- 1Coastal Geology Laboratory, Department of Oceanography, Texas A&M University at Galveston, Galveston, TX, United States
- 2Department of Marine and Coastal Environmental Science, Texas A&M University at Galveston, Galveston, TX, United States
The winds associated with the passage of meteorological fronts cause waves that induce sediment remobilization/resuspension, especially within shallow estuaries such as Galveston Bay. The passage of cold fronts, collectively, on an annual to decadal basis, generate more sediment resuspension than most hurricanes and tropical storms. With a warming climate, the intensity of all meteorological events is shifting toward having greater impacts on these biologically productive environments. To better understand sediment resuspension within the bay, water samples were collected during frontal passages at two locations in Galveston Bay, including one location in the middle portion of the bay and another closer to the mouth of the bay. By collecting precipitation, water samples in both the middle and lower bay, and measuring the ratio of 7Be/210Pbxs in these samples; we quantified the residence times of total suspended sediment (TSS) in middle and lower Galveston Bay. Our results showed that suspended sediment age increased and percent of new suspended sediment decreased along the axis from the middle bay to the lower bay. This results from the initial introduction of newly labeled isotopes and suspended load coming from fluvial discharges which enter at the top of the bay and travel through the bay. The age of suspended sediment from the first sampling event was 70 ± 10 days, whereas the age in the second event was 16 ± 3 days greater. In the last sampling event, the age of suspended sediment event was 35 ± 7.4 days younger than the second, suggesting that the majority of suspended sediments was likely transported entirely out of the bay by the second cold front, prior to the final sampling event. This indicates that there are longer suspended sediment residence times when the water is trapped within the bay. Our estimated residence time of suspended sediments (51–105 days) suggest the particle-bound contaminants adsorb to suspended sediment may spend months suspended in the bay before exiting the bay or being accreted into the bay sediment column, increasing the exposure time of living organisms to various particle-bound contaminants.
Introduction
Sediment remobilization/resuspension plays a role in many estuarine processes; especially associated with the cycling of nutrients and pollutants in and out of an estuary (Baskaran and Santschi, 1993). Wind-induced currents and wave resuspension are important sources of energy for sediment transport within an ecosystem and can be dominant in shallow, microtidal estuaries, affecting a large portion, if not all of the water column (Booth et al., 2000). Sediment resuspension associated with strong weather events can reintroduce trace elements and pollutants back into the water column, which can have significant environmental impacts in an estuary (e.g., Dellapenna et al., 2006, 2020). These constituents can be stored in the sediment during periods of sediment deposition and are resuspended during wind-induced wave resuspension, in addition to any other resuspension events (Dellapenna et al., 2006). Particle reactive contaminants generally move slowly through an estuarine system, transported through the innumerous cycles of deposition and resuspension during various hydrological stages (Saari et al., 2010). Therefore, evaluating how long such innumerous cycles last will help us better monitor the transport of suspended sediments and their associated pollutants and trace elements, such as examining the residence time of suspended sediment, defined as the average length of time during which the sediment resides within the bay as suspended sediment.
Two naturally occurring radionuclides, i.e., beryllium-7 (7Be) and lead-210 (210Pb) can be paired and used to quantify the time that the particles surfaces sorbed the isotopes from the water column, which occurs when the particle became suspended in the water column (Matisoff et al., 2005). 7Be is a cosmogenic radionuclide produced in both the stratosphere and troposphere as a result of cosmic ray spallation of nitrogen and oxygen (Brost et al., 1991). Following atmospheric fallout, 7Be rapidly adsorbs to fine sediment particles (Taylor et al., 2013). Similar to 7Be, atmospherically derived 210Pb (210Pbxs), produced from the decay of its parent nuclide, radon-222, are also delivered to the waters via wet and dry fallout. Fallout patterns of 7Be and 210Pb are tightly correlated leading to these nuclides being useful for dependent tracers (Baskaran et al., 1993). Once radionuclides adsorb to sediment particles, they are strongly and nearly irreversibly bound to these particles, making it possible to study the movement and obtain the age of suspended sediment (Taylor et al., 2013). Short half-lives for 7Be (t1/2 = 53 days) relative to 22.3 years for 210Pb not only provide an advantage when considering recent events (e.g., winter cold front) that cause sediment redistribution (Taylor et al., 2013), but also allow us to use the ratio of 7Be /210Pbxs to quantify the proportion of resuspended bottom material in the water column, e.g., higher values indicating younger particles (Olsen et al., 1989).
Galveston Bay, the second largest estuarine system in Texas, United States, is a shallow and microtidal estuary, with an average depth of 2.1 m and water residence time of ∼40 days (Solis and Powell, 1999). The surrounding area of Galveston Bay are heavily industrialized, dominated by petroleum, petrochemical, and chemical industries, collectively hosting nearly 50% of total United States chemical production and oil refineries (Santschi et al., 2001). This makes Galveston Bay an important area to investigate sediment dynamics. The purpose of the present study is to understand the relationship between meteorological fronts, sediment resuspension and suspended sediment residence times. In the context of this study, we operationally define suspended sediment residence time as the amount of time a short-lived radio-isotope labeled sediment particle is actively involved within the water column sediment load prior to either being incorporated into the seabed or exported from the bay. These particles may be resuspended from the bay bottom and newly radio-isotope labeled or it may have been advected into the bay either from offshore or from the drainage basin.
Along the northern Gulf of Mexico, most meteorologically driven sediment resuspension occurs during the passage of northern cold fronts which mainly occur during winter months (Henry, 1979; Hardy and Henderson, 2003). Besides, meteorological events are necessary to collect precipitation along with suspended sediments as cold fronts are accompanied by precipitation and greater wind speeds, which allow us accessible to the near-bottom suspended sediments and their associated 7Be/210Pbxs. Therefore, together with rainwater samples, water samples in the middle and lower Galveston Bay during each weather event were collected at early 2020 for the measurement of 7Be and 210Pbxs to test and apply 7Be/210Pb pair to quantify the residence times of suspended sediment in the bay. We hypothesize that the age of the sediment should be younger in mid-Galveston Bay and older toward the mouth of Galveston Bay. Additionally, with the abundance of clay dominated mud in the middle bay and coarser mud (e.g., higher silt content, with sand) in the lower bay, total suspended sediment (TSS) concentrations will be higher in middle Galveston Bay versus closer to the mouth, allowing for deposition of coarser mud to occur at a faster rate than finer muds. Understanding physical processes, such as sediment resuspension and residence times, allows for proper management strategies to be developed to ensure a stable and productive ecosystem in the estuary (Walker and Hammack, 2000).
Materials and Methods
Background
Galveston Bay is a shallow, microtidal estuary and is the second largest estuarine system in Texas with a surface area of approximately 1,360 km2 (Dellapenna et al., 2006). The average depth of the bay is 2.1 m and contains a ship channel with dimensions of 150 m in width and 10–15 m deep, oriented along the main axis of the bay (50 km long) (Du and Park, 2019). The exchange of tidal water flows through Bolivar Roads, which is the tidal inlet between Bolivar Peninsula and Galveston Island. An additional inlet exists 47 km to the west and provides gulf flow into Christmas Bay and the western half of West Galveston Bay. Average water residence time within Galveston Bay is approximately 40 days (Solis and Powell, 1999). Trinity Bay comprises the northeastern portion of Galveston Bay (Figure 1), has depths generally ranging between 3 and 4 m (Dellapenna et al., 2006) and the Trinity River flows into the head northeastern end of the bay, The Trinity River accounts for approximately 90% of the freshwater input and is the largest sediment source into Galveston Bay [United States Geological Survey [USGS], 2005]. Another significant sediment load within the bay is the ongoing maintenance of the Houston Ship Channel. In waters deeper than 1.5 m, Trinity Bay bottom sediment is mud dominated (approx. 40% of total bay area) (Dellapenna et al., 2006). Mud is the dominant sediment composition of the majority of the Galveston Bay system (Figure 1).
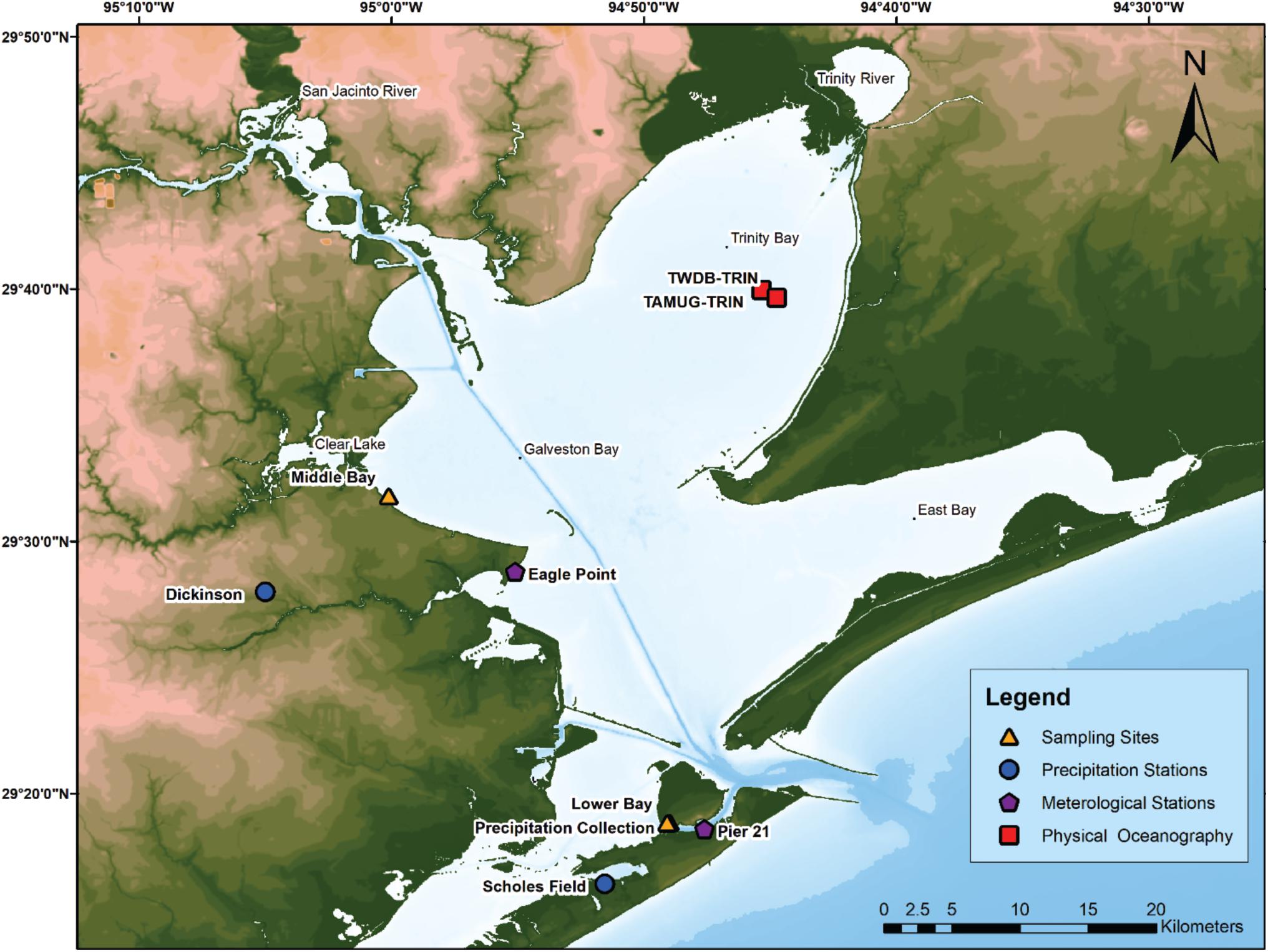
Figure 1. Map of Study Area and Sampling Stations, including Meteorological Stations (pentagon), Precipitation stations (circle), suspended sediment/precipitation sampling sites (triangle), and physical oceanography sites (square).
The watershed of Galveston Bay contains both metropolitan Houston as well as the Clear Lake-Texas City-Galveston area and the Port of Houston. Houston, Texas, is the fifth-largest metropolitan area (population of 7 million), is the fourth-largest city in the United States, and hosts the second-largest petrochemical complex in the world (Morse et al., 1993; Santschi et al., 2001). The Port of Houston is the second-largest seaport in the United States in terms of total shipping tonnage (Chambers et al., 2018) and services the 80 km long Houston Ship Channel, which extends up the axis of Galveston Bay from Bolivar Roads at its entrance to the San Jacinto Estuary and Buffalo Bayou. Further south of Houston is the Clear Lake-Texas City-Galveston area which is also heavily industrialized, dominated by petroleum, petrochemical, and chemical industries as well as shipyards in Galveston. Collectively, the shores and watershed of Galveston Bay host nearly 50% of total United States chemical production and oil refineries (Santschi et al., 2001). Galveston Bay also receives a significant amount of wastewater discharges for the state from surrounding facilities. Galveston Bay provides nursing habitat for multiple valuable fisheries, including white and brown shrimp (Stunz et al., 2010) and provides approximately 14% of the United States wild catch of oysters (Haby et al., 2009). A key process in shallow estuaries is the frequency of sediment resuspension. An increased amount of sediment resuspension and deposition in an estuary may cause smothering of benthic aquatic organisms and the clogging of water intakes (Winterwerp and Van Kesteren, 2004).
Sample Collection
Sampling occurred during three meteorological fronts from January 2020 to February 2020. 60–80 L of bay waters in two sites of the Galveston Bay (Figure 1), depending upon the turbidity of bay waters, were sampled within 1–2 days after each storm event, conducted by attaching a bilge pump to a 4 m long, 3.81 cm diameter aluminum pole, with the bilge pump being mounted 30 cm above the bottom of the pole to prevent penetration into the sediment while still collecting suspended sediment from the bottom-water. The pump rate of the bilge pump is about 1.5 L/min, which is slow enough that additional bottom sediment was not eroded during sample collection. This flow rate calculation was based upon information provided by the bilge pump company; therefore, the rate may be slower with the hose being attached to the pump and the distance required to pump water into the carboys.
Aliquots of the samples were filtered in the lab through a 0.45 μm polycarbonate filter for the measurement of total suspended particle (TSS) concentrations. The remaining samples were placed on the bench for a few days until the water is visibly clear of particles to allow the suspended particles sinking to the bottom of the containers, followed by the centrifugation to separate water from suspended sediments. Collected particulate matter from the centrifugation were dried in an oven at 50°C. The dried particles were grounded and transferred into the gamma counting tubes for the measurement of 7Be and 210Pb.
Precipitation was collected on the roof of the Ocean and Coastal Studies Building (OCSB) at Texas A&M Galveston Campus, where was close to the lower bay water sampling site (Figure 1). Although there is a distance of 30 km between middle bay water sampling site and precipitation sampling location, the 7Be/210Pb ratios in precipitation vary little in one study area (Baskaran et al., 1993; Koch et al., 1996), regardless of variable precipitation amounts between sites. The rainwater was collected using a 20 L jug with a large funnel attached to the opening along with a 2 L bucket of water next to it for more collection. This was deployed before each rain event (Supplementary Table 1). At the end of each storm event, the collected rainwater in all containers will be combined for the extraction and analysis of 7Be and 210Pb (Section “Measurements of 7Be and 210Pb”). Activity measurements of 210Pb and 7Be from rainwater is necessary to perform the Matisoff method, to determine the “initial” age of the two radionuclides for each frontal passage event.
Measurements of 7Be and 210Pb
For the rainwater samples, 7Be and 210Pb were be extracted from the collected precipitation based on the published method from Olsen et al. (1985) and Wang et al. (2013). After the adjustment of pH to < 2, a certain amount of Fe3+ carrier solution (FeCl3, 5 mg Fe per L of sample) is added under stirring. After homogenization and equilibration for overnight, pH is adjusted to 9 with ammonia solution to precipitate the iron, and Fe(OH)3 containing 7Be and 210Pb are left to stand overnight. The Fe(OH)3 precipitate will be collected through centrifugation and dissolved by 3M HCl solution. Extracted sample from precipitation and the ground sediments from bay waters will be placed into gamma counting tubes for analysis by a Canberra ultrahigh-purity germanium well gamma detector at the decay energies of 46.5 kev for 210Pb and 477.6 kev for 7Be. The sediment samples will be counted again after 3 weeks to allow secular equilibrium ingrowth of gaseous 222Rn from the decay of 226Ra, the parent nuclide of 210Pb. The supported 210Pb will be determined from the activity of the 214Bi from the 222Rn, at the decay energy of 609.3 kev. The atmospherically derived 210Pb in suspended sediments (210Pbxs) will be determined based on the difference between total activity of 210Pb and the supported 210Pb.
All samples will be counted for enough time to obtain the counting errors < 10%. Counting efficiencies will be determined, using the standards that are prepared with the same geometries as the samples. Activities concentrations of 7Be and 210Pbxs will be decay-corrected to the date of collection before the calculation of residence times of suspended sediments or the percentage of newly labeled sediments/particles.
Estimate of Residence Time and Percentage of Newly Labeled Sediments/Particles of Suspended Sediments
Due to the significant difference in half-life between two radionuclides (53.3 days for 7Be vs. 22.3 years for 210Pb) and their strong binding to particles after their delivery from the atmosphere to the surface, the ratio of 7Be over the atmospherically derived 210Pb (210Pbxs) can be an indicator of the extent of mixing of freshly derived (7Be enriched) suspended sediment with old (7Be deficient) sediment resuspended from the bed of the Galveston Bay. Therefore, the 7Be/210Pbxs ratio in the atmospheric deposition during the storm events is defined as the atmospheric tag received by the “newly tagged sediment” in the Galveston Bay (Matisoff et al., 2005). Based on the variability of 7Be/210Pbxs ratio in suspended sediments relative to the atmospheric 7Be/210Pbxs ratio, the residence time or the age of suspended sediments can be estimated, across the middle Galveston Bay to the lower bay, based on the following equation:
where T is the age of suspended sediment; A and B is the activity concentration of 7Be and 210Pb in the suspended sediments, respectively; Ao and Bo is the activity concentration of 7Be and 210Pb in the precipitation, respectively.
On the other hand, the 7Be/210Pbxs ratio can be alternatively used to estimate the percentage “new” particles in suspended sediments, which is defined as the sediment particles that have a 7Be/210Pbxs ratio equal to precipitation, as following shown:
Errors are provided throughout the manuscript for residence times and percentage of “new” particles. These are based on errors associated with the radioisotope counting method, addressed in Section “Measurements of 7Be and 210Pb.”
Results and Discussion
7Be/210Pbxs Ratios in Precipitation and Suspended Sediments
Activities of 210Pbxs and 7Be, shown in Table 1, were obtained for suspended sediment samples in both middle and lower bay along with precipitation samples during three sampling events. Generally, 7Be activities in all samples were higher than 210Pb. During January sampling, activities of 7Be and 210Pbxs were both higher in the lower bay than those in the middle bay, whereas, during late February sampling, the opposite occurred. The highest activities of 7Be for both bay waters and precipitation can be seen during middle February sampling (Table 1), which also coincides with an ebb tide. The ebb tide water mass is derived from upper Galveston Bay within estuarine tributaries, where this water is in more direct contact with drainage basin derived water. Therefore, newly deposited radioisotopes are rich, especially enriched in 7Be within this water mass. In contrast, the flood tide water mass is marine derived, only receiving drainage basin water after mixing with the ebb tidal water mass. For 210Pbxs, compared to the bay water samples, precipitation samples had lower 210Pbxs activities for all sampling events, ranging from 17 ± 1.5 Bq/kg for late February to 44 ± 5.2 Bq/kg for early February (Table 1). This could be due to the entirety of 210Pb flux in rainfall being solely from wet fallout, whereas in suspended sediment samples 210Pb becomes remobilized from bottom sediment (Baskaran et al., 1993). Overall, activities of both radionuclides fluctuated throughout sampling events at all locations.
For the 7Be/210Pb ratios of precipitation, the maximum value (11 ± 1.2) was detected at middle February 2020 (Table 2), where the greatest amount of rainfall was collected, but not recorded at Scholes Field (Supplementary Table 2). January samples had a close ratio (9.4 ± 0.8) to that collected in middle February 2020, but there was less rain collected and higher rainfall measurement from Scholes Field. The lowest 7Be/210Pb ratio in precipitation samples was found in late February 2020 (3.7 ± 0.4, Table 2), which had the least amount of rainfall collected for sampling. When looking at the activities in precipitation of all three events, the lowest 7Be/210Pb ratio in precipitation samples at late February were mostly caused by its low 7Be activity, showing more than 5 times decreasing activity concentrations compared with first two storms (Figure 2 and Table 1). This may be due to the relatively low precipitation amounts and probably weak stratosphere/troposphere exchange (Duenas et al., 2002) during our late February sampling period. Nevertheless, our reported 7Be/210Pb ratios of precipitation and its temporal change in the short period were comparable to and consistent with some previous observation in other study area (2–16, Caillet et al., 2001) or in the Galveston Bay, reported by Baskaran et al. (1993), which 7Be/210Pb ratios in precipitation ranged from 8.6 to 15.4 on average between Jan and March (1990−1992).

Table 2. 7Be/210Pbxs ratios ± standard error calculated from activities of precipitation, suspended sediment samples with calculated suspended sediment age and percentage new sediment.
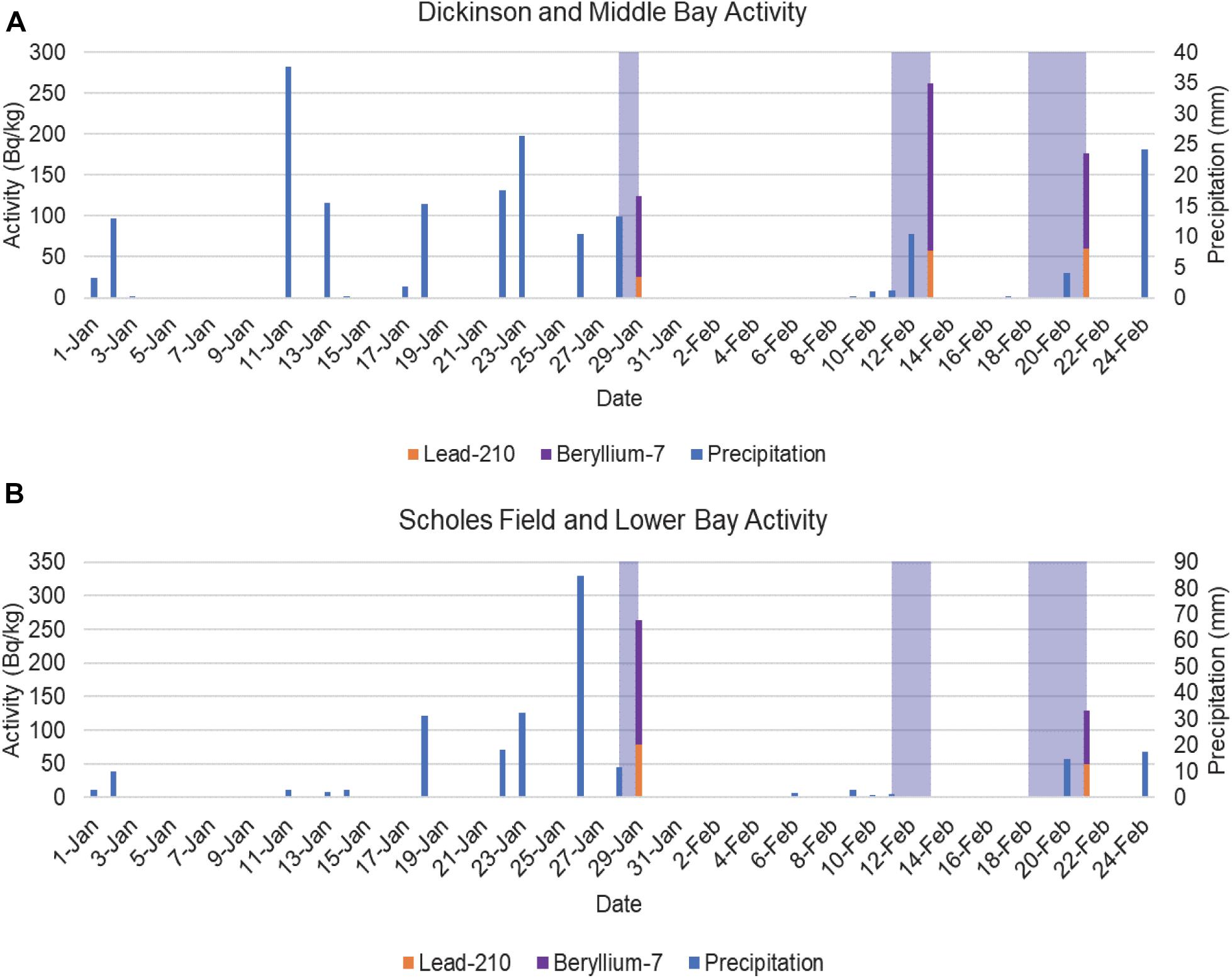
Figure 2. Rainfall vs. Isotope Activity. Rainfall data (blue) from Dickinson (A) and Scholes Field (B) Station (NWS) and Isotopic Activity of 210Pb (orange) and 7Be (purple) collected at the middle and lower bay site. Shading represents our precipitation sampling period.
When considering the variations in the 7Be/210Pbxs ratios in suspended sediment (Table 2), the January sample had a ratio of 3.8 ± 0.5 for the middle bay, higher than that (2.4 ± 0.4) in the lower bay. In contrast, for the late February samples, the middle and lower bay had relatively closer 7Be/210Pbxs ratios in suspended sediments (2.0 ± 0.4 vs. 1.6 ± 0.2). In comparison, the ratios of suspended sediment previously recorded in the Gironde estuary had comparable activity ratios of bottom sediment (averaging at 1.5, Saari et al., 2010) with our reported values, especially for the lower Galveston Bay site (Table 2). Both the Gironde (Supplementary Figure 1) and the lower Galveston Bay sites (Figure 1) were proximal to the respective bay mouths and the ocean. Lower ratios in suspended sediment can be from the reintroduction of 7Be-dead sediment, which is more commonly found below 2 cm into sediment column. During times of strong sediment resuspension, this can be reintroduced into the water column along with older 210Pb. This can be possible when measuring suspended sediment. For the middle and lower bay, this is not as likely even during frontal events, unless measurements were taken from where scouring may occur (i.e., wooden pilings). It is expected that the highest ratios would be found in rainfall followed by suspended sediment in “upstream” areas, like the middle bay, followed by suspended sediment in “downstream” areas (e.g., lower bay). All three-sampling events follow this trend throughout the Galveston Bay system (Table 2).
Age or Freshness of Suspended Sediments
Based on the 7Be/210Pbxs ratios in suspended sediments, our results show that age of suspended sediments during our sampling events increased along the axis from the upper/middle bay to the lower bay (Figure 3), corresponding to the decreasing abundance of newly labeled suspended sediments/particles, which were also derived from 7Be/210Pbxs ratios (Table 2). Throughout the three events, the age of the suspended sediment from the middle bay in middle February 2020 was the highest at 86 ± 13 days, followed by late January 2020 at 70 ± 10 days, and then late February 2020 at 50 ± 8.0 days. The abundance or percentage of newly labeled particles in the total suspended sedimentary pool were correspondingly to this, with the lowest percent new occurring with the middle February sample was 32 ± 5.0%, followed by late January 2020 (40 ± 5.6%), and the highest abundance was found to be 52 ± 8.0% at late February 2020, out of the three samples.
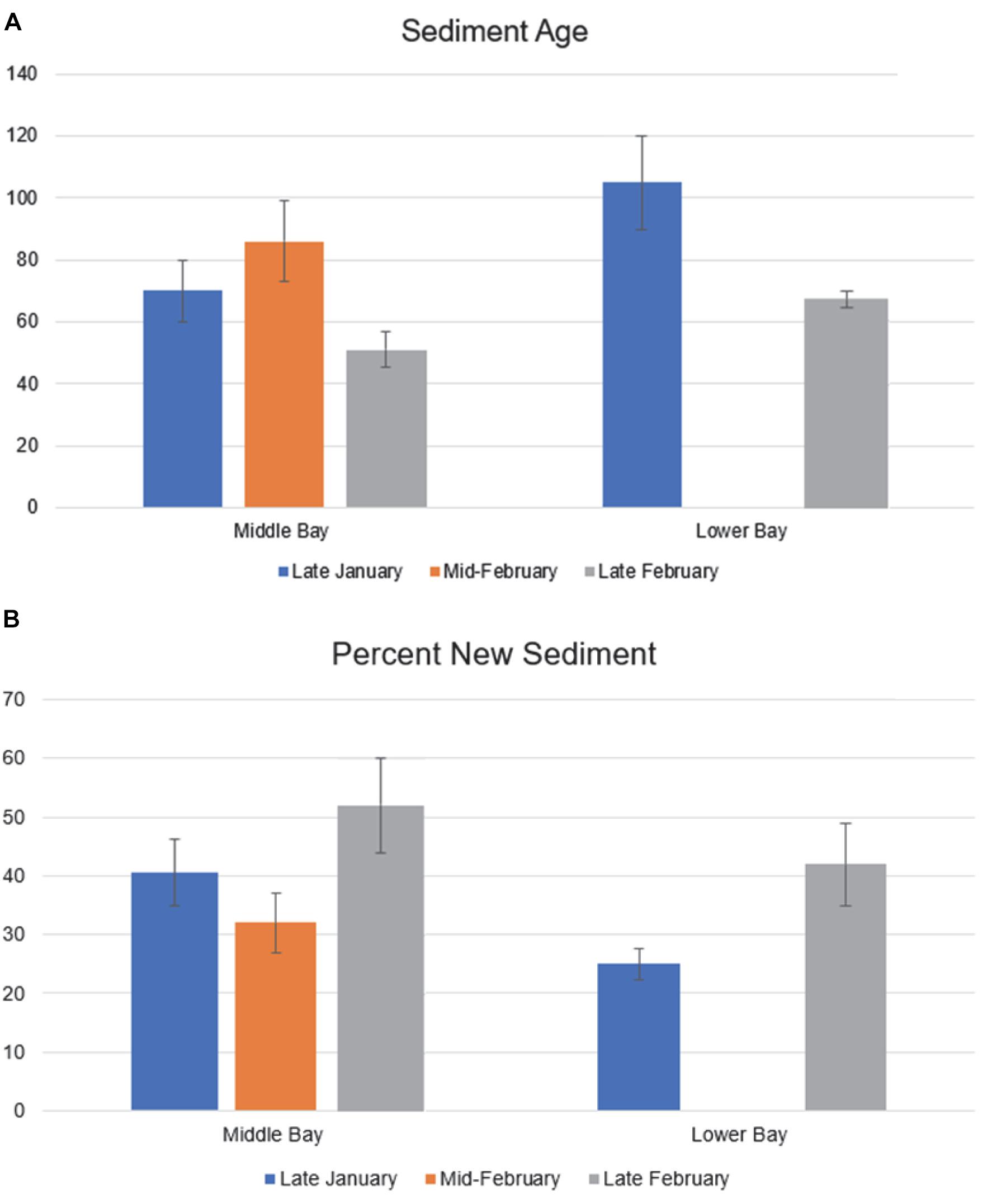
Figure 3. Age of suspended sediments (A) and Percent “New” sediments (B) in middle and lower Galveston Bay during three weather events at 2020.
Matisoff et al. (2005) measured the ages of suspended sediment or the percent new for three catchments (Table 3), all within the United States National Estuarine Research Reserves, they are: (1) Weeks Bay which is small bay embayed into the eastern shore of Mobile Bay; (2) South Slough, a small estuary off of Coos Bay in OR, United States; and (3) Old Woman Creek, a lake/fluvial system (lacustrine equivalent to an estuary) that empties into Lake Erie along its southern shore, in Ohio, United States. Among them, Weeks Bay is most comparable to Galveston Bay in terms of environments, both are shallow estuaries along the northern Gulf of Mexico. In addition to bay characteristics, water residence time in Galveston Bay has been measured to be about 40 days (Du et al., 2019), much longer than the water residence time in Weeks Bay (13 days, Solis and Powell, 1999; Novoveská and MacIntyre, 2019) and in Old Woman Creek (8.5 days, Matisoff et al., 2005). Therefore, it would be expected that the sediment would be older. However, the age of suspended sediments from both bays (i.e., Weeks Bay and Old Woman Creek) are actually falling within our reported range in the Galveston Bay (51–105 days, Table 3), with the age of the suspended sediment in Weeks Bay estimated to be 79 days; in Old Woman Creek, 104 days; South Slough, 93 days (Matisoff et al., 2005). It should be noted that the age of the suspended sediment is not the duration of time that the sediment has remained suspended in the water column, but rather the duration of time that the sediment remains an active component of the suspended sediment load. Being active within the sediment load means it is either suspended or sitting at or very near the seabed surface and maintaining a 7Be label comparable to the suspended sediment. An example of this is sediment that settles out during slack tide, when the tidal currents are below the critical shear stress velocity, but which is resuspended as the tidal current increase and exceed the critical shear stress. This would be an example of ephemeral deposition. If the water column residence time is longer than the age of the suspended sediment, then the suspended sediment is either buried to a depth for which it is no longer ephemerally resuspended or it is exported out of the bay within a single residence time. If, in contrast, the suspended sediment age is longer than the residence time of the bay water, then that means that the sediment would be trapped within the bay, maintained as part of the suspended sediment load for multiple water column residence time cycles. This trend was found to be the case in all samples throughout the sampling period at both locations in our study area (Table 2). If we divide the age of the suspended sediment by the water column residence time, the ratio produced is an estimate of the retention/export efficiency of the suspended sediment within the estuary. A higher value indicates that the suspended sediment is retained and available for sediment resuspension for a longer time. Whereas, a lower value indicates either more rapid burial to below a depth of resuspension or a more rapid export from the bay. The ratio of suspended sediment age over water residence time ranged from 1.28 to 2.15 in the middle bay and from 1.68 to 2.63 in the lower bay.
Among three estuarine systems (Galveston Bay vs. Weeks Bay vs. Old Woman Creek) the suspended sediment of Galveston Bay was available for resuspension for 1.28–2.63 water column residence times (Table 3), whereas, the suspended sediment within Weeks Bay was available for 6.1 water column residence times. If we divide the number of residence times needed to trap or export sediment for Weeks Bay by the same parameter for Galveston Bay, we can see that Galveston Bay is 2.3 to 4.7 times more efficient in trapping its sediment than Weeks Bay. At this point, we could not find any additional published literature where this method has been used to estimate suspended sediment residence within estuaries, so it is difficult to say whether the results so far for Galveston Bay are typical of other large, shallow, coastal plain estuaries. Additionally, this study is of only three events in a single year, so it is difficult to say whether these results are typical for Galveston Bay. However, the results show, for the period of time for which this study was conducted, that residence times for suspended sediment within Galveston Bay were much longer than the residence time of the water within the bay and suggest that this is a line of research that is both in its infancy and also warrants further investigation, both seasonally and also of other bay systems.
Sediment Transport in the Galveston Bay
Following precipitation and fluvial runoff, younger isotopes are introduced into the bay where the suspended load of sediment becomes isotopically labeled with a younger age. In contrast, precipitation in the bay resides within the fresher surface water while the bottom waters are isotopically older and have a higher salinity. As a result, resuspended sediment within the bay will, in general, have an older age than newly introduced suspended load from the fluvial systems. Because the head of the bay is where the largest fluvial systems discharge, the 7Be/210Pbxs ratio is expected to decrease as isotopes travel through a system (middle bay to lower bay). This statement assumes that suspended sediment is being advected through the bay along the salinity gradient. This is true for late January and late-February 2020, during each of these time periods there was a decreasing 7Be/210Pbxs ratio (Table 2) but increasing sediment age (Figure 3A) from middle to lower bay. These observations confirm the hypothesis that sediment age should increase as suspended sediment moves through an estuarine system. Alternatively, from the prospective of the percentage of newly labeled sediments/particles, an increase in the abundance of old particles in the suspended sediments pool can be observed from the middle bay to the bay mouth (Figure 3B) for both the late January and February sampling events. An older age in the lower bay versus the middle bay is likely due to the distal location of freshly labeled sediment along with the mixing with older resuspended bay sediment when they travel through the system. With the variability of water mass transport and trapping within Galveston Bay, the values obtained can only be used to observe the bigger picture of the overall sediment transport. It should be noted that the time of year and characteristics of meteorological events could potentially produce much different results than those observed here.
In order to understand what these trends mean in Galveston Bay, water levels and salinities were examined during the weeks preceding the sampling (Figure 4). It should be noted that the salinity data was collected from the Trinity Bay, part of the Galveston Bay (Figure 1). Although the data collected in Trinity bay may not completely reflect the salinity for the entirety of the bay but can provide inside on average salinity within the bay and how rainfall has an effect on changes in salinity in a shallow estuary. Based on the analyses of the water levels when compared to the predicted tides, we found that there was a large influx of flood water trapped within the bay during much of January 2020 before our sampling during late January 2020 (Figure 4). When we considered the variation in salinity (monitored in Trinity Bay, part of the Galveston Bay, Figure 1), for the month of January 2020, it ranged between 19 and 10 PSU. Nevertheless, combined with the total amount of rainfall for the month of January 2020 being 200 mm, it would imply that the salinity would be much lower than this range (10–19 of salinity). Therefore, such a phenomenon indicates that there was a greater mass of high-salinity marine-derived water trapped within the bay.
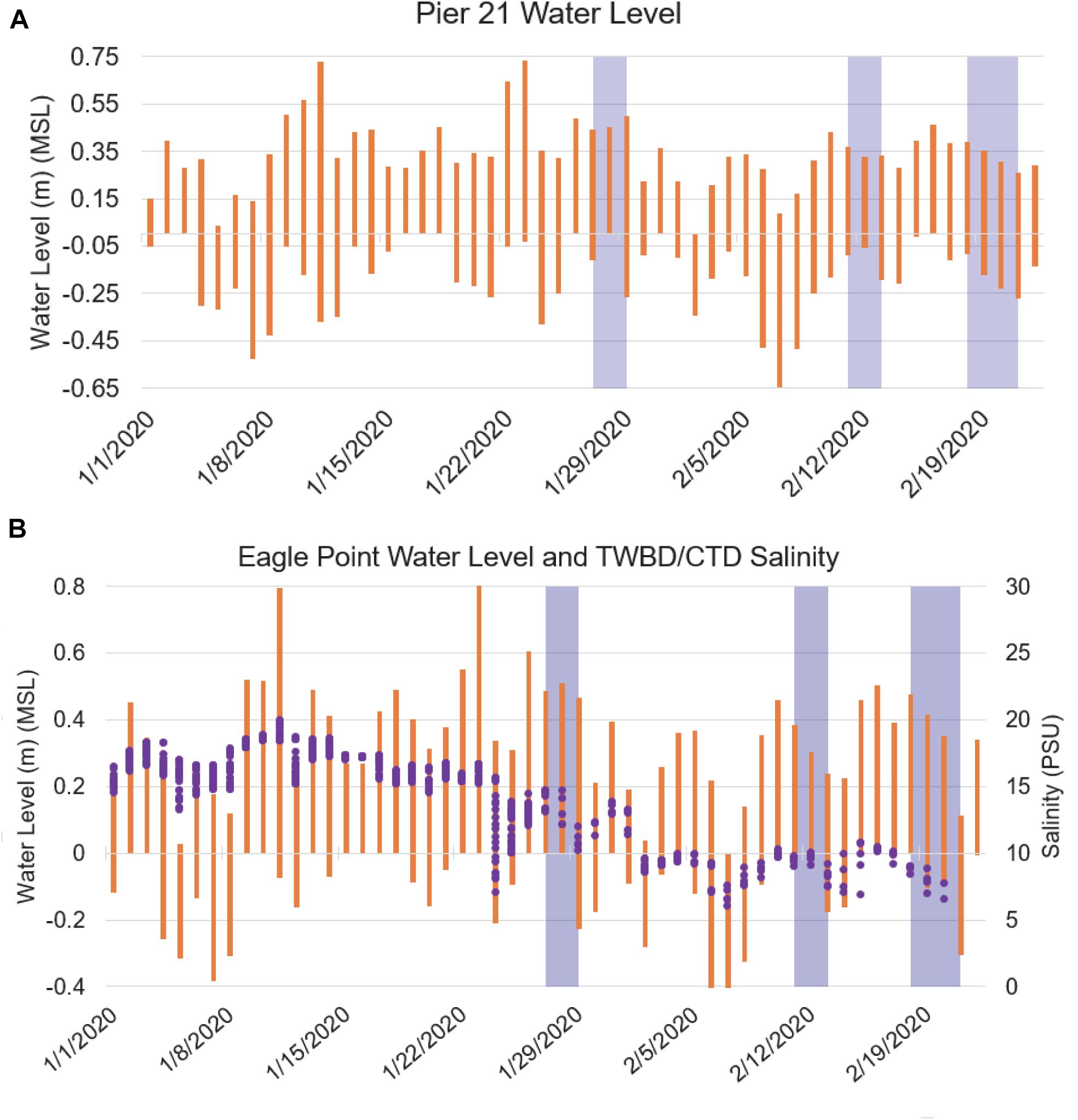
Figure 4. Water levels from NOAA Pier 21 (A) and Eagle Point Stations (B) for the months of January and February 2020, as well as the salinity (PSU) obtained from Texas Water Development Board (TWBD-TRIN) station (1/1/2020-2/5/2020) and from the TAMUG-TRIN CTD station (2/6/2020-2/20/2020). Shading represents our precipitation sampling period.
During our sampling on late January 2020 (Figure 4), salinity was observed to be between 13 and 14 PSU and progressively decreased to below 10 PSU following the cold front in late January 2020. After this rain event, the salinity does not rise above 11 PSU for the remainder of sampling period. This indicates that high salinity water mass was flushed following the weather event in late January 2020 toward the mouth of the bay. By the time of the water sampling on middle February 2020, the age of sediment was older than late January samples (Figure 3 and Table 2), implying that the high salinity water mass had yet to exit the bay but had moved further toward the mouth of the bay. Additionally, it is possible that the water pass may have passed the mouth of the bay, where suspended sediments had yet to arrive in middle February due to the longer transport time of suspended sediments (51–105 days, Table 3) than water masses (40 days, Table 3) within the bay. According to a 3D modeling study of the hydrodynamic circulation showing the weak tidal currents in the bay (Du et al., 2020), water masses can be trapped within the bay for prolonged periods of time, which appeared to be the case for much of January 2020 and middle February 2020. Based on this observation, there are longer residence times of unflushed water, leading to longer residence times of suspended sediment within these water masses. Older suspended sediment from January 2020 was still present in middle February 2020 where the age was 16 ± 3 days greater than late January 2020 (Figure 3 and Table 2). Whereas, in late February 2020, it appears that the majority of the sediment measured in middle February 2020 was transported entirely out of the bay from this cold front, as a result, the age in late February 2020 was 35 ± 7.4 days younger (Figure 3). Correspondingly, sediment dynamics with bay water masses can also be observed based on the variation in 7Be/210Pbxs-derived abundance of newly labeled particles in suspended sedimentary pool. For example, the percentage of new sediment in middle bay from middle February was about 8 ± 0.6% lower than that from late January 2020 (Figure 3), demonstrating the weather event in middle February 2020 resulted in more 7Be-depleted particles resuspended from deeper bay sediments to the water column. In comparison, more newly labeled suspended particles were found for both sampling sites from late February 2020 (e.g., 20% increase in middle bay compared with middle February 2020, Figure 3). This also suggests that the majority of 7Be-depleted old sediments may have been flushed out of the bay during the meteorological front in middle February 2020. The majority of suspended sediments during late February 2020 was thus mainly composed by newly 7Be-labeled particles. Thus, our results generally displayed that meteorological fronts have the ability to resuspend and transport sediments, but with the hydrodynamic trapping of water masses within the bay (e.g., Trinity River), the length of time sediment reside within the bay may vary significantly.
Implications to the Galveston Bay Environment
Estuaries and coasts are known to be the primary filter between the land-sea margin, where rivers and estuaries serve as sources of particulate contaminants to coastal environments (Huang et al., 2011). With Galveston Bay being a microtidal environment, residence times can be longer due to less water mass transport from tides, especially during low flow/water discharge periods. The heavy metals and different organic pollutants that quickly sorb to settling particles become the “sink” within an estuary. With much of the western shore of Galveston Bay and the watershed of many of its smaller tributaries heavily industrialized, there is an abundance of pollutants/chemical constituents which reside within the bottom sediment. For example, surface sediment was measured in Galveston Bay, and it was found that various particle reactive metals (e.g., lead, barium, mercury, copper, manganese, zinc, etc.) and polycyclic aromatic hydrocarbons (PAHs) sorbet onto the sediment, meaning that they have a great chance of resuspending during resuspension events (Santschi et al., 2001; Dellapenna et al., 2020; Camargo et al., 2021). Therefore, our estimated residence time of suspended sediments (51–105 days, Table 2) suggest these pollutants that adsorb to suspended sediment may spend months before they leave the Galveston Bay. The long residence times of sediments in Galveston Bay will increase the exposure time of living organisms to various pollutants. Along with the trapping of water masses that potentially allow for the accumulation in organisms will affect the health of Galveston Bay. Additionally, although cold fronts or other storm events can enhance sediment transport (i.e., reduce the residence time of suspended sediment), their induced sediment resuspension will result in the continuous interaction of the sedimentary pollutants with the water column of Galveston Bay, which loosely adsorbed pollutants in sediments may be released back to the Galveston Bay waters. In contrast to the potential negative impact from long resident time and resuspension of bay sediments, some positive feedback may be beneficial to the bay environments, such as the nutrients release from the sediment resuspension (e.g., phosphate, Lin et al., 2013; Chao et al., 2017; Guo et al., 2020), which can promote the phytoplankton growth and accelerate the ecosystem function in Galveston Bay. Thus, 7Be/210Pb-assisted investigation of sediment dynamics and age will help us better monitor the environmental quality in estuarine systems. Another factor this study brings to light is the ratio of the residence time of the water column to the residence time of the suspended sediment. The residence time of suspended sediment is an estimate of the duration of time that the sediment available for resuspension before either exiting the bay or being buried to a depth below which it can be resuspended. The more water column residence times that particle reactive contaminant labeled sediment resides within the estuary, the greater the opportunity that pelagic organisms have to be exposed to these particle reactive contaminants. It is not just the long residence time of the suspended sediment that is a factor, but also the amount of water column the particle reactive suspended sediment is exposed that is the risk to the environment.
Data Availability Statement
The original contributions presented in the study are included in the article/Supplementary Material, further inquiries can be directed to the corresponding author/s.
Author Contributions
TD provided the funding, designed and planned the project, and experimental process. NS carried out the experiments and data collection. PL performed the isotopic methods and analysis. NS, TD, and PL wrote the manuscript. All authors contributed to the article and approved the submitted version.
Funding
This work was also made possible in part by National Oceanic and Atmospheric Administration (NOAA) and the Texas General Land Office Texas Coastal Management Program under Grant Number NA18NOS4190153.
Conflict of Interest
The authors declare that the research was conducted in the absence of any commercial or financial relationships that could be construed as a potential conflict of interest.
Acknowledgments
We appreciate the assistance from undergraduate volunteers including Gregory Grimm, Ryland Lewis, Ryan Gage and Oscar Cavazos who assisted in collecting sample during frontal passages. All in-lab processing work conducted for this study was completed independently, with assistance from Josh Alarcon.
Supplementary Material
The Supplementary Material for this article can be found online at: https://www.frontiersin.org/articles/10.3389/fmars.2021.703945/full#supplementary-material
References
Baskaran, M., Coleman, C. H., and Santschi, P. H. (1993). Atmospheric depositional fluxes of 7Be and 210Pb at Galveston and College Station, Texas. J. Geophys. Res. 98, 20555–20571. doi: 10.1029/93jd02182
Baskaran, M., and Santschi, P. H. (1993). The role of particles and colloids in the transport of radionuclides in coastal environments of Texas. Mar. Chem. 43, 95–114. doi: 10.1016/0304-4203(93)90218-d
Booth, J. G., Miller, R. L., McKee, B. A., and Leathers, R. A. (2000). Wind-induced bottom sediment resuspension in a microtidal coastal environment. Cont. Shelf Res. 20, 785–806. doi: 10.1016/s0278-4343(00)00002-9
Brost, R. A., Feichter, J., and Heimann, M. (1991). Three-dimensional simulation of 7Be in a global climate model. J. Geophys. Res. 96, 22423–22445. doi: 10.1029/91jd02283
Camargo, K., Sericano, J. L., Bhandari, S., *Hoelscher, C., McDonald, T. J., Chiu, W. A., et al. (2021). Polycyclic aromatic hydrocarbon status in post-hurricane Harvey sediments: considerations for environmental sampling in the Galveston Bay / Houston Ship Channel region. Mar. Pollut. Bull. 162:111872. doi: 10.1016/j.marpolbul.2020.111872
Caillet, S., Arpagaus, P., Monna, F., and Dominik, J. (2001). Factors controlling 7Be and 210Pb atmospheric deposition as revealed by sampling individual rain events in the region of Geneva. Switzerland. J. Environ. Radio 53, 241–256. doi: 10.1016/s0265-931x(00)00130-2
Chambers, M., Mitchell, A., Fine, A., Mulder, K., Rainville, L., Hackett, D., et al. (2018). Port Performance Freight Statistics Program: Annual Report to Congress 2017. (Washington, DC: Department of Transportation. Bureau of Transportation Statistics).
Chao, J. Y., Zhang, Y. M., Kong, M., Zhuang, W., Wang, L. M., Shao, K. Q., et al. (2017). Long-term moderate wind induced sediment resuspension meeting phosphorus demand of phytoplankton in the large shallow eutrophic Lake Taihu. PLoS One 12:e0173477. doi: 10.1371/journal.pone.0173477
Dellapenna, T., Allison, M., Gill, G., Lehman, R., and Warnken, K. (2006). The impact of shrimp trawling and associated sediment resuspension in mud dominated, shallow estuaries. Estuar. Coast. Shelf Sci. 69, 519–530. doi: 10.1016/j.ecss.2006.04.024
Dellapenna, T. M., Hoelscher, C., Hill, L., Al Mukaimi, M. E., and Knap, A. (2020). How tropical cyclone flooding caused erosion and dispersal of mercury-contaminated sediment in an urban estuary: the impact of Hurricane Harvey on Buffalo Bayou and the San Jacinto Estuary, Galveston Bay, USA. Sci. Total Environ. 748:141226. doi: 10.1016/j.scitotenv.2020.141226
Du, J., and Park, K. (2019). Estuarine salinity recovery from an extreme precipitation event: hurricane Harvey in Galveston Bay. Sci. Total Environ. 670, 1049–1059. doi: 10.1016/j.scitotenv.2019.03.265
Du, J., Park, K., Shen, J., Zhang, Y. J., Yu, X., Ye, F., et al. (2019). A hydrodynamic model for Galveston Bay and the shelf in the northern Gulf of Mexico. Ocean Sci. 15, 951–966. doi: 10.5194/os-15-951-2019
Du, J., Park, K., Yu, X., Zhang, Y. J., and Ye, F. (2020). Massive pollutants released to Galveston Bay during Hurricane Harvey: understanding their retention and pathway using Lagrangian numerical simulations. Sci. Total Environ. 704:135364. doi: 10.1016/j.scitotenv.2019.135364
Duenas, C., Fernandez, M. C., Carretero, J., Liger, E., and Canete, S. (2002). Atmospheric deposition of 7Be at a coastal Mediterranean station. J. Geophy. Res. 106, 34059–34065. doi: 10.1029/2001jd000771
Guo, M., Li, X., Song, C., Liu, G., and Zhou, Y. (2020). Photo-induced phosphate release during sediment resuspension in shallow lakes: a potential positive feedback mechanism of eutrophication. Environ. Pollut. 258:113679. doi: 10.1016/j.envpol.2019.113679
Haby, M. G., Miget, R. J., and Falconer, L. L. (2009). Hurricane Damage Sustained by the Oyster Industry and the Oyster Reefs Across the Galveston Bay System With Recovery Recommendations. A Texas AgriLife Extension Service/Sea Grant Extension Program Staff Paper. Galveston, TX: The Texas A&M University System.
Hardy, J. W., and Henderson, K. G. (2003). Cold front variability in the southern United States and the influence of atmospheric teleconnection patterns. Phys. Geogr. 24, 120–137. doi: 10.2747/0272-3646.24.2.120
Henry, W. K. (1979). Some aspects of the fate of cold fronts in the Gulf of Mexico. Monthly Weather Rev. 107, 1078–1082. doi: 10.1175/1520-0493(1979)107<1078:saotfo>2.0.co;2
Herdendorf, C., Klarer, D. M., and Herdendorf, R. C. (2004). The Ecology of Old Woman Creek, Ohio: An Esturine and Watershed Profile. Columbus OH: Ohio Department of Natural Resources.
Huang, D., Du, J., and Zhang, J. (2011). Particle dynamics of 7Be, 210Pb and the implications of sedimentation of heavy metals in the Wenjiao/Wenchang and Wanquan River estuaries, Hainan, China. Estuar. Coast. Shelf Sci. 93, 431–437. doi: 10.1016/j.ecss.2011.05.013
Koch, D. M., Jacob, D. J., and Graustein, W. C. (1996). Vertical transport of troposheric aerosols as indicated by 7Be and 210Pb in a chemical tracer model. J. Geophy. Res. 101, 18651–18666. doi: 10.1029/96JD01176
Lin, P., Guo, L., Chen, M., and Cai, Y. (2013). Distribution, partitioning and mixing behavior of phosphorus species in the Jiulong River estuary. Mar. Chem. 157, 93–105. doi: 10.1016/j.marchem.2013.09.002
Matisoff, G., Wilson, C., and Whiting, P. (2005). The 7Be/210PbXS ratio as an indicator of suspended sediment age or fraction new sediment in suspension. Earth Surf. Process. Landf. 30, 1191–1201. doi: 10.1002/esp.1270
Morse, J. W., Presley, B. J., Taylor, R. J., Benoit, G., and Santschi, P. (1993). Trace metal chemistry of Galveston Bay: water, sediments and biota. Mar. Environ. Res. 36, 1–37. doi: 10.1016/0141-1136(93)90087-g
Novoveská, L., and MacIntyre, H. L. (2019). Study of the seasonality and hydrology as drivers of phytoplankton abundance and composition in a shallow estuary. Weeks Bay, Alabama (USA). J. Aquac. Mar. Biol. 8, 69–80.
Olsen, C. R., Larsen, I. L., Lowry, P. D., Cutshall, N. H., Todd, J. F., Wong, G. T. F., et al. (1985). Atmospheric fluxes and marsh-soil inventories of 7Be and 210Pb. J. Geophys. Res. 90, 10487–10495.
Olsen, C. R., Thein, M., Larsen, I. L., Lowry, P. D., Mulholland, P. J., Cutshall, N. H., et al. (1989). Plutonium, lead-210, and carbon isotopes in the Savannah estuary: riverborne versus marine sources. Environ. Sci. Technol. 23, 1475–1481. doi: 10.1021/es00070a004
Saari, H., Schmidt, S., Castaing, P., Blanc, G., Sautour, B., Masson, O., et al. (2010). The particulate 7Be/210Pbxs and 234Th/210Pbxs activity ratios as tracers for tidal-to-seasonal particle dynamics in the Gironde estuary (France): implications for the budget of particle-associated contaminants. Sci. Total Environ. 408, 4784–4794. doi: 10.1016/j.scitotenv.2010.07.017
Santschi, P. H., Presley, B. J., Wade, T. L., Garcia-Romero, B., and Baskaran, M. (2001). Historical contamination of PAHs, PCBs, DDTs, and heavy metals in Mississippi river Delta, Galveston bay and Tampa bay sediment cores. Mar. Environ. Res. 52, 51–79. doi: 10.1016/s0141-1136(00)00260-9
Solis, R. S., and Powell, G. (1999). Hydrography, Residence Times, and Physical Processes. Biogeochemistry of Gulf of Mexico Estuaries. New York NY: John Wiley, 29–61.
Stunz, G. W., Minello, T. J., and Rozas, L. P. (2010). Relative value of oyster reef as habitat for estuarine nekton in Galveston Bay, Texas. Mar. Ecol. Prog. Ser. 406:159.
Taylor, A., Blake, W. H., Smith, H. G., Mabit, L., and Keith-Roach, M. J. (2013). Assumptions\and challenges in the use of fallout beryllium-7 as a soil and sediment tracer in river basins. Earth Sci. Rev. 126, 85–95. doi: 10.1016/j.earscirev.2013.08.002
United States Geological Survey [USGS] (2005). US Geological Survey Suspended-Sediment Database, Daily Values of suspended Sediment And Ancillary Data. Reston, VA: USGS.
Walker, N. D., and Hammack, A. B. (2000). Impacts of winter storms on circulation and sediment transport: atchafalaya-Vermilion Bay region, Louisiana, USA. J. Coastal Res. 16, 996–1010.
Wang, Z., Yang, W., Chen, M., Lin, P., and Qiu, Y. (2013). Intra-annual deposition of atmospheric 210Pb, 210Po and the residence times of aerosol in Xiamen, China. Aerosol Air Qual. Res. 14, 1402–1410. doi: 10.4209/aaqr.2013.05.0170
Keywords: sediment resuspension, lead-210, residence times, beryllium-7, Galveston Bay
Citation: Schmidt N, Dellapenna T and Lin P (2021) 7Be/210Pbxs Ratio-Derived Age and Residence Time of Suspended Sediments in Galveston Bay. Front. Mar. Sci. 8:703945. doi: 10.3389/fmars.2021.703945
Received: 01 May 2021; Accepted: 28 June 2021;
Published: 20 July 2021.
Edited by:
Weifeng Yang, Xiamen University, ChinaReviewed by:
Huan Feng, Montclair State University, United StatesZiming Fang, Hong Kong University of Science and Technology, Hong Kong, SAR China
Copyright © 2021 Schmidt, Dellapenna and Lin. This is an open-access article distributed under the terms of the Creative Commons Attribution License (CC BY). The use, distribution or reproduction in other forums is permitted, provided the original author(s) and the copyright owner(s) are credited and that the original publication in this journal is cited, in accordance with accepted academic practice. No use, distribution or reproduction is permitted which does not comply with these terms.
*Correspondence: Timothy Dellapenna, ZGVsbGFwZXRAdGFtdWcuZWR1; Peng Lin, cGVuZ2wxMTA0QHRhbXVnLmVkdQ==