- 1Department of Environmental and Natural Resource Economics, University of Rhode Island, Kingston, RI, United States
- 2Department of Psychology, Rhode Island College, Providence, RI, United States
- 3Department of Marine Affairs, University of Rhode Island, Kingston, RI, United States
- 4Department of Fisheries, Animal, and Veterinary Science, University of Rhode Island, Kingston, RI, United States
- 5Graduate School of Oceanography, University of Rhode Island, Kingston, RI, United States
- 6Department of Economics and Finance, Rhode Island College, Providence, RI, United States
The socio-ecological systems (SESs) framework provides cross-disciplinary insight into complex environmental problems. Numerous studies have applied the SES framework to coastal and marine environments over the last two decades. We review and analyze 98 of those studies to (i) describe how SES concepts were examined and measured, (ii) describe how the studies included feedbacks and thresholds, and (iii) identify and analyze elements unique to coastal and marine SES frameworks. We find that progress has been made in understanding key SES properties in coastal and marine ecosystems, which include resilience, adaptive capacity, vulnerability, and governance. A variety of methods has been developed and applied to analyze these features qualitatively and quantitatively. We also find that recent studies have incorporated land-based stressors in their analyses of coastal issues related to nutrient runoff, bacterial pollution, and management of anadromous species to represent explicit links in land-to-sea continuums. However, the literature has yet to identify methods and data that can be used to provide causal evidence of non-linearities and thresholds within SES. In addition, our findings suggest that greater alignment and consistency are needed in models with regard to metrics and spatial boundaries between ecological and social systems to take full advantage of the SES framework and improve coastal and marine management.
Introduction
Coastal areas have supported human communities for centuries (Gari et al., 2015), and the environmental health of coastal areas depends on inter-connections between human social systems and ecological systems (Charles, 2012). In the past 60 years, however, coastal environments have been subjected to dramatic changes associated with human activities. Rapid population growth has had severe negative consequences for coastal landscapes in many parts of the world (Li et al., 2018). The damage primarily results from excessive nutrients deposited in coastal waters from wastewater discharges, stormwater runoff, and intensive agricultural activities such as plowing and fertilization. These contaminants lead to hypoxia (reduced dissolved oxygen in the water) that kills animals and plants and associated social side effects such as beach closures (Committee on Environment and Natural Resources, 2010). Other types of damage include historic overfishing of coastal resources around the world, resulting in the collapse of stocks of numerous species of fish (Myers and Worm, 2003; Perry et al., 2010). Marine creatures from plankton to whales are ingesting debris (particularly plastic waste) (Jambeck et al., 2015). When governments, coastal communities, and individuals react to these ecological changes and adapt their behaviors, there are almost certainly feedback effects on coastal and marine ecosystems, but it has been empirically challenging to study these relationships at relevant scales (Ferraro et al., 2019).
This study provides a review of the growing scientific literature on the environmental health of coastal and marine socio-ecological systems (SESs). We describe how four key concepts—resilience, vulnerability, adaptive capacity, and governance—have been examined and measured in studies of coastal and marine SESs, describe how the studies incorporated non-linear feedback and threshold processes to understand SES complexities, and identify and analyze elements unique to marine and coastal SESs. Resilience, vulnerability, and adaptive capacity are inter-related concepts from the natural sciences that have been adapted to applications in various contexts in the social sciences (Adger, 2006; Gallopin, 2006) and are commonly used to measure the quality of SESs (Adger, 2006; Liu et al., 2007; Charles, 2012; Li et al., 2018; Resilience Alliance – Resilience). After a systematic review of applications of these concepts in coastal and marine SESs, we identify areas where future work is needed.
Critical Elements of Social and Ecological Systems
Resilience, vulnerability, and adaptive capacity are three main concepts applied to understanding how SESs respond to change (Gallopin, 2006; Kaplan-Hallam et al., 2017). These concepts originally stem from different fields but have been widely used as lenses through which to analyze the linkages within and between social and ecological systems. Gallopin (2006) argues that despite “nontrivial” linkages between resilience, vulnerability, and adaptive capacity, there are no commonly accepted definitions of these terms across the social and natural sciences. Moreover, we find that only a handful of studies in our sample have comprehensively integrated the social and ecological components to study such coupled systems. These two observations drive our decision to treat resilience, vulnerability, and adaptive capacity as overlapping, yet distinct concepts in our review. Definitions within the coastal and marine SES as well as examples of each term are provided below. For a detailed review on the conceptual relationship and distinctions between these concepts, see Gallopin (2006).
For coastal and marine SESs, resilience refers to an internal property of a system and specifically its capacity to maintain the status quo in the face of a change, disturbance, or shock (Berkes and Seixas, 2005; Lebel et al., 2006; Renaud et al., 2010; Burkhard and Gee, 2012). Disturbance includes biophysical, social, economic, institutional, and political factors that affect human communities and their environment (Lozoya et al., 2015). A resilient system does not cross a threshold, keeps itself in the same stable state (Manuel-Navarrete et al., 2007; Larsen et al., 2011), and retains its essential functions, structure, identity, and feedbacks as the system recovers (Walker et al., 2004; Lozoya et al., 2015). A less resilient system is unable to maintain its identity, and disturbances instead lead to larger scale “regime change” radically altering the system (Scheffer et al., 2001). In biological, engineering, and decision-sciences this is sometimes also referred to as robustness (e.g., Mens et al., 2011). Often the term robustness is employed instead of resilience when an engineered component plays a crucial role in an SES such as polders (Ishtiaque et al., 2017). Resilience in the ecological system reflects how components respond to environmental changes, such as species' sensitivity to pollutants or changes in temperature, the number of links in an ecosystem's food web, or a marine environments' carbon storing capacity. The resilience of a social system depends on system members' ability to self-organize, learn and adapt (Folke et al., 2002; Berkes, 2017), and utilize social bonds or formal community planning to persist in the face of disturbances such as flooding or declining fish stocks (Trosper, 2003; Mens et al., 2011). Moreover, resilience of a socio-ecological system is increasingly understood to be a complex and dynamic concept. A resilient system is one that can respond to a range of probable as well as plausible or deep uncertainties and adapt toward transformative change (Polasky et al., 2011; Berkes, 2017).
A related but distinct concept is vulnerability, a composite property of the system consisting of three elements: exposure to disturbances, sensitivity to these disturbances, and capacity to adapt to the disturbances (Adger, 2006). While resilience and vulnerability are related, there is some debate about whether they are opposite concepts (Hu et al., 2018). Resilience is a property of the internal strength of a system while vulnerability partly relies on external factors such as exposure to disturbances (Adger, 2006; Gallopin, 2006). As such, vulnerability assessments gauge the impact of one or more disturbance events on ecological and/or social systems and potentially synergistic impacts multiple disturbances (Murphy, 2015). In both social and ecological systems, exposure to disturbances can vary in number, severity, or duration. In ecological systems, sensitivity characteristics could include, for instance, the temperature range at which a particular species of plant or animal can survive, or water flow which regulates an ecosystem. Similarly, sensitivity in a social system could refer to water availability which can support drinking water or livelihoods such as farming, or market shocks that affect income and asset values. Social and ecological systems can be exposed to common disturbances which can cause both systems to collapse (Barrett et al., 2011).
We treat adaptive capacity as its own concept rather than just as the third property of vulnerability since it is commonly investigated independently. Adaptive capacity denotes characteristics that determine whether and the degree to which a system can adjust to survive (Manuel-Navarrete et al., 2007). In ecological systems, adaptive capacity refers to species' capacity to persist despite changes in conditions (Whitney et al., 2017) and is strengthened by genetic, biological, or landscape diversity (Resilience Alliance—Resilience). Adaptive capacity may include whether organisms can withstand extreme weather events or reproduce rapidly enough to evolve to survive in a drier or wetter climate because of climate change (Lozoya et al., 2015). In contrast, studies can also address the effects of new system configurations, such as ecological system changes brought on by adaptive responses of coastal communities to reduce the negative effects of climate change (Charles, 2012). In social systems, it refers to characteristics that enable the system to respond effectively to changes in ecological systems and maintain the existing quality of life (Tiller et al., 2016), such as political structures that can enact large-scale infrastructure projects or formal and informal risk management strategies. For instance, coastal communities may be able to overcome their dependence on traditional species and take advantage of the availability of new biological resources due to climate change (Charles, 2012). In this way, adaptive capacity differs from resilience since a system with high adaptive capacity may still change significantly, but the assessment is more about the well-being of the social and ecological elements rather than about avoiding large changes to the system.
Another important concept that helps explain how coastal and marine SESs respond to or prevent changes is governance, the formal and informal arrangements that regulate both residents and resources. Governance is a broad concept that encompasses politics, policies, mutual trust, social knowledge (Barclay Frey and Berkes, 2014), stages of uncertainty about future outcomes (Walker et al., 2013), and voluntary actions that allow individuals to self-organize (Ban et al., 2017). The effectiveness of authorities' policies can strengthen or weaken the resilience, vulnerability, and adaptive capacity of SESs (Adger, 2006; Berkes, 2011; Barnett and Anderies, 2014). Governance of coastal and marine resources and communities must use a robust framework to integrate social, economic, and environmental dimensions (McClanahan et al., 2009) and manage the systems to address uncertainty and disturbances (Glaser et al., 2012).
Coastal governance is especially challenging in the face of deep uncertainty and evolving vulnerabilities from “cross-scalar,” multi-dimensional and inter-temporal impacts of global changes in climate, land use, biodiversity, technology, socio-economic conditions, and politics on coastal SES (Polasky et al., 2011; Walker et al., 2013; Maier et al., 2016; Gladstone-Gallagher et al., 2019; Groves et al., 2019; Naylor et al., 2019). Uncertainties can vary between the bounds of probable outcomes to multiple plausibly distinct scenarios (Maier et al., 2016). Walker et al. (2013) specifically outline five transitions in stages of uncertainty in decision-making ranging from complete certainty to ignorance. Deep uncertainty is reached in the last two stages when multiple plausible futures can either not be ranked or imagined. Governance of coastal SESs must therefore also evolve to embrace more adaptive decision-making strategies with the objective of strengthening system resilience to “surprises” (Berkes, 2017; Gladstone-Gallagher et al., 2019; Elsawah et al., 2020)1.
Non-linear feedback and threshold processes are also critical for understanding SES complexities. Feedback is an important mutual effect within or between elements of systems and covers long-term timeframes (Engie and Quiroga, 2014). It depicts the effect of an element A on B and vice versa, where A and B can be parts of the same system (both ecological or both social) or different systems (one ecological and one social). Feedback can stabilize a system by reducing unwanted changes or promote positive adaptive changes such as biotic interactions to reduce “undesirable” species of macroalgal blooms contributing to loss of coral reefs (Nyström et al., 2012; Engie and Quiroga, 2014; Kaplan-Hallam et al., 2017). Feedback can also amplify the consequences of undesirable changes making it difficult to break free from a destructive cycle, such as when new markets or technology increase the intensity of fishing efforts for a limited stock (Kittinger et al., 2012). Neglecting these types of feedback in a system can exacerbate existing problems and result in ineffective policy interventions (Perry et al., 2010). Some studies (Engie and Quiroga, 2014; Gladstone-Gallagher et al., 2019) have criticized the lack of attention paid to feedback and robust planning to incorporate multiple uncertain scenarios, such as climate and fisheries related collapse of coastal food webs around the world (Nyström et al., 2012), particularly when evaluating social systems.
Thresholds (also called tipping points) denote points at which changes push a system from one state to another, often irreversibly (Broderstad and Eythórsson, 2014; Hossain and Szabo, 2017). Biophysical or ecological thresholds are defined by physical, chemical, and biological characteristics of ecological systems (Renaud et al., 2010) while social thresholds are defined by the limits of an individual's and society's ability to learn and adapt (Renaud et al., 2010; Kaplan-Hallam et al., 2017). Thresholds are often treated as stable boundaries and/or as a maximum level of disturbance tolerated by a system while still able to maintain its original configuration. When the loss of a system's given function is too great, the system configuration changes (Renaud et al., 2010; Broderstad and Eythórsson, 2014; Bigagli, 2015). The threshold process in SESs has been characterized by Hossain and Szabo (2017) as beginning with a change in a system characteristic that exceeds the system's threshold and transitions to another state by positive feedback and lack of a governance response to the change early on.
We review each of these critical elements and methods devised to analyze them in studies of SESs published between 2003 and 2018.
Methods
In May 2018, we used two comprehensive databases of peer-reviewed research, BIOSIS on the Web of Science and Scopus, to identify studies of coastal and marine SESs from all years, all languages, and all document types. The search query used a set of wildcards to identify studies in which the title contained the phrases “socio-ecological system,” “socioecological,” or “human-environment system” and the title, abstract, and/or keywords contained the words coastal, ocean, marine, or sea.2 The query generated a list of 132 unique publications. We discarded 34 of the publications during the screening process because they did not include a coastal or marine SES, were only introduction or conclusion sections of the publications, or could not be retrieved (see Figure 1).
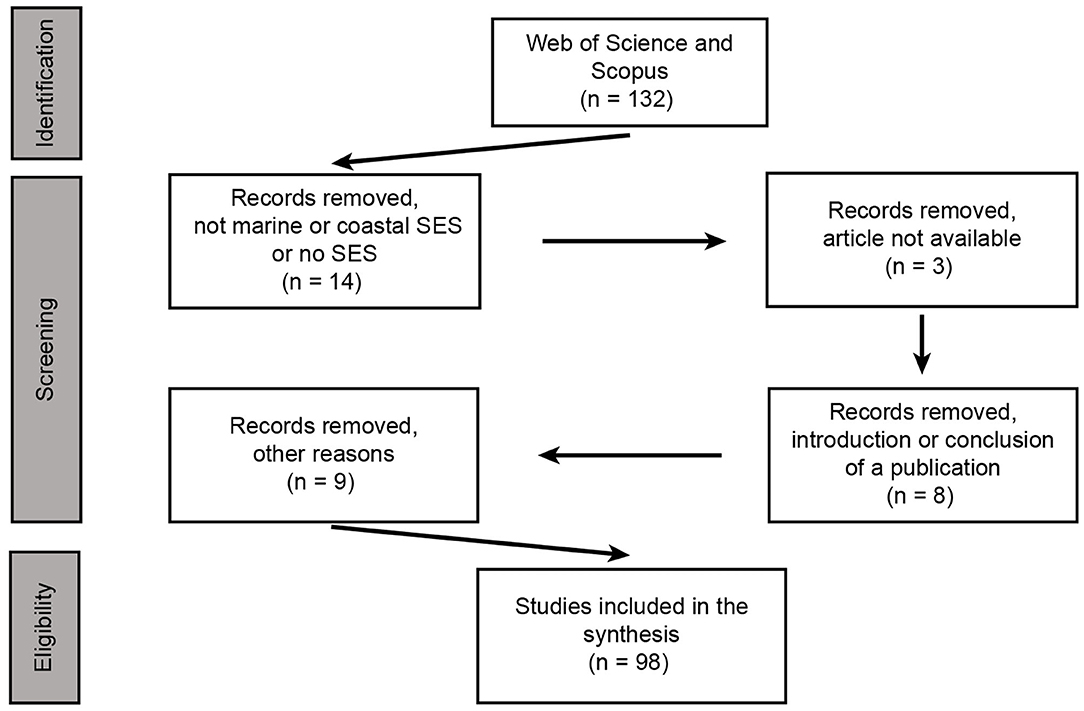
Figure 1. Screening process of the literature sample on coastal and marine socio-ecological systems.
We coded the 98 publications remaining in the final dataset by date of publication and geographical location (country and/or region) in which the study was conducted. Studies conducted in one country were coded as single. Studies conducted in more than one country or that included countries and regions at the same time were coded as multiple. Similarly, we recorded the regions in which studies were conducted with broader geographic designations such as ocean or portion of a continent. If no specific countries or region were identified, the study was coded as unspecified.
We next coded the studies using four criteria adapted from Binder et al. (2013) regarding inclusion of SESs: (1) inclusion of a clear description of its SES components (yes/no); (2) relative emphasis placed on the social and ecological systems (emphasized social, emphasized ecological, and equal emphasis); (3) inclusion of a coherent SES conceptual framework that introduced or advanced a new concept (yes/no); (4) and inclusion of an empirical analysis (case studies) in which the SESs were applied to a specific context (yes/no).
Finally, the publications were coded to identify whether the studies' keywords included any of the four key SES properties (resilience, vulnerability, adaptive capacity, and governance) and the two key processes (feedbacks and thresholds). The content of each publication was further reviewed qualitatively to establish how clearly the SES properties and complementary concepts were defined, the methodologies employed to study them, and the main findings (see Supplementary Table 1).
Results
Table 1 summarizes the characteristics identified for each of the 98 studies reviewed in terms of temporal and geographic coverage, criteria compliance, and incorporation of the four properties and two processes of particular interest in this review.
Changes in Temporal and Geographic Coverage
As shown in Figure 2, we find that the annual number of coastal and marine SES studies increased gradually. It increased from an average of 1.0 study per year between 2003 and 2006 to 3.9 for 2007 and 2013. Then, in 2014, the number of studies published nearly doubled to 17 and averaged 14.8 between 2014 and 2017 (As of May, there were nine in 2018). This rapid increase in research on SESs likely reflects both growing interest in the topic and advances in analytical methods and greater availability of data.
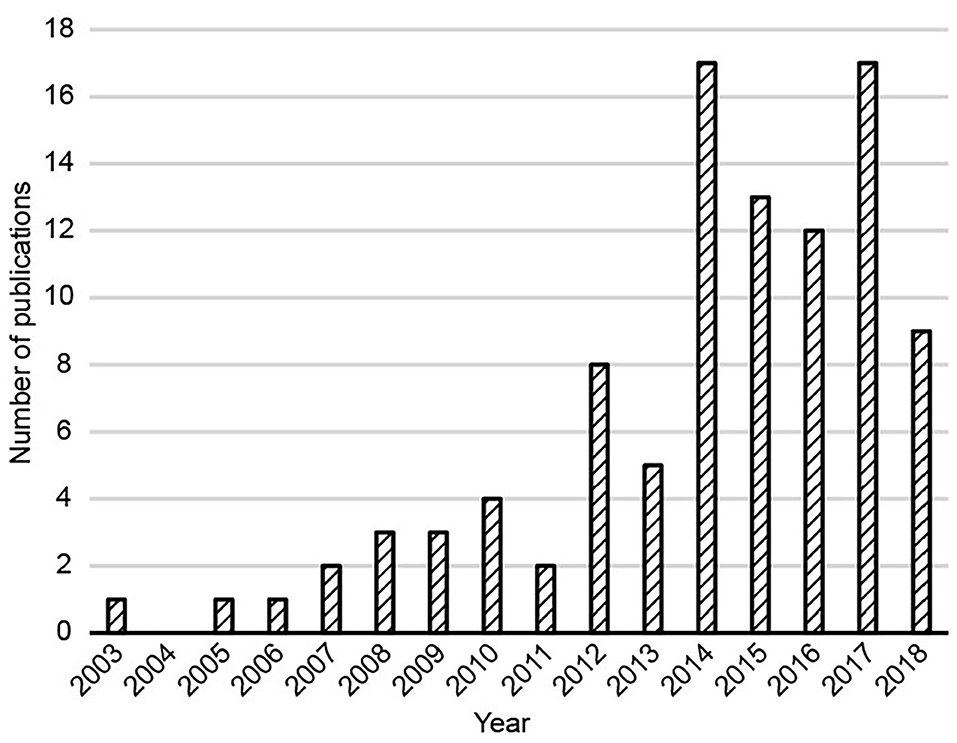
Figure 2. Number of publications per year. The figure shows the total number of hits classified according to the year of publication. Publications included journal articles and book chapters on coastal or marine SESs from 2003 to 5/14/2018.
The reviewed studies cover diverse geographical locations (Figure 3). Nearly two-thirds (62%) focused on a single country and almost one-fifth (21%) addressed systems in multiple countries. A small percentage addressed SESs on wider regional scales (9%). Only 7% of the studies did not specify a geographic location. The three most-studied countries are the United States (15), Canada (12), and Brazil (9), potentially reflecting relative greater availability of sufficient data about SESs in those countries.
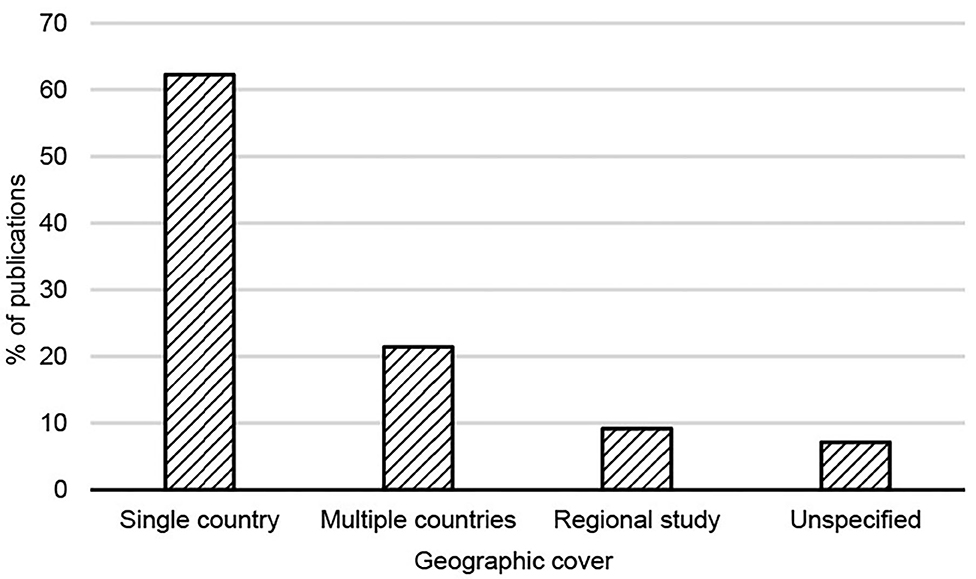
Figure 3. SES studies per geographic context. The figure shows the percentage of studies on coastal or marine SESs that took place in a single country (single), in more than one country (multiple countries), in a broader geographic area regardless of the country (regional), and in unspecified locations (unspecified).
Inclusion of Socio-Ecological System Components
Our review shows that 92% of the publications in the sample included clear descriptions of the SES components, though the balance of attention paid to social and ecological systems was sometimes uneven. We found that 67% incorporated both systems relatively equally, 31% almost exclusively emphasized social systems (e.g., Murray et al., 2008; Reenberg et al., 2008; Ferrol-Schulte et al., 2013; Roebeling et al., 2014; Villamor et al., 2014; Benham, 2017), and 2% strongly emphasized ecological systems (Ban et al., 2017; Hagenlocher et al., 2018). Among studies that analyzed both systems relatively equally, we found that Berkes (2011), Broderstad and Eythórsson (2014), Cullen-Unsworth et al. (2014), Engie and Quiroga (2014) analyzed the mutual influences of both systems, which can strengthen SES modeling (Davies et al., 2016; Melbourne-Thomas et al., 2017). Others (Glaser et al., 2012; Perry and Masson, 2013; Guillotreau et al., 2017; Hu et al., 2018; Li et al., 2018; Peña-Alonso et al., 2018) used indicators that integrated variables representing the social and ecological systems. For instance, Perry and Masson (2013) constructed indicators identifying changes in large-scale temporal patterns of variability in the Strait of Georgia. They applied multivariate statistical analysis to 37 variables related to ecological and social (human) systems, including the Oceanic Niño Index, spring phytoplankton blooms, and commercial herring hatchings, that were scaled to a unit variance. Guillotreau et al. (2017) normalized variables with different metrics by classifying natural, social, and governing resilience as low, medium, and high, and then scored all of them between one and three to calculate a multidimensional resilience index.
Approximately 21% of the studies that provided clear conceptual frameworks also advanced existing frameworks (e.g., Partelow and Boda, 2015; Crépin et al., 2017). Several made theoretical contributions (Berkes and Seixas, 2005; Whitney et al., 2017; Hagenlocher et al., 2018), introduced or reviewed new concepts (Berkes, 2011; Loring, 2016), and advanced an existing concept (Murphy, 2015). The vast majority of the literature sample (95%) included an empirical analysis based on case studies (e.g., Burbano et al., 2014; Chalcobsky et al., 2017).
Key Properties and Processes
Figure 4 briefly summarizes the studies' inclusion of each of the four key properties of SESs (resilience, vulnerability, adaptive capacity, and governance) and two key processes (feedbacks and thresholds). In general, we find that resilience and governance were frequently addressed, and that feedback and thresholds were rarely addressed. In the following sections, we explain how the SES properties and processes were defined and assessed in greater detail, review the primary findings, and discuss(Fuller et al., 2017)areas for future research.
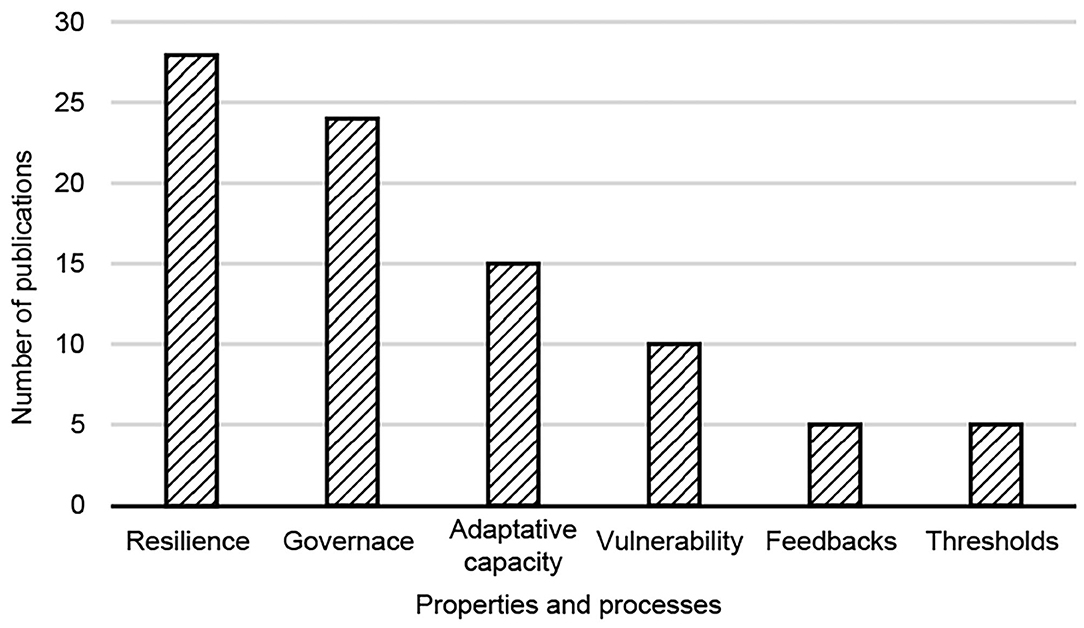
Figure 4. Analysis by properties and processes. The figure shows the number of publications on coastal or marine SESs that dealt with resilience, vulnerability, adaptive capacity, governance, feedbacks, and thresholds in this review.
Resilience
Using a variety of qualitative and quantitative research methods, SES studies have identified several factors that contribute to resilience of coastal and marine systems. Studies have indicated that resilient SES systems integrate multiple types and forms of knowledge (e.g., Berkes and Seixas, 2005; Faulkner et al., 2018). In one case, users' local knowledge of the coastal region led Bedouin breeders to reduce the size of their herds at the household level and migrate with them when facing drought, strengthening their resilience to ecosystem change (Daoud et al., 2016). Willingness to change has also been found to be a characteristic of resilient coastal and marine SES. Some communities prepare for change by diversifying livelihoods (Berkes and Seixas, 2005). For instance, along the US west coast, vessels capable of participating in multiple fisheries were able to reallocate effort to different fisheries in the face of disturbance (Fuller et al., 2017). In contrast, communities that are not willing to adjust practices in response to change can have weakened socio-ecological resilience. For example, immigrants who applied practices that they had used in their original localities to a different environment (ecological illiteracy) broke the link between their society's evolution and the environment's, reducing their resilience (Glaser et al., 2010). Attachments to place and cultural identities have also been found to strengthen social resilience in coastal and marine SES (González et al., 2008; Faulkner et al., 2018). In fact, place attachment served as the foundation for all other features of SES resilience in two coastal communities in the UK (Faulkner et al., 2018). Other studies have found that resilient SESs have institutional diversity where cross-scale linkages are encouraged (Berkes and Seixas, 2005), ability to self-organize and manage conflict (Berkes and Seixas, 2005), and capacity to implement short- and long-term interventions (Guillotreau et al., 2017). Modeling studies in Jiangsu Province, China that examined relationships between SES resilience and individual social, economic, and environmental factors highlighted the complex interactions among these factors and the dynamic nature of resilience (e.g., Hu et al., 2018; Li et al., 2018). Some factors affecting resilience that emerged in these studies included the capacity to make economic and policy re-investments in the region, implementation of environmental protection strategies, and participation in sustainable use practices (Hu et al., 2018; Li et al., 2018). All of the reviewed studies on coastal and marine SESs provide valuable insights into features associated with resilient systems and highlight the dynamic, multi-scalar, multi-dimensional, and complex nature of resilience.
Vulnerability
Both qualitative and quantitative methods to assess vulnerability were represented in the reviewed studies. Among the studies using qualitative methods, several compared multiple case studies to construct an SES framework and understand how and why coastal regions are more vulnerable than other regions (Manuel-Navarrete et al., 2007; Murphy, 2015). Manuel-Navarrete et al. (2007) identified three causal loops involving 13 symptoms of vulnerability, including lack of urban planning, occupation of hazardous areas, and ecosystem degradation. Their results are consistent with two other reviewed studies (Murphy, 2015; Rothenberger et al., 2018) that found that coastal regions are more vulnerable than other types of regions to the effects of climate change and hurricanes because coastal areas tend to (a) be densely populated; (b) be occupied by numerous wastewater treatment and landfill facilities, (c) lack naturally protective land functions, and (d) have poorly managed natural resources. Another qualitative approach found in the reviewed studies on vulnerability of a coastal SES is stakeholder participation. Wawo (2017) utilized community participation—by those who depend on the coastal ecosystem services and, in return, whose activities affect the ecosystem—to identify the vulnerability of coastal communities' livelihoods.
Vulnerability indices have been the most preferred quantitative method. For instance, Metcalf et al. (2015) measured coastal communities' socioeconomic vulnerability to climate change, using a set of indicators for climate exposure, species exposure, ecological vulnerability, resource dependence, and adaptive capacity. They found that climate exposure increases socioeconomic vulnerability, while diversity in employment opportunities and the restoration of local seafood markets are some of the factors reducing vulnerability. Hagenlocher et al. (2018) introduced the Global Delta Risk Index, which interacts hazard exposure and socio-ecological vulnerability to measure the risk of deltaic SESs to multiple hazards. This study identified that deltaic ecosystems are a SES component facing risk, and at the same time, an asset for risk reduction and adaptation. Other studies using indices have treated vulnerability and resilience as interrelated concepts (Renaud et al., 2010). For example, Hu et al. (2018) nested the vulnerability index in the social, economic, and ecological system resilience indices.
Other quantitative studies considered social vulnerability as a property of fishery connectivity networks. Fuller et al. (2017) estimated the social vulnerability of fishing communities using network-level metrics such as edge density, the universal resilience function, and network modularity Q. Edge density is inversely related to sensitivity and indicates how flexible fishers are to switch between fisheries to obtain income when they face a perturbation. The universal resilience function shows how sensitive human communities are to disturbances. Network modularity measures how connected a node is. A modular fishery has a reduced adaptive capacity. Overall, they found that a shrinking portfolio of species increases fishers' sensitivity to disturbances.
Adaptive Capacity
The reviewed studies of adaptive capacity vary in terms of the scale of the SESs addressed. Some focus on one scale alone, for example, focusing on how the social and ecological systems of small-scale pelagic fisheries respond to disturbances (Jarre et al., 2013). Others focus on multiple scales, for example, measuring adaptive capacity in ecological systems at the ocean basin, ecosystem, species, and population scales and in social systems at the international, national, community, and individual scale (Whitney et al., 2017).
We find that the most common method used to assess adaptive capacity over the last two decades is the adaptive cycle (e.g., González et al., 2008; Burkhard and Gee, 2012; Li et al., 2018), which comprises four phases: exploitation, conservation, release, and reorganization. The adaptive cycle method closely links adaptive capacity and resilience. Li et al. (2018), for example, showed that exploitation was the most appropriate phase in which to implement novel management measures designed to build resilience, the release phase was susceptible to loss of resilience, and that policy interventions were required in the exploitation and conservation phases for the systems to be efficient. Adaptive cycles can also be studied historically over time. For example, González et al. (2008) showed that Galapagos has gone through three adaptive cycles since the arrival of humans in 1,535 and was experiencing the beginning of a new renewal phase triggered by enactment of the Galapagos Special Law, the creation of the Galapagos Marine Reserve and associated tourism. Tourism facilitated entry of exotic species (leading to loss of biodiversity) and altered aspects of the economic system (fisheries, agriculture, and commerce). The adaptive cycle methodology has proven useful in both longitudinal and cross-sectional studies of adaptive capacity.
Adaptive capacity is also examined with the fuzzy cognitive mapping method, which analyzes causal feedbacks iteratively. For example, Tiller et al. (2016) analysis of different scenarios in Norway involving sea surface temperatures over 50 years demonstrated how the social system's response to changes in the ecological system can generate new types of feedbacks. The sustainable livelihood approach has also been used to examine adaptation of vulnerable or low-income communities in particular. Metcalf et al. (2015) utilized this method to assess the health of coastal communities finding that high levels of exposure to changes in rainfall due to climate change along with high resource dependency were a perilous combination that hindered adaptation to climate shocks. Fuzzy cognitive mapping is increasingly used in different socio-ecological contexts and shows promise for broad applications in future studies of adaptive capacity.
Governance
Several of the reviewed studies evaluated the relationships between human well-being and stakeholder preferences and the governance of coastal and marine SESs. Involving stakeholders can mitigate the tension between scientists and resource managers because it is possible to establish, for instance, the connection between human well-being and the marine food web (Levin et al., 2016). Participatory marine governance links community members' values and perceptions to the effect that the SESs have on their well-being, even though the outcomes are highly variable and context-specific (Murray et al., 2016). Likewise, a handful of studies (e.g., Villamor et al., 2014; Levin et al., 2015; Van Putten et al., 2016) found that incorporating stakeholder preferences and perceptions into the governance process improves their ability to establish conservation plans and management goals for SESs. Although participatory marine governance can bring consensus between parties by setting measurable conservation targets (Levin et al., 2015), it does not necessarily guarantee ecosystem services' optimal provision.
Several of the governance studies focused on identifying attributes of good governance and how those attributes influence resilience. Lebel et al. (2006) found that participation, representation, deliberation, social justice, and other attributes related to the role of actors characterized effective governance and built resilience. Larsen et al. (2011) showed that informal stakeholder arrangements can partially replace weak formal government institutions and effectively stimulate informal collective action that contributes to building resilience following an environmental shock. Moreover, two studies of ancient indigenous communities in Europe revealed that a flexible worldview (Forbes, 2013), solid stewardship (Forbes, 2013), and robust political representation (Lebel et al., 2006) facilitate effective management of natural resources and survival of community livelihoods and build social resilience in the face of ecological and governance-induced changes.
Other studies diagnosed factors that led to ineffective governance. Studies have identified a few of most critical drivers of poor governance outcomes such as the mismatch between ecological and administrative boundaries (Leslie et al., 2015) and of defined spatial resource boundaries (Barnett and Anderies, 2014; Bigagli, 2015). Bigagli (2015) noted that overlapping borders between systems, such as adjacent marine and freshwater environments, bring additional problems. Directly applying legislation aimed at continental lands to marine environments neglects unique characteristics of saltwater ecosystems. Two studies found that traditional top-down governance, which overlooks the role of customary institutions, discourages users from supporting the resulting policies (Charles, 2012; Barnett and Anderies, 2014). For example, fishers' perceptions of involvement in the decision-making process as having a high cost and providing little benefit made fisheries in Port Lameron, Nova Scotia, more vulnerable to social-ecological changes (Barnett and Anderies, 2014). Likewise, a case study of the Paraty fishery in Brazil (Begossi et al., 2012) identified interruption of feedback between local ecological knowledge and formal government institutions as the primary problem negatively affecting management of the fishery. Users' heterogeneous preferences have also caused conflicts and undermined trust between users (Fleischman et al., 2014), frustrating community-based resource management efforts. They found that better results could be obtained by establishing polycentric governance that involved all stakeholders and addressed all the economic activities within a resource system.
Few coastal and marine SES studies have addressed the complex influence of governance in large-scale environments, an issue that is important when addressing SESs that cross political boundaries (e.g., transboundary marine fisheries). One exception is Fleischman et al. (2014); their study applied Ostrom's design principles, which have typically been used for local studies, to large-scale SESs such as Great Barrier Reef Marine Park, the Rhine watershed, and the International Commission for Conservation of Atlantic Tunas (ICAAT). Overall, they found that successful governance cases for large SESs were characterized by common pool resources with well-defined biophysical and administrative boundaries and monitoring of ecological conditions and user behavior. Other design principles, such as monitoring related to accountability of the monitors to users, collective-choice arrangements, and recognition of rights of resource users to self-organize, did not prove to be successful in large-scale SESs. For example, management of Atlantic bluefin tuna by ICAAT has consistently failed to control overexploitation, primarily because the significant mobility of the fish resources has prevented ICAAT from developing government institutions that can regulate the large scale of the fishery.
Another area of research that is still largely absent in the reviewed literature is the connection between SES governance and uncertainty, especially in the context of coastal and marine management. Cascading stages of uncertainty from probability driven outcomes to higher stages of unrankable or even unimagined unknowns requires flexible governing principles that can adapt with evolving global stressors that can nonetheless unfold at local, regional and temporal scales (Walker et al., 2013; Berkes, 2017; Elsawah et al., 2020). Supplementing cause and effect quantitative modeling with a more holistic exploratory perspective helps multiple stakeholders consider, participate, collaborate and prepare for a robust response to unanticipated shocks (Berkes, 2017).
Non-linear Processes: Feedback and Thresholds
Though many SES studies have documented inter-connections within and between systems, few have incorporated measures of thresholds and feedback loops. Melbourne-Thomas et al. (2017), which addressed marine SESs in Australia, criticized prior studies for failing to include bio-geo-chemical and physical processes and higher trophic levels, oversimplifying social systems, and incorporating only limited representations of human-ecological interaction (primarily through fish catches) in modeling. This lack is likely partially explained by insufficient knowledge about many species' biological and social organizations, limiting researchers' ability to identify suitable connections to test (Martone et al., 2017). In this way, modeling feedback between social and ecological systems is more difficult and increasingly challenging when the SES faces a constant change (Engie and Quiroga, 2014).
Some of the reviewed studies used qualitative methods to describe and represent feedback loops. For example, Kittinger et al. (2012) introduced a heuristic conceptual framework for coral reefs that identified (i) environmental feedbacks in which an ecosystem responds to human activities and that response affects humans' social perceptions and (ii) institutional feedback in which governance responses to social or ecological stimuli alter individuals' interactions with the environment. Several studies examine how various social factors pressure fishers to increase their fishing efforts, creating a feedback loop (Jarre et al., 2013; Kaplan-Hallam et al., 2017). As more fish are caught, the more efforts must intensify leading fishers to expand their portfolios, work for longer periods, or adopt risky practices. These loops can increase the risk of resource collapse (Jarre et al., 2013) or of poaching and interpersonal violence (Kaplan-Hallam et al., 2017). Alternately, other researchers consider “missing feedbacks” a phenomenon in which a weak relationship between fishers and the government hinders the fishers' ability to self-regulate fishing practices in shared resources (Barnett and Anderies, 2014).
Several frameworks have been used in the reviewed studies to represent feedback loops graphically. Crépin et al. (2017) employed integrated ecosystem-based management in two scenarios—a decrease in availability of two zooplankton species and an increase in the population of invasive red king crabs—to represent strong, large-scale socio-ecological interactions that could lead to management failures. Though the framework cannot produce quantitative predictions of future outcomes, it has the advantage of providing a holistic view of an SES and uses well-grounded scientific information supporting the linkages despite scarce data. Vugteveen et al. (2015) used group model building and data visualization to explain the most critical feedback loops and their impacts to stakeholders involved in a participatory process to construct an SES framework of mussel fisheries and tourism. Manuel-Navarrete et al. (2007) also identified and represented causal loops associated with vulnerability to natural disasters using syndrome analysis. They found that ecosystem degradations and land conversions increased soil erosion, which, in turn, increased and intensified occurrences of meteorological phenomena that caused disasters. Subsequent economic losses increased the number of people in poverty, which exerted pressure on the ecosystems, completing the cycle.
Thresholds in marine SESs have not been fully studied despite being essential to understanding such systems, largely because of their complexity. Renaud et al. (2010) and Broderstad and Eythórsson (2014) contribute early efforts to identifying, modeling, and quantifying tipping points in coastal and marine SESs. Other studies addressed biophysical thresholds of biodiversity. Hossain and Szabo (2017), for example, analyzed biodiversity in the southwest coast of the Ganges-Brahmaputra Delta in Bangladesh and identified feedback in which shrimp farming increased the water's salinity to the point that the biodiversity threshold was exceeded and other species could not survive. The resulting loss of biodiversity was a benefit for shrimp producers.
Several reviewed studies addressed the relationship between social thresholds and ecological thresholds. Broderstad and Eythórsson (2014) showed how a series of ecological changes in Arctic Norway allowed invasive red king crabs to become a dominant species. This change in dominant species could have caused small fisheries there to collapse, but effective government interventions allowed fishers to harvest the red king crabs commercially while providing a complementary source of income to traditional cod fishers, preventing the ecological change in species from pushing fishing communities over the survivability threshold. In a qualitative study, Renaud et al. (2010) described how natural hazards such as tsunamis affected thresholds related to coastal community water supplies. Based on secondary information, they determined that the original quality of groundwater is not fully recovered after such natural disasters. In their case study, the water quality threshold was exceeded but the social threshold of the communities was not. The effect on the social threshold was more difficult to identify because of interventions by the national government and international community, which provided alternative sources of water that allowed the local communities to maintain their livelihoods.
Emerging Concept: Incorporating Land-Based Stressors
Our review of 98 coastal and marine SES studies reveals that land-based stressors associated with watersheds and watershed management are emerging as influential factors. Coastal systems are unique because of the interaction of land and sea environments, and human societies have been drawn to coastal resources in general and estuaries in particular and have extracted their resources for thousands of years (Gari et al., 2015). Zaucha et al. (2016), for example, found that the resilience of coastal systems benefits from cross-disciplinary land-to-sea watershed approaches to complex governance problems. To succeed, interventions must involve multiple stakeholders and address scale and temporal effects.
Several of the reviewed studies have noted that disturbances of coastal watersheds affect ocean resources to various extents. Several make tangential references to land-sea interactions to highlight the impacts of nutrient runoff caused by human activities (Lebel et al., 2006), the importance of freshwater environments to rural human societies (Forbes, 2013), connections between species coexistence and human resource allocations (Loring, 2016), and how human harvesting and recreational activities vary based on the flow of water between land and oceans (Berkes and Seixas, 2005). Other reviewed studies more fully incorporated land-based elements into their SES models. They found that freshwater scarcity stands out as a critical driver of ecosystem susceptibility (Hagenlocher et al., 2018) and that saline intrusion is a growing problem in coastal environments (Chapin et al., 2013). Ishtiaque et al. (2017), in a study of coastal Bangladesh, found that saline intrusions are severely affecting natural fish stocks and, consequently, human activities that rely on balanced watershed, marine, and estuarine ecosystems.
Zaucha et al. (2016) is by far the most comprehensive study of land-based stressors among studies reviewed in our synthesis. They acknowledged that land-sea interfaces involve particular characteristics, such as complex governance structures and high levels of uncertainty and were able to connect ecosystem services to the resilience of land-sea interfaces. They thus provide a way to integrate social and ecological processes into coastal management.
The studies of SESs in which land and sea systems interact have tended to focus on migrating species such as salmonids. Anadromous species require suitable marine and freshwater environments throughout their lives. Consequently, Hammer (2009), for example, found that restoring upstream habitats after severe incidents of pollution and large-scale land-use changes benefits these species and sport fisheries that rely on harvesting wild game fish.
Several of the reviewed studies highlight the challenges associated with governance at the land-sea interface since terrestrial and aquatic environments are typically covered by different authorities. This finding is congruent with Partelow et al. (2020), who criticized the traditional separation in land-sea regulation as well as the use of theories and applications grounded on some natural resources and specific sectors (see Partelow et al., 2020 for further information on governance theories applied to coastal environments). Bigagli (2016) described two global policies that address the unique characteristics of the land-sea interface: the Global Programme of Action for Protection of the Marine Environment from Land-based Activities (commonly referred to as the GPA) and the Water Framework Directive. Both take the connections between freshwater and coastal waters into account when developing sustainable management policies for coastal and marine systems. Two studies (Hammer, 2009; Vinueza et al., 2014) examined how ecosystem approaches, which address perturbations originating from both humans and the environment, were applied as a strategy to manage the terrestrial and marine interfaces. Likewise, Zaucha et al. (2016) proposed use of ecosystem services to establish links between environments and to ease decision-making processes in complex SESs. They showed that ecosystem services connect ecological systems to human welfare, providing long-term perspectives and collaboration by multiple stakeholders.
Discussion
Policymakers worldwide, in their efforts to address effects of human impacts on the environment, including species losses, water pollution and the effects of climate change, need reliable scientific information about the complex interactions between human social systems and ecological systems underlying coastal and marine resources. Policymakers are charged both with protecting and preserving healthy SESs and with establishing programs to remediate damaged systems. In this review of 98 studies of coastal and marine SESs spanning from 2003 through 2018, we analyze applications of four central properties of those systems—resilience, vulnerability, adaptive capacity, and governance—and non-linear processes of feedback and thresholds. Our purpose is to evaluate the progress of research into these critical, complex, and often compromised systems and to note areas that most need further study. In the process, we identified a fifth property, land-based stressors, increasingly used by researchers to assess coastal and marine systems and develop effective governance policies.
First, we find that methods must be developed to fully integrate social and ecological systems. Almost one-third of the sampled studies emphasized only one system (Table 1, C2). Only a small number focused more or less equally on the social and ecological systems and addressed them cohesively (e.g., Gordon, 2007; Manuel-Navarrete et al., 2007; Hu et al., 2018). Additional cohesive studies are required for development of a comprehensive understanding of coastal and marine SESs. However, our findings are limited to studies within the SES or human-environment literature. Other sets of related literature, such as work on integrated ecosystem assessment or decision making under deep uncertainty, may emphasize systems differently.
Second, we find that progress has been made in developing and applying qualitative and quantitative methods of analyzing resilience, vulnerability, adaptive capacity, and governance. The qualitative methods used in the reviewed studies include applying frameworks established to study other kinds of SESs, analyses of case studies, and heuristic models that can identify characteristics that weaken and strengthen socio-ecological properties in coastal and marine environments. Development of quantitative methods of measuring SES properties has been hampered by challenges associated with coupling variables from systems involving different metrics. Several of the reviewed studies addressed this difficulty by standardizing indicator values for each system to make them additive (Metcalf et al., 2015; Guillotreau et al., 2017; Li et al., 2018). Future studies are encouraged to develop improved methods with consistent metrics and spatial boundaries between ecological and social systems to take full advantage of the SES framework to improve coastal and marine management.
Several reviewed studies built on the knowledge that the key SES properties are interrelated and that estimating one SES property required evaluation of another SES property. For instance, Hu et al. (2018) used vulnerability and systems' adaptive capacity to measure socio-ecological resilience in coastal and marine SESs in mainland China. Fuller et al. (2017) computed the resilience of nodes of connectivity in fisheries and used those values as an input in a model measuring the sensitivity of human communities to disturbances such as the elimination of a fishery or a diminishing participation in a network because of ecological or management changes.
The results of several of the studies suggest that effective governance and policy and program interventions can blunt and even protect against adverse effects of natural disasters and disturbed ecosystems on coastal communities. For instance, Broderstad and Eythórsson (2014) showed in a study conducted in Norway that proliferation of red king crabs, an invasive species, pushed the ecosystems across a threshold, reducing the diversity of species in those waters. However, effective government interventions allowed fishers to harvest the red king crabs commercially while providing a complementary source of income to traditional cod fishers.
Conventional methods of governing and managing socio-ecological systems may work best when uncertainty is low, and management decisions can be largely about solving static optimization problems of sustaining fish stock or controlling point-source pollution to control nutrient run-off for example. Looking forward, defining and quantifying higher levels of deep uncertainties as states of multiple plausible futures is increasingly necessary as ever more vulnerable coastal SESs adapt to large global changes that have multiscalar and spatio-temporal impacts (Walker et al., 2013; Maier et al., 2016; Naylor et al., 2019; Elsawah et al., 2020). More research into integrating and explicitly characterizing the multi-dimensional nature of uncertainty in SES modeling is needed to inform the knowledge base and guide practice in the field (Elsawah et al., 2020). An emerging line of SES research, for example on managing watersheds (Carpenter et al., 2015) and marine ecosystems (Thrush et al., 2016) have begun to discuss pathways for integrating deep uncertainties into modeling resilient socio-ecological systems.
Third, we find that few of the studies identified and tested non-linear processes such as thresholds and feedback loops and the studies that did address these coupled systems mostly determine only the direction of changes and not the magnitude or the shape of the relationship in case of nonlinear response. Qualitative approaches used to identify feedbacks included using primary data to describe how elements interact (Kaplan-Hallam et al., 2017) and a literature review (e.g., Manuel-Navarrete et al., 2007). Two of the studies used loop analysis (Martone et al., 2017) and fuzzy cognitive mapping (Tiller et al., 2016) as semi-quantitative methods to identify feedbacks. Fuzzy cognitive mapping is useful for making consistent comparisons of different scenarios and concurrent disturbances because it considers only the initial states of critical variables involved in the feedback mechanisms. Loop analysis, on the other hand, circumvents the initial-state requirement by using only the effect one variable has on the rate of change of the other. Both methods represent biological and socio-economic variables mathematically, allowing one to define the qualitative effect of one variable on another. The disadvantage of these methods is that they can only determine the direction of the change—positive, negative, or neutral—but not the magnitude or the shape of the relationship. Thus, these studies have identified and described some feedback within and between social and ecological systems, but techniques are needed to measure the magnitude of such changes and thus how feedback loops change when additional variables are introduced.
Furthermore, we find that coastal and marine SES studies are progressively using quantitative methods to examine feedback and thresholds. However, similar to Ferraro et al. (2019), we also find that studies would greatly benefit from development of quantitative methods that can identify both the causality and the magnitude of the effects. Studies that incorporate methods that can examine bidirectional and non-linear effects through mathematical or computational simulation modeling can more reliably simulate the effects of policies and predict the direction of the effects of interventions on interacting elements of SESs.
Fourth and lastly, we identify a contextual gap in many coastal and marine SES studies: the effects of land-based stressors on coupled human social and ocean ecological systems. Though land-sea interactions have been integrated into coastal management regimes (e.g., Portman et al., 2015), few coastal SES studies reviewed here have explicitly taken the influence of watersheds and watershed management into account. Nutrient runoff, bacterial pollution, and management of anadromous species are likely to affect the resilience, vulnerability, and adaptive capacity of coastal systems and should be considered in future SES studies. This research can benefit from integrating the insights from the “decision-making under deep uncertainty” literature, which has created adaptive models with such linkages with the goal of preparing policy-makers for surprises and enabling more informed, robust decisions (e.g., Thrush et al., 2016; Gladstone-Gallagher et al., 2019; Groves et al., 2019).
Data Availability Statement
The original contributions presented in the study are included in the article/Supplementary Material, further inquiries can be directed to the corresponding author/s.
Author Contributions
SR-C contributed to the design of this study, extracted, analyzed and reviewed data from literature, prepared the figures and tables, and wrote the manuscript. EU conceived and designed this review article, contributed to reviewing data from the literature and writing the article. All authors participated in development of this review methodology, contributed to the article, and approved the submitted version.
Funding
This material is based on work supported in part by the National Science Foundation under EPSCoR Cooperatives Agreement #OIA-1655221.
Conflict of Interest
The authors declare that the research was conducted in the absence of any commercial or financial relationships that could be construed as a potential conflict of interest.
Supplementary Material
The Supplementary Material for this article can be found online at: https://www.frontiersin.org/articles/10.3389/fmars.2021.648006/full#supplementary-material
Footnotes
1. ^See Gladstone-Gallagher et al. (2019) for an example of adaptive decision-making under intermediate to deep uncertainty applied to coastal eutrophication.
2. ^There are some limitations to this search query. First, socio-ecological and human-environment systems are often described by a variety of terms; as a consequence, studies that do not explicitly characterize their systems as social-ecological, socioecological, or human-environment systems may not have been captured by the query. For instance, the literature in decision making under deep uncertainty and integrated ecosystem assessment both deal with SESs but may not use these terms explicitly. Also, we initially conducted a broader search using, for instance, (“soci* ecolog*” system*) and (coast* or ocean* or marin* or sea*) in the titles, abstracts, and keywords, and after excluding unrelated subject areas (Medicine, arts and humanities, nursing, immunology and microbiology, pharmacology, toxicology and pharmaceutics, chemical engineering, physics and astronomy, chemistry, health professions, neuroscience, veterinary, engineering, and biochemistry, genetics and molecular biology), we obtained 873 hits. However, a random check of the hits showed that many did not describe SES frameworks and their components. We therefore narrowed the search by limiting the search of the phrases to the title.
References
Adger, W. N. (2006). Vulnerability. Global Environ. Change 16, 268–281. doi: 10.1016/j.gloenvcha.2006.02.006
Ban, N. C., Whitney, C., Davies, T. E., Buscher, E., Lancaster, D., Eckert, L., et al. (2017). “Conservation actions at global and local scales in marine social-ecological systems: status, gaps, and ways forward,” in Conservation for The Anthropocene Ocean. Interdisciplinary Science in Support of Nature and People (Academic Press), 143–168. Available online at: https://www.sciencedirect.com/science/article/pii/B9780128053751000088?via%3Dihub
Barclay Frey, J., and Berkes, F. (2014). Can partnerships and community-based conservation reverse the decline of coral reef social-ecological systems? Int. J. Commons 8, 26–46. doi: 10.18352/ijc.408
Barnett, A. J., and Anderies, J. M. (2014). Weak feedbacks, governance mismatches, and the robutsness of social-ecological systems: an analysis of the Southwest Nova Scotia lobster fishery with comparison to Maine. Ecol. Soc. 19:39. doi: 10.5751/ES-06714-190439
Barrett, C. B., Travis, A. J., and Dasgupta, P. (2011). On biodiversity conservation and poverty traps. Proc. Natl. Acad. Sci. U. S. A. 108:13907. doi: 10.1073/pnas.1011521108
Begossi, A., Salyvonchyk, S., Nora, V., Lopes, P. F., and Silvano, R. A. M. (2012). The paraty artisanal fishery (southeastern Brazilian coast): ethnoecology and management of a social-ecological system. J. Ethnobiol. Ethnomed. 8:22. doi: 10.1186/1746-4269-8-22
Benham, C. F. (2017). Aligning public participation with local environmental knowledge in complex marine social-ecological systems. Mar. Policy 82, 16–24. doi: 10.1016/j.marpol.2017.04.003
Berkes, F. (2011). “Restoring Unity: the concept of marine social-ecological systems,” in World Fisheries: A Social-Ecological Analysis Fish and Aquatic Resources Series, eds R. E. Ommer, R. Ian Perry, K. Cochrane, and P. Cury (Oxford: Blackwell Publishing Ltd.), 9–25.
Berkes, F. (2017). Environmental Governance for the Anthropocene? Social-Ecological Systems, Resilience, and Collaborative Learning. Sustainability 9:1232. doi: 10.3390/su9071232
Berkes, F., and Seixas, C. S. (2005). Building resilience in lagoon social-ecological systems: a local-level perspective. Ecosystems 8, 967–974. doi: 10.1007/s10021-005-0140-4
Bigagli, E. (2015). The EU legal framework for the management of marine complex social-ecological systems. Mar. Policy 54, 44–51. doi: 10.1016/j.marpol.2014.11.025
Bigagli, E. (2016). The international legal framework for the managemetn of the global oceans social-ecological system. Mar. Policy 68, 155–164. doi: 10.1016/j.marpol.2016.03.005
Binder, C. R., Hinkel, J., Bots, P. W. G., and Pahl-Wostl, C. (2013). Comparison of frameworks for analyzing social-ecological systems. Ecol. Soc. 18:26. doi: 10.5751/ES-05551-180426
Broderstad, E. G., and Eythórsson, E. (2014). Resilient communities? Collapse and recovery of a social-ecological system in Arctic Norway. Ecol. Soc. 19:1. doi: 10.5751/ES-06533-190301
Burbano, D. V., Mena, C. F., Guarderas, P., Vinueza, L., and Reck, G. (2014). “Shifting baselines in the Galapagos White Fin Fishery, using fisher's Anecdotes to Reassess Fisheries Management: the case of the Galapagos Grouper,” in The Galapagos Marine Reserve: A Dynamic Social-Ecological System, eds. J. Denkinger and L. Vinueza (Cham: Springer International Publishing), 227–246.
Burkhard, B., and Gee, K. (2012). Establishing the Resilience of a Coastal-marine Social-ecological System to the Installation of Offshore Wind Farms. Ecol Soc. 17:32. doi: 10.5751/ES-05207-170432
Carpenter, S. R., Booth, E. G., Gillon, S., Kucharik, C. J., Loheide, S., Mase, A. S., et al. (2015). Plausible futures of a social-ecological system: Yahara watershed, Wisconsin, USA. Ecol. Soc. 20:10. doi: 10.5751/ES-07433-200210
Chalcobsky, B. A., Crespo, E. A., and Coscarella, M. A. (2017). Whale-watching in Patagonia: what regulation scheme should be implemented when the socio-ecological system is changing? Mar. Policy 75, 165–173. doi: 10.1016/j.marpol.2016.11.010
Chang, C.-T., Vadeboncoeur, M. A., and Lin, T.-C. (2018). Resistance and resilience of social-ecological systems to recurrent typhoon disturbance on a subtropical island: Taiwan. Ecosphere 9:e02071. doi: 10.1002/ecs2.2071
Chapin, F. S. III, Robards, M. D., Johnstone, J. F., Lantz, T. C., and Kokelj, S. V. (2013). “Case Study: novel socio-ecological systems in the north: potential pathways toward ecological and societal resilience,” in Novel Ecosystems In the New Ecological World Order, eds R. J. Hobbs, E. S. Higgs, and C. M. Hall (Chichester: John Wiley and Sons, Ltd.), 334–344.
Charles, A. (2012). People, oceans and scale: governance, livelihoods and climate change adaptation in marine social-ecological systems. Curr. Opin. Environ. Sustain. 4, 351–357. doi: 10.1016/j.cosust.2012.05.011
Cinner, J. E., McClanahan, T. R., MacNeil, M. A., Graham, N. A. J., Daw, T. M., Mukminin, A., et al. (2012). Comanagement of coral-reef social-ecological systems. Proc. Natl. Acad. Sci. U.S.A. 109, 5219–5222. doi: 10.1073/pnas.1121215109
Committee on Environment and Natural Resources (2010). Scientific Assessment of Hypoxia in U.S. Coastal Waters. Washington, DC: Interagency Working Group on Harmful Algal Blooms, Hypoxia, and Human Health of the Joint Subcommittee on Ocean Science and Technology.
Crépin, A.-S., Gren, Å., Engström, G., and Ospina, D. (2017). Operationalising a social-ecological system perspective on the Arctic Ocean. Ambio 46, 475–485. doi: 10.1007/s13280-017-0960-4
Cullen-Unsworth, L. C., Nordlund, L. M., Paddock, J., Baker, S., McKenzie, L. J., and Unsworth, R. K. F. (2014). Seagrass meadows globally as a coupled social-ecological system: Implications for human wellbeing. Mar. Pollut. Bull. 83, 387–397. doi: 10.1016/j.marpolbul.2013.06.001
D'Anna, L. M., and Murray, G. D. (2015). Perceptions of shellfish aquaculture in British Columbia and implications for well-being in marine social-ecological systems. Ecol. Soc. 20:57. doi: 10.5751/ES-07319-200157
Daoud, I., Oman, M. A.-E.-Z., Alary, V., Moselhy, N., Salal, E., Naga, A. A., et al. (2016). “Adaptation and resilience in pastoral management of the Mediterranean Bedouin social-ecological system in the northwestern coastal Zone of Egypt,” in Building Resilience of Human-Natural Systems of Pastoralism in the Developing World: Interdisciplinary Perspectives, eds. S. Dong, K.-A. S. Kassam, J. F. Tourrand, and R. B. Boone (Cham: Springer International Publishing), 209–250. doi: 10.1007/978-3-319-30732-9_6
Davies, N., Field, D., Gavaghan, D., Holbrook, S. J., Planes, S., Troyer, M., et al. (2016). Simulating social-ecological systems: the Island Digital Ecosystem Avatars (IDEA) consortium. Gigascience 5:14. doi: 10.1186/s13742-016-0118-5
De Andrès, M., Barragán, J. M., and García Sanabria, J. (2018). Ecosystem services and urban development in coastal social-ecological systems: The Bay of Cádiz case study. Ocean & Coastal Management. 154, 155–167. doi: 10.1016/j.ocecoaman.2018.01.011
Denkinger, J., Quiroga, D., and Murillo, J. C. (2014). “Assessing human-wildlife conflicts and benefits of galápagos sea lions on San Cristobal Island, Galápagos,” in The Galapagos Marine Reserve. Social and Ecological Interactions in the Galapagos Islands (Heidelberg; New York, NY; Dordrecht; London; Cham: Springer).
Elsawah, S., Filatova, T., Jakeman, A. J., Kettner, A. J., Zellner, M. L., Athanasiadis, I. N., et al. (2020). Eight grand challenges in socio-environmental systems modeling. SESMO 2:16226. doi: 10.18174/sesmo.2020a16226
Engie, E., and Quiroga, D. (2014). “The emergence of recreational fishing in the Galapagos Marine Reserve: adaptation and complexities,” in The Galapagos Marine Reserve: A Dynamic Social-Ecological System, eds. J. Denkinger and L. Vinueza (Cham: Springer), 203–226.
Faulkner, L., Brown, K., and Quinn, T. (2018). Analyzing community resilience as an emergent property of dynamic social-ecological systems. Ecol. Soc. 23:24. doi: 10.5751/ES-09784-230124
Ferraro, P. J., Sanchirico, J. N., and Smith, M. D. (2019). Causal inference in coupled human and natural systems. Proc. Natl. Acad. Sci. U. S. A. 116:5311. doi: 10.1073/pnas.1805563115
Ferrol-Schulte, D., Wolff, M., Ferse, S., and Glaser, M. (2013). Sustainable Livelihoods Approach in tropical coastal and marine social-ecological systems: a review. Mar. Policy 42, 253–258. doi: 10.1016/j.marpol.2013.03.007
Fleischman, F. D., Ban, N. C., Evans, L. S., Epstein, G., Garcia-Lopez, G., and Villamayor-Tomas, S. (2014). Governing large-scale social-ecological systems: lessons from five cases. Int. J. Commons 8, 428–456. doi: 10.18352/ijc.416
Folke, C., Carpenter, S., Elmqvist, T., Gunderson, L., Holling, C. S., and Walker, B. (2002). Resilience and sustainable development: building adaptive capacity in a world of transformations. AMBIO J. Hum. Environ. 31, 437–440. doi: 10.1579/0044-7447-31.5.437
Forbes, B. C. (2013). Cultural resilience of social-ecological systems in the Nenets and Yamal-Nenets Autonomous Okrugs, Russia: a focus on Reindeer Nomads of the Tundra. Ecol. Soc. 18:36. doi: 10.5751/ES-05791-180436
Fuller, E. C., Samhouri, J. F., Stoll, J. S., Levin, S. A., and Watson, J. R. (2017). Characterizing fisheries connectivity in marine social-ecological systems. ICES J. Mar. Sci. 74, 2087–2096. doi: 10.1093/icesjms/fsx128
Gallopin, G. C. (2006). Linkages between vulnerability, resilience, and adaptive capacity. Glob. Environ. Change 16, 293–303. doi: 10.1016/j.gloenvcha.2006.02.004
Gari, S. R., Newton, A., and Icely, J. D. (2015). A review of the application and evolution of the DPSIR framework with an emphasis on coastal social-ecological systems. Ocean Coast. Manag. 103, 63–77. doi: 10.1016/j.ocecoaman.2014.11.013
Gladstone-Gallagher, R. V., Hope, J. A., Bulmer, R. H., Clark, D. E., Stephenson, F., Mangan, S., et al. (2019). Old tools, new ways of using them: harnessing expert opinions to plan for surprise in marine socio-ecological systems. Front. Mar. Sci. 6:696. doi: 10.3389/fmars.2019.00696
Glaeser, B. (2016). From global sustainability research matrix to typology: a tool to analyze coastal and marine social-ecological systems. Reg. Environ. Change 16, 367–383. doi: 10.1007/s10113-015-0817-y
Glaser, M., Christie, P., Diele, K., Dsikowitzky, L., Ferse, S., Nordhaus, I., et al. (2012). Measuring and understanding sustainability-enhancing processes in tropical coastal and marine social-ecological systems. Curr. Opin. Environ. Sustain. 4, 300–308. doi: 10.1016/j.cosust.2012.05.004
Glaser, M., and Glaeser, B. (2014). Towards a framework for cross-scale and multi-level analysis of coastal and marine social-ecological systems dynamics. Reg. Environ. Change 14, 2039–2052. doi: 10.1007/s10113-014-0637-5
Glaser, M., Krause, G., Oliveira, R. S., and Fontalvo-Herazo, M. (2010). “Mangroves and people: a social-ecological system,” in Mangrove Dynamics and Management in North Brazil, eds. U. Saint-Paul and H. Schneider (Berlin; Heidelberg: Springer Berlin Heidelberg), 307–351.
González, J. A., Montes, C., Rodríguez, J., and Tapia, W. (2008). Rethinking the Galapagos Islands as a complex social-ecological system: implications for conservation and management. Ecol. Soc. 13:13. doi: 10.5751/ES-02557-130213
Gordon, I. J. (2007). Linking land to ocean: feedbacks in the management of socio-ecological systems in tge Great Barrier Reef catchments. Hydrobiologia 591, 25–33. doi: 10.1007/s10750-007-0781-8
Groves, D. G., Molina-Perez, E., Bloom, E., and Fischbach, J. R. (2019). “Robust Decision Making (RDM): application to water planning and climate policy,” in Decision Making under Deep Uncertainty: From Theory to Practice, eds. V. A. W. J. Marchau, W. E. Walker, P. J. T. M. Bloemen, and S. W. Popper (Cham: Springer International Publishing), 135–163.
Guillotreau, P., Allison, E. H., Bundy, A., Cooley, S. R., Defeo, O., Le Bihan, V., et al. (2017). A comparative appraisal of the resilience of marine social-ecological systems to mass mortalities of bivalves. Ecol. Soc. 22:46. doi: 10.5751/ES-09084-220146
Hagenlocher, M., Renaud, F. G., Haas, S., and Sebesvari, Z. (2018). Vulnerability and risk of deltaic social-ecological systems exposed to multiple hazards. Sci. Total Environ. 631–632, 71–80. doi: 10.1016/j.scitotenv.2018.03.013
Hammer, M. (2009). “Whose fish? managing salmonids and humans in complex social-ecological systems: examples from the Baltic Sea Region,” in Challenges for Diadromous Fishes in a Dynamic Global Environment American Fisheries Society Symposium (American Fisheries Society), 663–675.
Hossain, M. S., and Szabo, S. (2017). “Understanding the social-ecological system of wetlands,” in Wetland science: Perspectives from South Asia, eds Prusty, B. Anjan Kumar, C. Rachna, and P. A. Azeez, (New Delhi: Springer India), 285–301.
Hu, M., Zhang, J., and Huang, J. (2018). Assessing social-ecological system resilience in mainland China. Pol. J. Environ. Stud. 27, 1085–1096. doi: 10.15244/pjoes/76242
Ishtiaque, A., Sangwan, N., and Yu, D. J. (2017). Robust-yet-fragile nature of partly engineered social-ecological systems: a case study of coastal Bangladesh. Ecol. Soc. 22:5. doi: 10.5751/ES-09186-220305
Jambeck, J. R., Geyer, R., Wilcox, C., Siegler, T. R., Perryman, M., Andrady, A., et al. (2015). Plastic waste inputs from land into the ocean. Science 347:768. doi: 10.1126/science.1260352
Jarre, A., Ragaller, S. M., and Hutchings, L. (2013). Long-term, ecosystem-scale changes in the southern Benguela marine pelagic social-ecological system: interaction of natural and human drivers. Ecol. Soc. 18:55. doi: 10.5751/ES-05917-180455
Jarre, A., Shannon, L. J., Cooper, R., Duggan, G. L., Gammage, L. C., Lockerbie, E. M., et al. (2018). Untangling a Gordian knot that must not be cut: social-ecological systems research for management of southern Benguela fisheries. J. Mar. Syst. 188, 149–159. doi: 10.1016/j.jmarsys.2018.01.004
Kaplan-Hallam, M., Bennett, N. J., and Satterfield, T. (2017). Catching sea cucumber fever in coastal communities: conceptualizing the impacts of shocks versus trends on social-ecological systems. Glob. Environ. Change 45, 89–98. doi: 10.1016/j.gloenvcha.2017.05.003
Kittinger, J. N., Finkbeiner, E. M., Glazier, E. W., and Crowder, L. B. (2012). Human dimensions of coral reef social-ecological systems. Ecol. Soc. 17:17. doi: 10.5751/ES-05115-170417
Lade, S. J., and Niiranen, S. (2017). Generalized modeling of empirical social-ecological systems. Nat. Res. Model. 30:e12129. doi: 10.1111/nrm.12129
Larsen, R. K., Calgaro, E., and Thomalla, F. (2011). Governing resilience building in Thailand's tourism-dependent coastal communities: Conceptualising stakeholder agency in social-ecological systems. Glob. Environ. Change 21, 481–491. doi: 10.1016/j.gloenvcha.2010.12.009
Lazarus, E. D. (2014). Threshold effects of hazard mitigation in coastal human-environmental systems. Earth Surf. Dyn. 2, 35–45. doi: 10.5194/esurf-2-35-2014
Lebel, L., Anderies, J. M., Campbell, B., Folke, C., Hatfield-Dodds, S., Hughes, T. P., et al. (2006). Governance and the capacity to manage resilience in regional social-ecological systems. Ecol. Soc. 11:19. doi: 10.5751/ES-01606-110119
Leslie, H. M., Basurto, X., Nenadovic, M., Leila, S., Cavanaugh, K. C., Cota-Nieto, J. J., et al. (2015). Operationalizing the social-ecological systems framework to assess sustainability. Proc. Natl. Acad. Sci. U. S. A. 112, 5979–5984. doi: 10.1073/pnas.1414640112
Leslie, H. M., Schlüter, M., Cudney-Bueno, R., and Levin, S. A. (2009). Modeling responses of coupled social-ecological systems of Gulf of California to anthropogenic and natural perturbations. Ecol. Res. 24, 505–519. doi: 10.1007/s11284-009-0603-8
Levin, P. S., Francis, T. B., and Taylor, N. G. (2016). Thirty-two essential questions for understanding the social-ecological system of forage fish: the case of pacific herring. Ecosyst. Health Sustain. 2:e01213. doi: 10.1002/ehs2.1213
Levin, P. S., Williams, G. D., Rehr, A., Norman, K. C., and Harvey, C. J. (2015). Developing conservation targets in social-ecological systems. Ecol. Soc. 20:6. doi: 10.5751/ES-07866-200406
Li, Y., Kappas, M., and Li, Y. (2018). Exploring the coastal urban resilience and transformation of coupled human-environment systems. J. Clean. Prod. 195, 1505–1511. doi: 10.1016/j.jclepro.2017.10.227
Liu, J., Dietz, T., Carpenter, S. R., Alberti, M., Folke, C., Moran, E., et al. (2007). Complexity of coupled human and natural systems. Science 317:1513. doi: 10.1126/science.1144004
London, S., Rojas, M. L., Ibáñez Martin, M. M., Scordo, F., Huamantinco Cisneros, M. A., Bustos, M. L., et al. (2017). Characterization of an artisanal fishery in Argentina using the social-ecological systems framework. Int. J. Commons 11, 1–69. doi: 10.18352/ijc.534
López-Angarita, J., Moreno-Sánchez, R., Maldonado, J. H., and Sánchez, J. A. (2014). Evaluating linked social-ecological systems in marine protected areas. Conserv. Lett. 7, 241–252. doi: 10.1111/conl.12063
Loring, P. A. (2016). Toward a Theory of Coexistence in Shared Social-Ecological Systems: The case of Cool Inlet Salmon Fisheries. Hum Ecol 44, 123–165. doi: 10.1007/s10745-016-9806-0
Lozoya, J. P., Conde, D., Asmus, M., Polette, M., Píriz, C., Martins, F., et al. (2015). “Linking social perception and risk analysis to assess vulnerability of coastal socio-ecological systems to climate change in Atlantic South America,” in Handbook of Climate Change Adaptation, ed. W. Leal Filho (Berlin, Heidelberg: Springer), 373–399.
Maier, H. R., Guillaume, J. H. A., van Delden, H., Riddell, G. A., Haasnoot, M., and Kwakkel, J. H. (2016). An uncertain future, deep uncertainty, scenarios, robustness and adaptation: how do they fit together? Environ. Model. Softw. 81, 154–164. doi: 10.1016/j.envsoft.2016.03.014
Manuel-Navarrete, D., Gómez, J. J., and Gallopín, G. (2007). Syndromes of sustainability of development for assessing the vulnerability of coupled human-environmental systems. The case of hydrometeorological disasters in Central America and the Caribbean. Glob. Environ. Change 17, 207–217. doi: 10.1016/j.gloenvcha.2006.07.002
Marín, A., Gelcich, S., and Castilla, J. C. (2014). Ecosystem services and abrupt transformations in a coastal wetland social-ecological system: tubul-raqui after the 2010 earthquake in chile. Ecol. Soc. 19:22. doi: 10.5751/ES-05633-190122
Martone, R. G., Bodini, A., and Micheli, F. (2017). Identifying potential consequences of natural perturbations and management decisions on a coastal fishery social-ecological system using qualitative loop analysis. Ecol. Soc. 22:34. doi: 10.5751/ES-08825-220134
Mauerhofer, V., Ichinose, T., Blackwell, B. D., Willig, M. R., Flint, C. G., Krause, M. S., et al. (2018). Underuse of social-ecological systems: a research agenda for addressing challenges to biocultural diversity. Land Use Policy 72, 57–64. doi: 10.1016/j.landusepol.2017.12.003
McClanahan, T. R., Castilla, J. C., White, A. T., and Defeo, O. (2009). Healing small-scale fisheries by facilitating complex socio-ecological systems. Rev. Fish Biol. Fisheries 19, 33–47. doi: 10.1007/s11160-008-9088-8
Mee, L., Cooper, P., Kannen, A., Gilbert, A. J., and O'Higgins, T. (2015). Sustaining Europe's seas as coupled social-ecological systems. Ecol. Soc. 20:101. doi: 10.5751/ES-07143-200101
Melbourne-Thomas, J., Constable, A. J., Fulton, E. A., Corney, S. P., Trebilco, R., Hobday, A. J., et al. (2017). Integrated modelling to support decision-making for marine social-ecological systems in Australia. ICES J. Mar. Sci. 74, 2298–2308. doi: 10.1093/icesjms/fsx078
Mens, M. J. P., Klijn, F., de Bruijn, K. M., and van Beek, E. (2011). The meaning of system robustness for flood risk management. Environ. Sci. Policy 14, 1121–1131. doi: 10.1016/j.envsci.2011.08.003
Metcalf, S. J., van Putten, E. I., Frusher, S., Marshall, N. A., Tull, M., Caputi, N., et al. (2015). Measuring the vulnerability of marine social-ecological systems: a prerequisite for the identification of climate change adaptations. Ecol. Soc. 20:35. doi: 10.5751/ES-07509-200235
Murphy, D. W. A. (2015). Theorizing climate change, (im)mobility and socio-ecological systems resilience in low-elevation coastal zones. Clim. Dev. 7, 380–397. doi: 10.1080/17565529.2014.953904
Murray, G., and D'Anna, L. (2015). Seeing shellfish from the seashore: the importance of values and place in perceptions of aquaculture and marine social-ecological system interactions. Mar. Policy 62, 125–133. doi: 10.1016/j.marpol.2015.09.005
Murray, G., D'Anna, L., and MacDonald, P. (2016). Measuring what we value: the utility of mixed methods approaches for incorporating values into marine social-ecological system management. Mar. Policy 73, 61–68. doi: 10.1016/j.marpol.2016.07.008
Murray, G., Neis, B., Schneider, D. C., Ings, D., Gosse, K., Whalen, J., et al. (2008). “Opening the black box: methods, procedures, and challenges in the historical reconstruction of marine social-ecological systems,” in Making and Moving Knowledge Interdisciplinary and Community-based Research in a World on the Edge, eds. B. Neis and J. S. Lutz (McGill-Queen's University Press), 100–120.
Myers, R. A., and Worm, B. (2003). Rapid worldwide depletion of predatory fish communities. Nature 423, 280–283. doi: 10.1038/nature01610
Naylor, L. A., Brady, U., Quinn, T., Brown, K., and Anderies, J. M. (2019). A multiscale analysis of social-ecological system robustness and vulnerability in Cornwall, UK. Reg. Environ. Change 19, 1835–1848. doi: 10.1007/s10113-019-01530-7
Nyström, M., Norström, A. V., Blenckner, T., de la Torre-Castro, M., Eklöf, J. S., Folke, C., et al. (2012). Confronting feedbacks of degraded marine ecosystems. Ecosystems 15, 695–710. doi: 10.1007/s10021-012-9530-6
Partelow, S., and Boda, C. (2015). A modified diagnostic social-ecological system framework for lobster fisheries: case implementation and sustainability assessment in Southern California. Ocean Coast. Manage. 114, 204–217. doi: 10.1016/j.ocecoaman.2015.06.022
Partelow, S., Schlüter, A., Armitage, D., Bavinck, M., Carlisle, K., Gruby, R. L., et al. (2020). Environmental governance theories: a review and application to coastal systems. Ecol. Soc. 25:19. doi: 10.5751/ES-12067-250419
Peña-Alonso, C., Ariza, E., Hernández-Calvento, L., and Pérez-Chacón, E. (2018). Exploring multi-dimensional recreational quality of beach socio-ecological systems in the Canary Islands (Spain). Tourism Manag. 64, 303–313. doi: 10.1016/j.tourman.2017.09.008
Perry, R. I., and Masson, D. (2013). An integrated analysis of the marine social-ecological system of the Strait of Georgia, Canada, over the past four decades, and development of a regime shift index. Prog. Oceanogr. 115, 14–27. doi: 10.1016/j.pocean.2013.05.021
Perry, R. I., Ommer, R. E., Barange, M., and Werner, F. (2010). The challenge of adapting marine social-ecological systems to the additional stress of climate change. Curr. Opin. Environ. Sustain. 2, 356–363. doi: 10.1016/j.cosust.2010.10.004
Polasky, S., Carpenter, S. R., Folke, C., and Keeler, B. (2011). Decision-making under great uncertainty: environmental management in an era of global change. Trends Ecol. Evol. 26, 398–404. doi: 10.1016/j.tree.2011.04.007
Pollnac, R., Christie, P., Cinner, J. E., Dalton, T., Daw, T. M., Forrester, G. E., et al. (2010). Marine reserves as linked social-ecological systems. Proc. Natl. Acad. Sci. U.S.A. 107:18262. doi: 10.1073/pnas.0908266107
Portman, M. E., Dalton, T. M., and Wiggin, J. (2015). Revisiting integrated coastal zone management: is it past its prime? Environ. Sci. Policy Sustain. Dev. 57, 28–37. doi: 10.1080/00139157.2015.1001693
Quinn, C. H., Stringer, L. C., Berman, R. J., Le, H. T. V., Msuya, F. E., Pezzuti, J. C. B., et al. (2017). Unpacking changes in mangrove social-ecological systems: lessons from Brazil, Zanzibar, and Vietnam. Resources 6. doi: 10.3390/resources6010014
Reenberg, A., Birch-Thomsen, T., Mertz, O., Fog, B., and Christiansen, S. (2008). Adaptation of human coping strategies in a small island society in the SW Pacific- 50 Years of change in the coupled human-environment system on Bellona, Solomon Islands. Hum. Ecol. 36, 807–819. doi: 10.1007/s10745-008-9199-9
Renaud, F. G., Birkmann, J., Damm, M., and Gallopín, G. C. (2010). Understanding multiple thresholds of coupled social-ecological systems exposed to natural hazards as external shocks. Nat. Hazards 55, 749–763. doi: 10.1007/s11069-010-9505-x
Resilience Alliance – Resilience. Available online at: https://www.resalliance.org/resilience (accessed March 27, 2020).
Roebeling, P., Alves, H., Rocha, J., Brito, A., Almeida, P., and Mamede, J. (2014). Gains from trans-boundary water quality management in linked catchment and coastal socio-ecological systems: a case study for the Minho region. Water Resour. Econ. 8, 32–42. doi: 10.1016/j.wre.2014.10.002
Rothenberger, M., Armstrong, A., and Spitz, M. (2018). Social-ecological system responses to Hurricane Sandy in the Hudson-Raritan Estuary. Ambio 47, 284–297. doi: 10.1007/s13280-017-0949-z
Scheffer, M., Carpenter, S., Foley, J. A., Folke, C., and Walker, B. (2001). Catastrophic shifts in ecosystems. Nature 413, 591–596. doi: 10.1038/35098000
Schimel, D., Hibbard, K., Costa, D., Cox, P., and Van Der Leeuw, S. (2015). Analysis, integration and modeling of the earth system (AIMES): advancing the post-disciplinary understanding of coupled human-environment dynamics in the Anthropocene. Anthropocene 12, 99–106. doi: 10.1016/j.ancene.2016.02.001
Stevenson, T. C., and Tissot, B. N. (2014). Current trends in the analysis of co-management arrangements in coral reef ecosystems: a social-ecological systems perspective. Curr. Opin. Environ. Sustain. 7, 134–139. doi: 10.1016/j.cosust.2014.02.002
Sun, X., He, J., Shi, Y., Zhu, X., and Li, Y. (2012). Spatiotemporal change in land use patterns of coupled human-environment system with an integrated monitoring approach: a case study of Lianyungang, China. Ecol. Compl. 12, 23–33. doi: 10.1016/j.ecocom.2012.09.002
Thrush, S. F., Lewis, N., Le Heron, R., Fisher, K. T., Lundquist, C. J., and Hewitt, J. (2016). Addressing surprise and uncertain futures in marine science, marine governance, and society. Ecol. Soc. 21:44. doi: 10.5751/ES-08574-210244
Tiller, R., De Kok, J.-L., Vermeiren, K., Richards, R., Van Ardelan, M., and Bailey, J. (2016). Stakeholder perceptions of links between environmental changes to their socio-ecological system and their adaptive capacity in the region of Troms, Norway. Front. Mar. Sci. 3:267. doi: 10.3389/fmars.2016.00267
Trosper, R. L. (2003). Resilience in pre-contact pacific northwest social ecological systems. Conserv. Ecol. 7:6. doi: 10.5751/ES-00551-070306
Usseglio, P., Schuhbauer, A., and Friedlander, A. (2014). “Collaborative approach to fisheries management as a way to increase the effectiveness of future regulations in the Galapagos Archipelago,” in The Galapagos Marine Reserve. Social and Ecological Interactions in the Galapagos Islands (Heidelberg; New York, NY; Dordrecht; London; Cham: Springer).
Van Dolah, E. R., Paolisso, M., Sellner, K., and Place, A. (2016). Employing a socio-ecological systems approach to engage harmful algal bloom stakeholders. Aquat. Ecol. 50, 577–594. doi: 10.1007/s10452-015-9562-z
Van Putten, I. E., Dichmont, C. M., Dutra, L. X. C., Thébaud, O., Deng, R. A., Jebreen, E., et al. (2016). Objectives for management of socio-ecological systems in the Great Barrier Reef region, Australia. Reg. Environ. Change 16, 1417–1431. doi: 10.1007/s10113-015-0867-1
Villamor, G. B., Palomo, I., López Santiago, C. A., Oteros-Rozas, E., and Hill, J. (2014). Assessing stakeholders' perceptions and values towards social-ecological systems using participatory methods. Ecol. Process. 3:22. doi: 10.1186/s13717-014-0022-9
Villasante, S. (2012). The management of the blue whiting fishery as complex social-ecological system; The Galician case. Mar. Policy 36, 1301–1308. doi: 10.1016/j.marpol.2012.02.013
Vinueza, L., Post, A., Guarderas, P., Smith, F., and Idrovo, F. (2014). “Ecosystem-based management for rocky shores of the Galapagos Islands,” in The Galapagos Marine Reserve: A Dynamic Social-Ecological System, eds. J. Denkinger and L. Vinueza(Cham: Springer), 81–106.
Vugteveen, P., Rouwette, E., Stouten, H., van Katwijk, M. M., and Hanssen, L. (2015). Developing social-ecological system indicators using group model building. Ocean Coast. Manag. 109, 29–39. doi: 10.1016/j.ocecoaman.2015.02.011
Walker, B., Holling, C. S., Carpenter, S. R., and Kinzig, A. (2004). Resilience, adaptability and transformability in social-ecological systems. Ecol. Soc. 9:5. doi: 10.5751/ES-00650-090205
Walker, W. E., Lempert, R. J., and Kwakkel, J. H. (2013). “Deep uncertainty,” in Encyclopedia of Operations Research and Management Science, eds. S. I. Gass and M. C. Fu (Boston, MA: Springer US), 395–402.
Wawo, M. (2017). Social-ecological system in seagrass ecosystem management at Kotania Bay Waters, Western Seram, Indonesia. IOP Conf. Ser. Earth Environ. Sci. 89:012023. doi: 10.1088/1755-1315/89/1/012023
Whitney, C. K., Bennett, N. J., Ban, N. C., Allison, E. H., Armitage, D., Blythe, J. L., et al. (2017). Adaptive capacity: from assessment to action in coastal social-ecological systems. Ecol. Soc. 22:22. doi: 10.5751/ES-09325-220222
Keywords: adaptive capacity, feedback, governance, resilience, thresholds, vulnerability
Citation: Refulio-Coronado S, Lacasse K, Dalton T, Humphries A, Basu S, Uchida H and Uchida E (2021) Coastal and Marine Socio-Ecological Systems: A Systematic Review of the Literature. Front. Mar. Sci. 8:648006. doi: 10.3389/fmars.2021.648006
Received: 31 December 2020; Accepted: 19 March 2021;
Published: 23 April 2021.
Edited by:
Di Jin, Woods Hole Oceanographic Institution, United StatesReviewed by:
Mary S. Wisz, World Maritime University, SwedenYaqin Liu, Woods Hole Oceanographic Institution, United States
Copyright © 2021 Refulio-Coronado, Lacasse, Dalton, Humphries, Basu, Uchida and Uchida. This is an open-access article distributed under the terms of the Creative Commons Attribution License (CC BY). The use, distribution or reproduction in other forums is permitted, provided the original author(s) and the copyright owner(s) are credited and that the original publication in this journal is cited, in accordance with accepted academic practice. No use, distribution or reproduction is permitted which does not comply with these terms.
*Correspondence: Sonia Refulio-Coronado, c21yZWZ1bGlvY0B1cmkuZWR1