- 1Oceans Graduate School, The UWA Oceans Institute, The University of Western Australia, Crawley, WA, Australia
- 2Wildlife Trust of India, Noida, India
- 3School of Biological Sciences, The UWA Oceans Institute, The University of Western Australia, Crawley, WA, Australia
- 4Australian Institute of Marine Science, Indian Ocean Marine Research Centre, The University of Western Australia, Crawley, WA, Australia
Whale sharks off the western coast of India have suffered high levels of fishing pressure in the past, and today continue to be caught in small-scale fisheries as by-catch. Additionally, coastlines in this region host very large and growing human populations that are undergoing rapid development. This exacerbates ongoing anthropogenic threats to this species such as pollution, habitat loss, and ship traffic. For these reasons, there is an urgent need for data on movement patterns of whale sharks in this region of the Indian Ocean. Here, we address this issue by providing the first data on the horizontal movements of whale sharks tagged in the northern Arabian Sea off the western coast of the Indian state of Gujarat. From 2011 to 2017, eight individuals, ranging from 5.4 to 8 m were tagged and monitored using satellite telemetry. Tag retention varied from 1 to 137 days, with the sharks traveling distances of 34 – ∼2,230 km. Six of the eight individuals remained close to their tagging locations, although two sharks displayed wide ranging movements into the Arabian Sea, following frontal zones between water masses of different sea surface temperatures. We explore the relationship between the movement patterns of these whale sharks and the physical and biological processes of the region.
Introduction
The whale shark (Rhincodon typus, Smith 1828) is found in shallow and open ocean locations throughout the world’s tropical and subtropical environments, with known aggregations occurring from the Gulf of Mexico (Hueter et al., 2013) to Ningaloo Reef, Western Australia (Norman et al., 2016). Although there are predictable coastal occurrences of these sharks, they often transit large (1000s km) distances, spending most of their time in surface waters (<20 m depth), with regular dives to depths of 300–500 m (Brunnschweiler et al., 2009). Advances in satellite telemetry techniques have expanded our knowledge of the migratory movements of the species and assisted in identifying key drivers of these complex movements. Of these drivers, water temperatures (Sequeira et al., 2012; Meekan et al., 2015), frontal systems (Ryan et al., 2017), bathymetry and continental reef slopes (Copping et al., 2018), have been predicted to have the highest influence on the spatial use patterns of this species by contributing to enhanced primary productivity (Sleeman et al., 2010a).
The migratory behavior of whale sharks, in combination with their slow growth rates (Meekan et al., 2020) and K-selected life history, make populations highly vulnerable to anthropogenic pressures, such as ship strikes (Speed et al., 2008; Lester et al., 2020), bycatch and targeted fishing (Capietto et al., 2014) and pollution (Boldrocchi et al., 2020). These threats are of particular concern as the species is classified as “Endangered” on the IUCN Red List (Pearce and Norman, 2016). Ultimately, this has motivated satellite tagging programs that seek to identify movement patterns and assist in identifying areas of potential threats to the species (Reynolds et al., 2017; Robinson et al., 2017; Araujo et al., 2018; Rohner et al., 2020) in order to target effective conservation planning (Sequeira et al., 2019).
Several programs have deployed tags on sharks at various aggregation sites in the Indian Ocean, including Ningaloo, Madagascar, Mozambique and Seychelles (Supplementary Table 1). Despite this sampling effort, there have been no tagging studies of populations of whale sharks along the Indian coast in the northern Arabian Sea. This is important because the western coast of India has been predicted to be a location where a high probability of whale sharks occur (Sequeira et al., 2014) and a key aggregation site (Pravin, 2000; Bloch et al., 2018). Prior to 2001, whale sharks in the Arabian Sea were the subject of a targeted fishery that hunted these animals for their fins, skin and meat (Kumari and Raman, 2010). This fishery largely ceased operation after May 2001 following the inclusion of whale sharks into the Schedule I of the Indian Wildlife (Protection) Act, 1972. However, accidental entanglements during fishing and unintentional landings are common along the Indian coastline (Akhilesh et al., 2013; Retheesh et al., 2020) and whale sharks in this region may also face pressures from increased levels of pollution in nearby waters (Jabado et al., 2018). Data on the movement patterns of whale sharks along this coastline is now required to understand patterns of residency, identify the range of the population for conservation planning and document and aid any population recovery.
Here, we report the results of a satellite tagging study of whale sharks in the northern Arabian Sea along the coast of Gujarat, India. We describe horizontal patterns of movement of these animals in relation to remote sensing data of water temperature and sea surface colour (Chl-a) to identify key drivers of this species’ movement patterns in this region.
Materials and Methods
Whale sharks caught as by-catch in purse seine fishing nets were tagged in the northern Arabian Sea off the fishing town of Veraval (Figure 1a) from April 2011 to November 2017 using Smart Positioning Tags (SPOT tags; Wildlife Computers). Shark size was estimated using a tape measure and sex was determined based on the presence or absence of claspers (Norman and Stevens, 2007). Eight satellite tags were deployed, with two being fin-mounted (WS-1 and WS-2) and six tags tethered to the dorsal fins of sharks (WS-3–WS-8). Transmitted locations of each of the eight sharks tagged were obtained through the ARGOS satellite tracking system. To maximize battery life, tags transmitted every day for the first week and then every second day after that. Tags only transmit when the tag clears the surface long enough to locate and transmit to an ARGOS satellite. The transmitted positions were determined by Doppler-estimated calculations and assigned quality numbers or letters depending on associated errors (3, 2, 1, 0, A, B, and Z), which range inaccuracy from <250 m to >5 km. Locations with “Z” classes are considered unreliable (Argos, 2011), and they were removed from the datasets before analysis.
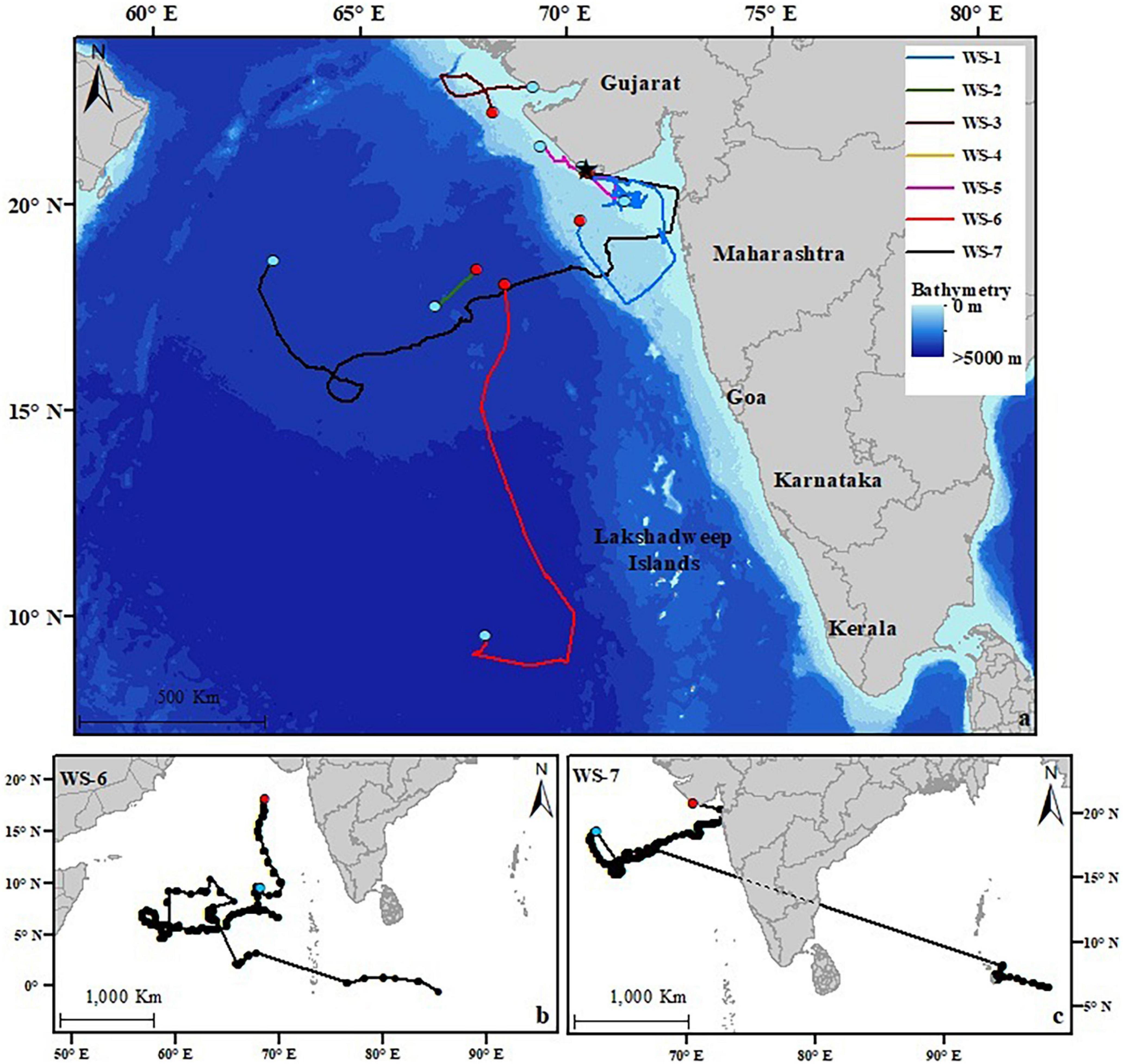
Figure 1. (a) Study area for the tagged whale sharks in the Arabian Sea. The star indicates the fishing town of Veraval, the port of tagging operations. Colored tracks show the estimated track lengths of the seven whale shark tracks (WS-1–WS-7). WS-8 was excluded from analysis as the tag failed to report. Red dots show the first geolocation and the blue dots show the estimated point of detachment. (b,c) The full length of transmissions for WS-6 and WS-7. Red dots show the first location and the blue dots show the estimated point of detachment.
We analyzed the tracks in R (R Core Team, 2013) to produce histograms of the quality of transmissions of each day. We removed duplicated messages and applied a 2 ms–1 speed filter to remove inaccurate locations based on the maximum speeds of whale sharks (Rowat and Gore, 2007; Rohner et al., 2018). Once filtered, tracks with alternating days missing satellite positions were interpolated to give estimated locations. When points in the tracks had greater gaps, they were fitted with a state-space model with the “bsam” package (Jonsen et al., 2005) in R to model the movement process. To determine if some of the tags detached before they ceased reporting, we analyzed the timing between points, the quality of the message and oceanographic data. We then assumed tags had detached from sharks when the following characteristics were observed: (i) speed increased to average current flows, (ii) direction of travel followed current patterns, and (iii) contact with satellites became regular and predictable (Hearn et al., 2013).
We obtained sea surface temperature (SST) data from the HYCOM + NCODA Global 1/120 analysis and the reanalysis database (Chassignet et al., 2007). Using MATLAB (2010), we extracted daily water temperature, salinity, surface elevation and water velocity data in the vicinity of each track. The daily SST were averaged to produce a single SST plot overlaid on the satellite track. We did not include the longest track (WS-7) in this part of the analysis, as the fine-scale resolution of the oceanographic features might have been lost, instead, we analyzed each daily plot for this track. The SST plots were visually examined to identify links between movements and features of physical (fronts etc.) or biological (phytoplankton etc.) oceanography. The daily SST plots were also used to produce animated movies showing the changes in water temperature in relation to the track for all sharks (see Supplementary Materials). Daily chlorophyll-a (Chl-a) concentrations were derived from the MODIS-Aqua, a product of the NASA satellite system1 at a 4.6 km resolution through ArcGIS (ESRI, 2011). Records for day and night were merged into a 24-h time series of the average concentration of Chl-a and overlaid over each track. Bathymetry data were obtained from the 2015 General Bathymetric Charts of the Oceans (GEBCO, 2015), at a resolution of 30 arc-s interval grids and analyzed in ArcGIS.
Results
Of the eight sharks tagged with SPOT tags from 2011 to 2017, the seven tags that transmitted locations (WS-1–WS-7; Table 1) are reported here. Two tags started transmitting the day of tagging, with the rest transmitting 1–8 days after tagging. Tags remained on the sharks from 6 to 137 days, however, transmissions from two tags (WS-6 and WS-7) continued for several months after detachment. Sharks traveled a mean distance of 29.45 km day–1 (0.34 ms–1; 1.46–60 km day–1 = 0.02–0.69 ms–1), covering distances of 33–2,229 km (Figure 1a). Five of the sharks tagged were females, whereas the remaining two were immature males (Table 1). The smallest shark tagged was a 5.8 m female and the largest an 8 m female.
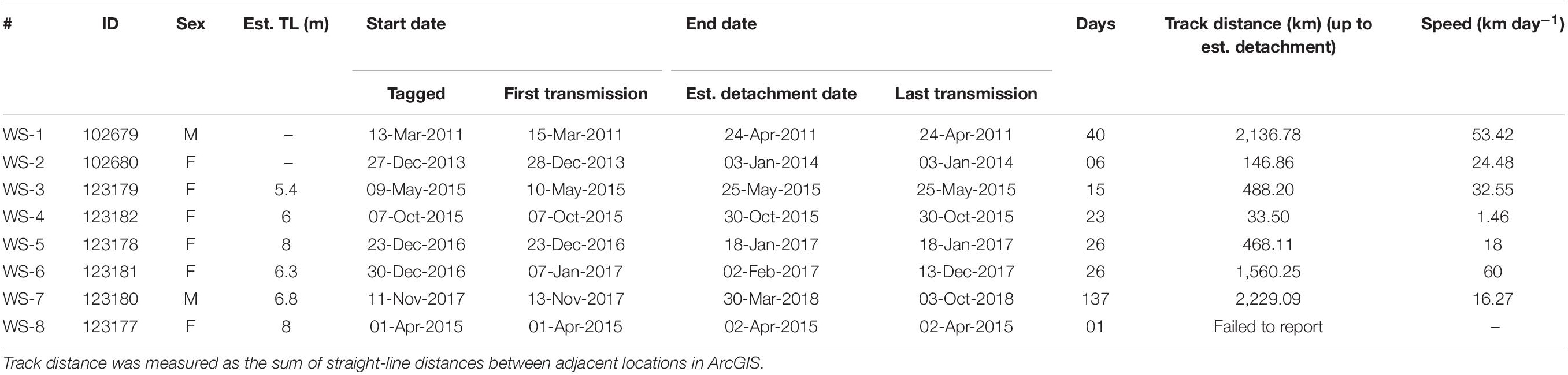
Table 1. Whale shark tagging information equipped with SPOT tags, with track number, shark ID, sex, estimated total length [TL (m)], tagging date, first transmission, estimated pop-up date, last transmission, track duration (starting from the first transmission date to estimated pop-up date), track distance (km), and speed (km day–1).
Six sharks had tag retentions of ≤40 days, and of these, five (WS-1–WS-5) mostly stayed on the continental shelf off the coast of Gujarat and Maharashtra (Figure 1a). WS-1 was tagged in the coastal waters off Veraval and traveled 2,136 km over 40 days, initially moving approximately 270 km south, then traveling north-east, coming within 40 km of the Maharashtra coastline, before heading back toward Gujarat. The tag attached to WS-2 transmitted for only six days during which time the shark covered a distance of 147 km. WS-3 was tagged off the northern coastline of Gujarat and over 15 days while the tag was attached moved north traveling ∼490 km to the Indus Canyon. WS-4 traveled 34 km in the 23 days of tag transmissions, remaining close to the Gujarat coast. WS-5 remained in close proximity (<20 km) to the coastline, moving 468 km before the tag stopped transmitting after 26 days. In contrast to the other sharks, WS-6 moved south into the Arabian Sea toward the latitudes of the Lakshadweep Islands approximately 370 km from the coast until the tag detached on the 2nd February 2017 (Figure 1b).
A 6.8 m female (WS-7) provided the longest track, traveling 2,229 km over 137 days (Figure 1c). This shark was tagged off the coast of Veraval and moved east, staying in shelf waters and coming within ∼20 km of the Daman and Diu coastline before heading west toward the middle of the Arabian Sea, inhabiting oceanic waters for the rest of the track. The shark stayed in SSTs ranging from 22 to 29°C while following frontal systems between water masses and cold-water eddies (Supplementary Video 7) until the tag probably detached on the 31st March 2018.
Sea surface temperatures through the area of the tracks ranged from 18.4 to 33.4°C, with a mean of 26.4 ± 4.77°C. The mean water temperature each shark experienced range from 27.32 to 29.91 (WS-1: 28.74 ± 1.13°C; WS-2: 27.32 ± 1.35°C; WS-3: 29.91 ± 0.55°C; WS-4: 29.37 ± 0.33°C; WS-5: 27.49 ± 1.69°C; WS-6: 27.35 ± 1.25°C; WS-7: 27.33 ± 1.28°C), with sharks spending most time within a SST range of 24–31°C (Figures 2a–f). Four of the seven sharks stayed close to the Gujarat coastline, where water temperatures of <27°C were observed in most years except for 2015 when temperatures were between 29 and 33°C (Figures 2c,d). WS-7 showed the greatest spatial extent of movements remaining in a temperature band of 24–28°C and close to frontal systems, a prominent oceanographic feature in the Arabian Sea (Madhupratap et al., 2001; Supplementary Video 7). Movements of the sharks remained in moderate to high levels of Chl-a ranging from 0.5 to 4.47 mg/m2, with the highest levels observed close to Veraval (WS-1: 1.82 ± 1.1 mg/m2; WS-2: 1.67 ± 0.1 mg/m2; WS-3: 1.63 ± 1.09 mg/m2; WS-5: 1.81 ± 0.27 mg/m2; WS-6: 1.53 ± 1.12 mg/m2; and WS-7: 1.64 ± 1 mg/m2). Due to the location of WS-4 being too close to the coastline, Chl-a data were unavailable for the geolocations of this shark (Figures 3a–g).
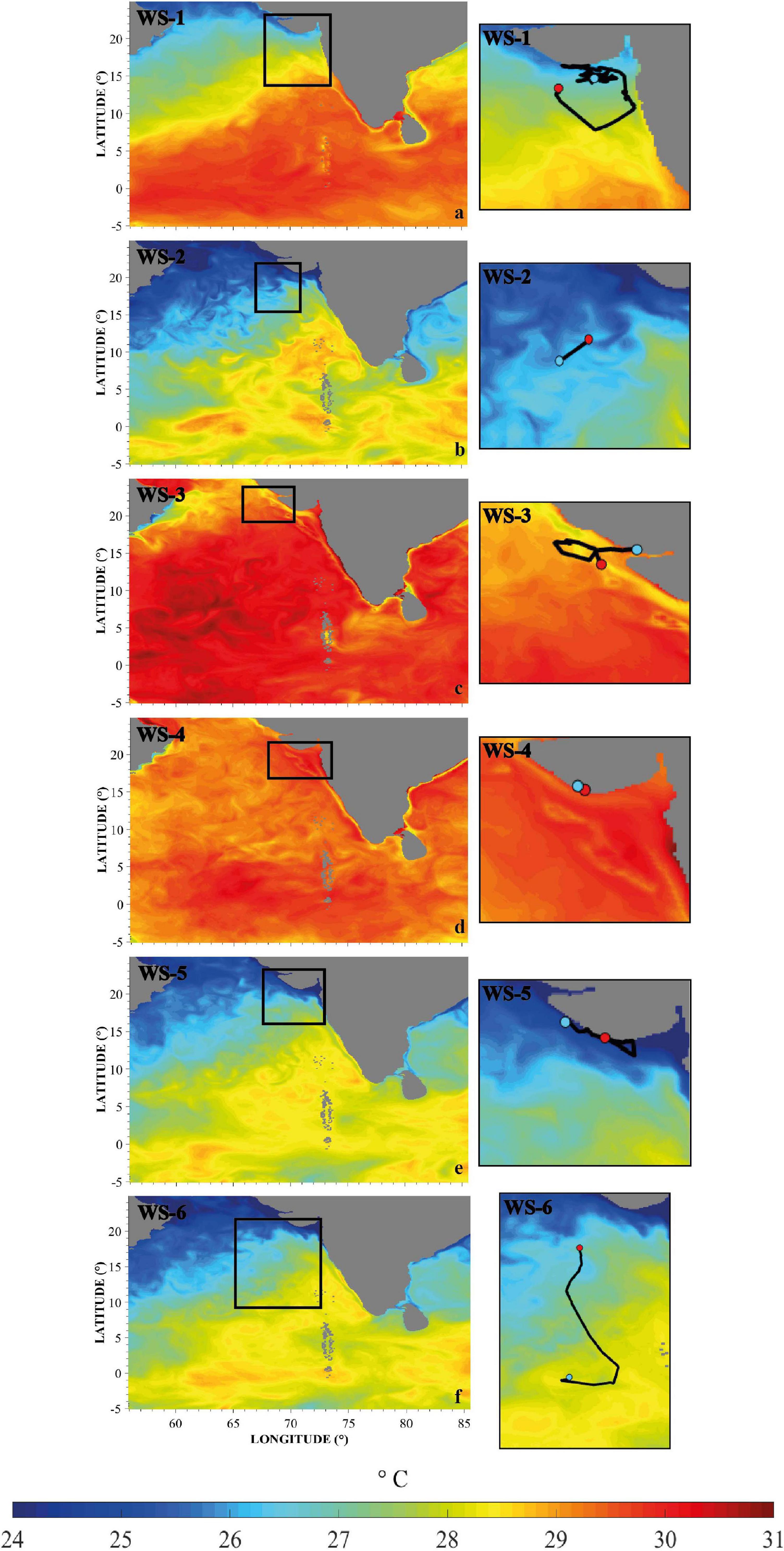
Figure 2. (a–f) Daily mean sea surface temperature plots in the Arabian Sea and northeastern Indian Ocean for WS-1–WS-6. Figures on the left-hand side show the overview of the area during the tagging period of each shark. Black boxes correspond to the right-hand plots, illustrating a subsection of the area where the whale shark tracks are shown. SST range from 24 to 31°C. The SST plot for WS-7 is not included here as it was analyzed separately and can be found in the Supplementary Materials (Supplementary Video 7).
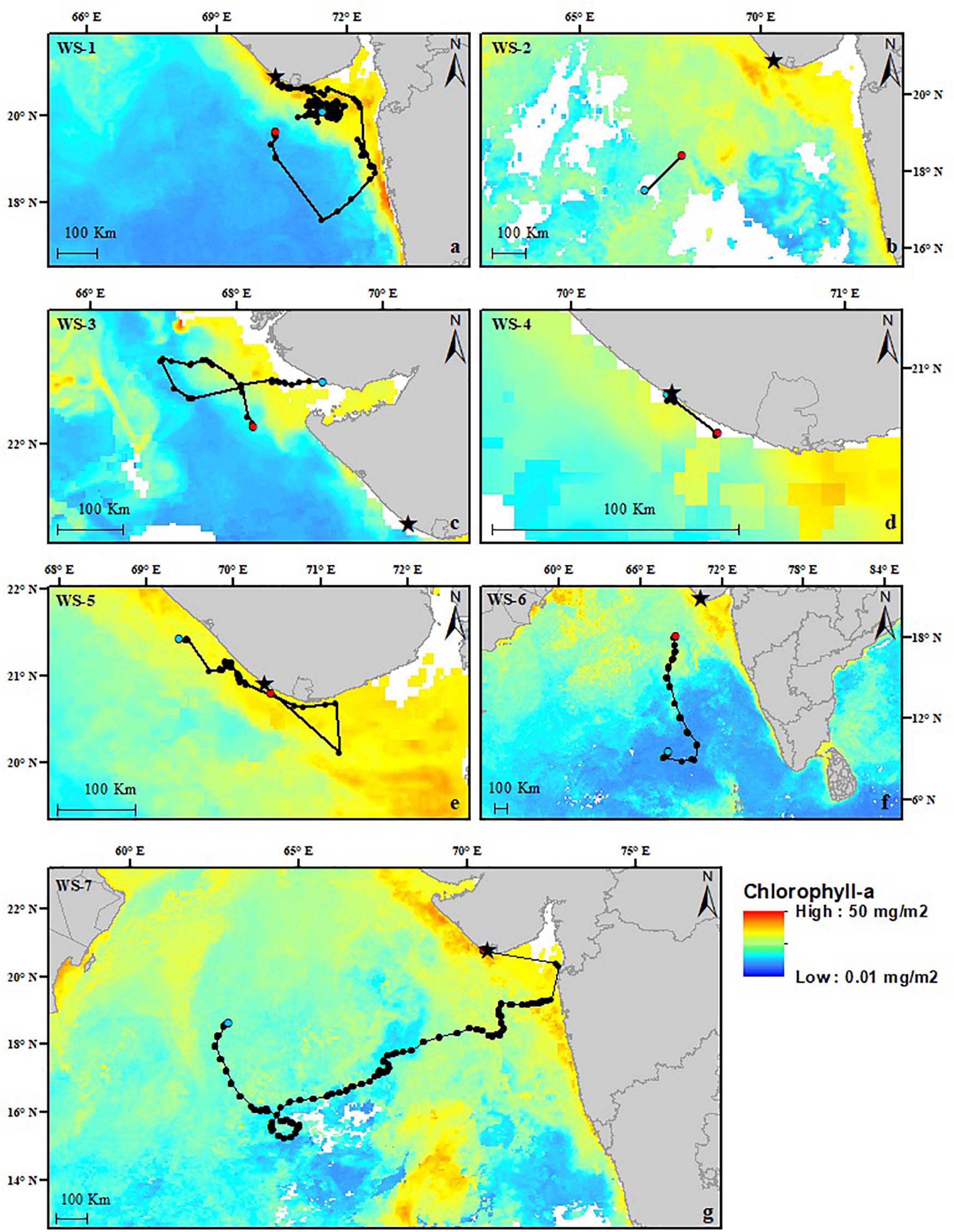
Figure 3. (a–g) Daily mean chlorophyll-a (Chl-a) plots from 2011–2018 corresponding to each whale shark track, shown in black. Red dots show the first locations, and the blue dots show the last locations, either from the tag ceasing reporting locations or determined by possible tag detachment locations. The star shows the location of the fishing town of Veraval, India. Chl-a ranged from 0.01 to 50 mg/m2.
Discussion
Our tagging resulted in tracks of different durations, with most lasting <40 days, however, one tag remained successfully attached to a whale shark for up to 137 days allowing a more detailed examination of the oceanographic context of their movement patterns in this region. The path of this longer track was consistent with suggestions that whale sharks tend to occupy water masses with temperatures in the range of 24–29°C and are associated with frontal zones (Sequeira et al., 2012; Ryan et al., 2017). When the tracks were relatively short, due to tag retention times, whale sharks tended to remain close to the coast where the coolest waters in the region were found, although this result may have also reflected the tagging location, especially for sharks with less than 1-week tagging duration.
Filter feeding on nekton and plankton provides access to food that is abundant and very widely distributed, but such prey is also patchy in pelagic environments. In the warm, oligotrophic tropical ocean, the need to search and locate prey requires strategies for cost-efficient foraging (Gleiss et al., 2013; Meekan et al., 2015). The focus of whale shark movements on frontal zones is consistent with cost-effective foraging behaviors since these oceanographic features in those locations lead to the accumulation of planktonic and small nektonic prey (Ramirez-Macias et al., 2017; Ryan et al., 2017). This minimizes the cost of foraging for this filter-feeder, which tends to have an energetic budget that is very finely balanced (Meekan et al., 2015), similar to basking sharks (Sims and Quayle, 1998). The association of whale sharks with frontal zones is consistent across various locations. For example, tagging studies in the Galapagos found that these sharks inhabited boundary systems between warm and cool water currents (Ryan et al., 2017). Indeed, selective foraging within such oceanographic features is a behavior common to a very wide range of oceanic taxa, including other sharks (Sims and Quayle, 1998; Queiroz et al., 2017; Andrzejaczek et al., 2018), turtles (Polovina et al., 2003), birds (Shaffer et al., 2009), and teleost fishes (Fiedler and Bernard, 1987). In addition to frontal zones, whale sharks have also been observed in areas of higher Chl-a levels (Sleeman et al., 2007; Rohner et al., 2018). When data were available, we found that the sharks in our study all remained in areas of relatively moderate to high Chl-a levels. Here, we suggest that the whale sharks may stay close to the coastline and on the shelf to take advantage of possible higher food availability than elsewhere in the northern Arabian Sea. This phenomenon is also thought to occur for juvenile whale sharks in Mozambique, where the coast has similar oceanographic characteristics (Rohner et al., 2018). Although Chl-a has been used as a proxy for zooplankton biomasses (Jaine et al., 2012), previous studies have suggested it to be poor for such observations (Sleeman et al., 2010b; Lyngsgaard et al., 2017), thus using sonar tags to detect prey fields (Goulet et al., 2019) might be a more appropriate method in investigating the foraging preferences of this species in the northern Arabian Sea.
Recent studies show that frontal systems between warm and cold-water masses may also offer the opportunity for ectothermic sharks to extend feeding opportunities while maintaining metabolic efficiency. Braun et al. (2019) showed that blue sharks (Prionace glauca) used warm core eddies to feed on otherwise inaccessible prey in the cool waters of the mesopelagic. By descending at frontal zones, whale sharks may be using a similar mechanism to forage on prey in the deep scattering layer (Ryan et al., 2017). Our study also shows that whale sharks tended to avoid waters that were too warm, with our tagged sharks moving to cooler waters when SSTs increased in this region. Higher SSTs were observed during 2015 (WS-3 and WS-4), at a time when the El Nino Southern Oscillation (ENSO) phenomenon was one of the strongest for the past four decades (Vidya and Kurian, 2018), resulting in higher SSTs in comparison to other years. Despite this, we observed these sharks remaining in cooler water temperatures for the duration of the track. Avoidance of very warm surface waters may be necessary to maintain optimal metabolic rates and limit energetic demands in an oligotrophic tropical ocean. Studies have shown that whale sharks spend a considerable amount of time in a preferred temperature range (24–29°C) (Wilson et al., 2006; Sequeira et al., 2012; Tyminski et al., 2015; Diamant et al., 2018), potentially using water temperatures to thermoregulate their bodies (Thums et al., 2013; Meekan et al., 2015). Here, we also observed a higher occurrence of sharks in a narrow band of SSTs, supporting the literature on the habitat preferences of this species. Identifying the key drivers of these movement patterns will require studies that deploy tags that provide high resolution and accurate records of temperature and depth use by whale sharks.
Our study provides some of the first information on the movement patterns of whale sharks in the eastern part of the northern Arabian Sea. Subpopulations of whale sharks in the southern and central-western Indian Ocean have been suggested to be separated from the northwestern Indian Ocean and Arabian Sea region based on photo identification and isotopic studies (Prebble et al., 2018; Boldrocchi et al., 2020). Additionally, Sequeira et al. (2013) hypothesized that whale sharks could potentially travel between Gujarat and the Maldives, indicating the connectivity between a subpopulation. In our study, WS-6 moved south toward the latitudes of Lakshadweep Islands, a pattern of movement consistent with the possibility some whale sharks migrate from Gujarat toward the Maldives. Additionally, the migration of this shark toward the Southern Equatorial Counter Current before traveling westward could support the theory that the current may form a southern boundary for Indian whale shark populations. One of the tracks of the sharks we tagged (WS-7) showed the animal crossing two-thirds of the northern Arabian Sea, heading toward Oman before the tag detached. Tagging of whale sharks in the Red Sea has shown some animals exiting the Gulf of Aden into the Arabian Sea (Berumen et al., 2014), whereas tagging of sharks in the Persian Gulf has recorded sharks exiting the Gulf of Oman and traveling southward toward the Gulf of Aden (Robinson et al., 2017). Furthermore, whale sharks have also been found along neighbouring coasts of the Persian Gulf, suggesting a wide distribution of these animals in this area (Gore et al., 2019). Given these long-distance movements of sharks in the northern Arabian Sea and the lack of major oceanographic boundaries in this region, there is little evidence (for the moment) to suggest that the populations of whale sharks off the coast of India are likely to form a separate subpopulation from those in other parts of the Arabian Sea. More tagging combined with high resolution genetic studies will be required to resolve this issue.
Sharks are under considerable threats from fishing (Queiroz et al., 2019) and ship strikes in the Indian Ocean (Speed et al., 2008; Lester et al., 2020). For whale sharks, fishing poses by-catch and ship strike threats as they spend a large part of daylight hours basking on the surface of the ocean (Thums et al., 2013; Meekan et al., 2015). On the shelf and close to the Gujarat coast, mechanized fishing vessels account for 89% of all fishing boats (CMFRI, 2019), and based on AIS data, there is intense fishing activity off the coast of Maharashtra (Murua et al., 2019). For this reason, it seems likely that there would be considerable overlap in distributions of fishing vessels and whale sharks in this region. In the northern Arabian Sea, tuna fishing is a major industry (Varghese et al., 2014; CMFRI, 2019) and higher catches have been reported in the vicinity of frontal zones (Anand et al., 2005). We showed that whale sharks moved into the open ocean of the Arabian Sea and given that some of these sharks focused their movements on frontal zones, that are often associated with tuna schools, an interaction between tuna fishers and whale sharks seems likely. Despite the prohibition of the intentional setting of seine nets on whale sharks in 2013 by regional fisheries management organisations (RMFOs) (e.g., Indian Ocean Tuna Commission; IOTC, 2013), and bycatch of whale sharks in purse seine nets being relatively low (Fontes et al., 2020), further work, particularly with juvenile animals, is required to identify the level of risk of mortality of whale sharks following interactions with fishing vessels.
Our study provides the first data on whale shark movements in the eastern part of the northern Arabian Sea and highlights movements linked to frontal systems and narrow temperature bands. We provide baseline information regarding the movements of this species, put in the context of potential threats that these animals face in this area. However, due to limited tag retention times and lack of environmental data recorded by the tags, our results require further validation through more deployments of satellite tags, to gain a better understanding of the behavior and oceanographic context of movements of the species. The deployment of tags that have capabilities to record temperature and depth to describe both horizontal and vertical axes of movement will be essential to optimize and implement effective conservation strategies for whale sharks in the Arabian Sea.
Data Availability Statement
The raw data supporting the conclusions of this article will be made available by the authors, without undue reservation.
Ethics Statement
The animal study was reviewed and approved by the Forest Department, Government of Gujarat (GFD).
Author Contributions
BC, RK, and MM conceived the study. CKP, FB, and SJ collected the data. LA analyzed the data. LA and MM wrote the manuscript with critical input from all authors.
Funding
The fieldwork funding for this study was from TATA Chemicals Limited. Tags and tagging equipment were provided by the Forest Department, Government of Gujarat (GFD). LA was supported by the Research Training Scholarship (RTP) at UWA. AS was funded by a 2020 Pew Fellowship in Marine Conservation and an Australian Research Council Discovery Early Career Research Award (Grant DE170100841).
Conflict of Interest
The authors declare that this study received funding from TATA Chemicals Limited. The funder was not involved in the study design, collection, analysis, interpretation of the data, the writing of this article or the decision to submit it for publication.
Acknowledgments
We would like to thank the Government of Gujarat Forest Department (GFD) for the necessary permissions to tag the whale sharks and to TATA Chemicals Limited for funding the study. We would like to thank Diresh Joshi, Prem Jothi, Gautham Sambath, and Prakash Doriva for their support in the field. We are also grateful to the fishermen for reporting the accidental captures of these animals and for assisting their release after tagging occurred.
Supplementary Material
The Supplementary Material for this article can be found online at: https://www.frontiersin.org/articles/10.3389/fmars.2021.682730/full#supplementary-material
Supplementary Table 1 | List of published Rhincodon typus studies using tagging technologies in the Indian Ocean. Table shows the years data were collected; the types of tags and the number (n) used in the study; the number (n) of individuals and sex of individuals (“M” represent males, “F” represents females, and “U” represents unidentified); size (m); the track duration in days; the track distance (km), and speed (km day–1).
Supplementary Video 1 | Animation of the changes in sea surface temperatures of WS-1. Black “X” indicates the daily whale shark locations, using a combination of recorded positions from the tag and locations from the state-space model when geolocations were unavailable. Sea surface temperatures were set to range from 24 to 31°C.
Supplementary Video 2 | Animation of the changes in sea surface temperatures of WS-2. Black “X” indicates the daily whale shark locations, using a combination of recorded positions from the tag and locations from the state-space model when geolocations were unavailable. Sea surface temperatures were set to range from 24 to 31°C.
Supplementary Video 3 | Animation of the changes in sea surface temperatures of WS-3. Black “X” indicates the daily whale shark locations, using a combination of recorded positions from the tag and locations from the state-space model when geolocations were unavailable. Sea surface temperatures were set to range from 24 to 31°C.
Supplementary Video 4 | Animation of the changes in sea surface temperatures of WS-4. Black “X” indicates the daily whale shark locations, using a combination of recorded positions from the tag and locations from the state-space model when geolocations were unavailable. Sea surface temperatures were set to range from 24 to 31°C.
Supplementary Video 5 | Animation of the changes in sea surface temperatures of WS-5. Black “X” indicates the daily whale shark locations, using a combination of recorded positions from the tag and locations from the state-space model when geolocations were unavailable. Sea surface temperatures were set to range from 24 to 31°C.
Supplementary Video 6 | Animation of the changes in sea surface temperatures of WS-6. Black “X” indicates the daily whale shark locations, using a combination of recorded positions from the tag and locations from the state-space model when geolocations were unavailable. Sea surface temperatures were set to range from 24 to 31°C.
Supplementary Video 7 | Animation of the changes in sea surface temperatures of WS-7. Black “X” indicates the daily whale shark locations, using a combination of recorded positions from the tag and locations from the state-space model when geolocations were unavailable. Sea surface temperatures were set to range from 24 to 31°C.
Footnotes
References
Akhilesh, K. V., Shanis, C. P. R., White, W. T., Manjebrayakath, H., Bineesh, K. K., Ganga, U., et al. (2013). Landings of whale sharks rhincodon typus smith, 1828 in Indian waters since protection in 2001 through the Indian Wildlife (Protection) Act, 1972. Environ. Biol. Fishes 96, 713–722. doi: 10.1007/s10641-012-0063-9
Anand, A., Kumari, B., Nayak, S. R., and Krishnamurthy, Y. V. N. (2005). Locating oceanic tuna resources in the eastern arabian sea using remote sensing. J. Indian Soc. Remote Sensing 33, 511–520. doi: 10.1007/BF02990736
Andrzejaczek, S., Gleiss, A., Jordan, L., Pattiaratchi, C., Howey, L., Brooks, E., et al. (2018). Temperature and the vertical movements of oceanic whitetip sharks, Carcharhinus longimanus. Sci. Rep. 8:8351. doi: 10.1038/s41598-018-26485-3
Araujo, G., Rohner, C. A., Labaja, J., Conales, S. J., Snow, S. J., Murray, R., et al. (2018). Satellite tracking of juvenile whale sharks in the Sulu and Bohol Seas, Philippines. PeerJ 6:18. doi: 10.7717/peerj.5231
Argos, C. (2011). ARGOS User’s Manual: Worldwide Tracking and Environmental Monitoring by Satellite. France: Argos/CLS.
Berumen, M., Braun, C., Cochran, J., Skomal, G., and Thorrold, S. (2014). Movement patterns of juvenile whale sharks tagged at an aggregation site in the red sea. PLoS One 9:e103536. doi: 10.1371/journal.pone.0103536
Bloch, F. H., Premjothi, P. V. R., Choudury, B. C., Kaul, R., and John, S. (2018). An assessment of the past and present distribution status of the Whale Shark (Rhincodon typus) along the west coast of India based on traditional ecological knowledge (TEK). Perspect. Biodiveristy India 400. ∗page,Google Scholar
Boldrocchi, G., Omar, M., Azzola, A., and Bettinetti, R. (2020). The ecology of the whale shark in Djibouti. Aquat. Ecol. 54, 535–551. doi: 10.1007/s10452-020-09758-w
Braun, C. D., Gaube, P., Sinclair-Taylor, T. H., Skomal, G. B., and Thorrold, S. R. (2019). Mesoscale eddies release pelagic sharks from thermal constraints to foraging in the ocean twilight zone. Proc. Natl. Acad. Sci. U.S.A. 116, 17187–17192. doi: 10.1073/pnas.1903067116
Brunnschweiler, J., Baensch, H., Pierce, S., and Sims, D. (2009). Deep-diving behaviour of a whale shark Rhincodon typus during long-distance movement in the western Indian Ocean. J. Fish Biol. 74, 706–714. doi: 10.1111/j.1095-8649.2008.02155.x
Capietto, A., Escalle, L., Chavance, P., Dubroca, L., Delgado de Molina, A., Murua, H., et al. (2014). Mortality of marine megafauna induced by fisheries: insights from the whale shark, the world’s largest fish. Biol. Conservation 174, 147–151. doi: 10.1016/j.biocon.2014.03.024
Chassignet, E. P., Hurlburt, H. E., Smedstad, O. M., Halliwell, G. R., Hogan, P. J., Wallcraft, A. J., et al. (2007). The hycom (hybrid coordinate ocean model) data assimilative system. J. Mari. Syst. 65, 60–83. doi: 10.1016/j.jmarsys.2005.09.016
Copping, J., Stewart, B., Mcclean, C., Hancock, J., and Rees, R. (2018). Does bathymetry drive coastal whale shark (Rhincodon typus) aggregations? PeerJ 6:e4904. doi: 10.7717/peerj.4904
Diamant, S., Rohner, C. A., Kiszka, J. J., Guillemain d’echon, A., Guillemain, d’echon, T., et al. (2018). Movements and habitat use of satellite-tagged whale sharks off western Madagascar. Endangered Spec. Res. 36, 49–58. doi: 10.3354/esr00889
Fiedler, P. C., and Bernard, H. J. (1987). Tuna aggregation and feeding near fronts observed in satellite imagery. Continental Shelf Res. 7, 871–881. doi: 10.1016/0278-4343(87)90003-3
Fontes, J., Mcginty, N., Machete, M., and Afonso, P. (2020). Whale shark-tuna associations, insights from a small pole-and-line fishery from the mid-north Atlantic. Fish. Res. 229:105598. doi: 10.1016/j.fishres.2020.105598
Gleiss, A., Wright, S., Liebsch, N., Wilson, R., and Norman, B. (2013). Contrasting diel patterns in vertical movement and locomotor activity of whale sharks at ningaloo reef. Mari. Biol. 160, 2981–2992. doi: 10.1007/s00227-013-2288-3
Gore, M., Waqas, U., Khan, M. M., Ahmad, E., Baloch, A. S., and Baloch, A. R. (2019). A first account of the elasmobranch fishery of Balochistan, south-west Pakistan. Western Indian Ocean J. Mari. Sci. 18, 95–105. doi: 10.4314/wiojms.v18i1.9
Goulet, P., Guinet, C., Swift, R., Madsen, P. T., and Johnson, M. (2019). A miniature biomimetic sonar and movement tag to study the biotic environment and predator-prey interactions in aquatic animals. Deep Sea Res. Part I Ocean. Res. Papers 148, 1–11. doi: 10.1016/j.dsr.2019.04.007
Hearn, A. R., Green, J. R., Espinoza, E., Peñaherrera, C., Acuña, D., and Klimley, A. P. (2013). Simple criteria to determine detachment point of towed satellite tags provide first evidence of return migrations of whale sharks (Rhincodon typus) at the galapagos islands, ecuador. Animal Biotelemetry 1:11. doi: 10.1186/2050-3385-1-11
Hueter, R., Tyminski, J., and de la Parra, R. (2013). Horizontal movements, migration patterns, and population structure of whale sharks in the gulf of mexico and northwestern caribbean sea. PLoS One 8:e71883. doi: 10.1371/annotation/491b9b6c-7f77-4fb0-b336-572078aec830
IOTC (2013). Resolution 13/05 on the Conservation of Whale Sharks (Rhincodon typus). Seychelles: IOTC.
Jabado, R. W., Kyne, P. M., Pollom, R. A., Ebert, D. A., Simpfendorfer, C. A., Ralph, G. M., et al. (2018). Troubled waters: threats and extinction risk of the sharks, rays and chimaeras of the Arabian sea and adjacent waters. Fish and Fisheries 19, 1043–1062. doi: 10.1111/faf.12311
Jaine, F. R. A., Couturier, L. I. E., Weeks, S. J., Townsend, K. A., Bennett, M. B., Fiora, K., et al. (2012). When giants turn up: sighting trends, environmental influences and habitat use of the manta ray manta alfredi at a coral reef. PLoS One 7:e46170. doi: 10.1371/journal.pone.0046170
Jonsen, I. D., Flemming, J. M., and Myers, R. A. (2005). Robust state–space modeling of animal movement data. Ecology 86, 2874–2880. doi: 10.1890/04-1852
Kumari, B., and Raman, M. (2010). Whale shark habitat assessments in the northeastern Arabian Sea using satellite remote sensing. Int. J. Remote Sensing 31, 379–389. doi: 10.1080/01431160902893444
Lester, E., Meekan, M. G., Barnes, P., Raudino, H., Rob, D., Waples, K., et al. (2020). Multi-year patterns in scarring, survival and residency of whale sharks in ningaloo marine park, Western Australia. Mari. Ecol. Prog. Series 634, 115–125. doi: 10.3354/meps13173
Lyngsgaard, M. M., Markager, S., Richardson, K., Møller, E. F., and Jakobsen, H. H. (2017). How well does chlorophyll explain the seasonal variation in phytoplankton activity? Estuar. Coasts 40, 1263–1275. doi: 10.1007/s12237-017-0215-4
Madhupratap, M., Nair, K. N. V., Gopalakrishnan, T. C., Haridas, P., Nair, K. K. C., Venugopal, P., et al. (2001). Arabian Sea oceanography and fisheries of the west coast of India. Curr. Sci. 81, 355–361.
Meekan, M., Fuiman, L., Davis, R., Berger, Y., and Thums, M. (2015). Swimming strategy and body plan of the world’s largest fish: implications for foraging efficiency and thermoregulation. Front. Mari. Sci. 2:64. doi: 10.3389/fmars.2015.00064
Meekan, M. G., Taylor, B. M., Lester, E., Ferreira, L. C., Sequeira, A. M. M., Dove, A. D. M., et al. (2020). Asymptotic growth of whale sharks suggests sex-specific life-history strategies. Front. Mari. Sci. 7:575683. doi: 10.3389/fmars.2020.575683
Murua, H., Granado, I., Gee, J., Kroodsma, D., Miller, N., Taconet, M., et al. (2019). FAO Area 51 - AIS-Based Fishing Activity in the Western Indian Ocean. ∗city pub.
Norman, B., Reynolds, S., and Morgan, D. (2016). Does the whale shark aggregate along the Western Australian coastline beyond Ningaloo reef? Pacific Conservation Biol. 22, 72–80. doi: 10.1071/PC15045
Norman, B. M., and Stevens, J. D. (2007). Size and maturity status of the whale shark (Rhincodon typus) at ningaloo reef in Western Australia. Fisheries Research 84, 81–86. doi: 10.1016/j.fishres.2006.11.015
Pearce, S., and Norman, B. (2016). Rhincodon typus. The IUCN Red List of Threatened Species 2016 [Online]. Available online at: http://dx.doi.org/10.2305/IUCN.UK.2016-1.RLTS.T19488A2365291.en (accessed 01/08/2018)
Polovina, J., Balazs, G., Howell, E., Parker, D., Seki, M., and Dutton, P. (2003). Foraging and migration habitat of loggerhead (Caretta caretta) and olive ridley (Lepidochelys olivacea) sea turtles in the central north pacific ocean. Fish. Oceanogr. 13, 36–51. doi: 10.1046/j.1365-2419.2003.00270.x
Pravin, P. C. F. P. D. A. (2000). Whale shark in the Indian coastneed for conservation. Curr. Sci. 79, 310–315.
Prebble, C. E. M., Rohner, C. A., Pierce, S. J., Robinson, D. P., Jaidah, M. Y., Bach, S. S., et al. (2018). Limited latitudinal ranging of juvenile whale sharks in the western indian ocean suggests the existence of regional management units. Mari. Ecol. Prog. Series 601, 167–183. doi: 10.3354/meps12667
Queiroz, N., Humphries, N. E., Couto, A., Vedor, M., da Costa, I., Sequeira, A. M. M., et al. (2019). Global spatial risk assessment of sharks under the footprint of fisheries. Nature 572, 461–466. doi: 10.1038/s41586-019-1444-4
Queiroz, N., Vila-Pouca, C., Couto, A., Southall, E., Mucientes, G., Humphries, N., et al. (2017). Convergent foraging tactics of marine predators with different feeding strategies across heterogeneous ocean environments. Front. Mari. Sci. 4:239. doi: 10.3389/fmars.2017.00239
Ramirez-Macias, D., Queiroz, N., Pierce, S., Humphries, N., Sims, D., and Brunnschweiler, J. (2017). Oceanic adults, coastal juveniles: tracking the habitat use of whale sharks off the Pacific coast of Mexico. PeerJ 5, 32–71. doi: 10.7717/peerj.3271
Retheesh, T., Jacob Peter, P., and Kishore, T. G. (2020). Whale shark landed in sakthikulangara fisheries harbour. Mari. Fish. Inform. Service Technical Extens. Series 2020, 37–37.
Reynolds, S., Norman, B., Beger, M., and Franklin, C. (2017). Movement, distribution and marine reserve use by an endangered migratory giant. J. Conservation Biogeog. 23, 1268–1279. doi: 10.1111/ddi.12618
Robinson, D., Jaidah, M., Bach, S., Rohner, C., Jabado, R., Ormond, R., et al. (2017). Some like it hot: repeat migration and residency of whale sharks within an extreme natural environment. PLoS One 12:e0185360. doi: 10.1371/journal.pone.0185360
Rohner, C., Richardson, A., Jaine, F., Bennett, M., Weeks, S., Cliff, G., et al. (2018). Satellite tagging highlights the importance of productive Mozambican coastal waters to the ecology and conservation of whale sharks. PeerJ 6, 41–61. doi: 10.7717/peerj.4161
Rohner, C. A., Cochran, J. E. M., Cagua, E. F., Prebble, C. E. M., Venables, S. K., Berumen, M. L., et al. (2020). No place like home? High residency and predictable seasonal movement of whale sharks off tanzania. Front. Mari. Sci. 7:2296–7745. doi: 10.3389/fmars.2020.00423
Rowat, D., and Gore, M. (2007). Regional scale horizontal and local scale vertical movements of whale sharks in the Indian Ocean off Seychelles. Fish. Res. 84, 32–40. doi: 10.1016/j.fishres.2006.11.009
Ryan, J., Green, J., Espinoza, E., and Hearn, A. (2017). Association of whale sharks (Rhincodon typus) with thermo-biological frontal systems of the eastern tropical Pacific. PLoS One 12:e0182599. doi: 10.1371/journal.pone.0182599.g002
Sequeira, A., Mellin, C., Fordham, D., Meekan, M., and Bradshaw, C. (2014). Predicting current and future global distributionsof whale sharks. Global Change Biol. 20, 778–789. doi: 10.1111/gcb.12343
Sequeira, A., Mellin, C., Meekan, M., Sims, D., and Bradshaw, C. (2013). Inferred global connectivity of whale shark, Rhincodon typus, populations. J. Fish Biol. 82, 367–389. doi: 10.1111/jfb.12017
Sequeira, A., Mellin, C., Rowat, D., Meekan, M., and Bradshaw, C. (2012). Ocean-scale prediction of whale shark distribution. Diversity Distribut. 18, 504–518. doi: 10.1111/j.1472-4642.2011.00853.x
Sequeira, A. M. M., Hays, G. C., Sims, D. W., Eguíluz, V. M., Rodríguez, J. P., Heupel, M. R., et al. (2019). Overhauling ocean spatial planning to improve marine megafauna conservation. Front. Mari. Sci. 6:639. doi: 10.3389/fmars.2019.00639
Shaffer, S. A., Weimerskirch, H., Scott, D., Pinaud, D., Thompson, D. R., Sagar, P. M., et al. (2009). Spatiotemporal habitat use by breeding sooty shearwaters Puffinus griseus. Mari. Ecol. Prog. Series 391, 209–220. doi: 10.3354/meps07932
Sims, D. W., and Quayle, V. A. (1998). Selective foraging behaviour of basking sharks on zooplankton in a small-scale front. Nature 393, 460–464. doi: 10.1038/30959
Sleeman, J., Meekan, M., Fitzpatrick, B., Steinberg, C., Ancel, R., and Bradshaw, C. (2010a). Oceanographic and atmospheric phenomena influence the abundance of whale sharks at Ningaloo Reef, Western Australia. J. Exp. Mari. Biol. Ecol. 382, 77–81. doi: 10.1016/j.jembe.2009.10.015
Sleeman, J., Meekan, M., Wilson, S., Jenner, C., Jenner, M., Boggs, G., et al. (2007). Biophysical correlates of relative abundances of marine megafauna at Ningaloo Reef, Western Australia. Mari. Fresh. Res. 58, 608–623. doi: 10.1071/MF06213
Sleeman, J., Meekan, M., Wilson, S., Polovina, J., Stevens, J., Boggs, G., et al. (2010b). To go or not to go with the flow: environmental influences on whale shark movement patterns. J. Exp. Mari. Biol. Ecol. 390, 84–98. doi: 10.1016/j.jembe.2010.05.009
Speed, C. W., Meekan, M. G., Rowat, D., Pierce, S. J., Marshall, A. D., and Bradshaw, C. J. A. (2008). Scarring patterns and relative mortality rates of Indian Ocean whale sharks. J. Fish Biol. 72, 1488–1503. doi: 10.1111/j.1095-8649.2008.01810.x
Thums, M., Meekan, M., Stevens, J., Wilson, S., and Polovina, J. (2013). Evidence for behavioural thermoregulation by the world’s largest fish. J. R. Soc. Int. 10, 1–5. doi: 10.1098/rsif.2012.0477
Tyminski, J., Parra-Venegas, R., Cano, J., and Hueter, R. (2015). Vertical movements and patterns in diving behaviour in whale sharks as revealed by pop-up satellite tags in the Eastern Gulf of Mexico. PLoS One 10:142–156. doi: 10.1371/journal.pone.0142156
Varghese, S. P., Somvanshi, V. S., and Dalvi, R. S. (2014). Diet composition, feeding niche partitioning and trophic organisation of large pelagic predatory fishes in the eastern Arabian Sea. Hydrobiologia 736, 99–114. doi: 10.1007/s10750-014-1895-4
Vidya, P. J., and Kurian, S. (2018). Impact of 2015–2016 ENSO on the winter bloom and associated phytoplankton community shift in the northeastern Arabian Sea. J. Mari. Syst. 186, 96–104. doi: 10.1016/j.jmarsys.2018.06.005
Keywords: satellite tags, migration, movement ecology, tagging, oceanography
Citation: Arrowsmith LM, Paidi CK, Bloch FH, John S, Choudhury BC, Kaul R, Sequeira AMM, Pattiaratchi CB and Meekan MG (2021) First Insights Into the Horizontal Movements of Whale Sharks (Rhincodon typus) in the Northern Arabian Sea. Front. Mar. Sci. 8:682730. doi: 10.3389/fmars.2021.682730
Received: 19 March 2021; Accepted: 10 June 2021;
Published: 06 July 2021.
Edited by:
Nuno Queiroz, Centro de Investigacao em Biodiversidade e Recursos Geneticos (CIBIO-InBIO), PortugalReviewed by:
Marisa Vedor, Centro de Investigacao em Biodiversidade e Recursos Geneticos (CIBIO-InBIO), PortugalOrnella Céline Weideli, Labormedizinische Zentrum Dr. Risch, Liechtenstein
Ginevra Boldrocchi, University of Insubria, Italy
Copyright © 2021 Arrowsmith, Paidi, Bloch, John, Choudhury, Kaul, Sequeira, Pattiaratchi and Meekan. This is an open-access article distributed under the terms of the Creative Commons Attribution License (CC BY). The use, distribution or reproduction in other forums is permitted, provided the original author(s) and the copyright owner(s) are credited and that the original publication in this journal is cited, in accordance with accepted academic practice. No use, distribution or reproduction is permitted which does not comply with these terms.
*Correspondence: Lucy M. Arrowsmith, bHVjeS5hcnJvd3NtaXRoQHJlc2VhcmNoLnV3YS5lZHUuYXU=
†ORCID: Lucy M. Arrowsmith, orcid.org/0000-0003-4558-6650; Charan Kumar Paidi, orcid.org/0000-0001-8608-5022; Farukhkha Husenkha Bloch, orcid.org/0000-0002-5062-6605; Sajan John, orcid.org/0000-0001-8885-6222; Binod Chandra Choudhury, orcid.org/0000-0001-5959-1261; Rahul Kaul, orcid.org/0000-0001-9930-7309; Ana M. M. Sequeira, orcid.org/0000-0001-6906-799X; Charitha B. Pattiaratchi, orcid.org/0000-0003-2229-6183; Mark G. Meekan, orcid.org/0000-0002-3067-9427