- 1Postgraduate Program in Tropical Biodiversity, Federal University of Amapá, Macapá, Brazil
- 2Marine and Environmental Sciences Centre, Faculty of Sciences, University of Lisbon, Lisbon, Portugal
- 3Department of Plant Biology, Faculty of Sciences, University of Lisbon, Lisbon, Portugal
Analyzing the presence and quantifying trace elements is of paramount importance to understand natural environmental processes and monitor the degree of anthropogenic disturbance to mitigate impacts already caused. Here, we aimed to establish a baseline of the trace elements profile and concentrations in sandy sediments of intertidal areas of three Amazonian beaches (Brazil). For each beach, sediments were collected from three different sectors (south, center, and north) and five shoreline distance levels (from the high- to the low-water mark), totalizing 15 samples per beach. The concentration of the different trace elements (Mg, Al, P, S, Ca, Ti, V, Cr, Mn, Fe, Co, Ni, Cu, Zn, As, Br, Rb, Sr, Y, Zr, Cd, Sn, I, Hg, and Pb) was determined by Total reflection X-ray Fluorescence spectrometry. Sediment was also characterized for its grain size, organic matter, and pH. To assess possible enrichment due to anthropogenic activities we compared trace element levels with the values for the Earth’s crust and calculated pollution indexes: geoaccumulation index (Igeo), ecological risk index (RI), contamination factor (CF), pollution load index (PLI), and sediment quality guideline (SQG), threshold effects level (TEL) and probable effects level (PEL). Individual trace metal concentrations did not vary significantly between beaches, sectors, or sample levels, evidencing a homogeneity of trace elements composition and concentrations across this environment. Igeo indicated 62.2% of the sampling stations uncontaminated, 20.0% from uncontaminated to moderately contaminated, and 4.44% (two sampling stations) strongly contaminated, the same two areas classified as high ecological risk by RI. Most of the sampling points presented low CF. Cadmium and Hg were the only elements that showed moderate to very high values of CF. According to the SQGs, 77.7 and 8.8% of the sampling points presented values above the moderate threshold effect level (SQG-TEL) and probable effect level (SQG-PEL), respectively. All points were classified as non-polluted according to the PLI. Our results show that the three beaches present safe levels of almost of the elements demonstrating the good state of preservation. Most of the indexes classified the sampling points as non-polluted, except for Cd and Hg in a few specific sampling points.
Introduction
Intertidal sediment characteristics are major drivers of its fauna and flora diversity, and thus affect the resources available for human use. Determining which trace elements, and in which extent are present in the sediment, allow a greater understanding of environmental processes, whether its composition pattern stems from a natural source, the matrix rock, or due to human activities, and identify eventual harmful levels, due to bioaccumulation and potential toxicity effects (Selvi et al., 2019).
Studies of geochemical background aim to characterize and record these environmental features ideally in its natural form, that is to say before anthropogenic impacts (Gałuszka and Migaszewski, 2011), supporting the elaboration of guidelines for environmental legislation, allowing monitoring programs to rely on a pre-established baseline assessment (Dung et al., 2013). There are studies carried out with sediments and soils from different parts of the world and of rocks from different origins, which provide overall, global reference values (Turekian and Wedepohl, 1961; Hans Wedepohl, 1995; Rudnick and Gao, 2013). Regional backgrounds, and their correspondence to proper reference values, reveal variations within- and between habitats, which includes the detection of environments that are naturally enriched with some elements, and therefore should not be interpreted as polluted by anthropogenic actions (Rasmussen, 1998). The use of interpretative tools, such as indexes and quality guidelines, provides guidance for decision-making and management strategies regarding the analyzed area (Macdonald et al., 1996; Liu et al., 2017).
Given its importance, the characterization of the sediment that makes up the intertidal zones has been the object of study for a long time in many countries of all over the world, mostly because sandy beaches are the most extensive coastal physiognomy across the globe, and of utmost touristic importance as well. However, in tropical areas, studies of this nature are still scarce, including the Amazon region. The Amazon Coastal Zone (ACZ) is very peculiar and covers a substantial extent of the Brazilian coastal ecosystem, about 35%, and encompasses three federal units, namely: Amapá, Pará, and Maranhão (de Menezes et al., 2008; Szlafsztein, 2012). The ACZ is one of the most preserved and extensive coastal areas in the world, composed of beaches, intertidal flats, mangroves, estuaries, and forests (de Menezes et al., 2008; Szlafsztein, 2012). This area has unique features, such as the presence of the mouth of the world’s largest river, which discharges massive amounts of solid suspended matter and freshwater to the adjacent marine ecosystem as far as Venezuela, thus shaping its turbidity, water column nutrient availability, and salinity (Anthony et al., 2010). The South Equatorial Current leads this river flow mainly westwards, where is located the Amapá Coastal Zone of all other Brazilian coastal provinces. This coast has been subjected to a great Holocene progradation and is divided into two sub-provinces, south- and a northern one, the latter where marine processes prevail over fluvial processes, and where all sandy beaches and flats occur. These beaches are fine-grained, have a large, flat slope, and are submitted to tidal ranges on the order of 9 m. Except for one beach, they lack land accesses; human settling in these areas there are only a few, small communities, whose economy is based primarily on fishing and rearing of livestock. These beaches are, therefore, highly preserved, free from activities such as tourism, industries, and intense urbanization. There are, though, gas and oil exploitation plans to the adjacent continental shelf of this region, which are likely to compromise its environmental quality.
There are only two studies on the sediment characteristics for this entire west ACZ: one that evaluated mercury concentrations on the continental shelf (Siqueira et al., 2018) and another one that evaluated 23 elements in the estuary of the Cunani River (Xavier et al., 2020). To fill this knowledge gap for such a vast and peculiar region, this study aimed to establish a baseline of the trace elements profile and concentrations, including metals and metalloids in the intertidal sediments of sandy beaches north to the Amazon river mouth. This baseline will support monitoring action in the ACZ, future studies on geochemical backgrounds in sandy beaches in the area and elsewhere.
Materials and Methods
Study Area
Three sandy beaches were sampled, Samaúma (2°48′4.50″N; 50°55′13.73″W), Goiabalinho (2°40′19.41″N; 50°51′52.91″W), and Goiabal (2°37′28.28″N; 50°50′43.11″W) located in the ACZ, west to the Amazon river mouth, specifically within the oceanic subprovince, northernmost Brazilian shore (Figure 1). These beaches are dissipative, macro to mega-tidal beaches (about 9 m tidal amplitude) (Santos et al., 2016), heavily influenced by the Amazon River plume, which reaches up to the Orinoco River in Venezuela and has an average annual sediment discharge of about 754 × 106 tonnes y–1 (Anthony et al., 2013). Several other rivers drain through the region, shaping homogeneous, concave beaches. Climate is humid to super humid tropical, with average annual temperatures ranging from 26 to 28°C. The region has abundant rainfall, with records above 3,000 mm y–1, making it the region with the highest rainfall in Brazil. The region is sparsely populated and comprises an area of 14,117,297 km2 (IBGE, 2004d). Its main use is for livestock breeding and fishing; upstream adjacent areas have mining activities for over 100 years. The Samaúma beach is 1.88 km long and the sampled intertidal zone ranged from 0.95 to 1.43 km. Goiabalinho beach is 2.08 km long and ranged from 0.76 to 1.40 km in intertidal extension. Goiabal beach is 4.07 km long and its intertidal zone ranged from 1.43 to 1.97 km (Figure 1).
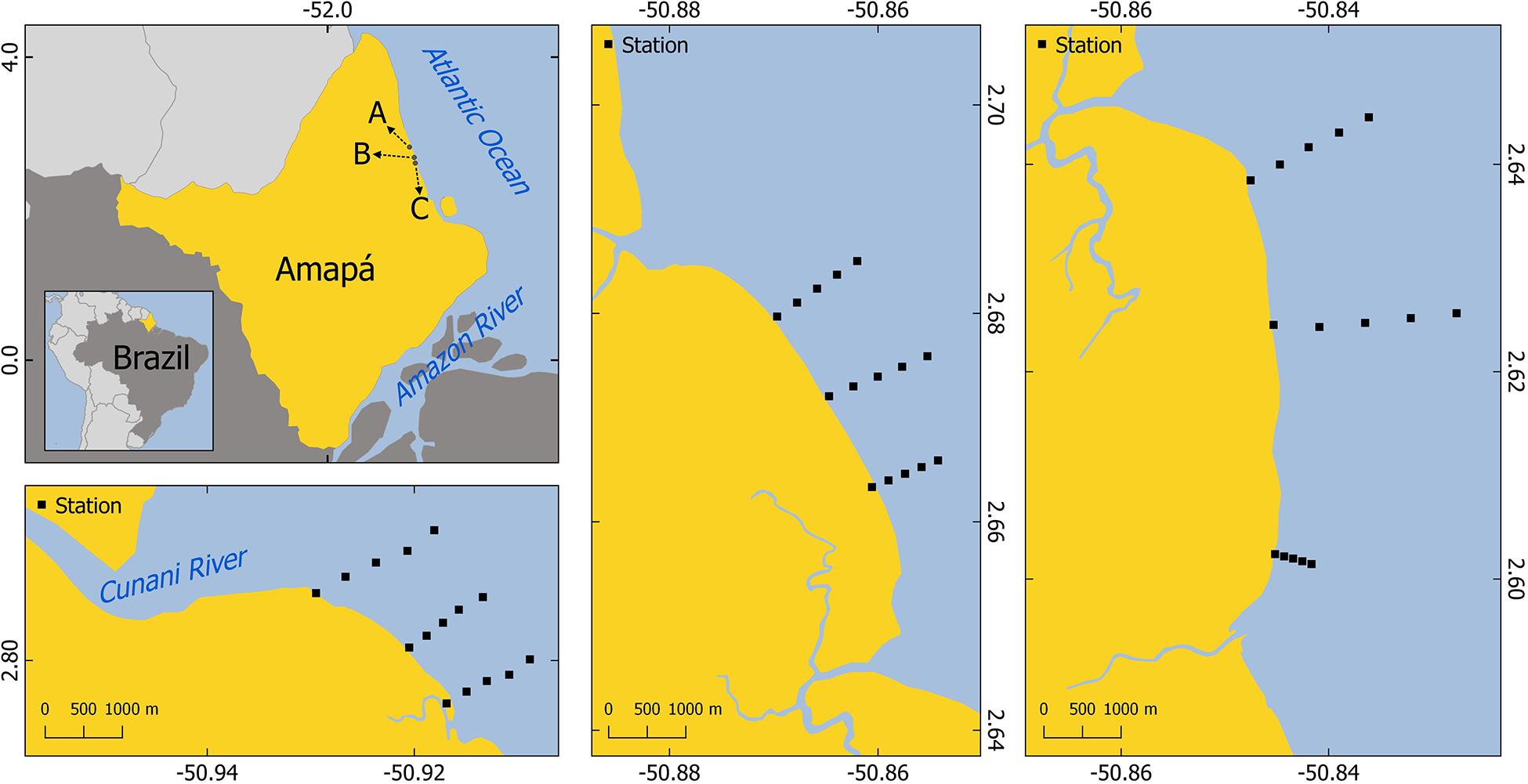
Figure 1. Map of the study area and sampling sites. (A) Samaúma, (B) Goiabalinho, and (C) Goiabal. Sampling stations are pinpointed with black dots.
The regional geological structure consists of Holocene fluvio-marine deposits, composed of sand, silt, clay, and gravel accretion in river mouth plains in the coastal region which are subject to tidal influence. It also may contain pre-Holocene terraces (IBGE, 2004a,b,c). Regarding the local geomorphology, the area is characterized by unconsolidated sedimentary deposits that form fluvio-marine and fluvio-lacustral plains, thus establishing a flat area resulting from marine accumulation, comprising beach ridge systems, high tide sandy beaches, and tidal flats. The soil in the region of the beaches has not been characterized so far, but the entire area around it is formed by a typical Plinthosol Argiluvic Dystrophic and a typical Dystrophic Haplic Gleisol (IBGE, 2004a,b,c).
Sampling
Samples were collected in September and November 2018 in the intertidal zone during the spring tide on three beaches. The studied beaches are north- and south-limited by rivers of with discharges that flow to the west by the strong oceanic current. To cover spatial differences within beaches, three sampling transects, 100 m long each, were equidistantly set in each area, and are herein named as sectors south, center, and north. South and North transects were set at least 300 m from beach extremes to ensure homogeneity between sectors. Within each sampling transect, five equidistant levels were defined, from the high- to the low-water mark (Figure 2). At each level, two samples were collected from a randomly selected sampling point within the 100 m. For one of the samples, we took 3 cm3 (3 × 3 × 3) of superficial sediment with the aid of a polyethylene spatula and placed it in a polyethylene container. This sample was used to assess pH, water content (%) and trace elements. The other sample was collected in the immediate adjacent area of the previous sample and was used to assess the granulometry. In this sample we took about 100 g of the superficial sediment (up to 3 cm) with a plastic shovel and put it in a plastic bag. The samples were taken to the laboratory and kept at 4°C.
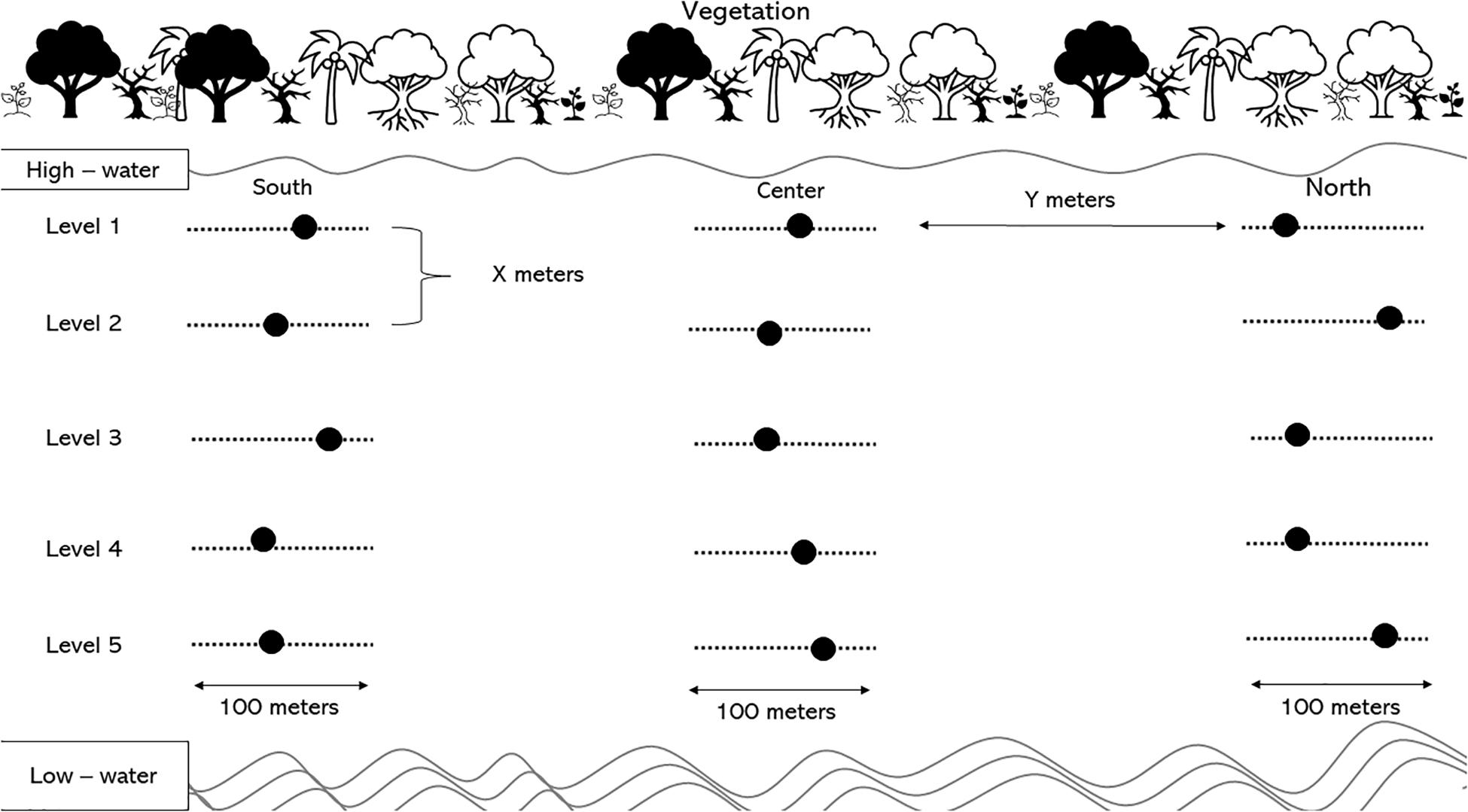
Figure 2. Graphical representation of the sample collection scheme applied to each beach. X meters: distance between levels, defined from the high-water to low-water line of each beach divided by 4; Y meters: distance between the South, Center, and North regions, defined by the size of each beach divided by 3. The black circles indicate where the samples were collected.
Physical-Chemical Sediment Parameters
The grain-size was obtained using the method of sequential sediment-sieving. The sediment samples were dried in a drying oven for 72 h at 60°C and sieved in analytical sieves (>355 mm, 355–250, 250–180, 180–125, 125–90, 90–63, >63 μ) to assess the size-fractions of the grain. The total organic matter was obtained by weight loss after combustion at 550°C for 4 h. The pH was obtained according to Forster (1995) using 1 g of sediment sample mixed with 10 ml of KCl 1M, left at room temperature for 1–2 h and the pH value was measured with a pH meter. The water content (%) of the samples was estimated as the difference between the wet and dry weight of the sediment, after freeze-drying.
Element Analysis
Samples were freeze-dried, and a sub-sample (≈100 mg) was mineralized using an acid mixture of HCl:HNO3 (7:1 v/v) at 110°C for 3 h in a closed Teflon vessel. After cooling, the mineralization products were filtered by Whatman 42 filter and added with an internal standard (Gallium, final concentration 1 mg/L) and stored at 4°C until analysis. Elemental quantification was performed through Total X-Ray Fluorescence spectroscopy (TXRF) element analysis, which is a reliable, environmentally friendly and efficient method (Klockenkämper et al., 2001; Towett et al., 2013; Al Maliki et al., 2017). The cleaning and preparation of the TXRF quartz glass sample carriers were performed according to Towett et al. (2013) and 5 μL of the digested sample was added to the center of the quartz carrier. The carriers with the samples were aligned in a sample holder containing a carrier with arsenic for gain correction (mono-element standard, Bruker Nano GmbH), a carrier with a nickel standard for sensitivity and detection limit (mono-element standard, Bruker Nano GmbH), and a carrier with a multi-element kraft for quantification accuracy (Kraft 10, Bruker Nano GmbH). Element measurements were made in a portable benchtop TXRF instrument (S2 PICOFOXTM spectrometer, Bruker Nano GmbH) for 800 s per sample (Human et al., 2020). The accuracy and precision of the analytical methodology for elemental determinations were assessed by replicate analysis of certified reference material BCR-146. The normalization was done according to Loring and Rantala (1992) through the ratio of the reference metal Aluminum and the element Yttrium (Turekian and Wedepohl, 1961; Prokisch et al., 2000; Bau et al., 2018). Blanks and the concurrent analysis of the standard reference material were used to detect possible contamination/losses during analysis. The TXRF spectra and data evaluation interpretation were performed using the Spectra 7.8.2.0 software (Bruker Nano GmbH, Germany).
Pollution Indexes
Five pollution indexes were calculated having as basis the trace element profiles obtained: geoaccumulation index (Igeo), ecological risk index (RI), sediment quality guideline (SQG), contamination factor (CF), and pollution load index (PLI), in order to facilitate the comparison with other published studies.
For these calculations were used the elements that typically correspond to have anthropogenic driven increases: Cr, Ni, Cu, Zn, As, Cd, Hg, and Pb (Sousa et al., 2012; Human et al., 2020).
Geoaccumulation index was calculated according to the following equation:
where, Cx represents the concentration of the element x in the sample and Bx is the geochemical background value of the element in sedimentary rocks (sandstones). The constant 1.5 is included to accommodate for possible variations in the background values due to lithologic variations in the sediments. Table 1 contains the classification of the sediment according to the result of Igeo (Müller, 1969).
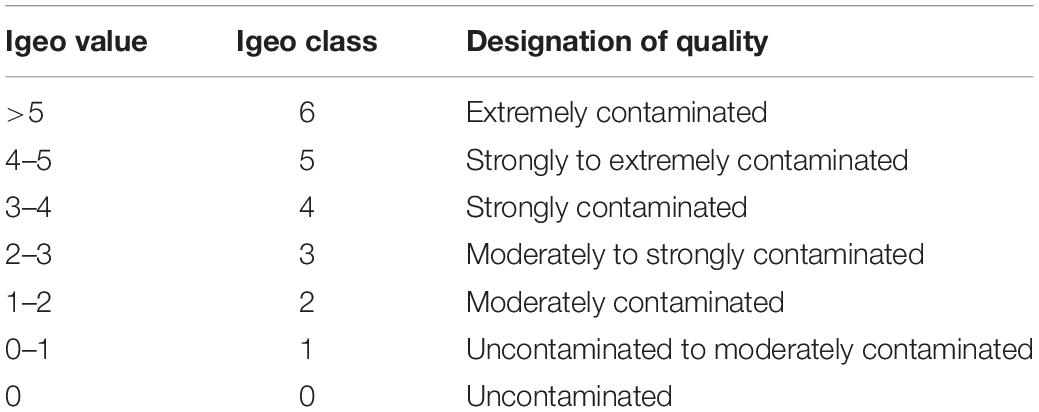
Table 1. Categories of sediment characterization according to the result of the value of Igeo and its classes.
The ecological risk index (RI) is defined as the sum of the proportion of the trace elements concentrations in the sediment in relation to the background, multiplied by a pre-established toxicological factor (Hakanson, 1980; Yang et al., 2009). RI is calculated by the following equation:
where n is the number of heavy metals, Ti is the toxic-response factor for a given substance (elements), Ci represents the trace element content in the sediment and C0 is the regional background value for the same elements. As there are no regional background values available for the heavy metals we assessed, we used the average metal concentrations for the world sandstones (Turekian and Wedepohl, 1961). According to the RI result, the sediment sample is classified into three categories: RI < 300 = ecological risk low to moderate, 300 ≤ RI ≤ 600 = ecological risk high, RI > 600 = ecological risk extremely high.
Contamination factor is the ratio between the trace element concentration and the background value. This was calculated according to Hakanson (1980) using the following equation:
Where represents the measured value of element x and represents the corresponding background value. To evaluate the pollution of each metal, we categorized the CF in four classes: Low degree CF < 1, moderate degree 1 ≤ CF < 3, considerable degree 3 ≤ CF < 6 and high degree CF≥.
Pollution load index is used to assess the toxicity status of each site, which was calculated using the concentration of trace elements in the samples and the trace elements concentration for the world sandstones average as background value (Turekian and Wedepohl, 1961). The PLI is obtained as a function of the CF of each metal by using the following equation:
where n is the number of trace elements and CF is as defined above. According to the PLI value, we classified the sample into two categories: (1) value <1 indicating no metal pollution and (2) >1 indicating pollution.
Sediment quality guideline were calculated for the minimum and maximum values of each contaminant, per sampling point, according to Long et al. (1998). Two SQGs were analyzed: The threshold effects level (TEL) and probable effects level (PEL) (Macdonald et al., 1996). These two values defined three ranges of chemical concentrations: (1) no impact, (2) moderately impacted, or (3) highly impacted.
The indexes have different peculiarities. The CF uses background values, which in dynamic environments, such as estuaries, may not represent inputs by land (Brady et al., 2015). PLI, which uses a combination of CFs, presents the same problem and is, therefore, oversensitive. On the other hand, the IGEO that multiplies the background value by 1.5 in an attempt to correct this problem. However, this generalization can lead to a lack of sensitivity in some cases (Birch, 2017). Both SQG and RI are indices that consider changes in the sediment’s biotic community. However, the fact that RI is unable to differentiate between lithological and sedimentary inputs is a negative aspect of this index. Despite of this, both SQG and RI offer important answers about possible damages that can be caused to the biotic community, which includes an important aspect in the environmental assessments of the sediment. Indexes are tools used to help interpret data and assist in mitigating future environmental problems. All indexes have particularities that must be assessed on a case-by-case basis by the researcher so that the best approach can be taken.
Statistical Analysis
The statistical analyses were performed in the R software, version 3.6.2 (R Core Team, 2019). We used Pearson correlations between all pairs of metals evaluated, and a principal component analysis – PCA to analyze correlation patterns between the variables, followed by an analysis of variance – ANOVA, which is robust to normality violations (Schmider et al., 2010; Blanca et al., 2017), to verify differences in the values of the extracted principal components between beaches, sectors, and levels. We used Ordinary Least Squares models to evaluate whether the first axis of the principal component analysis, which summarizes the profile of trace elements in the sediment, is related to sediment granulometry (proportion of sand and silt), organic matter, and pH. We ran a null model and every combination between these predictors and compared the models based on their AICc (Akaike Information Criterion corrected for small samples). We also used ANOVA with Tukey post hoc tests to verify differences in the granulometry, organic matter and pH of the sediment between beaches, sectors, and levels.
Results
Physical-Chemical Sediment Parameters
The granulometry showed that the sediment of all sampling points throughout the three beaches is composed mostly of very fine sand (63–125 μm), with an overall average of 97.93 ± 0.37% of sand. Silts (<63 μm) and organic matter were unrepresentative in the samples, with averages of 1.54 ± 0.40 and 0.85 ± 0.21%, respectively. The average pH recorded was 5.98 ± 0.17 and the average water content in the samples was 19.82 ± 1.80%.
The proportion of sand in the sediment varied between the levels of the beaches (p = 0.036), but not between beaches (p = 0.564) or beach sectors (p = 0.264). The proportion of sand was higher in the first level compared with the second level but was similar between all other levels. Similarly, the proportion of silt also varied between levels (p = 0.003), but not between beaches (p = 0.836) or sectors (p = 0.223). The proportion of silt was higher in the second level compared to the first, fourth and fifth levels, but was similar between the other levels. The amount of organic matter differed between beaches (p = 0.015), but not between sectors (p = 0.738) or levels (p = 0.375). The beach of Goiabal presented lower amounts of organic matter than the other two beaches. The pH did not vary between beaches, sectors, or levels (p > 0.05).
Sediment Trace Element Profile
Strong correlations between several trace elements could be assessed. Among the 300 possible correlations between the 25 metals, 97 (32.3%) presented r > 0.6 (MS1). Due to these strong correlations, the first two axes of the PCA captured 61.3% of the variance contained in the data (Figure 3). The first axis alone captured 50.5% of the variance and showed non-zero positive loads for almost all elements (except As, Cd, Co, and I), that is, points that have high amounts of a given metal present high amounts of almost all the other metals, while points that had low amounts of a given metal, had low amounts of almost all other metals. The values for this axis (principal component) did not vary between beaches, sectors, and levels (Table 2). The trace element profile, represented by the first axis of the principal component analysis, was not related to sediment granulometry, organic matter, or pH, i.e., the null model was the model with higher support (MS2).
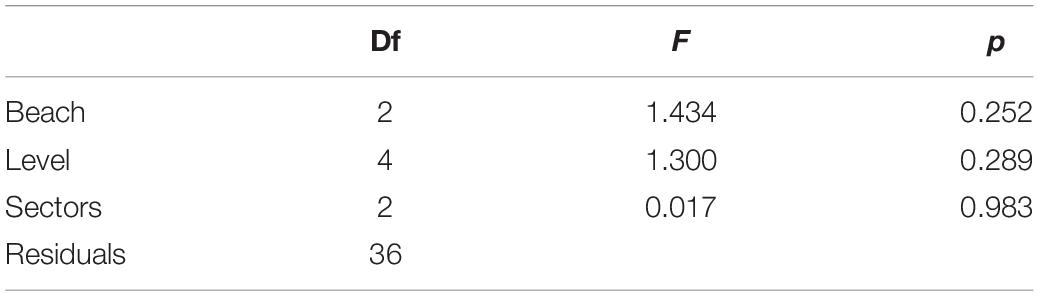
Table 2. Results of the analysis of variance (ANOVA) assessing if the first principal component that summarizes the profile of metals in the sediment varied between beaches, sectors (south, center, and north), and levels.
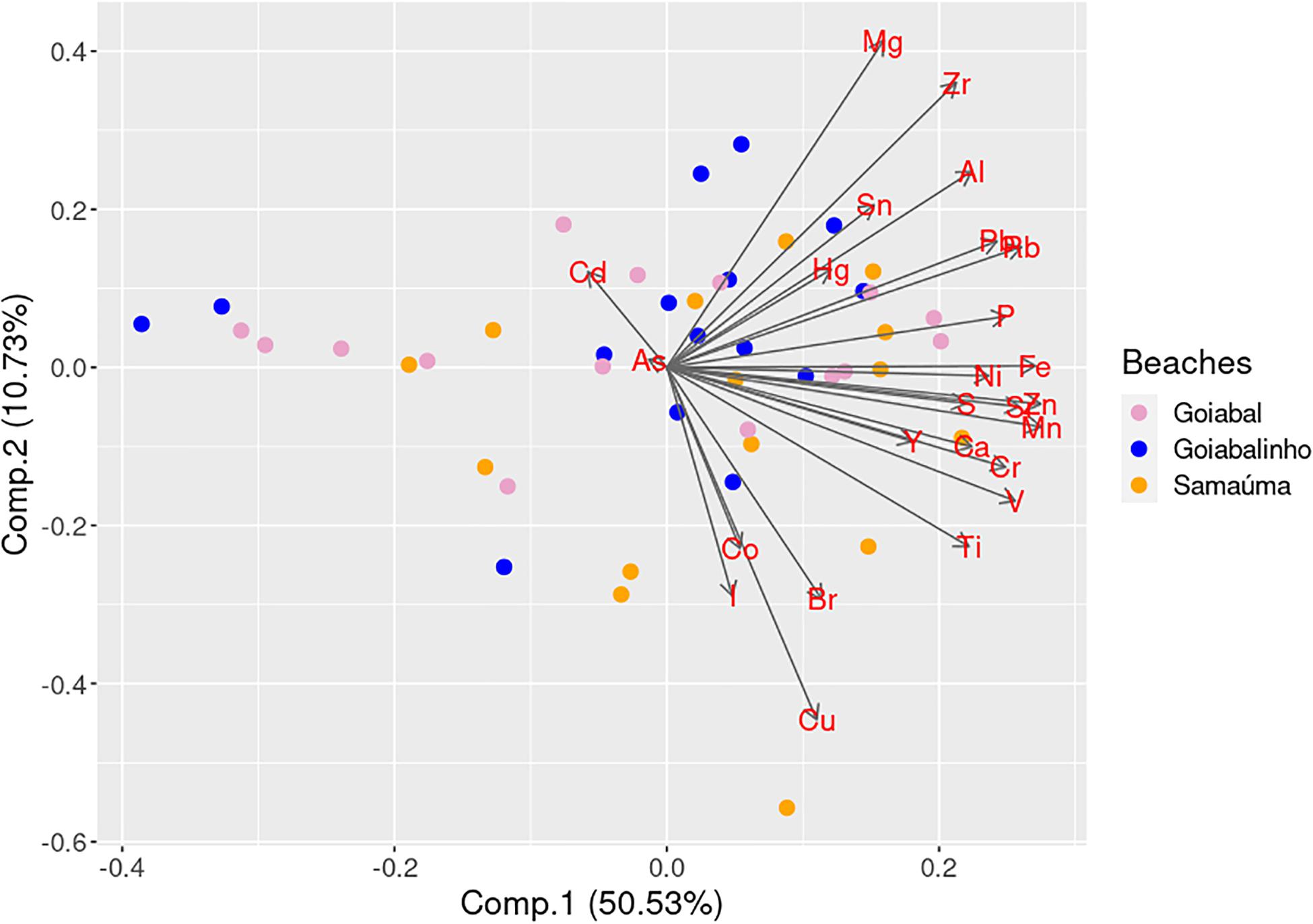
Figure 3. Principal component analysis biplot showing the elements (arrows) and sampling points (dots) of sediment samples from three beaches: Goiabal, Goiabalinho, and Samaúma.
The results of the ratio between the levels of the elements recorded on the beaches and the background values are shown in Figure 4. Cadmium, Sn, and Zr presented high concentrations on all three beaches. Cobalt and Pb concentrations were found to be higher in the sediments collected at Goiabalinho and Samaúma beaches in relation to the Earth’s crust background. Samaúma beach sediments also had a slightly high concentration for Br and Hg.
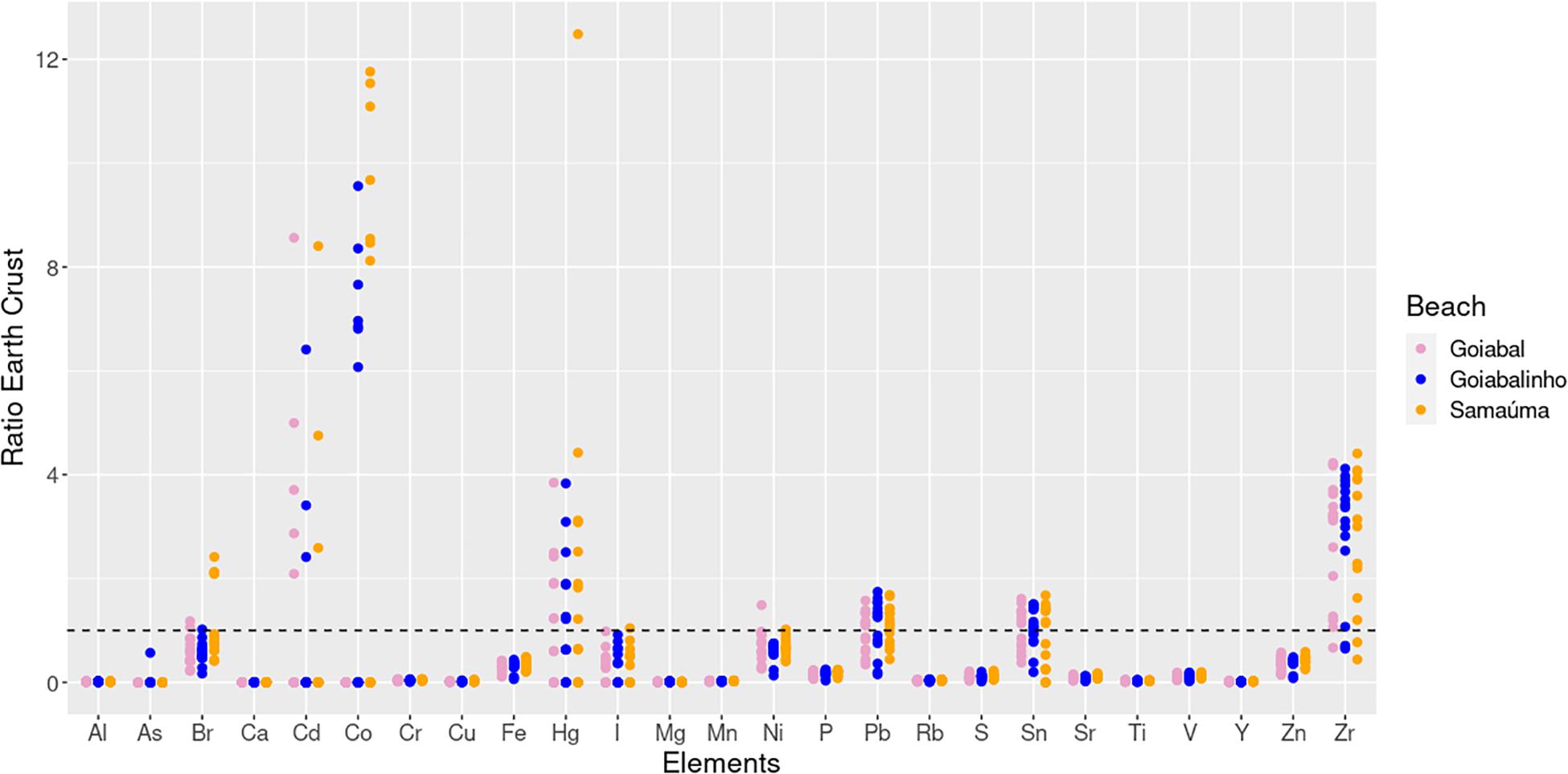
Figure 4. Ratio between the recorded levels of 25 elements of sediment samples from three beaches (Goiabal, Goiabalinho, and Samaúma) and the Earth Crust’s levels. The dashed line represents a ratio of 1, indicative of sediment concentrations near the ones determined for the Earth’s crust.
The average concentration per beach and standard deviation of each element are presented in Table 3 and the comparison of this results with other studies is presented in MS3.
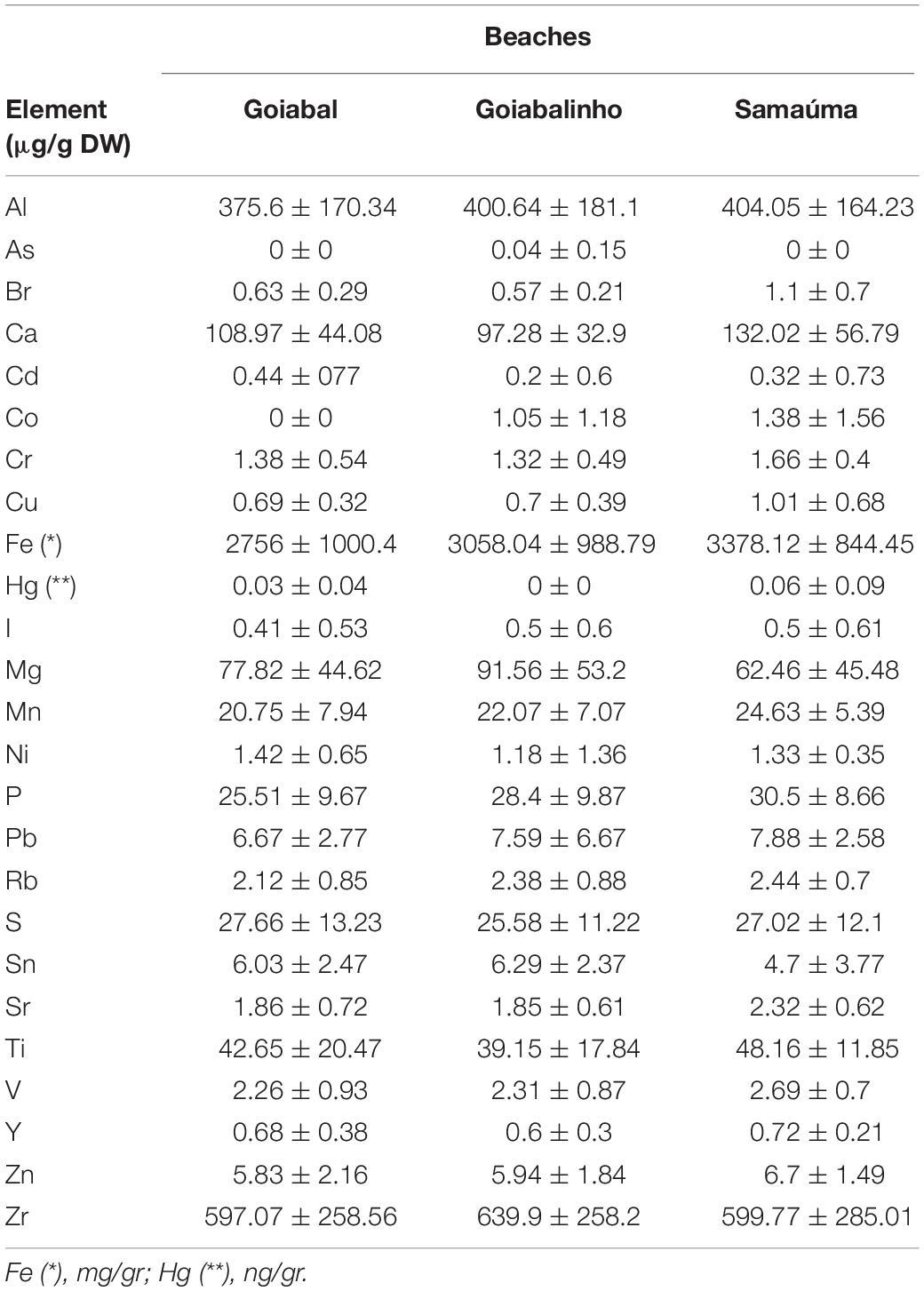
Table 3. Average concentration per beach and standard deviation of each element analyzed through (TXRF).
Igeo
The results obtained for the Igeo showed that 62.2% of the sampling points of the three beaches were classified as uncontaminated, 20.0% were classified as uncontaminated to moderately contaminated, 13.3% moderately contaminated, and 4.4% strongly contaminated (Figure 5). All three beaches had between 60.0 and 66.7% of the points classified as uncontaminated. Cadmium and Hg were the elements that had a high concentration in all three beaches, thus raising the Igeo value found in the sediment samples. Igeo’s pattern of values was similar between the three beaches.
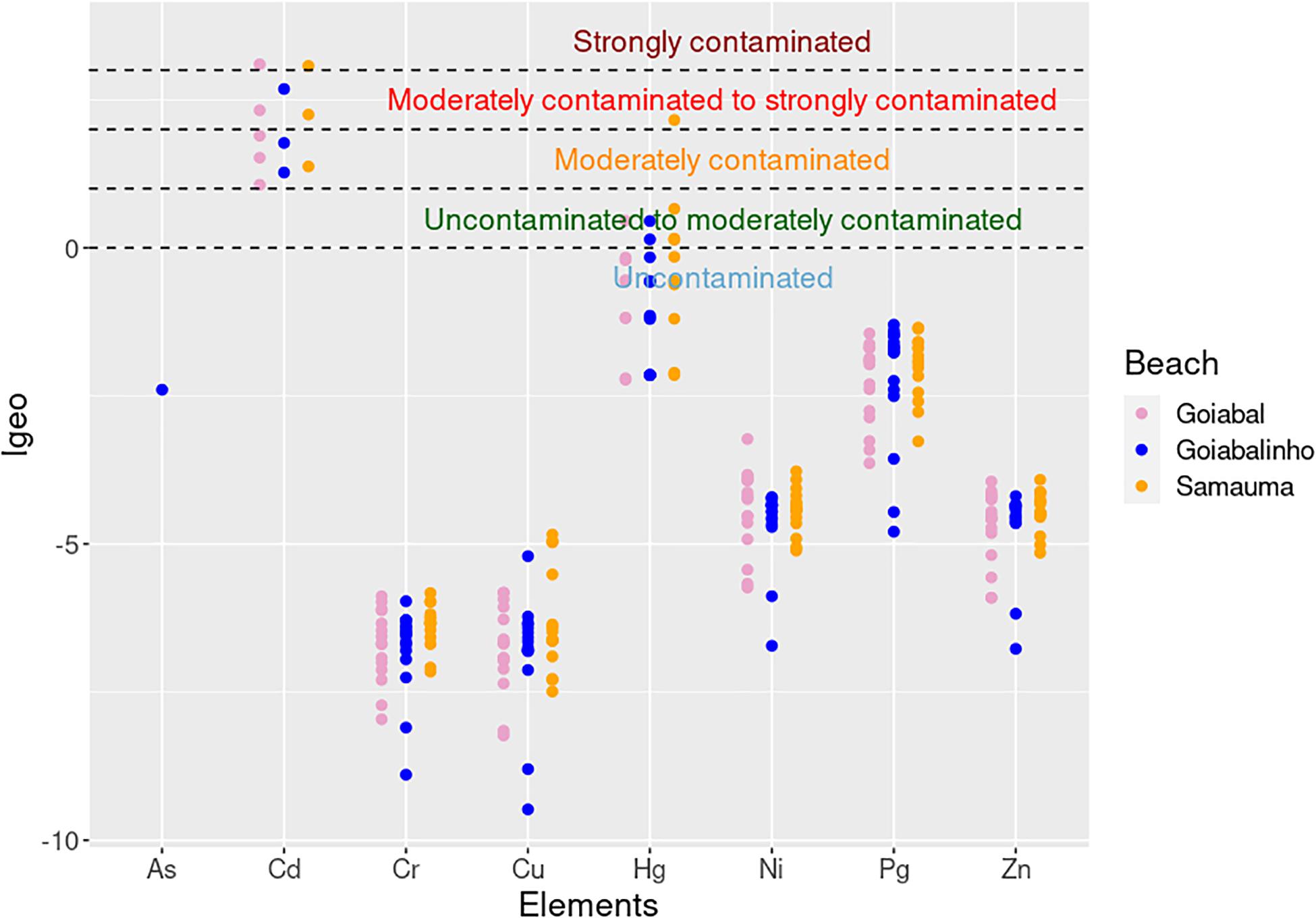
Figure 5. Geoaccumulation index (Igeo) of eight elements from sediment samples from three beaches: Goiabal, Goiabalinho, and Samaúma. The dots represent element levels for each sampling point. The dashed lines divide Igeo classes.
RI
The result for the RI of the samples indicated that only two sampling points (one at Goiabal and another at Samaúma) were classified as high ecological risk (Figure 6). All other sample points were classified as low to moderate ecological risk. This index did not show any variation pattern between beaches, sectors, or levels.
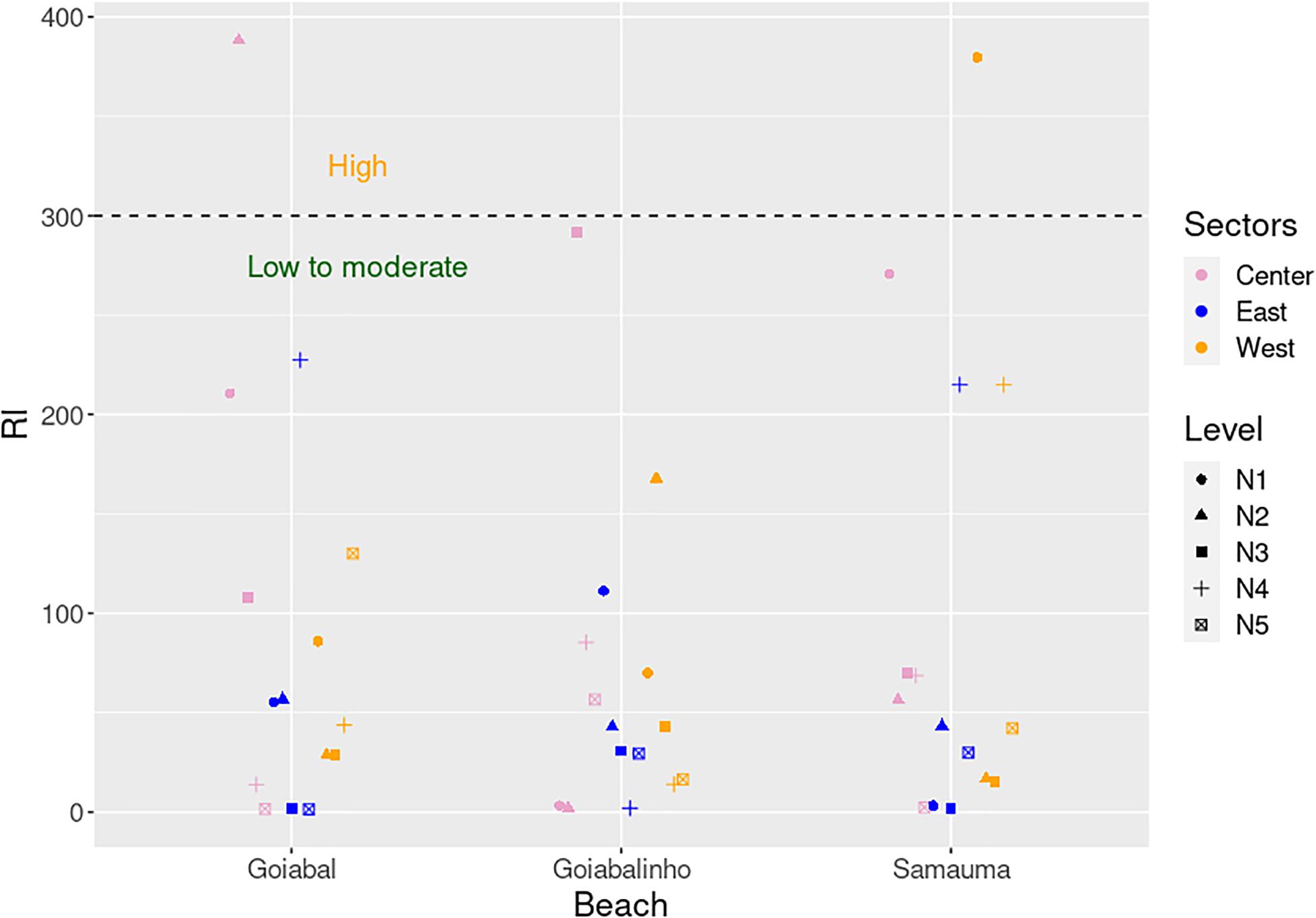
Figure 6. Ecological risk index (RI) of eight elements from sediment samples from three beaches: Goiabal, Goiabalinho, and Samaúma. The different shapes and colors represent levels and sectors, respectively. The dashed lines divide RI classes.
PLI
The PLI values ranged from 0.02 to 0.20. Thus, all points of the three beaches were classified as non-polluted (Figure 7). As with other indices, PLI did not show divergent patterns between beaches, sectors, or levels.
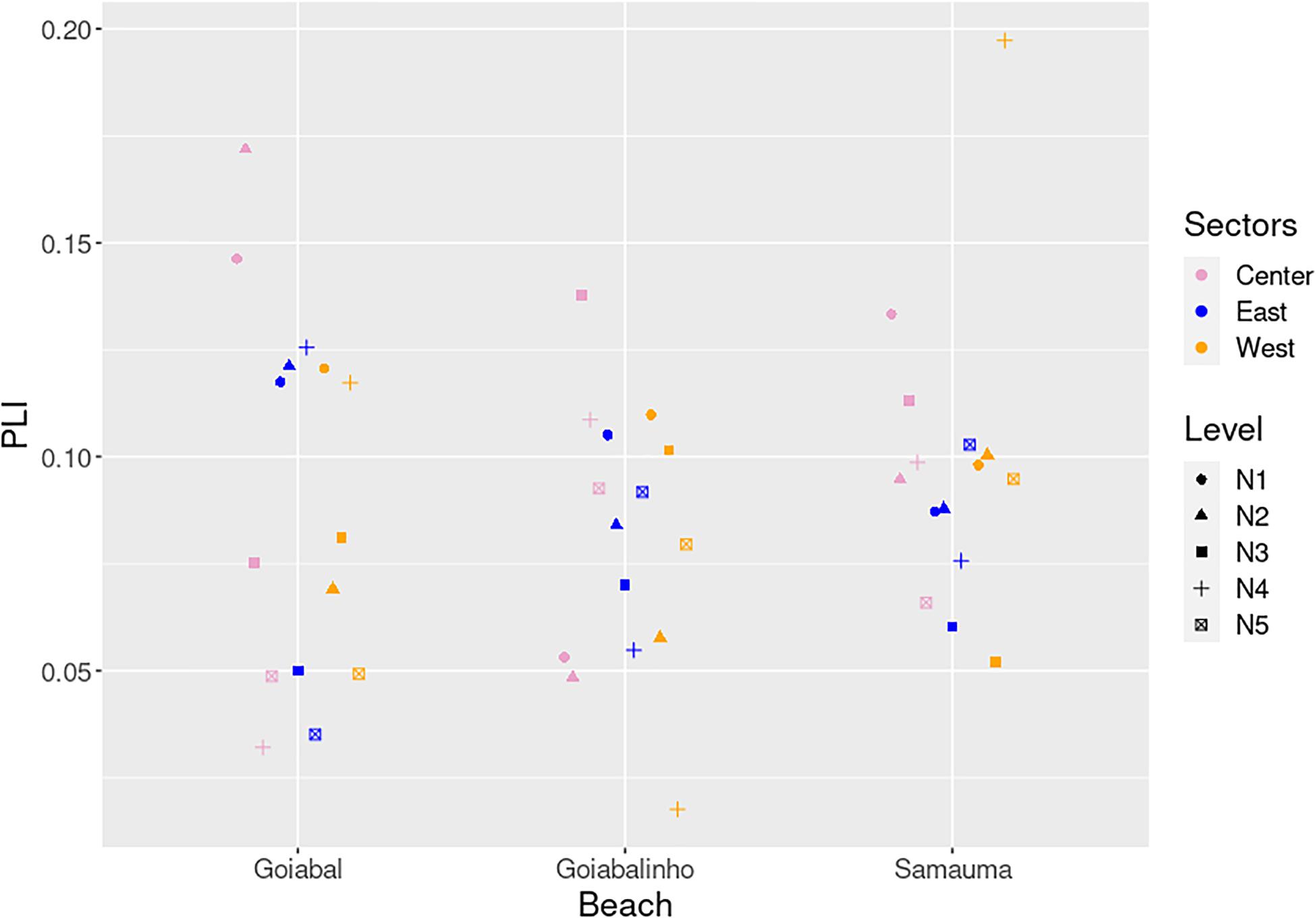
Figure 7. Pollution load index (PLI) of eight elements from sediment samples from three beaches: Goiabal, Goiabalinho, and Samaúma. The different forms and colors represent levels and sectors, respectively.
CF
Most of the assessed trace elements presented low CF in all sampling points. Cadmium and Hg were the only elements that showed moderate to very high values of CF in the three beaches (Figure 8). In fact, five out of the 11 points where Cd was detected had very high CF for this element, and another six had considerable CF. For Hg, one point had very high CF, whereas the other 15 points presented moderate CF. At 13 points the calculated CF for Hg was assessed as low. As with the other indexes, the CF values did not present different patterns between the beaches.
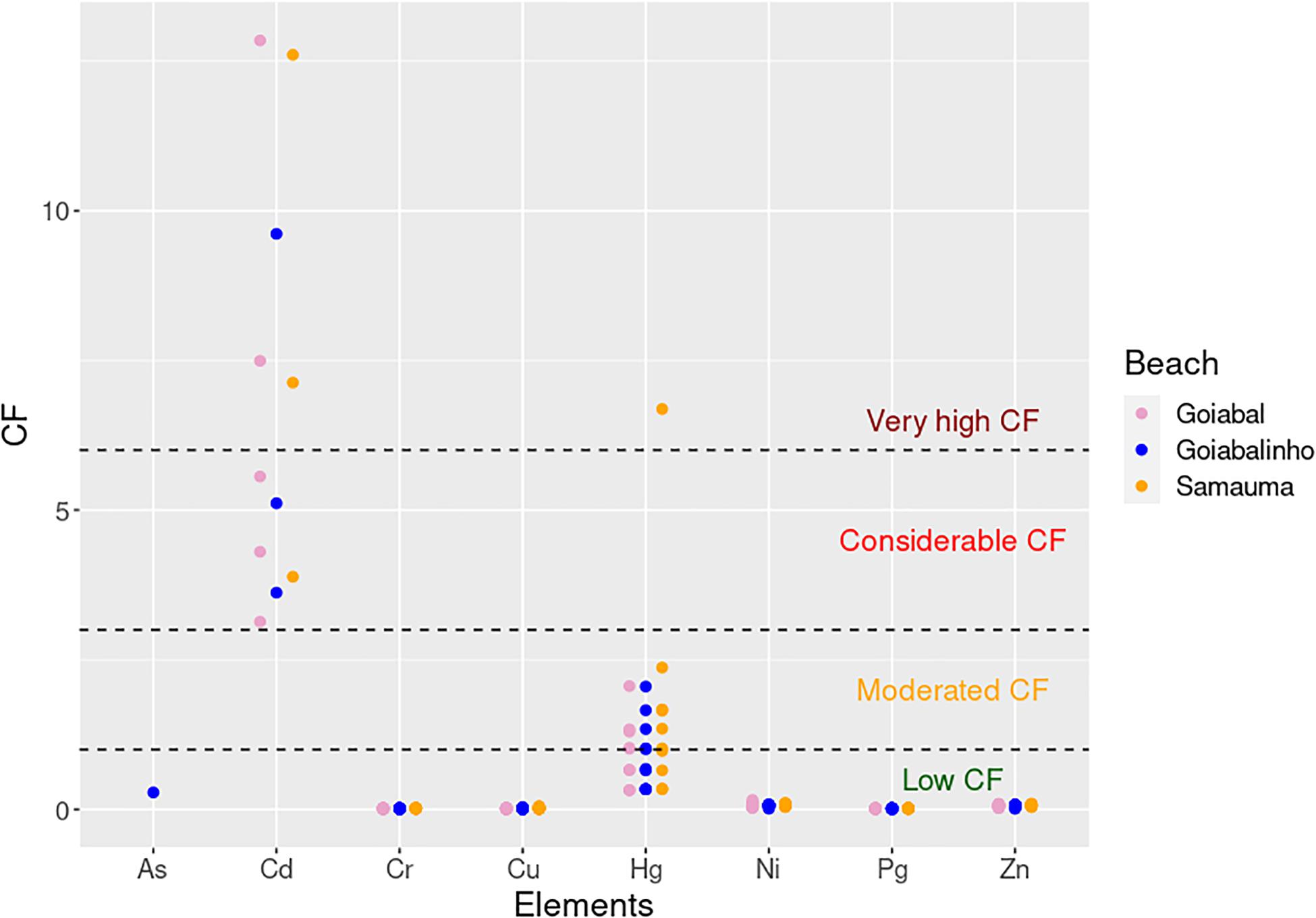
Figure 8. Contamination factor (CF) of eight elements from sediment samples from three beaches: Goiabal, Goiabalinho, and Samaúma. The dots represent element levels for each sampling point. The dashed lines divide CF classes.
SQG
The SQG showed that 22.2% of the 45 analyzed points were classified as not impacted and 77.7% as moderately impacted in relation to the TEL. The analysis of the PEL classified 91.1% of the points as not impacted and 8.9% as moderately impacted.
These results demonstrate that although the majority of the points were classified as moderately impacted in relation to TEL, the fact that the PEL classification for most points was not impacted demonstrates the low probability that toxic effects will happen in this environment.
Discussion
This first study of trace elements in ACZ beaches showed strong, positive correlations between most elements, which indicates a unique source and a single transport route in the environment (Pereira et al., 2015; Ahmad et al., 2020). The homogeneity in the distribution of these elements, across and within beaches, and the overall ratios with background values close to 1 or lower, indicate that the origin of trace metals is from natural weathering of the bedrock and that the form of release or the source of weather is the same.
The relatively homogeneous distribution of trace elements in these beaches denotes biological communities, inhabiting the oceanic coastal zone in northern Brazil, are exposed to very similar conditions on what concerns these elements (Sun et al., 2017). Beaches were chosen based on their similarity, but some difference in trace elements composition among sectors was expected, due to the influence of the rivers that limit these beaches: they flow to the west, thus affecting more strongly the south sectors of the beaches, with a marked influence on the composition of sediments in the bordering regions of the beaches. According to our data, sediment composition characteristics driven from the Amazon river plume influence, overcome the effects of smaller water-courses. Among levels (across-shore) some differences could also be expected however only minor differences were identified in the granulometry, but not in the metal profile, indicating that metal leach occurs equally through this dynamic ecosystem, constantly submitted to high temperatures, high wave energy, and high tidal influence.
One of the factors that probably contributed to the presence of low levels of the trace elements in the study area is the fact that the sediment is composed mostly of sand (fine grains) with low amounts of organic matter (Duarte et al., 2008). The gentle topography and high energy of the studied beaches results in a fine sand composition of the substrate (Wright and Short, 1984; Bramha et al., 2014), which has an acknowledged negative correlation with trace metals (Siqueira et al., 2018). Quartz has negative charges causing low adsorption of organic matter, decreasing its retention, and leaching out the associated metals (Mondal et al., 2021). In the studied beaches, most points presented a good sediment quality in relation to the background levels of the Earth’s, in agreement with the low human use of these areas, and with no or little anthropogenic inputs in terms of the trace elements surveyed.
Ratio Between the Recorded Levels and the Earth Crust’s Levels
The ratio between the levels of the assessed elements and the most suitable background available for this environment showed that the elements Al, As, Ca, Cr, Cu, Fe, I, Mg, Mn, P, Rb, S, Sr, Ti, V, Y, and Zn did not exceed the background values and did not increase in relation to the ratio/background, indicating its natural origin and reduced influence of anthropogenic activities. Bromine and Ni showed slightly increased levels. Seawater is the main source of bromides for coastal sediments (Mansouri et al., 2020) and Br generally has high correlations with iodine and organic matter (Leri et al., 2010). Although some points showed an increased level of Br in the sediment, they still presented low concentrations when compared to other areas (Mansouri et al., 2020).
The higher observed ratios in relation to the background levels were, in descending order, Cd, Co, Hg, Zr, Pb, and Sn, indicating possible anthropogenic contamination. Cadmium showed, along with Co, the highest relative levels among all assessed elements, so some environmental pollution by these elements may be in course. The recorded Cd concentrations were similar to other studies conducted on beaches along of the coasts of China (Zhao et al., 2016), India (Bramha et al., 2014), and Cameroon (Ekoa Bessa et al., 2020), where such levels were related to the presence of pollution caused by sewage, fertilizers, and industrial activities.
Cobalt values assessed were also indicative of some degree of contamination. However, the highest concentrations recorded by our study (2.87 and 3.53 μg/g DW) are lower than those recorded in a nearby estuary (13.82 μg/g DW: Xavier et al., 2020). Also, the values recorded in the present work are 5–30 times lower compared to other beaches already assessed (Vidinha et al., 2006; Vetrimurugan et al., 2016). Cobalt has an affinity with Fe (Ekoa Bessa et al., 2020), however, no correlation between these elements was observed in our study, which together with high relative levels of Co, could configure anthropogenic input of this element into the environment. But again, the lack of a specific background of the area hinders a conclusive diagnosis. When associated with human pressures, high Co levels have already been related to oil spills, industrial waste, and boat traffic (Vetrimurugan et al., 2016; Ekoa Bessa et al., 2020).
Mercury concentrations detected are assignable to pollution, but do not characterize necessarily an anthropogenic source of contamination. In beaches from the coast of Spain, values of Hg were higher than in the present study and attributed to local environmental characteristics (Sanz-Prada et al., 2020). The studies carried out in areas closer to ours, a nearby estuary (Xavier et al., 2020) and continental shelf (Siqueira et al., 2018), had similar Hg values. There are, indeed, gold mining activities in surrounding upstream areas, but the scale of these activities and the amount of mercury they generate would hardly influence the adjacent continental shelf in a similar way. There are also other environmental particularities, such as soil type (Roulet and Lucotte, 1995), intense fluvial discharge of organic matter (Anthony et al., 2013; Siqueira et al., 2018), and mining activity (Lacerda and Pfeiffer, 1992; Siqueira et al., 2018) that may affect the levels of Hg in Amazonian soils (Marchand et al., 2006; Siqueira et al., 2018; Xavier et al., 2020). Seasonal analyses that quantify the different forms of this element are necessary to answer properly the questions about Hg source and environmental risk (Lacerda and Pfeiffer, 1992; Siqueira et al., 2018).
We recorded high levels of Zr in our samples in comparison to the ratio/background, indicating an increase in its concentration in relation to the levels of the Earth’s crust. Our results, on average (597.07, 599.77, and 639.9 μg/g DW), are slightly above the results found for another beach in southern Brazil (419–499 μg/g: de Barros et al., 2010). These higher values found in our study may indicate intense natural sedimentation of the bedrock.
The Pb levels recorded were slightly above the background values, but much lower than those found in other coastal environments, beaches included, especially compared to places with large industries, intense tourism, and high population density (Vidinha et al., 2006; Bramha et al., 2014; Zhao et al., 2016; Salam et al., 2021). The concentrations recorded in the present study are below the values found in the nearby estuary, Cunani (Xavier et al., 2020), which recorded roughly twice the value found here. Thus, despite the lack of background values, the available information supports that the Pb local levels are most likely from natural origin.
Similarly, relative levels of Sn were only slightly increased. Compared to the results found by the study conducted in the Cunani estuary (3.38 μg/g: Xavier et al., 2020), the levels found in our study were higher. However, when compared to other locations, such as an estuary and a gulf on the coast of Spain (11–113 μg/g: Arambarri et al., 2003; 8.1–24 μg/g: DelValls et al., 2002), they were much lower. Tin is found in its organic and inorganic forms in the environment, being its organic form the most toxic to living organisms (Arambarri et al., 2003; Selvi et al., 2019). We evaluated the total form of the element, and it would be necessary to quantify, separately, its organic and inorganic fractions to determine if, and to which extent, there is an effective level of Sn toxicity in the environment.
Indexes
The geoaccumulation load index, CF, and RI indicated that most of the points among the three beaches were in the uncontaminated class, low CF, and low to moderate ecological risk, respectively. With this record, we can infer that sediment of the three beaches is mostly in good condition, in agreement with the beaches low use and environmental preservation, more specifically in relation to the level of contamination by metals As, Cr, Cu, Ni, Pb, and Zn. However, Cd and Hg reached high levels in some points, causing these indexes to reach levels that indicate contamination for these elements (Igeo, CF, and IR). Cadmium levels were classified as high in nearly half of the samples, across sampling areas, while Hg was detected in a concerning amount in only one sampling point, in Samaúma beach. Studies in the same region found similar results of Igeo, CF, and IR (Siqueira et al., 2018; Xavier et al., 2020), where most metal elements were classified into categories that indicated an unpolluted environment, except Hg, which as well as in our study presented high levels.
Cadmium levels were not assessed by the studies conducted in nearby areas (Siqueira et al., 2018; Xavier et al., 2020). The classification recorded for Cd by Igeo and CF demonstrates anthropic contamination of the aforementioned sampling points (Nobi et al., 2010; Bramha et al., 2014; Orani et al., 2018). The most frequent sources of Cd contamination are domestic sewage, industrial waste, fertilizers, paint industries, and ship paint (Nobi et al., 2010; Watts et al., 2017; Radomirović et al., 2020). Unlike regions in which Cd contamination is caused by industries and residential areas in their surroundings (Bramha et al., 2014; Salam et al., 2021), the possible contamination cause is not clear in our study area, since the region has low population density and does not have large industries or large agricultural areas that use fertilizers. Although the flow of vessels exists (Brasil, 2010; Kaluza et al., 2010), it is uncertain whether their intensity is sufficient to cause these Cd levels. Therefore, there is a need to monitor Cd levels in the region and investigative possible sources of contamination.
Cadmium behaved differently than most other metals, which indicates that this element may have a more specific source of contamination than the remaining evaluated elements (Pereira et al., 2015). This combined with the fact that Cd presented a high concentration in relation to the background reinforces its possible anthropic origin. Due to its concentration and the possibility of bioaccumulation, this increased level of Cd concentration can be toxic to the aquatic biota, mainly to benthic organisms, which live in close association with substrates (Chakraborty et al., 2012). Therefore, its monitoring should be considered in this environment.
Most of our sampling points had low to moderate ecological risk, which is favorable for the biotic community that inhabits the sediment and for the other animals in higher levels in the trophic chain. Only two points showed worrying results (high ecological risk) from high levels of Cd and Hg. An analysis of the biomagnification of Hg metalloid in the trophic chain of an estuary showed that concentrations increase with the trophic level (Fonseca et al., 2019). The presence of Hg in fish has already been recorded in rivers that flow near the studied beaches (Viana et al., 2020).
Cadmium and Hg are among the most widespread metals in the environment and in the group of non-essential and highly toxic metals (Selvi et al., 2019). The presence of high levels in the environment is worrying, as these metals have bioaccumulation capacity that can lead to contamination of polychaetes (Castiglioni et al., 2018), fish (Amirah et al., 2013; Bosch et al., 2016), birds (Hosseini et al., 2013), and even humans (Bosch et al., 2016). The fishing activity reinforces the risk to human beings in our study area (Brasil, 2010). Only some chemical forms of metals are able to bioaccumulate in living beings, and high levels of bioavailable forms are necessary for this contamination to occur (Bosch et al., 2016). Our results highlight the importance of investigating how many of these elements, focusing on Cd and Hg, are bioavailable to the benthic community.
Similar to the other calculated indexes, the PLI classified all three beaches as not contaminated, despite the results for the elements Cd and Hg. The PLI is a more robust index, encompassing higher threshold levels of all elements evaluated. The available studies for areas in this same region did not focus on this index, hindering such information comparison. Actually, geochemical baseline studies in areas that are similar to ours, i.e., sandy beaches preserved from direct impacts, are very few, as most beaches suffer intense anthropogenic pressure (Orani et al., 2018; Ekoa Bessa et al., 2020). Only two beaches had results that indicated an unpolluted environment: one on the coast of Turkey (Yalcin, 2020) and another on the coast of India (Vineethkumar et al., 2020).
The SQG also confirms this diagnosis, since all the points were within the TEL, where toxic effects are unlikely and did not exceed the PEL values, which could represent probable toxic effects to biota. Regarding Hg only, this result is similar to the analysis performed in sediments of the sediment of the continental shelf for this same region, where 86% of the analyzed samples were below the TEL (Siqueira et al., 2018). In a study conducted off the coast of Yemen, for the elements Cu, Zn, Cd, Pb, and Ni, all points were below the TEL, except for Ni, for which some points exceeded PEL (Saleh, 2021). In sediments from coastal China, a more impacted area, high levels of Cd were observed, exceeding the PEL in relation to the elements Ni and Cr (Bramha et al., 2014). Despite the conservation status, Cd showed the highest relative values in our study areas. According to the SQG/TEL analysis, though, there is a low probability of Cd toxicity to the local biota. The lack of a local background evaluation for the region prevents more assertive inferences about whether some of the trace elements evaluations are not in accordance with primary conditions. Further regional evaluations can now rely on the present data to assess eventual changes over space and time. At a global scale these results will also be useful for comparison with similar sandy dissipative beaches with low human impact.
Conclusion
Trace element profiles are essential to evaluate the anthropogenic impact in a certain area. This information was lacking for the Amazonian beaches and thus the need for a baseline study, such as the one presented here is reinforced. Most of the samples collected presented safe levels of the trace elements evaluated when compared with the composition of the Earth’s crust. Due to the lack of bibliography regarding similar environments and/or reference values for this specific beach typology the application of contamination indexes is of utmost relevance for the classification of the sampling sites and comparison with published data from bibliographic references. The application of these indexes allowed us to assess that the sampled beaches are in most cases classified as non-polluted, with only Cd and Hg showing some values of concern in specific sampling points, indicating punctual contamination by these elements. Thus, this baseline study in the Amazonian beaches is of key relevance for future monitoring and impact assessment studies, providing baseline reference trace element concentrations and indexes of environmental chemical quality for future studies in these important ecosystems.
Data Availability Statement
The raw data supporting the conclusions of this article will be made available by the authors, without undue reservation.
Author Contributions
BD and MP contributed to the conception and design of the study. JV and MP collected the samples. BD, JV, AA, and LR carried out the analyses. JV, AA, LR, BD, and MP collaborated with the drafts of the manuscript revision, read, and approved the submitted version.
Funding
This study had the support of Fundação para a Ciência e a Tecnologia, I.P. (FCT), Portugal, via UIDB/04292/2020. BD was funded by FCT researcher contract (CEECIND/00511/2017). This work was also supported by funding from the European Union’s Horizon 2020 Research and Innovation Programme under grant agreement N 810139: Project Portugal Twinning for Innovation and Excellence in Marine Science and Earth Observation – PORTWIMS. JV acknowledges the support from the Marine and Environmental Sciences Centre, Portugal, during her stay at the Faculdade de Ciências Universidade Lisboa. JV thanks the scholarship from CNPq (142613/2016-9).
Conflict of Interest
The authors declare that the research was conducted in the absence of any commercial or financial relationships that could be construed as a potential conflict of interest.
Acknowledgments
JV thanks Eduardo Feijão for helping with the analysis of the samples.
Supplementary Material
The Supplementary Material for this article can be found online at: https://www.frontiersin.org/articles/10.3389/fmars.2021.671390/full#supplementary-material
References
Ahmad, K., Muhammad, S., Ali, W., Jadoon, I. A. K., and Rasool, A. (2020). Occurrence, source identification and potential risk evaluation of heavy metals in sediments of the Hunza River and its tributaries, Gilgit-Baltistan. Environ. Technol. Innov. 18:100700. doi: 10.1016/j.eti.2020.100700
Al Maliki, A., Al-lami, A. K., Hussain, H. M., and Al-Ansari, N. (2017). Comparison between inductively coupled plasma and X-ray fluorescence performance for Pb analysis in environmental soil samples. Environ. Earth Sci. 76:433. doi: 10.1007/s12665-017-6753-z
Amirah, M. N., Afiza, A. S., Faizal, W. I. W., Nurliyana, M. H., and Laili, S. (2013). Human health risk assessment of metal contamination through consumption of fish. J. Environ. Pollut. Hum. Heal. 1, 1–5. doi: 10.12691/jephh-1-1-1
Anthony, E. J., Gardel, A., Gratiot, N., Proisy, C., Allison, M. A., Dolique, F., et al. (2010). The Amazon-influenced muddy coast of South America: a review of mud-bank-shoreline interactions. Earth Science Rev. 103, 99–121. doi: 10.1016/j.earscirev.2010.09.008
Anthony, E. J., Gardel, A., Proisy, C., Fromard, F., Gensac, E., Peron, C., et al. (2013). The role of fluvial sediment supply and river-mouth hydrology in the dynamics of the muddy, Amazon-dominated Amapá-Guianas coast, South America: a three-point research agenda. J. South Am. Earth Sci. 44, 18–24. doi: 10.1016/j.jsames.2012.06.005
Arambarri, I., Garcia, R., and Millán, E. (2003). Assessment of tin and butyltin species in estuarine superficial sediments from Gipuzkoa, Spain. Chemosphere 51, 643–649. doi: 10.1016/S0045-6535(03)00154-1
Bau, M., Schmidt, K., Pack, A., Bendel, V., and Kraemer, D. (2018). The European Shale: an improved data set for normalisation of rare earth element and yttrium concentrations in environmental and biological samples from Europe. Appl. Geochemistry 90, 142–149. doi: 10.1016/j.apgeochem.2018.01.008
Birch, G. F. (2017). Determination of sediment metal background concentrations and enrichment in marine environments – A critical review. Sci. Total Environ. 580, 813–831. doi: 10.1016/j.scitotenv.2016.12.028
Blanca, M. J., Alarcón, R., Arnau, J., Bono, R., and Bendayan, R. (2017). Datos no normales: ¿es el ANOVA una opción válida? Psicothema 29, 552–557. doi: 10.7334/psicothema2016.383
Bosch, A. C., O’Neill, B., Sigge, G. O., Kerwath, S. E., and Hoffman, L. C. (2016). Heavy metals in marine fish meat and consumer health: a review. J. Sci. Food Agric. 96, 32–48. doi: 10.1002/jsfa.7360
Brady, J. P., Ayoko, G. A., Martens, W. N., and Goonetilleke, A. (2015). Development of a hybrid pollution index for heavy metals in marine and estuarine sediments. Environ. Monit. Assess. 187:306. doi: 10.1007/s10661-015-4563-x
Bramha, S. N., Mohanty, A. K., Satpathy, K. K., Kanagasabapathy, K. V., Panigrahi, S., Samantara, M. K., et al. (2014). Heavy metal content in the beach sediment with respect to contamination levels and sediment quality guidelines: a study at Kalpakkam coast, southeast coast of India. Environ. Earth Sci. 72, 4463–4472. doi: 10.1007/s12665-014-3346-y
Castiglioni, D., Rezende, C. E., Muniz, P., Muir, A. I., and García-Alonso, J. (2018). Trace metals bioavailability approach in intertidal estuarine sediments and bioaccumulation in associated nereidid polychaetes. Bull. Environ. Contam. Toxicol. 100, 472–476. doi: 10.1007/s00128-018-2301-0
Chakraborty, P., Babu, P. V. R., and Sarma, V. V. (2012). A study of lead and cadmium speciation in some estuarine and coastal sediments. Chem. Geol. 29, 217–225. doi: 10.1016/j.chemgeo.2011.11.026
de Barros, C. E., Nardi, L. V. S., Dillenburg, S. R., Ayup, R., Jarvis, K., and Baitelli, R. (2010). Detrital minerals of modern beach sediments in Southern Brazil: a provenance study based on the chemistry of zircon. J. Coast. Res. 26, 80–93. doi: 10.2112/06-0817.1
de Menezes, M. P. M., Berger, U., and Mehlig, U. (2008). Mangrove vegetation in Amazonia: a review of studies from the coast of Pará and Maranhão States, north Brazil. Acta Amaz. 38, 403–419. doi: 10.1590/S0044-59672008000300004
DelValls, T. Á, Forja, J. M., and Gómez-Parra, A. (2002). Seasonality of contamination, toxicity, and quality values in sediments from littoral ecosystems in the Gulf of Cádiz (SW Spain). Chemosphere 46, 1033–1043. doi: 10.1016/S0045-6535(01)00176-X
Duarte, B., Reboreda, R., and Caçador, I. (2008). Seasonal variation of extracellular enzymatic activity (EEA) and its influence on metal speciation in a polluted salt marsh. Chemosphere 73, 1056–1063. doi: 10.1016/j.chemosphere.2008.07.072
Dung, T. T. T., Cappuyns, V., Swennen, R., and Phung, N. K. (2013). From geochemical background determination to pollution assessment of heavy metals in sediments and soils. Rev. Environ. Sci. Biotechnol. 12, 335–353. doi: 10.1007/s11157-013-9315-1
Ekoa Bessa, A. Z., Ngueutchoua, G., Kwewouo Janpou, A., El-Amier, Y. A., Njike Njome Mbella Nguetnga, O. A., Kankeu Kayou, U. R., et al. (2020). Heavy metal contamination and its ecological risks in the beach sediments along the Atlantic Ocean (Limbe coastal fringes, Cameroon). Earth Syst. Environ. doi: 10.1007/s41748-020-00167-5
Fonseca, V. F., França, S., Duarte, B., Caçador, I., Cabral, H. N., Mieiro, C. L., et al. (2019). Spatial variation in mercury bioaccumulation and magnification in a temperate estuarine food web. Front. Mar. Sci. 6:1–11. doi: 10.3389/fmars.2019.00117
Forster, J. C. (1995). “Soil sampling, handling, storage and analysis,” in Methods in Applied Soil Microbiology and Biochemistry, eds K. Alef and P. Nannipieri (London: Academic Press), 49–121. doi: 10.1016/B978-012513840-6/50018-5
Gałuszka, A., and Migaszewski, Z. (2011). Geochemical background-an environmental perspective. Mineralogia 42, 7–17. doi: 10.2478/v10002-011-0002-y
Hakanson, L. (1980). An ecological risk index for aquatic pollution control.a sedimentological approach. Water Res. 14, 975–1001. doi: 10.1016/0043-1354(80)90143-8
Hans Wedepohl, K. (1995). The composition of the continental crust. Geochim. Cosmochim. Acta 59, 1217–1232. doi: 10.1016/0016-7037(95)00038-2
Hosseini, M., Nabavi, S. M. B., and Parsa, Y. (2013). Bioaccumulation of trace mercury in trophic levels of benthic, benthopelagic, pelagic fish species, and sea birds from Arvand River, Iran. Biol. Trace Elem. Res. 156, 175–180. doi: 10.1007/s12011-013-9841-2
Human, L. R. D., Feijão, E., Cruz de Carvalho, R., Caçador, I., Reis-Santos, P., Fonseca, V., et al. (2020). Mediterranean salt marsh sediment metal speciation and bioavailability changes induced by the spreading of non-indigenous Spartina patens. Estuar. Coast. Shelf Sci. 243:106921. doi: 10.1016/j.ecss.2020.106921
IBGE (2004a). Mapa exploratório de solos-Pedologia. Available online at: https://geoftp.ibge.gov.br/informacoes_ambientais/pedologia/mapas/unidades_da_federacao/ap_pedologia.pdf (accessed August 14, 2020).
IBGE (2004b). Geologia. Available online at: https://geoftp.ibge.gov.br/informacoes_ambientais/geologia/levantamento_geologico/mapas/unidades_da_federacao/ap_geologia.pdf (accessed August 14, 2020).
IBGE (2004c). Geomorfologia. Available online at: https://geoftp.ibge.gov.br/informacoes_ambientais/geomorfologia/mapas/unidades_da_federacao/ap_geomorfologia.pdf (accessed August 14, 2020).
IBGE (2010d). IBGE Cidades. Available online at: https://cidades.ibge.gov.br/brasil/ap/calcoene/panorama (accessed January 14, 2021).
Kaluza, P., Kölzsch, A., Gastner, M. T., and Blasius, B. (2010). The complex network of global cargo ship movements. J. R. Soc. Interface 7, 1093–1103. doi: 10.1098/rsif.2009.0495
Klockenkämper, R., Alt, F., Brandt, R., Jakubowski, N., Messerschmidt, J., and Von Bohlen, A. (2001). Results of proficiency testing with regard to sediment analysis by FAAS, ICP-MS and TXRF. J. Anal. At. Spectrom. 16, 658–663. doi: 10.1039/b101481l
Lacerda, L. D., and Pfeiffer, W. C. (1992). Mercury from Gold Mining in the Amazon Environment - an overview. Quim. Nova 15, 155–160.
Leri, A. C., Hakala, J. A., Marcus, M. A., Lanzirotti, A., Reddy, C. M., and Myneni, S. C. B. (2010). Natural organobromine in marine sediments: new evidence of biogeochemical Br cycling. Global Biogeochem. Cycles 24, 1–15. doi: 10.1029/2010GB003794
Liu, H., Zhang, K., Chai, L., Yang, Z., Yang, W., Liao, Q., et al. (2017). A Comparative evaluation of different sediment quality guidelines for metal and metalloid pollution in the Xiangjiang River, Hunan, China. Arch. Environ. Contam. Toxicol. 73, 593–606. doi: 10.1007/s00244-017-0436-3
Long, E. R., Field, L. J., and MacDonald, D. D. (1998). Predicting toxicity in marine sediments with numerical sediment quality guidelines. Environ. Toxicol. Chem. 17, 714–727. doi: 10.1897/1551-5028(1998)017<0714:PTIMSW>2.3.CO;2
Loring, D. H., and Rantala, R. T. T. (1992). Manual for the geochemical analyses of marine sediments and suspended particulate matter. Earth Sci. Rev. 32, 235–283. doi: 10.1016/0012-8252(92)90001-A
Macdonald, D. D., Carr, R. S., Calder, F. D., Long, E. R., and Ingersoll, C. G. (1996). Development and evaluation of sediment quality guidelines for Florida coastal waters. Ecotoxicology 5, 253–278. doi: 10.1007/BF00118995
Mansouri, B., Gzam, M., Souid, F., Telahigue, F., Chahlaoui, A., Ouarrak, K., et al. (2020). Assessment of heavy metal contamination in Gulf of Gabès coastland (southeastern Tunisia): impact of chemical industries and drift currents. Arab. J. Geosci. 13:1180. doi: 10.1007/s12517-020-06163-3
Marchand, C., Lallier-Vergès, E., Baltzer, F., Albéric, P., Cossa, D., and Baillif, P. (2006). Heavy metals distribution in mangrove sediments along the mobile coastline of French Guiana. Mar. Chem. 98, 1–17. doi: 10.1016/j.marchem.2005.06.001
Mondal, P., Lofrano, G., Carotenuto, M., Guida, M., Trifuoggi, M., Libralato, G., et al. (2021). Health risk and geochemical assessment of trace elements in surface sediment along the Hooghly (Ganges) River Estuary (India). Water 13:110. doi: 10.3390/w13020110
Nobi, E. P., Dilipan, E., Thangaradjou, T., Sivakumar, K., and Kannan, L. (2010). Geochemical and geo-statistical assessment of heavy metal concentration in the sediments of different coastal ecosystems of Andaman Islands. India. Estuar. Coast. Shelf Sci. 87, 253–264. doi: 10.1016/j.ecss.2009.12.019
Orani, A. M., Vassileva, E., Wysocka, I., Angelidis, M., Rozmaric, M., and Louw, D. (2018). Baseline study on trace and rare earth elements in marine sediments collected along the Namibian coast. Mar. Pollut. Bull. 131, 386–395. doi: 10.1016/j.marpolbul.2018.04.021
Pereira, T. S., Moreira, ÍT. A., de Oliveira, O. M. C., Rios, M. C., Filho, W. A. C. S., de Almeida, M., et al. (2015). Distribution and ecotoxicology of bioavailable metals and As in surface sediments of Paraguaçu estuary, Todos os Santos Bay, Brazil. Mar. Pollut. Bull. 99, 166–177. doi: 10.1016/j.marpolbul.2015.07.031
Prokisch, J., Kovács, B., and Palencsár, A. J. (2000). Yttrium normalisation? A new tool for detection of chromium contamination in soil samples The scandium group elements and lanthanides, which are also called rare earth elements (REE), are not rare in soil compared to the other often measured elements. Environ. Geochem. Health 22, 317–323. doi: 10.1023/A:1006799715897
R Core Team (2019). R: A Language and Environment for Statistical Computing. Available online at: http://www.r-project.org/.
Radomirović, M., Ćirović, Ž, Maksin, D., Bakić, T., Lukić, J., Stanković, S., et al. (2020). Ecological risk assessment of heavy metals in the soil at a former painting industry facility. Front. Environ. Sci. 8:560415. doi: 10.3389/fenvs.2020.560415
Rasmussen, P. E. (1998). Long-range atmospheric transport of trace metals: the need for geoscience perspectives. Environ. Geol. 33, 96–108. doi: 10.1007/s002540050229
Roulet, M., and Lucotte, M. (1995). Geochemistry of mercury in pristine and flooded ferralitic soils of a tropical rain forest in French Guiana, South America. Water Air Soil Pollut. 80, 1079–1088. doi: 10.1007/BF01189768
Rudnick, R. L., and Gao, S. (2013). Composition of the Continental Crust, 2nd Edn. Amsterdam: Elsevier Ltd., doi: 10.1016/B978-0-08-095975-7.00301-6
Salam, M. A., Paul, S. C., Rahman, F. N. B. A., Iqbal, M. A., Siddiqua, S. A., Rak, A., et al. (2021). Trace metals concentration and associated risk assessment in sediment of Kelantan Coastline Area Estuaries, Malaysia. Soil Sediment Contam. 30, 1–20. doi: 10.1080/15320383.2020.1867503
Saleh, Y. S. (2021). Evaluation of sediment contamination in the Red Sea coastal area combining multiple pollution indices and multivariate statistical techniques. Int. J. Sediment Res. 36, 243–254. doi: 10.1016/j.ijsrc.2020.07.011
Santos, V. F., Short, A. D., and Mendes, A. C. (2016). “Beaches of the Amazon Coast: Amapá and West Pará,” in Brazilian Beach Systems. Coastal Research Library Coastal Research Library, eds A. Short and A. Klein (Cham: Springer), 67–93. doi: 10.1007/978-3-319-30394-9_3
Sanz-Prada, L., García-Ordiales, E., Roqueñí, N., Grande Gil, J. A., and Loredo, J. (2020). Geochemical distribution of selected heavy metals in the Asturian coastline sediments (North of Spain). Mar. Pollut. Bull. 156:111263. doi: 10.1016/j.marpolbul.2020.111263
Schmider, E., Ziegler, M., Danay, E., Beyer, L., and Bühner, M. (2010). Is It Really Robust: reinvestigating the robustness of ANOVA against violations of the normal distribution assumption. Methodology 6, 147–151. doi: 10.1027/1614-2241/a000016
Selvi, A., Rajasekar, A., Theerthagiri, J., Ananthaselvam, A., Sathishkumar, K., Madhavan, J., et al. (2019). Integrated remediation processes toward heavy metal removal/recovery from various environments-A review. Front. Environ. Sci. 7:66. doi: 10.3389/fenvs.2019.00066
Siqueira, G. W., Aprile, F., Irion, G., and Braga, E. S. (2018). Mercury in the Amazon basin: human influence or natural geological pattern? J. South Am. Earth Sci. 86, 193–199. doi: 10.1016/j.jsames.2018.06.017
Sousa, A. I., Lillebo, A. I., Risgaard-Petersen, N., Pardal, M. A., and Cacador, I. (2012). Denitrification: an ecosystem service provided by salt marshes. Mar. Ecol. Prog. Ser. 448, 79–92. doi: 10.3354/meps09526
Sun, Z., Li, J., He, T., Ren, P., Zhu, H., Gao, H., et al. (2017). Spatial variation and toxicity assessment for heavy metals in sediments of intertidal zone in a typical subtropical estuary (Min River) of China. Environ. Sci. Pollut. Res. 24, 23080–23095. doi: 10.1007/s11356-017-9897-1
Szlafsztein, C. F. (2012). The Brazilian Amazon coastal zone management: implementation and development obstacles. J. Coast. Conserv. 16, 335–343. doi: 10.1007/s11852-012-0184-5
Towett, E. K., Shepherd, K. D., and Cadisch, G. (2013). Quantification of total element concentrations in soils using total X-ray fluorescence spectroscopy (TXRF). Sci. Total Environ. 463–464, 374–388. doi: 10.1016/j.scitotenv.2013.05.068
Turekian, K. K., and Wedepohl, K. H. (1961). Ages of batholithic intrusions of northern and central Chile. Bull. Geol. Soc. Am. 72, 1551–1559.
Vetrimurugan, E., Jonathan, M. P., Roy, P. D., Shruti, V. C., and Ndwandwe, O. M. (2016). Bioavailable metals in tourist beaches of Richards Bay. Kwazulu Natal South Africa. Mar. Pollut. Bull. 105, 430–436. doi: 10.1016/j.marpolbul.2016.01.045
Viana, L. F., Cardoso, C. A. L., Lima-Junior, S. E., Súarez, Y. R., and Florentino, A. C. (2020). Bioaccumulation of metal in liver tissue of fish in response to water toxicity of the Araguari-Amazon River, Brazil. Environ. Monit. Assess. 192:781. doi: 10.1007/s10661-020-08696-2
Vidinha, J. M., Rocha, F., Patinha, C., Silva, E., and Andrade, C. (2006). Heavy metals contents on beach and dune sediments from Espinho to Mondego Cape (Portugal) - Influence of human activities. J. Geochemical Explor. 88, 404–407. doi: 10.1016/j.gexplo.2005.08.085
Vineethkumar, V., Sayooj, V. V., Shimod, K. P., and Prakash, V. (2020). Estimation of pollution indices and hazard evaluation from trace elements concentration in coastal sediments of Kerala, Southwest Coast of India. Bull. Natl. Res. Cent. 44:198. doi: 10.1186/s42269-020-00455-0
Watts, M. J., Mitra, S., Marriott, A. L., and Sarkar, S. K. (2017). Source, distribution and ecotoxicological assessment of multielements in superficial sediments of a tropical turbid estuarine environment: a multivariate approach. Mar. Pollut. Bull. 115, 130–140. doi: 10.1016/j.marpolbul.2016.11.057
Wright, L. D., and Short, A. D. (1984). Morphodynamic variability of surf zones and beaches: a synthesis. Mar. Geol. 56, 93–118. doi: 10.1016/0025-3227(84)90008-2
Xavier, D. A., Santos, V. F., Miranda, A. G. O., and Berrêdo, J. F. (2020). Determination of background geochemistry of an Amazon estuary: the Cuñaní Estuary – Amapá. Mar. Pollut. Bull. 155, 111144. doi: 10.1016/j.marpolbul.2020.111144
Yalcin, F. (2020). Data analysis of beach sands’ chemical analysis using multivariate statistical methods and heavy metal distribution maps: the case of Moonlight Beach sands, Kemer, Antalya, Turkey. Symmetry (Basel) 12:1538. doi: 10.3390/SYM12091538
Yang, Z., Wang, Y., Shen, Z., Niu, J., and Tang, Z. (2009). Distribution and speciation of heavy metals in sediments from the mainstream, tributaries, and lakes of the Yangtze River catchment of Wuhan, China. J. Hazard. Mater. 166, 1186–1194. doi: 10.1016/j.jhazmat.2008.12.034
Keywords: trace elements, Amazonian beaches, contamination indexes, intertidal sediment, baseline, pollution indexes
Citation: Vilhena JCE, Amorim A, Ribeiro L, Duarte B and Pombo M (2021) Baseline Study of Trace Element Concentrations in Sediments of the Intertidal Zone of Amazonian Oceanic Beaches. Front. Mar. Sci. 8:671390. doi: 10.3389/fmars.2021.671390
Received: 24 February 2021; Accepted: 26 April 2021;
Published: 21 May 2021.
Edited by:
Dawei Pan, Yantai Institute of Coastal Zone Research (CAS), ChinaReviewed by:
Periyadan K. Krishnakumar, King Fahd University of Petroleum and Minerals, Saudi ArabiaEr HUA, Ocean University of China, China
Copyright © 2021 Vilhena, Amorim, Ribeiro, Duarte and Pombo. This is an open-access article distributed under the terms of the Creative Commons Attribution License (CC BY). The use, distribution or reproduction in other forums is permitted, provided the original author(s) and the copyright owner(s) are credited and that the original publication in this journal is cited, in accordance with accepted academic practice. No use, distribution or reproduction is permitted which does not comply with these terms.
*Correspondence: Jéssica C. E. Vilhena, dmlsaGVuYS5qZXNzaWNhQGdtYWlsLmNvbQ==