- 1Department of Biological Sciences, School of Life Sciences and the Environment, Royal Holloway University of London, Egham, United Kingdom
- 2Department of Life Sciences, Natural History Museum, London, United Kingdom
Mesopelagic fishes were sampled around Tristan da Cunha and St Helena in the South Atlantic from the RRS Discovery at depths down to 1000 m. Sampling was part of the Blue Belt Programme, a marine survey of British Overseas Territories funded by the United Kingdom Government. Thirteen species of mesopelagic fishes identified from 30 specimens were compared with two species (two specimens) collected from rock pools or surface water near the shore. The digestive tracts of all fishes were examined for microplastics. Additionally, one specimen of Opostomias micripnus (Günther, 1878) was analyzed after recovery from the stomach of a commercially fished species, Hyperoglyphe antarctica (Carmichael, 1819). One specimen of Anoplogaster cornuta was found to have ingested a bearded sea devil (Linophryne sp.), a cock-eyed squid (Histioteuthis sp.), a bolitaenid octopus, Japetella diaphana, remains of unidentifiable fish, crustaceans, and possibly salps. These prey items were also examined for microfibres. Both Histioteuthis sp. and Linophryne sp. had ingested fibers and these were considered “ingested particles” for A. cornuta. Neither shallow water dwelling species had ingested microplastics, whilst 11 of the 13 studied mesopelagic species were found to be contaminated. Overall, 66.7% of mesopelagic fishes were found to contain microfibres. Anthropogenic fibers were common especially viscose, a semi-synthetic material which is associated with sanitary products as well as other items.
Introduction
Plastic production has continued to increase since its development in the 1970s. In 2018 alone, 359 million tonnes of plastic (not including synthetic fibers) was produced (PlasticsEurope, 2019), with an additional 63 million tonnes of synthetic fibers produced per annum (Lebreton and Andrady, 2019). For many reasons, plastic can enter the aquatic environment, creating a global problem (Shim et al., 2018) which has been recorded in the ocean for decades (Carpenter and Smith, 1972; Carpenter et al., 1972). It is estimated that 5.25 trillion pieces of plastic are floating on the surface of the ocean (Eriksen et al., 2014). Some of this plastic eventually descends from surface waters with the ocean floor proposed as a major sink. Consequently, plastic is increasingly reported in the deep sea, in both sediment and fauna (Woodall et al., 2014; Courtene-Jones et al., 2017; Chiba et al., 2018; Amon et al., 2020; Kane et al., 2020). In the Tyrrhenian Sea, Mediterranean, hotspots of up to 1.9 million microplastics per m2 have been recorded (Kane et al., 2020). Moreover, elasmobranchs caught at 500 m in these waters had a higher prevalence of microplastics in the digestive tract than sharks obtained from other areas (Valente et al., 2019). Since 2001, relatively large pieces of plastic have been found in 60% of the stomachs of lancetfish, Alepisaurus ferox, examined in Madeira (Manuel Biscoito, pers. comm., 2020).
Tristan da Cunha and St Helena are isolated islands with small populations (St Helena: 4800 residents, St Helena Government, 2015; Tristan: 270 residents, Scott, 2017). Respectively, the islands have an area of ca. 96 and 122 km2. The islands steeply descend into deep ocean, reaching depths of 3000 m within a few km of the coast (Scott, 2017). Fishing is the main source of income on Tristan with tourism from cruises and scientific expeditions providing additional revenue (Scott, 2017). As a larger island, St Helena receives more tourism but is also dependent on exporting fish and coffee (St Helena Government, 2015). Dependent on imports of food and resources, St Helena is regularly visited by cargo ships, with many vessels passing through the area (St Helena Government, 2015). Indeed, some of these vessels wreck in the area (Scott, 2017).
Microplastics in the environment are readily ingested by fish. Many articles focus on fishes due to their ease of sampling and consequently the literature is biased toward this group (de Sá et al., 2018). Deep-water species are underrepresented despite being highly abundant (Wieczorek et al., 2018), with the exception of lanternfish (Myctophidae) which are included in several studies (Boerger et al., 2010; Davidson and Asch, 2011; Lusher et al., 2016; Romeo et al., 2016; Wieczorek et al., 2018; Zhu et al., 2019). Regardless of foraging depth and geographical location ca. one third of fishes in any sampled population ingest plastic (Boerger et al., 2010; Lusher et al., 2013; McGoran et al., 2018). But this can vary, with mesopelagic myctophids reported to have contamination levels as low as 11% (Davidson and Asch, 2011; Lusher et al., 2016) whilst other studies have demonstrated ubiquitous contamination in deep-water species (Wieczorek et al., 2018; Zhu et al., 2019). It is therefore hypothesized that between ca. 10 and 30% of fishes sampled will be contaminated with microplastics. It is also hypothesized that active predators will ingest more microplastics than species implementing other feeding strategies due to trophic transfer and biomagnification of microplastics, which has been suggested in the literature (Courtene-Jones et al., 2017; Fang et al., 2018; Nelms et al., 2018).
Although utilizing a small sample size, this study highlights that mesopelagic fishes, which are relatively understudied, can ingest microplastics. The results from the present study provide a valuable insight into the potential impacts in an ecosystem known to be a significant sink for microplastics.
Materials and Methods
Sampling
Onboard RRS Discovery, between 11th March and 13th April 2019, pelagic sampling was carried out at night using a 25 m2 rectangular mid-water trawl (RMT25). The RMT25 consists of two nets that can be opened and closed remotely to sample discrete depth layers (e.g., 1000–700 m then 700–400 m). The nets were fitted with a reinforced “cod-end” container approximately 10 L in volume, which kept captured animals in good condition. The cod-end had a mesh of 5 mm and the net was divided in three with a 10 mm mesh near the cod-end and a 19 mm mesh toward the mouth of the net. Net hauls were undertaken on a total of 44 occasions around the islands of the Tristan da Cunha and St Helena (Figure 1) and their associated seamounts at depths between 0 and 1000 m. Stomachs had previously been removed from specimens of Hyperoglyphe antarctica (Carmichael, 1819) caught commercially by the Tristan da Cunha fishery. These were frozen and then later collected from Tristan during the cruise for examination onboard the RRS Discovery. The gut contents used in the present study came from an individual caught near Yakhont Seamount using a demersal longline at a depth of around 400 m (Laptikhovsky et al., 2020). Opportunistic sampling of shallow water fishes was undertaken, though this was outside the scope of the expedition and was not the focus of the present study.
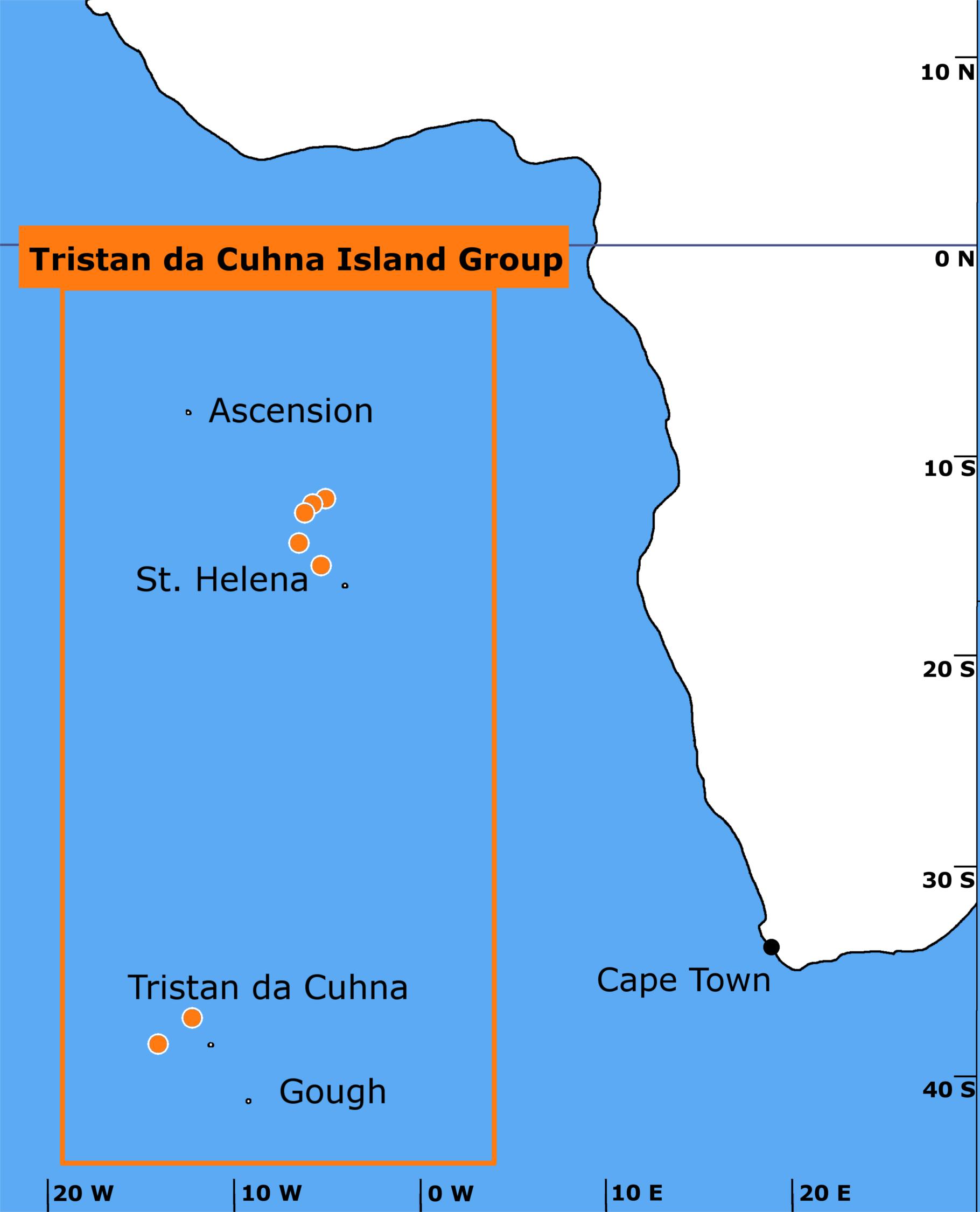
Figure 1. Trawls (marked as orange dots with white borders) were conducted in the South Atlantic off St. Helena and Tristan da Cunha. A total of 44 trawls were implemented. One fish (Gaidropsarus novaezealandiae) was also collected from a rockpool on Tristan da Cunha and another (Patagonotothen guntheri) was caught in surface waters near the Falkland Islands.
Material
A total of 30 South Atlantic mesopelagic fishes comprising 13 species were examined including: Anoplogaster cornuta (n = 2, active predator), Argyropelecus gigas (n = 1, planktivorous), Borostomias elucens (n = 1, active predator), Chauliodus sloani (n = 4, active predator), Ectreposebastes imus (n = 2, planktivorous), Idiacanthus atlanticus (n = 1, active predator), Lampanyctus australis (n = 4, planktivorous), Macrouroides inflaticeps (n = 1, benthic feeder), Melanonus zugmayeri (n = 1, planktivorous), Serivomer beanii (n = 6, planktivorous), Sigmops elongatus (Günther, 1878) (n = 5, active predator), Snyderidia canina (n = 1, planktivorous), and Opostomias micripnus (Günther, 1878) (obtained from the stomach of H. antarctica) (n = 1, active predator). In addition, two fishes collected from surface waters near the shore at Tristan da Cunha and the Falkland Islands were chosen for comparison, namely Gaidropsarus novaezealandiae (n = 1, feeds on amphipods in shallow water) and Patagonotothen guntheri (n = 1, feeds on small amphipods in shallow water).
For this study the above species were examined for microplastics (Supplementary Table 1) before being deposited in the collections of the Natural History Museum, London along with the other specimens caught during the Discovery survey.
Contamination Controls
For all laboratory work, a clean cotton laboratory coat and non-sterile, single-use gloves were worn. Work areas were cleaned prior to processing with filtered (32 μm nylon mesh) industrial methylated spirit (IMS, 80%). Equipment was rinsed with filtered IMS prior to dissection and in between samples. Scalpels, forceps, scissors, and mounted pins were inspected for plastics under a Leica MZ 6 microscope prior to use. Filtered (32 μm nylon mesh) distilled water was used to prepare a potassium hydroxide (KOH, 10%) solution, which was kept in glass bottles that had been rinsed three times with filtered distilled water.
Airborne contamination was recorded by empty Petri dishes placed next to samples at all stages of processing. These controls were implemented one per day dissecting or searching. Searching and dissection controls were always collected separately (i.e., a separate blank for each process). Instead of removing an average across all controls during dissection or searching, an average was collected per session. For example, if 10 fish were dissected in one session, one tenth of the recorded contamination, rounded to the nearest whole number, would be removed from the total number of items recorded for each individual in that session. This was repeated for all sessions. Procedural blanks of filtered distilled water were implemented during digestion. An average number of items resulting from contamination was recorded across digestion controls and the appropriate value removed from the counts for samples.
Plastic Extraction
Fish samples were identified on board RRS Discovery and initially preserved in 5% formalin before being transferred to 70% ethanol for long-term storage. Prior to dissection, standard length, to the caudal peduncle (to nearest mm), total length, to the end of the caudal fin (to nearest mm) and mass (Ohaus ranger 3000 balance, nearest 0.1 g) were recorded. The entire digestive tract was carefully removed through a small incision in the ventral surface and sectioned along its length to reveal any intact prey items which were then also examined for microplastics. Prey items and the digestive tract were placed in separate 15 ml Falcon tube. Twice the volume of 10% KOH compared to the sample volume was added to the tube and heated at 60°C overnight. The solution was filtered through a 32 μm nylon mesh with a vacuum pump. The tubes were rinsed with filtered distilled water a minimum of three times or until all visible material had been removed. Filters were stored in glass Petri dishes and dried at 60°C. Subsequently, filters were examined under a Leica MZ 6 microscope using mounted pins and forceps under 16–64 times magnification with a detection limit of 32 μm. The morphology, including shape and color, of all recovered items was described, and items measured (length and width) using ImageJ. Morphology was informed by Rochman et al. (2019) and Lusher et al. (2020).
FTIR Spectroscopy
FTIR spectroscopy is well documented for microplastic analysis (Lusher et al., 2017). Analysis of plastic pieces recovered from Sigmops elongatus, Lampanyctus australis, Ectreposebastes imus, Chauliodus sloani, and Borostomias elucens was undertaken using a PerkinElmer Spectrum One FTIR spectrometer, with an AutoIMAGE FTIR Microscope System PerkinElmer attachment. All fibers from fish were individually analyzed. A background spectrum was made before analysis and updated between samples. A total of 16 scans were collected for each item, with the average result being used to generate an absorption spectrum between 500 and 4000 cm–1. The output was visually compared to the “NHM Plastic Collection” spectra library. All the samples from the other fish species were analyzed with a Nicolet iN10 MX Infrared Microscope in OMNIC Picta. Absorption spectra were collected with an MCT-A detector over 16 scans at a resolution of 4 cm–1 in the range 650–4000 cm–1. The output was visually compared to the “NHM Plastic Collection” spectral library as well as commercially available libraries (Supplementary Table 2). No confidence threshold was used to determine polymer identity; instead, the identity was confirmed visually by the presence of matching key peaks in the spectrum.
Statistical Analysis
Statistical analysis was conducted using R version 3.4.2 with R version R-4.0.3 (R Core Team, 2020). Statistical analysis was limited due to the small sample size. To compensate for this, species were grouped into the feeding types (n = 4) listed above (see section “Material”), which included a separate group for shallow water fishes. This allowed for comparison with the mesopelagic groups. Plastic ingestion between feeding type was compared with a generalised linear model (GLM) with negative binomial error distribution (p-value threshold <0.05). Feeding type and standard length were included in initial models and factors were removed in a stepwise manner if not significant as determined by p-values. Models were also compared with Akaike information criterion (AIC) numbers to find the optimal fit. The model with the lowest AIC score was considered the best fit. Packages “multcomp” (Hothorn et al., 2008), “mvabund” (Wang et al., 2020), “lme4” (Bates et al., 2015), and “ggplot2” (Wickham, 2016) were used for analysis.
Results
Contamination controls contained on average one blue fiber, one clear fiber, one black fiber, <1 clear film, and <1 purple fiber per fish processed. Objects in samples matching those from contamination controls were removed for all fish. All potential plastics recovered from fish were analyzed by FTIR. Spectroscopy produced identifiable spectra for 45 items. Viscose was the most common material identified (51.1%), with cotton fibers also abundant (35.6%). The remaining items matched polyester (8.9%), with one item producing a match with calcium carbonate, one matching tissue and another matching wool. After accounting for contamination, 62.5% of individuals (n = 20 of n = 32) contained items in the GIT, with an average of 1.3 ± 2.2 SD pieces per fish. Overall, 73.3% of species (n = 11 of n = 15) were contaminated with microplastics. Length was not a significant factor influencing microplastic ingestion (GLM, p > 0.05) whilst feeding type was significantly different (GLM, p < 0.05). On average, active predators ingested 2.1 ± 3 SD microplastics compared to 0.8 ± 0.9 SD microplastics is planktivores, 1 ± 0 SD in benthic feeders and 0 ± 0 SD in shallow water species. Limited sample size restricts the conclusions which can be drawn from this analysis, but indicates possible trends for future study. Figure 2 depicts all recorded values of plastic ingestion and demonstrates that Anoplogaster cornuta and B. elucens ingested the most plastic on average, however, both are only represented by one individual. Both species are active predators, which ingested more microplastics on average than other feeding types (Figure 3). One individual of A. cornuta was found to have ingested a bearded sea devil (Linophryne sp.), a cock-eyed squid (Histioteuthis sp.), a bolitaenid octopus, Japetella diaphana, remains of unidentifiable fish, crustaceans, and possibly salps, almost certainly an example of “net feeding.” Both Linophryne sp. and Histioteuthis sp. had ingested fibers, one and eight items, respectively, and these were considered “ingested particles” for A. cornuta. A total of seven colors and three shapes of plastic were recovered: blue fiber, blue film, blue fragment, black fiber, gray fiber, red fiber, clear fiber, white fiber, and purple fiber. Microplastics were 97.4 μm to 16.9 mm in length and 7.5 to 92.3 μm wide. Fibers were the most abundant form of microplastics (93%) and blue was the most common color of plastic (39%) followed by black (28%).
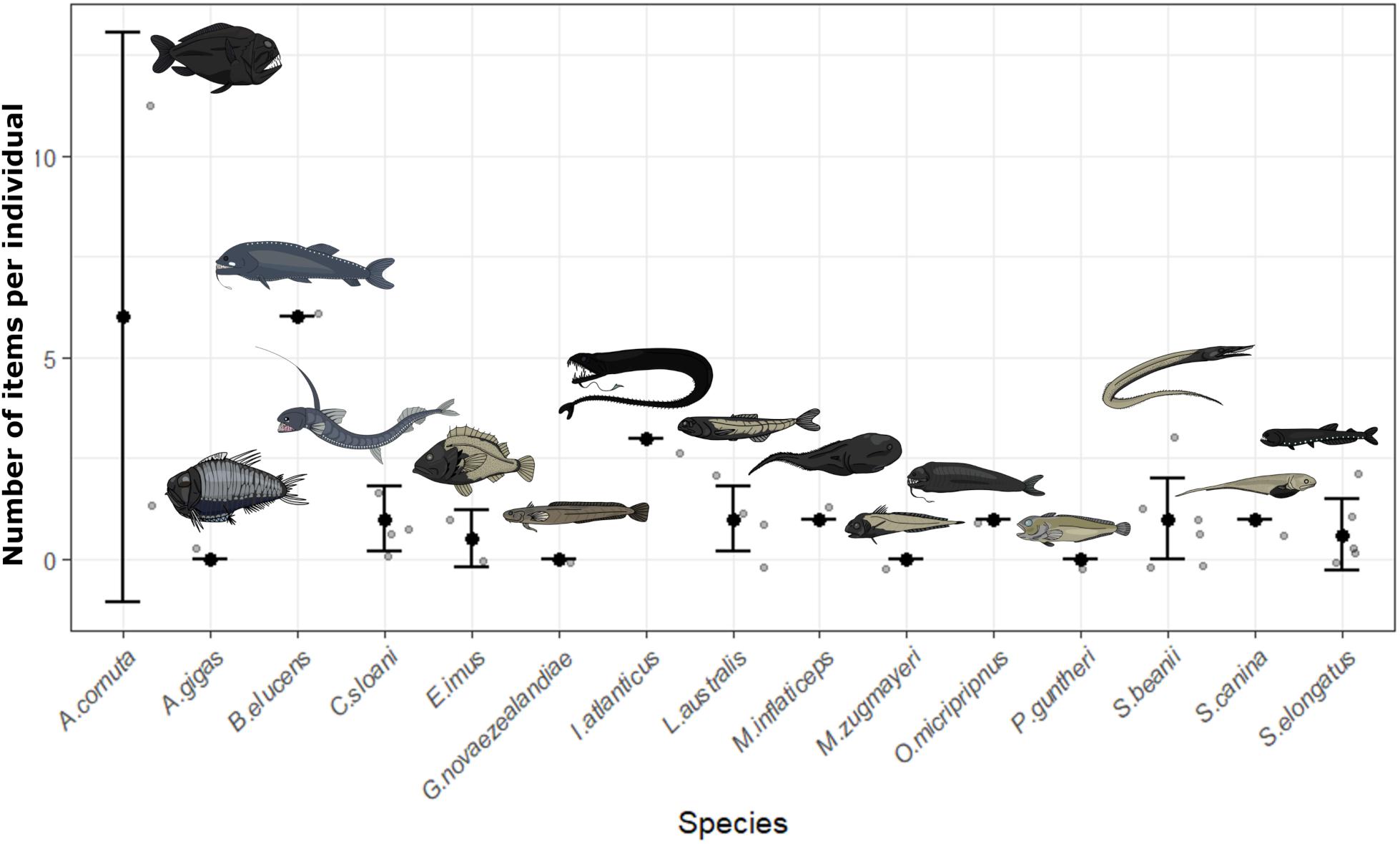
Figure 2. The number of fibers ingested by 13 mesopelagic fish species and two species collected from rockpools and surface waters (Gaidropsarus novaezealandiae, Patagonotothen guntheri). Gray points represent data for individual fish whilst black dots are the mean with whiskers of ± one standard deviation.
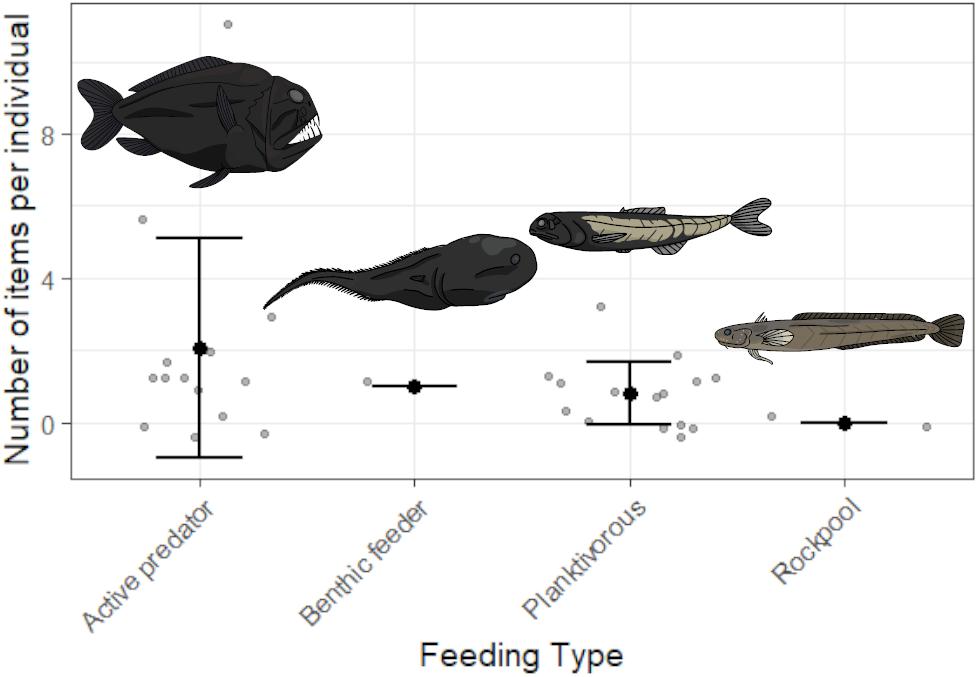
Figure 3. The number of fibers ingested by four distinct groups of species separated by their feeding type. Gray points represent data for individual fish whilst black dots are the mean with whiskers of ± one standard deviation. Active predators include: Borostomias elucens, Anoplogaster cornuta, Chauliodus sloani, Sigmops elongatus, Opostomias micripnus, Idiacanthus atlanticus; benthic feeders: Macrouroides inflaticeps; planktivorous species: Ectreposebastes imus, Lampanyctus australis, Argyropelecus gigas, Melanonus zugmayeri, Snyderidia canina, Serivomer beanii; non-mesopelagic species collected from rockpools or surface waters: Gaidropsarus novaezealandiae, Patagonotothen guntheri.
Discussion
A greater proportion of fishes were contaminated than expected, e.g., 62.5% compared to a third of fishes hypothesized to have ingested microplastic). Many factors, for example, foraging location can influence plastic exposure. Davidson and Asch (2011) noted that vertically migrating fishes (including myctophids) ingested higher concentrations of plastic than non-vertically migrating species from the same area, indicating that feeding depth significantly impacted plastic exposure. But the same was not observed by Lusher et al. (2016), Wieczorek et al. (2018) or Zhu et al. (2019), who all found no significant difference in ingestion with depth. Sathish et al. (2020) proposed proximity to anthropogenic sources of contamination has greater influence, with epipelagic fishes living in coastal waters exposed to more plastic than mesopelagic species as they were closer to wastewater outfalls. Yet, in the present study, two species collected from shallow water, which therefore might be expected to be feeding on local sources of plastic pollution, had not ingested any microplastics.
Feeding strategy can also impact plastic ingestion as seen in the present study. Two species ingested more plastic than the others studied: A. cornuta and B. elucens. Both are active predators, as are C. sloani and Sigmops elongatus. These predators feed on relatively large prey, such as fishes, cephalopods and crustaceans (Battaglia et al., 2018; Luna and Sampang-Reye, 2020; Torres and Kesner-Reye, 2020), with C. sloani preying almost exclusively on fishes, including myctophids, gonostomatids and other stomiids (Williams et al., 2001; Battaglia et al., 2018), all represented in the present study. Indeed, one of the two studied A. cornuta specimens was found to have ingested an octopus, squid and anglerfish. It is clear that plastic can potentially be transferred from prey to predator, with A. cornuta ingesting at least two contaminated prey items. This has also been reported in the literature (Courtene-Jones et al., 2017; Fang et al., 2018; Nelms et al., 2018). Other species feed on smaller prey, e.g., E. imus feeds primarily on amphipods and shrimp (Luna and Ortañez, 2020) and L. australis on zooplankton, such as copepods and euphausiids (Williams et al., 2001). Planktivorous fishes may selectively feed on plastic that resembles their prey (Boerger et al., 2010; Ory et al., 2017). Often blue plastic is targeted, as was seen by Ríos et al. (2020). The same study reported that smaller fishes ingested more plastic than larger individuals. It is possible that these fish, which might be feeding on smaller prey, could actively ingest plastic through mistaken identity. It is also possible that suction feeding (e.g., M. inflaticeps) could cause plastic ingestion with prey capture (Bermúdez-Guzmán et al., 2020). Some studies, however, demonstrate that feeding mechanics is not a significant factor (Sathish et al., 2020). If a larger sample size was available, other factors might need to be investigated to further determine why some species may be more affected than others. It is likely that microplastics are ubiquitous in the marine environment and small enough that all fishes are exposed.
Few studies have investigated plastic pollution in the South Atlantic, with most studies focussed on the coasts of South Africa and South America. In Brazil, chips of paint and fragments of fibers, thought to have originated from fishing vessels, were found in the surface water (Ivar do Sul et al., 2014). Fishes from Argentina were also contaminated and had ingested an average 1.6 microplastics, mostly fibers, per gram wet weight (Ríos et al., 2020). On the South African coast, sub-surface water samples contained microfibres, mostly semi-synthetic polymers (Kanhai et al., 2017). These fibers were though to have originated from clothing, fishing gear and ropes. Commercial fishes in the area had consumed microplastics. In total, 87% of fishes were contaminated and contained 3.72 ± 2.73 items per fish (Sparks and Immelman, 2020).
South Africa is one of the greatest contributors to ocean plastic globally (Ryan, 2020) and models of plastic release predicted that a third of plastic released there is exported to the open ocean. Low density polymers were carried to the Atlantic (e.g., 80% of low density plastic released from Cape Town) and high density polymers accumulated on the continental shelf (Collins and Hermes, 2019). The model did not include tides, wave action or vertical mixing and thus beached plastic may be resuspended and high density polymers may not sink as rapidly as proposed. Models have predicted that more plastic should be beached than has been recovered. Thus, resuspension is an important consideration (Ryan, 2020). As well as currents, plastic can be transported to remote environments by animals, such as seabirds which can feed great distances from where they roost and breed. Large colonies of seabirds, such as albatrosses, are present on Tristan (Scott, 2017). Plastic does not remain bouyant indefinitely and the seafloor is often considered the final sink for plastic (Ryan, 2020). Additionally, seamounts can increase the retention and accumulation of plastic debris (Woodall et al., 2015) and many seamounts surround Tristan (Scott, 2017). Similarly, wrecks surrounding the islands could be trapping plastic as well as being a potential source. This could expose mesopelagic fishes to more microplastics than surface-dwelling species.
It can be difficult to distinguish cellulosic fibers with FTIR, those identified in this present study most commonly matched viscose in the spectral library. Viscose is a semi-synthetic fiber consisting of modified cellulose. Whilst primarily consisting of organic, plant-based material its production is not always sustainable, can result in chemical pollution and the end product does not biodegrade in all environments, including landfill (Council of Fashion Designers of America (CFDA), 2016). The material is used in both the fashion textile industry (Changing Markets Foundation and The Forest Trust, 2018) and in sanitary products (Always, 2020; Bodyform, 2020). Landfill on St Helena could be a source of plastic runoff into the marine environment. Few recycling facilities are available on the island and a large amount of waste is sent to landfill, 10% of which is plastic (St Helena Government, 2015).
As in many studies, fibers were the most common microplastics recorded here. In most studies in the Atlantic, fishing gear and ropes are thought to be main source of plastic in the Atlantic. Indeed, Monteiro et al. (2018) reported that fishing gear was commonly recovered from island shorelines. Longline and rock lobster fishing are major sources of income in the Tristan da Cunha island group (Scott, 2017) and could be a major source of fibers in the environment. It is also an issue for the seabirds colonizing Tristan and the surrounding islands, which are often fatally entangled in longlines (Scott, 2017). Yet, in the present study, nylon and polypropylene, polymers commonly used in the fishing industry were not recovered. Polyester, however, was and a possible source could be effluent from washing machines on the island and mainland. Washing machine outfalls release average of between 700 thousand fibers (Napper and Thompson, 2016) and 6 million fibers (De Falco et al., 2018) per 5–6 kg load. Estimates even reach as high as 13 million fibers per kg of washing (Sillanpää and Sainio, 2017). Fortunately, wastewater treatment plants can recover 95% of fibers (Talvitie et al., 2017) preventing the direct release of many fibers into the environment. Accumulations of fibers, not recovered during treatment, still enter the environment and the sludge from treatment which retains many fibers is often used as fertilizer on agricultural land and run off from fields to water courses is still possible (Hurley et al., 2018). The introduction of improved filters in washing machines and treatment plants may reduce this source of contamination.
Additionally, billions of feminine hygiene products and wet wipes are flushed down toilets and can enter aquatic environments (O’Neill, 2019; McCoy et al., 2020; Women’s Environmental Network., 2020) and rapidly fragment (Williams and Simmons, 1996). The resulting fibers can be ingested (McCoy et al., 2020; McGoran et al., 2020). The authors recommend that communication between producers and consumers be improved, perhaps through improved product labeling.
Whilst there are attempts to remove plastic waste from aquatic environments (e.g., McCarthy and Sanchez, 2019; The Ocean Clean Up, 2020) there are concerns over the impact on organisms as a result of these techniques. Certainly, filters capable of retaining microplastics have the potential to trap and remove plankton; thus, having a cascade effect through the food chain. In short, stopping plastic at the source is a more effective and attainable target for management.
Microplastics can affect different levels in an ecosystem, from cellular impacts and individual mortality to population wide effects (Galloway et al., 2017). Once ingested, microplastics can cause physical and chemical damage, with additives and adsorbed persistent organic pollutants (POPs) potentially leaching into tissues (Moore, 2008; Wright et al., 2013). Reviews by Lusher et al. (2017) and Foley et al. (2018) have noted that microplastic ingestion can negatively impact growth, immune response, food consumption, fecundity and energy levels as well as having generational effects. The effects, however, varied between taxa. The growth and feeding rate of juvenile fishes are negatively impacted by exposure to microplastics (Critchell and Hoogenboom, 2018). Studies on the effects of microplastic ingestion have focused on microbeads despite the abundance of fibers, such as those recorded in the present study, in the environment. Ziajahromi et al. (2017) demonstrated that microfibres cause greater detrimental effects than microbeads. Additionally, POPs leached from microplastics can have additional effects such as endocrine disruption (Wright et al., 2013; Lusher et al., 2017). Moreover, if plastic ingestion causes mortality in lower trophic level organisms, thereby affecting prey abundance, there may be broader implications for oceanic food webs (Foley et al., 2018; Nelms et al., 2018). Indeed, if commercial species of fish ingest microplastics there is the potential for reduced growth and fitness to adversely affect the quality of the product and potentially pose a risk to human health.
Conclusion
Microfibres are abundant in the marine environment and persist at great depths below the surface. These fibers are readily ingested by mesopelagic fishes and their prey and have the potential to pass plastic to larger predators if they are then consumed. Thus there is the risk that commercial species of fish may be contaminated with microplastics or the chemicals associated with them. As yet, management of microplastic waste is insufficient to prevent leaks into the environment with the consequence that many remote habitats are impacted.
Data Availability Statement
The datasets presented in this study can be found in online repositories. The names of the repository/repositories and accession number(s) can be found below: Natural History Museum Repository (http://hdl.handle.net/10141/622875).
Author Contributions
AM: conceptualization, formal analysis, funding acquisition, investigation, methodology, visualization, writing – original draft, writing, review, and editing. JM: methodology, resources, writing – original draft, writing, review, and editing. PC: funding acquisition, methodology, supervision, writing, review, and editing. DM: funding acquisition, methodology, supervision, writing, review, and editing. All authors contributed to the article and approved the submitted version.
Funding
This work was supported by the National Environment Research Council (Grant Number NE/L002485/1) with co-sponsorship from a Fishmongers’ Company’s Fisheries Charitable Trust CASE Partnership. Specimens were collected onboard RRS Discovery as part of the Blue Belt Programme, a marine survey of British Overseas Territories, which is funded by the United Kingdom Government in collaboration with CEFAS and BAS.
Conflict of Interest
The authors declare that the research was conducted in the absence of any commercial or financial relationships that could be construed as a potential conflict of interest.
Acknowledgments
The authors would like to acknowledge the skipper and crew of RRS Discovery for their assistance with sampling, and would also to thank the scientists from BAS, CEFAS and St Helena with their help in processing the specimens. In addition, the authors thank Jon Ablett and Kirsty Lloyd, Natural History Museum for their assistance whilst on board RRS Discovery and also thank Jon for identifying the cephalopods ingested by Anoplogaster cornuta. Thanks also to Dr. Alexander Arkhipkin for providing the Falklands specimen. Alex McGoran would like to acknowledge Dr. Wren Montgomery and Dr. Emma Humphreys-Williams for setting up the FTIR spectrometer and their help and training with FTIR analysis.
Supplementary Material
The Supplementary Material for this article can be found online at: https://www.frontiersin.org/articles/10.3389/fmars.2021.633478/full#supplementary-material
References
Always (2020). What ingredients are in Always pads?. Available online at: https://always.com/en-us/about-us/what-ingredients-are-in-always-pads. (accessed 20, October 2020).
Amon, D. J., Kennedy, B. R. C., Cantwell, K., Suhre, K., Glickson, D., Shank, T. M., et al. (2020). Deep-sea debris in the central and western Pacific Ocean. Front. Mar. Sci. 7:369. doi: 10.3389/fmars.2020.00369
Bates, D., Maechler, M., Bolker, B., and Walker, S. (2015). Fitting linear mixed-effects models using lme4. J. Stat. Softw. 67, 1–48.
Battaglia, P., Ammendolia, G., Esposito, V., Romeo, T., and Andaloro, F. (2018). Few but relatively large prey: trophic ecology of Chauliodus sloani (Pisces: Stomiidae) in deep waters of the central Mediterranean Sea. J. Ichthy. 58, 8–16. doi: 10.1134/S0032945218010034
Bermúdez-Guzmán, L., Alpízar-Villalobos, C., Gatgens-García, J., Jiménez-Huezo, G., Rodríguez-Arias, M., Molina, H., et al. (2020). Microplastic ingestion by a herring Opisthonema sp. in the Pacific coast of Costa Rica. Reg. Stud. Mar. Sci. 38:101367. doi: 10.1016/j.rsma.2020.101367
Bodyform (2020). Ingredients. Available online at: https://www.bodyform.co.uk/ingredients/. (accessed 20, October 2020).
Boerger, C. M., Lattin, G. L., Moore, S. L., and Moore, C. J. (2010). Plastic ingestion by planktivorous fishes in the North Pacific Central Gyre. Mar. Pollut. Bull. 60, 2275–2278. doi: 10.1016/j.marpolbul.2010.08.007
Carpenter, E. J., Anderson, S. J., Harvey, G. R., Miklas, H. P., and Peck, B. B. (1972). Polystyrene spherules in coastal waters. Sci. 178, 749–750. doi: 10.1126/science.178.4062.749
Carpenter, E. J., and Smith, K. L. Jr. (1972). Plastics on the Sargasso Sea. Sci. 175, 1240–1241. doi: 10.1126/science.175.4027.1240
Changing Markets Foundation and The Forest Trust. (2018). Roadmap towards responsible viscose and modal fibre manufacturing. Available online at: https://changingmarkets.org/wp-content/uploads/2018/03/Roadmap-towards-responsible-viscose-and-modal-fibre-manufacturing.pdf. (accessed 20, October 2020).
Chiba, S., Saito, H., Fletcher, R., Yogi, T., Kayo, M., Miyagi, S., et al. (2018). Human footprint in the abyss: 30 year records of deep-sea plastic debris. Mar. Policy 96, 204–212. doi: 10.1016/j.marpol.2018.03.022
Collins, C., and Hermes, J. C. (2019). Modelling the accumulation and transport of floating marine micro-plastics around South Africa. Mar. Pollut. Bull. 139, 46–58. doi: 10.1016/j.marpolbul.2018.12.028
Council of Fashion Designers of America (CFDA) (2016). Rayon (viscose). Available online at: https://cfda.com/resources/materials/detail/rayon-viscose. (accessed 20, October 2020).
Courtene-Jones, W., Quinn, B., Gary, S. F., Mogg, A. O., and Narayanaswamy, B. E. (2017). Microplastic pollution identified in deep-sea water and ingested by benthic invertebrates in the Rockall Trough. North Atlantic Ocean. Environ. Pollut. 231, 271–280. doi: 10.1016/j.envpol.2017.08.026
Critchell, K., and Hoogenboom, M. O. (2018). Effects of microplastic exposure on the body condition and behaviour of planktivorous reef fish (Acanthochromis polyacanthus). PLoS One 13:e0193308. doi: 10.1371/journal.pone.0193308
Davidson, P., and Asch, R. G. (2011). Plastic ingestion by mesopelagic fishes in the North Pacific Subtropical gyre. Mar. Ecol. Prog. Ser. 432, 173–180. doi: 10.3354/meps09142
De Falco, F., Gullo, M. P., Gentile, G., Di Pace, E., Cocca, M., Gelabert, L., et al. (2018). Evaluation of microplastic release caused by textile washing processes of synthetic fabrics. Environ. Pollut. 236, 916–925. doi: 10.1016/j.envpol.2017.10.057
de Sá, L. C., Oliveira, M., Ribeiro, F., Rocha, T. L., and Futter, M. N. (2018). Studies of the effects of microplastics on aquatic organisms: what do we know and where should we focus our efforts in the future? Sci. Total Environ. 645, 1029–1039. doi: 10.1016/j.scitotenv.2018.07.207
Eriksen, M., Lebreton, L. C. M., Carson, H. S., Thiel, M., Moore, C. J., Borerro, J. C., et al. (2014). Plastic pollution in the world’s oceans: more than 5 trillion plastic pieces weighing over 250,000 tons afloat at sea. PLoS One 9:e111913. doi: 10.1371/journal.pone.0111913
Fang, C., Zheng, R., Zhang, Y., Hong, F., Mu, J., Chen, M., et al. (2018). Microplastic contamination in benthic organisms from the Arctic and sub-Arctic regions. J. Chemospher. 209, 298–306. doi: 10.1016/j.chemosphre.2018.06.101
Foley, C. J., Feiner, Z. S., Malinich, T. D., and Hook, T. O. (2018). A meta-analysis of the effects of exposure to microplastics on fish and aquatic invertebrates. Sci. Total Environ. 63, 550–559. doi: 10.1016/j.scitotenv.2018.03.046
Galloway, T. S., Cole, M., and Lewis, C. (2017). Interactions of microplastic debris throughout the marine ecosystem. Nat. Ecol. Evolut. 1:0116. doi: 10.1038/s41559-017-0116
Hothorn, T., Bretz, F., and Westfall, P. (2008). Simultaneous inference in general parametric models. Biom. J. 50, 346–363. doi: 10.1002/bimj.200810425
Hurley, R., Schell, T., Crossman, J., Rico, A., Nawrocki, B., Lavoy, M., et al. (2018). Presented in part at Micro 2018, Lanzarote, Canary Islands, Spain, November 2018. available online at: https://micro2018.sciencesconf.org/data/pages/micro2018_proceedings_book_1.pdf (accessed 3, December 2018).
Ivar, do Sul, J. A., Costa, M. F., and Fillmann, G. (2014). Microplastics in the pelagic environment around oceanic islands of the Western Tropical Atlantic Ocean. Water Air Soil Pollut. 225:2004. doi: 10.1007/s11270-014-2004-z
Kane, I. A., Clare, M. A., Miramontes, E., Wogelius, R., Rothwell, J. J., Garreau, P., et al. (2020). Seafloor microplastic hotspots controlled by deep-sea circulation. Sci. 2020:eaba5899. doi: 10.1126/science.aba5899
Kanhai, L. D. K., Officer, R., Lyashevska, O., Thompson, R. C., and O’Connor, I. (2017). Microplastic abundance, distribution and composition along a latitudinal gradient in the Atlantic Ocean. Mar. Pollut. Bull. 115, 307–314. doi: 10.1016/j.marpolbul.2016.12.025
Laptikhovsky, V., Bell, J. B., Benedet, R., Glass, J., Glass, W., Green, R., et al. (2020). Feeding habits of bluenose warehou, Hyperoglyphe antarctica (Carmichael, 1819) (Centrolophidae) at seamounts of the Southern Atlantic. Deep-Sea Res. Part I: Oceanog. Res. Pap. 156:103182. doi: 10.1016/j.dsr.2019.103182
Lebreton, L., and Andrady, A. (2019). Future scenarios of global plastic waste generation and disposal. Palgrave Comm. 5:6. doi: 10.1057/s41599-018-0212-7
Luna, S. M., and Ortañez, A. K. (2020). Fishbase: Ectreposebactes imus Garman, 1899). Available online at: https://www.fishbase.se/summary/Ectreposebastes-imus.html. (accessed 14, July 2020).
Luna, S. M., and Sampang-Reye, A. G. (2020). Fishbase Borostomias antarcticus (Lönnberg, 1905). Available online at: https://www.fishbase.se/summary/5098. (accessed 14, July 2020).
Lusher, A. L., Bråte, I. L. N., Munno, K., Hurley, R. R., and Welden, N. A. (2020). Is it or isn’t it: the importance of visual classification in microplastic characterization. Applied Spectro. Special issue early view 2020:0003702820930733. doi: 10.1177/0003702820930733
Lusher, A. L., Welden, N. A., Sobral, P., and Cole, M. (2017). Sampling, isolating and identifying microplastics ingested by fish and invertebrates. Anal. Methods 9, 1346–1360. doi: 10.1039/C6AY02415G
Lusher, A. L., McHugh, M., and Thompson, R. C. (2013). Occurrence of microplastics in the gastrointestinal tract of pelagic and demersal fish from the English Channel. Mar. Pollut. Bull. 67, 94–99. doi: 10.1016/j.marpolbul.2012.11.028
Lusher, A. L., O’Donnell, C., Officer, R., and O’Connor, I. (2016). Microplastic interactions with North Atlantic mesopelagic fish. ICES J. Mar. Sci. 73, 1214–1225. doi: 10.1093/icesjms/fsv241
McCarthy, J., and Sanchez, E. (2019). Activists remove 40 tons of plastic waste from Pacific Ocean. Available online at: https://www.globalcitizen.org/en/content/40-tons-plastic-removed-ocean/. (accessed 20, October 2020).
McCoy, K. A., Hodgson, D. J., Clark, P. F., and Morritt, D. (2020). The effects of wet wipe pollution on the Asian clam, Corbicula fluminea (Mollusca: Bivalvia) in the River Thames, London. Environ. Pollut. 264:114577. doi: 10.1016/j.envpol.2020.114577
McGoran, A. R., Clark, P. F., Smith, B. D., and Morritt, D. (2020). High prevalence of plastic ingestion by Eriocheir sinensis and Carcinus maenas (Crustacea: Decapoda: Brachyura) in the Thames Estuary. Environ. Pollut. 265:114972. doi: 10.1016/j.envpol.2020.114972
McGoran, A. R., Cowie, P. R., Clark, P. F., McEvoy, J. P., and Morritt, D. (2018). Ingestion of plastic by fish: a comparison of Thames Estuary and Firth of Clyde populations. Mar. Pollut. Bull. 137, 12–23. doi: 10.1016/j.marpolbul.2018.09.054
Monteiro, R. C. P., Ivar, do Sul, J. A., and Costa, M. F. (2018). Plastic pollution in islands of the Atlantic Ocean. Environ. Pollut. 238, 103–110. doi: 10.1016/j.envpol.2018.01.096
Moore, C. J. (2008). Synthetic polymers in the marine environment: a rapidly increasing, long-term threat. Environ. Res. 108, 131–139. doi: 10.1016/j.envres.2008.07.025
Napper, I. E., and Thompson, R. C. (2016). Release of synthetic plastic fibres from domestic washing machines: effects of fabric type and washing conditions. Mar. Pollut. Bull. 112, 39–45. doi: 10.1016/j.marpolbul.2016.09.025
Nelms, S. E., Galloway, T. S., Godley, B. J., Jarvis, D. S., and Lindeque, P. K. (2018). Investigating microplastic trophic transfer in marine top predators. Environ. Pollut. 238, 999–1007. doi: 10.1016/j.envpol.2018.02.016
O’Neill, E. (2019). Campaigning for plastic-free periods. Available online at: https://www.mcsuk.org/news/period-plastic. (accessed 21, October 2020).
The Ocean Clean Up (2020). Oceans: cleaning up the garbage patches. Available online at: https://theoceancleanup.com/oceans/. (accessed 20, October 2020).
Ory, N. C., Sobral, P., Ferreira, J. L., and Thiel, M. (2017). Amberstripe scal Decapterus muroadsi (Carangidae) fish ingest blue microplastics resembling their copepod prey along the coast of Rapa Nui (Easter Island) in the South Pacific subtropical gyre. Sci. Total Environ. 586, 430–437. doi: 10.1016/j.scitotenv.2017.01.175
PlasticsEurope (2019). Plastics – the facts 2019: an analysis of European plastics production, demand and waste data. PlasticsEurope, Brussels 2019, 1–42.
R Core Team (2020). R: A language and environment for statistical computing. R Foundation for Statistical Computing. Vienna: R Core Team.
Ríos, M. F., Hernández-Moresino, R. D., and Galván, D. E. (2020). Assessing urban microplastic pollution in a benthic habitat of Patagonia Aregentina. Mar. Pollut. Bull. 159:111491. doi: 10.1016/j.marpolbul.2020.111491
Rochman, C. M., Brookson, C., Bikker, J., Djuric, N., Earn, A., Bucci, K., et al. (2019). Rethinking microplastics as a diverse contaminant suite. Environ. Toxicol. Chem. 38, 703–711. doi: 10.1002/etc.4371
Romeo, T., Peda, C., Fossi, M. C., Andaloro, F., and Battaglia, P. (2016). First record of plastic debris in the stomach of Mediterranean lanternfishes. Acta Adriatica 57, 115–124. doi: 10.32582/aa
Ryan, P. G. (2020). The Transport and fate of marine plastics in South Africa and adjacent oceans. S. Afr. J. Sci. 116, 7677. doi: 10.17159/sajs.2020.7677
Sathish, M. N., Jeyasanta, I., and Patterson, J. (2020). Occurrence of microplastics in epipelagic and mesopelagic fishes from Tuticorin. Southeast coast of India. Sci. Total Environ. 720:137614. doi: 10.1016/j.scitotenv.2020.137614
Scott, S. (2017). A biophysical profile of the Tristan da Cunha Archipelago. Philadelphia, PA:The Pew Charitable Trusts, 191.
Shim, W. J., Hong, S. H., and Eo, S. (2018). “Marine microplastics: abundance, distribution, and composition,” in Microplastic contamination in aquatic environments: an emerging matter of environmental urgency, ed. E. Y. Zeng (Amsterdam: Elsevier), 1–26. doi: 10.1016/B978-0-12-813747-5.00001-1
Sillanpää, M., and Sainio, P. (2017). Release of polyester and cotton fibres from textiles in machine washings. Environ. Sci. Pollut. Res. 24, 19313–19321. doi: 10.1007/s11356-017-9621-1
Sparks, C., and Immelman, S. (2020). Microplastics in offshore fish from the Agulhas Bank. South Africa. Mar. Pollut. Bull. 156:111216. doi: 10.1016/j.marpolbul.2020.111216
St Helena Government (2015). State of the Island 2015. Available online at: https://www.sainthelena.gov.sh/wp-content/uploads/2017/10/State-of-the-Island-2015.pdf. (accessed 18, December 2020).
Talvitie, J., Mikola, A., Koistinen, A., and Setälä, O. (2017). Solutions to microplastic pollution – removal of microplastics from wastewater effluent with advanced wastewater treatment technologies. Water Res. 123, 401–407. doi: 10.1016/j.watres.2017.07.005
Torres, A. G., and Kesner-Reye, K. (2020). Fishbase: Sigmops elongatus (Günther, 1878). Available online at: https://www.fishbase.se/summary/Gonostoma-elongatum.html. (accessed 14, July 2020).
Valente, T., Sbrana, A., Scacco, U., Jacomini, C., Bianchi, J., Palazzo, L., et al. (2019). Exploring microplastic ingestion by three deep-water elasmobranch species: a case study from the Tyrrhenian Sea. Environ. Pollut. 253, 342–350. doi: 10.1016/j.envpol.2019.07.001
Wang, Y., Naumann, U., Eddelbuettel, D., Wilshire, J., and Warton, D. (2020). Mvabund: statistical methods for analysing multivariate abundance data. R package version 4.1.3.
Wieczorek, A. M., Morrison, L., Croot, P. L., Allcock, A. L., MacLoughlin, E., Savard, O., et al. (2018). Frequency of microplastics in mesopelagic fishes from the Northwest Atlantic. Front. Mar. Sci. 5:39. doi: 10.3389/fmars.2018.00039
Williams, A., Koslow, J., Terauds, A., and Haskard, K. (2001). Feeding ecology of five fishes from the mid-slope micronekton community off southern Tasmania. Australia. Mar. Biol. 139, 1177–1192. doi: 10.1007/s002270100671
Williams, A. T., and Simmons, S. L. (1996). The degradation of plastic litter in rivers: implications for beaches. J. Coastal Conserv. 2, 63–72. doi: 10.1007/BF02743038
Women’s Environmental Network. (2020). Environmenstrual. Available online at: https://www.wen.org.uk/our-work/environmenstrual/. (accessed 21, October 2020).
Woodall, L. C., Robinson, L. F., Rogers, A. D., Narayanaswamy, B. E., and Paterson, G. L. (2015). Deep-sea litter: a comparison of seamounts, banks and a ridge in the Atlantic and Indian Oceans reveals both environmental and anthropogenic factors impact accumulation and composition. Front. Mar. Sci. 2:3. doi: 10.3389/fmars.2015.00003
Woodall, L. C., Sanchez-Vidal, A., Canals, M., Paterson, G. L. J., Coppock, R., Sleight, V., et al. (2014). The deep sea is a major sink for plastic debris. R. Soc. Open Science 1:140317. doi: 10.1098/rsos.140317
Wright, S. L., Thompson, R. C., and Galloway, T. S. (2013). The physical impacts of microplastics on marine organisms: a review. Environ. Pollut. 178, 483–492. doi: 10.1016/j.envpol.2013.02.031
Zhu, L., Wang, H., Chen, B., Sun, X., Qu, K., and Xia, B. (2019). Microplastic ingestion in deep-sea fish from the South China Sea. Sci. Total Environ. 677, 493–501. doi: 10.1016/j.scitotenv.2019.04.380
Keywords: microplastic, mesopelagic, fibers, microfibres, semi-synthetic, viscose
Citation: McGoran AR, Maclaine JS, Clark PF and Morritt D (2021) Synthetic and Semi-Synthetic Microplastic Ingestion by Mesopelagic Fishes From Tristan da Cunha and St Helena, South Atlantic. Front. Mar. Sci. 8:633478. doi: 10.3389/fmars.2021.633478
Received: 25 November 2020; Accepted: 19 January 2021;
Published: 12 February 2021.
Edited by:
James Bell, Centre for Environment, Fisheries and Aquaculture Science (CEFAS), United KingdomReviewed by:
Sarah Nelms, University of Exeter, United KingdomWinnie Courtene-Jones, University of Plymouth, United Kingdom
Copyright © 2021 McGoran, Maclaine, Clark and Morritt. This is an open-access article distributed under the terms of the Creative Commons Attribution License (CC BY). The use, distribution or reproduction in other forums is permitted, provided the original author(s) and the copyright owner(s) are credited and that the original publication in this journal is cited, in accordance with accepted academic practice. No use, distribution or reproduction is permitted which does not comply with these terms.
*Correspondence: Alexandra R. McGoran, YWxleGFuZHJhLm1jZ29yYW4uMjAxMkBsaXZlLnJodWwuYWMudWs=