- 1Department of Institute of Fisheries Science, National Taiwan University, Taipei, Taiwan
- 2Department of Veterinary Medicine, National Taiwan University, Taipei, Taiwan
This study aims to evaluate the effect of light-emitting diodes (LEDs) of different wavelengths on the embryonic development, covering behavior, righting behavior, and phototaxis of collector urchins (Tripneustes gratilla). The collector urchins were divided into three groups according to the type of LED illumination they received: full-spectrum (400–750 nm wavelength), red light (630 nm), or blue light (450 nm). The results of the embryonic development experiment indicated that the blue LED group had the highest proportion of embryos reaching the prism stage at the 24th hour and the highest proportion of embryos entering the 4-arm pluteus stage, but it also had the highest death rate at the 48th hour. The full-spectrum and red LED groups exhibited similar speeds of embryonic development. In the experiment on covering behavior performed on adult urchins, our findings indicated that the blue LED group gripped the most acrylic sheets for cover, exhibiting the most covering behavior, followed by the full-spectrum group and then the red LED group. Moreover, behavior varied with coloration, as collector urchins with a lower level of melanin exhibited more covering behavior than those with a higher melanin level. In addition, the righting behavior experiments demonstrated that the blue LED group spent the longest time righting themselves. It is possible that the relatively strong stimulation from the blue LED illumination led to a higher level of stress in the collector urchins and hence slowed their righting. The phototaxis experiment revealed the most significant negative phototactic response in collector urchins when they were under the blue LED light, followed by the full-spectrum light; the red LED light did not induce any positive or negative phototactic response in the collector urchins. This experimental result verified collector urchins’ high sensitivity to and dislike of the blue LED light. The study results confirmed that the blue LED light environment accelerated the embryonic development of collector urchins; however, the relatively strong stimulation from that light also caused them to engage in covering behavior or move away from the light. These results indicate that short-wavelength irradiation significantly affects the embryonic development and behavior pattern of this species.
Introduction
Sea urchins are invertebrates with a crucial role in the marine benthic ecosystem as well as high value as an aquaculture product in the international market. The demand for high-quality sea urchins over the last three decades has led to the intensive harvest and exploitation of natural sea urchins; in light of this overharvesting and the decreasing numbers of sea urchins in various countries, sea urchin farming has become an industry with high potential. Sea urchins have a complicated life cycle. They undergo in vitro fertilization where male and female sea urchins fertilize and ovulate into the water, respectively, to complete insemination. Sea urchins hatch into blastulas approximately 9 h after gametes are fertilized, then enter the gastrula stage at 16 h, the prism stage at 22 h, the 2-arm pluteus stage at 34 h, and the 4-arm pluteus stage at 48 h (Parvez et al., 2018). Previous studies have pointed out that UVB radiation can cause sea urchin embryo malformations, changes in specific gene expression and the expression of pressure proteins (Bonaventura et al., 2005), and even embryonic cell apoptosis (Lesser et al., 2003). It has been confirmed that light wavelength affects the embryonic development of sea urchins. All kinds of sea urchins living in the intertidal zone, shallow sea, and deep-sea exhibit covering behavior, i.e., they use their spines and tube feet to move objects in the surrounding environment (such as gravel, shells, seaweed, seaweed, etc.) onto their exposed body surfaces. At present, proposed explanations for this sea urchin behavior can be summarized into six hypotheses: defense against natural enemies, reflex behavior, prevention of dehydration, ancillary feeding behavior, protection against damage from waves and suspended solids in the water, and to avoid over-exposure to light (Barros et al., 2020).
Righting behavior refers to the sea urchin using its tube feet and spines to turn itself into a normal position with the mouth facing downward. This behavior allows the sea urchin to recover and continue to escape when it is overturned into an abnormal position by a predator or wave (Percy, 1973). The results of previous studies are limited and show that when sea urchins encounter environmental pressure, their correction rate will decrease, or the probability of successful correction will decrease. Therefore, righting behavior can be used to judge whether the sea urchin is under pressure.
Light-emitting diodes (LEDs) are semiconductor electronic components that are energy efficient, environmentally friendly, small, and durable. By changing the chemical composition of LED semiconductor materials, the resulting LEDs can emit light of a single color. The discontinuous wavelength spectrum enables LEDs to emit near-UV, visible, or infrared light. Therefore, LED lighting fixtures are appropriate and necessary equipment for studying the effect of a single wavelength on sea urchin embryos development, covering behavior, righting behavior, and phototaxis. The previous research also suggests that albino sea urchins showed the greater covering response to susceptibility to solar radiation than wild-type urchins. These results indicate that the levels of melanin in sea urchins influence the response to light illumination (Kehas et al., 2004).
The present study regulated the light environment of a water body through the use of LED lights with different wavelengths to investigate the effects of light wavelength on the growth rate, death rate, and presence of malformations in sea urchin embryos as well as the various behaviors of adult sea urchins. The sea urchin Tripneustes gratilla, which exhibits obvious and representative covering behavior, was chosen as the subject of experiment. The present study sought to identify suitable lighting, in addition to natural light, for the embryonic development of sea urchins. Furthermore, this study aimed to determine the light wavelength to which adult sea urchins are most sensitive as indicated by the experimental results for covering behavior and phototaxis. These findings may be extended and applied for farming, managing, or during harvest gathering sea urchins.
Materials and Methods
Experimental Design and Sampling
Sea urchins were purchased from a sea urchin farm in Yilan, Taiwan. Sea urchins with a test size of 6–8 cm aged approximately 12 months old. Sea urchins were transported live to a laboratory and kept in a 70-L fish tank. The sea urchins were fed chayote vine (Sechium edule) or cabbage (Brassica oleracea var. capitata) once every 3 days, and the seawater was kept at 25°C. After the sea urchins had acclimatized for 2 weeks, we randomly selected several specimens (2 females and 3 males), injected 2 mL of 0.5M KCl into their body cavity, and shook them for a few seconds until sperm (white liquid) or eggs (yellow liquid) exuded from the genital pores on the aboral surface. The sperm was collected using a plastic dropper, deposited into a 1.5-mL centrifuge tube, and placed on 4°C ice for storage; the collection of seawater left on the sea urchin was avoided during the sperm collection process. When eggs exuded from the sea urchin, we positioned the sea urchin upside down (i.e., the genital pores were facing down) above a 100-mL beaker filled with filtered seawater to induce egg laying for 10–30 min. The sperm and eggs were subjected to artificial insemination immediately after collection.
The sperm and eggs must be diluted before artificial insemination in a Petri dish. The gamete dilution and artificial insemination methods used in this study were proposed by previous research and revised according to the needs of the present study (Rahman et al., 2012). The collected sperm was first placed under a dissecting microscope to observe sperm cell motility; once the cells had been confirmed to show a normal level of motility, 50 μL of the sperm was diluted in 5 mL filtered seawater. The collected eggs were also observed using a dissecting microscope to confirm that they had a normal shape (i.e., round), after which 1 mL of eggs at the bottom of the beaker were collected using a dropper without disturbing the seawater and added to 100 mL filtered seawater. In the artificial insemination, 15 mL of filtered seawater, 3 mL of the diluted egg-containing solution, and 1 mL of the diluted sperm-containing solution were added to a Petri dish and placed in a constant-temperature cabinet at 25°C. The embryos in the Petri dish were divided into three groups that were illuminated using LED light fixtures with three different wavelengths (EVERLIGHT Electronics Co., Ltd., Taiwan), as follows: full-spectrum (4500K 400–750 nm wavelength; 940 lux at 30 cm), red (630-nm wavelength; 360 lux at 30 cm) LED, and blue (450-nm wavelength; 30 lux at 30 cm) LED groups. The light: dark cycle was set to 12:12 h. LED lights were used to illuminate the embryos for a total of 48 h; 1-mL samples of the embryo-containing solution were collected at the 24th and 48th hours of illumination using a dropper and placed under a dissecting microscope to record the number of embryos at each stage of development. A total of 100 embryos were recorded for each group, and the experiments were conducted in triplicate.
Covering Behavior
The design of experiment was modify from the previous study (Ziegenhorn, 2016). A plastic box (33 × 25.5 × 9 cm) full with seawater slightly above the height of the sea urchin, placed 15 acrylic sheets (red, blue, or transparent) that were 3 cm2 and 0.1 cm thick into the box and let them sink to the bottom naturally. The black color sea urchins (black interambulacral- and ambulacral area) or the white color sea urchins (white interambulacral- and black ambulacral area) were randomly selected for the behavior test. The sea urchins were irradiated with full-spectrum, 630-nm, and 450-nm LED lights in a random order. The LED lighting device was used to illuminate the three sea urchins in the box for 60 min; the number of acrylic sheets gripped by the sea urchin for cover was recorded every 10 min. After 60 min of illumination, the LED lighting was turned off for 40 min so that the urchins could rest. This part of experiment is divided into four parts: (1) to test the influence of different wavelength light illumination on the covering behavior of sea urchin, the total number of acrylic sheets grabbed by three randomly selected black or white sea urchins was recorded after illuminating three different light sources; (2) the number of each color acrylic sheets grabbed by three randomly selected sea urchins were recorded after illuminating by three different light sources; (3) to compared the covering behavior of different color sea urchins, the total number of three different color acrylic sheets were recorded by three randomly selected white- or black sea urchins after illuminating three different light sources; (4) to test the choice of shelter, the number of each color acrylic sheets grabbed by three randomly selected sea urchins were recorded after illuminating by three different light sources. All experiments were conducted to three biologically replicates.
Righting Behavior
A (45 × 30 × 30 cm) glass tank was filled with seawater to 80% of its volume. Sea urchins were placed upside down (i.e., aboral side down and oral side up) in the glass tank for each for all groups (namely full-spectrum, red LED, and blue LED groups; sample size n = 3 required in each group). The time (in seconds) required for the urchins to right themselves was recorded; a value of 10 min was assigned to urchins that had not righted themselves after 10 min. Three biologically replicates were recorded for each group. The upper limit of the observation period was determined according to the previous study (Ding et al., 2020).
Phototaxis
A (60 × 30 × 36 cm) glass tank filled with seawater to slightly above the height of the sea urchin, placed a ruler at the bottom of the tank, and randomly selected one urchin for the experiment. The sea urchin was positioned at the center of the tank. The phototaxis of sea urchins (three black and three white) are tested according to the lighting group: three single-light-source groups (red LED, blue LED, and full spectrum) and three combined-light-source groups (red LED and blue LED, red LED and full spectrum, and blue LED and full spectrum). In the single-light-source experiment, one monochromatic or full-spectrum LED light tube was placed on one side of the tank, with no light source on the other side; for the combined-light-source experiment, two light tubes of different wavelengths were placed on opposite sides of the tank. The glass tank was covered using black plastic sheets to prevent other light sources from affecting the experimental results. The lights were switched on for 2 min, during which the direction and distance of the sea urchin’s movement were recorded. The experiment was conducted in four biological replicates for each group.
Statistical Analysis
Results are presented as the mean ± standard deviation (SD) of three replicates. All data were subjected to ANOVA followed by Duncan’s multiple range tests. A p < 0.05 was considered significant.
Results
Embryonic Development of the Sea Urchin Under Different Light Sources
Under different wavelengths of illumination, Figures 1A–E shows the changes in the embryonic development of sea urchin s within 24–48 h. Figure 1F depicts sea urchin embryos fertilized in percentage bar chart under LED illumination of varying wavelengths in a 25°C constant-temperature cabinet. Among those illuminated by a blue LED light for 24 h, 13.59% reached the blastula stage (Figure 1A), and 18.12% reached the gastrula stage (Figure 1B), 67.96% reached the prism stage (Figure 1C); the death rate was 0.33%. As shown in Figure 1F, among the embryos under 24 h of illumination by a full-spectrum LED light, 35.13, 44.71, and 19.16% reached the blastula, gastrula, and prism stages, respectively, with a death rate of 1%. Figure 1F presents that, after illumination by a red LED light for 24 h, 7.43% of the embryos were at the blastula stage, 56.93% at the gastrula stage, and 34.64% at the prism stage; the death rate was 1%. After 48 h of illumination by the blue LED light, 38.98% of the embryos reached the 2-arm pluteus stage (Figure 1D) and 27.84% reached the 4-arm pluteus stage (Figure 1E). However, the death rate was high at 31.33%. Figure 1F indicates that after full-spectrum light illumination for 48 h, 65.32% of the embryos entered the 2-arm pluteus stage and only 18.68% entered the 4-arm pluteus stage, with a death rate of 16%. Figure 1F reveals the percentages of embryos at the 2-arm and 4-arm pluteus stages to be 74.60 and 20.73%, respectively, after having been illuminated by the red LED light for 48 h, and the death rate was low at 4.67% (Figure 1F).
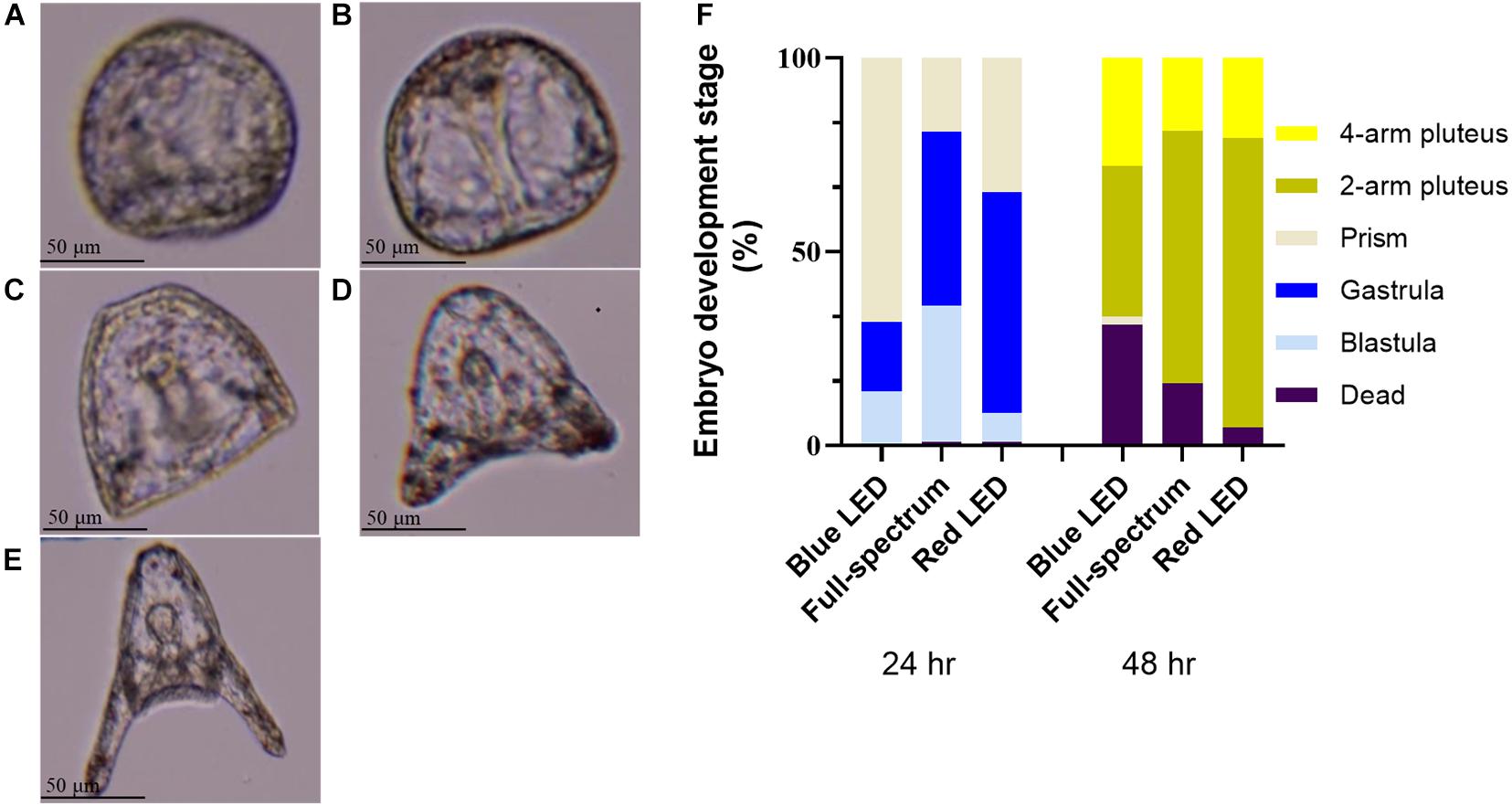
Figure 1. Embryo developmental stages of T. gratilla under the compound microscopy. (A) blastula, (B) gastrula, (C) prism, (D) 2-arm pluteus, (E) 4-arm pluteus. (F) The percentage bar chart showing the proportion of embryo development stage at 24 and 48 h after fertilization under blue-, full-spectrum- and red LED illumination.
Effects of Different Light Sources on Covering Behavior in Sea Urchins Covering Behavior in Different LED Light Environments
In the experiment on covering behavior, we divided the sea urchins into two types according to their level of melanin: white (Figure 2A, white interambulacral areas and black ambulacral areas) and black (Figure 2B, black interambulacral and ambulacral areas).
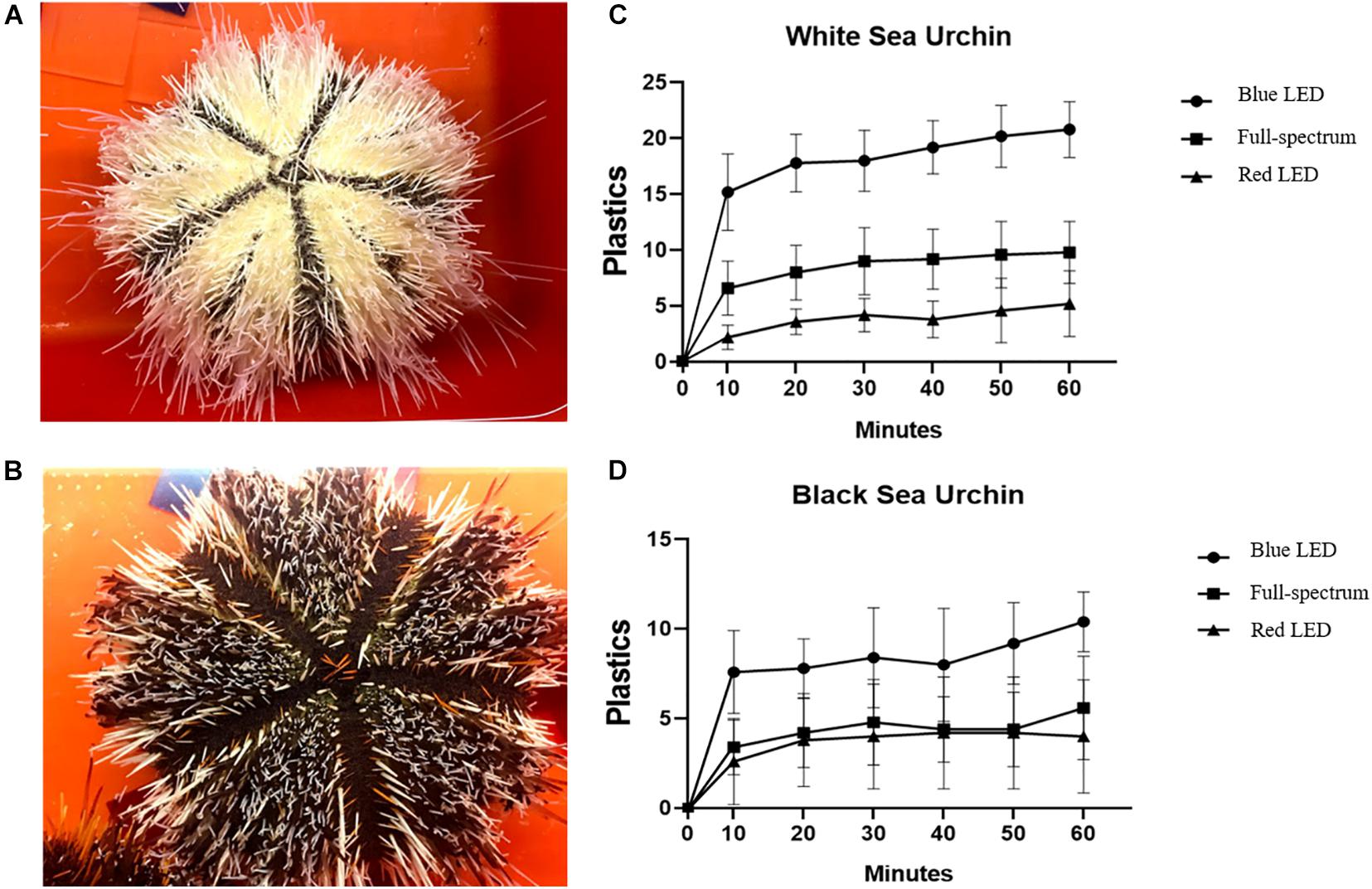
Figure 2. Numbers of plastics cover on (A,C) white and (B,D) black T. gratilla under blue-, full-spectrum- and red LED illumination. Each value represents mean ± SD.
Each sea urchin was illuminated using LED lighting devices with different wavelengths for 60 min, and the number of acrylic sheets gripped by the urchin for cover was recorded every 10 min. Figures 2C,D illustrate the covering behaviors of the white and black sea urchins, respectively; the urchins used their tube feet to move several acrylic sheets toward their aboral surfaces to cover themselves. For both white and black urchins, the number of acrylic sheets gripped increased with time and was the highest under blue LED illumination.
Covering Behavior With Acrylic Sheets of Different Colors
As shown in Figure 3A, when the sea urchins were provided with blue acrylic sheets, the blue LED group gripped significantly more acrylic sheets than did the full-spectrum and red LED groups in both the 10 and 60-min intervals. The number of acrylic sheets gripped by the full-spectrum group was significantly higher than that gripped by the red LED group after 10 min; however, after 60 min, the number was significantly higher in the red LED group (Figure 3A and Supplementary Table 1). Figure 3B presents the number of transparent acrylic sheets gripped by each group (Supplementary Table 1); the blue LED group, in both the 10 and 60-min observations, grabbed significantly more acrylic sheets than did the full-spectrum and red LED groups. Figure 3C presents the number of red acrylic sheets gripped by each group (Supplementary Table 1). The number of acrylic sheets gripped by the blue LED group was significantly higher than that gripped by the red LED group after 10 min; the number grew to be significantly higher than those of both the full-spectrum and red LED groups after 60 min. Accordingly, the number of acrylic sheets gripped by the urchins exhibited similar trends across the three colors of acrylic sheets. Specifically, the number of acrylic sheets, regardless of the color, gripped by the sea urchin was the highest in the blue LED group, followed by the full-spectrum group and then the red LED group.
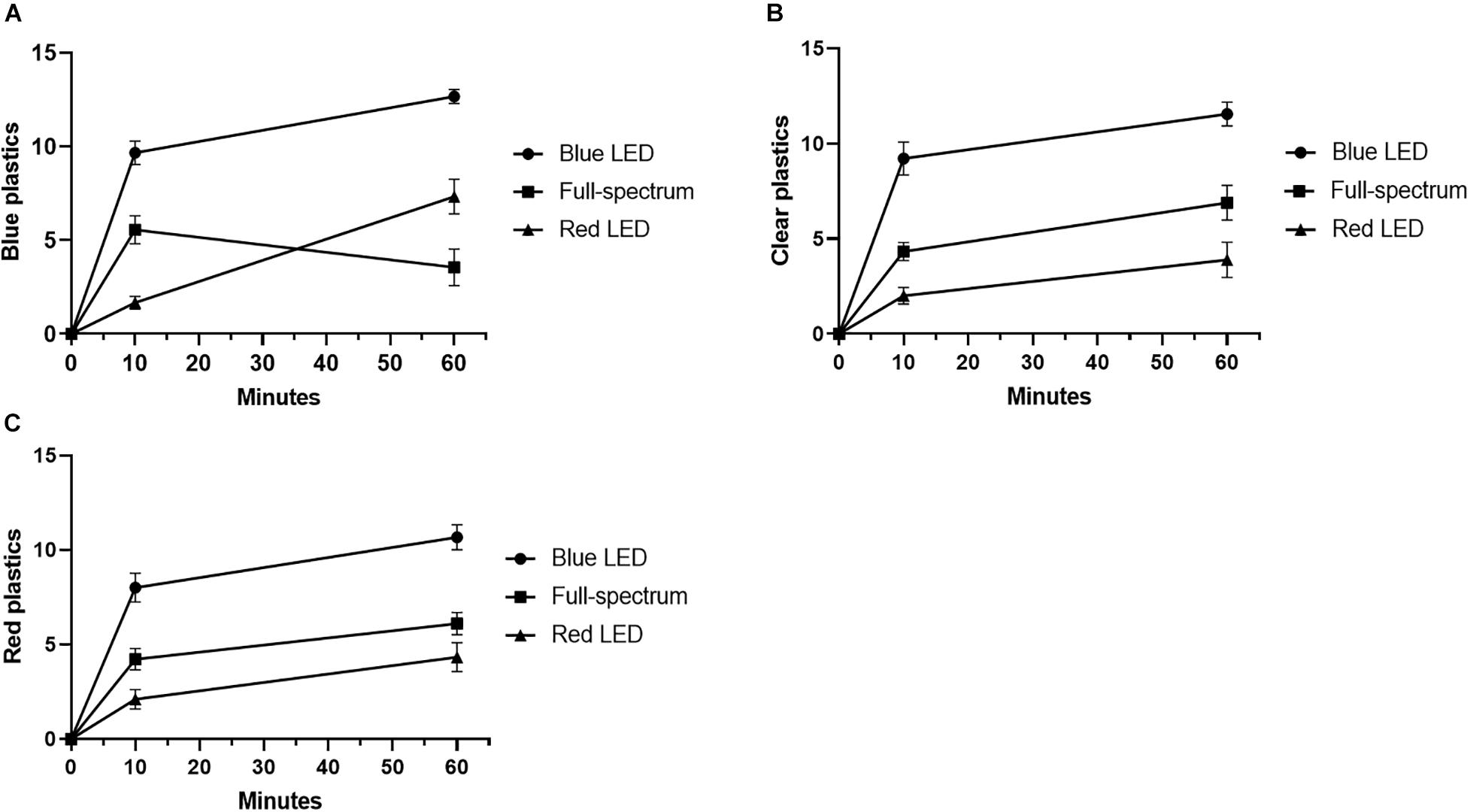
Figure 3. The covering behavior of acrylic sheets in different colors. (A) The covering behavior of T. gratilla with (A) blue-, (B) transparent- and (C) red plastics at 10 and 60 min under different wavelength LED illumination. Each value represents mean ± SD.
Comparison Between the Covering Behavior in White and Black Sea Urchin
The pattern of sea urchins grabbing acrylic sheet are shown in Figures 4A,B (Supplementary Table 2). The white sea urchins gripped significantly more acrylic sheets than did the black urchins under the illumination of both the blue LED (Figure 4C and Supplementary Table 2) and full-spectrum (Figure 4D and Supplementary Table 2) lights. As shown in Figure 4E (Supplementary Table 2), the numbers of acrylic sheets gripped by the white and black sea urchins were not significantly different when the urchins were illuminated by the red LED light. Furthermore, the white and black sea urchins demonstrated more covering behavior under the blue LED light than under the full-spectrum and red LED lights, and the white urchins exhibited more covering behavior under the full-spectrum illumination than under the red LED illumination.
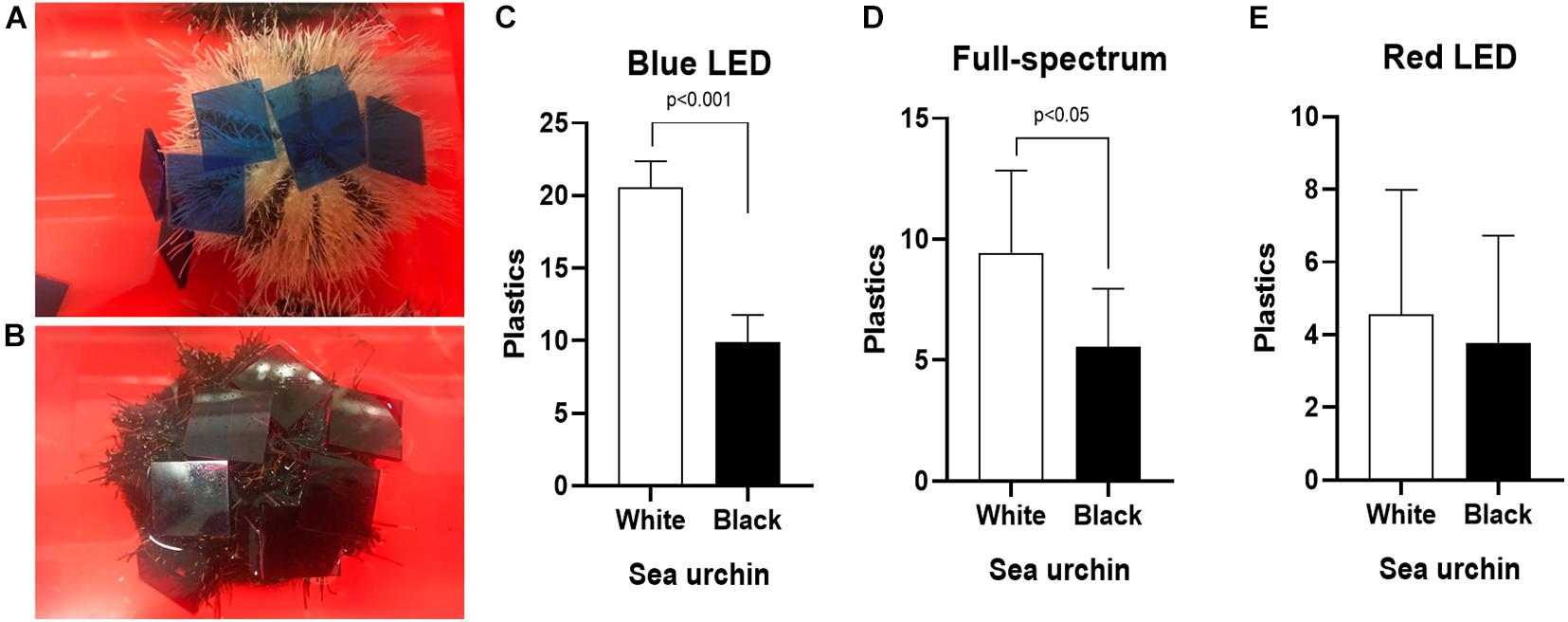
Figure 4. Effects of different wavelength LED illumination on the covering behavior of (A) white and (B) black T. gratilla. The numbers of acrylic sheets gripped by sea urchin were counted after 60 min test period under (C) blue-, (D) full- spectrum-, and (E) red light source. Each value represents mean ± SD.
The Choice of Cover
As shown in Figure 5, the numbers of blue, red, and transparent acrylic sheets gripped by the sea urchin did not differ significantly, regardless of the LED wavelengths (Supplementary Table 3). Hence, the sea urchins did not have any preferences for the colors of their covers.
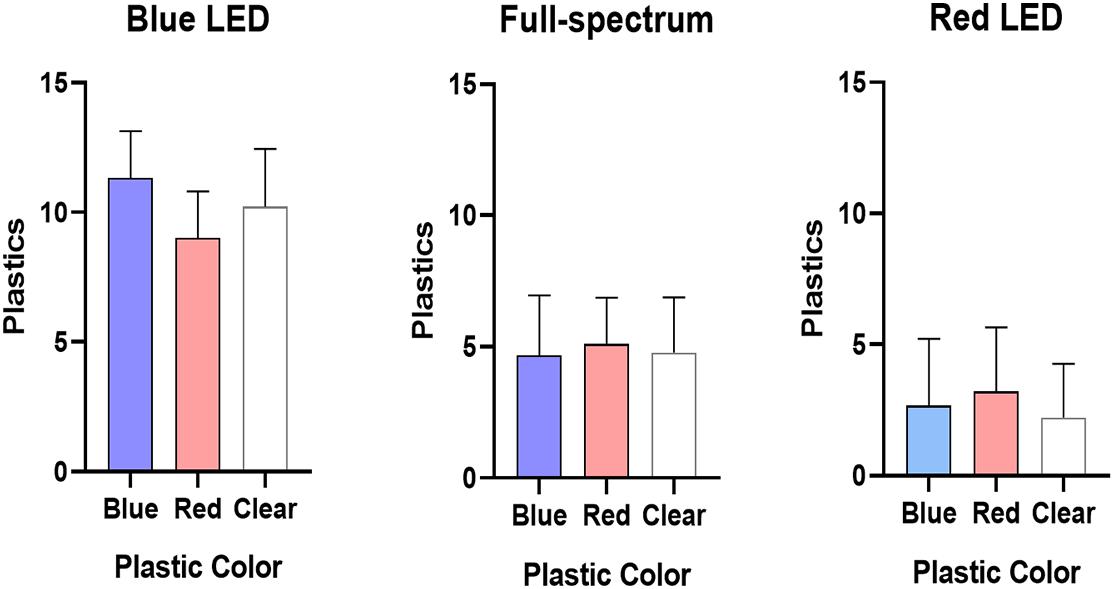
Figure 5. The numbers of blue, red, and transparent acrylic sheets gripped by T. gratilla under different wavelength LED illumination. Each value represents mean ± SD.
Effect of Different Light Sources on Righting Behavior in Sea Urchin
As illustrated in Figure 6, the blue LED group required longer times to right themselves than did the full-spectrum and red LED groups, but the differences were not statistically significant (Supplementary Table 4). According to the trend of righting behavior, the urchins were under higher environmental stress when illuminated by the blue LED light and thus required longer times to right themselves. Thus, the wavelength of light affected the behavioral capacities of the sea urchin.
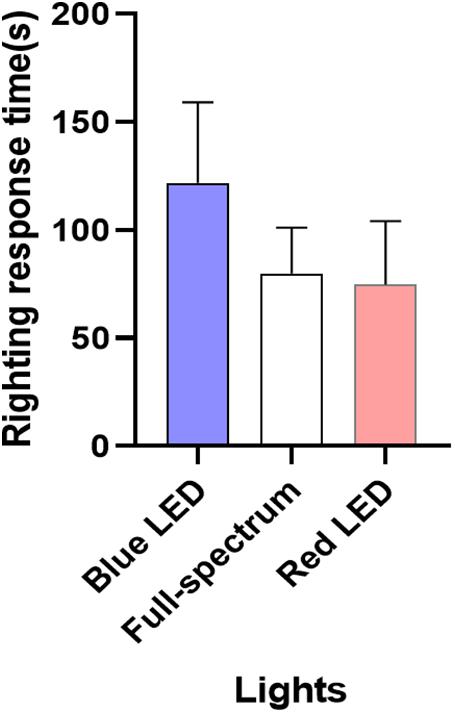
Figure 6. Righting response time of T. gratilla under different wavelength LED illumination. Each value represents mean ± SD.
Effect of Different Light Sources on Phototaxis in sea Urchins
As illustrated in Figures 7A,B, the sea urchins exhibited negative phototaxis under the blue LED and full-spectrum lights, whereas no noticeable movement was observed under the red LED light. When illuminated by the blue LED light on one side and red LED light on the other side, the sea urchin moved away from the blue LED light source and toward the red LED light source. With blue LED light on one side and full-spectrum light on the other side, the sea urchin also moved away from the blue LED light source and toward the full-spectrum light source. When the sea urchin was illuminated by the full-spectrum light on one side and the red LED light on the other side, they moved toward the red LED light source, but only slightly. When only one light source was provided, the sea urchin was the most negatively phototactic, i.e., it moved the farthest away from the light source, under the blue LED, followed by the full-spectrum light. The sea urchin moved similar distances away from the blue LED light when it was accompanied by the full-spectrum or red LED light on the other side.
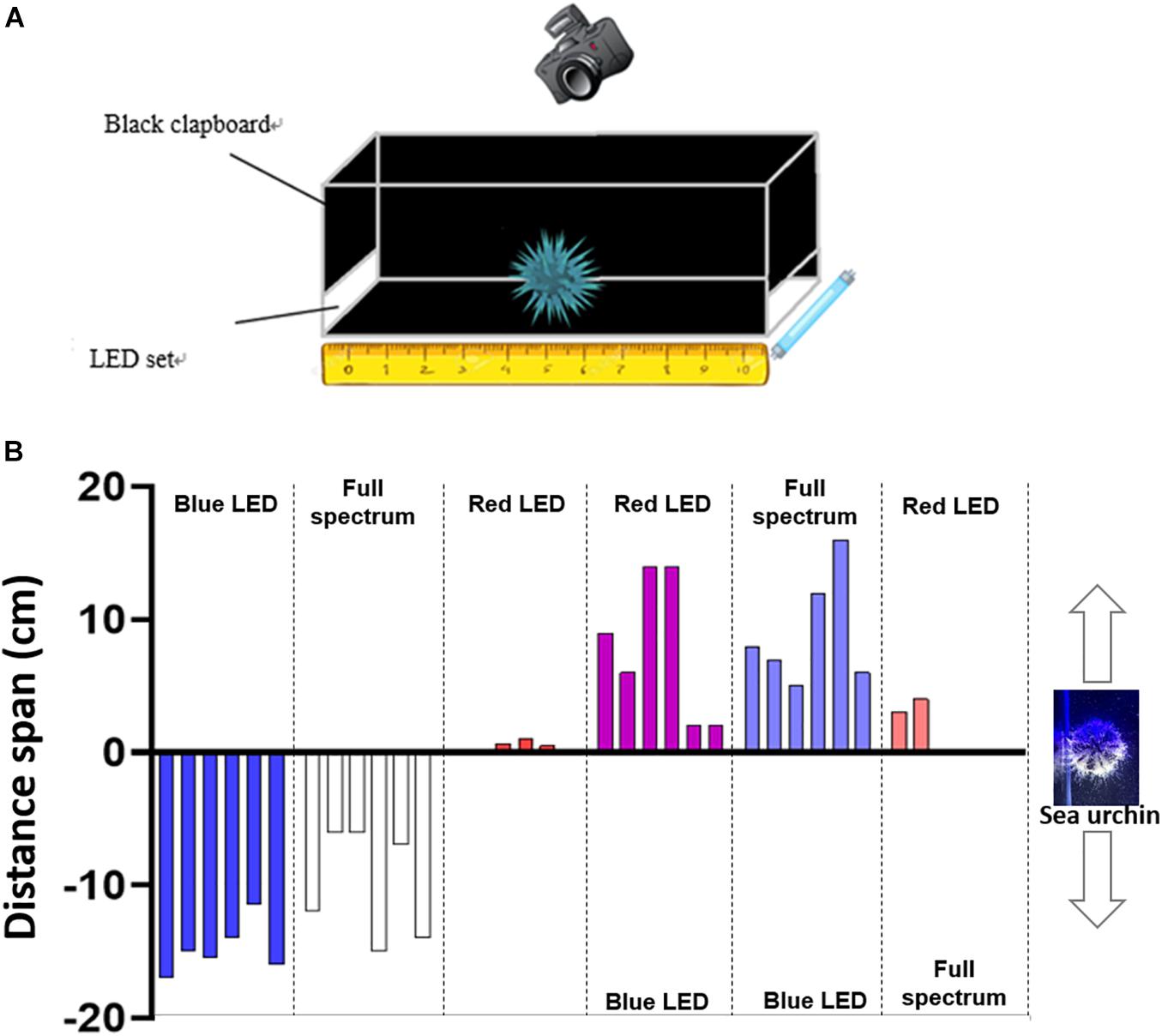
Figure 7. The phototaxis of T. gratilla. (A) Schematic diagram of the tank and LED light sources used in phototaxis experiment. (B) Measured distance span for each sea urchin tested in different light conditions. Measured distances were taken as positive (+) or negative (–) depending upon whether the sea urchin was moving toward or away from the light source.
Discussion
Studies have investigated the effects of various environmental factors, such as light (Belleza et al., 2012), temperature (Byrne et al., 2009; Abdul Wahab et al., 2016; Mejía-Gutiérrez et al., 2019; Ding et al., 2020), and metal content in a waterbody (Bielmyer et al., 2005; Bonaventura et al., 2018; Di Natale et al., 2019), on the embryonic development and behavior of sea urchins. For light, studies have predominantly employed natural light and UV light in their experiments, and few of them have included light with different wavelengths in the experiments. LEDs hold great potential for indoor aquaculture due to their light weight, low energy consumption, and ability to change the light environment of a waterbody through emitting monochromatic light (Shen et al., 2013). Therefore, the present study used LED devices to illuminate fertilized sea urchin embryos and adult sea urchins to examine the effects of light wavelength on the urchins. This study aimed to accelerate the embryonic development of sea urchins through the use of LED devices and thus increase the production of sea urchins. By changing the wavelengths of LED illumination, this study also hoped to understand the behavioral changes in sea urchin under different light wavelengths, thereby determining the wavelength to which sea urchins were the most sensitive and by extension the most suitable wavelength for sea urchin farming. According to the experimental results, the sea urchin embryos grew the fastest under the blue LED light; the adult sea urchin were the most sensitive to and most negatively phototactic under the blue LED light. The following discusses separately the embryonic development, covering behavior, righting behavior, and phototaxis of the sea urchin.
According to the proportion of embryos at each developmental stage, this study revealed that embryos cultured under LED lights of varying wavelengths grew at substantially different speeds. Previous research has reported that sea urchin embryos in a UV light environment exhibit slow growth and malformations (Bonaventura et al., 2005). In the present study, no embryonic malformation was observed under the LED lights at any of the tested wavelengths.
Twenty-four hours following fertilization, more than half of the embryos under the blue LED light had reached the prism stage; however, among those receiving the full-spectrum and red LED lights, approximately half of the embryos were still at the gastrula stage. Relative to the full-spectrum group, which served as the control group, the blue LED wavelength could accelerate embryonic development considerably, whereas the red LED wavelength had no noticeable effect on development rate. The three lights all yielded embryonic death rates of 1% or lower. Forty-eight hours following fertilization, the blue LED group demonstrated slightly faster development but a considerably higher death rate than did the other two groups. According to the study of Lesser et al., exposure to UV light contributes to higher expression of p53 and p21 proteins; the expressions of both proteins increase when DNA is damaged, and both are associated with apoptosis (Lesser et al., 2003). Despite its promotion of sea urchin embryonic development, the blue LED wavelength, which was the shortest wavelength used in this study, may have caused excessive stress on the embryos and thus led to death for the embryos that failed to withstand the stress. However, this study focused only on the embryonic development result; in-depth molecular biology experiments on genes and proteins are required to determine the causes of such differing development speeds and death rates. Bonaventura et al. (2005) observed that a UVB light slowed down the development of some embryos and found no effect of the UVB light in accelerating embryonic development (Lesser et al., 2003). According to the experimental results of this study, the blue LED increased the embryonic death rate but concurrently accelerated embryonic development. This accelerating effect, in comparison with the other groups, was particularly prominent within the first 24 h after fertilization. Therefore, among the LED lights tested, the blue LED light demonstrated the optimal performance at accelerating the embryonic development of sea urchins and was associated with a low death rate within the first 24 h following fertilization. Nevertheless, further research is warranted to investigate whether the use of a blue LED light source for accelerating embryonic development caused DNA damage or oxidative stress to the embryos or despite the faster embryonic growth led to incomplete embryonic development within the first 24 h following fertilization.
Previous studies have mostly focused on the effect of natural light on the covering behavior of sea urchins (Kehas et al., 2004; Ziegenhorn, 2016). According to Ziegenhorn found that among all environmental factors, light exerts the greatest influence on covering behavior in sea urchins, which engage in such behavior to shield themselves from light radiation. Their experiment result revealed that under natural light, sea urchins tended to grip red acrylic sheets rather than transparent sheets, indicating that the urchins may be able to determine whether an object can offer effective cover (Ziegenhorn, 2016). Different from this result, the present study demonstrated that under LED lights of three different wavelengths, sea urchins did not exhibit any preference for the color of acrylic sheets to grip (Figure 5). However, this discrepancy regarding the ability of sea urchins to distinguish different covers may be attributable to the different illumination distance and illuminance of the LED light and natural light used, respectively, in the present study and the study of Ziegenhorn et al. In addition, this study used acrylic sheets of one single color for each round of the experiment, which may have led the sea urchins to still grip the acrylic sheets even if they had a poor covering effect. Consistent with the literature, this study revealed that the number of acrylic sheets gripped by the sea urchin increased with the time of illumination (Figure 2), which again verified that sea urchins engage in covering behavior for the purpose of hiding from light radiation.
Kehas et al. (2004) discovered that albino West Indian sea urchins (Tripneustes ventricosus, which is in the same genus of sea urchin as the species studied here) gripped more covers than did the wild-type black sea urchins in both a dim environment with sunlight and an environment with direct sunlight (Kehas et al., 2004). This result was similar to the finding of the present study that sea urchins with a lower level of melanin gripped more covers than did their high-melanin counterparts under lights of all wavelengths, with the greatest difference found under the blue LED light. This result demonstrates that the pigmentation of a sea urchin affects its covering behavior; compared with sea urchins with high melanin, those with low melanin were more sensitive to light, particularly to light of shorter wavelengths. According to Dumont et al. (2007), green sea urchins (Strongylocentrotus droebachiensis) engage in covering behavior mainly to protect themselves from physical hazards such as radiation and waves (Dumont et al., 2007). Therefore, this study inferred that the sea urchin was the most threatened and stimulated by the blue LED light, followed by the full-spectrum light and then the red LED light, which was the closest to a dark environment. Moreover, studies have reported that sea urchins demonstrate covering behavior only in a light environment and remove all covers from themselves at night (Millott, 1966; Yoshida, 1966). In the present study, the sea urchins also removed acrylic sheets from their bodies when the LED light was turned off after having been on for 60 min.
The righting behavior of sea urchins refers to a sea urchin the use of tube feet and spines to return itself to its normal position (i.e., aboral surface up). This behavior enables a sea urchin to—after being positioned upside down by a predator or waves—orient itself to the normal position to escape from hazards or for survival (Percy, 1973; Collard et al., 2015). Therefore, the behavior is a critical survival skill of sea urchins and also an indicator of a capacity of sea urchin to protect itself under environmental stress (Brothers and McClintock, 2015). Righting behavior is a coordinated and reflexive movement of the neuromuscular system of a sea urchin after a drastic change in the environment (Kleitman, 1941). The behavior has also been used to examine the physiological state of sea urchins exposed to environmental stress, such as chemical pollutants (Böttger et al., 2001), genotoxins (Canty et al., 2009), low salinity (Lawrence, 1975), high partial pressure of carbon dioxide (Challener and McClintock, 2013), high temperature (Brothers and McClintock, 2015), and high light intensity (Sun et al., 2019). According to the literature, when environmental factors cause stress in sea urchins, the urchins become slower in righting, are less likely to right themselves, or even fail to do so, indicating that sea urchins are less able to right themselves in the presence of environmental stress. Therefore, the righting speed of sea urchins can be used to determine whether they perceive the environment as stressful. To better understand the physiological state of sea urchins exposed to different wavelengths, we recorded the time taken by sea urchins to right themselves under LED lights of three different wavelengths. According to the results, the sea urchins spent the most time righting themselves under the blue LED light, which although no significant difference was observed among the three lights caused the greatest stress to the sea urchin. Nevertheless, given the absence of significant difference among the three lights, the blue LED light may simply be an unpleasant light to the sea urchin without causing them any serious stress or damage.
Ullrich-Luter et al. (2011) conducted a phototaxis experiment on purple sea urchins (Strongylocentrotus purpuratus); they observed only negative phototaxis in the sea urchins illuminated on one side by a blue LED or full-spectrum light source and discovered both positive and negative phototaxis in sea urchins illuminated on one side by a red LED light source (Ullrich-Luter et al., 2011). In contrast, the present study revealed that sea urchins illuminated on one side by a red LED light source did not exhibit noticeable positive or negative phototaxis; however, those illuminated on one side by a blue LED or full-spectrum light source engaged in similar behavior as that found by Ullrich-Luter et al. Because the red LED light source was close to a dark environment, it may not have provided enough light to induce any positive or negative phototactic response in the sea urchins, thereby resulting in random movement of the sea urchin. To study sea urchins’ response when illuminated on both sides by different light sources that induced negative phototaxis, we included three light-source combinations in the phototaxis experiment: blue LED and full-spectrum lights, blue LED and red LED lights, and full-spectrum and red LED lights. According to the results, when illuminated by blue LED and full-spectrum lights on the sides, both of which provoked negative phototaxis, the sea urchin moved away from the blue LED light source and toward the full-spectrum light source. This result is consistent with the finding from the covering behavior experiment that the blue LED light caused stronger negative stimulation of the sea urchins than did the full-spectrum one. Furthermore, the sea urchins stretched their tube feet facing the direction of their movement and retracted their tube feet facing the opposite direction. Previous research has observed rhabdomeric cells in the tube feet of sea urchins; these cells are the photoreceptor cells in invertebrates and contain various genes and proteins associated with photoreceptor development and function, including Opsin4 and pax6. Hence, tube feet are seen as the photoreceptor organ of sea urchins; tube feet exhibit negative phototaxis (Sharp and Gray, 1962; Holmes, 1912; Burke et al., 2006; Ullrich-Luter et al., 2011) and have a neuromuscular system (Agca et al., 2011). Therefore, tube feet are able to coordinate the neuromuscular system and generate a series of behavioral responses (e.g., covering behavior and negative phototactic response) after receiving light stimuli.
Conclusion
In conclusion, the present study demonstrated that the embryonic development and behavior pattern of collector urchins are affected by the stimuli of short-wavelength irradiation. Replacing conventional lighting equipment with LEDs may benefit indoor sea urchin cultivation yields. These results will provide the basis for applications in the conservation and breeding of sea urchins in the future.
Data Availability Statement
The raw data supporting the conclusions of this article will be made available by the authors, without undue reservation.
Ethics Statement
Ethical review and approval was not required for the animal study because the present study does not involve any vertebrate or specified invertebrate animals.
Author Contributions
FS, YH, and YW designed the experiments and wrote the manuscript. YL and TH performed the experiments. YL and FS analyzed the data and wrote the original draft of the manuscript. YW supervised and validated the manuscript. All authors contributed to the article and approved the submitted version.
Funding
This article was subsidized for English editing by National Taiwan University under the Excellence Improvement Program for Doctoral Students (Grant No. 108-2926-I-002-002-MY4), sponsored by the Ministry of Science and Technology, Taiwan.
Conflict of Interest
The authors declare that the research was conducted in the absence of any commercial or financial relationships that could be construed as a potential conflict of interest.
Supplementary Material
The Supplementary Material for this article can be found online at: https://www.frontiersin.org/articles/10.3389/fmars.2021.684330/full#supplementary-material
References
Abdul Wahab, M., Rahman, M., Yusoff, F., Arshad, A. B., and Tan, S. S. (2016). Effects of temperature on the embryonic and early larval development in tropical species of black sea urchin, Diadema setosum (Leske, 1778). J. Environ. Biol. 37, 657–668.
Agca, C., Elhajj, M. C., Klein, W. H., and Venuti, J. M. (2011). Neurosensory and neuromuscular organization in tube feet of the sea urchin Strongylocentrotus purpuratus. J. Comparative Neurol. 519, 3566–3579. doi: 10.1002/cne.22724
Barros, F., Santos, D., Reis, A., Martins, A., Dodonov, P., and Nunes, J. (2020). Choosing trash instead of nature: sea urchin covering behavior. Mar. Pollut. Bull. 155:111188. doi: 10.1016/j.marpolbul.2020.111188
Belleza, D. F., Abao, S., Taguba, C., and Dy, D. (2012). Effects of UV-C on the masking behavior of the green urchin salmacis sphaeroides (Linnaeus, 1758). Philippine Scientist 49, 34–43.
Bielmyer, G., Brix, K., Capo, T., and Grosell, M. (2005). The effects of metals on embryo-larval and adult life stages of the sea urchin, Diadema antillarum. Aquatic Toxicol. (Amsterdam, Netherlands) 74, 254–263. doi: 10.1016/j.aquatox.2005.05.016
Bonaventura, R., Poma, V., Costa, C., and Matranga, V. (2005). UVB radiation prevents skeleton growth and stimulates the expression of stress markers in sea urchin embryos. Biochem. Biophys. Res. Commun. 328, 150–157. doi: 10.1016/j.bbrc.2004.12.161
Bonaventura, R., Zito, F., Chiaramonte, M., Costa, C., and Russo, R. (2018). Nickel toxicity in P. lividus embryos: dose dependent effects and gene expression analysis. Mar. Environ. Res. 139, 113–121. doi: 10.1016/j.marenvres.2018.05.002
Böttger, S. A., Mcclintock, J. B., and Klinger, T. S. (2001). Effects of inorganic and organic phosphates on feeding, feeding absorption, nutrient allocation, growth and righting responses of the sea urchin Lytechinus variegatus. Mar. Biol. 138, 741–751. doi: 10.1007/s002270000476
Brothers, C. J., and McClintock, J. B. (2015). The effects of climate-induced elevated seawater temperature on the covering behavior, righting response, and Aristotle’s lantern reflex of the sea urchin Lytechinus variegatus. J. Exp. Mar. Biol. Ecol. 467, 33–38. doi: 10.1016/j.jembe.2015.02.019
Burke, R. D., Angerer, L. M., Elphick, M. R., Humphrey, G. W., Yaguchi, S., Kiyama, T., et al. (2006). A genomic view of the sea urchin nervous system. Dev. Biol. 300, 434–460. doi: 10.1016/j.ydbio.2006.08.007
Byrne, M., Ho, M., Selvakumaraswamy, P., Nguyen, H. D., Dworjanyn, S. A., and Davis, A. R. (2009). Temperature, but not pH, compromises sea urchin fertilization and early development under near-future climate change scenarios. Proc. Biol. Sci. 276, 1883–1888. doi: 10.1098/rspb.2008.1935
Canty, M. N., Hutchinson, T. H., Brown, R. J., Jones, M. B., and Jha, A. N. (2009). Linking genotoxic responses with cytotoxic and behavioural or physiological consequences: differential sensitivity of echinoderms (Asterias rubens) and marine molluscs (Mytilus edulis). Aquatic Toxicol. 94, 68–76. doi: 10.1016/j.aquatox.2009.06.001
Challener, R. C., and McClintock, J. B. (2013). Exposure to extreme hypercapnia under laboratory conditions does not impact righting and covering behavior of juveniles of the common sea urchin Lytechinus variegatus. Mar. Freshw. Behav. Physiol. 46, 191–199. doi: 10.1080/10236244.2013.800759
Collard, M., Rastrick, S. P. S., Calosi, P., Demolder, Y., Dille, J., Findlay, H. S., et al. (2015). The impact of ocean acidification and warming on the skeletal mechanical properties of the sea urchin Paracentrotus lividus from laboratory and field observations. ICES J. Mar. Sci. 73, 727–738. doi: 10.1093/icesjms/fsv018
Di Natale, M., Bennici, C., Biondo, G., Masullo, T., Monastero, C., Tagliavia, M., et al. (2019). Aberrant gene expression profiles in Mediterranean sea urchin reproductive tissues after metal exposures. Chemosphere 216, 48–58. doi: 10.1016/j.chemosphere.2018.10.137
Ding, J., Zheng, D., Sun, J., Hu, F., Yu, Y., Zhao, C., et al. (2020). Effects of water temperature on survival, behaviors and growth of the sea urchin Mesocentrotus nudus: new insights into the stock enhancement. Aquaculture 519:734873. doi: 10.1016/j.aquaculture.2019.734873
Dumont, C. P., Drolet, D., Deschênes, I., and Himmelman, J. H. (2007). Multiple factors explain the covering behaviour in the green sea urchin, Strongylocentrotus droebachiensis. Anim. Behav. 73, 979–986. doi: 10.1016/j.anbehav.2006.11.008
Holmes, S. J. (1912). Phototaxis in the sea urchin, Arbacia punctulata. J. Anim. Behav. 2, 126–136. doi: 10.1037/h0076037
Kehas, A. J., Theoharides, K. A., and Gilbert, J. J. (2004). Effect of sunlight intensity and albinism on the covering response of the Caribbean sea urchin Tripneustes ventricosus. Mar. Biol. 146, 1111–1117. doi: 10.1007/s00227-004-1514-4
Kleitman, N. (1941). The effect of temperature on the righting of echinoderms. Biol. Bull. 80, 292–298. doi: 10.2307/1537716
Lawrence, J. M. (1975). The effect of temperature-salinity combinations on the functional well-being of adult Lytechinus variegatus (Lamarck) (Echlnodermata, Echinoldea). J. Exp. Mar. Biol. Ecol. 18, 271–275. doi: 10.1016/0022-0981(75)90111-2
Lesser, M. P., Kruse, V. A., and Barry, T. M. (2003). Exposure to ultraviolet radiation causes apoptosis in developing sea urchin embryos. J. Exp. Biol. 206, 4097–4103. doi: 10.1242/jeb.00621
Mejía-Gutiérrez, L. M., Benítez-Villalobos, F., and Díaz-Martínez, J. P. (2019). Effect of temperature increase on fertilization, embryonic development and larval survival of the sea urchin Toxopneustes roseus in the Mexican south Pacific. J. Thermal Biol. 83, 157–164. doi: 10.1016/j.jtherbio.2019.05.011
Millott, N. (1966). “Coordination of spine movements in echinoids,” in Physiology of Echinoderms, ed. B. Ra (New York, NY: John Wiley & Sons), 187–220.
Parvez, M. S., Rahman, M. A., Yusoff, F. M., Arshad, A., and Lee, S.-G. (2018). Studies on the ontogenic development of a high-valued tropical sea urchin, tripneustes gratilla (Linnaeus, 1758) for seed production and commercial aquaculture. Int’l. J. Adv. Chemical Engg. Biol. Sci. 5, 6–14. doi: 10.1016/j.aquaculture.2013.02.030
Percy, J. A. (1973). Thermal adaptation in the boreo-arctic echinoid strongylocentrotus droebachiensis (O. F. müller, 1776). II. seasonal acclimatization and urchin activity. Physiol. Zool. 46, 129–138. doi: 10.1086/physzool.46.2.30155593
Rahman, M. A., Yusoff, F. M., Arshad, A., Shamsudin, M. N., and Amin, S. (2012). Embryonic, larval, and early juvenile development of the tropical sea urchin, Salmacis sphaeroides (Echinodermata: echinoidea). Sci. World J. 2012:938482.
Sharp, D. T., and Gray, I. E. (1962). Studies on factors affecting local-distribution of two sea-urchins, arbacia-punctulata and lytechinus-variegatus. Ecology 43, 309–313. doi: 10.2307/1931986
Shen, S. C., Kuo, C. Y., and Fang, M.-C. (2013). Design and analysis of an underwater white LED fish-attracting lamp and its light propagation. Int. J. Adv. Robotic Syst. 10:183. doi: 10.5772/56126
Sun, J., Chi, X., Yang, M., Ding, J., Shi, D., Yu, Y., et al. (2019). Light intensity regulates phototaxis, foraging and righting behaviors of the sea urchin Strongylocentrotus intermedius. PeerJ 7:e8001. doi: 10.7717/peerj.8001
Ullrich-Luter, E. M., Dupont, S., Arboleda, E., Hausen, H., and Arnone, M. I. (2011). Unique system of photoreceptors in sea urchin tube feet. Proc. Natl. Acad. Sci. U.S.A. 108, 8367–8372. doi: 10.1073/pnas.1018495108
Yoshida, M. (1966). “Photosensitivity,” in Physiology of Echinoderms, ed. B. Ra (New York, NY: John Wiley & Sons), 435–464.
Keywords: Tripneustes gratilla, light emitting diodes condition, behavior, embryo development, phototaxis
Citation: Li Y-Y, Su F-J, Hsieh Y-J, Huang T-C and Wang Y-S (2021) Embryo Development and Behavior in Sea Urchin (Tripneustes gratilla) Under Different Light Emitting Diodes Condition. Front. Mar. Sci. 8:684330. doi: 10.3389/fmars.2021.684330
Received: 23 March 2021; Accepted: 01 June 2021;
Published: 23 June 2021.
Edited by:
Valerio Matozzo, University of Padua, ItalyReviewed by:
Antonia Chiarore, University of Naples Federico II, ItalyMarco Munari, Anton Dohrn Zoological Station, Italy
Giovanna Romano, Anton Dohrn Zoological Station, Italy
Ricardo Beiras, University of Vigo, Spain
Copyright © 2021 Li, Su, Hsieh, Huang and Wang. This is an open-access article distributed under the terms of the Creative Commons Attribution License (CC BY). The use, distribution or reproduction in other forums is permitted, provided the original author(s) and the copyright owner(s) are credited and that the original publication in this journal is cited, in accordance with accepted academic practice. No use, distribution or reproduction is permitted which does not comply with these terms.
*Correspondence: Yung-Song Wang, eXVuZ3Nvbmd3YW5nQG50dS5lZHUudHc=
†These authors have contributed equally to this work