- 1Faculty of Biology, “Alexandru Ioan Cuza” University of Iaşi, Iaşi, Romania
- 2National Institute for Research and Development on Marine Geology and Geoecology - GeoEcoMar, Bucharest, Romania
- 3Department of Biology, University of Prince Edward Island, Charlottetown, PE, Canada
Ecosystem engineers create habitat and provide conditions otherwise unavailable for the development of diverse communities. In marine soft-bottoms in particular, the biodiversity sustained by a matrix of relatively uniform sediments can be drastically enhanced by the presence of ecosystem engineers such as seagrasses. Unfortunately, the influence of seagrass meadows on the diversity of surrounding sediments is often unrecognized in spite of its importance, especially in coastlines exposed to multiple sources of pollution. This study examined composition and diversity associated with a bed of Zostera noltei Hornemann, 1832, and its surrounding bare sediments in a highly urbanized coastal area of the Romanian Black Sea. Dissimilarity levels were quantified and key species driving the differences between uniform (bare) and complex (eelgrass) sedimentary habitats were identified. 48 taxa were collected and counted, with epifaunal and infaunal species each accounting for nearly half of that diversity. Abundance, richness and diversity were strikingly higher in eelgrass-associated sediments, a difference driven primarily by various species of snails, crustaceans, polychaetes and bivalves. Between-habitat differences remained significant even after the removal of epifaunal species and each dataset undergoing strong data transformation. These results suggest that even small eelgrass beds, located in the vicinity of multiple sources of stress, can act as hotspots and make a substantial contribution to local benthic diversity.
Introduction
Ecosystem engineers (sensu Jones et al., 1994) create or alter local-scale conditions to provide critical habitat that allows the establishment of diverse communities (Wright and Jones, 2006). Coastal engineers are diverse and include, among others, corals, oysters, mussels, macroalgae, and seagrasses (e.g., Gutiérrez et al., 2003). The latter develop into distinct meadows embedded within the matrices of relatively uniform sediments associated with estuaries, lagoons, and sheltered bays (Lee et al., 2001; Bos et al., 2007). Seagrasses provide food resources, add live surface for colonization, attenuate currents, stabilize shorelines, buffer the negative effect of eutrophication, and help with carbon sequestration (Bos et al., 2007; Baeta et al., 2009; Bouma et al., 2009; Duarte et al., 2010; Marin-Diaz et al., 2020). Most studies on seagrass meadows show that they sustain and enhance local-scale diversity of communities (Attrill et al., 2000; Włodarska-Kowalczuk et al., 2014; Davis et al., 2017; Hyman et al., 2019; Rodil et al., 2021), or conspicuous groups of epifaunal organisms (Sánchez-Jerez et al., 1999; Hovel and Lipcius, 2002), polychaetes (Somaschini et al., 1994; Gambi et al., 1998) or fish (Guidetti and Bussotti, 2002). However, these meadows are not always recognized as hotspots of diversity and we argue that this is likely due to two main reasons: (1) there are well-known exceptions in which species composition or diversity measures within and outside seagrass beds do not differ (Den Hartog, 1983; Asmus and Asmus, 2000; Polte et al., 2005; Barnes, 2014; Barnes and Barnes, 2014) and (2) where striking between-habitat diversity differences occur, sometimes we have failed to highlight their potential influence on surrounding marine sediments (Hyman et al., 2019). The latter is particularly true in some regions of the Black Sea in which seagrasses have faced historical declines (Zaitsev and Mamaev, 1997; Uzunova, 2010), and where studies linking seagrasses with benthic diversity have lagged behind.
Seven phanerogam segrasses are known to occur in the Black Sea: Zostera (Zostera) marina Linnaeus, 1753, Zostera (Zosterella) noltei Hornemann, 1832, Stuckenia pectinata (L.) Börner, 1912, Zannichelia major (Hartman) Boenn. ex Reichenb., 1835, Ruppia maritima Linnaeus, 1753, Ruppia cirrhosa (Petagna) Grande, 1918, and Cymodocea nodosa (Ucria) Ascherson, 1870 (Milchakova, 1999; Milchakova and Phillips, 2003; Aysel et al., 2005). Five of these species (Z. noltei, Z. major, S. pectinata, R. maritima, and R. cirrhosa) occur in the Romanian Black Sea (Surugiu, 2008). However, data on their macrobenthic assemblages remain limited. Early studies by Borcea (1924, 1926, 1931) documented macrobenthic species composition from Zostera meadows, especially from the Agigea area. Four decades later, Manoleli (1967) described polychaetes associated to Zostera in the same area, and Băcescu et al. (1971) documented full macrobenthic assemblages. Since then, Zostera beds disappeared from the Romanian coast due to eutrophication and pollution in the 1980s (Surugiu, 2008). No further studies were published until a small Z. noltei bed was reported from the coast of Mangalia (Surugiu, 2008), and the composition of the species associated with this phanerogam was documented again (Surugiu et al., 2013).
Data from 2015 to 2018 (V. Surugiu and I. Şvedu; unpublished data) suggests that roughly the same number of species has prevailed in the Mangalia Z. noltei meadow. However, key comparisons with assemblages associated with nearby sedimentary bottoms have not been attempted. This study fulfills that gap in our knowledge by quantifying the differences between the bed of Z. noltei established in the vicinity of Mangalia, southern Romania (Surugiu, 2008) and surrounding bare sediments. Our working hypothesis was that habitat differences promote changes in macrobenthic species composition and abundance, beyond the natural variation associated with seasonality. Such hypothesis is based on two premises: (1) epifaunal species colonizing eelgrass should be absent in bare sediments (Duffy, 2006), and (2) infaunal species present in eelgrass-associated sediments would likely differ from those on structurally less complex bare sediments (Rodil et al., 2021). Results in support of that hypothesis were to be considered evidence of an eelgrass-driven enhancement of diversity that would critically contribute to local biodiversity.
Materials and Methods
Study Site and Sampling Procedure
The fauna inhabiting a bed of Z. noltei was sampled in the immediate vicinity of the highly urbanized coastal area of Mangalia (43°48′18.0′N, 028°35′31.9′E; Figure 1). This single eelgrass bed developed in sandy sediments located between 0.8 and 3.3 m of depth in a small embayment formed by a dyke. Surveys of the sediments associated with the eelgrass bed and adjacent (2 m away) bare sediments were conducted in summer and fall 2010 (July 28th and October 2nd, respectively). The timing of the surveys aimed to get a snapshot of seasonal macrobenthic communities during the main periods of eelgrass growth and development, following spring/early summer faunal recruitment. On each survey date, seven random sediment samples were collected from the central area of the eelgrass bed by SCUBA diving, while other seven were also randomly collected from the nearby bare sediments. Replication levels were deemed appropriate considering prior studies in the area (Surugiu et al., 2013) and elsewhere (e.g., Włodarska-Kowalczuk et al., 2014; Bououarour et al., 2021). Samples were collected with a 10 cm-diameter hand-held corer (inserted up to 15 cm deep) and transferred underwater to labeled jars. Samples were subsequently rinsed over 500 μm sieves, and the retained organisms were placed in 10% buffered seawater-formaldehyde solution. In the laboratory, samples were sorted in major taxonomic groups, preserved in 70% ethanol, and subsequently identified to the lowest possible taxonomic level under stereomicroscope (Mordukhai-Boltovskoi, 1968, 1969, 1972).
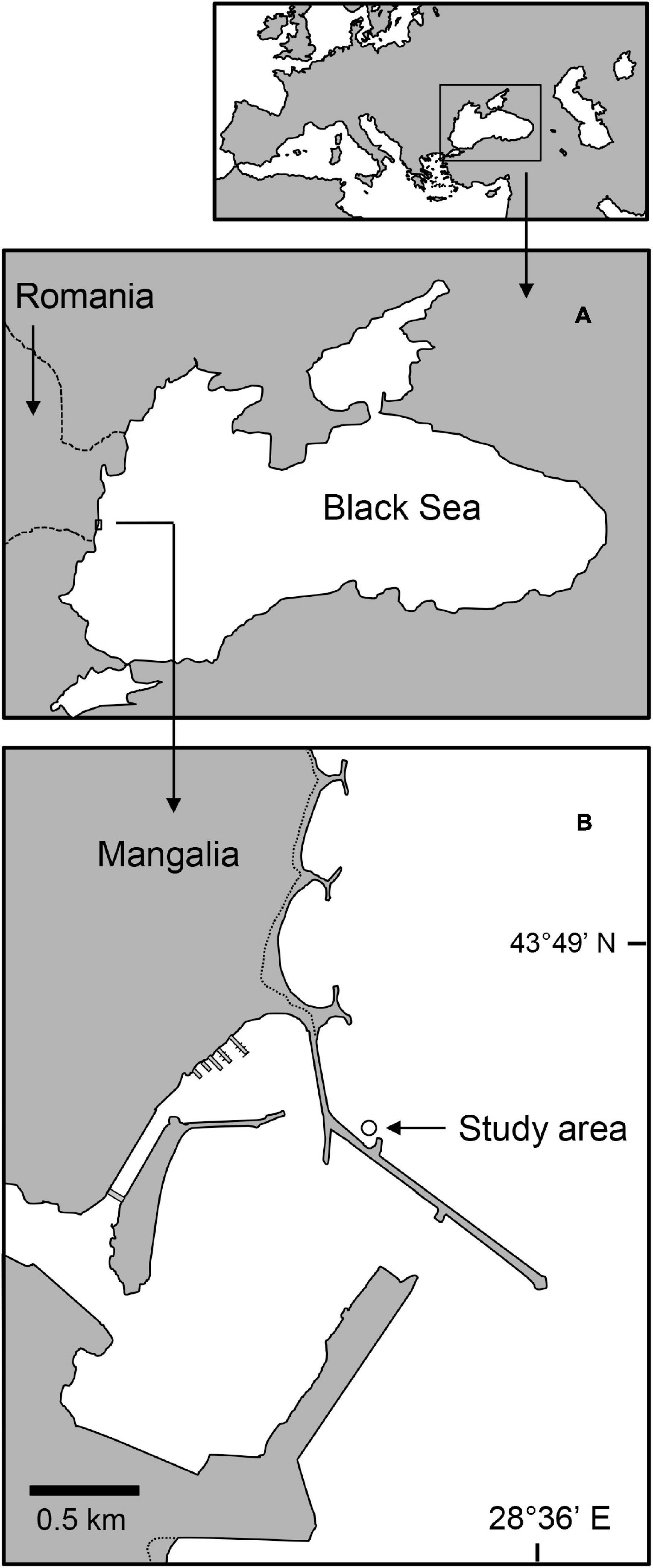
Figure 1. Map outlining the Black Sea (A) and the approximate location of the study area (B), immediately adjacent to the city of Mangalia, on the Romanian southern coast.
Data Analyses
Individual species abundances were used to calculate total abundance, species richness and Shannon-Wiener diversity (H’) from the full dataset of samples collected in eelgrass and bare sediments during summer and fall. A subset of that data, including only infaunal species was then used to calculate the same variables (i.e., abundance, species richness, and diversity of infaunal communities). The variables obtained from both datasets were subsequently used for between-habitat comparisons (eelgrass vs. bare sediments) using t-tests. Given assumption violations in 10 out of the 12 comparisons, these analyses were run using non-parametric Mann-Whitney U Statistic tests.
Both datasets (full data and infauna alone) were then analyzed with multivariate routines in the PRIMER 6 software. Specifically, species composition and abundance were used to create two-dimensional non-metric Multidimensional Scaling (MDS) plots using the Bray-Curtis Similarity index. Stress values assessed whether the distance between samples in the MDS plot was a reliable depiction of the actual differences between samples based on composition and abundance (values under 0.2 are considered a fairly reliable representation; Clarke et al., 2014). Potential between-habitat differences were then assessed with Analysis of Similarity (ANOSIM) which provided Global-R and p-values to assess significance. The same data was further examined with SIMPER analyses, which were used to estimate average levels of dissimilarity (%) and to generate a ranking of the species contributing the most to the difference between habitats. MDS, ANOSIM, and SIMPER analyses were all conducted with fourth-root transformed data, which removes the often overriding influence of numerically dominant species. Then, the same analyses were conducted with raw (untransformed) data and with data treated with the most severe transformation (presence-absence). The output of those parallel analyses is presented in detail in Supplementary Tables.
Results
Overall, 48 invertebrate taxa were collected from eelgrass and bare sediment habitats, predominantly including gastropods (13 species), crustaceans (11), polychaetes (10), and bivalves (6). Based on their life history, nearly half of these taxa were categorized as primarily infaunal and the other half as epifaunal species. Both subsets contributed to the abundance and diversity quantified in each habitat during summer and fall (see full species composition per habitat and date in Supplementary Table 1). For the full dataset (epifauna and infauna combined) and during both seasons (Figures 2A–C), median abundance, species richness and diversity (H’) were all significantly higher in sediments associated with eelgrass (Mann-Whitney U Statistic’s p < 0.001 in each of the six between-habitat comparisons; Figures 2A–C) than in nearby bare sediments. With regard to the subset of infaunal species alone (Figures 2D–F), with one exception (total abundance in the summer; Mann-Whitney U Statistic p = 0.805), the remaining five comparisons found again significantly higher median values of abundance, species richness and diversity in communities associated with eelgrass beds (Mann-Whitney U Statistic’s p < 0.001 to p = 0.027; Figures 2D–F).
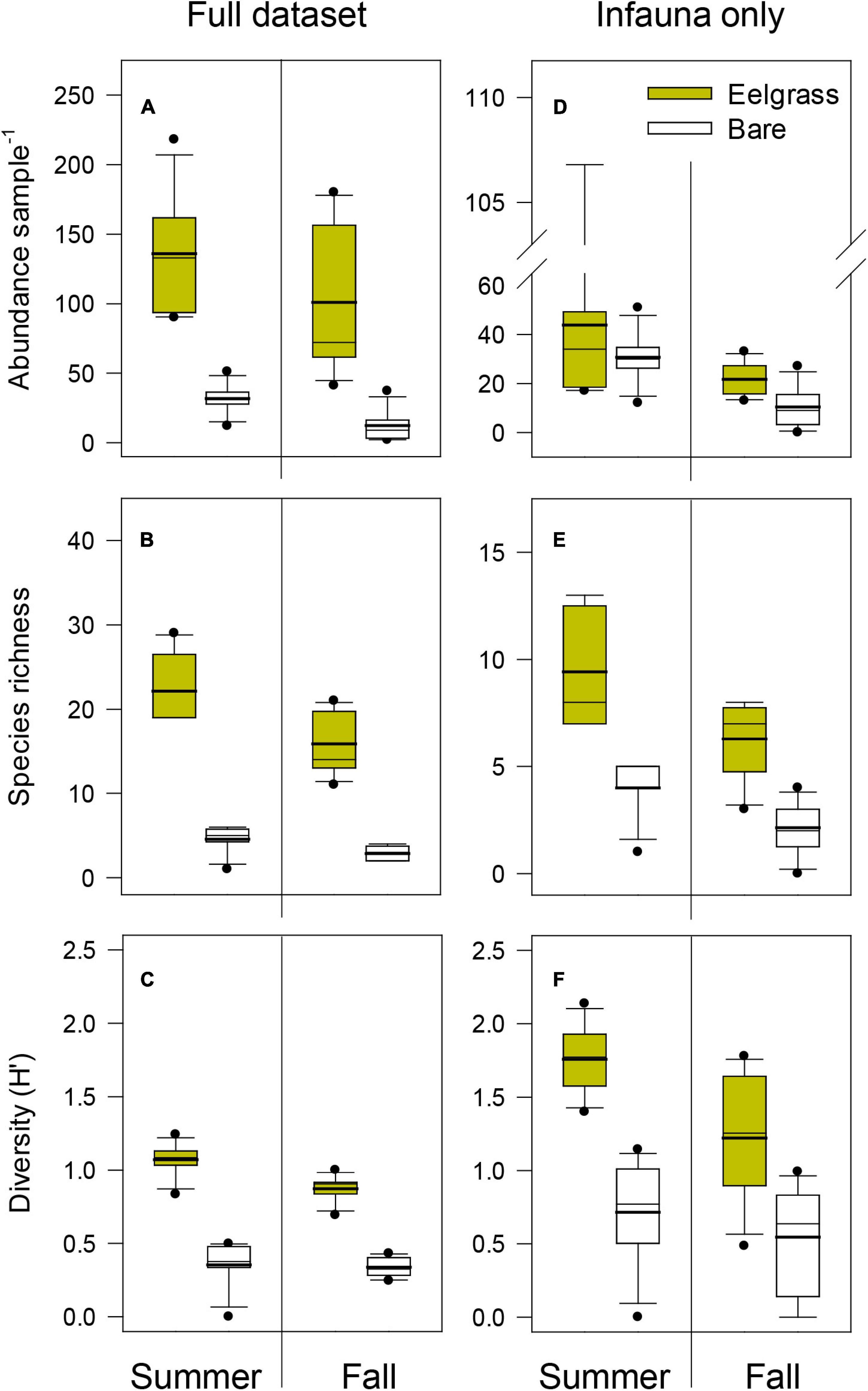
Figure 2. Boxplots illustrating abundance, species richness and Shannon-Wiener diversity of communities associated with eelgrass and bare sediment habitats during the 2010 summer and fall surveys. A distinction is made between results obtained from the full dataset (A–C) and a subset including infaunal species only (D–F). Mean values (thick black lines) are included in the boxplots. Between-habitat differences were significant (Mann-Whitney U Statistic’s p < 0.05) in all 12 comparisons except in the abundance of infauna during the summer (D; p > 0.05).
MDS plots (Figure 3) identified clear community differences between habitats, whereby samples associated with eelgrass clustered and segregated away from samples associated with bare sediments. Between-habitat ANOSIM comparisons confirmed that differences observed in the full dataset (Figure 3A) and in the subset of infaunal species (Figure 3B) were both significant: ANOSIM Global-R = 0.677 and 0.480, respectively; p = 0.001 in both analyses. Some of the most abundant species collected in this study were identified by SIMPER as those contributing the most to the differences between habitats (Table 1). When the full dataset was analyzed (86.31% average dissimilarity between habitats), the first seven taxa included five epifaunal and two infaunal species, which contributed up to near 39% of the dissimilarity measured. These include snails (e.g., Ecrobia maritima), bivalves (Mytilaster lineatus) and a spionid polychaete (Spio decorata) (Table 1). By comparison, when the subset of infaunal species was used (75.77% average dissimilarity), the first seven infaunal species explained near 60% of the between-habitats dissimilarity and included polychaetes (S. decorata) and various bivalves (e.g., Abra segmentum, Cerastoderma glaucum, Macomangulus tenuis). Similar between-habitat differences were detected using ANOSIM and SIMPER when full dataset and infaunal dataset were independently analyzed using untransformed (raw) data and presence-absence transformed data (see Supplementary Table 2).
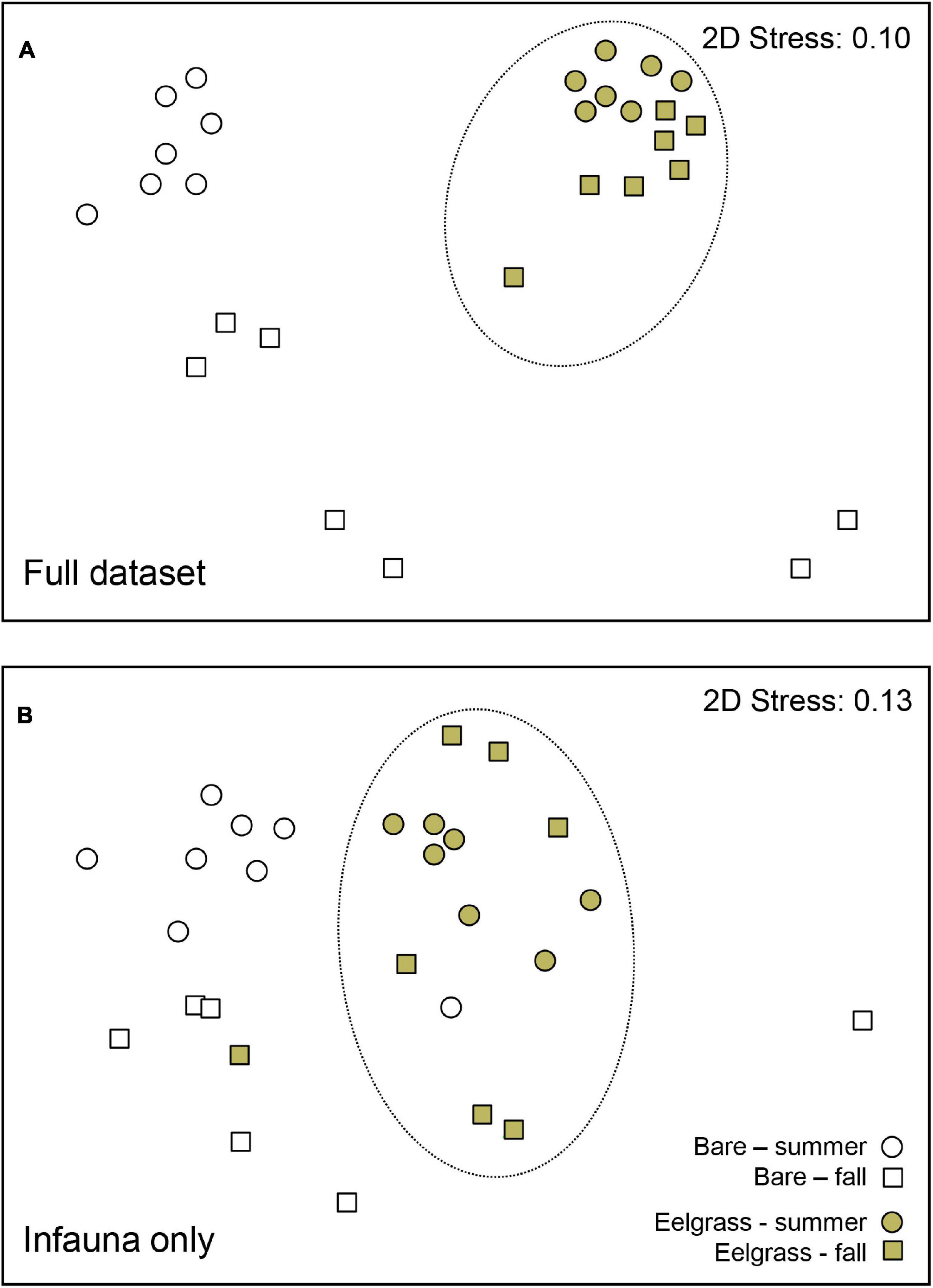
Figure 3. MDS plots illustrating community similarity among samples collected in eelgrass and bare sediment habitats for the 2010 summer and fall surveys combined. Separate plots are presented for the full dataset (A) and for a subset including infaunal species only (B). Dotted lines encircle primarily eelgrass samples to denote that they are significantly different from bare sediments according to ANOSIM (R-Global = 0.677 and 0.480 for (A,B), respectively) (p = 0.001 in both analyses).
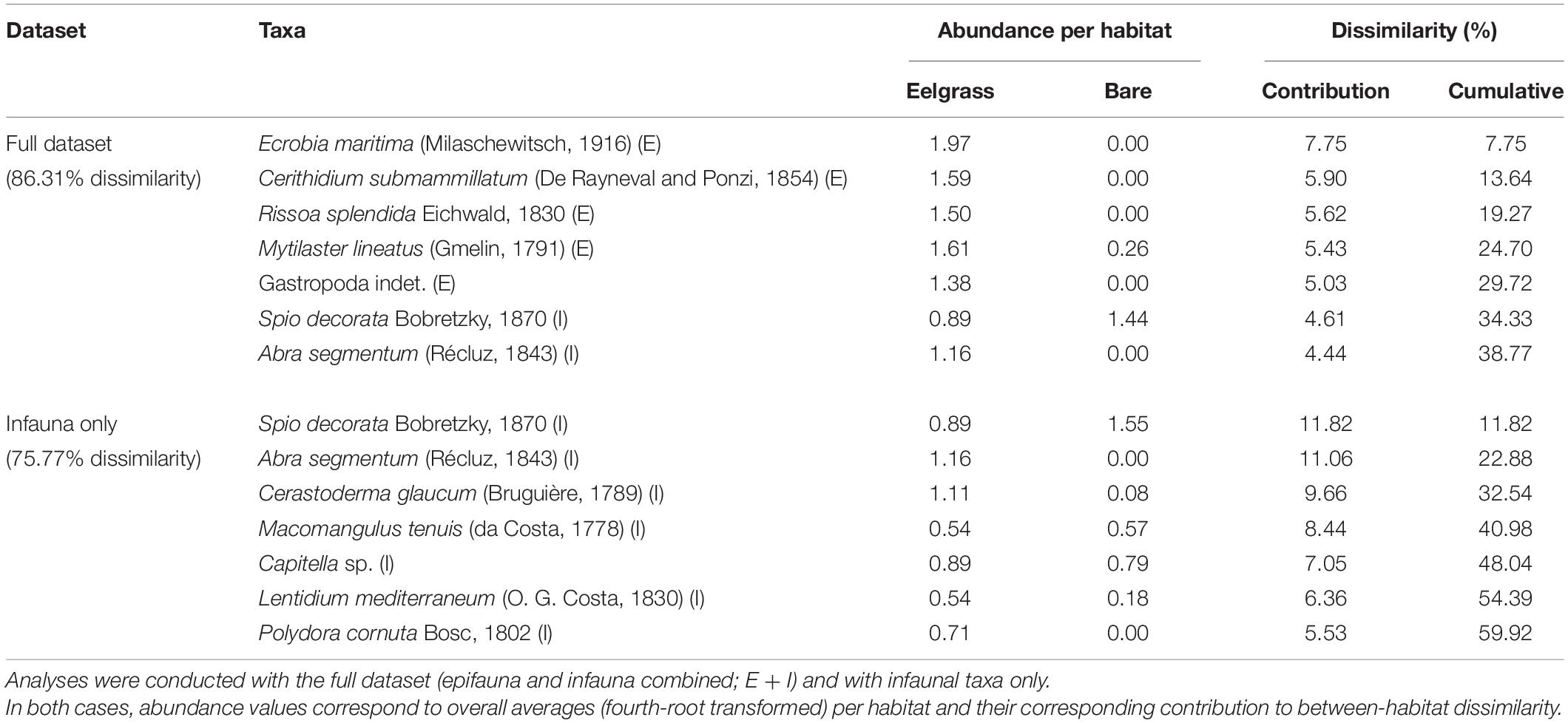
Table 1. Summary of SIMPER analyses identifying the (seven) main taxa contributing to the dissimilarity between communities associated with eelgrass and bare sediment habitats for summer and fall surveys combined.
Discussion
In this study, we demonstrate that eelgrass beds enhance local-scale diversity and abundance. Compared to bare (unstructured) sediments, those associated with Z. noltei were populated by significantly more diverse and abundant assemblages. In fact, multivariate analyses and almost every univariate comparison conducted (11 out of 12) identified striking between-habitat community differences. We therefore suggest that these beds act as hotspots of biodiversity that may in fact influence the sedimentary habitat well beyond the meadows’ physical edge. These results provide support to our working hypothesis and add to the broader body of literature (e.g., Frost et al., 1999; Hirst and Attrill, 2008; Klayn et al., 2013; Boyé et al., 2017), suggesting that seagrass meadows enhance local macrobenthic diversity.
Comparisons with prior studies provide a context for better understanding the diversity levels documented here. The number of taxa collected from Z. noltei bottoms (up to 37 in each seasonal survey) was above the historical range reported from the Romanian Black Sea (12–27 taxa in total; Borcea, 1924, 1926, 1931; Manoleli, 1967; Băcescu et al., 1971; see Supplementary Table 3). Such diversity was also fairly similar to the levels reported from Z. noltei meadows in the Crimean (37; Arnoldi, 1949) and Bulgarian Black Sea (39; Uzunova, 2010). Outside this region, these diversity levels also compare with those reported from Z. noltei in Galicia, Spain (36; Junoy and Viéitez, 1992) but are well above the ones reported from beds located in south west Netherlands (22; Bouma et al., 2009) and Portugal (21; Baeta et al., 2009). Our diversity levels also compare with those reported from Z. noltei meadows in the south-western coast of France (means of 27–39 species per site, with a total of 126 species; Blanchet et al., 2004), the Morocco coast (8–29 species per site, 69 species in total; Bououarour et al., 2021), or the diversity of peracaridans in the northwestern Iberian Peninsula (33–42 peracarid species; Esquete et al., 2011). Together, these records suggest that the diversity levels described here are at the upper end of those reported for this eelgrass in the region and elsewhere. Arguably, this is a remarkable result considering the small size of the eelgrass bed (940 m2 in 2010 and 1,132 m2 in 2020), its fairly recent re-establishment in the area (Surugiu, 2008), and its close proximity to a large urban center (Mangalia). The influence of eelgrass bed size has been addressed in habitat fragmentation studies which have found a positive relationship between size (combined with other features) and diversity levels (e.g., Rodil et al., 2021). The young age of the bed along with its proximity to Mangalia are also noteworthy because eelgrass beds are known to be susceptible to the various sources of pollution (Unsworth et al., 2014) normally associated with city port areas. Despite the odds, the diversity levels documented here were high, and as indicated before, strikingly different to those measured in the surrounding sediments.
Beyond measures of diversity and abundance, between-habitat differences were also driven by species composition, with several taxa strongly contributing to the clustering of samples by habitat. As expected, small grazing gastropods (e.g., Rissoa splendida, E. maritima, and Cerithidium submammillatum) were among the most prevalent in eelgrass-associated bottoms. Among them, R. splendida and other species of that genus (Rissoa spp.) had been in the past found abundantly in the region associated with Z. noltei beds (Arnoldi, 1949; Uzunova, 2010), Z. marina beds (Uzunova, 2010) and in beds where both Z. noltei and Z. marina were present (Makkaveeva, 1976; Klayn et al., 2013). With regards to infaunal organisms, the polychaete S. decorata was consistently linked with bare sediments, whereas cockles (C. glaucum), clams (A. segmentum), and mussels (M. lineatus) were all among the most dominant in eelgrass-associated bottoms. These species of clams and mussels had also been identified in Z. noltei beds before (Arnoldi, 1949), whereas M. lineatus, in particular, had been associated with beds of Z. noltei and Z. marina combined (Klayn et al., 2013). Above and beyond the scope of this study, the co-occurrence of two or more species of Zostera, or more generally, two or more ecosystem engineers, often entails added benefits to diversity (Rodil et al., 2021) and other ecosystem services (e.g., Tummon Flynn et al., 2020). Such benefits remain to be thoroughly explored in single and combined Zostera beds in the Black Sea and elsewhere.
Community differences documented here are likely linked with the obvious structural differences between bare sediments and bottoms associated with complex eelgrass beds (Heck and Wetstone, 1977; Duarte et al., 2010). This is not surprising considering the number of organisms that naturally depend on this type of vegetation for food, attachment, shelter, or other services (e.g., Möller et al., 2014), but contrasts with the lack of between-habitat differences found by e.g., Barnes (2014) and Polte et al. (2005). Among the latter studies, Barnes and Barnes (2014) suggested that where diversity differences occur, they may not be related to the benefits provided by the seagrass but to disruptive processes operating in the unvegetated bottoms (e.g., bioturbation by Callianassa shrimps). That is a plausible explanation for some coastal regions, but it is clearly not the case in the study area. Nonetheless, further studies here and elsewhere should consider the strong role of this and other bioturbators (e.g., green crabs; Lutz-Collins et al., 2016), when attempting to assess the role of eelgrass beds. Given that these benthic communities not only include epifauna, it is noteworthy to mention two novel elements in our methodological approach: (1) the analysis of infaunal organisms separately, by artificially excluding epifaunal species, and (2) the use of distinct data transformations. To our surprise, significant between-habitat differences were still found by both univariate and multivariate analyses when the more plant-dependent epifaunal component was removed and only infaunal species were compared. In addition, the initial use of fourth-root data transformation, which aimed to remove the influence of overly abundant species (Clarke et al., 2014), produced the same results than analyses conducted with raw data and arguably the most severe form of transformation (presence-absence). The consistent enhancement of local-scale diversity in soft-bottoms associated with eelgrass beds suggests that these isles of highly structured habitats do contribute to local-scale diversity (Hirst and Attrill, 2008; Fredriksen et al., 2010). Hence, despite the regional decline that seems to be affecting these habitats (Uzunova, 2010), even small eelgrass beds as those studied here should be deservedly highlighted as hotspots of benthic diversity (Thomsen et al., 2018). It follows that seagrass beds in general (Waycott et al., 2009) and eelgrass beds in the southern coast of the Romanian Black Sea (Surugiu, 2008) in particular, should be the focus of much needed conservation efforts.
Data Availability Statement
The original contributions presented in the study are included in the article/Supplementary Material, further inquiries can be directed to the corresponding author.
Author Contributions
VS and PQ conceived the study. VS, IŞ, and AT conducted the data acquisition. PQ performed multivariate and statistical analysis and interpretation. All authors contributed to the writing of the manuscript, its critical review, and approved the final version submitted.
Funding
This research received a partial financial support from the Romanian Ministry of Research in the framework of the CORE Programme projects PN 19200302 and PN 19200101.
Conflict of Interest
The authors declare that the research was conducted in the absence of any commercial or financial relationships that could be construed as a potential conflict of interest.
Publisher’s Note
All claims expressed in this article are solely those of the authors and do not necessarily represent those of their affiliated organizations, or those of the publisher, the editors and the reviewers. Any product that may be evaluated in this article, or claim that may be made by its manufacturer, is not guaranteed or endorsed by the publisher.
Acknowledgments
VS acknowledges Ciprian-Claudiu Mânzu, Irina Roşca, and Aurelian-Nicolae Roman for their help in sample collection and processing. PQ acknowledges the Natural Sciences and Engineering Research Council, Canada (NSERC) for its continued support during the preparation of the manuscript. We also thank Paula Tummon Flynn (UPEI) and two reviewers for their edits and suggestions on a preliminary version of the manuscript.
Supplementary Material
The Supplementary Material for this article can be found online at: https://www.frontiersin.org/articles/10.3389/fmars.2021.745137/full#supplementary-material
References
Arnoldi, L. V. (1949). Materials on the quantitative study of the Black Sea zoobenthos. II. Karkinitsky Bay. Tr. Sevast. Biol. St. 7, 127–192.
Asmus, H., and Asmus, R. (2000). Material exchange and food web of seagrass beds in the Sylt-Rømø Bight: how significant are community changes at the ecosystem level? Helgol. Mar. Res. 54, 137–150. doi: 10.1007/s101520050012
Attrill, M. J., Strong, J. A., and Rowden, A. A. (2000). Are macroinvertebrate communities influenced by seagrass structural complexity? Ecography 23, 114–121. doi: 10.1111/j.1600-0587.2000.tb00266.x
Aysel, V., Erduğan, H., Dural-Tarakçi, B., and Okudan, E. ş (2005). Marine algae and Seagrasses of Giresun Shores (Black Sea, Turkey). J. Black Sea Mediterr. Environ. 11, 241–255.
Băcescu, M., Müller, G. I., and Gomoiu, M.-T. (1971). Ecologie Marină. Cercetări De Ecologie Bentală În Marea Neagră. Analiza Cantitativă, Calitativă şi Comparată A Faunei Bentale Pontice, Vol. 4. Bucharest: Editura Academiei R.S.R.
Baeta, A., Valiela, I., Rossi, F., Pinto, R., Richard, P., Niquil, N., et al. (2009). Eutrophication and trophic structure in response to the presence of the eelgrass Zostera noltii. Mar. Biol. 156, 2107–2120. doi: 10.1007/s00227-009-1241-y
Barnes, R. S. K. (2014). Is spatial uniformity of soft-sediment biodiversity widespread and, if so, over what scales? Mar. Ecol. Prog. Ser. 504, 147–158. doi: 10.3354/meps10770
Barnes, R. S. K., and Barnes, M. K. (2014). Biodiversity differentials between the numerically-dominant macrobenthos of seagrass and adjacent unvegetated sediment in the absence of sandflat bioturbation. Mar. Environ. Res. 99, 34–43. doi: 10.1016/j.marenvres.2014.05.013
Blanchet, H., de Montaudouin, X., Lucas, A., and Chardy, P. (2004). Heterogeneity of macrozoobenthic assemblages within a Zostera noltii seagrass bed: diversity, abundance, biomass and structuring factors. Estuar. Coast. Shelf Sci. 61, 111–123. doi: 10.1016/j.ecss.2004.04.008
Borcea, I. (1926). Données sommaires sur la faune de la Mer Noire (Littoral de Roumanie). Ann. Sci. Univ. Jassy 14, 536–581.
Borcea, I. (1931). Nouvelles contributions à l’étude de la faune bentonique dans la Mer Noire, près du littoral roumain. Ann. Sci. Univ. Jassy 16, 655–750.
Bos, A. R., Bouma, T. J., de Korta, G. L. J., and van Katwijk, M. M. (2007). Ecosystem engineering by annual intertidal seagrass beds: sediment accretion and modification. Estuar. Coast. Shelf Sci. 74, 344–348. doi: 10.1016/j.ecss.2007.04.006
Bouma, T. J., Olenin, S., Reise, K., and Ysebaert, T. (2009). Ecosystem engineering and biodiversity in coastal sediments: posing hypotheses. Helgol. Mar. Res. 63, 95–106. doi: 10.1007/s10152-009-0146-y
Bououarour, O., El Kamcha, R., Boutoumit, S., Pouzet, P., Maanan, M., and Bazairi, H. (2021). Effects of the Zostera noltei meadows on benthic macrofauna in North Atlantic coastal ecosystems of Morocco: spatial and seasonal patterns. Biologia 1–13. doi: 10.1007/s11756-021-00718-9
Boyé, A., Legendre, P., Grall, J., and Gauthier, O. (2017). Constancy despite variability: local and regional macrofaunal diversity in intertidal seagrass beds. J. Sea Res. 130, 107–122. doi: 10.1016/j.seares.2017.06.004
Clarke, K. R., Gorley, R. N., Somerfield, P. J., and Warwick, R. M. (2014). Change In Marine Communities: An Approach To Statistical Analysis And Interpretation, 3rd Edn. Plymouth: PRIMER-E.
Davis, T. R., Harasti, D., Kelaher, B., and Smith, S. D. A. (2017). Spatial and temporal variation in subtidal molluscan diversity amongst temperate estuarine habitats. Mar. Ecol. 38:e12428. doi: 10.1111/maec.12428
Den Hartog, C. (1983). Structural uniformity and diversity in Zostera-dominated communities in Western Europe. Mar. Technol. Soc. J. 17, 6–14. doi: 10.1016/0304-3770(83)90022-0
Duarte, C. M., Marbá, N., Gacia, E., Fourqurean, J. W., Beggins, J., Barrón, C., et al. (2010). Seagrass community metabolism: assessing the carbon sink capacity of seagrass meadows. Glob. Biogeochem. Cycles 24, 1–8. doi: 10.1029/2010GB003793
Duffy, J. E. (2006). Biodiversity and the functioning of seagrass ecosystems. Mar. Ecol. Prog. Ser. 311, 233–250. doi: 10.3354/meps311233
Esquete, P., Moreira, J., and Troncoso, J. S. (2011). Peracarid assemblages of Zostera meadows in an estuarine ecosystem (O Grove inlet. NW Iberian Peninsula): spatial distribution and seasonal variation. Helgol. Mar. Res. 65, 445–455. doi: 10.1007/s10152-010-0234-z
Fredriksen, S., De Backer, A., Boström, C., and Christie, H. (2010). Infauna from Zostera marina L. meadows in Norway. Differences in vegetated and unvegetated areas. Mar. Biol. Res. 6, 189–200. doi: 10.1080/17451000903042461
Frost, M. T., Rowden, A. A., and Attrill, M. J. (1999). Effect of habitat fragmentation on the macroinvertebrate infaunal communities associated with the seagrass Zostera marina L. Aquat. Conserv. Mar. Freshw. Ecosyst 9, 255–263. doi: 10.1002/(SICI)1099-0755(199905/06)9:3<255::AID-AQC346<3.0.CO;2-F
Gambi, M. C., Conti, G., and Bremec, C. (1998). Polychaete distribution, diversity and seasonality related to seagrass cover in shallow soft bottoms of the Tyrrhenian Sea (Italy). Sci. Mar. 62, 1–17. doi: 10.3989/scimar.1998.62n1-21
Guidetti, P., and Bussotti, S. (2002). Effects of seagrass canopy removal on fish in shallow Mediterranean seagrass (Cymodocea nodosa and Zostera noltii) meadows: a local scale approach. Mar. Biol. 140, 445–453. doi: 10.1007/s00227-001-0725-1
Gutiérrez, J. L., Jones, C. G., Strayer, D. L., and Iribarne, O. O. (2003). Mollusks as ecosystem engineers: the role of shell production in aquatic habitats. Oikos 101, 79–90. doi: 10.1034/j.1600-0706.2003.12322.x
Heck, Jr. K. L., and Wetstone, G. S. (1977). Habitat complexity and invertebrate species richness and abundance in tropical seagrass meadows. J. Biogeogr. 4, 135–142. doi: 10.2307/3038158
Hirst, J. A., and Attrill, M. J. (2008). Small is beautiful: an inverted view of habitat fragmentation in seagrass beds. Estuar. Coast. Shelf Sci. 78, 811–818. doi: 10.1016/j.ecss.2008.02.020
Hovel, K. A., and Lipcius, R. N. (2002). Effects of seagrass habitat fragmentation on juvenile blue crab survival and abundance. J. Exp. Mar. Biol. Ecol. 271, 75–98. doi: 10.1016/S0022-0981(02)00043-6
Hyman, A. C., Frazer, T. K., Jacoby, C. A., Frost, J. R., and Kowalewski, M. (2019). Long-term persistence of structured habitats: seagrass meadows as enduring hotspots of biodiversity and faunal stability. Proc. R. Soc. B. 286:20191861. doi: 10.1098/rspb.2019.1861
Jones, C. G., Lawton, J. H., and Shachak, M. (1994). Organisms as ecosystem engineers. Oikos 69, 373–386. doi: 10.2307/3545850
Junoy, J., and Viéitez, J. M. (1992). Macrofaunal abundance analyses in the Ría de Foz (Lugo. Northwest Spain). Cah. Biol. Mar. 33, 331–345.
Klayn, S., Deyanova, D., Vasilev, V., and Karamfilov, V. (2013). “Effect of seagrass meadows (Zostera spp.) on the structure, abundance and diversity of macrozoobenthic communities in Sozopol Bay, South-Western Black Sea coast,” in Proceedings of Seminar of Ecology-2013 with international participation, eds S. Chankova, S. Gateva, and G. Jovtchev (Sofia: Bulgarian Academy of Sciences), 83–89.
Lee, S. Y., Fong, C. W., and Wu, R. S. S. (2001). The effects of seagrass (Zostera japonica) canopy structure on associated fauna: a study using artificial seagrass units and sampling of natural beds. J. Exp. Mar. Biol. Ecol. 259, 23–50. doi: 10.1016/S0022-0981(01)00221-0
Lutz-Collins, V., Cox, R., and Quijón, P. A. (2016). Habitat disruption by a coastal invader: local community change and recovery in Atlantic Canada sedimentary habitats. Mar. Biol. 163:177. doi: 10.1007/s00227-016-2947-2
Makkaveeva, E. B. (1976). The dynamics of mass species populations of eelgrass biocenoses. Biol. Morya 36, 25–40.
Manoleli, D. (1967). Date ecologice asupra polichetelor din dreptul Staţiunii marine de la Agigea. St. Cerc. Biol. Ser. Zool. 19, 509–515.
Marin-Diaz, B., Bouma, T. J., and Infantes, E. (2020). Role of eelgrass on bed-load transport and sediment resuspension under oscillatory flow. Limnol. Oceanogr. 65, 426–436. doi: 10.1002/lno.11312
Milchakova, N. A. (1999). On the status of seagrass communities in the Black Sea. Aquat. Bot. 65, 21–32. doi: 10.1016/S0304-3770(99)00028-5
Milchakova, N. A., and Phillips, R. C. (2003). Black Sea seagrasses. Mar. Pollut. Bull. 46, 695–699. doi: 10.1016/S0025-326X(02)00361-2
Möller, T., Kotta, J., and Martin, G. (2014). Spatiotemporal variability in the eelgrass Zostera marina L. in the northeastern Baltic Sea: canopy structure and associated macrophyte and invertebrate communities. Estonian J. Ecol. 63, 90–108. doi: 10.3176/eco.2014.2.03
Mordukhai-Boltovskoi, F. D. (ed.) (1968). Key to Fauna of The Black Sea and The Azov Sea, Vol. 1. Kiev: Naukova Dumka.
Mordukhai-Boltovskoi, F. D. (ed.) (1969). Key to Fauna of The Black Sea and The Azov Sea, Vol. 2. Kiev: Naukova Dumka.
Mordukhai-Boltovskoi, F. D. (ed.) (1972). Key to Fauna of The Black Sea and The Azov Sea, Vol. 3. Kiev: Naukova Dumka.
Polte, P., Schanz, A., and Asmus, H. (2005). The contribution of seagrass beds (Zostera noltii) to the function of tidal flats as a juvenile habitat for dominant, mobile epibenthos in the Wadden Sea. Mar. Biol. 147, 813–822. doi: 10.1007/s00227-005-1583-z
Rodil, I. F., Lohrer, A. M., Attard, K. M., Hewitt, J. E., Thrush, S. F., and Norkko, A. (2021). Macrofauna communities across a seascape of seagrass meadows: environmental drivers, biodiversity patterns and conservation implications. Biodivers. Conserv. doi: 10.1007/s10531-021-02234-3
Sánchez-Jerez, P., Barberá Cebrián, C., and Ramos Esplá, A. A. (1999). Comparison of the epifauna spatial distribution in Posidonia oceanica, Cymodocea nodosa and unvegetated bottoms: Importance of meadow edges. Acta Oecol. 20, 391–405. doi: 10.1016/S1146-609X(99)00128-9
Somaschini, A., Gravina, M. F., and Ardizzone, G. D. (1994). Polychaete depth distribution in a Posidonia oceanica bed (rhizome and matte strata) and neighbouring soft and hard bottoms. Mar. Ecol. 15, 133–151. doi: 10.1111/j.1439-0485.1994.tb00049.x
Surugiu, V. (2008). On the occurrence of Zostera noltei Hornemann at the Romanian coast of the Black Sea. An. Şt. Univ. “Al. I. Cuza”, Iaşi, ser. Biol. Veget. 54, 122–127.
Surugiu, V., Mânzu, C. C., Roşca, I., and Teacă, A. (2013). Community structure of macrozoobenthos associated with Zostera noltei Hornem. meadows in the southern Romanian Black Sea coast. Rapp. Comm. Int. Mer Médit. 40:696.
Thomsen, M. S., Altieri, A. H., Angelini, C., Bishop, M. J., Gribben, P. E., Lear, G., et al. (2018). Secondary foundation species enhance biodiversity. Nat. Ecol. Evol. 2, 634–639. doi: 10.1038/s41559-018-0487-5
Tummon Flynn, P., McCarvill, K., Lynn, K. D., and Quijón, P. A. (2020). The positive effect of coexisting ecosystem engineers: a unique seaweed-mussel association provides refuge for native mud crabs against a non-indigenous predator. PeerJ 8:e10540. doi: 10.7717/peerj.10540
Unsworth, R. K. F., Van Keulen, M., and Coles, R. (2014). Seagrass meadows in a globally changing environment. Mar. Pollut. Bull. 83, 383–386. doi: 10.1016/j.marpolbul.2014.02.026
Uzunova, S. (2010). The zoobenthos of eelgrass populations from Sozopol Bay (Black Sea). Bulg. J. Agric. Sci. 16, 358–363.
Waycott, M., Duarte, C. M., Carruthers, T. J. B., Orth, R. J., Dennison, W. C., Olyarnik, S., et al. (2009). Accelerating loss of seagrasses across the globe threatens coastal ecosystems. PNAS 106, 12377–12381. doi: 10.1073/pnas.0905620106
Włodarska-Kowalczuk, M., Jankowska, E., Kotwicki, L., and Balazy, P. (2014). Evidence of season-dependency in vegetation effects on macrofauna in temperate seagrass meadows (Baltic Sea). PLoS One 9:e100788. doi: 10.1371/journal.pone.0100788
Wright, J. P., and Jones, C. G. (2006). The concept of organisms as ecosystem engineers ten years 395 on: Progress, limitations, and challenges, BioScience 56, 203–209. doi: 10.1641/0006-3963568(2006)056[0203:TCOOAE]2.0.CO;2
Keywords: Zostera noltei, ecosystem engineers, benthic macrofauna, biodiversity hotspot, Black Sea
Citation: Surugiu V, Teacă A, Şvedu I and Quijón PA (2021) A Hotspot in the Romanian Black Sea: Eelgrass Beds Drive Local Biodiversity in Surrounding Bare Sediments. Front. Mar. Sci. 8:745137. doi: 10.3389/fmars.2021.745137
Received: 21 July 2021; Accepted: 12 August 2021;
Published: 01 September 2021.
Edited by:
Stefania Klayn, Bulgarian Academy of Sciences, BulgariaReviewed by:
Maria Flavia Gravina, University of Rome “Tor Vergata”, ItalySonya Uzunova, Institute of Fish Resources, Varna, Bulgaria
Copyright © 2021 Surugiu, Teacă, Şvedu and Quijón. This is an open-access article distributed under the terms of the Creative Commons Attribution License (CC BY). The use, distribution or reproduction in other forums is permitted, provided the original author(s) and the copyright owner(s) are credited and that the original publication in this journal is cited, in accordance with accepted academic practice. No use, distribution or reproduction is permitted which does not comply with these terms.
*Correspondence: Victor Surugiu, dnN1cnVnaXVAdWFpYy5ybw==
†These authors have contributed equally to this work and share senior authorship