Enhanced Growth Performance Physiological and Biochemical Indexes of Trachinotus ovatus Fed With Marine Microalgae Aurantiochytrium sp. Rich in n-3 Polyunsaturated Fatty Acids
- 1Guangdong Key Laboratory of Plant Epigenetics, Guangdong Technology Research Center for Marine Algal Bioengineering, College of Life Sciences and Oceanography, Shenzhen University, Shenzhen, China
- 2Shenzhen Key Laboratory of Marine Biological Resources and Ecology Environment, Shenzhen Key Laboratory of Microbial Genetic Engineering, College of Life Sciences and Oceanography, Shenzhen University, Shenzhen, China
- 3Longhua Innovation Institute for Biotechnology, Shenzhen University, Shenzhen, China
- 4School of Biomedical Sciences, The Chinese University of Hong Kong, Hong Kong, China
- 5Guangdong Research Center on Reproductive Control and Breeding Technology of Indigenous Valuable Fish Species, Fisheries College, Guangdong Ocean University, Zhanjiang, China
- 6Shenzhen Base of South China Sea Fisheries Research Institute, Chinese Academy of Fishery Sciences, Shenzhen, China
Aurantiochytrium sp. is a major source of n-3 polyunsaturated fatty acids (n-3 PUFAs), which are essential nutrients for marine fish. n-3 PUFAs have drawn increasing attention because of their great potential for improving the biological functions of fish. The growth performance and immune response of Trachinotus ovatus were studied by applying diets with various microalgae content from 1.00 to 11.00% for 8 weeks. The results showed that, with the addition of Aurantiochytrium sp., the survival rate, weight gain rate, and specific growth rate of fish increased by a maximum of 1.02, 1.16, and 1.08 times, respectively, indicating that the intake of marine microalgae Aurantiochytrium sp. was beneficial for fish growth. As the microalgae content increased, the feed utilization efficiency index feed conversion ratio decreased (maximum 15.00%) and feed efficiency increased (maximum 1.17 times), showing that the addition of Aurantiochytrium sp. contributed to the assimilation of fish feed. Furthermore, our results showed that as the addition of Aurantiochytrium sp. increased (from 1.00 to 11.00%), the glucose content increased in the blood (from 9.04 to 27.80%). The content of fatty acids ARA, ALA, DHA, and EPA in fish was significantly increased after adding Aurantiochytrium sp. in diets. In liver, ARA content increased from 1.17 to 1.63%, ALA increased from 0.56 to 0.85%, DHA increased from 14.44 to 20.61%, and EPA increased from 1.86 to 4.40%. In muscle, ARA content increased from 0.97 to 1.24%, ALA increased from 0.59 to 0.81%, DHA increased from 14.63 to 14.82%, and EPA increased from 4.58 to 5.19%. Positive changes were observed in the blood cell count of immune related cells (white blood cells, lymphocytes, monocytes, neutrophils, and red blood cells). These results indicated that microalgae rich in n-3 PUFAs could increase the number of immune cells, thus helping to improve fish immunity and disease resistance.
Introduction
The demand for fish feed is rapidly increasing globally. Improving the functionality of feed through the proper addition of nutrients could not only reduce the overuse of antibiotics but also promote the immunity and growth of aquatic products (Wang et al., 2020). Unsaturated fatty acids (FAs), which are essential nutrients for marine fish, show a wide range of important biological functions (Jin et al., 2019). n-3 polyunsaturated FAs (n-3 PUFAs) are an important category of unsaturated FAs, whose first unsaturated double bond appears between the third and fourth carbon atoms from the methyl end (ω terminus) of the FA. N-6 PUFAs arachidonic acid (20:4 ω6, ARA) and the several principal species of n-3 PUFAs eicosapentaenoic acid (20:5 ω3, EPA), linolenic acid (18:3 ω3, ALA), and docosahexaenoic acid (22:6 ω3, DHA), play an essential role in promoting the growth or boosting the immunity of fish (Leshno et al., 2018). Previous research has shown that n-3 PUFAs can increase leukocyte phagocytosis, T cell proliferation, and enhance cellular immunity in fish (Thompson et al., 1996; Wu et al., 2003). In addition, fish can also increase lectin activity and improve non-specific immune function by ingesting n-3 PUFAs (Pilarczyk, 1995). A previous study found that EPA and DHA help fish reduce inflammatory factors and reduce inflammation (Zhao et al., 2007). Moreover, as essential nutrients for the development of marine fish (Tocher, 2010), EPA and DHA can enrich the components of the mitochondrial phospholipid membrane and promote the growth of fish (Duda et al., 2009).
However, as a traditional source of unsaturated FAs, the extraction of n-3 PUFAs from fish oil is costly and inefficient. Therefore, finding novel, inexpensive, and edible sources of n-3 PUFAs is important (Tocher, 2015). In recent years, marine microalgae has been reported as a vital source of unsaturated FAs (Yadav et al., 2020) and have been increasingly used as a fish feed additive (Yin, 2014). Aurantiochytrium sp., a marine protist with confirmed food security benefits as determined by the Food and Drug Administration (FDA), has drawn increasing attention due to its rich production of n-3 PUFAs (Ren et al., 2020). Aurantiochytrium sp. can be mass-produced at low cost through industrial fermentation (Scott et al., 2011), and is a stable source of n-3 PUFAs (Li et al., 2013). The total FA content of Aurantiochytrium sp. reached 389 mg/g (Chang et al., 2015) with 68–74% PUFA and 42–44% essential PUFAs (EPA, ARA, and DHA) (Chang et al., 2011). Aurantiochytrium sp. has been used as a fish feed additive, indicating that the addition of Aurantiochytrium sp. can increase the content of n-3 PUFAs, especially DHA (Miller et al., 2007) in marine fish (Yamasaki et al., 2007). Kissinger et al. (2016) found that Aurantiochytrium sp. can be a suitable substitute for establishing protein in fish feed. Kousoulaki et al. (2016) reported that, with the increase in Aurantiochytrium sp. content in feed, the fat level in the fish liver decreased, and the FA accumulation and meat quality of fish continued to increase (García-Ortega et al., 2016). Some studies have shown that adding Aurantiochytrium sp. to fish feed can reduce energy and FA apparent digestibility (Tibbetts et al., 2020), and improve the growth performance of marine fish (Xie et al., 2019). Trachinotus ovatus is an carnivorous hard-bony marine fish whose meat is highly regarded by consumers, and it is a valuable edible fish in the southeast coastal areas of China (Liao, 2017). Nevertheless, the effects of Aurantiochytrium sp. as a feed supplement on the development and immunity of T. ovatus have barely been reported (Li Y. et al., 2019).
The purpose of the present study was to evaluate the effects of dietary Aurantiochytrium sp. on the growth performance and immune response of T. ovatus. Different proportions of Aurantiochytrium sp. powder were added to the feed, and the growth index, whole body FA content, blood composition, and other indicators of T. ovatus were measured.
Materials and Methods
Fish Feed Preparation
Aurantiochytrium sp. SZU445 was isolated from mangroves (22°31′13.044″ N, 113°56′58.560″ E) in the coastal waters of southern China. Aurantiochytrium sp. SZU 445 (used name as SW7-7) was used for powder preparation and was screened by our laboratory. Various percentages of Aurantiochytrium sp. powder (Diet 1: 0.00%, Diet 2: 1.00%, Diet 3: 3.00%, Diet 4: 5.00%, Diet 5: 7.00%, Diet 6: 9.00%, and Diet 7: 11.00%) were added to the fish feeds. Fish feeds were prepared according to the method presented by Ma et al. (2009). All the feed materials were crushed through an 80-mesh steel sieve. After mixing, the feed was formed into hard pellets with a diameter of 3 min. Fish feed was dried at 30°C for 72 h. Feed was stored in a refrigerator at −20°C for later use. The composition of the fish feed is shown in Tables 1, 2. The nutrient composition of the feed is shown in Table 3.
Animal Rearing
The T. ovatus fry used in this experiment had an initial weight of 8.00 ± 1.00 g and were purchased from Shenzhen Taifeng Oriental Marine Biotechnology Co., Ltd. Prior to the start of the experiment, T. ovatus were bred for 14 days. At the beginning of feeding, the fish were starved for 24 h, weighed, and then fish of similar size (initial body weight 8.00 ± 1.00 g) were randomly allocated to 14 sea cages (0.7 m × 0.5 m × 1.0 m; two cages per diet treatment); each cage was stocked with 50 fish. The total weight of 50 fish in each repetition was similar to ensure that there were no significant differences.
The cages had an overflow system, and the water speed was ∼19 L/rain. An aeration system was set in each cage, and the amount of dissolved oxygen in the water was maintained above 5.0 mg/L. During the test, the water temperature was controlled at 28 ± 1°C, and the salinity of the breeding water body was 29–31 g/L. The water quality was regularly monitored to ensure that it met breeding standards throughout the whole experiment.
Feeding occurred twice daily at 10:00 a.m. and 18:00 p.m., and lasted for 8 weeks. Each feeding weight was the percentage of the total weight. The body length and body weight were recorded for each fish every week to observe the weight gain of the fish. The number of fish deaths per day was recorded, and whether the fish exhibited pathological symptoms, such as n-3 PUFA deficiency, was observed.
Sample Collection
At the end of the feeding trial, fish were starved for 24 h. The fish were anesthetized with anesthetics (MS222, 60 mg/L) before sample collection. The number of fish in each cage and the length and weight of the fish were recorded. To collect whole fish, three fish were randomly selected from each cage, and the body surface was dried. Samples were quick-frozen with liquid nitrogen and stored at −20°C for later analysis. To collect plasma, we used the tail arterial blood sampling method. Three test fish were randomly selected from each cage. The side of the test fish was placed on an anatomic table. The vacuum blood collection needle was inserted into the posterior part of the gluteal fin and moved the blood to the ventral side of the vertebral body where to was collected. Blood samples were centrifuged at 900 × g (4°C) for 10 min to obtain plasma samples. Samples were stored at −20°C for later analysis. To collect liver samples, three fish were randomly selected from each cage. The liver of the fish was frozen immediately with liquid nitrogen and then placed in a sealed bag. Samples were stored at −20°C for later analysis. To collected back muscle samples, three fish were randomly selected from each cage. The back muscles of the fish were frozen immediately with liquid nitrogen and put into a sealed bag. Samples were stored at −20°C for later analysis.
Growth Performance Analysis
The following variables were calculated:
Survival rate (SR,%) = 100 × (final number of fish)/(initial number of fish);
Weight gain rate (WGR,%) = 100 × (final body weight – initial body weight)/initial body weight;
Specific growth rate (SGR,% day – 1) = 100 × (Ln final individual weight – Ln initial individual weight)/number of days;
Hepatosomatic index (HSI,%) = 100 × (liver weight, g)/(whole body weight, g);
Feed conversion ratio (FCR,%) = dry diet fed/wet weight gain;
Feed efficiency (FE,%) = wet weight gain/dry diet fed;
Condition factor (CF, g/cm3) = 100 × (body weight, g)/(body length, cm3).
Determination of Plasma Biochemical Indicators
The health status of fish was determined by analyzing plasma biochemical indicators. The content of protein, glucose, triglyceride (TG), and glutamic oxaloacetate (GOT) in fish plasma were determined using a kit provided by Nanjing Jiancheng Biotechnology Research Institute.
Fatty Acid Composition Analysis
The water content in the tissue samples was measured using the drying weight loss method. The protein content of tissue was determined using a quantitative protein kit after the tissue was ground and broken by ultrasound. FAs were extracted from tissue samples by Soxhlet extraction, and FA composition and content were determined by GC/MS. The detailed method was as follows: before the experiment, filter paper bags (Hanjiang Road, Shuncheng District, Fushun City, Liaoning Province, China) were pretreated with a solvent mixture [hloroform:methanol = 2:1 (v/v)] for 48 h and dried at 50°C. Five hundred milligrams of dried cells were placed in a pretreated filter paper bag as a filter paper package and extracted in a Soxhlet extractor at 70°C for 48 h (solvent as described above). Then, the filter paper package was dried and weighed. The weight of FAs was determined by subtracting the weight of FAs extracted from the weight before extraction. The remaining liquid was evaporated to dryness at 70°C using a rotary evaporator. The FAs were completely rinsed with 5 mL of n-hexane and placed in a 10-ml glass tube. Three biological replicates were examined for each sample.
Immune Related Cell Count in Fish
The number of red (RBC) and white (WBC) blood cells can be used as a reference indicator of immunity (Köllner and Kotterba, 2002). Fish blood cells were counted using an HB7510 five classification hemocytometer (Jiangsu China SINNOWA Medical Technology Co., Ltd.).
All data are presented as the mean ± standard deviation (SD) and were subjected to one-way ANOVA, independent-sample f-test, and Duncan multiple comparisons to test the effects of experimental diets using SPSS (v.16.0, United States). Statistical significance was considered at P < 0.05 unless otherwise noted.
Results
Trachinotus ovatus Growth Performance
Growth performance, feed utilization, and biometric parameters of T. ovatus fed different dietary levels are shown in Table 4. The high SR of fish in this experiment (SR was the lowest in Diets 3 and 7 at 86%, and the highest in Diet 2 at 92%) indicated that the test subjects barely suffered from the adverse stress caused by the feeding operation. In fish feeding experiments, the WGR and SGR of fish can reflect their growth performance. The results showed that adding Aurantiochytrium sp. rich in n-3 PUFAs to feed had a significant effect on the WGR and SGR of T. ovatus (P < 0.05). Furthermore, with increased addition of Aurantiochytrium sp. (from 1.00 to 11.00%), the WGR of fish also increased (from 264.95 to 306.65%). The SGR increased from 2.31 to 2.50% with increasing addition of Aurantiochytrium sp. This indicated that the addition of n-3 PUFAs had a positive effect on the growth of T. ovatus.
FCR and FE are often used to measure the ability of animals to absorb feed. A higher FE indicates that fish can more effectively absorb energy from feed. In contrast, a higher FE indicates that fish need more feed to accumulate the same weight. The present results indicated that T. ovatus fed diets supplemented with Aurantiochytrium sp. showed higher FE (from 73.63 to 79.28%) and lower FCR (from 126.14 to 148.39%) than the control (P < 0.05). With increasing n-3 PUFAs in the feed, FE increased (Diet 7 was 1.18 times that of the control), whereas FCR decreased [that in Diet 7 (126.14%) was lower than that in Diet 2 (135.96%)]. This indicated that the addition of n-3 PUFAs improved feed utilization in fish.
The CF is an indicator of fish weight and growth, and the higher the CF, the higher the nutritional level of fish. When Aurantiochytrium sp. was added to the feed, the CF of T. ovatus was significantly higher (from 290.76 to 302.32%) (P < 0.05). This indicates that n-3 PUFAs improved the nutritional level of fish.
Based on the above indices and dynamic characteristics, the growth and development of fish and their feed utilization capacity were summarized. With the addition of Aurantiochytrium sp. to the feed, the growth performance and feed utilization ability of T. ovatus significantly increased. Aurantiochytrium sp. as a feed additive has a positive effect on the growth and development of T. ovatus and can replace traditional fish oil to become a benign source of essential FAs.
Trachinotus ovatus Plasma Biochemical Index
The plasma biochemical index of T. ovatus fed different levels of dietary Aurantiochytrium sp. are shown in Figure 1. Compared with the control group, T. ovatus fed with n-3 PUFAs as a supplement showed a significant increase in blood glucose level (P < 0.05). The results showed that, as the addition of Aurantiochytrium sp. increased (from 1.00 to 11.00%), the glucose content in the blood increased (from 9.04 to 27.80%). Notably, the blood glucose of the fish fed Diet 6 (468.15 mg/L) increased the most among the experimental groups (by 28.47%). Adding 9.00% of Aurantiochytrium sp. significantly stimulated glucose absorption in the blood.
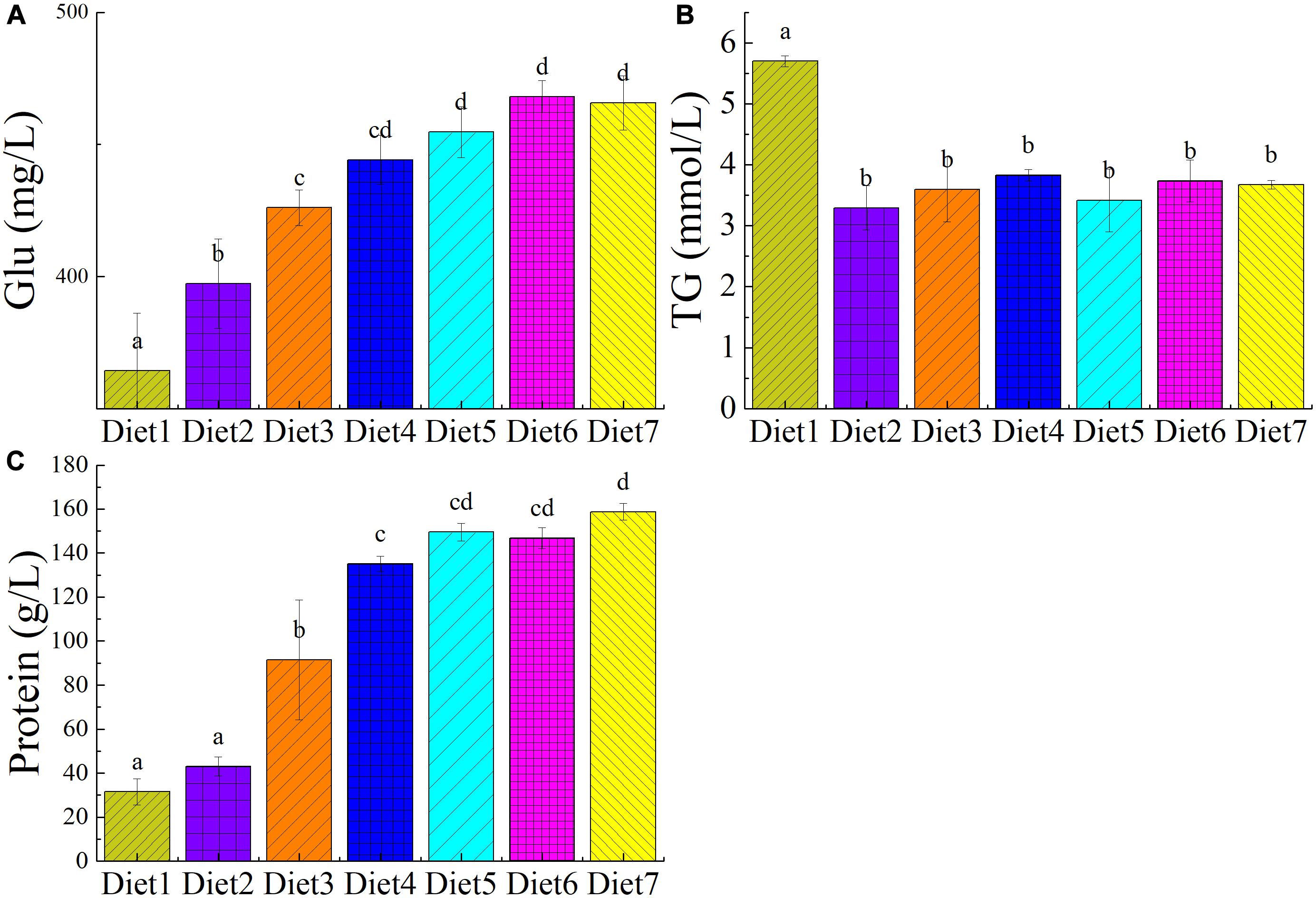
Figure 1. The plasma biochemical indicators of T. ovatus sp. The plasma biochemical index of T. ovatus sp. fed with diets in different levels of n-3 PUFAs Diet1∼7 for Fish feed with different PUFA contents in 7 groups. Glucose (A), triglyceride (B), and protein (C) content in blood. The amount of Aurantiochytrium sp. powder in feed is 0.00%, 1.00%, 3.00%, 5.00%, 7.00%, 9.00%, and 11.00% respectively.
When n-3 PUFAs were added to the T. ovatus feed, the TG content in the blood of the experimental group significantly decreased (5.70–3.29%) compared with that in the control group (P < 0.05). Among them, the TG content in the blood of the fish fed Diet 2 decreased by 42.28%, whereas the Diet 4 fish had the lowest TG content (32.80%). n-3PUFAs regulate blood lipids and are often used to treat cardiovascular diseases. The results showed that n-3 PUFAs could decrease TG content in blood, regulate blood lipid composition, and enhance the health of T. ovatus.
Adding n-3 PUFAs to the feed had a significant effect on the protein content of blood (P < 0.05). With increasing n-3 PUFAs in the feed, the protein content in fish blood significantly increased from 31.50 to 158.75 g/L (P < 0.05). Compared with the control group, when 3.00% Aurantiochytrium sp. was added to the feed (Diet 3), the blood protein content increased by almost two times (190.06%). Furthermore, when 11.18% n-3 PUFAs was added (Diet 7), the blood protein content increased by nearly four times (403.96%). The results showed that the amount of Aurantiochytrium sp. in the feed significantly influenced the blood protein content, with higher n-3 PUFA content significantly increasing blood protein content.
The addition of dietary Aurantiochytrium sp. had no significant effect on blood GOT content of T. ovatus (P > 0.05). This indicates that using Aurantiochytrium sp. to supplement T. ovatus diets will maintain liver function without causing pathological damage.
Trachinotus ovatus Fatty Acid Composition
The FA composition of the back muscles and liver of T. ovatus fed with the different diets is shown in Figure 2 and Table 5. The changes in FA content in the liver and muscles can directly reflect the effect of n-3PUFAs on the accumulation of FAs in T. ovatus. The content of ALA in liver FAs of each experimental group significantly increased (from 0.56 to 0.85%) after adding dietary Aurantiochytrium sp. (P < 0.05). Diet 2 exhibited the highest increase (by 51.78%), whereas Diet 3 exhibited the lowest increase (by 37.50%). The ARA content in the liver of all experimental groups decreased with increasing dietary Aurantiochytrium sp. The results showed that the content of ARA was 0.46% when the amount of Aurantiochytrium sp. was 1.00%, and 0.03% when the amount of Aurantiochytrium sp. was 3.00%.
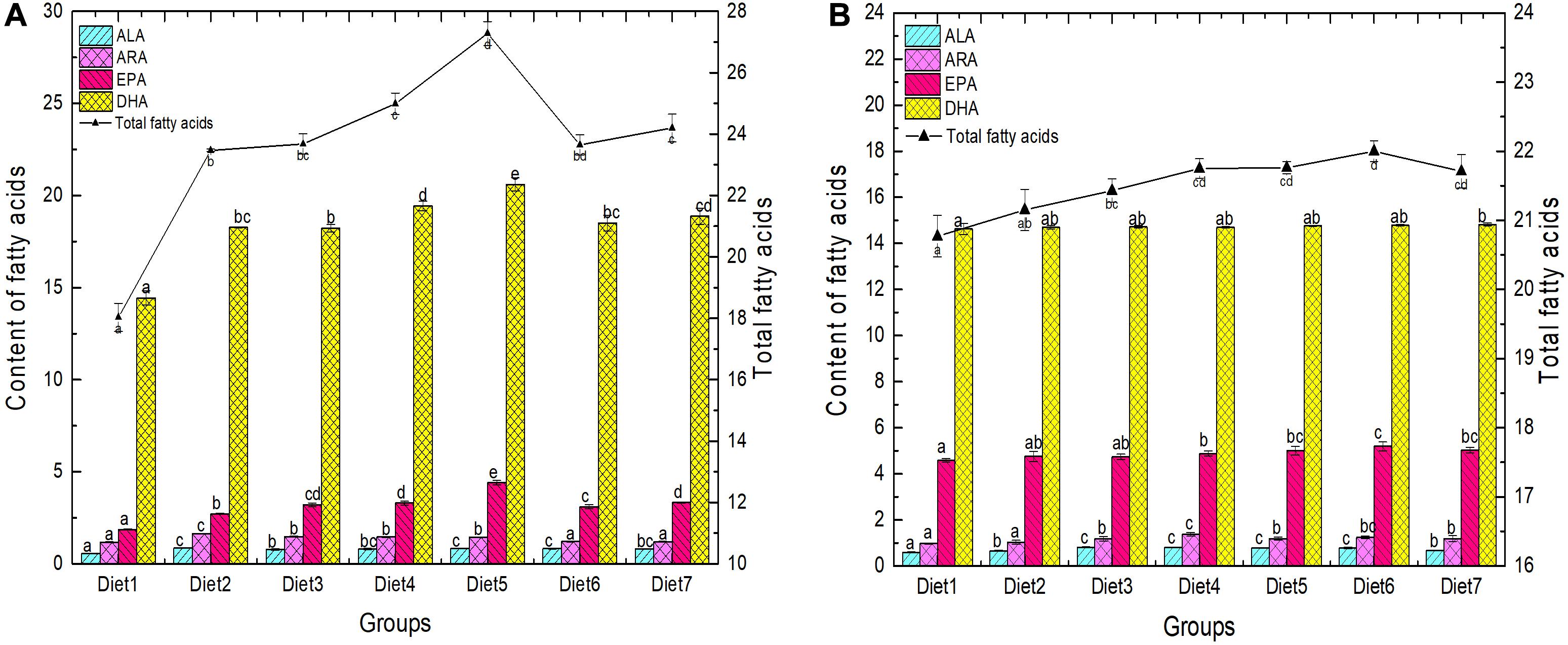
Figure 2. Fatty acid composition in liver (A) and muscle (B) of T. ovatus sp. Diet1∼7 for Fish feed with different n-3 PUFAs contents in 7 groups. The amount of Aurantiochytrium sp. powder in feed is 0.00%, 1.00%, 3.00%, 5.00%, 7.00%, 9.00%, and 11.00%, respectively.
EPA and DHA are essential FAs for the growth of marine fish. After adding Aurantiochytrium sp. to the diet, the content of EPA in each experimental group was significantly higher than that in the control. Compared with the group without Aurantiochytrium sp., the group with the lowest increase (Diet 2) increased by 46.23%, whereas the group with the highest increase (Diet 5) increased by 136.56%. Similar to EPA, the content of DHA in the liver of each group also increased initially followed by a decrease. The lowest DHA content was observed in Diet 1 (14.44%), and the highest was observed in Diet 5 (20.61%). The results showed that the content of the four main FAs (ALA, ARA, EPA, and DHA) in the liver significantly increased with the addition of n-3 PUFAs. Moreover, with the increase in Aurantiochytrium sp., the four FAs showed a general trend of first increasing and then decreasing, which indicated that Aurantiochytrium sp. intake could increase the accumulation of FAs in the liver, but excessive intake of Aurantiochytrium sp. inhibited the accumulation of FAs.
The effect of dietary Aurantiochytrium sp. on FA content in muscles was non-significant, but the proportion of the main n-3 PUFAs still increased. Among the groups with added Aurantiochytrium sp., the highest proportion of ALA was observed in Diets 3 and 4 (0.81%), and the lowest was observed in Diet 2 (0.66%). The highest ratio of ARA was observed in Diet 4 (1.37%), and the lowest was observed in Diet 1 (0.97%). With the increase in FA content in feed, the proportion of EPA in muscles increased until peaking at 5.19% (Diet 6), and then decreasing to a certain extent (Diet 7, 5.03%). With increased addition of the Aurantiochytrium sp. (from 0.00 to 11.00%), the DHA content in muscles increased (from 14.63 to 14.82%), but there was no significant difference among the groups.
The results showed that T. ovatus fed a diet supplemented with Aurantiochytrium sp. showed more n-3 PUFA accumulation than those in the control. However, the changes in n-3 PUFA composition in muscle tissue were more subtle. Moreover, with the increase in algae powder content, ALA, ARA, and EPA content increased initially, and then decreased gradually, but EPA and DHA showed an increasing trend. The results showed that a particular Aurantiochytrium sp. intake can promote the accumulation of ALA and ARA in muscles, while DHA accumulation in the fish required higher Aurantiochytrium sp. content in the feed.
Immune Related Cell Count in Fish
The numbers of RBCs, WBCs, lymphocytes, and granulocytes in the plasma of T. ovatus fed different levels of dietary Aurantiochytrium sp. are shown in Figure 3. When the amount of dietary Aurantiochytrium sp. reached 1.00% (Diet 2), the number of WBCs in T. ovatus blood began to significantly increase (P < 0.05). The number of WBCs in the blood of the fish fed Diet 3 increased by 8.64% and those fed Diet 7 increased by 30.27% compared with those in the control. In addition, the two main types of WBCs, lymphocytes and granulocytes, exhibited the same trend as the total number of WBCs in the blood. The number of lymphocytes in the fish fed Diet 4 was 1.13 times that of those in the control, and those fed Diet 7 had 1.30 times that of the fish in the control. When the fish were fed with 5.00% dietary Aurantiochytrium sp. (Diet 4), the number of granulocytes in T. ovatus blood reached 1.15 times that in the blood of those in the control. When the Aurantiochytrium sp. content was increased to 11.00% (Diet 7), the number of granulocytes was 1.37 times that of the control. The ratio of the number of lymphocytes and granulocytes to the total number of WBCs in the blood of each group fed with different feeds was normal, and there was no significant difference (P > 0.05). This result showed that, after adding Aurantiochytrium sp. to the feed, the number of WBCs increased, and the ratio of WBCs did not change outside of the normal range. Experimental results showed that adding Aurantiochytrium sp. to feed increased the number of immune cells and improved fish immunity and disease resistance.
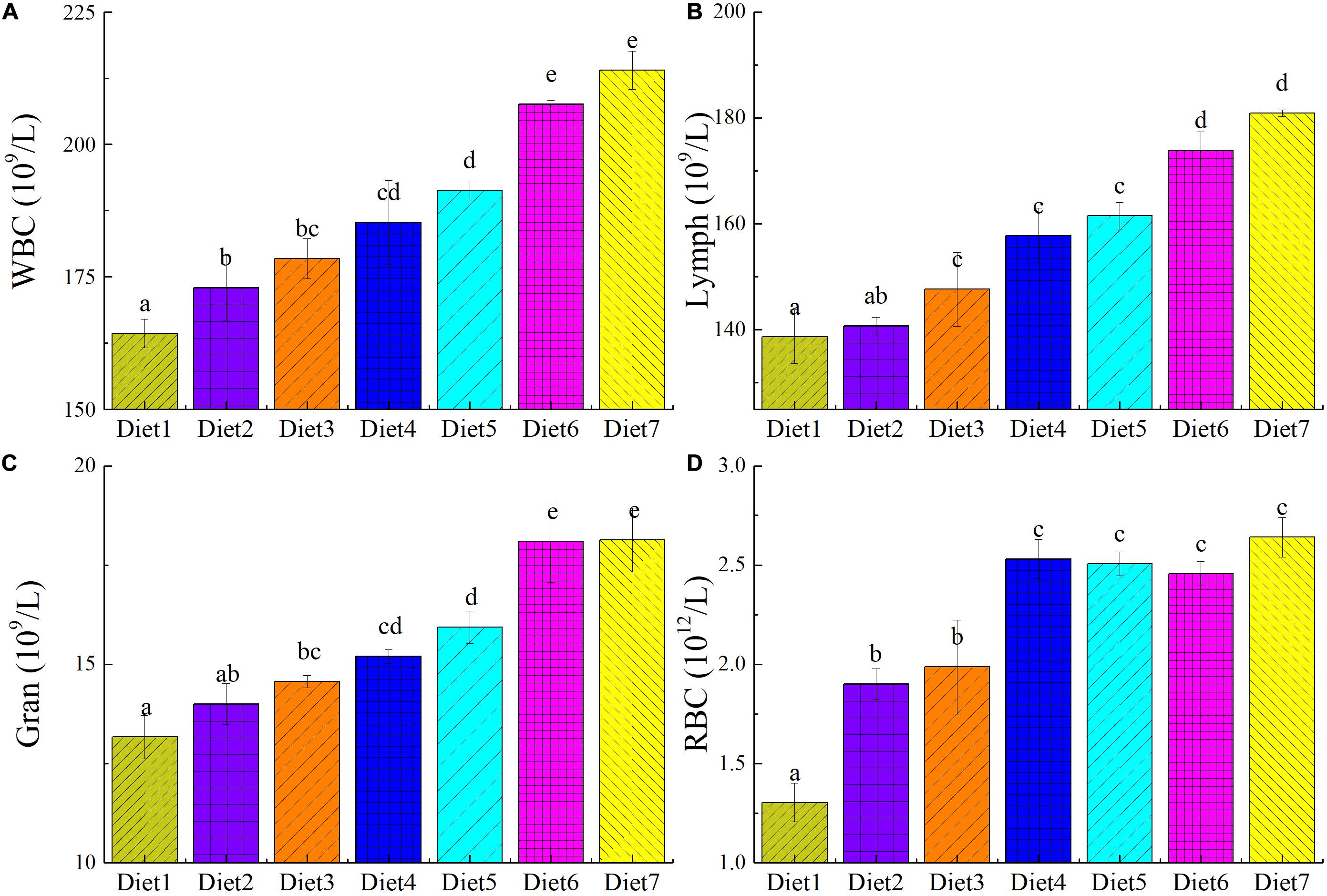
Figure 3. The blood cell count of T. ovatus sp. fed diets with different levels of n-3 PUFAs Diet1∼7 for Fish feed with different n-3 PUFAs contents in 7 groups. The number of white blood cells (A), lymphocyte (B), granulocyte (C), and red blood cells (D) in blood. The amount of Aurantiochytrium sp. powder in feed is 0.00%, 1.00%, 3.00%, 5.00%, 7.00%, 9.00%, and 11.00%, respectively.
With the increase in Aurantiochytrium sp. content in the feed, RBCs in the blood of T. ovatus significantly increased (P < 0.05). The RBC content of the fish fed Diet 3 was 1.53 times that of those in the control group. When the content of Aurantiochytrium sp. in the feed reached 11.00% (Diet 7), the number of RBCs peaked (2.64 × 1012/L, 2 times that of the control), and it no longer significantly changed with increasing Aurantiochytrium sp. content. This showed that the addition of Aurantiochytrium sp. increased the number of RBCs in T. ovatus. In addition to the function of transporting oxygen, teleost fish RBCs also have immune functions, such as enhanced phagocytosis, immune adhesion, and defense against infection. The increase in the number of RBCs indicated that after adding Aurantiochytrium sp., T. ovatus aerobic respiration capacity and RBC immunity-related functions increased.
Discussion
Growth Performance of Trachinotus ovatus
n-3 PUFAs are essential FAs that play important roles in fish growth and metabolism. However, the minimum requirements for PUFAs differ for different types of fish (Chen et al., 2018). Fish lacking PUFAs exhibit growth retardation and lack of vitality, and will suffer from seriously reduced feed-conversion efficiency (Xu et al., 2010). Growth performance parameters, such as WGR and SGR, are the most direct reflection of fish growth and nutritional status. The SR and FE can also reflect health status and energy-use efficiency.
Dietary Aurantiochytrium sp. supplementation significantly improved the WGR and SGR of T. ovatus compared with the supplemented group. The FE and other results related to energy-conversion efficiency also showed that the ability of T. ovatus to absorb energy from their diet improved after supplementation with dietary Aurantiochytrium sp. As seen from the results, Aurantiochytrium sp. provides sufficient DHA and other FAs necessary for the growth of T. ovatus. In addition, the algal-derived DHA can also increase the FE rate (Li et al., 2009) and stimulate the growth performance of aquatic animals (Glencross and Smith, 2001; Glencross and Rutherford, 2011). These results indicate that Aurantiochytrium sp. addition promotes the growth of T. ovatus by improving its energy-conversion efficiency.
Trachinotus ovatus Plasma Biochemical Index
Fish plasma is closely involved in fish metabolism, nutrition, and health status. Blood biochemical indicators are widely used to evaluate fish health, nutrition, and environmental adaptation (Abdel-Tawwab et al., 2010). Plasma protein concentration can be affected by dietary protein intake and can be used as an indicator of individual nutritional status to a certain extent. The serum total protein content can reflect protein metabolism in animals (Baldi et al., 1999). The higher the total protein content in fish serum, the higher the fish’s absorption and metabolism levels, and the higher the efficiency of protein synthesis and nitrogen deposition (Sun et al., 2014). In the present study, as dietary Aurantiochytrium sp. increased from 0.00 to 11.00%, the protein content in the blood also significantly increased and was maintained at a high level. When dietary Aurantiochytrium sp. was at 11.00% the weight of the feed, the total amount of protein in the blood was 4 times that in the control. Previous research has shown that adding Aurantiochytrium sp. to feed can increase the protein content in juvenile black sea bream blood (Ma et al., 2009). These results indicate that adding Aurantiochytrium sp. to feed can improve protein efficiency and increase the nutritional status of T. ovatus.
Blood glucose and triglyceride levels reflect energy and fat metabolism in fish. Generally, when the blood glucose level is high, the fish feed has a higher energy level and greater vitality (Imsland et al., 2001), but excess glucose is more likely to be converted into fat for storage in the body (Guo et al., 2006). TG content is an important indicator of blood fat levels. When the body fat level increases, the blood TG content also increases. n-3 PUFAs can affect insulin sensitivity and regulate blood glucose levels (Luo et al., 1998), and, as the main type of n-3 PUFAs, ARA can reduce the TG level in the blood (Kris-Etherton et al., 2003). The results of the present study showed that, as the n-3 PUFA content in the feed increased, the glucose content in the blood increased from 9.00 to 27.00%. In addition, after adding Aurantiochytrium sp. powder to the feed, compared with the control, the blood TG content in each experimental group significantly decreased. These results indicate that n-3 PUFAs can reduce the energy expenditure index and improve the energy absorption efficiency of T. ovatus (Tibbetts et al., 2020).
GOT mainly exists in the cytoplasm of hepatocytes, and is an indispensable enzyme for hepatocytes to synthesize proteins (Zhong et al., 2020). This enzyme is released into the blood when hepatocytes degenerate, and necrosis or cell membrane permeability increases, so blood GOT levels can well reflect the functional status of liver cells (Li B. et al., 2019). Results showed that adding Aurantiochytrium sp. to the diet well-maintained T. ovatus liver function.
Fatty Acid Composition of Trachinotus ovatus
The liver is an organ rich in FAs and is more sensitive to changes in FA content in animals (Castaño-Moreno et al., 2020). Therefore, the variety of FA components in the liver was more significant with the increase in n-3 PUFA intake. This result could be due to ALA being the precursor of EPA/DHA synthesis in the process of FA metabolism (Deng et al., 2017). Compared with fish oil, marine microalgae as a source of fatty acids can inhibit the desaturation pathway in the liver and increase the accumulation of fatty acids (Carvalho et al., 2020a). The present study indicated that oils from microalgae, when engineered to contain high levels of EPA and DHA can replace fish oil in feeds for sea bream juveniles and allowed complete replacement of fish oil in combination with more cost-effective lipid sources, such as poultry and rapeseed oils (Carvalho et al., 2020b). Furthermore, more EPA-DHA synthesis will lead to a decrease in ALA content. We speculated that with the increase in DHA content, the conversion process of ALA to DHA may be inhibited by feedback. In addition, the direct absorption and deposition of DHA may be inhibited by increased DHA conversion. However, the increase in FA content can protect the fish liver (Zirnheld et al., 2019) and increase the economic value of T. ovatus.
Immune Related Cell Count in Fish
The blood composition of fish is affected by a variety of endogenous factors, including nutrition status, individual size, and exogenous factors, including light, temperature, and dissolved oxygen. Some blood indicators (RBCs and WBCs) are used to assess the physiological and pathological conditions of fish (Harikrishnan et al., 2011). In addition, RBC, WBC, and lymphocytes are also important components of the cellular and humoral immunity of fish (Köllner and Kotterba, 2002).
As an important component of the RBC membrane, PUFAs can maintain a stable number of RBCs in the blood through the ingestion of dietary n-3 PUFAs (Ma et al., 2004). In the present study, compared with the control, the RBCs in the feed groups was significantly increased, which indicates that Aurantiochytrium sp. can indeed enhance the RBC immunity of T. ovatus. Leukocytes mainly function in defense. On the basis of traditional immunology, granulocytes are mainly responsible for host defense, immune regulation, and tissue damage (Kruger et al., 2015). Recent studies have shown that granulocytes exhibit a defense response to tumor cells (Fridlender and Albelda, 2012). Lymphocytes are a large group of immune cells that mainly participate in the specific immune response of the body. In the present study, the number of WBCs, granulocytes, and lymphocytes in the blood of the experimental group significantly increased compared with the control group, and the overall trend in growth remained stable. Generally, the number of WBCs in fish will increase due to environmental stress (Zuo et al., 2019), but there was no significant difference in the proportion of lymphocytes and granulocytes in the total number of WBCs in each experimental group. This shows that the increase in leukocyte number was not caused by external environmental stress, but rather by improvements in immunity (Köllner and Kotterba, 2002). Through a comprehensive analysis of the number of immune cells, it can be seen that the supplementation of dietary n-3 PUFAs can increase immunity in T. ovatus.
n-3 PUFAs have been widely used as health products owing to their ability to regulate blood lipids. The mechanism of PUFAs, as components of cell membranes, involves changing the composition of cell membranes (Gawrisch et al., 2008), but also changing the process of intracellular messenger transmission and cytokines. All phospholipids and some secondary messengers, such as triglycerides and ceramide, contain FA chains, so their functions can be changed by altering FA composition (Issazadeh-Navikas et al., 2012). In the field experiment, n-3 PUFAs can enhance natural killer cell activity, CD8+ T cell (a cytotoxic T lymphocyte) activation, and interleukin-γ and tumor necrosis factor-α production in tumor-bearing animals (Robinson et al., 2001). Previous studies have shown that appropriate intake of n-3 PUFAs can stimulate lymphocyte proliferation and enhance immune function (Hedelin et al., 2007; Gawrisch et al., 2008). This is consistent with the increase in the number of leukocytes and lymphocytes in T. ovatus blood after the addition of dietary Aurantiochytrium sp. in the present study. These results indicate that the immune function of T. ovatus was indeed increased by adding Aurantiochytrium sp. to its feed.
Data Availability Statement
The original contributions presented in the study are included in the article/supplementary material, further inquiries can be directed to the corresponding author/s.
Ethics Statement
The animal study was reviewed and approved by Experimental Animal Ethics Committee of Medical Department of Shenzhen University.
Author Contributions
LL, BW, XZ, YL, and CL analyzed the data and wrote the manuscript. XY and SL designed the experimental concept. CC, HC, and YS checked and revised the details of the manuscript. XY was responsible for critical reading and finalization of the manuscript. All authors have read and approved the final manuscript.
Funding
This study was supported by the National Key Research and Development Project (Grant No. 2018YFA0902500), the Natural Science Foundation of Guangdong Province (Grant No. 2018A030313139), Joint R&D Project of Shenzhen-Hong Kong Innovation (Grant No. SGLH20180622152010394), Hong Kong Innovation and Technology Commission TCFS (GHP/087/18SZ), and sponsored by the Shenzhen Taifeng East Marine Biotechnology Co., Ltd., Natural Science Foundation of Shenzhen (Grant No. KQJSCX20180328093806045), Shenzhen Science and Technology Application Demonstration Project (Grant No. KJYY20180201180253571). Shenzhen Taifeng East Marine Biotechnology Co. was not involved in the study design, collection, analysis, interpretation of data, the writing of this article, or the decision to submit it for publication.
Conflict of Interest
The authors declare that the research was conducted in the absence of any commercial or financial relationships that could be construed as a potential conflict of interest.
Acknowledgments
Instrument Analysis Center, Shenzhen University, Shenzhen, PR China; Shenzhen Taifeng Dongfang Marine Biotechnology Co., Ltd.
References
Abdel-Tawwab, M., Ahmad, M. H., Khattab, Y. A., and Shalaby, A. M. (2010). Effect of dietary protein level, initial body weight, and their interaction on the growth, feed utilization, and physiological alterations of Nile tilapia, Oreochromis niloticus (L.). Aquaculture 298, 267–274. doi: 10.1016/j.aquaculture.2009.10.027
Baldi, A., Bontempo, V., Dell’Orto, V., Cheli, F., Fantuz, F., and Savoini, G. (1999). Effects of dietary chromium-yeast in weaning-stressed piglets. Can. J. Anim. Sci. 79, 369–374. doi: 10.4141/a98-117
Carvalho, M., Montero, D., Torrecillas, S., Castro, P., Zamorano, M., and Izquierdo, M. (2020a). Effective complete replacement of fish oil by combining poultry and microalgae oils in practical diets for gilthead sea bream (Sparus aurata) fingerlings. Aquaculture 529:735696. doi: 10.1016/j.aquaculture.2020.735696
Carvalho, M., Montero, D., Torrecillas, S., Castro, P., Zamorano, M., and Izquierdo, M. (2020b). Hepatic biochemical, morphological and molecular effects of feeding microalgae and poultry oils to gilthead sea bream (Sparus aurata). Aquaculture 532:736073. doi: 10.1016/j.aquaculture.2020.736073
Castaño-Moreno, E., Castillo, V., Peñailillo, R., Llanos, M. N., Valenzuela, R., and Ronco, A. M. (2020). Fatty acid and lipid metabolism in liver of pregnant mice and their offspring is influenced by unbalanced folates/vitamin B12 diets. Prostaglandins Leukot. Essent. Fatty Acids 154:102057. doi: 10.1016/j.plefa.2020.102057
Chang, K. J. L., Mansour, M. P., Dunstan, G. A., Blackburn, S. I., Koutoulis, A., and Nichols, P. D. (2011). Odd-chain polyunsaturated fatty acids in thraustochytrids. Phytochemistry 72, 1460–1465. doi: 10.1016/j.phytochem.2011.04.001
Chang, K. J. L., Paul, H., Nichols, P. D., Koutoulis, A., and Blackburn, S. I. (2015). Australian thraustochytrids: potential production of dietary long-chain omega-3 oils using crude glycerol. J. Funct. Food 19, 810–820. doi: 10.1016/j.jff.2015.01.039
Chen, C., Guan, W., Xie, Q., Chen, G., He, X., Zhang, H., et al. (2018). n-3 essential fatty acids in Nile tilapia, Oreochromis niloticus: bioconverting LNA to DHA is relatively efficient and the LC-PUFA biosynthetic pathway is substrate limited in juvenile fish. Aquaculture 495, 513–522. doi: 10.1016/j.aquaculture.2018.06.023
Deng, B., Men, X. M., Zhu, D. R., Tao, X., Yao, Y. X., and Xu, Z. W. (2017). Effects of dietary flaxseed and fish oil on n-3 polyunsaturated fatty acid content in egg yolk and fatty acid metabolism in liver of hens. Chin. J. Anim. Nutr. 09:38.
Duda, M. K., O’Shea, K. M., and Stanley, W. C. (2009). ω-3 polyunsaturated fatty acid supplementation for the treatment of heart failure: mechanisms and clinical potential. Cardiovasc. Res. 84, 33–41. doi: 10.1093/cvr/cvp169
Fridlender, Z. G., and Albelda, S. M. (2012). Tumor-associated neutrophils: friend or foe? Carcinogenesis 33, 949–955. doi: 10.1093/carcin/bgs123
García-Ortega, A., Kissinger, K. R., and Trushenski, J. T. (2016). Evaluation of fish meal and fish oil replacement by soybean protein and algal meal from Schizochytrium limacinum in diets for giant grouper Epinephelus lanceolatus. Aquaculture 452, 1–8. doi: 10.1016/j.aquaculture.2015.10.020
Gawrisch, K., Soubias, O., and Mihailescu, M. (2008). Insights from biophysical studies on the role of polyunsaturated fatty acids for function of G-protein coupled membrane receptors. Prostaglandins Leukot. Essent. Fatty Acids 79, 131–134. doi: 10.1016/j.plefa.2008.09.002
Glencross, B., and Rutherford, N. (2011). A determination of the quantitative requirements for docosahexaenoic acid for juvenile barramundi (Lates calcarifer). Aquacult. Nutr. 17, e536–e548.
Glencross, B. D., and Smith, D. M. (2001). Optimizing the essential fatty acids, eicosapentaenoic and docosahexaenoic acid, in the diet of the prawn. Penaeus monodon. Aquacult. Nutr. 7, 101–112. doi: 10.1046/j.1365-2095.2001.00158.x
Guo, R., Liu, Y. J., Tian, L. X., and Huang, J. W. (2006). Effect of dietary cornstarch levels on growth performance, digestibility and microscopic structure in the white shrimp, Litopenaeus vannamei reared in brackish water. Aquacult. Nutr. 12, 83–88. doi: 10.1111/j.1365-2095.2006.00384.x
Harikrishnan, R., Kim, M. C., Kim, J. S., Balasundaram, C., and Heo, M. S. (2011). Protective effect of herbal and probiotics enriched diet on haematological and immunity status of Oplegnathus fasciatus (Temminck & Schlegel) against Edwardsiella tarda. Fish Shellfish Immun. 30, 886–893. doi: 10.1016/j.fsi.2011.01.013
Hedelin, M., Chang, E. T., Wiklund, F., Bellocco, R., Klint, Å, Adolfsson, J., et al. (2007). Association of frequent consumption of fatty fish with prostate cancer risk is modified by COX-2 polymorphism. Int. J. Cancer 120, 398–405. doi: 10.1002/ijc.22319
Imsland, A. K., Foss, A., Gunnarsson, S., Berntssen, M. H., FitzGerald, R., and Bonga, S. W. (2001). The interaction of temperature and salinity on growth and food conversion in juvenile turbot (Scophthalmus maximus). Aquaculture 198, 353–367. doi: 10.1016/s0044-8486(01)00507-5
Issazadeh-Navikas, S., Teimer, R., and Bockermann, R. (2012). Influence of dietary components on regulatory T cells. Mol. Med. 18, 95–110. doi: 10.2119/molmed.2011.00311
Jin, M., Lu, Y., Pan, T., Zhu, T., Yuan, Y., Sun, P., et al. (2019). Effects of dietary n-3 LC-PUFA/n-6 C-18 PUFA ratio on growth, feed utilization, fatty acid composition and lipid metabolism related gene expression in black seabream. Acanthopagrus schlegelii. Aquaculture 500, 521–531. doi: 10.1016/j.aquaculture.2018.10.056
Kissinger, K. R., García-Ortega, A., and Trushenski, J. T. (2016). Partial fish meal replacement by soy protein concentrate, squid and algal meals in low fish-oil diets containing Schizochytrium limacinum for longfin yellowtail Seriola rivoliana. Aquaculture 452, 37–44. doi: 10.1016/j.aquaculture.2015.10.022
Köllner, B., and Kotterba, G. (2002). Temperature dependent activation of leucocyte populations of rainbow trout, Oncorhynchus mykiss, after intraperitoneal immunisation with Aeromonas salmonicida. Fish Shellfish Immunol. 12, 35–48. doi: 10.1006/fsim.2001.0352
Kousoulaki, K., Mørkøre, T., Nengas, I., Berge, R. K., and Sweetman, J. (2016). Microalgae and organic minerals enhance lipid retention efficiency and fillet quality in Atlantic salmon (Salmo salar L.). Aquaculture 451, 47–57. doi: 10.1016/j.aquaculture.2015.08.027
Kris-Etherton, P. M., Harris, W. S., and Appel, L. J. (2003). Fish consumption, fish oil, omega-3 fatty acids, and cardiovascular disease. Circulation 23, e20–e30.
Kruger, P., Saffarzadeh, M., Weber, A. N., Rieber, N., Radsak, M., von Bernuth, H., et al. (2015). Neutrophils: between host defence, immune modulation, and tissue injury. PLoS Pathog. 11:e1004651. doi: 10.1371/journal.ppat.1004651
Leshno, M., Goldbourt, U., Pinchuk, I., and Lichtenberg, D. (2018). The cardiovascular benefits of indiscriminate supplementation of omega-3 fatty acids; meta-analysis and decision-making approach. Int. J. Food Sci. Nutr. 69, 549–556. doi: 10.1080/09637486.2017.1402868
Li, B., Wang, J., Huang, Y., Hao, T., Wang, S., Huang, B., et al. (2019). Effects of replacing fish oil with wheat germ oil on growth, fat deposition, serum biochemical indices and lipid metabolic enzyme of juvenile hybrid grouper (Epinephelus fuscoguttatus♀×Epinephelus lanceolatus♂). Aquaculture 505, 54–62. doi: 10.1016/j.aquaculture.2019.02.037
Li, J. J., Liu, Y., and Ma, J. (2013). Perspectives in DHA production of Thraustochytrids. Sci. Technol. Food Ind. 34, 367–371.
Li, M. H., Robinson, E. H., Tucker, C. S., Manning, B. B., and Khoo, L. (2009). Effects of dried algae Schizochytrium sp., a rich source of docosahexaenoic acid, on growth, fatty acid composition, and sensory quality of channel catfish Ictalurus punctatus. Aquaculture 292, 232–236. doi: 10.1016/j.aquaculture.2009.04.033
Li, Y., Li, M., Wang, M., Zhang, M., Ma, Y., Zhang, G., et al. (2019). Research advances in nutritional requirement and feed of Trachinotus ovatus. Prog. Fish. Sci. 40, 167–177. doi: 10.19663/j.issn2095-9869.20180314001
Liao, J. (2017). Golden catfish “industrial revolution” is about to start. Mar. Fish. 4, 24–25. doi: 10.3969/j.issn.1672-4046.2017.04.003
Luo, J., Rizkalla, S. W., Vidal, H., Oppert, J. M., Colas, C., Boussairi, A., et al. (1998). Moderate intake of n-3 fatty acids for 2 months has no detrimental effect on glucose metabolism and could ameliorate the lipid profile in type 2 diabetic men: Results of a controlled study. Diabetes Care 21, 717–724. doi: 10.2337/diacare.21.5.717
Ma, D. W., Seo, J., Switzer, K. C., Fan, Y. Y., McMurray, D. N., Lupton, J. R., et al. (2004). n-3 PUFA and membrane microdomains: a new frontier in bioactive lipid research. J. Nutr. Biochem. 15, 700–706. doi: 10.1016/j.jnutbio.2004.08.002
Ma, J., Shao, Q., Xu, Z., Zhou, F., Zhong, G., and Song, W. (2009). Effects of n-3 high unsaturated fatty acids on the growth and fat metabolism of juvenile black snapper. J. Fish. China 4, 639–649.
Miller, M. R., Nichols, P. D., and Carter, C. G. (2007). Replacement of fish oil with thraustochytrid Schizochytrium sp. L oil in Atlantic salmon parr (Salmo salar L) diets. Comp. Biochem. Phys. A 148, 382–392. doi: 10.1016/j.cbpa.2007.05.018
Pilarczyk, A. (1995). Changes in specific carp immune reaction caused by addition of fish oil to pellets. Aquaculture 129, 425–429. doi: 10.1016/0044-8486(94)00281-r
Ren, L., Sun, X., Zhang, L., Huang, H., and Zhao, Q. (2020). Exergy analysis for docosahexaenoic acid production by fermentation and strain improvement by adaptive laboratory evolution for Schizochytrium sp. Bioresource Technol. 298:122562. doi: 10.1016/j.biortech.2019.122562
Robinson, L. E., Clandinin, M. T., and Field, C. J. (2001). R3230AC rat mammary tumor and dietary long-chain (n-3) fatty acids change immune cell composition and function during mitogen activation. J. Nutr. 131, 2021–2027. doi: 10.1093/jn/131.7.2021
Scott, S. D., Armenta, R. E., Berryman, K. T., and Norman, A. W. (2011). Use of raw glycerol to produce oil rich in polyunsaturated fatty acids by a thraustochytrid. Enzyme Microb. Tech. 48, 267–272. doi: 10.1016/j.enzmictec.2010.11.008
Sun, H., Ye, Y., Yao, X., Wu, Y., Wang, X., Liu, Y., et al. (2014). Effects of partial replacement of fish meal by fermented cottonseed meal on growth performance, body composition and plasma biochemical indices of juvenile black sea bream. Chin. J. Anim. Nutr. 5, 1238–1245.
Thompson, K. D., Tatner, M. F., and Henderson, R. J. (1996). Effects of dietary (n-3) and (n-6) polyunsaturated fatty acid ratio on the immune response of Atlantic salmon, Salmo salar L. Aquacult. Nutr. 2, 21–31. doi: 10.1111/j.1365-2095.1996.tb00004.x
Tibbetts, S. M., Scaife, M. A., and Armenta, R. E. (2020). Apparent digestibility of proximate nutrients, energy and fatty acids in nutritionally-balanced diets with partial or complete replacement of dietary fish oil with microbial oil from a novel Schizochytrium sp. (T18) by juvenile Atlantic salmon (Salmo salar L.). Aquaculture 520:735003. doi: 10.1016/j.aquaculture.2020.735003
Tocher, D. R. (2010). Fatty acid requirements in ontogeny of marine and freshwater fish. Aquac. Res. 41, 717–732. doi: 10.1111/j.1365-2109.2008.02150.x
Tocher, D. R. (2015). Omega-3 long-chain polyunsaturated fatty acids and aquaculture in perspective. Aquaculture 449, 94–107. doi: 10.1016/j.aquaculture.2015.01.010
Wang, S., Wang, M., Zhang, H., Yan, X., Guo, H., You, C., et al. (2020). Long-chain polyunsaturated fatty acid metabolism in carnivorous marine teleosts: insight into the profile of endogenous biosynthesis in golden pompano Trachinotus ovatus. Aquac. Res. 51, 623–635. doi: 10.1111/are.14410
Wu, F. C., Ting, Y. Y., and Chen, H. Y. (2003). Dietary docosahexaenoic acid is more optimal than eicosapentaenoic acid affecting the level of cellular defence responses of the juvenile grouper Epinephelus malabaricus. Fish. Shellfish Immun. 14, 223–238. doi: 10.1006/fsim.2002.0433
Xie, J., Fang, H., Liao, S., Guo, T., Yin, P., Liu, Y., et al. (2019). Study on Schizochytrium sp. improving the growth performance and non-specific immunity of golden pompano (Trachinotus ovatus) while not affecting the antioxidant capacity. Fish Shellfish Immunol. 95, 617–623. doi: 10.1016/j.fsi.2019.10.028
Xu, Y. Q., Zhong, M., and Ding, Z. K. (2010). Influence and mechanism of polyunsaturated fatty acids on fish feed conversion ratio. Feed Ind. 8, 50–54.
Yadav, G., Meena, D. K., Sahoo, A. K., Das, B. K., and Sen, R. (2020). Effective valorization of microalgal biomass for the production of nutritional fish-feed supplements. J. Clean. Prod. 243:118697. doi: 10.1016/j.jclepro.2019.118697
Yamasaki, T., Aki, T., Mori, Y., Yamamoto, T., Shinozaki, M., Kawamoto, S., et al. (2007). Nutritional enrichment of larval fish feed with thraustochytrid producing polyunsaturated fatty acids and xanthophylls. J. Biosci. Bioeng. 104, 200–206. doi: 10.1263/jbb.104.200
Yin, Z. (2014). Towards sustainable sources forω-3 fatty acids production. Cereals Oils 11, 5–7. doi: 10.3969/j.issn.1008-9578.2014.11.002
Zhao, G., Etherton, T. D., Martin, K. R., Gillies, P. J., West, S. G., and Kris-Etherton, P. M. (2007). Dietary alpha-linolenic acid inhibits proinflammatory cytokine production by peripheral blood mononuclear cells in hypercholesterolemic subjects. Am. J. Clin. Nutr. 85, 385–391. doi: 10.1093/ajcn/85.2.385
Zhong, Y. F., Shi, C. M., Zhou, Y. L., Chen, Y. J., Lin, S. M., and Tang, R. J. (2020). Optimum dietary fiber level could improve growth, plasma biochemical indexes and liver function of largemouth bass, Micropterus salmoides. Aquaculture 518:734661. doi: 10.1016/j.aquaculture.2019.734661
Zirnheld, K. H., Warner, D. R., Warner, J. B., Hardesty, J. E., McClain, C. J., and Kirpich, I. A. (2019). Dietary fatty acids and bioactive fatty acid metabolites in alcoholic liver disease. Liver Res. 3, 206–217. doi: 10.1016/j.livres.2019.10.001
Keywords: n-3 polyunsaturated fatty acids, docosahexaenoic acid, polyunsaturated fatty acids, Aurantiochytrium sp., Trachinotus ovatus
Citation: Li S, Wang B, Liu L, Song Y, Lv C, Zhu X, Luo Y, Cheng CHK, Chen H, Yang X and Li T (2021) Enhanced Growth Performance Physiological and Biochemical Indexes of Trachinotus ovatus Fed With Marine Microalgae Aurantiochytrium sp. Rich in n-3 Polyunsaturated Fatty Acids. Front. Mar. Sci. 7:609837. doi: 10.3389/fmars.2020.609837
Received: 24 September 2020; Accepted: 08 December 2020;
Published: 24 January 2021.
Edited by:
Anthony Lee Dellinger, Kepley BioSystems, Inc., United StatesReviewed by:
Dizhi Xie, South China Agricultural University, ChinaMahdi Ebrahimi, Shahid Beheshti University, Iran
Copyright © 2021 Li, Wang, Liu, Song, Lv, Zhu, Luo, Cheng, Chen, Yang and Li. This is an open-access article distributed under the terms of the Creative Commons Attribution License (CC BY). The use, distribution or reproduction in other forums is permitted, provided the original author(s) and the copyright owner(s) are credited and that the original publication in this journal is cited, in accordance with accepted academic practice. No use, distribution or reproduction is permitted which does not comply with these terms.
*Correspondence: Xuewei Yang, yangxw@szu.edu.cn