- 1Departamento de Ecología y Biodiversidad, Facultad de Ciencias de la Vida, Universidad Andres Bello, Santiago, Chile
- 2Departamento de Biología Marina, Facultad de Ciencias del Mar, Universidad Católica del Norte, Coquimbo, Chile
- 3Millennium Nucleus for Ecology and Sustainable Management of Oceanic Islands, Coquimbo, Chile
- 4Ryan Institute and School of Natural Sciences, National University of Ireland Galway, Galway, Ireland
- 5Programa de Doctorado en Sistemática y Biodiversidad, Departamento de Zoología, Facultad de Ciencias Naturales y Oceanográficas, Universidad de Concepción, Concepción, Chile
- 6Laboratorio de cefalópodos, Instituto de Biología de Organismos Marinos, Consejo Nacional de Investigaciones Científicas y Técnicas, Puerto Madryn, Argentina
- 7Departamento de Ecología y Biología Animal, Universidade de Vigo and Centro de Investigación Mariña, BA2, Edifício Ciencias Experimentales, Campus de Vigo As Lagoas-Marcosende, Vigo, Spain
- 8Center for Marine Studies, University of Parana, Pontal do Paraná, Brazil
One of the major mechanisms responsible for the animals’ fitness dynamics is fecundity. Fecundity as a trait does not evolve independently, and rather interacts with other traits such as body and egg size. Here, our aim was to correctly infer the macroevolutionary trade-offs between body length, egg length, and potential fecundity, using cephalopods as study model. The correlated evolution among those traits was inferred by comparative phylogenetic methods. Literature data on biological and reproductive traits (body length, egg length, and potential fecundity) was obtained for 90 cephalopod species, and comparative phylogenetic methods based on a previous molecular phylogeny were used to test the correlated evolution hypothesis. Additionally, we estimated the phylogenetic signal and fitted five different evolutionary models to each trait. All traits showed high phylogenetic signal, and the selected model suggested an evolutionary trend toward increasing body length, egg length, and fecundity in relation to the ancestral state. Evidence of correlated evolution between body length and fecundity was observed, although this relationship was not detected between body length and egg length. The robust inverse relationship between fecundity and egg length indicates that cephalopods evolved a directional selection that favored an increase of fecundity and a reduction of egg length in larger species, or an increase in egg length with the concomitant reduction of fecundity and body length in order to benefit offspring survival. The use of phylogenetic comparative methods allowed us to properly detect macroevolutionary trade-offs.
Introduction
Life history traits of marine organisms are not only important for ecological and evolutionary studies as components of Darwinian fitness, but are also of crucial relevance in fisheries and aquaculture. Tracing trade-offs through evolution allows us to better estimate future changes of life history traits under different pressures, such as the incipient climate change and fisheries exploitation.
Life history theory predicts biological trade-offs between two or more traits defining reproduction and development patterns in the species. Traits do not evolve independently, but rather under a compensatory association that results in trade-offs between a series of physical and biological mechanisms that try to maximize the reproduction and survival of the offspring (Stearns, 1989; Garland, 2014). A prominent example is the evolutionary relationship between body size and number of eggs found in different taxonomic groups and hierarchical levels (e.g., insects, fishes, marine invertebrates, birds, and reptiles, Elgar, 1990; Stearns, 1992; Zera and Harshman, 2001; Ramirez-Llodra, 2002; Garland, 2014; Futuyma and Kirkpatrick, 2017). Indeed, there are multiple examples of micro- and macroevolutionary trade-offs (e.g., Stearns, 1992; Badyaev, 2002; Roff, 2002; Arendt, 2003; Poulin and Mouillot, 2004) including for instance, phenotypic correlations between offspring size and fecundity or survival, and between body size and longevity, although their interpretation has been controversial. This is due to uncertainties in determining whether the increase of performance in one trait causes a decrease of performance in another trait, or if traits interact functionally (Zera and Harshman, 2001). To solve this problem, by comparing species under a macroevolutionary context, Stephens and Wiens (2008) defined an “evolutionary trade-off” as an evolutionary increase in one aspect of performance or fitness (relative to the ancestral state) that is associated with an evolutionary decline in a related aspect of performance or fitness in the same branch of a given phylogeny. Since then, authors have emphasized the use of phylogenies to evaluate trade-offs among traits at a macroevolutionary scale (e.g., Stephens and Wiens, 2008; Vanhooydonck et al., 2014), an approach that has not been previously used to test hypotheses concerning evolutionary trade-offs of reproductive traits directly associated with fitness (e.g., fecundity and survival). In addition, a trade-off in life history traits could be the result of an evolutionary trend caused by clades evolving separately, as was detected in mammals, where different groups display convergent evolution in body size and active trends of size at maturity and longevity (Lartillot and Delsuc, 2012).
Among mollusks, cephalopods stand out due to their great diversity of life history strategies (Boyle, 1983; Hanlon and Messenger, 1996; Rocha et al., 2001). Body length can range from less than 1 cm in pygmy squids (Idiosepius spp.) with short life spans, to more than 20 m in total length (including tentacles) in the giant squid (Architeuthis dux), with a lifespan of around 3 years (Wood and O’Dor, 2000; Jereb and Roper, 2005; Perales-Raya et al., 2020). Similarly, variability in egg size can range from approximately 0.5 to 80 mm, while fecundity estimates vary from one or a few dozen eggs (e.g., cirrates, Nautilus, Sepiola, and Bathypolypus) to several millions (e.g., Architeuthis and Dosidicus), depending on body size (Calow, 1987; Boyle and Rodhouse, 2005; Laptikhovsky et al., 2018). Given that the energy invested in reproduction is limited, a trade-off between egg size and fecundity is expected (Calow, 1987; Boyle and Rodhouse, 2005). Hence, the number and size of eggs that a species can produce are determined by its energetic capability (Calow, 1987; Boyle and Rodhouse, 2005; Jereb and Roper, 2005). Nonetheless, despite acknowledging these evolutionary restrictions, no attempts have been made to explore possible trade-offs among body size, egg size, and fecundity under a phylogenetic perspective.
Cephalopods constitute monophyletic groups composed of species with either large eggs and low fecundity (e.g., Sepia and Graneledone) or small eggs and high fecundity (e.g., ommastrephid squids). However, it has been noted that parental investment in egg mass to ensure offspring survival, is not firmly linked to phylogeny, as closely related species can exhibit different parental investments. This is particularly detectable in morphologically similar octopus species that produce small or large eggs with planktonic or benthic offspring mode of development, respectively (Hochberg et al., 1992; Ibáñez et al., 2018). For marine mollusks, including cephalopods, fecundity and body size correlate positively (Jablonski et al., 1996; Laptikhovsky et al., 2007), although it has been detected that for cephalopods in particular, egg size covaries strongly with fecundity (Calow, 1987: Boyle and Rodhouse, 2005). Hence, the reproductive traits of cephalopods are expected to have a strong phylogenetic signal, evolving in a correlated manner with body size and showing evidence of macroevolutionary trade-offs. Moreover, paleontological records indicate that Mesozoic coleoid cephalopods produced small eggs, and some lineages evolved from these ancestors to produce larger egg sizes, while others advanced to produce a variety of large and small eggs (mixed strategies, Fuchs et al., 2020). Therefore, we suggest that both body size and reproductive traits of cephalopods evolved toward an increasing trend along the phylogeny. Currently, in terms of representativity, a considerable amount of data on life history traits is available from literature and public databases, depicting biological information within major groups (i.e., representatives of all orders within subclasses Nautiloidea and Coleoidea). In this context, our aim is to use this information to compare a large number of species with different body lengths, fecundities, and egg sizes under an explicit evolutionary context using phylogenetic comparative methods to solve this evolutionary puzzle.
Materials and Methods
Database
Ninety cephalopod species belonging to 7 orders, 31 families, and 60 genera were considered in this study. We performed an exhaustive literature review to obtain data of cephalopod lifestyle (pelagic vs. benthic), body length (BL, maximum mantle length for coleoids and maximum shell diameter for nautiloids, mm) as indicators of body size, egg length (EL, mm), and potential fecundity (PF, number of mature oocytes in the ovary prior to spawning) (see Supplementary Table 1). This database was combined with a previously published molecular phylogeny that included 173 species (Lindgren et al., 2012). This published phylogeny was selected over other phylogenetic hypotheses (for example, Ibáñez et al., 2021), because it was constructed with the largest number of species, thus, enabling to combine the tree topology and life history traits (BL, EL, and PF). It is worth noting that other authors in the field (e.g., Marian, 2015; Ponte et al., 2021) have also employed the phylogenetic reconstruction published by Lindgren et al. (2012) to evaluate macroevolutionary hypotheses of cephalopods according to other traits such as spermatophores and brains. For comparative purposes, all species lacking reproductive data (i.e., EL, PF, Supplementary Table 2) or species determination (21 tree tips, e.g., Bathypolypus sp., Gonatopsis sp., Notonykia sp., Benthoctopus sp.) were removed from the tree using drop.tip function in “APE” package (Paradis et al., 2004) implemented in R core (R Development Core Team, 2020). The new phylogram was transformed into an ultrametric tree using the Grafen’s (1989) method. A heatmap was employed to explore the association between the standardized database and the phylogeny using the phylo.heatmap function in “Phytools” package (Revell, 2012).
Phylogenetic Signal
The ultrametric tree was used to estimate the phylogenetic signal of each trait denoted as Pagel’s λ (Pagel, 1999). This parameter ranges between 0 and 1, and quantifies the amount of phylogenetic signal in the studied trait. When λ = 0 means that the trait distribution (across species) is independent of the phylogeny, whereas a λ = 1 indicates that the distribution of trait values fits as expected according to the Brownian motion model (BM; Pagel, 1999). The analyses were conducted using phylosig function implemented in “Phytools.” The likelihood value of λ estimated for each trait was contrasted to the likelihood value of λ equal to 0 by means of a likelihood ratio test (LRT).
Correlated Evolution
Phylogenetic Generalized Least Squares models (PGLS; Pagel, 1999) were used to establish the existence of a linear relationship between BL, EL, and PF. These models were performed using the corPagel function in “APE” package implemented in R. The correlation structure of PGLS was carried out under the assumption of BM, multiplying the off-diagonal elements (i.e., the covariances) by lambda (λ). Additionally, ordinary least squares (OLS) were performed in R to estimate linear relationships among BL, EL, and PF without using the phylogeny. These results were used to compare differences in coefficient estimation with the analysis mentioned above.
In order to avoid allometric effects on traits, we explored the relationship between potential fecundity and egg length corrected by body length (PF/BL and EL/BL) using PGLS. To evaluate the influence of lifestyle (pelagic vs. benthic) over PF, including BL and EL as covariates, we performed a phylogenetic analysis of covariance (ANCOVA) by means of PGLS.
Ancestral States
We fitted univariate models of trait evolution using the fitContinuous function implemented in the “Geiger” package (Harmon et al., 2008). The five models fitted to each trait were: (1) BM model (Felsenstein, 1973), which assumes that the correlation structure between traits is proportional to the extent of shared ancestry between pairs of species; (2) Early-Burst model (EB; Harmon et al., 2010), in which the change in the character tends to be concentrated toward the base of the tree; (3) Ornstein-Uhlenbeck model (OU; Butler and King, 2004), which models evolution of the trait with a tendency toward a central value, such as constant stabilizing selection; (4) Rate trend diffusion model (RT; Harmon et al., 2020), which models with a linear trend on trait rates through time (toward larger or smaller rates); and (5) Drift model (DF; Harmon et al., 2020), which simulates the evolution of the trait assuming a directional component (i.e., toward smaller or larger values through time). All these models were run using the new ultrametric tree, except for DF, which was run using a phylogram with branch lengths. Later, the corrected Akaike information criterion (AICc) and a LRT were used to select the best-fit model for the dataset. Finally, ancestral states for each trait using the fastAnc function were implemented in Phytools. This function performs a fast estimation of the maximum likelihood of ancestral states for a continuous trait using Felsenstein’s (1985) contrasts algorithm.
Results
Biological Data
The body length of the 90 cephalopod species ranged from 20 mm in Idiosepius pygmaeus to 3,000 mm in A. dux (mean species BL = 313.8 mm), whereas egg length ranged from 0.5 mm in Onychoteuthis banksii to 70 mm in Sepioteuthis australis (mean species EL = 8.32 mm) (Supplementary Table 1). Potential fecundity ranged from 20 eggs in Nautilus macromphalus, Nautilus pompilius, and Thaumeledone rotunda to 32,000,000 eggs in Dosidicus gigas (mean species PF = 871,139.6 eggs, Supplementary Table 1). Under a phylogenetic perspective, oegopsid squids evolved toward the largest BL and PF, while benthic octopuses and nautiloids adapted toward the largest eggs and smallest PF (Figure 1).
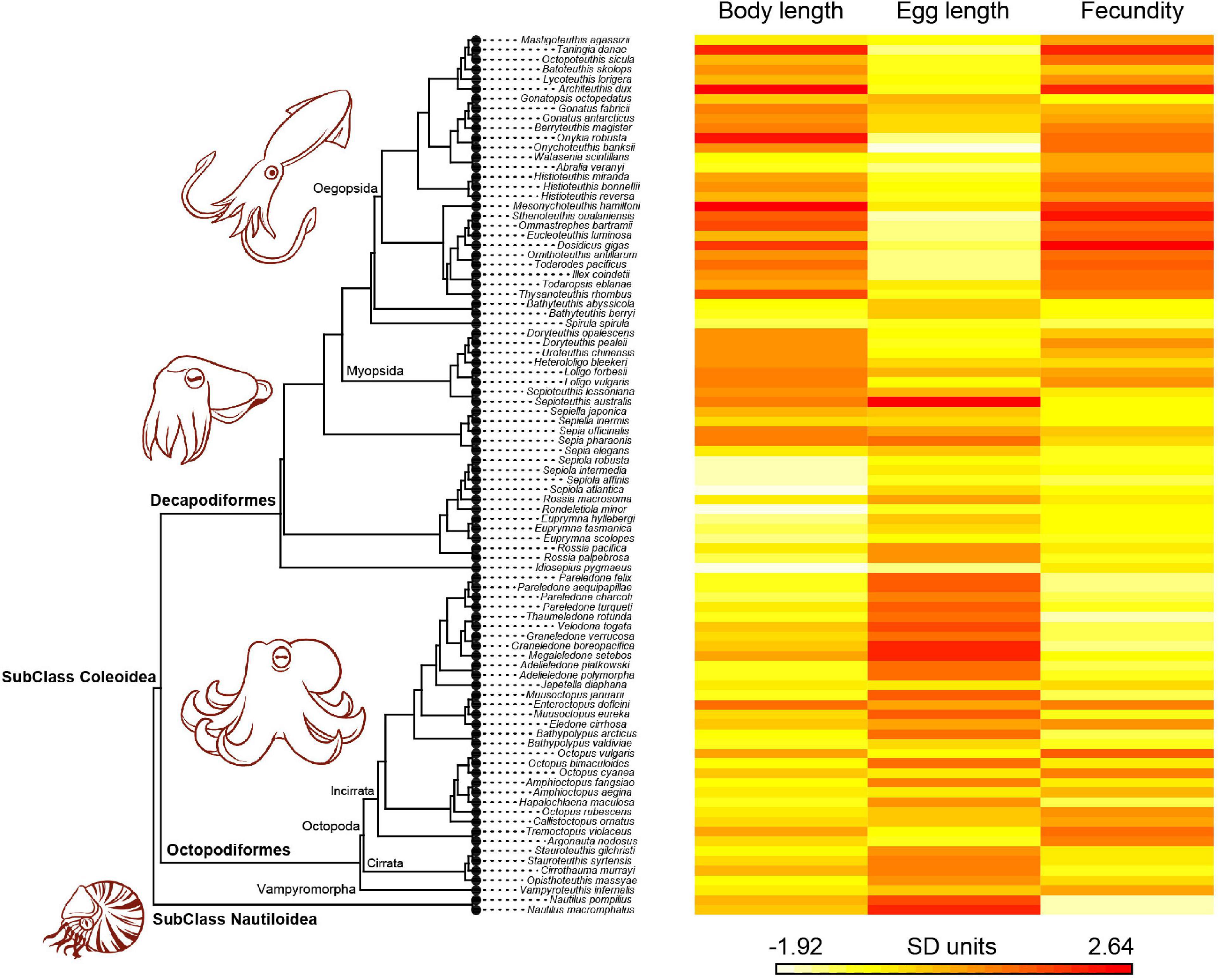
Figure 1. Phylogenetic heatmap tree of cephalopods traits (body length, egg length, and potential fecundity) across the 90 studied species.
Phylogenetic Signal
Cephalopod BL, EL, and PF were clearly structured across the phylogeny in the heatmap (Figure 1), with all traits exhibiting a highly significant phylogenetic signal (λ > 0.75, P < 0.001; Table 1). These results show evidence that there is a high degree of concordance between cephalopod phylogeny and the biological traits evaluated.
Correlated Evolution
The PGLS analysis showed no correlation between BL and EL (β = 0.036, Table 2 and Figure 2A). Whereas a positive correlation was found between BL and PF, as the main effects differed significantly from zero (β = 1.8, Table 2 and Figure 2B); however, PF was inversely correlated with EL (β = −1.5, Table 2 and Figure 2C). The OLS analysis showed a negative correlation between BL and EL (β = −0.26, Table 2) and an inverse correlation between PF and EL (β = −2.25, Table 2). Conversely, a positive relationship was found between BL and PF (β = 2.27, Table 2). The allometric correction on traits resulted in a negative relationship between EL/BL and PF/BL (Table 2 and Figure 3), showing evidence of this trade-off and enabling to avoid the body length scaling.
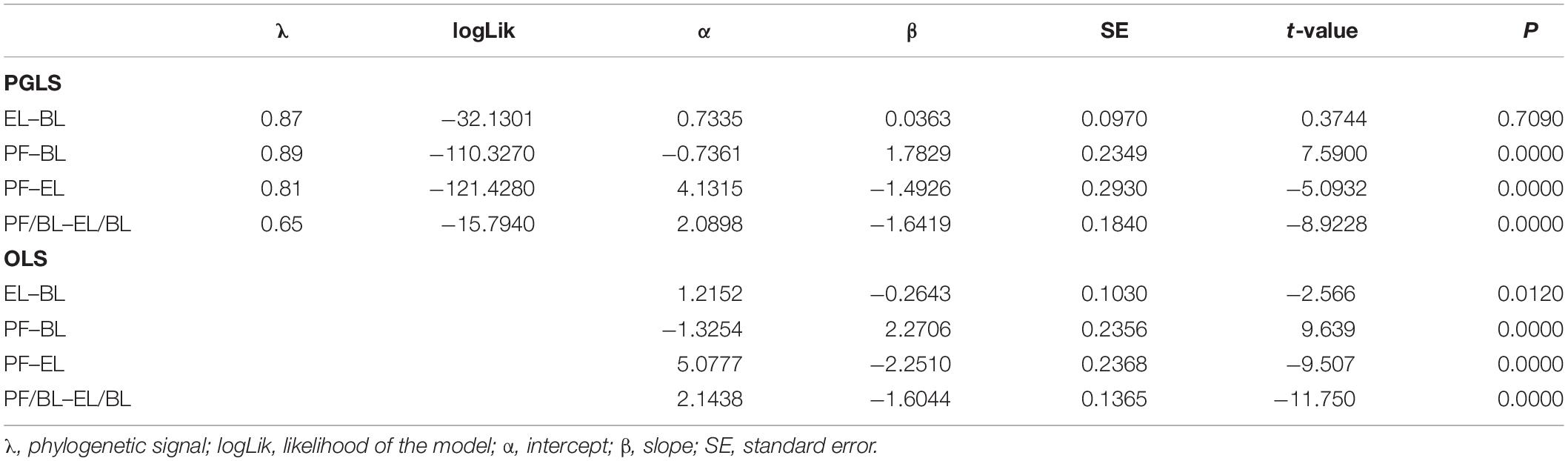
Table 2. Parameters of the relationship among body length (BL), egg length (EL), and potential fecundity (PF) of cephalopods obtained by phylogenetic generalized least squares (PGLS) and ordinary least squares (OLS) analyses.
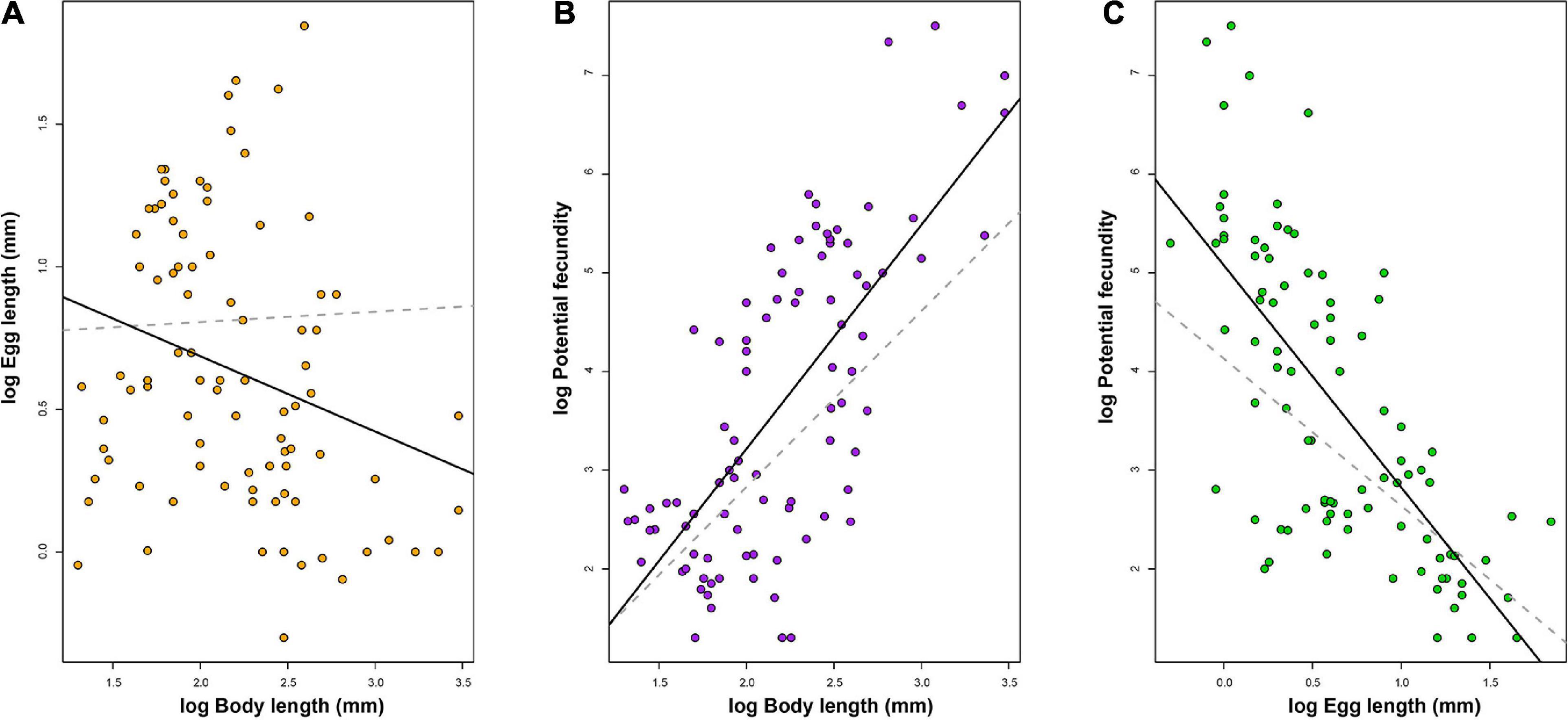
Figure 2. Relationships between reproductive traits of 90 cephalopod species, where (A) body length and egg length, (B) body length and potential fecundity, (C) egg length and potential fecundity. Dashed lines represent regressions from PGLS. Solid lines represent regressions from OLS.
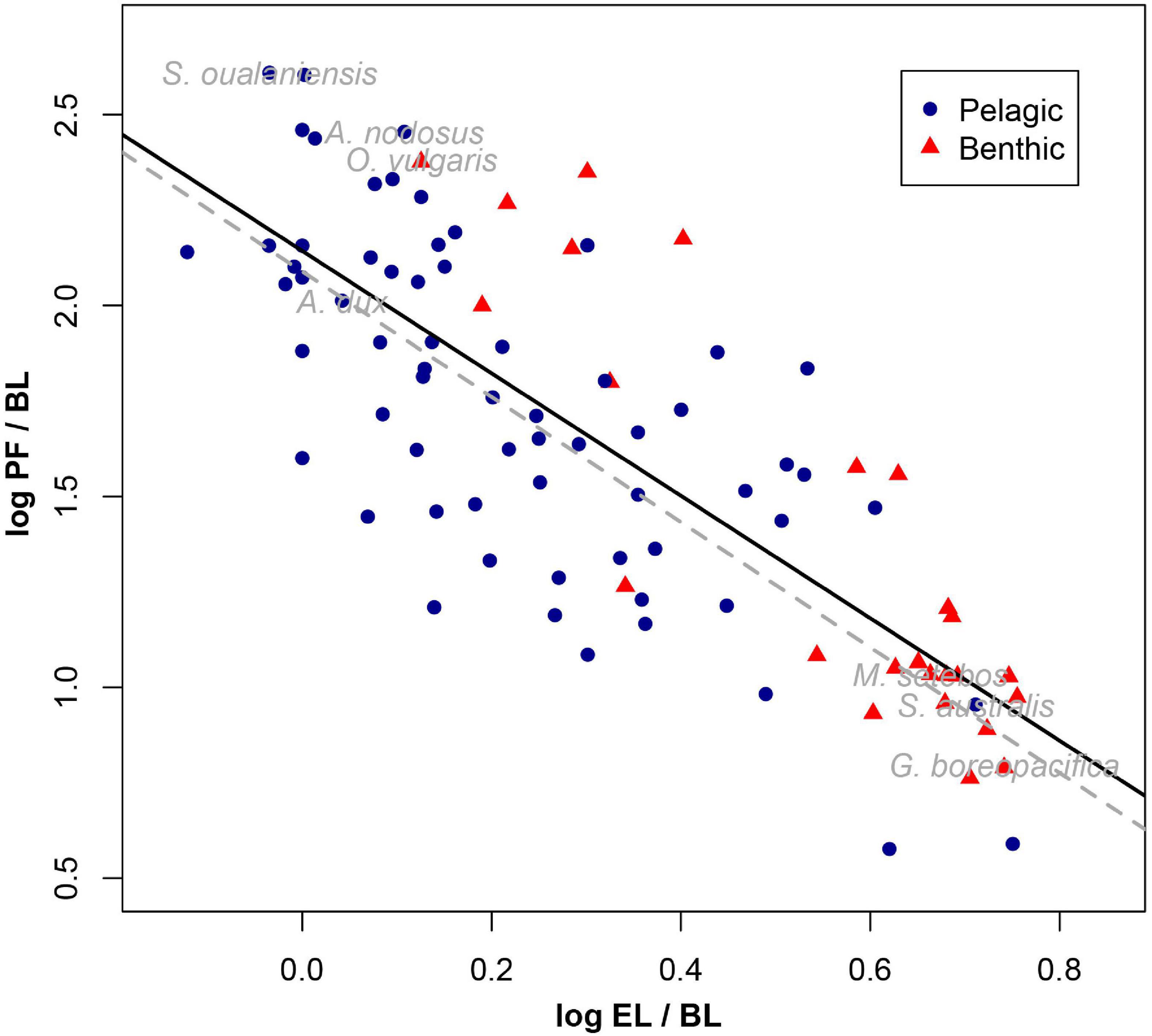
Figure 3. Relationships between reproductive traits of 90 cephalopod species corrected by body length. Dashed lines represent regressions from PGLS. Solid lines represent regressions from OLS.
Pelagic squids (e.g., A. dux and Sthenoteuthis oualaniensis) and pelagic octopuses (e.g., Argonauta nodosus and Tremoctopus violaceus) showed similar EL/BL and PF/BL, contrasting with deep-sea benthic species (e.g., Megaleledone setebos and Graneledone boreopacifica). In turn, lifestyle (pelagic vs. benthic) did not show a significant influence (P = 0.8724) in the phylogenetic ANCOVA (Figure 3 and Table 3). This analysis confirmed the significant influence of BL and EL on PF, as predicted based on EL/BL and PF/BL ratios (Table 3 and Figure 3).
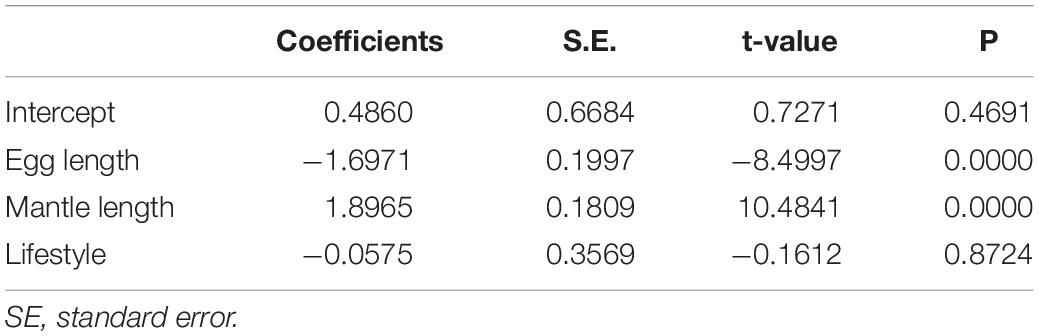
Table 3. Parameters of the phylogenetic analysis of covariance (phylogenetic ANCOVA) of cephalopods obtained by phylogenetic generalized least squares (PGLS).
Ancestral States
For all traits evaluated, the DF model was the best fitted as it reached the highest likelihood, lowest AICc (Table 4) and significant LRT values (Supplementary Table 3). The highest rate of change (σ2) values for all traits were detected in the OU model, although the ancestral state (z0) values at the root of the tree according to the OU model were the highest only for BL and PF (Table 4). The DF model showed the lowest ancestral state (z0, Table 4) values, suggesting an increase of BL, EL, and PF along the phylogeny, although at a low rate (σ2, Table 4). The OU model showed a high alpha parameter (α = 2.718) and a good fit (Table 4); therefore, evolution under an OU process cannot be discarded in the traits evaluated.
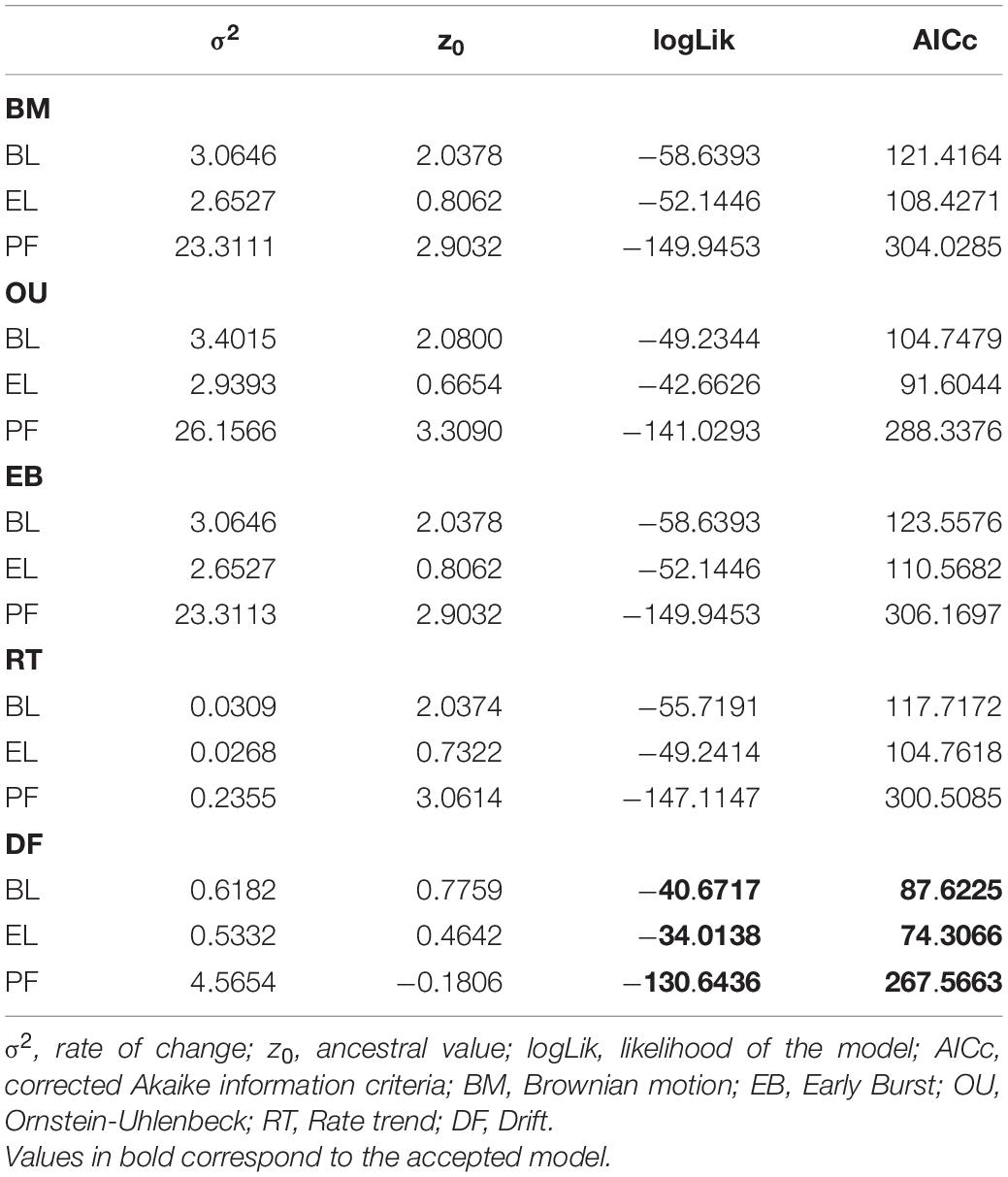
Table 4. Parameters of fitContinuos models for body length (BL), egg length (EL), and potential fecundity (PF) of cephalopods.
The ancestral values shown by the BM model for each trait were close to the mean values (BL = 2.1, ES = 0.68, and PF = 3.6) (see Figure 4). Under the BM model, within the clade containing Decapodiformes, some lineages evolved toward small BL, such as sepiolids, whereas others evolved in the opposite direction by increasing BL, such as oegopsid squids (Figure 4A). Egg length evolved to larger sizes in benthic octopuses (Octopodiformes clade) and Nautilus, compared with oegopsid squids, which showed the smallest egg sizes (Figure 4B). Consistently, PF evolved toward the production of few eggs in benthic octopuses and toward producing thousands to millions of eggs in oegopsid squids (Figure 4C).
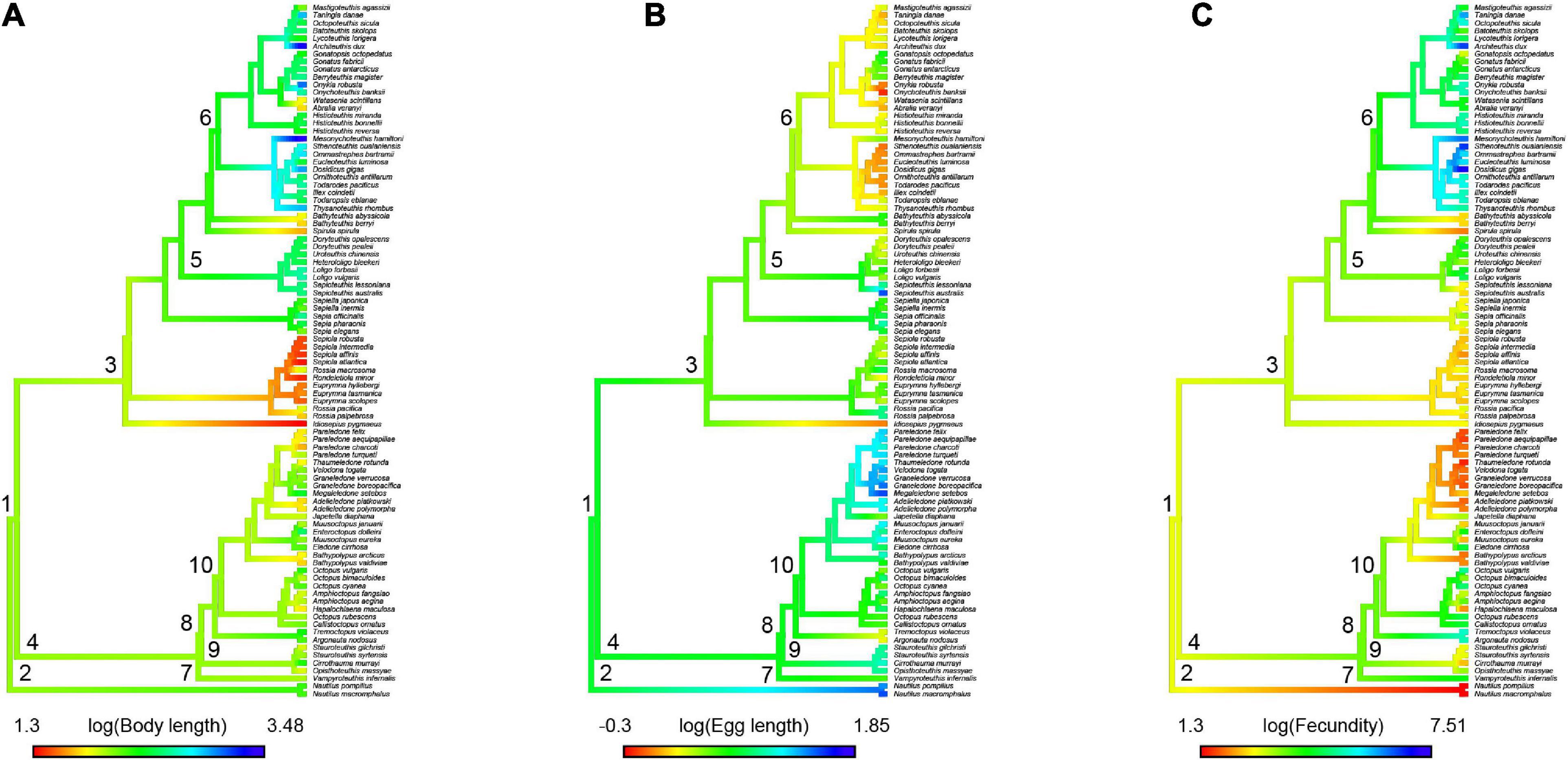
Figure 4. Density map of ancestral reconstruction of 90 cephalopod species, where (A) body length, (B) egg length, and (C) potential fecundity. The number in each node correspond to: (1) subclass Coleoidea, (2) subclass Nautiloidea, (3) superorder Decapodiformes, (4) superorder Octopodiformes, (5) order Myopsida, (6) order Oegopsida, (7) order Cirrata, and (8) order Incirrata, (9) superfamily Argonautoidea, (10) superfamily Octopodoidea.
Discussion
In this comparative study, we evaluated the life history traits of cephalopods considering their phylogenetic relationships, as suggested by Felsenstein (1985). This approach has been scarcely used in comparative studies of cephalopods, and particularly, the assessment of phylogenetic signal has only been conducted in five publications (i.e., Ibáñez et al., 2018, 2019, 2021; Anderson and Marian, 2020; Ponte et al., 2021). In this sense, our research is among the few studies correctly addressing trait comparisons for cephalopods, suggesting that further research should incorporate this approach in comparative biology of cephalopods.
The macroevolutionary analysis conducted in this study suggests an evident trade-off between reproductive traits of cephalopods—even including BL correction—, either by evolving toward large eggs and low fecundity, or toward small eggs and high fecundity. Several studies suggest r and k strategies for cephalopod mollusks (e.g., Nigmatullin and Laptikhovsky, 1994; Nesis, 1996; Ibáñez et al., 2018), whereas others invoke a continuum of different strategies that covary with environmental gradients (Rocha et al., 2001). Despite the strategies outlined above, some flexibility is expected due to the different lifestyles and geographic areas inhabited by cephalopods, where an important environmental component contributes to this observed variability in the evolution of life history traits (Rocha et al., 2001; Ibáñez et al., 2021). Indeed, it has been detected that ovulation patterns are correlated with environment (i.e., stable and unstable) (Ibáñez et al., 2021), hence, given our results here, it is possible that both EL and PF are also associated with the latter feature. Consistently, the effect of temperature over EL (and thus, over fecundity) has been evidenced at least for benthic octopods (Ibáñez et al., 2018). In this context, natural selection could benefit some of these strategies under fluctuating environmental conditions, for example, increasing the fitness of squids by enabling the production of millions of eggs (e.g., A. dux, D. gigas) owing to the energetic costs and strong selective pressures (Ramirez-Llodra, 2002). In this context, evidence also suggests phenotypic selection on sexual traits in octopuses driven by sexual selection via sperm competition and cryptic female choice (Ibáñez et al., 2019). Hence, it is expected that the copulatory organs of squids, sepiolids and other cephalopods also exhibit phenotypic selection, suggesting that not only the environment can exert selective pressures on cephalopods, as on other marine ectotherms, but also the intraspecific interactions associated with biological fitness.
This study is the first to directly relate reproductive traits associated with fitness in cephalopods under a phylogenetic context. Although it was possible to match phylogenetic and reproductive information for 90 species, a great amount of information on EL and PF is still lacking for several cephalopod species. In addition, life history trait data and phylogenies have historically been evaluated more extensively for commercial squids than the rest of cephalopod groups, which is also observable in the data and phylogenetic tree employed here, as Decapodiformes was better represented than Octopodiformes in both aspects (see Supplementary Tables 1, 2). Therefore, more efforts should be made to increase knowledge of these and other reproductive traits in order to better support further macroevolutionary inferences.
Phylogenetic Comparative Methods
Over 30 years ago, Felsenstein (1985) suggested that every study addressing comparative biology should consider the phylogenetic relationships of the group being evaluated, as most biological features are associated with the evolutionary history of living organisms. For this reason, several studies have shown the importance of including phylogenetic relationships in comparative studies of either physiological (Rezende and Diniz-Filho, 2012), reproductive (Ibáñez et al., 2018, 2019, 2021), or biogeographic traits (Hernández et al., 2013). In this context, comparative studies not including phylogeny violate data independency assumptions, being susceptible to spurious associations between traits (type I errors) or to mask true associations (type II errors) (Felsenstein, 1985; Rezende and Diniz-Filho, 2012). In the few comparative studies regarding the evolution of life history traits of cephalopods (e.g., Calow, 1987; Wood and O’Dor, 2000; Voight, 2001, 2009; Laptikhovsky et al., 2018), this type of correction was not conducted (e.g., independent contrast) to correctly infer true association between traits. Therefore, the approach presented here avoids type I and II errors, showing evidence that different results are obtained when incorporating the phylogeny to infer association between traits. Consistently, coefficients were significantly different when the phylogeny was not included, and under this scenario, we detected a false positive correlation between BL and EL of cephalopods (Table 2 and Figure 2), confirming that without using phylogenetic relationships coefficient estimates are biased.
We highlight a couple of studies that constituted a framework to conduct interspecific comparisons among traits (Rezende and Diniz-Filho, 2012; Hernández et al., 2013) and that can also be useful in further studies to correctly infer macroevolutionary relationships among life history traits of cephalopods and other marine species.
Egg Length
The lack of correlation between BL and EL observed here for cephalopod mollusks contrasts with previous studies on other invertebrates and vertebrates, including hymenopterans, lepidopterans, crustaceans, fishes, and birds, where correlated evolution between both traits was detected under a phylogenetic perspective (Blackburn, 1991; Poulin and Hamilton, 1997; Crespi and Teo, 2002; Dyke and Kaiser, 2010; Davis et al., 2012). However, it should be noted that cephalopod eggs are rich in yolk reserves, which are expected to have high energetic content (Boletzky, 1989). In this sense, the parental investment in egg production of cephalopods could be leaned toward energetic content rather than size, which would partially explain the lack of correlation found between BL and EL. Unfortunately, there is not enough data on the energetic content of cephalopod eggs to test this hypothesis. Additionally, for cephalopods, it could be expected that BL and EL are uncorrelated traits, as EL depends on their development span and mode (i.e., merobenthic, holobenthic, holopelagic and meropelagic) (Boyle and Rodhouse, 2005; Villanueva et al., 2021), however, it was worth evaluating the relationship between BL and EL under a phylogenetic context to correctly confirm that they are uncorrelated traits.
Cephalopods are globally distributed in all marine ecosystems, and egg size is one the most relevant reproductive traits for this group of mollusks due to its significant relationship with environmental variation (e.g., Ibáñez et al., 2018, 2021; Laptikhovsky et al., 2018). In general, highly fertile species that produce small eggs are more vulnerable to extreme climate or environmental conditions, such as high temperature (Pecl and Jackson, 2008), while species with large eggs and low fecundity are more vulnerable to ecological and biotic effects, such as the appearance of new predators (Packard, 1972; Aronson, 1991; Laptikhovsky et al., 2013). Furthermore, the relationship between environmental conditions and the egg size was detected in extinct cephalopod groups, such as Mesozoic ammonites and nautiluses, as dramatic changes in sea temperature were key factors influencing their egg size (Laptikhovsky et al., 2013; Fuchs et al., 2020). By specifically evaluating egg size in cephalopods, it was possible to assess the evolution of reproduction type in relation to biotic and abiotic events such as glaciations, global warming, expansions and setbacks of the continental shelf, as well as the origin and extinction of competitors and predators (Laptikhovsky et al., 2018). Ammonites, for example, possibly released floating masses of small eggs that hatched into planktonic larvae, favoring their dispersal and the colonization of new habitats, although subjected to high size-selective predation and offspring mortality (Packard, 1972; Aronson, 1991; Mapes and Nützel, 2009; Wani et al., 2011; Laptikhovsky et al., 2013). In contrast, nautiloids produced large eggs and hatchlings without planktonic larval phase, which probably allowed them to persist throughout geological history and survive great extinctions (e.g., Cretaceous-Tertiary mass extinction "K/T") at the expense of reduced dispersal capabilities and the mortality associated with size-selective predation (Mapes and Nützel, 2009; Wani et al., 2011; Laptikhovsky et al., 2013).
Fecundity and Life History Trade-Offs
The inverse phylogenetic correlation between EL and PF found in the present study is interpreted as a macroevolutionary trade-off that limits the Darwinian fitness in some species. Our results considering 90 cephalopod species strongly corroborate the pattern described by Calow (1987), who showed evidence of the inverse relationship between EL and PF for 23 cephalopod species including nautiloids and coleoids. This author found that this trend was more evident when planktonic versus benthic hatchlings are compared, which also concurs with our findings (Figure 3). Following the general pattern observed for most marine invertebrates, large eggs and low fecundity are generally linked to the production of benthic juveniles, while small eggs and high fecundity are associated with the production of planktonic hatchlings (Boletzky, 1977; Boyle and Rodhouse, 2005; Villanueva et al., 2016; Laptikhovsky et al., 2018). Evolution toward large eggs is directly associated with the increase of size, fitness/competence, and survival of the offspring, as large eggs contain sufficient energetic reserves to produce large and well-developed hatchlings (Villanueva and Norman, 2008). This increase in egg size necessarily entails reducing the number of eggs as a trade-off due to the limited reproductive reserves and anatomic restrictions. Consistently, in this study, species that favored the reduction of egg size increased the number of eggs, which is typical for species with planktonic paralarvae, where the low survival is compensated by the large number and high dispersal potential of offspring (Boletzky, 1989; Villanueva et al., 2016). In the case of pelagic species (squids and octopuses, Figure 3, Supplementary Table 1), these possibly evolved in a convergent manner toward small egg size and high fecundity in order to ensure higher fitness in low productivity habitats, such as the oceanic zone.
Our results showed a positively correlated evolution between BL and PF of cephalopods, as increments in body size accounted for an increase in egg/oocyte production. The most relevant aspect of this relationship is that it appears to be closely associated with both, habitats and hatching modes, which ultimately influence evolutionary traits at the population and species levels. Indeed, large-sized and highly fecund species that produce planktonic paralarvae with high dispersal potential (as many Oegopsida) show large geographical ranges (Jereb and Roper, 2010; Villanueva et al., 2016) and homogeneity in their population genetic structure (Carvalho et al., 1992; Ibáñez et al., 2011). In fact, our results suggest an evolutionary trend of directional selection toward increasing fecundity and body length. This strategy would be reflected in the high recruitment variability of some species that produce huge amounts of eggs during years with optimal environmental conditions, allowing high reproductive success and offspring survival.
From a behavioral perspective, Hanlon and Messenger (1996) suggested that cephalopods evolved contrasting life history strategies related to their reproductive success. In this context, and according to the macroevolutionary trade-off between PF and EL described here, two generalized paths can be observed through cephalopod evolution. The first is the development of a high fecundity at the expense of small egg sizes in species inhabiting the open ocean or temperate coastal areas (e.g., oegopsid squids and shallow-water benthic octopuses, Figure 3). The second is the evolution toward large eggs and low fecundity in species inhabiting the deep sea and cold-water areas (e.g., deep-sea octopuses and loliginid squids, Figure 3). Exceptions, at least in octopuses, are related to unbalanced speciation and extinction rates associated with development modes (Ibáñez et al., 2014).
Evolutionary Trends
An evolutionary trend is a bias in the direction of changes in a trait within one or multiple lineages through time (Futuyma and Kirkpatrick, 2017). In this sense, our results suggest an evolutionary trend for BL, EL, and PF in cephalopods, despite the fact that EL has a tendency to decrease while BL and PF to increase. This tendency is supported with the fossil record, which shows evidence of small egg sizes in Mesozoic coleoid cephalopods, with some lineages evolving toward larger egg size (Fuchs et al., 2020). Models of trait evolution are compatible with different evolutionary processes (e.g., selection, genetic drift; Harmon et al., 2010), and therefore, in the drift model, genetic drift, and directional selection fluctuate randomly through time (Felsenstein, 1988; Harmon, 2018) with a tendency to increase from low to high values. Considering that fecundity is one of the key components of fitness, and that the drift model was the best fit to our data, a directional selection process is more plausible than a stabilizing selection in the traits evaluated. This directional trend could be a result of species selection, associated with a trait correlation with speciation or extinction rates (Futuyma and Kirkpatrick, 2017), in this case, life history traits.
Data Availability Statement
The original contributions presented in the study are included in the article/Supplementary Material, further inquiries can be directed to the corresponding author.
Ethics Statement
The animal study was reviewed and approved by Andres Bello University.
Author Contributions
CI and MP-G designed the study. CI carried out analysis with support from CC and DL-C. CI, FF-Á, FR, EV, and MP-G compiled data. CI, MD-S-I, SC, FF-Á, CC, NO, FR, EV, and MP-G wrote the manuscript and approved the manuscript for publication. All authors contributed to the article and approved the submitted version.
Funding
This work was partially funded by FONDECYT grants #3110152 and #11170617 awarded to CI and SC, respectively. The additional support from the INACH research grant RG 50-18 awarded to MP-G, CI, and MD-S-I is also appreciated. FF-Á was supported by an Irish Research Council–Government of Ireland Postdoctoral Fellowship Award (ref. GOIPD/2019/460). CC was supported by a ANID, ANID-PCHA/Doctorado Nacional/2019-21191261, and EDPG LPR-161 project from Dirección de Postgrado de la Universidad de Concepción. NO was supported by National Scientific and Technical Research Council of Argentina. EV thanks the Brazilian National Research Council (CNPq – # 312332/2018-1).
Conflict of Interest
The authors declare that the research was conducted in the absence of any commercial or financial relationships that could be construed as a potential conflict of interest.
Publisher’s Note
All claims expressed in this article are solely those of the authors and do not necessarily represent those of their affiliated organizations, or those of the publisher, the editors and the reviewers. Any product that may be evaluated in this article, or claim that may be made by its manufacturer, is not guaranteed or endorsed by the publisher.
Acknowledgments
Thanks to Annie Lindgren for providing her published phylogenetic tree. Thanks to Jocelyn López-Córdova, who drew the cephalopod illustrations. MD-S-I wishes to thank CONACYT for the grant provided during the postdoctoral stay within the framework of the call Estancias Posdoctorales en el Extranjero 2019 and 2020.
Supplementary Material
The Supplementary Material for this article can be found online at: https://www.frontiersin.org/articles/10.3389/fmars.2021.707825/full#supplementary-material
References
Anderson, F. E., and Marian, J. E. A. R. (2020). The grass squid Pickfordiateuthis pulchella is a paedomorphic loliginid. Mol Phylogenet. Evol. 147:106801. doi: 10.1016/j.ympev.2020.106801
Arendt, J. D. (2003). Reduced burst speed is a cost of rapid growth in anuran tadpoles: problems of autocorrelation and inferences about growth rates. Funct. Ecol. 17, 328–334. doi: 10.1046/j.1365-2435.2003.00737.x
Aronson, R. B. (1991). Ecology, paleobiology and evolutionary constraint in the octopus. Bull. Mar. Sci. 49, 245–255.
Badyaev, A. V. (2002). Species divergence in sexually selected traits: increase in song elaboration is related to decrease in plumage ornamentation in finches. Evolution 56, 412–419. doi: 10.1111/j.0014-3820.2002.tb01350.x
Blackburn, T. M. (1991). Evidence for a fast slow continuum of life-history traits among parasitoid Hymenoptera. Funct. Ecol. 5, 65–74. doi: 10.2307/2389556
Boletzky, S. (1977). Post-hatching behaviour and mode of life in cephalopods. Symp. Zool. Soc. Lond. 38, 557–567.
Boletzky, S. (1989). Recent studies on spawning, embryonic development, and hatching in the Cephalopoda. Adv. Mar. Biol. 25, 85–115. doi: 10.1016/S0065-2881(08)60188-1
Boyle, P. R., and Rodhouse, P. G. (2005). Cephalopods, Ecology and fisheries. Oxford: Blackwell Publishing.
Butler, M. A., and King, A. A. (2004). Phylogenetic comparative analysis: a modeling approach for adaptive evolution. Am. Nat. 164, 683–695. doi: 10.1086/426002
Calow, P. (1987). “Fact and theory: an overview,” in Cephalopod life cycles: comparative reviews. Vol. 2, ed. P. R. Boyle (London: Academic Press), 351–366.
Carvalho, G. R., Thompson, A., and Stoner, A. L. (1992). Genetic diversity and population differentiation of the shortfin squid Illex argentinus in the south-west Atlantic. J. Exp. Mar. Biol. Ecol. 158, 105–121. doi: 10.1016/0022-0981(92)90311-W
Crespi, B. J., and Teo, R. (2002). Comparative phylogenetic analysis of the evolution of semelparity and life history in salmonid fishes. Evolution 56, 1008–1020. doi: 10.1111/j.0014-3820.2002.tb01412.x
Davis, R. B., Javois, J., Pienaar, J., Unap, E. O., and Tammaru, T. (2012). Disentangling determinants of egg size in the Geometridae (Lepidoptera) using an advanced phylogenetic comparative method. J. Evol. Biol. 26, 1379–1219. doi: 10.1111/j.1420-9101.2011.02420.x
Dyke, G. J., and Kaiser, G. W. (2010). Cracking a developmental constraint: egg size and bird evolution. Rec. Aust. Mus. 62, 207–216. doi: 10.3853/j.0067-1975.62.2010.1547
Elgar, M. A. (1990). Evolutionary compromise between a few large and many small eggs: comparative evidence in teleost fish. Oikos 59, 283–287. doi: 10.2307/3545546
Felsenstein, J. (1973). Maximum likelihood estimation of evolutionary trees from continuous characters. Am. J. Hum. Genet. 25, 471–492.
Felsenstein, J. (1985). Phylogenies and the comparative method. Am. Nat. 125, 1–15. doi: 10.2307/2461605
Felsenstein, J. (1988). Phylogenies and quantitative characters. Annu. Rev. Ecol. Syst. 19, 445–471. doi: 10.1146/annurev.es.19.110188.002305
Fuchs, D., Laptikhovsky, V., Nikolaeva, S., Ippolitov, A., and Rogov, M. (2020). Evolution of reproductive strategies in coleoid mollusks. Paleobiology 46, 82–103. doi: 10.1017/pab.2019.41
Grafen, A. (1989). The phylogenetic regression. Philos. Trans. R. Soc. Lond. B Biol. Sci. 326, 119–157. doi: 10.1098/rstb.1989.0106
Hanlon, R. T., and Messenger, J. B. (1996). Cephalopod Behaviour. Cambridge: Cambridge University Press.
Harmon, L. J. (2018). Phylogenetic comparative methods: learning from trees, Creative Commons Attribution 4.0 International License.
Harmon, L. J., Losos, J. B., Davies, T. J., Gillespie, R. G., Gittleman, J. L., Jennings, W. B., et al. (2010). Early bursts of body size and shape evolution are rare in comparative data. Evolution 64, 2385–2396. doi: 10.1111/j.1558-5646.2010.01025.x
Harmon, L. J., Pennell, M., Brock, C., Brown, J., Challenger, W., Eastman, J., et al. (2020). GEIGER: investigating evolutionary radiations. Available Online at: https://github.com/mwpennell/geiger-v2. (accessed 11, 2020).
Harmon, L. J., Weir, J. T., Brock, C. D., Glor, R. E., and Challenger, W. (2008). GEIGER: investigating evolutionary radiations. Bioinformatics 24, 129–131. doi: 10.1093/bioinformatics/btm538
Hernández, C. E., Rodríguez-Serrano, E., Avaria-Llautureo, J., Inostroza-Michael, O., Morales-Pallero, B., Boric-Bargetto, D., et al. (2013). Using phylogenetic information and the comparative method to evaluate hypotheses in macroecology. Methods Ecol. Evol. 4, 401–415. doi: 10.1111/2041-210X.12033
Hochberg, F. G., Nixon, M., Toll, R. B., and Boletzky, S. V. (eds) (1992). “Octopoda,” in Larval” and Juvenile Cephalopods: A Manual for Their Identification, eds M. J. Sweeney, C. F. E. Roper, K. M. Mangold, and M. R. Clarke (Washington: Smithsonian Contributions to Zoology), 213–280.
Ibáñez, C. M., Cubillos, L. A., Tafur, R., Argüelles, J., Yamashiro, C., and Poulin, E. (2011). Genetic diversity and demographic history of Dosidicus gigas (Cephalopoda: ommastrephidae) in the Humboldt Current System. Mar. Ecol. Progr. Ser. 431, 163–171. doi: 10.3354/meps09133
Ibáñez, C. M., Díaz-Santana-Iturrios, M., López-Córdova, D. A., Carrasco, S. A., Pardo-Gandarillas, M. C., Rocha, F., et al. (2021). A phylogenetic approach to understand the evolution of reproduction in coleoid cephalopods. Mol. Phylogenet. Evol. 155:106972. doi: 10.1016/j.ympev.2020.106972
Ibáñez, C. M., Peña, F., Pardo-Gandarillas, M. C., Méndez, M. A., Hernández, C. E., and Poulin, E. (2014). Evolution of development type in benthic octopuses: holobenthic or pelago-benthic ancestor? Hydrobiologia 725, 205–214. doi: 10.1007/s10750-013-1518-5
Ibáñez, C. M., Pérez-Álvarez, J., Catalán, J., Carrasco, S. A., Pardo-Gandarillas, M. C., and Rezende, E. L. (2019). Sexual Selection and the Evolution of Male Reproductive Traits in Benthic Octopuses. Front. Physiol. 10:1238. doi: 10.3389/fphys.2019.01238
Ibáñez, C. M., Rezende, E. L., Sepúlveda, R. D., Avaria-Llautureo, J., Hernández, C. E., Sellanes, J., et al. (2018). Thorson’s rule, life history evolution and diversification of benthic octopuses (Cephalopoda: octopodoidea). Evolution 7, 1829–1839. doi: 10.1111/evo.13559
Jablonski, D., Erwin, D. H., and Lipps, J. H. (1996). Body size and macroevolution. Evolutionary paleobiology. Chicago: University of Chicago Press.
Jereb, P., and Roper, C. F. E. (2005). Cephalopods of the world. An annotated and illustrated catalogue of cephalopod species known to date. Volume 1. Chambered nautiluses and sepioids (Nautilidae, Sepiidae, Sepiolidae, Sepiadariidae, Idiosepiidae and Spirulidae). FAO Species Catalogue for Fishery Purposes. No. 4, Vol. 1. Rome: FAO.
Jereb, P., and Roper, C. F. E. (2010). Cephalopods of the world. An annotated and illustrated catalogue of cephalopod species known to date. Volume 2. Myopsid and Oegopsid Squids. FAO Species Catalogue for Fishery Purposes. No. 4, Vol. 2. Rome: FAO.
Laptikhovsky, V. V., Arkhipkin, A. I., and Hoving, H. J. T. (2007). Reproductive biology in two species of deep-sea squids. Mar. Biol. 152, 981–990. doi: 10.1007/s00227-007-0749-2
Laptikhovsky, V. V., Nikolaeva, S., and Rogov, M. (2018). Cephalopod embryonic shells as a tool to reconstruct reproductive strategies in extinct taxa. Biol. Rev. 93, 270–283. doi: 10.1111/brv.12341
Laptikhovsky, V. V., Rogov, M. A., Nikolaeva, S. E., and Arkhipkin, A. I. (2013). Environmental impact on ectocochleate cephalopod reproductive strategies and the evolutionary significance of cephalopod egg size. Bull. Geosci. 88, 83–93. doi: 10.3140/bull.geosci.1351
Lartillot, N., and Delsuc, F. (2012). Joint reconstruction of divergence times and life-history evolution in placental mammals using a phylogenetic covariance model. Evolution 66, 1773–1787. doi: 10.1111/j.1558-5646.2011.01558.x
Lindgren, A. R., Pankey, M. S., Hochberg, F. G., and Oakley, T. H. (2012). A multi-gene phylogeny of Cephalopoda supports convergent morphological evolution in association with multiple habitat shifts in the marine environment. BMC Evol. Biol. 12:129. doi: 10.1186/1471-2148-12-129
Mapes, R. H., and Nützel, A. (2009). Late Palaeozoic mollusc reproduction: cephalopod egg-laying behavior and gastropod larval palaeobiology. Lethaia 42, 341–356. doi: 10.1111/j.1502-3931.2008.00141.x
Marian, J. E. A. R. (2015). Evolution of spermatophore transfer mechanisms in cephalopods. J. Nat. Hist. 49, 1423–1455. doi: 10.1080/00222933.2013.825026
Nesis, K. N. (1996). Mating, spawning, and death in oceanic cephalopods: a review. Ruthenica 6, 23–64.
Nigmatullin, C. M., and Laptikhovsky, V. V. (1994). Reproductive strategies in the squids of the family Ommastrephidae (preliminary report). Ruthenica 4, 79–82.
Packard, A. (1972). Cephalopods and fish: the limits of convergence. Biol. Rev. 47, 241–307. doi: 10.1111/j.1469-185X.1972.tb00975.x
Pagel, M. (1999). Inferring the historical patterns of biological evolution. Nature 401, 877–884. doi: 10.1038/44766
Paradis, E., Claude, J., and Strimmer, K. (2004). APE: analyses of phylogenetics and evolution in R language. Bioinformatics 20, 289–290. doi: 10.1093/bioinformatics/btg412
Pecl, G., and Jackson, G. (2008). The potential impacts of climate change on in shore squid: biology, ecology and fisheries. Rev. Fish. Biol. Fish. 18, 373–385. doi: 10.1007/s11160-007-9077-3
Perales-Raya, C., Bartolomé, A., Hernández-Rodríguez, E., Carrillo, M., Martín, V., and Fraile-Nuez, E. (2020). How old are giant squids? First approach to aging Architeuthis beaks. Bull. Mar. Sci. 96, 357–374. doi: 10.5343/bms.2019.0041
Ponte, G., Taite, M., Borrelli, L., Tarallo, A., Allcock, A. L., and Fiorito, G. (2021). Cerebrotypes in cephalopods: brain diversity and its correlation with species habits, life history, and physiological adaptations. Front. Neuroanat. 14:565109. doi: 10.3389/fnana.2020.565109
Poulin, R., and Hamilton, W. J. (1997). Ecological correlates of body size and egg size in parasitic Ascothoracida and Rhizocephala (Crustacea). Acta Oecol. 18, 621–635. doi: 10.1016/S1146-609X(97)80047-1
Poulin, R., and Mouillot, D. (2004). The relationship between specialization and local abundance: the case of helminth parasites of birds. Oecologia 140, 372–378. doi: 10.1007/s00442-004-1593-4
R Development Core Team. (2020). R: a language and environment for statistical computing. Vienna: R Foundation for Statistical Computing.
Ramirez-Llodra, E. R. (2002). Fecundity and life-history strategies in marine invertebrates. Adv. Mar. Biol. 43, 87–170. doi: 10.1016/s0065-2881(02)43004-0
Revell, L. J. (2012). phytools: an R package for phylogenetic comparative biology (and other things). Methods Ecol. Evol. 3, 217–223. doi: 10.1111/j.2041-210X.2011.00169.x
Rezende, E. L., and Diniz-Filho, J. A. (2012). Phylogenetic Analyses: comparing Species to Infer Adaptations and Physiological Mechanisms. Compr. Physiol. 2, 639–674. doi: 10.1002/cphy.c100079
Rocha, F., Guerra, A., and González, A. F. (2001). A review of reproductive strategies in cephalopods. Biol. Rev. 76, 291–304. doi: 10.1017/s1464793101005681
Stearns, S. C. (1989). Trade-offs in life-history evolution. Funct. Ecol. 3, 259–268. doi: 10.2307/2389364
Stephens, P. R., and Wiens, J. J. (2008). Testing for evolutionary trade-offs in a phylogenetic context: ecological diversification and evolution of locomotor performance in emydid turtles. J. Evol. Biol. 21, 77–87. doi: 10.1111/j.1420-9101.2007.01467.x
Vanhooydonck, B., James, R. S., Tallis, J., Aerts, P., Tadic, Z., Tolley, K. A., et al. (2014). Is the whole more than the sum of its parts? Evolutionary trade-offs between burst and sustained locomotion in lacertid lizards. Proc. Biol. Sci. 281:20132677. doi: 10.1098/rspb.2013.2677
Villanueva, R., Coll-Lladó, M., Bonnaud-Ponticelli, L., Carrasco, S. A., Escolar, O., Fernández-Álvarez, FÁ., et al. (2021). Born with bristles: new insights on the Kölliker’s organs of octopus skin. Front. Mar. Sci. 8:645738. doi: 10.3389/fmars.2021.645738
Villanueva, R., and Norman, M. D. (2008). Biology of the planktonic stages of benthic octopuses. Oceanogr. Mar. Biol. 46, 105–202. doi: 10.1201/9781420065756.CH4
Villanueva, R., Vidal, E. A. G., Fernández-Álvarez, F. A., and Nabhitabhata, J. (2016). Early mode of life and hatchling size in cephalopod molluscs: influence on the species distributional ranges. PLoS One 11:e0165334. doi: 10.1371/journal.pone.0165334
Voight, J. R. (2001). The relationship between sperm reservoir and spermatophore length in benthic octopuses (Cephalopoda: octopodidae). J. Mar. Biol. Assoc. U. K. 81, 983–986. doi: 10.1017/S0025315401004945
Voight, J. R. (2009). Differences in spermatophore availability among octopodid species (Cephalopoda: octopoda). Malacologia 51, 143–153. doi: 10.4002/040.051.0110
Wani, R., Kurihara, K. I., and Ayyasami, K. (2011). Large hatchling size in Cretaceous nautiloids persists across the end-Cretaceous mass extinction: new data of Hercoglossidae hatchlings. Cretac. Res. 32, 618–622. doi: 10.1016/j.cretres.2011.01.001
Wood, J. B., and O’Dor, R. K. (2000). Do larger cephalopods live longer? Effects of temperature and phylogeny on interspecific comparisons of age and size at maturity. Mar. Biol. 136, 91–99. doi: 10.1007/s002270050012
Keywords: Cephalopoda, phylogeny, comparative biology, life histories, egg size, fecundity
Citation: Ibáñez CM, Díaz-Santana-Iturrios M, Carrasco SA, Fernández-Álvarez FA, López-Córdova DA, Cornejo CF, Ortiz N, Rocha F, Vidal EAG and Pardo-Gandarillas MC (2021) Macroevolutionary Trade-Offs and Trends in Life History Traits of Cephalopods Through a Comparative Phylogenetic Approach. Front. Mar. Sci. 8:707825. doi: 10.3389/fmars.2021.707825
Received: 10 May 2021; Accepted: 16 August 2021;
Published: 03 September 2021.
Edited by:
Jin Sun, Ocean University of China, ChinaReviewed by:
Graziano Fiorito, Zoological Station Anton Dohrn, ItalyPamela Imperadore, Zoological Station Anton Dohrn, Italy
Copyright © 2021 Ibáñez, Díaz-Santana-Iturrios, Carrasco, Fernández-Álvarez, López-Córdova, Cornejo, Ortiz, Rocha, Vidal and Pardo-Gandarillas. This is an open-access article distributed under the terms of the Creative Commons Attribution License (CC BY). The use, distribution or reproduction in other forums is permitted, provided the original author(s) and the copyright owner(s) are credited and that the original publication in this journal is cited, in accordance with accepted academic practice. No use, distribution or reproduction is permitted which does not comply with these terms.
*Correspondence: Christian M. Ibáñez, aWJhbmV6LmNocmlzdGlhbkBnbWFpbC5jb20=; María Cecilia Pardo-Gandarillas, cGFyZG9nYW5kYXJpbGxhc0BnbWFpbC5jb20=