- Marine Turtle Research, Ecology and Conservation Group, Department of Earth, Ocean and Atmospheric Science, Florida State University, Tallahassee, FL, United States
While widely applied in fisheries science, acoustic telemetry remains an underutilized method in the field of marine turtle biotelemetry. However, with the ability to provide fine-scale spatial data (tens to hundreds of meters, depending on array setup and receiver range) at a low cost, acoustic telemetry presents an important tool for obtaining key information on marine turtle ecology. We present a comprehensive and systematic review acknowledging how acoustic telemetry has been used to advance the field of marine turtle ecology and conservation. We identify the extent of current studies and discuss common and novel research approaches while addressing specific limitations of acoustic telemetry. Forty-eight studies were reviewed, representing six of the seven marine turtle species and all life stages, with most individuals identified as juveniles (45%) and hatchlings (36%). Most studies (83%) focused on the spatial distribution of marine turtles, including estimating home ranges, investigating drivers of habitat use, and identifying horizontal movement patterns and vertical space use. Additionally, acoustic telemetry has been used to study hatchling dispersal and marine turtle exposure and response to threats, as well as to monitor physiological parameters. We identified that acoustic telemetry directly or indirectly informs 60% of the top questions and research priorities related to marine turtles identified by experts in the field. With an increase in acoustic telemetry receiver networks and collaborations across taxa, the applicability of acoustic telemetry is growing, not only for marine turtles but for a wide array of marine species. Although there are limitations that need to be considered at a site/project-level, acoustic telemetry is an important, low-cost technology able to address key questions related to marine turtle ecology that can aid in their conservation, and therefore should be considered by researchers as they develop their projects.
Introduction
Marine turtles are charismatic species that have garnered support due to their listing on the International Union for Conservation of Nature Red List of Threatened Species (Campbell and Smith, 2006; IUCN, 2021). Recently, some populations have demonstrated stabilization and even growth, while others are still facing declines (Wallace et al., 2011; Mazaris et al., 2017; Barrios-Garrido et al., 2020). To promote the recovery of declining populations as well as the continued success of growing populations, integrated conservation and management strategies that protect both individuals and key habitats are needed (IUCN/SSC Marine Turtle Specialist Group, 1995; National Marine Fisheries Service and U.S. Fish and Wildlife Service, 2008). In an effort to determine the strategies that will be most beneficial for aiding in this recovery, experts have identified key questions, research priorities, and knowledge gaps that need to be addressed. Priority topics range from demographics to nesting strategies to health parameters. Of particular importance is the topic of spatial distribution (Hamann et al., 2010; Hays et al., 2016; Wildermann et al., 2018). Indeed, understanding how individuals utilize space throughout time is imperative for identifying key areas to protect, as well as for assessing whether current and proposed protective measures are well placed to conserve species (Cooke, 2008; Gredzens et al., 2014; Lea et al., 2016; Santos et al., 2021).
Several research approaches have been used to study the spatial distribution and habitat use of marine turtles, such as mark-recapture studies (Blumenthal et al., 2009a; Llamas et al., 2017), visual transect surveys (Gorham et al., 2016), submersible camera recordings (Auster et al., 2020), drone surveys (Sykora-Bodie et al., 2017), stable isotope analyses (Bean and Logan, 2019; Pearson et al., 2019), epibiont identification (Silver-Gorges et al., 2021), biotelemetry studies (Berube et al., 2012; Santos et al., 2021), and a combination of the aforementioned techniques (Scales et al., 2011; Haywood et al., 2020; Siegwalt et al., 2020). Biotelemetry in particular, or the tracking of individuals, has emerged as a common method for obtaining habitat use information of species (Cooke et al., 2004; Hussey et al., 2015; Hays and Hawkes, 2018). Most marine turtle tracking studies have utilized satellite telemetry, with hundreds of studies tracking thousands of individuals (Hussey et al., 2015; Hays and Hawkes, 2018). Less common and perhaps underutilized in marine turtle biotelemetry is acoustic telemetry (Hussey et al., 2015). This method is widely used in fisheries and has the ability to provide fine-scale spatial information on a scale of tens to hundreds of meters at a lower cost than other biotelemetry technologies, depending on receiver set up and detection ranges (Heupel et al., 2006; Hussey et al., 2015; Zeh et al., 2015; Matley et al., 2021b).
Acoustic transmitters are attached to or implanted within an individual and emit coded ultrasonic signals which are logged by receivers. Individuals can be actively followed and tracked with a single receiver or tracked passively via an array of receivers moored throughout the study site. Because individuals must pass within the detection range of a receiver to be detected, acoustic telemetry provides presence-only data and studies are spatially constrained by the receiver array, limiting their applicability for highly migratory species. Passive array designs as well as study site characteristics will dictate what questions can be answered and how the data is analyzed and interpreted (Heupel et al., 2006; Heupel and Webber, 2012; Whoriskey and Hindell, 2016). However, since signals transverse through water easily, acoustic telemetry allows for near-continuous monitoring of marine turtles, who spend 91–97% of their time submerged (Renaud et al., 1995; Schmid et al., 2002), As a result, acoustic telemetry provides higher numbers of detections, improved resolution, and lower positional errors than other tracking techniques, such as satellite telemetry, which only transmits location data when turtles surface (Hazel, 2009; McClellan and Read, 2009; Hart et al., 2012; Whoriskey and Hindell, 2016). Therefore, when within the range of receivers, acoustic telemetry presents an opportunity for effectively collecting spatial information for marine turtles (Matley et al., 2021a; Pillans et al., 2021).
While acoustic transmitters themselves are low-cost compared to other biotelemetry devices, installing and maintaining an array of receivers and facilitating data retrieval can be quite costly (Whoriskey, 2015; Zeh et al., 2015). However, acoustic receivers are not species specific (Lea et al., 2016; Matley et al., 2019), which allows multiple taxa to be tracked simultaneously within the same receiver array, enabling cost-sharing among projects to reduce the financial burden to any one study (Selby et al., 2019; Bangley et al., 2020; Reubens et al., 2021). Tens of thousands of passive receivers exist throughout the world and as more are deployed, opportunities for collaborations and data sharing emerge (Hussey et al., 2015; Whoriskey, 2015; Whoriskey and Hindell, 2016). Organizations such as the Ocean Tracking Network (OTN) and FACT Network create regional and global networks that facilitate data sharing among members and encourage standardized data collection and analysis protocols. While challenges still exist in creating compatibility between transmitters and receivers of different manufacturers, these networks are working toward increasing the spatial scale of studies as well as the overall capacity of acoustic telemetry to address questions related to the ecology of marine species, such as marine turtles (Whoriskey, 2015; Bangley et al., 2020; Young et al., 2020; Matley et al., 2021b; Reubens et al., 2021).
To provide marine turtle researchers and managers with a comprehensive understanding of how acoustic telemetry can contribute to marine turtle conservation, we conducted a systematic literature review to investigate how acoustic telemetry has been used to study the ecology of marine turtles. We document the extent of marine turtle acoustic telemetry studies, identifying the regions, species, life stages, and approaches that have received the most attention. We detail the contribution of acoustic telemetry studies to the knowledge of marine turtle ecology and its application toward understanding complex concepts important to aiding their conservation, while also highlighting challenges and identifying knowledge gaps associated with acoustic telemetry. The approaches and limitations discussed here are applicable to many marine taxa and present a broad overview of the relevancy of acoustic telemetry to marine conservation.
Materials and Methods
A systematic literature search was conducted on the 28th of January 2021 following the Preferred Reporting Items for Systematic Reviews and Meta-Analyses (PRISMA) standard (Moher et al., 2009) to identify studies that have utilized acoustic telemetry to obtain information on marine turtles. The search was performed within EBSCO Host and Google Scholar using the below search string:
(“chelonia mydas” OR “caretta caretta” OR “eretmochelys imbricata” OR “lepidochelys kempii” OR “lepidochelys olivacea” OR “natator depress∗” OR “dermochelys coriacea”) AND (“acoustic telemetry” OR “acoustic transmitter∗” OR “sonic telemetry” OR “sonic transmitter∗”).
The above string was also searched in Web of Science (“All Databases”), prefaced with “TS = ” per the database’s search requirements. An additional, broader search was conducted in Web of Science using the search string:
TS = [(“marine turtle∗” OR “sea turtle∗”) AND (“acoustic telemetry” OR “acoustic transmitter∗” OR “sonic telemetry” OR “sonic transmitter∗”)].
For inclusion, papers must have been published in peer-reviewed academic journals and have utilized acoustic telemetry (also referred to as sonic/ultrasonic telemetry) to gain insight and knowledge on marine turtles during any life stage. Studies in laboratory settings and/or those focused solely on the efficacy of acoustic tag use were excluded, as well as reviews. The original search strings returned 316 unique results. After applying the selection criteria, 39 published journal articles fell within the scope of our review (Supplementary Figure 1). Backward and forward searches were conducted to identify additional papers for inclusion, which required scanning the reference sections of all 39 original papers, as well as scanning the titles of all articles that cited the selected papers. The same selection criteria were applied when determining whether additional articles should be added. In total, 48 studies were selected for inclusion in this review.
To understand the spatial, temporal, and demographic trends of existing marine turtle acoustic telemetry studies, we extracted the following information from each article: date of study and publication, study location, type of tracking method used, number of individuals successfully tracked, species, life stage, sex, duration of tracking, transmission interval, and transmitter attachment method, fate, and retention. It was also noted whether studies conducted range tests to estimate the detection probabilities of the receivers used in the study. Occasionally, the same tracking data was used in multiple published studies for different analyses. Individual turtle parameters that were the same between studies were omitted from our analysis, such as species, sex, life stage, and transmitter details. We used the life stage specified by the study; if the authors did not identify the life stage, individuals were classified as “unknown” due to varying growth rates and age at sexual maturity across species and populations (Avens and Snover, 2013). The study period was defined as the overall time between the first and last tracking events included in a study’s analysis. For active telemetry studies, tracking duration was considered to be the cumulative time an individual was actively followed, while for passive studies it was defined as the time between which the individual was released or the study began and when the study ended or the transmitter was evidenced as being dislodged. Some studies limited their analyses to a specific period of time (e.g., a period when all passive receivers were operable). For these studies, we included only the tracking duration used in analyses. Transmitter retention was only determined for those known to be lost.
Additionally, the research question(s), study goal(s), and major findings of all 48 studies were reviewed and grouped to identify the overarching broad research topics that acoustic telemetry studies have addressed, as well as to explore common and novel approaches utilized. Findings were synthesized to illustrate what information acoustic telemetry has provided for marine turtle ecology thus far. To assess the applicability of acoustic telemetry in eliciting high-priority information on marine turtle ecology and conservation, we reviewed three publications that employed expert opinions to identify top research priorities and key questions: Hamann et al. (2010), Hays et al. (2016), and Wildermann et al. (2018). These studies focused on marine turtles on a global scale, marine megafauna movement ecology, and immature marine turtles, respectively. After removing similarities, we critically reviewed the questions presented in these publications to assess whether acoustic telemetry could address key topics of importance for further marine turtle research.
Results
The 48 studies that met our criteria for inclusion in this review (Supplementary Table 1) were published between January 1983 and January 2021. The number of studies published per year has increased, with an evident shift from active to passive tracking methods (Figure 1). While 63% of the studies utilized active tracking methods, these studies generally had smaller sample sizes (avg: 16 individuals; range: 2–94) than passive studies (avg: 28; range: 3–89). Therefore, of 870 individuals tracked with acoustic telemetry, 66% were tracked passively. Studies were found to be globally distributed with most (46%) conducted in the Caribbean and Gulf of Mexico, followed by 31% in the Pacific, 13% in the Indian Ocean, and 10% in the North Atlantic (Figure 2).
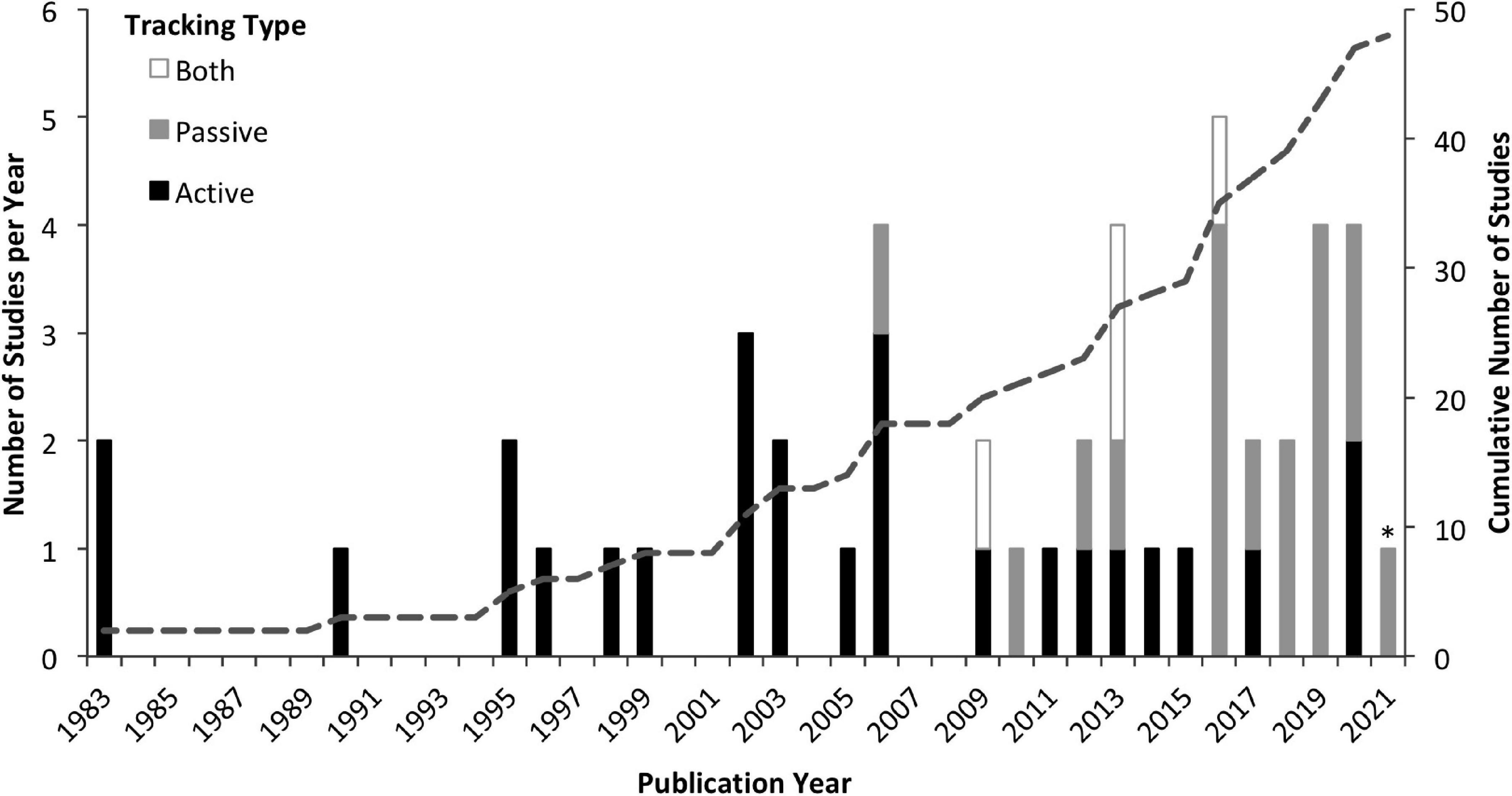
Figure 1. Number of acoustic telemetry studies published per year utilizing active tracking (black), passive tracking (gray), or both types of tracking (white). Cumulative number of studies shown by dotted line. ∗Data from 2021 only includes studies published prior to January 28th and therefore is not comparable to other years.
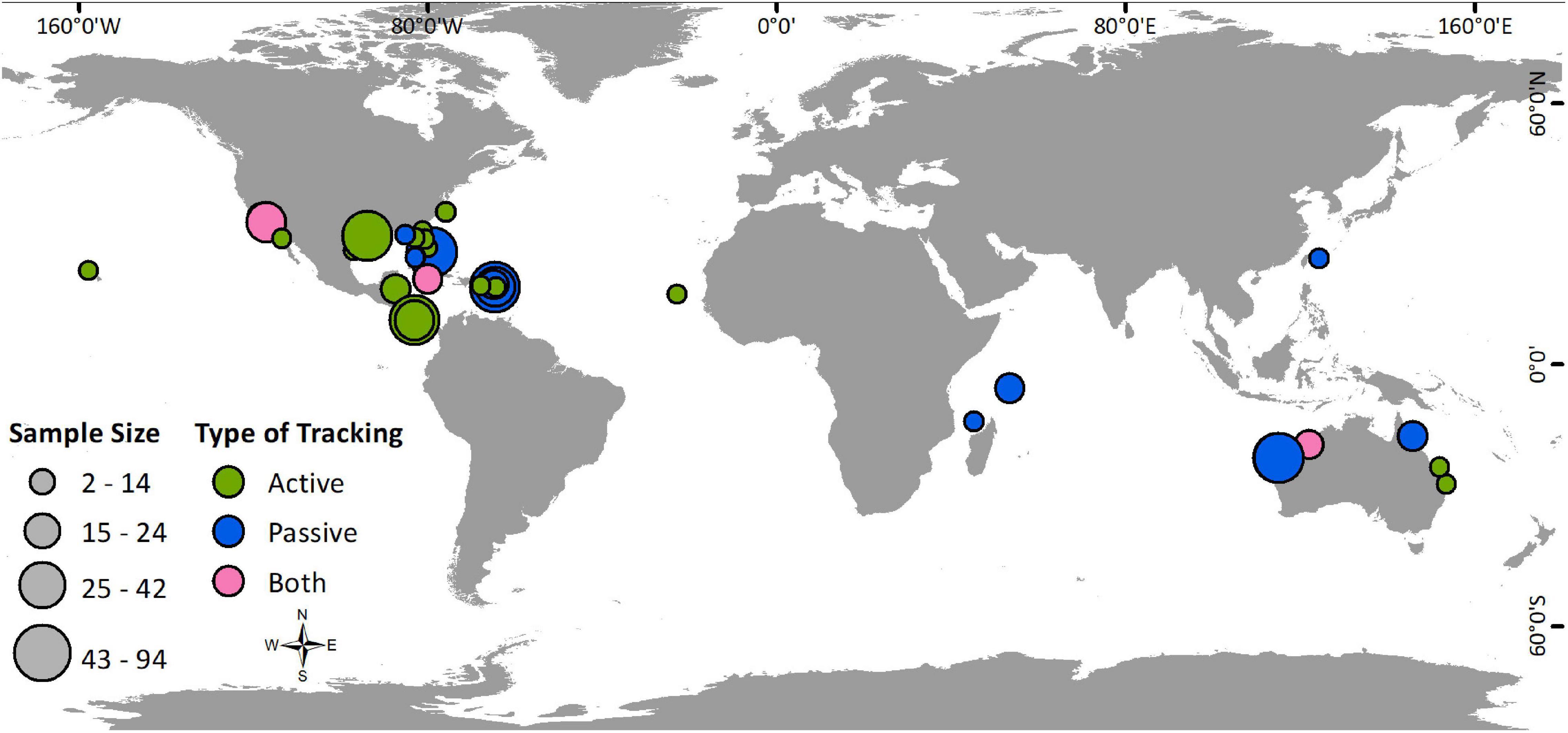
Figure 2. Locations of studies utilizing active tracking (green), passive tracking (blue), or both types of tracking (pink), with study sample sizes represented by the size of the marker.
All marine turtle species except the olive ridley (Lepidochelys olivacea) have been tracked acoustically, with most studies focusing on green (Chelonia mydas) turtles (Figure 3). Green and hawksbill turtles (Eretmochelys imbricata) are the only species that have been tracked with both active and passive telemetry (Figure 4A). Individuals from all life stages have been tracked, although the vast majority have been juveniles (45%) and hatchlings (36%; Figure 4B). Transmitters were most often (78%) applied to the dorsal posterior marginal scutes (3% to the ventral posterior marginal scutes) of juvenile and adult individuals. Attachment methods included wiring or bolting the transmitter through the marginal scutes (34%), using an epoxy, putty, or glue to affix the transmitter (25%), or a combination of both methods (36%). For hatchlings, acoustic transmitters were attached to the plastron with adhesive (71%) or attached via a tether (29%).
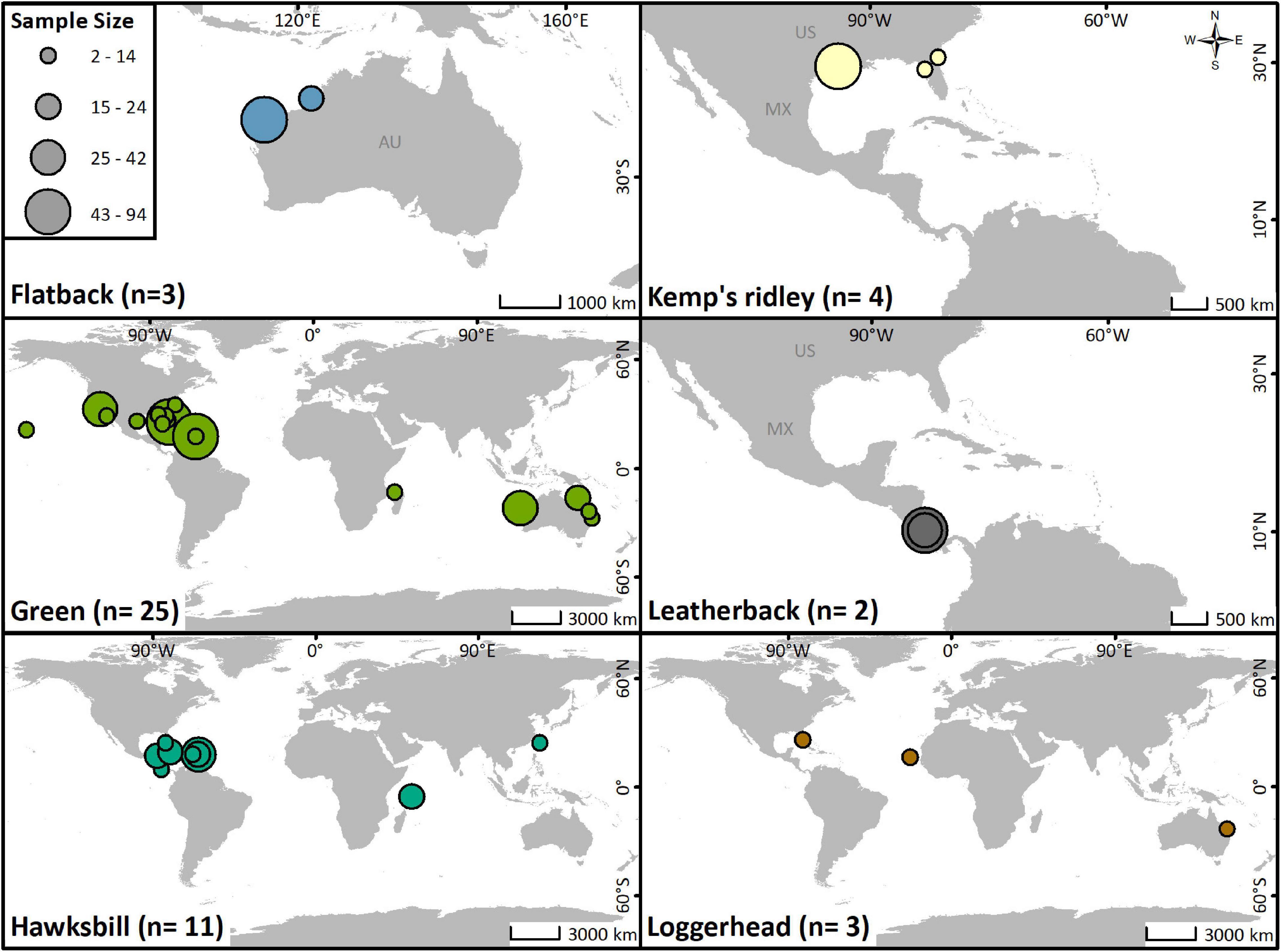
Figure 3. Locations of acoustic telemetry studies for each species of marine turtle, with study sample sizes represented by the size of the marker.
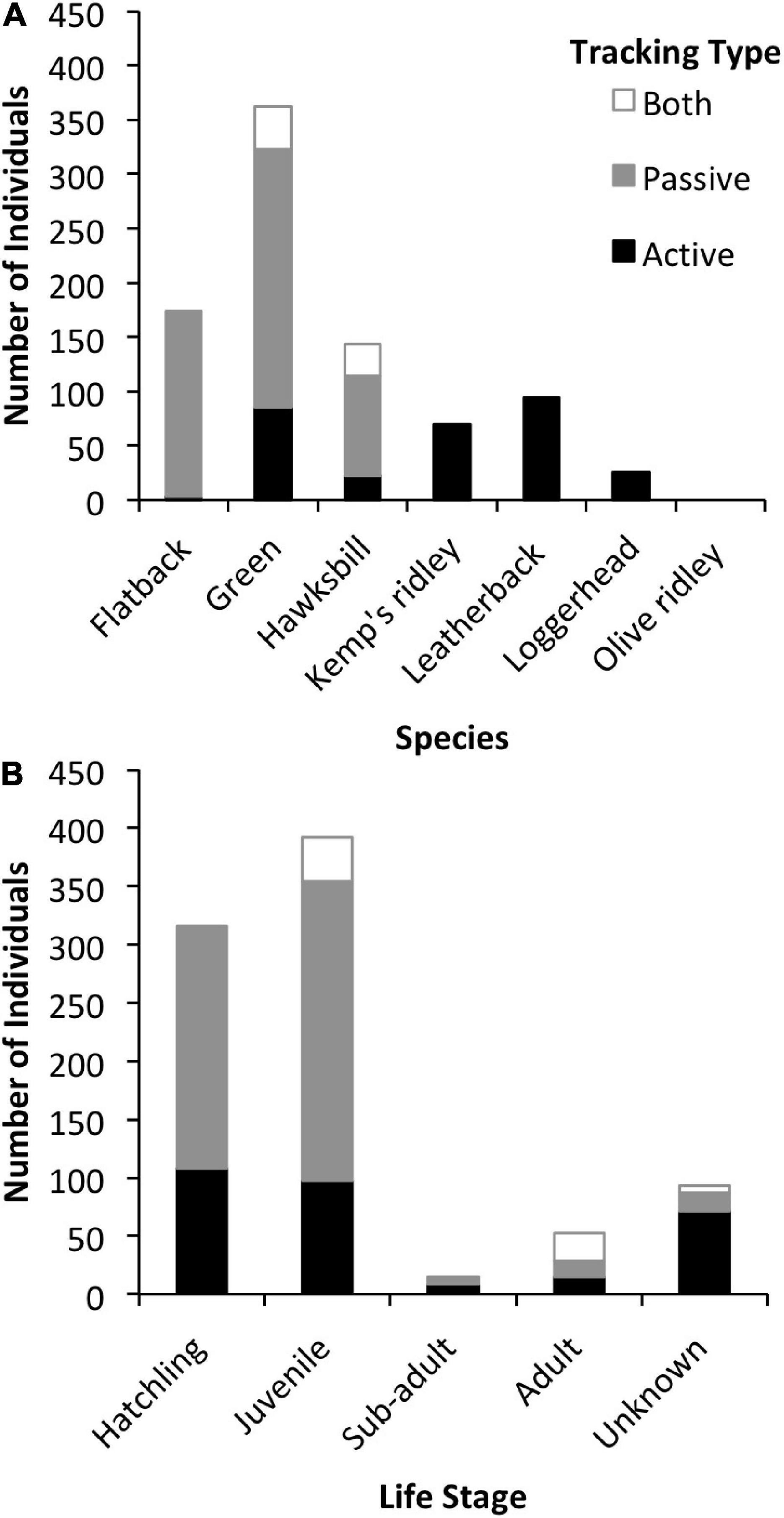
Figure 4. (A) Number of individuals per species tracked with active tracking (black), passive tracking (gray), or both types of tracking (white). (B) Number of individuals per life stage tracked actively (black), passively (gray), or with both tracking types (white).
Overall study periods ranged from 4 days to 8 years, with longer studies typically tagging new individuals throughout the study or replacing individuals’ transmitters periodically to increase tracking time. On average, individual tracking durations were less than 1 day (range: 5 min to 3.5 days) for active studies and 186 days (range: 7 min to 1,414 days) for passive studies. Short tracking durations were mostly those of hatchlings that traversed the study site quickly during their dispersal. Transmitter retention was largely unknown since in many cases (72%) it could not be discerned whether the transmitter was lost, the turtle never returned to the study area, or tracking ceased due to the end of the study. There were 55 known instances of tags becoming dislodged or lost from an individual, of which the average tag retention was approximately 255 days (range: 1–1,414 days).
Acoustic telemetry has been used to obtain information on a variety of topics related to marine turtle ecology and conservation. Most studies (83%) to date have focused on the spatial distribution of juvenile, sub-adult, and adult marine turtles and have addressed questions related to their home range and site fidelity, movement, vertical space use, and the drivers that influence these (Figure 5). Additionally, acoustic telemetry has been used to explore hatchling dispersal, assess spatial proximity and response to threats, and monitor turtle health and physiological parameters (Figure 5). Several of these topics fall within the research priorities identified by experts in the field, with acoustic telemetry directly or indirectly addressing 60% of key questions identified by Hamann et al. (2010), Hays et al. (2016), and Wildermann et al. (2018) (Supplementary Table 2).
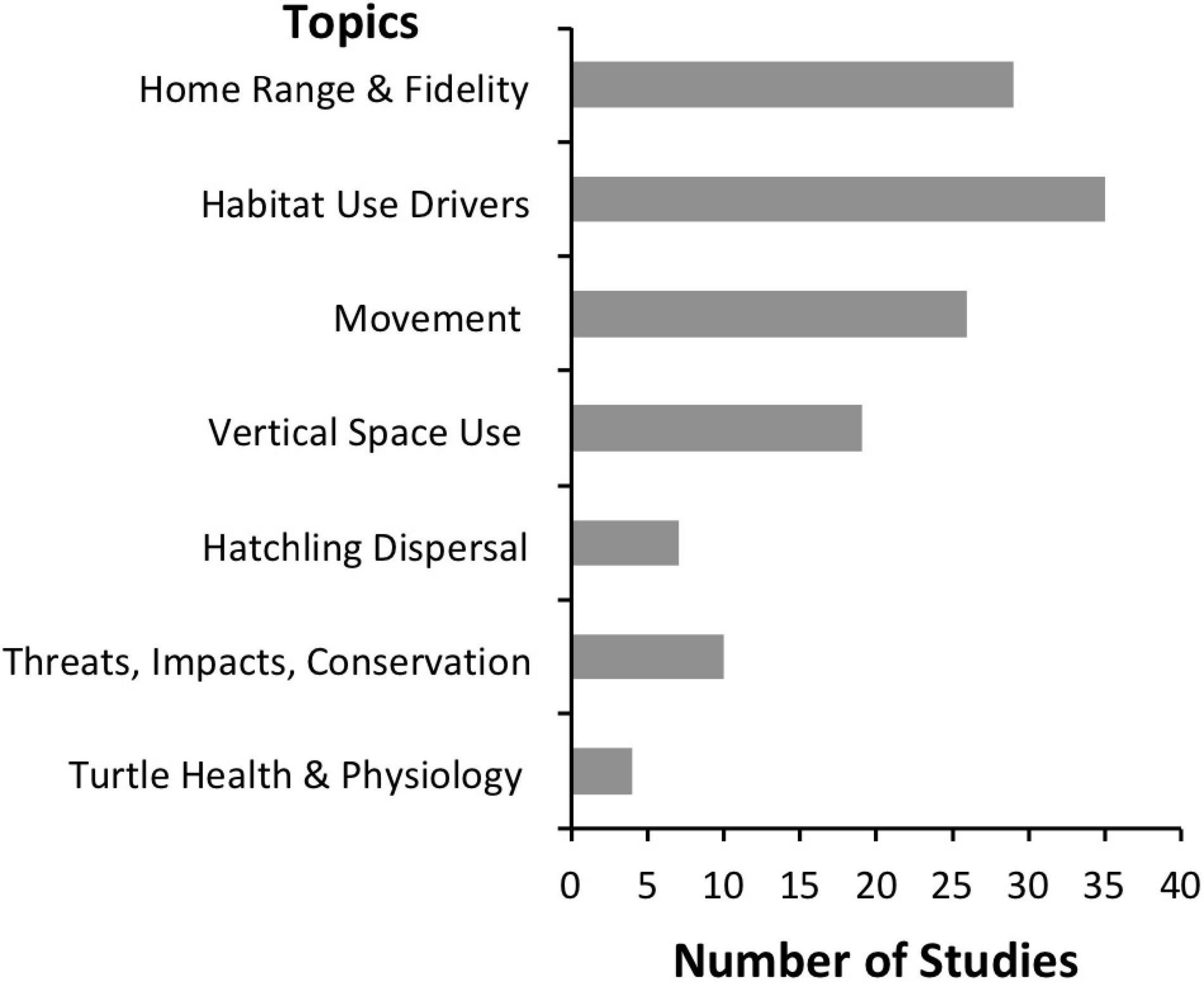
Figure 5. Broad topics investigated by marine turtle acoustic telemetry studies with the number of studies (n = 48) that explored or discussed each topic indicated.
Discussion
While acoustic telemetry has been utilized by a relatively small number of marine turtle studies, the technology has provided a wealth of information regarding marine turtle ecology, particularly regarding spatial distribution and movement within study sites. Here, we detail the contribution of acoustic telemetry to our knowledge of marine turtle ecology and explore both commonly used approaches as well as those less routinely used. We discuss the application of acoustic telemetry toward marine turtle conservation, while also acknowledging its limitations.
Spatial Distribution
Home Range and Site Fidelity
Acoustic telemetry was utilized by over a third of studies to estimate the space use of individual marine turtles, including minimum complex polygons, home ranges, and core use areas (Supplementary Table 3). In addition to identifying and quantifying these areas of regular use (Supplementary Table 1), studies also investigated the overlap among individuals, providing insight into simultaneous habitat use among marine turtles (Mendonca, 1983; Ogden et al., 1983; Schmid et al., 2003; Makowski et al., 2006; Scales et al., 2011; MacDonald et al., 2012; Hazel et al., 2013; Lamont et al., 2015; Fujisaki et al., 2016; Crear et al., 2017). Nearly half of all studies used acoustic telemetry to explore site fidelity (Supplementary Table 3), which, when combined with information on home ranges and overlap between individuals, can distinguish areas of high importance for marine turtles (Renaud et al., 1995; Blumenthal et al., 2009b; Hazel et al., 2013; Selby et al., 2019). Space use data from acoustic telemetry studies should be interpreted with care since detections are only possible when an individual is within the range of a receiver (Heupel et al., 2006; Whoriskey and Hindell, 2016). In our review, passively tracked marine turtles were detected on an average of 85% (n = 152) of the days they were tracked, indicating that individuals may utilize areas beyond the bounds of passive receiver arrays (Blumenthal et al., 2009b; Hart et al., 2012). Consideration of the spatial extent and design of arrays is necessary to avoid misinterpretation of results and underestimations of home ranges and fidelity indices (Heupel et al., 2006; McClellan and Read, 2009; Hart et al., 2012; Kessel et al., 2014; Whoriskey and Hindell, 2016). For home range analyses, receiver arrays should ideally be designed with overlapping detection ranges to avoid gaps in coverage, but costs of additional receivers and site characteristics prevent this in some cases (Heupel et al., 2006; Hazel et al., 2013; Chevis et al., 2017). Multiple factors (e.g., bathymetry, habitat type and rugosity, background noise) affect the detection probabilities of receivers and tests should be conducted to ensure that coverage is even across the study site to reduce spatial bias (Heupel et al., 2006; Kessel et al., 2014; Selby et al., 2016; Swadling et al., 2020). Of the passive studies included in this review, 73% conducted receiver range tests or modeled detection probability based on study site characteristics. With a well designed and tested array, or with the incorporation of analytical methods to account for inconsistencies in receiver detection ranges, acoustic telemetry is extremely useful in providing fine-scale spatial information to estimate home ranges (Heupel et al., 2006; Kessel et al., 2014). For marine turtles, it is best utilized in areas where turtles are known to aggregate and where arrays can be designed to cover the entire study area, such as juvenile and adult foraging areas where fidelity is high either within or across years.
Habitat Use Drivers
Identifying the factors that drive home range size and selection can assist managers in predicting current and future habitat use, which can guide management decisions (Hazen et al., 2013; Schofield et al., 2013). Seventy-three percent of acoustic studies coupled space use data with additional data sets (e.g., habitat type, water temperature, diel patterns) to investigate the factors that drive marine turtle habitat use (Supplementary Table 3). For example, several studies examined habitat type associations (Supplementary Table 3) and found benthic substrate to be a main predictor of marine turtle spatial distribution (Renaud et al., 1995; Seminoff et al., 2002; Schmid et al., 2003; McClellan and Read, 2009; Lea et al., 2016; Griffin et al., 2019, 2020; Selby et al., 2019). Acoustic telemetry was used to further investigate patterns of habitat type use throughout the day to understand the influence of diel patterns and behavior on space use (Supplementary Table 3). Acoustic telemetry has also been used to analyze the influence of food availability and selection on marine turtle habitat use by pairing spatial data with esophageal lavages (Mendonca, 1983; Brand-Gardner et al., 1999; Makowski et al., 2006; Carrión-Cortez et al., 2013).
However, care should be taken when interpreting habitat type usage results derived from acoustic telemetry, as studies typically used broad habitat classifications and considered the habitat type to be homogenous within the entire detection zone of a receiver (Hart et al., 2012; Selby et al., 2019). Since detection ranges potentially reach distances > 500 m, this may not always be true or representative of the habitat type the individual was using at the time of the detection (Heupel et al., 2006; McClellan and Read, 2009; Crear et al., 2016; Selby et al., 2019). If fine-scale information on habitat type associations is desired, geographic coordinates of an individual’s location can be derived if it is simultaneously detected by three receivers (Wilson et al., 2019). While passive receiver arrays must be designed in a tight grid to enable this, specific habitat types at the individual’s coordinates can be confirmed (Hart et al., 2012). Some habitat types, such as reefs and rocky structures, can also block acoustic signals leading to lower detections that underrepresent actual use of that habitat (Okuyama et al., 2010; Selby et al., 2016; Chevis et al., 2017). Regardless of these limitations, acoustic telemetry is commonly regarded as an appropriate tool for identifying general habitat type associations (Donaldson et al., 2014; Selby et al., 2019).
Other environmental habitat use drivers that have been investigated are season (Mendonca, 1983; Renaud and Williams, 2005; McClellan and Read, 2009; MacDonald et al., 2012, 2013; Carrión-Cortez et al., 2013; Crear et al., 2016), water temperature (Mendonca, 1983; Lamont et al., 2015; Madrak et al., 2016; Matley et al., 2020), moon index (Taquet et al., 2006), and air pressure (Matley et al., 2020). Individual metrics have also been studied, with a third of studies analyzing the relationship of turtle size and habitat use (Supplementary Table 3). Fujisaki et al. (2016) investigated how sex mediates space use among marine turtles which provided insight into attraction and territoriality between individuals (Fujisaki et al., 2016). Since sex can only be determined in the field for mature individuals by the presence of a large tail in males or the absence of such in females, this application is limited to specific populations of sexually mature individuals (Wibbels, 2003). However, this topic should be explored further as it has important implications for mate encounter frequency and levels of multiple paternity and their effects on populations (Uller and Olsson, 2008; Hays and Hawkes, 2018).
Movement Patterns
Over half (54%) of marine turtle acoustic studies identified movement patterns, including displacement, rate of movement (Supplementary Table 1), movements between habitats, and the drivers that influence those movements (Supplementary Table 3). Additionally, Addison et al. (2002) utilized active tracking to explore the movement patterns of post-nesting females up to 12 km offshore. An advantage of active telemetry is that location points can be taken as often as desired as an individual is followed, allowing researchers to discern even small variations in movement paths (MacDonald et al., 2013; Crear et al., 2017). With passive telemetry, movement patterns within a study site are inferred as an individual is detected on various receivers along its path through an array (Heupel et al., 2006; Whoriskey, 2015). Some studies have also placed receivers in a linear fashion to monitor upstream and downstream movements of marine turtles in rivers (Crear et al., 2016). Movement between locations and the strength of those connections has been explored through network analyses, which have found that even when links to other suitable habitats exist, turtles rarely travel between sites (Lea et al., 2016; Griffin et al., 2019). Within sites, movements are predominately driven by behavior, diel patterns, and environmental factors, such as temperature and tide (Mendonca, 1983; Renaud et al., 1995; van Dam and Diez, 1998; Schmid et al., 2002; Renaud and Williams, 2005; Seminoff and Jones, 2006; McClellan and Read, 2009; MacDonald et al., 2013; Crear et al., 2016, 2017; Griffin et al., 2019; Matley et al., 2020). Studying movement with acoustic telemetry is limited by short temporal durations of active tracking and the unknown path and direction of an individual between detections during passive tracking, both of which can affect displacement and rate of movement measurements (Heupel et al., 2006; Chevis et al., 2017). The interval at which transmitters send signals should be taken into consideration, as this will affect the timestep of location points used for movement analysis. Short intervals will increase the likelihood of signal collisions and autocorrelation of the data, but longer intervals decrease the temporal resolution of the data (Heupel et al., 2006). For the studies in this review, transmission intervals ranged from 1 s to 3 min. Compared to other technologies such as satellite telemetry, acoustic telemetry provides a higher number of detections for marine species and therefore may present a more representative picture of movement patterns within the extent of the receiver array (Hart et al., 2012; Dwyer et al., 2015). If very detailed movement patterns, displacement measurements, or rates of movement are of interest, active telemetry should be considered (Seminoff and Jones, 2006; Lamont et al., 2015; Crear et al., 2017).
Vertical Space Use
With information of passive receiver depths or with depth measurements taken during active tracking, acoustic telemetry has been used to infer vertical space use of marine turtles. With the incorporation of depth sensors or radio telemetry devices, further insights into submergence and surfacing patterns, dive patterns, and depth usage can be explored. Indeed, 40% of studies have investigated vertical space use in coastal habitats (Supplementary Table 3). This topic is important to understanding exposure to certain threats, such as boating and fishing, as well as energetics associated with diving and depth usage (Southwood et al., 2003, 2006; Blumenthal et al., 2009b; McClellan and Read, 2009). Since radio signals do not transmit well through water, the addition of radio transmitters to the acoustic transmitter can provide information of how often a turtle surfaces, and for how long (Addison et al., 2002; Schmid et al., 2002). Depth sensors can also be easily incorporated into the acoustic transmitter to provide timed depth information (Gitschlag, 1996; Blumenthal et al., 2009b). Exploring depth usage is vital to understanding habitat use in the three-dimensional space that turtles occupy. It has been suggested that vertical habitat partitioning may occur, which has implications for individuals’ access to food and shelter (Makowski et al., 2006; Blumenthal et al., 2009b; Hart et al., 2012; Carrión-Cortez et al., 2013). Further exploration of how depth mediates space use is necessary, but the cost of additional sensors may be a limitation to incorporating this data into acoustic telemetry studies (Matley et al., 2021a).
Beyond Spatial Distribution
Information obtained by acoustic telemetry can help answer more complex questions beyond spatial distribution of marine turtles, such as whether certain behaviors are learned or innate and the role of marine turtles within the ecosystem (Hays et al., 2016). Habitat use and movement patterns between groups of individuals can be easily compared with acoustic telemetry, an approach used by Okuyama et al. (2010) to gain insight into marine turtle behavior. By comparing acoustic telemetry data of wild-caught and head-started turtles, the study provided evidence that turtles may exhibit learned behaviors, such as the ability to navigate to previous locations and utilize rocky ledges for assisted resting (Okuyama et al., 2010). The role of marine turtles within the ecosystem also remains an understudied area and is a complex combination of habitat use, movement, foraging ecology, and predation (Hamann et al., 2010; Heithaus, 2013; Rees et al., 2016). Marine turtles affect seagrass growth and coral reef ecology through foraging and are responsible for small scale transportation of nutrients throughout their environment (Hill, 1998; Moran and Bjorndal, 2007; Schmitz et al., 2010; Heithaus, 2013; Rodriguez, 2018). Acoustic telemetry has been essential in identifying the extent of movement between foraging and resting sites, and provides insight into turtles’ role in nutrient cycling (Taquet et al., 2006; Schmitz et al., 2010; Heithaus, 2013; MacDonald et al., 2013). Additionally, mapping the spatial overlap of turtles and their predators can inform trophic ecology and community dynamics (Heithaus et al., 2008; Hammerschlag et al., 2015). While studies on ecosystem role remain limited (Rees et al., 2016), acoustic telemetry may present an effective tool to gain the spatial information needed for future research in this area.
Hatchling Dispersal
With the smallest transmitters weighing just under 0.4 g (Thums et al., 2013; Whoriskey and Hindell, 2016), acoustic telemetry offers a novel approach to gain in situ insights into the factors affecting nearshore marine turtle hatchling dispersal. It has been used to monitor movements of hatchlings to study the influence of oceanic conditions (Scott et al., 2014; Thums et al., 2016; Wilson et al., 2018; Barbour et al., 2020; Hoover et al., 2020), artificial light (Thums et al., 2013, 2016; Wilson et al., 2018, 2019), swimming speed and fitness (Scott et al., 2014; Barbour et al., 2020), and predation (Wilson et al., 2019) on dispersal patterns. These studies have found that while hatchlings exhibit active, directed swimming, oceanic conditions and anthropogenic factors (e.g., artificial lights, increased predation due to jetties) greatly affect their trajectories (Thums et al., 2013, 2016; Scott et al., 2014; Wilson et al., 2018; Barbour et al., 2020; Hoover et al., 2020), which has important implications for dispersal patterns, especially when considering the short- and long-term variability of oceanic conditions (Scott et al., 2014; Barbour et al., 2020). Data from acoustic studies can contribute to more accurate models and simulations of hatchling dispersal to better predict how changes in global climate, ocean currents, and coastal development will affect the survival and oceanic distributions of marine turtles (Scott et al., 2014; Thums et al., 2016; Wildermann et al., 2017; Putman et al., 2019).
Tracking hatchlings with acoustic telemetry comes with limitations. Passive studies are short in duration and distance, as hatchlings quickly transverse the receiver array (range: 5–35 min) and leave the nearshore area (Thums et al., 2013, 2016). Active telemetry allows for longer tracking durations (range: 20 min to 8 h) over greater distances (12 km), but is labor intensive and can be affected by weather conditions (Heupel et al., 2006; Scott et al., 2014). While acoustic telemetry can only track hatchlings in the nearshore environment and does not allow tracking over the entirety of their dispersal pathway, tracking hatchlings is a unique benefit of acoustic telemetry. Other tracking approaches, such as satellite telemetry, currently do not have tags small enough to accommodate hatchlings (Mansfield et al., 2017).
Threats, Impacts, and Conservation
Marine turtle spatial data can be coupled with information on threats, such as boating and fisheries, to identify and map areas of high exposure (Hart et al., 2018; Fuentes et al., 2020). However, only 10% of acoustic studies have taken advantage of this approach, possibly due to increased probability of loss or damage to receivers and decreased detection probability in areas of high vessel traffic (Clements et al., 2005; Heupel et al., 2006). Regardless, McClellan and Read (2009) used acoustic telemetry to analyze the overlap of small-scale fisheries operations with green turtle habitat, while four other studies assessed the presence of turtles within boating channels (Brill et al., 1995; Renaud et al., 1995; MacDonald et al., 2012, 2013). Findings from these studies can inform management strategy decisions, such as implementation of “go-slow” zones or marine protected areas (MPAs) (Schofield et al., 2013; Shimada et al., 2017; Fuentes et al., 2020). These strategies require planning and evaluation to ensure they are reaching their intended goals (Day, 2008). The effectiveness of MPAs at protecting marine turtle habitat has been evaluated with acoustic telemetry by assessing the percentage of habitat use that occurs within current or proposed MPA boundaries (Blumenthal et al., 2009b; Carrión-Cortez et al., 2013; Lea et al., 2016; Fuentes et al., 2018; Griffin et al., 2020). Acoustic telemetry has also facilitated a multispecies approach to MPA evaluation by tracking multiple species simultaneously (Lea et al., 2016). Effective evaluation of MPA success requires that passive receiver arrays overlap completely with the extent of the MPA.
Acoustic telemetry data can also help assess and predict the response of marine turtles to climate change (Madrak et al., 2016; Matley et al., 2020). Data from acoustic studies has shown that marine turtles make fine-scale changes in habitat use, movement patterns, and speed in response to changes in water temperature (McClellan and Read, 2009; Lamont et al., 2015; Madrak et al., 2016; Matley et al., 2020). As climate change progresses, distributions may shift as turtles adjust their habitat use to fit their thermal range and foraging preferences (Patel et al., 2021; Patrício et al., 2021). In addition, acoustic telemetry has shown that some juvenile marine turtles actively select for manmade structures over natural habitats, which could prompt small-scale shifts in habitat use as the number of jetties and other shoreline stabilization structures increase with rising sea levels (Renaud et al., 1995; MacDonald et al., 2013; Crear et al., 2017; Matley et al., 2019; Oppenheimer et al., 2019). As storm frequency and intensity increase, knowledge of marine turtles’ response and survivability to storms is important (Fuentes and Abbs, 2010; Fuentes et al., 2019; Crowe et al., 2020). Matley et al. (2020) and Lamont et al. (2021) assessed this response when separate Category 5 hurricanes moved over their passive array study sites. While turtles showed short-term changes in habitat use, all turtles survived and no long-term changes were observed (Matley et al., 2019; Lamont et al., 2021). An important caveat in analyzing data collected during storms is that wave action likely caused significant background noise and drastically reduced the detection probability of receivers (Heupel et al., 2006; Kessel et al., 2014; Matley et al., 2019; Lamont et al., 2021). Swells associated with extreme weather can also displace passive receivers, affecting spatial data collection. Indeed, Matley et al. (2019) noted the permanent loss of three receivers during the storm event.
Health and Physiological Parameters
While less commonly utilized, acoustic telemetry has also been used to provide insights to turtle health and physiological parameters, with 8% of studies tackling this topic. Brill et al. (1995) compared the spatial distribution, movement, and depth use of turtles with fibropapillomas (FP) tumors and those without, noting no differences between the two groups. Additionally, three acoustic telemetry studies took advantage of active tracking to recapture individuals for subsequent physiological sampling. Wibbels et al. (1990) relocated and collected blood samples from adult female loggerhead turtles up to 9 times each over 4 months to assess reproductive hormone production. Southwood et al. (2006) used acoustic telemetry to recapture individuals injected with doubly labeled water to measure water flux and field metabolic rates. The same team also relocated and resampled turtles to determine differences in body and water temperatures across seasons (Southwood et al., 2003). While this approach is limited to areas where turtles can be reliably recaptured (e.g., foraging grounds where turtles display high site fidelity), these studies illustrate how acoustic telemetry can provide information beyond spatial distribution.
Future Directions
Future marine turtle acoustic telemetry studies can build upon the approaches already established to develop novel ways to answer outstanding research questions. For example, further use of active tracking to recapture individuals can increase studies on physiological parameters over time (Wibbels et al., 1990; Southwood et al., 2003, 2006). Subsequent sampling of individuals can also inform epibiont colonization rates (Silver-Gorges et al., 2021), as well as provide additional data for growth rate curves (Patrício et al., 2014). Further, active tracking can be used to retrieve additional bio-logging equipment and sensors from individuals, allowing incorporation of in situ environmental data or video footage into studies (Harcourt et al., 2019; Jeantet et al., 2020; Smulders et al., 2021). Improved demographic information, including survivorship and timing of ontogenetic habitat use shifts, could be achieved with increases in acoustic studies investigating long-term home ranges and site fidelity (Hamann et al., 2010; Wildermann et al., 2018). Additionally, further studies analyzing predation and storm response could inform survivorship rates. Acoustic arrays placed offshore of known nesting beaches could provide insight into the movement patterns of internesting females (Addison et al., 2002). While only 10% of current acoustic telemetry studies analyzed the spatial overlap of marine turtles with threats, this approach can be expanded upon to further conduct threat assessments of chemical spills, pollution (e.g., plastic, light, noise) and a variety of human activities (e.g., boating, snorkeling, fishing) (Hamann et al., 2010; Sequeira et al., 2019).
As the field of acoustic telemetry grows, it will be important to look to other taxa to learn new approaches. For example, fisheries and elasmobranch studies have utilized tightly gridded arrays to triangulate locations of individuals for more fine-scale location data and have analyzed social interactions among individuals (Armansin et al., 2016; Binder et al., 2018; Becker et al., 2020). While differences in life histories, morphology, and behavior may deem some approaches inapplicable to marine turtles, there is much to gain from reviewing studies from fields where acoustic telemetry has been more widely utilized, such as fisheries (see reviews: Heupel and Webber, 2012; Donaldson et al., 2014; Hussey et al., 2015; Matley et al., 2021b). Currently, one of the main limitations to marine turtle acoustic studies is that transmitters are applied externally, which reduces the retention time compared to transmitters surgically implanted in fish and shark species (Smith et al., 2019; Smukall et al., 2019; Kennedy et al., 2020). Although marine turtle transmitter retention varies by species, the longest recorded duration (hawksbill) was just under 4 years (Chevis et al., 2017; Smith et al., 2019). Further research is needed to identify the effect of transmitter application techniques on tag retention rates. Meanwhile, active telemetry can be used to recapture individuals and proactively replace transmitters that are loose or near the end of their battery life in order to increase tracking durations (Selby et al., 2019).
Despite its limitations, acoustic telemetry’s capacity for answering important research questions is growing due to its advantages in providing spatial information on hard-to-monitor species and with the proliferation of acoustic tracking networks (Bangley et al., 2020; Matley et al., 2021b). With data-sharing within networks, acoustic studies can potentially span coastal regions and even ocean basins and inform migration paths and long-distance movements of marine species (Guttridge et al., 2017; Young et al., 2020). Networks, such as the OTN, are dedicating resources toward establishing standardization of metadata and analysis protocols and are working with manufacturers to resolve differences between transmitter models and encourage open decoding protocols (Whoriskey, 2015; Bangley et al., 2020; Reubens et al., 2021). New technologies are being developed to aid in data retrieval, such as autonomous underwater vehicles (AUVs) that can retrieve data from receivers, as well as systems that can transmit detection data to the surface with a modem and hydrophone. Advancements are also being made in utilizing marine organisms and AUVs as roving receiver platforms to increase detection probabilities (Whoriskey, 2015; Davis et al., 2018; Bangley et al., 2020; Ennasr et al., 2020). A proposed framework for standardization of data across all biotelemetry technologies will increase compatibility of data and improve biotelemetry’s ability to inform spatial distribution of marine species (Sequeira et al., 2021).
The combination of these advances provides opportunities for the exploration of a wider scope of topics related to marine species ecology and conservation. Data sharing can lead to studies that track multiple species to identify global hotspots for biodiversity and examine both intra-and interspecies interactions to understand social behavior among individuals, as well as predator-prey relationships. The advancement of multispecies studies can lead to ecological rather than species-level approaches to conservation and management (Lea et al., 2016; Brodie et al., 2018; Bangley et al., 2020).
Data Availability Statement
The original contributions presented in the study are included in the article/Supplementary Material, further inquiries can be directed to the corresponding author/s.
Author Contributions
MF developed the initial concept for this review and provided critical edits to the final manuscript. EH designed and conducted the systematic search, extracted and analyzed the data, and wrote the original manuscript. Both authors contributed to the article and approved the submitted version.
Funding
Funding in part for Open Access publication provided by the National Academy of Sciences.
Conflict of Interest
The authors declare that the research was conducted in the absence of any commercial or financial relationships that could be construed as a potential conflict of interest.
Publisher’s Note
All claims expressed in this article are solely those of the authors and do not necessarily represent those of their affiliated organizations, or those of the publisher, the editors and the reviewers. Any product that may be evaluated in this article, or claim that may be made by its manufacturer, is not guaranteed or endorsed by the publisher.
Acknowledgments
We would like to thank Alexa Putillo and Jonathan Lefcheck for their guidance while conducting the systematic review and data extraction portion of this publication, and Jeffrey Seminoff for introducing MF to acoustic telemetry.
Supplementary Material
The Supplementary Material for this article can be found online at: https://www.frontiersin.org/articles/10.3389/fmars.2021.765418/full#supplementary-material
References
Addison, D. S., Gore, J. A., Ryder, J., and Worley, K. (2002). Tracking post-nesting movements of loggerhead turtles (Caretta caretta) with sonic and radio telemetry on the southwest coast of Florida, USA. Mar. Biol. 141, 201–205. doi: 10.1007/s00227-002-0811-z
Armansin, N. C., Lee, K. A., Huveneers, C., and Harcourt, R. G. (2016). Integrating social network analysis and fine-scale positioning to characterize the associations of a benthic shark. Anim. Behav. 115, 245–258. doi: 10.1016/j.anbehav.2016.02.014
Auster, P. J., Campanella, F., Kurth, R., Muñoz, R. C., and Taylor, J. C. (2020). Identifying habitat associations of sea turtles within an area of offshore sub-tropical reefs (NW Atlantic). Southeast. Nat. 19, 460–471. doi: 10.1656/058.019.0302
Avens, L., and Snover, M. L. (2013). “Age and age estimation in sea turtles,” in The Biology of Sea Turtles, eds J. Wyneken, K. J. Lohmann, and J. A. Musick (Boca Raton: CRC Press), 97–133.
Bangley, C. W., Whoriskey, F. G., Young, J. M., and Ogburn, M. B. (2020). Networked animal telemetry in the Northwest Atlantic and Caribbean waters. Mar. Coast. Fish. Dyn. Manag. Ecosyst. Sci. 12, 339–347. doi: 10.1002/mcf2.10128
Barbour, N., Shillinger, G. L., Hoover, A. L., Williamson, S. A., Coles, V. J., Liang, D., et al. (2020). Environmental and biological factors influencing dispersal of neonate leatherback turtles (Dermochelys coriacea) from an endangered Costa Rican nesting population. Front. Mar. Sci. 7:582933. doi: 10.3389/fmars.2020.582933
Barrios-Garrido, H., Shimada, T., Diedrich, A., and Hamann, M. (2020). Conservation and Enforcement Capacity index (CECi): integrating human development, economy, and marine turtle status. J. Environ. Manage. 262:110311. doi: 10.1016/j.jenvman.2020.110311
Bean, S. B., and Logan, J. M. (2019). Stable isotope analyses of cold-stunned Kemp’s ridley (Lepidochelys kempii) sea turtles at the northern extent of their coastal range. Mar. Biol. 166:64. doi: 10.1007/s00227-019-3516-2
Becker, S. L., Finn, J. T., Novak, A. J., Danylchuk, A. J., Pollock, C. G., Hillis-Starr, Z., et al. (2020). Coarse- and fine-scale acoustic telemetry elucidates movement patterns and temporal variability in individual territories for a key coastal mesopredator. Environ. Biol. Fishes 103, 13–29. doi: 10.1007/s10641-019-00930-2
Berube, M. D., Dunbar, S. G., Rutzler, K., and Hayes, W. K. (2012). Home range and foraging ecology of Juvenile Hawksbill sea turtles (Eretmochelys imbricata) on Inshore Reefs of Honduras. Chelonian Conserv. Biol. 11, 33–43. doi: 10.2744/CCB-0898.1
Binder, T. R., Farha, S. A., Thompson, H. T., Holbrook, C. M., Bergstedt, R. A., Riley, S. C., et al. (2018). Fine-scale acoustic telemetry reveals unexpected lake trout, Salvelinus namaycush, spawning habitats in northern Lake Huron, North America. Ecol. Freshw. Fish 27, 594–605. doi: 10.1111/eff.12373
Blumenthal, J. M., Austin, T. J., Bell, C. D. L., Bothwell, J. B., Broderick, A. C., Ebanks-Petrie, G., et al. (2009a). Ecology of Hawksbill Turtles, Eretmochelys imbricata, on a Western Caribbean foraging ground. Chelonian Conserv. Biol. 8, 1–10. doi: 10.2744/CCB-0758.1
Blumenthal, J. M., Austin, T. J., Bothwell, J. B., Broderick, A. C., Ebanks-Petrie, G., Olynik, J. R., et al. (2009b). Diving behavior and movements of juvenile hawksbill turtles Eretmochelys imbricata on a Caribbean coral reef. Coral Reefs 28, 55–65. doi: 10.1007/s00338-008-0416-1
Brand-Gardner, S. J., Lanyon, J. M., and Limpus, C. J. (1999). Diet selection by immature green turtles, Chelonia mydas, in subtropical Moreton Bay, south-east Queensland. Aust. J. Zool. 47, 181–191. doi: 10.1071/ZO98065
Brill, R. W., Balazs, G. H., Holland, K. N., Chang, R. K. C., Sullivan, S., and George, J. C. (1995). Daily movements, habitat use, and submergence intervals of normal and tumor-bearing juvenile green turtles (Chelonia mydas L.) within a foraging area in the Hawaiian islands. J. Exp. Mar. Bio. Ecol. 185, 203–218. doi: 10.1016/0022-0981(94)00146-5
Brodie, S., Lédée, E. J. I., Heupel, M. R., Babcock, R. C., Campbell, H. A., Gledhill, D. C., et al. (2018). Continental-scale animal tracking reveals functional movement classes across marine taxa. Sci. Rep. 8:3717. doi: 10.1038/s41598-018-21988-5
Campbell, L. M., and Smith, C. (2006). What makes them pay? values of volunteer tourists working for Sea Turtle conservation. Environ. Manage. 38, 84–98. doi: 10.1007/s00267-005-0188-0
Carrión-Cortez, J., Canales-Cerro, C., Arauz, R., and Riosmena-Rodríguez, R. (2013). Habitat use and diet of Juvenile Eastern Pacific Hawksbill turtles (Eretmochelys imbricata) in the North Pacific Coast of Costa Rica. Chelonian Conserv. Biol. 12, 235–245. doi: 10.2744/CCB-1024.1
Chevis, M. G., Godley, B. J., Lewis, J. P., Lewis, J. J., Scales, K. L., and Graham, R. T. (2017). Movement patterns of juvenile hawksbill turtles Eretmochelys imbricata at a Caribbean coral atoll: long-term tracking using passive acoustic telemetry. Endanger. Species Res. 32, 309–319. doi: 10.3354/esr00812
Clements, S., Jepsen, D., Karnowski, M., and Schreck, C. B. (2005). Optimization of an acoustic telemetry array for detecting transmitter-implanted fish. North Am. J. Fish. Manag. 25, 429–436. doi: 10.1577/m03-224.1
Cooke, S. J. (2008). Biotelemetry and biologging in endangered species research and animal conservation: relevance to regional, national, and IUCN Red List threat assessments. Endanger. Species Res. 4, 165–185. doi: 10.3354/esr00063
Cooke, S. J., Hinch, S. G., Wikelski, M., Andrews, R. D., Kuchel, L. J., Wolcott, T. G., et al. (2004). Biotelemetry: a mechanistic approach to ecology. Trends Ecol. Evol. 19, 334–343. doi: 10.1016/j.tree.2004.04.003
Crear, D. P., Lawson, D. D., Seminoff, J. A., Eguchi, T., LeRoux, R. A., and Lowe, C. G. (2016). Seasonal shifts in the movement and distribution of green sea turtles Chelonia mydas in response to anthropogenically altered water temperatures. Mar. Ecol. Prog. Ser. 548, 219–232. doi: 10.3354/meps11696
Crear, D. P., Lawson, D. D., Seminoff, J. A., Eguchi, T., LeRoux, R. A., and Lowe, C. G. (2017). Habitat Use and Behavior of the East Pacific Green Turtle, Chelonia mydas, in an Urbanized System. Bull. South. Calif. Acad. Sci. 116, 17–32.
Crowe, L. M., Hatch, J. M., Patel, S. H., Smolowitz, R. J., and Haas, H. L. (2020). Riders on the storm: loggerhead sea turtles detect and respond to a major hurricane in the Northwest Atlantic Ocean. Mov. Ecol. 8:32. doi: 10.1186/s40462-020-00218-6
Davis, R., Comeau, A., L’Orsa, S., Van der Meer, J., Covey, B., Pye, J., et al. (2018). Lessons learned in developing a canadian operational glider fleet. Mar. Technol. Soc. J. 52, 13–18. doi: 10.4031/MTSJ.52.3.20
Day, J. (2008). The need and practice of monitoring, evaluating and adapting marine planning and management-lessons from the Great Barrier Reef. Mar. Policy 32, 823–831. doi: 10.1016/j.marpol.2008.03.023
Donaldson, M. R., Hinch, S. G., Suski, C. D., Fisk, A. T., Heupel, M. R., and Cooke, S. J. (2014). Making connections in aquatic ecosystems with acoustic telemetry monitoring. Front. Ecol. Environ. 12, 565–573. doi: 10.1890/130283
Dwyer, R. G., Campbell, H. A., Irwin, T. R., and Franklin, C. E. (2015). Does the telemetry technology matter? Comparing estimates of aquatic animal space-use generated from GPS-based and passive acoustic tracking. Mar. Freshw. Res. 66, 654–664. doi: 10.1071/MF14042
Ennasr, O., Holbrook, C., Hondorp, D. W., Krueger, C. C., Coleman, D., Solanki, P., et al. (2020). Characterization of acoustic detection efficiency using a gliding robotic fish as a mobile receiver platform. Anim. Biotelemetry 8:32. doi: 10.1186/s40317-020-00219-7
Fuentes, M. M. P. B., and Abbs, D. (2010). Effects of projected changes in tropical cyclone frequency on sea turtles. Mar. Ecol. Prog. Ser. 412, 283–292. doi: 10.3354/meps08678
Fuentes, M. M. P. B., Gillis, A. J., Ceriani, S. A., Guttridge, T. L., Van Zinnicq Bergmann, M. P. M., Smukall, M., et al. (2018). Informing marine protected areas in Bimini, Bahamas by considering hotspots for green turtles (Chelonia mydas). Biodivers. Conserv. 28, 197–211. doi: 10.1007/s10531-018-1647-2
Fuentes, M. M. P. B., Godfrey, M. H., Shaver, D., Ceriani, S., Gredzens, C., Boettcher, R., et al. (2019). Exposure of marine turtle nesting grounds to named storms along the continental USA. Remote Sens. 11:2996. doi: 10.3390/rs11242996
Fuentes, M. M. P. B., Wildermann, N., Gandra, T. B. R., and Domit, C. (2020). Cumulative threats to juvenile green turtles in the coastal waters of southern and southeastern Brazil. Biodivers. Conserv. 29, 1783–1803. doi: 10.1007/s10531-020-01964-0
Fujisaki, I., Hart, K. M., and Sartain-Iverson, A. R. (2016). Habitat selection by green turtles in a spatially heterogeneous benthic landscape in Dry Tortugas National Park, Florida. Aquat. Biol. 24, 185–199. doi: 10.3354/ab00647
Gitschlag, G. R. (1996). Migration and diving behavior of Kemp’s ridley (Garman) sea turtles along the U.S. southeastern Atlantic coast. J. Exp. Mar. Bio. Ecol. 205, 115–135. doi: 10.1016/S0022-0981(96)02602-0
Gorham, J. C., Bresette, M. J., Guertin, J. R., Shamblin, B. M., and Nairn, C. J. (2016). Green turtles (Chelonia mydas) in an urban estuary system: lake Worth Lagoon, Florida. Florida Sci. 79, 14–27.
Gredzens, C., Marsh, H., Fuentes, M. M. P. B., Limpus, C. J., Shimada, T., and Hamann, M. (2014). Satellite tracking of sympatric marine megafauna can inform the biological basis for species co-management. PLoS One 9:e98944. doi: 10.1371/journal.pone.0098944
Griffin, L. P., Finn, J. T., Diez, C., and Danylchuk, A. J. (2019). Movements, connectivity, and space use of immature green turtles within coastal habitats of the Culebra Archipelago, Puerto Rico: implications for conservation. Endanger. Species Res. 40, 75–90. doi: 10.3354/esr00976
Griffin, L. P., Smith, B. J., Cherkiss, M. S., Crowder, A. G., Pollock, C. G., Hillis-Starr, Z., et al. (2020). Space use and relative habitat selection for immature green turtles within a Caribbean marine protected area. Anim. Biotelemetry 8:22. doi: 10.1186/s40317-020-00209-9
Guttridge, T. L., Van Zinnicq Bergmann, M. P. M., Bolte, C., Howey, L. A., Finger, J. S., Kessel, S. T., et al. (2017). Philopatry and regional connectivity of the great Hammerhead Shark, Sphyrna mokarran in the U.S. and Bahamas. Front. Mar. Sci. 4:3. doi: 10.3389/fmars.2017.00003
Hamann, M., Godfrey, M. H., Seminoff, J. A., Arthur, K., Barata, P. C. R., Bjorndal, K. A., et al. (2010). Global research priorities for sea turtles: informing management and conservation in the 21st century. Endanger. Species Res. 11, 245–269. doi: 10.3354/esr00279
Hammerschlag, N., Broderick, A. C., Coker, J. W., Coyne, M. S., Dodd, M., Frick, M. G., et al. (2015). Evaluating the landscape of fear between apex predatory sharks and mobile sea turtles across a large dynamic seascape. Ecology 96, 2117–2126. doi: 10.1890/14-2113.1
Harcourt, R., Sequeira, A. M. M., Zhang, X., Roquet, F., Komatsu, K., Heupel, M., et al. (2019). Animal-borne telemetry: an integral component of the ocean observing toolkit. Front. Mar. Sci. 6:326. doi: 10.3389/fmars.2019.00326
Hart, K. M., Iverson, A. R., Fujisaki, I., Lamont, M. M., Bucklin, D., and Shaver, D. J. (2018). Marine threats overlap key foraging habitat for two imperiled sea turtle species in the Gulf of Mexico. Front. Mar. Sci. 5:336. doi: 10.3389/fmars.2018.00336
Hart, K. M., Sartain, A. R., Fujisaki, I., Pratt, H. L. Jr., Morley, D., and Feeley, M. W. (2012). Home range, habitat use, and migrations of hawksbill turtles tracked from Dry Tortugas National Park, Florida, USA. Mar. Ecol. Prog. Ser. 457, 193–207. doi: 10.3354/meps09744
Hays, G. C., Ferreira, L. C., Sequeira, A. M. M., Meekan, M. G., Duarte, C. M., Bailey, H., et al. (2016). Key questions in marine megafauna movement ecology. Trends Ecol. Evol. 31, 463–475. doi: 10.1016/j.tree.2016.02.015
Hays, G. C., and Hawkes, L. A. (2018). Satellite tracking sea turtles: opportunities and challenges to address key questions. Front. Mar. Sci. 5:432. doi: 10.3389/fmars.2018.00432
Haywood, J. C., Fuller, W. J., Godley, B. J., Margaritoulis, D., Shutler, J. D., Snape, R. T. E., et al. (2020). Spatial ecology of loggerhead turtles: insights from stable isotope markers and satellite telemetry. Divers. Distrib. 26, 368–381. doi: 10.1111/ddi.13023
Hazel, J. (2009). Evaluation of fast-acquisition GPS in stationary tests and fine-scale tracking of green turtles. J. Exp. Mar. Bio. Ecol. 374, 58–68. doi: 10.1016/j.jembe.2009.04.009
Hazel, J., Hamann, M., and Lawler, I. R. (2013). Home range of immature green turtles tracked at an offshore tropical reef using automated passive acoustic technology. Mar. Biol. 160, 617–627. doi: 10.1007/s00227-012-2117-0
Hazen, E. L., Jorgensen, S., Rykaczewski, R. R., Bograd, S. J., Foley, D. G., Jonsen, I. D., et al. (2013). Predicted habitat shifts of Pacific top predators in a changing climate. Nat. Clim. Chang. 3, 234–238. doi: 10.1038/nclimate1686
Heithaus, M. R. (2013). “Predators, Prey, and the Ecological Roles of Sea Turtles,” in The Biology of Sea Turtles, eds J. Wyneken, K. J. Lohmann, and J. A. Musick (Boca Raton: CRC Press), 249–284.
Heithaus, M. R., Wirsing, A. J., Thomson, J. A., and Burkholder, D. A. (2008). A review of lethal and non-lethal effects of predators on adult marine turtles. J. Exp. Mar. Bio. Ecol. 356, 43–51. doi: 10.1016/j.jembe.2007.12.013
Heupel, M. R., Semmens, J. M., and Hobday, A. J. (2006). Automated acoustic tracking of aquatic animals: scales, design and deployment of listening station arrays. Mar. Freshw. Res. 57, 1–13. doi: 10.1071/MF05091
Heupel, M. R., and Webber, D. M. (2012). “Trends in acoustic tracking: where are the fish going and how will we follow them? in advances in fish tagging and marking technology,” in American Fisheries Society Symposium, eds J. R. McKenzie, B. Parsons, A. C. Seitz, R. K. Kopf, M. G. Mesa, and Q. Phelps (Bethesda: American Fisheries Society Symposium), 219–231.
Hill, M. S. (1998). Spongivory on Caribbean reefs releases corals from competition with sponges. Oecologia 117, 143–150. doi: 10.1007/s004420050642
Hoover, A. L., Shillinger, G. L., Williamson, S. A., Reina, R. D., and Bailey, H. (2020). Nearshore neonate dispersal of Atlantic leatherback turtles (Dermochelys coriacea) from a non-recovering subpopulation. Sci. Rep. 10:18748. doi: 10.1038/s41598-020-75769-0
Hussey, N. E., Kessel, S. T., Aarestrup, K., Cooke, S. J., Cowley, P. D., Fisk, A. T., et al. (2015). Aquatic animal telemetry: a panoramic window into the underwater world. Science 348:1221. doi: 10.1126/science.1255642
IUCN (2021). The IUCN Red List of Threatened Species. Version 2021-1. Available Online at: http://www.iucnredlist.org [accessed May 1, 2021].
IUCN/SSC Marine Turtle Specialist Group (1995). A Global Strategy for the Conservation of Marine Turtles. Gland: IUCN.
Jeantet, L., Planas-Bielsa, V., Benhamou, S., Geiger, S., Martin, J., Siegwalt, F., et al. (2020). Behavioural inference from signal processing using animal-borne multi-sensor loggers: a novel solution to extend the knowledge of sea turtle ecology. R. Soc. Open Sci. 7:200139. doi: 10.1098/rsos.200139
Kennedy, R. J., Evans, D., and Allen, M. (2020). Long-term retention of dummy acoustic transmitters in adult brown trout. J. Fish Biol. 97, 1281–1284. doi: 10.1111/jfb.14498
Kessel, S. T., Cooke, S. J., Heupel, M. R., Hussey, N. E., Simpfendorfer, C. A., Vagle, S., et al. (2014). A review of detection range testing in aquatic passive acoustic telemetry studies. Rev. Fish Biol. Fish. 24, 199–218. doi: 10.1007/s11160-013-9328-4
Lamont, M. M., Fujisaki, I., Stephens, B. S., and Hackett, C. (2015). Home range and habitat use of juvenile green turtles (Chelonia mydas) in the northern Gulf of Mexico. Anim. Biotelemetry 3, 1–12. doi: 10.1186/s40317-015-0089-9
Lamont, M. M., Johnson, D., and Catizone, D. J. (2021). Movements of marine and estuarine turtles during Hurricane Michael. Sci. Rep. 11:1577. doi: 10.1038/s41598-021-81234-3
Lea, J. S. E., Humphries, N. E., von Brandis, R. G., Clarke, C. R., and Sims, D. W. (2016). Acoustic telemetry and network analysis reveal the space use of multiple reef predators and enhance marine protected area design. Proc. R. Soc. B Biol. Sci. 283:20160717. doi: 10.1098/rspb.2016.0717
Llamas, I., Flores, E. E., Abrego, M. E., Seminoff, J. A., Hart, C. E., Donadi, R., et al. (2017). Distribution, size range and growth rates of hawksbill turtles at a major foraging ground in the eastern Pacific Ocean. Lat. Am. J. Aquat. Res. 45, 597–605. doi: 10.3856/VOL45-ISSUE3-FULLTEXT-9
MacDonald, B. D., Lewison, R. L., Madrak, S. V., Seminoff, J. A., and Eguchi, T. (2012). Home ranges of East Pacific green turtles Chelonia mydas in a highly urbanized temperate foraging ground. Mar. Ecol. Prog. Ser. 461, 211–221. doi: 10.3354/meps09820
MacDonald, B. D., Madrak, S. V., Lewison, R. L., Seminoff, J. A., and Eguchi, T. (2013). Fine scale diel movement of the east Pacific green turtle, Chelonia mydas, in a highly urbanized foraging environment. J. Exp. Mar. Bio. Ecol. 443, 56–64. doi: 10.1016/j.jembe.2013.02.033
Madrak, S. V., Lewison, R. L., Seminoff, J. A., and Eguchi, T. (2016). Characterizing response of East Pacific green turtles to changing temperatures: using acoustic telemetry in a highly urbanized environment. Anim. Biotelemetry 4:22. doi: 10.1186/s40317-016-0114-7
Makowski, C., Seminoff, J. A., and Salmon, M. (2006). Home range and habitat use of juvenile Atlantic green turtles (Chelonia mydas L.) on shallow reef habitats in Palm Beach, Florida, USA. Mar. Biol. 148, 1167–1179. doi: 10.1007/s00227-005-0150-y
Mansfield, K. L., Mendilaharsu, M. L., Putman, N. F., dei Marcovaldi, M. A. G., Sacco, A. E., Lopez, G., et al. (2017). First satellite tracks of South Atlantic sea turtle ‘lost years’: seasonal variation in trans-equatorial movement. Proc. R. Soc. B Biol. Sci. 284:20171730. doi: 10.1098/rspb.2017.1730
Matley, J. K., Eanes, S., Nemeth, R. S., and Jobsis, P. D. (2019). Vulnerability of sea turtles and fishes in response to two catastrophic Caribbean hurricanes, Irma and Maria. Sci. Rep. 9, 14254. doi: 10.1038/s41598-019-50523-3
Matley, J. K., Klinard, N. V., Barbosa Martins, A. P., Aarestrup, K., Aspillaga, E., Cooke, S. J., et al. (2021b). Global trends in aquatic animal tracking with acoustic telemetry. Trends Ecol. Evol. doi: 10.1016/j.tree.2021.09.001 [Epub Online ahead of print].
Matley, J. K., Johansen, L. K., Klinard, N. V., Eanes, S. T., and Jobsis, P. D. (2021a). Habitat selection and 3D space use partitioning of resident juvenile hawksbill sea turtles in a small Caribbean bay. Mar. Biol. 168:120. doi: 10.1007/s00227-021-03912-0
Matley, J. K., Jossart, J., Johansen, L., and Jobsis, P. D. (2020). Environmental drivers of diving behavior and space-use of juvenile endangered Caribbean hawksbill sea turtles identified using acoustic telemetry. Mar. Ecol. Prog. Ser. 652, 157–171. doi: 10.3354/meps13466
Mazaris, A. D., Schofield, G., Gkazinou, C., Almpanidou, V., and Hays, G. C. (2017). Global sea turtle conservation successes. Sci. Adv. 3:e1600730. doi: 10.1126/sciadv.1600730
McClellan, C. M., and Read, A. J. (2009). Confronting the gauntlet: understanding incidental capture of green turtles through fine-scale movement studies. Endanger. Species Res. 10, 165–179. doi: 10.3354/esr00199
Mendonca, M. T. (1983). Movements and feeding ecology of immature green turtles (Chelonia mydas) in a Florida Lagoon. Copeia 1983, 1013–1023.
Moher, D., Liberati, A., Tetzlaff, J., Altman, D. G., and Group, T. P. (2009). Preferred reporting items for systematic reviews and meta-analyses: the PRISMA statement. J. Clin. Epidemiol. 62, 1006–1012. doi: 10.1016/j.jclinepi.2009.06.005
Moran, K. L., and Bjorndal, K. A. (2007). Simulated green turtle grazing affects nutrient composition of the seagrass Thalassia testudinum. Mar. Biol. 150, 1083–1092. doi: 10.1007/s00227-006-0427-9
National Marine Fisheries Service, and U.S. Fish and Wildlife Service (2008). Recovery Plan for the Northwest Atlantic Population of the Loggerhead Sea Turtle (Caretta caretta), Second Revision. Silver Spring: NOAA.
Ogden, J. C., Robinson, L., Whitlock, K., Daganhardt, H., and Cebula, R. (1983). Diel foraging patterns in juvenile green turtles (Chelonia mydas L.) in St. Croix United States Virgin Islands. J. Exp. Mar. Bio. Ecol. 66, 199–205. doi: 10.1016/0022-0981(83)90160-0
Okuyama, J., Shimizu, T., Abe, O., Yoseda, K., and Arai, N. (2010). Wild versus head-started hawksbill turtles Eretmochelys imbricata: post-release behavior and feeding adaptions. Endanger. Species Res. 10, 181–190. doi: 10.3354/esr00250
Oppenheimer, M., Glavovic, J., va de Wal, R., Magnan, A. K., Abd-Elgawad, A., Cai, R., et al. (2019). “Sea level rise and implications for low-lying islands, coasts and communities,” in IPCC Special Report on the Ocean and Cryosphere in a Changing Climate, eds H.-O. Pörtner, D. C. Roberts, V. Masson-Delmotte, P. Zhai, M. Tignor, and E. Poloczanska (Cambridge: Cambridge University Press), 126.
Patel, S. H., Winton, M. V., Hatch, J. M., Haas, H. L., Saba, V. S., Fay, G., et al. (2021). Projected shifts in loggerhead sea turtle thermal habitat in the Northwest Atlantic Ocean due to climate change. Sci. Rep. 11:8850.
Patrício, A. R., Hawkes, L. A., Monsinjon, J. R., Godley, B. J., and Fuentes, M. M. P. B. (2021). Climate change and marine turtles: recent advances and future directions. Endanger. Species Res. 44, 363–395. doi: 10.3354/esr01110
Patrício, R., Diez, C. E., and van Dam, R. P. (2014). Spatial and temporal variability of immature green turtle abundance and somatic growth in Puerto Rico. Endanger. Species Res. 23, 51–62. doi: 10.3354/esr00554
Pearson, R. M., van de Merwe, J. P., Gagan, M. K., Limpus, C. J., and Connolly, R. M. (2019). Distinguishing between sea turtle foraging areas using stable isotopes from commensal barnacle shells. Sci. Rep. 9:6565. doi: 10.1038/s41598-019-42983-4
Pillans, R. D., Fry, G. C., Haywood, M. D. E., Rochester, W., Limpus, C. J., Patterson, T., et al. (2021). Residency, home range and tidal habitat use of Green Turtles (Chelonia mydas) in Port Curtis, Australia. Mar. Biol. 168:88. doi: 10.1007/s00227-021-03898-9
Putman, N. F., Seney, E. E., Verley, P., Shaver, D. J., López-Castro, M. C., Cook, M., et al. (2019). Predicted distributions and abundances of the sea turtle ‘lost years’ in the western North Atlantic Ocean. Ecography 43, 506–517. doi: 10.1111/ecog.04929
Rees, A. F., Alfaro-Shigueto, J., Barata, P. C. R., Bjorndal, K. A., Bolten, A. B., Bourjea, J., et al. (2016). Are we working towards global research priorities for management and conservation of sea turtles? Endanger. Species Res. 31, 337–382. doi: 10.3354/esr00801
Renaud, M. L., Carpenter, J. A., Williams, J. A., and Manzella-Tirpak, S. A. (1995). Activities of juvenile green turtles, Chelonia mydas, at a jettied pass in South Texas. Fish. Bull. 93, 586–593.
Renaud, M. L., and Williams, J. A. (2005). Kemp’s Ridley Sea Turtle Movements and Migrations. Chelonian Conserv. Biol. 4, 808–816.
Reubens, J., Aarestrup, K., Meyer, C., Moore, A., Okland, F., and Afonso, P. (2021). Compatibility in acoustic telemetry. Anim. Biotelemetry 9, 1–6. doi: 10.1186/s40317-021-00253-z
Rodriguez, A. R. (2018). Juvenile Green Sea Turtle Grazing on Seagrass Meadows of St. Joseph Bay, Florida. Ann Arbor: ProQuest Publishing Company.
Santos, A. J. B., Bellini, C., Santos, E. A. P., Sales, G., Ramos, R., Vieira, D. H. G., et al. (2021). Effectiveness and design of marine protected areas for migratory species of conservation concern: a case study of post-nesting hawksbill turtles in Brazil. Biol. Conserv. 261:109229. doi: 10.1016/j.biocon.2021.109229
Scales, K. L., Lewis, J. A., Lewis, J. P., Castellanos, D., Godley, B. J., and Graham, R. T. (2011). Insights into habitat utilisation of the hawksbill turtle, Eretmochelys imbricata (Linnaeus, 1766), using acoustic telemetry. J. Exp. Mar. Bio. Ecol. 407, 122–129. doi: 10.1016/j.jembe.2011.07.008
Schmid, J. R., Bolten, A. B., Bjorndal, K. A., and Lindberg, W. J. (2002). Activity patterns of Kemp’s ridley turtles, Lepidochelys kempii, in the coastal waters of the Cedar Keys, Florida. Mar. Biol. 140, 215–228. doi: 10.1007/s002270100708
Schmid, J. R., Bolten, A. B., Bjorndal, K. A., Lindberg, W. J., Percival, H. F., and Zwick, P. D. (2003). Home range and habitat use by Kemp’s Ridley turtles in West-Central Florida. J. Wildl. Manage. 67, 196–206.
Schmitz, O. J., Hawlena, D., and Trussell, G. C. (2010). Predator control of ecosystem nutrient dynamics. Ecol. Lett. 13, 1199–1209. doi: 10.1111/j.1461-0248.2010.01511.x
Schofield, G., Scott, R., Dimadi, A., Fossette, S., Katselidis, K. A., Koutsoubas, D., et al. (2013). Evidence-based marine protected area planning for a highly mobile endangered marine vertebrate. Biol. Conserv. 161, 101–109. doi: 10.1016/j.biocon.2013.03.004
Scott, R., Biastoch, A., Roder, C., Stiebens, V. A., and Eizaguirre, C. (2014). Nano-tags for neonates and ocean-mediated swimming behaviours linked to rapid dispersal of hatchling sea turtles. Proc. R. Soc. B Biol. Sci. 281:20141209. doi: 10.1098/rspb.2014.1209
Selby, T. H., Hart, K. M., Fujisaki, I., Smith, B. J., Pollock, C. J., Hillis-Starr, Z., et al. (2016). Can you hear me now? Range-testing a submerged passive acoustic receiver array in a Caribbean coral reef habitat. Ecol. Evol. 6, 4823–4835. doi: 10.1002/ece3.2228
Selby, T. H., Hart, K. M., Smith, B. J., Pollock, C. G., Hillis-Starr, Z., and Oli, M. K. (2019). Juvenile hawksbill residency and habitat use within a Caribbean marine protected area. Endanger. Species Res. 40, 53–64. doi: 10.3354/esr00975
Seminoff, J. A., and Jones, T. T. (2006). Diel movements and activity ranges of green turtles (Chelonia mydas) at a temperate foraging area in the Gulf of California, Mexico. Herpetol. Conserv. Biol. 1, 81–86.
Seminoff, J. A., Resendiz, A., and Nichols, W. J. (2002). Home range of green turtles Chelonia mydas at a coastal foraging area in the Gulf of California, Mexico. Mar. Ecol. Prog. Ser. 242, 253–265. doi: 10.3354/meps242253
Sequeira, A. M. M., Hays, G. C., Sims, D. W., Eguíluz, V. M., Rodríguez, J. P., Heupel, M. R., et al. (2019). Overhauling ocean spatial planning to improve marine Megafauna conservation. Front. Mar. Sci. 6:639. doi: 10.3389/fmars.2019.00639
Sequeira, A. M. M., O’Toole, M., Keates, T. R., McDonnell, L. H., Braun, C. D., Hoenner, X., et al. (2021). A standardisation framework for bio-logging data to advance ecological research and conservation. Methods Ecol. Evol. 12, 996–1007. doi: 10.1111/2041-210X.13593
Shimada, T., Limpus, C., Jones, R., and Hamann, M. (2017). Aligning habitat use with management zoning to reduce vessel strike of sea turtles. Ocean Coast. Manag. 142, 163–172. doi: 10.1016/j.ocecoaman.2017.03.028
Siegwalt, F., Benhamou, S., Girondot, M., Jeantet, L., Martin, J., Bonola, M., et al. (2020). High fidelity of sea turtles to their foraging grounds revealed by satellite tracking and capture-mark-recapture: new insights for the establishment of key marine conservation areas. Biol. Conserv. 250:108742. doi: 10.1016/j.biocon.2020.108742
Silver-Gorges, I., Ingels, J., dos Santos, G. A. P., Valdes, Y., Pontes, L. P., Silva, A. C., et al. (2021). Epibionts Reflect Spatial and Foraging Ecology of Gulf of Mexico Loggerhead Turtles (Caretta caretta). Front. Ecol. Evol. 9:696412. doi: 10.3389/fevo.2021.696412
Smith, B. J., Selby, T. H., Cherkiss, M. S., Crowder, A. G., Hillis-Starr, Z., Pollock, C. G., et al. (2019). Acoustic tag retention rate varies between juvenile green and hawksbill sea turtles. Anim. Biotelemetry 7:15. doi: 10.1186/s40317-019-0177-3
Smukall, M. J., Kessel, S. T., Franks, B. R., Feldheim, K. A., Guttridge, T. L., and Gruber, S. H. (2019). No apparent negative tagging effects after 13 years at liberty for lemon shark, Negaprion brevirostris implanted with acoustic transmitter. J. Fish Biol. 94, 173–177. doi: 10.1111/jfb.13856
Smulders, F. O., O’Shea, O. R., and Christianen, M. J. (2021). Animal-borne video reveals atypical behaviour in provisioned green turtles: a global perspective of a widespread tourist activity. Glob. Ecol. Conserv. 25:e01417. doi: 10.1016/j.gecco.2020.e01417
Southwood, A. L., Reina, R. D., Jones, V. S., and Jones, D. R. (2003). Seasonal diving patterns and body temperatures of juvenile green turtles at Heron Island, Australia. Can. J. Zool. 81, 1014–1024. doi: 10.1139/z03-081
Southwood, A. L., Reina, R. D., Jones, V. S., Speakman, J. R., and Jones, D. R. (2006). Seasonal metabolism of juvenile green turtles (Chelonia mydas) at Heron Island, Australia. Can. J. Zool. 84, 125–135. doi: 10.1139/z05-185
Swadling, D. S., Knott, N. A., Rees, M. J., Pederson, H., Adams, K. R., Taylor, M. D., et al. (2020). Seagrass canopies and the performance of acoustic telemetry: implications for the interpretation of fish movements. Anim. Biotelemetry 8:8. doi: 10.1186/s40317-020-00197-w
Sykora-Bodie, S. T., Bezy, V., Johnston, D. W., Newton, E., and Lohmann, K. J. (2017). Quantifying nearshore sea turtle densities: applications of unmanned aerial systems for population assessments. Sci. Rep. 7:17690. doi: 10.1038/s41598-017-17719-x
Taquet, C., Taquet, M., Dempster, T., Soria, M., Ciccione, S., Roos, D., et al. (2006). Foraging of the green sea turtle Chelonia mydas on seagrass beds at Mayotte Island (Indian Ocean), determined by acoustic transmitters. Mar. Ecol. Prog. Ser. 306, 295–302. doi: 10.3354/meps306295
Thums, M., Whiting, S. D., Reisser, J., Pendoley, K. L., Pattiaratchi, C. B., Proietti, M., et al. (2016). Artificial light on water attracts turtle hatchlings during their near shore transit. R. Soc. open Sci. 3:160142. doi: 10.1098/rsos.160142
Thums, M., Whiting, S. D., Reisser, J. W., Pendoley, K. L., Pattiaratchi, C. B., Harcourt, R. G., et al. (2013). Tracking sea turtle hatchlings - A pilot study using acoustic telemetry. J. Exp. Mar. Bio. Ecol. 440, 156–163. doi: 10.1016/j.jembe.2012.12.006
Uller, T., and Olsson, M. (2008). Multiple paternity in reptiles: patterns and processes. Mol. Ecol. 17, 2566–2580. doi: 10.1111/j.1365-294X.2008.03772.x
van Dam, R. P., and Diez, C. E. (1998). Home range of immature hawksbill turtles (Eretmochelys imbricata (Linnaeus)) at two Caribbean islands. J. Exp. Mar. Bio. Ecol. 220, 15–24. doi: 10.1016/S0022-0981(97)00080-4
Wallace, B. P., DiMatteo, A. D., Bolten, A. B., Chaloupka, M. Y., Hutchinson, B. J., Abreu-Grobois, F. A., et al. (2011). Global conservation priorities for marine turtles. PLoS One 6:e24510. doi: 10.1371/journal.pone.0024510
Whoriskey, F., and Hindell, M. (2016). Developments in tagging technology and their contributions to the protection of marine species at risk. Ocean Dev. Int. Law 47, 221–232. doi: 10.1080/00908320.2016.1194090
Whoriskey, F. G. (2015). “The ocean tracking network: a global partnership uses electronic tagging technologies to track the movements of aquatic animals, answer science questions, stimulate new technology development and assist with sustainable development of the ocean,” in OCEANS 2015 - MTS/IEEE Washington (MTS) (Manhattan: IEEE), 1–5.
Wibbels, T. (2003). “Critical approaches to sex determination in sea turtles,” in The Biology of Sea Turtles, eds P. L. Lutz, J. A. Musick, and J. Wyneken (Boca Raton: CRC Press), 103–134.
Wibbels, T., Owens, D. W. M., Limpus, C. J., Reed, P. C., and Amoss, M. S. J. (1990). Seasonal changes in serum gonadal steroids associated with migration, mating, and nesting in the Loggerhead Sea Turtle (Caretta caretta). Gen. Comp. Endocrinol. 79, 154–164.
Wildermann, N., Critchell, K., Fuentes, M. M. P. B., Limpus, C. J., Wolanski, E., and Hamann, M. (2017). Does behaviour affect the dispersal of flatback post-hatchlings in the Great Barrier Reef? R. Soc. Open Sci. 4:170164. doi: 10.1098/rsos.170164
Wildermann, N. E., Gredzens, C., Avens, L., Barrios-Garrido, H. A., Bell, I., Blumenthal, J., et al. (2018). Informing research priorities for immature sea turtles through expert elicitation. Endanger. Species Res. 37, 55–76. doi: 10.3354/esr00916
Wilson, P., Thums, M., Pattiaratchi, C., Meekan, M., Pendoley, K., Fisher, R., et al. (2018). Artificial light disrupts the nearshore dispersal of neonate flatback turtles Natator depressus. Mar. Ecol. Prog. Ser. 600, 179–192. doi: 10.3354/meps12649
Wilson, P., Thums, M., Pattiaratchi, C., Whiting, S., Pendoley, K., Ferreira, L. C., et al. (2019). High predation of marine turtle hatchlings near a coastal jetty. Biol. Conserv. 236, 571–579. doi: 10.1016/j.biocon.2019.04.015
Young, J. M., Bowers, M. E., Reyier, E. A., Morley, D., Ault, E. R., Pye, J. D., et al. (2020). The FACT Network: philosophy, evolution, and management of a collaborative coastal tracking network. Mar. Coast. Fish. Dyn. Manag. Ecosyst. Sci. 12, 258–271. doi: 10.1002/mcf2.10100
Keywords: acoustic telemetry, spatial distribution, habitat use, sea turtle, tracking, biotelemetry, conservation
Citation: Hardin EE and Fuentes MMPB (2021) A Systematic Review of Acoustic Telemetry as a Tool to Gain Insights Into Marine Turtle Ecology and Aid Their Conservation. Front. Mar. Sci. 8:765418. doi: 10.3389/fmars.2021.765418
Received: 27 August 2021; Accepted: 18 October 2021;
Published: 05 November 2021.
Edited by:
Ana M. M. Sequeira, University of Western Australia, AustraliaReviewed by:
Jérôme Bourjea, Institut Français de Recherche pour l’Exploitation de la Mer (IFREMER), FranceGail Schofield, Queen Mary University of London, United Kingdom
Copyright © 2021 Hardin and Fuentes. This is an open-access article distributed under the terms of the Creative Commons Attribution License (CC BY). The use, distribution or reproduction in other forums is permitted, provided the original author(s) and the copyright owner(s) are credited and that the original publication in this journal is cited, in accordance with accepted academic practice. No use, distribution or reproduction is permitted which does not comply with these terms.
*Correspondence: Emily E. Hardin, ZWVoMjB3QG15LmZzdS5lZHU=