- 1Programa de Pós-graduação em Desenvolvimento e Meio Ambiente, Laboratório de Etnoconservação e Áreas Protegidas (LECAP), Universidade Estadual de Santa Cruz, Ilhéus, Brazil
- 2Grupo de Investigação Biológica Integrada (GIBI), Centro deEstudos Avançados da Biodiversidade (CEABIO), Universidade Federal do Pará, Belém, Brazil
- 3Laboratório de Ciências da Pesca (LabPESCA), Instituto do Mar, Universidade Federal de São Paulo, Santos, Brazil
- 4Natural History Museum, London, United Kingdom
- 5Laboratório de Etnoconservação e Áreas Protegidas (LECAP), Departamento de Ciências Agrárias e Ambientais, Universidade Estadual de Santa Cruz, Ilhéus, Bahia, Brazil
- 6Investigador Asociado CESIMAR/CENPAT, Puerto Madryn, Argentina
Shallow-water marine invertebrate fauna is diverse in tropical latitudes but generally poorly known. This is in part due the remoteness of many of these regions, and a lack of locally trained taxonomists. In such cases, the ethnoknowledge (i.e., information acquired from the sociocultural references of a given social group) of traditional human populations may be a valuable tool to elucidate gaps in the occurrence of some taxa. In this study, we used a combined approach of ethnoknowledge, classic taxonomy and molecular techniques to describe and diagnose an unsettled species of shallow-water octopus of the genus Callistoctopus. A neotype for the Brazilian eastern octopus Callistoctopus furvus (Gould, 1852) is described along with some ecological notes. Octopuses were collected between April and May 2018 during field trips guided by artisanal octopus fishers of Bahia State (Brazil). A linear discriminant analysis showed that the morphology of C. furvus overlapped poorly with Callistoctopus sp. from the Caribbean and Macaronesia (Madeira Archipelago) as well as with Callistoctopus macropus stricto sensu from Mediterranean Sea. Analysis of mitochondrial large ribosomal subunit (rrnL, also known as 16S) gene and cytochrome C oxidase I (COI) showed that C. furvus differs genetically from European C. macropus and the other species in the genus. In general, C. furvus differs from other Atlantic/Mediterranean Callistoctopus species in having a slender body shape and longer mantle lengths. As in other species of the genus, C. furvus is nocturnal and inhabits sandy bottoms, seagrass beds and/or low-profile reefs. Interestingly, C. furvus burrows itself into the sand as a defense mechanism. In conclusion, we showed the support of ethnoknowledge for integrative biodiversity assessments in poorly surveyed remote areas in the western tropical Atlantic.
Introduction
Modern taxonomy has benefited greatly from advances in molecular techniques and tools in the last few decades (Radulovici et al., 2010; White and Last, 2012). At the same time, traditional taxonomy–that based in meticulous and methodical descriptions of morphology, color patterns and other characters–have experienced an unjustified and unfair decline in prestige (Wheeler, 2004; Wheeler et al., 2004; Agnarsson and Kuntner, 2007; Chen et al., 2011). Traditionally less valued than these two taxonomic schools, is the use of traditional folk knowledge, or ethnoknowledge, which is a precious but underappreciated tool that can allow us to improve and complement our taxonomic knowledge, particularly in remote or isolated geographic areas (see Alves and Souto, 2015, for a comprehensive review).
Shallow-water marine invertebrate fauna is believed to be diverse in tropical latitudes, but this biodiversity remains generally poorly known (Briggs and Bowen, 2013). This is in part due the remoteness of many of these regions, and a generalized lack of locally trained taxonomists. As expected, knowledge on tropical shallow-water octopus fauna remains rudimentary (Voight, 1998; Leite et al., 2008; Lima et al., 2020), particularly in the southwestern Atlantic Ocean, including the South American mainland and oceanic islands (Voss and Toll, 1998). Among these poorly known octopuses, the genus Callistoctopus comprises a speciose monophyletic group supported by both morphological and genetic analyses (Norman and Hochberg, 2005; Kaneko et al., 2011). This genus likely includes many cryptic species and occurs in tropical and temperate waters of the Atlantic, Indian and Pacific Oceans (Norman, 2000; Norman et al., 2016). Typically, live Callistoctopus are brick red or bright red in color with white spots or blotches forming distinctive patterns on the body (Norman, 2000).
The white-spotted octopus Callistoctopus macropus (Risso, 1826) is cited to be present in the western Atlantic waters (including the Brazilian coast) and has long been considered an amphi-Atlantic species (Voight, 1998; Haimovici et al., 2009). However, this species is restricted to the Mediterranean Sea and northeastern Atlantic Ocean down to Senegal, and therefore considered an eastern Atlantic species (Norman et al., 2016). Brazilian occurrences were based on a lot deposited at the University of São Paulo Zoology Museum, comprising specimens collected in the shallow-waters off Bahia state (∼15°S) (Perez and Haimovici, 1991), plus data from a cephalopod biogeography study that was conducted in the late 1970s (Palacio, 1977). Two newer lots (one from the same region and another from Fernando de Noronha Island) were deposited at the University of Rio Grande Oceanographic Museum (Leite and Haimovici, 2006). Leite et al. (2008) urged a critical revision on the taxonomic status of “Brazilian” C. macropus, and recent genetic analysis suggest that the western Atlantic Callistoctopus is distinct form C. macropus stricto sensu (Ritschard et al., 2019; Lima et al., 2020).
The American malacologist August Addison Gould (1805–1866) established Callistoctopus furvus as a distinct species from C. macropus some 168 years ago. He acquired the specimen at a fish market in Rio de Janeiro (Brazil) and, according to his original description, “Its proportions are much like those of Octopus macropus, Risso, but is coloration is very different.” Thus, the taxonomic validity of C. furvus remained disputed (taxon inquirendum) for a long time, and the species was treated as a junior synonym of C. macropus (WoRMS Editorial Board, 2020). To complicate things further, the holotype is missing, and the original description has been considered too ambiguous to allow for reliable taxonomic identification (Voss and Toll, 1998). During recent morphological and genetic studies on shallow-water octopuses off the Mexican and Colombian Caribbean coasts, samples representing this genus have been variably named as C. cf. macropus (Flores-Valle et al., 2018), C. furvus (Cedillo-Robles and Pliego-Cardenas, 2018), or as an undefined species of Callistoctopus requiring description (Ritschard et al., 2019).
Molecular techniques and tools have been widely applied to elucidate cephalopod systematics and biogeography, allowing for the identification of distinct genetic linages, full descriptions of new species and genera, and the revision and more accurate delimitation of distribution ranges (Cheng et al., 2014; Sales et al., 2014, 2019; Amor et al., 2016, 2017; González-Gómez et al., 2018). Such molecular studies have also been used to validate genetic linages, or to clarify the taxonomic status of unsettled species (Söller et al., 2000; Sales et al., 2013; Anderson and Marian, 2020; Costa et al., 2021). For instance, Anderson and Marian (2020) established the grass squid Pickfordiateuthis pulchella as a genetically valid species with affinities to the new world genera Doryteuthis and Lolliguncula, whereas the subsequent papers of Sales et al. (2014, 2017) revised the accepted distribution range of these two loliginids, showing the influence of past biogeographic barriers and events on the emergence of specific genetic linages. Costa et al. (2021) has proven the molecular validity of Lolliguncula argus Brackoniecki and Roper (1985), suggesting that the recent speciation between L. argus and Lolliguncula diomedeae is associated to oceanic environmental changes associated with past glaciation, deep sea cooling and tropical upwelling.
Considering (1) the increasing body of evidence on the validity of C. furvus as a species and, (2) the fact that the missing holotype was collected in Brazil, we used an integrated, multidisciplinary taxonomic approach (as recommended by Dayrat, 2005) to designate a neotype for the species. Our methods combine traditional taxonomy and molecular techniques with ethnoknowledge to provide a comprehensive description of C. furvus, an updated distribution range, and notes on its ecology, as a contribution to the difficult taxonomy of the C. macropus-group complex.
Materials and Methods
Ethnoecological Survey
Based on earlier results (i.e., Martins et al., 2011; Jesus et al., 2015) that indicated the existence of an “unusual” shallow-water octopus off Bahia State, an ethnoknowledge-based survey was designed to establish a baseline on the occurrence of Callistoctopus in Brazilian waters. Data-collection took place from March 2018 to August 2019 at 17 locations on the Brazilian coast (Figure 1). To maximize the likelihood of finding specimens, surveyed locations encompassed localities nested within seven marine protected areas (MPAs) with different limits of use and protection, plus two non-protected locations (Table 1). Participants included octopus fishers, spearfishers and commercial SCUBA divers contacted via fishing associations, fishing colonies, and diving schools. Permission to conduct interviews was granted by the study participants and approved by the UESC Human Ethics committee (clearance number # 2.593.218).
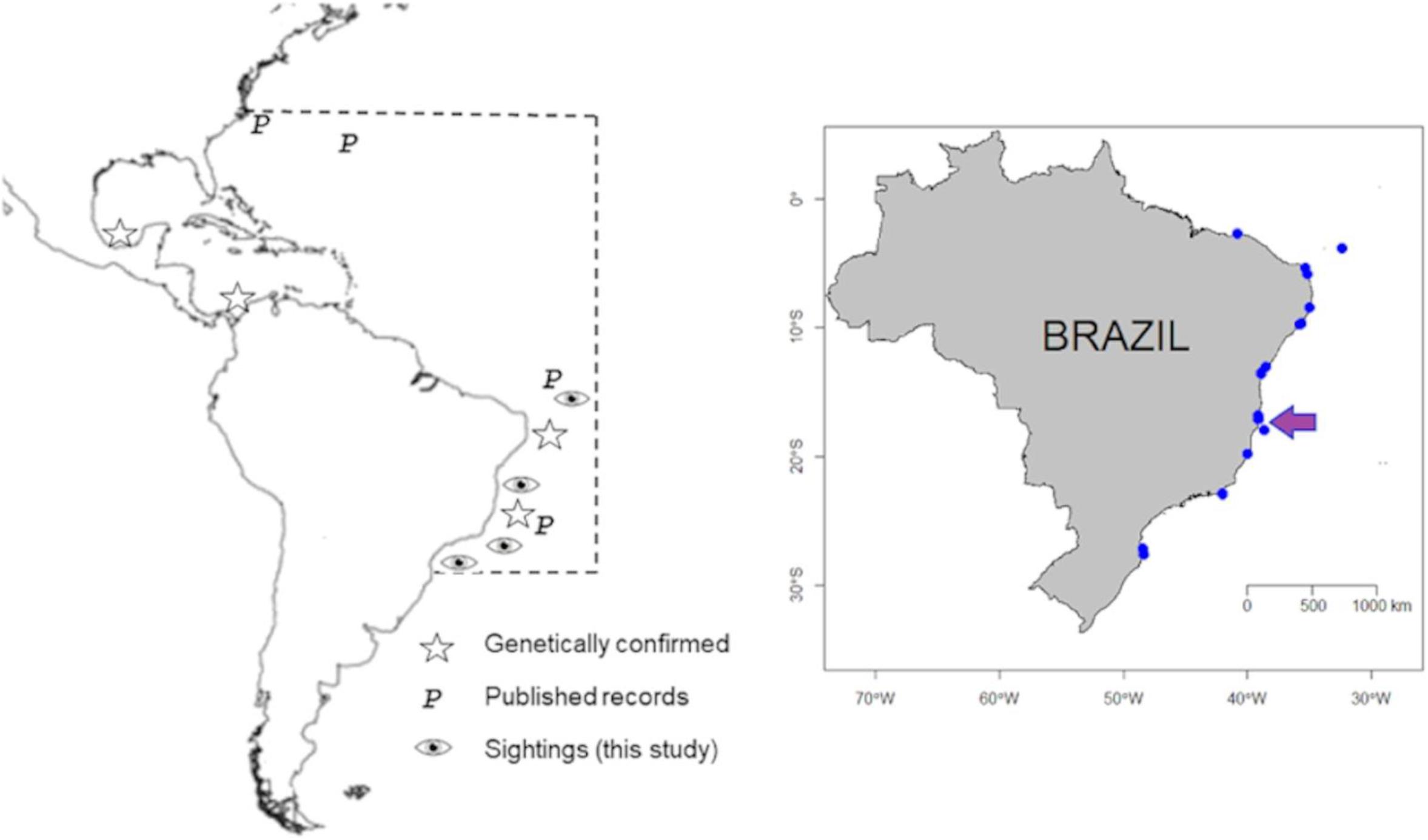
Figure 1. Callistoctopus furvus. Left: putative occurrence area on the western Atlantic (boxed). Right: surveyed area, including neotype and voucher specimens site locations (arrowed). Blue dots refer to localities where personal interviews were conducted.
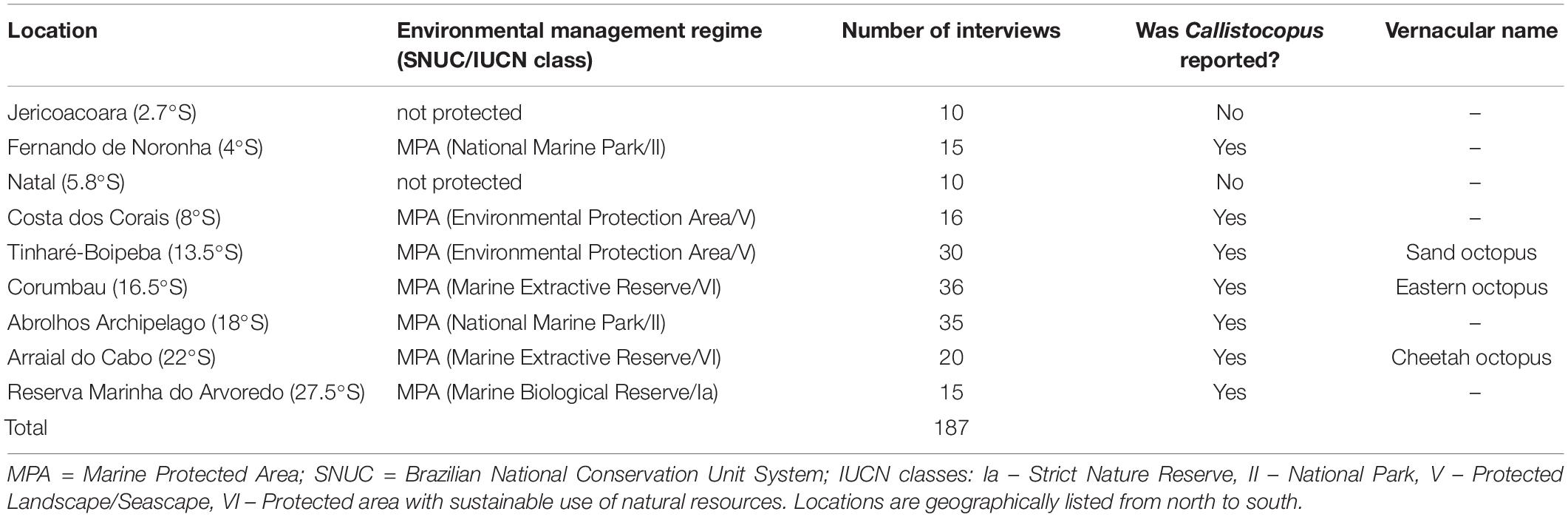
Table 1. Summary of Callistoctopus ethnobiological survey areas, including protected and unprotected locations.
Participants were personally interviewed either during fishing trips, or elsewhere (households, cooperatives, markets, restaurants, etc.) We used the direct observation technique with the fishers during fishing trips (Minayo, 2016) to investigate Callistoctopus occurrence, identification, and ecological characteristics. Some participants also voluntarily shared photos and video clips before, during and after interviews, and those visual records were used to substantiate the geographic distribution of Callistoctopus (Jesus et al., 2020).
A semi-structured questionnaire was used for interviews with the participants (Supplementary File 1). Questions sought to obtain information about Callistoctopus fishing and ecology. Fishing-related questions included information on fishing period (day/night), fishing duration (in hours), fishing frequency, type of gear used and tide level, plus data on consumption and marketing. The ecological component of the survey included questions on Callistoctopus frequency of occurrence, color pattern, behavior and the co-occurrence of other octopus species on the fishing grounds. SCUBA divers and spearfishers were also questioned about the most frequent depths of Callistoctopus sightings.
For practical purposes, we only use the name C. furvus for ethnoecological data gathered close to or on the neotype locality (see below). For results based on sights reported during interviews elsewhere, octopuses are referred to as Callistoctopus.
Collection Sites, Sampling and Laboratory Procedures
Samples were acquired during guided fishing trips with artisanal fishers at Morro de São Paulo (13°24′24″S 38°54′09″W) (Bahia State, Brazil), carried out between 12 and 15 April 2018 (Figure 1). Octopuses were collected at night (07:00 to 11:00 PM) on the mosaic of sandy bottoms/seagrass beds among reef flats. Fishing was conducted by foot at low tide, and the octopuses were captured either by hand or hooks and stored in plastic buckets. Live animals were photographed and filmed in situ to record color and body patterns and the behavior. Samples were frozen on site and airfreighted several days later to the laboratory elsewhere for measurement and genetic analysis. Authorization to take samples was granted by the Brazilian Institute of the Environment and Renewable Natural Resources (IBAMA) (SISBIO clearance number # 60468-2).
On arrival, samples were thawed at room temperature, and fresh octopuses were weighed to the nearest gram. Beaks and radula were extracted from the buccal mass and stored in a mixture of 70% ethanol and glycerin to prevent dehydration. Tissue samples were taken from the inner side of the mantle and stored in 5 ml Eppendorf flasks with absolute ethanol for genetic analyses (see below). Fresh octopuses were fixed in 10% buffered formalin solution for 72 h and then transferred to 70% ethanol. Each specimen was identified with a numbered plastic tag.
Morphometric measurements (millimeters), counts, illustrations and indices were obtained from the ethanol-preserved specimens following Roper and Voss (1983); Norman et al. (1997) and Huffard and Hochberg (2005). Sucker counts were totaled for each arm (Huffard and Hochberg, 2005). Abbreviations for depositories, measurements, counts and indices are given in Table 2. Maturity stage was assigned following the dissection of ventral mantle according to Norman (1993) three-point macroscopic scale: immature (sex indeterminate or reproductive organs minute), submature (reproductive organs distinct but poorly developed) and mature (distinct developed spermatophores or eggs).
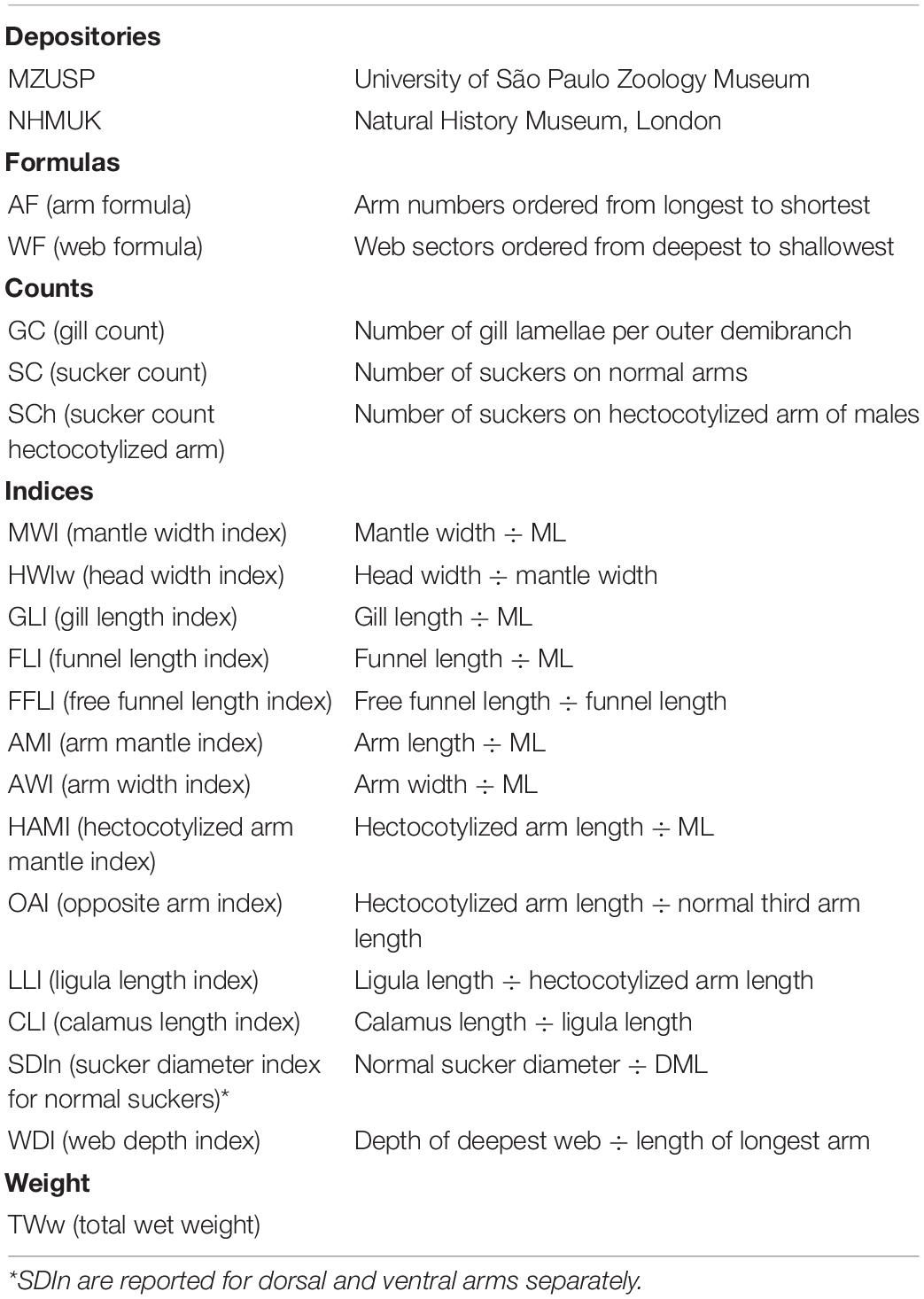
Table 2. Abbreviation for depositories, formulas, counts, indices and weight measurements used in the present study.
Shape Variation Analysis
To test our hypothesis of a distinct species for Brazilian Callistoctopus on a morphological basis, we followed the methodology employed by Voight (1998) to analyze morphologic variation in shallow-water octopuses. In short, we ran a Principal Component Analysis (PCA) using standard measurements made on the octopuses. According to the author, body size variability is captured by the first Principal Component (PC1), whereas the second (PC2) and third (PC3) Principal Components account for shape variability. Plots of specimen scores on PC2 and PC3 allowed visual comparison of shape differences.
Following the PCA analyses, we used a size-free Linear Discriminant Analysis (LDA) to maximally separate groups defined a priori. We removed the body size influence by using the residuals resulting from the regression between each morphological character measured against the PC1 loadings as input data. Groups were considered significantly different if they did not overlap on discriminant plots (Voight, 1998).
Morphological data of Atlantic/Mediterranean Callistoctopus groups included the neotype, voucher specimens and animals not selected for the taxonomic description (n = 13), octopuses from the Caribbean (Barbados, n = 1, and Haiti, n = 3), Mediterranean Sea (Italy, n = 2, France, n = 1, and “Mediterranean,” n = 1), and Macaronesia (Madeira Archipelago, n = 8). Details on the specimens used are given in Supplementary Table 1. Since complete datasets for each standard measurement were not available for all individuals, we used only the total length (TL), dorsal mantle length (DML), mantle width (MW), and head width (HW) in this analysis. Data were normalized (zero mean and unit standard deviation) prior to analysis (Allcock et al., 2008).
Genetic Analysis
We used six tissue samples for genetic procedures. Samples were kept in a freezer at −4°C until the extraction of the DNA. The genomic DNA was extracted with a Wizard Genomic DNA Purification kit (Madison, WI, United States), using the mouse-tail protocol. The fragments of mitochondrial 16S rDNA and Cytochrome Oxidase I (COI) gene were amplified by PCR following Sales et al. (2013), including the same primers for the markers 16S (Palumbi et al., 1991) and COI (Folmer et al., 1994). To sequence the fragments, the PCRs were first purified using the ExoSAP-IT enzyme (Amersham Pharmacia Biotech Inc.), and the sequencing reactions were done with a BigDye Terminator kit (Applied Biosystems) before being processed on an ABI 3500 automatic sequencer (Applied Biosystems).
The sequences of the two markers analyzed here were aligned in Geneious 9.0 (Kearse et al., 2012), using the MUSCLE tool (Edgar, 2004) in default mode. The automatically aligned sequences were inspected visually to verify possible incongruities. We utilized three data sets for the present study: A-16S (544 pb), B-COI (588 bp) and C-16S + COI (1132 bp). Additional sequences of Callistoctopus species were obtained from GenBank. We used Grimpella thaumastocheir Robson (1928) as an outgroup for our analysis (Supplementary Table 2). Given that some sequences were just available for one of the markers, we completed all unknown sequences with N for the concatenate database.
The best evolutionary model for each data set was selected using ModelTest 2 (Darriba et al., 2012). We used the AIC criteria to determine the model for subsequent Maximum Likelihood (ML) analyses and BIC criteria for subsequent Bayesian Inference (BI) analyses. Phylogenetic trees (ML and BI) were obtained from PhyML 3.0 (Guidon et al., 2010) and Mr. Bayes v. 3.2 (Ronquist et al., 2012), respectively. For the ML tree, branch support values were obtained using 1000 bootstrap pseudo-replicates (Felsenstein, 1985). The BI trees were based on MCMC (Markov Chain Monte Carlo) sampling, with four simultaneous runs of 10 million generations, each consisting of four chains (one cold and three hot). Bayesian posterior probabilities were defined using a 60% consensus rule, random seeds, and sampling every 1000 generations, with 25% of the first trees sampled in each MCMC run discarded as burn-in. The log-likelihood files generated by each run were then visualized in Tracer v. 1.4 (Rambaut and Drummond, 2007) and only runs with ESS values equal to or higher than 200 for all marginal parameters.
Results
Distribution and Notes on Ethnoecology
One hundred and eighty-seven interviews were carried out during this study, covering nearly 24° of latitude on the Brazilian coast. Most surveyed areas were located on the Brazilian northeastern and eastern coasts (2.7–19°S), and only two on the southeastern and south coasts (22–27°S) (Table 1). Among the surveyed areas, Callistoctopus was not reported/recognized in only two locations. The northernmost and easternmost record came from the Fernando de Noronha National Park (4°S; an oceanic island) and the southernmost was near Arvoredo Biological Reserve (27.5°S). Interestingly, the two localities where Callistoctopus was not recorded/recognized are located in the region where this octopus has been previously frequently reported (Table 1).
According to SCUBA divers and spearfishers, whenever spotted underwater, Callistoctopus is readily recognized as a slender, long armed red/orange octopus with distinctive white spots. Reported underwater observations of live specimens in the wild occurred mostly between 8 and 15 meters, but the deepest bathymetric limit for these octopuses remains unknown. Shore-based artisanal octopus fishers, on the other hand, could not answer questions regarding Callistoctopus bathymetric distribution, as they only interact with these octopuses on reef flats/seagrass beds/sandy bottoms during low tide.
Octopus fishers at Morro de São Paulo (13°S) report that at least four aspects can easily tell C. furvus apart from Octopus insularis (a sympatric species exploited in the same fishing grounds; Jesus et al., 2015). These include: (1) capture time (C. furvus nocturnal vs. O. insularis diurnal), (2) color pattern (brick red color body in C. furvus), (3) total body length and thickness of the arms (smaller and slender in C. furvus) and (4) escape strategy. As regarding the latter, fishers’ accounts indicated that C. furvus buries itself in the sand to escape from predators, while O. insularis takes refuge in holes and crevices on the reef. Because of this observation, fishers name C. furvus as the “sand octopus” whereas O. insularis is called the “stone octopus.”
Morro de São Paulo fishers capture C. furvus exclusively at night during low tides, describing that the species is more abundant during new moon nights. They capture octopuses that traverse shallow pools among reef flats, or areas of exposed reef, using an iron rod and a bucket, or sometimes without any fishing gear (i.e., by hand). C. furvus has no commercial value and, whenever caught, is either used as bait for subsistence fishing, or as food in the fisher’s households. In contrast, fishers interviewed at Porto Seguro (16°S) had less information on the species, because they rarely catch C. furvus, as their fishing operations take place during the day, targeting the sympatric O. insularis.
Systematic Description
Family Octopodidae d’Orbigny, 1840
Subfamily Octopodinae d’Orbigny, 1840
Genus Callistoctopus Taki, 1964.
Synonym: “macropus species-group” sensu Norman (1993, 2000).
Callistoctopus furvus (Gould, 1852).
Common Names
Brazilian eastern octopus (English), Polvo-de-leste (Brazilian Portuguese).
Material Examined
All specimens from Morro de São Paulo (13°24′24″S 38°54′09″W, Bahia State, Brazil). Neotype, here designed, submature male (ML 97 mm), MZUSP 152154, April 2018, M.D. Jesus.
Other Material Examined
All samples collected with the neotype at the same location and date by M.D. Jesus. 1 submature male (ML 113 mm), MZUSP 152155; 1 submature male (ML 111 mm), NHMUK 20200275; 1 submature female (ML 135 mm), MZUSP 152156; 1 submature female (ML 110 mm), MZUSP 152157; 1 submature female (ML 98 mm), NHMUK 20200276; 1 submature female (ML 94 mm), NHMUK 20200277, 1 immature female (ML 85 mm), NHMUK 20200278 1 immature female (ML 80 mm), MZUSP 152158.
Amended Diagnosis
Taki (1964) established the genus Callistoctopus to accommodate two new species from Japanese waters. According to the author, Callistoctopus is very similar to Octopus Cuvier, 1797, but differs from the latter by including large and robust octopuses with reduced ink sacs and a characteristic color pattern. However, apart from the color, the remaining characters are not reliable for Callistoctopus species identification (Voss, 1981). The genus Callistoctopus includes medium to large benthic octopuses (mantle to 190 mm and total weight up to 4.2 kg). Mantle muscular, ovoid to elongate cylindrical in shape, lacking a skin ridge around the lateral margin. Arms muscular, long (5–8 × mantle length), bearing two rows of suckers with no augmented suckers in either sex. Dorsal arms longest. Hectocotylized arm (third right arm) in males is remarkably shorter than the opposite arm. A well-developed, deeply grooved cylindrical ligula present, along with a calamus. Interbrachial web shallow to moderate, with dorsal webs deepest. Multicuspid radula with nine elements, namely seven rows of teeth plus two marginal plates. Skin smooth or with scattered low papillae. Conspicuous primary papillae present over each eye. Live animals brick red to bright red in color, with white spots, blotches or bars forming distinctive patterns over the whole body surface. Large funnel organ W- or U-shaped. Ink sac and anal flaps present. Gills with 10–15 lamellae per outer demibranch. Octopuses of this genus are typically nocturnal, inhabiting reefs, seagrass beds and soft bottoms from the intertidal zone up to 200 m. Diet consists of crustaceans and shelled mollusks.
Description
The following description is based on three males (all submature) and six females (four submature and two immature). Counts, measurements and indexes for this material are given in Tables 3, 4. Indices are included in the text as ranges in parentheses. Since we found no mature individuals, egg and spermatophore descriptions are not reported. Notwithstanding, dissection of ovaries of submature females yielded high numbers of tiny oocytes, implying that mature eggs would certainly be small.
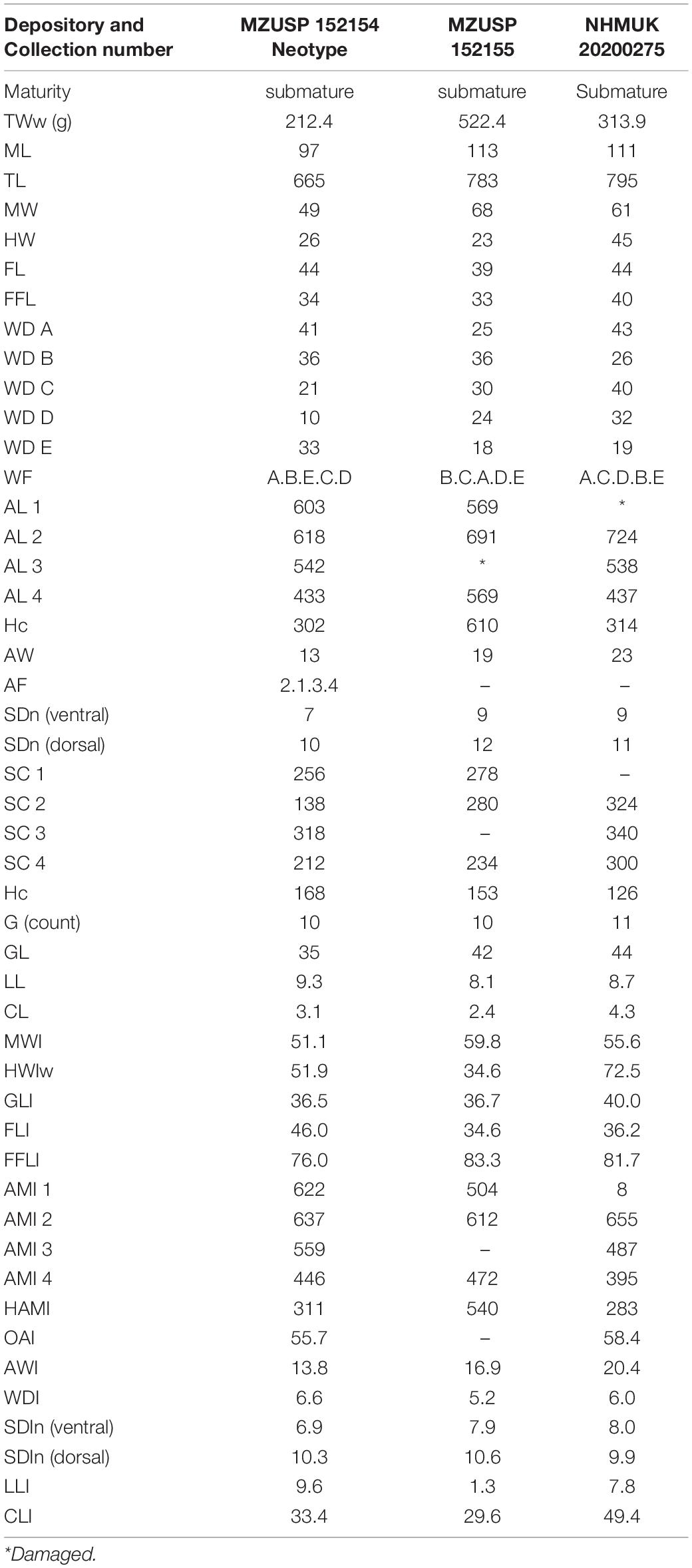
Table 3. Callistoctopus furvus measurements (mm), counts and indices for preserved males (neotype and voucher specimens).
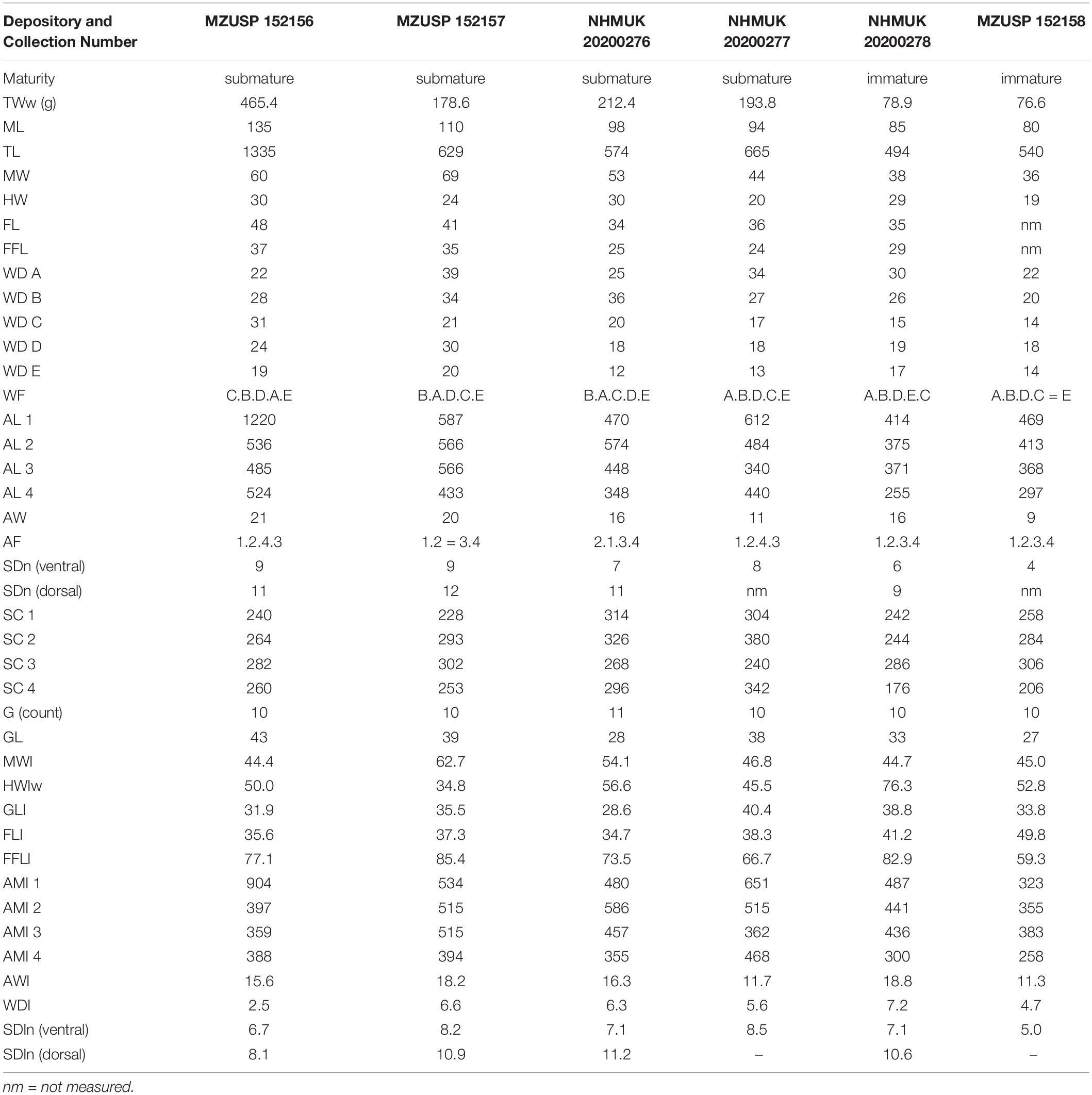
Table 4. Callistoctopus furvus measurements (mm), counts and indices for preserved females (voucher specimens).
Preserved animals pinkish gray in color on the dorsal side and creamy-colored on the ventral side, with a smooth skin texture. The typical white spots of live animals remain in preserved specimens as darkish spots. Body with a remarkably slender outline. Medium to large species (Figure 2). Submature males to at least 110 mm ML, submature females to at least 135 mm ML, TL up to 1335 mm and fresh weight up to 530 g. Saccular mantle with thin, muscular wall, widest medially (MWI 44.4–62.7), tapering to a blunt point in the posterior end. Lateral mantle skin ridge absent. Head typically narrower (HWI 34.6–72.5) than the mantle. Eyes not prominent. Stylets absent. Funnel tubular, moderately long (FLI 34.6–46.0), broad-based, with a large U-shaped funnel organ (Figure 2). Gills with 10 to 11 lamellae per demibranch.
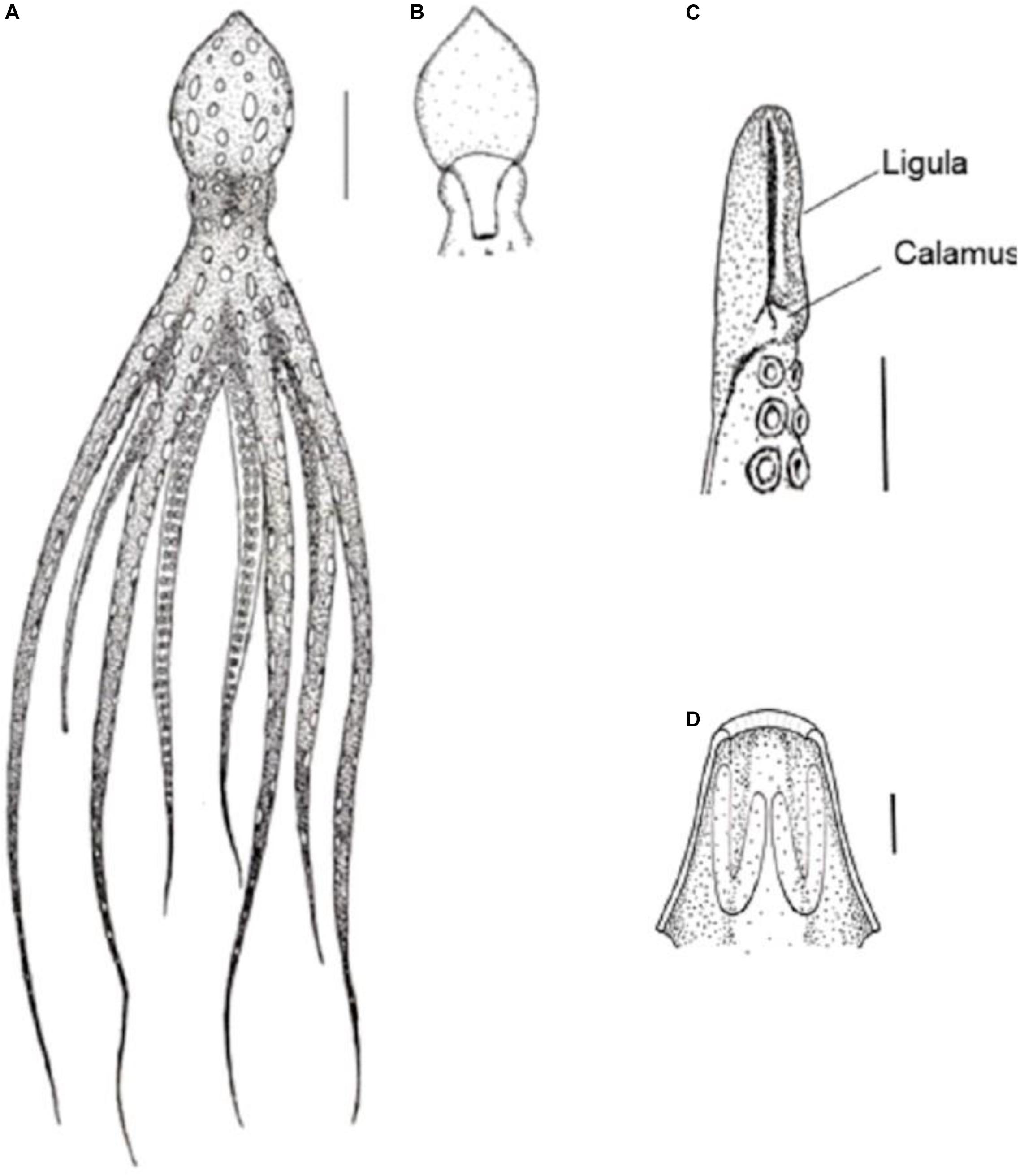
Figure 2. Callistoctopus furvus. (A–C) Neotype (95 mm ML MZUSP 152154); (D) Voucher specimen (85 mm ML NHMUK 20200278). (A) Dorsal view. (B) Ventral view (mantle). (C) Copulatory organ (ligula and calamus) on tip of right third arm. (D) Funnel organ on dorsal surface of funnel. Scale bars: (A,B) = 50 mm, (C) = 5 mm, (D) = 10 mm.
Arms long, unequal in length, longest arms typically 4.6 to 9 times mantle length. Dorsal arms longest (typically AF 1 > 2 > 3 > 4). Arms narrow (AMI 11.7–20.4) tapering evenly along length. Normal arm tips very fine, long and delicate. Dorsal arms thicker than ventral arms. Two rows of suckers on each arm. Sucker counts, normal arms 138–380 per intact arm in immature and submature animals (males: 138–340; females: 228–380), 126–168 on hectocotylized arms of submature males. Suckers smaller (SDI 6.7–8.0) on ventral arms and larger on dorsal arms (SDI 8.1–11.2), none especially enlarged in either sex. Web very shallow (WI 2.5–6.6). Web formula variable, but dorsal webs (A and B) typically deepest, and web E generally the shallowest (33–64% as deep as the deepest web). Web may reach between 1/3 and 1/4 of arm length in live animals (Figure 3). Third right arm of males hectocotylized, relatively shorter than opposite arm (OAI 55.7–58.4). Ligula medium-sized (LLI 6.3–9.6), with a long calamus (CLI 29.6–49.4). Ligula blunt, roughly cylindrical and deeply grooved (Figure 2).
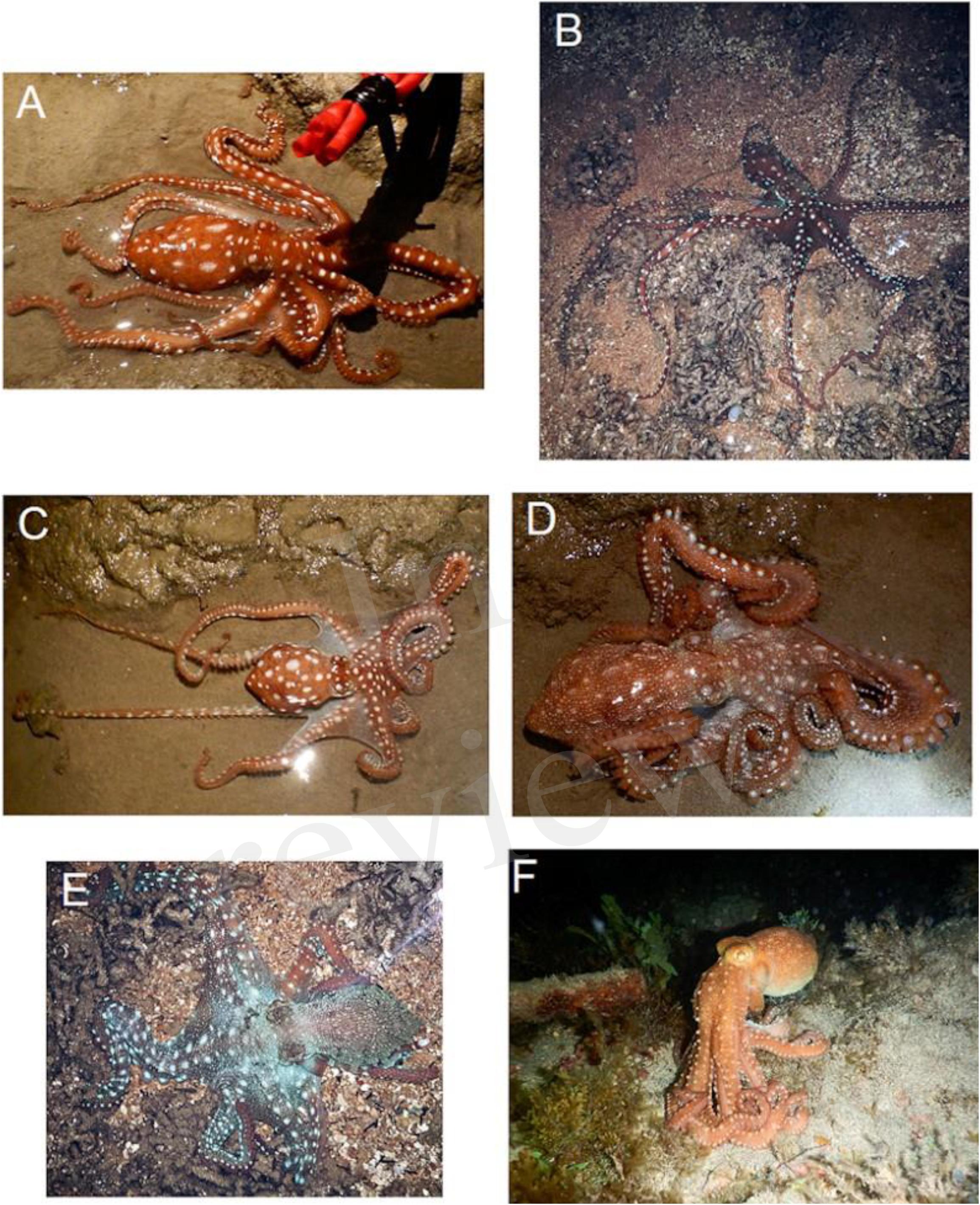
Figure 3. Callistoctopus furvus. Examples of color patterns of living specimens recorded (except F) at Morro de São Paulo (Bahia State). (A,B) Typically brick red color with white spots associated to smooth skin. (C) Red brick color associated to smooth skin with numerous minuscule white spots dotting the superior half of arms and the expanded web. (D) Brick red pattern dotted with numerous minuscule with spots associated to a knobbed texture of the larger white spots. (E) Green-grayish associated with a rugose texture over the whole body and maximally extended rounded and flat dorsal mantle papillae. (F) Uniformly red color associated to distinguishable rows of knobbed white spots on the arms (Abrolhos, Bahia State).
Beaks and radula are depicted in Figure 4. The narrow hooded upper beak has a distinct but rather short, slightly hooked pointed rostrum, and an obtuse jaw angle. Lower beak with a short and sharp rostrum, obtuse jaw angle, hood narrow, wings broadly spread with flaring lateral walls, split in the posterior half. Radula with seven teeth in each transverse row. Rhachidian teeth long, broad-based with three cusps on each side, basal cusps largest, decreasing in size distally. First lateral tooth short, cusp absent. Second lateral larger than first, broad-based and triangular, with concave margins and a single cusp point toward the midline. First marginal teeth curved, scythe-like, nearly as long as second laterals, with tooth directed toward midline. Marginal plates flat, almost rectangular and slightly curved.
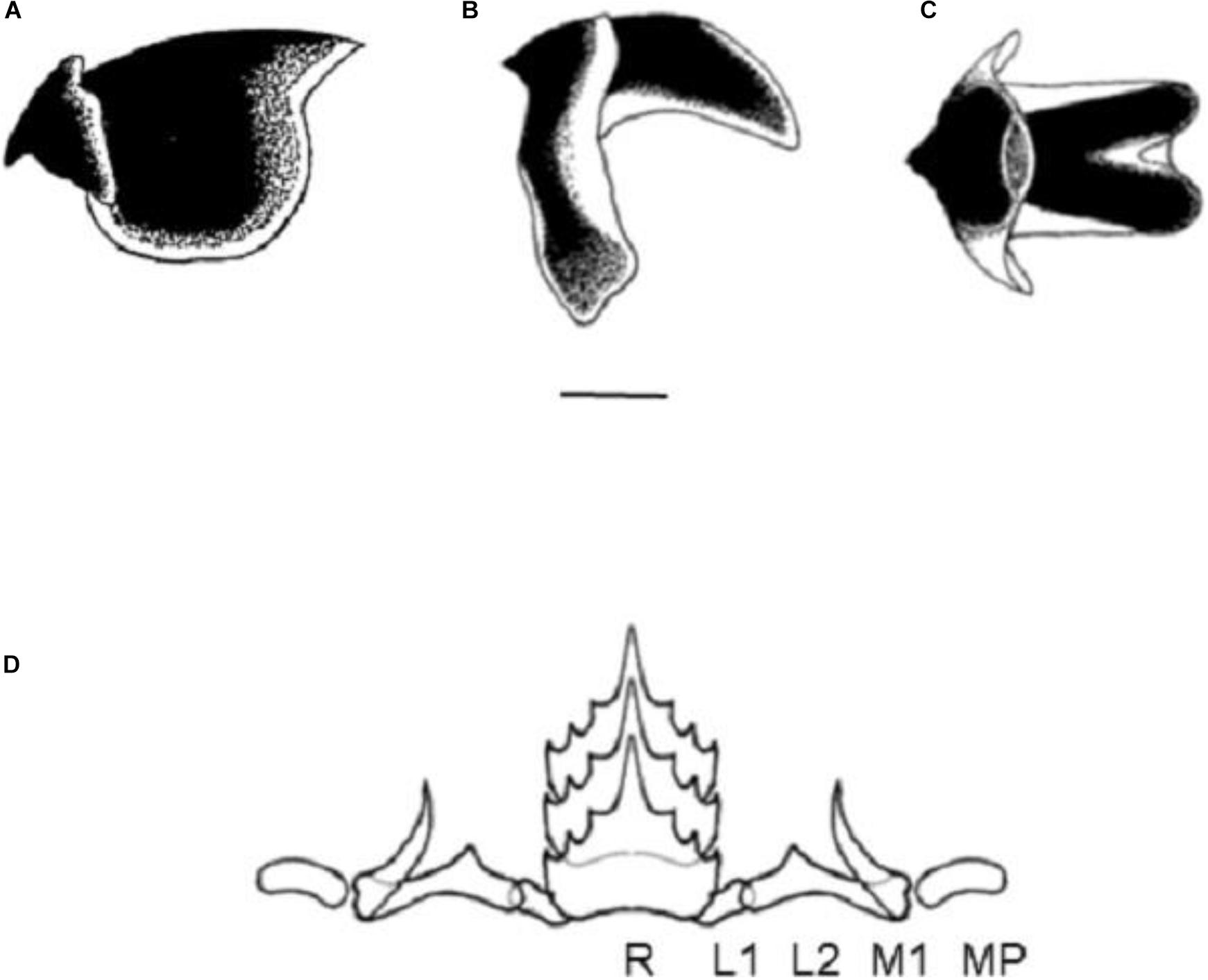
Figure 4. Callistoctopus furvus. (A–D) Neotype (95 mm ML MZUSP 152154). (A) Upper beak, lateral view of. (B) Lower beak, lateral. (C) Lower beak, tripod orientation. (D) Radula. Abbreviations: L1, first lateral tooth; L2, second lateral tooth; M1, first marginal tooth; MP, marginal plate; R, Rachidian tooth. Scale bars (A–C): 5 mm.
Color, Skin Sculpture and Body Patterns of Live Animals
These octopuses are typically brick red in color, with numerous white spots forming distinct patterns on dorsal mantle, head, and arms, most often associated to a smooth skin texture over the whole body (Figures 3A,B). A slightly similar color pattern, but with numerous small white dots scattered on the whole body, but denser on the arm’s bases and extended webs, was associated to a rugose skin texture (Figure 3C) and sometimes to knobbed white spots on the mantle (Figure 3D). A distinctive pattern included green-grayish color over the mantle concealing its white spots, plus dark brick red arms with evident white spots and a dense white dotted network. This color pattern was associated with a strikingly rugose texture over the whole body and distinct rounded and flat skin papillae on the mantle (Figure 3E). On several specimens, the white spots on the mantle were barely noticeable, and they were almost uniformly red in color across the whole body, except for rows of knobbed white spots on the arms. In these situations, the body skin texture is rugose, and raised flattish papillae are present on the upper half of the arms (Figure 3F). Another color pattern worthy of note was a gray coloration over a flattened body shape that allowed the animal to blend with the substrate (see Supplementary Movie 1).
Morphological Comparison Between Atlantic and Mediterranean Callistoctopus spp.
Principal Component Analysis results showed that the three axes explained over 99% of the total morphological variance. The size-related first principal axis had equal contributions of ML and MW component loadings, accounted for nearly 92.49% of the total variance. Shape variation, as explained by the second and third axes, had the HW (5.37%) and MW (1.62%) as the most important loadings for the explained variance (Table 3). Callistoctopus of all geographic origins overlapped in shape, but it is worthwhile to note that Brazilian representatives tended to show narrower heads and mantles, and Mediterranean animals had the broadest heads among all samples analyzed. Interestingly, Caribbean and Macaronesian Callistoctopus overlapped strongly in terms of shape (Figure 5A).
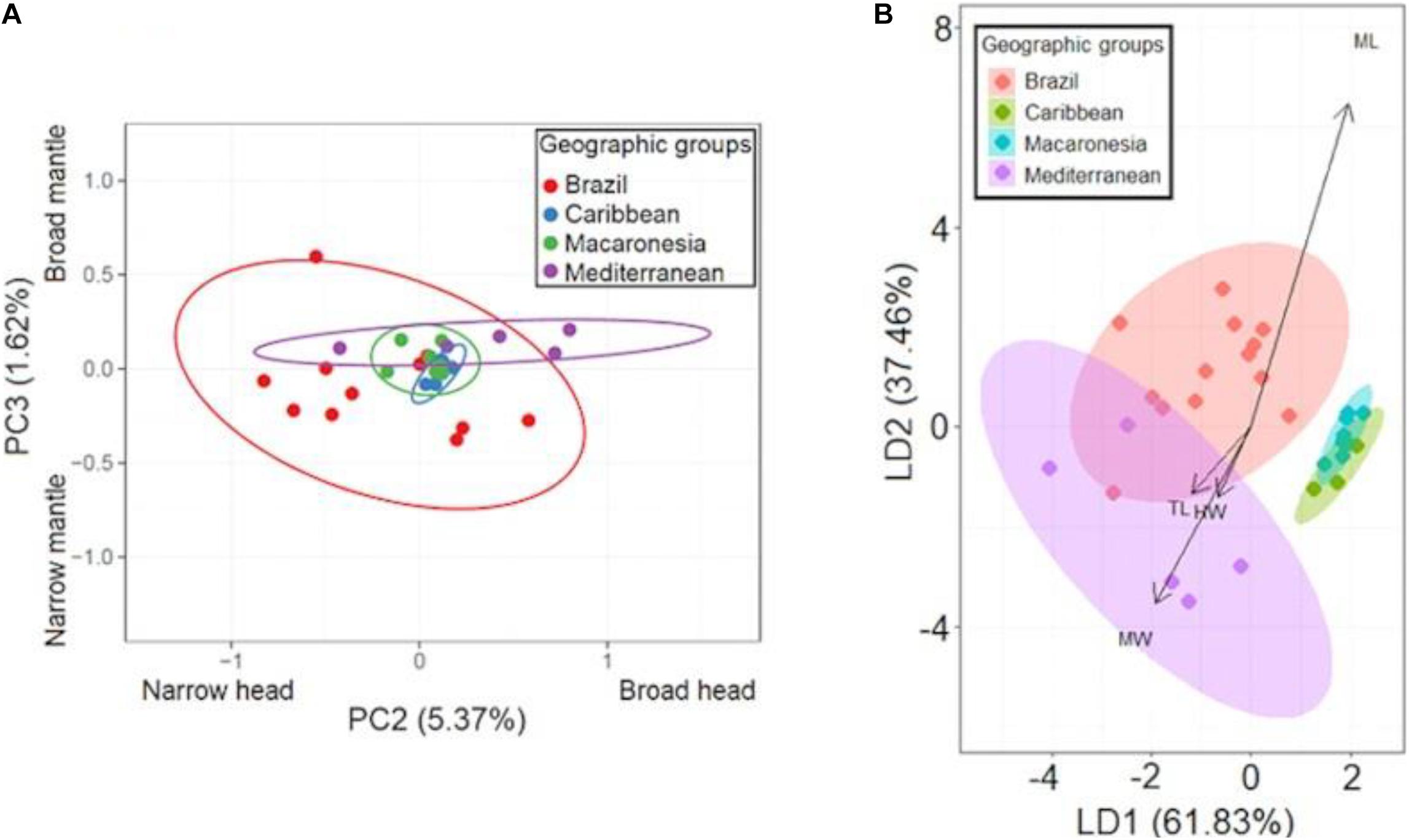
Figure 5. Morphological variation between regional populations of Callistoctopus, including C. furvus and C. macropus. (A) Plot of individual scores of PC2 vs. PC3 depicting shape variation among geographic samples. (B) Size-free LDA plot depicting the degree of morphological separation among geographic samples. Prediction ellipses in both plots are such that with probability 0.95, a new observation from the same group will fall inside the ellipse.
Size-free LDA results reflected the PCA outputs showed some degree of overlap, although the centroid for most groups were clearly well separated. Brazilian Callistoctopus had the longest ML and shortest TL, and the narrowest HW and MW, overlapping poorly with Mediterranean specimens, and completely separated from Caribbean and Macaronesian animals. Again, these two latter groups had the highest degree of overlap, clearly split from Mediterranean and Brazilian specimens. Mediterranean octopuses were considerably shorter in ML than their counterparts elsewhere, and had the largest dimensions for all the remaining measures taken in our analysis (Figure 5B).
Callistoctopus furvus vs. C. macropus stricto sensu
Historically, C. furvus has been misidentified as C. macropus (i.e., Voss and Toll, 1998). Thus, it was most instructive to compare basic indexes of male and female octopuses of both species (Table 5). Of note, it is clear that C. furvus mantle and head are narrower and the funnel is shorter than those of C. macropus. It is also remarkable that C. macropus suckers are proportionally larger, and the dimensions of suckers on dorsal and ventral arms are not as marked as in C. furvus (in fact, those differences in sucker dimensions were even not noted on arms of C. macropus females). Unfortunately, we had just one C. macropus male for comparison. Nonetheless, we found that the hectocotylus length was about half of the length of the opposite arm in C. macropus, but slightly longer in C. furvus material. In contrast, the ligula and calamus indexes were considerably larger in C. macropus. Finally, it is also worth noting that, despite similar coloration in live animals (brick red) and smooth skin texture in both species, the single, large supraocular papilla often reported in C. macropus was not present in C. furvus.
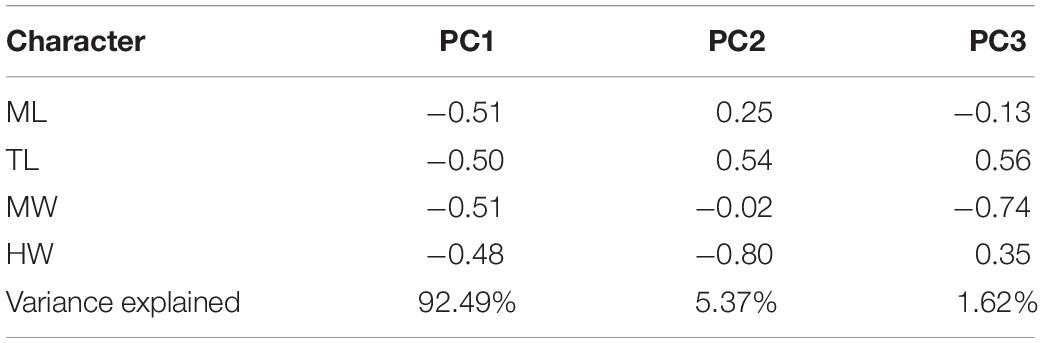
Table 5. Character loadings on the principal components analysis applied for morphological variation of Callistoctopus spp. in Brazil, the Caribbean, Macaronesia and Mediterranean Sea.
Callistoctopus furvus Neotype/Voucher Specimens vs. Gould (1852) Original Description
Other authors have reported that Gould’s original description of C. furvus was not sufficiently detailed to allow for a clear separation from the morphologically similar C. macropus (e.g., Voss and Toll, 1998). A general comparison between our and Gould’s original description is given in Table 6. Overall, the slender, elongated body shape, smooth skin, relatively long funnel, narrow head, long arms and short webs reported in Gould’s description conform to the general morphology in our neotype and voucher specimens. However, we also found two noticeable differences, namely: (i) the absence of three distinct cirri on each eye as reported by Gould (not present in our samples; either in preserved or fresh specimens; in fact, it was not discernible even in live animals; see Figure 3) and (ii) differing colorations (varying from dark to ash to ash-mottled in Gould’s description) (Table 7).
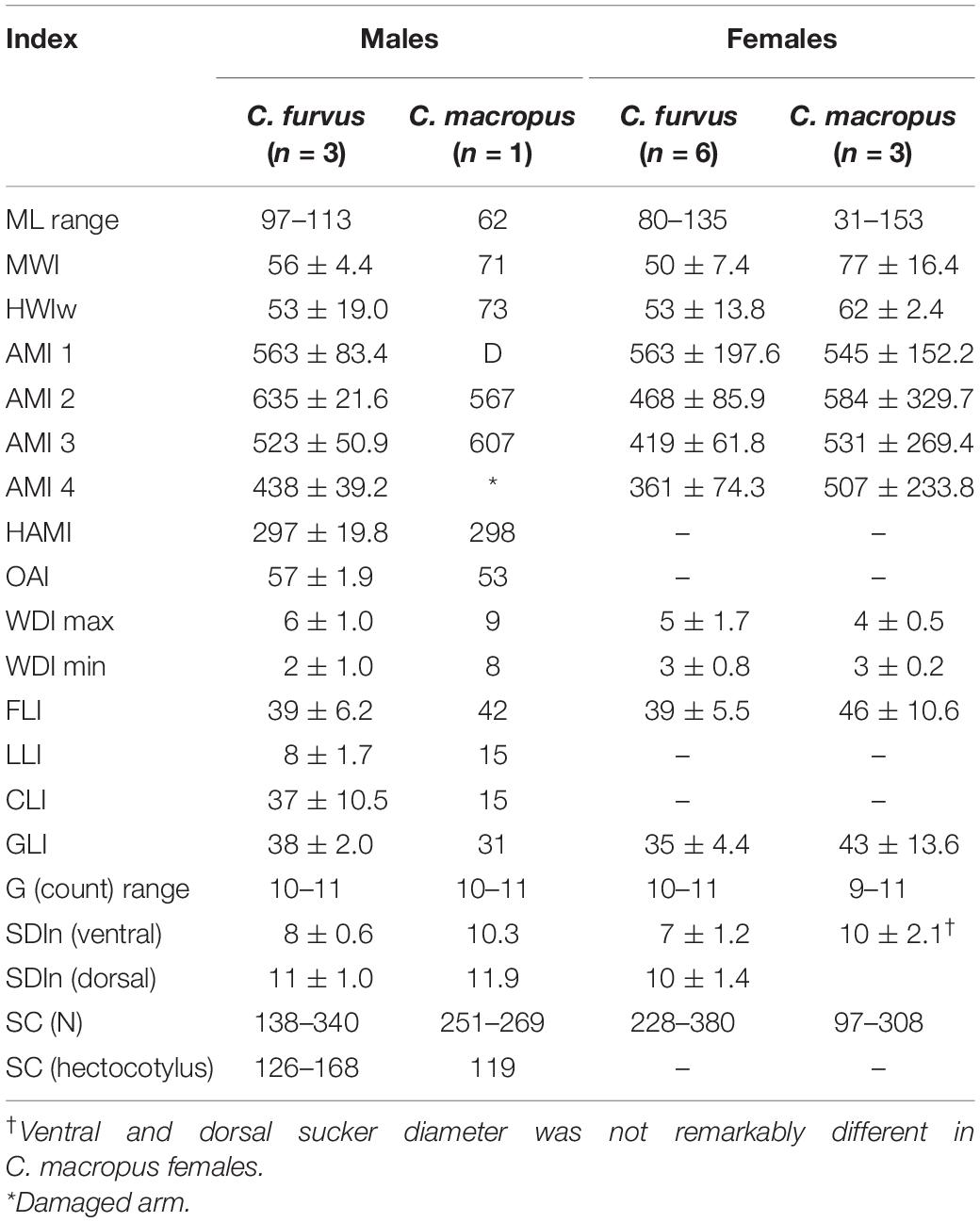
Table 6. Comparison of basic measurement indices for male and female Callistoctopus furvus and Mediterranean C. macropus stricto sensu (male: 62 mm ML, Villefranche-sur-Mer, France, NHMUK 1952.4.1.43; females: 30 mm ML, Naples, Italy, NHMUK Reg TBC; 47 mm ML, Zoological Station Naples, Italy, NHMUK 1898.5.21.344; and 153 mm ML, “Mediterranean,” NHMUK 1908.12.22.20).
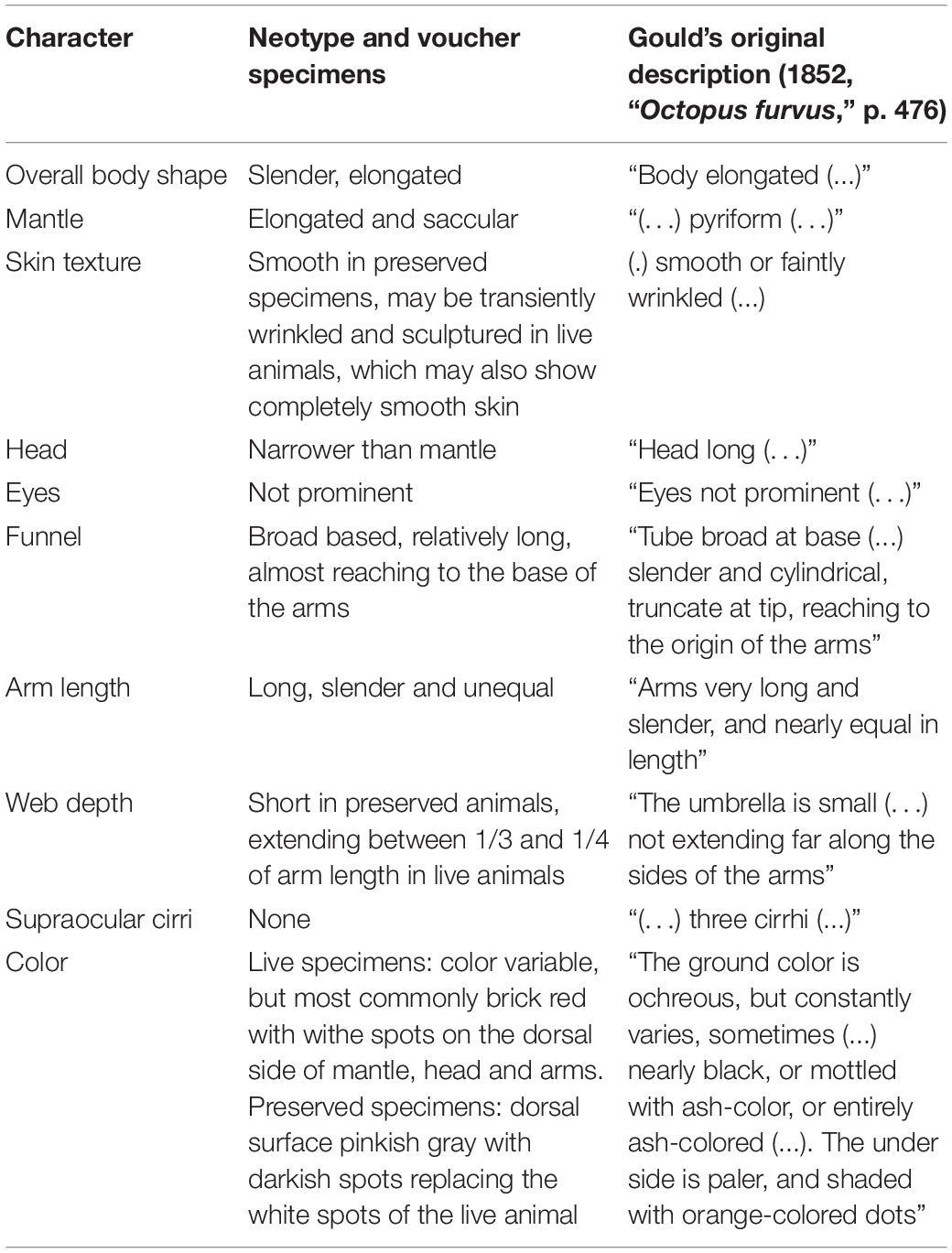
Table 7. Comparison of some selected characters between Callistoctopus furvus new (present study) and original (Gould, 1852) description.
Molecular Results
The ML and BI evolutionary models for the concatenated database recovered extremely similar topologies, with strong statistical support. Thus, for the sake of simplicity, we only present the BI topology (Figure 6). The Callistoctopus lineage present in the western Atlantic Ocean (Brazil, Colombia and Mexico) comprises a genetically well-supported clade (ML = 93%, BI = 1). This indicates that C. furvus is evolutionarily distinct from C. macropus, with the two linages clustered as sister species.
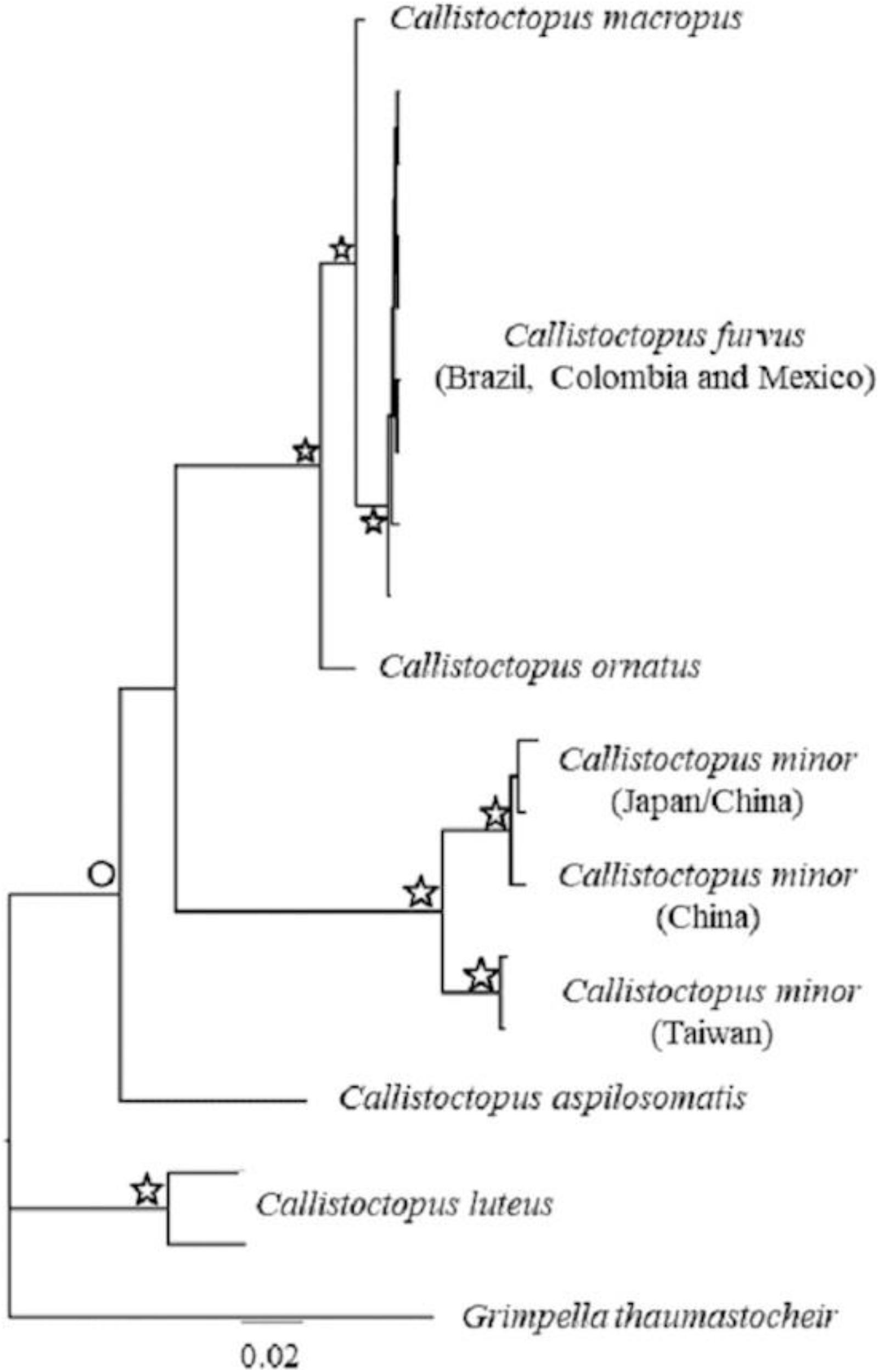
Figure 6. Bayesian consensus phylogenetic tree of Callistoctopus species based on two molecular markers (16S + COI). Star denotes support values between 95% (0.95) and 100 (1) for Maximum Likelihood (ML) and Bayesian Inference (BI), respectively. Circles, denotes support values between 85% ML (0.85 BI) and 90% ML (0.9 BI).
Callistoctopus ornatus was recovered as the closest species to C. furvus and C. macropus clade (ML = 100%, BI = 1). The two Callistoctopus minor linages recovered were closely related to Callistoctopus aspilosomatis (ML = 89%, BI = 0.92). The position of Callistoctopus luteus could not be defined within our phylogeny, probably due to the absence of other species of the genus in our database. Nonetheless, this species was found to be phylogenetically valid and monophyletic (ML = 100%, BI = 1) (Figure 6).
Discussion
Overview
To the best of our knowledge, this is the first octopus neotype described with the aid of fishers’ traditional knowledge. Previous ethnoecological surveys indicated that artisanal octopus fishers exploited an “unusual” shallow-water octopus species (e.g., Martins et al., 2011; Jesus et al., 2015), and served as a baseline for our taxonomic study. Thus, our collaborative approach highlights the importance and usefulness of coupling ethnoknowledge with traditional and molecular taxonomy for producing and refining biodiversity assessments. Neotype designation is often necessary and desirable, particularly in octopuses with missing type specimens belonging to widespread and “catchall” species names such as “vulgaris,” “macropus,” and “defilippi,” for which superficial morphological similarities often lead to misidentification (Norman and Hochberg, 2005; Gleadall, 2016).
The taxonomic status of the western Atlantic Callistoctopus has long been problematic and, along with C. furvus, at least two other described species [i.e., Callistoctopus bermudensis (Hoyle, 1885) and Callistoctopus chromatus (Heilprin, 1888)] were initially recognized in the region but later synonymized within C. macropus (Voss and Toll, 1998). However, earlier authors did not have access to modern multivariate statistics and molecular tools that allowed us to recognize C. furvus as a valid species distinct from C. macropus, a conclusion also supported by genetic studies of tropical western Atlantic shallow-water octopus fauna recently conducted by Ritschard et al. (2019) and Lima et al. (2020).
Ethnoknowledge and Callistoctopus furvus
Systematic classification was one of the main goals of scientists in the 19th century, and at that time, taxonomists relied heavily on morphological/morphometric data along with other characters (color, behavior, etc.) to describe and classify species (Mayr, 1971). Like trained “classical” taxonomists, traditional fishers systematically record morphological, chromatic and behavioral characters and habitats to recognize their target species (Begossi and Figueiredo, 1995; Johannes et al., 2000; Carvalho et al., 2009), and octopuses are no exception. In our study, it was shown that artisanal octopus fishers, spearfishers and SCUBA divers alike use accumulated sets of perceived characteristics to identify and name sympatric octopuses.
In the region of Porto Seguro (16°S, Bahia State), where the ethnoecological aspects of C. furvus were firstly addressed (e.g., Martins et al., 2011; Jesus et al., 2015), octopus fishers associate the occurrence of the species to strong and persistent easterly winds, using the name “eastern octopus” to identify the species. The species is deemed rare amongst local fishers. However, this is most likely because fishers work during daylight hours, and the species is nocturnal. Thus, the infrequent encounters with C. furvus–often in early mornings–may represent the last individuals foraging at low tide before seeking daylight refuge in the reef (Jesus et al., 2015).
According to Marques (1991), the identification process takes place through an information set, where specific morphological characteristics are added up to more general characters, as the shape and/or coloration of the animal, through analogies with other animals. In the region of Cabo Frio (22°S, Rio de Janeiro State), Callistoctopus is called the “cheetah octopus” by SCUBA divers, based on similarity to the color pattern of the white-spotted “cheetah stingray” (Aetobatus narinari). Interestingly, Callistoctopus had no vernacular names in six out of nine of our surveyed locations.
At Morro de São Paulo (13°S, Bahia State), fishers’ adopted ecological criteria refer mainly to the usual habitat or substrate to name two exploited sympatric octopus species. “Sand octopus” (C. furvus) and “stone octopus” (O. insularis) are the names that indicate the usual habitat of each octopus species. Behavioral perceived characteristics were another set of information used for systematization. Fishers correctly recognize the stone octopus as a diurnal species, and the sand octopus as nocturnal (Jesus et al., 2015). They also had a clear perception that frightened stone octopuses seek refuge in existing holes and crevices on the reef environment where it is commonly found (Batista and Leite, 2016), whereas sand octopuses burrows themselves into the sand as an escape response from predators or immediate danger.
Burrowing behavior (i.e., active movement through soft substrates; Dorgan, 2015) is found in very few cephalopod species (Hanlon and Messenger, 2018) and, as far as we known, it has never been described for any Callistoctopus species. In addition, it seems that it is only the third instance in which burrowing behavior is reported for western Atlantic shallow-water octopuses (this was firstly reported in southern Caribbean for Amphioctopus burryi and Macrotritopus beatrixi; see Guerrero-Kommritz and Rodriguez-Bermudez, 2019). Whether C. furvus burrows itself by displacing sediments using sweeping movements of the arms and suckers, or by sediment-fluidization akin to quicksand formation (the two recognized burrowing mechanisms in octopuses; Montana et al., 2015), is currently unknown.
Thus, fishers at Morro de São Paulo correctly identified habitat use and behaviors that allow direct competition avoidance and therefore the coexistence of these two sympatric octopuses in the same environment. This method of distinction is clearly as valid, reliable and accurate as scientifically based surveys. For instance, Bennice et al. (2019), using in situ observations, marking locations of octopus-occupied dens, and photoquadrats, observed that the use of different types of substrate allow fine-scale habitat partitioning between Macrotritopus defilippi (associated to sandy bottoms) and Octopus vulgaris (now Octopus americanus; Avendaño et al., 2020) (associated to hard substrate) in a shallow-water lagoon on the Florida coast.
Distribution and Taxonomic Remarks
Our data points to a wide latitudinal range for the genus Callistoctopus along the Brazilian coast, with records spanning from Fernando de Noronha National Park (4°S) to the Arvoredo Biological Reserve (27.5°S). Thus, in accordance with previous studies (i.e., Haimovici and Perez, 1991; Leite and Haimovici, 2006; Haimovici et al., 2009; Martins et al., 2011; Jesus et al., 2015, 2020) our data indicate that these octopuses occur on both nearshore and oceanic island waters off Brazil. Despite the lack of morphological and genetic confirmation for most of our records (largely based on interviews and photographic records) in the surveyed area, the high dispersal potential of small-egged C. furvus (see below) suggests that most–if not all–recorded octopuses may belong to the same species.
On the continental scale, combining genetic studies in southern Caribbean (Ritschard et al., 2019) and northeastern Brazil (Lima et al., 2020 and our results), plus published occurrences in the western Atlantic (Hanlon, 1988; Voss and Toll, 1998; Cedillo-Robles and Pliego-Cardenas, 2018; Flores-Valle et al., 2018) results in a broad, cross-hemisphere distribution range for the species on the western Atlantic Ocean, including the Caribbean Sea (∼19°N–28°S). However, Schwartz (1992) reported the occurrence of “Octopus macropus” off North Carolina (34°N; United States), implying that C. furvus northernmost range limit may extend farther north than the Gulf of Mexico (Figure 1).
The precise bathymetric horizon for C. furvus off the Brazilian coast is poorly established. Accounts of Callistoctopus reported by SCUBA divers and spearfishers alike are relatively rare along the entire studied area, which may be due to the shallow-water and nocturnal habits of these octopuses (Norman, 2000), as both recreational and professional/fishing dives are often conducted during daylight hours in deeper (>10 m) waters. The same is true for northwestern Atlantic records, as published accounts invariably describe C. furvus as a shallow-water species (e.g., Ritschard et al., 2019).
Callistoctopus-like octopuses are most commonly recognized by traditional fishers in northeast and eastern Brazilian shores, and apparently less further south on the Brazilian coast. This may partially be explained by the presence of easily accessible intertidal reef flats at these latitudes, the typical Callistoctopus habitat (Norman, 2000), where traditional fishers often exploit shellfish at low tide using a number of fishing techniques (e.g., Hauzer et al., 2013; Pratchett et al., 2020). On the other hand, similar shallow-water environments are virtually absent on the southeastern Brazilian coast, which may partially account for the lower awareness of this species by local fishers in this region. In fact, southern Callistoctopus records were reported exclusively by SCUBA divers (Jesus et al., 2020).
The present study is the first to direct molecular research specifically to the genus Callistoctopus in the western Atlantic. Thus far, the taxonomic status of Callistoctopus in this region remained dubious, as previous reviews based purely on morphology indicated the presence of C. macropus on both sides of the Atlantic (Voight, 1998; Voss and Toll, 1998; Leite and Haimovici, 2006; Haimovici et al., 2009). However, Gould (1852) stressed that the southwestern Atlantic Callistoctopus differed from eastern Atlantic/Mediterranean C. macropus in color and arm formula. Our results not only support this morphological differentiation, but also validate C. furvus as the genetic lineage of the genus in the western Atlantic Ocean.
The genetic homogeneity between specimens from Brazil and the Caribbean/Gulf of Mexico can possibly be explained by the high dispersal potential of C. furvus as the species is small-egged, and their paralarvae may be transported over long distances by the action of ocean currents (Villanueva et al., 2016). It has long been thought that the presence of the low salinity Amazon–Orinoco plume (AOP) in the North Brazil Shelf may act as a barrier to larval dispersal and population connectivity between the Caribbean and Brazilian biogeographic provinces (Muller-Karger et al., 1988; Floeter et al., 2008). However, recent studies indicated that the AOP does not represent a barrier for both squid and octopus species (Lima et al., 2017; Sales et al., 2017; Flores-Valle et al., 2018; González-Gómez et al., 2018; Pratt et al., 2020). The same is also true for some fish, crustaceans and fire coral species (Luiz et al., 2012; Laurenzano et al., 2013; Souza et al., 2017; Buranelli et al., 2019).
Shape Variation in Atlantic and Mediterranean Callistoctopus
Allcock et al. (2008) criticized the use of multivariate analysis of morphological datasets in coleoid cephalopods as, according to their results, this methodology does not provide good enough discrimination at species level. Notwithstanding, these authors suggest that multivariate analysis may be useful at the generic level. At least in our case, multivariate analysis yielded excellent shape discrimination among Callistoctopus sampled at several locations in the Atlantic Ocean and Mediterranean Sea. Similar results using multivariate analysis allowed for the identification of subtle morphological differences between O. insularis sampled off Vera Cruz (19°N, Mexico) and Brazil, despite the lack of genetic differences (i.e., González-Gómez et al., 2018).
The poor morphological overlap among different geographic samples found in our discriminant analysis reinforces the distinction of Callistoctopus-like octopuses between the eastern and western Atlantic. Brazilian C. furvus differs morphologically from Mediterranean Sea C. macropus by the overall slender shape (i.e., relatively narrower head and MWs), shorter TLs, and longer mantle lengths. Since Mexican and southern Caribbean specimens (sequences produced by Flores-Valle et al., 2018; Ritschard et al., 2019) were identified as C. furvus belonging to the same linage as the Brazilian octopuses, the shape separation may be due to phenotypic variability, as also seen in the case of subtle morphological differences between Mexican and Brazilian O. insularis (González-Gómez et al., 2018).
The morphological overlap between Caribbean and Macaronesian Callistoctopus warrants further investigation, since these octopuses inhabit very distinct oceanographic environments (i.e., the tropical waters of the Caribbean and warm temperate waters of Macaronesia). Although reliable lineage assignments require (currently lacking) genetic studies, we hypothesize that Macaronesian Callistoctopus may potentially belong to the western Atlantic clade. While the pelagic larval duration in C. furvus is still unknown, the eastward, fast-flowing Gulf Stream (average core velocity of ∼ 1 m s–1; Rossby et al., 2014) and its associated filaments and meanders (Caldeira and Reis, 2017) may maintain larval connectivity between the Caribbean and Macaronesian Islands. A similar mechanism (i.e., long-distance transport by fast-flowing ocean currents) seems to connect O. insularis between the Brazilian mainland and the mid-Atlantic islands of Ascension and St Helena (Amor et al., 2017).
Phylogenetic Relationships
Our results suggest a close phylogenetic affinity among C. furvus, C. macropus, and C. ornatus, where each of those constitutes well-supported phylogenetic lineages. In addition, the three species share behavioral and morphological similarities (Lima et al., 2020). The recently estimated time divergence between C. macropus and C. furvus (identified as ‘Callistoctopus sp.’ in Lima et al., 2020) indicates that the two lineages probably separated some ∼ 5 MYA, when the Panama seaway played an important role in the colonization of the western Atlantic Ocean from the western Pacific Ocean (Lima et al., 2020). However, their results had low statistical support, suggesting that the addition of more species of the genus would improve the reconstruction of the dispersion route of the common ancestor of the two species. Taking into account the diversity of species in the genus (greater in Asia and Oceania, smaller in Africa, Europe and in the Americas), it is likely the ancestor of the two species also followed a similar route from the Pacific toward the Atlantic, as other neritic cephalopod species (e.g., Anderson, 2000; Ulloa et al., 2017).
In our phylogenetic reconstructions, the position of C. luteus was not well defined, probably due to the lack of sequences from other species of the genus. The position of this species was also found to be unclear in previous studies (Kaneko et al., 2011; Acosta-Jofré et al., 2012). Increasing the taxonomic and geographic coverage of sequences for a target group should improve the internal phylogenetic resolution, yielding more accurate indications about the evolution of a given group (Sales et al., 2014; Anderson and Marian, 2020).
Reconciling Contemporary and Original Callistoctopus furvus Descriptions
The taxonomic status of C. furvus remained controversial for many decades. This may be not only due the morphological, behavioral and chromatic resemblance with eastern Atlantic C. macropus, but also because the holotype was missing (Voss and Toll, 1998). In addition, Callistoctopus records in the western Atlantic Ocean have been relatively infrequent (e.g., Haimovici et al., 2009), and Gould’s original description was not sufficiently detailed enough to allow for reliable identification to species level. Increasing recent research efforts on western Atlantic shallow-water octopus fauna, undoubtedly driven and boosted by the development, diffusion and popularization of molecular tools (Radulovici et al., 2010; White and Last, 2012), renewed the interest of regional teuthologists in these species (e.g., Flores-Valle et al., 2018; Ritschard et al., 2019; Lima et al., 2020).
On a morphological basis, the most controversial difference between our neotype description and Gould’s original work is the alleged existence of three distinct cirri on each eye in C. furvus. No supraocular cirri were observed in preserved, fresh or even in live specimens analyzed in this study. In addition, the sole contemporary C. furvus drawing found (i.e., Cedillo-Robles and Pliego-Cardenas, 2018) also show no cirri on the eyes. Unfortunately, this dispute will remain open, at least in the short term, as the most recently published accounts (e.g., Leite and Haimovici, 2006; Flores-Valle et al., 2018, Lima et al., 2020 and others) do not describe the morphology in sufficient detail for useful comparisons. Nonetheless, distinct morphotypes in genetically homogeneous species populations (either sympatric or allopatric) have been reported in marine mollusks (Saad et al., 2014), including cephalopods (van der Vyver et al., 2016; González-Gómez et al., 2018). This suggests that the lack of supraocular cirri in our specimens may be the result of phenotypic variability. It is also reasonable to assume that the different coloration described by Gould could well be an artifact caused by the preservation methods used by the author in the mid-19th century.
Conclusion
In summary, in line with contemporary best practices in taxonomy (see Dayrat, 2005), we have redescribed and designated a neotype for C. furvus using an integrative approach, combining scientifically orthodox methods for diagnosis with ethnoknowledge gathered from traditional fishers. This work highlights the reliability of information furnished by traditional people that are scientifically untrained but nonetheless seasoned observers of their surrounding nature (e.g., Ogar et al., 2020). Undoubtedly, this kind of collaborative approach may be useful in the case of cryptic invertebrates such as octopuses, particularly in remote coastal locations such as those studied here. Thus, our integrative taxonomic approach supports biodiversity assessments in poorly surveyed and/or difficult-to-access coastal regions, and may help in the development of management plans of small-scale fisheries and marine conservation areas (Johannes et al., 2000; Hauzer et al., 2013; Carvalho et al., 2009).
Data Availability Statement
The datasets presented in this study can be found in online repositories. The names of the repository/repositories and accession number(s) can be found in the article/Supplementary Material.
Ethics Statement
Ethical review and approval was not required for this animal study, in accordance with the local legislation and institutional requirements. Brazilian law on ethics in the use of animals in research (“Lei n° 11.794, de 8 de outubro de 2008” – an online version of this law, in Portuguese, can be accessed at https://www.planalto.gov.br/ccivil_03/_ato2007-2010/2008/lei/l11794.htm) does not consider invertebrates among the organisms that require consideration in protocols, recommendations and regulations in their use. According to Section 02 of the referred law: “The provisions of this Law apply to animals of classified species as phylum Chordata, subphylum Vertebrata, subject to environmental legislation.”
Author Contributions
MDJ, AS, RSM, and JBLS contributed to conception and design of the study. MDJ collected the biological samples. JBLS, JSR, and TASC conducted the genetic analyses. RSM, MDJ, and JDA carried out the morphological measurements. RSM and JBLS performed the statistical analysis. RSM wrote the first draft of the manuscript. All authors contributed to manuscript revision, read, and approved the submitted version.
Conflict of Interest
The authors declare that the research was conducted in the absence of any commercial or financial relationships that could be construed as a potential conflict of interest.
Acknowledgments
We acknowledge the Coordenação de Aperfeiçoamento de Pessoal de Nível Superior (CAPES; Funding Code 001) for the doctoral grant awarded to MJ, and the Conselho Nacional de Desenvolvimento Científico e Tecnológico (CNPq) for the productivity grant awarded to AS. We are indebted with Arieli Tristão Rézio (UNIFESP’s Graduate Program in Marine and Coastal Biodiversity and Ecology) and Caroline Marinho Mano (São Paulo State Public Attorney’s Office) for the aid in recording body measurements and counts. We extend our gratitude to Dr. Camila Marinho Mano (S o Paulo State Scientific Police – SPTC), who took photos that helped in the taxonomic description and drawings. Finally, we also thank Dr. Richard Schwarz (Universidade do Vale do Itajaí) for facilitating our collaboration with JDA (Natural History Museum, London).
Supplementary Material
The Supplementary Material for this article can be found online at: https://www.frontiersin.org/articles/10.3389/fmars.2020.595244/full#supplementary-material
References
Acosta-Jofré, M. S., Sahade, R., Laudien, J., and Chiappero, M. B. (2012). A contribution to the understanding of phylogenetic relationships among species of the genus Octopus (Octopodidae: Cephalopoda). Sci. Mar. 76, 311–318. doi: 10.3989/scimar.03365.03B
Agnarsson, I., and Kuntner, M. (2007). Taxonomy in a changing world: seeking solutions for a science in crisis. Syst. Biol. 56, 531–539. doi: 10.1080/10635150701424546
Allcock, A. L., Strugnell, J. M., and Johnson, M. P. (2008). How useful are the recommended counts and indices in the systematics of the Octopodidae (Mollusca: Cephalopoda). Biol. J. Linn. Soc. Lond. 95, 205–218. doi: 10.1111/j.1095-8312.2008.01031.x
Alves, R. R. N., and Souto, W. M. S. (2015). Ethnozoology: a brief introduction. Ethnobiol. Conserv. 4:1. doi: 10.15451/ec2015-1-4.1-1-1
Amor, M. D., Laptikhovsky, V. V., Norman, M. D., and Strugnell, J. M. (2017). Genetic evidence extends the known distribution of Octopus insularis to the mid-Atlantic islands Ascension and St Helena. J. Mar. Biol. Assoc. UK 97, 753–758. doi: 10.1017/S0025315415000958
Amor, M. D., Norman, M. D., Roura, A., Leite, T. S., Gleadall, I. G., Reid, A., et al. (2016). Morphological assessment of the Octopus vulgaris species complex evaluated in light of molecular-based phylogenetic inferences. Zool. Scr. 46, 275–288. doi: 10.1111/zsc.12207
Anderson, F. E. (2000). Phylogeny and historical biogeography of the loliginid squids (Mollusca: Cephalopoda) based on mitochondrial DNA sequence data. Mol. Phylogenet. Evol. 15, 191–214. doi: 10.1006/mpev.1999.0753
Anderson, F. E., and Marian, J. E. (2020). The grass squid Pickfordiateuthis pulchella is a paedomorphic loliginid. Mol. Phylogenet. Evol. 147:106801. doi: 10.1016/j.ympev.2020.106801
Avendaño, O., Roura, Á, Cedillo-Robles, C. E., González, ÁF., Rodríguez-Canul, R., Velaázquez-Abunader, I., et al. (2020). Octopus americanus: a cryptic species of the O. vulgaris species complex redescribed from the Caribbean. Aquat. Ecol. 54, 909–925. doi: 10.1007/s10452-020-09778-6
Batista, A. T., and Leite, T. S. (2016). Octopus insularis (Cephalopoda: Octopodidae) on the tropical coast of Brazil: where it lives and what it eats. Braz. J. Oceanogr. 64, 353–364. doi: 10.1590/s1679-87592016123406404
Begossi, A., and Figueiredo, J. L. (1995). Ethnoichthyology of southern coastal fishermen: cases from Búzios Island and Sepetiba Bay (Brazil). Bull. Mar. Sci. 56, 710–717.
Bennice, C. O., Rayburn, A. P., Brooks, W. R., and Hanlon, R. T. (2019). Fine-scale habitat partitioning facilitates sympatry between two octopus species in a shallow Florida lagoon. Mar. Ecol. Prog. Ser. 609, 151–161. doi: 10.3354/meps12845
Briggs, J., and Bowen, B. (2013). Marine shelf habitat: Biogeography and evolution. J. Biogeogr. 40, 1023–1035. doi: 10.2307/23463644
Buranelli, R. C., Felder, D. L., and Mantelatto, F. L. (2019). Genetic diversity among populations of the western Atlantic mangrove crab Ucides cordatus (Linnaeus, 1763) (Decapoda: Brachyura: Ocypodidae): evidence for panmixia and useful data for future management and conservation. J. Crust. Biol. 39, 386–395. doi: 10.1093/jcbiol/ruz036
Caldeira, R. M. A., and Reis, J. C. (2017). The Azores confluence zone. Front. Mar. Sci. 4:37. doi: 10.3389/fmars.2017.00037
Carvalho, A. R., Williams, S., January, M., and Sowman, M. (2009). Reliability of community-based data monitoring in the Olifants River estuary (South Africa). Fish. Res. 96, 119–128. doi: 10.1016/j.fishres.2008.08.017
Cedillo-Robles, C. E., and Pliego-Cardenas, R. (2018). “Shallow water octopuses from off Mexico,” in Cephalopod Research Across Scales: From Molecules to Ecosystems, ed. H. Judkins (St Petersburg, FL: Cephalopod International Advisory Council Symposium), 246.
Chen, J., Li, Q., Kong, L., and Yu, H. (2011). How DNA Barcodes complement taxonomy and explore species diversity: the case study of a poorly understood marine fauna. PLoS One 6:e21326. doi: 10.1371/journal.pone.0021326
Cheng, S. H., Anderson, F. E., Bergman, A., Mahardika, G. N., Muchilsin, Z. A., Dang, B. T., et al. (2014). Molecular evidence for co-occurring cryptic lineages within the Sepioteuthis cf. lessoniana species complex in the Indian and Indo-West Pacific Oceans. Hydrobiologia 725, 165–188. doi: 10.1007/s10750-013-1778-0
Costa, T. A. S., Sales, J. B. L., Markaida, U., Granados-Amores, J., Serrão, S. G., Sampaio, I., et al. (2021). Revisiting the phylogeny of the genus Lolliguncula Steenstrup 1881 improves understanding of their biogeography and proves the validity of Lolliguncula argus Brakoniecki & Roper, 1985. Mol. Phylogenet. Evol. 154:106968. doi: 10.1016/j.ympev.2020.106968
Darriba, D., Taboada, G. L., Doallo, R., and Posada, D. (2012). jModelTest 2: more models, new heuristics and parallel computing. Nat. Methods 9:772. doi: 10.1038/nmeth.2109
Dayrat, B. (2005). Towards integrative taxonomy. Biol. J. Linn. Soc. 85, 407–417. doi: 10.1111/j.1095-8312.2005.00503.x
Dorgan, K. M. (2015). The biomechanics of burrowing and boring. J. Exp. Biol. 218, 176–183. doi: 10.1242/jeb.086983
Edgar, R. C. (2004). MUSCLE: multiple sequence alignment with high accuracy and high throughput. Nucleic Acids Res. 32, 1792–1797. doi: 10.1093/nar/gkh340
Felsenstein, J. (1985). Confidence limits on phylogenies: an approach using the bootstrap. Evolution 39, 783–791. doi: 10.2307/2408678
Floeter, S. R., Rocha, L. A., Robertson, D. R., Joyeux, J. C., Smith-Vaniz, W. F., Wirtz, P., et al. (2008). Atlantic reef fish biogeography and evolution. J. Biogeogr 35, 22–47. doi: 10.1111/j.1365-2699.2007.01790.x
Flores-Valle, A., Pliego-Cárdenas, R., Jimenéz-Badillo, M. D. L., Arredondo-Figueroa, J. L., and Barriga-Sosa, I. Á (2018). First record of Octopus insularis Leite and Haimovici, 2008 in the octopus fishery of a Marine Protected Area in the Gulf of Mexico. J. Shellfish Res. 37, 221–227. doi: 10.2983/035.037.0120
Folmer, O., Black, M., Hoech, W., Lutz, R., and Vrienhock, R. (1994). DNA primers for amplification of mitochondrial cytochrome C subunit I from diverse metazoan ivertebrates. Mol. Mar. Biol. Biotechnol. 3, 294–299.
Gleadall, I. G. (2016). Octopus sinensis d’Orbigny, 1841 (Cephalopoda: Octopodidae): valid species name for the commercially valuable East Asian Common Octopus. Species Divers. 21, 31–42. doi: 10.12782/sd.21.1.031
González-Gómez, R., Barriga-Sosa, I. A., Pliego-Cárdenas, R., Jiménez-Badillo, L., Markaida, U., Meiners-Mandujano, C., et al. (2018). An integrative taxonomic approach reveals Octopus insularis as the dominant species in the Veracruz Reef System (southwestern Gulf of Mexico). PeerJ 6:e6015. doi: 10.7717/peerj.6015
Gould, A. A. (1852). “Mollusca and Shells”, in United States Exploring Expedition During the Years 1838, 1839, 1840, 1841, 1842 Under the Command of Charles Wilkes. Boston, MA: Gould & Lincoln, 475–476.
Guerrero-Kommritz, J., and Rodriguez-Bermudez, A. (2019). Soft-bottom octopods (Cephalopoda: Octopodidae) of the southern Caribbean with the description of a new species of Macrotritopus. Mar. Biodivers. 49, 1197–1215. doi: 10.1007/s12526-018-0903-8
Guidon, S., Dufayard, J. F., Lefort, V., Anisimova, M., Hordjik, W., and Gascuel, O. (2010). New algorithms and methods to estimate maximum-likelihood phylogenies: assessing the performance of PhyML 3.0. Syst. Biol. 59:307. doi: 10.1093/sysbio/syq010
Haimovici, M., and Perez, J. A. A. (1991). Abundância e distribuição de cefalópodes em cruzeiros de prospecção pesqueira demersal na plataforma externa e talude continental do sul do Brasil. Atlântica, Rio Grande 13, 189–200. Aviable online at: https://demersais.furg.br/images/producao/1991_haimovici_cephalopod_fauna_southern_brazil_bull_mar_sci.pdf
Haimovici, M., Santos, R. A., and Fischer, L. G. (2009). “Class Cephalopoda,” in Compendium of Brazilian Sea Shells, ed. E. C. Rios (Rio Grande, RS: Evangraf), 610–649.
Hanlon, R. T. (1988). Behavioral and body patterning characters useful in taxonomy and field identification of cephalopods. Malacologia 29, 247–265.
Hanlon, R. T., and Messenger, J. B. (2018). Cephalopod Behaviour, 2nd Edn. Cambridge: Cambridge University Press.
Hauzer, M., Dearden, P., and Murray, G. (2013). The fisherwomen of Ngazidja island, Comoros: fisheries livelihoods, impacts, and implications for management. Fish. Res. 140, 28–35. doi: 10.1016/j.fishres.2012.12.001
Huffard, C. L., and Hochberg, F. G. (2005). Description of a new species of the genus Amphioctopus (Mollusca: Octopodidae) from the Hawaiian Islands. Molluscan Res. 25, 113–128.
Jesus, M. D., Gouveia, M. T. J., Zapelini, C., and Schiavetti, A. (2015). Pesca artesanal e cadeia produtiva de Octopus insularis: o caso dos ambientes recifais do sul da Bahia, Brasil. Gaia Sci. 9, 195–204.
Jesus, M. D., Zapelini, C., and Schiavetti, A. (2020). Can citizen Science Help Delimit the Geographical Distribution of a Species? The Case of the Callistoctopus sp. (“Eastern Octopus”) on the Brazilian Coast. Ethnobiol. Conserv. 10. Available at: https://ethnobioconservation.com/index.php/ebc/article/view/413
Johannes, R. E., Freeman, M. M. R., and Hamilton, R. J. (2000). Ignore fishers’ knowledge and miss the boat. Fish Fish. 1, 257–271. doi: 10.1111/j.1467-2979.2000.00019.x
Kaneko, N., Kubodera, T., and Iguchis, A. (2011). Taxonomic study of shallow-water octopuses (Cephalopoda: Octopodidae) in Japan and adjacent waters using mitochondrial genes with perspectives on octopus DNA barcoding. Malacologia 54, 97–108. doi: 10.4002/040.054.0102
Kearse, M., Moir, R., Wilson, A., Stones-Havas, S., Cheung, M., Sturrock, S., et al. (2012). Geneious basic: an integrated and extendable desktop software platform for the organization and analysis of sequence data. Bioinformatics 28, 1647–1649. doi: 10.1093/bioinformatics/bts199
Laurenzano, C., Mantelatto, F. L., and Schubart, C. D. (2013). South American homogeneity versus Caribbean heterogeneity: population genetic structure of the western Atlantic fiddler crab Uca rapax (Brachyura, Ocypodidae). J. Exp. Mar. Biol. Ecol. 449, 22–27. doi: 10.1016/j.jembe.2013.08.007
Leite, T. S., and Haimovici, M. (2006). “Presente conhecimento da biodiversidade e habitat dos polvos (Cephalopoda: Família Octopodidae) de águas rasas das ilhas oceânicas do nordeste brasileiro,” in Ilhas Oceânicas Brasileiras – da Pesquisa ao Manejo, eds R. J. V. Alves and J. W. A. Castro (Brasília: Ministério do Meio Ambiente), 199–214.
Leite, T. S., Haimovici, M., Molina, W., and Warnke, K. (2008). Morphological and genetic description of Octopus insularis. a new cryptic species in the Octopus vulgar is complex (Cephalopoda: Octopodidae) from the tropical southwestern Atlantic. J. Molluscan Stud. 74, 63–74. doi: 10.1093/mollus/eym050
Lima, F. D., Berbel-Filho, W. M., Leite, T. S., Rosas, C., and Lima, S. M. Q. (2017). Occurrence of Octopus insularis Leite and Haimovici, 2008 in the tropical northwestern Atlantic and implications of species misidentification to octopus fisheries management. Mar. Biodivers. 47, 723–734. doi: 10.1007/s12526-017-0638-y
Lima, F. D., Strugnell, J. M., Leite, T. S., and Lima, S. M. Q. (2020). A biogeographic framework of octopod species diversification: the role of the Isthmus of Panama. PeerJ 8:e8691. doi: 10.7717/peerj.8691
Luiz, O. J., Madin, J. S., Robertson, D. R., Rocha, L. A., Wirtz, P., and Floeter, S. R. (2012). Ecological traits influencing range expansion across large oceanic dispersal barriers: insights from tropical Atlantic reef fishes. Proc. R. Soc. B 279, 1033–1040. doi: 10.1098/rspb.2011.1525
Marques, J. G. W. (1991). Aspectos Ecológicos na Etnoictiologia dos Pescadores do Complexo Estuarino-Lagunar Mundaú-Manguaba, Alagoas. Dissertation. Campinas, SP: UNICAMP.
Martins, V. S., Schiavetti, A., and Souto, F. J. B. (2011). Ethnoecological knowledge of the artisan fishermen of octopi (Octopus spp.) in the community of Coroa Vermelha (Santa Cruz de Cabrália, Bahia). An. Acad. Bras. Ciênc. 83, 513–522. doi: 10.1590/S0001-37652011000200011
Mayr, E. (1971). Methods and strategies in taxonomic research. Syst. Biol. 20, 426–433. doi: 10.2307/2412118
Montana, J., Finn, J. K., and Norman, M. D. (2015). Liquid sand burrowing and mucus utilisation as novel adaptations to a structurally-simple environment in Octopus kaurna Stranks, 1990. Behaviour 152, 1871–1881. doi: 10.1163/1568539X-00003313
Muller-Karger, F. E., McClain, C. R., and Richardson, P. (1988). The dispersal of Amazon’s water. Nature 333, 56–59. doi: 10.1038/333056a0
Norman, M. D. (1993). Four new octopus species of the Octopus macropus group (Cephalopoda: Octopodidae) from the Great Barrier Reef. Australia. Mem. Mus. Vic. 53, 267–308. doi: 10.24199/j.mmv.1992.53.13
Norman, M. D., Finn, J. K., and Hochberg, F. G. (2016). “Family Octopodidae,” in Cephalopods of the World. An Annotated and Illustrated Catalogue of Cephalopod Species Known to Date. Octopods and Vampire Squids. FAO Species Catalogue for Fishery Purposes, Vol. 3, eds P. Jereb, C. F. E. Roper, M. D. Norman, and J. K. Finn (Rome: FAO), 36–215.
Norman, M. D., and Hochberg, F. G. (2005). The current state of octopus taxonomy. Phuket Mar. Biol. Cent. Res. Bull. 66, 127–154.
Norman, M. D., Hochberg, F. G., and Lu, C. C. (1997). Mollusca Cephalopoda: mid-depth octopuses (200–1000 m) of the Banda and Arafura Seas (Octopodidae and Alloposidae). Résult. Camp. Musorstom 16, 357–383.
Ogar, E., Pecl, G., and Mustonen, T. (2020). Science must embrace traditional and indigenous knowledge to solve our biodiversity crisis. One Earth 3, 162–165. doi: 10.1016/j.oneear.2020.07.006
Palacio, J. F. (1977). A Study of the Coastal Cephalopods From Brazil with Reference to Brazilian Zoogeography. dissertation. Miami, FL: University of Miami.
Palumbi, S., Martin, A., Romano, S., McMillan, W. O., Stice, L., and Grabowski, G. (1991). The Simple Fool’s Guide to PCR. Honolulu: University of Hawaii.
Perez, J. A. A., and Haimovici, M. (1991). Cephalopod collection of the Museu de Zoologia da Universidade de São Paulo, São Paulo, Brazil. Pap. Avulsos de Zool. 37, 251–258.
Pratchett, M. S., Munday, P. L., Graham, N. A. J., Kronen, M., Pinca, S., Friedman, K., et al. (2020). “Chapter 9. Vulnerability of coastal fisheries in the tropical Pacific to climate change,” in Climate Change and Impacts in the Pacific, ed. L. Kumar (Switzerland: Springer Climate), 359–402.
Pratt, A., Baldwin, C., and Vecchione, M. (2020). Octopods of deep reefs off Curaçao, southern Caribbean, including description of one newly discovered species. Bull. Mar. Sci. 96, 297–308. doi: 10.5343/bms.2019.0040
Radulovici, A. E., Archambault, P., and Dufresne, F. (2010). DNA Barcodes for marine biodiversity: moving fast forward? Diversity 2, 450–472. doi: 10.3390/d2040450
Rambaut, A., and Drummond, A. J. (2007). Tracer v.1.5. Available at: http://beast.bio.ed.ac.uk/LogCombiner (accessed on 07 July 2020)
Ritschard, E. A., Guerrero-Kommritz, J., and Sanchez, J. A. (2019). First molecular approach to the octopus fauna from the southern Caribbean. PeerJ 7:e7300. doi: 10.7717/peerj.7300
Ronquist, F., Teslenko, M., van der Mark, P., Ayres, D. L., Darling, A., Hohn, S., et al. (2012). MrBayes 3.2: efficient Bayesian phylogenetic inference and model choice across a large model space. Syst. Biol. 22, 539–542. doi: 10.1093/sysbio/sys029
Roper, C. F. E., and Voss, G. L. (1983). “Guidelines for taxonomic description of cephalopod species,” in Proceedings of the Workshop on the Biology and Resource Potential of Cephalopods, eds C. F. E. Roper, C. C. Lu, and F. G. Hochberg (Melbourne: National Museum of Victoria), 48–64. doi: 10.24199/j.mmv.1983.44.03
Rossby, T., Flagg, C., Donohue, K., Sanchez-Franks, A., and Lillibridge, J. (2014). On the long-term stability of Gulf Stream transport based on 20 years of direct measurements. Geophys. Res. Lett. 41, 114–120. doi: 10.1002/2013GL058636
Saad, L. O., Cunha, C. M., Colpo, K. D., and Valdés, Á (2014). It’s not what it looks like: molecular data fails to substantiate morphological differences in two sea hares (Mollusca, Heterobranchia, Aplysiidae) from southern Brazil. Helgol. Mar. Res. 68, 523–530. doi: 10.1007/s10152-014-0408-1
Sales, J. B. L., Haimovici, M., Ready, J. S., Souza, R. F., Ferreira, Y., Pinon, J. C. S., et al. (2019). Surveying cephalopod diversity of the Amazon reef system using samples from red snapper stomachs and description of a new genus and species of octopus. Sci. Rep. 9:5956. doi: 10.1038/s41598-019-42464-8
Sales, J. B. L., Markaida, U., Shaw, P. W., Haimovici, M., Ready, J. S., Figueredo-Ready, W. M., et al. (2014). Molecular phylogeny of the Genus Lolliguncula Steenstrup, 1881 based on nuclear and mitochondrial DNA sequences indicates genetic isolation of populations from North and South Atlantic, and the possible presence of further cryptic species. PLoS One 9:e88693. doi: 10.1371/journal.pone.0088693
Sales, J. B. L., Rego, P. S., Hilsdorf, A. W. S., Moreira, A. A., Haimovici, M., Tomás, A. R., et al. (2013). Phylogeographical features of Octopus vulgaris and Octopus insularis in the southwestern Atlantic based on the analysis of mitochondrial markers. J. Shellfish Res. 32, 325–339. doi: 10.2983/035.032.0211
Sales, J. B. L., Rodrigues-Filho, L. F. D. S., Ferreira, Y. D. S., Carneiro, J., Asp, N. E., Shaw, P. W., et al. (2017). Divergence of cryptic species of Doryteuthis plei Blainville, 1823 (Loliginidae, Cephalopoda) in the Western Atlantic Ocean is associated with the formation of the Caribbean Sea. Mol. Phylogenet. Evol. 106, 44–54. doi: 10.1016/j.ympev.2016.09.014
Schwartz, O. M. (1992). Octopus macropus, a new occurrence for North Carolina. J. Elisha Mitchell Sci. Soc. 180, 35–37.
Söller, R., Warnke, K., Saint-Paul, U., and Blohm, D. (2000). Sequence divergence of mitochondrial DNA indicates cryptic biodiversity in Octopus vulgaris and supports the taxonomic distinctiveness of Octopus mimus (Cephalopoda: Octopodidae). Mar. Biol. 136, 29–35. doi: 10.1007/s002270050004
Souza, J. N., Nunes, F. L. D., Zilberberg, C., Sanchez, J. A., Migotto, A. E., Hoeksema, B. W., et al. (2017). Contrasting patterns of connectivity among endemic and widespread fire coral species (Millepora spp.) in the tropical southwestern Atlantic. Coral Reefs 36, 701–716. doi: 10.1007/s00338-017-1562-0
Taki, I. (1964). On eleven new species of the Cephalopoda from Japan, including two new genera of Octopodinae. J. Fac. Fish. Anim. Husb. 5, 277–330.
Ulloa, P. M., Hernández, C. E., Rivera, R. J., and Ibáñez, C. M. (2017). Biogeografía histórica de los calamares de la familia Loliginidae (Teuthoidea: Myopsida). Lat. Am. J. Aquat. Res. 45, 113–129. doi: 10.3856/vol45-issue1-fulltext-11
van der Vyver, F., Sauer, W. H. H., McKeown, N. J., Yemane, D., Shaw, P. W., and Lipinski, M. (2016). Phenotypic divergence despite high gene flow in chokka squid Loligo reynaudii (Cephalopoda: Loliginidae): implications for fishery management. J. Mar. Biol. Assoc. UK 96, 1507–1525. doi: 10.1017/S0025315415001794
Villanueva, R., Vidal, É. A. G., Fernández-Álvarez, F. Á, and Nabhitabhata, J. (2016). Early mode of life and hatchling size in cephalopod molluscs: influence on the species distributional ranges. PLoS One 11:e0165334. doi: 10.1371/journal.pone.0165334
Voight, J. R. (1998). “An overview of shallow-water octopus biogeography,” in Systematics and Biogeography of Cephalopods. II, eds N. A. Voss, M. Vecchione, and R. B. Toll (Washington, DC: Smithsonian Contributions to Zoology), 549–559.
Voss, G. L. (1981). A redescription of Octopus ornatus Gould, 1952 (Octopoda: Cephalopoda) and the status of Callistoctopus Taki, 1964. Proc. Biol. Soc. Wash. 94, 525–534.
Voss, G. L., and Toll, R. B. (1998). “The systematics and nomenclatural status of the octopodinae described from the western Atlantic Ocean,” in Systematics and Biogeography of Cephalopods. II, eds N. A. Voss, M. Vecchione, and R. B. Toll (Washington, DC: Smithsonian Contributions to Zoology), 456–474.
Wheeler, Q. D. (2004). Taxonomic triage and the poverty of phylogeny. Phil. Trans. R. Soc. Lond. B 359, 571–583. doi: 10.1098/rstb.2003.1452
Wheeler, Q. D., Raven, P. H., and Wilson, E. O. (2004). Taxonomy: impediment or expedient? Science 303:285. doi: 10.1126/science.303.5656.285
White, W. T., and Last, P. R. (2012). A review of the taxonomy of chondrichthyan fishes: a modern perspective. J. Fish Biol. 80, 901–917. doi: 10.1111/j.1095-8649.2011.03192.x
WoRMS Editorial Board (2020). World Register of Marine Species. Available at: http://www.marinespecies.org (accessed on 26 July 2020)
Keywords: Octopoda, cryptic species, neotype, ethnoknowledge, western Atlantic Ocean, Brazil
Citation: Jesus MD, Sales JBL, Martins RS, Ready JS, Costa TAS, Ablett JD and Schiavetti A (2021) Traditional Knowledge Aids Description When Resolving the Taxonomic Status of Unsettled Species Using Classical and Molecular Taxonomy: The Case of the Shallow-Water Octopus Callistoctopus furvus (Gould, 1852) From the Western Atlantic Ocean. Front. Mar. Sci. 7:595244. doi: 10.3389/fmars.2020.595244
Received: 15 August 2020; Accepted: 18 November 2020;
Published: 21 January 2021.
Edited by:
Rachel Ann Hauser-Davis, Oswaldo Cruz Foundation (Fiocruz), BrazilReviewed by:
Luis Fernando Da Silva Rodrigues-Filho, Federal Rural University of the Amazon, BrazilAcacio Tomas, Agência Paulista de Tecnologia dos Agronegócios (APTA), Brazil
Copyright © 2021 Jesus, Sales, Martins, Ready, Costa, Ablett and Schiavetti. This is an open-access article distributed under the terms of the Creative Commons Attribution License (CC BY). The use, distribution or reproduction in other forums is permitted, provided the original author(s) and the copyright owner(s) are credited and that the original publication in this journal is cited, in accordance with accepted academic practice. No use, distribution or reproduction is permitted which does not comply with these terms.
*Correspondence: Alexandre Schiavetti, YWxlc2NoaUB1ZXNjLmJy