- 1Department of Biological Sciences, Macquarie University, North Ryde, NSW, Australia
- 2Institute for Antarctic and Marine Studies, University of Tasmania, Hobart, TAS, Australia
- 3IMOS Animal Tagging, Sydney Institute of Marine Science, Mosman, NSW, Australia
- 4NIWA, Wellington, New Zealand
- 5National Oceanic and Atmospheric Administration, National Marine Fisheries Service, Alaska Fisheries Science Center, National Marine Mammal Laboratory, Seattle, WA, United States
- 6Sorbonne Université, CNRS/IRD/MNHN, LOCEAN-IPSL, Paris, France
- 7Institute of Marine Sciences, University of California, Santa Cruz, Santa Cruz, CA, United States
- 8Woods Hole Oceanographic Institution, Woods Hole, MA, United States
- 9CSIRO Oceans and Atmosphere, CSIRO, Hobart, TAS, Australia
- 10Te Kura Aronukurangi, Gateway Antarctica, University of Canterbury, Canterbury, New Zealand
- 11Wellington Zoo, Wellington, New Zealand
The relative importance of intrinsic and extrinsic determinants of animal foraging is often difficult to quantify. The most southerly breeding mammal, the Weddell seal, remains in the Antarctic pack-ice year-round. We compared Weddell seals tagged at three geographically and hydrographically distinct locations in East Antarctica (Prydz Bay, Terre Adélie, and the Ross Sea) to quantify the role of individual variability and habitat structure in winter foraging behaviour. Most Weddell seals remained in relatively small areas close to the coast throughout the winter, but some dispersed widely. Individual utilisation distributions (UDi, a measure of the total area used by an individual seal) ranged from 125 to 20,825 km2. This variability was not due to size or sex but may be due to other intrinsic states for example reproductive condition or personality. The type of foraging (benthic vs. pelagic) varied from 56.6 ± 14.9% benthic dives in Prydz Bay through 42.1 ± 9.4% Terre Adélie to only 25.1 ± 8.7% in the Ross Sea reflecting regional hydrographic structure. The probability of benthic diving was less likely the deeper the ocean. Ocean topography was also influential at the population level; seals from Terre Adélie, with its relatively narrow continental shelf, had a core (50%) UD of only 200 km2, considerably smaller than the Ross Sea (1650 km2) and Prydz Bay (1700 km2). Sea ice concentration had little influence on the time the seals spent in shallow coastal waters, but in deeper offshore water they used areas of higher ice concentration. Marine Protected Areas (MPAs) in the Ross Sea encompass all the observed Weddell seal habitat, and future MPAs that include the Antarctic continental shelf are likely to effectively protect key Weddell seal habitat.
Introduction
Where and how animals forage is fundamental to almost all aspects of their biology. Animal foraging strategies are determined by a complex mix of intrinsic (e.g., personality, sex, age, and reproductive status) and extrinsic (e.g., topography/bathymetry, resource availability, habitat) factors which shape the distribution and availability of prey and presence of predators (McNamara and Houston, 1986; Stephens and Krebs, 1986; Daunt et al., 2005; Stephens, 2008; Russell et al., 2015). The relative importance of these factors varies within and among species, but how a population or species responds to change, whether short- or long-term, will depend on a complex interplay of the relative importance of these factors and the advantages that they confer. Quantifying the role of intrinsic and extrinsic factors in determining foraging strategies can be difficult due to the dynamic nature of individual behaviour and environmental conditions (Humphreys et al., 2006). One way to disentangle competing factors is to apply a comparative approach wherein different demographic classes or geographic regions are compared (Catry et al., 2009). For example, regional comparisons of foraging behaviour in Northern fur seals, Callorhinus ursinus, (Nordstrom et al., 2013), and southern elephant seals, Mirounga leonina, (Hindell et al., 2016) have revealed habitat partitioning and distinct individual foraging strategies that differ by sex and/or location.
In the marine environment, megafauna often exhibit different foraging behaviours over continental shelves compared to open ocean environments, with more directed movement in the latter, presumably a function of its less complex, if not more dynamic, features (Sequeira et al., 2018). The Southern Ocean is one of Earth’s most extreme marine environments, and its physical structure is highly variable seasonally and spatially (Constable et al., 2003). Coastal bathymetry in the Southern Ocean is complex, with shallow banks and deep canyons compounding dynamic and static aspects of the shelf environment, as illustrated by the inflow of Modified Circumpolar Deep water (mCDW) onto the shelf and the outflow of Antarctic Bottom water to the abyssal ocean (Williams et al., 2016). This is further confounded by the formation and movement of ice (Cavalieri et al., 1999) which influences primary production and food web dynamics (Nicol et al., 2000). At the same time, the Southern Ocean is a highly productive environment that attracts multiple predator species including migratory (e.g., southern elephant seals, killer whales, Orcinus orca) and resident species such as Weddell seals (Leptonychotes weddellii), Adélie (Pygoscelis adeliae), and emperor (Aptenodytes forsteri) penguins (Hindell et al., 2020). Although migratory species forage voraciously during spring and summer, they are excluded from the continental shelf regions around the Antarctic continent as the winter ice expands by up to 300% (Cavalieri et al., 1999; Brierley and Thomas, 2002). Of the resident species, only Weddell seals and Emperor penguins appear capable of surviving in situ year-round without obligative dispersal (Burns and Kooyman, 2001). Emperor penguins winter-over with males fasting while incubating their single egg, and females dispersing to forage (Kirkwood and Robertson, 1997), but Weddell seals continue to forage under the sea ice and actively maintain breathing holes throughout the winter (Kooyman et al., 1981).
There is limited understanding of the extrinsic factors influencing Weddell seal distribution and foraging behaviour during winter. Pioneering investigations showed that an isolated colony at White Island, Ross Archipelago (78°8′S 167°24′E) foraged by diving to the sea floor, primarily feeding upon benthic prey (Castellini et al., 1984). Similarly, free-ranging Weddell seals off Terre Adélie and in Prydz Bay, stayed within 5 km of breathing holes, moving only as local food became depleted (Heerah et al., 2016). Heerah et al. (2017) also reported regional differences, with seals in Prydz Bay travelling up to three times the distance and spending half the time in hunting mode than seals off Terre Adélie. Hunting dives at both locations were pelagic, concentrated in areas of high ice concentration, over areas of relatively complex bathymetry and favouring water masses such as Antarctic Surface Water and mCDW (Heerah et al., 2013, 2016). However, other studies from the Ross Sea (Testa, 1994) and the Weddell Sea (Nachtsheim et al., 2019; Photopoulou et al., 2020; Labrousse et al., 2021) reported dispersal of several hundred kilometres, albeit for the most part staying close to or over the continental shelf.
Intrinsic factors that influence foraging strategies of marine predators include age, sex, body-size, condition, reproductive status (Weise et al., 2010; Votier et al., 2017; Salton et al., 2019), intra-specific competition (Kuhn et al., 2014), and individual personality traits. Within a dynamic environment, distinct foraging strategies (e.g., epipelagic, mesopelagic or benthic) may coexist in a population (Cherel and Hobson, 2007); or be state-dependent with animals in poorer condition adopting riskier foraging behaviours (Beltran et al., 2021). Diverse foraging strategies are likely to arise because within a diverse prey field, different individuals will have greater foraging success depending on local conditions, and no single individual strategy will dominate under all conditions.
Weddell seal movements are constrained during winter by heavy pack-ice and access to open water to breathe. This study quantifies factors influencing the distribution and foraging behaviour of Weddell seals, by for the first time comparing three geographically discrete locations in Antarctica during the austral winter. We consider three aspects of Weddell seal foraging ecology: (i) individual differences in benthic vs. pelagic foraging and foraging trip characteristics; (ii) the relationship between winter distribution of individual seals and bathymetry and sea ice concentration; and (iii) comparing these physical characteristics with the overall utilisation distributions for each deployment location.
Materials and Methods
Deployment Locations (Refer to Figures 1, 3)
Prydz Bay (Davis Station)
Prydz Bay is the third largest embayment on the Antarctic coast and is located in the southern Indian Ocean sector. Prydz Bay is broadly similar to its larger counterparts in the Weddell and Ross Seas. The bay is generally 500–600 m deep, but there are areas that are over 1000 m in depth (1085 m) adjacent to the Amery Ice Shelf. The bathymetry within Prydz Bay is complex and there are numerous shallow banks rising to 200 m depth e.g., Fram Bank and Four Ladies Bank (Nunes Vaz and Lennon, 1996).
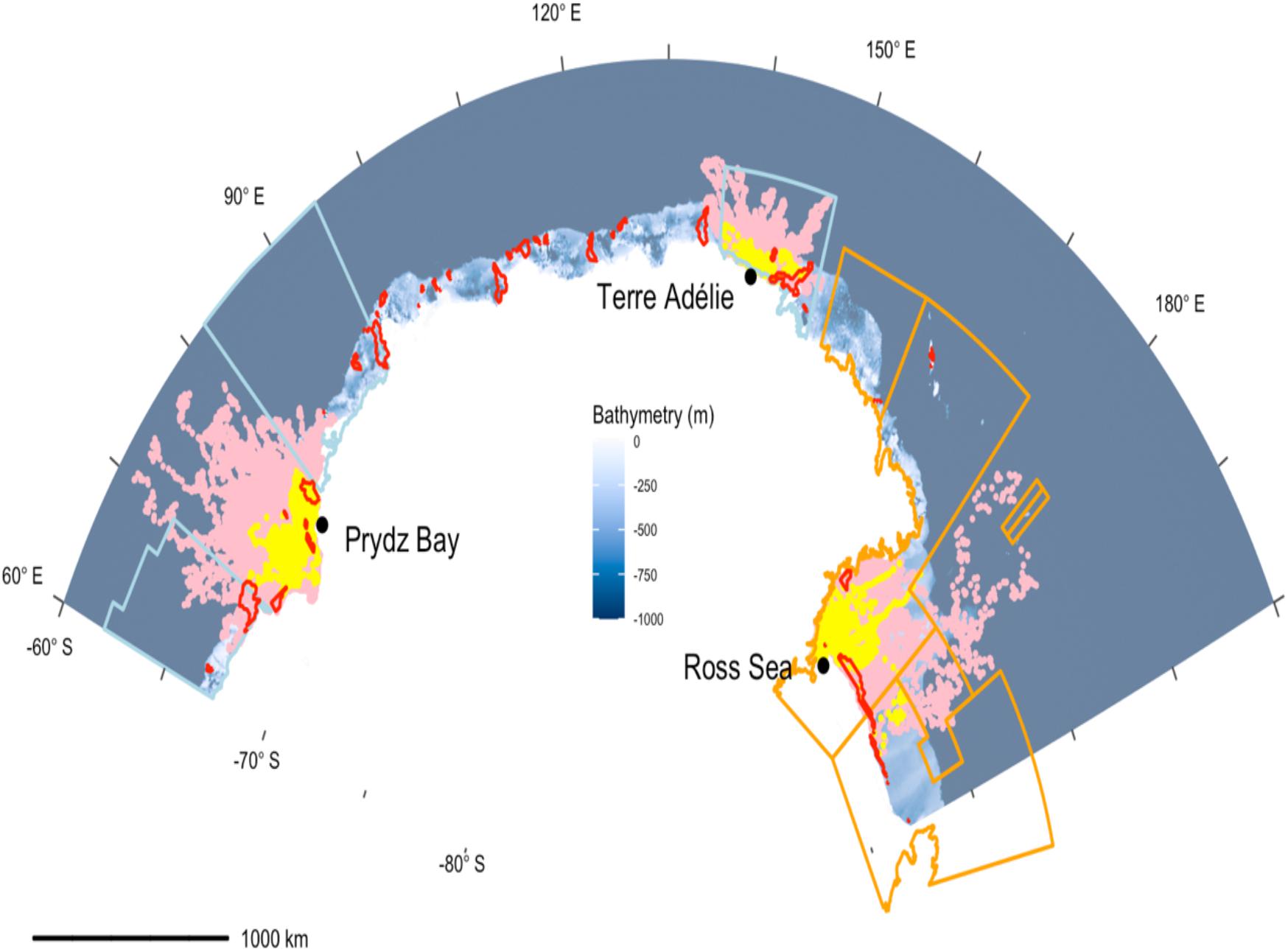
Figure 1. Maps of the estimated daily locations (yellow points) of 78 Weddell seals tracked over the winter months in three regions (Prydz Bay, Terre Adélie, and Ross Sea). The blue background represents the GEBCO19 bathymetry. Recurrent polynyas (from Arigo et al., 2003) are outlined in red. The orange line outlines the existing Ross Sea Marine Protected Area and the light blue line outlines the proposed East Antarctica Marine Protected Area (MPA). The pink dots indicate the regions accessible to the seals based on simulated tracks.
Terre Adélie (Dumont d’Urville Station, DDU)
Our study site in Terre Adélie was the only one not situated in major embayment resulting in a relatively narrow shelf, 120–130 km wide. There is a complex network of submarine canyons with the Jussieu and Cuvier canyons connecting the shelf break and inner-shelf depressions. Within the canyons, there is a steep corrugated slope with deep-sea channels between 2000 and 3000 m in depth. At the base of the slope is a gentler lower rise that in areas exceeds 3000 m deep. Over the shelf, shallow banks of 200 m (Adélie and Mertz Banks) alternate with deep inner-shelf depressions, some of which are large e.g., the D’Urville Trough, the George V and Adélie Basins (Koubbi et al., 2010).
Ross Sea (Scott Base)
The Ross Sea continental shelf is extensive, unusually deep (mean depth 600 m) with a complex bathymetry which includes numerous deep troughs (up to 1200 m deep) and shallow banks (less than 200 m). Where the seals were tagged in the south, the region is dominated by the deep McMurdo Sound (up to 700 m). An important feature of the Ross Sea is the high rates of tidal current flow that occurs across the region which results in the resuspension of phytodetritus, in turn providing benthic suspension feeders with enhanced concentrations of food and transporting organic matter over large distances. This enhanced benthic productivity plays an important role in structuring the ecosystem, including the distribution of larger predator species such as Weddell seals (Smith et al., 2007).
Tag Deployment and Data Processing
Weddell seals were equipped with either Satellite-Relayed Data Loggers (SRDLs) or Conductivity-Temperature-Depth SRDLs (CTD-SRDLs) manufactured by the Sea Mammal Research Unit, University of St Andrews (Boehme et al., 2009). Deployments took place at two sites in East Antarctica: in Prydz Bay near Davis Station (−68.58°, 77.97°, n = 27, years: 2006, 2007, and 2011) and Terre Adélie near Dumont d’Urville Station, (DDU) (−66.66°, 140.00°, n = 22, years: 2006, 2007, 2008, 2009, and 2019) and one site in the Ross Sea near Scott Base (−77.85°, 166.76°, n = 29, years: 2014, 2016, and 2019). Note that the Prydz Bay and the Terre Adélie data sets (excluding 2019) formed the basis of Heerah et al. (2017). Only females were tagged in Prydz Bay and the Ross Sea, in Terre Adélie both sexes were tagged. The tags were all deployed following the annual moult in February. The capture, sedation and tagging procedures followed those described in detail elsewhere (Mellish et al., 2010; Heerah et al., 2013; Shero et al., 2018), with the tags glued directly on the head of the seal. The tags recorded data on a seal’s diving behaviour as well as in situ hydrographic conditions. Summaries of these data were transmitted via the polar-orbiting Argos satellite constellation whenever seals surfaced to breathe (Harcourt et al., 2019). Only seals for which the tag transmitted for longer than 50 days were included in the analyses.
Diving Behaviour and Bathymetry
The dive data underwent an initial quality control to remove anomalously deep dives. This was done by calculating a lower edge for the dive duration vs. dive depth scatter plot (using a quantile regression set at 0.05). Dives that fell below this edge were deemed to be too deep for that duration (thereby requiring unrealistic rates of travel) and were flagged for removal. We also removed dives of less than 5 min duration as these were regarded as unlikely to be foraging, and dives deeper than −1000 m as these were deeper than the Antarctic continental shelf (Padman et al., 2010). A location was recorded for each remaining dive by using a state-space-model with a 2 h time step and interpolating from the start time of each dive (Jonsen et al., 2020). Each dive was then allocated a bathymetric depth using GEBCO19 (Gebco Compilation Group., 2020).
A scatter plot of bathymetric depth against the maximum dive depth of each dive indicated that 23.6% of the total 171,201 dives were more than 20 m deeper than the GEBCO19 bathymetry at that point (Supplementary Figure 1). The three sources of error that contribute to this mismatch are (1) errors in dive location estimates, (2) errors in the tag’s depth sensors and (3) errors in the bathymetry. These uncertainties were problematic for allocating individual dives to a behavioural type (e.g., hunting “benthic” vs. “pelagic” prey). Therefore, we calculated the difference in depth between the GEBCO19 bathymetry for those dives that exceeded the bathymetry. We then calculated the 30 percentile of these differences, as this approximates 1 Standard Error of a distribution centred on the red line representing equivalence of a dives depth and estimated bathymetry at that point. This value was ∼100 m. Assuming that these mismatches were symmetrical about the 1:1 line in Supplementary Figure 1, we then categorised any dives that were within 100 m of the GEBCO bathymetry as “benthic” dives and those that were >100 m from the bottom as “pelagic” dives. Dives more than 100 m deeper than the bottom was classified as “unknown” (Supplementary Figure 1).
We compared simple metrics of diving behaviours (maximum depth and dive duration) for benthic and pelagic dives between the three study sites using generalised linear mixed models (GLMMs) with individual seal as a random term and deployment location as the main effect. We also modelled the probability of making a benthic dive relative to the bathymetry at the location of that dive using a logistic GLMM, again with individual seal as a random term. We then used this model to make spatial predictions of where seals were likely to make benthic dives through the entire domain (defined by the extent of seal tracks) at each deployment location.
Calculation of Utilisation Distributions
Utilisation Distributions are a common way of quantifying spatial use by individuals and populations (Laver and Kelly, 2008). We used a raster-based approach to calculate the utilisation distributions (UD), in which we recognised two types of Utilisation Distributions: (i) Individual utilisation distributions (UDi), that is the total area used by an individual seal and (ii) Sample utilisation distributions (UDs) representing the spatial use by the population of seals tagged at each deployment location. We used a raster with a 5 × 5 km grid cell. This resolution corresponds to the estimated accuracy (± 5 km) of state-space-model modelled ARGOS-based elephant seal locations using the same trackers (Jonsen et al., 2020). The UDs analysis required three rasters (Supplementary Figure 2); (i) the density (number) of seals that visited each 5 × 5 km cell, (ii) the mean time spent by each seal in each cell (i.e., the overall mean of the individual UDi raster stack) (iii) the product of these two rasters provides a measure of overall habitat utilisation in terms of seal days per cell. This value is a close analogue of kernel density analysis but has the advantage of not imposing a smoother over the data. With sufficient data this smoothing is unnecessary as we have an empirical, quantitatively assessed, measure of the degree of use per cell. To estimate the degree of representativeness of the sample of seals compared to the actual population of seals at each location, we calculated the cumulative area occupied by increasing numbers of seals (Supplementary Figure 3) (Hindell et al., 2003). Finally, we calculated the percent usage contours by sorting the UDs values in each cell, taking the cumulative sum, and using that to identify user defined contours. While there are many methods for summarising utilisation distributions there is broad agreement on the use of 50% contours (i.e., enclosing 50% of locations) to define the core usage areas and 90% to indicate overall usage (Laver and Kelly, 2008).
Individual Utilisation Distributions Statistics
First, we calculated the area (km2) of each UDi by counting the number of 5 × 5 km cells visited by each seal and compared these among the three deployment locations using a GLMM which included deployment duration as a random term to account for animals with longer durations potentially having larger UD areas. Seal standard length (m) was included in the model to account for animal size potentially being an important source of individual variation in space use (Hindell et al., 2021). We used length as it was the only morphometric measure common to all studies. We also compared UDi areas between sexes at DDU, the only site where males were also instrumented, to ascertain if there were differences in habitat use and foraging by sex (Photopoulou et al., 2020).
The Role of Bathymetry and Sea-Ice Concentration on the Winter Distribution of Individual Seals
We fit GLMMs relating the number of days that an individual spent in a 5 × 5 km cell to the bathymetry and ice concentration in that cell. Ice concentration in a cell varied little over the winter months, so we took the mean of the ice concentrations over the study period in each cell. While this may lose some fine-scale detail, the Antarctic continental shelf is fully covered by sea-ice by April (Eayrs et al., 2019) and so inconsequential (study lasts March–October). Prior to analysis, outliers (1 and 99% percentiles) for both the response and predictor variables were trimmed from the data set. Predictor variables were also centred and scaled to unit variance to facilitate efficient optimisation of the GLMMs. The distributions of each variable were checked for normality and the response variable (seal days per cell) was subsequently log-transformed. Inter-correlation between the predictor variables were checked with cross-correlation analyses, comparing Pearson Correlation coefficients. The full model GLMMs (with individual seal as a random term) were initially run with and without a spatial autocorrelation term to test its effect on model performance. We found that the spatial autocorrelation term did not affect model performance and so all subsequent analyses were run excluding it. Comparison of log-likelihoods and corrected Akaike Information Criterion (AICc) indicated that the model performed best without spatial autocorrelation, so this was not used in the final model assessment. Overall model fit was assessed with the conditional r-squared which provides the variance explained by the entire model, i.e., both fixed effects and random effects. Finally, we ranked the full suite of 19 models by AICc to identify the best performing model (Burnham and Anderson, 2002, 2004).
The Role of Bathymetry and Sea-Ice Concentration on the Winter Distribution of the Populations of Weddell Seals
We assessed qualitatively the physical characteristics of the seals’ habitats at the population level by contrasting the distribution of bathymetry and sea-ice concentrations within three locations, (i) the core UDs (50%) the overall UDs (90%) and the regions potentially available to the seals. The UDs derived from observed locations only provide information about where animals occur, not about where they could have gone. To estimate potentially available geographic space, we simulated sets of tracks for each observed track using the availability R package1 simulating 10 tracks per seal. This yielded simulated tracks with movement characteristics (distributions of step length and turning angle) similar to observed tracks, but tracks were random and independent of environmental effects. These simulated tracks provide an estimate of the geographic space that each animal could have occupied (given its movement characteristics and track length) if it had no habitat preferences (Raymond et al., 2014; Hindell et al., 2020). We compared the ice concentration on July 30 (averaged for all years of the study) as this was the time when ice extent was nearing its maximum, and when most tags were still transmitting.
Analyses were conducted in R 4.0.2 (R Core Team, 2020). FoieGras was used to filter the seal tracks (Jonsen et al., 2020). Descriptive statistics presented as mean (X) and standard deviation (±s.d) unless indicated. All models were Generalised Linear Mixed Effects Models in the R package LME4. The models were assessed by ranking candidate models using AICc, and evidence ratios calculated for each model comparison to provide a2n indication of the importance relative to the top model (Burnham and Anderson, 2004).
Results
Overview of Seal Distributions
Tags transmitted for 150.1 ± 72 days (mean ± s.d. Table 1 and Supplementary Figure 3) and this did not vary between sites (Supplementary Table 1a). Seals from all three deployment locations occurred almost exclusively on the Antarctic continental shelf (seafloor >−1000 m) with seals staying within 200 km of the central point of their distribution (Figure 1). This central point was not where they were tagged, because they were tagged when aggregated to moult. Post-moult, the animals moved to other areas as the sea-ice grew. The seals then remained relatively local with individuals moving on average 138.2 ± 117.8 km from that central point. This differed among the three deployment locations; seals tagged in the Ross Sea travelled further than seals in Prydz Bay (mean maximum distance = 178.0 ± 147.3 km compared to Prydz Bay mean max = 151.0 ± 89.1 km) and Terre Adélie (mean max = 70.5 ± 70.4 km) (Table 1).
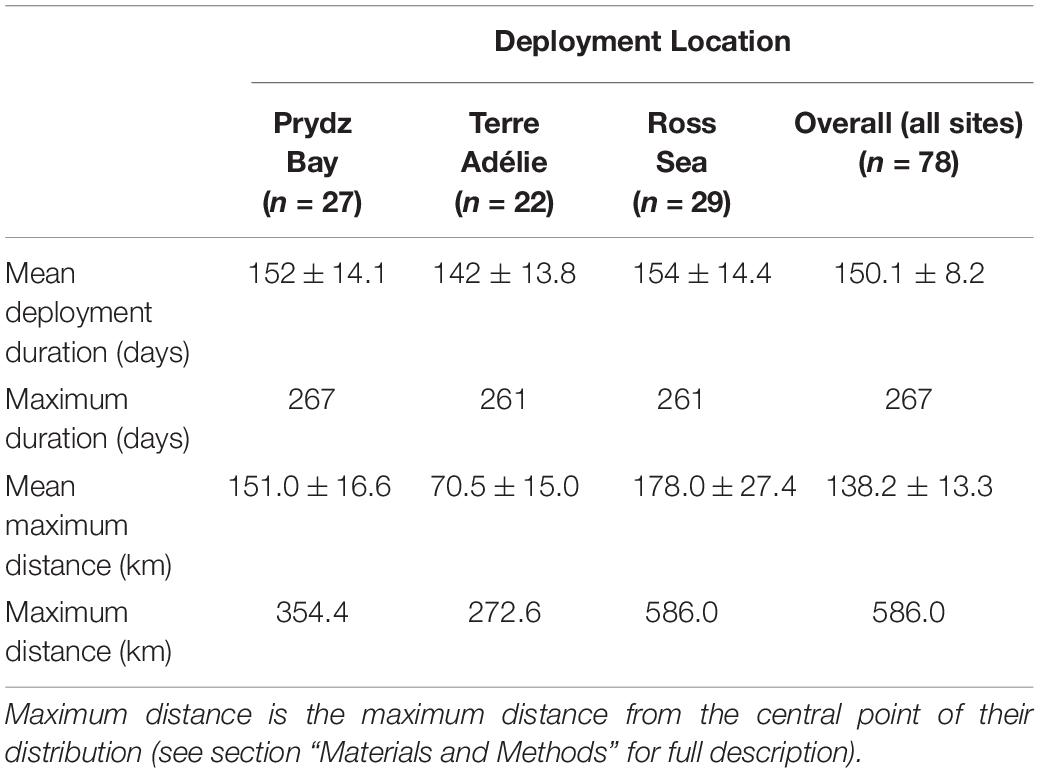
Table 1. Summary statistics (mean ± s.d.) of tracking data from Weddell seals and three deployment locations during the winter months.
Diving Behaviour
All seals made at least some benthic dives. Nearly 50% of dives were pelagic, 40% benthic and 12% unknown. Seals apportioned dives differently between deployment locations, with 60% benthic dives in Prydz Bay, 42% in Terre Adélie and 25% in the Ross Sea (Table 2).
Benthic dives were deeper than pelagic dives (Table 2). The mean ± s.e. depth of a benthic dive was deepest in the Ross Sea (216 ± 40.0 m), followed by Prydz Bay at 205 ± 67.9 m and Terre Adélie at 122 ± 36.6 m (Table 2). For pelagic dives, the deepest were in the Ross Sea (148 ± 27.4 m) followed by Prydz Bay (86.9 ± 23.7 m) and the shallowest in Terre Adélie (64.9 ± 18.9 m) (Table 2). The proportion of dives classified as unknown varied with Prydz Bay and Terre Adélie low (7.2 and 8.5%, respectively), but in the Ross Sea 18.1% were classified as unknown, reflecting the poorer understanding of its bathymetry.
The probability of a seal making a benthic dive decreased with increasing ocean depth (Supplementary Table 1g), but varied among the regions (Figure 2A and Supplementary Table 1g). In Prydz Bay and the Ross Sea, benthic dives were highly likely (probability = 0.75) at ocean depths of around −250 m, while the 0.75 likelihood was approximately −125 m in Terre Adélie.
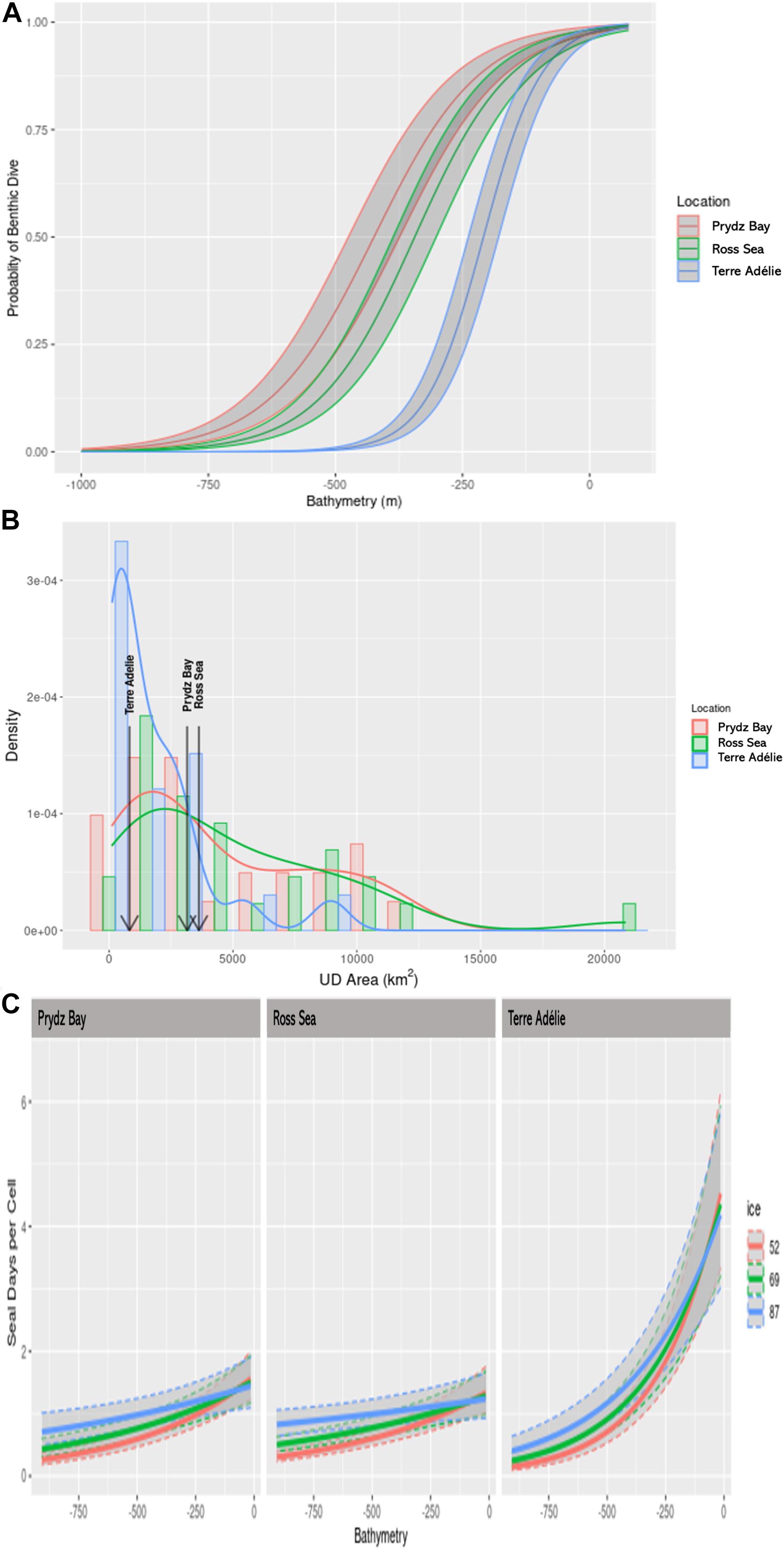
Figure 2. (A) The predicted probability of a dive being benthic with respect to bathymetry, based on a binomial GLMM. Shaded areas represent 95% confidence intervals. (B) Frequency distribution (bars) and density (curves) of the area of individual Utilisation Distributions of Weddell seals from three regions in Antarctica. The arrows indicate the median areas for each of the locations. (C) Predicted values of seal days per cell from the best model using location, bathymetry and sea-ice concentration (day∼location + bathymetry, ice, bathymetry:location, bathymetry:ice). The figure illustrates the interaction between bathymetry and sea-ice concentration. In this case values were predicted for high (87%) medium (69%), and low (52%) ice concentrations (intervals set by the R package ggeffects).
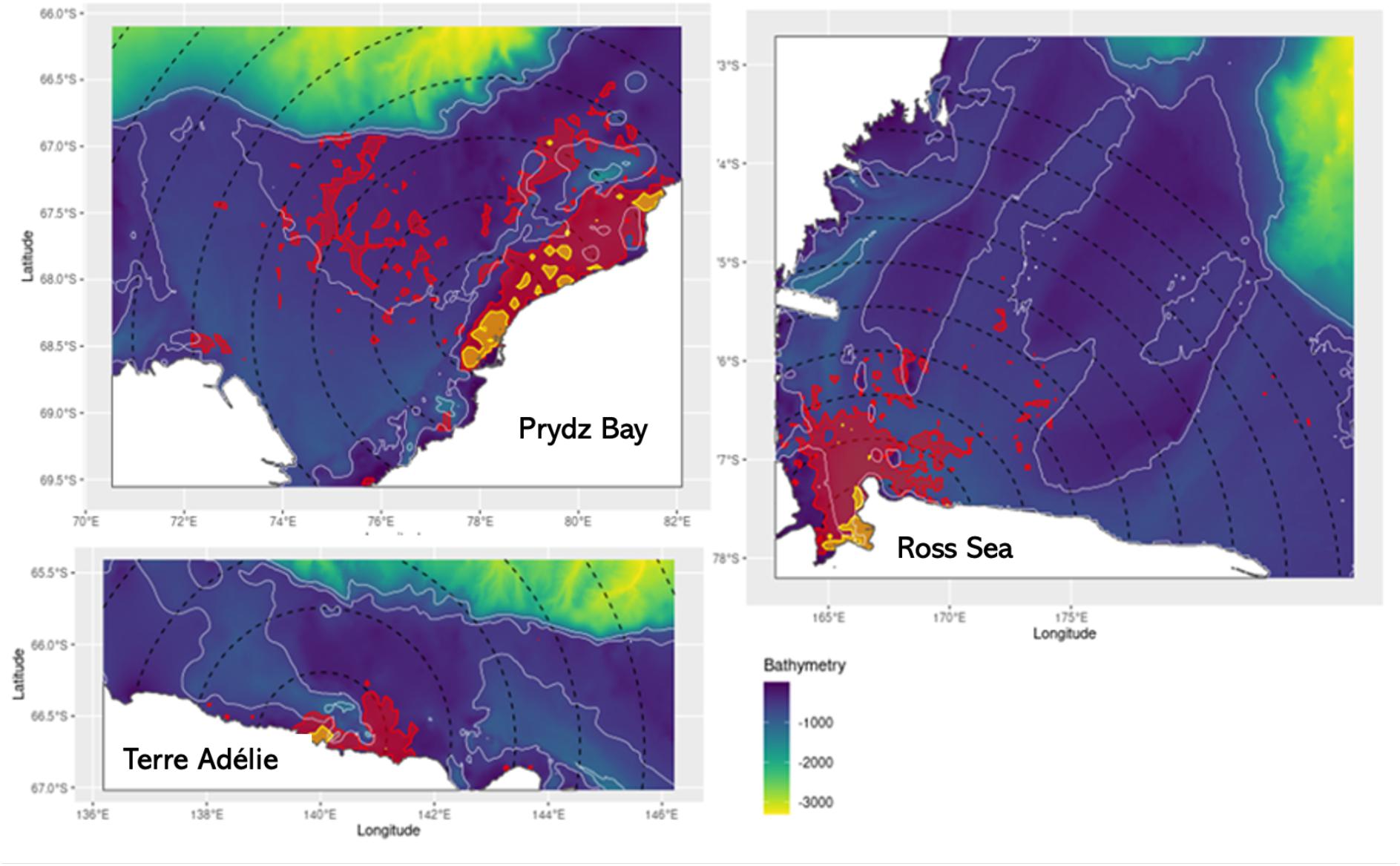
Figure 3. The sample Utilisation Distributions for Weddell seals at each location. These represent the number seal days per 5 × 5 km pixel and were calculated as the product of the mean of the individual UDs (mean days per pixel) and the total number of seals that used each pixel. The yellow areas enclose the cells in which 50% of all seal days occurred (which we regard as the core UD for each population) and the red polygons enclose the cells in which 90% of all seal days occurred. The black dotted lines indicate 50 km bands from the centre of the overall distribution (defined as the most commonly used 5 × 5 km pixel). The white contours represent the –1000 and –500 m bathymetric contours.
Utilisation Distributions
Individual Utilisation Distributions
Individual Utilisation Distributions (UDis) varied among individuals and locations (Figure 2B), but individual size (seal length) was not influential (Supplementary Table 1e). The median area of the UDi for seals in Terre Adélie was 825 km2, less than 1/3 of the 3150 km2 in Prydz Bay and 3625 km2 in the Ross Sea. There were no differences in the size of the UDis for male and female seals in Terre Adélie (Supplementary Table 1d). Consequently, we did not discriminate male and female seals in subsequent analyses.
The Role of Bathymetry and Sea-Ice Concentration on the Winter Distribution of Individual Seals
The best model relating the number of seal days per 5 × 5 km pixel included location, bathymetry, ice concentration and the interactions of deployment location with bathymetry and bathymetry with ice concentration, an AICc value of 12538.1 and an AICc weight of 0.996 (Supplementary Table 1f). When considering main effects, bathymetry was best of the single term models with an AICc of 12670.7, followed by ice concentration (12776.0) and then location (12784.3). The null model had an AICc of 12794.9. Intensity of use of a cell was negatively related to bathymetry with the areas of most intensive use in shallowest waters, and this was most pronounced in Terre Adélie (Figure 2C). However, when considering the interaction terms, the intensity of use was slightly higher in high ice concentration over deep water (Figure 2C).
The Role of Bathymetry and Sea-Ice Concentration on the Winter Distribution of the Populations of Weddell Seals
Our sample sizes, while not large enough to capture the estimated maximum area used by a theoretical population of seals (Supplementary Figure 4), did cover more than 85% of the estimated asymptote. In Prydz Bay, the Gompertz model fitted to the saturation curve had an asymptote of 58335 km2, of which our 26 seals occupied (91.8%). In Terre Adélie the 22 seals occupied 86.7% of the estimated 21185 km2, and in the Ross Sea the 28 seals occupied 91.0% of the estimated 66724 km2.
The core (50%) population UDs were in shallow waters within 200 km of the central pixel (Figure 3) at all locations. In Prydz Bay, the core UDs extended northward along the coast, largely contained within the −500 m bathymetric contour. The seals in Terre Adélie had the smallest core UDs mostly within 50 km of the central point and also within the −500 m bathymetric contour. The core UDs for seals in the Ross Sea was concentrated on the western edge of McMurdo Sound extending 50 km north of Ross Island. In Prydz Bay the core 50% UDs with an area of 1700 km2 occupied 12.6% of the overall 90% UDs of the sample of seals at that site. In Terre Adélie the core UD (200 km2) occupied 10% of the overall 90% UDs, and in the Ross Sea (core = 1650 km2) 11.1% of the 90% UDs.
The bathymetry available to the seals differed from those in the overall and core UDs, indicating a strong preference for shelf waters, with randomised tracks venturing off continental shelf break, with mean values deeper than −1000 m for seals from Prydz Bay and Terre Adélie (Figure 4 and Supplementary Table 2). Seals from the Ross Sea showed a different pattern, with available habitat almost exclusively contained to shelf. The bathymetry within the core UDs also differed from the overall (90%) UDs (Figure 4 and Supplementary Table 2). At all three locations, the core areas were in considerably shallower water than the overall area (Supplementary Table 2), reflecting the coastal positions of the core UDs. In the Ross Sea overall UDs had the deepest bathymetry (mean = −608 ± 147 m) followed by Prydz Bay (−370 ± 112 m) and then Terre Adélie (−262 ± 118 m) reflecting the topographic characteristics of the locations. Ice characteristics varied between available, core and overall areas (Figure 4 and Supplementary Table 2). Ice concentrations were consistently higher within regions available to the seals (Figure 4) compared to regions they actually used. Further, ice concentration was slightly lower in core UDs than overall UDs, although the differences were relatively minor, being largest in the Ross Sea (85.0 ± 3.7 [90%] compared to 79.4 ± 2.2 [50%]).
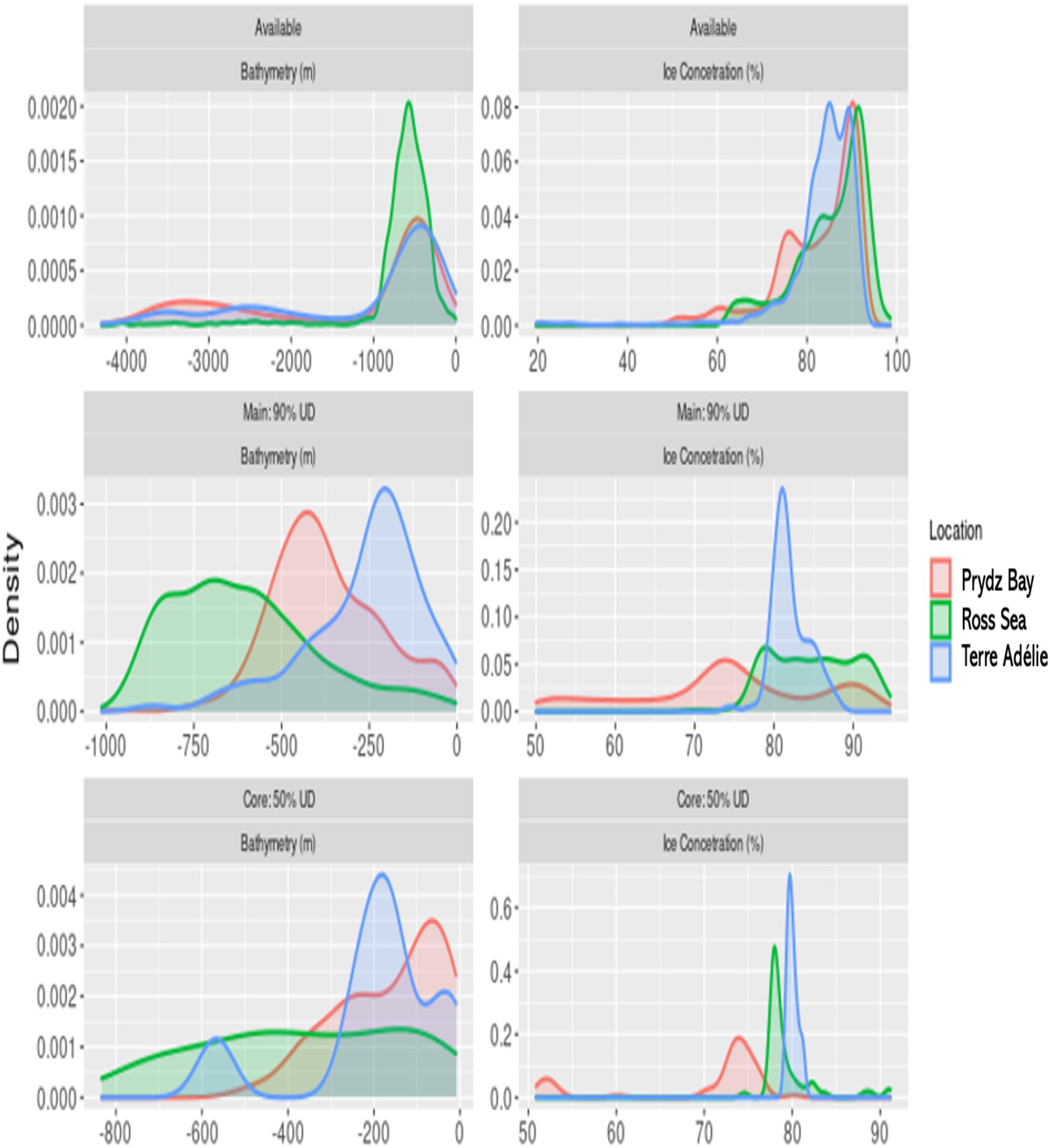
Figure 4. Frequency distributions (expressed as densities) of the bathymetry (m) and ice concentrations (%) on July 30 comparing (i) the region potentially available to the seals (see Methods), (ii) the full sample Utilisation Distributions (UDs) and (iii) the core UDs.
The area of shallow water where benthic foraging was most likely [i.e., ≥−250 m (Figure 2A)] constituted 85% of the core UD in Terre Adélie, 85% in Prydz Bay and only 36% in the Ross Sea (Table 2).
Discussion
By comparing data from three different regions, we found that Weddell seal foraging behaviour during the winter months is strongly influenced by extrinsic factors, but individual variability still plays a key role. Of the extrinsic factors, bathymetry played the most important role in determining the distribution and foraging behaviour of Weddell seals. The seals made on average more pelagic dives than benthic dives, and benthic foraging behaviour was more likely in shallower waters less than −250 m. Off Terre Adélie and in Prydz Bay individual seals spent 60% of their time in waters less than −250 m, making predominantly benthic dives. By contrast, seals in the Ross Sea spent less time in benthic habitats, and we attribute this to the relatively small area of benthic habitat within their core area dominated by the deep McMurdo Sound. However, individual variation in foraging behaviour persisted at each site, reflecting differences in intrinsic factors. Some individuals remained very local, foraging predominantly on the benthos throughout the winter, while others moved further afield, foraging more pelagically.
A potential limitation in this study is that bathymetry is poorly known in remote regions like the Southern Ocean (Millan et al., 2020). The Weddell seal dive data reflects this inaccuracy with 24% of the dives recorded being deeper than the ocean floor provided by GEBCO19. To account for this potential source of error, we designated foraging behaviours conservatively. Many studies have reported that seal dive depths recorded by SRDLs and CTD-SRDLs are accurate (Boehme et al., 2009; Costa et al., 2010; Padman et al., 2010; Roquet et al., 2014) and the locations of diving seals have at least a relatively well-derived error component (Jonsen et al., 2020). Given the paucity of direct ship-borne measurement for many of these areas of the Southern Ocean, integrating seal dive depths with those collected by more traditional means may help to constrain and therefore improve the confidence of many bathymetry models (Padman et al., 2010).
Foraging Behaviour
Weddell seal foraging occurs in a mix of habitat types (benthic, epipelagic, and mesopelagic) and they have a catholic diet taking benthic fish, crustaceans and cephalopods as well as mesopelagic prey such as Pleurogramma spp. (Supplementary Table 3). The likelihood of benthic foraging increased in shallower water. To reach the benthos in these areas, seals traverse the entire water column rather than stopping to feed in the pelagic zone, either because the mesopelagic prey are not there or because the seals get a greater energetic return from benthic prey. Harcourt et al. (2007) found that males that dived deeply during the breeding season in McMurdo Sound lost weight at a slower rate than those remaining shallow. This suggests that deeper prey resources are especially profitable or at least predictable (Photopoulou et al., 2020). Making diet inference based simply on predator dive behaviour is potentially confounded by the knowledge that some key prey species such as silverfish and toothfish can occupy multiple habitats (O’Driscoll et al., 2011). Feeding studies and acoustic observations of silverfish are consistent with a predominantly pelagic niche (Pinkerton, 2017; O’Driscoll et al., 2018), but adult silverfish are sometimes found close to the bottom over the Ross Sea shelf (O’Driscoll et al., 2011). In contrast, toothfish over the Ross Sea shelf are likely to be predominantly demersal (Pinkerton et al., 2016), but pelagically feeding in McMurdo Sound (Ainley et al., 2013).
As seals venture into deeper water, the benefits of benthic foraging are likely to decline due to the energetic trade-offs. These are associated with deeper diving, such as reduced time available in the foraging zone, greater cost of transport to and from deeper depths (Thompson and Fedak, 2001; Williams et al., 2004), physiologic costs associated with exceeding the aerobic dive limit or even potentially cardiac anomalies (Williams et al., 2015). This may explain why the seals in all three regions used predominantly shallower habitats in accordance with their relative availability.
Regional Variation in Habitat Use Is Explained by Site Variability Not Inherent Population Level Behavioural Differences
Across the three sites dive behaviour differed due to regional differences in bathymetry. For example, off Terre Adélie there was more shallow benthic diving than in Prydz Bay or the Ross Sea. In the Ross Sea Weddell seals foraged more pelagically, presumably because water is deeper in McMurdo Sound. Overall, the seals in all three regions remained close to the continental land mass in areas of heavy ice concentration (75–85% in core areas) over relatively shallow waters (<200 m). This corroborates previous observations of Weddell seal distributions and their preference for relatively shallow water areas (Stirling, 1969) in areas of dense ice (Lake et al., 2005; Boehme et al., 2016; Heerah et al., 2016; Nachtsheim et al., 2019). We observed differences between sites in the areas used by the seals, most likely due to the differences in physical habitats. Prydz Bay and Terre Adélie seals were close to regions where productive circumpolar deep water may reduce their need to forage further afield. In the Ross Sea, the seals were further from areas of influx of circumpolar deep water, with bathymetry influencing the advection of circumpolar deep water (Williams et al., 2016).
A recent study in the Weddell Sea observed differences in diving behaviour between males and females (Photopoulou et al., 2020), but we did not, which is possibly due to the similar body size of the males and females we studied. Photopoulou et al. (2020) reported that male Weddell seals spent more time in high-salinity shelf water masses at depth, benthically diving, while females ventured off the continental shelf and visited warmer, shallower water masses while undertaking a mix of benthic and pelagic dives. Our sample did not include sub-adults or juveniles, age classes which have very different distributions in other Southern Ocean pinnipeds (e.g., elephant seals, Hindell et al., 2021). Burns et al. (1999) reported that some weaned Weddell seal pups from McMurdo Sound in the southern Ross Sea dispersed widely, others did not, but all stayed relatively close to the Antarctic coastline.
Individual Variability
In this study there was a high degree of inter-individual variation in winter foraging behaviour. Some individuals were notably sedentary, did not move far from tagging location and had small home ranges. Others had large home ranges, travelled several hundred kilometres, and made more pelagic dives. These differences may be due to divergent behavioural traits among individual seals (Toscano et al., 2016; Troxell-Smith and Mella, 2017). Individual behavioural traits under-pins differences in foraging behaviour in other species (Patrick et al., 2014; Toscano et al., 2016; Krüger et al., 2019; DiNuzzo and Griffen, 2020; Steinhoff et al., 2020), and distinct, stable foraging strategies have been identified in many pinnipeds [e.g., Australian sea lions, Neophoca cinerea (Lowther et al., 2011); Galapagos sea lions, Zalophus wollebaeki (Villegas-Amtmann et al., 2008)], consistent with individual traits (Schwarz et al., 2021). In dynamic environments, multiple foraging strategies within a population may reduce resource competition (Lewis et al., 2006; Baylis et al., 2015). We found no relationship between home range area and seal size. In northern elephant seals, Mirounga angustirostris, body condition influences foraging behaviour, with animals in poor condition adopting riskier foraging strategies (Beltran et al., 2021) but, post-tagging we have no information on individual condition on our animals. Development of in situ proxies of condition (e.g., buoyancy) in Weddell seals similar to those well established in elephant seals (Biuw et al., 2003) could help to resolve whether these differences result from intrinsic strategies or arise from variance in foraging success. Finally, pre- and post-tagging reproductive status may both be important. The majority of these animals were females and at the time of tagging some would have just weaned their pup from the preceding breeding season, while others were not reproductive that season. Animals that skip breeding have different energetic demands as they have avoided the high cost incurred by birthing and lactation, where females lose about 30% of their body mass (Wheatley et al., 2006; Shero et al., 2015). Females that skipped breeding are in much better condition and so may have the luxury of adopting a safer foraging strategy as with fat northern elephant seals (Beltran et al., 2021). Conversely, not all females are pregnant each year. Pregnancy incurs increased energetic demands which translates to increased winter foraging effort (Shero et al., 2018), and this may well influence space use.
Our results differ from studies of Weddell seal distributions in the Weddell Sea (Boehme et al., 2016; Nachtsheim et al., 2019; Photopoulou et al., 2020; Labrousse et al., 2021), where tracked individuals showed diverse and wide-ranging movements including moving off the Antarctic continental shelf. In those studies, seals were often captured from ships some distance from the coast and later in the winter. In our study, all seals were captured just after their annual moult (∼2–3 months after the breeding season) at or near their breeding sites on coastal fast ice. We hypothesise that the seals tagged offshore in the Weddell Sea may contain a higher proportion of those individuals that are predisposed to make longer excursions, and so represent a different sample from those tagged near their breeding areas. Supportive evidence arises from Nachtsheim et al. (2019) who were forced to tag four of their six animals in a sheltered inlet due to bad weather. They found that the four Weddell seals they tagged on the fast ice stayed close to their colony, while the two tagged on the pack ice ventured further afield.
Bathymetry and ice concentration were important determinants of Weddell seal distribution. Sea-ice concentration on the continental shelf in east Antarctica is dynamic both within and among years (Massom et al., 2013), but in most years ice concentration reaches a maximum in late winter, around August (Eayrs et al., 2019), while still exhibiting considerable spatial variation due to recurrent coastal polynyas (Arrigo et al., 2015). These seals did not concentrate in polynyas, but instead those individuals that made excursions beyond the core areas moved into areas of relatively high ice concentrations (80% plus). Inhabiting high sea-ice concentration areas may improve access to prey or reduce the risk of attack by predators such as killer whales (Lauriano et al., 2020). Similarly, sedentary individuals remaining in the fast ice may reduce predation risk.
Implications
Antarctica and the surrounding Southern Ocean, like many regions across the globe, are changing in response to changes in climate (Rogers et al., 2020) and anthropogenic activities such as increased exploitation (e.g., fisheries), and these can profoundly affect endemic biota (Bestley et al., 2020). To quantify how these broad scale changes affect individuals and animal populations requires information on animal distribution and behaviour, and the structure and dynamics of the physical environment (Hindell et al., 2020). Quantifying movement and habitat use by predators for conservation and management strategies is particularly pertinent given the recent designation of the Ross Sea region Marine Protected Area (MPA), and the ongoing debate for new MPAs in east Antarctica and elsewhere in the Southern Ocean (Hays et al., 2019; Hindell et al., 2020). It is especially important in the management context to develop policies that protect the endemic biota over the long-term while regulating the human behaviours like fishing within the broader framework of a changing climate, over which it is much harder to effect change (Hays et al., 2019; Hindell et al., 2020). In the Southern Ocean, animal conservation and fisheries regulation is generally managed by the Commission for the Conservation of Antarctic Marine Living Resources (CCAMLR) under an ecosystem-based management approach which includes establishing a network of MPAs in the Southern Ocean. Information on where, when and how animals use their habitats is critical for the design and evaluation of these MPAs.
Conclusion
We found that Weddell seals in the Eastern Antarctic are relatively sedentary, preferring to forage in shallow water on the continental shelf. We detected regional differences in the proportion of benthic and foraging dives that accord with the available habitat. Within populations, we identified significant individual variability, ranging from little dispersal, and foraging within a relatively small core area, to individuals that ventured further afield, diving pelagically when in deeper waters but keeping to areas of high ice concentration. All the animals tagged in the Ross Sea remained within the boundaries of the Ross Sea MPA. The proposed MPAs in East Antarctica, Weddell Sea and Western Antarctic Peninsula also encompass potential core Weddell seal habitat (LaRue et al., 2019; Teschke et al., 2020). However, none of the areas used by seals in Prydz Bay are inside any current or proposed MPAs and this is obviously an important region to consider. This study suggests that protecting shelf areas will protect Weddell seals within that prescribed area, but local populations outside MPA’s will only indirectly benefit.
Data Availability Statement
The datasets presented in this study can be found in online repositories. The names of the repository/repositories and accession number(s) can be found below: https://portal.aodn.org.au/.
Ethics Statement
The animal study was reviewed and approved by Macquarie University ARA 2014_057.
Author Contributions
RH, MH, and CM lead the writing. MH, CM, and IJ conducted the analysis. RH, CM, MH, KG, J-BC, and MP organised the funding. RH, MH, CM, KG, KH, RHo, XH, MS, RF, BL, and ET conducted field work. All contributed writing and final edits.
Funding
Field support was provided in the Ross Sea by Malcolm O’Toole, Rupert Woods, and Antarctica New Zealand and in Prydz Bay by Malcolm O’Toole, Andrew Doube, Iain Field, and the Australian Antarctic Division. The tagging study in Terre Adélie had logistical support from IPEV (Institut Paul Emile Victor) and the French Polar Institute. New Zealand funding was provided by the Ministry for Business, Innovation and Employment Endeavour Fund C01 × 1710: “RAMPing-up protection of the Ross Sea”. The 2014 field event was funded by NZARI (NZ Antarctic Research Institute) and Fisheries New Zealand (respectively), with Regina Eisert as CI, and tags and some field personnel funded by IMOS. The IMOS deployments in Prydz Bay were supported logistically by the Australian Antarctic Division through the Australian Antarctic Science Grant Scheme (AAS Projects 2794 & 4329). The tagging study in Terre Adélie was supported by the Program Terre-Océan-Surface Continentale-Atmosphère from Centre National d’Etudes Spatiales (TOSCA-CNES). The ARGOS seal tracking and dive data were sourced and are available from the Integrated Marine Observing System (IMOS), NIWA, and LOCEAN. IMOS is a national collaborative research infrastructure, supported by the Australian Government. It is operated by a consortium of institutions as an unincorporated joint venture, with the University of Tasmania as lead agent.
Conflict of Interest
The authors declare that the research was conducted in the absence of any commercial or financial relationships that could be construed as a potential conflict of interest.
Publisher’s Note
All claims expressed in this article are solely those of the authors and do not necessarily represent those of their affiliated organizations, or those of the publisher, the editors and the reviewers. Any product that may be evaluated in this article, or claim that may be made by its manufacturer, is not guaranteed or endorsed by the publisher.
Acknowledgments
We thank all expeditioners at Dumont d’Urville who provided field assistance over the years. Bathymetry was from the Gebco Compilation Group. (2020) GEBCO 2020 Grid (10.5285/a29c5465-b138-234d-e053-6c86abc040b9).
Supplementary Material
The Supplementary Material for this article can be found online at: https://www.frontiersin.org/articles/10.3389/fmars.2021.720335/full#supplementary-material
Footnotes
References
Ainley, D. G., Nur, N., Eastman, J. T., Ballard, G., Parkinson, C. L., Evans, C. W., et al. (2013). Decadal trends in abundance, size and condition of Antarctic toothfish in McMurdo Sound. Antarctica, 1972-2011. Fish. Fish. 14, 343–363. doi: 10.1111/j.1467-2979.2012.00474.x
Arrigo, K. R., van Dijken, G. L., and Strong, A. L. (2015). Environmental controls of marine productivity hot spots around Antarctica. J. Geophys. Res. 120, 5545–5565. doi: 10.1002/2015JC010888
Baylis, A. M., Orben, R. A., Arnould, J. P., Peters, K., Knox, T., Costa, D. P., et al. (2015). Diving deeper into individual foraging specializations of a large marine predator, the southern sea lion. Oecologia 179, 1053–1065. doi: 10.1007/s00442-015-3421-4
Beltran, R. S., Kendall-Bar, J. M., Pirotta, E., Adachi, T., Naito, Y., Takahashi, A., et al. (2021). Lightscapes of fear: How mesopredators balance starvation and predation in the open ocean. Sci. Adv. 2021:7. doi: 10.1126/sciadv.abd9818
Bestley, S., Ropert-Coudert, Y., Bengtson Nash, S., Brooks, C. M., Cotté, C., Dewar, M., et al. (2020). Marine Ecosystem Assessment for the Southern Ocean: Birds and Marine Mammals in a Changing Climate. Front. Ecol. Evol. 8:566936. doi: 10.3389/fevo.2020.566936
Biuw, M., McConnell, B. J., Bradshaw, C. J. A., Burton, H. R., and Fedak, M. A. (2003). Blubber and buoyancy: monitoring the body condition of free- ranging seals using simple dive characteristics. J. Exp. Biol. 206, 3405–3423. doi: 10.1242/jeb.00583
Boehme, L., Baker, A., Fedak, M., Arthun, M., Nicholls, K., Robinson, P., et al. (2016). Bimodal winter haul-out patterns of adult Weddell seals (Leptonychotes weddellii) in the Southern Weddell Sea. Plos One 2016, 11. doi: 10.1371/journal.pone.0155817
Boehme, L., Lovell, P., Biuw, M., Roquet, F., Nicholson, J., Thorpe, S. E., et al. (2009). Animal-borne CTD-Satellite Relay Data Loggers for real-time oceanographic data collection. Ocean Sci. 5, 685–695. doi: 10.5194/os-5-685-2009
Brierley, A. S., and Thomas, D. N. (2002). Ecology of Southern Ocean pack ice. Adv. Mar. Biol. 43, 171–276. doi: 10.1016/S0065-2881(02)43005-2
Burnham, K. P., and Anderson, D. R. (2002). Model selection and multimodal inference: a practical information-theoretic approach. New York, USA: Springer-Verlag.
Burnham, K. P., and Anderson, D. R. (2004). Multimodel inference - understanding AIC and BIC in model selection. Sociolog. Methods Res. 33, 261–304. doi: 10.1177/0049124104268644
Burns, J. M., Castellini, M. A., and Testa, J. W. (1999). Movements and diving behaviour of weaned Weddell seal (Leptonychotes weddellii) pups. Polar Biol. 21, 23–36. doi: 10.1007/s003000050329
Burns, J. M., and Kooyman, G. L. (2001). Habitat Use by Weddell Seals and Emperor Penguins Foraging in the Ross Sea, Antarctica1. Am. Zool. 41, 90–98. doi: 10.1668/0003-1569(2001)041[0090:HUBWSA]2.0.CO;2
Castellini, M. A., Davis, R. W., Davis, M., and Horning, M. (1984). Antarctic marine life under the McMurdo Ice Shelf at White Island: A link between nutrient influx and seal population. Polar Biol. 2, 229–231. doi: 10.1007/BF00263629
Catry, T., Ramos, J. A., Jaquemet, S., Faulquier, L., Berlincourt, M., Hauselmann, A., et al. (2009). Comparative foraging ecology of a tropical seabird community of the Seychelles, western Indian Ocean. Mar. Ecol. Prog. Ser. 374, 259–272. doi: 10.3354/meps07713
Cavalieri, D. J., Parkinson, C. L., Gloersen, P., Comiso, J. C., and Zwally, H. J. (1999). Deriving long-term time series of sea ice cover from satellite passive-microwave multisensor data sets. J. Geophys. Res. Oceans 104, 15803–15814. doi: 10.1029/1999JC900081
Cherel, Y., and Hobson, K. A. (2007). Geographical variation in carbon stable isotope signatures of marine predators: a tool to investigate their foraging areas in the Southern Ocean. Mar. Ecol. Prog. Series 329, 281–287. doi: 10.3354/meps329281
Constable, A. J., Nicol, S., and Strutton, P. G. (2003). Southern Ocean productivity in relation to spatial and temporal variation in the physical environment. J. Geophys. Res. 108:8079. doi: 10.1029/2001JC001270
Costa, D. P., Huckstadt, L. A., Crocker, D. E., McDonald, B. I., Goebel, M. E., and Fedak, M. A. (2010). Approaches to Studying Climatic Change and its Role on the Habitat Selection of Antarctic Pinnipeds. Integr. Compar. Biol. 50, 1018–1030. doi: 10.1093/icb/icq054
Daunt, F., Afanasyev, V., Silk, J. R. D., and Wanless, S. (2005). Extrinsic and intrinsic determinants of winter foraging and breeding phenology in a temperate seabird. Behav. Ecol. Sociobiol. 59, 381–388. doi: 10.1007/s00265-005-0061-4
DiNuzzo, E. R., and Griffen, B. D. (2020). The effects of animal personality on the ideal free distribution. Proc. Biol. Sci. 287:20201095. doi: 10.1098/rspb.2020.1095
Eayrs, C., Holland, D., Francis, D., Wagner, T., Kumar, R., and Li, X. (2019). Understanding the Seasonal Cycle of Antarctic Sea Ice Extent in the Context of Longer-Term Variability. Rev. Geophys. 57, 1037–1064. doi: 10.1029/2018RG000631
Harcourt, R., Sequeira, A. M. M., Zhang, X., Roquet, F., Komatsu, K., Heupel, M., et al. (2019). Animal-Borne Telemetry: an integral component of the ocean observing toolkit. Front. Mar. Sci. 6:326. doi: 10.3389/fmars.2019.00326
Harcourt, R. G., Kingston, J. J., Waas, J. R., and Hindell, M. A. (2007). Foraging while breeding: alternative mating strategies by male Weddell seals? Aquat. Conserv. Mar. Freshw. Ecosyst. 17, S68–S78. doi: 10.1002/aqc.915
Hays, G. C., Bailey, H., Steven, J., Bograd, S. J., Don, W., Bowen, W. D., et al. (2019). Translating marine animal tracking data into conservation policy and management. Trends Ecol. Evol. 34, 459–473. doi: 10.1016/j.tree.2019.01.009
Heerah, K., Andrews-Goff, V., Williams, G., Sultan, E., Hindell, M., Patterson, T., et al. (2013). Ecology of Weddell seals during winter: Influence of environmental parameters on their foraging behaviour. Deep-Sea Res. Part I-Top. Stud. Oceanogr. 88-89, 23–33. doi: 10.1016/j.dsr2.2012.08.025
Heerah, K., Hindell, M. A., Andrews-Goff, V., Field, I. C., McMahon, C. R., and Charrassin, J. B. (2016). Contrasting behaviour between two populations of an ice-obligate predator in East Antarctica. Ecol. Evol. 2016:2652. doi: 10.1002/ece1003.2652
Hindell, M. A., Bradshaw, C. J. A., Sumner, M. D., Michael, K. J., and Burton, H. R. (2003). Dispersal of female southern elephant seals and their prey consumption during the austral summer: relevance to management and oceanographic zones. J. Appl. Ecol. 40, 703–715. doi: 10.1046/j.1365-2664.2003.00832.x
Hindell, M. A., McMahon, C. R., Bester, M. N., Boehme, L., Costa, D., Fedak, M. A., et al. (2016). Circumpolar habitat use in the southern elephant seal: implications for foraging success and population trajectories. Ecosphere 7:e01213. doi: 10.1002/ecs2.1213
Hindell, M. A., McMahon, C. R., Harcourt, R., Arce, F., Guinet, C., and Jonsen, I. (2021). Inter- and intra-sex habitat partitioning in the highly dimorphic southern elephant seal. Ecol. Evol. 2021:7147. doi: 10.1002/ece1003.7147
Hindell, M. A., Reisinger, R. R., Ropert-Coudert, Y., Hückstädt, L. A., Trathan, P. N., Bornemann, H., et al. (2020). Tracking of marine predators to protect Southern Ocean ecosystems. Nature 580, 87–92.
Humphreys, E. M., Wanless, S., and Bryant, D. M. (2006). Stage-dependent foraging in breeding black-legged kittiwakes Rissa tridactyla: distinguishing behavioural responses to intrinsic and extrinsic factors. J. Avian Biol. 37, 436–446. doi: 10.1111/j.2006.0908-8857.03594.x
Jonsen, I., Patterson, T. A., Doherty, P. D., Godley, B. J., Grecian, W. J., Guinet, C., et al. (2020). A continuous-time state-space model for rapid quality-control of Argos locations from animal-borne tags. Move. Ecol. 8:31. doi: 10.1186/s40462-020-00217-7
Kirkwood, R., and Robertson, G. (1997). The foraging ecology of female emperor penguins in winter. Ecolog. Monogr. 67, 155–176. doi: 10.1890/0012-9615(1997)067[0155:TFEOFE]2.0.CO;2
Kooyman, G. L., Castellini, M. A., and Davis, R. W. (1981). Physiology of diving in marine mammals. Ann. Rev. Physiol. 43, 343–356. doi: 10.1146/annurev.ph.43.030181.002015
Koubbi, P., Ozouf-Costaz, C., Goarant, A., Moteki, M., Hulley, P.-A., Causse, R., et al. (2010). Estimating the biodiversity of the East Antarctic shelf and oceanic zone for ecoregionalisation: Example of the ichthyofauna of the CEAMARC (Collaborative East Antarctic Marine Census) CAML surveys. Polar Sci. 4, 115–133.
Krüger, L., Pereira, J. M., Paiva, V. H., and Ramos, J. A. (2019). Personality influences foraging of a seabird under contrasting environmental conditions. J. Exp. Mar. Biol. Ecol. 516, 123–131. doi: 10.1016/j.jembe.2019.04.003
Kuhn, C. E., Baker, J. D., Towell, R. G., and Ream, R. R. (2014). Evidence of localized resource depletion following a natural colonization event by a large marine predator. J. Anim. Ecol. 83, 1169–1177. doi: 10.1111/1365-2656.12202
Labrousse, S., Ryan, S., Roquet, F., Picard, B., McMahon, C. R., Harcourt, R., et al. (2021). Weddell seal behaviour during an exceptional oceanographic event in the Filchner-Ronne Ice Shelf in 2017. Antar. Sci. 2021, 1–13. doi: 10.1017/S0954102021000092
Lake, S., Wotherspoon, S., and Burton, H. R. (2005). Spatial utilisation of fast-ice by Weddell seals Leptonychotes weddelli during winter. Ecography 28, 295–306. doi: 10.1111/j.0906-7590.2005.03949.x
LaRue, M. A., Salas, L., Nur, N., Ainley, D. G., Stammerjohn, S., Barrington, L., et al. (2019). Physical and ecological factors explain the distribution of Ross Sea Weddell seals during the breeding season. Mar. Ecol. Progr. Series 612, 193–208. doi: 10.3354/meps12877
Lauriano, G., Pirotta, E., Joyce, T., Pitman, R. L., Borrell, A., and Panigada, S. (2020). Movements, diving behaviour and diet of type−C killer whales (Orcinus orca) in the Ross Sea, Antarctica. Aquat. Conserv. 30, 2428–2440. doi: 10.1002/aqc.3371
Laver, P. N., and Kelly, M. J. (2008). A Critical Review of Home Range Studies. J. Wildlife Manag. 72, 290–298. doi: 10.2193/2005-589
Lewis, R., O’Connell, T. C., Lewis, M., Carnpagna, C., and Hoelzel, A. R. (2006). Sex-specific foraging strategies and resource partitioning in the southern elephant seal (Mirounga leonina). Proc. R. Soc. B-Biol. Sci. 273, 2901–2907. doi: 10.1098/rspb.2006.3642
Lowther, A. D., Harcourt, R. G., Hamer, D. J., and Goldsworthy, S. D. (2011). Creatures of habit: foraging habitat fidelity of adult female Australian sea lions. Mar. Ecol. Progr. Series 443:249. doi: 10.3354/meps09392
Massom, R., Reid, P., Stammerjohn, S., Raymond, B., Fraser, A., and Ushio, S. (2013). Change and Variability in East Antarctic Sea Ice Seasonality, 1979/80-2009/10. PLoS One 2013:8. doi: 10.1371/journal.pone.0064756
McNamara, J. M., and Houston, A. I. (1986). The Common Currency for Behavioral Decisions. Am. Natural. 127, 358–378. doi: 10.1086/284489
Mellish, J. A., Tuomi, P. A., Hindle, A. G., and Horning, M. (2010). Chemical immobilization of Weddell seals (Leptonychotes weddellii) by ketamine/midazolam combination. Vet. Anaesth. Anal. 37, 123–131. doi: 10.1111/j.1467-2995.2009.00517.x
Millan, R., St-Laurent, P., Rignot, E., Morlighem, M., Mouginot, J., and Scheuchl, B. (2020). Constraining an Ocean Model Under Getz Ice Shelf, Antarctica, Using A Gravity-Derived Bathymetry. Geophysical Research Letters 2020, 47. doi: 10.1029/2019GL086522
Nachtsheim, D. A., Ryan, S., Schröder, M., Jensen, L., Oosthuizen, W. C., Bester, M. N., et al. (2019). Foraging behaviour of Weddell seals (Leptonychotes weddellii) in connection to oceanographic conditions in the southern Weddell Sea. Progr. Oceanogr. 173, 165–179. doi: 10.1016/j.pocean.2019.02.013
Nicol, S., Pauly, T., Bindoff, N. L., Wright, S., Thiel, D., Hosie, G. W., et al. (2000). Ocean circulation off east Antarctica affects ecosystems structure and sea-ice extent. Nature 406, 504–507. doi: 10.1038/35020053
Nordstrom, C. A., Battaile, B. C., Cotte, C., and Trites, A. W. (2013). Foraging habitats of lactating northern fur seals are structured by thermocline depths and submesoscale fronts in the eastern Bering Sea. Deep-Sea Res. Part I-Top. Stud. Oceanogr. 88-89, 78–96. doi: 10.1016/j.dsr2.2012.07.010
Nunes Vaz, R. A., and Lennon, G. W. (1996). Physical oceanography of the Prydz Bay region of Antarctic waters. Deep Sea Res. Part I: Oceanogr. Res. Papers 43, 603–641. doi: 10.1016/0967-0637(96)00028-3
O’Driscoll, R. L., Canese, S., Ladroit, Y., Parker, S. J., Ghigliotti, L., Mormede, S., et al. (2018). First in situ estimates of acoustic target strength of Antarctic toothfish (Dissostichus mawsoni). Fish. Res. 206, 79–84. doi: 10.1016/j.fishres.2018.05.008
O’Driscoll, R. L., Macaulay, G. J., Gauthier, S., Pinkerton, M., and Hanchet, S. (2011). Distribution, abundance and acoustic properties of Antarctic silverfish (Pleuragramma antarcticum) in the Ross Sea. Deep Sea Res. Part II: Top. Stud. Oceanogr. 58, 181–195. doi: 10.1016/j.dsr2.2010.05.018
Padman, L., Costa, D. P., Bolmer, S. T., Goebel, M. E., Huckstadt, L. A., Jenkins, A., et al. (2010). Seals map bathymetry of the Antarctic continental shelf. Geophys. Res. Lett. 37:L21601. doi: 10.1029/2010GL044921
Patrick, S. C., Bearhop, S., Grémillet, D., Lescroël, A., Grecian, W. J., Bodey, T. W., et al. (2014). Individual differences in searching behaviour and spatial foraging consistency in a central place marine predator. Oikos 123, 33–40. doi: 10.1111/j.1600-0706.2013.00406.x
Photopoulou, T., Heerah, K., Pohle, J., and Boehme, L. (2020). Sex-specific variation in the use of vertical habitat by a resident Antarctic top predator. Proc. R. Soc. B. 287:20201447. doi: 10.1098/rspb.2020.1447
Pinkerton, M. H. (2017). Diet and trophic ecology of adult Antarctic silverfish (Pleuragramma antarctica) Antarctic silverfish. Adv. Polar Ecol. Series 2017, 93–111. doi: 10.1007/978-3-319-55893-6_5
Pinkerton, M. H., Lyver, P. O. B., Stevens, D. W., Forman, J., Eisert, R., and Mormede, S. (2016). Increases in Adélie penguins in the Ross Sea: Could the fishery for Antarctic toothfish be responsible? Ecolog. Model. 337, 262–271. doi: 10.1016/j.ecolmodel.2016.07.007
Raymond, B., Lea, M.-A., Patterson, T., Andrews-Goff, V., Sharples, R., Charrassin, J.-B., et al. (2014). Important marine habitat off east Antarctica revealed by two decades of multi-species predator tracking. Ecography 2014:1021. doi: 10.1111/ecog.01021
Rogers, A. D., Frinault, B. A. V., Barnes, D. K. A., Bindoff, N. L., Downie, R., Ducklow, H. W., et al. (2020). Antarctic Futures: An Assessment of Climate-Driven Changes in Ecosystem Structure, Function, and Service Provisioning in the Southern Ocean. Annu. Rev. Mar. Sci. 12, 87–120. doi: 10.1146/annurev-marine-010419-011028
Roquet, F., Williams, G. D., Hindell, M., Harcourt, R., McMahon, C. R., Charrassin, J. B., et al. (2014). A Southern Indian Ocean database of hydrographic profiles obtained with instrumented elephant seals. Nat. Sci. Data 1:140028. doi: 10.1038/sdata.2014.28
Russell, D. J. F., McClintock, B. T., Matthiopoulos, J., Thompson, P. M., Thompson, D., Hammond, P. S., et al. (2015). Intrinsic and extrinsic drivers of activity budgets in sympatric grey and harbour seals. Oikos 124, 1462–1472. doi: 10.1111/oik.01810
Salton, M., Kirkwood, R., SLip, D., and Harcourt, R. (2019). Mechanisms for sex-based segregation in foraging behaviour by a polygynous marine carnivore. Mar. Ecol. Progr. Ser. 624, 213–226. doi: 10.3354/meps13036
Schwarz, J. F. L., Mews, S., DeRango, E. J., Langrock, R., Piedrahita, P., Paez-Rosas, D., et al. (2021). Individuality counts: A new comprehensive approach to foraging strategies of a tropical marine predator. Oecologia 195, 313–325. doi: 10.1007/s00442-021-04850-w
Sequeira, A. M. M., Rodriguez, J. C., Eguiìluza, V. M., Harcourt, R., Hindell, M., Sims, D. W., et al. (2018). Convergence of marine megafauna movement patterns in coastal and open oceans. Proc. Natl. Acad. Sci. 2018:1716137115. doi: 10.1073/pnas.1716137115
Shero, M. R., Goetz, K. T., Costa, D. P., and Burns, J. M. (2018). Temporal changes in Weddell seal dive behavior over winter: Are females increasing foraging effort to support gestation? Ecol. Evol. 8, 11857–11874. doi: 10.1002/ece3.4643
Shero, M. R., Krotz, R. T., Costa, D. P., Avery, J. P., Burns, J. M., and Konarzewski, M. (2015). How do overwinter changes in body condition and hormone profiles influence Weddell seal reproductive success? Funct. Ecol. 2015:12434. doi: 10.1111/1365-2435.12434
Smith, W. O., Ainley, D. G., and Cattaneo-Vietti, R. (2007). Trophic interactions within the Ross Sea continental shelf ecosystem. Philosoph. Transac. R. Soc. B-Biol. Sci. 362, 95–111. doi: 10.1098/rstb.2006.1956
Steinhoff, P. O. M., Warfen, B., Voigt, S., Uhl, G., and Dammhahn, M. (2020). Individual differences in risk−taking affect foraging across different landscapes of fear. Oikos 129, 1891–1902. doi: 10.1111/oik.07508
Stephens, D. W. (2008). Decision ecology: foraging and the ecology of animal decision making. Cogn. Affect. Behav. Neurosci. 8, 475–484. doi: 10.3758/CABN.8.4.475
Stirling, I. (1969). Ecology of the Weddell Seal in McMurdo Sound, Antarctica. Ecology 50, 573–586. doi: 10.2307/1936247
Teschke, K., Pehlke, H., Siegel, V., Bornemann, H., Knust, R., and Brey, T. (2020). An integrated compilation of data sources for the development of a marine protected area in the Weddell Sea. Earth Syst. Sci. Data 12, 1003–1023. doi: 10.5194/essd-12-1003-2020
Testa, J. W. (1994). Over-winter movements and diving behaviour of female Weddell seals (Leptonychotes weddellii) in the soutwestern Ross Sea, Antarctica. Can. J. Zool. 72, 1700–1710. doi: 10.1139/z94-229
Thompson, D., and Fedak, M. A. (2001). How long should a dive last? A simple model of foraging decisions by breath-hold divers in a patchy environment. Anim. Behav. 61, 287–296. doi: 10.1006/anbe.2000.1539
Toscano, B. J., Gownaris, N. J., Heerhartz, S. M., and Monaco, C. J. (2016). Personality, foraging behavior and specialization: integrating behavioral and food web ecology at the individual level. Oecologia 182, 55–69. doi: 10.1007/s00442-016-3648-8
Troxell-Smith, S. M., and Mella, V. S. A. (2017). “You Are What You Eat: The Interplay Between Animal Personality and Foraging Ecology,” in Personality in Nonhuman Animals, eds J. Vonk, A. Weiss, and S. Kuczaj (Cham: Springer), 295–305. doi: 10.1007/978-3-319-59300-5_15
Villegas-Amtmann, S., Costa, D. P., Tremblay, Y., Salazar, S., and Aurioles-Gamboa, D. (2008). Multiple foraging strategies in a marine apex predator, the Galapagos sea lion Zalophus wollebaeki. Mar. Ecol. Progr. Ser. 363, 299–309. doi: 10.3354/meps07457
Votier, S. C., Fayet, A. L., Bearhop, S., Bodey, T. W., Clark, B. L., Grecian, J., et al. (2017). Effects of age and reproductive status on individual foraging site fidelity in a long-lived marine predator. Proceedings of the Royal Society B 2017, 284. doi: 10.1098/rspb.2017.1068
Weise, M. J., Harvey, J. T., and Costa, D. P. (2010). The role of body size in individual-based foraging strategies of a top marine predator. Ecology 91, 1004–1015. doi: 10.1890/08-1554.1
Wheatley, K. E., Bradshaw, C. J. A., Davis, L. S., Harcourt, R. G., and Hindell, M. A. (2006). Influence of maternal mass and condition on energy transfer in Weddell seals. J. Anim. Ecol. 75, 724–733. doi: 10.1111/j.1365-2656.2006.01093.x.
Williams, G. D., Herraiz-Borreguero, L., Roquet, F., Tamura, K., Ohshima, K. I., Fukamachi, Y., et al. (2016). The suppression of Antarctic Bottom Water formation by melting ice shelves in Prydz Bay. Nat. Comm. 2016:12577. doi: 10.1038/NCOMMS12577
Williams, T. M., Fuiman, L. A., Horning, M., and Davis, R. W. (2004). The cost of foraging by a marine predator, the Weddell seal Leptonychotes weddellii: pricing by the stroke. J. Exp. Biol. 207, 973–982. doi: 10.1242/jeb.00822
Keywords: marine protected areas, Antarctica, marine ecosystems, bathymetry, ecosystem monitoring, Weddell seals
Citation: Harcourt R, Hindell MA, McMahon CR, Goetz KT, Charrassin J-B, Heerah K, Holser R, Jonsen ID, Shero MR, Hoenner X, Foster R, Lenting B, Tarszisz E and Pinkerton MH (2021) Regional Variation in Winter Foraging Strategies by Weddell Seals in Eastern Antarctica and the Ross Sea. Front. Mar. Sci. 8:720335. doi: 10.3389/fmars.2021.720335
Received: 04 June 2021; Accepted: 16 August 2021;
Published: 22 September 2021.
Edited by:
David M. P. Jacoby, Lancaster University, United KingdomReviewed by:
Mia Wege, University of Pretoria, South AfricaNatalie Elizabeth Wildermann, Texas A&M University Corpus Christi, United States
Copyright © 2021 Harcourt, Hindell, McMahon, Goetz, Charrassin, Heerah, Holser, Jonsen, Shero, Hoenner, Foster, Lenting, Tarszisz and Pinkerton. This is an open-access article distributed under the terms of the Creative Commons Attribution License (CC BY). The use, distribution or reproduction in other forums is permitted, provided the original author(s) and the copyright owner(s) are credited and that the original publication in this journal is cited, in accordance with accepted academic practice. No use, distribution or reproduction is permitted which does not comply with these terms.
*Correspondence: Clive R. McMahon, Y2xpdmUubWNtYWhvbkB1dGFzLmVkdS5hdQ==