- 1College of Ocean and Earth Sciences, Xiamen University, Xiamen, China
- 2State Key Laboratory of Marine Environmental Science, Xiamen University, Xiamen, China
- 3Fujian Provincial Key Laboratory for Coastal Ecology and Environmental Studies, Xiamen University, Xiamen, China
- 4Fujian Institute of Oceanography, Xiamen, China
- 5Second Institute of Oceanography, Ministry of Natural Resources, Hangzhou, China
- 6Coastal and Ocean Management Institute, Xiamen University, Xiamen, China
- 7College of Chemistry and Environment, Minnan Normal University, Zhangzhou, China
Riverine outflow is one of the major pathways for microplastic transportation to coastal environments. Research on the output of microplastics in small- or medium-sized rivers will help accurately understand the status of their marine loads. In this study, we used both trawling and pumping methods to collect microplastics of different sizes in the Jiulong River Estuary and Xiamen Bay. We found that the abundance of small microplastics (44 μm–5.0 mm) was at least 20 times higher than the large particles (0.33–5.0 mm). The abundance of the large particles ranges from 4.96 to 16.3 particles/m3, and that of the small particles ranged from 82.8 to 918 particles/m3. Granule was the dominant shape (>60%), and polyethylene (PE), polypropylene (PP), and polyethylene terephthalate (PET) were the most common components. The riverine flux of small microplastics (44 μm–5 mm, 472 ± 230 t/y) was at a medium level and was eight times greater than that of large particles (0.33–5.0 mm, 61.2 ± 2.6 t/y). The behavior of the large microplastics was relatively conservative, whose abundance had a significant correlation with salinity (R2 = 0.927) and was mainly influenced by physical factors. In contrast, results of statistical analysis revealed that more complicated factors influenced the small microplastics.
Introduction
Microplastics have become a global concern due to their ecological threats and ubiquitous existence in marine environments (Cole et al., 2015; Galloway and Lewis, 2016; Pagter et al., 2020; Qi et al., 2020). In addition to direct ingestion and physical damage, microplastic may increase the bioaccumulation of persistent organic pollutants and heavy metals along the food chain (Zettler et al., 2013; Bakir et al., 2014; Brennecke et al., 2016; Fu et al., 2020). Rivers connect most land surfaces, and estuarine areas are the most significant socio-economic development belts around the world (Zayen et al., 2020). As for marine plastics, they are mainly from the land-based sources, thus estuarine-coastal environments act as important converters (Schmidt et al., 2017). Riverine outflow is regarded as one of the major pathways for plastics entering coastal environments, and the annual flux reaches 57000 MT worldwide (Mai et al., 2020).
Several studies have estimated the microplastic flux of riverine flows into the sea. The flux of plastic waste into marine waters globally is estimated to range between 4.8 and 12.7 Mt in 2010, 46.7–126.4 in 2015 on model predictions (Yonkos et al., 2014; Jambeck et al., 2015; Lebreton et al., 2017; Mai et al., 2019; Zhao et al., 2019). These data can help estimate the real value of the reserves of plastics and the plastic pollution in the ocean. However, the results could be more accurate if combined with field data representing the actual situation of watershed-offshore microplastic transportation. Although small- and medium-sized rivers have become essential parts of freshwater systems, most estimations currently focus on major rivers, and outflows in small- or medium-sized rivers are less well known. Therefore, to better study riverine discharges of microplastics and provide robust suggestions on microplastic management, it is essential to consider more small- or medium-sized rivers (Huang et al., 2012).
Xiamen Bay is located in one of the fastest-growing areas in Southeast Asia, the southeast coast of China, and borderers the Jiulong River in the west and the Taiwan Strait in the east. It is a semi-enclosed bay with a normal semi-diurnal tide, and which is divided into the Western Harbor and the Eastern Sea (Chen et al., 2021). Xiamen Bay receives considerable domestic, agricultural, and industrial wastes, and undergoes increasing threats to its environments and ecosystem (Chen et al., 2013). The levels of various contaminants, such as nutrients, persistent organic pollutants, heavy metals, and microplastics, have been reported (Klumpp et al., 2002; Ya et al., 2014; Tang et al., 2018; Wu et al., 2021). Especially, it has been verified that the abundance of microplastics has a relationship with socio-economic development and human activities along the Taiwan Strait Coast (Wu et al., 2021). Because of the intense pollutant release and weak hydrological conditions, more studies are focusing on the transport and fate of contaminants in the Xiamen Bay.
Jiulong River represents a small- or medium-sized river, whose lower reach is connected with Xiamen Bay and further flows into the Taiwan Strait (Li et al., 2015). From the first study on microplastics in this area, the abundance of microplastics by trawling ranges from 103 to 2017 particles/m3 (Tang et al., 2018). Under the influence of different water exchange dynamics, foam is the most common shape, and the compositions of PP and PE occupied the highest proportions. According to a study on microplastics in seawater and two sides of the Taiwan Strait, particles from the west coast, such as Xiamen Bay, are more prone to transport to the central Taiwan Strait (Wu et al., 2021). Since microplastics with different sizes usually have various buoyancy and vertical transport behavior, further research is needed on the export dynamics of microplastics to support the future management and control (Poulain et al., 2019).
This study focuses on the outflow of microplastics from the Jiulong River. The objectives are (1) to obtain the current status of microplastic pollution in the Jiulong River Estuary and Xiamen Bay, (2) to estimate the flux of microplastics from the river into the sea, and (3) to analyze the factors affecting microplastics of different particle sizes with the help of a variety of correlation analysis methods.
Materials and Methods
Microplastic Sampling
Microplastic sampling was conducted at 22 sites in the Jiulong River Estuary and Xiamen Bay by R/V Haiyang II in April 2019 (Figure 1 and Supplementary Table 1). We both used trawling and pumping methods to collect microplastics in different sizes (trawling: 0.33–5.0 mm, pumping: 44 μm–5.0 mm); pumping was conducted at all sites, and trawling was conducted at 10 sites.
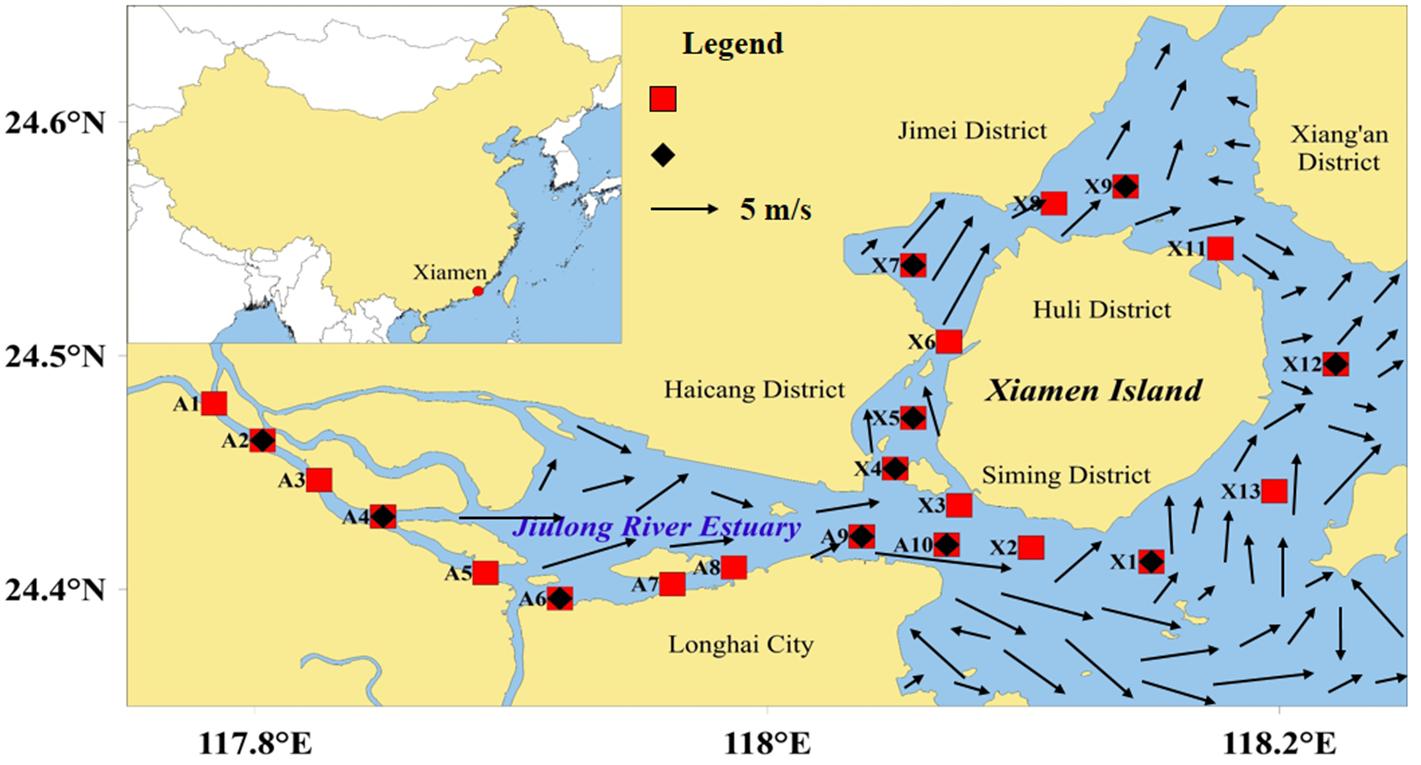
Figure 1. Map of the sampling sites. X1-X9 and X11-X13 are located in Xiamen Bay, and A1-A10 are located in the Jiulong River Estuary. Pumping samples were collected at all sites, and trawling samples were collected at half of the sites, marked in black.
Surface trawling samples were collected with a 1 m wide and 0.25 deep Manta net with a mesh size of 0.33 mm, at a speed of approximately 2 knots for 15 min. The passing water volume was calculated by the following function:
where V is the volume of the passing water; v is the shipping speed; t is the time of trawling; and S is the cross-sectional area, which is related to the width and depth of the net.
After trawling, the contents were transferred into a precleaned glass bottle with Milli-Q water, fixed in 2.5% formaldehyde solution, and kept in the dark until further processing was conducted in the laboratory (Lattin et al., 2004).
The particles, which include smaller ones (44 μm–5.0 mm), were collected from the surface water of all 22 sites by pumping. The pumping machine extracted surface water and filtered it in the field. The passing water volume was recorded by a calibrated flow meter (HYDRO-BIOS in Germany) at the mesh port (150–200 L/sample). Relying on a centrifugal pump made of titanium and stainless steel connected to a dual water meter and water hose (Cai et al., 2018). The seawater pump was located 0.5 m below the quarterdeck and bow waterline. The substances remaining on the 5 mm mesh were discarded. Those remaining on the 44 μm mesh were obtained, wrapped, and isolated with aluminum foil, and then stored at a low temperature for further analysis. In addition, according to the data recorded in the two water meters and the sampling duration, the water discharge could be estimated.
Microplastic Pretreatment
The pretreatment method used in this study was the same as that used in our previous studies (Cai et al., 2018; Tang et al., 2018) and is briefly described here. The trawling samples on nets and the pumping samples on meshes were washed three times with Milli-Q water using an ultrasonic cleaning instrument for 2 min. Then all washing solutions were transferred to a precleaned 1 L beaker and prefiltered through a 5 mm metallic mesh to discard the large debris (>5.0 mm). The remaining samples were collected by passing them through a filter consisting of a sand core sandwiched between nylon filter papers (47 mm) and connected to a vacuum pump (Millipore, 20 μm).
The particles on the nylon filters (20 μm) were disposed of with digestion and flotation to eliminate non-plastic components. Next, the filter contents were treated with a 30% H2O2 solution and placed on a 75°C heating plate for 24–48 h for oxidation and digestion (Nuelle et al., 2014; Zhao et al., 2014). After filtering the digestion solution again with a nylon filter (20 μm), the filter contents were density-separated by a saturated NaCl solution (with a density of 1.2 g/cm3). The upper liquid was collected through nylon filters. Then, the obtained filter membranes and solid retention were placed in capsules for further observation and analysis (Hidalgo-Ruz et al., 2012).
Observation and Identification
The particles collected on the filter papers from the trawling and pumping samples were observed with a microscope (ZEISS, Scope A1, Germany) at approximately 40× magnification, and the microscope was connected with a computer to take photos in “zigzag” mode until every position had been covered and photographed. The number of microplastics in each photo was calculated manually. The possible number of microplastics in each sample was obtained by integration, and they were classified according to their different colors and shapes. According to the color, microplastics can be classified into five categories: black, white, brown, transparent, and others; and the shapes of microplastics can be categorized into four groups: fibers, foams, films, and granules. The size of pumping samples is 44 μm–5.0 mm, and the size of trawling samples is 0.33–5.0 mm.
For identification of polymer type, 10 particles were picked randomly from each filter sample. Three different sections were detected for each particle with a micro-Fourier Transform Infrared Spectroscopy (micro-FTIR) (Nicolet iN10, Thermo Fisher Scientific, United States). Then the obtained spectra were processed by OMNIC Picta software, and were compared to the OMNIC polymer reference spectra library. The sample was regarded as plastics only when the matching rate to a plastic component was higher than 70%, and the polymer type was identified as the type with the maximum matching rate. Finally, statistical data was used to calculate the proportion of particles in each sample.
Quality Assurance and Quality Control
All the sampling devices were thoroughly cleaned with Milli-Q water before use. At each sampling site, the meshes and nets were immediately folded and sealed with aluminum foil in a compact bag, or their contents were directly transferred to glass bottles for further processing. In addition, blank samples were collected at selected sites by filtering 20 L Milli-Q water to analyze potential contamination, e.g., from the air and workers’ clothes. The mean abundance of the blank microplastic samples was 0 particles/m3.
During the laboratory pretreatment and analysis, all researchers wore non-plastic coats and gloves to prevent external pollution. The laboratory platform was cleaned, each instrument was washed with Milli-Q water at least three times before use. All analysis devices and sample containers were covered by aluminum foil when not in use. In addition, after each microscopic examination was complete, we immediately placed the lid on the sample (Nuelle et al., 2014).
The lab blanks, consisting of 250 mL Milli-Q water in place of seawater samples, were treated the same as other samples during the whole process. No particles were found on the filters during the examination, which meant that contamination from the containers, operations, and laboratories could be ignored.
Data Processing
Estimation of the Flux of Microplastics Into the Sea
The flux of microplastics into the sea indicates a situation of microplastic pollution. The following equation is used to calculate the flux of microplastics per unit time (Zhao et al., 2019):
where Flux (t/year) is the annual plastic flux input to the ocean; Σwt. (g/m3) is the average weight of microplastics in the Jiulong River per square meter. Discharge (m3/s) is the average annual discharge of the river, and Dischargeratio–i is the proportional discharge rate through sampling depth. The discharge rate is assumed to be the same for all depths, h is the depth of sampling, and H is the average water depth of each sampling station. Dischargeratio–i is calculated by the ratio of h to H (Miller et al., 2017).
To estimate the microplastics flux of the Jiulong River estuary, we used the abundance of microplastic from our data and the average weight of microplastic in different components (Eriksen et al., 2013) to calculate Σwt. (Supplementary Table 7). Flux of the Jiulong River could be obtained from the previous record, which is 469.3 m3/s. Moreover, the h for the trawl is 0.25 m deep, and for the pump is 0.5 m deep, and H is different in each site, which shows in Supplementary Table 2. Therefore, the Dischargeratio−i can be got in this study.
Data Analysis and Graphing
Cluster analysis, Pearson correlation analysis, and principal component analysis were performed to study the correlations between appearance and composition characteristics of microplastics and other water quality parameters by SPSS version 22.0 (IBM Corporation). The characteristics of microplastics include their abundance, shapes, components, and the water quality parameters include salinity, PH, DO, nutrients, chlorophyll-α, and so on. Microsoft Excel (2019), Golden Software Grapher, and Surfer were used to record and calculate data, and graph.
Results and Discussion
Microplastic Abundance and Spatial Distribution
Microplastics were widely detected in water samples collected from the Jiulong River and Xiamen Bay, and there were significant spatial variations in distributions of the microplastics (Figure 2 and Supplementary Figure 1).
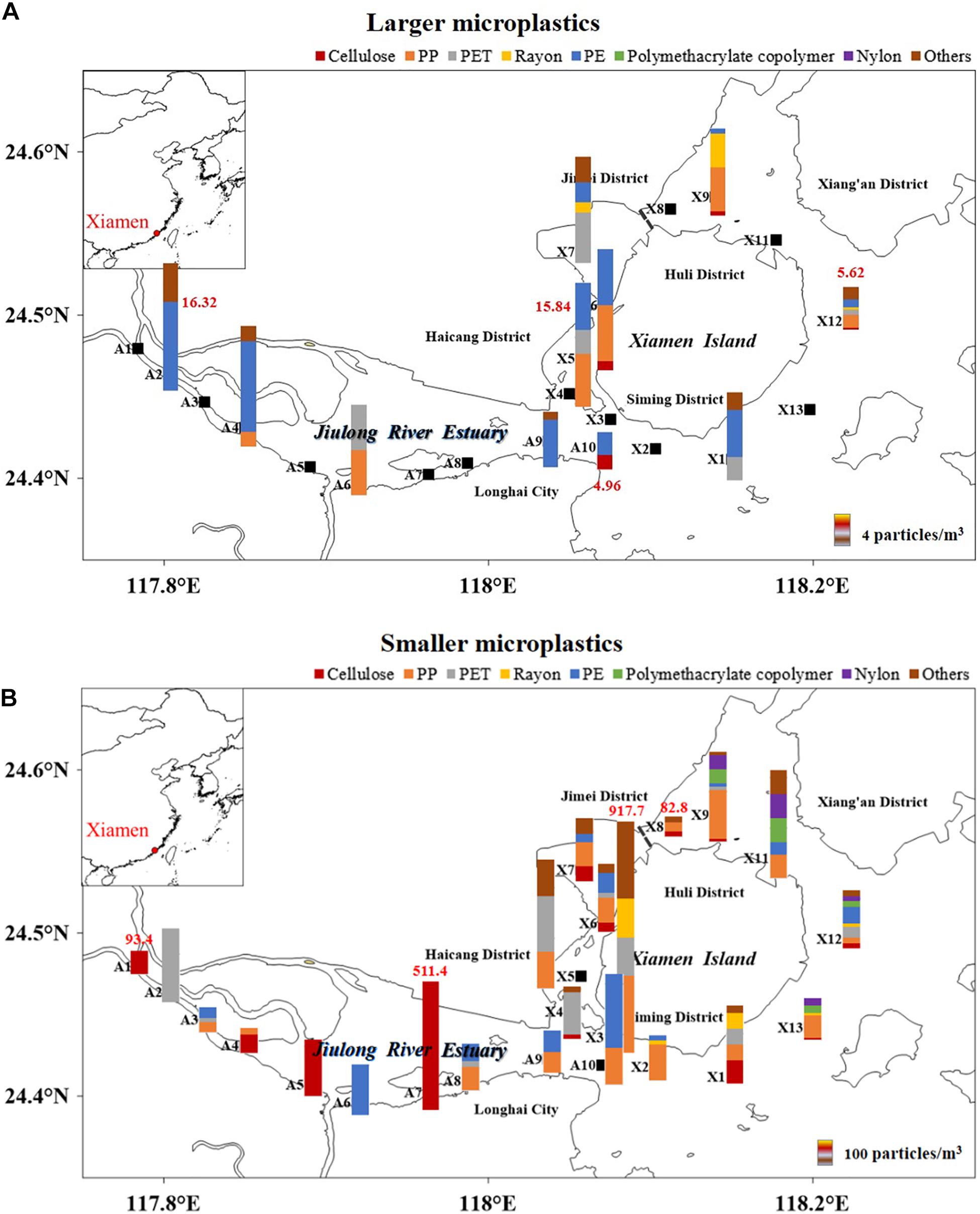
Figure 2. The abundances of larger (A) or smaller (B) microplastics in Xiamen Bay and the Jiulong River Estuary. The length of vertical bars indicates microplastic abundance, and different colors in the figures represent different compositions of microplastics.
Xiamen Bay
The abundances of large microplastics collected by trawling varied from 5.52 to 14.8 particles/m3, and the average value was 12.2 particles/m3. Microplastic abundance decreased along with river flow, and the abundance in the populated western harbor was higher than those in the eastern area (p < 0.05). It was also found in a previous study that there is a significant positive correlation between the abundance of microplastics and population density (Browne et al., 2011).
For the small microplastics collected by pumping, the abundances varied from 82.8 to 918 particles/m3, and the average value was 340 particles/m3. Most of the high values were also concentrated in the western harbor, far away from the sea. The abundance of the northwestern island was higher than that of the southwestern island, which indicates that terrestrial inputs near industrialized and populated coastal areas may transport more microplastics in the northwest of the island, while gathering of streams may provide fewer microplastics in the southwest of the island (Browne et al., 2011; Cheung et al., 2016).
The results of other studies can be compared with our data: the average value was 0.490 ± 0.430 particles/m3 by neuston net with 333μm mesh size in Chabahar Bay, Iran (Aliabad et al., 2019), 8.90 ± 4.70 particles/m3 collected by plankton net tows with 330 μm in Xiangshan Bay, China (Chen et al., 2018), 0.240 ± 0.350 particles/m3 by using a Manta trawl with a 335 μm mesh net in the Bay of Brest, France (Frere et al., 2017), 0.140 ± 0.120 particles/m3 by trawl nets with 330 μm mesh size in Hangzhou Bay, China (Wang et al., 2020), 514 ± 520 particles/m3 by a manta trawl with 330 μm mesh in Xiamen Bay, China (Tang et al., 2018); the range of abundance was 0.0100–0.700 particles/m3 by a manta trawl with 333 μm in Todos Santos Bay, Mexico (Ramirez-Alvarez et al., 2020) and 20.0–120 particles/m3 collected by a 20μm mesh in Jiaozhou Bay, China (Zheng et al., 2019). It can be found that most previous research used the trawl to collect the microplastic, and the sizes of them mainly larger than 330 μm, and that is why the data we obtained by pumping was high than others. The data we obtained by trawling was at the middle level among the abundance observed in other bays with a nearly similar sea.
Jiulong River Estuary
The abundance of large microplastics varied from 4.96 to 16.3 particles/m3, and the average was 11.1 particles/m3, which was slightly lower than that observed in Xiamen Bay. Compared with those collected from in large-scale rivers, microplastic abundance observed in the Jiulong River Estuary was lower than that in the Yangtze River Estuary, which was 79.4 ± 60.8 particles/m3 (0.3–5 mm) (Zhao et al., 2019). This difference may be due to human activities and the scales of these two rivers. In addition, as samples were collected further downstream in the Jiulong River, the abundances decreased gradually due to the dilution effect.
For small microplastics, the distribution varied significantly and irregularly. The abundances ranged from 93.4 to 511 particles/m3, and the average was 232 particles/m3, at a moderate level compared with the results of other estuaries with similar sampling methods. For example, the average abundance was 157 ± 75.8 particles/m3 in the Yangtze River Estuary (60 μm–5.0 mm) (Zhao et al., 2019), 8.90 × 103 particles/m3 (50 μm–5.0 mm) in the Pearl River Estuary (Yan et al., 2019), and 30.0 particles/m3 (80 μm–5.0 mm) in the Qin River Estuary (Zhang et al., 2020).
The abundance of small microplastics (44 μm–0.33 mm) accounted for a much large proportion of the total collected microplastics than that of large microplastics. This result was in accordance with previous studies, that the dominant size of microplastics in the Saigon River was found to be <0.25 mm (Lahens et al., 2018), and Lindeque et al. (2020) found that United Kingdom data revealed a 2.5-fold increase in microplastics using 100 μm nets compared to 333 μm nets. Different environmental conditions influenced the microplastic abundance with different sizes. For the Manta trawling, the turbulence caused by the vessel’s wake could influence the data, while for the pumping, air can get sucked in when the waves are higher, therefore affecting the certainty of the sampled volume.
Appearance Characteristics and Chemical Compositions
Colors and Shapes
In this study, the colors and shapes of both the large and small microplastics were recorded. Color has been considered an essential aspect affecting the ingestion of microplastics by organisms (Abayomi et al., 2017). Therefore, the various colors of microplastics found in the samples were divided into five categories: brown, black, white, transparent, and others.
In Xiamen Bay, transparent (29.5%) and white (26.6%) were the dominant colors of large microplastics. For small particles, the proportion of each color was almost the same, except for the “others” category. However, in the Jiulong River Estuary, transparent (28.0%) and white (33.2%) colors were the most prominent in the large and small microplastics (Supplementary Figure 2 and Supplementary Table 3).
In addition, the abundance of large microplastics of “other” colors was much higher than that of small microplastics, while the opposite trend occurred on black particles. Brown, transparent, and white particles did not display apparent differences between the two particle sizes. This result indicated that compared to the black microplastics, colored particles had more difficulty physically breaking into small pieces.
Microplastics of different shapes were observed: films, foams, fibers, and granules. A granule is a spherical or cylindrical piece of plastic debris or fragment, and when a particle could not be defined as one of the other shapes, it was identified as a granule (Yan et al., 2019). Supplementary Figure 3 and Supplementary Table 4 show the proportions of all shapes in our study. Granule particles were the most common at all sites (>60%), followed by film particles (>15%). However, small microplastics with fiber shapes were few. The shape pattern founded in this study was not in accordance with the typical pattern described in the literature, where fiber and fragment are the most abundant, implying the strong sinking and export processes in the estuarine-coastal area (Kooi and Koelmans, 2019).
Chemical Composition Patterns
Ten components of microplastics in Xiamen Bay and the Jiulong River were identified by micro-FTIR spectral analysis (Supplementary Tables 5, 6). For the large microplastics, polyethylene (PE), polypropylene (PP), and polyethylene terephthalate (PET) accounted for over 80% of the samples. Among them, PE was of the highest proportion (43%), followed by PP (22%) and PET (22%). The other component types included Rayon, cellulose, nitrocellulose (NC), acrylics (ACR), polyacrylonitrile (PAN), polystyrene (PS), and phenoxy resin.
For the small microplastics, 13 kinds of components were identified; this number was greater than that of the large microplastics. PP (27%), cellulose (19%), PE (18%), and PET (17%) were the major components, and a few items were found to be composed of ACR, polyacrylic acid (PAA), polyvinyl chloride (PVC), NC, and PS.
No significant difference was observed when comparing the component results between the large and small microplastics (Figure 2, p = 0.05). PE, PP, and PET particles were the most common component in all samples. The densities of PP and PE were approximately 0.90 and 0.95 g/cmł, respectively, so most of them remained on the seawater surface. Previous studies also reported that PP and PE could be treated as the most common microplastic types due to the wide application and ease of transport (Hidalgo-Ruz et al., 2012; Cozar et al., 2014). However, cellulose accounted for a high proportion of small microplastics but a relatively low proportion of large microplastics, which may mean that it is easy for cellulose plastic to break into small pieces physically. In addition, there were 14 component types found in Xiamen Bay and only 8 types collected in the Jiulong River, and the composition of microplastics in Xiamen Bay was rather complicated. Xiamen Bay is more influenced by intensive human activities, such as tourism, port transportation, and domestic discharge. Therefore there are more multiple pollution sources than the Jiulong River Estuary. Besides, compared to the estuarine area, the weak hydrological dynamics would lead to plastic accumulation in the inner bay (Tang et al., 2018).
Moreover, oxidation decomposition and density separation are the steps of the pretreatment to remove organic and non-plastic inorganic matters, which will inevitably affect the original characteristics of the collected microplastics, including the amount, size, color, shape, and composition. Moreover, it has been confirmed that using 30% H2O2 solution for treatment can alter the characteristics of microplastics, especially for fibers, or even dissolve the polymers (Crawford and Quinn, 2017). Thus, these steps were only undertaken when the impurities of samples had high degrees of non-plastics.
Flux Estimation of Riverine Outflow
In the Jiulong River Estuary, the flux of microplastics was estimated based on the field data (Supplementary Table 7). The flux of microplastics that includes the smaller microplastics (44 μm–5.0 mm) was 472 ± 230 t/y (River section S < 5), while that for the large particles (0.33–5.0 mm), the flux was 61.2 ± 2.6 t/y (River section S < 5). Thus, the flux of small particles was approximately 8 times greater than that of large particles, which can be explained by the fact that a few large particles can be physically broken into a large number of small particles. In addition, different sampling methods may cause changes in the observed concentrations (Cai et al., 2018), so the observed gap between the sizes between the two kinds of particles is acceptable.
In addition, the small-sized particle data were used to calculate the flux of microplastics from the Jiulong River Estuary into the sea. Compared with the other rivers (Supplementary Figure 4 and Supplementary Table 8), the Jiulong River is a typical small- or medium-sized river whose flux of microplastics (472 ± 230 t/y) is at the medium level for large-sized rivers. For example, the microplastic flux is 538–906 t/y in the Yangtze River Estuary according to samples collected by pumping (60 μm–5.0 mm) (Zhao et al., 2019); 2.83 × 103 t/y from the Qiantang River to the Hangzhou Bay (45 μm–5.0 mm) (Zhao et al., 2020); 66.0 t/y in the Pearl River delta, as measured by a Manta trawl (0.3 mm–5 mm) (Mai et al., 2019); 22.0 t/y in the Rhone River (0.3–5 mm) (Constant et al., 2020); and 120 t/y in the Po River (0.3–5 mm) (Van der Wal et al., 2015).
Three main factors affect flux values and give rise to the estimation uncertainties: The first is the size of the sampling net. The minimum net size in our pumping method (44 μm) is small than those in other studies, but particles with a small size (<44 μm) are still neglected in this study. Hence, the actual value of the total amount of microplastics is less than the real value. The second factor is the flow of the river, which varies among different seasons and in the rainy and dry seasons. For example, our sampling took place during the rainy season in summer when there is great runoff. The rain washes away many microplastics, which may lead to the calculated result being higher than the actual value. Last but not least, the representativeness of the field data is an important factor influencing flux values as well. Microplastic abundance was influenced by rainfall, tide and other environmental factors, and it has daily and monthly fluctuations. Since field sampling was carried out only once, attention should be paid to the representativeness of microplastic abundance.
Moreover, it is inaccurate to equate a high flux with a high abundance because the components and sizes of microplastics vary greatly among different rivers. The average weight estimated in the study (Eriksen et al., 2013) is much higher than that used for the Yangtze River (0.00000330 g/particles) (Zhao et al., 2019) or other rivers. The measured average weight per particle was significantly different from that obtained by modeling (Lebreton et al., 2017). Hence, there would be some inevitable errors in the modeled flux results.
The Relationship Between Microplastic Abundance and Hydrology
The Relationship Between Changes in Microplastic Abundance and Salinity
Here we further analyzed the correlation between salinity (shown in Supplementary Table 9) and the abundance of microplastics in the Jiulong River Estuary, and the result is shown in Figure 3. The abundance of large microplastics decreased as the river flowed into the sea, while the salinity gradually increased (R2 = 0.927). As large microplastics are affected by light, wind, and waves, they could gradually decompose into small microplastics. Some of these microplastics are too small-sized for their surface features to be distinguished, so it is difficult to identify their sources (Zhao et al., 2019). The Jiulong River Estuary is dominated by semi-diurnal tides. The water columns were approximately well-mixed during the last flood and were stratified during the early ebb, leading to distinct differences in the magnitude and vertical structure (Cheng et al., 2020). The mixture and stratification processes between the saline and freshwater would influence the distribution of buoyant plastics, and the strong tidal currents could influence their residence time and transport processes between high and low tides (Browne et al., 2011; Sadri and Thompson, 2014). Based on the analysis, the dilution effect and physical weathering were the main reasons for the loss of large microplastics in the Jiulong River Estuary; moreover, physical processes occurred more often along coastlines or in marine environments (Corcoran et al., 2009).
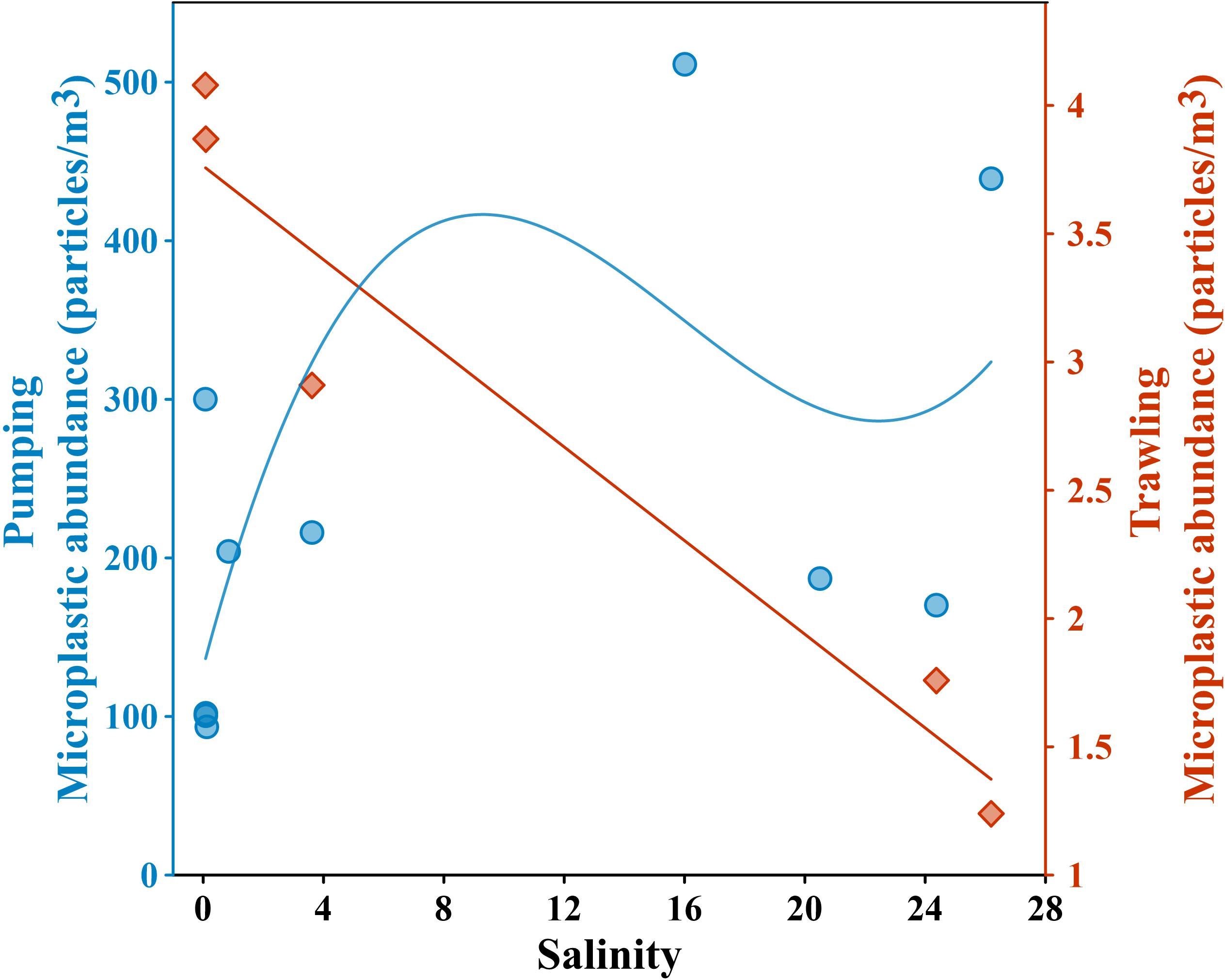
Figure 3. The relationship between the abundance of microplastics and the salinity of the water from which they were sampled in the Jiulong River Estuary. The red squares refer to the large particles that were collected by trawling, and the blue dots refer to the small particles that were collected by pumping.
The Small Particles Are Affected by a Variety of Factors
The linear relationship between small microplastics and salinity was not significant but showed a slightly positive trend, and its variation was much more complicated in the estuary. On the one hand, the physical properties of microplastics, such as density, shape, and size, determine their bio-fouling celerity and residence time, influencing their transport and fate in the marine environments (Chubarenko et al., 2016). When exposed to ultraviolet light for a long time, plastic could be less elastic and more brittle (Torikai et al., 2000). In addition, the smaller microplastics may be absorbed by microorganisms and ingested by fish, and the diversity of organisms that can ingest plastic particles would increase with the particle size being smaller (Auta et al., 2017; Jabeen et al., 2018). On the another hand, the environmental variation and extra discharge would change microplastic abundances. The fragmentation of microplastics could be accelerated where winds, waves, and currents are strong. An additional input source of microplastics (e.g., fibers in wastewater might be from domestic sewage), leading to abnormally high abundances at stations A2 and A7.
Correlation of Characteristics With Water Quality Parameters
Principal component analysis (PCA) and Pearson correlation analysis were performed to explore the potential relationship between the microplastic characteristics and the physicochemical properties of the estuarine waters.
Principal Component Analysis (PCA)
Three principal components were extracted in this study, and their cumulative variance was 90% (Supplementary Table 10). The first principal component (PC1) showed more non-conservative properties dominated by nutrients, while the second component (PC2) showed more conservative properties (Figure 4). PC1 explained 60% of the data variability and was positively related to temperature, NO2–, NH4+, PO43–, SiO43–, and Chl-α and negatively related to pH and salinity. The parameters that had maximums in the PC1 showed decreasing trends from the Jiulong River Estuary to the sea, suggesting that they were mainly associated with riverine inputs. Abundant nutrients in the Jiulong River are mostly from the land-derived runoffs and release from sediments, leading to frequent blooms in the estuarine-coastal area (Li, 2019; Lin et al., 2010). Microplastics could be present in the natural aggregates, increasing their sinking rates and enhancing their vertical export.
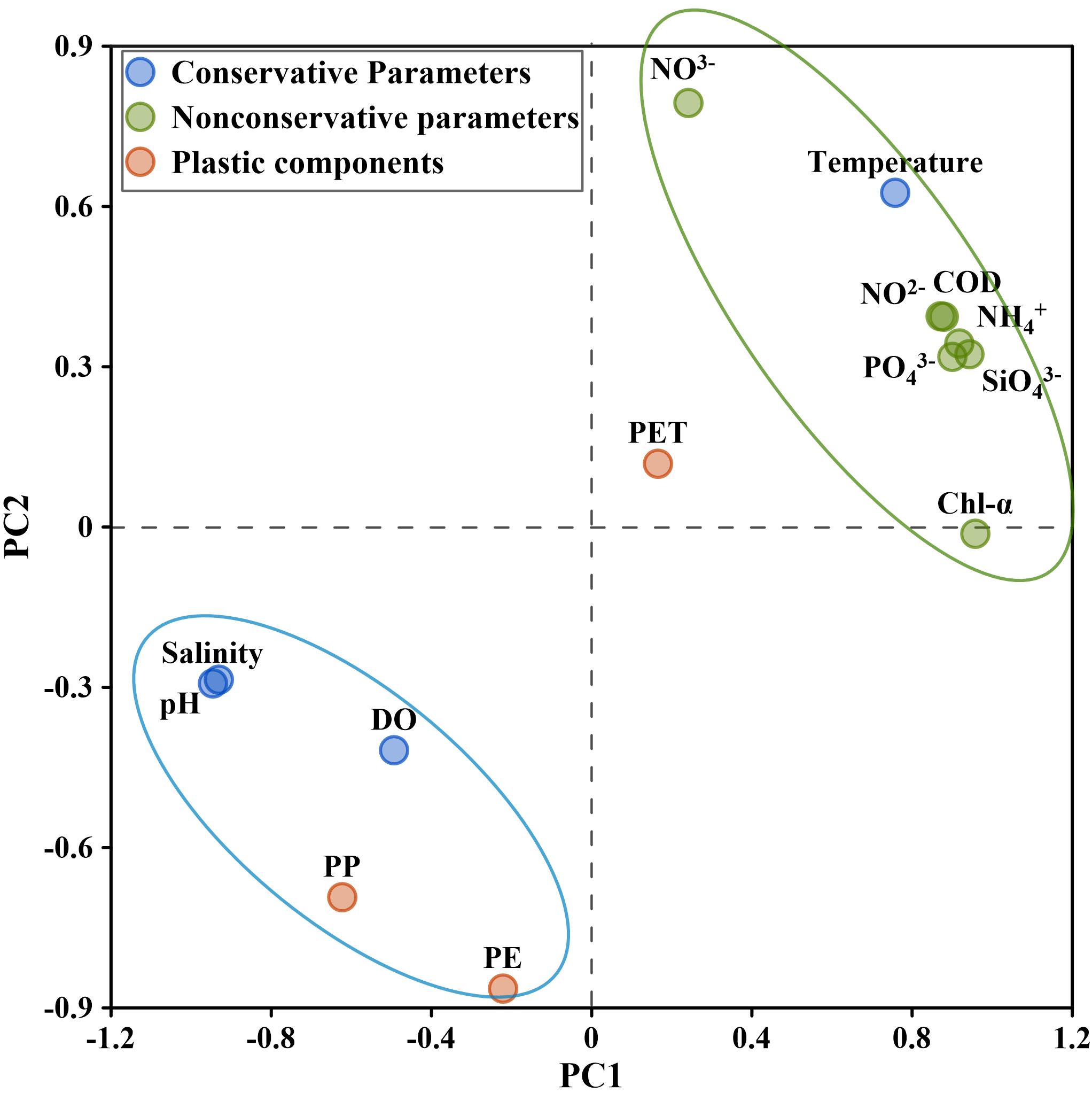
Figure 4. Loadings of microplastic parameters on principal component 1 (PC1) and principal component 2 (PC2). The parameters were classified into 3 types: conservative (such as temperature and salinity), nonconservative (such as nutrients), and plastic components (including PET, PP, and PE).
PC2, which explained 20% of the data variability, was positively related to NO3– and was negatively related to PP and PE. NO3– is a typical pollutant and mainly originates from outfalls. Domestic sewage, offshore aquaculture, and fertilizer are the potential sources of NO3– in Zhanjiang Bay (Zhang et al., 2019), and manure, sewage, and soil are the sources of NO3– in surface waters. Therefore, it can be speculated that part of PP and PET, which have remarkable correlations with NO3– potentially come from sewage in outfalls.
PC3, explaining 10% of the data variability, was positively related to dissolved oxygen and PET. Dissolved oxygen in water has two primary sources, air and photosynthesis. Air-sea exchange process can bring or take away dissolved oxygen in seawater, which is influenced by temperature, wind, and some physical factors. And Photosynthesis is a process capture and store solar energy on a massive scale and produces O2 by plants, algae, cyanobacteria, and anoxygenic photosynthetic bacteria (Hoganson and Babcock, 1997). Therefore, it is speculated that biological and chemical factors (oxidation) are the main factors affecting PET.
Pearson Correlation Analysis
Pearson correlation analysis shows that PP and foam were significantly correlated with some water quality parameters (Supplementary Table 11). PP had negative correlations with temperature, NO2–, NO3–, NH4+, PO43–, SiO43– and chemical oxygen demand (COD) and positively correlated with pH and salinity. The foam was positively correlated with pH and negatively correlated with NO2–, NH4+, PO43– and Chl-α.
After the Jiulong River entering the sea, the seawater salinity gradually rose, and the nutrient concentration gradually decreased. It has been revealed that NO3–, SiO43, and dissolved inorganic nitrogen (DIN) behave conservatively in the predominantly P-limited Jiulong River Estuarine (Chen et al., 2013). The results of the Pearson correlation analysis showed that there are new sources of PP and foam occurring during the transmission process, and PP has a new source near the river estuary. For the foam, it is more likely that large particles are broken into small particles during transport from the estuary to the sea, leading to the increase of small particles’ abundance along the direction of flow.
Conclusion
Microplastics were studied in water samples collected from Xiamen Bay and the Jiulong River Estuary. Microplastic pollution is mainly caused by the smaller microplastics, whose abundance is considered high (for the large particles) and medium (for the small particles) compared with other bays globally. Furthermore, the abundance of the Jiulong River is considered medium to low levels compared with other rivers worldwide. The flux of the small particles was approximately 8 times greater than that of the large particles, which are 472 ± 230 t/y and 61.2 ± 2.6 t/y, respectively. It means that the transportation of microplastic in the ocean by small and medium-sized rivers cannot be ignored, and the management of plastic discharge in these kinds of rivers should also be strengthened. In addition, the behavior of large microplastics is more conservative. In contrast, that of small microplastics is more complex, because various components of small microplastics have different sources and influencing factors, which also provides theoretical support for the treatment of microplastics pollution.
Data Availability Statement
The original contributions presented in the study are included in the article/Supplementary Material, further inquiries can be directed to the corresponding author/s.
Author Contributions
YFL: writing—original draft. SL: field investigation. ML: graphing, writing—editing, and revising. WH: instrumental analysis. KC: statistical analysis. YD: field sampling and pretreatment. FW: instrumental analysis. HK: supervision. LL: field investigation. YL: consulting. MZ: pretreatment. FL: funding support. CW: lab management. MC: supervision. All authors contributed to the article and approved the submitted version.
Funding
This work was financially supported by the National Natural Science Foundation of China (U2005207, 41776088, 41976216, and 41961144011), Natural Science Foundation of Fujian Province (2020J0141 and 2019Y4010), Dean’s Research Funding of Xiamen University (20720190105), and Training Program of Innovation and Entrepreneurship for Undergraduates, Xiamen University (S202010384579 and S202010384769).
Conflict of Interest
The authors declare that the research was conducted in the absence of any commercial or financial relationships that could be construed as a potential conflict of interest.
Publisher’s Note
All claims expressed in this article are solely those of the authors and do not necessarily represent those of their affiliated organizations, or those of the publisher, the editors and the reviewers. Any product that may be evaluated in this article, or claim that may be made by its manufacturer, is not guaranteed or endorsed by the publisher.
Acknowledgments
We thank the crew of R/V Haiyang II for their help during the sampling process. We are thankful to Wenlu Zhao and Xuan Ni for their help during the instrumental analysis and Peng Huang for his assistance with data processing.
Supplementary Material
The Supplementary Material for this article can be found online at: https://www.frontiersin.org/articles/10.3389/fmars.2021.712727/full#supplementary-material
References
Abayomi, O. A., Range, P., Al-Ghouti, M. A., Obbard, J. P., Almeer, S. H., and Ben-Hamadou, R. (2017). Microplastics in coastal environments of the Arabian Gulf. Mar. Pollut. Bull. 124, 181–188. doi: 10.1016/j.marpolbul.2017.07.011
Aliabad, M. K., Nassiri, M., and Kor, K. (2019). Microplastics in the surface seawaters of Chabahar Bay, Gulf of Oman (Makran Coasts). Mar. Pollut. Bull. 143, 125–133. doi: 10.1016/j.marpolbul.2019.04.037
Auta, H. S., Emenike, C. U., and Fauziah, S. H. (2017). Distribution and importance of microplastics in the marine environment: a review of the sources, fate, effects, and potential solutions. Environ. Int. 102, 165–176. doi: 10.1016/j.envint.2017.02.013
Bakir, A., Rowland, S. J., and Thompson, R. C. (2014). Enhanced desorption of persistent organic pollutants from microplastics under simulated physiological conditions. Environ. Pollut. 185, 16–23. doi: 10.1016/j.envpol.2013.10.007
Brennecke, D., Duarte, B., Paiva, F., Cacador, I., and Canning-Clode, J. (2016). Microplastics as vector for heavy metal contamination from the marine environment. Estuar. Coast. Shelf Sci. 178, 189–195. doi: 10.1016/j.ecss.2015.12.003
Browne, M. A., Crump, P., Niven, S. J., Teuten, E., Tonkin, A., Galloway, T., et al. (2011). Accumulation of microplastic on shorelines woldwide: sources and sinks. Environ. Sci. Technol. 45, 9175–9179. doi: 10.1021/es201811s
Cai, M., He, H., Liu, M., Li, S., Tang, G., Wang, W., et al. (2018). Lost but can’t be neglected: huge quantities of small microplastics hide in the South China Sea. Sci. Total Environ. 633, 1206–1216. doi: 10.1016/j.scitotenv.2018.03.197
Chen, B. H., Ji, W. D., Chen, J. M., Lin, C., Huang, H. N., Huo, Y. L., et al. (2013). Characteristics of nutrients in the Jiulong River and its impact on Xiamen Water, China. Chin. J. Oceanol. Limnol. 31, 1055–1063. doi: 10.1007/s00343-013-2263-3
Chen, B., Kang, W., Xu, D., and Hui, L. (2021). Long-term changes in red tide outbreaks in Xiamen Bay in China from 1986 to 2017. Estuar. Coast. Shelf Sci. 249:107095. doi: 10.1016/j.ecss.2020.107095
Chen, M., Jin, M., Tao, P., Wang, Z., Xie, W., Yu, X., et al. (2018). Assessment of microplastics derived from mariculture in Xiangshan Bay, China. Environ. Pollut. 242, (Pt B), 1146–1156. doi: 10.1016/j.envpol.2018.07.133
Cheng, P., Yu, F. L., Chen, N. W., and Wang, A. J. (2020). Observational study of tidal mixing asymmetry and eddy viscosity-shear covariance-induced residual flow in the Jiulong River estuary. Cont. Shelf Res. 193:104035. doi: 10.1016/j.csr.2019.104035
Cheung, P. K., Cheung, L. T. O., and Fok, L. (2016). Seasonal variation in the abundance of marine plastic debris in the estuary of a subtropical macro-scale drainage basin in South China. Sci. Total Environ. 562, 658–665. doi: 10.1016/j.scitotenv.2016.04.048
Chubarenko, I., Bagaev, A., Zobkov, M., and Esiukova, E. (2016). On some physical and dynamical properties of microplastic particles in marine environment. Mar. Pollut. Bull. 108, 105–112. doi: 10.1016/j.marpolbul.2016.04.048
Cole, M., Lindeque, P., Fileman, E., Halsband, C., and Galloway, T. S. (2015). The impact of polystyrene microplastics on feeding, function and fecundity in the marine copepod Calanus helgolandicus. Environ. Sci. Technol. 49, 1130–1137. doi: 10.1021/es504525u
Constant, M., Ludwig, W., Kerherve, P., Sola, J., Charriere, B., Sanchez-Vidal, A., et al. (2020). Microplastic fluxes in a large and a small Mediterranean river catchment: the Tet and the Rhone, Northwestern Mediterranean Sea. Sci. Total Environ. 716:136984. doi: 10.1016/j.scitotenv.2020.136984
Corcoran, P. L., Biesinger, M. C., and Grifi, M. (2009). Plastics and beaches: a degrading relationship. Mar. Pollut. Bull. 58, 80–84. doi: 10.1016/j.marpolbul.2008.08.022
Cozar, A., Echevarria, F., Ignacio Gonzalez-Gordillo, J., Irigoien, X., Ubeda, B., Hernandez-Leon, S., et al. (2014). Plastic debris in the open ocean. Proc. Natl. Acad. Sci. U.S.A. 111, 10239–10244.
Crawford, C. B., and Quinn, B. (eds.). (2017). “Microplastic identification techniques” in Microplastic Pollutants, (Elsevier) 219–267. doi: 10.1016/B978-0-12-809406-8.00010-4
Eriksen, M., Cummins, A., Maximenko, N., Thiel, M., Lattin, G., Wilson, S., et al. (2013). Plastic pollution in the South Pacific subtropical gyre. Mar. Pollut. Bull. 68, 71–76. doi: 10.1016/j.marpolbul.2012.12.021
Frere, L., Paul-Pont, I., Rinnert, E., Petton, S., Jaffre, J., Bihannic, I., et al. (2017). Influence of environmental and anthropogenic factors on the composition, concentration and spatial distribution of microplastics: a case study of the Bay of Brest (Brittany, France). Environ. Pollut. 225, 211–222. doi: 10.1016/j.envpol.2017.03.023
Fu, Z., Chen, G., Wang, W., and Wang, J. (2020). Microplastic pollution research methodologies, abundance, characteristics and risk assessments for aquatic biota in China. Environ. Pollut. 266:115098. doi: 10.1016/j.envpol.2020.115098
Galloway, T. S., and Lewis, C. N. (2016). Marine microplastics spell big problems for future generations. Proc. Natl. Acad. Sci. U.S.A. 113, 2331–2333. doi: 10.1073/pnas.1600715113
Hidalgo-Ruz, V., Gutow, L., Thompson, R. C., and Thiel, M. (2012). Microplastics in the marine environment: a review of the methods used for identification and quantification. Environ. Sci. Technol. 46, 3060–3075. doi: 10.1021/es2031505
Hoganson, C. W., and Babcock, G. T. (1997). A metallo radical mechanism for the generation of oxygen from water in photosynthesis. Science 277, 1953–1956. doi: 10.1126/science.277.5334.1953
Huang, J., Li, Q., Huang, L., Wang, J., Hu, Y., and Feng, Y. (2012). Preliminary delineation and classification of estuarine drainage areas for major coastal rivers in China. Acta Ecol. Sin. 32, 3516–3527.**** journal wrong doi: 10.5846/stxb201105100610
Jabeen, K., Li, B. W., Chen, Q. Q., Su, L., Wu, C. X., Hollert, H., et al. (2018). Effects of virgin microplastics on goldfish (Carassius auratus). Chemosphere 213, 323–332. doi: 10.1016/j.chemosphere.2018.09.031
Jambeck, J. R., Geyer, R., Wilcox, C., Siegler, T. R., Perryman, M., Andrady, A., et al. (2015). Plastic waste inputs from land into the ocean. Science 347, 768–771. doi: 10.1126/science.1260352
Klumpp, D. W., Hong, H. S., Humphrey, C., Wang, X. H., and Codi, S. (2002). Toxic contaminants and their biological effects in coastal waters of Xiamen, China. I. Organic pollutants in mussel and fish tissues. Mar. Pollut. Bull. 44, 752–760. doi: 10.1016/s0025-326x(02)00053-x
Kooi, M., and Koelmans, A. A. (2019). Simplifying microplastic via continuous probability distributions for size, shape, and density. Environ. Sci. Technol. Lett. 6, 551–557. doi: 10.1021/acs.estlett.9b00379
Lahens, L., Strady, E., Kieu-Le, T.-C., Dris, R., Boukerma, K., Rinnert, E., et al. (2018). Macroplastic and microplastic contamination assessment of a tropical river (Saigon River, Vietnam) transversed by a developing megacity. Environ. Pollut. 236, 661–671. doi: 10.1016/j.envpol.2018.02.005
Lattin, G. L., Moore, C. J., Zellers, A. F., Moore, S. L., and Weisberg, S. B. (2004). A comparison of neustonic plastic and zooplankton at different depths near the southern California shore. Mar. Pollut. Bull. 49, 291–294. doi: 10.1016/j.marpolbul.2004.01.020
Lebreton, L. C. M., Van der Zwet, J., Damsteeg, J. W., Slat, B., Andrady, A., and Reisser, J. (2017). River plastic emissions to the world’s oceans. Nat. Commun. 8:15611.
Li, G. (2019). Fast acclimation of phytoplankton assemblies to acute salinity stress in the Jiulong River estuary. Acta Oceanol. Sin. 38, 78–85. doi: 10.1007/s13131-019-1389-3
Li, Y., Chen, Y. N., Ruan, M. N., and Chen, J. W. (2015). The Jiulong River plume as cross-strait exporter and along-strait barrier for suspended sediment: evidence from the endmember analysis of in-situ particle size. Estuar. Coast. Shelf Sci. 166, 146–152. doi: 10.1016/j.ecss.2015.03.002
Lin, H., Zhang, C. H., Qi, W. D., Zhou, Q. L., Lin, L. B., Lu, M. L., et al. (2010). Changing trends of DIN and PO4-P content in Xiamen Seawaters. J. Ocean. Taiw. Strait. 29, 314–319.
Lindeque, P. K., Cole, M., Coppock, R. L., Lewis, C. N., Miller, R. Z., Watts, A. J. R., et al. (2020). Are we underestimating microplastic abundance in the marine environment? A comparison of microplastic capture with nets of different mesh-size. Environ. Pollut. 265:114721. doi: 10.1016/j.envpol.2020.114721
Mai, L., He, H., Bao, L. J., Liu, L. Y., and Zeng, E. Y. (2020). Plastics are an insignificant carrier of riverine organic pollutants to the coastal oceans. Environ. Sci. Technol. 54, 15852–15860. doi: 10.1021/acs.est.0c05446
Mai, L., You, S. N., He, H., Bao, L. J., Liu, L. Y., and Zeng, E. Y. (2019). Riverine microplastic pollution in the Pearl River Delta, China: are modeled estimates accurate? Environ. Sci. Technol. 53, 11810–11817. doi: 10.1021/acs.est.9b04838
Miller, R. Z., Watts, A. J. R., Winslow, B. O., Galloway, T. S., and Barrows, A. P. W. (2017). Mountains to the sea: River study of plastic and non-plastic microfiber pollution in the northeast USA. Mar. Pollut. Bull. 124, 245–251. doi: 10.1016/j.marpolbul.2017.07.028
Nuelle, M. T., Dekiff, J. H., Remy, D., and Fries, E. (2014). A new analytical approach for monitoring microplastics in marine sediments. Environ. Pollut. 184, 161–169. doi: 10.1016/j.envpol.2013.07.027
Pagter, E., Frias, J., Kavanagh, F., and Nash, R. (2020). Differences in microplastic abundances within demersal communities highlight the importance of an ecosystem-based approach to microplastic monitoring. Mar. Pollut. Bull. 160:111644. doi: 10.1016/j.marpolbul.2020.111644
Poulain, M., Mercier, M. J., Brach, L., Martignac, M., Routaboul, C., Perez, E., et al. (2019). Small microplastics as a main contributor to plastic mass balance in the North Atlantic subtropical gyre. Environ. Sci. Technol. 53, 1157–1164. doi: 10.1021/acs.est.8b05458
Qi, R., Jones, D., Li, Z., Liu, Q., and Yan, C. (2020). Behavior of microplastics and plastic film residues in the soil environment: a critical review. Sci. Total Environ. 703:134722. doi: 10.1016/j.scitotenv.2019.134722
Ramirez-Alvarez, N., Mendoza, L. M. R., Vinicio Macias-Zamora, J., Oregel-Vazquez, L., Alvarez-Aguilar, A., Augusto Hernandez-Guzman, F., et al. (2020). Microplastics: sources and distribution in surface waters and sediments of Todos Santos Bay, Mexico. Sci. Total Environ. 703:134838. doi: 10.1016/j.scitotenv.2019.134838
Sadri, S. S., and Thompson, R. C. (2014). On the quantity and composition of floating plastic debris entering and leaving the Tamar Estuary, Southwest England. Mar. Pollut. Bull. 81, 55–60. doi: 10.1016/j.marpolbul.2014.02.020
Schmidt, C., Krauth, T., and Wagner, S. (2017). Export of plastic debris by rivers into the sea. Environ. Sci. Technol. 51, 12246–12253. doi: 10.1021/acs.est.7b02368
Tang, G., Liu, M., Zhou, Q., He, H., Chen, K., Zhang, H., et al. (2018). Microplastics and polycyclic aromatic hydrocarbons (PAHs) in Xiamen coastal areas: implications for anthropogenic impacts. Sci. Total Environ. 634, 811–820. doi: 10.1016/j.scitotenv.2018.03.336
Torikai, N., Matsushita, Y., Langridge, S., Bucknall, D., Penfold, J., and Takeda, M. (2000). Interfacial structures of block and graft copolymers with lamellar microphase-separated structures. Physica B 283, 12–16. doi: 10.1016/s0921-4526(99)01882-7
Van der Wal, M., Van del Meulen, M., Tweehuijsen, G., Peterlin, M., Palatinus, A., Virsek, M. K., et al. (2015). Identification and Assessment of Riverine Input of (Marine) Litter. Final Report for the European Commission DG Environment under Framework Contract No ENV. D (Vol. 25). 2/FRA/2012. Bristol: Eunomia Research & Consulting Ltd.
Wang, T., Hu, M., Song, L., Yu, J., Liu, R., Wang, S., et al. (2020). Coastal zone use influences the spatial distribution of microplastics in Hangzhou Bay, China. Environ. Pollut. 266(Pt 2):115137. doi: 10.1016/j.envpol.2020.115137
Wu, Q., Liu, S., Chen, P., Liu, M., Cheng, S. Y., Ke, H., et al. (2021). Microplastics in seawater and two sides of the Taiwan Strait: reflection of the social-economic development. Mar. Pollut. Bull. 169:112588.
Ya, M. L., Wang, X. H., Wu, Y. L., Ye, C. X., and Li, Y. Y. (2014). Enrichment and partitioning of polycyclic aromatic hydrocarbons in the sea surface microlayer and subsurface water along the coast of Xiamen Island, China. Mar. Pollut. Bull. 78, 110–117. doi: 10.1016/j.marpolbul.2013.10.053
Yan, M., Nie, H., Xu, K., He, Y., Hu, Y., Huang, Y., et al. (2019). Microplastic abundance, distribution and composition in the Pearl River along Guangzhou city and Pearl River estuary, China. Chemosphere 217, 879–886. doi: 10.1016/j.chemosphere.2018.11.093
Yonkos, L. T., Friedel, E. A., Perez-Reyes, A. C., Ghosal, S., and Arthur, C. D. (2014). Microplastics in four estuarine rivers in the Chesapeake Bay, USA. Environ. Sci. Technol. 48, 14195–14202. doi: 10.1021/es5036317
Zayen, A., Sayadi, S., Chevalier, C., Boukthir, M., Ben Ismail, S., and Tedetti, M. (2020). Microplastics in surface waters of the Gulf of Gabes, southern Mediterranean Sea: distribution, composition and influence of hydrodynamics. Estuar. Coast. Shelf Sci. 242:106832. doi: 10.1016/j.ecss.2020.106832
Zettler, E. R., Mincer, T. J., and Amaral-Zettler, L. A. (2013). Life in the “Plastisphere”: microbial communities on plastic marine debris. Environ. Sci. Technol. 47, 7137–7146. doi: 10.1021/es401288x
Zhang, L., Liu, J., Xie, Y., Zhong, S., Yang, B., Lu, D., et al. (2020). Distribution of microplastics in surface water and sediments of Qin River in Beibu Gulf, China. Sci. Total Environ. 708:135176. doi: 10.1016/j.scitotenv.2019.135176
Zhang, P., Wei, L., Lai, J., Dai, P., Chen, Y., and Zhang, J. (2019). Concentration, compoition and fluxes of land-based nitrogen and phosphorus source pollutants input into Zhanjiang Bay in summer. J. Guangdong Ocean Univ. 039, 63–72.
Zhao, S., Wang, T., Zhu, L., Xu, P., Wang, X., Gao, L., et al. (2019). Analysis of suspended microplastics in the Changjiang estuary: implications for riverine plastic load to the ocean. Water Res. 161, 560–569. doi: 10.1016/j.watres.2019.06.019
Zhao, S., Zhu, L., Wang, T., and Li, D. (2014). Suspended microplastics in the surface water of the Yangtze estuary system, China: first observations on occurrence, distribution. Mar. Pollut. Bull. 86, 562–568. doi: 10.1016/j.marpolbul.2014.06.032
Zhao, W., Huang, W., Yin, M., Huang, P., Ding, Y., Ni, X., et al. (2020). Tributary inflows enhance the microplastic load in the estuary: a case from the Qiantang River. Mar. Pollut. Bull. 156:111152. doi: 10.1016/j.marpolbul.2020.111152
Keywords: microplastics, Xiamen Bay, Jiulong River, riverine outflow, export dynamics
Citation: Li Y, Liu S, Liu M, Huang W, Chen K, Ding Y, Wu F, Ke H, Lou L, Lin Y, Zhang M, Liu F, Wang C and Cai M (2021) Mid-Level Riverine Outflow Matters: A Case of Microplastic Transport in the Jiulong River, China. Front. Mar. Sci. 8:712727. doi: 10.3389/fmars.2021.712727
Received: 21 May 2021; Accepted: 29 June 2021;
Published: 23 July 2021.
Edited by:
Xiaoshan Zhu, Tsinghua University, ChinaReviewed by:
Jianxiang Feng, Sun Yat-sen University Zhuhai Campus, ChinaXiaoshou Liu, Ocean University of China, China
Jingli Mu, Minjiang University, China
Copyright © 2021 Li, Liu, Liu, Huang, Chen, Ding, Wu, Ke, Lou, Lin, Zhang, Liu, Wang and Cai. This is an open-access article distributed under the terms of the Creative Commons Attribution License (CC BY). The use, distribution or reproduction in other forums is permitted, provided the original author(s) and the copyright owner(s) are credited and that the original publication in this journal is cited, in accordance with accepted academic practice. No use, distribution or reproduction is permitted which does not comply with these terms.
*Correspondence: Minggang Cai, bWdjYWlAeG11LmVkdS5jbg==
†These authors share first authorship