- Israel Oceanographic and Limnological Research, National Institute of Oceanography, Haifa, Israel
Long-term trends in oxygen, salinity, and nutrients were followed in the Southeastern Mediterranean (SEMS) deep waters from 2002 to 2020. Results show a net decrease in oxygen since 2008 of −0.5 ± 0.1 μmol kg−1 yr−1 in the bathypelagic depths (1,200–2,000 m). Multiannual variability in oxygen levels superimposed this trend, and is likely associated with variations in thermohaline fluxes. The 2020 mean oxygen concentration of 179.5 ± 2.3 μmol kg−1 is comparable to the pre-Eastern Mediterranean Transient (EMT) mean value. The post-EMT signature is clearly demonstrated in both oxygen and salinity over the period of 2002–2013, but since 2014 it diminished, mainly due to mixing of the Aegean deep water (AegDW) mass with the overlying old Adriatic water mass. This trend reflects a switch back to the pre-EMT regime, characterized by thermohaline homogeneity of the deep water column in the SEMS. The long-term decline of deep water oxygen levels is also accompanied by a corresponding increase in dissolved inorganic nutrients, supporting aging of the deep water masses. Our results suggest that ventilation of the SEMS deep water is currently occurring at a lower, pre-EMT rate, probably as a result of moderated deep water formation in recent time.
Introduction
The ocean interior plays a major role in regulating Earth’s climate, acting as a sink for anthropogenic CO2 produced by fossil fuels burning and the heat produced by the emitted greenhouse gases (e.g., Sarmiento et al., 1998; Reid et al., 2009; Glecker et al., 2016). The evaluation of long-term changes in the ocean interior biogeochemistry is, thus, essential for better understanding of the ocean-atmosphere coupling and feedbacks that are routinely simulated in many models. Unlike the upper ocean surface layer, which is tightly related to the atmosphere, deep layers of the oceans are assumed to be constant over a much longer time frame (e.g., Sarmiento et al., 1992, 1998). However, recent studies have shown a strong coupling between atmospheric oscillations and those occurring in intermediate and deep water masses (Watanabe et al., 2003; Ozer et al., 2017). Moreover, many observational studies have shown links between present-day climate changes and those occurring in deep-sea environments, primarily increased deep-sea temperature (Purkey and Johnson, 2010), and deoxygenation (e.g., Stramma et al., 2008; Keeling et al., 2010; Helm et al., 2011). Unfortunately, long time series with high temporal resolution of deep-sea observations are relatively sparse and thus, the effect of long and short term disturbances on the ocean interior biogeochemistry remains relatively unexplored and unconfirmed.
The Eastern Mediterranean, in particular the Levantine basin, is one of the most evaporative marginal seas in the world – a crucial area in the larger framework of the Mediterranean conveyor belt (Pinardi and Masetti, 2000; Millot and Taupier-Letage, 2005; Tanhua et al., 2013). The water body in this region is considered ultra-oligotrophic, due to the advection of low salinity, nutrient depleted Modified Atlantic Water (MAW), limited freshwater input (Hecht, 1992; Béthoux et al., 1999), and shallow vertical mixing. Moreover, the deep water residence time in the SEMS is relatively short, around 100 years (Stöven and Tanhua, 2014; Li and Tanhua, 2020). Hence, this region is an ideal location to investigate water column temporal and vertical changes, occurring over relatively short time scales, annual to decadal (Kress et al., 2014; Sisma-Ventura et al., 2016; Ozer et al., 2017, 2020). Analysis of regional deep-sea time series in the SEMS could provide strong evidence that can confirm or validate broader-based studies and modeling efforts of similar processes on oceanic scales. One such example is the Eastern Mediterranean Transient (EMT) that took place in the early 1990s, during which the source of deep water formation shifted from the Adriatic to the Aegean and resulted in significant deep water ventilation and increased oxygen levels (Roether et al., 1996; Malanotte-Rizzoli et al., 1997; Klein et al., 1999). Before the EMT, the primary source of deep water formation was the Adriatic Sea (Roether et al., 2007). Following the EMT, denser, more saline, but warmer water from the southern Aegean formed a new water mass at the bottom of the SEMS that displaced upward the old Adriatic water mass (Gertman et al., 2006; Kress et al., 2014; Ozer et al., 2020).
The massive influx of oxygen-rich surface water from the Aegean was followed by accelerated oxygen consumption (AOC) rates in the deep layers, likely due to the transport of large amounts of highly labile dissolved organic carbon, which, in turn, enhanced bacterial production/respiration, and hence oxygen consumption (Klein et al., 2003). Such perturbations of the biological carbon pump due to changes in export production or re-mineralization efficiency can explain short term trends in oxygen (Keller et al., 2002), such as the AOC episode (Klein et al., 2003). However, longer trends can be driven by a variety of other mechanisms. One important mechanism is a change in ventilation rates, which shows a considerable decadal variability (Watanabe et al., 2003; Vilibić et al., 2013) and is expected to vary in response to global warming and the increased stratification of the ocean surface layer (Keeling et al., 2010).
A recent study based on an oceanic general circulation model has shown that the meridional overturning circulation of the SEMS has multiple equilibria states under present-day-like conditions that are closely regulated by the water exchange between the Aegean and the Adriatic Seas (Amitai et al., 2017). It describes a passive mode of the Adriatic deep water (ADW) formation and ventilation, when the Aegean stops supplying saline intermediate water to the Adriatic, due to warm steady state, resulting in minor to no deep water outflow from both basins. Indeed, observational studies show that the eastern Mediterranean Sea, including the Aegean basin is undergoing a fast warming trend (Shaltout and Omstedt, 2014; Ozer et al., 2017), which could dramatically affect deep water formation and ventilation of the deep layers (Bensi et al., 2013). Simulations also show that the Adriatic-Ionian Bimodal Oscillating System (BiOS; Gačić et al., 2010, 2011) can rapidly change oceanographic properties in the middle and south Adriatic Sea (e.g., also during the EMT; Mihanović et al., 2015) where deep waters are formed. The dynamics of the Southern Adriatic and Ionian Sea are linked by the BiOS mechanism that changes the circulation of the North Ionian Gyre from cyclonic to anti-cyclonic, on a decadal time scale (Civitarese et al., 2010), effecting the circulation of the SEMS intermediate water (Ozer et al., 2017). Moreover, a thermohaline coupling between the Aegean-Levantine Seas as well as between the Adriatic-Ionian Seas was suggested by Theocharis et al. (2014) and Velaoras et al. (2014), as a driving force that regulates the observed quasi-decadal thermohaline circulation changes effecting the SEMS deep water masses.
Evidence for an abrupt, transient halt of the Mediterranean deep zonal overturning circulation under a decreased meridional temperature gradient are found in geological records of the Sapropel episodes (Rohling et al., 2015) and were simulated using models forced by paleo-atmospheric conditions (Meijer and Dijkstra, 2009). Observations report evidence of recent long-term weakening of the northwestern part of the Adriatic−Ionian thermohaline cell, possibly affecting the deep water ventilation rate (Bensi et al., 2013). This coincides with recent observations from the southeast Mediterranean indicating that the EMT signal is gradually eroding, largely due to vertical diffusional processes (Ozer et al., 2020). However, climate-driven changes in the thermohaline cell remain highly uncertain, resulting in different scenarios regarding the future of oxygen in the deep layers of the SEMS. For example, a model by Powley et al. (2016), could not predict severe dissolved oxygen (DO) depletion of the deep waters of the SEMS in the foreseeable future and other studies foresee an increase in EMT-like conditions (Adloff et al., 2015). Here, we compiled time series data of DO, salinity, and nutrients between 2002 and 2020, obtained during 35 hydrographic cruises in the southeast Levantine basin deepest water layer (>1,200–2,000 m), to elucidate long-term trends and oscillations, related to deep water formation and ventilation since the EMT.
Materials and Methods
Data of DO, salinity, and nutrients were compiled from 35 research cruises held between 2002 and 2020 in the Levantine basin (Eastern Mediterranean Sea) as part of the National Monitoring Program of Israel’s Mediterranean waters conducted by the Israel Oceanographic and Limnological Research (IOLR). The hydrographic raw data are available in ISRAMAR data center: https://isramar.ocean.org.il/isramar2009/ and presented by Ozer et al. (2017, 2020). Here, we focus on the post-EMT DO, nutrients, and bacterial production (BP) trends at depths >1,200–1,950 m measured at the hydrographic stations G05 and H06 with bottom depths of 1,950 and 1,750 m, respectively (Figure 1). This study presents new data from stations H06 and G05 for the period 2011–2020, which is combined with previous years (2002–2010) published data of DO and nutrients from H06 (Kress et al., 2014). The study area represents the southeastern Levantine Basin offshore (bathyal zone) water.1 The old ADW uplifted by the Cretan Sea Outflow water (CSOW) was identified by Kress et al. (2014) as the mid-depth minimum salinity and temperature centered at 1,000–1,200 m depth. We analyzed the time series data of DO, salinity, and nutrients for depth range below the ADW to elucidate long-term trends, related to deep water formation and ventilation. Station H06 was visited twice a year, usually during the height of summer (August–September) and winter–spring (March–April) for a total of 35 times over the entire observational period. In contrast, station G05 was visited only three times during the monitoring period (2013, 2017, and 2019) and provides snapshots of the deep layer closest to 2,000 m depth.
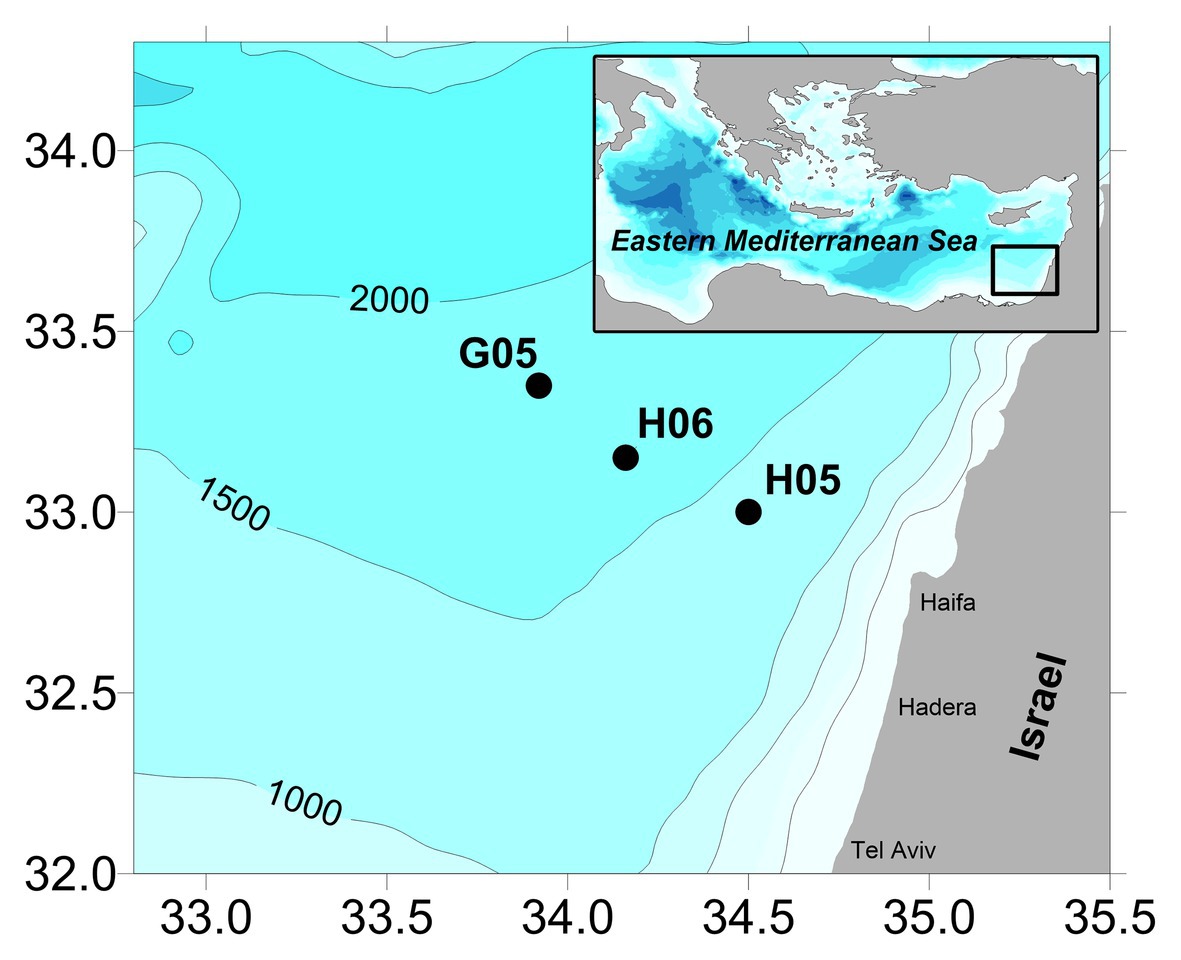
Figure 1. A map showing the study site in the southeastern Mediterranean (SEMS) Sea and the long-term monitoring stations.
Seawater samples were collected using a Rosette sampler (SeaBird profiler SBE911plus). CTD oxygen electrode data were calibrated to discrete depth DO measurements using the Winkler titration method immediately upon collection (Kress et al., 2014). Dissolved inorganic nutrients [NOx, PO4, and Si(OH)4] were determined using a Seal auto-analyzer system with colorimetric detection as described in detail in Kress et al. (2014). Quality control of the nutrient results along the years was performed with the use of internal and certified reference standards and by the participation in international laboratory performance exercises (QUASIMEME). All nutrient measurements were well beyond the limit of detection and quantification.
Bacterial production measurements were collected at discrete depths between 2013 and 2020 at station H06 using the 3H-Leucine incorporation method. For detailed methodology, see Rahav et al. (2019).
Results and Discussion
The DO levels in the oldest water mass of the Adriatic (800–1,100 m) remained largely unchanged (176 ± 3.0 μmol kg−1) during the period 2002–2020, but significantly decreased below depths >1,200 m (Figures 2, 3A). During the period 2008–2020, a long-term decreasing trend in oxygen levels at a rate of −0.5 ± 0.1 μmol kg−1 yr−1 (r2 = 0.82; p < 0.001) was observed in the water layer between 1,200 and the bottom (1,750 m) at station H06 (Figure 2). Prior to this, there was a short period of increasing levels since 2004, following a very steep decline within a year of 5 μmol kg−1 (Figure 2). Similar DO inter-annual variability was also observed in a time series of the entire Eastern Mediterranean Sea (1960–2011), across the depth layer of 600–2,000 m, and was associated with dynamical processes (deep-water formation variability, transients, etc.) of the thermohaline properties (Mavropoulou et al., 2020). The decreasing rate since 2008 agrees well with the pre-EMT oxygen utilization rate of −0.5 ± 0.2 μmol kg−1 yr−1 for depths >1,000 m that was determined on the basis of a two-dimensional kinematic model calibrated using hydrographic and tracer data (Roether and Well, 2001). Much higher utilization rates of approximately −1.3 μmol kg−1 yr−1 were observed for a few years following the EMT by Klein et al. (2003). It was proposed that this rate was driven by a large amount of dissolved organic carbon with an unusually high fraction of labile material, delivered by the Aegean deep water (AegDW) inflow. In accordance with the hypothesis made by Klein et al. (2003), integrated bacterial production measurements at H06 (1,200–1,750 m) from 2013 to 2020 show a gradual and linear increase (Figure 4D), suggesting high dissolved organic carbon availability.
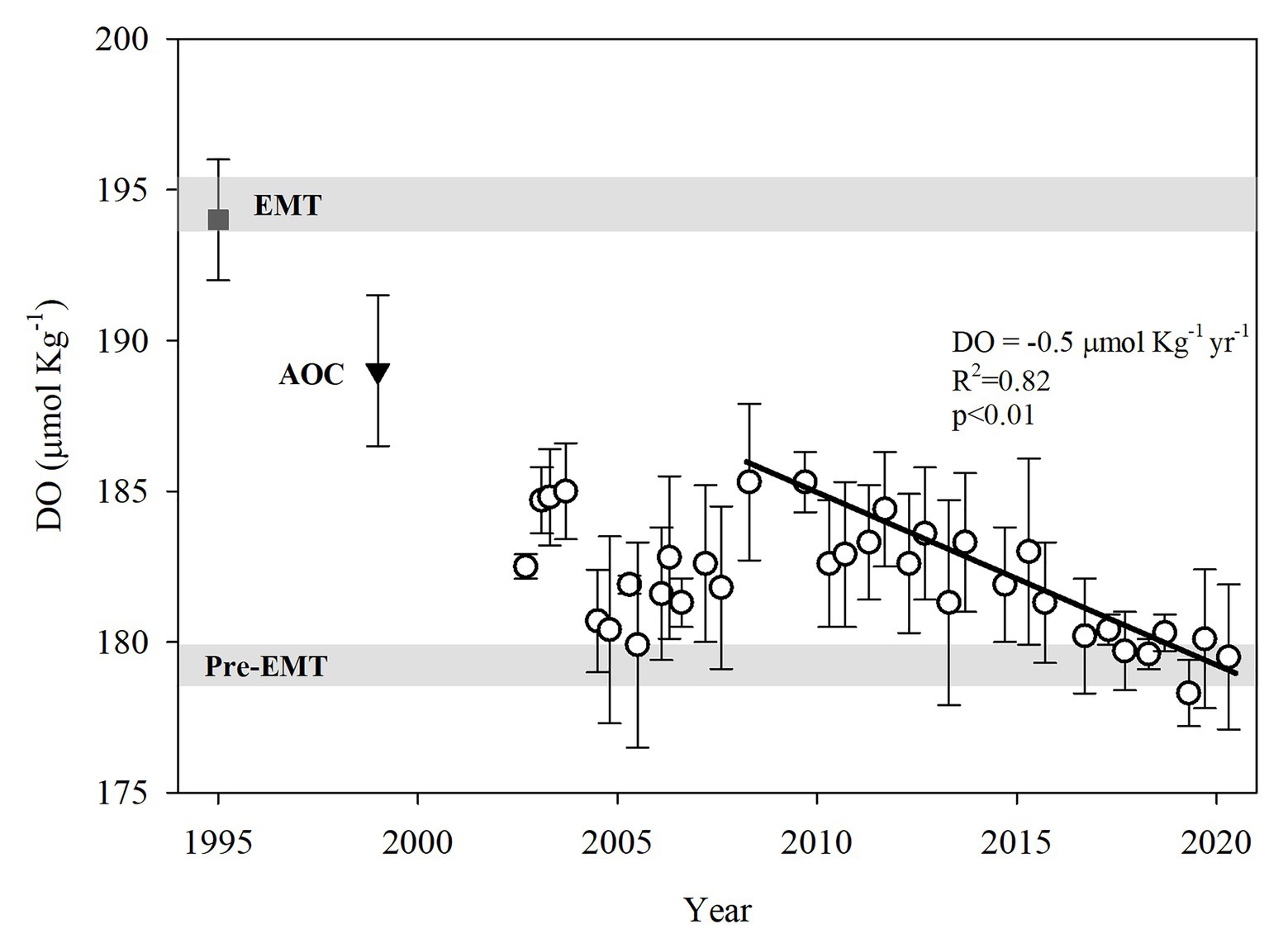
Figure 2. Temporal variation of the mean oxygen concentrations measured in the deep layer (1200–1750 m) at station H06, during the period 2002–2020 (black circles). The error bars are the SDs of all the measurements made within this depth range in each cruise. The thick gray line indicates the pre-eastern mediterranean transient (EMT) oxygen concentration. The EMT and accelerated oxygen consumption (AOC) oxygen levels in the EMS, indicated by ■ and ▲, respectively, are from Klein et al. (2003). The black trend line denotes the long-term decreasing trend in oxygen levels since 2008.
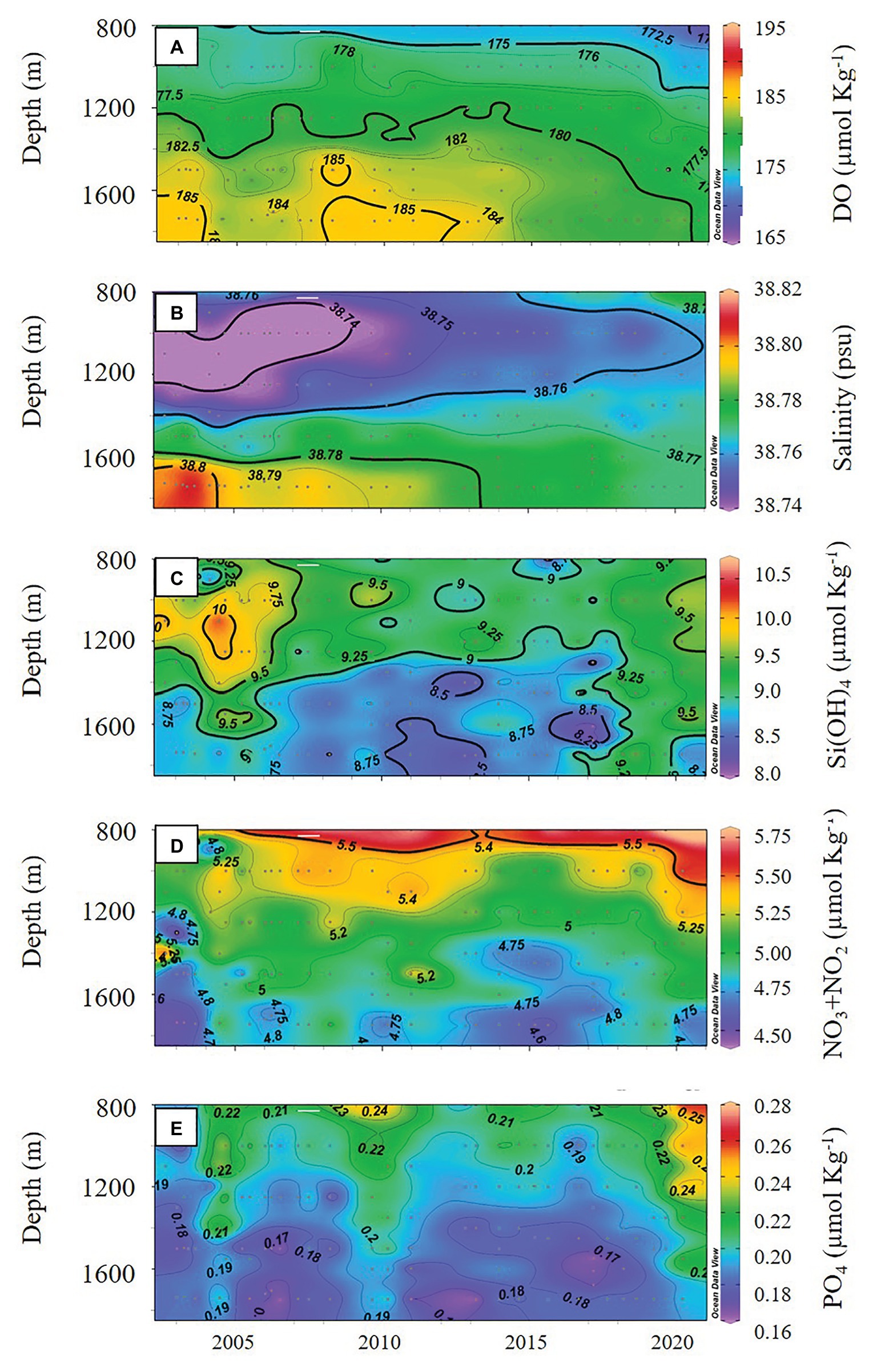
Figure 3. Temporal variation in: (A) oxygen, (B) salinity, (C) Si(OH)4, (D) NO3 + NO2 (NOx), and (E) PO4, measured in the deep layer (1200–1750 m) at station H06.
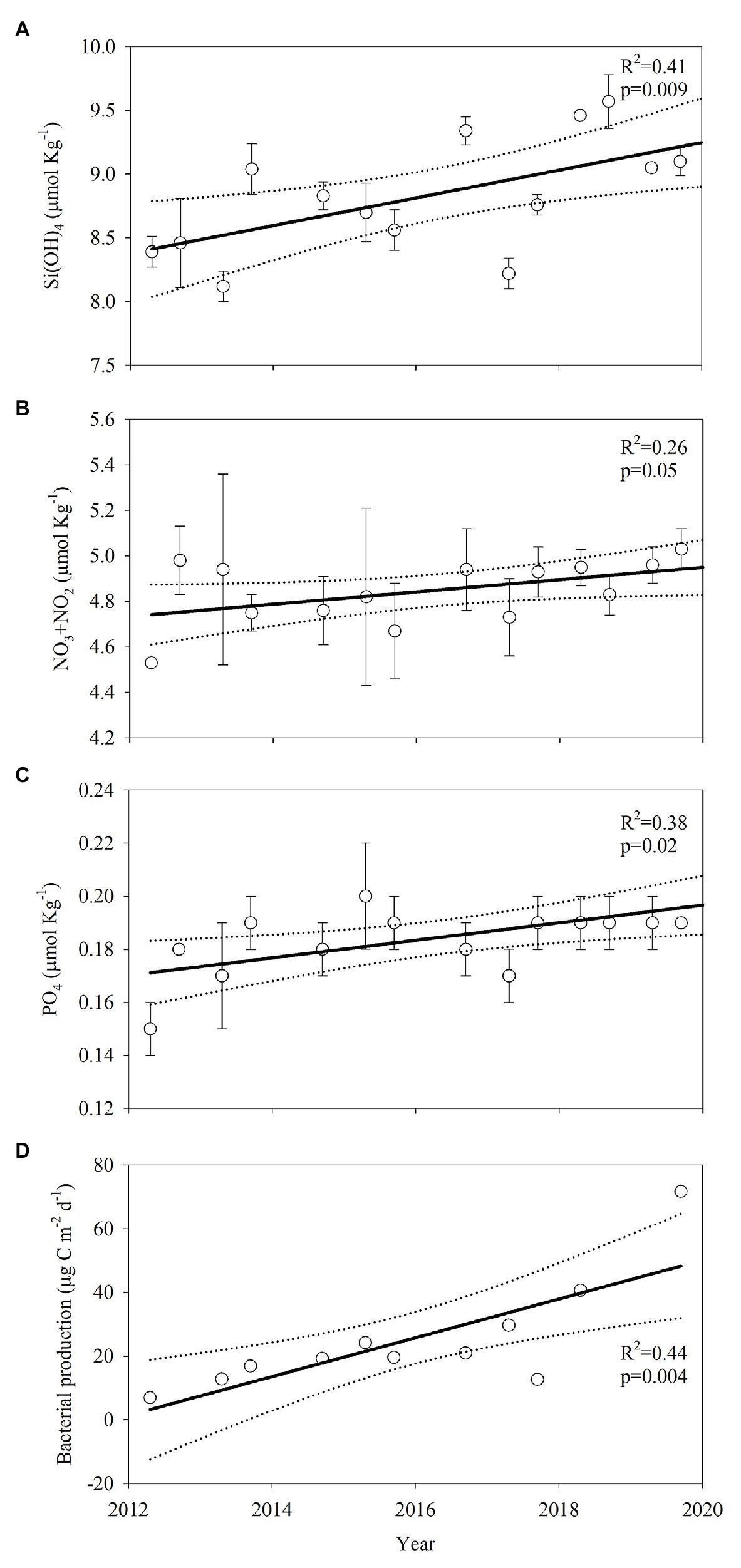
Figure 4. Long term trends of mean Si(OH)4 (A), NO3 + NO2 (B), and PO4 (C) at depths of 1,200–1750 m during 2013–2020, and the integrated bacterial production (D). The solid line shows the regression trend-line while the dotted lines show the 95% CI. R2 and p denote the correlation coefficient and the corresponding significance.
Our data set also presents some inter-annual and multi-annual variability, with sharp changes in DO levels as large as 5.0 μmol kg−1 yr−1. These variations are likely associated with thermohaline flux variations and delivery of organic matter, as was found in a longer time series of the Eastern Mediterranean’s deep-water DO, based on positive correlation with water density (Mavropoulou et al., 2020). The peak in DO levels in 2003, ~185 μmol kg−1 on average, is associated by a salinity increase (Figure 3B). The increase in oxygen levels (ca. +3 μmole kg−1) during 2007–2008 can be related to the new ADW mass, which was observed close to Crete in September 2008 and is slightly less saline and colder than the CSOW. This water mass has been observed since 2001 in the Ionian basin (Manca et al., 2006; Rubino and Hainbucher, 2007).
However, despite the evidence for short-term ventilation events of the deep water between 2001 and 2008, oxygen is gradually decreasing since 2008. Thus, the 2020 mean oxygen concentration in the depth range of 1,200–1,950 m of 179.5 ± 2.3 μmol kg−1 is comparable to the pre-EMT mean value (Klein et al., 2003; Kress et al., 2003). The significant change in deep water DO concentrations can be driven by two main mechanisms: (1) changes in the recycling rates driven by changes in export of labile organic carbon via the biological carbon pump and/or by lateral transport of POM in marginal seas (i.e., Katz et al., 2020) and (2) changes in deep water formation and ventilation rates, as well as deep advection and admixture (Keller et al., 2002). Advection of dense Aegean overflow would tend to increase both salinity and oxygen (Kress et al., 2014). This holds, for the inflow dating back to the EMT or occurring more recently, because this inflow is always comparatively high in both oxygen and salinity (Klein et al., 2003). The influx of new ADW mass, which is slightly less saline and colder compared to the CSOW, can explain inter-annual and multi-annual variations near the bottom that can amount to 5.0 μmol kg−1. However, net admixture with overlying old deep Adriatic water will tend to lower both oxygen and salinity (Ozer et al., 2020). The post-EMT signature is well-observed by the vertical gradient in both oxygen and salinity (Figures 3A,B). These vertical gradient results from the EMT newly added AegDW higher in oxygen and salinity and the overlaying old Adriatic water with lower oxygen and salinity (Kress et al., 2003, 2014). Our observations indicate an erosion of the EMT associated vertical gradient and an increase in vertical homogeneity of salinity between 2002 and 2020 at a rate of 0.016–0.019 psu yr−1 (Figure 3B). This process is accompanied by a similar increase in vertical homogeneity of DO levels since 2013 (Figure 3A). Thus, the vertical gradients in both salinity and oxygen, associated with the EMT, are gradually eroded. Mixing with the overlying old Adriatic water mass can, thus, explain the convergence of salinity and oxygen (Figures 3A,B) – also indicating minor replenishment of oxygen to the deep layers of the Levantine basin since the EMT. These findings are in agreement with a monotonic salinity rise in the deep layers of the SEMS (stations H06 and H05) during the period 2012–2019, recently observed by Ozer et al. (2020) that can be largely attributed to vertical diffusional processes, gradually eroding the EMT signal.
The reduction in oxygen variability of the SEMS deep water layer (1,200–2,000 m), between 2008 and 2020, further supports a switch back into pre-EMT regime, which is defined by a homogeneous deep water column (Figure 3B). The recent warming of the Aegean surface water (Shaltout and Omstedt, 2014), which could lead to low production of dense water as suggested by Amitai et al. (2017), could also be responsible for the constant depth profile (from 1,250 to down 1,950 m) of DO levels. This recent weakening of ventilation rates was reported for the shallow middle Adriatic (Vilibić et al., 2013) and the southern Adriatic, where the final ADW forms (i.e., Bensi et al., 2013; Mihanović et al., 2013; Gačić et al., 2014) and is further supported by our data. We note, however, that climate-driven changes in the thermohaline circulation remains highly uncertain, and a significant DO depletion of the deep water masses of the SEMS was not predicted by near future model simulations (Adloff et al., 2015; Powley et al., 2016).
Silica concentrations at H06 increased with time since 2013 at a rate of 0.11 μmol kg−1 yr−1 (r2 = 0.41; p = 0.010; Figure 4A). During the first stage of the time series, silica variability corresponded to changes in water salinity (Figure 3C), further supporting deep water egressing from the Adriatic Sea probably replacing the CSOW, as has been seen since 2001 in the Ionian basin (Manca et al., 2006; Rubino and Hainbucher, 2007). The increase in concentrations from 2013 can be largely explained by mixing with water masses with higher concentration, namely the old ADW, but also due to the aging of the AegDW (Kress et al., 2014).
Long-term trends in nutrient levels (Figures 3D,E) also reflect changes in deep water re-mineralization rates (Klein et al., 2003). Here, changes in the re-mineralization rate were assessed using the data from G05, the deepest station visited in the SEMS during 2013 and 2019 (Figure 5). We note that the structure of the 2013 profile at G05 is still consistent with that of post-EMT, where an extended deep-water oxygen maximum is centered in 1,200–2,000 m depth, resulting from mixing with the oxygen–enriched AegDW during the EMT (Klein et al., 1999; Roether and Well, 2001; Kress et al., 2003, 2014). We observed an average oxygen reduction of 4.2 ± 1.3 μmol/kg at G05 between 2013 and 2019 (Figure 5A), similar in magnitude to the oxygen reduction at H06 of ~5 μmol/kg during the period 2013–2020. During the same period, the oxygen reduction was coupled with increasing concentrations of Si(OH)4, PO4, and NO3 (Figures 5B–D). A similar positive-linear increase in NOx (NO2 + NO3) or PO4 was also confirmed in a time series of station H06 (Figures 4B,C). Assuming that the production of nutrients is the result of aerobic remineralization of organic matter, the estimated changes due to the oxygen decline applying the classical Redfield ratios, although not ideal for the SEMS (Kress and Herut, 2001), are ΔNO3 = +0.6 μmol kg−1 and ΔPO4 = +0.04 μmol kg−1. However, the observed increase in NOx and PO4 at G05 between 2013 and 2019 accounts for about 50% of the oxygen consumption at most. Similar estimates were obtained using the time series data of H06, about 60% (PO4) and 40% (NOx) due to aerobic re-mineralization of organic matter. We note, however, that the measured DO:NO3 ratio at intermediate depths (nutricline, 200–600 m) is only slightly lower (7%) than the classical Redfield ratio (Figure 6), and using the local ratio, the increase in NO3 in GO5 accounts for 55% of oxygen consumption due to re-mineralization. The local DO:PO4 of −162:1 ratio is higher than the classical Redfield ratios (by 17%), again accounting for about 60% of the PO4 release due to re-mineralization. This agrees well with estimates by Kress and Herut (2001), indicating re-mineralization of N, and in particular, P-poor sinking organic matter in the SEMS. Our estimates support that about half of the decrease in oxygen in the deepest layer between 2013 and 2020 is due to oxygen utilization. Assuming averaged bacterial production of ~0.01 μmol C Kg−1 at 1,200–1,750 m (Rahav et al., 2019; Supplementary Figure 1) and DO:C ratio of ~1.3 (Redfield, 1934), DO uptake by microbial processes account for the period of 2009–2020 is ~1.5 μg O2 Kg−1. This suggests that microbial processes can explain ~30–40% of the average oxygen reduction at these respective depths (~1.5 μmol kg−1 out of 4–5 μmol kg−1), similar to the estimates discussed above for nutrients. Moreover, this calculation confirms previous studies from the Mediterranean Sea indicating that the deep water is a hotspot for microbial activity (Luna et al., 2012; Rahav et al., 2019).
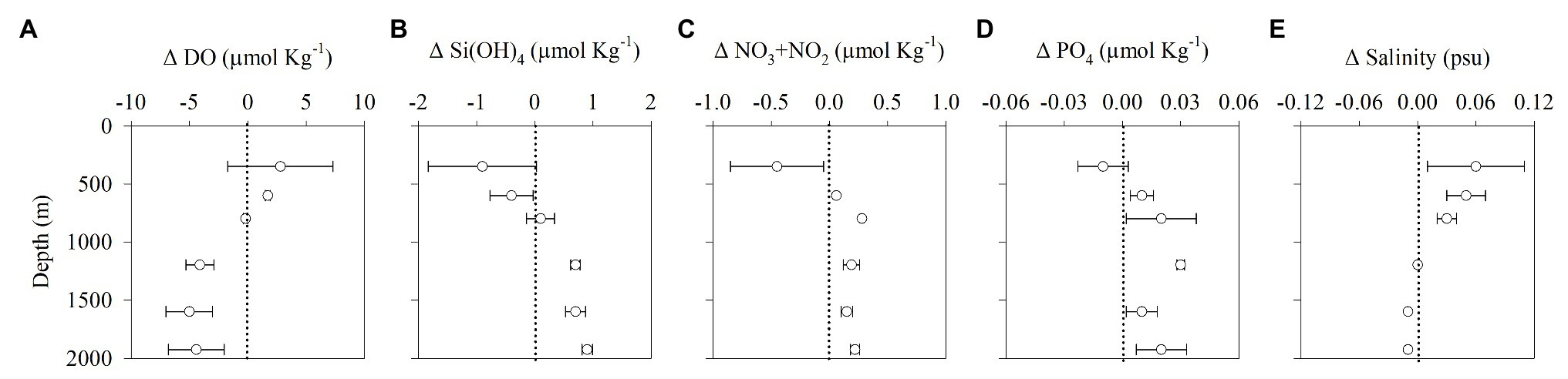
Figure 5. The vertical net difference in (A) DO, (B) Si(OH)4, (C) NOx, (D) PO4, and (E) salinity at G05 between 2013 and 2019.
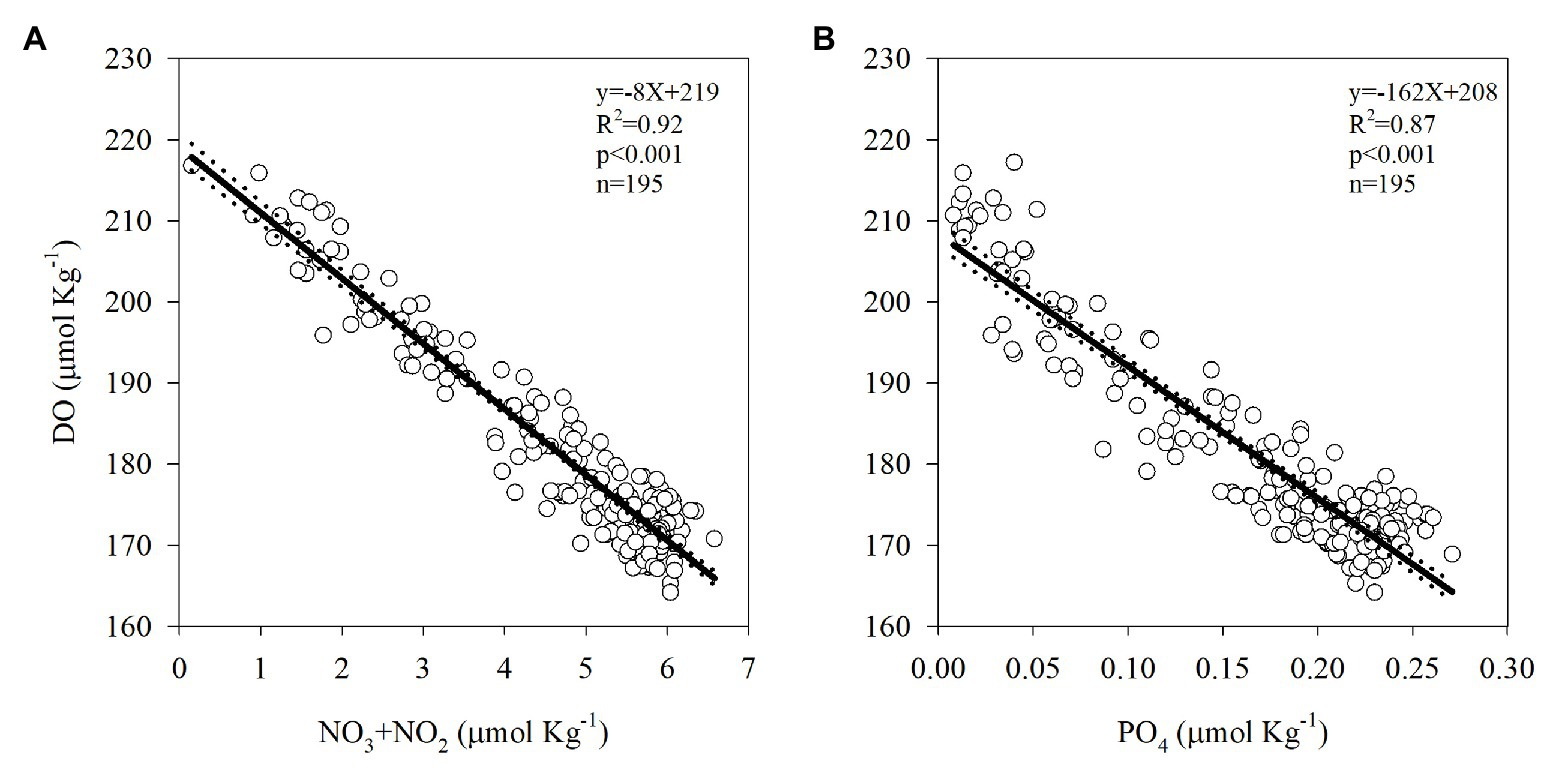
Figure 6. Correlations between (A) DO:NO3 + NO2 and (B) DO:PO4 within the depth range of the nutricline (200–600 m), where the majority of the organic matter sinking from the surface layer is re-mineralized in the SEMS. The solid line shows the regression trend-line while the dotted lines show the 95% CI. The data compiling this figure are from stations H05, H06, and G05.
Our long-term record from 2002 to 2020 of oxygen levels in the deep water of the SEMS yields an overall decreasing trend −0.5 μmol kg−1 yr−1 since 2008, which agrees well with pre-EMT rates (Roether and Well, 2001). Furthermore, our results indicate that oxygen replenishment of the SEMS deep waters since the EMT has been limited. A short-term ventilation event was observed in 2008, but since then oxygen has been declining monotonically. These observations support (or at least do not oppose) the prediction that deep water ventilation rates in the Mediterranean Sea will decline due to global warming (Powley et al., 2016), as was already observed in the Adriatic Sea (Bensi et al., 2013; Mihanović et al., 2013; Vilibić et al., 2013; Gačić et al., 2014), which is a source of deep water in the SEMS. Assuming that the present trend is the result of changes in the Mediterranean thermohaline circulation associated with global warming, hypoxic conditions in the SEMS will not develop in the foreseeable future (~200 years). However, it has been suggested that even relatively small depletions in oxygen levels may impact deep water ecosystems and their services (Sweetman et al., 2017) and therefore, should be monitored and assessed regularly.
Conclusion
The temporal and vertical distribution of oxygen and nutrients were studied in the deepest water mass of the southeast Mediterranean Sea (Levantine basin) to evaluate long term trends during the last 20 years. The data were also evaluated in the context of the EMT ventilation event that occurred during the early 1990s, as well as bacterial activity measurements from the last ~10 years. Our time series show a significant decline in DO since the EMT and a net decrease in oxygen since 2008 of −0.5 ± 0.1 μmol kg−1 yr−1 at the bathypelagic depths (1,200–2,000 m). This was followed by an increase in dissolved inorganic nutrients and bacterial production rates, indicating an upsurge in re-mineralization rates. This trend was also accompanied by an increase in vertical homogeneity of the deep water masses, reflecting gradual erosion of the EMT core, attributed to vertical diffusional processes. Our results support the mechanism suggested by Klein et al. (2003) indicating that ventilation events transport highly labile dissolved organic carbon to the deep water masses of the SEMS, which, in turn, enhance bacterial production/respiration and hence oxygen consumption. This study highlights the physical and biological processes impacting the oxygen variability in deep water masses of the SEMS. We surmise that although oxygen levels are not likely to decline drastically in the near future (based on model simulations), the current reduction, and potential influences of climate change should be addressed in future studies.
Data Availability Statement
The datasets presented in this study can be found in online repositories. The names of the repository/repositories and accession number(s) can be found in ISRAMAR data center: https://isramar.ocean.org.il/isramar2009.
Author Contributions
All co-authors contributed to the data acquiring and interpretation. Manuscript drafting was led by GS-V with the help of all co-authors. All authors contributed to the article and approved the submitted version.
Funding
This work was partly supported by the National Monitoring Program of Israel’s Mediterranean waters.
Conflict of Interest
The authors declare that the research was conducted in the absence of any commercial or financial relationships that could be construed as a potential conflict of interest.
Acknowledgments
We would like to thank the IOLR chemistry department for their help in sampling and analyses, and the R.V. Shikmona and R.V. Bat-Galim captains and crew for help at sea.
Supplementary Material
The Supplementary Material for this article can be found online at: https://www.frontiersin.org/articles/10.3389/fmars.2020.598686/full#supplementary-material
Supplementary Figure 1 | Box Whisker plot showing the interquartile range (25–75th percentile) of the bacterial production measurements. The mean value is shown as a solid line.
Footnotes
References
Adloff, F., Somot, S., Sevault, F., Jordà, G., Aznar, R., Déqué, M., et al. (2015). Mediterranean Sea response to climate change in an ensemble of twenty first century scenarios. Clim. Dyn. 45, 2775–2802. doi: 10.1007/s00382-015-2507-3
Amitai, Y., Ashkenazy, Y., and Gildor, H. (2017). Multiple equilibria and overturning variability of the Aegean-Adriatic seas. Glob. Planet. Chang. 151, 49–59. doi: 10.1016/j.gloplacha.2016.05.004
Bensi, M., Rubino, A., Cardin, V., Hainbucher, D., and Mancero-Mosquera, I. (2013). Structure and variability of the abyssal water masses in the Ionian Sea in the period 2003-2010. J. Geophys. Res. Oceans 118, 931–943. doi: 10.1029/2012jc008178
Béthoux, J. P., Gentili, B., Morin, P., Nicolas, E., Pierre, C., and Ruiz-Pino, D. (1999). The Mediterranean Sea: a miniature ocean for climatic and environmental studies and a key for the climatic functioning of the North Atlantic. Prog. Oceanogr. 44, 131–146. doi: 10.1016/s0079-6611(99)00023-3
Civitarese, G., Gačić, M., Lipizer, M., and Eusebi-Borzelli, G. L. (2010). On the impact of the bimodal oscillating system (BiOS) on the biogeochemistry and biology of the Adriatic and Ionian seas (eastern Mediterranean). Biogeosciences 7, 3987–3997. doi: 10.5194/bg-7-3987-2010
Gačić, M., Borzelli, G. L. E., Civitarese, G., Cardin, V., and Yari, S. (2010). Can internal processes sustain reversals of the ocean upper circulation? The Ionian Sea example. Geophys. Res. Lett. 37:L09608. doi: 10.1029/2010gl043216
Gačić, M., Civitarese, G., Eusebi Borzelli, G. L., Kovacevic, V., Poulain, P. -M., Theocharis, A., et al. (2011). On the relationship between the decadal oscillations of the northern Ionian Sea and the salinity distributions in the eastern Mediterranean. J. Geophys. Res. 116:C12002. doi: 10.1029/2011jc007280
Gačić, M., Civitarese, G., Kovačević, V., Ursella, L., Bensi, M., Menna, M., et al. (2014). Extreme winter 2012 in the Adriatic: an example of climatic effect on the BiOS rhythm. Ocean Sci. 10, 513–522. doi: 10.5194/os-10-513-2014
Gertman, I., Pinardi, N., Popov, Y., and Hecht, A. (2006). Aegean Sea water masses during the early stages of the eastern Mediterranean climatic transient (1988–90). Phys. Oceanogr. 36, 1841–1859. doi: 10.1175/jpo2940.1
Glecker, P. J., Durack, P. J., Stouffer, R. J., Johnson, G. C., and Forest, C. E. (2016). Industrial-era global ocean heat uptake doubles in recent decades. Nat. Clim. Chang. 6, 394–398. doi: 10.1038/nclimate2915
Hecht, A. (1992). Abrupt changes in the characteristics of Atlantic and Levantine intermediate waters in the south-eastern Levantine Basin. Oceanol. Acta 15, 25–42.
Helm, K. P., Bindoff, N. L., and Church, J. A. (2011). Observed decreases in oxygen content of the global ocean. Geophys. Res. Lett. 38:L23602. doi: 10.1029/2011GL049513
Katz, T., Weinstein, Y., Alkalay, R., Biton, E., Toledo, Y., Lazar, A., et al. (2020). The first deep-sea mooring station in the eastern Levantine basin (DeepLev), outline and insights into regional sedimentological processes. Deep-Sea Res. II 171:104663. doi: 10.1016/j.dsr2.2019.104663
Keeling, R. F., Körtzinger, A., and Gruber, N. (2010). Ocean deoxygenation in a warming world. Annu. Rev. Mar. Sci. 2, 199–229. doi: 10.1146/annurev.marine.010908.163855
Keller, K., Slater, R. D., Bender, M., and Key, R. M. (2002). Possible biological or physical explanations for decadal scale trends in North Pacific nutrient concentrations and oxygen utilization. Deep-Sea Res. II 49, 345–362. doi: 10.1016/s0967-0645(01)00106-0
Klein, B., Roether, W., Kress, N., Manca, B. B., Ribera dAlcala, M., Souvermezoglou, E., et al. (2003). Accelerated oxygen consumption in eastern Mediterranean deep waters following the recent changes in thermohaline circulation. J. Geophys. Res. 108:8107. doi: 10.1029/2002JC001454
Klein, B., Roether, W., Manca, B. B., Bregant, D., Beitzel, V., Kovacevic, V., et al. (1999). The large deep water transient in the eastern Mediterranean. Deep-Sea Res. I Oceanogr. Res. Pap. 46, 371–414. doi: 10.1016/s0967-0637(98)00075-2
Kress, N., Gertman, I., and Herut, B. (2014). Temporal evolution of physical and chemical characteristics of the water column in the easternmost Levantine basin (eastern Mediterranean Sea) from 2002 to 2010. J. Mar. Syst. 135, 6–13. doi: 10.1016/j.jmarsys.2013.11.016
Kress, N., and Herut, B. (2001). Spatial and seasonal evolution of dissolved oxygen and nutrients in the southern Levantine Basin (eastern Mediterranean Sea): chemical characterisation of the water masses and inferences on the N:P ratios. Deep-Sea Res. I 48, 2347–2372. doi: 10.1016/s0967-0637(01)00022-x
Kress, N., Manca, B. B., Klein, B., and Deponte, D. (2003). Continuing influence of the changed thermohaline circulation in the eastern Mediterranean on the distribution of dissolved oxygen and nutrients: physical and chemical characterization of the water masses. J. Geophys. Res. 108:8109. doi: 10.1029/2002jc001397
Li, P., and Tanhua, T. (2020). Recent changes in deep ventilation of the Mediterranean Sea, evidence from long-term transient tracer observations. Front. Mar. Sci. 7:594. doi: 10.3389/fmars.2020.00594
Luna, G. M., Bianchelli, S., Decembrini, F., De Domenico, E., Danovaro, R., and Dell’Anno, A. (2012). The dark portion of the Mediterranean Sea is a bioreactor of organic matter cycling. Glob. Biogeochem. Cycles 26, 1–14. doi: 10.1029/2011gb004168
Malanotte-Rizzoli, P., Manca, B. B., d’Alcala, M. R., Theocharis, A., Bergamasco, A., Bregant, D., et al. (1997). A synthesis of the Ionian Sea hydrography, circulation and water mass pathways during POEM-phase I. Prog. Oceanogr. 39, 153–204.
Manca, B. B., Ibello, V., Pacciaroni, M., Scarazzato, P., and Giorgetti, A. (2006). Ventilation of deep waters in the Adriatic and Ionian seas following changes in thermohaline circulation of the eastern Mediterranean. Clim. Res. 31, 239–256. doi: 10.3354/cr031239
Mavropoulou, A. M., Vervatis, V., and Sofianos, S. (2020). Dissolved oxygen variability in the Mediterranean Sea. J. Mar. Syst. 208:103348. doi: 10.1016/j.jmarsys.2020.103348
Meijer, P., and Dijkstra, H. A. (2009). The response of Mediterranean thermohaline circulation to climate change: a minimal model. Clim. Past 5, 713–720. doi: 10.5194/cp-5-713-2009
Mihanović, H., Vilibić, I., Carniel, S., Tudor, M., Russo, A., Bergamasco, A., et al. (2013). Exceptional dense water formation on the Adriatic shelf in the winter of 2012. Ocean Sci. 9, 561–572. doi: 10.5194/os-9-561-2013
Mihanović, H., Vilibić, I., Dunić, N. J., and Sepić, J. (2015). Mapping of decadal middle Adriatic oceanographic variability and its relation to the BiOS regime. J. Geophys. Res. Oceans 120, 5615–5630. doi: 10.1002/2015jc010725
Millot, C., and Taupier-Letage, I. (2005). “Circulation in the mediterranean sea” in The handbook of environmental chemistry. Vol. 5K. Berlin, Heidelberg: Springer, 29–66.
Ozer, T., Gertman, I., Gildor, H., Goldman, R., and Herut, B. (2020). Evidence for recent thermohaline variability and processes in the deep water of the southeastern Levantine basin, Mediterranean Sea. Deep-Sea Res. II 171:104651. doi: 10.1016/j.dsr2.2019.104651
Ozer, T., Gertman, I., Kress, N., Silverman, J., and Herut, B. (2017). Interannual thermohaline (1979-2014) and nutrient (2002–2014) dynamics in the Levantine surface and intermediate water masses SE Mediterranean Sea. Glob. Planet. Chang. 151, 60–67. doi: 10.1016/j.gloplacha.2016.04.001
Pinardi, N., and Masetti, E. (2000). Variability of the large scale general circulation of the Mediterranean Sea from observations and modelling: a review. Palaeogeogr. Palaeoclimatol. Palaeoecol. 158, 153–173. doi: 10.1016/s0031-0182(00)00048-1
Powley, H. R., Krom, M. D., and Van Cappellen, P. (2016). Circulation and oxygen cycling in the Mediterranean Sea: sensitivity to future climate change. J. Geophys. Res. Oceans 121, 8230–8247. doi: 10.1002/2016jc012224
Purkey, S. G., and Johnson, G. C. (2010). Warming of global abyssal and deep Southern Ocean waters between the 1990s and 2000s: contributions to global heat and sea level rise budgets. J. Clim. 23, 6336–6351. doi: 10.1175/2010jcli3682.1
Rahav, E., Silverman, J., Raveh, O., Hazan, O., Rubin-Blum, M., Zeri, C., et al. (2019). The deep water of eastern Mediterranean Sea is a hotspot for bacterial activity. Deep-Sea Res. II 164, 135–143. doi: 10.1016/j.dsr2.2019.03.004
Redfield, A. C. (1934). “On the proportions of organic derivatives in sea water and their relation to the composition of plankton” in James Johnstone memorial volume. University Press of Liverpool, 176–192.
Reid, P. C., Fischer, A. C., Lewis-Brown, E., Meredith, M. P., Sparrow, M., Andersson, A. J., et al. (2009). Impacts of the oceans on climate change. Adv. Mar. Biol. 56, 1–150. doi: 10.1016/S0065-2881(09)56001-4
Roether, W., Klein, B., Manca, B. B., Theocharis, A., and Kioroglou, S. (2007). Transient eastern Mediterranean deep waters in response to the massive dense-water output of the Aegean Sea in the 1990s. Prog. Oceanogr. 74, 540–571. doi: 10.1016/j.pocean.2007.03.001
Roether, W., Manca, B. B., Klein, B., Bregant, D., Georgopoulos, D., Beitzel, V., et al. (1996). Recent changes in eastern Mediterranean deep waters. Science 271, 333–335. doi: 10.1126/science.271.5247.333
Roether, W., and Well, R. (2001). Oxygen consumption in the eastern Mediterranean. Deep-Sea Res. I 48, 1535–1551. doi: 10.1016/s0967-0637(00)00102-3
Rohling, E. J., Marino, G., and Grant, K. (2015). Mediterranean climate and oceanography, and the periodic development of anoxic events (sapropels). Earth-Sci. Rev. 143, 62–97. doi: 10.1016/j.earscirev.2015.01.008
Rubino, A., and Hainbucher, D. (2007). A large abrupt change in the abyssal water masses of the eastern Mediterranean. Geophys. Res. Lett. 34:L23607. doi: 10.1029/2007gl031737
Sarmiento, J. L., Hughes, T. M. C., Stouffer, R. J., and Manabe, S. (1998). Simulated response of the ocean carbon cycle to anthropogenic climate warming. Nature 393, 245–249. doi: 10.1038/30455
Sarmiento, J. L., Orr, J. C., and Siegenthaler, U. (1992). A perturbation simulation of CO2 uptake in an ocean general circulation model. J. Geophys. Res. 97, 3621–3645. doi: 10.1029/91jc02849
Shaltout, M., and Omstedt, A. (2014). Recent sea surface temperature trends and future scenarios for the Mediterranean Sea. Oceanologia 56, 411–443. doi: 10.5697/oc.56-3.411
Sisma-Ventura, G., Yam, R., Kress, N., and Shemesh, A. (2016). Water column distribution of stable isotopes and carbonate properties in the south-eastern Levantine basin (eastern Mediterranean): vertical and temporal change. J. Mar. Syst. 158, 13–25. doi: 10.1016/j.jmarsys.2016.01.012
Stöven, T., and Tanhua, T. (2014). Ventilation of the Mediterranean Sea constrained by multiple transient tracer measurements. Ocean Sci. 10, 439–457. doi: 10.5194/os-10-439-2014
Stramma, L., Johnson, G. C., Sprintall, J., and Mohrholz, V. (2008). Expanding oxygen-minimum zones in the tropical oceans. Science 320, 655–658. doi: 10.1126/science.1153847
Sweetman, A. K., Thurber, A. R., Smith, C. R., Levin, L. A., Mora, C., Wei, C. -L., et al. (2017). Major impacts of climate change on deep-sea benthic ecosystems. Elementa Sci. Anthr. 5:4. doi: 10.1525/elementa.203
Tanhua, T., Hainbucher, D., Schroeder, K., Cardin, V., Álvarez, M., and Civitarese, G. (2013). The Mediterranean Sea system: a review and an introduction to the special issue. Ocean Sci. 9, 789–803. doi: 10.5194/os-9-789-2013
Theocharis, A., Krokos, G., Velaoras, D., and Korres, G. (2014). “An internal mechanism driving the alternation of the eastern Mediterranean dense/deep water sources” in The Mediterranean Sea: Temporal variability and spatial patterns. eds. G. L. E. Borzelli, M. Gačić, P. Lionello, and P. Malanotte-Rizzoli (Oxford, UK: John Wiley), 113–137.
Velaoras, D., Krokos, G., Nittis, K., and Theocharis, A. (2014). Dense intermediate water outflow from the Cretan Sea: a salinity driven, recurrent phenomenon, connected to thermohaline circulation changes. J. Geophys. Res. 119, 4797–4820. doi: 10.1002/2014JC009937
Vilibić, I., Sepić, J., and Proust, N. (2013). Weakening of thermohaline circulation in the Adriatic Sea. Clim. Res. 55, 217–225. doi: 10.3354/cr01128
Keywords: East Mediterranean, deep water, EMT, time series, oxygen decline, nutrients
Citation: Sisma-Ventura G, Kress N, Silverman J, Gertner Y, Ozer T, Biton E, Lazar A, Gertman I, Rahav E and Herut B (2021) Post-eastern Mediterranean Transient Oxygen Decline in the Deep Waters of the Southeast Mediterranean Sea Supports Weakening of Ventilation Rates. Front. Mar. Sci. 7:598686. doi: 10.3389/fmars.2020.598686
Edited by:
Katrin Schroeder, National Research Council (CNR), ItalyReviewed by:
Dimitris Velaoras, Hellenic Centre for Marine Research (HCMR), GreeceVedrana Kovacevic, National Institute of Oceanography and Experimental Geophysics (OGS), Italy
Copyright © 2021 Sisma-Ventura, Kress, Silverman, Gertner, Ozer, Biton, Lazar, Gertman, Rahav and Herut. This is an open-access article distributed under the terms of the Creative Commons Attribution License (CC BY). The use, distribution or reproduction in other forums is permitted, provided the original author(s) and the copyright owner(s) are credited and that the original publication in this journal is cited, in accordance with accepted academic practice. No use, distribution or reproduction is permitted which does not comply with these terms.
*Correspondence: Guy Sisma-Ventura, Z3V5LnNpdkBvY2Vhbi5vcmcuaWw=; Eyal Rahav, ZXlhbC5yYWhhdkBvY2Vhbi5vcmcuaWw=