- Centre for Environment, Fisheries and Aquaculture Science (Cefas), Lowestoft, United Kingdom
Maritime states are faced with the challenge of effectively managing their marine spaces to use resources sustainably, maximise economic potential and simultaneously protect their marine environments. Anthropogenic activities, whether in isolation or combination, all have effects on the natural environment. Each of these effects has a footprint in time and space. Assessing the distribution and intensity of human activities and their effects on marine biodiversity, and all other human uses and users is necessary for effective spatial planning, as well as to harmonise conservation with sustainable development. Assessing and managing combined pressures from human activities can be achieved using risk assessment and risk management processes. There are multiple examples of environmental risk assessments which propose a similar formula. However, standardised approaches to ecological risk assessment in data-limited locations that relate to sand extraction are limited. Also most assessments require a certain level of information to produce meaningful outcomes, that enable subsequent management action to appropriately reflect the identified level of risk. Here we outline an approach to assess the risk to the marine environment of sand extraction activity within the Exclusive Economic Zone and Marine Protected Area of St Helena Island in the Atlantic. The proposed risk assessment tool has supported the development of a sand extraction management strategy on St Helena, and will be used to inform future management plans and policies that allow anthropogenic activities to take place in a way that balances local management, monitoring and enforcement capability, in line with the International Union for Conservation of Nature (IUCN) Category VI designation. Both the tool and strategy promote sustainable use of resources and protection of the marine environment, which are key objectives stated in the St Helena Marine Management Plan.
Introduction
The marine environment provides a set of ecosystem goods and services that are critical for societal wellbeing, cultural importance and prosperity, whether for food, transportation, tourism and recreation, provision of natural materials, management of blue carbon budget or waste disposal, to name a few (Beaumont et al., 2007; Liquete et al., 2013; Cabral et al., 2015; Schuhmann and Mahon, 2015; Rees et al., 2016). These services are particularly important for remote and isolated island communities, whose economies are often reliant on the jobs, food and raw materials afforded by their local marine environments (Teelucksingh et al., 2013; Forster et al., 2014; Baker et al., 2015; Techera and Appadoo, 2020). There is a growing demand for marine space and resources which leads to marine activities overlapping and applying cumulative pressures to the marine environment, thus challenging the ambition for sustainable development and use (Koss et al., 2011; Goodsir et al., 2015; Austen et al., 2018). Complex spatial management considerations arise where the policy objectives aim to achieve sustainable development. Integral to effective spatial management, including sustainable use, is a clear process supported by scientific evidence (Rosenberg and McLeod, 2005).
The sustainable development of the marine environment, as well as the wider environment, is high on the global political and scientific agenda (UN, 2016). However, the vision of achieving and maintaining sustainable development is threatened by the increasing number and frequency of different types of activities in the marine environment (Judd et al., 2015; Elliott et al., 2020; Lonsdale et al., 2020; Caswell et al., 2020; Jouffray et al., 2020). To attempt to reach this global vision (for a suite of complex ecosystems), marine managers need to be able to robustly and consistently assess the potential risks an activity could have on the marine environment, and balance the environment, social and economic needs with the legal system (Dawe and Ryan, 2003; Rogers et al., 2007; Elliott, 2013).
For developed States such as the United Kingdom that share seas with similar neighbouring States, and have an historic and rapidly expanding marine sector, there is often an established process for ocean governance. This includes access to tools and/or methods to assess the interactions of new and emerging activities on the marine environment (Boyes and Elliott, 2014, 2015; Lonsdale et al., 2015). These developed States with established offshore industries also tend to have greater access to funds and equipment, which improves their ability to generate environmental data over time and space, and consequently, affords higher confidence in the evidence available to inform decision-making. Assessments based on sound data and evidence, collected and analyzed based on standard practices, are typically less influenced by the subjectivity of expert judgement, and are typically more robust.
The global vision requires all States, including small island and remote States, to undertake robust, consistent and transparent assessments to predict, manage and where applicable, mitigate impacts to ensure sustainable development (UN, 2016). Whilst these small islands and remote States are often unable to access the funds, equipment, personnel, and capability necessary to conduct extensive monitoring required for such assessments, they do have extensive knowledge of the local human activities and marine environment. This can enable an environmental assessment to be undertaken, but requires a standardised approach to facilitate the process. Moreover, seeking to capture all the relevant data would be time and resource intensive (Lonsdale et al., 2017). Nevertheless, a paucity of data does not remove the need for a State to manage its waters, nor should it stop or delay progress towards sustainable development.
Risk-based decision-making is a process of choice, based on identification of the likely consequences of different options and selection of the best course of action to minimise and manage environmental risk (Gormley et al., 2011). Risk assessments that link relationships between environmental receptors and human pressures can assist with the evaluation of effects. They can also help to understand the nexus between the magnitude of a pressure and sensitivity of a marine receptor, and are increasingly included in the process of managing human activities. Risk assessments can be both quantitative and qualitative (Holsman et al., 2017) and often include a level of expert judgement. However, their reliability is often challenged by a multitude of complex interactions that are present within marine and coastal ecosystems.
There are multiple examples of environmental risk assessments which propose a similar formula: (1) formulating the problem; (2) carrying out an assessment of the risk; (3) identifying and appraising the management options available; and (4) addressing the risk with the chosen risk management strategy (see Gormley et al., 2011; Cains and Henshel, 2021). An international standard exists for risk management, the International Organisation for Standardisation (ISO) 31000. Globally, this standard has been employed alongside other risk-based methodologies for marine management purposes, for example, as a risk-based approach to implementing the ecosystem approach to fisheries management (Fletcher, 2005, 2015).
However, standardised approaches that apply quantitative and qualitative evidence in data-limited situations or locales are scarce and/or may require investment (to purchase tools or implement wider standards/organisational schemes). This can lead to an under- or overestimation of threats to the marine environment and potentially effects on marine receptors. A reliable means of assessing the risks from different human activities is essential to a management programme seeking to reduce environmental risk to as low as reasonably possible, whilst sustaining the integrity of marine ecosystems. There is little specificity in terms of data-limited environmental risk assessment methodologies, at either national or international levels. Furthermore, information on case studies that utilise the available methods are not widely publicised [e.g., ISO 31000; Bowtie analysis (Cormier et al., 2019) CHARM model (Thatcher et al., 2017)] and it is, therefore, difficult to determine whether these are fit for purpose.
Environmental risk assessments can, and need to be applied in situations/locations where data may be limited to ensure a consistent approach to marine management where, otherwise, data paucity may be used as a reason to allow a development with high uncertainty and low confidence assessment outcome to progress (e.g., Gilman et al., 2014; Krishnakumar et al., 2017; Caballero-Gallardo et al., 2020; Pratap et al., 2020). One activity which is established throughout most of the world, with generally known impacts is marine aggregate extraction (Velegrakis et al., 2010). The impacts of marine aggregate extraction are well documented (Newell et al., 2004; Kaikkonen et al., 2018; Anbleyth-Evans, 2018) with impacts ranging from local (increased water depth at the dredge site) to wide reaching (plumes from screening of material). Whilst the impacts of aggregate dredging can be mitigated for, they cannot be eliminated completely, yet with marine aggregate a major source for developments both in the coastal areas (e.g., marinas) and on land (e.g., in buildings or roads) it is likely the demand will remain for some time (Velegrakis et al., 2010). In England (United Kingdom), a novel approach to maintaining benthic communities has been established (Cooper, 2013). Given the finite viable resource and the high likelihood of ongoing impacts, the activity itself is not deemed sustainable, but steps can be taken to work towards wider sustainable marine development.
This paper presents a standardised approach to environmental risk assessment for extraction of sand (used in small scale construction projects) in a data-limited situation. It outlines the first of a multi-stage methodology for risk assessment which builds on the strengths of previous risk assessments, but is specifically tailored to the needs of a small, remote island with limited resources and a paucity of extraction-specific monitoring data. The approach was developed for and tested on a case study for a small island State, St Helena, to consider the potential impacts and management of sand extraction within a designated MPA. The step-wise process was devised to facilitate robust, consistent and transparent management of the ongoing (and future) activity, and to ensure sustainable use of resources and protection of the marine environment (and features of the MPA). Whilst it has been developed predominantly for a data limited case, the process should ideally be accompanied by data collection to address gaps in the evidence base, decrease uncertainty and increase confidence in the assessment.
Method
Using definitions (pressures; effects; pathway; impact) described by Judd et al. (2015), we describe an approach that is considered to be aligned with existing methods of environmental risk assessment (e.g., Gormley et al., 2011; NIWA, 2012; Judd et al., 2013; Rowden et al., 2015).
The chosen ecological risk assessment in this study has been presented in the form of a step-wise decision tree (Figure 1). The method draws on the principles of environmental impact assessment and ecological risk assessment approaches, with a measure of certainty for communication to managers and decision-makers. It is intended that the decision tree would be utilised by marine managers/regulatory staff in the geographic location where the assessment will be undertaken, since these personnel typically have knowledge of the local geographic environment and marine activities.
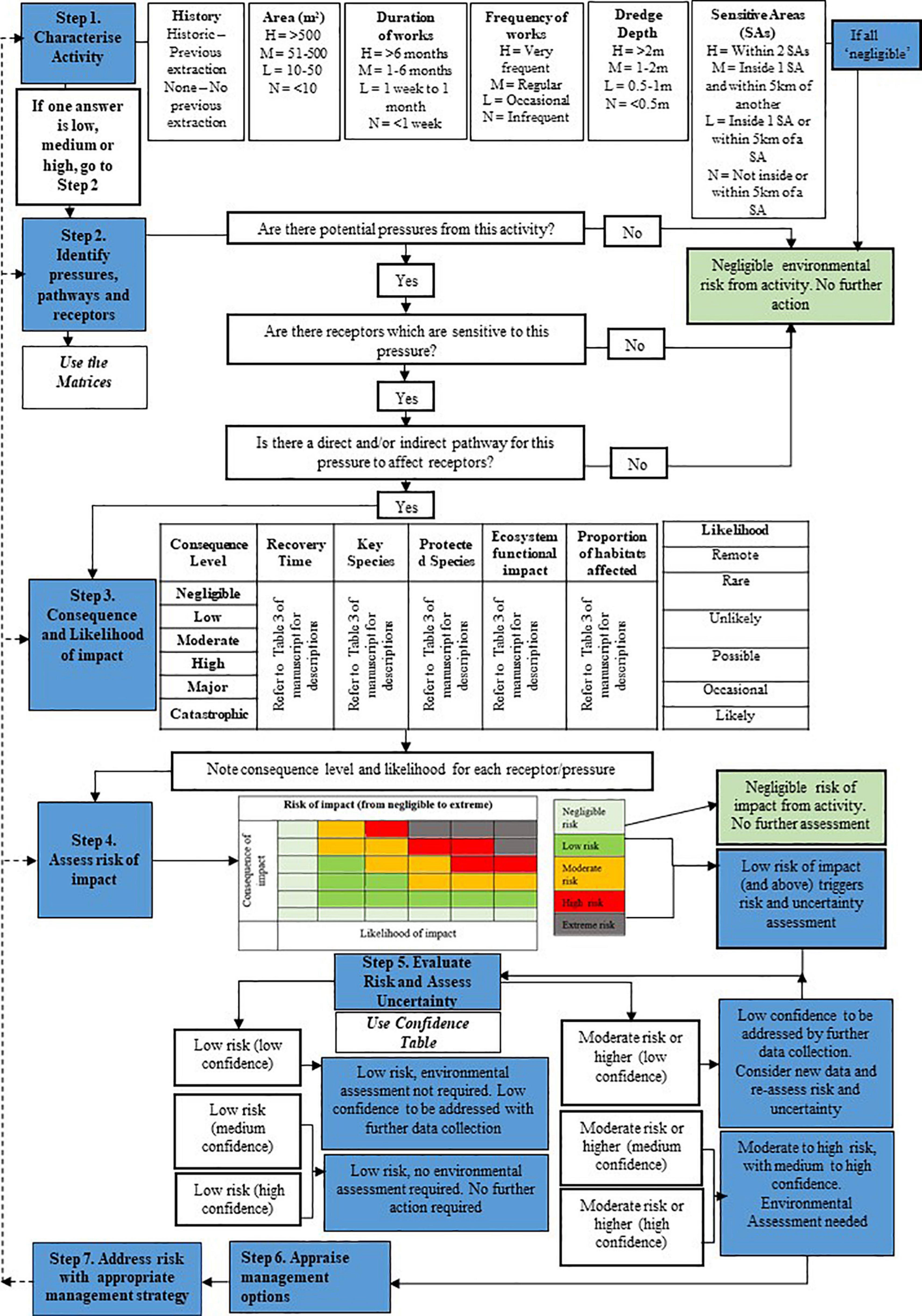
Figure 1. Decision tree for assessing potential risks posed by sand extraction and actions required. ‘H’ High risk; ‘M’ medium risk; ‘L’ low risk; ‘N’ negligible risk. Dashed lines represent feedback loops following the collection and assessment of data.
Step 1: Characterise the Activity
The purpose of Step 1 is to enable a relatively quick characterisation of the activity, by answering questions about the nature of the activity and surrounding environment (Table 1). The questions and scores were modified from Judd et al. (2013) and Lonsdale et al. (2015). The criteria were agreed by experts in regulatory science and the application of risk assessments in marine activities (including aggregate extraction) and in consultation with St Helena Government.
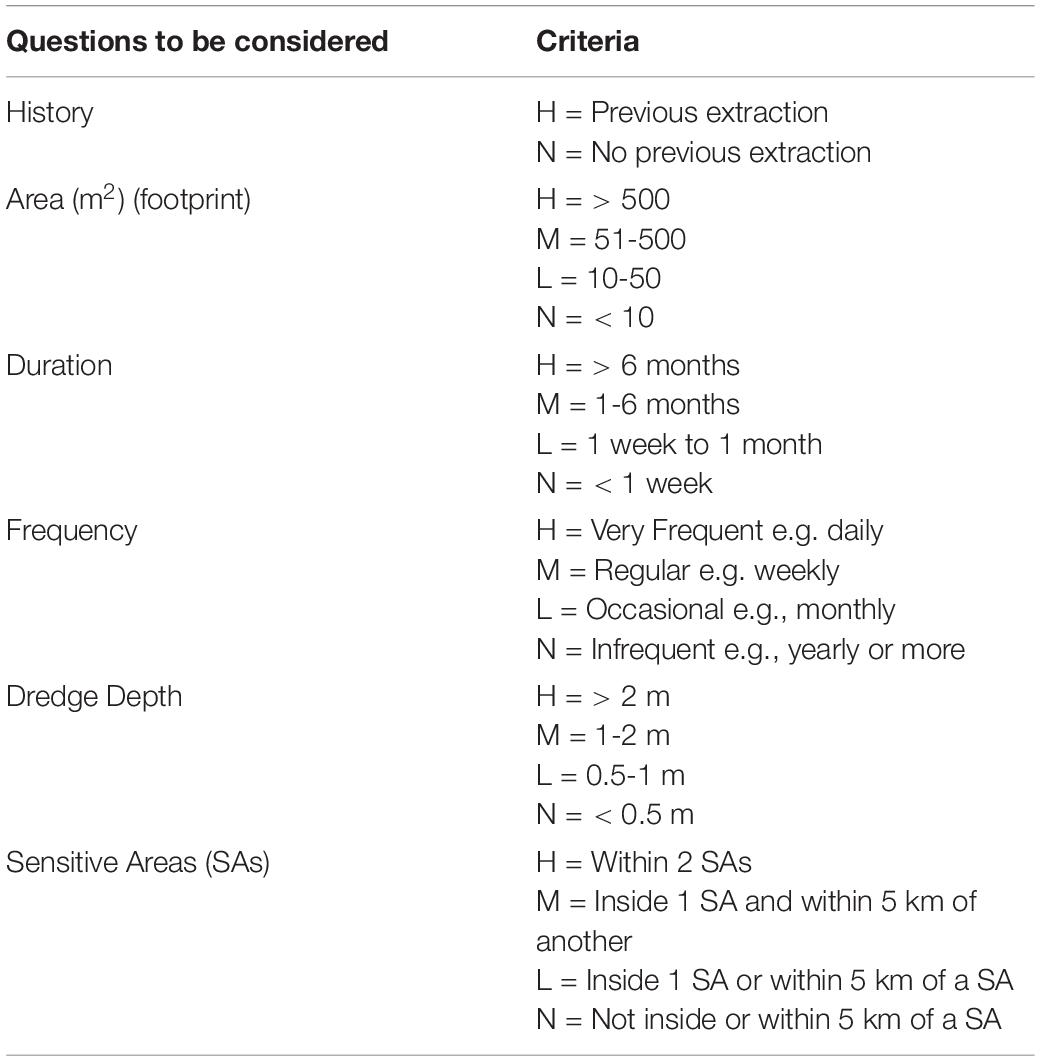
Table 1. Questions and criteria to be considered when characterising an extraction activity. ‘H’ High risk; ‘M’ medium risk; ‘L’ low risk; ‘N’ negligible risk.
History of the Activity: Has It Occurred Previously or Is It a New Activity?
If an activity is new, any potential impacts which may present a risk to the marine environment may not have been identified or quantified. If the activity has been carried out before, on a similar scale and in the same location, potential impacts are likely to have already occurred. If no significant adverse impacts have been identified at a previously impacted site, the continuation of activity at a similar scale and magnitude is unlikely to result in additional impacts and the proposed activity may be considered lower risk.
However, concluding that an activity is low risk because it is existing or has occurred previously will produce lower confidence in the assessment and introduces the potential for indirect, or direct impacts to remain unidentified. This scenario is particularly relevant for small island states that do not routinely monitor the activity. Assessment of the impacts of an existing activity with no prior monitoring data will provide a new baseline against which an assessment can be made, and this new baseline may not necessarily reflect the original baseline. The assessment should account for this shift in baseline and identify evidence gaps to be addressed through additional data collection. A feedback loop is, therefore, included in the approach to link the collation of data (either through baseline surveys or monitoring), to a review of the assessment where the risk can be re-assessed.
What Is the Scale of the Activity Based on the Activity Footprint (Primary Area of Seabed Affected Plus Secondary Area)?
Level of risk at this stage can be associated with the footprint of the activity. For the sand extraction activity, this translates to the area of seabed directly affected by the extraction (Primary Impact Zone or PIZ), but can also consider the wider area within which indirect and secondary impacts can occur (the Secondary Impact Zone or SIZ). Hence, the extraction footprint comprises the combined effects of the directly affected area (PIZ) and adjacent areas in the SIZ that may be exposed, for instance, to overflow of sediment. Extraction areas of 500 m2 are likely to present highest risk to the marine environment whilst extraction areas <10 m2 are likely to present negligible risk (Table 1). It should be noted that the numerical values for the dredging area and depth criteria (Table 1 and Figure 1) are from a dredging risk screening tool, that was developed to assess risk from dredging in United Kingdom waters (Judd et al., 2013).
In general, the larger the footprint, the higher the likelihood that the activity will impact on receptors and, therefore, the higher the likelihood that an impact assessment will be required. Additionally, consideration of size of the potentially impacted habitat relative to the scale of the activity footprint can be important, notably where habitats are rare in occurrence.
What Is the Duration and Frequency of the Works?
Frequency and duration of extraction can directly influence significance of impact. The activity may coincide with important ecological times for receptors e.g., migration, nursery or spawning periods which may have been determined if there are data available for the geographic area of interest. Information may be available for the receptor group in other geographic locations. This could be used a proxy in the absence of data for receptors in the area of interest. It is recognised that a level of knowledge may thus be required about ecological receptors, sensitive timings and whether other information for receptors/receptor group could be used as a proxy.
Risk can be considered along a scale, with highest risk indicated for activity occurring very frequently and/or for >6 months duration, whereas at the lower end of the scale, negligible risk is indicated for work <1 week, and/or occurring very infrequently (Table 1).
What Is the Proposed or Existing Extraction Depth?
Dredge depth is considered in this initial step because it may lead to changes in sediment size distributions of seabed habitats and consequently, changes in benthic communities and their ability to recolonise an area (Newell et al., 1998; Seiderer and Newell, 1999; Foden et al., 2009; Cooper et al., 2011; de Jong et al., 2015). Deeper sediments tend to have different physical properties to the uppermost layers due to a variety of physical factors e.g. compaction and oxygen availability. In general, different benthic organisms prefer different sediment size fractions (e.g., Cooper, 2013).
Is the Proposed Activity Located in or Near to Any Sensitive Areas?
Designated sites/sensitive areas (SAs) are identified in relation to the activity. In this study, SA are defined as either a marine protected area where the activity is >0.1% of the protected area, or a spawning ground, migration route or vulnerable marine ecosystem. Risk can be considered in terms of highest risk indicated for activity within 2 SAs, whilst at the lower end of the scale, negligible risk is indicated for activity that is not inside or within 5 km of a SA (Table 1). Whilst all receptors should be considered in an assessment, SAs represent the habitats and species that are considered most vulnerable and/or rare, and may play a key role in protecting wider habitats, communities and environmental function. These areas may be designated as protected areas or simply consist of an area of functional or ecological importance, such as a spawning or nursery area. These areas may warrant additional protection and should, therefore, be considered as part of the assessment.
Outcome of Step 1
If all answers to step 1 are reported as ‘negligible’, no further assessment is required because the level of environmental risk associated with the project is considered negligible. If any of the answers are categorised as ‘low’, ‘medium’ or ‘high’, proceed to Step 2.
Step 2: Identify Pressure Pathways and Environmental Receptors
In this step, there is a review of the potential pressures from sand extraction. A pressure is a mechanism by which an activity can have effect on a part of the ecosystem. Noise, removal of seabed substrate and generation of suspended sediment are all examples of pressures.
Are there any receptors (habitats and species) present that are considered sensitive to these pressures? Benthic invertebrates are examples of receptors. Is there a direct or indirect pathway that would enable the pressure to affect the receptor directly or indirectly? In the example provided, the surface layers of sediment removed within the extraction area, can destroy benthic habitats (like burrows) and alter sediment particle size distribution.
There are several ways to display the information and outputs of step 2. Activity pressure-matrices are one such method. An exert of the benthic receptors pressure-activity matrix is shown in Table 2, with the full matrix in the Supplementary Materials. The matrix contains a list of activities relevant to the sand extraction case study, that have been identified from existing knowledge of the activity. A list of pressures are shown in the matrix and the pressures are based on the standardised marine pressure descriptions, originating from work in the North East Atlantic by the Oslo-Paris Convention Intercessional Correspondence Group on Cumulative Effects (OSPAR ICG-C) in 20111.
The Joint Nature Conservation Committee (JNCC) pressures-activities database (PAD)2 was consulted in step 2 to help identify potential pressures from aggregate dredging (in a UK context); this activity was considered to be comparable to the sand pumping at St Helena. The PAD is a compilation of evidence on the relationships of marine-based human activities and their associated pressures (based on the OSPAR pressure list) and is UK-based. The PAD gives an indication of the general risk the pressures pose to the environment under normal conditions, and all activity-pressure relationships, supported by available evidence with confidence scoring (Robson et al., 2018). In the present study, the activity-pressure table in the JNCC PAD was filtered to display aggregate dredging and associated pressures. The corresponding information on the types of pressures and accompanying evidence was used to inform the development of receptor-specific matrices.
For each activity-pressure combination in the matrix, potential for an activity-pressure pathway is appraised using expert knowledge and peer-reviewed literature. Receptor presence and ecology (including potential sensitivity) have been drawn from published studies (including: Newell et al., 1998, 2004; Seiderer and Newell, 1999; Boyd et al., 2005), monitoring datasets (where available for St Helena), and analogous assessments for an activity (which may be in a different geographic locale), as well as expert judgement. By working through each pressure-activity combination, it is possible to ‘screen’ in or out the activity-pressure pathway for/from further assessment.
The identification of potential impact pathways through a screening process is a common approach applied within Environmental Impact Assessment (EIA), because it helps to identify which activities, pressures and receptors are linked by pathways that might eventually result in impacts. These are the interactions that can be carried forward for further assessment in steps 3 to 7; the remainder can be scoped out of the assessment on the basis no impact pathway exists.
Outcome of Step 2
Screening results for pressures and pathways for impacts to receptors that will be taken forward for further consideration in step 3.
Step 3: Determine Consequence and Likelihood of Impact(s)
Where a pressure directly or indirectly interacts with a receptor/receptor group, an assessment of the consequence of that interaction and the likelihood of effect should be made (Tables 3, 4, respectively). The focus of step 3 is to establish whether the scale and duration of the activity is sufficient to cause harm.
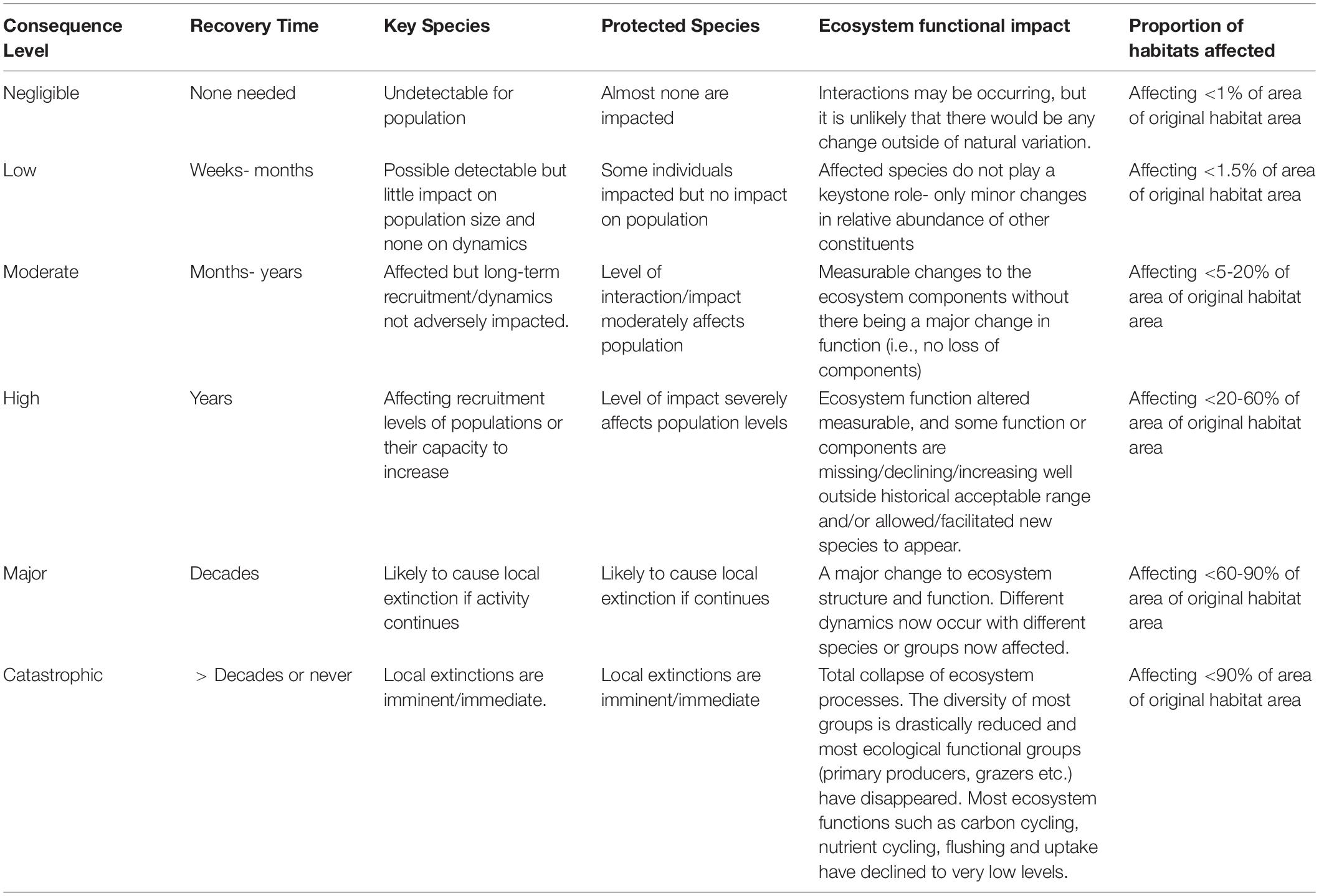
Table 3. Consequence levels with descriptions. Adapted from Fletcher (2005).
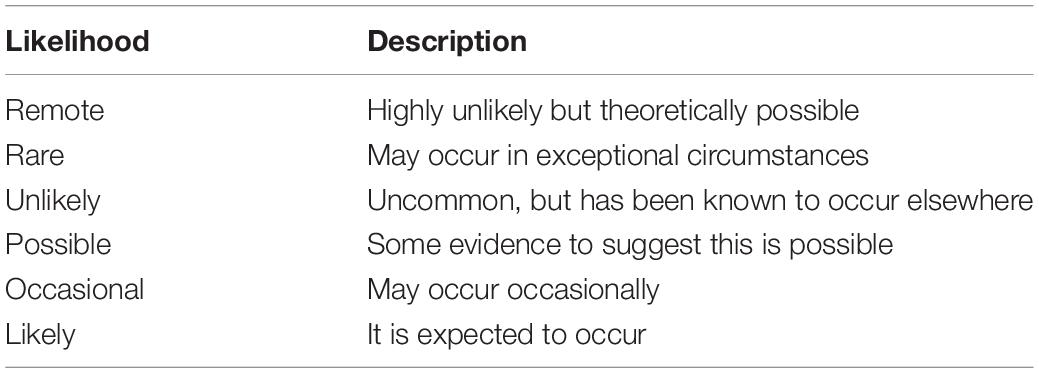
Table 4. Likelihood of occurrence level with description. Adapted from Fletcher (2005).
To assign consequence, there needs to be consideration of resilience and recovery of a given receptor to a given pressure, and the potential consequences resulting from exposure at a given magnitude. This could mean estimating the magnitude of the hazard at the receptor or estimating the probability that a hazard will be realised at a level large enough to cause harm.
Outcome of Step 3
Consequence level and likelihood of impact for each receptor/pressure have been identified and are taken forward for assessment of risk in step 4.
Step 4: Assess Risk of Impact
Risk is characterised by evaluating a combined measure of consequence and likelihood to determine likelihood of harm. It is possible to assign a level of significance to the risk that a given impact will occur (Table 5).
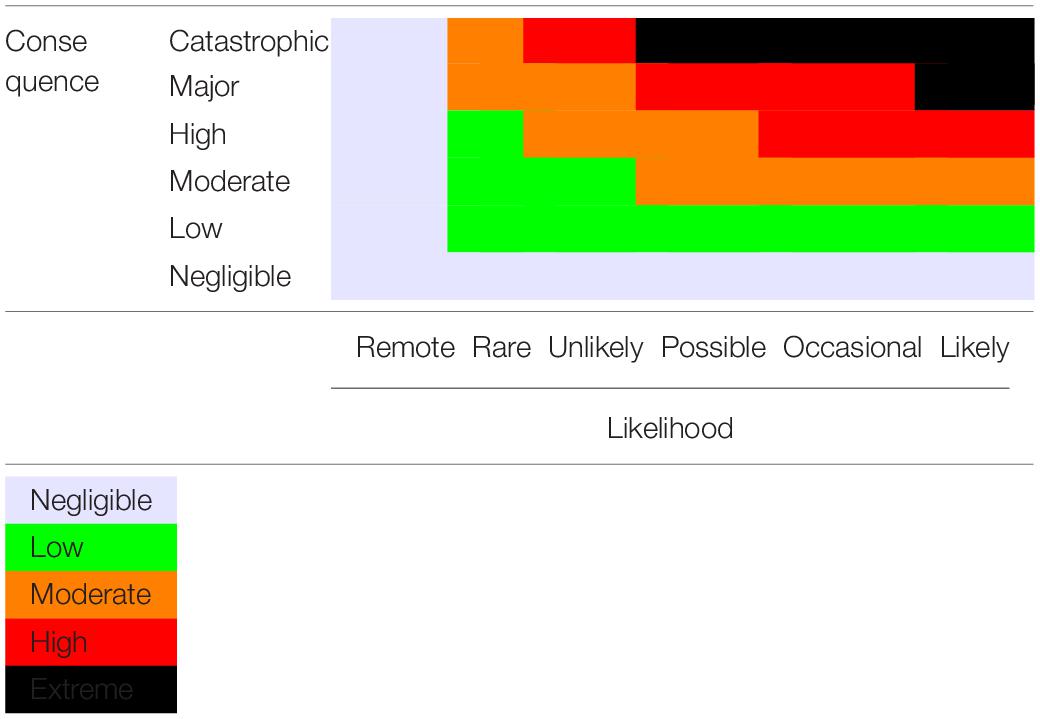
Table 5. Consequence and likelihood combined to characterise risk (negligible, low, moderate, high or extreme risk).
Outcome of Step 4
For impacts from an activity that are assessed as negligible risk, there is no further assessment made. Low, moderate, high or extreme risk triggers a more extensive assessment of risk and uncertainty (step 5).
Step 5: Evaluate Risk and Assess Uncertainty
The level of risk and associated level of uncertainty (or ‘confidence’) are evaluated with regard to the assigned level of risk.
A level of uncertainty can be associated with any assessment and expression of this uncertainty can differ when using qualitative (rather than quantitative) inputs to inform the assessment(s). This is because qualitative assessments can be more subjective and open to interpretation. Rather than utilise quantitative measures of uncertainty for the overall assessment, ratings of high, medium and low confidence are proposed, with definitions given in Table 6 (from Goodsir et al., 2019).
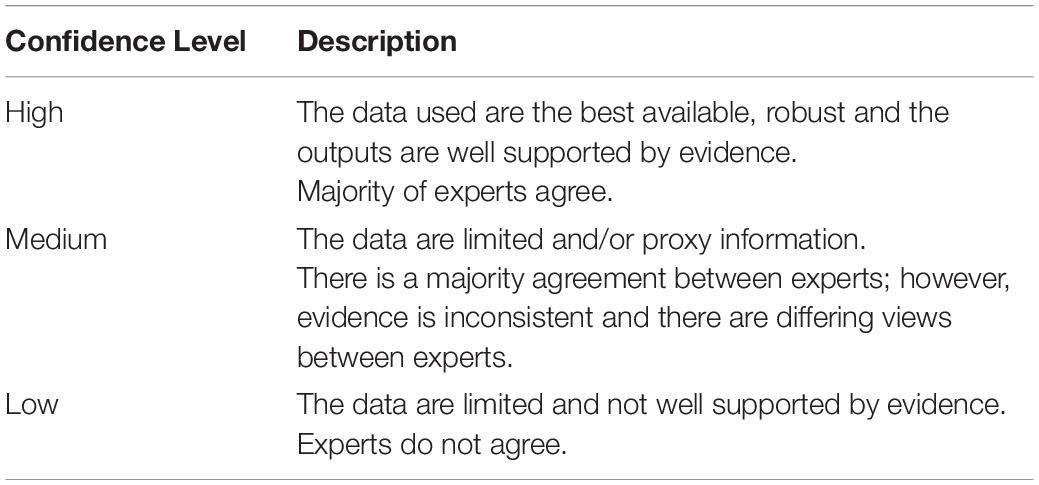
Table 6. Confidence levels with descriptions [from Goodsir et al. (2019)].
Within the decision tree (Figure 1), a distinction has been made between the levels of risk and confidence, and the next steps:
• Low risk (low confidence) means an environmental assessment would not be required. But low confidence should be addressed with further data collection, where resources and capacity allow.
• Low risk (medium to high confidence) means that no environmental assessment would be required. No further action would be required.
• Moderate risk or higher (low confidence) means that low confidence should be addressed with further data collection. The new data should be examined and then risk and uncertainty re-assessed.
• Moderate to high risk (medium to high confidence) means that an Environmental Assessment or EIA would be needed.
Outcome of Step 5
An assessment of risk and confidence are made during step 5. Management options can then be appraised in step 6 and risk addressed with the chosen, appropriate management strategy during step 7.
Step 6: Appraise Options and Step 7: Address Risk With Chosen Management Strategy
The final steps are to identify, appraise and implement the chosen management options to address risk and thus prevent, minimise or reduce any adverse and unwanted effects identified. These two steps are, however, outside the scope of this paper and so no further consideration is made of management options and implementation to address risk.
We applied the risk assessment approach outlined in Section 2 to a small island state; St Helena Island, which is part of the UK Overseas Territory of Ascension, St Helena and Tristan da Cunha, located in the tropical south Atlantic (Section 3 case study).
Case Study: Sand Extraction at St Helena
Background
St Helena is an isolated, tropical island located in the South Atlantic Ocean (Figure 2). The United Kingdom Overseas Territory is administered by the Government of St Helena and the entire 200 nm Exclusive Economic Zone (EEZ) is designated as an IUCN Category VI (Sustainable Use) MPA. The St Helena MPA aims to protect biodiversity and ensure sustainable use of its natural resources (SHG, 2016b).
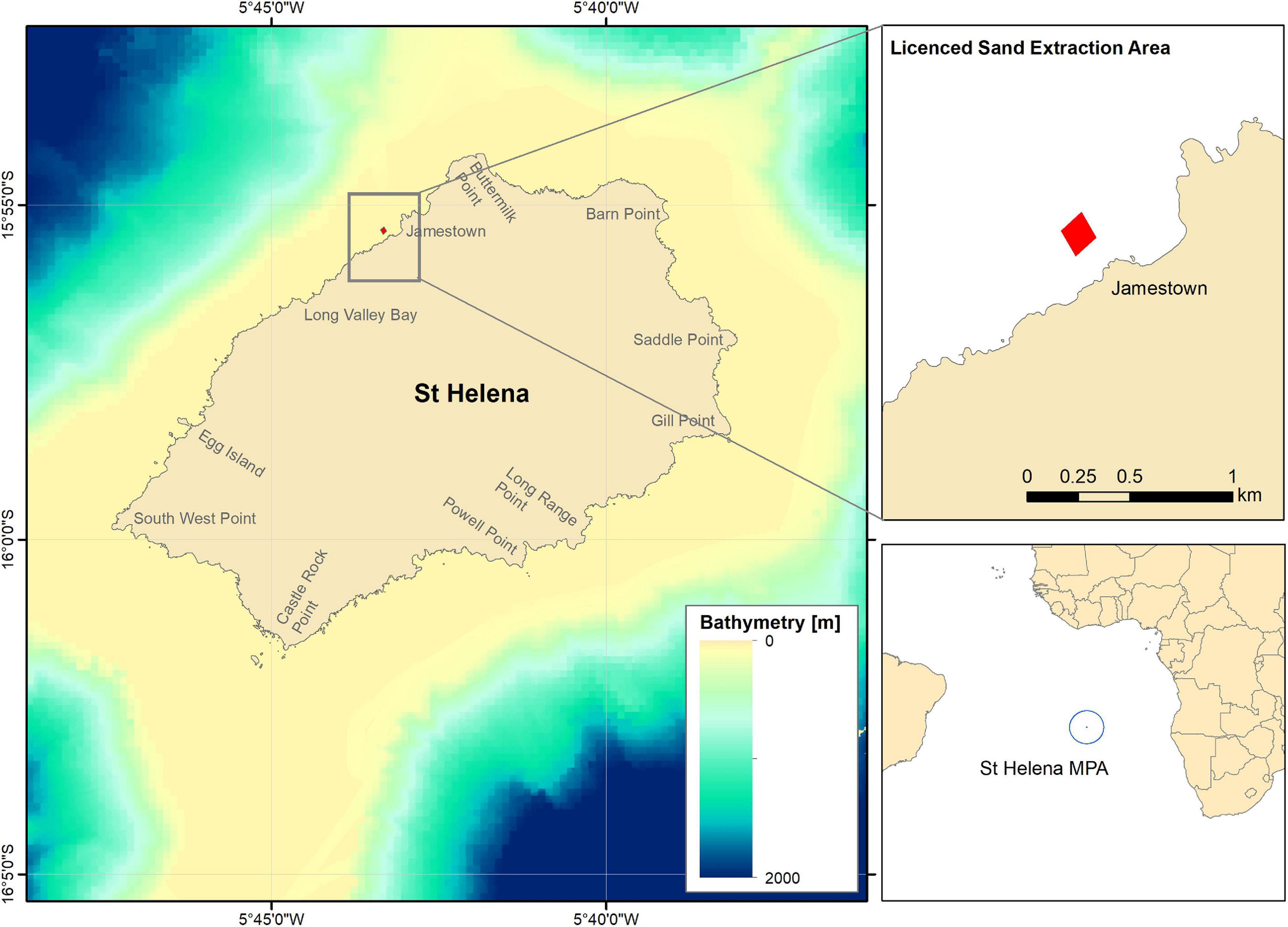
Figure 2. Map of St Helena island (left insert) with a zoom into James Bay on the north coast of the island (upper right insert), where the majority of sand extraction activity takes place. St Helena is located in the mid-South Atlantic Ocean, and the entire 200 nm Exclusive Economic Zone is designated as an IUCN Category VI (Sustainable Use) MPA (lower right insert). Bathymetry data sources: Cefas, British Antarctic Survey and St Helena Government (2018 and 2019).
The assessment of the case study was carried out by UK marine scientists with expert knowledge of regulatory processes, environmental assessment of marine activities, fisheries and fish biology, benthic ecology and physical processes. The assessors worked closely with St Helena Government to share expert, local knowledge.
Marine Environment of St Helena
Following the MPA designation, the St Helena Marine Management Plan (MMP) was adopted in 2016 (SHG, 2016b). The 2016 MMP highlights that sand (mineral) extraction is a concern because the potential impacts on the marine environment and MPA have not been assessed. The MMP refers to the development of a licensing or permit system to regulate current and future sand extraction.
The overarching goal of the St Helena MPA is to conserve the marine environment and its associated biodiversity, habitats and ecosystems and ensure sustainable use of its marine resources (SHG, 2016b). The risk assessment method proposed in this study, is considered relevant to the following MPA objectives specified in the MMP:
• “To protect natural ecosystems and use natural resources sustainably”; and
• “To sustainably manage the marine natural resources of St Helena including fisheries and mineral extraction with minimum impact on species abundance, diversity and habitats”.
There is high importance placed on the marine environment around St Helena, with significant socio-economic and cultural value associated with ecosystem goods and services sourced from the marine environment (Rees et al., 2016). Marine life such as whale sharks (Rhincodon typus) supports a growing eco-tourism sector. Natural resources like finfish and marine sand support the island economy through fishing and provision of building materials. Appropriate management of human activities that have a high risk of negatively impacting the marine ecosystem is considered essential for long-term sustainable development on the island.
The St Helena marine environment (intertidal areas, near and offshore waters) are considered biologically diverse. Some species are endemic to the island, with an estimated 50 endemic species recorded to date (SHG, 2016b). A diversity of marine species including algae; corals; crustaceans; fish; sharks and rays; sea turtles; seabirds; cetaceans (dolphins and whales); and seabirds have been recorded, either as residential or seasonal migrants. The St Helena Environmental Protection Ordinance (EPO) (SHG, 2016a) lists over 60 marine species protected around St Helena. The EPO makes provision for the protection of the environment at St Helena and has a schedule of marine species that are protected under the St Helena MPA.
Existing Sand Extraction
Sand extraction from the subtidal shelf has been ongoing since 1979. Extraction has mainly been from James Bay, next to the island capital of Jamestown (Figures 2, 3). The method involves pumping sand from a barge anchored in nearshore waters. The barge is moved between permanent anchors, and anchors can be dragged to a new position if needed. The sand is transported through an 8 cm suction pipe with a 5 cm delivery end. The sand is filtered to remove any non-target material e.g., larger rock/stone, organic matter, litter etc. The filtered material is disposed of away from the extraction sites. The extracted and filtered sand is stored on land to dry. This marine sand is used mostly for mortar and plastering by local businesses. The existing level of extraction activity is estimated to be relatively low e.g., average of 1,500 m3 pumped per annum, and stable. From habitat mapping work (information shown in Figure 3), the total area of the sand habitat in the inshore area of the island is considered to be approximately 43.2 km2, representing 26.6% of the mapped area (162.4 km2) and 19.5% of the whole shelf area. However, this value may be an underestimate because the whole inshore area (22 km2) was not mapped at the time of the survey.
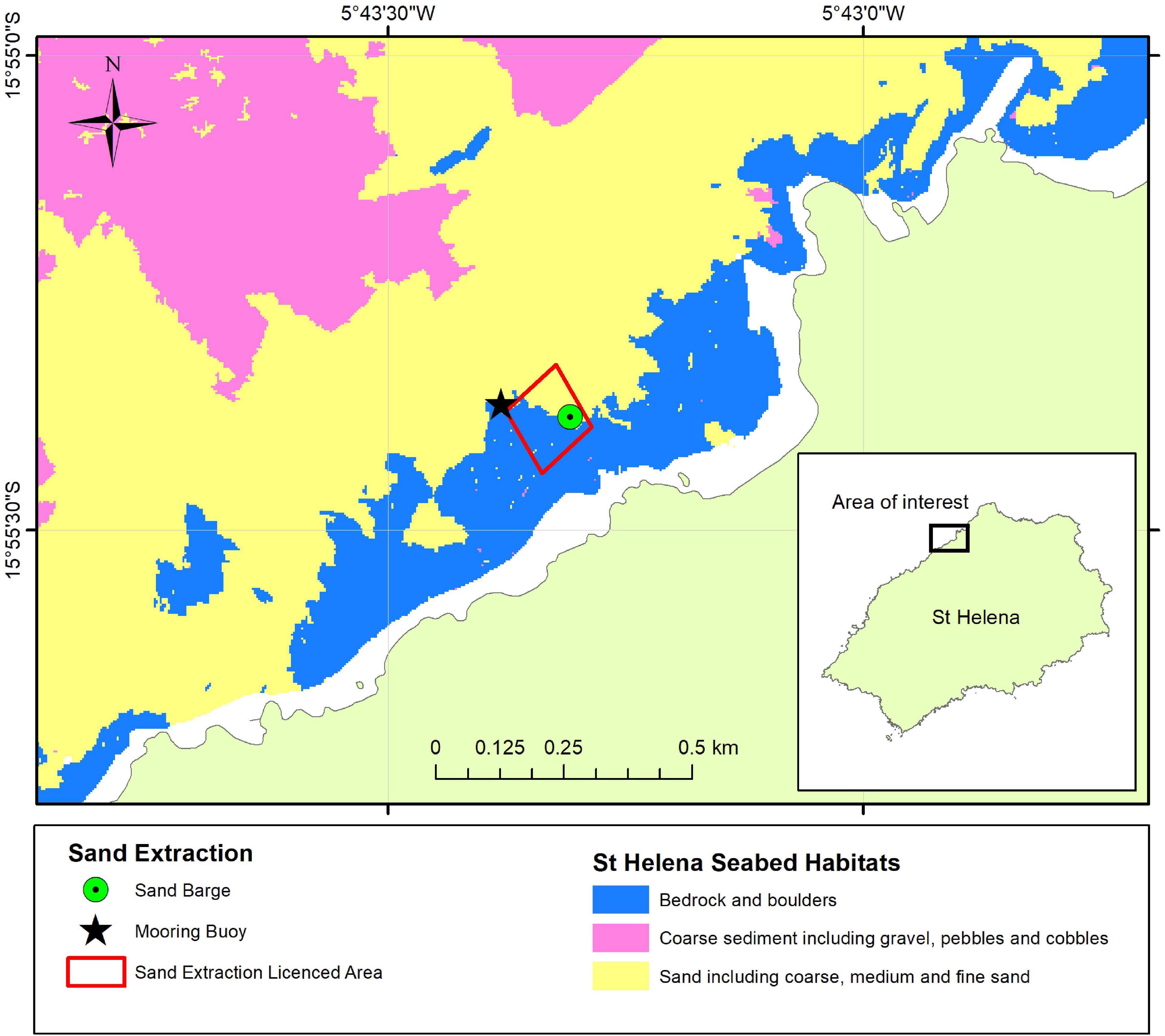
Figure 3. Habitat map of the St Helena subtidal area showing the location of the sand extraction licenced area (red square).
Assessment of Existing Activity
Here we present a case study based on existing sand extraction activity, in a scenario where an operator has requested a permit/licence for the continuation of sand extraction. The steps in the decision tree (Figure 1) have been followed to appraise generic risk, using an example receptor group of benthos (infauna and epifauna). The study seeks to determine whether current levels of extraction pose a risk to the marine environment and are sustainable. Extraction of more resource than would be sustainable is undesirable and could potentially have a significant adverse impact on the marine environment and MPA.
Step 1: Characterise the Activity
• Previous extraction activity.
• Area of extraction estimated to be 50 m2. Figure 3 shows the licenced area for sand extraction and the seabed habitat that dredging can directly and indirectly affect.
• Duration of works between 1 week and 1 month, but occurring sporadically throughout a year, depending on resource need.
• Occasional frequency of the activity.
• Dredge depth estimated to be 0.5-1 m.
• Within the St Helena IUCN Category VI (Sustainable Use) MPA.
Step 1 outcome: low-high rating and so proceed to step 2
Step 2: Identify Pressure Pathways and Environmental Receptors
To identify potential benthic receptors in the extraction area, the EPO list was consulted and supplemented with information about non-EPO benthic species identified from monitoring data, from, for example fish and benthic epifauna surveys (Brown, 2014, 2015). Examples of sand-associated species (primarily epifauna) at St Helena include:
• Annelida: Devil worm (Lygdamis wirtzi), a tube forming bristle worm (polychaete).
• Arthropoda: Crustaceans such as Lesser spotted shame-faced crab (Cryptosoma cristatum) that lives buried in sand substrata and has been recorded in shallow waters of James Bay.
• Mollusca: Scallop (Euvola turtoni) and bivalve mollusc (Semele modesta).
• Echinodermata: Sand sea cucumber (Thymiosycia spp.), St Helena sea star (Astropecten sanctaehelenae), Hairy pincushion urchin (Pseudoboletia atlantica) and Slate pencil urchin (Eucidaris tribuloides).
In addition to the available site-specific evidence, the sand biotope classification (infralittoral mobile clean sand with sparse fauna) was considered. Within the location of existing dredging, the analogous marine biotope from the 2019 EUNIS habitat Classification (for European habitats)3, is considered to be the biotope ‘Sparse fauna in Atlantic infralittoral mobile clean sand’. A list of common phyla associated with sand habitats were developed to assess the likely impacts of sand extraction on benthic communities associated with sand habitats.
As shown in Table 2 (and the Supplementary Materials), the activities considered are anchor dredging with suction pipe, disposal of screened material from sand pumping/anchor dredging, and movement between existing anchors or deployment of new anchors. In this St Helena example, benthic invertebrates present in the dredge area may suffer mortality or physical damage from being drawn into the intake pipe and passed through screens on board the vessel; hence these may be removed from the local population. The seabed area in James Bay where sand extraction occurs is coarse sandy substrate, as indicated from drop-down images obtained in the inshore areas (SHG data, 2013-2019 unpublished). Analysis of photos from a diver based subtidal benthic monitoring programme (2013-2019) also indicates the sandy substrate to be species-poor and with an absence of any benthic species listed in the EPO (Cefas, unpublished). Detailed benthic infaunal data, notably within the extraction area, were not available at the time of assessment.
The spatial extent of the known extraction (estimated to be 50 m2) is a very limited area compared with known sand substrata around St Helena (estimated at 43.2 km2) and potentially, a small proportion of the benthos are likely to be affected. Taking in consideration the current levels of extraction activity and species-poor substrate in the seabed area exposed to dredging (as evidenced from analysis of subtidal monitoring data from quadrats and drop down cameras), impacts are unlikely to be observed at the population level. The spatial extent of the extraction is a very limited area compared with known sand substrata around St Helena and potentially, a small proportion of the benthos are likely to be affected relative to the total population. Also, the biotope (‘Sparse fauna in Atlantic infralittoral mobile clean sand’) is characterised by benthic macrofauna considered to be mobile, abundant and possessing biological traits such as rapid reproduction, that generally facilitate recolonisation and thus high recoverability.
Step 2 outcome: pressures and pathways identified for benthic receptors, so proceed to step 3 to assess consequences and likelihood
Step 3: Consequences and Likelihood of Impact
A ‘low’ level of consequence can, therefore, be assigned (Table 3) to this example. The likelihood of occurrence can be deemed ‘likely’ (Table 4), because the activity itself (sand extraction) is known to occur frequently.
Step 3 outcome: consequence level noted and proceed to assess risk in step 4
Step 4: Assess Risk
The ‘low’ level of consequence and likelihood of occurrence as ‘likely’ means that it is ‘likely’ that the local benthic invertebrate fauna is affected by the sand extraction activity, hence the predicted level of consequence is ‘low’.
Step 4 outcome: risk level noted and proceed to include confidence rating in step 5
Step 5: Evaluate Risk and Assess Uncertainty/Confidence
It can be concluded that with these factors combined, a ‘low’ level of risk is indicated. However, low confidence is assigned due to limited quantitative data on receptors affected and the nature and characteristics of the activity itself. A low confidence, low risk conclusion means a more detailed environmental assessment may not be required. But low confidence should be addressed with further data collection, where resources and capacity allow.
Step 5 outcome: risk level with confidence indicated, and potential for further data collection to address low confidence. New data that are collected would trigger a new ecological risk assessment
With the completion of ‘step 5’, and where moderate or high risk has been identified then management options would need to be appraised in ‘step 6’ for mitigation and monitoring and applied during ‘step 7’. However, appraisal and implementation of management options (steps 6 and 7) are not considered further, as they are outside the scope of this study.
Discussion
This study has developed a novel approach to environmental impact assessments in the marine environment. The St Helena MMP (SHG, 2016b) identified the potential for sand extraction to have negative impacts on the marine environment, noting the requirement for a tailored assessment to identify, minimise and prevent potential impacts. The tool we developed focused on the second step of a risk assessment and provided a detailed decision tree/step wise process that allows a consistent approach to be taken for these environmental impact studies, and the screening and scoping thereof. Whilst there are guidance documents that provide either specific or generic guidance for the different steps (e.g., Fletcher, 2005, 2015; Gormley et al., 2011; Koss et al., 2011; Lonsdale et al., 2015, 2020; European Union, 2017a,b,c; Cains and Henshel, 2021), this is the first of its kind, that the authors are aware of, that brings all of these together to deliver a consistent and comprehensive assessment of a development in the marine environment.
Evaluation of Proposed Approach/Tool
A pragmatic, robust and cost-effective risk-based approach has been developed and presented as a step-wise decision tree. The steps and requirements have been applied to assess potential ecological risk from existing sand extraction at St Helena. To the best of our knowledge, this is the first instance of a risk-based marine ecological assessment method, developed for a marine activity (sand extraction) at an overseas island.
The case study was based on existing activity at St Helena; however, the approach is considered adaptable in that new data/evidence could be incorporated when these become available. The approach could be adapted for assessing risk from different scenarios of sand extraction activity. For instance, increased extraction volume within an existing site, or even an assessment for a new proposed extraction site.
Furthermore, the approach could be applied to strategic planning of resource exploitation, for example, by identifying marine areas that offer the least environmental impact (with or without mitigation/monitoring). Operators/applicants can then be directed towards those areas selected for exploitation to minimise potential impacts on the marine environment and the MPA (e.g., see Polido et al., 2014).
Aside from sand extraction, the criteria and requirements in our approach could be adapted to help assess potential environmental risk from other types of marine activity, like capital or maintenance dredging (e.g., Manap and Voulvoulis, 2014). This framework does not explicitly determine spatial or temporal scales, but these are implicit within the framework when determining the impact magnitude e.g., in Steps 1, 2, and 3 of Figure 1.
There is an opportunity to develop the decision tree further to incorporate a management stage (step 7) relating to licensing/permitting by a marine manager/regulatory body. The management stage could consist of a table of agreed mitigation measures (activity specific or more generic), and a table for environmental monitoring options. The marine manager or regulatory body could consult the table when specifying conditions to be attached to a licence/permit for an activity.
The approach could also be further adapted to incorporate socio-economic and cultural considerations, thereby enabling a more holistic assessment.
More broadly, the approach proposed could be adapted for use by marine decision makers/regulators in other overseas States, where environmental data are lacking, resources to support assessment and marine management are limited, yet regulatory drivers exist for sustainable management and development. Therefore, the approach in this study could have a wider application.
Moreover, the proposed approach is noteworthy as it potentially aligns with recommendations from a global perspective report on sand resource (marine and terrestrial), sand extraction and sustainability (United Nations Environment Programme, 2019):
• “Customise existing standards and best practices to national circumstances [and extend where necessary to curb irresponsible and illegal extraction]…. Guidelines for governing, planning and managing sand extraction at the regional and international legal scale are needed. So is support to countries for customising these guidelines in national policy, law and regulation where these do not currently exist.”
• “Invest in sand production and consumption measurement, monitoring and planning…International community organisations with mandates and access to relevant data need to collaborate on a rapid information synthesis, design a long-term monitoring programme and produce a rapid rapid assessment tool in the context of existing processes in EIA, SEA, Responsible Mining and water governance…”.
Strengths
The risk-based approach developed and presented in the form of a decision tree has several strengths. The framework can be applied in remote areas and/or data-limited situations to arrive at an assessment of risk and confidence (in conclusions) quite quickly, and with relatively limited resources. The presented approach provides a way to evaluate risk of multiple effects on multiple ecosystem components and even to a degree, considers the complex interactions of direct and indirect effects. Whilst the framework has been developed to support decision makers (and developers) in data limited areas, it also provides a consistent and transparent method that could be applied anywhere in the world, either as a screening tool or where an interim decision is needed to manage an activity until the required data is collected.
The risk-based approach provides a mechanism to filter the parameters included in the assessment and narrows the scope of any further assessment by considering the pathways between activities, pressures and ecosystem components, alongside the likelihood (risk) of exposure. This serves to (1) eliminate parameters from the assessment and conversely, highlight parameters that should form part of the assessment; (2) recognise where data are sufficient but identify the need to collect additional information where there are gaps in data, knowledge or understanding; and (3) identify the need for more extensive environment assessment and/or monitoring and/or management intervention where the level of risk is assessed as high.
The presented approach could be developed into structured guidance for regulators and industry for use in local scale evaluations. Once published, the method can be accessible to marine managers, without any associated fees, charges or commitments to ISO accreditation.
This tool could provide a clearer pathway for science advice in the ecological assessment process, ensuring the evidence-base for management measures and decisions are sound, defensible, informed by best available information and derived using a systematic process. The approach introduces transparency of process, clearer communication at all levels and cultivates public and stakeholder trust (and potential for engagement) in the decision-making process.
The tool also facilitates improved understanding of the marine system through a feedback loop, which prompts repetition and review of the assessment as new data are collected, thus implementing an adaptive management approach. This is particularly important for small Island States, such as St Helena, where activities are ongoing, but a robust baseline or monitoring regime are lacking. Conversely, the tool could be used in data rich areas to ensure a consistent and transparent process is followed to assess and licence all activities and developments in the marine environment.
The approach can be used as a mechanism to communicate confidence in an assessment to decision-makers and stakeholders. It should also increase decision maker’s confidence when conducting an assessment of evidence, which has been submitted by an operator for a permit/licence application.
Limitations
The risk-based approach can be subjective and does have basic data/knowledge requirements to understand and apply the approach. Awareness of evidence sources and having a level of ecological knowledge, or having access to specialists with the knowledge, would be important for the assessment of pressures, pathways and impacts to marine receptors, when interpreting and using matrices in the approach. Regardless of the assessment method employed, including any data collection methods, the limitations, confidence, and uncertainty must be clearly described in the assessment report (Lonsdale et al., 2017). The level of confidence (including uncertainty and taking into account the limitations) will be a consideration for the decision maker when determining an application, and whether any additional surveys or monitoring are required either before, during or following the development. Assessment of uncertainty is included in Step 5 of the framework (Figure 1), but specifics are not provided (and are out of scope) in this study.
The approach does not account for traditional, cultural, social, ecological, technical, and economic policy objectives, as is recommended by Cormier et al. (2019). Also, there is not a full consideration of what is deemed to be ‘acceptable’ impact/change by a regulator/manager (Berger and Hodge, 1998; Fletcher, 2005; Aubry and Elliott, 2006; Tett et al., 2007; Cooper, 2013; Elliott and Quintino, 2019).
As per other environmental assessments, not all impacts and receptors can be assessed at the same level of detail or the same spatial or temporal scales (Hobday et al., 2011; Lonsdale et al., 2017). This may be due to data or time constraints, or due to the nature of the impacts and/or receptors e.g., short versus long lived species will react to pressures differently. This approach assumes that if an activity has occurred in the past, and no impacts have been identified, that it is low risk. However, given limited resources, there may be a risk that impacts have occurred but due to no, little or ineffectual monitoring, have not been observed. A feedback loop is, therefore, included in the approach to link the collation of data (either through baseline surveys or monitoring), to a review of the assessment to check and where necessary, revise the level of risk.
Leading on from the above, a current limitation (but also a driver for the development) of the tool is the lack of robust data for small Island states to inform such assessments. St Helena, for example, has a high level of endemism due to its isolation. Species with restricted ranges and genetic separation or geographical isolation may be more vulnerable to the direct and indirect impacts of human activities. An absence of spatial and temporal data to support decision making can put such species at higher risk and so an initial step prior to any environmental or risk assessment should be to better understand the marine system (Ostrom, 2009, 2011); this might include cataloguing the data available. Around St Helena, knowledge of the ecology and distribution of endemic fish species is constantly expanding, but less is known about their reproductive behaviour and wider movement. Similarly, little is known about the seabed infauna. A gap analysis is therefore a useful precursor to a risk assessment and in an MPA, enables prioritisation of data for vulnerable species; species that share the same spatial footprint as the activity or it’s predicted impacts; species that are afforded international conservation status; or species with little or no information on their life stages or resilience to human activity induced stressors.
Whilst providing generic mitigation measures, the risk-based approach does not assess the efficacy of such measures, as these are dependent on the magnitude of the impact, the vulnerability of the receptor and natural prevailing conditions.
Conclusion
Here a specific approach to assessing environmental risk in data-limited locations is proposed. The suggested multi-step process is based on existing concepts of risk and environmental assessment and is paired with an assessment of uncertainty. Although, the application is at an early stage, several of its uses and limitations can already be identified.
There are many challenges associated with monitoring and managing human activities within maritime areas, and especially within MPAs. Information is not always available at the relevant spatial or temporal scale for management and management measures need to be aligned so that they address objectives from local to regional scales. This mismatch of scales makes it difficult for managers to account for future combined human-natural systems in marine planning processes (Lubchenco and Petes, 2010; Stelzenmüller et al., 2013).
We hope that our approach will drive forward practical guidance on marine spatial management in remote areas. Delivered with structured guidance, we believe the approach developed would be beneficial for remote island States with limited resources, human activity data and environmental data. The risk-based approach could also support States seeking to achieve the UN Sustainable Development Goal 14: ‘Conserve and sustainably use the oceans, seas and marine resources’.
In the future, feedback from St Helena Government on tool use and application will be used to inform its refinement. It is also anticipated that the tool could be tested on other cases so that the lessons learned provide insights into the approaches required. This should support the long-term management of marine spaces to ensure sustainable use of resources and marine protection.
Data Availability Statement
The data analyzed in this study is subject to the following licenses/restrictions: Data on sand extraction activity from St Helena is commercially sensitive. Some of the video analysis data for the island subtidal benthic monitoring programme is unpublished. Requests to access these datasets should be directed to TS, dGFtbXkuc3RhbWZvcmRAY2VmYXMuY28udWs=.
Author Contributions
All authors listed have made a substantial, direct and intellectual contribution to the work, and approved it for publication.
Funding
The work described in the manuscript was enabled through the UK Blue Belt Programme 2016–2020, which supports delivery of the United Kingdom Government’s commitment to provide long-term protection for the marine environment of the United Kingdom Overseas Territories. The programme was delivered through a partnership between the Centre for Environment, Fisheries and Aquaculture Science (Cefas) and the Marine Management Organisation (MMO), in support of the UK Overseas Territories, and on behalf of the Foreign and Commonwealth Office and the Department for Environment, Food and Rural Affairs.
Conflict of Interest
The authors declare that the research was conducted in the absence of any commercial or financial relationships that could be construed as a potential conflict of interest.
Acknowledgments
The assessment method was developed by the Blue Belt Programme partners (MMO and Cefas) in 2018–2020 to support the St Helena Government with the sustainable management of its marine resources. Thanks to Cefas staff who assisted with the collation of evidence, input to the evidence review, assessment, and recommendations during project delivery (Paul Whomersley, Steve Wallbridge, and Serena Wright). We thank staff at the MMO (Emily Hardman and Joanna Stockill), St Helena Environment and Natural Resources Directorate (ENRD) Marine Section (Rhys Hobbs and Leeann Henry), and St Helena Foreign Commonwealth and Development Office (Elizabeth Clingham) for support in collection and collation of evidence and review of the assessment method. We also thank Simeon Archer-Rand (Cefas) for assistance with the map production and to Adrian Judd (Cefas) for reviewing the draft manuscript. We acknowledge that the bathymetry data sources for Figure 2 are Cefas, British Antarctic Survey, and St Helena Government (2018 and 2019). We also acknowledge that the underlying bathymetry data sources for Figure 3 also include the UK Hydrographic Office.
Supplementary Material
The Supplementary Material for this article can be found online at: https://www.frontiersin.org/articles/10.3389/fmars.2021.645225/full#supplementary-material
Footnotes
- ^ https://data.jncc.gov.uk/data/1e136107-1396-4c67-b755-dc9f43bf3bb1/20110328-ICG-C-Pressures-list-v4.pdf
- ^ https://jncc.gov.uk/our-work/marine-activities-and-pressures-evidence/. Database contains JNCC data © copyright and database right 2018.
- ^ https://www.eea.europa.eu/data-and-maps/data/eunis-habitat-classification
References
Anbleyth-Evans, J. (2018). Aggregate dredging impacts in South East England: Improving ecological health by integrating fisher ecological knowledge with scientific research. Mar. Pollut. Bull. 135, 129–138.
Aubry, A., and Elliott, M. (2006). The use of environmental integrative indicators to assess seabed disturbance in estuaries and coasts: Application to the Humber Estuary, UK. Mar. Pollut. Bull. 53, 175–185. doi: 10.1016/j.marpolbul.2005.09.021
Austen, M. C., Crowe, T. P., Elliott, M., Paterson, D. M., Peck, M. A., and Piraino, S. (2018). VECTORS of change in the marine environment: Ecosystem and economic impacts and management implications. Estuar. Coast. Shelf Sci. 201, 1–6. doi: 10.1016/j.ecss.2018.01.009
Baker, S., Paddock, J., Smith, A. M., Unsworth, R. K. F., Cullen-Unsworth, L. C., and Hertler, H. (2015). An ecosystems perspective for food security in the Caribbean: Seagrass meadows in the Turks and Caicos Islands. Ecosyst. Serv. 11, 12–21. doi: 10.1016/j.ecoser.2014.07.011
Beaumont, N. J., Austen, M. C., Atkins, J. P., Burdon, D., Degraer, S., Dentinho, T. P., et al. (2007). Identification, definition and quantification of goods and services provided by marine biodiversity: Implications for the ecosystem approach. Mar. Pollut. Bull. 54, 253–265. doi: 10.1016/j.marpolbul.2006.12.003
Berger, A. R., and Hodge, R. A. (1998). Natural Change in the Environment: A Challenge to the Pressure-State-Response Concept. Soc. Indic. Res. 44, 255–265. doi: 10.1023/A:1006888532080
Boyd, S. E., Limpenny, D. S., Rees, H. L., and Cooper, K. M. (2005). The effects of marine sand and gravel extraction on the macrobenthos at a commercial dredging site (results 6 years post-dredging). ICES J. Mar. Sci. 62, 145–162. doi: 10.1016/j.icesjms.2004.11.014
Boyes, S. J., and Elliott, M. (2014). Marine legislation – The ultimate ‘horrendogram’: International law, European directives & national implementation. Mar. Pollut. Bull. 86, 39–47. doi: 10.1016/j.marpolbul.2014.06.055
Boyes, S., and Elliott, M. (2015). The excessive complexity of national marine governance systems – Has this decreased in England since the introduction of the Marine and Coastal Access Act 2009? Mar. Policy 51, 57–65. doi: 10.1016/j.marpol.2014.07.019
Brown, J. (2014). Marine life abundance and diversity surveys for long term monitoring Environmental Management Division. Available online at: https://www.sainthelena.gov.sh/wp-content/uploads/2013/07/Marine-Life-Abundance-and-Diversity-Survey-for-Long-Term-Monitoring.pdf (accessed August 01, 2014)
Caballero-Gallardo, K., Alcala-Orozco, M., Barraza-Quiroz, D., De la Rosa, J., and Olivero-Verbel, J. (2020). Environmental risks associated with trace elements in sediments from Cartagena Bay, an industrialized site at the Caribbean. Chemosphere 242:125173. doi: 10.1016/j.chemosphere.2019.125173
Cabral, H., Marques, J., Guilhermino, L., Jonge, V. N., and Elliott, M. (2015). Coastal systems under change: Tuning assessment and management tools. Estuar. Coast. Shelf Sci. 167, 1–3. doi: 10.1016/j.ecss.2015.11.022
Cains, M. G., and Henshel, D. (2021). Parameterization framework and quantification approach for integrated risk and resilience assessments. Integrat. Environ. Assess. Manage. 17, 131–146.
Caswell, B. A., Klein, E. S., Alleway, H. K., Ball, J. E., Botero, J., Cardinale, M., et al. (2020). Something old, something new: Historical perspectives provide lessons for blue growth agendas. Fish Fisher. 21, 774–796.
Cooper, K. M. (2013). Setting limits for acceptable change in sediment particle size composition: Testing a new approach to managing marine aggregate dredging. Mar. Pollut. Bull. 73, 86–97. doi: 10.1016/j.marpolbul.2013.05.034
Cooper, K. M., Curtis, M., Wan Hussin, W. M. R., Barrio Froján, C. R. S., Defew, E. C., Nye, V., et al. (2011). Implications of dredging induced changes in sediment particle size composition for the structure and function of marine benthic macrofaunal communities. Mar. Pollut. Bull. 62, 2087–2094. doi: 10.1016/j.marpolbul.2011.07.021
Cormier, R., Elliott, M., and Rice, J. (2019). Putting on a bow-tie to sort out who does what and why in the complex arena of marine policy and management. Sci. Total Environ. 648, 293–305. doi: 10.1016/j.scitotenv.2018.08.168
Dawe, N. K., and Ryan, K. L. (2003). The Faulty Three-Legged-Stool Model of Sustainable Development. Conserv. Biol. 17, 1458–1460.
de Jong, M. F., Baptist, M. J., Lindeboom, H. J., and Hoekstra, P. (2015). Short-term impact of deep sand extraction and ecosystem-based landscaping on macrozoobenthos and sediment characteristics. Mar. Pollut. Bull. 97, 294–308. doi: 10.1016/j.marpolbul.2015.06.002
Elliott, M. (2013). The 10-tenets for integrated, successful and sustainable marine management. Mar. Pollut. Bull. 74, 1–5. doi: 10.1016/j.marpolbul.2013.08.001
Elliott, M., and Quintino, V. (2019). “The Estuarine Quality Paradox Concept ★,” in Reference Module in Earth Systems and Environmental, ed. S. Elias (Oxford: Elsevier), 78–85. doi: 10.1016/B978-0-12-409548-9.11054-1
Elliott, M., Borja, A., and Cormier, R. (2020). Activity-footprints, pressures-footprints and effects-footprints – Walking the pathway to determining and managing human impacts in the sea. Mar. Pollut. Bull. 155:111201. doi: 10.1016/j.marpolbul.2020.111201
European Union (2017a). Environmental Impact Assessment of Projects Guidance on Screening (Directive 2011/92/EU as amended by 2014/52/EU). Brussels: European Union, 84.
European Union (2017b). Environmental Impact Assessment of Projects Guidance on Scoping (Directive 2011/92/EU as amended by 2014/52/EU. Brussels: European Union, 81.
European Union (2017c). Environmental Impact Assessment of Projects Guidance on the preparation of the Environmental Impact Assessment Report (Directive 2011/92/EU as amended by 2014/52/EU). Brussels: European Union, 130.
Fletcher, W. J. (2005). The application of qualitative risk assessment methodology to prioritize issues for fisheries management. ICES J. Mar. Sci. 62, 1576–1587. doi: 10.1016/j.icesjms.2005.06.005
Fletcher, W. J. (2015). Review and refinement of an existing qualitative risk assessment method for application within an ecosystem-based management framework. ICES J. Mar. Sci. 72, 1043–1056. doi: 10.1093/icesjms/fsu142
Foden, J., Rogers, S., and Jones, A. (2009). Recovery rates of UK seabed habitats after cessation of aggregate extraction. Mar. Ecol. Prog. Ser. 390, 15–26. doi: 10.3354/meps08169
Forster, J., Lake, I. R., Watkinson, A. R., and Gill, J. A. (2014). Marine dependent livelihoods and resilience to environmental change: A case study of Anguilla. Mar. Policy 45, 204–212. doi: 10.1016/j.marpol.2013.10.017
Gilman, E., Owens, M., and Kraft, T. (2014). Ecological risk assessment of the Marshall Islands longline tuna fishery. Mar. Policy 44, 239–255. doi: 10.1016/j.marpol.2013.08.029
Goodsir, F., Bloomfield, H. J., Judd, A. D., Kral, F., Robinson, L. A., and Knights, A. M. (2015). A spatially resolved pressure-based approach to evaluate combined effects of human activities and management in marine ecosystems. ICES J. Mar. Sci. 72, 2245–2256. doi: 10.1093/icesjms/fsv080
Goodsir, F., Lonsdale, J. A., Mitchell, P. J., Suehring, R., Farcas, A., Whomersley, P., et al. (2019). A standardised approach to the environmental risk assessment of potentially polluting wrecks. Mar. Pollut. Bull. 142, 290–302. doi: 10.1016/j.marpolbul.2019.03.038
Gormley, A., Pollard, S., Rocks, S., and Black, E. (2011). Guidelines for Environmental Risk 671 Assessment and Management - Green leaves III. United Kingdom: DEFRA.
Hobday, A. J., Smith, A. D. M., Stobutzki, I. C., Bulman, C., Daley, R., Dambacher, J. M., et al. (2011). Ecological risk assessment for the effects of fishing. Fish. Res. 108, 372–384. doi: 10.1016/j.fishres.2011.01.013
Holsman, K., Samhouri, J., Cook, G., Hazen, E., Olsen, E., Dillard, M., et al. (2017). An ecosystem-based approach to marine risk assessment. Ecosyst. Heal. Sustain. 3:e01256. doi: 10.1002/ehs2.1256
Jouffray, J. B., Blasiak, R., Norström, A. V., Österblom, H., and Nyström, M. (2020). The blue acceleration: the trajectory of human expansion into the ocean. One Earth 2, 43–54.
Judd, A. D., Backhaus, T., and Goodsir, F. (2015). An effective set of principles for practical implementation of marine cumulative effects assessment. Environ. Sci. Policy 54, 254–262. doi: 10.1016/j.envsci.2015.07.008
Judd, A., Hart, A., Prpich, G., Whelan, M., and Jude, S. (2013). “Guidelines for Marine Environmental Risk Assessment,” in Final Report by Cefas, (Bedford: Fera and Cranfield University).
Kaikkonen, L., Venesjärvi, R., Nygård, H., and Kuikka, S. (2018). Assessing the impacts of seabed mineral extraction in the deep sea and coastal marine environments: current methods and recommendations for environmental risk assessment. Mar. Pollut. Bull. 135, 1183–1197.
Koss, R. S., Knights, A. M., Eriksson, A., and Robinson, L. A. (2011). ODEMM Linkage Framework Userguide. ODEMM Guidance Document Series No.1. EC FP7 project (244273) ‘Options for Delivering Ecosystem-based Marine Management’. Liverpool: ODEMM.
Krishnakumar, S., Ramasamy, S., Chandrasekar, N., Peter, T. S., Godson, P. S., Gopal, V., et al. (2017). Spatial risk assessment and trace element concentration in reef associated sediments of Van Island, southern part of the Gulf of Mannar. Ind. Mar. Pollut. Bull. 115, 444–450. doi: 10.1016/j.marpolbul.2016.10.067
Liquete, C., Piroddi, C., Drakou, E. G., Gurney, L., Katsanevakis, S., Charef, A., et al. (2013). Current Status and Future Prospects for the Assessment of Marine and Coastal Ecosystem Services: A Systematic Review. PLoS One 8:e67737. doi: 10.1371/journal.pone.0067737
Lonsdale, J., Weston, K., Blake, S., Edwards, R., and Elliott, M. (2017). The Amended European Environmental Impact Assessment Directive: UK marine experience and recommendations. Ocean Coast. Manag. 148, 131–142. doi: 10.1016/j.ocecoaman.2017.07.021
Lonsdale, J.-A., Nicholson, R., Judd, A., Elliott, M., and Clarke, C. (2020). A novel approach for cumulative impacts assessment for marine spatial planning. Environ. Sci. Policy 106, 125–135. doi: 10.1016/j.envsci.2020.01.011
Lonsdale, J.-A., Weston, K., Barnard, S., Boyes, S. J., and Elliott, M. (2015). Integrating management tools and concepts to develop an estuarine planning support system: A case study of the Humber Estuary. Eastern Engl. Mar. Pollut. Bull. 100, 393–405. doi: 10.1016/j.marpolbul.2015.08.017
Lubchenco, J., and Petes, L. E. (2010). The Interconnected Biosphere: Science at the Ocean’s Tipping Points. Oceanography 23, 115–129.
Manap, N., and Voulvoulis, N. (2014). Risk-based decision-making framework for the selection of sediment dredging option. Sci. Total Environ. 496, 607–623. doi: 10.1016/j.scitotenv.2014.07.009
Newell, R. C., Seiderer, L. J., and Hitchcock, D. R. (1998). The impact of dredging works in coastal waters: a review of the sensitivity to disturbance and subsequent recovery of biological resources on the seabed. Annu. Rev. 36, 127–178.
Newell, R. C., Seiderer, L. J., Simpson, N. M., and Robinson, J. E. (2004). Impacts of Marine Aggregate Dredging on Benthic Macrofauna off the South Coast of the United Kingdom. J. Coast. Res. 20, 115–125.
NIWA (2012). Expert risk assessment of activities in the New Zealand Exclusive Economic Zone and Extended Continental Shelf. Prepared for the Ministry for the Environment. Auckland: NIWA.
Ostrom, E. (2009). A general framework for analyzing sustainability of social-ecological systems. Science 325, 419–422. doi: 10.1126/science.1172133
Ostrom, E. (2011). Background on the institutional analysis and development framework. Policy Stud. J. 39, 7–27. doi: 10.1111/j.1541-0072.2010.00394.x
Polido, A., João, E., and Ramos, T. B. (2014). Sustainability approaches and strategic environmental assessment in small islands: An integrative review. Ocean Coast. Manag. 96, 138–148. doi: 10.1016/j.ocecoaman.2014.05.005
Pratap, A., Mani, F. S., and Prasad, S. (2020). Heavy metals contamination and risk assessment in sediments of Laucala Bay, Suva, Fiji. Mar. Pollut. Bull. 156:111238. doi: 10.1016/j.marpolbul.2020.111238
Rees, S., Clingham, E., Rodwell, L., Glegg, G. A., and Collins, M. (2016). Marine Ecosystem Services of St Helena. Part 2: Ecosystem Service Valuations, Future Development Thresholds and Management. Saint Helena: St Helena Government.
Robson, L. M., Fincham, J., Peckett, F. J., Frost, N., Jackson, C., Carter, A. J., et al. (2018). UK Marine Pressures-Activities Database “PAD”: Methods Report. JNCC Report No. 624. Peterborough. Peterborough: JNCC.
Rogers, S. I., Tasker, M. L., Earll, R., and Gubbay, S. (2007). Ecosystem objectives to support the UK vision for the marine environment. Mar. Pollut. Bull. 54, 128–144. doi: 10.1016/j.marpolbul.2006.11.015
Rosenberg, A., and McLeod, K. L. (2005). Implementing ecosystem-based approaches to management for the conservation of ecosystem services. Mar. Ecol. Ser. 300, 270–274. doi: 10.3354/meps300270
Rowden, A. A., Clark, M. R., Lundquist, C. J., Guinottte, J. M., Anderson, O. F., Julian, K. A., et al. (2015). “Developing spatial management options for the protection of vulnerable marine ecosystems in the South Pacific Ocean region,” in Report for the Ministry for Primary Industries, New Zealand Government, (New Zealand: New Zealand Aquatic Environment and Biodiversity).
Schuhmann, P. W., and Mahon, R. (2015). The valuation of marine ecosystem goods and services in the Caribbean: A literature review and framework for future valuation efforts. Ecosyst. Serv. 11, 56–66. doi: 10.1016/j.ecoser.2014.07.013
Seiderer, L. J., and Newell, R. C. (1999). Analysis of the relationship between sediment composition and benthic community structure in coastal deposits: Implications for marine aggregate dredging. ICES J. Mar. Sci. 56, 757–765. doi: 10.1006/jmsc.1999.0495
Stelzenmüller, V., Breen, P., Stamford, T., Thomsen, F., Badalamenti, F., and Borja, Á, et al. (2013). Monitoring and evaluation of spatially managed areas: A generic framework for implementation of ecosystem based marine management and its application. Mar. Policy 37, 149–164. doi: 10.1016/j.marpol.2012.04.012
Techera, E. J., and Appadoo, K. A. (2020). “Achieving SDG 14 in the African Small Island Developing States of the Indian Ocean,” in Africa and the Sustainable Development Goals, eds M. Ramutsindela and D. Mickler (Cham: Springer International Publishing), 219–227. doi: 10.1007/978-3-030-14857-7_21
Teelucksingh, S., Nunes, P., and Perrings, C. (2013). Biodiversity-based development in Small Island Developing States. Environ. Dev. Econ. 18, 381–391.
Tett, P., Gowen, R., Mills, D., Fernandes, T., Gilpin, L., Huxham, M., et al. (2007). Defining and detecting undesirable disturbance in the context of marine eutrophication. Mar. Pollut. Bull. 55, 282–297. doi: 10.1016/j.marpolbul.2006.08.028
Thatcher, M., Robson, M., Henriquez, L. R., Karman, C. C., Payne, G., and Robinson, N. (2017). CHARM Chemical Hazard Assessment and Risk Management for the use of chemicals used offshore User Guide Version 1.5.
UN (2016). Transforming our World: The 2030 Agenda for Sustainable Development. New York, NY: UN, doi: 10.1201/b20466-7
United Nations Environment Programme (2019). Sand and sustainability: finding new solutions for environmental governance of global sand resources. Nairobi: United Nations Environment Programme, 56.
Keywords: environmental, risk, assessment, extraction, sustainable, MPA (Marine Protected Area), St Helena
Citation: Mynott F, Lonsdale J-A and Stamford T (2021) Developing an Ecological Risk Assessment to Effectively Manage Marine Resources in Data-Limited Locations: A Case Study for St Helena Sand Extraction. Front. Mar. Sci. 8:645225. doi: 10.3389/fmars.2021.645225
Received: 22 December 2020; Accepted: 14 April 2021;
Published: 02 June 2021.
Edited by:
Sam Weber, University of Exeter, United KingdomReviewed by:
Judith Brown, Limpet Blue Ltd., United KingdomLaura Kaikkonen, University of Helsinki, Finland
Copyright © 2021 Mynott, Lonsdale and Stamford. This is an open-access article distributed under the terms of the Creative Commons Attribution License (CC BY). The use, distribution or reproduction in other forums is permitted, provided the original author(s) and the copyright owner(s) are credited and that the original publication in this journal is cited, in accordance with accepted academic practice. No use, distribution or reproduction is permitted which does not comply with these terms.
*Correspondence: Frances Mynott, ZnJhbmNlcy5teW5vdHRAY2VmYXMuY28udWs=
†These authors have contributed equally to this work and share first authorship