- 1Key Laboratory of Groundwater Resources and Environment of the Ministry of Education, College of Environment and Resources, Jilin University, Changchun, China
- 2Northeast Institute of Geography and Agricultural Ecology, Chinese Academy of Sciences, Changchun, China
Due to their toxicity, non-biodegradability, and biological amplification in the food chain, heavy metal accumulation in humans via the consumption of fishes has become a problem. In this study, we analyzed the concentrations of six trace metals (Cd, Cr, Cu, Ni, Pb, Zn) in 12 marine fish species collected from Liaodong Bay, China, from 2015 to 2020, to understand the pollution status of the bay and evaluate the impact of fish consumption on human health. In addition, 5 fish species with the potential to serve as bioindicators of metal contamination were identified. In general, the average concentrations were Zn > Pb ≈ Cr > Ni ≈ Cu > Cd. There were significant differences in the concentrations in the muscle tissues of fish with different feeding strategies. The total target hazard quotient values of some fish were greater than 1 in 2015, suggesting that people were susceptible to high health risks. The year 2017 had the lowest values, which suggests that metal pollution in Liaodong Bay may have been decreasing at that time. Monitoring of metal concentrations in marine fish from Liaodong Bay warrants more study.
Introduction
The marine environment is a sink of various inorganic and organic pollutants, which even at very low levels can have adverse effects on ecosystems (Traven et al., 2015; Briaudeau et al., 2019). Among such pollutants, trace metals pose a serious threat to the marine environment (Polak-Juszczak, 2009; Liu et al., 2021). They are persistent pollutants (Cunningham et al., 2019) that can be absorbed and accumulated by marine organisms from the surrounding seawater, sediment, and food chain after entering the marine environment through river transport, atmospheric deposition, and/or local sewage (Jonathan et al., 2015; Wang et al., 2017; Hao et al., 2019). Some metals (such as Cd, Pb, Hg) are highly toxic and may damage marine species diversity and ecosystems (Hao et al., 2019). Some metals (such as Hg) can bioaccumulate and biomagnify in aquatic food webs (Liu et al., 2014). Fish are usually at the top of the aquatic food chain and may accumulate large amounts of certain metals (Ge et al., 2020). They have become an important part of a balanced human diet because they are rich in high-quality protein, vitamins, essential minerals, omega 3 fatty acids, and other essential nutrients (Domingo, 2016). The American Heart Association recommends eating two servings of fish per week to reduce the risk of heart attack (Neff et al., 2014; Varol et al., 2017). However, certain metals (such as Cd, Pb, Hg) have no known nutritional or beneficial effects on human health (Varol et al., 2017). Trace metals in fish can be transferred to human tissues, thereby posing potential health risks, particularly in sensitive populations (e.g., pregnant and nursing women, infants, and children) and coastal residents who consume large amounts of seafood (Liu et al., 2018; Hao et al., 2019; Koker et al., 2020). Diet is one of the important ways of human exposure to pollutants. Muscle is the main edible part of fish. Although many studies have reported the accumulation of trace metals in fish muscle, the determination of their prevalence will help evaluate the risk of eating certain fish.
The Bohai Sea in China is a semi-enclosed inland sea. Since China implemented the reform and opening policy in the late 1970s, the economy in the coastal areas around the Bohai Sea has developed rapidly, and river discharges have brought high concentrations of trace metals (Gao et al., 2014). As the largest bay in this sea, Liaodong Bay has 13 heavily polluted rivers flowing into it. Gulf environments have poor water circulation (Cunningham et al., 2019), and because of the nearly enclosed structure of Liaodong Bay, it takes 15 years to complete one water-exchange cycle (Wan et al., 2008; Guo et al., 2020). This worsens pollution pressure and ecological destruction, and the ecosystem is in a sub-healthy or unhealthy state (State Oceanic Administration of China [SOA], 2012). It has been confirmed that metal pollution has contributed to a significant decline in the population growth rates of common fishery species in Liaodong Bay (Gao et al., 2014). Therefore, it is particularly important to monitor the trace metals concentration in this marine environment.
The concentration of metals in aquatic animals is much higher than that in the surrounding environment (Malik et al., 2010), but reflects the degree of environmental pollution to a certain extent (Gao et al., 2014). Some marine organisms, such as gastropods, bivalves, and cephalopods, have been used as bioindicators of certain trace metals in marine environments (Rabaoui et al., 2017; Savorelli et al., 2017; Ubrihien et al., 2017). Fishes are an important part of most aquatic ecosystems, and the accumulation of trace metals in fishes is affected by many factors, including internal differences among species (trophic level and feeding strategy, age, sex, size) and external environmental conditions (chemical forms of trace metals, the severity of pollution) (Cunningham et al., 2019). In the aquatic food chain, fishes are also good biomarkers for long-term monitoring of metal pollution due to their accumulation of high concentrations of metals in tissues (Rahman et al., 2012; Jonathan et al., 2015; Briaudeau et al., 2019). Using fishes as biological indicators can help detect the temporal and spatial changes in trace metals and help understand pollution trends in water bodies (Azizi et al., 2018).
In this study, we investigated and evaluated trace metal pollution in the muscle tissue of marine fish in Liaodong Bay to determine whether metal levels are within the permissible limits for human consumption. We measured the concentration of trace metals from 2015 to 2020 and analyzed overall trends. We recommend fish species that can be used as bioindicator for monitoring metal pollution in the bay, and describe the health risks to women and children in coastal areas exposed to these trace metals through fish consumption. Our results provide biological data for long-term and systematic investigation of metal pollution in Liaodong Bay and can be used to help formulate guidelines for human consumption of marine fish from this body of water.
Materials and Methods
Study Area and Sample Collection
Liaodong Bay is the largest bay in the Bohai Sea and the deepest point is about 32 m (Gao et al., 2021). The cities along the coast include Huludao, Jinzhou, Panjin, and Yingkou. The industrial effluents of petrochemical production enterprises, chlor-alkali facilities, and non-ferrous metal smelting industries bring large amounts of trace metals to Liaodong Bay (Pan and Wang, 2012). Liaodong Bay was previously an important fishing ground for small yellow croaker, hairtail, and prawns, among others. In recent years, the above-mentioned resources have declined and the fisheries are much less robust. We chose Huludao as the city for collecting marine fish samples. The study area and sampling points are shown in Figure 1. Huludao is adjacent to Liaodong Bay in the south, forming a modern industrial system with petrochemical, non-ferrous metals, machinery and shipbuilding, energy, and electric power as the pillar industries.
From 2015 to 2020, 12 species of fresh marine fish (Table 1) were purchased at the end of September each year, directly from Huludao fishermen, in Liaoning Province, northern China. All of the collected samples were packaged and sealed in a clean polyethylene bag. After freezing, they were sent to the laboratory immediately and rinsed in deionized water to remove surface adherents, and then the species were identified and the body length and weight were measured. After the samples were dissected, the wet weights of the marine fish muscle tissue were recorded, and then dry weights were obtained after freeze-drying (freeze dryer of FD-1-50, Beijing Boyikang Experimental Instrument Co., Ltd.) to calculate the moisture content. The samples were ground into powder and stored in polyethylene self-sealing bags for further analysis.
Sample Processing and Analysis
Samples were digested using the method of Anandkumar et al. (2018). Briefly, 1 g freeze-dried sample was accurately weighed in a digestion container, and then 10 mL HNO3 (65%) and H2O2 (30%) (1:1, v/v) were added to the digestion container and kept overnight. Then the digestible container was heated to 130°C on a hot plate until the volume of the mixture was reduced to 2–3 mL. After digestion, samples were cooled, diluted to 50 mL, and filtered. Finally, the digested samples were analyzed via inductively coupled plasma mass spectrometry (Agilent 7500C) to determine the contents of Cd, Cr, Cu, Pb, Zn, and Ni. Quality assurance and quality control throughout the measurement process were accomplished by comparison to standard reference material GBW10049 (GSB-27, Institute of Geophysical and Geochemical Exploration, Chinese Academy of Geological Sciences) prepared using the same procedures as samples. The overall average recovery of trace metals in standard substances was between 87 and 104%.
Health Assessment Methods
The estimated daily intake (EDI) of trace metals ingested by humans through the consumption of marine fish was calculated according to the following formula (Gu et al., 2018b), expressed in units of μg/kg bw/day:
where IR is the intake rate of fish, consumption by children is 16 g/day for 2- to 5-year-olds and 37.9 g/day for 6- to 11-year-olds, consumption by women is 55 g/day and 131.8 g/day (in extreme cases) (Guo et al., 2010; Wang et al., 2019); C is the content of trace metals in the fish (μg/g w.w.); AR is the contaminant intestinal absorption rate (AR) of trace metals in raw fish muscle tissue in the human gastrointestinal tract. The values of AR for Cd, Pb, Cr, Ni, Cu, and Zn are 51.9%, 35.1%, 3.8%, 58.4%, 30.2%, and 46.8%, respectively (Gu et al., 2018b); BW is body weight (59 kg for women, 16.6 kg for children aged 2–5, and 29.8 kg for children aged 6–11) (Guo et al., 2010; CRNTCD, 2020).
The target hazard quotient (THQ) was used to assess the non-carcinogenic health hazards of each trace metal. The reference dose (RfD) for Cu, Pb, Zn, Cd, Ni, and Cr was 0.04, 0.004, 0.3, 0.001, 0.02, and 1.5 mg/kg/day, respectively (USEPA, 2015), and the formula as follows (Varol et al., 2017):
where EF is the exposure frequency (365 days/year), ED is the exposure duration (70 years for women, 3.5 years for children aged 2–5 years old, and 8.5 years for children aged 6–11 years old), AT is the average time (365 days/year multiplied by the number of exposure years), and 10–3 is a conversion factor. A value of less than 1 suggests that lifetime exposure to that metal will not cause non-carcinogenic effects on humans (such as reproductive toxicity, teratogenicity, or liver toxicity). However, there are uncertainties in these calculations. Therefore, a THQ value between 1 and 10 indicates that there may be a chance of such effects. As the THQ value increases, the possibility of these effects also increases (Zheng et al., 2007; Hang et al., 2009).
The TTHQ was obtained by the sum of the individual metal THQ values (Varol et al., 2017).
Statistical Analysis
Statistical software SPSS 24 was used for data analysis. Kolmogorov-Smirnov test (K-S) and Shapiro-Wilk test (S-W) were used to test the normality of large sample data larger than 30 rows and small sample data smaller than 30 rows, respectively. A one-way ANOVA and non-parametric test were used to determine whether there were significant differences in the trace metal content of muscle tissue in different species, habitats (demersal, reef-associated, and pelagic-neritic) and dietary strategies (carnivorous, planktivorous, benthivorous, and omnivorous). A p-value < 0.05 was considered significant. Pearson correlation was used to explore associations among trace metals and length and weight. Graphics were drawn using Origin Pro 8 and ArcGIS 10.2.
Results and Discussion
Concentrations of Trace Metals in Fish Tissue
Huludao is well known for being polluted by metals. Huludao Zinc Plant in Huludao City, China, is the largest zinc plant in Asia and a major source of pollution in the city. Pb, Cd, Zn, and Cu in Zn ore are discharged into the environment through waste gas, general waste residue, and waste residue during the zinc smelting process, and they flow into Liaodong Bay mainly through river transport (Zheng et al., 2007; Gao et al., 2014). As a result, marine animals in Liaodong Bay could accumulate large amounts of trace metals from the marine environment. Figure 2 shows the average concentration of the studied trace metals in the 12 species of fish considered in this study. To facilitate comparison with various limit standards, the metal concentrations were all converted from dry weight to wet weight based on the water content.
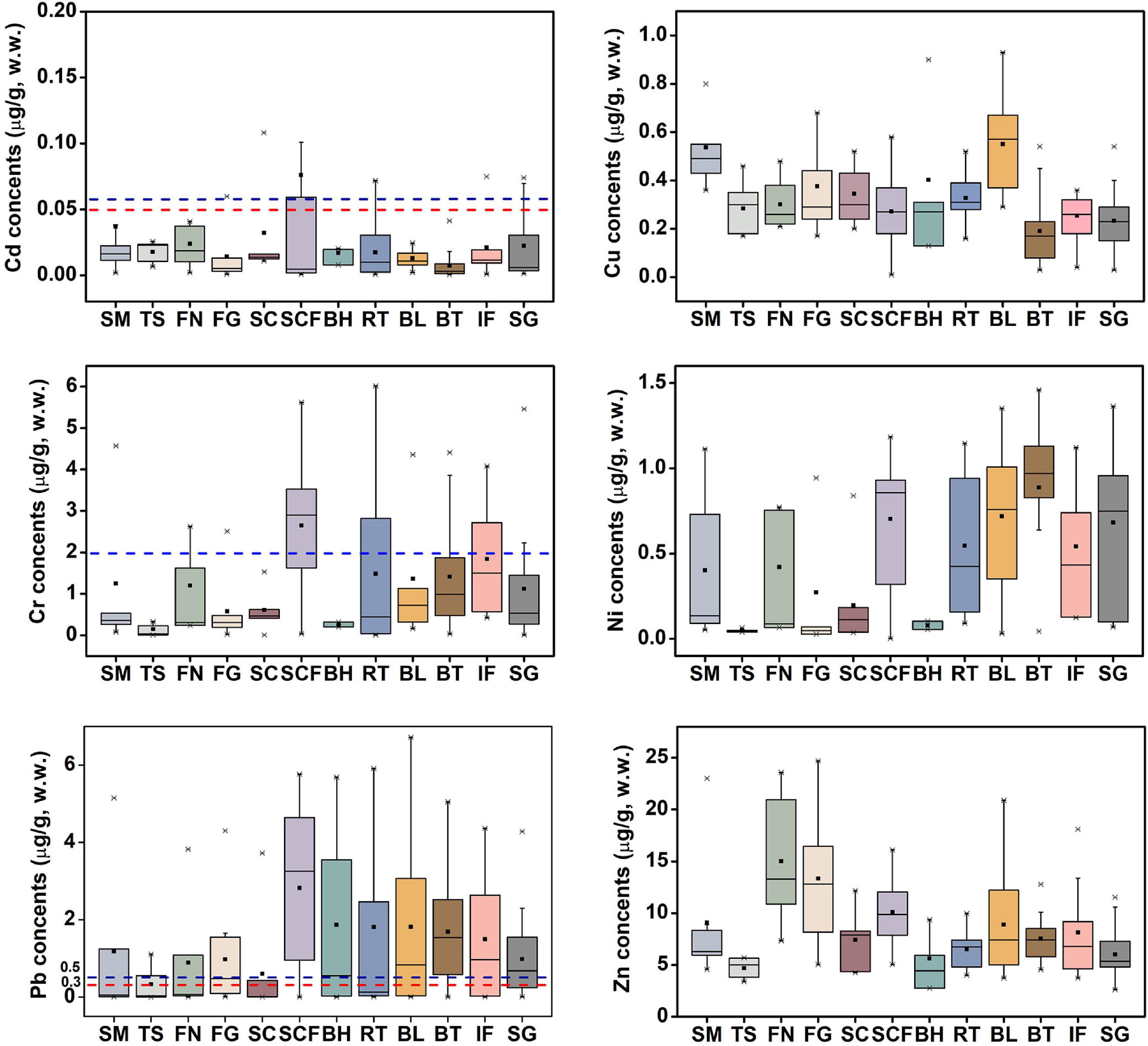
Figure 2. Concentrations (μg/g, w.w.) of trace metals in muscle tissue of marine fish from Liaodong Bay. Red dotted line – the maximum permissible levels for fish proposed by European Union Commission Regulation; Blue dotted line – the maximum permissible levels for fish proposed by Ministry of Health of the People’s Republic of China (Ministry of Health of the People’s Republic of China [MHPRC], 2017). SM: Spanish mackerel; TS: Tongue Sole; FN: Flat needlefish; FG: Fang’s gunnel; SC: Sea chub; SCF: Sea catfish; BH: Bastard halibut; RT: Robust tonguefish; BL: Bluefin leatherjacket; BT: Black tetra; IF: Indian flathead; SG: Shokihaze goby.
Metal levels ranged from 0.01 to 6.02 μg/g for Cr, “not detected” (hereafter, 0) to 1.46 μg/g for Ni, 0.03–0.91 μg/g for Cu, 0–6.72 μg/g for Pb, 2.75–24.71 μg/g for Zn, and 0–0.11 μg/g for Cd. Except for Cd (p > 0.05), there were significant differences among fish species. For example, the concentration of Cu in bluefin leatherjacket (0.55 ± 0.21) was significantly higher than that of black tetra (0.19 ± 0.12) (Mann-Whitney U test, p < 0.001), and the level of Zn in flat needlefish (15.02 ± 6.15) was significantly higher than that of the tongue sole (4.68 ± 0.96) (ANOVA, p < 0.001). This is related to feeding strategy, and may also be affected by differences in trophic position, habitat, chemical absorption and elimination kinetics (Varol et al., 2017; Hao et al., 2019). In general, the hierarchy of trace metals was: Zn > Pb ≈ Cr > Ni ≈ Cu > Cd. Liu J. H. et al. (2019) and Zhang et al. (2020) have, respectively, reported that the mean concentration of trace metals in marine organisms in the coastal areas of Guangzhou and Laizhou Bay in China was as follows: Zn > Cu > Ni > Cr > Cd > Pb, which is slightly different from the results of this study. However, the hierarchy of trace metals in marine fish from Saint Martin Island, Bangladesh, was Cd > Zn > Pb > Cu > Cr (Baki et al., 2018). This may be due to differences in metal concentrations in the local marine environment.
Cd and Pb are generally considered to be toxic, and there are differences between international regulations on the maximum allowable concentration of Cd and Pb in fishes. The maximum limits of Cd and Pb in Chinese national standards are 0.1 and 0.5 μg/g, respectively (Ministry of Health of the People’s Republic of China [MHPRC], 2017). Here we used the maximum allowable concentrations of Cd and Pb in edible fish stipulated by the stricter European Commission Regulations, which are 0.05 and 0.3 μg/g, respectively (European Union Commission Regulation, 2014, 2015). To intuitively compare the contents of trace metals in the muscle tissue of marine fish from Liaodong Bay and other regions see Table 2.
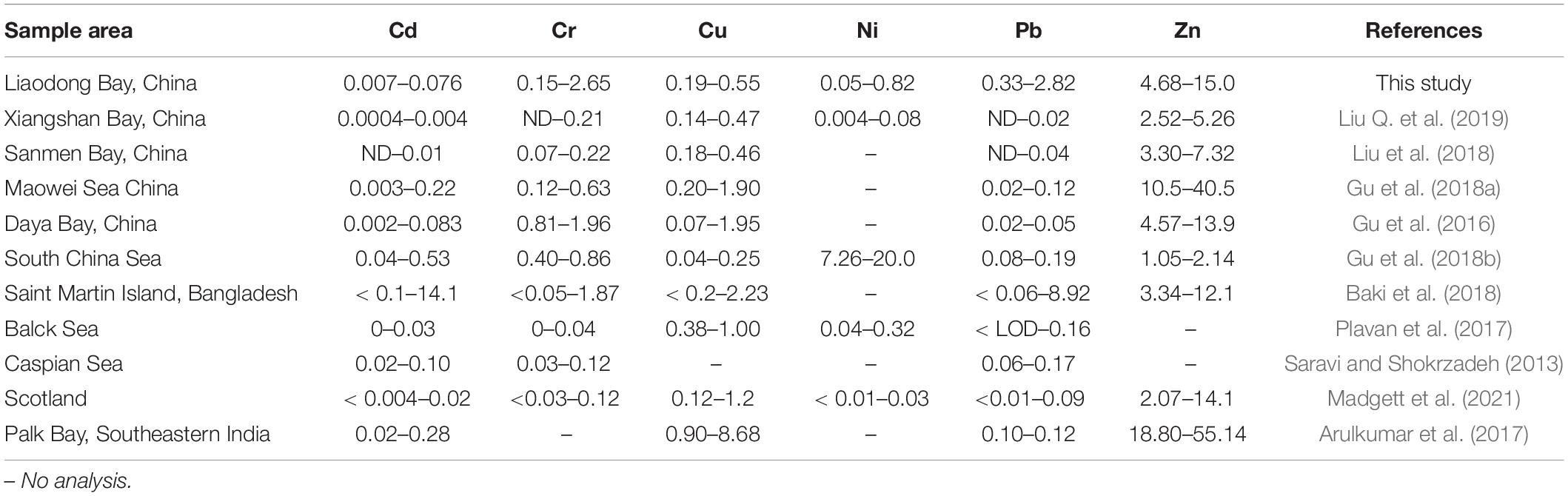
Table 2. Comparison of trace metal concentrations (μg/g, wet weight) in marine fish collected from Liaodong Bay and other areas.
For Cd, the average concentration (0.076 ± 0.149 μg/g) was the highest in sea catfish, and black tetra had the lowest (0.007 ± 0.012 μg/g). Among the 447 samples tested, we detected Cd contents in 11 (6.3%) shokihaze goby, 1 (1.5%) robust tonguefish, 1 (8.3%) fang’s gunnel, 13 (44.8%) indian flathead, and 1 (6.7%) sea chub: these exceeded the maximum allowable concentration (0.05 μg/g). Yet, the average concentration in each species did not exceed the maximum limit, except in sea catfish. These Cd levels are similar to those reported for fish from the Daya Bay of China (0.002–0.083 μg/g; Gu et al., 2016) and the Black Sea (0–0.03 μg/g; Plavan et al., 2017), but lower than those reported for the South China Sea (0.04–0.53 μg/g; Gu et al., 2018b) and Saint Martin Island (< 0.1–14.1 μg/g; Baki et al., 2018).
For Pb, the average concentration was 0.33–2.82 μg/g, and the maximum level (6.72 μg/g) was observed in the bluefin leatherjacket. The maximum average value was detected in sea catfish, and the tongue sole had the lowest levels. Ribeiro et al. (2005) pointed out that Pb can lead to decreased fertility and cell and tissue damage of Anguilla anguilla. Pb exposure can also cause body deformities in juvenile Chinese sturgeon (Hou et al., 2011). Deformities can be serious and fatal problems for fish, which can greatly reduce their free locomotion and effective foraging, thereby reducing their survival rate. In natural environments, Pb has been proven to be one of the reasons for the decrease of trophic level and population growth rate of common species in Liaodong Bay. In this study, the mean values of Pb were higher than the maximum limit (0.3 μg/g) for all fish species. These values are comparable to the Pb concentration in edible fishes (< 0.06–8.90 μg/g) in Saint Martin Island (Baki et al., 2018), but higher than the Pb concentration in marine fish in most other regions, such as the Caspian Sea (0.06–0.17 μg/g; Saravi and Shokrzadeh, 2013) and Xiangshan Bay, China (ND–0.02; Liu Q. et al., 2019). Considering the possible health risks of Pb caused by the consumption of marine fishes, the monitoring of Pb content in the muscle tissue of marine fishes in Liaodong Bay should continue to be strengthened.
Zn levels were the highest in all species, with a range from 2.62 to 24.71 μg/g. Flat needlefish had the highest levels (15.02 ± 6.15 μg/g), followed by fang’s gunnel (13.34 ± 5.49 μg/g) and sea catfish (10.09 ± 2.72). Tongue sole had the lowest levels (4.68 ± 0.96 μg/g). The average concentration was 8.69 μg/g. The observed values of Zn in marine fish muscles were similar to the values reported for Saint Martin Island (3.34–12.1 μg/g; Baki et al., 2018) and Scotland (2.07–14.1 μg/g; Madgett et al., 2021) but higher than those in fish from Xiangshan Bay, China (2.52–5.26 μg/g; Liu Q. et al., 2019) and South China (1.05–2.04 μg/g; Gu et al., 2018b). The maximum allowable Zn content in edible fishes by the European Union is 30 μg/g (Food and Agriculture Organization [FAO], 1983). No samples in this study exceeded this limit.
The Cu concentration varied between 0.01 and 0.93 μg/g, and the average value was 0.31 μg/g. Levels were lowest in black tetra (0.19 ± 0.12 μg/g) and highest in spanish mackerel (0.54 ± 0.15 μg/g). Our Cu concentrations did not exceed the maximum Cu content (30 μg/g) regulated by the Food and Agriculture Organization [FAO] (1983). Liu et al. (2018) reported similar values (0.18–0.46 μg/g) in different fish species from the Sanmen Bay, China, whereas our levels are lower than those reported in fishes from Palk Bay, India (0.90–8.68 μg/g; Arulkumar et al., 2017). As can be seen in Table 2, the range of Cu in marine fishes from different regions is not as high as other metals.
The data for Cr were as follows: range 0–6.02 μg/g; average 1.46 ± 1.47 μg/g; maximum level in sea catfish (2.65 ± 1.61 μg/g); lowest level in tongue sole (0.15 ± 0.16 μg/g). According to Chinese national standards, the maximum limit of Cr in edible fish is 2.0 μg/g (Ministry of Health of the People’s Republic of China [MHPRC], 2017). In all the samples in this study, except tongue sole and bastard halibut, individuals for each fish species had levels that exceeded the maximum allowable concentration. However, except for sea catfish, their mean concentration was all within the allowable level. Our levels are similar to those reported by Gu et al. (2016) in the Daya Bay, China (0.81–1.96 μg/g), but higher than those in fish species from Scotland (< 0.03–0.12; Madgett et al., 2021), Sanmen Bay (0.07–0.22; Liu et al., 2018), and the Black Sea (0–0.04; Plavan et al., 2017).
The data for Ni were as follows: range 0–1.46 μg/g; maximum value and maximum average concentration (0.82 ± 0.45 μg/g) in black tetra; lowest level (0.05 ± 0.01 μg/g) in tongue sole. Compared with other trace metals, reports of Ni in marine fishes are relatively few. Ni concentrations in fish from the South China Sea were 7.26–13.92 μg/g (Gu et al., 2018b), much higher than the values measured in this study. However, the Ni concentrations in marine fishes in Liaodong Bay were higher than the values reported in Xiangshan Bay, China (0.004–0.08; Liu Q. et al., 2019) and Scotland (< 0.01–0.03; Madgett et al., 2021). There is no limit on the amount of Ni for seafood by Chinese standards.
Time Series of Trace Metal Concentrations
Time series analysis of Cr, Ni, and Pb levels in the studied fishes showed a statistically significant historical trend of decline whereas Cd fluctuated between 2015 and 2019 and increased in 2020; no obvious changes were observed in Zn and Cu. Nevertheless, the content of Zn in some marine fishes, such as spanish mackerel, sea chub, indian flathead, and black tetra, have declined in recent years compared with 2015 (Figure 3).
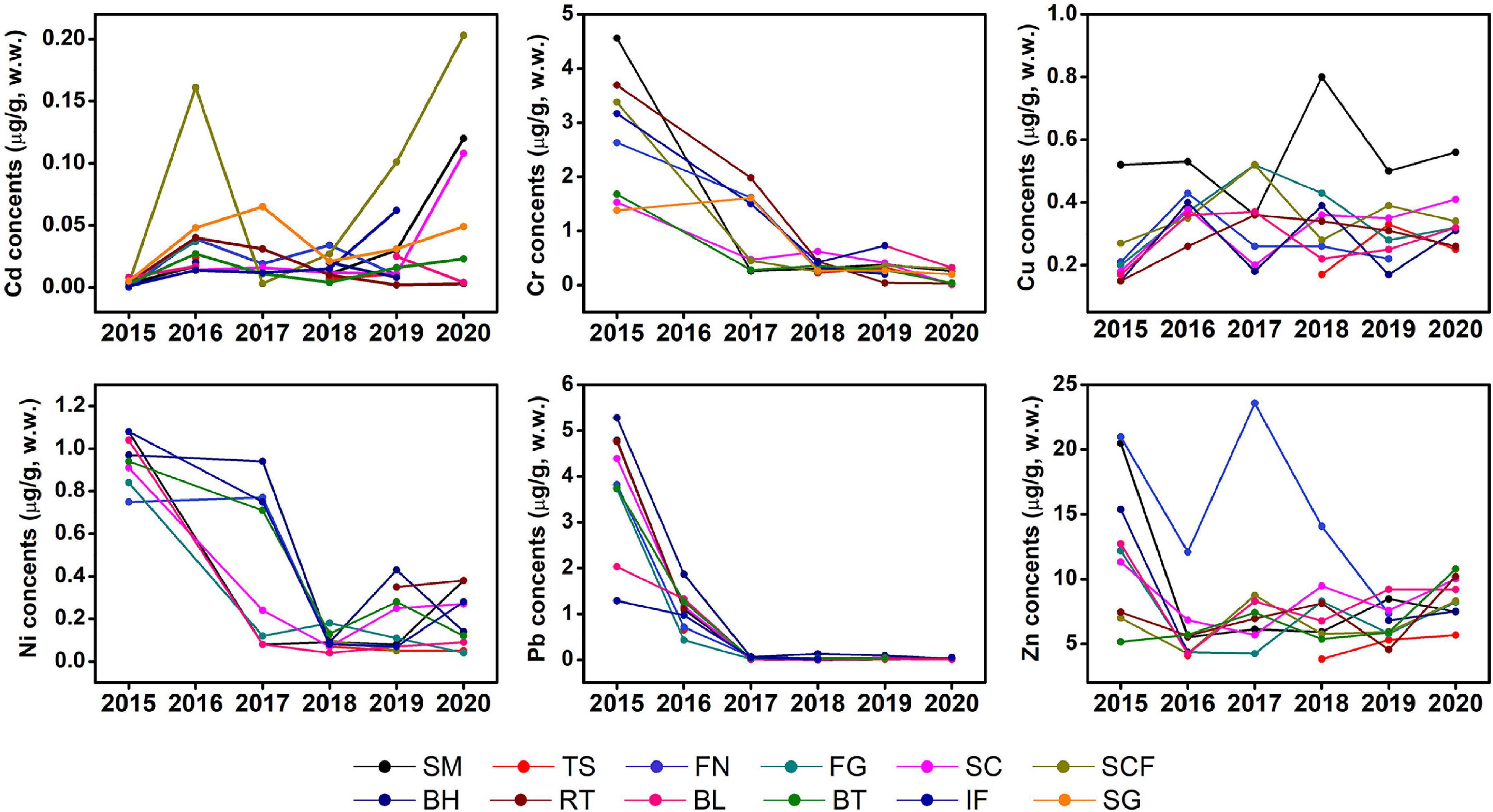
Figure 3. Interannual variations of mean concents of trace metals (μg/g, w.w.) in muscle tissues of marine fish in Liaodong Bay from 2015 to 2020. SM: Spanish mackerel; TS: Tongue Sole; FN: Flat needlefish; FG: Fang’s gunnel; SC: Sea chub; SCF: Sea catfish; BH: Bastard halibut; RT: Robust tonguefish; BL: Bluefin leatherjacket; BT: Black tetra; IF: Indian flathead; SG: Shokihaze goby.
Liaodong Bay has poor water exchange and self-purification capabilities, and the ecosystem is extremely fragile. Since China adopted a policy of reform and opening-up at the end of the 1970s, the long-term increase and accumulation of pollutants have led to a sharp deterioration in its environmental quality. In 2001, China launched a 15-year “Bohai Blue Ocean Action Plan,” but it failed due to unsatisfactory governance effects and insufficient funding. But even so, the study of Wang and Wang (2007) showed that compared with earlier data the average concentration of dissolved trace metals in the Bohai Sea has decreased, and the trace metals in the sediments have as well (North Sea Branch of the State Oceanic Administration, 2016).
Land-sourced pollution is the main source of environmental pollution in the Bohai Sea (Gao et al., 2014). In 2018, China carried out the “Action Plan for the Comprehensive Treatment of the Bohai Sea,” and breakthroughs were also made in land-based pollution control. Trace metals can enter the fish body through gills, skin, and the digestive tract (Ahmed et al., 2016). There is a close relationship between the concentration of trace metals in seawater, sediments, and organisms, and the concentration of trace metals in sediments is much higher than that in seawater and marine organisms (Gao et al., 2014). Coastal sediment is an important sink and reservoir of trace elements (Eggleton and Thomas, 2004), which is a good indicator of marine environmental pollution. A large number of studies have investigated the content of trace metals in sediments of Liaodong Bay. To further examine trends of trace metals in marine fishes in this study, we refer to the concentration of trace metals in the sediments of the Liaodong Bay in recent years (Supplementary Table 1). We found that there was an obvious downward trend in Cr and Pb, and Zn also shows a variable downward trend. However, the data of Ni in the sediments are insufficient and more study is needed, but the fluctuation range is not large. In recent years, the concentration of Cd and Cu also fluctuated over years; It can be seen from these data that the decrease of Cr and Pb concentration in liaodong Bay is the main reason for the decrease of Cr and Pb concentration in fish species, and further study is warranted. The concentration of trace metals in the marine environment affects the concentration of trace metals in marine organisms, and further study is warranted.
Effect of Feeding Strategy on Trace Metal Levels in Marine Fish
The metal content in fish tissue may be affected by fish characteristics such as dietary strategy, habitat, metabolism, body length, and weight (Ali and Khan, 2018). In this study, no significant correlation was found between metal concentration and fish length, nor in weight (p > 0.05). Due to direct contact with sediments, feeding on benthic organisms, and metal-benthic interactions, benthic fish may have higher concentrations of trace metals than fishes that frequent the water column (Gu et al., 2015; Anandkumar et al., 2018). Velusamy et al. (2014) reported that the accumulation of trace metals in benthic fishes is the highest, followed by shallow water and pelagic fish. However, in our study, no significant differences (p > 0.05) were found in the concentration of trace metals among different habitats except for Cu. The study of Signa et al. (2017) also shown that Cd bioaccumulation in fish was not affected by habitat. This may be the reason that the trace elements in fish muscle tissues are more affected by feeding strategies and other factors than the habitat.
Studies have also shown that dietary uptake is the most influential factor for the accumulation of trace metals in marine organisms (Jakimska et al., 2011). Hence, we divided the dietary strategy of all studied fish species into four categories: carnivorous, planktivorous, benthivorous, and omnivorous. As shown in Figure 4, except for Cu, metal levels significantly varied by feeding habit. Generally speaking, the order was as follows: omnivorous > carnivorous > benthivorous (except for benthivorous > omnivorous for Cd). Moreover, Cu and Zn levels were highest in zooplankton feeders whereas the other metals were lowest in such species. Previous studies differed from this study in the effect of feeding strategy on the accumulation of trace metals in fishes. The bioaccumulation of Cd and Pb in three fish species from Nansi Lake, China was the highest among carnivorous species, followed by omnivorous and filter species (Li et al., 2015). However, Liu et al. (2015) found that the highest concentrations of Cr, Zn, and Pb were in omnivorous fishes, but the Cu content was highest in benthivorous fish, and filter feeder fish had the lowest Cr, Cu, Zn, and Pb content. Yousafzai et al. (2010) also reported that omnivorous fish may accumulate higher concentrations of trace metals than carnivorous fish, which is consistent with our results. These suggest that there is no significant relationship between nutrient levels and bioaccumulation of trace metals. The trophic transfer of trace metals in marine food webs has always been controversial. Previous studies have shown that metal concentrations in organisms may not be related to trophic levels in the food chain (Wang, 2002). Recently, however, Sun et al. (2020) found that Pb and Zn were biomagnified in the food webs, and Cd, Cr, and Cu did not show obvious biomagnification or biodilution trends. The study of Laizhou Bay in the Bohai Sea showed that Cr was bioamplified and Cu was biodiluted in the food web (Liu J. H. et al., 2019). These differences may be related to many factors such as community structure, environmental conditions, and temporal and spatial differences (Sun et al., 2020).
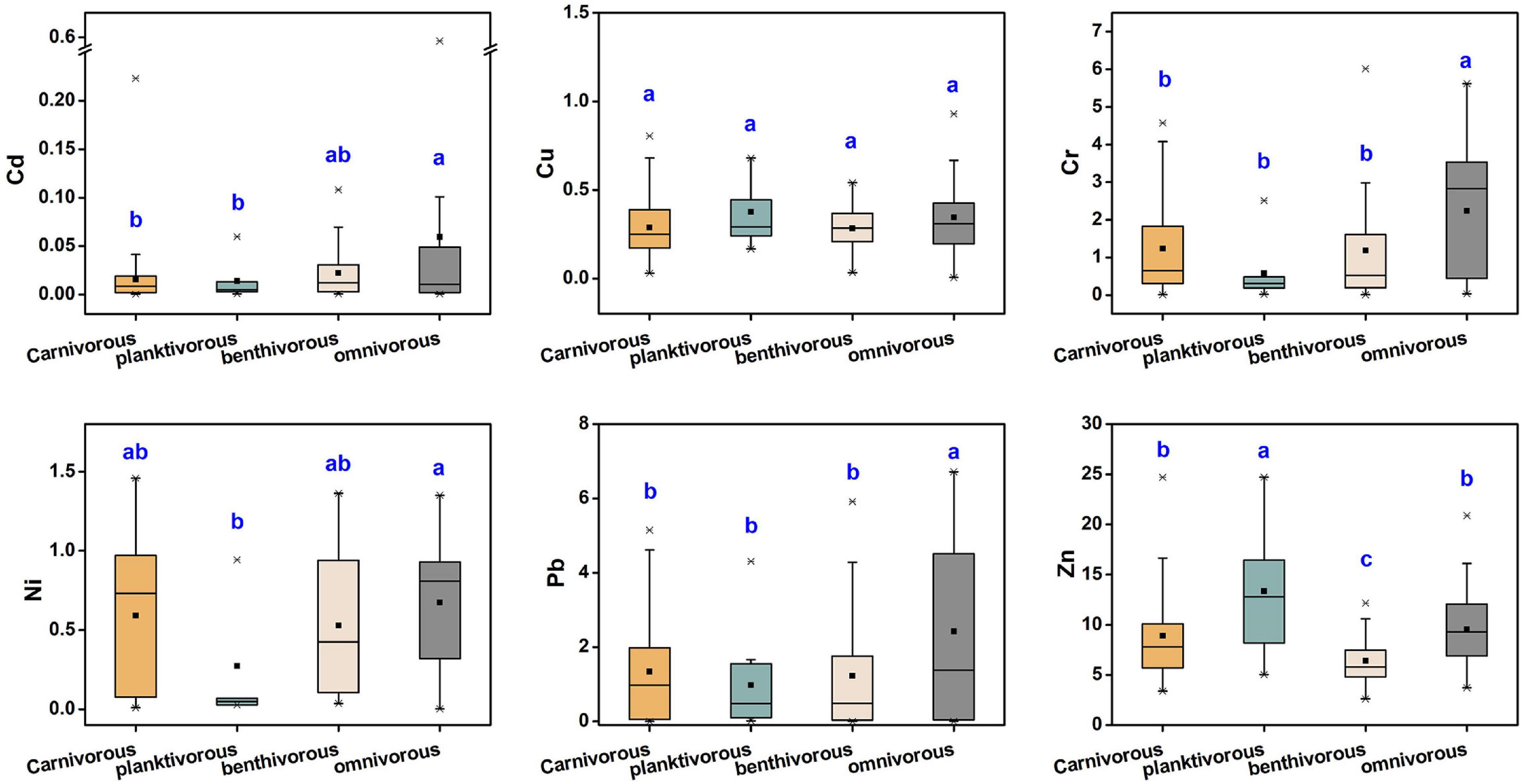
Figure 4. The difference of trace metal concentrations (μg/g, w.w.) in marine muscle tissue with different feeding strategies.
Selection of Potential Bioindicator Species in Liaodong Bay
Fishes are sensitive to toxic substances in water (Jarić et al., 2011), and many studies have used fishes as bioindicators of pollutants in aquatic ecosystems. For example, horse mackerel is an appropriate bioindicator for microplastic monitoring in the pelagic Iberian ecosystem (Lopes et al., 2020). Santos et al. (2021) suggested that Orthopristis ruber could be used as a sensitive species for monitoring mercury accumulation. At the top of the aquatic food chain, fish accumulate high concentrations of trace metals in their tissues. Toxic metals can have some toxic effects on fishes, such as affecting reproduction, reducing developmental growth, weakening the immune system, and causing pathological changes in tissue or cellular structure (Luczynska et al., 2018; Cunningham et al., 2019). Long-term exposure to toxic metals can also reduce population sizes and the biodiversity of fish communities. For example, Pb is considered the direct or indirect cause of the decline of fish trophic levels in the Bohai Sea (Gao et al., 2014). Therefore, fishes can be good biological indicators of the effects of metals. There are three main criteria when selecting bioindicator species in fishes: the species should be widely consumed in the area, geographically widely distributed, and have the potential to accumulate high concentrations of metals (Cunningham et al., 2019). Based on this concept, we constructed a matrix list based on the methods of Cunningham et al. (2019) to evaluate various species characteristics, such as the accumulation of toxic metals, habitat, feeding strategy, commercial status, IUCN Red List ratings, and vulnerability (Table 3).
Because all fish analyzed in this study were obtained from fishing boats and were traded in large quantities in the local fish market, the lowest IUCN Red List rating was of least concern, which would meet the standards of use as a bioindicator. When selecting biological indicator species, we considered three habitats (demersal, reef-associated, and pelagic) and four feeding strategies (omnivorous, carnivorous, benthivorous, and planktivorous). Their ability to accumulate Cd and Pb was also considered. Bluefin leatherjacket and sea catfish are omnivorous fish. Although their IUCN Red List ratings are both of least concern, during our sampling period, the market abundance of bluefin leatherjacket was not as abundant as that of sea catfish. Sea catfish has flexible requirements for habitat water quality and depth. There are also large numbers of sea catfish in lightly polluted and shallow waters where other marine fish cannot survive. In addition, the accumulation of toxic metals in sea catfish is relatively high, and the average concentration can exceed the maximum allowable levels. Therefore, sea catfish was identified as a bioindicator for demersal-omnivorous species. Sea chub and robust tonguefish are benthivorous fish, and they have the same commercial status and IUCN Red List rating. However, the accumulated Pb concentration in robust tonguefish is much higher than that of sea chub, so robust tonguefish was selected as the bioindicator for demersal-benthivorous species. Fang’s gunnel and spanish mackerel are the only planktivorous and pelagic-neritic species, respectively. Although the commercial status of the fang’s gunnel has not been evaluated, it is widely sold in the local market, so they were also selected as bioindicators of metal pollution in Liaodong Bay. Reef-associated fishes include flat needlefish and the indian flathead, both of which are commercially valuable species. The commercial status, IUCN Red List rating, and accumulated Pb content of flat needlefish are lower than that of indian flathead. Therefore, we choose the indian flathead as a bioindicator of reef-associated carnivorous species. Finally, five potential bioindicator species were identified, as shown in bold font in Table 3. The combination of these five bioindicators represents a wide range of habitat types and feeding strategies, and the species are relatively abundant in the study area.
Human Health Risk Assessment
Among the metals studied, Cd and Pb are the most concerning contaminants. These toxic metals are both nephrotoxic, particularly damaging to the renal cortex (Wilk et al., 2017). Cd accumulates in the human body, which can induce renal injury accompanied by proximal tubular reabsorption dysfunction (Järup and Åkesson, 2009). Children are more sensitive to Pb poisoning due to their growth and metabolism. Pb poisoning has adverse effects on the psychological development and behavior of children aged 2–4 years (Hou et al., 2013). Zn is one of the most important trace elements in the human body, involved in most metabolic pathways. A lack of Zn can lead to loss of appetite, growth inhibition, skin changes, and immune abnormalities. However, excessive Zn intake is harmful to human health and can cause poisoning, nausea, acute stomach pain, diarrhea, and fever (Chi et al., 2007; Tuzen, 2009). Cu is an essential trace mineral that plays an important role in enzymatic processes and is necessary for the synthesis of hemoglobin. However, excessive intake can also lead to adverse health problems, such as liver and kidney damage (Ikem and Egiebor, 2005; Satheeshkumar et al., 2011). Cr is an important trace element that plays a crucial role in glucose metabolism (Mertz, 1969). Insufficient dietary intake of Cr is related to increased risk for diabetes and cardiovascular disease (Calabrese et al., 1985; Mertz, 1993; Anderson, 2000).
To assess the effects of the consumption of contaminated fishes on human health, it is essential to first gather fish consumption information. People living along the coast often consume extremely high amounts of seafood. For example, the estimated daily consumption of marine fishes by residents in Hainan Province is 131.8 g/day according to Wang et al. (2019). In this study, we only calculated the health risks for women and children because of their sensitivity to trace metals. Women and children were divided into two groups according to consumption and age, namely, 131 g/day, 55 g/day for women and ages 2–5 (16 g/day), and 6–11 (37.9 g/day) for children. The potential health risks associated with fish consumption in 2015–2016 are shown in Figure 5. The TTHQ values decreased in the order: women (131.8 g/day) > children (6–11 years old) > children (2–5 years old) > women (55 g/day); this indicates that children face higher health risks than women (except for extreme consumption scenarios, 131.8 g/day). Corresponding to the time trend of concentration of trace metals, the THQ values of Cr, Pb, and Ni showed a downward trend, Cd showed an upward trend, and Zn and Cu fluctuated between years. The TTHQ value of each population group was the highest in 2015. In addition, under the extreme consumption scenario, the TTHQ values of some marine fish were greater than 1, such as spanish mackerel, tobust tonguefish, and bluefin leatherjacket. This is due to the high concentration of Pb in fish tissue in 2015, with the highest THQ value being 1.03 in robust tonguefish. Coastal women may be exposed to potential non-carcinogenic health risks associated with Pb through the consumption of this marine fish, the consumption of these marine fish should be controlled. However, the TTHQ values of all studied species were less than 1 from 2016 to 2020. Except for 2016, Zn and Cd had the highest contribution to the TTHQ values, followed by Ni and Cu. This is due to the decrease in the contribution of Pb to TTHQ, and it also proves that Pb pollution has been more effectively controlled and coastal-living women may not suffer any significant health risks related to this metal.
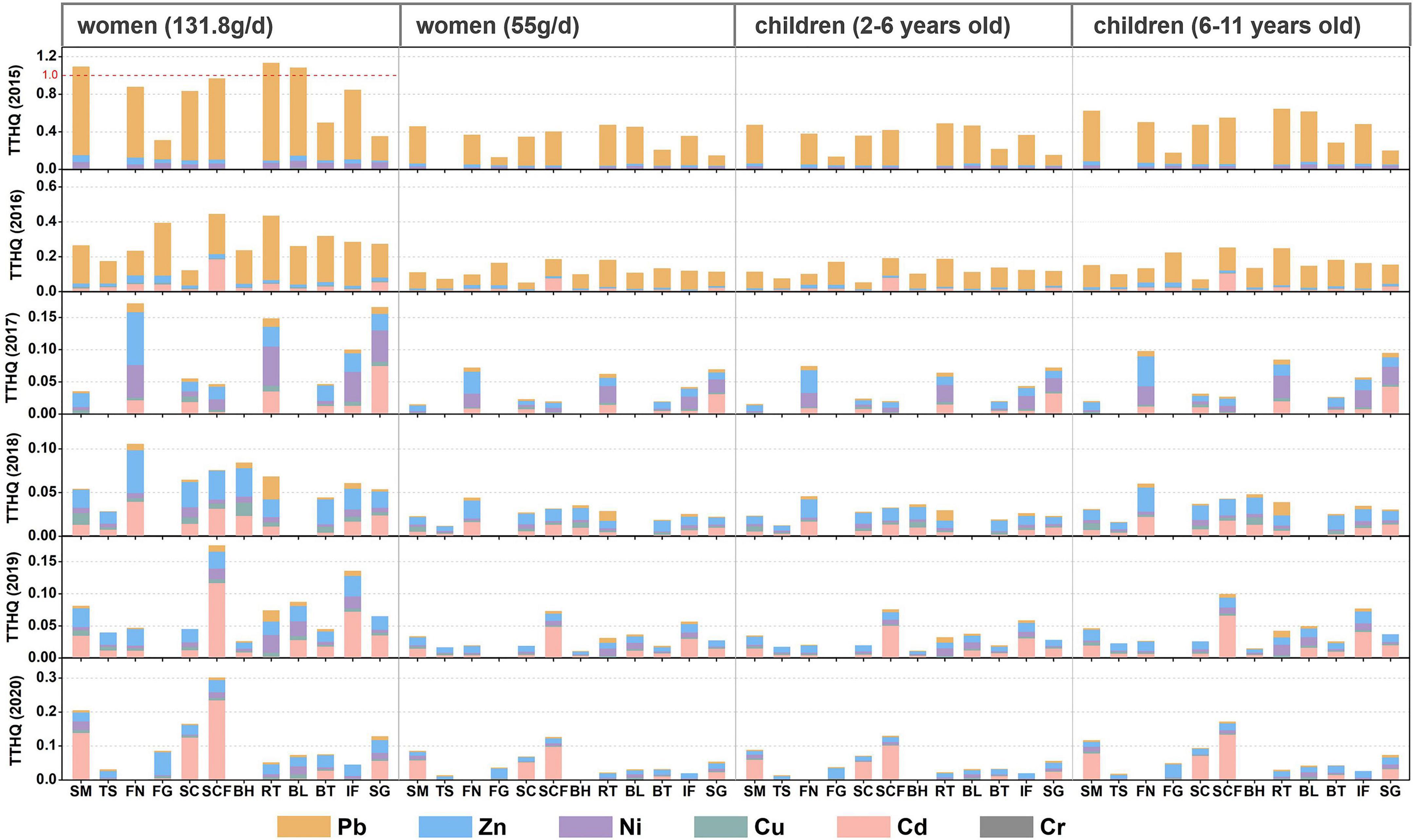
Figure 5. Total target hazaed quotients (TTHQ) of metals in marine fish from Liaodong Bay. SM: Spanish mackerel; TS: Tongue Sole; FN: Flat needlefish; FG: Fang’s gunnel; SC: Sea chub; SCF: Sea catfish; BH: Bastard halibut; RT: Robust tonguefish; BL: Bluefin leatherjacket; BT: Black tetra; IF: Indian flathead; SG: Shokihaze goby.
Conclusion
The concentrations of trace metals (Cd, Cr, Cu, Ni, Pb, and Zn) in 12 marine fish species from Liaodong Bay from 2015 to 2020 were investigated. The concentrations of Pb and Cd were relatively high and exceeded the maximum allowable concentrations (0.3 and 0.05 μg/g, respectively) in some species. Except for Cu, trace metal levels significantly differed by diet strategy. Interannual variation in Cd and Cu levels in fish showed an upward trend, whereas Cr, Ni, and Pb showed a downward trend, with no obvious changes in Zn. Our data suggest that fang’s gunnel, robust tonguefish, spanish mackerel, indian flathead, and sea catfish could serve as good bioindicators of metal concentrations in Liaodong Bay; these species represent different habitats and feeding strategies. We also found that excessive consumption of certain fish, such as sea catfish, can have a non-carcinogenic health risk in women in 2015. However, as the Pb concentration in fish in Liaodong Bay has declined in recent years, the consumption of fish will no longer poses non-carcinogenic health risks to humans.
Data Availability Statement
The original contributions presented in the study are included in the article/Supplementary Material, further inquiries can be directed to the corresponding author.
Ethics Statement
The animal study was reviewed and approved by Ethics Committee of the first Hospital of Jilin University Clinical Trial and Study Approval.
Author Contributions
SW: performing most of the experiments, data curation, and writing – original draft. NZ: funding acquisition, writing – review and editing, and supervision. SS and QA: software. PL: formal analysis. XL: conceptualization. ZL: validation. WZ: investigation. All authors contributed to the article and approved the submitted version.
Conflict of Interest
The authors declare that the research was conducted in the absence of any commercial or financial relationships that could be construed as a potential conflict of interest.
Publisher’s Note
All claims expressed in this article are solely those of the authors and do not necessarily represent those of their affiliated organizations, or those of the publisher, the editors and the reviewers. Any product that may be evaluated in this article, or claim that may be made by its manufacturer, is not guaranteed or endorsed by the publisher.
Acknowledgments
We would like to acknowledge the support of the National Natural Science Foundation of China (No. 42177413). Leading talents and Team Project of Scientific and Technological Innovation for young and Middle-aged Group in Jilin Province (20200301015RQ). Major Special Program of Scientific and Technological of Jilin Province (20200503003SF), and Research Fund Program of Guangdong Provincial Key Laboratory of Environmental Pollution Control and Remediation Technology (2020B1212060022).
Supplementary Material
The Supplementary Material for this article can be found online at: https://www.frontiersin.org/articles/10.3389/fmars.2021.789572/full#supplementary-material
References
Ahmed, M., Ahmad, T., Liaquat, M., Abbasi, K. S., Farid, I. B. A., and Jahangir, M. (2016). Tissue specific metal characterization of selected fish species in Pakistan. Environ. Monit. Assess. 188:212. doi: 10.1007/s10661-016-5214-6
Ali, H., and Khan, E. (2018). Bioaccumulation of non-essential hazardous heavy metals and metalloids in freshwater fish. Risk to human health. Environ. Chem. Lett. 163, 903–917. doi: 10.1007/s10311-018-0734-7
Anandkumar, A., Nagarajan, R., Prabakaran, K., Bing, C. H., and Rajaram, R. (2018). Human health risk assessment and bioaccumulation of trace metals in fish species collected from the Miri coast, Sarawak, Borneo. Mar. Pollut. Bull. 133, 655–663. doi: 10.1016/j.marpolbul.2018.06.033
Anderson, R. A. (2000). Chromium in the prevention and control of diabetes. Diabetes Metab. 26, 22–27.
Arulkumar, A., Paramasivam, S., and Rajaram, R. (2017). Toxic heavy metals in commercially important food fishes collected from Palk Bay, Southeastern India. Mar. Pollut. Bull. 119, 454–459. doi: 10.1016/j.marpolbul.2017.03.045
Azizi, G., Layachi, M., Akodad, M., Yanez-Ruiz, D. R., Martin-Garcia, A. I., Baghour, M., et al. (2018). Seasonal variations of heavy metals content in mussels (Mytilus galloprovincialis) from Cala Iris offshore (Northern Morocco). Mar. Freshwater Res. 137, 688–694. doi: 10.1016/j.marpolbul.2018.06.052
Baki, M. A., Hossain, M. M., Akter, J., Quraishi, S. B., Shojib, M. F. H., Ullah, A. K. M. A., et al. (2018). Concentration of heavy metals in seafood (fishes, shrimp, lobster and crabs) and human health assessment in Saint Martin Island, Bangladesh. Ecotoxi. Environ. Safety 159, 153–163. doi: 10.1016/j.ecoenv.2018.04.035
Briaudeau, T., Zorita, I., Cuevas, N., Franco, J., Marigomez, I., and Izagirre, U. (2019). Multi-annual survey of health status disturbance in the Bilbao estuary (Bay of Biscay) based on sediment chemistry and juvenile sole (Solea spp.) histopathology. Mar. Pollut. Bull. 145, 126–137. doi: 10.1016/j.marpolbul.2019.05.034
Calabrese, E. J., Canada, A. T., and Sacco, C. (1985). Trace elements and public health. Annu. Rev. Pub. Health 6, 131–146.
Chi, Q. Q., Zhu, G. W., and Alan, L. (2007). Bioaccumulation of heavy metals in fishes from Taihu Lake, China. J. Environ. Sci. 19, 1500–1504. doi: 10.1016/S1001-0742(07)60244-7
CRNTCD (2020). Chinese Residents and Nutrition Report on Chronic Diseases. Beijing: National Health Commission of the People’s Republic of China (National Health Commission, PRC).
Cunningham, P. A., Sullivan, E. E., Everett, K. H., Kovach, S. S., Rajan, A., and Barber, M. C. (2019). Assessment of metal contamination in Arabian/Persian Gulf fish: a review. Mar. Pollut. Bull. 143, 264–283. doi: 10.1016/j.marpolbul.2019.04.007
Domingo, J. L. (2016). Nutrients and chemical pollutants in fish and shellfish. Balancing health benefits and risks of regular fish consumption. Crit. Rev. Food Sci. 56, 979–988. doi: 10.1080/10408398.2012.742985
Eggleton, J., and Thomas, K. V. (2004). A review of factors affecting the release and bioavailability of contaminants during sediment disturbance events. Environ. Int. 30, 973–980. doi: 10.1016/j.envint.2004.03.001
European Union Commission Regulation (2014). EU 488/2014 of 12 May 2014, Amending Regulation EC 1881/2006 as Regards Maximum Levels of Cadmium in Foodstuffs. Brussels: The European Commission. Available online at: http://extwprlegs1.fao.org/docs/pdf/eur133613.pdf
European Union Commission Regulation (2015). EU 2015/1005 of 25 June 2015, Amending Regulation EC 1881/2006 as Regards Maximum Levels of Lead in Certain Foods. Brussels: The European Commission. Available online at: http://extwprlegs1.fao.org/docs/pdf/eur146524.pdf
Food and Agriculture Organization [FAO] (1983). Compilation of Legal Limits for Hazardous Substances in Fish and Fishery Products. FAO Fishery Circular No. 464. Rome: Food and Agriculture Organization of the United Nations.
Froese, R., and Pauly, D. (2017). FishBase — A Global Information System on Fishes. Available online at: http://fishbase.org/search.php (accessed October 2021).
Gao, X. L., Zhou, F. X., and Chen, C. T. A. (2014). Pollution status of the Bohai Sea: an overview of the environmental quality assessment related trace metals. Environ. Int. 62, 12–30. doi: 10.1016/j.envint.2013.09.019
Gao, Y. F., Wang, R. Y., Li, Y. Y., Ding, X. B., Jiang, Y. M., Feng, J. F., et al. (2021). Trophic transfer of heavy metals in the marine food web based on tissue residuals. Sci. Total. Environ. 772:145064. doi: 10.1016/j.scitotenv.2021.145064
Ge, M., Liu, G. J., Liu, H. Q., and Liu, Y. (2020). Levels of metals in fish tissues of Liza haematocheila and Lateolabrax japonicus from the Yellow River Delta of China and risk assessment for consumers. Mar. Pollut. Bull. 157:111286. doi: 10.1016/j.marpolbul.2020.111286
Gu, Y. G., Huang, H. H., and Lin, Q. (2016). Concentrations and human health implications of heavy metals in wild aquatic organisms captured from the core area of Daya Bay’s Fishery Resource Reserve, South China Sea. Environ. Toxicol. Pharmacol. 45, 90–94. doi: 10.1016/j.etap.2016.05.022
Gu, Y. G., Huang, H. H., Liu, Y., Gong, X. Y., and Liao, X. L. (2018a). Non-metric multidimensional scaling and human risks of heavy metal concentrations in wild marine organisms from the Maowei Sea, the Beibu Gulf, South China Sea. Environ. Toxicol. Pharmacol. 59, 119–124. doi: 10.1016/j.etap.2018.03.002
Gu, Y. G., Ning, J. J., Ke, C. L., and Huang, H. H. (2018b). Bioaccessibility and human health implications of heavy metals in different trophic level marine organisms: a case study of the South China Sea. Ecotoxicol. Environ. Saf. 163, 551–557. doi: 10.1016/j.ecoenv.2018.07.114
Gu, Y. G., Lin, Q., Wang, X. H., Du, F. Y., Yu, Z. L., and Huang, H. H. (2015). Heavy metal concentrations in wild fishes captured from the South China Sea and associated health risks. Mar. Pollut. Bull. 96, 508–512. doi: 10.1016/j.marpolbul.2015.04.022
Guo, J., Wu, F., Shen, R., and Zeng, E. Y. (2010). Dietary intake and potential health risk of DDTs and PBDEs via seafood consumption in South China. Ecotoxicol. Environ. Saf. 73, 1812–1819. doi: 10.1016/j.ecoenv.2010.08.009
Guo, Y. D., Mei, X., Meng, X. J., Lan, X. H., Chen, H. J., and Yang, H. (2020). Benthic foraminifera and its response to heavy metal pollution: a case study from Liaodong Bay, China. Mar. Pollut. Bull. 154:111084. doi: 10.1016/j.marpolbul.2020.111084
Hang, X. S., Wang, H. Y., Zhou, J. M., Ma, C. L., Du, C. W., and Chen, X. Q. (2009). Risk assessment of potentially toxic element pollution in soils and rice (Oryza sativa) in a typical area of the Yangtze River Delta. Environ. Pollut. 157, 2542–2549. doi: 10.1016/j.envpol.2009.03.002
Hao, Z., Chen, L. H., Wang, C. L., Zou, X. Q., Zheng, F. Q., Feng, W. H., et al. (2019). Heavy metal distribution and bioaccumulation ability in marine organisms from coastal regions of Hainan and Zhoushan, China. Chemosphere 226, 340–350. doi: 10.1016/j.chemosphere.2019.03.132
Hou, J. L., Zhuang, P., Zhang, L. Z., Feng, L., Zhang, T., Liu, J. Y., et al. (2011). Morphological deformities and recovery, accumulation and elimination of lead in body tissues of Chinese sturgeon, Acipenser sinensis, early life stages: a laboratory study. J. Appl. Ichthyol. 27, 514–519. doi: 10.1111/j.1439-0426.2011.01703.x
Hou, S. X., Yuan, L. F., Jin, P. P., Ding, B. J., Qin, N., Li, L., et al. (2013). A clinical study of the effects of lead poisoning on the intelligence and neurobehavioral abilities of children. Theor. Biol. Med. Model. 10:13. doi: 10.1186/1742-4682-10-13
Ikem, A., and Egiebor, N. O. (2005). Assessment of trace elements in canned fishes (mackerel, tuna, salmon, sardines and herrings) marketed in Georgia and Alabama (United States of America). J. Food Compos. Anal. 18, 771–787. doi: 10.1016/j.jfca.2004.11.002
Jakimska, A., Konieczka, P., Skora, K., and Namiesnik, J. (2011). Bioaccumulation of metals in tissues of marine animals, part II: metal concentrations in animal tissues. Pol. J. Environ. Stud. 10, 1127–1146.
Jarić, I., Višnjić-Jeftić, Z., Cvijanović, G., Gačić, Z., Jovanović, L., Skorić, S., et al. (2011). Determination of differential heavy metal and trace element accumulation in liver, gills, intestine and muscle of sterlet (Acipenser ruthenus) from the Danube River in Serbia by ICP-OES. Microchem. J. 98, 77–81. doi: 10.1016/j.microc.2010.11.008
Järup, L., and Åkesson, A. (2009). Current status of cadmium as an environmental health problem. Toxicol. Appl. Pharmacol. 238, 201–208. doi: 10.1016/j.taap.2009.04.020
Jonathan, M. P., Aurioles-Gamboa, D., Villegas, L. E. C., Bohorquez-Herrera, J., Hernandez-Camacho, C. J., and Sujitha, S. B. (2015). Metal concentrations in demersal fish species from Santa Maria Bay, Baja California Sur, Mexico (Pacific coast). Mar. Pollut. Bull. 99, 356–361. doi: 10.1016/j.marpolbul.2015.07.032
Koker, L., Aydin, F., Gaygusuz, O., Akcaalan, R., Camur, D., Ilter, H., et al. (2020). Heavy metal concentrations in Trachurus Mediterraneus and Merlangius merlangus captured from Marmara Sea, Turkey and associated health risks. Environ. Manage. 67, 522–553. doi: 10.1007/s00267-020-01352-y
Li, P., Zhang, J., Xie, H., Liu, C., Liang, S., Ren, Y., et al. (2015). Heavy metal bioaccumulation and health hazard assessment for three fish species from Nansi Lake, China. Bull. Environ. Contam. Toxicol. 94, 431–436. doi: 10.1007/s00128-015-1475-y
Liu, J. H., Gao, L., and Dou, S. Z. (2019). Trophic transfer, biomagnification and risk assessments of four common heavy metals in the food web of Laizhou Bay, the Bohai Sea. Sci. Total. Environ. 670, 508–522. doi: 10.1016/j.scitotenv.2019.03.140
Liu, J. L., Xu, X. R., Ding, Z. H., Peng, J. X., JIn, M. H., Wang, Y. S., et al. (2015). Heavy metals in wild marine fish from South China Sea: levels, tissue- and species-specific accumulation and potential risk to humans. Ecotoxicology 24, 1583–1592. doi: 10.1007/s10646-015-1451-7
Liu, J. L., Xu, X. R., Yu, S., Cheng, H. F., Peng, J. X., Hong, Y. G., et al. (2014). Mercury contamination in fish and human hair from Hainan Island, South China Sea: Implication for human exposure. Environ. Res. 135, 42–47. doi: 10.1016/j.envres.2014.08.023
Liu, Q., Liao, Y. B., and Shou, L. (2018). Concentration and potential health risk of heavy metals in seafoods collected from Sanmen Bay and its adjacent areas, China. Mar. Pollut. Bull. 131, 356–364. doi: 10.1016/j.marpolbul.2018.04.041
Liu, Q., Xu, X. Q., Zeng, J. N., Shi, X. L., Liao, Y. B., Du, P., et al. (2019). Heavy metal concentrations in commercial marine organisms from Xiangshan Bay, China, and the potential health risks. Mar. Pollut. Bull. 141, 215–226. doi: 10.1016/j.marpolbul.2019.02.058
Liu, Y. H., Xu, J. Y., Wang, Y., and Yang, S. (2021). Trace metal bioaccumulation in oysters (Crassostrea gigas) from Liaodong Bay (Bohai Sea, China). Environ. Sci. Pollut. Res. 28, 20682–20689. doi: 10.1007/s11356-020-11968-6
Lopes, C., Raimundo, J., Caetano, M., and Garrido, S. (2020). Microplastic ingestion and diet composition of planktivorous fish. Limnol. Oceanogr. Lett. 5, 103–112. doi: 10.1002/lol2.10144
Luczynska, J., Paszczyk, B., and Luczynski, M. J. (2018). Fish as a bioindicator of heavy metals pollution in aquatic ecosystem of Pluszne Lake, Poland, and risk assessment for consumer’s health. Ecotoxicol. Environ. Saf. 153, 60–67. doi: 10.1016/j.ecoenv.2018.01.057
Madgett, A. S., Yates, K., Webster, L., McKenzie, C., and Moffat, C. F. (2021). The concentration and biomagnification of trace metals and metalloids across four trophic levels in a marine food web. Mar. Pollut. Bull. 173:112929. doi: 10.1016/j.marpolbul.2021.112929
Malik, N., Biswas, A. K., Qureshi, T. A., Borana, K., and Virha, R. (2010). Bioaccumulation of heavy metals in fish tissues of a freshwater lake of Bhopal. Environ. Monit. Assess. 160, 267–276. doi: 10.1007/s10661-008-0693-8
Mertz, W. (1993). Chromium in human nutrition: a review. J. Nutr. 123, 626–633. doi: 10.1093/jn/123.4.626
Ministry of Health of the People’s Republic of China [MHPRC] (2017). National Food Safety Standard, Maximum Levels of Contaminants in Foods (GB2762-2017). Beijing: Ministry of Health of the People’s Republic of China.
Neff, M. R., Bhavsar, S. P., Ni, F. J., Carpenter, D. O., Drouillard, K., Fisk, A. T., et al. (2014). Risk-benefit of consuming Lake Erie fish. Environ. Res. 134, 57–65. doi: 10.1016/j.envres.2014.05.025
North Sea Branch of the State Oceanic Administration (2016). Bulletin on The State of Marine Ecology and Environment in China. Beijing: Ministry of Ecology and Environment of the People’s Republic of China.
Pan, K., and Wang, W. X. (2012). Trace metal contamination in estuarine and coastal environments in China. Sci. Total Environ. 421–422, 3–16. doi: 10.1016/j.scitotenv.2011.03.013
Plavan, G., Jitar, O., Teodosiu, C., Nicoara, M., Micu, D., and Strungaru, S. A. (2017). Toxic metals in tissues of fishes from the Black Sea and associated human health risk exposure. Environ. Sci. Pollut. Res. 24, 7776–7787. doi: 10.1007/s11356-017-8442-6
Polak-Juszczak, L. (2009). Temporal trends in the bioaccumulation of trace metals in herring, sprat, and cod from the southern Baltic Sea in the 1994–2003 period. Chemospere 76, 1334–1339. doi: 10.1016/j.chemosphere.2009.06.030
Rabaoui, L., El Zrelli, R., Balti, R., Mansour, L., Courjault-Radé, P., Daghbouj, N., et al. (2017). Metal bioaccumulation in two edible cephalopods in the Gulf of Gabes, South-Eastern Tunisia: environmental and human health risk assessment. Environ. Sci. Pollut. Res. 24, 1686–1699. doi: 10.1007/s11356-016-7945-x
Rahman, M. S., Molla, A. H., Saha, N., and Rahman, A. (2012). Study on heavy metals levels and its risk assessment in some edible fishes from Bangshi River, Savar, Dhaka, Bangladesh. Food Chem. 134, 1847–1854. doi: 10.1016/j.foodchem.2012.03.099
Ribeiro, C. A. O., Vollaire, Y., Sanchez-chardi, A., and Roche, H. (2005). Bioaccumulation and the effects of organochlorine pesticides, PAH and heavy metals in the Eel (Anguilla anguilla) at the Camargue nature reserve, France. Aquat. Toxicol. 74, 53–69. doi: 10.1016/j.aquatox.2005.04.008
Santos, L. N., Neves, R. A. F., Koureiche, A. C., and Lailson-Brito, J. (2021). Mercury concentration in the sentinel fish species Orthopristis ruber: effects of environmental and biological factors and human risk assessment. Mar. Pollut. Bull. 169:112508. doi: 10.1016/j.marpolbul.2021.112508
Saravi, S. S. S., and Shokrzadeh, M. (2013). Heavy metals contamination in water and three species of most consumed fish sampled from Caspian Sea. Environ. Monit. Assess. 185, 10333–10337. doi: 10.1007/s10661-013-3335-8
Satheeshkumar, P., Senthilkumar, D., Ananthan, G., Soundarapandian, P., and Khan, A. B. (2011). Measurement of hematological and biochemical studies on wild marine carnivorous fishes from Vellar estuary, Southeast Coast of India. Comp. Clin. Path. 20, 127–134.
Savorelli, F., Manfra, L., Croppo, M., Tornambe, A., Palazzi, D., Canepa, S., et al. (2017). Fitness evaluation of Ruditapes philippinarum exposed to Ni. Biol. Trance Elem. Res. 177, 384–393. doi: 10.1007/s12011-016-0885-y
Signa, G., Mazzola, A., Tramati, C. D., and Vizzini, S. (2017). Diet and habitat use influence Hg and Cd transfer to fish and consequent biomagnification in a highly contaminated area: Augusta Bay (Mediterranean Sea). Environ. Pollu. 230, 394–404. doi: 10.1016/j.envpol.2017.06.027
State Oceanic Administration of China [SOA] (2012). Marine Environmental Quality Bulletin of China. Beijing: State Oceanic Administration of China.
Sun, T., Wu, H. F., Wang, X. Q., Ji, C. L., Shan, X. J., and Li, F. (2020). Evaluation on the biomagnification or biodilution of trace metals in global marine food webs by meta-analysis. Environ. Pollut. 264:113856. doi: 10.1016/j.envpol.2019.113856
Traven, L., Furlan, N., and Cenov, A. (2015). Historical trends (1998–2012) of nickel (Ni), copper (Cu) and chromium (Cr) concentrations in marine sediments at four locations in the Northern Adriatic Sea. Mar. Pollut. Bull. 98, 289–294. doi: 10.1016/j.marpolbul.2015.07.001
Tuzen, M. (2009). Toxic and essential trace elemental contents in fish species from the Black Sea, Turkey. Food Chem. Toxicol. 47, 1785–1790. doi: 10.1016/j.fct.2009.04.029
Ubrihien, R. P., Taylor, A. M., and Maher, W. A. (2017). Bioaccumulation, oxidative stress and cellular damage in the intertidal gastropod Bembicium nanum exposed to a metal contamination gradient. Mar. Freshwater Res. 68, 922–930. doi: 10.1071/MF16026
USEPA (2015). Human Health Risk Assessment, Risk-Based Screening Table, Regional Screening Level (Rsl) Summary Table. Washington, DC: USEPA.
Varol, M., Kaya, G. K., and Alp, A. (2017). Heavy metal and arsenic concentrations in rainbow trout (Oncorhynchus mykiss) farmed in a dam reservoir on the Firat (Euphrates) River: risk-based consumption advisories. Sci. Total. Environ. 599–600, 1288–1296. doi: 10.1016/j.scitotenv.2017.05.052
Velusamy, A., Satheesh Kumar, P., Ram, A., and Chinnadurai, S. (2014). Bioaccumulation of heavy metals in commercially important marine fishes from Mumbai Harbor, India. Mar. Pollut. Bull. 81, 218–224. doi: 10.1016/j.marpolbul.2014.01.049
Wan, L., Wang, N. B., Li, Q. B., Zhou, Z. C., Sun, B., Xue, K., et al. (2008). Estival distribution of dissolved metal concentrations in Liaodong Bay. Bull.. Environ. Contam. Toxicol. 80, 311–314. doi: 10.1007/s00128-008-9376-y
Wang, C. Y., and Wang, X. L. (2007). Spatial distribution of dissolved Pb, Hg, Cd, Cu and As in the Bohai Sea. J. Environ. Sci. 19, 1061–1066. doi: 10.1016/S1001-0742(07)60173-9
Wang, J., Ye, S. Y., Laws, E. A., Yuan, H. M., Ding, X. G., and Zhao, G. M. (2017). Surface sediment properties and heavy metal pollution assessment in the Shallow Sea wetland of the Liaodong Bay, China. Mar. Pollut. Bull. 120, 347–354. doi: 10.1016/j.marpolbul.2017.05.051
Wang, P., Chen, S. W., Chen, Z. H., Huo, W. L., Huang, R., Huang, W. X., et al. (2019). Benefit risk assessment of commonly consumed fish species from South China Sea based on methyl mercury and DHA. Environ. Geochem. Health 41, 2055–2066. doi: 10.1007/s10653-019-00254-1
Wang, W. X. (2002). Interactions of trace metals and different marine food chains. Mar. Ecol. Prog. Ser. 243, 295–309. doi: 10.3354/meps243295
Wilk, A., Kalisinska, E., Kosik-Bogacka, D. I., Romanowski, M., Rozanski, J., Ciechanowski, K., et al. (2017). Cadmium, lead and mercury concentrations in pathologically altered human kidneys. Environ. Geochem. Health 39, 889–899. doi: 10.1007/s10653-016-9860-y
Yousafzai, A. M., Chivers, D. P., Khan, A. R., Ahmad, I., and Siraj, M. (2010). Comparison of heavy metals burden in two freshwater fishes Wallago attu and Labeo dyocheilus with regard to their feeding habits in natural ecosystem. Pak. J. Zool. 42, 537–544.
Zhang, L. L., Yan, W., Xie, Z. Y., Cai, G. Q., Mi, W. Y., and Xu, W. H. (2020). Bioaccumulation and changes of trace metals over the last two decades in marine organisms from Guangdong coastal regions, South China. Sci. Total. Environ. 98, 103–108. doi: 10.1016/j.jes.2020.05.007
Keywords: trace metals, marine fish, bioindicator, risk-assessment, interannual trend
Citation: Wang S, Zheng N, Sun S, An Q, Li P, Li X, Li Z and Zhang W (2022) Trends and Health Risk of Trace Metals in Fishes in Liaodong Bay, China, From 2015 to 2020. Front. Mar. Sci. 8:789572. doi: 10.3389/fmars.2021.789572
Received: 05 October 2021; Accepted: 23 December 2021;
Published: 31 January 2022.
Edited by:
Sílvia C. Gonçalves, Polytechnic Institute of Leiria, PortugalReviewed by:
Manal El-Sadaawy, National Institute of Oceanography and Fisheries (NIOF), EgyptGeraldina Signa, University of Palermo, Italy
Ghada El-Said, National Institute of Oceanography, Egypt
Copyright © 2022 Wang, Zheng, Sun, An, Li, Li, Li and Zhang. This is an open-access article distributed under the terms of the Creative Commons Attribution License (CC BY). The use, distribution or reproduction in other forums is permitted, provided the original author(s) and the copyright owner(s) are credited and that the original publication in this journal is cited, in accordance with accepted academic practice. No use, distribution or reproduction is permitted which does not comply with these terms.
*Correspondence: Na Zheng, emhlbmduYWx6ekBuZWlnYWUuYWMuY24=, emhlbmduYWx6ekBqbHUuZWR1LmNu