with a 5 cm deep layer of sediment and a 4 cm water layer. The experiment consisted of 20 containers for each treatment, each stocked with eight female worms. Seawater was continuously aerated by pressurized air supplied through air stone and replaced completely with new seawater every other day. Feed residues were removed, and new feed was added after each water exchange.
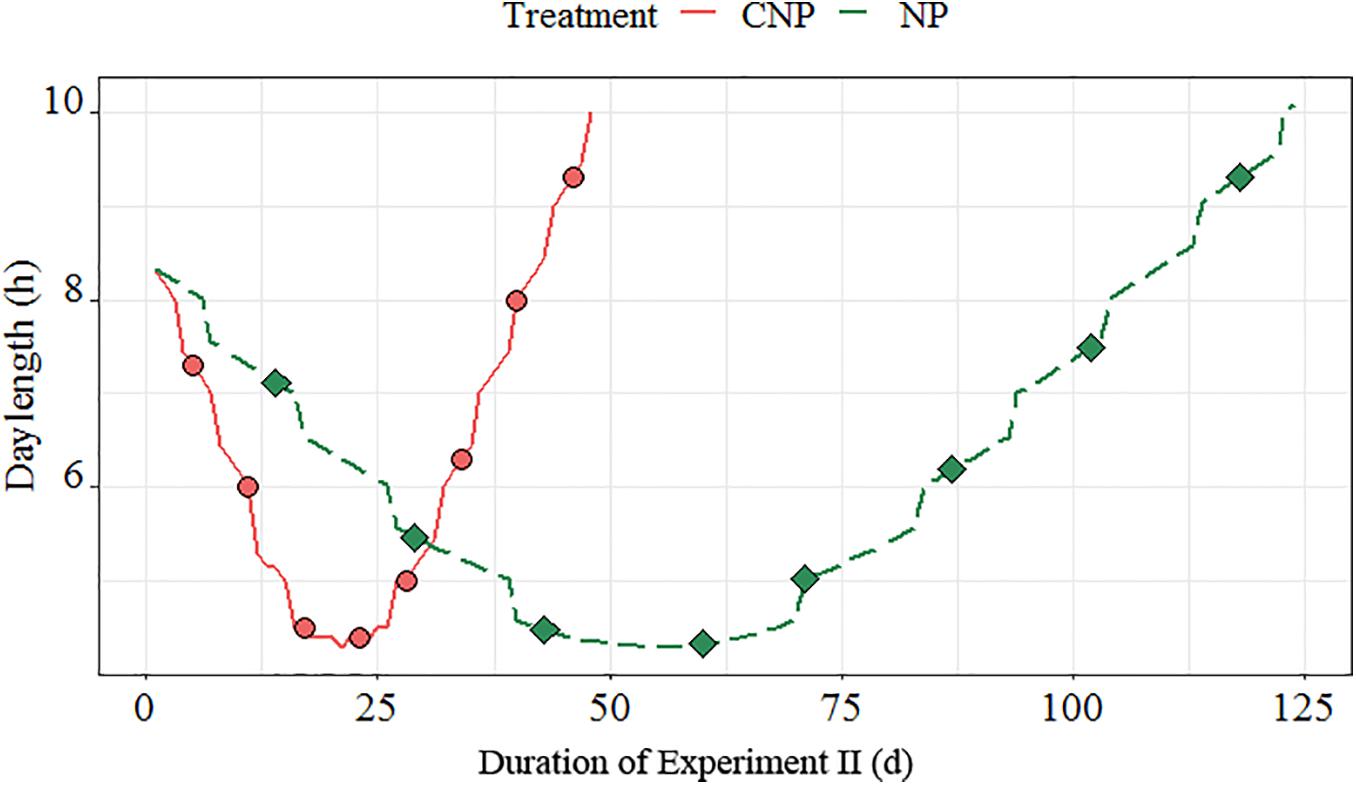
Figure 2. Light regimes for the compressed (CNP) and natural (SNP) photoperiods used in the experiment. Dots represent the photoperiod at each sampling date.
Sixteen worms (two containers with eight worms each) were sampled at each sampling point and anesthetized using a 4% magnesium chloride (MgCl2) solution. Pictures were taken after anaesthetization for length and segment measurement. Finally, the worms were fixed in 4% formaldehyde (CH2O) solution for later examination. Half of the worms (n = 8) were used for tissue sectioning, and the rest (n = 8) were used to record oocyte count and size. In these worms, the coelom was opened longitudinally and chopped into 10 mm pieces with scalpel and forceps to release the oocytes from the coelom and parapodia. All the eggs were washed out from the whole body, diluted in seawater, and adjusted to a volume of 20 mL. Ten subsamples (50 μL) each were used for counting the number of oocytes. Meanwhile, worms were sampled from the natural population (hereafter NP) and processed according to the methods described above. Sediment temperatures (from 0 to 20 cm depth) were measured while sampling the NP (Figure 3).
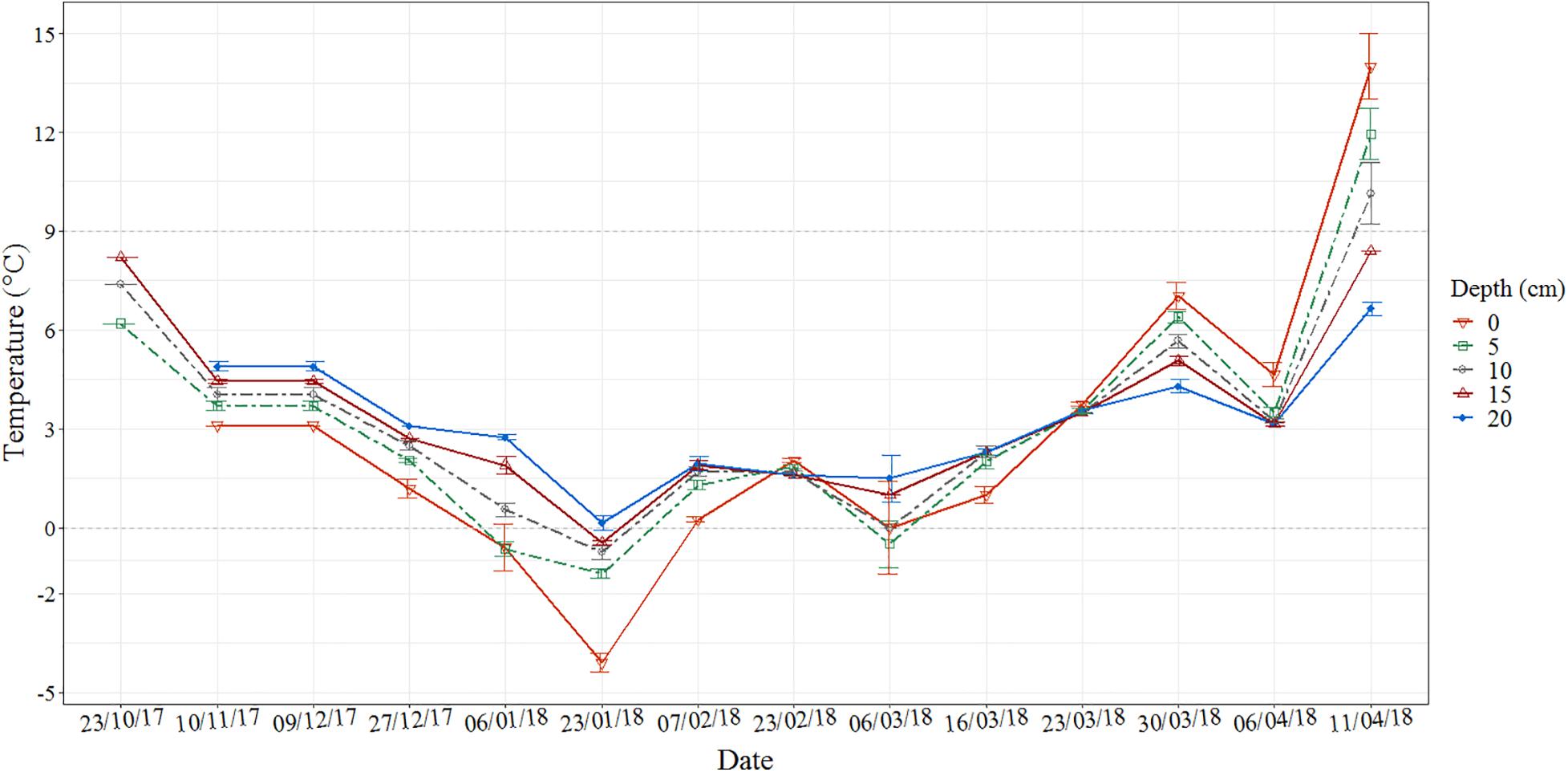
Figure 3. Sediment temperatures at Leangen Bay (Trondheim, Norway) during the whole experimental period, reference points for sampling dates of the natural population (NP) and laboratory experiments (Experiments II and III).
Histological Sectioning and Analyses
The worm tissues were dehydrated in a graded series of ethonal with a tissue processor (Leica TP1020, Germany) and embedded with paraffin wax. At least two longitudinal and cross sections were taken for each polychaete. The sections were cut at 4 μm thickness using a microtome (RM2255, Leica, Germany) with a CoolClamp RM (Leica, Germany). And the distance between each section was a minimum of 200 μm. In total, 201 individuals (116 females) were sectioned for histological analyses.
The sections were stained with HE staining (Fischer et al., 2008; Ross and Pawlina, 2017), and were scanned at 40x magnifications using a digital slide scanner (Hamamatsu NanoZoomer, Japan) and examined with an image viewing software (Hamamatsu NDP.view2) afterward. To quantify oocyte development, the circumference (μm) and area (μm2) of the oocytes in each individual were measured in the sections where the nucleolus was visible. The measurements were conducted using NanoZoomer and by retracing the cell membrane using a drawing tablet (Wacom Bamboo, CTH-670). About 25–50 oocytes were measured for each polychaete. Females with oocytes less than 70 μm were considered not mature this year and were excluded in the calculation.
Effect of Increasing Temperature on Final Stages of Oocyte Maturation (Experiment III)
Worms, collected on 6 March, were exposed to two artificial temperature regimes in the laboratory: low temperature (2°C), resembling the sediment temperature at the time the worms were sampled (hereafter LT) vs. high temperature, progressively increasing temperature from 2 to 8°C raised by 1°C per day (hereafter HT). In situ samplings from the NP were sampled as the control collected simultaneously as LT and HT (Table 1). The sediment temperature was recorded (Figure 3) during each sampling. The worms (n = 5) were sampled on days 10, 17, 24, 31, 36, and on the final sampling because most died as they were spawning. Oocytes were extracted from two to five female worms from each of the three treatments. The wet weight of these female worms varied from 180 to 550 mg, length ranged between 49.98 and 101.88 mm.
Statistics
A linear mixed-effect model was used to assess the influence of light and temperature on oocyte development, expressed as oocyte diameter. In our model, we hypothesized oocytes were sphere, and recorded the perimeter. Then diameter of the oocytes was calculated based on the perimeter. The residual standard error is used to measure the distance between the observed data and fitted regression curve based on the model. The model allowed for within-group errors being correlated and/or having unequal variances. Therefore, we added “worm” at each treatment as a random effect. The linear mixed effect model was Model = lme (Diameter∼Date∗Treatment, random = ∼1| Individual Worm, method = “ML”). In the model, oocyte dimeter was correlated with date, and date was added as a continuous fixed effect (analysis of covariance). Akaike’s information criterion (AIC) was used to select the best model. Fulton’s condition factor was calculated from weight and length, expressed by the equation (where W was wet weight of the worm (mg) and L was the length (mm) (Datta et al., 2013). All analyses were performed in Rstudio (version 1.0.143) and lme() functions from the lme4 package was used.
Results
Combined Effects of Temperature and Photoperiod on Oogenesis (Experiment I)
The average growth in oocyte diameter was highest in worms reared at short photoperiod and constant temperature (SDT16, 0.76 μm⋅day–1), followed by worms reared at long photoperiod and decreasing temperature (LDT16–7, 0.66 μm⋅day–1). Oocytes of worms exposed to long photoperiod and constant temperature (LDT16, 0.51 μm⋅day–1) as well as short photoperiod and decreasing temperature (SDT16–7, 0.49 μm⋅day–1) (Figure 4) grew slowest. Between-treatment variation was most pronounced (Figure 4 and Table 2), except for no significant difference found in mean oocyte diameter growth between LDT16 and SDT16–7. The distribution of oocyte size within a worm changed over time as shown in Supplementary Figure 1.
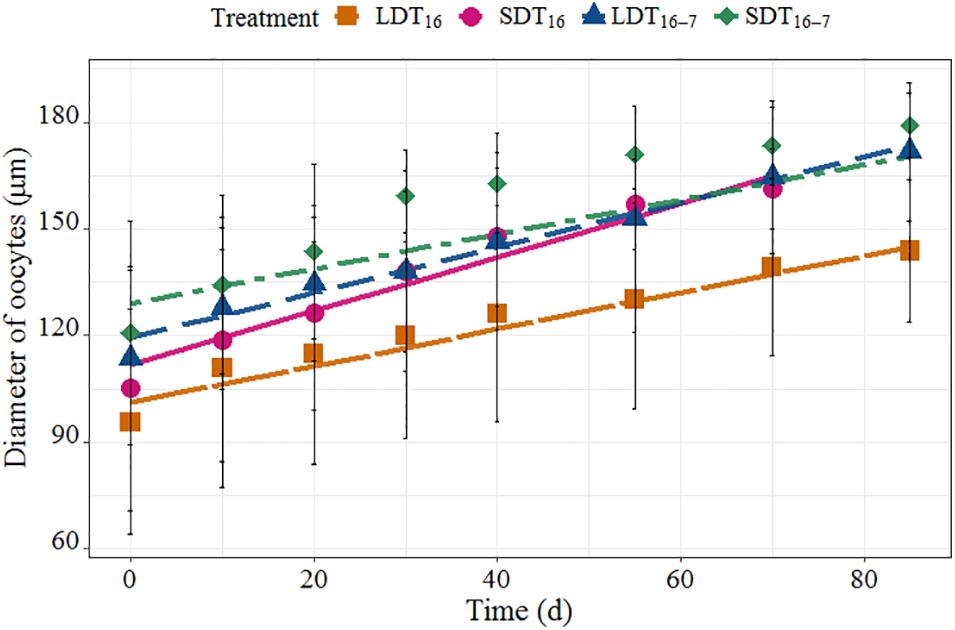
Figure 4. Fitted linear mixed regression showing the growth curve of oocyte diameter under four different treatments. The line was fitted regression line, and the dot was the mean oocyte diameter at four treatments (±1 SD).
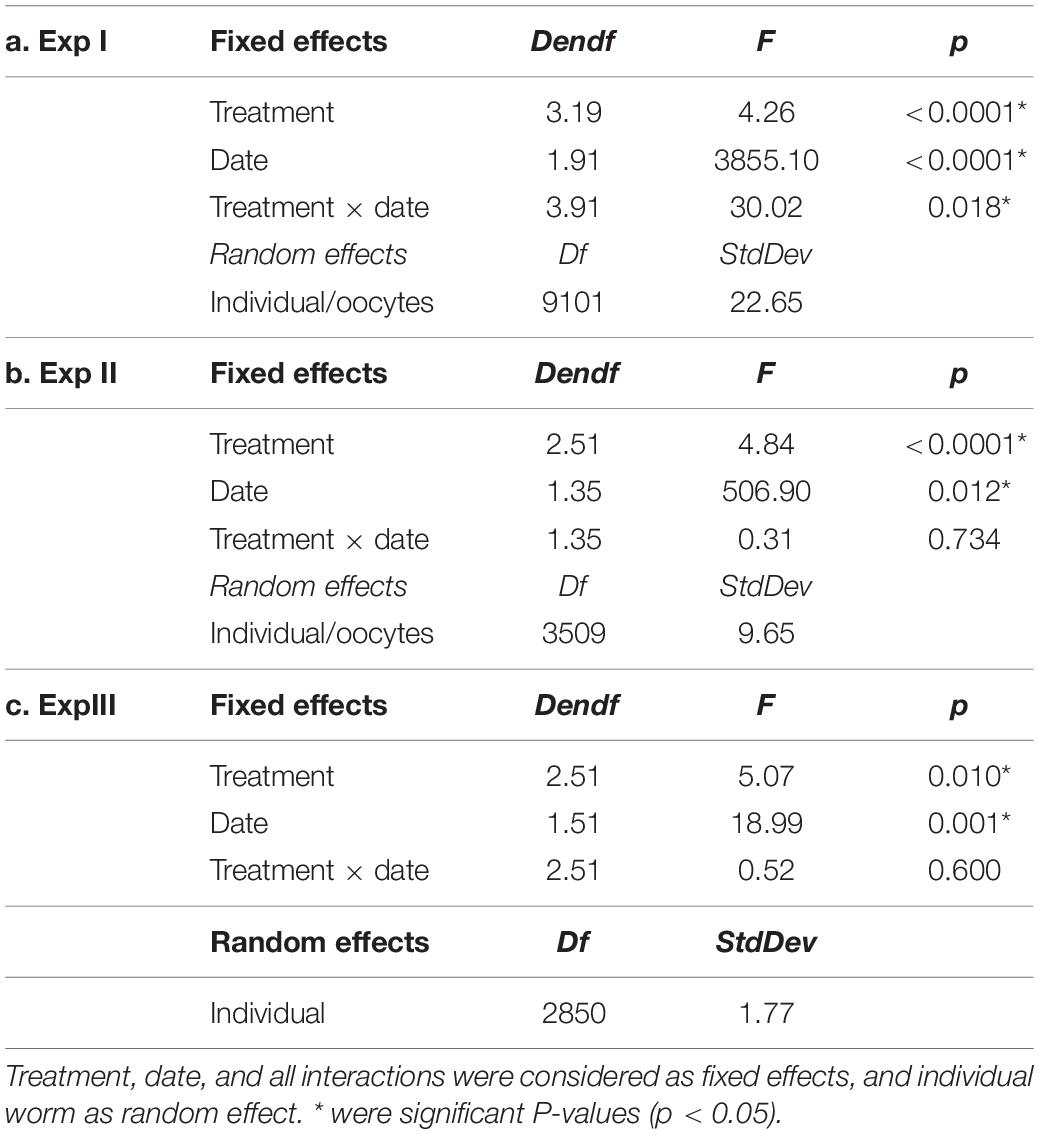
Table 2. Results of linear mixed model analysis on photoperiod and temperature treatment–manipulated oocyte diameter over time in three experiments (Exp I, Exp II, Exp III).
Compressed Rate of Winter Photoperiod Changes on Oocyte Growth (Experiment II)
Oocytes grew faster in the CNP regime compared to the SNP treatment, whereas both laboratory treatments showed higher oocyte growth compared to the NP (0.72, 0.52, and 0.34 μm⋅day–1, respectively) (Figure 5A). At the third sampling, the oocyte diameter for the CNP-treatment was significantly larger than for the NP (p < 0.05, Figure 5B and Table 2), while oocyte size became larger at the SNP treatment from the fourth sampling onward.
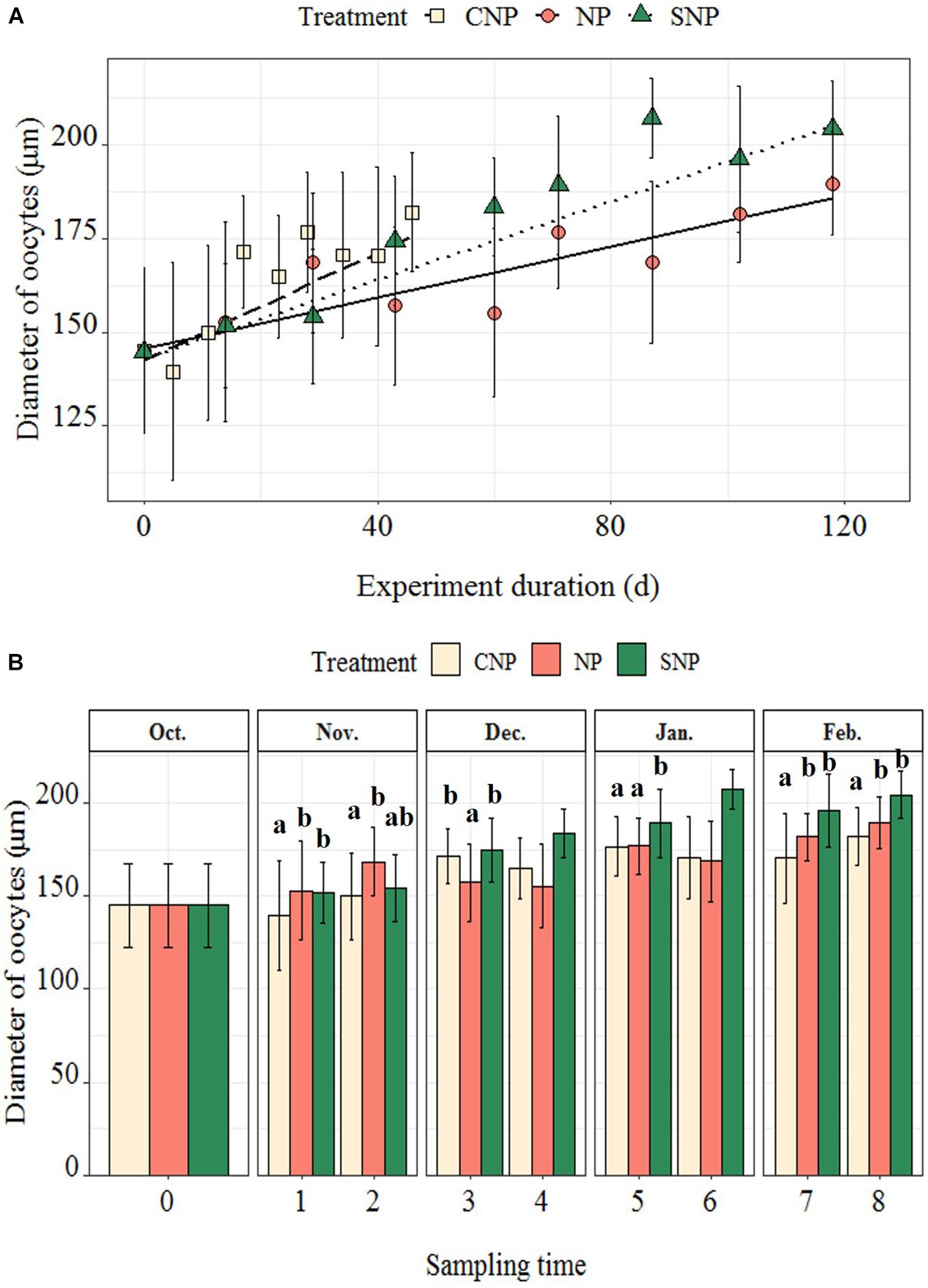
Figure 5. (A) Fitted linear mixed regression showing the general growth curve of oocyte diameter under three photoperiod regimes; the dots were mean oocyte diameters at each sampling time (±1 SD). (B) Bar plot showing oocyte diameters at each sampling time. The differences in oocyte diameter among three regimes at each sampling time were shown with superscripts. There was just a single individual at the fourth and sixth samplings at SNP treatment, so they were not included in the comparisons. The number of individuals in each treatment at each sampling time was recorded in Supplementary Table 2.
Histological samples were used to assess morphological changes during oocyte development (Figure 6). Oocytes in previtellogenic phase were found in young females (Figure 6A), while other oocytes were in vitellogenic phase. The shape of the oocytes became more spherical when they approached maturation, and the size and number of lipid droplets the oocytes contained increased. The yolk granules became bigger, and some melted together with the lipid droplets when approaching maturation. A detailed description of the histological sections containing different sizes of oocytes is shown in Table 3.
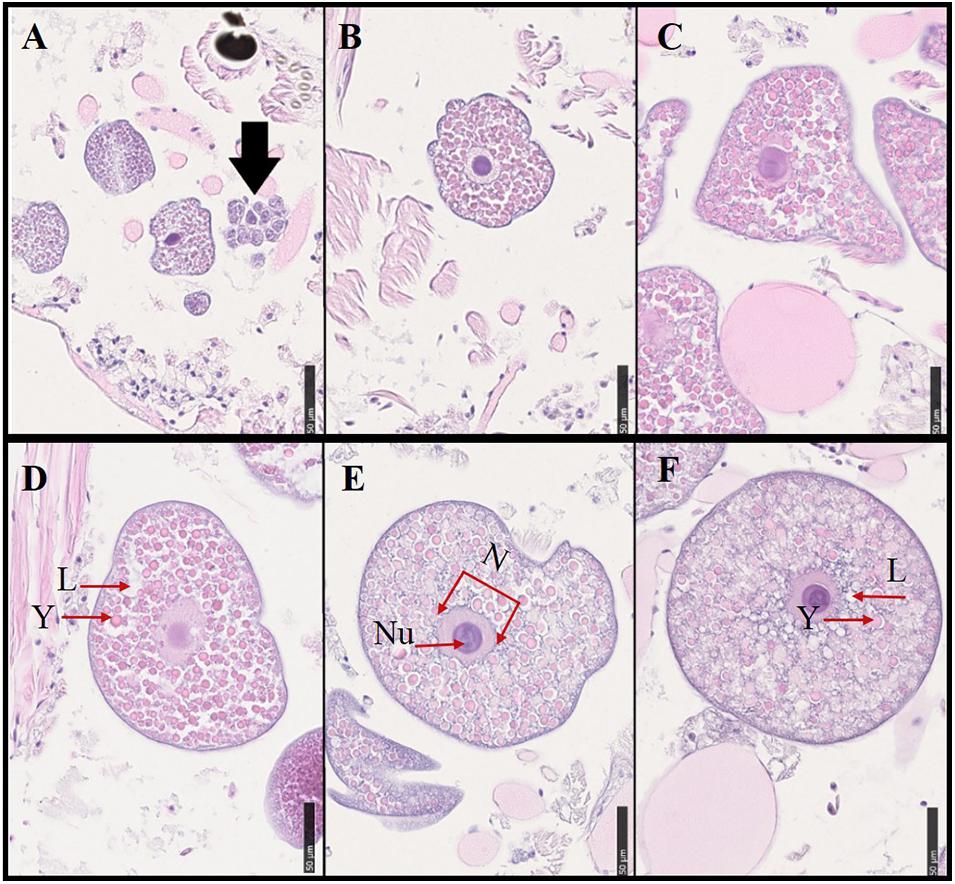
Figure 6. Histological samples showing oocyte development in the polychaetes sampled from the natural population. Various sizes of oocytes from different individuals were selected. Oocyte sizes, sampling dates, and descriptive characteristics for oocyte samples from the natural population were described in Table 3. Scale bar = 50 μ m. (A) The black arrow showed oogonia. L, lipid droplets; Y, yolk granules; N, nucleus; Nu, nucleolus.
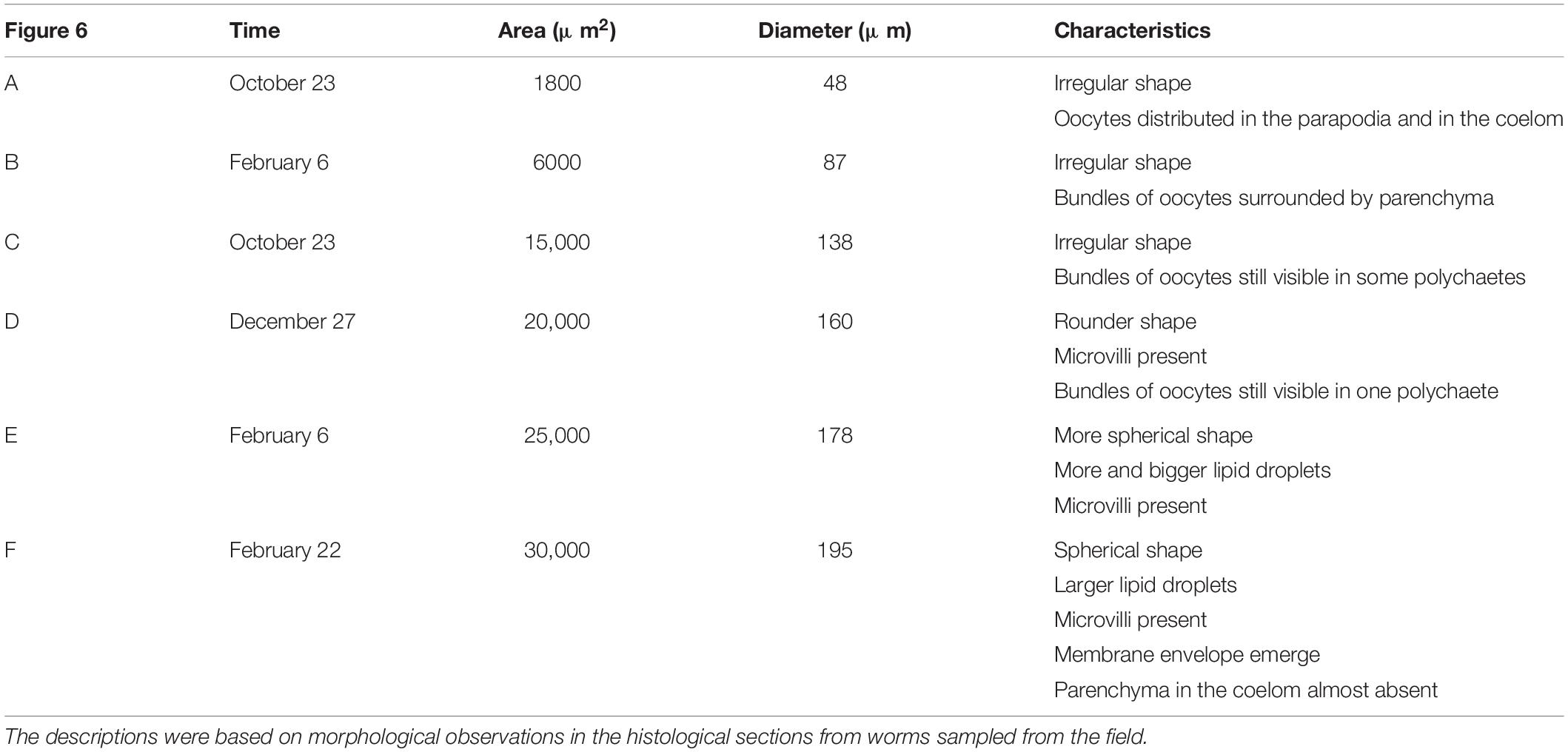
Table 3. Oocyte development of H. diversicolor based on the different area and size of oocytes. (A–F) was corresponded to the various-size oocytes in Figure 6.
Effect of Increasing Temperature on the Final Stages of Oocyte Maturation (Experiment III)
Oocyte size increased the most at higher constant temperature (HT, 8°C); meanwhile, no significant difference was observed between oocyte growth in the lower-temperature treatment (LT, 2°C) and worms collected from the field (NP) (Tables 1, 2 and Figure 7). The oocyte size at HT treatment was significantly larger than that of field specimens at 17 and 24 days (23 and 30 March, p = 0.028, 0.019). At the first sampling, oocyte at the NT treatment became spherical, while in all later samplings the natural specimens had irregular oocytes. The average oocyte size was assumed at around 196 μm (measured without cover slip; Supplementary Table 1). The Futon’s condition factor (K value) in the mature female ranged from 0.03 to 0.24 (Figure 8). The K value showed a generally declining trend over the following worm length range (r2 = 0.62, p < 0.05).
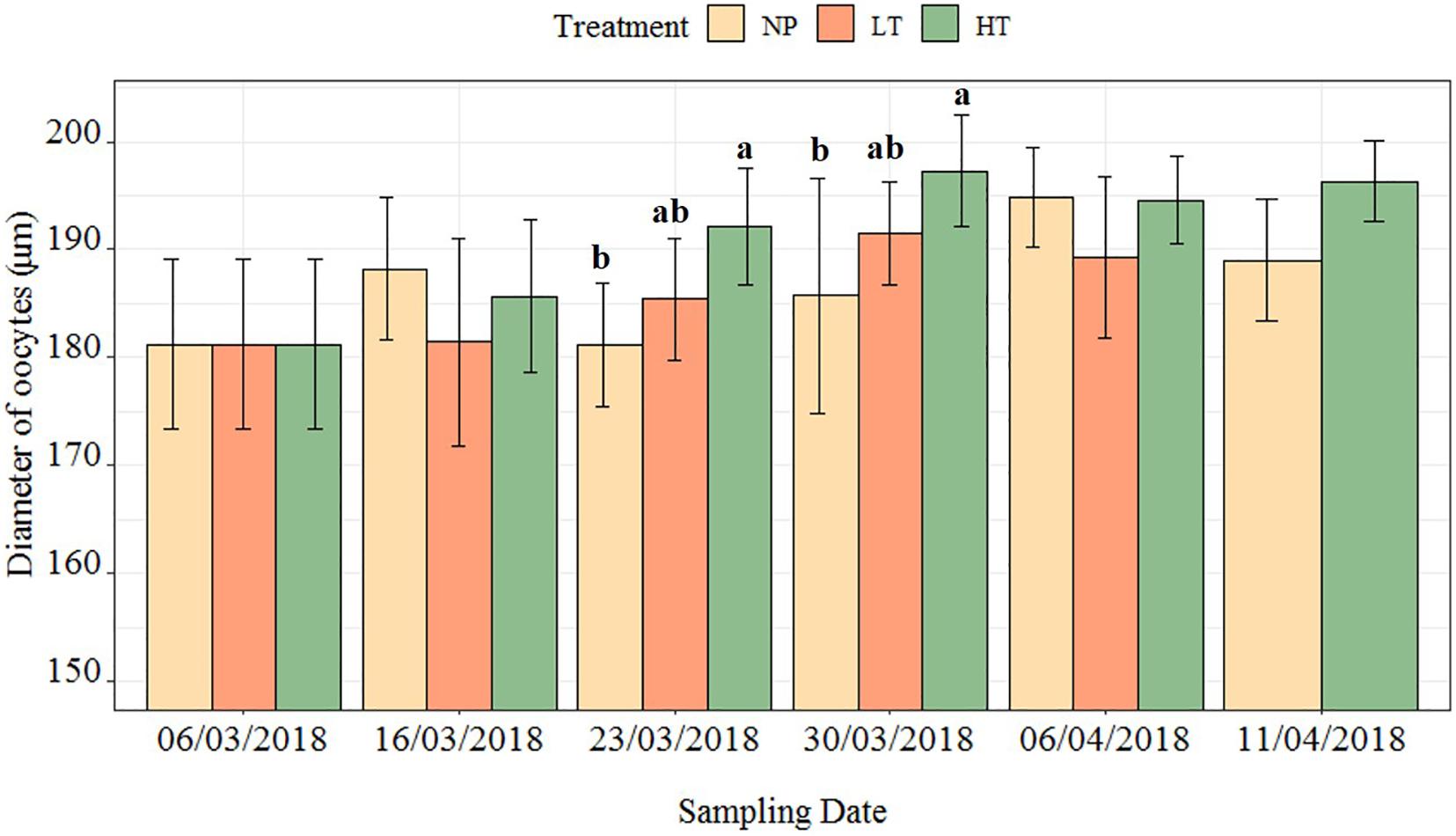
Figure 7. The change in oocyte size of polychaete H. diversicolor at three treatments over time. The letters mark the differences between three treatments at one time point. The number of worms used at each time point of each treatment was documented in Supplementary Table 2. In each worm, 50 oocytes were measured.
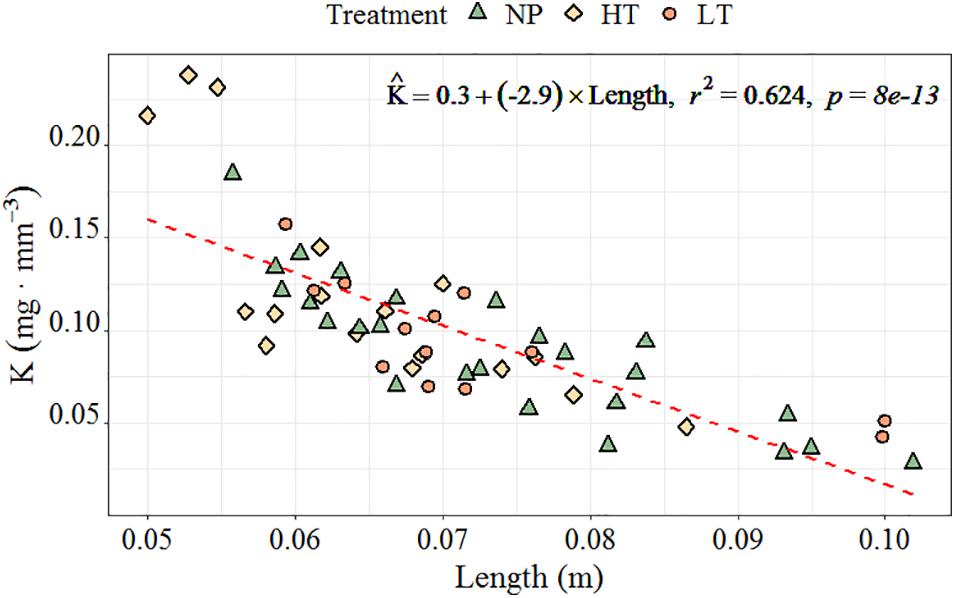
Figure 8. The relationship between the Fulton’s condition factor (K, mg⋅mm– 3) and total body length (cm) with regression equation in H. diversicolor. Calculations were based on pooled individuals from all three treatments (n = 55).
Discussion
Reproduction in many marine species usually occurs at specific times of the year, and often there is only one well-defined reproductive season per year. This reproductive season can be determined by endogenous or environmental cues, or combinations of both. The sexes of H. diversicolor in this study could be differentiated from January onward (observation from 2017 to 2019) by color assessment, and we observed that the proportion of mature worms started to decrease in March. Hardly any mature worms were found after mid-April. Therefore, spawning season seemed in the period between March and April at the study site. Chambers and Milne (1975) found that N. diversicolor in Scotland had two spawning seasons: January to March, and June to August. Kristensen (1984) also noted two offspring recruitment events per year of N. diversicolor in Denmark: one from March to May, and another from July to August. At our sampling site, in relation to observations done in 2018, maturing worms were found during the summer season. Hence, we conclude that H. diversicolor breed only once per year in spring in Leangen Bay, probably to take advantage of significantly warmer temperatures for fast growth during the summer and autumn seasons. Further investigations are needed before clarifying the life cycle of H. diversicolor in middle Norway.
Reproduction of polychaete can be influenced by both external factors such as photoperiod, temperature, and food availability (Bentley and Pacey, 1992), and internal endocrine regulation (Lawrence and Soame, 2009). Photoperiod and temperature influences energy partitioning toward either somatic growth or oogenesis, and further determines life span (Olive, 1995; Olive et al., 1997). The results from Experiment I showed that constant appropriate temperature combined with decreasing photoperiod promoted oocyte growth rates. Meanwhile, worms reared at constant temperature (16°C) and long photoperiod, or at decreasing temperature and decreasing photoperiod, also increased their oocyte diameter during the experiment. However, the increase was significantly lower than for treatments where just one environmental factor was changed. This is consistent with Last and Olive (1999)’s finding that a long photoperiod (>16 h light) may inhibit the oocyte growth of N. virens. Suitable temperatures and long photoperiod promote somatic growth (Chu and Levin, 1989; Last and Olive, 1999), and while low temperatures may help synchronize gamete release, short photoperiod might stabilize early oogenesis, enhance fecundity, and advance the onset of sexual maturity (Clark, 1988; Olive, 1995). Our experiment provides information on a vitellogenic process that oocyte growth can be promoted by a transfer from long photoperiod to short at suitable temperature or decreasing temperatures while upholding long photoperiod. Northern species have been observed to have more restricted and earlier breeding seasons than southern ones (Olive, 1995). However, exposure to low temperatures or short photoperiod may be a necessary cue in the gametogenic cycle, where the minimum duration of such cues and the timing for induction must be addressed in further studies.
In some cases, it is a change rate in photoperiod rather than the actual photoperiod that manipulates maturation (Cabrita et al., 2008). In Experiment II, the influence of an increased rate of change in photoperiod on oocyte growth was evaluated. Basically, we compressed the light regime of the winter season by 2.5 times. It showed that a compressed winter photoperiod can accelerate oocyte growth rate. These worms exposed to compressed natural photoperiod were assumed to mature 2.1 times earlier than those in the field, while the worms under simulated natural photoperiod at constant 8°C matured 1.5 times earlier than the NP. It was speculated that an increase in the rate of photoperiod shift during the autumn–winter period will to some extent increase oocyte growth. The worms in the laboratory had faster oocyte growth but never experienced harsh winter temperatures, so temperature was considered to compensate oocyte growth. Bartels-Hardege and Zeeck (1990) stimulated oogenesis by raising the temperature from 7 to 16°C outside the normal spawning season, although this resulted in unsynchronized spawning, which considered that a certain minimum exposure to winter temperatures would be necessary to synchronize spawning. In our study, because of a low temperature during the 2017 winter, the worms matured late, and spawning season lasted from the end of March until mid-April. At the end of our experiment, only one matured worm was found for the simulated natural photoperiod treatment; hence, whether different photoperiods with or without experiencing winter temperatures will synchronize oocyte maturation remains unknown.
Oocyte size becomes more homogeneous during maturation (Clark and Ruston, 1963). Experiment III was carried out to verify if oocyte development of overwintered worms could be synchronized by increasing the temperature before the onset of maturation. Oocyte were observed to be spherical at first sampling (∼10 days) at higher temperature treatment, while irregular oocytes were observed till spawning season at mid-April for the NP. Therefore, after experiencing winter conditions, increasing temperature promoted oocyte homogeneity and growth. The mean oocyte diameter of H. diversicolor near spawning was observed to be approximately 196 μm in our study, which was similar to the 220 μm reported by Dales (1950). Clark and Ruston (1963) reported that oocytes appear in the coelom of N. diversicolor already when they are as small as 18 μm in body length. In this study, we observed oocytes from 20 to 60 μm diameter in some females and we expect these females to mature in the upcoming breeding season (early oocytes in several females were measured in Experiment II). Chu and Levin (1989) found that females of Streblospio benedicti appear to funnel all their energy into reproduction when favorable temperatures and photoperiods co-occur. Studies on fish also proposed that photoperiod may be the most prominent factor determining breeding seasons, providing a “data” signal, while temperature plays a role in cueing the precise timing of gamete maturation and spawning (Pankhurst and King, 2010). Therefore, providing a suitable temperature after a winter season can help shorten the duration and provide a precise time cue toward final maturity.
Female fecundity (total number of oocytes per worm) varied from 6 × 103 to 2.9 × 104 ova⋅ind–1 (Supplementary Figure 8). In polychaeta, fecundity has been reported to be influenced mainly by environmental factors such as food quantity, quality, and temperature (Chu and Levin, 1989; Qian and Chia, 1991). The fecundity in our experiment was about two times more than what was found by Amiard-Triquet and Rainbow (2009). Long photoperiods have a negative effect on fecundity in H. imbricate, and early oogenesis may be aborted if the polychaete does not experience a minimum of 42 days of photoperiods below the critical photoperiod (Clark, 1988; Olive, 1995). Therefore, to have healthy broodstock and obtain a high-quality and quantity production of H. diversicolor offspring, studies on the effect of photoperiod and temperature transitions on female fecundity and offspring survival for cohorts are needed to provide information for closing the life cycle and enabling large-scale production.
The Futon’s condition factor (K value) can be used to estimate changes in nutritional condition in fishery research (Froese, 2006). In our study, K value W = 22.98L0.61W = 0.016L2.36 was found to decrease with increased length, which means longer worms are relatively smaller in girth. The K value was obtained from the formula in Nesto et al. (2018), which decreased from 0.56 to 0.08 as worm body length increased from 5 to 105 mm, which had the same trend but larger values than our worms in the range of 50–150 mm. As the worms in Nesto et al. (2018) were reared at lab, ours were from the field. We speculated that the body volume of the worm could increase relatively higher when growing at an optimal condition, which may then result in larger size and higher fecundity of puberty.
Conclusion
Exerting one stimulus of transition to short photoperiods or decreasing temperatures can elevate the size growth of eggs in the vitellogenic process after effective somatic growth during long photoperiod exposure. Shorten the change in winter photoperiod can increase the growth rate of oocytes. After low temperatures, increasing temperature can trigger a more homogenous oocyte size and growth near the final maturity. Understanding the regulation of photoperiod, temperature, and their combinations will contribute to trigger oocyte growth, and further for control of breeding out of season in H. diversicolor.
Data Availability Statement
The raw data supporting the conclusions of this article will be made available by the authors, without undue reservation, to any qualified researcher.
Author Contributions
AM, AnH, KR, AlH, and HW conceived and designed the experiment. HW and MU carried out the implementation. HW drafted the manuscript. AM, AnH, KR, AlH, MU, and EK aided with the writing. All authors agreed with the final version submitted for publication.
Funding
This work was carried out with the support of the Research Council of Norway project POLYCHAETE (#280836). The experiments were carried out within the framework of the national research infrastructure “Norwegian Center for Plankton Technology” (#245937/F50).
Conflict of Interest
AM, AnH, AlH, and HW were employed by the company SINTEF Ocean.
The remaining authors declare that the research was conducted in the absence of any commercial or financial relationships that could be construed as a potential conflict of interest.
Acknowledgments
The authors would like to acknowledge the laboratory histological instruction by Tora Bardal (NTNU) and acknowledge financial support from China Scholarship Council for HQW (201506220184).
Supplementary Material
The Supplementary Material for this article can be found online at: https://www.frontiersin.org/articles/10.3389/fmars.2020.544061/full#supplementary-material
References
Amiard-Triquet, C., and Rainbow, P. S. (2009). Environmental Assessment of Estuarine Ecosystems: A Case Study. Boca Raton, FL: CRC press.
Bartels-Hardege, H., and Zeeck, E. (1990). Reproductive behaviour of Nereis diversicolor (Annelida: Polychaeta). Mar. Biol. 106, 409–412. doi: 10.1007/BF01344320
Batista, F., Fidalgo e Costa, P., Matias, D., Joaquim, S., Massapina, C., Passos, A., et al. (2003). Preliminary results on the growth and survival of the polychaete Nereis diversicolor (OF Muller, 1776), when fed with faeces from the carpet shell clam Ruditapes decussatus (L., 1758). Boletin Instit. Espanol. Oceanografia 19, 443–446.
Bentley, M., and Pacey, A. (1992). Physiological and environmental control of reproduction in polychaetes. Oceanogr. Mar. Biol.Annu. Rev. 30, 443–481.
Bischoff, A. A., Fink, P., and Waller, U. (2009). The fatty acid composition of Nereis diversicolor cultured in an integrated recirculated system: possible implications for aquaculture. Aquaculture 296, 271–276. doi: 10.1016/j.aquaculture.2009.09.002
Cabrita, E., Robles, V., and Herráez, P. (2008). Methods in Reproductive Aquaculture: Marine and Freshwater Species. Boca Raton, FL: CRC Press.
Chambers, M., and Milne, H. (1975). Life cycle and production of Nereis diversicolor OF Müller in the Ythan Estuary, Scotland. Estuar. Coast. Mar. Sci. 3, 133–144. doi: 10.1016/0302-3524(75)90016-X
Chu, J., and Levin, L. A. (1989). Photoperiod and temperature regulation of growth and reproduction in Streblospio benedicti (Polychaeta: Spionidae). Inverteb. Reprod. Dev. 15, 131–142. doi: 10.1080/07924259.1989.9672033
Clark, R. B., and Ruston, R. (1963). The influence of brain extirpation on oogenesis in the polychaete Nereis diversicolor. Gen. Comp. Endocrinol. 3, 529–541. doi: 10.1016/0016-6480(63)90087-X
Clark, S. (1988). A two phase photoperiodic response controlling the annual gametogenic cycle in Harmothoe imbricata (L.)(Polychaeta: Polynoidae). Int. J. Inverteb. Reprod. Dev. 14, 245–265. doi: 10.1080/01688170.1988.10510383
Dales, R. P. (1950). The reproduction and larval development of Nereis diversicolor OF Müller. J. Mar. Biol. Assoc. U.K. 29, 321–360. doi: 10.1017/S0025315400055405
Datta, S. N., Kaur, V. I., Dhawan, A., and Jassal, G. (2013). Estimation of length-weight relationship and condition factor of spotted snakehead Channa punctata (Bloch) under different feeding regimes. SpringerPlus 2:436. doi: 10.1186/2193-1801-2-436
Fischer, A. H., Jacobson, K. A., Rose, J., and Zeller, R. (2008). Hematoxylin and eosin staining of tissue and cell sections. Cold Spring Harbor Prot. 2008:db.rot4986.
Food and Agriculture Organization [FAO] (2017). The State of the World Fisheries and Aquaculture 2016. Rome: FAO Fisheries and Aquaculture Department, Food and Agriculture Organization of the United Nations.
Froese, R. (2006). Cube law, condition factor and weight–length relationships: history, meta-analysis and recommendations. J. Appl. Ichthyol. 22, 241–253. doi: 10.1111/j.1439-0426.2006.00805.x
Garwood, P., and Olive, P. (1982). The influence of photoperiod on oocyte growth and its role in the control of the reproductive cycle of the polychaete Harmothoe imbricata (L.). Int. J. Inverteb. Reprod. 5, 161–165. doi: 10.1080/01651269.1982.10553465
Kristensen, E. (1984). Life cycle, growth and production in estuarine populations of the polychaetes Nereis virens and N. diversicolor. Ecography 7, 249–250. doi: 10.1111/j.1600-0587.1984.tb01128.x
Last, K., and Olive, P. (1999). Photoperiodic control of growth and segment proliferation by Nereis (Neanthes) virens in relation to state of maturity and season. Mar. Biol. 134, 191–199. doi: 10.1007/s002270050537
Lawrence, A., and Soame, J. (2009). The endocrine control of reproduction in Nereidae: a new multi-hormonal model with implications for their functional role in a changing environment. Philos. Trans. Royal Soc. B Biol. Sci. 364, 3363–3376. doi: 10.1098/rstb.2009.0127
Lawrence, A. J., and Soame, J. M. (2004). The effects of climate change on the reproduction of coastal invertebrates. Ibis 146, 29–39. doi: 10.1111/j.1474-919X.2004.00325.x
Mandario, M. A. E. (2018). Addressing gaps in the culture of pathogen-free polychaetes as feed in shrimp hatcheries. Fish People 16, 19–23.
Müller, O. F. (1776). Zoologiae Danicae Prodromus: Seu Animalium Daniae et Norvegiae Indigenarum; Characteres, Nomina, et Synonyma Imprimis Popularium, Havniae: Typis Hallageriis. 1730–1784. doi: 10.5962/bhl.title.13268
Nesto, N., Simonini, R., Prevedelli, D., and Da Ros, L. (2012). Effects of diet and density on growth, survival and gametogenesis of Hediste diversicolor (OF Müller, 1776)(Nereididae. Polychaeta). Aquaculture 362, 1–9. doi: 10.1016/j.aquaculture.2012.07.025
Nesto, N., Simonini, R., Prevedelli, D., and Da Ros, L. (2018). Evaluation of different procedures for fertilization and larvae production in Hediste diversicolor (OF Muller, 1776) (Nereididae. Polychaeta). Aquacult. Res. 49, 1396–1406. doi: 10.1111/are.13589
Olive, P., and Pillai, G. (1983). Reproductive biology of the polychaete Kefersteinia cirrata Keferstein (Hesionidae). II. The gametogenic cycle and evidence for photoperiodic control of oogenesis. Int. J. Inverteb. Reprod. 6, 307–315. doi: 10.1080/01651269.1983.10510056
Olive, P. J. W. (1995). Annual breeding cycles in marine invertebrates and environmental temperature: probing the proximate and ultimate causes of reproductive synchrony. J. Ther. Biol. 20, 79–90. doi: 10.1016/0306-4565(94)00030-M
Olive, P. J. W. (1999). Polychaete aquaculture and polychaete science: a mutual synergism. Hydrobiologia 402, 175–183. doi: 10.1023/a:1003744610012
Olive, P. J. W., Fletcher, J., Rees, S., and Desrosiers, G. (1997). Interactions of environmental temperature with photoperiod in determining age at maturity in a semelparous polychaete Nereis (Neanthes) virens Sars. J. Ther. Biol. 22, 489–497. doi: 10.1016/S0306-4565(97)00067-3
Olive, P. J. W., Rees, S. W., and Djunaedi, A. (1998). Influence of photoperiod and temperature on oocyte growth in the semelparous polychaete Nereis (Neanthes) virens. Mar. Ecol. Prog. Ser. 172, 169–183. doi: 10.3354/meps172169
Pankhurst, N., and King, H. (2010). Temperature and salmonid reproduction: implications for aquaculture. J. Fish Biol. 76, 69–85. doi: 10.1111/j.1095-8649.2009.02484.x
Pfannenstiel, H. (1978). Endocrinology of polychaete reproduction and sexual development. Italian J. Zool. 45, 171–188. doi: 10.1080/11250007809440126
Qian, P. Y., and Chia, F. S. (1991). Fecundity and egg size are mediated by food quality in the polychaete worm Capitella sp. J. Exp. Mar. Biol. Ecol. 148, 11–25. doi: 10.1016/0022-0981(91)90143-k
Ross, M., and Pawlina, W. (2017). Histology, A Text and Atlas, With Correlated Cell and Molecular Biology. Philadelphia, PA: Lippincott Williams & Wilkins.
Wang, H., Seekamp, I., Malzahn, A., Hagemann, A., Carvajal, A. K., Slizyte, R., et al. (2019). Growth and nutritional composition of the polychaete Hediste diversicolor (OF Müller, 1776) cultivated on waste from land-based salmon smolt aquaculture. Aquaculture 502, 232–241. doi: 10.1016/j.aquaculture.2018.12.047
Keywords: daylength, temperature, change, polychaete, oocyte growth
Citation: Wang H, Hagemann A, Malzahn AM, Handå A, Uhre M, Kjørsvik E and Reitan KI (2020) The Role of Manipulating Photoperiod and Temperature in Oocyte Development of the Polychaete Hediste diversicolor (O.F. Müller, 1976). Front. Mar. Sci. 7:544061. doi: 10.3389/fmars.2020.544061
Received: 19 March 2020; Accepted: 17 November 2020;
Published: 08 December 2020.
Edited by:
Liping Liu, Shanghai Ocean University, ChinaReviewed by:
Pedro M. Costa, Faculdade de Ciências e Tecnologia da Universidade Nova de Lisboa, PortugalDazuo Yang, Dalian Ocean University, China
Copyright © 2020 Wang, Hagemann, Malzahn, Handå, Uhre, Kjørsvik and Reitan. This is an open-access article distributed under the terms of the Creative Commons Attribution License (CC BY). The use, distribution or reproduction in other forums is permitted, provided the original author(s) and the copyright owner(s) are credited and that the original publication in this journal is cited, in accordance with accepted academic practice. No use, distribution or reproduction is permitted which does not comply with these terms.
*Correspondence: Haiqing Wang, hqwang@hainanu.edu.cn; haiqing.wang1@gmail.com