- 1Department of Marine Sciences, University of Puerto Rico, Mayagüez, Puerto Rico
- 2Caribbean Coral Reef Institute, University of Puerto Rico, Mayagüez, Puerto Rico
- 3Department of Botany, U.S. Museum of Natural History, Smithsonian Institution, Washington, DC, United States
- 4National Oceanic and Atmospheric Administration (NOAA) Restoration Center, Aguadilla, Puerto Rico
- 5HJR Reefscaping, Hormigueros, Puerto Rico
There is limited information on the intra-annual variability of mesophotic coral ecosystems (MCEs), worldwide. The benthic communities, measured as % cover, of two geomorphologically different mesophotic sites (El Hoyo and Hole-in-the-Wall) were examined during 2009–2010 in southwest Puerto Rico. Depths sampled were 50 and 70 m. At each site/depth combination, two permanent transects, measuring 10-m long by 40-cm wide, were surveyed by successive photoquadrants, 0.24 m2 in area. Scleractinian corals, octocorals, macroalgae, crustose coralline algae (CCA), sponges and unconsolidated sediment were the main components along the transects. Significant community differences were observed both among sites and among depths. Differences among sites were greater at 50 m than at 70 m. The El Hoyo site at 50 m was the most divergent, and this was due to a lower coral and sponge cover and a higher algal cover (Amphiroa spp., Peyssonnelia iridescens, turf) relative to the other site/depth combinations. As a consequence, the differences in community structure with depth were larger at El Hoyo than at Hole-in-the-Wall. The communities at 70 m were distinguished from those at 50 m by the greater proportion of the corals Agaricia undata, Madracis pharensis and CCA, and a reduced cover of the cyanobacterium Schizothrix. Temporal variation in the benthic assemblages was documented throughout the year. For both mesophotic sites, the magnitude of change at 50 m was significantly greater than at 70 m. For both depths, the magnitude of change at El Hoyo was significantly greater than at Hole-in-the-Wall. All assemblages experienced almost the same temporal patterns, despite the differences in species composition across sites and depths. Changes in temporal patterns are driven by an increase in the percent cover of the macroalgae Dictyota spp., and a decrease in the percent cover of non-colonized substrata (sand, pavement or rubble). Relatively rapid, intra-annual changes are dictated by the negative correlation between cyclic Dictyota spp. cover and open substrata cover. Other observed mechanisms for rapid community changes in the photoquadrants were diseases and collapses of substrata along with their associated fauna indicating that small-scale disturbance processes may play an important role within MCEs.
Introduction
Mesophotic coral ecosystems (MCEs) occur in tropical waters where light levels are reduced but where benthic photosynthesis is still important for community development. They were initially defined operationally to start at a depth of 30 m, although that was based more on the technical limits of conventional scuba diving, and to extend to approximately 150 m (Hinderstein et al., 2010) depending on the degree of light penetration. From a community and functional perspective, the shallow limits of MCEs are equally dependent on light penetration (Kahng et al., 2019) and in most cases the shallower mesophotic depths have similar community compositions to reef areas shallower than 30 m (Laverick et al., 2018). With increasing depth, there is a gradual change in community composition, with depth restricted species increasing in abundance and some shallow species dropping out. Some studies suggest that this change is sufficient enough to define separate upper and deep MCEs at about 60 m (Slattery et al., 2011; Rocha et al., 2018; Bongaerts and Smith, 2019; Lesser et al., 2019).
With shallow reefs under stress (Hoegh-Guldberg, 1999; Gardner et al., 2003; Carpenter et al., 2008; Jackson et al., 2014), it was initially thought that MCEs may act as a refuge for some species (Bongaerts et al., 2010), but more recent work suggests that role, if verified, is limited only to upper MCEs (Rocha et al., 2018; Slattery et al., 2018). Yet, mesophotic reefs are ecologically important and provide a number of ecological services, including biodiversity, critical habitat supporting fisheries and endangered species and as a sentinel for climate change (Bridge et al., 2013; Holstein et al., 2016; Rocha et al., 2018), so questions concerning the stability and vulnerability of MCEs are still of paramount importance. While there has been a dramatic increase in our knowledge of MCEs (see Hinderstein et al., 2010; Loya et al., 2016, 2019) these questions largely remain unresolved.
The most significant study on short and long-term changes in MCEs is centered in Curaçao, but only extends into the upper mesophotic range to a depth of 40 m. Sampling at annual and occasionally subannual time periods was conducted by Bak and Luckhurst (1980), who reported no significant change in benthic communities at 40 m over 5 years. They concluded that at these depths, natural processes, such as rocky surfaces being continuously covered/exposed by loose sediment, are important but generally small in scale, and some catastrophic loss, e.g., substrate collapse, would be necessary to generate substantial change. Even after 20 (Bak and Nieuwland, 1995) and 30 (Bak et al., 2005) years, little change had been observed at 40 m, although they did observe cold-water induced bleaching. Yet, data after 40 years clearly suggested that the benthic communities at 40 m had changed in response to anthropogenic stress, showing a marked decline in species diversity, and that this change had begun over a decade before (De Bakker et al., 2016).
Less work on temporal change has been done within lower MCEs. Liddell and Avery (2000) conducted four surveys over 3 years at depths of 50 and 75 m in the Bahamas and found that community changes were small and declined with depth. Lesser and Slattery (2011), also based on research in the Bahamas, found no change in benthic communities after 2 years. However, a re-evaluation 3 years later found substantial changes they attributed to the arrival of invasive lionfish (Pterois volitans) and their impact on the fish herbivore community, leading to a significant increase in algal cover at depths of 46 and 61 m. Lastly, Slattery et al. (2018) reported widespread changes over a decade at Pulley Ridge, off west Florida, but without sampling at shorter time intervals no influencing factors could be proposed. Unlike the work of Bak and colleagues at their 40 m sites, there has been no study within lower MCEs to quantify community change at short temporal scales (i.e., sub-annual intervals), which is paramount for understanding the relative importance of large-scale temporal changes. Since conducting manipulative experiments in these systems is logistically difficult, we must rely on describing patterns of change at different spatial and temporal scales in order to identify potential processes affecting these communities (Underwood et al., 2000).
Studies to date show that the rate of change in community composition within both upper and lower MCEs declines with depth and that changes over short time periods are slight (relative to those observed within shallow reefs), except when there is a catastrophic or disruptive event. Suggested drivers of this slow rate of change are varied. Bak and Luckhurst (1980) initially argued that natural processes were responsible for these slow rates of change, with the dominant factor being sediment flow from shallow depths into MCEs. Sherman et al. (2016) supported this view, showing that downslope bedload transport was much greater than siltation from the water column and that this transport was possibly driven by surface waves. Yet, it has been proposed that over the longer-term declines in coral species richness occurred (Bak and Nieuwland, 1995) due in part to the competitive dominance of Agaricia species (Bak et al., 2005). Similarly, Liddell and Avery (2000) argued that biological processes were important, such as competition and fish grazing (at least down to 50 m). The drastic changes observed by Lesser and Slattery (2011) clearly support the latter view. Bak et al. (2005) saw cold-water bleaching as another potentially catastrophic event, and later observations show that warm-water bleaching can significantly and frequently impact MCEs and occur down to at least 50 m and even to 120 m in some localities (McClanahan et al., 2018; Weil, 2019). The expectation is that with warming seas, such bleaching events will become more frequent and intensive. Other potential drivers of catastrophic events include infectious diseases, invasive species, increased intensity and frequency of storms, ocean acidification, and anthropogenic effects (Smith et al., 2019; Weil, 2019).
The purpose of this study was to assess the temporal variability and dynamics within lower MCEs off the southwest coast of Puerto Rico through multiple samplings over a 1-year time frame, at two sites differing in geomorphology, and two depths (nominally 50 and 70 m) at each site. Geomorphology (and related physical and geological processes) are known to be important in structuring MCEs (Sherman et al., 2010, 2016; Appeldoorn et al., 2019), but how this translates into temporal community dynamics is not fully appreciated. For this assessment, major components (e.g., algae, corals, octocorals, sponges) of the benthic community were quantified. The objectives where: (i) to describe patterns of temporal variation of benthic assemblages at two different sites and at two depths, (ii) identify species/taxa responsible for those changes and (iii) propose potential processes responsible for those short-term changes. Furthermore, and given that the study incorporates any seasonal variability, it also serves as a baseline against which future changes can be assessed.
Location
This study was conducted on the insular slope of southwest Puerto Rico 8–9 km offshore of La Parguera (Figure 1). A long band of submerged reefs occurs at the shelf edge at 18–25 m depth after which the slope drops to oceanic depths.
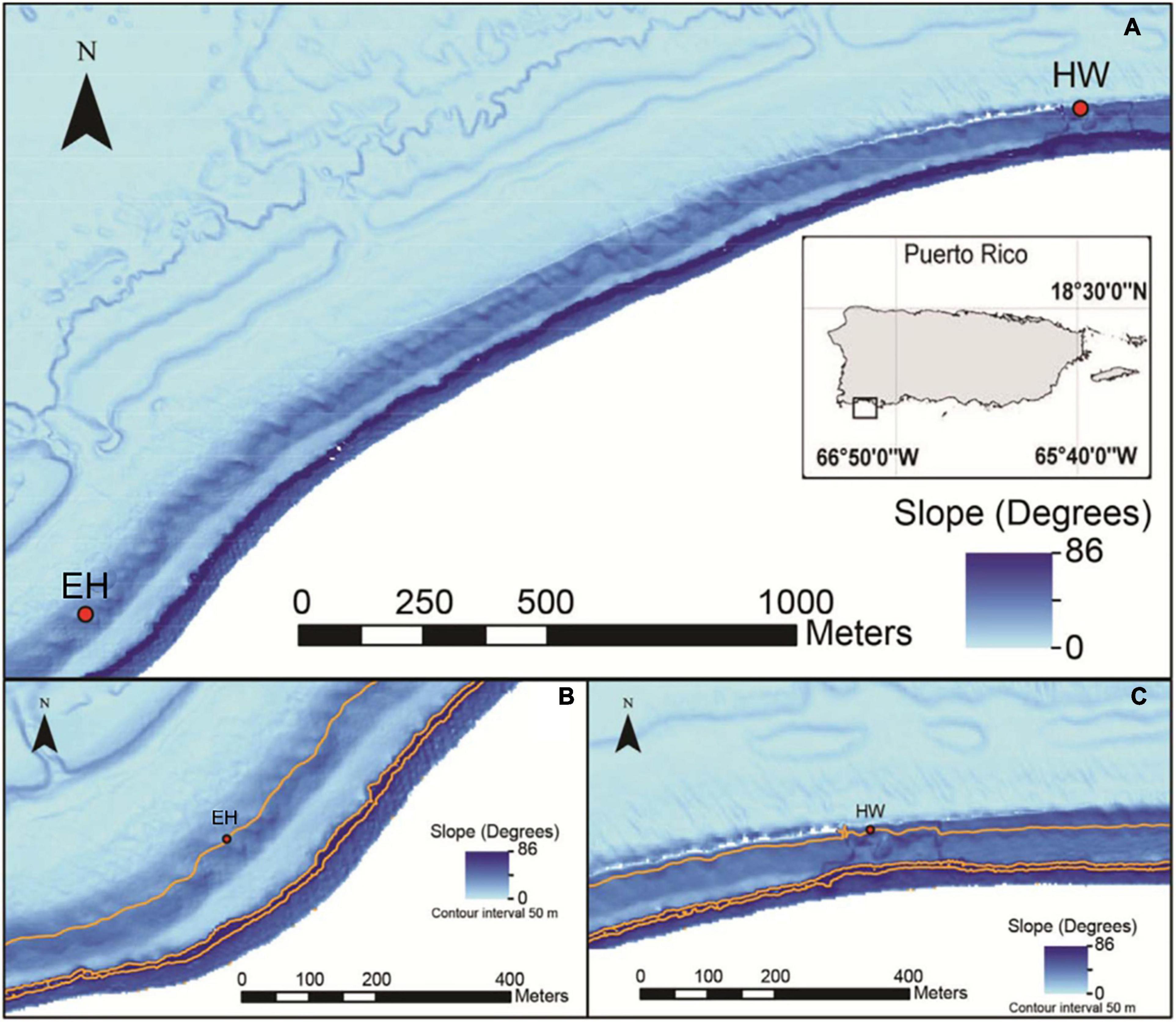
Figure 1. Composite multibeam and Lidar imagery of the study area. (A) Insular shelf margin off southwest Puerto Rico showing relative locations of the two study sites, El Hoyo (EH), and Hole-in-the-Wall (HW). (B) Close-up view of El Hoyo, a low-gradient, southeast-facing site. (C) Close-up view of Hole-in-the-Wall, a steep south-southwest-facing site. Orange lines in (B,C) are the 50, 100, and 150 m isobaths (adopted from Sherman et al., 2016).
The general area and the two specific study sites, El Hoyo (EH) and Hole-in-the-Wall (HW), including general species composition, have previously been described in detail (Sherman et al., 2010, 2016; Appeldoorn et al., 2019). In the area, winds are predominantly easterly with average speeds of ∼5–6 m s–1, with resulting currents being generally westward, averaging ∼0.5 m s–1 in the upper 3 m. Flow at deeper depths is predominately tidally driven. Tides are diurnal and of low amplitude (mean range < 30 cm). Waves are from the southeast with average heights of ∼1 m and periods of ∼6 s. At 50 and 70 m depths, modeled near-bottom orbital velocities at the study sites average 0.02 and 0.01 m s–1, respectively, which are considered to be greater than erosional threshold velocities for skeletal carbonate sand.
The water column along the steep insular slope below 40 m is weakly stratified and is characterized by a seasonally varying (∼20–80 m) and energetic thermocline, with high-amplitude incident internal waves and frequent mesoscale eddy impingements. At 40 m, temperatures vary between 26 and 29.8°C, tracking seasonal surface temperatures. At 80 m, temperatures are almost always lower than surface temperatures, particularly during the summer. In contrast, at 60 m depth the bottom is routinely subjected to large amplitude internal waves, occasionally resulting in rapid temperature changes of up to 3°C. Particularly large internal waves may penetrate up to 40 m and down to 80 m causing sharp temperature decreases/increases, respectively.
The two selected sites are 2.25 km apart and differ in their geomorphologies (Sherman et al., 2010, 2016). El Hoyo is a more exposed, southeast facing slope with an average gradient of ∼25° (range: 18–34°) from 20 to 90 m and of low-relief topography consisting of broad, low-gradient buttresses separated by open slopes. The site is characterized by sparsely colonized hardground with low coral cover and thin, discontinuous sheets of unconsolidated sand. In contrast, HW represents a more sheltered southwest facing slope characterized by a steeper gradient (∼44°; range: 33°–64°) with an irregular topography consisting of steep-sided buttresses separated by narrow sand chutes. The buttresses support a well-developed and diverse benthic assemblage consisting of algae, corals, sponges, and other components.
Materials and Methods
At each site and depth, five quantitative benthic surveys were made. Sampling was at 3-month intervals from June 2009 to June 2010 (Table 1). Actual intervals ranged from 72 to 103 days (average = 90.6 days). For each time period at site/depth, two technical divers, using Inspiration (Silent Diving Systems) rebreathers (Sherman et al., 2009), conducted two continuous high-resolution benthic phototransects, each 10 m long by 40 cm wide (Sherman et al., 2009, 2010; Appeldoorn et al., 2016). Each phototransect consisted of sequential quadrat photographs, each 40 × 60 cm, taken with a 10.2-MP digital camera (Nikon D200) in an underwater housing (Titan D200) fitted with two Inon Z-240 strobes. The number of photoquadrats per transect ranged between 16 and 21 depending upon the degree of quadrat overlap.
Percent cover for identified taxa was estimated for each 40 × 60 cm digital photograph by overlaying 100 random points using Coral Point Count with an Excel extension (CPCe) (Kohler and Gill, 2006). To reduce clustering, each photograph was divided into a 10 × 10 grid and one point was randomly positioned within each grid cell. Identifications associated with the random points were classified into 36 taxa/categories (Table 2) that focused primarily on the algae (15 taxa) and scleractinian corals (17 spp.). One category for open substrata combined sand, pavement and rubble. Algal identifications and nomenclature followed Wynne (2011) as updated by Guiry and Guiry (2011). Scleractinian coral identification followed Wells (1973); Wells and Lang (1973), Stemann (1991), and Humann and DeLoach (2001a) with nomenclature from www.reefbase.org, while those for the lumped invertebrate categories followed Colin (1978) and Humann and DeLoach (2001b).
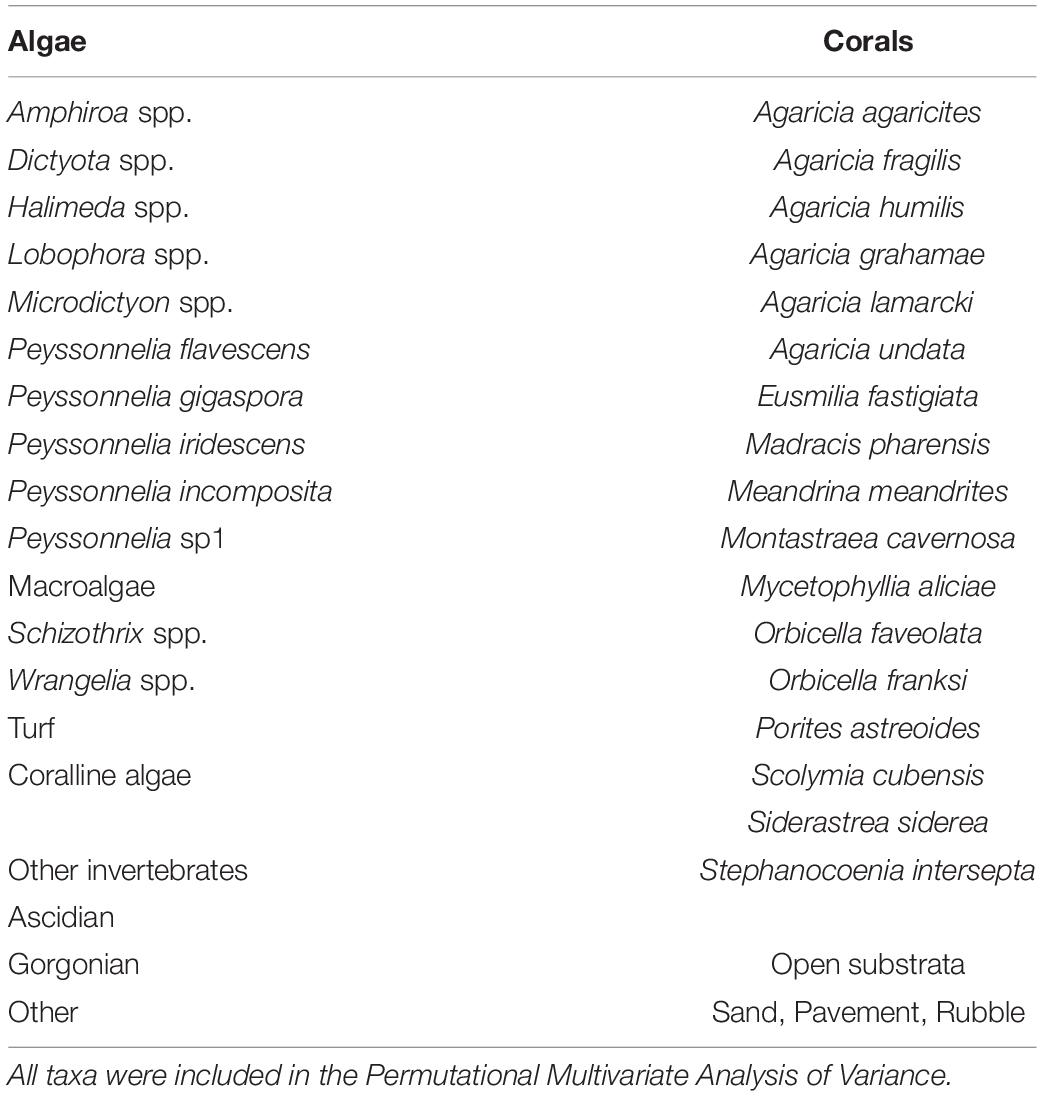
Table 2. Pooled list of taxa/categories identified from all photoquadrats at El Hoyo and Hole-in-the-Wall for both 50 and 70 m depths.
At each site and depth, temperature was recorded at 2-h intervals with an accuracy of ± 0.2°C using a HOBO® Water Temp Pro V2 data logger (Onset Computer Corporation). These were placed at the end of one of the transects at each site shortly prior to the first benthic sampling. Temperature records ran until the end of April/mid-May 2010, except for HW 70 m, where a second deployment recorded temperatures until after the last sampling period. Photosynthetically active radiation (broadband PAR—400–700 nm) at the surface was measured using a Biospherical Instruments GUV-511 temperature-controlled radiometer located in La Parguera at the Magueyes Island Marine Laboratory of the Department of Marine Sciences, University of Puerto Rico. Measurements were in μE cm–2 s–1 and were recorded at 1-min intervals. These values were summed over each day as an estimate of total daily PAR.
To test the hypothesis of no temporal variation or spatial differences in benthic community structure we used a mixed multifactorial model that considered three factors: Sampling time (random with five levels), Site (random with two levels) and Depth (fixed with two levels). All factors were orthogonal to each other. This model was analyzed using a Permutational Multivariate Analysis of Variance (PERMANOVA) based on Bray-Curtis similarities (Anderson et al., 2008). Data were pre-treated with a square-root transformation to weight down dominant taxa that could mask potential patterns in community structure explained by cryptic and/or rare species. Decisions on transformations were based on visual explorations of shade plots that were constructed with various transformations. For the PERMANOVA analyses, p-values were obtained using 9999 permutations using unrestricted permutation of residuals.
The distance among centroids for levels of significant sources of variation [factors] and their interactions were obtained and plotted in Euclidean space using a Principal Coordinates Analysis (PCO) for visualization of complex spatiotemporal patterns (Guerra-Castro and Cruz-Motta, 2014; Guerra-Castro et al., 2016). Variables contributing to spatiotemporal variability on community structure were identified using the similarity percentage analysis (SIMPER) (Clarke, 1993). A cut-off of 50% of the total dissimilarity between levels of significant factors and their interactions was selected to get the top contributors. These key variables in explaining spatiotemporal patterns were selected to overlay Pearson correlation vectors in the PCO plots. These multivariate statistical routines were carried out with the software PRIMER v.7 with PERMANOVA add-on (Anderson et al., 2008; Clarke and Gorley, 2015).
Results
Physical Characterization
Temperatures at all sites reflected an annual cycle of lower temperatures in the winter and warmer temperatures in the late summer/early fall (Figure 2), but seasonal differences in the depth of the thermocline and the effect of internal wave traveling along the thermocline add complexity and greatly alter the temperature records among depths. Temperature records at the same depth across sites were almost identical (Supplementary Figure 1). When temperatures are highest, the water column is more stratified, and the depth of the thermocline is shallowest, such that during this period the sites at 50 m were more affected by large short-term temperature fluctuations caused by internal waves. At other times of the year the thermocline is deeper and internal wave induced temperature fluctuations more greatly impacted the sites at 70 m. Thus, during the winter at 50 m, temperatures were fairly stable, staying above 27°C, with only the occasional internal wave peak extending up to this depth, rapidly exposing the site to short-term temperature declines of up to 1.5°C lower (e.g., early March 2010). Maximum seasonal temperatures at this depth (>29°C) occur in the late summer/early fall, but this period is also characterized by frequent internal waves resulting in highly dynamic short-term temperature declines of up to 4.0°C. Maximum temperatures at 70 m during the winter are similar to those at 50 m, but while temperatures at the latter depth are fairly stable, those at 70 m were frequently exposed to lower temperatures from the passage of internal wave peaks. During the summer, base temperatures at 70 m were ≤ 26°C, but while temperatures were fairly stable at 50 m (e.g., July–August), at 70 m there were frequent passages of internal wave troughs and resulting in sudden short-term temperature increases, again up to 3.7°C.
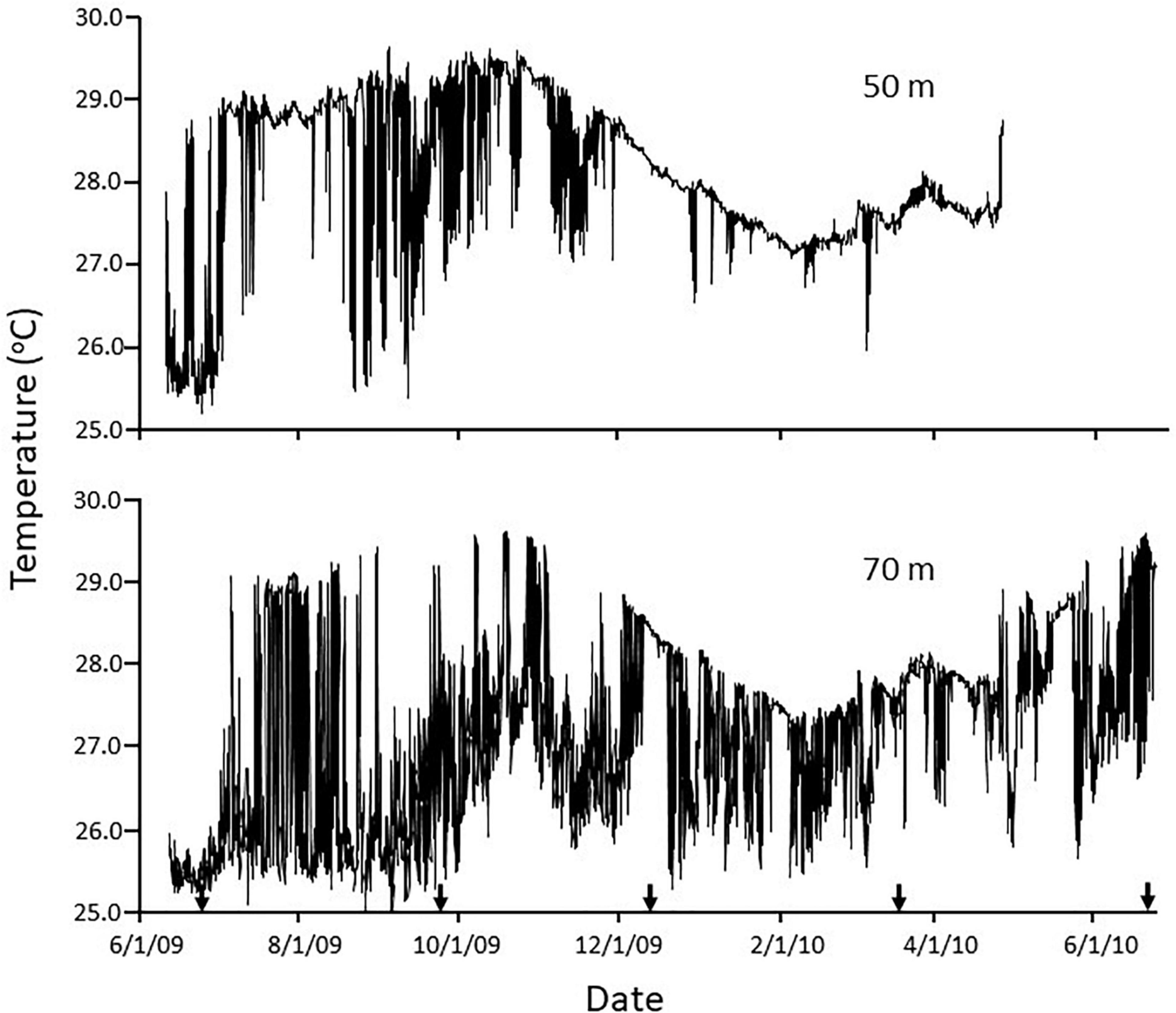
Figure 2. Temperature vs. time at 50 m (top) and 70 m (bottom) at Hole-in-the-Wall on the insular slope off La Parguera, Puerto Rico. Arrows indicate dates of phototransects.
Total daily PAR fluctuated seasonally with minimum and maximum values occurring around the winter and summer solstice, respectively (Figure 3). Substantial deviations to lower values (e.g., maximum 88% reduction, June 19, 2010) occur periodically due to cloudy periods, which may occur over several days.
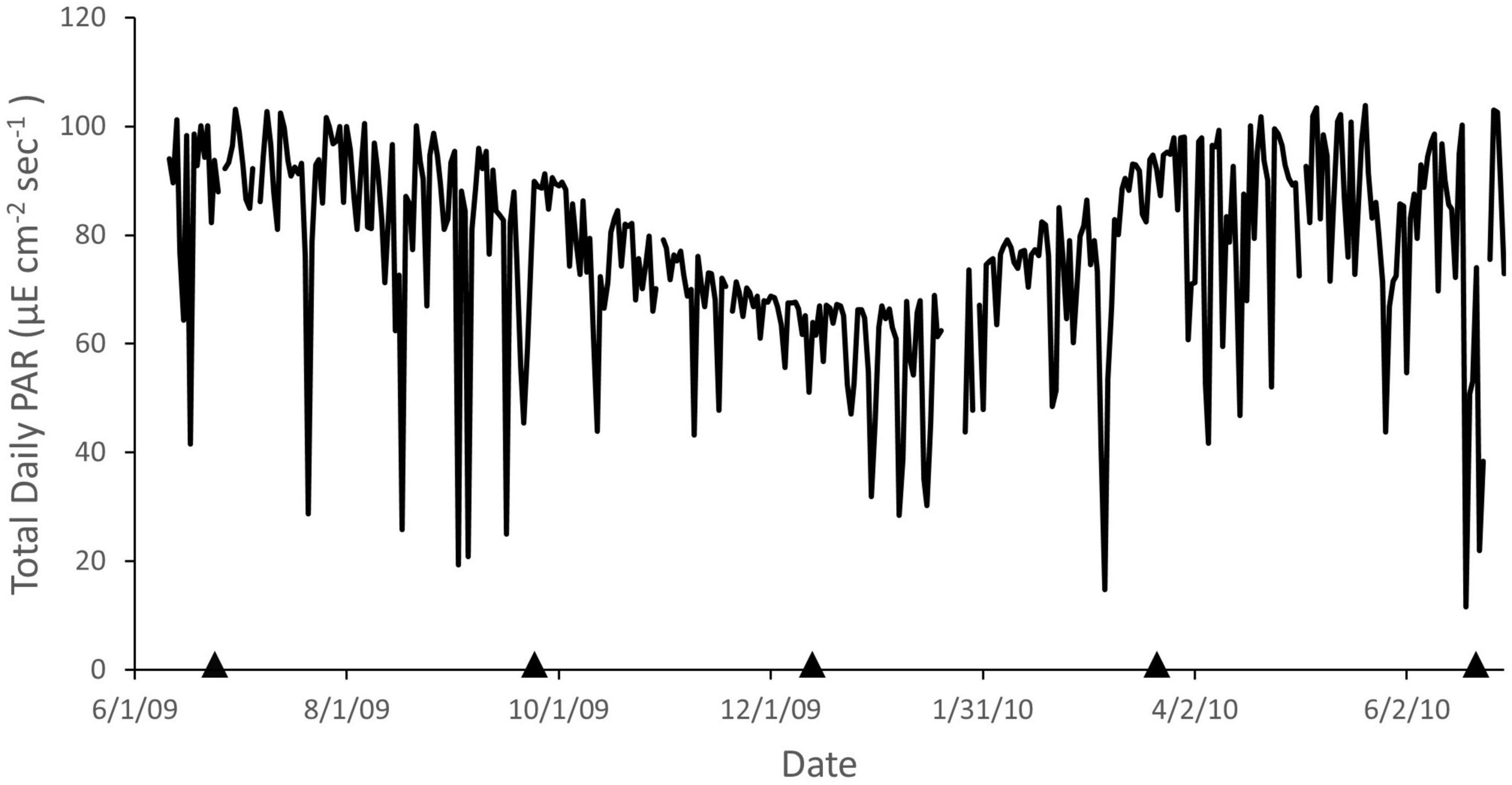
Figure 3. Daily total photosynthetically active radiation (PAR) recorded at La Parguera, Puerto Rico from June 2009 through June 2010. Arrows indicate dates of phototransects. Data from the University of Puerto Rico Bio-optical Oceanography Laboratory.
Benthic Community Composition
Hypothesis testing analyses (PERMANOVA) showed a second order interaction between the three factors considered in this study: time, location and depth (Table 3), indicating that changes of the structure and composition of benthic assemblages were not the same at each location and time (Figure 4). In general, among sites the communities at 70 m are more similar than those at 50 m. The deeper sites are characterized by a greater proportion of Agaricia undata, Madracis pharensis and coralline algae, and a decrease in algal cover overall. relative to the shallower sites (Figure 5). The shallow EH site diverges most from the other sites/depths, and this is due to a lower proportion of coral and sponge cover and a higher algal cover (Amphiroa spp., Peyssonnelia iridescens, turf) relative to the other site/depth combinations (Figure 5). As a consequence, the differences in community structure with depth were larger at EH than at HW.
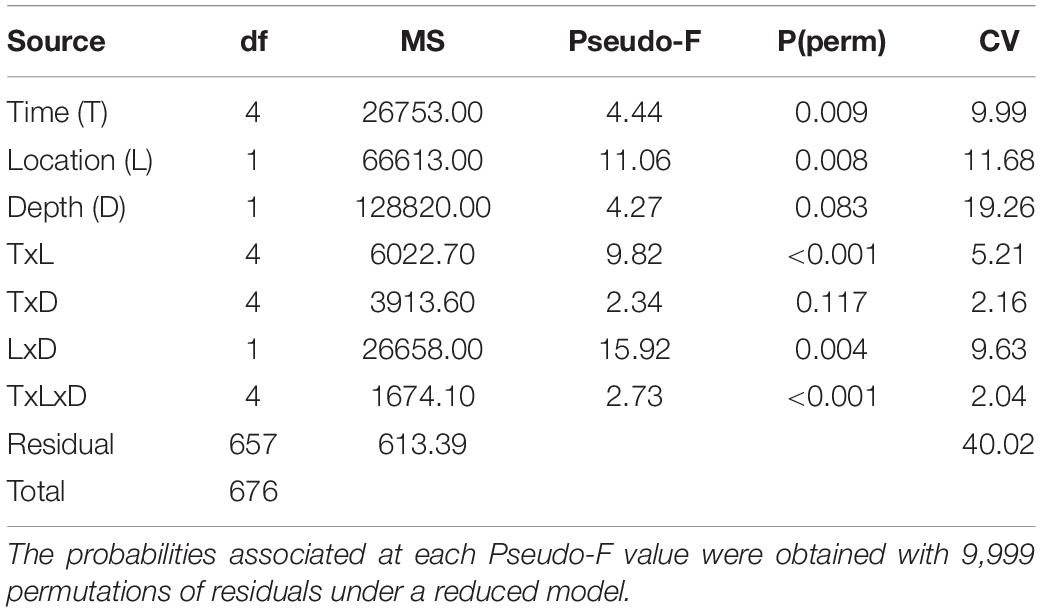
Table 3. Permutational multivariate analyses of variance based on Bray-Curtis dissimilarities for square-root transformed percentage cover data, on the basis of a mixed multifactorial model (Time: random, Location: random, Depth: fixed).
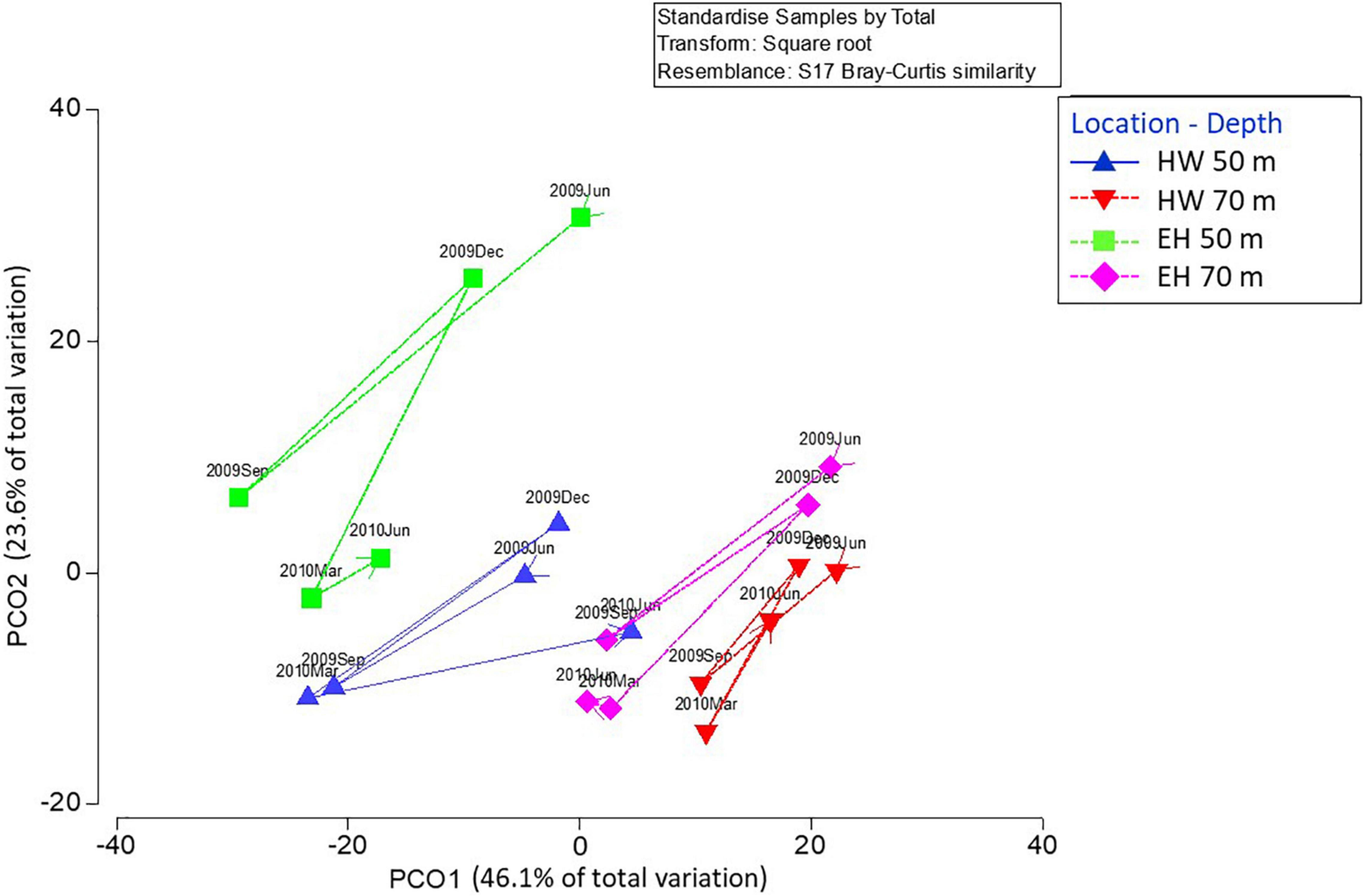
Figure 4. Multivariate ordination (PCO) of centroids representing the structure and composition of benthic assemblages at two locations and depths. Interconnecting lines represent temporal changes at each location and depth. Percentages of variation explained by each axis are shown. HW, Hole-in-the-Wall, EH, El Hoyo.
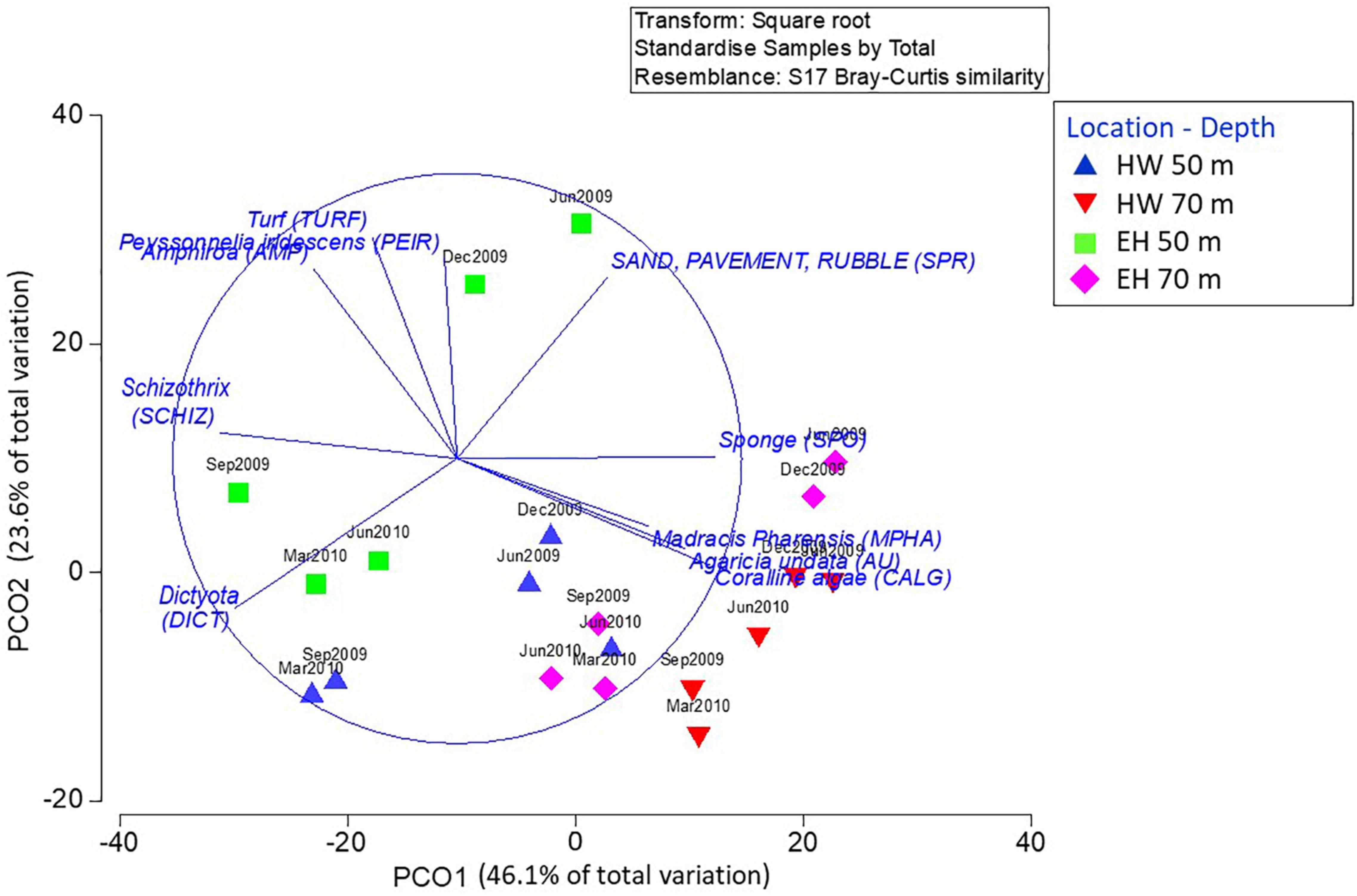
Figure 5. Multivariate ordination as per the previous figure, but vectors indicate species/taxa most correlated with the first two axis, which can be used to interpret spatial differences and or temporal changes. HW, Hole-in-the-Wall, EH, El Hoyo.
Variations in the benthic assemblages are noted throughout the year. For both sites, the magnitude of change at 50 m was significantly greater than at 70 m as indicated by the multivariate dispersion values among sampling times for each combination of location and depth (EH: shallow = 19.65 ± 2.54 (std. error) vs. deep = 14.05 ± 0.89; HW: shallow = 15.87 ± 1.54 vs. deep = 7.26 ± 1.15). Despite composition differences across sites and depths, all assemblages experienced the same temporal patterns, characterized by a general diagonal shift in unison (upper right vs. lower left) in Figure 4. Positions to the lower left (September 2009, March 2010) are due primarily to an increase in the percent cover of Dictyota spp., and a decrease in the percent cover of non-colonized substrata (sand, pavement, or rubble) (Figure 5). The only departure from this pattern occurred in the last period, where at EH at both depths there was little change, primarily due to a sustained high cover of Dictyota spp. (Figure 6). These oscillations are illustrated for a single photoquadrat at HW (Figure 7), where repetitive increases and decreases in the cover of Dictyota spp. are evident. Other algal taxa also varied substantially in % cover over the year (Figure 6). Notable was the increase in Schizothrix sp. at EH 50 m, which peaked at 30% cover in March 2010. These variations in the % cover of other algal taxa were independent of the variations in Dictyota spp.
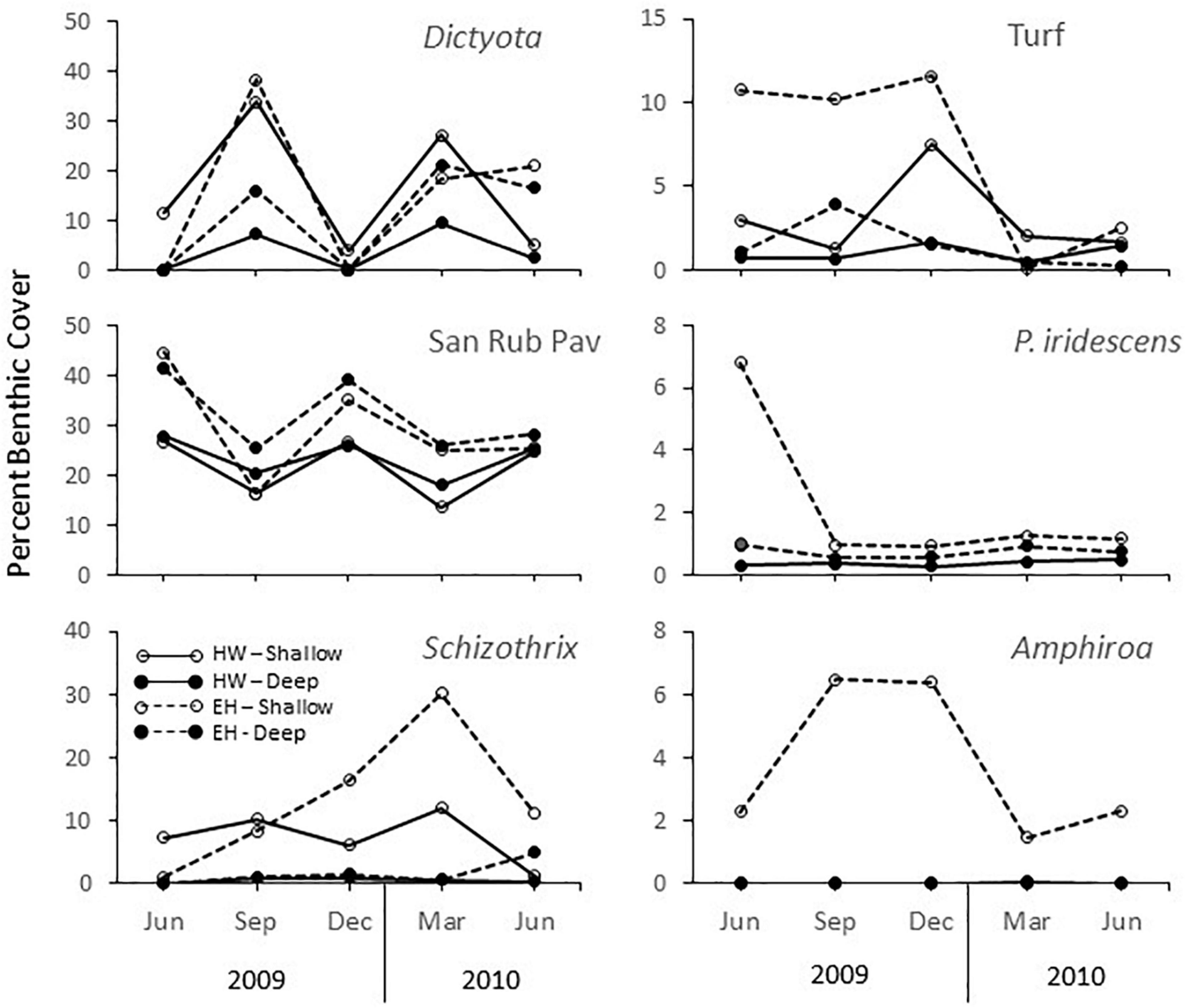
Figure 6. Average percent cover key algal taxa and open substrata (sand-rubble-pavement) in phototransect at El Hoyo (EH) and Hole-in-the-Wall (HW), 50 and 70 m off La Parguera, Puerto Rico.
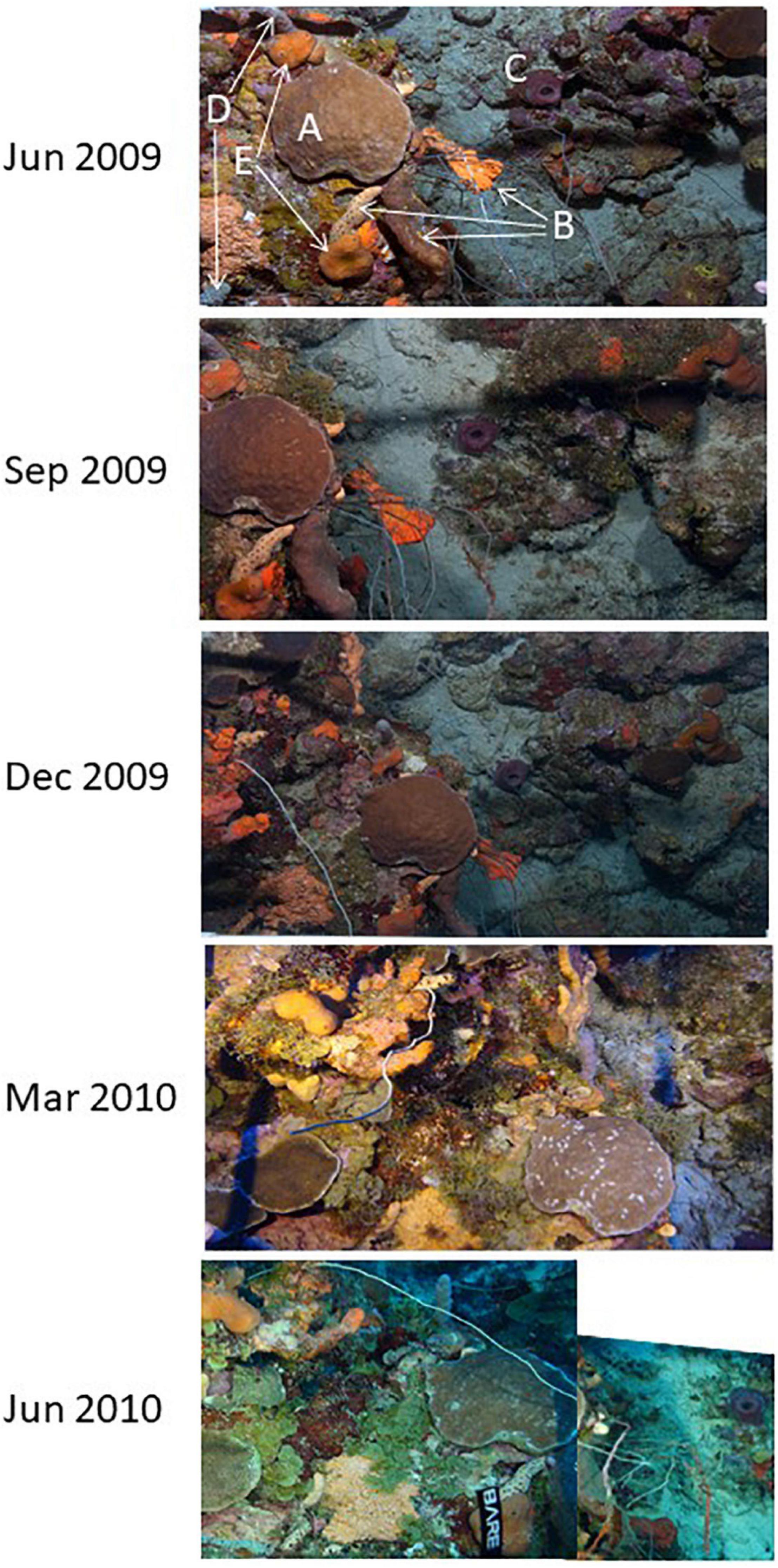
Figure 7. Change in one photoquadrat over 1 year at 50 m at Hole-in-the-Wall (HW) off La Parguera, Puerto Rico. Each photograph is 60 cm wide. Within the photoquadrat are a colony of Porites astreoides (A), several species of sponge, including three species of Agelas (B), a juvenile barrel sponge Xestospongia muta (C), Niphates sp. (D), and Plakortis sp. (E). Note recent sediment flow evident in September 2010 and the appearance of numerous bite marks on the P. astreoides colony in March 2010.
After 1 year, there was little net change in community composition at HW, with the magnitude of change at 50 m being 53% of the average seasonal change and only 45% of the largest seasonal change. At HW 70 m, the respective numbers are 24 and 21%. Without the large decrease in Dictyota spp. in the last period at EH, the magnitudes of change after 1 year were greater. At 50 m the annual change was 131% that of the average seasonal change, but only 83% of the largest seasonal change. At 70 m the relative annual changes were larger, being 160 and 123%, respectively.
Discussion
The benthic assemblages at each site and depth location were different, as was reported earlier (Appeldoorn et al., 2019) albeit with a less rigorous statistical approach. Differences between depths is an expected result, observed over many studies (e.g., Bak and Luckhurst, 1980; Liddell et al., 1997; Slattery et al., 2011) including initial work off La Parguera (Sherman et al., 2010). Differences with respect to location, and specifically with respect to geomorphology are less appreciated (e.g., Liddell et al., 1997), yet this has been a focus of studies off La Parguera (Sherman et al., 2010, 2016; Appeldoorn et al., 2019). To date, however, this work has focused only on two sites (EL, HW), so the comparative sample size is limited.
Despite the limited sample size, the differences observed among site and depth combinations allow temporal changes to be placed in context. While changes in the overall assemblages were observed throughout the year, these changes were small, especially when compared to the differences among sites and depths. Only for one sample did the assemblage from one site-time fall within the cluster of points of another (HW 50 m June 2010 overlapped with the EH 70 m assemblage of points; Figure 4).
For both sites, the magnitude of variation was greater at the shallowest depth. Greater stability with depth is a core assumption of the Deep Reef Refuge Hypothesis (Bongaerts et al., 2010). However, this is predicated on the presumed reduced exposure of MCEs from land-based sources of stress, while the variations observed in this study are most likely the response of natural processes that would be expected to have a greater impact at shallower depths, such as the role of higher light levels fueling more rapid algal growth or surface waves inducing sediment resuspension and down-slope bedload transport (but see below).
The magnitude of change (in any direction) among sampling periods was similar to that observed after 1 year, although the relative change varied by site. At HW, annual changes were less than observed within sampling periods, while at EH annual changes were larger. There was no consistent pattern with depth. That intra-annual changes can be as large or larger than the observed annual change indicates that caution needs to be given to the significance of change when assessing community differences over annual (and perhaps longer) time frames. Knowledge of the magnitude of intra-annual variations can be used to assess such significance, such that any future sampling that shows the benthic community structure having a magnitude of change greater than seen within a year would be more likely to represent significant change.
The most noteworthy result was that, despite the differences in assemblages across sites and depths, all varied in a similar pattern during the year except during the last sampling period, when there was reduced change at EH. This pattern reflected an oscillation between periods of high Dictyota spp./low open substrata cover and low Dictyota spp./high open substrata cover indicating repetitive cycles of Dictyota spp. presence and removal. This pattern was not observed within other algal taxa. While Amphiroa spp. and Peyssonnelia iridescens are slower growing taxa, turf species and Schizothrix sp. are capable of rapid growth (with the latter showing a pronounced increase in % cover at EH 50 m) yet they did not respond in a manner similar to Dictyota spp. On shallow reefs, both Herron et al. (2006) and Renken et al. (2010) found that Dictyota spp. can fragment and then reattach and coalesce, which allow it to respond quickly to perturbations, causing it to be characteristically variable. This suggests Dictyota spp. can more easily be dislodged upon disturbance, but also can more easily recover as well. Ruiz and Ballantine (2009) studied the dynamics of algal species at 6-month intervals over 3.5 years within two shelf-edge sites off La Parguera and found no seasonal patterns in the algal community and no coherence among or within species at multiple spatial scales. They attributed variations to small scale recruitment and extinction events, which in turn, were driven by life history characteristics, in particular individual frond half-life. Nevertheless, this lack of coherence across samples and sites occurred within a broad-scale pattern of steady increase in the % algal cover that they partially attributed to the availability of new space following the bleaching and disease-induced mass mortality (53% loss of coral cover) associated with the thermal event in 2005–6 (Weil et al., 2009). Furthermore, an additional analysis at one of these sites showed that within this overall increase in algal cover, there were distinct community changes year to year that were consistent across replicate samplings (Ruiz Torres, 2012), suggesting that temporal variations at these shallow depths were greater than at mesophotic depths but also suggesting a broader scale driver. In effect, within a context of algal dynamics consisting of short-term recruitment and extension events with little spatial coherence, emergent larger scale patterns of change can be seen.
That coherence in temporal patterns at mesophotic depths was observed across 20-m depth at sites 2.2 km apart suggests that these patterns were the result of some large-scale driver(s) affecting either Dictyota spp. growth and removal, sediment dynamics, or an interaction between the two. With respect to growth, it is difficult to ascribe general causal factors to this pattern, at least with the available data. Dictyota spp. cover was low in December, when solar irradiance was at a minimum, but was also low when solar irradiance was at a maximum (Figure 3). Similarly, there is no apparent pattern with respect to temperature (Figure 2) nor the position of the thermocline, which should affect the availability of nutrients (Corredor and Morell, 2001).
Sherman et al. (2016) studied the wave environment and sediment dynamics at EH and HW and found that trap accumulation rates of suspended sediment and bed-load sediment show a similar temporal pattern both within and between sites. This suggested a uniform driving mechanism, which they postulated as surface wave-induced resuspension and subsequent downslope bedload transport. The highest orbital velocities from surface waves occurred from September to November, the same period in which suspended-sediment trap accumulation rates peaked at both sites, and bed-load trap accumulation peaked at HW. This is consistent with the observed peak in open substrata and low cover of Dictyota spp. observed in December, suggesting that increased sediment flow in the prior months resulted in dislodgement of Dictyota spp. (relative to other algal species). However, this leaves open what would have driven the similar values observed in June of both years. Furthermore, increases and decreases in Dictyota spp. cover occur at elevated sites not subject to sediment flows, which at HW are channeled along narrow sand chutes. Additionally, peaks in Dictyota spp. cover can occur at the same time that sediment is actively flowing along the bottom. Both of these situations are illustrated in Figure 7. On the left side of each image is a raised area that shows the pattern of Dictyota spp. increases and decreases, while on the right side is a clear sediment chute. Yet, sediment appears to be actively moving through the chute in September, when open substrate should be low. At the same time, patches of Dictyota spp. are clearly evident to the right of the sediment chute, indicating that the flow had not removed the algae, at least within the time frame depicted. That there is an increase in bedload transport at this time is still consistent with the findings of Sherman et al. (2016) as they found sediment accumulation occurs throughout the year and rates were highly variable across time and trap locations, yet with emergent large-scale trends apparent.
The lack of large magnitude changes occurring over the annual sampling period supports the contention that small-scale natural processes are dominant and that mesophotic communities are stable over short time frames absent any catastrophic event or increase in anthropogenic stress (Bak and Luckhurst, 1980; Liddell and Avery, 2000). Bak and Luckhurst (1980) in particular emphasized the role of sedimentary processes leading to a continuous pattern of hard bottom burial/exposure. This was clearly supported by Sherman et al.’s (2016) study of sediment dynamics off La Parguera, which recorded oscillations between sand and Dictyota spp. % cover. In this context, the magnitude of these oscillations was found to be greater at 50 m than at 70 m. However, site differences were observed and ascribed to differences in geomorphology, with the magnitude of these oscillations being less at the steeper sloped, more rugose HW, where bedload transport can more easily be channeled into chutes and away from benthic organisms growing in elevated areas.
Liddell and Avery (2000) emphasized the importance of biological processes, such as competition and herbivory, and it is clear that these can be important over larger time frames (Bak et al., 2005; Lesser and Slattery, 2011). Within the present study, the repeated ability of Dictyota spp. to colonize and cover a significant portion of the bottom within a 3-month period indicates the importance of biological processes affecting mesophotic benthic communities, at least in the short term. Additional evidence was given by Weil (2019), who reviewed the role of bleaching and disease in tissue and colony loss within mesophotic corals and quantified disease rates within the La Parguera transects. Overall disease prevalence within the transects was low (∼6%), with little variability over time. However, disease progressions within individual colonies were noted. At the extreme, one medium sized Mycetophyllia aliciae colony at HW 50 m suffered full mortality within 6 months, while a ∼20-cm wide colony of Agaricia grahamae at HW 70 m underwent rapid and total mortality in 2 months after showing only a slow progression and partial mortality during the preceding 9 months. Invasive lionfish were not present within mesophotic depths at the time of the transect study and were still uncommon 2 years later (Bejarano et al., 2014).
What was also evident in this study is the potential importance of small-scale physical disturbance processes in affecting change. This is demonstrated in Figure 8, where a small portion of the substratum has broken off, taking with it an area colonized by algae, sponges and a colony of Agaricia grahamae. Another example is given in Figure 9 from HW 50 m. In the first time period, a colony of Agaricia agaricites can be seen covering over a colony of Stephanocoenia intersepta. The A. agaricites colony has a large white patch indicating recent mortality. Although speculative, given the abundance of sand in the photo and the size and condition of the S. intersepta colony (see last two time periods) it may have been that the U. agaricites colony recently slid downslope due to some unknown disturbance, coming to rest within the transect and on top of the S. intersepta colony. Between December 2009 and March 2010, another unknown disturbance led to the disappearance of the original A. agaricites colony, thus fully uncovering the colony of S. intersepta, which shows partial mortality at the top and partial bleaching in the center—areas that were completely covered by the A. agaricites colony for at least 6–9 months. At the same time, a different A. agaricites colony appears (center left) in the transect. Unfortunately for this colony, it came to rest in a position such that bedload transport from above would be deposited on top, leading to extensive tissue mortality after 3 months. In contrast, the bleached portion of the S. intersepta colony appears to have recovered. As illustrated, cumulative small-scale disturbances may lead to significant changes in benthic communities over time. Agariciid corals, in particular, are loosely attached and may be especially susceptible to displacement on steep insular slopes. Hughes (1999), in shallow reefs quantified the magnitude of the downslope transfer of live corals and hard substrata in the absence of storm events and discussed the ecological significance of this processes in promoting reef extension. Small-scale disturbance processes may play a similar role within MCEs. While the sources of disturbance in these examples are unknown, such disturbances can be either biological or non-biological in origin. Of the former, large megafauna such as hawksbill turtles (Eretmochelys imbricata), Caribbean reef (Carcharhinus perezi) and other sharks, and black (Mycteroperca bonaci) and goliath (Epinephelus itajara) groupers have been observed at mesophotic depths, and all are capable of causing small disturbances when feeding on or near the bottom.
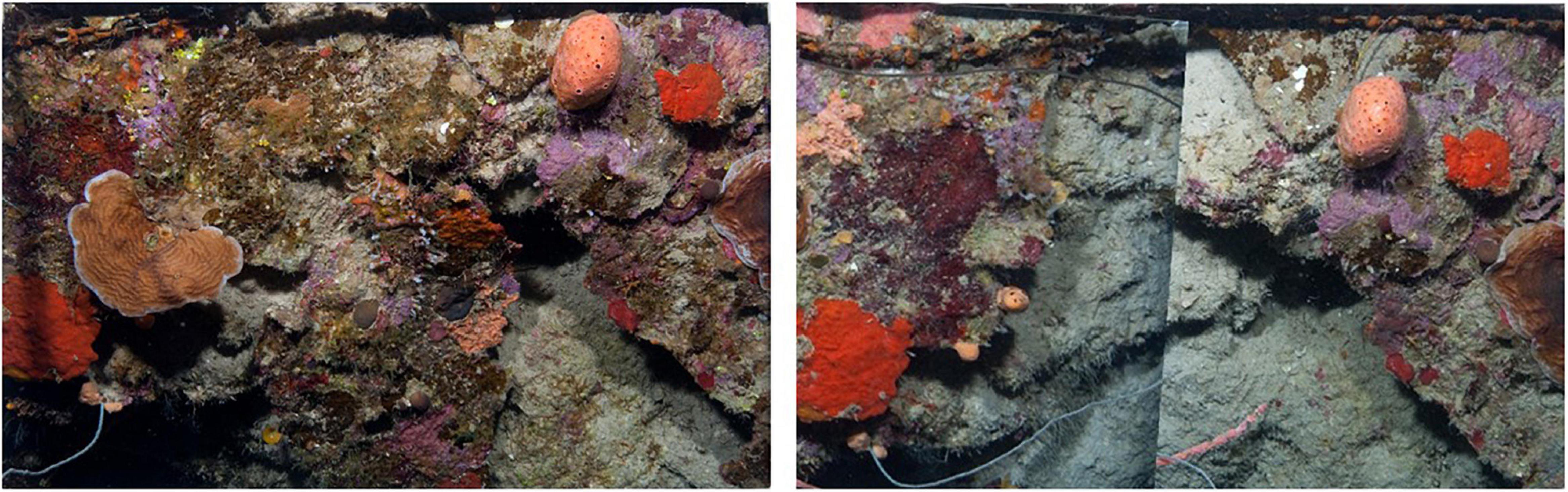
Figure 8. Sequence from Hole-in-the-Wall 70 m off La Parguera, Puerto Rico showing a section of the substratum breaking off between September 29, 2009 (left) and December 14, 2009 (right). Note the colony of Agaricia grahamae (center left in September) that was lost in the event. Photos are approximately 60 cm wide.
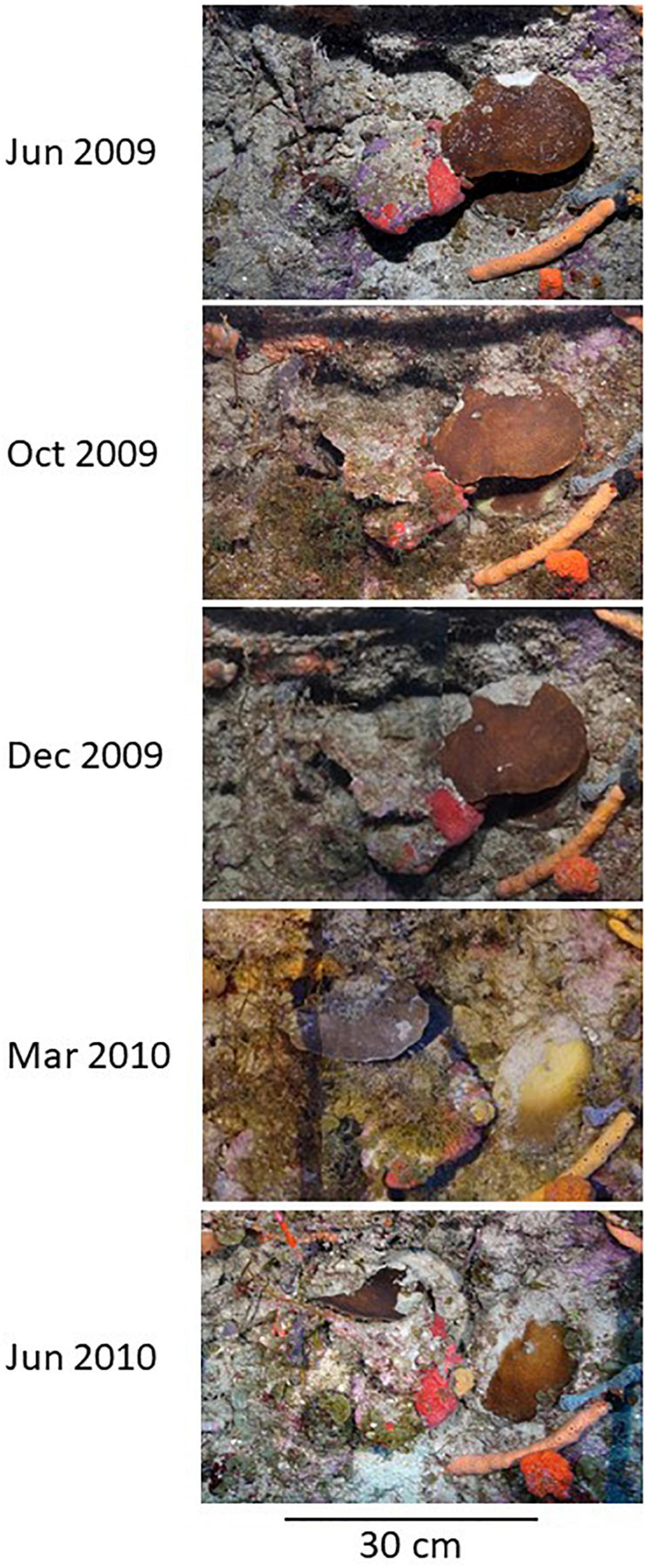
Figure 9. Photosequence from Hole-in-the-Wall 50 m off La Parguera, Puerto Rico from June 2009 to June 2010. Between December 2009 and March 2010, the colony of Agaricia agaricites (center right) is lost, uncovering a colony of Stephanocoenia intercepta. At the same time, a different colony of A. agaricites appears (center left).
Off La Parguera, the most noticeable community changes observed in benthic cover were among the algae, whose cover can vary markedly over short time frames due to their potential for rapid response. This is best exemplified by the dynamics of Schizothrix sp. at EH 50 m, which increased from near 0% cover to 30% cover over 9 months before declining to 10% in the final period. In shallower waters, cyanophytes such as Schizothrix sp., are generally ephemeral species capable of dramatic, but short-term blooms (Ruiz and Ballantine, 2009; Sangil et al., 2012), and these high coverages may not necessarily affect the underlying biota unless coverage is extensive and of long duration (e.g., Stielow and Ballantine, 2003). In this study, neither the Schizothrix sp. bloom nor the oscillations in cover of Dictyota spp. seem to affect the larger, longer-lived structural components. Thus, this study generally supports previous observations indicating that in the absence of significant physical or biological disturbances, or sustained changes in environmental drivers (e.g., climate change), MCEs will change slowly overtime either through the cumulative effects of small-scale disturbances, or through biological interactions, such as competitive dominance (Bak et al., 2005). Understanding change across the time scales of these various processes will allow for determining their relative significance in the future.
Data Availability Statement
The raw data supporting the conclusions of this article will be made available by the authors, without undue reservation.
Author Contributions
RA, DB, JC, NS, CS, EW, and PY: experimental design. MN, HR, and CS: data collection. RA, JC, and HR: data analysis. RA, DB, JC, HR, NS, CS, and EW: interpretation of the data. RA: drafting work. RA, DB, HR, NS, CS, and EW: critical revision. All authors agreed to be accountable for all aspects of the work.
Funding
This work was supported by the National Oceanic and Atmospheric Administration’s National Centers for Coastal and Ocean Science (NOAA/NCCOS) (NA06NOS4780190), through the Caribbean Coral Reef Institute. Partial funding was provided by the National Science Foundation OCE Award No. 1809878. This project was conducted at the Magueyes Island Marine Laboratory of the Department of Marine Sciences, University of Puerto Rico—Mayagüez.
Conflict of Interest
The authors declare that the research was conducted in the absence of any commercial or financial relationships that could be construed as a potential conflict of interest.
Publisher’s Note
All claims expressed in this article are solely those of the authors and do not necessarily represent those of their affiliated organizations, or those of the publisher, the editors and the reviewers. Any product that may be evaluated in this article, or claim that may be made by its manufacturer, is not guaranteed or endorsed by the publisher.
Acknowledgments
We thank Anibal Santiago, Marcos Rosado, and Ubaldo Lopez for support in boat and diving operations. Maria Cardona of the Bio-optical Oceanography Laboratory recovered the PAR data for 2009-10.
Supplementary Material
The Supplementary Material for this article can be found online at: https://www.frontiersin.org/articles/10.3389/fmars.2021.732926/full#supplementary-material
Supplementary Figure 1 | Temperature vs. time at 50 m (top) and 70 m (bottom) at El Hoyo on the insular slope off La Parguera, Puerto Rico. Arrows indicate dates of phototransects.
References
Anderson, M., Gorley, R. N., and Clarke, K. R. (2008). PERMANOVA + for PRIMER User Manual. Plymouth: PRIMER-E Ltd.
Appeldoorn, R., Ballantine, D., Bejarano, I., Carlo, M., Nemeth, M., Otero, E., et al. (2016). Mesophotic coral ecosystems under anthropogenic stress: a case study at Ponce, Puerto Rico. Coral Reefs 35, 63–75. doi: 10.1007/s00338-015-1360-5
Appeldoorn, R. S., Alfaro, M., Ballantine, D. L., Bejarano, I., Ruíz, H. J., Schizas, N. V., et al. (2019). “Puerto Rico,” in Mesophotic Coral Ecosystems of the World, eds Y. Loya, K. Puglise, and T. Bridge (New York, NY: Springer), 111–129.
Bak, R. P. M., and Luckhurst, B. (1980). Constancy and change in coral reef habitats along depth gradients at Curacao. Oecologia 47, 145–155. doi: 10.1007/BF00346812
Bak, R. P. M., and Nieuwland, G. (1995). Long-term change in coral communities along depth gradients over leeward reefs in the Netherlands Antilles. Bull. Mar. Sci. 56, 609–619.
Bak, R. P. M., Nieuwland, G., and Meesters, E. H. (2005). Coral reef crisis in deep and shallow reefs: 30 years of constancy and change in reefs of Curacao and Bonaire. Coral Reefs 24, 475–479. doi: 10.1007/s00338-005-0009-1
Bejarano, I., Appeldoorn, R. S., and Nemeth, M. (2014). Fishes associated with mesophotic coral ecosystems in La Parguera, Puerto Rico. Coral Reefs 33, 313–328. doi: 10.1007/s00338-014-1125-6
Bongaerts, P., Ridgway, T., Sampayo, E. M., and Hoegh-Guldberg, O. (2010). Assessing the ‘deep reef refugia’ hypothesis: focus on Caribbean reefs. Coral Reefs 29, 309–327. doi: 10.1007/s00338-009-0581-x
Bongaerts, P., and Smith, T. B. (2019). “Beyond the “Deep Reef Refuge” hypothesis: a conceptual framework to characterize persistence at depth,” in Mesophotic Coral Ecosystems of the World, eds Y. Loya, K. Puglise, and T. Bridge (New York, NY: Springer), 881–895. doi: 10.1007/978-3-319-92735-0_45
Bridge, T. C. L., Hughes, T. P., Guinotte, J. M., and Bongaerts, P. (2013). Call to protect all coral reefs. Nat. Clim. Change 3, 528–530. doi: 10.1038/nclimate1879
Carpenter, K., Abrar, M., Aeby, G., Aronson, R. B., Banks, S., Bruckner, A., et al. (2008). One third of reef-building corals face elevated extinction risk from climate change and local impacts. Science 321, 560–563. doi: 10.1126/science.1159196
Clarke, K. R. (1993). Nonparametric multivariate analyses of changes in community structure. Aust. J. Ecol. 18, 117–143. doi: 10.1111/j.1442-9993.1993.tb00438.x
Colin, P. I. (1978). Caribbean Reef Invertebrates and Plants. Neptune, NJ: T.H.F. Publications, Inc.
Corredor, J. E., and Morell, J. M. (2001). Seasonal variation of physical and biogeochemical features in eastern Caribbean surface water. J. Geophys. Res. 106, 4517–4525. doi: 10.1029/2000jc000291
De Bakker, D. M., Meesters, E. H., Bak, R. P. M., Nieuwland, G., and Van Duyl, F. C. (2016). Long-term shifts in coral communities on shallow to deep reef slopes of Curaçao and Bonaire: are there any winners? Front. Mar. Sci. 3:247. doi: 10.3389/fmars.2016.00247
Gardner, T., Côté, I. M., Gill, J., Grant, A., and Watkinson, A. R. (2003). Long term region-wide declines in Caribbean corals. Science 301, 958–960. doi: 10.1126/science.1086050
Guerra-Castro, E., and Cruz-Motta, J. J. (2014). Ecology of fouling assemblages associated with mangrove’s roots: an artificial substrate for manipulative experiments. J. Exp. Mar. Biol. Ecol. 457, 31–40.
Guerra-Castro, E. J., Conde, J. E., and Cruz-Motta, J. J. (2016). Scales of spatial variation in tropical benthic assemblages and their ecological relevance: epibionts on Caribbean mangrove roots as a model system. Mar. Ecol. Prog. Ser. 548, 97–110. doi: 10.3354/meps11693
Guiry, M. D., and Guiry, G. M. (2011). AlgaeBase. Galway: World-wide electronic publication, National University of Ireland.
Herron, L. W., Walters, L. J., and Beach, K. S. (2006). Fragment generation, survival, and attachment of Dictyota spp. at Conch Reef in the Florida Keys, USA. Coral Reefs 25, 287–295. doi: 10.1007/s00338-006-0096-7
Hinderstein, L., Marr, J. C. A., Martinez, F. A., Dowgiallo, M. J., Puglise, K. A., Pyle, R. L., et al. (2010). Theme section on “mesophotic coral ecosystems: characterization, ecology, and management”. Coral Reefs 29, 247–251. doi: 10.1007/s00338-010-0614-5
Hoegh-Guldberg, O. (1999). Climate change, coral bleaching and the future of the world’s coral reefs. Mar. Freshw. Res. 50, 839–866.
Holstein, D. M., Paris, C. B., Vaz, A. C., and Smith, T. B. (2016). Modeling vertical coral connectivity and mesophotic refugia. Coral Reefs 35, 23–37. doi: 10.1007/s00338-015-1339-2
Hughes, T. P. (1999). Off-reef transport of coral fragments at Lizard Island, Australia. Mar. Geol. 157, 1–6. doi: 10.1016/s0025-3227(98)00187-x
Humann, P., and DeLoach, N. (2001a). Reef Coral Identification: Florida Caribbean Bahamas, 2nd Edn. Jacksonville, FL: New World Publications Inc.
Humann, P., and DeLoach, N. (2001b). Reef Creature Identification: Florida Caribbean Bahamas, 2nd Edn. Jacksonville, FL: New World Publications Inc.
Jackson, J. B. C., Donovan, M. K., Cramer, K. L., and Lam, V. Y. (2014). Status and Trends of Caribbean Coral Reefs: 1970–2012. Gland: Global Coral Reef Monitoring Network, IUCN.
Kahng, S. E., Akkaynak, D., Shlesinger, T., Hochberg, E. J., Wiedenmann, J., Tamir, R., et al. (2019). “Light, temperature, photosynthesis, heterotrophy, and the lower depth limits of mesophotic coral ecosystems,” in Mesophotic Coral Ecosystems of the World, eds Y. Loya, K. Puglise, and T. Bridge (New York, NY: Springer), 801–828.
Kohler, K. E., and Gill, S. M. (2006). Coral Point Count with excel extensions (CPCe): a visual basic program for the determination of coral and substrate coverage using random point count methodology. Comp. Geosci. 32, 1259–1269. doi: 10.1016/j.cageo.2005.11.009
Laverick, J. H., Piango, S., Andradi-Brown, D. A., Exton, D. A., Bongaerts, P., Bridge, T. C. L., et al. (2018). To what extent do mesophotic coral ecosystems and shallow reefs share species of conservation interest? A systematic review. Environ. Evid. 7:15. doi: 10.1186/s13750-018-0127-1
Lesser, M. P., and Slattery, M. (2011). Phase shift to algal dominated communities at mesophotic depths associated with lionfish (Pterois volitans) invasion on a Bahamian coral reef. Biol. Invasions 13, 1855–1868. doi: 10.1007/s10530-011-0005-z
Lesser, M. P., Slattery, M., Laverick, J. H., Macartney, K. J., and Bridge, T. C. (2019). Global community breaks at 60 m on mesophotic coral reefs. Glob. Ecol. Biogeogr. 12, 1403–1416. doi: 10.7717/peerj.2475
Liddell, W. D., and Avery, W. E. (2000). “Temporal change in hard substrate communities 10-250 m, the Bahamas,” in Proceedings of the 9th International Coral Reef Symposium, Vol. 2, Bali, 1053–1058.
Liddell, W. D., Avery, W. E., and Ohlhorst, S. L. (1997). “Patterns of benthic community structure, 10-250 m, the Bahamas,” in Proceedings of the 8th International Coral Reef Symposium, Vol. 1, Panama City, FL, 437–442.
Loya, Y., Eyal, G., Treibitz, T., Lesser, M. P., and Appeldoorn, R. (2016). Theme section on mesophotic coral ecosystems: advances in knowledge and future perspectives. Coral Reefs 35, 1–9. doi: 10.1007/s00338-016-1410-7
Loya, Y., Puglise, K. A., and Bridge, T. C. L. (2019). Mesophotic Coral Ecosystems. New York, NY: Springer.
McClanahan, T. R., Weil, E., and Baird, A. H. (2018). “Consequences of coral bleaching for sessile reef organisms,” in Coral Bleaching. Ecological Studies (Analysis and Synthesis), Vol. 233, eds M. van Oppen and J. Lough (Cham: Springer), 231–263.
Renken, H., Mumby, P. J., Matsikis, I., and Edwards, H. J. (2010). Effects of physical environmental conditions on the patch dynamics of Dictyota pulchella and Lobophora variegata on Caribbean coral reefs. Mar. Ecol. Prog. Ser. 403, 63–74. doi: 10.3354/meps08441
Rocha, L. A., Pinheiro, H. T., Shepherd, B., Papastamatiou, Y. P., Luiz, O. J., Pyle, R. L., et al. (2018). Mesophotic coral ecosystems are threatened and ecologically distinct from shallow water reefs. Science 361, 281–284. doi: 10.1126/science.aaq1614
Ruiz, H., and Ballantine, D. L. (2009). Dynamics of shelf edge coral reef-associated macroalgae at La Parguera, Puerto Rico. Caribb. J. Sci. 45, 260–268.
Ruiz Torres, H. J. (2012). Spatial and Temporal Variations in the Relative Abundances of Coral Reef Algae in Southwest Puerto Rico. Ph.D. dissertation. Mayagüez, PR: University of Puerto Rico at Mayagüez.
Sangil, C., Sansón, M., Afonso-Carrillo, J., Herrera, R., Rodríguez, A., Martín-García, L., et al. (2012). Changes in subtidal assemblages in a scenario of warming: proliferations of ephemeral benthic algae in the Canary Islands (eastern Atlantic Ocean). Mar. Environ. Res. 77, 120–128. doi: 10.1016/j.marenvres.2012.03.004
Sherman, C., Appeldoorn, R., Carlo, M., Nemeth, M., Ruiz, H., and Bejarano, I. (2009). “Use of technical diving to study deep reef environments in Puerto Rico,” in Proceedings of the 28th Annual AAUS Scientific Symposium, Atlanta, Georgia, ed. N. W. Pollock (Dauphin Island, AL: American Academy of Underwater Sciences), 58–65.
Sherman, C., Nemeth, M., Ruíz, H., Bejarano, I., Appeldoorn, R., Pagán, F., et al. (2010). Geomorphology and benthic cover of mesophotic coral ecosystems of the upper insular slope of southwest Puerto Rico. Coral Reefs 29, 347–360. doi: 10.1007/s00338-010-0607-4
Sherman, C., Schmidt, W., Appeldoorn, R., Hutchinson, Y., Ruiz, H., Nemeth, M., et al. (2016). Sediment dynamics and their potential influence on insular-slope mesophotic coral ecosystems. Cont. Shelf Res. 129, 1–9.
Slattery, M., Lesser, M., Brazeau, D., Stokes, M., and Leichter, J. (2011). Connectivity and stability of mesophotic coral reefs. J. Exp. Mar. Biol. Ecol. 408, 32–41. doi: 10.1016/j.jembe.2011.07.024
Slattery, M., Moore, S., Boye, L., Whitney, S., Woolsey, A., and Woolsey, M. (2018). The Pulley Ridge deep reef is not a stable refugia through time. Coral Reefs 37, 391–396. doi: 10.1002/ece3.6340
Smith, T. B., Holstein, D. M., and Ennis, R. S. (2019). “Disturbance in mesophotic coral ecosystems and linkages to conservation and management,” in Mesophotic Coral Ecosystems of the World, eds Y. Loya, K. Puglise, and T. Bridge (New York, NY: Springer), 911–929.
Stemann, T. A. (1991). Evolution of the Reef-Coral Family Agariciidae (Anthozoa; Scleractinia) in the Neogene Through Recent of the Caribbean. Ph.D. dissertation. Iowa City IA: University of Iowa.
Stielow, S., and Ballantine, D. L. (2003). Benthic cyanobacterial, Microcoleus lyngbyaceus, blooms in shallow, inshore Puerto Rican seagrass habitats, Caribbean Sea. Harmful Algae 2, 127–133. doi: 10.1016/s1568-9883(03)00007-6
Underwood, A. J., Chapman, M. G., and Connell, S. D. (2000). Observations in ecology: you can’t make progress on processes without understanding the patterns. J. Exp. Mar. Biol. Ecol. 250, 97–115. doi: 10.1016/s0022-0981(00)00181-7
Weil, E. (2019). “Disease problems,” in Mesophotic Coral Ecosystems of the World, eds Y. Loya, K. Puglise, and T. Bridge (New York, NY: Springer), 779–800.
Weil, E., Croquer, A., and Urreiztieta, I. (2009). Temporal variability and impact of coral diseases and bleaching in La Parguera, Puerto Rico from 2003-2007. Caribb. J. Sci. 45, 221–246. doi: 10.18475/cjos.v45i2.a10
Wells, J. W., and Lang, J. (1973). Systematic list of Jamaican shallow-water Scleractinia. Bull. Mar. Sci. 23, 55–58.
Keywords: mesophotic coral ecosystems, benthic assemblages, intra-annual variation, depth variation, Puerto Rico
Citation: Appeldoorn RS, Ballantine DL, Carlo M, Cruz Motta JJ, Nemeth M, Ruiz HJ, Schizas NV, Sherman CE, Weil E and Yoshioka PM (2021) Intra-Annual Variation in Mesophotic Benthic Assemblages on the Insular Slope of Southwest Puerto Rico as a Function of Depth and Geomorphology. Front. Mar. Sci. 8:732926. doi: 10.3389/fmars.2021.732926
Received: 29 June 2021; Accepted: 27 October 2021;
Published: 14 December 2021.
Edited by:
Daniel Wangpraseurt, University of California, San Diego, United StatesReviewed by:
Patricia Briones-Fourzan, National Autonomous University of Mexico, MexicoGretchen Goodbody-Gringley, Central Caribbean Marine Institute, Cayman Islands
Copyright © 2021 Appeldoorn, Ballantine, Carlo, Cruz Motta, Nemeth, Ruiz, Schizas, Sherman, Weil and Yoshioka. This is an open-access article distributed under the terms of the Creative Commons Attribution License (CC BY). The use, distribution or reproduction in other forums is permitted, provided the original author(s) and the copyright owner(s) are credited and that the original publication in this journal is cited, in accordance with accepted academic practice. No use, distribution or reproduction is permitted which does not comply with these terms.
*Correspondence: Richard S. Appeldoorn, cmljaGFyZC5hcHBlbGRvb3JuQHVwci5lZHU=
†Deceased