Marine Heatwaves, Sewage and Eutrophication Combine to Trigger Deoxygenation and Biodiversity Loss: A SW Atlantic Case Study
- 1Graduate Program in Ecology, Federal University of Santa Catarina, Florianópolis, Brazil
- 2Graduate Program in Oceanography, Federal University of Santa Catarina, Florianópolis, Brazil
- 3Department of Marine Sciences, University of Gothenburg, Gothenburg, Sweden
- 4Department of Oceanography, Federal University of Santa Catarina, Florianópolis, Brazil
- 5Department of Botany, Federal University of Santa Catarina, Florianópolis, Brazil
- 6Department of Mechanical Engineering, Federal University of Santa Catarina, Florianópolis, Brazil
- 7School of Biological and Marine Sciences, University of Plymouth, Plymouth, United Kingdom
- 8Shimoda Marine Research Center, University of Tsukuba, Tsukuba, Japan
Marine heatwaves (MHWs) are a major concern worldwide due to their increasing impacts in recent years, and these extreme events may trigger deoxygenation of coastal waters affected by sewage and eutrophication. Here we investigate the combined effects of MHWs and nutrient enrichment on the water quality and biodiversity of the Bay of Santa Catarina Island (Brazil). We used historical (1994–2020) sea surface temperature data from satellites and in situ physical, chemical and biological parameters to assess temporal trends. Oxygen levels have been decreasing whilst phosphorus levels have been increasing in the bay. During the austral summer of 2020 a regional sea surface heatwave was detected by satellite, lasting for 9 days and coinciding with our research cruise. During this period, seawater temperatures reached 29.8°C and anoxia was detected for the first time in the bay. A decrease in macrobenthic and phytoplankton community richness correlated with decreases in oxygen both through time and towards more urbanized areas. Overall, poor wastewater treatment is a key stressor that combined with MHWs to degrade coastal waters. Mitigation strategies are needed to minimize the impact of MHWs, including improved sewage treatment, restoration and conservation of wetlands and the use of nature-based technologies to promote coastal ecosystem recovery.
Introduction
Much attention has been given to climate change and ocean warming projections for the end of this century (Bestion et al., 2020). However, marine heatwaves (MHWs) are already causing major biodiversity loss and are having socio-environmental consequences worldwide. These events can be defined as when sea surface temperature (SST) exceeds a threshold for more than five consecutive days (Hobday et al., 2016). On a global scale, MHW intensity, duration and frequency are increasing (Frölicher et al., 2018; Oliver et al., 2019; Rodrigues et al., 2019; Smale et al., 2019). Some of the consequences are well known, such as widespread mortality of marine organisms, shifts in species distributions, increases in biological invasions, coral bleaching, and the loss of blue carbon storage (Garrabou et al., 2009; Cavole et al., 2016; Arias-Ortiz et al., 2018; Smale et al., 2019). In the South West Atlantic, MHWs have started to occur due to atmospheric blocking and droughts during summer (Rodrigues et al., 2019), but their effects on marine life had not previously been studied.
Organic enrichment and eutrophication are major environmental problems worldwide, especially in South America due to an exponential increase in urbanization of coastlines (Scherner et al., 2013) and inadequate sewage treatment (Barletta et al., 2019). Nutrient inputs often boost algal blooms in systems with long water residence time, such as lagoons and bays (Bricker et al., 2008). In South Brazil, harmful algal blooms, deoxygenation and human diseases (e.g., gastroenteritis, mycosis, conjunctivitis) have frequently been associated with sewage pollution and nutrient enrichment from agricultural land run-off (Silva and Fonseca, 2016; Alves and Mafra, 2018; Cabral and Fonseca, 2019). One of the few studies about the interactive effects of eutrophication and high temperatures in Brazil showed a major decline in the physiological performance of a macroalga, indicating that individuals inhabiting densely urbanized areas are more susceptible to warm water episodes (Gouvêa et al., 2017). Here, we present the first field study of the interaction between MHWs and the pollution caused by organic enrichment and eutrophication.
Hypoxic and anoxic events are defined as when the dissolved oxygen (DO) is less than 2.0 mg L–1 and equal to 0 mg L–1, respectively, and are symptomatic of organic enrichment and eutrophication (Bricker et al., 2008). Global warming and high organic matter loads are the primary cause of deoxygenation, altering biogeochemical cycles and lowering marine species diversity (Breitburg et al., 2018). Coastal hypoxia has exponentially increased “dead zones” worldwide since the 1960s, mainly as a result of human activities (Diaz and Rosenberg, 2008). In South America, seasonal and permanent dead zones in several estuaries are linked to human population growth and lack of basic sanitation (Barletta et al., 2019). The cumulative effects of dead zones and MHWs is likely to reduce environmental resilience and biodiversity (Scherner et al., 2013) although our knowledge of this is limited due to a lack of temporal and spatial in situ data.
Temperature is a key driver that effects the physiology, behavior and ecology of marine fauna and flora. Initially, temperature increases usually increase metabolism and energy consumption but can eventually kill marine organisms. Shallow water species tolerate a much wider temperature range than the stenothermal inhabitants of the deep-sea. However, an increase of 2–3°C above normal peak temperature quickly compromises the physiology of coastal organisms, and can be lethal (Peck et al., 2009; Nguyen et al., 2011). For this reason, the distribution of benthic organisms is shifting in response to ocean warming, causing marine community reconfigurations world-wide (Wernberg et al., 2013; Sanford et al., 2019). Experimental tests suggest that Anomalocardia flexuosa, a socio-economically important bivalve in South America, is overly sensitive to temperature increases (Carneiro et al., 2020). In Brazil, higher temperatures and pollution can be expected to change the community structure and productivity of benthic marine species (Bernardino et al., 2015), although the effects of MHWs remain a key knowledge gap (Intergovernmental Panel on Climate Change, 2019).
Improving urban planning and sanitation in South America would build resilience to the cumulative effects of global stressors, helping to achieve the UN Sustainable Development Goals (Jacobi and Sulaiman, 2016). Sustainable Development Goal 14 advocates for reductions in marine pollution from all sources, including sewage, although empirical evidence of the benefits of mitigation and management actions is often lacking. Santa Catarina Island is an area of exceptionally high socioeconomic importance in South Brazil, but it suffers from multiple anthropogenic pressures, including poor wastewater treatment (Pagliosa et al., 2006; Garbossa et al., 2017; Cabral et al., 2019). This region has experienced an increase in MHW frequency, intensity and duration (Rodrigues et al., 2019). However, there are no studies addressing the interactive effects of poor water quality and MHWs. Here, we assess the long-term relationships between the physicochemical properties of the water column and phytoplanktonic and benthic communities. We hypothesize that effects of MHWs on oxygen levels are made worse by organic and inorganic enrichment degrading ecosystem state, decreasing water quality and causing biodiversity loss. We hope that the results described here will guide better management of wastewater treatment in the region, for the benefit of all.
Materials and Methods
Study Area
Santa Catarina Island, south Brazil, has a 50-km long microtidal embayment (Figure 1) with a surface area of 430 km2 and an average depth of 3 m (Bonetti et al., 2007). The water residence time is around 18 days during summer (Cabral et al., 2020) mixing with the open Atlantic at the North and the South ends of the bay and with low circulation in the central region (Garbossa et al., 2014). The semi-diurnal tides have daily amplitudes of less than 2 m (Garbossa et al., 2014). The adjacent watersheds cover an area of 1875 km2, where around 1 million people reside permanently. The main economic activities in the region are summer tourism (1.5 million people/year) and mollusc aquaculture (70% of the Brazilian bivalve production) (Garbossa et al., 2017). The system is moderately urbanized and only ∼30% of the wastewater is treated (Garbossa et al., 2017).
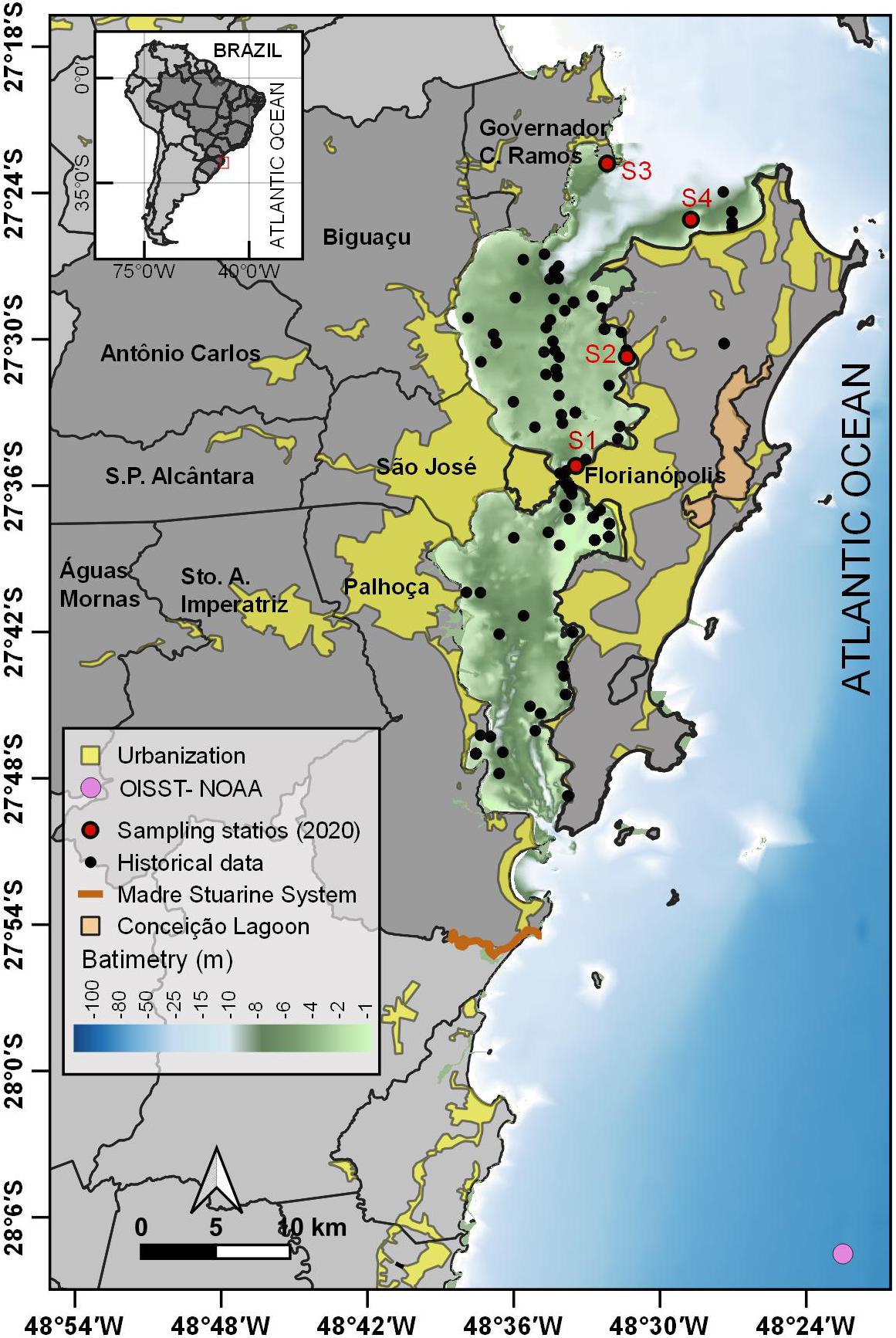
Figure 1. Bay of Santa Catarina Island off Brazil showing coastal water sampling points (black and red circles) and urbanized areas (yellow). The pink circle represents NOAA–OISST: the Optimum Interpolation Sea Surface Temperature from the National Oceanic and Atmospheric Administration.
Sampling and Laboratory Procedures
In situ data were collected in January 2020 at four sampling stations: Station 1 (near the island’s bridge at 27.59°S 48.56°W), Station 2 (Santo Antônio de Lisboa at 27.51°S 48.52°W), Station 3 (Armação da Piedade at 27.38°S 48.54°W), and Station 4 (Ilha do Francês at 27.42°S 48.48°W) (Figure 1). Temperature and DO were measured using a YSI Pro 2030 probe. Water samples were collected and filtered to determine the dissolved inorganic phosphorus (DIP) concentration, following the colorimetric method (described in Grasshoff et al., 1999). Data from 1994 to 2019 on water temperature (°C), DO (mg L-), and DIP (μM) were obtained from Cabral et al. (2020) for the Austral summer (December, January, February, and March) (see Figure 1 for sampling details).
To assess the MHW event in this study, we used SST data obtained from the National Oceanic and Atmospheric Administration, the Optimum Interpolation Sea Surface Temperature V2.1 (OISST) for 1982–2020, which provided better temporal coverage compared to the in situ data available for the study area (Reynolds et al., 2007). We calculated the climatology for a pixel at 28.13°S 48.38°W (Figure 1). A MHW event occurs when the SST exceeds the 90th percentile for 5 or more days (Hobday et al., 2016). Time series of MHW frequency, maximum intensity, and cumulative intensity were obtained for the whole period. Frequency is the number of MHW days per summer (December–March), maximum intensity is the maximum SST anomaly for all MHW days per summer, and cumulative intensity is the cumulative sum of the intensity for all MHW days within the summer. Number of inhabitants of the nine municipalities around the bays (Água Mornas, Antônio Carlos, Biguaçu, Florianópolis, Governador Celso Ramos, Palhoça, Santa Amaro da Imperatriz, São José, and São Pedro de Alcântara) were retrieved from the Brazilian Institute of Geography and Statistics website (Instituto Brasileiro de Geografia e Estatística – IBGE) for the period of 1994–2019.
Benthic fauna was sampled in triplicate at all stations by scuba-divers using a PVC core of 15 cm diameter and 10 cm high (0.018 m–2). Samples were then washed using a 0.5 mm granulometric sieve and fixed in 70% alcohol for subsequent identification. In the laboratory, the benthic fauna was identified to the lowest possible taxonomic level under a stereoscopic microscope. Historical data of the benthos sampled during summer (December–March) were selected based on the proximity to the stations and the use of the same field and laboratory procedures. Our search returned data from summer 2001, 2006 (available in Souza, 2008) and from 2014 to 2017, corresponding to a total of 22 stations (Supplementary Table 2). For phytoplankton analysis, sampling was carried out at two stations (S1 and S4), using a 20 μm net towed at ∼1 m s–1. Samples were fixed in 4% formaldehyde and then identified using keys (Tomas, 1997; Tenenbaum et al., 2004; Tenenbaum and Gomes, 2006).
Statistical Analyses
The significance of trends in temperature, oxygen, DIP and macrobenthic taxonomic richness were obtained using the non-parametric test of Mann–Kendall (MK; p < 0.05) (Harding et al., 2019). The test is calculated by paired comparisons of each data value with the previous one, determining the number of increases, decreases and draws (Gilbert, 1987). Mann–Kendall are shown by the strength of association (-1 < τ < 1), where positive values indicate significant increases over the years and negative values a decrease. Kruskal–Wallis (KW) test was applied to verify significant univariate differences among groups (p < 0.05). When KW was significant, the Dunn’s post-hoc test was used to obtain pairwise comparison results. Pearson linear regression was applied to find significant correlations (p < 0.05). To test which variables (DO, in situ temperature, DIP, MHWs frequency, maximum intensity, and cumulative intensity) better explained the temporal variations in the benthic richness of the Bays, we used individual Generalized Linear Models (GLM) under negative binomial distribution (Zuur et al., 2009), that is known to address overdispersion (which was the case for our data sets). All analyses were performed in the “R” software using packages “wq” (Jassby and Cloern, 2017), “ggplot2” (Wickham, 2016) and “MASS” (Venables and Ripley, 2013).
Results
Physical and Chemical Long-Term Variability
The average SST detected by satellite was 25.6 ± 1.1 for the period of 1982–2020, ranging from a minimum of 21.5°C in December 1984 to a maximum of 29.5°C in January 2019. The average for the in situ data was 26.2 ± 2.3 and ranged widely from 17.0°C in March 2008 to 34.1°C in March 2007. There was a statistically significant positive trend (τ = 0.10, p < 0.0001) for the OISST data time series but not for in situ data. Marine heatwaves frequency, intensity, and cumulative intensity have progressively increased since the 2000’s (Figure 2). These trends are respectively 0.4, 0.025, and 0.8°C days per summer each year. Rodrigues et al. (2019) showed that the trends are statistically significant considering a large area adjacent to Santa Catarina Island. Our survey coincided with the 7th day of a MHW event, which lasted 9 days and reached a maximum SST of 26.4°C. The maximum intensity was 1.6°C and cumulative intensity was 12.7°C-Days. In the bays (in situ data), water temperature (29.3 ± 0.5°C) was 3°C higher than the values found in the shelf (OISST) during the MHW event. Overall, water temperature was significantly higher (p < 0.05) in the bays than in the shelf (OISST) in 8 of the 13 comparable years (Figure 3A). Temperatures were similar (p > 0.05) between these two data sets in the summer of 2000, 2008, 2014, 2015, and 2018.
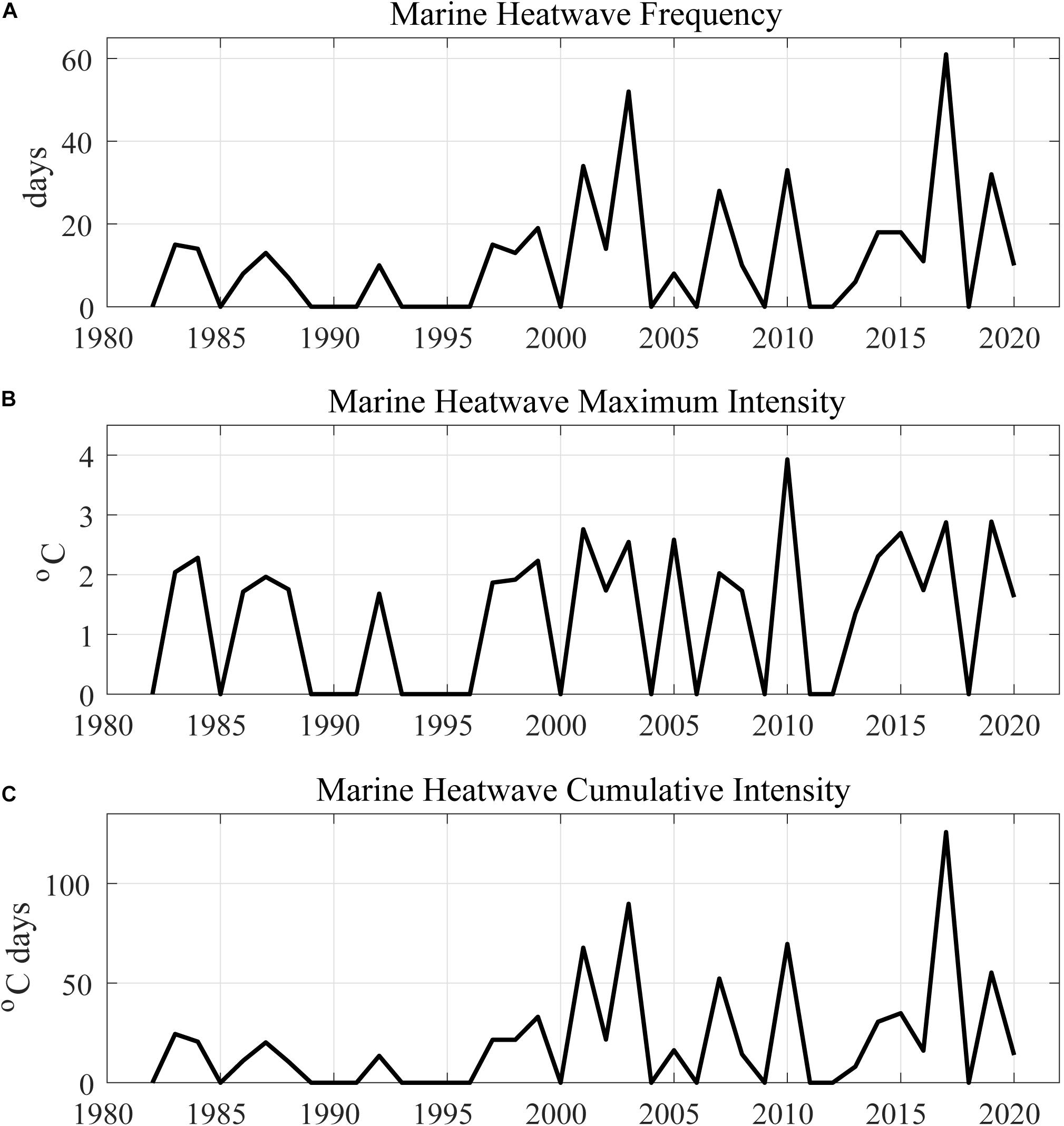
Figure 2. Time series with marine heatwave metrics detected at 28.125°S 48.375°W for the Austral summer months (December–March). (A) Frequency in days per summer; (B) Maximum intensity in °C per summer; and (C) Cumulative intensity in °C Days per summer.
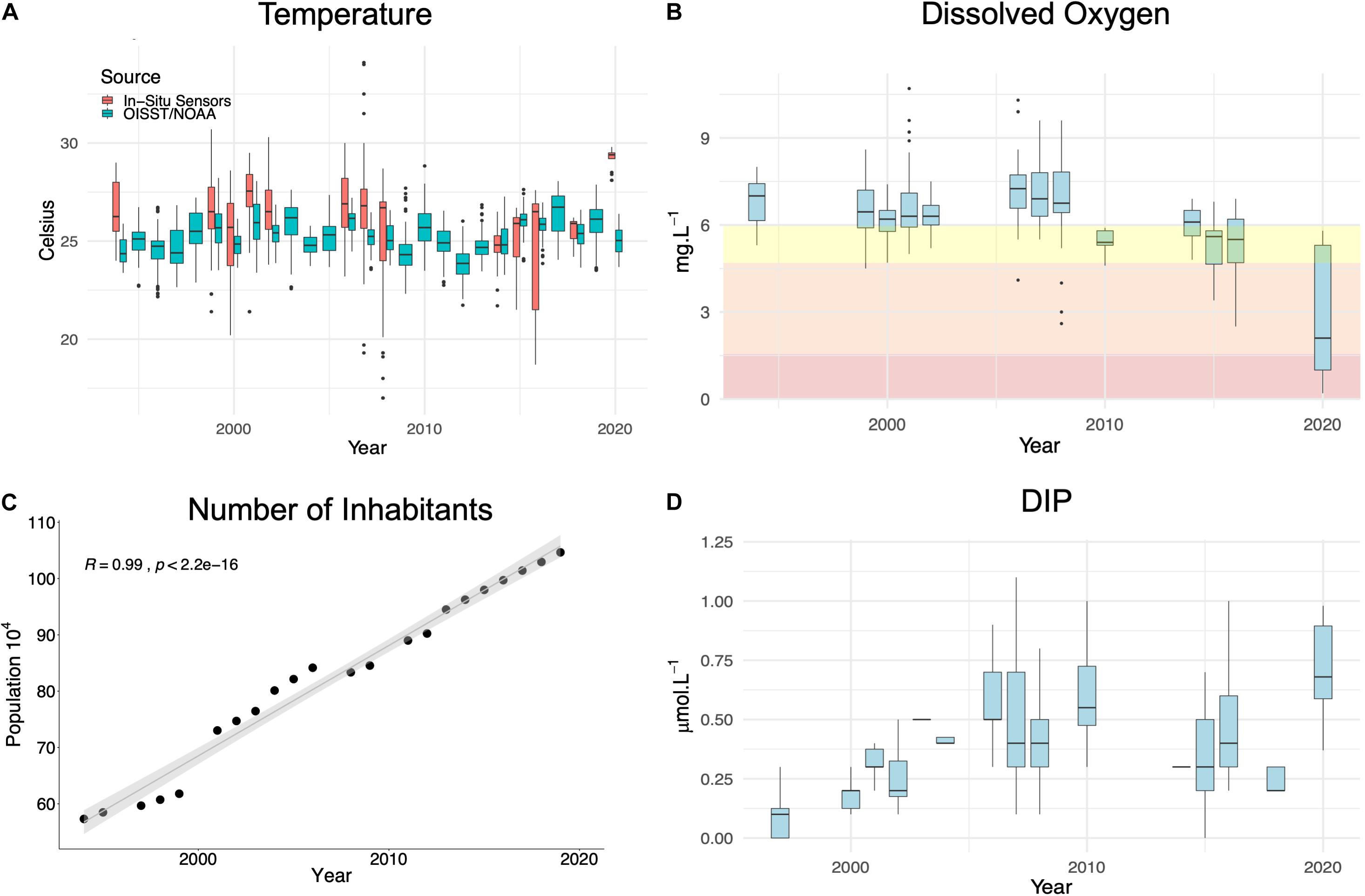
Figure 3. Interannual variability (1994–2020) of (A) temperature (in situ and OISST), (B) dissolved oxygen (DO), (C) number of inhabitants, and (D) dissolved inorganic phosphorus (DIP) in the Bay of Santa Catarina Island during the summer seasons. The number of human inhabitants per year (C) was calculated considering all nine municipalities around the bay. In the DO graph, red shows hypoxia (DO < 2.0 mg L–1, Breitburg et al., 2018), orange indicates biological stress conditions (DO < 5.0 mg L–1, Devlin et al., 2011) and yellow indicates a warning boundary considering the benthic and phytoplanktonic richness losses found in this study.
Dissolved oxygen concentration in seawater (mean 6.4 ± 1.3 mg L–1) varied from 0.0 to 10.7 mg L–1. The lowest values were observed during the summer of 2020 (mean 4.0 ± 2.3 mg L–1), considering all the summer season time-series (Figure 3B). The MK test (τ = -0.51, p < 0.01) also showed that DO is decreasing over the years. Dissolved inorganic phosphorus (0.5 ± 0.4 μM) ranged from 0.1 to 4.4 μM. The MK test (τ = 0.33, p < 0.05) indicated that DIP is increasing over the time series (Figure 3D), from 0.1 ± 0.1 μM in the 1990’s to 0.7 ± 0.2 in 2020. Demographic data show that the human population has continuously increased in the nine municipalities around the bay (Figure 3C).
Biological Long-Term Variability
Species richness of the benthic community has declined significantly over the years (MK test; τ = −0.62, p < 0.05) (Figure 4A). There was a 67% loss, from an average of 10 (± 9.69 taxa/0.018 m–2) species in summer 2001 to the lowest average of 3.5 (± 3.52 taxa/0.018 m–2) species in summer 2020. In general, spatial patterns of richness were lower in the innermost parts of the bay, increasing to the outer reaches of the bay throughout the time series (Figure 4B).
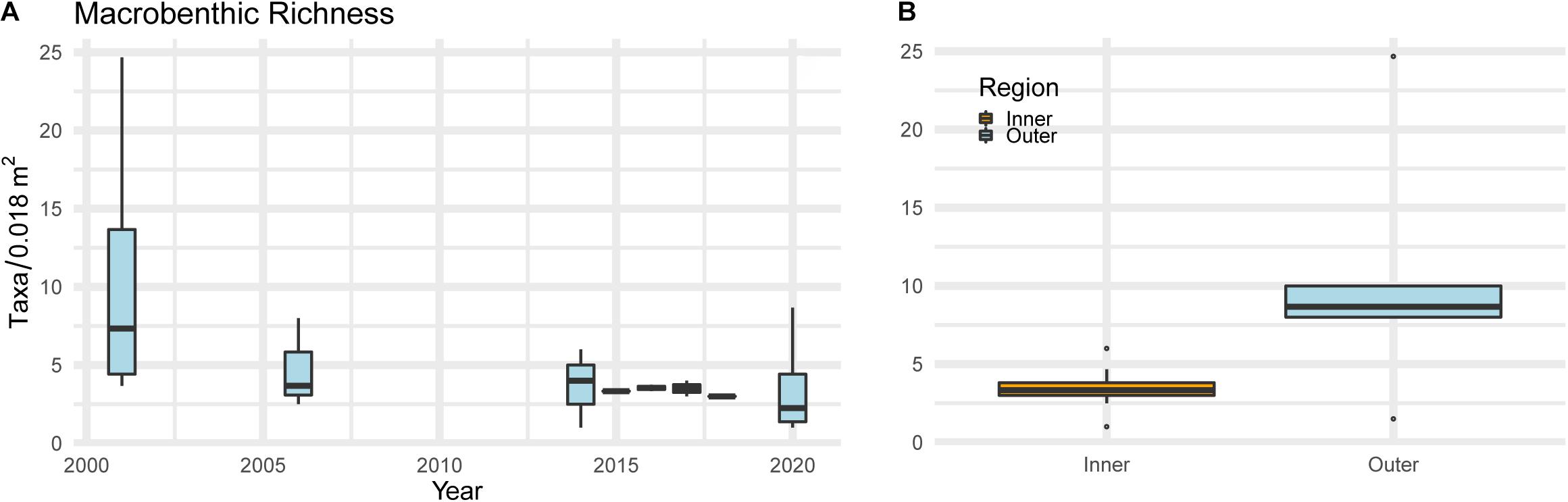
Figure 4. Interannual variability (2001–2020) of macrobenthic species richness (A) and within inner and outer areas (B) of the Bay of Santa Catarina Island during the summer seasons.
General linear model results showed that variations in the richness of the macrobenthic communities were significantly affected by DO, DIP, and MHW maximum intensity between 2001 and 2020 (Table 1). In situ temperature, MHW frequency and cumulative intensity showed no influence on the benthic communities within this period. Data were adequately represented by the negative binomial models, with estimates of dispersion parameters generally close to one (range: 0.97 – 1.16).
Qualitative analysis of phyto- and protozooplankton showed differences between station 2 and 4 (Supplementary Table 1). In station 2, which is shallower and located inside the Bay of Santa Catarina Island, the taxon richness was lower, with 42 units, 81% of Bacillariophyceae, 7% of Dinophyceae, 10% of ciliate (protozooplankton) and 2% of other phytoplankton groups. In station 4, deeper and located outside the bay, the taxon richness was greater, with 58 units, 72% of which were Bacillariophyceae, 21% of Dinophyceae, 3.5% of ciliate and 3.5% of other phytoplankton groups. Station 2 also showed a greater number of organisms of benthic (or ticopelagic) origin, with 24% against 13% in station 4. In both stations potentially toxic taxa such as Pseudo-nitzschia spp., Trichodesmium sp. and the dinoflagellate Dinophysis acuminata were found.
Discussion
Marine Heatwaves and Eutrophication
Our data show that MHW events are increasing both in frequency and intensity in South Brazil, as described by Rodrigues et al. (2019) for a wider area of the South Atlantic. We did not find an increasing temperature trend in the inshore waters of Santa Catarina Island due to the temporal sparseness of the in situ data. However, in 63% of simultaneous measurements of OISST/NOAA satellite data and in situ temperatures (N = 128), the inshore area had significantly higher temperatures than the shelf waters, indicating that heatwave events impacts are likely to be stronger in coastal regions, as observed by Schlegel et al. (2017). During a MHW in summer 2020, the inshore waters were up to 3°C warmer than offshore, and this occurred at the same time as tourism and sewage discharge peaked in the region (Silva et al., 2016). In addition, extreme rainfall events have become more frequent during summer (Ávila et al., 2016), boosting nutrient and organic matter input from the rivers and triggering eutrophication. The water residence time of the bays in the summer (∼18 days) is the lowest among all seasons (∼83 days during winter), due to high rainfall and the offshore transport of coastal waters by the northerly winds, and yet we found elevated nutrient concentrations and hypoxia in the summer as this coastal system is unable to process current sewage loads (Cabral et al., 2020).
The watershed of our study area is now experiencing ∼60% human population growth, two times Brazil’s average (IBGE, 2020). At the same time, sewage treatment is inadequate throughout the region, resulting in high organic matter and nutrient loads (Garbossa et al., 2017; Cabral et al., 2019). We show that DIP has been increasing over the years, probably related to human population increase and inadequate wastewater treatment. The pressure on sewage systems increases in summer, when the number of inhabitants triples in some watersheds because of mass tourism (Silva et al., 2016). Moreover, only 30% of the urban population in the region is serviced by sewerage; most households use cesspits or dump the sewage in natura, polluting the coastal zone. Human adenoviruses have been detected in streams that drain to the bays (Rigotto et al., 2010), and this is directly correlated to the elevated trophic status of its waters (Silva and Fonseca, 2016). Santa Catarina Island bays have moderate to high eutrophication pressure (Cabral et al., 2020). The combination of organic enrichment and eutrophication (Bricker et al., 2008) can cause low oxygen levels in the rivers (Pagliosa et al., 2006) and harmful algal blooms in marine shelf waters (Alves and Mafra, 2018).
Our plankton analyses showed ecological differences between the inner and outer areas of the bay. The highest proportion of diatoms was found inside the bay, indicating that the system is enriched with nutrients and affected by continuous resuspension of sediments. Previous studies also showed that the relative importance of diatoms in the bays increased during dredging in the late 1990’s, leading to more sediment resuspension and, possibly, nutrients and pollutants turnover (Rörig et al., 2010, 2011). Our data show that DIP concentrations after (2001) dredging (1994) were significantly (p < 0.05) higher, never returning to the same levels again (Figure 3). This suggests that, besides sewage inputs, P fluxes from the sediment might contribute to eutrophication, especially during hypoxic events due to P desorption, as already shown for the estuaries that drain to the bay (Pagliosa et al., 2005). The greater taxon richness outside the bay, also with greater relative importance of dinoflagellates (Dinophyceae), shows a less restrictive environment, with more diversity of ecological niches. Moreover, some studies have also shown an increase in harmful algal blooms in the region, possibly related to the degradation of water quality and anomalous meteo-oceanographic events (Proença et al., 2017; Rörig et al., 2018).
Dead Zones and Loss of Biodiversity
Dead zones are spreading worldwide mainly because of increased wastewater discharge from untreated sewage and effluents from agriculture (Diaz and Rosenberg, 2008; Breitburg et al., 2018). The interactive effects of this pollution and MHWs may generate multiple impacts in coastal ecosystems. Ocean warming can contribute to the spread of dead zones, especially in estuaries and bays, where proximity to urban areas and higher water residence times contribute to elevated water temperature and organic matter mineralization rates (Altieri and Gedan, 2014). Our data show that the shallow bays inshore of Santa Catarina Island experience these processes; DO levels in this enclosed system have been decreasing for years, at the same time as sewage and nutrient inputs have been increasing, and MHWs have become more frequent and intense. Monitoring data from 1994 to 2020 show that the first recorded episode of hypoxia and anoxia during summer occurred in 2020, concomitant with a MHW. Monitoring programs and solutions are needed in the Bay of Santa Catarina Island since the interaction of local and climate stressors might also threaten important economic activities around the region, such as aquaculture, fisheries and tourism.
A lack of monitoring data in the coastal ecosystems of the South Atlantic makes it hard to assess and control the deoxygenation problem. Two systems close to the bays have periodic dead zones due to sewage discharge (Conceição lagoon) and agricultural runoff (Madre estuarine system) (Fontes et al., 2011; Barros et al., 2017; Cabral and Fonseca, 2019). These dead zones were developed due to strong vertical stratification (saline or/and thermal) that prevents reoxygenation of the benthos. Water in the Bay of Santa Catarina Island is not stratified as it is tidal and shallow (<3 m depth) and yet we observed hypoxia and anoxia during the MHW event of 2020. Also, sediment in the northern bay is mostly mud enriched with organic matter boosting benthic respiration and deoxygenation (Bonetti et al., 2007). Limited oxygen influx into sediments during hypoxic and anoxic events decreases denitrification and stimulates P desorption from ferric complex, releasing nutrients to the water column and enhancing eutrophication in a positive feedback (Fennel and Testa, 2019).
Biological stress from low DO (< 5.0 mg L–1) was first reported in 2007 and 2008 as outliers. However, concentrations below this threshold started to increase in 2015 and, in summer 2020, dead zones (<2.0 mg L–1) were found. Following long-term (2001–2020) DO reductions and water temperature increases, the benthic communities also lost richness. The innermost region and most urbanized area of the bay was particularly adversely affected. The faunal responses show overlapping effects of high temperatures and low DO linked to the increase of sewage discharges. Our results reinforce the perspective that the biological stress threshold for benthic communities could be 6.0 mg L–1, 1.0 mg L–1 more than the threshold generally used in the literature (Vaquer-Sunyer and Duarte, 2008; Devlin et al., 2011). This highlights that new coastal water quality standards should be pursued in mitigation and restoration programs.
The richness of the macrobenthos in the most urbanized areas around Santa Catarina Island have long been poor, with a more recent deterioration in the outer reaches of the bay between 2001 and 2020. Evidence of macrofaunal negative responses to higher contents of trace metals (copper and lead), suspended particulate matter, nitrogen and phosphorus have been previously found in urbanized estuaries that drain to the bay (Pagliosa et al., 2006; Netto et al., 2018). Richness, abundance, and productivity decrease in virtue of bottom hypoxia, hampering larval settlement, and community recovery (Kodama and Horiguchi, 2011; Sturdivant et al., 2014). The outer areas of the bay have higher exchange with shelf waters (Garbossa et al., 2014), promoting dilution of contaminants. Although these areas had higher macrobenthic richness than the inner bay, their decline between 2001 and 2020 suggests that even the well flushed outer bay is polluted. Carbon and nutrient content over the past 100 years have constantly increased in the bay with population growth (Lamego et al., 2017), likely affecting the benthos.
Local pressures frequently exacerbate global stressors, such as ocean warming (Todgham and Stillman, 2013), increasing the chances of damage to local ecosystem functions. Extreme summer temperatures in the Bay of Santa Catarina Island, such as observed in 2007 (34.1°C) and 2020 (29.8°C), can negatively impact the macrobenthic fauna (Bernardino et al., 2015) since DO levels fall as water temperature increases. The effects of a MHW are superimposed upon the effects of other existing stressors, such as deoxygenation and nutrient enrichment. For example, many polychaete species, the dominant faunal group in our dataset, have declined in abundance in outer parts of the bay over the years. Assemblages subjected to sewage and low DO become dominated by a few tolerant and opportunistic species (Souza et al., 2013; Brauko et al., 2016; Weis et al., 2017). Benthic productivity mediates both biogeophysical and biogeochemical feedbacks to climate change via degradation of organic matter, trophic transfer, denitrification and nutrient remineralization (Bernardino et al., 2015). Long-term monitoring efforts allied to experimental evidence are still needed for a better comprehension of their threshold limits and future scenarios aiming to maintain ecosystem functions.
Local Solutions to Mitigate Local and Global Stressors in Coastal Areas
The increase of remote techniques and studies of coastal regions have revealed spreading degradation of natural ecosystems in the last decade, associated with an increasing human population and deficient wastewater treatment. Transdisciplinary solutions are needed to address this problem, working on regional solutions to global stressors. Islands have scarce water resources for sewage treatment and frequently use crude submarine outfalls. However, given the sensitivity of coastal environments, often within nature conservation and multiple-use zones, oceanic disposal requires tertiary treatment, i.e., nutrient, pathogen and micropollutant removal. Conventional tertiary treatment technologies are costly to implement and maintain (Mota and Von Sperling, 2009), so nature-based technologies are gaining prominence, as they integrate relatively low costs with sustainability. Examples include constructed wetlands (mainly based on aquatic plants) and phycoremediation systems (based on algae).
Wetlands and coastal vegetation can help to improve water quality, by reducing pathogen and nutrient concentrations and increasing oxygen availability (Zedler, 2000; Duarte et al., 2017). Restoration of these systems is a good option to improve effluent treatment in coastal areas. In addition, artificial wetlands can also help water quality restoration. Wetland parks, which combine treatment units in a multifunctional setting (Haron and Feisal, 2020) can be built at the land-water interface, reducing pollution and stimulating recovery of ecological functions. Another option is to use algal-based solutions (Young et al., 2017), such as High-Rate Algal Ponds that generate intense algal growth. Although this technology requires lot of space, it can be an alternative to manage macroalgae growth and to increase nutrient removal and biomass growth. Algal Turf Scrubbers have algal communities growing on screens, with effluent pumped into the system and released with lower nutrient and higher oxygen concentrations (Adey and Loveland, 2007). In Santa Catarina Island, this technology could be useful since the sewage treatment goes up to just the secondary phase and it is not efficient to control eutrophication (Cabral et al., 2019).
Perhaps the greatest challenge to reduce pollution in the coastal zone is not technological, given the sustainable options available. There is a major difficulty in convincing authorities and economic sectors that managing wastewater is an affordable priority (Chrispim et al., 2019) to build resilience to extreme events. Conservation and restoration of coastal wetlands and blue carbon stores, such as mangroves and salt marshes, also enhance CO2 sequestration capacity and biodiversity recovery (Serrano et al., 2019). We propose a conceptual model considering the current scenario of the bays and an improved perspective, where some of the local solutions discussed were applied (Figure 5). The aim of the mitigation strategies is to build resilience against eutrophication and oxygen depletion due to MHWs. Moreover, those measures could help to recover biodiversity, aquaculture and fisheries damaged by MHW events (Carneiro et al., 2020).
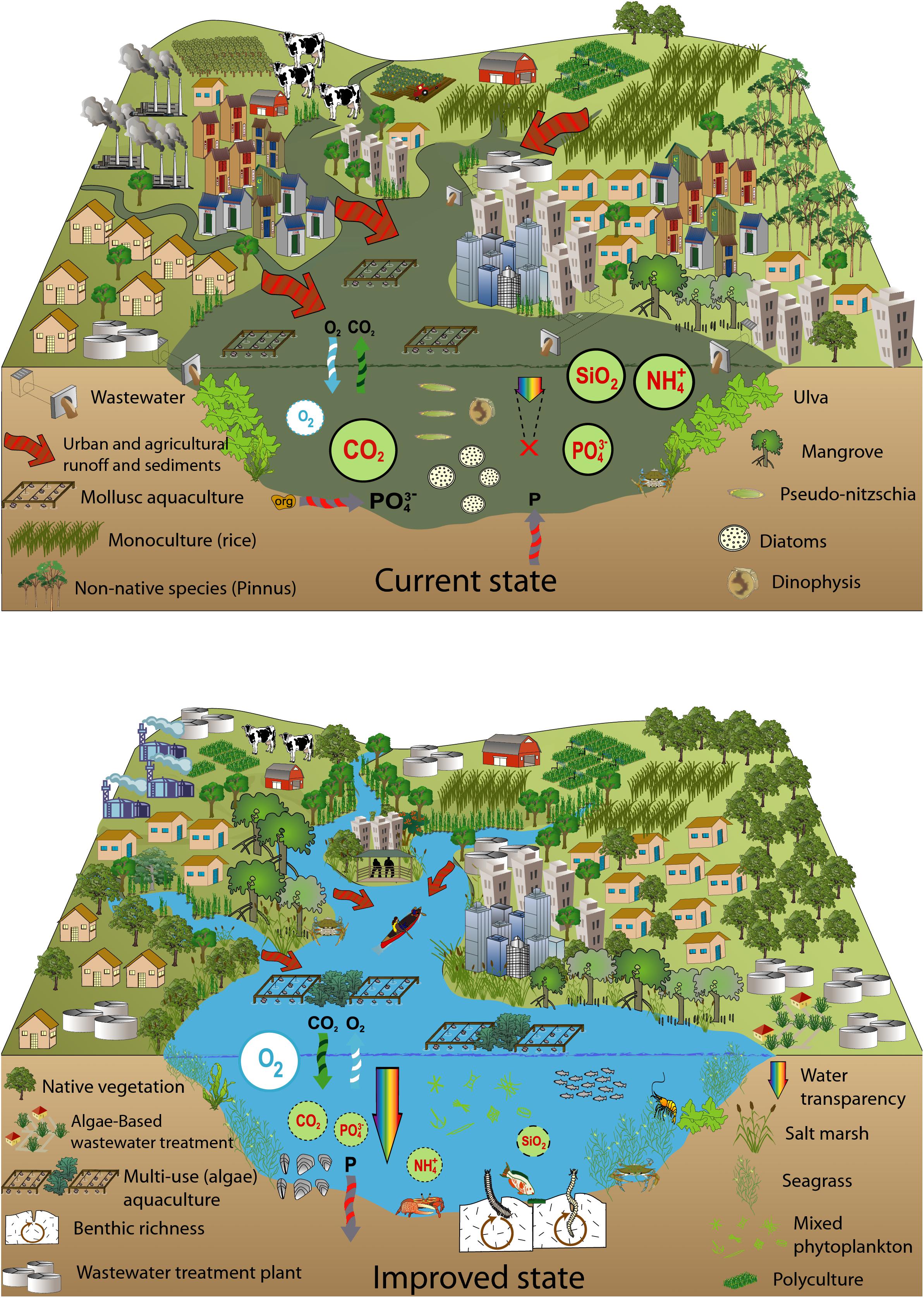
Figure 5. Current state of the Bay of Santa Catarina Island, polluted by organic and inorganic enrichment, and a future scenario after improved long-term wastewater treatment and wetlands restoration.
Conclusion
We show an increase in frequency and intensity of MHWs in southern Brazil and recorded temperatures much higher than those registered using satellites in a socioeconomically important enclosed water body off Santa Catarina Island. Marine heatwaves are more intense in coastal systems with limited water exchange and this emphasizes the importance of monitoring coastal waters. The watersheds of the region are under great pressure due to the combined effect of a recent 60% increase in human population and inadequate sewage systems. This is especially problematic in the summer when the temperatures are high and the number of inhabitants triples due to tourism. Increasing phosphorus concentrations and dominance by diatoms show that the enclosed water body is eutrophic. Dissolved oxygen levels have been decreasing in this enclosed system for years, causing dead zones to appear for the first time, during a MHW in 2020. Spatio-temporal losses in the richness of benthic communities suggest overlapping effects of high temperatures and low oxygen linked to sewage discharge. Currently used thresholds of coastal pollution and related hypoxia are not conservative enough to avoid losses of biodiversity and ecosystems goods and services. Levels of permitted organic and inorganic compounds need to take ocean warming into consideration. We highlight the potential benefits of restoration efforts and relatively low-cost nature-based wastewater treatment technologies. Marine management and mitigation options are also discussed to build local resilience to global-scale warming events.
Data Availability Statement
Macrobenthic richness data can be found in the Supplementary Material. Physical and chemical in situ datasets are available at http://dx.doi.org/10.17632/458xcpxwbh.1. Sea surface temperature were obtained from the National Oceanic and Atmospheric Administration at https://www.ncdc.noaa.gov/oisst/optimum-interpolation-sea-surface-temperature-oisst-v21.
Author Contributions
KB and AC: conception of the work, data analysis and interpretation, and wrote and reviewed the article. NC: conception of the work, data collection, and heatwave analysis and recommendations. JH: phytoplankton analysis and interpretation. CD, EL, RW, and CM: conception of the work and data collection and interpretation. JH-S: conception of the work, data collection and interpretation, and article review. RR: heatwave analysis and article review. LR: data analysis and interpretation and article review. PP: benthic analysis and interpretation and article review. AF: provided historical chemical data and article review. OA: conception of the work and data collection. PH: conception of the work, data collection, and article review. All authors contributed to the article and approved the submitted version.
Funding
This study was funded by grants from CAPES (Higher Education Personnel Improvement Coordination) – CAPES Senior Visitor, CAPES PrInt 310793/2018-01; CNPq (National Council for Scientific and Technological Development) – CNPq PVE 407365/2013-3, CNPq Universal 426215/2016-8 and CNPq PQ 308537/2019-0; ICNT-TMCOcean 573.601/2008-9); FINEP (Brazilian Innovation Agency); the Boticario Foundation; and FAPESC – Foundation for Support of Research and Innovation, Santa Catarina.
Conflict of Interest
The authors declare that the research was conducted in the absence of any commercial or financial relationships that could be construed as a potential conflict of interest.
Acknowledgments
PH and KB thank CAPES – Senior Visitor, CAPES-PrInt 310793/2018-01, CNPq-PVE 407365/2013-3, CNPq-Universal 426215/2016-8, and CNPq-PQ- 308537/2019-0. OA thanks FINEP for Veleiro ECO funding. All authors thank J.C. Simonassi for the assistance with chemical analyses.
Supplementary Material
The Supplementary Material for this article can be found online at: https://www.frontiersin.org/articles/10.3389/fmars.2020.590258/full#supplementary-material
References
Adey, W. H., and Loveland, K. (2007). Dynamic Aquaria: Building Living Ecosystems, 3rd Edn. New York: Academic Press.
Altieri, A. H., and Gedan, K. B. (2014). Climate change and dead zones. Glob. Change Biol. 21, 1395–1406. doi: 10.1111/gcb.12754
Alves, T. P., and Mafra, L. L. (2018). Diel variations in cell abundance and trophic transfer of diarrheic toxins during a massive dinophysis bloom in Southern Brazil. Toxins 10:232. doi: 10.3390/toxins10060232
Arias-Ortiz, A., Serrano, O., Masqué, P., Lavery, P. S., Mueller, U., Kendrick, G. A., et al. (2018). A marine heatwave drives massive losses from the world’s largest seagrass carbon stocks. Nat. Clim. Change 8:338. doi: 10.1038/s41558-018-0096-y
Ávila, A., Justino, F., Wilson, A., Bromwich, D., and Amorim, M. (2016). Recent precipitation trends, flash floods and landslides in southern Brazil. Environ. Res. Lett. 11:114029. doi: 10.1088/1748-9326/11/11/114029
Barletta, M., Lima, A. R. A., and Costa, M. F. (2019). Distribution, sources and consequences of nutrients, persistent organic pollutants, metals and microplastics in South American estuaries. Sci. Total Environ. 651, 1199–1218. doi: 10.1016/j.scitotenv.2018.09.276
Barros, G. D., Fonseca, A. L. D. O., Santos, A. C. D., Fontes, M. L. S., Varela, A. R. D., and Franco, D. (2017). Nutrient distribution in a shallow subtropical lagoon, south Brazil, subjected to seasonal hypoxic and anoxic events. Braz. J. Oceanogr. 65, 116–127. doi: 10.1590/s1679-87592017101206502
Bernardino, A. F., Netto, S. A., Pagliosa, P. R., Barros, F., Christofoletti, R. A., Rosa Filho, J. S., et al. (2015). Predicting ecological changes on benthic estuarine assemblages through decadal climate trends along Brazilian Marine Ecoregions. Estuar. Coast. Shelf Sci. 166, 74–82. doi: 10.1016/j.ecss.2015.05.021
Bestion, E., Barton, S., García, F. C., Warfield, R., and Yvon−Durocher, G. (2020). Abrupt declines in marine phytoplankton production driven by warming and biodiversity loss in a microcosm experiment. Ecol. Lett. 23, 457–466. doi: 10.1111/ele.13444
Bonetti, C., Bonetti, J., and Barcelos, R. L. (2007). “Caracterização sedimentar e geoquímica de sistemas costeiros com ênfase na avaliação da influência de sítios de cultivo de moluscos,” in Sistemas de Cultivos Aquícolas Costeiros no Brasil: Recursos, Tecnologias e Aspectos Ambientais e Sócio-Econômicos, eds G. F. Barroso, L. H. S. Poersch, R. O. Cavalli, and A. O. Galvez (Rio de Janeiro: Museu Nacional), 139–149.
Brauko, K. M., Muniz, P., Martins, C. C., and Lana, P. C. (2016). Assessing the suitability of five benthic indices for environmental health assessment in a large subtropical South American estuary. Ecol. Indicators 64, 258–265. doi: 10.1016/j.ecolind.2016.01.008
Breitburg, D., Levin, L. A., Oschlies, A., Gregoire, M., Chavez, F. P., Conley, D. J., et al. (2018). Declining oxygen in the global ocean and coastal waters. Science 359, 46–57.
Bricker, S. B., Longstaff, B., Dennison, W., Jones, A., Boicourt, K., Wicks, C., et al. (2008). Effects of nutrient enrichment in the nation’s estuaries: a decade of change. Harmful Algae 8, 21–32. doi: 10.1016/j.hal.2008.08.028
Cabral, A., Bercovich, M. V., and Fonseca, A. (2019). Implications of poor-regulated wastewater treatment systems in the water quality and nutrient fluxes of a subtropical coastal lagoon. Region. Stud. Mar. Sci. 29:100672. doi: 10.1016/j.rsma.2019.100672
Cabral, A., Bonetti, C., Garbossa, L., Pereira-Filho, J., Besen, K., and Fonseca, A. (2020). Water masses seasonality and meteorological patterns drive the biogeochemical processes of a subtropical and urbanized watershed-bay-shelf continuum. Sci. Total Environ. 749:141553. doi: 10.1016/j.scitotenv.2020.141553
Cabral, A., and Fonseca, A. (2019). Coupled effects of anthropogenic nutrient sources and meteo-oceanographic events in the trophic state of a subtropical estuarine system. Estuar. Coast. Shelf Sci. 225:106228. doi: 10.1016/j.ecss.2019.05.010
Carneiro, A. P., Soares, C. H. L., Manso, P. R. J., and Pagliosa, P. R. (2020). Impact of marine heat waves and cold spell events on the bivalve Anomalocardia flexuosa: a seasonal comparison. Mar. Environ. Res. 156:104898. doi: 10.1016/j.marenvres.2020.104898
Cavole, L. M., Demko, A. M., Diner, R. E., Giddings, A., Koester, I., Pagniello, C. M., et al. (2016). Biological impacts of the 2013–2015 warm-water anomaly in the Northeast Pacific: winners, losers, and the future. Oceanography 29, 273–285.
Chrispim, M. C., Scholz, M., and Nolasco, M. A. (2019). Phosphorus recovery from municipal wastewater treatment: Critical review of challenges and opportunities for developing countries. J. Environ. Manag. 248, 109268. doi: 10.1016/j.jenvman.2019.109268
Devlin, M., Bricker, S., and Painting, S. (2011). Comparison of five methods for assessing impacts of nutrient enrichment using estuarine case studies. Biogeochemistry 106, 177–205. doi: 10.1007/s10533-011-9588-9
Diaz, R. J., and Rosenberg, R. (2008). Spreading dead zones and consequences for marine ecosystems. Science 321, 926–929. doi: 10.1126/science.1156401
Duarte, I. D., Silva, N. H. V. F., da Costa Souza, I., de Oliveira, L. B., Rocha, L. D., Morozesk, M., et al. (2017). Water quality of a coastal lagoon (ES, Brazil): abiotic aspects, cytogenetic damage, and phytoplankton dynamics. Environ. Sci. Pollut. Res. 24, 10855–10868. doi: 10.1007/s11356-017-8721-2
Fennel, K., and Testa, J. M. (2019). Biogeochemical controls on coastal hypoxia. Annu. Rev. Mar. Sci. 11, 105–130. doi: 10.1146/annurev-marine-010318-095138
Fontes, M. L. S., Suzuki, M. T., Cottrell, M. T., and Abreu, P. C. (2011). Primary production in a subtropical stratified coastal lagoon—contribution of anoxygenic phototrophic bacteria. Microb. Ecol. 61, 223–237. doi: 10.1007/s00248-010-9739-x
Frölicher, T. L., Fischer, E. M., and Gruber, N. (2018). Marine heatwaves under global warming. Nature 560, 360–364. doi: 10.1038/s41586-018-0383-9
Garbossa, L. H. P., Souza, R. V., Campos, C. J. A., Vanz, A., Vianna, L. F. N., and Rupp, G. S. (2017). Thermotolerant coliform loadings to coastal areas of Santa Catarina (Brazil) evidence the effect of growing urbanization and insufficient provision of sewerage infrastructure. Environ. Monit. Assess. 189:27.
Garbossa, L. H. P., Vanz, A., Fernandes, L. D. F., Souza, R. V. D., Vianna, L. F., and Rupp, G. S. (2014). “Modelling and validation of the Santa Catarina Island Bays hydrodynamics based on astronomical tides and measured tides,” in Proceedings of the 11th International Conference on Hydroinformatics HIC 2014, (New York. NY: Curran Associates, Inc).
Garrabou, J., Coma, R., Bensoussan, N., Bally, M., Chevaldonné, P., Cigliano, M., et al. (2009). Mass mortality in Northwestern Mediterranean rocky benthic communities: effects of the 2003 heat wave. Glob. Change Biol. 15, 1090–1103. doi: 10.1111/j.1365-2486.2008.01823.x
Gilbert, R. O. (1987). Statistical Methods for Environmental Pollution Monitoring. Hoboken, NJ: John Wiley & Sons.
Gouvêa, L. P., Schubert, N., Martins, C. D. L., Sissini, M., Ramlov, F., Rodrigues, E. R. O., et al. (2017). Interactive effects of marine heatwaves and eutrophication on the ecophysiology of a widespread and ecologically important macroalga. Limnol. Oceanogr. 62, 2056–2075. doi: 10.1002/lno.10551
Grasshoff, K., Kremling, K., and Ehrhardt, M. (1999). Methods of Seawater Analysis, Methods of Seawater Analysis: Third, Completely Revised and Extended Edition. Hoboken, NJ: Wiley.
Harding, L. W., Mallonee, M. E., Perry, E. S., Miller, W. D., Adolf, J. E., Gallegos, C. L., et al. (2019). Long-term trends, current status, and transitions of water quality in Chesapeake Bay. Sci. Rep. 9, 1–19.
Haron, A., and Feisal, Z. (2020). Constructed wetland parks: a pathway to sustainability for Cairo. J. Urban Res. 36, 61–81.
Hobday, A. J., Alexander, L. V., Perkins, S. E., Smale, D. A., Straub, S. C., Oliver, E. C. J., et al. (2016). A hierarchical approach to defining marine heatwaves. Prog. Oceanogr. 141, 227–238. doi: 10.1016/j.pocean.2015.12.014
IBGE (2020). Instituto Brasileiro de Geografia e Estatística. Available online at: https://www.ibge.gov.br/ (accessed July, 2020)
Intergovernmental Panel on Climate Change (2019). Special Report on the Ocean and Cryosphere in a Changing Climate. Geneva: IPCC.
Jacobi, P. R., and Sulaiman, S. N. (2016). Governança ambiental urbana em face das mudanças climáticas. Revist. USP 109, 133–142. doi: 10.11606/issn.2316-9036.v0i109p133-142
Jassby, A. D., and Cloern, J. E. (2017). wq: Some Tools for Exploring Water Quality Monitoring Data. R Package Version 0.4.8. Available online at: https://cran.r-project.org/package=wq (accessed October 17, 2020).
Kodama, K., and Horiguchi, T. (2011). Effects of hypoxia on benthic organisms in Tokyo Bay, Japan: a review. Mar. Pollut. Bull. 63, 215–220. doi: 10.1016/j.marpolbul.2011.04.022
Lamego, F., da Silva, A. L. G., Simonassi, J. C., and Nepomuceno, A. (2017). Reconstructing recent land–ocean changes in the Brazilian Southern Coast using sedimentary proxies and tracking airborne 210 Pb. J. Radioanal. Nuclear Chem. 314, 2281–2299. doi: 10.1007/s10967-017-5623-1
Mota, S., and Von Sperling, M. (2009). Nutrientes de Esgoto Sanitário: Utilização e Remoção, Vol. 1. Rio de Janeiro: FINEP, 430.
Netto, S. A., Pagliosa, P. R., Colling, A., Fonseca, A. L., and Brauko, K. M. (2018). “Benthic estuarine assemblages from the Southern Brazilian marine ecoregion,” in Brazilian Estuaries. Brazilian Marine Biodiversity, eds P. Lana and A. Bernardino (Cham: Springer).
Nguyen, K. D. T., Morley, S. A., Lai, C.-H., Clark, M. S., Tan, K. S., Bates, A. E., et al. (2011). Upper temperature limits of tropical marine ectotherms: global warming implications. PLoS One 6:e29340. doi: 10.1371/journal.pone.0029340
Oliver, E. C. J., Burrows, M. T., Donat, M. G., Sen Gupta, A., Alexander, L. V., Perkins-Kirkpatrick, S. E., et al. (2019). Projected marine heatwaves in the 21st century and the potential for ecological impact. Front. Mar. Sci. 6:734. doi: 10.3389/fmars.2019.00734
Pagliosa, P. R., Fonseca, A., Barbosa, F. A. R., and Braga, E. (2006). Urbanization impact on subtropical estuaries: a comparative study of water properties in urban areas and in protected areas. J. Coast. Res. 39, 731–735.
Pagliosa, P. R., Fonseca, A., Bosquilha, G. E., Braga, E. S., and Barbosa, F. A. R. (2005). Phosphorus dynamics in water and sediments in urbanized and non-urbanized rivers in Southern Brazil. Mar. Pollut. Bull. 50, 965–974. doi: 10.1016/j.marpolbul.2005.04.005
Peck, L., Massey, A., Thorne, M., and Clark, M. (2009). Lack of acclimation in Ophionotus victoriae: brittle stars are not fish. Polar Biol. 32, 399–402. doi: 10.1007/s00300-008-0532-y
Proença, L. A. O., Schramm, M. A., Alves, T. P., and Piola, A. R. (2017). “The extraordinary 2016 autumn DSP outbreak in Santa Catarina, Southern Brazil, explained by large-scale oceanographic processes,” in Marine and Fresh-Water Harmful Algae. Proceedings of the 17th International Conference on Harmful Algae, eds L. A. O. Proença and G. M. Hallegraeff (Washington, DC: International Society for the Study of Harmful Algae), 42–45.
Reynolds, R. W., Smith, T. M., Liu, C., Chelton, D. B., Casey, K. S., and Schlax, M. G. (2007). Daily high-resolution-blended analyses for sea surface temperature. J. Clim. 20, 5473–5496. doi: 10.1175/2007jcli1824.1
Rigotto, C., Victoria, M., Moresco, V., Kolesnikovas, C. K., Corrêa, A. A., Souza, D. S. M., et al. (2010). Assessment of adenovirus, hepatitis A virus and rota-virus presence in environmental samples in Florianopolis, South Brazil. J. Appl. Microbiol. 109, 1979–1987. doi: 10.1111/j.1365-2672.2010.04827.x
Rodrigues, R. R., Taschetto, A. S., Gupta, A. S., and Foltz, G. R. (2019). Common cause for severe droughts in South America and marine heatwaves in the South Atlantic. Nat. Geosci. 12, 620–626. doi: 10.1038/s41561-019-0393-8
Rörig, L. R., Pereira Filho, J., and Almeida, T. C. M. (2010). “O fitoplâncton no diagnóstico de mudanças ambientais: O Caso da Baía Sul - Florianópolis, SC,” in III Congresso Brasileiro de Oceanografia, 2010, (Rio Grande, RS: CBO).
Rörig, L. R., Pereira Filho, J., Silva, R. L., Horta, P. A., and Almeida, T. C. M. (2011). “O fitoplâncton no diagnóstico de mudanças ambientais: O Caso da Baía Sul - Florianópolis,” in Proceedings of the XIV Congresso Latino-Americano de Ciências do Mar - XIV COLACMAR, (Balneário Camboriú: COLACMAR).
Rörig, L. R., Tamanaha, M. S., Persich, G. R., Schettini, C. A. F., and Truccolo, E. C. (2018). “Phytoplankton patterns and processes in a tropical-subtropical transition region: Santa Catarina Coast, Southern Brazil,” in Plankton Ecology of the Southwestern Atlantic, eds M. S. Hoffmeyer, M. E. Sabatini, F. P. Brandini, D. L. Calliari, and N. H. Santinelli (Cham: Springer International), 269–287. doi: 10.1007/978-3-319-77869-3_13
Sanford, E., Sones, J. L., García-Reyes, M., Goddard, J. H., and Largier, J. L. (2019). Widespread shifts in the coastal biota of northern California during the 2014–2016 marine heatwaves. Sci. Rep. 9, 1–14.
Scherner, F., Horta, P. A., Oliveira, E. C., Simonassi, J. C., Hall Spencer, J. M., Chow, F., et al. (2013). Coastal urbanization leads to remarkable seaweed species loss and community shifts along the SW Atlantic. Mar. Pollut. Bull. 76, 106–115. doi: 10.1016/j.marpolbul.2013.09.019
Schlegel, R. W., Oliver, E. C., Wernberg, T., and Smit, A. J. (2017). Nearshore and offshore co-occurrence of marine heatwaves and cold-spells. Prog. Oceanogr. 151, 189–205. doi: 10.1016/j.pocean.2017.01.004
Serrano, O., Kelleway, J. J., Lovelock, C., and Lavery, P. S. (2019). “Conservation of blue carbon ecosystems for climate change mitigation and adaptation,” in Coastal Wetlands, eds G. M. E. Perillo, E. Wolanski, D. R. Cahoon, and C. S. Hopkinson (Amsterdam: Elsevier), 965–996. doi: 10.1016/b978-0-444-63893-9.00028-9
Silva, A. R., and Fonseca, A. L. D. (2016). Eutrofização dos recursos hídricos como ferramenta para a compreensão das doenças de vinculação hídrica. Geosul 31:247. doi: 10.5007/2177-5230.2016v31n62p247
Silva, A. R., Fonseca, A. L. D., Rodrigues, C. J., and Beltrame, A. V. (2016). Application of ecological indicators in coastal watershed under high pressure during summer period. RBRH 21, 537–548. doi: 10.1590/2318-0331.011615106
Smale, D. A., Wernberg, T., Oliver, E. C., Thomsen, M., Harvey, B. P., Straub, S. C., et al. (2019). Marine heatwaves threaten global biodiversity and the provision of ecosystem services. Nat. Clim. Change 9, 306–312. doi: 10.1038/s41558-019-0412-1
Souza, F. M., Brauko, K. M., Lana, P. C., Muniz, P., and Camargo, M. G. (2013). The effect of urban sewage on benthic macrofauna: a multiple spatial scale approach. Mar. Pollut. Bull. 67, 234–240. doi: 10.1016/j.marpolbul.2012.10.021
Souza, R. S. D. (2008). Efeitos dos Cultivos de Bivalves (Mollusca, Bivalvia) sobre as associações bênticas macrofaunais na baía da ilha de Santa Catarina (SC), Brasil. Ph. D. Dissertation, Universidade Federal do Paraná, Curitiba.
Sturdivant, S. K., Díaz, R. J., Llansó, R., and Dauer, D. M. (2014). Relationship between hypoxia and macrobenthic production in Chesapeake Bay. Estuar. Coasts 37, 1219–1232. doi: 10.1007/s12237-013-9763-4
Tenenbaum, D. R., and Gomes, E. A. T. (2006). Dinoflagelados e Tintinídeos da Região Central da Zona Econômica Exclusiva Brasileira: Guia de Identificação. Rio de Janeiro: Museu Nacional Rio de Janeiro.
Tenenbaum, D. R., Villac, M. C., Viana, S. C., Matos, M., Lima, I. V., and Menezes, M. (2004). Phytoplankton Atlas of Sepetiba Bay, Rio de Janeiro, Brasil. London: Global Ballast Water Management Programme.
Todgham, A. E., and Stillman, J. H. (2013). Physiological responses to shifts in multiple environmental stressors: relevance in a changing world. Integr. Comp. Biol. 53, 539–544. doi: 10.1093/icb/ict086
Vaquer-Sunyer, R., and Duarte, C. M. (2008). Thresholds of hypoxia for marine biodiversity. Proc. Natl. Acad. Sci. U.S.A. 105, 15452–15457. doi: 10.1073/pnas.0803833105
Venables, W. N., and Ripley, B. D. (2013). Modern Applied Statistics with S-PLUS. Berlin: Springer Science & Business Media.
Weis, W. A., Soares, C. H. L., de Quadros, D. P. C., Scheneider, M., and Pagliosa, P. R. (2017). Urbanization effects on different biological organization levels of an estuarine polychaete tolerant to pollution. Ecol. Indic. 73, 698–707. doi: 10.1016/j.ecolind.2016.10.029
Wernberg, T., Smale, D. A., Tuya, F., Thomsen, M. S., Langlois, T. J., De Bettignies, T., et al. (2013). An extreme climatic event alters marine ecosystem structure in a global biodiversity hotspot. Nat. Clim. Change 3, 78–82. doi: 10.1038/nclimate1627
Young, P., Taylor, M., and Fallowfield, H. J. (2017). Mini-review: high rate algal ponds, flexible systems for sustainable wastewater treatment. World J. Microbiol. Biotechnol. 33:117.
Keywords: benthos, Brazil, climate change, coastal management, dead zones, extreme events
Citation: Brauko KM, Cabral A, Costa NV, Hayden J, Dias CEP, Leite ES, Westphal RD, Mueller CM, Hall-Spencer JM, Rodrigues RR, Rörig LR, Pagliosa PR, Fonseca AL, Alarcon OE and Horta PA (2020) Marine Heatwaves, Sewage and Eutrophication Combine to Trigger Deoxygenation and Biodiversity Loss: A SW Atlantic Case Study. Front. Mar. Sci. 7:590258. doi: 10.3389/fmars.2020.590258
Received: 31 July 2020; Accepted: 09 November 2020;
Published: 15 December 2020.
Edited by:
Richard Alan Feely, Pacific Marine Environmental Laboratory (NOAA), United StatesReviewed by:
Laura Carrillo, The South Border College (ECOSUR), MexicoAlvise Finotello, Ca’ Foscari University of Venice, Italy
Copyright © 2020 Brauko, Cabral, Costa, Hayden, Dias, Leite, Westphal, Mueller, Hall-Spencer, Rodrigues, Rörig, Pagliosa, Fonseca, Alarcon and Horta. This is an open-access article distributed under the terms of the Creative Commons Attribution License (CC BY). The use, distribution or reproduction in other forums is permitted, provided the original author(s) and the copyright owner(s) are credited and that the original publication in this journal is cited, in accordance with accepted academic practice. No use, distribution or reproduction is permitted which does not comply with these terms.
*Correspondence: Kalina M. Brauko, kalinabio@gmail.com; Alex Cabral, alex.cabral@gu.se
†These authors share first authorship