- 1Department of Oceanography, National Sun Yat-sen University, Kaohsiung, Taiwan
- 2Department of Applied Science, Republic of China Naval Academy, Kaohsiung, Taiwan
- 3Department of Fisheries and Aquaculture, Faculty of Fisheries and Marine Sciences & Technology, University of Ruhuna, Matara, Sri Lanka
- 4Center for Marine Science and Technology, University of Sri Jayewardenepura, Nugegoda, Sri Lanka
Hypoxic events are becoming frequent in some estuaries and coastal waters due to over-enrichment of anthropogenic nutrients, organic matter, and/or due to restricted water circulation. The coastal lagoons and estuaries of Sri Lanka are facing high population pressure and lacking sufficient infrastructure. Coastal lagoons may receive high anthropogenic inputs of natural or untreated nitrogen and phosphorus wastes, and consequently result in hypoxic conditions while sluggish circulation occurred. In this study, we examined the spatiotemporal variability of eutrophication and hypoxia in the Negombo Lagoon, one of the most productive and sensitive coastal ecosystems in Sri Lanka. Based on seasonal measurements of dissolved oxygen, nutrients, chlorophyll-a (Chl-a), particulate and dissolved organic carbon (POC and DOC), we concluded that eutrophication and hypoxia occurred in both the dry and wet seasons. The main contributing factors were high seawater temperature and poor water circulation in the dry season and high nutrient loading combined with elevated POC and DOC inputs in the wet season.
Introduction
Sensitive coastal ecosystems such as wetlands, lagoons, and barrier-built estuaries are threatened by a combination of both marine and/or river discharge factors such as elevated seawater temperature, nutrient pollution, organic matter loading, and/or sediment inputs from turbid river waters (Hoegh-Guldberg and Smith, 1989; Hoegh-Guldberg et al., 2007; Mayfield et al., 2013; Breitburg et al., 2018; Cziesielski et al., 2019). Anthropogenic loading of nitrogen and phosphorus in particular has led to the proliferation of pelagic and benthic algal species that are not readily consumed and thus represent a burden in terms of their eutrophication potential (Andersen et al., 2006; Yan et al., 2021). In turn, the microbial respiration and decomposition of this biomass leads to increasing rates of oxygen consumption (Kemp et al., 2005) and oxygen concentrations decrease to less than 2 mg L–1, the level not suitable for fish, shrimp, or crab (Renaud, 1986). In many of these ecosystems, hypoxia has indeed become a frequent seasonal or persistent feature.
Most hypoxic zones in coastal habitats can be directly linked to population growth and increasing industrial and tourism activity which generates industrial and municipal wastes and results in increased terrestrial fluxes of nutrients (Diaz and Rosenberg, 1995; Steckbauer et al., 2011). This type of eutrophication is most commonly seen in estuaries, coastal and marginal sea areas such as the Changjiang estuary (Wei et al., 2007), the East China Sea (Chen, 2008; Chen and Guo, 2020; Chen et al., 2020, 2021), the Shenzhen Bay (Yan et al., 2021) and the Gulf of Mexico (Rabalais et al., 2001; Bianchi et al., 2010). In most of these regions, anthropogenic eutrophication has results in hypoxia.
The Negombo Lagoon is a shallow basin estuary running parallel to the west coast of Sri Lanka. It has an average water depth of less than 2 m and covers an area of approximately 32 km2. At its northern end, it connects to the Indian Ocean through a narrow channel (Figure 1). At its southern end, it is connected to the Muthurajawela marsh (Samarakoon and Van Zon, 1991; IUCN, 2013). Negombo Lagoon receives freshwater from Attanagalu Oya as well as from Ja-Ela. In addition, the Hamilton canal brings freshwater from the Kelani River into the lagoon. The total freshwater discharge ranges from 20 to 225 m3 s–1, with the Attanagalu Oya contributing over 70% (Rajapaksha, 1997; Amarasinghe et al., 1999). Negombo receives rainfall from the South-western monsoon from April to June and September to December (>200 mm/month, wet season) and there is little precipitation (less than 100 mm/month) during the remaining months (dry season) (Department of Meteorology, Sri Lanka and Devendra, 2002; Figure 2A).
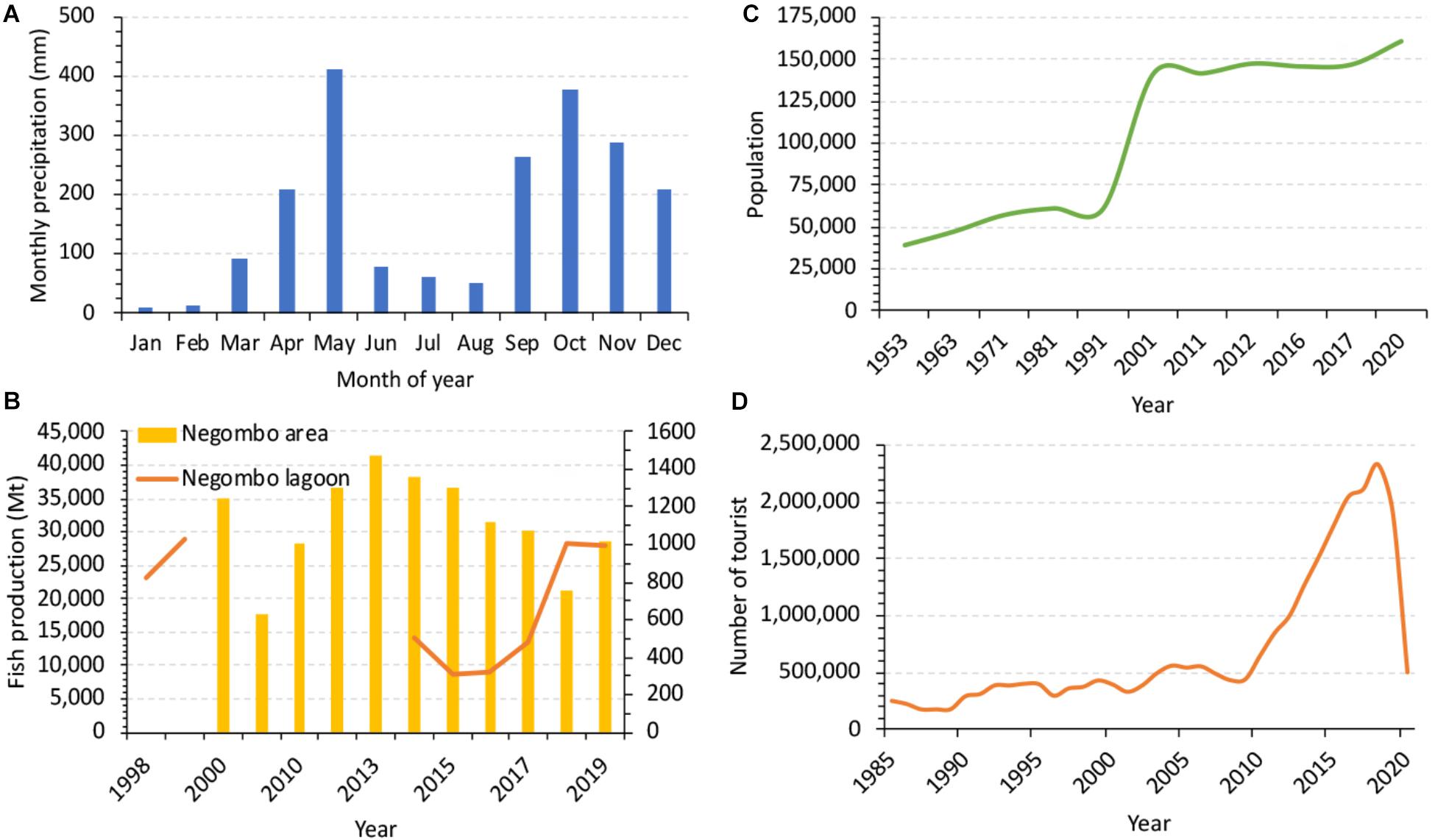
Figure 2. Information of (A) monthly precipitation, (B) fish production, (C) population, and (D) tourists in the Negombo lagoon and the adjacent area.
Negombo Lagoon is a part of a larger coastal wetland along with Muthurajawela marsh. The lagoon and its associated sensitive habitats such as mangrove and sea grass beds provide an array of ecosystem services also serves as a shrimp and fish nursery and a nutrient source for the coastal waters. The Negombo lagoon has been one of the most productive coastal ecosystems which support important fishery (DFAR, 2012a). Negombo has an active fishery industry and it contributes 16% for Sri Lanka fish production with 80% of marine fish and 20% of lagoon fish (Urban Development Authority, 2019; Figure 2B). The Negombo fish market, situated in the lagoon, is a potential pollution source within the lagoon, but organic wastes from the fish market are discharged directly into the estuary and the data are not measured.
The population in Negombo area is continuously increasing from 60,000 in 1950 to 161,484 in 2020 (Figure 2C). The area around the Negombo Lagoon attracts nearly 350,000 tourists annually and concentrates 32% of all domestic tourist visits in Sri Lanka (Urban Development Authority, 2019; Figure 2D). Food waste and untreated human waste from heavily populated areas as well as from hotels situated in the Negombo Lagoon catchment (Malawaraaratchi, 2003) add up to very significant inputs of nutrients and organic matter which contribute to the overall degradation of water quality.
The presence of these pollutants and the subsequent eutrophic conditions may result in depletion of dissolved oxygen which is thought to be responsible for the fish kill events reported in the lagoon and surrounded waters (Dahanayaka et al., 2012; Narangoda et al., 2015; National Aquatic Resources Research and Development Agency, 2019). Dahanayaka et al. (2012) reported elevated chlorophyll-a (Chl-a) concentrations in the dry season as evidence for the above sequence of events, but nutrient and dissolved oxygen (DO) data were not available.
Here we report on the results of three seasonal surveys conducted along the main axis of the lagoon and extending into the adjacent coastal zone. We examined hydrodynamics, dissolved oxygen, nutrients, dissolved and particulate organic carbon across the full salinity mixing gradient between freshwater and seawater in order to investigate the eutrophication and hypoxia events in the dry and wet seasons. This study was focused on studying potential factors currently responsible for the development of eutrophication and hypoxia in the Negombo Lagoon and to assess the extent to which long-term, global changes may affect the lagoon in the future.
Materials and Methods
Sampling Site and Hydrographic Parameter Analysis
Water samples were collected from 18 stations chosen to encompass the full salinity gradient from freshwater to coastal seawater. The only exception was Station 11, a private deck of a waterfront hotel (Figure 1) selected for real-time data recording purposes. Sampling took place in two dry seasons (February 2019 and 2020) and one wet season (October 2019). Precipitation was major in April to June and September to December, two rain seasons in southwest of Sri Lanka. The higher precipitation was shown in May in first rain season, and shown in October in second wet season. Dry season was regarded in inter wet season period, which monthly precipitation was lower than 100 mm day–1. Sampling was conducted in the morning and repeated in the evening in the wet season. Salinity, pH, dissolved oxygen, and temperature were measured by conductivity meter (WTW Cond 3310, Germany), a pH meter (WTW pH 3110, Germany) and a dissolved oxygen meter (WTW Oxi 3310, Germany). Subsamples were filtered immediately in the shore-based laboratory and stored until analysis. Nitrite (NO2), nitrate (NO3), silicate (SiO3), phosphate (PO4), total suspended matter (TSM), chlorophyll-a (Chl-a), particulate organic carbon (POC), and dissolved organic carbon (DOC) were determined according to the methods described in Hung et al. (2000, 2013). The methods used for the determination of nutrients (nitrite, nitrate, phosphate, and silicate) were based on the standard dye methods which have been adapted for use with a flow injection analyzer by Gong et al. (1996). Chl-a concentrations were measured with a Turner Designed 10-AU-005 fluorometer using the non-acidification method (Gong et al., 1996; Shih et al., 2019). Concentrations of TSM and POC were measured following the methods of Hung et al. (2013) while DOC was measured by the method of Hung et al. (2016). The ecological water quality state (European Commission, 2000) was used to classify the conditions of water in Negombo lagoon. Different levels of nutrients were corresponded to different state from “very good” to “bad,” the threshold of each category was presented in Table 1.
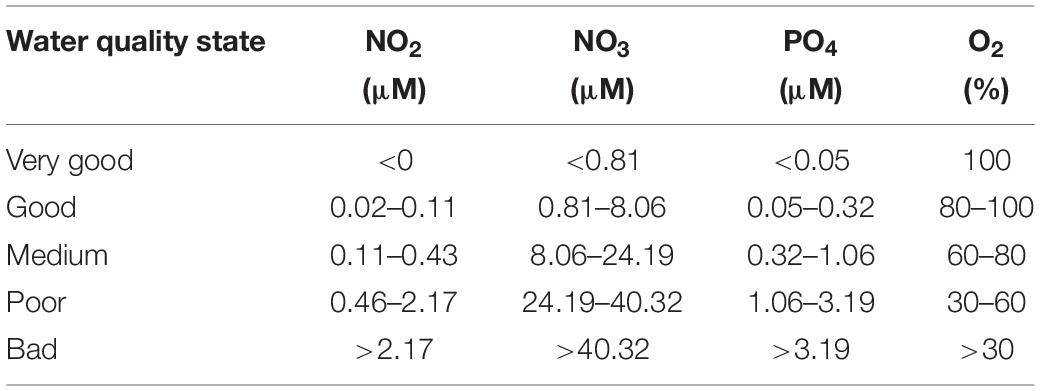
Table 1. Classification of ecological water quality state by concentration inorganic nutrients, this table was modified from European Commission (2000).
Statistical Analysis
A trophic state index (TSI) for brackish water lagoon (Kratzer and Brezonik, 1981) was used in this study to understand the trophic state in Negombo lagoon in dry and wet seasons. TSI of the lagoon water was calculated using TSITP, TSITN, and TSIChl–a. The equations are described as follows:
The units of TP and Chl-a were in μg L–1, and TN was in mg L–1. According to Kratzer and Brezonik (1981), TSI below 40 represents to oligotrophic state, TSI between 40 and 50 represents to mesotrophic, TSI from 50 to 70 represents to eutrophic, TSI above 70 represents to hypereutrophic state of the water.
A principal components analysis (PCA) was used for identifying the data similarities and differences. For example, projections considered the principal components 1 and 2 for hydrographic parameters (temperature, salinity, DO, nutrients, Chl-a, DOC, and POC etc.) and sampling locations (located in river, lagoon, and ocean) in dry and wet seasons in the Negombo lagoon.
Results
Hydrographic Settings, DO, DOC, and POC in the Dry Season
Sea surface temperatures showed a relatively uniform distribution along the entire transect and fell within the previously reported range of 27.5–31.7°C (Malawaraaratchi, 2003). Concentrations of dissolved and particulate chemicals measured in the surface waters are listed in Table 1 and their distribution along the salinity gradient is shown in Figure 2. Surface salinity gradually increased from the southern part (stations 1–3) of the lagoon toward the lagoon inlet and the Indian Ocean (station 14) (Table 1) except one lower salinity (Sal = 28.5) at station 11 compared to station 10 (Sal = 30.0) in February 2019, suggesting an input of fresh water from hotels along the northeast lagoon. This depressed salinity at station 11 was associated with hypoxia (Figure 2B), even though DO concentrations at all other stations were above 4 mg l–1 (Table 2). In February 2020, however, DO values at stations 1–3 fell below 3 mg l–1 suggesting that oxygen deficient conditions were more widespread in February 2020 than in February 2019 (Table 2).
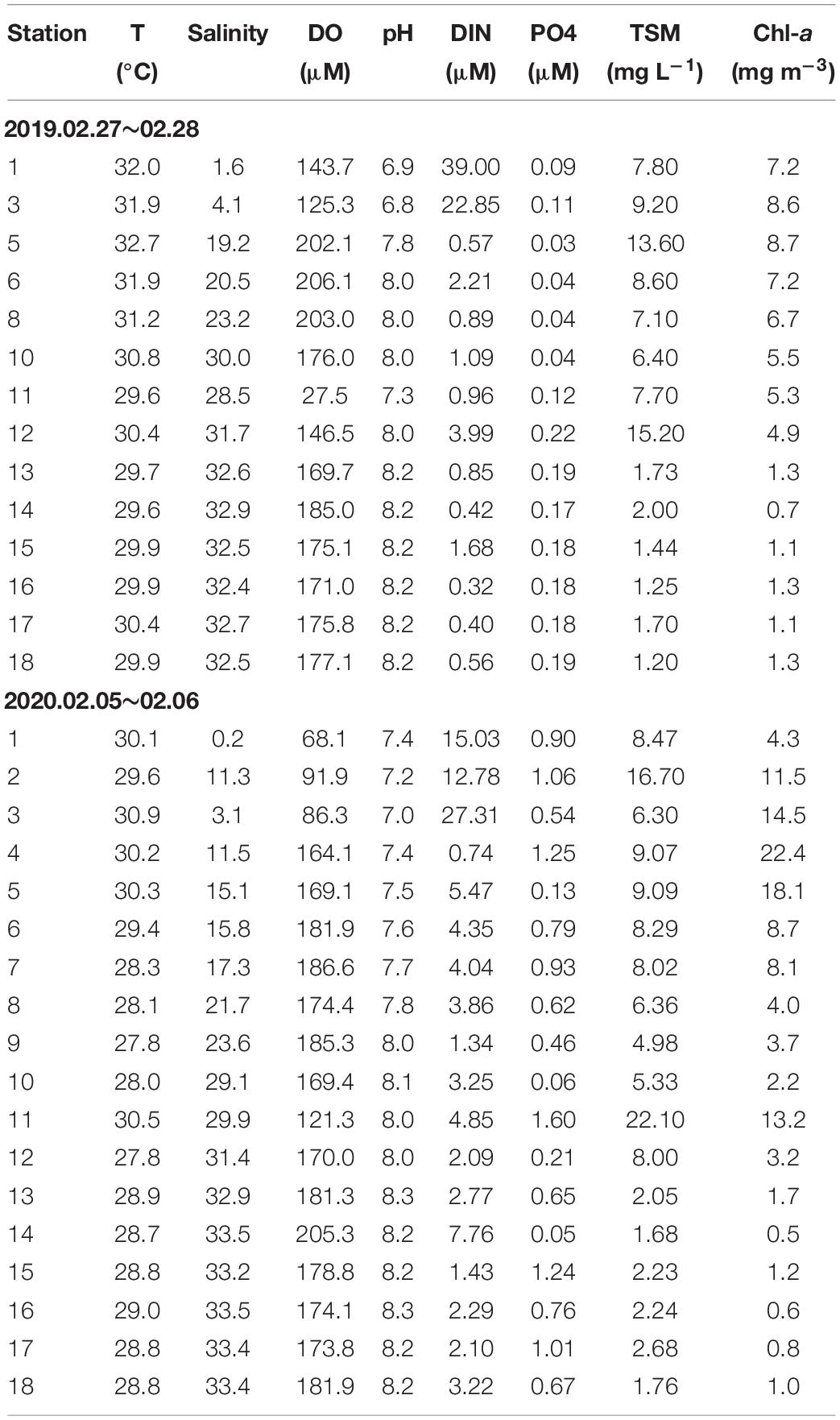
Table 2. Water temperature, salinity, dissolved oxygen (DO), pH, dissolved nitrogen (NO3+NO2), total suspended matter (TSM) and chlorophyll-a (Chl-a) in the Negombo Lagoon in the dry season 2019 and 2020.
As with DO, pH values were lowest in the southern portion of the lagoon (inner lagoon, stations 1–3) and gradually increased to typical oceanic values (8.0–8.2) toward the lagoon inlet (Figure 3C), following a typical trend.
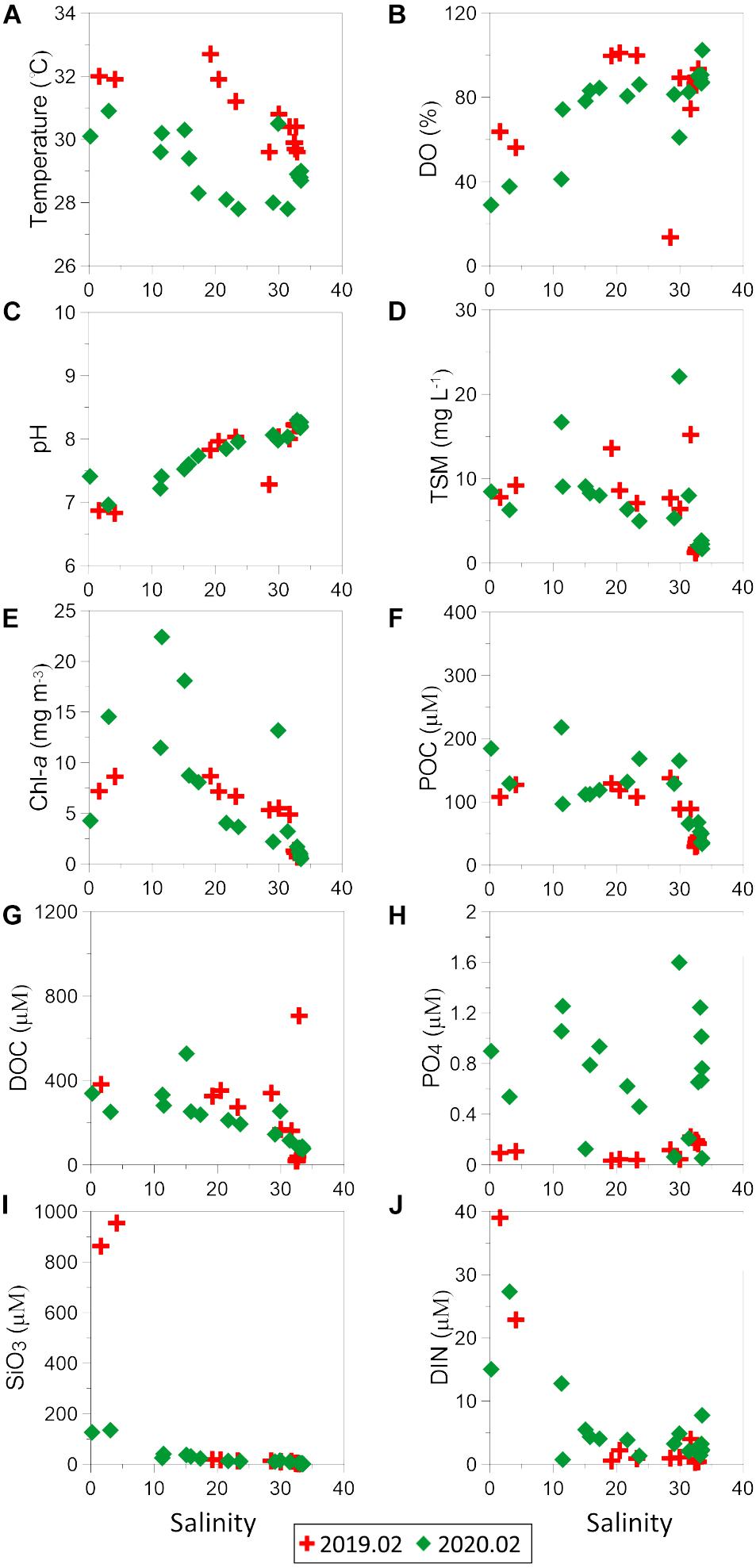
Figure 3. The distribution of (A) surface temperature, (B) DO, (C) pH, (D) TSM, (E) Chl-a, (F) POC, (G) DOC, (H) PO4, (I) SiO3, and (J) DIN along the salinity gradient in dry season.
Concentrations of TSM and chlorophyll-a (Chl-a) were highest at low salinities as well as in freshwater, i.e., inner lagoon, Ja-Ela and Dandugam Oya rivers, and decreased steadily toward the lagoon inlet (Figures 3D,E). The elevated Chl-a concentrations (0.5–22.4 mg m–3) were comparable to previously reported values in the same lagoon by Gammanpila (2010).
Concentrations of dissolved inorganic phosphate, silicate and nitrogen (=nitrite + nitrate) were elevated throughout the inner lagoon (Figures 3H–J). Some dissolved nitrogen with high concentration values (13–39 μM) were turn the water to “poor” in ecological water quality state, and this occurred in both 2019 and 2020.
The cumulative explanation of PCA variables in PC1 and PC2 axis was 69.9% in dry season (Figure 4A). Different characteristic of water was separated by PC1 and PC2 axis. For example, positive part of PC1 was related to high salinity, pH and DO in lagoon water. Nutrients and biogenic parameters (Chl-a, POC, PN, and DOC) were in negative part in lagoon water. On PC2 axis, nitrite, nitrate and silicate were predominate in positive part and phosphate, chlorophyll, POC, PN, and DOC were on the opposite side (Figure 4A).
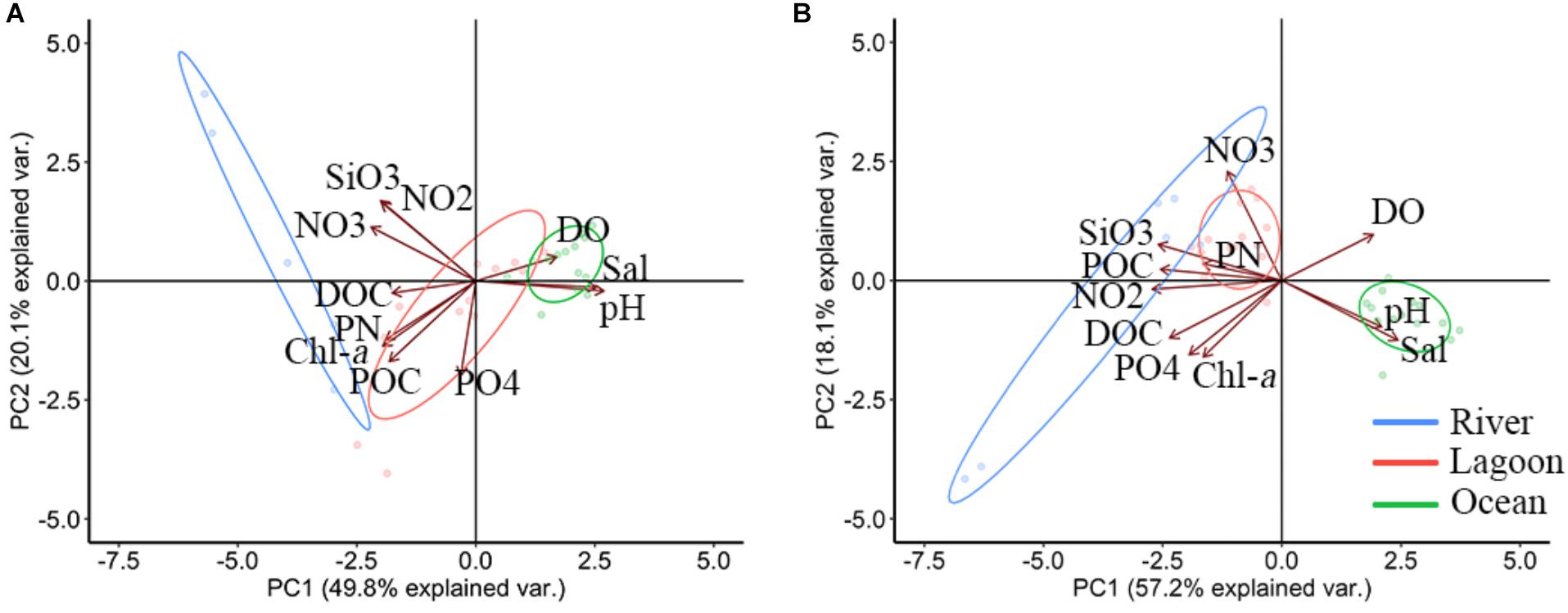
Figure 4. Results from the principal component analysis (PCA) analysis, showing the principal components 1 and 2 for hydrographic parameters and sampling locations in dry season (A) and wet season (B) in Negombo lagoon from 2019 to 2020.
The molar ratio (Si:N:P) in different waters was presented in dry season (Figure 5A). Highly biotic activity of phosphate in phytoplankton growth was evaluated as a P limitation in dry season in 2019 according to Redfield molar ratio, but it was N limitation in dry season in 2020 (Table 1 and Figure 5A).
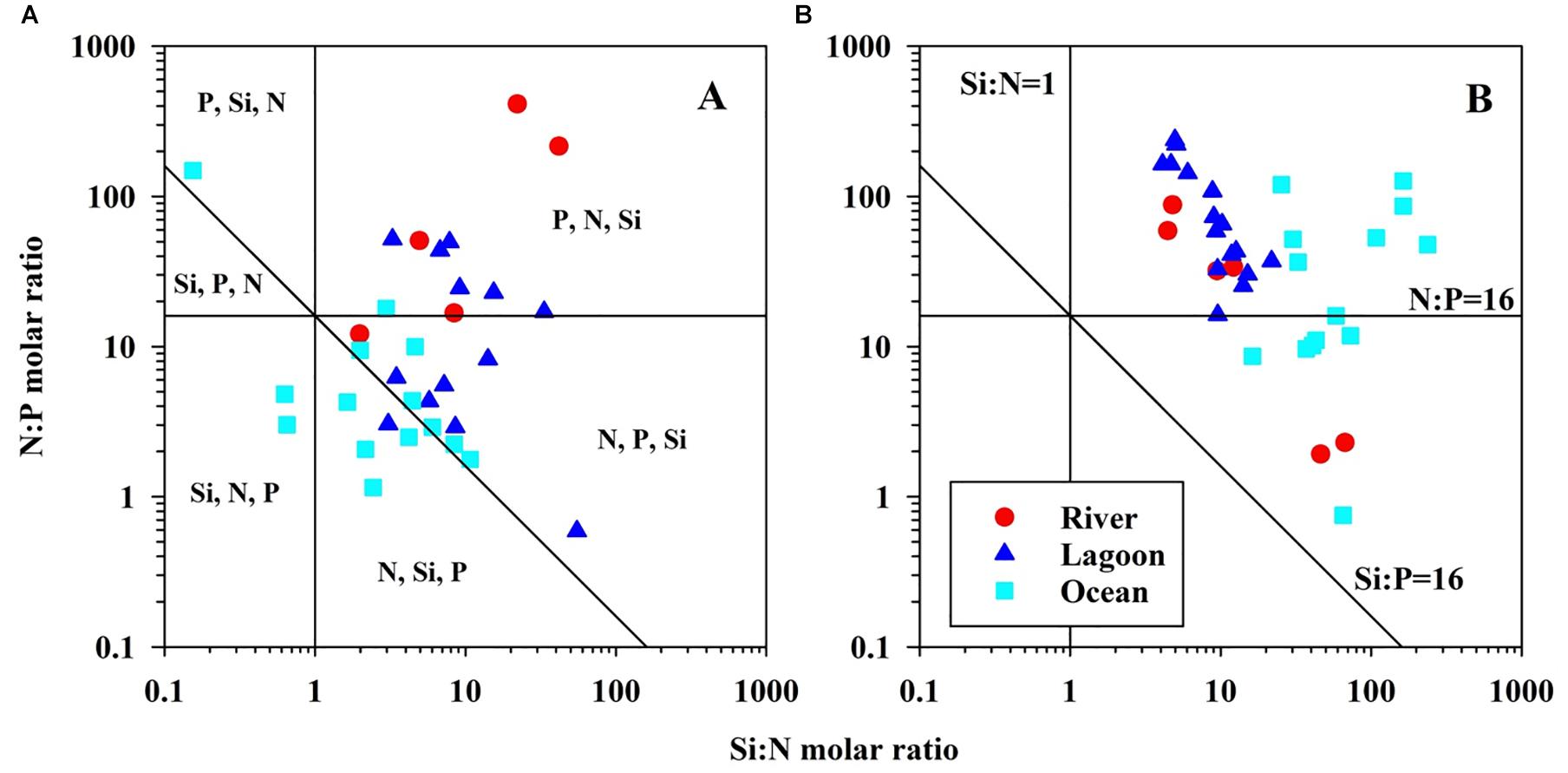
Figure 5. Molar ratio of Si:N:P in Negombo lagoon in dry season (A) and wet season (B). The potentially limited nutrients are divided from Redfield ratio (Si:N:P = 16:16:1). Different area in the plot was characterized by potentially limited nutrients in order of priority (Rocha et al., 2002).
The TSI in Negombo lagoon was decreased from river mouth to lagoon mouth in both 2019 and 2020 (Figure 6). Overall, water nature was shown as the mesotrophic state overall in 2019, but the trophic state in some sampling stations turned into the eutrophic state in 2020 (Figure 6).
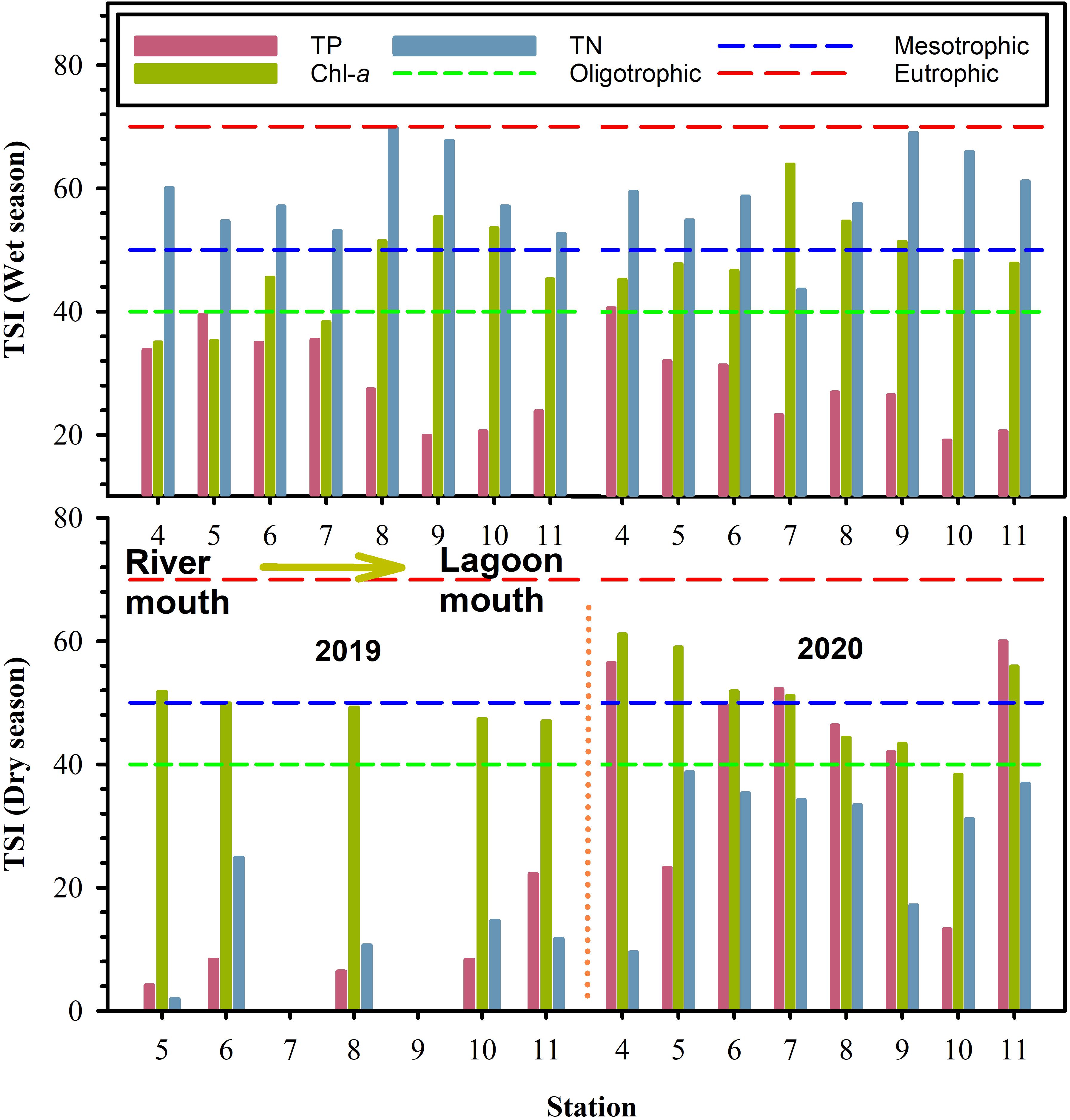
Figure 6. Trophic state index from TP, TN, and Chl-a in dry season and wet season in Negombo lagoon. Short dash line represents oligotrophic, medium dash line represents transition from oligotrophic to mesotrophic, long dash line represents transition from mesotrophic to eutrophic.
Hydrographic Settings, DO, DOC, and POC in the Wet Season
The distributions of surface temperature, DO, pH, TSM, Chl-a, POC, and DOC along the salinity gradient are shown in Figures 3A–G which combines data of the two surveys conducted in the wet season of 2019. Salinity values at all stations were generally lower than those recorded in the dry season due to seasonal precipitation. Sea surface temperature was relatively uniform and lower than in the dry season. In the first survey, DO values were above 5 mg l–1 at most stations (Table 3) although lower values were recorded in the inner lagoon (0.7–3.8 mg l–1 at stations 1–4) and also at station 11 (4.8 mg l–1). The same pattern was observed in the second survey, i.e., DO values were generally above 5 mg l–1, but four lower values (<3.5 mg l–1) were also recorded in the inner lagoon and at station 11 (Table 3). These results show that oxygen deficient conditions arise mainly in the inner lagoon but also along the periphery of the lagoon.
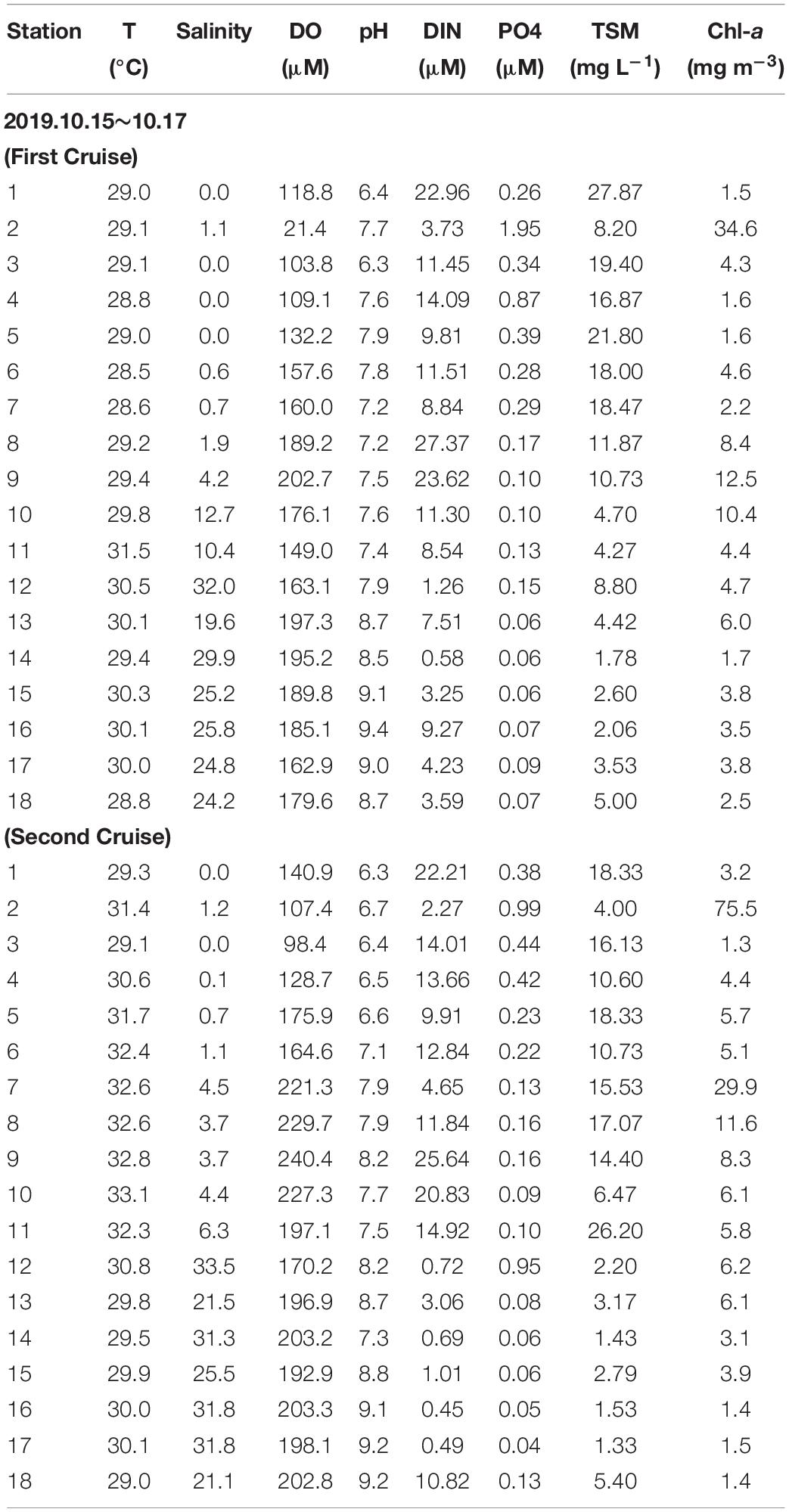
Table 3. Water temperature, salinity, dissolved oxygen (DO), pH, dissolved nitrogen (NO3+NO2), total suspended matter (TSM) and chlorophyll-a (Chl-a) in the Negombo Lagoon in the wet season 2019.
Surface pH values gradually increased from inner lagoon to lagoon inlet. Some anomalously high values (8.7–9.3) were recorded in the coastal waters outside the lagoon, shortly after a heavy rainfall event. Similar to the trend observed in the dry season, nitrogen concentrations decreased steadily from the inner to the outer part of the lagoon and beyond. However, the entire concentration gradient developed across the inlet itself whereas in the dry season the concentration gradient extended across the whole length of the lagoon. Dissolved nitrogen concentration values above the threshold level (24.6 μM) used to classify ecological water quality status as “poor” were recorded in nine stations. This suggests that flood water, storm water runoff and effluent discharge into the inner lagoon provided additional sources of biologically available nutrients and organic matter in the wet season.
As in the dry season, concentrations of TSM and chlorophyll-a (Chl-a) were highest in low salinity waters, i.e., inner lagoon, Ja-Ela and Dandugam Oya, and decreased toward the lagoon inlet (Figures 7D,E). POC and DOC followed a similar trend, but their values were higher than in the dry season, giving weight to the notion that they had been washed off the land by freshwater runoff, like the nutrients.
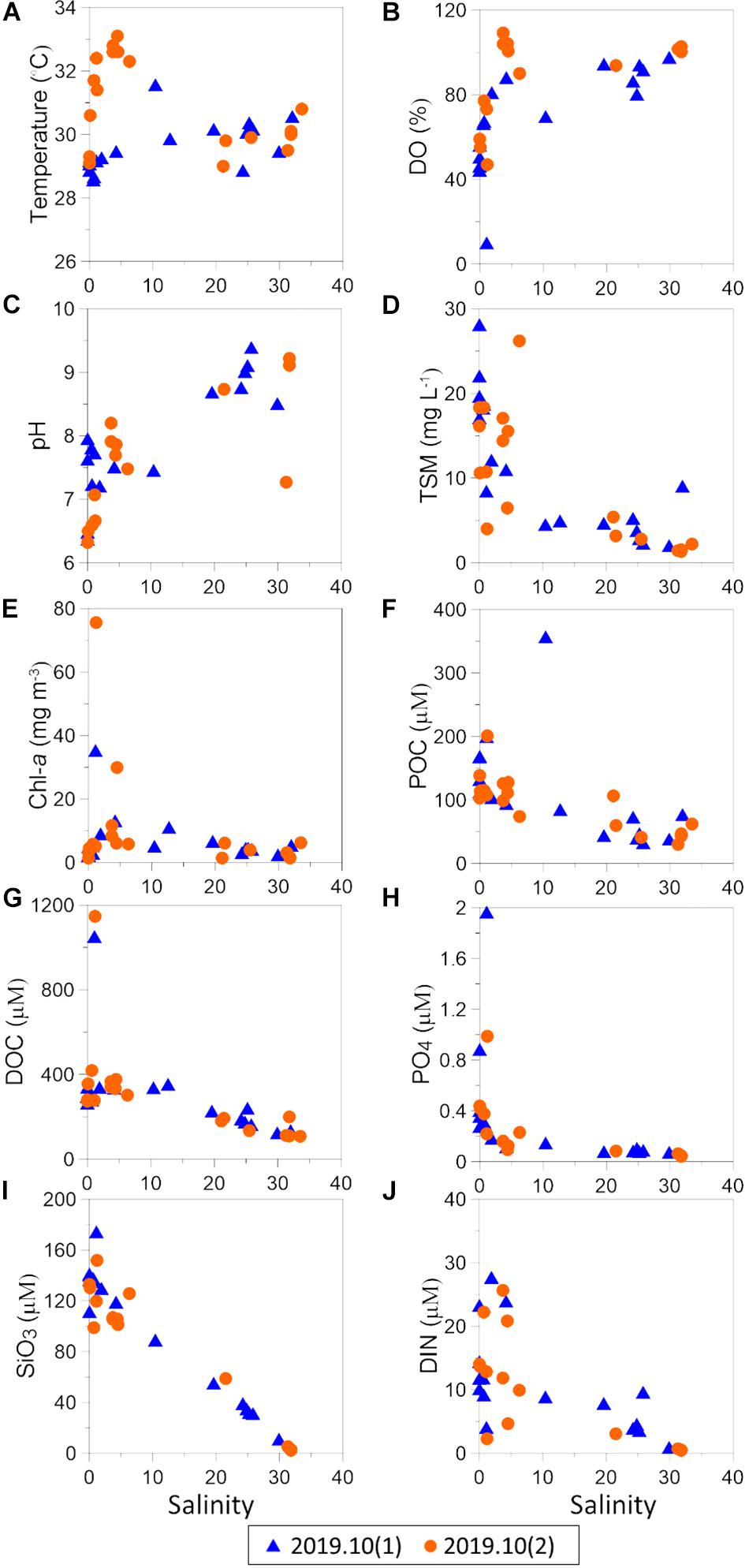
Figure 7. The distribution of (A) surface temperature, (B) DO, (C) pH, (D) TSM, (E) Chl-a, (F) POC, (G) DOC, (H) PO4, (I) SiO3, and (J) DIN along the salinity gradient in wet season.
The cumulative explanation of variables of PCA in PC1 and PC2 axis in wet season was 75.3% (Figure 4B). PC1 and PC2 axes were similar to those at dry season. However, the POC and PN were shown in positive part of PC2, and they (POC and PN) were not related to observed Chl-a suggesting there was external source of particulate matters.
The molar ratio (Si:N:P) in different waters in wet season was presented in Figure 5B. Similar to in dry season, phosphate in both PCA result and Si:N:P plot was evaluated as a P limitation in wet season. One can easily see elevated N:P ratios in wet season (Figure 5B).
The TSI in Negombo lagoon in wet season was between mesotrophic and eutrophic conditions (Figure 6) and the trophic state suggesting that nutrient supply is quite sufficient for marine organisms in wet season as compared to in dry season.
Discussion
Nutrient Dynamics the Negombo Lagoon
We observed a negative relationship between dissolved nitrogen and salinity (Figures 3J, 7J) during both the dry and wet seasons, which suggests that the sources of nitrogen were mainly from freshwater sources. This is consistent with previous work (Malawaraaratchi, 2003) indicating that Negombo Lagoon receives large quantities of wastewater containing high levels of nutrients and organic matter originating from its heavily populated catchment. At the same time, Negombo is rated as the third most popular tourist destination in Sri Lanka due to its gorgeous lagoon, bountiful fishery resources and attractive coastal scenery. Nearly 32% of tourists from Sri Lanka visit the Negombo lagoon every year. Most of the hotels are located along the shore and the lagoon. Hotels may become point source if wastewater is discharged to the lagoon without advance treatment systems. This is a matter of great concern, not least because there is growing evidence (Halkos and Matsiori, 2018; Schuhmann et al., 2019) that water quality is the single most important factor contributing to the decision of tourists to revisit a particular seaside location.
Furthermore, it has been reported that unproper management planning, fecal pollution and solid waste pollution may be important problems in Negombo lagoon due to direct discharge of sewage, solid waste into water (Panagoda et al., 2016). Joseph (2011) estimated that nearly 250 kg/day of raw faces are released to the lagoon through channel segment and neatly 50 % uncollected solid waste are discharge to the surrounding environment illegally (Karunarathana et al., 2019). Therefore, the water quality of Negombo Lagoon is critical for the coastal communities and lagoon ecosystem.
Moreover, brackish water fishery is one of the major activity in the Negombo lagoon by using engine FRP boats which are anchored along the estuary bank (Joseph, 2011; DFAR, 2012b). Some of the pollutions are from these FRP boats and pollutants are directly released to the estuary or the lagoon. In addition, waste water discharge from the small scale shrimp farms, crab fattening in estuary and the waste from the dry fish production have estimated negative effect to the lagoon water quality (Pahalawattaarachchi and Siriwardena, 2003; Pathmi et al., 2003).
A negative relationship between phosphate and salinity was also observed within the lagoon (Figures 3H, 7H). However, phosphorus as a strong bioactive element, it usually depleted in the water. A remarkable difference of N:P ratio altered nitrogen become limited factor in dry season in 2020 (Figure 5B). High concentration of phosphate shown in Negombo lagoon in dry season in 2020, might result from remineralization of sediment or discharge of anthropogenic wastewater from coastal of lagoon. Besides, some elevated phosphate values were also found at high salinity stations revealing other sources of phosphate input, but it is difficult to trace their sources based on limited information.
It is worth noting that the main freshwater sources, i.e., the Dandugam Oya and Ja-Ela, brought enormous amounts of dissolved silicate and TSM to the lagoon in both seasons, while the Hamilton canal also brought large amounts of silicate in wet season only.
Nutrient supply to coastal lagoon habitats tends to play an important role in their primary production which in turn plays an indirect role in providing a variety of habitats such as feeding and nursery ground for juvenile marine organisms (Vasconcelos et al., 2011). However, tropical lagoon worldwide has been damaged by human activity such as shoreline modifications and coastal works for tourists (Pérez-Ruzafa et al., 2019), sea salt extraction (Pérez-Ruzafa et al., 2011), fisheries and aquaculture, water sports etc. All of these factors affect nutrient and organic matter inputs as well as sediment resuspension in such a way as to shift the ecosystems and the lagoon waters toward eutrophication (Pérez-Ruzafa et al., 2019).
Eutrophication in the Negombo Lagoon
According to the European Commission (2000), nitrate concentrations in excess of 24.2 μM indicate bad quality water. Based on these criteria, the water quality status of the lagoon was “poor” in the wet season but switched to “good” in the dry season when concentrations were less than 8 μM. With respect to phosphorus concentrations, the status of lagoon waters was consistently “good” to “medium,” with concentrations less than 1 μM. These findings are in agreement with those of Mendis et al. (2015). Only in the Hamilton canal could the phosphorus quality status be rated as “bad,” with concentrations consistently higher than 1.7 μM.
In this study, Chl-a concentrations were persistently higher than 2 μg L–1 throughout the entire lagoon and Chl-a was always slightly higher in the dry season than that in the wet season (Figures 3E, 7E). These observations are likely the result of plankton blooms fueled by the abundance of nutrients coming from freshwater inputs. Negombo lagoon was between mesotrophic and eutrophic status in both dry and wet seasons based on the trophic index (Figure 7). In comparison our result to previous investigations, we observed that water quality in Negombo lagoon has been declined since 2009 (Table 4). We cannot give a real answer what mechanisms result in mesotrophic and eutrophic status in Negombo lagoon because previous sampling locations and time period might be different from this study. However, water quality in the wet season is more worsen than dry season based on current study. The observations may be affected by temporal-spatial variation in wet season. It is likely that flood water, storm water runoff and effluent discharge into the inner lagoon provided additional sources of biologically available nutrients and organic matter from watershed of main rivers. However, it needs further investigations to trace their sources of nutrients and organic matter at different time scales in the future.
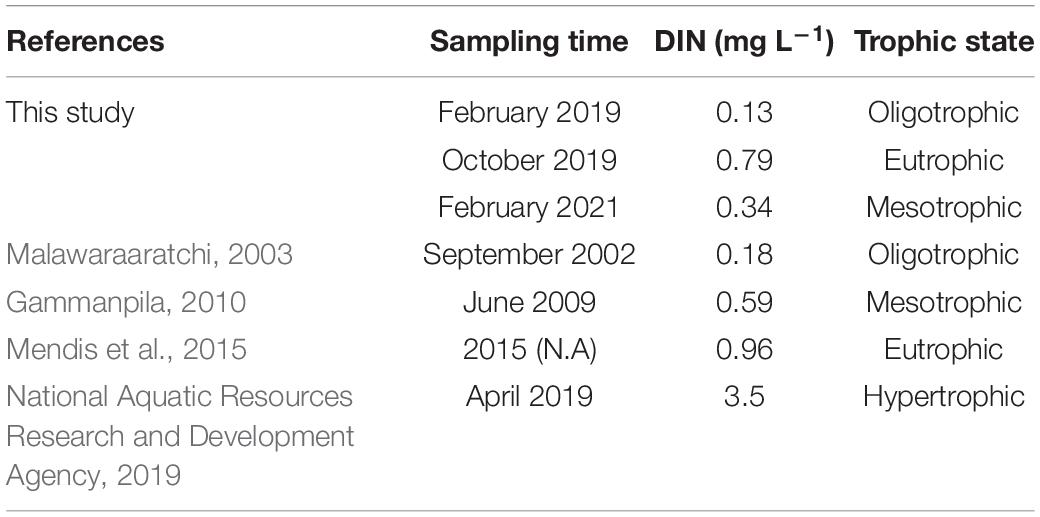
Table 4. Concentrations of dissolved inorganic nitrogen (DIN) and trophic state from previous studies and this study in Negombo lagoon.
Hypoxia and pH Variation in the Negombo Lagoon
The metabolic activity of all aquatic organisms increases exponentially with temperature. The main consequence of these elevated rates of physiological processes in the context of water quality is the consumption of oxygen by aerobic heterotrophs, mainly bacteria (Sokolova and Lannig, 2008). Another effect of increasing temperature is the decreasing solubility of oxygen in seawater (Garcia and Gordon, 1992). Higher sea surface temperatures also lead to density stratification which may severely reduce vertical mixing and hence prevent the transport of oxygen into the subsurface layer (Howarth et al., 2011). In the present study, surface water temperatures were in the range 27.8–33.1°C, with a mean value of 30.1 ± 1.0, i.e., similar to previously reported results for this lagoon (Gammanpila, 2010). Such high temperatures, coupled with the slow water circulation resulting from the very narrow connection to the Indian Ocean (Dahanayaka et al., 2012), mean that the Negombo Lagoon is at risk of experiencing hypoxic events (Hearn and Robson, 2001; Tyler et al., 2009). Moreover, the mean DO concentration we recorded in the Dandugam Oya river outflow (2.76 mg l–1) was considerably lower than that recorded by Gammanpila (2010) 10 years earlier (6.7 ± 2.1 mg l–1), which suggests that the lagoon is prone to hypoxic events in the near future. Likewise, the DO concentrations we recorded in the Hamilton canal, albeit a very small contributor to the total freshwater input to the lagoon, occasionally dropped below 1 mg l–1.
Shallow coastal lagoons where the mean daily water temperature is above 26°C often exhibit a significant decrease in oxygen concentration with depth, coupled with intense dynamics of the daily cycle of oxygen concentration, with highest concentrations occurring in the late afternoon and lowest concentrations occurring around dawn. While our surface water measurements were all above 4 mg l–1, the above considerations make it likely that some parts of the Negombo Lagoon must have been experiencing hypoxia in the waters immediately above the sediments on a daily or seasonal basis. Besides, the fish would avoid low DO water in usual (Claireaux and Chabot, 2016), however, shallow water restrict movement of fish in Negombo lagoon result in fish kill events.
High level of nutrients discharged into Negombo lagoon can trigger microorganism growth in both dry and wet seasons. During dry season, phytoplankton in Negombo lagoon produce large amounts of particulate organic carbon and consumed nutrients and oxygen in the water column. Oxygen depletion (<70% of saturated oxygen) in wet season was more remarkable. As mentioned above, heavy rainfall brought turbid water with high concentration nitrogenous nutrient to Negombo lagoon may stimulate phytoplankton growth, however, high concentration of POC and DOC were also discharged into the lagoon in wet season. Negative correlations between DO and DOC, and DO and POC can be observed in both seasons (Figure 8) suggesting that heterotrophic bacteria may largely use dissolved oxygen in the water column. Similar phenomenon about heterotrophic microorganism growth consumed more oxygen has been reported in the coastal water of the East China Sea (Chen et al., 2007, 2021).
Some unusually high pH values (8.5–9.3) were recorded in the coastal plume that formed off the lagoon’s inlet in the wet season. The large freshwater outflow during the wet season meant that the mean salinity of the coastal water samples was only 27.0 ± 4.6 as compared to 32.8 ± 0.6 in the dry season. Therefore, it seems counterintuitive that seawater pH should be so much higher in the wet (pH = 8.5–9.3) than in the dry season (pH = 8.0–8.2). Two possible factors may account for the unusually high pH phenomenon: (1) photosynthetic activity by marine phytoplankton (2) discharge of septic wastewater effluents or industrial effluents through runoff or sea outfalls (Schneeberger et al., 2015). Photosynthetic activity has the potential to raise the pH of seawater significantly by fixing CO2. Under high discharge conditions, plankton blooms are more likely to happen in the offshore waters than in the lagoon because of the short residence time and high turbidity of water inside the lagoon. Photosynthetic activity may thus explain in part the high pH values in the coastal zone. Chou et al. (2018) reported that large variation of pH in seagrass-dominated coastal region. On the other hand, the discharge of effluents cannot be discounted as a contributing factor, especially in view of the documented evidence for unregulated and untreated waste discharge along the shore of the lagoon. The main sources of septic wastewater effluent to the Negombo lagoon are local settlements, municipal sewage carried with the freshwater inflows and tourist industry along its coastal area (Malawaraaratchi, 2003). Finally, the illegal dumping of solid wastes along the banks of the populated area and shoreline closer to the mouth (Malawaraaratchi, 2003) as well as waste from fish processing and fish landing sites may also be a contributing factor (DFAR, 2012a).
Driving Forces of Eutrophication in the Negombo Lagoon
Water exchange between the Negombo Lagoon and the Indian Ocean occurs through a channel which is 150 m wide and less than 2 m deep on average. The mean tidal range on the ocean side of the inlet is 30 cm and that inside the lagoon is only 10 cm. This decrease across the inlet is due to tidal choking (Rydberg and Wickbom, 1996; Rajapaksha and Jayasiri, 2000; Sivanantha et al., 2016). The extent to which choking occurs depends on the inlet structure, freshwater supply, size of the basin, and spring neap tidal ranges. For example, work conducted in another west coast lagoon (Wijeratne and Rydberg, 2007) suggests that tidal choking across the inlet is likely to be more pronounced during spring tides than during neap tides.
In recent years, mangroves have been planted around the lagoon by local residents for the purpose of subsequent landfill. The area of mangrove vegetation in some areas has increased to form new islets consequently narrowing the channels (Dahanayaka et al., 2012). This has also led to sediment deposition, further enhance choking the channels. If left unchecked, it will ultimately lead to the destruction of the Negombo estuarine lagoon as a productive ecosystem (IUCN, 2011). Besides encouraging sedimentation and restricting water exchange, mangrove forests can naturally lead to bottom water hypoxia during low tide (Dubuc et al., 2019). The major cause of hypoxia in mangrove swamps is the buildup of monosulfides and pyrites in the organic-rich sediment, creating a monosulfidic black ooze (Knight et al., 2013). Therefore, this may be another possible cause for very low DO concentrations in the Negombo lagoon, as it comprises several mangrove swamps and borders on the largest saline coastal salt marsh in Sri Lanka, called Muthurajawela, which is underlain by extensive peat deposits.
Dissolved oxygen recorded continuously for 24 h at station 11 (from the outdoor deck of a waterfront hotel) revealed that severe hypoxic episodes occurred at nighttime during the dry season (personal communication, W-JH). This may be due to the poor circulation of water in that part of the lagoon, coupled with the long residence time of water in the central part of the lagoon in the dry season. In contrast, during the wet season, nighttime DO concentrations did not fall drastically as water replenished at much higher rates in the lagoon due to shorter residence time. Rajapaksha and Jayasiri (2000) calculated a mean residence time for the lagoon as a whole which ranged from 2 to 10 days, the shortest time corresponding to the highest recorded river flow (160 m3 s–1) and the longest time to the dry season (20 m3 s–1). Based on the above considerations and a case study from another tropical estuarine lagoon (Hearn and Robson, 2001), it can be anticipated that dredging the main channel of the Negombo Lagoon inlet, or a new channel, will a good option to increase ocean exchange and improve water quality in the lagoon.
High nutrient loading into the lagoon is a problem that also needs to be addressed. The anthropogenic sources are well identified and are associated with a range of intense activities around the lagoon such as local population, tourism, fishery activities, discharge of poorly treated water, pollution from both point sources and non-point sources. We provided investigations in both dry and wet seasons, but it indeed provides valuable data sets about eutrophication and hypoxia formation. It is worthy to note that such a short term study on this unique system may not be adequate to describe its whole scenario of Negombo lagoon and may still miss some considerations. For example, Sivanantha et al. (2016) reported that most of the water discharge to the lagoon does not go through a proper purification system to reduce nutrient pollution. This high nutrient loading has contributed to a 96% decline of the standing crop of the seagrass in northern, eastern, and western parts of the Negombo Lagoon between 1997 and 2004 (De Silva and Amarasinghe, 2007). In turn, the reduction of the seagrass may also contribute to the present-day low dissolved oxygen concentrations in certain parts of the lagoon.
In summary, elevated levels of nutrients, Chl-a, POC, and DOC were found in the water bodies emptying into the Negombo lagoon as well as in the inner lagoon, in both dry and wet seasons. The inner lagoon and probably some stretches of coast isolated from the main circulation (e.g., Station 11) is facing hypoxia, not only because of the in situ processes triggered by the abundance of nutrients and organic matter from sewage, but also because of the already low levels of dissolved oxygen in river discharge. Therefore, it would be good to significantly reduce nutrient loading while at the same time improving the existing mouth or new channel to improve water quality and water circulation. Going forward, regular hydrographic, biological oxygen demand, heterotrophic respiration, nutrient monitoring and phytoplankton assemblages will be essential for eutrophication and hypoxia trend analysis. If any modification to the lagoon’s outline and physiography is to take place, it will be important to characterize the new estuarine mixing behavior of the lagoon as a function of river discharge variability in order to correctly interpret the monitoring data.
Data Availability Statement
The original contributions presented in the study are included in the article/Supplementary Material, further inquiries can be directed to the corresponding author/s.
Author Contributions
C-CH conceived the idea. C-CH, H-HH, and WW wrote the manuscript. H-HH, M-HC, Y-YS, WW, W-JH, C-CH, RR, and DW performed the experiments and created the figures. C-CH and H-HH revised the manuscript. All authors reviewed the manuscript.
Funding
This research was supported by Sun Yat-sen University and the Ministry of Sciences and Technology (MOST 107-2911-I-110-301, MOST 108-2911-I-110-301, and MOST 109-2911-I-110-301) of Taiwan.
Conflict of Interest
The authors declare that the research was conducted in the absence of any commercial or financial relationships that could be construed as a potential conflict of interest.
Publisher’s Note
All claims expressed in this article are solely those of the authors and do not necessarily represent those of their affiliated organizations, or those of the publisher, the editors and the reviewers. Any product that may be evaluated in this article, or claim that may be made by its manufacturer, is not guaranteed or endorsed by the publisher.
Acknowledgments
We appreciate the assistance of people of the Environmental Change Sciences and Technology Innovation Center at University of Sri Jayewardenepura in collaboration with National Sun Yat-sen University.
Supplementary Material
The Supplementary Material for this article can be found online at: https://www.frontiersin.org/articles/10.3389/fmars.2021.678832/full#supplementary-material
References
Amarasinghe, U. A., Mutuwatta, L., and Sakthivadivel, R. (1999). Water Scarcity Variations within a Country: A Case Study of Sri Lanka. IWMI Research Reports H024897. Colombo: International Water Management Institute.
Andersen, J. H., Schlüter, L., and Ærtebjerg, G. (2006). Coastal eutrophication: recent developments in definitions and implications for monitoring strategies. J. Plankton Res. 28, 621–628. doi: 10.1093/plankt/fbl001
Bianchi, T. S., DiMarco, S. F., Cowan, J. H. Jr., Hetland, R. D., Chapman, P., Day, J. W., et al. (2010). The science of hypoxia in the Northern Gulf of Mexico: a review. Sci. Total Environ. 408, 1471–1484. doi: 10.1016/j.scitotenv.2009.11.047
Breitburg, D., Levin, L. A., Oschlies, A., Gregoire, M., Chavez, F. P., Conley, D. J., et al. (2018). Declining oxygen in the global ocean and coastal waters. Science 359:eaam7240. doi: 10.1126/science.aam7240
Chen, C.-C., Gong, G.-C., Chiang, K.-P., Shiah, F.-K., Chung, C.-C., and Hung, C.-C. (2021). Scaling effects of a eutrophic river plume on organic carbon consumption. Limnol. Oceanogr. 66, 1867–1881. doi: 10.1002/Ino.11729
Chen, C.-C., Gong, G.-C., Chou, W.-C., and Shiah, F.-K. (2020). Hypoxia in autumn of the East China Sea. Mar. Pollut. Bull. 152:110875. doi: 10.1016/j.marpolbul.2019.110875
Chen, C.-C., Gong, G.-C., and Shiah, F.-K. (2007). Hypoxia in the East China Sea: one of the largest coastal low-oxygen areas in the world. Mar. Environ. Res. 64, 399–408. doi: 10.1016/j.marenvres.2007.01.007
Chen, C.-T. A. (2008). Distributions of nutrients in the East China Sea and the South China Sea connection. J. Oceanogr. 64, 737–751. doi: 10.1007/s10872-008-0062-9
Chou, W.-C., Chu, H.-C., Chen, Y.-H., Syu, R.-W., Hung, C.-C., and Soong, K. (2018). Short-term variability of carbon chemistry in two contrasting seagrass meadows at Dongsha Island: implications for pH buffering and CO2 sequestration. Estuar. Coast. Shelf Sci. 210, 36–44. doi: 10.1016/j.ecss.2018.06.006
Claireaux, G., and Chabot, D. (2016). Responses by fishes to environmental hypoxia: integration through Fry’s concept of aerobic metabolic scope. J. Fish Biol. 88, 232–251. doi: 10.1111/jfb.12833
Cziesielski, M. J., Schmidt-Roach, S., and Aranda, M. (2019). The past, present, and future of coral heat stress studies. Ecol. Evol. 9, 10055–10066. doi: 10.1002/ece3.5576
Dahanayaka, D., Tonooka, H., Wijeyaratne, M., Minato, A., and Ozawa, S. (2012). Monitoring land use changes and their impacts on the productivity of Negombo Estuary, Sri Lanka using time series satellite data. Asian Fish. Sci. 25, 97–112.
De Silva, K., and Amarasinghe, M. (2007). Substrate characteristics and species diversity of marine angiosperms in a micro tidal basin estuary on the west coast of Sri Lanka. Sri Lanka J. Aquat. Sci. 12, 103–114. doi: 10.4038/sljas.v12i0.2217
Devendra, A. (2002). Hydrodynamics of Muthurajawela Marsh & Negombo lagoon coastal wetland ecosystem. Effective Management for Biodiversity Conservation in Sri Lankan Coastal Wetlands. Portsmouth: University of Portsmouth, 9–10.
DFAR (2012a). Fisheries Development and Management Plan of Negombo lagoon. Regional Fisheries Livelihoods Programme for South and Southeast Asia (GCP/RAS/237/SPA): Field Project Document 2012/LKA/CM/12. Colombo: Department of Fisheries and Aquatic Resources.
DFAR (2012b). Rapid Fisheries Frame Survey Report of Coastal and Brackish Water Fisheries in the Fisheries District of Negombo. Regional Fisheries Livelihoods Programme for South and Southeast Asia (GCP/RAS/237/SPA): Field Project Document 2012/LKA/CM/01. Colombo: Department of Fisheries and Aquatic Resources.
Diaz, R. J., and Rosenberg, R. (1995). Marine benthic hypoxia: a review of its ecological effects and the behavioural responses of benthic macrofauna. Oceanogr. Mar. Biol. 33, 245–303.
Dubuc, A., Baker, R., Marchand, C., Waltham, N. J., and Sheaves, M. (2019). Hypoxia in mangroves: occurrence and impact on valuable tropical fish habitat. Biogeosciences 16, 3959–3976. doi: 10.5194/bg-16-3959-2019
European Commission (2000). Water framework directive, directive 2000/60/EEC of 22 December 2000 establishing a framework for community action in the field of water policy. Off. J. Eur. Communities 327, 1–72.
Gammanpila, M. (2010). Hydrography, nutrients and abundance and distribution of zooplankton in Negombo Lagoon, Sri Lanka. Sri Lanka J. Aquat. Sci. 15, 13–24. doi: 10.4038/sljas.v15i0.5447
Garcia, H. E., and Gordon, L. I. (1992). Oxygen solubility in seawater: better fitting equations. Limnol. Oceanogr. 37, 1307–1312. doi: 10.4319/lo.1992.37.6.1307
Gong, G.-C., Lee Chen, Y.-L., and Liu, K.-K. (1996). Chemical hydrography and chlorophyll a distribution in the East China Sea in summer: implications in nutrient dynamics. Cont. Shelf Res. 16, 1561–1590. doi: 10.1016/0278-4343(96)00005-2
Halkos, G., and Matsiori, S. (2018). Environmental attitudes and preferences for coastal zone improvements. Econ. Anal. Policy 58, 153–166. doi: 10.1016/j.eap.2017.10.002
Hearn, C. J., and Robson, B. J. (2001). Inter-annual variability of bottom hypoxia in shallow mediterranean estuaries. Estuar. Coast. Shelf Sci. 52, 643–657. doi: 10.1006/ecss.2001.0773
Hoegh-Guldberg, O., Mumby, P. J., Hooten, A. J., Steneck, R. S., Greenfield, P., Gomez, E., et al. (2007). Coral reefs under rapid climate change and ocean acidification. Science 318, 1737–1742. doi: 10.1126/science.1152509
Hoegh-Guldberg, O., and Smith, G. J. (1989). The effect of sudden changes in temperature, light and salinity on the population density and export of zooxanthellae from the reef corals Stylophora pistillata Esper and Seriatopora hystrix Dana. J. Exp. Mar. Biol. Ecol. 129, 279–303. doi: 10.1016/0022-0981(89)90109-3
Howarth, R., Chan, F., Conley, D. J., Garnier, J., Doney, S. C., Marino, R., et al. (2011). Coupled biogeochemical cycles: eutrophication and hypoxia in temperate estuaries and coastal marine ecosystems. Front. Ecol. Environ. 9, 18–26. doi: 10.1890/100008
Hung, C. C., Tseng, C. W., Gong, G. C., Chen, K. S., Chen, M. H., and Hsu, S. C. (2013). Fluxes of particulate organic carbon in the East China Sea in summer. Biogeosciences 10, 6469–6484. doi: 10.5194/bg-10-6469-2013
Hung, C. C., Wong, G. T., Liu, K. K., Fuh-Kwo, S., and Gwo-Ching, G. (2000). The effects of light and nitrate levels on the relationship between nitrate reductase activity and 15NO3− uptake: field observations in the East China Sea. Limnol. Oceanogr. 45, 836–848. doi: 10.4319/lo.2000.45.4.0836
Hung, C. W., Huang, K. H., Shih, Y. Y., Lin, Y. S., Chen, H. H., Wang, C. C., et al. (2016). Benthic fluxes of dissolved organic carbon from gas hydrate sediments in the northern South China Sea. Sci. Rep. 6:29597. doi: 10.1038/srep29597
IUCN (2011). An Appraisal of Mangrove Management in Micro tidal Estuaries and Lagoons in Sri Lanka, Vol. viii. Colombo: IUCN Sri Lanka Office, 116.
IUCN (2013). Proceedings of the Workshop on Ecological Considerations in Coastal Development, Held at the Sequel, Cinnamon Grand, Colombo, Sri lanka, 22 November 2013. Colombo: IUCN, xiv–80.
Joseph, L. (2011). Fisheries and Environmental profile of Negombo lagoon, Sri Lanka: A Literature Review. Regional Fisheries Livelihoods Programme for South and Southeast Asia (GCP/RAS/237/SPA) Field Project Document 2011/LKA/CM/04. Rome: FAO.
Karunarathana, A., Singh, R. K., Rajapaksha, T., Gamaralalage, D., Premakumara, J., Onogawa, K., et al. (2019). State of Municipal Solid Waste Management in Negombo, Sri Lanka. Hayama: Institute for Global Environmental Strategies.
Kemp, W. M., Boynton, W. R., Adolf, J. E., Boesch, D. F., Boicourt, W. C., Brush, G., et al. (2005). Eutrophication of Chesapeake Bay: historical trends and ecological interactions. Mar. Ecol. Prog. Ser. 303, 1–29. doi: 10.3354/meps303001
Knight, J. M., Griffin, L., Dale, P. E. R., and Sheaves, M. (2013). Short-term dissolved oxygen patterns in sub-tropical mangroves. Estuar. Coast. Shelf Sci. 131, 290–296. doi: 10.1016/j.ecss.2013.06.024
Kratzer, C. R., and Brezonik, P. L. (1981). A carlson-type trophic state index for nitrogen in florida lakes. Water Resour. Bull. 17, 713–715. doi: 10.1111/j.1752-1688.1981.tb01282.x
Malawaraaratchi, R. (2003). A Study of the Negombo Lagoon with Respect to the Salinity Variation and Pollution of the Lagoon Water and Effects of Proposed Dredging Activities. Moratuwa: University of Moratuwa.
Mayfield, A. B., Chen, M. N., Meng, P. J., Lin, H. J., Chen, C. S., and Liu, P. J. (2013). The physiological response of the reef coral Pocillopora damicornis to elevated temperature: results from coral reef mesocosm experiments in Southern Taiwan. Mar. Environ. Res. 86, 1–11. doi: 10.1016/j.marenvres.2013.01.004
Mendis, B., Najim, M., Kithsiri, H., and Azmy, S. (2015). “Assessment of water quality and identification of pollution status of Negombo estuary in Sri Lanka,” in Proceedings of the 21st Scientific Sessions of the Sri Lanka Association for Fisheries and Aquatic Resources, May, 2015. Sri Lanka Association for Fisheries and Aquatic Resources, Colombo.
Narangoda, S. R. C., Maddumage, M. D. S. R., Wickramaarachchi, D. N., Weerasekara, K. A. W. S., Hettige, N. D., et al. (2015). “Water quality status of selected aquatic environments in Sri Lanka during fish kill incidents,” in Proceedings of 21st Annual Scientific Sessions of Sri Lanka Association for Fisheries and Aquatic Resources (SLAFAR), 22 May 2015, Nara, 17.
National Aquatic Resources Research and Development Agency (2019). Preliminary Report on Investigation of Fish in Hamilton Canal. Nara: National Aquatic Resources Research and Development Agency.
Pahalawattaarachchi, V., and Siriwardena, P. (2003). “Effect of shrimp farm effluent on seagrass beds in Negombo Lagoon,” in Proceeding of the 1st Scientific Sessions on Inland Aquatic Resources and Aquaculture, National Aquatic Resources Research and Development Agency, (Colombo).
Panagoda, P. A. B. G., Dissanayake, D. M. S. N., Nawalage, N. M. S. K., Silva, H. K. A. D., Tharaka, L. K. D. N., Weerasinghe, H. W. G. A. S., et al. (2016). “Sustainable tourism development in Negombo coastline: an option for co-management,” in Proceedings of the International Research Symposium on Pure and Applied Sciences (IRSPAS 2016), Faculty of Science, University of Kelaniya, Kelaniya, 106.
Pathmi, A., Jayamanne, S., Siriwardena, P., and Hirimuthugoda, Y. (2003). “Impact of crab fattening on the lagoon environment and the live hood of fishers in Negombo Lagoon,” in Proceeding of the 1st scientific sessions on Inland Aquatic Resources and Aquaculture, National Aquatic Resources Research and Development Agency, Colombo.
Pérez-Ruzafa, A., Campillo, S., Fernández-Palacios, J. M., García-Lacunza, A., García-Oliva, M., Ibañez, H., et al. (2019). Long-term dynamic in nutrients, chlorophyll a, and water quality parameters in a coastal Lagoon during a process of eutrophication for decades, a sudden break and a relatively rapid recovery. Front. Mar. Sci. 6:26. doi: 10.3389/fmars.2019.00026
Pérez-Ruzafa, A., Marcos, C., Pérez-Ruzafa, I. M., and Pérez-Marcos, M. (2011). Coastal lagoons:“transitional ecosystems” between transitional and coastal waters. J. Coast. Conserv. 15, 369–392. doi: 10.1007/s11852-010-0095-2
Rabalais, N. N., Turner, R. E., and Wiseman, W. J. Jr. (2001). Hypoxia in the Gulf of Mexico. J. Environ. Qual. 30, 320–329. doi: 10.2134/jeq2001.302320x
Rajapaksha, J. K. (1997). Low Frequency Tidal Response and Water Exchange in a Restricted Lagoon: The Negombo Lagoon, Sri Lanka. M.Sc. thesis. Gothenburg: University of Gothenburg.
Rajapaksha, J. K., and Jayasiri, H. B. (2000). Restricted water exchange in the Negombo Lagoon on the west coast of Sri Lanka. J. Natl. Aquat. Resour. Res. Dev. Agency 36, 35–46.
Renaud, M. L. (1986). Detecting and avoiding oxygen deficient sea water by brown shrimp, Penaeus aztecus (Ives), and white shrimp Penaeus setiferus (Linnaeus). J. Exp. Mar. Biol. Ecol. 98, 283–292. doi: 10.1016/0022-0981(86)90218-2
Rocha, C., Galvao, H., and Barbosa, A. (2002). Role of transient silicon limitation in the development of cyanobacteria blooms in the Guadiana estuary, south-western Iberia. Mar. Eco. Prog. Ser. 228, 35–45. doi: 10.3354/meps228035
Rydberg, L., and Wickbom, L. (1996). Tidal choking and bed friction in Negombo Lagoon, Sri Lanka. Estuaries 19, 540–547. doi: 10.2307/1352516
Samarakoon, J., and Van Zon, H. (1991). Environmental Profile of Muthurajawela and Negombo Lagoon. Colombo: Euroconsult/Greater Colombo Economic Commission.
Schneeberger, C. L., O’Driscoll, M., Humphrey, C., Henry, K., Deal, N., Seiber, K., et al. (2015). Fate and transport of enteric microbes from septic systems in a coastal watershed. J. Environ. Health 77, 22–30.
Schuhmann, P., Skeete, R., Waite, R., Bangwayo-Skeete, P., Casey, J., Oxenford, H. A., et al. (2019). Coastal and marine quality and tourists’ stated intention to return to Barbados. Water 11:1265. doi: 10.3390/w11061265
Shih, Y.-Y., Lin, H.-H., Li, D., Hsieh, H.-H., Hung, C.-C., and Chen, C. T. A. (2019). Elevated carbon flux in deep waters of the South China Sea. Sci. Rep. 9:1496. doi: 10.1038/s41598-018-37726-w
Sivanantha, N., Wijesinghe, M., and Wijesekara, R. (2016). Distribution of five toxic heavy metals in biotic and abiotic constituents of the Negombo Lagoon, Sri Lanka. Sri Lankan J. Biol. 1, 1–14. doi: 10.4038/sljb.v1i1.1
Sokolova, I. M., and Lannig, G. (2008). Interactive effects of metal pollution and temperature on metabolism in aquatic ectotherms: implications of global climate change. Clim. Res. 37, 181–201. doi: 10.3354/cr00764
Steckbauer, A., Duarte, C. M., Carstensen, J., Vaquer-Sunyer, R., and Conley, D. J. (2011). Ecosystem impacts of hypoxia: thresholds of hypoxia and pathways to recovery. Environ. Res. Lett. 6:025003. doi: 10.1088/1748-9326/6/2/025003
Tyler, R. M., Brady, D. C., and Targett, T. E. (2009). Temporal and spatial dynamics of biel-cycling hypoxia in estuarine tributaries. Estuar. Coast 32, 123–145. doi: 10.1007/s12237-008-9108-x
Urban Development Authority (2019). Negombo Development Plan – 2019 - 2030, Vol. I., Colombo: Urban Development Authority.
Vasconcelos, R. P., Reis-Santos, P., Costa, M. J., and Cabral, H. N. (2011). Connectivity between estuaries and marine environment: integrating metrics to assess estuarine nursery function. Ecol. Indic. 11, 1123–1133. doi: 10.1016/j.ecolind.2010.12.012
Wei, H., He, Y., Li, Q., Liu, Z., and Wang, H. (2007). Summer hypoxia adjacent to the Changjiang Estuary. J. Mar. Syst. 67, 292–303. doi: 10.1016/j.jmarsys.2006.04.014
Wijeratne, E. M. S., and Rydberg, L. (2007). Modelling and observations of tidal wave propagation, circulation and residence times in Puttalam Lagoon, Sri Lanka. Estuar. Coast. Shelf Sci. 74, 697–708. doi: 10.1016/j.ecss.2007.05.012
Keywords: hypoxia, eutrophication, organic pollution, nutrients pollution, Negombo Lagoon
Citation: Hsieh H-H, Chuang M-H, Shih Y-Y, Weerakkody WS, Huang W-J, Hung C-C, Muller FLL, Ranatunga RRMKP and Wijethunga DS (2021) Eutrophication and Hypoxia in Tropical Negombo Lagoon, Sri Lanka. Front. Mar. Sci. 8:678832. doi: 10.3389/fmars.2021.678832
Received: 11 March 2021; Accepted: 05 August 2021;
Published: 06 September 2021.
Edited by:
Jesper H. Andersen, NIVA Denmark Water Research, DenmarkReviewed by:
Helena Galvão, Universidade do Algarve, PortugalMichelle Jillian Devlin, Centre for Environment, Fisheries and Aquaculture Science (CEFAS), United Kingdom
Copyright © 2021 Hsieh, Chuang, Shih, Weerakkody, Huang, Hung, Muller, Ranatunga and Wijethunga. This is an open-access article distributed under the terms of the Creative Commons Attribution License (CC BY). The use, distribution or reproduction in other forums is permitted, provided the original author(s) and the copyright owner(s) are credited and that the original publication in this journal is cited, in accordance with accepted academic practice. No use, distribution or reproduction is permitted which does not comply with these terms.
*Correspondence: Chin-Chang Hung, Y2NodW5nQG1haWwubnN5c3UuZWR1LnR3