- 1National Institute of Aquatic Resources, Technical University of Denmark, Lyngby, Denmark
- 2AZTI, Marine Research Division, Basque Research and Technology Alliance, Sukarrieta, Spain
- 3MRAG Ltd., London, United Kingdom
- 4Wageningen Marine Research, Wageningen University & Research, IJmuiden, Netherlands
- 5ILVO, Research Institute for Agriculture, Fisheries and Food, Oostende, Belgium
- 6Institute of Sea Fisheries, Bremerhaven, Germany
- 7IEO Spanish Institute of Oceanography, Madrid, Spain
- 8Division of Modelling and Management of Fishery Resources, Portuguese Institute for the Ocean and Atmosphere, Algés, Portugal
- 9Marine Institute, Oranmore, Ireland
The impacts of fisheries on ocean resources are no longer considered in isolation but should account for broader ecosystem effects. However, ongoing ecosystem-wide changes added to the inherent dynamics of marine ecosystems, create challenges for fisheries and fisheries management by affecting our ability to ensure future fishing opportunities and sustainable use of the seas. By reviewing a corpus of fisheries science literature, we contribute to informing managers and policymakers with considerations of the various threats to fisheries and the marine ecosystems that support them. We identify and describe 25 ecosystem challenges and 7 prominent families of management options to address them. We capture the challenges acting within three broad categories: (i) fishing impacts on the marine environments and future fishing opportunities, (ii) effects of environmental conditions on fish and fishing opportunities, and (iii) effects of context in terms of socioeconomics, fisheries management, and institutional set-up on fisheries. Our review shows that, while most EU fisheries are facing a similar array of challenges, some of them are specific to regions or individual fisheries. This is reflected in selected regional cases taking different perspectives to exemplify the challenges along with fishery-specific cases. These cases include the dramatic situation of the Baltic Sea cod, facing an array of cumulative pressures, the multiple and moving ecosystem interactions that rely on the North Sea forage fish facing climate change, the interaction of fishing and fish stocks in a fluctuating mixed fishery in the Celtic Sea, the bycatch of marine mammals and seabirds and habitat degradation in the Bay of Biscay, and finally the under capacity and lack of fundamental knowledge on some features of the EU Outermost Regions. In addition to these ecoregion specific findings, we discuss the outcomes of our review across the whole of European waters and we conclude by recognizing that there are knowledge gaps regarding the direction of causality, nonlinear responses, and confounding effects. All of the challenges we identify and characterize may guide further data collection and research coordination to improve our fundamental understanding of the system and to monitor real changes within it, both of which are required to inform an Ecosystem Approach to Fisheries Management (EAFM). An European EAFM could build upon an array of management measures currently tailored for fisheries management only, including promoting funding interdisciplinary research and ecosystem monitoring. Such integrative management should reduce uncertainties in environmental, social and economic trends, and lower the risk for disruptive events or ecosystem effects with far-reaching consequences, including a shift toward less productive marine ecosystems.
Introduction
The Ecosystem Approach to Fisheries Management (EAFM) can be defined as a systematic approach to fisheries management in a geographically specified area that contributes to the resilience and sustainability of the marine ecosystem (Long et al., 2015). This recognizes the ecological, economic, and social dimensions of sustainability affecting all fishery-related components of the ecosystem, including humans, and seeks to balance benefits among a diverse set of societal goals (e.g., Cowan et al., 2012; O’Hagan et al., 2020). One of those goals is to maintain ecosystems in healthy, productive, and resilient conditions so that they can support sustainable use in an equitable way, for example, employment opportunities or fish for human consumption, as well as providing habitats as supportive ecosystem services that will maintain long-term fishing opportunities and increase system biodiversity. Hence, EAFM is an integrated approach that recognizes the full array of interactions within an ecosystem, rather than considering single issues, species, or ecosystem services in isolation (see for example, the numerous integrated ecosystem assessment working groups in place in ICES1).
The overarching aim of the European Commission’s revised Common Fisheries Policy (CFP; EC, 2013) is to ensure that fishing is environmentally, economically, and socially sustainable throughout European waters and that an EAFM should be implemented to achieve this through a reduction of the environmental impacts of fishing activities. Thus, an EAFM should aim to supply the same level of provisioning services and their socio-economic benefits while reducing environmental impacts. The CFP requires an integrated approach to managing fisheries within ecologically meaningful boundaries; taking account of fishing and other human activities, while preserving both the biological wealth and the ecological processes necessary to safeguard the composition, structure, and functioning of the habitats within affected ecosystems. This requires fisheries scientists to utilize knowledge of, and to account for, uncertainties in biotic, abiotic, and human components of ecosystems (EU CFP Art 4.9).
In this study we reviewed the ecosystem challenges that might impede an EAFM’s goal of achieving sustainability across the ecological, social, and economic dimensions. An “ecosystem challenge” can then be defined in an EAFM context as causing some degree of impact on the ecological, social, and economic sustainability of a given ecosystem. We aim to identify and categorize all relevant ecosystem challenges that arise when addressing EAFM principles. These can come from scientific and gray literature or be identified as knowledge gaps. In order to systematically break down ecosystem challenges as expressions combining an action (some aspect of fishing), an ecosystem component potentially under threat, and a target (e.g., Good Environmental Status, Maximum Sustainable Yields). This should include all ecosystem components subject to environmental policy goals (e.g., Marine Strategy Framework Directive-MSFD) and that may be directly affected by specific fisheries and their management. It should also include indirect, or second order effects on the exploited stocks and possible effects of routine fisheries management. In addition, the stocks and fisheries may also be affected by wider environmental changes (e.g., climate). When considering the linkages between management, fisheries and the environment which work differently, we distinguish between an ecosystem effect, an ecosystem impact and an ecosystem challenge. An ecosystem effect is the potential that a change in one part of the link affects another. An ecosystem impact is an actual change in state of an ecosystem component (i.e., receptor) caused by one or more pressures (i.e., stressors). These may, or may not, be subject to management aimed at mitigating these impacts. An ecosystem challenge represents a specific suite of ecosystem effects that are expected to cause a significant ecosystem impact and result from a specific suite of changes in one or more of the pressures. The significance of the impact is determined by the magnitude of the pressure or the degree of change it causes on the ecosystem components.
The aim of this review of ecosystem challenges is to categorize the many possible impacts of fishing on the wider ecosystem, and the governance required by an EAFM to mitigate them. Fisheries operate in a full marine ecosystem context, which implies that we should take account of any mechanism through which fisheries impact any aspect of the ecosystem and vice versa. This would include both target and non-target species, but also habitats and food web functioning. We aim to capture this complexity through a simple conceptual model that splits the overall review into three sub-reviews: (i) fishing impacts on marine ecosystems including fishing opportunities, (ii) environmental impacts on habitats, marine resource and fishing opportunities, and (iii) framing conditions in socioeconomic drivers, fisheries management and institutional factors which impact upon fisheries, and then potentially modulate fishery impacts on the ecosystem.
The conceptual model forms the basis for subsequent work to link identified ecosystem challenges to specific fisheries and the possible management measures to address them. Because EAFM recognizes that fisheries are part of a social-ecological system and that exploited fish and shellfish stocks are not isolated entities, we consider all biological, environmental, economic, social, and institutional factors and interactions likely to influence fishery impacts on ecosystem integrity. More specifically, we expect these factors and interactions to influence the short and long-term stock productivity of the commercial stocks with fishing levels safeguarding reproductive capacity while maximizing yield.
Methods
The review methodology for this study followed a three-step approach, the first being record identification, the second screening and the third data extraction. Scientific article identification was based on three different sets of database search terms (Supplementary Appendix A); each of which corresponded to links in our conceptual model of the relationships between the environment, fisheries, and society at large, as described in the introduction. The search was undertaken using the Scopus literature database for scientific peer-reviewed literature on the 15th of February, 2020 and returned 462 results.
We undertook two levels of screening, coarse and fine. Initial coarse screening removed records that were duplicated within each review (i.e., 1, 2 and 3) but not those duplicated across the different reviews. This allowed individual articles to contribute to each of the relevant links in the conceptual model (Figure 1). Four of the identified articles were not retrievable or not accessible due to language barriers. Initial screening removed 96 articles out the geographic scope of this study; namely, European waters excluding the Mediterranean Sea and the Black Sea.
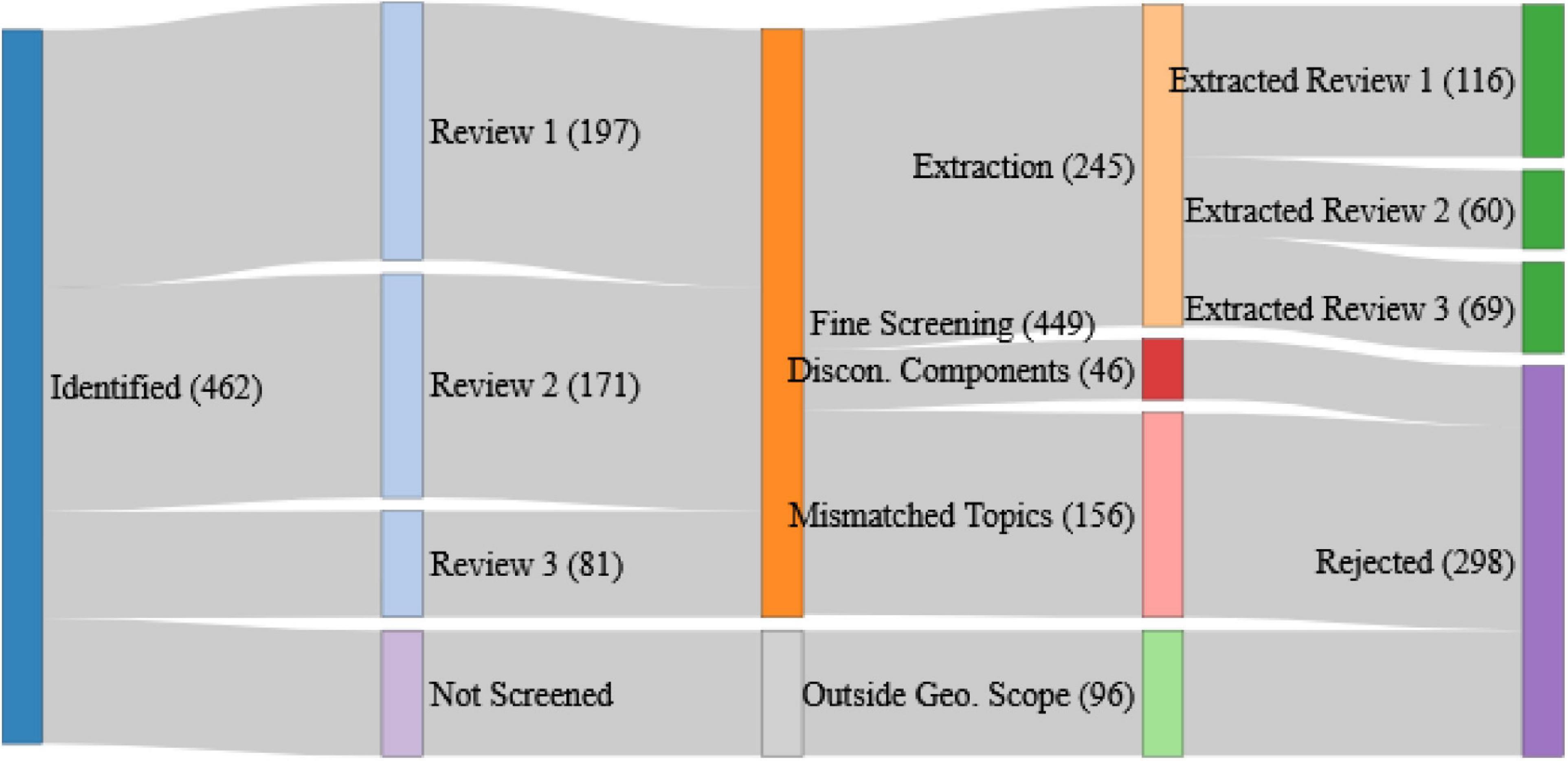
Figure 1. The fate of peer-reviewed articles identified from the defined search terms. Blue represents the pathway toward data extraction, dark green are the articles retained for extraction broken down by component review, red, pink, and light green represent the different reasons for article rejection at each of the course and fine screening (disconnected ecosystem components, mismatched topics, and studies outside the geographical scope, respectively), and dark purple represents all rejections across the whole process.
Data extraction was carried out in parallel to the fine level screening. The largest cause for article rejection was “Mismatched Review and Article Topics” (158), which was only detectable during the fine-scale screening when the detail in the article was being examined. The reasons for these mismatches were diverse but were often due to articles using terminology like our search terms but in a different context. We also experienced a substantial number of articles where relationships between ecosystem components were discussed but were neither qualitatively nor quantitatively described (46).
Of the 245 retained articles, data extractions were undertaken for 116, 60, and 69 for review one, two, and three, respectively. Data extractions were made against a fixed set of criteria (in Supplementary Material) covering the setting and scale of the study, the methodological approach, the ecosystem components considered, the magnitude and direction of any detected effects, as well as any remaining challenges, management suggestions, or links to advice that were discussed. These extractions were undertaken by one author per component review to ensure continuity within each review.
To supplement the three primary literature reviews, we also conducted reviews of recent gray-literature reports and surveyed a network of European based fisheries researchers. The supplementary gray literature came from the International Council for Exploration of the Seas (ICES), Scientific Technical and Economic Committee for Fisheries (STECF), and European Parliament Committee on Fisheries (PECH). We selected the latest (2019-20) reports from 17 ICES working groups that provide a scientific basis for advice. Likewise, seven of the most recent PECH reports (in 2019), twelve STECF (2017-20) plenary reports and one STECF expert working group report were selected. The survey of fisheries researchers (Supplementary Material Annex 1) was a simple spreadsheet survey, emailed directly to, and undertaken independently by, 13 partners from a pre-organized consortium of researchers, all of whom had project funding to contribute to this task. The purpose in asking individual institutions was to collect case specific information and obtain an up-to-date list of ecosystem challenges the local fisheries in Europe are facing. Using our ad hoc network of experts was our best source of expert knowledge to inform local issues beyond the usually more generic ones found in peer-reviewed literature.
The extracted data from all three sources (the three primary literature reviews, the gray literature review, and the survey) were summarized by combining the individual works’ descriptions of explicit challenges into challenge categories; first, according to the original literature searches (fishing impacts, environment, and societal context), and eventually into sub-categories.
Results
We present the findings of our investigation on ecosystem challenges in an overview conceptual figure (Figure 2), and we list the reference to each challenge in Table 1. The references that support each ecosystem challenge we identified are stored in this table therefore not repeated in the below text.
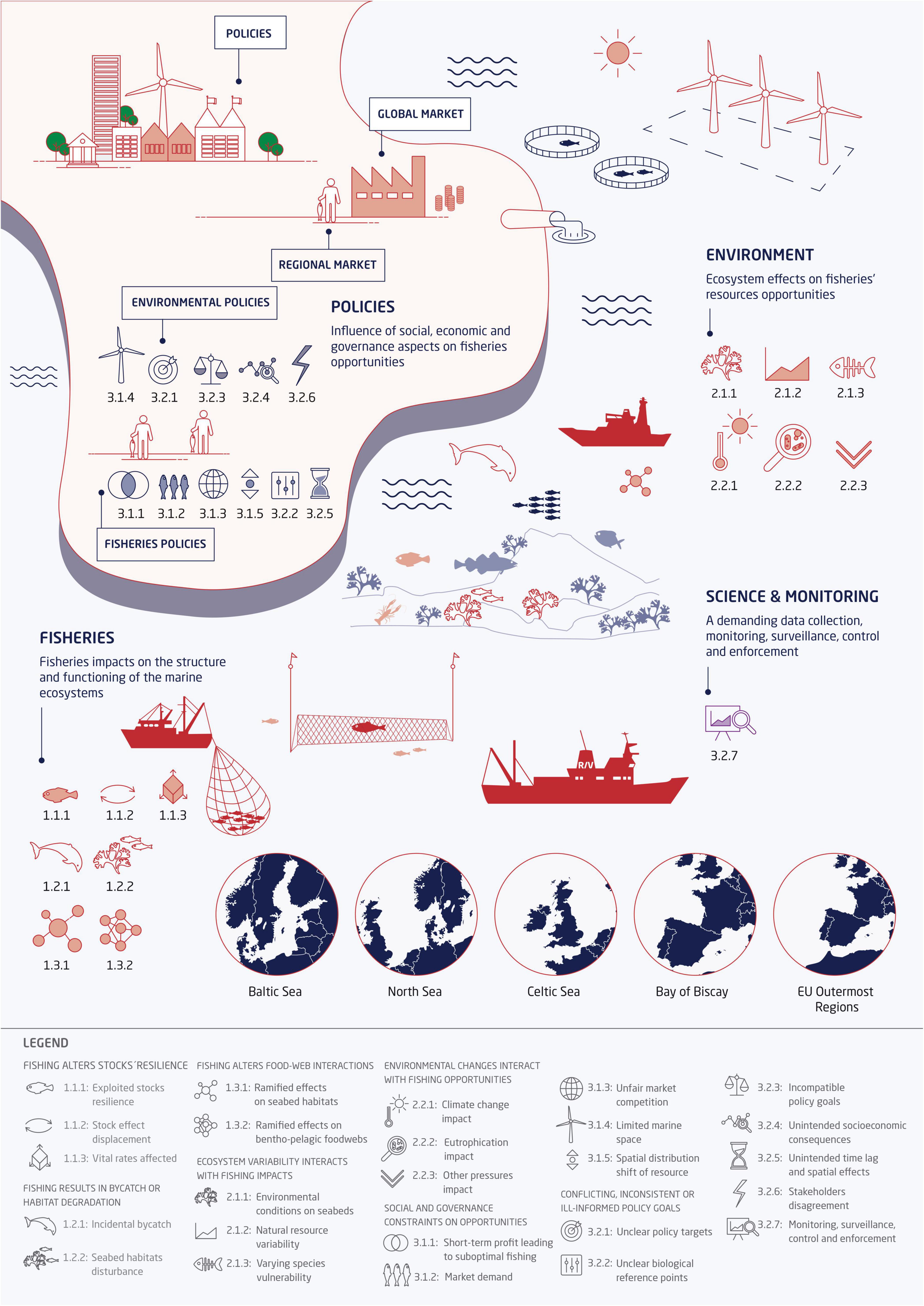
Figure 2. Conceptual model of fisheries as a component of an ecosystem that both affects, and is affected by, other components. These other components are categorized under societal benefits and imposition, as well as environmental components, which encompass natural variation as well as changes brought about indirectly by human activity. Reviews indicate the different sets of search terms used at the review’s identification stage. Each review covers a single direction of action; fisheries effects on society was not a part of this review.
Ecosystem Challenges
Fish Extraction Alters Exploited Stocks’ Resilience
Fishing is an economic activity that is intrinsically dependent on the productivity of the exploited stocks. Altered stock size or size composition from selective fishing (challenge 1.1.1 Exploited stocks resilience) may have far-reaching consequences by altering the resilience of the exploited populations with long-term stock productivity at stake. By selectively extracting fish over a certain size threshold, many fisheries targeting long-lived species reduce the relative biomass of larger, more fecund fish, reducing the population’s ability to renew itself. Fishing changes stock structure, body size condition and stock abundance, sometimes to the point that the stock ceases to be a viable fishing resource. Besides this, poorly or incorrectly defined stock identities put the stocks at risk when fishing activity is sanctioned to remove fish according to their catch allowance but disproportionately from the component sub-stocks (challenge 1.1.2 Stock effect displacement).
Over a long period of exploitation, size-selective extraction can lead to a change in vital rates (challenge 1.1.3 Vital rates affected) and adaptations, such as the earlier onset of maturation, smaller maximum size and lower growth rates. All of these can reduce the resilience of a population to internal-stochastic pressures (e.g., lower population-level reproductive output) and external, natural pressures (e.g., higher predation pressure on smaller sizes). Truncating the size structure of a population, fisheries can reduce both the geographical range and accessibility of different habitats available to the population, reducing its access to refugia and resilience to localized perturbations. The productivity of a population with a higher proportion of its biomass in smaller, younger individuals is also more sensitive to inter-annual environmental fluctuations.
Fishing Results in Loss of Biodiversity (Bycatch and Habitat Degradation)
Fisheries exploitation does not only affect target species populations. It also affects the fish community structure and diversity of non-target species through bycatch, i.e., the incidental catch of undersized classes of the target species and other non-target species or unwanted low-priced fish, changing the total biomass and species composition of the ecosystems (challenge 1.2.1 Incidental bycatch). There are some indications that the composition of fish communities in any given area have changed over long time periods, leading to long-lasting impacts on marine ecosystems. Demersal trawling causes disturbance to the seabed, altering benthic communities’ structure as well as the habitats’ physical structure, both of which impact upon ecosystem functioning, including the habitat suitability for the fish community being targeted (challenge 1.2.2 Seabed habitats disturbance). The impact mainly depends on the fishing gear used, the type of habitat and the fishing intensity. These impacts also depend on the level of natural disturbance and the degree of species sensitivity (see environmental context challenges). In the long run, bottom trawling may affect the recovery time of production or biomass of benthic habitat.
Concerning the protection of vulnerable species, there is an inherent challenge in raising the effects of observed fishery effects to the population level. This is because knowledge of how by-catch affects the by-caught species’ ecology and population size is difficult to attain. Reliable bycatch data are only available from sparse fisheries observer reports and are rarely found in fishers’ logbooks. Population size is also difficult to obtain, and usually comes from sight surveys that also involve many assumptions in raising. The lack of reference points (i.e., for numbers or removals) for many by-catch species is a direct consequence of these knowledge gaps and represent a fundamental problem in managing impacts on vulnerable species. Hence, currently, there are typically no guidelines defining bycatch limits for elasmobranchs, marine mammals, or marine seabirds for mortality levels that could be deemed as sustainable.
On the seafloor, the direction of causality is sometimes ambiguous, several explanatory factors may be confounded, and while correlations may be observed between environmental factors and trends in relative abundance, establishing causal relationships in such data sets is problematic. The impact of trawling on benthic habitats is nonlinear. Some habitats are affected much more strongly than other habitats equal trawling intensities. The practice of studying fishing disturbance spatially, does not provide adequate knowledge to overcome this challenge because a common problem is the lack of proper control sites with which chronically disturbed areas of the seabed can be compared. Equally, a baseline shift in benthic communities due to chronic fishing disturbance has probably already occurred in some areas.
Fishing Alters Food-Web Interactions
Fishing can provoke a change in trophic interactions among predators and prey in benthic, demersal and pelagic systems – changing the balance between predator and prey abundance, or leading to prey switching. These changes can also interact with multiple other pressure effects to drive change in the abundance and density of not just the targeted living marine resources but also, the associated communities. This includes the diversity of responses to fishing perturbations from different communities due to the inherent biological variation in resilience and vulnerabilities. A well-documented example of such a perturbation is the impact of fishing activities upon benthos communities and their habitats (challenge 1.3.1 Ramified effects on seabed habitats), which can create unexpected outcomes, for example, the release of predation on r-selected species, the loss of functional redundancy that contribute to the resilience of the benthic habitats, etc. Such changes can result from direct impacts of extraction and habitat modifications or indirect impacts such as modified predation pressure.
While fishing has well-known direct effects on the size-structure of targeted fish populations, by contrast, the indirect effect of changing predator-prey balance ratios is documented to a lesser degree. At the same time, it may induce a diverse set of responses including indirectly reducing primary production, the energy transferred to fish and hence, the carrying capacity or the maximal possible productivity of the impacted ecosystem (challenge 1.3.2 Ramified effects on bentho-pelagic food webs). Hence, fishing can change trophic interactions with possible far-reaching indirect changes. By selective removal of species (targeted or by-catch), fishing can cause trophic cascades and change the species composition of the marine ecosystem. Under such circumstances, some ecosystems are likely to switch to an alternative stable state dominated by small pelagics and marine mammals with unanticipated consequences on other commercially and/or ecologically important species.
Some species can be very sensitive to fishing while being the keystone species, i.e., low abundance species which have a high disproportional impact in directing foodweb and ecosystem functioning. Key ecosystem species also include large biomass pelagic stocks typically in upwelling systems with a critical role in both structure and function of the ecosystems. Depleting these species poses an ecosystem challenge by causing substantial changes compared to unexploited ecosystem functioning. These effects of fishing on keystone species and important habitats occur in both coastal and offshore fisheries, nor are they limited to just benthic habitats, as they impact upon ecosystem functioning in the pelagic realm too.
The importance of benthic versus pelagic pathways is also poorly studied. However, a change in the strength of these pathways would lead to change in the relative biomass of stocks and therefore, the long-term sustainable yields of the fisheries they support. In some areas, the ramification effect through trophic cascades and multiple pressure interactions is proven to be weak and change in the ecosystem likely reflects selective removals and thus a direct effect of fishing. There are challenges in identifying the direction of the causality and disentangling these confounding effects. At the same time, there are signs of non-linearity and sometimes evidence that fishing pressure is not the main effect causing impacts on the measured ecosystem components. Most time series of data began long after the development of fisheries and, therefore, do not integrate long periods of overfishing. The lack of contrast due to the relative shortness of time-series likely impairs the detection of ecosystems effects. Using historical data as a reference state might not be sufficient to reveal effects when the lasting impact keeps the system in an altered state.
Inherent Ecosystem Variability and Ecosystem Component Attributes Interact With Fishing Impacts Affecting Fishing Opportunities
Changes in the biophysical environment can occur naturally, at fine and large spatial and temporal scales – from days to geological time scales. Any such change in environmental conditions can affect the abundance, density or vital rates of exploited marine living resources, and hence, affect their accessibility or productivity for exploitation in fisheries. In seabed habitats, fishing and environmental conditions can combine to indirectly contribute to reducing the abundance of benthic food for commercial fish in a wide range of habitats and hence lowering the carrying capacity of the habitats for exploited benthivorous species (challenge 2.1.1 Environmental conditions on seabeds).
The effect of environmental changes on exploited species is difficult to discern because fishing pressure is so ubiquitous that partitioning off these effects must be done without an unexploited population as a control. Almost all articles under the environmental context to fisheries review used fishing pressure as a covariate in their analyses or included strong caveats in their discussion regarding the unavoidable effects of fishing on their results. In some circumstances, the fishing disturbance may have minimal effect compared to natural disturbance (e.g., Baltic Sea), while in others fishing may cause long-lasting or irreversible changes to marine ecosystems (e.g., Vulnerable Marine Ecosystems; VMEs). Similarly, changing environmental conditions may mitigate some effects of fishing pressure through so-called counteracting or compensatory effects (challenge 2.1.2 Natural resource variability), or these changing conditions may exacerbate the effect of fishing pressure through synergistic or cumulative impacts. There are, however, examples where the direction of the effect is not ambiguous. Fish stock-recruitment is sometimes heavily influenced by environmental drivers, where changes in primary productivity affect recruitment success.
There are many specific knowledge gaps regarding individual species’ vulnerability to exploitation. Intra-specific variation in life-history traits or strategies can have different effects on the vulnerability across stocks. In particular, slow-growing species can be more challenging to obtain appropriate data with which to estimate stock size, while they are at the same time likely to be more vulnerable to exploitation (challenge 2.1.3 Varying species vulnerability). The interactive effect of fishing and environmental change is poorly understood, partly because of the lack of non-exploited stocks or areas to consider the effect of environmental changes in the absence of fishing pressure.
Anthropogenic and Environmental Changes Interact With Fishing Opportunities Individually and Collectively
Large-scale changes, such as those caused by climate change (challenge 2.2.1 Climate change impact), can act directly or indirectly on exploited species. Direct effects of increasing temperature include changes in growth rate, the timing of biological processes, or forcing poleward or deeper water shifts in distribution, reduced ranges due to other geographic boundaries limiting distribution shifts, as well as changes in local assemblage structure and the productivity of different species in those assemblages. Indirect effects arise when climate change affects the prey availability and quality for exploited species, which also may influence their spatial distribution and productivity. Generally, current changes caused by the global warming benefits southern warm-water species against northern cold-water species.
In some cases, the overall trend may not be a directional change in the mean conditions, but an increasing inter-annual or seasonal variability. Phenomena such as marine heat-waves, or periodic fluxes in regional hydrology can exclude keystone species from an otherwise productive area, reduce primary productivity in areas of upwelling or mixing, or even prevent populations from completing their life cycle.
Where species and communities may have a high tolerance and/or low exposure to these environmental changes, the additive or synergistic effect of fishing may nevertheless increase their vulnerability and force a shift in the ecosystem structure past some tipping point, which prevents a return to the previous state even after the total cumulative pressure is reduced or removed.
Eutrophication and fishing pressure combine to alter the dynamic of the marine ecosystems (challenge 2.2.2 Eutrophication impact) via changed oxygen conditions on the seabed with changed biological interactions between functional groups. Eutrophication encourages phytoplankton blooms and promotes the growth of other ephemeral algae that die, sink and decompose, consuming the oxygen of bottom-waters. This is especially pronounced during summertime in coastal habitats. Areas of low-oxygen reduce the habitat available to many species by directly excluding them or by causing reduced benthic productivity or die-off of prey species. The loss of potential habitat also reduces the access to potential refugia from fishing.
Fishing is not the only disturbance factor for exploited species. As defined specifically for climate change and eutrophication above, fishing interacts with other pressures, both natural and anthropogenic, to form complex mediating, additive or synergistic effects on different exploited species (challenge 2.2.3, Other pressures impact). A diverse array of pressures affects the ecosystem, including interactions with land use, coastal development and pollution of coastal waters with chemicals, pesticides, noise pollution, marine litter, and micro-plastics. European seas are now subject to massive development of marine infrastructure including energy facilities, e.g., offshore wind farms, exploitation of wave energy, and development and implementation of marine aquaculture. Pathogens can be introduced via aquaculture sites. Invasive species in some parts of the world can appear to restructure the benthic invertebrate community with a more substantial effect than bottom trawling. The stocking and transfer of non-native species may have many unintended effects. They may threaten native aquatic biodiversity at all levels including genes, populations, species, and ecosystems, with subsequent loss of yield and the reduction or even extinction of native species or populations.
Social and Governance Constraints on Fishing Opportunities
Suboptimal fishing (challenge 3.1.1 Short-term profit leading to suboptimal fishing), arise where higher yield or revenue might be obtained with a different level of fishing pressure. This often arises from mismanagement, sometimes originating from unforeseen and unintended socioeconomic consequences in a situation where a tradeoff exists between resource productivity and fleets’ immediate demands. Suboptimal fishing arises from imbalanced fleet capacity when there is a mismatch between the scale of the resource exploited and the scale of the fleets involved in a single stock fishery which can then lead to higher TACs to support these overlarge fleets. In many cases, suboptimal fishing can occur due to quotas being set higher than scientifically advised, because of the social pressure to keep fishing vessels in business. Suboptimal fishing also arises in multispecies and multi-gear fisheries, whenever different nations or fishing métiers can interact to the detriment of each other, the target species of one activity being the bycatch of another. Fisheries are often focused on immediate short-term profit that can overwhelm any longer-term consideration and by-product. Hence, even when seeking the maximum sustainable yield based on assumed stable past conditions, setting the catch allowances for the year to come may largely ignore potential long-term effects that would reduce the chances of meeting the CFP objectives. The decisions on TACs are often made based on perceived short-term socioeconomic impacts to the detriment of long-term sustainability goals, primarily for TACs outside an adopted long-term management plan. The search for immediate profit, or merely economic sustainability, and securing jobs may lead to an escalation in fishing power or the maintenance of an oversized fleet that has possible far-reaching consequences on both the ecosystem and the economy of the fishing sector (e.g., overcapitalization).
The market demand especially for non-regulated fish species partly influences which species assemblage fishers choose to target and what might be considered optimum yields, and with which fishing methods, with possible far-reaching effects on marine foodwebs and a changing exploitation pattern and footprint on marine habitats (challenge 3.1.2 Market demand). There are several factors that individual fishers have limited or no control over, such as, among others, stock size and fish movement, fisheries management including quota allocation or other regulations, weather, operating costs, fish prices, and labor. Inshore fleets are particularly challenged when average prices of the harvest might not increase in line with operating costs, leading to decreased profitability.
In a globalization context, there are market forces such as differential competitiveness, market vertical integration, consolidation, and product substitution that pose a challenge to some fisheries (challenge 3.1.3 Unfair market competition). Market substitution may also occur as a side effect of implementing “best practices” for EU fleets, leading potentially to an inevitable loss in competitiveness when all fishing activities do not operate under strictly similar social conditions that would push for lowering labor costs and social standards. This is especially the case between small-scale and large-scale vessels targeting the same stock or species where larger vessels usually fish with lower net costs.
Fisheries may experience competition for limited marine space (challenge 3.1.4 Limited marine space), including competition among different fishing practices (passive versus active gears), with recreational fisheries, or with different users at sea beyond the fishing sector (offshore windmill farms, aquaculture, etc.). Fishers are also challenged to be part of the “problem-solving process” that aims for coexistence with other marine users. The governance challenge is expanded from optimum yield to optimum combination of ecosystem services. Displacing fishing activities protecting areas or reserving to other marine sectors may lead to increasingly congested areas, as open space is getting increasingly scarce. Congested areas are the ground for conflicts and can further induce safety concerns, whenever mutually exclusive activities conflict for occupying the same marine space. Spatial displacement of fisheries may also induce costs when vessels need to spend more time steaming to reach a productive fishing ground. Displacement may also result in damage to previously unfished grounds with unknown ecological impacts.
Shared or straddling stocks (such as widely distributed pelagic stocks) are of particular concern, as they can change in distribution or productivity and rapidly encourage conflict between states and destabilize international agreements when moving in or out of territorial waters of multiple nations or even neighboring communities with a state due to changes in species distributions caused by climate change (challenge 3.1.5 Spatial distribution shift of resource). The “Brexit” challenge is another good illustration of uncertainties regarding the way shared stocks may be managed in the future outside EU waters, with possibly contradicting policy goals across borders.
Conflicting, Inconsistent or Ill-Informed Policy Goals Across Industries and Stakeholders
Fisheries or ecosystem policy targets are not always defined at the operational level (challenge 3.2.1 Unclear policy targets), which leads to different interpretations. One example is the lack of threshold levels for pressure and impact indicators that relate to the GES of seafloor integrity to be derived. Another example arises when fishing one species may indirectly affect other species and ecosystem components. In such a situation, the EU CFP further lack of agreed guidance on priority to be given to objectives when tradeoffs have to be made between the return from fishing and the acceptable environmental impact. In particular, social objectives tend to be simplified or are downplayed within fisheries management. There might also be some balance, but that might be more in terms of acceptable environmental impact (which has a social dimension) than social objectives.
Fisheries management is generally based on the assessment of the fishing impact on a single stock but achieving MSY for an individual stock can hamper the achievement of MSY for other stocks (challenge 3.2.2 Unclear biological reference points). In addition to species interactions, the biological reference points should not ignore environmental determinants.
There is a risk for fisheries management or fisheries policy goals to ignore conservation issues or other ecosystem considerations besides the targeted stocks, or otherwise account for some but only partially mitigating the fishing impacts. A fishery policy not addressing conservation issues will contribute to degrading ecosystem components without commercial fishery interest through, e.g., bycatch or habitat degradation (challenge 3.2.3 Incompatible policy goals). Objectives prioritizations is a way forward; however, sometimes, tradeoffs are inevitable. This is because “saving fish or habitat” can be a reward in the long-term but costly for the fisheries exploiting them in the short-term, or to the society at large that has to bear the costs for conservation. To rationalize these tradeoffs, the focus should be on the design of institutions to deliver knowledge to both environmental and fisheries policies integrated.
Mismanagement with technical measures (gear modifications or closed areas to fishing) can arise both on the measures failing to reach its goals for protecting the ecosystem, and on the measures affecting the fishing opportunities and economy adversely (challenge 3.2.4 Unintended socioeconomic consequences). Some fisheries management such as the landing obligation could have a limited effect for ensuring future fishing opportunities, given the reluctance of fishers to take actions, e.g., for improving selectivity in responding to regulation. However, the decision to include a lot of possible exemptions in CFP Art. 15 of the basic regulation made it easy for the fishing sector to lobby for prolonging the status quo.
A challenge is posed by time lag for changes to occur or effects to be measured given the ecosystem inertia, possibly creating misunderstanding or mismanagement (challenge 3.2.5 Unintended time lag and spatial effects). On the other hand, rapid changes in the ecosystems can create a rapid mismatch between the component to protect and the protection, making the management irrelevant. The time required to translate knowledge to policy is also contributing to the delay.
There are conflicting opinions among stakeholders inducing lack of social acceptance with/non-compliance to the rules or a possible cause for management failure (challenge 3.2.6 Stakeholders disagreement). Finding the compromises needed to build common ground is a challenging exercise. For lowering the level of distrust and fears of patronization, the challenge is to leave some room for regional divergence while maintaining some fundamental CFP principles and objectives applicable to all fisheries. Unfortunately, regional groups are facing a lack of scientific expertise. However, it appears that social acceptance of the rules is critical to management success if the performance of management is affected by the social, economic, and institutional dimensions. For example, the successful use of technical measures appears to depend mainly on their acceptance by industry. Some rules, such as closed areas or seasons, are particularly prone to low acceptance.
All these ecosystem challenges require a reporting system to collect data on an array of aspects (biological, environmental, socioeconomics, and human behavior data) and a routine basis to acquire new knowledge and understanding of the ecosystems but also to monitor the change (challenge 3.2.7 Monitoring, surveillance, control, and enforcement). Monitoring fishing impacts suppose developing fisheries-oriented indicators for measuring the fishing impacts and indicators for measuring the performance of the management measures taken to oppose the fishing impacts. There is equally a need for indicators that would reflect the fish and fish communities dynamics, and the oceanographic changes. Sustainably managing natural resources requires a greater understanding of community dynamics and ecosystem functioning under changing environmental conditions. A changing environment would suggest studying the ecosystem dynamics at the right time and scale resolution.
Management Measures for an EAFM
There are numerous management measures in place to manage European fisheries (e.g., the Technical Measures EC Reg 2019/1241). Among them, there are measures as part of an EAFM aimed at resolving the ecosystem challenges we have identified (Figure 3). Additionally, there are other European environmental policies (Habitat Directive, Water Framework Directive, Marine Strategy Framework Directive) which an EAFM should consider to bridge the historical divide between conservation and socio-economic goals. Below we list proposals put forward by studies we reviewed:
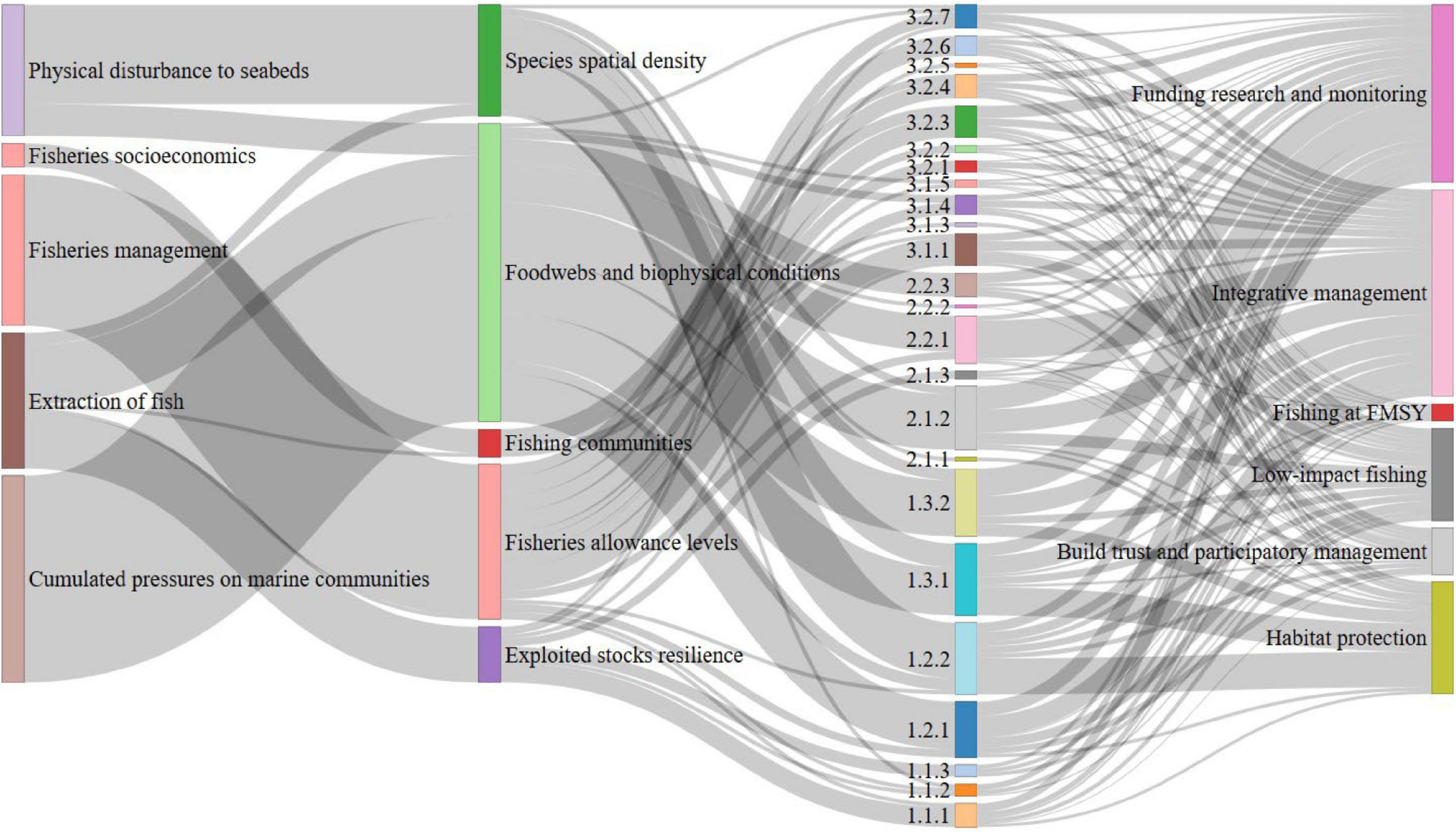
Figure 3. The links between the impacting pressures, the impacted ecosystem components, the ecosystem challenges identified from the review that arise from the combination of pressures, and the suggested management actions to oppose the challenges. The width of the links represents the frequencies of occurrences cited in the screened studies.
Promoting integrative management. An EAFM should set the desired exploitation level acknowledging potential conflicts between fisheries (e.g., one fishing on a species that serves as prey to other fisheries) or other maritime sectors, and synergies with the environmental protection (Kraufvelin et al., 2018). In addition to this, the harvest control rules should be responsive to, and account for, changing environmental conditions. Mitigation measures should be strategically implemented to preventing adverse effects on critical habitats of fish, and any vulnerable or protected marine species (Christensen et al., 2015; Costalago et al., 2019). Different options should be presented to stakeholders, and policymakers prioritize in regard to these tradeoffs (Lynam et al., 2017; Chagaris et al., 2019) (for selecting different closed areas, etc.).
Ensuring habitat protection of essential and productive fish habitats, seabeds, and sensitive habitats. Protected areas should be developed together with specific spatial management plans (allowance for certain fishing practices, etc.) and identify priority areas for management (Mérillet et al., 2018). In addition to such spatial (and/or temporal) closures, other conservation measures such as gear substitution or shifting to low seabed impact gear, should be further developed (e.g., replacement of bottom contacting trawl doors with pelagic trawl doors, Krag et al., 2016). Utilizing knowledge on the distribution of Vulnerable Marine Ecosystems (VMEs) in setting spatial restrictions will aid in maximizing biodiversity preservation by restricting any future fishing to the historic footprint. It could also be important to prioritize the protection of areas that have been found so far unsuitable for trawling and hence, keep them untrawled before new gears could develop to exploit them. Finally, areas identified as lightly fished are often those with high recovery potential (especially of the benthic community) and could be protected with both minimal harms to current fishing activities but the maximum effect for ecosystem function. For ensuring an acceptable tradeoff with the economic return, some management plans should aim at displacing the fishing effort from marginal (typically low catch rates) fishing grounds to core fishing grounds (high catch rate areas) (Bastardie et al., 2020).
Promoting low-impact fishing with adaptive, or precautionary management. Fishing catch levels should both adapt to changes in productivity and survival of the exploited stocks (e.g., arising from background environmental changes) and to the bycatch levels of unwanted fish. The management should help at changing the level of catch to balance and adapt to the fishing opportunities as well as balancing with impact associated with a given level of catch. Low impact fishing should be promoted by developing technical measures that include gear modifications and innovations (Wallace et al., 2008; Žydelis et al., 2009), and spatial management with demonstrated performance in reducing bycatch (e.g., sorting grids, benthos release panels, closed areas, and seasons) for both the commercial and recreational fisheries. Some actions should be taken to improve the registration of lost gears and limit ghost fishing (Maes et al., 2018).
Fishing at FMSY. Fishing at FMSY should rebuild the overexploited stocks (Rindorf et al., 2017). Meanwhile, fishing at FMSY will limit the effort spent to catch the fish and therefore limit the adverse effects of repeated disturbance or the extending of the footprint from searching the fish over a larger area. If fishing at a balanced level with the fishing opportunities should contribute to avoiding suboptimal fishing in mixed fisheries, this could also contribute to reducing fuel consumption in fisheries along with fuel-efficient gear innovations or substitutions (Ziegler and Hornborg, 2014).
Funding research and monitoring. Research should help include multispecies, and ecosystem model approaches in fisheries management (Chagaris et al., 2019). Exploitation levels for stocks should be developed in consideration with other stocks. EAFM requires more mechanistic knowledge, for example, about how environmental variables relate to climate change and affect the stocks at all life stages and spatial distribution. Monitoring is necessary to determine whether management objectives are achieved, and monitoring, for example, habitat protection, requires first knowledge acquisition (e.g., mapping conflicts areas for delineating spatial reservation, impact assessment). Funding should promote innovation for developing selective gears that help to avoid unwanted fish. In EAFM, innovative technical measures could oppose indirect fishing effects on marine food webs. Social science research should help to identify the social and cultural significance of commercial fishing for different coastal regions, including community dependence on fishing, and climate vulnerability assessment. Support for capacity building can be essential in some regions (the ORs) to collect the data required by a proper stock assessment, and eventually support indicator-based management (Casini et al., 2011; González-Irusta et al., 2014). Strategic perspective with science-based evidence and scenario testing may be able to anticipate some effects of fishing pressure under background environmental change. Case studies could be the basis for scaling-up local knowledge of structure and functioning of the marine ecosystems and fisheries, combined with a bio-economic modeling approach at the national or regional level investigating future scenarios, to illustrate to what extent natural resources could contribute to society through various possible spatial management plans and across different social, economic, and governance contexts. Finally, alternatives should be explored where data-poor or low-cost EAFM methods would need to be identified to address the particular (e.g., small-scale fishing) challenges of OR fisheries.
Build mutual trust with a participatory, regional approach. Results-based management system put the focus on the local fishers to develop gears that will avoid unwanted catches (Alzorriz et al., 2016) including, e.g., the development of seal safe fishing gears. Co-management or participatory approaches could help for better compliance (Haapasaari et al., 2007; Röckmann et al., 2012) by increasing the mutual trust between the local fishing communities and the public administration and prevent the imposition of issues for conservation only (Ramírez-Monsalve et al., 2016; Maya Jariego et al., 2018). It should also promote the involvement of small-scale fishers that are typically poorly organized. There has recently been more of an emphasis on “co-design” as an aspect of co-management where challenges and objectives are agreed, and measures are developed together with scientists or by fishers and reviewed by scientists.
Develop market instruments. Along with promoting low-impact fishing, creating a brand or a certification is advised to maintain and receive a price premium on the catches or at least keep market access in case, for example, wholesalers demand only certified fish. Vessels that are members must comply with several criteria for the catch activity among short trip duration, the gear to use, the period for fishing, etc., all kind of criteria that the fisheries and marine science could have demonstrated to be efficient at ensuring sustainable use of the exploited seas (e.g., Floc′h et al., 2008).
Discussion
Our review identified a collection of generic ecosystem challenges that result from the analysis of a corpus of scientific studies focusing on ecosystem effects in fisheries science, supported by advisory reports. The list arises from topics that justified the scientific investigation in the reviewed articles but also the remaining issues that the authors mentioned for future work. In the discussion, we move transversely through the list of challenges, with illustrations within selected case studies across EU waters. This approach illustrates some of the different regional perspectives that a pan-European EAFM should take into account. We selected the following cases: (i) Baltic cod, from the single-stock perspective toward working on a fully fledged EAFM, (ii) the exploitation of forage fish in the North Sea, a demonstration of close benthopelagic coupling, (iii) the Celtic Sea mixed fisheries, where suboptimal fishing and conflicting policy objectives dominate the issue, (iv) Bycatch and habitat degradation in the Bay of Biscay, and (v) the current under-capacity and lack of scientific studies in some of the EU Outermost Regions. To complete the perspective, we provide Supplementary Material for referring to the described challenges per fishery obtained by collecting fisheries and marine ecosystem local expertise.
Case Study: Baltic Sea Cod
In the Baltic Sea, there are two genetically distinct cod stocks with considerable mixing of stocks (Hüssy et al., 2016). Such a stock mixing between eastern and western Baltic cod can jeopardize the reliability of fish stock assessments and the fishery’s economy by blurring the area-based management of catch limits (challenge 1.1.2). Ignoring such bio-complexity could lead to overexploitation of one of the component stocks in any given management area by setting imbalanced and higher catch levels, based on the productivity of the stocks in combination, than the individual component stock would be able to support (Bastardie et al., 2017). A vicious circle on the exploited population’s capacity to renew can significantly jeopardize future fishing opportunities (challenge 1.1.4). Concurrent to these fisheries derived challenges, environmental conditions can change radically jumping from a long stable regime to an alternative transient or steady-state, unpredictably (challenge 2.1.2) changing long-term vital rates (challenge 1.1.2). Eastern Baltic cod recovery capacity may deteriorate under these new environmental regimes and predicting the capacity of the stock to recover via improved recruitment may become more challenging. A clear example of such a limitation is the effect of increasing temperature in the Baltic Sea on Baltic cod larvae; reducing the spatial and temporal window for survival (Voss et al., 2019). Increasing areas of hypoxia and anoxia (challenge 2.2.2) also directly affect the available suitable habitat sizes (Voss et al., 2019). Such environmentally induced reductions of suitable habitat increase exposure to fishing by amplifying the effect of the environment when the same fishing pressure is applied to a reduced productive capacity (Isomaa et al., 2013). One primary source of uncertainty arises from predictions of environmental change, including but not limited to the magnitude of future climate change (challenge 2.1.3) and future water salinity concentration. The uncertainty in the predictions of future environmental conditions drive uncertainty in Baltic cod recruits, and hence, future fishing opportunities for cod (Thøgersen et al., 2015). So far, the water volume with suitable conditions for cod reproduction has remained relatively constant from 1993 to 2017 (von Schuckmann et al., 2019). However, a change in water salinity would perturb the usual patterns of water stratification and hence, cod reproductive success through the survival of eggs. Climate and fishing opportunities projections should account for the significant Baltic inflow events shaping a suitable environment for cod reproduction (Röckmann et al., 2007). Projecting future fishing opportunities should also account for eutrophication effects (challenge 2.2.2), because the effects of ambient oxygen conditions affecting benthic organisms, especially in the coastal zones, will cascade to adversely impact the growth and development of their predators, including the Baltic juvenile cod (Bossier et al., 2018).
The Baltic cod population dynamics are determined by competition and predator-prey interactions, depending on the spatiotemporal overlap between Baltic cod, herring and sprat (Lindegren et al., 2014). Because of the relatively low complexity of trophic interactions in the Baltic Sea, fisheries have possible far-reaching consequences when extracting single species or functional groups. A good example of this is the fishery for sprat in the southern Baltic, which may impact food availability for cod, thereby limiting its growth and body condition (challenge 1.3.2). The exploitation of cod now also competes with exploitation from top-predators such as seals (challenge 2.2.3). The growing gray seal population leads to dramatic increases in both catch losses and damage to fishing gear (Königson et al., 2015; Dinesen et al., 2019). Gray seals affect cod with parasite infestation, affecting fish body growth, condition, and natural mortality (Horbowy et al., 2016). Differing views on the environment and the need for protection (challenge 3.2.6) of, e.g., the gray seals, are a source of conflicts among stakeholders, including scientists (Meek et al., 2011). In addition to these cod specific issues, native species must now compete with the invasive species, round goby (Ojaveer et al., 2018). The round goby competes for space and food resources with species of commercial value such as flatfish and juvenile cod (challenge 2.2.3).
Fisheries management, including catch allowance levels, has historically not been effective in controlling Baltic cod fishing mortality (challenge 3.2.6), due to poor enforcement before 2008. In recent history, the actual catches have been significantly lower than the allowed limits (Aranda et al., 2019). It is also the result of a lack of compliance to the rules arising from conflicts between different groups of fishers who view the measures as more or less credible and acceptable (challenge 3.2.6). Other sources of fish removals are at play in the Baltic Sea, and recreational fishing makes a significant portion of the removals (challenge 2.2.3), as high as 30% of total catches (ICES, 2019b). However, catches from recreational fishing is challenging the monitoring of commercial stocks (challenge 3.2.7). Recreational fisheries may be a significant proportion of the mortality of those fish owing to their coastal distribution. Surveillance and monitoring from indicators are tremendously important, and a robust science should accompany their interpretation (challenge 3.2.7). For example, if the Baltic cod stock abundance was perceived to have recovered, there were indications for the cod growth (as reported by averages of individual body weight) to have sharply declined in recent years (Svedäng and Hornborg, 2015). The seemingly positive sign in increased abundance resulted in the certification of fisheries targeting Eastern Baltic cod by the Marine Stewardship Council in 2014. It is obvious now that such as certification was premature regarding the dramatic 2020 Baltic cod status. Biological reference points (challenge 3.2.2) are not adequately defined if not considering the state of the interlinked cod, sprat, and herring species in the Baltic ecosystem (Casini et al., 2011). Finally, the relationship between the recruitment and large-scale climate indices (challenge 2.2.1) stresses the need to take account of the influence of climate on fish recruitment to improve the management of fish stocks (challenge 3.2.4). The recent availability of open-source environmental data provides an opportunity to test a variety of predictors that may have an influential role in stock-recruitment (Smoliñski, 2019). It is known that an array of pressures in the Baltic Sea affects fish population dynamics and their essential fish habitats (Kraufvelin et al., 2018). Fisheries managers must consider the broader ecosystem and environmental context driving changes in individual stocks (challenge 3.2.7). While many stock status reports include such information, managers should explicitly request this context to give advisory bodies the mandate to investigate and deliver such information.
Case Study: Ecosystem Interactions With North Sea Forage Fish
Planktivorous forage fish play a key role in energy transfer from secondary production to top predators, and care should be taken to keep these stocks within safe biological limits (challenge 1.3). The cost of overfishing is twofold; the lower abundance constrains the energy transfer function described above, but there is also an internal stock cost given that forage fish show higher individual survival when abundance is high (challenge 1.1.3) (Dickey-Collas et al., 2014). Changes in the impact of fisheries on forage fish may have complex and unanticipated consequences on other commercially and ecologically important species (challenge 1.3.2). Forage fish link bottom-up and top-down processes (challenge 1.3.2) and a fishery induced reduction in predation pressure on plankton can cascade up to impact demersal fish and predatory seabirds (Lynam et al., 2017). The dependence on forage fish for seabirds, marine mammals, and predatory fish species in the North Sea makes these species sensitive to variations in forage fish abundance. Because of their specialized diets and limited foraging range seabirds are the most sensitive. Among the fishery exploited forage fish, sandeel is the most “universally important” as prey (Engelhard et al., 2014). Many forage fish species are exposed to large-scale industrial fisheries while simultaneously impacted by climate change. This interaction of fishing and climate change (challenge 2.2.1) can have profound effects on prey availability for seabirds and other top predators (Anderson et al., 2014; Church et al., 2019). The importance of benthic versus pelagic energy flows to fish pathways is poorly studied. However, a change in the strength of these pathways (challenge 1.3.1) would lead to change in the relative biomass of stocks and the long-term yield that is considered sustainable (challenge 3.2.2). Weak interactions among species may tend to stabilize food webs, whereas strong interactions may destabilize them (challenge 2.1.3). North Sea herring, anchovy, and sardine are three species that illustrate there will be winners and losers from projected climatic warming (challenge 2.2.1). The North Sea is close to the southern boundary for herring; hence this species will likely be adversely affected by global warming (Baudron et al., 2020). The North Sea is the current northern boundary for anchovy and sardine, and warmer water is likely to affect these stocks positively (Engelhard et al., 2014).
The commercial exploitation of forage fish removes large amounts of fish biomass from ecosystems and the fish populations. A shortage of sandeel resulting from overfishing may well change the food chains of the North Sea ecosystem (challenge 1.3.2). Predatory fish species critical to human consumption fisheries will target suboptimal prey with a lower calorific value, or net energy gain, leading to reduced growth and condition which in turn is likely to impair reproduction and survival (Engelhard et al., 2008; Dickey-Collas et al., 2014). These cascading effects may have far-reaching ecosystem consequences, right up to the economy of the entire fleet of the North Sea region. A precautionary forage fish exploitation rate might also well be economically more optimal for the industrial fleet due to the resulting benefits for the other fleet-segments (challenge 3.1.1). The higher market value of top predator fish can constrain forage fish catch allowance when trying to recover the top predator stocks and increase yields (challenge 3.1.2). Because of bycatch, it is also not possible to reach FMSY for the North Sea forage fish without also fishing more on human consumption species such as mackerel and herring (challenge 1.2.1). While currently not considered in the management, exploitation of both forage fish and the top predator species at individually determined MSY levels is not realistic without accounting for species interactions (challenge 3.2.2). Strongly interlinked ecosystem interactions could cause time lag effects (challenge 3.2.5). The fishery induced decline in top predator fish in the North Sea has likely reduced pressure on small forage fish, which might impair the recovery of the predatory species because their juveniles will experience increased competition with small fish (Greenstreet et al., 2011).
Case Study: Mixed Demersal Fisheries in the Celtic Sea
Demersal fishing in the Celtic Sea is characterized as a mixed fishery, where fleets target a range of commercial demersal species while deploying different gears. This type of fishery introduces a range of different challenges for management, including initially challenge 3.1.1. Because the vessels will catch multiple species in a normal fishing operation the fishing pattern (relative catch rates of the main species) will not generally map onto the fishing opportunities, in terms of TAC and quota. These fisheries can then cause unwanted changes in stock structures and stock levels (challenge 1.1.1). For example, both cod and whiting in the Celtic Sea are well below MSYbtrigger, both are fished over FMSY, and cod has a recommended zero catch. The stocks are all managed on a single species basis, although with some consideration of the mixed fishery issue. Implementing single stock MSY can then lead to compromises in fishing practices (challenge 3.2.2) and outcomes (Rindorf et al., 2017). This issue is particularly important where it would lead to the potential of early closure of the fishery as is the case in the Celtic Sea (Aranda et al., 2019). The central management issue is that when low or even zero catch is recommended for one of the species caught in the mixed fishery, the Landing Obligation (LO) requires the closing of the fishery. As a result of this, the LO creates a strong risk of large quota under-utilization (challenge 3.2.3). This could also be considered as an example of challenge 3.2.4.
The above challenges are all related to mainstream fishery management issues. However, the mixed fishery in the Celtic Sea also introduces a number of more ecological challenges. One challenge (challenge 2.1.3) concerns impacts on vulnerable or sensitive species. Possibly the most important would be the impacts on a number of sensitive elasmobranch species of which there at least ten species found in the Celtic Sea including the critically endangered Dipturus spp. (Shephard et al., 2012b). The demersal fishing in the Celtic Sea also has the potential to cause habitat damage to both sensitive, and less vulnerable habitats as well as bycatch of non-target species, e.g., crustacean, or age classes of fish, linking to challenges 1.2.1 and 1.2.2. The Celtic Sea also lies at the biogeographical border between two faunal assemblages, i.e., warm-water Lusitanian species and colder-water boreal species. Climate change has already led to possible changes in distributions and fishing opportunities (challenge 3.1.5), with Lusitanian species moving into the Celtic Sea, and potentially Boreal species moving out (Lynam et al., 2010). Boreal species in the Celtic Sea including cod, haddock and herring are at the southern limit of their distribution, and there is likely to be an interaction between climate-induced temperature changes and fishing pressure (Mérillet et al., 2020) (challenge 2.2.1).
Finally, the Celtic Sea may also show other social-ecological challenges. The market strongly influences the choice of targeted species (challenge 3.1.2). Policy targets for EAFM are not very well defined (challenge 3.2.1), and there is significant stakeholder disagreement with enacted policies and a relatively low level of co-management (challenge 3.2.6). It is also arguable that the science could be improved, particularly in terms of species interactions, and that better control and monitoring, and data collection is needed, particularly onboard observers (challenge 3.2.7).
Case Study: Bycatch, Climate Change, and Habitat Degradation in the Bay of Biscay
The fishery management with the landing obligation will inevitably affect the fishing opportunities in the Bay of Biscay (challenge 3.2.4), at least in the short-term. Fisheries economy will be hit if fisheries do not adapt by improving gear selectivity to avoid unwanted catches of undersized or immature individuals in the bottom fishing fisheries (Alzorriz et al., 2016). Besides this, the pelagic purse-seining fisheries are affected by inherent large fluctuations in pelagic species density and abundance. These fluctuations arise from background environmental fluctuation now trending toward new levels. For pelagics, species may shift their distribution in response to long-term changes in the environment (challenge 3.1.5). Anchovy egg abundance in the Bay of Biscay is also expected to increase along with changing climate (challenge 2.2.1), which could further induce an expansion of the spawning area (Erauskin-Extramiana et al., 2019). Mean body length and weight of sardines have been decreasing since the early 2000s. If these trends continue, they could severely impact the fishing and seafood industry sector, even if these trends may have no apparent link with fishing pressure (Véron et al., 2020), whereas the effects of fishing are generally expected to be of equivalent magnitude, and on a more immediate time scale (Bell et al., 2015).
Future fisheries economy in the Bay of Biscay is hence difficult to foresee, and the fish market response is uncertain, even more in a globalized market (challenge 3.1.4). Responses of prices to variations in volumes landed would likely differ among species (challenge 3.1.2). In the Bay of Biscay, high priced species such as seabass may have a price to raise if volume decreases, whereas low price species such as anchovy or sardines, will be substituted with import if volume decreases, therefore creating a lost for fisheries operating on them (Floc′h et al., 2008).
Besides affecting target species, fisheries in the Bay of Biscay are responsible for incidental catch of birds, notably when lines are set in coastal and estuarine areas when these species are migrating, and of bycatch of cetaceans such as common dolphins (challenge 1.2.1). Fishing contributes to the decline of marine mammals along with catching anchovy, sardine, and hake. Fishing also causes major disturbance to benthic communities (challenge 1.2.2) on deeper bottoms, i.e., >100 m of the continental shelf and no fragile species are anymore present in the most exploited areas (Blanchard et al., 2004). This prevents recolonization from adjacent areas or originates from a lack of redundant species available locally or in adjacent areas (Ramalho et al., 2018). These fishing impacts create concerns for environmentalists, and public opinion may be mobilized with bad press on certain damaging fishing practices both on seabed habitats impacted and emblematic species. In South Bay of Biscay, narrow space of continental shelf increases the risk for technical interactions and social conflicts (challenge 3.1.4) beside the safety challenges caused by congested marine areas on the daily fishing operations.
Case Study: Undercapacity and Better Science and Opportunities in Outermost Regions
The purse-seining for tuna adversely affects other marine populations including bycatching other tuna fish, sharks, rays, billfish, and sea turtles (challenge 2.1.1). By-catch species from tropical tuna purse seine fishery have been affected by fishery pressure since the last century (Torres-Irineo et al., 2014). In the ORs, bycatch rates are mostly unknown given the existence of illegal, unreported, and unregulated (IUU) fisheries in the southern oceans, and the non-systematic presence of onboard observers (Thiebot et al., 2016). Because of this, the overall removals of exploited fish and other species are not known (challenge 3.2.7). Combat the IUU fishing requires cooperative efforts amongst the diverse countries. At the meantime, there is a lack of resource to enforce and control, and the lack of scientific capacities to collect and analyze data, for both commercial and well-represented sport fishing. Lack of resource leads to a lack of suitable data from issues in quality, quantity, and timely data reporting for target and non-target species to support sound assessment and management advice, even for the very valuable and catch-limit regulated tuna fisheries (challenge 3.2.7). The habitat distribution, the stock identities and spatial connectivity, and the climate change impacts on these species are also poorly known (Lezama-Ochoa et al., 2016). Fishing adversely affects elasmobranch populations with unwanted catches along with an increasing trend when the habitat suitability area is moving (challenge 3.1.5) in response to changing climate that provokes an increased overlap with fishing grounds for tuna (Lezama-Ochoa et al., 2016) or swordfish that follow a window for preferred temperature (Schirripa et al., 2017). Longline fishing could adversely affect a broad range of teleost populations of different lifestyles, from smaller, fast growth, to larger animals (Lucena Frédou et al., 2016). The risk for incidental catches was evaluated to increase along with the increasing fishing power over time (Milessi and Defeo, 2002).
In ORs, the fishing is very diverse, from numerous small boats doing mixed fisheries, with many different gears and landing sites, to a large polyvalent fleet under effort control, to well-established large seiners fisheries for tuna and swordfish under quota management. Some public fundings are generally justified to renew an aging EU fleet (STECF, 2018d) with new vessels gaining in efficiency and safety, to the extent that the overall national fleets capacity should keep the same. The efficacy of the capacity constraint is in practice always questioned (challenge 3.1.1), and strong voices argue against any modernization of fishing vessels through engine replacement or other capacity enhancing change given the risk it hides, especially in areas where catch allowances are not in place for most species, e.g., in the ORs (e.g., ClientEarth Policy Briefing June 2018).
Concerning the marine habitats, there are knowledge gaps that jeopardize the management of deep-sea habitats (challenge 1.3.1), present in the ORs. The environment of isolated deep seamounts is rather difficult to explore due to their remote position in combination with a lack of appropriate sampling and observation tools (challenge 3.2.7). The application of sophisticated video-supported scientific equipment such as ROVs can obtain in situ information about the characteristics of a seamount by visual observation and dedicated sampling (Wienberg et al., 2013). Mapping vulnerable habitats is an issue and most VMEs locations are currently based on fisheries-independent data while these habitats are widespread on a large area. Hence, the actual survey coverage is so low that it is uncertain how to differentiate between the absence of VMEs and not sampled areas. Commercial data only used to estimate the abundance on location, and it is not advised to use fishery because fishing would be destructive on these habitats (ICES, 2019a). Hence, we should recognize that many unsurveyed areas in the ORs may contain important biogenic habitats (Hourigan, 2009). However, in recent years the Azores OR was a world pioneer in MPA’s development and the coastal habitats, seamounts, hydrothermal vents are now very well studied and protected. The stakeholder’s interaction and governance pillar of an EAFM can be less problematic when compared to the mainland, and some very good examples can also be found.
In the ORs, habitat and environmental factors also impact fisheries (challenge 2.2.3), such as coastal development and pollution of coastal waters with chemicals, including pesticides (such as chlordecone), noise pollution as a repellent of marine mammals, marine litter, and microplastics. Marine litter presents a global problem, with increasing quantities documented in recent decades (Maes et al., 2018). Massive Sargassum inflows is also an issue throughout the Caribbean region (STECF, 2020b). In the EU MSFD, policy targets are not operationally defined, i.e., are defined qualitatively (i.e., the Good Environmental Status GES that ecosystem must reach) likely on purpose so that the policy can ground on future science, but therefore creating immediate room for interpretation (challenge 3.2.1). For example, threshold levels for the fishing pressure on the seabed and impact indicators that relate to the GES of the habitat still need to be derived.
Conclusion
Fisheries can cause changes from natural ecosystem functioning through to human activities, and conversely, changes in the marine ecosystem and human activities likely affect fisheries. This relationship places fisheries at the convergence of human and natural worlds. Essentially, fisheries represent one of the last examples in the developed world of an industry based on a hunter-gatherer strategy, where the human elements interact so directly with the ecosystem upon which they depend. The complexity of these systems and the feedback of different processes within them makes predicting changes in the whole system very difficult. Acting with imperfect information, fisheries policymakers and managers must try to balance tradeoffs of fisheries short-term productivity against long-term environmental, economic and social sustainability as well as the provision of cultural services. The drive-in fisheries science to recognize and reflect these diverse pressures in advice to managers is the essence of an Ecosystem Approach to Fisheries Management. This change places heavy demands on data collection, modeling approaches, and the scientist’s abilities to integrate a wide diversity of factors, often with only qualitative data and where data is often found with highly diverse time and spatial scales, uncertainty, precision and quality. In this review of primary and gray literature, we have categorized and highlighted the key challenges to implementing an EAFM. Furthermore, we have provided regional examples of the complex interplay between these challenges across European waters and have described specific management options that have the potential to overcome them.
Fishing impacts interact with other ecological forcings (temperature, primary productivity) and other anthropogenic pressures to increase the susceptibility of marine ecosystems, habitats, species and exploited stocks to external pressure. This “cumulative pressure” effect can increase uncertainty in predictions used to manage these fishery resources. This would be true whether the “cumulative effects” were additive, synergistic or antagonistic, or even beneficial. Such cumulative impacts make it more difficult to accurately predict stock trajectories needed for management. They also make it far more difficult to assume that a particular pressure will lead to a particular outcome. As simple illustration, a certain amount of fishing mortality that may be sustainable under certain conditions of stock productivity may become excessive if there is an increase in natural mortality due to unfavorable environmental conditions, pollution or the emergence of pathogens, threatening the future fishing opportunities.
We also found that this complexity of interaction in finding ecosystem function sustainability also needs to include the human factors (need for food, income, employment – ecosystem goods, and services generally). This adds another layer of complex interactions that challenge prediction. Our initial purpose was to evaluate if a change in any pressure would induce a disproportionate impact on the marine ecosystem and its components posing an ecosystem challenge. The primary striking outcome of our study is that there is no unambiguous, quantitative evidence to assess if pressure or pressures pose a challenge or not. Instead, we found that changes are often unpredictable in both magnitude and effects, and also possibly direction. The possibility for compensatory effects arising from change being absorbed along with effect propagation in the ecosystem makes any prediction very challenging. Causal links and the direction of effects are often not clear, particularly because challenges are bidirectional – fishing can affect the ecosystem functioning, which in turn can affect the sustainability of the fishing. A particularly difficult challenge emerges when an impact (or mix of impacts) is strong enough to cause a radical change pushing the ecosystem past some tipping point. This manifests as a regime change toward a new alternative state equilibrium which is not easily reversible.
To avoid undesirable ecosystem states or declining trends in those states, we need to develop advice on strategies, i.e., management measures, to address ecosystem challenges. In particular, developing a precautionary approach is a way in uncertain situations and where the magnitude of the effects is poorly known. The obvious first measure would be to prioritize transdisciplinary research and monitoring programs to fill the knowledge gaps, also making the bridge to management for practical implementation (Christie, 2011). This could include promoting the development of new technologies (e.g., for field data collection, or data from modeling) that could build up the scale and detail of our knowledge and understanding of the marine ecosystem and the fisheries systems. As a different, complementary approach, we could also aim to design novel management approaches that were designed for the purpose (i.e., EAFM), rather than ad hoc modifications of existing fisheries management. All these challenges would require us to collect routine monitoring data (ideally) and to coordinate research on many ecosystem indicators (e.g., benthic status, food web interactions, etc.). Ecological Risk Assessment methodologies (Zhou et al., 2016) can be deployed to identify and prioritize the main risks, either by their scale, likelihood or societal importance. Research in oceanography has advanced well in recent years, while the collection of biological data with current observation systems is lagging these physical, geological, and biogeochemical observations (von Schuckmann et al., 2019). However, we should not expect a “one size fits all” solution to be appropriate for mitigation of the status of all degraded ecosystems. In many cases, local solutions and variants would be best and local governance will be needed as such as current aim for CFP regionalization. Solutions may need to be even more local, depending on the challenges being considered.
When looking at social-ecological systems and the challenges, we need to keep a strong focus on the “social” part as well as the “ecosystem” part. Social sciences have a crucial role to play here in identifying metrics and thresholds appropriate to community well-being, beyond the current default of “employment.” They can also identify how fishing communities interact with the rest of society, and how to use that relationship to help the fishing communities understand and accept the EAFM management measures in place. Again here, there is a trade-off, whether it is acceptable to degrade social sustainability to improve ecological sustainability, and to what extent. We also need to recognize that in many fisheries the level of social capital has been severely eroded by fisheries management that is considered heavy-handed and often inappropriate, and that social scientists may be able to help reverse that pattern. To operationalize an EAFM, there are also practical implementation challenges to address. Identifying and prioritizing the challenges and candidate management measures for an EAFM is a necessary first step. The following step would be to start collecting practical knowledge on the best implementation pathway to achieving our policy goals. This should be addressed in further review and analysis work targeted at implementing EAFM approaches.
Author Contributions
EA, RA, EB, JD, RD, OE, IG-B, ML, HM, GP, and DR provided materials to identify ecosystem challenges in local European fisheries and contributed to the revision of the final draft. All authors contributed to the article and approved the submitted version.
Funding
The information and views set out in this article were based on scientific data and information collected under the Specific Contracts EASME/EMFF/2018/1.3.2.4/Lot1/SI2.818390-SC01 and EASME/EMFF/2018/1.3.2.4/Lot2/SI2.818388-SC03, signed with the Executive Agency for Small and Medium-sized Enterprises (EASME) and funded by the European Union. The information and views set out in this publication were those of the authors and do not necessarily reflect the official opinion of EASME or of the European Commission. Neither EASME, nor the European Commission can guarantee the accuracy of the scientific data/information collected under the above Specific Contract or the data/information included in this publication. Neither EASME nor the European Commission or any person acting on their behalf may be held responsible for the use which may be made of the information contained therein.
Conflict of Interest
RA was employed by the company MRAG Ltd.
The remaining authors declare that the research was conducted in the absence of any commercial or financial relationships that could be construed as a potential conflict of interest.
Acknowledgments
We thank the other participants of the consultations, Didzis Ustups (BIOR), Christopher Lynam (CEFAS), Margit Eero, Marie Storr-Paulsen Mikael van Deurs, Anna Rindorf (DTU), Hans Polet, Klaas Sys, Noémi Van Bogaert, and Gert Van Hoey (ILVO), Johanna Ferretti (vTi), Jan Horbowy (MIR), Valerio Bartolino, and Håkan Wennhage (SLU).
Supplementary Material
The Supplementary Material for this article can be found online at: https://www.frontiersin.org/articles/10.3389/fmars.2020.629186/full#supplementary-material
Footnotes
References
Aas, Ø, Cucherousset, J., Fleming, I. A., Wolter, C., Höjesjö, J., Buoro, M., et al. (2018). Salmonid stocking in five North Atlantic jurisdictions: identifying drivers and barriers to policy change. Aquat. Conserv. Mar. Freshw. Ecosyst. 28, 1451–1464. doi: 10.1002/aqc.2984
Allen, J. I., and Clarke, K. R. (2007). Effects of demersal trawling on ecosystem functioning in the North Sea: a modelling study. Mar. Ecol. Prog. Ser. 336, 63–75. doi: 10.3354/meps336063
Alzorriz, N., Arregi, L., Herrmann, B., Sistiaga, M., Casey, J., and Poos, J. J. (2016). Questioning the effectiveness of technical measures implemented by the Basque bottom otter trawl fleet: implications under the EU landing obligation. Fish. Res. 175, 116–126. doi: 10.1016/j.fishres.2015.11.023
Andersen, K. H., and Brander, K. (2009). Expected rate of fisheries-induced evolution is slow. Proc. Natl. Acad. Sci. 106:11657. doi: 10.1073/pnas.0901690106
Anderson, H. B., Evans, P. G. H., Potts, J. M., Harris, M. P., and Wanless, S. (2014). The diet of Common Guillemot Uria algae chicks provides evidence of changing prey communities in the North Sea. Ibis 156, 23–34. doi: 10.1111/ibi.12099
Aranda, M., Ulrich, C., Le Gallic, B., Borges, L., Metz, S., Prellezo, R., et al. (2019). Research for PECH Committee — EU Fisheries Policy – Latest Developments and Future Challenges. Brussels: European Parliament, Policy Department for Structural and Cohesion Policies.
Asch, R. G., and Collie, J. S. (2008). Changes in a benthic megafaunal community due to disturbance from bottom fishing and the establishment of a fishery closure. Fish. Bull. 106, 438–456.
Asci, S. C., Langton, R. W., and Stokesbury, K. D. E. (2018). Estimating similarity in benthic communities over decades and in areas open and closed to fishing in the central gulf of Maine, USA. Mar. Ecol. Prog. Ser. 595, 15–26. doi: 10.3354/meps12563
Auber, A., Travers-Trolet, M., Villanueva, M. C., and Ernande, B. (2015). Regime shift in an exploited fish community related to natural climate oscillations. PLoS One 10:e0129883. doi: 10.1371/journal.pone.0129883
Bailey, D. M., Collins, M. A., Gordon, J. D. M., Zuur, A. F., and Priede, I. G. (2009). Long-term changes in deep-water fish populations in the northeast Atlantic: a deeper reaching effect of fisheries? Proc. R. Soc. B Biol. Sci. 276, 1965–1969. doi: 10.1098/rspb.2009.0098
Bartolino, V., Margonski, P., Lindegren, M., Linderholm, H. W., Cardinale, M., Rayner, D., et al. (2014). Forecasting fish stock dynamics under climate change: baltic herring (Clupea harengus) as a case study. Fish. Oceanogr. 23, 258–269. doi: 10.1111/fog.12060
Bastardie, F., Danto, J., Rufener, M.-C., van Denderen, P. D., Eigaard, O. R., Dinesen, G. E., et al. (2020). Reducing fisheries impacts on the seafloor: a bio-economic evaluation of policy strategies for improving sustainability in the Baltic Sea. Fish. Res. 230:105681. doi: 10.1016/j.fishres.2020.105681
Bastardie, F., Nielsen, J. R., Eero, M., Fuga, F., and Rindorf, A. (2017). Effects of changes in stock productivity and mixing on sustainable fishing and economic viability. ICES J. Mar. Sci. 74, 535–551. doi: 10.1093/icesjms/fsw083
Batsleer, J., Marchal, P., Vaz, S., Vermard, V., Rijnsdorp, A. D., and Poos, J. J. (2018). Exploring habitat credits to manage the benthic impact in a mixed fishery. Mar. Ecol. Prog. Ser. 586, 167–179. doi: 10.3354/meps12392
Baudron, A. R., Brunel, T., Blanchet, M. A., Hidalgo, M., Chust, G., Brown, E. J., et al. (2020). Changing fish distributions challenge the effective management of European fisheries. Ecography 43, 494–505. doi: 10.1111/ecog.04864
Beare, D., Rijnsdorp, A. D., Blaesberg, M., Damm, U., Egekvist, J., Fock, H., et al. (2013). Evaluating the effect of fishery closures: lessons learnt from the Plaice Box. J. Sea Res. 84, 49–60. doi: 10.1016/j.seares.2013.04.002
Bell, R. J., Collie, J. S., Branch, T. A., Fogarty, M. J., Minto, C., and Ricard, D. (2018). Changes in the size structure of marine fish communities. ICES J. Mar. Sci. 75, 102–112. doi: 10.1093/icesjms/fsx118
Bell, R. J., Richardson, D. E., Hare, J. A., Lynch, P. D., and Fratantoni, P. S. (2015). Disentangling the effects of climate, abundance, and size on the distribution of marine fish: an example based on four stocks from the Northeast US shelf. ICES J. Mar. Sci. 72, 1311–1322. doi: 10.1093/icesjms/fsu217
Bellanger, M., Macher, C., Merzéréaud, M., Guyader, O., and Le Grand, C. (2018). Investigating trade-offs in alternative catch share systems: an individual-based bio-economic model applied to the Bay of Biscay sole fishery. Can. J. Fish. Aquat. Sci. 75, 1663–1679. doi: 10.1139/cjfas-2017-0075
Bennecke, S., and Metaxas, A. (2017). Effectiveness of a deep-water coral conservation area: evaluation of its boundaries and changes in octocoral communities over 13 years. Deep Sea Res. II Top. Stud. Oceanogr. 137, 420–435. doi: 10.1016/j.dsr2.2016.06.005
Bergström, L., Karlsson, M., Bergström, U., Pihl, L., and Kraufvelin, P. (2019). Relative impacts of fishing and eutrophication on coastal fish assessed by comparing a no-take area with an environmental gradient. Ambio 48, 565–579. doi: 10.1007/s13280-018-1133-9
Beukhof, E., Dencker, T. S., Pecuchet, L., and Lindegren, M. (2019). Spatio-temporal variation in marine fish traits reveals community-wide responses to environmental change. Mar. Ecol. Prog. Ser. 610, 205–222. doi: 10.3354/meps12826
Bigné, M., Rasmus Nielsen, J., and Bastardie, F. (2019). Opening of the Norway pout box: will it change the ecological impacts of the North Sea Norway pout fishery? ICES J. Mar. Sci. 76, 136–152. doi: 10.1093/icesjms/fsy121
Bjørndal, T., and Ekerhovd, N.-A. (2014). Management of pelagic fisheries in the North East Atlantic: norwegian spring spawning herring, mackerel, and blue whiting. Mar. Resour. Econ. 29, 69–83. doi: 10.1086/676286
Blanchard, F., and Boucher, J. (2001). Temporal variability of total biomass in harvested communities of demersal fishes. Fish. Res. 49, 283–293. doi: 10.1016/s0165-7836(00)00203-4
Blanchard, F., LeLoc’h, F., Hily, C., and Boucher, J. (2004). Fishing effects on diversity, size and community structure of the benthic invertebrate and fish megafauna on the Bay of Biscay coast of France. Mar. Ecol. Prog. Ser. 280, 249–260. doi: 10.3354/meps280249
Borges, L. (2018). Setting of total allowable catches in the 2013 EU Common Fisheries Policy reform: possible impacts. Mar. Policy 91, 97–103. doi: 10.1016/j.marpol.2018.01.026
Bossier, S., Palacz, A. P., Nielsen, J. R., Christensen, A., Hoff, A., Maar, M., et al. (2018). The Baltic sea Atlantis: an integrated end-to-end modelling framework evaluating ecosystem-wide effects of human-induced pressures. PLoS One 13:e0199168. doi: 10.1371/journal.pone.0199168
Boyd, R., Roy, S., Sibly, R., Thorpe, R., and Hyder, K. (2018). A general approach to incorporating spatial and temporal variation in individual-based models of fish populations with application to Atlantic mackerel. Ecol. Model. 382, 9–17. doi: 10.1016/j.ecolmodel.2018.04.015
Bradshaw, C., Veale, L. O., Hill, A. S., and Brand, A. R. (2001). The effect of scallop dredging on Irish Sea benthos: experiments using a closed area. Hydrobiologia 465, 129–138. doi: 10.1007/978-94-010-0434-3_13
Brown, E. J., Vasconcelos, R. P., Wennhage, H., Bergström, U., Stottrup, J. G., Van De Wolfshaar, K., et al. (2018). Conflicts in the coastal zone: human impacts on commercially important fish species utilizing coastal habitat. ICES J. Mar. Sci. 75, 1203–1213. doi: 10.1093/icesjms/fsx237
Brunel, T., and Boucher, J. (2007). Long-term trends in fish recruitment in the north-east Atlantic related to climate change. Fish. Oceanogr. 16, 336–349. doi: 10.1111/j.1365-2419.2007.00435.x
Burgess, M. G., McDermott, G. R., Owashi, B., Peavey Reeves, L. E., Clavelle, T., Ovando, D., et al. (2018). Protecting marine mammals, turtles, and birds by rebuilding global fisheries. Science 359:1255. doi: 10.1126/science.aao4248
Burthe, S. J., Wanless, S., Newell, M. A., Butler, A., and Daunt, F. (2014). Assessing the vulnerability of the marine bird community in the western North Sea to climate change and other anthropogenic impacts. Mar. Ecol. Prog. Ser. 507, 277–295. doi: 10.3354/meps10849
Callaway, R., Alsvåg, J., De Boois, I., Cotter, J., Ford, A., Hinz, H., et al. (2002). Diversity and community structure of epibenthic invertebrates and fish in the North Sea. ICES J. Mar. Sci. 59, 1199–1214. doi: 10.1006/jmsc.2002.1288
Callaway, R., Engelhard, G. H., Dann, J., Cotter, J., and Rumohr, H. (2007). A century of North Sea epibenthos and trawling: comparison between 1902-1912, 1982-1985 and 2000. Mar. Ecol. Prog. Ser. 346, 27–43. doi: 10.3354/meps07038
Casini, M., Mollmann, C., and Osterblom, H. (2011). “Food-web and climate-related dynamics in the Baltic Sea: present and potential future applications in fish stock assessment and management,” in Ecosystem-Based Management for Marine Fisheries: An Evolving Perspective, eds A. Belgrano and C. W. Fowler (Cambridge: Cambridge University Press), 9–31. doi: 10.1017/cbo9780511973956.004
Chagaris, D., Sagarese, S., Farmer, N., Mahmoudi, B., de Mutsert, K., VanderKooy, S., et al. (2019). Management challenges are opportunities for fisheries ecosystem models in the Gulf of Mexico. Mar. Policy 101, 1–7. doi: 10.1016/j.marpol.2018.11.033
Cheung, W. W. L., Jones, M. C., Reygondeau, G., and Frölicher, T. L. (2018). Opportunities for climate-risk reduction through effective fisheries management. Global Change Biol. 24, 5149–5163. doi: 10.1111/gcb.14390
Christensen, E. D., Stuiver, M., Guanche, R., Møhlenberg, F., Schouten, J.-J., Svenstrup Pedersen, O., et al. (2015). Go Offshore -Combining Food and Energy Production. Kgs. Lyngby: Technical University of Denmark. Department of Mechanical Engineering.
Christensen-Dalsgaard, S., Anker-Nilssen, T., Crawford, R., Bond, A., Sigurðsson, G. M., Glemarec, G., et al. (2019). What’s the catch with lumpsuckers? A North Atlantic study of seabird bycatch in lumpsucker gillnet fisheries. Biol. Conserv. 240:108278. doi: 10.1016/j.biocon.2019.108278
Christie, P. (2011). Creating space for interdisciplinary marine and coastal research: five dilemmas and suggested resolutions. Environ. Conserv. 38, 172–186. doi: 10.1017/s0376892911000129
Christou, M., Haralabous, J., Stergiou, K. I., Damalas, D., and Maravelias, C. D. (2017). An evaluation of socioeconomic factors that influence fishers’ discard behaviour in the Greek bottom trawl fishery. Fish. Res. 195, 105–115. doi: 10.1016/j.fishres.2017.07.003
Church, G. E., Furness, R. W., Tyler, G., Gilbert, L., and Votier, S. C. (2019). Change in the North Sea ecosystem from the 1970s to the 2010s: great skua diets reflect changing forage fish, seabirds, and fisheries. ICES J. Mar. Sci. 76, 925–937. doi: 10.1093/icesjms/fsy165
Coates, D. A., Kapasakali, D.-A., Vincx, M., and Vanaverbeke, J. (2016). Short-term effects of fishery exclusion in offshore wind farms on macrofaunal communities in the Belgian part of the North Sea. Fish. Res. 179, 131–138. doi: 10.1016/j.fishres.2016.02.019
Costalago, D., Bauer, B., Tomczak, M. T., Lundström, K., and Winder, M. (2019). The necessity of a holistic approach when managing marine mammal–fisheries interactions: environment and fisheries impact are stronger than seal predation. Ambio 48, 552–564. doi: 10.1007/s13280-018-1131-y
Cowan, J. H. Jr., Rice, J. C., Walters, C. J., Hilborn, R., Essington, T. E., and Day, J. W. Jr., et al. (2012). Challenges for implementing an ecosystem approach to fisheries management. Mar. Coast. Fish. 4, 496–510.
Crilly, R., and Esteban, A. (2013). Small versus large-scale, multi-fleet fisheries: the case for economic, social and environmental access criteria in European fisheries. Mar. Policy 37, 20–27. doi: 10.1016/j.marpol.2012.04.018
Daan, N., Gislason, H., Pope, J. G., and Rice, J. C. (2005). Changes in the North Sea fish community: evidence of indirect effects of fishing? ICES J. Mar. Sci. 62, 177–188. doi: 10.1016/j.icesjms.2004.08.020
Daewel, U., Peck, M. A., and Schrum, C. (2011). Life history strategy and impacts of environmental variability on early life stages of two marine fishes in the North Sea: an individual-based modelling approach. Can. J. Fish. Aquat. Sci. 68, 426–443. doi: 10.1139/f10-164
Daly, E., Johnson, M. P., Wilson, A. M., Gerritsen, H. D., Kiriakoulakis, K., Allcock, A. L., et al. (2018). Bottom trawling at Whittard Canyon: evidence for seabed modification, trawl plumes and food source heterogeneity. Prog. Oceanogr. 169, 227–240. doi: 10.1016/j.pocean.2017.12.010
Dannheim, J., Brey, T., Schröder, A., Mintenbeck, K., Knust, R., and Arntz, W. E. (2014). Trophic look at soft-bottom communities – Short-term effects of trawling cessation on benthos. J. Sea Res. 85, 18–28. doi: 10.1016/j.seares.2013.09.005
Datta, S., and Blanchard, J. L. (2016). The effects of seasonal processes on size spectrum dynamics. Can. J. Fish. Aquat. Sci. 73, 598–610. doi: 10.1139/cjfas-2015-0468
Dickey-Collas, M., Engelhard, G. H., Rindorf, A., Raab, K., Smout, S., Aarts, G., et al. (2014). Ecosystem-based management objectives for the North Sea: riding the forage fish rollercoaster. ICES J. Mar. Sci. 71, 128–142. doi: 10.1093/icesjms/fst075
Diesing, M., Stephens, D., and Aldridge, J. (2013). A proposed method for assessing the extent of the seabed significantly affected by demersal fishing in the Greater North Sea. ICES J. Mar. Sci. 70, 1085–1096. doi: 10.1093/icesjms/fst066
Dinesen, G. E., Neuenfeldt, S., Kokkalis, A., Lehmann, A., Egekvist, J., Kristensen, K., et al. (2019). Cod and climate: a systems approach for sustainable fisheries management of Atlantic cod (Gadus morhua) in coastal Danish waters. J. Coast. Conserv. 23, 943–958. doi: 10.1007/s11852-019-00711-0
Dinmore, T. A., Duplisea, D. E., Rackham, B. D., Maxwell, D. L., and Jennings, S. (2003). Impact of a large-scale area closure on patterns of fishing disturbance and the consequences for benthic communities. ICES J. Mar. Sci. 60, 371–380. doi: 10.1016/s1054-3139(03)00010-9
Dos Santos Schmidt, T. C., Slotte, A., Kennedy, J., Sundby, S., Johannessen, A., Óskarsson, G. J., et al. (2017). Oogenesis and reproductive investment of Atlantic herring are functions of not only present but long-ago environmental influences as well. Proc. Natl. Acad. Sci. U.S.A. 114, 2634–2639. doi: 10.1073/pnas.1700349114
Drabble, R. (2012). Monitoring of East Channel dredge areas benthic fish population and its implications. Mar. Pollut. Bull. 64, 363–372. doi: 10.1016/j.marpolbul.2011.10.035
Duineveld, G. C. A., Bergman, M. J. N., and Lavaleye, M. S. S. (2007). Effects of an area closed to fisheries on the composition of the benthic fauna in the southern North Sea. ICES J. Mar. Sci. 64, 899–908. doi: 10.1093/icesjms/fsm029
Duplisea, D. E., Jennings, S., Warr, K. J., and Dinmore, T. A. (2002). A size-based model of the impacts of bottom trawling on benthic community structure. Can. J. Fish. Aquat. Sci. 59, 1785–1795. doi: 10.1139/f02-148
EC (2013). Regulation (EU) No 1380/2013 of the European Parliament and of the Council of 11 December 2013 on the Common Fisheries Policy, Amending Council Regulations (EC) No 1954/2003 and (EC) No 1224/2009 and Repealing Council Regulations (EC) No 2371/2002 and (EC) No 639/2004 and Council Decision 2004/585/EC. Brussels: European Council.
EEA (2016). Seafood in Europe. A Food System Approach for Sustainability. Technical Report No 25/2016. Copenhagen: European Environment Agency, 56.
Eero, M., Lindegren, M., and Köster, F. W. (2012). The state and relative importance of drivers of fish population dynamics: an indicator-based approach. Ecol. Indic. 15, 248–252. doi: 10.1016/j.ecolind.2011.09.035
Elliott, S. A. M., Allan, B. A., Turrell, W. R., Heath, M. R., and Bailey, D. M. (2018). Survival of the fittest: explanations for gadoid imbalance in heavily fished seas. Aquat. Conserv. Mar. Freshw. Ecosyst. 28, 1192–1199. doi: 10.1002/aqc.2926
Embling, C. B., Illian, J., Armstrong, E., van der Kooij, J., Sharples, J., Camphuysen, K. C. J., et al. (2012). Investigating fine-scale spatio-temporal predator-prey patterns in dynamic marine ecosystems: a functional data analysis approach. J. Appl. Ecol. 49, 481–492. doi: 10.1111/j.1365-2664.2012.02114.x
Engelhard, G. H., Peck, M. A., Rindorf, A., Smout, C. S., Van Deurs, M., Raab, K., et al. (2014). Forage fish, their fisheries, and their predators: who drives whom? ICES J. Mar. Sci. 71, 90–104. doi: 10.1093/icesjms/fst087
Engelhard, G. H., Van Der Kooij, J., Bell, E. D., Pinnegar, J. K., Blanchard, J. L., Mackinson, S., et al. (2008). Fishing mortality versus natural predation on diurnally migrating sandeels Ammodytes marinus. Mar. Ecol. Prog. Ser. 369, 213–227. doi: 10.3354/meps07575
EP (2014). The Conflict Between Static Gear and Mobile Gear in Inshore Fisheries. Directorate General For Internal Policies. Policy Department B: Structural an Cohesion Policies, Fisheries, IP/B/PECH/IC/2014-018, July 2014. Brussels: European Parliament, doi: 10.2861/11432
Erauskin-Extramiana, M., Alvarez, P., Arrizabalaga, H., Ibaibarriaga, L., Uriarte, A., Cotano, U., et al. (2019). Historical trends and future distribution of anchovy spawning in the Bay of Biscay. Deep Sea Res. II Top. Stud. Oceanogr. 159, 169–182. doi: 10.1016/j.dsr2.2018.07.007
Estrella-Martínez, J., Schöne, B. R., Thurstan, R. H., Capuzzo, E., Scourse, J. D., and Butler, P. G. (2019). Reconstruction of Atlantic herring (Clupea harengus) recruitment in the North Sea for the past 455 years based on the δ13C from annual shell increments of the ocean quahog (Arctica islandica). Fish Fish. 20, 537–551. doi: 10.1111/faf.12362
Faulks, L. K., and Östman, Ö (2016). Adaptive major histocompatibility complex (MHC) and neutral genetic variation in two native Baltic Sea fishes (perch Perca fluviatilis and zander Sander lucioperca) with comparisons to an introduced and disease susceptible population in Australia (P. fluviatilis): assessing the risk of disease epidemics. J. Fish Biol. 88, 1564–1583. doi: 10.1111/jfb.12930
Ferro, R. S. T., Özbilgin, H., and Breen, M. (2008). The potential for optimizing yield from a haddock trawl fishery using seasonal changes in selectivity, population structure and fish condition. Fish. Res. 94, 151–159. doi: 10.1016/j.fishres.2008.08.018
Floc′h, P. L., Poulard, J.-C., Thébaud, O., Blanchard, F., Bihel, J., and Steinmetz, F. (2008). Analyzing the market position of fish species subject to the impact of long-term changes: a case study of French fisheries in the Bay of Biscay. Aquat. Living Resour. 21, 307–316. doi: 10.1051/alr:2008047
Floor, J. R., van Koppen, C. S. A., and Lindeboom, H. J. (2013). A review of science-policy interactions in the Dutch Wadden Sea – the cockle fishery and gas exploitation controversies. J. Sea Res. 82, 165–175. doi: 10.1016/j.seares.2012.06.001
Fock, H. (2011). Integrating multiple pressures at different spatial and temporal scales: a concept for relative ecological risk assessment in the European marine environment. Hum. Ecol. Risk Assess. 17, 187–211. doi: 10.1080/10807039.2011.538634
Fock, H. O., Kloppmann, M. H. F., and Probst, W. N. (2014). An early footprint of fisheries: changes for a demersal fish assemblage in the German Bight from 1902-1932 to 1991-2009. J. Sea Res. 85, 325–335. doi: 10.1016/j.seares.2013.06.004
Frederiksen, M., Wanless, S., Harris, M. P., Rothery, P., and Wilson, L. J. (2004). The role of industrial fisheries and oceanographic change in the decline of North Sea black-legged kittiwakes. J. Appl. Ecol. 41, 1129–1139. doi: 10.1111/j.0021-8901.2004.00966.x
Frelat, R., Orio, A., Casini, M., Lehmann, A., Mérigot, B., Otto, S. A., et al. (2018). Contribution to the symposium: ‘sustainable use of Baltic sea resources’ original article a three-dimensional view on biodiversity changes: spatial, temporal, and functional perspectives on fish communities in the Baltic sea. ICES J. Mar. Sci. 75, 2463–2475. doi: 10.1093/icesjms/fsy027
Frid, C. L. J., Clark, R. A., and Percival, P. (2001). How far have the ecological effects of fishing in the North Sea ramified? Senckenb. Mar. 31, 313–320. doi: 10.1007/bf03043039
Froese, R., Stern-Pirlot, A., Winker, H., and Gascuel, D. (2008). Size matters: how single-species management can contribute to ecosystem-based fisheries management. Fish. Res. 92, 231–241. doi: 10.1016/j.fishres.2008.01.005
Fujii, T. (2015). Temporal variation in environmental conditions and the structure of fish assemblages around an offshore oil platform in the North Sea. Mar. Environ. Res. 108, 69–82. doi: 10.1016/j.marenvres.2015.03.013
Furness, R. W. (2002). Management implications of interactions between fisheries and sandeel-dependent seabirds and seals in the North Sea. ICES J. Mar. Sci. 59, 261–269. doi: 10.1006/jmsc.2001.1155
Gacutan, J., Galparsoro, I., and Murillas-Maza, A. (2019). Towards an understanding of the spatial relationships between natural capital and maritime activities: a bayesian belief network approach. Ecosyst. Serv. 40:101034. doi: 10.1016/j.ecoser.2019.101034
Gasche, L., Mahévas, S., and Marchal, P. (2013). Supporting fisheries management by means of complex models: can we point out isles of robustness in a sea of uncertainty? PLoS One 8:e77566. doi: 10.1371/journal.pone.0077566
Gatti, P., Cominassi, L., Duhamel, E., Grellier, P., Le Delliou, H., Le Mestre, S., et al. (2018). Bioenergetic condition of anchovy and sardine in the Bay of Biscay and English Channel. Prog. Oceanogr. 166, 129–138. doi: 10.1016/j.pocean.2017.12.006
Gerritsen, H. D., Minto, C., and Lordan, C. (2013). How much of the seabed is impacted by mobile fishing gear? Absolute estimates from vessel monitoring system (VMS) point data. ICES J. Mar. Sci. 70, 523–531. doi: 10.1093/icesjms/fst017
Girardin, R., Vermard, Y., Thébaud, O., Tidd, A., and Marchal, P. (2015). Predicting fisher response to competition for space and resources in a mixed demersal fishery. Ocean Coast. Manag. 106, 124–135. doi: 10.1016/j.ocecoaman.2015.01.017
Godbold, J. A., Bailey, D. M., Collins, M. A., Gordon, J. D. M., Spallek, W. A., and Priede, I. G. (2013). Putative fishery-induced changes in biomass and population size structures of demersal deep-sea fishes in ICES Sub-area VII, Northeast Atlantic Ocean. Biogeosciences 10, 529–539. doi: 10.5194/bg-10-529-2013
Goikoetxea, N., and Irigoien, X. (2013). Links between the recruitment success of northern European hake (Merluccius merluccius L.) and a regime shift on the NE Atlantic continental shelf. Fish. Oceanogr. 22, 459–476. doi: 10.1111/fog.12033
Goñi, N., Didouan, C., Arrizabalaga, H., Chifflet, M., Arregui, I., Goikoetxea, N., et al. (2015). Effect of oceanographic parameters on daily albacore catches in the northeast Atlantic. Deep Sea Res. II Top. Stud. Oceanogr. 113, 73–80. doi: 10.1016/j.dsr2.2015.01.012
González-Irusta, J. M., Preciado, I., López-López, L., Punzón, A., Cartes, J. E., and Serrano, A. (2014). Trawling disturbance on the isotopic signature of a structure-building species, the sea urchin Gracilechinus acutus (Lamarck, 1816). Deep Sea Res. II Top. Stud. Oceanogr. 106, 216–224. doi: 10.1016/j.dsr2.2013.09.036
González-Irusta, J. M., and Wright, P. J. (2017). Spawning grounds of whiting (Merlangius merlangus). Fish. Res. 195, 141–151. doi: 10.1016/j.fishres.2017.07.005
Goti-Aralucea, L. (2019). Assessing the social and economic impact of small-scale fisheries management measures in a marine protected area with limited data. Mar. Policy 101, 246–256. doi: 10.1016/j.marpol.2017.10.039
Goti-Aralucea, L., Fitzpatrick, M., Döring, R., Reid, D., Mumford, J., and Rindorf, A. (2018). Overarching sustainability objectives overcome incompatible directions in the Common Fisheries Policy. Mar. Policy 91, 49–57. doi: 10.1016/j.marpol.2018.02.006
Graziano, M., Fox, C. J., Alexander, K., Pita, C., Heymans, J. J., Crumlish, M., et al. (2018). Environmental and socio-political shocks to the seafood sector: what does this mean for resilience? Lessons from two UK case studies, 1945–2016. Mar. Policy 87, 301–313. doi: 10.1016/j.marpol.2017.10.014
Greenstreet, S. P. R., Fraser, H. M., and Piet, G. J. (2009). Using MPAs to address regional-scale ecological objectives in the North Sea: modelling the effects of fishing effort displacement. ICES J. Mar. Sci. 66, 90–100. doi: 10.1093/icesjms/fsn214
Greenstreet, S. P. R., Rogers, S. I., Rice, J. C., Piet, G. J., Guirey, E. J., Fraser, H. M., et al. (2011). Development of the EcoQO for the North Sea fish community. ICES J. Mar. Sci. 68, 1–11. doi: 10.1093/icesjms/fsq156
Gröger, J. P., Hinrichsen, H.-H., and Polte, P. (2014). Broad-scale climate influences on spring-spawning herring (Clupea harengus, L.) recruitment in the Western Baltic Sea. PLoS One 9:e87525. doi: 10.1371/journal.pone.0087525
Haapasaari, P., Michielsens, C. G. J., Karjalainen, T. P., Reinikainen, K., and Kuikka, S. (2007). Management measures and fishers’ commitment to sustainable exploitation: a case study of Atlantic salmon fisheries in the Baltic Sea. ICES J. Mar. Sci. 64, 825–833. doi: 10.1093/icesjms/fsm002
Harma, C., Brophy, D., Minto, C., and Clarke, M. (2012). The rise and fall of autumn-spawning herring (Clupea harengus L.) in the Celtic Sea between 1959 and 2009: temporal trends in spawning component diversity. Fish. Res. 12, 31–42. doi: 10.1016/j.fishres.2012.01.005
Hatchard, J. L., and Gray, T. S. (2014). From RACs to advisory councils: lessons from north sea discourse for the 2014 reform of the European Common Fisheries Policy. Ma. Policy 47, 87–93. doi: 10.1016/j.marpol.2014.02.015
Hawkins, J. P., and Roberts, C. M. (2004). Effects of artisanal fishing on caribbean coral reefs. Conserv. Biol. 18, 215–226. doi: 10.1111/j.1523-1739.2004.00328.x
Hawkins, S. J., Evans, A. J., Mieszkowska, N., Adams, L. C., Bray, S., Burrows, M. T., et al. (2017). Distinguishing globally-driven changes from regional- and local-scale impacts: the case for long-term and broad-scale studies of recovery from pollution. Mar. Pollut. Bull. 124, 573–586. doi: 10.1016/j.marpolbul.2017.01.068
Hedd, A., Montevecchi, W. A., Davoren, G. K., and Fifield, D. A. (2009). Diets and distributions of Leach’s storm-petrel (Oceanodroma leucorhoa) before and after an ecosystem shift in the Northwest Atlantic. Can. J. Zool. 87, 787–801. doi: 10.1139/z09-060
Heikinheimo, O. (2011). Interactions between cod, herring and sprat in the changing environment of the Baltic Sea: a dynamic model analysis. Ecol. Model. 222, 1731–1742. doi: 10.1016/j.ecolmodel.2011.03.005
Hélias, A., Langlois, J., and Fréon, P. (2018). Fisheries in life cycle assessment: operational factors for biotic resources depletion. Fish Fish. 19, 951–963. doi: 10.1111/faf.12299
Henriques, F. F., Serrão, E. A., and González-Wangüemert, M. (2016). Novel polymorphic microsatellite loci for a new target species, the sea cucumber Holothuria mammata. Biochem. Systemat. Ecol. 66, 109–113. doi: 10.1016/j.bse.2016.03.012
Hermsen, J. M., Collie, J. S., and Valentine, P. C. (2003). Mobile fishing gear reduces benthic megafaunal production on Georges Bank. Mar. Ecol. Prog. Ser. 260, 97–108. doi: 10.3354/meps260097
Hernvann, P.-Y., and Gascuel, D. (2020). Exploring the impacts of fishing and environment on the Celtic Sea ecosystem since 1950. Fish. Res. 225:105472. doi: 10.1016/j.fishres.2019.105472
Hidalgo, M., Olsen, E. M., Ohlberger, J., Saborido-Rey, F., Murua, H., Piñeiro, C., et al. (2014). Contrasting evolutionary demography induced by fishing: the role of adaptive phenotypic plasticity. Ecol. Appl. 24, 1101–1114. doi: 10.1890/12-1777.1
Hiddink, J. G., and Coleby, C. (2012). What is the effect of climate change on marine fish biodiversity in an area of low connectivity, the Baltic Sea? Global Ecol. Biogeogr. 21, 637–646. doi: 10.1111/j.1466-8238.2011.00696.x
Hiddink, J. G., Hutton, T., Jennings, S., and Kaiser, M. J. (2006a). Predicting the effects of area closures and fishing effort restrictions on the production, biomass, and species richness of benthic invertebrate communities. ICES J. Mar. Sci. 63, 822–830. doi: 10.1016/j.icesjms.2006.02.006
Hiddink, J. G., Jennings, S., and Kaiser, M. J. (2007). Assessing and predicting the relative ecological impacts of disturbance on habitats with different sensitivities. J. Appl. Ecol. 44, 405–413. doi: 10.1111/j.1365-2664.2007.01274.x
Hiddink, J. G., Jennings, S., Kaiser, M. J., Queirós, A. M., Duplisea, D. E., and Piet, G. J. (2006b). Cumulative impacts of seabed trawl disturbance on benthic biomass, production, and species richness in different habitats. Can. J. Fish. Aquat. Sci. 63, 721–736. doi: 10.1139/f05-266
Hiddink, J. G., Moranta, J., Balestrini, S., Sciberras, M., Cendrier, M., Bowyer, R., et al. (2016). Bottom trawling affects fish condition through changes in the ratio of prey availability to density of competitors. J. Appl. Ecol. 53, 1500–1510. doi: 10.1111/1365-2664.12697
Hinz, H., Moranta, J., Balestrini, S., Sciberras, M., Pantin, J. R., Monnington, J., et al. (2017). Stable isotopes reveal the effect of trawl fisheries on the diet of commercially exploited species. Sci. Rep. 7:6334.
Holmgren, N. M. A., Norrström, N., Aps, R., and Kuikka, S. (2012). MSY-orientated management of Baltic Sea herring (Clupea harengus) during different ecosystem regimes. ICES J. Mar. Sci. 69, 257–266. doi: 10.1093/icesjms/fsr202
Horbowy, J., Podolska, M., and Nadolna-Ałtyn, K. (2016). Increasing occurrence of anisakid nematodes in the liver of cod (Gadus morhua) from the Baltic Sea: does infection affect the condition and mortality of fish? Fish. Res. 179, 98–103. doi: 10.1016/j.fishres.2016.02.011
Hourigan, T. F. (2009). Managing fishery impacts on deep-water coral ecosystems of the USA: emerging best practices. Mar. Ecol. Prog. Ser. 397, 333–340. doi: 10.3354/meps08278
Hüssy, K., Mosegaard, H., Albertsen, C. M., Nielsen, E. E., Hemmer-Hansen, J., and Eero, M. (2016). Evaluation of otolith shape as a tool for stock discrimination in marine fishes using Baltic Sea cod as a case study. Fish. Res. 174, 210–218. doi: 10.1016/j.fishres.2015.10.010
ICES (2016). AORAC-SA FAO Workshop: Making the Ecosystem Approach Operational, 21-22 January. Copenhagen: ICES Headquarters, 55.
ICES (2018a). Interim Report of the Working Group on SOCIAL Indicators (WGSOCIAL). 25-29 June 2018. Copenhagen: ICES Headquarters, 19.
ICES (2018b). Interim Report of the Working Group on Economics (WGECON), 11–15 June 2018. Copenhagen: ICES Headquarters, 45.
ICES (2020a). Working Group on Fisheries Benthic Impact and Trade-offs (WGFBIT; outputs from 2019 meeting). ICES Scientific Reports. 2:6. Copenhagen: ICES Headquarters, 101. doi: 10.17895/ices.pub.5955
ICES (2020b). Working Group on Bycatch of Protected Species (WGBYC). ICES Scientific Reports. 2:81. Copenhagen: ICES Headquarters, 209. doi: 10.17895/ices.pub.7471
Isomaa, M., Kaitala, V., and Laakso, J. (2013). Baltic cod (Gadus morhua callarias) recovery potential under different environment and fishery scenarios. Ecol. Model. 266, 118–125. doi: 10.1016/j.ecolmodel.2013.06.015
Jansen, T. (2014). Pseudocollapse and rebuilding of North Sea mackerel (Scomber scombrus). ICES J. Mar. Sci. 71, 299–307. doi: 10.1093/icesjms/fst148
Jenkins, S. R., Mullen, C., and Brand, A. R. (2004). Predator and scavenger aggregation to discarded by-catch from dredge fisheries: importance of damage level. J. Sea Res. 51, 69–76. doi: 10.1016/j.seares.2003.05.002
Jennings, S., and Blanchard, J. L. (2004). Fish abundance with no fishing: predictions based on macroecological theory. J. Anim. Ecol. 73, 632–642. doi: 10.1111/j.0021-8790.2004.00839.x
Jennings, S., Dinmore, T. A., Duplisea, D. E., Warr, K. J., and Lancaster, J. E. (2001a). Trawling disturbance can modify benthic production processes. J. Anim. Ecol. 70, 459–475. doi: 10.1046/j.1365-2656.2001.00504.x
Jennings, S., Greenstreet, S. P. R., Hill, L., Piet, G. J., Pinnegar, J. K., and Warr, K. J. (2002). Long-term trends in the trophic structure of the North Sea fish community: evidence from stable-isotope analysis, size-spectra and community metrics. Mar. Biol. 141, 1085–1097. doi: 10.1007/s00227-002-0905-7
Jennings, S., Pinnegar, J. K., Polunin, N. V. C., and Warr, K. J. (2001b). Impacts of trawling disturbance on the trophic structure of benthic invertebrate communities. Mar. Ecol. Prog. Ser. 213, 127–142. doi: 10.3354/meps213127
Jentoft, S., and Knol, M. (2014). Marine spatial planning: risk or opportunity for fisheries in the North Sea? Marit. Stud. 13, 1–16.
Johnson, A. F., Gorelli, G., Jenkins, S. R., Hiddink, J. G., and Hinz, H. (2015). Effects of bottom trawling on fish foraging and feeding. Proc. R. Soc. B Biol. Sci. 282:20142336.
Josefson, A. B., Loo, L.-O., Blomqvist, M., and Rolandsson, J. (2018). Substantial changes in the depth distributions of benthic invertebrates in the eastern Kattegat since the 1880s. Ecol. Evol. 8, 9426–9438. doi: 10.1002/ece3.4395
Kabat, P., Bazelmans, J., van Dijk, J., Herman, P. M. J., van Oijen, T., Pejrup, M., et al. (2012). The Wadden Sea Region: towards a science for sustainable development. Ocean Coast. Manag.68, 4–17.
Kadin, M., Blenckner, T., Casini, M., Gårdmark, A., Torres, M. A., and Otto, S. A. (2019). Trophic interactions, management trade-offs and climate change: the need for adaptive thresholds to operationalize ecosystem indicators. Front. Mar. Sci. 6:249. doi: 10.3389/fmars.2019.00249
Kaiser, M. J., and Spence, F. E. (2002). Inconsistent temporal changes in the megabenthos of the English Channel. Mar. Biol. 141, 321–331. doi: 10.1007/s00227-002-0826-5
Kaplan, K. A., Hart, D. R., Hopkins, K., Gallager, S., York, A., Taylor, R., et al. (2018). Invasive tunicate restructures invertebrate community on fishing grounds and a large protected area on Georges Bank. Biol. Invasions 20, 87–103. doi: 10.1007/s10530-017-1517-y
Kempf, A., Stelzenmüller, V., Akimova, A., and Floeter, J. (2013). Spatial assessment of predator-prey relationships in the North Sea: the influence of abiotic habitat properties on the spatial overlap between 0-group cod and grey gurnard. Fish. Oceanogr. 22, 174–192. doi: 10.1111/fog.12013
Kenchington, E. L., Kenchington, T. J., Henry, L.-A., Fuller, S., and Gonzalez, P. (2007). Multi-decadal changes in the megabenthos of the Bay of Fundy: the effects of fishing. J. Sea Res. 58, 220–240. doi: 10.1016/j.seares.2007.04.001
Kirby, R. R., Beaugrand, G., Lindley, J. A., Richardson, A. J., Edwards, M., and Reid, P. C. (2007). Climate effects and benthic-pelagic coupling in the North Sea. Mar. Ecol. Prog. Ser. 330, 31–38. doi: 10.3354/meps330031
Kokkonen, E., Heikinheimo, O., Pekcan-Hekim, Z., and Vainikka, A. (2019). Effects of water temperature and pikeperch (Sander lucioperca) abundance on the stock–recruitment relationship of Eurasian perch (Perca fluviatilis) in the northern Baltic Sea. Hydrobiologia 841, 79–94. doi: 10.1007/s10750-019-04008-z
Königson, S. J., Fredriksson, R. E., Lunneryd, S.-G., Strömberg, P., and Bergström, U. M. (2015). Cod pots in a Baltic fishery: are they efficient and what affects their efficiency? ICES J. Mar Sci. 72, 1545–1554. doi: 10.1093/icesjms/fsu230
Krag, L. A., Herrmann, B., Feekings, J., and Karlsen, J. D. (2016). Escape panels in trawls-a consistent management tool? Aquat. Living Resour. 29:306. doi: 10.1051/alr/2016028
Kraufvelin, P., Pekcan-Hekim, Z., Bergström, U., Florin, A.-B., Lehikoinen, A., Mattila, J., et al. (2018). Essential coastal habitats for fish in the Baltic Sea. Estuar. Coast. Shelf Sci. 204, 14–30.
Le Bris, A., Mills, K. E., Wahle, R. A., Chen, Y., Alexander, M. A., Allyn, A. J., et al. (2018). Climate vulnerability and resilience in the most valuable North American fishery. Proc. Natl. Acad. Sci. U.S.A. 115, 1831–1836. doi: 10.1073/pnas.1711122115
Leitão, F., Maharaj, R. R., Vieira, V. M. N. C. S., Teodósio, A., and Cheung, W. W. L. (2018). The effect of regional sea surface temperature rise on fisheries along the Portuguese Iberian Atlantic coast. Aquat. Conserv. Mar. Freshw. Ecosyst. 28, 1351–1359. doi: 10.1002/aqc.2947
Lezama-Ochoa, N., Murua, H., Chust, G., Van Loon, E., Ruiz, J., Hall, M., et al. (2016). Present and future potential habitat distribution of Carcharhinus falciformis and Canthidermis maculata by-catch species in the tropical tuna purse-seine fishery under climate change. Front. Mar. Sci. 3:34. doi: 10.3389/fmars.2016.00034
Lindegren, M., Andersen, K. H., Casini, M., and Neuenfeldt, S. (2014). A metacommunity perspective on source-sink dynamics and management: the Baltic Sea as a case study. Ecol. Appl. 24, 1820–1832. doi: 10.1890/13-0566.1
Lindegren, M., and Eero, M. (2013). Threshold-dependent climate effects and high mortality limit recruitment and recovery of the Kattegat cod. Mar. Ecol. Prog. Ser. 490, 223–232. doi: 10.3354/meps10437
Lindley, J. A., and Kirby, R. R. (2010). Climate-induced changes in the North Sea Decapoda over the last 60 years. Clim. Res. 42, 257–264. doi: 10.3354/cr00917
Link, J. S., and Garrison, L. P. (2002). Changes in piscivory associated with fishing induced changes to the finfish community on Georges Bank. Fish. Res. 55, 71–86. doi: 10.1016/s0165-7836(01)00300-9
Long, R. D., Charles, A., and Stephenson, R. L. (2015). Key principles of marine ecosystem-based management. Mar. Policy 57, 53–60. doi: 10.1016/j.marpol.2015.01.013
Lucena Frédou, F., Frédou, T., Gaertner, D., Kell, L., Potier, M., Bach, P., et al. (2016). Life history traits and fishery patterns of teleosts caught by the tuna longline fishery in the South Atlantic and Indian Oceans. Fish. Res. 179, 308–321. doi: 10.1016/j.fishres.2016.03.013
Luzeñczyk, A. (2017). Change in biological reference points under different biological, fishery, and environmental factors. Acta Ichthyol. Piscat. 47, 41–51. doi: 10.3750/aiep/02111
Lynam, C. P., Cusack, C., and Stokes, D. (2010). A methodology for community-level hypothesis testing applied to detect trends in phytoplankton and fish communities in Irish waters. Estuar. Coast. Shelf Sci.87, 451–462. doi: 10.1016/j.ecss.2010.01.019
Lynam, C. P., Llope, M., Möllmann, C., Helaouët, P., Bayliss-Brown, G. A., and Stenseth, N. C. (2017). Interaction between top-down and bottom-up control in marine food webs. Proc. Natl. Acad. Sci. U.S.A. 114, 1952–1957. doi: 10.1073/pnas.1621037114
MacKenzie, B. R., Eero, M., and Ojaveer, H. (2011). Could seals prevent cod recovery in the Baltic Sea? PLoS One 6:e18998. doi: 10.1371/journal.pone.0018998
Mackenzie, C. L., Bell, M. C., Birchenough, S. N. R., Culloty, S. C., Sanderson, W. G., Whiteley, N. M., et al. (2013). Future socio-economic and environmental sustainability of the Irish Sea requires a multi-disciplinary approach with industry and research collaboration, and cross-border partnership. Ocean Coast. Manag. 85, 1–6. doi: 10.1016/j.ocecoaman.2013.08.012
Mackinson, S. (2014). Combined analyses reveal environmentally driven changes in the North Sea ecosystem and raise questions regarding what makes an ecosystem model’s performance credible? Can. J. Fish. Aquat. Sci. 71, 31–46. doi: 10.1139/cjfas-2013-0173
Mackinson, S., Platts, M., Garcia, C., and Lynam, C. (2018). Evaluating the fishery and ecological consequences of the proposed North Sea multi-annual plan. PLoS One 13:e0190015. doi: 10.1371/journal.pone.0190015
Maes, T., Barry, J., Leslie, H. A., Vethaak, A. D., Nicolaus, E. E. M., Law, R. J., et al. (2018). Below the surface: twenty-five years of seafloor litter monitoring in coastal seas of North West Europe (1992–2017). Sci. Total Environ. 630, 790–798. doi: 10.1016/j.scitotenv.2018.02.245
Marshall, A. M., Bigg, G. R., van Leeuwen, S. M., Pinnegar, J. K., Wei, H.-L., Webb, T. J., et al. (2016). Quantifying heterogeneous responses of fish community size structure using novel combined statistical techniques. Global Change Biol. 22, 1755–1768. doi: 10.1111/gcb.13190
Martins, G. M., Arenas, F., Neto, A. I., and Jenkins, S. R. (2012). Effects of fishing and regional species pool on the functional diversity of fish communities. PLoS One 7:e44297. doi: 10.1371/journal.pone.0044297
Maya Jariego, I., Florido del Corral, D., Sáez, J., and Sobrado Llompart, F. (2018). A tale of two coasts: Networks of artisanal boat skippers in a fishing reserve in the Gulf of Cádiz and a recreational port in Islas Baleares. Ocean Coast. Manag. 161, 105–116. doi: 10.1016/j.ocecoaman.2018.05.002
McLaverty, C., Eigaard, O. R., Gislason, H., Bastardie, F., Brooks, M. E., Jonsson, P., et al. (2020). Using large benthic macrofauna to refine and improve ecological indicators of bottom trawling disturbance. Ecol. Indic. 110:105811. doi: 10.1016/j.ecolind.2019.105811
McLean, M., Mouillot, D., and Auber, A. (2018). Ecological and life history traits explain a climate-induced shift in a temperate marine fish community. Mar. Ecol. Prog. Ser. 606, 175–186. doi: 10.3354/meps12766
McLean, M., Mouillot, D., Lindegren, M., Villéger, S., Engelhard, G., Murgier, J., et al. (2019). Fish communities diverge in species but converge in traits over three decades of warming. Global Change Biol. 25, 3972–3984. doi: 10.1111/gcb.14785
Meek, C. L., Lauren Lovecraft, A., Varjopuro, R., Dowsley, M., and Dale, A. T. (2011). Adaptive governance and the human dimensions of marine mammal management: implications for policy in a changing North. Mar. Policy 35, 466–476. doi: 10.1016/j.marpol.2010.10.021
Meier, H. E. M., Andersson, H. C., Arheimer, B., Blenckner, T., Chubarenko, B., Donnelly, C., et al. (2012). Comparing reconstructed past variations and future projections of the Baltic Sea ecosystem – first results from multi-model ensemble simulations. Environ. Res. Lett. 7:034005. doi: 10.1088/1748-9326/7/3/034005
Mellett, M., Curtin, C., Hennessey, T., and O’Hagan, A. M. (2011). Attainment of ecosystem based governance in European waters-a state property rights regime approach for Ireland. Mar. Policy 35, 739–747. doi: 10.1016/j.marpol.2011.01.011
Mendez, M. M., Robledo, J., and van der Molen, S. (2017). Infaunal communities associated to the razor clam Ensis macha: water-jet fishing impact. Ecol. Austral 27, 474–481.
Mérillet, L., Kopp, D., Robert, M., Mouchet, M., and Pavoine, S. (2020). Environment outweighs the effects of fishing in regulating demersal community structure in an exploited marine ecosystem. Global Change Biol. 26, 2106–2119. doi: 10.1111/gcb.14969
Mérillet, L., Mouchet, M., Robert, M., Salaün, M., Schuck, L., Vaz, S., et al. (2018). Using underwater video to assess megabenthic community vulnerability to trawling in the Grande Vasière (Bay of Biscay). Environ. Conserv. 45, 163–172. doi: 10.1017/s0376892917000480
Milessi, A. C., and Defeo, O. (2002). Long-term impact of incidental catches by tuna longlines: The black escolar (Lepidocybium flavobrunneum) of the southwestern Atlantic Ocean. Fish. Res. 58, 203–213. doi: 10.1016/s0165-7836(01)00365-4
Morgan, R. (2016). Exploring how fishermen respond to the challenges facing the fishing industry: a case study of diversification in the english channel fishery. Reg. Stud. 50, 1755–1768. doi: 10.1080/00343404.2015.1057892
Moritz, C., Gravel, D., Savard, L., McKindsey, C. W., Brethes, J.-C., and Archambault, P. (2015). No more detectable fishing effect on Northern Gulf of St Lawrence benthic invertebrates. ICES J. Mar. Sci. 72, 2457–2466. doi: 10.1093/icesjms/fsv124
Mullon, C., Steinmetz, F., Merino, G., Fernandes, J. A., Cheung, W. W. L., Butenschön, M., et al. (2016). Quantitative pathways for Northeast Atlantic fisheries based on climate, ecological-economic and governance modelling scenarios. Ecol. Model. 320, 273–291. doi: 10.1016/j.ecolmodel.2015.09.027
Murillo, F. J., Weigel, B., Bouchard Marmen, M., and Kenchington, E. (2020). Marine epibenthic functional diversity on Flemish Cap (north-west Atlantic)—Identifying trait responses to the environment and mapping ecosystem functions. Divers. Distrib. 26, 460–478. doi: 10.1111/ddi.13026
Myers, R. A., Baum, J. K., Shepherd, T. D., Powers, S. P., and Peterson, C. H. (2007). Cascading effects of the loss of apex predatory sharks from a coastal ocean. Science 315, 1846–1850. doi: 10.1126/science.1138657
Nicholson, M. D., and Jennings, S. (2004). Testing candidate indicators to support ecosystem-based management: the power of monitoring surveys to detect temporal trends in fish community metrics. ICES J. Mar. Sci. 61, 35–42. doi: 10.1016/j.icesjms.2003.09.004
Niiranen, S., Blenckner, T., Hjerne, O., and Tomczak, M. T. (2012). Uncertainties in a baltic sea food-web model reveal challenges for future projections. Ambio 41, 613–625. doi: 10.1007/s13280-012-0324-z
Nilsson, H. C., and Rosenberg, R. (2003). Effects on marine sedimentary habitats of experimental trawling analyzed by sediment profile imagery. J. Exp. Mar. Biol. Ecol. 28, 453–463. doi: 10.1016/s0022-0981(02)00543-9
Oberle, F. K. J., Swarzenski, P. W., Reddy, C. M., Nelson, R. K., Baasch, B., and Hanebuth, T. J. J. (2016). Deciphering the lithological consequences of bottom trawling to sedimentary habitats on the shelf. J. Mar. Syst. 159, 120–131. doi: 10.1016/j.jmarsys.2015.12.008
O’Hagan, A. M., Paterson, S., and Tissier, M. L. (2020). Addressing the tangled web of governance mechanisms for land-sea interactions: assessing implementation challenges across scales. Mar. Policy 112:103715. doi: 10.1016/j.marpol.2019.103715
O’Higgins, T., and Roth, E. (2011). “Integrating the common fisheries policy and the marine strategy for the baltic: discussion of spatial and temporal scales in the management and adaptation to changing climate,” in Global Change and Baltic Coastal Zones. Coastal Research Library. Vol. 1, eds G. Schernewski, J. Hofstede, and T. Neumann (Dordrecht: Springer), 275. doi: 10.1007/978-94-007-0400-8_16
Ojaveer, H., Neuenfeldt, S., Dierking, J., Eek, L., Haldin, J., Martin, G., et al. (2018). Sustainable use of baltic sea resources. ICES J. Mar. Sci. 75, 2434–2438. doi: 10.1093/icesjms/fsy133
Olsen, E. M., Ottersen, G., Llope, M., Chan, K.-S., Beaugrand, G., and Stenseth, N. C. (2011). Spawning stock and recruitment in North Sea cod shaped by food and climate. Proc. R. Soc. B Biol. Sci. 278, 504–510. doi: 10.1098/rspb.2010.1465
ORFISH (2019). Development of Innovative, Low-impact Offshore Fishing Practices for Small-scale Vessels in Outermost Regions - MARE/2015/06. WP2. Raising Awareness of the Opportunities to Develop Innovative Fishing Techniques. Task 2.1 Status and Evolution of Small-scale Fisheries in Outermost Regions (ORs). Deliverable #7. Available online at: https://archimer.ifremer.fr/doc/00628/74011/ (accessed February 15, 2020).
Östman, Ö, Karlsson, O., Pönni, J., Kaljuste, O., Aho, T., and Gårdmark, A. (2014). Relative contributions of evolutionary and ecological dynamics to body size and life-history changes of herring (Clupea harengus) in the Bothnian Sea. Evol. Ecol. Res. 16, 417–433.
Papaioannou, E. A., Vafeidis, A. T., Quaas, M. F., Schmidt, J. O., and Strehlow, H. V. (2014). Using indicators based on primary fisheries’ data for assessing the development of the German Baltic small-scale fishery and reviewing its adaptation potential to changes in resource abundance and management during 2000-09. Ocean Coast. Manag. 98, 38–50. doi: 10.1016/j.ocecoaman.2014.06.005
Pecuchet, L., Reygondeau, G., Cheung, W. W. L., Licandro, P., van Denderen, P. D., Payne, M. R., et al. (2018). Spatial distribution of life-history traits and their response to environmental gradients across multiple marine taxa. Ecosphere 9:e02460. doi: 10.1002/ecs2.2460
Pecuchet, L., Törnroos, A., and Lindegren, M. (2016). Patterns and drivers of fish community assembly in a large marine ecosystem. Mar. Ecol. Prog. Ser. 546, 239–248. doi: 10.3354/meps11613
Pennino, M. G., Vilela, R., and Bellido, J. M. (2019). Effects of environmental data temporal resolution on the performance of species distribution models. J. Mar. Syst. 189, 78–86. doi: 10.1016/j.jmarsys.2018.10.001
Perkins, A., Ratcliffe, N., Suddaby, D., Ribbands, B., Smith, C., Ellis, P., et al. (2018). Combined bottom-up and top-down pressures drive catastrophic population declines of Arctic skuas in Scotland. J. Anim. Ecol. 87, 1573–1586. doi: 10.1111/1365-2656.12890
Pita, P., Artetxe, I., Diogo, H., Gomes, P., Gordoa, A., Hyder, K., et al. (2017). Research and management priorities for Atlantic marine recreational fisheries in Southern Europe. Mar. Policy 86, 1–8. doi: 10.1016/j.marpol.2017.08.030
Pitois, S. G., Lynam, C. P., Jansen, T., Halliday, N., and Edwards, M. (2012). Bottom-up effects of climate on fish populations: data from the continuous plankton recorder. Mar. Ecol. Prog. Ser. 456, 169–186. doi: 10.3354/meps09710
Planque, B., Fromentin, J. M., Cury, P., Drinkwater, K. F., Jennings, S., Perry, R. I., et al. (2010). How does fishing alter marine populations and ecosystems sensitivity to climate? J. Mar. Syst. 79, 403–417. doi: 10.1016/j.jmarsys.2008.12.018
Ramalho, S. P., Almeida, M., Esquete, P., Génio, L., Ravara, A., Rodrigues, C. F., et al. (2018). Bottom-trawling fisheries influence on standing stocks, composition, diversity and trophic redundancy of macrofaunal assemblages from the West Iberian Margin. Deep Sea Res. I Oceanogr. Res. Pap. 138, 131–145. doi: 10.1016/j.dsr.2018.06.004
Ramírez-Monsalve, P., Raakjær, J., Nielsen, K. N., Laksá, U., Danielsen, R., Degnbol, D., et al. (2016). Institutional challenges for policy-making and fisheries advice to move to a full EAFM approach within the current governance structures for marine policies. Mar. Policy 69, 1–12. doi: 10.1016/j.marpol.2016.03.016
Ramos, S., Vázquez-Rowe, I., Artetxe, I., Moreira, M. T., Feijoo, G., and Zufía, J. (2011). Environmental assessment of the Atlantic mackerel (Scomber scombrus) season in the Basque Country. Increasing the timeline delimitation in fishery LCA studies. Int. J. Life Cycle Assess. 16, 599–610. doi: 10.1007/s11367-011-0304-8
Raoux, A., Dambacher, J. M., Pezy, J.-P., Mazé, C., Dauvin, J.-C., and Niquil, N. (2018). Assessing cumulative socio-ecological impacts of offshore wind farm development in the Bay of Seine (English Channel). Mar. Policy 89, 11–20. doi: 10.1016/j.marpol.2017.12.007
Ravard, D., Brind’Amour, A., and Trenkel, V. M. (2014). Evaluating the potential impact of fishing on demersal species in the Bay of Biscay using simulations and survey data. Fish. Res. 157, 86–95. doi: 10.1016/j.fishres.2014.03.007
Reiss, H., Greenstreet, S. P. R., Robinson, L., Ehrich, S., Jørgensen, L. L., Piet, G. J., et al. (2010). Unsuitability of TAC management within an ecosystem approach to fisheries: an ecological perspective. J. Sea Res. 63, 85–92. doi: 10.1016/j.seares.2009.10.003
Reiss, H., Greenstreet, S. P. R., Sieben, K., Ehrich, S., Piet, G. J., Quirijns, F., et al. (2009). Effects of fishing disturbance on benthic communities and secondary production within an intensively fished area. Mar. Ecol. Prog. Ser. 394, 201–213. doi: 10.3354/meps08243
Rijnsdorp, A. D., Bastardie, F., Bolam, S. G., Buhl-Mortensen, L., Eigaard, O. R., Hamon, K. G., et al. (2016). Towards a framework for the quantitative assessment of trawling impact on the seabed and benthic ecosystem. ICES J. Mar. Sci. 73, i127–i138.
Rijnsdorp, A. D., Bolam, S. G., Garcia, C., Hiddink, J. G., Hintzen, N. T., van Denderen, P. D., et al. (2018). Estimating sensitivity of seabed habitats to disturbance by bottom trawling based on the longevity of benthic fauna. Ecol. Appl. 28, 1302–1312. doi: 10.1002/eap.1731
Rindorf, A., Dichmont, C. M., Levin, P. S., Mace, P., Pascoe, S., Prellezo, R., et al. (2017). Food for thought: pretty good multispecies yield. ICES J. Mar. Sci. 74, 475–486. doi: 10.1093/icesjms/fsw071
Robinson, L. A., and Frid, C. L. J. (2008). Historical marine ecology: examining the role of fisheries in changes in North Sea Benthos. Ambio 37, 362–371. doi: 10.1579/07-a-300.1
Rochet, M.-J., Collie, J. S., and Trenkel, V. M. (2013). How do fishing and environmental effects propagate among and within functional groups? Bull. Mar. Sci. 89, 285–315. doi: 10.5343/bms.2011.1138
Rochet, M.-J., Trenkel, V. M., Carpentier, A., Coppin, F., de Sola, L. G., Léauté, J.-P., et al. (2010). Do changes in environmental and fishing pressures impact marine communities? An empirical assessment. J. Appl. Ecol. 47, 741–750. doi: 10.1111/j.1365-2664.2010.01841.x
Röckmann, C., St. John, M. A., Schneider, U. A., and Tol, R. S. J. (2007). Testing the implications of a permanent or seasonal marine reserve on the population dynamics of Eastern Baltic cod under varying environmental conditions. Fish. Res. 85, 1–13. doi: 10.1016/j.fishres.2006.11.035
Röckmann, C., Ulrich, C., Dreyer, M., Bell, E., Borodzicz, E., Haapasaari, P., et al. (2012). The added value of participatory modelling in fisheries management – what has been learnt? Mar. Policy 36, 1072–1085. doi: 10.1016/j.marpol.2012.02.027
Rouyer, T., Fromentin, J.-M., Hidalgo, M., and Stenseth, N. C. (2014). Combined effects of exploitation and temperature on fish stocks in the Northeast Atlantic. ICES J. Mar. Sci. 71, 1554–1562. doi: 10.1093/icesjms/fsu042
Rubio, I., Ganzedo, U., Hobday, A. J., and Ojea, E. (2020). Southward re-distribution of tropical tuna fisheries activity can be explained by technological and management change. Fish Fish. 21, 511–521. doi: 10.1111/faf.12443
Sánchez, F., and Olaso, I. (2004). Effects of fisheries on the Cantabrian Sea shelf ecosystem. Ecol. Model. 172, 151–174. doi: 10.1016/j.ecolmodel.2003.09.005
Sangil, C., Clemente, S., Martín-García, L., and Hernández, J. C. (2012). No-take areas as an effective tool to restore urchin barrens on subtropical rocky reefs. Estuar. Coast. Shelf Sci. 112, 207–215. doi: 10.1016/j.ecss.2012.07.025
Schirripa, M. J., Abascal, F., Andrushchenko, I., Diaz, G., Mejuto, J., Ortiz, M., et al. (2017). A hypothesis of a redistribution of North Atlantic swordfish based on changing ocean conditions. Deep Sea Res. II Top. Stud. Oceanogr. 140, 139–150. doi: 10.1016/j.dsr2.2016.08.002
Schratzberger, M., and Jennings, S. (2002). Impacts of chronic trawling disturbance on meiofaunal communities. Mar. Biol. 141, 991–1000. doi: 10.1007/s00227-002-0895-5
Seara, T., Pollnac, R. B., Poggie, J. J., Garcia-Quijano, C., Monnereau, I., and Ruiz, V. (2017). Fishing as therapy: impacts on job satisfaction and implications for fishery management. Ocean Coast. Manag. 141, 1–9. doi: 10.1016/j.ocecoaman.2017.02.016
Selim, S. A., Blanchard, J. L., Bedford, J., and Webb, T. J. (2016). Direct and indirect effects of climate and fishing on changes in coastal ecosystem services: a historical perspective from the North Sea. Reg. Environ. Change 16, 341–351. doi: 10.1007/s10113-014-0635-7
Sguotti, C., Lynam, C. P., García-Carreras, B., Ellis, J. R., and Engelhard, G. H. (2016). Distribution of skates and sharks in the North Sea: 112 years of change. Global change Biol. 22, 2729–2743. doi: 10.1111/gcb.13316
Sguotti, C., Otto, S. A., Frelat, R., Langbehn, T. J., Ryberg, M. P., Lindegren, M., et al. (2018). Catastrophic dynamics limit Atlantic cod recovery. Proc. R. Soc. B Biol. Sci. 286:20182877. doi: 10.1098/rspb.2018.2877
Shephard, S., Gerristen, H., Kaiser, M. J., and Reid, D. G. (2012a). Spatial heterogeneity in fishing creates de facto refugia for endangered Celtic Sea Elasmobranchs. PLoS One 7:e49307. doi: 10.1371/journal.pone.0049307
Shephard, S., Fung, T., Houle, J. E., Farnsworth, K. D., Reid, D. G., and Rossberg, A. G. (2012b). Size-selective fishing drives species composition in the Celtic Sea. ICES J. Mar. Sci. 69, 223–234. doi: 10.1093/icesjms/fsr200
Shin, Y. J., Rochet, M. J., Jennings, S., Field, J. G., and Gislason, H. (2005). Using size-based indicators to evaluate the ecosystem effects of fishing. ICES J. Mar. Sci. 62, 384–396. doi: 10.1016/j.icesjms.2005.01.004
Simpson, A. W., and Watling, L. (2006). An investigation of the cumulative impacts of shrimp trawling on mud-bottom fishing grounds in the Gulf of Maine: effects on habitat and macrofaunal community structure. ICES J. Mar. Sci. 63, 1616–1630. doi: 10.1016/j.icesjms.2006.07.008
Sköld, M., Göransson, P., Jonsson, P., Bastardie, F., Blomqvist, M., Agrenius, S., et al. (2018). Effects of chronic bottom trawling on soft-seafloor macrofauna in the Kattegat. Mar. Ecol. Prog. Ser. 586, 41–55. doi: 10.3354/meps12434
Smith, M. D., Fulton, E. A., and Day, R. W. (2014). An investigation into fisheries interaction effects using Atlantis. ICES J. Mar. Sci. 72, 275–283. doi: 10.1093/icesjms/fsu114
Smoliñski, S. (2019). Incorporation of optimal environmental signals in the prediction of fish recruitment using random forest algorithms. Can. J. Fish. Aquat. Sci. 76, 15–27. doi: 10.1139/cjfas-2017-0554
Soma, K., Nielsen, J. R., Papadopoulou, N., Polet, H., Zengin, M., Smith, C. J., et al. (2018). Stakeholder perceptions in fisheries management - Sectors with benthic impacts. Mar. Policy 92, 73–85. doi: 10.1016/j.marpol.2018.02.019
Soma, K., Ramos, J., Bergh, Ø, Schulze, T., VanOostenbrugge, H., VanDuijn, A. P., et al. (2014). The ‘mapping out’ approach: effectiveness of marine spatial management options in European coastal waters. ICES J. Mar. Sci. 71, 2630–2642. doi: 10.1093/icesjms/fst193
Stallings, C. D. (2009). Predator identity and recruitment of coral-reef fishes: indirect effects of fishing. Mar. Ecol. Prog. Ser. 383, 251–259. doi: 10.3354/meps08004
STECF (2017a). Scientific, Technical and Economic Committee for Fisheries (STECF) – 55th Plenary Meeting Report (PLEN-17-02). Luxembourg: Publications Office of the European Union, doi: 10.2760/53335
STECF (2017b). Scientific, Technical and Economic Committee for Fisheries (STECF) – 56th Plenary Meeting Report (PLEN-17-03). Luxembourg: Publications Office of the European Union, doi: 10.2760/605712
STECF (2017c). Scientific, Technical and Economic Committee for Fisheries (STECF) – 54th Plenary Meeting Report (PLEN-17-01). Luxembourg: Publications Office of the European Union, doi: 10.2760/33472
STECF (2018a). Scientific,Technical and Economic Committee for Fisheries (STECF) – 59th Plenary Meeting Report (PLEN-18-01). Luxembourg: Publications Office of the European Union. doi: 10.2760/088784,JRC111800
STECF (2018b). Scientific, Technical and Economic Committee for Fisheries (STECF) – 58th Plenary Meeting Report (PLEN-18-02). Luxembourg: Publications Office of the European Union, doi: 10.2760/74942
STECF (2018c). Scientific, Technical and Economic Committee for Fisheries (STECF) – 59th Plenary Meeting Report (PLEN-18-03). Luxembourg: Publications Office of the European Union, doi: 10.2760/335280
STECF (2018d). Scientific, Technical and Economic Committee for Fisheries (STECF) –The 2018 Annual Economic Report on the EU Fishing Fleet (STECF-18-07). Luxembourg: Publications Office of the European Union, doi: 10.2760/56158
STECF (2019a). Scientific, Technical and Economic Committee for Fisheries (STECF) – 61st Plenary Meeting Report (PLEN-19-02). Luxembourg: Publications Office of the European Union, doi: 10.2760/31279
STECF (2019b). Scientific, Technical and Economic Committee for Fisheries (STECF) –62nd Plenary Meeting Report (PLEN-19-03). Luxembourg: Publications Office of the European Union, doi: 10.2760/1597
STECF (2019c). Scientific, Technical and Economic Committee for Fisheries (STECF) – 60th Plenary Meeting Report (PLEN-19-01). Luxembourg: Publications Office of the European Union, doi: 10.2760/56785
STECF (2020a). Scientific, Technical and Economic Committee for Fisheries (STECF) –Outermost Regions (OR) (STECF-19-19). Luxembourg: Publications Office of the European Union, doi: 10.2760/834602
STECF (2020b). Scientific, Technical and Economic Committee for Fisheries (STECF) –63rd Plenary Report–Written Procedure (PLEN-20-01). Luxembourg: Publications Office of the European Union, doi: 10.2760/465398
Svedäng, H., and Hornborg, S. (2015). Waiting for a flourishing Baltic cod (Gadus morhua) fishery that never comes: Old truths and new perspectives. ICES J. Mar. Sci. 72, 2197–2208. doi: 10.1093/icesjms/fsv112
Szostek, C. L., Murray, L. G., Bell, E., Rayner, G., and Kaiser, M. J. (2016). Natural vs. fishing disturbance: drivers of community composition on traditional king scallop, Pecten maximus, fishing grounds. ICES J. Mar. Sci. 73, i70–i83.
Ter Hofstede, R., and Rijnsdorp, A. D. (2011). Comparing demersal fish assemblages between periods of contrasting climate and fishing pressure. ICES J. Mar. Sci. 68, 1189–1198. doi: 10.1093/icesjms/fsr053
Thiebot, J.-B., Delord, K., Barbraud, C., Marteau, C., and Weimerskirch, H. (2016). 167 individuals versus millions of hooks: bycatch mitigation in longline fisheries underlies conservation of Amsterdam albatrosses. Aquat. Conserv. Mar. Freshw. Ecosyst. 26, 674–688. doi: 10.1002/aqc.2578
Thøgersen, T., Hoff, A., and Frost, H. S. (2015). Fisheries management responses to climate change in the Baltic Sea. Clim. Risk Manag. 10, 51–62. doi: 10.1016/j.crm.2015.09.001
Tidd, A. N., Vermard, Y., Marchal, P., Pinnegar, J., Blanchard, J. L., and Milner-Gulland, E. J. (2015). Fishing for space: fine-scale multi-sector maritime activities influence fisher location choice. PLoS One 10:e0116335. doi: 10.1371/journal.pone.0116335
Tillin, H. M., Hiddink, J. G., Jennings, S., and Kaiser, M. J. (2006). Chronic bottom trawling alters the functional composition of benthic invertebrate communities on a sea-basin scale. Mar. Ecol. Prog. Ser. 318, 31–45. doi: 10.3354/meps318031
Tomczak, M. T., Heymans, J. J., Yletyinen, J., Niiranen, S., Otto, S. A., and Blenckner, T. (2013). Ecological network indicators of ecosystem status and change in the Baltic Sea. PLoS One 8:e75439. doi: 10.1371/journal.pone.0075439
Tomczak, M. T., Müller-Karulis, B., Järv, L., Kotta, J., Martin, G., Minde, A., et al. (2009). Analysis of trophic networks and carbon flows in south-eastern Baltic coastal ecosystems. Prog. Oceanogr. 81, 111–131. doi: 10.1016/j.pocean.2009.04.017
Torres, M. T., Coll, M., Heymans, J. J., Christensen, V., and Sobrino, I. (2013). Food-web structure of and fishing impacts on the Gulf of Cadiz ecosystem (South-western Spain). Ecol. Model. 265, 26–44. doi: 10.1016/j.ecolmodel.2013.05.019
Torres-Irineo, E., Amandè, M. J., Gaertner, D., de Molina, A. D., Murua, H., Chavance, P., et al. (2014). Bycatch species composition over time by tuna purse-seine fishery in the eastern tropical Atlantic Ocean. Biodivers. Conserv. 23, 1157–1173. doi: 10.1007/s10531-014-0655-0
Trifonova, N., Kenny, A., Maxwell, D., Duplisea, D., Fernandes, J., and Tucker, A. (2015). Spatio-temporal Bayesian network models with latent variables for revealing trophic dynamics and functional networks in fisheries ecology. Ecol. Inform. 30, 142–158. doi: 10.1016/j.ecoinf.2015.10.003
Trifonova, N., Maxwell, D., Pinnegar, J., Kenny, A., and Tucker, A. (2017). Predicting ecosystem responses to changes in fisheries catch, temperature, and primary productivity with a dynamic Bayesian network model. ICES J. Mar. Sci. 74, 1334–1343. doi: 10.1093/icesjms/fsw231
Trimmer, M., Petersen, J., Sivyer, D. B., Mills, C., Young, E., and Parker, E. R. (2005). Impact of long-term benthic trawl disturbance on sediment sorting and biogeochemistry in the southern North Sea. Mar. Ecol. Prog. Ser. 298, 79–94. doi: 10.3354/meps298079
Tulp, I., Glorius, S., Rippen, A., Looije, D., and Craeymeersch, J. (2020). Dose-response relationship between shrimp trawl fishery and the macrobenthic fauna community in the coastal zone and Wadden Sea. J. Sea Res. 156:101829. doi: 10.1016/j.seares.2019.101829
van Denderen, P. D., Bolam, S. G., Hiddink, J. G., Jennings, S., Kenny, A., Rijnsdorp, A. D., et al. (2015a). Similar effects of bottom trawling and natural disturbance on composition and function of benthic communities across habitats. Mar. Ecol. Prog. Ser. 541, 31–43. doi: 10.3354/meps11550
van Denderen, P. D., Hintzen, N. T., Rijnsdorp, A. D., Ruardij, P., and van Kooten, T. (2014). Habitat-specific effects of fishing disturbance on benthic species richness in marine soft sediments. Ecosystems 17, 1216–1226. doi: 10.1007/s10021-014-9789-x
van Denderen, P. D., Hintzen, N. T., Van Kooten, T., and Rijnsdorp, A. D. (2015b). Temporal aggregation of bottom trawling and its implication for the impact on the benthic ecosystem. ICES J. Mar. Sci. 72, 952–961. doi: 10.1093/icesjms/fsu183
van der Molen, J., Aldridge, J. N., Coughlan, C., Parker, E. R., Stephens, D., and Ruardij, P. (2013). Modelling marine ecosystem response to climate change and trawling in the North Sea. Biogeochemistry 113, 213–236. doi: 10.1007/s10533-012-9763-7
van der Reijden, K. J., Hintzen, N. T., Govers, L. L., Rijnsdorp, A. D., and Olff, H. (2018). North Sea demersal fisheries prefer specific benthic habitats. PLoS One 13:e0208338. doi: 10.1371/journal.pone.0208338
Varela-Lafuente, M. M., Garza-Gil, M. D., and Surís-Regueiro, J. C. (2019). Evolution of management in the Celtic Sea fishery: economic effects on the Galician fleet. Ocean Coast. Manag. 167, 229–235. doi: 10.1016/j.ocecoaman.2018.10.021
Varisco, M., and Vinuesa, J. H. (2007). Diet of Munida gregaria (Fabricius, 1793) (Crustacea: Anomura: Galatheidae) in fishing beds of the San Jorge Gulf, Argentina. Rev. Biol. Mar. Oceanogr. 42, 221–229.
Vergnon, R., and Blanchard, F. (2006). Evaluation of trawling disturbance on macrobenthic invertebrate communities in the Bay of Biscay, France: abundance biomass comparison (ABC method). Aquat. Living Resour. 19, 219–228. doi: 10.1051/alr:2006022
Véron, M., Duhamel, E., Bertignac, M., Pawlowski, L., and Huret, M. (2020). Major changes in sardine growth and body condition in the Bay of Biscay between 2003 and 2016: temporal trends and drivers. Prog. Oceanogr. 182:102274. doi: 10.1016/j.pocean.2020.102274
Verschueren, B., Lenoir, H., Soetaert, M., and Polet, H. (2019). Revealing the by-catch reducing potential of pulse trawls in the brown shrimp (crangon crangon) fishery. Fish. Res. 211, 191–203. doi: 10.1016/j.fishres.2018.11.011
Vilela, R., and Bellido, J. M. (2015). Fishing suitability maps: helping fishermen reduce discards. Can. J. Fish. Aquat. Sci. 72, 1191–1201. doi: 10.1139/cjfas-2013-0522
Villasante, S., Pita, P., Antelo, M., and Neira, J. A. (2019). Socio-economic impacts of the landing obligation of the european union common fisheries policy on galician (NW Spain) small-scale fisheries. Ocean Coast. Manag. 170, 60–71. doi: 10.1016/j.ocecoaman.2018.12.029
Vinther, M., and Eero, M. (2013). Quantifying relative fishing impact on fish populations based on spatio-temporal overlap of fishing effort and stock density. ICES J. Mar. Sci. 70, 618–627. doi: 10.1093/icesjms/fst001
Voerman, S. E., Llera, E., and Rico, J. M. (2013). Climate driven changes in subtidal kelp forest communities in NW Spain. Mar. Environ. Res. 90, 119–127. doi: 10.1016/j.marenvres.2013.06.006
von Nordheim, L., Kotterba, P., Moll, D., and Polte, P. (2018). Impact of spawning substrate complexity on egg survival of atlantic herring (Clupea harengus, L.) in the Baltic Sea. Estuar. Coasts 41, 549–559. doi: 10.1007/s12237-017-0283-5
von Schuckmann, K., Le Traon, P. Y., Smith, N., Pascual, A., Djavidnia, S., Gattuso, J. P., et al. (2019). Copernicus marine service ocean state report, issue 3. J. Operat. Oceanogr. 12(suppl. 1), S1–S123. doi: 10.1080/1755876X.2019.1633075
Voss, R., Quaas, M. F., Schmidt, J. O., Tahvonen, O., Lindegren, M., and Möllmann, C. (2014). Assessing social – ecological trade-offs to advance ecosystem-based fisheries management. PLoS One 9:e107811. doi: 10.1371/journal.pone.0107811
Voss, R., Quaas, M. F., Stiasny, M. H., Hänsel, M., Stecher Justiniano Pinto, G. A., Lehmann, A., et al. (2019). Ecological-economic sustainability of the Baltic cod fisheries under ocean warming and acidification. J. Environ. Manag. 238, 110–118. doi: 10.1016/j.jenvman.2019.02.105
Wallace, B. P., Heppell, S. S., Lewison, R. L., Kelez, S., and Crowder, L. B. (2008). Impacts of fisheries bycatch on loggerhead turtles worldwide inferred from reproductive value analyses. J. Appl. Ecol. 45, 1076–1085.
Ward, I., and Larcombe, P. (2008). Determining the preservation rating of submerged archaeology in the post-glacial southern North Sea: a first-order geomorphological approach. Environ. Archaeol. 13, 59–83. doi: 10.1179/174963108x279229
Wienberg, C., Wintersteller, P., Beuck, L., and Hebbeln, D. (2013). Coral patch seamount (NE Atlantic) – a sedimentological and megafaunal reconnaissance based on video and hydroacoustic surveys. Biogeosciences 10, 3421–3443. doi: 10.5194/bg-10-3421-2013
Wikström, A., Knape, J., Casini, M., Gårdmark, A., Cardinale, M., Hjelm, J., et al. (2016). Fishing, reproductive volume and regulation: population dynamics and exploitation of the eastern Baltic cod. Popul. Ecol. 58, 199–211. doi: 10.1007/s10144-015-0520-3
Wright, P. J., Palmer, S. C. F., and Marshall, C. T. (2014). Maturation shifts in a temperate marine fish population cannot be explained by simulated changes in temperature-dependent growth and maturity. Mar. Biol. 161, 2781–2790. doi: 10.1007/s00227-014-2543-2
Zhou, S., Hobday, A. J., Dichmont, C. M., and Smith, A. D. M. (2016). Ecological risk assessments for the effects of fishing: a comparison and validation of PSA and SAFE. Fish. Res. 183(Suppl. C), 518–529. doi: 10.1016/j.fishres.2016.07.015
Ziegler, F., and Hornborg, S. (2014). Stock size matters more than vessel size: the fuel efficiency of Swedish demersal trawl fisheries 2002-2010. Mar. Policy 44, 72–81. doi: 10.1016/j.marpol.2013.06.015
Zimmermann, F., Claireaux, M., and Enberg, K. (2019). Common trends in recruitment dynamics of north-east Atlantic fish stocks and their links to environment, ecology and management. Fish Fish. 20, 518–536. doi: 10.1111/faf.12360
Keywords: cumulative impacts, fishing opportunities, integrative management, marine policy, Atlantic Europe, EU Outermost Regions, Baltic Sea, lack of knowledge
Citation: Bastardie F, Brown EJ, Andonegi E, Arthur R, Beukhof E, Depestele J, Döring R, Eigaard OR, García-Barón I, Llope M, Mendes H, Piet G and Reid D (2021) A Review Characterizing 25 Ecosystem Challenges to Be Addressed by an Ecosystem Approach to Fisheries Management in Europe. Front. Mar. Sci. 7:629186. doi: 10.3389/fmars.2020.629186
Received: 13 November 2020; Accepted: 24 December 2020;
Published: 22 January 2021.
Edited by:
Cornelia E. Nauen, Mundus Maris, BelgiumReviewed by:
Fabio Fiorentino, National Research Council (CNR), ItalyClaudio Vasapollo, Independent Researcher, Ancona, Italy
Copyright © 2021 Bastardie, Brown, Andonegi, Arthur, Beukhof, Depestele, Döring, Eigaard, García-Barón, Llope, Mendes, Piet and Reid. This is an open-access article distributed under the terms of the Creative Commons Attribution License (CC BY). The use, distribution or reproduction in other forums is permitted, provided the original author(s) and the copyright owner(s) are credited and that the original publication in this journal is cited, in accordance with accepted academic practice. No use, distribution or reproduction is permitted which does not comply with these terms.
*Correspondence: Francois Bastardie, ZmJhQGFxdWEuZHR1LmRr; Elliot J. Brown, ZWxickBhcXVhLmR0dS5kaw==
†These authors have contributed equally to this work