- 1Department of Ichthyology, Natural History Museum of Los Angeles County, Los Angeles, LA, United States
- 2National Museum of Natural History, Smithsonian Institution, Washington, DC, United States
Disjunct distributions of flora and fauna have intrigued biogeographers for centuries and have been central to debates over the roles that dispersal and vicariance have in speciation and evolutionary biology. One of the most notable disjunct distributions is when a single species or closely related taxa are present in temperate or polar regions of both hemispheres, but absent near the equator. Generally referred to as an anti-tropical distribution, this pattern is prevalent in marine taxa, occurring across the Tree of Life from single-celled bacteria and radiolarians, to algae, many invertebrates, fishes and marine mammals. The abundance of different species that exhibit this distribution pattern has led to a large number of theories on how it is formed and maintained. Here, seven central hypotheses that recur throughout the literature regarding the mechanisms responsible for this pattern are described and discussed in light of both historic and contemporary data. Recent advances in paleoclimate modeling and phylogenetic approaches have revealed that three of these hypotheses lack support. However, these data also highlight that a single driver of anti-tropicality is unlikely and that multiple mechanisms are responsible for this observed distribution pattern. The importance of using a multifaceted approach in future studies that incorporates phylogenetic, physiological, life history and ecological data is discussed, as there is a gap in our knowledge regarding adult and larval behavior, as well as thermal tolerances of many anti-tropical species. While recent studies have helped reveal some commonalities between anti-tropical groups, only by using an integrative approach will we be able to more fully understand which mechanisms are most prevalent in driving this pattern across the oceans.
Introduction
As early scientific explorers began traveling the world, they were intrigued when they came across the same, or closely related, species at two locations separated by thousands of kilometers (Hooker, 1847; Ross, 1847). Disjunct distributions have inspired a variety of questions in the field of biogeography, which have led to many debates in the literature regarding potential shared mechanisms that may influence these patterns. One of the most striking disjunct distributions is that of species which are found in the temperate or polar zones of each hemisphere, but which are absent from the tropics (Figure 1). These latitudinally disjunct distributions have gone by a variety of names over time, some of which signify subtle distinctions (Table 1). For simplicity, in this review these distributions will be collectively encompassed by the term “anti-tropical” (as per Hubbs, 1952). This distribution pattern is observed at multiple taxonomic scales, including populations of a single species, sister-species, genera, or families separated by the equator. While anti-tropical distributions are found in terrestrial systems (particularly in both vascular and non-vascular plants: Thorne, 1972; Smissen et al., 2003; Villaverde et al., 2015), it is more common in marine taxa, with examples found across the Tree of Life (see Briggs, 1995).
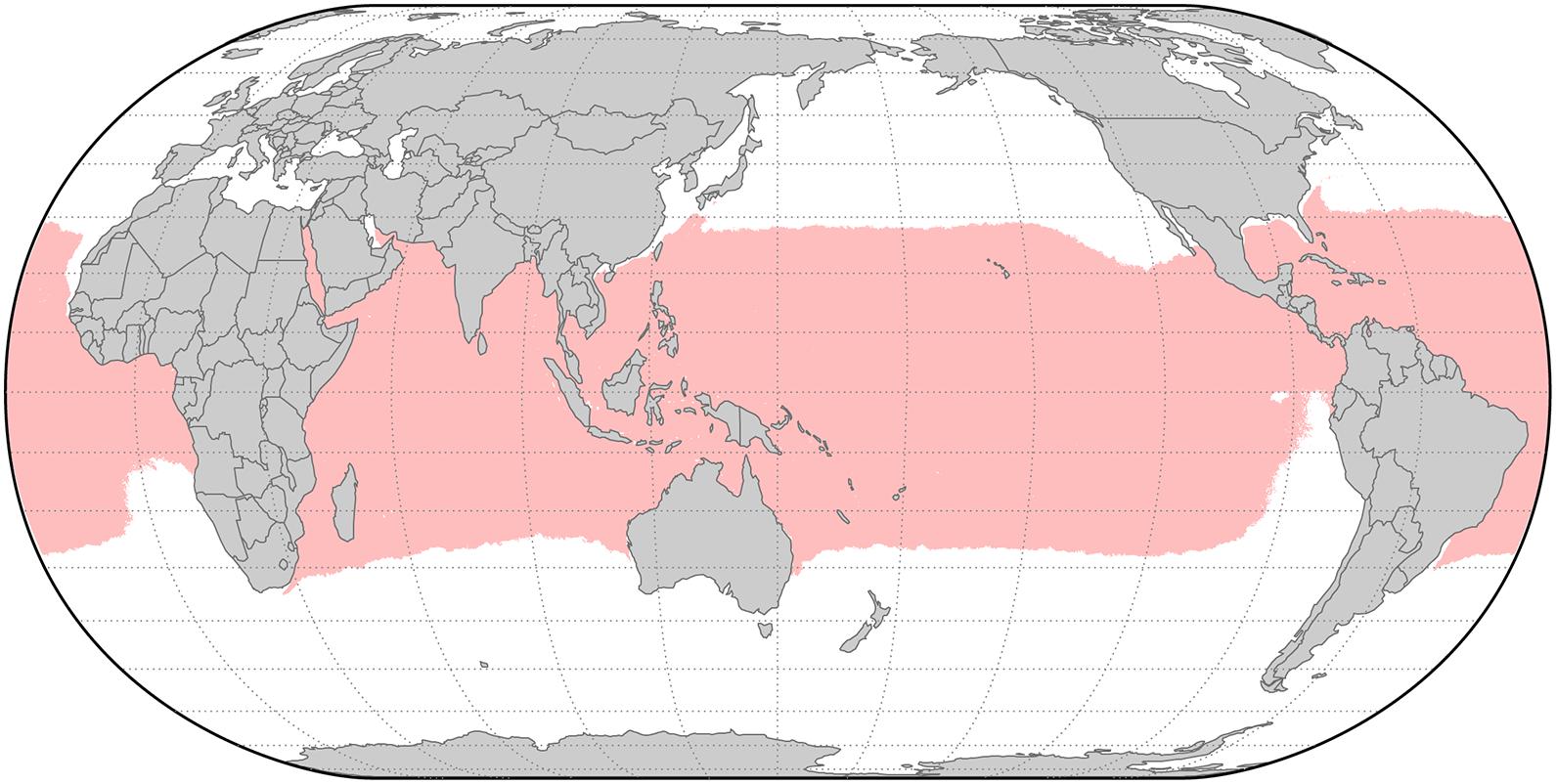
Figure 1. The tropics (shown in red), as defined by a 20°C isocryme during the coldest period of the year. Anti-tropical taxa are generally distributed on opposite sides of this zone (with Hawai’i as a notable exception), although some authors use latitudinal lines to define anti-tropicality instead of temperatures. Data shown is the SST from the coldest ice-free month from the MARSPEC database (Sbrocco and Barber, 2013) plotted using a Robinson projection in ArcMap v10.8.1 (ESRI).
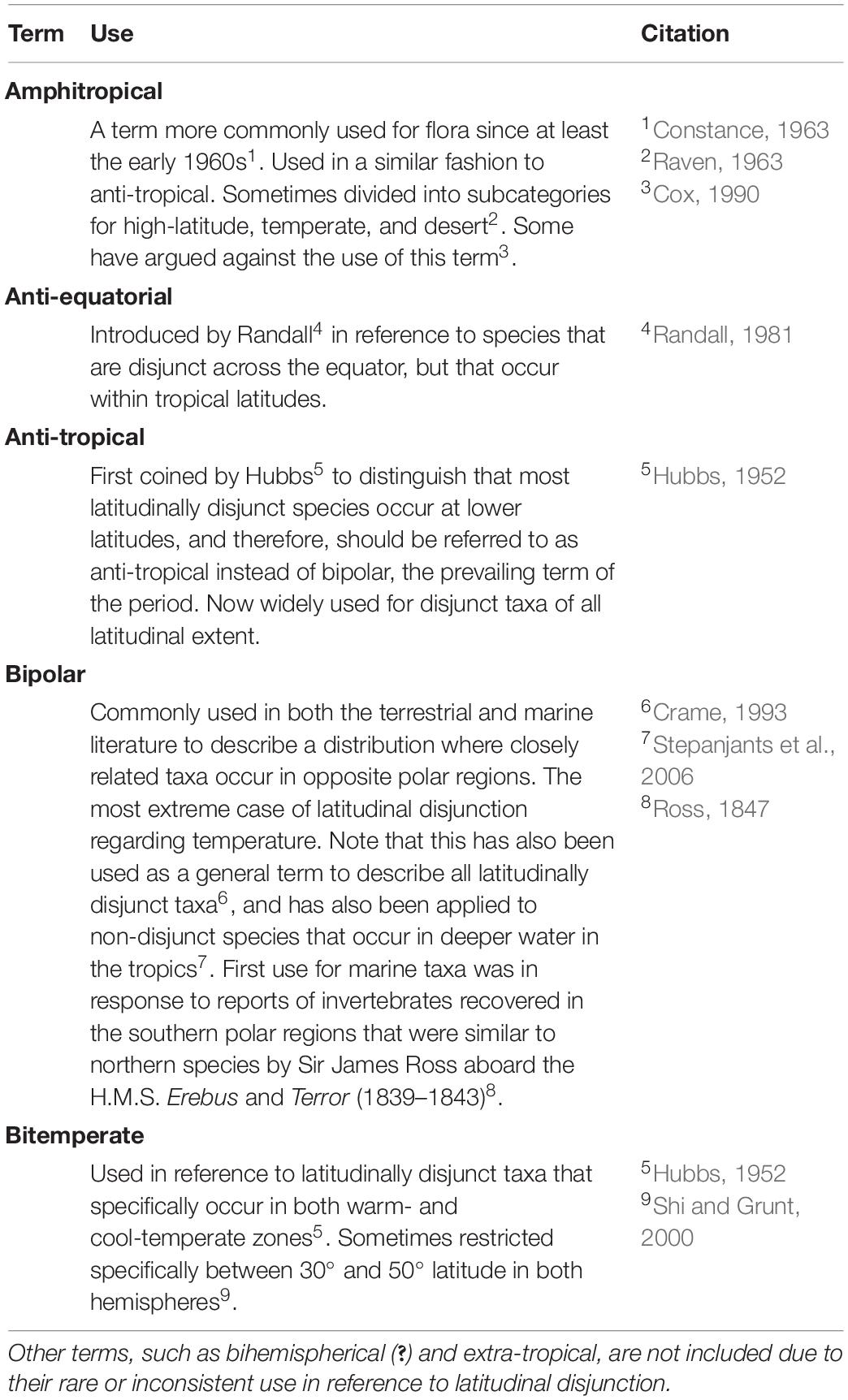
Table 1. Common terms used to describe terms used to describe various degrees of latitudinal disjunct taxa over time and how they have been applied.
It is perhaps the aquatic medium of the world’s oceans that allows for such a distribution to form repeatedly. Many marine organisms disperse by means of a pelagic larval stage, where larvae can stay in the water column anywhere from days, to months on end, traveling with the currents. Dispersal potentials can also be high for species that lack a pelagic larval dispersal stage, as some species, such as cetaceans, can disperse or migrate long distances as adults. Throughout the Cenozoic there have been few hard barriers to gene flow and movement of these larvae or adults of highly mobile species, especially latitudinally. In concert, this lack of barriers gives many marine taxa extremely high dispersal potentials over evolutionary time scales, allowing them to either disperse across the tropics, or to expand and occupy large latitudinal ranges that could be subsequently split into anti-tropical distributions.
This biogeographic pattern has been noted for over a century, with numerous hypotheses proposed for its formation. These hypotheses generally fall into two overarching categories: dispersal and vicariance. Each hypothesis is further distinguished by the timing of disjunction, or by the mechanisms operating within these two broader categories. However, it is whether or not species disperse from one hemisphere to the other, or whether species were once widespread, and have subsequently been extirpated from lower latitudes that mainly distinguish these hypotheses. While other reviews on anti-tropicality have been published, these have either generally listed anti-tropical species for certain groups (e.g., Randall, 1981; Shi and Grunt, 2000; Stepanjants et al., 2006) or argued for one specific mechanism using observed species distributions (e.g., Briggs, 1987a). Creative approaches and new types of data have allowed researchers to test which mechanisms are ultimately responsible for this pattern, and are quickly changing the general perception of how anti-tropical distributions can form in a marine setting. Here, I aim to give a broad overview of the history of anti-tropicality by focusing on seven central hypotheses (Table 2). This review is not meant to be an exhaustive explanation of all potential hypotheses that have been proposed over the past century, but is meant to lay a general framework of explanations that have been recurrent throughout the literature over time. The historical background, associated assumptions and expectations, and example studies that either support or contradict each hypothesis are outlined below. Relevant recent research, outstanding questions, and open avenues for research are discussed at the end. Ultimately, it is my goal that the reader understands the history behind this pattern, and gains enough background to assess recent and future studies regarding this fascinating distribution.
Vicariance and Anti-Tropicality
Continental Fragmentation
The hypothesis operating at the oldest time scales for anti-tropicality is continental fragmentation, which suggests that anti-tropical species were restricted to the continental margins of the supercontinent Pangea (Nur and Ben-Avraham, 1982; Nelson, 1985). As this continent separated during the early Mesozoic, species moved with the continents and were split by the tropics, resulting in an anti-tropical distribution. For continental fragmentation to be a mechanism that has resulted in extant anti-tropical taxa, there are several assumptions that must be met. First, it assumes that anti-tropical species are not restricted by thermal tolerances, or at least were not originally restricted due to thermal tolerances, as strong latitudinal thermal gradients were not established until ice-sheets formed in the Southern hemisphere during the late Eocene/early Oligocene (Zachos et al., 2001; Liu et al., 2009). Second, it assumes that evolutionary lineages are dispersal limited, even over the time period of 175 million years (myr). Fragmentation occurred over such a long period that under this hypothesis, anti-tropical lineages should only be found at higher extant taxonomic levels or only be found in extinct clades. If lineages restricted to coastlines could not disperse between hemispheres, then these lineages would have over 100 myr to diversify in their respective hemispheres.
While this may be true for taxa restricted to continental landmasses (e.g., McCulloch et al., 2016), over these time periods most marine lineages are unlikely to be dispersal limited. Evidence for this hypothesis is limited primarily due to the fact that many extant anti-tropical species, genera, and families originated after the start of the Cenozoic. Therefore, the timing of this splitting event does not correspond to many current anti-tropical distributions at the population, sister-species, or sister-genera taxonomic levels (e.g., in fishes: Burridge, 2002; for fossil mollusks: Lindberg, 1991). Evidence is limited for this hypothesis even at higher taxonomic levels, such as freshwater fish families on opposite sides of the tropics, which date to approximately the Cretaceous–Palaeogene transition (Chen et al., 2014). It is possible that this mechanism could have resulted in anti-tropical distributions for taxa that are now extinct. Crame (1993) proposed this as a possible explanation for the distribution of extinct mollusks of the Mesozoic, for which there is a decent fossil record. Unfortunately, the fossil record is rather incomplete for many marine lineages making it difficult to confidently identify anti-tropical distributions. Further, fossil evidence for this explanation may be spurious, with some suggesting that phenotypically similar anti-tropical fossils may actually represent distantly related taxa that were subject to convergent evolution, making this hypothesis difficult to confidently assess in some extinct groups (see Lee et al., 2016).
Island Integration
Islands have been used as natural experiments over the last century. Understanding the impact that isolation, colonization, and geological history have on terrestrial island biotas has resulted in seminal papers in biogeography (for example the theory of island biogeography; MacArthur and Wilson, 1967). Dispersal is prominent in discussions of island biogeography, but came under scrutiny when vicariance gained popularity in the latter half of the 20th century. Originally postulated by Rotondo et al. (1981) as a vicariant counter-point to the founder principle (the dispersal and colonization of remote islands), island integration suggests that species do not need to undergo long-distance dispersal to reach isolated areas. Instead, this theory suggests that the islands themselves are dispersal vectors that follow the movements of the oceanic tectonic plates (Rotondo et al., 1981). Under this scenario, species reach isolated islands if the paths of the islands they are currently on intersect other island arcs.
Rotondo et al. (1981) originally used island integration to explain the taxonomic similarities between the Hawaiian Islands and islands in southeast Oceania by noting that some of the seamounts surrounding the Hawaiian Islands are substantially older than their nearby Hawaiian counterparts. These ages suggest that they originated elsewhere, and moved with the Pacific plate to their current location, intersecting the islands formed by the Hawaiian anomaly zone (hot spot). The authors also suggest, based on the ages estimated for these older islands and sea mounts, that they could have originated south of the equator near the East Pacific Rise and traveled approximately 30° in latitude to their current locations (Figure 2 in Rotondo et al., 1981). Springer (1982) later explicitly used this hypothesis to explain anti-tropicality in the Pacific. A community forming on an isolated island could conceivably travel this distance between 33 and 66 myr (assuming a rapid plate movement of 10 cm year–1 or the average oceanic plate movement of 5 cm year–1), respectively placing endemic biota in radically different ecosystems (see Ali, 2017).
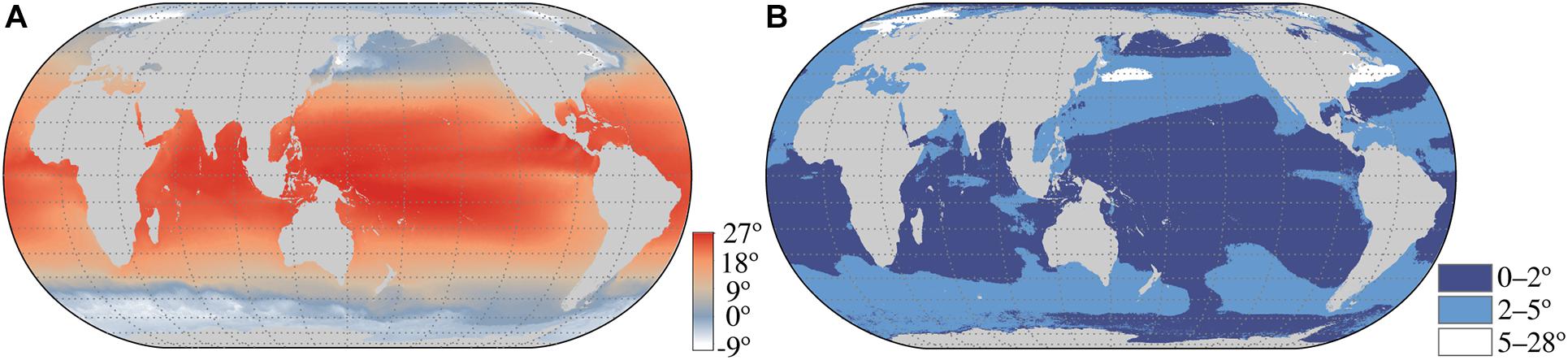
Figure 2. Pleistocene glacial sea surface temperature (SST) during the coldest month of the year (A), and the temperature difference between current SST and Pleistocene LGM SST (B). Data shown is the SST from the coldest ice-free month from the MARSPEC database (Sbrocco and Barber, 2013) plotted using a Robinson projection in ArcMap v10.8.1 (ESRI).
There are several assumptions associated with island integration. Primarily, it assumes that anti-tropical taxa are dispersal limited across evolutionary time scales, and that these species are not physiologically restricted to cooler environments, but could cross the warmer tropics without extirpation. It also assumes that the islands could traverse this distance while either remaining subaerial, or staying shallow enough to support many of the anti-tropical species that do not inhabit deeper waters. Divergence estimates between Hawaiian and southeast Pacific taxa dating between the late Eocene/early Oligocene and the onset of the Cenozoic (or older), approximately 33–66 million years ago (mya), would provide some support for this hypothesis.
On the scale of 71–77.6 myr [Clague and Dalrymple (1975); the original age estimates used in Rotondo et al. (1981)], Wentworth Seamount and Necker Island would have experienced significant erosion and sinking due to lithospheric cooling (Parsons and Sclater, 1977; Stein and Stein, 1992; Hillier and Watts, 2005). Updated age estimates for this sea mount of 90 myr (Pringle and Dalrymple, 1993) place it on the older range of time frames for the island integration hypothesis. And while there are anti-tropical sister-species that occur in the Hawaiian islands and southeast Pacific islands like Rapa Nui, one study examining the biogeographic history of reef fishes from several families recovered molecular data that suggest Miocene to Pleistocene connections between these locations, contradicting this hypothesis (Delrieu-Trottin et al., 2019). Furthermore, while this proposal addresses anti-tropical species between Hawaii and the southeast Pacific, it does not explain anti-tropical taxa that occur along continental margins. The myriad of anti-tropical taxa associated with continental margins, and the younger dates recovered between southeastern Pacific islands and the Hawaiian islands suggest that this is not a likely mechanism that generates anti-tropical distributions.
Island Submergence
Island submergence, another island-centric vicariant scenario, suggests that widespread oceanic species are split into northern and southern hemisphere populations when suitable habitat in the tropics, represented by islands, are submerged and lost. Originally proposed by Rehder (1980), this hypothesis does not have any defined time period on which it is acting, and therefore there are no expectations about which taxonomic level we would expect to be anti-tropical. However, this hypothesis implies several things. First, it assumes again that species are restricted to their current anti-tropical distributions due to a lack of available habitat in the tropics, and not due to physiological restrictions or species interactions. Secondly, this hypothesis assumes that there is a current lack of tropical oceanic habitat associated with islands. However, numerous examples of tropical islands can be found in the Indian (e.g., the Seychelles, Chagos, and Maldives), Pacific (e.g., the Caroline, Line, Marshall, Solomon, and Cook Islands), and Atlantic Oceans (e.g., the Antilles, St. Paul’s Rock, and Sao Tome and Principe). All of these examples contradict a current lack of tropical habitat assumed by this hypothesis.
Mid-Miocene Warming
Climate has varied considerably throughout the Cenozoic, with several peaks in global temperatures. From an early Eocene maximum ∼52 mya when ocean sea surface temperatures (SST) were 4–10° warmer than current values (Miller et al., 1987; Zachos et al., 1994, 2003), SST gradually cooled until the early Oligocene when Antarctic ice sheets formed ∼34 mya, strengthening the latitudinal thermal gradient (Zachos et al., 1994, 2001; Liu et al., 2009). Following the cooler temperatures in the Oligocene, temperatures gradually rose from the late Oligocene until the mid-Miocene climatic optimum ∼17–15 mya, when temperatures reached their highest since the early Eocene (Flower and Kennett, 1994; Zachos et al., 2001). Following this peak in global temperatures, SST cooled throughout the remainder of the Miocene, Pliocene, and Pleistocene (Zachos et al., 2001; Zhang et al., 2014).
This thermal history likely helped shape the distribution of organisms across the planet, including anti-tropical marine taxa. Links between anti-tropicality and Cenozoic temperature fluctuations were first proposed by Valentine (1984), who suggested that many species may have adapted to cooler temperatures during the Oligocene, only to be split into disjunct distributions by rapid warming during the mid-Miocene climatic optimum. This was further expanded by White (1986) as the main driver of anti-tropicality due to the fact that it “requires nothing unusual of modern anti-tropical taxa, only a mutual intolerance of modern tropical conditions.” In this view, even the most distantly related anti-tropical taxa with contradictory life history strategies or habitat requirements could be explained by the same mechanism.
The mid-Miocene warming hypothesis explicitly links anti-tropical distributions to physiological tolerances of marine organisms and to a definitive time period (White, 1986). Therefore, anti-tropical divergence events between clades should date to approximately 17–15 mya for this hypothesis to be supported in the strictest sense. A secondary assumption that anti-tropical marine taxa resulting from the mid-Miocene climatic optimum event should be physiologically restricted to cooler water temperatures may also be valid if niche conservatism operates on extended time scales (Romdal et al., 2013; Chiu et al., 2020).
This all-encompassing hypothesis has received criticism. Anti-tropical distributions are found across a variety of taxonomic scales, from populations of the same species separated by the tropics, to sister-species, genera, and higher taxonomic levels (Briggs, 1987a). The assortment of taxonomic scales for this pattern suggests that the mechanisms driving it span a variety of time-scales as well, and that they are not centered around a single event, such as the mid-Miocene warming period (Briggs, 1987b). Furthermore, advances in our understanding of paleoclimate have revealed that SST at the beginning of the Oligocene were not as cold as previously thought, and while there was substantial cooling at higher latitudes, the tropics only cooled ∼3°C when Southern hemisphere ice sheets formed (Liu et al., 2009). This thermal regime does not create a scenario where there were widespread temperate taxa prior to global warming that occurred in the late Oligocene and continued to the mid-Miocene envisioned by White (1986). The discrepancies between SST reconstructions, and between the taxonomic levels of anti-tropicality suggest that the mid-Miocene climatic optimum did not drive this pattern for all anti-tropical taxa.
However, the mid-Miocene hypothesis may remain a viable hypothesis for at least some anti-tropical species. Several studies have recovered divergence dates for anti-tropical taxa split by the tropics that date to the Miocene across a variety of marine groups, including deep-sea corals (Herrera et al., 2012), bryozoans (Schwaninger, 2008), echinoderms (Naughton et al., 2014), fishes (Stepien et al., 1997; Burridge, 2002; Ludt et al., 2015), and even higher taxonomic levels of dolphins (Banguera-Hinestroza et al., 2014; Supplementary Table 1). While not all of these dates exactly coincide with the mid-Miocene climatic optimum (with many dating to the middle to late Miocene), shifting temperature regimes and the strengthening of latitudinal thermal gradients before and after this time period likely influenced the strength and direction of currents, possibly affecting the distribution of species in the oceans more generally (Herrera et al., 2012; see discussion on Miocene currents below).
Biotic Exclusion From the Tropics
A central tenet in evolution is the observation that every species has a center of origin from which they expand (Darwin, 1859). This principle forms the basis of the “Center of Origin” hypothesis for marine species, which explains the latitudinal, and longitudinal, biodiversity gradients in the Indo-Pacific by stating that the tropics, and in particular the Indo-Australian Archipelago (IAA), is where species originate and later expand their ranges, outcompeting other species (Ekman, 1953; Briggs, 1999, 2000). If new species originate in the tropics, and outcompete older, widespread species that have expanded into the temperate zones of both hemispheres, then this could result in anti-tropical distributions. Originally coined the “relict theory” (Theéel, 1885), this hypothesis was later refined and championed by Briggs (1987a).
Unlike many of the other hypotheses proposed for anti-tropical species, this is not contingent on any one time period. An older, widespread species could be excluded from the tropics to form a disjunct distribution at any period. This flexibility has been used as support for the relict theory, as anti-tropical distributions occur at multiple taxonomic scales, and therefore conceivably form at different time periods (Briggs, 1987a). However, what excludes these species from the tropics? Is it competition from younger species, as originally proposed? If so, then are they outcompeted by younger species in the same family, or unrelated, but functionally equivalent species? Biotic exclusion may also not be related to competition, but could be the result of some other biotic interaction, such as the loss of food sources or mutualists, or the introduction of predators, parasites, or diseases in the tropics.
As such, biotic exclusion is notoriously difficult to test, but it does provide three central predictions. First, it assumes that anti-tropical species are old, and should not have formed recently. Second, it assumes that species are not restricted to temperate areas due to physiological constraints. That is, they are excluded from the tropics by biotic factors (competition, predation, disease, etc.), but if only abiotic factors are considered, then they should have available habitat. Third, it assumes that anti-tropical taxa originated in the tropics. Younger coalescent times for anti-tropical taxa, physiological restrictions to colder temperatures, and biogeographic ancestral range reconstructions or fossil evidence suggesting species formation in temperate regions would question the validity of this hypothesis.
Support for this hypothesis remains mixed. In general, the evolutionary history and latitudinal diversity gradients across several marine groups support a scenario where species originate in the tropics and subsequently expand into temperate regions as predicted by the biotic exclusion hypothesis (Kiessling et al., 2010; Cowman and Bellwood, 2011; Jablonski et al., 2013; Siqueira et al., 2016). Yet, at broader taxonomic scales an inverse latitudinal diversification gradient, with higher speciation rates outside of the tropics, has been observed in marine fishes (Rabosky et al., 2018), which runs counter to the biotic exclusion hypothesis. While these broad patterns may not strictly apply to all temperate taxa, ancestral area reconstructions focusing specifically on anti-tropical fishes like sawtail surgeonfishes and stripeys, and also herbivorous sea chubs that are a mix of temperate and tropical species, have suggested species origination in temperate areas, not tropical ones (Ludt et al., 2015; Knudsen et al., 2019; Tea et al., 2019). While this runs counter to one of the assumptions of the biotic exclusion hypothesis, suggesting that it might not apply to all anti-tropical taxa, the occurrence of contemporary suitable abiotic habitat in the tropics across a wide diversity of anti-tropical fishes suggests that biotic exclusion may be a plausible explanation for some taxa (Ludt and Myers, 2021; see discussion below).
Dispersal and Anti-Tropicality
Isothermal Submergence
Dispersal hypotheses for anti-tropicality involve individuals dispersing across the tropics during cooler time periods, and generally assume that these species are physiologically restricted to temperate regions (although this is often untested). These thermal limits presumably prevent anti-tropical taxa from dispersing across the warmer surface waters in the tropics. One parsimonious way to explain the inter-hemisphere connections between anti-tropical taxa is that they merely cross the tropics in deeper, cooler water. This avenue of dispersal was perhaps first suggested by Sir James Ross during the Ross expeditions to the Antarctic Ocean (Ross, 1847), where Ross noticed certain invertebrate species that resembled species he had personally seen in the artic, and postulated that they must have migrated in abyssal waters (Murray et al., 1897). This idea was later formalized into the “migration theory” of Ekman (1953), and is still considered a plausible explanation for certain taxa (Hubbs, 1952; Poortvliet et al., 2013).
For species that are adapted to shallow-water habitats, Ekman (1953) considered this process a historical one, rather than an ongoing connection between hemispheres. Randall (1981) furthered this by suggesting that shallow water species may only disperse in deeper waters during glacial periods when they would not have to go as deep to access cooler water. Wide, shallow continental shelves were largely exposed during the Last Glacial Maximum (LGM), restricting many shallow water species to thin continental margins, and closer to steep drop offs (Ludt and Rocha, 2015). Restricting isothermal submergence to glacial periods makes this hypothesis similar to glacial dispersal (see below), and could be an explanation if SST during the Pleistocene were not cool enough in the tropics for temperate taxa to cross. Pleistocene glacial restrictions, however, would not be necessary for species that occupy a wide depth range and that disperse longer distances as adults, such as some sharks (e.g., Veríssimo et al., 2010), or for species that are only known from deeper waters. While there are many contemporary shallow-water anti-tropical taxa that do not occupy deep water habitats, and do not disperse far as adults, it is still plausible that larvae of these species may use deeper waters to cross the tropics during a pelagic larval phase, as many of the specific larval life history traits of anti-tropical taxa are unknown.
Population genetic studies of intra-specific anti-tropical taxa known to disperse long-distances in deep water that reveal contemporary gene flow between hemispheres would support this as a possible mechanism. In many cases this hypothesis is only applied to shallow water species that may use deeper, cooler water to disperse, but anti-tropical distributions in clades that aren’t known to occur near the surface highlight that possible deep-water connections between hemispheres can form latitudinally disjunct distributions. Anti-tropical taxa such as the mesopelagic fish genus Caristius (Stevenson and Kenaley, 2013), the deep-sea coral Paragorgia arborea (Herrera et al., 2012), and the recent occurrence of deep-sea northern hemisphere species in certain southern hemisphere locations, such as deep-sea grenadiers (Arkhipkin et al., 2010; Laptikhovsky et al., 2013), king crabs (Pérez-Barros et al., 2020), and several cephalopods (Laptikhovsky et al., 2011), emphasize these deep-water inter-hemispheral connections. If species with a wide depth range dispersed between hemispheres in deeper water during the Pleistocene, then this hypothesis is difficult to disentangle from the glacial dispersal hypothesis, and many of the assumptions and predictions will be similar (see below), but some studies suggest isothermal submergence due to species-specific life history traits such as dispersal ability and depth ranges (e.g., Poortvliet et al., 2013).
Glacial Dispersal
The Pleistocene epoch is defined by the onset of repeated glacial cycles. These cycles altered global temperatures and shifted sea levels. During glacial periods, sea levels were up to ∼125 m lower than current levels, and shallow water habitat was reduced to narrow bands on the continental shelves, in many cases reducing population sizes, and restricting gene flow among marine taxa (Ludt and Rocha, 2015). While these fluctuations may have negatively impacted many marine species, SST during glacial maxima may have been cool enough to allow anti-tropical species to disperse between hemispheres (Darwin, 1859; Berg, 1933). Glacial dispersal is a natural conclusion to make in marine systems where there are not many barriers to dispersal and many species have a dispersive larval stage that can last for weeks to months. One may think that the only thing preventing gene-flow across the tropics currently would be physiological constraints that may disappear during cooler glacial periods.
The glacial dispersal hypothesis assumes that species are currently unable to inhabit tropical waters due to physiological limits, and that anti-tropical divergence events occurred during the Pleistocene. Phylogenetic studies that date anti-tropical sister-species pairs to the Pleistocene, or population genetic studies of intra-specific anti-tropical taxa that reveal genetic diversity signatures consistent with founder events, or limited contemporary gene flow dating to this time period may support this hypothesis. The assumptions associated with the glacial dispersal and isothermal submergence hypotheses differ from the only alternative hypothesis that may operate on the same time scale, the vicariant biotic exclusion hypothesis, with regards to physiological restrictions of species to cooler waters, which is assumed in the former two hypotheses, but not the latter. Therefore, physiological studies of thermal tolerances suggesting that tropical temperatures exceed maximum critical temperatures in anti-tropical taxa would also support this hypothesis.
This conceptual model has been challenged with critics stating that SST did not cool enough during glacial periods to allow temperately restricted species to cross the tropics (Briggs, 1987a). SST during the LGM were cooler at higher latitudes, but tropical temperatures did not dramatically change (Figure 2). This likely compressed temperate communities into narrower latitudinal bands, and may have reduced the overall distance between disjunct populations, but even during the coldest month of the year, it is possible that tropical temperatures were not cool enough to allow anti-tropical species to cross in surface waters. An additional challenge of the glacial dispersal hypothesis, including glacial isothermal submergence, is determining whether taxa disperse across the tropics, or if they underwent range expansions across the tropics during glacial periods followed by subsequent vicariance as tropical temperatures rose after the LGM. While this is difficult to assess for many taxa, fossil distributions shed some light on this question. Radiolarians examined from seafloor sediments document at least one species, Cycladophora davisiana davisiana, that expanded its range from the northern hemisphere, to a widespread distribution that traversed the tropics into the southern hemisphere in the early Pleistocene, to an anti-tropical distribution in the late Pleistocene with rare occurrence points in tropical latitudes (Motoyama, 1997). This supports the notion of Pleistocene cosmopolitan distributions followed by vicariance after the LGM. However, radiolarians may not be representative of many coastal taxa. Fossil mollusks in the eastern Pacific, for example, first appear in northern or southern temperate areas, followed by appearances in the opposite hemisphere without intervening widespread cosmopolitan distributions (several examples reviewed in Lindberg, 1991). Unfortunately, the majority of anti-tropical species have a poor fossil record, making this is difficult to assess.
Despite the above challenges, there are several lines of evidence suggesting that many equatorial divergence events occurred during this time period. By dating molecular data in a variety of fishes using molecular clocks, Burridge (2002) found that many intra-specific, and sister-species equatorial divergences dated to either the Pleistocene or Pliocene epochs, which has been found in subsequent studies as well (Supplementary Table 1). Using a different approach, Lindberg (1991) demonstrated that a variety of fossil marine invertebrates in the Eastern Pacific also show bidirectional movement across the tropics during the Pleistocene and Pliocene epochs. Critical thermal maximum temperature studies, while limited in number for anti-tropical species, have also supported the notion that many of these taxa are near their critical temperature thresholds at their lowest latitudes, and therefore would experience thermal stress in tropical waters (Walther et al., 2013; Payne et al., 2016). Additionally, using ecological niche models across 29 anti-tropical fishes, Ludt and Myers (2021) found that some species lack current suitable abiotic habitat in the tropics, but that suitable habitat and dispersal corridors between hemispheres were available for some species during the LGM despite only minor tropical temperature shifts during this time period. Together, these studies provide compelling evidence that glacial dispersal is one mechanism that produced anti-tropical distributions.
Discussion
The variety of hypotheses proposed for anti-tropicality all have different levels of support and criticism. Historically researchers argued for, or against, these hypotheses only using distributional records of various species. Advancements in modeling and phylogenetic approaches now allow for robust testing of these hypotheses. To identify the hypotheses that could potentially drive anti-tropicality, a multi-faceted approach needs be taken. Perhaps most importantly, one needs to show that species are indeed anti-tropical. While this may be straightforward in cases of intra-specific anti-tropicality, where populations of the same species are disjunct across the tropics, at higher taxonomic levels this needs explicit testing. Strongly supported systematic hypotheses with complete taxonomic sampling are required, therefore, to confirm anti-tropical distributions, and are ideally obtained using molecular approaches for dating purposes. If taxa are missing, either due to incomplete taxonomic sampling or due to the incomplete nature of the fossil record, or if relationships are unclear, then it is impossible to tell if the species of interest are actually anti-tropical. Once a strongly supported phylogeny is obtained, many of these proposed hypotheses can be separated by the timing of anti-equatorial divergences (Table 2). Determining the timing of anti-tropical divergences in extinct taxa can be estimated from the strata associated with the fossils. Molecular phylogenetic hypotheses of extant taxa, however, should be rigorously dated. This is ideally done with fossil calibration points (as opposed to molecular clocks) from multiple fossil formations if possible (to avoid any bias associated with the dating of a single formation). These time-calibrated phylogenies can then be examined within a historical biogeographic context to estimate how species shifted their distributions over time.
The studies that have examined the timing of these events have found interesting results. The oldest potential mechanism, continental fragmentation, is only partially supported by fossil evidence (Crame, 1993; Shi and Grunt, 2000). However, care should be taken in interpreting these data, as some taxa previously considered as anti-tropical may actually represent unrelated taxa with convergent phenotypes (Lee et al., 2016), and extinct taxa that appear anti-tropical in the fossil record may actually have had wider distributions that were not recovered due to the incomplete nature of the fossil record. Molecular evidence of anti-tropicality largely dates to within the Cenozoic, even for families separated by the tropics (Chen et al., 2014), suggesting that continental fragmentation is not a common mechanism forming anti-tropical distributions. For species that are anti-tropical within a genus (i.e., each species is only in one hemisphere, but in total the genus is anti-tropical), early divergences across the tropics have been dated to the Miocene in a few studies (Stepien et al., 1997; two species in Stillman and Reeb, 2001; Burridge, 2002; Banguera-Hinestroza et al., 2014; Naughton et al., 2014; Ludt et al., 2015; Pozzobon et al., 2021). However, the majority of studies date divergence events between anti-tropical species to the Pliocene and Pleistocene (Bowen and Grant, 1997; most datasets used in Burridge, 2002; Hilbish et al., 2000; Mabuchi et al., 2004; Poortvliet et al., 2013; Hoover et al., 2017; Beldade et al., 2021). Intra-specific anti-tropical species (those with populations in both hemispheres) interestingly have also been found with varying divergence dates, ranging from the Miocene in some species (Veríssimo et al., 2010; Herrera et al., 2012), to the last 100,000 years (Tea et al., 2019). While the oldest intra-specific divergence dates likely represent distinct species (discussed in Veríssimo et al., 2010 and Schwaninger, 2008), additional intra-specific population genetic studies are needed across a variety of taxa.
While the timing of molecular and fossil findings link divergence events to the Pleistocene, and therefore may be used to support the glacial dispersal hypothesis, they also support Pliocene divergences as well (Lindberg, 1991; Burridge, 2002; Colgan, 2018). However, the tropics during the Pliocene extended to higher latitudes than modern limits, and lacked high-amplitude climate cycles (Dowsett et al., 1994). If the tropics did not decrease in temperature during this time period, then how could temperately restricted species cross this barrier? Both the Pliocene and Pleistocene have been regarded as periods of dramatic change in marine systems due to the closure of the Isthmus of Panama, which impacted global currents, and altered regional ecosystems (Karas et al., 2017). These changes may have created cold-water upwelling zones along continental margins, allowing anti-tropical species to cross the tropics in a stepping stone manner (Lindberg, 1991; Poortvliet et al., 2013). While the tropics maintained warm temperatures during these periods, the tropical eastern Pacific had higher cooling rates during the Pliocene and Pleistocene than the western tropical Pacific and had a shallow thermocline and active upwelling (Figure 2; Zhang et al., 2014), leading to the suggestion that the eastern Pacific may be an important corridor for anti-tropical taxa (Lindberg, 1991; Bowen and Grant, 1997; Burridge, 2002; Grant and Bowen, 2006; Dawson et al., 2011; Marchant et al., 2015; Bringloe et al., 2019).
Similar global changes occurred in the Miocene, such as the narrowing of the Indonesian Seaway and the closure of the Tethyan Seaway, that may have impacted global circulation patterns, altering the strength of major currents (Kennett et al., 1985; Nathan and Leckie, 2009; Eberli et al., 2010; Bialik et al., 2020). The dating of several anti-tropical divergence events to the Miocene, in both the Pacific (Veríssimo et al., 2010; Ludt et al., 2015) and Atlantic (Pozzobon et al., 2021), that do not correspond to the timelines of the mid-Miocene warming hypothesis raise the possibility that changes in current patterns may have also been important in the formation of anti-tropical distributions. Although, it should be noted that brief periods of rapid cooling and warming occurred in the middle to late Miocene, after the mid-Miocene climatic optimum, that may have also facilitated the formation of anti-tropical distributions (Naughton et al., 2014). Relaxing the assumption that anti-tropical divergence dates have to correspond to the mid-Miocene climatic optimum for the mid-Miocene warming hypothesis to be valid may lead to a more realistic understanding of how a dynamic Miocene climate facilitated the formation of anti-tropical species.
Of the hypotheses listed above, the glacial dispersal, isothermal submergence, and biotic exclusion hypotheses could all potentially operate on the same temporal scales, and cannot be distinguished solely by using a dated phylogeny. Therefore, interdisciplinary studies that use phylogenetic data in conjunction with physiological or environmental data are needed to tease these hypotheses apart. Both the glacial dispersal and isothermal submergence hypotheses revolve around the assumption that anti-tropical species are restricted to their current habitats due to physiological restrictions (Table 2). While thermal tolerances have not been explicitly tested in many cases, there is some support for physiological restrictions driving this distribution pattern. The Pacific Goose barnacle (Pollicipes elegans) is largely considered an anti-tropical species, despite small, genetically isolated populations occurring within tropical latitudes of the eastern Pacific (Van Syoc, 1994). Interestingly, thermal tolerances in temperate populations of P. elegans larvae approach their optimal thermal limits during warm summer months, suggesting that warmer, tropical water likely acts as a barrier to dispersal between populations (Walther et al., 2013). Likewise, Payne et al. (2016) examined temperature thresholds in several adult fish species with varying latitudinal trends, including Parore (Girella tricuspidata), a species in a largely anti-tropical genus. Their findings suggest that for all the species they examined, maximum performance values optimized nearest to the equator for all species, but that each species approached their critical temperature values near the extent of their current ranges (Payne et al., 2016). For G. tricuspidata, this result suggests that the species is absent from tropical waters in part due to physiological restrictions, and further that even small temperature shifts during the LGM could allow distributions of this species (and potentially other anti-tropical congeners) to expand nearer to the equator, lessening the distance needed to disperse across the tropics into another hemisphere. Small temperature changes could also have an impact on the success of pelagic larvae, as current tropical temperatures exceed optimal temperatures for larval growth and settlement size in some species (McLeod et al., 2015), and cooler water temperatures may result in a greater dispersal potential (Raventos et al., 2021; although see Leis et al., 2013 on the need for more temperate larval studies in general). Together, these findings are consistent with several of the dispersal hypotheses for anti-tropicality, but additional physiological studies examining a greater number of anti-tropical taxa during both larval and adult stages are needed to better understand the influence that temperature limits have on driving this distribution pattern.
Another direction that studies have taken is ecological niche modeling to examine the suitability of habitats in the Pleistocene (Waltari and Hickerson, 2013; Ludt and Myers, 2021). This approach has the potential to distinguish between some of the hypotheses that are notoriously hard to test. Ludt and Myers (2021) used niche modeling to distinguish between glacial dispersal and biotic exclusion in intra-specific anti-tropical marine fishes by examining the amount of suitable abiotic habitat in the tropics for both the LGM and contemporary time periods, and found potential support for both hypotheses in different species. However, these approaches are more informative if combined with other sources of data. For example, Waltari and Hickerson (2013) used a niche modeling approach in north Atlantic intertidal invertebrates to largely corroborate predictions from a prior population genetic analysis (Ilves et al., 2010). This approach could be applied to anti-tropical taxa in future studies.
Conclusion
The last two decades have seen studies on anti-tropicality advance from lists of potentially disjunct species/populations and hypothetical mechanisms, to a more solid understanding of paleoclimates, how anti-tropical distributions may have shifted with these climate changes, and evidence of specific divergence times associated with this distribution pattern. While some early hypotheses (such as continental fragmentation, island integration and island submergence) seem inadequate to describe this pattern in a general sense, divergences due to Miocene climate fluctuations, biotic interactions, and dispersal in shallow or deep water are likely still plausible explanations given the range of equatorial divergence dates recovered in recent studies. Therefore, it is likely that these mechanisms have caused this distribution to repeatedly form over time. And while equatorial SST may not have changed dramatically during the LGM, the few physiological studies that have included anti-tropical taxa suggest that even minor temperature fluctuations could result in range expansions toward the tropics. However, combined datasets including population genetic/phylogenetic divergence times, habitat data, and physiological data are lacking for the vast majority of anti-tropical species. These data will be critical for understanding the prevalence of each mechanism in driving this distribution pattern, and future studies should take a multifaceted approach. Lastly, future studies should also examine anti-tropicality in the same context as other disjunct distributions, as anti-tropicality may not be due to its own particular circumstances, but might be associated with general ocean-wide changes in current patterns that occurred in the Miocene, Pliocene, and Pleistocene. By taking a wholistic approach, instead of examining this pattern on its own, we may gain a better understanding of the major drivers of marine biogeographic patterns over geologic time.
Author Contributions
The author confirms being the sole contributor of this work and has approved it for publication.
Funding
Support for this work was provided by Louisiana State University and the Natural History Museum of Los Angeles County.
Conflict of Interest
The author declares that the research was conducted in the absence of any commercial or financial relationships that could be construed as a potential conflict of interest.
Acknowledgments
I would like to thank Prosanta Chakrabarty, Michael Hellberg, and Jeremy Brown for reviewing earlier versions of this manuscript, Jessie F. Salter for reviewing later versions, and also Corinne E. Myers for fruitful discussions on anti-tropicality. I would also like to thank ESRI for providing software to the Natural History Museum of Los Angeles County that was used to design the figures presented here. Two reviewers and W-JC provided valuable feedback that substantially improved this manuscript.
Supplementary Material
The Supplementary Material for this article can be found online at: https://www.frontiersin.org/articles/10.3389/fmars.2021.660984/full#supplementary-material
References
Ali, J. R. (2017). Islands as biological substrates: classification of the biological assemblage components and the physical island types. J. Biogeogr. 44, 984–994. doi: 10.1111/jbi.12872
Arkhipkin, A. I., Laptikhovsky, V. V., and Brickle, P. (2010). An antipodal link between the north Pacific and south Atlantic Oceans? Deep Sea Res. Part I 57, 1009–1011. doi: 10.1016/j.dsr.2010.05.004
Banguera-Hinestroza, E., Hayano, A., Crespo, E., and Hoelzel, A. R. (2014). Delphinid systematics and biogeography with a focus on the current genus Lagenorhynchus: multiple pathways for antitropical and trans-oceanic radiation. Mol. Phylogenet. Evol. 80, 217–230. doi: 10.1016/j.ympev.2014.08.005
Beldade, R., Longo, G. C., Clements, K. D., Robertson, D. R., Perez-Matus, A., Itoi, S., et al. (2021). Evolutionary origin of the Atlantic Cabo Verde nibbler (Girella stuebeli), a member of a primarily Pacific Ocean family of antitropical herbivorous reef fishes. Mol. Phylogenet. Evol. 156:107021. doi: 10.1016/j.ympev.2020.107021
Berg, L. S. (1933). Die bipolare verbreitung der organisman und die eiszeit. Zoogeographica 1, 444–484.
Bialik, O. M., Auer, G., Ogawa, N. O., Kroon, D., Waldmann, N. D., and Ohkouchi, N. (2020). Monsoons, upwelling, and the deoxygenation of the northwestern Indian Ocean in response to middle to late Miocene global climatic shifts. Paleoceanogr. Paleoclimatol. 35:e2019A003762.
Bowen, B. W., and Grant, W. S. (1997). Phylogeography of the sardines (Sardinops spp.): assessing biogeographic models and population histories in temperate upwelling zones. Evolution 51, 1601–1610. doi: 10.2307/2411212
Briggs, J. C. (1987a). Anti-tropical distribution and evolution in the Indo-West Pacific Ocean. Syst. Biol. 36, 237–247. doi: 10.2307/2413064
Briggs, J. C. (1987b). Antitropicality and vicariance. Syst. Zool. 36, 206–207. doi: 10.2307/2413269
Briggs, J. C. (1999). Coincident biogeographic patterns: Indo-West Pacific Ocean. Evolution 53, 326–335. doi: 10.2307/2640770
Briggs, J. C. (2000). Centrifugal speciation and centres of origin. J. Biogeogr. 27, 1183–1188. doi: 10.1046/j.1365-2699.2000.00459.x
Bringloe, T. T., Macaya, E. C., and Saunders, G. W. (2019). The phylogeographic history of amphitropical Callophyllis variegata (Florideophyceae, Rhodophyta) in the Pacific Ocean. Algae 34, 91–97. doi: 10.4490/algae.2019.34.5.26
Burridge, C. P. (2002). Anti-tropicality of pacific fishes: molecular insights. Environ. Biol. Fishes 65, 151–164. doi: 10.1023/a:1020040515980
Chen, W.-J., Lavoué, S., Beheregaray, L. B., and Mayden, R. L. (2014). Historical biogeography of a new antitropical clade of temperate freshwater fishes. J. Biogeogr. 41, 1806–1818. doi: 10.1111/jbi.12333
Chiu, W.-T. R., Yasuhara, M., Cronin, T. M., Hunt, G., Gemery, L., and Wei, C.-L. (2020). Marine latitudinal diversity gradients, niche conservatism, and out of the tropics and Arctic: climatic sensitivity of small organisms. J. Biogeogr. 47, 817–828. doi: 10.1111/jbi.13793
Clague, D. A., and Dalrymple, G. B. (1975). Cretaceous K-Ar ages of volcanic rocks from the Musicians Seamounts and the Hawaiian Ridge. Geophys. Res. Lett. 2, 305–308. doi: 10.1029/gl002i007p00305
Colgan, D. J. (2018). Genetic studies of Australian Trichomya hirsuta (Bivalvia: Mytilidae) suggest antitropical divergence of this species. J. Asia Pacific Biodivers. 11, 146–150. doi: 10.1016/j.japb.2017.12.003
Cowman, P. F., and Bellwood, D. R. (2011). Coral reefs as drivers of cladogenesis: expanding coral reefs, cryptic extinction events, and the development of biodiversity hotspots. J. Evol. Biol. 24, 2543–2562. doi: 10.1111/j.1420-9101.2011.02391.x
Cox, C. B. (1990). Guest essay: new geological theories and old biogeographical problems. J. Biogeogr. 17, 117–130. doi: 10.2307/2845321
Crame, J. A. (1993). Bipolar molluscs and their evolutionary implications. J. Biogeogr. 20, 145–161. doi: 10.2307/2845668
Dawson, M. N., Barber, P. H., González-Guzmán, L. I., Toonen, R. J., Dugan, J. E., and Grosberg, R. K. (2011). Phylogeography of Emerita analoga (Crustacea, Decapoda, Hippidae), an eastern Pacific Ocean sand crab with long-lived pelagic larvae. J. Biogeogr. 38, 1600–1612. doi: 10.1111/j.1365-2699.2011.02499.x
Delrieu-Trottin, E., Brosseau-Acquaviva, L., Mona, S., Neglia, V., Giles, E. C., Rapu-Edmunds, C., et al. (2019). Understanding the origin of the most isolated endemic reef fish fauna of the Indo-Pacific: coral reef fishes of Rapa Nui. J. Biogeogr. 46, 723–733. doi: 10.1111/jbi.13531
Dowsett, H., Thompson, R., Barron, J., Cronin, T., Fleming, F., Ishman, S., et al. (1994). Joint investigations of the Middle Pliocene climate I: PRISM paleoenvironmental reconstructions. Global Planetary Change 9, 169–195. doi: 10.1016/0921-8181(94)90015-9
Eberli, G. P., Anselmetti, F. S., Isern, A. R., and Delius, H. E. I. K. E. (2010). “Timing of changes in sea-level and currents along Miocene platforms on the Marion Plateau, Australia,” in Cenozoic Carbonate Systems of Australasia, Vol. 95, eds W. A. Morgan, A. D. George, P. M. Harris, J. A. Kupecz, and J. F. Sarg, (Tulsa, OK: SEPM Society for Sedimentary Geology Special Publication), 219–242. doi: 10.2110/sepmsp.095.219
Flower, B. P., and Kennett, J. P. (1994). The middle Miocene climatic transition: East Antarctic ice sheet development, deep ocean circulation and global carbon cycling. Palaeogeogr. Palaeoclimatol. Palaeoecol. 108, 537–555. doi: 10.1016/0031-0182(94)90251-8
Grant, W. S., and Bowen, B. W. (2006). Living in a tilted world: climate change and geography limit speciation in Old World anchovies (Engraulis; Engraulidae). Biol. J. Linnean Soc. 88, 673–689. doi: 10.1111/j.1095-8312.2006.00651.x
Herrera, S., Shank, T. M., and Sánchez, J. A. (2012). Spatial and temporal patterns of genetic variation in the widespread antitropical deep-sea coral Paragorgia arborea. Mol. Ecol. 21, 6053–6067. doi: 10.1111/mec.12074
Hilbish, T. J., Mullinax, A., Dolven, S. I., Meyer, A., Koehn, R. K., and Rawson, P. D. (2000). Origin of the antitropical distribution pattern in marine mussels (Mytilus spp.): routes and timing of transequatorial migration. Mar. Biol. 136, 69–77. doi: 10.1007/s002270050010
Hillier, J. K., and Watts, A. B. (2005). Relationship between depth and age in the North Pacific Ocean. J. Geophys. Res. 110:B02405
Hooker, S. J. D. (1847). The Botany of the Antarctic Voyage of HM Discovery Ships’ Erebus’ and ‘Terror’, in the Years 1839–1843. London: Reeve.
Hoover, C. A., Padula, V., Schrödl, M., Hooker, Y., and Valdés, Á (2017). Integrative taxonomy of the Felimare californiensis and F. ghiselini species complex (Nudibranchia: Chromodorididae), with description of a new species from Peru. J. Molluscan Stud. 83, 461–475. doi: 10.1093/mollus/eyx035
Hubbs, C. L. (1952). “Antitropical distribution of fishes and other organisms,” in Proceedings of the Seventh Pacific Science Congregation. Symposium on Problems of Bipolarity and of Pantemperate Faunas, Vol. 3, (Honolulu, HI: Pacific Science Association), 324–329.
Ilves, K. L., Huang, W. E. N., Wares, J. P., and Hickerson, M. J. (2010). Colonization and/or mitochondrial selective sweeps across the North Atlantic intertidal assemblage revealed by multi-taxa approximate Bayesian computation. Mol. Ecol. 19, 4505–4519. doi: 10.1111/j.1365-294x.2010.04790.x
Jablonski, D., Belanger, C. L., Berke, S. K., Huang, S., Krug, A. Z., Roy, K., et al. (2013). Out of the tropics, but how? Fossils, bridge species, and thermal ranges in the dynamics of the marine latitudinal diversity gradient. Proc. Natl. Acad. Sci. U.S.A. 110, 10487–10494. doi: 10.1073/pnas.1308997110
Karas, C., Nürnberg, D., Bahr, A., Groeneveld, J., Herrle, J. O., and Tiedemann, R. (2017). Pliocene oceanic seaways and global climate. Sci. Rep. 7:39842.
Kennett, J. P., Keller, G., and Srinivasan, M. (1985). “Miocene planktic foraminifer biogeography and paleoceanographic development of the Indo-Pacific region”, in The Miocene Ocean: Paleoceanography and Biogeography. Memoir, Vol. 163, ed. J. P. Kennett (Boulder, CO: Geological Society of America, 197–236.
Kiessling, W., Simpson, C., and Foote, M. (2010). Reefs as cradles of evolution and sources of biodiversity in the Phanerozoic. Science 327, 196–198. doi: 10.1126/science.1182241
Knudsen, S. W., Choat, J. H., and Clements, K. D. (2019). The herbivorous fish family Kyphosidae (Teleostei: Perciformes) represents a recent radiation from higher latitudes. J. Biogeogr. 46, 2067–2080. doi: 10.1111/jbi.13634
Laptikhovsky, V., Arkhipkin, A., Brickle, P., Hearne, S., and Neely, K. (2011). Species range shifts due to environmental changes in scaled squid, Pholidoteuthis massyae and bathyal octopus, Muusoctopus eureka. Mar. Biodivers. Records 4:E34.
Laptikhovsky, V., Gaither, M. R., and Black, A. (2013). A Pacific grenadier Coryphaenoides acrolepis in the south-west Atlantic and environmental changes in the Falkland deep seas. Mar. Biodivers. Records 6:E126.
Lee, S., Shi, G. R., Park, H., and Tazawa, J. I. (2016). Antitropicality and convergent evolution: a case study of Permian neospiriferine brachiopods. Palaeontology 59, 109–138. doi: 10.1111/pala.12213
Leis, J. M., Caselle, J. E., Bradbury, I. R., Kristiansen, T., Llopiz, J. K., Miller, M. J., et al. (2013). Does fish larval dispersal differ between high and low latitudes? Proc. R. Soc. B Biol. Sci. 280:20130327. doi: 10.1098/rspb.2013.0327
Lindberg, D. R. (1991). Marine biotic interchange between the Northern and Southern hemispheres. Paleobiology 17, 308–324. doi: 10.1017/s0094837300010629
Liu, Z., Pagani, M., Zinniker, D., DeConto, R., Huber, M., Brinkhuis, H., et al. (2009). Global cooling during the Eocene-Oligocene climate transition. Science 323, 1187–1190. doi: 10.1126/science.1166368
Ludt, W. B., and Myers, C. E. (2021). Distinguishing between dispersal and vicariance: a novel approach using anti-tropical taxa across the fish Tree of Life. J. Biogeogr. 48, 577–589. doi: 10.1111/jbi.14021
Ludt, W. B., and Rocha, L. A. (2015). Shifting seas: the impacts of Pleistocene sea-level fluctuations on the evolution of tropical marine taxa. J. Biogeogr. 42, 25–38. doi: 10.1111/jbi.12416
Ludt, W. B., Rocha, L. A., Erdmann, M. V., and Chakrabarty, P. (2015). Skipping across the tropics: the evolutionary history of sawtail surgeonfishes (Acanthuridae: Prionurus). Mol. Phylogenet. Evol. 84, 166–172. doi: 10.1016/j.ympev.2014.12.017
Mabuchi, K., Nakabo, T., and Nishida, M. (2004). Molecular phylogeny of the anti-tropical genus Pseudolabrus (Perciformes: Labridae): evidence for a Southern Hemisphere origin. Mol. Phylogenet. Evol. 32, 375–382. doi: 10.1016/j.ympev.2004.01.008
MacArthur, R. H., and Wilson, E. O. (1967). The Theory of Island Biogeography. Princeton: NJ: The University of Chicago Press.
Marchant, S., Moran, A. L., and Marko, P. B. (2015). Out-of-the tropics or trans-tropical dispersal? The origins of the disjunct distribution of the gooseneck barnacle Pollicipes elegans. Front. Zool. 12:39. doi: 10.1186/s12983-015-0131-z
McCulloch, G. A., Wallis, G. P., and Waters, J. M. (2016). A time-calibrated phylogeny of southern hemisphere stoneflies: testing for Gondwanan origins. Mol. Phylogenet. Evol. 96, 150–160. doi: 10.1016/j.ympev.2015.10.028
McLeod, I. M., McCormick, M. I., Munday, P. L., Clark, T. D., Wenger, A. S., Brooker, R. M., et al. (2015). Latitudinal variation in larval development of coral reef fishes: implications of a warming ocean. Mar. Ecol. Prog. Ser. 521, 129–141. doi: 10.3354/meps11136
Miller, K. G., Janecek, T. R., Katz, M. E., and Keil, D. J. (1987). Abyssal circulation and benthic foraminiferal changes near the Paleocene/Eocene boundary. Paleoceanography 2, 741–761. doi: 10.1029/pa002i006p00741
Motoyama, I. (1997). Origin and evolution of Cycladophora davisiana Ehrenberg (Radiolaria) in DSDP Site 192, Northwest Pacific. Mar. Micropaleontol. 30, 45–63. doi: 10.1016/s0377-8398(96)00047-3
Murray, J., Hooker, J. D., Neumayer, G., Markham, C., Geikie, A., and D’Arcy, W. T. (1897). The scientific advantages of an Antarctic expedition. Proc. R. Soc. London 62, 424–451.
Nathan, S. A., and Leckie, R. M. (2009). Early history of the Western Pacific Warm Pool during the middle to late Miocene (~ 13.2–5.8 Ma): role of sea-level change and implications for equatorial circulation. Palaeogeogr. Palaeoclimatol. Palaeoecol. 274, 140–159. doi: 10.1016/j.palaeo.2009.01.007
Naughton, K. M., O’Hara, T. D., Appleton, B., and Cisternas, P. A. (2014). Antitropical distributions and species delimitation in a group of ophiocomid brittle stars (Echinodermata: Ophiuroidea: Ophiocomidae). Mol. Phylogenet. Evol. 78, 232–244. doi: 10.1016/j.ympev.2014.05.020
Nelson, G. (1985). A decade of challenge the future of biogeography. Earth Sci. History 4, 187–196. doi: 10.17704/eshi.4.2.c347xp1671w4m0n0
Nur, A., and Ben-Avraham, Z. (1982). Oceanic plateaus, the fragmentation of continents, and mountain building. J. Geophys. Res. 87, 3644–3661. doi: 10.1029/jb087ib05p03644
Parsons, B., and Sclater, J. G. (1977). An analysis of the variation of ocean floor bathymetry and heat flow with age. J. Geophys. Res. 82, 803–827. doi: 10.1029/jb082i005p00803
Payne, N. L., Smith, J. A., van der Meulen, D. E., Taylor, M. D., Watanabe, Y. Y., Takahashi, A., et al. (2016). Temperature dependence of fish performance in the wild: links with species biogeography and physiological thermal tolerance. Funct. Ecol. 30, 903–912. doi: 10.1111/1365-2435.12618
Pérez-Barros, P., Albano, M., Diez, M. J., and Lovrich, G. A. (2020). Pole to pole: the deep-sea king crab Lithodes couesi (Decapoda: Lithodidae) in the Burdwood Bank, Southwestern Atlantic Ocean. Polar Biol. 43, 81–86. doi: 10.1007/s00300-019-02609-x
Poortvliet, M., Longo, G. C., Selkoe, K., Barber, P. H., White, C., Caselle, J. E., et al. (2013). Phylogeography of the California sheephead, Semicossyphus pulcher: the role of deep reefs as stepping stones and pathways to anti-tropicality. Ecol. Evol. 3, 4558–4571. doi: 10.1002/ece3.840
Pozzobon, A. P. B., Gonçalves, P. R., Anderson, J. D., Rocha, L. A., de Astarloa, J. M. D., and Di Dario, F. (2021). Phylogenetic relationships, genetic diversity and biogeography of menhadens, genus Brevoortia (Clupeiformes, Clupeidae). Mol. Phylogenet. Evol. 160:107108. doi: 10.1016/j.ympev.2021.107108
Pringle, M. S., and Dalrymple, G. B. (1993). Geochronological constraints on a possible hot spot origin for Hess Rise and the Wentworth Seamount Chain. Mesozoic Pacific 77, 263–277. doi: 10.1029/gm077p0263
Rabosky, D. L., Chang, J., Title, P. O., Cowman, P. F., Sallan, L., Friedman, M., et al. (2018). An inverse latitudinal gradient in speciation rate for marine fishes. Nature 559, 392–395. doi: 10.1038/s41586-018-0273-1
Randall, J. E. (1981). Examples of antitropical and antiequatorial distribution of Indo-West-Pacific fishes. Pacific Sci. 35, 197–209.
Raven, P. H. (1963). Amphitropical relationships in the floras of North and South America. Q. Rev. Biol. 38, 151–177. doi: 10.1086/403797
Raventos, N., Torrado, H., Arthur, R., Alcoverro, T., and Macpherson, E. (2021). Temperature reduces fish dispersal as larvae grow faster to their settlement size. J. Anim. Ecol. doi: 10.1111/1365-2656.13435
Rehder, H. A. (1980). The Marine Mollusks of Easter Island (Isla de Pascua) and Sala y Gómez. Smithsonian Contributions to Zoology, Washington, DC.
Romdal, T. S., Araújo, M. B., and Rahbek, C. (2013). Life on a tropical planet: niche conservatism and the global diversity gradient. Global Ecol. Biogeogr. 22, 344–350. doi: 10.1111/j.1466-8238.2012.00786.x
Ross, J. C. (1847). A Voyage of Discovery and Research in the Southern and Antarctic Regions During the Years 183–1843, Vol. 2. London: J. Murray.
Rotondo, G. M., Springer, V. G., Scott, G. A., and Schlanger, S. O. (1981). Plate movement and island integration—a possible mechanism in the formation of endemic biotas, with special reference to the Hawaiian Islands. Syst. Biol. 30, 12–21. doi: 10.2307/2992298
Sbrocco, E. J., and Barber, P. H. (2013). MARSPEC: ocean climate layers for marine spatial ecology. Ecology 94:979. doi: 10.1890/12-1358.1
Schwaninger, H. R. (2008). Global mitochondrial DNA phylogeography and biogeographic history of the antitropically and longitudinally disjunct marine bryozoan Membranipora membranacea L. (Cheilostomata): another cryptic marine sibling species complex? Mol. Phylogenet. Evol. 49, 893–908. doi: 10.1016/j.ympev.2008.08.016
Shi, G. R., and Grunt, T. A. (2000). Permian Gondwana–Boreal antitropicality with special reference to brachiopod faunas. Palaeogeogr. Palaeoclimatol. Palaeoecol. 155, 239–263. doi: 10.1016/s0031-0182(99)00118-2
Siqueira, A. C., Oliveira-Santos, L. G. R., Cowman, P. F., and Floeter, S. R. (2016). Evolutionary processes underlying latitudinal differences in reef fish biodiversity. Global Ecol. Biogeogr. 25, 1466–1476. doi: 10.1111/geb.12506
Smissen, R. D., Garnock-Jones, P. J., and Chambers, G. K. (2003). Phylogenetic analysis of ITS sequences suggests a Pliocene origin for the bipolar distribution of Scleranthus (Caryophyllaceae). Aust. Syst. Bot. 16, 301–315. doi: 10.1071/sb01032
Springer, V. G. (1982). Pacific Plate Biogeography, With Special Reference to Shore Fishes. Smithsonian Contributions to Zoology, Washington, DC.
Stein, C. A., and Stein, S. (1992). A model for the global variation in oceanic depth and heat flow with lithospheric age. Nature 359:123. doi: 10.1038/359123a0
Stepanjants, S. D., Cortese, G., Kruglikova, S. B., and Bjørklund, K. R. (2006). A review of bipolarity concepts: history and examples from Radiolaria and Medusozoa (Cnidaria). Mar. Biol. Res. 2, 200–241. doi: 10.1080/17451000600781767
Stepien, C. A., Dillon, A. K., Brooks, M. J., Chase, K. L., Hubers, A. N., and Kocher, T. (1997). “The evolution of blennioid fishes based on an analysis of mitochondrial 12S RDNA,” in Molecular Systematics of Fishes, eds T. D. Kocher, and C. A. Stepien, (San Diego, CA: Academic Press), 245–270. doi: 10.1016/b978-012417540-2/50016-6
Stevenson, D. E., and Kenaley, C. P. (2013). Revision of the manefish genera Caristius and Platyberyx (Teleostei: Percomorpha: Caristiidae), with descriptions of five new species. Copeia 2013, 415–434. doi: 10.1643/ci-12-086
Stillman, J. H., and Reeb, C. A. (2001). Molecular phylogeny of eastern Pacific porcelain crabs, genera Petrolisthes and Pachycheles, based on the mtDNA 16S rDNA sequence: phylogeographic and systematic implications. Mol. Phylogenet. Evol. 19, 236–245. doi: 10.1006/mpev.2001.0924
Tea, Y. K., Van Der Wal, C., Ludt, W. B., Gill, A. C., Lo, N., and Ho, S. Y. (2019). Boomeranging around Australia: historical biogeography and population genomics of the anti-equatorial fish Microcanthus strigatus (Teleostei: Microcanthidae). Mol. Ecol. 28, 3771–3785. doi: 10.1111/mec.15172
Theéel, H. (1885). Report on the Holothuroidea. Part II. Voyage of H.M.S. Challenger. Zoology 14, 1–290.
Thorne, R. F. (1972). Major disjunctions in the geographic ranges of seed plants. Q. Rev. Biol. 47, 365–411. doi: 10.1086/407399
Valentine, J. W. (1984). Neogene marine climate trends: implications for biogeography and evolution of the shallow-sea biota. Geology 12, 647–650. doi: 10.1130/0091-7613(1984)12<647:nmctif>2.0.co;2
Van Syoc, R. J. (1994). Genetic divergence between subpopulations of the eastern Pacific goose barnacle Pollicipes elegans: mitochondrial cytochrome c subunit 1 nucleotide sequences. Mol. Mar. Biol. Biotechnol. 3, 338–346.
Veríssimo, A., McDowell, J. R., and Graves, J. E. (2010). Global population structure of the spiny dogfish Squalus acanthias, a temperate shark with an antitropical distribution. Mol. Ecol. 19, 1651–1662. doi: 10.1111/j.1365-294x.2010.04598.x
Villaverde, T., Escudero, M., Luceño, M., and Martín-Bravo, S. (2015). Long-distance dispersal during the middle–late Pleistocene explains the bipolar disjunction of Carex maritima (Cyperaceae). J. Biogeogr. 42, 1820–1831. doi: 10.1111/jbi.12559
Waltari, E., and Hickerson, M. J. (2013). Late Pleistocene species distribution modelling of North Atlantic intertidal invertebrates. J. Biogeogr. 40, 249–260. doi: 10.1111/j.1365-2699.2012.02782.x
Walther, K., Crickenberger, S. E., Marchant, S., Marko, P. B., and Moran, A. L. (2013). Thermal tolerance of larvae of Pollicipes elegans, a marine species with an antitropical distribution. Mar. Biol. 160, 2723–2732. doi: 10.1007/s00227-013-2265-x
Whatley, R., and Ballent, S. C. (1994). Bi-Hemispherical distribution of Jurassic Ostracoda: paleogeographical implications. Canad. Soc. Petrol. Geol. Memoir, 17, 961–966.
White, B. N. (1986). The isthmian link, antitropicality and American biogeography: distributional history of the Atherinopsinae (Pisces: Atherinidae). Syst. Biol. 35, 176–194. doi: 10.2307/2413429
Zachos, J. C., Stott, L. D., and Lohmann, K. C. (1994). Evolution of early Cenozoic marine temperatures. Paleoceanography 9, 353–387. doi: 10.1029/93pa03266
Zachos, J. C., Wara, M. W., Bohaty, S., Delaney, M. L., Petrizzo, M. R., Brill, A., et al. (2003). A transient rise in tropical sea surface temperature during the Paleocene-Eocene thermal maximum. Science 302, 1551–1554. doi: 10.1126/science.1090110
Zachos, J., Pagani, M., Sloan, L., Thomas, E., and Billups, K. (2001). Trends, rhythms, and aberrations in global climate 65 Ma to present. Science 292, 686–693. doi: 10.1126/science.1059412
Keywords: anti-tropical, amphitropical, bitemperate, bipolar, dispersal, vicariance, biogeography
Citation: Ludt WB (2021) Missing in the Middle: A Review of Equatorially Disjunct Marine Taxa. Front. Mar. Sci. 8:660984. doi: 10.3389/fmars.2021.660984
Received: 30 January 2021; Accepted: 16 April 2021;
Published: 19 May 2021.
Edited by:
Wei-Jen Chen, National Taiwan University, TaiwanReviewed by:
Joseph D. DiBattista, Australian Museum, AustraliaSébastien Lavoué, National Taiwan University, Taiwan
Copyright © 2021 Ludt. This is an open-access article distributed under the terms of the Creative Commons Attribution License (CC BY). The use, distribution or reproduction in other forums is permitted, provided the original author(s) and the copyright owner(s) are credited and that the original publication in this journal is cited, in accordance with accepted academic practice. No use, distribution or reproduction is permitted which does not comply with these terms.
*Correspondence: William B. Ludt, d2x1ZHRAbmhtLm9yZw==