- 1Ecology & Evolution, Department of Biological Science, Florida State University, Tallahassee, FL, United States
- 2Coastal and Marine Laboratory, Florida State University, St. Teresa, FL, United States
Nematodes are among the most abundant organisms on Earth, and have important roles in terrestrial, freshwater, and marine ecosystems. Free-living marine nematodes have been used successfully as indicators of biological health and ocean pollution for at least the past 40 years, but their use as bioindicators is not ubiquitous. They have been most often used specifically as indicators of heavy metal and hydrocarbon pollution, with far fewer instances of their use as indicators of biological, environmental, or physical perturbations. Although free-living marine nematodes are among the best bioindicators owing to their worldwide distributions, abundances, and genus- and species-specific responses to environmental pollution, there are still some challenges that prevent their use globally. Here, we present a review of characteristics that make free-living marine nematodes excellent bioindicators, recent studies that have used them as bioindicators, and suggestions for future directions in the use of these fauna as indicators in the marine environment. Specifically, we consider the use of marine nematodes for microplastics (an understudied class of pollutants that are a future threat to global biodiversity), the value of current nematode indices as measures of ecosystem health, and the importance of improved and continued international collaboration in the field of marine nematology.
Introduction
Anthropogenic impacts on the global environment span hundreds of years, even predating European colonization of the Americas (Oldfield and Dearing, 2003). Those impacts, concomitant with climate change, have accelerated in recent years owing to insufficient efforts to mitigate contamination by many different pollutants, reducing fossil fuel consumption, and limiting the release of greenhouse gases. In parallel, biomes, biota, and biodiversity have suffered increased loss with extinction rates 50–500 times that of background levels in the wake of inadequate environmental conservation efforts (Woodruff, 2001). Consequences of cumulative carbon emissions include warming, changing weather patterns, rising sea levels, ocean acidification, de-oxygenation and changes in biogeochemistry, changing nutrient loads, and shifts in ocean currents and circulation. Anthropogenic activities, such as fisheries, pollution, aggregate and mineral mining, can exert significant pressures on marine ecosystems in addition to climate change, and may act additively or synergistically on the marine environment (see Cabral H. et al., 2019 for review). In situ and laboratory-based studies have shown multiple interaction effects in marine ecosystems (Duplisea et al., 2001, 2002; Halpern et al., 2008; Foden et al., 2011; Martín et al., 2014). These interactions affect local ecosystems but can extend beyond the directly impacted area (Lenihan et al., 2001). In addition, the effects of growing human populations in coastal areas and an increased reliance on ocean resources to sustain humanity (Claudet and Fraschetti, 2010; Rombouts et al., 2013) exacerbate an already distressed ecosystem. Central in dealing with the urgent need of assessing marine ecosystems health is doing so in an integrative way, using various tools, such as sentinel organisms or indicators, and clearly identifying what good health or environmental status is and how we know when it has been attained (Borja et al., 2013; Tett et al., 2013; Borja, 2014). An integrative long-term monitoring effort linking different ecosystem components and biotic and abiotic aspects is required to assess communities and ecosystems, and their responses to environmental and anthropogenic changes (Borja, 2014).
A major challenge, however, with monitoring anthropogenic changes to the marine environment is that our understanding of the history of environmental health is limited to what has been recorded in books and journals. Pauly (1995) coined the phrase shifting baseline syndrome to describe the mismanagement of fisheries stocks, but the phrase has become a standard in marine conservation sciences to explain that conservation efforts are limited by available data; the baseline for recovery is determined using data from recent history which does not convey environmental health and status predating human interference. This underscores the importance of setting reference conditions against which to measure change with the appropriate tools (Borja et al., 2012). We can assess current environmental statuses and try to either regress anthropogenic impacts or use current environmental health as a baseline to which future measurements are compared.
Assessing the status of marine environmental health can be achieved in various ways. In the past, measures of environmental quality have focused on effects of specific stressors on ecosystem components. These components can be assessed on various levels of biotic or abiotic organization, including genetic, biochemical, physiological, pathological, behavioral, and from species, over populations, to communities (Harding, 1992). More recently, environmental monitoring efforts have approached the issues more broadly, in recognition that assessment at the ecosystem level must include attributes of whole ecosystems, including productivity, instability, resistance and resilience, nutrient cycling, and diversity and function across taxa or functional groups. Yet, of great importance is finding ways to simplify how ecosystem health is evaluated (Rombouts et al., 2013). Three ways that have proven to be successful are oceanographic modeling, using ecological indicators, or using key functional attributes that can be expressed in quantitative metrics; all three encompass a range of suitable methods (Harding, 1992; Rombouts et al., 2013). Oceanographic modeling may use a combination of biological, chemical, and physical data from the ocean and assumptions and empirically assessed parameters about biogeochemical processes to hypothesize the effects of various anthropogenic activities, including for instance overfishing and pollution, on ecosystem services and community composition in the study environments (Doney et al., 2011). Models can be validated post hoc using field observations to assess how effectively the model explains what is observed in reality. However, the use of oceanographic models to explore how humans are affecting the marine environment can be limited by computing power, suitability and temporal and spatial coverage of the available data, and, even more so, by the decision-making of the researchers in how to configure their models (Holt et al., 2014). Furthermore, as evidenced by Holt et al. (2014), modeling ecosystem processes is challenging because of the coupling of physical, chemical, and biological ocean components.
Ecological indicators on the other hand are taxa used to signify the biological quality of a system and to monitor the environmental changes in the area (Siddig et al., 2016). Indicator species, rather than physical or chemical metrics, can be used to monitor ecosystem health because the organisms’ health or abundances may reflect effects of environmental perturbation and provide a less variable approach to environmental monitoring. The effects of pollutants and xenobiotics have been documented for over 2,000 years [see for instance Zong et al. (2010) and Wan et al. (2015) for earliest records of pollution and Shaw et al. (1983), Hodda and Nicholas (1986), Lambshead (1986), Warwick (1986), and Sandulli and De Nicola-Giudici (1990) for more recent examples]. However, it wasn’t until the past few decades that environmental monitoring programs were established (Markert et al., 2003). Macrofauna are often used as biological indicators in ocean health assessments (Borja et al., 2000), but using meiofauna may prove to be equally or even more useful and efficient, owing to their community responsiveness, high abundance, short life cycles, and limited sampling requirements. However, there is still an open debate on the importance of macrofauna versus meiofauna as bioindicators, with support for the use of meiofauna (Semprucci et al., 2015; Ingels et al., 2021), macrofauna (Danovaro et al., 2020; McLaverty et al., 2020), and a few instances of support for using both size classes together (Denoyelle et al., 2010; Patrício et al., 2012), sometimes depending on the specific pollutant or disturbance assessed. The use of biological indicators to assess ecosystem health requires the use of well-studied, widely-distributed indicator species. Alternatively, indicator taxa may be replaced by using assigned metrics based on functional characteristics that are related to important processes and functions performed in the ecosystem.
Free-living marine nematodes may serve as one of the most cost-efficient and effective biological indicators of all fauna, as they are ubiquitous and sensitive to environmental change (Balsamo et al., 2012; Semprucci et al., 2015). Moreover, nematodes exhibit characteristics that make them one of the most successful metazoans on Earth, including in systems with extremely challenging conditions (Sapir, 2021). Collecting nematodes in coastal ecosystems and even deep-sea sediments requires relatively small sample sizes, but even non-quantitative samples may yield reliable community structure and diversity data. Sediments generally contain large numbers of nematodes, comprising up to 99% of the entire meiofauna community in terms of abundance, and densities which can exceed 10,000 individuals per 10 cm2. Multiple studies have shown that nematodes can be used as ecosystem or environmental health indicators owing to described characteristics above (Moreno et al., 2011). However, slow progress in (1) research to identify conservation priorities, (2) research to support monitoring approaches (including indicator development), and (3) research to advance ecosystem understanding that can support (1) and (2) may hamper generally accepted use of meiofauna or nematodes for ecosystem monitoring (Ingels et al., 2021).
To date, several frameworks exist that support the ecosystem approach in assessing environmental quality status of an ecosystem, requiring several essential components (including indicator taxa) to be assessed and quantified. A good example is the European Union’s Water Framework Directive (WFD, Directive 2000/60/EC) and Marine Strategy Framework Directive, which identified the importance of biological indicators in assessing environmental health and Good Environmental Status. The WFD also established an Ecological Quality Status scale as a way of categorizing ecosystem health, which when combined with nematode community and biodiversity indices (see Moreno et al., 2011; Semprucci and Balsamo, 2014), can serve as a guideline for the use of nematodes as biological indicators.
In the present study, we provide a review of existing nematode ecological indication literature with the goals of: (1) identifying reasons to use free-living nematodes as ecological indicators; (2) synthesizing recent works that use nematode abundances and assemblage compositions as proxies for environmental health and resilience; and (3) evaluating the guidelines and providing considerations for the future use of free-living nematodes as ecological indicators in the face of changing anthropogenic impacts on marine environments.
Methodological Approach
To assist in our review and assess the suitability of marine free-living nematodes as biological indicators, we applied the environmental impact assessment framework of Kennedy and Jacoby (1999), to which we fitted relevant nematode characteristics. To add to our own knowledge, we conducted a literature review of peer-reviewed studies. We conducted a Thomson Reuters Web of Knowledge search using the terms “marine AND nematod∗ AND bioindic∗”, querying all available databases for the period 1980–2020. This approach does not necessarily exclude general works and reviews on ecological or biological indicator work that mentioned nematodes, or if the work focused on terrestrial or riverine systems. Therefore, search results were assessed individually to include relevant information. At the same time, we are aware that our search may exclude those works that have used free-living marine nematodes to study changes in nematode assemblages but were not associated with “bioindic∗” as reference term in the search. The search was conducted on October 09, 2020, and returned a total of 94 publications (Web of Science Core Collection; Supplementary Table 1), covering an array of study fields and disturbances, the effects of which were investigated with nematodes as indicators.
To assess the differences in nematode taxa (species and genera) responses to pollution and other disturbances, we searched for specific mentions in the literature beyond the literature search mentioned above; based on our own knowledge of the literature. A total of 35 different categories of pollution and disturbances were identified. When a taxon was characterized as resistant or tolerant to a certain impact a value of +1 was awarded; when a taxon was designated as sensitive, a value of −1 was awarded. Total scores were calculated for each taxon and disturbance category (Figure 1). To synthesize the information, we summarized scores per genus. This gave us a comprehensive table of nematode taxa (genus and species level, which were then pooled to genus level) which, based on literature, we can conclude are indicative of a nematode community stress response and environmental impact as a consequence of specific types of disturbance.
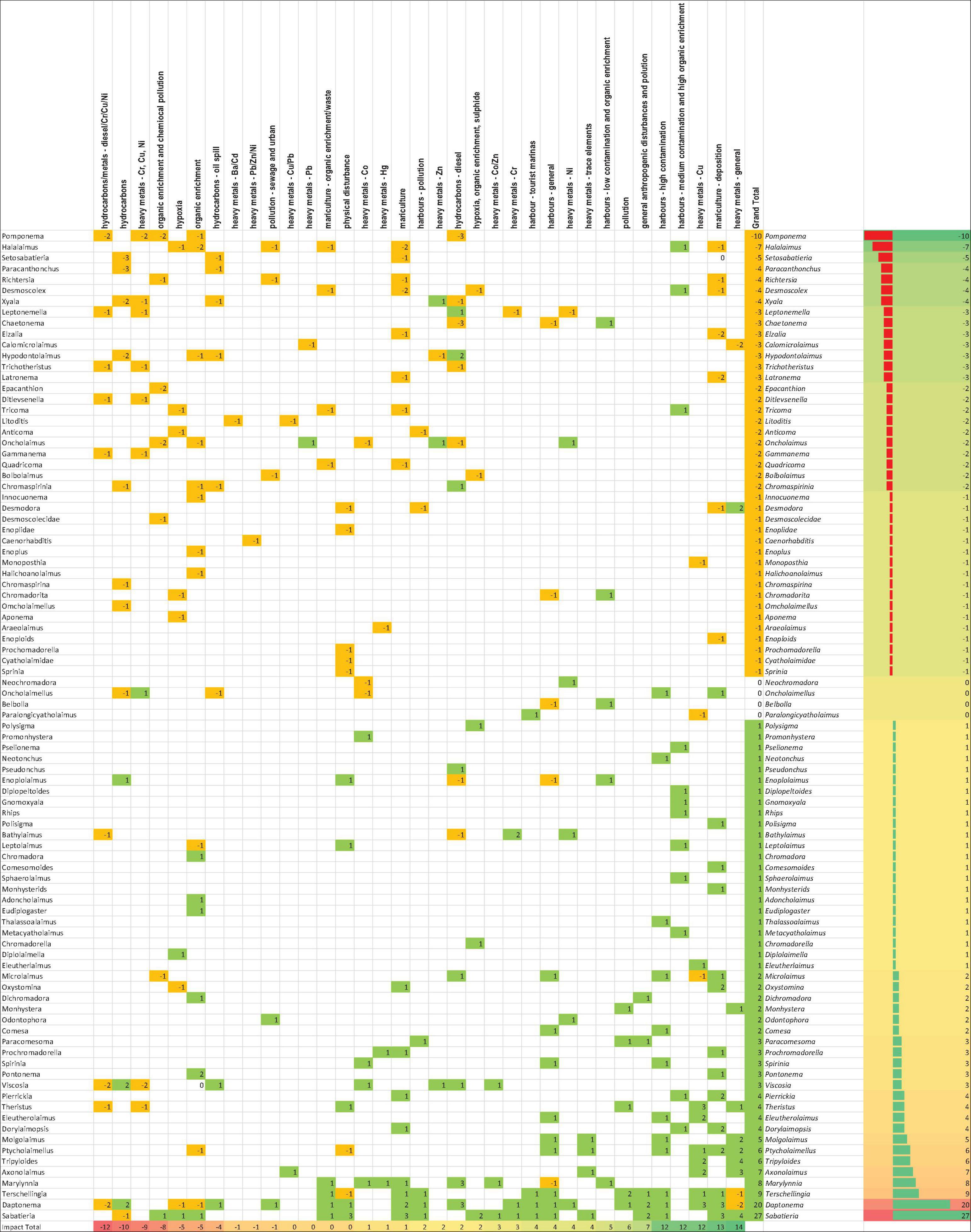
Figure 1. Nematode taxa responses to disturbances and perturbations. 35 different categories of pollution and disturbances were identified from papers specifically referencing the disturbance types. All taxa were scored based on a resistance/sensitivity framework. When a taxon was identified as resistant to a certain disturbance, a value of +1 was awarded, and when a taxon was denoted as sensitive it was awarded a value of −1. Positive and negative values were color-coded (green for positive, yellow for negative), and scores were summed for all taxa. Taxa with scores well above 0 are the most resistant to anthropogenic stressors, while scores below 0 denote taxa that are very much sensitive to disturbance.
Why Nematodes as Bioindicators?
Criteria for Use as Bioindicators
Kennedy and Jacoby (1999) proposed a six-part system for qualifying an organism as a bioindicator that was condensed by Holt and Miller (2010) into four parts: the fauna must (1) have a good indicator ability, which includes responses that are representative of the ecosystem components; (2) be abundant and common, as well as present in undisturbed areas; (3) be well studied, easy and inexpensive to study; and (4) be economically or commercially important. Meiofauna have been shown to meet all four of the qualifiers by Kennedy and Jacoby (1999), and most of the satisfaction statements in their work, though stated as valid for the entire meiofaunal size-class, are based on research on nematodes. Here we provided an updated assessment on why nematodes meet each criterion for use as ecological indicators.
Biological Indicators Must Have a Good Indicator Ability, Which Includes Responses That Are Representative of the Ecosystem Components
Nematodes have been used to assess the effects of a wide range of environmental disturbances, as evident by the breadth of literature on nematodes as bioindicators. Of the 94 papers, assessments of chemical perturbations were the most common, while papers that focused on biological and environmental disturbances were scarce (Figure 2). Common environmental impact assessments using nematodes as indicator species focused on heavy metals, hydrocarbon pollution, organic enrichment, xenobiotics, combinations of these pollutants (Figure 2). Predictable shifts in fauna life history characteristics, including reproductive potential (Boufahja et al., 2012), and community compositions (see Meadows et al., 2015; Pérez-García et al., 2019) occur when benthic systems are perturbed, and the severity of the perturbations modify the benthic communities, as a factor of both the novelty of the disturbance type and the nematode taxa present (Schratzberger and Warwick, 1998). Early work by Warwick et al. (1988) demonstrated that nematode responses to pollutants may be genera- or species-specific, illustrating that free-living nematode indicator ability is present at different taxonomic resolutions.
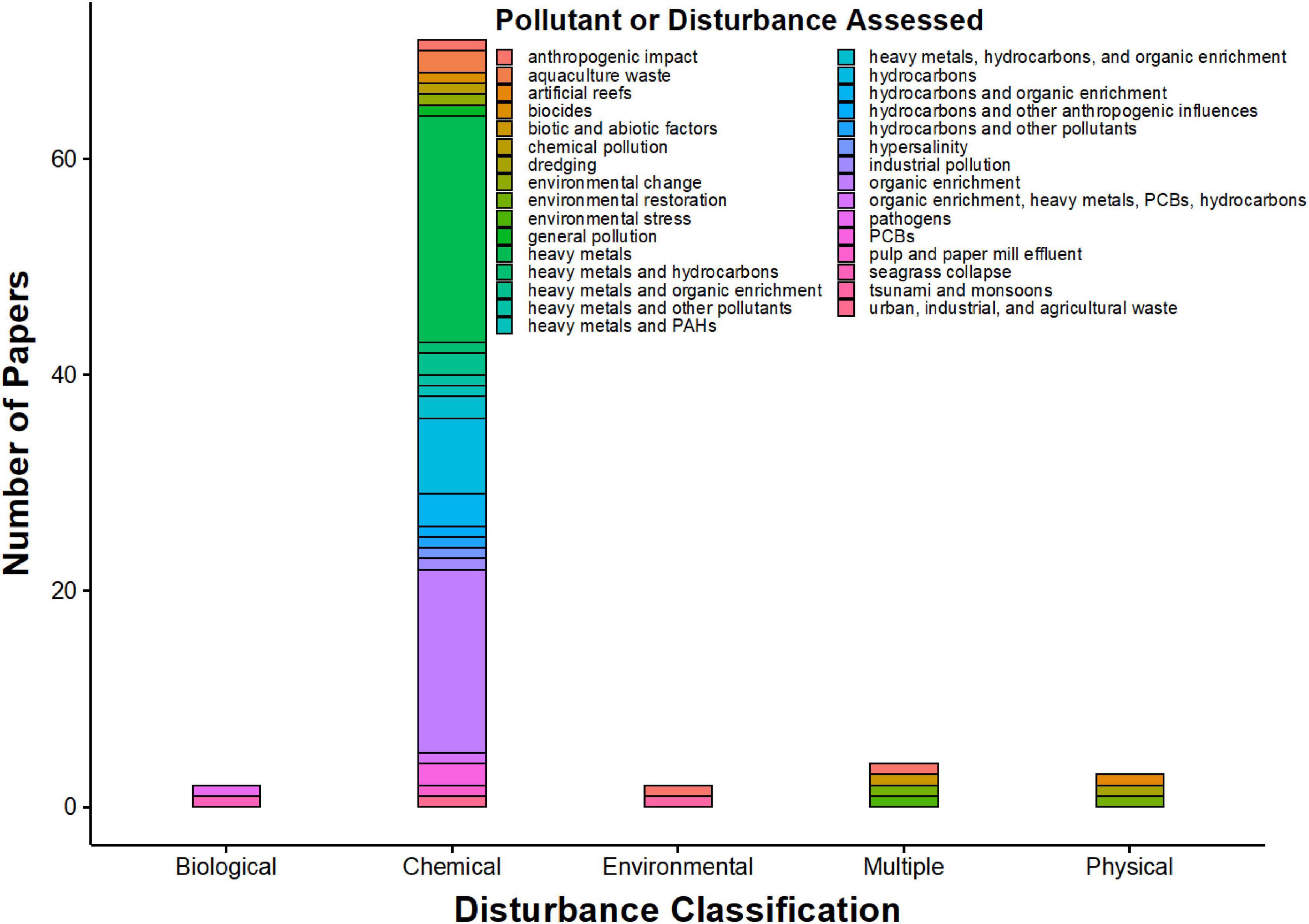
Figure 2. Frequency of disturbance classifications and disturbances assessed in marine nematode bioindication literature from 1980-October 2020 based on an ISI Web of Knowledge search using the terms “marine AND nematod* AND bioindic*.” Papers were only considered if they explicitly featured nematodes as bioindicators or if they were reviews on nematode bioindication. Most of the papers addressed a combination of heavy metals and other pollutants as well as a combination of hydrocarbons and other pollutants.
Biological Indicators Must Be Abundant and Common, and Present in Undisturbed Areas
The number of global marine nematode species has been estimated between about 50,000 and one million or more (Poinar Jr, 1983; Lambshead, 2004; Appeltans et al., 2012). This would mean that at the upper end of the estimation, about 97% of the marine nematode species are yet unidentified or undiscovered. Nematodes also comprise the largest amount of biomass and production within the meiofauna (Giere, 2009) and in marine sediments, nematode density can be more than 10 million individuals/m2 (Giere, 2009; Soetaert et al., 2009). Free-living marine nematodes are present in pretty much every viable habitat type on Earth, including all of the deep sea and extreme habitats such as mud volcanoes, hydrothermal vents, hadal trenches, Polar Regions, Oxygen Minimum Zones and hypoxic environments, as well as hypersaline water bodies (Wetzel et al., 2001; Vanreusel et al., 2010; Rose et al., 2015; Sergeeva et al., 2019). In conclusion, free-living nematodes are ubiquitous, which allows for their assessment in the widest range of habitats possible, from pristine to the most disturbed and challenging.
Biological Indicators Must Be Well Studied, Easy, and Inexpensive to Study
Nematodes have been widely studied since the mid-nineteenth century. The World Register of Marine Species reports 6,247 accepted species. As previously stated, 94 primary literature articles have been written on marine nematodes as bioindicators since 1980, and a search using the terms “marine AND nematod∗ NOT parasit∗” on Web of Knowledge returns 5,337 results. Furthermore, marine nematology is a rapidly growing field; between January and October 2020, 298 papers have been published using the terms marine AND nematod∗. Free-living nematodes are not exceptionally easy to study due to their small size and the limited taxonomic expertise in the field of nematology. However, owing to their high abundances in sediments, and presence in each kind of substratum, marine nematodes are potentially easier to sample than macrofauna. Free-living marine nematodes can number five million individuals per square meter (Soetaert et al., 2009) and may represent 50–90% of biomass in shallow sediments (Semprucci and Balsamo, 2014). The high abundance confers a streamlined sampling process and the persistence of nematodes within the top layer of marine sediments, especially with increasing water depth (Soetaert et al., 1997), makes large-scale sampling a cost-effective possibility. Barcoding and next generation sequencing can offer a partial solution to the lack of taxonomic expertise and the requirement of rapid biodiversity assessment using nematodes (Bhadury et al., 2006; Bhadury, 2016), but expansion of DNA libraries to support identification of sequences is required to move beyond operational taxonomic units and diversity numbers and achieve systematic and functional trait data. In addition, new developments in visualization techniques and automated image recognition certainly offers promise in enhancing the speed of identification (Kitahashi et al., 2018). These new advances will greatly enhance more time-efficient and effective assessment of small eukaryotes such as nematodes in the very near future (Macheriotou et al., 2019).
Biological Indicators Must Be Economically or Commercially Important
Researchers have not focused explicitly on the economic value of free-living nematodes, largely because it is challenging to measure nematode importance at the megafauna and macromolecule scale. Papers on nematode contributions to ecosystem goods and services, which include bioturbation and bio-irrigation (Cullen, 1973), nutrient cycling through stimulation of nitrifying and denitrifying microbes (Bonaglia et al., 2014), and energy flow processes (van Oevelen et al., 2006; Rzeznik-Orignac et al., 2008), however, describe ecosystem processes contributed to by nematodes that have socioeconomic benefits (see Schratzberger and Ingels, 2018). The benefits that free-living marine nematodes provide can be separated into two categories: sedimentary processes and trophic processes, both of which are linked to supporting other organisms that may have more tangible economic value, such as in the context of fisheries. Sedimentary processes denote nematode behaviors that affect the physical and biochemical properties of ocean substrate, and include bioturbation, which increases solute exchange (Middelburg and Meysman, 2007) and often results in higher bacterial abundance and activity compared to surrounding sediments (Bird et al., 2000; Papaspyrou et al., 2005). Trophic processes refer to energy exchange dynamics facilitated by marine nematodes. In coastal areas meiofauna can play a dominant role in metazoan energy transfer (Villares and de Ward, 2020) and nematodes represent the largest portion of the meiofaunal biomass, often exceeding macro- or megafaunal biomass per seafloor area (Giere, 2009). Within the marine nematodes traditionally four different buccal structure morphologies are identified (Wieser, 1953), later enhanced by Moens and Vincx (1997) who suggested that nematodes may control multiple energy transfer pathways in benthic systems. A prime example of meiofauna (including nematodes) importance to higher consumers that have direct economic value as human food source, are fish (Gee, 1989; Coull, 1990). However, measuring energy flow and assessing metabolic costs of nematodes can be challenging, and research attempting to quantify meiobenthic energy transfer across trophic levels varies (van Oevelen et al., 2006, 2011a,b; Ingels et al., 2010, 2011) and may not be supported by theoretical assumptions (see Leduc and Probert, 2009) or microbial data (Schuelke et al., 2018). There is still much work to be done to quantify the economic value of marine nematodes or even to achieve a (semi-) quantitative assessment of their contributions to ecosystem processes, functions, and services. However, we posit that the numerous processes and functions of nematodes that lead into essential ecosystem goods and services meet the economic importance standard set by Ward and Jacoby (1992) and Holt and Miller (2010).
Current Status of the Use of Nematodes as Bioindicators
Literature from the past decade on free-living marine nematodes as ecological indicators and their responses to perturbation display distinct, though not surprising, trends. First, the majority of studies on nematodes as ecological indicators have occurred in European and Asian coastal and marine systems, specifically in Italy, Portugal, and China (based on sampling locations in literature analysis; Supplementary Table 1), likely related to the presence of strong meiofauna research groups in those countries. Work has even been completed in Antarctica (Stark et al., 2017, 2020), which suggests the worldwide viability of free-living marine nematodes as ecological indicators. The majority of the literature from the past decade, based on the search criteria, has focused on heavy metal pollution, hydrocarbons, and organic enrichment (Figure 2). Owing to the impact of the Deepwater Horizon oil spill in the Gulf of Mexico in 2010, all studies using nematodes as ecological indicators in North America, post 2010, have assessed the health of the Gulf of Mexico, a total of four studies (Supplementary Table 1). These results suggest much greater potential and applicability of using nematodes as ecological indicators for coastal and marine systems worldwide, although the specific literature search terms may have omitted some relevant studies.
Nematode Indices for Environmental Health Assessments
A multitude of diversity and taxonomic indices or metrics are currently used—to varying degrees—enable the use of free-living nematodes as ecological indicators in marine environments, but the issue of which metric is most appropriate remains debated and subject to further study. While multivariate community structure and traditional diversity indices based on taxonomic classification and abundance [e.g., Shannon diversity, Pielou evenness, etc. cf. Heip et al. (1998)] are recognized as valuable indicators of pollution or other anthropogenic impacts (e.g., Warwick, 1988; Somerfield and Clarke, 1995), changes in taxonomic diversity may modify ecosystem processes that are not accounted for by such indices alone (Bremner et al., 2003). Owing to criticisms of the simplicity and lack of robustness provided by taxonomic-based methods of nematode community structure (Maurer, 2000; Schratzberger et al., 2007) and its relation to environmental health, a number of other metrics are frequently used. A long-standing example is the nematode-copepod ratio, developed by Raffaelli and Mason (1981). Although justifiably criticized by Coull et al. (1981), its use can be demonstrated under certain conditions (Warwick, 1981; Rubal et al., 2009). Another valuable approach is based on the colonizer-persister (c-p) ratio (drawing comparison with r-k strategies in ecology), developed by Bongers (1990), which uses life history characteristics of nematodes and the presence or absence of such fauna to provide information on the environmental health of a system. These ratios were cast in the single-value metric termed the Maturity Index (Bongers, 1990). Bongers et al. (1991) presents that a high proportion of colonizers represents resource availability and/or recent environmental perturbation, whereas a high proportion of persisters represents system stability, food web complexity, and connectivity. Originally designed to be used in terrestrial soil and freshwater systems, the Maturity Index has also been applied successfully in marine and brackish-water environments (Bongers et al., 1991), as an ecological monitoring tool in marine systems (Moreno et al., 2011; Balsamo et al., 2012), and in the assessment of ecological quality status (Semprucci et al., 2018). The Maturity Index has been used recently to describe environmental health in the Mediterranean Sea (Moreno et al., 2008; Semprucci et al., 2013, 2018), the Black Sea (Ürkmez et al., 2014), and the Caribbean Sea (Armenteros et al., 2009). However, the impact of some disturbances, such as those physical in nature, may not be captured accurately by using the Maturity Index, possibly owing to adaptation of nematodes to recurring disturbance (Semprucci et al., 2016). In addition, multiple anthropogenic and environmental factors may obscure interpretation of a single index in the absence of comprehensive information on differential responses to various disturbance types (e.g., Semprucci et al., 2016).
One way to address the variable performance of different metrics is to employ combinations of faunal parameters to evaluate ecological health. For instance, Semprucci et al. (2018) used five parameters, including nematode taxon richness, the Shannon Index, maturity index, and two sets of c–p values, based on guidance from the European Ecological Quality (EcoQ) directive, to evaluate the health along the coast of southern Italy. Furthermore, Balsamo et al. (2012) believe that a combination of taxonomic metrics (presence or absence of specific genera) and diversity indices provide a more well-rounded understanding of the environmental health of an area. As scientists gather more information on the life-history characteristics of marine nematodes from coastal waters to the deep sea, and responses of nematode taxa (individually and in connection with other abiotic and biotic components) to stressors and forms of pollution, we expect further developments and uses of metrics of ecological or environmental quality based on nematodes will become optimized to address a wide range of disturbance and pollution impacts.
Nematodes as Indicators of Oil Spills and Hydrocarbon Pollution
Some of the most frequent uses of nematodes as ecological indicators are to assess the health of coastal and deep-sea benthic systems perturbed by oil spills and hydrocarbon pollution. Egres et al. (2019) showed that nematode communities are more sensitive to PAH pollution than macrobenthic communities. Whether through microcosm experimentation or environmental impact assessments, researchers have shown that nematode taxa and assemblages show predictable trends when encountering crude oil, motor oil, petroleum, or polycyclic aromatic hydrocarbons (PAHs). Nematode sensitivities to oil and PAHs are species-specific, with some species persisting in the presence of the pollutants and others displaying increased mortality (Balsamo et al., 2012; Monteiro et al., 2018). Species belonging to the genera Sabateria, Dorylaimopsis, Cheironchus, Rhabditis, and Sphaerolaimus gracilis, Calamicrolaimus honestus, and Oncholaimus campylocercoides were found to be tolerant to oil or PAH pollution (Elarbaoui et al., 2015; Soto et al., 2017; Allouche et al., 2020), while Odontophora villoti, Parasphaerolaimus paradoxus, Trichotheristus mirabilis, Theristus pertenuis, and members of the family Encheliidae were extremely sensitive (Boufahja et al., 2012; Allouche et al., 2020). The other notable trend in literature is that nematode assemblages can recover from oil and hydrocarbon perturbation events. Two years after the Deepwater Horizon oil spill in the Gulf of Mexico, nematode assemblages increased in the number of genera by approximately 150% (Soto et al., 2017), and Stark et al. (2017) found that nematodes in polluted Antarctic sediments showed functional recovery in terms of increased trophic diversity and maturity index scores 5 years after hydrocarbon pollution. Nematode assemblage shifts due to oil and hydrocarbon pollution and recovery after the perturbations suggest that nematodes can be used as effective ecological indicators of these disturbances.
Nematodes as Indicators of Organic Enrichment
Other works on the effects of xenobiotics and organic pollutants on benthic communities can be placed into three general categories: organic enrichment and sewage wastes, aquaculture wastes, and heavy metal pollutants. Of the three categories, the effects of organic enrichment and sewage pollutants on nematode communities are the least consistent. While some works have found that organic enrichment increases nematode abundances (Dal Zotto et al., 2016; Soltwedel et al., 2018), there is evidence to suggest that nematode responses to organic pollutants may not be consistent (see Bertocci et al., 2019). Results from environmental monitoring works even suggest that organic enrichment and sewage sludge are too broad for categorizing environmental pollutants, as individual components of organic enrichment or sewage pollutants lead to unique responses in nematode assemblages (see Xu et al., 2014; Caswell et al., 2018; Kandratavicius et al., 2018; Sahraeian et al., 2020). Therefore, it may be challenging to assert specific conclusions about the ecological quality status of an estuary or bay using standard nematode community metrics. Instead, researchers suggest that functional metrics, such as the index of trophic diversity (Kandratavicius et al., 2018) or biomass spectra measures (Losi et al., 2013), may be more appropriate to assess the effects of organic enrichment in marine systems in some cases as taxonomic patterns may not always be consistent (Sahraeian et al., 2020).
Nematodes as Indicators of Aquaculture Pollution
Aquaculture practices across the world introduce excess nitrogen and phosphorus to local ecosystems, leading to eutrophication and oxygen depletion (Cole et al., 2009). The release of excess nitrogen to waterways is often well-documented due to large algal blooms in coastal areas, but current aquaculture practices also generate waste through the introduction of medications and cleaning chemicals to the fish farms, as well as through inconsistent removal of animal waste products that can affect the surrounding environment (Dauda et al., 2019). However, the effects of aquaculture waste in benthic systems are dependent on the type of farming, and the accuracy of assessment depends on the organisms being studied and used as indicators. Della Patrona et al. (2016) postulated that the abundance of phytoplankton within shrimp farm effluent may promote epistrate-feeding nematode abundance, and work done by Riera et al. (2012) and Mirto et al. (2014) found that nematode abundances increased in sediments below fish cages. However, work by Mirto et al. (2010) in the Mediterranean found that meiofaunal abundances may increase or decrease based on the site and farm characteristics, which was later supported by Mirto et al. (2012). Nematode abundance has also shown no change due to aquaculture perturbance in other areas of the world (see Venekey and de Melo, 2016). Research has suggested, however, that shifts in nematode assemblage compositions may be more informative than generalized abundance data. Two separate works found that nematode communities shifted from being dominated by long-lived, slow growing genera to dominance of short-lived genera with fast generation times in response to aquaculture wastes (Mirto et al., 2014; Lacoste et al., 2020).
Nematodes as Indicators of Heavy Metal Pollution
Heavy metal pollution sourced from sewage and industrial wastes or through offshore drilling activities can leach into sediments and affect the meiobenthos. Recent works have reported that not all metal pollution affects nematodes equally. Boufahja et al. (2011) found that increased chromium concentrations in sediments increased the prevalence of four nematode species, while eliminating a fifth species. Similarly, Nasira et al. (2010) demonstrated that high levels of mercury in the Arabian sea led to the highest nematode abundance among sampling sites. Large-scale works assessing nematode community compositions influenced by multiple metal pollutants have shown that shifting meiobenthic compositions may indicate the presence of metal-tolerant as well as metal-intolerant nematode species (Bastami et al., 2017; Stark et al., 2020). In general, studies on how metal pollution affects nematode assemblage composition report lower species diversity and lower abundances of community members and suggest the need for future work to assess which species are tolerant to specific heavy metals (Nasira et al., 2010; Sedano et al., 2014; Bastami et al., 2017).
Nematodes as Indicators of Physical Disturbances
Although much of the bioindication literature based on free-living nematodes has focused on xenobiotics in the marine environment, there are good examples of scientists assessing marine nematode responses to physical disturbances. Notably, multiple microcosm studies, field experiments, and literature analyses have been conducted on the topic of nematode responses to physical disturbance (see Schratzberger and Warwick, 1998; Schratzberger et al., 2009; Schratzberger and Somerfield, 2020) and include work that demonstrates that benthic trawling frequency affects nematode community structure (Schratzberger and Jennings, 2002). Two recent works have assessed changes in the meiobenthos in response to the presence of artificial reefs (Semprucci et al., 2017; Yang et al., 2019), and there is a wealth of literature on the effects of urbanization on the benthos (see Felix et al., 2016; Pereira et al., 2018; Muresan et al., 2019). The construction of artificial reefs, though done with conservation purposes in mind, can be detrimental to meiofauna community structure. Semprucci et al. (2017) found that artificial reefs were the lead contributors to differences in environmental quality between sites, and similarly, Yang et al. (2019) found that artificial reef presence modified hydrodynamics of the surrounding area and disturbed sediment structure, which is the major driver of nematode community assemblages in the marine environment (Hong et al., 2020). Works assessing the effects of urbanization of coastal areas show that coastal construction causes decreases in nematode densities due to increased organic enrichment (Carugati et al., 2018), heavy metal pollution (Yen et al., 2020), and altered sediment grain size and topography (Pereira et al., 2018; Muresan et al., 2019). In many of these studies, however, maturity indices were not calculated, even though the maturity index has been identified as an effective metric in determining human impacts on marine systems (Hong et al., 2020).
Environmental disturbances relating to wave activity and storm systems, as well as physical modifications to the marine environment and their effects on meiobenthos have also been investigated in the past decade or so. Most of the bioindication literature on the effects of environmental disturbances on nematode assemblages concerns the large storm systems and wave activities, in part because of how sedimentation and hydrology drive nematode assemblage composition. Works on the effect of monsoons and associated climate events have shown that nematode community structures are well-adapted to physical stressors in the environment (Semprucci et al., 2016). Nematode assemblages were dominated by a combination of colonizing and persisting genera during the early and late monsoon phases (Ghosh et al., 2018), suggesting that physical environmental perturbation may not be a strong driving factor in structuring nematode assemblages. The low effect size of physical impacts on nematode community structure is supported by others who found that physical modifications to ecosystems did little to modify nematode assemblages; rather, the secondary effects of physical disturbances, including the amount of organic matter, nitrate availability, and food quality, play a more prominent role in determining nematode community composition (Sabeel and Vanreusel, 2015; Branco et al., 2018).
Nematodes as Indicators of Biological Changes in Communities
Few works in the past decade have assessed the effect of megaflora loss or gain on nematode community structure. Lack of literature on the effect of invasive species on free-living nematodes can be attributed to the nature of invasive species research. As outlined by Hanley and Roberts (2019), research on invasive species often supports an economic rationale. Research on the effect of invasive species does not support a singular effect of invasive plants and algae on nematodes; Chen et al. (2015) found that Spartina alterniflora (an invasive seagrass) presence in salt marshes in the Yangtze River estuary increased epifaunal (incl. nematodes) abundance five-fold compared to native seagrass presence. Cvitković et al. (2017), however, reported that there was no difference in meiofauna composition in the presence of the invasive alga Caulerpa taxifolia compared to the native seagrass Posidonia oceanica, but that meiofauna density (mostly nematodes; 50–80%) was ten times higher in the native seagrass communities than in the invasive algae communities. Both works did claim that the changes to the nematode and meiofaunal communities are more likely due to changes in abiotic factors caused by the presence or absence of different flora in the area.
Research on the effects of megaflora collapse in the marine environment on meiobenthic community composition is also sparse. Two separate works have been conducted on the effects of Zostera noltii, a native seagrass to the coast of Western Europe. Materatski et al. (2015) assessed shifts in nematode assemblages prior to and following the collapse of Z. noltii beds in Portugal. The authors found that there was a higher nematode density in assemblages before the collapse, but the assemblages were more diverse after the seagrass beds collapsed. Work assessing the potential of nematode biomass and morphometric attributes as ecological indicator measures in the face of Z. noltii collapse also found that while nematode communities were very similar, they were denser before the seagrass beds collapsed, but exhibited greater biomass after the collapse (Materatski et al., 2018). Recalculation of the maturity index based on 75% of the nematode community suggests that environmental health in the estuary did not differ after seagrass collapse and may have been low to start with.
Specific Nematode Responses to Different Pollution Sources and Disturbances
Across studies, investigations have shown that while nematode community structure shifts are an effective indicator of pollution or disturbance in marine systems, the responsible underlying taxa responses (usually genera or species) can be very specific depending on the source of pollution or disturbance. For instance, certain metals may evoke a species-specific response [e.g., Araeolaimus bioculatus is sensitive to Hg, while Prochromadorella neapolitana is resistant to Hg (Hermi et al., 2009)]. To explore this phenomenon further, we assessed across literature how nematode taxa were observed to be tolerant or sensitive to specific types of disturbance in marine systems.
Our investigation highlighted that many marine nematode species and genera (91) have been put forward as indicative of a stress response or resistance to environmentally damaging factors (Figure 1). The two most tolerant genera in our analysis were Sabatieria and Daptonema. For Sabatieria, four species were mentioned in the literature, Sabatieria breviseta, Sabatieria longisetosa, Sabatieria punctata, and Sabatieria pulchra. Other mentions were either at the genus level or as Sabatieria morphospecies. In the case of Daptonema, Daptonema normandicum, Daptonema tenuispiculum, Daptonema longiseta, and Daptonema fallax were mentioned, or identification was limited to morphospecies or to genus level. Within the top 10 of tolerant genera, we further find Terschellingia, Marylynnia, Axonolaimus, Tripyloides, Ptycholaimellus, Molgolaimus, Dorylaimopsis, and Eleutherolaimus. The list of sensitive genera is led by Pomponema, Halalaimus, Setosabatieria, Paracanthonchus, Richtersia, Desmoscolex, and Xyala.
To better understand which types of pollution and other disturbances were reported most often in the context of resistant or sensitive nematode taxa, we ranked them accordingly. The four disturbances that yielded most mentions of sensitive taxa were the combinations of hydrocarbons and heavy metals (Cr, Cu, and Ni) and more general organic enrichment and chemical pollution. In the context of tolerant genera being mentioned most often, harbor pollution, organic enrichment, heavy metals (Cu and general), and mariculture were the top-four disturbances.
Future Considerations for the Use of Nematodes in Ecological Assessment
Assessment of Nematodes as Indicators of Microplastics Pollution
Microplastics pollution is a burgeoning topic in studies on anthropogenic impacts on the marine environment and researchers estimate that there may be 14.4 million metric tons of microparticles in the top 9 cm of ocean sediments (Barrett et al., 2020). Currently, marine nematodes are not used as indicators of plastics pollution, but scientists are conducting laboratory microcosm studies and feeding experiments to assess how micro- and nanoplastics may modify terrestrial nematode life history traits. Recent work by Lei et al. (2018) found that 1.0-μm polystyrene particles caused strong lethality in nematodes and that microplastic particles also decreased nematode brood size and embryo number. Microplastics are of special concern in the marine environment due to their ability to accumulate xenobiotics (Müller et al., 2018; Wang et al., 2018; Xu et al., 2018), and to act as substrate for bacterial aggregations (Arias-Andres et al., 2018). Antibiotics have also been found to adsorb to microplastics, although physical modifications to the plastic particles and environmental factors may influence the sorption pathways (Li et al., 2018). We currently have little information on how such modifications may occur in the benthic environment, through exposure, degradation or via trophic pathways. Therefore, we propose the use of nematodes as indicators of new, previously unassessed pollutants in the ocean, such as microplastics.
Plastics pollution may affect mankind’s ability to conserve biodiversity in the near future (Sutherland et al., 2010) and is now considered an indicator of the Anthropocene (Duis and Coors, 2016; Zalasiewicz et al., 2016). Villarrubia-Gómez et al. (2018) present that plastics pollution is also irreversible and ubiquitous across the world’s oceans, meeting two of the three conditions for a chemical pollution planetary boundary threat. Therefore, assessing the effects of microplastics pollution on meiofauna that may comprise key species in ecological processes is necessary to determine conservation needs. We suggest two different lines of inquiry are investigated to assess the effects of microplastics on free-living nematode health and behavior: the first is an assessment of how microplastics affect nematode vitality and reproductive behaviors. Work done by Fueser et al. (2019) found that some deposit-feeding nematodes will ingest microplastics and that most of the consumed particles will aggregate in the intestine. Recent work conducted by Shang et al. (2020) also showed that Caenorhabditis elegans life span decreased by 52.4% when exposed to microplastics for 10 days, as compared to the control group. However, it is important to note that the lowest concentrations of microplastics used in both the Fueser et al. (2019) and the Shang et al. (2020) studies may not be reflective of in situ microplastic abundances. A comprehensive study of the microplastic concentrations in wastewater effluent in the United States found that, on average, a wastewater treatment plant releases 1.53 × 106 microparticles/m2 (Mason et al., 2016), which is 50% less than the concentration used by Fueser et al. (2019) and almost 94% less than the concentration used by Shang et al. (2020).
The second investigation path should assess how microplastics pollution affects nematode community composition. As observed with most chemical pollutants, nematode community composition changes in response to xenobiotics in benthic systems, shifting from communities dominated by genera with slow reproductive cycles and long life-histories to communities dominated by colonizer genera and opportunists. We expect that nematode community responses to microplastics pollution are likely species-specific due to buccal cavity morphologies. Fueser et al. (2019) demonstrated that nematodes with buccal cavities smaller than microplastic particles did not ingest the particles, suggesting that there is a size-structured effect to how microplastics may affect nematodes. Species-specific effects of microplastics on nematodes have also been demonstrated by Fueser et al. (2020) and Mueller et al. (2020). However, there is very limited information on in situ relationships between microplastic concentrations and meiofauna diversity (see Haegerbaeumer et al., 2019 for review), nor are there studies—modeling or otherwise—on how microplastic particles elicit changes in the species diversity of an ecosystem.
As marine nematode community compositions and colonizer-persister ratios have been effective indicators of other xenobiotic pollution in the ocean, they may be successful in detecting impact at potential microplastics hotspots such as near wastewater treatment plants. Research that quantifies microparticles concentrations in such areas and investigates the influence these have on indicators species is urgently needed. Although an argument can be made that nematode community composition data in polluted environments may be biased by confounding pollutants or nutrients, mesocosm studies in pristine areas where the scientist adds known concentrations of microplastics may provide a complementary, more rigorous data set.
The Use of Singular Nematode Indices for All Classes of Pollutants
The status of nematodes as ecological indicators, and the detailed response reports of sensitivity or resistance to environmental disturbances, suggests the need for global environmental health management standards, and widespread guidelines for appropriate ecological indicator metrics that include nematodes. Rather than treating nematode indices as holistically appropriate metrics, indices should be assessed individually to determine which class of environmental pollutant or disturbance the index is best suited for. Validation on whether a holistic and standardized nematode bioindicator approach is suitable for all disturbance types is needed. A recent example of this issue is presented by Sahraeian et al. (2020), whereby the maturity index was found to perform better when detecting organic pollution compared to polychlorinated biphenyls. It is also necessary to determine which classes of pollutants can be addressed simultaneously, since a combination of physical, chemical, and biological perturbations likely interact in a marine affected area (Cabral J.S. et al., 2019).
The Need for Improved International Collaborations in the Field of Marine Nematology
Studies that specifically use marine nematodes as ecological indicators are rather limited in geographic scope. European nations and Asian countries seem at the forefront of publishing research focusing on nematodes as bioindicators, while relatively much less work in this specific field has been conducted in Australia and New Zealand, Africa, North America, along the western coast of South America, and along the northern coast of Eurasia (Figure 3). It is of course important to be reminded that these results are based on the search terms used. We also note that geographical representation does not reflect other meiofauna and nematode research, neither in terms of quantity nor quality, nor does it necessarily reflect the use of meiofauna or nematodes in research that generally addresses environmental impacts of pollution—the limitations of our focus on nematode bioindicator research must be considered. The generally low cost associated with nematode sampling and the increasing availability of taxonomic identification resources, next generation sequencing techniques and automated recognition technologies, support larger-scale environmental health assessments. International partnerships between nations should be encouraged and facilitated, particularly since few governmental, environmental management frameworks support a collaborative and holistic approach to marine conservation and sustainability, such as the European Union’s Marine Strategy Framework Directive. Due to the global presence of anthropogenic pollution and the wide geographic ranges of nematode taxa, international collaboration is essential in understanding the effects of pollutants on the environment and recognizing the responses of nematode communities to such threats. Future efforts could, therefore, focus on international knowledge exchange and multinational workshops or courses in nematology, especially with regards to taxonomy of free-living nematodes, identification and classification techniques, as well as ways to help identify impacts through indicators metrics. The current lack of wide-spread expertise, and/or incentive to use nematodes as indicator organisms in marine systems could be a contributing factor to the lack of such focused research efforts in Australia, Africa, and North America. We therefore suggest international partnerships and collaborations that spread knowledge of the nematode taxa beyond researchers’ local study systems, and joint efforts in environmental monitoring schemes. In addition, moving beyond the research questions sensu stricto and raising awareness in science, but also in environmental and conservation management circles, about the use of nematodes as efficient ecological indicator organisms may enhance national and international efforts in this field. We acknowledge that groups like the International Association of Meiobenthologists support such information sharing, but meiobenthology covers an extensive field of researchers, not limited to nematologists.
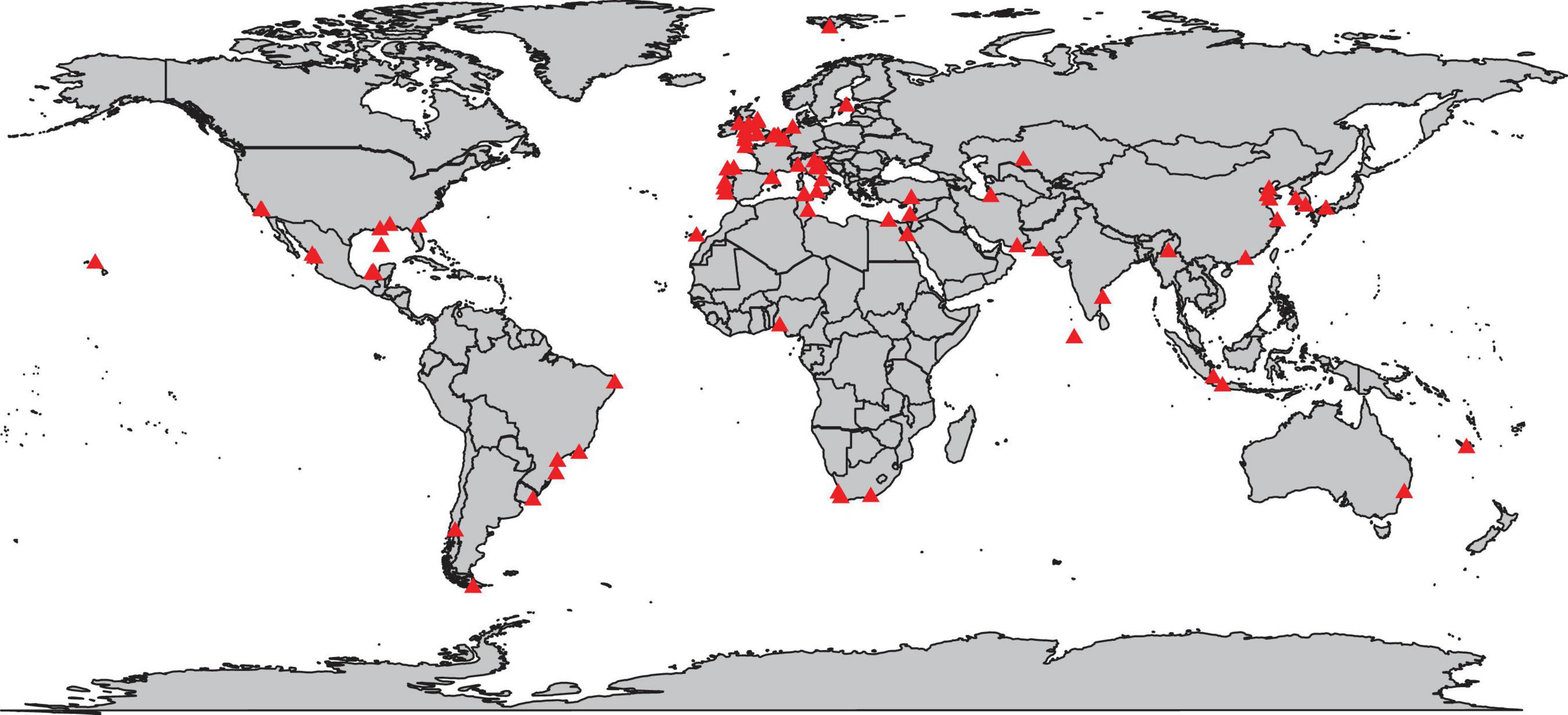
Figure 3. Geographic distribution of marine nematode bioindication studies conducted between 1980 and October 2020. Individual points are based on the geographic space, rather than the number of studies conducted in the associated waterway.
Conclusion
There are some counterarguments to consider using nematodes as widespread ecological indicators. Firstly, there is a relative paucity of genomics work or metabarcoding conducted on marine nematodes compared to nematodes in an agricultural or medical context. Instead, most studies identify nematodes based on morphological data and life history characteristics (Derycke et al., 2013). There is a relatively even divide between the number of described terrestrial species (15,600) and the number of described marine species (11,400) (Hugot et al., 2001; Appeltans et al., 2012; Holterman et al., 2019), which suggests a drastically underexplored and currently undervalued potential of free-living marine clades considering estimations of the total number of marine species; most remain unknown or undiscovered. Avó et al. (2017) described the need for the development of a robust database of genomics for the nematode phylum and the importance of molecular techniques in assessments of marine health, but the size of the nematode phylum makes the completion of such a database untimely. Current research using nematodes as ecological indicators in marine systems have sometimes addressed the lack of information by identifying nematodes at higher taxonomic levels and relying on characteristics that are descriptive of families and genera to make determinations about environmental health; an approach that has been validated for pollution effects on marine benthic communities (Warwick, 1988).
Coupled with the lack of genomic information on the phylum is an absence of literature on individual nematode species. Ecological indication studies with nematodes focus on generalities of higher taxonomic levels, which may overlook subtle differences that exist at the species level. This can be deducted from our analysis as well, where different species within the same genus have been observed to respond differently to either the same or different types of pollution. Given current estimates that at least 10,000 species of free-living marine nematodes (and potentially 100,000s) have not yet been discovered (Appeltans et al., 2012; Holterman et al., 2019), the severe underestimation of the potential numbers of free-living marine nematodes, and the large discrepancies in estimates of the size of the phylum (Appeltans et al., 2012; Semprucci and Balsamo, 2012), more work needs to be done on the discovery of marine nematodes so that ecological indication results and interpretations can be provided with more certainty.
A third challenge we recognize with nematode-based bioindication research is the limited number of experts in marine nematology. The lack of expertise in the field could be attributed to two factors: (1) The large number of estimated marine nematode species and the presumed difficulty of identification, creates a misinformed threshold that limits involvement from students and researchers, feeding the taxonomic impediment, and (2) The relative lack of current research on free-living nematodes as a result of more focus on larger organisms. The latter suggests more work and resources are partitioned away from the study of the marine meiofauna. A notable improvement in marine nematode expertise has been the development and integration of the World Database of Free-Living Marine Nematodes (NeMys) into the World Register of Marine Species (WoRMS), which provides open access to field guides, taxonomy resources, and relevant literature. However, there is still a need for more global expertise in the field and strengthened international collaborative efforts to further the spread of information about the importance of nematodes and the services they can and do provide.
There is clearly more work to be done before free-living marine nematodes are appropriately valued and used as ubiquitous biological indicators, but current evidence suggests that nematodes have and continue to show characteristic and identifiable responses to perturbations in the marine environment. Although there are some challenges to overcome, increasing the use of nematodes as biological indicators in marine systems will likely start by increasing the number of researchers, laboratories, and programs worldwide that use nematodes as focal fauna and increasing expertise in nematode taxonomy, biology, and ecology in the diverse fields of marine sciences.
Author Contributions
AR and JI conceived the idea for this study. AR and JI wrote and revised the manuscript. AR conducted the literature search and summarized the findings, while JI conducted the calculation of nematode species/genus responses to different disturbance types. Both authors approved the submitted and revised versions.
Funding
AR acknowledges support from the PADI Foundation, Friends of Gumbo-Limbo Nature Center, and the William R. and Lenore MOTE Eminent Scholar in Marine Biology Research Assistantship to study the effects of microplastics on nematode communities and the potential use of nematodes as indicators of microplastics pollution, which has helped develop some of the ideas for this paper. JI acknowledges partial support from NOAA OER (Grant Nr. NA180AR0110285) to study the use of meiofauna and nematodes as indicators of disturbance, which has helped formulate some of the information of this manuscript.
Conflict of Interest
The authors declare that the research was conducted in the absence of any commercial or financial relationships that could be construed as a potential conflict of interest.
Acknowledgments
We gratefully acknowledge Federica Semprucci for helpful comments and discussion on earlier versions of the manuscript. We thank the reviewers and the editor, XL, for their helpful comments and suggestions.
Supplementary Material
The Supplementary Material for this article can be found online at: https://www.frontiersin.org/articles/10.3389/fmars.2021.685327/full#supplementary-material
References
Allouche, M., Nasri, A., Harrath, A. H., Mansour, L., Beyrem, H., and Boufahja, F. (2020). Migratory behavior of free-living marine nematodes surrounded by sediments experimentally contaminated by mixtures of polycyclic aromatic hydrocarbons. J. King Saud Univ. 32, 1339–1345. doi: 10.1016/j.jksus.2019.11.025
Appeltans, W., Ahyong, S. T., Anderson, G., Angel, M. V., Artois, T., Bailly, N., et al. (2012). The magnitude of global marine species diversity. Curr. Biol. 22, 2189–2202. doi: 10.1016/j.cub.2012.09.036
Arias-Andres, M., Klümper, U., Rojas-Jimenez, K., and Grossart, H. P. (2018). Microplastic pollution increases gene exchange in aquatic ecosystems. Environ. Pollut. 237, 253–261. doi: 10.1016/j.envpol.2018.02.058
Armenteros, M., Ruiz-Abierno, A., Fernández-Garcés, R., Pérez-García, J. A., Díaz-Asencio, L., Vincx, M., et al. (2009). Biodiversity patterns of free-living marine nematodes in a tropical bay: cienfuegos, Caribbean Sea. Estuar. Coast. Shelf Sci. 85, 179–189. doi: 10.1016/j.ecss.2009.08.002
Avó, A. P., Daniell, T. J., Neilson, R., Oliveira, S., Branco, J., and Adão, H. (2017). DNA barcoding and morphological identification of benthic nematodes assemblages of estuarine intertidal sediments: advances in molecular tools for biodiversity assessment. Front. Mar. Sci. 4:66. doi: 10.3389/fmars.2017.00066
Balsamo, M., Semprucci, F., Frontalini, F., and Coccioni, R. (2012). “Meiofauna as a tool for marine ecosystem biomonitoring,” in Marine Ecosystem, ed. A. Cruzado (London: INTECH).
Barrett, J., Chase, Z., Zhang, J., Holl, M. M. B., Willis, K., and Williams et al. (2020). Microplastic pollution in deep-sea sediments from the Great Australian Bight. Front. Mar. Sci. 7:576170. doi: 10.3389/fmars.2020.576170
Bastami, K. D., Taheri, M., Foshtomi, M. Y., Haghparast, S., Hamzehpour, A., Bagheri, H., et al. (2017). Nematode community structure in relation to metals in the southern of Caspian Sea. Acta Oceanol. Sin. 36, 79–86. doi: 10.1007/s13131-017-1051-x
Bertocci, I., Dell’Anno, A., Musco, L., Gambi, C., Saggiomo, V., Cannavacciuolo, M., et al. (2019). Multiple human pressures in coastal habitats: variation of meiofaunal assemblages associated with sewage discharge in a post-industrial area. Sci. Total Environ. 655, 1218–1231. doi: 10.1016/j.scitotenv.2018.11.121
Bhadury, P. (2016). “DNA barcoding in marine nematodes: successes and pitfalls BT,” in DNA Barcoding in Marine Perspectives: Assessment and Conservation of Biodiversity, eds S. Trivedi, A. A. Ansari, S. K. Ghosh, and H. Rehman (Cham: Springer), 131–145. doi: 10.1007/978-3-319-41840-7_8
Bhadury, P., Austen, M. C., Bilton, D. T., Lambshead, P. J. D., and Rogers, A. D. (2006). Development and evaluation of a DNA-barcoding approach for the rapid identification of nematodes. Mar. Ecol. Prog. Ser. 320, 1–9. doi: 10.3354/meps320001
Bird, F. L., Boon, P. I., and Nichols, P. D. (2000). Physicochemical and microbial properties of burrows of the deposit-feeding thalassinidean ghost shrimp Biffarius arenosus (Decapoda: Callianassidae). Estuar. Coast. Shelf Sci. 51, 279–291. doi: 10.1006/ecss.2000.0676
Bonaglia, S., Nascimento, F. J. A., Bartoli, M., Klawonn, I., and Brüchert, V. (2014). Meiofauna increases bacterial denitrification in marine sediments. Nat. Commun. 5:5133. doi: 10.1038/ncomms6133
Bongers, T. (1990). The maturity index: an ecological measure of environmental disturbance based on nematode species composition. Oecologia 83, 14–19. doi: 10.1007/BF00324627
Bongers, T., Alkemade, R., and Yeates, G. W. (1991). Interpretation of disturbance-induced maturity decrease in marine nematode assemblages by means of the Maturity Index. Mar. Ecol. Prog. Ser. 76, 135–142. doi: 10.3354/meps076135
Borja, Á (2014). Grand challenges in marine ecosystems ecology. Front. Mar. Sci. 1:1. doi: 10.3389/fmars.2014.00001
Borja, Á, Dauer, D. M., and Grémare, A. (2012). The importance of setting targets and reference conditions in assessing marine ecosystem quality. Ecol. Indic. 12, 1–7. doi: 10.1016/j.ecolind.2011.06.018
Borja, Á, Elliott, M., Andersen, J. H., Cardoso, A. C., Carstensen, J., Ferreira, J. G., et al. (2013). Good Environmental Status of marine ecosystems: what is it and how do we know when we have attained it? Mar. Pollut. Bull. 76, 16–27. doi: 10.1016/j.marpolbul.2013.08.042
Borja, Á, Franco, J., and Pérez, V. (2000). A marine biotic index to establish the ecological quality of soft bottom benthos within European estuarine and coastal environments. Mar. Pollut. Bull. 40, 1100–1114. doi: 10.1016/s0025-326x(00)00061-8
Boufahja, F., Hedfi, A., Amorri, J., Aïssa, P., Beyrem, H., and Mahmoudi, E. (2011). An assessment of the impact of chromium-amended sediment on a marine nematode assemblage using microcosm bioassays. Biol. Trace Elem. Res. 142, 242–255. doi: 10.1007/s12011-010-8762-6
Boufahja, F., Hedfi, A., Essid, N., Aïssa, P., Mahmoudi, E., and Beyrem, H. (2012). An observational study on changes in biometry and generation time of Odontophora villoti (Nematoda, Axonolaimidae) related to petroleum pollution in Bizerte bay, Tunisia. Environ. Sci. Pollut. Res. 19, 646–655. doi: 10.1007/s11356-011-0609-y
Branco, J., Pedro, S., Alves, A. S., Ribeiro, C., Materatski, P., Pires, R., et al. (2018). Natural recovery of Zostera noltii seagrass beds and benthic nematode assemblage responses to physical disturbance caused by traditional harvesting activities. J. Exp. Mar. Bio. Ecol. 502, 191–202. doi: 10.1016/j.jembe.2017.03.003
Bremner, J., Rogers, S. I., and Frid, C. L. J. (2003). Assessing functional diversity in marine benthic ecosystems: a comparison of approaches. Mar. Ecol. Prog. Ser. 254, 11–25. doi: 10.3354/meps254011
Cabral, H., Fonseca, V., Sousa, T., and Costa Leal, M. (2019). Synergistic effects of climate change and marine pollution: an overlooked interaction in Coastal and Estuarine Areas. Int. J. Environ. Res. Public Health 16:2737. doi: 10.3390/ijerph16152737
Cabral, J. S., Whittaker, R. J., Wiegand, K., and Kreft, H. (2019). Assessing predicted isolation effects from the general dynamic model of island biogeography with an eco-evolutionary model for plants. J. Biogeogr. 46, 1569–1581.
Carugati, L., Martire, M. L., Gambi, C., and Danovaro, R. (2018). Impact of breakwater relocation on benthic biodiversity associated with seagrass meadows of northern Adriatic Sea. Rend. Lincei. Sci. Fis. e Nat. 29, 571–581. doi: 10.1007/s12210-018-0720-9
Caswell, B. A., Paine, M., and Frid, C. L. J. (2018). Seafloor ecological functioning over two decades of organic enrichment. Mar. Pollut. Bull. 136, 212–229. doi: 10.1016/j.marpolbul.2018.08.041
Chen, H., Zhang, P., Li, B., and Wu, J. (2015). Invasive cordgrass facilitates epifaunal communities in a Chinese marsh. Biol. Invasions 17, 205–217. doi: 10.1007/s10530-014-0720-3
Claudet, J., and Fraschetti, S. (2010). Human-driven impacts on marine habitats: a regional meta-analysis in the Mediterranean Sea. Biol. Conserv. 143, 2195–2206. doi: 10.1016/j.biocon.2010.06.004
Cole, D. W., Cole, R., Gaydos, S. J., Gray, J., Hyland, G., Jacques, M. L., et al. (2009). Aquaculture: environmental, toxicological, and health issues. Int. J. Hyg. Environ. Health 212, 369–377. doi: 10.1016/j.ijheh.2008.08.003
Coull, B. C. (1990). Are members of the meiofauna food for higher trophic levels? Trans. Am. Microsc. Soc. 3, 233–246. doi: 10.2307/3226794
Coull, B. C., Hicks, G. R. F., and Wells, J. B. J. (1981). Nematode/copepod ratios for monitoring pollution: a rebuttal. Mar. Pollut. Bull. 12, 378–381. doi: 10.1016/0025-326x(81)90408-2
Cullen, D. J. (1973). Bioturbation of superficial marine sediments by interstitial meiobenthos. Nature 242, 323–324. doi: 10.1038/242323a0
Cvitković, I., Despalatović, M., Žuljević, A., Matijević, S., Bogner, D., Lušić, J., et al. (2017). Structure of epibiontic and sediment meiofauna in the area invaded by invasive alga Caulerpa taxifolia. Mar. Biol. 164, 1–15.
Dal Zotto, M., Santulli, A., Simonini, R., and Todaro, M. A. (2016). Organic enrichment effects on a marine meiofauna community, with focus on Kinorhyncha. Zool. Anzeiger-A J. Comp. Zool. 265, 127–140. doi: 10.1016/j.jcz.2016.03.013
Danovaro, R., Fanelli, E., Aguzzi, J., Billett, D., Carugati, L., Corinaldesi, C., et al. (2020). Ecological variables for developing a global deep-ocean monitoring and conservation strategy. Nat. Ecol. Evol. 4, 181–192. doi: 10.1038/s41559-019-1091-z
Dauda, A. B., Ajadi, A., Tola-Fabunmi, A. S., and Akinwole, A. O. (2019). Waste production in aquaculture: sources, components and managements in different culture systems. Aquac. Fish. 4, 81–88. doi: 10.1016/j.aaf.2018.10.002
Della Patrona, L., Marchand, C., Hubas, C., Molnar, N., Deborde, J., and Meziane, T. (2016). Meiofauna distribution in a mangrove forest exposed to shrimp farm effluents (New Caledonia). Mar. Environ. Res. 119, 100–113. doi: 10.1016/j.marenvres.2016.05.028
Denoyelle, M., Jorissen, F. J., Martin, D., Galgani, F., and Miné, J. (2010). Comparison of benthic foraminifera and macrofaunal indicators of the impact of oil-based drill mud disposal. Mar. Pollut. Bull. 60, 2007–2021. doi: 10.1016/j.marpolbul.2010.07.024
Derycke, S., Backeljau, T., and Moens, T. (2013). Dispersal and gene flow in free-living marine nematodes. Front. Zool. 10:1. doi: 10.1186/1742-9994-10-1
Doney, S. C., Ruckelshaus, M., Emmett Duffy, J., Barry, J. P., Chan, F., English, C. A., et al. (2011). Climate change impacts on marine ecosystems. Ann. Rev. Mar. Sci. 4, 11–37. doi: 10.1146/annurev-marine-041911-111611
Duis, K., and Coors, A. (2016). Microplastics in the aquatic and terrestrial environment: sources (with a specific focus on personal care products), fate and effects. Environ. Sci. Eur. 28, 1–25. doi: 10.1186/s12302-015-0069-y
Duplisea, D. E., Jennings, S., Malcolm, S. J., Parker, R., and Sivyer, D. B. (2001). Modelling potential impacts of bottom trawl fisheries on soft sediment biogeochemistry in the North Sea†. Geochem. Trans. 2:112. doi: 10.1186/1467-4866-2-112
Duplisea, D. E., Jennings, S., Randall, K., and Dinmore, T. (2002). A size-based model of the impacts of bottom trawling on benthic community structure. Can. J. Fish. Aquat. Sci. 59, 1785–1795. doi: 10.1139/f02-148
Egres, A. G., Hatje, V., Miranda, D. A., Gallucci, F., and Barros, F. (2019). Functional response of tropical estuarine benthic assemblages to perturbation by polycyclic aromatic hydrocarbons. Ecol. Indic. 96, 229–240. doi: 10.1016/j.ecolind.2018.08.062
Elarbaoui, S., Richard, M., Boufahja, F., Mahmoudi, E., and Thomas-Guyon, H. (2015). Effect of crude oil exposure and dispersant application on meiofauna: an intertidal mesocosm experiment. Environ. Sci. Process. Impacts 17, 997–1004. doi: 10.1039/c5em00051c
Felix, G., Marenzi, R. C., Polette, M., and Netto, S. A. (2016). Landscape visual quality and meiofauna biodiversity on sandy beaches. Environ. Manage. 58, 682–693. doi: 10.1007/s00267-016-0735-x
Foden, J., Rogers, S. I., and Jones, A. P. (2011). Human pressures on UK seabed habitats: a cumulative impact assessment. Mar. Ecol. Prog. Ser. 428, 33–47. doi: 10.3354/meps09064
Fueser, H., Mueller, M.-T., and Traunspurger, W. (2020). Ingestion of microplastics by meiobenthic communities in small-scale microcosm experiments. Sci. Total Environ. 746:141276. doi: 10.1016/j.scitotenv.2020.141276
Fueser, H., Mueller, M. T., Weiss, L., Höss, S., and Traunspurger, W. (2019). Ingestion of microplastics by nematodes depends on feeding strategy and buccal cavity size. Environ. Pollut. 255:e113227. doi: 10.1016/j.envpol.2019.113227
Gee, J. M. (1989). An ecological and economic review of meiofauna as food for fish. Zool. J. Linn. Soc. 96, 243–261. doi: 10.1111/j.1096-3642.1989.tb01830.x
Ghosh, M., Mandal, S., and Chatterjee, M. (2018). Impact of unusual monsoonal rainfall in structuring meiobenthic assemblages at Sundarban estuarine system, India. Ecol. Indic. 94, 139–150. doi: 10.1016/j.ecolind.2018.06.067
Giere, O. (2009). Meiobenthology: The Microscopic Motile Fauna of Aquatic Sediments, 2nd Edn. Berlin: Springer.
Haegerbaeumer, A., Mueller, M.-T., Fueser, H., and Traunspurger, W. (2019). Impacts of micro-and nano-sized plastic particles on benthic invertebrates: a literature review and gap analysis. Front. Environ. Sci. 7:17. doi: 10.3389/fenvs.2019.00017
Halpern, B. S., Walbridge, S., Selkoe, K. A., Kappel, C. V., Micheli, F., D’Agrosa, C., et al. (2008). A global map of human impact on marine ecosystems. Science 319, 948–952.
Hanley, N., and Roberts, M. (2019). The economic benefits of invasive species management. People Nat. 1, 124–137. doi: 10.1002/pan3.31
Harding, L. E. (1992). Measures of marine environmental quality. Mar. Pollut. Bull. 25, 23–27. doi: 10.1016/0025-326x(92)90178-9
Heip, C. H. R., Herman, P. M. J., and Soetaert, K. (1998). Indices of diversity and evenness. Oceanis 24, 61–88.
Hermi, M., Mahmoudi, E., Beyrem, H., Aïssa, P., and Essid, N. (2009). Responses of a free-living marine nematode community to mercury contamination: results from microcosm experiments. Arch. Environ. Contam. Toxicol. 56, 426–433. doi: 10.1007/s00244-008-9217-3
Hodda, M., and Nicholas, W. L. (1986). Nematode diversity and industrial pollution in the Hunter River estuary, NSW, Australia. Mar. Pollut. Bull. 17, 251–255. doi: 10.1016/0025-326X(86)90058-5
Holt, E. A., and Miller, S. W. (2010). Bioindicators: using organisms to measure environmental impacts. Nat. Educ. Knowl. 3:8.
Holt, J., Icarus Allen, J., Anderson, T. R., Brewin, R., Butenschön, M., Harle, J., et al. (2014). Challenges in integrative approaches to modelling the marine ecosystems of the North Atlantic: physics to fish and coasts to ocean. Prog. Oceanogr. 129, 285–313. doi: 10.1016/j.pocean.2014.04.024
Holterman, M., Schratzberger, M., and Helder, J. (2019). Nematodes as evolutionary commuters between marine, freshwater and terrestrial habitats. Biol. J. Linn. Soc. 128, 756–767. doi: 10.1093/biolinnean/blz107
Hong, J.-H., Semprucci, F., Jeong, R., Kim, K., Lee, S., Jeon, D., et al. (2020). Meiobenthic nematodes in the assessment of the relative impact of human activities on coastal marine ecosystem. Environ. Monit. Assess. 192, 1–13.
Hugot, J.-P., Baujard, P., and Morand, S. (2001). Biodiversity in helminths and nematodes as a field of study: an overview. Nematology 3, 199–208. doi: 10.1163/156854101750413270
Ingels, J., Billett, D. S. M., Van Gaever, S., and Vanreusel, A. (2011). An insight into the feeding ecology of deep-sea canyon nematodes — Results from field observations and the first in-situ 13C feeding experiment in the Nazaré Canyon. J. Exp. Mar. Bio. Ecol. 396, 185–193. doi: 10.1016/j.jembe.2010.10.018
Ingels, J., Van den Driessche, P., De Mesel, I., Vanhove, S., Moens, T., and Vanreusel, A. (2010). Preferred use of bacteria over phytoplankton by deep-sea nematodes in polar regions. Mar. Ecol. Prog. Ser. 406, 121–133. doi: 10.3354/meps08535
Ingels, J., Vanreusel, A., Pape, E., Pasotti, F., Macheriotou, L., Arbizu, P. M., et al. (2021). Ecological variables for deep-ocean monitoring must include microbiota and meiofauna for effective conservation. Nat. Ecol. Evol. 5, 27–29. doi: 10.1038/s41559-020-01335-6
Kandratavicius, N., de Ward, C. P., Venturini, N., Giménez, L., Rodriguez, M., and Muniz, P. (2018). Response of estuarine free-living nematode assemblages to organic enrichment: an experimental approach. Mar. Ecol. Prog. Ser. 602, 117–133. doi: 10.3354/meps12699
Kennedy, A. D., and Jacoby, C. A. (1999). Biological indicators of marine environmental health: meiofauna–a neglected benthic component? Environ. Monit. Assess. 54, 47–68.
Kitahashi, T., Watanabe, H. K., Tsuchiya, M., Yamamoto, H., and Yamamoto, H. (2018). A new method for acquiring images of meiobenthic images using the FlowCAM. MethodsX 5, 1330–1335. doi: 10.1016/j.mex.2018.10.012
Lacoste, É, Boufahja, F., Pelaprat, C., Le Gall, P., Berteaux, T., Messiaen, G., et al. (2020). First simultaneous assessment of macro-and meiobenthic community response to juvenile shellfish culture in a Mediterranean coastal lagoon (Thau, France). Ecol. Indic. 115:106462. doi: 10.1016/j.ecolind.2020.106462
Lambshead, P. (1986). Sub-catastrophic sewage and industrial waste contamination as revealed by marine nematode faunal analysis. Mar. Ecol. Prog. Ser. 29, 247–259. doi: 10.3354/meps029247
Lambshead, P. J. D. (2004). “Marine nematode biodiversity,” in Nematology: Advances and Perspectives, eds Z. X. Chen, S. Y. Chen, and D. W. Dickson (Wallingford: CABI Publishing), 436–467.
Leduc, D., and Probert, P. K. (2009). The effect of bacterivorous nematodes on detritus incorporation by macrofaunal detritivores: a study using stable isotope and fatty acid analyses. J. Exp. Mar. Bio. Ecol. 371, 130–139. doi: 10.1016/j.jembe.2009.01.011
Lei, L., Wu, S., Lu, S., Liu, M., Song, Y., Fu, Z., et al. (2018). Microplastic particles cause intestinal damage and other adverse effects in zebrafish Danio rerio and nematode Caenorhabditis elegans. Sci. Total Environ. 619–620, 1–8. doi: 10.1016/j.scitotenv.2017.11.103
Lenihan, H. S., Peterson, C. H., Byers, J. E., Grabowski, J. H., Thayer, G. W., and Colby, D. R. (2001). Cascading of habitat degradation: oyster reefs invaded by refugee fishes escaping stress. Ecol. Appl. 11, 764–782. doi: 10.1890/1051-0761(2001)011[0764:cohdor]2.0.co;2
Li, J., Zhang, K., and Zhang, H. (2018). Adsorption of antibiotics on microplastics. Environ. Pollut. 237, 460–467. doi: 10.1016/j.envpol.2018.02.050
Losi, V., Moreno, M., Gaozza, L., Vezzulli, L., Fabiano, M., and Albertelli, G. (2013). Nematode biomass and allometric attributes as indicators of environmental quality in a Mediterranean harbour (Ligurian Sea, Italy). Ecol. Indic. 30, 80–89. doi: 10.1016/j.ecolind.2013.01.034
Macheriotou, L., Guilini, K., Bezerra, T. N., Tytgat, B., Nguyen, D. T., Phuong Nguyen, T. X., et al. (2019). Metabarcoding free-living marine nematodes using curated 18S and CO1 reference sequence databases for species-level taxonomic assignments. Ecol. Evol. 9, 1211–1226. doi: 10.1002/ece3.4814
Markert, P. D. B., Breure, A., and Zechmeister, H. (2003). Chapter 1 Definitions, strategies and principles for bioindication/biomonitoring of the environment. Trace Met. other Contam. Environ. 6, 3–39. doi: 10.1016/S0927-5215(03)80131-5
Martín, J., Puig, P., Palanques, A., and Ribó, M. (2014). Trawling-induced daily sediment resuspension in the flank of a Mediterranean submarine canyon. Deep Sea Res. Part II Top. Stud. Oceanogr. 104, 174–183. doi: 10.1016/j.dsr2.2013.05.036
Mason, S. A., Garneau, D., Sutton, R., Chu, Y., Ehmann, K., Barnes, J., et al. (2016). Microplastic pollution is widely detected in US municipal wastewater treatment plant effluent. Environ. Pollut. 218, 1045–1054. doi: 10.1016/j.envpol.2016.08.056
Materatski, P., Ribeiro, R., Moreira-Santos, M., Sousa, J. P., and Adão, H. (2018). Nematode biomass and morphometric attributes as descriptors during a major Zostera noltii collapse. Mar. Biol. 165, 1–17.
Materatski, P., Vafeiadou, A.-M., Ribeiro, R., Moens, T., and Adão, H. (2015). A comparative analysis of benthic nematode assemblages from Zostera noltii beds before and after a major vegetation collapse. Estuar. Coast. Shelf Sci. 167, 256–268. doi: 10.1016/j.ecss.2015.07.001
Maurer, D. (2000). The dark side of taxonomic sufficiency (TS). Mar. Pollut. Bull. 40, 98–101. doi: 10.1016/s0025-326x(99)00235-0
McLaverty, C., Eigaard, O. R., Gislason, H., Bastardie, F., Brooks, M. E., Jonsson, P., et al. (2020). Using large benthic macrofauna to refine and improve ecological indicators of bottom trawling disturbance. Ecol. Indic. 110:105811. doi: 10.1016/j.ecolind.2019.105811
Meadows, A. S., Ingels, J., Widdicombe, S., Hale, R., and Rundle, S. D. (2015). Effects of elevated CO2 and temperature on an intertidal meiobenthic community. J. Exp. Mar. Bio. Ecol. 469, 44–56. doi: 10.1016/j.jembe.2015.04.001
Middelburg, J. J., and Meysman, F. J. R. (2007). Burial at sea. Science 316, 1294–1295. doi: 10.1093/nq/s12-VI.107.174
Mirto, S., Arigò, C., Genovese, L., Pusceddu, A., Gambi, C., and Danovaro, R. (2014). Nematode assemblage response to fish-farm impact in vegetated (Posidonia oceanica) and non-vegetated habitats. Aquac. Environ. Interact. 5, 17–28. doi: 10.3354/aei00091
Mirto, S., Bianchelli, S., Gambi, C., Krzelj, M., Pusceddu, A., Scopa, M., et al. (2010). Fish-farm impact on metazoan meiofauna in the Mediterranean Sea: analysis of regional vs. habitat effects. Mar. Environ. Res. 69, 38–47. doi: 10.1016/j.marenvres.2009.07.005
Mirto, S., Gristina, M., Sinopoli, M., Maricchiolo, G., Genovese, L., Vizzini, S., et al. (2012). Meiofauna as an indicator for assessing the impact of fish farming at an exposed marine site. Ecol. Indic. 18, 468–476. doi: 10.1016/j.ecolind.2011.12.015
Moens, T., and Vincx, M. (1997). Observations on the feeding ecology of estuarine nematodes. Oceanogr. Lit. Rev. 44:739.
Monteiro, L., Traunspurger, W., Roeleveld, K., Lynen, F., and Moens, T. (2018). Direct toxicity of the water-soluble fractions of a crude and a diesel-motor oil on the survival of free-living nematodes. Ecol. Indic. 93, 13–23. doi: 10.1016/j.ecolind.2018.04.066
Moreno, M., Ferrero, T. J., Gallizia, I., Vezzulli, L., Albertelli, G., and Fabiano, M. (2008). An assessment of the spatial heterogeneity of environmental disturbance within an enclosed harbour through the analysis of meiofauna and nematode assemblages. Estuar. Coast. Shelf Sci. 77, 565–576. doi: 10.1016/j.ecss.2007.10.016
Moreno, M., Semprucci, F., Vezzulli, L., Balsamo, M., Fabiano, M., and Albertelli, G. (2011). The use of nematodes in assessing ecological quality status in the Mediterranean coastal ecosystems. Ecol. Indic. 11, 328–336. doi: 10.1016/j.ecolind.2010.05.011
Mueller, M. T., Fueser, H., Höss, S., and Traunspurger, W. (2020). Species-specific effects of long-term microplastic exposure on the population growth of nematodes, with a focus on microplastic ingestion. Ecol. Indic. 118:106698. doi: 10.1016/j.ecolind.2020.106698
Müller, A., Becker, R., Dorgerloh, U., Simon, F.-G., and Braun, U. (2018). The effect of polymer aging on the uptake of fuel aromatics and ethers by microplastics. Environ. Pollut. 240, 639–646. doi: 10.1016/j.envpol.2018.04.127
Muresan, M., Teaca, A., Popa, A., and Begun, T. (2019). Free-living marine nematodes community structural changes within a post-dredging site at the Romanian shelf. J. Environ. Prot. Ecol. 20, 753–760.
Nasira, K., Shahina, F., and Kamran, M. (2010). Response of free living marine nematode community to heavy metal contamination along the coastal areas of Sindh and Baluchistan, Pakistan. Pakistan J. Nematol. 28, 263–278.
Oldfield, F., and Dearing, J. A. (2003). “The role of human activities in past environmental change,” in Paleoclimate, Global Change and the Future. Global Change — The IGBP Series, eds K. D. Alverson, T. F. Pedersen, and R. S. Bradley (Berlin: Springer), 143–162. doi: 10.1007/978-3-642-55828-3_7
Papaspyrou, S., Gregersen, T., Cox, R. P., Thessalou-Legaki, M., and Kristensen, E. (2005). Sediment properties and bacterial community in burrows of the ghost shrimp Pestarella tyrrhena (Decapoda: Thalassinidea). Aquat. Microb. Ecol. 38, 181–190. doi: 10.3354/ame038181
Patrício, J., Adão, H., Neto, J. M., Alves, A. S., Traunspurger, W., and Marques, J. C. (2012). Do nematode and macrofauna assemblages provide similar ecological assessment information? Ecol. Indic. 14, 124–137. doi: 10.1016/j.ecolind.2011.06.027
Pauly, D. (1995). Anecdotes and the shifting baseline syndrome of fisheries. Trends Ecol. Evol. 10:430. doi: 10.1016/s0169-5347(00)89171-5
Pereira, T. J., Gingold, R., Villegas, A. D. M., and Rocha-Olivares, A. (2018). Patterns of spatial variation of meiofauna in sandy beaches of northwestern Mexico with contrasting levels of disturbance. Thalass. An Int. J. Mar. Sci. 34, 53–63. doi: 10.1007/s41208-017-0038-x
Pérez-García, J. A., Marzo Perez, D., and Armenteros, M. (2019). Spatial scale influences diversity patterns of free-living nematode assemblages in coral degradation zones from the Caribbean Sea. Mar. Biodivers. 49, 1831–1842. doi: 10.1007/s12526-019-00945-z
Raffaelli, D. G., and Mason, C. F. (1981). Pollution monitoring with meiofauna, using the ratio of nematodes to copepods. Mar. Pollut. Bull. 12, 158–163. doi: 10.1016/0025-326x(81)90227-7
Riera, R., Sanchez-Jerez, P., Rodríguez, M., and Monterroso, Ó, and Ramos, E. (2012). Long-term monitoring of fish farms: application of Nematode/Copepod index to oligotrophic conditions. Mar. Pollut. Bull. 64, 844–850. doi: 10.1016/j.marpolbul.2012.01.014
Rombouts, I., Beaugrand, G., Artigas, L. F., Dauvin, J.-C., Gevaert, F., Goberville, E., et al. (2013). Evaluating marine ecosystem health: case studies of indicators using direct observations and modelling methods. Ecol. Indic. 24, 353–365. doi: 10.1016/j.ecolind.2012.07.001
Rose, A., Ingels, J., Raes, M., Vanreusel, A., and Arbizu, P. M. (2015). Long-term iceshelf-covered meiobenthic communities of the Antarctic continental shelf resemble those of the deep sea. Mar. Biodivers. 45, 743–762. doi: 10.1007/s12526-014-0284-6
Rubal, M., Veiga, P., and Besteiro, C. (2009). Nematode/copepod index: importance of sedimentary parameters, sampling methodology and baseline values. Thalassas 25, 9–18. doi: 10.1007/978-3-662-05052-1_2
Rzeznik-Orignac, J., Boucher, G., Fichet, D., and Richard, P. (2008). Stable isotope analysis of food source and trophic position of intertidal nematodes and copepods. Mar. Ecol. Prog. Ser. 359, 145–150. doi: 10.3354/meps07328
Sabeel, R. A. O., and Vanreusel, A. (2015). Potential impact of mangrove clearance on biomass and biomass size spectra of nematode along the Sudanese Red Sea coast. Mar. Environ. Res. 103, 46–55. doi: 10.1016/j.marenvres.2014.11.003
Sahraeian, N., Sahafi, H. H., Mosallanejad, H., Ingels, J., and Semprucci, F. (2020). Temporal and spatial variability of free-living nematodes in a beach system characterized by domestic and industrial impacts (Bandar Abbas, Persian Gulf, Iran). Ecol. Indic. 118:106697. doi: 10.1016/j.ecolind.2020.106697
Sandulli, R., and De Nicola-Giudici, M. (1990). Pollution effects on the structure of meiofaunal communities in the Bay of Naples. Mar. Pollut. Bull. 21, 144–153. doi: 10.1016/0025-326x(90)90550-r
Sapir, A. (2021). Why are nematodes so successful extremophiles? Commun. Integr. Biol. 14, 24–26. doi: 10.1080/19420889.2021.1884343
Schratzberger, M., and Ingels, J. (2018). Meiofauna matters: the roles of meiofauna in benthic ecosystems. J. Exp. Mar. Biol. Ecol. 502, 12–25. doi: 10.1016/j.jembe.2017.01.007
Schratzberger, M., and Jennings, S. (2002). Impacts of chronic trawling disturbance on meiofaunal communities. Mar. Biol. 141, 991–1000. doi: 10.1007/s00227-002-0895-5
Schratzberger, M., Lampadariou, N., Somerfield, P., Vandepitte, L., and Vanden Berghe, E. (2009). The impact of seabed disturbance on nematode communities: linking field and laboratory observations. Mar. Biol. 156, 709–724. doi: 10.1007/s00227-008-1122-9
Schratzberger, M., and Somerfield, P. J. (2020). Effects of widespread human disturbances in the marine environment suggest a new agenda for meiofauna research is needed. Sci. Total Environ. 728:138435. doi: 10.1016/j.scitotenv.2020.138435
Schratzberger, M., Warr, K., and Rogers, S. I. (2007). Functional diversity of nematode communities in the southwestern North Sea. Mar. Environ. Res. 63, 368–389. doi: 10.1016/j.marenvres.2006.10.006
Schratzberger, M., and Warwick, R. (1998). Effects of physical disturbance on nematode communities in sand and mud: a microcosm experiment. Mar. Biol. 130, 643–650. doi: 10.1007/s002270050286
Schuelke, T., Pereira, T. J., Hardy, S. M., and Bik, H. M. (2018). Nematode-associated microbial taxa do not correlate with host phylogeny, geographic region or feeding morphology in marine sediment habitats. Mol. Ecol. 27, 1930–1951. doi: 10.1111/mec.14539
Sedano, F., Marquina, D., and Espinosa Torre, F. (2014). Usefulness of meiofauna at high taxonomic levels as a tool to assess harbor quality status. Marina del Este Harbor (Granada, Spain) as a case study. Rev. Ciencias Mar. Y Costeras 6, 103–113.
Semprucci, F., and Balsamo, M. (2012). Free-living marine nematodes as bioindicators: past, present and future perspectives. Environ. Res. J. 6, 17–35.
Semprucci, F., and Balsamo, M. (2014). Free-living marine nematodes as bioindicators: past, present and future perspectives. Trends Environ. Sci. 6, 17–36.
Semprucci, F., Balsamo, M., Appolloni, L., and Sandulli, R. (2018). Assessment of ecological quality status along the Apulian coasts (eastern Mediterranean Sea) based on meiobenthic and nematode assemblages. Mar. Biodivers. 48, 105–115. doi: 10.1007/s12526-017-0745-9
Semprucci, F., Colantoni, P., and Balsamo, M. (2016). Is maturity index an efficient tool to assess the effects of the physical disturbance on the marine nematode assemblages?—A critical interpretation of disturbance-induced maturity successions in some study cases in Maldives. Acta Oceanol. Sin. 35, 89–98. doi: 10.1007/s13131-016-0832-y
Semprucci, F., Losi, V., and Moreno, M. (2015). A review of Italian research on free-living marine nematodes and the future perspectives on their use as Ecological Indicators (EcoInds). Mediterr. Mar. Sci. 16:352. doi: 10.12681/mms.1072
Semprucci, F., Moreno, M., Sbrocca, S., Rocchi, M., Albertelli, G., and Balsamo, M. (2013). The nematode assemblage as a tool for the assessment of marine ecological quality status: a case-study in the Central Adriatic Sea. Mediterr. Mar. Sci. 14, 48–57. doi: 10.12681/mms.366
Semprucci, F., Sbrocca, C., Baldelli, G., Tramontana, M., and Balsamo, M. (2017). Is meiofauna a good bioindicator of artificial reef impact? Mar. Biodivers. 47, 511–520. doi: 10.1007/s12526-016-0484-3
Sergeeva, N., Shadrin, N., and Anufriieva, E. (2019). Long-term changes (1979-2015) in the nematode fauna in Sivash Bay (Sea of Azov), Russia, worldwide the largest hypersaline lagoon, during salinity transformations. Nematology 21, 337–347. doi: 10.1163/15685411-00003217
Shang, X., Lu, J., Feng, C., Ying, Y., He, Y., Fang, S., et al. (2020). Microplastic (1 and 5 μm) exposure disturbs lifespan and intestine function in the nematode Caenorhabditis elegans. Sci. Total Environ. 705:135837. doi: 10.1016/j.scitotenv.2019.135837
Shaw, K. M., Lambshead, P. J. D., and Platt, H. M. (1983). Detection of pollution-induced disturbance in marine benthic assemblages with special reference to nematodes. Mar. Ecol. Prog. Ser. Oldend. 11, 195–202. doi: 10.3354/meps011195
Siddig, A. A. H., Ellison, A. M., Ochs, A., Villar-Leeman, C., and Lau, M. K. (2016). How do ecologists select and use indicator species to monitor ecological change? Insights from 14 years of publication in ecological indicators. Ecol. Indic. 60, 223–230. doi: 10.1016/j.ecolind.2015.06.036
Soetaert, K., Franco, M. A., Lampadariou, N., Muthumbi, A., Steyaert, M., Vandepitte, L., et al. (2009). Factors affecting nematode biomass, length and width from the shelf to the deep sea. Mar. Ecol. Prog. Ser. 392, 123–132. doi: 10.3354/meps08202
Soetaert, K., Vanaverbeke, J., Heip, C., Herman, P. M. J., Middelburg, J. J., Sandee, A., et al. (1997). Nematode distribution in ocean margin sediments of the Goban Spur (northeast Atlantic) in relation to sediment geochemistry. Deep Sea Res. Part I Oceanogr. Res. Pap. 44, 1671–1683. doi: 10.1016/s0967-0637(97)00043-5
Soltwedel, T., Guilini, K., Sauter, E., Schewe, I., and Hasemann, C. (2018). Local effects of large food-falls on nematode diversity at an arctic deep-sea site: results from an in situ experiment at the deep-sea observatory HAUSGARTEN. J. Exp. Mar. Bio. Ecol. 502, 129–141. doi: 10.1016/j.jembe.2017.03.002
Somerfield, P. J., and Clarke, K. R. (1995). Taxonomic levels, in marine community studies, revisited. Mar. Ecol. Prog. Ser. 127, 113–119. doi: 10.3354/meps127113
Soto, L. A., Salcedo, D. L., Arvizu, K., and Botello, A. V. (2017). Interannual patterns of the large free-living nematode assemblages in the Mexican Exclusive Economic Zone, NW Gulf of Mexico after the Deepwater Horizon oil spill. Ecol. Indic. 79, 371–381. doi: 10.1016/j.ecolind.2017.03.058
Stark, J. S., Mohammad, M., McMinn, A., and Ingels, J. (2017). The effects of hydrocarbons on meiofauna in marine sediments in Antarctica. J. Exp. Mar. Bio. Ecol. 496, 56–73. doi: 10.1016/j.jembe.2017.07.009
Stark, J. S., Mohammad, M., McMinn, A., and Ingels, J. (2020). Diversity, abundance, spatial variation, and human impacts in marine meiobenthic nematode and copepod communities at Casey Station, East Antarctica. Front. Mar. Sci. 7:480. doi: 10.3389/fmars.2020.00480
Sutherland, W. J., Clout, M., Côté, I. M., Daszak, P., Depledge, M. H., Fellman, L., et al. (2010). A horizon scan of global conservation issues for 2010. Trends Ecol. Evol. 25, 1–7. doi: 10.1016/j.tree.2009.10.003
Tett, P., Rj, G., Sj, P., Elliott, M., Forster, R., Dk, M., et al. (2013). Framework for understanding marine ecosystem health. Mar. Ecol. Prog. Ser. 494, 1–27.
Ürkmez, D., Sezgin, M., and Bat, L. (2014). Use of nematode maturity index for the determination of ecological quality status: a case study from the Black Sea. J. Black Sea Mediterranean Environ. 20:96.
van Oevelen, D., Bergmann, M., Soetaert, K., Bauerfeind, E., Hasemann, C., Klages, M., et al. (2011a). Carbon flows in the benthic food web at the deep-sea observatory HAUSGARTEN (Fram Strait). Deep Sea Res. Part I Oceanogr. Res. Pap. 58, 1069–1083. doi: 10.1016/j.dsr.2011.08.002
van Oevelen, D., Soetaert, K., Garcia, R., de Stigter, H. C., Cunha, M. R., Pusceddu, A., et al. (2011b). Canyon conditions impact carbon flows in food webs of three sections of the Nazaré canyon. Deep Sea Res. Part II Top. Stud. Oceanogr. 58, 2461–2476. doi: 10.1016/j.dsr2.2011.04.009
van Oevelen, D., Soetaert, K., Middelburg, J. J., Herman, P. M. J., Moodley, L., Hamels, I., et al. (2006). Carbon flows through a benthic food web: integrating biomass, isotope and tracer data. J. Mar. Res. 64, 453–482. doi: 10.1357/002224006778189581
Vanreusel, A., De Groote, A., Gollner, S., and Bright, M. (2010). Ecology and biogeography of free-living nematodes associated with chemosynthetic environments in the deep sea: a review. PLoS One 5:e12449. doi: 10.1371/journal.pone.0012449
Venekey, V., and de Melo, T. P. G. (2016). Nematodes as indicators of shrimp farm impact on an amazonian estuary (Curuçá, Pará, Brazil). Braz. J. Oceanogr. 64, 75–87. doi: 10.1590/s1679-87592016108206401
Villares, G., and de Ward, C. P. (2020). Increased biomass of free-living marine nematodes may be indicative of disturbances in the ecosystem of the San Antonio Bay. Open J. Environ. Biol. 5, 1–6.
Villarrubia-Gómez, P., Cornell, S. E., and Fabres, J. (2018). Marine plastic pollution as a planetary boundary threat – The drifting piece in the sustainability puzzle. Mar. Policy 96, 213–220. doi: 10.1016/j.marpol.2017.11.035
Wan, S., Toucanne, S., Clift, P. D., Zhao, D., Bayon, G., Yu, Z., et al. (2015). Human impact overwhelms long-term climate control of weathering and erosion in southwest China. Geology 43, 439–442. doi: 10.1130/g36570.1
Wang, F., Wong, C. S., Chen, D., Lu, X., Wang, F., and Zeng, E. Y. (2018). Interaction of toxic chemicals with microplastics: a critical review. Water Res. 139, 208–219. doi: 10.1016/j.watres.2018.04.003
Ward, T., and Jacoby, C. (1992). A strategy for assessment and management of marine ecosystems: baseline and monitoring studies in Jervis Bay, a temperate Australian embayment. Mar. Pollut. Bull. 25, 163–171. doi: 10.1016/0025-326x(92)90220-z
Warwick, R. (1986). A new method for detecting pollution effects on marine macrobenthic community. Mar. Biol. 92, 557–562. doi: 10.1007/BF00392515
Warwick, R., Clarke, K., Gee, J. M., and Green, R. (1988). A mesocosm experiment on the effects of hydrocarbon and copper pollution on a sublittoral soft-sediment meiobenthic community. Mar. Ecol. Ser. 46, 181–191. doi: 10.3354/meps046181
Warwick, R. M. (1981). The nematode/copepod ratio and its use in pollution ecology. Mar. Pollut. Bull. 12, 329–333. doi: 10.1016/0025-326x(81)90105-3
Warwick, R. M. (1988). The level of taxonomic discrimination required to detect pollution effects on marine benthic communities. Mar. Pollut. Bull. 19, 259–268. doi: 10.1016/0025-326x(88)90596-6
Wetzel, M. A., Fleeger, J. W., and Powers, S. P. (2001). Effects of hypoxia and anoxia on meiofauna: a review with new data from the gulf of mexico. Coast. Hypoxia Consequences Living Resour. Ecosyst. 58, 165–184. doi: 10.1029/CE058p0165
Wieser, W. (1953). Die beziehung zwischen mundhohlengestalt, emahrungsweise und vorkommen bei freilebenden marinen nematoden. Eine skologisen-morphologische studie. Ark. Zool. 4, 439–484.
Woodruff, D. S. (2001). Declines of biomes and biotas and the future of evolution. Proc. Natl. Acad. Sci. U.S.A. 98, 5471–5476. doi: 10.1073/pnas.101093798
Xu, B., Liu, F., Brookes, P. C., and Xu, J. (2018). Microplastics play a minor role in tetracycline sorption in the presence of dissolved organic matter. Environ. Pollut. 240, 87–94. doi: 10.1016/j.envpol.2018.04.113
Xu, W. Z., Cheung, S. G., and Shin, P. K. S. (2014). Structure and taxonomic composition of free-living nematode and macrofaunal assemblages in a eutrophic subtropical harbour, Hong Kong. Mar. Pollut. Bull. 85, 764–773. doi: 10.1016/j.marpolbul.2014.01.023
Yang, X., Lin, C., Song, X., Xu, M., and Yang, H. (2019). Effects of artificial reefs on the meiofaunal community and benthic environment-A case study in Bohai Sea, China. Mar. Pollut. Bull. 140, 179–187. doi: 10.1016/j.marpolbul.2018.12.031
Yen, N. T. M., Vanreusel, A., Lins, L., Thai, T. T., Nara Bezerra, T., and Quang, N. X. (2020). The effect of a dam construction on subtidal nematode communities in the Ba Lai Estuary, Vietnam. Diversity 12:137. doi: 10.3390/d12040137
Zalasiewicz, J., Waters, C. N., Ivar do Sul, J. A., Corcoran, P. L., Barnosky, A. D., Cearreta, A., et al. (2016). The geological cycle of plastics and their use as a stratigraphic indicator of the Anthropocene. Anthropocene 13, 4–17. doi: 10.1016/j.ancene.2016.01.002
Keywords: nematode, bioindicator, marine, pollution, microplastic (MP)
Citation: Ridall A and Ingels J (2021) Suitability of Free-Living Marine Nematodes as Bioindicators: Status and Future Considerations. Front. Mar. Sci. 8:685327. doi: 10.3389/fmars.2021.685327
Received: 25 March 2021; Accepted: 15 June 2021;
Published: 15 July 2021.
Edited by:
Xiaoshou Liu, Ocean University of China, ChinaReviewed by:
Punyasloke Bhadury, Indian Institute of Science Education and Research Kolkata, IndiaWenzhe Xu, Tianjin University of Science and Technology, China
Copyright © 2021 Ridall and Ingels. This is an open-access article distributed under the terms of the Creative Commons Attribution License (CC BY). The use, distribution or reproduction in other forums is permitted, provided the original author(s) and the copyright owner(s) are credited and that the original publication in this journal is cited, in accordance with accepted academic practice. No use, distribution or reproduction is permitted which does not comply with these terms.
*Correspondence: Aaron Ridall, YXJpZGFsbEBiaW8uZnN1LmVkdQ==