- 1Centre for Environment, Fisheries and Aquaculture Science, Lowestoft, United Kingdom
- 2British Antarctic Survey, High Cross, Cambridge, United Kingdom
Seamounts have long been recognised as hotspots for pelagic productivity and diversity in the world’s open ocean habitats. Recent studies have suggested that productivity may vary greatly between different seamounts, depending on complex interactions between the bathymetric features and local oceanography. These processes may enhance local primary production which support elevated biomass at higher trophic levels. In addition to enhancing local biomass, seamounts may also act as aggregative features, attracting pelagic species from the surrounding waters. Such characteristics make seamounts attractive targets for fisheries. However, as these unique habitats are localised and relatively small, they are vulnerable to overexploitation, which may have detrimental impact on the wider region. Mapping and quantitative assessments of the fish biomass at different seamounts are crucial prerequisites to identifying vulnerable seamounts and will aid toward understanding the dynamics of these important ecosystems and their vulnerability to fishing pressures. We used fisheries acoustics during two expeditions in 2018 and 2019, to investigate the distribution and abundance of fish and micronekton on and around five little studied seamounts of Tristan da Cunha, a remote archipelago in the South Atlantic Ocean. The results confirmed increased productivity at the seamounts, compared to the surrounding open ocean with higher acoustic backscatter values, a proxy for biomass, particularly at the shallower (~200 m depth) seamounts. Fish largely dominated the backscatter on most of the seamounts especially over the plateau areas where large densities of prey fish, primarily the mesopelagic Maurolicus inventionis, were detected. Very large aggregations, thought to consist of bentho-pelagic fish, were also observed over the slope of McNish Seamount that resulted in very high biomass estimates. Aggregations of this size and magnitude, have, to our knowledge, never been mapped or quantified on seamounts, using acoustic methods. Specific physical processes, such as enhanced retention and vertical mixing that were identified by an oceanographic model, may be some of the drivers of the enhanced fish biomass detected at McNish. The characteristics of the seamounts observed in this work suggest that these habitats are highly suitable for the presence of large predatory fish that can utilise these areas as their primary habitat or as important foraging grounds.
Introduction
Seamounts are topographically distinct features that rise from the seafloor with most being of volcanic origin that are widely distributed in all ocean basins. The number of seamounts that have an elevation of more than 1,000 m above the seafloor was estimated to be between 10,000 and 100,000, a number that increases considerably if the smaller features are included (Kitchingman et al., 2007).
Seamounts often support unique ecosystems and have been recognised as hotspots for pelagic productivity and diversity in many areas of the world (Morato et al., 2010a). Enhanced fish densities at seamounts include small mesopelagic fish (e.g., pearlside—Maurolicus sp.) (Kalinowski and Linkowski, 1983; Parin and Kobylianski, 1996), bentho-pelagic species (orange roughy—Hoplostethus atlanticus, alfonsino—Beryx splendens, rockfish—Sebastes sp.) (Koslow, 1996; Koslow et al., 2000), elasmobranchs and large pelagic predators (Morato et al., 2008, 2010b). The seamounts can act as primary habitat for resident fish populations or as foraging grounds for other species that will spend only a limited amount of time of their life cycles associated with the seamount (Morato and Clark, 2007). It has also been hypothesised that certain highly migratory species such as tuna can use the seamounts as navigational aids in large movement patterns (Holland et al., 1999; Klimley et al., 2003; Holland and Grubbs, 2007).
The increased productivity around seamounts is likely the result of multiple factors. Firstly, the coupling of topographic characteristics of the seafloor with local hydrodynamics enhances local primary productivity: the interaction generates physical features such as eddies, isopycnal doming, Taylor column/cone, and internal waves, creating a unique environment, different from the surrounding open ocean (Owens and Hogg, 1980). These processes can produce biological responses, for instance by increasing vertical fluxes of nutrient-rich waters, enhancing primary productivity, facilitating the retention of nutrients and planktonic organisms over the seamount with a direct positive effect on the higher trophic levels such as fish (Comeau et al., 1995; Goldner and Chapman, 1997; Mohn and Beckmann, 2002; Genin, 2004; White et al., 2007). These processes are characterised by high spatial and temporal variability, dependent on the specific topographic characteristics of the seamounts. Secondly, in other cases, high plankton and fish biomass detected at the seamounts does not correspond to an increase in the local primary production (Genin, 2004; Genin and Dower, 2007). The enhanced secondary production may have an allochthonous origin, instead. For example, the seamount seafloor can act as a trap for vertically migrating plankton and micronekton advected from the surrounding waters that are unable to complete their descent to deeper waters during the day making them more visible and vulnerable to fish predation (Genin et al., 1988, Genin, 2004; Fock et al., 2002).
The presence of high densities of fish in these ecosystems makes them attractive targets to fisheries. Moreover, as these unique habitats are highly localised and relatively small, they are vulnerable to overexploitation. Mapping and quantitatively assessing the (relative) biomass at seamounts is therefore a crucial prerequisite to understanding the dynamics of these important ecosystems, identifying their vulnerability to fishing pressures and their role in the wider ocean systems. Despite the increasing number of works focused on seamounts, few seamounts have been studied in detail. Most remain unexplored primarily due to the remoteness of these areas. It is crucial to describe and characterise the processes that occur on the un-studied seamounts to better understand and identify the large variability of these habitats.
One of the main objectives of the UK Government funded Blue Belt programme is to provide a better understanding of these environments and develop efficient marine protection strategies in the UK Overseas Territories. Tristan da Cunha, a remote archipelago that consists of four islands located in the Southern Atlantic, was one of the main areas of interest to this programme. The EEZ (Exclusive Economic Zone) of Tristan da Cunha includes several seamounts with very different topographic characteristics that, to date, have not been studied in detail. These seamounts are exploited by fisheries, primarily targeting bluenose warehou (Hyperoglyphe antarctica) and, to a lesser extent, bluefin tuna (Thunnus maccoyii). Even though fishing effort has been relatively low, very high catch rates (catch per unit effort, CPUE) have been recorded, suggesting the presence of enhanced fish biomass (Bell et al., 2021). In 2020, the environmental and ecological importance of this area was recognised by the designation of the entire Tristan da Cunha EEZ as Marine Protected Area (MPA). Covering an area of ∼700,000 km2, this represents the largest MPA in the Atlantic Ocean and fourth largest in the world. The results of the work conducted under the Blue Belt programme helped underpin the designation of the MPA, and will serve as a baseline that will help the evaluation and monitoring of the effectiveness of the MPA in the long term.
In this study we used fisheries acoustic methods and pelagic trawl sampling to investigate the distribution, (relative) biomass and diversity of fish and micronekton on and around five seamounts located in the south of Tristan da Cunha’s EEZ during two expeditions (in 2018 and 2019) conducted under the Blue Belt programme. The main objectives of this study were to (i) investigate whether the seamounts in Tristan da Cunha support enhanced productivity compared to the surrounding open ocean, (ii) identify any differences in micronekton and fish distribution between the surveyed seamounts, (iii) provide estimates of fish biomass where possible, and (iv) investigate the physical and biological processes that drive any differences between seamounts.
Materials and Methods
Study Area
Tristan da Cunha is a remote archipelago that consists of four islands (Tristan da Cunha, Gough Island, Inaccessible Island, Nightingale Island) located between 37° and 41°S (9–13°W) in the South Atlantic and positioned 2,816 km from the nearest land (South Africa) and 3,360 km from South America. Five seamounts located within the Tristan da Cunha EEZ were surveyed during two expeditions, one conducted on the RRS James Clark Ross (2018) and the second on RRS Discovery (2019): Crawford and Yakhont in 2018 and RSA, McNish and an un-named seamount in 2019 (Figure 1). Crawford is made up of a pair of seamounts (Esk Guyot and Crawford Seamount) situated in the centre of the Tristan da Cunha EEZ, between the northern islands and Gough. The shallowest points of Esk and Crawford are at approximately 250 and 380 m deep, respectively. Only two small areas of the Crawford complex (east and west ends), both characterised by plateaus of about 15 km in diameter, were surveyed in March 2018. McNish is a small dome-shaped seamount, located 116 km east of Gough Island, which rises to less than 90 m below the sea surface at its shallowest point. The plateau is 15 km across in a north-south direction and 12 km across east-west. RSA Seamount, in the far south-east, is the largest feature in the Tristan da Cunha EEZ at almost 111 km long. Owing largely to its size, this feature remains poorly mapped, but the plateau is thought to be mostly at 300–350 m deep with a shallow region with depth <100 m located at the west side of the seamount. Yakhont is a large, flat-topped feature, around 426 km south-east of Tristan da Cunha island that rises to around 270 m deep. The un-named seamount is the smallest of the seamounts surveyed with a diameter of only 11 km (plateau diameter ∼5 km) rising to a depth of 500–600 m.
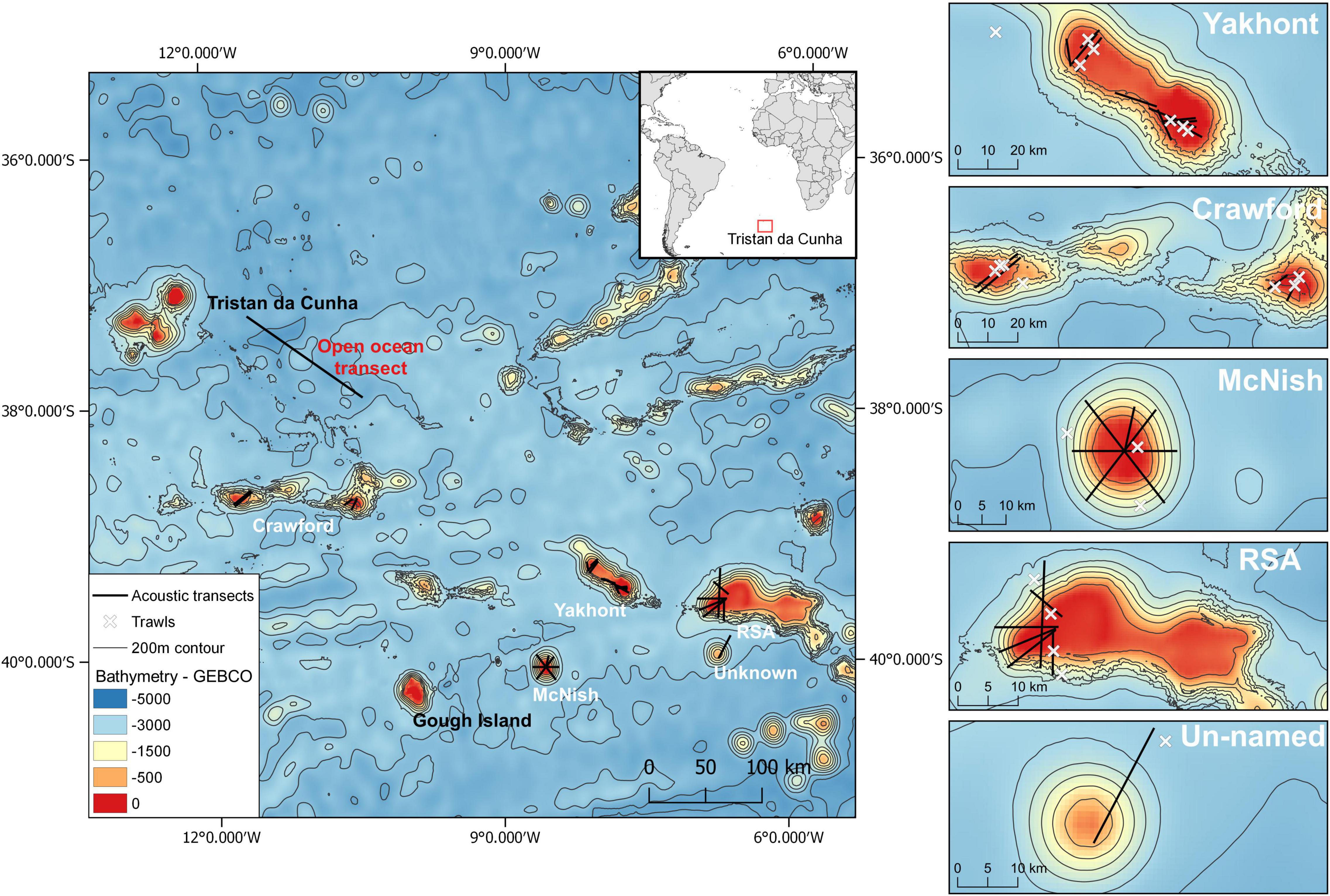
Figure 1. Overview of study area (left), with detailed maps of the seamounts (right), including acoustic transects and net trawls (see legend), conducted during the 2018 (Yakhont and Crawford seamounts) and 2019 expeditions (McNish, RSA and un-named seamounts, open ocean transect).
Acoustic Sampling and Processing
Acoustic transects were carried out over five seamounts to gather information on the horizontal and vertical distribution, abundance and behaviour of fish aggregations and micronekton. Acoustic data were also collected during the transit between Tristan da Cunha and the seamounts to obtain information about fish and micronekton distribution in the open ocean. In particular, a 100 km transect starting 20 km from Tristan da Cunha heading south-east, with depths ranging from 3,000 to 4,000 m, was carried out during the 2019 expedition. Multifrequency acoustic data were collected from the vessel-mounted Simrad EK60 echosounders (at 18, 38, 70, 120, 200, and 333 kHz). The echosounders were calibrated using a 38.1 mm tungsten carbide sphere following the standard sphere method (Demer et al., 2015). The calibration performed on the RRS Discovery in 2019 highlighted a malfunctioning of the 18 and 38 kHz transducers precluding the use of those frequencies for quantitative analysis. For this reason, the main frequency used for the quantitative analysis was 70 kHz (70 kHz was also used for the 2018 data for consistency). The settings and calibration parameters of the acoustic equipment used in the two expeditions are listed in the Supplementary Tables 1,2. The original survey design for the seamounts consisted of a star-shaped transect pattern centred at the peak of the seamount (sensu Godø et al., 2012). This was not consistently achieved across all the seamounts due to time limitation, topography and size of the seamounts, rough weather that limited the choice of the ship’s heading, and location of the net samplings (Figure 1). The acoustic data were collected primarily during the night with some opportunistic daytime data also collected.
The acoustic data from the surface to a maximum depth of 600 m (below which the 70 kHz data were affected by absorption) were cleaned and processed using the software Echoview v10. The first step involved editing the bottom line and removing noise and unwanted targets. The area immediately below the transducers (∼10 m below) was excluded from the analysis because it was affected by the near-field effect and surface noise (e.g., surface bubbles). Background noise, pulse noise and attenuated signals were removed using a series of tools integrated in the Echoview software. To reduce the stochastic variability, the data were resampled from the original horizontal (10–20 m depending on vessel speed) and vertical (0.2 m) sampling resolution to a lower resolution (20 m H × 2 m V cell) before further processing steps were applied. The Nautical Area Backscattering Coefficient (NASC), was exported from the “clean” echograms and used for further analyses. While NASC is often converted to a measure of biomass, the required details on the species composition, size and on the acoustic target strengths (see next section) were not available for the broader geographic area. Therefore, NASC, also referred to as backscatter, was considered a proxy for relative biomass. While several assumptions were made, including on the homogeneity of the scattering properties and of the composition of the acoustic targets, other studies investigating the meso-pelagic fish community have applied similar methods (Irigoien et al., 2014; Proud et al., 2017). The horizontal sampling unit used to export the NASC, integrated for the whole water column (maximum depth: 600 m), was 500 m. The NASC was exported at 70 kHz using a minimum Sv (Volume backscattering strength, dB re 1 m–1) threshold value of −65 dB. This threshold was chosen based on visual inspection of the echogram and excluded the weak scatterers caused by planktonic organisms (e.g., copepods, euphausiids). The exported NASC was mainly associated with fish but it could also have included gas-filled organisms such as siphonophores. The resulting data on the horizontal distribution of fish densities (NASC) were then used for two further analyses: firstly, NASC data for the whole study area, were used to investigate the potential topographic drivers affecting fish distribution (“Statistical Analysis” section). Secondly, for a small area for which good groundtruth data were available, NASC was converted to relative biomass (“Fish Biomass Estimation” section).
Statistical Analysis
To investigate the potential effects of the topographic features of different seamounts on the micronekton and fish distribution, Generalised Additive Models (GAM) with a gaussian distribution and identity link function were used (Wood, 2006). Fish backscatter data (NASC) for the whole study area were used as the response variable and bottom depth, slope, distance from the plateau edges and distance from the centre of gravity of the plateau and location as explanatory variables. A stepwise forward selection was applied to select the best model based on the minimisation of Akaike’s Information Criterion (AIC) and maximisation of deviance explained (Anderson et al., 1998). To avoid potential overfitting of the smoothing function, the maximum degrees of freedom (measured as number of knots k) were limited to k = 5. The explanatory variables were derived from multibeam data that were collected at the seamounts during the two expeditions (Morley et al., 2018; Whomersley et al., 2019). Given the differences in backscatter observed between the shallow and deep seamounts, the modelling dataset was split into two parts based on the average depth of the seamounts and two separate models were created: one for the shallow seamounts (RSA, McNish, Crawford-west, Yakhont) and one for the deeper seamounts (Un-named, Crawford-East). The GAM analysis was conducted using the “mgcv” library in the R statistical software (Wood, 2006).
Fish Biomass Estimation
At two seamounts, McNish and RSA, an attempt was made to convert acoustic backscatter (NASC) to biomass. These two seamounts were selected, because of their considerable importance to commercial fisheries, the high backscatter encountered, and better coverage achieved during the survey. A multifrequency analysis (Ballón et al., 2011) confirmed that the aggregations identified consisted of fish with a swimbladder. However, the analysis was limited to the top 200 m of the water column because of the limited observation range of the higher frequencies (due to sound absorption). The conversion of acoustic backscatter to biomass, requires a species-specific target strength (TS) length equation. No in situ Target Strength could be obtained during the survey, due to the density of the aggregations. Previous studies suggested the mesopelagic community in the area was dominated by pearlside (Maurolicus inventionis) a small swimbladdered fish of the Sternoptychidae family (Kalinowski and Linkowski, 1983). Therefore, biomass of pearlside was estimated based on a Target Strength/Length relationship for a similar species of the same genus, Maurolicus muelleri (Sobradillo et al., 2019). Bluenose warehou (Hyperoglyphe antarctica), was selected as the likely species dominating a bentho-pelagic aggregation observed. It was one of the main targets of local commercial fisheries and was the focus of recent research conducted in the area (Laptikhovsky et al., 2020; Bell et al., 2021). Since no TS-length equation was published for bluenose and little was known about its morphology, specifically the presence and type of swimbladder, we calculated two different biomass estimates based on two separate TS/length equations: a generalised equation for fish with physoclist swimbladders (Foote, 1987) and a TS-length equation derived for orange roughy (Hoplostethus atlanticus) (McClatchie et al., 1999), a deep-sea species with a regressed swimbladder invested with wax esters (Phleger and Grigor, 1990) that might be similar to bluenose swimbladder. A study on stromatoed fish anatomy, which included bluenose, indicated that a swimbladder is present in the juvenile stage but that it can regress in the adulthood (Horn, 1975). Further details of parameters used in the calculations are provided in Table 1.
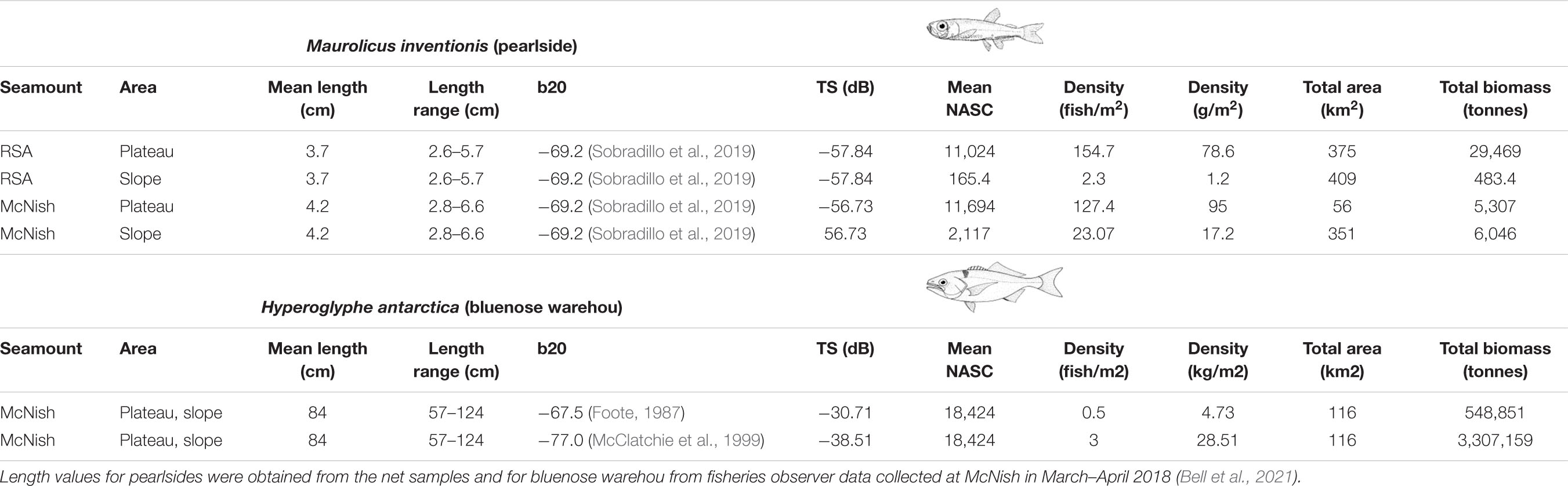
Table 1. Details of the acoustic-based density and biomass estimates for pearlside at RSA and McNish and for the bluenose warehou aggregation at McNish, including parameters used in the equations.
Net Sampling
The species composition of the micronekton community was investigated (qualitatively) using an 8 m2 Rectangular Midwater Trawl (RMT8, during the 2018 expedition) and a 25 m2 rectangular midwater trawl (RMT25, during the 2019 expedition). Both systems consisted of two nets that are opened and closed remotely from the ship to sample discrete depth layers (Roe and Shale, 1979; Piatkowski et al., 1994), with a nominal mouth opening of 8 and 25 m2, respectively, and with a cod-end mesh of 5 mm. The nets were monitored and controlled in real-time using a custom-built net monitoring system which also logged depth. All nets were deployed at night to minimise potential avoidance by the mobile micronekton, in particular fish, and to take advantage of the shallower distribution of the vertically migrating organisms that, at night, are generally more concentrated in surface layers. Tows were undertaken to (i) target acoustically detected aggregations and (ii) sample discrete depth layers, typically 0–200 and 200–400, although some deeper deployments were undertaken with the RMT25 in 2019 (Supplementary Table 3) to characterise oceanic and seamount micro-nekton communities. Nets were towed obliquely at 2.5–3 knots for 30–60 min in each depth horizon, with a protected cod-end fitted to keep captured animals in good condition. Once back onboard, the catches from each cod-end were collected in separate buckets to ensure catches from the different depth bands remained separate. The catches were then sorted and individuals identified to the lowest taxonomic level possible, enumerated, measured (only fish species), weighed and photographed before being preserved. Due to the absence of motion compensated scales during the 2018 cruise, total catch and species composition were recorded in volume rather than weight. While the ability of different species (and life stages) to avoid the trawl varies and will have affected catch composition, conducting the trawls at night was thought to have reduced this effect (Collins et al., 2008, 2012) and, in the absence of detailed knowledge of the avoidance behaviour, we assumed the catch composition was representative of the acoustically observed micronekton. We point out that the catch composition was only used to provide qualitative information about the species composition of the acoustically observed backscatter.
Hydrodynamic Modelling
The hydrographic characteristics of two seamounts, McNish and RSA, were investigated to enable a qualitative comparison between the physical processes and the biological characteristics observed over these two topographically different seamounts. A high-resolution oceanographic model of the Tristan da Cunha region provided the physical context. The regional ocean model is an implementation of the Nucleus for European Modelling of the Ocean (NEMO) modelling framework (Young et al., in prep). The model domain extended from 24 to 0°W, and from 45 to 31°S, with a horizontal resolution of 1/30° longitude by 1/40° latitude (∼2.75 km), and with 75 levels in the vertical arranged on a partial-step z-coordinate. This configuration allowed good representation of the complex regional bathymetry whilst capturing large-scale features of the wider oceanic circulation. Surface atmospheric forcing was derived from the DFS5.2 reanalysis (Dussin et al., 2016). At the open boundaries, tides were imposed using the Oregon State University global ocean tide model, TPXO7.2 (Egbert and Erofeeva, 2002). Three-dimensional temperature and salinity, barotropic flux and sea surface height at the open boundaries were derived from a global 1/12° implementation of NEMO, provided by the National Oceanography Centre, Southampton, United Kingdom. The oceanographic model has been verified by comparing outputs with a combination of historic in situ (conductivity, temperature, depth) and satellite-derived (sea surface temperature) data and was found to provide good representation of observed oceanographic properties in the region (Young et al., in prep). The model was used as an explanatory tool to explore potential physical mechanisms that could provide the underlying physical conditions for enhanced productivity at seamounts in the region. Analyses were concentrated on two seamounts with the most comprehensive survey coverage: McNish and RSA. Existing model outputs for the period 2000–2009 were analysed, focussing on model outputs for the Austral autumn 2006, when the ATL3 index for Atlantic Niño was comparable to that during the 2019 expedition to McNish and RSA seamounts. Although model outputs were not available for the period of the observations, the time period chosen had the same large-scale forcing conditions as the observational period, and results are interpreted in this context. Mean horizontal and vertical velocities for 2000–2009 at 10 and 200 m depth were calculated to visualise the dominant circulation patterns around McNish and RSA seamounts. To examine the oceanography at McNish seamount in more detail, cross-sections of 5-day mean oceanographic properties (potential temperature, salinity, density, horizontal and vertical velocities) were extracted along 40°8”S, and 8°36”W.
The possible effect of the circulation on local retention of biota at McNish Seamount was investigated using Lagrangian particle tracking. Passive particles were released every 5 days for 1 year (2000) at all model grid cells with depth <500 m. At each release event, 50 particles per grid cell were released at a depth of 10 m with subsequent advection in the 3D velocity fields simulated using a 2nd order Runge-Kutta scheme. Particle movements were restricted to the upper 200 m to capture the main depth range of the micronekton and mesopelagic fish communities, and their positions were tracked for 180 days. Local retention was represented by the e-folding time (time taken for the number of particles within the 500 m isobath to reduce to 1/e of the original number), with particles considered to have left the seamount if they did not return within 5 days. E-folding times were calculated for each 5-day release to resolve temporal variability in retention.
Results
General Patterns and Topographic Drivers of SSL at and Around Five Seamounts
The average acoustic backscatter detected in the top 600 m of the water column was significantly higher at the seamounts compared to the open ocean area (Figure 2). All the seamounts, except for Crawford East and un-named, were characterised by the presence of a strong Sound Scattering Layer (SSL) with similar characteristics across the seamounts (examples of echograms are shown in Figure 3). The SSL was mainly concentrated over the summit of the seamounts, occupying almost the entire water column with decreasing density and height at the seamount’s flanks. McNish and Yakhont had the highest average backscatter, followed by Crawford-West and RSA (Figures 3, 4). These seamounts were characterised by relatively shallow summit depths of less than 400 m with McNish being the shallowest (∼150 m). These seamounts also showed higher backscatter on the plateau compared to the slope area (Figure 5). The deeper seamounts (Un-named and Crawford East, summit depth ∼500 m) were characterised by very low levels of backscatter and a very weak SSL (Figures 3, 4) with no clear differences between plateau and slope (Figure 5).
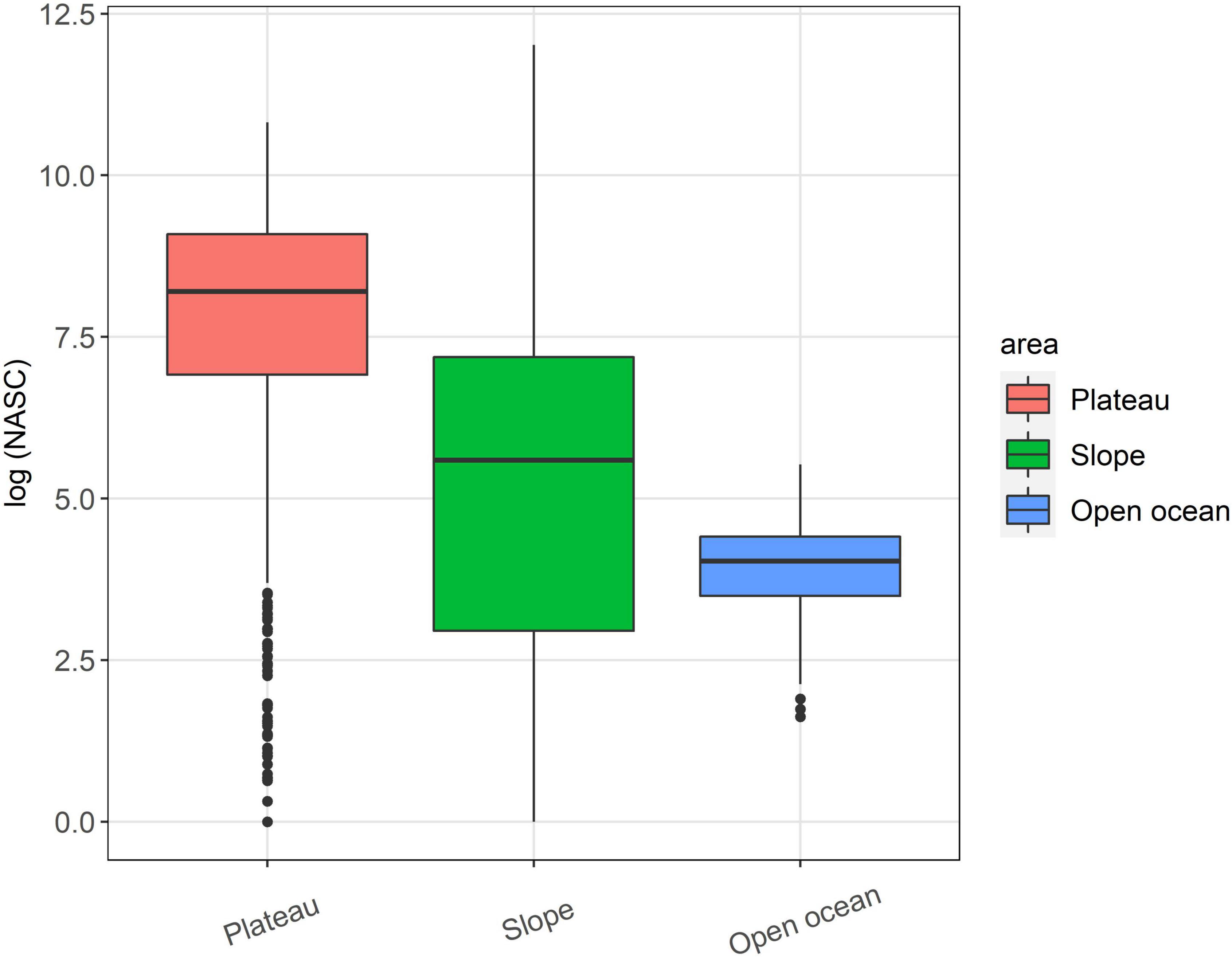
Figure 2. Boxplot of the log-transformed Nautical Area Backscattering Coefficient (NASC) calculated over the plateau and slope of the seamounts and at the open ocean area. Lower and the upper hinges correspond to the first and third quartiles. Dots at the end of the boxplot represent outliers.
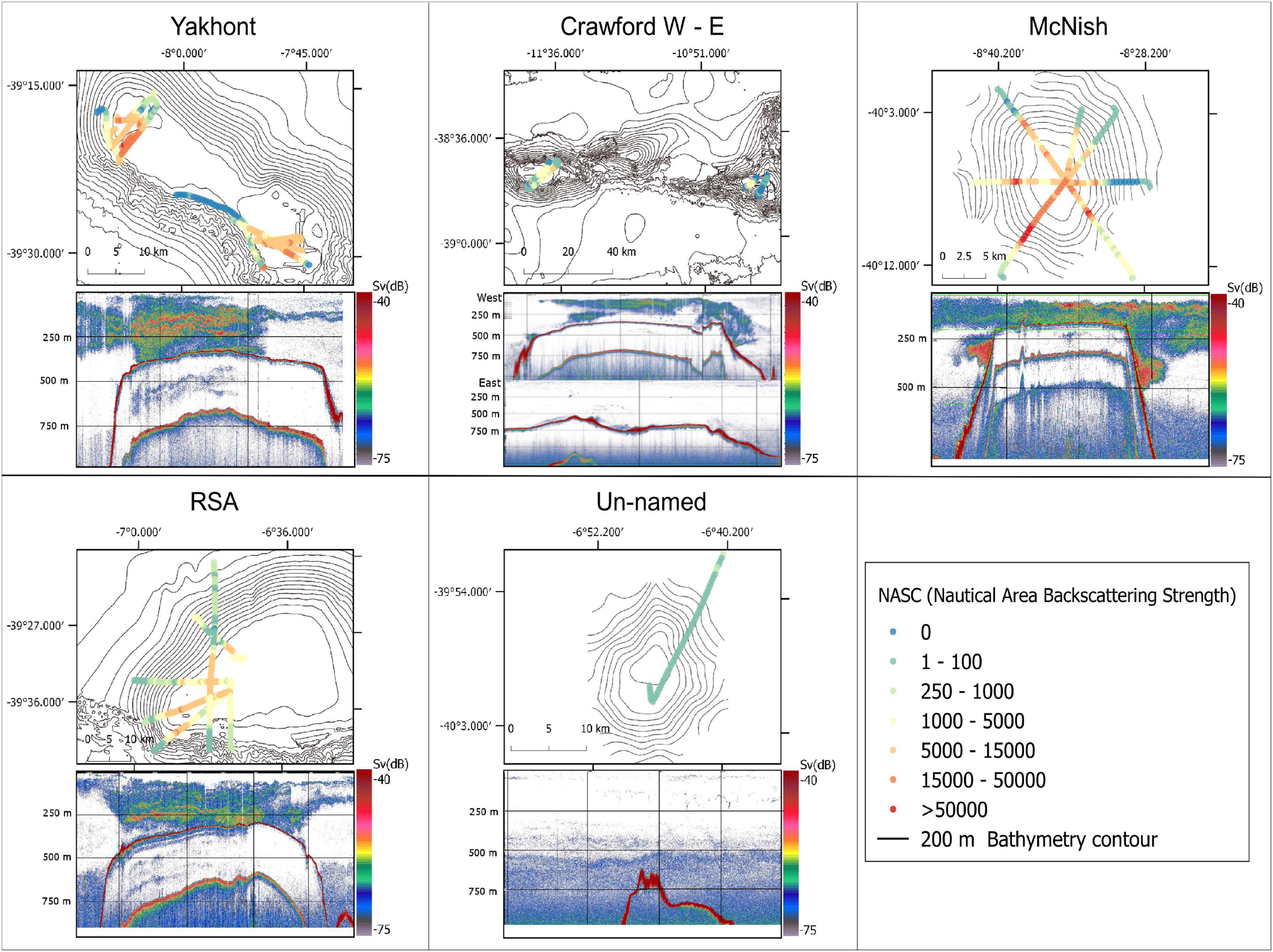
Figure 3. Maps of the Nautical Area Backscattering Coefficient (NASC) at 70 kHz for all the Tristan da Cunha seamounts. An example echogram (Sv, volume backscattering strength) representing one full transect of the corresponding seamount, is shown at the bottom of each panel. The vertical and horizontal black lines on the echograms are 2.5 nautical miles and 250 m spaced, respectively.
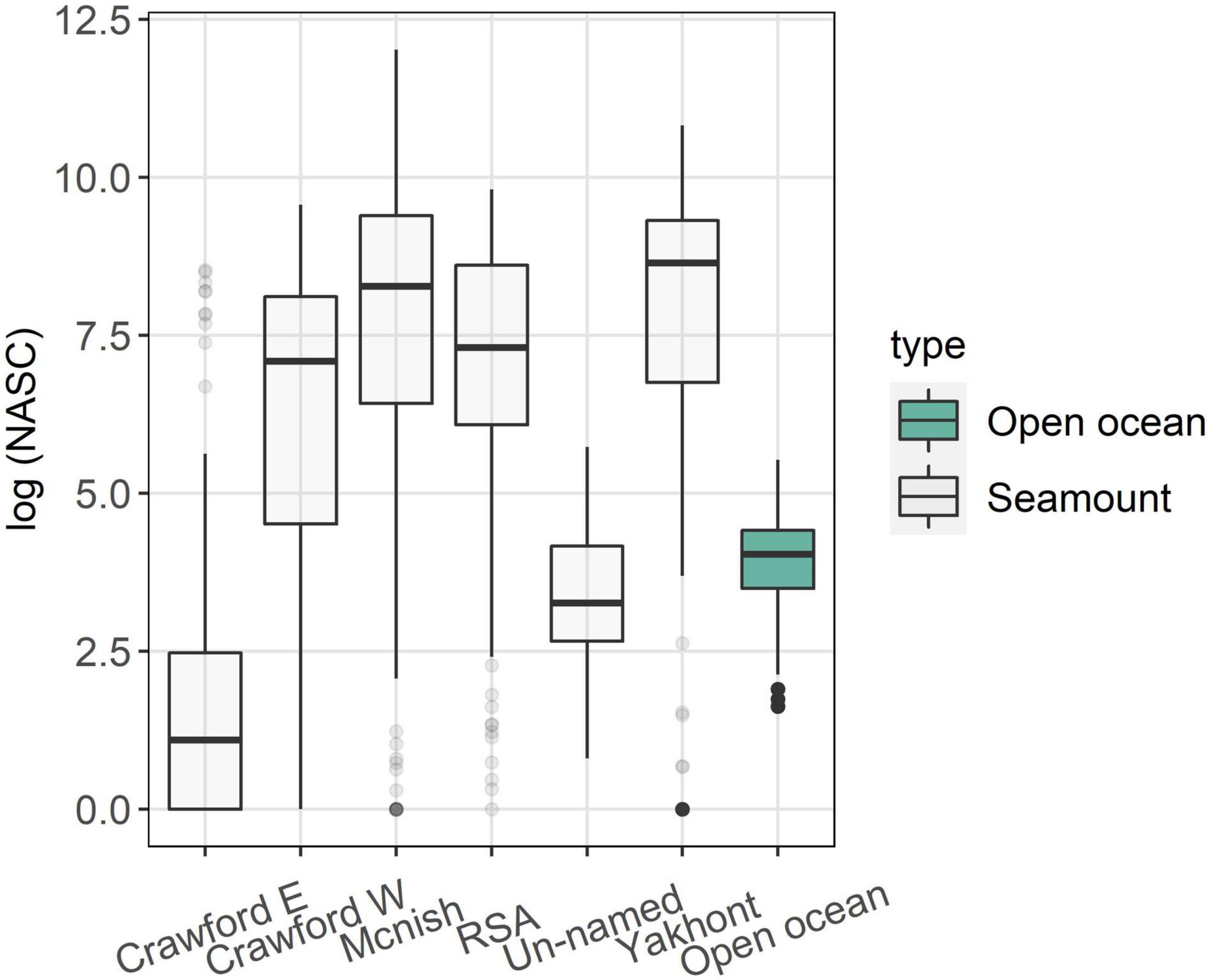
Figure 4. Boxplot of the log-transformed Nautical Area Backscattering Coefficient (NASC), a proxy for biomass, calculated for the area above each of the seamounts and for the open ocean area. Lower and upper hinges correspond to the first and third quartiles. Dots at the end of the boxplot represent outliers.
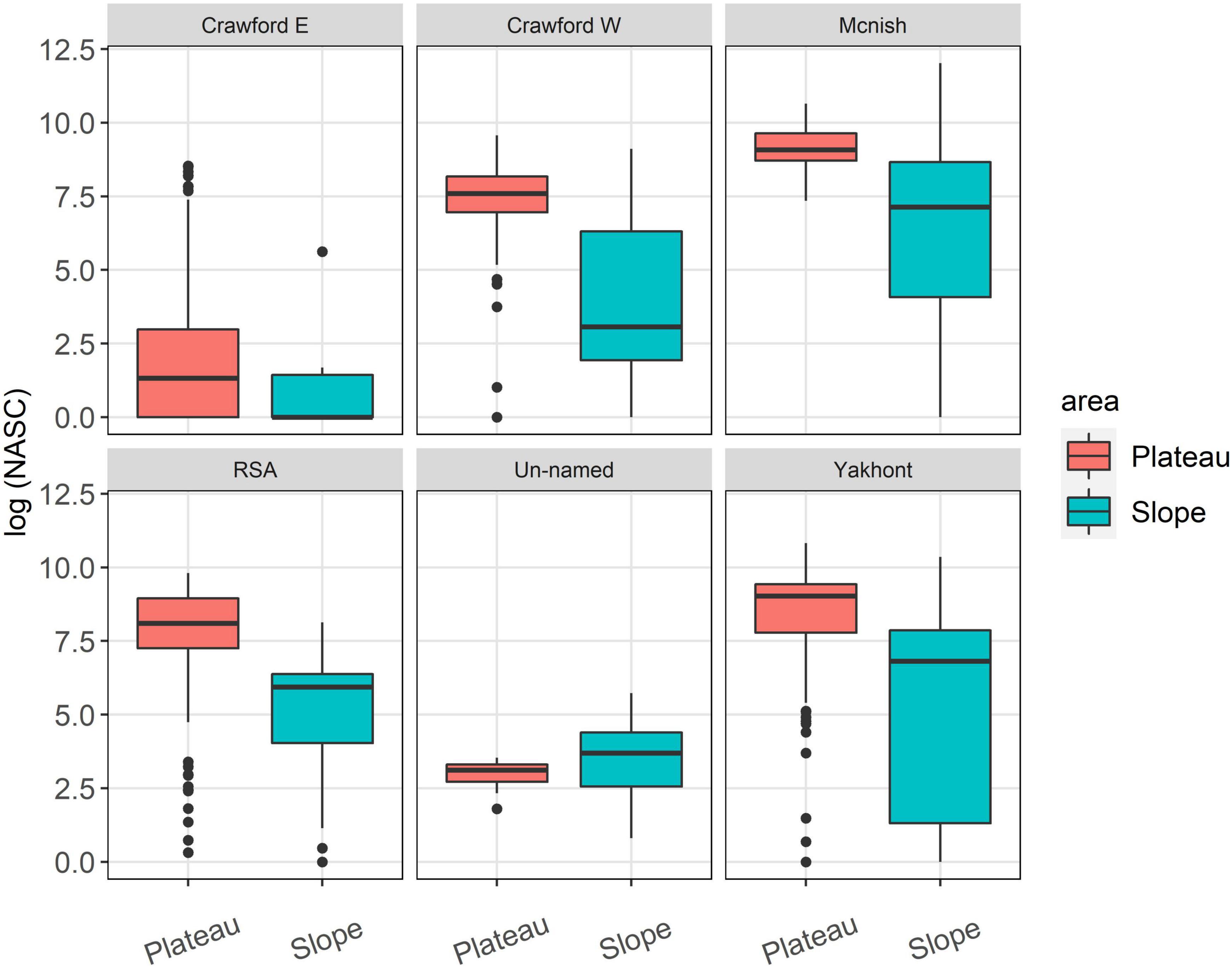
Figure 5. Boxplot of the log-transformed Nautical Area Backscattering Coefficient (NASC) calculated at the plateau and slope for each of the seamounts. Lower and the upper hinges correspond to the first and third quartiles. Dots at the end of the boxplot represent outliers.
GAM models for both the shallow and deep seamount datasets selected the same explanatory variables:
where “s” are the smoothing terms, “slope” is the seabed slope in degrees and “distance_to_plateau” is the distance from the plateau edge (in metres). The variable seabed depth was not included in the models because it was highly collinear with “distance_to_plateau.” The location was used as a categorical variable to account for the variability between the different seamounts. The deviance explained for the shallow and deep seamount models were 35 and 14.5%, respectively. The results highlighted the importance of the distance from the plateau on the distribution of the backscatter, which was the covariate that explained most of the variation in the shallow seamount model. The areas over the plateaus positively affected the backscatter density at the shallow seamounts, while increasing distances away from the plateau had a negative effect (Figure 6). In addition, the model indicated the positive effect of both low and high values of slope, which correspond to the plateau and the steep seamount flanks, respectively. The deep seamount model showed opposite patterns with a positive effect on the backscatter density of increasing distances from the plateau and low values of slope.
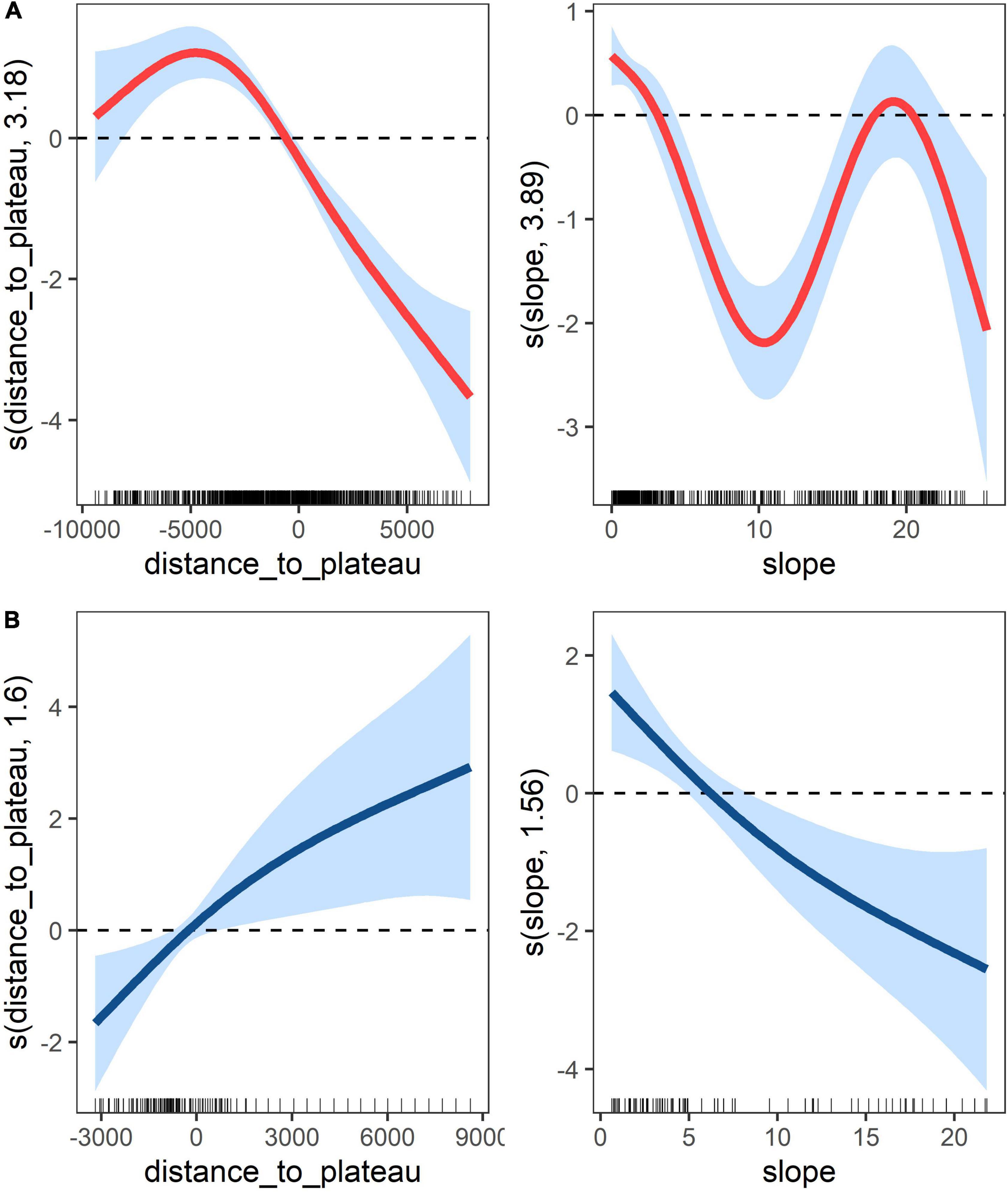
Figure 6. Smooths of generalised additive model terms showing the effect of slope (degrees) and distance from the plateau edges (metre; the negative values are distances from the edges of the plateau to the centre of the seamount and the positive values are distances from the edges of the plateau going toward the open ocean) on the backscattering at 70 kHz for the shallow seamounts (McNish, RSA, Yakhont, Crawford West; (A) and the deeper seamounts (un-named, Crawford east; (B). The solid lines are the estimates of the smooths, the thick marks on the x-axis are observed datapoints and the shaded regions indicate 95% confidence bounds.
Even though the acoustic surveys were conducted during the night, some opportunistic data were collected during the day and the crepuscular period. These data highlighted the presence of a strong Diel Vertical Migration (DVM) of the SSL that occurred at dawn and dusk. At sunrise the SSL became denser and moved to deeper waters forming a strong and homogeneous layer close to the seafloor (Figure 7). The inverse migration occurred at sunset where the SSL moved vertically to the upper water column and maintained that behaviour throughout the night. The night and crepuscular data were mainly collected at the McNish seamount.
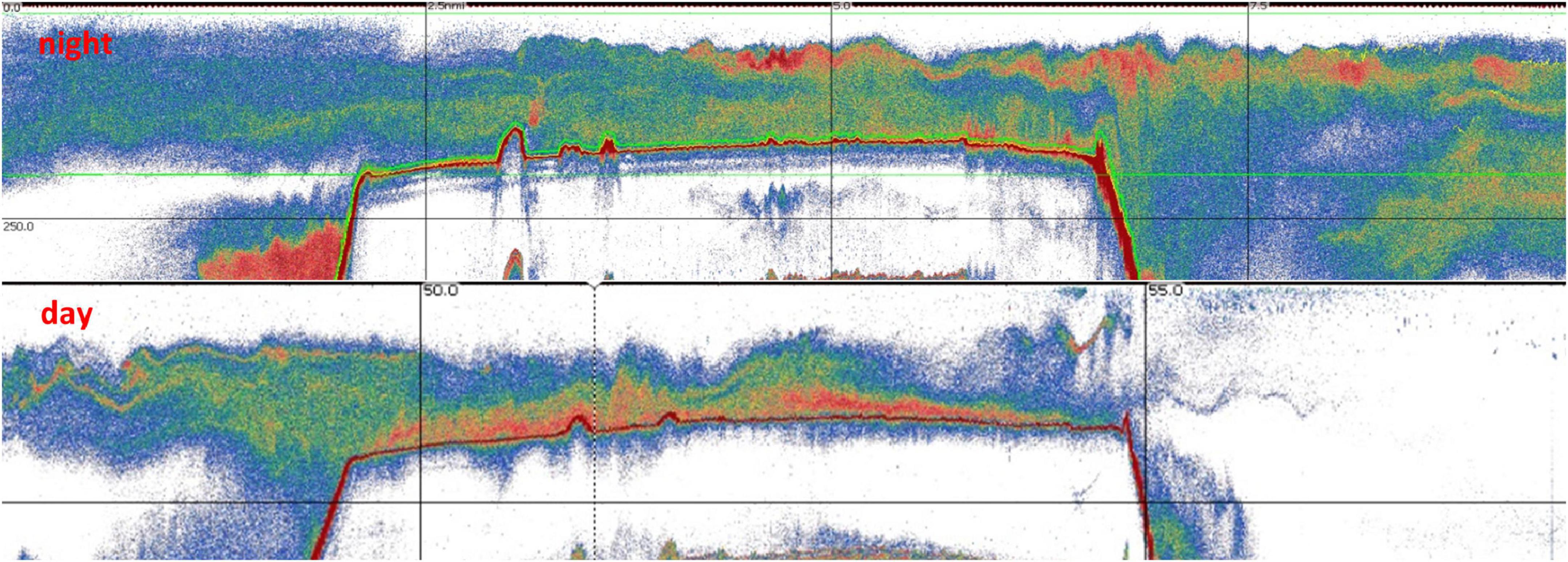
Figure 7. Sv (volume backscattering strength) echograms at 70 kHz of two adjacent transects conducted at night (top) and day (bottom) at the McNish seamount.
Biomass Estimation at McNish and RSA
The catches of the RMT25 and RMT8 pelagic nets performed over the summit of these seamounts (below), confirmed our assumption that the main component of the SSL to be pearlside (Maurolicus inventionis), although small numbers of siphonophores and several species of euphausiids were also caught. Because the acoustic contribution of the other organisms was not known we converted the backscatter of the SSL at McNish and RSA into biomass based on pearlside TS equations. The pearlside density for McNish (mean length estimated from net samples: 4.2 cm) was estimated to be 127.4 fish/m2 for the plateau area and 23.07 fish/m2 for the slope area resulting in a total biomass of 113,503 tonnes on the slope and plateau of the seamount (area: 407 km2). The pearlside density for RSA (using a mean length of 3.7 cm based on net samples) was estimated to be 154.7 fish/m2 for the plateau area and 2.3 fish/m2 for the slope area resulting in a total biomass of 29,952 tonnes (area: 784 km2) (Table 1).
A large and very dense discrete aggregation, which was not part of the SSL, was also detected on McNish (Figure 8). This aggregation was distributed over the slope of the seamount near the seafloor at depths ranging approximately from 200 to 500 m. It was detected on every pass performed over the seamount with the highest density and size detected at the south-west side of the seamount where it reached a length of about 4 km and a height of 200 m. No net deployment could be conducted due to risks of damaging the gear on the slope and the species composition of the school could therefore not be validated. However, based on the high backscattering strength, the morphology that characterised the aggregation and the outcome of the multifrequency analysis (limited to the shallower part of the aggregation), we attributed the aggregation to fish with swimbladders. The aggregation could be ascribed to different bentho-pelagic fish species that are known to inhabit seamounts in this area such as alfonsino (Beryx splendens), rockfish (Sebastes sp.), jacopever (Helicolenus mouchezi) and bluenose warehou (Hyperoglyphe antarctica). As mentioned previously, we considered it was most likely a bluenose warehou spawning or feeding aggregation. In the absence of a dedicated TS equation for this species and limited information on the morphology of the swimbladder, we used two existing but contrasting equations, to estimate the minimum and maximum biomass. Using a conservative physoclist fish target strength equation, the acoustic backscatter of the total aggregation was estimated to represent a biomass of 548,851 tonnes with a density of 0.5 fish/m2. The biologically more comparable target strength equation of the orange roughy, suggested a biomass of 3,307,159 tonnes with a density of 3 fish/m2. For both estimates we used a mean bluenose length of 84 cm (range 57–124 cm) and mean weight of 9.4 kg that were derived from fisheries observer data collected at McNish in March-April 2018 (Bell et al., 2021). Other discrete, but considerably smaller, schools were also detected on Yakhont at a similar depth range to that observed at McNish.
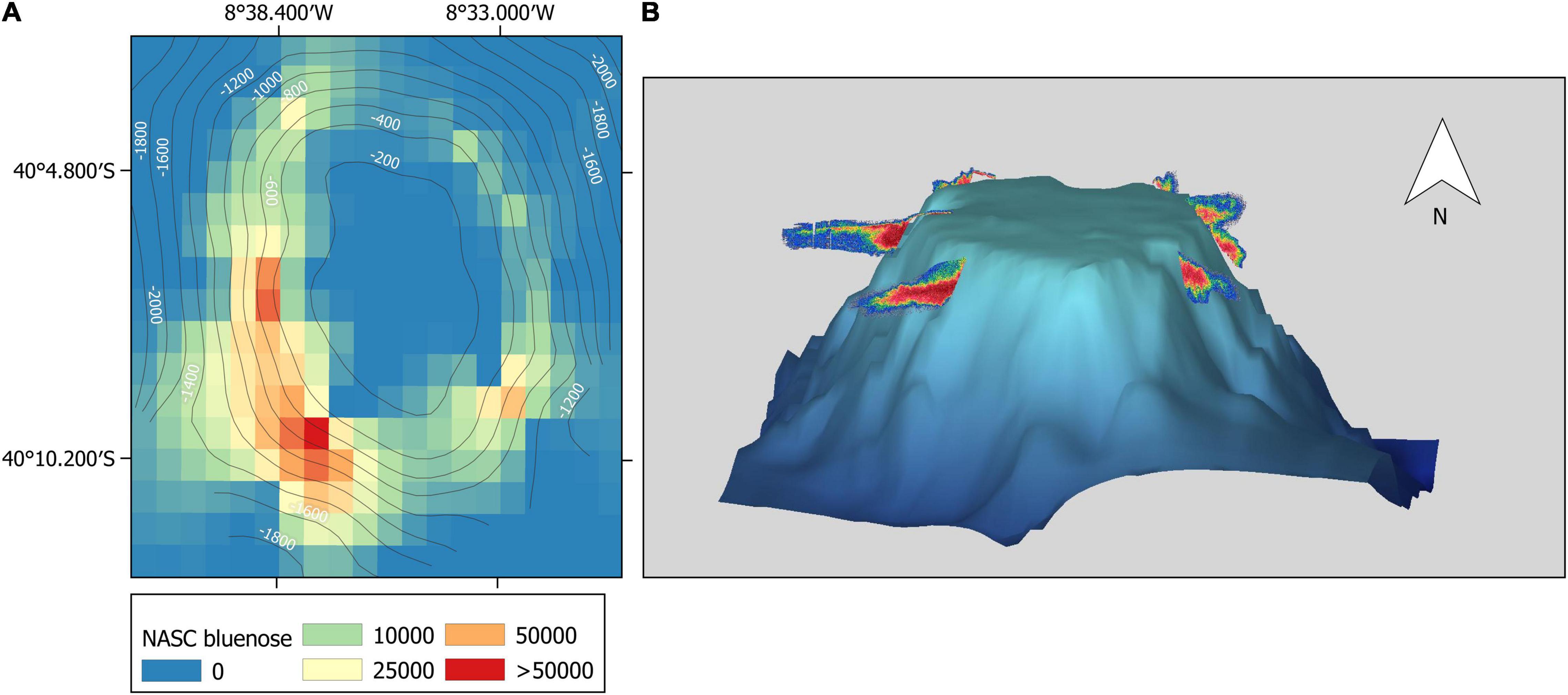
Figure 8. Presumed bluenose warehou (Hyperoglyphe antarctica) aggregation at McNish seamount visualised in two ways: (A) Interpolated map of NASC of the bluenose aggregation at McNish. (B) 70 kHz Sv (volume backscattering strength) echogram curtain of the bluenose aggregation overlaid on the 3D McNish elevation.
Micronekton and Fish Community
Eighteen net hauls were undertaken to target acoustically detected aggregations over the seamounts (Supplementary Table 3) and of these, fourteen were dominated by catches of Maurolicus inventionis (pearlside) (37–90% of the catch). The only target hauls that did not catch pearlside were those targeting layers in the upper 100 m, which had catches dominated by siphonophores and salps (Supplementary Table 3). Over Yakhont Seamount (2018) two size modes of pearlsides (25–28 mm and 44–46 mm SL) were caught, with both modes only found in deeper layers (∼200 M), but only the smaller mode caught during shallower (<150 m) deployments. Twenty-eight non-target, depth stratified RMT deployments were undertaken in the vicinity of the seamounts and in open ocean in the Tristan EEZ. Of these only the nets that fished over the seamount plateaus or very close (Net 21-2 near Yakhont) to the seamounts caught substantial numbers of pearlsides (Supplementary Table 3). The small numbers of fish caught in nets on the slope (Supplementary Table 3) may have been stuck in the net, undetected when large catches were taken over the seamounts. Of the RMT nets that fished open ocean areas, only one caught pearlsides and that was near RSA Seamount. Across all deployments, pearlside was caught primarily between 100 and 400 m depths with size ranging from 19 to 66 mm. Away from the seamounts, the mesopelagic fish fauna was much more diverse, with the genera Cyclothone, Diaphus, Lampanyctus, Argyropelecus, Protomyctophum, Bathylagus and Ceratoscopelus well represented in catches. Siphonophores were another important group present in almost all stations, with higher densities caught especially in shallow waters (10–100 m). Similar to the catches from open oceanic waters, catches over the seamount slopes showed high variability within seamounts and stations.
Hydrodynamics
The 10-year mean horizontal velocities revealed a tendency for anticyclonic flows around McNish Seamount, with strongest flows on the south and east flanks (Figure 9A). These were particularly clear in the flows at 10 m depth, but were also evident at 200 m, albeit weaker (not shown). Associated with the anticyclonic circulation were enhanced vertical velocities, with upwelling in the centre of the seamount, and downwelling on the southeast and northwest flanks (Figure 9C). The simulated mean circulation patterns thus suggested a tendency for local retention on the seamount through the convergent nature of anticyclonic flows, and the potential for increased local productivity through vertical mixing of nutrients associated with enhanced vertical velocities. By comparison, the 10-year mean flows at RSA seamount showed a weak anticyclonic circulation at 10 m depth, centred over the shallower western part of the seamount (Figure 9B). At 200 m, an additional weak anticyclonic flow was centred over the eastern part of the seamount (not shown). Whilst the model suggested enhanced vertical velocities over the RSA seamount relative to the surrounding open ocean (Figure 9D), they were weaker than those at McNish seamount. Thus, whilst the mean flow fields suggested an increase in productivity at RSA Seamount relative to the open ocean, and some local retention, the patterns were weaker than at McNish Seamount.
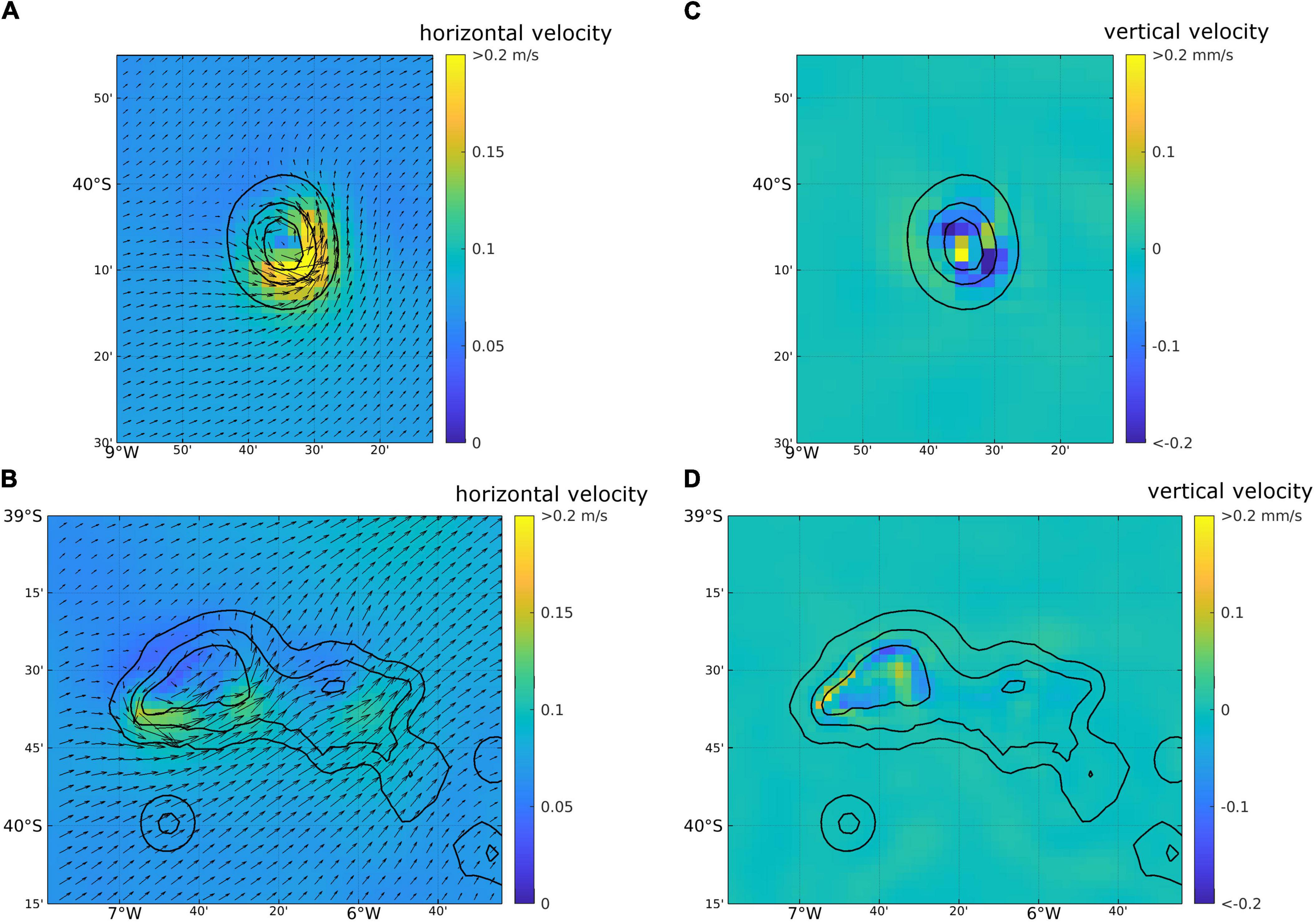
Figure 9. Simulated 10-year mean horizontal velocities (A,B) and vertical velocities (C,D) at 10 m depth from the regional oceanographic model: McNish seamount (A,C), RSA seamount (B,D). Vectors in (A,B) indicate the direction of flow [every 2nd flow vector shown in (B)], and shading represents current speed.
A more detailed picture of the oceanography around McNish Seamount was provided in cross-sections of 5-day mean oceanographic properties (Figure 10). Considering a west-east section along 40°8”S for the 5-day period 31st January to 4th February 2006, and focussing on the upper 300 m, the isotherms, isohalines and isopycnals were inclined downward toward the flanks of the seamount below ∼70 m, with an upward doming above the seamount itself (Figures 10A–C). The associated horizontal flows (Figure 10D) were strongest at a depth of ∼50 m, and were southward (northward) on the west (east) side of the seamount. A north-south section across the seamount for the same time period showed westward (eastward) flows on the north (south) side of the seamount (not shown); such flows are consistent with an anticyclonic circulation around the seamount, in accord with the mean circulation pattern described earlier. However, detailed examination of the 5-day mean fields for 2000–2009 suggested that, although the anticyclonic circulation was a frequent occurrence, it is not a persistent feature. There may therefore be temporal variability in any associated local productivity and retention.
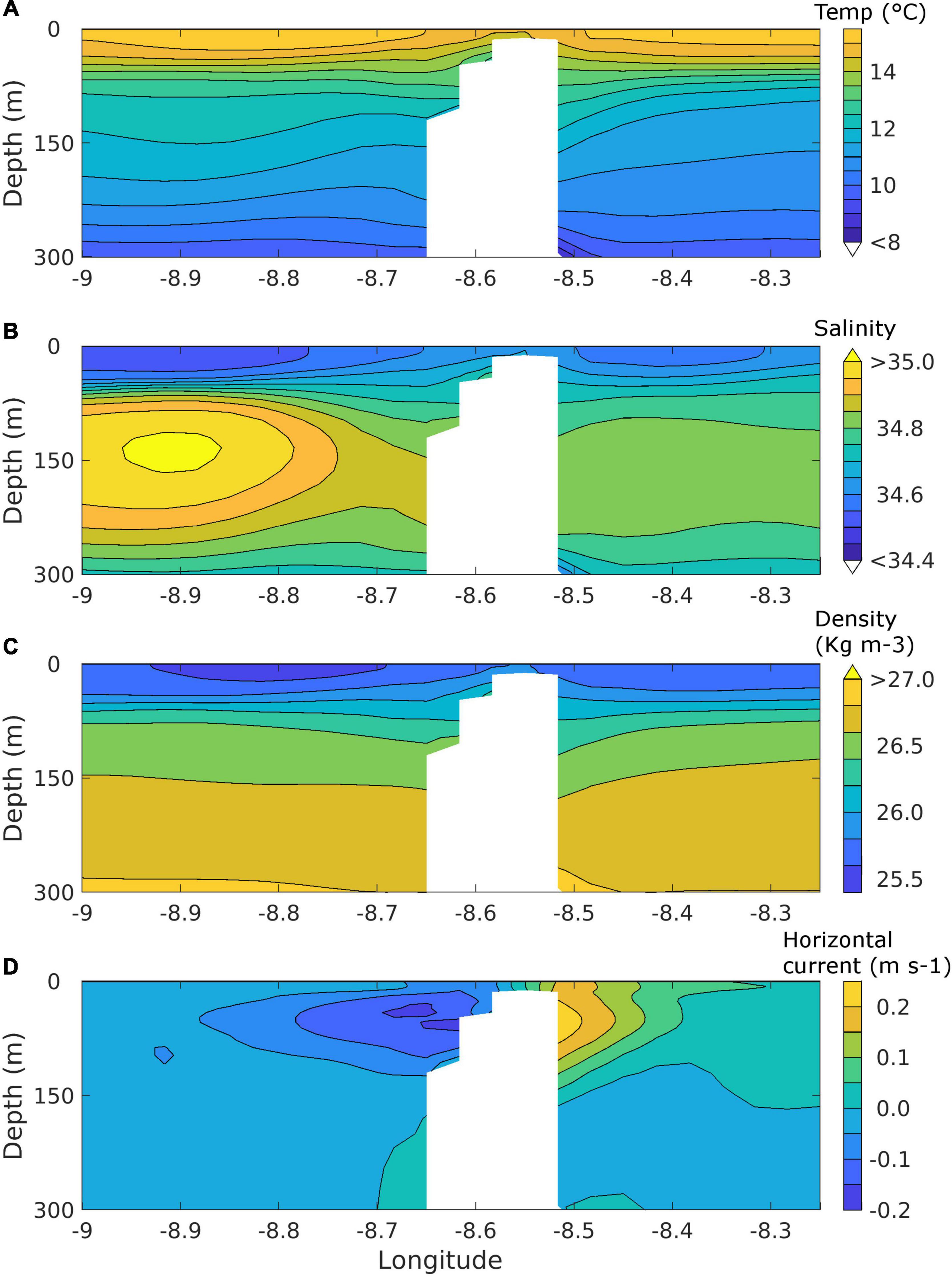
Figure 10. Cross-section of 5-day mean (31/1/2006–4/2/2006) ocean properties along 40°8”S from the regional oceanographic model: (A) potential temperature (°C), (B) salinity, (C) potential density (σ, kg m−3), (D) horizontal current (m s−1). Positive (negative) horizontal currents are northward (southward). Model grid cells below the seabed are shaded white.
The effect of the localised circulation patterns on time scales of retention of marine biota at McNish Seamount was illustrated by calculating the e-folding time for passive particles released above the seamount in the model. By simulating particle releases for a year of oceanographic model output, the potential impact of the predicted anticyclonic circulation features on local retention was shown within a wider temporal context. Simulations of particles released at 5-day intervals for a year (2000) suggested a mean e-folding time of 17 days, albeit with considerable temporal variability (ranging from 1 to 37 days). Such temporal variability likely introduces variability in the retention of passive marine biota. However, the long mean e-folding time suggests that the local oceanography associated with McNish Seamount is able to support enhanced productivity, and potentially to allow marine biota such as fish larvae sufficient time to develop active behaviour that would enable them to maintain their position at the seamount.
Discussion
The results of this study provide a first insight into the Sound Scattering Layers around several little-known seamounts of Tristan da Cunha in the south Atlantic Ocean. They confirm previous observations from other areas, that these relatively small structures have a significant role in supporting high quantities of fish, and other organisms, at different trophic levels, compared to the surrounding waters. Only shallow seamounts, with plateau depths of less than 400 m below the surface, exhibited this increased production (Genin and Dower, 2007) in contrast to the two deeper seamounts. In general, the plateau areas supported higher biomass (backscatter) than the slope.
McNish exhibited some of the highest biomass (backscatter) in the area and modelling outputs, presented in this study, suggested that this might be linked to a specific hydrographic regime. Frequent anticyclonic flows, with isopycnal doming and enhanced vertical velocities, may positively affect retention and vertical mixing of nutrients, which propagate to the higher trophic levels. McNish’s regular shape and homogenous topography are likely contributors to this process, as the hydrographic patterns at RSA, a larger seamount characterised by a more complex topography, were much weaker and more variable. However, variability was also predicted in the hydrodynamic processes at McNish that adversely influenced the long-term persistence of these features. The processes linking the increased productivity and hydrodynamics, even though possible and detected over some seamounts (Genin and Boehlert, 1985; Dower et al., 1992), are difficult to quantify without extensive measurements.
All seamounts, except the deeper un-named and Crawford (east side), featured layers of strong backscatter. The acoustic properties and the catch composition of the targeted trawl hauls, suggested these consisted of micronekton, dominated by pearlsides (Maurolicus inventionis). Small mesopelagic fish of the genus Maurolicus are abundant in all oceans (Kalinowski and Linkowski, 1983; Boehlert et al., 1994; Sassa et al., 2002; Wilson and Boehlert, 2004), and M. inventionis (Parin and Kobylianski, 1996) is endemic to the southern Atlantic, notably around the Discovery seamounts. Pearlsides are considered “pseuodoceanic” (sensu Hulley and Lutkeharms, 1989), and prefer continental shelf-slope breaks to open ocean habitats. This matches the results from this study where they were not observed away from the seamount plateaus and flanks and suggests they were part of a seamount mesopelagic boundary community (Reid et al., 1991; Benoit-bird et al., 2001). The absence of other mesopelagic species over the seamounts may be due to the shallow depths (<250 m) of the plateaus, which prevented lateral advection of diel migrating micronekton from the surrounding mesopelagic zones.
The results of this study could not confirm whether the pearlsides observed at the seamounts were part of resident seamount populations or whether they were reliant on periodic replenishment through advection of eggs, larvae and juveniles from larger populations elsewhere. Most of the pearlside sampled over the Tristan da Cunha seamounts during this study and previously (Linkowski, 1983) were juveniles and sexually immature specimens. The absence of adults could suggest that the juveniles were advected from a different location and were not spawned locally. However, it cannot be excluded that adults were present but were not caught by the trawl because they occupied a different habitat on the seamount (e.g., close to the seafloor) or avoided the RMT nets. In addition, biological data were only collected during the limited temporal window of the survey and may have missed the spawning period. For these reasons, the presence of a self-recruiting resident populations cannot be excluded. Pearlside would benefit from the enhanced productivity of the seamounts and the specific hydrographic regimes, such as the ones simulated at McNish, that could favour retention of eggs and larvae at the seamounts. Seasonal information about length, age and maturity of pearlside and an analysis of the connectivity between seamounts and other potential sources of recruitment is needed to confirm the role of these seamounts in this species’ ecology.
Another notable observation, which further confirmed the unique properties of McNish Seamount and its likely important ecological role in the area, was the presence of a large acoustic aggregation on its slopes, locally as large as 200 m tall and 4 km long. We were unable to confirm the identity of the taxa contributing to this aggregation, but we have presented evidence that it most likely consisted of bentho-pelagic fish with candidate species including bluenose warehou, alfonsino (Beryx spp.) and rockfish (Sebastes spp.), all of which have been caught commercially in the vicinity of the Tristan seamounts (Marecol, 2017) and were sampled during longline surveys close to the timing of the survey (Laptikhovsky et al., 2020). In recent years, the principal commercially caught species was bluenose warehou, known to form large feeding and spawning aggregations at seamounts, including on McNish. It is a long-lived, slow-growing fish that is widely distributed in the southern oceans although little is known about its ecology in the southern Atlantic Ocean.
On the basis that there is a high probability that the aggregation consisted of bluenose, we attempted to estimate the biomass from the acoustic data using two different Target Strength (TS) equations, in the absence of a dedicated bluenose TS value. The resulting density and biomass estimates ranged from 0.5 to 3 fish/m2 and 548,851 to 3,307,159 tonnes, respectively, representing fish with well-developed swim-bladder vs. those with a regressed swimbladder. Both scenarios were explored as the size and physiology of a swimbladder is the most significant variable affecting the TS (Simmonds and Maclennan, 2005) and no detailed information on bluenose swimbladders was available. For context, we point out that if fish species without a swimbladder were present in the aggregation, the resulting biomass estimate would be higher than the (conservative) value provided. Also, if the aggregation consisted (partly) of other bentho-pelagic species like alfonsino (Beryx spp.) or rockfish (Sebastes spp.), the same physoclist swimbladder target strength equations would have been applied. Regardless of the taxa concerned (and swim-bladder form), this study presents evidence of significant bentho-pelagic fish biomass at McNish. If the aggregation is bluenose, our data suggest that the stock size of this species at McNish could be very high compared to other bluenose stocks for which stock assessments have been produced (Cordue and Pomaréde, 2012; Williams et al., 2016). However, given the aforementioned uncertainties and the resulting large variability in the estimates, the results on the biomass of presumed bluenose reported in this paper are only preliminary and should not be used, in isolation, for any management decisions such as the status and size of bluenose warehou stock.
It is unclear if the population of bluenose at McNish is self-recruiting at the seamount or if it receives external fluxes of eggs and larvae. The specific hydrodynamic conditions at McNish that seem to allow a relatively persistent retention, could facilitate self-recruitment at the seamount in different ways. Firstly, a portion of the planktonic eggs and larvae produced at the seamount could be retained. Accumulation of larval stages has been observed on other seamounts characterised by persistent hydrographic features (Mullineaux and Mills, 1997; Dower and Ian Perry, 2001). Secondly, the hydrographic conditions may also act to create an optimal habitat for bluenose juveniles with flotsam and drift algae, known to provide suitable habitat conditions for its early pelagic life stages (Blackwell and Gilbert, 2003), accumulating over the seamounts and juvenile bluenose prey, such as salps and pyrosomes, advecting by prevailing currents (Laptikhovsky et al., 2020).
The results of this study suggested the presence of potentially high densities of bentho-pelagic fish, which matched reported spawning behaviour of bluenose warehou (as well as several other species). If that is the case, it would mean that McNish could act as the main hub for these bentho-pelagic species by providing fluxes of eggs and larvae to replenish the other seamounts in the area. Similar to pearlside, we cannot confirm this hypothesis without a thorough analysis of the connectivity between seamounts. Moreover, since the coverage of our surveys was spatially and temporally limited, especially over the larger seamounts such as RSA, we could not confirm if other large aggregations, such as the one observed at McNish, were present at other seamounts. Other factors, such as larval stage duration, larval swimming ability and spatio-temporal patterns of the spawning activity would need to be investigated as they may largely contribute to the self-recruitment success (Sponaugle et al., 2002).
This study implies the presence of high mesopelagic and bentho-pelagic biomass at McNish Seamount, and would support the important role of seamounts in betho-pelagic coupling. The mesopelagic community, including pearlsides, transfer energy from the productive pelagic zone to the benthic zone by diel vertical migration (DVM) (Trueman et al., 2014). Mesopelagic fish such as pearlside, inhabit shallower waters at dusk and during the night (Staby et al., 2011) to feed on plankton and descend to the seabed where they reside during the day and are predated on by benthic and bentho-pelagic predators. The limited daytime acoustic observations at the seamounts confirmed that the pelagic pearlside scattering layers observed at night, descended tight to the seafloor where they were visible on the echogram as a dense layer.
The diet of bluenose from these seamounts (McNish, RSA and Yakhont) is known to include pearlside (Laptikhovsky et al., 2020), especially over McNish where it constituted more than 60% of the total diet, confirming the important trophic interactions between benthopelagic and pelagic environments. High pearlside densities, estimated to range from 78.6 to 95 g/m2, were similar to previous estimates (Kalinowski and Linkowski, 1983). The highest estimates were recorded over the flanks at McNish (compared to RSA), which might explain the higher importance of pearlside in the bluenose diet at McNish compared to other seamounts. The large bentho-pelagic fish aggregation was mainly located over the slope of the seamount, likely because of a higher availability of migrating prey. The higher backscatter values of both aggregations were located on the western flanks of the seamount, which, according to the oceanographic model, were generally characterised by weaker currents. It is possible that pearlside occupied these calmer habitats during non-feeding intervals to preserve energy (feed-rest hypothesis; Genin, 2004), and bluenose would target these high concentrations of prey. It is unclear whether the quantity of meso-pelagic prey at McNish would be able to support such a potentially high benthopelagic fish biomass, particularly for a prolonged period. For this reason, another hypothesis that could explain the presence of the large amount of fish at McNish is migration. Tagging studies on bluenose carried out in New Zealand have shown that adults can travel up to 490 km along the continental margin (Horn, 2003). Considering that the period of the survey (March–April) overlaps with the spawning season reported in the South Atlantic (Piotrovsky, 1994), bluenose might have migrated from the nearby seamounts and islands to McNish to spawn. Even though bluenose spawning migration has not been observed, this hypothesis cannot be excluded and more work is needed to investigate this further.
To our knowledge, this work represents the first scientific study combining fisheries acoustic methods, qualitative trawls and hydrodynamic modelling to explore the ecological role of the seamount ecosystems around the Tristan da Cunha archipelago. Despite several caveats, discussed in this study, the multi-disciplinary approach enabled us to describe patterns in the micronekton dynamics, analyse the influence of seamount topographic features on these observations and interpret them in context of the ecology of the region. This study contributes toward a better understanding of the relative importance of the newly established MPA, its role in the wider region and, more broadly, to the general understanding of the role of seamount systems in the open oceans. The suggested observations of enhanced (mesopelagic and benthopelagic) fish biomass detected near seamounts (compared to the surrounding ocean) corroborated the role of seamounts as ecological “oasis” (sensu McClain, 2007). As this enhanced productivity was only observed over specific seamounts, possibly as a result of complex interactions between topography, hydrodynamics and environmental factors, it further highlighted the heterogeneity of these habitats with differing ecological roles. More scientific efforts are needed to disentangle the complex interactions operating at seamounts by expanding the research focus to other components of the ecosystems such as planktonic and benthic organisms and a more comprehensive analysis on the physical forcing. Although further questions remain about the large bentho-pelagic aggregation on McNish, including on the species composition, this study has helped identify priority areas for future monitoring surveys which will be important to underpin sustainable management.
Data Availability Statement
The raw data supporting the conclusions of this article will be made available by the authors, without undue reservation.
Author Contributions
FC, MC, and JK designed the acoustic surveys. FC, MC, VL, and PW collected the acoustic data, performed the net sampling, and process the samples on board the research vessels. FC conducted the acoustic analysis. EY performed the analysis of the oceanographic model data. All authors contributed to the article and approved the submitted version.
Conflict of Interest
The authors declare that the research was conducted in the absence of any commercial or financial relationships that could be construed as a potential conflict of interest.
Funding
The surveys were funded by the UK Government funded Blue Belt Programme and the British Antarctic Survey through the Natural Environment Research Council (RSS Discovery Survey: Grant NE/R000107/1) NC-ODA project. The participation of EY was funded by the Natural Environment Research Council through award NE/R000107/1. The oceanographic modelling used the ARCHER UK National Supercomputing Service (http://www.archer.ac.uk).
Acknowledgments
We are grateful to the masters and crew of the RRS James Clark Ross and RRS Discovery for their cooperation during the fisheries surveys, and to Tristan da Cunha Government for their support during this research.
Supplementary Material
The Supplementary Material for this article can be found online at: https://www.frontiersin.org/articles/10.3389/fmars.2021.663278/full#supplementary-material
References
Anderson, D. A., Burnham, K. P., and Anderson, D. R. (1998). Model Selection and Inference: a Practical Information-Theoretic Approach. Berlin: Springer.
Ballón, M., Bertrand, A., Lebourges-Dhaussy, A., Gutiérrez, M., Ayón, P., Grados, D., et al. (2011). Is there enough zooplankton to feed forage fish populations off Peru? An acoustic (positive) answer. Prog. Oceanogr. 91, 360–381. doi: 10.1016/j.pocean.2011.03.001
Bell, J., Laptikhovsky, V., Barnes, D. K. A., Benedet, R., Bridges, E., Glass, J., et al. (2021). Life history and ecology of bluenose warehou (Hyperoglyphe antarctica, Centrolophidae) in the southern Atlantic. Front. Mar. Sci. 8:610172. doi: 10.3389/fmars.2021.610172
Benoit-bird, K. J., Au, W. W. L., Brainard, R. E., and Lammers, M. O. (2001). Diel horizontal migration of the Hawaiian mesopelagic boundary community observed acoustically. Mar. Ecol. Prog. Ser. 217, 1–14. doi: 10.3354/meps217001
Blackwell, R. G., and Gilbert, D. J. (2003). Review of methods for the estimation of relative biomass of bluenose. New Zeal. Fish. Assess. Rep. 14:57.
Boehlert, G., Wilson, C., and Mizuno, K. (1994). Populations of the Sternoptychid Fish Maurolicus muelleri on Seamounts in the Central North Pacific. Pacific Sci. 48, 57–69.
Collins, M. A., Stowasser, G., Fielding, S., Shreeve, R., Xavier, J. C., Venables, H. J., et al. (2012). Latitudinal and bathymetric patterns in the distribution and abundance of mesopelagic fish in the Scotia Sea. Deep. Res. II 59–60, 189–198. doi: 10.1016/j.dsr2.2011.07.003
Collins, M. A., Xavier, J. C., Johnston, N. M., North, A. W., Enderlein, P., Tarling, G. A., et al. (2008). Patterns in the distribution of myctophid fish in the northern Scotia Sea ecosystem. Polar Biol. 31, 837–851. doi: 10.1007/s00300-008-0423-2
Comeau, L. A., Vézina, A. F., Bourgeois, M., and Juniper, S. K. (1995). Relationship between phytoplankton production and the physical structure of the water column near Cobb Seamount, northeast Pacific. Deep Sea Res. Part I Oceanogr. Res. Pap. 42, 993–1005. doi: 10.1016/0967-0637(95)00050-G
Cordue, P. L., and Pomaréde, M. (2012). A 2011 stock assessment of bluenose (Hyperoglyphe antarctica). New Zeal. Fish. Assess. Rep. 06:56.
Demer, D. A., Berger, L., Bernasconi, M., Bethke, E., Boswell, K. M., Chu, D., et al. (2015). Calibration of Acoustic Instruments. ICES Cooperative Research Report 326. London: ICES.
Dower, J., Freeland, H., and Juniper, K. (1992). A strong biological response to oceanic flow past Cobb Seamount. Deep Sea Res. Part A. Oceanogr. Res. Pap. 39, 1139–1145. doi: 10.1016/0198-0149(92)90061-W
Dower, J. F., and Ian Perry, R. (2001). High abundance of larval rockfish over Cobb Seamount, an isolated seamount in the Northeast Pacific. Fish. Oceanogr. 10, 268–274. doi: 10.1046/j.1365-2419.2001.00162.x
Dussin, R., Barnier, B., and Brodeau, L. (2016). The making of Drakkar forcing set DFS5. DRAKKAR/MyOcean Rep. 01-04–16. Gütersloh: Bertelsmann.
Egbert, G. D., and Erofeeva, S. Y. (2002). Efficient Inverse Modeling of Barotropic Ocean Tides. J. Atmos. Ocean. Technol. 19, 183–204. doi: 10.1175/1520-0426(2002)019<0183:eimobo>2.0.co;2
Fock, H. O., Matthiessen, B., Zidowitz, H., and Westernhagen, H. V. (2002). Diel and habitat-dependent resource utilisation by deep-sea fishes at the Great Meteor seamount: Niche overlap and support for the sound scattering layer interception hypothesis. Mar. Ecol. Prog. Ser. 244, 219–233. doi: 10.3354/meps244219
Foote, K. G. (1987). Fish target strengths for use in echo integrator surveys. J. Acoust. Soc. Am. 82, 981–987. doi: 10.1121/1.395298
Genin, A. (2004). Bio-physical coupling in the formation of zooplankton and fish aggregations over abrupt topographies. J. Mar. Syst. 50, 3–20. doi: 10.1016/j.jmarsys.2003.10.008
Genin, A., and Boehlert, G. W. (1985). Dynamics of temperature and chlorophyll structures above a seamount: an oceanic experiment. J. Mar. Res. 43, 907–924. doi: 10.1357/002224085788453868
Genin, A., and Dower, J. F. (2007). “Seamount plankton dynamics,” in Seamounts: Ecology, Fisheries & Conservation, eds T. J. Pitcher, T. Morato, P. J. B. Hart, M. R. Clark, N. Haggan, and R. S. Santos (Oxford: Blackwell Publishing), 85–100.
Genin, A., Haury, L., and Greenblatt, P. (1988). Interactions of migrating zooplankton with shallow topography: predation by rockfishes and intensification of patchiness. Deep Sea Res. Part A. Oceanogr. Res. Pap. 35, 151–175. doi: 10.1016/0198-0149(88)90034-9
Godø, O. R., Samuelsen, A., Macaulay, G. J., Patel, R., and Hjøllo, S. S. (2012). Mesoscale Eddies Are Oases for Higher Trophic Marine Life. PLoS ONE 7:e30161. doi: 10.1371/journal.pone.0030161
Goldner, D. R., and Chapman, D. C. (1997). Flow and particle motion induced above a tall seamount by steady and tidal background currents. Deep Sea Res. Part I Oceanogr. Res. Pap. 44, 719–744. doi: 10.1016/S0967-0637(96)00131-8
Holland, K. N., and Grubbs, R. D. (2007). “Fish visitors to seamounts: tunas and bill fish at seamounts,” in Seamounts Ecol. Fish. Conserv, eds T. J. Pitcher, T. Morato, P. J. B. Hart, M. R. Clark, N. Haggan, and R. S. Santos (Oxford: Blackwell Publishing), 189–201. doi: 10.1002/9780470691953.ch10a
Holland, K. N., Kleiber, P., and Kajiura, S. M. (1999). Different residence times of yellowfin tuna, Thunnus albacares, and bigeye tuna, T. obesus, found in mixed aggregations over a seamount. Fish. Bull. 97, 392–395.
Horn, M. H. (1975). Swim-bladder state and structure in relation to behavior and mode of life in stromateoid fishes. Fish. Bull. 73, 95–109.
Horn, P. L. (2003). Stock structure of bluenose (Hyperoglyphe antarctica) off the north-east coast of New Zealand based on the results of a detachable hook tagging programme. New Zeal. J. Mar. Freshw. Res. 37, 623–631. doi: 10.1080/00288330.2003.9517193
Hulley, P. A., and Lutkeharms, J. R. (1989). Lanternfishes of the Southern Benguela Region Part 3. The Pseudoceanic-oceanic interface. Ann. South African Museum 98, 645–658.
Irigoien, X., Klevjer, T. A., Røstad, A., Martinez, U., Boyra, G., Acuña, J. L., et al. (2014). Large mesopelagic fishes biomass and trophic efficiency in the open ocean. Nat. Commun. 5:3271. doi: 10.1038/ncomms4271
Kalinowski, J., and Linkowski, T. B. (1983). Hydroacoustic observations on Maurolicus muelleri (Sternoptychidae) over R.S.A. and Discovery Seamounts (South Atlantic). London: ICES.
Kitchingman, A., Lai, S., Morato, T., and Pauly, D. (2007). “How many seamounts are there and where are they located?,” in Seamounts: Ecology, Fisheries & Conservation, eds T. J. Pitcher, T. Morato, P. J. B. Hart, M. R. Clark, N. Haggan, and R. S. Santos (Oxford: Blackwell Publishing), 26–40. doi: 10.1002/9780470691953.ch2
Klimley, A. P., Jorgensen, S. J., Muhlia-Melo, A., and Beavers, S. C. (2003). The occurrence of yellowfin tuna (Thunnus albacares) at Espiritu Santo Seamount in the Gulf of California. Fish. Bull. 101, 684–692.
Koslow, J. A. (1996). Energetic and life-history patterns of deep-sea benthic, benthopelagic and seamount-associated fish. J. Fish Biol. 49, 54–74. doi: 10.1111/j.1095-8649.1996.tb06067.x
Koslow, J. A., Boehlert, G. W., Gordon, J. D. M., Haedrich, R. L., Lorance, P., and Parin, N. (2000). Continental slope and deep-sea fisheries: implications for a fragile ecosystem. ICES J. Mar. Sci. 57, 548–557. doi: 10.1006/jmsc.2000.0722
Laptikhovsky, V., Bell, J. B., Benedet, R., Glass, J., Glass, W., Green, R., et al. (2020). Feeding habits of bluenose warehou, Hyperoglyphe antarctica (Carmichael, 1819) (Centrolophidae) at seamounts of the Southern Atlantic. Deep. Res. Part I Oceanogr. Res. Pap. 156:103182. doi: 10.1016/j.dsr.2019.103182
Linkowski, T. B. (1983). Some Aspects of the Biology of Maurolicus muelleri/Sternoptychidae from the South Atlantic. London: ICES.
Marecol (2017). South Atlantic Overseas Territories Commercial Fisheries Management Review. A Report Commissioned by the RSPB. Available online at: https://www.rspb.org.uk/globalassets/downloads/documents/conservation-projects/ukots-hidden-treasures/2017/summary-south-atlantic-overseas-territories-fisheries-sustainability-report-2017.pdf
McClain, C. R. (2007). Seamounts: Identity crisis or split personality? J. Biogeogr. 34, 2001–2008. doi: 10.1111/j.1365-2699.2007.01783.x
McClatchie, S., Macaulay, G., Coombs, R. F., Grimes, P., and Hart, A. (1999). Target strength of an oily deep-water fish, orange roughy (Hoplostethus atlanticus) I. Experiments. J. Acoust. Soc. Am. 106, 131–142. doi: 10.1121/1.427042
Mohn, C., and Beckmann, A. (2002). The upper ocean circulation at Great Meteor Seamount. Ocean Dyn. 52, 179–193. doi: 10.1007/s10236-002-0017-4
Morato, T., and Clark, M. R. (2007). “Seamount fishes: ecology and life histories,” in Seamounts: Ecology, Fisheries & Conservation, eds T. J. Pitcher, T. Morato, P. J. B. Hart, M. R. Clark, N. Haggan, and R. S. Santos (Oxford: Blackwell Publishing), 170–188. doi: 10.1002/9780470691953.ch9
Morato, T., Hoyle, S. D., Allain, V., and Nicol, S. J. (2010a). Seamounts are hotspots of pelagic biodiversity in the open ocean. Proc. Natl. Acad. Sci. U. S. A. 107, 9707–9711. doi: 10.1073/pnas.0910290107
Morato, T., Hoyle, S. D., Allain, V., and Nicol, S. J. (2010b). Tuna longline fishing around west and central pacific seamounts. PLoS ONE 5:e0014453. doi: 10.1371/journal.pone.0014453
Morato, T., Varkey, D. A., Damaso, C., Machete, M., Santos, M., Prieto, R., et al. (2008). Evidence of a seamount effect on aggregating visitors. Mar. Ecol. Prog. Ser. 357, 23–32. doi: 10.3354/meps07269
Morley, S. A., Collins, M. A., Barnes, D. K. A., Sands, C., Bell, J. B., Walmsley, S., et al. (2018). Helping Tristan da Cunha and St Helena manage their marine environments. Report of James Clark Ross cruise 17-004. Liverpool: BODC.
Mullineaux, L. S., and Mills, S. W. (1997). A test of the larval retention hypothesis in seamount-generated flows. Deep. Res. Part I Oceanogr. Res. Pap. 44, 745–770. doi: 10.1016/S0967-0637(96)00130-6
Owens, W. B., and Hogg, N. G. (1980). Oceanic observations of stratified Taylor columns near a bump. Deep Sea Res. Part A. Oceanogr. Res. Pap. 27, 1029–1045. doi: 10.1016/0198-0149(80)90063-1
Parin, N. V., and Kobylianski, S. G. (1996). Diagnoses and distribution of fifteen species recognized in genus Maurolicus cocco (Sternoptychidae, Stomiiformes) with a key to their identification. Cybium 20, 185–195.
Phleger, C. F., and Grigor, M. R. (1990). Role of wax esters in determining buoyancy in Hoplostethus atlanticus (Beryciformes: Trachichthyidae). Mar. Biol. 105, 229–233. doi: 10.1007/bf01344291
Piatkowski, U., Rodhouse, P. G., White, M. G., Bone, D. G., and Symon, C. (1994). Nekton community of the Scotia Sea as sampled by the RMT 25 during austral summer. Mar. Ecol. Prog. Ser. 112, 13–28. doi: 10.3354/meps112013
Piotrovsky, A. S. (1994). Stromateoidei in the southern part of Africa and adjacent areas (distribution, biology and fisheries). Main results of YugNIRO complex research in Azov-Black Seas region and the World Ocean in 1993. Proc. South. Aust. Sci. Res. Inst. Mar. Fish. Ocean. 40, 69–77. doi: 10.15407/gpimo2020.01.069
Proud, R., Cox, M. J., and Brierley, A. S. (2017). Biogeography of the Global Ocean’s Mesopelagic Zone. Curr. Biol. 27, 113–119. doi: 10.1016/j.cub.2016.11.003
Reid, S. B., Hirota, J., Young, R. E., and Hallacher, L. E. (1991). Mesopelagic-boundary community in Hawaii: Micronekton at the interface between neritic and oceanic ecosystems. Mar. Biol. 109, 427–440. doi: 10.1007/BF01313508
Roe, H. S. J., and Shale, D. M. (1979). A new multiple rectangular midwater trawl (RMT 1+ 8M) and some modifications to the Institute of Oceanographic Sciences’ RMT 1+ 8. Mar. Biol. 50, 283–288. doi: 10.1007/bf00394210
Sassa, C., Kawaguchi, K., Kinoshita, T., and Watanabe, C. (2002). Assemblages of vertical migratory mesopelagic fish in the transitional region of the western North Pacific. Fish. Oceanogr. 11, 193–204. doi: 10.1046/j.1365-2419.2002.00199.x
Simmonds, E. J., and Maclennan, D. N. (2005). Fisheries Acoustics: Theory and Practice. Oxford, UK: Blackwell Publishing.
Sobradillo, B., Boyra, G., Martinez, U., Carrera, P., Pe, M., and Irigoien, X. (2019). Target Strength and swimbladder morphology of Mueller’s pearlside. Sci. Rep. 9:17311. doi: 10.1038/s41598-019-53819-6
Sponaugle, S., Cowen, R. K., Shanks, A., Morgan, S. G., Leis, J. M., Pineda, J., et al. (2002). Predicting self-recruitment in marine populations: Biophysical correlates and mechanisms. Bull. Mar. Sci. 70, 341–375.
Staby, A., Røstad, A., and Kaartvedt, S. (2011). Long-term acoustical observations of the mesopelagic fish Maurolicus muelleri reveal novel and varied vertical migration patterns. Mar. Ecol. Prog. Ser. 441, 241–255. doi: 10.3354/meps09363
Trueman, C. N., Johnston, G., O’Hea, B., and MacKenzie, K. M. (2014). Trophic interactions of fish communities at midwater depths enhance long-term carbon storage and benthic production on continental slopes. Proc. R. Soc. B Biol. Sci. 281:20140669. doi: 10.1098/rspb.2014.0669
White, M., Bashmachnikov, I., Arístegui, J., and Martins, A. (2007). “Physical processes and seamount productivity,” in Seamounts Ecol. Fish. Conserv, eds T. J. Pitcher, T. Morato, P. J. B. Hart, M. R. Clark, N. Haggan, and R. S. Santos (Oxford: Blackwell Publishing), 62–84. doi: 10.1002/9780470691953.ch4
Whomersley, P., Morley, S., Bell, J., Collins, M. A., Pettafor, A., Campanella, F., et al. (2019). Blue Belt Programme: RRS Discovery DY100 survey report.
Williams, A., Hamer, P., Haddon, M., Robertson, S., Althaus, F., Green, M., et al. (2016). Determining Blue-eye Trevalla Stock Structure and Improving Methods for Stock Assessment. Fisheries Research and Development Corporation Report 05. Washington, DC: NOAA.
Wilson, C. D., and Boehlert, G. W. (2004). Interaction of ocean currents and resident micronekton at a seamount in the central North Pacific. J. Mar. Syst. 50, 39–60. doi: 10.1016/j.jmarsys.2003.09.013
Keywords: seamount, Tristan da Cunha, Maurolicus, bentho-pelagic fishes, Blue Belt, fisheries acoustics, UK Overseas Territories, oceanographic modelling
Citation: Campanella F, Collins MA, Young EF, Laptikhovsky V, Whomersley P and van der Kooij J (2021) First Insight of Meso- and Bentho-Pelagic Fish Dynamics Around Remote Seamounts in the South Atlantic Ocean. Front. Mar. Sci. 8:663278. doi: 10.3389/fmars.2021.663278
Received: 02 February 2021; Accepted: 18 May 2021;
Published: 17 June 2021.
Edited by:
Rochelle Diane Seitz, William & Mary’s Virginia Institute of Marine Science, United StatesReviewed by:
Réka Domokos, National Oceanic and Atmospheric Administration (NOAA), United StatesAndrew M. Fischer, University of Tasmania, Australia
Copyright © 2021 Campanella, Collins, Young, Laptikhovsky, Whomersley and van der Kooij. This is an open-access article distributed under the terms of the Creative Commons Attribution License (CC BY). The use, distribution or reproduction in other forums is permitted, provided the original author(s) and the copyright owner(s) are credited and that the original publication in this journal is cited, in accordance with accepted academic practice. No use, distribution or reproduction is permitted which does not comply with these terms.
*Correspondence: Fabio Campanella, ZmFiaW8uY2FtcGFuZWxsYUBjZWZhcy5jby51aw==