- 1Laboratorio Costero de Recursos Acuáticos de Calfuco, Instituto de Ciencias Marinas y Limnológicas, Universidad Austral de Chile, Valdivia, Chile
- 2Centro de Investigación Dinámica de Ecosistemas Marinos de Altas Latitudes, Valdivia, Chile
- 3Département de Biologie and Québec-Océan, Université Laval, Quebec City, QC, Canada
- 4DLR Projektträger, Bonn, Germany
Sea stars often function as keystone predators in food webs of intertidal and subtidal communities, especially in temperate and sub-polar regions. In South America the sea star Cosmasterias lurida is distributed along both the Atlantic and Pacific coasts of Patagonia and is one of the most conspicuous and abundant benthic predators in the shallow subtidal zone (<25 m). Its feeding strategy and prey selection are, however, still poorly known. This study describes the feeding behavior of C. lurida at a site in the Seno del Reloncaví (Chile), assessing its abundance, size and prey selection in the field relative to observed prey abundance and size along a bathymetric gradient. We hypothesized that C. lurida is a generalist predator, feeding on suitable prey according to their availability. However, we found that this predator only consumed a limited number (7 of 48) of potential prey species, primarily the slipper limpets Crepipatella spp. and the mussels Aulacomya ater and Mytilus chilensis. Electivity analysis revealed a clear preference for one mussel (A. ater) but not the other (M. chilensis) as well as depth-dependent selectivity for the slipper limpets, which changed from avoidance to preference with increasing depth. Sea star densities varied with depth, peaking between depths of 5 and 10 m, but the size of sea stars and the size of their prey did not vary significantly along a depth gradient. No significant correlations were found with the most commonly selected prey. These results would indicate that while this predator may be a generalist–opportunist, its feeding behavior is context-dependent and its high selectivity for certain species suggests that this sea star plays a key role structuring subtidal benthic communities in Patagonia.
Introduction
Predators strongly affect populations of their prey, but in turn, the availability of prey also regulates the behavior of predators (Sih et al., 1985; Gaymer and Himmelman, 2002; Ross et al., 2003; Navarrete and Manzur, 2008; Skein et al., 2018). In benthic marine communities, sea stars are one of the most active predators and control both directly and indirectly the abundance and distribution of numerous species (Paine, 1966; McClintock, 1994; Saier, 2001; Manzur and Navarrete, 2011; Calderwood et al., 2016; Gianguzza et al., 2016). They have thus been recognized as important components of intertidal and subtidal communities, at times even being considered keystone species (Paine, 1966; Dayton, 1985; Gaymer and Himmelman, 2008; Menge and Sanford, 2013).
The effect of sea stars on benthic communities depends on their feeding strategy and the trophic level of their prey in the community (Ross et al., 2003; Menge and Sanford, 2013; Motti et al., 2018). Many sea stars are opportunistic omnivores [e.g., Oreaster reticulatus (Linnaeus, 1758), Martín et al. (2001)], but some species are specialized predators [e.g., Heliaster helianthus (Lamarck, 1816), Meyenaster gelatinosus (Meyen, 1834), Urriago et al. (2011)], herbivores [e.g., Phataria unifascialis (Gray, 1840), Pharia pyramidatus (Gray, 1840), Salguero and Bonilla (2010)], or detritus feeders [e.g., Pentaceraster cumingi (Gray, 1840), Salguero and Bonilla (2010) and Hyphalaster inermis (Sladen, 1883), Mironov et al. (2016)]. The prey of sea stars consist of a wide range of organisms including sponges, sea anemones, mollusks, polychaetes, crustaceans, and even other echinoderms (Mutschke and Mah, 2009). Further, cannibalism as well as ontogenetic changes in diet can occur (Verling et al., 2003; Urriago et al., 2012; Baeta and Ramon, 2013; Fernandez et al., 2017; Deaker et al., 2020). For example, Heliaster helianthus (Lamarck, 1822) a keystone predator in rocky intertidal habitats of central Chile, showed ontogenetic changes in habitat and diet composition of prey as it grew. That is, when individuals were recruits inhabit boulders and crevices in the high or mid-high intertidal zones preying on small species, mostly on the periwinkle Austrolittorina araucana (d’Orbigny, 1840) while adults prey on more species including mussels and limpets in the lower intertidal zone (Manzur et al., 2010).
In southern South America, the sea star Cosmasterias lurida (Philippi 1858) is one of the most abundant benthic predators in shallow subtidal habitats and can be found on both soft sediments and rocky bottoms (Figure 1). C. lurida is widely distributed along the temperate shores of South America, ranging from La Serena (29° 56′ S) on the Pacific coast of Chile to Golfo de San Matias (38° 00′ S) on the Atlantic coast of Argentina (Madsen, 1956; Hernández and Tablado, 1985; Clark and Downey, 1992) as well as around the Falkland (Malvinas) Islands, Burdwood Bank, and South Georgia (Vásquez and Castilla, 1984; Hernández and Tablado, 1985; Fraysse et al., 2018). Although its bathymetric distribution is large, ranging between the lower intertidal zone and 650 m in depth (Madsen, 1956; Clark and Downey, 1992), highest abundances have been recorded in shallow water habitats (Vásquez and Castilla, 1984; Pastor-de-Ward et al., 2007). Despite its wide geographic and bathymetric distribution, previous work on this species has focused mainly on its reproductive biology (Pastor-de-Ward et al., 2007; Cossi et al., 2015, 2017; Fraysse et al., 2020) and biochemistry (Seldes and Gros, 1985; Maier et al., 1993, 1998; Roccatagliata et al., 1994). However, a wide range of prey items in its diet has been also recorded (Castilla and Moreno, 1982; Vásquez and Castilla, 1984; Pastor-de-Ward et al., 2007; Gordillo and Archuby, 2012), and C. lurida is thought to be an important consumer in the shallow benthic food webs along the Patagonian coast (Adami and Gordillo, 1999; Schejter et al., 2008; Gordillo and Archuby, 2012; Amsler et al., 2014; Cossi et al., 2015; Fraysse et al., 2018). For example, C. lurida within kelp beds [Macrocystis pyrifera (L.) C. Agardh, 1820] in Tierra del Fuego (Chile) mainly preyed on barnacles [Balanus spp. (Costa, 1778)] and slipper limpets [Crepipatella dilatata (Lamarck, 1822)] but also consumed 25 other species, including other gastropods, other crustaceans, bivalves, ascidians, brachiopods, fish, priapulids, sea urchins, and carrion (Vásquez and Castilla, 1984). In contrast, the main prey items of C. lurida in the shallow soft sediment environments of the Magellan Strait (Chile) were endobenthic bivalves, primarily Ameghinomya antiqua (P. P. King, 1832) (Garrido, unpublished data). These differences in diet suggest that this species behaves as a generalist-opportunist, being able to use different resources depending on the prey availability in the habitat (Ross et al., 2003). However, understanding trophic relationships between predators and prey requires information on both the availability of prey and the preference of the predator. Although spatial associations between predators and prey have been interpreted as preferences, true preference requires an explicit behavior (Singer, 2000; Underwood et al., 2004) where the predator selects a particular prey over others. A proxy of preference, known as electivity, can be estimated as the difference of the relative proportion of prey in the diet compared to the available relative proportion in the local environment (Singer, 2000; Underwood et al., 2004).
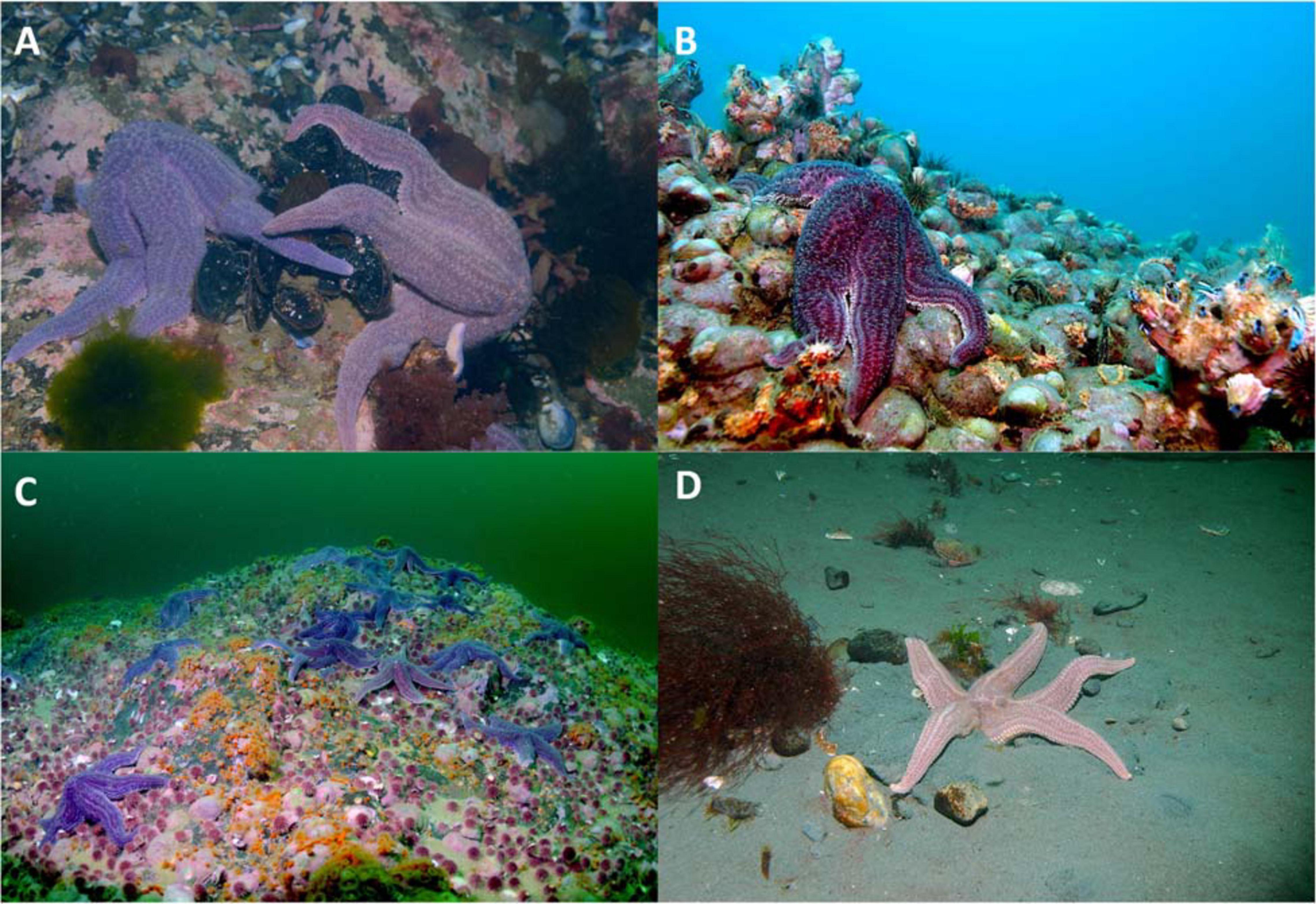
Figure 1. The sea star Cosmasterias lurida on different substrata in southern Chile. (A) Feeding on mussels on a rocky bottom at 7-m depth; (B) feeding on Crepipatella spp. on a rocky bottom at 8-m depth; (C) occurring at high densities on a rocky bottom at 10-m depth; (D) foraging on a sandy bottom at 9-m depth (A–C – Yerbas Buenas Bay, Seno del Reloncaví; (D) – Faro San Isidro, Magellan Strait, Chile).
Although previous studies (Madsen, 1956; Vásquez and Castilla, 1984; Gordillo and Archuby, 2012) have observed C. lurida feeding on different prey items, Vásquez and Castilla (1984) suggested that this predator is an opportunistic-generalist with little selectivity of prey. In the northern Patagonian zone of Chile, C. lurida, like many higher trophic level sea stars, feeds on a wide range of prey in shallow rocky environments. In this study we test the hypothesis that the diet of C. lurida would reflect prey availability in the environment, i.e., no selectivity of prey. We tested this hypothesis by quantifying sea star abundance, prey abundance, and prey electivity across a depth gradient where pronounced changes in the prey availability.
Materials and Methods
Study Site
Field observations using SCUBA were conducted in 2010 from May to July (austral winter) at Yerbas Buenas Bay (41° 40′ 20″ S; 72° 39′ 25″ W), a cove located in Seno del Reloncaví, a large bay extending 34 km south from Puerto Montt, Chile (Figure 2). Seno del Reloncaví geographically marks the end of Chile’s central valley and the beginning of the Patagonian region and is the first of several large extensions of channels and fjords of the Golfo de Ancud (Soto-Mardones et al., 2009). During our research, tidal level was measured continuously every 10 min at depths of 5 and 20 m with SOLINST pressure sensors, where maximum measured difference between low and high tide was approximately 7 m. All reported depths were corrected to the level of the annual mean tide to be able to compare data taken by SCUBA divers during different tide levels.
Predator and Prey Abundance
To quantify sea star densities and assess prey availability at this site, we selected five transects perpendicular to the coastline from the lowest intertidal level to a depth of 30 m and separated from one another by 10 m. Using a subaquatic GPS connected to a surface buoy with a GPS antenna (Schories and Niedzwiedz, 2012), we georeferenced these transects, allowing future studies to precisely relocate the study area without the need for physical markers in the field. For this study, transects were censused only once, each on a different day between May 1 and June 29 with two objectives: (1) determine the density of C. lurida and (2) assess the availability of potential prey (see details below). For each transect, two divers connected by a rope of 3-m length worked in parallel. While one diver handled the subaquatic GPS and measured depths, the other diver counted all C. lurida between them and recorded photo-quadrats (0.12 m2) using an underwater camera (Nikon D300 inside a Sea and Sea underwater housing) mounted over an aluminum frame that assured that all photo-quadrats were taken from the same distance and were perpendicular to the bottom.
Cosmasterias lurida Density in Relation to Depth
As described above, C. lurida were counted by one of the divers along each transect within the area separating the divers for the length of the transect within each 5-m depth intervals, starting from the surface down to 30 m (i.e., six depth intervals in total: 0–5, >5–10, >10–15, >15–20, >20–25, >25–30 m). The actual area surveyed within each depth range depended on the bottom slope and was calculated using the starting and ending points of the GPS positions at depths of 0, 5, 10, 15, 20, 25, and 30 m and the difference in depths between each interval. In this way following the Pythagorean theorem we calculated the total distance traveled for each depth interval and multiplied it by the transect width (3 m). The density of C. lurida was then calculated as the number of individuals per m2 (ind./m2).
Prey Availability in Relation to Depth
Along each transect, three photo-quadrants of the bottom (see above), separated by at least 2 m from each other, were taken at each 1 m depth interval along the transects to estimate the prey field (i.e., the relative abundances of epibenthic invertebrates considered to be potential prey items of C. lurida). A total 360 photo-quadrats were thus recorded along the five transects. Photographs were analyzed using the software Coral Point Count with Excel extensions [CPCe 3.6; (Kohler and Gill, 2006)] to superimpose a uniform 10 × 10 grid of points on each image after which the benthic component (algae, sessile and mobile invertebrates, bare substratum) under each point was determined (Figure 3). Nearly all organisms were able to be identified at the species level, and only a few images of poor quality (due to shade, excess particles in the water column or distortion) could not be identified either at the species level or the taxonomic group. In these cases, they were classified as unknown. Any organisms that were identifiable in the photo were also noted. Empty shells of the most abundant mollusks [the mussels Aulacomya atra (Molina, 1782) and Mytilus chilensis (Hupe, 1854) and the slipper limpets Crepipatella spp.] could be easily identified in the images. They were not included in estimates of the relative abundances of epibenthic invertebrates but gave additional evidence of possible important prey items for C. lurida. Crepipatella spp. included two cryptic species, Crepipatella peruviana (Lamarck, 1822) and C. dilatata that could not be distinguished in the field.
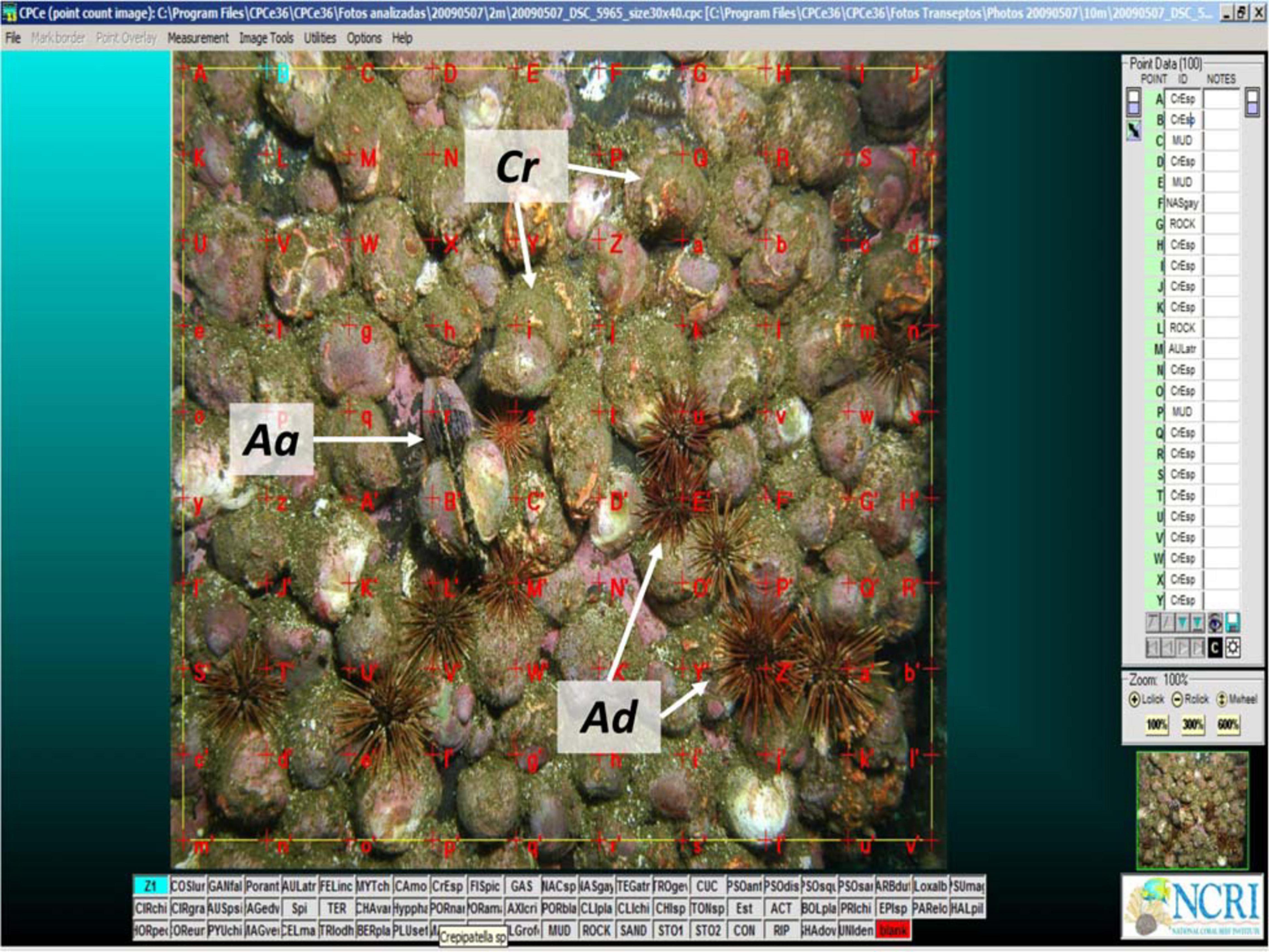
Figure 3. Image analysis of photo-quadrats (example from 10-m depth) with red crosses indicating the matrix of uniform 10 × 10 points used to assess potential prey abundance. The mussel Aulacomya atra (Aa), the sea urchin, Arbacia dufresnii (Ad), and the slipper limpets Crepipatella spp. (Cr) are easily identified in the image.
In situ Feeding of Cosmasterias lurida
Four transects running perpendicular from the shore to a depth of 30 m were also surveyed to observe in situ feeding behavior of C. lurida and determine if there were differences in the prey consumption across this depth gradient. Each transect was again divided into five intervals of 5 m of depth (see above). Within each depth interval we turned over every C. lurida encountered and recorded if the sea star was feeding and if so, what prey was being consumed. Observations were divided into five behavioral classes: (1) feeding on mussels (Aulacomya ater or Mytilus chilensis); (2) feeding on the slipper limpets Crepipatella spp.; (3) feeding on other species; (4) stomach extended but without any retained prey (with activity – “W/activity”); and (5) no activity (no feeding – “N/activity”). As the number of observations was not identical between each transect and depth interval, it was standardized as a percentage. In addition, the first 20 sea stars that were observed feeding (i.e., with stomach everted and a prey trapped within) within each depth interval of a given transect were collected and placed in separate mesh bags together with the prey item. The wet weight and disk diameter were measured for each sea star and body length for the associated prey item.
Statistical Analysis
Cosmasterias lurida Density in Relation to Depth
Differences in density of sea stars among depth intervals were analyzed with the non-parametric Kruskal–Wallis test and pair-wise comparisons by a Dunn test, because the data did not comply with the assumptions of normality and homogeneity of the variances, even after testing various transformations.
In situ Feeding of Cosmasterias lurida
Feeding activity data of C. lurida fulfilled the assumption of normality (Shapiro Wilk) and homogeneity of variance (Levene’s test). Thus, a two-way ANOVA was used to compare differences in feeding activity with depth interval. We performed a posteriori test (Tukey HSD) for cases that were significant (p < 0.05). The proportion of the species preyed upon by sea stars among depth intervals was visually examined using a Principal Coordinate Analysis (PCO) performed on a Bray-Curtis dissimilarity index matrix estimated from the relative abundance (%) of prey item at each depth. Then, we use a One-way PERMANOVA to test differences in prey proportions along the depth gradient. The data were not transformed to calculate the resemblance matrix. A Pearson correlation was used to determine the relationship between length of the predator and the three most frequently observed prey species consumed by the sea star. Univariate analyses were performed on R software (version 4.0.2; R Development Core Team, 2020) and multivariate analyses were performed on PRIMER software (Version 7.0).
The Dietary Electivity of Cosmasterias lurida
The dietary electivity analysis was performed using a selection index of relative prey availability (Pearre, 1982). The coefficient “C” [which is a correction of V coefficient of Yule (X2)] (Kendall, 1952; Kendall and Stuart, 1973) was calculated for each item by depth interval using the following formulae:
where:
n: Number of total individuals feeding,
ad: Number of a given prey item in the diet,
be: Available number of the other prey items in the environment,
bd: Number of other prey items in the diet,
ae: Available number of a particular prey item in the environment,
a: Sum of ad and ae,
b: Sum of bd and be,
d: Sum of ad and bd,
c: Sum of ae and be.
The C index ranges from −1 to +1 with the value 0 indicating no selection, i.e., the prey was consumed according to its availability. A value of 1 is absolute preference (maximum electivity), whereas a value of −1 indicates that the predator avoids the prey completely. Those extreme values are called absolute associations (Kendall, 1952). The significance of prey selectivity index C was tested using a Chi-square test (Pearre, 1982).
Results
Distribution and Density of Cosmasterias lurida
Cosmasterias lurida was restricted from 2 to 25 m of depth at Yerbas Buenas Bay (Figure 4) and was not observed deeper even though rocky substratum continued to a depth of 35 m. Densities reached peak abundance in the >5–10-m interval (0.93 ± 0.56 ind/m2) where they were over twice the levels observed in any other interval. In contrast, densities were very low in the >20–25-m interval, less than 10% observed overall. Sea star abundance was significantly different among depth intervals [Kruskal–Wallis, H (5, n = 140) = 39.4; p < 0.001; Dunn method].
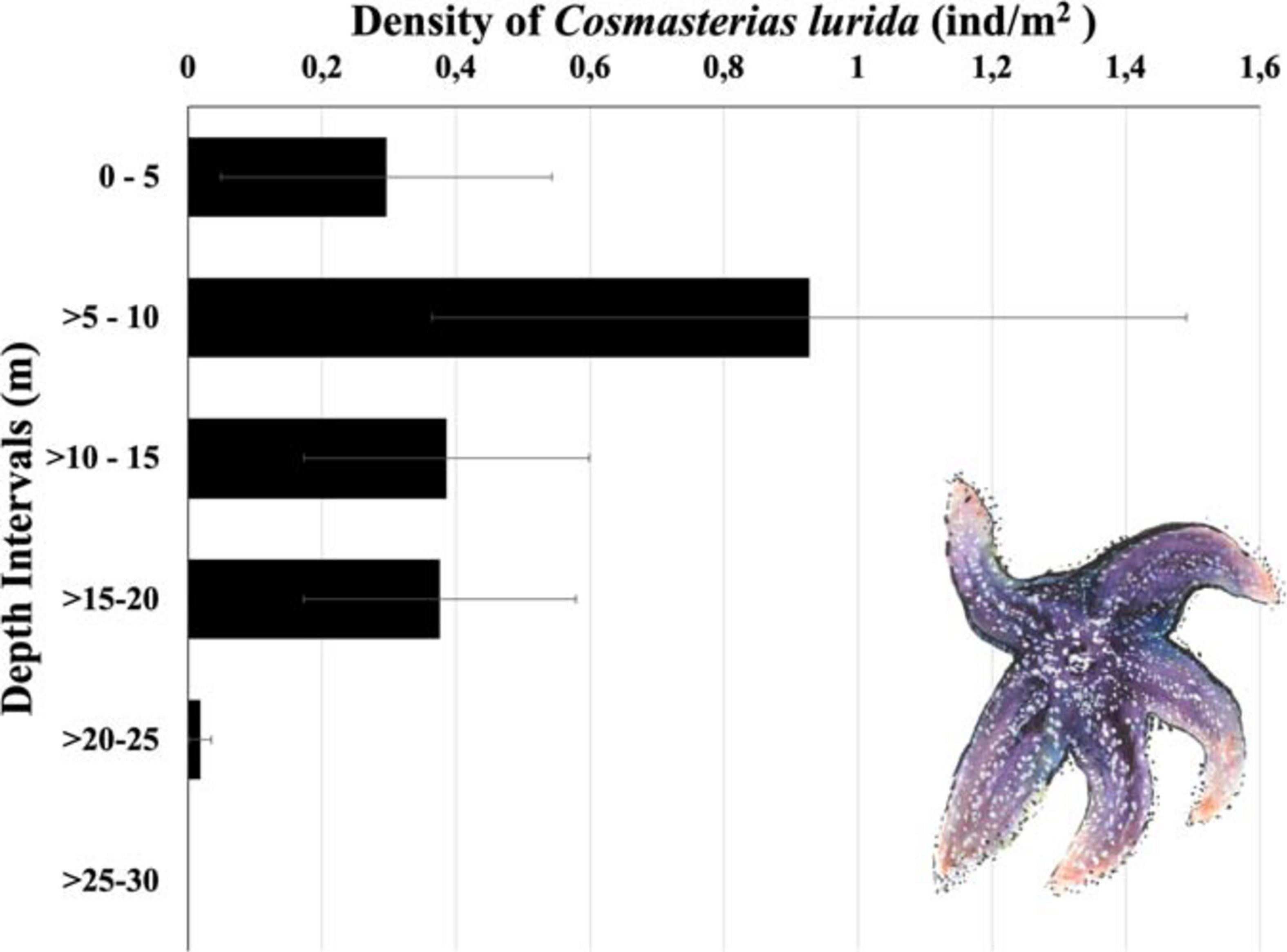
Figure 4. Densities [mean (ind/m2), SE] of the sea star Cosmasterias lurida at different depth intervals. The shallowest depth at which an individual was found 2.4 m (illustration of C. lurida by Fernanda Pardo).
Bathymetric Distribution of Potential Prey Species
Forty-eight species of benthic invertebrates considered as potential prey items were identified in the survey (Supplementary Material) and covered 56% of the bottom at this site with the remaining surface being shell fragments and sand (31%), bare rock (10%, including bedrock and cobbles), and mud (4%). The percent covers of the general taxa were 16% sessile gastropods, 15% echinoids, 11% algae, 3% bivalves, 3% holothuroids, 3% crustaceans, 2% anthozoa, 1% polychaetes, 1% porifera, <1% polyplacophores, <1% ascidians, <1% brachiopods, <1% bryozoans, <1% hydrozoans, and <1% asteroids.
The most abundant potential prey species at the site were the slipper limpets Crepipatella spp., the mussels Aulacomya ater and Mytilus chilensis, the barnacles Notobalanus flosculus (Darwin, 1854), Elminius kingii (Gray, 1831), Balanus laevis (Bruguière, 1789), and Austromegabalanus psittacus (Molina 1788), and the sea urchins Pseudechinus magellanicus (Philippi, 1857), Arbacia dufresnii (Blainville, 1825), and Loxechinus albus (Molina, 1782).
Unlike most gastropods, Crepipatella spp. are sessile. They were most abundant between depths of 0 and 10 m (18–20% cover) but decreased thereafter, falling to 3% within the deepest interval where sea stars occurred (>20–25 m; Figure 5). Other sessile species were mussels and barnacles. They were also more abundant in shallow depth intervals and, indeed, were not observed below 15 m, except for the barnacle A. psittacus, which was recorded only in some transects below the 15 m but in low abundance. The two mussel species, A. ater and M. chilensis, were less abundant than Crepipatella spp., reaching only 1% and 11% cover, respectively, in the shallowest depth interval (Figure 5). Barnacles were relatively abundant (13% in this same depth interval) but mainly consisted of small individuals (<1-cm diameter). Among mobile animals, the sea urchins P. magellanicus, A. dufresnii, and L. albus were the most abundant and were recorded in all depth intervals (Figure 5). P. magellanicus was the most abundant sea urchin species and occurred primarily in depths from 10 to 15 m. A diverse group of mobile small gastropods (<1 cm) was observed at the site but even collectively they were not abundant (Figure 5, Supplementary Material, and Table 1).
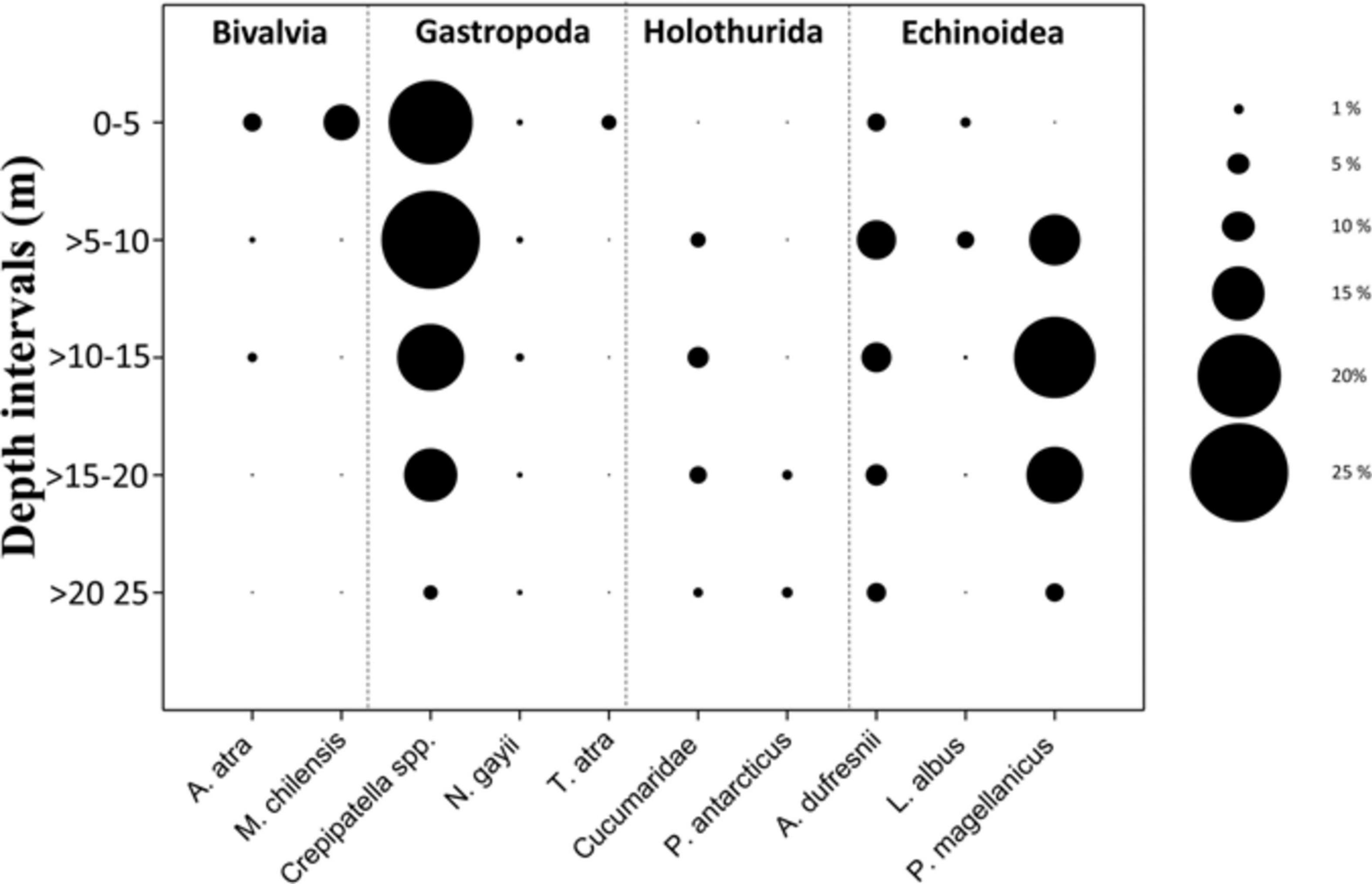
Figure 5. Prey availability – Abundance (percent cover) at different depth intervals of common invertebrates that are potential prey of the sea star Cosmasterias lurida in Yerbas Buenas Bay.
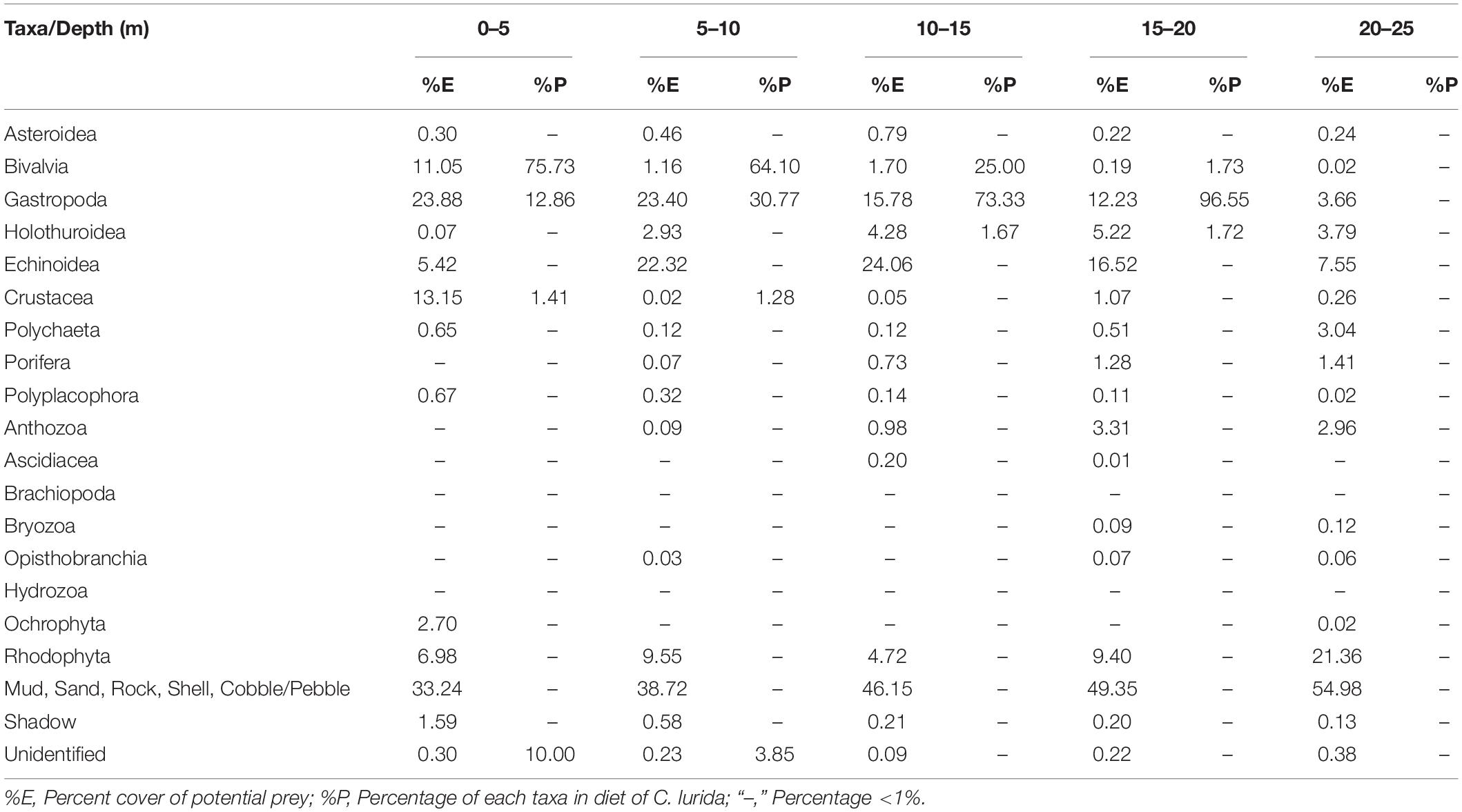
Table 1. Abundance (percent cover) of the potential prey of the sea star Cosmasterias lurida in photo-quadrats at depths down to 25 m (no sea stars were observed below this depth).
In situ Feeding Activity of Cosmasterias lurida
Regarding the feeding activity survey of C. lurida, significant differences were found in the interaction between the prey items and the depth intervals (F = 8.512; df = 9, 48; P < 0.001) (Table 2). Regardless of the depth interval, approximately half the sea stars sampled were not feeding, and no individuals of C. lurida were found feeding at depths below 20 m (0–5 m = 55%; >5–10 m = 46%; >10–15 m = 43%; >15–20 m = 57%; >20–25 = 0%; Figure 6). Among those that were feeding (0–5 m = 42%; >5–10 m = 45%; >10–15 m = 52%; >15–20 m = 31%; Figure 6), only seven species of the 48 taxonomic groups recorded in the photo-quadrats were observed as prey (Supplementary Material). Moreover, the principal prey observed were limited to just four species [the two species of Crepipatella (51%), Aulacomya ater (36%), and Mytilus chilensis (8%)] with three other taxa making up the balance (holothuroid, crustacean, gastropod – each <2%; Figure 6). Regarding the proportion of the species preyed upon by C. lurida PERMANOVA showed significant differences among depth intervals (Table 3). The posteriori Pair-wise test indicated that the most similar depth intervals were >10–15 and >15–20 dissimilar depth intervals were 0–5 and >15–20 m (Table 3). Those differences are better visualized in the PCO analysis, where the shift in the importance of the major prey items (A. ater and Crepipatella spp.) shift with depth can be seen (upper panels of Figure 7) as well as the lack of importance of the other mussel (M. chilensis) and other prey items (lower panels of Figure 7). With regards to the size of sea stars [mean disk diameter ± SD: 31.0 ± 4.5 mm and the size of prey sizes (mean length ± SD: 57.0 ± 26.0 mm)] that they consumed, neither varied significantly with depth, and there were no significant correlations between predator and prey size for the three most commonly selected prey: A. ater [Pearson (r) = 0.238, n = 99, p > 0.05]; M. chilensis [Pearson (r) = −0.383, n = 25, p > 0.05]; Crepipatella spp. [Pearson (r) = 0.207, n = 134, p > 0.05].
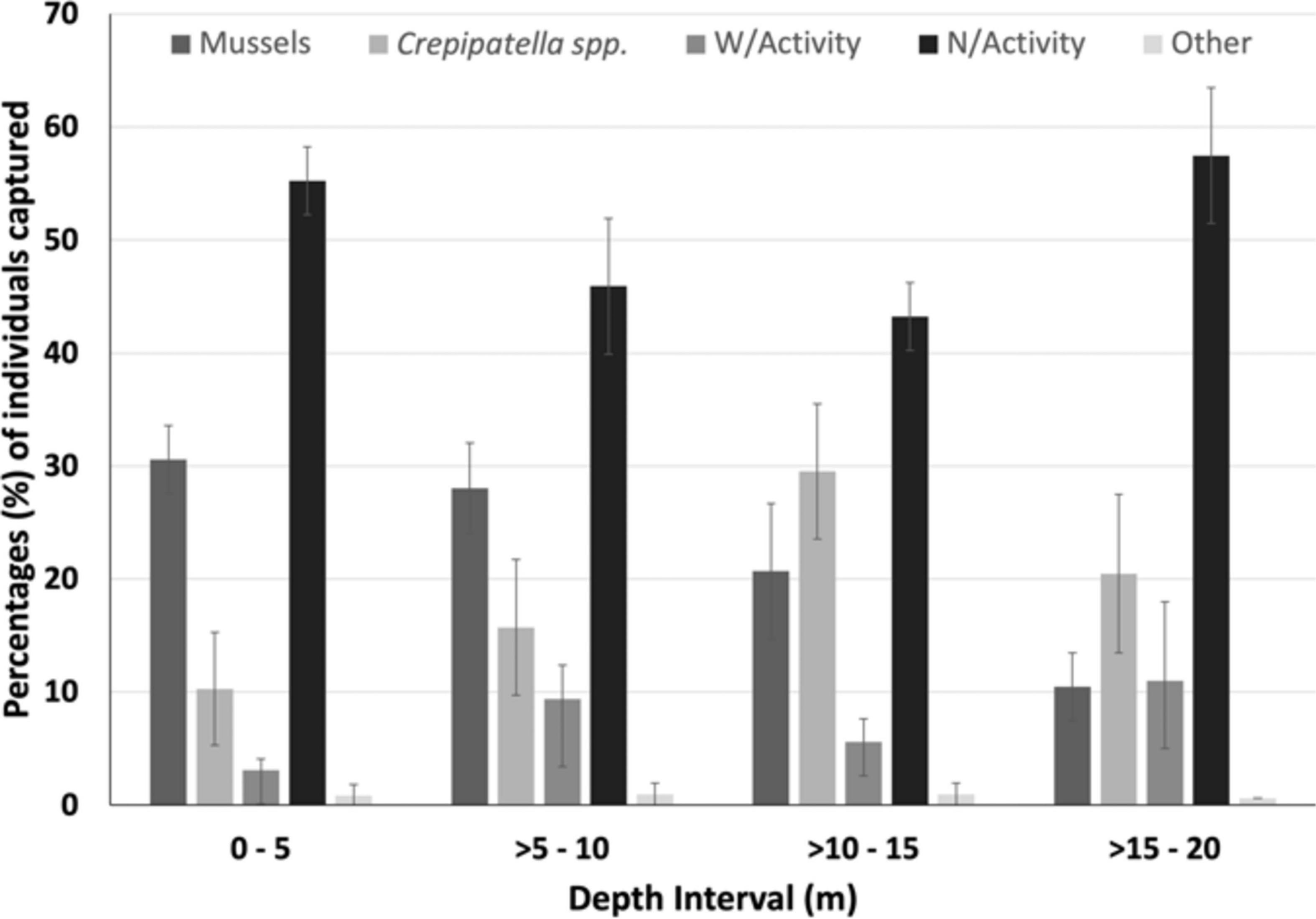
Figure 6. Five categories of feeding activity of the sea star Cosmasterias lurida in Yerbas Buenas Bay: 1, feeding on mussels; 2, feeding on Crepipatella spp.; 3, with activity (W/activity; stomachs out but no associated prey); 4, no activity (N/activity); and 5, feeding on other prey species. No sea stars were observed feeding below 20 m in depth. Bars represent standard errors.
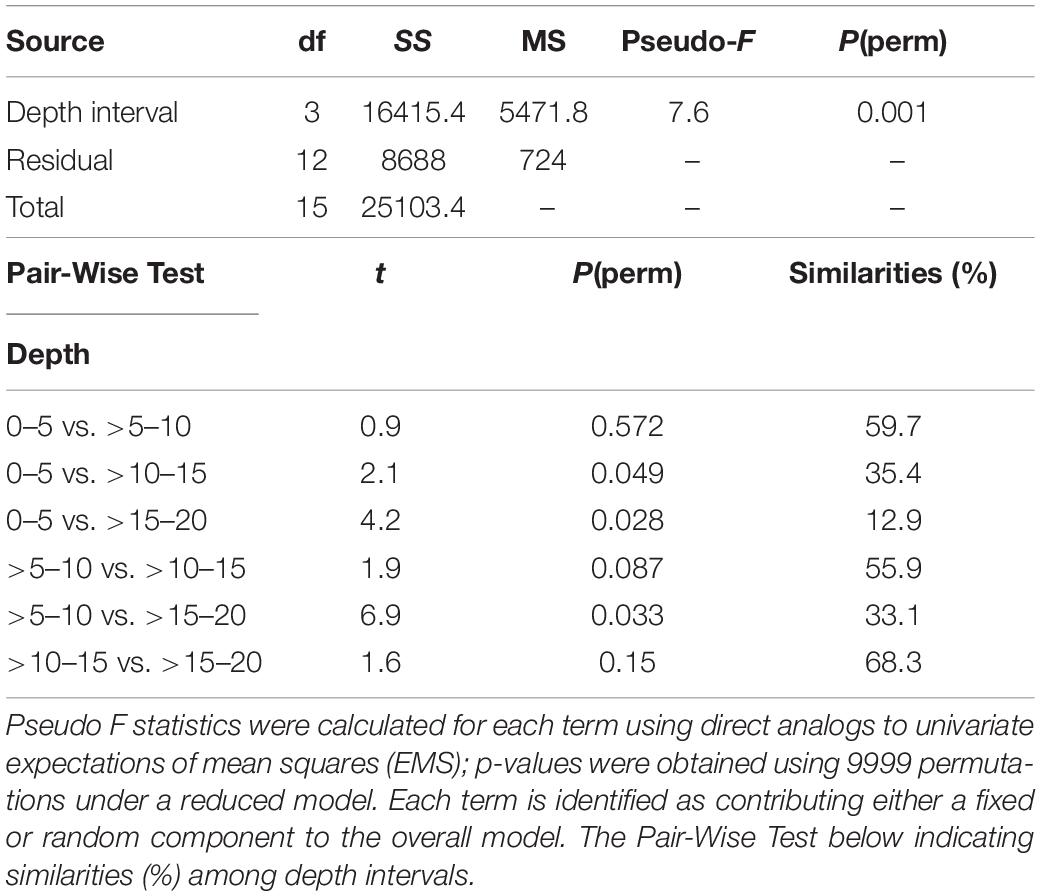
Table 3. PERMANOVA partitioning and analysis of prey item per depth intervals (4) from Yerbas Buenas Bay, based Bray–Curtis dissimilarities.
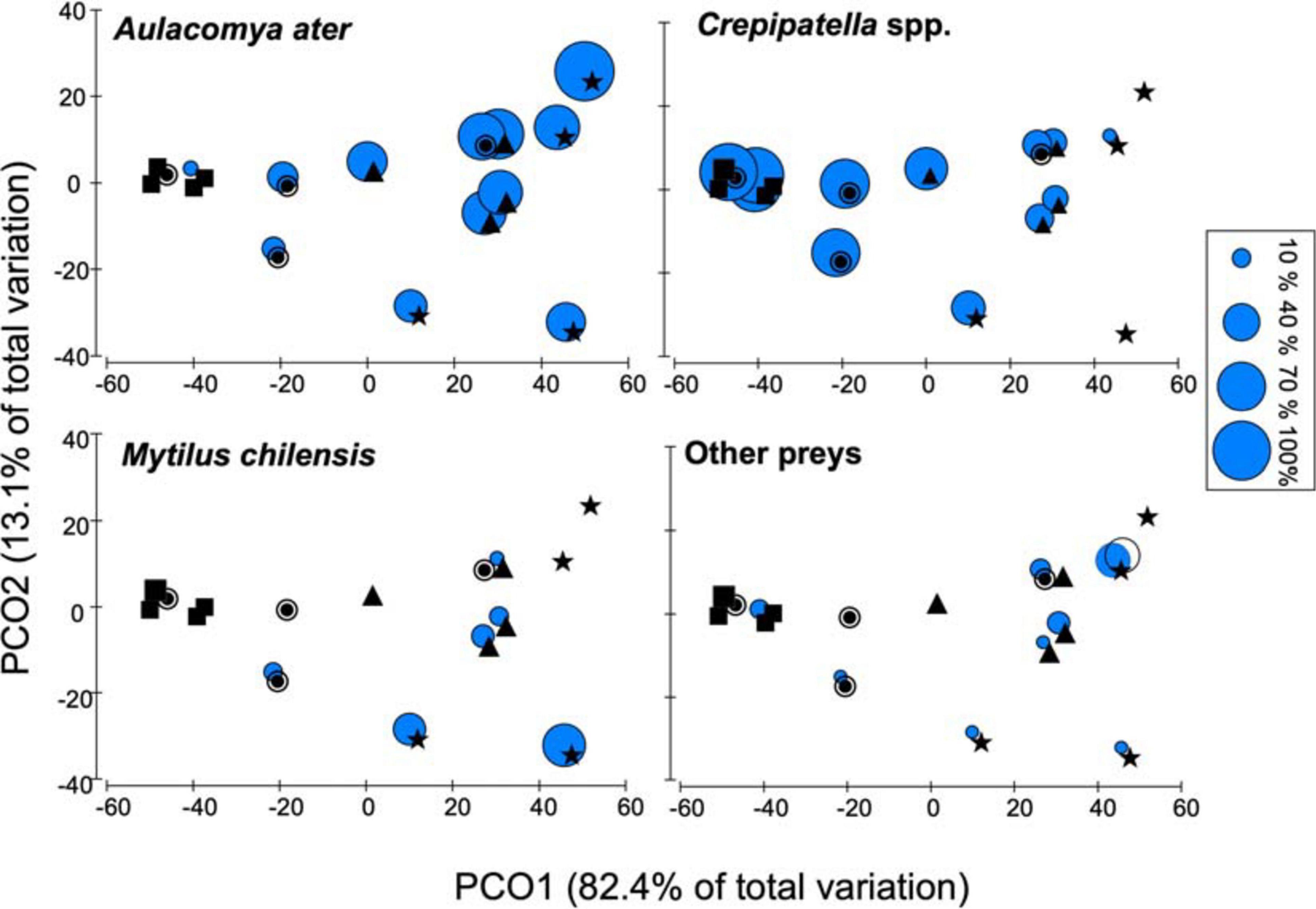
Figure 7. Principal Coordinates Analysis (PCO) showing prey distinctly selected according to depth. Symbols represent different depth intervals (m); ★ = 0–5, ▲ = >5–10, = >10–15, ■ = >15–20. The size of the circles represent the proportion of the main species preyed upon by Cosmasterias lurida.
Prey Electivity
The electivity (C) of different prey types changed by depth interval (Table 4 and Figure 8). In shallower depth intervals, the sea star had a high positive electivity for the mussel A. ater in depths of 0–15 m, especially in the >5–10-m depth interval (C = 0.72). It also had a slight but not significant positive electivity for the other mussel, M. chilensis, but only in the >5–10-m depth interval. Electivity changed dramatically below 10 m where there was a moderately high electivity for Crepipatella spp., which increased with depth (C = 0.28 and 0.44 in the >10–15-m and >15–20-m depth intervals, respectively). This contrasts sharply with the shallowest depth intervals with the significantly negative electivity value (C = −0.33) in the 0–5-m interval, which indicated that Crepipatella spp. was avoided there, and C = 0.062 for the >5–10-m depth interval, where this gastropod was not selected by the sea star. With regard to other prey, the index was close to 0 in the shallowest depth interval, indicating that they were consumed to the degree of their availability in the environment, but in all other depth intervals, the indices were negative as these other prey were avoided to a moderate degree (Table 4 and Figure 8). If we compare the most common prey with their availability in the environment (Figure 8), there is a dramatic shift in prey choice from mussels in shallow depths (where they are rare relative to all other potential prey) to Crepipatella spp. in deeper depth intervals. Crepipatella spp. was abundant in the 0–5-m depth interval (19%) but made up only 10% of prey consumed in this interval. In the next deeper interval (>5–10-m) the cover was similar (22% cover), but consumption increased to 31%. In the deeper intervals of >10–15 m and >15–20 m, Crepipatella spp. were less abundant (14% and 12% cover, respectively) but were observed as prey items 67% and 96% of the time, respectively. In the 0–5-m depth interval, M. chilensis and A. ater occurred at low percent cover (8% and 3%, respectively) but were frequently consumed (21% and 58%, respectively). In the >5–10-m depth interval, abundance of both species decreased dramatically (0.17% and 1%, respectively), but 8% and 57% of the sea star diet was M. chilensis and A. ater, respectively. In the deeper intervals of >10–15 m and >15–20 m, M. chilensis and A. ater were present at very low cover, so not part of the main diet of C. lurida. Other prey items collectively occurred at high cover in all depth intervals studied with 26% (0–5 m), 29% (>5–10 m), 36% (>10–15 m), 29% (>15–20 m), and 18% (>20–25 m). They were, however, less commonly observed as prey [11% (0–5 m), 5% (>5–10 m), 4% (>10–15 m), 3% (>15–20 m), and 0% (>20–25)].
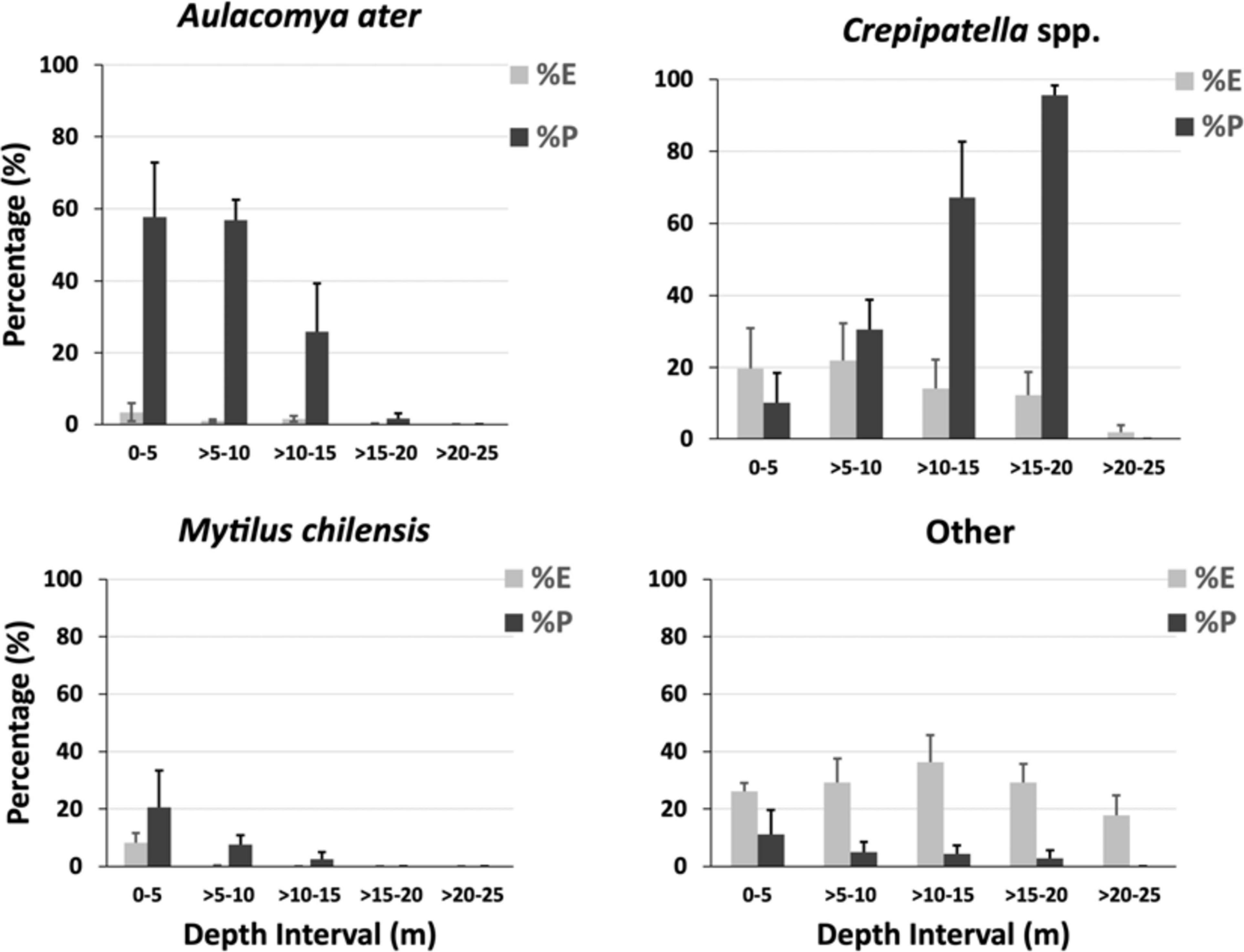
Figure 8. Availability of potential prey (percent cover) in the environment compared to their occurrence in the diet (percentage) of the sea star Cosmasterias lurida in Yerbas Buenas Bay.
Discussion
The impact of any predator on community structure and organization depends on multiple factors, for example, the degree of selectivity (generalist vs. selective predators) when choosing their prey, prey diversity, trophic level of the prey together with their defense mechanisms or prey recruitment dynamics. Predator behavior is especially relevant because selective predators can have strong indirect effects on competition among prey, producing drastic changes in community structure, especially when having preferences for competitive dominants (Paine et al., 1985; Castilla, 1999; Rettig and Smith, 2021). In many marine ecosystems sea stars are considered to be keystone species (Paine, 1966, 1969; McClintock and Lawrence, 1985; Menge and Sanford, 2013), and indeed, this general ecological concept arose from early studies on sea star ecology (Paine, 1966, 1969). However, this original concept has evolved into a holistic vision and is now used in a more complex conceptual framework to be able to define an entire ecosystem as a “keystone species complex” (Hermosillo-Nunez et al., 2018). In many cases, prey diversity (species and size), system productivity, prey recruitment dynamics, and predator preferences have all been important factors determining their impacts (Menge and Sanford, 2013). Because sea stars prey strongly on bivalves, mussels are often a principal component of the diet of many asteroids, especially in shallow waters (Castilla and Crisp, 1970; Tokeshi, 1991; Tokeshi and Romero, 1995; Gaymer et al., 2001a, b; Gaymer and Himmelman, 2002; Gil and Zaixso, 2008; Lamare et al., 2009). For example, Heliaster helianthus, described as a keystone predator in intertidal communities along the central coast of Chile, limits the lower distribution of the mussel Perumytilus purpuratus (Lamarck, 1819), which can monopolize the rocky surface when H. helianthus is absent (Paine et al., 1985). The dominance of filter-feeding gastropods, the slipper limpets Crepipatella spp. (52% of all feeding observations), in the diet would then seem, at first glance, to go against this trend, suggesting that C. lurida does not appear to be a predator that specializes on mussels but is instead a generalist. However, the electivity analysis across depth showed clearly that one of the mussel species, Aulacomya ater, was preferred in shallower zones. Strikingly, the other mussel species, Mytilus chilensis, was never preferentially consumed by this sea star, and this difference could possibly explain why this mussel occurred in higher abundance than the preferred species. Regardless, this pattern goes against the generalization that sea stars invariably prefer feeding on mussels. The subtle differences that might drive the distinction between these two very similar species remain unknown and worthy of future investigation.
The large proportion of gastropod prey in the diet of C. lurida was, however, not surprising for several reasons. First, unlike most gastropods, slipper limpets are sessile and thus cannot move to escape predators as observed in many other systems where mobile gastropods are common (McClintock, 1985; Bryan et al., 1997; San-Martin et al., 2009). Second, it was the most abundant substratum occupier at this site and thus was the most available item in the prey field. Therefore, there was a high overall electivity index for this group, especially at greater depths (5–20 m) where alternative invertebrate prey were either scarce (e.g., mussels) or highly mobile (e.g., sea urchins).
Selective removal of species by sea stars can structure benthic communities (Paine, 1966; Gaymer and Himmelman, 2008; Manzur et al., 2010), and C. lurida may be acting as a keystone predator in Patagonian rocky subtidal ecosystems. Indeed, the dominance of the slipper limpets Crepipatella spp. at our study site (reaching 100% in some photo-quadrats) may rely on the selective predation of C. lurida on the mussel A. atra, a potential competitor for space. Manipulative experiments would be needed to establish any such role of this predator. For example, the very low abundance of mussels below depths of 5 m could be due simply to competitive displacement by Crepipatella spp. or by predation from C. lurida, which occurred at its highest local densities at depths between 5 and 15 m. Either mechanism could allow the slipper limpets to monopolize the substratum. Alternatively, physiological restrictions could limit mussels to inhabit shallower subtidal environments. However, both A. ater and M. chilensis have been recorded at depths up to 25 m in other localities (Solís and Lozada, 1971; Lorenzen et al., 1979; Cazzaniga, 1990; Zagal et al., 2001), making this latter possibility unlikely.
The decrease in abundance of Crepipatella spp. below a depth of 20 m corresponded to the low abundance of sea stars also observed at these depths, suggesting that it is not predation that is determining the lower limit of these species. However, the spatial distribution and abundance of asteroids often tracks that of their prey (Gaymer and Himmelman, 2002; Himmelman et al., 2005), and the low abundance of all invertebrates indicates that other ecological processes (e.g., low recruitment) are structuring benthic assemblages at deeper depths, which were dominated primarily by octocorals, mainly Primnoella sp. (Garrido, unpublished data), and another calyptraeid gastropod, Calyptraea aurita (Reeve, 1859) (Holtheuer et al., 2018), below depths of 20 m.
The depth-dependent selectivity that we observed in our study contrasts strikingly with the only other detailed study of the feeding ecology of this species (Vásquez and Castilla, 1984). That study, conducted in southern Patagonia, concluded that C. lurida was a generalist, consuming prey species according to their availability in the environment. Their assessment of selectivity was, however, based on a much simpler, non-parametric analysis, which may have been unable to resolve smaller differences. For example, consistent with our results, A. ater, the preferred prey in our study, was ranked higher as a prey species (#3) than its availability (#5) at their study site, but their selectivity analysis only assessed the ensemble of the prey available and not individual species. In addition, they did not stratify their sampling by depth [likely because of the limited bathymetric distribution (2–12 m) of C. lurida at their site], which again may have limited resolution. A major ecological difference was, however, that barnacles were both the most abundant prey species at their site and the most common prey species (27%). In contrast, we saw almost no predation on barnacles even though they represented 13% of the available prey in the shallowest depth interval. Interestingly, they also recorded many more prey species (27) than we did (7), in spite of similar sampling efforts (132 vs. 136 observations of feeding associated with a prey species, respectively) and the high diversity of potential prey species at our study site (48 species). One consistency between the two studies was the high percentage of sea stars not associated with any prey (both approximately 50%). The underlying causes in both cases remains unknown, but it is surprising to see almost half the population of this predator was not feeding even when potential prey (e.g., slipper limpets) were readily available (e.g., shallow depth intervals).
An additional explanation for the differences between our results and those of Vásquez and Castilla (1984) is the difference in the timing of sampling which was done in the austral summer in their study (January and April) but in the austral winter in our study (May to July). Species can exhibit seasonal changes in their feeding behavior in response to temporal variation in biotic parameters (e.g., density, energy content of prey) and abiotic factors (e.g., substratum, temperature) (Christensen, 1970; Kreiling et al., 2020). Such changes in diet, feeding preferences and foraging behavior have been reported for other sea stars [e.g., Asterias rubens (Castilla, 1972); Luidia clathrate (McClintock and Lawrence, 1985); Astropecten marginatus (Guilherme and Rosa, 2014)], due possibly to seasonal variations in the nutritional value of prey (e.g., reproductive condition) that might lead to prey switching by predators (Krebs et al., 1977; Ostfeld, 1982) or seasonal changes in energetic requirements of sea star predators (Aguera et al., 2012). Such possibilities certainly exist, but both studies were only conducted over 4-month periods, and thus temporal variability in the diet could not be assessed. Regardless, the differences between the two studies caution against making generalizations from studies restricted to one place and one time. Spatial and temporal variation in patterns and processes are, of course, the norm for ecological studies, even when involving the same suite of species, but the contrasting results from these two studies emphasize the need for greater spatiotemporal sampling to better understand the feeding behavior of C. lurida. Another limitation of our study is that in situ observations of the feeding behavior only provide a “snapshot” of an individual’s diet and cannot determine if individuals are specialists or generalists. In contrast, stable isotope analysis (Wang et al., 2014; Zenteno et al., 2019) does integrate past feeding activity and could be used as a complementary approach to confirm and extend the patterns observed in this study. Finally, as all our observations were made during daylight hours, we also do not know if these sea stars were feeding differently at night although given that they are not visual predators, this possibility is unlikely. Regardless, these questions can and should be addressed in future studies on the feeding behavior of this and other predatory sea stars.
Overall, we found that C. lurida does not feed on prey in direct proportion to their availability in the habitat, showing selectivity of certain prey species (in particular, the mussel A. ater). Thus, our original hypothesis that this species is an opportunist-generalist is not supported as C. lurida appears to be a flexible specialist, one with strong preferences but also an ability to change its prey selection according to the environmental context. However, it appears to limit its prey selection mostly to sessile species because the sea urchins Loxechinus albus, Arbacia dufresnii, and Pseudechinus magellanicus were never recorded in the diet of C. lurida even though they occurred in relatively high abundance at our study site. One explanation is that sea urchins, including one of the species in our study (Loxechinus albus), can have effective risk-avoidance behaviors to sea star attacks (Urriago et al., 2011, 2012).
Prey electivity could also change depending on the size of predators and prey (Paine, 1976; Barbeau and Scheibling, 1994). However, we did not find a predator–prey size relationship between C. lurida and any of its three primary prey species. Similarly, observations on feeding behavior in another Chilean sea star, Meyenaster gelatinosus, did not indicate preferences for any particular size of the sea urchin L. albus, the dominant invertebrate species at that study site (Dayton et al., 1977). In contrast, Leptasterias polaris (Müller and Troschel, 1842) and Asterias vulgaris (Packard, 1863), two common subtidal sea stars in eastern Canada, had differences in their use of prey (species and size of bivalves) that may facilitate their coexistence (Gaymer et al., 2001a). On the Pacific coast of North America, the selection of different sizes of prey (mussels) appears to maximize the energy intake in the competing sea stars Leptasterias hexactis (Stimpson, 1862) and Pisaster ochraceus (Brandt, 1835) (Menge and Menge, 1974). Thus, the presence of competitors might select for intraspecific differences in size to partition the trophic niche. This is not the case, however, for our study system, because no other predatory sea stars co-occur with C. lurida (Garrido, unpublished data).
This study shows the importance of assessing the environmental context to describe the trophic behavior of predators. Laboratory studies about dietary preference indicate that asteroids show preferences for some prey offered, but they did not consider the relative abundance of prey in the environment (Castilla and Crisp, 1970; Castilla, 1972; Rochette et al., 1994; Pratchett, 2007). On the other hand, field studies usually show generalist behaviors regardless of environmental variability. Species that have been considered as generalists are frequently much more selective when the environmental offer is evaluated (Gaymer and Himmelman, 2008). Detailed and realistic knowledge of the trophic habits of species is a critical element for constructing food webs (Martinez, 1993), which, in turn, help to predict effects of perturbations on communities (Pérez-Matuz et al., 2017). In addition, anthropogenic activity, such as coastal urbanization that results in the destruction of habitats, needs to be considered as it can significantly affect sea star populations and their impact on the trophic structure and function of subtidal communities (Chan et al., 2018). In this sense, this study is a key contribution to understanding food web dynamics of the benthic marine communities of northern Patagonia.
Data Availability Statement
The original contributions presented in the study are included in the article/Supplementary Material, further inquiries can be directed to the corresponding author.
Author Contributions
IG: conceptualization, validation, formal analysis, writing, and visualization. LP: conceptualization, investigation, and writing – review and editing. LJ: conceptualization, validation, and writing – review and editing. DS: conceptualization, investigation, validation, formal analysis, and writing. All authors contributed to the article and approved the submitted version.
Funding
This work was supported by the International Bureau, Bonn, Federal Republic of Germany (FRG) (project #CHL 07/001 and CHL 07/007), the Direction of Investigation and Development of the Universidad Austral de Chile, Valdivia, Chile (S-2008-14), and FONDAP-IDEAL Center (Program 15150003).
Conflict of Interest
DS was employed by the Universidad Austral de Chile during the time the field data for this research was collected. DS is employed by DLR Project Management Agency.
The remaining authors declare that the research was conducted in the absence of any commercial or financial relationships that could be construed as a potential conflict of interest.
Acknowledgments
We are grateful for the assistance of the Scientific Diving Group of the Universidad Austral de Chile in the field, especially to Jose Luis Kappes, Maria José Díaz, Isabel del Moral, and Jorge Holtheuer.
Supplementary Material
The Supplementary Material for this article can be found online at: https://www.frontiersin.org/articles/10.3389/fmars.2021.636208/full#supplementary-material
References
Adami, M. L., and Gordillo, S. (1999). Structure and dynamics of the biota associated with Macrocystis pyrifera (Phaeophyta) from the Beagle Channel, Tierra del Fuego. Sci. Mar. 63, 183–191. doi: 10.3989/scimar.1999.63s1183
Aguera, A., Trommelen, M., Burrows, F., Jansen, J. M., Schellekens, T., and Smaal, A. (2012). Winter feeding activity of the common starfish (Asterias rubens L.): the role of temperature and shading. Journal of Sea Research 72, 106–112. doi: 10.1016/j.seares.2012.01.006
Amsler, C. D., McClintock, J. B., and Baker, B. J. (2014). Chemical mediation of mutualistic interactions between macroalgae and mesograzers structure unique coastal communities along the Western Antarctic Peninsula. J. Phycol. 50, 1–10. doi: 10.1111/jpy.12137
Baeta, M., and Ramon, M. (2013). Feeding ecology of three species of Astropecten (Asteroidea) coexisting on shallow sandy bottoms of the northwestern Mediterranean Sea. Mar. Biol. 160, 2781–2795. doi: 10.1007/s00227-013-2270-0
Barbeau, M. A., and Scheibling, R. E. (1994). Procedural effects of prey tethering experiments: predation of juvenile scallops by crabs and sea stars. Mar. Ecol. Prog. Ser. 111, 305–310. doi: 10.3354/meps111305
Bryan, P. J., McClintock, J. B., and Hamann, M. (1997). Behavioral and chemical defenses of marine prosobranch gastropod Calliostoma canaliculatum in response to sympatric seastars. J. Chem. Ecol. 23, 645–658. doi: 10.1023/b:joec.0000006401.97339.b9
Calderwood, J., O’connor, N. E., and Roberts, D. (2016). Efficiency of starfish mopping in reducing predation on cultivated benthic mussels (Mytilus edulis Linnaeus). Aquaculture 452, 88–96. doi: 10.1016/j.aquaculture.2015.10.024
Castilla, J. C. (1972). Responses of Asterias rubens to bivalve prey in a Y-maze. Mar. Biol. 12, 222–228. doi: 10.1007/bf00346770
Castilla, J. C. (1999). Coastal marine communities: trends and perspectives from human exclusion experiments. Trends Ecol. Evol. 14, 280–283. doi: 10.1016/s0169-5347(99)01602-x
Castilla, J. C., and Crisp, D. J. (1970). Responses of Asterias rubens to olfactory stimuli. J. Mar. Biol. Assoc. U.K. 50, 829–847.
Castilla, J. C., and Moreno, C. A. (1982). “Sea urchins and Macrocystis pyrifera: experimental test of their ecological relations in southern Chile,” in Echinoderms, ed. J. M. Lawrence (Rotterdam: Balkema), 257–263.
Cazzaniga, N. J. (1990). A concern for grammar: the name of the Magellanic mussel (Bivalvia: Mytilidae). Malacol. Rev. 27:110.
Chan, Y. K. S., Toh, T. C., and Huang, D. (2018). Distinct size and distribution patterns of the sand-sifting sea star, archaster typicus, in an urbanised marine environment. Zool. Stud. 57:11.
Christensen, A. M. (1970). Feeding biology of the sea-star Astropecten irregularis Pennant. Ophelia 8, 1–134.
Cossi, P. F., Boy, C. C., Gimenez, J., and Perez, A. F. (2015). Reproductive biology and energy allocation of the sea star Cosmasterias lurida (Echinodermata: Asteroidea) from the Beagle Channel, Tierra del Fuego, Argentina. Polar Biol. 38, 1321–1333. doi: 10.1007/s00300-015-1696-x
Cossi, P. F., Boy, C. C., and Perez, A. F. (2017). Pattern of energy allocation during the gametogenesis of the asteroid Cosmasterias lurida (Forcipulatida: Stichasteridae) from the Beagle Channel, Ushuaia, ArgentinaPaula. Rev. Biol. Trop. 65, S197–S206.
Dayton, P. K. (1985). The structure and regulation of some south american kelp communities. Ecol. Monogr. 55, 447–468. doi: 10.2307/2937131
Dayton, P. K., Rosental, R. J., Mahen, L. C., and Antezana, T. (1977). Population structure and foraging biology of the predaceus chilean asteroid Meyenaster gelatinosus and the escape biology of its prey. Mar. Biol. 39, 361–370. doi: 10.1007/bf00391939
Deaker, D. J., Aguera, A., Lin, H.-A., Lawson, C., Budden, C., Dworjanyn, S. A., et al. (2020). The hidden army: corallivorous crown-of-thorns seastars can spend years as herbivorous juveniles. Biol. Lett. 16:20190849. doi: 10.1098/rsbl.2019.0849
Fernandez, W. S., Dias, G. M., Majer, A. P., Delboni, C. G., Denadai, M. R., and Turra, A. (2017). Resource partitioning between sympatric starfish from tropical unconsolidated substrate: implications for coexistence and top-down control on benthic prey. Estuar. Coast. Shelf Sci. 196, 141–149. doi: 10.1016/j.ecss.2017.06.039
Fraysse, C., Calcagno, J., and Perez, A. F. (2018). Asteroidea of the southern tip of South America, including Namuncura Marine Protected Area at Burdwood Bank and Tierra del Fuego Province, Argentina. Polar Biol. 41, 2423–2433. doi: 10.1007/s00300-018-2377-3
Fraysse, C. P., Perez, A. F., Calcagno, J. A., and Boy, C. C. (2020). Energetics and development modes of Asteroidea (Echinodermata) from the Southwestern Atlantic Ocean including Burdwood Bank/MPA Namuncura. Polar Biol. 43, 175–186. doi: 10.1007/s00300-020-02621-6
Gaymer, C., and Himmelman, J. (2002). Mussel beds in deeper water provide an unusual situation for competitve interactions between the seastars Leptasterias polaris and Asterias vulgaris. J. Exp. Mar. Biol. Ecol. 277, 13–24. doi: 10.1016/s0022-0981(02)00234-4
Gaymer, C. F., and Himmelman, J. H. (2008). A keystone predatory sea star in the intertidal zone is controlled by a higher-order predatory sea star in the subtidal zone. Mar. Ecol. Prog. Ser. 370, 143–153. doi: 10.3354/meps07663
Gaymer, C. F., Himmelman, J. H., and Johnson, L. E. (2001a). Distribution and feeding ecology of the seastars Leptasterias polaris and Asterias vulgaris in the northern Gulf of St Lawrence, Canada. J. Mar. Biol. Assoc. U.K. 81, 827–843. doi: 10.1017/s0025315401004660
Gaymer, C. F., Himmelman, J. H., and Johnson, L. E. (2001b). Use of prey resources by the seastars Leptasterias polaris and Asterias vulgaris: a comparison between field observations and laboratory experiments. J. Exp. Mar. Biol. Ecol. 262, 13–30. doi: 10.1016/s0022-0981(01)00264-7
Gianguzza, P., Di Trapani, F., Bonaviri, C., Agnetta, D., Vizzini, S., and Badalamenti, F. (2016). Size-dependent predation of the mesopredator Marthasterias glacialis (L.) (Asteroidea). Mar. Biol. 163:65.
Gil, D. G., and Zaixso, H. E. (2008). Feeding ecology of subantarctic sea star Anasterias minuta within tide pools in Patagonia, Argentina. Biol. Trop. 56, 311–328.
Gordillo, S., and Archuby, F. (2012). Predation by drilling gastropods and asteroids upon mussels in rocky shallow shores of southernmost South America: paleontological implications. Acta Palaeontol. Polon. 57, 633–646. doi: 10.4202/app.2010.0116
Guilherme, P. D. B., and Rosa, L. C. (2014). Seasonal variation in body size and diet of the sea star Astropecten marginatus (Paxillosida, Astropectinidae) off coast of Parana, Southern Brazil. Rev. Biol. Trop. 62, 59–68. doi: 10.15517/rbt.v62i1.7888
Hermosillo-Nunez, B. B., Ortiz, M., and Rodriguez-Zaragoza, F. A. (2018). Keystone species complexes in kelp forest ecosystems along the northern Chilean coast (SE Pacific): improving multispecies management strategies. Ecol. Indic. 93, 1101–1111. doi: 10.1016/j.ecolind.2018.06.014
Hernández, D. A., and Tablado, A. (1985). Asteroidea de Puerto Deseado (Santa Cruz, Argentina). Argentina: Centro Nacional Patagónico.
Himmelman, J. H., Dutil, C., and Gaymer, C. F. (2005). Foraging behavior and activity budgets of sea stars on a subtidal sediment bottom community. J. Exp. Mar. Biol. Ecol. 322, 153–165. doi: 10.1016/j.jembe.2005.02.014
Holtheuer, J., Aldea, C., Schories, D., and Gallardo, C. S. (2018). The natural history of Calyptraea aurita (Reeve, 1859) from Southern Chile (Gastropoda, Calyptraeidae). Zookeys 798, 1–22. doi: 10.3897/zookeys.798.25736
Kendall, M. G., and Stuart, A. (1973). The Advanced Theory of Statistics. Vol 2. Inference and Relationship, 3rd Edn. New York, NY: Hafner.
Kohler, K. E., and Gill, S. M. (2006). Coral point count with excel extensions (CPCe): a visual basic program for the determination of coral and substrate coverage using random point count methodology. Comput. Geosci. 32, 1259–1269. doi: 10.1016/j.cageo.2005.11.009
Krebs, J. R., Erichsen, J. T., Webber, M. I., and Charnov, E. L. (1977). Optimal prey selection in the great tit (Parus major). Anim. Behav. 25, 30–38. doi: 10.1016/0003-3472(77)90064-1
Kreiling, A. K., O’gorman, E. J., Palsson, S., Benhaim, D., Leblanc, C. A., Olafsson, J. S., et al. (2020). Seasonal variation in the invertebrate community and diet of a top fish predator in a thermally stable spring. Hydrobiologia 848, 531–545. doi: 10.1007/s10750-020-04409-5
Lamare, M. D., Channon, T., Cornelisen, C., and Clarke, M. (2009). Archival electronic tagging of a predatory sea star: testing a new technique to study movement at the individual level. J. Exp. Mar. Biol. Ecol. 373, 1–10. doi: 10.1016/j.jembe.2009.02.010
Lorenzen, S., Gallardo, C., Jara, C., Clasing, E., Pequeño, G., and Moreno, C. A. (1979). Mariscos y Peces de Importancia Comercial en el SUR DE CHILE. Valdivia: Universidad Austral de Chile.
Madsen, J. (1956). Asteroidea with a survey of the asteroidea of the Chilean Shelf. Lunds Univ. Arsskrift. N. F. Avd. 52, 1–53.
Maier, M. S., Kuriss, A., and Seldes, A. M. (1998). Isolation and structure of glucosylceramides from the starfish Cosmasterias lurida. Lipids 33, 825–827. doi: 10.1007/s11745-998-0277-8
Maier, M. S., Roccatagliata, A., and Seldes, A. M. (1993). 2 Novel steroidal glycoside sulfates from the starfish Cosmasterias lurida. J. Nat. Prod. 56, 939–942. doi: 10.1021/np50096a020
Manzur, T., Barahona, M., and Navarrete, S. A. (2010). Ontogenetic changes in habitat use and diet of the sea star Heliasther helianthus on the coast of central Chile. J. Mar. Biol. Assoc. U.K. 90, 537–546. doi: 10.1017/s0025315409990786
Manzur, T., and Navarrete, S. A. (2011). Scales of detection and escape of the sea urchin Tetrapygus niger in interactions with the predatory sun star Heliaster helianthus. J. Exp. Mar. Biol. Ecol. 407, 302–308. doi: 10.1016/j.jembe.2011.06.025
Martín, A., Penchaszadeh, P., and Atienza, D. (2001). Densidad y hábitos alimentarios de Oreaster reticulatus (Linnaeus, 1758) (Echinodermata, Asteroidea) en praderas de fanerógamas marinas de Venezuela. Bol. Instit. Español Oceanogr. 17, 203–208.
Martinez, N. D. (1993). Effect of scale on food web structure. Science 260, 242–243. doi: 10.1126/science.260.5105.242
McClintock, J. B. (1985). Avoidance and escape responses of the Sub-Antarctic limpet Nacella edgari (powell) (mollusca, gastropoda) to the sea star Anasterias perrieri (smith) (echinodermata, asteroidea). Polar Biol. 4, 95–98. doi: 10.1007/bf00442906
McClintock, J. B. (1994). Trophic biology of Antarctic shallow-water echinoderms. Mar. Ecol. Prog. Ser. 111, 191–202. doi: 10.3354/meps111191
McClintock, J. B., and Lawrence, J. M. (1985). Characteristics of foraging in the soft bottom benthic starfish Luidia clathrata (echinodermata: Asteroidea): prey selectivity, switching behavior, functional responses and movement patterns. Oecologia 66, 291–298. doi: 10.1007/bf00379867
Menge, B. A., and Sanford, E. (2013). “Ecological role of sea stars from populations to meta-ecosystems,” in Starfish: Biology and Ecology of the Asteroidea, ed. J. M. Lawrence (Baltimore: Johns Hopkins University Press), 67–80.
Menge, J. L., and Menge, B. A. (1974). Role of resource allocation, aggression and spatial heterogeneity in coexistence of two competing intertidal starfish. Ecol. Monogr. 44, 189–209. doi: 10.2307/1942311
Mironov, A. N., Dilman, A. B., Vladychenskaya, I. P., and Petrov, N. B. (2016). Adaptive strategy of the Porcellanasterid sea stars. Biol. Bull. 43, 503–516. doi: 10.1134/s106235901606011x
Motti, C. A., Bose, U., Roberts, R. E., Mcdougall, C., Smith, M. K., Hall, M. R., et al. (2018). Chemical ecology of chemosensation in asteroidea: insights towards management strategies of pest species. J. Chem. Ecol. 44, 147–177. doi: 10.1007/s10886-018-0926-4
Mutschke, E., and Mah, C. (2009). “Asteroidea-starfish,” in Marine Benthic Fauna of Chilean Patagonia. Santiago, eds V. Häussermann and G. Fösterra (Cham: Springer), 802–830.
Navarrete, S. A., and Manzur, T. (2008). Individual and population level responses of a keystone predator to geographic variation in prey. Ecology 89, 2005–2018. doi: 10.1890/07-1231.1
Ostfeld, R. S. (1982). Foraging strategies and prey switching in the California sea otter. Oecologia 53, 170–178. doi: 10.1007/bf00545660
Paine, R. T. (1966). Food web complexity and species diversity. Am. Nat. 100, 65–87. doi: 10.1086/282400
Paine, R. T. (1969). A note on trophic complexity and community stability. Am. Nat. 103, 91–104. doi: 10.1086/282586
Paine, R. T. (1976). Size-limited predation: an observational and experimental approach with the mytilus-pisaster interaction. Ecology 57, 858–873. doi: 10.2307/1941053
Paine, R. T., Castilla, J. C., and Cancino, J. (1985). Perturbation and recovery patterns of starfish dominated intertidal assemblages in Chile, New Zealand and Washington State. Am. Nat. 125, 679–691. doi: 10.1086/284371
Pastor-de-Ward, C. T., Rubilar, T., Díaz-De-Vivar, M. E., Gonzalez-Pisani, X., Zarate, E., Kroeck, M., et al. (2007). Reproductive biology of Cosmasterias lurida (Echinodermata: Asteroidea) an anthropogenically influenced substratum from Golfo Nuevo, Northern Patagonia (Argentina). Mar. Biol. 151, 205–217. doi: 10.1007/s00227-006-0479-x
Pearre, S. (1982). Estimating prey preference by predators: uses of various indices, and a proposal of another based on X2. Can. J. Fish. Aquat. Sci. 39, 914–923. doi: 10.1139/f82-122
Pérez-Matuz, A., Ospina-Alvarez, A., Camus, P. A., Carrasco, S. A., Fernandez, M., Gelcich, S., et al. (2017). Temperate rocky subtidal reef community reveals human impacts across the entire food web. Mar. Ecol. Prog. Ser. 567, 1–16. doi: 10.3354/meps12057
Pratchett, M. S. (2007). Feeding preferences of Acanthaster planci (Echinodermata: Asteroidea) under controlled conditions of food availability. Pac. Sci. 61, 113–120. doi: 10.1353/psc.2007.0011
R Development Core Team (2020). R: A Language and Environment for Statistical Computing. Vienna: R Foundation for Statistical Computing.
Rettig, J. E., and Smith, G. R. (2021). Relative strength of top-down effects of an invasive fish and bottom-up effects of nutrient addition in a simple aquatic food web. Environ. Sci. Pollut. Res. Int. 28, 5845–5853. doi: 10.1007/s11356-020-10933-7
Roccatagliata, A. J., Maier, M. S., Seldes, A. M., Iorizzi, M., and Minale, L. (1994). Starfish saponins .2. Steroidal oligoglycosides from the starfish Cosmasterias lurida. J. Nat. Product. 57, 747–754. doi: 10.1021/np50108a010
Rochette, R., Hamel, J.-F., and Himmelman, J. H. (1994). Foraging strategy of the asteroid Leptasterias polaris: role of prey odors, current and feeding status. Mar. Ecol. Prog. Ser. 106, 93–100. doi: 10.3354/meps106093
Ross, D. J., Johnson, C. R., and Hewitt, C. L. (2003). Variability in the impact of an introduced predator (Asterias amurensis : Asteroidea) on soft-sediment assemblages. J. Exp. Mar. Biol. Ecol. 288, 257–278. doi: 10.1016/s0022-0981(03)00022-4
Saier, B. (2001). Direct and indirect effects of seastars Asterias rubens on mussel beds (Mytilus edulis) in the Wadden Sea. J. Sea Res. 46, 29–42. doi: 10.1016/s1385-1101(01)00067-3
Salguero, B. M. L., and Bonilla, H. R. (2010). Estructura comunitaria y trófica de las estrellas de mar (Echinodermata; Asteroidea) en arrecifes rocosos de Loreto, Golfo de California, México. Hidrobiológica 20, 127–134.
San-Martin, A., Rovirosa, J., Gaete, K., Olea, A., and Ampuero, J. (2009). Mantle defensive response of marine pulmonate Trimusculus peruvianus. J. Exp. Mar. Biol. Ecol. 376, 43–47. doi: 10.1016/j.jembe.2009.06.005
Schejter, L., Bremec, C. S., and Hernandez, D. (2008). Comparison between disturbed and undisturbed areas of the Patagonian scallop (Zygochlamys patagonica) fishing ground “Reclutas” in the Argentine Sea. J. Sea Res. 60, 193–200. doi: 10.1016/j.seares.2008.04.007
Schories, D., and Niedzwiedz, G. (2012). Precision, accuracy, and application of diver-towed underwater GPS receivers. Environ. Monit. Assess. 184, 2359–2372. doi: 10.1007/s10661-011-2122-7
Seldes, A. M., and Gros, E. G. (1985). Main sterols from the starfish Comasterias lurida. Comp. Biochem. Physiol. Part B Comp. Biochem. 80, 337–339. doi: 10.1016/0305-0491(85)90215-9
Sih, A., Crowley, P., Mcpeek, M., Petranka, J., and Strohmeier, K. (1985). Predation, competition and prey communities: a review of field experiments. Annu. Rev. Ecol. Syst. 16, 269–311. doi: 10.1146/annurev.es.16.110185.001413
Singer, M. C. (2000). Reducing ambiguity in describing plant - insect interactions: “Preference”, “acceptability” and “electivity”. Ecol. Lett. 3, 159–162. doi: 10.1046/j.1461-0248.2000.00136.x
Skein, L., Robinson, T. B., and Alexander, M. E. (2018). Impacts of mussel invasions on the prey preference of two native predators. Behav. Ecol. 29, 353–359. doi: 10.1093/beheco/arx172
Solís, I. U., and Lozada, E. L. (1971). Algunos aspectos biológicos de la cholga de Magallanes Aulacomya ater Molina. Biol. Pesquera 5, 109–144.
Soto-Mardones, L., Letelier, J., Salinas, S., Pinillas, E., and Belmar, J. P. (2009). Analysis of oceanographic and atmospheric parameters of Seno de Reloncaví. Gayana 73, 141–155.
Tokeshi, M. (1991). Extraoral and intraoral feeding: flexible foraging tactics in South American sun-star, Heliaster helianthus. J. Zool. 225, 439–447. doi: 10.1111/j.1469-7998.1991.tb03827.x
Tokeshi, M., and Romero, L. (1995). Quantitative analysis of foraging behaviour in a field popolation of the South American sun-star Heliaster helianthus. Mar. Biol. 122, 297–303.
Underwood, A. J., Chapman, M. G., and Crowe, T. P. (2004). Identifying and understanding ecological preferences for habitat or prey. J. Exp. Mar. Biol. Ecol. 300, 161–187. doi: 10.1016/j.jembe.2003.12.006
Urriago, J. D., Himmelman, J. H., and Gaymer, C. F. (2011). Responses of the black sea urchin Tetrapygus niger to its sea star predators Heliaster helianthus and Meyenaster gelatinosus under field conditions. J. Exp. Mar. Biol. Ecol. 399, 17–24. doi: 10.1016/j.jembe.2011.01.004
Urriago, J. D., Himmelman, J. H., and Gaymer, C. F. (2012). Sea urchin Tetrapygus niger distribution on elevated surfaces represents a strategy for avoiding predatory sea stars. Mar. Ecol. Prog. Ser. 444, 85–95. doi: 10.3354/meps09396
Vásquez, J. A., and Castilla, J. C. (1984). Some aspects of the biology and trophic range of Cosmasterias lurida (Asteroidea, Asteriinae) in belts of Macrocystis pyrifera at Puerto Toro, Chile. Ambientes Acuaticos 7, 47–51.
Verling, E., Crook, A. C., Barnes, D. K. A., and Harrison, S. S. C. (2003). Structural dynamics of a sea star (Marthasterias glacialis) population. J. Mar. Biol. Assoc. U.K. 83, 583–592. doi: 10.1017/s0025315403007513h
Wang, T. W., Chan, T. Y., and Chan, B. K. K. (2014). Trophic relationships of hydrothermal vent and non-vent communities in the upper sublittoral and upper bathyal zones off Kueishan Island, Taiwan: a combined morphological, gut content analysis and stable isotope approach. Mar. Biol. 161, 2447–2463. doi: 10.1007/s00227-014-2479-6
Zagal, C., Hermosilla, C., and Riedemann, A. (2001). Guía de Invertebrados Marinos del Litoral Valdiviano. Chile: Santiago de Chile.
Keywords: Cosmasterias lurida, benthic ecology, feeding behavior, starfish, predation, dietary preference
Citation: Garrido I, Pardo LM, Johnson LE and Schories D (2021) Selective Feeding by a Predatory Sea Star Across a Depth Gradient in Northern Patagonia, Chile. Front. Mar. Sci. 8:636208. doi: 10.3389/fmars.2021.636208
Received: 01 December 2020; Accepted: 19 March 2021;
Published: 09 April 2021.
Edited by:
Eduardo Joel Quiroga Jamett, Pontifical Catholic University of Valparaíso, ChileReviewed by:
Benny K. K. Chan, Academia Sinica, TaiwanAldo S. Pacheco, National University of San Marcos, Peru
Copyright © 2021 Garrido, Pardo, Johnson and Schories. This is an open-access article distributed under the terms of the Creative Commons Attribution License (CC BY). The use, distribution or reproduction in other forums is permitted, provided the original author(s) and the copyright owner(s) are credited and that the original publication in this journal is cited, in accordance with accepted academic practice. No use, distribution or reproduction is permitted which does not comply with these terms.
*Correspondence: Ignacio Garrido, ignacio.garrido@uach.cl; ignacio.garrido-iriondo.1@ulaval.ca