Effects of Low Temperature on Shrimp and Crab Physiology, Behavior, and Growth: A Review
- 1Key Laboratory for Sustainable Utilization of Marine Fisheries Resources, Ministry of Agriculture, Yellow Sea Fisheries Research Institute, Chinese Academy of Fishery Sciences, Qingdao, China
- 2Function Laboratory for Marine Fisheries Science and Food Production Processes, Qingdao National Laboratory for Marine Science and Technology, Qingdao, China
- 3College of Fisheries and Life Science, Shanghai Ocean University, Shanghai, China
- 4Jiangsu Key Laboratory of Marine Bioresources and Environment/Jiangsu Key Laboratory of Marine Biotechnology, Jiangsu Ocean University, Lianyungang, China
As important aquaculture species worldwide, shrimps and crabs are thermophilic animals with a feeble thermoregulation ability. Changes in environmental factors are the main reason for the decrease in the immunity and disease resistance ability of cultured organisms. Water temperature is one of the most common abiotic stress factors for aquatic ectotherms. It influences nearly all biochemical and physiological processes in crustaceans, resulting in an imbalance in ion and water homeostasis, neuromuscular function loss, cellular dehydration, and altered metabolic pathways. The present review summarizes the current knowledge on the effects of low temperature on the physiological response, and the behavior, development, and growth of shrimp and crab. We suggest a deeper research to understand the physiological processes involved in thermoregulation; this knowledge could be used to reduce the adverse effects in the shrimps and crabs during the culture.
Introduction
As important aquaculture species worldwide, crustaceans such as shrimp and crab have very weak cold regulation abilities. Since the 1980s, various diseases have caused huge losses in the aquaculture industry. Epidemiological surveys showed that shrimp and crab diseases mainly occurred in spring and summer, and the peak of the disease often occurred after drastic changes in environmental conditions. Changes in environmental factors are the main reason for the decline of biological immunity and disease resistance (Le Moullac and Haffner, 2000). For crustaceans such as shrimp and crab, the water temperature is an important survival-related environmental factor, which not only directly influences their metabolism, growth, molting, and survival, but also affects other environmental factors (e.g., dissolved oxygen) (Chen et al., 1995; Hennig and Andreatta, 1998; Saucedo et al., 2004). Therefore, the temperature has become an essential factor restricting shrimp and crab culture.
There has been significant research progress on how temperature affects crustacean growth, physiology, survival, energy metabolism, and biochemistry. In shrimps and crabs, cold shock can be discussed in the context of the general stress response. The present review used the definition of stress reported by Donaldson et al. (2008), which described stress as a cascade of physiological responses occurring in an organism that tries to re- disturbance its homeostasis after an insult. There are three broad categories of responses to environmental stress: primary (e.g., the release of corticosteroids and catecholamine and the neuroendocrine response), secondary (e.g., immunological, osmoregulatory, hematological, cellular, and metabolic changes), and tertiary (e.g., behavioral and physiological stress responses in the whole organism). This paper reviews the research progress of the effects of temperature from the three aspects (see Figure 1), which will enrich the basic physiological data of shrimp and crab, and to guide the artificial culture of shrimp and crab, providing a reference for related research in the future.
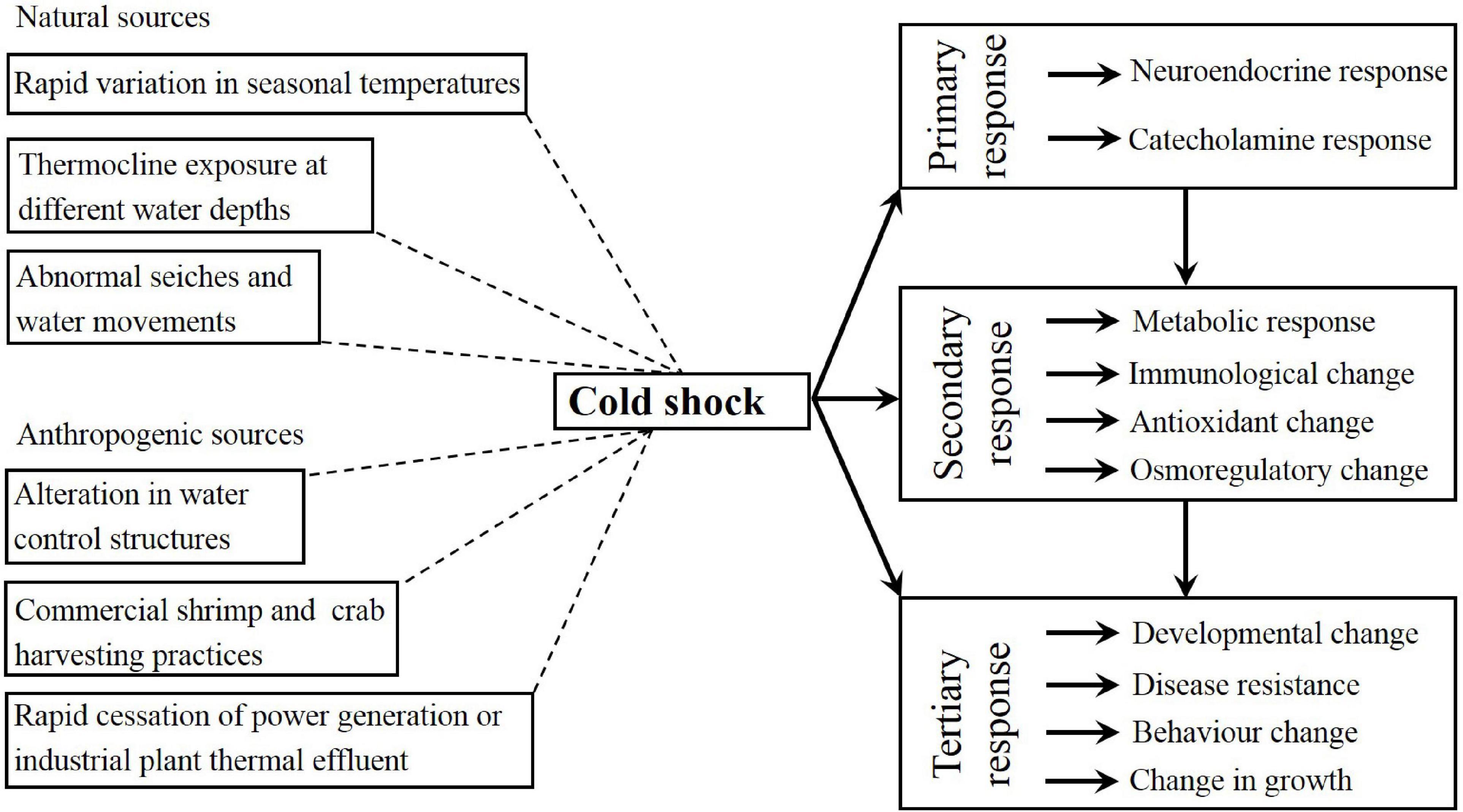
Figure 1. Schematic representation of natural and anthropogenic sources of cold shock and the primary, secondary and tertiary responses to cold shock.
Primary Responses – The Neuroendocrine Response
The endocrine and nervous systems function synchronously to regulate many physiological processes and to maintain balanced organism-wide homeostasis in both normal and stressful conditions, via a process, termed neuroendocrine integration (Adamski et al., 2019). The neuroendocrine system and its related signaling molecules (e.g., biogenic amines (BAs) and neuropeptides) regulate many crustacea behavioral and physiological processes; therefore, they might also affect cold tolerance (Chen et al., 2014).
BAs identified in crustaceans include catecholamines [dopamine (DA), norepinephrine (NE), and epinephrine (E)] and indoleamine [5-hydroxytryptamine (5-HT)] (Chang et al., 2009, 2015). The stress response involves BAs (Zhao et al., 2016). For instance, low temperatures alter BA concentrations, allowing insects to survive in, or prepare for, unfavorable conditions such as prolonged stress. BAs have important functions in the regulation of fundamental life processes (Sinakevitch et al., 2018). Not only do BAs function as neuromodulators and neurotransmitters in nervous tissues, but also can act as neurohormones after their release into body fluids (Sinakevitch et al., 2018). According to the target tissue, BAs bind to different types of G protein-coupled receptors (GPCRs), resulting in the stimulation of various secondary messengers, such as Ca2+ or cyclic adenosine monophosphate (cAMP) (Farooqui, 2012). Research has identified four DA and five 5-HT receptor subtypes in crustaceans to date (Northcutt et al., 2016; Pang et al., 2019). Most of these receptors are member of a GPCR superfamily that activates cascades of second messengers, mainly protein kinase A (PKA) and cAMP (Costa et al., 2016). In crayfish (Procambarus clarkii), agonistic behavior, such as the loser and winner effects is mediated by the cAMP-PKA signaling pathway (Momohara et al., 2016).
BAs’ neuroprotective role in supporting muscle activity in various crustaceans in response to low temperature has been studied (Hamilton et al., 2007). In lobster and crayfish muscles, increased haemolymph 5-HT levels in response to cold resulted in an increase in the amplitude of the excitatory postsynaptic potential (EPSP). BAs’ effects are frequently temperature-dependent; e.g., 5-HT-induced alterations of the EPSP occur only at suboptimal temperatures, which might aid the function of neuromuscular junctions under low temperature stress (Hamilton et al., 2007; Zhu and Cooper, 2018). This hypothesis was supported partially by the observation that in Drosophila melanogaster larval heart exposed to cold, only 5-HT had a strong excitatory effect (Zhu et al., 2016).
In crustaceans subjected to cold stress, the BA levels are altered. For example, in the giant prawn Macrobrachium rosenbergii, variations in NE levels in the haemolymph, eyestalk, and thoracic ganglion, suggested that NE mediates cold shock-induced hyperglycemia (Hsieh et al., 2006). Higher haemolymph levels of DA were detected in 24°C-acclimated white shrimp (Litopenaeus vannamei) when shifted to a lower temperature (18 or 21°C) (Pan et al., 2008).
The crustacean hyperglycaemic hormone (CHH) family is an important endocrine hormone, comprising CHH, molt-inhibiting hormone (MIH), gonad-inhibiting hormone (GIH), and mandibular organ-inhibiting hormone (MOIH) (Chen et al., 2020). In particular, CHH, which mainly regulates the release of glucose, is involved in the mediation of stress responses. CHH is probably the most widely studied neuroendocrine mechanism that mediates the crustacean stress response (Wanlem et al., 2011). CHH is a neurohormone produced by the X-organ sinus gland complex, which is located in the eyestalk, and is regulated by several neuromodulators, e.g., catecholamines (Liu et al., 2008; Aparicio-Simón et al., 2010). DA’s hyperglycemic effects involve CHH (Webster et al., 2012). A hyperglycemic response is also elicited by NE and E and to NE and E also elicit a hyperglycemic response; however, this effect is not dependent on the eyestalk, suggesting that this effect is not mediated by CHH or is mediated by non-eyestalk produced CHH (Si et al., 2019). A significant increase in CHH levels in the haemolymph in response to cold stress have been reported in several crustaceans, including the L. vannamei (Lago-Lestón et al., 2007) and the freshwater crayfish, Cherax quadricarinatus (Prymaczok et al., 2016).
Secondary Responses – Changes in Metabolism, the Immune System, and Osmoregulation
Low temperature is closely related to the immune and antioxidant system of shrimps and crabs (see Table 1), and is the most important stress factor in aquaculture (Xu et al., 2019). Low temperature not only causes a disorder of free radical metabolism, damage the normal physiological function and immune defense ability of cells and tissues, and directly affects the metabolism of aquatic animals, but also affect dissolved oxygen and other environmental factors, thus leading to the susceptibility of shrimps and crabs to pathogens.
Effects of Low Temperature on Metabolism
Temperature can directly affect the respiration and energy metabolism of crustaceans. Under low temperature stress, on the one hand, energy consumption increases. On the other hand, neurohormone secretion and digestive enzyme activity decrease, and energy metabolism-related enzyme activity and metabolic modes are altered, resulting in crustacean metabolic disorder (Anestis et al., 2008). Low temperatures are believed to have widespread effects on marine organisms’ behavioral and physical traits, including their metabolism. Generally, crustaceans lack efficient regulators, making them sensitive to reduced temperatures.
In shrimp and crab, proteins are the primary energy source (Cuzon et al., 2010). In cold-adapted L. vannamei, fat absorption and digestion, and the protein pathways were enhanced significantly (He et al., 2018). Similarly, under cold stress (23°C), plasma lipids (especially total cholesterol and triglycerides) and total proteins increased significantly; there were no significant changes in glucose levels (Wu et al., 2020). Therefore, it was speculated that in crustaceans under acute cold-stress, lipids and proteins are the main energy sources (Wang et al., 2019). A metabolic study of the black tiger shrimp (Penaeus monodon) cultured under low temperature revealed that its amino acid and trehalose contents increased significantly (Jiang et al., 2019). Under low temperature stress, in addition to the fatty acid composition of tissues and cells, the content of free amino acids (FAA) in tissues also changed. The content of total FAA increased in spring and autumn, but decreased rapidly in winter. As an important nutrient in the body, protein may be automatically decomposed into amino acids under low temperature stimulation. On the one hand, amino acids are used for protein synthesis and turnover, and on the other hand, they might have anti-stress functions. To improve the metabolic rate and oxygen carrying capacity of the body, or to meet the needs of protein synthesis, the structure and synthesis rate of hemocyanin in shrimp and crab will change significantly in response to stress.
The fatty acid metabolism of crustaceans is sensitive to temperature. Cold temperature mainly affects membrane fluidity by affecting the saturation of fatty acids in the cell membrane. Membrane fatty acid desaturation is considered an important mechanism by which crustaceans adapt to low temperature, and is crucial to maintain membrane fluidity, enzyme activity, and normal cell function (Pruitt, 1990; Suprayudi et al., 2004). Cold stress leads to a change in the fatty acid (FA) composition in crustacean cells, which usually leads to the decrease in the saturated fatty acid (SFA) ratio and a rapid increase in the unsaturated fatty acid (UFA) ratio, which is conducive to the maintenance of cell membrane fluidity (Azra et al., 2020a, b). In Scylla serrata, Cancer pagurus, and Carcinus maenas, the SFA content decreased significantly at low temperature (Cuculescu et al., 1995; Wang et al., 2007). In the crayfish cultured at low temperatures, the haemolymph cholesterol and triglyceride contents were reduced significantly, suggesting that under cold stress, these two substances are consumed to release energy (Wu et al., 2020). UFAs are important components of cellular membranes and participate in energy metabolism (Nemeth et al., 2014). Under cold stress, UFA levels increased in L. vannamei (Fan et al., 2019), the Chinese fleshy shrimp (Fenneropenaeus chinensis) (Meng et al., 2019), and the kuruma shrimp (Marsupenaeus japonicas) (Ren et al., 2020). Desaturase enzymes play an important role in the synthesis of unsaturated fatty acids. In C. quadricarinatus low temperature treatment increased Δ6 desaturase mRNA expression and enzyme activity with decreasing water temperature (Wu et al., 2018). However, the mechanisms for the induction of Δ6 desaturases at low temperature remain unclear.
As an important energy source, sugar plays a vital role in the low temperature stress of shrimp and crab. A decrease in temperature led to an increased blood glucose content and a decreased glycogen content in M. rosenbergii, S. serrata, Pachygrapus crassipesran Dall, Paranephrops planfrons, and L. vannamei (Hsieh et al., 2006; Kong et al., 2008; Valle et al., 2009; Zhou et al., 2011). This change in sugar levels in shrimp and crab is an adaptation to low temperature. During cold stress, glucose is consumed as a fast energy source, and the hepatopancreas continuously decomposes glycogen to meet the needs of maintaining the metabolic energy supply. When the temperature rises, or the crustacean adapts to low temperature, the haemolymph glucose level will gradually recover.
Effects of Low Temperature on Immune System
The crustacean immune system mainly functions via innate immune mechanisms comprising humoral and cellular responses. Cellular innate immunity comprises all hemocyte-mediated reactions (e.g., phagocytosis, nodule formation, and encapsulation). Humoral innate immunity comprises mainly lysozyme, phosphatases, antimicrobial peptides (AMPs), protease inhibitors, agglutinins, and the prophenoloxidase-activating system (Kenneth and Lage, 1992; Kulkarni et al., 2020). In the humoral response, AMPs, lysozyme, or phenoloxidase (PO) concentrations increase markedly under stress conditions, e.g., invasive pathogens, disease outbreaks, and environmental hazards. Hemocytes comprise the major component of the crustacean cellular immune system, and their levels will change according to the condition of the organism and the environment (Wang and Chen, 2006). Thus, stress-induced immune system activity is conveniently assessed using the total haemocyte count (THC) (Xu et al., 2019). Fan et al. (2013) found that the THC in L. vannamei was reduced when the temperature decreased from 28 to 13°C. These results indicated that the THC of crustaceans is closely related to temperature. The lower the temperature, the lower the enzyme activity and the lower the THC. In lobsters, the hemocyte phagocytic activity was affected negatively by low temperature (Steenbergen et al., 1978). The evolutionarily conserved cellular process of autophagy involves maintaining homeostasis by recycling damaged or excess cellular components (e.g., misfolded proteins, intracellular pathogens, damaged organelles, and damaged DNA) (Bolliet et al., 2017). In L. vannamei, autophagy is associated with low temperatures (Liang et al., 2020).
In invertebrates, the important innate immune response mechanism, melanization, functions via the prophenoloxidase (proPO)-activating system and is catalyzed by PO (Amparyup et al., 2013). In shrimp, melanization has been suggested to be an antiviral response (Zhao et al., 2020). Meanwhile, PO functions in cellular defense in association with phagocytosis-enhancing factors; therefore, PO is used frequently to assess the effect of environmental stress on the invertebrate immune system (Ellis et al., 2011). In brown shrimp (Penaeus californiensis) exposed to increasing temperature (18−32°C), the hemocyte proPO system activity decreased at 32°C (Vargas-Albores et al., 2008). In the crab (Carcinus aestuarii), when incubated at 4°C, the PO activity in cell-free haemolymph was significantly higher than that in the control crabs incubated at 17°C (p < 0.05) (Matozzo et al., 2011).
In addition, immune parameters, such as antibacterial activity, are suppressed by low temperature. Taken together, these previous studies show that low temperature has important effects on shrimp disease tolerance and survival. However, to date, there have been few studies investigating the immune regulatory mechanisms in shrimp exposed to low temperature. Lysozyme (LSZ), as a kind of hydrolase, is the basis of phagocyte sterilization, existing widely in different tissues, body fluids, and secretions of various organisms, and can be used to measure the non-specific immune capacity of organisms (Mock and Peters, 1990). Low temperature can affect the activity of LSZ. Ding et al. (2010) reported that temperature change could inhibit the LSZ activity of S. serrata. In the red claw crayfish, LSZ was inhibited significantly following low temperature exposure (Wu et al., 2019). Hemocyanins are extracellular negatively charged proteins that are involved in numerous physiological functions, such as protein storage, osmoregulation, oxygen transport, and enzyme activities (Ishwarya et al., 2018; Coates and Costa-Paiva, 2020). In the crayfish P. clarkii and P. zonangulus, the acclimation temperature directly affected the hemocyanin binding affinity (Powell and Watts, 2006). Thus, it is believed that shrimp are more susceptible to pathogens under low temperature conditions.
Effects of Low Temperature on the Antioxidant System
In healthy organisms, the production and elimination of free radicals are in a dynamic balance; however, in adversity, stress will induce a reaction from the enzyme systems and non-enzyme systems of mitochondria, microsomes, and the cytoplasm, resulting in the production of excess reactive oxygen species (ROS) and oxygen free radicals, thus breaking the balance of reactive oxygen metabolism (Wade et al., 2017). In cells and tissues, oxidative stress’s effects on cellular damage can be indicated by the level of lipid peroxidation (Mensah et al., 2012). To reduce oxidative stress and repair damaged cells, the primary defense response comprises the production of enzymatic and non-enzymatic antioxidants to scavenge ROS and free radicals (El-Gendy et al., 2010). In all organisms, the main antioxidative enzymes that detoxify ROS are glutathione S-transferase (GST), glutathione reductase (GR), catalase (CAT), glutathione peroxidase (GPx), and superoxide dismutase (SOD), in addition to the non-enzymatic antioxidant molecule, reduced glutathione (GSH) (Lesser, 2006; Zheng et al., 2019).
In mud crabs subjected to cold stress, the CAT, SOD, and GPX activities increased over 2 h, and then decreased gradually; the content of malondialdehyde (MDA) also increased gradually under cold stress (Kong et al., 2007). In S. paramamosain acclimated at 5, 10, 15, and 27°C (control group), the SOD, CAT, and GPx activities, and the MDA content decreased gradually with lowering temperatures and were significantly reduced at 5 and 10°C compared with those in crabs incubated at 27°C (Kong et al., 2012). Qiu et al. (2011) evaluated the physiological effects of continuous temperature decrease on L. vannamei. The MDA level increased when water temperature decreased from 23 to 12°C.
It has become clear that organisms share a common adaptation mechanism, termed the heat shock response (HSR), to cope with temperature-induced stress, which results in a dramatic change in gene expression patterns and leads to the elevated synthesis of a range of molecular chaperones and the induction of other cell-protective pathways (Richter et al., 2010). The heat shock protein (HSP) and heat shock factor (HSF)- mediated regulation pathways play crucial roles in the HSR, and have been studied intensively in terms of HSR mechanisms and the cold-tolerance of organisms (Gbotsyo et al., 2020).
HSPs are regulated by heat shock elements (HSEs), HSFs, and other factors to control their cellular levels (Morimoto and Santoro, 1998). HSF1 is an important transcription factor that regulates the heat shock response, and is expressed widely in eukaryotes, playing an important role in maintaining intracellular homeostasis during heat stress (Anckar and Sistonen, 2011). When the body is subjected to cold stress, it combines with HSE. In addition, HSPs are conserved at the evolutionary level. In a study of high temperature stress of Penaeus monodon, PmHSF1 expression was elevated. The expression levels of HSPs and other heat tolerance related genes in P. monodon changed significantly after the PmHSF1 gene was knocked down (Sornchuer et al., 2018). In M. japonicas, MjHSF1 transcription was upregulated under heat stress (Zheng et al., 2020). To date, most of the studies on the related functions of HSF1 have focused on the interaction between HSF1 and HSPs, and there are few studies on the expression of immune related factors associated with HSF1. Several HSP genes are downstream targets of HSF1, which are involved in crustacean resistance to adverse environments.
HSPs comprise molecular chaperones that are produced during the exposure to, and recovery from environmental or physiological stress, including cold stress (Johnston et al., 2018). HSPs, also referred to as molecular chaperones or stress proteins, comprise a group of highly conserved proteins that are present ubiquitously in both prokaryotic and eukaryotic organisms (Roberts et al., 2010). HSPs protect cellular functions and structures and from the effects of stress and have important functions in the maintenance of cellular homeostasis (Morimoto and Santoro, 1998). Based on their molecular weight, HSPs are generally classified into five families, HSP100, HSP90, HSP70, HSP60, and small HSPs (Ahn and Im, 2020). In F. chinensis, the levels of FcHSP90 mRNA were induced sensitively in response to heat shock (from 25 to 35°C), reaching a maximum level after 6 h of heat shock (Li et al., 2009). In other crustaceans (S. serrata and L. vannamei) mRNA levels of HSP40, HSP70, or HSP90 were increased in response to cold or heat shock (Fu et al., 2013; Chen et al., 2018; Sung et al., 2018; Fan et al., 2019).
Apoptosis, a cell death process, has a crucial function in maintaining tissue hemostasis and disease protection. As a component of inflammatory reactions, the physiological function of apoptosis helps to remove damaged or harmful cells from immune tissues (Johnstone et al., 2002). Li et al. (2014) evaluated the effect of continuous temperature decrease on hemocyte apoptosis of L. vannamei, which showed an increase in the apoptotic cell ratio and a decrease in caspase-3 activity when the water temperature was reduced from 27 to 17°C. Cold temperature led to increase caspase-3 expression in the swimming crab (Portunus trituberculatus) (Meng et al., 2014). A previous study from our group demonstrated that in M. japonicus, the expression of p53 increased significantly under cold stress, which suggested that cold-induced apoptosis might involve p53 (Ren et al., 2020). Significant changes in p53 signaling pathways under cold stress were also observed in the hepatopancreas of the red claw crayfish under cold stress (Wu et al., 2019).
Low Temperature’s Effects on Osmoregulation
During cold acclimation (or low temperature adaptation), shrimps and crabs change the composition and concentration of intracellular ions by regulating the number and distribution of various ion channels on the cell membrane and changing the composition and concentration of intracellular ions to maintain normal physiological activities (Masroor et al., 2018). On the gill cell membrane of S. serrata, four kinds of adenosine triphosphatases (Ca2+/Mg2+-ATPase, Ca2+-ATPase, Mg2+-ATPase, and Na+/K+-ATPase), which are involved in ion uptake and osmotic pressure regulation, were upregulated during the process of adaptation to a lower temperature (Kong et al., 2012). In the hepatopancreas of M. nipponense, the Na+-K+ ATPase activity in the temperature range 16−22°C was enhanced by 1.38-fold compared with that in the temperature range 25−32°C (Wang et al., 2006). In Procambarus clarkia, exposure from room temperature (23°C) to 4°C for 28 days resulted in a significant increase in Ca2+-ATPase activity (Gao et al., 2009). Thus, in a cold environment, shrimps and crabs can reduce heat loss by adjusting the ionic concentration and osmotic pressure of their body fluid to reduce the difference between their body temperature and that of the outside water.
Level Three – Changes in Behavioral and Growth Responses
Temperature is a basic environmental factor that limits species distribution, affecting individual growth and determining the reproductive cycle. How shrimps and crabs adapt to temperature change and maintain a steady state of life process is a long-term scientific problem. Low temperature has adverse effects on the growth and development of organisms (Shields, 2019). The temperature adaptation range of an organism is an important character in aquaculture. Improving tolerance to temperature stress is a challenging problem in aquaculture breeding. In the rock crab (Cancer irroratus), progressive temperature increase caused their heart rate to increase between 12 and 26°C, peaking at 153 ± 27 beats min–1 at 26°C (Frederich et al., 2009). The molting and reproduction of crustaceans are also affected by temperature. The lower the taxonomic position of the organism, the more susceptible it is to temperature. Therefore, to regulate the reproductive physiology of crustaceans, water temperature is an important factor.
Effect of Temperature on Shrimp and Crab Embryonic Development
The embryonic development of crustaceans is a dynamic physiological process. In addition to the influence of the parents, the external environmental conditions also have an important impact on embryonic development. In particular, the temperature not only affects the time of embryonic development, but also affects the quality and speed of embryo development (Yamamoto et al., 2017). Studies have shown that only when the temperature of organisms is above zero can they begin to develop and grow (Hartnoll and Abele, 1982). The biological zero of embryonic development of Exopalaemon carinicauda, S. serrata, and P. clarkii are 12.18°C, 11.70°C, and 5.60°C, respectively (Wu, 1991; Lv et al., 2004; Liang et al., 2013).
In the suitable temperature range, the higher the temperature, the faster the embryo develops. Wang et al. (1998) found that the embryonic development time of Thenus orientalis was shortened from 43 to 21 days with an increase in temperature from 22 to 31°C. Liang et al. (2013) found that in the ridgetail white prawn E. carinicauda, the incubation time of embryos shortened with the increase in temperature when the temperature was between 18 and 28°C. Cooler water retards growth and delays maturity, causing crabs to begin maturation when they are at larger sizes (Azra et al., 2020a).
Gonadal Development of Crustaceans in Response to Temperature
During evolution, crustaceans have formed a relatively perfect reproductive regulation system, involving neuropeptides, hormones, neurotransmitters, and other hormones (Nguyen et al., 2016). The levels of these hormone are adjusted with the changes in temperature, salinity, and other environmental factors, such that crustaceans can reproduce under the best environmental conditions. Among them, temperature is involved in gonadal maturation by regulating hormone synthesis and secretion (Qian et al., 2015).
Xu et al. (2008) studied P. clarkii and found that an increase in water temperature from 22 to 28°C could promote gonadal maturation. Carmona-Osalde et al. (2004) found that in the range of 16–25°C, the ovary development of P. llamasir could be promoted by increasing the temperature. When the water temperature was between 15 and 25°C, the egg holding rate of S. serrata increased as the temperature increased (Yao et al., 2005). These studies confirmed that the water temperature is a major factor that influences crustacean gonadal development. In a certain temperature range, the higher the water temperature, the better the quality of gonadal development of crustaceans.
Effects of Low Temperature on Behavior and Growth of Crustaceans
Behavioral modifications comprise changes in microhabitat use, abundance and distribution, feeding, predation, migration and spawning behaviors. In crustaceans grown under low temperatures, decreased activity and a decrease or cessation of feeding are the most frequently observed in behavior (Matheson and Gagnon, 2012). Fighting behavior increases the heart rate and metabolic rate of animals, and has a certain impact on their ability to withstand high temperature (Wang et al., 2020). Crustaceans are intolerant to low temperature and lack the ability to regulate their body temperature. In a low temperature environment of 9°C, the body of L. vannamei lost its balance and was slow to respond to external stimuli. Temperature has more complicated effects on locomotor activities (e.g., swimming or walking), which form part of the normal behavior of an animal, and are thus controlled by the central nervous system (Lagerspetz and Vainio, 2006).
Temperature is a growth limiting factor for all living things, but especially for aquatic organisms (Lushchak, 2011). All shrimps and crabs have a temperature tolerance range (see Table 2). When the water temperature exceeds the regulatory capacity of shrimp and crab, low temperatures will slow down their growth rate and even cause death. Temperature optima can be defined as the temperature at which shrimp grow fastest and most efficiently (González et al., 2010). The tolerance of different crustaceans to temperature is shown in Table 1. At low temperatures, shrimp and crabs need more energy to cope with stress, resulting in a significant reduction of reserves used for the growth process. Studies have shown that temperature is closely related to the growth of L. vannamei (Wyban et al., 1995) P. monodon (Deering et al., 1995), and Macrobrachium nipponense (Wang et al., 2006).
Temperature can affect the growth of crustaceans by altering two factors, the molt increment (the increase in duration between successive molts) and the intermolt period (the time interval between successive molts). Increasing temperature usually decreases the intermolt period; however, its effect on the molt increment is unknown. In early juvenile mud crabs, S. paramamosain, temperature-induced autotomy influenced the molting of early juvenile mud crabs, and changes in the levels of mRNA encoding the ecdysone receptor (EcR) seemed to play an important regulatory role in the molting process (Gong et al., 2015). Juvenile dungeness crabs (Metacarcinus magister) at different stages of molting (12, 19, or 26 days post-molting) were moved from ambient temperature (15°C) to temperatures of 5°C and 20°C for 14 days. From 5 to 20°C, survival ranged from 97 to 100% Molt stage progression increased from 5 to 15°C, but not at 20°C (Wittmann et al., 2018). L. vannamei incubated at 13°C showed significant reductions in swimming and feeding behaviors, and more deaths were observed at this temperature (Huang et al., 2017).
Perspectives
Climate change is causing alterations to oceans, rivers, and lakes; therefore, it is vital to determine the mechanism by which crustaceans tolerate low temperatures, to gain a deeper understanding of the effects environmental fluctuation on biology. This will allow us to implement the required measures to conserve aquatic organisms. However, we lack sufficient detail of the biological responses of crustaceans to low temperatures. To gather these data, it is important to study the expression and functions of genes and proteins that are influenced by temperature changes. The temperature adaptation range of an organism is an important agricultural character of an aquaculture variety. Improving the tolerance to low temperature stress is an important issue in aquaculture breeding. However, it is precisely because of the wide range of physiological effects of low temperature that involves many genes, which important genes determine the temperature tolerance is obviously a question that needs to be answered first. With the completion of the whole genome sequencing of shrimp and crab (Zhang et al., 2019; Tang et al., 2020; Jin et al., 2021; Yuan et al., 2021; Zhao et al., 2021), it has become an important research method to mine the key regulatory genes from the temperature responsive gene regulatory network.
Conclusion
Short- and in long-term temperature fluctuation has become a major stress factor responsible for altering the distribution patterns of marine crustaceans. The accumulated literature shows that the physiological parameters of crustaceans are influenced significantly by temperature changes. To adapt to environmental temperature alteration, crustaceans must invoke endocrine responses, changes in their metabolic rate, immune responses, and antioxidant responses. Despite having a good general grasp of the effects of temperature on crustaceans’ responses, there are still gaps in our knowledge. However, obtaining a complete understanding of crustaceans’ temperature adaptation mechanisms will permit us to predict future changes and will augment our knowledge of their physiological and ecological requirements. Current research provides a basis for future studies of the responses of crustaceans to low temperatures. The specific FAA metabolism pathways and ROS signal transduction pathways that are triggered in response to low temperature variation should be investigated in the future.
Author Contributions
XR, QW, and JL conceived the idea, performed the literature search, and wrote the manuscript, with input and suggestions from the other authors. All authors contributed to the article and approved the submitted version.
Funding
This research was supported by the project of National Key R&D program of China (grant number 2019YFD0900403), the Projects of the agricultural improved variety project in Shandong Province-Breeding of breakthrough new prawn varieties with high quality and resistance (grant number 2019LZGC014), the basic scientific research fund of Yellow Sea Fisheries Research Institute of Chinese Academy of Fishery Sciences (20603022018024) and basic scientific research business expenses of Chinese Academy of Fishery Sciences of “Innovation Team Project of Ecological Aquaculture in Seawater Pond” (2020td46).
Conflict of Interest
The authors declare that the research was conducted in the absence of any commercial or financial relationships that could be construed as a potential conflict of interest.
Publisher’s Note
All claims expressed in this article are solely those of the authors and do not necessarily represent those of their affiliated organizations, or those of the publisher, the editors and the reviewers. Any product that may be evaluated in this article, or claim that may be made by its manufacturer, is not guaranteed or endorsed by the publisher.
Acknowledgments
The authors thank the contribution of several reviewers of earlier versions, who have greatly improved the quality of this manuscript.
References
Adamski, Z., Bufo, S. A., Chowański, S., Falabella, P., Lubawy, J., Marciniak, P., et al. (2019). Beetles as model organisms in physiological, biomedical and environmental studies -a review. Front. Physiol. 10:319. doi: 10.3389/fphys.2019.00319
Ahn, Y. J., and Im, E. (2020). Heterologous expression of heat shock proteins confers stress tolerance in Escherichia coli, an industrial cell factory: a short review. Biocatal. Agric. Biotechnol. 29:101833.
Amparyup, P., Charoensapsri, W., and Tassanakajon, A. (2013). Prophenoloxidase system and its role in shrimp immune responses against major pathogens. Fish Shellfish Immunol. 34, 990–1001. doi: 10.1016/j.fsi.2012.08.019
Anckar, J., and Sistonen, L. (2011). Regulation of HSF1 function in the heat stress response: implications in aging and disease. Annu. Rev. Biochem. 80, 1089–1115. doi: 10.1146/annurev-biochem-060809-095203
Anestis, A., Pörtner, H. O., Lazou, A., and Michaelidis, B. (2008). Metabolic and molecular stress responses of sublittoral bearded horse mussel Modiolus barbatus to warming sea water: implications for vertical zonation. J. Exp. Biol. 211, 2889–2898. doi: 10.1242/jeb.016782
Aparicio-Simón, B., Piñón, M., Racotta, R., and Racotta, I. S. (2010). Neuroendocrine and metabolic responses of Pacific whiteleg shrimp Litopenaeus vannamei exposed to acute handling stress. Aquaculture 298, 308–314.
Azra, M. N., Aaqillah-Amr, M. A., Ikhwanuddin, M., Ma, H. Y., Waiho, K., Ostrensky, A., et al. (2020a). Effects of climate-induced water temperature changes on the life history of brachyuran crabs. Rev. Aquacult. 12, 1211–1216.
Azra, M. N., Chen, J. C., Ikhwanuddin, M., and Abol-Munafi, A. B. (2018). Thermal tolerance and locomotor activity of blue swimmer crab Portunus pelagicus instar reared at different temperatures. J. Therm. Biol. 74, 234–240. doi: 10.1016/j.jtherbio.2018.04.002
Azra, M. N., Tavares, C. P. D. S., Abol-Munafi, A. B., and Ikhwanuddin, M. (2020b). Growth rate and fatty acid composition of orange mud crab instars, Scylla olivacea, reared at different temperatures. Egypt. J. Aquat. Res. 46, 97–102.
Baylon, J., and Suzuki, H. (2007). Effects of changes in salinity and temperature on survival and development of larvae and juveniles of the crucifix crab Charybdis feriatus (Crustacea: Decapoda: Portunidae). Aquaculture 269, 390–401. doi: 10.1016/j.aquaculture.2007.03.024
Bolliet, V., Labonne, J., Olazcuaga, L., Panserat, S., and Seiliez, I. (2017). Modeling of autophagy-related gene expression dynamics during long term fasting in European eel (Anguilla anguilla). Sci. Rep. 7:17896. doi: 10.1038/s41598-017-18164-6
Carmona-Osalde, C., Rodriguez-Serna, M., Olvera-Novoa, M. A., and Gutierrez-Yurrita, P. J. (2004). Gonadal development, spawning, growth and survival of the crayfish Procambarus llamasi at three different water temperatures. Aquaculture 232, 305–316. doi: 10.1016/S0044-8486(03)00527-1
Chang, C. C., Jiang, J. R., and Cheng, W. T. (2015). A first insight into temperature stress-induced neuroendocrine and immunological changes in giant freshwater prawn, Macrobrachium rosenbergii. Fish Shellfish Immunol. 47, 528–534. doi: 10.1016/j.fsi.2015.09.041
Chang, C. C., Yeh, M. M., and Cheng, W. T. (2009). Cold shock-induced norepinephrine triggers apoptosis of haemocytes via caspase-3 in the white shrimp, Litopenaeus vannamei. Fish Shellfish Immunol. 6, 695–700.
Chen, H. Y., Toullec, J. Y., and Lee, C. Y. (2020). The crustacean hyperglycemic hormone superfamily: progress made in the past decade. Front. Endocrinol. 11:578958. doi: 10.3389/fendo.2020.578958
Chen, J. C., Lin, M. N., Ting, Y. Y., and Lin, J. N. (1995). Survival, haemolymph osmolality and tissue water of Penaeus chinensis juveniles acclimated to different salinity and temperature levels. Comp. Biochem. Phys. A 110, 253–258. doi: 10.1016/0300-9629(94)00164-O
Chen, R. B., Xiao, M. M., Buchberger, A., and Li, L. J. (2014). Quantitative neuropeptidomics study of the effects of temperature change in the crab Cancer borealis. J. Proteome Res. 13, 5767–5776. doi: 10.1021/pr500742q
Chen, T., Lin, T., Li, H., Lu, T., Li, J., Huang, W., et al. (2018). Heat shock protein 40 (HSP40) in Pacific white shrimp (Litopenaeus vannamei): molecular cloning, tissue distribution and ontogeny, response to temperature, acidity/alkalinity and salinity stresses, and potential role in ovarian development. Front. Physiol. 9:1784. doi: 10.3389/fphys.2018.01784
Cheng, W., and Chen, J. C. (2000). Effects of pH, temperature and salinity on immune parameters of the freshwater prawn Macrobrachium rosenbergii. Fish Shellfish Immunol. 10, 387–391. doi: 10.1006/fsim.2000.0264
Coates, C. J., and Costa-Paiva, E. M. (2020). “Multifunctional roles of hemocyanins,” in Vertebrate and Invertebrate Respiratory Proteins, Lipoproteins and other Body Fluid Proteins, eds U. Hoeger and J. R. Harris (Berlin: Springer), 233–250.
Costa, J. R., Dalosto, M. M., Palaoro, A. V., and Santos, S. (2016). Contest duration and dynamics are affected by body size in a potentially subsocial crayfish (Crustacea: decapoda). Ethology 122, 502–512. doi: 10.1111/eth.12496
Cuculescu, M., Hyde, D., and Bowler, K. (1995). Temperature acclimation of marine crabs, changes in plasma membrane fluidity and lipid composition. J. Therm. Biol. 20, 207–222. doi: 10.1016/0306-4565(94)00058-Q
Cuzon, G., Cahu, C., Aldrin, J. F., Messager, J. L., Stephan, G., and Mevel, M. (2010). Starvation effect on metabolism of Penaeus japonicus. J. World Aquacult. Soc. 11, 410–423.
de Souza, D. M., Borges, V. D., Furtado, P., Romano, L. A., Wasielesky, J. W., Monserrat, J. M., et al. (2016). Antioxidant enzyme activities and immunological system analysis of Litopenaeus vannamei reared in biofloc technology (BFT) at different water temperatures. Aquaculture 451, 436–443.
Deering, M. J., Fielder, D. R., and Hewitt, D. R. (1995). Effects of temperature on growth and protein assimilation in juvenile leader prawns Penaeus monodon. J. World Aquacult. Soc. 26, 465–468.
Ding, X. F., Yang, Y. J., Jin, S., and Wang, G. L. (2010). The stress effects of temperature fluctuation on immune factors in crab Scylla serrata. Fish. Sci. 29, 1–6.
Donaldson, M. R., Cooke, S. J., Patterson, D. A., and Macdonald, J. S. (2008). Cold shock and fish. J. Fish Biol. 73, 1491–1530.
Dong, H. B., Mao, Y., Duan, Y. F., Su, Y. Q., Wang, J., and Zhang, J. S. (2020). Physiological and molecular differences in the thermal tolerance of two varieties of kuruma prawn Marsupenaeus japonicus: critical thermal maximum and heat shock protein 70. Fish. Sci. 86, 163–169. doi: 10.1007/s12562-019-01383-3
El-Gendy, K. S., Aly, N. M., Mahmoud, F. H., Kenawy, A., and El-Sebae, A. K. H. (2010). The role of vitamin C as antioxidant in protection of oxidative stress induced by imidacloprid. Food Chem. Toxicol. 48, 215–221. doi: 10.1016/j.fct.2009.10.003
Ellis, R. P., Parry, H., Spicer, J. I., Hutchinson, T. H., Pipe, R. K., and Widdicombe, S. (2011). Immunological function in marine invertebrates: responses to environmental perturbation. Fish Shellfish Immunol. 30, 1209–1222.
Fan, L. F., Wang, A. L., and Wu, Y. X. (2013). Comparative proteomic identification of the hemocyte response to cold stress in white shrimp, Litopenaeus vannamei. J. Proteomics 80, 196–206. doi: 10.1016/j.jprot.2012.12.017
Fan, L. F., Wang, L., and Wang, Z. L. (2019). Proteomic characterization of the hepatopancreas in the Pacific white shrimp Litopenaeus vannamei under cold stress: revealing the organism homeostasis mechanism. Fish Shellfish Immunol. 92, 1209–1222. doi: 10.1016/j.fsi.2019.06.037
Farooqui, T. (2012). Review of octopamine in insect nervous systems. Open Access Insect Physiol. 4, 1–17. doi: 10.2147/OAIP.S20911
Frederich, M., O’Rourke, M. R., Furey, N. B., and Jost, J. A. (2009). AMP-activated protein kinase (AMPK) in the rock crab, Cancer irroratus: an early indicator of temperature stress. J. Exp. Biol. 212, 722–730.
Fu, W., Zhang, F., Liao, M., Liu, M., Zheng, B., Yang, H., et al. (2013). Molecular cloning and expression analysis of a cytosolic heat shock protein 70 gene from mud crab Scylla serrata. Fish Shellfish Immunol. 34, 1306–1314.
Gao, Y. P., Gillen, C. M., Whalen, D. R., Francieli Vigo, F. M., Golshani, A. E., and Wheatlya, M. G. (2009). Expression of genes encoding Ca2+ exporting proteins in freshwater crayfish Procambarus clarkii during cold exposure. J. Therm. Biol. 34, 144–151. doi: 10.1016/j.jtherbio.2009.01.002
Gbotsyo, Y. A., Rowarth, N. M., Weir, L. K., and MacRae, T. H. (2020). Short-term cold stress and heat shock proteins in the crustacean Artemia franciscana. Cell Stress Chaperon 25, 1083–1097. doi: 10.1007/s12192-020-01147-4
Gong, J., Yu, K., Shu, L., Ye, H. H., Li, S. J., and Zeng, C. S. (2015). Evaluating the effects of temperature, salinity, starvation and autotomy on molting success, molting interval and expression of ecdysone receptor in early juvenile mud crabs, Scylla paramamosain. J. Exp. Marine Biol. Ecol. 464, 11–17.
González, R. A., Díaz, F., Licea, A., Re, A. D., Sánchez, L. N., and García-Esquivel, Z. (2010). Thermal preference, tolerance and oxygen consumption of adult white shrimp Litopenaeus vannamei (Boone) exposed to different acclimation temperatures. J. Therm. Biol. 35, 218–224. doi: 10.1016/j.jtherbio.2010.05.004
Hamilton, J. L., Edwards, C. R., Holt, S. R., and Worden, M. K. (2007). Temperature dependent modulation of lobster neuromuscular properties by serotonin. J. Exp. Biol. 210, 1025–1035. doi: 10.1242/jeb.02717
Hartnoll, R. G., and Abele, L. G. (1982). The biology of Crustacea. Embryol. Morphol. Genet. 2, 111–196.
Haubrock, P. J., Oficialdegui, F. J., Zeng, Y. W., Patoka, J., Yeo, D. C. J., and Kouba, A. (2021). The redclaw crayfish: a prominent aquaculture species with invasive potential in tropical and subtropical biodiversity hotspots. Rev. Aquacult. 13, 1488–1530.
He, P., Wei, P., Zhang, B., Zhao, Y., Li, Q., Chen, X., et al. (2018). Identification of microRNAs involved in cold adaptation of Litopenaeus vannamei by high-throughput sequencing. Gene 677, 24–31. doi: 10.1016/j.gene.2018.07.042
Hennig, O. L., and Andreatta, E. R. (1998). Effect of temperature in an intensive nursery system for Penaeus paulensis (Pérez Farfante, 1967). Aquaculture 164, 167–172. doi: 10.1016/S0044-8486(98)00184-7
Hsieh, S. L., Chen, S. M., Yang, Y. H., and Kuo, C. M. (2006). Involvement of norepinephrine in the hyperglycemic responses of the freshwater giant prawn, Macrobrachium rosenbergii, under cold shock. Comp. Biochem. Phys. A 143, 254–263. doi: 10.1016/j.cbpa.2005.12.009
Huang, H., Huang, C., Guo, L., Zeng, C., and Ye, H. (2019). Profiles of calreticulin and Ca2+ concentration under low temperature and salinity stress in the mud crab, Scylla paramamosain. PLos One 14:e0220405. https://doi.org/10.1371/journal.pone.0220405
Huang, W., Ren, C., Li, H., Huo, D., Wang, Y., Jiang, X., et al. (2017). Transcriptomic analyses on muscle tissues of Litopenaeus vannamei provide the first profile insight into the response to low temperature stress. PLoS One 12:e178604. doi: 10.1371/journal.pone.0178604
Ishwarya, R., Vaseeharan, B., Jayakumar, J., Ramasubramanian, V., Govindarajan, M., Alharbi, N. S., et al. (2018). Bio-mining drugs from the sea: high antibiofilm properties of haemocyanin purified from the haemolymph of flower crab Portunus pelagicus (L.) (Decapoda: Portunidae). Aquaculture 489, 130–140.
Jesus, P. P., Martinez-Palacios, C. A., and Ross, L. G. (1997). The effects of salinity and temperature on the growth and survival rates of juvenile white shrimp, Penaeus vannamei, Boone, 1931. Aquaculture 157, 107–115. doi: 10.1016/S0044-8486(97)00148-8
Jiang, S., Zhou, F. L., Yang, Q. B., Huang, J. H., Yang, L. S., and Jiang, S. G. (2019). Impact of temperature stress on oxygen and energy metabolism in the hepatopancreas of the black tiger shrimp, Penaeus monodon (Crustacea: Decapoda: Penaeidae). Pak. J. Zool. 51, 141–148.
Jin, S. B., Bian, C., Jiang, S. F., Han, K., Xiong, Y. W., Zhang, W. Y., et al. (2021). A chromosome-level genome assembly of the oriental river prawn, Macrobrachium nipponense. GigaScience 10:giaa160. doi: 10.1093/gigascience/giaa160
Johnston, C. L., Marzano, N. R., van Oijen, A. M., and Ecroyd, H. (2018). Using single-molecule approaches to understand the molecular mechanisms of heat-shock protein chaperone function. J. Mol. Biol. 430, 4525–4546.
Johnstone, R. W., Ruefli, A. A., and Lowe, S. W. (2002). Apoptosis: a link between cancer genetics and chemotherapy. Cell 108, 153–164.
Kenneth, S., and Lage, C. (1992). Crustacean immunity. Annu. Rev. Fish Dis. 2, 3–23. doi: 10.1016/0959-8030(92)90053-Z
Kong, X. H., Wang, G. Z., and Li, S. J. (2012). Effects of low temperature acclimation on antioxidant defenses and ATPase activities in the muscle of mud crab (Scylla paramamosain). Aquaculture 12, 144–149.
Kong, X. H., Wang, G. Z., and Li, S. L. (2007). Antioxidation and ATPase activity in the gill of mud crab Scylla serrata under cold stress. Chin. J. Oceanol. Limn. 25, 221–226. doi: 10.1007/s00343-007-0221-7
Kong, X. H., Zhan, H. X., Wang, G. Z., Li, S. J., and Pei, D. S. (2008). Seasonal changes of soluble proteins and soluble saccharide in mud crab (Scylla serrata). J. Henan Norm. Univ. 36, 99–102.
Kulkarni, A., Krishnan, S., Anand, D., Kokkattunivarthil Uthaman, S., Otta, S. K., Karunasagar, I., et al. (2020). Immune responses and immunoprotection in crustaceans with special reference to shrimp. Rev. Aquacult. 13, 431–459. doi: 10.1111/raq.12482
Lagerspetz, K. H. Y., and Vainio, L. A. (2006). Thermal behaviour of crustaceans. Biol. Rev. 81, 237–258. doi: 10.1017/S1464793105006998
Lago-Lestón, A., Ponce, E., and Muñoz, M. E. (2007). Cloning and expression of hyperglycemic (CHH) and molt-inhibiting (MIH) hormones mRNAs from the eyestalk of shrimps of Litopenaeus vannamei grown in different temperature and salinity conditions. Aquaculture 270, 343–357.
Le Moullac, G., and Haffner, P. (2000). Environmental factors affecting immune responses in Crustacea. Aquaculture 191, 121–131.
Lesser, M. P. (2006). Oxidative stress in marine environments: biochemistry and physiological ecology. Annu. Rev. Physiol. 68, 253–278.
Li, B., Xian, J. A., Guo, H., Wang, A. L., Miao, Y. T., Ye, J. M., et al. (2014). Effect of temperature decrease on hemocyte apoptosis of the white shrimp Litopenaeus vannamei. Aquacult. Int. 22, 761–774. doi: 10.1007/s10499-013-9704-z
Li, F. H., Luan, W., Zhang, C. S., Zhang, J. Q., Wang, B., and Xie, Y. S. (2009). Cloning of cytoplasmic heat shock protein 90 (FcHSP90) from Fenneropenaeus chinensis and its expression response to heat shock and hypoxia. Cell Stress Chaperon. 14, 161–172. doi: 10.1007/s12192-008-0069-6
Liang, J. P., Li, J., Li, J. T., Liu, P., Zhao, F. Z., Liu, D. Y., et al. (2013). Effects of water temperature on the embryonic development, survival and development period of larvae of ridgetail white prawn (Exopalaemon carinicauda) reared in the laboratory. Acta Ecol. Sin. 33, 1142–1152.
Liang, Q. J., Ou, M. F., Li, Z. H., Ren, Y. H., Wei, W., Qiao, L., et al. (2020). Functional analysis target of rapamycin (TOR) on the Penaeus vannamei in response to acute low temperature stress. Fish Shellfish Immunol. 96, 53–61.
Liu, H. Y., Pan, L. Q., and Zheng, D. B. (2008). Injection of biogenic amines modulates osmoregulation of Litopenaeus vannamei: response of hemolymph osmotic pressure, ion concentration and osmolality effectors. Comp. Biochem. Phys. A 151, 191–197. doi: 10.1016/j.cbpa.2008.06.021
Lushchak, V. I. (2011). Environmentally induced oxidative stress in aquatic animals. Aquat. Toxicol. 101, 13–30. doi: 10.1016/j.aquatox.2010.10.006
Lv, B., Liu, B., Zhou, Q. L., Song, C. Y., Sun, C. X., Zhang, H. M., et al. (2021). Effects of different temperatures and protein levels on growth performance, physiological response and expression of immune-related genes of juvenile oriental river prawn (Macrobrachium nipponense). Aquaculture 536:736435.
Lv, J., Song, S. L., Tang, J. Q., Ge, J. C., and Pan, J. L. (2004). Analysis on temperature factor in hatching of Procambarus clarkia. J. Nanjing Univ. 40, 226–231.
Masroor, W., Farcy, E., Gros, R., and Lorin-Nebel, C. (2018). Effect of combined stress (salinity and temperature) in European sea bass Dicentrarchus labrax osmoregulatory processes. Comp. Biochem. Phys. A 215, 45–54.
Matheson, K., and Gagnon, P. (2012). Temperature mediates non-competitive foraging in indigenous rock (Cancer irroratus Say) and recently introduced green (Carcinus maenas L.) crabs from Newfoundland and Labrador. J. Exp. Mar. Biol. Ecol. 414, 6–18. doi: 10.1016/j.jembe.2012.01.006
Matozzo, V., Gallo, C., and Marin, M. G. (2011). Effects of temperature on cellular and biochemical parameters in the crab Carcinus aestuarii (Crustacea, Decapoda). Mar. Environ. Res. 71, 351–356.
Meng, X. H., Dong, L. J., Shi, X. L., Li, X. P., Sui, J., Luo, K., et al. (2019). Screening of the candidate genes related to low-temperature tolerance of Fenneropenaeus chinensis based on high-throughput transcriptome sequencing. PLoS One 14:e0211182. doi: 10.1371/journal.pone.0211182
Meng, X. L., Liu, P., Li, J., Gao, B. Q., and Chen, P. (2014). Physiological responses of swimming crab Portunus trituberculatus under cold acclimation: antioxidant defense and heat shock proteins. Aquaculture 434, 11–17.
Mensah, P. K., Palmer, C. G., and Muller, W. J. (2012). Lipid peroxidation in the freshwater shrimp Caridina nilotica as a biomarker of Roundup® herbicide pollution of freshwater systems in South Africa. Water Sci. Technol. 65, 1660–1666. doi: 10.2166/wst.2012.060
Mock, A., and Peters, G. (1990). Lysozyme activity in rainbow trout Omorhynchus mykiss (Walbaum), stressed by handling, transport and water pollution. J. Fish Biol. 37, 873–885. doi: 10.1111/j.1095-8649.1990.tb03591.x
Momohara, Y., Minami, H., Kanai, A., and Nagayama, T. (2016). Role of cAMP signalling in winner and loser effects in crayfish agonistic encounters. Eur. J. Neurosci. 44, 1886–1895. doi: 10.1111/ejn.13259
Morimoto, R. I., and Santoro, M. G. (1998). Stress-inducible responses and heat shock proteins: new pharmacologic targets for cytoprotection. Nat. Biotechnol. 16, 833–838. doi: 10.1038/nbt0998-833
Nemeth, M., Millesi, E., Wagner, K. H., and Wallner, B. (2014). Effects of diets high in unsaturated fatty acids on socially induced stress responses in guinea pigs. PLoS One 9:e116292. doi: 10.1371/journal.pone.0116292
Nguyen, T. V., Cummins, S. F., Elizur, A., and Ventura, T. (2016). Transcriptomic characterization and curation of candidate neuropeptides regulating reproduction in the eyestalk ganglia of the Australian crayfish, Cherax quadricarinatus. Sci. Rep. 6, 1–19. doi: 10.1038/srep38658
Northcutt, A. J., Lett, K. M., Garcia, V. B., Diester, C. M., Lane, B. J., Marder, E., et al. (2016). Deep sequencing of transcriptomes from the nervous systems of two decapod crustaceans to characterize genes important for neural circuit function and modulation. BMC Genomics 17:868. doi: 10.1186/s12864-016-3215-z
Pan, L. Q., Hu, F. W., Jing, F. T., and Liu, H. J. (2008). The effect of different acclimation temperatures on the prophenoloxidase system and other defence parameters in Litopenaeus vannamei. Fish Shellfish Immunol. 25, 137–142.
Pang, Y. Y., Song, Y. M., Zhang, L., Song, X. Z., Zhang, C., Lv, J. H., et al. (2019). 5-HT2B, 5-HT7, and DA2 receptors mediate the effects of 5-HT and DA on agonistic behavior of the Chinese mitten crab (Eriocheir sinensis). ACS Chem. Neurosci. 10, 4502–4510. doi: 10.1021/acschemneuro.9b00342
Powell, M. L., and Watts, S. A. (2006). Effect of temperature acclimation on metabolism and hemocyanin binding affinities in two crayfish, Procambarus clarkii and Procambarus zonangulus. Comp. Biochem. Phys. A 144, 211–217.
Pruitt, N. L. (1990). Adaptations to temperature in the cellular membranes of crustacea: membrane structure and metabolism. J. Therm. Biol. 15, 1–8.
Prymaczok, N. C., Pasqualino, V. M., Viaul, V. E., Rodríguez, E. M., and Medesan, D. A. (2016). Involvement of the crustacean hyperglycemic hormone (CHH) in the physiological compensation of the freshwater crayfish Cherax quadricarinatus to low temperature and high salinity stress. J. Comp. Phys. B 186, 181–191. doi: 10.1007/s00360-015-0954-0
Qian, H., Xiong, Y. W., Zhang, W. Y., Fu, H. T., Liang, S. F., Sun, S. M., et al. (2015). Characterization, expression, and function analysis of gonad-inhibiting hormone in Oriental River prawn, Macrobrachium nipponense and its induced expression by temperature. Comp. Biochem. Phys. A 185, 1–8.
Qiu, J., Wang, W. N., Wang, L. J., Liu, Y. F., and Wang, A. L. (2011). Oxidative stress, DNA damage and osmolality in the Pacific white shrimp, Litopenaeus vannamei exposed to acute low temperature stress. Comp. Biochem. Phys. C 154, 36–41.
Qyli, M., Aliko, V., and Faggio, C. (2020). Physiological and biochemical responses of Mediterranean green crab, Carcinus aestuarii, to different environmental stressors: evaluation of hemocyte toxicity and its possible effects on immune response. Comp. Biochem. Phys. C 231:108739. doi: 10.1016/j.cbpc.2020.108739
Ren, X. Y., Yu, Z. X., Xu, Y., Zhang, Y. B., Mu, C. M., Liu, P., et al. (2020). Integrated transcriptomic and metabolomic responses in the hepatopancreas of kuruma shrimp (Marsupenaeus japonicus) under cold stress. Ecotox. Environ. Saf. 206:111360. doi: 10.1016/j.ecoenv.2020.111360
Richter, K., Haslbeck, M., and Buchner, J. (2010). The heat shock response: life on the verge of death. Mol. Cell 40, 253–266. doi: 10.1016/j.molcel.2010.10.006
Roberts, R. J., Agius, C., Saliba, C., Bosier, P. B., and Sung, Y. Y. (2010). Heat shock proteins (chaperones) in fish and shellfish and their potential role in relation to fish health: a review. J. Fish Dis. 33, 789–801.
Saucedo, P. E., Ocampo, L., Monteforte, M., and Bervera, H. (2004). Effect of temperature on oxygen consumption and ammonia excretion in the Calafia mother-of-pearl oyster, Pinctada mazatlanica (Hanley, 1856). Aquaculture 229, 377–387. doi: 10.1016/S0044-8486(03)00327-2
Shields, J. D. (2019). Climate change enhances disease processes in crustaceans: case studies in lobsters, crabs, and shrimps. J. Crustacean Biol. 39, 673–683. doi: 10.1093/jcbiol/ruz072
Si, L. J., Pan, L. Q., Zhang, X., Wang, H. D., and Wei, C. (2019). Evidence that dopamine is involved in neuroendocrine regulation, gill intracellular signalling pathways and ion regulation in Litopenaeus vannamei. J. Exp. Biol. 222:jeb204073. doi: 10.1242/jeb.204073
Sinakevitch, I. T., Wolff, G. H., Pfluger, H. J., and Smith, B. H. (2018). Editorial: biogenic amines and neuromodulation of animal behavior. Front. Syst. Neurosci. 12:31. doi: 10.3389/fnsys.2018.00031
Song, L. S., Li, Y. B., Cai, Z. H., Fang, M., Su, J. G., and Cui, Z. X. (2004). The Immunochemical variation of mitten hand crab Eriocheir Sinensis after the increment of temperature. Oceanol. Limnol. Sin. 35, 74–77.
Sornchuer, P., Junprung, W., Yingsunthonwattana, W., and Tassanakajon, A. (2018). Heat shock factor 1 regulates heat shock proteins and immune-related genes in Penaeus monodon under thermal stress. Dev. Comp. Immunol. 88, 19–27. doi: 10.1016/j.dci.2018.06.013
Steenbergen, J. F., Steenbergen, S. M., and Schapiro, H. C. (1978). Effects of temperature on phagocytosis in Homarus americanus. Aquaculture 14, 23–30. doi: 10.1016/0044-8486(78)90137-0
Sung, Y. Y., Rahman, N. A., Shazili, N., Chen, S., Lv, A., Sun, J., et al. (2018). Non-lethal heat shock induces Hsp70 synthesis and promotes tolerance against heat, ammonia and metals in postlarvae of the white leg shrimp Penaeus vannamei (Boone, s1931). Aquaculture 483, 21–26.
Suprayudi, M. A., Takeuchi, T., and Hamasaki, K. (2004). Essential fatty acids for larval mud crab Scylla serrata: implications of lack of the ability to bioconvert C18 unsaturated fatty acids to highly unsaturated fatty acids. Aquaculture 231, 403–416.
Tang, B. P., Zhang, D. Z., Li, H. R., Jiang, S. H., Zhang, H. B., Xuan, F. J., et al. (2020). Chromosome-level genome assembly reveals the unique genome evolution of the swimming crab (Portunus trituberculatus). GigaScience 9:giz161.
Valle, S. C., Eichler, P., Maciel, J. E., Machado, G., Kucharski, L. C., and Da Silva, R. S. (2009). Seasonal variation in glucose and neutral amino acid uptake in the estuarine crab Neohelice granulata. Comp. Biochem. Physiol. A 153, 252–257. doi: 10.1016/j.cbpa.2009.02.033
Vargas-Albores, F., Baltazar, P. H., Portillo-Clark, G., and Magallon-Barajas, F. (2008). Influence of temperature and salinity on the yellowlegs shrimp, Penaeus californiensis Holmes, prophenoloxidase system. Aquacult. Res. 29, 549–553.
Wade, N. M., Gabaudan, J., and Glencross, B. D. (2017). A review of carotenoid utilisation and function in crustacean aquaculture. Rev. Aquacult. 9, 141–156. doi: 10.1111/raq.12109
Wang, F. I., and Chen, J. C. (2006). The immune response of tiger shrimp Penaeus monodon and its susceptibility to Photobacterium damselae subsp. damselae under temperature stress. Aquaculture 258, 34–41.
Wang, G. Z., Kong, X. H., Wang, K. J., and Li, S. J. (2007). Variation of specific proteins, mitochondria and fatty acid composition in gill of Scylla serrata (Crustacea, Decapoda) under low temperature adaptation. J. Exp. Mar. Biol. Ecol. 352, 129–138. doi: 10.1016/j.jembe.2007.07.017
Wang, G. Z., Zhu, D. F., and Li, S. J. (1998). Preliminary study on the temperature-specific rates of embryonic development of the flathead lobster Thenus orientalis (Lund.). Mar. Sci. Bull. 17, 39–44.
Wang, W. N., Wang, A. L., Liu, Y., Liu, Z. B., and Sun, R. Y. (2006). Effects of temperature on growth, adenosine phosphates, ATPase and cellular defense response of juvenile shrimp Macrobrachium nipponense. Aquaculture 256, 624–630.
Wang, Z. L., Qu, Y. X., Zhuo, X. L., Li, J. Y., Zou, J. X., and Fan, L. L. (2019). Investigating the physiological responses of Pacific white shrimp Litopenaeus vannamei to acute cold-stress. Peer J 7:e7381. doi: 10.7717/peerj.7381
Wang, Z. L., Zhou, J., Li, J. Y., Zou, J. X., and Fan, L. L. (2020). The immune defense response of Pacific white shrimp (Litopenaeus vannamei) to temperature fluctuation. Fish Shellfish Immunol. 103, 103–110. doi: 10.1016/j.fsi.2020.04.053
Wanlem, S., Supamattaya, K., Tantikitti, C., Prasertsan, P., and Graidist, P. (2011). Expression and applications of recombinant crustacean hyperglycemic hormone from eyestalks of white shrimp (Litopenaeus vannamei) against bacterial infection. Fish Shellfish Immunol. 30, 877–885. doi: 10.1016/j.fsi.2011.01.014
Webster, S. G., Keller, R., and Dircksen, H. (2012). The CHH-superfamily of multifunctional peptide hormones controlling crustacean metabolism, osmoregulation, moulting, and reproduction. Gen. Comp. Endocr. 175, 217–233.
Wittmann, A. C., Benrabaa, S. A. M., Loìpez-Ceroìn, D. A., Chang, E. S., and Mykles, D. L. (2018). Effects of temperature on survival, moulting, and expression of neuropeptide and mTOR signalling genes in juvenile Dungeness crab (Metacarcinus magister). J. Exp. Biol. 221:jeb187492. doi: 10.1242/jeb.187492
Wu, D. L., Huang, Y. H., Chen, Q., Jiang, Q. C., Li, Y. M., and Zhao, Y. L. (2019). Effects and transcriptional responses in the hepatopancreas of red claw crayfish Cherax quadricarinatus under cold stress. J. Therm. Biol. 85:102404.
Wu, D. L., Liu, Z. Q., Huang, Y. H., Lv, W. W., Chen, M. H., Li, Y. M., et al. (2018). Effects of cold acclimation on the survival, feeding rate, and non-specific immune responses of the freshwater red claw crayfish (Cherax quadricarinatus). Aquacult. Int. 26, 557–567. doi: 10.1007/s10499-018-0236-4
Wu, D. L., Liu, Z. Q., Yu, P., Huang, Y. H., Cai, Y. H., Zhang, M., et al. (2020). Cold stress regulates lipid metabolism via AMPK signalling in Cherax quadricarinatus. J. Therm. Biol. 92:102693. doi: 10.1016/j.jtherbio.2020.102693
Wu, H. X. (1991). A preliminary study on the embryonic development of mud crab Scylla serrata. J. Zhejiang College Fish. 10, 97–101.
Wyban, J., Walsh, W. A., and Godin, D. M. (1995). Temperature effects on growth, feeding rate and feed conversion of the Pacific white shrimp (Penaeus vannamei). Aquaculture 138, 267–279. doi: 10.1016/0044-8486(95)00032-1
Xu, J. Y., Yue, C. F., Dai, Y., and Wang, Y. F. (2008). Effects of water temperature, photoperiod and diet on survival rate and ovarian development of the crawfish, Procambarus clarkill. J. Hangzhou Norm. Univ. 42, 97–101.
Xu, Z. H., Guan, W. L., Xie, D. D., Lu, W. J., Ren, X. C., Yuan, J. J., et al. (2019). Evaluation of immunological response in shrimp Penaeus vannamei submitted to low temperature and air exposure. Dev. Comp. Immunol. 100:103413.
Xu, Z. H., Regenstein, J. M., Xie, D. D., Lu, W. J., Ren, X. C., Yuan, J. J., et al. (2018). The oxidative stress and antioxidant responses of Litopenaeus vannamei to low temperature and air exposure. Fish Shellfish Immunol. 72, 564–571.
Yamamoto, T., Jinbo, T., and Hamasaki, K. (2017). Intrinsic optimum temperature for the development of decapod crustacean larvae based on a thermodynamic model. J. Crustacean Biol. 37, 272–277. doi: 10.1093/jcbiol/rux016
Yang, Q. B., Wang, Z. W., Zhou, F. L., Wen, W. G., and Su, T. F. (2013). Analysis on growth and characteristic of immune enzymes activity of Penaeus monodon family under environment-stress. J. Trop. Oceanogr. 33, 78–85.
Yao, H. F., Shi, H. D., and Mao, G. M. (2005). Experiment on the effect of different temperature and different salinity on hatching eggs of Scylla serrate. J. Zhejiang Ocean Univ. 24, 41–42.
Yuan, J. B., Zhang, X. J., Wang, M., Sun, Y. M., and Liu, C. Z. (2021). Simple sequence repeats drive genome plasticity and promote adaptive evolution in penaeid shrimp. Comms. Biol. 4:186. doi: 10.1038/s42003-021-01716-y
Zhang, X. J., Yuan, J. B., Sun, Y. M., Li, S. H., and Gao, Y. (2019). Penaeid shrimp genome provides insights into benthic adaptation and frequent molting. Nat. Commun. 10, 1–14. doi: 10.1038/s41467-018-08197-4
Zhao, M., Wang, W., Zhang, F. Y., Ma, C. Y., Liu, Z. Q., Yang, M. H., et al. (2021). A chromosome-level genome of the mud crab (Scylla paramamosain Estampador) provides insights into the evolution of chemical and light perception in this crustacean. Mol. Ecol. Resour. 02, 1–19. doi: 10.1111/1755-0998.13332
Zhao, M. M., Yao, D. F., Li, S. K., Zhang, Y. L., and Aweya, J. J. (2020). Effects of ammonia on shrimp physiology and immunity: a review. Rev. Aquac. 12, 2194–2211. doi: 10.1111/raq.12429
Zhao, Q., Pan, L. Q., Ren, Q., Wang, L., and Miao, J. J. (2016). Effect of salinity on regulation mechanism of neuroendocrine-immunoregulatory network in Litopenaeus vannamei. Fish Shellfish Immunol. 49, 396–406.
Zheng, J. B., Cao, J. W., Mao, Y., Su, Y. Q., and Wang, J. (2019). Effects of thermal stress on oxidative stress and antioxidant response, heat shock proteins expression profiles and histological changes in Marsupenaeus japonicus. Ecol. Indic. 101, 780–791. doi: 10.1016/j.ecolind.2018.11.044
Zheng, J. B., Mao, Y., Su, Y. Q., and Wang, J. (2020). Cross talk between heat shock protein 10 and a heat shock factor identified from Marsupenaeus japonicas. Int. J. Biol. Macromol. 147, 1041–1052.
Zhou, M., Wang, A. L., and Xian, J. A. (2011). Variation of free amino acid and carbohydrate concentrations in white shrimp, Litopenaeus vannamei: effects of continuous cold stress. Aquaculture 317, 182–186.
Zhu, Y. C., and Cooper, R. L. (2018). Cold exposure effects on cardiac function and synaptic transmission at the neuromuscular junction in invertebrates. Int. J. Zool. Res. 14, 49–60. doi: 10.3923/ijzr.2018.49.60
Keywords: behavior, cold stress, crab, growth, physiology, shrimp
Citation: Ren X, Wang Q, Shao H, Xu Y, Liu P and Li J (2021) Effects of Low Temperature on Shrimp and Crab Physiology, Behavior, and Growth: A Review. Front. Mar. Sci. 8:746177. doi: 10.3389/fmars.2021.746177
Received: 23 July 2021; Accepted: 20 September 2021;
Published: 21 October 2021.
Edited by:
Shengming Sun, Shanghai Ocean University, ChinaReviewed by:
Changkao Mu, Ningbo University, ChinaMario Alberto Burgos-Aceves, University of Salerno, Italy
Copyright © 2021 Ren, Wang, Shao, Xu, Liu and Li. This is an open-access article distributed under the terms of the Creative Commons Attribution License (CC BY). The use, distribution or reproduction in other forums is permitted, provided the original author(s) and the copyright owner(s) are credited and that the original publication in this journal is cited, in accordance with accepted academic practice. No use, distribution or reproduction is permitted which does not comply with these terms.
*Correspondence: Jian Li, lijian@ysfri.ac.cn
†These authors have contributed equally to this work