- 1Halmos College of Natural Sciences and Oceanography, Nova Southeastern University, Dania Beach, FL, United States
- 2Florida Fish and Wildlife Research Institute, St. Petersburg, FL, United States
- 3Keys Marine Laboratory, Layton, FL, United States
- 4Department of Biological Sciences, Florida International University, Miami, FL, United States
- 5National Operations Center, Bureau of Land Management, Denver, CO, United States
Coral reefs worldwide are in a state of decline, but the population status and impacts of stressors for rare species are generally not well documented using broad-scale monitoring protocols. We fate-tracked all known colonies of the pillar coral, Dendrogyra cylindrus, on the Florida Reef Tract (FRT) from 2013 to 2020 to assess population condition and trend, and to document the relative impacts of chronic and acute stressors. Large average colony size, an absence of juveniles, and large geographic distances between genotypes suggest that the Florida D. cylindrus population has been reproductively extinct for decades. During the study period, low-intensity chronic stressors were balanced by regrowth, while back-to-back years of coral bleaching and thermally-exacerbated disease led to declines that the subsequent recovery rates suggest would require 11 uninterrupted years to overcome. The most recent stressor on Florida’s D. cylindrus population is “stony coral tissue loss disease” (SCTLD). Following the appearance of the disease in Florida in 2014, unrecoverable losses occurred within the D. cylindrus population as tissue, colonies, and whole genotypes suffered complete mortality. Losses of 94% of coral tissue, 93% of colonies, and 86% of genotypes between 2014 and the end of 2020 have led to functional extinction of D. cylindrus on the FRT.
Introduction
The pillar coral Dendrogyra cylindrus is a conspicuous stony coral described as uncommon or rare in reef environments throughout the Caribbean (Szmant, 1986). It is taxonomically unique as the only member of its genus, structurally unique as the only Caribbean columnar coral, and behaviorally unique as a species whose polyps are extended during light as well as dark hours.
Dendrogyra cylindrus is listed as Vulnerable on the IUCN Red List (Aronson et al., 2008) and Threatened under the U.S. Endangered Species Act (USFWS, 2014). It was also listed as Threatened by the state of Florida in 2011 and, like all stony corals on the Florida Reef Tract (FRT), is protected under Florida’s Coral Reef Protection Act and the Florida Fish and Wildlife Conservation Commission’s Marine Life Rule which prohibits take, injury, or destruction of stony corals. However, population information on D. cylindrus throughout its range has been scarce, and with one exception in Colombia (Bernal-Sotelo et al., 2019), population assessments, stressors, and trends have remained largely undocumented.
The FRT represents the northernmost extent of most Caribbean reef corals. Declines in coral cover over decadal scales on this Florida barrier reef system are well documented (Porter and Meier, 1992; Ruzicka et al., 2013). Many of these losses are the result of short-term stressors such as bleaching or disease events causing the demise of long-lived colonies. Decades of these acute stressors on the FRT include historic bleaching events (Causey, 2008), outbreaks of white band disease in Acroporids (Aronson and Precht, 2001), and outbreaks of white plague, black band, and yellow-band diseases on a wide range of species (Goreau et al., 1998; Richardson et al., 1998; Sutherland et al., 2004). Chronic declines in health related to predation (Baums et al., 2003) and water quality (Thurber et al., 2014; Lapointe et al., 2019) have also occurred. Beginning in 2014, a series of high-intensity stressors included two consecutive hyperthermal bleaching events (summer 2014 and summer 2015) and the outbreak of a temporally and geographically extensive disease termed “stony coral tissue loss disease” (SCTLD) (Precht et al., 2016; Florida Coral Disease Response Research and Epidemiology Team, 2018).
The impact of stressors may vary by event, region, and species, but declines in rare species are more likely to lead to reproductive isolation or extinction (Courchamp et al., 1999; Chan et al., 2019). Population status of rare species is also more difficult to assess because traditional survey techniques are ineffective at capturing them. D. cylindrus, for example, is seldom seen in randomized survey techniques in Florida and must be systematically targeted to attain quantitative information. Such targeting allows for fate-tracking of individuals in order to document status and trends over time and through various environmental events.
This study assessed the population demographics of D. cylindrus colonies on the FRT and documented their fate through chronic and acute stressors from 2014 to 2020. It followed the decline of a rare species into near-extinction in the region.
Materials and Methods
Groundtruthing and Monitoring
Dendrogyra cylindrus is particularly uncommon on Florida reefs, and random sampling cannot capture the abundance of the species nor be used to extrapolate total numbers. As such, beginning in 2013, all known and suspected locations of D. cylindrus colonies on the FRT were cataloged by requesting and collating databases and observations from various government agencies (FKNMS, Florida FWC), monitoring programs (CREMP, SECREMP, SCREAM, FRRP-DRM, and FWC RVC), and restoration projects (Mote Marine Lab), as well as local knowledge solicited from dive shops and other resource users. As new reports of colonies came in throughout the time period of the study, they were frequently and increasingly identical to already known colonies within the collated database. The exception is the westernmost area of the Lower Keys region, which has largely been ignored by monitoring programs and likely contains unknown D. cylindrus colonies. However, as the vast majority of the FRT is highly visited and well surveyed, we present the colonies identified here as largely comprehensive of the D. cylindrus population in Florida.
Each reported location was searched, including a surrounding area of approximately 700 m2, for the presence of D. cylindrus colonies; the goal of this search was to find the reported colony if location data were inaccurate and also to find any asexually produced fragments within the region. Data on the 68 colonies north of Biscayne National Park were collected by the Gilliam lab (Nova Southeastern University) and published in Kabay (2016); these data were added to the assessments from Dry Tortugas through Biscayne to provide Florida-wide information on population structure (locations, number of colonies, colony size, and depth). Across the FRT, a total of 542 colonies were identified between October 2013 and May 2014. From June 2014 through December 2020, an additional 277 colonies were identified and assessed. At each site, corals were surveyed for condition and monumented with cattle tags or by maps and photographs so that each distinct colony could be accurately identified in future surveys.
In addition to the initial baseline surveys, all known live colonies within the Florida Keys National Marine Sanctuary (FKNMS) and Biscayne National Park were visited in spring 2017, spring 2018, summer 2019, summer 2020, and winter 2020 for full colony assessments. Other assessments occurred opportunistically. Additionally, beginning in 2014, a subset of 27 sites was selected for tri-annual monitoring to document fine-scale temporal trends and seasonality of various stressors. These sites were selected to include a range of depths, geographic regions (Biscayne, Upper Keys, Middle Keys, Lower Keys, and Dry Tortugas), and clustering of colonies within sites. These sites included 15 individual isolated colonies, four sites with two to three colonies, and eight sites with four or more colonies. All colonies at each site were monitored three times each year with the exception of three sites that each contained more than 50 colonies. At each of these, a subset of 24 colonies was selected for tri-annual fate-tracking. These 24 colonies were selected to spatially cover the site and to incorporate both large and small colonies. At 10 of the regularly monitored sites, data loggers (Onset HOBO Inc., Bourne, MA, United States) placed at the base of a colony recorded hourly temperatures. Additional data on thermal stress were acquired using NOAA’s Coral Reef Watch indices of degree heating weeks, which sum thermal stress (number of degrees Celsius above the highest summertime mean sea surface temperature) across rolling 12-week periods (NOAA, 2013-2019).
Genetic Diversity
During the 2013–2015 D. cylindrus monitoring events, sampling to determine genetic variability was conducted in collaboration with the Baums lab (Pennsylvania State University). Briefly, tissue clips and/or syringe tissue biopsy samples (Kemp et al., 2008) were taken from 161 colonies spanning the full FRT; sample selection was stratified by depth and by the number of colonies at the sites. Full sampling and analysis protocols, as well as results from these samples are presented in Chan et al. (2019). The 17 genotypes with multiple ramets (colonies that were asexually produced and genetically identical to surrounding colonies) analyzed by Chan et al. had a maximum geographic distance of 90 m (meaning that ramets were all within 90 m of their clones). Of these, 94% (16/17) had a maximum geographic difference of less than 70 m. We extrapolated these results across all known Florida D. cylindrus colonies to estimate the total number of genotypes in the population. We assumed that any non-genotyped colonies within 70 m of each other were clonal, while any non-genotyped coral at least 70 m from a conspecific was a unique genotype. We note that at some sites analyzed by Chan et al., multiple genets were located within 70 m of each other, so our extrapolations to the full Florida population are likely conservative.
Demographics
During the first visit to every colony, linear measurements of maximum length, width, and height were taken with a 50 cm measuring stick. Surface area was estimated as that of a half ovoid using length, width, and height measurements as axes; live surface area was then calculated by multiplying the surface area by the percent live tissue on the colony. Tissue gains or losses over time were calculated by subtracting the live surface area at each monitoring event from the live surface area at the baseline. Volume estimates were calculated as a half ovoid using the same axes measurements. Depth at the base of each colony was identified using a dive computer.
For analyses on colony depth, size, and distances between neighboring genotypes, only the largest colony from each known or presumed genotype was used. It is presumed that the largest colony best represents the original recruited coral, with all smaller ramets resulting from stochastic fragmentation events.
Health Status and Mortality
During every monitoring event (baseline as well as any subsequent visit), the percentages of each colony covered with live tissue, old mortality, and recent mortality (classified as bare white skeleton with defined polyp structure) were visually estimated in the field. The vast majority of percentage assessments were conducted by the same diver to minimize observer noise, but in a few instances, an alternate diver recorded percentages. Informal quality control assessments between the two divers were done periodically and found to be in agreement. When recent mortality was observed, the cause(s) were determined and recorded to the extent possible (Figure 1). Such determinations were based on the observers’ extensive experience in coral monitoring and advised by published descriptions of stressors (Weil and Hooten, 2008; Humann and DeLoach, 2013; Florida Coral Disease Response Research and Epidemiology Team, 2018; Neely, 2018). If multiple stressors had caused mortality, the percent attributable to each was differentiated. Bleaching/paling was also recorded categorically as “paling,” “partial bleaching,” and “bleaching.”
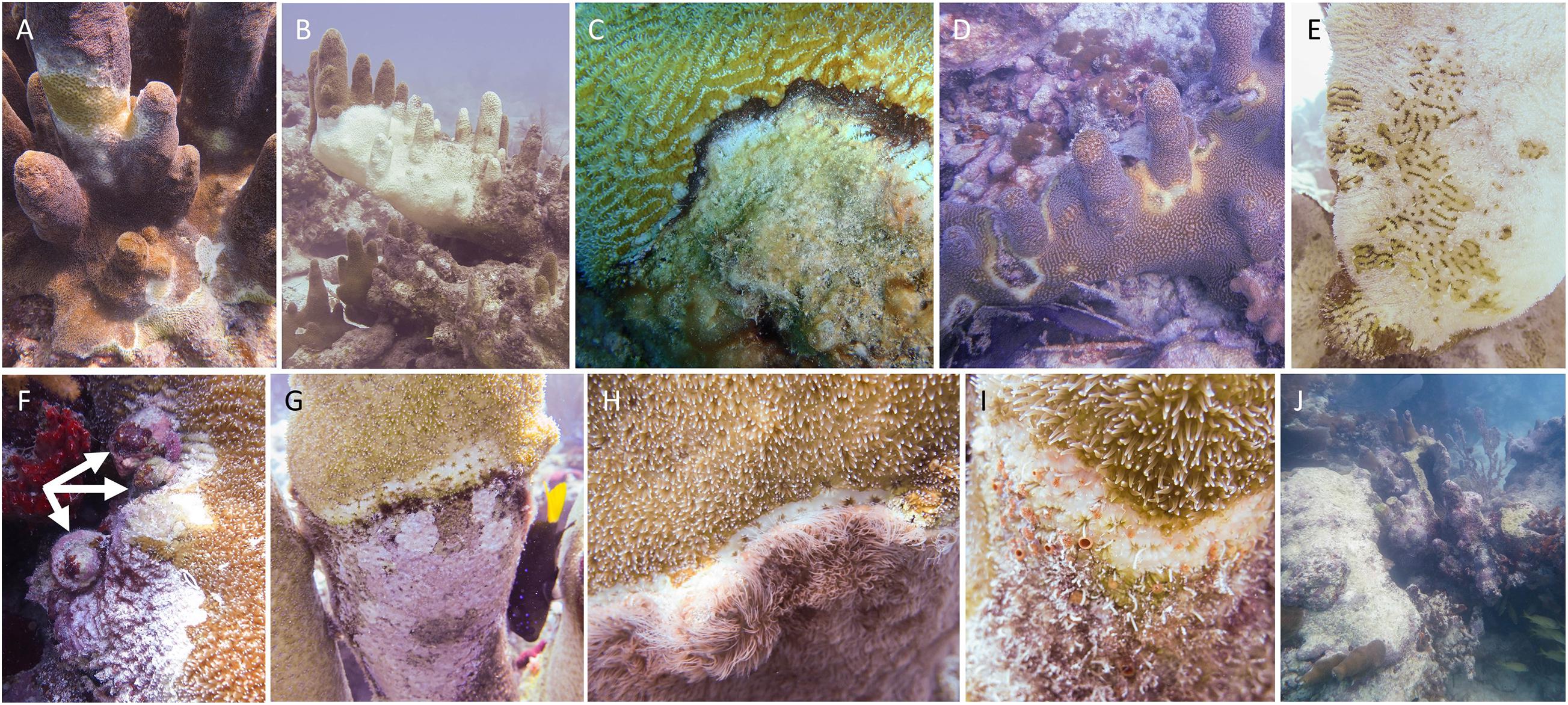
Figure 1. Appearance of stressors affecting Florida Dendrogyra cylindrus. (A) Stony coral tissue loss disease, characterized by acute multifocal lesions occasionally including sloughing tissue. (B) White plague, characterized by acute focal lesions generally generating from the bottom of the colony. (C) Black band disease. (D) Unidentified yellow-band disease. (E) Bleaching-related mortality, characterized by sloughing tissue following many months of bleaching. (F) Predation by Coralliophila abbreviata snails (indicated by arrows). (G) Damselfish-related mortality on the edge of territories or nests. (H) Competition with encrusting octocorals. (I) Mortality-related to the boring sponge Cliona sp. (orange oscules visible). (J) Breakage from storms, anchors, etc. (pictured is a large colony toppled by hurricane Irma in 2017).
The number of observations of each stressor was compared to the total number of D. cylindrus monitoring records to identify prevalence. The amount of recent mortality attributed to each stressor was averaged to quantify the severity.
Analyses
Using GIS layering, colony locations were overlaid on the Disturbance Response Monitoring zone map (FRRP, 2015) to analyze inshore to offshore reef distribution. Colonies were also classified to habitat by overlaying locations on the Florida Unified Reef Map (FWRI, 2014).
We compared maximum colony diameter, height, surface area, and volume between the Florida geographic regions, and also compared colony height of the Florida population with a Colombian population using t-tests or ANOVAs when normality and equal variance were met, and by using Kruskal–Wallis ANOVA on ranks when they were not. We used a linear regression to compare the same size metrics with depth. We also compared the prevalence of stressors between regions using z-tests for independent proportions. Tests were conducted using SigmaPlot 14.0. All analyses of significance are reported with α = 0.05.
Results
Population Distribution
Between October 2013 and December 2020, a total of 819 D. cylindrus colonies were recorded along the FRT (Figure 2). Of these total known colonies, 11 were located within Dry Tortugas National Park, 713 were located within the FKNMS, 27 were located within Biscayne National Park, and 68 were located north of Biscayne National Park in the Southeast Florida region. Management zones within these regions offered varying levels of resource protection and usage patterns. Of the 819 colonies, 144 (18%) were located within no-take marine protected areas (143 in FKNMS Sanctuary Preservation Areas and one within the Dry Tortugas Research Natural Area), 36 (4%) were within zones that limit fishing to catch-and-release trolling, and 639 (78%) were in commercially and recreationally fishable waters.
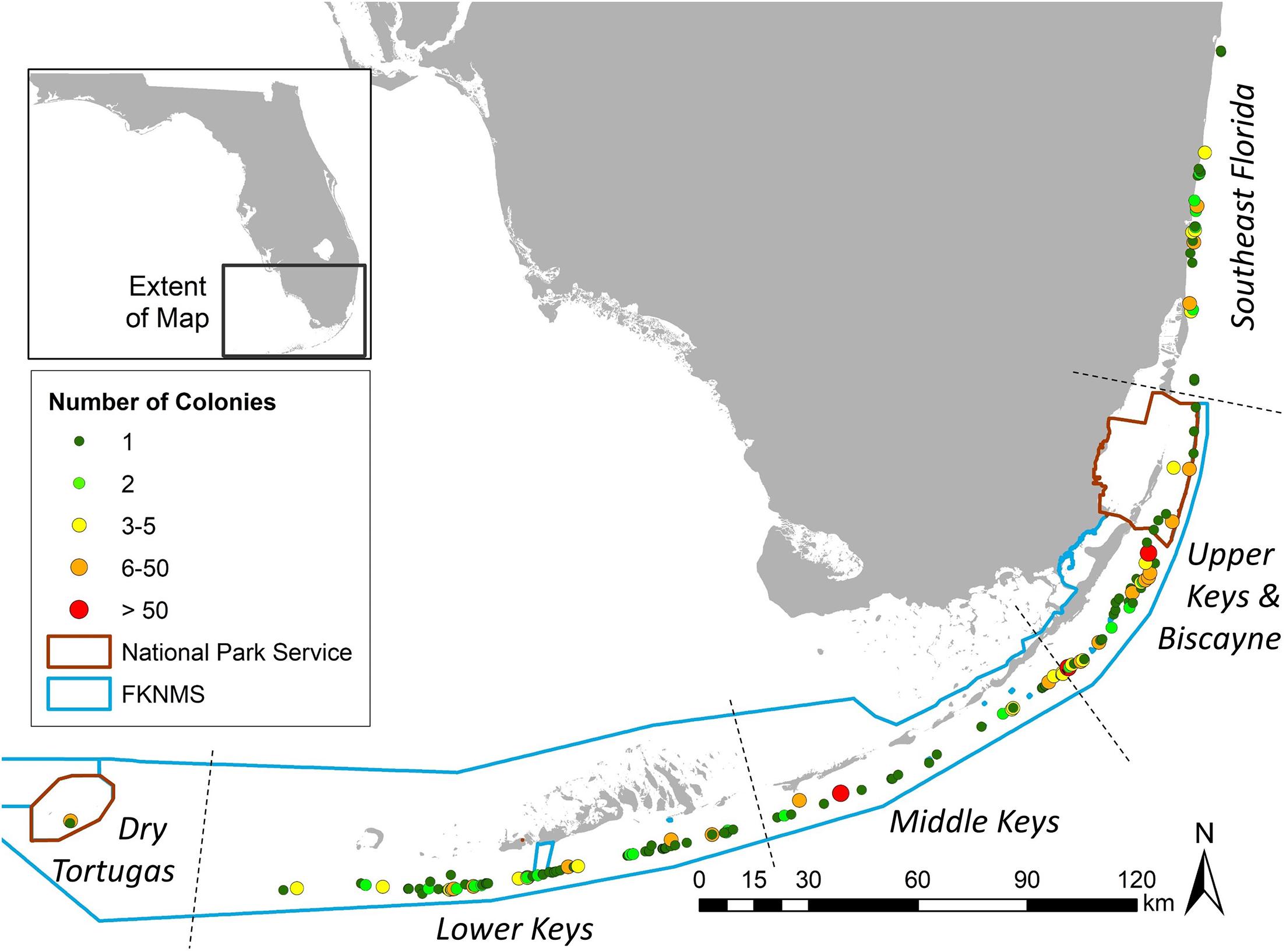
Figure 2. Location of Dendrogyra cylindrus colonies along the Florida Reef Tract. Color/size differences represent the number of colonies at each site. National Park and Florida Keys National Marine Sanctuary boundaries are shown.
Colony depth averaged 7.4 m (±2.5 SD). The shallowest site was 2.4 m, and the deepest was 19.5 m (Supplementary Figure 1). The two deepest colonies were observed at the two northernmost sites.
Within the Southeast Florida region, the Disturbance Response Monitoring map delineated inshore, inner reef, middle reef, and outer reef zones. The distribution of D. cylindrus colonies on these was: 21 inshore, 40 inner reef, 3 middle reef, and 2 outer reef (Figure 3A). Within the Biscayne to Dry Tortugas area, the map classifications include mid-channel patch reef, offshore patch reef, and forereef areas. The number of D. cylindrus colonies on each was: 20 mid-channel patch reef, 82 offshore patch reef, and 648 forereef (Figure 3B). Overlays from the Unified Reef Map across the full FRT identified 362 colonies located on spur and groove or high-relief linear reef, 107 on patch reef/isolated reef structures, 13 on ridges, and 338 on low-relief contiguous reef/colonized pavement (Figure 3C).
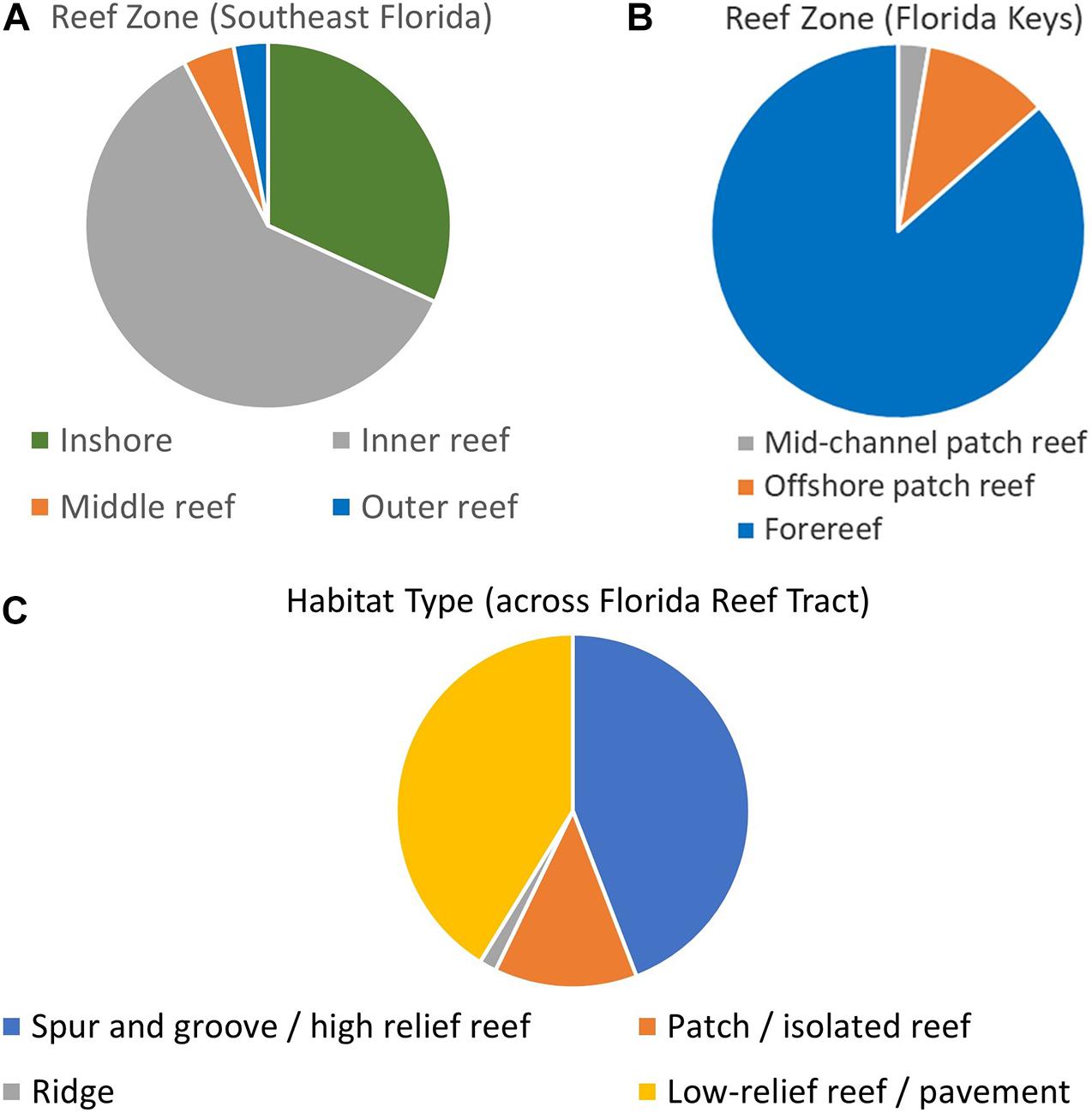
Figure 3. Dendrogyra cylindrus zone and habitat distribution. Maps from the Disturbance Monitoring Program identified reef zone distribution of colonies within southeast Florida (A) and the Florida Keys (B). The Florida Unified Reef Map identified the nearest habitat type across the whole of the Florida Reef Tract (C).
Genetic Diversity
Microsatellite analysis of sampled D. cylindrus colonies identified clonality at 12 out of 16 sites containing multiple colonies (Chan et al., 2019). The maximum distance between ramets in Florida was identified at an Upper Keys site, where 110 colonies were distributed across a maximum diameter of 84 m. Three other sites had distances between individual ramets that exceeded 60 m; the greatest was 69.9 m.
In order to estimate the number of genotypes within the FRT to include colonies that were not genetically sampled, all unsampled colonies or groups of colonies that were located within 70 m of other colonies were presumed to be of the same genotype. All colonies or groups of colonies that were located greater than 70 m from any other colonies were presumed genetically distinct. These assumptions resulted in an estimated 190 unique genotypes on the FRT.
Colony numbers were not evenly distributed across the genotypes. Fifty percent of Florida colonies were represented by only five genotypes (Supplementary Figure 2). Of the 190 genotypes, 62% (118) were represented by a single colony each. Overall, asexual reproduction accounted for 77% (629/819) of Florida D. cylindrus colonies.
Distance between genotypes ranged from 2.5 to 6596 m. The average distance was 1037 m (±1344 SD) (Supplementary Figure 3). Of the 190 distinct genotypes, 36% (69 genotypes) were greater than 1 km from their nearest neighbor, 42% (79 genotypes) were between 100 and 1000 m, 20% (38 genotypes) were between 10 and 100 m, and 2% (four genotypes) were less than 10 m.
Demographics
Colony sizes were assessed using all known D. cylindrus genotypes on the FRT (including those north of Biscayne published in Kabay, 2016). For genotypes with multiple colonies, only the largest colony was included in analyses. Colony size averaged 168 cm in diameter and 124 cm in height. Estimated surface area averaged 15.6 m2 and estimated volume averaged 10.9 m3 (Table 1). The largest colonies exceeded 5 m in length, 3 m in height, and 114 m3 in volume. In 2014, a single juvenile colony with a diameter of 7 cm was found in the Upper Keys, but perished within a year of first siting.
No measure of size (maximum diameter, height, surface area, and volume) correlated significantly with depth (linear regressions: p > 0.13). However, size differences were observed within different regions of the FRT. The average volumetric size of D. cylindrus colonies in the Lower Keys was 7.8 m3, while average size in all other regions exceeded 10 m3 (Figure 4). Volumetric distributions did not pass Shapiro–Wilk normality, and ANOVA on Ranks determined that differences between regions were not significant (p = 0.14). However, when Lower Keys colonies and Upper Keys colonies were compared, volumetric differences were significant (Kruskal–Wallis ANOVA on ranks: p = 0.04).
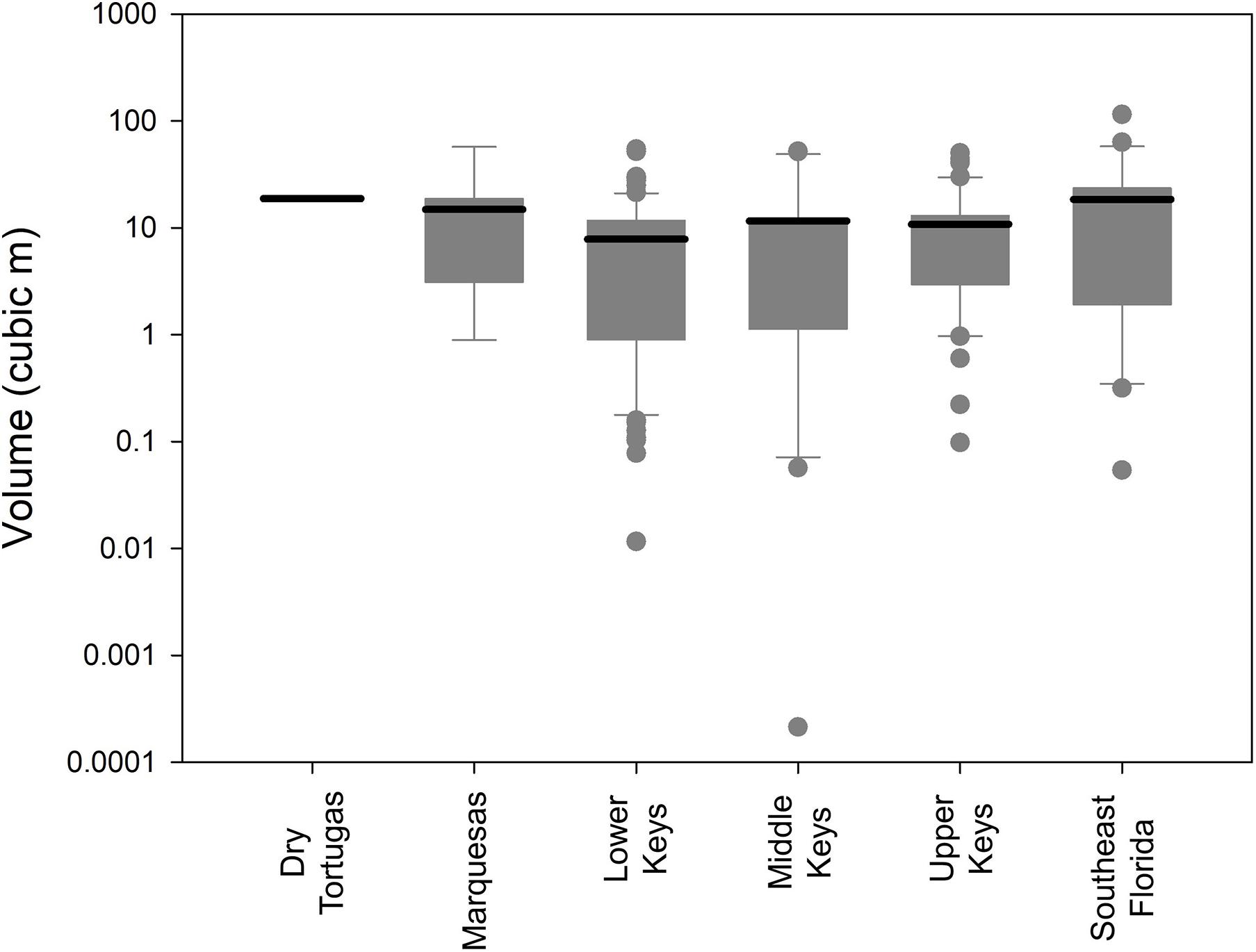
Figure 4. Box plots (logarithmic) of Dendrogyra cylindrus colony volumetric estimates separated by reef region. Boxes show the quartiles with the mean. Whiskers incorporate the 10th–90th percentiles. Dots are outliers. Size analyses include only the largest colony within each genotype.
Baseline Status
During baseline surveys in 2013–2014, a total of 542 colonies were identified and surveyed; 533 of these were alive. The average live coral cover on all colonies (including the dead ones) was 70%, and the average recent mortality was 0.5%. Twenty-two percent (117/542) of the baseline colonies exhibited recent mortality. On those colonies, the average recent mortality was 2.2%.
From 2015 to December 2020, an additional 277 colonies were identified and surveyed, increasing the total known colonies to 819. If we parsimoniously assume no gains or losses in live tissue from the time of “new” colony identification back to the baseline surveys, the total amount of live D. cylindrus tissue estimated across the FRT in 2013–2014 was 2767 m2. However, this estimate is conservative, as most colonies identified from 2015 onward had already suffered from mild to complete mortality.
Minor and Chronic Sources of Mortality
Dendrogyra cylindrus colonies were subject to a variety of minor, chronic sources of mortality including damselfish gardens and nests, predation by the corallivorous snail Coralliophila abbreviata, competition with other benthic organisms, and physical damage from abrasion or burial.
A total of 2845 monitoring events on live colonies from Dry Tortugas through Biscayne National Park were conducted from 2013 to 2020. Out of these, 28 observations (<1%) recorded recent mortality from damselfish gardens (primarily from the threespot damselfish Stegastes planifrons) or damselfish nests (primarily from the sergeant major Abudefduf saxatilis). Recent mortality on colonies affected by these gardens or nests averaged 0.6% of the surface area of the colony. Mortality as a result of damsel gardens was observed year-round, but mortality associated with damsel nests was seen only in March through May (Figure 5).
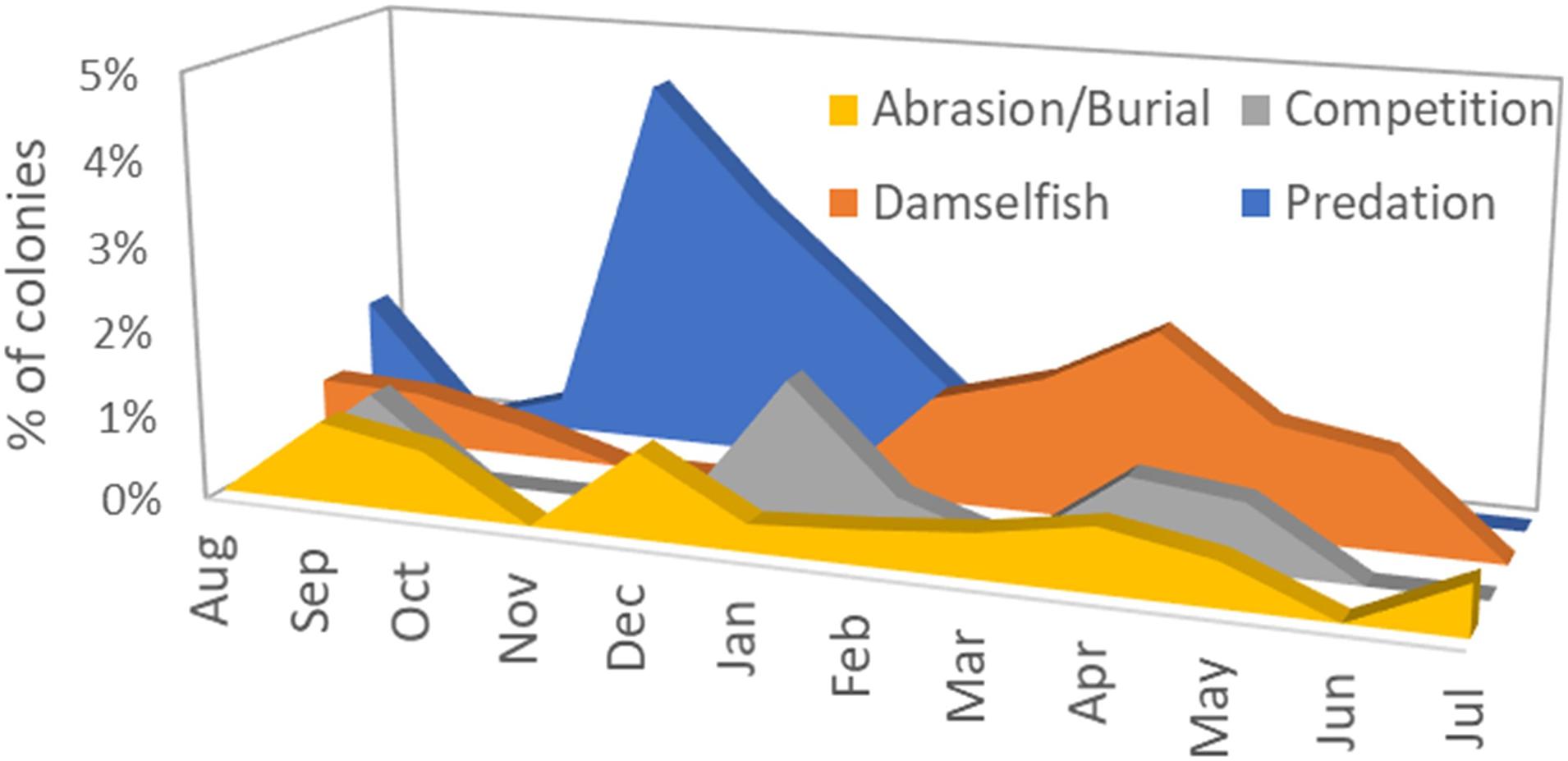
Figure 5. Percentage of observations on live Dendrogyra cylindrus colonies in which recent mortality could be attributed to four chronic stressors: abrasion/burial, competition with other benthic species, damselfish nests or gardens, and predation by the corallivorous snail Coralliophila abbreviata.
Mortality as a result of predation on D. cylindrus by the corallivorous snail C. abbreviata was recorded in 27 of the 2845 observations (<1%). The amount of recent mortality attributed to these snails on affected colonies averaged 1.1%. Mortality caused by snail predation was seen across all seasons, but spiked in November to January (Figure 5).
Recent mortality as a result of competition with the encrusting octocoral Erythropodium caribaeorum or with boring sponges in the genus Cliona was recorded in 17 of the 2845 observations (<1%). Average recent mortality on affected colonies was 0.7% and was observed across all seasons (Figure 5).
Of the 2845 observations, 16 (<1%) identified recent mortality associated with tissue that had been abraded or buried. Two instances of tissue mortality were attributed to abrasion by marine debris (polyethylene trap rope). Average recent mortality on colonies experiencing abrasion or burial was 1.4%. These stressors were observed across all seasons (Figure 5).
Bleaching Events
Of the 2845 colony monitoring observations, 79 (2.8%) documented recent mortality attributable to bleaching; the recorded severity of such recent mortality averaged 7.2% of colony surface area. In 27 observations, bleaching-related recent mortality exceeded 5%, and in three of those, it exceeded 60%. The majority (70%) of bleaching-related mortality observations resulted from the 2014 summer hyperthermal event and were seen at eight sites from the Upper Keys to the Lower Keys. The remaining observations (30%) followed the 2015 hyperthermal event and were documented at two sites, one in the Upper Keys and one in the Lower Keys. More detailed information on bleaching-related patterns of Florida D. cylindrus colonies during these events can be found in Lewis et al. (2019).
Hourly in situ water temperatures from D. cylindrus sites across the Florida Keys recorded elevated summer temperatures in 2014 and 2015. Analyses of NOAA Degree Heating Week indices also identified 2014 and 2015 as high thermal stress years (NOAA, 2013-2019). D. cylindrus bleaching events followed these warm-water events (Supplementary Figure 4). During other years (2013, 2016–2019), some paling was recorded on some colonies during the late summer months, but bleaching and/or bleaching-related mortality was not observed on D. cylindrus. Thermal stress, as measured by Degree Heating Weeks, was high in 2019 as well, but no bleaching beyond some instances of paling was observed on the species.
Disease (Excluding SCTLD)
A variety of diseases were observed on D. cylindrus colonies: black band disease, an unidentified yellow band disease, white plague, and SCTLD. All had deleterious effects on the population.
Black band disease was identified in 14 of the 2845 (<1%) monitoring observations. All of these events were observed in September through December. Recent mortality on black-band diseased colonies averaged 1.3% of colony surface area. More detailed information on the prevalence and temporal dynamics of black band disease on these D. cylindrus colonies is published in Lewis et al. (2017).
An unknown disease was observed on Upper Keys D. cylindrus during periods of cooler water in 2014. The disease presented similarly to yellow-band disease, with a pale/yellow band of tissue often accompanied by slow tissue loss (Figure 1 and Supplementary Figure 5). This was observed in 18 of the 2845 (<1%) monitoring records. The disease was documented at eight sites, all in the Upper Keys. Observations were confined to winter/spring months, with 10 of the observations in January/February and the other eight in late April/early May. Average recent mortality on affected colonies was less than 1%.
White plague was the most common and deleterious disease impacting Florida D. cylindrus before the arrival of SCTLD (discussed below). White plague was documented in 329 of the 2845 observations (12%). Of affected colonies, the average recent mortality was 5.0% of colony surface area. Observations of white plague were distributed across the FRT. Prevalence of white plague in 2013–2015 (before the appearance of SCTLD) increased during and following periods of heat stress. Low levels of heat stress in the fall of 2013 were marked by peak white plague prevalence under 20%. High levels of heat stress in the autumns of 2014 and 2015 were marked by peak white plague prevalence levels exceeding 40% (Figure 6).
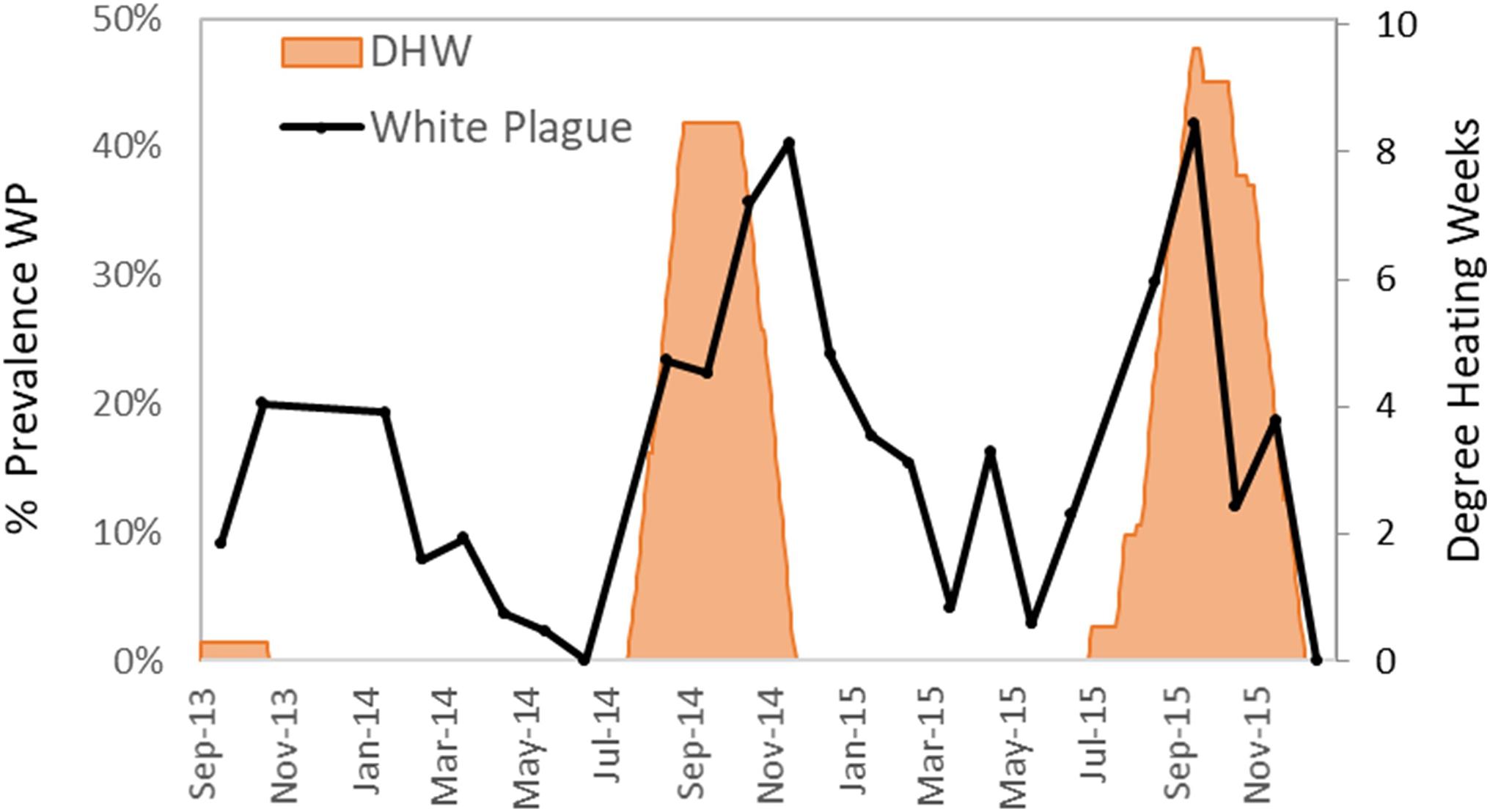
Figure 6. Percentage of surveyed Dendrogyra cylindrus with recent mortality caused by white plague during each month (solid line). Cumulative degree heating weeks (DHW), a measure of thermal stress as determined by NOAA, are shown in orange fill.
Stony Coral Tissue Loss Disease
Stony coral tissue loss disease was identified as distinct from white plague following raised awareness from Precht et al. (2016) and subsequent regional collaborative efforts that resulted in a case definition (Florida Coral Disease Response Research and Epidemiology Team, 2018). As a result, photos of colonies from 2015 to 2017 were reassessed to correctly separate observations of white plague and SCTLD that may have been combined during the field assessments. The first known SCTLD observation on Florida D. cylindrus occurred in February 2016 in Biscayne National Park. Infections occurred throughout the Upper Keys population in 2016. In the Middle Keys, SCTLD was first observed at Conch Reef in February 2017, progressed southwest through Long Key in December 2017, and reached the Sombrero Reef area in April 2018. The uppermost sites within the Lower Keys were also affected in April 2018, with southwestern progression continuing through all but the Dry Tortugas colonies by late 2020 (Figure 7).
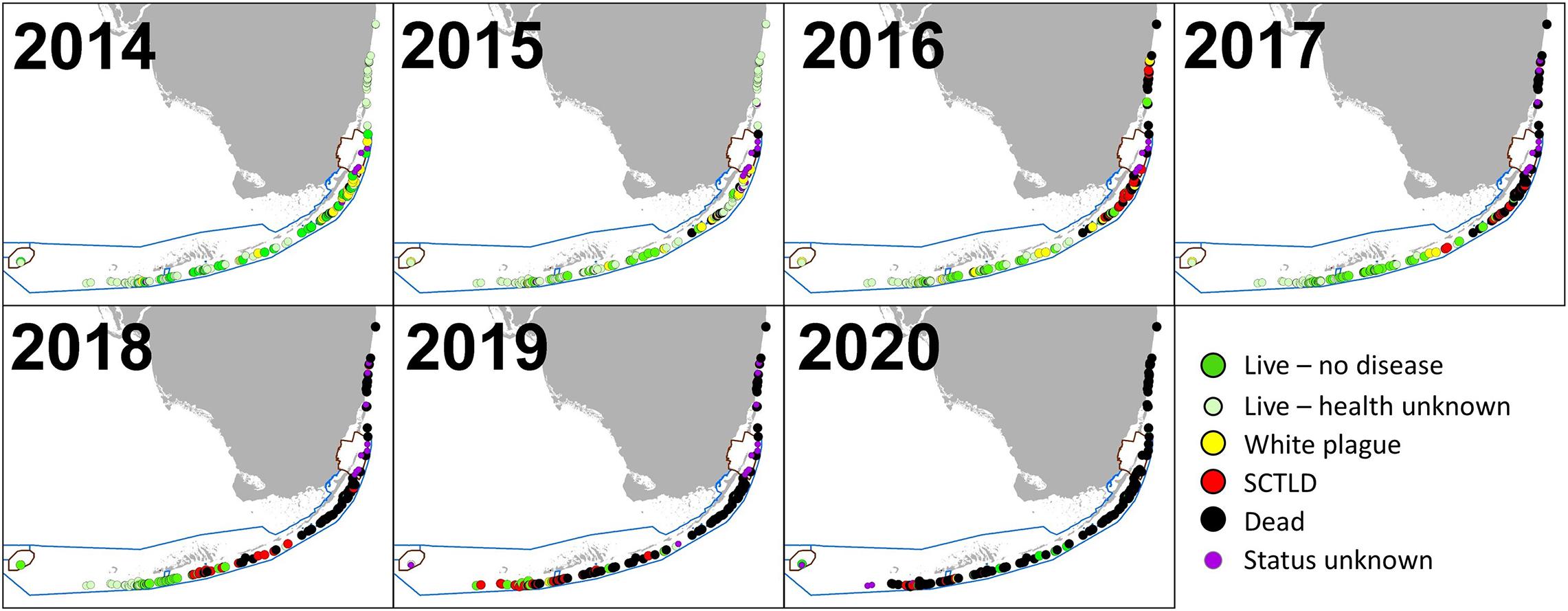
Figure 7. Status of Dendrogyra cylindrus colonies from 2014 through 2020. At sites with multiple observations within a calendar year, the most recent is indicated. For sites with multiple colonies, disease on any colony within a site resulted in the site being classified as diseased. Sites where the status of the colonies during a given year is unknown are classified as either “Live—no health info” if colonies were known to be alive during subsequent years or “Status unknown” if subsequent years’ data cannot confirm whether the colony was alive at the time. Stony coral tissue loss disease radiated from the area around Biscayne National Park, and in most cases resulted in complete colony mortality.
Quantifying the proportion of D. cylindrus colonies affected by SCTLD was complicated by spatial and temporal variation as the disease front progressed. SCTLD was observed in 14% of monitoring observations (410/2845), but its rapid spread across infected colonies (Figure 8) occasionally resulted in complete mortality between monitoring events leading to inconclusive causation. However, there were no colonies within the SCTLD-affected regions that did not experience catastrophic loss as the disease progressed along the FRT. In regions in which SCTLD had been present for at least 1 year (first observed before January 2019), 100% of colonies either (a) had active SCTLD, (b) were completely dead and assumed to be SCTLD-affected due to the rapid nature of the mortality, or (c) suffered severe tissue loss leaving only small patches of remaining tissue. Colonies observed with active SCTLD had an average recent mortality of 17.4% of the colony surface area.
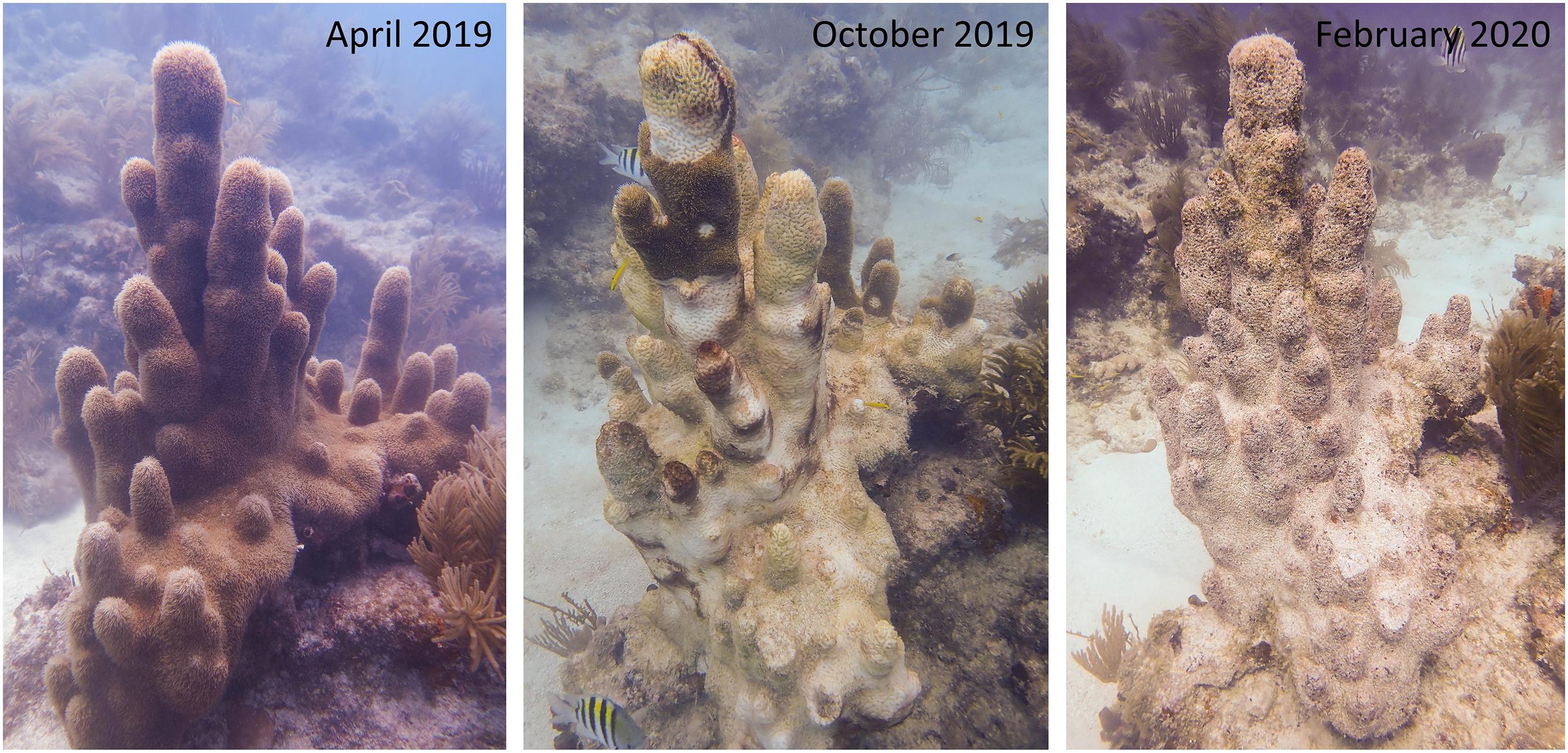
Figure 8. Rapid mortality of a Dendrogyra cylindrus colony as a result of stony coral tissue loss disease. The colony was unaffected in April 2019 and completely dead by February 2020.
Summary of Losses
Stressors varied in their prevalence (frequency of observation) and severity (% of recent mortality associated with each observation). SCTLD impacted the greatest number of D. cylindrus colonies, followed by white plague and bleaching-related mortality (Table 2). SCTLD also had the highest severity, followed by white plague, bleaching, and black band disease.
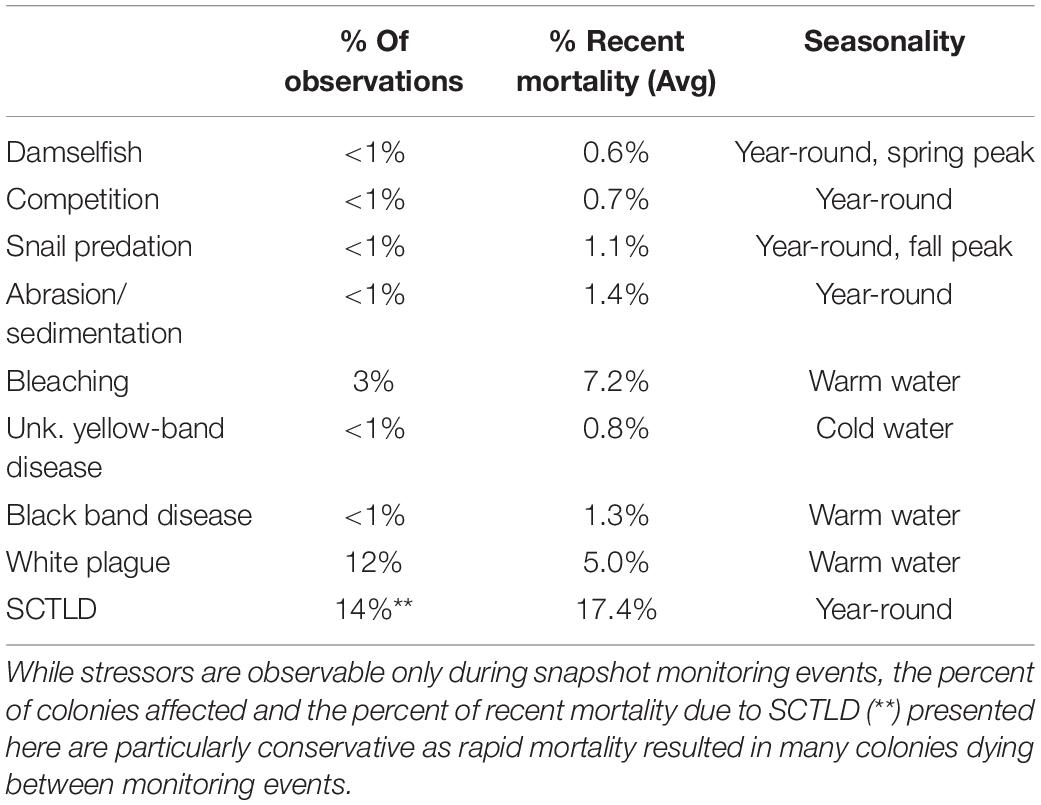
Table 2. Summary of stressors affecting D. cylindrus colonies across 2845 observations between 2013 and 2020.
Colonies with at least one annual monitoring event for each year from 2013/14 to 2020 were analyzed for tissue loss over time. Between 41 and 65 colonies representing 8–20 genotypes from the Upper, Middle, and Lower Keys were assessed (Figure 9). Heavy tissue losses (24–43%) occurred across all regions between the 2013/2014 baseline and the end of 2015. Those losses were dominated by white plague and bleaching-related mortality. As SCTLD reached each region, additional losses were rapid, generally leading to complete mortality with no possibility of recovery. Within the Upper Keys/Biscayne region, all analyzed colonies were dead by the end of 2018. Within the Middle Keys region, the amount of live tissue stabilized during 2016, but SCTLD impacts from 2017 through 2020 resulted in a 98.2% loss of tissue by the end of 2020. In the Lower Keys, the amount of tissue was stable through 2017 and 2018 (despite the direct overpass of a category 4 hurricane), but the arrival of SCTLD in 2018 led to a 99.8% loss of tissue by the end of 2020 (Figure 9).
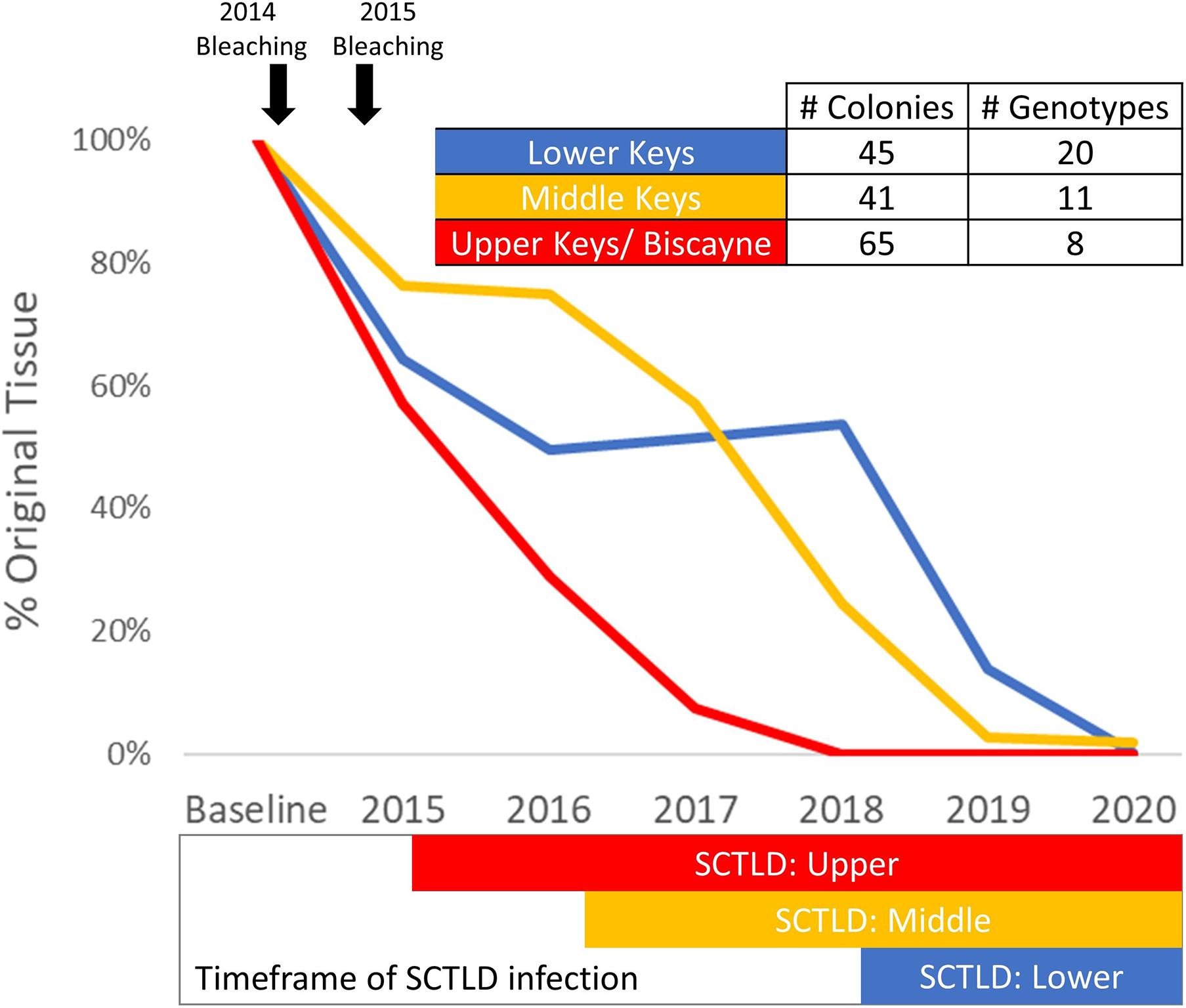
Figure 9. Percent of original tissue on a subset of Dendrogyra cylindrus colonies with at least one annual monitoring from 2013/14 through 2020. Total tissue surface area (cm2) is calculated from size measurements and percent live coral. Amount of tissue each year on the selected colonies within each region was summed and compared to baseline surveys. The timing of the 2014 and 2015 bleaching events is highlighted (top), as is the presence of stony coral tissue loss disease within each region (bottom).
Population Status (End of 2020)
Losses of D. cylindrus on the FRT from the baseline 2013/14 surveys through the end of 2020 are as follows:
• 86% loss of genotypes (not including the 6% with active SCTLD at the end of 2020)
• 93% loss of colonies
• 94% loss of tissue.
At the end of 2020, 25 known D. cylindrus genotypes remained on the FRT. Of these, 12 had declined to less than 2% live coral cover since the baseline surveys, and 11 were actively experiencing rapid tissue loss due to SCTLD. Five of the remaining genotypes had been treated with topical antibiotics to slow or halt SCTLD lesions. Only the two genotypes located in Dry Tortugas National Park where SCTLD had not yet reached remained unaffected.
Discussion
Demographics and Genetic Diversity
Demographic surveys of FRT D. cylindrus colonies indicate a population comprised of predominantly large and presumably old individuals. Most small colonies were very near larger colonies and had the appearance of fragmented pillars. This project documented only one small colony (<20 cm) that was greater than 70 m from another colony and presumed to be a sexual recruit. Similarly, ongoing Florida monitoring programs, which survey hundreds of sites per year, have not documented juvenile D. cylindrus (CREMP pers comm; Miller, 2000-2011). Growth rates of D. cylindrus are poorly understood, but measurements of transplanted pillars in Key Largo from 1993 to 1995 recorded an average vertical pillar extension of 1.8 (±0.3 SD) cm per year (Hudson and Goodwin, 1997). Making the liberal assumptions that the same growth rate exists in the development of the base in vertical and horizontal directions and that the rate is constant from settlement onward, only 11 of the FRT genotypes (6%) could potentially be younger than 20 years. Of note, one small colony (46 cm × 43 cm) without any vertical pillar development was found on an artificial substrate sunk in 1986 (FWC/DMF, 2020), indicating a maximum age of 31 years when first measured.
Demographic surveys conducted on a D. cylindrus population in Colombia (Acosta and Acevedo, 2006) allow for comparisons with a non-Florida population. The Florida population had significantly larger colonies (Mann–Whitney rank sum test p < 0.001; Figure 10). In the Colombian study, the population was comprised mostly (53%) of colonies less than 60 cm in height. In contrast, only 18% of Florida colonies fell within that size class. In other coral species, such a lack of juveniles has been suggested to correlate with poor recruitment resulting from reef degradation (Meesters et al., 2001).
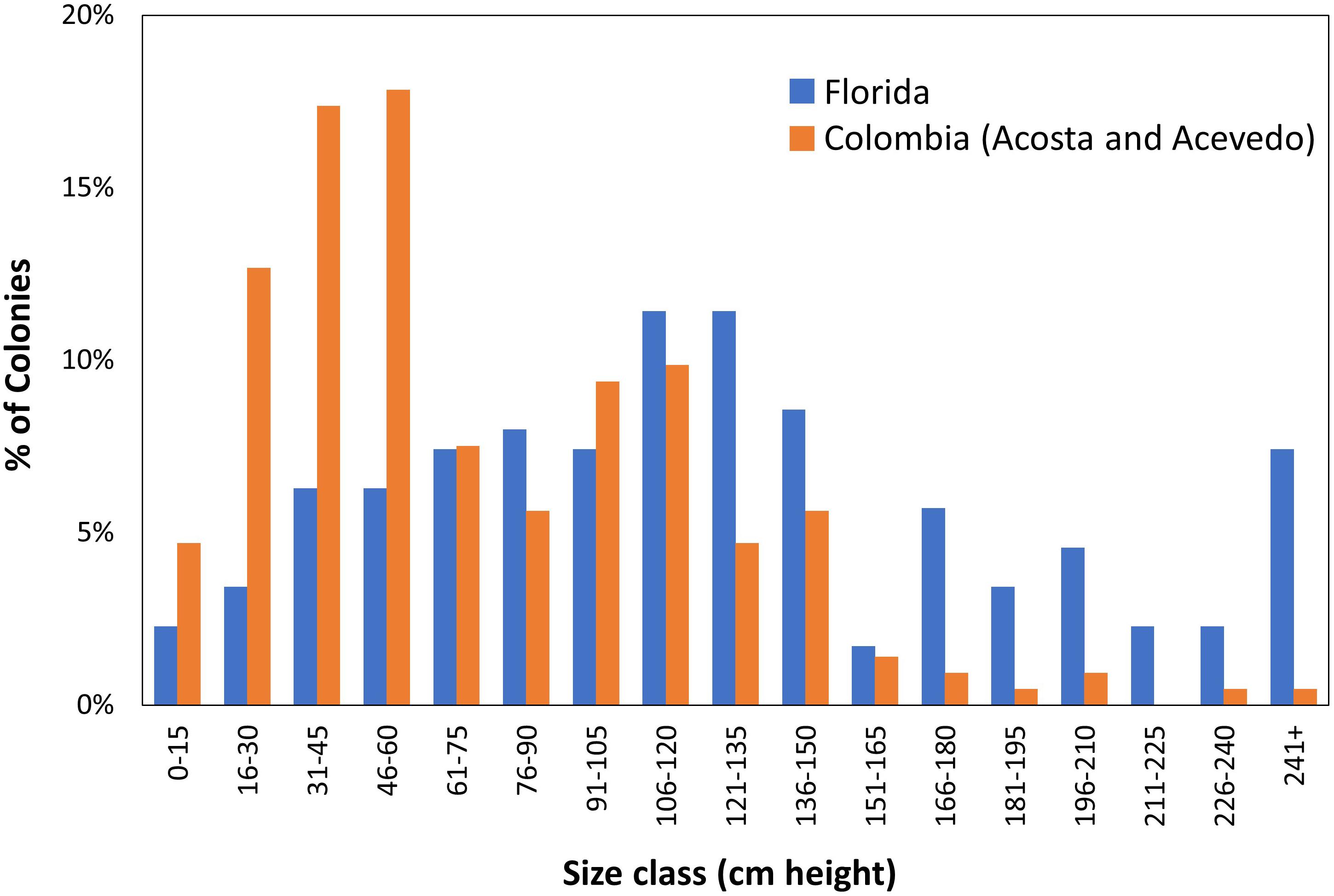
Figure 10. Demographic comparisons of Dendrogyra cylindrus height distributions in Florida and Colombia. Colombian data taken from Acosta and Acevedo (2006).
The near absence of juvenile D. cylindrus in Florida may be a result of (a) poor juvenile survival, (b) poor settlement rates, and/or (c) poor fertilization. We will examine each of these in turn based on the population demographics.
If low juvenile survival were causing the lack of observed juveniles, mortality would need to occur quite early to escape notice by various monitoring programs. The fixed-site and stratified random monitoring programs that have noted an absence of juveniles operate by recording all corals greater than 4 cm in diameter, and so mortality would have to occur before that size. While growth rates of juvenile D. cylindrus settlers have never been quantified in the wild, larvae that settled in land-based nurseries have remained smaller than 2 cm for up to 3 years (pers obs), which in the wild could be overlooked. However, coral recruitment studies conducted throughout the Florida Keys which focus on new settlers have also never documented juvenile D. cylindrus (CREMP, pers comm; Bartlett et al., 2018).
Poor larval settlement may be a possible bottleneck leading to the absence of juveniles. Coral recruitment rates in Florida were identified by Moulding (2005) to be highest in the Lower Keys, with decreasing recruitment in the Middle and Upper Keys. The smaller average size of D. cylindrus colonies in the Lower Keys could be a result of more common or more recently viable recruitment within that region. However, very little is known about settlement cues, growth rates, or mortality curves for D. cylindrus, and this hypothesis would require further testing.
The third hypothesis is that the number of larvae available for settlement is limited. Corals are generally considered r-selected species; millions of eggs are produced, and survival is extremely low through the early life stages. In the case of Florida’s D. cylindrus population, fertilization is likely to be non-existent due to several factors:
1. Of the 190 genotypes, only four were within 10 m of a genetically different neighbor. Diffusion and fertilization across even 10 m are unlikely given the obstacles of predation, physical barriers, and currents.
2. In contrast to most broadcast spawners that have positively buoyant gametes, eggs and sperm of D. cylindrus do not rapidly rise to the surface. Lab and field observations indicate that eggs are only slightly positively buoyant, while sperm is neutral or slightly negative. As such, fertilization success requires gametes navigating three dimensions rather than mixing in surface slicks, which adds an additional barrier to fertilization potential.
3. Dendrogyra cylindrus colonies are mostly gonochoric (but see Kabay, 2016; Neely et al., 2018, 2020). Thus, even if gametes from genetically-distinct nearby colonies do find each other, there is only a 50% chance of gender compatibility if 1:1 sex ratios are assumed.
Other Caribbean populations of D. cylindrus in which multiple genotypes are in close proximity to each other are likely to overcome these obstacles. However, we suggest that the population on the FRT, even before the losses of 2014–2020, was largely constrained by Allee effects (Levitan and McGovern, 2005) that appear to have rendered the population reproductively extinct for decades.
Sources of Mortality
Dendrogyra cylindrus colonies in Florida were subjected to a variety of stressors between 2014 and the end of 2020. Minor stressors included chronic and relatively low-impact factors such as predation by C. abbreviata, damselfish gardens and nests, competition with other benthic organisms, and abrasion or tissue burial. These stressors were uncommon (<1% of observations) and minor (≤1% recent mortality). Though not quantified, we observed many of these minor sources of mortality healing with tissue regrowth in subsequent surveys. We suggest that losses from these minor, chronic stressors can be balanced by tissue regrowth and do not necessarily lead to net tissue loss over time.
Acute stressors were more prevalent and detrimental. Four different diseases impacted the Florida D. cylindrus population during the survey period: an unidentified yellow-band disease, black band disease, white plague, and SCTLD. These diseases varied in prevalence, seasonality, and resulting mortality. Black-band disease and white plague are both known in other species and regions to increase in prevalence during seasonal hyperthermal events (Kuta and Richardson, 1996; Cróquer and Weil, 2009). Before the emergence of SCTLD, white plague had the greatest impact on the Florida population, was particularly associated with warm-water events, and contributed to unsustainable losses. White plague was documented during 12% of observations, with seasonal peaks of 40 and 42% prevalence during the autumns of 2014 and 2015, respectively. In comparison, observations on a D. cylindrus population in Colombia documented white disease prevalence of 9.4% in January 2002 and 1.5% in October 2012 (Bernal-Sotelo et al., 2019), and a study of a population in Venezuela identified white disease in only 0.2% of colonies (Cavada-Blanco et al., 2020).
The Florida D. cylindrus population was heavily impacted by hyperthermal bleaching events in 2014 and 2015 that resulted in high tissue mortality at some sites. During the 1983 Florida mass thermal bleaching, Jaap (1985) identified D. cylindrus as bleaching resistant. Maximum water temperatures in the Lower Keys region of study during that event were 32.3°C. During the 2014 and 2015 bleaching events, in situ water temperatures in the same region exceeded 32.3°C for 3 and 4 days, respectively. Single-day high temperatures are poor predictors of bleaching compared to compounded thermal stress as identified by Degree Heating Weeks (Kayanne, 2017), but high thermal stress or thermal stress compounded by other factors such as ultraviolet radiation (Gleason and Wellington, 1993) or degraded water quality (Lapointe et al., 2019) in 2014–2015 resulted in severe bleaching and associated mortality in a species historically thought to be bleaching-resistant.
The stressors documented from the baseline surveys through the end of 2015, which were predominantly white plague and two bleaching events, led to substantial losses of D. cylindrus, including the extirpation of two genotypes. Within the subset of colonies monitored every year (shown in Figure 9), 35% of live tissue died during this time frame; regional breakdowns were 43% loss in the Upper Keys, 24% loss in the Middle Keys, and 36% loss in the Lower Keys. However, in the Middle Keys and Lower Keys, tissue losses subsequently stabilized until SCTLD began impacting the regions. Using the same subset of colonies monitored every year, we calculated regeneration rates during non-stressor years. In the Lower Keys, the amount of live tissue increased 3.8% from 2016 to 2017 and 4.1% from 2017 to 2018. Thus, in the absence of severe stressors, regeneration of lost tissue on extant colonies slowly began rebuilding the population. Assuming a 4% annual regeneration rate, an 11-year interval would be required to replace the tissue losses that occurred as a result of the 2014–2015 mortality events. These values are similar to those predicted for the branching coral Acropora palmata in the Florida Keys, which suggested an 11-year recovery period following mortality from the 2005 hurricane season (Williams and Miller, 2012). Many factors are likely to influence the recovery rates of colonies, including the severity and pattern of tissue mortality, the capacity of the denuded skeleton to support recolonization, and the reef conditions suitable for coral growth (Lester and Bak, 1985). However, if colonies retain some live tissue, the Lower Keys data suggest that colony recovery would be possible if tissue loss did not result in complete colony mortality and if suitable conditions extended for long enough between acute mortality events.
Though recovery may be possible in suitable conditions, the intervals between acute mortality events including hurricanes, bleaching events, and disease outbreaks are currently shorter than the expected recovery time. Hurricane strikes are irregular, but based on historical data, the interval of a strike to the Key West region averages every 8 years (Blake et al., 2011). Lower Keys D. cylindrus colonies impacted by Hurricane Irma in 2017 exhibited some pillar breakage as well as lateral colony movements of up to 12 m. However, tissue loss resulting from these processes was not substantial. The extensive clonality of D. cylindrus colonies within sites, including areas with up to 150 clonal ramets, suggests that this species relies on asexual fragmentation as a form of colony production, and that storm-related breakage can have positive as well as negative impacts on the species’ success.
Other stressors that resulted in substantial tissue loss to the Florida D. cylindrus population are predicted to increase in frequency. Mass coral bleaching, which led to both tissue and whole colony losses in 2014 and 2015, is expected to become an annual event across much of the FRT by 2040 (van Hooidonk et al., 2016). Though bleaching does not necessarily result in total mortality, and some D. cylindrus have shown adaptability to bleaching through shuffled symbionts (Lewis et al., 2019), recovery of typical symbiont and microbial communities may take several years (Bourne et al., 2008; Edmunds et al., 2014; Kemp et al., 2014). Due to sub-optimal holobiont partnerships, bleaching stress is likely to alter long-term physiological functions, including reduced colony growth and reproductive output (Szmant and Gassman, 1990; Mendes and Woodley, 2002).
In considering the many stressors affecting Florida D. cylindrus, disease-related mortality has been the primary driver of population decline. SCTLD has been unprecedented in its temporal and spatial extent and is unusual in maintaining its virulence throughout multiple seasonal cycles. Prior to SCTLD, D. cylindrus colonies were mostly affected by white plague and black band disease, both associated with high thermal stress. This mirrors correlations between hyperthermal stress and disease prevalence in other regions and species (Cróquer and Weil, 2009). Maynard et al. (2015) modeled the timing of conditions favoring general coral diseases: host susceptibility, pathogen abundance, and pathogen virulence. In Florida, these models indicate that at least two of these three disease-enhancing scenarios will be met approximately 25 years before annual bleaching is predicted. With van Hooidonk et al. (2016) predicting annual bleaching throughout southeast Florida by 2040, this 25-year earlier disease prediction coincided with the rise of SCTLD and widespread loss of D. cylindrus to disease.
These model predictions have matched the demise of D. cylindrus in Florida, but future predictions are largely irrelevant for this now decimated population. Initial small population size and geographic distances between genotypes most likely rendered the Florida population reproductively extinct during the 20th century. Genetic analyses by Chan et al. (2019) indicate that the Florida D. cylindrus population is distinct from other Caribbean populations, so influx from other regions is neither historically significant nor likely to be an option for natural replenishment. The Florida colonies have suffered catastrophic loss, with the most recent and devastating cause of mortality being SCTLD. Losses in genetic diversity, colony numbers, and live tissue have rendered the species functionally extinct on the FRT, with no ability for natural recovery.
Future of the Species
In Florida, D. cylindrus is reproductively and functionally extinct. The few remaining bits of live tissue are small, geographically isolated, and vulnerable to chronic stressors as well as widespread acute stressors such as SCTLD infection or the next thermally-associated bleaching and disease events.
In response to D. cylindrus losses in 2014 and 2015, a genetic rescue program was initiated in late 2015. Fragments were removed from the wild for safekeeping at nursery and land-based facilities. Through this effort, the extinction of Florida genotypes was halted by maintaining genetic material and creating grow-out and broodstock. As of 2020, 543 collected fragments representing 123 genotypes were held in land-based and offshore nurseries. In addition to maintaining the Florida genetic stock, these fragments have also been used as the broodstock in the first successful controlled reproduction of an Atlantic species (Johnson, 2019) which has increased the genetic diversity of the population within the genetic bank. Much like the California condor (Wallace and Toone, 1992), the fate of D. cylindrus lies in captive breeding to produce diverse as well as resilient genotypes. Large-scale efforts to improve water quality, and curb climate change are also essential for creating the conditions that will allow for the successful future restoration, survival, and wild reproduction of this iconic and unique coral.
Data Availability Statement
The raw data supporting the conclusions of this article will be made available by the authors, without undue reservation.
Author Contributions
KL contributed to initial funding, project design, and gathering of baseline colony locations. LK primarily surveyed sites north of Biscayne National Park. CL assisted in data collection through monitoring and collaborative funding support and assisted with manuscript preparation. KN led data collection, secured subsequent funding, conducted analyses, and led manuscript preparation. All authors provided feedback on the manuscript.
Funding
This research was supported by Florida’s Wildlife Legacy Initiative (State Wildlife Grants CFDA #15.634 to KL), the United States Fish and Wildlife Service (Marine Projects Grant Cycle award #F13AF01085 to KL), the National Oceanographic and Atmospheric Administration (Coral Reef Conservation Program Award #NA18NOS4820206 to KN), the National Science Foundation [Rapid Response Research grant #1503483 to Rodriguez-Lanetty at Florida International University Integrative Marine Genomics and Symbiosis (IMaGeS) lab], and significant donation of time and resources by the authors as well as by the Florida Aquarium’s Center for Conservation. The work was conducted under FKNMS permits FKNMS-2013-085-A1, FKNMS-2014-004-A1, and FKNMS-2016-004-A1, Dry Tortugas Permit DRTO-2015-SCI-0018, Biscayne National Park Permits BISC-2013-SCI-018 and BISC-2015-SCI-0019, and State of Florida permit SAL-13-1451-SRP.
Conflict of Interest
The authors declare that the research was conducted in the absence of any commercial or financial relationships that could be construed as a potential conflict of interest.
Acknowledgments
We thank scientific divers from Florida Aquarium, Keys Marine Laboratory, Florida FWC/FWRI, Florida International University, and Nova Southeastern University, particularly Kevin Macaulay, Emily Hower, and the Coral Reef Restoration, Assessment and Monitoring Lab, for assistance with fieldwork. We are additionally grateful to individuals, dive shops, and research programs that provided site locations. This is contribution number 256 from the Coastal and Oceans Division of the Institute of the Environment at Florida International University.
Supplementary Material
The Supplementary Material for this article can be found online at: https://www.frontiersin.org/articles/10.3389/fmars.2021.656515/full#supplementary-material
References
Acosta, A., and Acevedo, A. (2006). Population structure and colony condition of Dendrogyra cylindrus (Anthozoa: Scleractinea) in Providencia Island, Colombian Caribbean. Proc. Int. Coral Reef Symp. 10, 1605–1610.
Aronson, R., Bruckner, A., Moore, J. A., Precht, B., and Weil, E. (2008). Dendrogyra cylindrus. The IUCN Red List of Threatened Species 2008: e.T133124A3582471. doi: 10.2305/IUCN.UK.2008.RLTS.T133124A3582471.en
Aronson, R. B., and Precht, W. F. (2001). White-band disease and the changing face of Caribbean coral reefs. Hydrobiologia 460, 25–38. doi: 10.1007/978-94-017-3284-0_2
Bartlett, L. A., Brinkhuis, V. I., Ruzicka, R. R., Colella, M. A., Lunz, K. S., Leone, E. H., et al. (2018). Dynamics of stony coral and octocoral juvenile assemblages following disturbance on patch reefs of the Florida Reef Tract. Mar. Sci. Publicat. 2018:1230.
Baums, I. B., Miller, M. W., and Szmant, A. M. (2003). Ecology of a corallivorous gastropod, Coralliophila abbreviata, on two scleractinian hosts. 1: Population structure of snails and corals. Mar. Biol. 142, 1083–1091. doi: 10.1007/s00227-003-1024-9
Bernal-Sotelo, K., Acosta, A., and Cortés, J. (2019). Decadal Change in the Population of Dendrogyra cylindrus (Scleractinia: Meandrinidae) in Old Providence and St. Catalina Islands, Colombian Caribbean. Front. Mar. Sci. 2019:5.
Blake, E. S., Landsea, C. W., and Gibney, E. J. (2011). The deadliest, costliest, and most intense United States tropical cyclones from 1851 to 2010 (and other frequently requested hurricane facts) NOAA Technical Memorandum NSW NHC-6. Washington, D.C: NOAA/NWS/NCEP/National Hurricane Center.
Bourne, D., Iida, Y., Uthicke, S., and Smith-Keune, C. (2008). Changes in coral-associated microbial communities during a bleaching event. ISME J. 2, 350–363. doi: 10.1038/ismej.2007.112
Causey, B. (2008). “Coral Reefs of the U.S. Caribbean: The History of Massive Coral Bleaching and other Perturbations in the Florida Keys,” in Status of Caribbean coral reefs after bleaching and hurricanes in 2005. Global Coral Reef Monitoring Network, eds C. Wilkinson and D. Souter (Townsville, QLD: Reef and Rainforest Research Center), 61–67.
Cavada-Blanco, F., Cappelletto, J., Agudo-Adriani, E., Martinez, S., Croquer, A., and Rodriguez, J. P. (2020). Status of the pillar coral Dendrogyra cylindrus in Los Roques National Park, Southern Caribbean. bioRxiv. doi: 10.1101/2020.09.15.297770
Chan, A. N., Lewis, C. L., Neely, K. L., and Baums, I. B. (2019). Fallen Pillars: The Past, Present, and Future Population Dynamics of a Rare, Specialist Coral–Algal Symbiosis. Front. Mar. Sci. 6:218.
Courchamp, F., Clutton-Brock, T., and Grenfell, B. (1999). Inverse density dependence and the Allee effect. Trends Ecol. Evol. 14, 405–410. doi: 10.1016/s0169-5347(99)01683-3
Cróquer, A., and Weil, E. (2009). Changes in Caribbean coral disease prevalence after the 2005 bleaching event. Dis. Aquatic Organ. 33–43. doi: 10.3354/dao02164
Edmunds, P. J., Pochon, X., Levitan, D. R., Yost, D. M., Belcaid, M., Putnam, H. M., et al. (2014). Long-term changes in Symbiodinium communities in Orbicella annularis in St. John, US Virgin Islands. Mar. Ecol. Prog. Ser. 506, 129–144. doi: 10.3354/meps10808
Florida Coral Disease Response Research and Epidemiology Team (2018). Case Definition: Stony Coral Tissue Loss Disease. Available online at: https://floridadep.gov/sites/default/files/Copy%20of%20StonyCoralTissueLossDisease_CaseDefinition%20final%2010022018.pdf (accessed date October 2, 2018).
FRRP (2015). Florida Reef Resilience Program’s Disturbance Response Monitoring Florida Keys Reef Zones GIS Shapefile (Version 2).
Gleason, D. F., and Wellington, G. M. (1993). Ultraviolet Radiation and Coral Bleaching. Nature 365, 836–838. doi: 10.1038/365836a0
Goreau, T. J., Cervino, J. M., Goreau, M., Hayes, R., Hayes, M., Richardson, L., et al. (1998). Rapid spread of diseases in Caribbean coral reefs. Rev. Biol. Trop 46, 157–171.
Hudson, J. H., and Goodwin, W. B. (1997). Restoration and growth rate of hurricane damaged pillar coral (Dendrogyra cylindrus) in the Key Largo National Marine Sanctuary, Florida. Proc. Int. Coral Reef Symp. 1, 567–570.
Humann, P., and DeLoach, N. (2013). Reef Coral Identification: Florida, Caribbean, Bahamas. Jacksonville, FL: New World Publications
Jaap, W. (1985). An epidemic zooxanthellae expulsion during 1983 in the lower Florida Keys coral reefs: hyperthermic etiology. Proc. Int. Coral. Reef. Symp. 6, 143–148.
Johnson, L. M. (2019). A scientific breakthrough at the Florida Aquarium could save “America’s Great Barrier Reef”, CNN.Available online at: https://www.cnn.com/2019/08/21/us/historic-coral-discovery-scn-trnd/index.html(accessed date 22 August 2019)
Kabay, L. (2016). Population Demographics and Sexual Reproduction Potential of the Pillar Coral, Dendrogyra cylindrus, on the Florida Reef Tract. Fort Lauderdale, FL: Nova Southeastern University.
Kayanne, H. (2017). Validation of degree heating weeks as a coral bleaching index in the northwestern Pacific. Coral Reefs 36, 63–70. doi: 10.1007/s00338-016-1524-y
Kemp, D., Fitt, W., and Schmidt, G. (2008). A microsampling method for genotyping coral symbionts. Coral Reefs 27, 289–293. doi: 10.1007/s00338-007-0333-8
Kemp, D. W., Hernandez-Pech, X., Iglesias-Prieto, R., Fitt, W. K., and Schmidt, G. W. (2014). Community dynamics and physiology of Symbiodinium spp. before, during, and after a coral bleaching event. Limnol. Oceanogr. 59, 788–797. doi: 10.4319/lo.2014.59.3.0788
Kuta, K., and Richardson, L. (1996). Abundance and distribution of black band disease on coral reefs in the northern Florida Keys. Coral Reefs 15, 219–223. doi: 10.1007/bf01787455
Lapointe, B. E., Brewton, R. A., Herren, L. W., Porter, J. W., and Hu, C. (2019). Nitrogen enrichment, altered stoichiometry, and coral reef decline at Looe Key, Florida Keys, USA: a 3-decade study. Mar. Biol. 166:108.
Lester, R. T., and Bak, R. P. M. (1985). Effects of environment on regeneration rate of tissue lesions in the reef coral Montastrea annularis (Scleractinia). Mar. Ecol. Prog. Ser. 24, 183–185. doi: 10.3354/meps024183
Levitan, D. R., and McGovern, T. M. (2005). The Allee effect in the sea. Marine conservation biology: the science of maintaining the sea’s biodiversity. Washington DC: Island Press, 47–57.
Lewis, C., Neely, K., and Rodriguez-Lanetty, M. (2019). Recurring Episodes of Thermal Stress Shift the Balance From a Dominant Host-Specialist to a Background Host-Generalist Zooxanthella in the Threatened Pillar Coral, Dendrogyra cylindrus. Front. Mar. Sci. 6:5.
Lewis, C. L., Neely, K. L., Richardson, L. L., and Rodriguez-Lanetty, M. (2017). Temporal dynamics of black band disease affecting pillar coral (Dendrogyra cylindrus) following two consecutive hyperthermal events on the Florida Reef Tract. Coral Reefs 36, 427–431. doi: 10.1007/s00338-017-1545-1
Maynard, J., van Hooidonk, R., Eakin, C. M., Puotinen, M., Garren, M., Williams, G., et al. (2015). Projections of climate conditions that increase coral disease susceptibility and pathogen abundance and virulence. Nat. Clim. Chang. 5, 688–694. doi: 10.1038/nclimate2625
Meesters, E. H., Hilterman, M., Kardinaal, E., Keetman, M., de Vries, M., and Bak, R. P. M. (2001). Colony size-frequency distributions of scleractinian coral populations: spatial and interspecific variation. Mar. Ecol. Prog. Ser. 209, 43–54. doi: 10.3354/meps209043
Mendes, J. M., and Woodley, J. D. (2002). Effect of the 1995-1996 bleaching event on polyp tissue depth, growth, reproduction and skeletal band formation in Montastraea annularis. Mar. Ecol. Prog. Ser. 235, 93–102. doi: 10.3354/meps235093
Moulding, A. (2005). Coral recruitment patterns in the Florida Keys. Rev. Biol. Trop. 53(Suppl. 1), 75–82.
Neely, K. L. (2018). Appearance of Stony Coral Tissue Loss Disease (SCTLD) on susceptible species. Available at https://www.agrra.org/coral-disease-outbreak/ (accessed March 26, 2021).
Neely, K. L., Lewis, C., Chan, A., and Baums, I. B. (2018). Hermaphroditic spawning by the gonochoric pillar coral Dendrogyra cylindrus. Coral Reefs 37, 1087–1092. doi: 10.1007/s00338-018-1730-x
Neely, K. L., Lewis, C. L., and Macaulay, K. A. (2020). Disparities in spawning times between in situ and ex situ pillar corals. Front. Mar. Sci. 2020:7. doi: 10.3389/fmars.2020.00643
NOAA (2013-2019). NOAA Coral Reef Watch Version 3.1 Daily Global 5-km Satellite Coral Bleaching Degree Heating Week Product. College Park, MD, USA.
Porter, J. W., and Meier, O. W. (1992). Quantification of Loss and Change in Floridian Reef Coral Populations. Am. Zool. 32, 625–640.
Precht, W. F., Gintert, B. E., Robbart, M. L., Fura, R., and van Woesik, R. (2016). Unprecedented Disease-Related Coral Mortality in Southeastern Florida. Sci. Rep. 6:31374.
Richardson, L., Goldberg, W., Carlton, R. G., and Halas, J. C. (1998). Coral disease outbreak in the Florida Keys: Plague Type II. Rev. Biol. Trop. 46, 187–198
Ruzicka, R. R., Colella, M. A., Porter, J. W., Morrison, J. M., Kidney, J. A., Brinkhuis, V., et al. (2013). Temporal changes in benthic assemblages on Florida Keys reefs 11 years after the 1997/1998 El Nino. Mar. Ecol. Prog. Ser. 489, 125–141. doi: 10.3354/meps10427
Sutherland, K. P., Porter, J. W., and Torres, C. (2004). Disease and immunity in Caribbean and Indo-Pacific zooxanthellate corals. Mar. Ecol. Prog. Ser. 266, 273–302. doi: 10.3354/meps266273
Szmant, A. M. (1986). Reproductive Ecology of Caribbean Reef Corals. Coral Reefs 5, 43–53. doi: 10.1007/bf00302170
Szmant, A. M., and Gassman, N. J. (1990). The effects of prolonged “bleaching” on the tissue biomass and reproduction of the reef coral Montastrea annularis. Coral Reefs 8, 217–224. doi: 10.1007/BF00265014
Thurber, R. L. V., Burkepile, D. E., Fuchs, C., Shantz, A. A., McMinds, R., and Zaneveld, J. R. (2014). Chronic nutrient enrichment increases prevalence and severity of coral disease and bleaching. Glob Change Biol 20, 544–554. doi: 10.1111/gcb.12450
USFWS (2014). Endangered and Threatened Wildlife and Plants: Adding 20 Coral Species to the List of Endangered and Threatened Wildlife: 50 CFR Part 17. Federal Registry 59, 67356–67359.
van Hooidonk, R., Maynard, J., Tamelander, J., Gove, J., Ahmadia, G., Raymundo, L., et al. (2016). Local-scale projections of coral reef futures and implications of the Paris Agreement. Sci. Rep. 2016:6.
Wallace, M., and Toone, W. (1992). Captive management for the long term survival of the California condor Wildlife 2001: populations. Berlin: Springer, 766–774.
Weil, E., and Hooten, A. J. (2008). Underwater Cards for Assessing Coral Health on Caribbean Reefs. Australia: Coral Reef Targeted Research & Capacity Building for Management.
Keywords: pillar coral, stony coral tissue loss disease, bleaching, population decline, regional extinction, Dendrogyra cylindrus
Citation: Neely KL, Lewis CL, Lunz KS and Kabay L (2021) Rapid Population Decline of the Pillar Coral Dendrogyra cylindrus Along the Florida Reef Tract. Front. Mar. Sci. 8:656515. doi: 10.3389/fmars.2021.656515
Received: 20 January 2021; Accepted: 31 March 2021;
Published: 22 April 2021.
Edited by:
William F. Precht, Dial Cordy and Associates, Inc., United StatesReviewed by:
J. P. Rippe, University of Texas at Austin, United StatesAldo Cróquer, The Nature Conservancy (Dominican Republic), Dominican Republic
Copyright © 2021 Neely, Lewis, Lunz and Kabay. This is an open-access article distributed under the terms of the Creative Commons Attribution License (CC BY). The use, distribution or reproduction in other forums is permitted, provided the original author(s) and the copyright owner(s) are credited and that the original publication in this journal is cited, in accordance with accepted academic practice. No use, distribution or reproduction is permitted which does not comply with these terms.
*Correspondence: Karen L. Neely, a25lZWx5MEBub3ZhLmVkdQ==