- 1Department of Environmental Sciences, Zoological Institute, University of Basel, Basel, Switzerland
- 2Bimini Biological Field Station Foundation, South Bimini, Bahamas
- 3Faculty of Life Sciences, Albrecht Daniel Thaer-Institute of Agricultural and Horticultural Sciences, Humboldt-Universität zu Berlin, Berlin, Germany
- 4Leibniz-Institute of Freshwater Ecology and Inland Fisheries, Berlin, Germany
- 5College of Fisheries and Ocean Sciences, University of Alaska Fairbanks, Fairbanks, AK, United States
- 6Rosenstiel School of Marine and Atmospheric Sciences, University of Miami, Miami, FL, United States
- 7Saving the Blue, Cooper City, FL, United States
Wildlife provisioning is popular, economically valuable, and a rapidly growing part of marine tourism, with great potential to benefit conservation. However, it remains controversial due to limited understanding of its implications on the behavior and ecology of target species. In this study, we modeled how various abiotic and biotic factors influenced great hammerhead sharks’ (Sphyrna mokarran) use of a recreational dive site in Bimini, the Bahamas, where shark-feeding has been conducted since 2012. Further, we calculated bioenergetic models to estimate their daily ration and examined if individual sharks fulfilled their daily energetic requirements from food uptake during dives. Between December 2016 and May 2017, we collected data during 104 provisioning dives in collaboration with a local dive operator. Twenty-eight individual great hammerhead sharks were observed, 11 were philopatric (i.e., identified at the dive site in previous years), and 17 were new (i.e., identified at the dive site for the first time during this study) individuals. On average, four sharks were observed daily, occasionally up to nine individuals, with some individuals spending more than 2 h attending each dive, consuming up to 4.75 kg of provisioned food per dive and returning repeatedly throughout the study period. When we grouped sharks based on their previous experience of the dive site (i.e., philopatric vs. new sharks), we found significantly higher attendance indices, i.e., the number of attended dives divided by the total number dives, and longer presence times during dives in philopatric sharks and different responses toward the number of boats and conspecifics between the two groups. Overall, great hammerhead sharks increased their bait uptake during longer dives and when more boats were present at the dive site. Finally, nine of 12 provisioned great hammerhead sharks were regularly able to fuel their daily energetic requirements from provisioned food alone, with two sharks doing so on 77.8% of all dives. Our study provides insights into how large-bodied marine predators react toward wildlife tourism associated provisioning and allows further discussion about daily energy uptake during provisioning dives, its potential impacts on the ecological role of the target species and associated management measures.
Introduction
Observing wildlife in its natural habitat has become increasingly popular, with a wide range of tourism opportunities from well-known terrestrial safaris to the more recent close-up dive encounters with sharks (MacDonald et al., 2017). This greater interest in shark dive tourism activities is supporting local and national economies (e.g., Huveneers et al., 2017; Zimmerhackel et al., 2019). Importantly, alongside such financial benefits, there is great potential to raise public awareness and to support conservation efforts for sharks (Apps et al., 2018; Vianna et al., 2018).
To ensure the industry remains popular, operators often need to increase the probability of encounters with the target species. Food attraction and provisioning (i.e., feeding of the target animal) are, therefore, becoming increasingly common as methods to attract megafauna that would otherwise be difficult to reliably observe in the wild (Orams, 2002; Brena et al., 2015). However, there is an ongoing debate if such activities have detrimental effects, and if they could outweigh the potential benefits and diminish the conservational value of wildlife tourism (Donaldson et al., 2012).
So far, studies of the effects of provisioning in sharks and rays have produced ambiguous results. Minimal impacts of food attraction have been documented in bull sharks (Carcharhinus leucas) that maintained their local-scale movement patterns despite year-round food availability (Brunnschweiler and Barnett, 2013). Furthermore, tiger (Galeocerdo cuvier) and Caribbean reef sharks (Carcharhinus perezi) did not show changes in their movement ranges or increased fidelity at dive sites (Maljković and Côté, 2011; Hammerschlag et al., 2012). But other results suggest that the mere presence of humans can elicit behavioral changes in sharks. For example, after cage diving, efforts were increased in 2007 at the Neptune Islands in Australia, local white shark (Carcharodon carcharias) numbers and individual residency times at the provisioning site significantly increased, with centers of activity spatially and temporally identical to provisioning activities (Bruce and Bradford, 2013). Ecotourism and feeding have also been shown to increase the activity of white sharks (Huveneers et al., 2018) and the daily field metabolic rate of white-tip reef sharks (Triaenodon obesus; Barnett et al., 2016). Further, they can modify sharks’ and stingrays’ natural dial activity patterns with an increase in activity during the daylight hours in normally nocturnally active species (T. obesus, Fitzpatrick et al., 2011; Hypanus americanus, Corcoran et al., 2013). Only a few studies have assessed the extent to which provisioning impacts the diet of fed sharks and rays (e.g., Semeniuk et al., 2007; Abrantes et al., 2018). Impact on the diet of the target species could have consequences for natural foraging behaviors (Gallagher et al., 2015), which in turn can impact ecosystem function, as sharks can occupy high trophic levels and have important ecological roles (Heupel et al., 2014; Roff et al., 2016).
The Bahamas is a globally recognized destination and industry leader for diving with sharks (e.g., Hammerschlag et al., 2012; Madigan et al., 2015), which contributes $114 million USD annually to the country’s economy (Haas et al., 2017). The Bimini Islands, located along the western edge of the Great Bahama Bank, are renowned for reliable and frequent encounters with the typically solitary great hammerhead shark (Sphyrna mokarran). An initial tracking and observation study found that some great hammerheads in Bimini displayed seasonal residency and high site fidelity to the islands during the winter months. This philopatric behavior was positively correlated with time spent at a local shark provisioning dive site, suggesting that frequent food rewards may be localizing the movements of great hammerhead sharks (Guttridge et al., 2017). However, despite these early findings, the daily use of the dive site and potential impacts of provisioning on the bioenergetic requirements of great hammerhead sharks remains unknown. Given the Critically Endangered conservation status of great hammerhead sharks, as assessed on the IUCN Red List (Rigby et al., 2019), and the increasing popularity of the dive site, information is needed regarding the potential effects of provisioning for this species.
In this study, we collaborated with a local shark dive operator in Bimini, The Bahamas, to monitor the dive site use and bait uptake of great hammerhead sharks during provisioning dives. Because provisioned sharks and rays have been found to show anticipatory responses (e.g., Meyer et al., 2009; Pini-Fitzsimmons et al., 2018), we expected to find a decrease in the arrival time of sharks over the season due to anticipatory responses toward provisioning dives. Further, we expected to find differences in the time spent and the bait uptake at the dive site between sharks that were experienced (i.e., philopatric) vs. sharks that were new to the dive site. Finally, based on Brunnschweiler et al. (2018) and the frequency and intensity of provisioning dives in Bimini, we expected that some great hammerhead sharks were likely fulfilling a considerable amount of their daily ration through provisioned food. Such information will be important to find a balance between sustainable ecotourism protocols that minimize the potential impacts on the sharks and their ecological role, while maintaining tourism activities that are important for the local economy.
Materials and Methods
Study Site
The study was conducted in the islands of Bimini (25° 44′ N, 79° 15′ W), the Bahamas (Figure 1A). The dive site was located approximately 1 km off the west coast, on sandy substrate, at an average depth of 8.78 ± 1.96 m (mean ± SD; Figure 1B for dive locations), where provisioning of great hammerhead sharks during commercial dive tourism activities has taken place during every winter (northern hemisphere) since 2012. The dive site was originally used for leisure time baiting of former Bimini Biological Field Station staff members between 2004 and 2012 with pole-tagging techniques used for scientific purposes starting in 2008. During these years, baiting trips were infrequent and sharks received very little to no food rewards. The start of commercial dive tourism activities was marked by a change from free‐ to self-contained underwater breathing apparatus (SCUBA) diving techniques, increased frequency, and regular food rewards and hand-feeding. The study period started on December 31, 2016 and ended on the May 3, 2017. Dive vessels from different operators, local and foreign, frequently anchor at the study site. The number of dive boats operating at the study site simultaneously ranged from 1 to 8 per day (2.77 ± 1.48, mean ± SD). During the study period of 124 days, no dive vessels were anchored at the study site on 28 days, typically due to adverse sea conditions for diving, and, therefore, no observations occurred on these days.
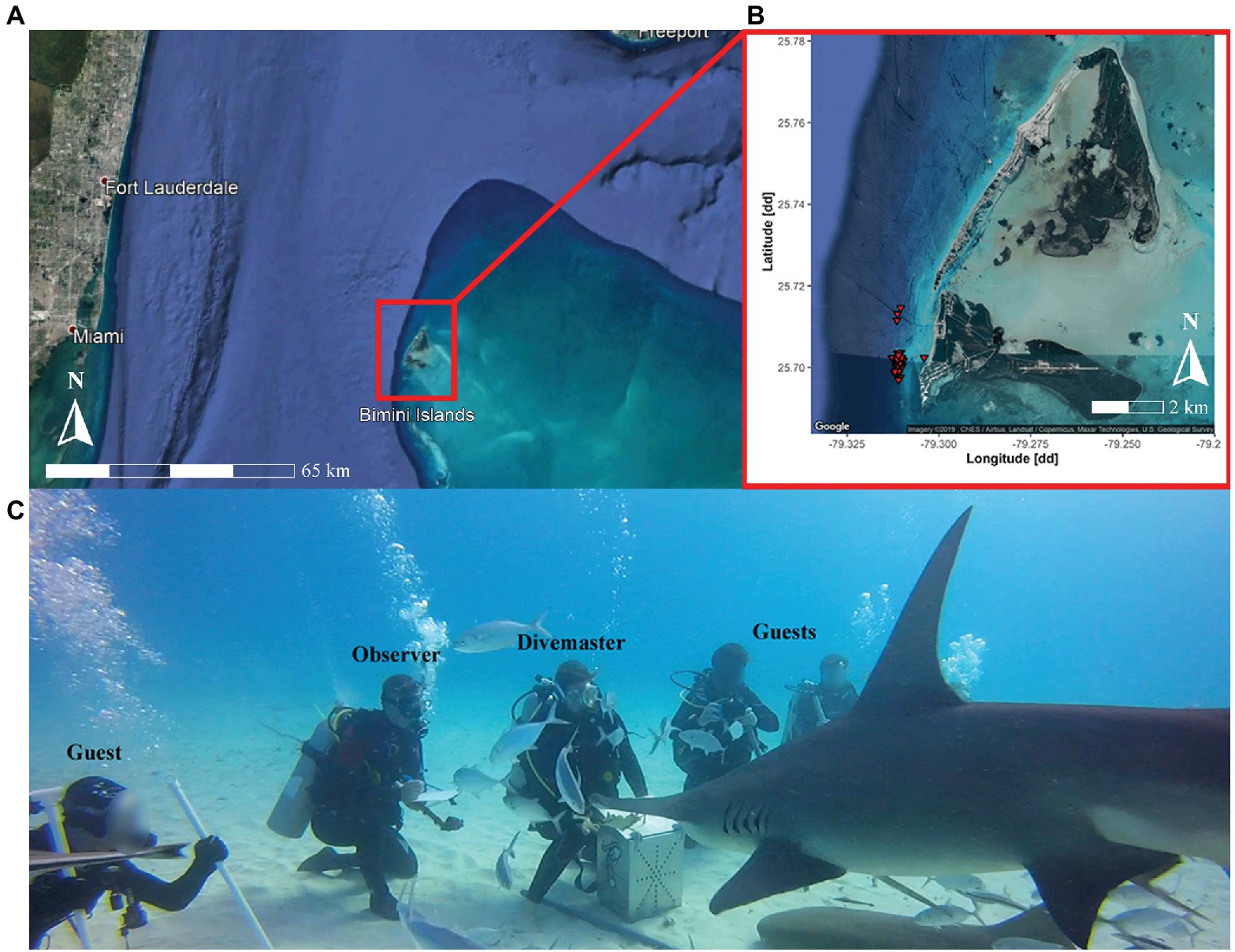
Figure 1. Study site (A), dive locations (B), and underwater dive set-up (C). The Bimini Islands are a group of islands on the western edge of the Great Bahama Bank that are approximately 85 km east of Miami (A). The surveyed provisioning dives all took place on the west side of South Bimini (B). The position of the observer combined with the underwater dive set-up used by the Neal Watson’s Bimini Scuba Center (NWBSC), allowed for a fast and reliable identification of individual sharks as well as quantification of the bait uptake (C). Map generated using Google Earth (version 7.3.3.7786, accessed October 22nd 2020), SIO, NOAA, U.S. Navy, NGA, GEBCO. Insert generated using the “ggmap” package (Kahle and Wickham, 2013) in R.
Dive Surveys
Data were collected during SCUBA dive surveys (n = 104) between December 31, 2016 and May 3, 2017, in collaboration with the Neal Watson’s Bimini Scuba Center (NWBSC). The NWBSC is a local shore-based operator that frequents the dive site the most regularly and offers daily hammerhead provisioning dives. Future mentions of provisioning dives where great hammerhead sharks have been provisioned (i.e., hand-fed) by the NWBSC will be referred to as dives. On average, the NWBSC conducted 25.50 ± 7.05 (mean ± SD) dives per month between January and April 2017 with only one dive each being surveyed in December 2016 and May 2017. A dive was planned as a two-tank dive conducted back-to-back with minimal surface time and was normally ended when either the dive master or all guests were low on air (<500 psi, <50 bar) for the second tank. Unpredictable events (e.g., drastic and sudden decrease in visibility) occasionally caused dives to be ended prematurely. During a dive, dive guests were free to surface and re-descend when their first SCUBA tank was empty at any time during the dive. Normally, one dive was conducted per day but on 8 days more than one dive using different dive vessels were conducted by the NWBSC. During these days, the dives were planned in sequence and the dive vessels anchored close to each other so that the observer was able to change to the next dive vessel and survey all conducted dives.
Prior to each day of diving, bait, which was imported frozen from the United States, was thawed, and cut into uniform sized pieces. Ten randomly selected pieces were weighed, and the average piece weight (g) calculated. The two main bait sources were Pacific bonito (Sarda chiliensis lineolate) and Atlantic herring (Clupea harengus), which were used on 89.4 and 76.0% of dives, respectively (see Supplementary Material 1, “a. Bait sources”).
On arrival to the dive site, the boat was anchored, and a perforated aluminum box filled with bait (bait box) hung from the boat at ~2–3 m depth to attract sharks. On sighting a great hammerhead shark, the divemaster, followed by the observer tasked to record data, and finally by guests, entered the water to commence the dive, with the bait box then placed on the seafloor. Dives were conducted with the divemaster kneeling on the substrate in the middle of a line of divers (range; 2–22 divers, 10.45 ± 0.46, mean ± SD) perpendicular to the current (Figure 1C). On four occasions, only the divemaster, a safety diver and the observer were on SCUBA and the guests snorkeled at the surface. Great hammerhead sharks were hand-fed by the divemaster with pieces from the bait box. This allowed the observer to accurately record the number of bait pieces consumed by each individual great hammerhead shark. Bait was only ever offered to great hammerhead sharks but no other shark species that might have been present.
Sharks at the dive site were identified through external markings such as fin notches, ID tags, and pigmentation patterns (see Guttridge et al., 2017). Two underwater cameras (GoPro, Inc., San Mateo, CA, U.S.A) were positioned to record the entire dive. If the observer surfaced to switch SCUBA tanks, data were extracted post-dive from the camera footage. If a previously unidentified individual was observed, the unknown shark was given a new unique ID. For this project, all sharks identified in Bimini during at least one previous season, were classified as “philopatric” (Guttridge et al., 2017), whereas individuals that were identified for the first time in Bimini during the study period were referred to as “new.” Water temperature (°C) was recorded using a HOBO Water Temperature Pro v2 Data Logger (Part # U22-001, accuracy: ±0.21°C from 0 to 50°C, resolution: 0.02°C at 25°C, Onset Computer Corporation, Bourne, MA, U.S.A.). The logger deployed directly at the dive site mechanically failed during the study and, therefore, we used temperature data from a surrogate logger at a nearby site (1.8 km away) located in similar habitat, depth, and proximity to prevailing currents. The water temperature was recorded at a 1-h interval and we calculated the average water temperature for each dive. If the recording time and the start of the dive did not match, the last temperature recording prior to the dive was used. The tide at arrival and departure of the dive vessel was retrieved for North Bimini from the National Oceanic and Atmospheric Administration (NOAA) of the U.S. Department of Commerce.1 The number of dives a shark attended was divided by the total number of dives surveyed to obtain an attendance index. The list of all data collected by the observer during dive events that was used in the statistical analyses is available in Table 1.
Daily Ration Calculation
Bioenergetic models were calculated for great hammerhead sharks to estimate the contribution of provisioned food to a shark’s daily energetic requirements. This enabled us to gain insights into how provisioning might result in a change of the natural daily ration, due to caloric differences between provisioned bait and natural prey items. We followed the steps outlined in Bethea et al. (2007). Based on the balanced energetic model described by Winberg (1960), the consumption (C) was calculated, in kcal∙day−1, as:
where Mr is the routine metabolic rate and Ms the energetic costs of digestion. The energy invested in growth (G) is the sum of somatic growth (GS ) and reproduction (GR ). The model includes the energy that is lost due to the production of waste (W), the sum of feces (WF ) and urine (Wu ).
As there is no species-specific metabolic data available for great hammerhead sharks, we used the mass-specific metabolic rate for the closely related bonnethead shark (Sphyrna tiburo) as described by Parsons (1990, p. 364; equation 2). The equation for the mass-specific metabolic rate described by Parsons (1990, p. 364) already included the oxycalorific coefficient for fish (3.25 cal mg O2−1; Brafield and Solomon, 1972) that is used to convert the relationship between weight-oxygen consumption to calories. Piercy et al. (2010) provided length conversions (Supplementary Material 1, “b. Length data to calculate daily ration of individual great hammerhead sharks”) and species-specific growth rates. Length values were converted to mass following Romanov and Romanova (2012). This allowed us to calculate the difference in weight between 2 consecutive years. Growth was then calculated as the difference in weight from 1 year to the next in grams per day and we used the energy density of juvenile scalloped hammerhead (Sphyrna lewini) tissue from Lowe (2002) to convert the obtained values for mass of growth into kilocalories. Given the biennial reproductive cycle of great hammerhead sharks and a gestation period of approximately 11 months, we accounted for potential metabolic costs for pregnancy for some of the sharks in this study by calculating the costs for reproduction as the average litter size (n = 23; Cortés et al., 2015) multiplied by the average offspring weight and the caloric content of juvenile scalloped hammerhead shark tissue (Lowe, 2002).
The first bioenergetic model we present was calculated for sharks that followed a natural diet, which considered prey items and their caloric values as defined in the literature (Supplementary Table 1; Supplementary Material 1, “c. Dietary items and their energy content used in the calculation of the bioenergetic model”). To estimate if great hammerheads met their daily energetic requirements following a provisioned diet, we calculated a second bioenergetic model that considered the caloric values of the two main bait sources only (Pacific bonito, S. chiliensis lineolate and Atlantic herring, C. harengus), which were used on more than 75% of all dives.
The daily ration (DR) for each shark was then calculated as previously described by Bethea et al. (2007) using the equation:
where C is expressed in kcal∙day−1, F is the calorific value of the food source (kcal), and W denotes the weight of the individual shark (g). The daily ration of each shark that consumed bait during at least one dive was expressed as percent bodyweight per day (% BW∙day−1).
During the study period, three female great hammerheads were caught and scanned with a portable ultrasound (Ibex Pro, E.I. Medical Imaging, United States of America). Of the three, only one individual (shark-ID #24; Table 2) was confirmed pregnant. Given the seasonal residency of great hammerhead sharks to Bimini (Guttridge et al., 2017) and their biennial reproductive cycle (Cortés et al., 2015) declaring all mature females as gravid in the model would have most likely resulted in an overestimation of the daily ration. We, therefore, used the obtained daily ration values for non-gravid sharks to estimate the contribution of provisioned food to meeting daily energetic requirements for sharks of which no information about their reproductive status was available. However, we used daily ration values that accounted for metabolic costs of pregnancy for the individual (shark-ID #24) that was confirmed pregnant.
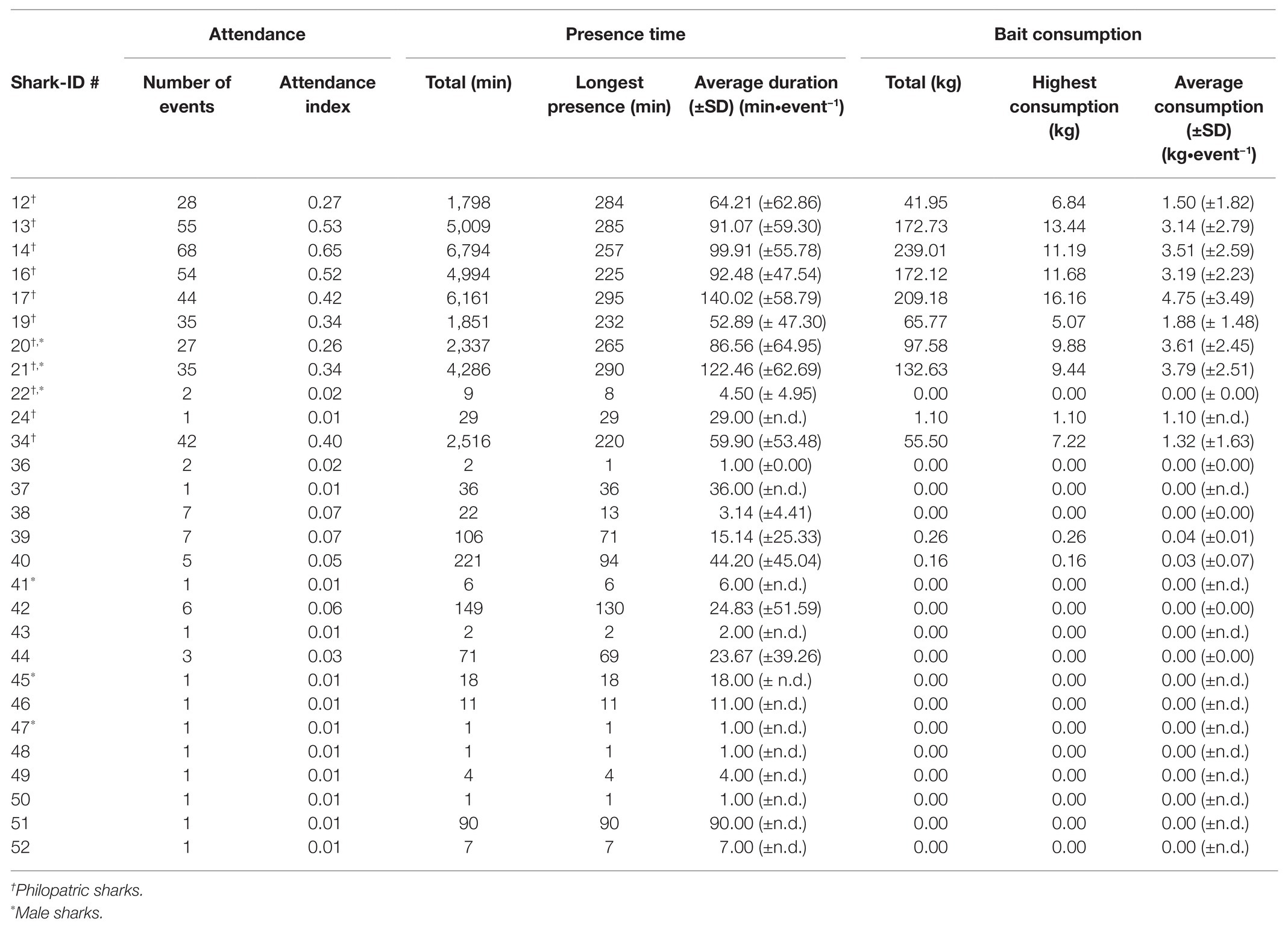
Table 2. Overview table with provisioning site measures for all identified great hammerhead sharks (S. mokarran).
The total number of pieces consumed during a dive multiplied by the daily average bait piece weight (g) allowed us to calculate the total weight of daily food uptake for each shark. The bait uptake of individual sharks was then expressed as % BW and compared to corresponding daily ration values. Lastly, we calculated the percentage of attended dives where sharks were able to fully meet their daily energetic requirements.
Statistical Analysis
All statistical analyses were performed in the programming language R (version 4.0.0; R Core Team, 2020) in the software R Studio (version 1.3.959; RStudio Team, 2020). The non-parametric Wilcoxon rank sum test was used to assess differences in the attendance indices between two groups (i.e., philopatric vs. new).
We computed four different statistical models. In model 1, we assessed what factors influenced the number of sharks present during dives. Model 2 was used to assess if there were changes in the time of the arrival of the first shark to the dive site over the study period. Models 3 and 4 evaluated factors that potentially influenced the sharks’ attendance and presence time as well as their bait uptake.
All statistical models used in this study were computed using the R package MCMCglmm (version 2.29; Hadfield, 2010). For each model, we chose a chain length of 9,200,000 iterations, a thinning interval of 9,000 and we discarded the first 200,000 iterations, resulting in Monte Carlo Markov chains with an effective sample size of 1,000 and low autocorrelation. Fixed effects were considered significant if the 95% confidence interval (95% CI) of their posterior mean excluded zero. Significance between the different levels of categorical effects was assessed by comparing the 95% CI of the posterior means of the effects. Non-overlapping intervals were considered significantly different. We considered random effects to be significant when the variance was not approximating 0. The posterior mean has the same unit as the coefficient of the corresponding model. For the binomial part of the zero-inflated Poisson models (models 3 and 4), the posterior mean represents an increase in the log-odds to be a 0 per unit increase of the fixed effect.
We used generalized linear models (GLMs) with a Poisson distribution and the scalar parameters of the inverse-Wishart prior distribution V = 1 and nu = 2, with V defining a point mass that the prior distribution tends toward and nu defining our belief parameter, to calculate models 1 and 2. In model 1, we used the number of sharks identified during a dive as a response variable. Two dives were removed from the dataset due to missing values in the predictor variables, resulting in a total of 102 dives incorporated into the models. As fixed effects, we included the total number of boats at the dive site during the dive (continuous), the baiting time in minutes (continuous), the number of days passed since the first dive of the study period (continuous), the water temperature (°C, continuous), and the tidal phase (categorical; Table 1). Apart from the NWBSC, other dive operators, local and foreign, frequented the dive site (see Study Site section). Varying number of baiting boats at the dive site could impact the number of sharks we identified, the time a shark spent attending dives as well as the amount of bait a shark consumed during the surveyed dive. We, therefore, controlled for potential consequences of other operators baiting at the dive site, by including the number of baiting vessels operating simultaneously around the dive site during a surveyed dive of the NWBSC.
In model 2, we estimated the effect of the water temperature (°C, continuous) and the number of days passed since the first dive of the study period (continuous) on the arrival time of the first shark (continuous). Here, we only considered days when the NWBSC was the first boat at the dive site (n = 36 days). If the NWBSC had two dives on the same day with different boats and non-overlapping dive times, only the data from the first boat were considered.
Because the sharks’ presence time and bait uptake were strongly zero-inflated, we estimated which variables influenced them using generalized linear mixed models (GLMMs) with a zero-inflated Poisson distribution (model 3 and model 4). MCMCglmm uses a log-link function for the Poisson part of the model and a logit-link function for the zero-inflated part. We decided to use a zero-inflated Poisson model over a hurdle model to account for the possibility that a 0 in the dataset was not only caused by the actual absence or lack of bait uptake of a shark, but also due to sharks being present but out of visual range, as well as sharks consuming bait at other dive vessels during days when more than one vessel was present (Zuur et al., 2009). We used parameter expanded priors that were informative toward small values for the variances with V = diag(2), nu = 2 for the R-matrix and V = diag(2), nu = 1,000, and alpha.V = diag(2) for the G-matrix. For both models, we added the ID of the shark and the dive ID as random effects to control for inter-individual and inter-dive differences.
In model 3, we tested which variables influenced a sharks’ presence time at the dive site. Here, we assigned an observation to each individual shark for each dive. When a shark was not visually identified, its presence time (minutes) was recorded as 0, otherwise, the presence time was calculated as the time between the arrival and the departure of the shark (or the end of the dive; Table 1). A shark was recorded to have departed the dive if it was not sighted for 15 min. This allowed us to test which factors were influencing the presence of sharks at the dive site, as well as their presence time. Due to missing values in the predictor variables, 140 observations were removed from the dataset resulting in 2,772 observations being entered into the model. Fixed effects included the total number of sharks visually identified during the dive (continuous), the dive time in minutes (continuous), the total number of boats at the dive site during the dive (continuous), the appearance number of a shark (continuous), the group of the shark (i.e., philopatric vs. new, categorical), and the water temperature (°C, continuous, Table 1). To account for different sharks arriving to the island at varying times during the study, we calculated an appearance number for each shark (Table 1). The appearance number was 0 until the first time the shark was identified attending a dive during the study period, at which point the appearance number was then recorded as 1. This value then increased by 1 for each additional dive, until the end of the study period, whether the shark was present during that dive or not. We included interaction terms between the group of a shark and; the total number of sharks, the total number of boats, the dive-time, and the appearance number of a shark, as we expected these variables to influence the shark groups differently. We did not include the number of divers in the model as an inclusion of this variable caused strong autocorrelation.
To assess the variables potentially impacting food consumption of sharks during the dives (model 4), we used bait uptake (in grams) as a response variable. As fixed predictors, we included the total number of sharks identified during the dive (continuous) and the total number of boats during the dive (continuous). In addition, we included the presence time of a shark in minutes (continuous) and the water temperature in °C (continuous) as fixed effects, but only within the Poisson part of the model. Only sharks that attended at least five dives were included in the model. We considered five dives to be a reasonable cut off as this gave every shark in the model at least five different occasions to make the decision whether to consume or not to consume bait. We were not able to include shark group as a fixed effect as new sharks rarely consumed bait and most of these sharks (n = 13; Table 2) attended less than five total dives. The number of divers was not included in the model as the inclusion of this variable caused strong autocorrelation in the data. Overall, 413 observations were included in the model. The full statistical analysis can be found in the Supplementary Material 2.
Results
Number and Arrival Time of Great Hammerhead Sharks at Provisioning Dives
From December 31, 2016 to May 3, 2017, 104 dives across 94 days were recorded. Great hammerhead sharks were sighted on 97% of these dives (n = 101 dives), resulting in 483 individual observations recorded from 28 different sharks (female = 22 and male = 6). Of these 28 individuals, 11 were confirmed to be philopatric to Bimini (Table 2). On average, 3.69 ± 1.83 (mean ± SD) female and 0.64 ± 0.76 (mean ± SD) male sharks attended each dive.
The number of great hammerhead sharks attending dives ranged from 0 to 9 individuals (4.33 ± 2.26 sharks, mean ± SD). Over the entire season, the number of sharks per dive decreased with time (Figure 2; Table 3). No other tested variables influenced the number of sharks present at the dive site (Table 3; Supplementary Figure 1; Supplementary Material 1, “d. Supplementary results”). On average, it took the first shark 41.78 ± 24.54 min (mean ± SD) to arrive at the dive site after bait entered the water. While the longest arrival times were recorded toward the end of the season, the time it took sharks to arrive at the dive site after the anchoring of the dive vessel did not change over the duration of the study [posterior mean = 0.001, 95% CI (−0.01, 0.01)] nor was it impacted by the water temperature [posterior mean = 0.12, 95% CI (−0.09, 0.34)].
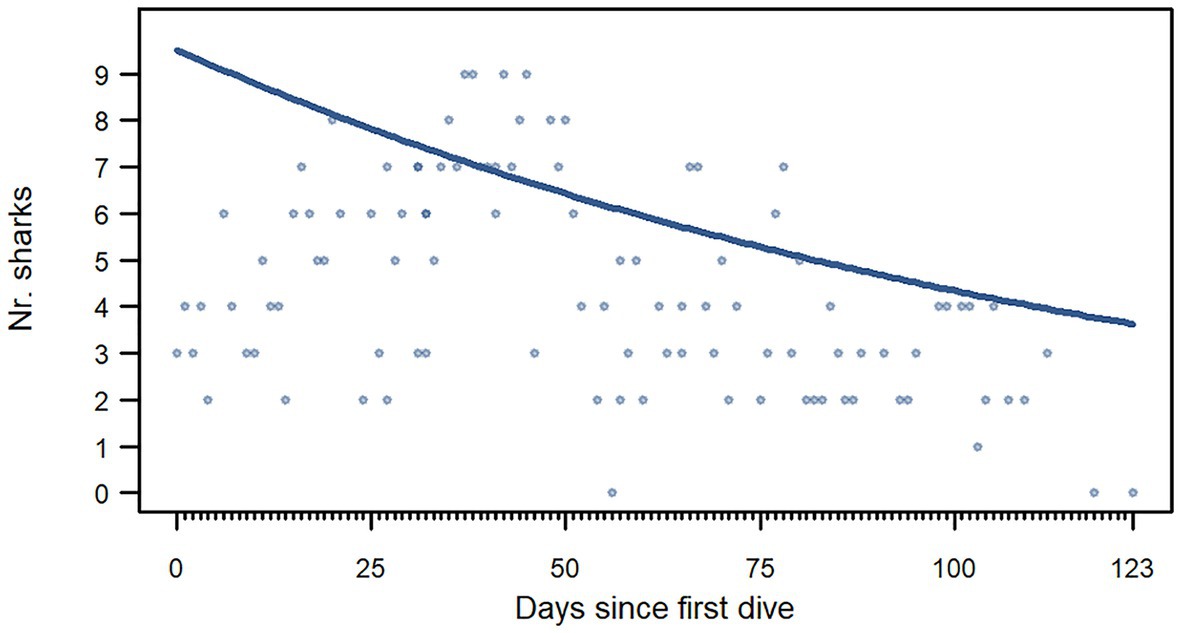
Figure 2. The number of great hammerhead sharks (Sphyrna mokarran) identified during provisioning dives in response to the number of days since the first dive. The plot shows a graphical representation of the significant effects of model 1 where the number sharks (y-axis) is shown as a function of the predictor variable (x-axis). The blue curve represents the significant regression extracted from the model (Table 3). The plots that contain a graphical representation of the non-significant effects for model 1 can be found in Supplementary Figure 1.
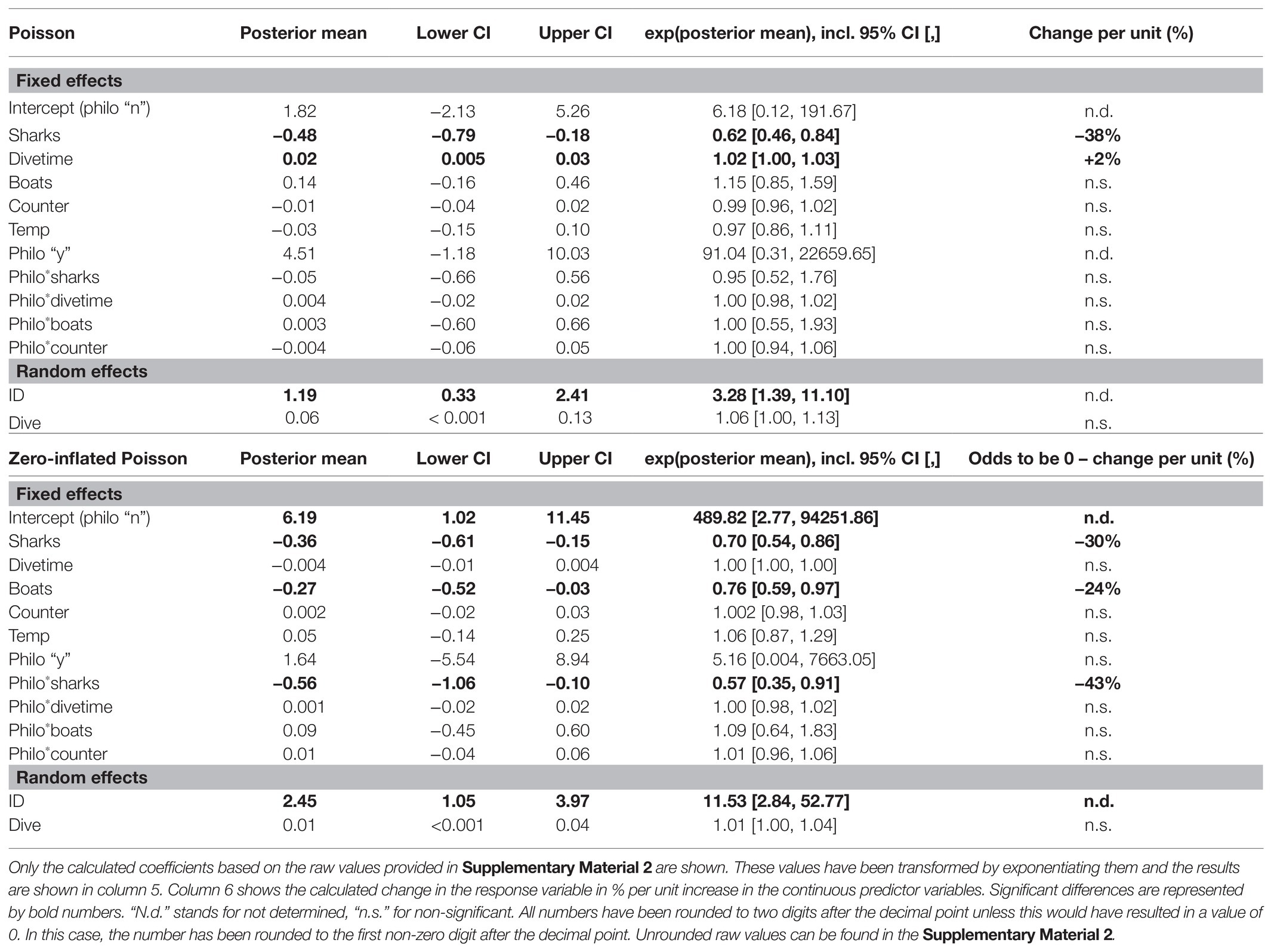
Table 3. Summary of the generalized linear model (GLM) for the number of great hammerhead sharks (S. mokarran) during provisioning dives (model 2).
Differences in Provisioning Dive Attendance and Bait Uptake Patterns
Individual attendance indices (i.e., number of dives attended/total number of dives) ranged from 0.01 to 0.65 (Table 2) with philopatric sharks (n = 11) having significantly higher attendance indices [Wilcoxon’s rank sum test; W = 17, 95% CI (0.25, 0.41), p < 0.0005] than new sharks (n = 17).
Dives lasted between 54 and 278 min (140.58 ± 54.44 min, mean ± SD). Overall great hammerhead sharks attended dives for 84.56 ± 63.31 min (mean ± SD). There was a wide range in attendance with some sharks (shark-ID #17 and #21) attending most dives for nearly the entire average dive duration while others attended only one dive for only 1 min (Table 2). Philopatric sharks attended dives for significantly longer (91.52 ± 61.74 min, mean ± SD, n = 391) than new sharks [18.24 ± 32.25 min, mean ± SD, n = 41, raw model output: posterior mean = 2.69, 95% CI (0.96, 4.77); see Table 4 for every calculated coefficient] and new sharks showed significantly higher odds of having a 0-min presence time during a dive (i.e., absent; Table 4) compared to philopatric sharks [raw model output: posterior mean = −4.55, 95% CI (−6.56, −2.51)]. A higher number of conspecifics resulted in a decreased odds of being absent for new and philopatric sharks (Figure 3A; Table 4). However, more conspecifics resulted in a decreased presence time for new sharks but did not impact how long philopatric sharks attended dives (Figure 3B; Table 4). The number of baiting boats significantly reduced the odds of being absent for new sharks, but did not change the odds of being absent for philopatric sharks (Figure 3C; Table 4), nor did it impact the presence time of either group of sharks during dives (Table 4; Supplementary Figure 2B; Supplementary Material 1, “d. Supplementary results”).
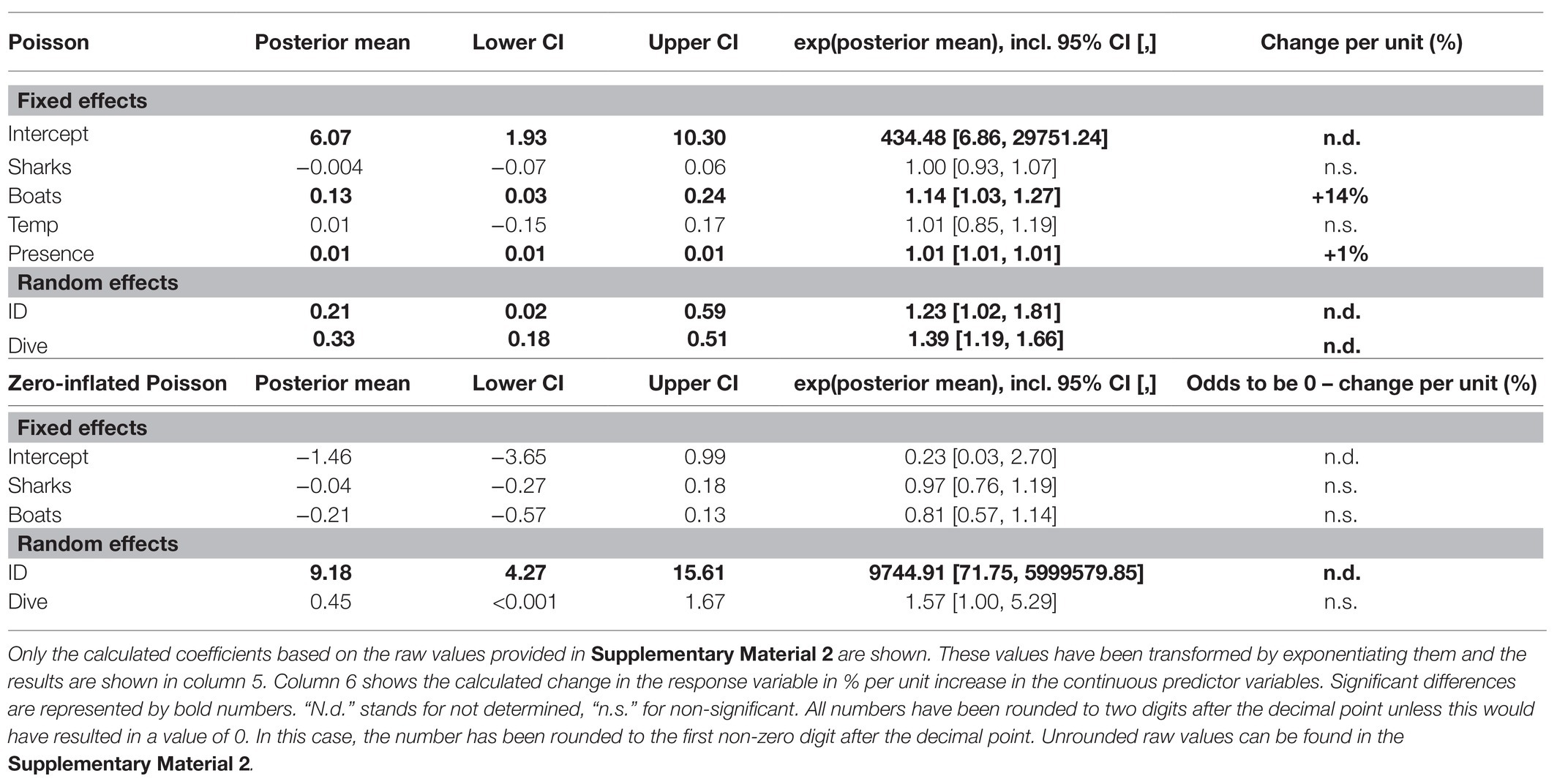
Table 4. Summary of the zero-inflated Poisson GLMM for the presence time of great hammerhead sharks (S. mokarran) during provisioning dives (model 3).
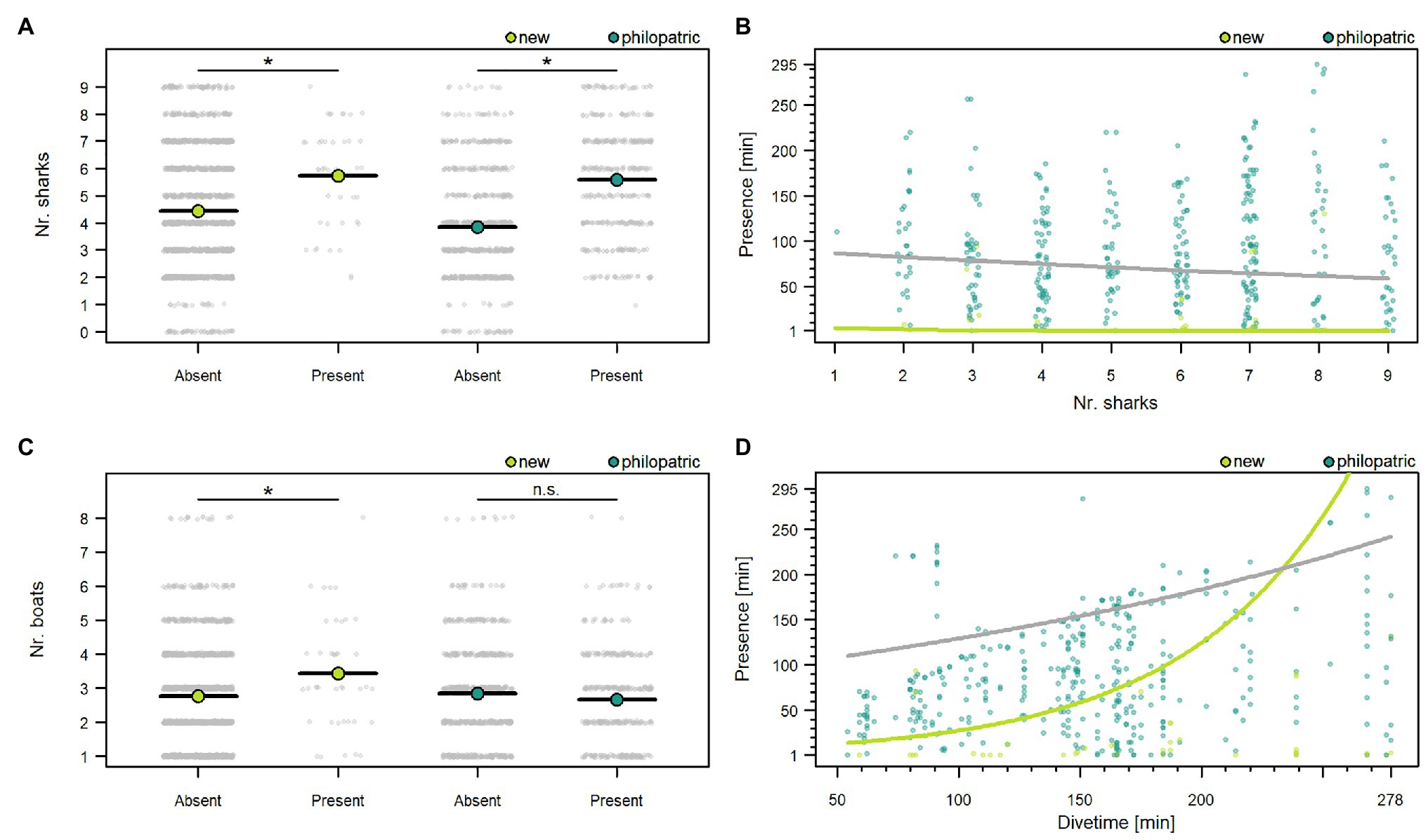
Figure 3. Effects of the number of sharks on the presence (A) and presence time (B), the number boats on the presence (C), and the dive time on the presence time (D), of great hammerhead sharks (S. mokarran) during provisioning dives. Plots (A,C) show a graphical representation of the Zero-Inflated Poisson generalized linear mixed model (GLMM; model 3). The x-axis contains the absence vs. presence data of individuals and the original data points are shown in light gray. The predictor variable is on the y-axis. Mean values in plots (A,C) are represented by the black bar containing a colored dot. Significance of a fixed effect in plots (A,C) is represented by “*,” whereas “n.s.” denotes a non-significant effect. Plots (B,D) show the Poisson part of model 3. The x-axis contains the predictor variables, and the response variable is on the y-axis. Original data for each group of sharks are shown in different colors as an interaction was entered. For the ease of representation only presence time values >0 min have been included in plots (B,D). Colored curves represent significant regressions extracted from model 3 (Table 4). Significant interactions but non-significant effects are shown as gray curves. The plots that contain a graphical representation of the non-significant effects for model 3 can be found in Supplementary Figure 2.
When dives were longer, new sharks spent more time attending dives (Figure 3D; Table 4). However, the dive time did not change the presence time of philopatric sharks (Figure 3D; Table 4), nor did it impact the odds of being absent for either group of sharks (Table 4; Supplementary Figure 2A; Supplementary Material 1, “d. Supplementary results”). The number of days sharks were present in Bimini during the season had no effect on the presence time during, nor the odds of being absent from dives for either group of sharks (Table 4; Supplementary Figures 2C,D; Supplementary Material 1, “d. Supplementary results”). Water temperature differences did not change the presence time of sharks or their odds of being absent (Table 4; Supplementary Figures 2E,F; Supplementary Material 1, “d. Supplementary results”). The variance in the amount of time sharks were present during dives and in their odds of being absent was different between individuals but not between dives (Table 4).
Great hammerhead sharks consumed a total of 1,189 kg bait throughout the season with individual bait pieces weighing 142.63 ± 33.80 g (mean ± SD). Sixteen sharks that attended dives did not consume any bait, with one of these individuals known to be philopatric to Bimini (shark-ID #22). The average amount of bait consumed per individual per dive ranged from 0 to 4.75 kg (±3.49 kg, mean ± SD; Table 2). Overall, philopatric individuals consumed 3.03 ± 2.64 kg (mean ± SD) per dive and new sharks 0.01 ± 0.04 kg (mean ± SD; Table 2).
The odds of great hammerhead sharks consuming no bait were neither impacted by the number of conspecifics attending the same event (Table 5; Supplementary Figure 3A; Supplementary Material 1, “d. Supplementary results”), nor by the number of other dive vessels present at the dive site on that date (Table 5; Supplementary Figure 3C; Supplementary Material 1, “d. Supplementary results”). Further, the number of conspecifics did not influence the amount of bait individuals consumed during dives (Table 5; Supplementary Figure 3B; Supplementary Material 1, “d. Supplementary results”). However, we found that the amount of bait great hammerhead sharks consumed during a dive was increased by the factor 1.14, i.e., 14%, per unit increase in the number of dive vessels at the dive site (Figure 4A; Table 5). An increase in the bait uptake of individuals was also found when the presence time of individuals during a dive was longer (Figure 4B; Table 5). The bait consumption of sharks was not impacted by the water temperature (Table 5; Supplementary Figure 3D; Supplementary Material 1, “d. Supplementary results”).
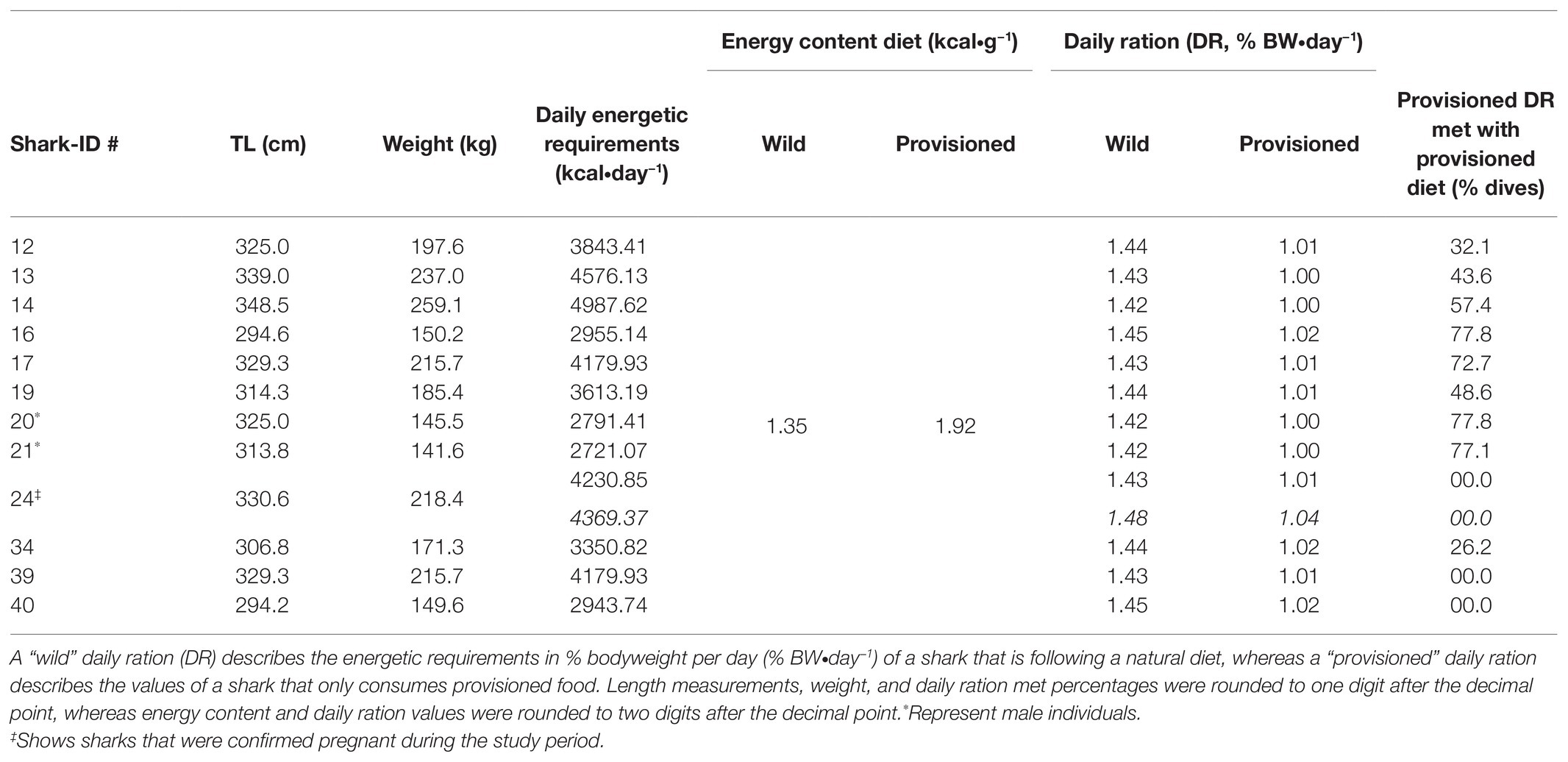
Table 5. Summary of the zero-inflated Poisson GLMM for the bait uptake of great hammerhead sharks (S. mokarran) during provisioning dives (model 4).
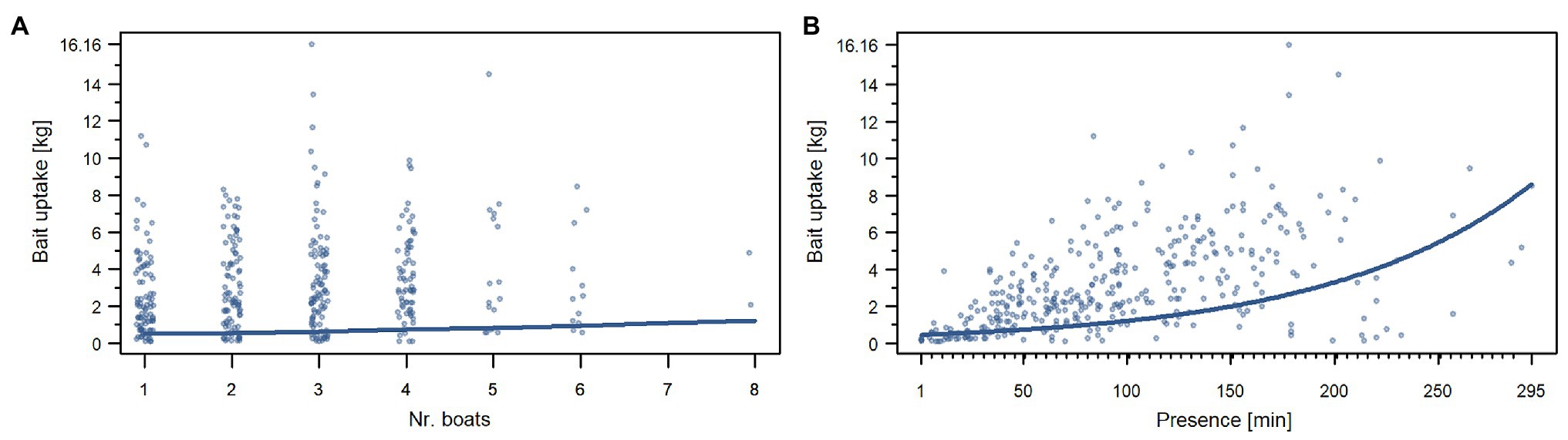
Figure 4. Effects of the number of boats (A) and the presence time (B) on the bait uptake of great hammerhead sharks (S. mokarran) during provisioning dives. Plots (A) and (B) show the Poisson part of model 4. The x-axis contains the predictor variables, and the y-axis contains the response variable. The y-axis has been transformed to show the bait uptake in kilograms. For the ease of representation, only bait uptake values >0 kg have been included in these plots. Colored curves represent significant regressions extracted from model 4 (Table 5). The plots that contain a graphical representation of the non-significant effects for model 4 can be found in Supplementary Figure 3.
We found a significant variance between the odds of consuming no bait between the individuals but not between the dives (Table 5). The variance of consumed bait between the individuals and between the dives (Table 5) was significant.
Daily Ration of Great Hammerhead Sharks and the Influence of Food Provisioning
Our bioenergetic model estimated that female great hammerhead sharks in this study have daily energetic requirements ranging from 2943.74 to 4987.62 kcal, with male sharks requiring between 2721.07 and 2791.41 kcal∙day−1. The daily ration values for non-gravid female sharks that consumed a natural diet ranged from 1.42 to 1.45% BW and the males had a daily ration of 1.42%. We found a decrease in the daily ration values when sharks consumed a provisioned diet, which has a higher caloric value (Table 6; Supplementary Table 1) with values ranging from 1 to 1.02% BW for females and 1% BW for males (Table 6). When comparing the daily ration values of provisioned (n = 12) great hammerhead sharks, i.e., sharks that consumed bait during at least one dive, to their bait uptake values in % BW, we found that three sharks did not meet their daily energetic requirements, five were able to on 26–57% of dives and four on 73–78% of all dives (Table 6).
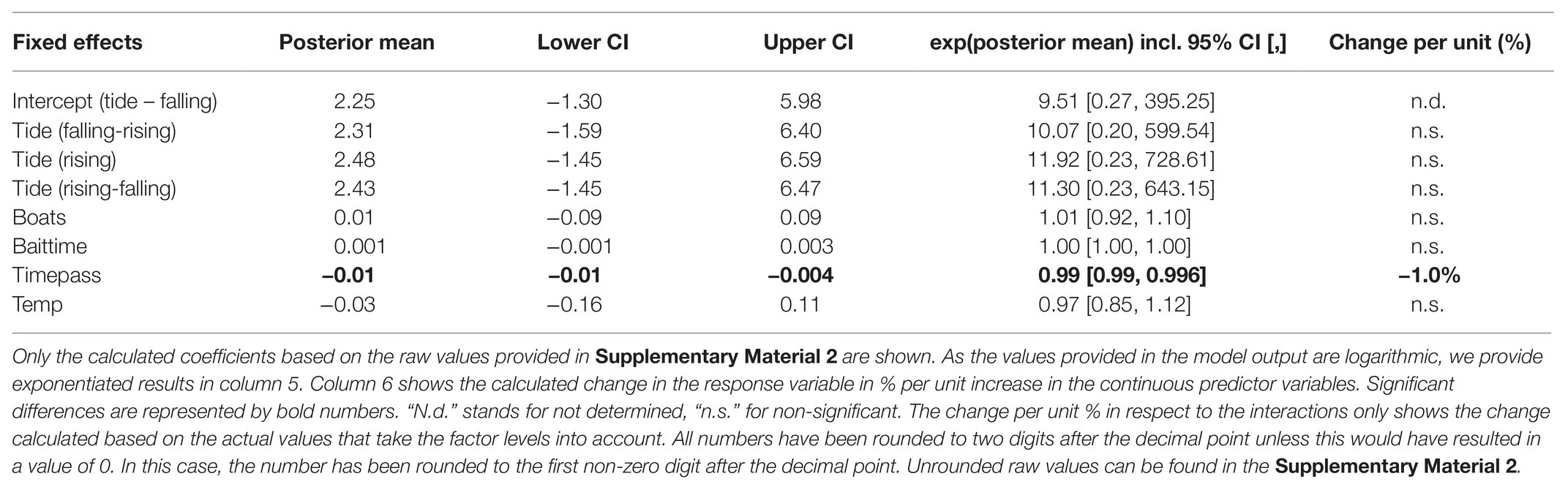
Table 6. Daily ration estimates for great hammerhead sharks (S. mokarran) following a wild or provisioned diet.
Discussion
In this study, we observed great hammerhead sharks at a popular dive site to understand how provisioning influenced their attendance and daily ration during dives. We found significant differences in how individual sharks responded, with results revealing that previous experience (i.e., philopatric vs. new) was an important factor when investigating which variables influenced the sharks’ presence, time spent, and consumption of food at provisioning dives. Individual great hammerhead sharks consumed up to 4.75 kg of bait per dive, and through bioenergetics modeling, we found that some were capable of fully fuelling their daily energetic requirements from provisioned food alone.
Number and Arrival Time of Great Hammerhead Sharks at Provisioning Dives
Our first objective was to understand which factors influenced the number of sharks attending provisioning dives. Great hammerhead sharks are generally recognized for being highly mobile and solitary (Miller et al., 2014; Rigby et al., 2019), but due to food provisioning, here, we observed an artificial aggregation of up to nine mature great hammerhead sharks. Similarly, large-bodied species such as bull (Brunnschweiler and Baensch, 2011) and tiger sharks (Hammerschlag et al., 2012) have been documented to form temporary, loose aggregations when resources are concentrated.
The number of great hammerhead sharks attending dives in our study was not impacted by how long the bait was in the water, tidal state, water temperature, nor the number of dive boats present in the area. We recorded the highest numbers of great hammerhead sharks at dives during the first 2 weeks of February, i.e., the mid-point of the hammerhead season in Bimini (Figure 3), and our model showed a decreasing number of individuals attending dives over the course of the study period. We believe that the lower numbers at the beginning and end of the season compared to the high numbers in February are a consequence of the previously documented annual migrations of great hammerhead sharks in this area (Guttridge et al., 2017). Guttridge et al. (2017) reported that the highest residency indices over a full year were recorded between water temperatures of ~24 and 27°C. The water temperature in this study ranged from 20.23 to 27.21°C and the mean water temperature was 25.70°C (±0.87, mean ± SD). Only five dives were recorded where the water temperature was higher than 27°C. Of those, three dives were at the very beginning of the season in January and the other two were the two last dives of the season when no great hammerhead sharks were recorded. Therefore, temperature may be impacting the presence of great hammerhead sharks around the islands in general but not their presence at dives. It is, however, unclear how much of the observed seasonal residency of great hammerheads is due to the provisioning, as local movement data were not collected until after the first commercial great hammerhead shark dives in Bimini in 2012.
Similar to our results, the mean counts of Galapagos (Carcharhinus galapagensis), sandbar (Carcharhinus plumbeus), and tiger sharks at a dive site in Hawaii did not change when one or two boats were present (Meyer et al., 2009). However, whale sharks (Rhincodon typus) tended to show a higher frequency of avoidance responses when more boats were present (Pierce et al., 2010). Such patterns were not found in the response of great hammerhead sharks in Bimini, where philopatric sharks were not influenced by the number of boats and new sharks were even more likely to be present when boat numbers increased (see below).
The majority (79%) of the 28 great hammerhead sharks identified at the provisioning site in Bimini were female, similar to studies from other provisioning sites on bull (Brunnschweiler and Baensch, 2011), Caribbean reef (Maljković and Côté, 2011), and tiger sharks (Hammerschlag et al., 2012). Sexual segregation (reviewed by Wearmouth and Sims, 2008) and a potentially more nomadic life-style in male sharks (Chapman et al., 2015) could explain this observed bias. Another explanation could be sex-driven behavioral differences, as male gray nurse sharks (Carcharias taurus) in Australia did not react to the presence of divers while females did (Barker et al., 2011). Given that both male and female great hammerheads attended and consumed food on dives in Bimini, we believe that sexual segregation is a more likely explanation.
Next, we examined whether sharks arrived at the dive site earlier as the season progressed, a measure we expected to reveal anticipatory behavior. However, despite repeated, sometimes nearly daily use of the dive site by some individuals (e.g., Shark-ID #14, attended 68 dives), we did not find a significant change in the time it took great hammerhead sharks to arrive at the site upon anchoring of the dive vessel. This is in contrast to other studies (e.g., Meyer et al., 2009; Fitzpatrick et al., 2011) that found sharks arriving faster, or waiting at the dive site prior to the arrival of the dive boat. This might be a direct effect of sharks being able to reach their daily ration on up to 77% of the dives. The dive master offered food to every great hammerhead shark approaching the bait box at the dive site. This means that any shark that approached the dive master could have received a piece of bait, which mitigated potential competition for resources between sharks as food was readily available. Additionally, we suspect our lack of evidence for anticipatory behavior could also be partly explained by conditioning occurring in previous years. All philopatric sharks had been observed to use the dive site for at least 1 year prior to this study, with some of these sharks being observed at the dive site every winter for 7 consecutive years (e.g., shark-ID #21; Supplementary Table 2). Due to other boats using the site, we were also only able to include approximately one-third of all dives in the model for this measure which renders firm conclusions difficult. Future research about potential effects of provisioning on the great hammerhead sharks in Bimini would benefit from the addition of telemetry techniques to assess the use of the dive site on non-feeding days, similar to studies with bull sharks in Fiji (see Brunnschweiler and Barnett, 2013) and white sharks in Australia (see Huveneers et al., 2013). This would provide useful additional insights into the anticipatory behavior of great hammerhead sharks.
Differences in Provisioning Dive Attendance and Bait Uptake Patterns
Attendance at provisioning dives varied greatly among individual great hammerhead sharks, with many making less than five appearances during the season, whereas others attended 52% and more of all dives (e.g., shark-ID #14, n = 68 dives). We could explain the differences in the dive site use by assigning sharks to the groups “philopatric” and “new” based on previous findings in Bimini (Guttridge et al., 2017). Philopatric sharks showed significantly higher attendance indices and spent significantly longer (on average 92 min) at the dive site than new sharks (on average 18 min). In addition, when we modeled this data using a zero-inflated Poisson GLMM, new sharks were found to have higher odds of a 0-presence time than philopatric sharks. The capacity of sharks to learn through classical and operant conditioning paradigms has been documented for many species (Schluessel, 2015; Guttridge et al., 2018). Repeated exposure to the dive set up with consistent food rewards for philopatric great hammerheads sharks, clearly provides an opportunity for learning (Guttridge et al., 2018). Indeed, recent experimental work using an operant conditioning paradigm showed that learning rates were increased when sharks were trained at a higher reinforcement frequency (Heinrich et al., 2020). Other studies have found that sharks can remember a conditioned response for nearly 12 months (Fuss and Schluessel, 2015; as reviewed in Guttridge et al., 2018). Thus, a likely explanation for our results is that philopatric sharks remembered the dive and feeding setup from previous seasons. Since 2012, NWBSC has used a standardized dive set-up and bait box (Figure 1; pers. communication NWBSC), where the divers in one line create a wall of air bubbles, which could serve as a conditioned stimulus toward being fed. Indeed, the potential of air bubbles to serve as a strong conditioning stimulus was previously demonstrated in Port Jackson sharks (Heterodontus portusjacksoni; Guttridge and Brown, 2013). Another conditioning stimulus could be boat noise (Meyer et al., 2009; Fitzpatrick et al., 2011) as sharks are known to be attracted to low-frequency sounds (Nelson and Gruber, 1963) as well as the near-standardized use of particular bait types that may lead to conditioning. Further, when philopatric sharks returned in subsequent years since this study they swam toward the feeder with apparent intent and oriented to food rewards rapidly (pers. observation V. Heim). This is in stark contrast to new sharks that typically spent their time in the periphery with limited approaches to the feeder or setup.
We found differences in how the presence of philopatric and new sharks were impacted by abiotic and biotic factors. Only new sharks reduced their presence time when more conspecifics were present and increased their presence time with increasing dive time. However, none of the tested variables impacted the duration philopatric sharks were present during dives. While both groups had decreased odds of being absent when more conspecifics were present, only new sharks showed reduced odds when more boats were present. These results suggest that great hammerhead sharks may initially be attracted to the activity of feeding conspecifics, through a social facilitation mechanism which is a common phenomenon in sharks and other animals (Dindo et al., 2009; Jacoby et al., 2012). However, new great hammerheads go on to reduce their time spent at the dive site with increasing numbers of conspecifics, which we suspect is in response to the presence of experienced and possibly dominant philopatric sharks (Ward et al., 2006; Brown et al., 2011). A further explanation could lie in the solitary nature of great hammerhead sharks (Rigby et al., 2019). Solitary behaviors in large predator species are often beneficial compared to group living (Bekoff et al., 1984; Elbroch and Quigley, 2017). For the great hammerhead sharks new to Bimini’s dive site, the artificially created aggregation of individuals could, therefore, present a novel and potentially stressful (e.g., Semeniuk and Dill, 2005; Semeniuk and Rothley, 2008) situation so that these individuals not only have to habituate to the stimulus of the dive, but also to sharing space with conspecifics. This potential stress factor would have been reduced during the first sightings of now philopatric sharks as during the early days, fewer great hammerhead sharks as well as fewer nurse sharks were present during dives (see Study Site section, pers. communication, Sean Williams, former Bimini Biological Field Station Foundation staff member 2005–2010). We did not observe any intraspecific aggression, suggesting that our results might also reflect other individual differences, such as personality and cognition (Carere and Locurto, 2011). Research on lemon sharks (Negaprion brevirostris) has found that individual sharks react differently toward novel situations (Finger et al., 2016). A stronger stimulus (i.e., olfactory stimulation) when more boats were baiting probably explains the reduced odds to be absent with an increasing number of boats. These will be important aspects to consider should sharks that were new during this study return in following years, and if the observed patterns change over time.
Philopatric great hammerhead sharks consumed up to 4.75 kg per individual per dive during this study, whereas only two out of 17 new sharks consumed bait at all. None of the modeled variables impacted the odds of not consuming any bait. However, all great hammerhead sharks consumed more bait when they attended dives for longer and when more dive vessels were present. Our first finding is a logical consequence of sharks simply having more time to approach the dive master and be provisioned more frequently. However, the increase in bait consumption with increasing dive vessel numbers (as above) is best explained by a greater olfactory corridor generated by more boats chumming the dive site. The increased olfactory stimulation might create a food pulse and thus a greater feeding motivation for sharks in the area. We believe that the unique dive set-up, boat noise, and protocols of the NBWSC (see Dive Surveys section) and the NWBSC being present at the dive site most frequently served as highly effective conditioning stimulus for the great hammerhead sharks, leading to the sharks attending primarily dives of the NWBSC even if other dive operators were baiting at the dive site simultaneously. We see this hypothesis partly supported by the unchanged numbers of sharks attending dives even when other dive operators were present (Table 3). Consequently, sharks attending NWBSC dives during days when multiple boats were at the dive site, received stronger provisioning cues but preferred to attend NWBSC dives, explaining the higher bait uptake values during these days.
The water temperature was not a significant effect in any of the models. While the water temperature is a decisive factor for the presence of great hammerhead sharks in Bimini during the winter months (Guttridge et al., 2017), once these sharks are in Bimini, our results show that other variables such as the group (i.e., philopatric vs. new) of a shark are more important in explaining the differences and observed presence time and bait uptake values at the dive site.
We also found that the random effect “shark-ID” was significant when modeling the presence time and the bait uptake of great hammerhead sharks. Furthermore, the random effect “dive” was significant when modeling the bait uptake. This highlights the differences in responses between individual sharks. However, we acknowledge that the time a shark spends at a dive and the bait it consumes is most likely influenced by a complex combination of individual measures such as feeding history and motivation, as well as environmental variables (Huveneers et al., 2013). We further believe that the group of a shark (i.e., philopatric vs. new) could be a main driver of the observed individual differences.
Daily Ration of Great Hammerhead Sharks and the Influence of Food Provisioning
Our bioenergetic model suggested daily calory requirements ranging from 2721.07 to 4987.62 kcal and daily ration values ranging from 1.00 to 1.02% BW when sharks were consuming provisioned food. The daily ration values calculated for a natural, less calory dense diet, were higher and ranged from 1.42 to 1.45% BW.
Most of the great hammerhead sharks that consumed provisioned food during this study were able to fully meet their daily energetic requirements, some of them on nearly 78% of all dives they attended. Only three sharks (two new and one philopatric) did not meet their daily ration. Our results support findings from bull sharks in Fiji (Brunnschweiler et al., 2018) suggesting that provisioning can be a considerable factor in fuelling attending sharks’ energetic requirements. The high energy content of the main bait sources resulted in lower daily ration values if the sharks followed an entirely provisioned diet, implying that dive tourism can make it easier for great hammerhead sharks to fuel their daily energetic requirements.
Great hammerhead sharks occupy a high trophic level within food webs (Raoult et al., 2019) and as such can have important ecological roles in the maintenance and function of coastal ecosystems (e.g., Heithaus et al., 2008; Roff et al., 2016). Should the high frequency of dives where great hammerhead sharks meet their daily ration result in these individuals ceasing their natural foraging behavior, this could have important consequences for local ecosystem dynamics. Using stable isotope analysis, Maljković and Côté (2011) and Abrantes et al. (2018) found, however, that such drastic changes did not occur in Caribbean reef and bull sharks, respectively. A similar approach with stable isotopes or fatty acids (e.g., Meyer et al., 2019) would be useful at our study site. Great hammerhead sharks are known to feed on mesopredatory elasmobranchs (e.g., gray reef shark, Carcharhinus amblyrhnchos; Mourier et al., 2013) and have been observed to actively predate on southern stingrays (H. americanus; Strong et al., 1990) and spotted eagle rays (Aetobatus narinari; Chapman and Gruber, 2002) in Bimini. The risk of a potential mesopredator release as a result of great hammerhead shark provisioning, and for a potential subsequent reduction in biodiversity on lower trophic levels (Ritchie and Johnson, 2009) should be explored. Further, the health implications of fuelling their daily ration with provisioned food, vs. with natural prey items, are still unknown, a concern that was already raised for provisioned stingrays in the Caribbean (Semeniuk et al., 2007).
Bimini is home to numerous great hammerhead sharks during the winter months every year. However, this seasonal residency (Guttridge et al., 2017) sees them feed only during the winter months. As summer approaches, most great hammerhead sharks naturally leave the islands migrating to the United States (Guttridge et al., 2017) which determines the end of each hammerhead diving season. Dive operators have attempted to bait at the provisioning site during the off season (May–October) with very limited success and unreliable great hammerhead shark sightings only. Our study shows the impacts of the provisioning on meeting daily energetic requirements of individual great hammerhead sharks. To what extent these findings apply to the ecosystem role of the great hammerhead population in Bimini is difficult to conclude. We identified 16 sharks that attended dives but did not consume any bait. Sightings of unknown great hammerhead sharks in other areas around the island without the use of bait could be an indication that only a small subset of the seasonally resident individuals is responsive to the provisioning, and that the impact on the wider population is limited. Although there is no published data estimating the effective population size of great hammerhead sharks in the United States Atlantic or the Bahamas between where these provisioned sharks migrate (Guttridge et al., 2017), thus it is difficult to make any firm conclusions on the implications on the overall great hammerhead population.
We acknowledge that our bioenergetics model was estimated based on approximations from other species, as some of the parameters were not yet available for the great hammerhead (e.g., species-specific metabolic rate). In addition, we recognize that other factors such as daily activity and water temperature could also impact these values (e.g., Lear et al., 2017). Indeed, studies that have used accelerometer loggers have found increased overall dynamic body acceleration, which can be considered as an approximation for the metabolic rate, via increased vertical movements during dives, suggesting that there is a cost to the reward (Barnett et al., 2016; Huveneers et al., 2018). Further, we used daily ration values of non-gravid females for females where the pregnancy status was uncertain. Some individuals, nevertheless, could have been pregnant during the study period, in which case the daily ration value used to calculate the percentage of dives where the DR was met might have been too low. However, we believe that even if more sharks were pregnant, the percentage of dives where the daily ration was met would not have changed considerably (Supplementary Table 3; Supplementary Material 1, “d. Supplementary results”).
Conclusion, Outlook, and Management Measures
Our results support that some (e.g., philopatric) great hammerhead sharks were conditioned to the dive site in Bimini, showing regular attendance throughout the winter months of up to 2 h daily and frequent consumption of enough provisioned food to fuel their daily ration. However, despite these obvious behavioral and feeding responses, it was not possible for us to examine how provisioning impacts great hammerhead sharks ecological role within the local ecosystem, or indeed if it affects their residency to Bimini and its surrounding environs. We believe that dives are localizing the sharks to the western edge of Bimini during the winter months, but it does not seem to be impacting their annual migration patterns (see Guttridge et al., 2017). Future studies should combine individual observations on dive attendance with bait uptake at the provisioning site, telemetry methods to monitor dive site residency as well as local space use patterns, and tissue sampling for stable isotope analysis to examine these potential impacts further. This is particularly important given the critically endangered status of great hammerheads globally (Rigby et al., 2019) and the biological significance of their migrations for reproductive purposes.
To our knowledge in the Bahamas there are currently no laws or guidelines to regulate shark dives. It is beyond the scope of this study to provide a framework; however, we do point toward other dive sites, such as in Australia or in a locally managed MPA in Fiji that have implemented successful management strategies (e.g., limiting the number of dive vessels as well as the amount of provisioning) to ensure the viability of impacted shark populations and sustainability of tourism (e.g., Brunnschweiler, 2010; Huveneers and Lloyd, 2017; Heinrich et al., 2020). Furthermore, other dive sites have successfully introduced a shark diving “code of conduct,” including measures such as limiting the number of divers or regulating diver behavior to reduce the potential disturbances to the sharks (e.g., Smith et al., 2010). Given the results of our study, the above could be considered for great hammerhead sharks in Bimini especially since provisioning activities have intensified further after the conclusion of the field work for this study (pers. communication, NWBSC).
In summary, shark provisioning activities are important as they can benefit local economies by generating income both directly via shark dives and indirectly through expenditure at local hotels, restaurants, and shops (Haas et al., 2017). Further, they offer a platform to raise awareness and for education. All three of these aspects can contribute to improved conservation plans for the target species. Our results show changes in presence and feeding patterns of great hammerhead sharks during provisioning dives as well as a considerable contribution of bait toward their daily ration. Albeit not yet tested in this system, such impacts could have consequences for the individuals as well as the ecosystem they live in. Our findings contribute to a better understanding of provisioning impacts on marine megafauna, future management considerations, and the potential ecosystem implications of provisioning activities as part of wildlife tourism.
Data Availability Statement
The raw data supporting the conclusions of this article will be made available by the authors, without undue reservation.
Ethics Statement
Ethical review and approval was not required for the animal study because all field work was conducted under the permit (no: MAF/LIA/22) issued by the Department of Marine Resources, Bahamas granted to the Bimini Biological Field Station Foundation (BBFSF). Written informed consent was obtained from the relevant individual(s) for the publication of any potentially identifiable images or data included in this article.
Author Contributions
VH, SHG, and TLG designed the study. VH collected and analyzed the data and led the writing of the manuscript. FD supervised the data analysis and statistics. MJS, SHG, and TLG supervised the study. FD, MJS, and TLG reviewed the manuscript. All authors contributed to the article and all authors but SHG approved the submitted version.
Funding
The field work for this study was supported by a Save Our Seas Foundation keystone special grant (SOS 260).
Conflict of Interest
The authors declare that the research was conducted in the absence of any commercial or financial relationships that could be construed as a potential conflict of interest.
Acknowledgments
We would like to acknowledge the Department of Fisheries of the Commonwealth of the Bahamas providing the necessary permits to work in the Bimini Islands. All field work was conducted under the permit (no: MAF/LIA/22) issued by the Department of Marine Resources, Bahamas. We wish to thank the editor and the two reviewers of this manuscript, who through critical but fair comments helped to significantly improve the quality and content of the presented manuscript. A special thanks to Dieter Ebert from the University of Basel, Switzerland, for his supervision, support, and for accepting the first author as an MSc-student of his research group. We would like to thank John K. Carlson (National Oceanic and Atmospheric Administration, NOAA, Panama City, United States) for his help and expertise to develop the bioenergetic model for the great hammerhead sharks and Maurits van Zinnicq Bergmann (Florida International University, FIU, Miami, United States) for his constructive comments regarding the field work protocols. Furthermore, we would like to thank Pierre de Villemereuil (National Museum of Natural History, Paris, France) for his suggestions of suitable priors for the GLMMs and his help and expertise with the Bayesian statistics of this manuscript. Similarly, we would like to thank Simon Dedman (Stanford University, Stanford, United States) for his support with the statistical analysis. This study would not have been possible without the Neal Watson’s Bimini Scuba Center and its entire crew, especially the crew members during the study period: Neal Watson Jr., Alonzo Curry, Billy, Jamie and Robin Ferguson, Carson Saunders, Gepetto Rolle, Kevion Knowles, and Sean Williams. We are grateful for all their exceptional help on the dive vessels and during the field days. Finally, we would like to thank you all the staff and volunteers at the Bimini Biological Field Station Foundation.
Supplementary Material
The Supplementary Material for this article can be found online at: https://www.frontiersin.org/articles/10.3389/fmars.2021.628469/full#supplementary-material
Footnotes
References
Abrantes, K. G., Brunnschweiler, J. M., and Barnett, A. (2018). You are what you eat: examining the effects of provisioning tourism on shark diets. Biol. Conserv. 224, 300–308. doi: 10.1016/j.biocon.2018.05.021
Apps, K., Dimmock, K., and Huveneers, C. (2018). Turning wildlife experiences into conservation action: can white shark cage-dive tourism influence conservation behavior? Mar. Policy 88, 108–115. doi: 10.1016/j.marpol.2017.11.024
Barker, S. M., Peddemors, V. M., and Williamson, J. E. (2011). A video and photographic study of aggregation, swimming and respiratory behavior changes in the grey nurse shark (Carcharias taurus) in response to the presence of SCUBA divers. Mar. Freshw. Behav. Physiol. 44, 75–92. doi: 10.1080/10236244.2011.569991
Barnett, A., Payne, N. L., Semmens, J. M., and Fitzpatrick, R. (2016). Ecotourism increases the field metabolic rate of whitetip reef sharks. Biol. Conserv. 199, 132–136. doi: 10.1016/j.biocon.2016.05.009
Bekoff, M., Daniels, T. J., and Gittleman, J. L. (1984). Life history patterns and the comparative social ecology of carnivores. Annu. Rev. Ecol. Syst. 15, 191–232. doi: 10.1146/annurev.es.15.110184.001203
Bethea, D. M., Hale, L., Carlson, J. K., Cortés, E., Manire, C. A., and Gelsleichter, J. (2007). Geographic and ontogenetic variation in the diet and daily ration of the bonnethead shark, Sphyrna tiburo, from the eastern Gulf of Mexico. Mar. Biol. 152, 1009–1020. doi: 10.1007/s00227-007-0728-7
Brafield, A. E., and Solomon, D. J. (1972). Oxy-calorific coefficients for animals respiring nitrogenous substrates. Comp. Biochem. Physiol. Part A Physiol. 43A, 837–841. doi: 10.1016/0300-9629(72)90155-7
Brena, P. F., Mourier, J., Planes, S., and Clua, E. (2015). Shark and ray provisioning functional insights into behavioral, ecological and physiological responses across multiple scales. Mar. Ecol. Prog. Ser. 538, 273–283. doi: 10.3354/meps11492
Brown, C., Laland, K., and Krause, J. (2011). Fish Cognition and Behavior. 2nd Edn. Oxford: Wiley-Blackwell.
Bruce, B. D., and Bradford, R. W. (2013). The effects of shark cage-diving operations on the behavior and movements of white sharks, Carcharodon carcharias, at the Neptune Islands, South Australia. Mar. Biol. 160, 889–907. doi: 10.1007/s00227-012-2142-z
Brunnschweiler, J. M. (2010). The Shark Reef Marine Reserve: a marine tourism project in Fiji involving local communities. J. Sustain. Tour. 18, 29–42. doi: 10.1080/09669580903071987
Brunnschweiler, J. M., and Baensch, H. (2011). Seasonal and long-term changes in relative abundance of bull sharks from a tourist shark feeding site in Fiji. PLoS One 6:e16597. doi: 10.1371/journal.pone.0016597
Brunnschweiler, J. M., and Barnett, A. (2013). Opportunistic visitors: long-term behavioral response of bull sharks to food provisioning in Fiji. PLoS One 8:e58522. doi: 10.1371/journal.pone.0058522
Brunnschweiler, J. M., Payne, N. L., and Barnett, A. (2018). Hand feeding can periodically fuel a major portion of bull shark energy requirements at a provisioning site in Fiji. Anim. Conserv. 21, 31–35. doi: 10.1111/acv.12370
Carere, C., and Locurto, C. (2011). Interaction between animal personality and animal cognition. Curr. Zool. 57, 491–498. doi: 10.1093/czoolo/57.4.491
Chapman, D. D., Feldheim, K. A., Papastamatiou, Y. P., and Hueter, R. E. (2015). There and back again: a review of residency and return migrations in sharks, with implications for population structure and management. Annu. Rev. Mar. Sci. 7, 547–570. doi: 10.1146/annurev-marine-010814-015730
Chapman, D. D., and Gruber, S. H. (2002). A further observation of the prey-handling behavior of the great hammerhead shark, Sphyrna mokarran: predation upon the spotted eagle ray, Aetobatus narinari. Bull. Mar. Sci. 70, 947–952.
Corcoran, M. J., Wetherbee, B. M., Shivji, M. S., Potenski, M. D., Chapman, D. D., and Harvey, G. M. (2013). Supplemental feeding for ecotourism reverses diel activity and alters movement patterns and spatial distribution of the southern stingray, Dasyatis americana. PLoS One 8:e59235. doi: 10.1371/journal.pone.0059235
Cortés, E., Domingo, A., Miller, P., Forselledo, R., Mas, F., Arocha, F., et al. (2015). Expanded ecological risk assessment of pelagic sharks caught in Atlantic pelagic longline fisheries. Collect Vol Sci. Pap. ICCAT 71, 2637–2688.
Dindo, M., Whiten, A., and De Waal, F. B. M. (2009). Social facilitation of exploratory foraging behavior in capuchin monkeys (Cebus apella). Am. J. Primatol. 71, 419–426. doi: 10.1002/ajp.20669
Donaldson, R., Finn, H., Bejder, L., Lusseau, D., and Calver, M. (2012). The social side of human-wildlife interaction: wildlife can learn harmful behaviors from each other. Anim. Conserv. 15, 427–435. doi: 10.1111/j.1469-1795.2012.00548.x
Elbroch, L. M., and Quigley, H. (2017). Social interactions in a solitary carnivore. Curr. Zool. 63, 357–362. doi: 10.1093/cz/zow080
Finger, J. S., Dhellemmes, F., Guttridge, T. L., Kurvers, R. H. J. M., Gruber, S. H., and Krause, J. (2016). Rate of movement of juvenile lemon sharks in a novel open field, are we measuring activity or reaction to novelty? Anim. Behav. 116, 75–82. doi: 10.1016/j.anbehav.2016.03.032
Fitzpatrick, R., Abrantes, K. G., Seymour, J., and Barnett, A. (2011). Variation in depth of whitetip reef sharks: does provisioning ecotourism change their behavior? Coral Reefs 30, 569–577. doi: 10.1007/s00338-011-0769-8
Fuss, T., and Schluessel, V. (2015). Something worth remembering: visual discrimination in sharks. Anim. Cogn. 18, 463–471. doi: 10.1007/s10071-014-0815-3
Gallagher, A. J., Vianna, G. M. S., Papastamatiou, Y. P., MacDonald, C., Guttridge, T. L., and Hammerschlag, N. (2015). Biological effects, conservation potential, and research priorities of shark diving tourism. Biol. Conserv. 184, 365–379. doi: 10.1016/j.biocon.2015.02.007
Guttridge, T. L., and Brown, C. (2013). Learning and memory in the Port Jackson shark, Heterodontus portusjacksoni. Anim. Cogn. 17, 415–425. doi: 10.1007/s10071-013-0673-4
Guttridge, T. L., Van Zinnicq Bergmann, M. P. M., Bolte, C., Howey, L. A., Finger, J. S., Kessel, S. T., et al. (2017). Philopatry and regional connectivity of the great hammerhead shark, Sphyrna mokarran in the U.S. and Bahamas. Front. Mar. Sci. 4:3. doi: 10.3389/fmars.2017.00003
Guttridge, T. L., Yopak, K. E., and Schluessel, V. (2018). “Sharks—elasmobranch cognition,” in Field and Laboratory Methods in Animal Cognition. eds. N. Bueno-Guerra and F. Amici (Cambridge: Cambridge University Press), 354–380.
Haas, A. R., Fedler, T., and Brooks, E. J. (2017). The contemporary economic value of elasmobranchs in The Bahamas: reaping the rewards of 25 years of stewardship and conservation. Biol. Conserv. 207, 55–63. doi: 10.1016/j.biocon.2017.01.007
Hadfield, J. D. (2010). MCMC methods for multi-response generalized linear mixed models: the MCMCglmm R Package. J. Stat. Softw. 33, 1–22. doi: 10.18637/jss.v033.i02
Hammerschlag, N., Gallagher, A. J., Wester, J., Luo, J., and Ault, J. S. (2012). Don’t bite the hand that feeds: assessing ecological impacts of provisioning ecotourism on an apex marine predator. Funct. Ecol. 26, 567–576. doi: 10.1111/j.1365-2435.2012.01973.x
Heinrich, D. D. U., Vila Pouca, C., Brown, C., and Huveneers, C. (2020). Effects of reward magnitude and training frequency on the learning rates and memory retention of the Port Jackson shark Heterodontus portusjacksoni. Anim. Cogn. 23, 939–949. doi: 10.1007/s10071-020-01402-2
Heithaus, M. R., Frid, A., Wirsing, A. J., and Worm, B. (2008). Predicting ecological consequences of marine top predator declines. Trends Ecol. Evol. 23, 202–210. doi: 10.1016/j.tree.2008.01.003
Heupel, M. R., Knip, D. M., Simpfendorfer, C. A., and Dulvy, N. K. (2014). Sizing up the ecological role of sharks as predators. Mar. Ecol. Prog. Ser. 495, 291–298. doi: 10.3354/meps10597
Huveneers, C., and Lloyd, M. (2017). Residency of white sharks, Carcharodon carcharias, at the Neptune Islands Group Marine Park (2016–17). Report to the Department of the Environment, Water and Natural Resources. Adelaide, South Australia, Flinders University.
Huveneers, C., Meekan, M. G., Apps, K., Ferreira, L. C., Pannell, D., and Vianna, G. M. S. (2017). The economic value of shark-diving tourism in Australia. Rev. Fish Biol. Fish. 27, 665–680. doi: 10.1007/s11160-017-9486-x
Huveneers, C., Rogers, P. J., Beckmann, C., Semmens, J. M., Bruce, B. D., and Seuront, L. (2013). The effects of cage-diving activities on the fine-scale swimming behavior and space use of white sharks. Mar. Biol. 160, 2863–2875. doi: 10.1007/s00227-013-2277-6
Huveneers, C., Watanabe, Y. Y., Payne, N. L., and Semmens, J. M. (2018). Interacting with wildlife tourism increases activity of white sharks. Conserv. Physiol. 6:coy019. doi: 10.1093/conphys/coy019
Jacoby, D. M. P., Croft, D. P., and Sims, D. W. (2012). Social behavior in sharks and rays: analysis, patterns and implications for conservation. Fish Fish. 13, 399–417. doi: 10.1111/j.1467-2979.2011.00436.x
Kahle, D., and Wickham, H. (2013). ggmap: spatial visualization with ggplot2. R J. 5, 144–161. doi: 10.32614/RJ-2013-014
Lear, K. O., Whitney, N. M., Brewster, L. R., Morris, J. J., Hueter, R. E., and Gleiss, A. C. (2017). Correlations of metabolic rate and body acceleration in three species of coastal sharks under contrasting temperature regimes. J. Exp. Biol. 220, 397–407. doi: 10.1242/jeb.146993
Lowe, C. G. (2002). Bioenergetics of free-ranging juvenile scalloped hammerhead sharks (Sphyrna lewini) in Kāne’ohe Bay, Ō’ahu, HI. J. Exp. Mar. Bio. Ecol. 278, 141–156. doi: 10.1016/S0022-0981(02)00331-3
MacDonald, C., Gallagher, A. J., Barnett, A., Brunnschweiler, J., Shiffman, D. S., and Hammerschlag, N. (2017). Conservation potential of apex predator tourism. Biol. Conserv. 215, 132–141. doi: 10.1016/j.biocon.2017.07.013
Madigan, D. J., Brooks, E. J., Bond, M. E., Gelsleichter, J., Howey, L. A., Abercrombie, D. L., et al. (2015). Diet shift and site-fidelity of oceanic whitetip sharks Carcharhinus longimanus along the Great Bahama Bank. Mar. Ecol. Prog. Ser. 529, 185–197. doi: 10.3354/meps11302
Maljković, A., and Côté, I. M. (2011). Effects of tourism-related provisioning on the trophic signatures and movement patterns of an apex predator, the Caribbean reef shark. Biol. Conserv. 144, 859–865. doi: 10.1016/j.biocon.2010.11.019
Meyer, C. G., Dale, J. J., Papastamatiou, Y. P., Whitney, N. M., and Holland, K. N. (2009). Seasonal cycles and long-term trends in abundance and species composition of sharks associated with cage diving ecotourism activities in Hawaii. Environ. Conserv. 36, 104–111. doi: 10.1017/S0376892909990038
Meyer, L., Pethybridge, H., Beckmann, C., Bruce, B., and Huveneers, C. (2019). The impact of wildlife tourism on the foraging ecology and nutritional condition of an apex predator. Tour. Manag. 75, 206–215. doi: 10.1016/j.tourman.2019.04.025
Miller, M. H., Carlson, J., Hogan, L., and Kobayashi, D. (2014). Status review report: great hammerhead shark (Sphyrna mokarran). Final Rep. to Natl. Mar. Fish. Serv. Off. Prot. Resour. 116.
Mourier, J., Planes, S., and Buray, N. (2013). Trophic interactions at the top of the coral reef food chain. Coral Reefs 32:285. doi: 10.1007/s00338-012-0976-y
Nelson, D. R., and Gruber, S. H. (1963). Sharks: attraction by low-frequency sounds. Science 142, 975–977. doi: 10.1126/science.142.3594.975
Orams, M. B. (2002). Feeding wildlife as a tourism attraction: a review of issues and impacts. Tour. Manag. 23, 281–293. doi: 10.1016/S0261-5177(01)00080-2
Parsons, G. R. (1990). Metabolism and swimming efficiency of the bonnethead shark Sphyrna tiburo. Mar. Biol. 104, 363–367. doi: 10.1007/BF01314338
Pierce, S. J., Méndez-Jiménez, A., Collins, K., Rosero-Caicedo, M., and Monadjem, A. (2010). Developing a code of conduct for whale shark interactions in Mozambique. Aquat. Conserv. Mar. Freshwat. Ecosyst. 20, 782–788. doi: 10.1002/aqc.1149
Piercy, A. N., Carlson, J. K., and Passerotti, M. S. (2010). Age and growth of the great hammerhead shark, Sphyrna mokarran, in the North-Western Atlantic Ocean and Gulf of Mexico. Mar. Freshw. Res. 61, 992–998. doi: 10.1071/MF09227
Pini-Fitzsimmons, J., Knott, N. A., and Brown, C. (2018). Effects of food provisioning on site use in the short-tail stingray Bathytoshia brevicaudata. Mar. Ecol. Prog. Ser. 600, 99–110. doi: 10.3354/meps12661
R Core Team (2020). R: a language and environment for statistical computing. R Foundation for Statistical Computing, Vienna, Austria. Available at: https://www.r-project.org/ (Accessed October 23, 2020).
Raoult, V., Broadhurst, M. K., Peddemors, V. M., Williamson, J. E., and Gaston, T. F. (2019). Resource use of great hammerhead sharks (Sphyrna mokarran) off eastern Australia. J. Fish Biol. 95, 1430–1440. doi: 10.1111/jfb.14160
Rigby, C. L., Barreto, R., Carlson, J., Fernando, D., Fordham, S., Francis, M. P., et al. (2019). Sphyrna mokarran. IUCN Red List Threat. Species 2019 e.T39386A2920499.
Ritchie, E. G., and Johnson, C. N. (2009). Predator interactions, mesopredator release and biodiversity conservation. Ecol. Lett. 12, 982–998. doi: 10.1111/j.1461-0248.2009.01347.x
Roff, G., Doropoulos, C., Rogers, A., Bozec, Y.-M., Krueck, N. C., Aurellado, E., et al. (2016). The ecological role of sharks on coral reefs. Trends Ecol. Evol. 31, 395–407. doi: 10.1016/j.tree.2016.02.014
Romanov, E. V., and Romanova, N. V. (2012). Size distribution and length-weight relationships for some large pelagic sharks in the Indian Ocean. Communication 2. Bigeye thresher shark, tiger shark, silvertip shark, sandbar shark, great hammerhead shark, and scalloped hammerhead shark.
RStudio Team (2020). RStudio: integrated development environment for R. RStudio, PBC, Boston, MA. Available at: http://www.rstudio.com/
Schluessel, V. (2015). Who would have thought that ‘Jaws’ also has brains? Cognitive functions in elasmobranchs. Anim. Cogn. 18, 19–37. doi: 10.1007/s10071-014-0762-z
Semeniuk, C. A. D., and Dill, L. M. (2005). Cost/benefit analysis of group and solitary resting in the cowtail stingray, Pastinachus sephen. Behav. Ecol. 16, 417–426. doi: 10.1093/beheco/ari005
Semeniuk, C. A. D., and Rothley, K. D. (2008). Costs of group-living for a normally solitary forager: effects of provisioning tourism on southern stingrays Dasyatis americana. Mar. Ecol. Prog. Ser. 357, 271–282. doi: 10.3354/meps07299
Semeniuk, C. A. D., Speers-Roesch, B., and Rothley, K. D. (2007). Using fatty-acid profile analysis as an ecologic indicator in the management of tourist impacts on marine wildlife: a case of stingray-feeding in the Caribbean. Environ. Manag. 40, 665–677. doi: 10.1007/s00267-006-0321-8
Smith, K., Scarr, M., and Scarpaci, C. (2010). Grey nurse shark (Carcharias taurus) diving tourism: tourist compliance and shark behaviour at Fish Rock, Australia. Environ. Manag. 46, 699–710. doi: 10.1007/s00267-010-9561-8
Strong, W. R., Snelson, F. F., and Gruber, S. H. J. (1990). Hammerhead shark predation on stingrays: an observation of prey handling by Sphyrna mokarran. Copeia 1990, 836–840. doi: 10.2307/1446449
Vianna, G. M. S., Meekan, M. G., Rogers, A. A., Kragt, M. E., Alin, J. M., and Zimmerhackel, J. S. (2018). Shark-diving tourism as a financing mechanism for shark conservation strategies in Malaysia. Mar. Policy 94, 220–226. doi: 10.1016/j.marpol.2018.05.008
Ward, A. J. W., Webster, M. M., and Hart, P. J. B. (2006). Intraspecific food competition in fishes. Fish Fish. 7, 231–261. doi: 10.1111/j.1467-2979.2006.00224.x
Wearmouth, V. J., and Sims, D. W. (2008). “Chapter 2 Sexual Segregation in Marine Fish, Reptiles, Birds and Mammals,” in Advances in Marine Biology. Vol. 54. ed. D. W. Sims (Academic Press), 107–170.
Winberg, G. G. (1960). Rate of metabolism and food requirements of fishes. Fish Res. Board Can. Trans. Ser. 194, 1–202.
Zimmerhackel, J. S., Kragt, M. E., Rogers, A. A., Ali, K., and Meekan, M. G. (2019). Evidence of increased economic benefits from shark-diving tourism in the Maldives. Mar. Policy 100, 21–26. doi: 10.1016/j.marpol.2018.11.004
Keywords: wildlife tourism, shark diving, bioenergetics, endangered species, elasmobranch
Citation: Heim V, Dhellemmes F, Smukall MJ, Gruber SH and Guttridge TL (2021) Effects of Food Provisioning on the Daily Ration and Dive Site Use of Great Hammerhead Sharks, Sphyrna mokarran . Front. Mar. Sci. 8:628469. doi: 10.3389/fmars.2021.628469
Edited by:
Juerg Michael Brunnschweiler, ETH Zürich, SwitzerlandReviewed by:
Katya Abrantes, James Cook University Singapore, SingaporeLuis Cardona, University of Barcelona, Spain
Copyright © 2021 Heim, Dhellemmes, Smukall, Gruber and Guttridge. This is an open-access article distributed under the terms of the Creative Commons Attribution License (CC BY). The use, distribution or reproduction in other forums is permitted, provided the original author(s) and the copyright owner(s) are credited and that the original publication in this journal is cited, in accordance with accepted academic practice. No use, distribution or reproduction is permitted which does not comply with these terms.
*Correspondence: Vital Heim, dml0YWwuaGVpbUB1bmliYXMuY2g=
†Deceased
‡ORCID: Vital Heim orcid.org/0000-0002-8492-9196
Félicie Dhellemmes orcid.org/0000-0002-2043-4653
Matthew J. Smukall orcid.org/0000-0003-3790-3644