- 1Ecology and Evolutionary Biology Department, University of California, Santa Cruz, Santa Cruz, CA, United States
- 2Chicago Zoological Society’s Sarasota Dolphin Research Program, c/o Mote Marine Laboratory, Sarasota, FL, United States
Marine mammal body composition has been an important tool that is used as a proxy for the health and condition of individuals within a population. Common bottlenose dolphin (Tursiops truncatus) body composition is influenced by variations in blubber thickness resulting from changes in temperature, prey availability, health, and life-history traits. We examined how environmental, ontogenetic, and reproductive variables influenced the body composition of common bottlenose dolphins in Sarasota Bay using data collected from a long-term monitoring project by the Sarasota Dolphin Research Program (SDRP). We found that both sea surface temperature (SST) and catch per unit effort (CPUE), used as a proxy for prey availability, influenced body composition. There was a high degree of seasonality in body composition, with higher values occurring in winter when SST and CPUE were both low. Ontogeny also greatly influenced body composition, as younger dolphins typically had thicker blubber than mature individuals. Interestingly, young females allocated more energy to allometric growth than deposition of blubber for body composition when compared to young males. However, as females matured and their growth slowed, they invested more in body composition. We found no significant difference in body composition of females of varying reproductive states, providing further evidence of their status as true income breeders. Our work highlights that changes in body composition result from fluctuations in environmental variables and that energy allocation to body composition changes with ontogeny.
Introduction
Blubber aids marine mammals in thermoregulation, energy storage, and locomotion (Parry, 1949; Struntz et al., 2004; Noren and Wells, 2009). This vascularized tissue, composed of adipocytes and collagen, helps form the streamlined shape of marine mammals, while also providing insulation against the harsh aquatic environment; a particularly important function in cetaceans, as it is their primary insulative layer (Parry, 1949). Blubber is metabolically active and can be mobilized during nutritional stress when energy intake falls below energy output. As a result, body composition, defined as the ratio of blubber mass to either total body mass or lean mass, is representative of the health and condition of an individual (Lockyer et al., 1985; Lockyer, 1986; Read, 1990; Ryg et al., 1990; Koopman et al., 2002).
Temperature has been shown to impact the depth and thermal properties of blubber, as species in colder climates typically have more insulative blubber (Worthy and Edwards, 1990; Koopman, 2007; Montie et al., 2008). This aids in heat retention and lowers the cost of thermoregulation. Seasonal trends in blubber depth are also observed due to fluctuations in temperature (Williams and Friedl, 1990; Meagher et al., 2008). Temperature also has indirect effects on blubber due to the relationship between oceanographic features and prey availability. Features such as thermal fronts and cold, deep-water upwelling typically increase prey abundance via input of nutrients to the surface ocean, thereby influencing the body composition of marine mammals (Miller et al., 2011; Øigård et al., 2013; Braithwaite et al., 2015).
Blubber, and therefore body composition, is also heavily influenced by age, sex, and reproductive status (Koopman et al., 1996; McLellan et al., 2002; Dunkin et al., 2005; Beck et al., 2007; Koopman, 2007). The ontogeny of blubber development has been well studied in cetaceans (Struntz et al., 2004; Dunkin et al., 2005; Noren and Wells, 2009); however, less is known about how body composition changes throughout an animal’s lifetime (although see McLellan et al., 2002; Mallette et al., 2016). Cetacean calves and juveniles tend to have thicker blubber layers compared to adults as more energy is allocated to blubber deposition at this stage in life, likely due to an increased need for insulation as a result of their small body size and concurrently high surface area to volume ratio (McLellan et al., 2002; Struntz et al., 2004; Dunkin et al., 2005; Noren and Wells, 2009; Mallette et al., 2016).
Understanding the ontogeny of blubber development has also provided insight into how male and female marine mammals differentially allocate resources to growth and body composition. Male and female cetaceans and pinnipeds exhibit differences in fatty acid composition (West et al., 1979; Aguilar and Borrell, 1990; Samuel and Worthy, 2004) and blubber deposition and development (Ackman et al., 1975). However, the factors driving these differences in blubber composition, morphology, and deposition (i.e., the insulative properties of blubber) are often difficult to tease apart due to variability in feeding ecology, energy expenditure, and reproductive strategies between the sexes. Sex-specific differences in body composition can be particularly challenging to detect in income breeders who continue to feed during gestation and lactation, and thereby respond to the elevated metabolic costs of gestation and lactation by increasing food consumption (Reddy et al., 1991).
We aimed to assess the abiotic, biotic, and life history stage-related factors influencing the body composition of an income breeding cetacean, the common bottlenose dolphin (Turiops truncatus), using data from the Sarasota Dolphin Research Program (SDRP) long-term monitoring effort in Sarasota Bay, Florida. This long-term monitoring effort began in 1970 and has resulted in detailed and high-quality data from the resident dolphin community, including information on age, sex, life history, and environmental features such as prey abundance and water temperature (Wells, 2009, 2014; Berens McCabe et al., 2010). Our objectives were to (1) assess the factors driving body composition for the resident common bottlenose dolphin community, (2) determine how these factors change based on age and sex, (3) examine whether the reproductive state alters body composition of sexually mature female dolphins, and (4) assess the long-term trends in body composition for this dolphin community.
Materials and Methods
Data Collection
Common bottlenose dolphin body condition, morphometrics, genetics, life history and health data were obtained through periodic capture-release research (Wells et al., 2004). The shallow, sheltered bay waters facilitated encircling small groups of selected dolphins with a 500 m long × 4 m deep seine net. Individual dolphins were placed in a sling, and lifted aboard a veterinary examination vessel. The animals were weighed, measured for standardized lengths and girths, and ultrasonic measurements of blubber thickness were made at standardized sites. Each individual was given a full examination, including ultrasonic imaging of internal organs, by experienced marine mammal veterinarians. Sex was confirmed through direct examination of the genital region. Upon completion of sampling, dolphins were marked with freeze brands or tagged as appropriate, photographed, and released. While the ages of most of the sampled dolphins were already known from observations since birth to identifiable mothers, occasionally ages needed to be determined from examination of growth layer groups in a tooth extracted under local anesthesia (Hohn et al., 1989). Reproductive status was evaluated through measurements of reproductive hormone concentrations, through ultrasonic examination of reproductive organs, and through observations of young calves believed to be the first calves of identifiable females.
Relative prey fish abundance of the 10 most common dolphin prey species (pinfish, Lagodon rhomboides; pigfish, Orthopristis chrysoptera; scaled sardine, Harengula jaguana; Atlantic threadfin herring, Opisthonema oglinum; ladyfish, Elops saurus; mullet, Mullet spp.; sheepshead, Archosargus probatocephalus; spot Leiostomus xanthurus; spotted seatrout, Cynoscion nebulosus; and Gulf toadfish, Opsanus beta; Wells et al., 2013) was estimated from systematic, seasonal purse seining in Sarasota Bay and vicinity (Gannon et al., 2009; Berens McCabe et al., 2010). Since 2004, multispecies fisheries surveys have been conducted during the months of June through September, and January through March, using a 183 × 6.7-m purse seine with 2.5-cm mesh, deployed from a 9-m flat-bottomed skiff in waters between 0.4 and 4 m in depth (Sarasota Bay’s maximum depth is 4 m), with the focus in recent years on sampling in seagrass meadows as an index habitat. The net sampled the entire water column, from surface to bottom. All fishes, cephalopods, and penaeid shrimps caught in each purse seine set were identified to species and counted, and a subsample was measured for length and weight. Catch per unit effort (CPUE), or the number of organisms caught per standardized purse seine set, was used as an index of prey availability.
Truncated Cones
Body composition was calculated as the proportion of blubber volume to total body volume following the truncated cones method (Gales and Burton, 1987) using lengths, girths, and ultrasound measurements of blubber depth. Blubber density was assumed to be 1.039 kg/m3 (Dunkin et al., 2010). The morphometric measurements taken varied across the time series, with three distinct methods and time periods, detailed in Figure 1.
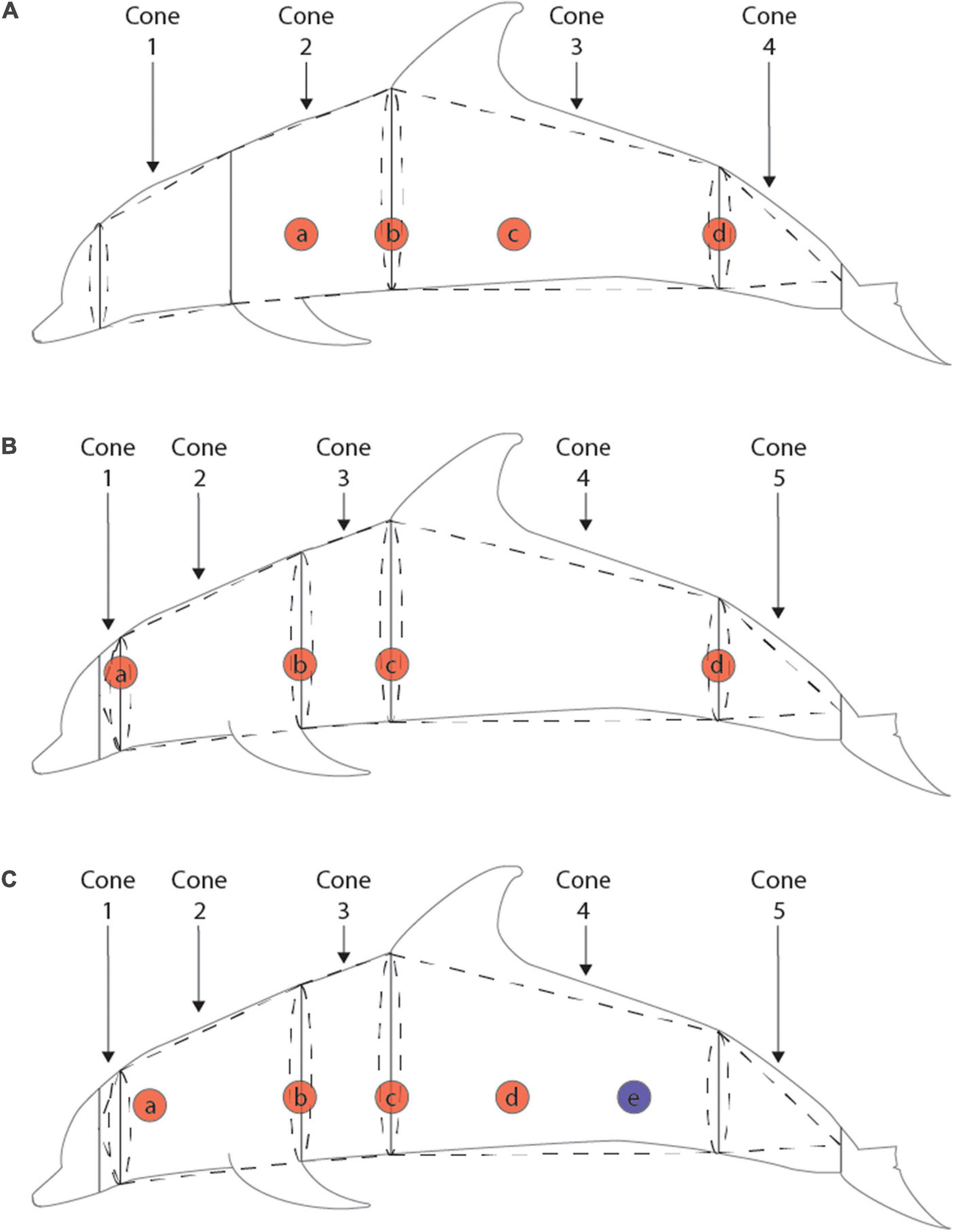
Figure 1. Girth, blubber depth, and truncated cones methods for three time periods: pre-1989 (A), 1989–2012 (B), and post-2012 (C). Solid lines indicate placement of girth measurements, red circles indicate lateral placement of ultrasound measurements of blubber depth, blue circles indicate approximate location of caudal biopsy of blubber depth, and dotted lines represent the breakdown of cones used in the truncated cones calculations. For the pre-1989 measurements, the average of blubber depths a and b were averaged for cones 1 and 2, the average of blubber depths b and c were used for cone 3, and blubber depth d was used for cone 4. For the 1989–2012 measurements, blubber depth a was used for cone 1, the average of blubber depths a and b were used for cone 2, the average of blubber depths b and c were used for cone 3, the average of blubber depths c and d were used for cone 4, and blubber depth d was used for cone 5. For the post-2012 measurements blubber depth a was used for cone 1, the average of blubber depths a, b, and c were used for cones 2 and 3, and the average of blubber depths c, d, and e were used for cones 4 and 5.
The first method of collected morphometrics occurred prior to 1989, when only four body segments were consistently measured for lengths and girths. Girth measurements were consistently taken at the blowhole, axillary, anus, and tail fluke insertion, and some individuals had measurements taken anterior to the pectoral flipper. To estimate anterior flipper girth in individuals missing this measurement, we used a linear regression to derive anterior flipper girth measurements from axillary girth based on individuals with both measurements (LM R2 = 0.9169, p < < 0.01). Ultrasound measurements were taken at four non-corresponding locations along the animals’ length, so the average of two appropriate locations was used for each body segment (see Figure 1). Between 1989 and 2012, five body segments were consistently measured at the eye, blowhole, posterior pectoral flipper, axillary, anus, and tail fluke insertion. Blubber depth was measured at five lateral positions along the body with 4–11 measurements taken in a ring around the girth of the animal at 10 cm intervals at each position. These ring measurements were averaged to give a single value for each lateral position. For each body segment, either a single ring measurement or the mean of two corresponding lateral ring measurements was used as the blubber depth (see Figure 1). For the final method used after 2012, morphometric measurements were the same as those taken from 1989 to 2012, however, ultrasound measurements were only taken at 3–5 individual points along the length of the body. Again, mean ultrasound values at appropriate lateral locations were used to calculate blubber depth for each segment.
The total body volume, which scales allometrically with mass, was calculated using the truncated cones method by summing the volume of each cone produced by an individual body segment. Lean body volume was then estimated using the truncated cones method and the girth measurements used here were estimated by subtracting blubber depth estimates from the estimated radius of the original girth measurement. Blubber volume was obtained by subtracting the lean volume from total body volume, and body composition was estimated as the ratio of blubber volume to total body volume.
To ensure that data were comparable across the time series, we used measurements from the 1989 to 2012 methods to calculate body composition for the same individuals using the pre-1989 and post-2012 methods. We then calculated regression coefficients comparing the body composition calculated using the 1989–2012 method to pre-1989 (LM R2 = 0.735, p << 0.01) and post-2012 (LM R2 = 0.780, p << 0.01) and used those coefficients to correct the pre-1989 and post-2012 calculations to the 1989–2012 method (Figure 2).
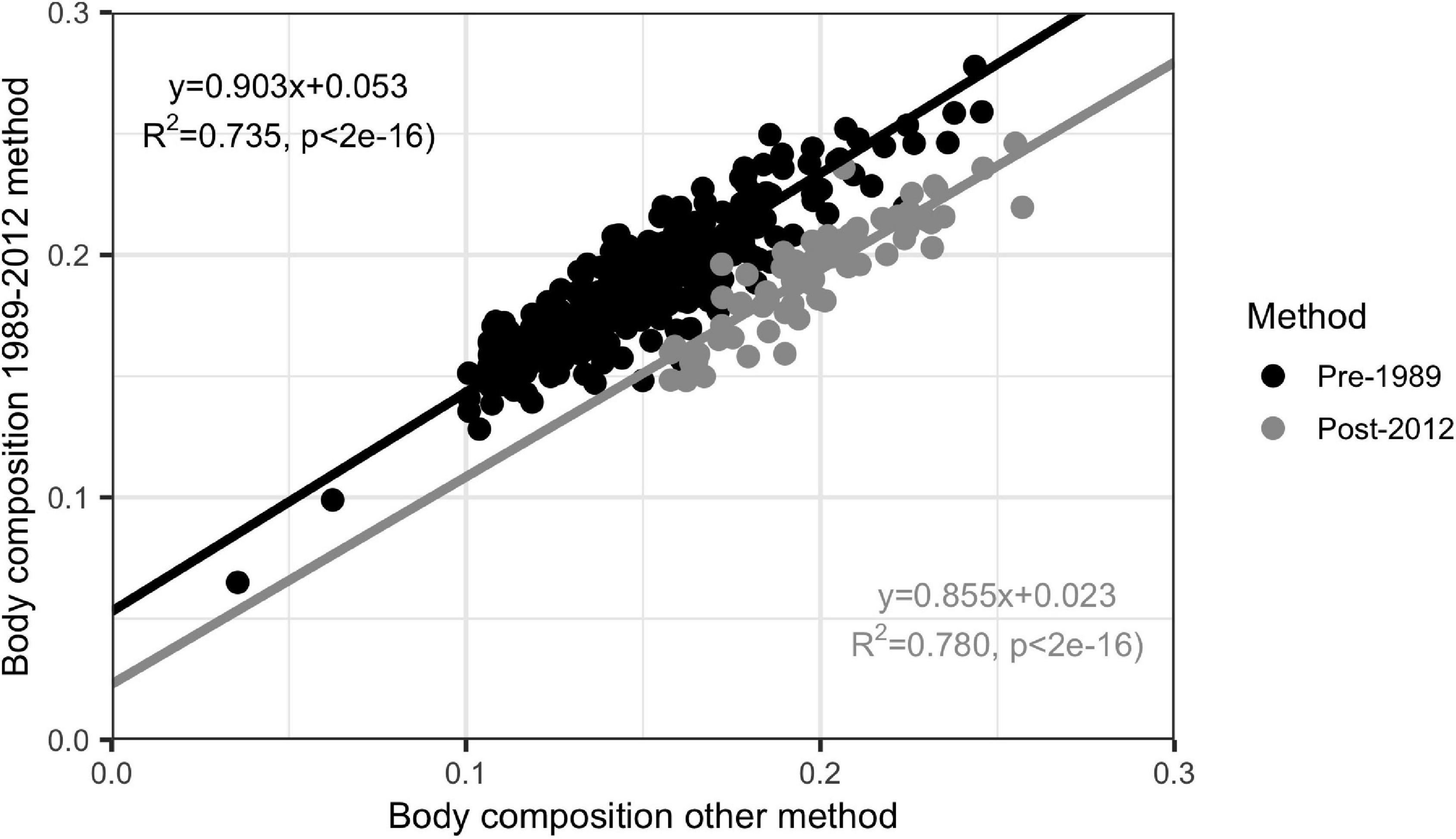
Figure 2. Linear regression of pre-1989 (n = 108) and post-2012 (n = 80) body composition calculation methods against 1989–2012 method. Regression coefficients shown were used to correct data to body compositions from before and after the 1989 to 2012 time period to the 1989–2012 method.
Statistics
All statistical analyses were conducted using R version 4.03 (R Core Team, 2020) with packages “lme4” (Bates et al., 2015) and “MuMIn” (Barton, 2009). Sea surface temperature (SST) for models was extracted from the HadISST dataset1 using the ‘‘rerddapXtracto’’ package in R2. The data consisted of 534 individuals across varying ages and reproductive statuses (Supplementary Table 1), with 324 repeated measures of individuals occurring throughout the long-term study. To account for this, we repeated statistical analyses without repeated measures to ensure that our results were robust.
We used generalized linear mixed models (GLMMs) to determine which factors influenced body composition (Breslow and Clayton, 1993). Fixed effects included standard body length, sex, season, year, monthly SST, and CPUE. Northern hemisphere seasons were used in these analyses and were defined as follows: Winter (December, January, and February), Spring (March, April, May), Summer (June, July, August), and Fall (September, October, November). Monthly SST data were extracted from HadISST Average Sea Surface Temperature at 1° spatial resolution for 1986–2016. Repeated measures of individual dolphins and year were regarded as random effects. Age was not included in the model due to the significant correlation between age and body length (Kendall correlation; z = 24.12, p < 2.2 × 10–16; Supplementary Figure 1). Models were fit for all (n = 534), young (less than or equal to 10 years of age, n = 254; Read et al., 1993), and mature (n = 280) dolphins because of the importance of body length and age in determining body composition (see “Results”). GLMMs that incorporated CPUE as a predictor of body composition were evaluated on a subsample of the data from 2004 onward, when CPUE measurements first became available (n = 73 and n = 84 for young and mature individuals, respectively). Best fit models were selected using Akaike’s Information Criteria corrections (AICc) following suggestions to use this method unless sample sizes are exceedingly large (Burnham and Anderson, 1994; Wagenmakers and Farrell, 2004). Because the GLMMs conducted here were performed on subsamples of the full dataset the sample size for each analysis was reduced, necessitating the use of AICc. Models with delta AICc values less than two were compared and selected based upon biological accuracy.
GLMMs indicated that season was a significant predictor of body composition in dolphins (see “Results”). We aimed to explore this relationship further using a Kruskal-Wallis one-way analysis of variance (ANOVA). The Kruskal-Wallis ANOVA is a non-parametric analysis that can test for variation amongst groups (McKnight and Najab, 2010). This test was applied to examine seasonal differences in body composition for both young and mature dolphins (n = 254 and n = 280, respectively). A Dunn post hoc analysis was performed to determine which groups significantly differed. Seasonal changes in body composition were further compared to monthly SST and seasonal CPUE from data collected in Sarasota Bay from 2006 to 2016 in winter (collected in January, February, and March) and summer (collected in June, July, August, and September).
We used generalized additive models (GAMs) to evaluate the non-linear relationship between dolphin age and body composition for all dolphins (Hastie and Tibshirani, 1987). GAMs were applied to data for both sexes (n = 260 and n = 274 for males and females, respectively) to account for sex-specific differences in body composition as found by GLMMs and the number of knots was restricted to 1 to avoid overfitting the model.
To examine how reproductive state influenced body composition in mature females of known reproductive status (n = 127), we used a Kruskal-Wallis ANOVA followed by the Dunn post hoc test. Analyses were run on mature females only, and then again with mature males (n = 150) to compare body composition in different reproductive states to body composition of males.
To assess long-term trends in body composition we applied a GAM to the relationship between year and body composition for all dolphins, incorporating covariates of annually averaged SST and annually averaged CPUE. Due to a sampling bias in the months of data collection, with 76% of data collected in May and June, average body composition collected in June from 1986 to 2006 and May from 2007 to 2016 were used for this analysis. Models were fit from 1986 to 2016 (n = 409) and from 2004 to 2016 (when CPUE data were available; n = 125) to ensure that the best fit model was not impacted by limitations of CPUE. All combinations of monthly SST, seasonal CPUE, and the interaction between monthly SST and seasonal CPUE were run and the best fit model was selected using AICc.
Results
GLMMs
In the GLMMs, monthly SST was an important determinant of body composition in both young and mature dolphins, as body composition decreased with increasing SST (Table 1, Supplementary Table 2, and Figure 3). For young individuals, body length, season, and sex also influenced body composition (Table 1). When applying the model to mature individuals, season and sex were important factors influencing body composition. However, body length was not a predictor of body composition (Table 1). Additionally, year impacted body composition of mature dolphins (Table 1). Models including CPUE (data from 2004 to 2016) mirrored the results stated above, however, CPUE was an important predictor of body composition for both young and mature dolphins (Table 2 and Figure 3).
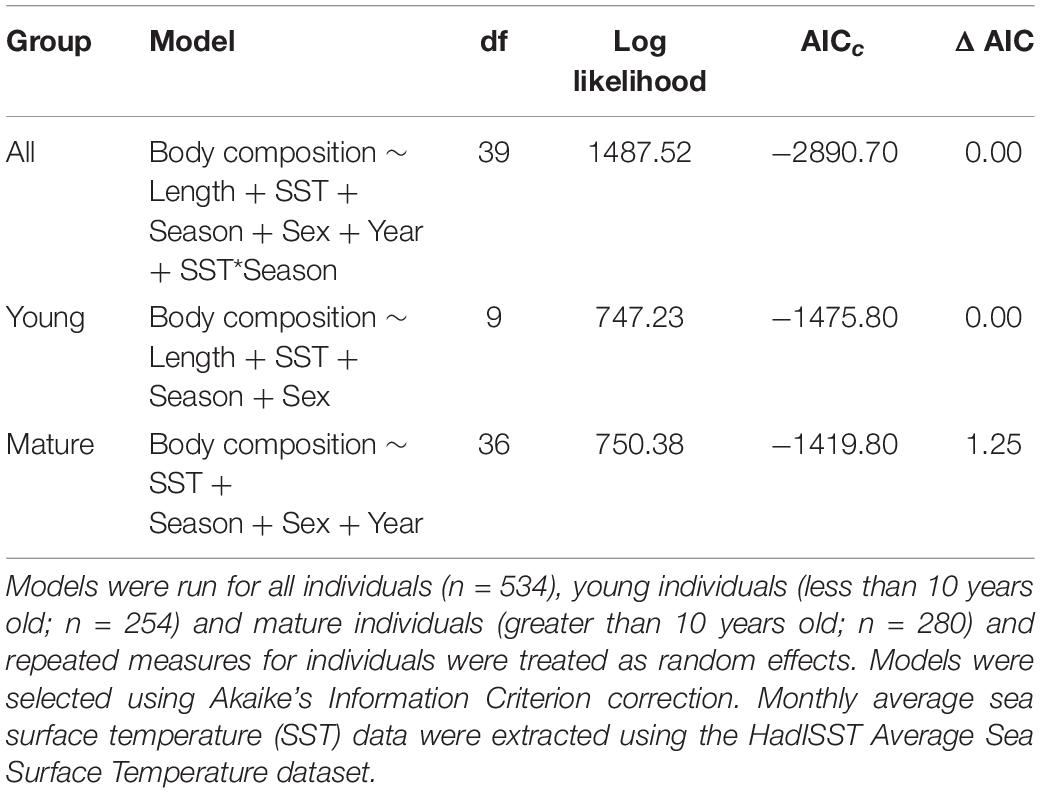
Table 1. Generalized linear mixed models predicting body composition of common bottlenose dolphin (Tursiops truncatus) in Sarasota Bay, Florida with fixed effects of body length (length), monthly SST (SST), season, sex, year from 1986 to 2016.
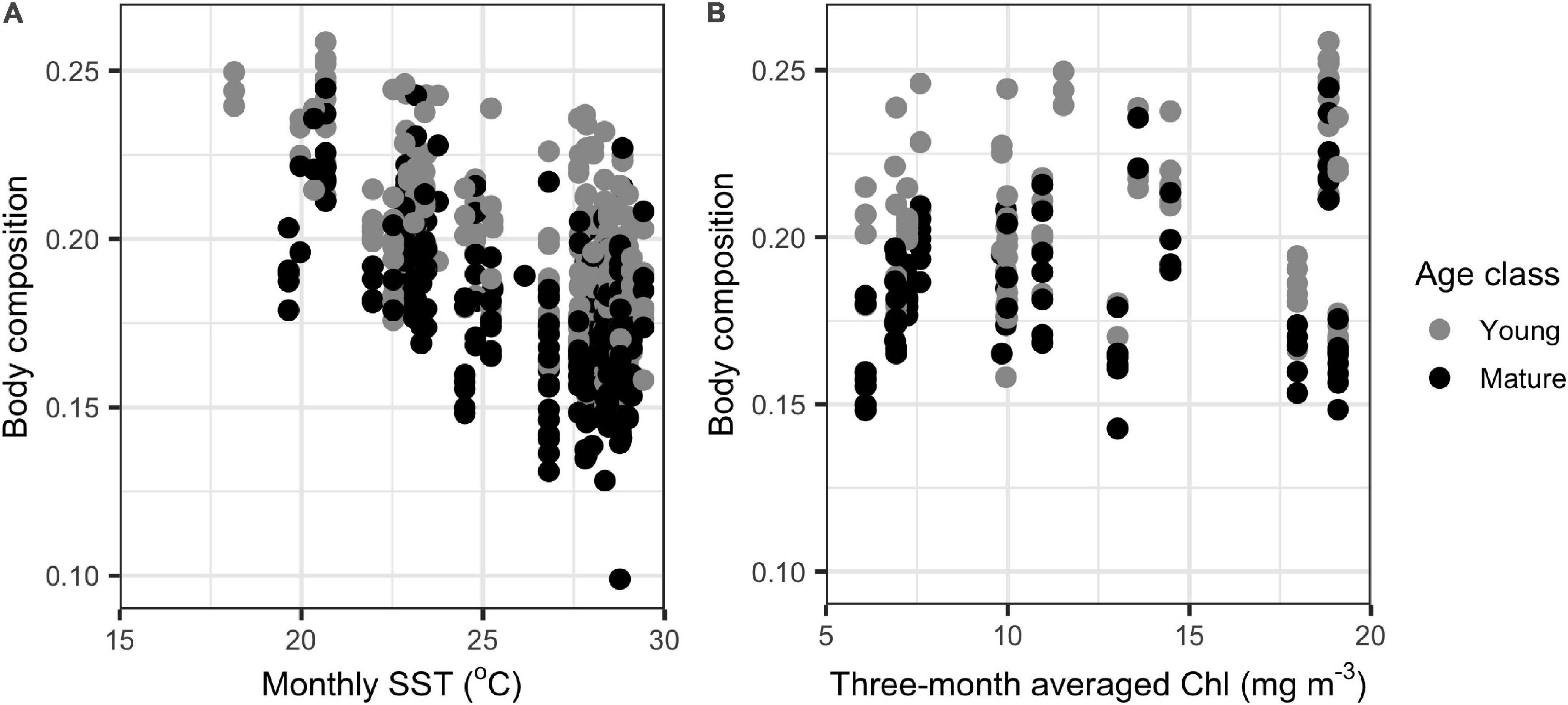
Figure 3. Comparison of the relationship between monthly sea surface temperature (SST) and body composition (n = 254 and n = 280 for young and mature individuals, respectively) (A) and CPUE and body composition (n = 73 and n = 84 for young and mature individuals, respectively) (B) for common bottlenose dolphins (Tursiops truncatus) in Sarasota Bay. Monthly SST data were extracted from HadISST Average Sea Surface Temperature at 1° spatial resolution.
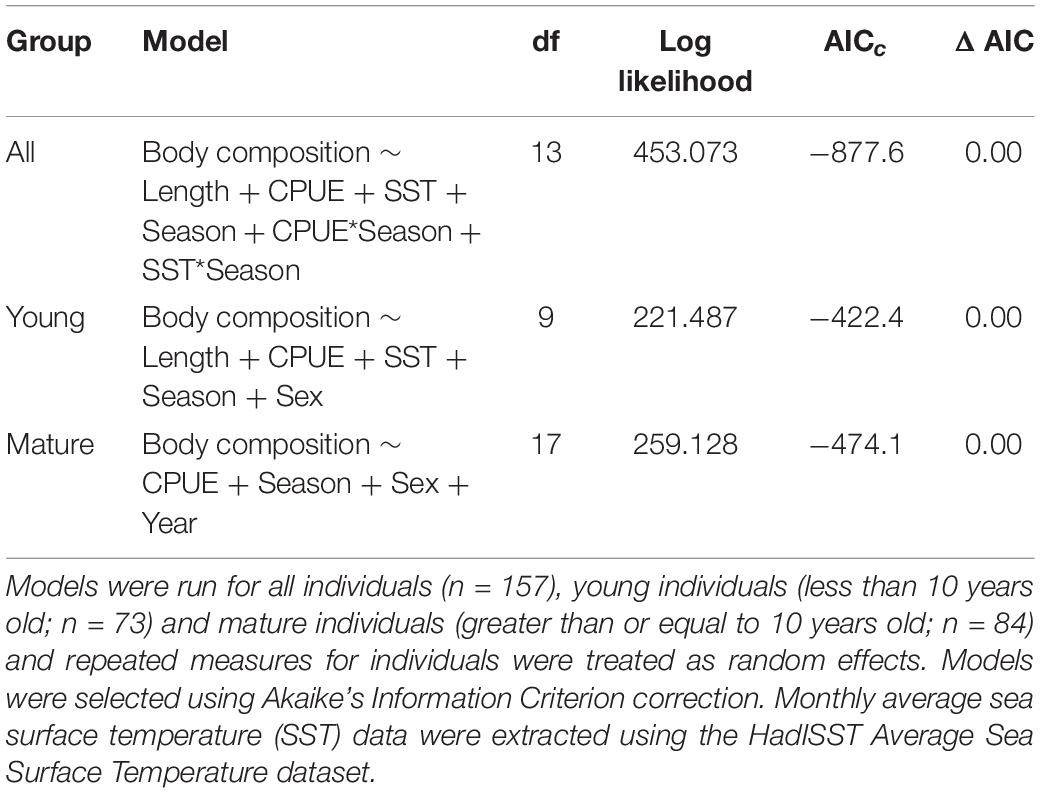
Table 2. Generalized linear mixed models predicting body composition of common bottlenose dolphin (Tursiops truncatus) in Sarasota Bay, Florida with fixed effects of body length (length), monthly SST (SST), catch per unit effort (CPUE), season, sex, and year from 2004 to 2016.
Seasonality
There was a significant difference in body composition between seasons for both young and mature dolphins (X2 = 88.75, df = 3, p < 2.22 × 10–16 and X2 = 96.81, df = 3, p < 2.22 × 10–16, respectively). The greatest difference in body composition in both mature and young dolphins occurred between summer, when body composition was the lowest, and winter, when body composition was the highest (Figure 4). Body composition in spring was significantly higher than body composition in summer and significantly lower than body composition in winter (Figure 4). Based on visual analyses, trends in body composition appear to follow an inverse pattern with seasonal CPUE, as body composition was highest in winter when CPUE was lowest (Figure 4). However, seasonal CPUE were only available for winter and summer, potentially obscuring patterns and trends between body composition and CPUE. Lower seasonally averaged SST co-occurred with high body composition values and vice versa; however, there did not appear to be a direct inverse relationship between seasonally averaged SST and body composition (Figure 4).
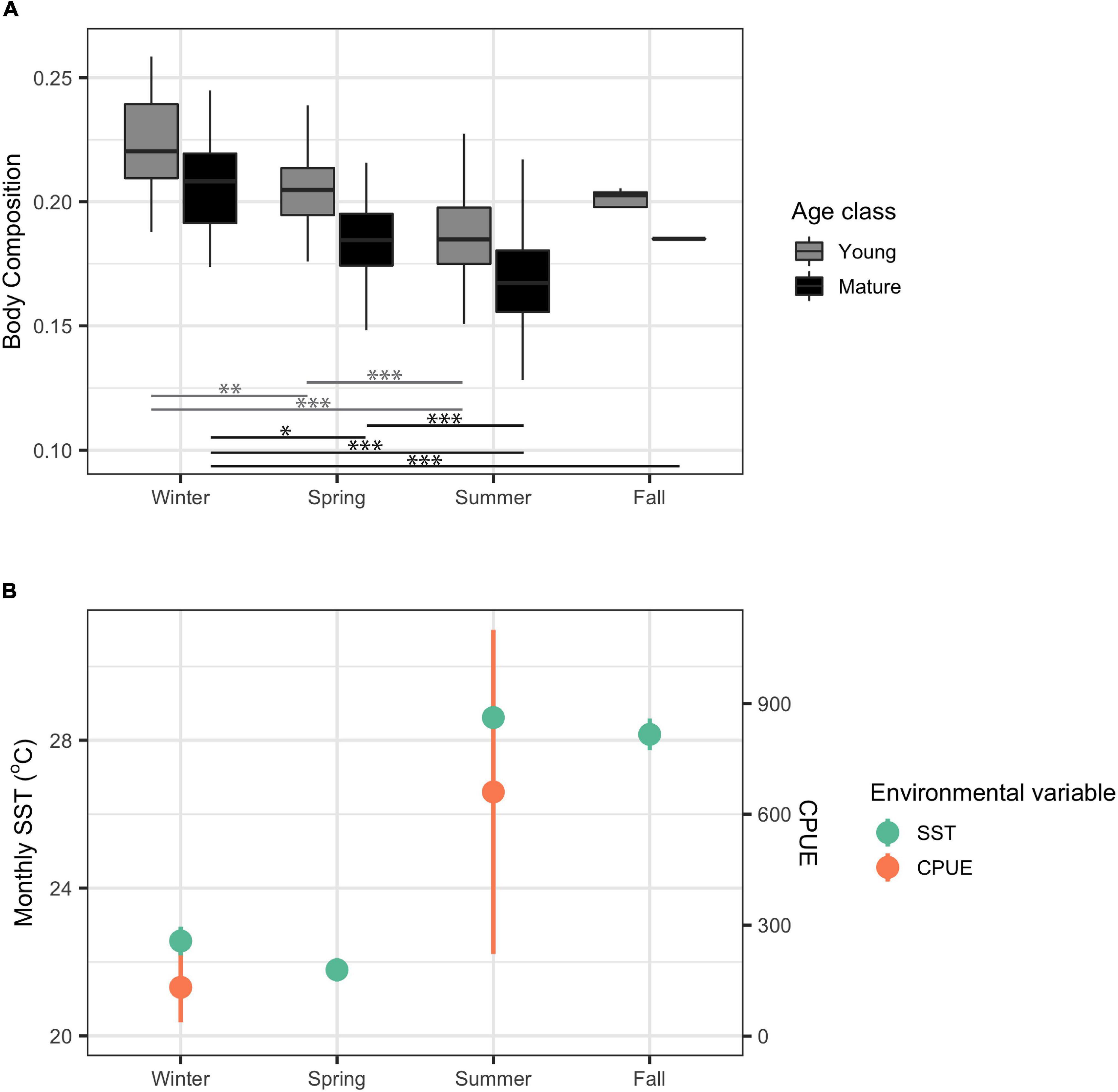
Figure 4. Body composition for young and mature (n = 254 and n = 280, respectively) common bottlenose dolphins (Tursiops truncatus) in Sarasota Bay by season from data collected from 1986 to 2016 (A) and monthly sea surface temperature (SST) and catch per unit effort (CPUE) in Sarasota Bay average by season during the years 1986–2016, 2003–2016, and 2006–2016, respectively (B). Significance from Dunn test results are indicated by one asterisk (p < 0.05), two asterisks (p < 0.001), and three asterisks (p < 0.0001). Monthly SST data were extracted from HadISST Average Sea Surface Temperature at 1° spatial resolution. CPUE data were collected from seine trawls in winter and spring in Sarasota Bay.
Age
Both male and female dolphins demonstrated statistically significant negative relationships between age and body composition; however, there was a better fit for males than females (GAMs: R2 = 0.39, p < 2.22 × 10–16 for males and R2 = 0.05, p = 0.001 for females; Figure 5). There was a high degree of variability in the relationship tested for females, resulting in poor model fit.
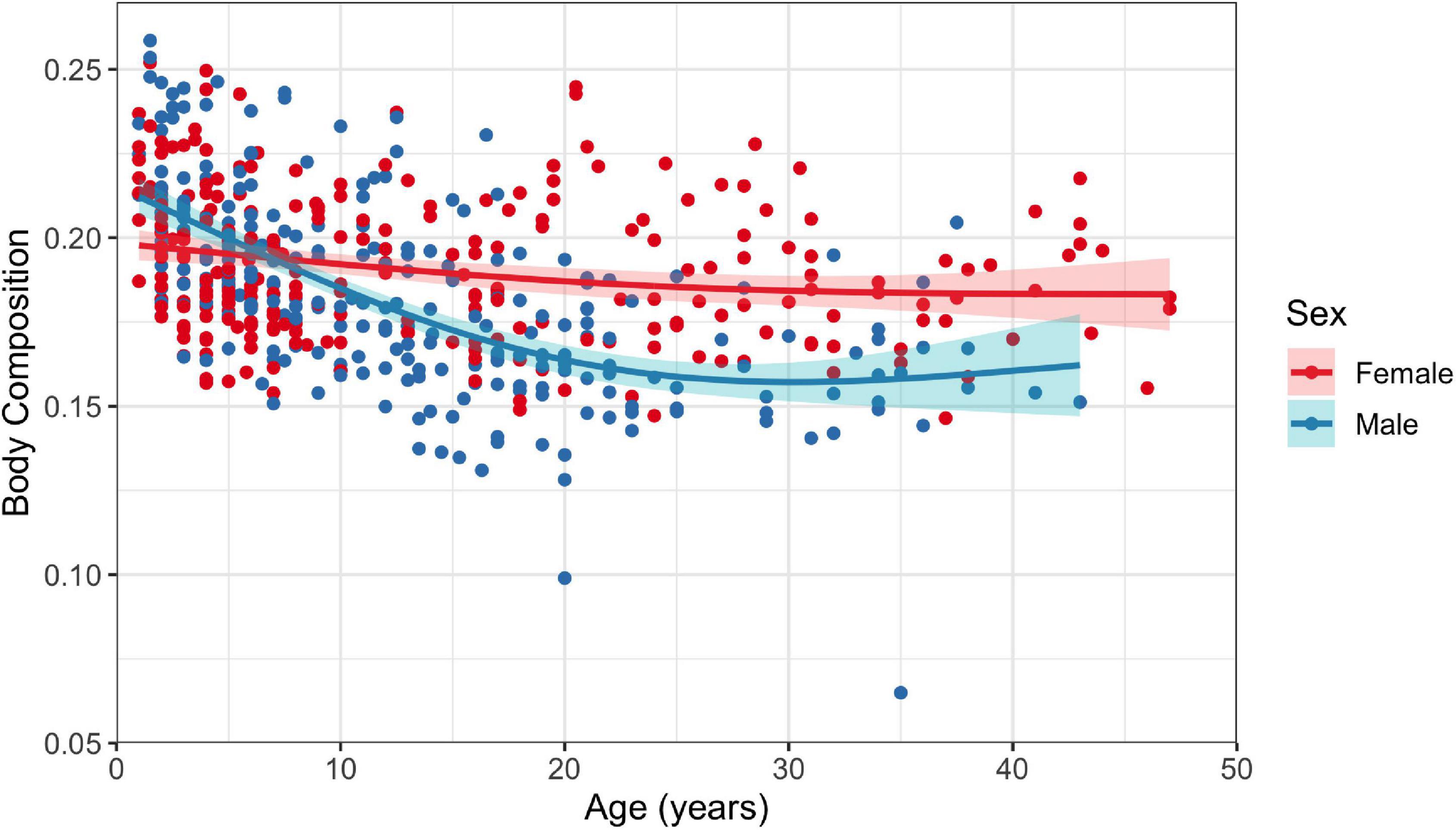
Figure 5. Fitted generalized additive model with 95% confidence interval for the relationship between age and body composition for female and male (n = 260 and n = 274, respectively) common bottlenose dolphins (Tursiops truncatus) in Sarasota Bay. Number of knots was restricted to one.
Reproductive State
We found no significant difference in female’s body composition in different reproductive states (Figure 6). However, when re-running the Kruskal-Wallis test including reproductively active males, both pregnant and lactating mature females had significantly higher body composition than that of mature males, while mature females that were simultaneously pregnant and lactating had a body composition similar to that of males (Figure 6).
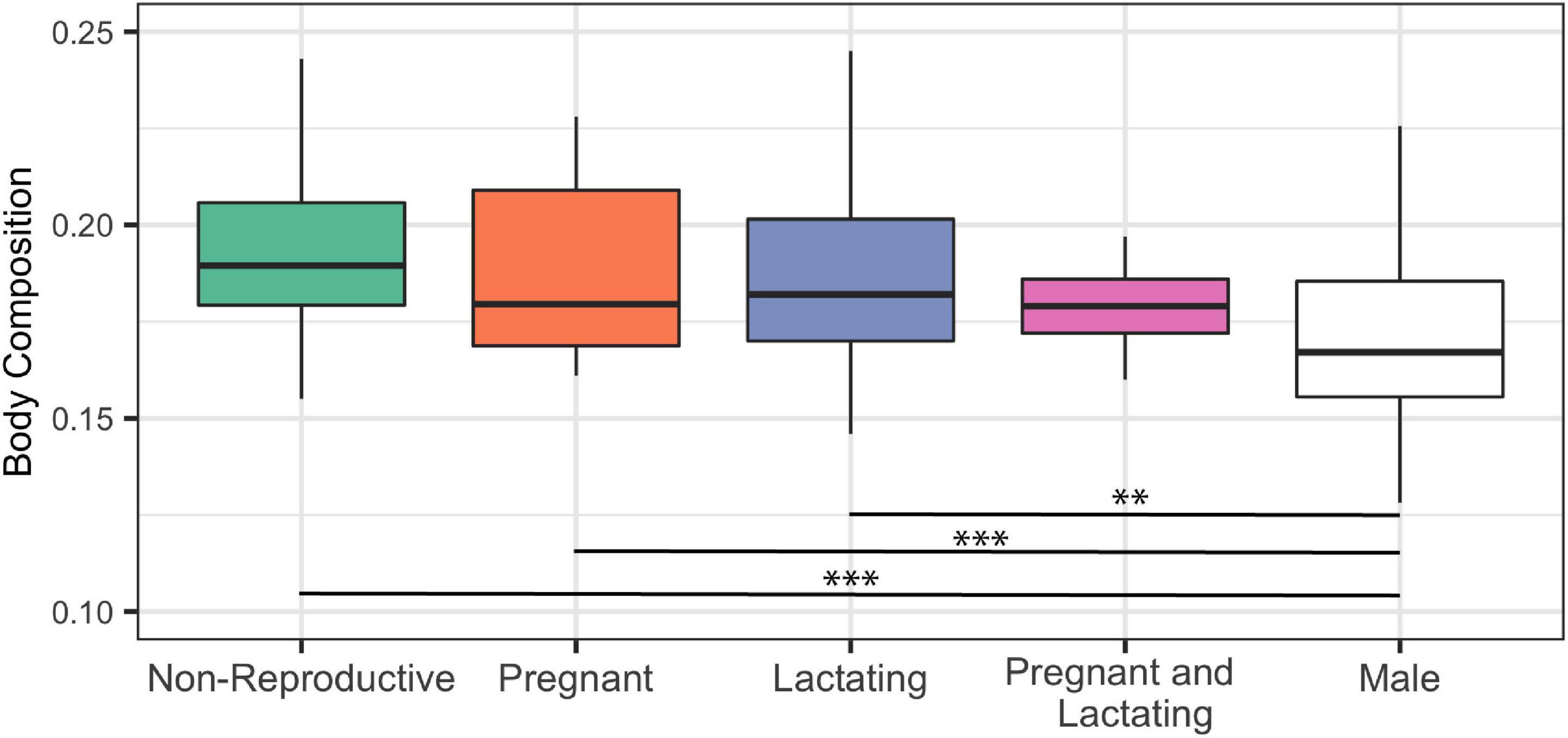
Figure 6. Body composition of mature female (n = 127) common bottlenose dolphins (Tursiops truncatus) of differing reproductive status and adult male (n = 150) common bottlenose dolphins in Sarasota Bay. Significance from Dunn test results are indicated by two asterisks (p < 0.001) and three asterisks (p < 0.0001).
Long-Term Trends
The best fit GAM for long-term trends in body composition incorporated annually averaged SST and described 55.2% of the deviance in the data (Table 2 and Figure 7). Adding annually averaged CPUE to the 1986–2016 and 2004–2016 models did not affect their output. Peaks in body composition occurred during a brief period from 1997 to 1999, and during a sudden spike in 2005. Although body composition appeared to increase over time, this is likely not a result of increasing water temperatures, as annual averages of SST in Sarasota Bay have remained relatively constant (Supplementary Figure 2).
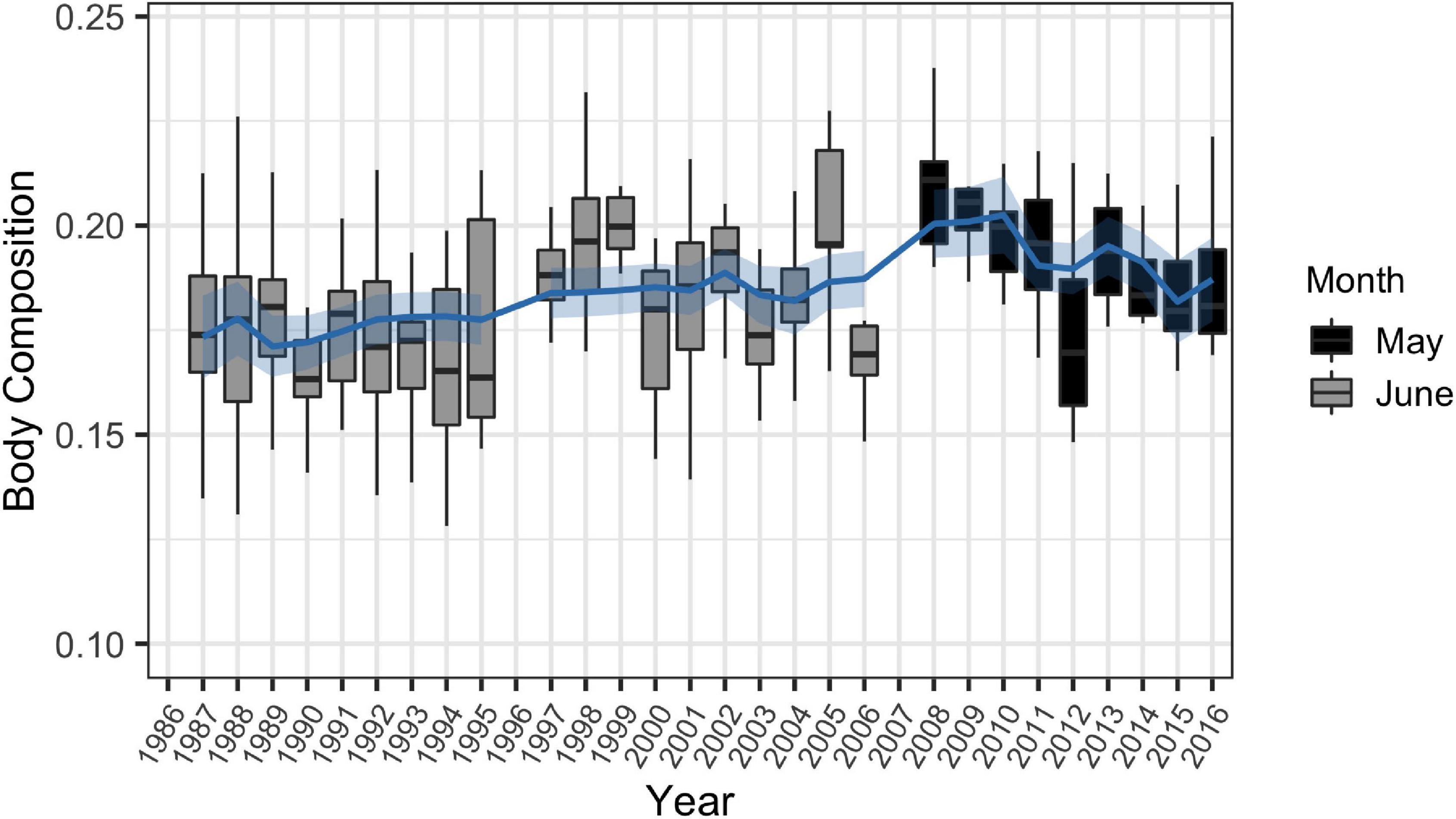
Figure 7. Fitted generalized additive model with 95% confidence interval for long term trends in body composition for male and female common bottlenose dolphins (Tursiops truncatus) of all ages in Sarasota Bay between 1986 and 2016 (n = 480). Analyses were restricted to May and June to reduce effects of seasonal sampling bias.
Discussion
Dolphin body composition is strongly linked to SST and seasonal changes in temperature. While the factors influencing body composition of common bottlenose dolphins varied by age and sex, SST was a significant variable in determining long-term trends in body composition and greatly affected the available energy stores of the Sarasota Bay common bottlenose dolphins. This pattern is common to marine mammals, as their blubber thickness and other characteristics typically vary in response to seasonal changes in water temperature (Beck et al., 1993; Kastelein et al., 1997; Meagher et al., 2008; Montie et al., 2008). Modifications in blubber thickness are especially important for dolphins as they rely solely on blubber for insulation, are unable to haul-out and use land as a thermal refuge, and are small in size when compared to other marine mammals, making them more vulnerable to heat loss in cold waters (Parry, 1949; Hokkanen, 1990; Ryg et al., 1993; Mauck et al., 2003; Beentjes, 2006). Water temperature impacts both the morphology and deposition of blubber in dolphins, as colder environments often result in larger adipocyte size (Montie et al., 2008), thicker blubber layers (Noren and Wells, 2009), and greater stratification in blubber layers (Koopman, 2007). As such, dolphin body composition is strongly linked to SST and seasonal changes in temperature.
CPUE also influenced the body composition of both young and mature dolphins. Although GLMM results indicate that SST is an important factor driving changes in body composition for dolphins in Sarasota Bay, CPUE was still a significant predictor of body composition when included in GLMMs (Supplementary Table 3). CPUE influenced the body composition of both young and mature dolphins. In the present study, CPUE was only available for winter and summer, which may have decreased the strength of the relationship between CPUE and body composition. Additionally, the effect of CPUE on body composition may be lagged and the seasonal CPUE averages used here may have been too coarse when paired with the fine-scale body composition values used in the GLMMs.
Body composition values were highest in winter, which coincided with low water temperatures but also low prey availability (Figure 4). Winter metabolic rates are typically lower for both male and female dolphins in Sarasota Bay when compared to other seasons, which may decrease the need to forage during the colder months of winter, decreasing the effects of low prey availability (Costa et al., 1993). This is particularly true for females, which decrease feeding rates in winter (Waples, 1995). The decreased metabolic rate of dolphins in winter may aid in maintaining high body composition, allowing for more insulation when water temperatures decrease. There may also be lagged effects of seasonal prey availability on the body composition of Sarasota Bay dolphins, as dolphins eat fish with higher lipid content in the fall, perhaps in preparation for the winter (Waples, 1995). Prey quality and availability control the fatty acid composition and thickness of blubber in marine mammals (Kirch et al., 2000; Bradshaw et al., 2003; Miller et al., 2011), so the higher fat content of fish consumed in the fall may heavily contribute to increased body composition in the following season. Our results mirror those of other studies that have documented the importance of seasonal trends in blubber quality, quantity, and composition (Aguilar and Borrell, 1990; Samuel and Worthy, 2004; Noren and Wells, 2009). Although our results suggest strong seasonal patterns in body composition, the seasonal bias in the present dataset toward spring and summer warrants further inquiry into the association between season and seasonally driven features, such as SST and CPUE, on the body composition of Sarasota Bay common bottlenose dolphins.
Long-term trends in body composition were strongly linked to annually averaged SST (Figure 7). Although body composition values appeared to increase throughout the study’s duration, this is likely due to changes over time from summer to spring health assessments, when water was cooler, rather than due to shifts in annual SST in Sarasota Bay. Although SST has been rising globally as a result of climate change, temperatures in the eastern Gulf of Mexico have slightly decreased within the past few decades (Lluch-Cota et al., 2013), and temperatures have remained static in Sarasota Bay (Supplementary Figure 2). As a result, body composition of dolphins in Sarasota Bay may not be impacted by climate change at the present moment. However, this dolphin community may be vulnerable to changes to oceanography resulting from climate change in the future as a result of their high site-fidelity, which may make it difficult for them to find a thermal refuge once water temperatures change (Wells, 2010). As a result, continued monitoring is critical to determine future SST trends in this region as body composition is heavily dependent upon this metric.
Ontogenetic patterns in body composition revealed that mature dolphins were leaner, and body composition was less variable as dolphins reached maturity (Figure 5). Mature dolphins are larger with lower surface area to volume ratios, which would improve their capacity to retain heat, reducing the need for thicker blubber (Worthy and Edwards, 1990; Noren and Wells, 2009; Adamczak et al., 2020). In contrast, as young dolphins are smaller, they have thicker, more insulative blubber to compensate for the additional thermoregulatory costs of being small (McLellan et al., 2002; Dunkin et al., 2005; Koopman, 2007; Montie et al., 2008; Noren and Wells, 2009).
In Sarasota Bay, young dolphins demonstrated sex-specific differences in resource allocation. Young females invest more heavily in growth than blubber deposition, yielding a trade-off between depositing blubber and reaching asymptotic length (Cockroft and Ross, 1990; Read et al., 1993; Calzada et al., 1997). The discrepancy in energy allocation early in life between females and males may result from the different reproductive strategies between the sexes and earlier sexual maturation of females (Read et al., 1993). Common bottlenose dolphins in Sarasota Bay typically have a promiscuous mating system, in which males prioritize competition for access to females and females prioritize maternal investment (Wells et al., 1987; Murphy et al., 2005). As a result of the differing strategies between the sexes, females prioritize early somatic growth and sexual maturation at the cost of body condition compared to males of the same age, allowing them to maximize fitness.
Reproductive costs are exceedingly high for females across marine mammal species (Fedak and Anderson, 1982; Oftedal, 1985; Yasui and Gaskin, 1986; Reddy et al., 1991; West et al., 2007; Hückstädt et al., 2018), necessitating elevated body composition values. In marine mammals, the energy density of fetal tissues approximately doubles during gestation, increasing pregnancy costs through time (Lockyer, 1987), and female energy consumption increases by nearly 150% during lactation (Oftedal, 1985; Worthy, 2001). Despite the elevated costs associated with reproduction, we found no significant difference in body composition between the reproductive states of females (Figure 6). As income breeders, female dolphins continue to forage while lactating, which may offset any potential declines in body composition. Both pregnant and lactating female dolphins increase prey intake and nutritional content of prey, which likely allows them to maintain body composition despite the high costs associated with these reproductive states (Bernard and Hohn, 1989; Reddy et al., 1991; Malinowski and Herzing, 2015).
Body composition was highly variable across all ages of mature females, but was not predicted by reproductive status. Female dolphins in Sarasota Bay limit competition by specializing on different prey types, decreasing overlap in diets between females (Rossman et al., 2015). This prey specialization may influence a female’s body composition and lead to high variability in these values, as prey selection can influence the composition and morphology of blubber in dolphins (Smith and Worthy, 2006).
Conclusion
Our results suggest that both SST and CPUE influence the body composition of Sarasota Bay dolphins. There is evidence that CPUE is a strong predictor of body composition, however, the long-term monitoring effort of the SDRP must continue to further elucidate the significance of these relationships. Further, this study suggests that young female and male dolphins in Sarasota Bay differentially allocate resources to growth and body composition. We found that reproductive state did not alter the body composition of female dolphins, providing further evidence of their status as true income breeders. This research was made possible by long-term monitoring of the resident dolphin community in Sarasota Bay, and highlights the importance of continuing this effort to better understand the factors influencing body composition.
Data Availability Statement
The data analyzed in this study is subject to the following licenses/restrictions: The data used in these analyses are a part of a long-term monitoring project conducted by the Chicago Zoological Society’s Sarasota Dolphin Research Program and, as such, are not publicly available. Requests to access these datasets should be directed to RW.
Ethics Statement
The Research in Sarasota Bay was conducted under a series of National Marine Fisheries Service Scientific Research Permits, and annual Mote Marine Laboratory Institutional Animal Care and Use Committee approvals.
Author Contributions
RW and DC conceived this study. RW and EB collected the morphometrics and blubber depth data used here. EB led the prey fish surveys. RH conceptualized and carried out the methodology necessary to convert the raw data to body composition. SA performed statistical analyses and drafted the manuscript. RW, DC, EB, and RH provided feedback on statistical analyses and manuscript drafts. All authors have read and approved the final manuscript.
Funding
Primary long-term funding for this research has come from Dolphin Quest, Inc., Earthwatch Institute, and the National Oceanic and Atmospheric Administration. SA was supported by the E&P Sound and Marine Life Joint Industry Project of the International Association of Oil and Gas Producers.
Conflict of Interest
The authors declare that this study received funding from Dolphin Quest, Inc. The funder had the following involvement in the study: They provided some of the funding support for the health assessments, and one of the principals in the company, Dr. Jay Sweeney, as one of the most experienced marine mammal veterinarians in the world, collected some of the samples.
The authors declare that the research was conducted in the absence of any commercial or financial relationships that could be construed as a potential conflict of interest.
Acknowledgments
We would like to thank the many individuals—staff, students, trained volunteers, collaborators–involved in data collection for the SDRP over the decades. Additionally, we would like to acknowledge Dr. Lisa Schwarz for her initial efforts on this body composition project and Drs. Roxanne Beltran and Pete Raimondi for providing feedback on the statistical methods. Research in Sarasota Bay is conducted under a series of National Marine Fisheries Service Scientific Research Permits, annual Mote Marine Laboratory Institutional Animal Care and Use Committee approvals (06-10-DG1 to 19-10-RW2), and Florida Fish and Wildlife Conservation Commission fishing permits (Special Activity License numbers 032r-809 to 19-0809-SR).
Supplementary Material
The Supplementary Material for this article can be found online at: https://www.frontiersin.org/articles/10.3389/fmars.2021.615773/full#supplementary-material
Supplementary Figure 1 | Relationship between age and body length for female and male (n = 260 and n = 274, respectively) common bottlenose dolphins (Tursiops truncatus) in Sarasota Bay fitted with Gompertz growth curve from parameters determined by Read et al. (1993).
Supplementary Figure 2 | Annual trends in sea surface temperature (SST) in Sarasota Bay from 1986 to 2016 and CPUE in Sarasota Bay from 2004 to 2016. Annual averages were calculated from monthly SST data extracted from HadISST Average Sea Surface Temperature at 1° spatial resolution.
Supplementary Table 1 | Sample sizes, mean values, and standard error for body composition, defined as the ratio of blubber volume to total body volume, of common bottlenose dolphins in Sarasota Bay, Florida.
Supplementary Table 2 | Chi-squared value, degrees of freedom, and p-value for fitted generalized linear mixed models with fixed effects of body length (length), monthly SST (SST), season, sex, and year from 1986 to 2016. Models were run for all individuals (n = 534), young individuals (less than 10 years old, n = 254) and mature individuals (n = 280, greater than or equal to 10 years old) and repeated measures for individuals were treated as random effects. Models were selected using Akaike’s Information Criterion correction. Monthly average sea surface temperature (SST) data were extracted using the HadISST Average Sea Surface Temperature dataset.
Supplementary Table 3 | Chi-squared value, degrees of freedom, and p-value for fitted generalized linear mixed models with fixed effects of body length (length), catch per unit effort (CPUE), monthly SST (SST), season, sex, and year from 2004 to 2016. Models were run for all individuals (n = 157), young individuals (n = 73, less than 10 years old) and mature individuals (n = 84, greater than or equal to 10 years old) and repeated measures for individuals were treated as random effects. Models were selected using Akaike’s Information Criterion correction. Monthly average sea surface temperature (SST) data were extracted using the HadISST Average Sea Surface Temperature dataset.
Footnotes
- ^ https://climatedataguide.ucar.edu/climate-data/sst-data-hadisst-v11
- ^ https://github.com/rmendels/rerddapXtracto
References
Ackman, R. G., Hingley, J. H., Eaton, C. A., Sipos, J. C., and Mitchell, E. D. (1975). Blubber fat deposition in mysteceti whales. Can. J. Zool. 53, 1332–1339. doi: 10.1139/z75-158
Adamczak, S. K., Pabst, D. A., McLellan, W. A., and Thorne, L. H. (2020). Do bigger bodies require bigger radiators? Insights into thermal ecology from closely related marine mammal species and implications for ecogeographic rules. J. Biogeogr. 47, 1193–1206. doi: 10.1111/jbi.13796
Aguilar, A., and Borrell, A. (1990). Patterns of lipid content and stratification in the blubber of fin whales (Balaenoptera physalus). J. Mammal. 71, 544–554. doi: 10.2307/1381793
Bates, D., MäHoer, M., Bolker, B., and Walker, S. (2015). Fitting linear mixed-effects models using lme4. J. Stat. Softw. 67, 1–48. doi: 10.18637/jss.v067.i01
Beck, C., Rea, L., Iverson, S., Kennish, J., Pitcher, K., and Fadely, B. (2007). Blubber fatty acid profiles reveal regional, seasonal, age-class and sex differences in the diet of young Steller sea lions in Alaska. Mar. Ecol. Prog. Ser. 338, 269–280. doi: 10.3354/meps338269
Beck, G. G., Smith, T. G., and Hammill, M. O. (1993). Evaluation of body condition in the Northwest Atlantic harp seal (Phoca groenlandica). Can. J. Fish. Aquat. Sci. 50, 1372–1381. doi: 10.1139/f93-157
Beentjes, M. P. (2006). Behavioral thermoregulation of the New Zealand Sea Lion (Phocarctos hookeri). Mar. Mamm. Sci. 22, 311–325. doi: 10.1111/j.1748-7692.2006.00022.x
Berens McCabe, E. J., Gannon, D. P., Barros, N. B., and Wells, R. S. (2010). Prey selection by resident common bottlenose dolphins (Tursiops truncatus) in Sarasota Bay, Florida. Mar. Biol. 157, 931–942. doi: 10.1007/s00227-009-1371-2
Bernard, H. J., and Hohn, A. A. (1989). Differences in feeding habits between pregnant and lactating spotted dolphins (Stenella attenuata). J. Mammal. 70, 211–215. doi: 10.2307/1381693
Bradshaw, C. J. A., Hindell, M. A., Best, N. J., Phillips, K. L., Wilson, G., and Nichols, P. D. (2003). You are what you eat: describing the foraging ecology of southern elephant seals (Mirounga leonina) using blubber fatty acids. Proc. R. Soc. Lond. B 270, 1283–1292. doi: 10.1098/rspb.2003.2371
Braithwaite, J. E., Meeuwig, J. J., Letessier, T. B., Jenner, K. C. S., and Brierley, A. S. (2015). From sea ice to blubber: linking whale condition to krill abundance using historical whaling records. Polar Biol. 38, 1195–1202. doi: 10.1007/s00300-015-1685-0
Breslow, N. E., and Clayton, D. G. (1993). Approximate inference in generalized linear mixed models. J. Am. Stat. Assoc. 88, 9–25. doi: 10.2307/2290687
Burnham, K. P., and Anderson, D. R. (1994). Model Selection and Multi-Model Inference: A Practical Information-Theoretic Approach. New York, NY: Springer Publishing.
Calzada, N., Aguilar, A., Grau, E., and Lockyer, C. (1997). Patterns of growth and physical maturity in the western Mediterranean striped dolphin, Stenella coeruleoalba (Cetacea: Odontoceti). Can. J. Zool. 75, 632–637.
Cockroft, V. G., and Ross, G. J. B. (1990). Age, growth and reproduction in bottlenose dolphins from the east coast of southern Africa. Fish. Bull. 88, 289–302.
Costa, D. P., Worthy, G. A. J., Wells, R. S., Read, A. J., Scott, M. D., Irvine, A. B., et al. (1993). “Seasonal changes in the field metabolic rate of bottlenose dolphins Tursiops truncatus,” in Proceedings of the Tenth Biennial Conference on the Biology of Marine Mammals, (Galveston, TX).
Dunkin, R. C., McLellan, W. A., Blum, J. E., and Pabst, D. A. (2005). The ontogenetic changes in the thermal properties of blubber from Atlantic bottlenose dolphin Tursiops truncatus. J. Exp. Biol. 208, 1469–1480. doi: 10.1242/jeb.01559
Dunkin, R. C., McLellan, W. A., Blum, J. E., and Pabst, D. A. (2010). The buoyancy of the integument of Atlantic bottlenose dolphins (Tursiops truncatus): effects of growth, reproduction, and nutritional state. Mar. Mamm. Sci. 26, 573–587. doi: 10.1111/j.1748-7692.2009.00353.x
Fedak, M. A., and Anderson, S. S. (1982). The energetics of lactation: accurate measurements from a large wild mammal, the grey seal (Halichoerus grypus). J. Zool. 198, 473–479. doi: 10.1111/jzo.1982.198.4.473
Gales, N. J., and Burton, H. R. (1987). Ultrasonic measurement of blubber thickness of the southern elephant seal, Mirounga-Leonina (Linn). Austr. J. Zool. 35, 207–217. doi: 10.1071/zo9870207
Gannon, D. P., Berens, E. J., Camilleri, S. A., Gannon, J. G., Brueggen, M. K., Barleycorn, A., et al. (2009). Effects of Karenia brevis harmful algal blooms on nearshore fish communities in southwest Florida. Mar. Ecol. Prog. Ser. 378, 171–186.
Hastie, T., and Tibshirani, R. (1987). Generalized additive models: some applications. J. Am. Stat. Assoc. 82, 371–386. doi: 10.1080/01621459.1987.10478440
Hohn, A. A., Scott, M. D., Wells, R. S., Sweeney, J. C., and Irvine, A. B. (1989). Growth layers in teeth from known-age, free-ranging bottlenose dolphins. Marine Mamm. Sci. 5, 315–342.
Hückstädt, L. A., Holser, R. R., Tift, M. S., and Costa, D. P. (2018). The extra burden of motherhood: reduced dive duration associated with pregnancy status in a deep-diving marine mammal, the northern elephant seal. Royal Soc. B 14:4. doi: 10.1098/rsbl.2017.0722
Kastelein, R. A., van der Sijs, S. J., Staal, C., and Nieuwstraten, S. H. (1997). “Blubber thickness in harbour porpoises (Phocoena phocoena),” in The Biology of the Harbour Porpoise, eds A. J. Read, P. R. Wiepkema, and P. E. Nachtigall (Woerden: De Spil Publishers), 179–199.
Kirch, P. E., Iverson, S. J., and Bowen, W. D. (2000). Effect of a low-fat diet on body composition and blubber fatty acids of captive juvenile harp seals (Phoca groenlandica). Physiol. Biochem. Zool. 73, 45–58. doi: 10.1086/316723
Koopman, H. N. (2007). Phylogenetic, ecological, and ontogenetic factors influencing the biochemical structure of the blubber of odontocetes. Mar. Biol. 151, 277–291. doi: 10.1007/s00227-006-0489-8
Koopman, H. N., Iverson, S. J., and Gaskin, D. E. (1996). Stratification and age-related differences in blubber fatty acids of the male harbour porpoise (Phocoena phocoena). J. Comp. Physiol. B 165, 628–639. doi: 10.1007/BF00301131
Koopman, H. N., Pabst, D. A., McLellan, W. A., Dillaman, R. M., and Read, A. J. (2002). Changes in blubber distribution and morphology associated with starvation in the harbor porpoise (Phocoena phocoena): evidence for regional references in blubber structure and function. Physiol. Biochem. Zool. 75, 498–512.
Lluch-Cota, S. E., Tripp-Valdez, M., Lluch-Cota, D. B., Bautista-Romero, J. J., Lluch-Belda, D., Verbesselt, J., et al. (2013). Recent trends in sea surface temperature off Mexico. Atmósfera 26, 537–546. doi: 10.1016/S0187-6236(13)71094-4
Lockyer, C. (1986). Body fat condition in Northeast Atlantic fin whales, Balaenoptera physalus, and its relationship with reproduction and food source. Can. J. Fish. Aquat. Sci. 43, 142–147.
Lockyer, C. (1987). The relationship between body fat, food resource and reproductive energy costs in North Atlantic fin whales (Balaeoptera physalus). Symp. Zool. Soc. Lond. 57, 343–361.
Lockyer, C. H., McConnell, L. C., and Waters, T. D. (1985). Body condition in terms of anatomical and biochemical assessment of body fat in North Atlantic fin and sei whales. Can. J. Zool. 63, 2328–2338. doi: 10.1139/z85-345
Malinowski, C. R., and Herzing, D. L. (2015). Prey use and nutritional differences between reproductive states and age classes in Atlantic spotted dolphins (Stenella frontalis) in the Bahamas. Mar. Mamm. Sci. 31, 1471–1493. doi: 10.1111/mms.12238
Mallette, S. D., McLellan, W. A., Scharf, F. S., Koopman, H. N., Barco, S. G., Wells, R. S., et al. (2016). Ontogenetic allometry and body composition of the common bottlenose dolphin (Tursiops truncatus) from the U.S. mid-Atlantic. Mar. Mamm. Sci. 32, 86–121. doi: 10.1111/mms.12253
Mauck, B., Bilgmann, K, Jones, DD, Eysel, U, and Dehnhardt, G. (2003). Thermal windows on the trunk of hauled-out seals: hot spots for thermoregulatory evaporation? J. Exp. Biol. 206, 1727–1738. doi: 10.1242/jeb.00348
McKnight, P. E., and Najab, J. (2010). “Kruskal-wallis test,” in The Corsini Encyclopedia of Psychology, eds W. E. Craighead and C. Nemeroff (Haboken: John Wiley & Sons), doi: 10.1002/9780470479216.corpsy0491
McLellan, W. A., Koopman, H. N., Rommel, S. A., Read, A. J., Potter, C. W., Nicolas, J. R., et al. (2002). Ontogenetic allometry and body composition of harbour porpoises (Phocoena phocoena, L.) from the western North Atlantic. J. Zool. 257, 457–471. doi: 10.1017/S0952836902001061
Meagher, E. M., McLellan, W. A., Westgate, A. J., Wells, R. S., Blum, J. E., and Pabst, D. A. (2008). Seasonal patterns of heat loss in wild bottlenose dolphins (Tursiops truncatus). J. Comp. Physiol. B 178, 529–543. doi: 10.1007/s00360-007-0245-5
Miller, C., Reeb, D., Best, P., Knowlton, A., Brown, M., and Moore, M. (2011). Blubber thickness in right whales Eubalaena glacialis and Eubalaena australis related with reproduction, life history status and prey abundance. Mar. Ecol. Ser. 438, 267–283. doi: 10.3354/meps09174
Montie, E. W., Garvin, S. R., Fair, P. A., Bossart, G. D., Mitchum, G. B., McFee, W. E., et al. (2008). Blubber morphology in wild bottlenose dolphins (Tursiops truncatus) from the Southeastern United States: influence of geographic location, age class, and reproductive state. J. Morphol. 269, 496–511. doi: 10.1002/jmor.10602
Murphy, S., Collet, A., and Rogan, E. (2005). Mating strategy in the male common dolphin (Delphinus delphis): what gonadal analysis tells us. J. Mammal. 86, 1247–1258.
Noren, S. R., and Wells, R. S. (2009). Blubber deposition during ontogeny in free-ranging bottlenose dolphins: balancing disparate roles of insulation and locomotion. J. Mammal. 90, 629–637. doi: 10.1644/08-MAMM-A-138R.1
Oftedal, O. T. (1985). “Pregnancy and lactation,” in Bioenergetics of Wild Herbivores, eds R. J. Hudson and R. G. White (Boca Raton, FL: CRC Press), 215–238.
Øigård, T. A., Lindstrøm, U., Haug, T., Nilssen, K. T., and Smout, S. (2013). Functional relationship between harp seal body condition and available prey in the Barents Sea. Mar. Ecol. Prog. Ser. 484, 287–301. doi: 10.3354/meps10272
Parry, D. A. (1949). The structure of whale blubber, and a discussion of its thermal properties. J. Cell Sci. 3, 13–25.
R Core Team (2020). R: A Language and Environment for Statistical Computing. Vienna, Austria: R Foundatin for Statistical Computing. Available online at: http.//www.R-project.org/.
Read, A. J. (1990). Estimation of body condition in harbour porpoises, Phocoena phocoena. Can. J. Zool. 68, 1962–1966. doi: 10.1139/z90-276
Read, A. J., Wells, R. S., Hohn, A. A., and Scott, M. D. (1993). Patterns of growth in wild bottlenose dolphins, Tursiops truncatus. J. Zool. 231, 107–123. doi: 10.1111/j.1469-7998.1993.tb05356.x
Reddy, M., Kamolnick, T., Skaar, D., Curry, C., and Ridgway, S. (1991). “Bottlenose dolphins: Energy consumption during pregnancy, lactation, and growth,” in Proceedings of the International Marine Animal Trainer’s Association Conference Proceedings 1991, (San Diego, CA), 30–37.
Rossman, S., McCabe, E. B., Barros, N. B., Gandhi, H., Ostrom, P. H., Stricker, C. A., et al. (2015). Foraging habits in a generalist predator: sex and age influence habitat selection and resource use among bottlenose dolphins (Tursiops truncatus). Mar. Mamm. Sci. 31, 155–168. doi: 10.1111/mms.12143
Ryg, M., Lydersen, C., Knutsen, L. Ø, Bjørge, A., Smith, T. G., and Øritsland, N. A. (1993). Scaling of insulation in seals and whales. J. Zool. 230, 193–206. doi: 10.1111/j.1469-7998.1993.tb02682.x
Ryg, M., Smith, T. G., and Øritsland, N. A. (1990). Seasonal changes in body mass and body composition of ringed seals (Phoca hispida) on Svalbard. Can. J. Zool. 68, 470–475. doi: 10.1139/z90-069
Samuel, A. M., and Worthy, G. A. J. (2004). Variability in fatty acid composition of bottlenose dolphin (Tursiops truncatus) blubber as a function of body site, season, and reproductive state. Can. J. Zool. 82, 1933–1942.
Smith, H. R., and Worthy, G. A. J. (2006). Stratification and intra- and inter-specific differences in fatty acid composition of common dolphin (Delphinus sp.) blubber: implications for dietary analysis. Comp. Biochem. Physiol. B 143, 486–499.
Struntz, D. J., McLellan, W. A., Dillaman, R. M., Blum, J. E., Kucklick, J. R., and Pabst, D. A. (2004). Blubber development in bottlenose dolphins (Tursiops truncatus). J. Morphol. 259, 7–20. doi: 10.1002/jmor.10154
Wagenmakers, E. J., and Farrell, S. (2004). AIC model selection using Akaike weights. Psychon. Bull. Rev. 11, 192–196. doi: 10.3758/BF03206482
Waples, D. M. (1995). Activity Budgets of Free-Ranging Bottlenose Dolphins (Tursiops truncatus) in Sarasota Bay, Florida. Master’s of Science thesis. Santa Cruz: University of California.
Wells, R. S. (2009). Learning from nature: bottlenose dolphin care and husbandry. Zoo Biol. 28, 635–651.
Wells, R. S. (2010). Feeling the heat – potential climate change impacts on bottlenose dolphins. Whalewatcher 39, 12–17.
Wells, R. S. (2014). “Social structure and life history of common bottlenose dolphins near Sarasota Bay, Florida: Insights from four decades and five generations,” in Primates and Cetaceans: Field Research and Conservation of Complex Mammalian Societies, Primatology Monographs, eds J. Yamagiwa and L. Karczmarski (Tokyo: Springer), 149–172. doi: 10.1007/978-4-431-54523-1_8
Wells, R. S., McHugh, K. A., Douglas, D. C., Shippee, S., Berens McCabe, E. J., Barros, N. B., et al. (2013). Evaluation of potential protective factors against metabolic syndrome in bottlenose dolphins: feeding and activity patterns of dolphin in Sarasota Bay, Florida. Front. Endocrinol. 4:16. doi: 10.3389/fendo.2013.00139
Wells, R. S., Rhinehart, H. L., Hansen, L. J., Sweeney, J. C., Townsend, F. I., Stone, R., et al. (2004). Bottlenose dolphins as marine ecosystem sentinels: developing a health monitoring system. EcoHealth 1, 246–254.
Wells, R. S., Scott, M. D., and Irvine, A. B. (1987). “The social structure of free-ranging bottlenose dolphins,” in Current Mammalogy, Vol. 1, ed. H. Genoways (New York, NY: Plenum Press), 247–305.
West, G. C., Burns, J. J., and Modafferi, M. (1979). Fatty acid composition of blubber from the four species of Bering Sea phocid seals. Can. J. Zool. 57, 189–195. doi: 10.1139/z79-017
West, K. L., Oftedal, O. T., Carpenter, J. R., Krames, B. J., Campbell, M., and Sweeney, J. C. (2007). Effect of lactation stage and concurrent pregnancy on milk composition in the bottlenose dolphin. J. Zool. 273, 148–160. doi: 10.1111/j.1469-7998.2007.00309.x
Williams, T. M., and Friedl, W. A. (1990). Heat flow properties of dolphin blubber: insulating warm bodies in cold water. Am. Zool. 30:191.
Worthy, G. A. J. (2001). “Nutrition and energetics,” in CRC Handbook of Marine Mammal Medicine, second Edn, eds L. A. Dierauf and F. M. D. Gulland (Boca Raton, FL: CRC Press), 791–827.
Worthy, G. A. J., and Edwards, E. F. (1990). Morphometric and biochemical factors affecting heat loss in a small temperate cetacean (Phocoena phocoena) and a small tropical cetacean (Stenella attenuata). Physiol. Zool. 63, 432–442.
Keywords: bottlenose dolphin, body composition, cetacean, long-term monitoring, ontogeny
Citation: Adamczak SK, Holser RR, Costa DP, Berens McCabe EJ and Wells RS (2021) Body Composition of Common Bottlenose Dolphins in Sarasota Bay, Florida. Front. Mar. Sci. 8:615773. doi: 10.3389/fmars.2021.615773
Received: 28 October 2020; Accepted: 27 April 2021;
Published: 15 June 2021.
Edited by:
Adrian C. Gleiss, Murdoch University, AustraliaReviewed by:
Nahiid Stephens, Murdoch University, AustraliaDelphine Brigitte Hélène Chabanne, Murdoch University, Australia
Copyright © 2021 Adamczak, Holser, Costa, Berens McCabe and Wells. This is an open-access article distributed under the terms of the Creative Commons Attribution License (CC BY). The use, distribution or reproduction in other forums is permitted, provided the original author(s) and the copyright owner(s) are credited and that the original publication in this journal is cited, in accordance with accepted academic practice. No use, distribution or reproduction is permitted which does not comply with these terms.
*Correspondence: Stephanie K. Adamczak, sadamcza@ucsc.edu