- 1Dauphin Island Sea Lab, Dauphin Island, AL, United States
- 2Department of Marine Sciences, University of South Alabama, Mobile, AL, United States
- 3Clearwater Marine Aquarium Research Institute, Clearwater, FL, United States
Partial migration provides a mechanism for species to shift their geographic ranges into new, environmentally favorable regions but has been poorly studied as a means to alleviate effects of climate change. Populations at the edge of their geographic range are ideal to investigate how migratory behaviors may enable range expansion as adjacent areas become more climatically favorable. We determined the contribution of partial migration to the range expansion of West Indian manatees (Trichechus manatus) using GPS data from tagged individuals that migrated between the northern Gulf of Mexico (nGoM) and primary habitat in peninsular Florida. Most of these manatees migrated to the nGoM annually and exhibited high site fidelity among years. Many individuals spent cumulatively more time in the nGoM than in peninsular Florida, indicating the nGoM is a regular part of their geographic range, and they returned to peninsular Florida to meet temperature-related physiological needs for survival. Autumn migrations most frequently terminated at Crystal River, Florida, and manatees that commenced migration late in the season stopped less frequently and had more directed movements. Spring migrations most frequently terminated at Mobile Bay, Alabama, and several manatees quickly and directly migrated from Florida to nGoM stopover sites. Migrations ranged from 10 to 133 days in length, and variation in duration was primarily driven by use of stopover sites and directedness of travel. These data confirm partial migration as an important component of manatee migratory behavior that has already enabled range shifts for manatees on the U.S.A. Gulf of Mexico coast and has potential to facilitate future responses to climate change. As the most common type of migration across the animal kingdom, partial migration may provide a global mechanism for a diverse variety of species to resist the range limiting effects of climate change.
Introduction
Migration is the directed, often seasonal movement of individuals among locations that provide important resources or refuges (Dingle and Drake, 2007; Shaw and Couzin, 2013; Cagnacci et al., 2016). Migration is often essential to species that must relocate because environmental conditions are not conducive to the species’s survival year-round (Dingle and Drake, 2007; Mysterud et al., 2011; Bischof et al., 2012). Partial migration, where only part of the population migrates and individuals can switch between resident and migratory tactics, is the most common type of migration among animals (Dingle and Drake, 2007; Chapman et al., 2011, 2012; Shaw and Couzin, 2013). Motivation to migrate, or to switch between migratory and residency tactics, is driven by interacting environmental factors, such as temperature, resource quality and availability, intra- and interspecific competition, and predation (Brodersen et al., 2011; Chapman et al., 2011; Mysterud et al., 2011; Eggeman et al., 2016). Many of these factors and their interactive effects are altered by anthropogenic disturbances and climate change (Winder and Schindler, 2004; Hoegh-Guldberg and Bruno, 2010). As a result, migration patterns in many species are changing or are likely to change in the coming decades (Cotton, 2003; Saino et al., 2011; Nelson et al., 2012). These changes will have negative and positive species-specific effects (Van Buskirk et al., 2009; Saino et al., 2011; Ruiz-Cooley et al., 2013), but most research has focused on how climate change may disrupt migratory patterns and phenological relationships (Van Buskirk et al., 2009; Visser et al., 2009; Saino et al., 2011; Batalden et al., 2014). Much less work has focused on how migration strategies such as partial migration may provide a mechanism for species to expand or shift their range as climate changes (but see Ruiz-Cooley et al., 2013; Hovick et al., 2016).
Partial migration may benefit populations under climate change scenarios because these migrations allow species to flexibly track favorable conditions through space and time (Naidoo et al., 2012; Hovick et al., 2016; Acker et al., 2021; Bright Ross et al., 2021). Through partial migration, species may be able to exploit new areas as they become available, residing in new areas while conditions are favorable, and retreating, often seasonally, when conditions become unfavorable (Naidoo et al., 2012; Bright Ross et al., 2021). If conditions in the areas of expansion remain favorable for longer periods throughout the year, individuals may be able to stay longer and eventually transition from migrants to residents (Bright Ross et al., 2021). Populations that are living at or near their geographic range limit will likely be the first to experience conditions that allow them to move into new areas (Perry et al., 2005; Parmesan, 2006), and it is likely easier to detect these movements in these areas. Populations that already exhibit migratory plasticity may be better able to respond to changing environmental conditions (Martin et al., 2018; Bright Ross et al., 2021), making them ideal study populations to examine the role of partial migration in facilitating climate driven range expansions.
West Indian manatees (Trichechus manatus; hereafter referred to as manatees) are a threatened and protected sirenian with the northern most populations occurring in the southeastern United States of America (U.S.A.). Some of these populations are potentially expanding into estuarine and tidal riverine habitats outside the known habitats of highest density on the southeastern U.S.A. coastline (Gannon et al., 2007; Marsh et al., 2011; Cummings et al., 2014). During winter, manatees in the U.S.A. depend on warm water refugia, such as natural warm springs or power plant effluents, primarily in peninsular Florida (Irvine, 1983; Deutsch et al., 2003; Laist and Reynolds, 2005). Manatee densities can be extremely high at refugia during cold periods when water temperatures are below 20°C (Laist et al., 2013), and most manatees leave refuge sites when water temperatures rise above this thermal threshold (Deutsch et al., 2003; Cummings et al., 2014; Hieb et al., 2017). Tagging and photo-identification studies provide evidence of partial migration, with most manatees migrating at least locally. One study of 48 satellite telemetry tagged manatees found 87% of manatees migrated short (50–150 km), medium (150–400 km), or long (>400 km) distances from refuge sites, with some manatees migrating to habitats outside of Florida (Deutsch et al., 2003). More recent studies indicate an increasing number of manatees make regular, seasonal migrations that span hundreds of kilometers (Cummings et al., 2014; Hieb et al., 2017; Cloyed et al., 2019; Rood et al., 2020). Habitat use by manatees that migrate, even short distances, can be defined in terms of the duration of time spent at specific locations (Deutsch et al., 2003). The resulting mosaic of partial migrations helps define manatee distributions and range through time and may confer resiliency to environmental variation (Mysterud et al., 2011; Martin et al., 2018; Purdon et al., 2018; Picardi et al., 2020). Furthermore, increased occurrence of manatees outside of Florida is likely driven by a combination of population growth, favorable temperatures, and potential changes in dietary resources associated with ecological and climatic changes (Marsh et al., 2011, 2017; DaCosta et al. Unpublished). These factors make manatees useful sentinels to test how partial migration may facilitate range shifts or expansion due to climate change.
While increasing geographical range can be encouraging for a threatened species (Neel et al., 2012), development of new patterns of movement across greater distances presents challenges for effective conservation and management (e.g., Neel et al., 2012; Cloyed et al., 2019). In the northern Gulf of Mexico (nGoM), manatee sightings have been documented since the early 1900s, but sightings have increased exponentially during the past several decades (Gunter, 1941; Powell and Rathbun, 1984; Fertl et al., 2005; Hieb et al., 2017). Similarly, manatee strandings and mortalities have recently increased in this region as manatees face diverse threats, such as cold stress during winter months and vessel collision throughout their range (Hieb et al., 2017; Cloyed et al., 2019). Manatees are also likely harbingers of climate driven, ecological changes in places like the nGoM, as other tropical associated species are becoming increasingly common (Fodrie et al., 2010; Heck et al., 2015; Scheffel et al., 2018). Understanding the ecologically meaningful components of manatee migrations will support ongoing range-wide management, conservation, and species recovery actions, as well as provide evidence for how other species that exhibit partial migration may be affected under future climate scenarios (Martin et al., 2018; Bright Ross et al., 2021).
Here, we expand on a previous study of satellite telemetry tagged manatees (Aven et al., 2016) using Global Positioning System (GPS) data from manatees that migrated between the nGoM and peninsular Florida to determine whether partial migration contributed to the expanding range of manatees. We defined spatial and temporal patterns of migration for manatees along the nGoM coast by identifying terminal (most easterly or westerly) and stopover locations, the latter based on duration of use by manatees. We defined movements among individuals with the net-squared displacement (NSD) method (Bunnefeld et al., 2011) and determined how factors such as number of stopovers, directedness of travel, season, and migration distance affected the duration of migrations among individuals. These data provide the most detailed information to-date on manatee movements in the U.S.A. outside of Florida to support ongoing assessments of manatee abundance, distribution, and habitat use across the species range. These data emphasize the ecological importance of partial migration and other flexible migration strategies to facilitate movements at the edge of a geographic range and the potential for these strategies to enable responses to climate change.
Materials and Methods
Study Area
We tracked manatee movements across the northern and eastern Gulf of Mexico, from Lake Pontchartrain, Louisiana, a brackish lake that is connected to Mississippi Sound (longitude ∼ 90.00° W), to Tampa Bay, Florida, a highly urbanized estuary (−82.45° W) (Figure 1). Seasonal variation in water temperatures during the time of study ranged from a daily average of ∼ 32°C in the summer (June - August) to ∼ 16°C in the winter (December – February) in the northern Gulf of Mexico (nGoM) and from a daily average of ∼ 32°C in the summer to ∼ 21°C in winter in the eastern Gulf of Mexico (Buckingham et al., 1999; Cloyed et al., 2019). Within the study area, natural, thermal springs at the Wakulla, Homosassa, and Crystal Rivers, Florida and power plant discharge sites in Crystal River, Big Bend, and Tampa Bay, Florida provided warm-water refuge sites used by manatees (Laist and Reynolds, 2005; Laist et al., 2013). The study area includes extensive tidal river and open water systems with highly diverse habitats, such as seagrass beds in St. George Sound, Apalachicola/St. Joseph Bay, and St. Andrew Bay, Florida, marshes, barrier islands, and active and remnant oyster reefs (Crassostrea virginica) (Livingston, 1984; Stout, 1984; Lewis, 1987). Several large rivers provide freshwater habitats and dietary resources preferred by manatees, including (west-to-east) the Mississippi, Pearl, Pascagoula, Mobile-Tensaw, Apalachicola, Ochlockonee, Wakulla, Suwannee, and Crystal Rivers. The Intracoastal Waterway runs the length of the study area and is used by manatees traveling along the nGoM coast (Aven et al., 2015; Cloyed et al., 2019).
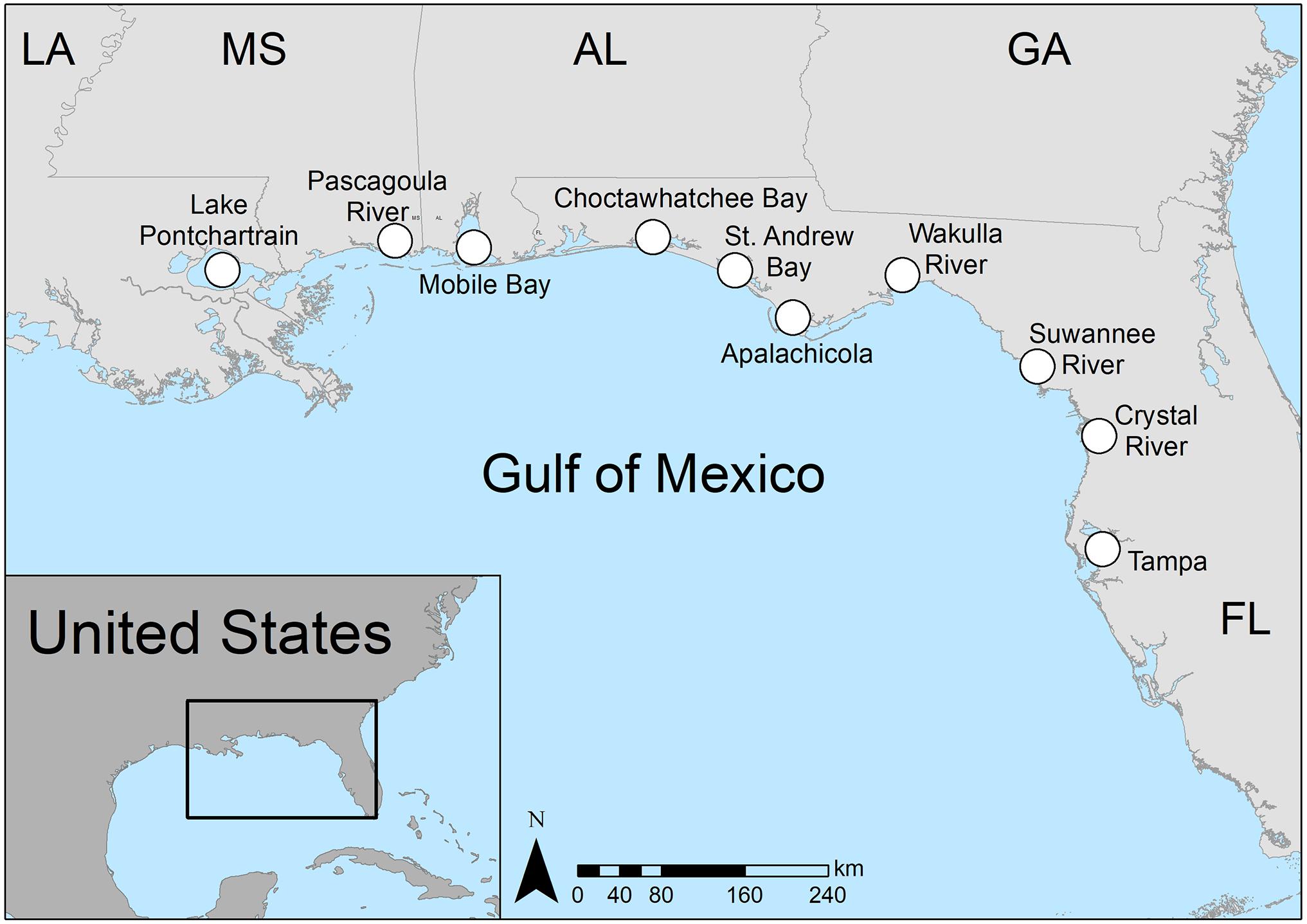
Figure 1. Major stopover sites and terminal locations used by manatees migrating in the study area, along the northern Gulf of Mexico and peninsular Florida coasts.
Data Collection
Manatee movement data were collected via GPS satellite telemetry from manatees between September 2009 and January 2015. Manatees were captured and tagged using established methods for manatee health assessments, and one manatee, TCR026, was tagged following rescue and rehabilitation (Supplementary Table 1; Bonde et al., 2012). Health assessments were part of an ongoing, multi-agency effort in which biologists and veterinarians determine the health and wellbeing of wild manatees by measuring morphometrics (i.e., straight-length), temperature, heart rate, and respiration rate and collecting blood samples for later analyses (Bonde et al., 2012). Individual manatees were located via an aerial observer and were targeted for capture in a net deployed from a specialized capture boat. Captured manatees were brought aboard the boat and underwent a veterinary evaluation prior to outfitting with a belt and floating, tow-behind tagging platform (Telonics Inc., Mesa, AZ, United States) (Deutsch et al., 2003; Aven et al., 2015). Each belt was outfitted with a Sonotronics CHP-87-L acoustic transmitter (Sonotronics, Inc., Tucson, AZ, United States) detectable by passive acoustic monitors (PAMs; Lotek WHS2000; Lotek Wireless Inc., Newmarket, ON, Canada). Tags were programmed to record GPS locations every 15 or 30 min, and locations were downloaded following tag recovery. Captures and releases occurred at the same location, and manatees were usually released within an hour. For TCR026, rescue capture occurred in Magnolia Springs, Alabama during January 2015 and release was in Crystal River, Florida during March 2015. Tags were exchanged near the end of their battery life or recovered after a designed breakaway released the tracking gear from the manatee to protect against entanglement. New tags or tag and tether combinations were attached by snorkeling or during a subsequent capture event (Supplementary Table 1). Tag recovery was 98% because lost tags were easily located in the shallow, coastal systems where tags were lost.
Manatee locations were monitored using Service ARGOS satellite system and by obtaining periodic visual observations (Aven et al., 2015). Data were directly downloaded from tagging platforms and included standard GPS locations or quick-fix pseudoranging (QFP) positions accurate within 10 or 75 m, respectively. All locations that had successful GPS fixes or resolved QFP positions were included in the dataset. GPS data were plotted in ArcMap 10.3 to verify accuracy of locations, and all locations on land were removed (0.00006%). To fill data gaps for manatee locations when satellite GPS data were unavailable, additional location data were provided from PAMs and photo-identification methods. PAMs were deployed at strategic locations in Mobile Bay, Alabama and Apalachicola, Florida (as described in Aven et al., 2015, in which a portion of these data were previously reported). Photo-identification data were obtained during field observations and from opportunistic, publicly reported manatee sightings to the Dauphin Island Sea Lab’s Manatee Sighting Network (DISL/MSN; Pabody et al., 2009; Hieb et al., 2017). Photos of individual manatees were submitted to the Manatee Individual Photo-identification System (Beck and Reid, 1995) to define and document the regional sighting history of each animal in collaboration with the U.S. Geological Survey Sirenia Project, Florida Fish and Wildlife Conservation Commission, Mote Marine Laboratory, and other researchers and managers who contributed to the database. All work on manatees was performed under U.S. Fish and Wildlife Service Research Permits MA107933-1 and MA37808A-0, Alabama Department of Conservation and Natural Resources Division of Wildlife and Freshwater Fisheries annual permits, and University of South Alabama (581568, 1038636) and Sea to Shore Alliance (S2S201003) IACUC protocols.
Movement Analysis
To determine the terminal and stopover locations of migrations and the duration manatees spent at each location, we calculated the mean daily locations of manatees from all recorded latitudes and longitudes per day for each manatee during each seasonal migration. Autumn migrations were eastward in direction and occurred August-December, while spring migrations were westward and occurred February-August. During the study period, we calculated the number of times sites were used as terminal locations (i.e., the most westerly or easterly site used), and the number of manatees that visited each terminal location. We did not count any terminal locations in cases where tags detached during migration unless there were PAM or photo-identification records at a site after the tag detached. We considered locations to be stopovers when manatees spent more than 7 consecutive days at that location (Deutsch et al., 2003). If manatees spent more than 7 days at a terminal location it was also considered a stopover. We examined the daily locations of manatees within stopover locations to delineate boundaries for each site. To be consistent with previous work, when location data were not available (i.e., non-continuous tagging duration due to tag loss, malfunction, etc.) for fewer than 25 days, and the animal was in the same location before and after the data-gap period, the animal was considered to occupy the same stopover location throughout that time (Deutsch et al., 2003). When location data were unavailable for more than 25 days, we considered the manatee’s location before and after the data-gap period to be separate stopover occurrences regardless of location (Deutsch et al., 2003).
To better understand manatee movement patterns during migration, we calculated total distance, duration and step lengths of migration segments during each migration season. We calculated total migration distance as the distance between two terminal sites using Geographic Information System (GIS) tools to delineate a continuous aquatic path following the contour of the nGoM coast and the most direct migratory travel routes used by most manatees. To estimate the total duration of migration, we considered migrations to have commenced when a manatee moved in a consistent direction, with differences between daily mean longitudes of > 0.02°, until the manatee reached its terminal location. Step lengths are segments of an animal’s trajectory that all occur in a similar direction (Edelhoff et al., 2016) and were calculated to better understand fine scale movements and directedness of travel during migration among terminal and stopover locations. We defined step length as the distance (calculated as the sum of distances between GPS locations) a manatee moved in a certain direction before making a turn ≥ 45° (Turchin, 1998). To obtain a measurement of overall directedness of travel during migration, we averaged step lengths for the total duration of each migration. Additionally, we determined the locations of long steps (i.e., step lengths >20 km).
Migration Models
We used net-squared displacement (NSD) models to evaluate migration patterns among individuals (Bunnefeld et al., 2011), where NSD is defined as the linear distance between two points in space. We calculated NSD as the shortest distance between the location of a manatee and a defined point within Mobile Bay (30.6284° N, −88.0318° W) using the haversine formula in the geosphere package in R (Hijmans et al., 2019). Mobile Bay was chosen as the starting point because it was a primary terminal and stopover location in the nGoM outside of peninsular Florida, and it was the general region where manatees were captured and outfitted with tags. NSD models use a collection of single- and double-logistic and linear equations to differentiate between fixed and mixed migration, dispersal, nomadism, and residency as originally developed by Bunnefeld et al. (2011). Fixed migration occurs when individuals return to the same location where migration commenced and is fit with a double-logistic equation
where δ is the asymptotic height (i.e., maximum NSD), θa and θs are the times, in days, that migration is half completed for the autumn and spring migrations, respectively (Bunnefeld et al., 2011). φa and φs are the times that between half and ∼3/4 of the migration is completed for the autumn and spring migrations, respectively, and t is time since 1 August, because most manatees were tagged in August or early September. Mixed migration occurs when individuals return to the same region but not the exact location where migration had commenced and is also modeled with a double-logistic equation
where the asymptotes δ can vary between the autumn (δa) and spring (δs) (Bunnefeld et al., 2011). Dispersal occurs when individuals permanently relocate to a new location and is modeled with a single-logistic equation
(Bunnefeld et al., 2011). Nomadism occurs when individuals disperse from their initial location and move continually to new locations but never settle permanently
where β is a constant (Bunnefeld et al., 2011). Finally, residency occurs when individuals never leave their initial location and is modeled with a linear equation that only has an intercept
where c is a constant (Bunnefeld et al., 2011).
We fit each equation to movement of individuals for whom we had reliable satellite telemetry data during both autumn and spring migrations. We estimated NSD model parameters using the nls() function of the nls2 package in R (Grothendieck, 2013). We used Akaike Information Criterion (AIC) to determine the best fitting model for each individual (Bunnefeld et al., 2011; Burnham et al., 2011), where the model with the lowest AIC value was considered the best-fitting model (Burnham et al., 2011). To measure the relative strength of each model, we calculated normalized Akaike weights, w, for each model i, where , which provides a probability that the given model is the best-fitting model: w > 0.9 indicate strong model support over others, and w > 0.1 indicate some model support and should be considered in the analysis (Burnham et al., 2011).
Statistical Analyses
To determine factors that mediated the duration of manatee migrations, we used generalized linear mixed effects models with an identity link (Zuur, 2009). The response variable of these models was the duration, in days, of manatee migrations. Fixed effects included average step length, season (autumn, spring), migration distance, and number of stopovers. Interactive terms included season∗step length and season∗migration distance. We also ran a null model and included manatee individual as a random effect in all models (Zuur, 2009), and all models were run with the lme() function in the R package lme4 using a maximum likelihood method (Bates et al., 2015). We performed a model selection analysis to determine which model best explained variation in the duration of migrations and used AIC values corrected for small sample sizes (AICc) to determine the best-fitting models. We calculated normalized Akaike weights, wi, for each model i and used those weights to calculate weighted parameter averages (Burnham et al., 2011). All statistical analyses were performed in R (R Core Team, 2013).
Results
Manatee Tagging
We tagged 11 manatees between September 2009 and March 2015. Eight of those tagged manatees were adult males and the remaining three were adult females (Supplementary Table 1). Continuous tagging duration ranged from 0.5 to 13 months, and non-continuous tagging duration (i.e., an individual tagged more than once) ranged from 4 to 22 months. Collection of GPS location data for tagged manatees ended in February 2016 (Figure 2).
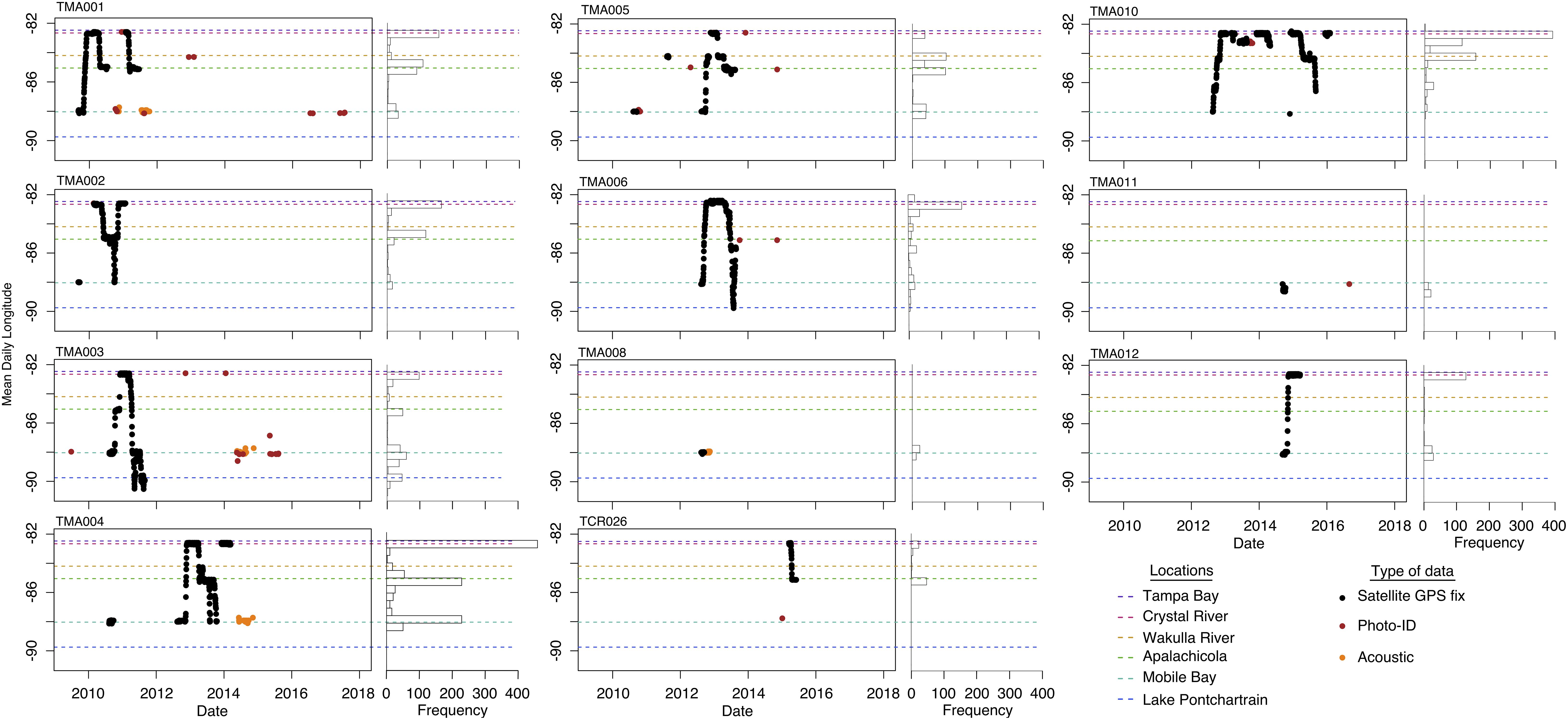
Figure 2. Mean daily longitudes through time and total frequency of longitudes for individual manatees during migrations from 2009 to 2018. Location data include satellite tag detections, passive acoustic monitoring, and photo-identification of individuals with known scar patterns.
Daily Mean Locations, Terminal Locations, and Stopover Locations
Daily mean locations revealed predictable, seasonal movements in which manatees migrated from the nGoM to peninsular Florida during autumn (eastward) and returned to the nGoM during spring (westward; Figure 2). Some manatees migrated directly back to peninsular Florida during autumn migrations and usually overwintered in Crystal River but also Suwannee River and Tampa Bay (Figure 2). Other manatees stopped at one or more stopover sites during migration between terminal locations (Figure 2). All manatees except one, TMA010, returned to the nGoM after overwintering in peninsular Florida (Figure 2).
Crystal River and Mobile Bay were the two most frequently used terminal locations for autumn/eastward and spring/westward migrations, respectively (Figures 2, 3A,B). Eight manatees used Crystal River, and ten manatees used Mobile Bay as a terminal location (Figures 2, 3A). Crystal River was used as a terminal location 14 times and Mobile Bay was used 16 times (Figures 2, 3B). Three individuals, TMA003, TMA006, and TMA010, were tracked over multiple years and possibly had different terminal locations among years (Figure 2). Suwannee River and Tampa Bay were each used once as terminal sites by two different manatees during autumn migration (Figures 2, 3A,B). These eastern terminal locations were overwintering sites (Figure 2). Apalachicola and Lake Pontchartrain were each used twice as terminal locations by two different manatees during spring migrations (Figures 2, 3A,B).
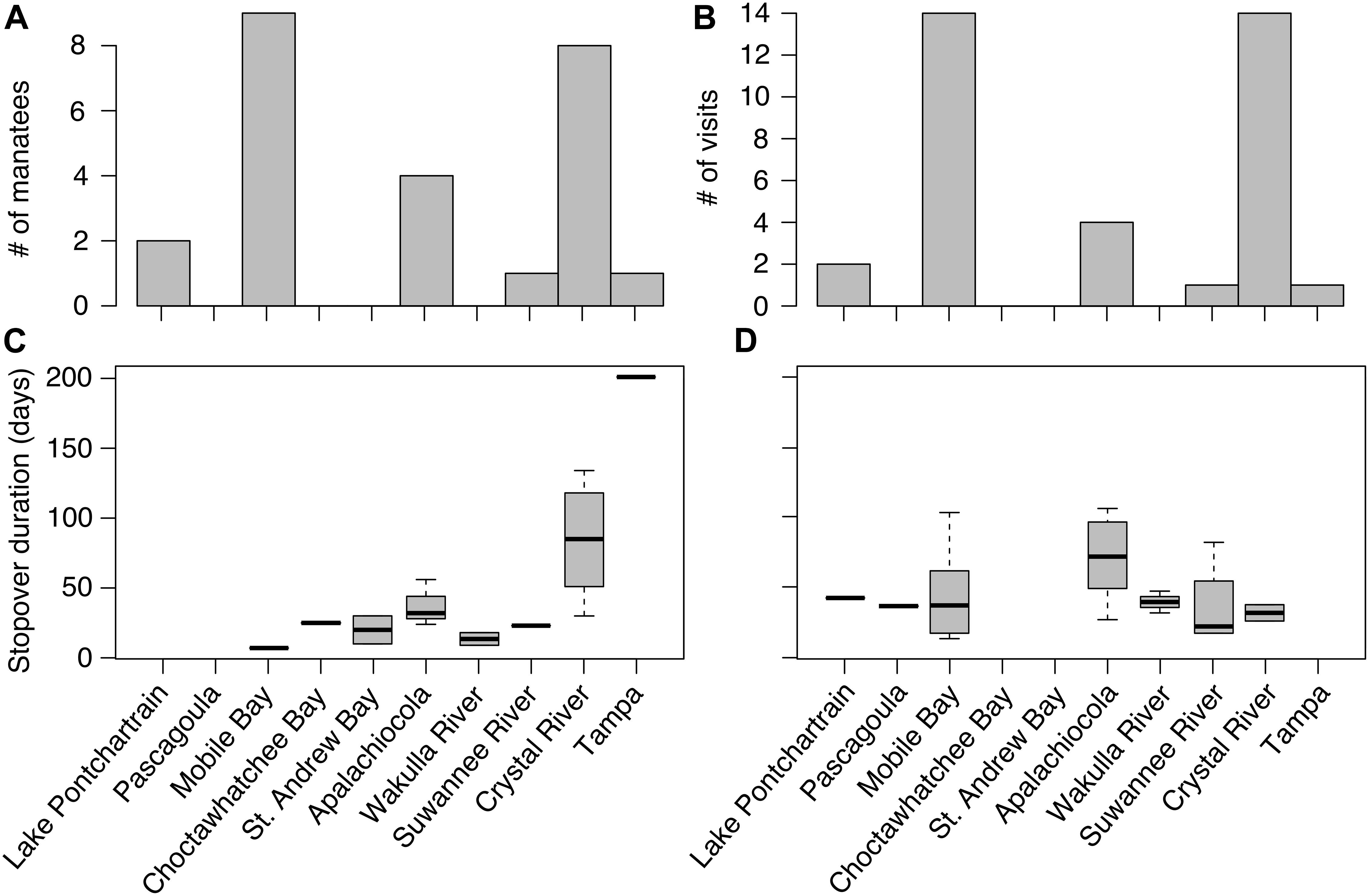
Figure 3. The number of satellite telemetry tagged manatees (A) and visits (B) at terminal locations along manatee migration routes in the northern Gulf of Mexico and peninsular Florida. Stopover duration (days) for satellite tagged manatees at each site during autumn (C) and spring (D) migrations. Tampa was used once as a terminal location and stopover.
We identified 10 stopover locations between, and including, Lake Pontchartrain and Tampa Bay. The stopover locations most used by tagged manatees were (west-east) Mobile Bay, Apalachicola/St. Joseph Bay, and Wakulla, Ochlocknee, and Crystal Rivers (Figure 2). Mobile Bay and Apalachicola were the most frequently used stopover locations along the nGoM coast (Figure 2). Additionally, manatees used Lake Pontchartrain, Pascagoula River, Choctawhatchee Bay, St. Andrews Bay, and Suwannee River as stopover locations (Figure 2). During autumn migrations, manatees stopped most frequently at sites farther east along the Florida panhandle (Figures 2, 3C) and made fewer, shorter stops at more westerly sites in Alabama and Mississippi. Manatees frequently stopped at Apalachicola for 30–60 days before continuing east (Figures 2, 3C). Manatees also frequently stopped at Wakulla River for 14–30 days, and one manatee stopped at Suwannee River for ∼30 days during autumn migration (Figure 3C). During spring/westward migrations, manatees stopped most frequently and for the longest periods of time in Apalachicola and Mobile Bay, where manatees spent 30–110 days (Figures 2, 3D). Some manatees (n = 3) also frequently used Suwannee and Wakulla Rivers for 30–60 days on their way west (Figure 2). Manatees (n = 2) that migrated west of Mobile Bay during spring/westward migrations most frequently used Pascagoula River and Lake Pontchartrain for 40–45 days each (Figures 2, 3D). Although manatees spent more time at Crystal River and Tampa Bay than other sites, most manatees (n = 8) spent more time cumulatively in the nGoM, between Wakulla River and Lake Pontchartrain than at any sites in peninsular Florida (Suwannee River, Crystal River, Tampa Bay; Figure 2). Cumulatively, manatees spent 200–250 days of the year in the nGoM and 100–150 days of the year in peninsular Florida.
Migration Models
We were able to fit all five net-squared displacement (NSD) models to satellite telemetry data for seven round-trip migrations by seven manatees (Figures 2, 4; Supplementary Tables 2–8). For five of those seven round trip migrations, mixed migration was the best-fitting model (Table 1; Figure 4), where manatees departed Mobile Bay, overwintered in Crystal River or Tampa Bay, and returned to the nGoM but not necessarily to Mobile Bay. TMA006 was best fit with a fixed model because TMA006 returned relatively close to Mobile Bay (Figure 4), but the mixed migration model also had a high AIC weight (Supplementary Table 6). The one manatee migration trip that was not best fit by the mixed or fixed migration model, TMA010, was best fit by a dispersal model. TMA010 did not return to the nGoM during 2013 but summered in Suwannee River in northern peninsular Florida (Table 1; Figure 4).
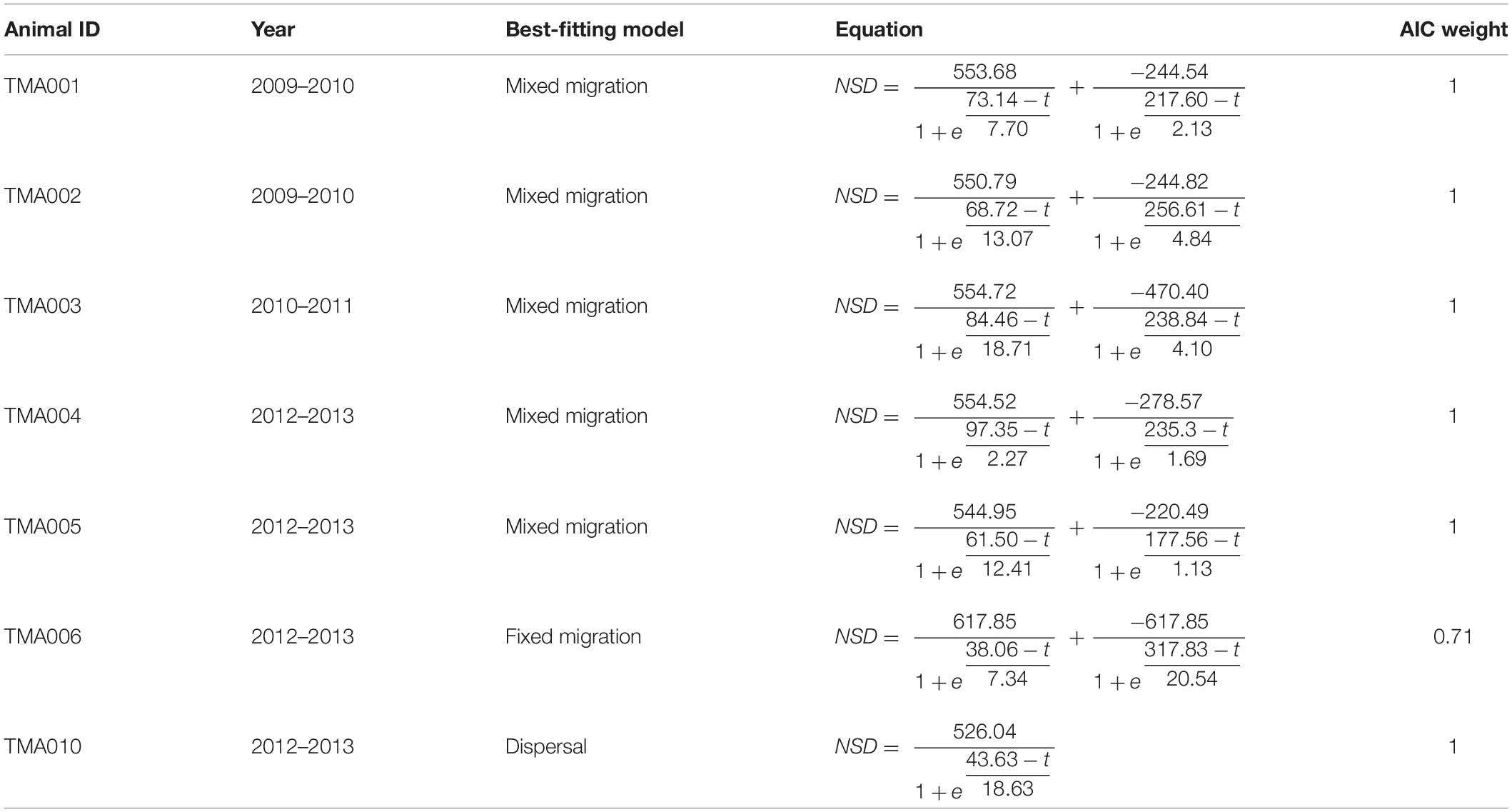
Table 1. Best-fitting net-squared displacement models for each manatee where satellite telemetry data were available for both autumn and spring migrations.
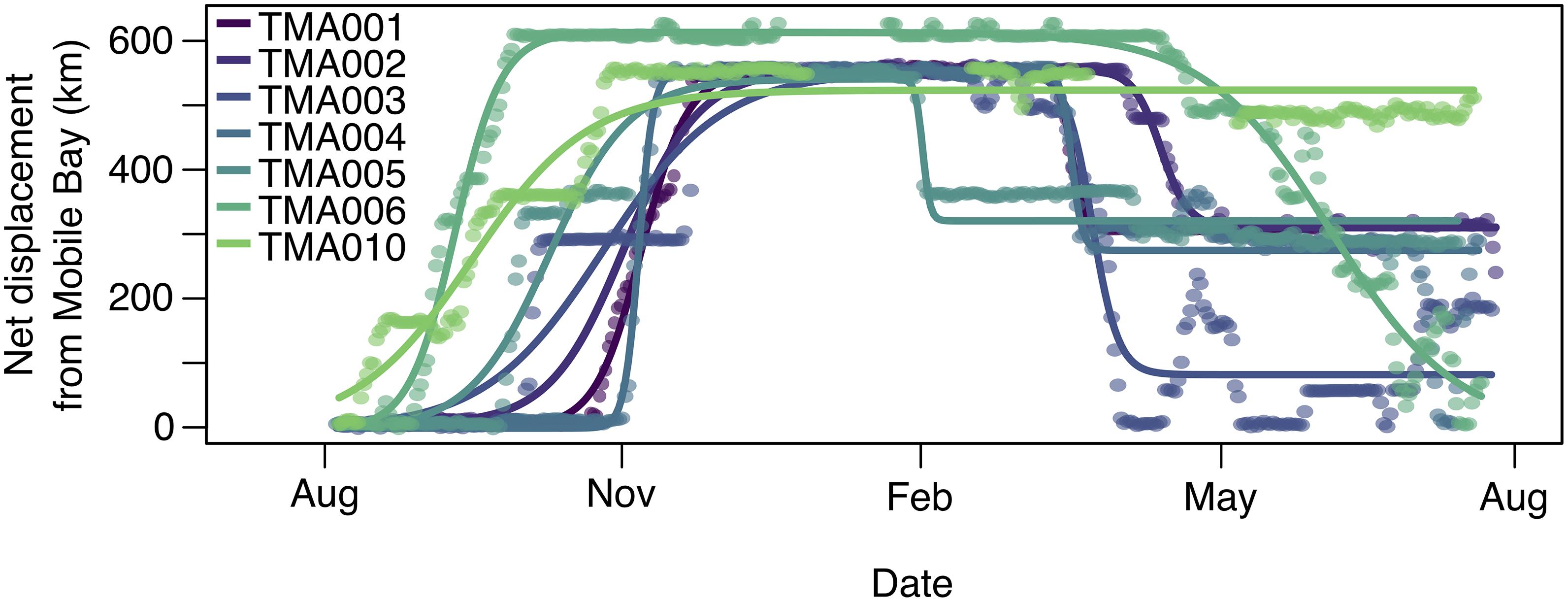
Figure 4. Best fitting net-squared displacement models of migratory movement for seven tagged manatees for which satellite telemetry data were available for both autumn and spring migrations. Displacement was measured as distance from Mobile Bay, Alabama.
Overall Migration Patterns and Durations
We documented eight autumn and eight spring migrations (Table 2). Autumn migrations commenced between August and November, ended between October and December, and ranged in length from 10 to 79 days (Table 2). In general, manatees that commenced autumn migrations earlier had longer migration durations and used more stopovers (Table 2; Figure 4). Spring migrations commenced as early as February and as late as May, ended between February and September, and ranged in length from 10 to 133 days (Table 2). For manatees that made the spring journey to the nGoM, those that went directly to Apalachicola made quick, direct migrations, and those that continued past Apalachicola had much longer migrations durations with more stopovers (Figures 2, 4). The duration of migrations was most affected by the number of stopovers and the directedness of travel, with a lesser effect of season; migrations with longer durations included more stopovers, had less direct travel (i.e., shorter average step lengths), and tended to occur in the spring (Table 3; Figure 5). The model that best fit the variation in the data supported these movement patterns and included the number of stopovers and average step lengths (Table 4). However, the model that included only the number of stopovers had a low AIC value and high weight (Table 4). The strength of the effects of these factors and the ordering of the models suggest that the number of stopovers had the greatest effect on migration duration, followed by average step length and season (Tables 3, 4; Figure 5). Migration distance had little effect on duration (Tables 3, 4).
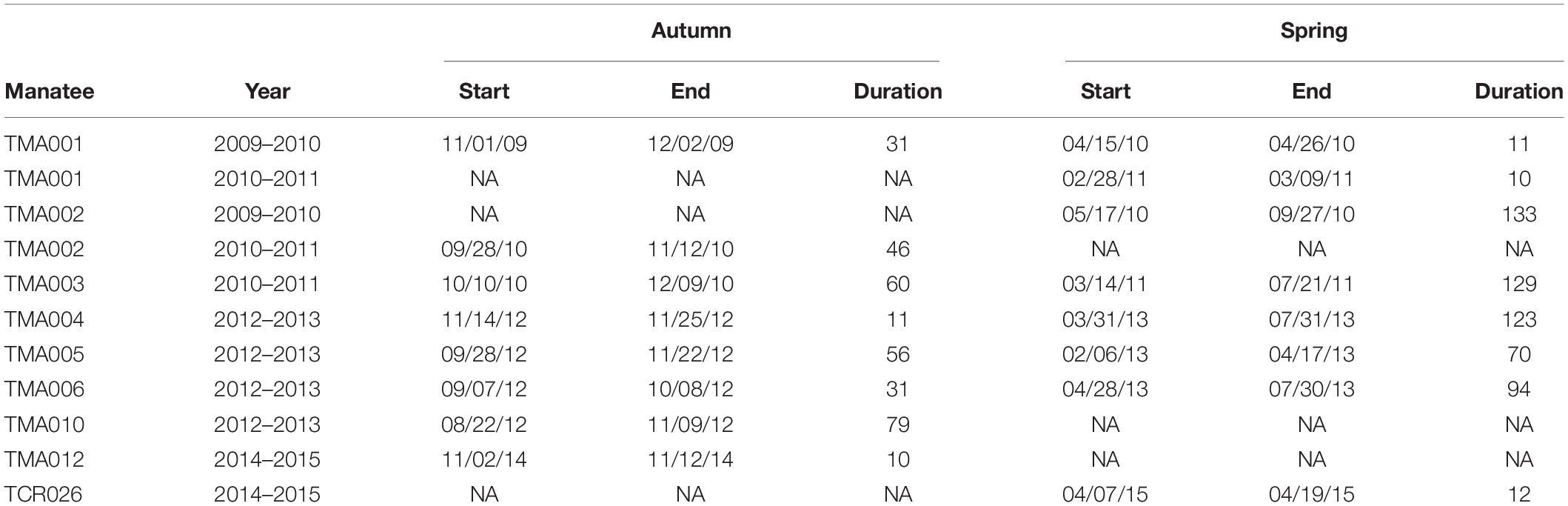
Table 2. Start and end dates and duration (days) of manatee seasonal migrations between the northern Gulf of Mexico and peninsular Florida.
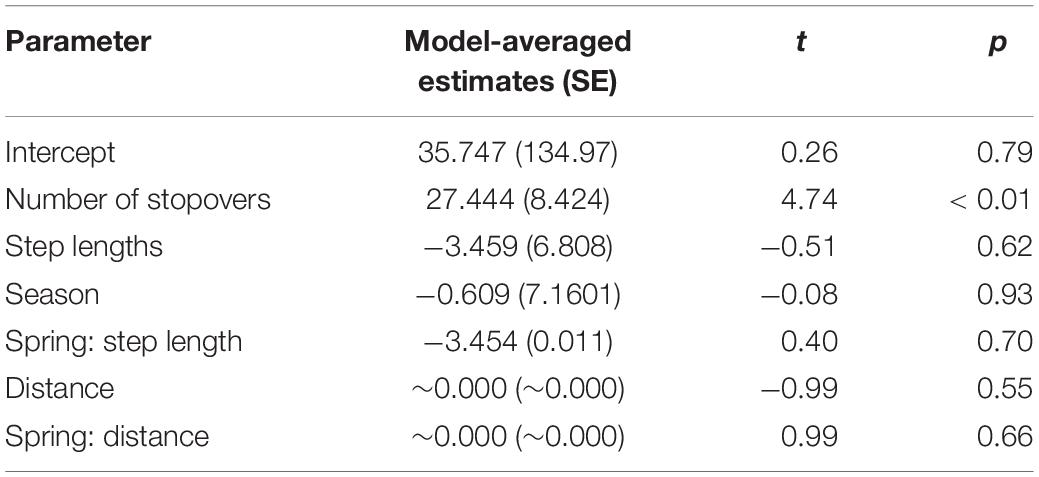
Table 3. Weighted estimates of parameter values from the selection analysis on modeling the duration of migrations.
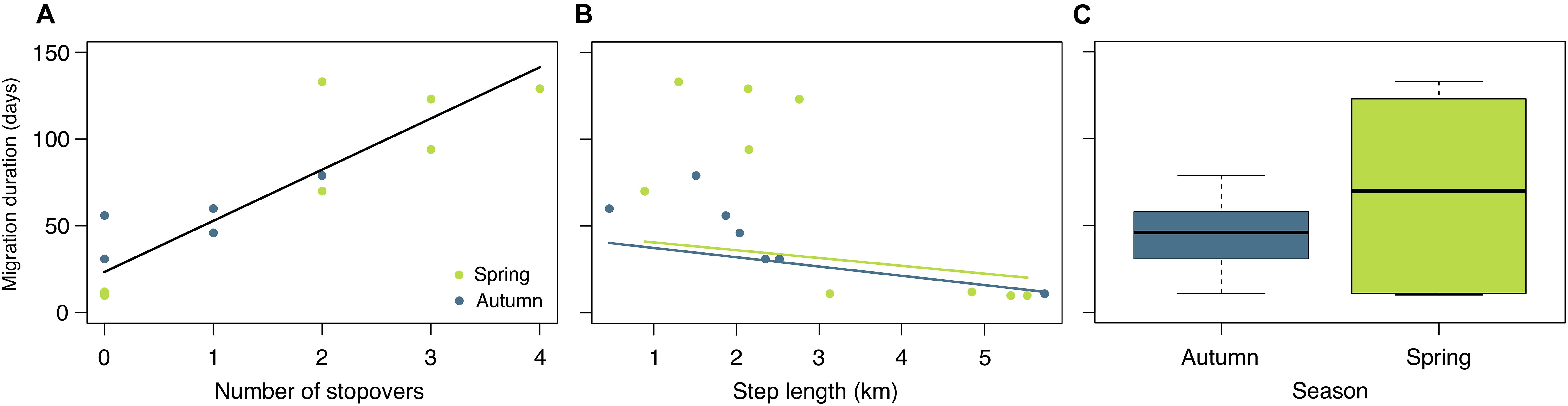
Figure 5. The effects of number of stopovers (A), step length (B), and season (C) on duration of manatee migrations.
Collectively, manatees made 179 steps that were longer than 20 km (i.e., long step lengths). The longest step lengths occurred between Mobile Bay and Apalachicola (Figure 6), and the longest single step (142 km) also occurred in this region (Figure 6). The next longest step lengths occurred between Wakulla and Crystal Rivers, with the longest steps in this region occurring just east of the Wakulla River (Figure 6). Some long steps also occurred between Apalachicola and Wakulla River as well as between Lake Pontchartrain and Mobile Bay, but step lengths in these areas were generally shorter (Figure 6).
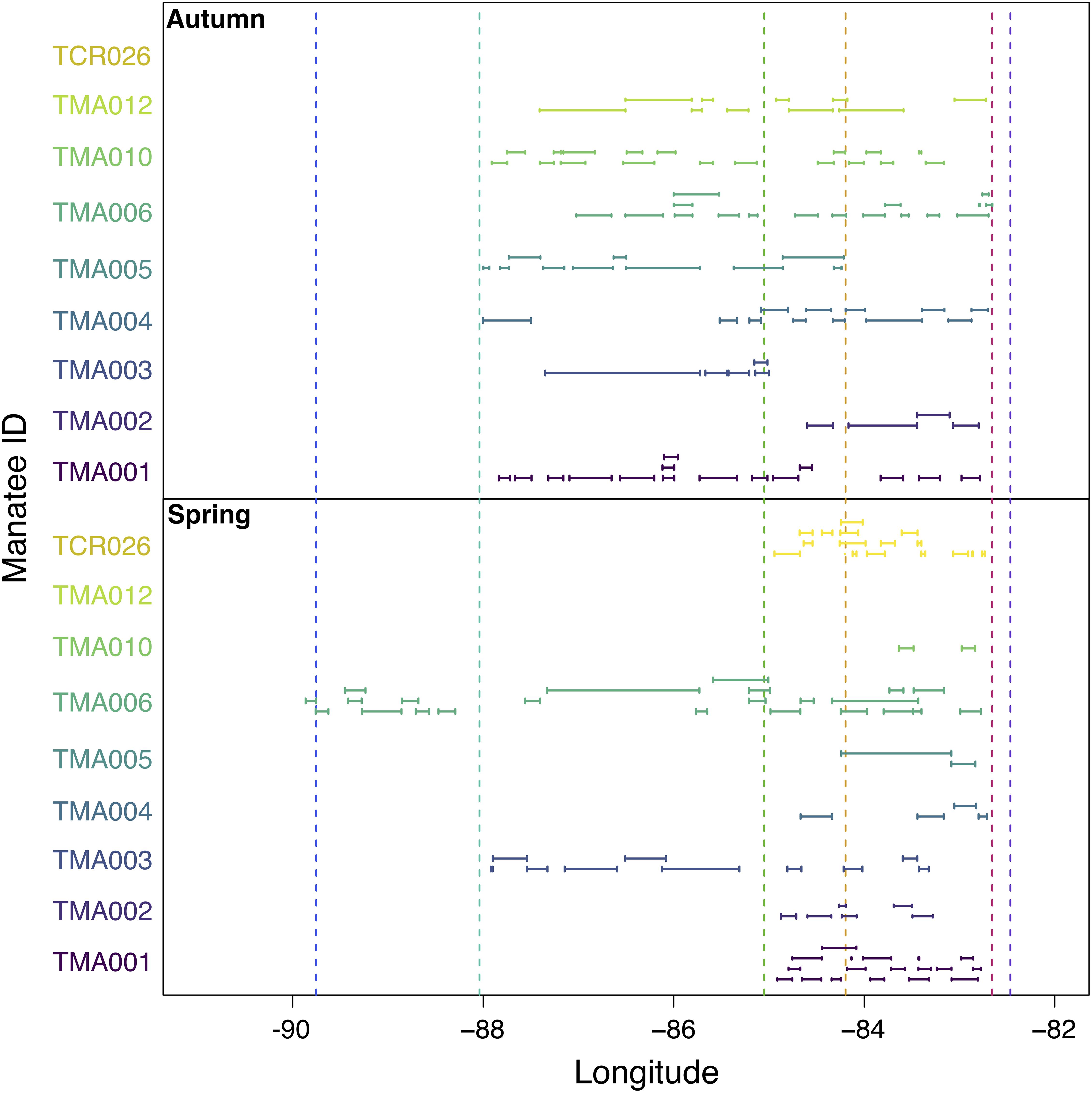
Figure 6. Location of long step lengths (>20 km) during autumn and spring migrations. Line caps indicate start and end longitudes of the step length. Colored dashed lines refer to locations shown in Figure 2.
Discussion
We found that West Indian manatees in the southeastern U.S.A. expanded their geographic range poleward through partial migrations. Tagged manatees made repeated, seasonal movements between the nGoM and peninsular Florida, extensively using and exhibiting high fidelity to multiple sites in the nGoM. Manatees are becoming increasingly common in the nGoM (Hieb et al., 2017), and the migratory patterns we found are supported by the timing and number of opportunistic manatee sightings in the nGoM (Pabody et al., 2009; Hieb et al., 2017), and similar in timing to migrations along the southeastern Atlantic coast (Deutsch et al., 2003; Cummings et al., 2014). These migrations enable manatees to track favorable environmental conditions as they expand poleward and into regions outside manatees’ more modern range (Moore, 1951a,b). Similar migratory patterns are driving range shifts in other species (McKilligan et al., 1993; Ruiz-Cooley et al., 2013; Hovick et al., 2016). The poleward range expansion of jumbo squid, Dosidicus gigas, into warming waters of the eastern Pacific is at least in part facilitated by migratory flexibility (Ruiz-Cooley et al., 2013). The cattle egret, Bubulcus ibis, a species that has experienced rapid global range expansion in the last century, performs migrations that exploit seasonal resources and likely contribute to their range expansion at least in southern Australia (McKilligan et al., 1993). Among North American terrestrial birds, those with partial and intra-continental migrations had greater latitudinal range shifts compared to residents and neotropical migrants, likely because the former can more easily track conditions in migratory regions (Hovick et al., 2016). Similar to poleward range shifts, altitudinal migration has enabled roe deer in central Europe to exploit decreased winter snowpack and shift the altitudinal limit of their winter range (Bright Ross et al., 2021). Partial migration may, therefore, be a general mechanism for climate driven range shifts among a diverse range of species as it is the most common type of migration and occurs in most animal phyla (Dingle and Drake, 2007; Chapman et al., 2011, 2012; Shaw and Couzin, 2013).
Although manatees observed in the nGoM and other areas outside peninsular Florida have long been assumed to be temporary or accidental visitors (Gunter, 1941; Fertl et al., 2005), our results indicate that the nGoM is part of their geographic range, and this region will likely become increasingly important under future climate projections. Most of the tagged manatees we studied spent cumulatively more time in the nGoM, between Wakulla River and Lake Pontchartrain, than in peninsular Florida. This novel finding suggests that some manatees use peninsular Florida primarily when they need to seasonally seek thermal refuge and reside in the nGoM for the remainder of the year. For example, of the 11 tagged manatees, nine showed repeated migration to many of the same locations annually, and TMA001 has been documented in the nGoM outside of Florida in nine of the past 12 years (Carmichael et al., 2021). As climate changes and sea temperatures warm in coming years, the need to return to refugia in Florida may diminish and manatees may spend more time in and ultimately transition residency from peninsular Florida to areas like the nGoM. Timing and locations of manatee sightings in the nGoM during recent cold seasons provides evidence that a few manatees have possibly stayed in the region into or through the cold season instead of promptly returning to refuge sites in peninsular Florida (Carmichael et al., 2021); from 2018 to 2021 live manatees were confirmed in waters along the Mississippi-Alabama coast from mid-November to March, and manatees have been sighted during March and April with symptoms of mild cold stress sustained at an unknown location during the cold season (Carmichael et al., 2021). The movement patterns of our tagged manatees along with these observations may therefore provide early evidence that some sites in the nGoM may become warm water, overwintering sites and that long-term changes in migratory tactics could include transitioning to periodic overwintering or residency.
Manatees use habitats at stopover sites within the nGoM for extended periods, making their motivation for stopovers different from other species. Manatees in this study often spent longer periods of time at stopovers than at terminal locations, sometimes staying only a few days at westernmost sites before returning eastward. For most other species, migration includes highly directed movements (Sheppard et al., 2006; Michelot et al., 2017), and the use of stopovers is often limited to replenishing energy reserves (Gómez et al., 2017). For example, many birds use particular sites to resupply energy reserves and recondition their bodies before or after arduous sections of migratory routes (Buchanan et al., 2012; Hasselquist et al., 2017). Terrestrial herbivores often track resource quality as they move from winter to summer ranges (i.e., the green wave hypothesis; Bischof et al., 2012; Aikens et al., 2017). For manatees, migration distance had little effect on the duration of migrations, and individuals made long distance migrations sometimes slowly (using many stopover locations) and sometimes quickly, demonstrating that no single stopover site was essential to replenishing energy reserves during migration. Although Mobile Bay and Crystal River were the most used western and eastern terminal locations, respectively, some animals opted to travel farther west to Lake Pontchartrain (TMA003, TMA006) or east to Tampa (TMA006). In some cases, terminal locations were the conservative end points because tags were lost, particularly during spring migration, resulting in shorter tracking durations, and animals may have traveled farther west than known. The similarity in phenology of annual plant cycles across sites in the nGoM along with ready access to freshwater and a variety of vegetation types known to be used by manatees may enable manatees to flexibly use stopover sites and endpoints along the migration route from the Florida panhandle to eastern Louisiana (Hillmann et al., 2019). These observations suggest that manatees have flexible migratory patterns for which the distance and duration are not strictly driven by environmental or physiological needs once the basic parameters for survival are met.
Multiple factors, including season, use of stopover sites, and directedness of travel, contributed to variation in the duration of nGoM migrations (Erni et al., 2002; Nilsson et al., 2013; Michelot et al., 2017). Manatee migrations tended to be shorter in duration during autumn when manatees used stopovers for shorter periods of time (∼30 days), and manatees that commenced autumn migration earlier made more stopovers. Manatees that left the nGoM late in the season (i.e., November) returned directly (indicated by long average step lengths) to peninsular Florida, suggesting that skipping stopovers may have been necessary to arrive in Florida before water temperatures in the nGoM became too cold (Irvine, 1983; Bossart et al., 2003; Deutsch et al., 2003). The somewhat longer but more variable duration of springtime migrations were likely possible because of the lack of a looming or eminent driver of migration such as the threat of cold temperatures. Manatees that migrate long distances along the southeast Atlantic coast of the U.S.A. also have shorter autumn than spring migrations that are faster when they leave later in the season (Deutsch et al., 2003). In contrast, several individual manatees in this study promptly left Crystal River in the early spring and swam with direct movements to Suwannee River or Apalachicola, suggesting that resource availability may become limited at high-density overwintering sites and affect the timing and duration of migration in some years (Littles et al., 2019). Of note, during 2020–2021 there has been an unusual mortality event for manatees on the U.S.A. Atlantic coast attributed largely to starvation from limited food resources in key peninsular habitats (Deutsch et al., 2021). Coincidentally, the first documented nGoM mortality of a manatee previously known only from the Atlantic coast occurred in Mississippi waters during February 2021 (Carmichael et al., 2021). Seasonal differences in the duration of migrations are found in a variety of species, typically due to variation in suitability of environmental conditions, including food availability and temperature (Nilsson et al., 2013; Martin et al., 2018; Deng et al., 2019). These findings suggest that seasonal and inter-annual variation in factors such as resource availability and water temperature affect immediate variation in the timing and duration of migration, with longer-term implications for range expansion.
Directed movements during migration likely reflect location-specific variation in habitat quality and structure. First, many segments in which movements were most directed (step lengths >20 km) were areas where freshwater and dietary resources were absent or uncommon. For many species, migration and movements between sites and resource patches are often very directed with long step lengths and small angles between steps (De Knegt et al., 2007; Michelot et al., 2017; Cloyed and Dell, 2019). Second, manatees often used the Intracoastal Waterway and inshore waters and rivers or followed the coastline during migrations, making their movements frequently constrained by land and conducive to long steps (Cloyed et al., 2019). Manatees also performed long step lengths across open sections of water such as crossing Apalachee Bay near Wakulla River and from Cedar Key, just south of Suwannee River, to Crystal River. This combination of traveling among resource-rich sites and in areas where movements were constrained to follow the coast or east-west waterways resulted in similar spatial patterns of directed movements among manatees.
The increased presence of manatees in the nGoM raises new conservation concerns. Our results revealed migration to a series of sites in the nGoM that are currently important for manatees, particularly Mobile and Apalachicola Bays. In populations of other species that are comprised of both migrants and residents, ecological conditions such as competition, predation, and resource abundance all contribute to switching among tactics (Brodersen et al., 2011; Chapman et al., 2011; Mysterud et al., 2011; Eggeman et al., 2016) and are likely to affect manatee migrations. TMA010 did not migrate to the nGoM during the summer of 2013, even though he migrated in years preceding and following 2013. Although more work is needed to determine the factors driving manatee geographic distributions and their specific habitat use in the nGoM into the future, this study makes an important contribution to the growing body of data needed to understand mechanisms of migration under heterogeneous and changing environmental conditions. Detailed information on species occurrence and habitat use will enable identifying critical resources along expanding migratory routes and implementing location-specific strategies to reduce injury and mortality (Hieb et al., 2017). For example, manatees may be at greater risk from vessel interactions and strikes, other types of human interaction (Hieb et al., 2017, 2021; Cloyed et al., 2019), and cold stress associated with migrations to the nGoM. An increasing number of tropical species, from primary producers to large vertebrates like manatees, have recently become established in the nGoM, leading to the hypothesis that these waters will continue to tropicalize, significantly impacting ecosystem functions (Heck et al., 2015). These observations have raised local management and conservation concerns and make manatees an excellent sentinel species to monitor migratory behaviors and help define the broader effects of climate change on tropicalization of the nGOM and similarly affected waters elsewhere (Bonde et al., 2004; Heck et al., 2015; Scheffel et al., 2018).
Data Availability Statement
The datasets presented in this study can be found in online repositories. The names of the repository/repositories and accession number(s) can be found below: http://cf.disl.org/datamanagement/metadata_folder/DISL-Carmichael-MSN-010-2016.xml.
Ethics Statement
The animal study was reviewed and approved by the University of South Alabama (581568 and 1038636) and Sea to Shore Alliance (S2S201003) IACUC protocols.
Author Contributions
CC analyzed the data, wrote the main draft of the manuscript, and participated in editing the manuscript. EH, KD, MR, and RC contributed to collection and analysis of data from tagged and sighted manatees and participated in writing and editing the manuscript. RC and MR conceived the project. All authors contributed to the article and approved the submitted version.
Funding
This project was funded in part by the Alabama Division of Wildlife and Freshwater Fisheries under traditional Section 6 of the U. S. Fish & Wildlife Service, the Northern Gulf Institute, Mobile Bay National Estuary Program, Seamen’s Foundation, the University of South Alabama, and the Dauphin Island Sea Lab.
Conflict of Interest
The authors declare that the research was conducted in the absence of any commercial or financial relationships that could be construed as a potential conflict of interest.
Publisher’s Note
All claims expressed in this article are solely those of the authors and do not necessarily represent those of their affiliated organizations, or those of the publisher, the editors and the reviewers. Any product that may be evaluated in this article, or claim that may be made by its manufacturer, is not guaranteed or endorsed by the publisher.
Acknowledgments
We thank Allen Aven for helping to conceive of this project, collect data, and generate the initial analyses. We also thank personnel at SeaWorld Orlando, Sea to Shore Alliance, and University of Florida Veterinary School, flight crews, DISL staff and volunteers, particularly Courtney Nelson Seely, Nicole Taylor, Rod Kellogg, and many others for their field work assistance related to capturing, tagging, and tracking manatees.
Supplementary Material
The Supplementary Material for this article can be found online at: https://www.frontiersin.org/articles/10.3389/fmars.2021.725837/full#supplementary-material
References
Acker, P., Daunt, F., Wanless, S., Burthe, S. J., Newell, M. A., Harris, M. P., et al. (2021). Strong survival selection on seasonal migration versus residence induced by extreme climatic events. J. Anim. Ecol. 90, 796–808. doi: 10.1111/1365-2656.13410
Aikens, E. O., Kauffman, M. J., Merkle, J. A., Dwinnell, S. P., Fralick, G. L., and Monteith, K. L. (2017). The greenscape shapes surfing of resource waves in a large migratory herbivore. Ecol. Lett. 20, 741–750. doi: 10.1111/ele.12772
Aven, A., Carmichael, R. H., Hieb, E. E., and Ross, M. (2016). West Indian manatee movements reveal novel occupancy and distribution patterns in the northern Gulf of Mexico. PeerJ Preprints 4, e2072v1. doi: 10.7287/peerj.preprints.2072v1
Aven, A. M., Carmichael, R. H., Ajemian, M. J., and Powers, S. P. (2015). Addition of passive acoustic telemetry mitigates lost data from satellite-tracked manatees. Mar. Freshw. Res. 66, 371–374. doi: 10.1071/MF14178
Batalden, R. V., Oberhauser, K., and Peterson, A. T. (2014). Ecological niches in sequential generations of eastern North American monarch butterflies (Lepidoptera: Danaidae): the ecology of migration and likely climate change implications. Environ. Entomol. 36, 1365–1373. doi: 10.1603/0046-225X(2007)36[1365:ENISGO]2.0.CO;2
Bates, D., Mächler, M., Bolker, B., and Walker, S. (2015). Fitting linear mixed-effects models using lme4. J. Stat. Softw. 67, 1–48. doi: 10.18637/jss.v067.i01
Beck, C. A., and Reid, J. P. (1995). An automated photo-identification catalog for studies of the life history of the Florida manatee. USGS Inf. Technol. Rep. 1, 120–134.
Bischof, R., Loe, L. E., Meisingset, E. L., Zimmermann, B., Van Moorter, B., and Mysterud, A. (2012). A migratory northern ungulate in the pursuit of spring: jumping or surfing the green wave? Am. Nat. 180, 407–424. doi: 10.1086/667590
Bonde, R. K., Aguirre, A. A., and Powell, J. (2004). Manatees as sentinels of marine ecosystem health: are they the 2000-pound canaries? EcoHealth 1, 255–262. doi: 10.1007/s10393-004-0095-5
Bonde, R. K., Garrett, A., Belanger, M., Askin, N., Tan, L., and Wittnich, C. (2012). Biomedical health assessments of the Florida manatee in Crystal River—providing opportunities for training during the capture, handling, and processing of this endangered aquatic mammal. J. Mar. Anim. Ecol. 5, 17–28.
Bossart, G. D., Meisner, R. A., Rommel, S., Ghim, S., and Jenson, A. B. (2003). Pathological features of the Florida manatee cold stress syndrome. Aquat. Mamm. 29, 9–17. doi: 10.1578/016754203101024031
Bright Ross, J., Peters, W., Ossi, F., Moorcraft, P., Cordano, E., Eccel, E., et al. (2021). Climate change and anthropogenic food manipulation interact in shifting the distribution of alarge herbivore at its altitudinal range limit. Sci. Rep. 11:7600. doi: 10.1038/s41598-021-86720-2
Brodersen, J., Nicolle, A., Nilsson, P. A., Skov, C., Brönmark, C., and Hansson, L. A. (2011). Interplay between temperature, fish partial migration and trophic dynamics. Oikos 120, 1838–1846. doi: 10.1111/j.1600-0706.2011.19433.x
Buchanan, J. B., Lyons, J. E., Salzer, L. J., Carmona, R., Arce, N., Wiles, G. J., et al. (2012). Among-year site fidelity of Red Knots during migration in Washington. J. Field Ornithol. 83, 282–289. doi: 10.1111/j.1557-9263.2012.00376.x
Buckingham, C. A., Lefebvre, L. W., Schaefer, J. M., and Kochman, H. I. (1999). Manatee response to boating activity in a thermal refuge. Wildlife Soc. Bull. 27, 514–522.
Bunnefeld, N., Börger, L., van Moorter, B., Rolandsen, C. M., Dettki, H., Solberg, E. J., et al. (2011). A model-driven approach to quantify migration patterns: individual, regional and yearly differences. J. Anim. Ecol. 80, 466–476. doi: 10.1111/j.1365-2656.2010.01776.x
Burnham, K. P., Anderson, D. R., and Huyvaert, K. P. (2011). AIC model selection and multimodel inference in behavioral ecology: some background, observations, and comparisons. Behav. Ecol. Sociobiol. 65, 23–35. doi: 10.1007/s00265-010-1029-6
Cagnacci, F., Focardi, S., Ghisla, A., Van Moorter, B., Merrill, E. H., Gurarie, E., et al. (2016). How many routes lead to migration? Comparison of methods to assess and characterize migratory movements. J. Anim. Ecol. 85, 54–68. doi: 10.1111/1365-2656.12449
Carmichael, R. H., Hieb, E. E., Aven, A., Taylor, N., Seely, C., Delo, J., et al. (2021). Dauphin Island Sea Lab’s Manatee Sighting Network Database (1912-2021). Dauphin Island, AL: Dauphin Island Sea Lab.
Chapman, B., Hulthén, K., Brodersen, J., Nilsson, P. A., Skov, C., Hansson, L. A., et al. (2012). Partial migration in fishes: causes and consequences. J. Fish Biol. 81, 456–478. doi: 10.1111/j.1095-8649.2012.03342.x
Chapman, B. B., Brönmark, C., Nilsson, J. Å, and Hansson, L. A. (2011). The ecology and evolution of partial migration. Oikos 120, 1764–1775. doi: 10.1111/j.1600-0706.2011.20131.x
Cloyed, C. S., and Dell, A. I. (2019). Resource distribution and internal factors interact to govern movement of a freshwater snail. Proc. R. Soc. B 286:20191610. doi: 10.1098/rspb.2019.1610
Cloyed, C. S., Hieb, E. E., Collins, M. K., DaCosta, K. P., and Carmichael, R. H. (2019). Linking use of ship channels by West Indian manatees (Trichechus manatus) to seasonal migration and habitat use. Front. Mar. Sci. 6:318. doi: 10.3389/fmars.2019.00318
Cotton, P. A. (2003). Avian migration phenology and global climate change. Proc. Natl. Acad. Sci. U.S.A. 100, 12219–12222. doi: 10.1073/pnas.1930548100
Cummings, E. W., Pabst, D. A., Blum, J. E., Barco, S. G., Davis, S. J., Thayer, V. G., et al. (2014). Spatial and temporal patterns of habitat use and mortality of the Florida manatee (Trichechus manatus latirostris) in the mid-Atlantic states of North Carolina and Virginia from 1991 to 2012. Aquat. Mamm. 40:126. doi: 10.1578/AM.40.2.2014.126
De Knegt, H., Hengeveld, G., Van Langevelde, F., De Boer, W., and Kirkman, K. (2007). Patch density determines movement patterns and foraging efficiency of large herbivores. Behav. Ecol. 18, 1065–1072. doi: 10.1093/beheco/arm080
Deng, X., Zhao, Q., Fang, L., Xu, Z., Wang, X., He, H., et al. (2019). Spring migration duration exceeds that of autumn migration in Far East Asian Greater White-fronted Geese (Anser albifrons). Avian Res. 10, 1–11. doi: 10.1186/s40657-019-0157-6
Deutsch, C. J., de Wit, M., Barlas, M. E., Greer, W., Howell, A. R., Garrett, A., et al. (2021). Unprecedented mortatlity of Florida manatees along the Atlantic coast. Sirenews Newslett. IUCN Sirenia Special. Group 73, 24–26.
Deutsch, C. J., Reid, J. P., Bonde, R. K., Easton, D. E., Kochman, H. I., and O’Shea, T. J. (2003). Seasonal movements, migratory behavior, and site fidelity of West Indian manatees along the Atlantic coast of the United States. Wildlife Monogr. 151, 1–77.
Dingle, H., and Drake, V. A. (2007). What is migration? Bioscience 57, 113–121. doi: 10.1641/B570206
Edelhoff, H., Signer, J., and Balkenhol, N. (2016). Path segmentation for beginners: an overview of current methods for detecting changes in animal movement patterns. Mov. Ecol. 4:21. doi: 10.1186/s40462-016-0086-5
Eggeman, S. L., Hebblewhite, M., Bohm, H., Whittington, J., and Merrill, E. H. (2016). Behavioural flexibility in migratory behaviour in a long-lived large herbivore. J. Anim. Ecol. 85, 785–797. doi: 10.1111/1365-2656.12495
Erni, B., Liechti, F., and Bruderer, B. (2002). Stopover strategies in passerine bird migration: a simulation study. J. Theor. Biol. 219, 479–493. doi: 10.1006/jtbi.2002.3138
Fertl, D., Schiro, A., Regan, G., Beck, C. A., Adimey, N., Price-May, L., et al. (2005). Manatee occurrence in the northern Gulf of Mexico, west of Florida. Gulf Caribb. Res. 17, 69–94. doi: 10.18785/gcr.1701.07
Fodrie, F. J., Heck, K. L. Jr., Powers, S. P., Graham, W. M., and Robinson, K. L. (2010). Climate-related, decadal-scale assemblage changes of seagrass-associated fishes in the northern Gulf of Mexico. Glob. Chang. Biol. 16, 48–59. doi: 10.1111/j.1365-2486.2009.01889.x
Gannon, J. G., Scolardi, K. M., Reynolds, J. E. III, Koelsch, J. K., and Kessenich, T. J. (2007). Habitat selection by manatees in Sarasota Bay, Florida. Mar. Mamm. Sci. 23, 133–143. doi: 10.1111/j.1748-7692.2006.00096.x
Gómez, C., Bayly, N. J., Norris, D. R., Mackenzie, S. A., Rosenberg, K. V., Taylor, P. D., et al. (2017). Fuel loads acquired at a stopover site influence the pace of intercontinental migration in a boreal songbird. Sci. Rep. 7, 1–11. doi: 10.1038/s41598-017-03503-4
Gunter, G. (1941). Occurrence of the manatee in the United States, with records from Texas. J. Mammal. 22, 60–64. doi: 10.2307/1374684
Hasselquist, D., Montràs-Janer, T., Tarka, M., and Hansson, B. (2017). Individual consistency of long-distance migration in a songbird: significant repeatability of autumn route, stopovers and wintering sites but not in timing of migration. J. Avian Biol. 48, 91–102. doi: 10.1111/jav.01292
Heck, K. Jr., Fodrie, F., Madsen, S., Baillie, C., and Byron, D. (2015). Seagrass consumption by native and a tropically associated fish species: potential impacts of the tropicalization of the northern Gulf of Mexico. Mar. Ecol. Prog. Ser. 520, 165–173. doi: 10.3354/meps11104
Hieb, E. E., Carmichael, R. H., Aven, A., Nelson-Seely, C., and Taylor, N. (2017). Sighting demographics of the West Indian manatee Trichechus manatus in the north-central Gulf of Mexico supported by citizen-sourced data. Endanger. Species Res. 32, 321–332. doi: 10.3354/esr00817
Hieb, E. E., Eniang, E. A., Keith-Diagne, L. W., and Carmichael, R. H. (2021). In-water bridge construction effects on manatees with implications for marine megafauna species. J. Wildlife Manag. 85, 674–685. doi: 10.1002/jwmg.22030
Hijmans, R. J., Williams, E., and Vennes, C. (2019). geosphere: spherical trigonometry. CRAN. Available online at: https://cran.r-project.org/web/packages/geosphere/index.html (accessed July 2020).
Hillmann, E. R., DeMarco, K., and La Peyre, M. K. (2019). Salinity and water clarity dictate seasonal variability in coastal submerged aquatic vegetation in subtropical estuarine environments. Aquat. Biol. 28, 175–186. doi: 10.3354/ab00719
Hoegh-Guldberg, O., and Bruno, J. F. (2010). The impact of climate change on the world’s marine ecosystems. Science 328, 1523–1528. doi: 10.1126/science.1189930
Hovick, T. J., Allred, B. W., McGranahan, D. A., Palmer, M. W., Elmore, R. D., and Fuhlendorf, S. D. (2016). Informing conservation by identifying range shift patterns across breeding habitats and migration strategies. Biodivers. Conserv. 25, 345–356. doi: 10.1007/s10531-016-1053-6
Irvine, A. B. (1983). Manatee metabolism and its influence on distribution in Florida. Biol. Conserv. 25, 315–334. doi: 10.1016/0006-3207(83)90068-X
Laist, D. W., and Reynolds, J. E. III (2005). Influence of power plants and other warm-water refuges on Florida manatees. Mar. Mamm. Sci. 21, 739–764. doi: 10.1111/j.1748-7692.2005.tb01263.x
Laist, D. W., Taylor, C., and Reynolds, J. E. III (2013). Winter habitat preferences for Florida manatees and vulnerability to cold. PLoS One 8:e58978. doi: 10.1371/journal.pone.0058978
Lewis, F. (1987). Crustacean epifauna of seagrass and macroalgae in Apalachee Bay, Florida, USA. Mar. Biol. 94, 219–229. doi: 10.1007/BF00392934
Littles, C. J., Bonde, R. K., Butler, S. M., Jacoby, C. A., Notestein, S. K., Reid, J. P., et al. (2019). Coastal habitat change and marine megafauna behavior: Florida manatees encountering reduced food provisions in a prominent winter refuge. Endanger. Species Res. 38, 29–43. doi: 10.3354/esr00933
Livingston, R. J. (1984). The Ecology of the Apalachicola Bay system: An Estuarine Profile. The Department. Washington, DC: The Service.
Marsh, H., Arraut, E. M., Diagne, L. K., Edwards, H., and Marmontel, M. (2017). “Impact of climate change and loss of habitat on sirenians,” in Marine Mammal Welfare, ed. A. Butterworth (Cham: Springer), 333–357. doi: 10.1007/978-3-319-46994-2_19
Marsh, H., O’Shea, T. J., and Reynolds, J. E. III (2011). Ecology and Conservation of the Sirenia: Dugongs and Manatees. Cambridge: Cambridge University Press. doi: 10.1017/CBO9781139013277
Martin, J., Tolon, V., Morellet, N., Santin-Janin, H., Licoppe, A., Fischer, C., et al. (2018). Common drivers of seasonal movements on the migration–residency behavior continuum in a large herbivore. Sci. Rep. 8, 1–14. doi: 10.1038/s41598-018-25777-y
McKilligan, N., Reimer, D., Seton, D., Davidson, D., and Willows, J. (1993). Survival and seasonal movements of the cattle egret in eastern Australia. Emu 93, 79–87. doi: 10.1071/MU9930079
Michelot, T., Langrock, R., Bestley, S., Jonsen, I. D., Photopoulou, T., and Patterson, T. A. (2017). Estimation and simulation of foraging trips in land-based marine predators. Ecology 98, 1932–1944. doi: 10.1002/ecy.1880
Moore, J. C. (1951b). The status of the manatee in the Everglades National Park, with notes on its natural history. J. Mammal. 32, 22–36. doi: 10.2307/1375409
Mysterud, A., Loe, L. E., Zimmermann, B., Bischof, R., Veiberg, V., and Meisingset, E. (2011). Partial migration in expanding red deer populations at northern latitudes–a role for density dependence? Oikos 120, 1817–1825. doi: 10.1111/j.1600-0706.2011.19439.x
Naidoo, R., Du Preez, P., Stuart-Hill, G., Jago, M., and Wegmann, M. (2012). Home on the range: factors explaining partial migration of African buffalo in a tropical environment. PLoS One 7:e36527. doi: 10.1371/journal.pone.0036527
Neel, M. C., Leidner, A. K., Haines, A., Goble, D. D., and Scott, J. M. (2012). By the numbers: how is recovery defined by the US Endangered Species Act? BioScience 62, 646–657. doi: 10.1525/bio.2012.62.7.7
Nelson, A. A., Kauffman, M. J., Middleton, A. D., Jimenez, M. D., McWhirter, D. E., Barber, J., et al. (2012). Elk migration patterns and human activity influence wolf habitat use in the Greater Yellowstone Ecosystem. Ecol. Appl. 22, 2293–2307. doi: 10.1890/11-1829.1
Nilsson, C., Klaassen, R. H., and Alerstam, T. (2013). Differences in speed and duration of bird migration between spring and autumn. Am. Nat. 181, 837–845. doi: 10.1086/670335
Pabody, C. M., Carmichael, R. H., Rice, L., and Ross, M. (2009). A new sighting network adds to 20 years of historical data on fringe West Indian manatee (Trichechus manatus) populations in Alabama waters. Gulf Mex. Sci. 27:6. doi: 10.18785/goms.2701.06
Parmesan, C. (2006). Ecological and evolutionary responses to recent climate change. Annu. Rev. Ecol. Evol. Syst. 37, 637–669. doi: 10.1146/annurev.ecolsys.37.091305.110100
Perry, A. L., Low, P. J., Ellis, J. R., and Reynolds, J. D. (2005). Climate change and distribution shifts in marine fishes. Science 308, 1912–1915. doi: 10.1126/science.1111322
Picardi, S., Frederick, P. C., Borkhataria, R. R., and Basille, M. (2020). Partial migration in a subtropical wading bird in the southeastern United States. Ecosphere 11:e03054. doi: 10.1002/ecs2.3054
Powell, J. A., and Rathbun, G. B. (1984). Distribution and abundance of manatees along the northern coast of the Gulf of Mexico. Gulf Mex. Sci. 7:1. doi: 10.18785/negs.0701.01
Purdon, A., Mole, M. A., Chase, M. J., and Van Aarde, R. J. (2018). Partial migration in savanna elephant populations distributed across southern Africa. Sci. Rep. 8, 1–11. doi: 10.1038/s41598-018-29724-9
Rood, K., Teague, A., Barton, S., Alvarez-Aleman, A., and Hieb, E. E. (2020). First documented round-trip movement between Cuba and the continental United States. Sirenews 71, 29–32.
Ruiz-Cooley, R. I., Ballance, L. T., and McCarthy, M. D. (2013). Range expansion of the jumbo squid in the NE Pacific: δ15N decrypts multiple origins, migration and habitat use. PLoS One 8:e59651. doi: 10.1371/journal.pone.0059651
Saino, N., Ambrosini, R., Rubolini, D., von Hardenberg, J., Provenzale, A., Hüppop, K., et al. (2011). Climate warming, ecological mismatch at arrival and population decline in migratory birds. Proc. R. Soc. B Biol. Sci. 278, 835–842. doi: 10.1098/rspb.2010.1778
Scheffel, W. A., Heck, K. L., and Johnson, M. W. (2018). Tropicalization of the northern Gulf of Mexico: impacts of salt marsh transition to black mangrove dominance on faunal communities. Estuaries Coasts 41, 1193–1205. doi: 10.1007/s12237-017-0334-y
Shaw, A. K., and Couzin, I. D. (2013). Migration or residency? The evolution of movement behavior and information usage in seasonal environments. Am. Nat. 181, 114–124. doi: 10.1086/668600
Sheppard, J. K., Preen, A. R., Marsh, H., Lawler, I. R., Whiting, S. D., and Jones, R. E. (2006). Movement heterogeneity of dugongs, Dugong dugon (Müller), over large spatial scales. J. Exp. Mar. Biol. Ecol. 334, 64–83. doi: 10.1016/j.jembe.2006.01.011
Stout, J. P. (1984). The Ecology of Irregularly Flooded Salt Marshes of the Northeastern Gulf of Mexico: A Community Profile. Washington, DC: National Coastal Ecosystems Team, Division of Biological Services, Research and Development, Fish and Wildlife Service, U.S. Department of the Interior.
Van Buskirk, J., Mulvihill, R. S., and Leberman, R. C. (2009). Variable shifts in spring and autumn migration phenology in North American songbirds associated with climate change. Glob. Chang. Biol. 15, 760–771. doi: 10.1111/j.1365-2486.2008.01751.x
Visser, M. E., Perdeck, A. C., Van Balen, J. H., and Both, C. (2009). Climate change leads to decreasing bird migration distances. Glob. Chang. Biol. 15, 1859–1865. doi: 10.1111/j.1365-2486.2009.01865.x
Winder, M., and Schindler, D. E. (2004). Climate change uncouples trophic interactions in an aquatic ecosystem. Ecology 85, 2100–2106. doi: 10.1890/04-0151
Keywords: climate change, ecosystem change, migration duration, step length, site fidelity, Trichechus manatus
Citation: Cloyed CS, Hieb EE, DaCosta K, Ross M and Carmichael RH (2021) West Indian Manatees Use Partial Migration to Expand Their Geographic Range Into the Northern Gulf of Mexico. Front. Mar. Sci. 8:725837. doi: 10.3389/fmars.2021.725837
Received: 15 June 2021; Accepted: 30 August 2021;
Published: 23 September 2021.
Edited by:
Ana M. M. Sequeira, University of Western Australia, AustraliaReviewed by:
Nataly Castelblanco-Martínez, National Council of Science and Technology (CONACYT), MexicoSiddhartha Pati, Association for Biodiversity Conservation and Research (ABC), India
Copyright © 2021 Cloyed, Hieb, DaCosta, Ross and Carmichael. This is an open-access article distributed under the terms of the Creative Commons Attribution License (CC BY). The use, distribution or reproduction in other forums is permitted, provided the original author(s) and the copyright owner(s) are credited and that the original publication in this journal is cited, in accordance with accepted academic practice. No use, distribution or reproduction is permitted which does not comply with these terms.
*Correspondence: Carl S. Cloyed, Y2Nsb3llZEBkaXNsLm9yZw==