- 1Graduate School of Oceanography, University of Rhode Island, Narragansett, RI, United States
- 2Center for Coastal Studies, Provincetown, MA, United States
- 3FitzPatrick Institute of African Ornithology, DST-NRF Centre of Excellence, University of Cape Town, Cape Town, South Africa
- 4Stellwagen Bank National Marine Sanctuary, National Oceanic and Atmospheric Administration, Scituate, MA, United States
- 5Woods Hole Oceanographic Institution, Woods Hole, MA, United States
- 6Center for Marine Sciences and Technology, North Carolina State University, Morehead City, NC, United States
- 7Waterbirds and Sea Turtles Laboratory, Universidade Federal do Rio Grande - FURG, Rio Grande, Brazil
- 8Northeast Fisheries Science Center, NOAA Fisheries, National Oceanic and Atmospheric Administration, Woods Hole, MA, United States
- 9Conservation Department, Government of Tristan da Cunha, Edinburgh of the Seven Seas, Tristan da Cunha, United Kingdom
Limited work to date has examined plastic ingestion in highly migratory seabirds like Great Shearwaters (Ardenna gravis) across their entire migratory range. We examined 217 Great Shearwaters obtained from 2008–2019 at multiple locations spanning their yearly migration cycle across the Northwest and South Atlantic to assess accumulation of ingested plastic as well as trends over time and between locations. A total of 2328 plastic fragments were documented in the ventriculus portion of the gastrointestinal tract, with an average of 9 plastic fragments per bird. The mass, count, and frequency of plastic occurrence (FO) varied by location, with higher plastic burdens but lower FO in South Atlantic adults and chicks from the breeding colonies. No fragments of the same size or morphology were found in the primary forage fish prey, the Sand Lance (Ammodytes spp., n = 202) that supports Great Shearwaters in Massachusetts Bay, United States, suggesting the birds directly ingest the bulk of their plastic loads rather than accumulating via trophic transfer. Fourier-transform infrared spectroscopy indicated that low- and high-density polyethylene were the most common polymers ingested, within all years and locations. Individuals from the South Atlantic contained a higher proportion of larger plastic items and fragments compared to analogous life stages in the NW Atlantic, possibly due to increased use of remote, pelagic areas subject to reduced inputs of smaller, more diverse, and potentially less buoyant plastics found adjacent to coastal margins. Different signatures of polymer type, size, and category between similar life stages at different locations suggests rapid turnover of ingested plastics commensurate with migratory stage and location, though more empirical evidence is needed to ground-truth this hypothesis. This work is the first to comprehensively measure the accumulation of ingested plastics by Great Shearwaters over the last decade and across multiple locations spanning their yearly trans-equatorial migration cycle and underscores their utility as sentinels of plastic pollution in Atlantic ecosystems.
Introduction
An estimated 5 to 125 trillion pieces of plastic greater than 100 μm in size are buoyant within the ocean surface layer, where these items are subject to oceanic scale circulation and varied biophysical interactions (Eriksen et al., 2014; Lindeque et al., 2020). The abundance and ready transport of small plastic pieces within ocean surface environments results in an increased probability of encounter between epipelagic marine fauna and plastic items, with subsequent opportunity for ingestion of plastic items. Ingestion of plastic may occur via direct ingestion of plastic pieces (Savoca et al., 2016), or ingestion of prey items containing plastic (Nelms et al., 2018). In vertebrate species, ingested plastics can result in impaction or perforations within the digestive tract (Pierce et al., 2004), reduced feeding due to false satiation (Santos et al., 2020), potential uptake of adsorbed chemical pollutants or innate chemical additives (Tanaka et al., 2015; Koelmans et al., 2016), and as-of-yet poorly characterized sublethal impacts on individual health and fitness (Roman et al., 2019c; Senko et al., 2020).
The accumulation of ingested plastics in seabirds has been documented since the 1960s (Kenyon and Kridler, 1969). Meta-analysis efforts conducted by Wilcox et al. (2015) found that 59% of seabird species examined between 1962 and 2012 had ingested plastic, and, within those studies, on average 29% of individuals had plastic in their gastrointestinal tract (Ryan et al., 2009; Wilcox et al., 2015). Seabirds in the order Procellariiformes (e.g., albatrosses, shearwaters, petrels, and storm-petrels) are thought to be most vulnerable to plastic ingestion (Moser and Lee, 1992; Provencher et al., 2014) related to their wide-ranging pelagic habits (Davies et al., 2010) and substantial use of olfactory and visual cues during foraging (Nevitt, 2008; Nishizawa et al., 2021), yet the frequency of plastic ingestion varies widely among members of this order. Some albatross species have been shown to infrequently ingest plastics or retain ingested plastics on shorter time-scales due to particular gut morphology that facilitates ready regurgitation of indigestible plastics (Furness, 1985; Ryan et al., 2016). In contrast, numerous research efforts spanning multiple ocean basins suggest that Ardenna and Calonectris shearwaters frequently ingest plastic. For example, ingested plastics were found in 65–90% of adult or juvenile Short-tailed Shearwaters (Ardenna tenuirostris) collected in Eastern Australia in 2010–2013 (Acampora et al., 2014; Roman et al., 2016). Similarly, 90% of Flesh-footed Shearwater (Ardenna carneipes) fledglings sampled in 2011 on Lord Howe Island in eastern Australia contained ingested plastics, with a mean plastic count of 18 items and a maximum count of 276 items in one individual (Lavers et al., 2014). In the Atlantic basin, Great Shearwaters (Ardenna gravis) have been found to ingest plastic at high rates for decades, with multiple studies documenting 70–95% ingestion rates by the species, sampled within their breeding or non-breeding ranges (Furness, 1983, 1985; Ryan, 1987a,b, 2008; Bond et al., 2014). However, several studies have noted a lower frequency of plastic ingestion in this species, ranging from 0–39% frequency of ingestion (Brown, 1981; Ryan, 2008; Haman et al., 2013; Bond et al., 2014).
Rates of plastic ingestion and subsequent accumulation are thought to vary based on a suite of interacting factors, including gut morphology, life stage, foraging strategy, and geographic location. In procellariiform seabirds, the isthmus connecting the proventriculus and ventriculus is angled and narrow, unlike the wider, more linear junctions in other seabirds (Furness, 1985; Ryan and Jackson, 1987). This unique configuration is thought to restrict the passage of materials from the ventriculus back into the proventriculus, leading to an increased proclivity to accumulate ingested plastics (Furness, 1985). Foraging strategy is also a primary driver of plastic ingestion, with trophic generalists and surface seizing birds reliant on crustacean diets considered the most vulnerable (Day et al., 1985; Roman et al., 2019a; Ryan, 1987a,2019). The incidence of plastic ingestion is also thought to increase with abundance of plastics in the environment and use of regions considered plastic hotspots such as subtropical gyres and the southern boundary of the Atlantic, Pacific, and Indian Oceans (Ryan et al., 2009; Van Franeker and Law, 2015; Wilcox et al., 2015). For example, Northern Fulmars (Fulmarus glacialis) found dead on beaches around the North Sea have been monitored for plastic ingestion since the 1970s. The ingestion of industrial pellets by fulmars has roughly tracked the measured abundance of industrial pellets in ocean surface water over time (Van Franeker and Law, 2015).
Despite a rapid increase in the number of published studies focused on plastic pollution in shearwaters and other seabirds over recent years, data gaps remain surrounding the dynamics of seabird plastic ingestion as influenced by migration, life stage, and polymer makeup. Existing studies often focus on samples collected from species of convenience or highly monitored species during the breeding season when chicks are stationary and adults are central place foragers (Rayner et al., 2010), though sample collection mechanisms employed in breeding colony studies vary widely (e.g., birds killed by fisheries, beached birds, unsuccessful fledglings, remains in predator regurgitations) (Provencher et al., 2019; Ryan, 2019).
The Great Shearwater is a trans-hemispheric migratory seabird, endemic to the Atlantic Ocean. Monitoring of this species provides an opportunity to assess and interrogate the ecological occurrence and impacts of plastic in the Atlantic; this stands as a continued need, given the projected increases in plastic leakage into the environment. Plastic assessment in this species is readily achievable and valuable due to their abundance, well-described habitat use, co-location with key marine megafauna, and status as an ecological indicator related to their compact representation of temperate and subtropical Atlantic regions (Cuthbert, 2005; Powers et al., 2017, 2020; Silva et al., 2020). They are also frequently bycaught across their migratory range, resulting in plentiful sample collection opportunities.
The accumulation of ingested plastics has been previously assessed in this seabird at discrete locations across its breeding and non-breeding range over the past 60 years, via studies covering a single year, or a span up to 14 years including variable numbers of individuals (n = 1 to 86) (Furness, 1985; Ryan, 1987a,b; Moser and Lee, 1992; Haman et al., 2013; Bond et al., 2014; González-Carman et al., 2021). The most recent monitoring effort focused on this species examined 84 individuals collected between 2000–2011 from Sable Island, Nova Scotia, Canada in the NW Atlantic, but did not include any formal time trend analysis due to highly variable yearly sample sizes (n = 0–25/year) (Bond et al., 2014). Alternatively, Ryan (2008) compared plastic in Great Shearwaters sampled in the South Atlantic from 1983–1985 (n = 33) and 2005–2006 (n = 53) and found major shifts in the composition but not the total amount of ingested plastics in this species, with decreased abundance of virgin industrial pellets over time (Ryan, 2008), similar to results observed in Short-tailed Shearwaters over time (Vlietstra and Parga, 2002). No efforts focused on this species have yet described the polymer signature of ingested plastics, the influence of life stage on plastic ingestion, trends of ingested plastics over the annual migratory cycle of this highly mobile marine species, or time trend analysis over the last decade.
Here we seek to remedy these data gaps, and comprehensively describe plastic ingestion in Great Shearwaters, using bycatch and beach-cast adult and juvenile samples obtained over 2008–2019 from multiple regions frequented in the annual cycle of this trans-equatorial migrant. The goals of this study were to (a) deduce temporal trends of ingested plastics, (b) compare plastic categories and polymer types found among different locations, age classes, and over time, and (c) explore the trophic or environmental origin of ingested plastics.
Materials and Methods
Study Species
The Great Shearwater is a long-lived, pelagic seabird that feeds on squid, crustaceans, and small forage fish including Sand Lance (Ammodytes spp.), and has regularly been observed attending fishing vessels seeking fisheries offal (Bugoni et al., 2008, 2010; Powers et al., 2020). Most foraging activities occur within the top 10 m of the water column via surface plunging and seizing behaviors, with most dives less than 2 m in depth (Ronconi et al., 2010). This species breeds during the boreal winter in the South Atlantic, on Gough Island, Inaccessible Island, and Nightingale Island in the Tristan da Cunha Island group and Kidney Island in the Falkland Islands (Cuthbert, 2005). Tracking data suggest use of large pelagic regions of the temperate South Atlantic between South Africa and South America during the breeding season (Ronconi et al., 2018; Schoombie et al., 2018). Great Shearwaters inhabit the NW Atlantic during their non-breeding period over the boreal summer; tracking and necropsy data suggest some degree of age-specific segregation in the Gulf of Maine and along NE Canada, with preferential use of Massachusetts Bay by juveniles (Powers et al., 2017, 2020).
Specimen Collection, Necropsy, and Sample Condition
A total of 217 Great Shearwaters were analyzed for plastics, from six locations including sites within breeding/wintering areas as well as places along migratory pathways (Figure 1). Samples obtained included both bycatch and beach-cast individuals, procured through a variety of collection and monitoring programs. Most individuals were frozen individually as a whole specimen upon collection and stored at −20°C until necropsy, while the chicks from Nightingale Island were killed, processed for edible parts, and stomach contents retained frozen for further plastic analysis. Supplementary Tables 1, 2 summarize the sample set and collection locations included in this study.
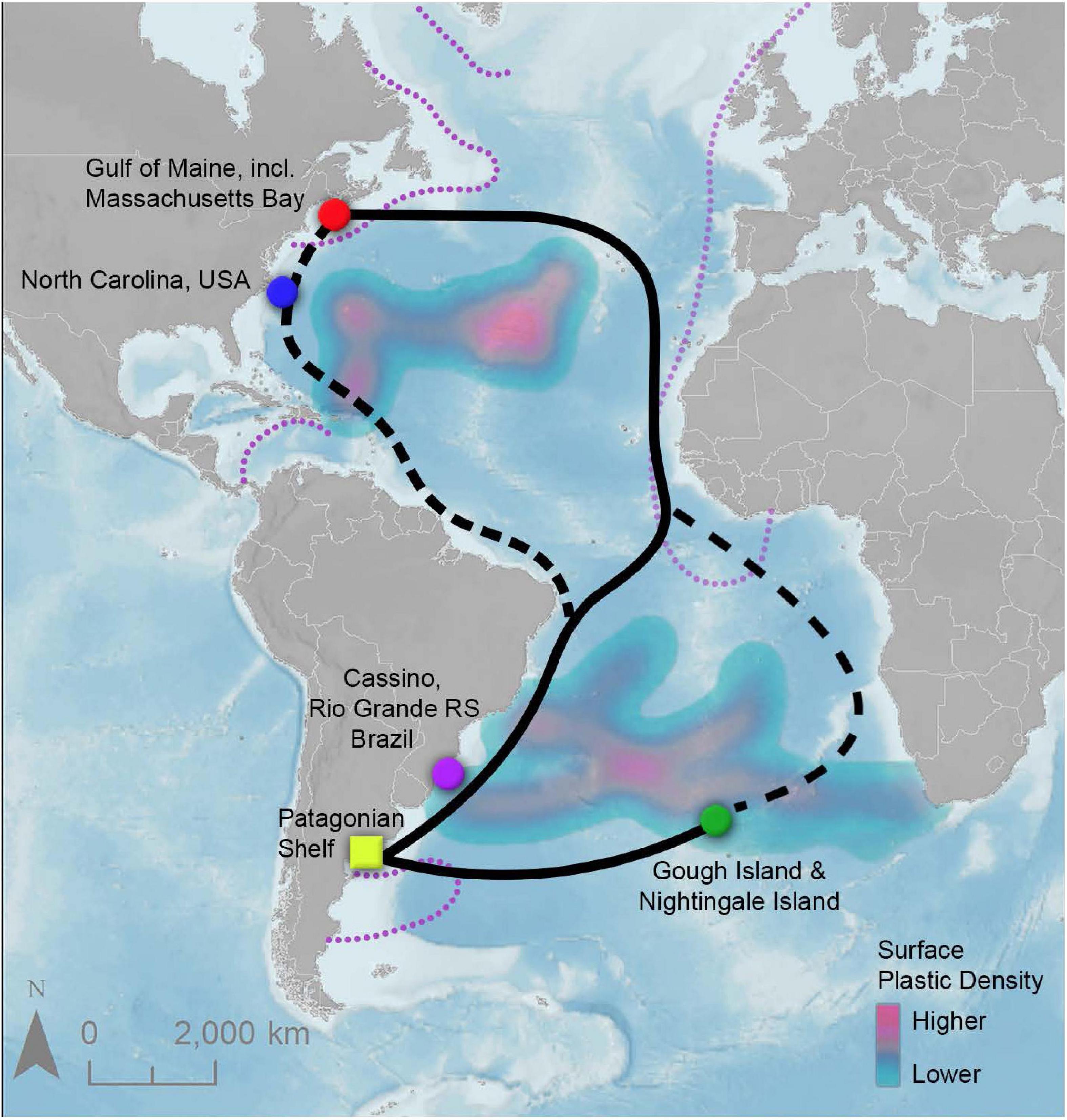
Figure 1. Map of Great Shearwater migratory routes (black and dashed lines) and sample collection locations (marked by colored circles). Solid lines are considered the dominant migratory route based on tracking data. No samples were collected off the Patagonian Shelf (marked by the yellow square), but this location is heavily used by both adult and juvenile Great Shearwaters as a staging and feather molt location52 surrounding trans-equatorial migration. Purple dashed lines indicate areas where fishing activity may contribute plastics to the environment. Surface plastic density data is derived from a compilation of literature sources curated by the NOAA Marine Debris Program, freely available online.
Most individuals were necropsied in a standardized manner, including measurements of morphometric characteristics, body condition, and organ mass (Van Franeker, 2004). Clinical necropsies were undertaken using pre-cleaned metal tools on stainless steel tables to minimize risk of environmental contamination. Age was determined following the age characterization of Great Shearwaters as defined previously considering gonad size and color, molt status, and presence of bursa of Fabricius (Supplementary Table 3), while sex determination was solely based upon gonad presentation (Powers et al., 2020). The entire gastrointestinal tract (GIT) was collected, wired shut using metal wire, and frozen at −15°C until analysis. The stomach contents of chicks from Nightingale Island were obtained without clinical necropsy of associated individuals as part of harvesting activities. Supplementary Table 2 summarizes data collection efforts associated with each set of birds.
Analysis of Accumulated, Ingested Plastics
Food debris and/or seawater were commonly found in the esophagus and mouth of sampled bycatch individuals, due to regurgitation during entanglement and drowning. Given the heavy reliance on bycatch samples in this study (155 of 217), the esophagus and proventriculus were not assessed for plastic content, assuming any assessment of plastic occurrence in these compartments was compromised by regurgitation. Plastics were instead only assessed in the ventriculus, or “gizzard,” a muscular organ used to grind up indigestible, hard diet materials. The angled and narrow isthmus between the ventriculus and proventriculus likely insulates measurements obtained from this organ from bias related to regurgitation. Plastics were assessed in both the ventriculus and proventriculus of 10 individuals with visually full stomachs to test the validity of this assumption. Results suggested our approach was appropriate, as >95% of accumulated plastics occurred in the ventriculus of bycatch birds; this is further supported in the literature (Ryan, 2015a). However, we note that by entirely omitting assessment of plastics in the proventriculus, we are potentially truncating the maximum count and mass of plastics found in the most heavily polluted individuals, given plastic is usually only in the proventriculus when the ventriculus is full, or the item is too large to pass into the ventriculus. Therefore, the maximum number of plastics observed in the individuals with higher plastic load herein may be underestimated. We also note that migration of plastics from the gizzard back into the proventriculus may be possible to some limited degree, as Northern Fulmars in rehabilitation have been documented to spontaneously regurgitate plastic, presumably from the gizzard, though lavage and reflexive regurgitation as a result of handling have not caused plastic or natural item offloading from the gizzard in Great Shearwaters and fulmars (Ryan, 1988; Terepocki et al., 2017).
Plastics were obtained from the ventriculus as previously described (Van Franeker and Meijboom, 2002; Van Franeker, 2004; Van Franeker and Law, 2015). Briefly, the organ was cut open using a solvent-cleaned metal scalpel and flushed thoroughly using deionized water over a metal sieve with 1 mm mesh. Plastic items were visually separated from natural items with the aid of a dissection microscope, magnifying lenses, and/or density separation techniques as required (Van Franeker and Meijboom, 2002; Provencher et al., 2017), and collected in solvent-cleaned glassware. Samples were dried in a clean and plastic-free fume hood for ∼2 days. Personnel wore cotton lab coats during sample preparation and analysis to avoid fiber contamination. Plastic items from chicks harvested for human consumption were obtained by Tristan Conservation staff with appropriate training. Items were cleaned with ethanol and shipped to the University of Rhode Island for measurements and Fourier transform infrared spectroscopy (FTIR) analysis with the rest of collected items. Among chicks, 26 of 29 that were identified to contain plastic were included in plastic characterization and FTIR analysis.
Plastic material was visually sorted into qualitative categories by two trained individuals, as outlined elsewhere (Van Franeker and Meijboom, 2002; Provencher et al., 2017). Each suspected plastic item was first classified as an industrial (nurdle or pellet) or user plastic (non-industrial items likely sourced from consumer use). User plastics were further differentiated into five subcategories including flexible sheet-like plastics (e.g., bag and film), thread-like plastics (e.g., microfibers, rope), foam (e.g., polystyrene), fragments (unknown hard plastics), and other (e.g., balloon, rubber, and melted plastic). Each item was weighed to the nearest 0.0001 g using an analytical balance, and length, width, and thickness measured to the nearest 0.01 mm using a calibrated electronic caliper at the largest extent of each dimension. Soft amorphous plastics (e.g. balloons, melted/liquified rubbers, and sheets) were weighed but dimensions were not measured. Each item was also classified into a size category, with microplastics defined as pieces 1–5 mm in their longest dimension, mesoplastics including pieces 5–25 mm, and macroplastics including items >25 mm (GESAMP, 2015). Plastic mass was compared to ecological quality objectives set for Northern Fulmars by the Convention for the Protection of the Marine Environment of the North-East Atlantic. This threshold, though specific to Northern Fulmars, suggests ecological integrity is sufficiently met when no more than 10% of a sample set containing >50 individuals contains a mass of plastic greater than 0.1 g or 100 mg (OSPAR Commission, 2008). Average mass and item size was calculated only based on those individuals containing plastic items. Length and width are reported instead of surface area given the irregular shape of most plastic items.
Plastics were visually assessed in the primary forage fish prey of Great Shearwaters as part of a larger diet analysis as described elsewhere (Suca et al., 2021); briefly, the stomach contents of 202 Sand Lance (Ammodytes spp.) were examined for prey species identification and visually apparent plastic items using a Leica 205C stereomicroscope.
Polymer Analysis
In total, 91% of items visually identified as plastic were processed for polymer identity; 207 items were omitted due to cost and COVID-19 limitations. Prior to analysis, each item was cleaned with deionized water and/or ethanol to remove biological debris and filed with a metal file. Items were scanned through the Center for Coastal Studies’ Thermo Scientific Nicolet TM iS5 FTIR spectrometer with an attenuated total reflection (ATR) accessory, using the Thermo Scientific Omnic software (Thermo Fisher Scientific, Waltham, MA, United States). A diamond crystal ATR plate was used for most analyses, however, for analysis of black plastic a germanium crystal ATR plate was used due to the crystal’s high refractive index and its capacity to accurately scan high absorbing substances such as carbon-black colored objects.
A spectrum was obtained for each plastic fragment and compared to spectral libraries. The principal spectral libraries searched included the Center for Coastal Studies (Provincetown, MA, United States) private library which includes the Center for Marine Debris Research Polymer Kit 1.0, the Aldrich Polymers library, and the Hummel Polymer and Additives library. Each result from the spectral library was verified by identifying the spectrum’s wavelength peaks and matching wavelength numbers to known wavelength ranges of the corresponding polymer. Only fragments with greater than a 70% confidence of assignment were reported (Lusher et al., 2013).
Each identified plastic fragment was categorized under one of 16 polymer groups; non-plastic items identified via FTIR were categorized as biological, vegetation, rock, glass, natural resin, wood, or natural fiber blend, and were not included in further count or polymer identity metrics. Polyethylene was further divided into low density polyethylene (LDPE), high density polyethylene (HDPE), and polyethylene (inconclusive on the type of polyethylene). Mixed polymers were labeled under the primary polymer, e.g., polyacrylate mix, polyethylene mix.
Only the first 50 suspected plastic items were analyzed in three outlier individuals that possessed over 50 suspected plastic items to maximize the number of individuals included in FTIR analyses. All items (n = 104 items) were analyzed for one adult individual from Gough Island found to contain an exceptional diversity of plastics that appeared to have died as a result of plastic ingestion; this individual was considered an outlier throughout statistical analysis. All plastic items found in all other individuals were assessed and included in statistical analyses.
Statistical Analysis
All data manipulation and statistical analyses were performed in R version 3.6.1 (R Core Team, 2020). Quantitative data were checked for normality and homoscedasticity using the Shapiro-Wilk test and Levene’s test. Plastic count and mass data were non-normal despite log transformation and therefore treated non-parametrically for statistical analyses; year groups displayed no significant differences in variance. Differences between years or groups were assessed using Kruskal-Wallis tests with post-hoc application of Dunn’s test with Bonferroni correction for multiple testing as needed. Relationships between continuous variables (specifically morphometric measurements, plastic count, and plastic mass data) were assessed using Spearman rank correlation coefficients (Rs2). Chi-squared tests of independence or Fisher’s exact tests were used to compare ratios of plastic category and polymer type between age groups, sexes, locations and sampling years. Effects observed within all statistical tests were considered significant when p < 0.05.
Results
Frequency and Accumulation of Ingested Plastics Over Time and Between Locations
Out of 2328 plastic-like items found in the ventriculus of 217 Great Shearwaters, 2121 items were assessed via FTIR, and 2035 items (95.9%) were confirmed as plastic via FTIR; the mean FTIR confidence score of identification was 96.8%. Overall, 84 items were of natural origin (primarily rock fragments and vegetation); FTIR analyses were inconclusive for 2 items. Frequency of occurrence (FO) of ingested plastic varied by location, sampling year, and age of birds, with the lowest FO observed in breeding phase individuals from Gough and Nightingale Island (∼60% in adults and 58% in chicks, respectively), ranging up to 100% in the NW Atlantic and off the coast of Brazil (Table 1). The mass and number of plastics found in each individual varied by location and year; only those individuals found to contain plastic were included in reported summary metrics describing the count and mass of ingested plastic. Considering all years of data and only those birds with plastics, Massachusetts Bay birds contained an average of 8 plastic items and 149 mg plastic (range = 1–42 items, 0.4–797 mg, n = 128 birds), Gough Island adult individuals contained an average of 10 plastic items and 345 mg plastic (range = 1–104 items, 1–1867 mg, n = 15 birds), Nightingale chicks contained an average of 22 items and 1294 mg (range = 3–93 items, 40–8047 mg, 26 birds), individuals collected off the coast of Brazil contained a mean of 8 pieces and 311 mg each (range = 1–40 items, 4–2330 mg, n = 13 birds), and individuals collected off the coast of North Carolina contained an average of 12 items and 181 mg plastic (range = 3–28 items, 19–445 mg, n = 15 birds) (Table 2 and Supplementary Figures 1, 2). Count and mass data were highly correlated across all locations (Rs2 = 0.88, p < 0.001).
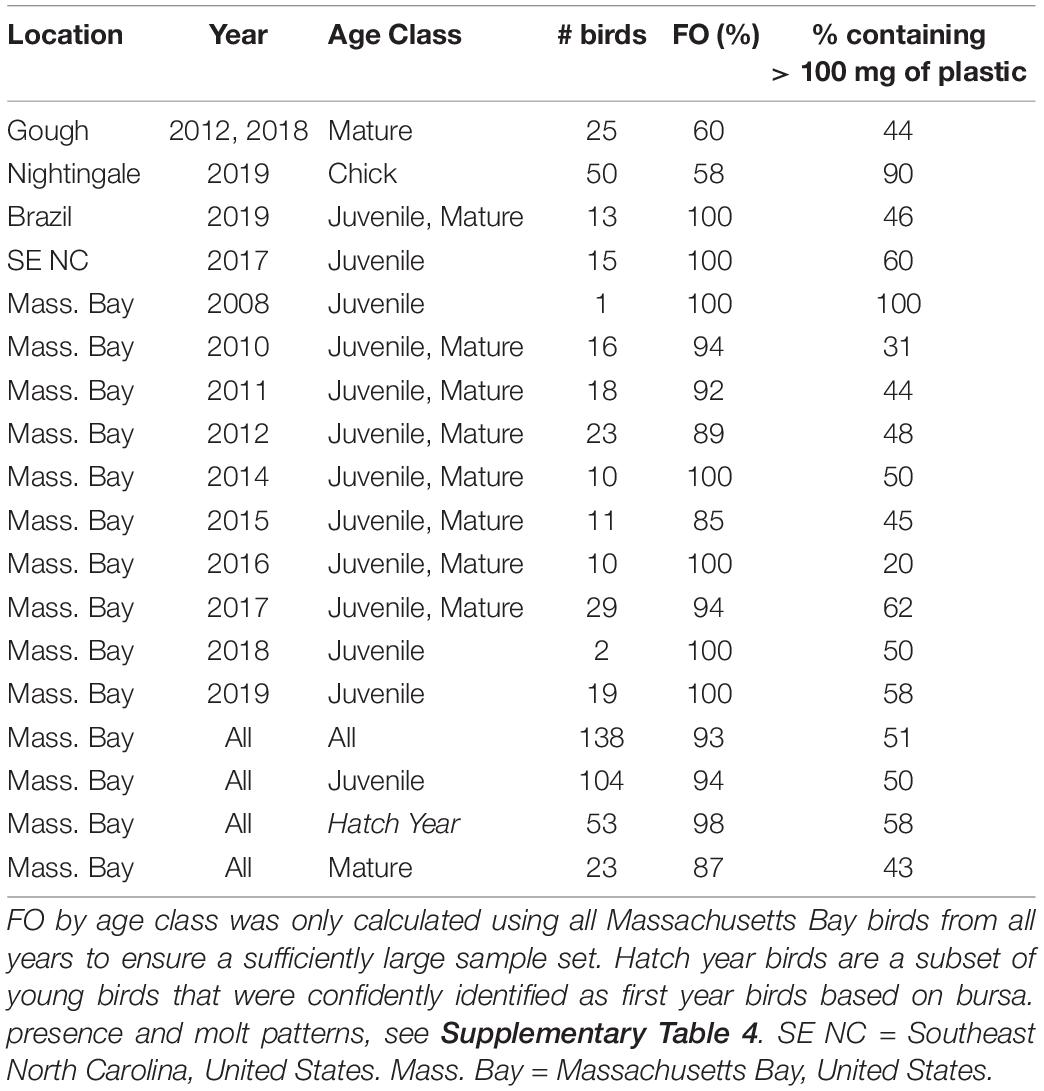
Table 1. Frequency of occurrence (FO) of plastic ingested by Great Shearwaters Ardenna gravis, by location, year, and age, along with percentage of individuals per collection location and year that contained greater than 100 mg of ingested plastic.
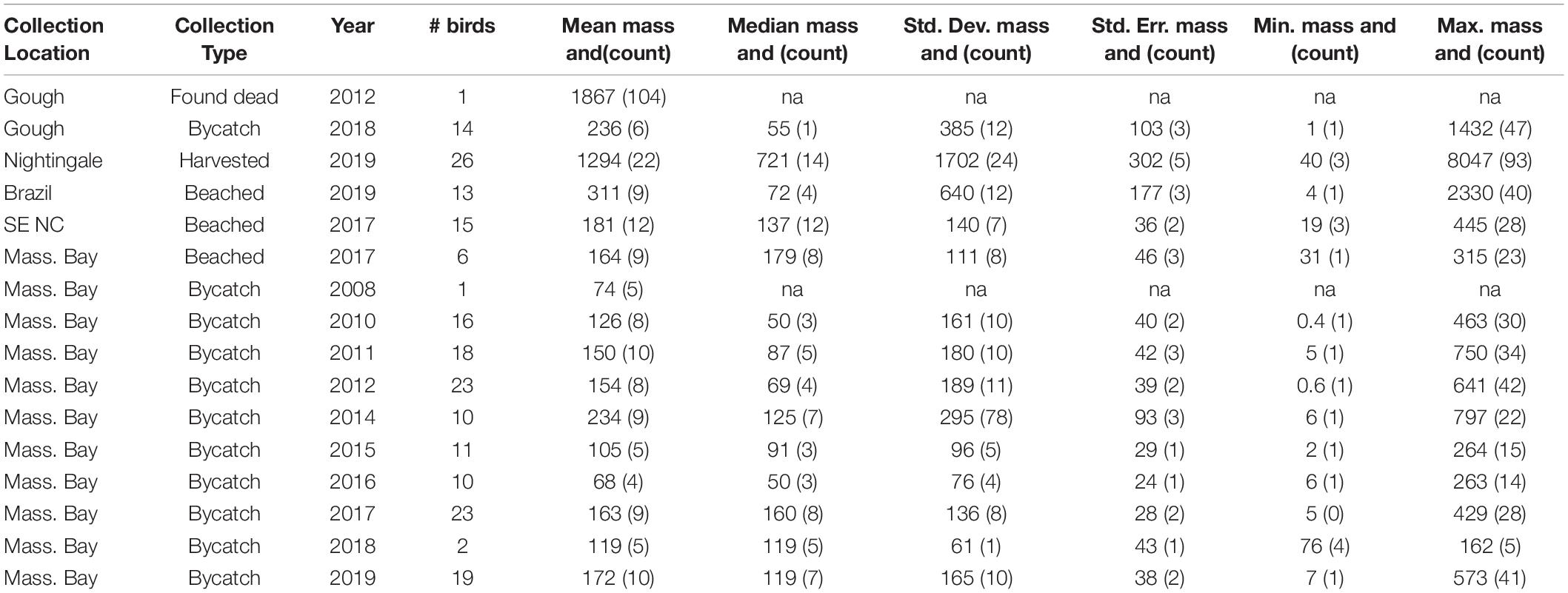
Table 2. Summary statistics describing accumulated ingested plastics by mass (in mg) and count (in parentheses) observed in Great Shearwaters Ardenna gravis obtained from different regions of the Atlantic Ocean in different years.
Plastic Item Morphology
The largest rigid ingested item, found in a harvested chick from Nightingale Island, measured 101.5 mm in its longest dimension. The largest plastic item by length and width was a flexible, sheet-like plastic found in a bycatch juvenile from Massachusetts Bay, measuring 1595 mm2 when fully extended. Consumer use plastics dominated, making up 91% of plastic items across the entire sample set (range 75–100%). Industrial plastics made up a small proportion of observed plastics from each year and location, with no significant changes in the percent of industrial plastics over time in Massachusetts Bay birds (mean for all locations = 10%, range for all locations = 5–25%, Figure 2).
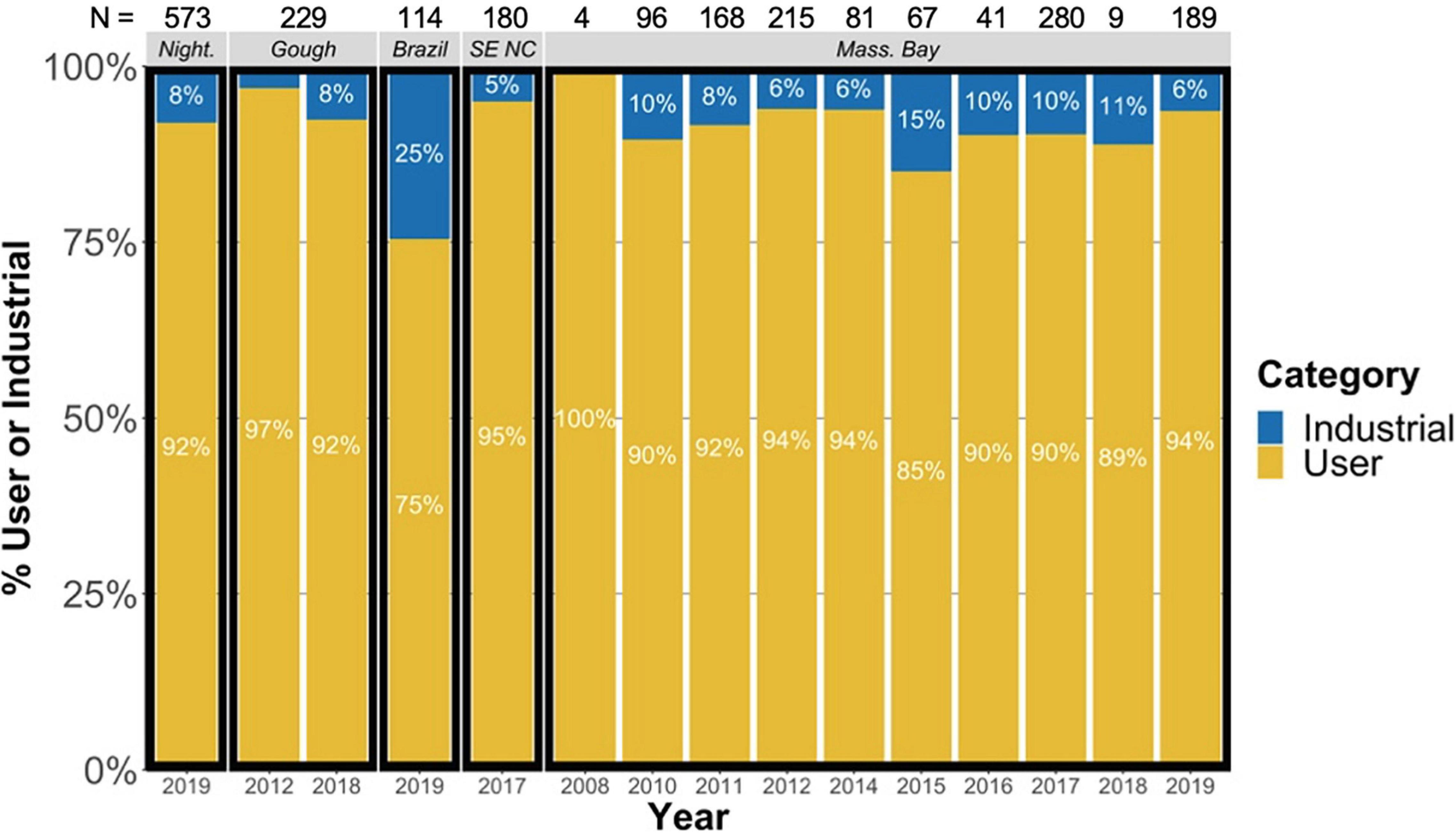
Figure 2. Proportions of user and industrial plastic observed in gastrointestinal samples of Great Shearwaters Ardenna gravis from each location, and across multiple years in Massachusetts Bay. Night. = Nightingale Island; SE NC = Southeast North Carolina, United States; Mass. Bay = Massachusetts Bay, United States; N = number of plastic items identified.
Proportions of each user plastic morphological category were assessed as a percentage of the total count of user plastic items measured within a given year and location. Category ratios differed between years in Massachusetts Bay (Fisher’s exact test, two-sided, p < 0.001), but were consistently dominated by fragments (mean = 85%, range = 70–100%, Figure 3). Fragments likewise made up the largest proportion of items across all locations (mean length and width = 7 × 5 mm). Category ratios did not differ significantly between all collection locations (χ216,1954 = 25.17, p = 0.067) when considering all plastic items identified from each location, excluding items found in one outlier from Gough Island (Figure 3). Removing fragments from the comparison, the remaining category ratios were significantly different between collection locations (χ212,231 = 24.42, p = 0.018), driven by a higher incidence of flexible sheet-like plastics in Massachusetts Bay and Brazil, as well as a higher incidence of thread-like and “other” plastics in Massachusetts Bay.
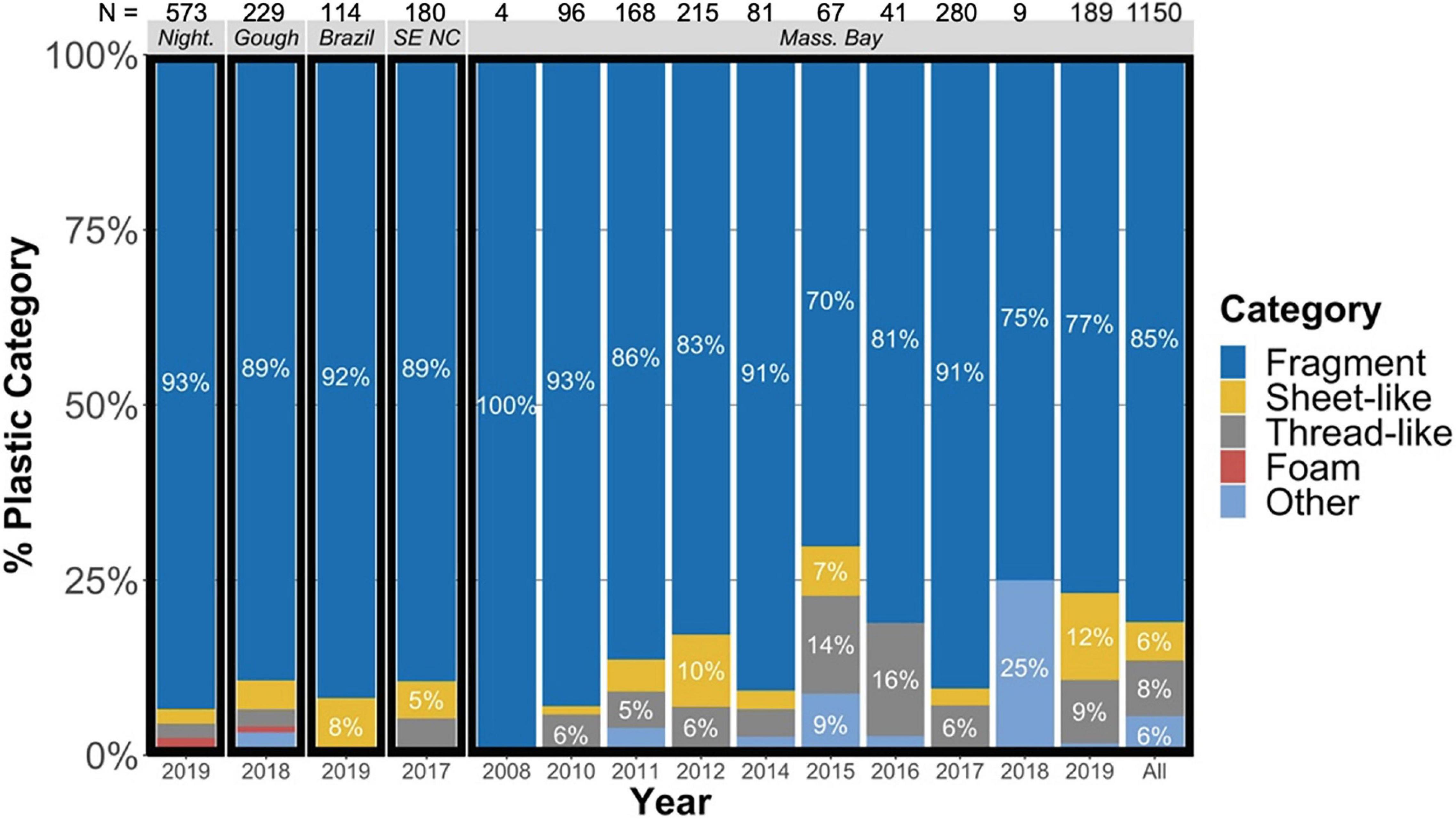
Figure 3. Proportions of each plastic morphology, as a percentage of the total count of plastic items measured within a given year and location. The summary of the Gough Island dataset presented herein omits one clear outlier that possessed 104 plastic pieces of greater category diversity than observed across other mature birds from Gough Island. Night. = Nightingale Island; SE NC = Southeast North Carolina, United States; Mass. Bay = Massachusetts Bay, United States; N = number of plastic items identified.
Spatial Differences in Plastic Item Size
The size of fragments differed between locations, as birds from the South Atlantic contained larger (by length and length × width) fragments compared to items found in individuals from the NW Atlantic (S. Atlantic mean length = 9.0 mm, NW Atlantic mean length = 6.8 mm, Kruskal-Wallis χ2 = 105.29, df = 1, p < 0.001 – S. Atlantic mean length × width = 67 mm2, NW Atlantic mean length × width = 30 mm2, Kruskal-Wallis χ2 = 171.5, df = 1, p < 0.001) (Figure 4). These differences were likewise apparent when the comparison was restricted to plastic items found in mature birds from Massachusetts Bay compared to items found in mature birds from Gough Island, with mature birds from Gough Island (mean length 8.0 mm; length × width = 40 mm2) containing larger items than mature birds from Massachusetts Bay (6.3 mm and 32.1 mm2, Kruskal-Wallis χ2 = 4.19 and 4.33, both df = 1, p = 0.040 and 0.037, respectively). Likewise, items found in hatch year birds from Massachusetts Bay were significantly shorter in their longest dimension (mean length = 7.0 mm) as well as length x width (mean length × width = 32.5 mm2) compared to items found in chicks from Nightingale Island (9.2 mm and 70.2 mm2, respectively, Kruskal-Wallis χ2 = 79.27 and 138.44, both df = 1, p < 0.001).
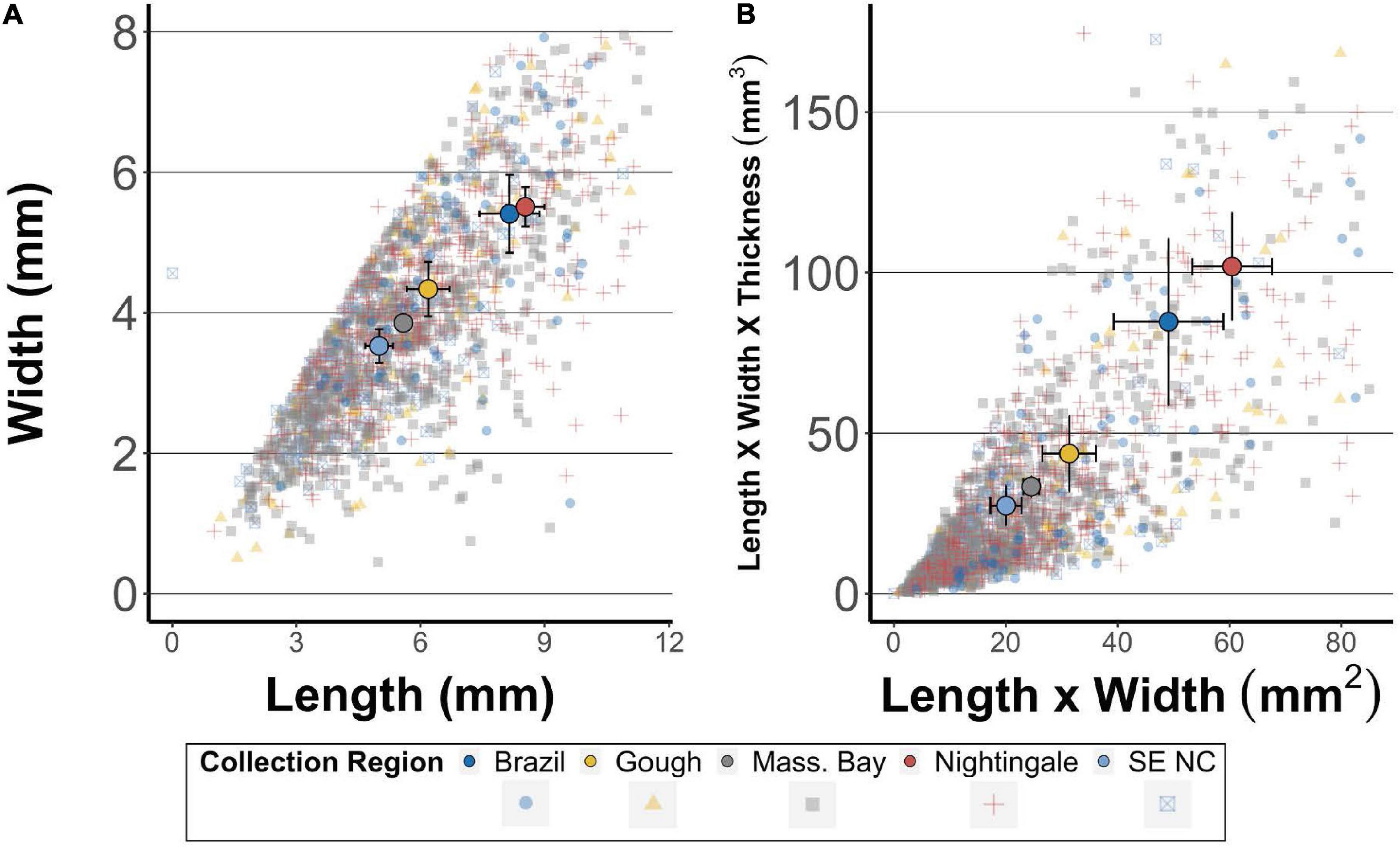
Figure 4. (A) Length and width and (B) length × width and length × width × thickness of plastic fragments found in digestive tracts of Great Shearwaters Ardenna gravis. Colors and shapes indicate collection region. 95% of the data is displayed, with the upper 5% omitted for data and axis legibility. Bold circles in each plot present the mean, and associated error bars present 95% confidence intervals. SE NC = Southeast North Carolina, United States. Mass. Bay = Massachusetts Bay, United States.
Patterns in Polymer Identity Between Regions and Over Time
Ingested plastic items from all locations were primarily composed of polyethylene (60–89% of items per location) (Figure 5), followed by polypropylene and polypropylene mixes. Polymer signatures of ingested plastic items varied between collection locations and age classes, with Massachusetts Bay birds generally containing more diverse plastic types (Figure 5 and Supplementary Table 4). The polymer make-up of plastic items found in mature individuals from Gough Island was significantly different compared to the polymer composition of plastic items found in mature individuals from Massachusetts Bay, driven by higher proportions of polypropylene and polypropylene mixes in Gough Island adults (Supplementary Table 4). Similarly, the composition of plastic items found in chicks from Nightingale Island was significantly different compared to hatch year individuals from Massachusetts Bay, driven by increased ingestion of polypropylene in Nightingale chicks coupled to increased abundance of rubber and resin polymers in Massachusetts Bay hatch year birds (Supplementary Table 4).
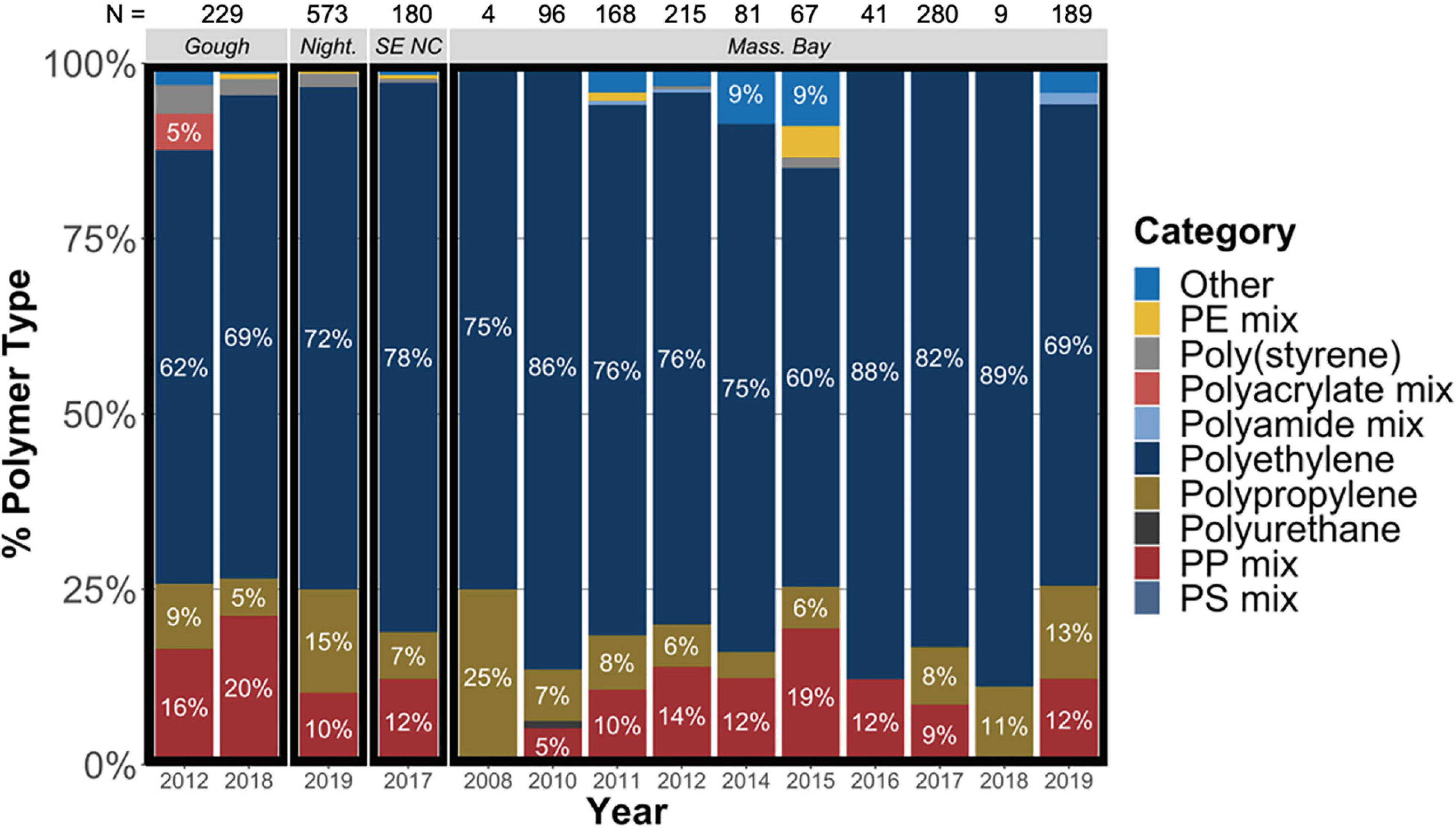
Figure 5. Polymer composition of plastic items ingested by Great Shearwater Ardenna gravis grouped by year and sampling location. Night. = Nightingale Island; SE NC = Southeast North Carolina, United States; Mass. Bay = Massachusetts Bay, United States; N = number of plastic items identified.
Demographic, Spatial, and Temporal Trends
Plastic count and mass did not significantly differ between males and females. In Massachusetts Bay, juveniles (mean = 9.3 items) contained more plastic pieces than adults (6.3 items, Dunn’s test, p = 0.034); juveniles also contained marginally higher masses of plastic (162 mg) compared to adults (149 mg, Dunn’s test, p = 0.073). Fully grown birds in the South Atlantic contained a significantly higher mass of plastic (mean = 236 mg) compared to adults from the North Atlantic (149 mg, Kruskal-Wallis χ2 = 6.020, df = 1, p = 0.049), but not a higher count of plastics. Chicks from Nightingale Island contained a larger number of plastic pieces (mean = 22 items, mass = 1294 mg) compared to hatch year birds from Massachusetts Bay (10 items, 171 mg, Kruskal-Wallis χ2 = 7.850 and 24.82, df = 1, p = 0.005 and >0.001). Considering the entire sample set, juvenile birds contained higher masses and counts of plastic (juvenile mean = 11 items, mass = 354 mg) than adults (8 items, 214 mg, Dunn’s tests, both p < 0.001). Overall, 51% of birds contained more than 100 mg of ingested plastic, including individuals from all sampled locations (Table 2).
No statistically significant trends in the number or mass of plastic items were detected among birds from Massachusetts Bay over 2010–2019, possibly due to modest sample sizes and resulting variability apparent in some years (e.g. 2014, 2016, 2018). Plastic mass increased over time if only years when more than 15 birds were sampled were included in the analysis (n = 5 years, Rs2 = 0.87, p = 0.044).
Plastic in Sand Lance
Sand Lance contained no visible plastic fragments of the same size range or morphology as those found in Great Shearwater stomachs. A single thread-like item was found in one fish; this item was not confirmed as plastic via FTIR due to COVID-19 limitations.
Discussion
Ingested Plastics Compared to Prior Studies
Dating back to the early 1980s, multiple studies have documented a high frequency of plastic ingestion in Great Shearwaters across the entirety of their range. For example, Furness (1983) documented a 90% FO in the Benguela Current, while Ryan (1987a) documented a 95% FO in individuals from Gough Island. Overall, Moser and Lee (1992) reported FO = 64% (n = 55 individuals) from the NW Atlantic Ocean, collected between 1975–1989 (Moser and Lee, 1992). More recently, Bond et al. (2014) documented a FO = 88% (n = 84) for beach-cast Great Shearwaters on Sable Island, Nova Scotia, Canada, from 2000 – 2011 (Bond et al., 2014). On a year-by-year basis we report similar or slightly higher FO values here (range = 58–100%) compared to prior work (range = 0–95%) (Supplementary Table 5), suggesting that Great Shearwaters continue to regularly ingest plastic. The range of FO values observed within this study is similar to FO values for Short-tailed Shearwaters (63–90%) (Acampora et al., 2014; Roman et al., 2016) and Flesh-footed Shearwaters in the Pacific (0–100%) (Hutton et al., 2008; Lavers et al., 2014), emphasizing the prevalence of plastic ingestion in Ardenna shearwaters across the globe. FO values reported in this study were higher than those observed for Wedge-tailed Shearwaters (Ardenna pacifica) in Australia (0–43%) (Hutton et al., 2008; Verlis et al., 2013; Roman et al., 2016; Lavers et al., 2018) and Sooty Shearwaters (Ardenna grisea) in the Pacific and Atlantic (0–72%) (Brown, 1981; Furness, 1983; Barbieri, 2009; Bond et al., 2014; Terepocki et al., 2017). The rationale behind variable FO between similar large shearwaters remains unclear. Hutton et al. hypothesized that variable FO between similar species was related to variability in foraging strategy and/or foraging range, with higher environmental abundance of plastics leading to higher rates of ingestion (Hutton et al., 2008). However, we acknowledge that sample collection and plastic analysis procedures varied between this study and previous studies (Supplementary Table 5), and therefore these comparisons of FO may be confounded to some degree.
The number of plastic pieces and the mass of ingested plastic varied with location, but the range observed generally overlapped with previously published metrics published for this species, suggesting consistent vulnerability to plastic ingestion (mean number of plastics range = 4–22 items, mean mass plastics range = 68–1294 mg, prior studies mean count range = 6–16 items, prior studies mass range = 236–2510 mg) (Ryan, 1987a,b; Moser and Lee, 1992; Bond et al., 2014) (Supplementary Table 5). Additionally, juveniles ingested more plastic by mass and count compared to adults as seen in prior work in Short-tailed Shearwaters in eastern Australia (Acampora et al., 2014), underscoring the increased sensitivity of chick and juvenile birds to plastic ingestion compared to adults, likely due to their naïve foraging habits and provisioning of plastic during parental care.
Prior work in this species has demonstrated changes in composition and number of plastics over time (Ryan, 2008). No trends were apparent in count or mass over time within our complete time series data set or when compared to prior work, but we note that temporal trends warrant continued investigation, as the mass of plastic increased over time in Massachusetts Bay birds when only considering those years with large sample sizes. This contrasts with the decrease in ingested plastic loads in Northern Fulmars from the North Sea over the last two decades (Van Franeker and Law, 2015).
Measurements of Plastics in Prey and Surface Water Do Not Explain the Composition of Ingested Plastics in Great Shearwaters
Limited data details the occurrence of different plastic categories in surface waters of the Gulf of Maine and South Atlantic, as most large-scale datasets present abundance by size rather than plastic piece category or polymer type (Eriksen et al., 2014). However, 2013 surface water surveys conducted near Isles of Shoals in the SW Gulf of Maine found microfibers dominated (84%) in the surface layer (∼1 m) compared to fragments (14%); these fibers were smaller in length and diameter than those found ingested in Great Shearwaters (Lindeque et al., 2020). Although limited in spatial coverage, this survey dataset suggests the composition of plastics found in the surface ocean does not align with the composition of plastics ingested and accumulated by Great Shearwaters in the region, which contained ∼85% hard fragments. This suggests that Massachusetts Bay birds do not inherit their consistent plastic category signature from their environment and instead seek out plastic fragments selectively, as opposed to accumulating plastics based on likelihood of encounter in the environment. This selectively has been inferred from selective patterns of ingestion in numerous other studies of petrels (e.g., Day et al., 1985; Ryan, 1987a; Acampora et al., 2014). This selection bias may be related to the complex interplay of visual and olfactory cues that inform seabird foraging at different scales (Vlietstra and Parga, 2002; Savoca et al., 2016, 2017; Roman et al., 2019a,d, 2020; Nishizawa et al., 2021).
Secondary ingestion also is unlikely given the very low incidence of ingested plastic in Sand Lance, the primary forage item of Great Shearwaters during their non-breeding period in the Gulf of Maine. This finding corroborates evidence from other seabirds suggesting that the transfer of plastics between fish prey and seabird predators is limited (Carbery et al., 2018; Nelms et al., 2018).
Size-Mediated Environmental Distribution of Plastics Is Reflected in Great Shearwater Plastic Ingestion
Chick and adult Great Shearwaters collected in the South Atlantic contained larger plastic pieces compared to their North Atlantic counterparts, with chicks containing the largest plastics overall, the highest counts of plastic, and the highest masses of ingested plastic. The increased abundance of larger plastic items in South Atlantic birds and chicks in particular is problematic as larger plastic items pose an increased risk of physical impairment or injury (Roman et al., 2019b); potential harm may be particularly deleterious to the migratory success of post-breeding adults in poor body condition or naïve chicks making their first northward migration.
This abundance of larger plastic fragments may be related to size-mediated distribution of plastic items in the surface ocean (Ryan, 2015b; Van Sebille et al., 2020), coupled to the distinct foraging ranges of Great Shearwaters in their breeding and non-breeding phases. While Great Shearwaters are highly mobile predators, tracking data reveals core foraging ranges within their respective breeding and non-breeding ranges. During the breeding season, adults utilize vast expanses of open ocean habitat, alternating between short and long foraging trips during incubation and chick-rearing (Schoombie et al., 2018). Utilization density estimates suggest these foraging activities center around Gough, Inaccessible, and Nightingale Islands in the remote South Atlantic Ocean and the Antarctic Polar Front, 1000s of km from major population centers and continental margins (Ronconi et al., 2018; Schoombie et al., 2018). Additionally, Great Shearwaters forage along the Patagonian Shelf during the breeding season and as a migratory staging area; the adjacent land area is sparsely populated, with a human population density of only 4 inhabitants⋅km–2 (Gil et al., 2019). By comparison, during the non-breeding season Great Shearwaters forage in the Gulf of Maine, frequenting Massachusetts Bay, George’s Bank, and the coastal margins of NW Canada. Tracking data indicates core foraging areas exist close to highly urbanized coastal margins of the northeast United States, near the major metropolitan center of Boston and the greater Washington – Boston megalopolis (Powers et al., 2017, 2020). Circulation in these key foraging regions and across the wider Gulf of Maine is driven by local and regional processes (Li et al., 2014; Townsend et al., 2014). Therefore surface water environments in the Gulf of Maine that support Great Shearwaters likely reflect a higher abundance of regionally sourced plastics from highly developed land margins (Brooks, 1992; Pershing et al., 2015; Lindeque et al., 2020). Additionally, coastal surface waters often host smaller, more polymerically diverse, and less buoyant plastic items compared to items found in open ocean environments (Lusher, 2015; Ryan, 2015b; Fazey and Ryan, 2016; Erni-Cassola et al., 2019). Smaller plastic items, often associated with coastal environments, typically possess higher surface area:volume ratios and settle out of the water column rapidly with increasing distance from coastal regions due to biofouling and other biophysical processes (Van Sebille et al., 2020); this size-mediated settling process has the potential to influence the distribution of plastic items in the size range ingested by Great Shearwaters (Kooi et al., 2017). Larger, more buoyant pieces preferentially remain in the surface ocean to circulate to more remote regions (Ryan, 2015b).
The starkly different environmental characteristics of breeding vs. non-breeding ranges have previously been demonstrated to expose Great Shearwaters to higher levels of human-derived pollutants compared to other seabirds from the same breeding location that solely rely on South Atlantic environments (Roscales et al., 2019; Robuck et al., 2020). We believe a similar dynamic is driving the physical characteristics of plastic items observed in this study. We hypothesize that Great Shearwaters are more likely to encounter large, rigid plastic items during their breeding and post-breeding season when they primarily forage in pelagic, remote environments in the South Atlantic; larger, low surface area:volume items are preferentially retained at the water surface away from coastal margins through size-mediated, oceanic-scale dispersal processes. Conversely, Great Shearwaters during their non-breeding phase forage adjacent to urbanized coastal margins in the NW Atlantic Ocean, and could ingest and accumulate smaller, more diverse, or less buoyant plastic items that have not yet settled out of the water column due to proximity to coastal sources. In our data, these hypotheses are supported by the distinctly large item size of plastics ingested by chicks and adults at the breeding colony and found beach-cast in Brazil (Figure 4), and to a lesser extent, the reduced FO seen in individuals from the breeding colony. Moreover, plastic item type and morphology has been observed to vary between seabirds collected inshore versus those collected offshore as bycatch, with flexible sheet-like plastics more common in beach-cast, inshore samples while rigid fragments and fibers dominated in individuals collected offshore (Roman et al., 2020). Ostensibly, such item distributions reflect size- and buoyancy mediated environmental distribution, with smaller items or items with high surface area:volume ratios less likely to migrate into offshore oceanic environments. Our data mirrors this, with a higher percentage of sheet-like, thread-like, balloons, and other non-fragment items in Massachusetts Bay individuals compared to individuals from Gough and Nightingale Islands (Massachusetts Bay mean FO of non-fragment items = 16%, Nightingale mean = 7%, Gough mean = 10%, - Dunn’s test, p < 0.01). We acknowledge that wear in the gizzard and intergenerational transfer could also play a role in the size and morphology of plastic items observed between locations. However, as described below, we believe our polymer and category observations strongly suggest turnover of the predominant plastic signature at rapid (4–6 month) time scales, suggesting these factors are not the dominant drivers of size dynamics discussed here. We also note that chick-provisioning behavior could influence the size of plastic items found in chicks and adults in the South Atlantic, with adults more likely to select larger items to provision chicks compared to non-breeding prey/item selection. More concerted research is required to rigorously explore this hypothesis and its ramifications for plastic ingestion and related risk assessment in remote and coastal marine fauna.
Differences in Polymer Signature Between Locations Suggest Plastic Items Likely Turn Over Within 4–6 Months in vivo
Despite an increasing amount of research documenting plastic ingestion by seabirds, the retention time of plastic items in different groups of seabirds remains unclear. Species- and item-specific factors confound general estimates of retention time, as this metric varies based on co-ingested diet items present in the GIT, species-specific size thresholds required to pass items through ventriculi to the lower GIT, initial item size, item composition, and rate of wear within the GIT (Ryan, 2015a). Fragments, the dominant morphology found in Great Shearwaters within this study, may also be prone to reduced retention times due to increased exposure to UV and other environmental stressors that may degrade structural integrity and resilience prior to ingestion (Ryan, 2015a). Observational evidence underscores the complex nature of this question. Van Franeker and Law (2015) suggested Northern Fulmars depurate approximately 75% of their plastic load within 1 month based on assessment of plastic loads in fulmars between their breeding and non-breeding locations (Van Franeker and Law, 2015). However, their inference did not account for possible transfer from adults to chicks during chick-rearing, which may impact the incidence of plastics in breeding phase adults via offloading to chicks. Alternatively, direct studies of plastic pellet breakdown in seabirds or in controlled environments suggest slow breakdown of plastics in vivo or in situ (Ryan, 2015a; Chamas et al., 2020); for example, virgin industrial pellets fed to White-chinned Petrel (Procellaria aequinoctialis) fledglings lost only ∼1% of their mass over 12 days within the GIT, making break down within a month improbable and suggesting a half-life of over a year (Ryan and Jackson, 1987). However, no studies to date have empirically measured the retention time of plastics in shearwaters or other procellariiform seabirds using weathered plastics, which are considerably more fragile compared to virgin plastic items of the same polymer type (Min et al., 2020).
While we are unable to account for all variables that may influence in vivo plastic retention time or directly measure this phenomena, our data further refines current hypotheses by suggesting that a significant proportion of plastic fragments retained in Great Shearwater ventriculi turnover within a few months, as items collected in Great Shearwaters from disparate locations approximately 4–6 months apart reflected significantly different polymer signatures and morphological categories (Supplementary Table 4 and Figure 5). For these signature differences to occur, a significant portion of accumulated ingested plastics must be replaced by new items of different morphology and polymer type, ostensibly via egestion and ingestion of new items. Changes in polymer and category signature between locations could not be explained by dilution of an existing plastic signature by accumulation of more plastic items (e.g., apparent lower proportion of PP mixes in North Atlantic birds due to accumulation of more plastics overall), because birds from most locations contained similar counts of plastic items (Table 2). Moreover, chicks contained some of the largest plastic items that would ostensibly take the longest to breakdown; however, the chick polymer signature is not retained in hatch year birds collected in the North Atlantic, which contained more polyethylene, rubber, and resin-based materials. Chicks also contained a higher number and mass of plastics that was not reflected in hatch year birds in Massachusetts Bay (Table 2). Moreover, if plastics were retained indefinitely, we would expect homogenous FO in comparable life stages between locations, which is not the case (Table 1). Crucially, this suggests mitigation of plastic sources within both the North and South Atlantic are key to reduce the high incidence of plastic ingestion in this species, which occurs at levels well above ecological quality objectives thresholds developed for other seabirds in the Northeast Atlantic. Considering all plastic items, 84% of all items were composed of polyethylene and polypropylene, and thus originated from plastic types widely recyclable in the United States and around the globe, indicating infrastructure and knowledge already exist to mitigate loads of these polymers into aquatic environments.
Industrial Plastics Generally Remain a Minor Component of Ingested Plastic Loads
The percentage of industrial plastics within this sample set (mean = 9%) was equal to or lower than the percentage of industrial plastics reported in previous studies in seabirds and specifically Great Shearwaters (Figure 2; Moser and Lee, 1992; Ryan, 2008; Bond et al., 2014; Van Franeker and Law, 2015; Avery-Gomm et al., 2017; Kühn et al., 2020). The reduced incidence of industrial plastics is likely related to decrease of industrial pellets in marine environments due to source reduction efforts over time (Van Franeker and Law, 2015). However, the ratio of industrial pellets ingested by Great Shearwaters found on the coast of Brazil in 2019 (25%) was higher than other locations and more comparable to historical occurrence of industrial pellets in Great Shearwaters examined in the South Atlantic in 1985–1986 (up to 64%) (Ryan, 2008).
Recommendations for Future Monitoring
Some evidence suggests the accumulation of ingested plastics can increase risk of mortality in seabirds, while the sublethal impacts of plastic ingestion are more poorly understood due to the range of factors that might influence the magnitude of harm (Roman et al., 2019b). To date, it is unclear how, if it all, plastic ingestion may be impacting the health of Great Shearwater populations, due to an overall lack of information about chronic adverse impacts, and the many confounding factors preventing clear investigation of impacts (Senko et al., 2020; Roman et al., 2021). However, our work highlights that plastic ingestion is a continued stressor of this species and likely other pelagic seabirds in the Atlantic Ocean. Great Shearwaters in particular offer an abundant, accessible platform to monitor future plastic dynamics in the wider Atlantic basin, as the subtropical North and South Atlantic are projected to experience continued accumulation of plastic pieces in the coming decades (Wilcox et al., 2020).
We recommend increased standardization of methodology and approach in studies evaluating plastics in Great Shearwaters, to leverage this species more fully as a compact and affordable indicator of plastics in subtropical and temperate Atlantic regions. Mass, count, and FO should be presented for each compartment of the GIT as a function of location, age, and sex given the highly variable mass, count, and FO reported between different ages and locations across time, following guidelines outlined previously for assessment of plastics in marine birds (Provencher et al., 2019). We particularly highlight the need for reporting plastic ingestion within each GIT compartment separately to facilitate increased use of bycatch samples within monitoring studies, as Great Shearwaters are the most abundantly bycaught species in the Gulf of Maine (Hatch et al., 2016; Hatch, 2018) and are also bycaught in the South Atlantic (Bugoni et al., 2008). Bycatch samples are also considered healthy prior to death and lack bias potentially associated with beach-cast samples which may have been sickly or compromised leading to death (Colabuono et al., 2009; Rodríguez et al., 2018).
Monitoring efforts focused on this species also present an opportunity to assess the efficacy of any future interventions to address plastic pollution, given the breadth of data presented here and in previous studies. In addition, our finding that much of the plastics consumed by Great Shearwaters is recyclable material and therefore preventable, provides an opportunity for education and intervention that can support the social messaging needed to remedy an ubiquitous problem in the marine environment.
Data Availability Statement
The raw data supporting the conclusions of this article will be made available by the authors, without undue reservation.
Author Contributions
AR designed the study, drafted the manuscript, and finalized through contributions of all authors. AR, CHu, GE, LA, JS, JP, VP, PR, LB, and DW contributed to sample analysis and data interpretation. PR, LB, CHu, CHa, KP, DW, and RL contributed significantly to the manuscript drafting and editing. All authors approved the final version of the manuscript.
Funding
This project was supported by the NOAA Fisheries National Seabird Program and the Volgenau Foundation. AR acknowledges support from the National Oceanic and Atmospheric Administration Dr. Nancy Foster Scholarship Program (NOAA Award Number NA17NOS4290028), the Robert and Patricia Switzer Foundation, the STEEP Superfund Research Program (NIEHS Award Number P42ES027706), and the Oak Ridge Institute for Science and Education (ORISE) program. LB was funded by INCT-Mar COI and PQ Grant No. 311409/2018-0, both by the Brazilian National Research Council (CNPq). JS was funded by the National Science Foundation Graduate Research Fellowship program.
Conflict of Interest
The authors declare that the research was conducted in the absence of any commercial or financial relationships that could be construed as a potential conflict of interest.
Publisher’s Note
All claims expressed in this article are solely those of the authors and do not necessarily represent those of their affiliated organizations, or those of the publisher, the editors and the reviewers. Any product that may be evaluated in this article, or claim that may be made by its manufacturer, is not guaranteed or endorsed by the publisher.
Acknowledgments
We are indebted to the observers of the Northeast Fisheries Observer Program and Stephanie DePasquale for their assistance obtaining samples used in this study. We also thank Maelle Connan (Nelson Mandela University) for assistance procuring samples. Although NOAA employees contributed to this article, this research was conceived, designed, and implemented by URI. Consequently, the views, interpretations, and conclusions expressed in the article are solely those of the authors and do not necessarily reflect or represent NOAA views or policies. We are also grateful to L. Furlanetto for preparing Brazilian samples and the team of the Waterbirds and Sea Turtles Lab at FURG for sampling birds on the beach.
Supplementary Material
The Supplementary Material for this article can be found online at: https://www.frontiersin.org/articles/10.3389/fmars.2021.719721/full#supplementary-material
References
Acampora, H., Schuyler, Q. A., Townsend, K. A., and Hardesty, B. D. (2014). Comparing plastic ingestion in juvenile and adult stranded Short-tailed Shearwaters (Puffinus tenuirostris) in eastern Australia. Mar. Pollut. Bull. 78, 63–68. doi: 10.1016/j.marpolbul.2013.11.009
Avery-Gomm, S., Provencher, J. F., Liboiron, M., Poon, F. E., and Smith, P. A. (2017). Plastic pollution in the Labrador Sea: an assessment using the seabird Northern Fulmar Fulmarus glacialis as a biological monitoring species. Mar. Pollut. Bull. 127, 817–822. doi: 10.1016/j.marpolbul.2017.10.001
Barbieri, E. (2009). Occurrence of plastic particles in procellariiforms, south of São Paulo state (Brazil). Braz. Arch. Biol. Technol. 52, 341–348. doi: 10.1590/S1516-89132009000200011
Bond, A. L., Provencher, J. F., Daoust, P.-Y., and Lucas, Z. N. (2014). Plastic ingestion by fulmars and shearwaters at Sable Island, Nova Scotia, Canada. Mar. Pollut. Bull. 87, 68–75. doi: 10.1016/j.marpolbul.2014.08.010
Brooks, D. A. (1992). “A brief overview of the physical oceanography of the Gulf of Maine,” in Proceedings of the Gulf of Maine Scientific Workshop, eds J. Wiggin and C. N. K. Mooers (Boston, MA: Urban Harbors Institute), 51–74.
Brown, R. G. B. (1981). Revised Atlas of Eastern Canadian Seabirds: Shipboard Surveys. Ottawa, ON: Canadian Wildlife Service.
Bugoni, L., Mancini, P. L., Monteiro, D. S., Nascimento, L., and Neves, T. S. (2008). Seabird bycatch in the Brazilian pelagic longline fishery and a review of capture rates in the southwestern Atlantic Ocean. Endanger. Species Res. 5, 137–147. doi: 10.3354/esr00115
Bugoni, L., McGill, R. A. R., and Furness, R. W. (2010). The importance of pelagic longline fishery discards for a seabird community determined through stable isotope analysis. J. Exp. Mar. Biol. Ecol. 391, 190–200. doi: 10.1016/j.jembe.2010.06.027
Carbery, M., O’Connor, W., and Palanisami, T. (2018). Trophic transfer of microplastics and mixed contaminants in the marine food web and implications for human health. Environ. Int. 115, 400–409. doi: 10.1016/j.envint.2018.03.007
Chamas, A., Moon, H., Zheng, J., Qiu, Y., Tabassum, T., Jang, J. H., et al. (2020). Degradation rates of plastics in the environment. ACS Sustain. Chem. Eng. 8, 3494–3511. doi: 10.1021/acssuschemeng.9b06635
Colabuono, F. I., Barquete, V., Domingues, B. S., and Montone, R. C. (2009). Plastic ingestion by Procellariiformes in southern Brazil. Mar. Pollut. Bull. 58, 93–96. doi: 10.1016/j.marpolbul.2008.08.020
Cuthbert, R. J. (2005). Breeding biology, chick growth and provisioning of Great Shearwaters (Puffinus gravis) at Gough Island, South Atlantic Ocean. Emu 105, 305–310. doi: 10.1071/MU05036
Davies, R. G., Irlich, U. M., Chown, S. L., and Gaston, K. J. (2010). Ambient, productive and wind energy, and ocean extent predict global species richness of procellariiform seabirds. Glob. Ecol. Biogeogr. 19, 98–110. doi: 10.1111/j.1466-8238.2009.00498.x
Day, R. H., Wehle, D. H. S., and Coleman, F. C. (1985). “Ingestion of Plastic Pollutants by Marine Birds,” in Proceedings of the Workshop on Fate and Impact of Marine Debris, 27 – 29 November 1984. NOAA Technical Memorandum, NMFS, SWFC 54. Washington, DC: NOAA, 344–386.
Eriksen, M., Lebreton, L. C. M., Carson, H. S., Thiel, M., Moore, C. J., Borerro, J. C., et al. (2014). Plastic pollution in the world’s oceans: more than 5 trillion plastic pieces weighing over 250,000 tons afloat at sea. PLoS One 9:e0111913. doi: 10.1371/journal.pone
Erni-Cassola, G., Zadjelovic, V., Gibson, M. I., and Christie-Oleza, J. A. (2019). Distribution of plastic polymer types in the marine environment: a meta-analysis. J. Hazard. Mater. 369, 691–698. doi: 10.1016/j.jhazmat.2019.02.067
Fazey, F. M. C., and Ryan, P. G. (2016). Biofouling on buoyant marine plastics: an experimental study into the effect of size on surface longevity. Environ. Pollut. 210, 354–360. doi: 10.1016/j.envpol.2016.01.026
Furness, B. L. (1983). Plastic particles in three procellariiform seabirds from the Benguela Current, South Africa. Mar. Pollut. Bull. 14, 307–308. doi: 10.1016/0025-326X(83)90541-6
Furness, R. W. (1985). Ingestion of plastic particles by seabirds at Gough Island, South Atlantic Ocean. Environ. Pollution. Ser. A Ecol. Biol. 38, 261–272. doi: 10.1016/0143-1471(85)90131-X
GESAMP (2015). Sources, Fate, and Effects of Microplastics in the Marine Environment: A Global Assessment. London: GESAMP.
Gil, M. N., Giarratano, E., Barros, V., Bortolus, A., Codignotto, J. O., Schenke, R. D., et al. (2019). “Southern Argentina: the Patagonian continental shelf,” in World Seas: An Environmental Evaluation, ed. C. Sheppard (San Diego, CA: Academic Press), 783–811. doi: 10.1016/B978-0-12-805068-2.00040-1
González-Carman, V., Denuncio, P., Vassallo, M., Berón, M. P., Álvarez, K. C., and Rodriguez-Heredia, S. (2021). Charismatic species as indicators of plastic pollution in the Río de la Plata Estuarine Area, SW Atlantic. Front. Mar. Sci. 8:699100. doi: 10.3389/fmars.2021.699100
Haman, K. H., Norton, T. M., Ronconi, R. A., Nemeth, N. M., Thomas, A. C., Courchesne, S. J., et al. (2013). Great shearwater (Puffinus gravis) mortality events along the eastern coast of the United States. J. Wildl. Dis. 49, 235–245. doi: 10.7589/2012-04-119
Hatch, J. M. (2018). Comprehensive estimates of seabird–fishery interactions for the US Northeast and mid-Atlantic. Aquat. Conserv. Mar. Freshw. Ecosyst. 28, 182–193. doi: 10.1002/aqc.2812
Hatch, J. M., Wiley, D., Murray, K. T., and Welch, L. (2016). Integrating satellite-tagged seabird and fishery-dependent data: a case study of Great Shearwaters (Puffinus gravis) and the U.S. New England sink gillnet fishery. Conserv. Lett. 9, 43–50. doi: 10.1111/conl.12178
Hutton, I., Carlile, N., and Priddel, D. (2008). Plasstic ingestion by Flesh-footed Shearwaters, Puffinus carneipes, and Wedge-tailed Shearwater, Puffinus pacificus. Papers Proc. R. Soc. Tasman. 142, 67–72.
Kenyon, K. W., and Kridler, E. (1969). Laysan Albatrosses swallow indigestible matter. Auk 86, 339–343. doi: 10.2307/4083505
Koelmans, A. A., Bakir, A., Burton, G. A., and Janssen, C. R. (2016). Microplastic as a vector for chemicals in the aquatic environment: critical review and model-supported reinterpretation of empirical studies. Environ. Sci. Technol. 50, 3315–3326. doi: 10.1021/acs.est.5b06069
Kooi, M., Van Nes, E. H., Scheffer, M., and Koelmans, A. A. (2017). Ups and downs in the ocean: effects of biofouling on vertical transport of microplastics. Environ. Sci. Technol. 51, 7963–7971. doi: 10.1021/acs.est.6b04702
Kühn, S., van Oyen, A., Bravo-Rebolledo, E. L., Ask, A. V., and Van Franeker, J. A. (2020). Polymer types ingested by Northern Fulmars (Fulmarus glacialis) and southern hemisphere relatives. Environ. Sci. Pollut. Res. 28, 1643–1655. doi: 10.1007/s11356-020-10540-6
Lavers, J. L., Bond, A. L., and Hutton, I. (2014). Plastic ingestion by Flesh-footed Shearwaters (Puffinus carneipes): implications for fledgling body condition and the accumulation of plastic-derived chemicals. Environ. Pollut. 187, 124–129. doi: 10.1016/j.envpol.2013.12.020
Lavers, J. L., Hutton, I., and Bond, A. L. (2018). Ingestion of marine debris by Wedge-tailed Shearwaters (Ardenna pacifica) on Lord Howe Island, Australia during 2005 – 2018. Mar. Pollut. Bull. 133, 616–621. doi: 10.1016/j.marpolbul.2018.06.023
Li, Y., He, R., and Manning, J. P. (2014). Coastal connectivity in the Gulf of Maine in spring and summer of 2004–2009. Deep. Res. Part II Top. Stud. Oceanogr. 103, 199–209. doi: 10.1016/j.dsr2.2013.01.037
Lindeque, P. K., Cole, M., Coppock, R. L., Lewis, C. N., Miller, R. Z., Watts, A. J. R., et al. (2020). Are we underestimating microplastic abundance in the marine environment? A comparison of microplastic capture with nets of different mesh-size. Environ. Pollut. 265, 114721. doi: 10.1016/j.envpol.2020.114721
Lusher, A. (2015). “Microplastics in the marine environment: distribution, interactions and effects,” in Marine Anthropogenic Litter, eds M. Bergmann, L. Gutow, and M. Klages (Cham: Springer International Publishing), 245–307. doi: 10.1007/978-3-319-16510-3_10
Lusher, A. L., McHugh, M., and Thompson, R. C. (2013). Occurrence of microplastics in the gastrointestinal tract of pelagic and demersal fish from the English Channel. Mar. Pollut. Bull. 67, 94–99. doi: 10.1016/j.marpolbul.2012.11.028
Min, K., Cuiffi, J. D., and Mathers, R. T. (2020). Ranking environmental degradation trends of plastic marine debris based on physical properties and molecular structure. Nat. Commun. 11:727. doi: 10.1038/s41467-020-14538-z
Moser, M. L., and Lee, D. S. (1992). A fourteen-year survey of plastic ingestion by western north Atlantic seabirds. Colon. Waterbirds 15, 83–94.
Nelms, S. E., Galloway, T. S., Godley, B. J., Jarvis, D. S., and Lindeque, P. K. (2018). Investigating microplastic trophic transfer in marine top predators. Environ. Pollut. 238, 999–1007. doi: 10.1016/j.envpol.2018.02.016
Nevitt, G. A. (2008). Sensory ecology on the high seas: the odor world of the procellariiform seabirds. J. Exp. Biol. 211, 1706–1713. doi: 10.1242/jeb.015412
Nishizawa, B., Thiebot, J. B., Sato, F., Tomita, N., Yoda, K., Yamashita, R., et al. (2021). Mapping marine debris encountered by albatrosses tracked over oceanic waters. Sci. Rep. 11:10944. doi: 10.1038/s41598-021-90417-x
OSPAR Commission (2008). Background Document for the EcoQO on Plastic Particles in Stomachs of Seabirds OSPAR Commission – Biodiversity Series. London: OSPAR.
Pershing, A. J., Alexander, M. A., Hernandez, C. M., Kerr, L. A., Le Bris, A., Mills, K. E., et al. (2015). Slow adaptation in the face of rapid warming leads to collapse of the Gulf of Maine cod fishery. Science 350, 809–812. doi: 10.1126/science.aac9819
Pierce, K. E., Harris, R. J., Larned, L. S., and Pokras, M. A. (2004). Obstruction and starvation associated with plastic ingestion in a Northern Gannet Morus bassanus and a Greater Shearwater Puffinus gravis. Mar. Ornithol. 32, 187–189.
Powers, K. D., Wiley, D. N., Allyn, A. J., Welch, L., and Ronconi, R. A. (2017). Movements and foraging areas of Great Shearwaters in the Gulf of Maine. Mar. Ecol. Prog. Ser. 574, 211–226.
Powers, K. D., Wiley, D. N., Robuck, A. R., Olson, Z. H., Welch, L. J., Thompson, M. A., et al. (2020). Spatiotemporal characterization of non-breeding Great Shearwaters Ardenna gravis within their wintering range. Mar. Ornithol. 48, 215–229.
Provencher, J. F., Bond, A. L., Avery-Gomm, S., Borrelle, S. B., Rebolledo, E. L. B., Hammer, S., et al. (2017). Quantifying ingested debris in marine megafauna: a review and recommendations for standardization. Anal. Methods 9, 1454–1469. doi: 10.1039/C6AY02419J
Provencher, J. F., Bond, A. L., Hedd, A., Montevecchi, W. A., Bin, S., Courchesne, S. J., et al. (2014). Prevalence of marine debris in marine birds from the North Atlantic. Mar. Pollut. Bull. 84, 411–417. doi: 10.1016/j.marpolbul.2014.04.044
Provencher, J. F., Borrelle, S. B., Bond, A. L., Lavers, J. L., Van Franeker, J. A., Kühn, S., et al. (2019). Recommended best practices for plastic and litter ingestion studies in marine birds: collection, processing, and reporting. FACETS 4, 111–130. doi: 10.1139/facets-2018-0043
R Core Team (2020). R: A Language and Environment for Statistical Computing. Vienna: R Foundation for Statistical Computing.
Rayner, M. J., Hartill, B. W., Hauber, M. E., and Phillips, R. A. (2010). Central place foraging by breeding Cook’s Petrel Pterodroma cookii: foraging duration reflects range, diet and chick meal mass. Mar. Biol. 157, 2187–2194. doi: 10.1007/s00227-010-1483-8
Robuck, A., Cantwell, M. G., McCord, J. P., Addison, L. M., Pfohl, M., Strynar, M. J., et al. (2020). Legacy and novel per- and polyfluoroalkyl substances (PFAS) in juvenile seabirds from the US Atlantic Coast. Environ. Sci. Technol. 54, 12938–12948. doi: 10.1021/acs.est.0c01951
Rodríguez, A., Ramírez, F., Carrasco, M. N., and Chiaradia, A. (2018). Seabird plastic ingestion differs among collection methods: examples from the Short-tailed Shearwater. Environ. Pollut. 243, 1750–1757. doi: 10.1016/j.envpol.2018.09.007
Roman, L., Lowenstine, L., Parsley, L. M., Wilcox, C., Hardesty, B. D., Gilardi, K., et al. (2019c). Is plastic ingestion in birds as toxic as we think? Insights from a plastic feeding experiment. Sci. Total Environ. 665, 660–667. doi: 10.1016/j.scitotenv.2019.02.184
Roman, L., Bell, E., Wilcox, C., Hardesty, B. D., and Hindell, M. (2019a). Ecological drivers of marine debris ingestion in procellariiform seabirds. Sci. Rep. 9:916. doi: 10.1038/s41598-018-37324-w
Roman, L., Paterson, H., Townsend, K. A., Wilcox, C., Hardesty, B. D., and Hindell, M. A. (2019d). Size of marine debris items ingested and retained by petrels. Mar. Pollut. Bull. 142, 569–575. doi: 10.1016/j.marpolbul.2019.04.021
Roman, L., Hardesty, B. D., Hindell, M. A., and Wilcox, C. (2019b). A quantitative analysis linking seabird mortality and marine debris ingestion. Sci. Rep. 9:3202. doi: 10.1038/s41598-018-36585-9
Roman, L., Gilardi, K., Lowenstine, L., Hardesty, B. D., and Wilcox, C. (2021). The need for attention to confirmation bias and confounding in the field of plastic pollution and wildlife impacts: comment on “clinical pathology of plastic ingestion in marine birds and relationships with blood chemistry”. Environ. Sci. Technol. 55, 801–804. doi: 10.1021/acs.est.0c02874
Roman, L., Hardesty, B. D., Hindell, M. A., and Wilcox, C. (2020). Disentangling the influence of taxa, behaviour and debris ingestion on seabird mortality. Environ. Res. Lett. 15:124071. doi: 10.1088/1748-9326/abcc8e
Roman, L., Schuyler, Q. A., Hardesty, B. D., and Townsend, K. A. (2016). Anthropogenic debris ingestion by avifauna in eastern Australia. PLoS One 11:e0158343. doi: 10.1371/journal.pone.0158343
Ronconi, R. A., Ryan, P. G., and Ropert-Coudert, Y. (2010). Diving of Great Shearwaters (Puffinus gravis) in cold and warm water regions of the South Atlantic Ocean. PLoS One 5:e0015508. doi: 10.1371/journal.pone.0015508
Ronconi, R. A., Schoombie, S., Westgate, A. J., Wong, S. N. P., Koopman, H. N., and Ryan, P. G. (2018). Effects of age, sex, colony and breeding phase on marine space use by Great Shearwaters Ardenna gravis in the South Atlantic. Mar. Biol. 165:58. doi: 10.1007/s00227-018-3299-x
Roscales, J. L., Vicente, A., Ryan, P. G., González-Solís, J., and Jiménez, B. (2019). Spatial and interspecies heterogeneity in concentrations of perfluoroalkyl substances (PFASs) in seabirds of the Southern Ocean. Environ. Sci. Technol. 53, 9855–9865. doi: 10.1021/acs.est.9b02677
Ryan, P. G. (1987a). The incidence and characteristics of plastic particles ingested by seabirds. Mar. Environ. Res. 23, 175–206. doi: 10.1016/0141-1136(87)90028-6
Ryan, P. G. (1987b). The effects of ingested plastic on seabirds: correlations between plastic load and body condition. Environ. Pollut. 46, 119–125. doi: 10.1016/0269-7491(87)90197-7
Ryan, P. G. (1988). Intraspecific variation in plastic ingestion by seabirds and the flux of plastic through seabird populations. Condor 90, 446–452. doi: 10.2307/1368572
Ryan, P. G. (2008). Seabirds indicate changes in the composition of plastic litter in the Atlantic and south-western Indian Oceans. Mar. Pollut. Bull. 56, 1406–1409. doi: 10.1016/j.marpolbul.2008.05.004
Ryan, P. G. (2015a). How quickly do albatrosses and petrels digest plastic particles? Environ. Pollut. 207, 438–440. doi: 10.1016/j.envpol.2015.08.005
Ryan, P. G. (2015b). Does size and buoyancy affect the long-distance transport of floating debris? Environ. Res. Lett. 10:84019. doi: 10.1088/1748-9326/10/8/084019
Ryan, P. G. (2019). “Ingestion of plastics by marine organisms,” in Hazardous Chemicals Associated with Plastics in the Marine Environment, eds H. Takada and H. K. Karapanagioti (Cham: Springer International Publishing), 235–266. doi: 10.1007/698_2016_21
Ryan, P. G., and Jackson, S. (1987). The lifespan of ingested plastic particles in seabirds and their effect on digestive efficiency. Mar. Pollut. Bull. 18, 217–219. doi: 10.1016/0025-326X(87)90461-9
Ryan, P. G., de Bruyn, P. J. N., and Bester, M. N. (2016). Regional differences in plastic ingestion among Southern Ocean fur seals and albatrosses. Mar. Pollut. Bull. 104, 207–210. doi: 10.1016/j.marpolbul.2016.01.032
Ryan, P. G., Moore, C. J., Van Franeker, J. A., and Moloney, C. L. (2009). Monitoring the abundance of plastic debris in the marine environment. Philos. Trans. R. Soc. B Biol. Sci. 364, 1999–2012. doi: 10.1098/rstb.2008.0207
Santos, R. G., Andrades, R., Demetrio, G. R., Kuwai, G. M., Sobral, M. F., Vieira, J. S., et al. (2020). Exploring plastic-induced satiety in foraging Green Turtles. Environ. Pollut. 265B:114918. doi: 10.1016/j.envpol.2020.114918
Savoca, M. S., Tyson, C. W., McGill, M., and Slager, C. J. (2017). Odours from marine plastic debris induce food search behaviours in a forage fish. Proc. R. Soc. Lond. B Biol. Sci. 284:20171000. doi: 10.1098/rspb.2017.1000
Savoca, M. S., Wohlfeil, M. E., Ebeler, S. E., and Nevitt, G. A. (2016). Marine plastic debris emits a keystone infochemical for olfactory foraging seabirds. Sci. Adv. 2:e1600395. doi: 10.1126/sciadv.1600395
Schoombie, S., Dilley, B. J., Davies, D., and Ryan, P. G. (2018). The foraging range of Great Shearwaters (Ardenna gravis breeding on Gough Island. Polar Biol. 41, 2451–2458. doi: 10.1007/s00300-018-2381-7
Senko, J., Nelms, S., Reavis, J., Witherington, B., Godley, B., and Wallace, B. (2020). Understanding individual and population-level effects of plastic pollution on marine megafauna. Endanger. Species Res. 43, 234–252. doi: 10.3354/esr01064
Silva, T. L., Wiley, D. N., Thompson, M. A., Hong, P., Kaufman, L., Suca, J. J., et al. (2020). High collocation of Sand Lance and protected top predators: implications for conservation and management. Conserv. Sci. Pract. 3:e274. doi: 10.1111/csp2.274
Suca, J. J., Wiley, D. N., Silva, T. L., Robuck, A. R., Richardson, D. E., Glancy, S. G., et al. (2021). Sensitivity of Sand Lance to shifting prey and hydrography indicates forthcoming change to the Northeast US shelf forage fish complex. ICES J. Mar. Sci. 78, 1023–1037. doi: 10.1093/icesjms/fsaa251
Tanaka, K., Takada, H., Yamashita, R., Mizukawa, K., Fukuwaka, M., and Watanuki, Y. (2015). Facilitated leaching of additive-derived PBDEs from plastic by seabirds’ stomach oil and accumulation in tissues. Environ. Sci. Technol. 49, 11799–11807. doi: 10.1021/acs.est.5b01376
Terepocki, A. K., Brush, A. T., Kleine, L. U., Shugart, G. W., and Hodum, P. (2017). Size and dynamics of microplastic in gastrointestinal tracts of Northern Fulmars (Fulmarus glacialis) and Sooty Shearwaters (Ardenna grisea). Mar. Pollut. Bull. 116, 143–150. doi: 10.1016/j.marpolbul.2016.12.064
Townsend, D. W., McGillicuddy, D. J., Thomas, M. A., and Rebuck, N. D. (2014). Nutrients and water masses in the Gulf of Maine-Georges Bank region: variability and importance to blooms of the toxic dinoflagellate Alexandrium fundyense. Deep. Res. Part II Top. Stud. Oceanogr. 103, 238–263. doi: 10.1016/j.dsr2.2013.08.003
Van Franeker, J. A. (2004). Save the North Sea Fulmar-Litter-EcoQO Manual – Part 1: Collection and Dissection Procedures. Alterra-rapport 672. Wageningen: Alterra, 1–38.
Van Franeker, J. A., and Law, K. L. (2015). Seabirds, gyres and global trends in plastic pollution. Environ. Pollut. 203, 89–96. doi: 10.1016/j.envpol.2015.02.034
Van Franeker, J. A., and Meijboom, A. (2002). LITTER NSV, Marine Litter Monitoring by Northern Fulmars: A Pilot Study. Wageningen: Alterra.
Van Sebille, E., Aliani, S., Law, K. L., Maximenko, N., Alsina, J. M., Bagaev, A., et al. (2020). The physical oceanography of the transport of floating marine debris. Environ. Res. Lett. 15:23003. doi: 10.1088/1748-9326/ab6d7d
Verlis, K. M., Campbell, M. L., and Wilson, S. P. (2013). Ingestion of marine debris plastic by the Wedge-tailed Shearwater Ardenna pacifica in the Great Barrier Reef, Australia. Mar. Pollut. Bull. 72, 244–249. doi: 10.1016/j.marpolbul.2013.03.017
Vlietstra, L. S., and Parga, J. A. (2002). Long-term changes in the type, but not amount, of ingested plastic particles in Short-tailed Shearwaters in the southeastern Bering Sea. Mar. Pollut. Bull. 44, 945–955. doi: 10.1016/S0025-326X(02)00130-3
Wilcox, C., Hardesty, B. D., and Law, K. L. (2020). Abundance of floating plastic particles is increasing in the Western North Atlantic Ocean. Environ. Sci. Technol. 54, 790–796. doi: 10.1021/acs.est.9b04812
Keywords: Ardenna gravis, migration, pollution, shearwaters, marine debris, microplastic, nurdles, bycatch
Citation: Robuck AR, Hudak CA, Agvent L, Emery G, Ryan PG, Perold V, Powers KD, Pedersen J, Thompson MA, Suca JJ, Moore MJ, Harms CA, Bugoni L, Shield G, Glass T, Wiley DN and Lohmann R (2022) Birds of a Feather Eat Plastic Together: High Levels of Plastic Ingestion in Great Shearwater Adults and Juveniles Across Their Annual Migratory Cycle. Front. Mar. Sci. 8:719721. doi: 10.3389/fmars.2021.719721
Received: 03 June 2021; Accepted: 02 November 2021;
Published: 05 January 2022.
Edited by:
Matthew Savoca, Stanford University, United StatesReviewed by:
Lauren Roman, Commonwealth Scientific and Industrial Research Organisation (CSIRO), AustraliaVeronica Padula, University of Alaska Fairbanks, United States
Copyright © 2022 Robuck, Hudak, Agvent, Emery, Ryan, Perold, Powers, Pedersen, Thompson, Suca, Moore, Harms, Bugoni, Shield, Glass, Wiley and Lohmann. This is an open-access article distributed under the terms of the Creative Commons Attribution License (CC BY). The use, distribution or reproduction in other forums is permitted, provided the original author(s) and the copyright owner(s) are credited and that the original publication in this journal is cited, in accordance with accepted academic practice. No use, distribution or reproduction is permitted which does not comply with these terms.
*Correspondence: Anna R. Robuck, YW5uYXJvYnVja0BnbWFpbC5jb20=
†These authors have contributed equally to this work and share last authorship