- 1Institute of Oceanography and Fisheries, Split, Croatia
- 2Instituto de Ciencias Naturales Alexander Von Humboldt, Universidad de Antofagasta, Antofagasta, Chile
- 3Universidad de Antofagasta Stable Isotope Facility, Instituto de Antofagasta, Universidad de Antofagasta, Antofagasta, Chile
- 4INVASAL, Concepción, Chile
We examined how the trophic ecology of nine economically important marine taxa varied across three distinct areas of the Adriatic Sea. These taxa included three species of demersal fishes (European hake Merluccius merluccius, red mullet Mullus barbatus, black-bellied angler Lophius budegassa) and two species of decapod crustaceans (Norway lobster Nephrops norvegicus, deep-water rose shrimp Parapenaeus longirostris) and four species of pelagic fishes (sardine Sardina pilchardus, anchovy Engraulis encrasicolus, Mediterranean horse mackerel Trachurus mediterraneus, Atlantic horse mackerel Trachurus trachurus). We used two complementary methods that differed in their temporal context to examine and compare diet. Stomach contents analysis was used to describe the short term diet while stable isotope analysis was used compare long-term assimilated diet. Results showed that although there were spatial differences in what each species consumed, and in their trophic and isotopic niches, each species fed at similar trophic position across locations, indicating similar ecological function. Comparisons of biomass-weighted trophic position (δ15N) and consumer body size (log2 mass) showed evidence for a common isotopic size spectrum across areas, indicating the existence of a size-structured food web. In turn this allowed us to provide a first estimate of the predator–prey body mass ratio (PPMR) for this area (655:1). Results obtained within this study, in future, could be used for ecological modeling and improved long-term management of the Adriatic Sea’s marine resources.
Introduction
Recent decades have seen marked reductions in the biomass of several commercially important fish stocks across the Mediterranean region (FAO, 2018), including the Adriatic Sea. Although the Adriatic Sea only represents ca. 5% of the total surface area of the Mediterranean Sea, several stocks of pelagic and demersal fishes important to Mediterranean fisheries are located in this semi-enclosed sea, and are shared between bordering countries (UNEP-MAP-RAC/SPA, 2014). Fisheries management relies on accurate information regarding the ecology and factors affecting the population dynamics of target stocks and how they vary over time and space.
In marine ecosystems, habitats and species are exposed to a wide range of natural and anthropogenic pressures. Over time, those can affect and drive shifts in marine ecosystems and taxa. The Adriatic Sea has experienced the combined effects of multiple stressors including climatic shift, pollution, and overexploitation in recent decades (Grbec et al., 2014; Ramírez et al., 2018). As such and reflecting patterns seen in other geographical areas (Burkhard, 2003; Nagelkerken et al., 2020) it is reasonable to expect that Adriatic food webs have also changed over the same period, with shifts in trophic structure, feeding patterns and nutritional relationships. Given the importance of commercial fisheries in the Adriatic Sea (mean annual yield (2000-2017) = 156 651 tonnes; FAO, 2020) there is a pressing need to provide an integrated characterization of the trophic ecology of key species as a first step toward future ecosystem-based management.
To date, the feeding habits of commercially exploited pelagic (sardine, anchovy, Atlantic horse mackerel, Mediterranean horse mackerel) and demersal (European hake, red mullet and black-bellied angler) fishes in the Adriatic have largely been studied at a species-specific level (Jardas et al., 2004; Šantić et al., 2004; Vrgoč et al., 2004; Tirelli et al., 2006; Borme et al., 2009; Zorica et al., 2017; Riccioni et al., 2018). However, a few comparative studies do exist (Zorica et al., 2016). Most existing information on trophic ecology is largely based on stomach content analysis (SCA), which provides information regarding the most frequent and abundant prey items consumed. Although this is a commonly used method in fish biology, it has its limitations. SCA provides information on prey that have been ingested most recently, while the contribution of digested prey items is neglected even though their assimilation has contributed to the energy budget of an individual (Hyslop, 1980; Wolf et al., 2009).
In order to provide a wider understanding of fish trophic ecology that counters some of the limitations of SCA, scientists have increasingly used alternative techniques, including stable isotope analysis (SIA) (Pethybridge et al., 2018). This method assumes that the ratio of heavy and light isotopes (e.g., 13C/12C, 15N/14N) in the tissues of an individual can indicate the relative contributions of prey items or prey characteristics (e.g., trophic position) assimilated over a longer time period than SCA. This time period depends on the tissues analyzed (e.g., liver, white muscle, blood), as their isotopic turnover rate can range from a few days to months (Hesslein et al., 1993; Bearhop et al., 2004).
Although SIA cannot provide the taxonomic resolution offered by SCA, it can reveal contributions of different energy sources, e.g., pelagic and benthic (Docmac et al., 2017) and it allows the estimation of consumer trophic position and food web length (Cabana and Rasmussen, 1996). Importantly, information can be gathered from individuals with empty or inverted stomachs, which can be a key limitation of SCA when examining the diet of demersal fishes. When interpreting the results of stable isotope analysis, one should be aware that consumer isotopic values may be the result of several combinations of possible food items. Both SCA and SIA have their advantages and disadvantages (reviewed by Majdi et al., 2018; Nielsen et al., 2018; Pethybridge et al., 2018). However, their combined use can provide a robust characterization of species diet and of food web structure (Winemiller et al., 2007; Fanelli and Cartes, 2010; Young et al., 2018). Recently, analysis of stable nitrogen isotopes in the context of marine food webs has attracted considerable attention (Jennings et al., 2001; Bode et al., 2003; Funes et al., 2019). This has proved particularly useful as a mean of examining different theories regarding how ecosystems function. At a functional level, consumer body size can be considered as a proxy for trophic position, meaning that body size rather than the species identity can be considered as a trophic position identifier in marine ecosystems (Jennings et al., 2008). This has proved a useful tool to examine food webs and how they vary over space and time, and to rapidly estimate important parameters that characterize ecosystem function such predator:prey mass ratios (PPMR) and that are commonly required for ecosystem-based models (Jennings et al., 2008; McCormack et al., 2019). By analyzing the stomach content and stable isotopes of nine ecologically and economically most important fisheries resources in the Adriatic Sea, we aimed to provide some important parameters (TP, PPMR) that might be used in future ecological modeling. We also aimed to examine how spatial differences in habitat characteristics affect trophic ecology at the species and community level. To achieve these general aims, we characterized trophic ecology using SCA and SIA across three distinct geographic areas of the Adriatic Sea (Northern, Middle and Southern areas) of which differed in terms of depth, distance from the shore, and productivity. We also estimated the community isotopic size spectrum of each area and estimated PPMR.
More specifically, we examined: (i) how the diet of each species varied spatially, (ii) how species-level estimates of trophic position varied; and (iii) how community level measures of food web structure differed across three areas of the Adriatic Sea. Our data provide a first comparative survey of the feeding habits in commercially important species in the Adriatic, and how these vary spatially. Our estimates of trophic position for the different species and the community-level estimate of PPMR can be used to parameterize Ecopath with Ecosim models that can provide predictions of stock biomass in response to fishing pressure and various environmental factors as recommended by the General Fisheries Commission for the Mediterranean (GFCM, 2018).
Materials and Methods
Study Area and Sampling Methods
The Adriatic Sea is the northernmost part of the Mediterranean Sea (Figure 1). It has a general cyclonic circulation driven by estuarine inputs, with pronounced seasonality induced by changing winds and thermal fluxes throughout the year (Cushman-Roisin et al., 2001). The water column is typically thermally stratified during summer, while substantial wind-mixing and winter surface cooling leads to stratification breaking down in the winter (Buljan and Zore-Armanda, 1974). The Adriatic Sea is characterized by varying oceanographic conditions, especially regarding nutrient status (Zavatarelli et al., 2000). The shallow northern basin, particularly its north-western area, is influenced by materials from the discharge of the River Po, and is considered as productive and potentially eutrophic area. Conversely, the deeper central and southern basins are considered as oligotrophic, particularly along the eastern margin (Kovač et al., 2018). In general, the Adriatic Sea supports a diverse marine community (Coll et al., 2010; Dulčić et al., 2017). This study was conducted in three distinct areas within the Croatian part of the Adriatic Sea – Northern, Middle and Southern (Figure 1).
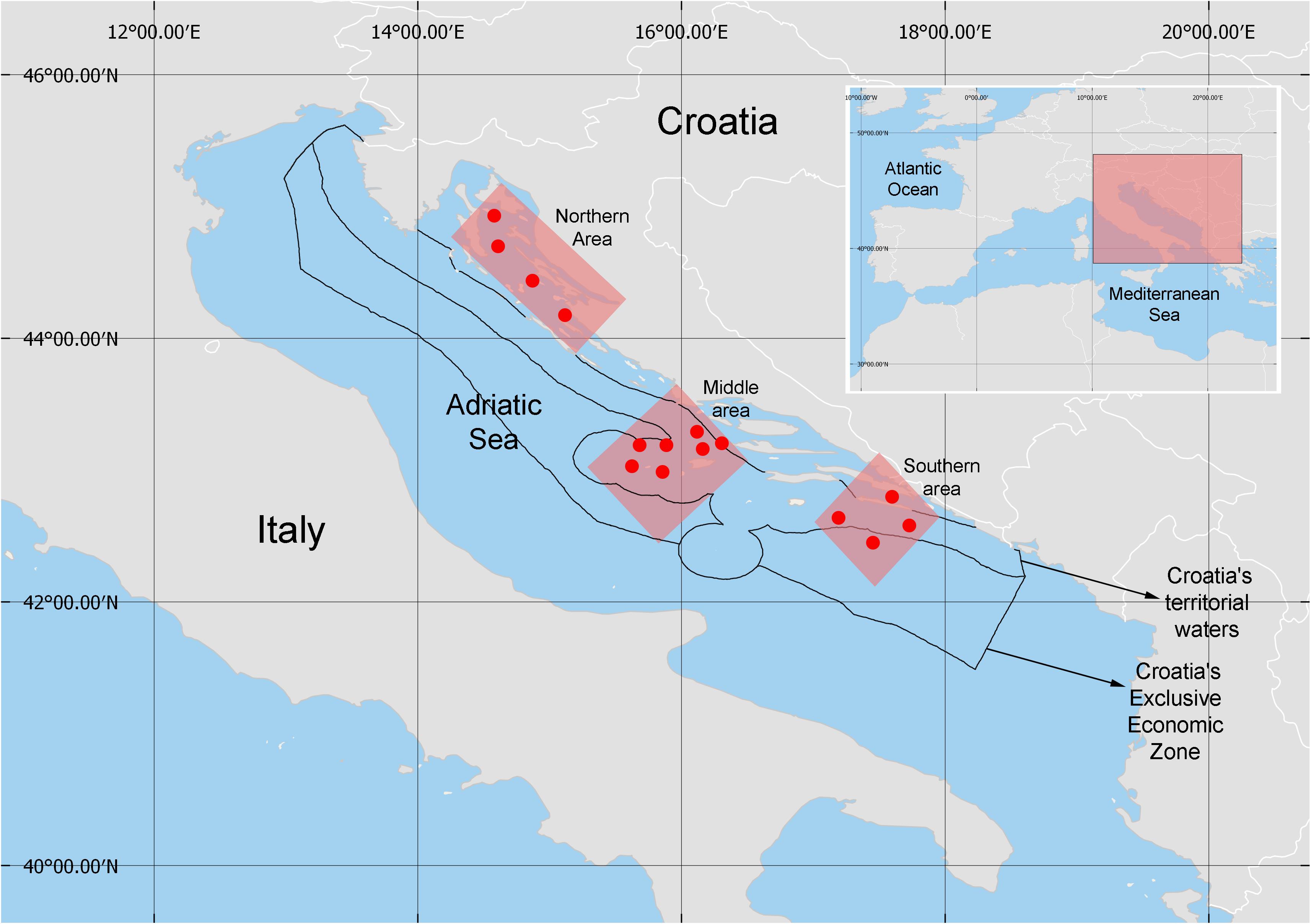
Figure 1. Sites sampled in the Northern, Middle and Southern areas located within the Croatian fishing grounds of the Adriatic Sea.
Here, we refer to the Northern area as the northern region of the eastern Adriatic Sea, where we sampled the channel area of Kvarnerić (four sampling stations; Figure 1). This area acts as a spawning and/or nursery ground of both pelagic and demersal species (Tičina et al., 2000; Sinovčić and Zorica, 2009; Piccinetti et al., 2012), associated with its productivity being enhanced by riverine inputs (Viličić et al., 2013).
The open Middle area (seven sampling stations; Figure 1) is characterized by a gradient of decreasing nutrient conditions from more productive coastal areas to oligotrophic open sea areas (Ninčević Gladan et al., 2006; Šantić et al., 2014). Moreover, the open sea is influenced by discharge of the nutrient-enriched river Po along the western coast, and it is also an area of upwelling (Bergamasco and Gačić, 1996; Gačić et al., 1997; Mauri and Poulain, 2001). The Middle area acts as spawning and/or nursing habitat for several marine fishes (European hake, black-bellied angler, Mediterranean poor cod, Mediterranean scaldfish) and crustaceans (Norway lobster, deep-water rose shrimp) (Županović and Jardas, 1989; Piccinetti et al., 2012). A Fisheries Restricted Area (FRA) in the Jabuka/Pomo Pit was recently established in this area (GFCM, 2018) to protect spawning habitats of commercially important demersal taxa (Vrgoč et al., 2004).
The Southern area (four sampling stations; Figure 1) is characterized both by deeper water and by low relative productivity compared to the Northern and Middle areas, the latter reflecting water exchange between the Adriatic and the northern Ionian Sea across the Otranto Strait (Civitarese et al., 2010).
In order to study food web structure in each of the three areas of the Adriatic Sea we sampled demersal fishes (European hake merluccius, red mullet Mullus barbatus, black-bellied angler Lophius budegassa) and decapod crustaceans (Norway lobster Nephrops norvegicus, deep-water rose shrimp Parapenaeus longirostris) as well as pelagic fishes (sardine Sardina pilchardus, anchovy Engraulis encrasicolus, Mediterranean horse mackerel Trachurus mediterraneus, Atlantic horse mackerel Trachurus trachurus). Samples were collected by bottom trawl (MEDITS trawl-net GOC73) during the scientific survey ‘Mediterranean International Bottom Trawl-Survey’ (MEDITS) carried out along the eastern Adriatic Sea (Croatian fishing ground, Figure 1) between 30 June and 21 July, 2018. The tows were carried out over the continental shelf between 40 and 800 m with duration of 30 to 60 minutes depending on the depth and a mean trawl speed of 3 knots.
Immediately after landing, the total length (TL ± 0.1 cm) and wet mass (W ± 0.01 g) of target specimens (up to 10 individuals from each area) were recorded onboard. Within these species, marked ontogenetic diet shifts have been reported for hake (Garrison and Link, 2000; Mahe et al., 2007). As such, hakes were sub-sampled by length classes: small (S: TL < 20.0 cm), medium (M: 20.1 cm < TL < 30.0 cm) and large (L: 30.1 cm > TL), while all other fishes were treated as a single category (Supplementary Table 1).
Stomach contents analysis were performed on 10 specimens of each fish species per area. Some European hake and black-bellied angler had inverted stomachs or had obviously regurgitated some prey following capture. These individuals were excluded from SCA. Fish visceral cavities were carefully opened prior to preservation in 10% buffered formalin. Stomachs were removed and weighed (± 0.01 g) before and after (total mass of full Wsf, g; and empty stomach Wse, g) prey items were removed. Prey items were identified under a stereomicroscope (Zeiss Discovery. V12; magnification 40–80x) to class or family systematic level using relevant identification keys (Trégouboff and Rose, 1957; Todd et al., 1996; Larink and Westheide, 2006) and counted.
We collected zooplankton to compare food availability in the water column with prey consumed by fish. Zooplankton samples were collected on same occasion as fish samples using a standard WP2 plankton net of 200 μm mesh size (mouth opening, 0.255 m2). The WP2 gear was hauled vertically from the near-bottom to the surface, performing double tows in on one sampling station in each of the three areas (Northern, Middle and Southern areas). Samples for SIA were frozen immediately after collection (−20°C), while samples for microscopic analysis of the zooplankton community were fixed with buffered 4% formaldehyde–seawater solution. Counting and species identification of zooplankton were performed using an inverted microscope (Olympus IX51), following standard laboratory practice. Subsamples for counting were obtained by the splitting method (1/32), while the entire sample was checked for rare species. Abundances were expressed as the number of individuals per cubic meter (ind. m–3). Taxonomic identification was generally performed to the species or genus level for holoplanktonic groups, while miscellaneous larvae were determined to lower taxonomic levels (class or phylum). Margalef’s index was calculated to express the diversity of zooplankton community at each sampling station (PRIMER v6 software package; Clarke, 1993; Clarke and Warwick, 1994).
For stable isotope analysis, a total of 241 individuals from 9 species were sampled (Supplementary Table 2). All individuals were frozen (−20°C) immediately after sampling. In the laboratory, samples were defrosted, and in fish, a small piece of dorsal-anterior muscle was dissected, and skin and bones removed. Samples were dried in an oven at 60°C for 48 h and afterward ground using a mortar and pestle to homogenize the sample. To avoid possible inorganic carbon contamination due to residual bones in all small fish (e.g., sardine, anchovy), acid fumigation treatment was performed (following the procedure applied in Lorrain et al., 2003 for suspended particulate material). After acidification samples were dried at 60°C for 24 h. For decapod crustaceans, exoskeletons were removed and a sample of tail muscle tissue was taken for analysis, dried and homogenized in a similar way to fish. Plankton samples used as baseline were sieved through a 200 μm sieve to remove smaller plankton size fraction and three subsamples were obtained from each sample. Each plankton subsample was dried in oven (60°C for 48 h), homogenized, exposed to acid fumigation treatment (Lorrain et al., 2003) and dried (at 60°C for 24 h). Due to potential acidification effects, δ15N was analyzed separately on non-treated samples, both for small fish and plankton samples. Acidified samples were packed into silver capsules to avoid corrosion and sample leakage.
Samples were analyzed at the Stable Isotope Facility at the University of California, Davis (United States). Stable isotope values of carbon (δ13C) and nitrogen (δ15N), and elemental%C and%N analyses were estimated using a PDZ Europa ANCA-GSL elemental analyzer interfaced to a PDZ Europa 20-20 isotope ratio mass spectrometer (Sercon Ltd., Cheshire, United Kingdom). The long-term standard deviation was ± 0.1‰ for δ13C and ± 0.3‰ for δ15N. The final delta values are expressed relative to international VPDB (Vienna PeeDee Belemnite) standards and air for carbon and nitrogen, respectively. Fish δ13C values were lipid-corrected following Kiljunen et al. (2006). Arithmetic lipid normalization was not applied to decapod crustaceans as Bodin et al. (2007) noted that it performed badly for these taxa. Although we not present lipid corrected δ13C values for decapod crustaceans, their mean muscle C:N was < 3.5, indicating a value close to that expected for protein, and as such there is unlikely a major lipid-effect on their δ13C values.
Data Analyses
To assess the diet composition of each species the following indices were calculated:
The fullness index %Jr = (Wp/W) × 100, where Wp is the mass of prey items calculated as the difference between the mass of full and empty stomachs (Wsf – Wse); W refers to a total body mass.
The vacuity index %V = E/N × 100, where E is the number of empty stomachs and N the total number of stomachs analyzed.
The frequency of occurrence %F = n/N × 100, where n is the number of stomachs containing a certain prey and N is the total number of analyzed stomachs containing any kind of prey.
Numerical abundance %N = np/Np × 100, where np is the number of prey specimens in a specific group and Np is the number of all determined prey groups. The niche width of each species in each area of sampling was calculated by Levins’ standardized index (Hurlbert, 1978; Krebs, 1989): Bi = [1/(n-1)](1/(∑ jpij2)-1) where Bi is Levins’ standardized index for predator i; pij the proportion of diet of predator i that is made up of prey j; n the number of prey categories. The values of Bi ranges from 0 to 1; lower values indicate diets dominated by few prey items (specialist predators) while higher values indicate generalist diets (Krebs, 1989).
Stomach contents abundance data (for the species that had 3 or more individuals) from individual fish were log(x + 1)-transformed, and a Bray–Curtis similarity matrix was constructed using PRIMER v6 (Clarke, 1993; Clarke and Warwick, 1994). In order to compare whether the observed diet varied within each species (or size classes in the case of hake) we used two factor PERMANOVA (fixed factors were area and species) (PRIMER-E Ltd.; Clarke and Warwick, 2001). Post hoc pairwise PERMANOVA was used to identify species among which the differences in diet were statistically significant. A one-way PERMANOVA test, followed by post hoc pairwise tests, was used to compare the diet of different European hake size classes. For species that had low number of permutations, Monte-Carlo P values were calculated. To visualize dietary variation, we used non-metric multidimensional scaling (nMDS) ordination based on a Bray–Curtis similarity matrix. Average dissimilarities between ambient mesozooplankton from the three study areas were determined using the SIMPER routine in PRIMER software package on a log(x + 1)-transformed abundance matrix (Clarke, 1993; Clarke and Warwick, 1994).
The size and overlap of isotopic niches between different species were estimated in the R package SIBER (Version 2.1.4, Jackson et al., 2011). Isotopic niches were estimated from each species (with N > 4 individuals) by calculating the Standard Ellipse Area corrected for small sample size (SEAc) which is based on the core 40% of δ15N and δ13C values. Furthermore, overlap of isotopic niches between species was assessed by calculating the percentage of the overlap of SEAc area.
We estimated and compared species (and for hake, size class) modal trophic position (TP) and 95% credibility interval (i.e., 95% of modeled estimates of TP) for each area using the R package tRophicPosition (version 0.7.7; Quezada-Romegialli et al., 2018). We used mesozooplankton δ15N values from the study area as the trophic baseline (λ = 2) and conducted comparisons using the oneBaseline model option. We used a trophic discrimination factor (TDF) based on aquatic consumers from McCutchan et al. (2003) (Δ15N = 2.9 ± 0.3‰).
We examined community isotopic size spectra and mean predator-prey body mass ratios (PPMR) for the three study areas to test the possible size-structuring of trophic relationships within each community. We followed Jennings et al. (2001) by using linear correlation to examine possible relations between biomass-weighted trophic position (δ15N) (Al-Habsi et al., 2008) and body size (log2 body mass) for each area of the Adriatic studied (Northern, Middle and Southern). Following equation was used:
where δ15N is the nitrogen isotopic values of individuals 1 to i and Wt is their respective mass. We compared the form of the relationships between each region using ANCOVA. We calculated mean PPMRs using the equation mass ratio = 2TDF/b, where TDF is the mean trophic discrimination factor and b is the slope of the relationship between biomass weighted δ15N and Log2 size class, and we used the mean TDF of 2.9 ‰ (McCutchan et al., 2003).
Results
Diet Composition Obtained by Stomach Content Analysis
Overall, we examined 183 individuals belonging to seven species collected from the three areas of the Adriatic Sea (Supplementary Table 1). Of these, 141 individuals, (77%) had food remains in their stomachs. The greatest number of empty stomachs was observed in hake, especially in large (TL > 30.0 cm) individuals. In contrast, all sardine specimens had at least one prey item in their stomach.
The estimated abundance and frequency of occurrence of different prey categories in each species are given in Tables 1–3. In the Northern area, 14 different taxonomic groups were identified among prey items of five fish species, while in the Middle and Southern areas SCA was conducted on six fish species in each area and revealed the presence of 14 and 10 different prey items, respectively. Since both animal prey and phytoplankton items were identified in four fish species (anchovy, sardine, small-bodied European hake and red mullet), we classified these taxa as omnivores. Mediterranean and Atlantic horse mackerel, medium and large hake as well as black-bellied angler, were classified as being carnivorous, as they only had animal prey in their stomachs.
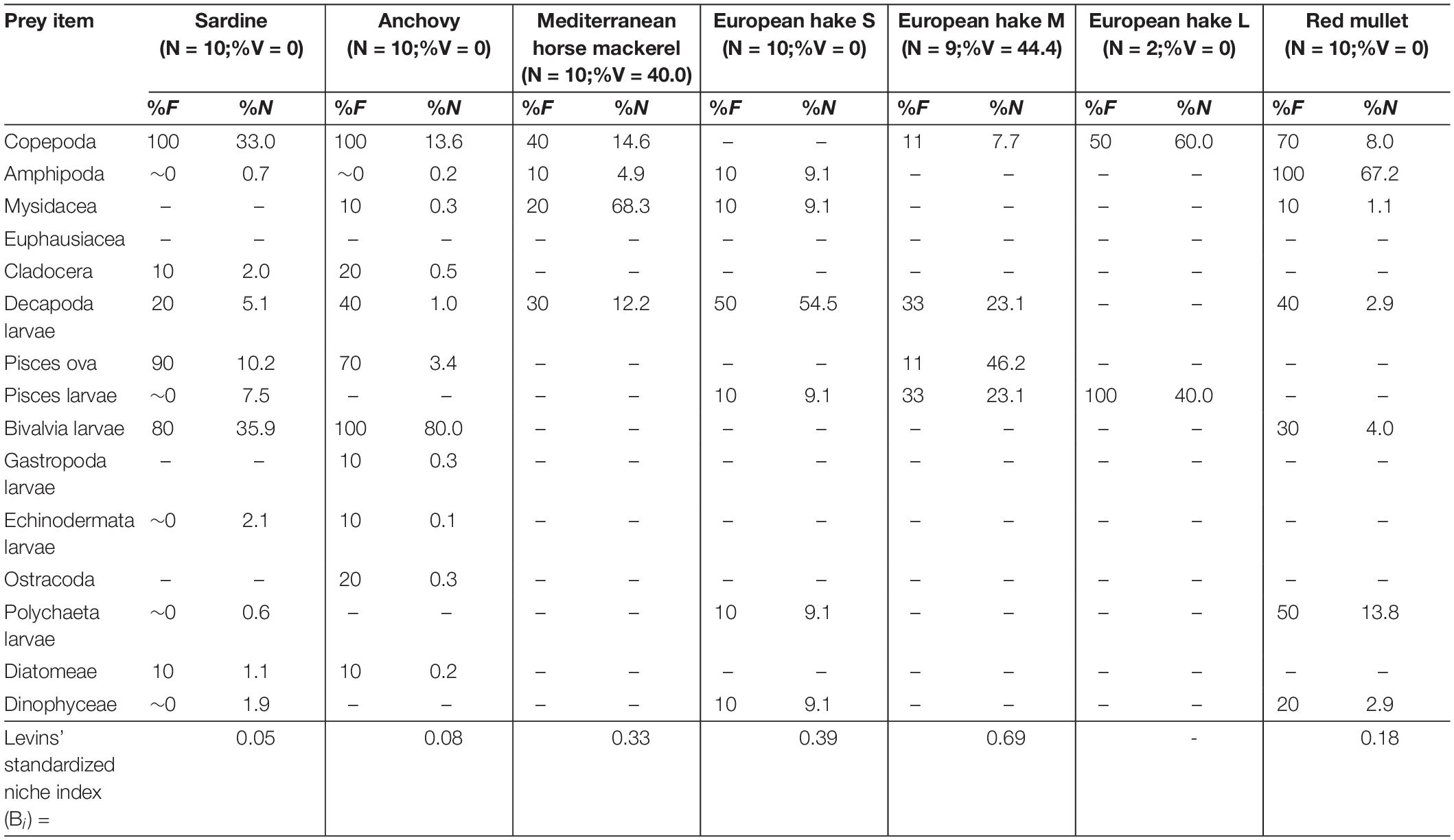
Table 1. Frequency of occurrence (%F) and abundance (%N) of prey items found in the sardine, anchovy, Mediterranean horse mackerel, European hake [by size classes: small (S: TL < 20.0 cm), medium (M: 20.1 cm < TL < 30.0 cm) and large (L: 30.1 cm > TL)] and red mullet collected in the Northern area (Kvarnerić Bay) of eastern Adriatic Sea during MEDITS survey (June–July, 2018) along with number of analyzed specimens (N), its vacuity index (%V) and Levins’ standardized index (Bi).
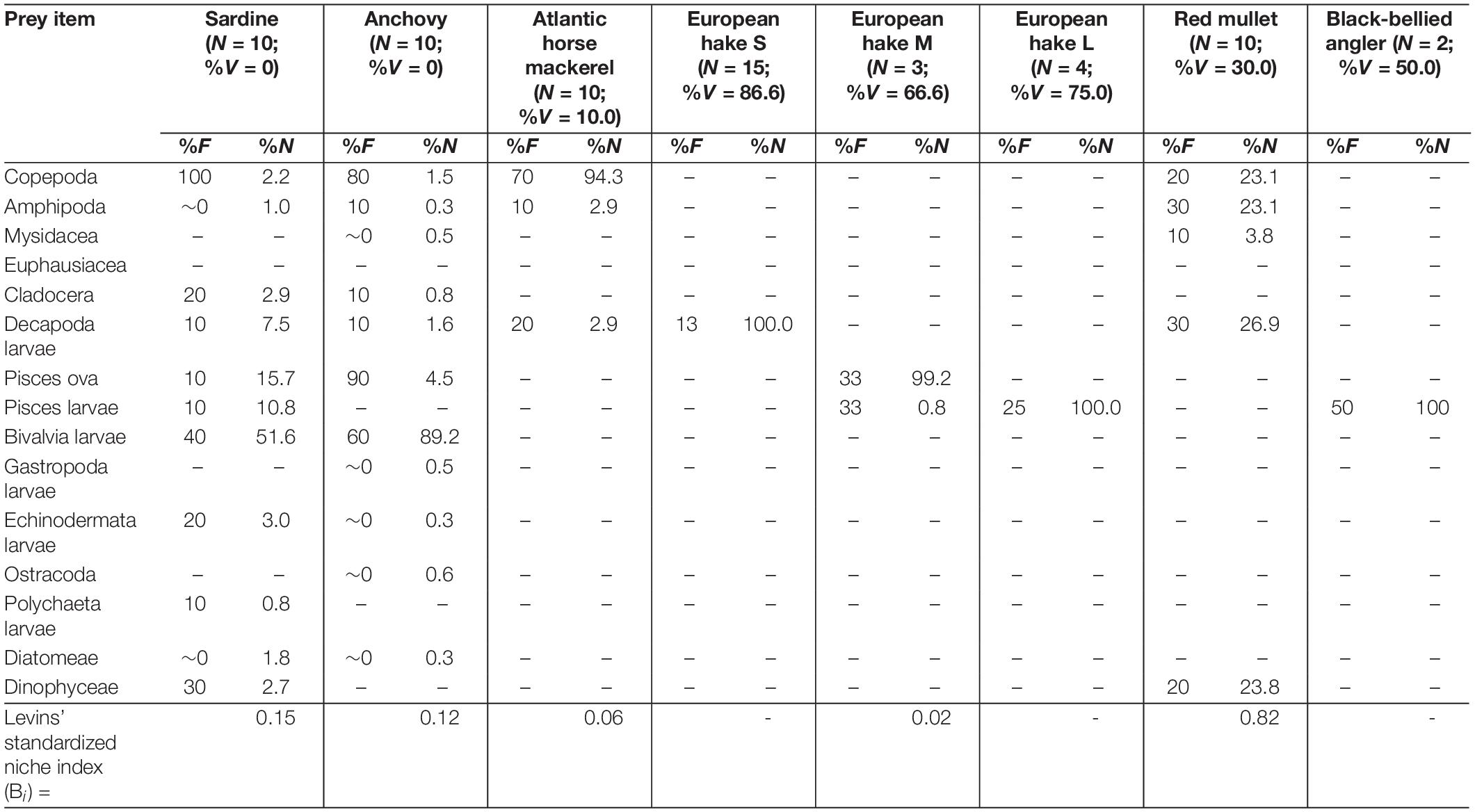
Table 2. Frequency of occurrence (%F) and abundance (%N) of prey items found in the sardine, anchovy, Atlantic horse mackerel, European hake [by size classes: small (S: TL < 20.0 cm), medium (M: 20.1 cm < TL < 30.0 cm) and large (L: 30.1 cm > TL)], red mullet and black-bellied angler collected in the Middle area of eastern Adriatic Sea during MEDITS survey (June–July, 2018) along with number of analyzed specimens (N), its vacuity index (%V) and Levins’ standardized index (Bi).
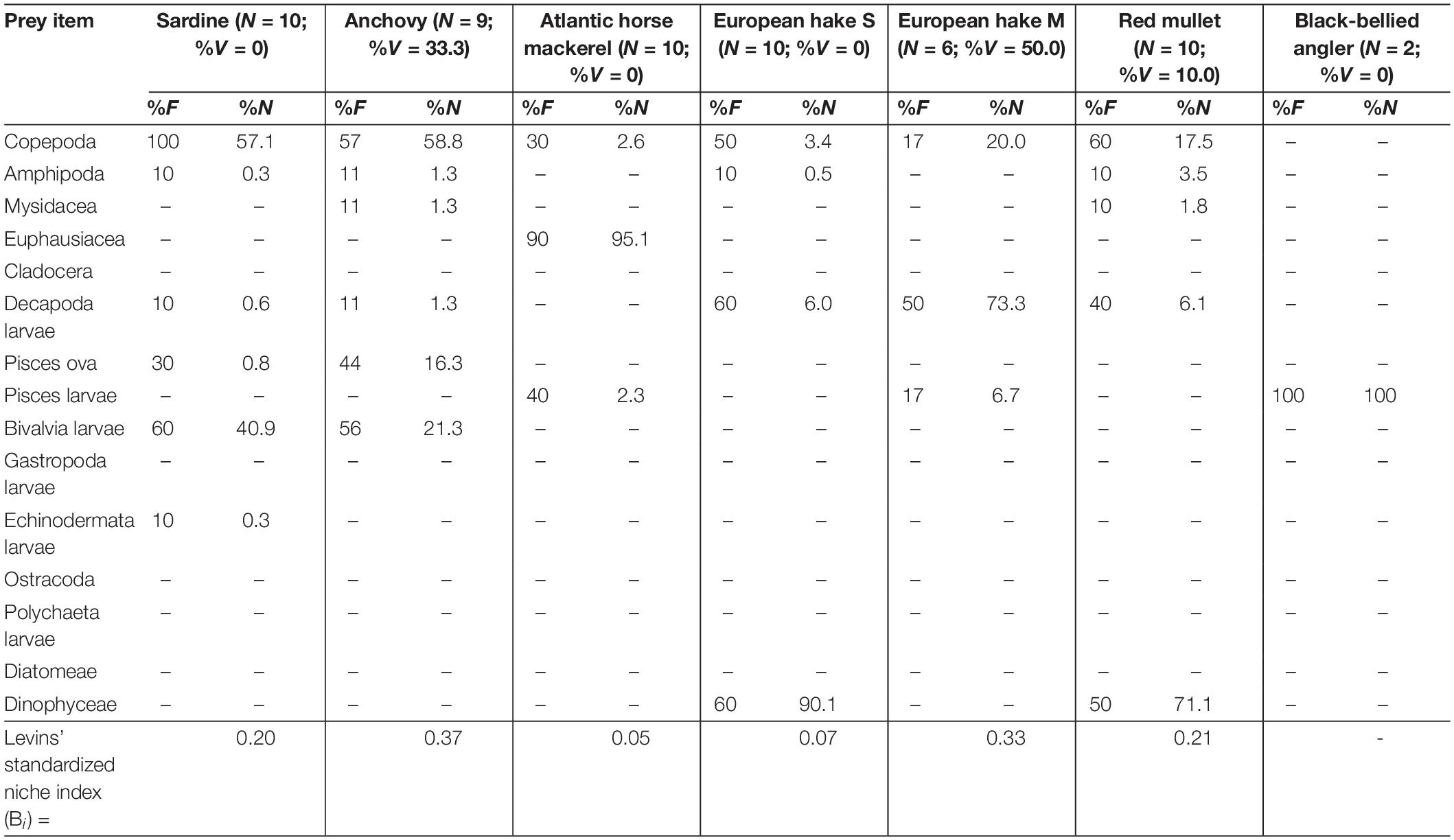
Table 3. Frequency of occurrence (%F) and abundance (%N) of prey items found in the sardine, anchovy, Atlantic horse mackerel, European hake [by size classes: small (S: TL < 20.0 cm), medium (M: 20.1 cm < TL < 30.0 cm) and large (L: 30.1 cm > TL)] and red mullet collected in the Southern area of eastern Adriatic Sea during MEDITS survey (June–July, 2018) along with number of analyzed specimens (N), its vacuity index (%V) and Levins’ standardized index (Bi).
Regardless of sampling area, copepods and bivalve larvae were the most frequent and abundant prey items in two small pelagic fish species (sardine and anchovy). Copepods, euphausiids and decapod larvae were the most frequent and abundant prey items in both mackerel species. Of the three demersal fish species, only the black-bellied angler was an entirely piscivorous species, while copepods and amphipods were the most frequent and abundant prey in red mullet. The most frequent and abundant prey items all size classes of European hake specimens were decapod larvae and fish eggs, respectively.
There was no obvious relationship between individual fish size and stomach fullness (%Jr) in the different fishes examined, with only European hake showing a statistically significant negative regression between %Jr and TL (%Jr = –0.22TL + 4.44; r2 = 0.33; p < 0.05), with large-size class individuals having a greater vacuity index than medium- or small-sized specimens. Diet content analysis between three size categories of European hake revealed a statistically significant difference (PERMANOVA, Pseudo-F = 3.04, df = 2, 30, p = 0.03) between size classes when locations were pooled. The most marked differences (PERMANOVA Pairwise test, p(MC) = 0.001) were observed between the diet of S- and L-sized classes of European hake. Smaller specimens consumed decapod larvae and dinoflagellates, which were their most frequent (35% and 21%, respectively) and abundant (8% and 88%, respectively) prey items, while large specimens consumed large amounts of fish larvae (%F = 43 and %N = 50, respectively). There was no evidence for a linear relationship between %Jr and TL in other species (r < 0.25; p > 0.05).
PERMANOVA results of SCA data showed significant differences both between areas and among species (Supplementary Table 3). Furthermore, interaction between those two factors was also significant. In general, only medium-sized European hake did not show significant differences between the three study areas. For all other species, some levels of diet dissimilarity were apparent between the three areas.
Pairwise PERMANOVA (Supplementary Table 3) and nMDS (Figure 2) showed some evidence for diet overlap between species within each area. In general, diet composition differed between pelagic and demersal fishes, as well as among pelagic species in all study areas. An exception was seen in mackerel, a putatively pelagic fish, but whose diet overlapped with that of demersal species. The diet of demersal fishes was similar across the three areas, with the exception of red mullet, where individuals from the Northern area differed significantly from other demersal species.
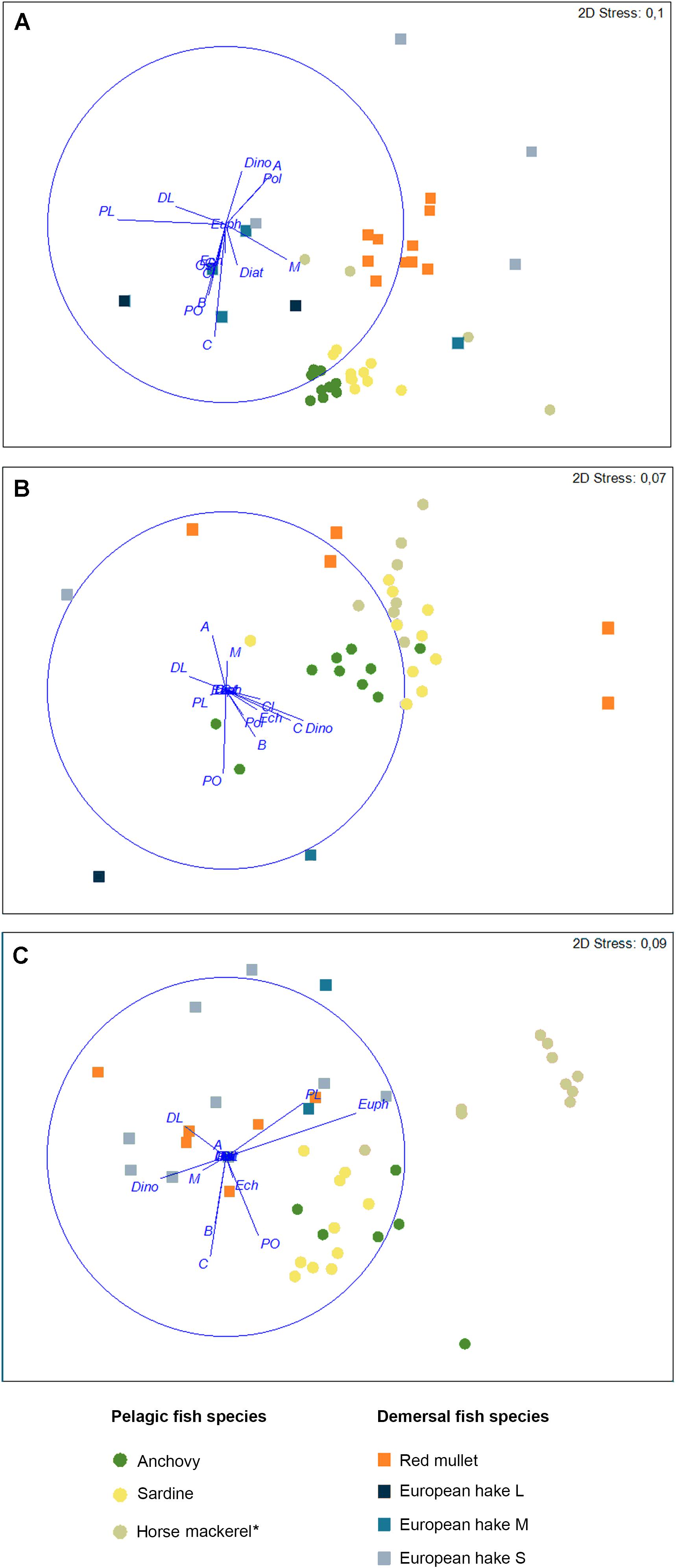
Figure 2. nMDS ordinations showing individual variation in stomach contents of the investigated fish species in the (A) Northern, (B) Middle and (C) Southern areas in the Croatian part of the Adriatic Sea. Vectors show the relative strength and direction of correlations between prey abundance and the nMDS axes. Taxa are identified as: C, Copepoda; A, Amphipoda; M, Mysidacea; Eup, Euphausiacea; Cl, Cladocera; DL, Decapoda larvae; PO, Pisces ova; PL, Pisces larvae; B, Bivalvia larvae; G, Gastropoda larvae; Ech, Echinodermata larvae; Ost, Ostracoda; Pol, Polychaeta larvae; Dia, Diatomeae; Dino, Dinophyceae. *Mediterranean horse mackerel in the Northern and Middle areas and Atlantic horse mackerel in the Southern area.
Levins’ standardized index (Bi) values indicated that sardine and anchovy had smaller trophic niche widths in the Northern area and larger niche widths in the Southern area. Conversely, other species showed the largest niche widths in the Northern area (Tables 1–3). Only red mullet had a larger trophic niche width in the Middle area.
Prey-Availability: Composition and Abundance of Zooplankton in the Water Column
The highest total zooplankton abundance was recorded in the Northern area (919 ind. m–3), while in the Middle and Southern areas values were 3- and 2.5-fold lower, respectively. Overall, we determined a high dominance of copepods in all samples, accounting for > 65% of the total community (Figure 3). Structure-wise, there was a distinct prevalence of small and medium-sized copepods, mostly belonging to families Oithonidae, Oncaeidae, Clausocalanidae and Paracalanidae (> 64%). In the Middle and Southern areas there was a higher contribution of Euchaetidae (20% and 7% respectively), while Acartiidae were better represented (6%) in the Northern areas. Ostracods and amphipods were not found in the Northern area, but were present, albeit in low abundances, in the Middle and Southern areas. Appendicularians and chaetognaths both occurred in very low abundances (< 5 ind. m–3), mostly represented by juvenile individuals and only contributed < 2% to the total community. Cladocerans were abundant in the Northern area (27%), but were very scarce in both the Middle and Southern areas (< 2%). Miscellaneous larvae were dominated mainly by Bivalvia larvae and showed a somewhat increased contribution in the Southern area (21%), mostly due to the abundance of molluscan veligers (Bivalvia and Gastropoda). Additionally, euphausiid furciliae were found in the Middle area, decapod larvae in the Southern and Northern areas, while Polychaeta larvae were apparent in the Northern area. Fish eggs were scarce (< 1%) in zooplankton samples, with anchovy eggs found in the Northern and Middle areas and sardine eggs in the Southern area. A SIMPER analysis showed that the Southern and Middle areas both showed higher average dissimilarity with the Northern area (55.1 and 67.9%, respectively) than between each other (44.2%), with Penilia avirostris (Cladocera) highlighted as the top discriminant species. Margalef’s index of species richness was lower in the Northern area (4.13), in comparison with the Middle (7.3) and Southern (5.7) areas.
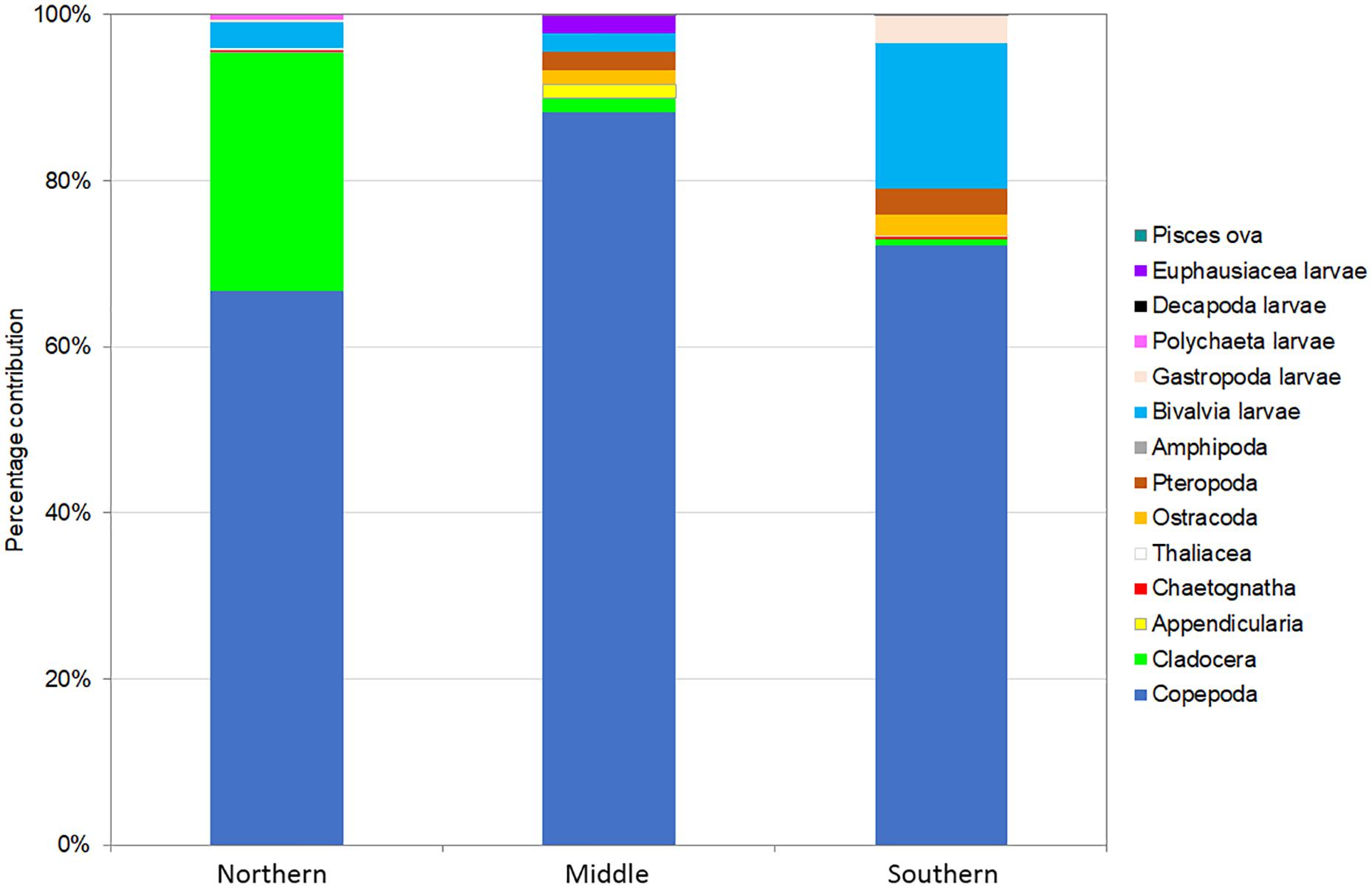
Figure 3. Abundance contribution (%) of zooplankton sampled from the three study areas of the Adriatic Sea.
Isotopic Niche Overlap and Trophic Position of Investigated Species
The SEAc values of the fish species from the Northern Adriatic Sea ranged from 0.2 ‰2 for the M-sized European hake to 2.1 ‰2 for the sardine (Table 4). Relatively high isotopic overlap was recorded between sardine and other pelagic species (Table 4 and Figure 4A). Furthermore, among the demersal fish species only European hake overlapped with pelagic fish species. The isotopic niches of small European hake overlapped with that of sardine and Mediterranean horse mackerel by 56% and 36%, respectively. Between demersal species, a high degree of niche overlap was also recorded between medium- and small-sized European hake (81%) while isotopic niche overlap was not recorded between other demersal species. Red mullet did not show isotopic overlap with any other species. The only crustacean species examined in this region was Norway lobster, and this species did not show isotopic niche overlap with any of the fish species.
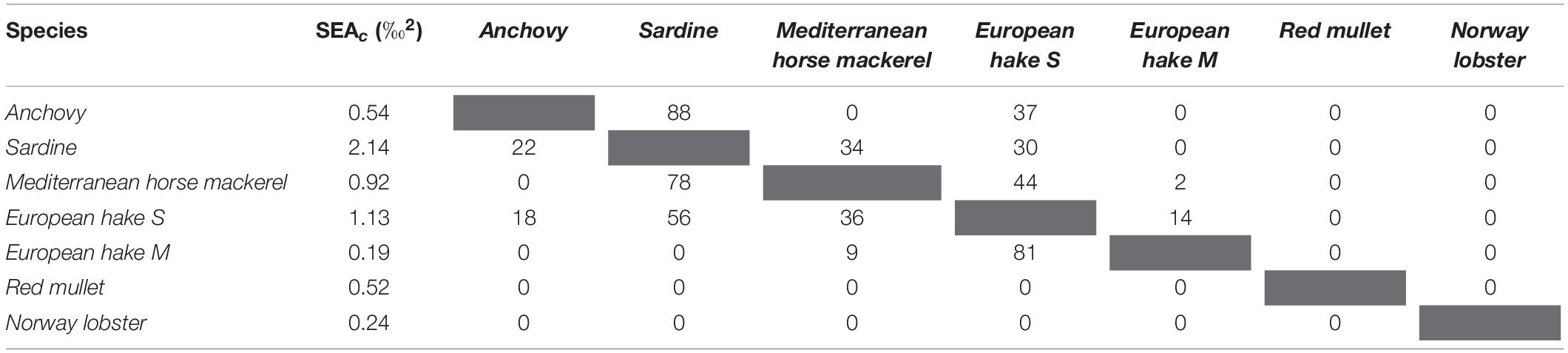
Table 4. Standard Ellipse Area corrected for small sample (SEAc) and SEAc overlap percentage between the species sampled in the Northern area of the Adriatic Sea (values indicate SEAc overlap of the species in the row with the species in the column).
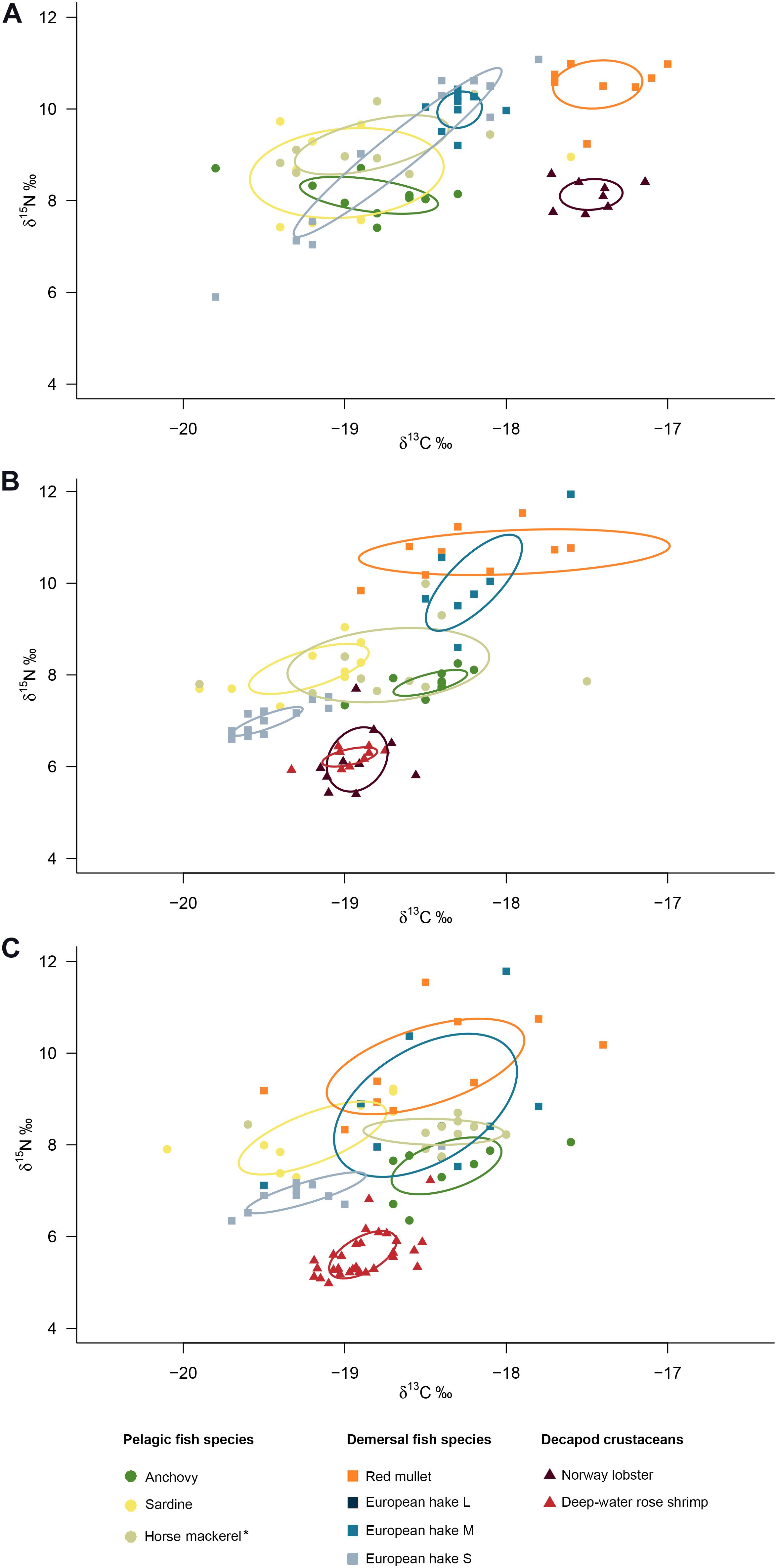
Figure 4. Variation in δ13C and δ15N, SEAc and isotopic niche overlap of the fish and crustacean species examined in the (A) Northern, (B) Middle and (C) Southern areas of the Croatian part of the Adriatic Sea. *Mediterranean horse mackerel in the Northern and Middle areas and Atlantic horse mackerel in the Southern area.
In the Middle area, fish SEAcs ranged from 0.1 ‰2 for small-sized European hake and deep-water rose shrimp to 1.8 ‰2 for Atlantic horse mackerel (Table 5). Among pelagic fish species, sardine and anchovy isotopic niches did not overlap, but both species showed a high degree of overlap with Atlantic horse mackerel. Demersal and pelagic fish species did not overlap (Table 5 and Figure 4B). The medium-sized European hake did not overlap with small-sized European hake. Furthermore, medium-sized European hake exhibited high overlap (42%) with red mullet. Among the crustacean species, deep-water rose shrimp had a smaller SEAc value (0.1 ‰2) in comparison with Norway lobster (0.5 ‰2), but they showed almost complete overlap (96%). However, their isotopic niches did not overlap with any of the fish species.
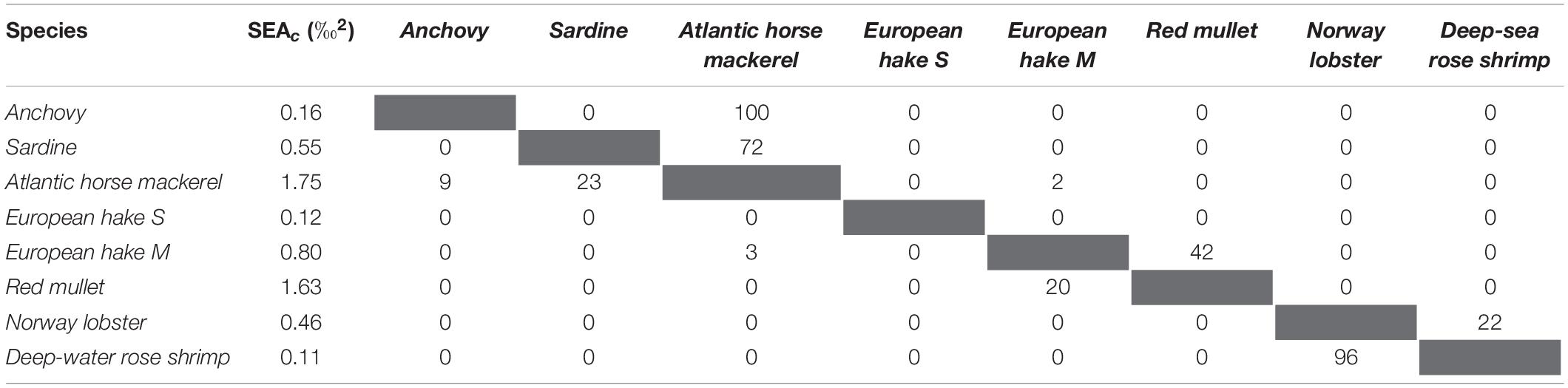
Table 5. Standard Ellipse Area corrected for small sample (SEAc) and SEAc overlap percentage between sampled species in the Middle area of the Adriatic Sea (values indicate SEAc overlap of the species in the row with the species in the column).
The species with the smallest isotopic niche in the Southern area (Table 6) was deep-water rose shrimp (SEAc: 0.3 ‰2), while the largest niche was recorded for medium-sized European hake (2.9 ‰2). Relatively small isotopic niche overlap was recorded between pelagic fish species (Table 6 and Figure 4C), with Atlantic horse mackerel and anchovy showing the largest overlap (13%). Demersal and pelagic fish species overlapped partially, and the highest overlaps were those of Atlantic horse mackerel with medium-sized European hake (93%) while lower overlap was between anchovy (38%) and sardine (35%) and medium-sized hake. Among demersal fish species, only the niches of red mullet and European hake medium size showed higher degrees of isotopic overlap (> 50%). The deep-water rose shrimp niche did not overlap with any fish species.
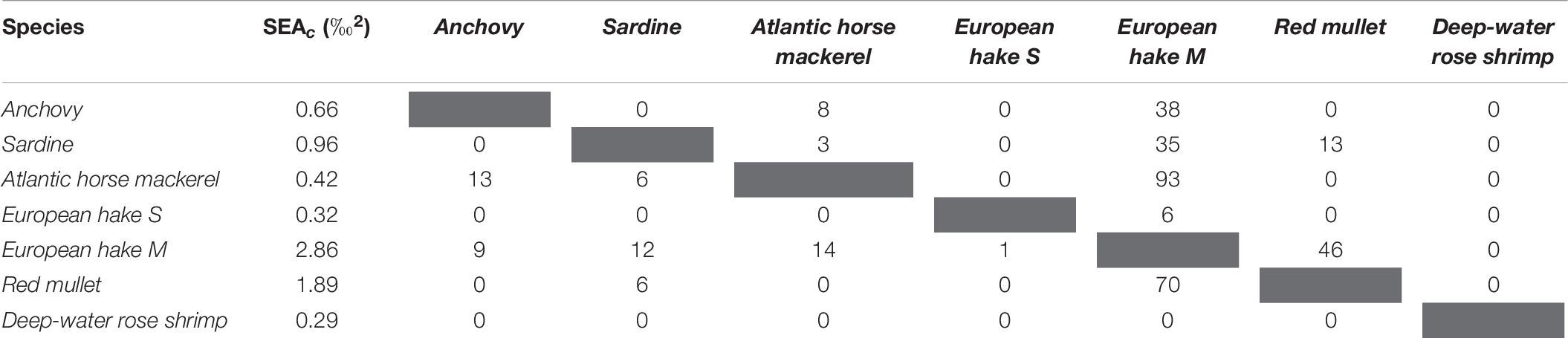
Table 6. Standard Ellipse Area corrected for small sample (SEAc) and SEAc overlap percentage between sampled species in the Southern Adriatic Sea (values indicate SEAc overlap of the species in the row with the species in the column).
We estimated that mean TP ranged between 2.5 and 4.4 in the species examined here (Supplementary Table 2), which indicates that the taxa sampled here consumed prey over approximately three different trophic levels. Trophic position increased from decapod crustaceans, followed by pelagic fishes, with demersal fish species at the highest trophic position (Figure 5). Generally, each species belonged to the same trophic position, regardless of the sampling area. However, in small-sized European hake and Norway lobsters, individuals from the Northern area fed at a higher trophic level compared to conspecifics from the Southern Adriatic and the Middle, respectively. This possibly reflects differences in consumer size between the different study areas as analyzed specimens of small-sized European hake and Norway lobsters in average were larger in the Southern and the Middle Adriatic, respectively.
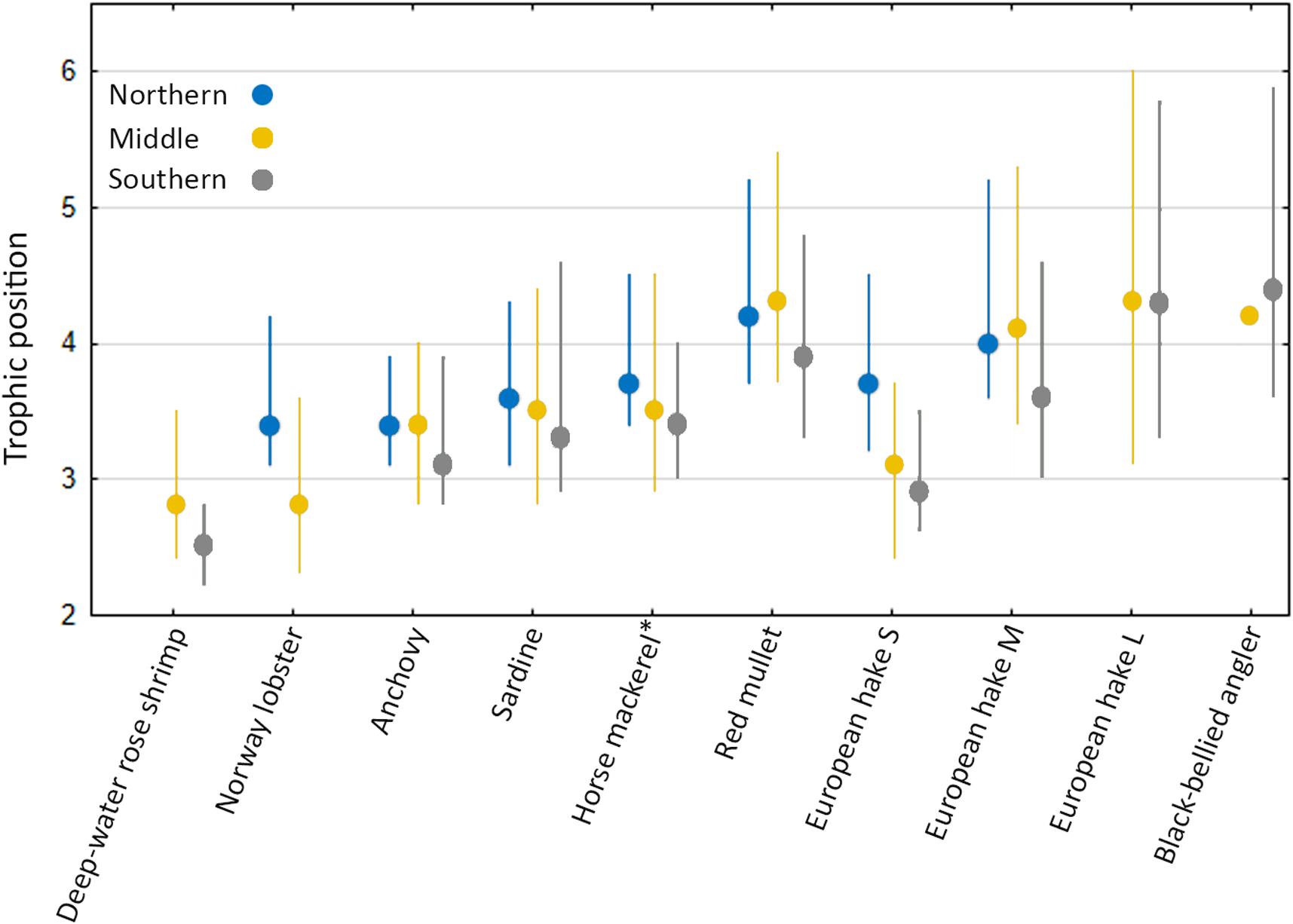
Figure 5. Modal (95% Bayesian credibility intervals) estimated trophic position for each of the fish species examined from the Northern, Middle and Southern areas of the Croatian part of the Adriatic Sea. *Mediterranean horse mackerel in theNorthern and Middle areas and Atlantic horse mackerel in the Southern area.
Predator–Prey Body Mass Ratio Estimates
Community isotopic size spectra are shown in Figure 6. All three linear regressions indicated that the component of the marine food web studied here were strongly size-based. According to ANCOVA results there was no significant difference in estimates for either the slope (area x Log2 Class: F2,27 = 1.01, p = 0.377) or the intercept (area: F2,29 = 2.05, p = 0.146) (Figure 6) between areas. As such, data were pooled and a single regression was conducted for the overall study area, which indicated a strong relationship between trophic position and consumer size (biomass-weighted δ15N = 6.59(SE ± 0.49) + 0.31(± SE 0.06) Log2 Mass Class; r2 = 0.49; p < 0.0001). Using a mean TDF of 2.9 ‰ and the value of the slope (b = 0.31) from this relationship, a mean predator-prey body-mass ratio for the Eastern part of the Adriatic Sea was estimated as 654.8:1.
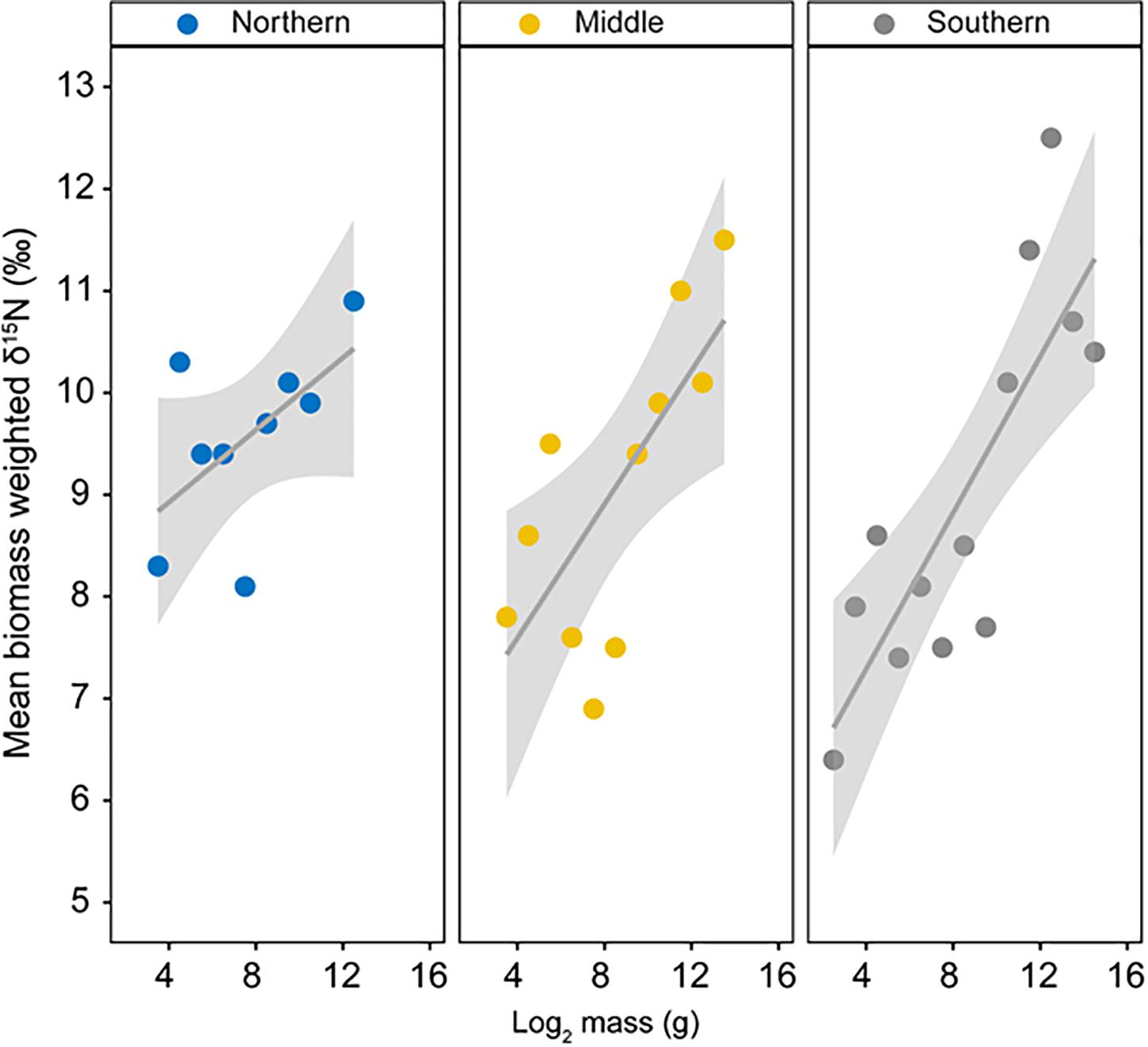
Figure 6. Variation in community isotopic size spectra (relationship between δ15N and log2W (W- body mass in g) for fishes from the Northern, Middle and Southern areas of the Croatian part of the Adriatic Sea.
Discussion
Diet Composition by the Stomach Content Analysis
The exploration and understanding of intra-/inter-species relationships are challenging within any marine ecosystem. Here, we examined spatial and taxonomic variation in the diet of seven commercially exploited species of fish across three different areas in the Eastern Adriatic Sea. Our SCA data were in line with the few available previous studies performed in the Adriatic Sea, while some slight differences were observed with studies from wider geographical areas (Mediterranean Sea, Atlantic Ocean). Our data from pelagic fishes (sardine, anchovy, Atlantic horse mackerel and Mediterranean horse mackerel) were in accordance with previously published data for the same area (sardine: Zorica et al., 2016, 2017; anchovy: Borme et al., 2009; Zorica et al., 2016; Atlantic horse mackerel: Jardas et al., 2004; Mediterranean horse mackerel: Šantić et al., 2004). Those data indicated that small pelagic fish species (sardine, anchovy) preferred to feed on animal food items, specifically on copepods, Bivalvia larvae and fish ova/larvae, although both fish species also had phytoplankton cells within their stomachs. Medium-sized pelagic fishes (Atlantic and Mediterranean horse mackerel) consumed larger animal prey including adult Euphausiacea and Mysidacea or Decapoda larvae (Tables 1–3).
Qualitatively, the diet of the Adriatic pelagic fish species examined in this study was quite similar to those reported for the same species distributed in the Mediterranean area (small pelagics: Costalago et al., 2015; Mazlum et al., 2017; medium-sized pelagics: Rumolo et al., 2016; Karachle, 2017; Georgieva et al., 2019; Saglam and Yıldız, 2019). The slight differences in their relative proportions reported between studies likely reflect spatial and temporal variation in availability. In general, our SCA data indicated that pelagic fish had narrow trophic niche widths (Levins’ index: Bi < 0.37) and consumed a limited range of prey species, potentially indicating trophic specialism. Alternatively, these species may have opportunistically consumed the most abundant food source (Figure 3) available (Castro Hernández and Ortega, 2000; Ganias, 2014).
Demersal trophic niche width values (i.e., based on SCA) were high (Tables 1–3) relative to those of pelagic species. This suggests that demersal fishes can be considered not only as generalist, but also as opportunistic feeders (Esposito et al., 2014; Mellon-Duval et al., 2017; Riccioni et al., 2018). Among the demersal fish species examined, only the black-bellied angler proved to be strictly piscivorous. This supports earlier studies from the Adriatic (Jardas, 1987), while in the area of Mediterranean sea some studies reported have noted that black-bellied anglers also consume other taxa, including crustaceans, cephalopods and molluscs (Negzaoui-Garali et al., 2008; Stagioni et al., 2013; López et al., 2016). The lack of these other prey in our dataset may reflect the limited number of individuals, especially larger size fishes, sampled here. This is most probably related to the fact that majority of the Adriatic fisheries occurs on the continental shelf and/or near its edge (sea depth < 200 – 250 m) where the abundance of those fishes is not high as their abundance is related to the bathymetry - larger fish inhabit greater depths according to Drazen and Haedrich (2012). Furthermore, the number of empty stomachs, which is also size related (Vinson and Angradi, 2011), and the fact that most black-bellied angler specimens, similar to the European hake specimens, had inverted stomachs affected our results. Reflecting this problem, our use of SIA provided a useful means to complement the study of the trophic ecology of European hake and black-bellied angler.
Red mullet largely preyed on copepods, amphipods, decapods and polychaete larvae. This result is in accordance with data previously reported from the Adriatic (Vrgoč et al., 2004) and Mediterranean populations of this species (Vassilopoulou and Papaconstantinou, 1993; Bautista-Vega et al., 2008; Chérif et al., 2011; Esposito et al., 2014; Boudraa et al., 2018; Onay and Dalgic, 2019). Our data on the diet of European hake were qualitatively similar to that reported in previous studies from both the Adriatic (Vrgoč et al., 2004; Riccioni et al., 2018) and Mediterranean seas (Bozzano et al., 2005; Carpentieri et al., 2005; Philips, 2012; Modica et al., 2015; Carrozzi et al., 2019). These studies have typically characterized European hake as an opportunistic feeder, exhibiting dietary shifts as the individuals grow (Vrgoč et al., 2004; Modica et al., 2015; Carrozzi et al., 2019). We obtained similar results, with hake showing a shift in diet from smaller zooplankton toward fishes as their total body length increased. The trophic niche of small-bodied European hake was smaller than that of their larger conspecifics, a pattern similar to that noted by Modica et al. (2013).
Our SCA data showed spatial differences in diet within all species apart from European hake and highlighted how individuals captured from the shallow Northern area (Kvarnerić Bay) differed in their diet from conspecifics from the Middle and Southern areas. This observation reflects earlier information from this and other eastern Adriatic channels (Vrgoč et al., 2004), which led to them being designated as key habitats for planktivorous pelagic fishes (Vučetić, 1963; Sinovčić and Zorica, 2009). Channels located along the eastern coast of the Northern Adriatic support higher zooplankton densities than the open waters of the Middle and Southern Adriatic, with productivity driven by the nutrient enrichment from freshwater sources (submarine springs, smaller rivers), high precipitation over the Kvarnerić Bay and strong vertical mixing due to katabatic ‘bora’ winds (Viličić, 2014; Vilibić et al., 2018). Variation in trophic niche width also reflected differences between functional groups and study area. Within the productive channel areas of the Northern area, pelagic fish trophic niche widths became increasingly smaller, indicating specialist feeding behavior. Conversely, demersal fish niches increased, indicating a more generalist strategy.
Potential Zooplankton Prey in the Water Column
We showed a distinct decrease in total zooplankton abundance through the water column toward the most southerly station, in line with the general longitudinal gradient observed in the Adriatic Sea (Fonda Umani, 1996; Hure and Kršinić, 1998). Overall, zooplankton abundance was very low, especially in the Middle and Southern area (> 350 ind. m–3). This may have reflected selectivity of the 200 μm mesh size, which does not effectively retain smaller-bodied mesozooplankton (e.g., copepodite stages, juvenile appendicularians and early larvae of benthic taxa). Alternatively, it may have reflected a temporal effect, given that the annual zooplankton peak is usually reported in spring (Hure et al., 1980), and we sampled in summer. Nevertheless, the presence and relative proportions of prey categories determined in fish guts (especially in sardine and anchovy) corresponded well with the abundance of zooplankton in the water column. Indeed, the most abundant prey items – copepods, cladocerans and bivalve larvae combined – represented > 90% of ambient mesozooplankton at all stations sampled. The overall mesozooplankton composition across the three different areas of the Adriatic was relatively uniform, with minor discrepancies reflecting the regular distribution patterns of individual mesozooplankton groups, e.g., an increased contribution of cladocerans and an absence of euphausiids in the Northern area (Lipej et al., 1997; Gangai et al., 2018). The least-represented groups of ambient zooplankton (e.g., chaetognaths, appendicularians, pteropods and thaliaceans) were absent from the fish guts. Conversely, planktivorous fish species are likely more efficient at capturing hyperbenthic mysids than the standard net vertical hauls, which generally do not sample the first few meters above the sea bottom. The observed concordance between prey categories and zooplankton composition/abundance in the water column points at opportunistic feeding behavior in the planktivorous fishes examined here, where individuals consume the most abundant prey, resulting in indiscriminate feeding on mesozooplankton. However, it is possible that further and subtler differences in prey composition per area might be revealed at zooplankton species, rather than group level.
Isotopic Niche Overlap and Trophic Position of Investigated Species
In most cases, SIA confirmed the results obtained through SCA in terms of niche overlap. However, some discrepancies were observed, which likely reflect the strengths and weaknesses of the two approaches (Majdi et al., 2018; Nielsen et al., 2018; Pethybridge et al., 2018). SCA provides information on recently consumed items, while bulk SIA of muscle tissue provides data about source utilization over a period of several months. In general, the isotopic niches of pelagic and demersal species did not show high degree of overlap. Among pelagic fish species, anchovy had the smallest isotopic niche, which rarely overlapped with the other pelagic species. Such a narrow isotopic niche for anchovy suggests a specialist diet. Furthermore, it might be related to the fact that the sampling time corresponded to the spawning time of this species (April – July; Zorica et al., 2020), when its individuals tend to feed on larger and therefore higher-energy-content prey (e.g., decapod larvae) according to Zorica et al. (2016), as we also confirmed through SCA (Tables 1–3). Apart from in the most productive Northern area, the isotopic niches of anchovy and sardine did not overlap. Anchovy frequently shifts from filter feeding to particulate feeding (Rumolo et al., 2016), meaning that they are probably capable of foraging in deeper layers and utilizing larger and more nutritious prey items. Although SCA data indicated that anchovy diet was distinct from demersal fishes, there was some overlap with small- and medium-sized European hake in the Northern and Southern areas, respectively. This indicates that over the longer temporal window afforded by SIA, anchovy and certain life stages of European hake may partially share a similar trophic niche. The isotopic niche of sardine overlapped with other pelagic fish species, particularly with the Atlantic horse mackerel. In areas of high (Northern area) and low (Southern area) prey availability, sardine isotopic niche overlapped with the niche of demersal fish (small and medium size European hake). Both mackerel species displayed overlap in their isotopic niche with sardine, except in the Southern area where its niche overlapped with medium-sized European hake. This likely reflects limitations of prey availability in this area, where mackerel species had the smallest isotopic niche that showed elevated overlap with medium-sized European hake. The isotopic niche of red mullet did not overlap with any pelagic fish species but showed certain degree of overlap with demersal European hake in the Middle and Southern areas. This was supported by stomach contents data, where PERMANOVA indicated similar prey composition in the stomachs of these species. Bautista-Vega et al. (2008) noted that red mullet with a TL > 11.0 cm are surface/sub-surface deposit feeders; hence, the slight overlap with other fish species can be explained by their different feeding mechanisms. Small- and medium-sized European hake showed isotopic overlap with some pelagic fishes (sardine and horse mackerel). This is in line with the fact that smaller hakes tend to move throughout the water column in order to capture prey (Bozzano et al., 2005; Carpentieri et al., 2005; Riccioni et al., 2018). The niche-overlap of medium European hake with red mullet was high in the Middle and Southern Adriatic, where isotopic niche overlap between demersal fish species was the most pronounced.
Comparisons showed that the isotopic niches of the two commercially important decapod crustaceans did not overlap with any of the fish examined: they did however show almost complete mutual isotopic overlap (Table 5). Both species were only encountered in sympatry in the Middle area. Here, the relatively small isotopic niche of the deep-water rose shrimp overlapped completely with the much wider niche of the Norway lobster. Although there are no comparative studies concerning the diets of those two species, our data are in accordance with studies dealing with each species separately, which revealed that their feeding habits are relatively similar. Norway lobster is a scavenger but also a predator capable of active feeding (Oakley, 1979; Parslow-Williams et al., 2002) and filter feeding (Loo et al., 1993). According to dietary analysis performed by Parslow-Williams et al. (2002), Norway lobster largely consumes molluscs, crustaceans, polychaetes and echinoderms. Furthermore, a recent isotopic study highlighted the importance of suspended particulate organic matter to Norway lobster during certain seasons, and noted that its contribution was likely underestimated in other studies (Santana et al., 2020). The deep-water rose shrimp has a similar prey range to the Norway lobster, and exhibits two feeding phases: hunting and digging (Tursi et al., 1999). Hunting is the more active phase, during which it preys on small fish, cephalopods and crustaceans, while during the digging phase it forages in the mud, consuming mainly polychaetes, bivalves, echinoderms and benthic foraminifera (Tursi et al., 1999). The larger isotopic niche of Norway lobster compared to the rose shrimp could be due to its ability to utilize various food sources including decomposing food, plankton and live prey. Analysis of the gut contents of decapod crustaceans would probably detect different prey taxonomic groups, but unfortunately those analyses were not included within this study. Future studies should consider such analyses in these taxa as a priority. Isotopic niche differentiation between the two demersal crustaceans and most of the fishes studied here likely reflects the consumption of benthic prey, which were not observed from fish stomachs.
Although the target species showed dietary differences across the three study areas of the Eastern Adriatic Sea, they had similar trophic position across locations, indicating equivalent ecological function. This suggests that although their diet may differ spatially, they feed at similar trophic levels regardless of location. However, a common community-level relationship was shown between δ15N and body weight across the three basins. Furthermore, it was obvious that smaller size classes of European hake feed at lower trophic levels than the larger size-class, as was confirmed by its diet analysis within this study. European hake, as they grow, change their feeding habits toward higher-energy-content prey such as fishes. In general, our estimates of trophic position for the species examined here were in accordance with those obtained by Coll et al. (2006) for the wider Mediterranean Sea and by Albo-Puigserver et al. (2016) for the North-western Mediterranean. The slight differences we observed are most probably due to differences in the environments sampled, but in general the trophic position of the study species did not show pronounced differences across the different areas. Mesozooplankton δ13C values shown in this study (mean δ13C −23.0 ‰) were slightly 13C depleted in comparison with those reported from other marine areas (e.g., the global mean ± SD for zooplankton δ13C included in Magozzi et al. (2017) is −21.0 ± 2.1 ‰), and compared to mesoplankton collected off Crete and Cyprus (e.g., Koppelmann et al. (2009) mean = −19.7 ± 0.8 ‰), further south in the Mediterranean. The reason for the apparent 13C depletion in mesozooplankton δ13C reported here possibly reflects terrestrial inputs (e.g., from the Po River), effects of local oceanographic conditions or even variation in mesozooplankton lipid contents. Our values do however coincide with the modeled isoscape δ13C values presented for the Adriatic Sea in McMahon et al. (2013). A more detailed study is required to resolve the effects of such influences on spatial variation in stable isotope values in different functional groups across our study region.
Since δ15N values reflect the trophic position at which species are feeding (Peterson and Fry, 1987), it is apparent that the species in question feed at different trophic levels; more precisely, that the food webs in each sampling area and overall were size-structured. This is also consistent with the findings of Jennings et al. (2001, 2008), who reported that in marine food webs based on phytoplankton the mentioned relationships are strong and might reflect the TP of each body mass class. Additionally, these authors estimated similar slope values for the North Sea community (b = 0.34) as we estimated for the Adriatic Sea (b = 0.31). In some other areas where community size structuring was studied, like the Western Arabian Sea (Al-Habsi et al., 2008) and the Galician upwelling area (Bode et al., 2003), slope values were somewhat lower (b = 0.26 and b = 0.28, respectively), most probably due to weak cross-species relationship or variation in food web length. The PPMR value (655:1) estimated for the Eastern Adriatic Sea was different from the PPMR reported for Western Arabian Sea (7762:1; Al-Habsi et al., 2008), Galician upwelling area (4500:1; Bode et al., 2003) and North Sea (1136:1; Jennings et al., 2001). Observed differences were expected since they used different TDF values (e.g., 2.5‰ and 3.4‰) and their studies covered wider and/or narrower range of trophic levels.
Data Availability Statement
The raw data supporting the conclusions of this article will be made available by the authors, without undue reservation.
Ethics Statement
Ethical review and approval was not required for the animal study because performed research involved sampling of marine animals including vertebrates (fishes) and invertebrates (crustaceans). Animals were collected through scientific surveys and caught with methodology normally used in the scientific sampling or in fishery practices and were not exposed to any unnecessary pain, injuries or suffering in this study. Furthermore, special attention was given to the avoidance of accidental catches of sensitive or endangered species (sea turtles, marine mammals, etc.).
Author Contributions
BZ did the conceptualization, data curation, formal analysis, funding acquisition, investigation, methodology, resources, supervision, validation, visualization, and writing. DE-B did the conceptualization, data curation, formal analysis, funding acquisition, investigation, methodology, resources, supervision, validation, visualization, and writing. OV did the zooplankton analysis, visualization, and writing. VV did the fieldwork. MŠ and VČK did the stomach content analysis. II did the resources and fieldwork. NV did the funding acquisition and investigation. CH did the conceptualization, visualization, and writing. All authors contributed to the article and approved the submitted version.
Conflict of Interest
The authors declare that the research was conducted in the absence of any commercial or financial relationships that could be construed as a potential conflict of interest.
Funding
This study was supported throughout the project “Exploration of ecologically sensitive areas with special emphasis on growth, development and protection of commercially important maritime organisms (ESAmar)” funded by Croatian Science Foundation (IP-2018-01-8013) and research project “Data Collection Framework” funded by the Ministry of Agriculture of the Republic of Croatia. CH was supported by Nucleo Milenio INVASAL funded by Chile’s government program, Iniciativa Cientifica Milenio from the Ministerio de Ciencia, Tecnología, Conocimiento e Innovación.
Supplementary Material
The Supplementary Material for this article can be found online at: https://www.frontiersin.org/articles/10.3389/fmars.2021.609432/full#supplementary-material
References
Albo-Puigserver, M., Navarro, J., Coll, M., Layman, C. A., and Palomera, I. (2016). Trophic structure of pelagic species in the northwestern Mediterranean Sea. J. Sea Res. 117, 27–35. doi: 10.1016/j.seares.2016.09.003
Al-Habsi, S. H., Sweeting, C. J., Polunin, N. V. C., and Graham, N. (2008). δ15N and δ13C elucidation of size structured food webs in a Western Arabian Sea demersal trawl assemblage. Mar. Ecol. Prog. Ser. 353, 55–63. doi: 10.3354/meps07167
Bautista-Vega, A. A., Letourneur, Y., Harmelin-Vivien, M., and Salen-Picard, C. (2008). Difference in diet and size-related trophic level in two sympatric fish species, the red mullets Mullus barbatus and Mullus surmuletus, in the Gulf of Lions (north-west Mediterranean Sea). J. Fish Biol. 73, 2402–2420. doi: 10.1111/j.1095-8649.2008.02093.x
Bearhop, S., Adams, C. E., Waldron, S., Fuller, R. A., and Macleod, H. (2004). Determining trophic niche width: a novel approach using stable isotope analysis. J. Anim. Ecol. 73, 1007–1012. doi: 10.1111/j.0021-8790.2004.00861.x
Bergamasco, A., and Gačić, M. (1996). Baroclinic response of the Adriatic sea to an episode of bora wind. J. Phys. Oceanogr. 26, 1354–1369.
Bode, A., Carrera, P., and Lens, S. (2003). The pelagic foodweb in the upwelling ecosystem of Galicia (NW Spain) during spring: natural abundance of stable carbon and nitrogen isotopes. ICES J. Mar. Sci. 60, 11–22. doi: 10.1006/jmsc.2002.1326
Bodin, N., Le Loc’h, F., and Hily, C. (2007). Effect of lipid removal on carbon and nitrogen stable isotope ratios in crustacean tissues. J. Exp. Mar. Biol. Ecol. 341, 168–175. doi: 10.1016/j.jembe.2006.09.008
Borme, D., Tirelli, V., Brandt, S., Fonda Umani, S., and Arneri, E. (2009). Diet of Engraulis encrasicolus in the northern Adriatic Sea (Mediterranean): ontogenetic changes and feeding selectivity. Mar. Ecol. Prog. Ser. 392, 193–209. doi: 10.3354/meps08214
Boudraa, I., Derbal, F., and Kara, H. (2018). Feeding habits of the red mullet, Mullus barbatus (Mullidae) of eastern coasts of Algeria. Vie Milieu 68, 213–220.
Bozzano, A., Sardà, F., and Ríos, J. (2005). Vertical distribution and feeding patterns of the juvenile European hake, Merluccius merluccius in the NW Mediterranean. Fish. Res. 73, 29–36. doi: 10.1016/j.fishres.2005.01.006
Buljan, M., and Zore-Armanda, M. (1974). Oceanographical properties of the Adriatic Sea. Oceanogr. Mar. Biol. Annu. Rev. 14, 11–98.
Burkhard, L. P. (2003). Factors influencing the design of bioaccumulation factor and biota-sediment accumulation factor field studies. Environ. Toxicol. Chem. 22, 351–360. doi: 10.1002/etc.5620220216
Cabana, G., and Rasmussen, J. B. (1996). Comparison of aquatic food chains using nitrogen isotopes. PNAS 93, 10844–10847. doi: 10.1073/pnas.93.20.10844
Carpentieri, P., Colloca, F., Cardinale, M., Belluscio, A., and Ardizzone, G. D. (2005). Feeding habits of European hake Merluccius merluccius in the Central Mediterranean Sea. Fish. Bull. Natl. Ocean. Atmos. Adm. 103, 411–416.
Carrozzi, V., Lorenzo, M. D., Massi, D., Titone, A., Ardizzone, G., and Colloca, F. (2019). Prey preferences and ontogenetic diet shift of European hake Merluccius merluccius (Linnaeus, 1758) in the central Mediterranean Sea. Reg. Stud. Mar. Sci. 25:100440. doi: 10.1016/j.rsma.2018.100440
Castro Hernández, J. J., and Ortega, A. T. S. (2000). Synopsis of Biological Data on the Chub Mackerel (Scomber Japonicus Houttuyn, 1782). Rome: FAO.
Chérif, M., Ben Amor, M. M., Selmi, S., Gharbi, H., Missaoui, H., and Carapé, C. (2011). Food and feeding habits of the red mullet, Mullus barbatus (Actinopterygii: Perciformes: Mullidae), off the northern Tunisian coast (central Mediterranean). Acta Icth. Piscat. 41, 109–116. doi: 10.3750/AIP2011.41.2.06
Civitarese, G., Gačić, M., Lipizer, M., and Borzelli, L. E. (2010). On the impact of the bimodal oscillating system (BIOS) on the biogeochemistry and biology of the Adriatic and Ionian Seas (Eastern Mediterranean). Biogeosciences Discuss. 7, 6971–6995.
Clarke, K., and Warwick, R. (2001). Clarke KR, Warwick RM.Change in Marine Communities: An Approach to Statistical Analysis and Interpretation. Plymouth: Primer-E Ltd.
Clarke, K. R. (1993). Non-parametric multivariate analyses of changes in community structure. Aust. J. Ecol. 18, 117–143.
Clarke, K. R., and Warwick, R. M. (1994). Changes in marine communities: an approach to statistical analysis and interpretation. Clim. Res. 31, 181–193.
Coll, M., Piroddi, C., Steenbeek, J., Kaschner, K., Ben Rais Lasram, F., Aguzzi, J., et al. (2010). The biodiversity of the Mediterranean Sea: estimates, patterns, and threats. PLoS One 5:e11842. doi: 10.1371/journal.pone.0011842
Coll, M., Shannon, L. J., Moloney, C. L., Palomera, I., and Tudela, S. (2006). Comparing trophic flows and fishing impacts of a NW Mediterranean ecosystem with coastal upwelling systems by means of standardized models and indicators. Ecol. Model. 198, 53–70. doi: 10.1016/j.ecolmodel.2006.04.009
Costalago, D., Garrido, S., and Palomera, I. (2015). Comparison of the feeding apparatus and diet of European sardines Sardina pilchardus of Atlantic and Mediterranean waters: ecological implications. J. Fish Biol. 86, 1348–1362. doi: 10.1111/jfb.12645
Cushman-Roisin, B., Gačić, M., Poulain, P.-M., and Artegiani, A. (2001). Physical Oceanography of the Adriatic Sea; Past, Present and Future, 1st Edn. Dordrecht: Springer Netherlands.
Docmac, F., Araya, M., Hinojosa, I. A., Dorador, C., and Harrod, C. (2017). Habitat coupling writ large: pelagic-derived materials fuel benthivorous macroalgal reef fishes in an upwelling zone. Ecology 98, 2267–2272. doi: 10.1002/ecy.1936
Drazen, J. C., and Haedrich, R. L. (2012). A continuum of life histories in deep-sea demersal fishes. Deep Sea Res. 1 Oceanogr. Res. Pap. 61, 34–42. doi: 10.1016/j.dsr.2011.11.002
Dulèić, J., Vrgoě, N., and Lipej, L. (2017). The Current Status of the Adriatic Sea Fish Biodiversity. Palermo: Carlo Saladino Editore.
Esposito, V., Andaloro, F., Bianca, D., Natalotto, A., Romeo, T., Scotti, G., et al. (2014). Diet and prey selectivity of the red mullet, Mullus barbatus (Pisces: Mullidae), from the southern Tyrrhenian Sea: the role of the surf zone as a feeding ground. Mar. Biol. Res. 10, 167–178. doi: 10.1080/17451000.2013.797585
Fanelli, E., and Cartes, J. E. (2010). Temporal variations in the feeding habits and trophic levels of three deep-sea demersal fishes from the western Mediterranean Sea, based on stomach contents and stable isotope analyses. Mar. Ecol. Prog. Ser. 402, 213–232. doi: 10.3354/meps08421
FAO (2018). The State of World Fisheries and Aquaculture 2018 - Meeting the Sustainable Development Goals. Rome: FAO.
FAO (2020). Fisheries and Aquaculture Software. FishStatJ - Software for Fishery Statistical Time Series. Rome: FAO Fisheries and Aquaculture Department.
Funes, M., Marinao, C., and Galván, D. E. (2019). Does trawl fisheries affect the diet of fishes? A stable isotope analysis approach. Isotopes Environ. Health Stud. 55, 327–343. doi: 10.1080/10256016.2019.1626381
Gačić, M., Marullo, S., Santoleri, R., and Bergamasco, A. (1997). Analysis of the seasonal and interannual variability of the sea surface temperature field in the Adriatic Sea from AVHRR data (1984–1992). J. Geophys. Res. Oceans 102, 22937–22946. doi: 10.1029/97JC01720
Gangai, B. Z., Lučić, D., Hure, M., Onofri, I., and Pestorić, B. (2018). Composition and diel vertical distribution of euphausiid larvae (calyptopis stage) in the deep southern Adriatic. Oceanologia 60, 128–138. doi: 10.1016/j.oceano.2017.09.001
Garrison, L. P., and Link, J. S. (2000). Diets of five hake species in the northeast United States continental shelf ecosystem. Mar. Ecol. Prog. Ser. 204, 243–255.
Georgieva, Y. G., Daskalov, G. M., Klayn, S. L., Stefanova, K. B., and Stefanova, E. S. (2019). Seasonal diet and feeding strategy of horse mackerel Trachurus mediterraneus (Steindachner, 1868) (Perciformes: Carangidae) in the South-Western Black Sea. Acta Zool. Bulg. 71, 201–210.
GFCM (2018). Report of the Twentieth Session of the Scientific Advisory Committee on Fisheries. Tangiers: Food and Agriculture Organization of the United Nations.
Grbec, B., Morović, M., Matić, F., Ninčević Gladan, Ž, Marasović, I., Vidjak, O., et al. (2014). Climate regime shifts and multi-decadal variability of the Adriatic Sea pelagic ecosystem. Acta Adriat. 55, 117–126.
Hesslein, R. H., Hallard, K. A., and Ramlal, P. (1993). Replacement of sulfur, carbon, and nitrogen in tissue of growing broad whitefish (Coregonus nasus) in response to a change in diet traced by δ34S, δ13C, and δ15N. Can. J. Fish. Aquat. Sci. 50, 2071–2076. doi: 10.1139/f93-230
Hure, J., Ianora, A., and di Carlo, B. S. (1980). Spatial and temporal distribution of copepod communities in the Adriatic Sea. J. Plankton Res. 2, 295–316. doi: 10.1093/plankt/2.4.295
Hure, J., and Kršinić, F. (1998). Planktonic copepods of the Adriatic Sea. Spatial and temporal distribution. Nat. Croat. 7, 1–135.
Hurlbert, S. H. (1978). The measurement of niche overlap and some relatives. Ecology 59, 67–77. doi: 10.2307/1936632
Hyslop, E. J. (1980). Stomach contents analysis—a review of methods and their application. J. Fish Biol. 17, 411–429. doi: 10.1111/j.1095-8649.1980.tb02775.x
Jackson, A. L., Inger, R., Parnell, A. C., and Bearhop, S. (2011). Comparing isotopic niche widths among and within communities: SIBER – Stable Isotope Bayesian Ellipses in R. J. Anim. Ecol. 80, 595–602. doi: 10.1111/j.1365-2656.2011.01806.x
Jardas, I. (1987). “On the biology and ecology of Lophius species (Teleostei, Lophiidae) in the Adriatic Sea,” in Proceedings of the V. Congress of European Ichthyologists (Stockholm: Department of Vertebrate Zoology, Sweden Museum of natural History), 181–185.
Jardas, I., Šantić, M., and Pallaoro, A. (2004). Diet composition and feeding intensity of horse mackerel, Trachurus trachurus (Osteichthyes: Carangidae) in the eastern Adriatic. Mar. Biol. 144, 1051–1056. doi: 10.1007/s00227-003-1281-7
Jennings, S., Maxwell, T., Schratzberger, M., and Milligan, S. (2008). Body-size dependent temporal variations in nitrogen stable isotope ratios in food webs. Mar. Ecol. Prog. Ser. 370, 199–206. doi: 10.3354/meps07653
Jennings, S., Pinnegar, J. K., Polunin, N. V. C., and Boon, T. W. (2001). Weak cross-species relationships between body size and trophic level belie powerful size-based trophic structuring in fish communities. J. Anim. Ecol. 70, 934–944. doi: 10.1046/j.0021-8790.2001.00552.x
Karachle, P. K. (2017). Diet composition and overlap for 43 fishes in the North Aegean Sea, Greece. Acta Adriat. 58, 125–136.
Kiljunen, M., Grey, J., Sinisalo, T., Harrod, C., Immonen, H., and Jones, R. I. (2006). A revised model for lipid-normalizing δ13C values from aquatic organisms, with implications for isotope mixing models. J. Appl. Ecol. 43, 1213–1222. doi: 10.1111/j.1365-2664.2006.01224.x
Koppelmann, R., Böttger-Schnack, R., Möbius, J., and Weikert, H. (2009). Trophic relationships of zooplankton in the eastern Mediterranean based on stable isotope measurements. J. Plankton Res. 31, 669–686. doi: 10.1093/plankt/fbp013
Kovač, Ž, Platt, T., Ninèević Gladan, Ž, Morović, M., Sathyendranath, S., Raitsos, D., et al. (2018). A 55-year time series station for primary production in the Adriatic Sea: data correction, extraction of photosynthesis parameters and regime shifts. Remote Sens. 10:1460. doi: 10.3390/rs10091460
Larink, O., and Westheide, W. (2006). Coastal Plankton Photo Guide for European Seas. Munchen: Verlag Dr. Friedrich Pfeil.
Lipej, L., Mozetič, P., Turk, V., and Malej, A. (1997). The trophic role of the marine cladoceran Penilia avirostris in the Gulf of Trieste. Hydrobiologia 360, 197–203. doi: 10.1023/A:1003180030116
Loo, L.-O., Baden, S. P., and Ulmestrand, M. (1993). Suspension feeding in adult Nephrops norvegicus (L.) and Homarus gammarus (L.) (decapoda). Neth. J. Sea Res. 31, 291–297. doi: 10.1016/0077-7579(93)90029-R
López, N., Navarro, J., Barría, C., Albo-Puigserver, M., Coll, M., and Palomera, I. (2016). Feeding ecology of two demersal opportunistic predators coexisting in the northwestern Mediterranean Sea. Estuar. Coast. Shelf Sci. 175, 15–23. doi: 10.1016/j.ecss.2016.03.007
Lorrain, A., Savoye, N., Chauvaud, L., Paulet, Y.-M., and Naulet, N. (2003). Decarbonation and preservation method for the analysis of organic C and N contents and stable isotope ratios of low-carbonated suspended particulate material. Anal. Chim. Acta 491, 125–133. doi: 10.1016/S0003-2670(03)00815-8
Magozzi, S., Yool, A., Vander Zanden, H. B., Wunder, M. B., and Trueman, C. N. (2017). Using ocean models to predict spatial and temporal variation in marine carbon isotopes. Ecosphere 8:e01763. doi: 10.1002/ecs2.1763
Mahe, K., Amara, R., Bryckaert, T., Kacher, M., and Brylinski, J. M. (2007). Ontogenetic and spatial variation in the diet of hake (Merluccius merluccius) in the Bay of Biscay and the Celtic Sea. ICES J. Mar. Sci. 64, 1210–1219. doi: 10.1093/icesjms/fsm100
Majdi, N., Hette-Tronquart, N., Auclair, E., Bec, A., Chouvelon, T., Cognie, B., et al. (2018). There’s no harm in having too much: a comprehensive toolbox of methods in trophic ecology. Food Webs 17:e00100. doi: 10.1016/j.fooweb.2018.e00100
Mauri, E., and Poulain, P. (2001). Northern Adriatic Sea surface circulation and temperature/pigment fields in September and October 1997. J. Mar. Syst. 29, 51–67.
Mazlum, R. E., Solak, E., and Biling, S. (2017). Size and seasonal diet variation of European anchovy Engraulis encrasicolus (Linnaeus, 1758) in the southeast Black Sea. Cah. Biol.Mar. 58, 251–260. doi: 10.21411/CBM.A.B2C2DBE2
McCormack, S. A., Trebilco, R., Melbourne-Thomas, J., Blanchard, J. L., Fulton, E. A., and Constable, A. (2019). Using stable isotope data to advance marine food web modelling. Rev. Fish Biol. Fish. 29, 277–296. doi: 10.1007/s11160-019-09552-4
McCutchan, J. H., Lewis, W. M., Kendall, C., and McGrath, C. C. (2003). Variation in trophic shift for stable isotope ratios of carbon, nitrogen, and sulfur. Oikos 102, 378–390.
McMahon, K. W., Hamady, L. L., and Thorrold, S. R. (2013). A review of ecogeochemistry approaches to estimating movements of marine animals. Limnol. Oceanogr. 58, 697–714. doi: 10.4319/lo.2013.58.2.0697
Mellon-Duval, C., Harmelin-Vivien, M., Métral, L., Loizeau, V., Mortreux, S., Roos, D., et al. (2017). Trophic ecology of the European hake in the Gulf of Lions, northwestern Mediterranean Sea. Sci. Mar. 81, 7–18. doi: 10.3989/scimar.04356.01A
Modica, L., Bozzano, A., and Velasco, F. (2013). The influence of body size on the foraging behaviour of European hake after settlement to the bottom. J. Exp. Mar. Biol. Ecol. 444, 46–54. doi: 10.1016/j.jembe.2013.03.001
Modica, L., Cartes, J. E., Velasco, F., and Bozzano, A. (2015). Juvenile hake predation on Myctophidae and Sternoptychidae: quantifying an energy transfer between mesopelagic and neritic communities. J. Sea Res. 95, 217–225. doi: 10.1016/j.seares.2014.05.004
Nagelkerken, I., Goldenberg, S. U., Ferreira, C. M., Ullah, H., and Connell, S. D. (2020). Trophic pyramids reorganize when food web architecture fails to adjust to ocean change. Science 369, 829–832. doi: 10.1126/science.aax0621
Negzaoui-Garali, N., Salem, M. B., and Capapé, C. (2008). Feeding habits of the black anglerfish, Lophius budegassa (Osteichthyes: Lophiidae), off the Tunisian coast (central Mediterranean). Cah. Biol.Mar. 49, 113–122.
Nielsen, J. M., Clare, E. L., Hayden, B., Brett, M. T., and Kratina, P. (2018). Diet tracing in ecology: method comparison and selection. Methods Ecol. Evol. 9, 278–291. doi: 10.1111/2041-210X.12869
Ninčević Gladan, Ž, Marasović, I., Kušpilić, G., Krstulović, N., Šolić, M., and Šestanović, S. (2006). Abundance and composition of picoplankton in the mid Adriatic Sea. Acta Adriat. 47, 127–140.
Oakley, S. G. (1979). “Diurnal and seasonal changes in the timing of peak catches of Nephrops norvegicus reflecting changes in behaviour,” in Cyclic Phenomena in Marine Plants and Animals, eds E. Naylor and R. G. Hartnoll (Oxford: Pergamon), 367–373. doi: 10.1016/B978-0-08-023217-1.50054-0
Onay, H., and Dalgic, G. (2019). Seasonal changes in the food spectrum and day-time rhythm of feeding in red mullet Mullus barbatus (Linnaeus, 1758) in the southeast Black Sea. Fresenius Environ. Bull. 28, 2671–2678.
Parslow-Williams, P., Goodheir, C., Atkinson, R. J. A., and Taylor, A. C. (2002). Feeding energetics of the Norway lobster, Nephrops norvegicus in the Firth of Clyde, Scotland. Ophelia 56, 101–120. doi: 10.1080/00785236.2002.10409493
Peterson, B. J., and Fry, B. (1987). Stable isotopes in ecosystem studies. Annu. Rev. Ecol. Evol. Syst. 18, 293–320. doi: 10.1146/annurev.es.18.110187.001453
Pethybridge, H. R., Choy, C. A., Polovina, J. J., and Fulton, E. A. (2018). Improving marine ecosystem models with biochemical tracers. Annu. Rev. Mar. Sci. 10, 199–228. doi: 10.1146/annurev-marine-121916-063256
Philips, A. E. (2012). Feeding behavior of the European hake Merluccius merluccius Linnaeus, 1758 (Family: Gadidae) from Egyptian Mediterranean waters off Alexandria. Egypt. J. Aquat. Res. 38, 39–44. doi: 10.1016/j.ejar.2012.09.002
Piccinetti, C., Vrgoè, N., Marèeta, B., and Manfredi, C. (eds). (2012). Recent State of Demersal Resources in the Adriatic Sea. Split: Institute of Oceanography and Fisheries.
Quezada−Romegialli, C., Jackson, A. L., Hayden, B., Kahilainen, K. K., Lopes, C., and Harrod, C. (2018). tRophicPosition, an r package for the Bayesian estimation of trophic position from consumer stable isotope ratios. Methods Ecol. Evol. 9, 1592–1599. doi: 10.1111/2041-210X.13009
Ramírez, F., Coll, M., Navarro, J., Bustamante, J., and Green, A. J. (2018). Spatial congruence between multiple stressors in the Mediterranean Sea may reduce its resilience to climate impacts. Sci. Rep. 8:14871. doi: 10.1038/s41598-018-33237-w
Riccioni, G., Stagioni, M., Piccinetti, C., and Libralato, S. (2018). A metabarcoding approach for the feeding habits of European hake in the Adriatic Sea. Ecol. Evol. 8, 10435–10447. doi: 10.1002/ece3.4500
Rumolo, P., Bonanno, A., Barra, M., Fanelli, E., Calabrò, M., Genovese, S., et al. (2016). Spatial variations in feeding habits and trophic levels of two small pelagic fish species in the central Mediterranean Sea. Mar. Environ. Res. 115, 65–77. doi: 10.1016/j.marenvres.2016.02.004
Saglam, H., and Yıldız, I. (2019). Temporal and ontogenetic variation in the diet of three small pelagic fish in the Black Sea of Turkey. Mar. Biodivers. 49, 1799–1812. doi: 10.1007/s12526-019-00944-0
Santana, C. A., da, S., Wieczorek, A. M., Browne, P., Graham, C. T., and Power, A. M. (2020). Importance of suspended particulate organic matter in the diet of Nephrops norvegicus (Linnaeus, 1758). Sci. Rep. 10:3387. doi: 10.1038/s41598-020-60367-x
Šantić, D., Šestanović, S., Šolić, M., Krstulović, N., Kušpilić, G., Ordulj, M., et al. (2014). Dynamics of picoplankton community from coastal waters to the open sea in the Central Adriatic. Mediterr. Mar. Sci. 15, 179–188. doi: 10.12681/mms.701
Šantić, M., Jardas, I., and Pallaoro, A. (2004). Diet composition and feeding intensity of Mediterranean horse mackerel, Trachurus mediterraneus (Osteichthyes: Carangidae), in the central Adriatic Sea. Acta Adriat. 45, 43–50.
Sinovčić, G., and Zorica, B. (2009). “Small pelagic fish resources in the Rijeka Bay, Kvarner and Kvarnerić,” in Natural History Researches of the Rijeka Region II, eds M. Arko-Pijevac and B. Surina (Rijeka: PMR Rijeka), 273–282.
Stagioni, M., Montanini, S., and Vallisneri, M. (2013). Feeding habits of anglerfish, Lophius budegassa (Spinola, 1807) in the Adriatic Sea, north-eastern Mediterranean. J. Appl. Ichthyol. 29, 374–380. doi: 10.1111/jai.12148
Tičina, V., Ivančić, I., and Emrić, V. (2000). Relation between the hydrographic properties of the northern Adriatic Sea water and sardine (Sardina pilchardus) population schools. Period. Biol. 102, 181–192.
Tirelli, V., Borme, D., Tulli, F., Cigar, M., Fonda Umani, S., and Brandt, S. B. (2006). Energy density of anchovy Engraulis encrasicolus L. in the Adriatic Sea. J. Fish Biol. 68, 982–989. doi: 10.1111/j.0022-1112.2006.00987.x
Todd, C. D., Laverack, M. S., and Boxshall, G. A. (1996). Coastal Marine Zooplankton, 2nd Edn. London: Cambridge University Press.
Trégouboff, G., and Rose, M. (1957). Manuel de Planctonologie Méditerranéenne. Paris: Centre National de la Recherche Scientifique.
Tursi, A., Matarrese, A., D’Onghia, G., Maiorano, P., Mastrotataro, F., Balanini, M., et al. (1999). “Parapenaeus longirostris,” in Synthesis of Knowledge on Bottom Fishery Resources in Central Mediterranean (Italy and Corsica) Biol. Mar. Medit., eds G. Relini, J. Bertrand, and A. Zamboni (Genova: SIBM), 541–553.
UNEP-MAP-RAC/SPA (2014). “Status and conservation of fisheries in the Adriatic Sea,” in Draft Internal Report for the Purposes of the Mediterranean Regional Workshop to Facilitate the Description of Ecologically or Biologically Significant Marine Areas, Malaga, Spain, 7-11 April 2014, eds H. Farrugio and Soldo Alen (Malaga: UNEP-MAP-RAC/SPA).
Vassilopoulou, V., and Papaconstantinou, C. (1993). Feeding habits of red mullet (Mullus barbatus) in a gulf in western Greece. Fish. Res. 16, 69–83. doi: 10.1016/0165-7836(93)90110-S
Vilibić, I., Mihanović, H., Janeković, I., Denamiel, C., Poulain, P.-M., Orlić, M., et al. (2018). Wintertime dynamics in the coastal northeastern Adriatic Sea: the NAdEx 2015 experiment. Ocean Sci. 14, 237–258. doi: 10.5194/os-14-237-2018
Viličić, D. (2014). Specifièna oceanološka svojstva hrvatskog dijela Jadrana. Hrvat. Vode 22, 297–314.
Viličić, D., Kuzmanić, M., Tomažić, I., Ljubešić, Z., Bosak, S., Precali, R., et al. (2013). Northern Adriatic phytoplankton response to short Po River discharge pulses during summer stratified conditions. Mar. Ecol. 34, 451–466.
Vinson, M. R., and Angradi, T. R. (2011). Stomach emptiness in fishes: sources of variation and study design implications. Rev. Fish. Sci. 19, 63–73. doi: 10.1080/10641262.2010.536856
Vrgoč, N., Arneri, E., Jukić-Peladić, S., Krstulović Šifner, S., Mannini, P., Marèeta, B., et al. (2004). Review of current knowledge on shared demersal stocks of the Adriatic Sea. FAO-MiPAF scientific cooperation to support responsible fisheries in the Adriatic Sea. GCP/RER/010/ITA/TD-12. AdriaMed Technical Documents, 12. Rome: FAO, 91.
Vučetić, T. (1963). Ishrana odrasle srdele (Sardina pilchardus Walb.) u srednjem Jadranu. Acta Adriat. 98, 1–47.
Winemiller, K. O., Akin, S., and Zeug, S. C. (2007). Production sources and food web structure of a temperate tidal estuary: integration of dietary and stable isotope data. Mar. Ecol. Prog. Ser. 343, 63–76. doi: 10.3354/meps06884
Wolf, N., Carleton, S. A., and Martínez del Rio, C. (2009). Ten years of experimental animal isotopic ecology. Funct. Ecol. 23, 17–26. doi: 10.1111/j.1365-2435.2009.01529.x
Young, T., Pincin, J., Neubauer, P., Ortega-García, S., and Jensen, O. P. (2018). Investigating diet patterns of highly mobile marine predators using stomach contents, stable isotope, and fatty acid analyses. ICES J. Mar. Sci. 75, 1583–1590. doi: 10.1093/icesjms/fsy025
Zavatarelli, M., Baretta, J. W., Baretta-Bekker, J. G., and Pinardi, N. (2000). The dynamics of the Adriatic Sea ecosystem: an idealized model study. Deep Sea Res. I Oceanogr. Res. Pap. 47, 937–970. doi: 10.1016/S0967-0637(99)00086-2
Zorica, B., Čikeš Keč, V., Vidjak, O., Kraljević, V., and Brzulja, G. (2017). Seasonal pattern of population dynamics, spawning activities, and diet composition of sardine (Sardina pilchardus Walbaum) in the eastern Adriatic Sea. Turk. J. Zool. 41, 892–900. doi: 10.3906/zoo-1609-27
Zorica, B., Čikeš Keč, V., Vidjak, O., Mladineo, I., and Ezgeta Balić, D. (2016). Feeding habits and helminth parasites of sardine (S. pilchardus) and anchovy (E. encrasicolus) in the Adriatic Sea. Mediterr. Mar. Sci. 17, 216–229. doi: 10.12681/mms.1467
Zorica, B., Čikeš Keč, V., Vrgoè, N., Isajlović, I., Piccinetti, C., Mandić, M., et al. (2020). A review of reproduction biology and spawning/nursery grounds of the most important Adriatic commercial fish species in the last two decades. Acta Adriat. 61, 89–100. doi: 10.32582/aa.61.1.7
Keywords: demersal fish, pelagic fish, food web, PPRM, trophic ecology, spatial variation, trophic position
Citation: Zorica B, Ezgeta-Balic´ D, Vidjak O, Vuletin V, Šestanovic´ M, Isajlovic´ I, Cˇ ikeš Kecˇ V, Vrgocˇ N and Harrod C (2021) Diet Composition and Isotopic Analysis of Nine Important Fisheries Resources in the Eastern Adriatic Sea (Mediterranean). Front. Mar. Sci. 8:609432. doi: 10.3389/fmars.2021.609432
Received: 23 September 2020; Accepted: 12 February 2021;
Published: 04 March 2021.
Edited by:
Tomaso Fortibuoni, Higher Institute for Environmental Protection and Research (ISPRA), ItalyReviewed by:
Marta Albo-Puigserver, Instituto de Ciencias del Mar (CSIC), SpainPaola Rumolo, Institute of Marine Science (CNR), Italy
Copyright © 2021 Zorica, Ezgeta-Balić, Vidjak, Vuletin, Šestanović, Isajlović, Čikeš Keč, Vrgoč and Harrod. This is an open-access article distributed under the terms of the Creative Commons Attribution License (CC BY). The use, distribution or reproduction in other forums is permitted, provided the original author(s) and the copyright owner(s) are credited and that the original publication in this journal is cited, in accordance with accepted academic practice. No use, distribution or reproduction is permitted which does not comply with these terms.
*Correspondence: Daria Ezgeta-Balić, ZXpnZXRhQGl6b3IuaHI=