- 1Department of Collective Behaviour, Max Planck Institute of Animal Behavior, Konstanz, Germany
- 2Centre for the Advanced Study of Collective Behaviour, University of Konstanz, Konstanz, Germany
- 3Department of Biology, University of Konstanz, Konstanz, Germany
A key aspect of understanding social interactions in marine animals is determining whether individuals freely interact in fission-fusion groups, or have spatially structured interactions, for example territories or home ranges. Territoriality can influence access to mates, food resources, or shelter sites, and may also impact conservation efforts, as the delineation of marine protected areas relies on knowledge of home ranges and movement patterns. However, accurately determining distribution and movement is challenging for many marine species, especially small and medium species, which cannot carry beacons or tags to automatically measure movement, and are also difficult for human observers to accurately follow. Yet these smaller species comprise the bulk of near-shore assemblages, and are essential conservation targets. As such, novel solutions for monitoring movement and behavior are required. Here we use a combination of tracking and environmental reconstruction to explore territoriality, aggression, and navigation in a small marine fish, explicitly applying this technique to questions of sociality in the marine environment. We use the Mediterranean Rainbow Wrasse, Coris julis, as a test case, but this approach can be extended to many other species and contexts. In contrast with previous reports for this species, we find that during our observation period, female C. julis occupy consistent territories over sand patches, and that they defend these territories against same-sex conspecifics. Displacement experiments revealed two further important social behavioral traits – first that displaced individuals were able to navigate back to their territory, avoiding almost all other female territories as they returned. Second that when displaced fish approached the territories of others, residents of these territories were often aggressive to the non-neighboring fish, in contrast with our observations of low aggression counts toward their natural neighbors. Resident fish therefore appear to show differing levels of aggressiveness depending on their social relationship with same-sex conspecifics. Overall, these results suggest a sophisticated degree of social behavior in this marine wrasse, dependent on social and structural environment, but which can only effectively be revealed by state-of-the-art tracking and environment reconstruction techniques.
Introduction
Animals interact dynamically with their environment and can develop specific relationships with their surroundings, as for example expressed through home ranges, defined as the areas where individuals spend most of their time with activities such as foraging, resting, or mating (Pearl, 2000). For many animals, the core of their home range is the most important area and is often considered the territory, and by defending such an area, individuals can monopolize resources, including food, shelter [e.g., in rodents Meriones unguiculatus: (Ågren et al., 1989), and mates (e.g., butterflies Papilio zelicaon: (Lederhouse, 1982)]. In the Tuatara, Sphenodon punctatus, territory structure also depends on the distribution of mates and competitors. Females and males of this species occupy independent territories, and for the males the size of territory scales with body size. Large males are more likely to own and defend larger territories that overlap with female home ranges, increasing their access to mates and providing a strong benefit (Moore et al., 2009).
Defending territories against intruders may involve displays or fights between residents and intruders, which come at a high cost to both winners and losers because of the energetic requirements and the possibility of physical damage and mortality (Dugatkin et al., 1998). From a game theoretic perspective, both individuals would benefit by avoiding confrontation if they could predict the outcome of the confrontation. However, it is often unclear which individual will win a fight, resulting in physical interactions. One way to avoid these costly interactions is to establish social relationships, the formation of which may lead to spatial structuring within populations as territory borders are established. In teleost fish, it has been suggested that spatial learning and social interactions are necessary prerequisites for territoriality (Bronstein, 1986). Territorial individuals might benefit from neighboring conspecifics and form integrated social groups within territorial neighborhoods (Stamps, 1988).
As well as the ecological impacts of territoriality, an understanding of the spatial structure of animal populations is essential for conservation efforts and management strategies. Protected areas, for example, can only be an effective conservation management tool if they are larger than the home ranges of the occurring species [as shown in a case study for the Mediterranean Sea: (Di Franco et al., 2018)]. To protect animals that are habitat specialists (for example the swift fox Vulpes velox in short-grass prairies), knowledge about their habitat preference and utilization are of primary importance (Kamler et al., 2003). In terrestrial systems, monitoring the movement and home ranges of animals can be achieved through tracking or remote telemetry, but this presents a major problem in marine habitats, where approaches such as Global Positioning System (GPS) or Pop-up Satellite Archival Tags (PSATs) are mostly applicable for larger, or in the case of acoustic telemetry, medium-sized aquatic animals (Hussey et al., 2015; Thys et al., 2015). For reasons of animal size, species abundance, and habitat complexity, many available tracking methods are poorly suited to these inshore regions and are not easily applied in smaller animals, despite these comprising the bulk of vertebrate species assemblages. To address this knowledge gap, alternative methods are necessary, and in the present study we employ a computer-vision based tracking methodology (Francisco et al., 2020) to track and analyse the movement and social interactions of a small inshore species, the Mediterranean rainbow wrasse (Coris julis). This study provides a demonstration of the potential for this type of approach to generate unprecedented quantitative insight into the behavior and movement of a class of marine vertebrates that was previously inaccessible to modern tracking approaches.
The Mediterranean rainbow wrasse belongs to the family Labridae and lives in coastal regions up to a depth of 120 m on rocky bottoms or seagrass (e.g., Posidonia oceanica) beds (Lejeune, 1987). As for many Labrids, it is a protogynous hermaphrodite, with a temporal separation of the sexes in one individual (Bentivegna et al., 1985). Sex change to the secondary male phase usually occurs at four years of age and is accompanied with a color change and testes function. Most C. julis are born as females but some are initially born as males (primary males) that phenotypically resemble females (Linde et al., 2011). The females live in harems and mate with the dominant and much larger secondary male, producing pelagic eggs (Lejeune, 1987). Lejeune (1987) states that only secondary phase males are territorial and that initial phase individuals have home ranges between 5 and 10 m. Males defend their territories because of the benefit of having multiple mates living in their home range.
No reports of female territoriality exist for C. julis, but harems have previously been suggested to form when females are defendable and thus site attached (Gladstone, 1987; Lejeune, 1987). Female territoriality could be adaptive because the defended area provides food resources or shelter sites (Bujalska and Saitoh, 2000). This may be particularly pertinent for this species, as C. julis rest in holes that they dig in the sand, so it may be advantageous for females to defend sandy areas (Videler et al., 1986). The question of female territoriality in C. julis is therefore important at many levels; to understand the social and breeding system of the species, to understand the home range and therefore efficacy of protection measures, and more generally to better understand sex-specific territoriality in fish, for example the assumed relationship that when one sex of a species is territorial, the other one is not (Ostfeld, 1985). This relationship may not always hold, for example in the haremic dwarf hawkfish Cirrhitichtys falco both males and females are territorial; territorial behavior in males depends on female access, whereas female territoriality is based on food resources (Kadota et al., 2011).
A theoretical approach of studying territoriality in a standardized way is to assume that the benefits and costs of defending a territory are measurable. Here, the territory is defined as the core area where an animal spends the majority of its time and which it actively defends against intruders, located in the potentially larger home range, the area that it frequently visits (Powell, 2000). The costs of holding such a territory are caused by the behavioral activities used to defend their area against con- or heterospecific individuals, such as aggressive behavior and patrols, while benefits are defined as the access to limited resources or mates (Stamps, 1994). The interaction between resource exploitability and exploration difficulty, which both directly link to costs and benefits, can drive territorial behavior when both the exploitation potential and the exploration difficulty are high (Monk et al., 2018). In contrast with the approach that concentrates on a focal resident and its responses to the costs and benefits of defense, alternative models exist that mainly consider the interactions with other individuals. One example is focusing on interactions among direct neighbors and how these interactions shape their use of space and another one defines territory size as the result of the interactions between residents and potential settlers seeking to gain territory (Adams, 2001). In this study, we combine both of these focal resident models by collecting information on direct neighbor interactions as well as interactions with unfamiliar conspecifics. We predict female C. julis will show territorial defense and have home ranges, because secondary males are territorial and are assumed to defend female harems. In order to test this, we use a novel method for underwater animal tracking to measure the home ranges of female C. julis in a non-invasive way. Furthermore, to test their spatial memory and their tendency to stay within their home ranges, we displaced focal fish from their territories and observed if and how they returned to their original putative territories.
Materials and Methods
Field Experiments
All experiments were conducted by scuba diving at the STARESO Field Station, Calvi, France from mid-June to mid-July 2019 at two sandy patches embedded in a P. oceanica seagrass bed (see Figure 1A). These patches were located approximately 100 m from the coast (42°34′48.4″N 8°43′31.8″E), at a depth of 13 to 15.3 m, spanning an area of 261 m2 with 60.4 m as the longest side (north-to-south).
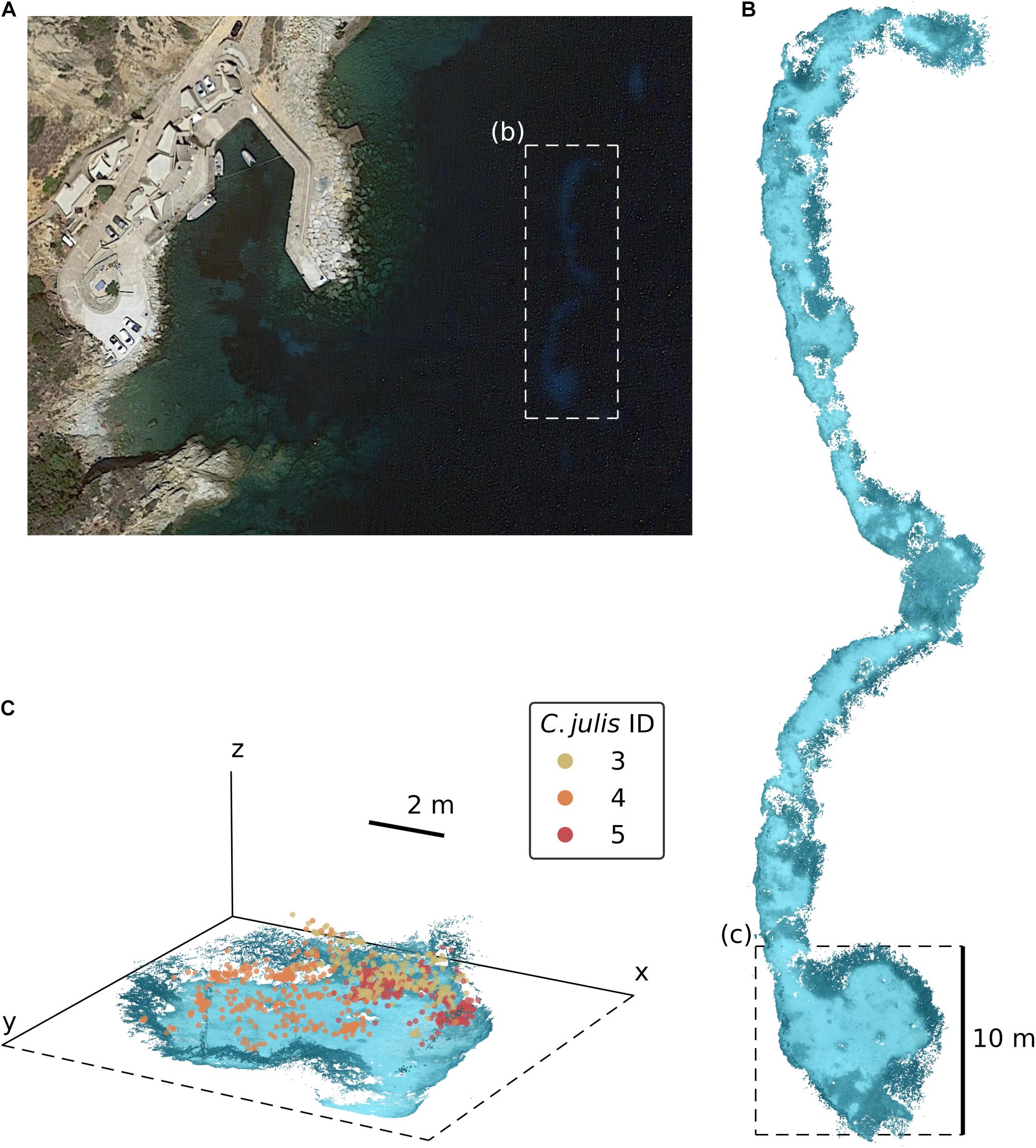
Figure 1. (A) Satellite image of the STARESO bay near Calvi on Corsica, France (image source: Google Earth). The dashed rectangle (b) marks the location of the experimental area, the two sandy patches embedded in a Posidonia oceanica seagrass bed. (B) Dense SfM reconstruction of the sandy patches. (C) Detailed 3D view of the environmental reconstruction [the dashed rectangle marked as (c) in panel (B)] with the triangulated positions of Coris julis 3, 4, and 5.
First, we aimed to capture and tag all phenotypically female C. julis in this area were with a visible, unique elastomer code (Northwest Marine Technology, Inc.) to maintain the identity of fish across all experiments and tracking observations. The least invasive way to determine sex in C. julis is measuring the body length and identifying the phenotype. Individuals bigger than 18 cm are considered to be secondary males (Bertoncini et al., 2009). Therefore, targeted fish for this study were phenotypic females ranging from 8.5 cm to 12.5 cm standard length. In total, 11 fish were captured, of which 10 were within this range. Much larger or smaller individuals were not used in the experiments to avoid including secondary males and juveniles. After capturing the fish, the elastomer was injected below the skin, parallel to the dorsal fin, to allow visual identification of the tagged individuals. During the tagging procedure, a picture was taken of each fish to measure the standard body length (for details on the sizes of all tagged fish see Supplementary Table 2). Afterward, the individuals were released at the location of capture. At no point were the fish removed from the water (nor their depth changed), and all procedures were conducted in accordance with the STARESO field station’s general scientific permit.
To determine if the tagged C. julis had territories or home ranges, we followed a repeated observation protocol in which each fish was observed for 10 min on a total of three days (repeated on day 3 and 5 after the first observation on day 1, see Supplementary Table 1 for a summary of all tracking observations). During these observations, a diver followed the focal fish at a distance of approximately 2 m and recorded from a top-down perspective using a T-shaped stereo-camera setup (2x GoPro Hero 7, see Supplementary Table 3 for further GoPro parameters and Supplementary Figures 1A,D for a picture of the setup). This setup ensured that the disturbance of the focal fish by the diver was minimized, apparent through the naturally behaving C. julis. Additionally, a video covering the sandy patches was recorded using the same setup for spatial reference.
In these observations, the female C. julis appeared to have defined home ranges. To further analyse their territorial behavior, each fish was captured, placed inside a transparent container (Supplementary Figures 1B,C) and displaced between 30 to 40 m from its roughly estimated core area to the core area of another tagged individual. The behavioral responses of the resident C. julis toward the displaced fish were recorded for 5 min. Then, the displaced fish were released and recorded by a diver with the stereo-camera setup until they entered their respective home range.
Video Analysis
A combination of tracking and structure-from-motion (SfM) was employed to determine the home ranges of individual C. julis. We mainly followed the methodology presented by Francisco et al. (2020), but implemented a few changes to tailor the technique to our specific use case. Firstly, the corresponding videos that resulted from the stereo-camera setup were temporally synchronized using their audio signals (Francisco et al., 2020). Then, one video frame was extracted every 2 s of the sandy patch footage and one frame every 3 s of each individual fish tracking observation. Secondly, we used COLMAP, an open-source SfM pipeline, to reconstruct the visual environment in 3D (Schönberger and Frahm, 2016; Schönberger et al., 2016). In addition to the reconstruction of environmental features, SfM also estimated camera positions and orientations for each of the extracted video frames. This resulted in one reconstruction for each tracking observation, all referenced within the reconstruction of the sandy patch. Finally, we used COLMAP to merge all reconstructions into a single one that was then used to triangulate 3D fish trajectories.
For the latter, the focal individuals were tracked in both videos from the stereo-camera setup for each observation. Diverging from Francisco et al. (2020), we chose to manually track the fish using a custom-written Python video interface to record the pixel coordinates at a sampling frequency of 0.33 Hz (in the same video frames that were extracted for SfM). This resulted in corresponding, stereo-view pixel coordinates for each observation of each fish. Subsequently, these coordinates were triangulated into 3D trajectories (again, with a temporal resolution of 0.33 Hz) using “multiviewtracks” (Francisco et al., 2020). For an overview of trajectory completeness, we calculated the track coverage of each trajectory as the percentage of successfully triangulated 3D positions.
Statistical Analyses
For further analysis of home ranges, the 2D kernel density utilization distribution (UD) of the trajectory points from all three tracking observations (using only X and Y components) was calculated for each fish using the “adehabitatHR” package in R (Calenge, 2006). Utilization distribution is a well-established, objective technique to estimate the home range area from location data, calculating the probability that an individual is found at a specific point in space. The kernel density estimator is one of the common non-parametric statistical methods for estimating these probabilities. Using the UD, we calculated the home range and territory area (the core area of the home range) as the commonly used UD95 and UD50 contours, respectively (Worton, 1989; Nicholls et al., 2005).
Since no detailed description of aggressive behavior was found for this species, we created an ethogram based on aggressive behavior described in other teleost species [see Table 1, derived from Balzarini et al. (2014)]. The interactions between the focal individual and other C. julis in the 10 min tracking observations were manually scored and then spatially referenced into the 3D reconstructions. We then used BORIS to further analyse the video recordings obtained from the displacement experiments (Friard and Gamba, 2016). Here, the same ethogram was used to analyse the interactions of territory holders and displaced fish. Additionally, the paths of all individuals returning to their home ranges from the release locations of respective displacement trials were manually estimated on the map of the sandy patches based on observations and key features recognized in the video. The lengths of these paths were measured, as well as the “beeline” (the Euclidean distance between release and arrival locations) and the shortest distance through the sandy patch (following a path consisting of linear segments between the release and arrival location, bounded by the sandy patch). Further, the interactions between the released fish and any other C. julis were scored using the same ethogram.
Using this data, we conducted a series of statistical analyses. First, we tested whether the standard length of a fish has an effect on the size of its home range area (UD95) or core area (UD50). Next, we tested if the fraction of UD95 and UD50 that an individual shares with neighbors is dependent on the difference in their body lengths. For both tests, we used linear models in R (Zeileis and Hothorn, 2002). The parametric assumptions were tested using the Shapiro-Wilk (for normality of residuals) and the Breusch-Pagan test (for homoscedasticity). If the model did not meet the parametric assumptions, the response variable was log-transformed. All proportional data (area overlaps) was logit-transformed.
Furthermore, equivalent models were used to test two behavioral hypotheses: (1) the body size difference between the resident and displaced fish affects the aggression presented by the resident individual and (2) the frequency of the territory holder’s aggression toward the displaced intruder is dependent on the size of its home range or core area (UD95 and UD50). Here, we modeled the behavior frequency as the response, dividing the count of observed behaviors by the time the resident fish showed attention toward the displaced individual. Lastly, we tested whether the released C. julis traveled a significantly longer distance than the beeline or the shortest way through the sandy patches, avoiding the territories of other individuals located in the patches. After testing that the differences of the sample pairs are normally distributed using the Shapiro-Wilk test, we used paired t-tests to compare the path length to the beeline and the shortest way through the sandy patch.
Results
Using the three repeated observations for each of the 10 tracked fish, we were able to obtain 27 SfM reconstructions and successfully reference them into a common reconstruction. 85.8% of all extracted images were reconstructed into this 3D scene (Figure 1B). The observations of two individuals (C. julis 1 and 8) were only partially reconstructed with 46.2% and 33.3% of the images, respectively. For the remaining individuals, the fraction of reconstructed images varied between 93% and 99.3%. Since both of the stereo-images needed to be reconstructed with the focal individual visible in both of them for a location to be successfully triangulated, the track coverage (percentage of time points with obtained 3D location) can be lower than the percentage of reconstructed images. The mean track coverage of the three observations per fish varied between a minimum of 27.6% (C. julis 1) and a maximum of 85.1% (C. julis 4), with an overall mean track coverage of 66.7%. See Figure 1C for a detailed 3D view of triangulated fish locations and Figure 2A for all locations embedded in the reconstruction of the sandy patches.
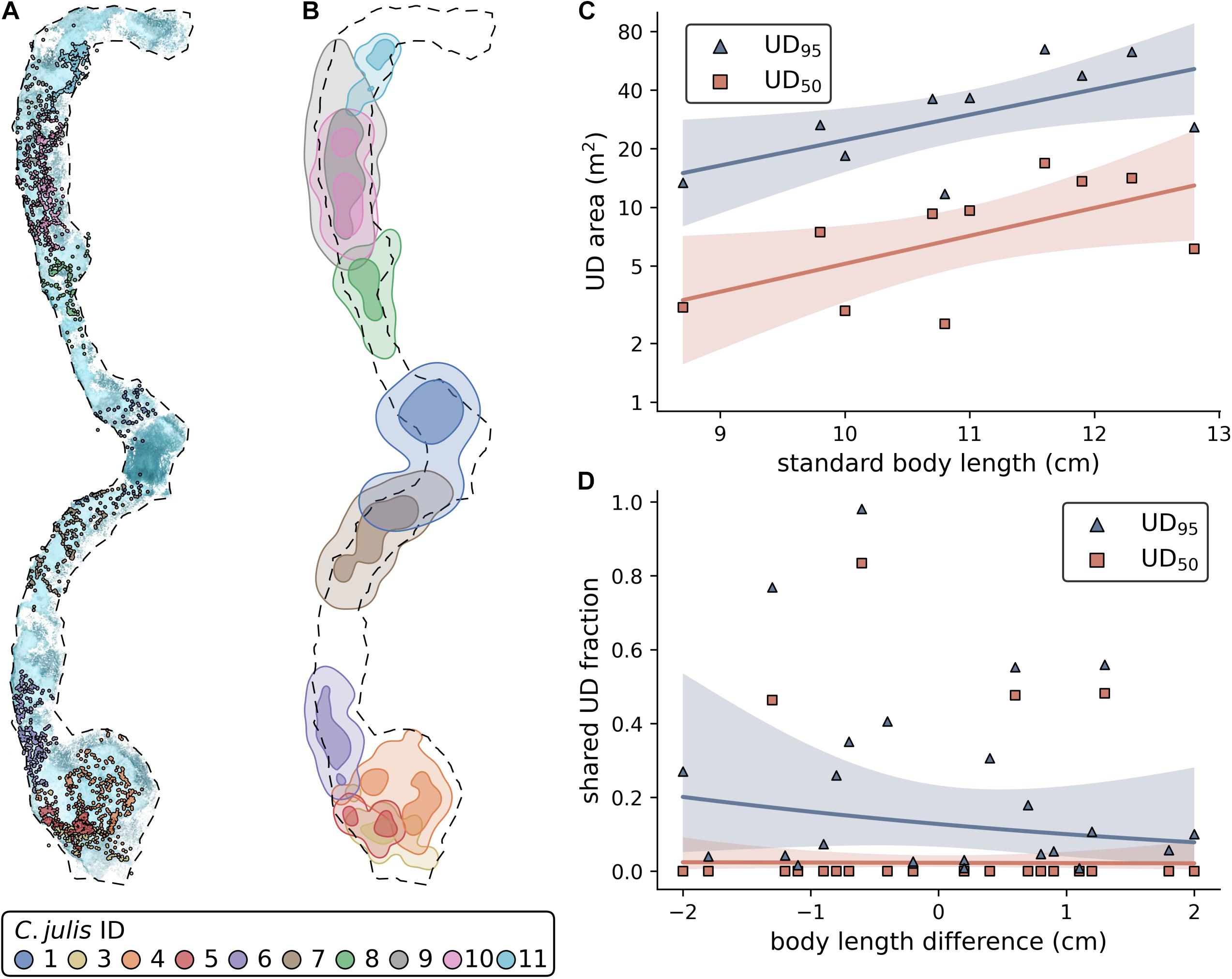
Figure 2. (A) All Coris julis locations embedded in the reconstruction of the sandy patches. The dashed outline represents a contour approximation of the sandy patches that was used to estimate the size of this area. (B) UD95 (light areas) and UD50 (darker areas) contours of each fish. (C) Effect of standard body length on UD area. Note the log-scale of the y-axis. (D) Effect of body length differences on the shared UD areas (shared fraction of focal fish area). (C,D) Solid lines represent non-significant model fits, shaded areas 90% confidence intervals.
Using the triangulated fish positions, we were able to estimate the UD95 and UD50 and shared areas for each fish (Figure 2B; Supplementary Tables 2, 4). The statistical models showed that fish size does not significantly affect UD95 (Figure 2C; estimate ± SE = 0.3 ± 0.13, t = 2.26, p = 0.054, N = 10) or UD50 (est. ± SE = 0.33 ± 0.16, t = 2.07, p = 0.073, N = 10). Further, we found that neither the shared fraction of UD95 or UD50 is affected by the pairwise size difference of the individuals (Figure 2D; UD95: est. ± SE = -0.271 ± 0.39, t = -0.696, p = 0.494, N = 24; UD50: est. ± SE = -0.319 ± 0.364, t = -0.088, p = 0.931, N = 24).
The behavioral scorings that were obtained with BORIS (Friard and Gamba, 2016) using the established ethogram (Table 1) and the displacement experiment recordings are summarized in Supplementary Table 5. With this data, we found that (i) the body length of the resident fish does not influence its aggression toward the presented individual (Figure 3A; overt behaviors: est. ± SE = 0.027 ± 0.023, t = 1.18, p = 0.28, N = 8; restrained behaviors: est. ± SE 0.004 ± 0.004, t = 1.026, p = 0.345, N = 8), (ii) the difference in body length between both fish does not influence the aggressive response of the resident fish (Figure 3B; overt behaviors: est. ± SE = 0.017 ± 0.016, t = 1.038, p = 0.339, N = 8; restrained behaviors: est. ± SE = 0.002 ± 0.003, t = 0.637, p = 0.5475, N = 8); and that (iii) neither UD95 nor UD50 of the resident fish have an effect on its aggressive response (Figures 3C,D; UD95: est. ± SE = 0.0008 ± 0.0027, t = 0.295, p = 0.778, N = 8; UD50: est. ± SE = 0.0027 ± 0.009, t = 0.283, p = 0.786, N = 8). Note that we could not successfully identify all individuals based on their elastomer tags during these experiments due to the distance between the cameras and the diver to the resident fish that was adhered to, minimizing the diver’s disturbance of the fish. Therefore, the sample size in these models was limited to 8 unique resident/presented fish pairs. Further, we were able to spatially map the behavioral interactions during the tracking observations, however, due to the small sample sizes within the different behaviors, we chose not to statistically analyse the relationship between behavior counts and C. julis density (Figure 3E).
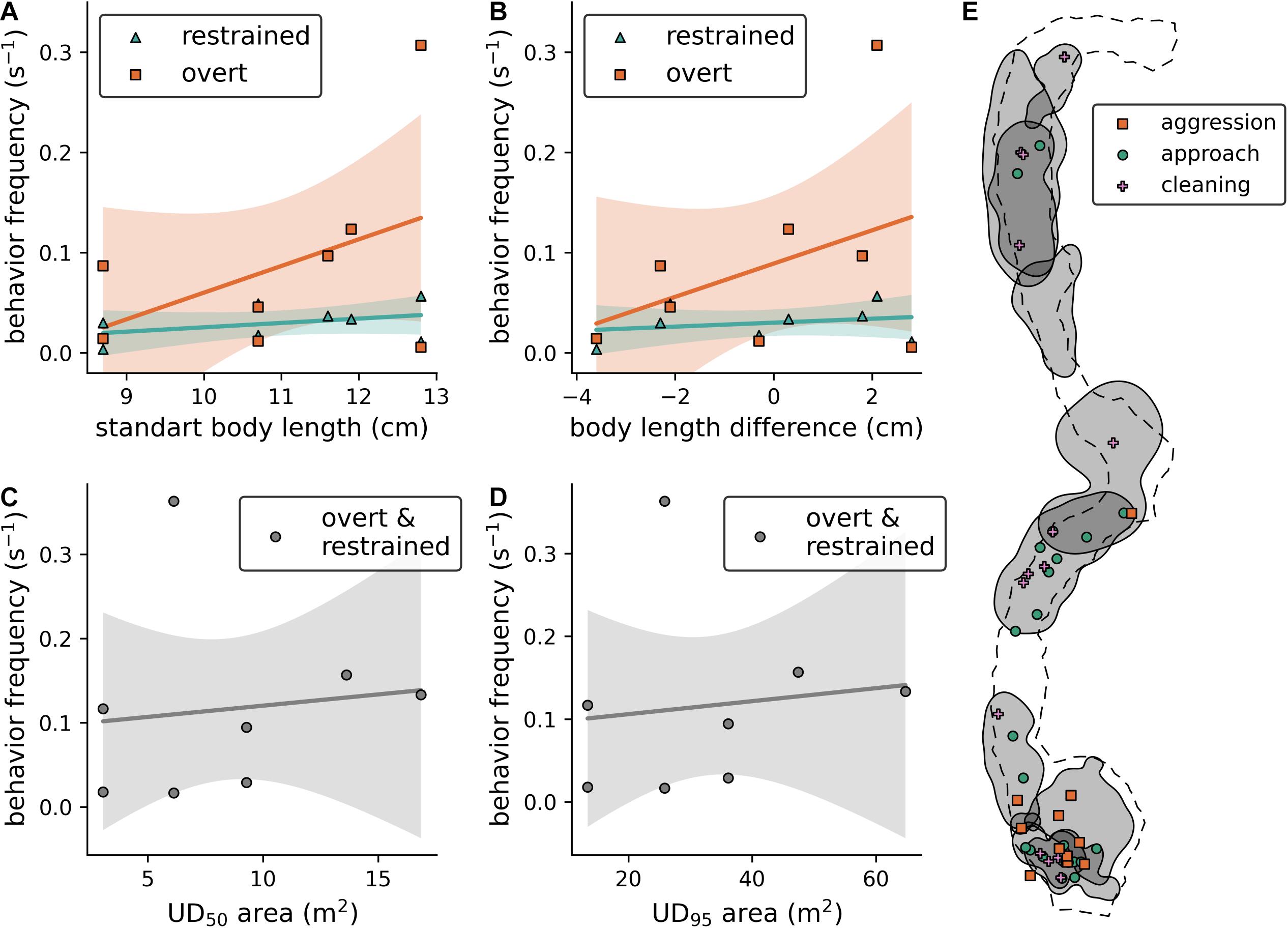
Figure 3. (A) Effect of resident fish size on the frequency of aggressive behaviors toward the presented intruder. (B) Effect of the size difference between resident and presented fish on the frequency of aggressive behaviors. (C,D) Effect of UD area (UD50 and UD95, respectively) on the frequency of aggressive behaviors (both overt and restrained). (A–D): Solid lines represent non-significant model fits, shaded areas 90% confidence intervals. (E) Visualization of UD95 contours (gray areas) with the locations of behavioral interactions of fish during the tracking observations.
The displacements were made at distances ranging from 30 to 46 m from each estimated core area. After the release from the transparent container, the fish took between 3.25 and 14.3 min to return to their respective home range, with average velocities of the fish varying from 0.09 m/s to 0.28 m/s (mean velocity 0.18 m/s, for more details see Supplementary Table 6). Coris julis 9 was lost directly after the release, but was found at its home range the day after. We manually mapped the path that the fish swam from their locations of displacement to their respective home ranges, and the interactions that they had during these observations (Figure 4A). All displaced individuals swam through the P. oceanica with little to no contact with the sand and other home ranges. Noticeably, all behavioral interactions between the returning C. julis and other individuals took place in the sandy patches. Using paired t-tests, we showed that the paths used by C. julis after they were released were significantly longer than the ‘beeline’ and the more direct paths over the sand (Figure 4B; “beeline”: p < 0.001, mean of differences = 26.6, N = 9; shortest path on sand: p < 0.001, mean of differences = 21.0, N = 9).
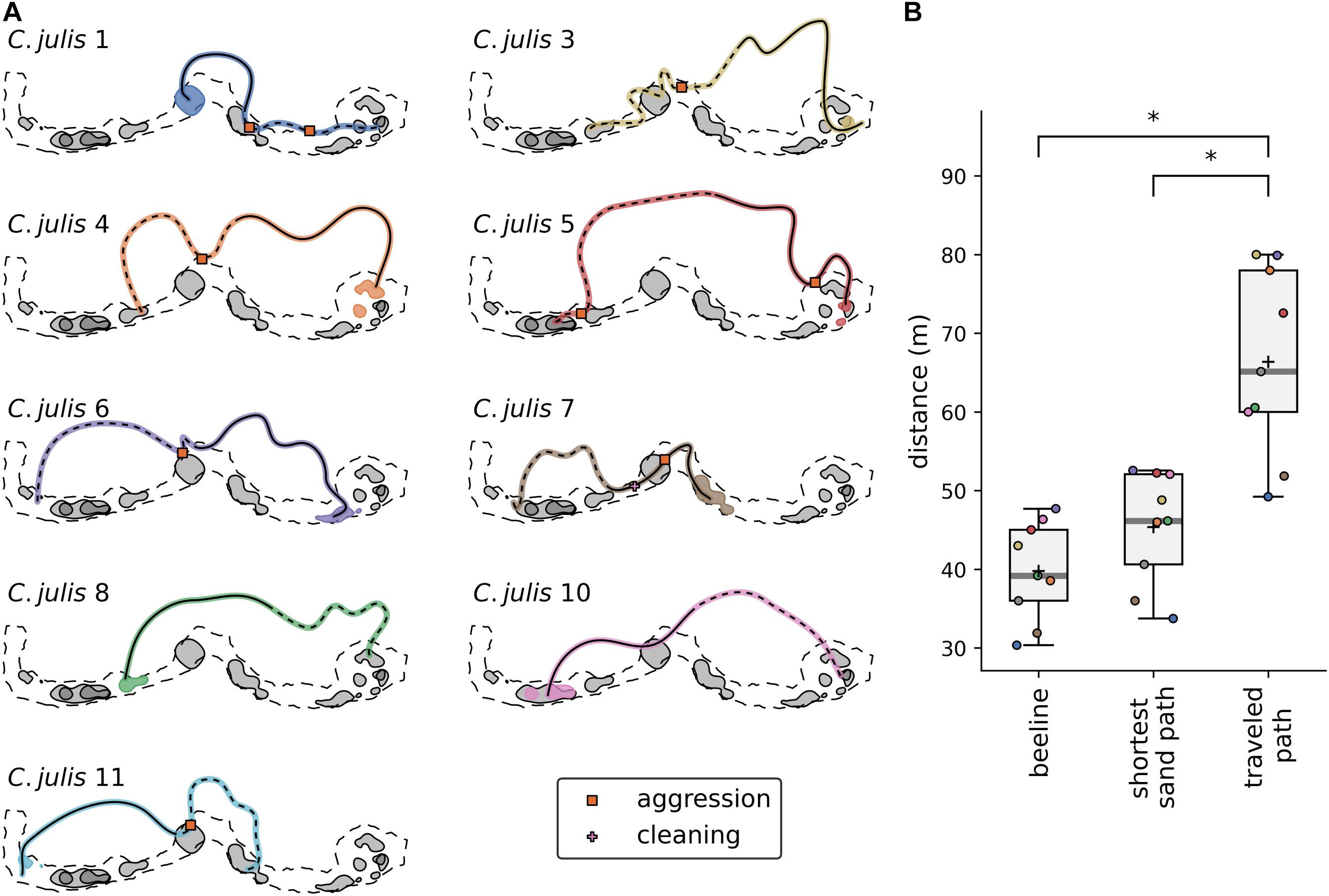
Figure 4. (A) Estimated paths of Coris julis after their release at the locations of the displacement experiments. The paths start with the dashed and end with the solid lines. Shaded areas represent the UD50 contours of each fish with the colored area marking the core area of the displaced individual. Coris julis 10 was lost directly after its release, so no path could be estimated. Note that visualizations were rotated 90°counter-clockwise. (B) Distances of the “beeline” (the Euclidean distance between the points of release and arrival), the shortest path on the sand (a path consisting of linear segments bounded within the sandy patch), and the estimated traveled path. Asterisks denote significant differences.
Discussion
Territoriality is an important aspect of animal behavior. Defending an area and therefore monopolizing its associated resources, such as food, cover, shelter or mates, may increase fitness if these resources are limiting. Territoriality is therefore not only expressed in the spatial relationship the individual has with its surroundings, but also the social relationship it has with its neighbors and intruders. In the case of C. julis, only second phase males were previously described as being territorial, potentially defending harems of females (Lejeune, 1987). Because of the potential site fidelity of females, we were also interested in testing whether females were territorial. In order to examine this apparent knowledge gap, we deployed a novel technique to measure the movement behavior of not only individual females, but also their interactions with neighbors and unknown conspecifics. Based on video imaging, this non-invasive method yielded highly detailed positional data and allowed the estimation of home ranges and territories. We were able to determine the home ranges and territories of ten female C. julis which were largely confined to sandy regions during our observations, in contrast to the more broadly ranging males. We showed that every individual returned to its home range when displaced, which took between 3 and 14 min. This result suggests these fish have good spatial memory or an existing cognitive map, although further experimental tests would be required to fully test for this possibility. Furthermore, we studied the social relationships that the individuals had with their neighbors and possible intruders. The interactions among neighbors were mostly non-aggressive, but when we presented a possible settler to territory holders, the behavioral response was overwhelmingly aggressive. This may be due to dear-enemy effects (Aires et al., 2015), providing further suggestions of long-term fixed territories in females of this species.
In addition, we found that the observed female C. julis were site attached. Each of the individuals was spotted within a relatively small, confined area in all of its respective observations. These areas were considered as home ranges and were estimated as UD95, varying between 11.7 and 64.8 m2, with a mean of 34.37 ± 19 m2. Most territories were of similar sizes and the observed C. julis barely left the sand (Figure 2B), as shown with the UD50 estimates, which range between 3 and 16.8 m2, with a mean of 8.6 ± 5 m2. A similar relationship was found in a previous study made with six different Caribbean wrasse species (Jones, 2005). Although C. julis has been reported to live on rocky bottoms or seagrass (Lejeune, 1987; Fruciano et al., 2011), our study suggests that the observed female individuals mostly live on sand. Presumably, sand is of importance because they feed during the day and dig themselves into the sand to rest during the night (Videler et al., 1986). Almost the entire area of the two sandy patches in our study site was part of an observed home range. We found only two spots in which no camera positions from the individual observations were reconstructed. In one case, we assume that it belongs to the home ranges of the adjacent individuals that were not completely reconstructed (missing 53.7% and 66.66% of the images, respectively, Supplementary Table 1). Structure-from-motion relies on a static background with detectable keypoints, and it is possible there was moving debris in this location, making it difficult to reconstruct. In the other case, only 2.6% and 0.74% of the adjacent territories did not get reconstructed, so this spot is either not habitable or already occupied by an individual that was not tagged and filmed.
From the video recordings, we not only calculated the home ranges and territories, but also scored the observed interactions (Figure 3E). Aggression was mainly observed in areas with at least two overlapping UD95, however, due to the small sample size we did not statistically test this potential effect of fish density. A general limitation of our study is the small sample size of observed individuals; although we aimed to tag and track the movement behavior of all female individuals in the experimental area, we only found 10 individuals that matched our target range of fish sizes within the sandy patches. We later observed one other female that also matched this range, but it is unclear whether it was a new settler in this area or if we missed it during the tagging dives. With this limited data, we did not find that larger individuals were significantly more aggressive toward intruders in the displacement experiments or that the aggressiveness of the resident depended on the size difference between the presented and the resident fish. The non-significant trend in the first case, however, implies that such an effect could exist if a larger sample of female C. julis with a broader range of body lengths were studied. In addition to aggressive interactions, we also observed cleaning behavior, which was only presented by juveniles (fish smaller than the ones targeted in this study) toward the focal fish. This substantiates the assumption that only juvenile C. julis are frequent cleaners in the Mediterranean (Vasco-Rodrigues and Cabrera, 2015). Some wrasses have fixed “cleaning stations,” for example Halichoeres cyanocephalus (Sazima et al., 1998) but our current dataset cannot fully investigate such site-specific cleaning behaviors in C. julis. However, cleaning was observed in almost every territory, and the spatial relationships between cleaning, territories and potential “cleaning stations” should be addressed in further research.
We found that body size does not significantly affect the UD95 or UD50, and that the shared fraction of the UD95 is not affected by the difference in body length of the individuals (Figures 2C,D). However, these results are likely to be influenced by the small sample size, as we only observed the interactions and territories of 10 individuals in this study. For example, it has been shown in a study on six different wrasse species that body length and territory size correlate (Jones, 2005). An alternative explanation for our results could be that territory size depends on the time and energy an animal has spent in a specific area, more than on its body size. For example, smaller individuals that hold a territory for a longer time might own larger territories than large individuals that have recently settled in a territorial neighborhood. Again, this is an area of fruitful future research. The territories (UD50) do not frequently overlap (Figures 2A,D), except between C. julis 3 and 5, and C. julis 9 and 10, where we observed almost complete overlaps in UD50. This indicates shared territories between these individuals and potential benefits from forming a social group within a territorial neighborhood. It might be less costly to cooperate at defending against intruders than to fight over the territory to decide which of the individuals keeps the area (Stamps, 1988). Close neighbors also might act as an early warning system to detect intruders [for example described in red-capped cardinals, Paroaria gularis (Eason and Stamps, 1993)]. However, it should be considered that initial phase males have the same phenotype as females, and thus, a small fraction of our focal rainbow wrasse are likely to be males. A previous study in the same study site showed that 14.7% of initial phase individuals were initial phase males, suggesting that even if some of the focal fish where initial phase males, the vast majority were likely to be females (Lejeune, 1987). This presents the alternative that the observed territory overlaps were the result of initial phase males courting females, although we did not observe courtship behavior between these individuals. Our observations were made in June and July, overlapping with the peak of sexual activity that was described to last from April to mid-September in C. julis (Lejeune, 1987). Furthermore, territories of initial phase males vary between 5 and 10 m2, which is in the range of area sizes that we estimated as territories (UD50, 8.6 ± 5 m2).
Summary
Here, we used a novel method to test for territoriality and to measure home ranges and territories in a highly quantitative manner based on video recordings and individual tracking. Our results show that female C. julis can be territorial, and that none of the observed fish changed its location throughout three consecutive trials in the course of seven days, demonstrating high site-specificity in this period. It is possible that at other periods of the day, or season, this species has different distributions for example outside of breeding periods, a possibility requiring further research attention. Interactions with neighbors were mostly non-aggressive, but territory holders defended their area against potential settlers. Moreover, when displaced, individuals immediately returned to their original territory, mostly avoiding territories of other individuals. When they did enter the territory of other individuals, they were frequently met with aggression. We assume that the observed female C. julis defended territories in sandy areas because they monopolize food resources and their presumed resting sites. Further, the territoriality of female C. julis during our observations suggests that they form harems in the broader territories of males. These two aspects are fruitful avenues for future research on how female C. julis use the habitat within their home ranges and which resources they defend inside their territories. When including other habitat types such as rocky reefs and considering the context of male territoriality, follow-up experiments could provide an in-depth description of the mating, homing and territorial behavior of one of the abundant species in the Mediterranean Sea using these approaches. This non-invasive, quantitative study of social interactions and movement in a small marine fish reveals the insight that can be gained in previously understudied systems, contributing further understanding species social systems, relationships with their environment, and ultimately, effective conservation measures.
Data Availability Statement
The original contributions presented in the study are included in the article/Supplementary Material, further inquiries can be directed to the corresponding author.
Ethics Statement
Ethical review and approval was not required for the animal study because all research was conducted under the general scientific permit of the STARESO Marine Station.
Author Contributions
ZG, PN, and AJ designed experiments and wrote the manuscript. ZG performed experiments and filming in the field. ZG and PN performed tracking and performed statistical analysis. All authors contributed to the article and approved the submitted version.
Funding
This research was funded by the Deutsche Forschungsgemeinschaft (DFG, German Research Foundation) under Germany’s Excellence Strategy – EXC 2117 – 422037984 and by the Max Planck Institute of Animal Behavior.
Conflict of Interest
The authors declare that the research was conducted in the absence of any commercial or financial relationships that could be construed as a potential conflict of interest.
Publisher’s Note
All claims expressed in this article are solely those of the authors and do not necessarily represent those of their affiliated organizations, or those of the publisher, the editors and the reviewers. Any product that may be evaluated in this article, or claim that may be made by its manufacturer, is not guaranteed or endorsed by the publisher.
Acknowledgments
We thank members of the Jordan lab for many fruitful discussions. We thank Myriam Knöpfle, Jakob Gübel, and Karina Weiler for their assistance in the field. We also thank the staff at STARESO Marine Station, in particular Cyril Steibel, for kindly supporting our research in the field.
Supplementary Material
The Supplementary Material for this article can be found online at: https://www.frontiersin.org/articles/10.3389/fmars.2021.695100/full#supplementary-material
References
Adams, E. S. (2001). Approaches to the study of territory size and shape. Annu. Rev. Ecol. Syst. 32, 277–303. doi: 10.1146/annurev.ecolsys.32.081501.114034
Ågren, G., Zhou, Q., and Zhong, W. (1989). Ecology and social behaviour of Mongolian gerbils, Meriones unguiculatus, at Xilinhot, Inner Mongolia, China. Anim. Behav. 37, 28–32. doi: 10.1016/0003-3472(89)90003-1
Aires, R. F., Oliveira, G. A., Oliveira, T. F., Ros, A. F. H., and Oliveira, R. F. (2015). Dear enemies elicit lower androgen responses to territorial challenges than unfamiliar intruders in a cichlid fish. PLoS One 10:e0137705. doi: 10.1371/journal.pone.0137705
Balzarini, V., Taborsky, M., Wanner, S., Koch, F., and Frommen, J. G. (2014). Mirror, mirror on the wall: the predictive value of mirror tests for measuring aggression in fish. Behav. Ecol. Sociobiol. 68, 871–878. doi: 10.1007/s00265-014-1698-7
Bentivegna, F., Cirino, P., and Rasotto, M. B. (1985). Further investigations into sex reversal of Coris julis L. (Pisces, Labridae). Bolletino di Zool. 52, 355–358. doi: 10.1080/11250008509440539
Bertoncini, ÁA., MacHado, L. F., Barreiros, J. P., Hostim-Silva, M., and Verani, J. R. (2009). Cleaning activity among Labridae in the Azores: the rainbow wrasse Coris julis and the Azorean blue wrasse Centrolabrus caeruleus. J. Mar. Biol. Assoc. U.K. 89, 859–861. doi: 10.1017/S002531540900040X
Bronstein, P. M. (1986). Socially mediated learning in male betta splendens. J. Comp. Psychol. 100, 279–284. doi: 10.1037/0735-7036.100.3.279
Bujalska, G., and Saitoh, T. (2000). Territoriality and its consequences. Polish J. Ecol. 48, 37–49.
Calenge, C. (2006). The package “adehabitat” for the R software: a tool for the analysis of space and habitat use by animals. Ecol. Modell. 197, 516–519. doi: 10.1016/j.ecolmodel.2006.03.017
Di Franco, A., Plass-Johnson, J. G., Di Lorenzo, M., Meola, B., Claudet, J., Gaines, S. D., et al. (2018). Linking home ranges to protected area size: the case study of the Mediterranean Sea. Biol. Conserv. 221, 175–181. doi: 10.1016/j.biocon.2018.03.012
Dugatkin, L. A., Reeve, H. K., and Mangel, M. (1998). Game theory and animal behaviour. Nature 395:32.
Eason, P. K., and Stamps, J. A. (1993). An early warning system for detecting intruders in a territorial animal. Anim. Behav. 46, 1105–1109. doi: 10.1006/anbe.1993.1300
Francisco, F. A., Nührenberg, P., and Jordan, A. (2020). High-resolution, non-invasive animal tracking and reconstruction of local environment in aquatic ecosystems. Mov. Ecol. 8:27. doi: 10.1186/s40462-020-00214-w
Friard, O., and Gamba, M. (2016). BORIS: a free, versatile open-source event-logging software for video/audio coding and live observations. Methods Ecol. Evol. 7, 1325–1330. doi: 10.1111/2041-210X.12584
Fruciano, C., Tigano, C., and Ferrito, V. (2011). Geographical and morphological variation within and between colour phases in Coris julis (L. 1758), a protogynous marine fish. Biol. J. Linn. Soc. 104, 148–162. doi: 10.1111/j.1095-8312.2011.01700.x
Gladstone, W. (1987). Role of female territoriality in social and mating systems of Canthigaster valentini (Pisces: Tetraodontidae): evidence from field experiments. Mar. Biol. 96, 185–191. doi: 10.1007/BF00427018
Hussey, N. E., Kessel, S. T., Aarestrup, K., Cooke, S. J., Cowley, P. D., Fisk, A. T., et al. (2015). Aquatic animal telemetry: a panoramic window into the underwater world. Science 348:1255642. doi: 10.1126/science.1255642
Jones, K. M. M. (2005). Home range areas and activity centres in six species of caribbean wrasses (Labridae). J. Fish Biol. 66, 150–166. doi: 10.1111/j.0022-1112.2005.00589.x
Kadota, T., Osato, J., Hashimoto, H., and Sakai, Y. (2011). Harem structure and female territoriality in the dwarf hawkfish Cirrhitichthys falco (Cirrhitidae). Environ. Biol. Fishes 92, 79–88. doi: 10.1007/s10641-011-9817-z
Kamler, J. F., Ballard, W. B., Fish, E. B., Lemons, P. R., Mote, K., and Perchellet, C. C. (2003). Habitat use, home ranges, and survival of swift foxes in a fragmented landscape: conservation implications. J. Mammal. 84, 989–995. doi: 10.1644/BJK-033
Lederhouse, R. C. (1982). Territorial defense and lek behavior of the black swallowtail butterfly, Papilio polyxenes. Behav. Ecol. Sociobiol. 10, 109–118. doi: 10.1007/BF00300170
Lejeune, P. (1987). The effect of local stock density on social behavior and sex change in the mediterranean labrid Coris julis. Environ. Biol. Fishes 18, 135–141. doi: 10.1007/BF00002601
Linde, M., Palmer, M., and Alós, J. (2011). Why protogynous hermaphrodite males are relatively larger than females? Testing growth hypotheses in mediterranean rainbow wrasse Coris julis (Linnaeus, 1758). Environ. Biol. Fishes 92, 337–349. doi: 10.1007/s10641-011-9844-9
Monk, C. T., Barbier, M., Romanczuk, P., Watson, J. R., Alós, J., Nakayama, S., et al. (2018). How ecology shapes exploitation: a framework to predict the behavioural response of human and animal foragers along exploration–exploitation trade-offs. Ecol. Lett. 21, 779–793. doi: 10.1111/ele.12949
Moore, J. A., Daugherty, C. H., and Nelson, N. J. (2009). Large male advantage: phenotypic and genetic correlates of territoriality in tuatara. J. Herpetol. 43, 570–578. doi: 10.1670/08-290.1
Nicholls, D. G., Robertson, C. J. R., and Naef-Daenzer, B. (2005). Evaluating distribution modelling using kernel functions for northern royal albatrosses (Diomedea sanfordi) at sea off South America. Notornis 52:223.
Ostfeld, R. S. (1985). Limiting resources and territoriality in microtine rodents. Am. Nat. 126, 1–15. doi: 10.1086/284391
Pearl, M. C. (2000). Research Techniques in Animal Ecology. New York, NY: Columbia University Press.
Powell, R. (2000). “Animal home ranges and territories and home range estimators,” in Research Techniques in Animal Ecology: Controversies and Consequences, eds M. C. Pearl, L. Boitani, and T. Fuller (New York, NY: Columbia University Press), 65–110.
Sazima, I., Moura, R. L., and Luiz Gasparini, J. (1998). The wrasse Halichoeres cyanocephalus (Labridae) as a specialized cleaner fish. Bull. Mar. Sci. 63, 605–610.
Schönberger, J. L., and Frahm, J. M. (2016). “Structure-from-motion revisited,” in Proceedings of the IEEE Computer Society Conference on Computer Vision and Pattern Recognition (CVPR) (Las Vegas, NV: IEEE), 4104–4113. doi: 10.1109/CVPR.2016.445
Schönberger, J. L., Zheng, E., Pollefeys, M., and Frahm, J.-M. (2016). “Pixelwise view selection for unstructured multi-view stereo,” in Proceedings of the 14th European Conference on Computer Vision (ECCV), Amsterdam.
Stamps, J. A. (1988). Conspecific attraction and aggregation in territorial species. Am. Nat. 131, 329–347. doi: 10.1086/284793
Thys, T. M., Ryan, J. P., Dewar, H., Perle, C. R., Lyons, K., O’Sullivan, J., et al. (2015). Ecology of the ocean sunfish, mola mola, in the southern California current system. J. Exp. Mar. Bio. Ecol. 471, 64–76. doi: 10.1016/j.jembe.2015.05.005
Vasco-Rodrigues, N., and Cabrera, P. M. (2015). Coris julis cleaning a mola mola, a previously unreported association. Cybium 39, 315–316.
Videler, J. J., Koella, W. P., Obál, F., and Schulz, H. (1986). “Sleep under sand cover of the labrid fish Coris julis,” in Sleep, eds W. Koella, F. Obal, H. Schulz, and P. Visser (Stuttgart, FRG: Fischer), 145–147.
Worton, B. J. (1989). Kernel methods for estimating the utilization distribution in home- range studies. Ecology 70, 164–168. doi: 10.2307/1938423
Keywords: territory, range, social, marine, wrasse
Citation: Goverts Z, Nührenberg P and Jordan A (2021) Environmental Reconstruction and Tracking as Methods to Explore Social Interactions in Marine Environments: A Test Case With the Mediterranean Rainbow Wrasse Coris julis. Front. Mar. Sci. 8:695100. doi: 10.3389/fmars.2021.695100
Received: 14 April 2021; Accepted: 28 June 2021;
Published: 23 July 2021.
Edited by:
David M. P. Jacoby, Zoological Society of London, United KingdomReviewed by:
Josep Alos, Mediterranean Institute for Advanced Studies (IMEDEA), SpainMichele Gristina, National Research Council (CNR), Italy
Copyright © 2021 Goverts, Nührenberg and Jordan. This is an open-access article distributed under the terms of the Creative Commons Attribution License (CC BY). The use, distribution or reproduction in other forums is permitted, provided the original author(s) and the copyright owner(s) are credited and that the original publication in this journal is cited, in accordance with accepted academic practice. No use, distribution or reproduction is permitted which does not comply with these terms.
*Correspondence: Alex Jordan, YWpvcmRhbkBhYi5tcGcuZGU=