- 1National Oceanography Centre, Southampton, United Kingdom
- 2Life Sciences Department, Natural History Museum, London, United Kingdom
- 3Center for Microbiome Innovation, University of California, San Diego, San Diego, CA, United States
- 4Department of Pediatrics, University of California, San Diego, San Diego, CA, United States
- 5Department of Genetics and Evolution, University of Geneva, Geneva, Switzerland
- 6Shirshov Institute of Oceanology Russian Academy of Sciences, Moscow, Russia
- 7Tokyo University of Science and Technology, Tokyo, Japan
- 8Keppel-NUS Corporate Laboratory, Tropical Marine Science Institute, National University of Singapore, Singapore, Singapore
- 9Institute of Oceanology, Polish Academy of Sciences, Sopot, Poland
- 10ID-Gene Ecodiagnostics, Campus Biotech Innovation Park, Geneva, Switzerland
- 11Institute of Marine and Environmental Sciences, University of Szczecin, Szczecin, Poland
Benthic foraminiferal research in the North Pacific has a long history, with works published over a century ago providing important information about the taxonomy and distribution of morphospecies. These studies focused mainly on areas outside the Clarion-Clipperton Zone (CCZ). Our knowledge of foraminiferal faunas within the CCZ originates largely from recent baseline investigations related to likely future seabed mining of the polymetallic nodule deposits. These have revealed highly diverse assemblages of sediment-dwelling morphospecies among the meiofauna and macrofauna, as well as megafaunal xenophyophores and nodule-attached fauna. Morphological analyses have been complemented by metabarcoding studies that yielded even higher numbers of molecular species (Operational Taxonomic Units - OTUs). Monothalamids, the vast majority undescribed, constitute a substantial proportion of both morphological and molecular datasets, with multichambered agglutinated and calcareous foraminifera being less common. Their importance in this abyssal (>4,000 m depth) habitat likely reflects food limitation combined with carbonate dissolution close to and below the carbonate compensation depth. Literature records, supported in a few cases by genetic data, suggest that many morphospecies found in the CCZ have wide geographical distributions across the Pacific abyss and in other oceans. At smaller spatial scales (several 100s of kilometers) there is a general uniformity in assemblage composition. Nevertheless, many morphospecies are too rare to conclude anything about their geographical distributions. Similarly, the part played by benthic foraminifera in CCZ ecosystems is largely a matter of speculation, although their abundance across different size classes suggests that it is significant. Meiofauna-sized taxa that consume freshly-deposited organic detritus may be important in carbon cycling, particularly at the shallower, more eutrophic eastern end of the CCZ. Megafaunal xenophyophores can provide habitat structure for other organisms, potentially enhancing benthic biodiversity. Foraminifera of all sizes could be among the earliest recolonisers of disturbed or redeposited sediments. Their potential contributions in terms of both ecology and biodiversity make these protists significant members of benthic communities in the CCZ.
Introduction
Foraminifera, shell-bearing protists in the supergroup Rhizaria, are common in all marine environments from the intertidal zone to the deepest ocean trenches and have also adapted to fresh water and damp terrestrial settings. They inhabit hard and soft substrates and flourish in the virtual absence of oxygen as well as in well-oxygenated environments. The extraordinary success of foraminifera may be attributable to unique physiological and ultrastructural adaptations, including their highly mobile system of reticulated pseudopodia that is involved in many life processes, including feeding, respiration and movement (Travis and Bowser, 1991). These protists become an increasingly important component of benthic communities with increasing water depth (Thiel, 1975, 1983), and on abyssal plains they may account for more than 50% of the meiofaunal and macrofaunal abundance and biomass (Gooday et al., 1992; Gooday, 2019). Interest in foraminifera is not confined to biologists. They have an outstanding fossil record and are widely used as indicators of seafloor conditions in ancient oceans (Jorissen et al., 2007). As a result, much of the more recent research on the ecology of modern deep-sea benthic foraminifera has been driven by efforts to refine their utility in palaeoceanography.
Foraminifera research in the Pacific Ocean has a long history, extending back well into the 19th century. This has generated a large body of information on species distributions, making foraminifera one of the better documented deep-sea benthic taxa. Much of this earlier research concerned species with relatively robust multichambered shells, composed either of secreted calcium carbonate or agglutinated particles. However, the true nature of foraminifera assemblages on Pacific abyssal plains, and the scale of their diversity, only started to become apparent as a result of research in the central North Pacific during the 1970s (Hessler, 1974). This revealed that the macrofauna is dominated by agglutinated foraminifera (Bernstein et al., 1978), many of them single-chambered monothalamids (class Monothalamea) including tubular forms and those with highly unusual test morphologies, for which Tendal and Hessler (1977) established the superfamily Komokiacea. A group of even larger monothalamids, the xenophyophores (Tendal, 1972), make a major contribution to the North Pacific megafauna (Gooday et al., 2017b; Simon-Lledó et al., 2019a), while largely undescribed sessile foraminifera are very abundant on polymetallic nodules (Veillette et al., 2007; Gooday et al., 2015).
Here, we focus on the Clarion-Clipperton Zone (CCZ). Spanning some 4.5 million km2 of seafloor from about 115° to 155° W and 03° to 19° N, the region hosts some of the ocean’s richest and most extensive seabed deposits of polymetallic nodules, a likely target for commercial mining in the near future (Hein et al., 2013; Washburn et al., 2019; Smith et al., 2020). The regulating body, the International Seabed Authority (ISA), has concluded contracts with 18 entities (states, enterprises and consortia) for polymetallic nodule deposit exploration in areas up to ∼75,000 km2, and designated nine Areas of Particular Environmental Interest (APEIs) that will be protected from mining. These developments have prompted a sharp rise in ecological and taxonomic research across the CCZ during the last decade, aimed at assessing the present status of biodiversity in areas designated for future nodule extraction, and at finding potential repositories (gene banks) of taxa capable of recolonizing mined areas. Much of this effort has focused on the eastern CCZ (Washburn et al., 2021) and foraminifera have featured prominently in it. Our synthesis combines information from classical taxonomic and distributional studies of benthic foraminifera with morphological and genetic data, both published and unpublished, obtained during this recent phase of mining-related research. We first consider the important earlier literature on abyssal North Pacific foraminifera, before summarizing data on the biodiversity, biogeography, and species ranges of foraminifera within the CCZ. Finally, we speculate about possible ecological roles for foraminifera and suggest some plausible scenarios for their recovery from seabed mining impacts.
Previous Research on Modern Benthic Foraminifera in the CCZ and Adjacent Areas
Foraminifera have been collected at a large number of sites across the Pacific Ocean at depths of > 3000 m. The locations of sites sampled during some of the more important recent studies, as well as some early reports, are shown in Figure 1. The map is not intended to be comprehensive. In particular, it omits the numerous Albatross and Nero sites, which yielded many of the records summarized by Cushman (1910–1917).
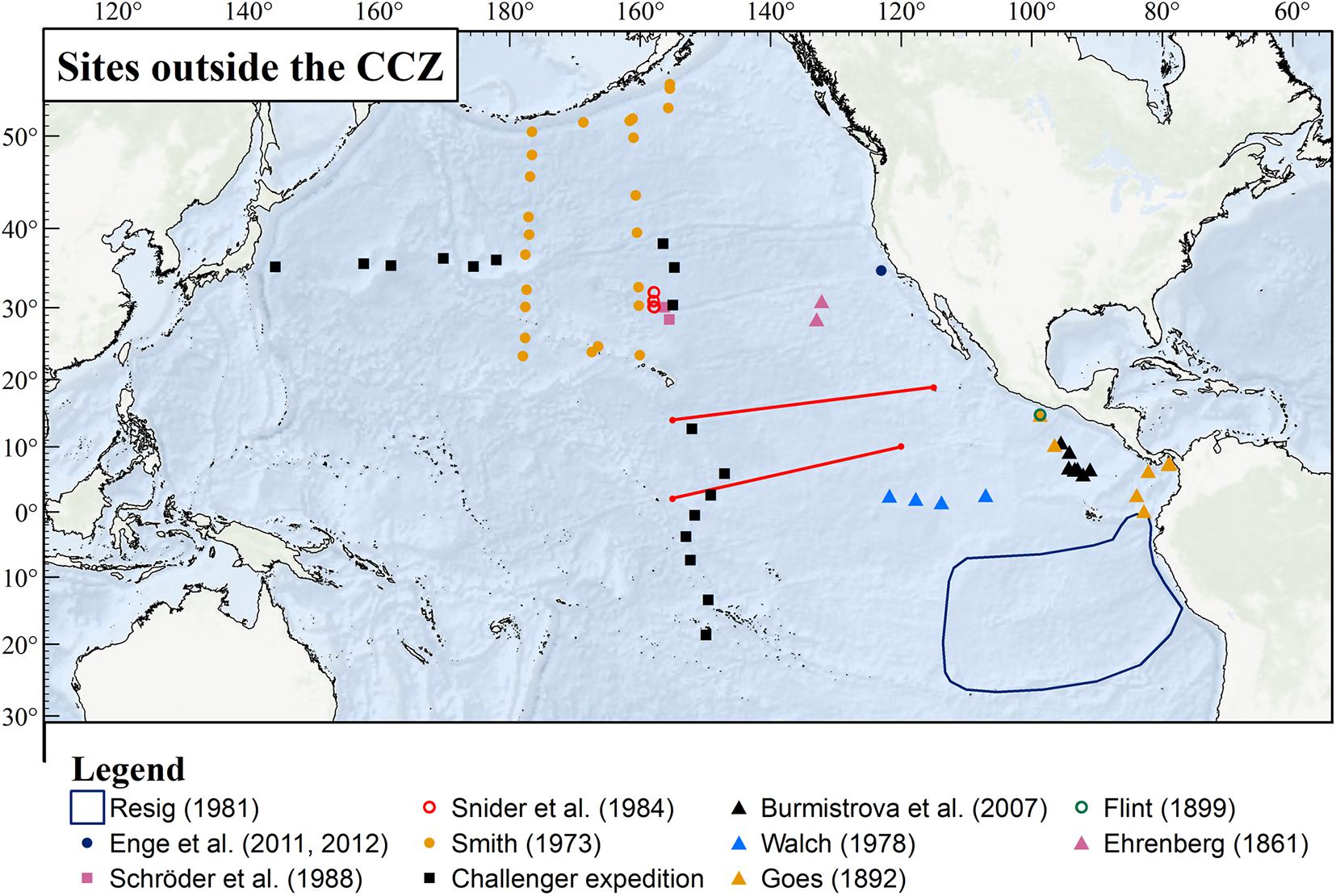
Figure 1. The location of selected studies and sampling sites in the Pacific Ocean. Apart from two Challenger stations at the western end of the CCZ, all are located outside of the CCZ. The Clarion and Clipperton Fractures that delimit the CCZ are marked with red lines; the dark blue line outlines the Resig (1981) study area.
The Megafaunal Xenophyophores
Earlier records of xenophyophores from within the CCZ and adjacent parts of the equatorial Pacific are summarized in Supplementary Table 3 in Gooday et al. (2017b). Gooday et al. (2020b) list the 24 described and 39 undescribed species reported from this region. Sampling sites are shown in Figure 2a. Xenophyophores from H.M.S. Challenger Station 270, located just to the south of APEI 4, and Stations 271, 272 and 274 to the south of the CCZ, were studied by Haeckel (1889), Schulze (1907a,b), and Tendal (1972). Fragments of Aschemonella ramuliformis, later shown to be a xenophyophore, were present in the sample from Station 272 (Brady, 1884), and two other Challenger stations (241, 244) in the NW Pacific to the east of Japan yielded species of Stannophyllum. Extensive Pacific collections were made during cruises in 1891, 1899, 1904, and 1905 of the American fisheries vessel Albatross. These included xenophyophores from sites located to the north, south and east of the CCZ (Goës, 1892; Schulze, 1907a,b; Tendal, 1972; Gooday et al., 2017b). More recently, Tendal (1980) described Stannophyllum setosum from a sample collected well within the CCZ (Figure 2a), Levin et al. (1986) and Levin and Thomas (1988) illustrated xenophyophores from seamounts located to the east and north of the CCZ (mostly from depths < 3000 m), Mullineaux (1987) and Veillette et al. (2007) reported undescribed species on polymetallic nodules from, respectively, the eastern and western CCZ, and Kamenskaya (2005) based the description of a new genus (Spiculammina) on material from the central CCZ. In the NW Pacific, a new species and genus, Shinkaiya lindsayi, was described by Lecroq et al. (2009c) from 5,435 m depth near the Japan Trench, and a new species, Syringammina limosa, was described by Voltski et al. (2018) from 3,351 to 3,366 m depth in the Sea of Okhotsk.
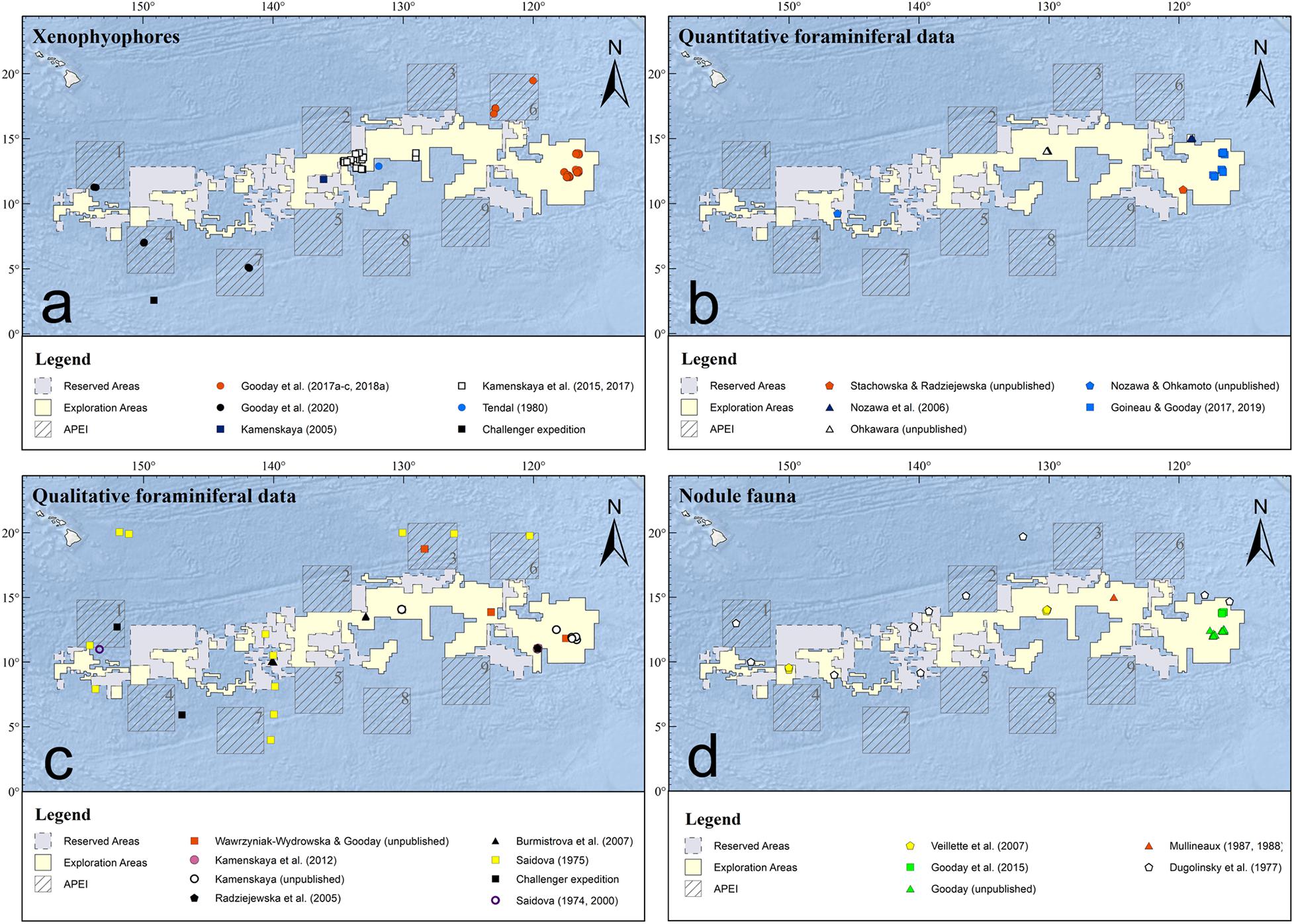
Figure 2. Location of study sites within and in the vicinity of the Clarion-Clipperton Zone. (a) Xenophyophores. (b) Quantitative studies. (c) Qualitative studies. (d) Nodule-attached faunas.
The latest and most intensive phase of xenophyophore research in the CCZ has been linked to mining-related baseline studies. This has resulted in the description of four new genera and 24 new species from the eastern (Gooday et al., 2017a,c, 2018a), central (Kamenskaya et al., 2015, 2017), and western (Gooday et al., 2020a) parts of the CCZ. Efforts to amplify DNA from xenophyophores collected in the eastern and western areas have had a high success rate (∼85% of extractions), a reflection of the multinucleate organization of these large foraminifera. As a result, sequence data have been obtained from 15 described and 10 undescribed species (Table 1 in Gooday et al., 2020b), more than for any other group of monothalamous foraminifera. At the same time, analyses of seafloor images have revealed xenophyophores to be a dominant component of the megafaunal assemblages across the CCZ nodule fields (Kamenskaya et al., 2013; Amon et al., 2016; Simon-Lledó et al., 2019a).
Other Foraminifera
The earliest record of benthic foraminifera in the deep Pacific Ocean is that of Ehrenberg (1861), who recorded a few species of “Polythalamien,” together with a long list of radiolarians and diatoms, in two small sounding samples, one from 3,658 m and the other from 4,755 m to the north of the CCZ (Figure 1). However, the first important study was based on material collected by H.M.S Challenger. She sailed south from Hawaii and sampled in a north to south direction along a line (approximately 150° W), crossing the western CCZ. The dredge sample from Challenger Station 265, one of eight (263-270) situated within the CCZ, was included in Brady’s (1884) Challenger Report on the Foraminifera, together with samples from the more southerly stations 271, 272, 274. Picaglia (1892) listed planktonic and benthic species present in five samples from the equatorial Pacific (3647–4670 m depth), mainly to the west and east of the CCZ. Albatross samples were an important source of Pacific foraminifera and formed the basis for a number of papers and monographs. Goës (1896) worked on material collected in 1891 on the Californian, Mexican and Central American margins and the Galapagos region, to the south and east of the CCZ; the three deepest samples were from between 3,279 and 4,081 m. Flint (1899) included foraminifera from one North Pacific Albatross station deeper than 3,000 m in his “descriptive catalog” of foraminifera, the first work to illustrate foraminifera photographically. Bagg (1908) described species in Albatross samples collected down to 2,557 m around the Hawaiian Islands. Cushman’s major monograph of North Pacific foraminifera, published in six parts between 1910 and 1917, was based on samples from the 1906 Albatross campaign obtained mainly from the NW Pacific, and those collected by the U.S.S. Nero during a 1899-1900 cable-laying survey west of Hawaii (Flint, 1905), together with a few other sources and literature records (Cushman, 1910–1917). Cushman’s later monograph of foraminifera from the tropical Pacific (Cushman, 1932, 1933, 1942; completed by Todd, 1965) utilized samples from depths down to 4512 m in the South Pacific, as well as a few samples from deep-water sites (maximum depth 4,804 m) to the north and west of the CCZ. More recently, an unpublished thesis by Walch (1978) described “live” (stained) and dead foraminifera (>63-μm fraction) in box core subsamples from 5 sites located to the east and south of the CCZ (3189–4583 m). Other important papers on modern deep-sea foraminifera in areas more distant from the CCZ include those of CCZ include those of Smith (1973; transects running between the Aleutian Islands and the latitude of Hawaii), Resig (1981; SE Pacific), Schröder et al. (1988; CLIMAX II site north of Hawaii, material of Bernstein et al., 1978), Szarek et al. (2007; Sulu Sea), Enge et al. (2012; Station M on the Californian margin), and Shi et al. (2020; seamounts and abyssal plans in the tropical western Pacific).
It is remarkable that, apart from Challenger Stations 265 and 269, none of the early studies mentioned above includes sites within the CCZ. Saidova’s (1975) monograph on Pacific foraminifera seems to be the first to include significant numbers of samples from this region. The bulk of her material came from the northeastern and particularly the northwestern margins, but some originated from more central parts of the North Pacific. Two transects crossed the western and central CCZ, although foraminiferal species were apparently not recorded by Saidova (1975) from all of the sites located between the two fracture zones. As in earlier Pacific studies, Saidova (1975) dealt mainly with “hard-shelled” multichambered taxa, both calcareous and agglutinated (mainly class Globothalamea). Appendix A (Supplementary Material) list 44 species and subspecies present at 12 of these stations within and immediately adjacent to the CCZ. Some have not been reported in the non-Russian foraminiferal literature. In other papers, Saidova (1965, 1976, 1981) compiled important syntheses of the geographical and bathymetric distribution of benthic foraminifera based on comprehensive species-level datasets from across the Pacific. These show large areas of the abyssal Pacific occupied entirely by agglutinated assemblages. Saidova (1974, 2000) also described different associations of foraminifera, related to seafloor topography within a 23 × 23 km area at the western end of the CCZ (Appendix B; Supplementary Material). A later Russian contribution (Burmistrova et al., 2007) summarizes the distribution of agglutinated species in samples from three areas, two in the central part of the CCZ (4918–4970 m depth) and the third to the east of the CCZ in the Guatemala Basin (3350–4040 m). Fifty of the 68 species listed are recorded from the two CCZ areas.
Renewed interest in seabed mining during the last two decades has generated an upsurge of research on benthic foraminifera within the CCZ (Figures 2b,c). The 1970s had seen the establishment of the Komokiacea and their recognition as an important component of the abyssal Pacific macrofauna (Tendal and Hessler, 1977), although this unusual group of what are presumed to be soft-bodied monothalamous foraminifera had been known to Russian scientists since the 1950s and a few were included in Saidova’s (1975) monograph (summarized in Gooday et al., 2007). Most recent foraminiferal studies in the CCZ have included these and other delicate monothalamids. Kamenskaya et al. (2012) presented a survey of macrofauna-sized foraminifera (> 300 μm) from the IOM contract area. These included many komokiaceans, as well as chain-like forms, tube fragments, and other kinds of monothalamids. Meiofaunal assemblages (> 32 μm), again mainly monothalamids, were analyzed by Nozawa et al. (2006) from the Kaplan East site in the eastern CCZ, and Radziejewska et al. (2006) from the nearby IOM contract area. Ohkawara et al. (2009) described a particularly abundant new species of tiny agglutinated sphere that is very common at the Kaplan Central site. More recently, Goineau and Gooday (2017, 2019) and Gooday and Goineau (2019) have described highly diverse monothalamid-dominated assemblages (> 150 μm, > 63 μm fraction respectively) from the UK-1 and OMS areas, also in the eastern CCZ.
Nodule-Encrusting Foraminifera
Although the presence of foraminifera on nodules was noted briefly during the Challenger Expedition (Murray and Renard, 1891), they only attracted serious attention during the 1970s (e.g., Greenslate et al., 1974). The main initial studies of these novel faunas were those of Dugolinsky et al. (1977), based on samples from different parts of the Pacific including the CCZ, and Mullineaux (1987, 1988) who studied nodules from within (15°N, 125°W) and to the north (30°N, 125°W) of the CCZ (Figure 2d). Later, Veillette et al. (2007) conducted detailed studies of nodule assemblages in the two French contract areas located in the western and central CCZ, and Gooday et al. (2015) added a preliminary survey from the UK-1 contract area.
Other Data
In addition to published data, we have consulted a number of theses and unpublished datasets, mainly from the eastern CCZ (Table 1). Quantitative data (abundance, species richness etc.) for meiofaunal foraminifera are available from the Kaplan East (KE; Fusae Nozawa, 2005, M.Sc thesis) and Kaplan Central (KC; Nina Ohkawara, 2011, PhD thesis) sites, and for macrofaunal foraminifera (> 250 μm) from the IOM contract area (Zofia Stachowska, 2020, M.Sc thesis) (Figure 2b). Two undergraduate theses based on samples from the Japan Deep-Sea Impact Experiment (JET) site provide the only quantitative data from the western CCZ (Okamoto, 1998; Nozawa, 2003). Unpublished qualitative information (species occurrences) for macrofaunal foraminifera (>300 μm) come from epibenthic sledge samples obtained in the UK-1, OMS, IOM, German, Belgium, French and Russian contract areas and APEI-3 (Kamenskaya, unpublished; Wawrzyniak-Wydrowska, unpublished) (Figure 2c). Numerous qualitative observations on sessile foraminifera encrusting nodules from the OMS and UK-1 areas were made during cruises AB01 and AB02 of the ABYSSal baseLINE (ABYSSLINE) project (Gooday unpublished). Genetic data (SSU rRNA gene sequences) for some meiofaunal foraminiferal species were obtained from OMS and UK-1 samples (Voltski, Holzmann and Pawlowski, unpublished). Finally, samples for environmental DNA and RNA (eDNA/eRNA) metabarcoding were collected during the AB02 cruise, with additional samples being obtained from three parts of the BGR area (MANGAN cruise) and French area (BIONOD cruise, Apothéloz-Perret-Gentil, unpublished) (Lejzerowicz et al., 2021).
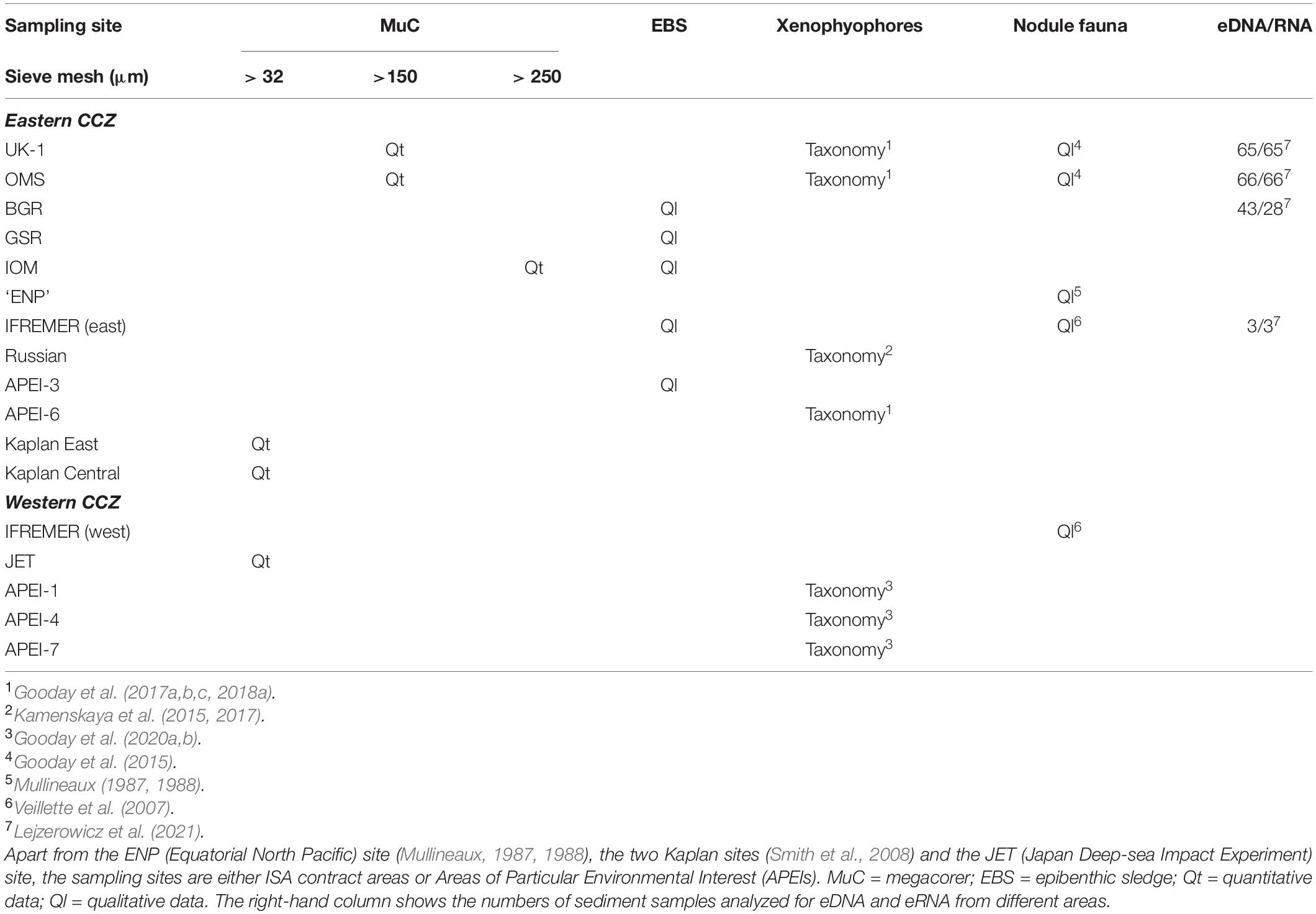
Table 1. Distribution of samples from the CCZ used for published and unpublished foraminiferal studies.
General Observations on Abyssal Pacific Assemblages
Smith et al. (1983) reported much lower Rose-Bengal-stained foraminiferal densities on central (5,755 to 5,904 m depth) and NE Pacific (4,392 to ∼4,800 m) abyssal plains than at shallower sites (1,300 and 3,815 m) on the Californian margin. Early studies also noted that foraminifera are very sparse in the deep North Pacific. Cushman (1910, p. 15 therein) found very few foraminifera (“practically nil”) in “very deep water in red clay areas” sampled by the U.S.S. Nero during 1899 and 1900. More than half (51.5%) of the 466 Nero soundings between Midway atoll and Guam were deeper than 5,000 m (Flint, 1905). Cushman (1910) also concluded (p. 16) that, with a very few exceptions, “almost no” calcareous foraminifera were present at depths below 2,500 fathoms’ (4,572 m) in the North Pacific generally, although some agglutinated taxa were present. Similarly, Saidova (1966) found Pacific foraminiferal assemblages to be almost entirely agglutinated below 3,500–4,500 m depth. Maps based on her comprehensive dataset showed large areas of the central North and South Pacific, including much of the western CCZ, devoid of calcareous foraminifera (Saidova, 1976, 1981). Within the area (0°–20°N, 115°–155° W) that encompasses the CCZ, Figure 1 in Saidova (1965) shows calcareous benthic foraminifera confined to the southeastern part. This reflects the influence of the carbonate compensation depth (CCD), below which calcareous foraminifera cannot easily maintain their tests in a corrosive environment. The CCD is situated around 4,500 m depth in the southern North Pacific, deepening to ∼5,000 m near the equator (Lisitzin, 1996). The shallower eastern end of the CCZ, where calcareous foraminifera are a minor but consistent faunal component (Goineau and Gooday, 2017, 2019), is located somewhat above the CCD. At the western end, Brady (1884) found no calcareous foraminifera, apart from 2–3 miliolids and a few globigerinids, in reddish-brown siliceous mud at Challenger Station 265, located at 5,304 m depth, well below the CCD just to the east of the area now covered by APEI-1. He reports miliolids from stations deeper than 5000 m elsewhere in the North Pacific, but their calcareous shells were either completely dissolved (Challenger Station 238; 7,224 m) or very thin (Challenger Stations 245 and 253; 5,073 m and 5,715 m, respectively). Other calcareous taxa (rotaliids and lageniids) were present at shallower Challenger stations (241, 242, 271, 272, 276; 4,206–4,758 m) in the Pacific (Brady, 1884). Saidova (1974) reports that 80–90% of benthic foraminiferal tests in sediments containing 10–23% carbonate on the crests of hills at 4,780–4,790 m depth at the western end of the CCZ (Figure 2b) were calcareous. Elsewhere in the North Pacific, “live” (stained) calcareous foraminifera are found occasionally at depths as great as 6,560 m and 7,230 m (Smith, 1973).
Biodiversity and Assemblage Composition
Morphological Data
Recent studies have shown foraminifera to be much more abundant and diverse in the CCZ than earlier data suggested. Eleven megacorer samples (0–1 cm layer, >150-μm size fraction) from three 30 × 30 km “strata” in the eastern CCZ, two in the UK-1 contract area and one in the OMS area, yielded a grand total of 580 meiofauna-sized morphospecies, including live and dead tests and fragments (Goineau and Gooday, 2019). Between 310 and 411 species were found in particular strata and 132–228 in individual cores or core splits (Table 2). The combined dataset included a few relatively common (>100 specimens) morphospecies but the majority were uncommon and 29% were represented by singletons (Supplementary Figure 1), a pattern that is typical for the deep sea (Rex and Etter, 2010; McClain, 2021), including for benthic foraminifera (Douglas and Woodruff, 1981). Species were still being added after 11 samples (Figure 3), and rarefaction curves for individual cores did not reach an asymptote (Supplementary Figure 2), suggesting that the total number of species (>150 μm) was higher. Estimates ranged from 690 (abundance-based estimator ACE) to 877 (incidence-based estimator Jacknife 2) (Goineau and Gooday, 2019). A subset of 5 samples sieved on a 63-μm mesh yielded 462 morphospecies, of which 170 were absent in the >150-μm fractions (Gooday and Goineau, 2019). The number in individual cores ranged from 133 to 209 (Table 2). Finally, 158–252 morphospecies were recognized in the fine fractions (all foraminifera larger than 32 μm) of small subsamples from megacores taken at the Kaplan East (KE), Kaplan Central (KC) and JET sites. Between 18 and 100 were picked from single subcores (Table 2).
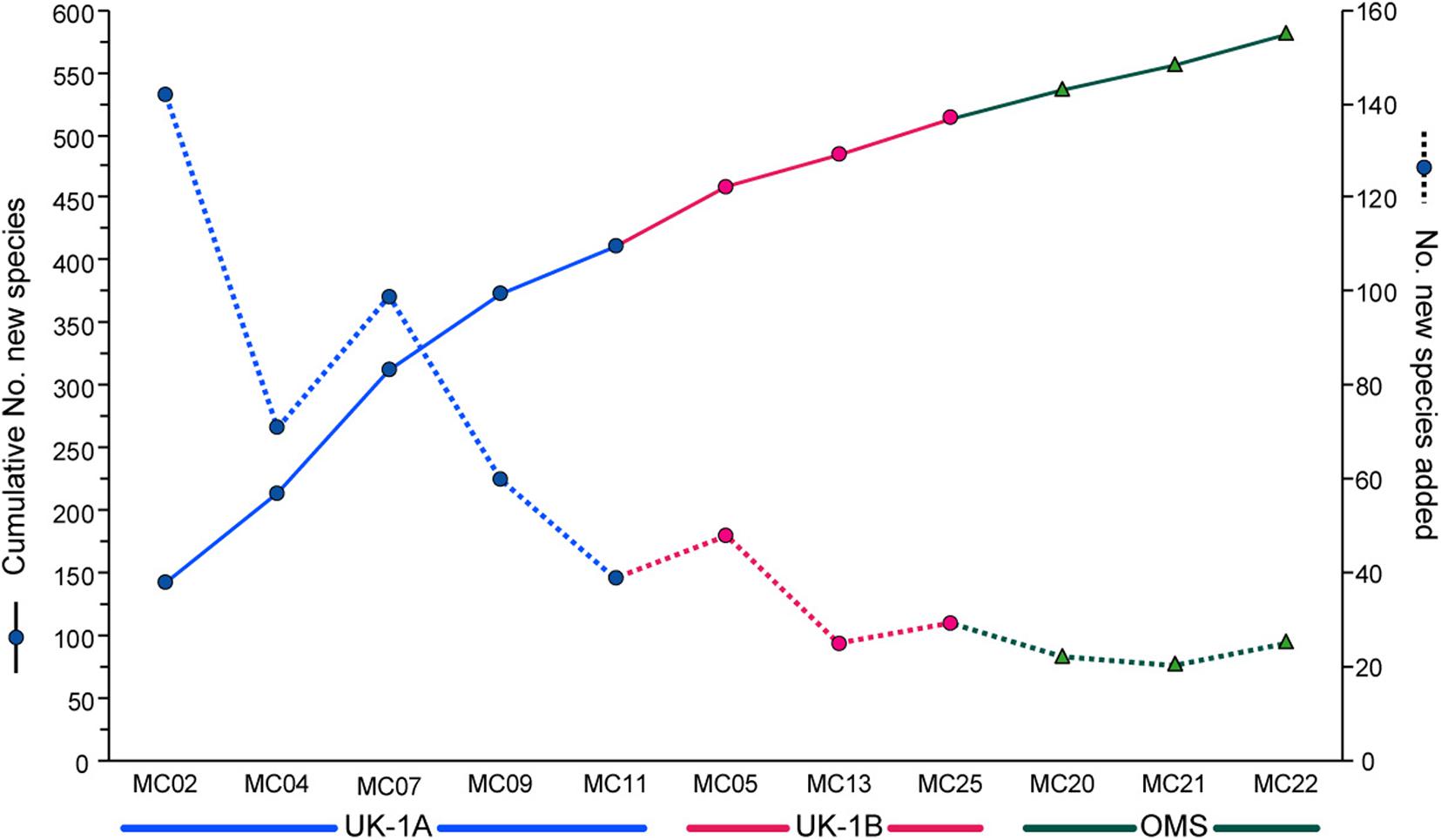
Figure 3. Species accumulation curve (solid line; left-hand axis) showing the increase in the number of species with the addition of new samples from Strata A and B in the UK-1 contract area and the single Stratum in the OMS area (the ‘strata’ are 30 × 30 km study areas). The dotted line shows the number of new species added with each new sample (right-hand axis). Redrawn from Deep-Sea Research I, vol. 149, A. Goineau and A.J. Gooday, Diversity and spatial patterns of foraminiferal assemblages in the eastern Clarion–Clipperton zone (abyssal eastern equatorial Pacific), Article 103036, 2019, with permission from Elsevier.
Monothalamids are always a dominant element of these meiofauna-sized assemblages, accounting for ∼75% of species in the two UK-1 strata and the nearby KE site, and almost 70% in the OMS stratum, irrespective of the size fraction analyzed (Table 2). They contribute an even higher proportion of species (>80%) in the fine fractions (larger than 32 μm) at the KC and JET sites. Most other complete foraminiferal tests in these samples belong to multichambered agglutinated taxa. The inclusion of this important monothalamid component is the main difference between these recent and earlier foraminiferal studies in the Pacific.
The comparison of data across wider areas of the CCZ is hampered by inconsistencies in the sizes and methods used to analyze the samples (notably sieve mesh sizes) (Table 2), as well as the research bias toward the eastern CCZ (Washburn et al., 2021). The only quantitative samples that can be compared directly are those obtained at the KE (4000 m, eastern CCZ) and JET (5300 m, western CCZ) sites, which were analyzed by the same person using the same methods (Nozawa, 2003, 2005; Table 2). At the JET site, a total of 179 species (mean 46.1 ± 20.5 per subcore) was recognized among 1,702 specimens (excluding fragments) compared to 168 species among 983 specimens (mean 53.4 ± 9.36 per sample) at the KE site. The rarefied species richness is slightly higher at KE than at JET (Figure 4).
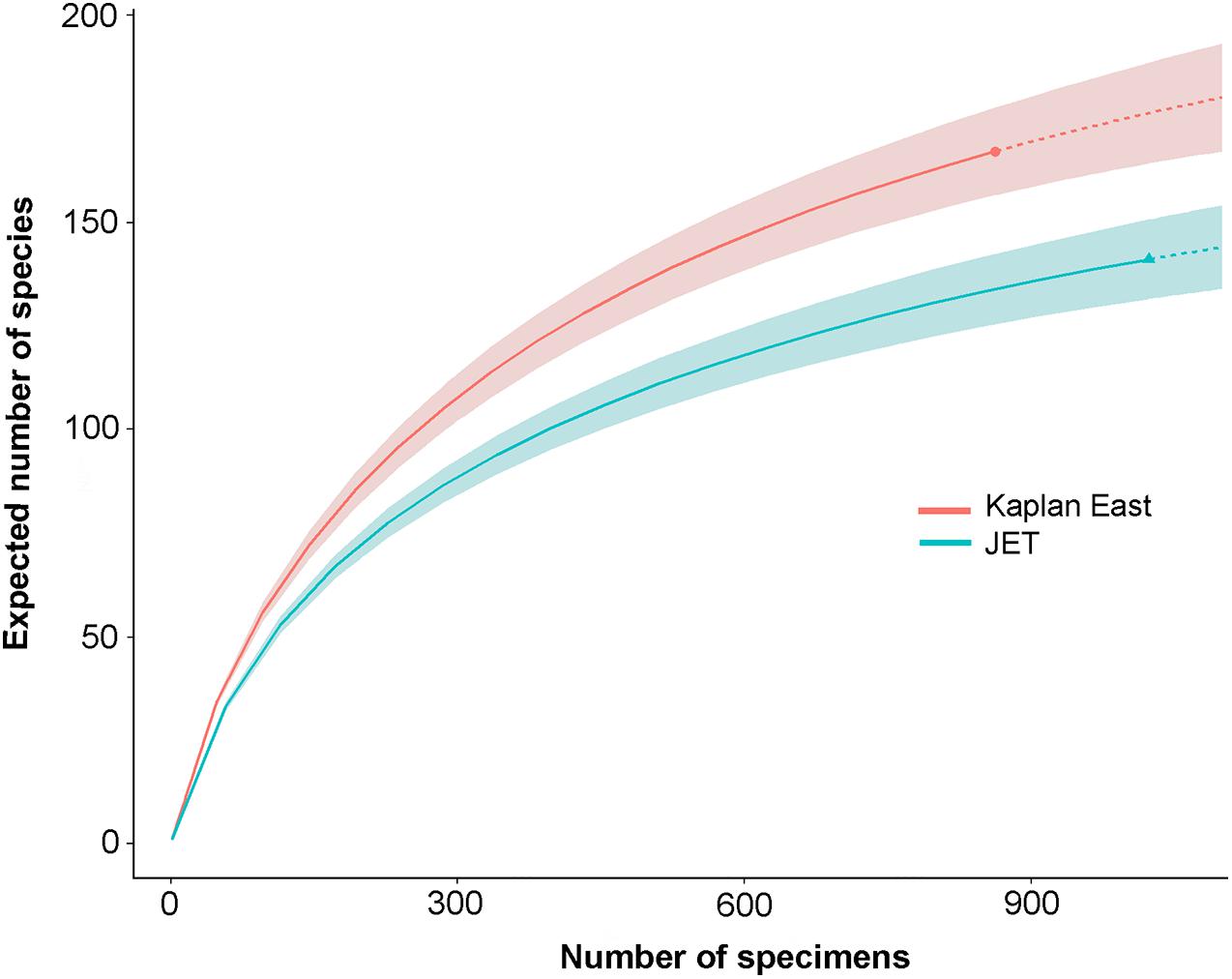
Figure 4. Rarefaction curves with 95% confidence limits for foraminifera from the Kaplan East (∼15°N, 119°W; ∼4100 m depth) and JET (09° 14′ N, 146° 15′ W; 5300 m depth) sites. The curves are based on data from the 0-1 cm layer of subsamples (6.6 cm2 surface area) of megacores, sieved on a 32-μm screen (Nozawa, 2003, 2005).
Macrofauna-sized (> 300 μm) monothalamids are very common in qualitative epibenthic sledge residues from the CCZ. Samples from five sites in the eastern CCZ (German, IOM, Belgium, French contract areas and APEI-3), sorted by the same people using consistent methods, yielded 224 monothalamid morphospecies (Wawrzyniak-Wydrowska and Gooday, unpublished). At least 30 additional forms have been recognized in samples analyzed by Kamenskaya (unpublished) from the French, German and Russian areas. Nine megacorer samples (> 250-μm fraction) from the IOM area have also yielded diverse foraminiferal assemblages, comprising more than 220 morphospecies, of which 78% are monothalamids (Stachowska, unpublished).
The eastern equatorial Pacific is notable for hosting diverse assemblages of megafaunal xenophyophores (Schulze, 1907a; Tendal, 1996; Gooday et al., 2017b). In total, 35 described species (>40% of the global total) have been reported from this region, of which 24 occur in the CCZ, where an additional 39 species have been recognized but not formally described (Gooday et al., 2020b). Most (52) of these 63 described and undescribed species have been found only in the eastern CCZ (i.e., east of 140° W), 8 only in the western CCZ, while three (Aschemonella monilis, Moanammina semicircularis, and Stannophyllum zonarium) span both sectors. Many xenophyophores species live attached to polymetallic nodules, suggesting that nodule occurrence may be a key driver of their diversity. However, since xenophyophores can colonize soft as well as hard substrates, the potential role of nodules in this respect has yet to be tested.
Other kinds of sessile foraminifera are also common on nodules (Table 3). Two early studies reported 28 (Dugolinsky et al., 1977) and 46 (Mullineaux, 1987, 1988) morphospecies from the CCZ and nearby areas (Figure 2d). In the eastern and western French contract areas, Veillette et al. (2007) distinguished 68 species attached to nodules, with a further 5 loosely associated species. In the UK-1 area seven nodules yielded a total of 75 attached species, with another eleven recognized during shipboard observations (Gooday et al., 2015). Further studies of nodule-attached foraminifera from the OMS area, as well as UK-1 area, would certainly increase this number further.
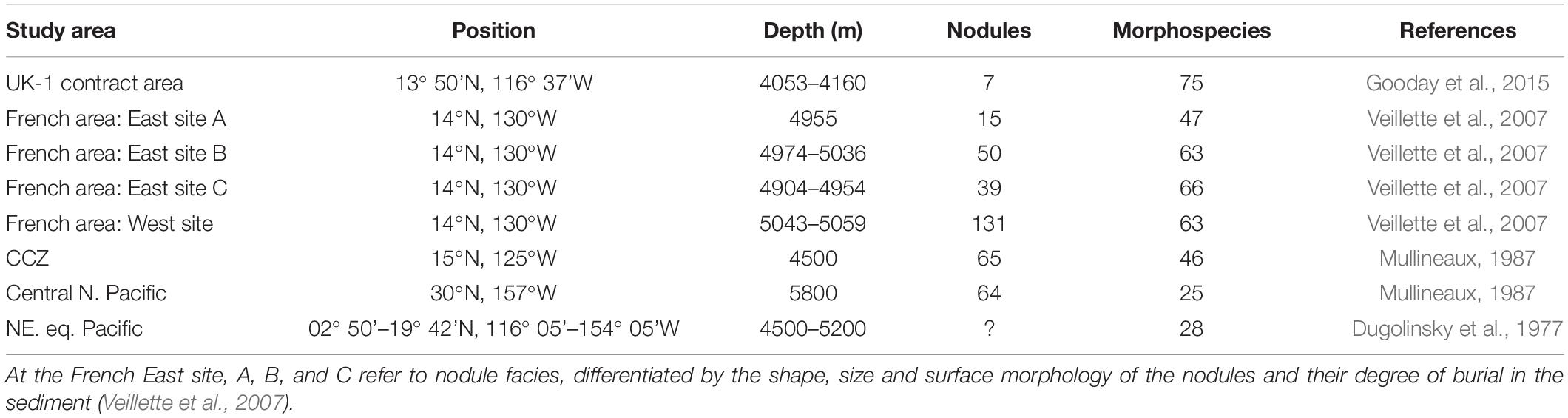
Table 3. Number of foraminiferal morphospecies associated with nodules within, and to the north of the CCZ.
Based on these limited morphological analyses from a relatively small number of study sites, we estimate that the total number of foraminiferal morphospecies across all size fractions and substrates could easily exceed 1,000. Samples from sites in other parts of the CCZ will probably yield additional species. In the western CCZ, Saidova (1974, 2000) reported species-level variations in foraminiferal assemblages at a 10-km scale, particularly in relation to topographic features (abyssal hills). Heterogeneity of this kind would undoubtedly inflate species numbers further.
Environmental DNA and RNA Metabarcoding Data
Metabarcoding studies based on samples from the UK-1, OMS, BGR and eastern French (IFREMER) contract areas (Lejzerowicz et al., 2021), as well as previous studies in other regions (Lecroq et al., 2011; Lejzerowicz et al., 2014; Shi et al., 2020), provide further evidence that deep-sea foraminifera are highly diverse and include a substantial proportion of monothalamids. The Operational Taxonomic Units (OTUs) recognized in the CCZ encompass most of the existing monothalamid clades, some known only from environmental samples (Lejzerowicz et al., 2021). Environmental DNA (eDNA) and RNA (eRNA) was extracted from 166 sediment samples collected according to a nested design in four areas. Only 3 samples were processed for the French site, which is situated in the more central part of the CCZ. Hence, the scope of the eDNA analyses is currently limited to faunal patterns in the UK-1 and OMS regions, which are relatively homogeneous in terms of nodule abundance and POC flux.
The processing of foraminiferal metabarcoding data was tailored for quality by using PCR replicates, cross-contamination filtering, four sequence assignment methods and compositional data analyses (Lejzerowicz et al., 2021). The eDNA and eRNA sequences clustered into 6,425 OTUs, including 21.7% assigned to the Monothalamea and 3.7% assigned to the multichambered Globothalamea (Figure 5). On average, the number of monothalamid OTUs obtained from samples at each site was 7.2 (± 3 standard deviation) times the number assigned to the Globothalamea. For both groups, the UK-1 and OMS areas yielded significantly higher richness than the well-sampled BGR South area (MANGAN16 cruise) (72 samples, Figure 5), and this is also true after normalizing sequencing read depths with rarefaction [Figure 6 in Lejzerowicz et al. (2021)]. Nevertheless, the majority of OTUs remained unassigned at lower taxonomic levels, suggesting that the foraminiferal genetic diversity in the CCZ is largely unknown and more effort is needed to complete the reference database necessary to assign the foraminiferal metabarcodes.
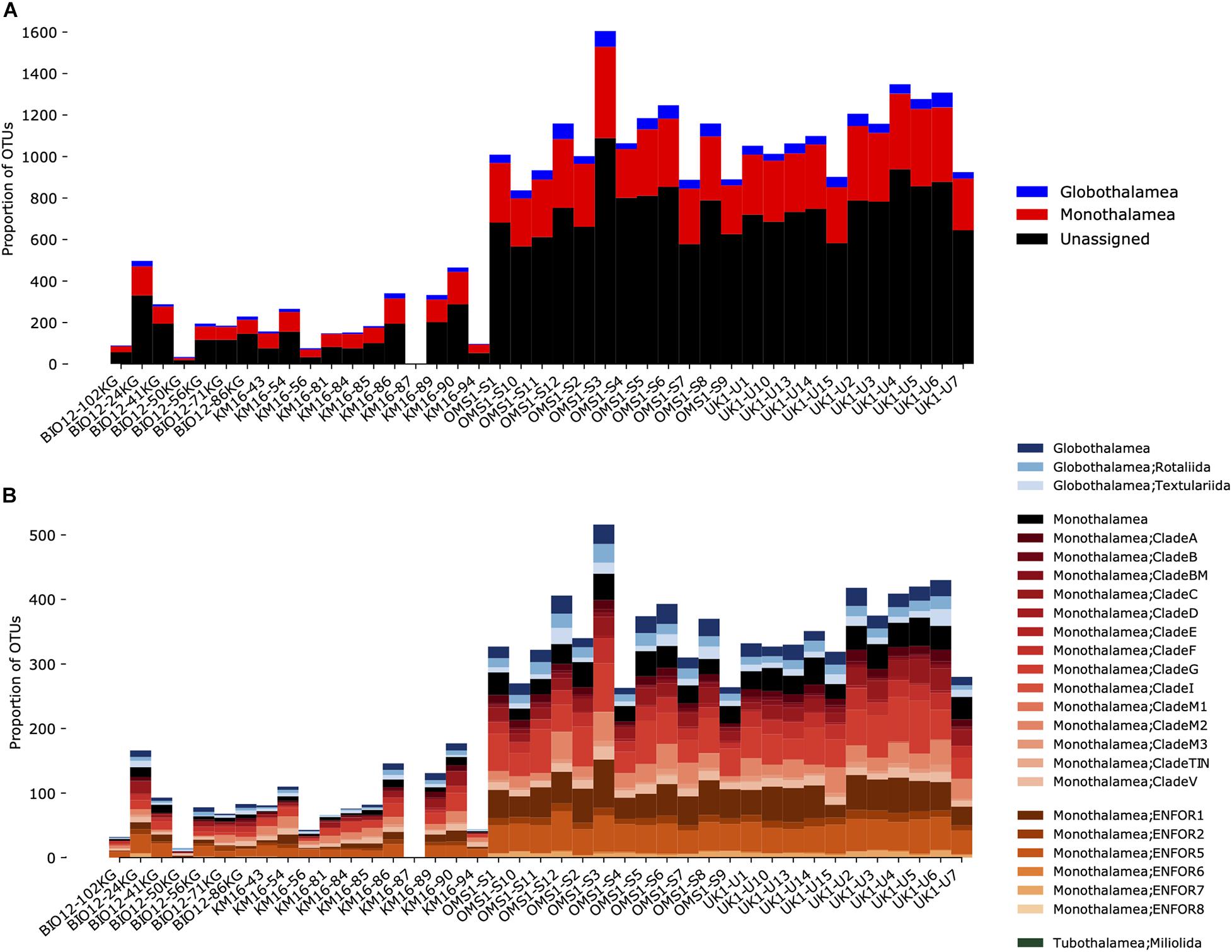
Figure 5. Taxonomic composition of the OTUs found in samples from CCZ sites. The top panel includes the unassigned OTUs (A), which are absent in the bottom panel where only OTUs that were assigned to Globothalamea, Tubulothalamea, and Monothalamea are shown, at a finer taxonomic level (B); note the high diversity of monothalamid clades.
We first identified localized OTUs that occurred in only one of the CCZ areas, and then investigated those area-specific OTUs that a) also occurred at only one station per area and/or b) in only one sediment core per station (Table 4). More than half (3457 = 53.8%) of the 6,425 OTUs met the first criterion. In the OMS and UK-1 areas, a large majority (82.0% and 84.9%, respectively) of these area-specific OTUs do tend to occur at only one station; this proportion approaches 100% for BGR- and IFREMER-specific OTUs. Since OTUs associated with very few sequence reads are necessarily rare and strongly contribute to localized occurrences, we also determined these single-station and single-core occurrences for the 685 OTUs (19.8% of the localized OTUs and 10.7% of all OTUs) that include at least 1% of the total read count for each sample (Table 5). This reveals that even these more highly-sequenced OTUs tend to be found locally. This is notably the case for 2 and 4 OTUs assigned to Textulariida and 2 and 5 assigned to the Rotaliida in the OMS and UK1 areas, respectively. Some of these were genus- or species-level assignments (Pullenia subcarinata, Cibicidoides wuellerstorfi, Eggerella sp., and Reophax sp.). Even more striking is the fact that a large majority of these highly-sequenced OTUs are also found in one of the two sediment cores taken at each station (100% in column D of Table 5), suggesting a higher degree of local patchiness.
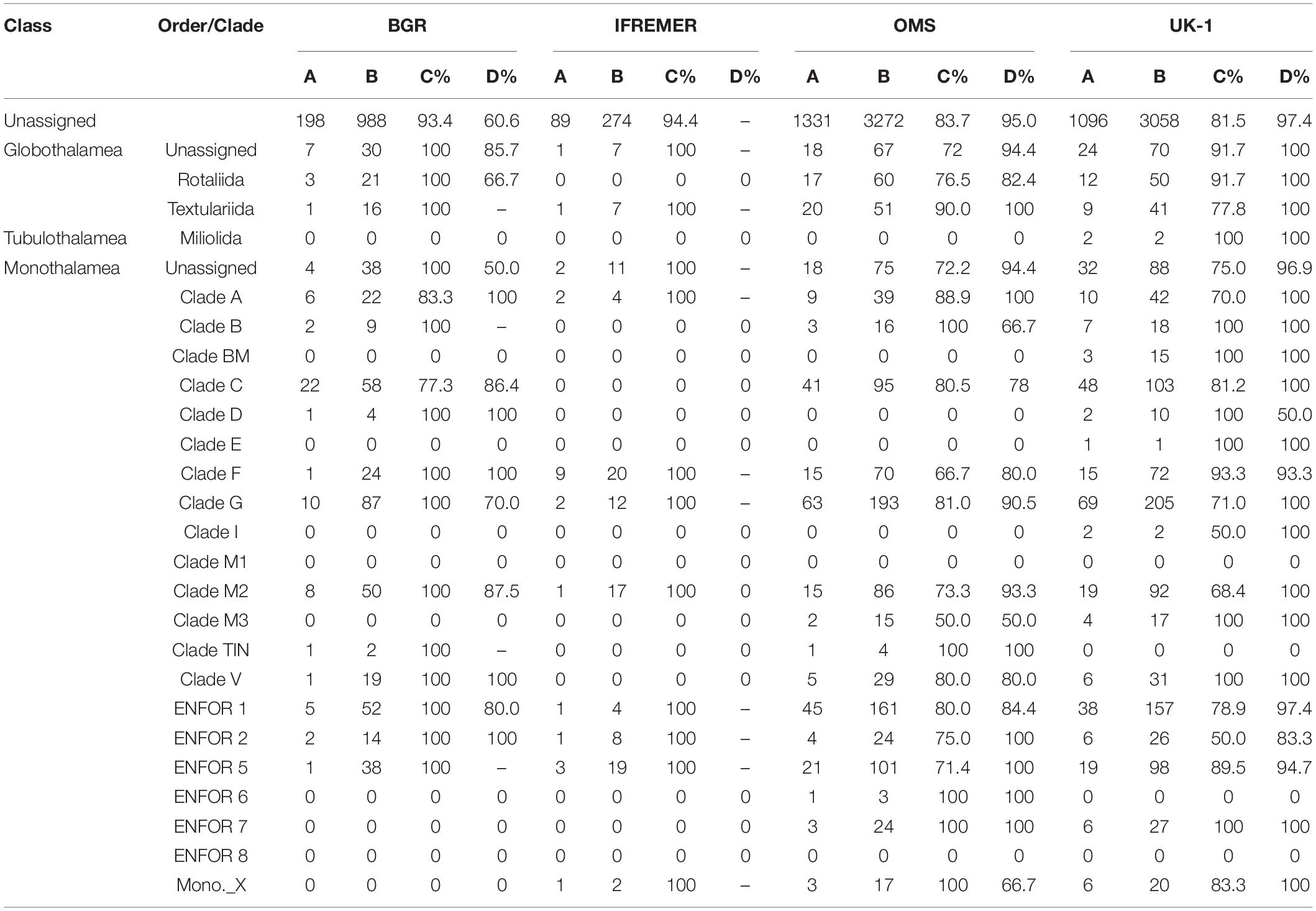
Table 4. Number of OTUs that are confined to one CCZ area, station or single core. Data are arranged according to foraminiferal orders or clades and include only OTUs that are confined to the CCZ. A = number of OTUs found only in this area; B = total number of OTUs in the full dataset; C = percent of ‘A’ found at only one station; D = percent of ‘A’ found in only one core from this station. Note that a dash in column D indicates that only one core was sampled for metabarcoding. Two cores per station were always sampled in the OMS and UK-1 areas, but only one core was sampled per station in the IFREMER area and at some of the BGR stations.
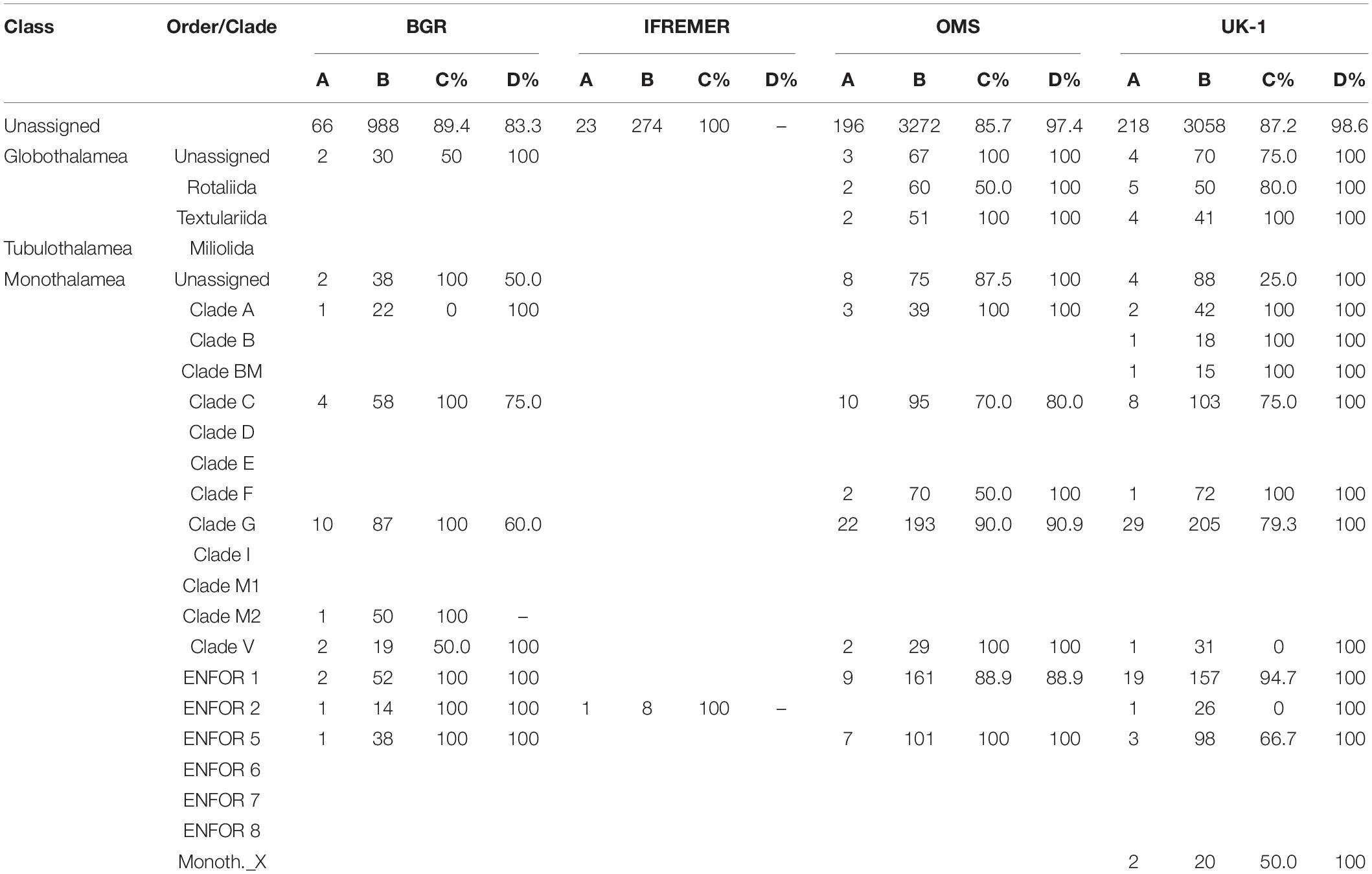
Table 5. Number of OTUs that are confined to one CCZ area, station or single core. Data are arranged according to foraminiferal orders or clades and include only OTUs that are specific to CCZ areas and present in samples with >1% of sequence reads. A = number of OTUs found only in this area. B = total number of OTUs in the full dataset. C = percent of ‘A’ found at only one station. D = percent of ‘A’ found in only one core from this station. Note that a dash in column D indicates that only one core was sampled for metabarcoding. Two cores per station were always sampled in the OMS and UK-1 areas, but only one core was sampled per station in the IFREMER area and at some of the BGR stations.
Faunal Trends and Species Distributions Across the CCZ
Overall Trends
Across the CCZ, latitudinal and longitudinal gradients in surface productivity and food flux to the seafloor (Smith et al., 2019), together with the east to west increase in water depth and carbonate dissolution, are likely to influence faunal composition and therefore species ranges. The scarcity of data from the western CCZ means that longitudinal trends in foraminiferal assemblage composition are not well known. Saidova (1981) recognized a number of assemblages on Pacific abyssal plains that were linked partly to productivity. In the southern part of the CCZ, a Cribrostomoides profundum assemblage in the east gives way to a Recurvoidatus spiculotestus assemblage in the west, while the northern part is occupied by a Rhabdammina inordita assemblage. Our only across-CCZ data based on consistent sieve fractions (> 32 μm) come from three Japanese studies at the Kaplan East (KE), Kaplan Central (KC) and Japan Deep-Sea Impact Experiment (JET) sites, a total east to west distance of more than 3,000 km (Table 6). These reveal similar proportions of major groups. Monothalamids (which we interpret to include Lagenammina species and Nodellum-like forms) are dominant, particularly at the KE and KC sites (>80%). If monothalamids are excluded, then the main groups are hormosinids, and at the JET site, other textulariids. Calcareous rotaliids, which might be expected to be less common in the deeper and less productive western CCZ, represent the highest proportion (22%) of the monothalamid-free assemblages at the KC site, and the lowest proportion (8.2%) at the JET site. However, they are relatively more important at the JET (8.53%) compared to the KC and KE (4.54 and 4.29%, respectively) sites when all foraminiferal groups are included. Rotaliids persist at depths below the CCD, despite the fact that their tests are often dissolved, presumably as a result of carbonate dissolution on the seafloor.
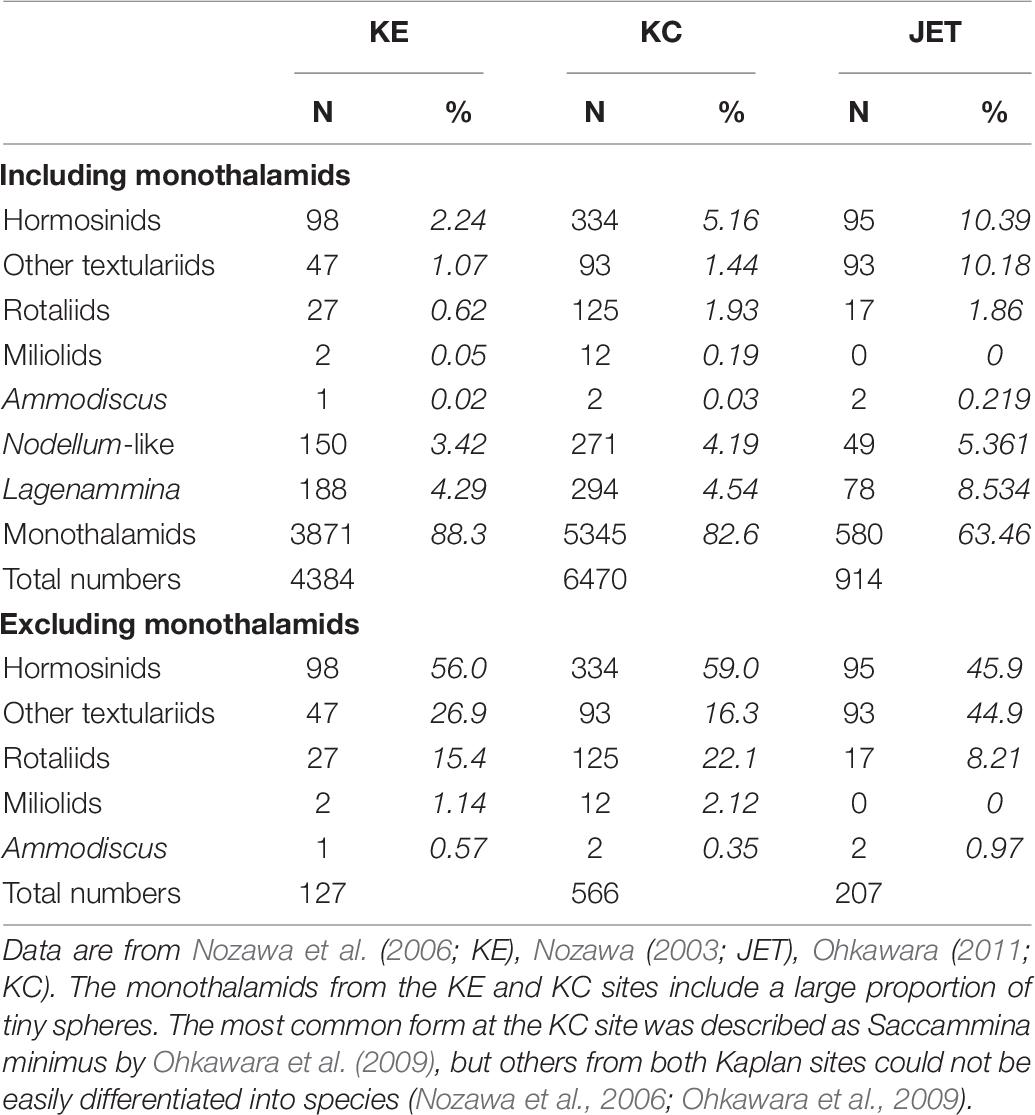
Table 6. Proportions of major foraminiferal groupings in > 32-μm fraction of subsamples (0-1 cm layer) from Kaplan East (KE), Kaplan Central (KC) and JET sites.
At smaller spatial scales, all samples from UK-1 and OMS fall within 95% confidence limits in MDS plots (data not shown), indicating a high degree of assemblage uniformity at the contract-area scale (Goineau and Gooday, 2019). A decay-distance analysis based on Bray-Curtis similarity vs distance showed only minor differences in the species composition of UK-1 and OMS samples separated by increasing distances of up to ∼220 km (Figure 6). However, there was a general tendency for differences in species composition to increase with distance, and this overall trend was significant (p = 0.006). Thus, although particular morphospecies may have wide ranges, there seem to be gradual shifts in the species composition of foraminiferal assemblages in relation to environmental gradients within the CCZ.
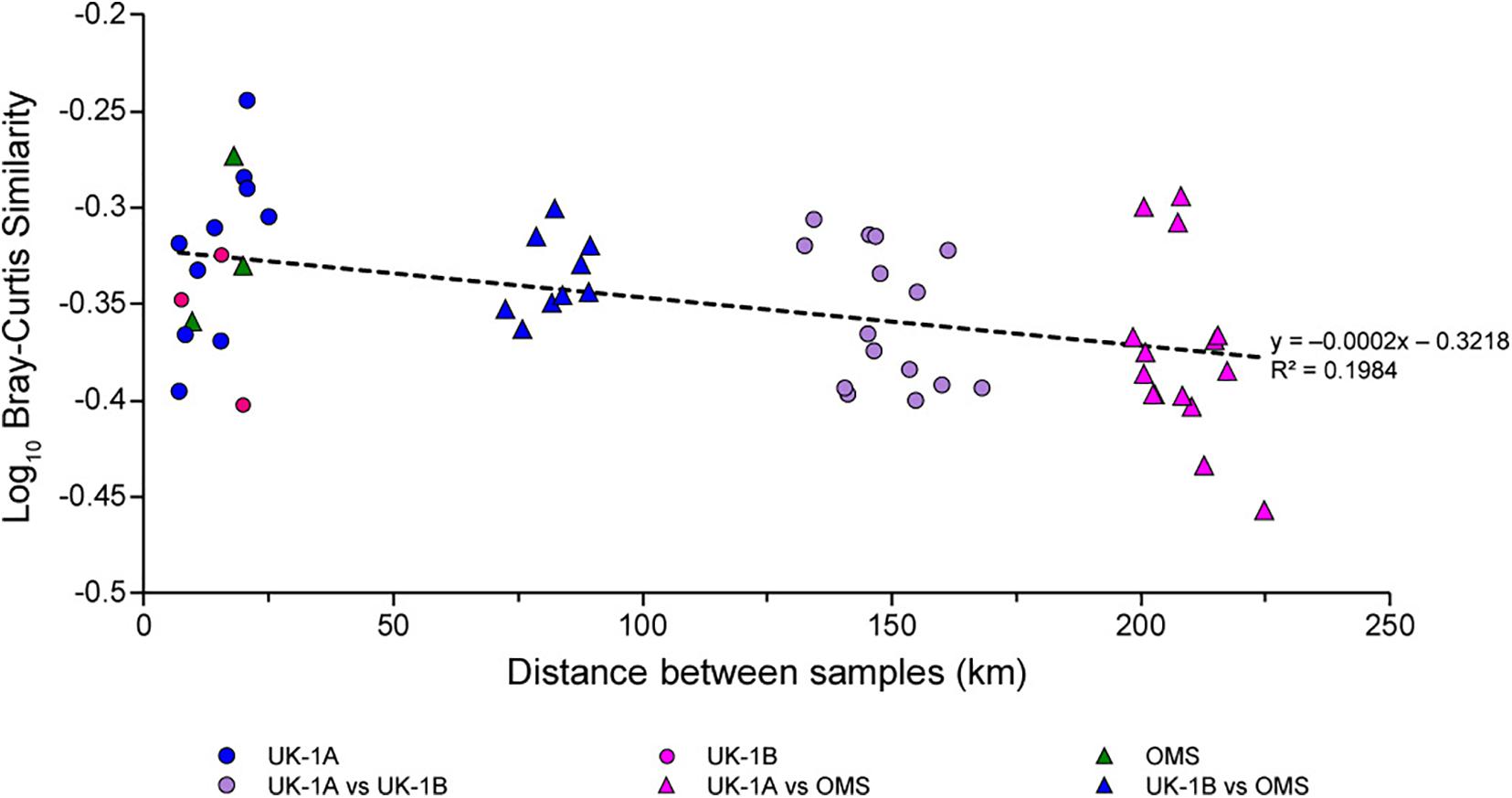
Figure 6. Similarity between samples compared across increasing distances in the UK-1 and OMS contract areas. Based on Bray-Curtis similarity indices computed with species presence-absence data. The cluster of symbols at the left-hand side refers to comparisons of samples within each of the 30 × 30 km ‘strata’ (UK-1A, UK-1B, and OMS; 5, 3, and 3 samples, respectively); the other clusters refer to comparisons between these three strata. Similarity values within and between strata are significantly different (t-test, p = 0.012) only when the two most widely spaced strata, UK-1A and OMS, are compared (cluster of purple triangles on right-hand side). However, the overall trend indicated by the dotted line is significant (Spearman’s rank correlation, rs = 0.373, p = 0.006). Redrawn from Deep-Sea Research I, vol. 149, A. Goineau and A.J. Gooday, Diversity and spatial patterns of foraminiferal assemblages in the eastern Clarion–Clipperton zone (abyssal eastern equatorial Pacific), Article 103036, 2019, with permission from Elsevier.
Species Distributions
Relatively little is known about species ranges across the CCZ. Saidova (1974, 2000) provides some information on species found at R/V Vityaz Station 5996, located at the western extremity of the CCZ (153–154° W; Figure 2c, Appendices A and B in Supplementary Material). Although different names are sometimes used in Russian and non-Russian literature, a number of species reported by Saidova from this western site are probably the same as those found in the eastern CCZ (Goineau and Gooday, 2017, 2019). They include Adercotryma glomeratum, Cyclammina trullissata [= Cyclammina. subtrullissata (Parr, 1950) of Saidova, 1975], Globocassidulina subglobosa [= Bradynella subglobosa] and Nuttallides umbonatus [likely = Osangulariella bradyi]. Some meiofauna-sized monothalamid morphospecies that span similar distances within the CCZ (146°–150° W to ∼116°–117° W) are illustrated in Figure 7.
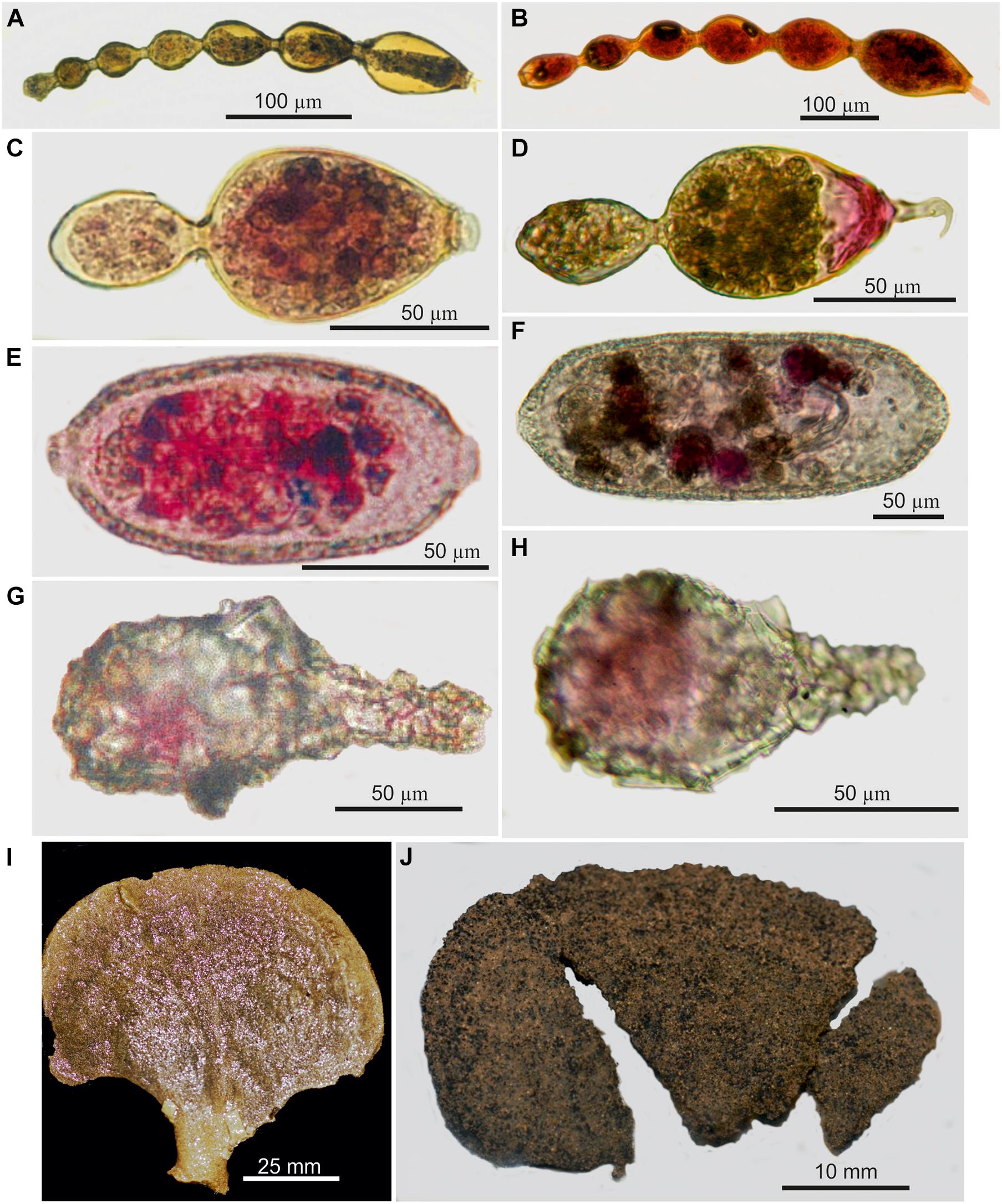
Figure 7. Morphospecies occurring at the western (A,C,E,G,I) and eastern (B,D,F,H,J) ends of the CCZ. (A,B) Resigella moniliforme (Resig, 1982) from the JET site (A) and UK-1 contract area (B). (C,D) Bilocular Resigella sp. from the JET (C) and KE (D) sites. (E,F) Saccamminid with terminal apertures from the JET site (E) and UK-1 contract area (F). (G,H) Lagenammina sp. from the JET site (G) and OMS contract area. (H) Moanammina semicircularis Gooday and Holzmann, 2020 in Gooday et al. (2020a) from APEI4 (I) and the OMS contract area (J); in this case, genetic data confirm that these two specimens are conspecific (Gooday et al., 2020a).
Five of the macrofaunal komokiacean morphospecies from epibenthic sledge samples taken in the German, IOM, Belgium, French, and APEI-3 areas (Wawrzyniak-Wydrowska and Gooday, unpublished) were originally described, either from the central North Pacific (30°N, 156°W; 6070 m depth) (Tendal and Hessler, 1977) or the North Atlantic (Shires et al., 1994), indicating wide distributions. Similarly, some of the nodule-encrusting macrofaunal morphospecies in the UK-1 and OMS areas (Gooday et al., 2015; unpublished data) are also reported from the eastern and western CCZ sites of Veillette et al. (2007). These include two species of the komokiacean genus Chrondrodapsis, originally described from within the CCZ (15°N, 125°W) (Mullineaux, 1988). Delicate net-like formations assigned to the genus Telammina have been found growing on firm substrates in the CCZ and North Atlantic (Gooday et al., 2015), as well as the Indian Ocean (Figure 6D in Aranda da Silva and Gooday, 2009). However, there are no reliable genetic data for any komokiaceans or indeed for any nodule-encrusting species.
Previous studies have provided a wealth of information on the distribution of foraminiferal morphospecies, mainly hard-shelled, at abyssal depths in the wider Pacific Ocean. Table 7 summarizes Pacific records for meiofauna-sized species, most of them with easily recognizable multichambered tests, recorded by Goineau and Gooday (2017, 2019) from the eastern CCZ. They all have wide geographical ranges at abyssal depths in the Pacific. There are also records of many of these species in other oceans, and some are considered to have “cosmopolitan” distributions (e.g., Holbourn et al., 2013).
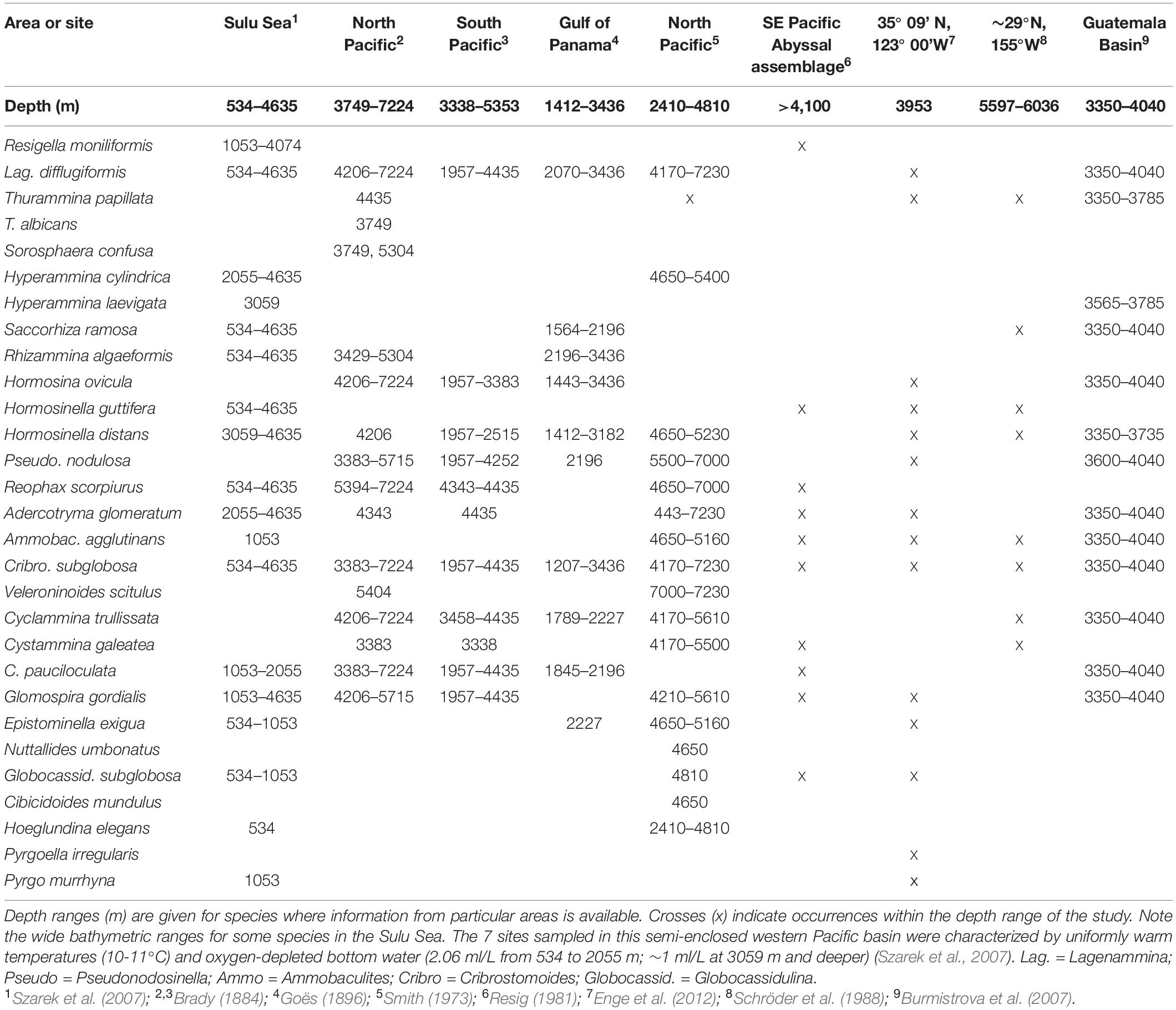
Table 7. Literature records from the Pacific Ocean of selected foraminiferal species that occur in the UK-1 and OMS contract areas.
Genetic studies (SSU rRNA sequences) provide some support for wide ranges. Those of two rotaliids, Cibicidoides wuellerstorfi and Epistominella exigua, span 17,000 km from the Arctic to the Southern Ocean (Pawlowski et al., 2007), extending into the NW Pacific in the case of E. exigua (Lecroq et al., 2009b) and the NE Pacific in the case of C. wuellerstorfi (Burkett et al., 2020). Sequences derived from E. exigua specimens collected in the UK-1 and OMS contract areas during the ABYSSLINE project were identical to those of Pawlowski et al. (2007) and Lecroq et al. (2009b) from the NW Pacific and other oceans. A SW Atlantic specimen of Nuttallides umbonatus was genetically identical to several from the UK-1 and OMS areas, where this is the most common rotaliid species. The greater degree of genetic differentiation reported in Arctic and Antarctic populations of other rotaliid species, Oridorsalis umbonatus (Pawlowski et al., 2007), however, suggests that some “cosmopolitan” morphospecies, particularly those found across a wide depth range, may encompass a number of cryptic species.
Many xenophyophores species (described and undescribed) are represented in the CCZ by 1-2 specimens and are known from a single site, so nothing can be said about their wider distributions (Gooday et al., 2020b). Psammina limbata, described morphologically from the Russian area in the central CCZ (Kamenskaya et al., 2015), may also be present in the UK-1 and OMS areas, although without genetic data from Russian specimens this cannot be confirmed (Gooday et al., 2018a). However, wide ranges across a distance of 3,800 km (from APEI-4 to UK-1/OMS sites) have been confirmed genetically for two xenophyophore species (Gooday et al., 2020a). One of these (Moanammina semicircularis) is illustrated in Figure 7I,J. The other, Aschemonella monilis, is known from the Russian area (Gooday et al., 2017a) and APEI-6 (Simon-Lledó et al., 2019a), in addition to UK-1, OMS and APEI-4.
Undersampling, Patchiness and Rarity
Biogeographic patterns, including endemism, can be discerned with some confidence among benthic foraminiferal species living in coastal and shelf environments (Culver and Buzas, 1999; Murray, 2013; Hayward et al., 2021). However, limited sampling, combined with small-scale faunal patchiness, makes establishing geographical ranges and recognizing species with restricted distributions a much more difficult task in the deep ocean. The problem is compounded, particularly in the abyss, by the vast extent of the environment and the apparent rarity of many species. Around 60% of the 547 unfragmented species (> 150-μm fraction) in UK-1 and OMS samples are found at only 1 or 2 out of 11 sites but are represented by a relatively small proportion (16.7%) of specimens (Figure 8). Many species confined to one site are singletons. On the other hand, the few species (4.2%) that are recorded across 10 or 11 sites account for a relatively large proportion (35.9%) of specimens. Similarly, > 80% of macrofaunal foraminiferal species in epibenthic sledge samples from the Belgian, German, IOM, and French areas and APEI-3 are confined to one area, and 74% are confined to one of the 2 replicate samples analyzed from each area (Wawrzyniak-Wydrowska and Gooday, unpublished). The metabarcoding data summarized above (Table 4) also indicate that many OTUs are confined to one station and in some cases to one core. These OTUs are often rare (represented by few reads) and this, together with patchiness and under-sampling, most likely accounts for their localized occurrence. A minority of OTUs found only at one station or in one core are represented by > 1% of reads (Table 5) and therefore are not particularly rare. In these cases, under-sampling and a patchy distribution could still explain why they are only found in one place. The unique sequences that are combined to create OTUs may exhibit even more localized spatial distributions. These morphological andmetabarcoding studies highlight the likely prevalence of ‘pseudo-endemism’ and the need for more sampling.
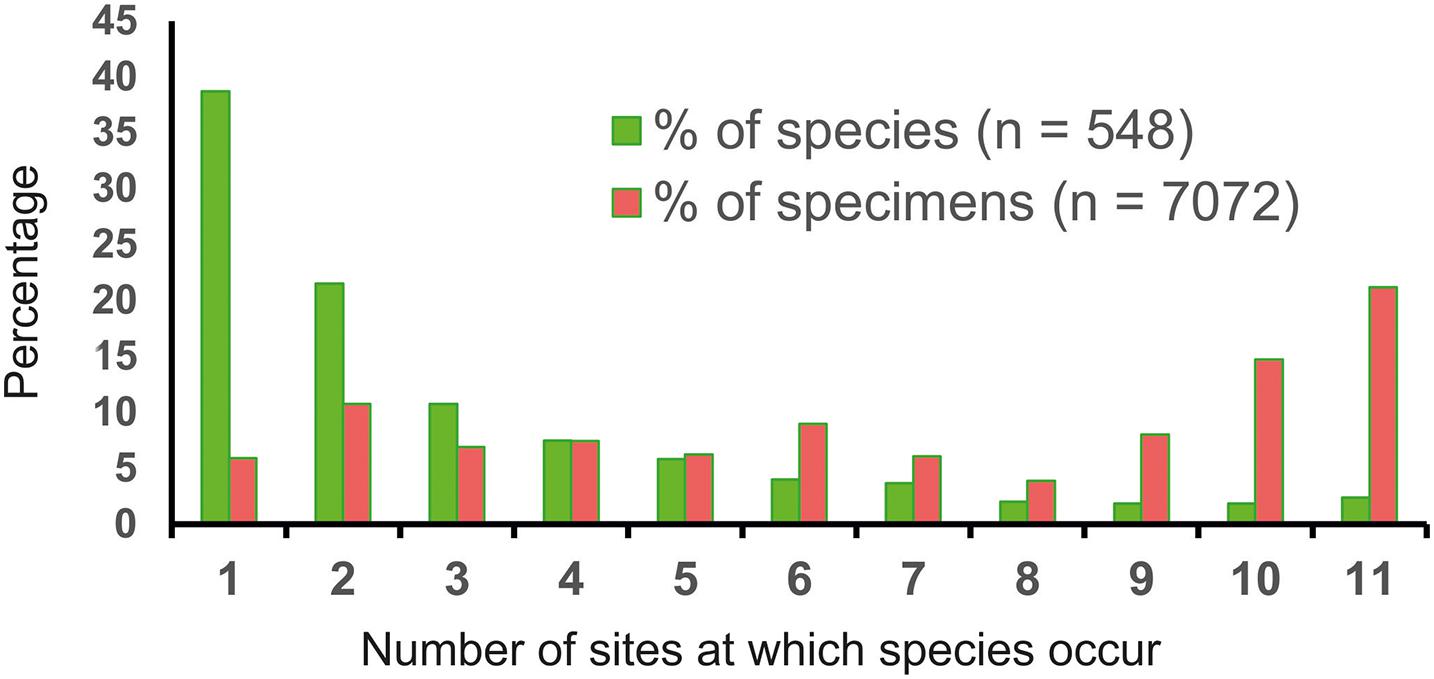
Figure 8. The percentage abundance of species (total = 548) represented by complete tests and specimens (total = 7072) occurring at different numbers of sites (from a single site on the left of the x axis to all 11 sites on the right) within the UK-1 and OMS areas in the eastern CCZ. On the left-hand side, 60.2% of species are confined to 1 or 2 sites but these represent only 16.6% of specimens (i.e., species confined to 1 or 2 sites tend to be rare). In contrast, only 4.2% of species occur at 10 or 11 sites, but these represent 35.9% of specimens (i.e., widely-distributed species tend to be abundant). The relatively high percentage (10.7%) of specimens occurring at 2 sites (second point on x axis) reflects the high abundance (n = 296) of a single species. If this is removed, then the contribution of species confined to 1 or 2 sites decreases from 16.6% to 13.0% of specimens and that of species occurring at 10 or 11 sites increases 35.9 to 37.5%. Based on data from Goineau and Gooday (2019).
Broad biogeographic patterns undoubtedly exist across the Pacific abyss. Several abyssal benthic provinces have been proposed for this ocean, most recently by Watling et al. (2013), while Saidova (1981) recognized a series of foraminiferal communities on Pacific abyssal plains related to surface productivity, combined with carbonate dissolution and bottom-water currents. Recent, metagenomic studies have revealed high number of OTUs that are confined to the CCZ (Lejzerowicz et al., 2021). However, whether some foraminiferal species are confined to smaller areas of the Pacific, including parts of the CCZ, and occur nowhere else in the global ocean, is difficult to establish. At present, it is not possible to distinguish strictly endemic distributions of this kind from artifacts resulting from rarity combined with under-sampling (i.e., ‘pseudo-endemism’). It is worth noting that the ability of foraminiferal species to reproduce asexually may allow them to persist at very low population densities (Murray, 2013) over large, oligotrophic areas of the deep sea, without the need for propagules to be introduced from more productive areas, as postulated for some metazoan macrofaunal taxa (“source-sink hypothesis”; Rex et al., 2005; Hardy et al., 2015). This may enable even rare species to maintain wide geographical ranges.
Genetic Connectivity
As indicated above, there is genetic evidence that some foraminiferal species have wide ranges across the CCZ and beyond. Lecroq et al. (2009b) analyzed the population genetics of Epistominella exigua (a calcareous species found in the UK-1 and OMS areas) from sites in the Arctic, North Atlantic, Southern Ocean, and the western Pacific off Japan, based on the complete ITS rDNA sequences. They found very little divergence between ITS haplotypes from different oceans. Whether or not these results for E. exigua, which is an unusually opportunistic species that exploits phytodetritus deposits (Gooday, 1988), are typical for other foraminifera living in the CCZ is an important question for the future.
Possible Ecological Roles for Benthic Foraminifera in the CCZ
The abundance and diversity of foraminifera across different size classes and microhabitats in the CCZ suggest that they play an important ecological role in benthic communities (reviewed by Gooday, 2019; Gooday et al., 2020b). As a group, deep-sea foraminifera have a wide range of diets (Gooday et al., 1992, 2008; Nomaki et al., 2006). Species of Globothalamea and Tubothalamea, notably the calcareous rotaliids and miliolids, respectively, probably depend more on inputs of labile organic matter derived from surface production than most abyssal monothalamids, particularly those that accumulate stercomata, the likely waste products of feeding on sedimentary particles and detritus. Many rotaliids have smooth test morphologies consistent with movement through the sediment and are probably relatively active metabolically, whereas deep-sea monothalamids often have test morphologies that must make movement difficult or impossible, suggesting relatively low metabolic rates. Foraminiferal ecology is complex and this distinction is certainly too simplistic. Not all deep-sea monothalamids accumulate stercomata (Turley et al., 1993; Gooday et al., 2004) and some rotaliids ingest sediment and associated bacteria (Goldstein and Corliss, 1994); some small monothalamids are quite mobile (Groos, 2000) while some rotaliids are sessile (Mullineaux, 1987). However, it may be useful to bear this distinction in mind, particularly in abyssal settings where food limitation, combined with carbonate dissolution, favours stercomata-bearing monothalamids.
Role in Food-Webs and Carbon Cycling
Research conducted since the 1970s has documented the potentially important contribution of foraminifera to deep-sea food webs and carbon cycling. By typically feeding at a low trophic level and being consumed by specialist and incidental predators, foraminifera represent an important trophic link at the base of deep-sea foodwebs (Lipps and Valentine, 1970; Gooday et al., 1992; Nomaki et al., 2008; Würzberg et al., 2011). Feeding and reproductive responses to phytodetritus are reported in the bathyal and abyssal NE Atlantic (Gooday, 1988; Gooday and Lambshead, 1989; Gooday and Hughes, 2002), the bathyal western Pacific (Kitazato et al., 2003), and at Station M (4000 m depth) on the Californian margin to the north of the CCZ (Drazen et al., 1998). In situ “pulse-chase” experiments using 13C-labelled diatoms as tracers of food uptake have likewise suggested a significant role for foraminifera in the short-term processing of fresh organic matter in the bathyal NW and NE Atlantic (Levin et al., 1999; Moodley et al., 2002), the bathyal NW Pacific (Nomaki et al., 2005), and at Station M (Enge et al., 2011). At the level of individual foraminifera, shipboard experiments and transmission electron microscopy have demonstrated the ingestion of fresh phytoplankton and zooplankton detritus, along with increases in the cellular carbon content and metabolic and enzymatic activity within days of artificial and natural food pulses (Heeger, 1990; Altenbach, 1992; Linke, 1992; Graf and Linke, 1992; Linke et al., 1995). Köster et al. (1991) found high levels of enzymatic activity associated with agglutinated foraminifera (Hyperammina and Reophax) in the Norwegian-Greenland Sea. They concluded that foraminifera can dominate the enzymatic hydrolysis of organic matter in areas where they are abundant.
How far are these observations, made mainly on eutrophic continental margins, applicable in the oligotrophic CCZ, where there is much less evidence for seasonality in food supply? Sweetman et al. (2018) carried out pulse-chase experiments to determine the uptake of 13C-labeled diatoms by bacteria and macrofauna (>300 μm) in the UK-1 and OMS areas. Uptake was dominated by bacteria, with macrofaunal foraminifera contributing very little. This is not surprising since this size fraction in the abyssal Pacific comprises mainly low-biomass, stercomata-bearing monothalamids (Tendal and Hessler, 1977; Bernstein et al., 1978). Nevertheless, phytodetritus deposition events have been observed occasionally in the CCZ (Radziejewska, 2002) and are likely to evoke a response from small species such as Epistominella exigua, which occurs in the eastern CCZ (Gooday and Goineau, 2019). This and other rotaliids, including the most common calcareous species Nuttallides umbonatus, contain green cytoplasm, an indication of feeding on fresh phytoplankton-derived material. Moreover, the macrofaunal fraction in this area includes some large species (miliolids, the textulariid Cribrostomoides subglobosa, and the monothalamous Crithionina hispida), which were among those to respond strongly to food pulses on the Norwegian margin (Heeger, 1990; Thies, 1991; Altenbach, 1992; Linke, 1992; Linke et al., 1995). According to Enge et al. (2011), Saccorhiza ramosa, a common nodule-attached species across the CCZ (Gooday et al., 2015), was responsible for 61 and 99% of tracer uptake in the 1-2 and 2-3 cm layers, respectively, at Station M. These observations suggest that foraminifera play some role in the processing of organic carbon in the CCZ, although the contribution of calcareous taxa will probably be greater at the shallower eastern end where carbonate dissolution is less of an issue.
Limited knowledge about the ecology of abyssal foraminifera, and monothalamids in particular, hampers understanding of the overall contribution that these benthic protists make to carbon cycling in the CCZ. Although saccamminids (flask-shaped, soft-walled monothalamids) were a major component of foraminiferal assemblages at Station M, their contribution to carbon uptake in 13C-tracer experiments was almost zero (Enge et al., 2011). Some small, organic-walled monothalamids have relatively featureless cytoplasm (Supplementary Figure 9A–C in Gooday and Goineau, 2019) and may consume bacteria (Turley et al., 1993), while gromiids are packed with waste pellets (stercomata) and often ingest cysts, fragments of other organisms, or mineral grains (Figures 3A,B in Renaud-Mornant and Gourbault, 1990). The storage of stercomata, which comprise mainly clay minerals, is typical of many abyssal monothalamids and probably reflects the ingestion of sediment and degraded organic matter. Lipid analyses suggest that bacteria may also be consumed by xenophyophores (Laureillard et al., 2004). Stercomata-retaining groups such as komokiaceans and xenophyophores, often have large tests but sparse cytoplasm. As a result, their biomass is much less than their macro- or mega-faunal sizes would suggest (Tendal, 1979; Levin and Gooday, 1992; Shires et al., 1994; Gooday et al., 2018b), a likely adaptation to severely energy-limited abyssal environments. This kind of organization also makes distinguishing “live” from dead specimens in fixed samples using Rose Bengal staining problematic, possibly leading to the over-estimation of “live” abundances. All of these factors suggest that the contribution of monothalamids to carbon cycling in the CCZ does not match their abundance and diversity although, given their large contribution to benthic communities, it may still be considerable. However, there are very few reliable data about their biomass or rates of metabolic activity, which are crucial parameters if foraminifera are to be included in carbon-based, food-web modeling studies (van Oevelen et al., 2011; de Jonge et al., 2020).
Provision of Heterogeneity
Gooday et al. (2020b) review the role of xenophyophores as a potentially important source of seafloor heterogeneity in parts of the deep sea where they are an abundant component of the megafauna. Briefly, their tests, whether alive or dead, may (1) enhance the deposition of fine sediment and labile material in their immediate vicinity, creating food-rich hotspots (a likely reason why ophiuroids are often seen coiled around test bases; Levin and Thomas, 1988); (2) provide habitat structure that is utilized for multiple purposes by a wide range of meiofaunal and macrofaunal metazoans (e.g., Levin et al., 1986; Levin, 1991; Levin and Gooday, 1992), foraminifera (Hughes and Gooday, 2004), and even fish (Levin and Rouse, 2019), and (3) host an enhanced and distinctive microflora (Hori et al., 2013). These heterogeneity-enhancing attributes are either known or likely to apply in the CCZ.
Although the large test sizes of many xenophyophores makes them especially important in this regard, smaller meio- and macro-faunal foraminifera can also create habitat structure that is relevant to smaller organisms (Thistle, 1979, 1982). A genetic analysis of komokiacean tests (Normanina conferta and Septuma ocotillo) from the Weddell Sea revealed an extraordinary diversity of fungal and protistan sequences (Lecroq et al., 2009a), suggesting that these structures are hotspots of microbial diversity. An X-ray radiograph of a core from the eastern equatorial Pacific Panama Basin (3912 m depth) shows the sediment permeated by a network of fine burrows attributed to Reophax (Kaminski et al., 1988), the most common multichambered agglutinated genus in the eastern CCZ (Goineau and Gooday, 2017, 2019). Based on an analysis of “vegematic” box cores (i.e., partitioned into subcores) at the CLIMAX II site north of Hawaii, Bernstein et al. (1978) found that certain groups of foraminifera exhibited possible correlations with biological variables, such as prevalence of surface or subsurface deposit feeders and carnivores. These examples suggest that the diverse agglutinated foraminifera, including morphologically complex komokiaceans, mudballs, branching tubes, and chain-like formations that are common in CCZ samples, may help to structure sedimentary habitats and serve as microhabitats for other organisms.
Possible Recolonization Scenarios
Some clues to possible foraminiferal responses to the blanketing of the seafloor by sediment redeposited from the plume created by mining may be gleaned from studies in the South China Sea, where a large area was covered by a layer of volcanic ash (1 to 90 mm thick at sampled sites) following an eruption in the Philippines in 1991. Different parts of the impacted area (2338–3322 m depth) were sampled on 5 occasions: April 1994, June 1996, November/December 1996 and June/July 1998, and April 1999 (Hess and Kuhnt, 1996; Hess et al., 2001; Kuhnt et al., 2005). Among the observations that may be relevant to seabed mining impacts were the following. (1) Impact of the ash layer depended on its thickness. All foraminifera were killed where it was 60–80 mm thick; mobile infaunal species were significantly reduced where it was 20 mm thick, and epifaunal suspension feeders were eliminated by ash deposits <10 mm thick. (2) Twenty agglutinated species survived the ash fall at a site where the thickness was 15 mm, as shown by their use of quartz grains and biogenic particles in early chambers (pre-dating ash fall) and ash particles in later chambers. Most were considered to be mobile infaunal species. Some (Ammobaculites agglutinans, Cribrostomoides subglobosum, Hormosinella distans, Hyperammina elongata, Reophax scorpiurus, Rhizammina algaeformis, Saccorhiza ramosa) have been identified in the eastern CCZ. 3) A first wave of pioneer recolonizing species was recognized in 1994 samples. These were followed by a second wave that dominated in 1996. Suspension feeders, including large xenophyophores, appeared in the 1998 samples. Xenophyophores not reliant on nodules as an attachment substrate could therefore be among the first large immobile organisms to reappear in areas where seabed mining has destroyed the fauna (Gooday et al., 2020b), although there is no evidence of this having happened yet at experimental disturbance sites such as DISCOL (Figure 1 in de Jonge et al., 2020). 4) The earliest recolonizers were small, mobile, agglutinated forms, mainly R. dentaliniformis and Textularia sp., that were not present in the original pre-eruption assemblage or in control samples from unaffected areas. They were succeeded by two other Reophax species (R. bilocularis, R. scorpiurus) and the miliolid Quinqueloculina seminula.
The ash-fall observations are suggestive, but the South China Sea is a very different environment from the CCZ and they should be extrapolated only with considerable caution. Apart from being shallower, the South China Sea experiences monsoonal winds, leading to seasonal changes in productivity, as well as much stronger current activity than the CCZ. The ash substrate is very different geochemically and mineralogically from CCZ sediments, and is much coarser-grained (Kuhnt et al., 2005). Nevertheless, in the absence of any real evidence for how foraminiferal recolonization will proceed following mining impacts, these observations could provide pointers for what might happen. They are also consistent with other observations. Two species of Reophax (R. dentaliniformis, R. excentricus), a genus that included some of the pioneer ash-fall recolonizers, were the most common foraminifera after 9 months in 30 × 30 cm trays of defaunated sediments deployed at a 3,912-m-deep site in the Panama Basin (> 297-μm fraction; Kaminski et al., 1988). Reophax dentaliniformis also recolonized disturbed sediments at 1,478 m in the axis of the Cap Breton Canyon in the NE Atlantic (Duros et al., 2017). We suggest that members of this important uniserial genus, which is the most abundant multichambered foraminiferal taxon in the eastern CCZ (Goineau and Gooday, 2017, 2019), may be among the earliest to reappear following direct or indirect mining impacts. They might be accompanied by small biserial agglutinated taxa, notably Textularia spp. and Spiroplectammina spp., that also occur in the CCZ. Dispersal of such species could be mediated by the transport of propagules (tiny 1-2 chambered juveniles) (Alve and Goldstein, 2003, 2010), although very low current speeds in the CCZ suggest that recolonization would proceed at a slower rate than in the South China Sea.
Concluding Remarks
Foraminifera are unique among marine eukaryotes, and protists generally, in spanning a size range from meiofauna to megafauna. They are also by far the most abundant benthic organisms to be preserved in the oceanic fossil record and important palaeoceanographic indicators of ancient seafloor environments. As a result, many deep-sea species have been described based on test morphology, and numerous publications record their distribution in modern oceans, making them one of the best documented of deep-sea benthic taxa and potential models for understanding biogeographic patterns in the abyss. The well-known, mainly multichambered foraminifera occur alongside delicate, monothalamous forms that have very little fossilization potential and include many undescribed species. In the CCZ and other parts of the abyssal Pacific, oligotrophic conditions, combined with increasing carbonate dissolution close to and below the carbonate compensation depth, favour the development of monothalamid-dominated assemblages. Some belong to known genera and species, or can be assigned to higher taxa like the Komokioidea and Xenophyophoroidea. However, other abyssal monothalamids are difficult to accommodate within current morphology-based taxonomic systems for agglutinated foraminifera (e.g., Kaminski, 2014).
The monothalamid component of CCZ assemblages poses further challenges. 1) Some tests, notably komokiaceans and tubular morphotypes, have a strong tendency to fragment, and are therefore difficult to quantify. The best solution is to count fragments separately from intact specimens (Bernstein et al., 1978; Nozawa et al., 2006) and include fragmented species in species numbers but not in calculations of species diversity. 2) Ecological studies of modern foraminifera need to distinguish between specimens that were living when collected and those that were dead. The simplest and most common method is Rose Bengal staining, but stercomata-bearing monothalamids have sparse cytoplasm and do not stain well, often making it difficult to discriminate between “live” and dead tests. 3) Although DNA sequences can be obtained easily from the megafaunal xenophyophores and smaller, more typical deep-sea monothalamids, including saccamminids (e.g., Conqueria) and allogromiids (e.g., Bathyallogromia, Micrometula), attempts to sequence the stercomata-bearing forms, such as komokiaceans, have often proved unsuccessful (e.g., Lecroq et al., 2009a). These difficulties limit understanding of the phylogenetic position and diversity of these problematic organisms, as well as hampering the description of new species. 4) Finally, although we can speculate that morphologically-complex, stercomata-bearing monothalamids probably consume sediment, degraded organic matter, and perhaps bacteria, and have lower energetic demands (metabolism) than multichambered foraminifera, we have very little direct information regarding their ecology. Experimental studies could help to address this important limitation. Because of difficulties such as these, there is a temptation to disregard monothalamids in surveys of the CCZ fauna. Given their importance, we would argue that they should be included, at least in assessments of seafloor biodiversity. As shown by several studies (Lecroq et al., 2011; Lejzerowicz et al., 2014; Cordier et al., 2019), metabarcoding of sediment DNA appears to be the best way to incorporate the smaller monothalamids in monitoring surveys. However, the biodiversity of komokiaceans and other enigmatic forms, which often dominate the abyssal Pacific macrofauna (Tendal and Hessler, 1977; Bernstein et al., 1978) and to a lesser extent the meiofauna (Goineau and Gooday, 2017, 2019), may still have to be evaluated morphologically.
In terms of data gaps, the most obvious is the scarcity of foraminiferal studies in the western CCZ. Apart from some species records, mainly in the works of Kh. Saidova (Supplementary Material), we have only some quantitative data from the JET site, some information on nodule-encrusting foraminifera from the western French site, and small collections of xenophyophores from APEIs 1, 4, 6 and 7. A transect of foraminiferal samples along east to west gradients of increasing depth and carbonate dissolution and decreasing surface productivity could prove very informative. Although xenophyophores have been collected (Gooday et al., 2017b, 2020a), and analyzed in seafloor images (Simon-Lledó et al., 2019a,b) in several APEIs, there are currently no quantitative morphological or metabarcoding data for foraminifera in samples from any of the nine present protected areas. This is an important gap to address given the importance of APEIs in protecting biodiversity and ecosystem functioning in the CCZ from future mining impacts. Finally, we agree with de Jonge et al. (2020) on the pressing need for more information about the biomass, metabolic activity, and ecology of abyssal foraminifera, particularly xenophyophores and other monothalamids, in order to include them in efforts to construct comprehensive carbon-flow models for the CCZ. Understanding the role of these remarkable protists in the functioning of deep-sea benthic ecosystems in the CCZ and across the globe remains a major challenge for the future.
Data Availability Statement
Data from the UK-1 and OMS sites are available in published supplementary materials (Goineau and Gooday, 2017, 2019; Gooday and Goineau, 2019). Data from the Kaplan East, Kaplan Central, and Japan Deep-Sea Impact Experiment (JET) sites are available on reasonable request from AJG and HK. Data from the IOM BIE site are available on reasonable request from ZS and TR.
Author Contributions
The original idea for a review was conceived by AJG and AG. AJG developed the idea, wrote much of the manuscript, and prepared Figures 7, 8. FL did the metabarcoding analyses, wrote the section “Environmental DNA and RNA Metabarcoding Data” contributed to the section on ‘Undersampling, Patchiness and Rarity’ and prepared Figure 5 and Tables 4, 5. AG prepared the original versions of Figures 3, 6 and was jointly responsible with AJG for the collection and analysis of some of the data summarized in the review. MH and JP were responsible for the barcoding sequence data. JP supervised the metabarcoding analyses. OK contributed faunal data and prepared Appendix A (Supplementary Material). S-CL, HK, TR, ZS, and BW-W contributed faunal data. JP, TR, and BW-W edited the manuscript. BW-W prepared Figures 1, 2, 4. All authors approved the final manuscript.
Funding
MH and JP were supported by Swiss National Science Foundation grants 31003A_179125 and 316030_150817 (JP). ZS, TR, and BW-W acknowledge support provided by the Polish National Science Center Grant No. 2014/13/B/ST10/02996 and by the JPI Oceans Pilot Action “Ecological Effects of Deep-Sea Mining”. Additional support was provided by the Institute of Marine and Environmental Sciences of the University of Szczecin, Poland. Research by OK was funded by the Ministry of Education and Science of the Russian Federation, State Assignment No. 0149-2019-0009 and Grant No. 13.1902.21.0012). FL was supported by an Early Postdoc Mobility Grant from the Swiss National Science Foundation (P2GEP3_171829). Research conducted in the UK-1 and OMS contract areas in the Clarion-Clipperton Zone were collected as part of the ABYSSLINE project, funded by UK Seabed Resources Development Ltd.
Conflict of Interest
JP was employed by ID-GENE Ecodiagnostics LTD.
The remaining authors declare that the research was conducted in the absence of any commercial or financial relationships that could be construed as a potential conflict of interest.
Acknowledgments
We thank Laure Apothéloz-Perret-Gentil, Ivan Voltski, and Alexandra Anh-Thu Weber who collected foraminiferal and eDNA samples from the CCZ during ABYSSLINE cruises. We thank the two reviewers for their comments that helped to improve the initial submission.
Supplementary Material
The Supplementary Material for this article can be found online at: https://www.frontiersin.org/articles/10.3389/fmars.2021.634726/full#supplementary-material
References
Altenbach, A. V. (1992). Short term processes and patterns in the foraminiferal response to organic flux rates. Mar. Micropaleontol. 19, 119–129. doi: 10.1016/0377-8398(92)90024-e
Alve, E., and Goldstein, S. T. (2003). Propagule transport as a key method of dispersal in benthic foraminifera. Limnol. Oceanogr. 48, 2163–2170. doi: 10.4319/lo.2003.48.6.2163
Alve, E., and Goldstein, S. T. (2010). Dispersal, survival and delayed growth of benthic foraminiferal propagules. J. Sea Res. 63, 36–51. doi: 10.1016/j.seares.2009.09.003
Amon, D. J., Ziegler, A. F., Dahlgren, T. G., Glover, A. G., Goineau, A., Gooday, A. J., et al. (2016). Insights into the abundance and diversity of abyssal megafauna in a polymetallic-nodule region in the eastern Clarion-Clipperton Zone. Sci. Rep. 6:30492. doi: 10.1038/srep30492
Aranda da Silva, A. A. S., and Gooday, A. J. (2009). Large organic-walled Protista (Gromia) in the Arabian Sea: density, diversity, distribution and ecology. Deep Sea Res. II 56, 422–433. doi: 10.1016/j.dsr2.2008.12.027
Bagg, R. M. (1908). Foraminifera collected near the Hawaiian Islands by the steamer Albatross in 1902. Proc. U.S. Nat. Mus. 34, 113–172. doi: 10.5479/si.00963801.34-1603.113
Bernstein, B. B., Hessler, R. R., Smith, R., and Jumars, P. A. (1978). Spatial dispersion of benthic Foraminifera in the abyssal central North Pacific. Limnol. Oceanogr. 23, 401–416. doi: 10.4319/lo.1978.23.3.0401
Brady, H. B. (1884). Report on the foraminifera dredged by H.M.S. Challenger during the Years 1873-1876. Report of the Scientific Results of the Voyage of H.M.S. Challenger during the years 1873–76. Zoology 9, (Pt 22) 1–814, pls 1–115.
Burkett, A., Rathburn, A., Pratt, R. B., and Holzmann, M. (2020). Insight into the ecology of epibenthic calcareous foraminifera from a colonization study at 4000 m (Station M) in the NE Pacific Ocean. Deep Sea Res. II 173:104709. doi: 10.1016/j.dsr2.2019.104709
Burmistrova, I. I., Khusid, T. A., Belyaeva, N. V., and Chekhovskaya, M. P. (2007). Agglutinated abyssal foraminifers of the equatorial Pacific. Oceanology 47, 824–832. doi: 10.1134/s0001437007060070
Cordier, T., Barrenechea, I., Lejzerowicz, F., Reo, E., and Pawlowski, J. (2019). Benthic foraminiferal DNA metabarcodes significantly vary along a gradient from abyssal to hadal depths and between each side of the Kuril-Kamchatka trench. Prog. Oceanogr. 178:102175. doi: 10.1016/j.pocean.2019.102175
Culver, S. J., and Buzas, M. A. (1999). “Biogeography of neritic benthic Foraminifera,” in Modern Foraminifera, ed. B. K. Sen Gupta (Dordrecht: Kluwer Academic Publisher), 93–102. doi: 10.1007/0-306-48104-9_6
Cushman, J. A. (1910). A monograph of the foraminifera of the North Pacific Ocean. Part 1. Astrorhizidae and Lituolidae. Bull. U.S. Natl. Mus. 71, 1–134. doi: 10.5479/si.03629236.104.1
Cushman, J. A. (1911). A monograph of foraminifera of the North Pacific Ocean. Pt. 2, Textulariidae. Bull. U.S. Natl. Mus. 71, 1–134.
Cushman, J. A. (1913). A monograph of foraminifera of the North Pacific Ocean. Pt. 3, Lagenidae. Bull. U.S. Natl. Mus. 71, 1–125, pls 1–47.
Cushman, J. A. (1914). A monograph of foraminifera of the North Pacific Ocean. Pt. 4, Chilostomellidae. Bull. U.S. Natl. Mus. 71, 1–46, pls 1–19.
Cushman, J. A. (1915). A monograph of foraminifera of the North Pacific Ocean. Pt. 5, Rotaliidae. Bull. U.S. Natl. Mus. 71, 1–81, pls 1–31.
Cushman, J. A. (1917). A monograph of foraminifera of the North Pacific Ocean. Pt. 6, Miliolidae. Bull. U.S. Natl. Mus. 71, 1–108, pls 1–39.
Cushman, J. A. (1932). The foraminifera of the tropical Pacific collections of the ‘Albatross’, 1899–1900. Pt. 1. Astrorhizidae to Trochamminidae. Bull. U.S. Nat. Mus. 161, 1–84, pls 1–17.
Cushman, J. A. (1933). The foraminifera of the tropical Pacific collections of the ‘Albatross’, 1899–1900. Pt. 2. Lagenidae to Alveolinellidae. Bull. U.S. Nat. Mus. 161, 1–74, pls 1–19.
Cushman, J. A. (1942). The foraminifera of the tropical Pacific collections of the ‘Albatross’, 1899–1900. Pt. 3. Heterohelicidae and Buliminidae. Bull. U.S. Nat. Mus. 161, 1–67, pls 1–15.
de Jonge, D. S. W., Stratmann, T., Lins, L., Vanreusel, A., Purser, A., Marcon, Y., et al. (2020). Abyssal food-web model indicates faunal carbon flow recovery and impaired microbial loop 26 years after a sediment disturbance experiment. Prog. Oceanogr. 189:102446. doi: 10.1016/j.pocean.2020.102446
Douglas, R. G., and Woodruff, F. (1981). “Deep-sea benthic foraminifera,” in The Sea: The Oceanic Lithosphere, Vol. 7, ed. E. Emiliani (New York, NY: John Wiley & Sons), 1233–1327.
Drazen, J. E., Baldwin, R. H., and Smith, K. L. (1998). Sediment community response to a temporally varying food supply at an abyssal station in the NE Pacific. Deep Sea Res. II 45, 893–913. doi: 10.1016/s0967-0645(98)00007-1
Dugolinsky, B. K., Margolis, S. V., and Dudley, W. C. (1977). Biogenic influence on growth of manganese nodules. J. Sediment. Petrol. 47, 428–445.
Duros, P., Silva Jacinto, R., Dennielous, B., Schmidt, S., Martinez Lamas, R., Gautier, E., et al. (2017). Benthic foraminiferal response to sedimentary disturbance in the Capbreton canyon (Bay of Biscay, NE Atlantic). Deep Sea Res. I 120, 61–75. doi: 10.1016/j.dsr.2016.11.012
Ehrenberg, C. G. (1861). Über den Tiefgrund des Stillen Oceans Zwischen Californien und den Sandwich-Inseln aus bis 15600’ Tiefe nach Lieutenant Brooke. Monatsberichte, Year 1860. Berlin: Königliche Preussische Akademie der Wissenschaften, 819–833.
Enge, A. J., Kucera, M., and Heinz, P. (2012). Diversity and microhabitats of living benthic foraminifera in the abyssal Northeast Pacific. Mar. Micropal. 96–97, 84–104. doi: 10.1016/j.marmicro.2012.08.004
Enge, A. J., Nomaki, H., Ogawa, N. O., Witte, U., Moeseneder, M. M., Lavik, G., et al. (2011). Response of the benthic foraminiferal community to a simulated short-term phytodetritus pulse in the abyssal North Pacific. Mar. Ecol. Prog. Ser. 438, 129–142. doi: 10.3354/meps09298
Flint, J. M. (1899). Recent Foraminifera. A descriptive catalogue of specimens dredged by the U.S. Fish Commission steamer Albatross. Rep. U.S. Natl. Mus. 1897, 251–349, pls 1–80.
Flint, J. M. (1905). A contribution to the oceanography of the Pacific. Bull U.S. Nat. Mus. 55, 1–62. doi: 10.1594/PANGAEA.847719
Goës, A. (1892). On a peculiar type of arenaceous foraminifer from the American tropical Pacific. Bull. Mus. Comp. Zool. Harv. 23, 195–198.
Goës, A. (1896). Report on the dredging operations off the west coast of Central America to the Galapagos, to the west coast of Mexico, and the Gulf of California, in charge of Alexander Agassiz, carried on by the U.S. Fish Commission steamer ‘Albatross’, during 1891, Lieu. Z.L. Tanner U.S.N., commanding. XX. The Foraminifera. Bull. Mus. Comp. Zool. Harv. 29, 1–105, pls 1–9.
Goineau, A., and Gooday, A. J. (2017). Novel benthic foraminifera are abundant and diverse in an area of the abyssal equatorial Pacific licensed for polymetallic nodule exploration. Sci. Rep. 7:45288. doi: 10.1038/srep45288
Goineau, A., and Gooday, A. J. (2019). Diversity and spatial patterns of foraminiferal assemblages in the eastern Clarion-Clipperton zone (abyssal eastern equatorial Pacific). Deep Sea Res. I 149:103036. doi: 10.1016/j.dsr.2019.04.014
Goldstein, S. T., and Corliss, B. H. (1994). Deposit feeding in selected deep-sea and shallow-water benthic foraminifera. Deep Sea Res. 41, 229–241. doi: 10.1016/0967-0637(94)90001-9
Gooday, A. J. (1988). A response by benthic foraminifera to phytodetritus deposition in the deep sea. Nature 332, 70–73. doi: 10.1038/332070a0
Gooday, A. J. (2019). “Deep-sea benthic foraminifera,” in Encyclopedia of Ocean Science, 3rd Edn, eds J. Cochran, J. Kirk, J. H. Bokuniewicz, and L. P. Yager (London: Elsevier), 684–705. doi: 10.1016/b978-0-12-409548-9.09071-0
Gooday, A. J., Cedhagen, T., Kamenskaya, O. E., and Cornelius, N. (2007). The biodiversity and biogeography of komokiaceans and other enigmatic foraminiferan-like protists in the deep Southern Ocean. Deep Sea Res. II 54, 1691–1719.
Gooday, A. J., Durden, J. M., Holzmann, M., Pawlowski, J., and Smith, C. R. (2020a). Xenophyophores (Rhizaria, Foraminifera), including four new species and two new genera, from the western Clarion-Clipperton Zone (abyssal equatorial Pacific). Eur. J. Protist. 75:125715. doi: 10.1016/j.ejop.2020.125715
Gooday, A. J., Durden, J. M., and Smith, C. R. (2020b). Giant, highly diverse protists in the abyssal Pacific: vulnerability to impacts from seabed mining and potential for recovery. Commun. Integr. Biol. 13, 189–197. doi: 10.1080/19420889.2020.1843818
Gooday, A. J., and Goineau, A. (2019). The contribution of fine sieve fractions (63–150 μm) to foraminiferal abundance and diversity in an area of the eastern Pacific Ocean licensed for polymetallic nodule exploration. Front. Mar. Sci. 6:114. doi: 10.3389/fmars.2019.00114
Gooday, A. J., Goineau, A., and Voltski, I. (2015). Abyssal foraminifera attached to polymetallic nodules from the eastern Clarion-Clipperton Fracture Zone: a preliminary description and comparison with North Atlantic dropstone assemblages. Mar. Biodiv. 45, 391–412. doi: 10.1007/s12526-014-0301-9
Gooday, A. J., Holzmann, M., Caulle, C., Goineau, A., Jones, D. O. B., Kamenskaya, O. E., et al. (2017a). New species of the xenophyophore genus Aschemonella (Rhizaria, Foraminifera) from areas of the abyssal eastern Pacific licensed for polymetallic nodule exploration. Zool. J. Linn. Soc. 182, 479–499. doi: 10.1093/zoolinnean/zlx052
Gooday, A. J., Holzmann, M., Caulle, C., Goineau, A., Kamenskaya, O. E., Weber, A. A.-T., et al. (2017b). Giant foraminifera (xenophyophores) are exceptionally diverse in parts of the abyssal eastern Pacific where seabed mining is likely to occur. Biol. Conserv. 207, 106–116. doi: 10.1016/j.biocon.2017.01.006
Gooday, A. J., Holzmann, M., Caulle, C., Goineau, A., Pearce, R. B., Voltski, I., et al. (2017c). Five new species and two new genera of xenophyophores (Foraminifera: Rhizaria) from part of the abyssal equatorial Pacific licensed for polymetallic nodule exploration. Zool. J. Linn. Soc. 183, 723–748. doi: 10.1093/zoolinnean/zlx093
Gooday, A. J., Holzmann, M., Goineau, A., Kamenskaya, O., Melnik, V. F., Pearce, R. B., et al. (2018a). Xenophyophores (Rhizaria, Foraminifera) from the Eastern Clarion-Clipperton Zone (equatorial Pacific): the genus Psammina. Protist 169, 926–957. doi: 10.1016/j.protis.2018.09.003
Gooday, A. J., Holzmann, M., Guiard, J., Cornelius, N., and Pawlowski, J. (2004). A new monothalamous foraminiferan from 100-6300 m water depth in the Weddell Sea: morphological and molecular characterisation. Deep Sea Res. II 51, 1603–1616. doi: 10.1016/j.dsr2.2004.06.025
Gooday, A. J., and Hughes, J. A. (2002). Foraminifera associated with phytodetritus deposits at a bathyal site in the northern Rockall Trough (NE Atlantic): seasonal contrasts and a comparison of stained and dead assemblages. Mar. Micropaleontol. 46, 83–110. doi: 10.1016/s0377-8398(02)00050-6
Gooday, A. J., and Lambshead, P. J. D. (1989). Influence of seasonally deposited phytodetritus on benthic foraminiferal populations in the bathyal northeast Atlantic: the species response. Mar. Ecol. Prog. Ser. 58, 53–67. doi: 10.3354/meps058053
Gooday, A. J., Levin, L. A., Linke, P., and Heeger, T. (1992). “The role of benthic foraminifera in deep-sea food webs and carbon cycling,” in Deep-Sea Food Chains and the Global Carbon Cycle, eds G. T. Rowe and V. Pariente (Dordrecht: Springer), 63–91. doi: 10.1007/978-94-011-2452-2_5
Gooday, A. J., Nomaki, H., and Kitazato, H. (2008). “Modern deep-sea benthic foraminifera: a brief review of their biodiversity and trophic diversity,” in Biogeochemical Controls on Palaeoceanographic Environmental Proxies, Vol. 303, eds W. E. N. Austin and R. H. James (London: Geological Society, Special Publications), 97–119. doi: 10.1144/SP303.8
Gooday, A. J., Sykes, D., Goral, T., Zubkov, M. V., and Glover, A. G. (2018b). Micro-CT 3D imaging reveals the internal structure of three abyssal xenophyophore species (Protista, Foraminifera) from the eastern equatorial Pacific Ocean. Sci. Rep. 8:12103. doi: 10.1038/s41598-018-30186-2
Graf, G., and Linke, P. (1992). “Adenosine nucleotides as indicators of deep-sea benthic metabolism,” in Deep-Sea Food Chains and the Global Carbon Cycle, eds G. T. Rowe and V. Pariente (Dordrecht: Kluwer Academic), 237–243. doi: 10.1007/978-94-011-2452-2_14
Greenslate, J., Hessler, R. R., and Thiel, H. (1974). “Manganese nodules are alive and well on the sea floor,” in Proceedings of the Marine Technology Society 10th Annual Conference, Washington, DC, 171–181.
Groos, O. (2000). The influence of temperature, oxygen and food availability on the migration activity of bathyal benthic foraminifera: evidence from microcosm experiments. Hydrobiologia 416, 123–137. doi: 10.1007/978-94-011-4148-2_12
Haeckel, E. (1889). Report on the Deep-Sea Keratosa collected by H.M.S. Challenger during the Years 1873-1876. Report of the Scientific Results of the Voyage of H.M.S. Challenger during the years 1873-76, Vol. 82, 1–92, pls 1–8.
Hardy, S. M., Smith, C. R., and Thurnherr, A. M. (2015). Can the source–sink hypothesis explain macrofaunal abundance patterns in the abyss? A modelling test. Proc. Roy. Soc. Lond. B 282:20150193. doi: 10.1098/rspb.2015.0193
Hayward, B. W., Holzmann, M., Pawlowski, J., Parker, J. H., Kaushik, T., Toyofuku, M. S., et al. (2021). Molecular and morphological taxonomy of living. Ammonia and related taxa (Foraminifera) and their biogeography. Micropaleontology 67, 109–313. doi: 10.47894/mpal.67.2-3.01
Heeger, T. (1990). Elektronmikrokopische untersuchungen zur ernährungsbiologie benthischer foraminiferen. Berichte Sondersforschungsbereich 313, 1–139.
Hein, J. R., Mizell, K., Koschinsky, A., and Conrad, T. A. (2013). Deep-ocean mineral deposits as a source of critical metals for high- and green-technology applications: comparison with land-based resources. Ore Geol. Rev. 51, 1–14. doi: 10.1016/j.oregeorev.2012.12.001
Hess, S., and Kuhnt, W. (1996). Deep-sea benthic foraminiferal recolonization of the 1991 Mt. Pinatubo ash layer in the South China Sea. Mar. Micropaleontol. 28, 171–197. doi: 10.1016/0377-8398(95)00080-1
Hess, S., Kuhnt, W., Hill, S., Kaminski, M. A., Holbourn, A., and de Leon, M. (2001). Monitoring the recolonization of the Mt. Pinatubo 1991 ash layer by benthic foraminifera. Mar. Micropaleontol. 43, 119–142. doi: 10.1016/s0377-8398(01)00025-1
Hessler, R. R. (1974). “The structure of deep-sea communities from central oceanic waters,” in The Biology of the Oceanic Pacific, ed. C. B. Miller (Corvallis, OR: Oregon State University Press), 79–93.
Holbourn, A., Henderson, A., and McLeod, N. (2013). Atlas of Benthic Foraminifera. Chichester: Wiley-Blackwell, doi: 10.1002/9781118452493
Hori, S., Tsuchiya, M., Nishi, S., Arai, W., Yoshidam, T., and Takami, H. (2013). Active bacterial flora surrounding foraminifera (Xenophyophorea) living on the deep-seafloor. Biosci. Biotechnol. Biochem. 77, 381–384. doi: 10.1271/bbb.120663
Hughes, J. A., and Gooday, A. J. (2004). The influence of dead Syringammina fragilissima (Xenophyophorea) tests on the distribution of benthic foraminifera in the Darwin Mounds region (NE Atlantic). Deep Sea Res. I 51, 1741–1758. doi: 10.1016/j.dsr.2004.06.004
Jorissen, F., Fontanier, C., and Thomas, E. (2007). “Paleoceanographical proxies based on deep-sea benthic foraminiferal assemblage characteristics,” in Proxies in Late Cenozoic Paleoceanography, eds C. Hillaire-Marcel and A. de Vernal (Amsterdam: Elsevier), 263–325. doi: 10.1016/s1572-5480(07)01012-3
Kamenskaya, O. E. (2005). Spiculammina delicata gen. et sp. n., a new xenophyophore from the eastern Pacific (Psamminidae). Invert. Zool. 2, 23–27. doi: 10.15298/invertzool.02.1.03
Kamenskaya, O. E., Gooday, A. J., Radziejewska, T., and Wawrzyniak-Wydrowska, B. (2012). Large, enigmatic foraminiferan-like protists in the eastern part of the Clarion-Clipperton Fracture Zone (abyssal eastern equatorial Pacific): biodiversity and vertical distribution in the sediment. Mar. Biodiv. 42, 311–327. doi: 10.1007/s12526-012-0114-7
Kamenskaya, O. E., Gooday, A. J., Tendal, O. S., and Melnik, V. F. (2015). Xenophyophores (Protista, Foraminifera) from the Clarion-Clipperton Fracture Zone with description of three new species. Mar. Biodiv. 45, 581–593. doi: 10.1007/s12526-015-0330-z
Kamenskaya, O. E., Gooday, A. J., Tendal, O. S., and Melnik, V. F. (2017). Xenophyophores (Rhizaria, Foraminifera) from the Russian license area of the Clarion-Clipperton Zone (eastern equatorial Pacific), with the description of three new species. Mar. Biodiv. 47, 299–306. doi: 10.1007/s12526-016-0595-x
Kamenskaya, O. E., Melnik, V. F., and Gooday, A. J. (2013). Giant protists (xenophyophores and komokiaceans) from the Clarion-Clipperton ferromanganese nodule field (Eastern Pacific). Biol. Bull. Rev. 3, 388–398. doi: 10.1134/s2079086413050046
Kaminski, M. (2014). The year 2010 classification of the agglutinated foraminifera. Micropaleontology 60, 89–108.
Kaminski, M. A., Grassle, J. F., and Whitlach, R. B. (1988). “Life history and recolonisation among agglutinated foraminifera in the Panama Basin,” in Proceedings of the 2nd Workshop on Agglutinated Foraminifera, Vol. 41, eds M. F. Gradstein and F. Rögl (Wien: Abh. Geol. Bundesanst.), 229–243.
Kitazato, H., Nakatsuka, T., Shimanaga, M., Kanda, J., Soh, W., Kato, Y., et al. (2003). Long-term monitoring of the sedimentary processes in the central part of Sagami Bay, Japan: rationale, logistics and overview of results. Prog. Oceanogr. 57, 3–16. doi: 10.1016/s0079-6611(03)00047-8
Köster, M., Jensen, P., and Meyer-Reil, L.-A. (1991). “Hydrolytic activities of organisms and biogenic structures in deep-sea sediments,” in Microbial Enzymes in Aquatic Environments, ed. R. J. Chrost (New York, NY: Springer-Verlag), 298–310. doi: 10.1007/978-1-4612-3090-8_19
Kuhnt, W., Hess, S., Holbourn, A., Paulsen, H., and Salomon, B. (2005). The impact of the 1991 Mt. Pinatubo eruption on deep-sea foraminiferal communities: A model for the Cretaceous–Tertiary (K/T) boundary? Palaeogeogr. Palaeocl. Palaeoecol. 224, 83–107. doi: 10.1016/j.palaeo.2005.03.042
Laureillard, J., Méjanelle, L., and Sibuet, M. (2004). Use of lipids to study the trophic ecology of deep-sea xenophyophores. Mar. Ecol. Prog. Ser. 270, 129–140. doi: 10.3354/meps270129
Lecroq, B., Gooday, A. J., Cedhagen, T., Sabbatini, A., and Pawlowski, J. (2009a). Molecular analyses reveal high levels of eukaryotic richness associated with enigmatic deep-sea protists (Komokiacea). Mar. Biodiv. 39, 45–55. doi: 10.1007/s12526-009-0006-7
Lecroq, B., Gooday, A. J., and Pawlowski, J. (2009b). Global genetic homogeneity in the deep-sea foraminiferan Epistominella exigua (Rotaliida: Pseudoparrellidae). Zootaxa 2096, 23–32. doi: 10.11646/zootaxa.2096.1.4
Lecroq, B., Gooday, A. J., Tsuchiya, M., and Pawlowski, J. (2009c). A new genus of xenophyophores (Foraminifera) from Japan Trench: morphological description, molecular phylogeny and elemental analysis. Zool. J. Linn. Soc. 156, 455–464. doi: 10.1111/j.1096-3642.2008.00493.x
Lecroq, B., Lejzerowicz, F., Bachar, D., Christen, R., Esling, P., Baerlocher, L., et al. (2011). Ultra-deep sequencing of foraminiferal microbarcodes unveils hidden richness of early monothalamous lineages in deep-sea sediments. Proc. Nat. Acad. Sci. U.S.A. 108, 13177–13182. doi: 10.1073/pnas.1018426108
Lejzerowicz, F., Esling, P., and Pawlowski, J. (2014). Patchiness of deep-sea benthic foraminifera across the Southern Ocean: insights from high-throughput DNA sequencing. Deep Sea Res. II 108, 17–26. doi: 10.1016/j.dsr2.2014.07.018
Lejzerowicz, F., Gooday, A. J., Barrenechea Angeles, I., Cordier, T., Morard, R., Apothéloz-Perret-Gentil, L., et al. (2021). Eukaryotic biodiversity and spatial patterns in the Clarion-Clipperton Zone and other abyssal areas: insights from sediment DNA and RNA metabarcoding. Front. Mar. Sci. doi: 10.3389/fmars.2021.671033
Levin, L. A. (1991). Interactions between metazoans and large, agglutinating protozoans: implications for the community structure of deep-sea benthos. Am. Sci. 31, 886–900. doi: 10.1093/icb/31.6.886
Levin, L. A., Blair, N. E., Martin, C. M., DeMaster, D. J., Plaia, G., and Thomas, C. J. (1999). Macrofaunal processing of phytodetritus at two sites on the Carolina margin: in situ experiments using 13C-labled diatoms. Mar. Ecol. Prog. Ser. 182, 37–54. doi: 10.3354/meps182037
Levin, L. A., DeMaster, D. J., McCann, L. D., and Thomas, C. L. (1986). Effects of giant protozoans (class: Xenophyophorea) on deep-seamount benthos. Mar. Ecol. Prog. Ser. 29, 99–104. doi: 10.3354/meps029099
Levin, L. A., and Gooday, A. J. (1992). “Possible roles for xenophyophores in deep-sea carbon cycling,” in Deep-Sea Food Chains and the Global Carbon Cycle, eds G. T. Rowe and V. Pariente (Dordrecht: Kluwer Academic Publishers), 93–104. doi: 10.1007/978-94-011-2452-2_6
Levin, L. A., and Rouse, G. W. (2019). Giant protists (xenophyophores) function as fish nurseries. Ecology 101:e02933. doi: 10.1002/ecy.2933
Levin, L. A., and Thomas, C. L. (1988). The ecology of the xenophyophores (Protista) on eastern Pacific seamounts. Deep Sea Res. 35, 2003–2027. doi: 10.1016/0198-0149(88)90122-7
Linke, P. (1992). Metabolic adaptations of deep-sea benthic foraminifera to seasonally varying food input. Mar. Ecol. Prog. Ser. 81, 51–63. doi: 10.3354/meps081051
Linke, P., Altenbach, A. V., Graf, G., and Heeger, T. (1995). Response of deep-sea benthic foraminifera to a simulated sedimentation event. J. Foram. Res. 25, 75–82. doi: 10.2113/gsjfr.25.1.75
Lipps, J. H., and Valentine, J. W. (1970). The role of foraminifera in the trophic structure of marine communities. Lethaia 3, 279–286. doi: 10.1111/j.1502-3931.1970.tb01271.x
Lisitzin, A. P. (1996). Oceanic Sedimentation: Lithology and Geochemistry. Washington, DC: American Geophysical Union.
Moodley, L., Middelburg, J. J., Boschker, H. T. S., Duineveld, G. C. A., Pel, R., Herman, P. M. J., et al. (2002). Bacteria and foraminifera: key players in a short-term deep-sea benthic response to phytodetritus. Mar. Ecol. Prog. Ser. 236, 23–29. doi: 10.3354/meps236023
Mullineaux, L. S. (1987). Organisms encrusting manganese nodules and crusts: distribution and abundance at three North Pacific sites. Deep Sea Res. 34, 165–184. doi: 10.1016/0198-0149(87)90080-x
Mullineaux, L. S. (1988). Taxonomic notes on large agglutinated foraminifers encrusting manganese nodules, including the description of a new genus, Chondrodapis (Komokiacea). J. Foram. Res. 18, 46–53. doi: 10.2113/gsjfr.18.1.46
Murray, J., and Renard, A. F. (1891). Report on Deep-Sea Deposits. Report of the Scientific Results of the Voyage of H.M.S. Challenger during the years 1873–76. 1–529, pls 1–29.
Murray, J. W. (2013). Living benthic foraminifera: biogeographical distributions and the significance of rare morphospecies. J. Micropalaeontol. 32, 1–58. doi: 10.1144/jmpaleo2012-010
Nomaki, H., Heinz, P., Nakatsuka, T., Shimanaga, M., and Kitazato, H. (2005). Species-specific ingestion of organic carbon by deep-sea benthic foraminifera and meiobenthos: In situ tracer experiments. Limnol. Oceanogr. 50, 134–146. doi: 10.4319/lo.2005.50.1.0134
Nomaki, H., Heinz, P., Nakatsuka, T., Shimanaga, M., Ohkouchi, N., Ogawa, N. O., et al. (2006). Different ingestion patterns of 13C-labeled bacteria and algae by deep-sea benthic foraminifera. Mar. Ecol. Prog. Ser. 310, 95–108. doi: 10.3354/meps310095
Nomaki, H., Ogawa, N. O., Ohkouchi, N., Suga, H., Toyofuku, T., Shimanaga, M., et al. (2008). Benthic foraminifera as trophic links between phytodetritus and benthic metazoans: carbon and nitrogen isotopic evidence. Mar. Ecol. Prog. Ser. 357, 153–164. doi: 10.3354/meps07309
Nozawa, F. (2003). Benthic Foraminifera from the JET Site, Equatorial Pacific Ocean. Bachelors thesis. Graduate School of Science. Chiba: University of Chiba. (In Japanese).
Nozawa, F. (2005). A deep-sea benthic foraminiferal assemblage in the equatorial Pacific Ocean, Masters thesis. Graduate School of Science. Chiba: University of Chiba. (In Japanese).
Nozawa, F., Kitazato, H., Tsuchiya, M., and Gooday, A. J. (2006). ‘Live’ benthic foraminifera at an abyssal site in the equatorial Pacific nodule province: abundance, diversity and taxonomic composition. Deep Sea Res. I 51, 1406–1422. doi: 10.1016/j.dsr.2006.06.001
Ohkawara, N. (2006). Deep-Sea Benthic Foraminiferal Distribution in the Central Pacific, Masters thesis, Hirosaki University, Japan.
Ohkawara, N. (2011). Deep-Sea Foraminiferal Fauna in the Equatorial Pacific: Abundance, Distribution and Species Diversity. Doctoral thesis. Yokohama: Yokohama National University.
Ohkawara, N., Kitazato, H., Uematsu, K., and Gooday, A. J. (2009). A minute new species of Saccammina (monothalamous Foraminifera; Protista) from the abyssal Pacific. J. Micropalaeontol. 28, 143–151. doi: 10.1144/jm.28.2.143
Okamoto, T. (1998). Responses of Deep-Sea Benthic Foraminiferal Distribution to Artificial Disturbance during JET. Bachelors thesis. Shizuoka: University of Shizuoka. (In Japanese).
Pawlowski, J., Fahrni, J., Lecroq, B., Longet, D., Cornelius, N., Excoffier, L., et al. (2007). Bipolar gene flow in deep-sea benthic foraminifera. Mol. Ecol. 16, 4089–4096. doi: 10.1111/j.1365-294x.2007.03465.x
Picaglia, L. (1892). Foraminiferi dei Saggi di Fondo dragati nel viaggio di circumnavazione della R. Nave ‘Vettor Pisani’ comandante G. Palumbo negli anni 1882–85. Atti. Soc. Nat. Modena Ser. 3 7, 152–155.
Radziejewska, T. (2002). Responses of deep-sea meiobenthic communities to sediment disturbance simulating effects of polymetallic nodule mining. Internat. Rev. Hydrobiol. 87, 457–477. doi: 10.1002/1522-2632(200207)87:4<457::aid-iroh457>3.0.co;2-3
Radziejewska, T., Gooday, A. J., Koltan, M., and Szyrwiel, E. (2006). Deep-sea non-calcareous foraminifera: some examples from the Pacific abyssal nodule field. Meiofauna Marina 15, 3–10.
Renaud-Mornant, J., and Gourbault, N. (1990). Evaluation of abyssal meiobenthos in the eastern central Pacific (Clarion-Clipperton fracture zone). Prog. Oceanogr. 24, 317–329. doi: 10.1016/0079-6611(90)90041-y
Resig, J. M. (1981). Biogeography of benthic foraminifera of the northern Nazca plate and adjacent continental margin. Geol. Soc. Am. Mem. 154, 619–666. doi: 10.1130/mem154-p619
Rex, M. A., and Etter, R. J. (2010). Deep-Sea Biodiversity: Pattern and Scale. Cambridge, MA: Harvard University Press.
Rex, M. A., McClain, C. R., Johnson, N. A., Etter, R. J., Allen, J. A., Bouchet, P., et al. (2005). A source-sink hypothesis for abyssal biodiversity. Am. Nat. 165, 163–178. doi: 10.2307/3473143
Saidova, Kh. M (1974). On large-scale facies confinement of deep-sea benthic foraminifera. Oceanologica 14, 534–540.
Saidova, Kh. M (1975). Benthonic Foraminifera of the Pacific Ocean. Moskva: Academy of Sciences of the USSR, Akademia Nauk, SSSR, Instituta Okeanologii, 1–875, pls 1–115. (in Russian).
Saidova, Kh. M (1976). Benthic Foraminifera of the World Ocean (Zonal and Quantitative Distribution). Moscow: Akademia Nauk, SSSR, Instituta Okeanologii, 1–159. (in Russian).
Saidova, Kh. M (1981). Recent foraminiferal communities of the Pacific abyssal plains. Oceanology 21, 259–262.
Saidova, Kh. M (2000). Facial variability of benthic foraminiferal commuities of the open regions of the ocean. Oceanology 40, 66–72.
Schröder, C. J., Scott, D. B., Medioli, F. S., Bernstein, B. B., and Hessler, R. R. (1988). Larger agglutinated foraminifera: comparison of assemblages from central Pacific and western North Atlantic (Nares Abyssal Plain). J. Foram. Res. 18, 25–41. doi: 10.2113/gsjfr.18.1.25
Schulze, F. E. (1907a). Die Xenophyophoren, eine besondere Gruppe der Rhizopoden. Wiss Ergebn. Dt. Tiefsee Expedit. Valdivia 11, 1–55. doi: 10.1515/9783486745320-005
Schulze, F. E. (1907b). Report on the dredging operations off the west coast of Central America to the Galapagos, to the west coast of Mexico, and the Gulf of California, in charge of Alexander Agassiz, carried on by the U.S. Fish Commission steamer ‘Albatross’, during 1891, Lieu. Z.L. Tanner U.S.N., commanding. XI. Die Xenophyophoren. Bull. Mus. Comp. Zool. Harv. 51, 143–162.
Shi, J., Lei, Y., Li, Q., Lyu, M., and Li, T. (2020). Molecular diversity and spatial distribution of benthic foraminifera of the seamounts and adjacent abyssal plains in the tropical Western Pacific Ocean. Mar. Micropaleontol. 156:101850. doi: 10.1016/j.marmicro.2020.101850
Shires, R., Gooday, A. J., and Jones, A. R. (1994). The morphology and ecology of an abundant new komokiacean mudball (Komokiacea, Foraminiferida) from the bathyal and abyssal NE Atlantic. J. Foram. Res. 24, 214–225. doi: 10.2113/gsjfr.24.4.214
Simon-Lledó, E., Bett, B. J., Huvenne, V. A. I., Schoening, T., Benoist, N. M., and Jones, D. O. B. (2019b). Ecology of a polymetallic nodule occurrence gradient: Implications for deep-sea mining. Limonol. Oceanogr. 64, 1883–1894. doi: 10.1002/lno.11157
Simon-Lledó, E., Bett, B. J., Huvenne, V. A. I., Schoening, T., Benoist, N. M. A., Jeffreys, R. M., et al. (2019a). Megafaunal variation in the abyssal landscape of the Clarion-Clipperton Zone. Prog. Oceanogr. 170, 119–133. doi: 10.1016/j.pocean.2018.11.003
Smith, C. R., Paterson, G. L. J., Lambshead, P. J. D., Glover, A. G., Rogers, A. D., Gooday, A. J., et al. (2008). Biodiversity, Species Ranges, and Gene Flow in the Abyssal Pacific Nodule Province: Predicting and Managing the Impacts of Deep Seabed Mining. ISA Technical Study. Kingston: International Seabed Authority, ISA Technical Study No. 3, 1–38.
Smith, C. R., Tunnicliffe, V., Colaço, A., Drazen, J. C., Gollner, S., Levin, L. A., et al. (2020). Deep-sea misconceptions cause underestimation of seabed-mining impacts. Trends Ecol. Evol. 35, 853–857. doi: 10.1016/j.tree.2020.07.002
Smith, C. R., Washburn, T., Menot, L., Boniface, P., Pape, E., Bribiesca-Contreras, L., et al. (2019). “Sediment macrofauna – A synthesis of sediment macrofaunal biodiversity in the Clarion-Clipperton Zone,” in Deep CCZ Biodiversity Synthesis Workshop Friday Harbor, Washington, USA, 1-4 October 2019, Workshop Report, Washington DC, 85–107.
Smith, K. L., Laver, M. B., and Brown, N. O. (1983). Sediment community oxygen consumption and nutrient exchange in the central and eastern North Pacific. Limnol. Oceanogr. 28, 882–898. doi: 10.4319/lo.1983.28.5.0882
Smith, P. B. (1973). Foraminifera of the North Pacific Ocean. Geol. Surv. Prof. Pap. 766. Washington, DC: United States Government Printing Office, 1–27.
Stachowska, Z. (2020). Deep-sea Foraminiferal Assemblages at a Former Experimental Sediment Disturbance Site in the Clarion-Clipperton Fracture Zone (Subequatorial NE Pacific): Diversity and Distribution of Larger Size Fractions. Masters thesis. Poland: University of Szczecin.
Sweetman, A. K., Smith, C. R., Shulse, C. N., Maillot, B., Lindt, M., Church, M. J., et al. (2018). Key role of bacteria in the short-term cycling of carbon at the abyssal seafloor in a low particulate organic carbon flux region of the eastern Pacific Ocean. Limnol. Oceanogr. 64, 694–713. doi: 10.1002/lno.11069
Szarek, R., Nomaki, H., and Kitazato, H. (2007). Living deep-sea benthic foraminifera from the warm and oxygen-depleted environment of the Sulu Sea. Deep Sea Res. II 54, 145–176. doi: 10.1016/j.dsr2.2006.02.017
Tendal, O. S. (1979). Aspects of the biology of Komokiacea and Xenophyophoria. Sarsia 64, 13–17. doi: 10.1080/00364827.1979.10411356
Tendal, O. S. (1980). Stannophyllum setosum sp. n., a remarkable xenophyophore (Rhizopodea, Protozoa) from the eastern Pacific. Cah. Biol. Mar. 21, 383–385.
Tendal, O. S. (1996). Synoptic checklist and bibliography of the Xenophyophorea (Protista), with a zoogeographical survey of the group. Galathea Rep 17, 79–101.
Tendal, O. S., and Hessler, R. R. (1977). An introduction to the biology and systematics of Komokiacea. Galathea Rep. 14, 165–194, pls 9–26.
Thiel, H. (1975). The size structure of the deep-sea benthos. Int. Rev. Ges. Hydrobiol. Hydrogr. 60, 575–606.
Thiel, H. (1983). “Meiobenthos and nanobenthos of the deep sea,” in The Sea, Vol. 8, ed. G. T. Rowe (New York, NY: Wiley Interscience), 167–230.
Thies, A. (1991). Die Benthos-Foraminiferen im Europäischen Nordmeer. Berichte aus dem Sondersforschungsbereich 313, Vol. 31. Kiel: Christian-Albrechts-Universität, 1–97.
Thistle, D. (1979). “Harpacticoid copepods and biogenic structures: implications for deep-sea diversity maintenance,” in Ecological Processes in Coastal and Marine Systems, ed. R. J. Livingston (New York, NY: Plenum Publishing Corporation), 217–231. doi: 10.1007/978-1-4615-9146-7_11
Thistle, D. (1982). Aspects of the natural history of the harpacticoid copepods of San Diego trough. Biol. Oceanogr. 1, 225–238. doi: 10.1080/00222935608655810
Todd, R. (1965). The foraminifera of the tropical Pacific collections of the ‘Albatross’, 1899–1900. Pt. 4. Rotaliform families and planktonic families. Bull U.S. Nat. Mus. 161, 1–127, pls 1–28.
Travis, J. L., and Bowser, S. S. (1991). “The motility of Foraminifera,” in Biology of Foraminifera, eds J. J. Lee and O. R. Anderson (London: Academic Press), 92–115.
Turley, C. M., Gooday, A. J., and Green, J. C. (1993). Maintenance of abyssal benthic foraminifera under high pressure and low temperature: some preliminary results. Deep Sea Res. I 40, 643–652. doi: 10.1016/0967-0637(93)90063-9
van Oevelen, D., Soetaert, K., Garcia, R., de Stigter, H. C., Cunha, M. R., Pusceddu, A., et al. (2011). Canyon conditions impact carbon flows in food webs of three sections of the Nazare canyon. Deep Sea Res. II 58, 2461–2476. doi: 10.1016/j.dsr2.2011.04.009
Veillette, J., Sarrazin, J., Gooday, A. J., Galéron, J., Caprais, J. C., Vangriesheim, A., et al. (2007). Ferromanganese nodule fauna in the equatorial north Pacific Ocean: species richness, faunal cover and spatial distribution. Deep Sea Res. I 54, 1912–1935. doi: 10.1016/j.dsr.2007.06.011
Voltski, I., Weiner, A., Tsuchiya, M., and Kitazato, H. (2018). Syringammina limosa sp. nov., the first xenophyophore from the deep Sea of Okhotsk: morphological and genetic description. Deep Sea Res. II. 154, 32–46. doi: 10.1016/j.dsr2.2017.12.001
Walch, C. C. (1978). Recent Abyssal Benthic Foraminifera from the Eastern Equatorial Pacific. Masters thesis. University of Southern California. Available online at: http://digitallibrary.usc.edu/cdm/compoundobject/collection/p15799coll37/id/383807/rec/304 (accessed April 9, 2021).
Washburn, T. W., Menot, L., Bonifácio, P., Pape, E., Błażewicz, M., Bribiesca-Contreras, G., et al. (2021). Patterns of macrofaunal biodiversity across the Clarion-Clipperton Zone: an area targeted for seabed mining. Front. Mar. Sci. 8:626571. doi: 10.3389/fmars.2021.626571
Washburn, T. W., Turner, P. J., Durden, J. M., Jones, D. O. B., Weaver, P., and Van Dover, C. L. (2019). Ecological risk assessment for deep-sea mining. Ocean Coast. Manag. 176, 24–39. doi: 10.1016/j.ocecoaman.2019.04.014
Watling, L., Guinotte, J., Clark, M. R., and Smith, C. R. (2013). A proposed biogeography of the deep ocean floor. Prog. Oceanogr. 111, 91–112. doi: 10.1016/j.pocean.2012.11.003
Keywords: polymetallic nodules, equatorial North Pacific Ocean, monothalamids, xenophyophores, metabarcoding analyses, biogeography, food webs, recolonization
Citation: Gooday AJ, Lejzerowicz F, Goineau A, Holzmann M, Kamenskaya O, Kitazato H, Lim S-C, Pawlowski J, Radziejewska T, Stachowska Z and Wawrzyniak-Wydrowska B (2021) The Biodiversity and Distribution of Abyssal Benthic Foraminifera and Their Possible Ecological Roles: A Synthesis Across the Clarion-Clipperton Zone. Front. Mar. Sci. 8:634726. doi: 10.3389/fmars.2021.634726
Received: 28 November 2020; Accepted: 19 April 2021;
Published: 28 June 2021.
Edited by:
Malcolm Ross Clark, National Institute of Water and Atmospheric Research (NIWA), New ZealandReviewed by:
Akkur Vasudevan Raman, Andhra University, IndiaAlessandra Asioli, Institute of Marine Science (CNR), Italy
Copyright © 2021 Gooday, Lejzerowicz, Goineau, Holzmann, Kamenskaya, Kitazato, Lim, Pawlowski, Radziejewska, Stachowska and Wawrzyniak-Wydrowska. This is an open-access article distributed under the terms of the Creative Commons Attribution License (CC BY). The use, distribution or reproduction in other forums is permitted, provided the original author(s) and the copyright owner(s) are credited and that the original publication in this journal is cited, in accordance with accepted academic practice. No use, distribution or reproduction is permitted which does not comply with these terms.
*Correspondence: Andrew J. Gooday, YW5nQG5vYy5hYy51aw==