- 1Center for Marine and Environmental Sciences (MARE), Lisbon, Portugal
- 2Dom Luiz Institute, Faculty of Sciences, University of Lisbon, Lisbon, Portugal
- 3Departamento de Biologia Vegetal, Faculdade de Ciências, Universidade de Lisboa, Lisbon, Portugal
- 4National Laboratory for Civil Engineering, Lisbon, Portugal
The Tagus Estuary is one of the largest estuaries in Europe and merges large urban and industrial areas. Understanding phytoplankton community variability is key for an appropriate assessment of the estuarine ecological status. The objective of the present study was to assess the importance of the tidal influence over the phytoplankton community and to evaluate its main drivers of variation. Weekly sampling was performed at two stations on the Tagus Estuary with different anthropogenic pressures (Alcântara and Barreiro). The sampling covered periods with different tidal amplitude. Alcântara presented both the lowest and highest concentrations of dissolved inorganic nitrogen (DIN) and orthophosphate concentration (DIP), depending on the tidal height. Such high variability in this sampling station is probably due to its proximity to a sewage treatment station outfall and to the estuary mouth. In the present study, both seasonal and tidal variations influenced the chlorophyll a concentration of which the tidal cycle explained up to 50% of the chlorophyll a variations. Chlorophyll a displayed a seasonal trend with two peaks of phytoplankton biomass between spring and mid-summer. The main drivers of chlorophyll a variation were radiation, water temperature, tidal amplitude, salinity, river discharge, and the inorganic nutrients DIN and DSi. The estuarine phytoplankton community was mainly dominated by Bacillariophyceae, especially at Alcântara. Bacillariophyceae were less important at Barreiro, where communities had a higher representation from other phytoplankton groups, such as Cryptophyceae and Prasinophyceae. The drivers of variability in the community composition were similar to those influencing the total biomass. In conclusion, the spring-neap tidal cycle strongly influenced the phytoplankton community, both in terms of biomass and community composition. Of the several tidal conditions, spring tides were the tidal condition that presented both higher biomass and higher Bacillariophyceae representativity in the community.
Introduction
Estuaries are among the most productive ecosystems in the world (Ketchum, 1967), acting as nursery areas (Cabral and Costa, 1999), habitat, and feeding grounds (Dias et al., 2008) for several species such as fish, marine mammals, and wading birds, which benefit from the high productivity of estuarine areas. Estuaries are often the focal point for coastal settlements and industrial areas suffering from several anthropogenic pressures, such as margin transformation, residential and industrial sewage discharge, boat traffic, and agriculture fertilizer runoff (Caeiro et al., 2005; Spatharis et al., 2007; McKinley et al., 2011). Eutrophication is a continuous threat to estuaries due to the continuous growth of the human population and consequent increase in food production (agriculture and animal farms) and energy demand (Pate et al., 2007; Bajželj et al., 2014).
The water exchange in estuaries is influenced by both the freshwater input and seawater dilution (Garcia-Soto et al., 1990), resulting in large spatial and temporal variations on the physicochemical parameters and the phytoplankton community. For instance, turbidity usually presents an increasing steady trend downward from riverine waters until salinity increases, generating a turbidity maximum at the landward limit of the salinity intrusion and greatly decreasing thereafter (Geyer, 1993). This also results in significant spatial variability in light availability, which in turn lead to different consumption and requirement of nutrients for the phytoplankton community (O'Donohue and Dennison, 1997). Nutrients, which are usually generated inland, tend to decrease from riverine to coastal waters (Cabeçadas et al., 1999; Wen et al., 2008). Still, such patterns can be altered by sewage discharges that are often present in estuaries. Moreover, tidal exchanges generate fluctuations in these parameters by continuously forcing coastal water (with higher salinity and, in general, lower nutrients and particles) upward or downward, contributing not only to the spatial but also to the temporal variability of both natural and anthropogenic drivers (Cabrita and Moita, 1995). Moreover, the confined waters of estuaries also have relatively low depths, being susceptible to atmospheric forcings, like wind and temperature variations, thus presenting great daily and seasonal variability (Kessarkar et al., 2009; Uncles and Stephens, 2010; Cereja et al., 2017). Also, coastal upwelling nearby to the estuary mouth can affect the estuarine waters through tidal mixing (Colbert and McManus, 2003; Davis et al., 2014).
Usually, the growth of estuarine phytoplankton communities is limited by nutrients, light availability, or both, depending on the water mass characteristics (Cloern, 1987, 2001; Nedwell et al., 2002; Nielsen et al., 2002 Gameiro et al., 2007). In several European turbid estuaries, located in temperate regions, light availability usually is the main limiting factor for phytoplankton growth (Goosen et al., 1999; Domingues et al., 2011). In general, these estuaries present higher concentrations of chlorophyll a in the upper and middle parts of the estuary. Spatially, chlorophyll a varies following two possible spatial patterns: (i) one in which chlorophyll decreases continuously throughout the salinity gradient and (ii) the other where chlorophyll maximum is located downstream to the turbidity maximum presenting a decreasing trend from there to the estuary mouth (Lemaire et al., 2002). Temperate estuaries usually present marked seasonal variations in the chlorophyll a concentrations, presenting maximum values between early spring and summer. Bacillariophyceae are usually the dominant group, representing high percentages of biomass (Lemaire et al., 2002; Lopes et al., 2007; Brito et al., 2015). Following the Water Framework Directive, community composition has been used as an indicator of the ecological status (Devlin et al., 2007, 2012). Bacillariophyceae dominance in estuarine waters has been considered a proxy of good ecological status since flagellate dominance is usually associated with eutrophic waters (Cloern, 1991). In mesotidal estuaries, tidal variations have been shown to influence parameters such as suspended solids and nutrients (Balls, 1990; Goosen et al., 1999). Fortnightly, tidal influence over chlorophyll a and community composition have been rarely analyzed, as most studies have focused on the low-water high-water daily cycle (Balls, 1994; Cabeçadas, 1999; Goosen et al., 1999).
The Tagus Estuary is a mesotidal estuary along the west coast of Portugal. It houses large cities, with around 2.3 million inhabitants in 2013 (INE.pt accessed on 17 July 2020), and industrial areas that discharge sewage (in general with secondary or more advanced treatment) into the estuarine waters (PGRH, Agência Portuguesa do Ambiente, 2016). It is a turbid estuary, with suspended particles varying between <12 and >500 mg/L along its longitudinal axis (Vale and Sundby, 1987). The Tagus Estuary presents vertical stratification in salinity and nutrients during high river discharges events (Neves, 2010; Rodrigues and Fortunato, 2017). Vale and Sundby (1987) also observed the existence of vertical stratification for turbidity during neap tides, not being registered during spring tides. The Tagus Estuary is one of the Portuguese estuaries with greater residence time (around 10 days) that has a high phytoplankton diversity (Ferreira et al., 2005). Tides have proven to influence the estuary conditions, in particular, water temperature, chlorophyll a, suspended particles, and nutrient concentrations, which are generally higher at low tides (Vale and Sundby, 1987; Cabrita and Moita, 1995). Nutrients present a seasonal trend, with maximal concentrations of dissolved inorganic nitrogen (DIN) and silicates during the winter–spring period (Gameiro et al., 2007; Borges et al., 2020). Gameiro and Brotas (2010) indicated that 15% of N input into the estuary originated from sewage discharges. Moreover, Gameiro et al. (2004) observed that the spatial distribution of ammonium was correlated with sewage distribution. The implementation of wastewater treatment in the Tagus Estuary started in the 1990s, and its efficiency greatly improved during the 2000s (Rodrigues et al., 2020). Although treatment began in the 1990s, Gameiro and Brotas (2010), sampling in an inner zone of the estuary, did not find any differences in nutrient concentrations between 1980 and 2007, but more recently, Rodrigues et al. (2020), sampling throughout the estuary, demonstrated a decline in all nutrients from the 1980s to 2019.
Chlorophyll a concentrations in Tagus Estuary are considered moderate to low when compared with other mesotidal estuaries (Gameiro et al., 2007). Its spatial, seasonal, and interannual variations have been intensely studied in the past. However, the variability induced by daily and fortnight tidal cycles has not yet been comprehensively studied. Cabrita and Moita (1995) reported daily differences in the phytoplankton biomass between high and low tides, with higher chlorophyll a, nutrients, and turbidity at low tide. Most of the previous monitoring works showed that seasonal variations in the chlorophyll a concentrations were mainly driven by air and water temperature, river flow, water retention time, and irradiance, i.e., seasonal-dependent variables (Cabeçadas, 1999 Gameiro et al., 2004, 2007, 2011; Gameiro and Brotas, 2010). Still, salinity and nutrients have also been referred to as important drivers for phytoplankton biomass (e.g., Brogueira et al., 2007), being associated with the retention time and river flow (Saraiva et al., 2007).
Phytoplankton community structure has changed in the last decades in comparison with the 1980s. From 1999 to 2007, Brito et al. (2015) reported lower chlorophyll a concentrations and higher total cell abundances than those registered in the 1980s, resulting from the higher importance of smaller organisms such as Cryptophyceae, Euglenophyceae, and Prasinophyceae (Brito et al., 2015). Generally, in terms of biomass, Bacillariophyceae is the dominant group in the estuary, with Cryptophyceae, Chlorophyceae, and Euglenophyceae contributing to the community (Gameiro and Brotas, 2010; and references herein). Gameiro et al. (2007) also reported a clear seasonal pattern within the community, where the Cryptophyceae contribution increased during autumn and winter.
Regarding spatial variability, although the phytoplankton community have been well-studied in the Tagus Estuary, such studies were mainly focused on the middle part of the estuary and during high-tide conditions (Gameiro et al., 2004, 2007, 2011; Gameiro and Brotas, 2010; Brito et al., 2015). Therefore, it is important to investigate the phytoplankton community dynamics in the water bodies of the lower part of the estuary, as well as to understand the influence of the tides over the community. Due to the rapid response of phytoplankton communities to several anthropogenic and environmentally driven factors, phytoplankton biomass, for which chlorophyll a is used as a universal proxy, is an important component for the assessment of the ecological quality of water bodies, under the Water Framework Directive (Devlin et al., 2012).
The main objective of the present study was to investigate the relationship between the Tagus estuarine phytoplankton community and environmental conditions in two superimposed temporal scales: tidal and seasonal. The specific questions were as follows: (i) What are the main drivers of seasonal variation of the phytoplankton community? and (ii) Is the tidal cycle a significant driver of variability? This work addresses the lack of information on how the community reacts to the tidal cycle and to better characterize the phytoplankton community in the lower parts of the estuary, using weekly data.
Materials and Methods
Sampling Campaigns
Weekly sampling campaigns were conducted from April 9, 2018 to April 18, 2019, in order to cover changes in the tidal cycle, being only one sampling event performed per week. Sampling was performed at two easily accessible land stations located at mid-lower estuary: (i) Alcântara (38.6978 N and 9.1754 W) and (ii) Barreiro (38.6839 N and 9.0583 W) (Figure 1). These stations were selected due to their easy access, with some distance into the water (docks), and located in an area of the estuary with little available data. Alcântara sampling station has a sewage treatment plant (STP) outfall nearby (at a distance of 115 m) and is possibly influenced by it. This is the largest STP in the estuary, receiving the sewage waters from the majority of Lisbon city area (David et al., 2015). There is an STP outfall near Barreiro station as well, however, it is located 550 m away and serves a much smaller population. All tidal phases were considered: high and low water and spring and neap tides, in a total of 44 sampling campaigns in Alcântara and 47 in Barreiro. Differences in the number of sampling campaigns were due to logistic constraints.
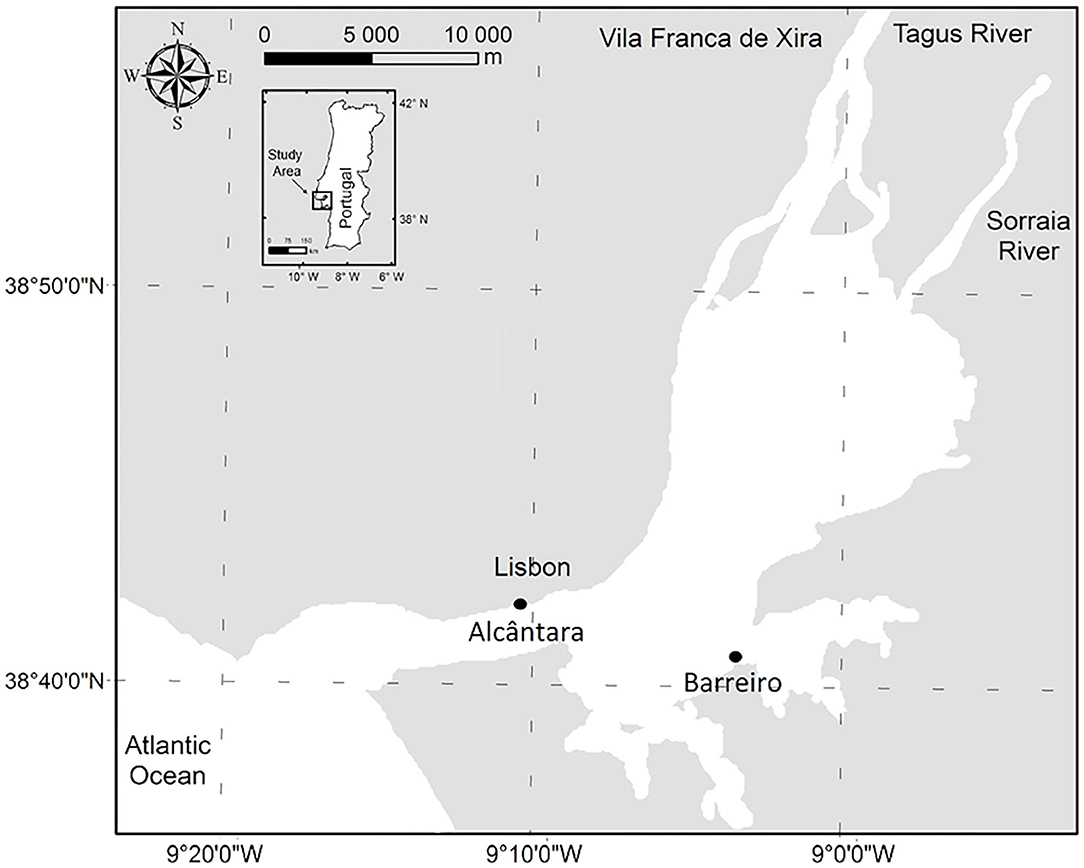
Figure 1. Geographic setting of the Tagus Estuary and the location of the sampling stations. Figure adapted from Brito et al. (2015).
A multiparameter probe (YSI EXO 2) was used to measure in situ temperature, salinity, pH, dissolved oxygen, and turbidity (in NTU) (Table 1). Vertical profiles were not performed as the Tagus Estuary is well mixed (Rodrigues and Fortunato, 2017), and these stations present low bottom depths (4–7 m). Secchi depth was assessed using a 50-cm white Secchi disk. Light extinction coefficient (Kd) was calculated by dividing 1.7 by the Secchi depth, as described in Tilzer (1988). A volume of 5 L of surface water was collected for analysis of (i) suspended particles, (ii) dissolved nutrients, and (iii) phytoplankton pigments. Water for nutrient analysis was filtered on-site with a hand filtration system using a precombusted (450°C for 4 h) 47-mm-diameter Whatman GF/C filter (Glass Fibber with 1.2 μm pore; Sigma Aldrich, St. Louis, MO, USA). These samples were then placed in a cooler, transported to the lab as soon as possible, and frozen at −20°C. Water for pigment analysis and suspended particulate matter (SPM) quantification were also placed in a cooler, protected from light and heat during transport to the lab, where they were processed.
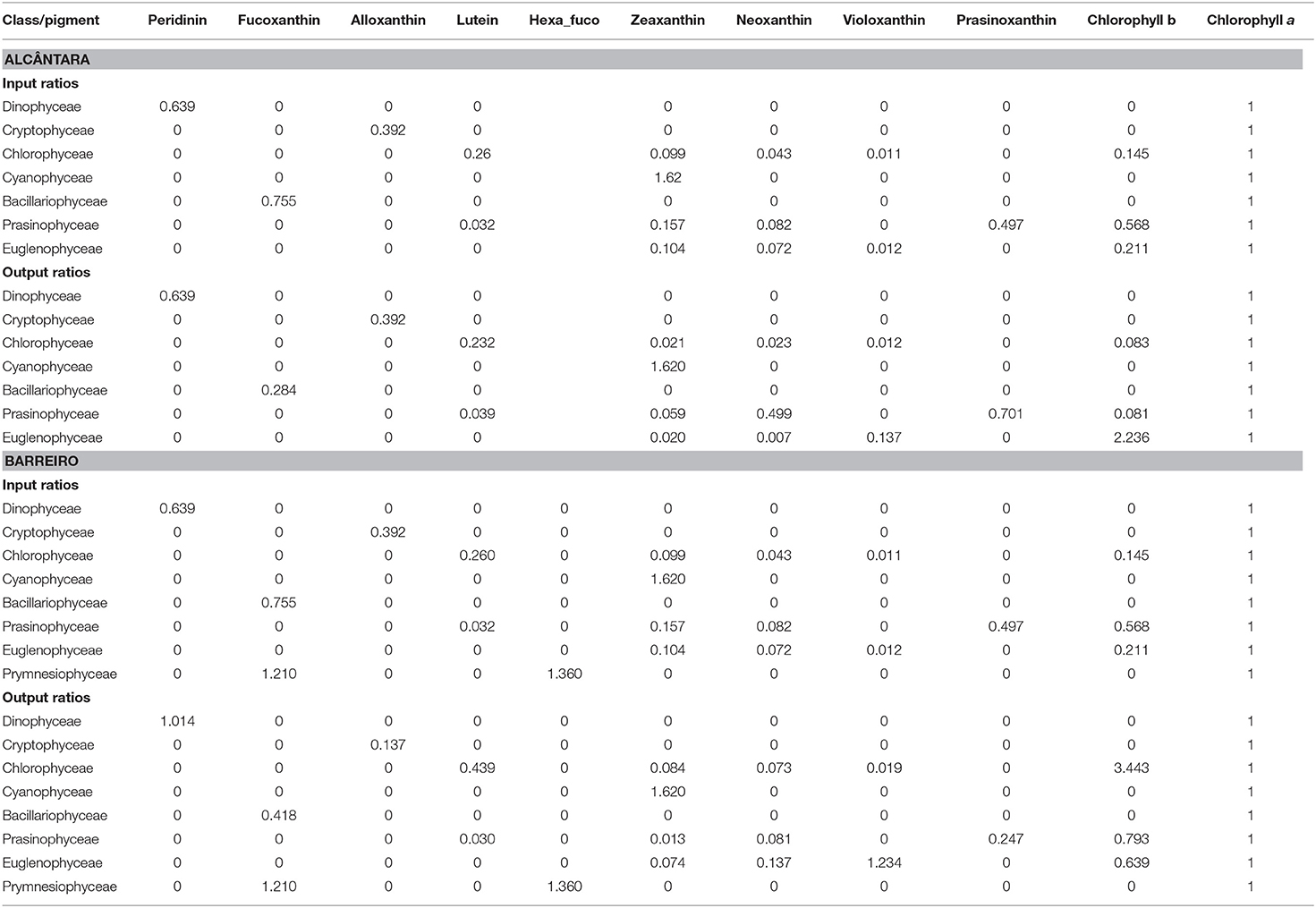
Table 1. Input and output ratios of marker pigments to chlorophyll a for Alcântara and Barreiro stations.
Weekly data on radiation (as weekly mean of total solar radiation per day), air temperature (both in Figure 2), and rainfall, as well as 5-day averages of river discharge (Figure 3) were gathered from Sistema Nacional de Informação de Recursos Hídricos [Sistema Nacional de Informação de Recursos Hídricos (SNIRH), 2019; www.snirh.pt].
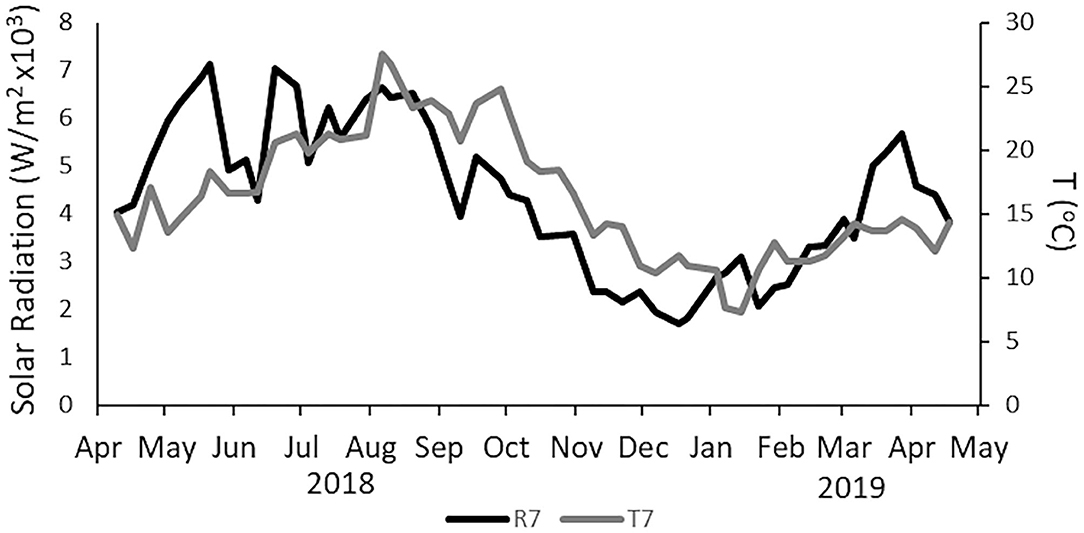
Figure 2. Weekly mean of total solar radiation per day (black line, responding to left side axis, on ×103 W/m2, radiation is expressed as the total radiation accumulated per day) and weekly mean temperature [light gray line, responding to right-side axis in degrees Celsius (°C)]. Data obtained from SNIRH (snirh.apambiente.pt) and measured at São João do Tojal meteorological station.
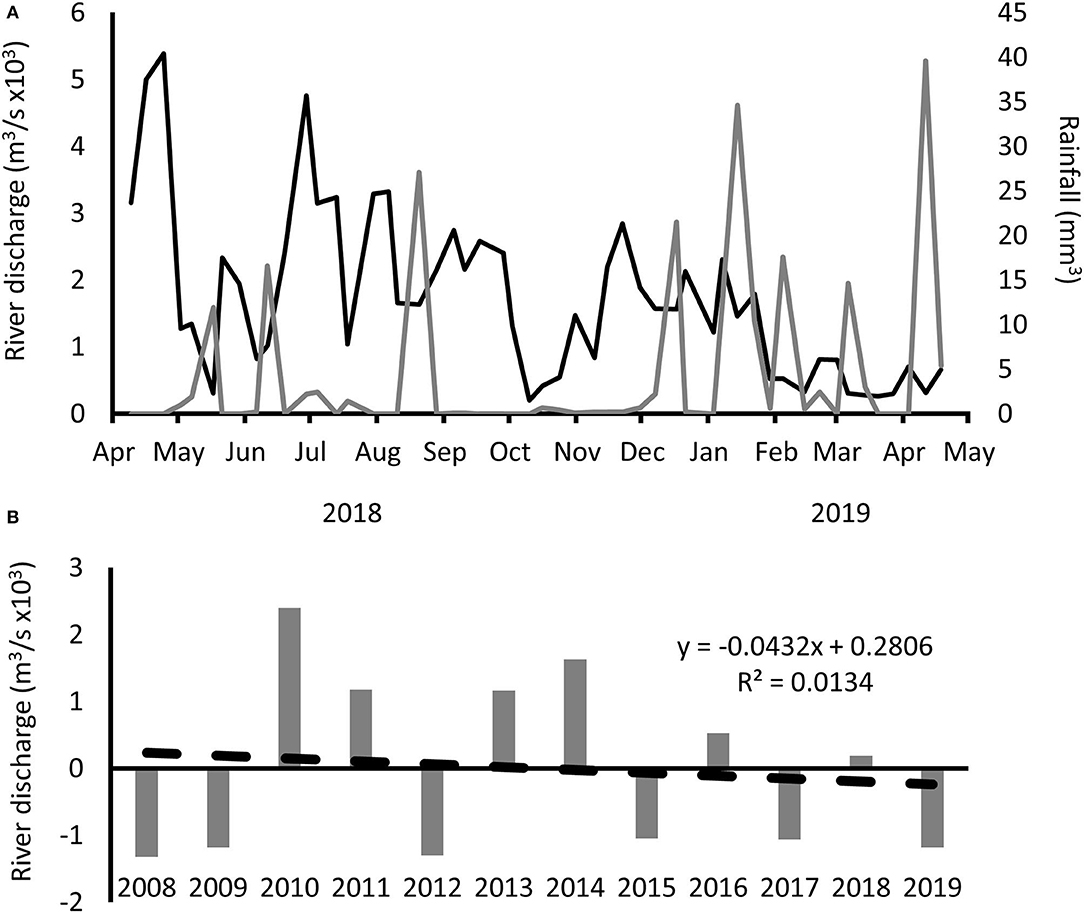
Figure 3. River discharge and rainfall in the Tagus Estuary. (A) Seven-day mean river discharge (black line) and 7-day total rainfall (gray line). (B) Yearly anomalies in relation to the global average using historical annual mean discharge from Almourol hydrometric station (data available since 1974) from SNIRH (snirh.apambiente.pt).
Analysis of Nutrient Concentrations
Triplicate samples for silicate, orthophosphate, nitrite, and nitrate concentrations were quantified through a TECATOR Flux Injection Analyzer FIA STAR 5000 (FOSS Tecator, Höganäs, Sweden) using the brand protocols. In the analyzer, nitrate was determined according to Grasshoff (1976), nitrite according to Bendschneider and Robinson (1952), and phosphates and silicates according to Murphy and Riley (1962) and Fanning and Pilson (1973), respectively. Ammonium concentrations were determined in triplicates, using manual colorimetric methods according to Koroleff (1969). DIN was computed as the sum of nitrite, nitrate, and ammonium concentrations.
Analysis of Suspended Particulate Matter and Organic Matter
For each sampling event, water for suspended particulate matter was filtered in triplicates using a precombusted (450°C for 4 h) 47-mm-diameter Whatman GF/C filter. After the sample filtration, 100 ml of ultra-pure water was passed through the filter to remove dissolved matter that would otherwise deposit in the filter, and the filter was stored in a drying oven at 60°C. Filters were allowed to dry for at least 24 h at 60°C and afterwards transferred to a desiccator to allow cooling. When resumed to room temperature, filters were weighted using a high precision scale. The weight of SPM (mg/L) is calculated by subtracting the weight of the filter before and after filtration. To quantify suspended organic (OM, mg/L) and inorganic matters (IM, mg/L), the filters were combusted at 450°C in order to extract the organic matter and weighed again. Organic matter is quantified by subtracting the weight of the filter after the last combustion to SPM. For inorganic matter, the weight of the combusted filter before filtration is subtracted to the weight of the filter after the last combustion.
Quantification of Phytoplankton Pigments
Phytoplankton pigments were quantified using two different methodologies: spectrophotometry and high-performance liquid chromatography (HPLC). HPLC analysis provided the full pigment signature of phytoplankton communities; however, only one sample was processed per station. For spectrophotometry, following the method proposed by Lorenzen (1967), to provide concentrations of chlorophyll a and phaeopigments, triplicate samples were used (for volumes, see Supplementary Table 1). Chlorophyll a data obtained from both methods presented the same temporal pattern and were almost identical (R2 = 0.90, m = 1.06 and R2 = 0.94, m = 1.03 for Alcântara and Barreiro, respectively). Given the different comparisons and statistical analyses conducted in this study, it was necessary to include both datasets. The spectrophotometric Chl a was used for the general phytoplankton biomass analysis while the HPLC chl a was only used for the community composition analysis. Water filtration was performed using 47 mm diameter (spectrophotometry) and 25 mm diameter (HPLC) GF/F Whatman filters (0.7 μm pore). Samples were stored at −80°C.
Quantification of Chlorophyll a and Phaeopigments by Spectrophotometry
Chlorophyll a and phaeopigment concentrations were estimated following the Lorenzen (1967) method. Extraction of pigments was performed by shredding the filters in 6 ml of acetone at 90% (v/v) dilution and left to rest at −20°C for 24 h. The samples were then centrifuged at 4,000 rpm for 15 min at 4°C. The supernatant was measured at 664 and 750 nm in a Shimadzu UV-260 UV-VIS spectrophotometer (Shimadzu, Kyoto, Japan). Measurements were taken before and after the addition of 12.5 μl of hydrochloric acid at 0.5 M to a 1-mL cuvette.
Quantification of Phytoplankton Pigments by HPLC and Chemotaxonomy
Pigment extraction and quantification was performed according to Gameiro et al. (2007) in a Shimadzu HPLC that is composed of a solvent delivery module (LC-10ADVP), a pump module (LC-10AD), a degasser module (DGU-14A), an oven module (CTO-10AS) and with system controller (SCL-10AVP), and a photodiode array (SPDM10AVP). Chromatography separation was performed using a C18 column for reversed-phase chromatography (Supelcosil, 0.46 25 cm, 5 mm particles; Sigma Aldrich, St. Louis, MO, USA). The solvent gradient followed Kraay et al. (1992) adapted by Brotas and Plante-Cuny (1996) with a flow rate of 0.6 m/min, an injection volume of 100 ml, and a duration of 35 min. Pigments were identified by comparing retention times and absorption spectra with pure crystalline standards from DHI. Pigment concentration was then calculated according to the following formula:
In which PP is the concentration of a specific phytoplankton pigment (μg/L), St is the internal standard theoretical area, Ss is the internal standard sample area (both Ss and St are measured at the same wavelength of each pigment being quantified), Vm is the volume (ml) of extraction solution, Vf is the water volume filtered by the filter being analyzed, AFP is the absorbance for the desired pigment, and m is the slope of the calibration curve.
The relative composition of the phytoplankton groups was calculated using HPLC pigment concentration data and CHEMTAX chemical taxonomy software, version 1.95 (Mackey et al., 1996). CHEMTAX uses factor analysis and the steepest-descent algorithm to find the best fit of the data on an initial pigment ratio matrix. The method proposed by Latasa (2007) was then applied. This consists of performing subsequent CHEMTAX runs using the optimized pigment ratios resulting from the previous run. The main objective is to reduce the associated error to the minimum. Generally, the pigment ratios used were adapted from Gameiro et al. (2007). Moreover, for Barreiro samples, the ratios for Prymnesiophyceae presented by Mendes et al. (2011) were also added to respond to the presence of 19′-hexanoyloxyfucoxanthin. This pigment was not identified in Alcântara. Groups were defined using the taxonomic level “Class” and following the World Register of Marine Species (worms, at marinespecies.org, accessed on April 21, 2021). Input and output ratios are presented in Table 1.
Statistical Analysis
General Additive Models
The effect of physicochemical parameters on chlorophyll a concentrations were assessed using a generalized additive model with a cubic regression spline with K = 3. Due to the traditional F-like distribution of chlorophyll a concentrations, a Gamma distribution with a “log” link function was used in these models. Also, to assess how relative community composition changes due to environmental forcing, generalized additive models were performed, for the dominant or most relevant groups (Bacillariophyceae, Cryptophyceae, and Dinophyceae in both sampling sites and also for Prasinophyceae in Barreiro), with cubic regression splines with K = 3 and beta regression family with “identity” link function. In both general additive model (GAM) analyses, variables that present a sparse distribution on higher values were logarithmized to avoid problems in the model fit due to the higher importance attributed to single values on the extreme of the distribution. To assess the presence of multicollinearity between predictor variables, Spearman correlations were performed. Variables were excluded from the analysis when correlation was higher than 0.7 or worst concurvity was higher than 0.8. Correlations and concurvity values are presented in Supplementary Table 2. When a variable had to be removed, variables that have proved, in previous works, to be important in explaining chlorophyll a variations were maintained. Tidal height (for which sampling campaigns were also set) was not included as it was highly concurve with tidal range and, in Alcântara, also with nutrients. DIN and DSi presented high concurvity in Barreiro but were both kept since both are important to explain either chlorophyll concentration or group relative abundance. R software (R Core Team, 2019) was used to compute the models.
Truncated Fourier Series
To investigate the temporal variation of chlorophyll a concentrations, and due to the existence of missing values and irregular sampling intervals, a truncated Fourier series with M sets of sine–cosine waves have been fitted to the chlorophyll a data in function with time as described in Brito et al. (2012b). In this model, the lowest frequency (n = 1) represents the annual wave while the highest frequency (n = M) was set as 26, which comply with the Nyquist frequency limit (see Brito et al., 2012b for more information) and represents a periodicity of 15 days, which correspond to the tidal neap spring cycle. Afterwards, an analysis of variance was carried out to assess the relative importance of the seasonal cycle (represented by the wave-pairs 1–3), the higher-frequency temporal variation (wave-pairs 4 through 26) and the within-day variability in each component.
This model has the advantage of dealing with irregular sampling, by not employing the usual, but older methods (Chatfield, 2003) involving the successive fitting of sine–cosine pairs of increasing frequency (Brito et al., 2009). The Fourier analysis was performed using Matlab 2019A.
Results
Physicochemical Parameters
Temperature and radiation presented the typical seasonal pattern for temperate latitudes. The temperature increased from April to August, with the maximum weekly mean temperature (27.5°C) registered on August 6, 2018, decreasing from this point until January 14, when the minimum temperature was registered (Figure 2). Table 2 presents a summary of the physicochemical parameters, and the raw data is presented in Supplementary Table 3. Radiation increased from April to June when its maximum was registered (mean daily radiation of 7,111 W/m2 on the week of May 21), and then decreased back until the minimum value registered on 21 of December (Figure 2). Alcântara presented the highest values for DIN, DIP, and suspended matter. Peaks in DIN concentrations, up to 200–500 μM, were frequently observed at Alcântara and were always related to peaks in DIP concentrations. At Barreiro, DIN concentrations varied between 15 and 35 μM, with two clear occasions, in May and August, where DIN concentrations decreased until almost 0 μM (Figures 4A,B). DIP concentrations in Alcântara were highly correlated with DIN (0.91, Supplementary Table 3; Figure 4C). At Barreiro, no correlation was found (0.39, Supplementary Table 3), although DIN reduction in August occurred simultaneously with a decrease of DIP and DSi concentrations to almost 0 μM (Figure 4D). Although the average concentrations of silicates were higher at Alcântara, observed values are relatively similar to the ones measured at Barreiro (Figures 4E,F, respectively). SPM was higher in Alcântara, and the concentration peaked during winter, from January to March. Regarding the Redfield ratios (Redfield, 1958; Brzezinski, 1985), Alcântara had most of the data points (73.8%) above the 16:1 DIN:DIP ratio, but only 7% over the 16:1 DSi:DIP ratio. Barreiro had 34% above the 16:1 DIN:DIP ratio, 9% over the 16:1 DSi:DIP ratio, and 4% above the 16:16:1 DSi:DIN:DIP (Supplementary Table 4; Figures 4I,J).
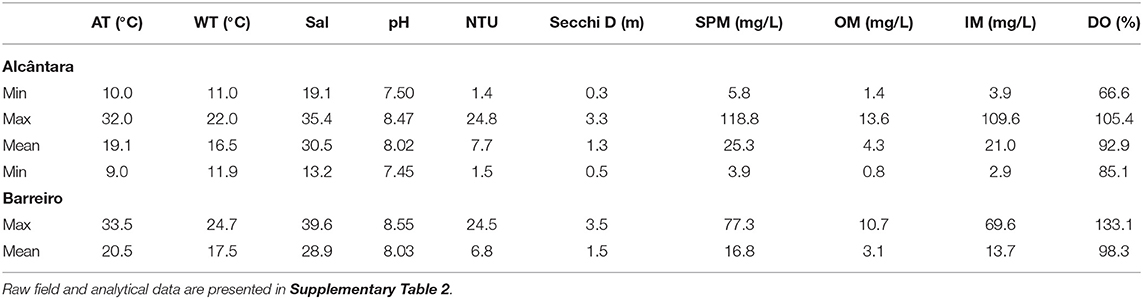
Table 2. Maximum, minimum, and mean for air temperature (AT), the water temperature at sampling (WT), salinity, pH, turbidity (NTU), Secchi depth (Secchi D), suspended particulate matter (SPM), suspended organic matter (OM), suspended inorganic matter (IM), dissolved oxygen saturation DO (%), and concentration of dissolved oxygen.
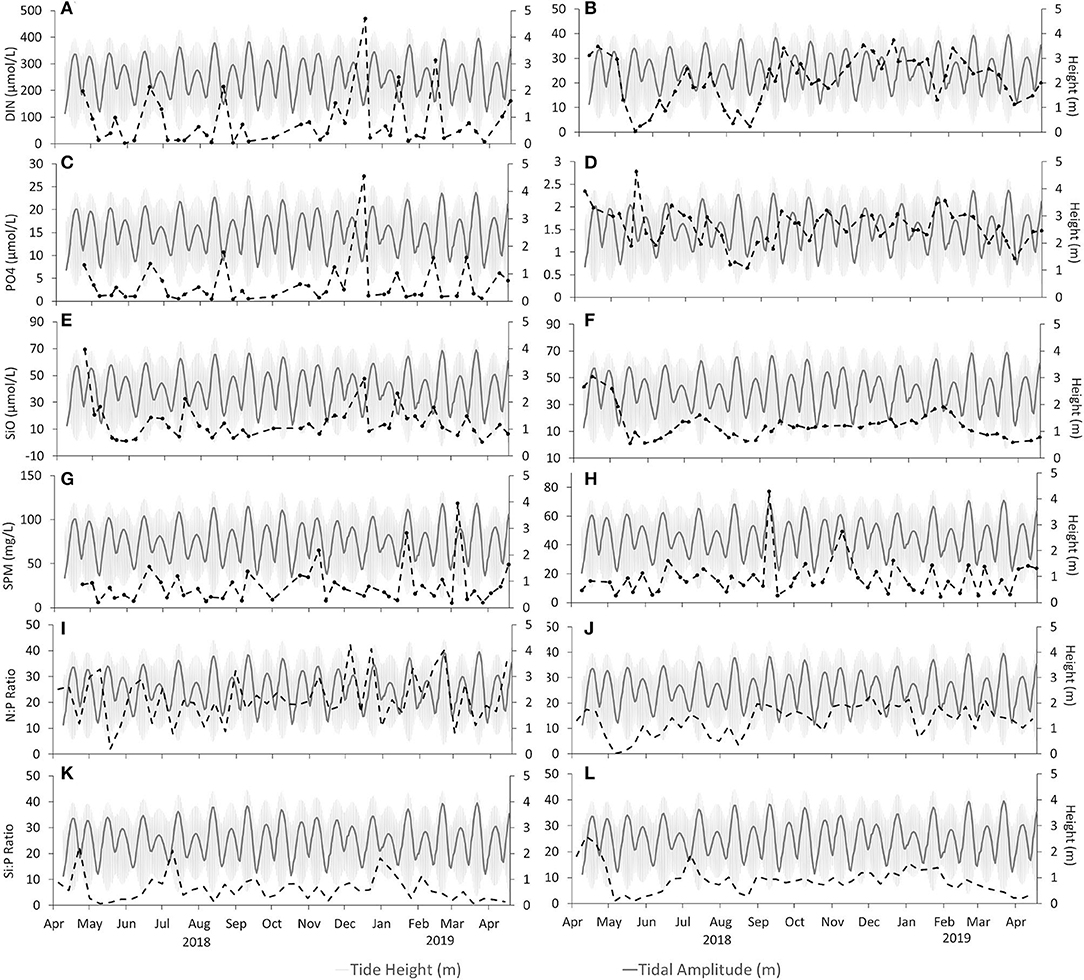
Figure 4. Nutrients and suspended particulate matter for the sampling period, plotted against tidal cycle (light gray shade on the back) and tidal range (gray line). The dotted lines represent, for Alcântara (left) and Barreiro (right), of which graphs (A,B) present dissolved inorganic nitrogen in micromoles per liter, (C,D) present the concentrations of orthophosphate (DIP) in micromoles per liter, (E,F) present the concentration of silicates (SiO) in micromoles per liter, (G,H) present the concentrations of suspended particulate matter (SPM) in milligrams per liter, and (I–L) present the nutrient ratio DIN:DIP and DSi:DIP.
Phytoplankton Biomass (Chlorophyll a)
Chlorophyll a concentrations varied between 0.5 and 6.2 μg/L in Alcântara and 0.6 and 11.3 μg/L in Barreiro (Figures 5A,B). The seasonal pattern observed at both stations has common features, namely two peaks of chlorophyll a: (i) the first observed in spring, starting in mid-May 2018 and lasting until mid-June 2018 at Barreiro and end of June at Alcântara and (ii) the second in summer, in early August. However, distinct features were registered between the two sites; in particular, there were two other peaks in Alcântara, not observed in Barreiro, in October 2018 and March 2019.
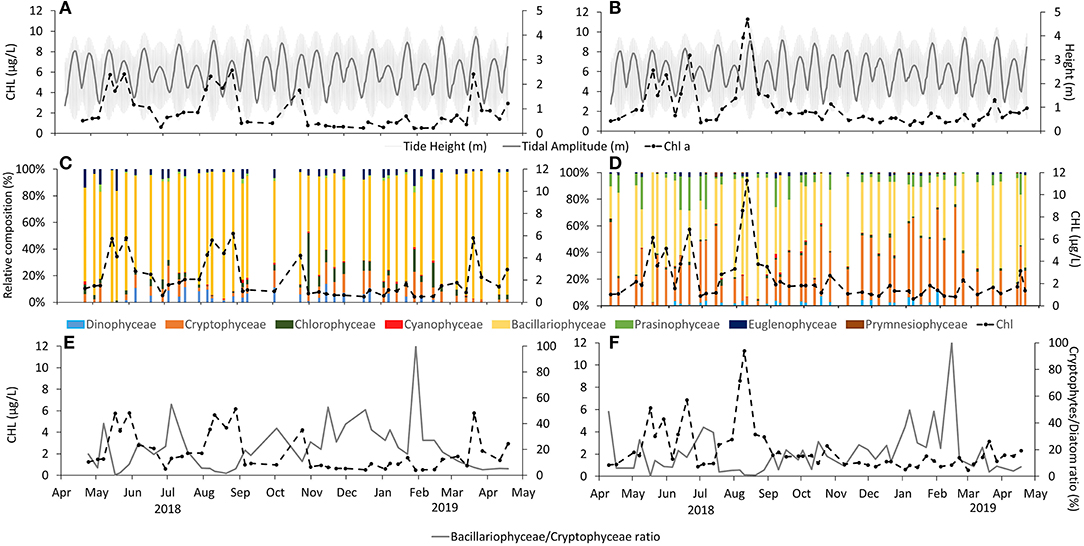
Figure 5. Chlorophyll a (quantified by spectrophotometer) distribution at (A) Alcântara (left side) and (B) Barreiro (right side) compared with tidal height (gray shade) and tidal range (gray line); in (C,D) with relative community composition quantified by HPLC and CHEMTAX and in (E,F) with Bacillariophyceae/Cryptophyceae ratio.
Phytoplankton Community Composition
In terms of phytoplankton community composition, it was possible to observe that Bacillariophyceae dominated the phytoplankton community in Alcântara throughout the year (up to 100% and a mean of 76% of the community, Figure 5). Other important groups in Alcântara were Chlorophyceae (up to 45% and mean of 5% of the community in October 2018), Cryptophyceae (up to 24% and mean of 8% of the community), and Dinophyceae with a maximum of 15% and mean of 4% of the community registered in September. In general, at Barreiro, Bacillariophyceae were also dominant (up to 97% and mean of 52% of the community, Figure 4), followed by Cryptophyceae (up to 74% and mean of 35% of the community) and Prasinophyceae (that represented up to 31% and a mean of 8% of the community). At both sampling sites, Bacillariophyceae clearly dominated the phytoplankton community when a chlorophyll a peak was observed (Figure 5).
In terms of tidal conditions, Bacillariophyceae presented stronger dominance during spring tides with up to 99 and 96% and a mean of 82 and 58% of the community at spring tides, in Alcântara and Barreiro, respectively. During neap tides, Bacillariophyceae represented up to 96% of the community in Alcântara and 91% at Barreiro, with associated means of 71 and 45% at Alcântara and Barreiro, respectively. Cryptophyceae presented the opposite trend with higher values during spring tides, representing up to 14 and 61% and means of 7 and 31% of the community for Alcântara and Barreiro. At neap tides, Cryptophyceae composed up to 24 and 74% and a mean of 9 and 40% of the community for Alcântara and Barreiro, respectively (Figure 6).
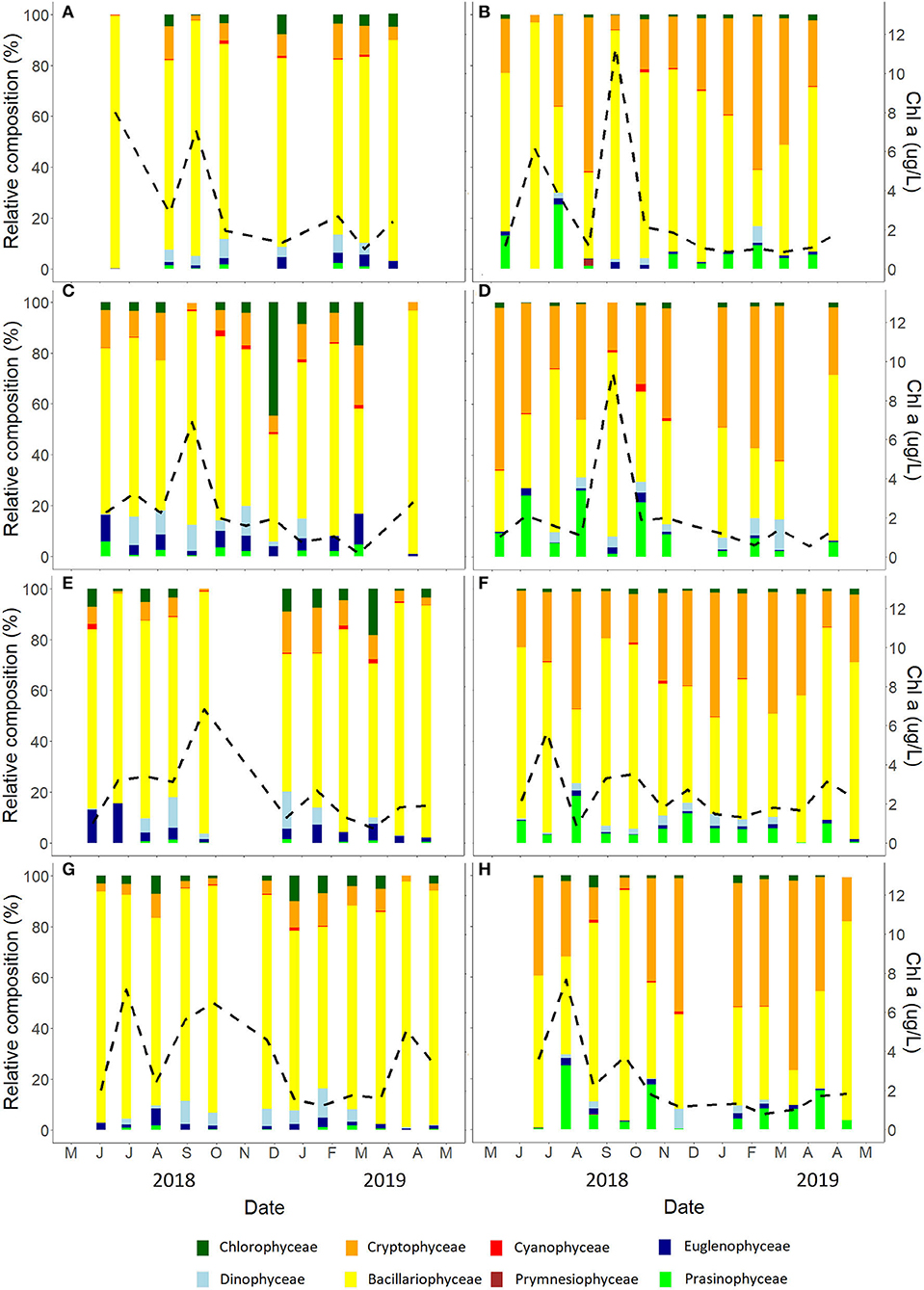
Figure 6. Community composition determined by CHEMTAX and expressed on micrograms per liter of chlorophyll a for Alcântara (left side) and Barreiro (right side) for spring high tide (A,B), neap high tide (C,D), neap low tide (E,F), and spring low tide (G,H).
Effect of the Spring-Neap Tidal Cycle on Phytoplankton Biomass
A Fourier analysis considering a maximum of 26 wave-pairs were found to be highly explicative for both Alcântara and Barreiro (92 and 90% of variance explained, respectively; Table 3; Figure 7). A good temporal agreement between model output, considering 26 wave-pairs, and data points were observed (Figure 7) for both sites. The seasonal cycle (1–3 waves) explained 37% of the variance at Alcântara. The higher-frequency temporal variation corresponding to a period down to 14 days, representing the spring vs. neap variation (4–26 waves) explained an additional 55% of the variance. At Barreiro, the results are very similar. The seasonal component explained 36% of the variance and an additional 54% were explained by fitting 4 to 26 waves (n). Fits were significant at a significance level of 0.05 (p < 0.001 for both Alcântara and Barreiro fits), which was used for all analyses.
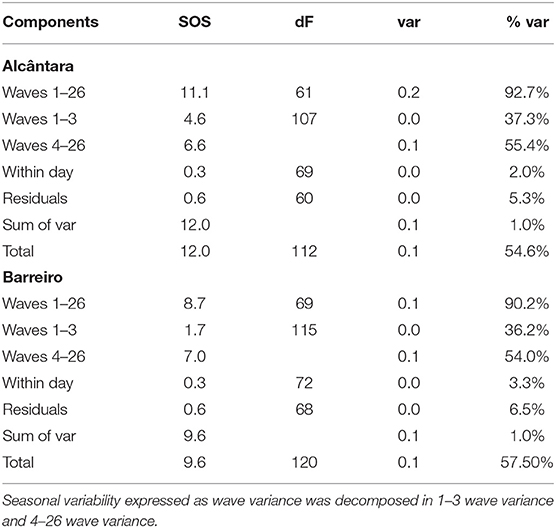
Table 3. Sum of squares (SOS), degrees of freedom (dF), variance (var), and percentage of explained variance (% var) of the three main components (waves, within day, and residuals) and totals.
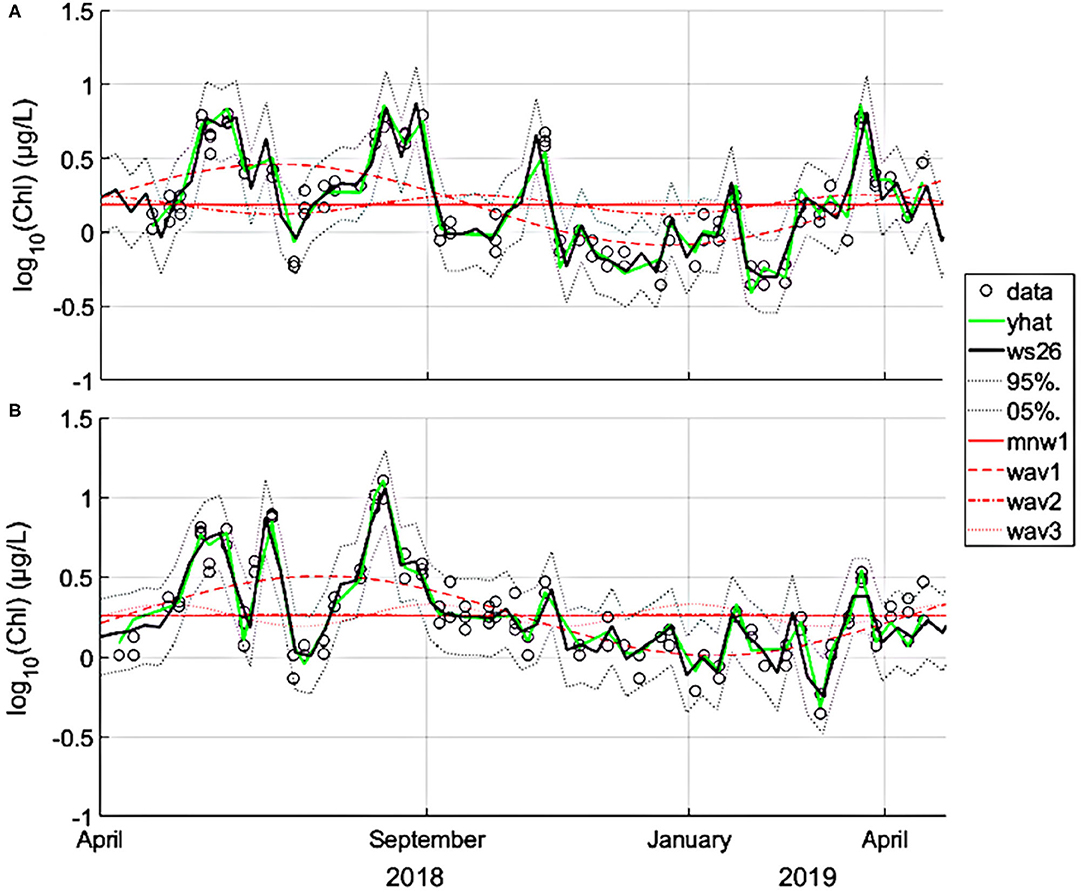
Figure 7. Seasonal pattern of chlorophyll a for Alcântara (A) and Barreiro (B), obtained by fitting 26 wave-pairs (sine–cosine) according to the truncated Fourier series approach. Log-transformed data are presented as dots. The 5 and 95% confidence intervals are represented as dotted black lines. The green line (yhat) is the equivalent to the sum of waves (ws) but adjusted for a time step that is coincident with the sampling date. Waves 1–3 (annual and seasonal variations) are presented as dashed and dotted red lines.
Assessment of Main Drivers of Phytoplankton Variability
The application of the GAM model to the chlorophyll a data, as a proxy of phytoplankton biomass, revealed a significant influence of several variables, such as the following: tidal range, water temperature, salinity, silicate concentration, DIN concentration, and river discharge. Salinity and river discharge values were higher during spring (Figure 3; Table 4). In Alcântara, chlorophyll a concentrations increased with tidal range and water temperature. Higher chlorophyll a concentrations were found to be associated with lower salinity (which was observed during spring), low silicate concentrations, low river discharges, and extreme values (both high and low) of DIN (Table 5; Supplementary Figure 1). For Barreiro, the main drivers of chlorophyll a concentrations were water temperature, pH, tidal range, silicate concentration, and solar radiation (Table 4). Of these, tidal range, water temperature, and salinity influenced the chlorophyll a concentrations with the same patterns observed in Alcântara (Table 5).
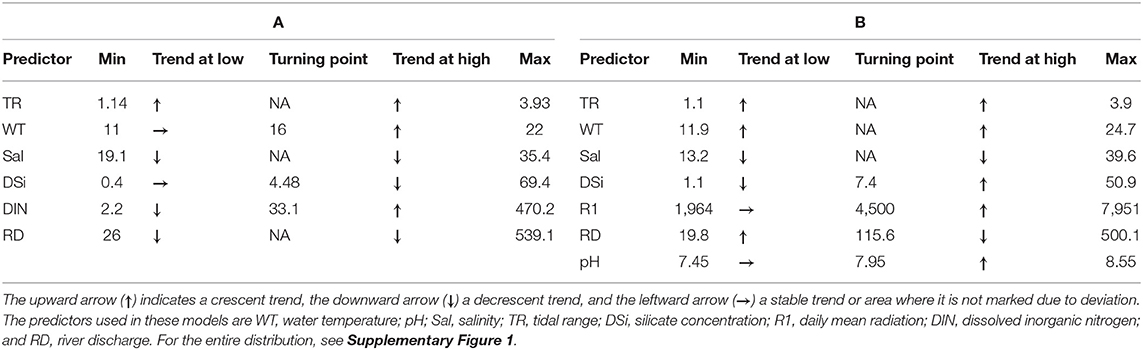
Table 5. Summary of the relationship between the predictor variables and chlorophyll a from minimum to a turning point in the trend (if any) and from there to maximum values of the predictor variable.
Additionally, high chlorophyll a concentrations were observed in association with high values of pH and solar radiation, particularly during late spring and summer (Table 5; Supplementary Figure 1).
Results from GAMs applied to groups are presented in Tables 6, 7, as well as in Supplementary Figure 2. General additive models show that, in Alcântara, Bacillariophyceae composition was positively related with tidal range and water temperature, while being negatively influenced by silicate concentration and river discharge. Cryptophyceae were significantly influenced by tidal range, water temperature, DIN, and silicate concentration and river discharge with all the variables presenting an inverse pattern to that found for the Bacillariophyceae. Dinophyceae presented significant differences only with river discharge, which increased their representativity from lower to medium values of river discharge but had no effect in higher values.
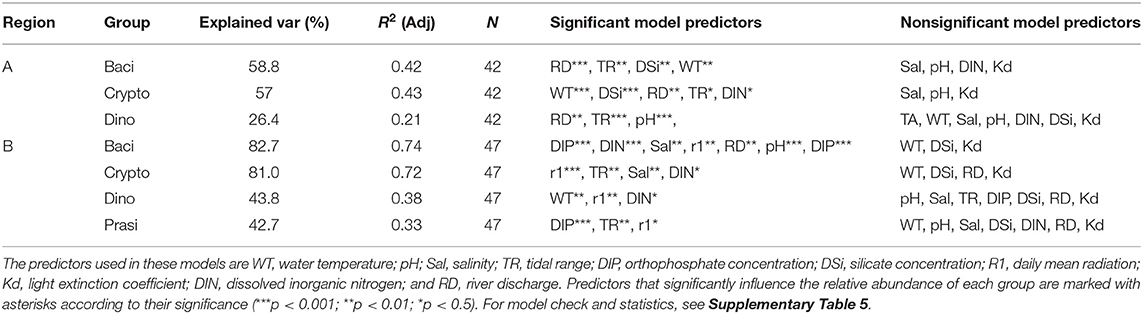
Table 6. Beta regression generalized additive model fit to each of the dominant groups (Bacillariophyceae, Cryptophyceae, and Prasinophyceae as Diato, Crypto, and Prasino, respectively) and Dinophyceae (as Dino) using cubic regression splines and K = 3.
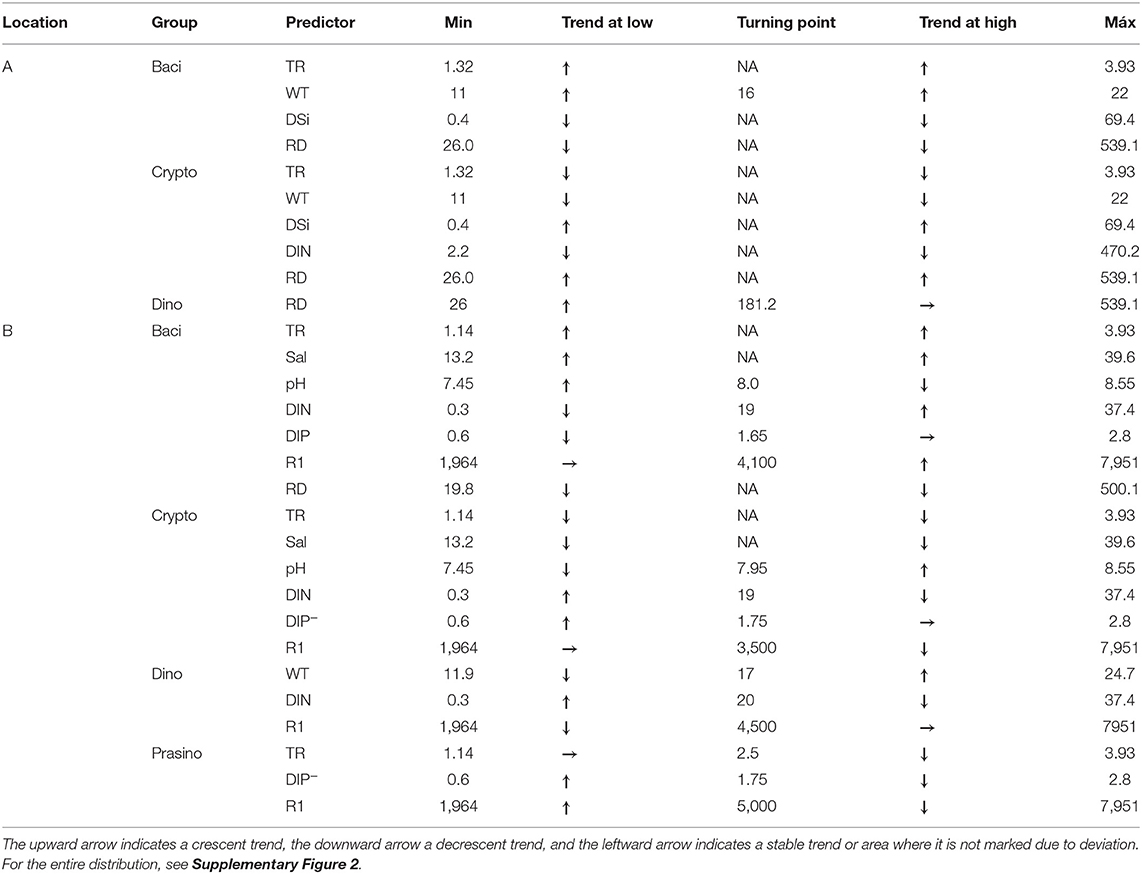
Table 7. Summary of the relation between the predictor variables and the phytoplankton groups from its minimum to a turning point in the trend (if any) and from there to the maximum of the predictor variable.
At Barreiro, the tidal range was the only variable that presented the same pattern of Alcântara for the different taxonomic groups. At Barreiro, Bacillariophyceae had a positive relationship with salinity, tidal range, and high values of solar radiation. Also, Bacillariophyceae were higher for median values of pH (maximum at around 8 of pH) and at extreme values of DIN (minimum at 20 μmol/L). In relation to DIP, Bacillariophyceae were higher when low concentrations of this nutrient were detected, presenting also a slight increase at high values (minimum at 1.7 μmol/L). Cryptophyceae were significantly influenced by water temperature, pH, salinity, tidal range, DIP, DIN, and solar radiation, from which all except water temperature (not significant for Bacillariophyceae) followed the opposite trend of that registered for Bacillariophyceae. In relation to water temperature, Cryptophyceae were negatively influenced by this variable, decreasing with warmer waters. Dinophyceae were significantly influenced by water temperature, tidal range, solar radiation, DIN, and SPM. From these, tidal range, solar radiation, and DIN presented an inverse pattern when compared with Bacillariophyceae. The importance of this phytoplankton class increased when the water temperature was higher than 17°C and lower than 16°C. Prasinophyceae representativity in the community was significantly influenced by tidal range, DIP, and solar radiation. Their importance decreased at high values of the tidal range being stable at ranges under 2.5 m. Also, higher percentages of Prasinophyceae were associated with medium values of both DIP and radiation (maxima at around 1.75 μmol/L of DIP and 5,000 W/m2 of radiation).
Discussion
Environmental Patterns in the Tagus Estuary
In previous studies, nutrient variability in the Tagus Estuary presented a seasonal trend, with maximal concentrations of dissolved inorganic nitrogen (DIN) and silicates during the winter–spring period. This is likely a consequence of their freshwater origin together with the decreased biomass of phytoplankton that is registered during winter, increasing again in the spring (Gameiro et al., 2007; Borges et al., 2020). Phosphates have also presented maximum values in summer, potentially due to the increase in water temperature during the summer that promotes the release of phosphate from the sediment (Gameiro et al., 2011; Borges et al., 2020). In estuaries, a decrease in turbidity, suspended matter, nutrients, and chlorophyll a from the turbidity maximum toward the estuary mouth is generally observed (conservative mixing) (Vale and Sundby, 1987; Cabeçadas et al., 1999; Garnier et al., 2010; Liang et al., 2013). Conservative mixing has been verified in several systems, such as the Scheldt (Soetaert et al., 2006), Lojn, Ems, and Douro estuaries, and occasionally also in the Girone Estuary (Lemaire et al., 2002; Azevedo et al., 2008). The Tagus Estuary presents its turbidity maximum around 30 to 50 km upstream of the estuary mouth, thus located upstream of the sampling stations of the present study (Vale and Sundby, 1987; Vale, 1990). In this work, the concentrations of nutrients, SPM and chlorophyll a observed at Barreiro were within the expected range for this location (SNIRH.pt accessed on February 18, 2021), being lower than the ones found in upstream areas (Gameiro et al., 2007; Gameiro and Brotas, 2010; Brito et al., 2012a). However, at Alcântara, a different situation was observed. The lowest values of chlorophyll a and DIP, as well as the maximum value for Secchi Depth, were observed at Alcântara. On the other hand, Alcântara also presented higher mean and maximum values of DIP, DIN, and suspended matter concentrations than those observed at the Barreiro sampling station. These values were also higher than the ones recorded in previous studies upstream in the estuary (Gameiro et al., 2007; Gameiro and Brotas, 2010; Brito et al., 2012a), which did not comply with the conservative mixing. Such higher concentrations were mainly detected during low tides while the lower nutrient concentrations were found during high tides. This divergence of the conservative mixing is probably a consequence of the proximity to a sewage treatment station outfall. Sewage discharges enhance both DIN and DIP while having a reduced effect on Si (Struyf et al., 2004; Caetano et al., 2016; Maguire and Fulweiler, 2016). Such associations between outfalls and the increase in inorganic forms of N and P have been verified in many European estuaries, such as the Ria de Aveiro (Lopes et al., 2007) and Forth estuary (Balls, 1990). In Alcântara, ammonium, nitrite, nitrate, and orthophosphate concentrations were highly correlated and presented high concentrations. Si, which is mainly originated by rock weathering (Treguer et al., 1995; Garnier et al., 2002), had concentrations that were within the range of values previously reported for the estuary (SNIRH.pt, accessed on February 1, 2020). In this case, during low tides, the water body is influenced by the water coming from the outfall. However, at high tide, the large volume of coastal water (poor in nutrients and turbidity) entering the estuary is bound to dissipate the outfall influence over this sampling location. Such effect may also have been magnified by the fact that the sampling was performed from land, and particularly, near the outfall. These stations had only 5–10 m depth in the low tide. Previous studies have verified that the sewage plume tends to be more noticeable closer to the margin (Costa et al., 2012). Therefore, if sampling is performed further away from the shoreline, where the bottom depth is higher, these differences are expected to be much smaller.
Temporal Variation of Phytoplankton Biomass as a Function of Its Drivers
Regarding annual variability, chlorophyll a presented a similar pattern in both sampling stations, yielding peaks in phytoplankton biomass from May to the end of June and in August. Afterwards, from October to March, chlorophyll a concentrations remained below 2 μg/L. Such temporal variability was identified by the first three waves of the Fourier analysis, explaining 37.2 and 36.2% of the data variability for Alcântara and Barreiro, respectively. The importance of seasonal effects on chlorophyll a is well-described in the literature as a common feature in estuarine and coastal waters (Iriarte and Purdie, 2004; Shen et al., 2011; Carstensen et al., 2015), as well as for the Tagus (Gameiro et al., 2007). In the Tagus Estuary, an increase in chlorophyll a is reported in spring and summer by several authors (Mateus and Neves, 2008; Gameiro and Brotas, 2010; Gameiro et al., 2011; Brito et al., 2012a). Still, as verified in the results presented herein, seasonal variations only accounted for a small part of the chlorophyll a variability, meaning that other periodic drivers were more relevant to chlorophyll a.
GAM analysis computed the relationship between chlorophyll a concentration and the variables that represented the seasonal variability: temperature and solar radiation (in Alcântara these parameters were highly correlated). Besides seasonal variables, also nutrients, salinity, and turbidity have been considered as important drivers of variation on phytoplankton biomass in the Tagus Estuary (Brogueira et al., 2007; Gameiro et al., 2007; Saraiva et al., 2007). In the present work, both salinity and nutrients were found to have a significant influence on chlorophyll a concentration at both sampling stations. Due to the location of these sampling stations in the lower estuary, silicate is probably being consumed upwards of these stations. Still, and although the correlation between discharges and silicate concentration was low, it is possible to observe that low silicate concentrations are coincident with low river discharge situations. In fact, although 2018 was not an extremely dry year for the area of the Tagus Estuary, 2019 was a dry year (Pordata.pt, accessed on February 2, 2020) (Base de dados de Portugal Contemporânio, 2020). As a consequence, a reduction in the river discharge in the final sampling months is clearly seen. The Tagus river discharges are greatly influenced by several dams along the river course. López-Moreno et al. (2009) noticed that dams reduce the seasonal effect of rain by decreasing the water flow in the winter and increasing it in the summer. In the same study, it is noticed that dams have the effect of increasing drought in areas downstream of their walls. Also, during the sampling period (spring 2018 to spring 2019), parts of the Iberian Peninsula were under drought, hence it is likely that large amounts of water could have been transferred from the Tagus to smaller rivers, a common procedure in dry years (Morote et al., 2019). Such reduction in freshwater discharges leads to a reduction in the entrance of silicates to the estuary (Rodrigues et al., 2019). As the Estuarine Turbidity Maximum is dependent on salinity, it may be forced upstream, which can promote phytoplankton growth in the lower areas of the estuary, leading to an increase in nutrient assimilation. The reduction in the freshwater input, and consequently in the DSi concentrations, can also lead to changes in the phytoplankton community (Rocha et al., 2002; Domingues et al., 2008, 2015). Effects of silicate reduction on the phytoplankton biomass and community succession has also been registered in several systems such as the Curonian Lagoon, in the Baltic Sea (Pilkaityte and Razinkovas, 2006), Moreton Bay (Glibert et al., 2006), and Pearl River Estuary (Yin et al., 2000).
The Fourier series analysis also indicated that high-frequency processes, up to 26 waves (i.e., 15-day variation) explained up to 55 and 58% of phytoplankton variability in Alcântara and Barreiro, respectively. The influence of the tidal range, which presents a periodicity of 15 days, on the phytoplankton dynamics has been well described in other estuaries and enclosed waters (Cloern, 1991; Gianesella et al., 2000; Azhikodan and Yokoyama, 2016). Generally, this relationship is driven by changes in the residence time and in the dilution effect due to the entrance of coastal waters, with higher chlorophyll a concentrations usually associated with neap tides. Monbet (1992) analyzed the influence of the neap-spring tidal cycle, conducting a metaanalysis with several estuaries around the world (including the Tagus Estuary) and suggested that the lower chlorophyll a concentrations should be observed during spring tides, as a consequence of the higher mixing during such tidal conditions that can drag the phytoplankton cells under the photic layer. In fact, the tidal range effect over chlorophyll was significant at both stations, presenting an increase in chlorophyll a at higher tidal range (spring tides). Given that higher tidal ranges are likely to be associated with lower residence times and higher turbidity, this seems to be in disagreement with what was previously described in the literature, i.e., an association between higher chlorophyll a concentration with higher residence times caused by neap tides (Cloern, 1991; Lucas et al., 1999; Trigueros and Orive, 2000). This is probably a combination of the well-flushed nature of the low and mid-Tagus Estuary, with the large extent of highly productive mudflats present in the mid-estuary (Brotas and Catarino, 1995; Rodrigues and Fortunato, 2017). Nevertheless, it is important to note that the residence time in the Tagus results from the joint action of several factors, such as tide, river flow and wind (Oliveira and Baptista, 1998; Braunschweig et al., 2003; Vaz and Dias, 2014) that adds complexity to the analysis. In addition, such an increase in chlorophyll a concentrations does not seem to result from changes in light availability during spring tides since turbidity increases during such tidal conditions due to the resuspension of sediments (Vale and Sundby, 1987). This resuspension is a common feature of highly turbid estuaries (De Jorge and Van Beusekom, 1995; Gianesella et al., 2000; Ubertini et al., 2012) and contributes to the increase in photosynthetic biomass by the resuspension of microphytobenthos (Brotas et al., 1995; Cabrita and Brotas, 2000; Jesus et al., 2009). The importance of tide in resuspending microphytobenthos and its association with SPM have been observed in other systems (Brito et al., 2012b, Redzuan and Underwood, 2021). In this case, the resuspension of sediments by the stronger currents of the spring tides could be the cause for the increase in the chlorophyll a in the water column. In the mid part of the Tagus Estuary, Brito et al. (2015) reported high numbers of several diatom species usually associated with microphytobenthos, such as Navicula sp. and Diploneis sp. In the future, additional microscopy analysis could provide valuable information to confirm such hypotheses for these locations.
Community Composition
General Patterns of Community Composition
In estuaries, the dominance of Bacillariophyceae over small flagellates is considered an indicator of a good environmental state (Devlin et al., 2012; Ferreira et al., 2017) and is found in many of the European estuaries as, the Krka Estuary (Cetinić et al., 2006), Schelde Estuary, Elbe Estuary (Muylaert and Sabbe, 1996), Ria de Aveiro (Lopes et al., 2007), and Guadiana Estuary (Rocha et al., 2002; Domingues et al., 2005). Bacillariophyceae has been recorded as the main bloomers in the mid-Tagus Estuary (Gameiro and Brotas, 2010). In a recent period (2006–2007), Brito et al. (2015) reported a reduction in Bacillariophyceae dominance in favor of Cryptophyceae. The authors suggested that this shift could be caused by a top–down effect due to the increase of the invasive clam Ruditapes philippinarum. Grazing is known for being able to shape the estuarine phytoplankton community (Lonsdale et al., 1996; Cloern, 2018). Bacillariophyceae are more edible for organisms at higher trophic levels, such as large fish and shellfish (Officer and Ryther, 1980; National Research Council, 1993). The influence of bivalve grazing over the community greatly varies with the system in analysis. Jones et al. (2017), analyzing the bivalve grazing effect on the food web in Whangateau Harbor, verified that its effect was higher over microphytobenthos than over pelagic species. On the other hand, Jiang et al. (2020) verified that grazing was one of the dominant drivers of phytoplankton spatial variability at Eastern Scheldt. Such relationships still need to be shown for the Tagus Estuary, but the continuous monitoring program that has been performed for 20 years in the mid-Tagus Estruary does not show a clear shift from Bacillariophyceae to Cryptophyceae in the latest years (Tracana and Brotas, 2019). In the present work, Bacillariophyceae were the dominant group in both sampling sites with Cryptophyceae being the second most important group, presenting small importance in Alcântara. Gameiro et al. (2007), which also used CHEMTAX to evaluate the community composition, observed a decrease in Bacillariophyceae and Chlorophyceae importance in the community from upstream to downstream with Cryptophyceae, Prasinophyceae, and Dinophyceae gaining importance toward downstream areas. Barreiro sampling station presented a similar trend with higher importance of Cryptophyceae and Prasinophyceae than that found in the central zone of the estuary by Gameiro et al. (2007). In contrast, the Alcântara community was almost entirely composed of Bacillariophyceae in most of the sampling occasions. Such high Bacillariophyceae dominance in comparison with the mid-estuary (Gameiro et al., 2007) is probably due to the higher influence of coastal waters dominated by Bacillariophyceae species, such as Detonula pumila and Thalassiosira sp. These taxa are dominant in the nearby Cascais Bay (Silva et al., 2009) and are carried to the estuary due to the strong currents observed in this area (Fortunato et al., 1997; Guerreiro et al., 2015). Chlorophyceae presented small importance in both Alcântara and Barreiro probably due to its freshwater origin (Cetinić et al., 2006; Gameiro et al., 2007).
Main Drivers of Variability in Community Composition
Seasonal drivers (i.e., radiation and water temperature), nutrients availability (Si and DIN in Alcântara and DIN and PO4 in Barreiro), tidal range, and river discharges explained variations in the phytoplankton community at both sampling stations. The Bacillariophyceae yielded a variation pattern that was similar to that described for chlorophyll a, indicating that Bacillariophyceae were the main group contributing to its variations. In many estuaries, phytoplankton seasonality is characterized as having a higher percentage of Bacillariophyceae from autumn to early spring with an increase in the flagellate fraction during summer, as seen in Ria de Aveiro (Lopes et al., 2007) and Guadiana Estuary (Rocha et al., 2002; Domingues et al., 2005). In this study, such a pattern was not observed as diatoms presented higher percentages during summer. In fact, Gameiro et al. (2007) has reported high flagellate contributions to the community at some stations during winter and autumn, presenting great annual variability. The Cryptophyceae presented a significant variation with almost all the same variables as Bacillariophyceae, but with opposite trends, meaning that they can benefit from conditions that are not the most adequate for Bacillariophyceae. Dinophyceae and Prasinophyceae were less represented, but, in general, also followed the opposite trend when compared with Bacillariophyceae.
The negative relationship between Bacillariophyceae and river discharge is a consequence of seasonality, due to higher river discharges in early spring. Nutrients were also important in explaining the changes in groups, with DSi concentrations presenting an inverse relationship with Bacillariophyceae in Alcântara while in Barreiro an inverse association with nutrients was detected with both DIN and DIP (MATLAB, 2019). Such trends may also be a consequence of seasonality due to lower nutrient concentrations during summer when nutrients are low due to high consumption and low riverine nutrient input. In Alcântara, the pattern is mostly influenced by the outfall introducing high concentrations of DIN and DIP with Bacillariophyceae responding only to DSi. Due to the association of nutrients with the river discharges, it is important to assess in future works what changes in the hydrologic regime can influence the community composition of the Tagus Estuary, as well as maintaining a concise monitoring of the phytoplankton community composition. Shifts in the phytoplankton community can facilitate the blooming of harmful algae bloom and influence phytoplankton biomass. Also, the change in the dominance from Bacillariophyceae to flagellate taxa can affect the estuarine food-web as diatoms are more easily digested by large filter feeders that usually are the link to higher trophic levels (Officer and Ryther, 1980; Managing Wastewater in Coastal Urban Areas, 1993).
The positive relation between Bacillariophyceae and tidal range follows the variations observed for chlorophyll and is likely to result from a combination of factors, in which microphytobenthos resuspension and the higher penetration of coastal water rich in Bacillariophyceae, should play a crucial role. In Barreiro, due to the extension of the mudflat areas in mid-estuary, microphytobenthos should make a relevant contribution to overall chlorophyll a during spring tides. The coupling between microphytobenthos and pelagic microalgae can be an important characteristic of the estuary, as indicated by the presence of microphytobenthos species in phytoplankton (Brotas and Catarino, 1995; Gameiro et al., 2011; Brito et al., 2012b). During spring tides, the strong currents registered in the estuary would not only resuspend microphytobenthos but also reduce the sinking rate, allowing heavier cells, such as Bacillariophyceae, to easily avoid sedimentation. In Alcântara the main factor contributing to the Bacillariophyceae dominance was probably the proximity to coastal waters and the higher water mixing during spring tides. Additionally, this is in accordance with the study of Margalef (1978) that indicated that Bacillariophyceae are positively influenced by high values of nutrients and mixing, while Cryptophyceae prefer more stratified low-nutrient waters.
Conclusions
In summary, the phytoplankton community in the Tagus Estuary was greatly influenced by two main factors: seasonality and tidal range. The fortnight tidal cycle explained most of the variability. This is a key finding for this study, indicating the relevance of taking into spring-neap tidal cycles in estuarine assessments of water quality. In addition, higher phytoplankton biomass, associated with higher dominance of Bacillariophyceae in the community, was mainly observed during spring tides. Bacillariophyceae seems to be the group that contributes the most to the overall phytoplankton biomass in the Tagus Estuary. In Alcântara, the physicochemical parameters (i.e., nutrients and SPM) presented strong variations in the spring-neap tidal cycle, probably due to the presence of an outfall. The results presented in this work reinforce the need to assess the influence of the tidal cycle to fully understand ecosystem functioning in estuaries. This is also key to evaluate how the tidal effect influences water quality classifications, as well as investigate how climate change will affect estuarine systems in the near future.
Data Availability Statement
The original contributions presented in the study are included in the article/Supplementary Materials, further inquiries can be directed to the corresponding author.
Author Contributions
RC performed the sampling campaigns and laboratory analysis, performed the statistical analysis and image formatting, and is the main writer of the document. VB contributed with the idea and together with AB and MR planed the sampling campaigns, acquired the necessary resources, and reviewed the document and statistics. JC helped on the sampling campaigns, performed laboratory analysis, and reviewed the CHEMTAX procedure and the document. All authors contributed to manuscript revision, read, and approved the submitted version.
Funding
The authors acknowledge Andreia Tracana, Pedro Oliveira, and Joshua Heumüller for their support in field campaigns and data analysis. In addition, the authors are grateful to Administração do Porto de Lisboa (APL) and Serviço de Estrageiros e Fronteiras (SEF) for allowing access to Alcântara harbor. RC and AB received funding from Fundação para a Ciência (FCT) e a Tecnologia (PD/BD/135064/2017 and CEECIND/00095/2017, respectively). This work received further support from the following projects: UBEST (PTDC/AAGMAA/6899/2014) funded by FCT; Infrastructure CoastNet (http://geoportal.coastnet.pt) funded by FCT, and the European Regional Development Fund (FEDER) through LISBOA2020 and ALENTEJO2020 regional operational programs, in the framework of the National Roadmap of Research Infrastructures of strategic relevance (PINFRA/22128/2016); MARE Center strategic grant (UIDB/04292/2020); and IDL strategic grant (UIDB/50019/2020), also granted by FCT. This work was funded by the Copernicus Evolution: Research for Harmonized Transitional Water Observation (CERTO) under the European Union's Horizon 2020 Research and Innovation Programme, Grant No. 870349 and was also supported by funding from the European Union's Horizon 2020 Research and Innovation Programme under grant agreement N810139: Project Portugal Twinning for Innovation and Excellence in Marine Science and Earth Observation (PORTWIMS).
Conflict of Interest
The authors declare that the research was conducted in the absence of any commercial or financial relationships that could be construed as a potential conflict of interest.
Supplementary Material
The Supplementary Material for this article can be found online at: https://www.frontiersin.org/articles/10.3389/fmars.2021.675699/full#supplementary-material
References
Agência Portuguesa do Ambiente (2016). Plano de Gestão da Região Hidrográfica, RH5, Parte 2 – Caracterização e Diagnostico. Lisbon: Agência Portuguesa do Ambiente.
Azevedo, I. C., Duarte, P. M., and Bordalo, A. A. (2008). Understanding spatial and temporal dynamics of key environmental characteristics in a mesotidal Atlantic estuary (Douro, NW Portugal). Estuar. Coast. Shelf Sci. 76, 620–633. doi: 10.1016/j.ecss.2007.07.034
Azhikodan, G., and Yokoyama, K. (2016). Spatio-temporal variability of phytoplankton (Chlorophyll-a) in relation to salinity, suspended sediment concentration, and light intensity in a macrotidal estuary. Cont. Shelf Res. 126, 15–26. doi: 10.1016/j.csr.2016.07.006
Bajželj, B., Richards, K. S., Allwood, J. M., Smith, P., Dennis, J. S., Curmi, E., et al. (2014). Importance of food-demand management for climate mitigation. Nat. Clim. Chang. 4:924. doi: 10.1038/nclimate2353
Balls, P. W. (1990). Distribution and composition of suspended particulate material in the Clyde estuary and associated sea lochs. Estuar. Coast. Shelf Sci. 30, 475–487. doi: 10.1016/0272-7714(90)90068-3
Balls, P. W. (1994). Nutrient inputs to estuaries from nine Scottish east coast rivers; influence of estuarine processes on inputs to the North Sea. Estuar. Coast. Shelf Sci. 39, 329–352. doi: 10.1006/ecss.1994.1068
Base de dados de Portugal Contemporânio (2020). Available online at: http://www.pordata.pt (accessed February 2, 2020).
Bendschneider, K., and Robinson, R. J. (1952). A New Spectrophotometric Method for the Determination of Nitrite in Sea Water. Washington, DC: University of Washington Oceanographic Laboratories.
Borges, C., da Silva, R. J. B., and Palma, C. (2020). Determination of river water composition trends with uncertainty: seasonal variation of nutrients concentration in Tagus river estuary in the dry 2017 year. Mar. Pollut. Bull. 158:111371. doi: 10.1016/j.marpolbul.2020.111371
Braunschweig, F., Martins, F., Chambel, P., and Neves, R. (2003). A methodology to estimate renewal time scales in estuaries: the Tagus Estuary case. Ocean Dyn. 53, 137–145. doi: 10.1007/s10236-003-0040-0
Brito, A., Newton, A., Tett, P., and Fernandes, T. F. (2009). Temporal and spatial variability of microphytobenthos in a shallow lagoon: Ria Formosa (Portugal). Estuar. Coast. Shelf Sci. 83, 67–76. doi: 10.1016/j.ecss.2009.03.023
Brito, A. C., Brotas, V., Caetano, M., Coutinho, T. P., Bordalo, A. A., Icely, J., et al. (2012a). Defining phytoplankton class boundaries in Portuguese transitional waters: an evaluation of the ecological quality status according to the Water Framework Directive. Ecol. Indic. 19, 5–14. doi: 10.1016/j.ecolind.2011.07.025
Brito, A. C., Fernandes, T. F., Newton, A., Facca, C., and Tett, P. (2012b). Does microphytobenthos resuspension influence phytoplankton in shallow systems? A comparison through a Fourier series analysis. Estuar. Coast. Shelf Sci. 110, 77–84. doi: 10.1016/j.ecss.2012.03.028
Brito, A. C., Moita, T., Gameiro, C., Silva, T., Anselmo, T., and Brotas, V. (2015). Changes in the phytoplankton composition in a temperate estuarine system (1960 to 2010). Estuaries Coasts 38, 1678–1691. doi: 10.1007/s12237-014-9900-8
Brogueira, M. J., do Rosário Oliveira, M., and Cabeçadas, G. (2007). Phytoplankton community structure defined by key environmental variables in Tagus estuary, Portugal. Mar. Environ. Res. 64, 616–628. doi: 10.1016/j.marenvres.2007.06.007
Brotas, V., Cabrita, T., Portugal, A., Serôdio, J., and Catarino, F. (1995). “Spatio-temporal distribution of the microphytobenthic biomass in intertidal flats of Tagus Estuary (Portugal),” in Space Partition Within Aquatic Ecosystems. Developments in Hydrobiology, ed G. Balvay (Dordrecht: Springer), 93–104. doi: 10.1007/978-94-011-0293-3_8
Brotas, V., and Catarino, F. (1995). Microphytobenthos primary production of Tagus estuary intertidal flats (Portugal). Netherland J. Aquatic Ecol. 29, 333–339. doi: 10.1007/BF02084232
Brotas, V., and Plante-Cuny, M. R. (1996). Identification and quantification of chlorophyll and carotenoid pigments in marine sediments. A protocol for HPLC analysis. Oceanol. Acta 19, 623–634.
Brzezinski, M. A. (1985). The Si: C: N ratio of marine diatoms: interspecific variability and the effect of some environmental variables 1. J. Phycol. 21, 347–357. doi: 10.1111/j.0022-3646.1985.00347.x
Cabeçadas, G., Nogueira, M., and Brogueira, M. J. (1999). Nutrient dynamics and productivity in three European estuaries. Mar. Pollut. Bull. 38, 1092–1096. doi: 10.1016/S0025-326X(99)00111-3
Cabeçadas, L. (1999). Phytoplankton production in the Tagus estuary (Portugal). Oceanol. Acta 22, 205–214. doi: 10.1016/S0399-1784(99)80046-2
Cabral, H., and Costa, M. J. (1999). Differential use of nursery areas within the Tagus estuary by sympatric soles, Solea solea and Solea senegalensis. Environ. Biol. Fishes. 56, 389–397. doi: 10.1023/A:1007571523120
Cabrita, M. T., and Brotas, V. (2000). Seasonal variation in denitrification and dissolved nitrogen fluxes in intertidal sediments of the Tagus estuary, Portugal. Mar. Ecol. Prog. Ser. 202, 51–65. doi: 10.3354/meps202051
Cabrita, M. T., and Moita, M. T. (1995). Spatial and temporal variation of physico-chemical conditions and phytoplankton during a dry year in the Tagus estuary (Portugal). Netherland J. Aquatic Ecol. 29, 323–332. doi: 10.1007/BF02084231
Caeiro, S., Costa, M. H., Ramos, T. B., Fernandes, F., Silveira, N., Coimbra, A., et al. (2005). Assessing heavy metal contamination in Sado Estuary sediment: an index analysis approach. Ecol. Indic. 5, 151–169. doi: 10.1016/j.ecolind.2005.02.001
Caetano, M., Raimundo, J., Nogueira, M., Santos, M., Mil-Homens, M., Prego, R., et al. (2016). Defining benchmark values for nutrients under the Water Framework Directive: application in twelve Portuguese estuaries. Mar. Chem. 185, 27–37. doi: 10.1016/j.marchem.2016.05.002
Carstensen, J., Klais, R., and Cloern, J. E. (2015). Phytoplankton blooms in estuarine and coastal waters: seasonal patterns and key species. Estuar. Coast. Shelf Sci. 162, 98–109. doi: 10.1016/j.ecss.2015.05.005
Cereja, R., Mendonça, V., Dias, M., Madeira, D., and Vinagre, C. (2017). Effect of Anilocra frontalis parasitization on the thermal tolerance, acclimation capacity and condition of its fish host Pomatoschistus microps (Krøyer. 1838). J. Appl. Ichthyol. 33, 829–831. doi: 10.1111/jai.13403
Cetinić, I., Viličić, D., Burić, Z., and Olujć, G. (2006). Phytoplankton seasonality in a highly stratified karstic estuary (Krka, Adriatic Sea). Hydrobiologia. 555, 31–40. doi: 10.1007/s10750-005-1103-7
Chatfield, C. (2003). The Analysis of Time Series: An Introduction. New York, NY: Chapman and Hall CRC, p. 352.
Cloern, J. E. (1987). Turbidity as a control on phytoplankton biomass and productivity in estuaries. Cont. Shelf Res. 7, 1367–1381. doi: 10.1016/0278-4343(87)90042-2
Cloern, J. E. (1991). Tidal stirring and phytoplankton bloom dynamics in an estuary. J. Mar. Res. 49, 203–221. doi: 10.1357/002224091784968611
Cloern, J. E. (2001). Our evolving conceptual model of the coastal eutrophication problem. Mar. Ecol. Prog. Ser. 210, 223–253. doi: 10.3354/meps210223
Cloern, J. E. (2018). Why large cells dominate estuarine phytoplankton. Limnol. Oceanogr. 63, S392–S409. doi: 10.1002/lno.10749
Colbert, D., and McManus, J. (2003). Nutrient biogeochemistry in an upwelling-influenced estuary of the Pacific Northwest (Tillamook Bay, Oregon, USA). Estuaries 26, 1205–1219. doi: 10.1007/BF02803625
Costa, A. C., Santos, J. A., and Pinto, J. G. (2012). Climate change scenarios for precipitation extremes in Portugal. Theor. Appl. Climatol. 108, 217–234. doi: 10.1007/s00704-011-0528-3
David, L. M., Rodrigues, M., Fortunato, A. B., Oliveira, A., Mota, T., Costa, J., et al. (2015). “Demonstration system for early warning of faecal contamination in recreational waters in Lisbon,” in Climate Change, Water Supply and Sanitation: Risk Assessment, Management, Mitigation and Reduction, Chapter 1- Demonstrations, subchapter 1.4, eds A. Hulsmann, G. Grützmacher, G. van den Berg, W. Rauch, A. L. Jensen, V. Popovych, et al. (IWA Publishing), 10.
Davis, K. A., Banas, N. S., Giddings, S. N., Siedlecki, S. A., MacCready, P., Lessard, E. J., et al. (2014). Estuary-enhanced upwelling of marine nutrients fuels coastal productivity in the US Pacific Northwest. J. Geophys. Res. Oceans 119, 8778–8799. doi: 10.1002/2014JC010248
De Jorge, V. N., and Van Beusekom, J. E. E. (1995). Wind-and tide-induced resuspension of sediment and microphytobenthos from tidal flats in the Ems estuary. Limnol. Oceanogr. 40, 776–778. doi: 10.4319/lo.1995.40.4.0776
Devlin, M., Best, M., Bresnan, E., Scanlan, C., and Baptie, M. (2012). Water Framework Directive: The Development and Status of Phytoplankton Tools for Ecological Assessment of Coastal and Transitional Waters. United Kingdom Water Framework Directive: United Kingdom Technical Advisory Group. Available online at: https://www.wfduk.org/sites/default/files/Media/Characterisation%20of%20the%20water%20environment/Biological%20Method%20Statements/Phytoplankton%20Technical%20Report_0.pdf
Devlin, M., Best, M., Coates, D., Bresnan, E., O'Boyle, S., Park, R., et al. (2007). Establishing boundary classes for the classification of UK marine waters using phytoplankton communities. Mar. Pollut. Bull. 55, 91–103. doi: 10.1016/j.marpolbul.2006.09.018
Dias, M. P., Peste, F., Granadeiro, J. P., and Palmeirim, J. M. (2008). Does traditional shellfishing affect foraging by waders? The case of the Tagus estuary (Portugal). Acta Oecol. 33, 188–196. doi: 10.1016/j.actao.2007.10.005
Domingues, R. B., Anselmo, T. P., Barbosa, A. B., Sommer, U., and Galvão, H. M. (2011). Light as a driver of phytoplankton growth and production in the freshwater tidal zone of a turbid estuary. Estuar. Coast. Shelf Sci. 91, 526–535. doi: 10.1016/j.ecss.2010.12.008
Domingues, R. B., Barbosa, A., and Galvao, H. (2005). Nutrients, light and phytoplankton succession in a temperate estuary (the Guadiana, south-western Iberia). Estuar. Coast. Shelf Sci. 64, 249–260. doi: 10.1016/j.ecss.2005.02.017
Domingues, R. B., Barbosa, A., and Galvão, H. (2008). Constraints on the use of phytoplankton as a biological quality element within the Water Framework Directive in Portuguese waters. Mar. Pollut. Bull. 56, 1389–1395. doi: 10.1016/j.marpolbul.2008.05.006
Domingues, R. B., Guerra, C. C., Barbosa, A. B., and Galvão, H. M. (2015). Are nutrients and light limiting summer phytoplankton in a temperate coastal lagoon? Aquatic Ecol. 49, 127–146. doi: 10.1007/s10452-015-9512-9
Fanning, K. A., and Pilson, M. (1973). On the spectrophotometric determination of dissolved silica in natural waters. Anal. Chem. 45, 136–140. doi: 10.1021/ac60323a021
Ferreira, J. G., Andersen, J. H., Borja, A., Bricker, S. B., Camp, J., Cardoso da Silva, M., et al. (2017). Marine Strategy Framework Directive—Task Group 5 Report: Eutrophication. Luxembourg: Office for Official Publications of the European Communities.
Ferreira, J. G., Wolff, W. J., Simas, T. C., and Bricker, S. B. (2005). Does biodiversity of estuarine phytoplankton depend on hydrology? Ecol. Modell. 187, 513–523. doi: 10.1016/j.ecolmodel.2005.03.013
Fortunato, A., Baptista, A. M., and Luettich, R. A. Jr. (1997). A three-dimensional model of tidal currents in the mouth of the Tagus estuary. Cont. Shelf Res. 17, 1689–1714. doi: 10.1016/S0278-4343(97)00047-2
Gameiro, C., and Brotas, V. (2010). Patterns of phytoplankton variability in Tagus Estuary (Portugal). Estuar. Coast. Shelf Sci. 33, 311–906. doi: 10.1007/s12237-009-9194-4
Gameiro, C., Cartaxana, P., and Brotas, V. (2007). Environmental drivers of phytoplankton distribution and composition in Tagus Estuary, Portugal. Estuar. Coast. Shelf Sci. 75, 21–34. doi: 10.1016/j.ecss.2007.05.014
Gameiro, C., Cartaxana, P., Cabrita, M. T., and Brotas, V. (2004). Variability in chlorophyll and phytoplankton composition in an estuarine system. Hydrobiologia 525, 113–124. doi: 10.1023/B:HYDR.0000038858.29164.31
Gameiro, C., Zwolinski, J., and Brotas, V. (2011). Light control on phytoplankton production in a shallow and turbid estuarine system. Hydrobiologia 669:249. doi: 10.1007/s10750-011-0695-3
Garcia-Soto, C., De Madariaga, I., Villate, F., and Orive, E. (1990). Day-to-day variability in the plankton community of a coastal shallow embayment in response to changes in river runoff and water turbulence. Estuar. Coast. Shelf Sci. 31, 217–229. doi: 10.1016/0272-7714(90)90102-W
Garnier, J., Billen, G., Hannon, E., Fonbonne, S., Videnina, Y., and Soulie, M. (2002). Modelling the transfer and retention of nutrients in the drainage network of the Danube River. Estuar. Coast. Shelf Sci. 54, 285–308. doi: 10.1006/ecss.2000.0648
Garnier, J., Billen, G., Némery, J., and Sebilo, M. (2010). Transformations of nutrients (N, P, Si) in the turbidity maximum zone of the Seine estuary and export to the sea. Estuar. Coastal Shelf Sci. 90, 129–141. doi: 10.1016/j.ecss.2010.07.012
Geyer, W. R. (1993). The importance of suppression of turbulence by stratification on the estuarine turbidity maximum. Estuaries 16, 113–125. doi: 10.2307/1352769
Gianesella, S. M. F., Saldanha-Corrêa, F. M. P., and Teixeira, C. (2000). Tidal effects on nutrients and phytoplankton distribution in Bertioga Channel, São Paulo, Brazil. Aquat. Ecosyst. Health Manag. 3, 533–544. doi: 10.1080/14634980008650690
Glibert, P. M., Heil, C. A., O'neil, J. M., Dennison, W. C., and O'Donohue, M. J. (2006). Nitrogen, phosphorus, silica, and carbon in Moreton Bay, Queensland, Australia: differential limitation of phytoplankton biomass and production. Estuar. Coasts 29, 209–221. doi: 10.1007/BF02781990
Goosen, N. K., Kromkamp, J., Peene, J., van Rijswijk, P., and van Breugel, P. (1999). Bacterial and phytoplankton production in the maximum turbidity zone of three European estuaries: the Elbe, Westerschelde and Gironde. J. Marine Syst. 22, 151–171. doi: 10.1016/S0924-7963(99)00038-X
Grasshoff, K., (ed.). (1976). “Determination of nitrate,” in Methods of Seawater Analysis (Veinheim: Verlag Chemie), 137–145.
Guerreiro, M., Fortunato, A. B., Freire, P., Rilo, A., Taborda, R., Freitas, M. C., et al. (2015). Evolution of the hydrodynamics of the Tagus estuary (Portugal) in the 21st century. Rev. Gestão Costeira Integrada J. Integrated Coastal Zone Manage. 15, 65–80. doi: 10.5894/rgci515
Iriarte, A., and Purdie, D. A. (2004). Factors controlling the timing of major spring bloom events in an UK south coast estuary. Estuar. Coast. Shelf Sci. 61, 679–690. doi: 10.1016/j.ecss.2004.08.002
Jesus, B., Brotas, V., Ribeiro, L., Mendes, C. R., Cartaxana, P., and Paterson, D. M. (2009). Adaptations of microphytobenthos assemblages to sediment type and tidal position. Continental Shelf Res. 29, 1624–1634. doi: 10.1016/j.csr.2009.05.006
Jiang, L., Gerkema, T., Kromkamp, J. C., van der Wal, D., Carrasco De La Cruz, P. M., and Soetaert, K. (2020). Drivers of the spatial phytoplankton gradient in estuarine–coastal systems: generic implications of a case study in a Dutch tidal bay. Biogeosciences 17, 4135–4152. doi: 10.5194/bg-17-4135-2020
Jones, H. F., Pilditch, C. A., Hamilton, D. P., and Bryan, K. R. (2017). Impacts of a bivalve mass mortality event on an estuarine food web and bivalve grazing pressure. N. Z. J. Mar. Freshwater Res. 51, 370–392. doi: 10.1080/00288330.2016.1245200
Kessarkar, P. M., Rao, V. P., Shynu, R., Ahmad, I. M., Mehra, P., Michael, G. S., et al. (2009). Wind-driven estuarine turbidity maxima in Mandovi Estuary, central west coast of India. J. Earth Syst. Sci. 118:369. doi: 10.1007/s12040-009-0026-5
Ketchum, B. H. (1967). “Phytoplankton nutrients in estuaries,” in Estuaries, ed G. H. Lauff (AAAS: Washington, D. C.), 329–335.
Koroleff, F. (1969). Determination of Ammonia as Indophenol Blue. Copenhagen: International Council for the Exploration of the Sea (ICES), 9.
Kraay, G. W., Zapata, M., and Veldhuis, M. J. (1992). Separation of chlorophylls c1c2, and c3 of marine phytoplankton by reversed-phase-C18-high-performance liquid chromatography 1. J. Phycol. 28, 708–712. doi: 10.1111/j.0022-3646.1992.00708.x
Latasa, M. (2007). Improving estimations of phytoplankton class abundances using CHEMTAX. Mar. Ecol. Prog. Ser. 329, 13–21. doi: 10.3354/meps329013
Lemaire, E., Abril, G., De Wit, R., and Etcheber, H. (2002). Distribution of phytoplankton pigments in nine European estuaries and implications for an estuarine typology. Biogeochemistry 59, 5–23. doi: 10.1023/A:1015572508179
Liang, D., Wang, X., Bockelmann-Evans, B. N., and Falconer, R. A. (2013). Study on nutrient distribution and interaction with sediments in a macro-tidal estuary. Adv. Water Resour. 52, 207–220. doi: 10.1016/j.advwatres.2012.11.015
Lonsdale, D. J., Cosper, E. M., and Doall, M. (1996). Effects of zooplankton grazing on phytoplankton size-structure and biomass in the lower Hudson River estuary. Estuaries 19, 874–889. doi: 10.2307/1352304
Lopes, C. B., Lillebø, A. I., Dias, J. M., Pereira, E., Vale, C., and Duarte, A. C. (2007). Nutrient dynamics and seasonal succession of phytoplankton assemblages in a Southern European Estuary: Ria de Aveiro, Portugal. Estuar. Coast. Shelf Sci. 71, 480–490. doi: 10.1016/j.ecss.2006.09.015
López-Moreno, J. I., Vicente-Serrano, S. M., Beguería, S., García-Ruiz, J. M., Portela, M. M., and Almeida, A. B. (2009). Dam effects on droughts magnitude and duration in a transboundary basin: the lower river Tagus, Spain and Portugal. Water Resour. Res. 45:W02405. doi: 10.1029/2008WR007198
Lorenzen, C. J. (1967). Determination of chlorophyll and pheo-pigments: spectrophotometric equations 1. Limnol. Oceanogr. 12, 343–346. doi: 10.4319/lo.1967.12.2.0343
Lucas, L. V., Koseff, J. R., Monismith, S. G., Cloern, J. E., and Thompson, J. K. (1999). Processes governing phytoplankton blooms in estuaries. II: The role of horizontal transport. Mar. Ecol. Prog. Ser. 187, 17–30. doi: 10.3354/meps187017
Mackey, M. D., Mackey, D. J., Higgins, H. W., and Wright, S. W. (1996). CHEMTAX-a program for estimating class abundances from chemical markers: application to HPLC measurements of phytoplankton. Mar. Ecol. Prog. Ser. 144, 265–283. doi: 10.3354/meps144265
Maguire, T. J., and Fulweiler, R. W. (2016). Urban dissolved silica: quantifying the role of groundwater and runoff in wastewater influent. Environ. Sci. Technol. 50, 54–61. doi: 10.1021/acs.est.5b03516
Margalef, R. (1978). Life-forms of phytoplankton as survival alternatives in an unstable environment. Oceanologica acta. 1, 493–509.
Mateus, M., and Neves, R. (2008). Evaluating light and nutrient limitation in the Tagus estuary using a process-oriented ecological model. J. Marine Eng. Technol. 7, 43–54. doi: 10.1080/20464177.2008.11020213
McKinley, A. C., Miskiewicz, A., Taylor, M. D., and Johnston, E. L. (2011). Strong links between metal contamination, habitat modification and estuarine larval fish distributions. Environ. Pollut. 159, 1499–1509. doi: 10.1016/j.envpol.2011.03.008
Mendes, C. R., Sá, C., Vitorino, J., Borges, C., Garcia, V. M. T., and Brotas, V. (2011). Spatial distribution of phytoplankton assemblages in the Nazaré submarine canyon region (Portugal): HPLC-CHEMTAX approach. J. Marine Syst. 87, 90–101. doi: 10.1016/j.jmarsys.2011.03.005
Monbet, Y. (1992). Control of phytoplankton biomass in estuaries: a comparative analysis of microtidal and macrotidal estuaries. Estuaries 15, 563–571. doi: 10.2307/1352398
Morote, Á. F., Olcina, J., and Hernández, M. (2019). The use of non-conventional water resources as a means of adaptation to drought and climate change in Semi-Arid Regions: South-Eastern Spain. Water 11:93. doi: 10.3390/w11010093
Murphy, J. A. M. E. S., and Riley, J. P. (1962). A modified single solution method for the determination of phosphate in natural waters. Anal. Chim. Acta. 27, 31–36. doi: 10.1016/S0003-2670(00)88444-5
Muylaert, K., and Sabbe, K. (1996). The diatom genus Thalassiosira (Bacillariophyta) in the estuaries of the Schelde (Belgium/The Netherlands) and the Elbe (Germany). Bot. Marina 39, 103–115. doi: 10.1515/botm.1996.39.1-6.103
National Research Council. (1993). Managing Wastewater in Coastal Urban Areas. Washington, DC: National Academies Press.
Nedwell, D. B., Dong, L. F., Sage, A., and Underwood, G. J. C. (2002). Variations of the nutrients loads to the mainland UK estuaries: correlation with catchment areas, urbanization and coastal eutrophication. Estuar. Coast. Shelf Sci. 54, 951–970. doi: 10.1006/ecss.2001.0867
Neves, F. S. (2010). Dynamics and hydrology of the Tagus estuary: results from in situ observations. PhD Thesis, Universidade de Lisboa, Lisbon, Portugal.
Nielsen, S. L., Sand-Jensen, K., Borum, J., and Geertz-Hansen, O. (2002). Phytoplankton, nutrients, and transparency in Danish coastal waters. Estuaries 25, 930–937. doi: 10.1007/BF02691341
O'Donohue, M. J., and Dennison, W. C. (1997). Phytoplankton productivity response to nutrient concentrations, light availability and temperature along an Australian estuarine gradient. Estuaries 20, 521–533. doi: 10.2307/1352611
Officer, C. B., and Ryther, J. H. (1980). The possible importance of silicon in marine eutrophication. Mar. Ecol. Prog. Ser. 3, 83–91. doi: 10.3354/meps003083
Oliveira, A., and Baptista, A. M. (1998). On the role of tracking on Eulerian-Lagrangian solutions of the transport equation. Adv. Water Resour. 21, 539–554. doi: 10.1016/S0309-1708(97)00022-5
Pate, R. C., Hightower, M. M., Cameron, C. P., and Einfeld, W. (2007). Overview of Energy-Water Interdependencies and the Emerging Energy Demands on Water Resources (No. SAND2007-1349C). Albuquerque, NM: Sandia National Lab. (SNL-NM).
Pilkaityte, R., and Razinkovas, A. (2006). “Factors controlling phytoplankton blooms in a temperate estuary: nutrient limitation and physical forcing,” in Marine Biodiversity. Developments in Hydrobiology, Vol 183, eds K. Martens, et al. (Dordrecht: Springer), 41–48. doi: 10.1007/1-4020-4697-9_4
R Core Team (2019). R: A Language and Environment for Statistical Computing. Vienna: R Foundation for Statistical Computing. Available online at: https://www.R-project.org/
Redfield, A. C. (1958). The biological control of chemical factors in the environment. Am. Scientist. 46, 230A–221.
Redzuan, N. S., and Underwood, G. J. (2021). The importance of weather and tides on the resuspension and deposition of microphytobenthos (MPB) on intertidal mudflats. Estuar. Coast. Shelf Sci. 251:107190. doi: 10.1016/j.ecss.2021.107190
Rocha, C., Galvão, H., and Barbosa, A. (2002). Role of transient silicon limitation in the development of cyanobacteria blooms in the Guadiana estuary, south-western Iberia. Mar. Ecol. Prog. Ser. 228, 35–45. doi: 10.3354/meps228035
Rodrigues, M., Cravo, A., Freire, P., Rosa, A., and Santos, D. (2020). Temporal assessment of the water quality along an urban estuary (Tagus estuary, Portugal). Marine Chem. 223:103824. doi: 10.1016/j.marchem.2020.103824
Rodrigues, M., and Fortunato, A. B. (2017). Assessment of a three-dimensional baroclinic circulation model of the Tagus estuary (Portugal). AIMS Environ. Sci. 4, 763–787. doi: 10.3934/environsci.2017.6.763
Rodrigues, M., Fortunato, A. B., and Freire, P. (2019). Saltwater intrusion in the upper Tagus Estuary during droughts. Geosciences 9:400. doi: 10.3390/geosciences9090400
Saraiva, S., Pina, P., Martins, F., Santos, M., Braunschweig, F., and Neves, R. (2007). Modelling the influence of nutrient loads on Portuguese estuaries. Hydrobiologia 587, 5–18. doi: 10.1007/s10750-007-0675-9
Shen, P. P., Li, G., Huang, L. M., Zhang, J. L., and Tan, Y. H. (2011). Spatio-temporal variability of phytoplankton assemblages in the Pearl River estuary, with special reference to the influence of turbidity and temperature. Cont. Shelf Res. 31, 1672–1681. doi: 10.1016/j.csr.2011.07.002
Silva, A., Palma, S., Oliveira, P. B., and Moita, M. T. (2009). Composition and interannual variability of phytoplankton in a coastal upwelling region (Lisbon Bay, Portugal). J. Sea Res. 62, 238–249. doi: 10.1016/j.seares.2009.05.001
Sistema Nacional de Informação de Recursos Hídricos (SNIRH) (2019). Available online at: http://snirh.ambiente.pt1075 (accessed November 26, 2019).
Soetaert, K., Middelburg, J. J., Heip, C., Meire, P., Van Damme, S., and Maris, T. (2006). Long-term change in dissolved inorganic nutrients in the heterotrophic Scheldt estuary (Belgium, The Netherlands). Limnol. Oceanogr. 51, 409–423. doi: 10.4319/lo.2006.51.1_part_2.0409
Spatharis, S., Danielidis, D. B., and Tsirtsis, G. (2007). Recurrent Pseudo-nitzschia calliantha (Bacillariophyceae) and Alexandrium insuetum (Dinophyceae) winter blooms induced by agricultural runoff. Harmful Algae 6, 811–822. doi: 10.1016/j.hal.2007.04.006
Struyf, E., Van Damme, S., and Meire, P. (2004). Possible effects of climate change on estuarine nutrient fluxes: a case study in the highly nutrified Schelde estuary (Belgium, The Netherlands). Estuar. Coast. Shelf Sci. 60, 649–661. doi: 10.1016/j.ecss.2004.03.004
Tilzer, M. M. (1988). Secchi disk—chlorophyll relationships in a lake with highly variable phytoplankton biomass. Hydrobiologia 162, 163–171. doi: 10.1007/BF00014539
Tracana, A. F., and Brotas, V. (2019). “Monitoring phytoplankton and nutrients in Tagus Estuary, Portugal, for 20 years,” in Frontiers in Marine Science Conference Abstract: IMMR'18| International Meeting on Marine Research 2018. doi: 10.3389/conf.FMARS.2018.06.00024
Treguer, P., Nelson, D. M., Van Bennekom, A. J., DeMaster, D. J., Leynaert, A., and Queguiner, B. (1995). The silica balance in the world ocean: a reestimate. Science 268, 375–379. doi: 10.1126/science.268.5209.375
Trigueros, J. M., and Orive, E. (2000). Tidally driven distribution of phytoplankton blooms in a shallow, macrotidal estuary. J. Plankton Res. 22, 969–986. doi: 10.1093/plankt/22.5.969
Ubertini, M., Lefebvre, S., Gangnery, A., Grangeré, K., Le Gendre, R., and Orvain, F. (2012). Spatial variability of benthic-pelagic coupling in an estuary ecosystem: consequences for microphytobenthos resuspension phenomenon. PLoS ONE 7:e44155. doi: 10.1371/journal.pone.0044155
Uncles, R. J., and Stephens, J. A. (2010). Turbidity and sediment transport in a muddy sub-estuary. Estuar. Coast. Shelf Sci. 87, 213–224. doi: 10.1016/j.ecss.2009.03.041
Vale, C. (1990). Temporal variations of particulate metals in the Tagus River Estuary. Sci. Total Environ. 97, 137–154. doi: 10.1016/0048-9697(90)90236-N
Vale, C., and Sundby, B. (1987). Suspended sediment fluctuations in the Tagus estuary on semi-diurnal and fortnightly time scales. Estuar. Coast. Shelf Sci. 25, 495–508. doi: 10.1016/0272-7714(87)90110-7
Vaz, N., and Dias, J. M. (2014). Residual currents and transport pathways in the Tagus estuary, Portugal: the role of freshwater discharge and wind. J. Coastal Res. 70, 610–615. doi: 10.2112/SI70-103.1
Wen, L. S., Jiann, K. T., and Liu, K. K. (2008). Seasonal variation and flux of dissolved nutrients in the Danshuei Estuary, Taiwan: a hypoxic subtropical mountain river. Estuar. Coast. Shelf Sci. 78, 694–704. doi: 10.1016/j.ecss.2008.02.011
Yin, K., Qian, P. Y., Chen, J. C., Hsieh, D. P., and Harrison, P. J. (2000). Dynamics of nutrients and phytoplankton biomass in the Pearl River estuary and adjacent waters of Hong Kong during summer: preliminary evidence for phosphorus and silicon limitation. Mar. Ecol. Prog. Ser. 194, 295–305. doi: 10.3354/meps194295
Keywords: transition waters, tidal range, seasonality, phytoplankton biomass and composition, spring neap tidal cycle
Citation: Cereja R, Brotas V, Cruz JPC, Rodrigues M and Brito AC (2021) Tidal and Physicochemical Effects on Phytoplankton Community Variability at Tagus Estuary (Portugal). Front. Mar. Sci. 8:675699. doi: 10.3389/fmars.2021.675699
Received: 03 March 2021; Accepted: 31 May 2021;
Published: 07 July 2021.
Edited by:
Janine Barbara Adams, Nelson Mandela University, South AfricaReviewed by:
Renzo Perissinotto, Nelson Mandela University, South AfricaRita B. Domingues, University of Algarve, Portugal
Daniel Alan Lemley, Nelson Mandela University, South Africa
Copyright © 2021 Cereja, Brotas, Cruz, Rodrigues and Brito. This is an open-access article distributed under the terms of the Creative Commons Attribution License (CC BY). The use, distribution or reproduction in other forums is permitted, provided the original author(s) and the copyright owner(s) are credited and that the original publication in this journal is cited, in accordance with accepted academic practice. No use, distribution or reproduction is permitted which does not comply with these terms.
*Correspondence: Rui Cereja, rfcereja@fc.ul.pt