- 1Cooperative Institute for Marine and Atmospheric Studies, University of Miami, Miami, FL, United States
- 2National Oceanographic and Atmospheric Administration (NOAA) Atlantic Oceanographic and Meteorological Laboratory, Ocean Chemistry and Ecosystems Division, Miami, FL, United States
- 3Department of Physics, University of Miami, Coral Gables, FL, United States
- 4Rosenstiel School of Marine and Atmospheric Studies, University of Miami, Miami, FL, United States
- 5Coral Reef Watch, U.S. National Oceanic and Atmospheric Administration, College Park, MD, United States
Since the appearance of stony coral tissue loss disease (SCTLD) on reefs off Miami in 2014, this unprecedented outbreak has spread across the entirety of Florida’s coral reef tract, as well as to many territories throughout the Caribbean. The endemic zone reached the upper Florida Keys by 2016, resulting in partial or complete mortality of coral colonies across numerous species. Disease was first observed at Cheeca Rocks (Islamorada, Florida) in the beginning of 2018, with reports of coral mortality peaking mid-year. The disease was still present at Cheeca Rocks as of March 2020, however, to a lesser degree compared to the initial outbreak. Annual monitoring efforts have been ongoing at Cheeca Rocks since 2012, including repeated benthic photomosaics of a 330 m2 survey zone, spanning six replicate sites. As such, a repository of coral community composition data exists for before and after the disease outbreak that was analyzed to assess the impacts of SCTLD on reef communities at an upper Florida Keys inshore reef. Cheeca Rocks is hypothesized to be a resilient reef due to its persistent high coral cover despite its inshore location, which subjects corals to fluctuating water quality and marginal environmental conditions. Coral populations here have been shown to recover from bleaching events and heat stress with minimal coral mortality. Though colonies of coral species characterized as highly and moderately susceptible to SCTLD (e.g., Colpophyllia natans, Diploria labyrinthiformis, Pseudodiploria strigosa, Orbicella annularis, and O. faveolata) suffered mortality as a result of the outbreak with an average loss of 16.42% relative cover by species, the overall impacts on coral cover and community structure were relatively low, contributing to a loss of total coral cover of only 1.65%. Comparison of photomosaic data to other studies indicate Cheeca Rocks may not have been affected as severely as other sites on Florida’s coral reef tract, underlying this site’s potential role in coral resilience to stressors including bleaching events, land-based pollution, and disease epizootics.
Introduction
Florida’s coral reef tract has seen near-continuous declines in coral cover since at least the late 1970’s, starting with a disease event affecting two key habitat-building coral species, Acropora cervicornis and Acropora palmata (Aronson and Precht, 2001), followed by precipitous population declines of a key herbivore, the urchin species Diadema antillarum (Mumby, 2006; Mumby et al., 2007). These two events are considered pivotal moments in the recent history of the reef tract, resulting in dramatic shifts in both species composition and ecosystem functionality toward lower coral cover and higher prevalence of macroalgae (Hughes, 1994; Gardner et al., 2003; Mumby, 2006; Maliao et al., 2008; Alvarez-Filip et al., 2019). In recent decades, anthropogenic stressors have continued to reduce coral cover across Florida’s coral reef tract, including increasing sea surface temperatures and associated coral bleaching events (Lang et al., 1992; Brandt and McManus, 2009; Manzello, 2015), overuse and overfishing of reefs (Ault et al., 2005), and nutrification which causes increased competition from macroalgae (Lapointe et al., 2004) and exacerbates disease and bleaching events (Voss and Richardson, 2006; Vega Thurber et al., 2013). In particular, coral disease outbreaks have increased in frequency and severity over this time period, including white plague, black band, and white band diseases, among others, all of which contributed to reduced live coral cover (Porter et al., 2001; Muller and van Woesik, 2012; Aeby et al., 2019; Alvarez-Filip et al., 2019).
In 2014, an unidentified disease characterized by rapid tissue loss with lesion progression rates as high as 9 mm day–1 appeared on the reefs surrounding Government Cut in the Port of Miami, Florida (Cunning et al., 2019; Gintert et al., 2019). The disease was discovered to affect a wide variety of scleractinian coral taxa spanning multiple genera and families, unlike previously known diseases which were largely species- or genus-specific (Precht et al., 2016; Rippe et al., 2018; Walton et al., 2018; Aeby et al., 2019; Alvarez-Filip et al., 2019). Due to this aggressive nature of rapid and high mortality, as well as its virulence across numerous species, it was characterized as a novel disease named stony coral tissue loss disease (SCTLD; NOAA, 2018). The endemic zone spread rapidly throughout Florida’s coral reef tract, reaching Broward County to the north and Biscayne National Park to the south in 2015; Palm Beach County and the upper Keys in 2016; Martin County and the middle Keys by 2017; and continuing to reach the lower Keys by 2018 and 2019 (NOAA, 2018; Sharp et al., 2020), and the Dry Tortugas in 2021 (Roth et al., 2020).
Little is known about SCTLD, including its etiology, pathogen(s), or modes of transmission. Currently, it is believed to be the result of transmissible bacteria due to some success in mitigation with the use of antibiotics (Neely et al., 2020), however, this is still unconfirmed experimentally (Aeby et al., 2019; Meyer et al., 2019; Landsberg et al., 2020; Rosales et al., 2020). It has been shown to affect over 20 species of stony coral, representing a significant threat to primary reef-building species in Florida (e.g., Orbicella faveolata, O. annularis, and Montastraea cavernosa; Precht et al., 2016), as well as to relatively rarer meandroid and pillar coral species. The resulting characteristics of the infection, including tissue loss rates, lesion morphology, and lesion occurrence, differ among species and reefs (Aeby et al., 2019; Alvarez-Filip et al., 2019; Meyer et al., 2019; Estrada-Saldívar et al., 2021; Meiling et al., 2021).
Thus far, monitoring and impact assessments of the disease in situ have largely been by conducted through fate-tracking of individual colonies through photographs (Precht et al., 2016; Aeby et al., 2019; Gintert et al., 2019), repetitive benthic transects (Walker, 2018; Walton et al., 2018), and prevalence surveys and reports by researchers and citizen scientists (Roth et al., 2020; Sharp et al., 2020). Although these methods are important for understanding the pace of the spread of the disease, both in terms of tissue loss and geospatial location, they tend to be time-consuming and cumbersome means of obtaining quantitative data due to the amount of diver time needed in-water. These limitations can translate to a reduced number of survey species and/or coral colonies (Aeby et al., 2019), or a relatively small spatial area used in data collection (Walton et al., 2018). Timed swim surveys can be conducted over a larger total area within a reasonable amount of time (Precht et al., 2016; Gintert et al., 2019), however, the survey area is generally confined to a linear swath of reef, relies on subjective in situ measurements, and only provides photographic data of specific colonies. One solution to these issues is through the implementation of photomosaicking approaches, wherein a large number (∼1,200–2,000) of individual photographs are stitched together to create a single mosaic image of a large area.
Photomosaics have been gaining traction in ecological studies over the past decade (Van Rein et al., 2011; Casella et al., 2016; Wood et al., 2016; Gintert et al., 2018; Carter et al., 2019), including with fate-tracking of SCTLD-infected colonies (Meiling et al., 2020; Combs et al., 2021; Shilling et al., 2021). In approximately 30 min of diving, and subsequent processing time that is dependent on computer resources, an area of roughly 10 m by 10 m (100 m2) can be surveyed, resulting in a permanent record in the form of a snapshot of any reef area, often with resolution high enough to identify individual polyps of certain species. Photomosaic analysis allows for consideration of all species and size classes present in the survey area, and provides the ability to collect quantitative data including percent cover, species composition, and percent mortality on hundreds to thousands of coral colonies (Gintert et al., 2018).
Photomosaics are an extremely informative quantitative approach to integrate into reef monitoring efforts. As part of NOAA’s Coral Reef Conservation Program’s (CRCP) National Coral Reef Monitoring Program (NCRMP; NOAA Coral Program, 2014), photomosaics have been collected for six long-term, ∼50 m2 plots at Cheeca Rocks at least annually since 2012 (NOAA Coral Reef Watch, 2014). Cheeca Rocks is an inshore patch reef off Islamorada, Florida in the upper Florida Keys, possessing anomalously high coral cover (∼25%) compared to other reefs throughout Florida’s coral reef tract (Gintert et al., 2018; Manzello et al., 2018). The reef community is dominated by large mounding corals, primarily of the species Orbicella faveolata and O. annularis, as well as meandroid corals including Colpophyllia natans, Diploria labyrinthiformis, and Pseudodiploria spp., all of which are susceptible to SCTLD (NOAA, 2018). SCTLD was first observed at Cheeca Rocks in February 2018 during ongoing monitoring of environmental parameters (pers. obs. by G. Kolodziej). Losses of many colonies of meandroid species were initially noted as partial or complete mortality, followed by similar instances of mortality from the orbicellids later in the year. Cheeca Rocks has otherwise been shown to be a robust and resilient reef with respect to bleaching severity and subsequent mortality (Manzello et al., 2015a,b, 2018, 2019; Gintert et al., 2018), as well as potential negative effects of ocean acidification (OA) due to its location inshore (Manzello et al., 2012). This monitoring effort provides annual benthic data of approximately 330 m2 at Cheeca Rocks, including from before the disease outbreak at the site (2017) and after most of the mortality had already occurred (2019).
Using photomosaic datasets, analyses were conducted to assess what impacts were visible at Cheeca Rocks within the surveyed areas. Pre-disease percent coral cover and species composition were analyzed for all six plots using methodology established by Gintert et al. (2018) from photomosaics taken in 2017. Colonies were then fate-tracked to assess mortality as a result of the disease, as well as overall disease prevalence and changes in species composition, using photomosaics taken in 2019 once the majority of SCTLD infections had subsided within the plots. While 2 years represents a substantial amount of time in the context of SCTLD progression rates along colonies, the intention was to quantify the downstream impacts of the SCTLD outbreak on coral cover and community structure, not fate-tracking of individual colonies.
Materials and Methods
Mosaic Capture and Build
Photomosaics were captured using dual GoPro Hero 4 Black cameras at each of six permanent plots (approximately 50 m2 each) located at Cheeca Rocks Reef (24.90°N, 80.62°W, Figure 1) on November 6, 2017 and September 18, 2019. These two time points were chosen to provide an estimate of the pre-disease, post-Hurricane-Irma (September 10–11, 2017) conditions of the plots, as well as the resulting conditions after the local outbreak had largely abated. Photomosaics from April 20, 2018 were also examined for presence of disease, but we did not find any active lesions within the plot areas at that time. Repeated qualitative observations later in 2018 indicated that the peak of the outbreak likely occurred during May–July 2018, but these data were not captured in the photomosaic datasets. For photomosaic generation, cameras were placed approximately 60 cm apart on a piece of aluminum square stock tube and secured via 3D printed mounts. Photo settings were 12MP wide angle, and the cameras were shooting in time lapse photo mode with 1 s intervals. Each plot had four corner pins permanently affixed which were relocated before each mosaic capture and temporarily marked with weighted plastic chains. The plots were swum in a lawnmower pattern back-and-forth in one direction, and again perpendicular to the original direction, to ensure full coverage of the area while maintaining ∼1.5 m height above the bottom. Each plot took ∼20–25 min to swim both directional transects and required roughly 5 min for both setup and recovery of scale bars and marker chains per plot.
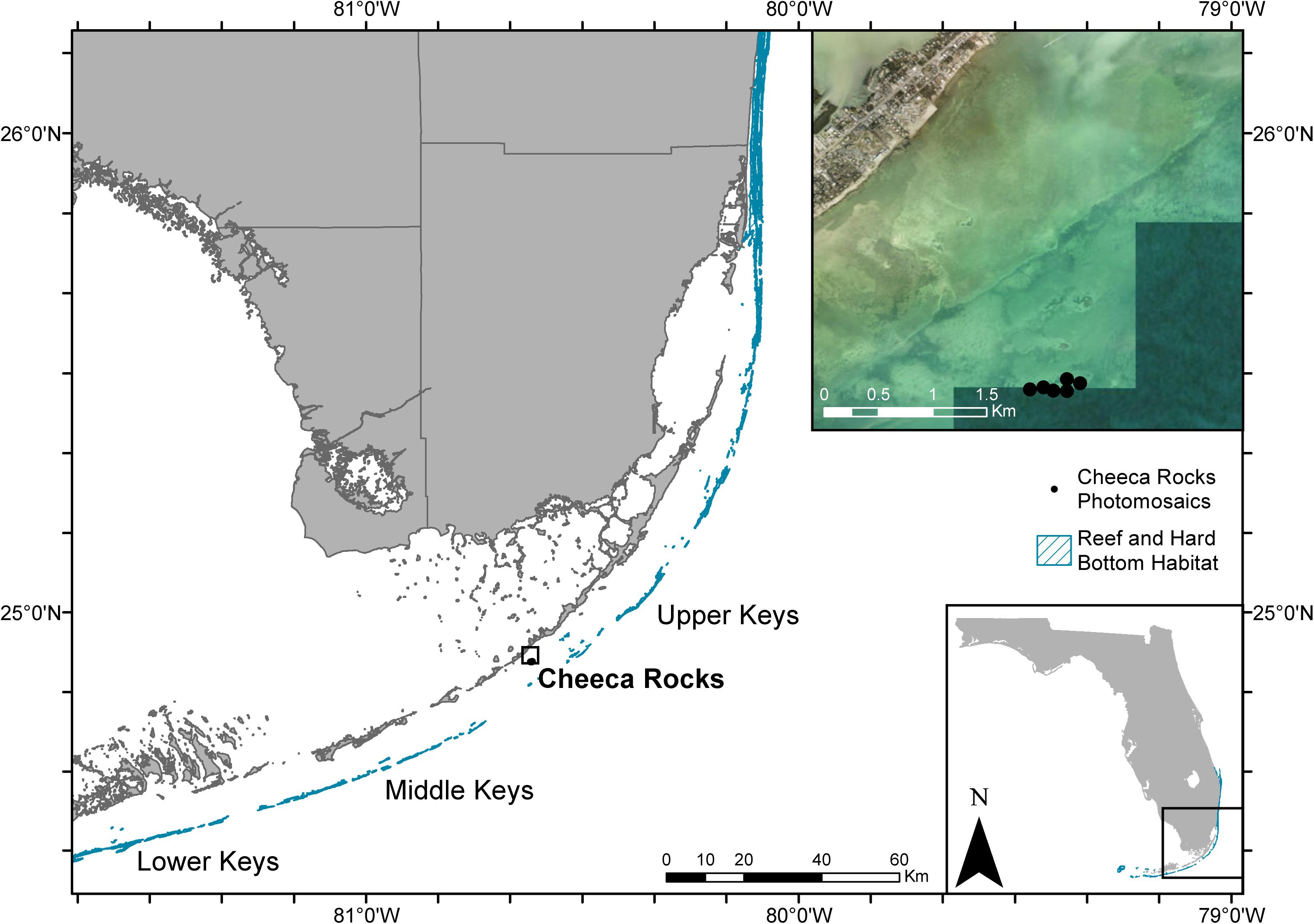
Figure 1. Map of the southern extent of Florida’s coral reef tract including the long-term monitoring plots at Cheeca Rocks. Reef and hard bottom habitat shapefile was sourced from the Florida Fish and Wildlife Conservation Commission-Fish and Wildlife Research Institute, and satellite imagery was sourced from ESRI.
Data from each plot consisted of 1,500–2,500 photos, depending on the plot area, swim speed, and number of passes made. Photo sets were uploaded onto a dedicated photomosaic build computer (Puget Systems) and imported into Agisoft Metashape (previously Photoscan v1.3.4 build 5067) software to be compiled into a single mosaic image. Software protocols and settings for the build are fully described in Supplementary Data Sheet 1, but briefly, are comprised of six main steps: (1) photo alignment, (2) alignment optimization, (3) dense point cloud generation, (4) mesh generation, (5) digital elevation model generation, and (6) orthomosaic generation.
Mosaic Data Collection
Orthomosaics were output from Metashape as TIFF images, then imported into ESRI ArcGIS software as raster datasets (FGDBR) and georeferenced to past Cheeca Rocks photomosaic datasets (Gintert et al., 2018) using known and obvious identifiable characteristics (e.g., corner pins, coral colonies) to obtain a scaled image of known area comparable to the previous study. A minimum of 10 characteristics were used per photomosaic for geo-referencing using the Add Control Points tool. Starting with the 2017 photomosaics, each plot’s four corner marking pins were located and used to define the spatial area for analysis (Figure 2a). Inside this boundary, all identifiable scleractinian coral colonies’ outlines were traced using the Create Feature Tool’s freehand tool to obtain pre-disease surface areas color-coded by species (Figure 2b). Similar to Gintert et al. (2018), adjacent colonies of the same species were treated as separate colonies when a visible gap of live tissue was present. Additionally, colonies were not included in the analyses if more than 5% of their surface area was outside the plot’s boundary.
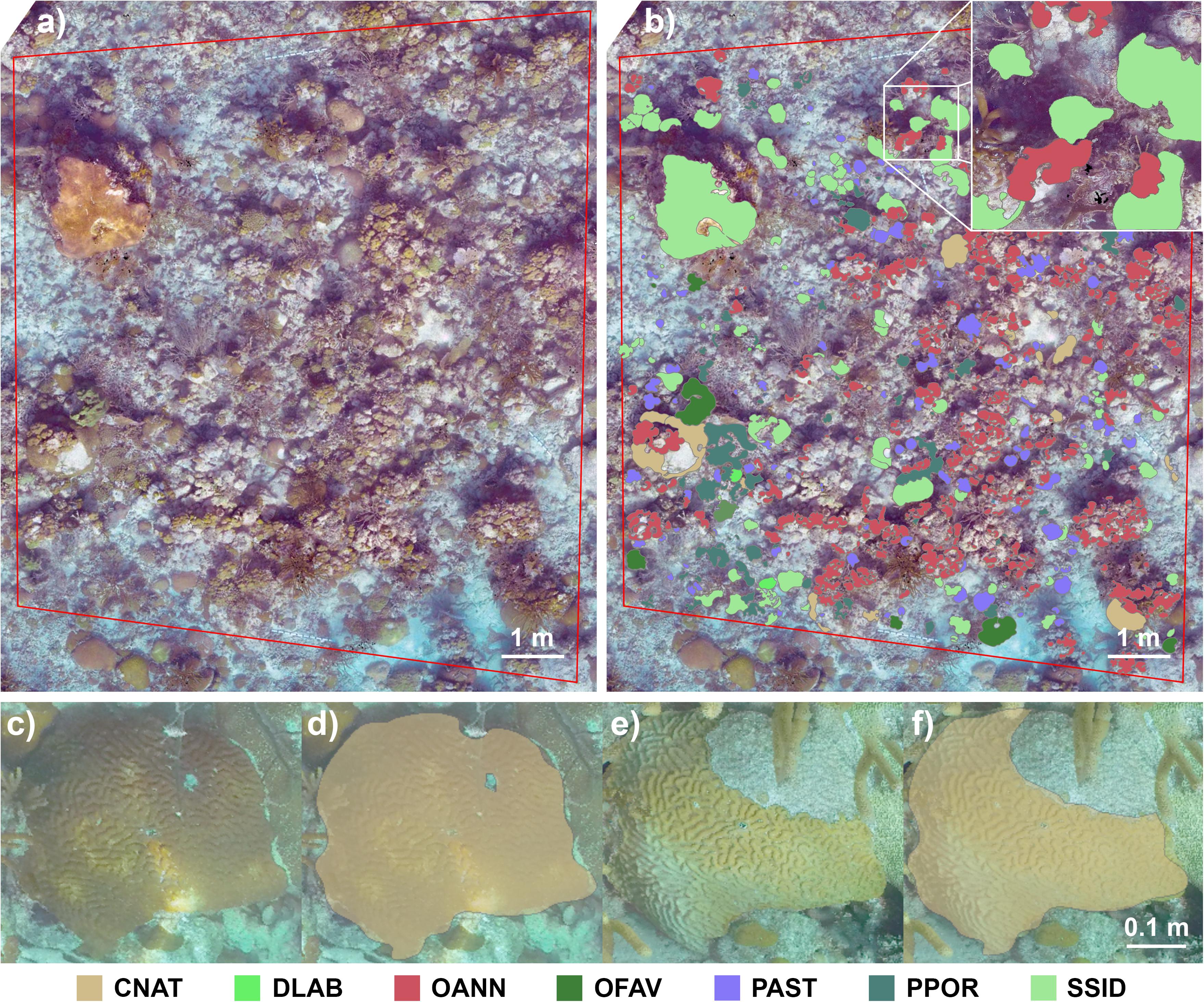
Figure 2. (a) Orthomosaic of one of the six plots analyzed in this study. The red boundary was defined by permanent markers at the site, and all scleractinian coral colonies within this area were included in the analysis. (b) The same orthomosaic with superimposed polygons representing the boundaries of individual coral colonies. A fate-tracked Colpophyllia natans colony in (c) November 2017, (d) with resulting analysis polygon, (e) and the same colony showing partial mortality in September 2019, (f) with its resulting edited polygon. CNAT, Colpophyllia natans; DLAB, Diploria labyrinthiformis; OANN, Orbicella annularis; OFAV, Orbicella faveolata; PAST, Porites astreoides; PPOR, Porites porites; SSID, Siderastrea siderea.
For data collection from 2019 mosaics, coral polygon layers from each of the 2017 plots were copied to create a new layer for editing (Figures 2c–f). Colonies were tracked only on mortality related to the SCTLD event; where new growth was not assessed, and any mortality seen unrelated to the disease event, such as physical damage, was noted but not quantified. This analysis method, rather than creating entirely new polygons for each coral colony in the 2019 plots, reduced potential impacts of minor discrepancies created during photomosaic builds. Every polygon was assessed for partial or complete mortality due to the magnitude of change in live tissue area. Colonies that experienced partial mortality had their polygons edited using the Reshape Feature tool to reflect the remaining live tissue area (Figure 2f), and polygons for colonies that perished completely were not edited but were noted as complete mortality. Colonies that were less visible in 2019 due to camera angle differences had their polygons edited only to the extent of visible mortality. Underlying polygon areas that were no longer visible, for instance due to obstruction by a gorgonian or macroalgal growth, were left intact even if mortality was suspected to reduce the possibility of overestimating mortality, though this was rare. Species IDs and areas for all coral polygons were captured from the respective layers’ attribute tables for subsequent statistical analyses.
Statistical Analyses
From the raw polygon areas for each coral colony in 2017 and 2019 plots, net change in area and type of mortality were calculated by the magnitude of change in area over time. Subsequent metrics were calculated by species on a per-plot basis unless otherwise noted, including proportion of complete and partial mortality, total disease prevalence, 2017 and 2019 absolute coral cover, and proportion of coral cover loss. Relative coral cover (species composition) was calculated after pooling data across plots. As the mortality and percent cover datasets did not meet the assumptions of normality, non-parametric statistics were conducted in the R statistical environment (R Core Team, 2019). The metrics for complete mortality, partial mortality, coral cover in 2017 and 2019, and coral cover loss were transformed using a log(x + 1) function prior to analyses to reduce impacts of data skew and zero values.
First, coral cover for all species within plots was tested for a significant decrease from 2017 to 2019 using a one-tailed Wilcoxon signed rank test. For all subsequent statistical analyses of proportional metrics (complete mortality, partial mortality, and coral cover loss), datasets were truncated to the eight most abundant, SCTLD-susceptible species found across at least four plots, including O. annularis, Siderastrea siderea, O. faveolata, C. natans, Montastraea cavernosa, Stephanocoenia intersepta, D. labyrinthiformis, and P. strigosa. Multivariate assessments of variation in the three rate metrics among species were conducted using a single-factor PERMANOVA with the package vegan (9999 permutations; Oksanen et al., 2015), including pairwise tests among pairs of species comparisons with the package pairwiseAdonis (Martinez Arbizu, 2020). Following a significant multivariate test result, univariate Kruskal-Wallis tests and pairwise Dunn’s tests were conducted for each of the three metrics in the packages ggpubr and FSA, respectively (Kassambara, 2017; Ogle, 2017).
Results
Coral Mortality and SCTLD Prevalence
A total of 2,251 colonies from 17 coral species were identified in the 2017 mosaic plots, while 2,197 live colonies remained in 2019, indicating mortality of 54 colonies between years due to SCTLD (Table 1). Of the 17 species, 10 were observed to be affected by the disease via tissue loss resulting in partial or complete mortality of individual colonies. Pseudodiploria strigosa saw the greatest proportion of colonies experiencing complete mortality (67.86%), as well as the highest disease prevalence (82.14%; Table 2 and Figure 3). Colpophyllia natans saw the greatest number of colonies affected in terms of partial mortality (25.12%). Other brain coral species, including D. labyrinthiformis and P. clivosa, experienced relatively high rates of mortality and disease prevalence, whereas star coral species, including O. annularis, S. siderea, O. faveolata, and M. cavernosa, conversely, had low rates of complete mortality and disease prevalence (Table 2 and Figure 3). Across all SCTLD-susceptible species observed in this study (excluding Porites astreoides and P. porites), 13.76% of all colonies experienced complete mortality, and 6.66% experienced partial mortality, corresponding to a global SCTLD prevalence of 20.42% (Table 2).
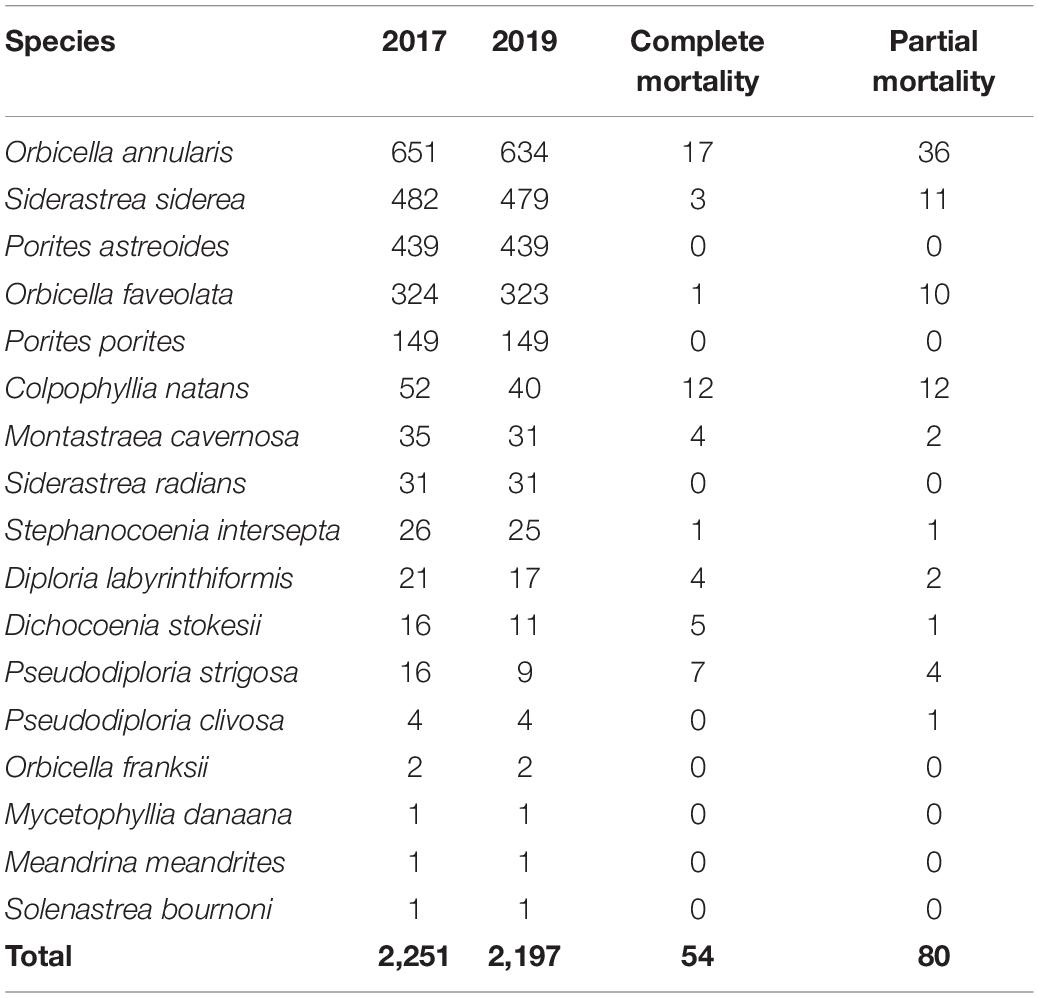
Table 1. The number of colonies analyzed per species for 2017 and 2019 plots, as well as the number of colonies which experienced complete or partial mortality during the same period. Species are ranked by their total abundance.
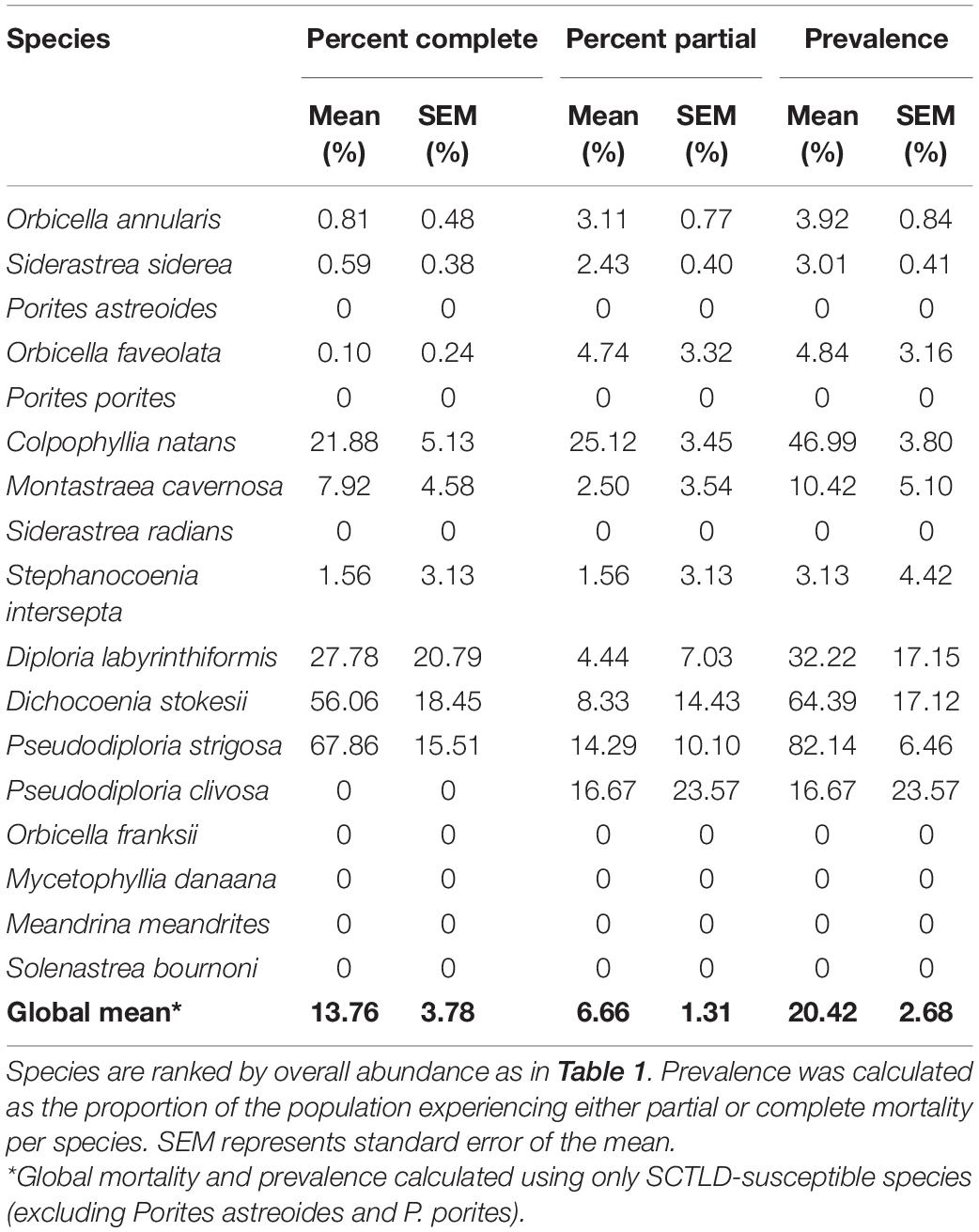
Table 2. Proportion of colonies experiencing partial or complete mortality, and overall disease prevalence across all six plots in 2019.
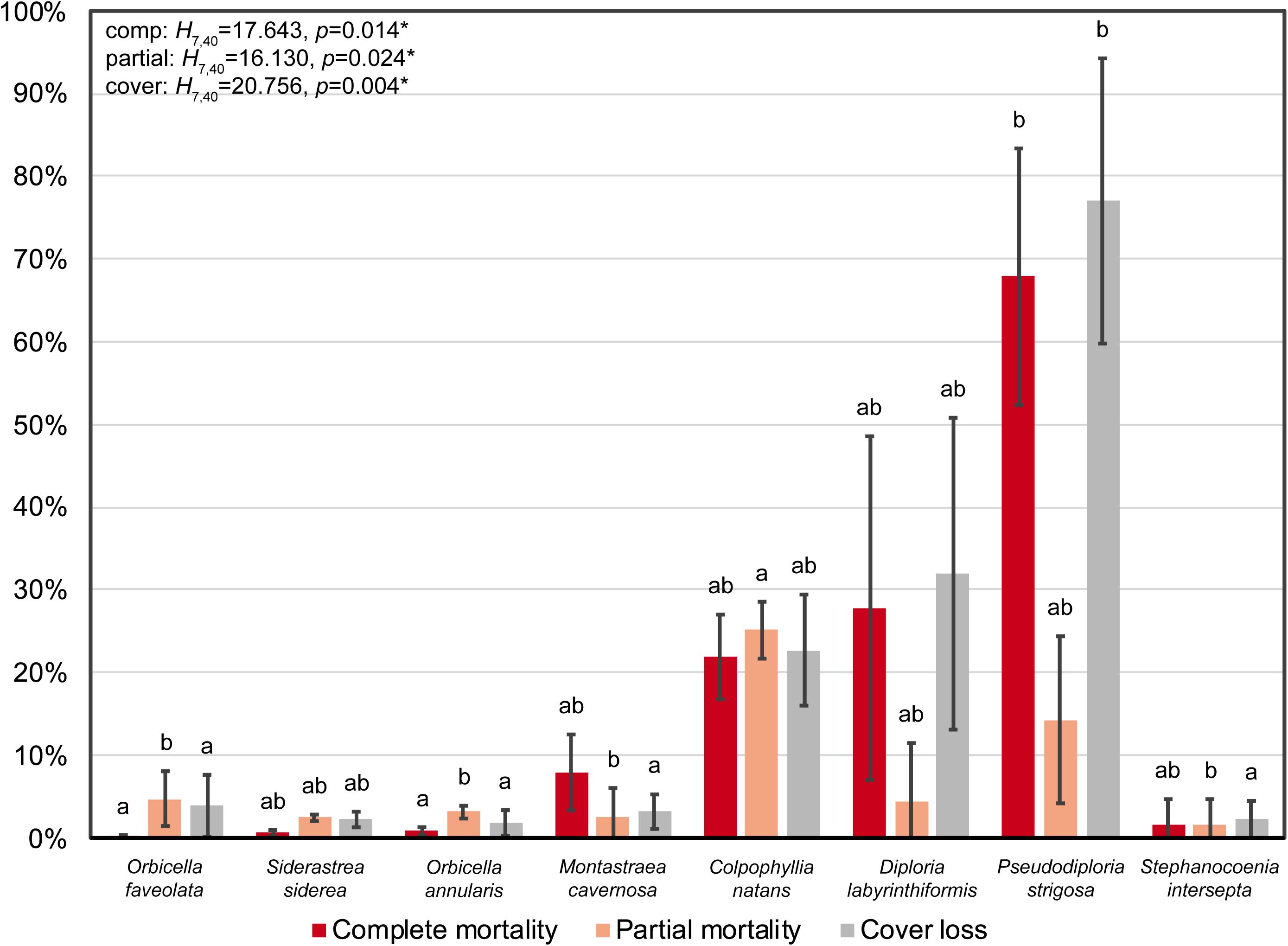
Figure 3. Proportion of complete mortality, partial mortality, and loss of coral cover for the eight most abundant, SCTLD-susceptible species across the photomosaic plots. Error bars represent standard error of the mean. Test results are from separate single-factor Kruskal-Wallis tests, and different letters denote significant Dunn’s pairwise comparisons between species pairs for each metric. Species ordered from left to right according to decreasing absolute percent cover.
The PERMANOVA indicated that there was significant variation in mortality and loss of coral cover among species [F(7, 40) = 5.129, p = 0.0001], which was primarily attributed to pairwise differences between brain corals and all other species (Supplementary Table 1 and Figure 3). Univariate Kruskal-Wallis tests demonstrated that rates of both complete and partial mortality were significantly different among species [complete: H(7, 40) = 17.643, p = 0.014; partial: H(7, 40) = 16.130, p = 0.024], with significant pairwise comparisons between Pseudodiploria strigosa and the orbicellids for complete mortality, and C. natans and the star corals for partial mortality (Supplementary Table 1 and Figure 3).
Coral Cover
Between 2017 and 2019, overall percent coral cover at Cheeca Rocks declined significantly from 26.98 to 25.33%, representing a 1.65% loss of live coral cover (Wilcoxon: V = 496, p = 1.67e–5; (Table 3). Although the brain coral species C. natans, D. labyrinthiformis, and P. strigosa made up only 1.86% of the total coral cover in 2017 (5.16% relative cover), they were disproportionately affected by SCTLD compared to star corals, with 22.71–77.02% reductions in coral cover. The star corals O. faveolata, S. siderea, O. annularis, and M. cavernosa comprised the majority of absolute (21.5%) and relative coral cover (76.0%) in 2017, and were largely unaffected by SCTLD with minimal losses of coral cover between 1.81–3.89% (Table 3 and Figure 3). Following the significant PERMANOVA result, a univariate Kruskal-Wallis test identified that loss of coral cover was significantly different among species [H(7, 40) = 20.756, p = 0.004], with significant pairwise differences between P. strigosa and the star corals M. cavernosa, O. annularis, O. faveolata, and Stephanocoenia intersepta (Supplementary Table 1 and Figure 3).
Species Composition
Since star coral species (O. faveolata, S. siderea, O. annularis, and M. cavernosa) and poritids (Porites astreoides and P. porites) dominated the coral community at Cheeca Rocks and were less affected by SCTLD (due to low mortality/prevalence for the former, and lack of susceptibility for the latter) than the highly susceptible, but relatively rarer/lower cover brain coral species (C. natans, D. labyrinthiformis, Pseudodiploria strigosa, and P. clivosa), species composition remained mostly unchanged from 2017 to 2019 (Figure 4). Slight reductions in the relative cover of C. natans, D. labyrinthiformis, and Pseudodiploria strigosa resulted in corresponding increases in the relative cover of star corals and poritids.
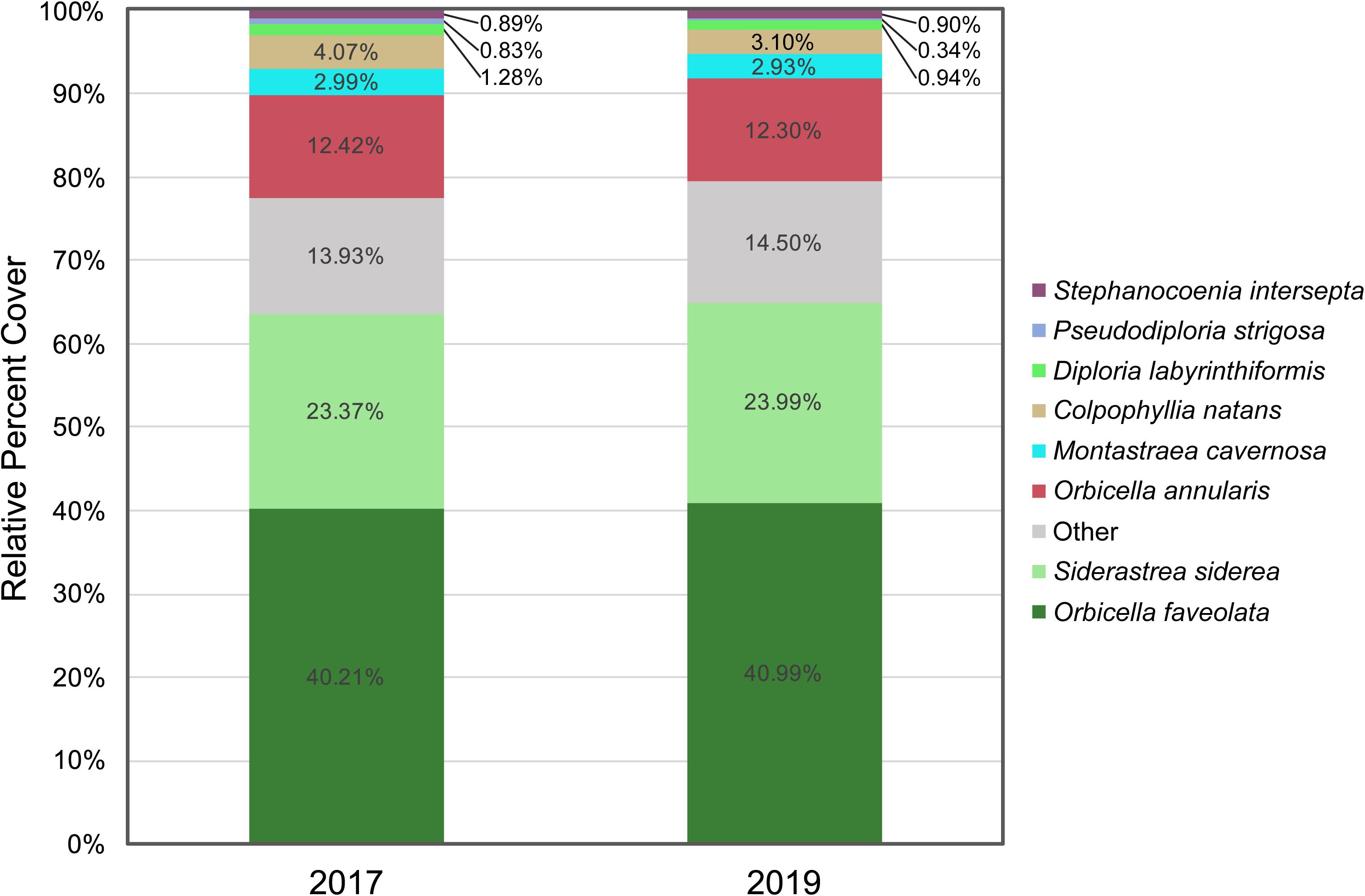
Figure 4. Species composition from 2017 to 2019, represented as relative percent cover of the top eight most abundant species, with all other species grouped as Other. Species ranked according to decreasing absolute percent cover.
Discussion
Cheeca Rocks has previously demonstrated resilience to thermal stress and bleaching events (Manzello et al., 2012, 2015a,2015b, 2018, 2019; Gintert et al., 2018). It was not, however, spared from the SCTLD outbreak, as complete and partial mortality of coral colonies were observed between photomosaic sampling events in 2017 and 2019. There was evidence of species-specific variation in mortality, disease prevalence, and loss of coral cover that follow the established rankings of species susceptibility to SCTLD observed among Florida reefs (NOAA, 2018; Aeby et al., 2019). It is important to note, however, that recent studies throughout the wider Caribbean, including the U.S. Virgin Islands (Meiling et al., 2020, 2021), the Mexican Caribbean (Alvarez-Filip et al., 2019; Estrada-Saldívar et al., 2021), and Turks and Caicos (Heres et al., 2021), suggest that there may be region-specific variation in species susceptibility to SCTLD, as well as disease prevalence, progression rates, and resulting impacts on community composition. Other site-specific environmental factors, such as depth, latitude, and eutrophication, and ecological factors such as species composition may also impact local SCTLD dynamics, but these trends are understudied at this point due to the rapid and seemingly inconsistent spread of the disease throughout the Caribbean (Roth et al., 2020).
Of the 17 species surveyed at Cheeca Rocks, 10 were affected by the disease, resulting in varying severity of mortality. Of the seven species that were not observed to be affected by the disease, two are suspected to be not susceptible to SCTLD (Porites astreoides and P. porites), while the other five (Siderastrea radians, O. franksii, Mycetophyllia danaana, Meandrina meandrites, and Solenastrea bournoni) were present in low abundance within the photomosaic plots, and therefore our observations may underestimate disease prevalence for these species at Cheeca Rocks. The previously reported highly susceptible species (C. natans, D. labyrinthiformis, P. strigosa, and Dichocoenia stokesii) showed the highest levels of complete and/or partial mortality due to SCTLD (Table 2 and Figure 3). Pseudodiploria clivosa, a species with comparable susceptibility to its congener P. strigosa, saw minimal disease prevalence (16.67%) compared to the latter (82.14%). Only four colonies of the former species were seen within the plots, however, thereby lacking the sample size to proceed with statistical analysis for this species.
Overall coral cover at Cheeca Rocks declined significantly by 1.65% from 26.98% in 2017 to 25.33% in 2019, with the reduction and/or loss of highly susceptible species being the primary driver (Table 3). As these species were already relatively rare at the outset of the study prior to SCTLD reaching the reef, the resulting impacts on overall coral community structure were minor. Coral communities at Cheeca Rocks were primarily composed of the star coral species O. faveolata, Siderastrea siderea, O. annularis, and to a lesser extent, Montastraea cavernosa, representing 21.50% of the total coral cover combined. Porites astreoides and P. porites also composed 2.86% of the total coral cover for the reef. Despite some observed mortality in the star coral species, the relative cover of live tissue remained similar between the sampling years (Figure 4). These observations suggest that while rarer species may continue to decline over time and may even lead to local extirpation, the primary reef building coral species appear to be remarkably persistent in the face of increasing frequency and intensity of stress events, including thermal anomalies and disease outbreaks (Manzello et al., 2012, 2015a,2015b, 2018, 2019; Gintert et al., 2018). The resilience of these primary reef building species at Cheeca Rocks, particularly O. faveolata and O. annularis, is perhaps the most important finding of this study given their effect on species composition at the site. Whereas other studies conducted beyond Florida identified these species as being highly impacted by SCTLD (Meiling et al., 2020; Estrada-Saldívar et al., 2021; Heres et al., 2021), the orbicellid populations surveyed at Cheeca Rocks saw disease prevalence rates below 5%. This low prevalence and resulting low mortality, combined with high abundance of these species, was a primary factor in the lack of significant impacts of SCTLD on species composition at Cheeca Rocks.
This study provided evidence that this site may have been largely spared the brunt of rapid mortality associated with the spread of SCTLD throughout Florida’s coral reef tract, perhaps due to reduced or late exposure to the disease, or perhaps inherent resistance and/or resilience in coral populations (particularly the dominant star coral species) to the disease. Coral communities at Cheeca Rocks saw only minimal reductions in coral cover from 2017 to 2019 following perhaps the most severe outbreak of a coral disease to date (1.65% reduction; this study), especially when compared to back-to-back bleaching years in 2014 and 2015 (3.5%; Gintert et al., 2018). Comparing our SCTLD prevalence data to other studies also suggests that the impacts of the disease were less severe at Cheeca Rocks relative to other areas of Florida’s coral reef tract. Aeby et al. (2019) reported that M. cavernosa colonies surveyed around Fort Lauderdale, Florida had a disease prevalence of 100%, whereas prevalence for this species was 10.42% at Cheeca Rocks. Their study incorporated fate-tracking of P. strigosa, Diploria labyrinthiformis, Dichocoenia stokesii, and Meandrina jacksoni colonies in the middle Florida Keys, which indicated a 61.9% overall initial disease prevalence (13 of 21 colonies), which rose to a final prevalence of 90.5% (19 of 21 colonies) at the end of the observation period. If Meandrina meandrites were substituted for its congener M. jacksoni due to the latter’s absence from our plots, our data would indicate a mean prevalence of 52.5% at Cheeca Rocks for the same species as in their study. Considering the above are some of the most highly susceptible species to SCTLD, this would suggest that Cheeca Rocks was largely spared from the disease outbreak, or possibly that coral populations here confer an inherent resistance or resilience to the disease.
Other studies surveying reefs in Miami, Florida (Precht et al., 2016; Gintert et al., 2019) also indicated higher SCTLD prevalence than at Cheeca Rocks. Precht et al. (2016) observed that 100% of fate-tracked D. stokesii, P. strigosa, and C. natans colonies suffered complete mortality, whereas only 43.9% of those species at Cheeca Rocks died, with a mean prevalence of 61.8%. That same study also had an overall disease prevalence range of 67–81%, compared to a mean prevalence of 13.75% reported here for all SCTLD-susceptible species. Disease prevalence was also lower for most star coral species at Cheeca Rocks compared to Miami, including with O. faveolata (4.84 versus 13%, respectively), M. cavernosa (10.42 versus 38%), O. annularis (3.92 versus 73%); however, prevalence was higher for S. siderea at Cheeca Rocks (3.01 versus 0%). Gintert et al. (2019) built on the aforementioned study (Precht et al., 2016) by expanding the dataset to include more colonies and sites, ultimately reporting that overall fatality rates (complete mortality) ranged from 31.7 to 33.7% across all species observed. This contrasts with Cheeca Rocks, which saw complete mortality in only 2.40% of colonies globally (11.29% averaged across all susceptible species) over a comparable length of time. It is important to note, however, that the sites observed off Miami were not dominated by orbicellids, and that Cheeca Rocks has higher species diversity.
When compared to other reefs throughout the Caribbean, Cheeca Rocks demonstrated similar or even higher rates of disease prevalence for meandroid and brain coral species, ranging from 18–40% along the northern Yucatan Peninsula (Alvarez-Filip et al., 2019) to ∼60–70% in Cozumel (Estrada-Saldívar et al., 2021). The converse was true for star coral species, including the foundational orbicellids, S. siderea, and M. cavernosa, with disease prevalence of 10–23% along the Yucatan and 20–50% in Cozumel. This reinforces the hypothesis that there are region-specific differences in SCTLD susceptibility and prevalence, and highlights the role of high-abundance star coral species in maintenance of coral cover at Cheeca Rocks. Whereas other sites have observed shifts in overall benthic community structure as a result of SCTLD outbreaks (Alvarez-Filip et al., 2019; Estrada-Saldívar et al., 2021; Heres et al., 2021), our data suggests the same has not occurred at Cheeca Rocks, perhaps largely due to resilience in the foundational coral species. While other taxa beyond coral species were not analyzed as part of the NCRMP objectives, qualitative observations suggest that algal cover has not increased visibly due to the SCTLD outbreak.
Finally, of note are the results from Rippe et al. (2018), which included Cheeca Rocks as one of its four study sites comparing disease prevalence among inshore and offshore reefs in the Florida Keys. No disease was seen at Cheeca Rocks by the end of their observation period in October 2017, whereas all three other study sites had SCTLD prevalence, with the majority of the colony mortality at the latter sites estimated to have occurred between 2016 and 2017. Photomosaics captured in April 2018, while not analyzed for this study, also indicated that the main outbreak had not occurred at the time of sampling, although qualitative observations made later that year confirmed relatively high prevalence of diseased colonies from May through July 2018. It is therefore likely that the delayed onset of disease at Cheeca Rocks relative to other sites in the region contributed to lower prevalence reported during our study period. No active disease lesions were seen in the 2019 mosaics as well, suggesting that the initial outbreak had run its course by September 2019 at Cheeca Rocks. Our observations of rapid spread within a few months are similar to those seen in the middle Florida Keys (Sharp et al., 2020) and Mexico (Alvarez-Filip et al., 2019; Estrada-Saldívar et al., 2021). Likewise, continued observations through 2020 and 2021 have found few diseased colonies, with estimates of SCTLD prevalence under 1%, suggesting that impacts of the disease on community dynamics remain low.
Despite a lack of active lesions in the 2019 photomosaics, the observed mortality and loss of coral cover were indicative of SCTLD infections, as the tissue area lost in less than 2 years was far greater than is expected from normal conditions, and there were no major disturbance events such as bleaching or major storms during the study period. This is perhaps best evidenced by the non-susceptible Porites astreoides and P. porites that experienced no discernable tissue mortality. Additionally, minimal physical disturbance was seen throughout the plots that would otherwise explain the mortality of other coral species. As the primary objective of this study, and the larger NCRMP monitoring project, was to compare the overall effects of SCTLD (and previously, other long-term disturbances) on coral communities at Cheeca Rocks, rather than quantifying rates of lesion progression on individual colonies, we recognize that these datasets are temporally limited. SCTLD is known to have a rapid progression rate within colonies (Cunning et al., 2019; Gintert et al., 2019), suggesting that more frequent monitoring efforts are warranted for fine-scale assessment of disease impacts at the individual level. Photogrammetric methods have been successfully applied to SCTLD fate-tracking studies on weekly or monthly scales (Meiling et al., 2020; Combs et al., 2021; Shilling et al., 2021), yet similar techniques remain equally informative over larger areas and annual temporal scales, capturing community-level impacts of SCTLD and other disturbance events as presented here.
Cheeca Rocks represents an outlier compared to contemporary reefs along Florida’s coral reef tract with respect to coral cover, even in the face of thermal and disease stressors (Manzello et al., 2012, 2015a,2015b, 2018, 2019; Gintert et al., 2018). Whereas reefs in many other regions of the Caribbean are facing declines in coral populations and even local extinction of certain species (Chan et al., 2019), Cheeca Rocks appears to maintain relatively resilient populations of its dominant coral species despite ongoing disturbance events (Manzello et al., 2015a, 2019; Gintert et al., 2018). This resilience is potentially the result of increased nutrient concentrations, turbidity, chlorophyll, and temperature variability seen in inshore reef environments (Lirman and Fong, 2007; Rippe et al., 2018) that may convey increased coral fitness to stressors including disease. Exposure to such extremes has shown to confer increased thermal stress tolerance (Kenkel et al., 2013) and calcification rates in inshore corals found in the Florida Keys (notably at Cheeca Rocks; Manzello et al., 2015b). Inshore sites throughout Florida’s coral reef tract also appeared to have lower rates of bleaching and disease prevalence relative to sites more characteristic of offshore environments (van Woesik and McCaffrey, 2017). There may also be hydrodynamic factors unique to Cheeca Rocks that perhaps delayed or minimized the onset of disease, but additional examination is needed to test this hypothesis (Dobbelaere et al., 2020).
Active SCTLD lesions have been reported once again at Cheeca Rocks as recently as March 2020, indicating a critical need to continue these analyses. Despite this, widespread mortality has still not been observed in the monitoring plots during recurring qualitative observations (pers. obs. by G. Kolodziej). Continued community analyses using photomosaic approaches at this and other comparable sites provide the ability to collect quantitative data on orders of magnitude more colonies than with traditional fate-tracking approaches (i.e., thousands versus dozens of colonies). Similarly, expanding upon these analyses to include additional benthic taxa beyond corals might yield greater insight into potential shifts on an ecosystem scale resulting from disease outbreaks and other disturbance events. Photogrammetric approaches enhance efforts to gather critical data needed to evaluate the impacts of SCTLD on reefs throughout Florida and the wider Caribbean, and to identify and characterize resilient coral populations for effective conservation and restoration strategies.
Data Availability Statement
The original contributions presented in the study are included in the article/Supplementary Material, further inquiries can be directed to the corresponding author/s.
Author Contributions
IE and DM secured funding for this research. GK and IE designed and performed the research and data collection. MS and GK conducted the data analyses. GK wrote the manuscript, with significant contributions and revisions by MS and all co-authors. All authors contributed to the article and approved the submitted version.
Funding
This study was supported by grants to DM and IE from the Florida Department of Environmental Protection (FDEP award B1C5EA) and NOAA’s Coral Reef Conservation Program (NCRMP award 743).
Conflict of Interest
The authors declare that the research was conducted in the absence of any commercial or financial relationships that could be construed as a potential conflict of interest.
Publisher’s Note
All claims expressed in this article are solely those of the authors and do not necessarily represent those of their affiliated organizations, or those of the publisher, the editors and the reviewers. Any product that may be evaluated in this article, or claim that may be made by its manufacturer, is not guaranteed or endorsed by the publisher.
Acknowledgments
We thank Nathan Formel, John Morris, Nicole Besemer, and Isabelle Basden for support with field activities, and Brooke Gintert for assistance with photomosaic capture and analysis. Karen Bohnsack provided valuable feedback on study design and implementation. We also thank Anderson Mayfield for feedback and review of the manuscript.
Supplementary Material
The Supplementary Material for this article can be found online at: https://www.frontiersin.org/articles/10.3389/fmars.2021.682163/full#supplementary-material
Supplementary Table 1 | Test results from the statistical analyses of multivariate (PERMANOVA/pairwise PERMANOVA) and univariate (Kruskal-Wallis/Dunn’s) tests of variation in coral mortality and loss of coral cover among species. Non-significant p-values are given as ns.
Supplementary Data Sheet 1 | Protocol for the generation and analysis of photomosaic images, using Agisoft Photoscan (Metashape) v1.3.4 build 5067.
Supplementary Data Sheet 2 | Raw data generated from the six photomosaic plots at Cheeca Rocks, including total plot area, colony and species IDs, 2017 and 2019 live tissue area, net change in live tissue area, and severity of mortality (complete, partial).
References
Aeby, G. S., Ushijima, B., Campbell, J. E., Jones, S., Williams, G. J., Meyer, J. L., et al. (2019). Pathogenesis of a tissue loss disease affecting multiple species of corals along the Florida Reef Tract. Front. Mar. Sci. 6:1–18. doi: 10.3389/fmars.2019.00678
Alvarez-Filip, L., Estrada-Saldívar, N., Pérez-Cervantes, E., Molina-Hernández, A., Gonzalez-Barrios, F. J., Alvarez-Filip, L., et al. (2019). A rapid spread of the Stony Coral Tissue Loss Disease outbreak in the Mexican Caribbean. PeerJ 7:e8069. doi: 10.7717/peerj.8069
Aronson, R. B., and Precht, W. F. (2001). White-band disease and the changing face of Caribbean coral reefs. Hydrobiologia 460, 25–38. doi: 10.1023/A:1013103928980
Ault, J. S., Bohnsack, J. A., Smith, S. G., and Luo, J. (2005). Towards sustainable multispecies fisheries in the Florida, USA, coral reef ecosystem. Bull. Mar. Sci. 76, 595–622.
Brandt, M. E., and McManus, J. W. (2009). Disease incidence is related to bleaching extent in reef-building corals. Ecology 90, 2859–2867. doi: 10.1890/08-0445.1
Carter, A. L., Edwards, C. B., Fox, M. D., Amir, C. G., Eynaud, Y., Johnson, M. D., et al. (2019). Changes in benthic community composition associated with the outbreak of the corallimorph, Rhodactis howesii, at Palmyra Atoll. Coral Reefs 38, 1267–1279. doi: 10.1007/s00338-019-01841-5
Casella, E., Collin, A., Harris, D., Ferse, S., Bejarano, S., Parravicini, V., et al. (2016). Mapping coral reefs using consumer-grade drones and structure from motion photogrammetry techniques. Coral Reefs 36, 269–275. doi: 10.1007/s00338-016-1522-0
Chan, A. N., Lewis, C. L., Neely, K. L., and Baums, I. B. (2019). Fallen pillars: the past, present, and future population dynamics of a rare, specialist coral–algal symbiosis. Front. Mar. Sci. 6:218. doi: 10.3389/fmars.2019.00218
Combs, I. R., Studivan, M. S., Eckert, R. J., and Voss, J. D. (2021). Quantifying impacts of stony coral tissue loss disease on corals in Southeast Florida through surveys and 3D photogrammetry. PLoS One 16:e0252593. doi: 10.1371/journal.pone.0252593
Cunning, R., Silverstein, R. N., Barnes, B. B., and Baker, A. C. (2019). Extensive coral mortality and critical habitat loss following dredging and their association with remotely-sensed sediment plumes. Mar. Pollut. Bull. 145, 185–199. doi: 10.1016/j.marpolbul.2019.05.027
Dobbelaere, T., Muller, E. M., Gramer, L. J., Holstein, D. M., and Hanert, E. (2020). Coupled epidemio-dydrodynamic modeling to understand the spread of a deadly coral disease in Florida. Front. Mar. Sci. 7:591881. doi: 10.3389/fmars.2020.591881
Estrada-Saldívar, N., Quiroga-García, B. A., Pérez-Cervantes, E., Rivera-Garibay, O. O., and Alvarez-Filip, L. (2021). Effects of the Stony Coral Tissue Loss Disease outbreak on coral communities and the benthic composition of Cozumel reefs. Front. Mar. Sci. 8:632777. doi: 10.3389/fmars.2021.632777
Gardner, T. A., Côté, I. M., Gill, J. A., Grant, A., and Watkinson, A. R. (2003). Long-term region-wide declines in Caribbean corals. Science 301, 958–960. doi: 10.1126/science.1086050
Gintert, B. E., Manzello, D. P., Enochs, I. C., Kolodziej, G., Carlton, R., Gleason, A. C. R., et al. (2018). Marked annual coral bleaching resilience of an inshore patch reef in the Florida Keys: a nugget of hope, aberrance, or last man standing? Coral Reefs 37, 533–547. doi: 10.1007/s00338-018-1678-x
Gintert, B. E., Precht, W. F., Fura, R., Rogers, K., Rice, M., Precht, L. L., et al. (2019). Regional coral disease outbreak overwhelms impacts from a local dredge project. Environ. Monitor. Assess. 191:630. doi: 10.1007/s10661-019-7767-7
Heres, M. M., Farmer, B. H., Elmer, F., and Hertler, H. (2021). Ecological consequences of Stony Coral Tissue Loss Disease in the Turks and Caicos Islands. Coral Reefs 40, 609–624. doi: 10.1007/s00338-021-02071-4
Hughes, T. P. (1994). Catastrophes, phase shifts, and large-scale degradation of a Caribbean coral reef. Science 265, 1547–1551.
Kassambara, A. (2017). ggpubr: “ggplot2” based publication ready plots. R package version 0.1.6. Vienna: R Core Team.
Kenkel, C. D., Goodbody-Gringley, G., Caillaud, D., Davies, S. W., Bartels, E., and Matz, M. V. (2013). Evidence for a host role in thermotolerance divergence between populations of the mustard hill coral (Porites astreoides) from different reef environments. Mol. Ecol. 22, 4335–4348. doi: 10.1111/mec.12391
Landsberg, J. H., Kiryu, Y., Peters, E. C., Wilson, P. W., Perry, N., Waters, Y., et al. (2020). Stony coral tissue loss disease in Florida is associated with disruption of host–zooxanthellae physiology. Front. Mar. Sci. 7:1090. doi: 10.3389/fmars.2020.576013
Lang, J. C., Lasker, H. R., Gladfelter, E. H., Hallock, P., Jaap, W. C., Losada, F. J., et al. (1992). Spatial and temporal variability during periods of “recovery” after mass bleaching on Western Atlantic coral reefs. Am. Zool. 32, 696–706. doi: 10.1093/icb/32.6.696
Lapointe, B. E., Barile, P. J., and Matzie, W. R. (2004). Anthropogenic nutrient enrichment of seagrass and coral reef communities in the Lower Florida Keys: discrimination of local versus regional nitrogen sources. J. Exp. Mar. Biol. Ecol. 308, 23–58. doi: 10.1016/j.jembe.2004.01.019
Lirman, D., and Fong, P. (2007). Is proximity to land-based sources of coral stressors an appropriate measure of risk to coral reefs? An example from the Florida Reef Tract. Mar. Pollut. Bull. 54, 779–791. doi: 10.1016/j.marpolbul.2006.12.014
Maliao, R. J., Turingan, R. G., and Lin, J. (2008). Phase-shift in coral reef communities in the Florida Keys National Marine Sanctuary (FKNMS), USA. Mar. Biol. 154, 841–853. doi: 10.1007/s00227-008-0977-0
Manzello, D. P. (2015). Rapid recent warming of coral reefs in the Florida Keys. Sci. Rep. 5:16762. doi: 10.1038/srep16762
Manzello, D. P., Enochs, I. C., Kolodziej, G., and Carlton, R. (2015a). Coral growth patterns of Montastraea cavernosa and Porites astreoides in the Florida Keys: the importance of thermal stress and inimical waters. J. Exp. Mar. Biol. Ecol. 471, 198–207. doi: 10.1016/j.jembe.2015.06.010
Manzello, D. P., Enochs, I. C., Kolodziej, G., and Carlton, R. (2015b). Recent decade of growth and calcification of Orbicella faveolata in the Florida Keys: an inshore-offshore comparison. Mar. Ecol. Prog. Ser. 521, 81–89. doi: 10.3354/meps11085
Manzello, D. P., Enochs, I. C., Kolodziej, G., Carlton, R., and Valentino, L. (2018). Resilience in carbonate production despite three coral bleaching events in 5 years on an inshore patch reef in the Florida Keys. Mar. Biol. 165:99. doi: 10.1007/s00227-018-3354-7
Manzello, D. P., Enochs, I. C., Melo, N., Gledhill, D. K., and Johns, E. M. (2012). Ocean acidification refugia of the Florida Reef Tract. PLoS One 7:e41715–e41715. doi: 10.1371/journal.pone.0041715
Manzello, D. P., Matz, M. V., Enochs, I. C., Valentino, L., Carlton, R. D., Kolodziej, G., et al. (2019). Role of host genetics and heat-tolerant algal symbionts in sustaining populations of the endangered coral Orbicella faveolata in the Florida Keys with ocean warming. Glob. Change Biol. 25, 1016–1031. doi: 10.1111/gcb.14545
Martinez Arbizu, P. (2020). pairwiseAdonis: Pairwise multilevel comparison using adonis. R package version 0.4. Vienna: R Core Team.
Meiling, S. S., Muller, E. M., Lasseigne, D., Rossin, A., Veglia, A. J., MacKnight, N., et al. (2021). Variable species responses to experimental stony coral tissue loss disease (SCTLD) exposure. Front. Mar. Sci. 8:670829. doi: 10.3389/fmars.2021.670829
Meiling, S., Muller, E. M., Smith, T. B., and Brandt, M. E. (2020). 3D photogrammetry reveals dynamics of stony coral tissue loss disease (SCTLD) lesion progression across a thermal stress event. Front. Mar. Sci. 7:1128. doi: 10.3389/fmars.2020.597643
Meyer, J. L., Castellanos-Gell, J., Aeby, G. S., Häse, C. C., Ushijima, B., and Paul, V. J. (2019). Microbial community shifts associated with the ongoing stony coral tissue loss disease outbreak on the Florida Reef Tract. Front. Microbiol. 10:2244. doi: 10.3389/fmicb.2019.02244
Muller, E. M., and van Woesik, R. (2012). Caribbean coral diseases: primary transmission or secondary infection? Glob. Change Biol. 18, 3529–3535. doi: 10.1111/gcb.12019
Mumby, P. J. (2006). Fishing, trophic cascades, and the process of grazing on coral reefs. Science 311, 98–101. doi: 10.1126/science.1121129
Mumby, P. J., Hastings, A., and Edwards, H. J. (2007). Thresholds and the resilience of Caribbean coral reefs. Nature 450, 98–101. doi: 10.1038/nature06252
Neely, K. L., Macaulay, K. A., Hower, E. K., and Dobler, M. A. (2020). Effectiveness of topical antibiotics in treating corals affected by Stony Coral Tissue Loss Disease. PeerJ 8:9289. doi: 10.7717/peerj.9289
NOAA Coral Program (2014). National Coral Reef Monitoring Plan. Silver Spring, MD: NOAA Coral Reef Conservation Program, 40.
NOAA Coral Reef Watch (2014). Coral Reef Watch—annual summaries of thermal conditions related to coral bleaching for U.S. National Coral Reef Monitoring Program (NCRMP) jurisdictions. Silver Spring, MD: NOAA, 16.
Oksanen, J., Blanchet, F. G., Kindt, R., Legendre, P., Minchin, P. R., O’Hara, R., et al. (2015). vegan: community ecology package. R package version 2.0-10. Vienna: R Core Team.
Porter, J. W., Dustan, P., Jaap, W. C., Patterson, K. L., Kosmynin, V., Meier, O. W., et al. (2001). “Patterns of spread of coral disease in the Florida Keys,” in The Ecology and Etiology of Newly Emerging Marine Diseases, ed. J. W. Porter (Dordrecht: Springer), 1–24. doi: 10.1007/978-94-017-3284-0_1
Precht, W. F., Gintert, B. E., Robbart, M. L., Fura, R., and Van Woesik, R. (2016). Unprecedented disease-related coral mortality in Southeastern Florida. Sci. Rep. 6:31374. doi: 10.1038/srep31374
R Core Team (2019). R: A language and environment for statistical computing. Vienna: R Foundation for Statistical Computing.
Rippe, J. P., Kriefall, N. G., Davies, S. W., and Castillo, K. D. (2018). Differential disease incidence and mortality of inner and outer reef corals of the upper Florida Keys in association with a white syndrome outbreak. Bull. Mar. Sci. 95, 305–316. doi: 10.5343/bms.2018.0034
Rosales, S. M., Clark, A. S., Huebner, L. K., Ruzicka, R. R., and Muller, E. M. (2020). Rhodobacterales and Rhizobiales are associated with stony coral tissue loss disease and its suspected sources of transmission. Front. Microbiol. 11:681. doi: 10.3389/fmicb.2020.00681
Roth, L., Kramer, P., Doyle, E., and O’Sullivan, C. (2020). Caribbean SCTLD Dashboard. Available online at: www.agrra.org. (accessed March 06, 2021)
Sharp, W. C., Shea, C. P., Maxwell, K. E., Muller, E. M., and Hunt, J. H. (2020). Evaluating the small-scale epidemiology of the stony-coral -tissue-loss-disease in the middle Florida Keys. PLoS One 15:e0241871. doi: 10.1371/journal.pone.0241871
Shilling, E. N., Combs, I. R., and Voss, J. D. (2021). Assessing the effectiveness of two intervention methods for stony coral tissue loss disease on Montastraea cavernosa. Sci. Rep. 11, 1–11. doi: 10.1038/s41598-021-86926-4
Van Rein, H., Brown, C. J., Schoeman, D. S., Quinn, R., and Breen, J. (2011). Fixed-station monitoring of a harbour wall community: the utility of low-cost photomosaics and scuba on hard-substrata. Aquat. Conservat. Mar. Freshw. Ecosyst. 21, 690–703. doi: 10.1002/aqc.1230
van Woesik, R., and McCaffrey, K. R. (2017). Repeated thermal stress, shading, and directional selection in the Florida reef tract. Front. Mar. Sci. 4:182. doi: 10.3389/fmars.2017.00182
Vega Thurber, R. L., Burkepile, D. E., Fuchs, C., Shantz, A. A., McMinds, R., and Zaneveld, J. R. (2013). Chronic nutrient enrichment increases prevalence and severity of coral disease and bleaching. Glob. Change Biol. 20, 544–554. doi: 10.1111/gcb.12450
Voss, J. D., and Richardson, L. L. (2006). Nutrient enrichment enhances Black Band Disease progression in corals. Coral Reefs 25, 569–576. doi: 10.1007/s00338-006-0131-8
Walker, B. K. (2018). Southeast Florida reef-wide Post-Irma coral disease surveys. Florida, FL: Florida Department of Environmental Protection, 1–37.
Walton, C. J., Hayes, N. K., and Gilliam, D. S. (2018). Impacts of a regional, multi-year, multi-species coral disease outbreak in Southeast Florida. Front. Mar. Sci. 5:323. doi: 10.3389/fmars.2018.00323
Keywords: Cheeca Rocks, photogrammetry, structure from motion, Florida Reef Tract, coral disease, reef resilience, Florida’s coral reef tract
Citation: Kolodziej G, Studivan MS, Gleason ACR, Langdon C, Enochs IC and Manzello DP (2021) Impacts of Stony Coral Tissue Loss Disease (SCTLD) on Coral Community Structure at an Inshore Patch Reef of the Upper Florida Keys Using Photomosaics. Front. Mar. Sci. 8:682163. doi: 10.3389/fmars.2021.682163
Received: 17 March 2021; Accepted: 06 July 2021;
Published: 06 August 2021.
Edited by:
William F. Precht, Dial Cordy and Associates, Inc., United StatesReviewed by:
Jason Croop, Consultant, Miami, FL, United StatesEdwin A. Hernandez-Delgado, University of Puerto Rico, Puerto Rico
Copyright © 2021 Kolodziej, Studivan, Gleason, Langdon, Enochs and Manzello. This is an open-access article distributed under the terms of the Creative Commons Attribution License (CC BY). The use, distribution or reproduction in other forums is permitted, provided the original author(s) and the copyright owner(s) are credited and that the original publication in this journal is cited, in accordance with accepted academic practice. No use, distribution or reproduction is permitted which does not comply with these terms.
*Correspondence: Graham Kolodziej, graham.kolodziej@noaa.gov; studivanms@gmail.com