- 1Mote Marine Laboratory & Aquarium, Sarasota, FL, United States
- 2Fish and Wildlife Research Institute, Florida Fish and Wildlife Conservation Commission, St. Petersburg, FL, United States
- 3Department of Environmental Science and Policy, George Mason University, Fairfax, VA, United States
During the last several decades, Florida’s Coral Reef (FCR) has been impacted by both global and local stressors that have devastated much of its living coral cover. Additionally, since 2014 FCR has experienced a lethal disease outbreak termed stony coral tissue loss disease (SCTLD). Here, we examined SCTLD spreading dynamics within and among fragmented coral colonies and quantified lesion progression rate of two intermediately susceptible species—Montastraea cavernosa and Orbicella faveolata—through induction experiments conducted in laboratory aquaria. M. cavernosa colonies showing subacute tissue loss were sequentially fragmented parallel to the lesion edge to determine whether isolated tissue that showed no tissue-loss signs, referred to as isolated apparently healthy (AH) donor fragments, would subsequently exhibit tissue loss. Additionally, AH M. cavernosa and O. faveolata fragments, referred to as recipient fragments, were placed in direct contact with the M. cavernosa donor fragments to assess incidence of new tissue-loss lesions. Finally, AH M. cavernosa donor fragments were placed in direct contact with recipient M. cavernosa and O. faveolata fragments to account for aggression from direct contact. Samples were collected for histopathology of the corals through time. Many isolated AH donor fragments developed tissue-loss lesions during the 60-day study, suggesting SCTLD may be systemic within small-sized colonies. Our results confirmed that physical contact between recipient fragments and subacute SCTLD-lesioned tissue often led to tissue loss in recipient fragments. None of the control recipient or donor fragments experienced tissue loss. Grossly, multifocal lesions started on or adjacent to the septal and costal basal body walls with tissue loss progressing across the polyp septa and coenenchyme, respectively, in both species. Histologically, initial tissue-loss lesions in both species exhibited characteristic lytic necrosis (LN) at the basal body wall of the gastrodermis. O. faveolata exhibited higher rates of lesion appearance and subsequent mortality compared to M. cavernosa, but once a lesion appeared, M. cavernosa lost tissue faster than O. faveolata. This work contributes to the growing knowledge of SCTLD dynamics and highlights the differences in lesion progression within susceptible species.
Introduction
Florida’s Coral Reef (FCR) has recently experienced a multi-year disease-related mortality event that has resulted in massive declines of live coral cover, particularly within major reef-building species (Precht et al., 2016; Walton et al., 2018). This disease has been termed stony coral tissue loss disease (SCTLD) and has been given its own case definition1. This disease impacts 24 coral species in Florida, including several major reef-building species and those listed under the Endangered Species Act. The first report of SCTLD was recorded off Virginia Key, Florida (Miller et al., 2016; Precht et al., 2016) in 2014. Since then the disease has continued to spread throughout FCR, following a spatial pattern consistent with a contagious model of transmission (Muller et al., 2020). The disease has now reached other areas of the Caribbean, changing the structure of coral communities within affected areas (Alvarez-Filip et al., 2019; Weil et al., 2019).
SCTLD appears as acute, subacute, or chronic tissue loss lesions that spread focally or multifocally throughout the colony, leaving freshly exposed skeleton; some species may have bleached tissue adjacent to tissue loss-lesions (Aeby et al., 2019; Landsberg et al., 2020). SCTLD was initially proposed as a ‘white plague-like disease or syndrome’ because its characteristics are similar to other tissue-loss diseases (Miller et al., 2016; Precht et al., 2016; Walton et al., 2018). To date, there are no existing diagnostic tools to positively identify SCTLD making it difficult to determine if all impacted species are suffering from the same disease. However, there appears to be a hallmark ecology of SCTLD, specifically the variation in species susceptibility (see Text Footnote 1). Highly susceptible species (typically first affected during an outbreak) demonstrate rapid disease progression, with total mortality ranging from 1 week for smaller colonies to 1–2 months for larger colonies. Onset of tissue loss in intermediately susceptible species occurs about 1 month after onset in highly susceptible species, with smaller colonies experiencing mortality over several months, and larger colonies experiencing mortality over several years with the possibility of developing new lesions (Sharp et al., 2020). The progression of SCTLD lesions within a colony is more rapid compared to other coral diseases (Alvarez-Filip et al., 2019; Estrada-Saldívar et al., 2020). This rapid progression and high incidences of mortality have led to significant decreases in overall coral cover, coral density, and biodiversity (Precht et al., 2016; Walton et al., 2018; Williams et al., 2021).
Like many coral diseases, definitive pathogen identification for SCTLD has been unsuccessful. Studies have indicated that lesion progression can be slowed down or halted on some coral species with topical antibiotics (Aeby et al., 2019; Neely et al., 2020; Shilling et al., 2021), suggesting that the presumptive pathogen(s) for SCTLD may have a bacterial component. Additionally, some studies have identified differences in bacterial communities between apparently healthy and SCTLD-affected corals, but whether identified bacteria are primary pathogens or secondary opportunistic infections is unknown (Meyer et al., 2019; Rosales et al., 2020; Ushijima et al., 2020).
Lab-based studies have already demonstrated that SCTLD-affected corals with acute lesions are capable of inducing disease in healthy corals through both physical contact and the water column (Aeby et al., 2019; Meiling et al., 2021). However, no studies to date have examined whether the apparently healthy (AH) tissue adjacent to or at increasing distances from an SCTLD lesion (when isolated from the active lesion) becomes affected by the disease. Additionally, no studies to date have determined whether this isolated tissue induces disease in recipient fragments. The goals of the present study were to (i) determine whether AH tissue developed disease signs after isolation from an SCTLD lesion, (ii) document if these fragments induce disease within recipient Montastraea cavernosa and Orbicella faveolata, (iii) compare the probability of disease signs appearing between each coral species and (iv) quantify the lesion progression within these two species if induction occurred. Lastly, histopathological samples were collected through time as corals exhibited grossly visible tissue loss to confirm the presence of characteristic lytic necrosis (LN), initially in the basal body wall (BBW) of the gastrodermis, a histopathological hallmark of SCTLD (Landsberg et al., 2020).
Materials and Methods
Coral Colony Collection for Experiments
Manipulative aquaria-based experiments were utilized to complete the two disease-induction studies, referred to as the (i) M. cavernosa to M. cavernosa and (ii) M. cavernosa to O. faveolata experiments. The first induction experiment was conducted from April 26–June 25, 2019 using AH and diseased (subacute tissue loss) M. cavernosa. The AH M. cavernosa colonies were collected on April 23, 2019. The second induction experiment was conducted from July 26–September 26, 2019 using AH O. faveolata and diseased (subacute tissue loss) M. cavernosa. The AH O. faveolata colonies were collected on July 23, 2019. The AH colonies for each experiment were collected at the Airport Coral Heads site off Key West (24.53919°, −81.77270°), a site that was outside of the known distribution of SCTLD within FCR at the time of collection. Seven AH colonies, approximately 30 cm in diameter, of each species were collected for each experiment via hammer and chisel. Field participants wore sterile gloves during the collection process. After the colonies were removed from the reef, they were transported by hand up to the boat and stored in a sterile cooler with ambient seawater-soaked bubble wrap. The AH colonies were then transferred to an isolated aquarium containing ambient saltwater from a deep-water well at Mote Marine Laboratory’s (MML) International Center for Coral Reef Research and Restoration in Summerland Key, FL and maintained under those conditions for 1–2 days while the diseased corals were collected. AH colonies were collected prior to diseased colony collections to minimize contamination and inadvertent spreading of the disease. Original plans to expose AH O. faveolata to diseased O. faveolata were not possible because diseased O. faveolata colonies were not available at the time of collection. Therefore, diseased M. cavernosa colonies for the first and second experiments were collected on April 25, 2019 and July 24, 2019, respectively, in the Looe Key reef area (24.54767°, −81.45697°). These colonies exhibited grossly visible signs consistent with SCTLD, were approximately 30 cm in diameter, and had approximately 30% disease progression. Each colony had a diffuse subacute tissue loss lesion, defined as a lesion with bare white skeleton less than five centimeters in width along the lesion edge (described in Aeby et al., 2019). At the time of collection (for both experiments), this lesion type was the most prevalent in the area of collection. This lesion type was also used in previous SCTLD induction studies (Aeby et al., 2019). A total of eight diseased M. cavernosa colonies were collected (four for each experiment). After diseased colony collections were completed for each study, the AH and diseased colonies were transported from Summerland Key, FL to MML’s Coral Health and Disease laboratory in Sarasota, Florida for testing. The diseased and AH colonies were transported in separate coolers, wrapped with seawater-soaked bubble wrap. On arrival the diseased and AH colonies were placed in separate (previously bleached) bins with ambient seawater (∼102 L volume) obtained from the Coral Health and Disease wetlab supply source overnight. Each bin was filled with seawater, equipped with multiple powerheads (songlong SL-381 submersible pump) to maintain circulation, and temperature was regulated by a recirculating temperature-controlled water bath.
Experimental Setup and Design
One 3 m × 1.2 m fiberglass raceway holding twenty 18.9 L aquaria was used for each experiment, to keep the temperature of the water within the aquaria constant. Temperature within the raceway was controlled by a heat exchanger that was set to a temperature of 27.5°C. Corals were held under a 10-h light: 14-h dark photoperiod using Radion XR30w Pro aquaria lights (EcoTech Marine, Allentown, PA, United States). The lights were set to a “traveling sunrise and sunset” feature, with fluctuating values of 50–300 PAR over the course of a day to mimic natural conditions as much as possible.
Using sterilized gloves, each diseased M. cavernosa colony (n = 4 × 2 experiments, identified as diseased donor colonies (DC) A to D [DCA–DCD]) was taken from the aquaria (∼24 h, including transportation time), and fragmented on a sterile table into four donor pieces, with each piece representing a sequential “distance” away from the active tissue-loss margin (Figure 1A). Coral colonies were fragmented using an angle grinder (Dewalt DWE402) and trimmed with a diamond blade band saw (Gryphon Corporation, C-40 CR Aquasaw XL). To minimize contamination, all blades and tools were sanitized with a 10% bleach solution between uses. Each diseased donor fragment in the induction experiments was approximately 16 cm long × 8 cm wide × 3–5 cm high. The diseased colonies were fragmented parallel to the lesion edge to determine whether isolated AH donor fragments that showed no obvious grossly visible tissue-loss signs, would subsequently exhibit tissue loss and induce SCTLD in recipient M. cavernosa or O. faveolata fragments. The donor fragment with active tissue loss was identified as “distance 1” (D1). The sequential donor fragments with no obvious grossly visible tissue-loss signs were approximately 4 cm (“distance 2” – D2), 12 cm (“distance 3” – D3) and 20 cm (“distance 4” – D4), respectively, away from the active tissue-loss margin (Figure 1A). One diseased M. cavernosa colony collected for the O. faveolata transmission experiment had multiple subacute lesions, therefore this colony (DCD) was fragmented into three D1 pieces only (i.e., no D2–D4 donors were used).
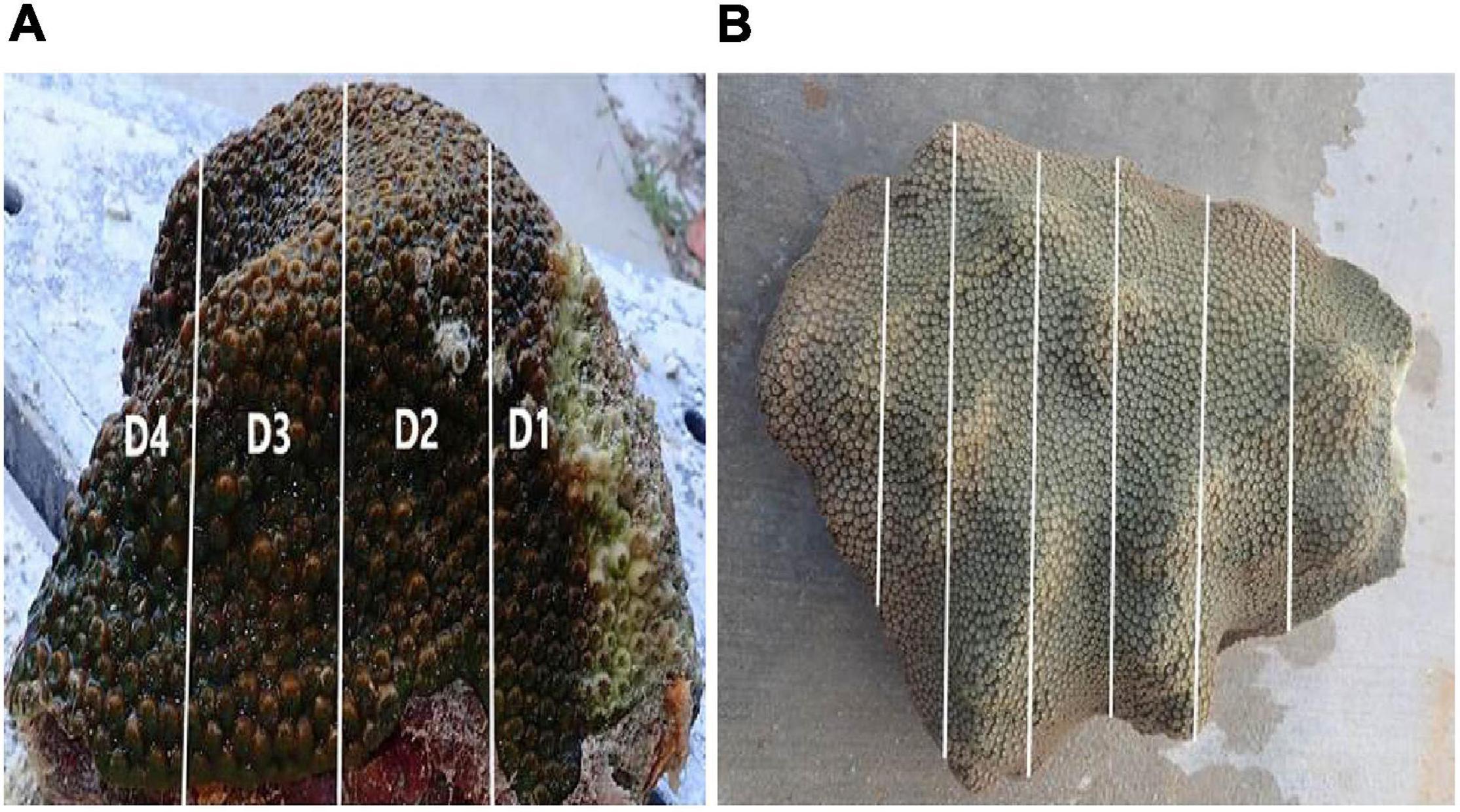
Figure 1. (A) Gross appearance of an SCTLD-affected diseased donor, Montastraea cavernosa, colony with a subacute lesion used for the induction studies. Representation of the longitudinal fragmentation of a donor colony cut into sequential “distances” ∼8 cm apart (Distance 1–Distance 4; D1–D4) from the lesion margin. (B) Representation of the longitudinal fragmentation of a recipient colony cut into fragments ∼10 cm long × 3 cm wide × 2 cm high.
For the M. cavernosa to M. cavernosa experiment, one AH M. cavernosa colony was randomly chosen to act as a donor control and was cut into four fragments of similar size as the diseased donor colony fragments. All AH colonies were fragmented first before fragmenting each diseased donor colony (and each treatment fragment within the donor colony) to reduce the possibility of cross contamination. The remaining six AH M. cavernosa colonies were fragmented into approximately ten pieces each, ∼10 cm long × 3 cm wide × 2 cm high, to act as recipient fragments.
For the M. cavernosa to O. faveolata experiment, an AH O. faveolata colony was randomly selected as a donor control and was fragmented into four fragments of similar size to the diseased donor colony fragments. Additionally, four AH M. cavernosa fragments of similar size were placed into control aquaria to account for inter-species aggression. The remaining six AH O. faveolata colonies were fragmented similarly to the M. cavernosa to M. cavernosa study (Figure 1B).
For each experiment, the recipient fragments were randomly distributed among the aquaria with equal distribution to each treatment and the controls. Each aquarium had one diseased donor fragment of a different treatment with three recipient fragments directly touching it. Some of the D1 donor fragments had to be cut in half so that each recipient fragment could be in contact with the active tissue-loss margin (Figure 2A). There was an excess of recipient fragments, so these fragments were randomly placed in aquaria, but not in direct contact with the donor fragment to assess the potential for waterborne transmission (Figure 2B). These fragments were designated as ‘non-touching.’ Four of the 20 aquaria were set up as controls to account for any aggression from direct contact. Recipient fragments were placed in direct contact with the AH donor colony that was randomly chosen as a control. The experimental design matrices for the aquaria set ups for the two induction experiments are summarized in Figures 3A,B (see Supplementary Tables 1, 2 for details).
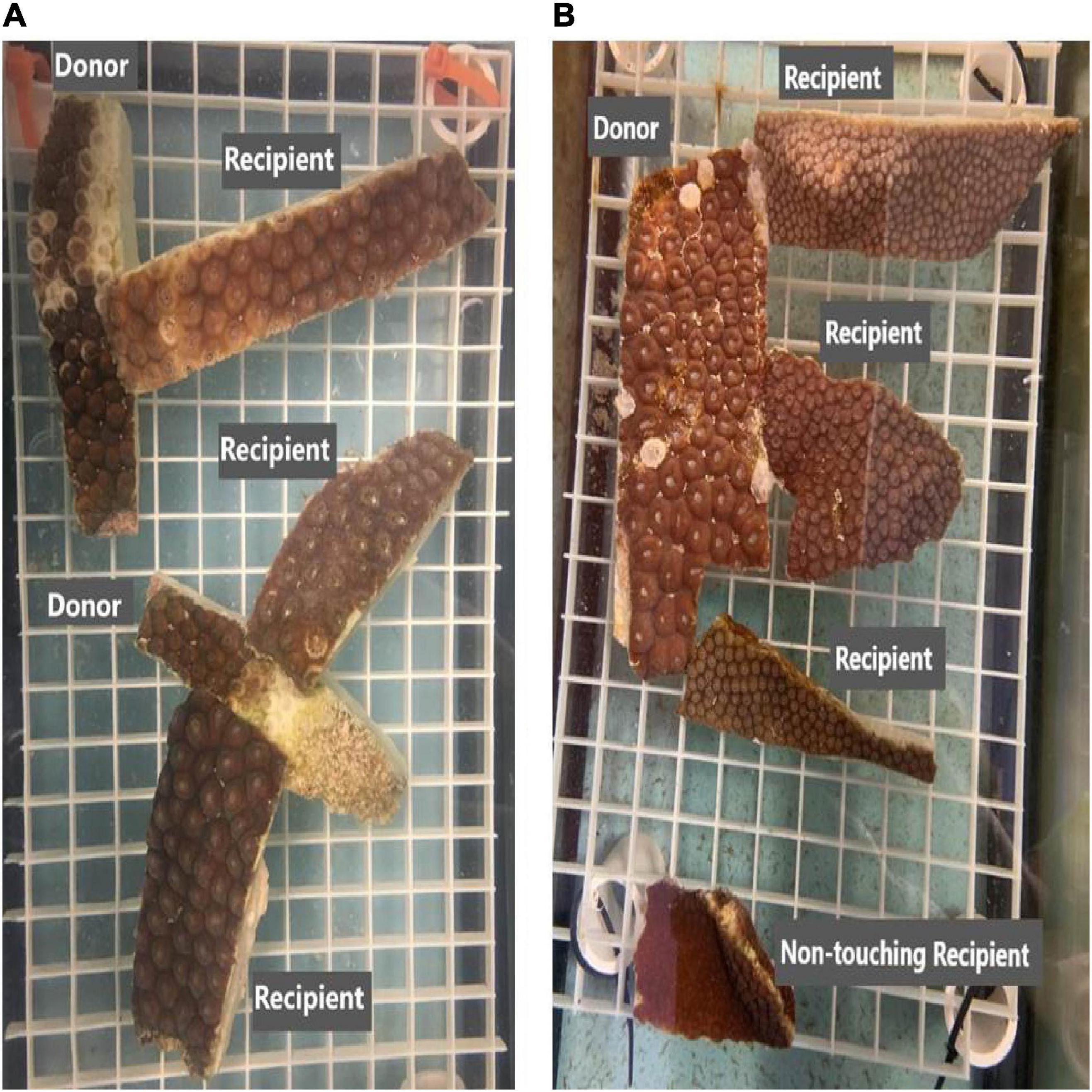
Figure 2. Examples of the experimental set up for the M. cavernosa to M. cavernosa and M. cavernosa to O. faveolata induction experiments, respectively. (A) Aquarium with one M. cavernosa disease donor fragment distance 1 (D1) cut in half, with three touching and one non-touching (NT, not shown) M. cavernosa recipient fragments. (B) Aquarium setup with three O. faveolata recipient fragments touching one M. cavernosa disease donor fragment distance 4 (D4) (exhibiting no grossly visible lesions) and one separate non-touching (NT) O. faveolata recipient fragment.
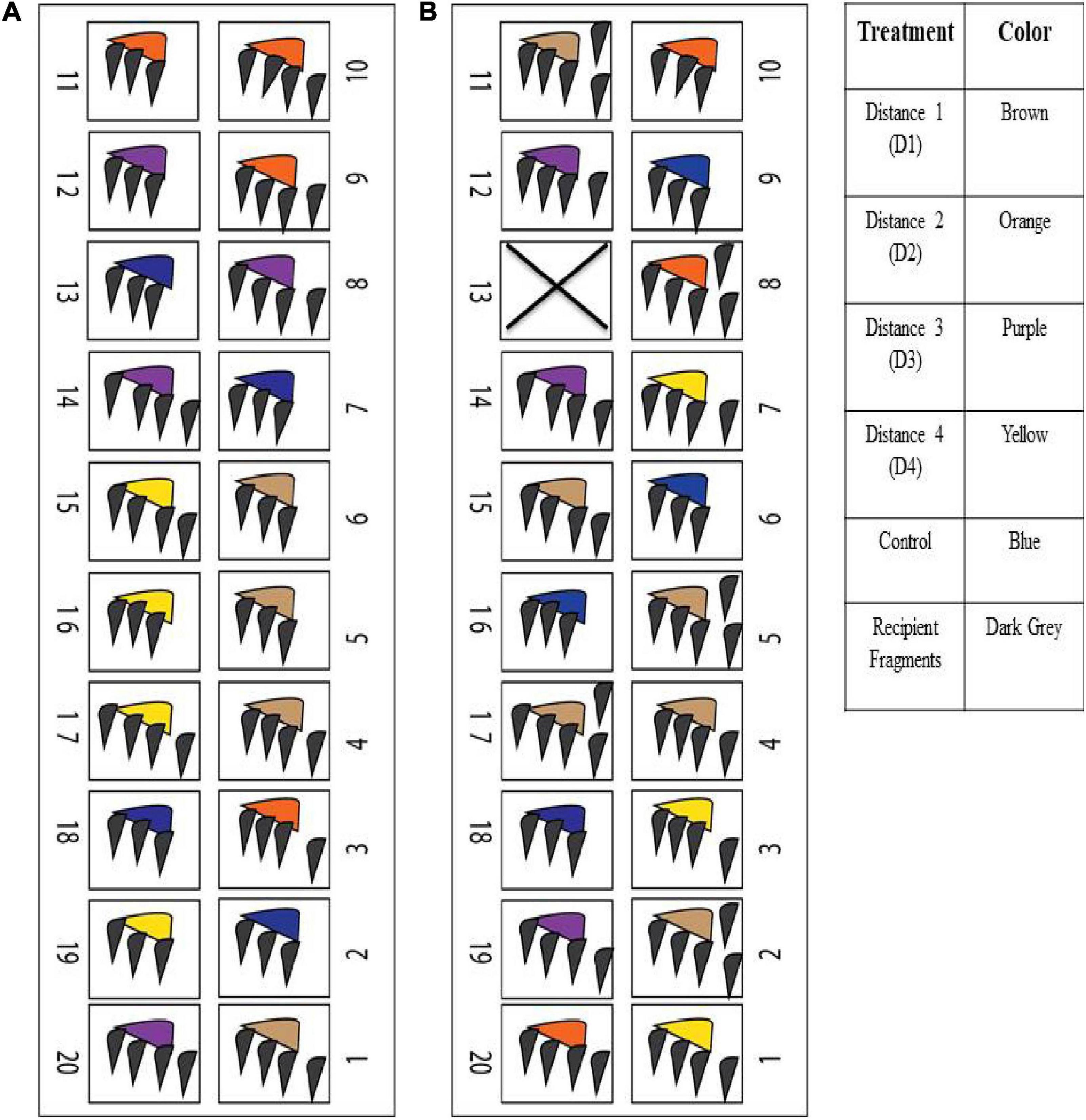
Figure 3. The experimental design matrices of the aquaria set ups for the two induction experiments. (A) M. cavernosa – M. cavernosa experiment, (B) M. cavernosa – O. faveolata experiment.
Aquaria Maintenance
All seawater at MML was pulled from the local Sarasota Bay, and went through several filtration and ozonation steps before entering the laboratory. Fifty percent water changes were completed daily on all aquaria, and a recirculating water pump (songlong SL-381 submersible pump) was used to maintain water motion within each aquarium. All contaminated seawater along with any exposed equipment was bleached (1:9 bleach to water ratio) and UV sterilized prior to disposal. Water quality parameters (including temperature, pH, salinity, and dissolved oxygen; Supplementary Table 3) of the seawater utilized for the 50% water changes were monitored daily using a YSI handheld instrument (YSI ProDSS, Yellow Springs, OH, United States).
Experiment Monitoring
Aquaria observations were recorded and photographs were taken daily to monitor tissue loss appearance and progression within the donor fragments and the touching and non-touching recipient fragments. When recipient fragments exhibited more than 5% tissue loss, they were closely monitored and prepared for histology sampling.
Collection of Samples for Histopathology
Baseline samples were collected for histopathological examination from the diseased and control donor fragments for both experiments before donors were assigned to experimental aquaria. In addition, recipient M. cavernosa and O. faveolata fragments were sampled at time zero and through time as tissue loss was grossly observed. Samples were collected when fragments had approximately 25, 50, and 75% tissue loss (so the same fragment could have been sampled up to three times, unless the fragment reached complete tissue loss before the sampling time point or the tissue loss had stopped progressing). Each sample collected from a recipient fragment within a treated disease aquarium was paired with a sample collected from a control recipient fragment. Control samples were taken from fragments of the same parent colony (A–B, D–G) as the recipient fragment being sampled from the treated aquarium.
When a recipient fragment was ready to be sampled, the fragment was removed with sterile gloves from its aquarium, photographed, and a 3 cm × 3 cm × 2 cm sample was extracted using a sanitized diamond-blade band saw (Gryphon Corporation, C-40 CR Aquasaw XL). After sampling, the coral fragment was re-photographed and placed back into the same aquarium in its original location touching the donor fragment, unless it was a non-touching fragment.
All histological samples were fixed with a solution of 1 part Z-Fix (zinc formalin; Z-Fix concentrate [18.5% formaldehyde; Anatech Ltd., Battle Creek, MI, United States]) mixed with 4 parts 0.2 μm-filtered, UV-sterilized natural seawater. Samples collected are summarized in Tables 1–4 (see Supplementary Tables 4–7 for details). All collected samples for histologic examination were processed, except that a subset of the paired sampled controls were randomly selected. All processing for histology was conducted at the Florida Fish and Wildlife Conservation Commission’s Fish and Wildlife Research Institute, St. Petersburg, FL, United States.

Table 1. Histological samples examined during the Montastraea cavernosa to M. cavernosa disease induction experiment.
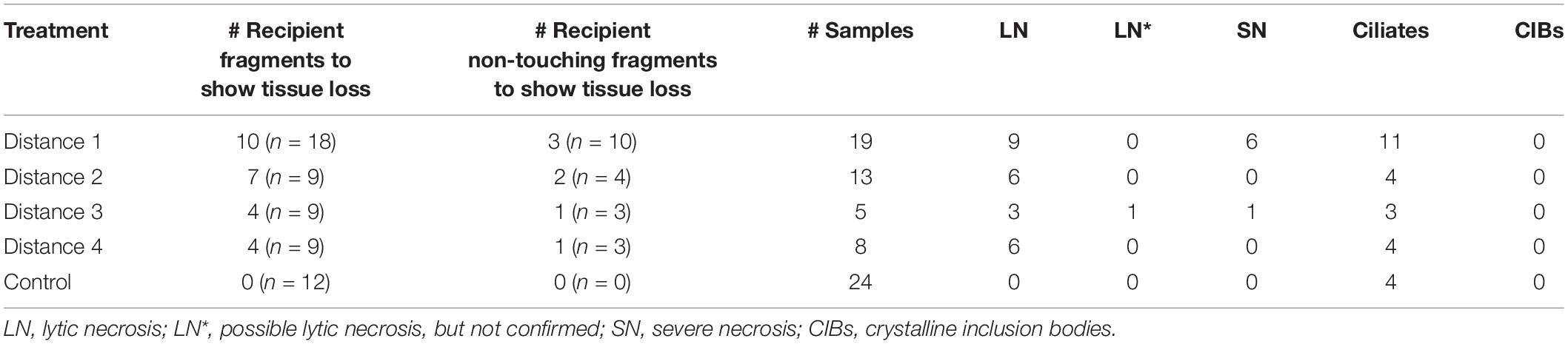
Table 2. Histological samples examined from the M. cavernosa to Orbicella faveolata disease induction experiment.
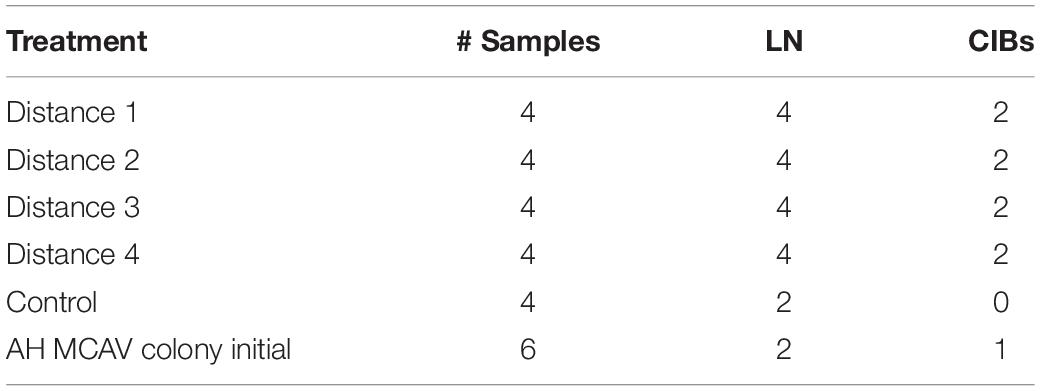
Table 3. Histological samples examined for baseline lytic necrosis (LN) and crystalline inclusion bodies (CIBs) in M. cavernosa donors prior to the M. cavernosa to M. cavernosa disease induction experiment.
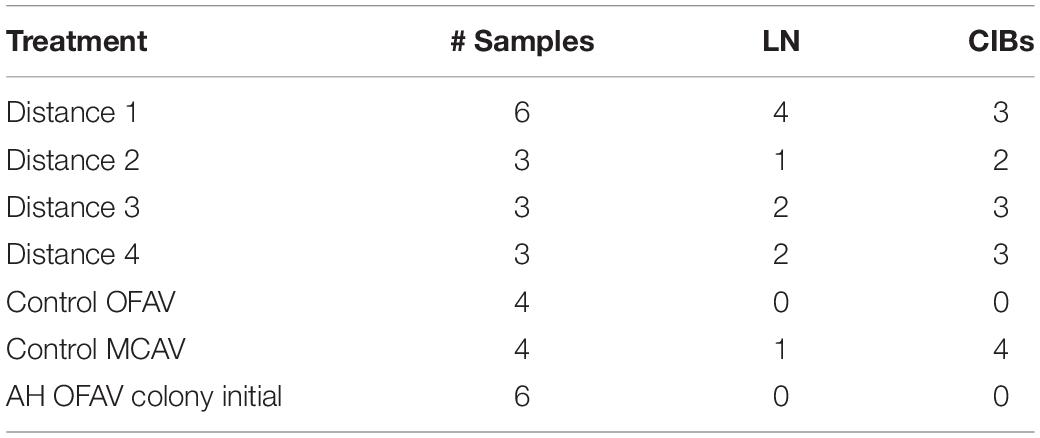
Table 4. Histological samples examined for baseline lytic necrosis (LN) and crystalline inclusion bodies (CIBs) in M. cavernosa donors prior to the M. cavernosa to O. faveolata disease induction experiment.
Before histopathology analyses, all samples were photographed using a digital camera fitted with a macro lens (Nikon, Tokyo, Japan). Diseased fragment samples exhibiting grossly visible lesions were further examined under higher magnification with a dissecting microscope attached to an Olympus DP72 digital camera (Tokyo, Japan), and photomicrographs taken. At this point folliculinid ciliate (heterotrich) infections (Verde et al., 2016), especially Halofolliculina sp., in the skeleton were also determined. These organisms were not detectable by histology because all skeleton, including these organisms, is removed during decalcification.
Coral tissue for routine histological processing followed Landsberg et al. (2020). Briefly, tissue was enrobed with agarose (for samples exhibiting gross lesions only), decalcified with 10% ethylenediaminetetraacetic acid (EDTA, Fisher Scientific, Na2⋅2H2O; MW = 372.1), and then processed for routine histology. After all the aragonite skeleton had dissolved away, decalcified soft tissues were also photographed microscopically prior to processing. Since the fragments were large enough to make both sagittal and radial cuts, tissues were trimmed this way – sagittally (perpendicular to polyp mouth) and radially (parallel to polyp mouth), embedded in paraffin (Paraplast Plus, Fisher Scientific, Waltham, PA, United States) or glycol methacrylate plastic resin (JB-4; Electron Microscopy Sciences, Hatfield, PA, United States). Embedded tissue blocks were sectioned at 4.0 μm with a rotary microtome and stained with Mayer’s hematoxylin and eosin (H&E for paraffin medium), Weigert’s H&E (for JB4 medium), thionin, or periodic acid Schiff/metanil yellow (PAS-MY for JB-4; Quintero-Hunter et al., 1991). Slides were examined with an Olympus BX51 light microscope equipped with an Olympus DP71 digital camera (Olympus Inc., Tokyo).
An initial screening of three slides (two paraffin and one JB-4 embedded medium as described above) from each specimen was conducted to assess if slides were suitable for further examination. For example, if the histological slide was not well prepared or missed the target tissue area for lesion evaluation (gastrodermis of basal body wall, BBW), then the specimen was censored from the histological examination.
Data Analysis
All statistical analyses were performed in R-4.0.2 (R Core Team, 2020), and figures were made using Prism (version 8.0.0 for Windows, GraphPad Software, San Diego, CA, United States). A Fisher’s Exact Test (Fisher, 1934) was used to examine the likelihood of tissue loss for the different treatment “distances” within the diseased donor colonies. The likelihood of the different treatment “distances” to exhibit tissue loss was examined for each experiment separately; and across both experiments.
A Fisher’s Exact Test was also used to identify statistical differences in the probability of touching vs. non-touching M. cavernosa recipient fragments to exhibit tissue loss, and a Chi-Square analysis (Pearson, 1900) was used to identify statistical significance of touching vs. non-touching O. faveolata recipient fragments to exhibit tissue loss.
A Fisher’s Exact Test was used to examine the likelihood of tissue loss in both M. cavernosa and O. faveolata recipient fragments exposed to different treatments. A Chi-Square analysis was used to compare the likelihood of tissue loss between species. A Fisher’s Exact Test was used to identify statistical significance of M. cavernosa vs. O. faveolata non-touching recipient fragments to exhibit tissue loss. Pearson’s correlation (Benesty et al., 2009) was used to assess the correlation between distance away from the lesion and time of tissue loss onset within the D2–D4 donor fragments.
Disease progression (i.e., tissue loss) within recipient-touching fragments was measured using ImageJ software (Abràmoff et al., 2004) on the photographs of the fragments taken in the aquaria during the experiment. Disease progression was measured only in recipient fragments that were affected by tissue loss that led to complete mortality of the tissue (i.e., all tissue had sloughed off of the skeleton). Disease progression was assessed by measuring the area of living tissue at the halfway point between initial grossly visible tissue loss signs and death (on average 3.5 days after initial tissue loss appearance for each species) and subtracting from the initial area of live AH (pigmented) tissue. One-way analysis of variance (ANOVA) tests were used to assess the statistical significance between species for timing of tissue-loss onset (i.e., disease induction) in recipient fragments among the treatments and lesion progression (cm2/day). Timing of tissue loss onset (i.e., disease induction) in recipient fragments among the treatments was measured after a grossly visible tissue loss lesion appeared on the donor colony. For the M. cavernosa experiment, this data was transformed using an aligned rank transformation (Conover and Iman, 1981).
A Cox proportional hazard model (Cox, 1972) was used to compare the survival times of M. cavernosa and O. faveolata touching recipient fragments. It was also used to compare survival times of touching recipient fragments among the four treatments within M. cavernosa and O. faveolata.
Results
M. cavernosa to M. cavernosa Experiment
Fragments Isolated From Diseased Donor Colonies Showed Signs of Tissue Loss
Over time, grossly observable tissue loss appeared within AH donor fragments after their isolation from the lesioned area. The likelihood of fragments showing signs of tissue loss was not significantly different among the four treatment distances (Fisher’s Exact, p = 0.41). Three out of four D2 fragments, two out of four D3, and two out of four D4 fragments exhibited gross signs of tissue loss (Supplementary Figure 1). It took an average of 7.7 days for initial signs of tissue loss to appear within D2 fragments, 2.5 days for D3 fragments, and 13 days for D4 fragments (Supplementary Figure 2). By the end of the experiment, all four D1 fragments, one D2, one D3, and one D4 fragment had reached complete mortality. None of the control donor fragments showed any grossly visible tissue loss.
Gross Appearance and Histopathology of Tissue Loss Within the Donor Fragments
All donor fragments (D1 – D4, n = 16) examined for baseline had LN prior to experimental start up, even though grossly visible lesions were absent in the D2–D4 fragments. Two donor control fragments had BBW LN lesions prior to start up (Table 3 and Supplementary Table 6). However, no donor control fragments or control recipient fragments showed macroscopic signs of tissue loss throughout the study.
Induction of Tissue Loss Occurred Within the Recipient Fragments
Grossly visible tissue loss did not occur in any control recipient fragments. However, grossly visible tissue loss occurred for both touching fragments and non-touching fragments in the disease treatments (Table 5). Disease induction did not occur in the recipient fragments unless there was corresponding grossly visible tissue loss on the donor treatment fragments, but not all aquaria with D2–D4 donor fragments induced disease in recipient fragments. Therefore, the touching and non-touching recipient fragments (n = 15) in aquaria with D2–D4 donor fragments (five out of 12) that did not exhibit tissue loss (see Table 5) were not included in the following analyses. Among all treatment distances where tissue loss did progress on the donor fragment (11 out of 16), tissue loss occurred in 13 (39.39%, n = 33) touching recipient fragments and one non-touching recipient fragment (16.66%, n = 6; Table 5), with nine (69.23%, n = 13) touching fragments exhibiting complete tissue loss (mortality) by the end of the study. Four (30.77%, n = 13) touching fragments experienced disease induction, but the tissue loss stopped progressing (Supplementary Table 1). There was no significant difference in the appearance of tissue loss for touching and non-touching fragments (Fisher’s Exact, p = 0.39). Non-touching fragments were not included in the following analyses for the M. cavernosa experiment since only one fragment exhibited tissue loss and this was not confirmed histologically with LN (Table 1).
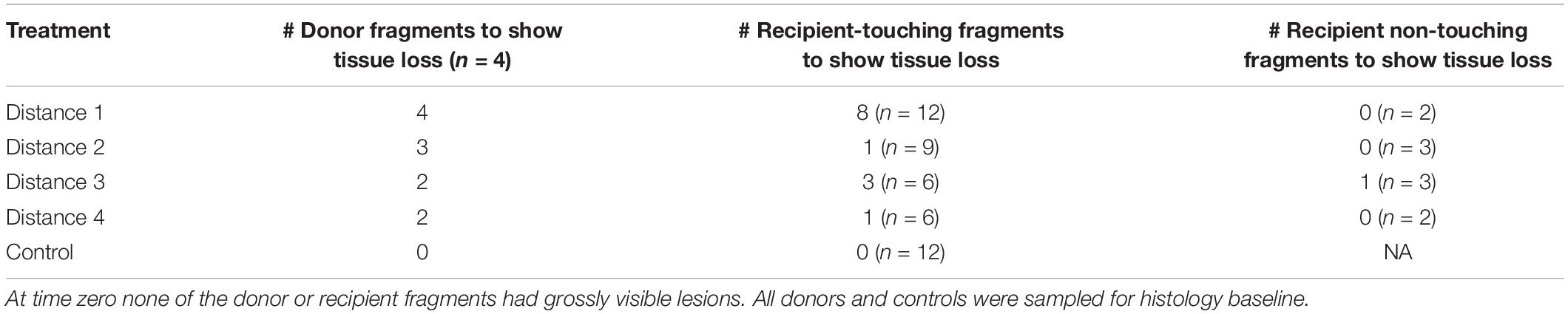
Table 5. Experimental design for the M. cavernosa to M. cavernosa experiment with monitored lesion progression on donor and recipient fragments.
Tissue loss in recipient fragments occurred less often in further distance treatment exposures than with D1. Eight out of 12 fragments (66.67%), one out of nine (11.11%), three out of six (50%), and one out of six fragments (16.66%) exposed to D1, D2, D3, and D4 donor fragments, respectively, showed signs of tissue loss (Figure 4A and Table 5). One donor colony (DCD) did not induce disease in any recipient fragments across all treatments, but the DCD D1 fragment was completely dead 2 days into the study. There was a significant difference in the probability of tissue loss occurring on recipient corals exposed to D1 compared with D2 fragments—recipient fragments touching D1 exhibited more tissue loss compared to recipient fragments touching D2 (Fisher’s Exact, p = 0.03). There were no significant differences in probability of tissue loss within recipient fragments for the rest of the treatment comparisons (D1 vs. D3, D1 vs. D4, D2 vs. D3, D2 vs. D4, D3 vs. D4).
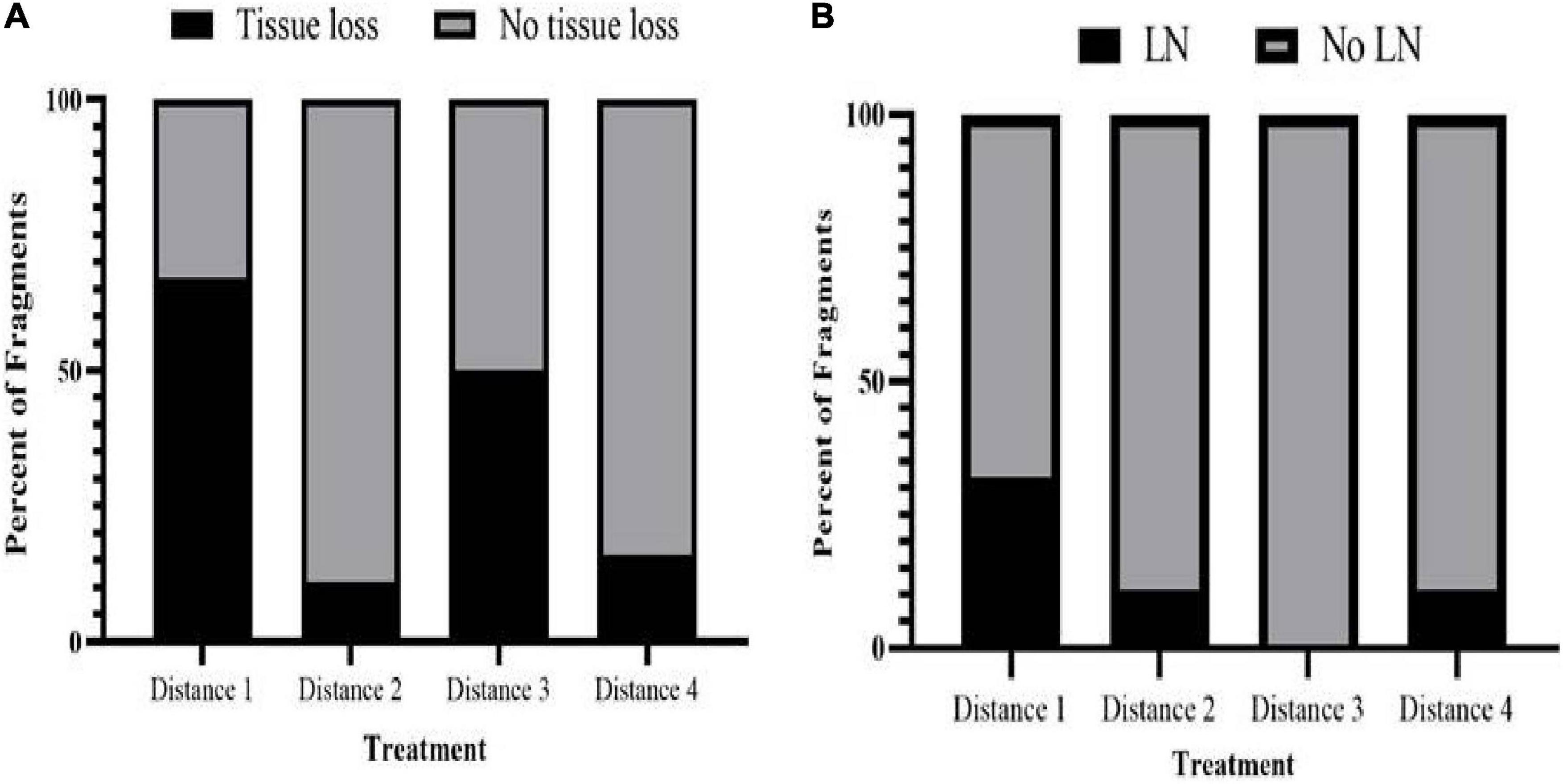
Figure 4. Comparison of disease induction in M. cavernosa recipient fragments touching different M. cavernosa donors (D1, n = 12; D2, n = 9; D3, n = 6; D4, n = 6). (A) Percent of touching M. cavernosa recipient fragments showing gross signs of tissue loss when in contact with different donor M. cavernosa treatment fragments. (B) Percent of touching M. cavernosa recipient fragments showing a histopathological parameter, lytic necrosis (LN) when in contact with different donor M. cavernosa treatment fragments.
There was no significant difference in survival among treatments of M. cavernosa recipient fragments. Six out of twelve fragments exposed to D1 survived, eight out of nine fragments exposed to D2 survived, and five out of six fragments exposed to both D3 and D4 survived (Supplementary Figure 4, p = 0.52).
There was no significant difference in the average number of days for first signs of tissue loss to appear in touching recipient fragments among D1 (23 ± 5.14 days) and D3 (34.5 ± 1.84 days) (ANOVA, F1,9 = 0.66, p = 0.44). Since only one recipient-touching fragment from both the D2 and D4 treatments exhibited tissue loss, these fragments were not included in this analysis.
Gross Appearance and Histopathology of Tissue Loss Within the Recipient Fragments
Samples from 14 diseased fragments [(15–90% lesion cover on recipient fragments) including one that was non-touching] and an additional 14 control M. cavernosa samples were collected for histology from 2 to 52 days after the experiment began (Table 1 and Supplementary Table 4). Of the 14 diseased fragments, six were sampled once only, four were sampled twice, and four were sampled three times as tissue loss progressed on each fragment (Supplementary Table 4).
After direct contact exposure to a D1 M. cavernosa fragment, the earliest sign of grossly observed tissue loss with histopathological confirmation of lytic necrosis (LN), was in one recipient fragment within 2 days of experimental startup (Figure 5 and Supplementary Table 4). Initiation appeared on the polyps’ individual septa with separation of the tissue away from the skeleton causing multifocal tissue loss lesioned areas (Figures 5C,D). Early lesions were also noted adjacent to the skeleton of the individual costa in the coenenchyme. Eventually, all the tissue sloughed off of the fragment revealing only the skeletal tissue.
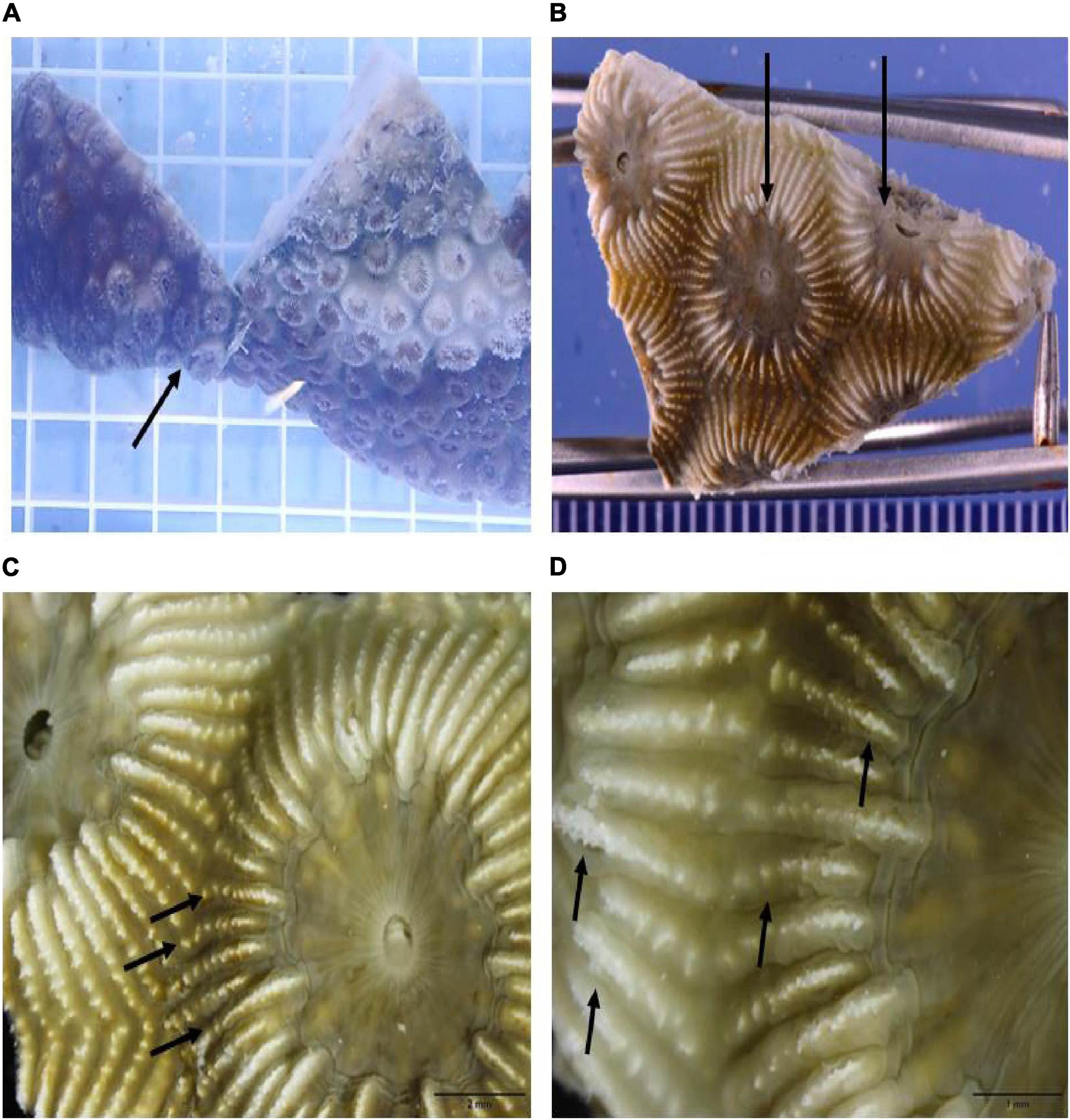
Figure 5. Gross appearance of tissue loss lesions induced after 2 days by direct contact of a M. cavernosa recipient fragment exposed to a D1 fragment. (A) In situ view of lesioned recipient fragment in direct contact showing appearance of gross lesions (arrow) adjacent to the lesion border on the donor fragment. (B) Excised recipient fragment taken for histology showing higher magnification tissue loss area at black arrows. (C) Macroscopic view of two polyps showing the initiation areas of tissue loss on individual septa and separation of the tissue away from the skeleton (on each septal ridge) at black arrows. Note tissue loss on the left polyp is more advanced. (D) Higher magnification of the septal ridges and initial loss and separation of tissue from the skeleton at black arrows.
Histologically, seven of 13 (53.84%) recipient touching fragments with disease exhibited tissue loss lesions characteristic of LN at the gastrodermal BBW (Figures 6A,B and Table 1). One fragment that was sampled three times for histology was confirmed with LN twice. One of 10 non-touching recipient samples in the disease treatment aquaria exhibited visibly obvious gross lesions, but this could not be definitively confirmed to be LN by histopathology (Table 1). Of the seven fragments that exhibited hallmark LN, five were from D1 treatment, one was from D2 treatment, and one was from D4 treatment (Figure 4B). Over the course of the experiment, six of seven fragments (85.71%) with confirmed LN and progressive grossly visible tissue loss exhibited complete mortality (Supplementary Table 1).
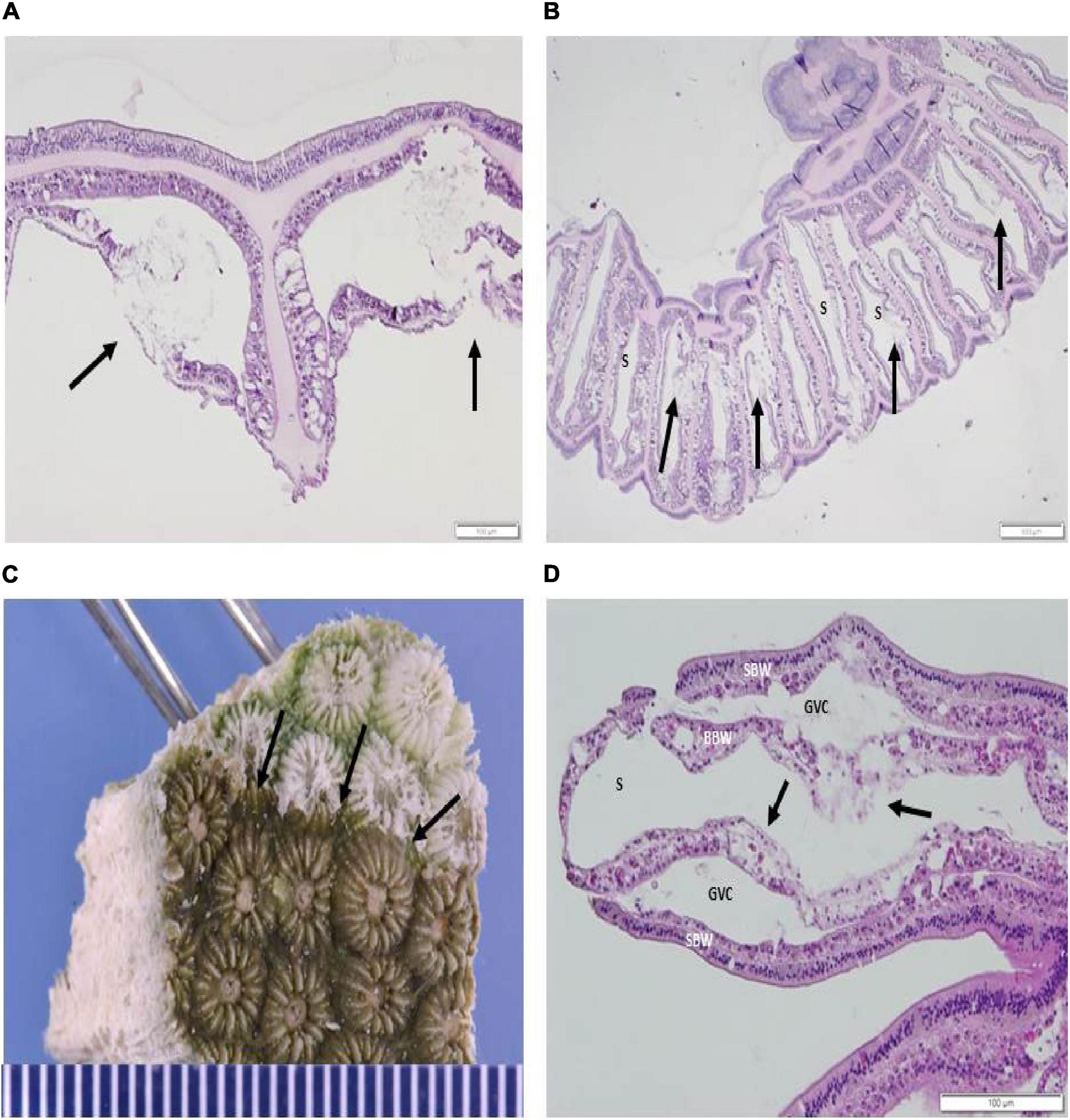
Figure 6. (A,B) Histopathology of M. cavernosa recipient fragment showing induced LN lesions (H&E). (A) Sagittal section of the coenenchyme showing two LN lesions (arrows) after 2 days exposure initiating in the basal body wall (BBW). (B) Radial section across the oral area showing LN lesions in the septa (arrows) adjacent to the skeleton (S) initiating in the BBW, 10 days post experimental start. (C,D) Induction of tissue loss in the M. cavernosa to O. faveolata induction experiment. (C) Gross appearance of tissue loss in an AH O. faveolata recipient fragment after 7 days exposure to a D1fragment. Tissue loss border shown at arrows. (D) Histopathology of O. faveolata recipient fragment [seen in (C)] in tangential section showing induced LN lesions in the BBW. Note deformed zooxanthellae, BBW necrosis, loss of architecture and vacuolation of the BBW gastrodermis (arrows). BBW, basal body wall; GVC, gastrovascular canal; SBW, surface body wall; S, skeleton (H&E).
All disease-induced samples had scattered pyknotic nuclei in the BBW gastrodermis. Nine samples had gastrodermal crystalline inclusion bodies (Table 1). Three samples (each in a D1, D2, and D4 treatment aquarium) were colonized by ciliates (hypotrichs) in the lesioned tissues surrounded by a bacterial mass (Table 1). No ciliate infections other than folliculinids were histologically confirmed in any of the controls examined. One fragment was sampled three times (within a D4 treatment aquarium) and collected 17–21 days post experiment start, exhibited severe diffuse necrosis. 12 of 14 control M. cavernosa samples did not exhibit LN at the gastrodermis nor present with any other prominent pathological changes (Table 1 and Figure 7A). Note that eight controls had Halofolliculina sp., folliculinid ciliate infections by 32 days from the experimental start, as confirmed with a dissecting microscope prior to decalcification in the skeletal tissue. Only one recipient fragment in the D1 treatment had Halofolliculina sp. (39 days post experimental start).
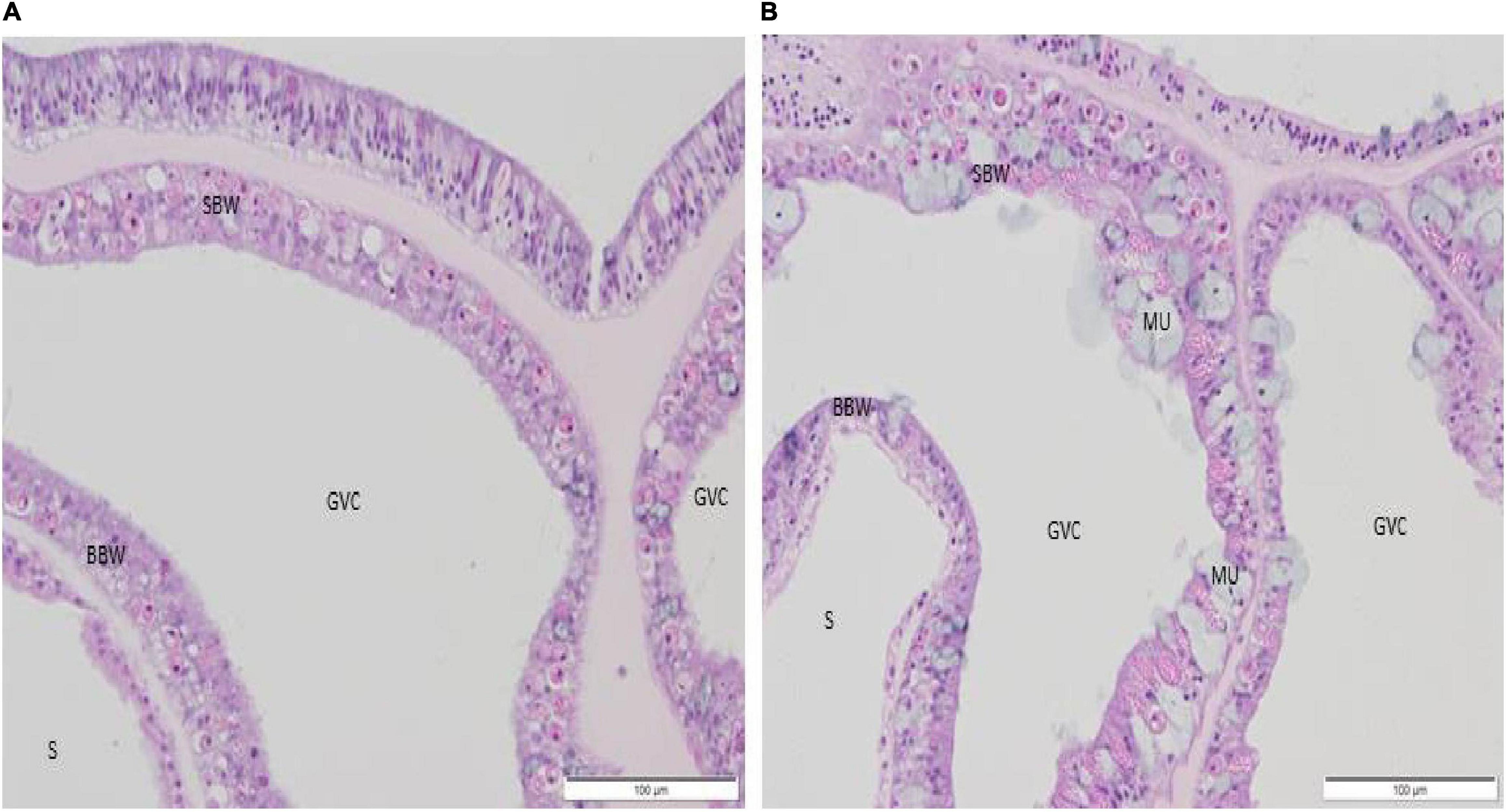
Figure 7. (A,B) Histological sagittal section of the coenenchyme in a control recipient fragment showing absence of lesions (H&E). (A) M. cavernosa, 17 days post experimental start. (B) O. faveolata, 9 days post experimental start. BBW, basal body wall; GVC, gastrovascular canal; MU, mucocyte; SBW, surface body wall; S, skeleton.
M. cavernosa to O. faveolata Disease Induction and Progression Experiment
Fragments Isolated From Diseased Donor Colonies Showed Signs of Tissue Loss
Over time, all D2–D4 fragments exhibited gross signs of SCTLD. It took an average of 17.3 days for initial signs of tissue loss to appear within D2 fragments, 14.7 days for D3 fragments, and 20.7 days for D4 fragments (Supplementary Figure 4). By the end of the experiment, 5 of 6 D1 fragments, two D2, one D3, and one D4 fragment had reached complete mortality (Supplementary Table 2). None of the control donor fragments showed any grossly visible tissue loss.
Gross Appearance and Histopathology of Tissue Loss Within the Donor Fragments
Histopathological evaluation of baseline disease and control donor fragments showed BBW or surface body wall LN in some specimens that were sampled before assignment to experimental aquaria (Table 4 and Supplementary Table 7). Four of six D1 fragments, one of three D2, two of three D3, and two of four D4 fragments examined for baseline had LN prior to experimental start up, even though grossly visible lesions were absent in the D2–D4 fragments. None of the healthy O. faveolata donors exhibited LN prior to the beginning of the experiment nor did macroscopic signs of tissue loss occur throughout the study. One apparently healthy M. cavernosa donor fragment had BBW LN lesions prior to aquarium assignment.
Induction of Tissue Loss Occurred Within the Recipient Fragments
Grossly visible tissue loss did not occur in any control recipient fragments. However, disease induction occurred in recipient O. faveolata fragments when there was grossly visible tissue loss on the donor fragment. All D2–D4 fragments exhibited tissue loss, so all recipient fragments were included in the analyses. Averaged across all distances, tissue loss occurred in 25 (55.56%, n = 45) recipient-touching fragments and seven (35%, n = 20) recipient non-touching fragments, with 14 (56%, n = 25) recipient-touching fragments and two (28.57%, n = 7) recipient non-touching fragments experiencing 100% area tissue loss (mortality). Eleven (44%, n = 25) recipient-touching fragments experienced disease induction, but the tissue loss stopped progressing. Note that not all 25 diseased fragment samples were processed for histology; six were not examined for LN. Similarly, only two of seven non-touching diseased fragments were examined (Table 2 and Supplementary Table 2). There was no significant difference in the probability of tissue loss occurring for touching and non-touching fragments [Chi Square, X2(1, N = 45) = 1.59, p = 0.21]. Since there was no significant difference, the sample size of non-touching fragments that exhibited grossly visible tissue loss was small, and no tissue loss was confirmed with LN, non-touching fragments were not included in the following analyses for the O. faveolata experiment.
Occurrence of tissue loss within recipient-touching fragments was similar for all donor treatments in O. faveolata. Ten out of 18 (56.0%), seven out of nine (77.78%), four out of nine (44.44%), and four out of nine (44.44%) recipient fragments exposed to D1, D2, D3, and D4 treatments, respectively, exhibited tissue loss (Figure 8A and Table 6). There was no significant difference in recipient O. faveolata tissue loss occurrence among the four distances (Fisher’s Exact, p = 0.46).
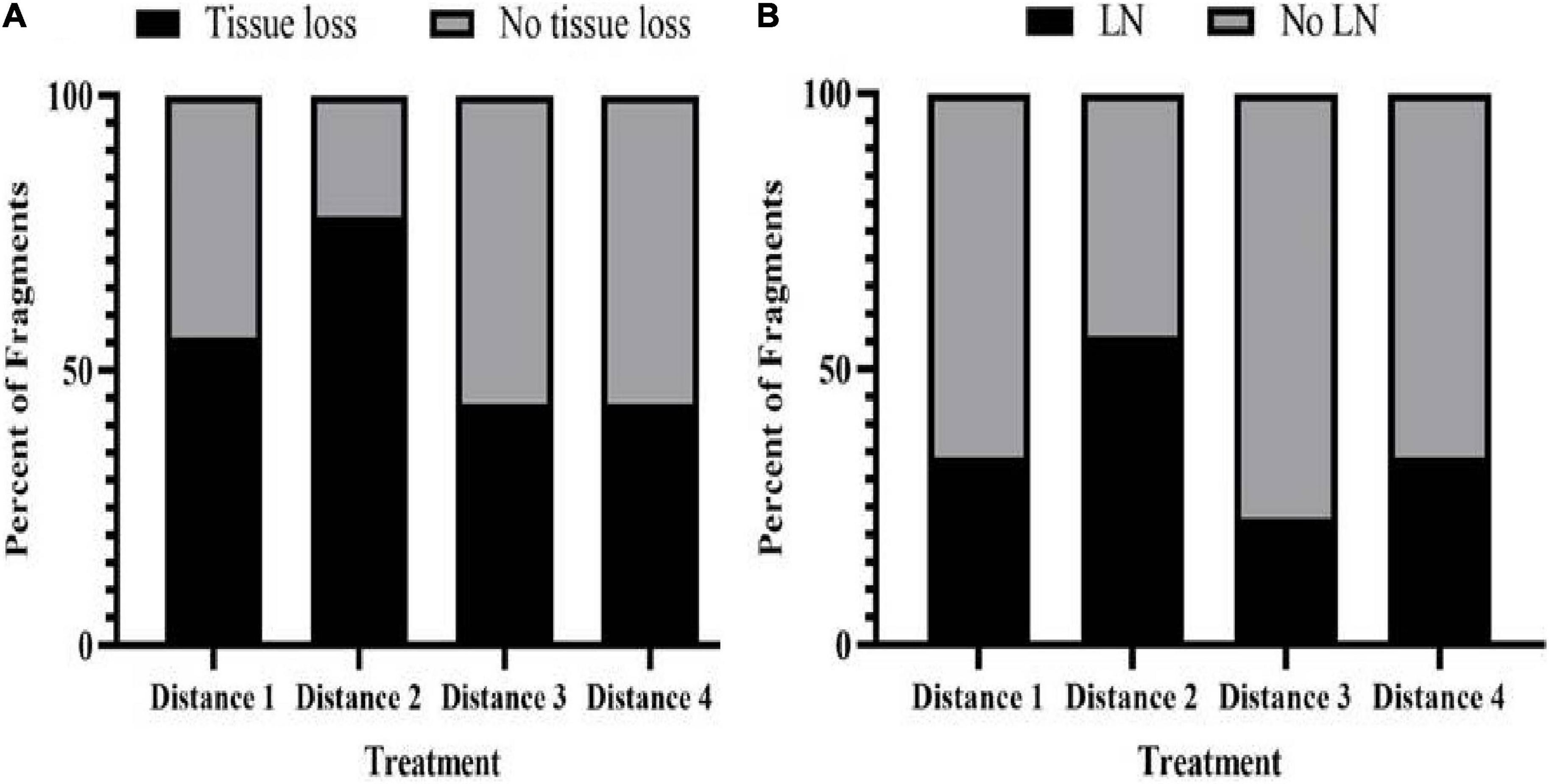
Figure 8. Comparison of disease induction in O. faveolata recipient fragments touching different M. cavernosa donors (D1, n = 18; D2, n = 9; D3, n = 9; D4, n = 9). (A) Percent of touching O. faveolata recipient fragments showing gross signs of tissue loss when in contact with different donor treatment fragments. (B) Percent of touching O. faveolata recipient fragments showing a histopathological parameter, lytic necrosis (LN) when in contact with different donor treatment fragments.
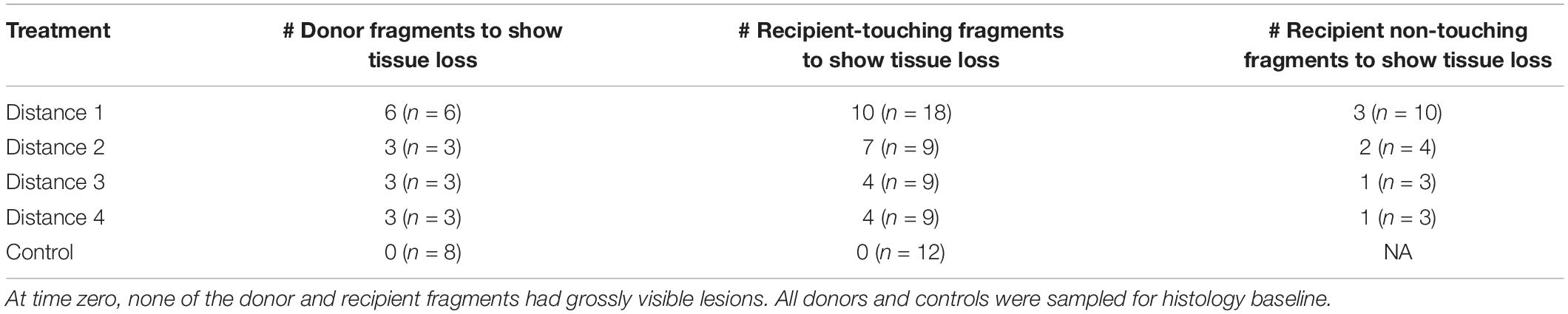
Table 6. Experimental design for the M. cavernosa to O. faveolata experiment with monitored lesion progression on donor and recipient fragments.
There was no significant difference in survival time when comparing O. faveolata touching recipient fragments among the different treatments (D1–D4). Ten out of 18 fragments exposed to D1 survived, five out of nine fragments exposed to D2 survived, nine out of nine fragments exposed to D3 survived, and six out of nine fragments exposed to D4 survived (Supplementary Figure 5, p = 0.29).
It took an average of 21.4 (±5.69) days, 21.7 (±3.70) days, 19 (± 6.65) days, and 32 (±2.65) days for initial signs of tissue loss to appear in fragments in contact with treatments D1, D2, D3, and D4, respectively. There was no significant difference in the average number of days for first signs of tissue loss to appear in touching fragments among the four distances (ANOVA, F3,21 = 0.72, p = 0.55).
Gross Appearance and Histopathology of Tissue Loss Within the Recipient Fragments
Histology samples were collected from a total of 19 diseased touching and two non-touching fragments, plus 9 paired control fragments. The histology samples were collected 5–55 days after the experiment began (Supplementary Table 5). Of the 19 diseased fragments, three were sampled once only, 10 were sampled twice, and six were sampled three times as tissue loss progressed on each fragment (Supplementary Table 5).
Grossly, recipient fragments exhibited tissue loss with a demarcated border with healthy tissue after exposure to a donor fragment (Figure 6C). Histologically, 16 (84.2%, n = 19) fragments with tissue-loss lesions were induced within all donor distance treatment fragments. A recipient O. faveolata fragment exposed to D1 exhibited characteristic LN as early as 7 days post experimental start (Figure 6 and Table 2). One of two non-touching recipient samples in a treatment aquarium exhibited a small putative LN lesion, but this could not be definitively confirmed (Table 2). Of the 16 fragments that exhibited hallmark LN, six had been exposed to the D1 fragments, five to D2, two to D3, and three to D4 donor fragments (Figure 8B). Over the course of the experiment, 12 fragments (75%, n = 16) with confirmed LN and progressive grossly visible tissue loss, exhibited complete mortality.
Twenty-two samples from recipient fragments had hypotrich ciliates in lesioned tissues (surrounded by a bacterial mass) that were exposed to D1 (n = 11), D2 (n = 4), D3 (n = 3), and D4 (n = 4) fragments over the course of the experiment. Ciliates were also detected in four control samples (aquarium #9, n = 3; aquarium #16, n = 1) as early as 13 days post experimental start, but they were found at the surface epidermis only and never extended into the deeper tissue (Table 2).
None of the control O. faveolata recipient fragments exhibited LN at the BBW gastrodermis or any other prominent pathological changes (Figure 7B). Note that two controls had Halofolliculina sp. infections on the skeleton as confirmed with a dissecting microscope at 49 and 54 days post experimental start. A total of 22 recipient samples exposed to D1, D2, and D4 treatments had Halofolliculina sp. as early as 32 days post experimental start (note that in the D3 treatment, lesions were noted earlier [day 9 through 27 days post experimental start; Table 2]); possibly a shorter time period than for ciliate infections to appear.
Experiment Comparison of M. cavernosa Fragments Isolated From a Diseased Donor Colony
Across both experiments, six out of the seven (85.7%) D2 M. cavernosa donor fragments exhibited gross signs of SCTLD, and five out of the seven (71.4%) D3 and D4 fragments exhibited signs of SCTLD (Supplementary Figure 6).
When reviewing data for both experiments combined, the correlation between the distance from the lesion border and time of tissue loss onset was not significant (Pearson’s correlation, coefficient = 0.82, p = 0.18). After collection from the field, it took an average of 12.5 (±2.64) days for initial signs of tissue loss to appear within D2 fragments, 9.8 (±3.61) days for D3 fragments, and 17.6 (±2.94) days for D4 fragments (Supplementary Figure 7).
Species Comparison
Probability of Induction and Survival of M. cavernosa and O. faveolata Recipient Fragments
There was no significant difference in the occurrence of tissue loss between M. cavernosa and O. faveolata touching and non-touching recipient fragments [Figures 4A, 8A, touching: Chi Square, X2(1, N = 78) = 1.40, p = 0.24; non-touching: Fisher’s Exact, p = 0.63]. There was no significant difference in survival when comparing M. cavernosa and O. faveolata touching recipient fragments. Thirty-nine out of 48 M. cavernosa fragments survived and 30 out of 45 O. faveolata fragments survived (Supplementary Figure 8, p = 0.29).
Disease Progression and Mortality of M. cavernosa and O. faveolata Touching Fragments
On average, there was approximately 7 days between initial disease appearance and 100% mortality of both M. cavernosa (n = 9) and O. faveolata (n = 14) recipient fragments. For the touching fragments, SCTLD progressed significantly faster in M. cavernosa recipient fragments (2.70 ± 0.35 cm2/day) compared with O. faveolata recipient fragments (1.32 ± 0.19 cm2/day) (Figure 9, ANOVA, F1,21 = 18.52, p < 0.01).
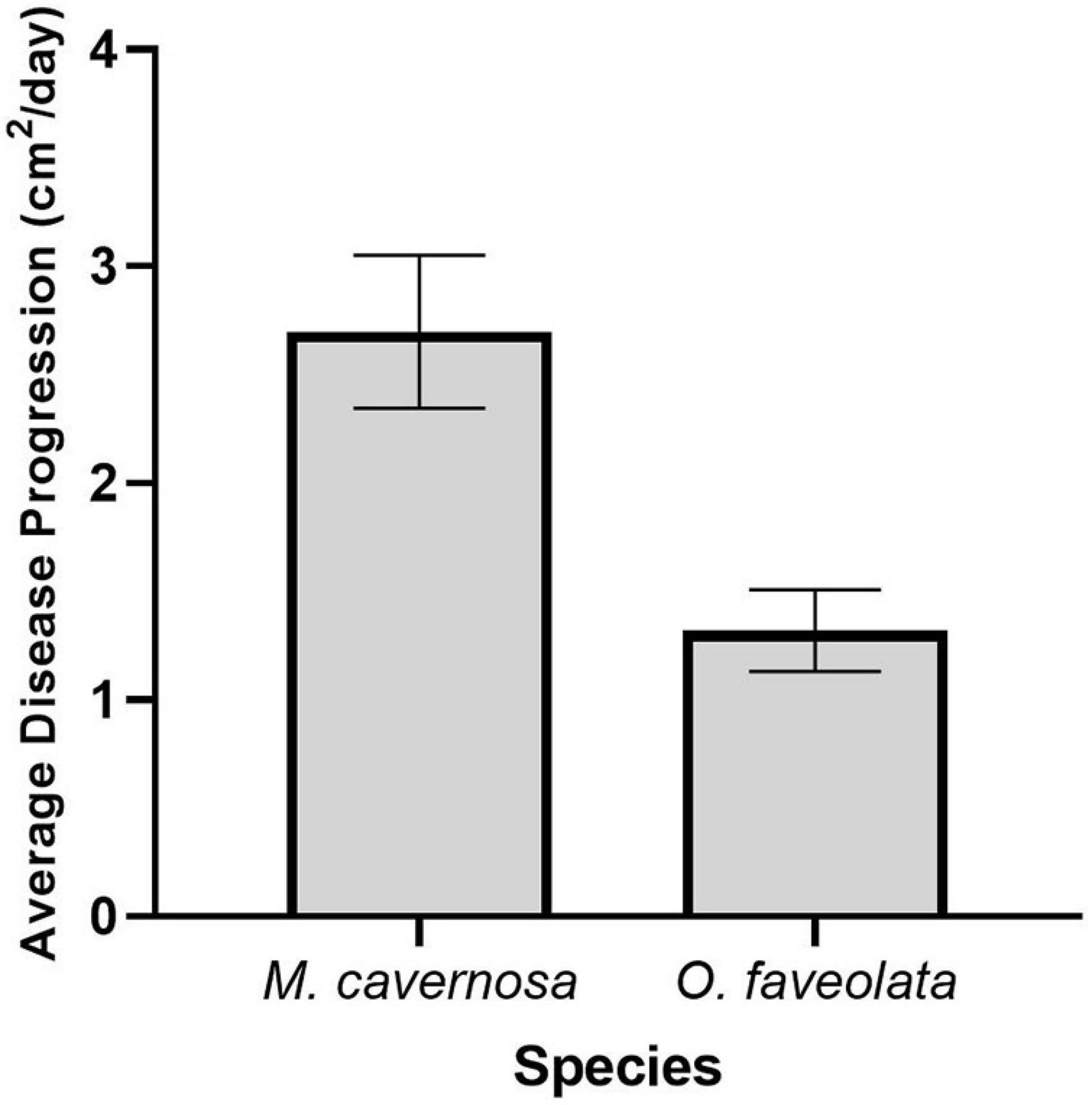
Figure 9. Disease progression in M. cavernosa (n = 9) and O. faveolata (n = 14) recipient fragments. This figure represents the average disease progression rate for each species. Measurements were taken at the halfway point between initial disease appearance and mortality, on average 3.5 days.
Discussion
Lesions Developed on Donor Coral Fragments Isolated From Tissue Loss
Results of this study indicate that tissue loss appears and progresses within isolated fragments even after their removal from the grossly visible lesioned area in diseased (subacute tissue loss) M. cavernosa colonies, approximately 30 cm in diameter. The likelihood of a donor fragment exhibiting tissue loss was not significantly different among the different donor disease treatment distances away from the active lesion, suggesting that SCTLD could be systemic within the colony. AH tissue in fragments taken as far as 20 cm from the grossly observable lesioned border area still became diseased even after fragment removal and isolation from the grossly visible diseased portion of the colony. It has been documented in the field that topical antibiotic applications are effective in halting SCTLD lesion progression (Aeby et al., 2019; Neely et al., 2020; Shilling et al., 2021); however, the effectiveness is likely to remain localized in the region of application (Neely et al., 2020), while new lesions can appear in other areas of the colony following treatment. Colony size may be a factor in lesion appearance among the different treatment distances. It is possible lesion appearance would not be observed grossly in the AH tissue directly adjacent to a subacute lesion within a larger colony, as new multifocal lesions typically appear throughout the colony with possible mortality occurring over several years in larger colonies of intermediately susceptible species (see Text Footnote 1). Additionally, the tissue could have already been diseased (sub-surface systemic lesions) upon collection but did not exhibit the grossly obvious appearance of SCTLD (active tissue loss and/or bleached tissue adjacent to the lesion border). Indeed, three donor colony samples showed LN within these sections of the colony at the time of collection and prior to experimentation. Results of Neely et al. (2020) and the present study suggest a colony-wide treatment may be necessary to effectively preserve remaining coral tissue. The present study suggest that SCTLD may be systemic within a 30 cm maximum diameter colony, but additional handling stress from transportation, fragmentation, or repeated sampling may have increased the susceptibility of the D2–D4 fragments to SCTLD in an experimental setting. Additionally, it is possible that tissue loss occurred in the D2–D4 fragments because there were other corals (i.e., recipient fragments) directly touching them. The D2–D4 fragments were already compromised before showing active tissue loss and the direct contact with recipient fragments could have exacerbated their condition. However, it is important to note that no control donor fragments experienced tissue loss in either experiment even though they were handled and treated similarly. Future studies should examine D2–D4 fragments in aquaria by themselves to assess whether tissue loss still occurs.
LN Was Observed Within Donor Coral Fragments and Recipient Fragments
In the present study, the successful SCTLD induction under controlled laboratory conditions was confirmed by histopathologic examinations for the presence of LN in the BBW (Landsberg et al., 2020) for recipient fragments in direct contact with donor fragments. LN was not confirmed in recipient fragments that did not have physical contact with a diseased fragment, although only a few of these samples were examined histologically. None of the recipient fragments exhibited tissue-loss lesions when donor treatments (D2–D4; i.e., at least 8 cm away from the grossly obvious SCTLD lesion) did not exhibit grossly visible SCTLD lesions. This suggests that it is necessary for the diseased donor fragments to exhibit grossly visible SCTLD lesions to induce disease in recipient fragments by physical contact. However, we did not evaluate by histological examination any recipient fragments that did not exhibit SCTLD lesions at the grossly visible level. Control recipient fragments were examined in parallel and showed LN histologically on two fragments (Table 2) but did not progress to SCTLD grossly visible lesions. Also, there were several recipient fragments that experienced disease induction but the active tissue loss stopped during the study. This may have been from a disruption to the disease etiology from sampling, as all of these recipient fragments were sampled at least once before the lesion stopped progressing.
The histological applications were a useful tool to couple with grossly visible tissue loss within this study, but it would have been useful to confirm the lack of LN in the BBW prior to the study beginning to ensure recipient corals were indeed healthy visibly and microscopically. However, histological processing involves time-consuming laborious work that includes decalcifying the skeleton. Histopathologic examination is a vital tool, but it may be important to develop more rapid, robust diagnostic tools for evaluating coral health status.
Induction of Tissue Loss Occurred on Recipient Fragments Touching D2–D4 Donor Corals
For both experiments, induction of tissue loss occurred on recipient fragments touching D2–D4 donor corals. However, the D2–D4 donor corals had to develop a grossly visible tissue-loss lesion in order for induction to occur. This has been documented in other transmission studies (Aeby et al., 2019; Meiling et al., 2021) — donor corals within these studies had a grossly visible tissue loss lesion when induction occurred in both touching and non-touching recipient fragments. However, this is the first study to document that the apparently healthy tissue adjacent to a grossly visible lesion can develop a lesion and ultimately induce disease in apparently healthy corals. Additionally, there was no significant difference in the average number of days prior to tissue-loss signs in recipient-touching fragments among those exposed to the different distances. Although tissue loss within recipient-touching fragments was observed less in further-distance exposures, on average it took the same amount of time for initial signs of tissue loss to appear within the recipient-touching fragments (after a grossly visible tissue-loss lesion appeared on the donor colony).
Touching Fragments Showed Similar Rates of Induction Compared With Non-touching Fragments
For both experiments, there was no significant difference in the gross appearance of tissue loss for touching and non-touching fragments. However, not all fragments were examined histologically for LN. Successful SCTLD induction was confirmed with histopathologic examinations by the presence of LN at the BBW of the gastrodermis only for touching recipient fragments. One non-touching O. faveolata recipient fragment was putatively confirmed with LN, but data were equivocal as the tissue on the histology section was minimal with only one small putative BBW LN lesion observed. A previous study by Aeby et al. (2019) indicated that 30% and 10% of M. cavernosa touching and non-touching fragments, respectively, exhibited tissue loss when placed in an aquarium with M. cavernosa colonies showing sub-acute tissue loss. In contrast, 100 and 60% of touching and non-touching M. cavernosa fragments, respectively, experienced tissue loss when exposed to Colpophyllia natans with acute tissue loss lesions. The present study showed that 39 and 10% of touching and non-touching M. cavernosa fragments and 56 and 35% of touching and non-touching O. faveolata fragments, respectively, developed tissue loss from diseased M. cavernosa. This supports that touching and non-touching fragments show similar rates of induction when exposed to diseased (subacute tissue loss) M. cavernosa.
Coinfection of Ciliates Was Observed Within the Study
Biotic factors possibly affecting the controlled laboratory experiments also need to be considered. It is interesting to note that there were only two cases of ciliate infections in the M. cavernosa to M. cavernosa experiment, but 26 cases (including 4 controls) were found in the M. cavernosa to O. faveolata study. Halofolliculina sp. was first detected at 32 days post experimental start for both experimental trials. This suggests that the Halofolliculina sp. propagated during the test period after 32 days and probably O. faveolata is more susceptible or weakened by experimental conditions compared to M. cavernosa. It may be possible that the M. cavernosa to O. faveolata study was affected by ciliate infections. Additionally, tissue loss occurred similarly for all distances in O. faveolata, and there was no significant difference in the amount of time for first signs of tissue loss to appear in touching fragments among the four distances. These results suggest that the visual signs and etiology (i.e., disease ecology) of the new lesions that appeared on the distances away from the disease lesion were comparable to the active lesion on coral collections. However, artificial laboratory conditions might have encouraged the proliferation of a ciliate coinfection that would have altered the probability of disease occurrence and rates of progression.
Orbicella faveolata Was More Susceptible to the Induction of Tissue Loss
Our results indicate that tissue loss occurred more often in O. faveolata compared to M. cavernosa, suggesting O. faveolata was more susceptible to SCTLD. Similar results have been documented in other SCTLD transmission studies (Aeby et al., 2019; Meiling et al., 2021); however, we did not detect any statistically significant differences between species in the present study. It is possible that concurrent infections by other organisms as discussed above may influence disease susceptibility among species. Also, different SCTLD pathogens or strains could affect M. cavernosa, which, in turn, could influence the susceptibility of O. faveolata to disease induction. We do acknowledge that the lack of diseased O. faveolata was a limitation for this study. However, this was unavoidable because there were no such colonies available in the field at the time of collection. Future studies should include an O. faveolata-O. faveolata trial to account for potentially different disease etiology between these two coral species. Additionally, it is important to note that these induction experiments were completed in an indoor controlled laboratory setting with artificial light conditions, and corals were fragmented immediately prior to exposure. Aeby et al. (2019) and Meiling et al. (2021) took place in outdoor systems, and Meiling et al. (2021) allowed for a 1-week acclimation period after fragging. Both of these studies showed Orbicella spp. had a greater susceptibility to SCTLD than M. cavernosa, similar to the present study. Regardless, results may differ under the influence of environmental factors that corals would encounter under natural conditions.
Montastraea cavernosa Tissue Loss Progressed Faster Than Orbicella faveolata
On average there was the same amount of time between initial disease appearance and complete mortality of both M. cavernosa and O. faveolata recipient-touching fragments. However, disease progressed significantly faster in M. cavernosa recipient-touching fragments compared with O. faveolata recipient-touching fragments. Interestingly, O. faveolata was more susceptible to SCTLD induction, but once disease was initiated, M. cavernosa lost tissue at a faster rate. These results have been observed in other studies. For example, Aeby et al. (2019) documented that M. cavernosa fragments touching diseased M. cavernosa developed lesions over a 2–6-days period, whereas O. faveolata developed lesions over a 2–12 days period. Meiling et al. (2020) measured absolute areal tissue loss rates in M. cavernosa and Orbicella annularis; and documented that M. cavernosa had a significantly higher rate of tissue loss when compared to O. annularis. This study observed average tissue loss of ∼7 cm2/day in M. cavernosa, and ∼2 cm2/day in O. faveolata, which is comparative to the present study (∼3 cm2/day in M. cavernosa, and ∼1.5 cm2/day in O. faveolata). Additionally, it is important to compare the disease induction susceptibility and lesion progression with species that are not considered to have intermediate susceptibility (i.e., high susceptibility and low susceptibility). Aeby et al. (2019) documented no disease induction when exposing a species with low susceptibility, Porites astreoides, to diseased (subacute tissue loss) M. cavernosa. They also documented 100% disease induction when exposing a species with high susceptibility, Meandrina meandrites, to a diseased coral; although the diseased coral was a different species with an acute tissue loss lesion.
Conclusion
The present study showed that AH tissue adjacent to the subacute lesion can exhibit tissue loss and induce disease in other AH coral fragments even after isolation from the original tissue-loss lesion. Histologically, initial tissue-loss lesions in both species exhibited characteristic LN at the BBW gastrodermis—a histopathological hallmark of SCTLD. Induction of SCTLD under controlled laboratory conditions was successful when recipient fragments were in physical contact with diseased donors. On average O. faveolata showed higher rates of induction compared with M. cavernosa, but the disease progressed at a faster rate in M. cavernosa recipient fragments compared to O. faveolata recipient fragments. Results for non-touching fragments were equivocal and we could not support or refute the potential for some type of waterborne disease induction process. This work contributes to the growing knowledge of SCTLD induction dynamics and highlights the potential for systemic disease within small-sized colonies. This study further identifies the need for additional multi-disciplinary research to gain a better understanding of SCTLD induction dynamics and lesion progression rates within species that are susceptible to the SCTLD outbreak.
Data Availability Statement
The raw data supporting the conclusions of this article will be made available by the authors, without undue reservation, to any qualified researcher.
Author Contributions
All authors designed the experiments. JL secured funding. Field collections were conducted by KE and mote colleagues. KE and EM set up and executed both transmission experiments and collected samples for histology. YK prepared samples for histology. JL, YK, and EP evaluated histology slides and interpreted histopathology. KE, JL, YK, and EM drafted the manuscript. All authors contributed to the writing of the manuscript.
Conflict of Interest
The authors declare that the research was conducted in the absence of any commercial or financial relationships that could be construed as a potential conflict of interest.
Publisher’s Note
All claims expressed in this article are solely those of the authors and do not necessarily represent those of their affiliated organizations, or those of the publisher, the editors and the reviewers. Any product that may be evaluated in this article, or claim that may be made by its manufacturer, is not guaranteed or endorsed by the publisher.
Funding
Funding for this study was provided by the United States Fish and Wildlife Service through the State Wildlife Grants program (award # FL-T-F18AF00492) awarded to JL at the Florida Fish and Wildlife Conservation Commission (FWC).
Acknowledgments
We would like to acknowledge Erich Bartels, Cory Walter, and Joe Keuhl for assistance with diseased and AH coral collections. We would also like to thank participating interns Nathan Martin, Kari Imhof, Bryce Corbett, Heidi Tate, Reagan Mason, Frannie Lach, Alex Macadam, Emma Martin, and Alec Lemus for assistance with the lab-based induction experiments. Noretta Perry, Michelle Franco, Yvonne Waters, and Patrick Wilson, FWRI-FWC conducted histology. Coral collections were completed under permit # FKNMS-2019-011.
Supplementary Material
The Supplementary Material for this article can be found online at: https://www.frontiersin.org/articles/10.3389/fmars.2021.717265/full#supplementary-material
Footnote
- ^ https://floridadep.gov/sites/default/files/Copy%20of%20StonyCoralTissueLossDisease_CaseDefinition%20final%2010022018.pdf
References
Abràmoff, M. D., Magalhães, P. J., and Ram, S. J. (2004). Image processing with image. J. Biophotonics Int. 11, 36–41. doi: 10.1201/9781420005615.ax4
Aeby, G. S., Ushijima, B., Campbell, J. E., Jones, S., Williams, G. J., Meyer, J. L., et al. (2019). Pathogenesis of a tissue loss disease affecting multiple species of corals along the Florida reef tract. Front. Mar. Sci. 6:678. doi: 10.3389/fmars.2019.00678
Alvarez-Filip, L., Estrada-Saldívar, N., Pérez-Cervantes, E., Molina-Hernández, A., and González-Barrios, F. J. (2019). A rapid spread of the stony coral tissue loss disease outbreak in the Mexican Caribbean. PeerJ 7:e8069. doi: 10.7717/peerj.8069
Benesty, J., Chen, J., Huang, Y., and Cohen, I. (2009). “Pearson correlation coefficient”, in Noise Reduction in Speech Processing, (Berlin, Germany: Springer), 1–4. doi: 10.1007/978-3-642-00296-0_5
Conover, W. J., and Iman, R. L. (1981). Rank transformations as a bridge between parametric and nonparametric statistics. Am. Statist. 35, 124–129. doi: 10.1080/00031305.1981.10479327
Estrada-Saldívar, N., Molina-Hernández, A., Pérez-Cervantes, E., Medellín-Maldonado, F., González-Barrios, F. J., and Alvarez-Filip, L. (2020). Reef-scale impacts of the stony coral tissue loss disease outbreak. Coral Reefs 39, 861–866. doi: 10.1007/s00338-020-01949-z
Fisher, R. A. (1934). Statistical Methods for Research Workers, 5th Edn. Edinburgh: Oliver and Boyd.
Landsberg, J. H., Kiryu, Y., Peters, E. C., Wilson, P. W., Perry, N., Waters, Y., et al. (2020). Stony coral tissue loss disease in Florida is associated with disruption of host–zooxanthellae physiology. Front. Mar. Sci. 7:576013. doi: 10.3389/fmars.2020.576013
Meiling, S., Muller, E. M., Lasseigne, D., Rossin, A., Veglia, A. J., MacKnight, N., et al. (2021). Variable species responses to experimental stony coral tissue loss disease (SCTLD) exposure. Front. Mar. Sci. 8:670829. doi: 10.3389/fmars.2021.670829
Meiling, S., Muller, E. M., Smith, T. B., and Brandt, M. E. (2020). 3D photogrammetry reveals dynamics of stony coral tissue loss disease (SCTLD) lesion progression across a thermal stress event. Front. Mar. Sci. 7:597643. doi: 10.3389/fmars.2020.597643
Meyer, J. L., Castellanos-Gell, J., Aeby, G. S., Häse, C. C., Ushijima, B., and Paul, V. J. (2019). Microbial community shifts associated with the ongoing stony coral tissue loss disease outbreak on the Florida reef tract. Front. Microbiol. 10:2244. doi: 10.3389/fmicb.2019.02244
Miller, M. W., Karazsia, J., Groves, C. E., Griffin, S., Moore, T., Wilber, P., et al. (2016). Detecting sedimentation impacts to coral reefs resulting from dredging the Port of Miami, Florida USA. PeerJ 4:e2711. doi: 10.7717/peerj.2711
Muller, E. M., Sartor, C., Alcaraz, N. I., and van Woesik, R. (2020). Spatial epidemiology of the stony-coral-tissue-loss disease in Florida. Front. Mar. Sci. 7:163. doi: 10.3389/fmars.2020.00163
Neely, K. L., Macaulay, K. A., Hower, E. K., and Dobler, M. A. (2020). Effectiveness of topical antibiotics in treating corals affected by Stony Coral Tissue Loss Disease. PeerJ 8, e9289. doi: 10.7717/peerj.9289
Pearson, K. (1900). X. On the criterion that a given system of deviations from the probable in the case of a correlated system of variables is such that it can be reasonably supposed to have arisen from random sampling. Lond. Edinb. Dubl. Phil. Mag. J. Sci. 50, 157–175. doi: 10.1080/14786440009463897
Precht, W. F., Gintert, B. E., Robbart, M. L., Fura, R., and Van Woesik, R. (2016). Unprecedented disease-related coral mortality in southeastern Florida. Sci. Rep. 6:31374. doi: 10.1038/srep31374
Quintero-Hunter, I., Grier, H., and Muscato, M. (1991). Enhancement of histological detail using metanil yellow as a counter-stain in periodic acid Schiff’s hematoxylin staining of glycol methacrylate tissue sections. Biotech. Histochem. 66, 169–172. doi: 10.3109/10520299109109964
R Core Team (2020). R: A Language and Environment for Statistical Computing. Vienna: R Foundation for Statistical Computing.
Rosales, S. M., Clark, A. S., Huebner, L. K., Ruzicka, R. R., and Muller, E. M. (2020). Rhodobacterales and Rhizobiales are associated with stony coral tissue loss disease and its suspected sources of transmission. Front. Microbiol. 11:681. doi: 10.3389/fmicb.2020.00681
Sharp, W. C., Shea, C. P., Maxwell, K. E., Muller, E. M., and Hunt, J. H. (2020). Evaluating the small-scale epidemiology of the stony-coral-tissue-loss-disease in the middle Florida Keys. PloS One 15:e0241871. doi: 10.1371/journal.pone.0241871
Shilling, E. N., Combs, I. R., and Voss, J. D. (2021). Assessing the effectiveness of two intervention methods for stony coral tissue loss disease on Montastraea cavernosa. Sci. Rep. 11:8566. doi: 10.1038/s41598-021-86926-4
Ushijima, B., Meyer, J. L., Thompson, S., Pitts, K., Marusich, M. F., Tittl, J., et al. (2020). Disease diagnostics and potential coinfections by Vibrio coralliilyticus during an ongoing coral disease outbreak in Florida. Front. Microbiol. 11:569354. doi: 10.3389/fmicb.2020.569354
Verde, A., Bastidas, C., and Croquer, A. (2016). Tissue mortality by Caribbean ciliate infection and white band disease in three reef-building coral species. PeerJ 4:e2196. doi: 10.7717/peerj.2196
Walton, C. J., Hayes, N. K., and Gilliam, D. S. (2018). Impacts of a regional, multi-year, multi-species coral disease outbreak in southeast Florida. Front. Mar. Sci. 5:323. doi: 10.3389/fmars.2018.00323
Weil, E., Hernández-Delgado, E. A., Gonzalez, M., Williams, S., Suleimán-Ramos, S., Figuerola, M., et al. (2019). Spread of the new coral disease “SCTLD” into the Caribbean: implications for Puerto Rico. Reef Encounter 34, 38–43.
Keywords: stony coral tissue loss disease, disease induction, Orbicella faveolata, Montastraea cavernosa, Florida’s coral reef
Citation: Eaton KR, Landsberg JH, Kiryu Y, Peters EC and Muller EM (2021) Measuring Stony Coral Tissue Loss Disease Induction and Lesion Progression Within Two Intermediately Susceptible Species, Montastraea cavernosa and Orbicella faveolata. Front. Mar. Sci. 8:717265. doi: 10.3389/fmars.2021.717265
Received: 30 May 2021; Accepted: 20 August 2021;
Published: 08 September 2021.
Edited by:
Sarah Annalise Gignoux-Wolfsohn, Smithsonian Environmental Research Center (SI), United StatesReviewed by:
Joshua Patterson, University of Florida, United StatesAdán Guillermo Jordán-Garza, Universidad Veracruzana, Mexico
Copyright © 2021 Eaton, Landsberg, Kiryu, Peters and Muller. This is an open-access article distributed under the terms of the Creative Commons Attribution License (CC BY). The use, distribution or reproduction in other forums is permitted, provided the original author(s) and the copyright owner(s) are credited and that the original publication in this journal is cited, in accordance with accepted academic practice. No use, distribution or reproduction is permitted which does not comply with these terms.
*Correspondence: Katherine R. Eaton, a2VhdG9uQG1vdGUub3Jn