- 1Yantai Institute of Coastal Zone Research, Chinese Academy of Sciences, Yantai, China
- 2National Marine Environmental Monitoring Center, Ministry of Ecology and Environment, Dalian, China
- 3College of Life Sciences, Yantai University, Yantai, China
Seawater acidification and warming have been found to affect the early life of many marine organisms, but their effects on the microbial community in the environment related to the early development stage of aquaculture species have been rarely investigated. To understand how seawater acidification and warming impact the microbial community in aquaculture systems, we designed four microcosms to monitor and characterize the microbial composition on the corrugated plates in the Apostichopus japonicus culture tanks during its post-settlement stage. High-throughput 16S rRNA sequencing revealed that the bacterial community composition varied significantly in different periods of incubation. The bacterial diversity and community composition were obviously changed by seawater acidification and warming in the early period and then tended to revert to the level of the control group. Acidification significantly increased the relative abundance of dominant families Rhodobacteraceae and Flavobacteriaceae in the early period, suggesting that microbiota could increase the abundance of predominant taxa to adapt to increased CO2 concentration and reconstruct a stable community structure. No interaction effect of both factors was observed in the combined group. Results reveal that the microbial communities on the corrugated plates in A. japonicus culture tank were affected in the early period of incubation, and could then acclimatize to the increased CO2 and temperature. This study provides new insights into the variation and adaptation responses of the microbiota in aquaculture systems to seawater acidification and warming.
Introduction
Increasing levels of atmospheric CO2 are causing changes in global climate (IPCC, 2021), and the ocean absorbs excessive CO2, resulting in ocean acidification and warming (OAW) (Lenz et al., 2019). The global average seawater pH was predicted to drop by 0.1 to 0.4 units by 2100 (Currie et al., 2017; Lenz et al., 2019). The global average seawater temperatures were predicted to increase between 1.5 and 2.0°C by the end of the 21st century (Rogelj et al., 2019; IPCC, 2021). OAW are stressors for numerous marine populations, which may affect their survival and growth, especially during critical life stages, such as fertilization and larval development. For instance, previous studies found that acidification could decrease the post-fertilization success and affect the growth, development, and physiological energetics of sea cucumbers (Yuan et al., 2015, 2016). Moreover, seawater acidification changes the seawater carbonate system, which could affect the marine ecological environment (Orr et al., 2005; Jian et al., 2019), and thus affect the marine primary productivity and community structure (Josiane et al., 2016) and the microbial composition and function (Lidbury et al., 2012; Kerfahi et al., 2020). Previous studies indicate that increased temperature is a driving abiotic factor in reshaping bacterial communities in the marine ecosystem (Smale et al., 2017; Mensch et al., 2020). Warming reduces commonly present and health-associated marine bacteria, but may lead to increased abundance of potential pathogenic bacteria and favors bacteria that play roles in microfouling processes (Mensch et al., 2020), which may drive fundamental shifts in ecosystem structure and function. The epibacterial community structure on Fucus vesiculosus forma mytili is strongly affected by increased temperature, and weakly affected by elevated pCO2, ultimately with potential consequences for host–microbe interactions (Birte et al., 2016).
In aquaculture systems, microorganisms play important roles in nutrient fluxes, water quality, and diseases (Blancheton et al., 2013), which are susceptible to environmental changes (e.g., seawater acidity and temperature). More importantly, the microbes in aquatic environment are as potential feed for the target marine species. For instance, the intestinal microbiota of the sea cucumber Apostichopus japonicus, which plays important roles in host health, growth, and immunity, is mainly derived from the habitat (Gao et al., 2014a). OAW may directly affect marine microbial abundance, diversity, and community composition (Currie et al., 2017), thereby indirectly affecting the growth and health condition of aquatic animals (Krause et al., 2012; Sunday et al., 2016). Base on the importance of microbiota in aquaculture systems, few studies have investigated how seawater temperature or acidification might affect them (Zhang et al., 2019c; Wang et al., 2021).
A. japonicus is a temperate holothurian and has been exploited as an economically valuable fishery resource in many Asian countries, especially in China (Yang et al., 2015). A. japonicus has an indirect life cycle, and planktonic larvae settle to benthic juveniles after 13–20 days of fertilization (Zhang and Liu, 1998). The post settlement stage starts with white juvenile (also named “white dot” in hatchery), followed by black juvenile (black dot). Post settlement juvenile is a key stage in the life cycle and shapes the sea cucumber’s morphology and structure, including the body color change, the ossicle development, and the tube foot formation (Qiu, 2013). Furthermore, benthic juveniles involve the digestive tract maturation and formation of intestinal microbiota (Li et al., 2019). In indoor hatchery practice, corrugated plates are used as substrates for culturing benthic juveniles. The microbial community on corrugated plates can be ingested as a dietary component, and may play an important role in the development of sea cucumber from “white dot” to “black dot” in terms of growth against seedling disease and abscission (Gao et al., 2014b; Zhang et al., 2019b). Our previous study discovered that seawater pH decreases growth, delay and slows the pigmentation of post-settlement juvenile sea cucumber A. japonicus, while seawater temperature increases the growth acceleration and pigmentation of the juveniles (Song et al., 2020). However, how increased CO2 and temperature impact the microbiota in the juvenile sea cucumber A. japonicus culture environment remains unclear. Understanding the regulatory mechanisms of the microbial community responses to seawater acidification and warming is an important objective in establishing effective microbial ecological strategies, and may help in the development of a theoretical basis for further understanding the microbiota characteristics in the aquatic ecosystem.
We set up four microcosms and analyzed the structure characteristics of the microbial community on the corrugated plates in the A. japonicus culture tank during the early developmental stages of animals. The results were used to address the following questions: How does the microbial diversity and community response to seawater acidification and warming? How does the microbial community composition change in different early developmental stages? This analysis allows us to pinpoint changes in the microbial composition and diversity that are likely associated with seawater acidification and warming. The results will be of great theoretical and practical significance to the understanding of the microbiota response to OAW in aquaculture systems and to the healthy aquaculture of sea cucumbers.
Materials and Methods
Experimental Set-Up
The seawater acidification and warming system used in this study consists of four artificial climate incubators (HP400G-D, Wuhan Ruihua Instrument and Equipment, Wuhan, China). Four glass aquariums (32L) were included in each incubator, and the seawater obtained from the Dalian coast was filtered using a composite sand filter. We set up four treatments, namely, the control (labeled as CK, with a seawater temperature similar to that of Dalian coast and an ambient pCO2 of 400 μatm), warming treatment (W, with a + 2°C temperature from that of the control and an ambient pCO2 of 400 μatm), acidification treatment (A, with the same seawater temperature as the control and a pCO2 of 1,000 μatm), and acidification and warming treatment (WA, with a + 2°C temperature and a pCO2 of 1,000 μatm), covering the present-day and end-of-century seawater temperatures and pH variability (Lenz et al., 2019; Rogelj et al., 2019; IPCC, 2021). During the experimentation, the daily water temperature of the Dalian coast was obtained from the Oceanguide website1, and the seawater temperatures in the incubators were adjusted weekly to match it (Supplementary Figure 1). Pure CO2 gas (99.9%) was pumped into the CO2-enrichment incubators and mixed with air to reach the desired atmospheric concentrations. The CO2 concentration was automatically controlled using a CO2 detector VC1008F with a sensitivity of 10 ppm [Sweden SenseAir (China), Chengdu, China]. A light/dark cycle of 12/12 h was set at a 342 Lx in the incubators.
Seawater total alkalinity (AT) was measured weekly during the experiment using a Total Alkalinity Gran Titration System (AS-ALK2, Appolo SciTech, Bogart, GA, United States). Seawater pHNBS was monitored daily using a S20P-K pH meter with a 0.001 -unit sensitivity [Mettler Toledo Instrument (China), Shanghai, China], which was calibrated with NBS buffers. The salinity and temperature were also monitored daily using a YSI 6600V2 Handheld Multiparameter Instrument (YSI Incorporated, Ohio, United States). Calcite and aragonite saturation states and the pCO2 were calculated using the CO2SYS software (Lewis et al., 1998). One-way ANOVA was used to analyze the seawater carbon chemistry, and the level applied for significance was 5%.
Experimental Juveniles and Sample Collection
We obtained mature eggs and sperms by stimulating parent sea cucumbers A. japonicus with flowing water (Yuan et al., 2015). Then, the fertilized eggs were developed for 24 days to “white dot” in seawater in the laboratory (indoor temperature). Corrugated plates (10 cm × 15 cm) were prepared to shelter the post-settlement juveniles. Before use, the plates were scrubbed in freshwater and then immersed in a KMnO4 solution (0.05%) for 48 h for sterilization, followed by cleaning with recirculating freshwater for 24 h. Four corrugated plates were placed in each aquarium as the substrate, and a density of 0.50 ind⋅L–1 “white dot” were gently placed. In total, sixty-four corrugated plates (four treatments × four aquariums × four plates) were used in this study to evaluate the effect of seawater acidification and warming on the microbial community in the sea cucumber A. japonicus culture tank. Post-settlement A. japonicus juveniles were reared in four treatments for 72 days until they all developed into “black dot.” During the experimental period, the juveniles were fed once a day (at 16:00) with a sea cucumber juvenile diet made with Sargassum thunbergii and spirulina powders (25% crude protein, 4% crude fat, 6% crude fiber, 55% ash, and 10% water). The powder diet is insoluble in seawater and fed juveniles through splashing with excess of feed. Feces and uneaten feed were gently siphoned every day. Daily water exchange by one-third or one-half.
Microbial samples were collected from the corrugated plates at the 20th, 43rd, and 72nd days as follows. The corrugated plates were gently taken out from each aquarium, and the residual feeds and feces were gently stripped off. Then, the surface biofilms at five sites were wiped with sterile cotton swabs on each plate. The samples from the same plate were placed in a sterile tube and preserved at −80°C until DNA extraction.
DNA Extraction and 16S rRNA Gene Sequencing
Total DNA was extracted from the surface biofilms on the corrugated plates by using a FastDNA SPIN Kit for Feces (MP Biomedicals, Santa Ana, CA, United States) according to the instructions provided by the manufacturer. The extracted DNA was dissolved in 50 μL of TE buffer, quantified using a NanoDrop spectrophotometer (NanoDrop, Thermo Scientific, United States), and stored at −20°C prior to analysis. The PCR amplification of the bacterial 16S rRNA hypervariable regions V4-V5 was conducted using the universal primer set 515f (GTGCCAGCMGCCGCGGTAA) and 907r (CCGTCAATTCMTTTRAGTTT), with 5-bp barcodes fused to the forward primer to allow sample multiplexing. The purified PCR products with different barcodes were normalized in equimolar amounts and then prepared using an NEB Next®UltraTMDNA Library Prep Kit for Illumina (NEB, United States) following the manufacturer’s protocol and sequenced on the Illumina HiSeq platform.
Deep Sequencing Data Processing
Raw deep sequencing data were processed using the Quantitative Insights Into Microbial Ecology software2 (QIIME version 1.9.0; Caporaso et al., 2010) with default parameters unless otherwise noted. After removing all chimeric and low-quality reads, the qualified sequences were clustered into operational taxonomic units (OTUs) at the 97% identity threshold level, and the most abundant sequence from each OTU was selected as the representative sequence for that OTU. The taxonomic classification of each OTU was assigned using the Ribosomal Database Project classifier. The average relative abundance (%) of the predominant genus-level taxonomic groups in each sample was estimated by comparing the number of sequences assigned to a specific taxon versus the number of total sequences obtained for that sample. Based on the OTU numbers, principal coordinates analysis (PCoA, unweighted) was performed using R version 3.0.2 (package stats) to test the differences in microbial community structures.
Statistical Analysis
The data were analyzed using the SPSS for Windows (Version 19.0) statistical package. Differences were determined through the LSD test, with P-values <0.05 having statistical significance.
Results
Seawater Carbonate Chemistry
The seawater carbonate chemistry measured and calculated for all treatments is shown in Table 1. The average seawater pH values in the CK and W groups were 8.08 ± 0.06 and 8.11 ± 0.05, while those of the A and WA groups were 7.79 ± 0.04 and 7.77 ± 0.04, respectively. The temperature variations in the CK and A groups were similar to that of the seawater temperature of Dalian offshore (Supplementary Figure 1), with the respective average values of 23.5 and 23.4°C, and the increased temperatures in the W and WA groups varied at + 2°C (Supplementary Figure 1), with respective averages of 25.5 and 25.4°C. The elevated pCO2 exerted no significant effect on salinity (F3,125 = 6.42, p = 0.389) and AT (F3,138 = 1.916, p = 0.130) but significantly affected all the other parameters of the carbonate system: pCO2 (F3,138 = 158.840, p < 0.0001), ΩCa (F3,138 = 148.453, p < 0.0001), Ωar (F3,138 = 148.400, p < 0.0001) (Table 1).
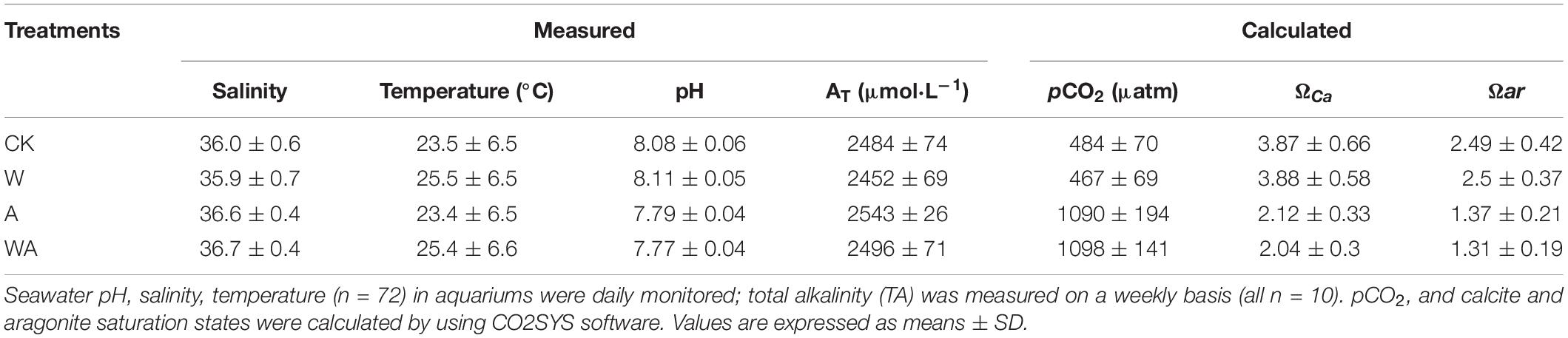
Table 1. Seawater carbonate chemistry in the seawater acidification and warming system during the experiment.
16S rRNA Gene Analysis Revealed a Temporal Pattern of Bacterial Diversity
At 97% sequence identity, a total of 7,511 OTUs were obtained across all samples. The samples from the CK group contained 4,195 OTUs (on average), while the W, A, and WA groups contained 5,044, 5,705, and 5,218 OTUs (on average), respectively (Table 2). We analyzed both the shared and unique OTUs in the bacterial communities of the four treatments in different periods to further identify the variation in bacterial composition. The samples from different groups shared 1,155, 1,651, and 1,772 OTUs in the 20-, 43-, and 72-day periods, respectively (Supplementary Figure 2). Low values for community richness were observed in the early period (20 days).
The alpha diversity of the bacterial community varied in different treatments and periods. The Shannon indices ranged from 6.888 to 8.986, and the Chao1 richness varied from 1,343 to 2,530 OTUs in all samples (Table 2). The A20 samples had the lowest Shannon index and Chao1 richness, with 6.888 and 1,343 OTUs, respectively, while the A72 samples had the highest Shannon index and Chao1 richness, with 8.986 and 2,530 OTUs, respectively. The alpha diversity and richness gradually increased in the later periods in all group samples. In particular, the samples from A group exhibited significant differences in alpha diversity between different periods.
Characterization of the Microbial Composition Responses
The bacterial communities on the A. japonicus culture plates were classified by breeding periods on the basis of the principal coordinates analysis (PCoA) of the OTUs, indicating differences in bacterial community compositions. Figure 1 shows that all the samples from the four treatments in the same period were grouped together, and the bacterial community structures in different periods exhibited temporal patterns that are distinct from one other. The samples from the middle stage (43 days) are close to the samples from the other two periods respectively, suggesting that the composition patterns of bacterial communities are associated with incubation period.
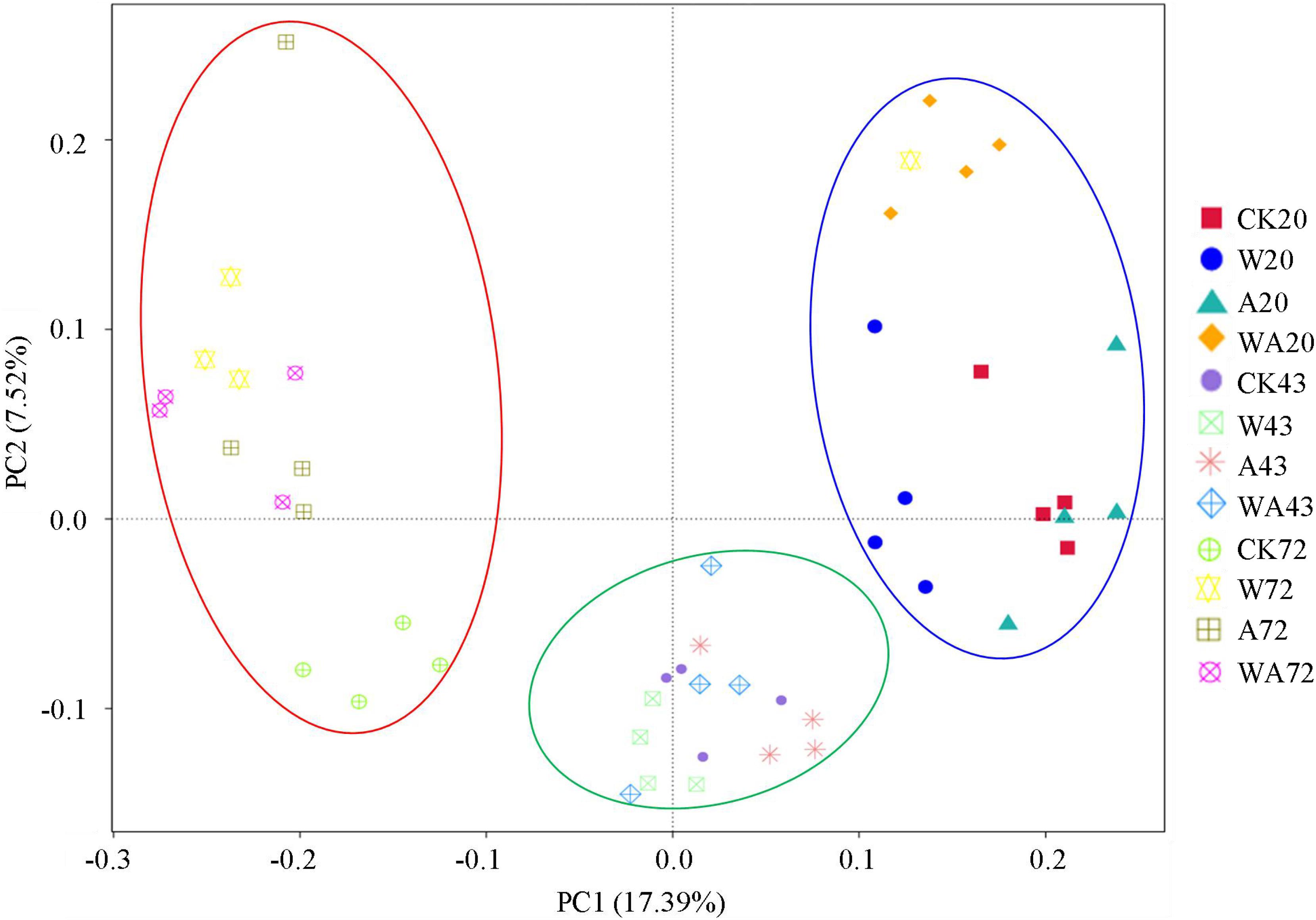
Figure 1. Principal coordinates analysis (PCoA) based on the relative abundance of 16S rRNA genes from 48 samples (4 treatments × 3 periods × 4 replicates per period). The scatterplot shows principal coordinate 1 (PC1) vs. principal coordinate 2 (PC2). The percentages of variation in the samples described by the plotted PCs are shown on the axes. The 4 replicates for each period are represented by a single color. CK, control; W, warming treatment; A, acidification treatment; WA, acidification and warming treatment. 20, 43, and 72: samples were collected at 20, 43, and 72 days.
Similar patterns of bacterial community composition were observed between the samples obtained from all the groups at the phylum and class levels (Supplementary Figure 3). Proteobacteria (51.29–64.66%) and Bacteroidetes (13.19–33.02%) were the predominant phyla in all the samples. Most of the Proteobacteria reads were assigned as Gammaproteobacteria (23.74–36.59%) and Alphaproteobacteria (16.51–33.64%). The Bacteroidetes phylum was represented mostly by the Bacteroidia (12.23–32.74%) class. Differences in bacterial compositions at the order and family levels were observed between the samples obtained from the different groups (CK, W, A, and WA).
The impact of elevated CO2 was more marked than increased temperature, with the relative sequence abundance of several predominant families showing significant changes at the different stages. As shown in Figure 2, compared to CK group, the relative abundance of the 10 most abundant families increased or decreased in the W, A, and WA groups. The WA group tended to revert to the level of the CK specimens in the middle and later periods (Supplementary Figure 4).
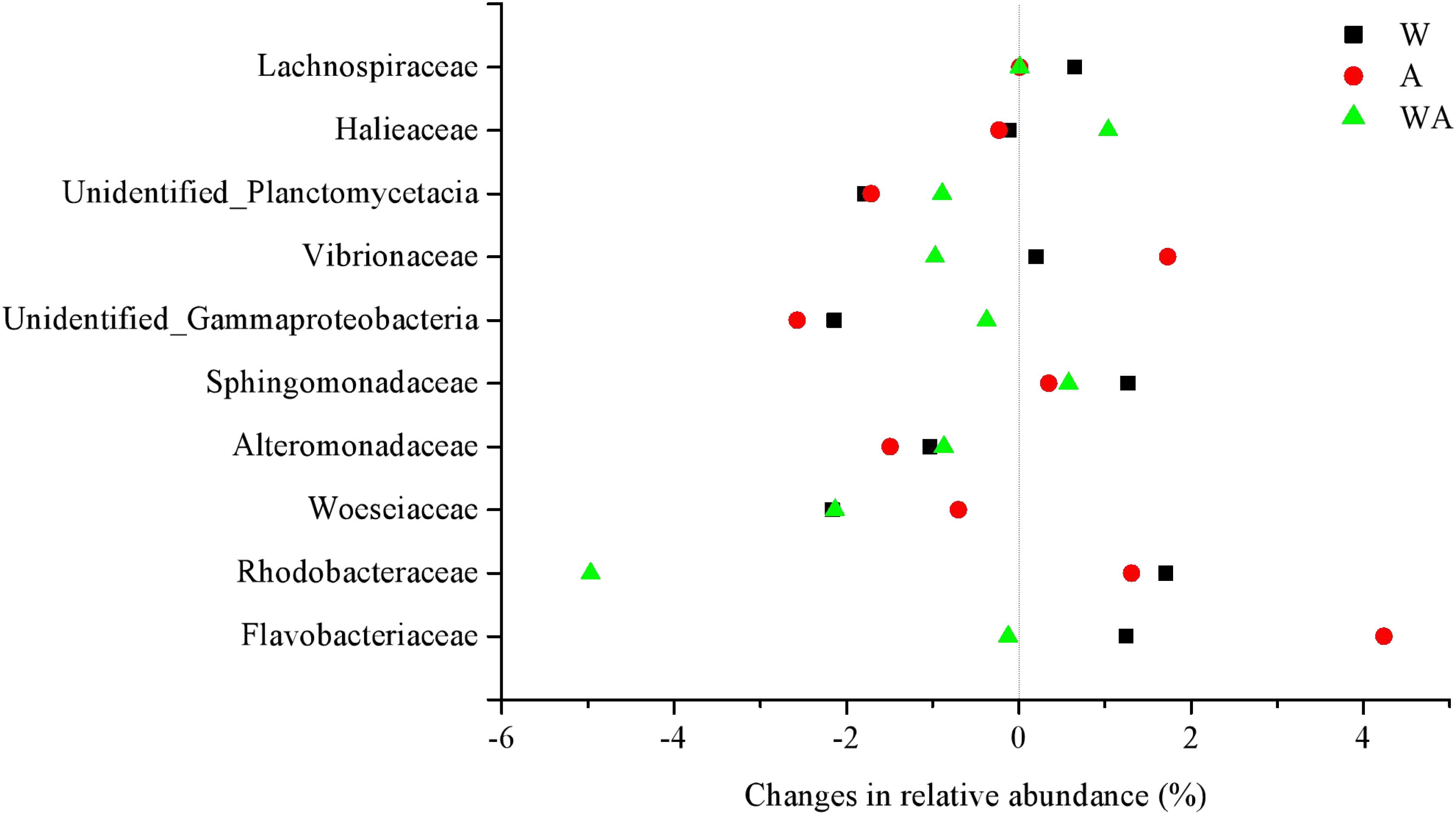
Figure 2. Changes in relative abundance of the dominant families compared to the control group in different treatments in all periods (on average,%). W, warming treatment; A, acidification treatment; WA, acidification and warming treatment. The data were on average from samples in all periods (20, 43, and 72 days).
The analysis of bacterial communities revealed distinct taxonomic compositions at the genus level between the samples obtained from different incubation periods (20, 43, and 72 days) (Figure 3). In the CK samples, the most abundant genera in the 20-day period were Marinicella (average 7.11%), Paraglaciecola (6.27%), and Rhodopirellula (5.97%), which changed to Woeseia (5.68%), Vibrio (4.14%), and Unidentified_Rhodobacteraceae (2.01%) in 43-day period. Woeseia (10.6%), Magnetospira (3.49%), and Unidentified_Gammaproteobacteria (1.91%) were the dominant genera in the 72-day period.
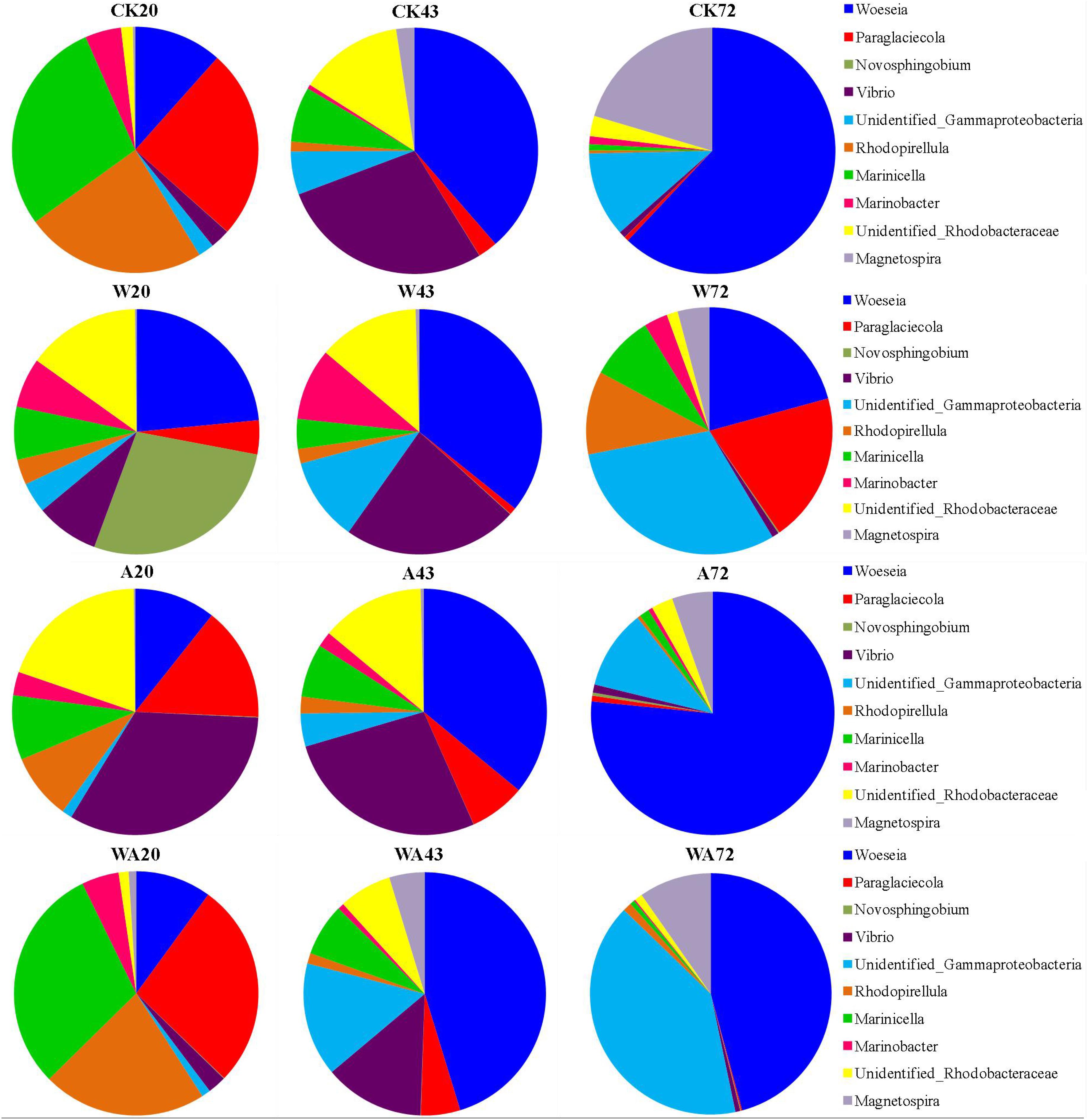
Figure 3. Changes in composition of bacterial communities in the Apostichopus japonicus culture tank at the genus level (%). CK, control; W, warming treatment; A, acidification treatment; WA, acidification and warming treatment. 20, 43, and 72: samples were collected at 20, 43, and 72 days.
In the W20 samples, the most abundant genera were Novosphingobium (average 3.63%), Woeseia (3.08%), and Unidentified_Rhodobacteraceae (1.915%). Woeseia (6.73%), Vibrio (4.34%), Unidentified_Rhodobacteraceae (2.51%), and Unidentified_ Gammaproteobacteria (2.08%) were the dominant genera in the W43 samples. In the W72 samples, the most abundant genera changed to Unidentified_Gammaproteobacteria (4.28%), Woeseia (2.92%), and Paraglaciecola (2.76%).
Compared to the CK samples, a recovery profile was detected in the A group (A43 and A72) samples (Figure 3). In the A20 samples, Vibrio (5.53%), Unidentified_Rhodobacteraceae (3.28%), Paraglaciecola (2.51%), and Woeseia (1.79%) were the dominant genera. In the A43 and CK43 samples, the most abundant genera were Woeseia (5.91%), Vibrio (4.45%), and Unidentified_Rhodobacteraceae (2.23%). Similar to the CK72 samples, the Woeseia (9.38%), Unidentified_Gammaproteobacteria (1.29%), and Magnetospira (0.66%) genera prevailed in the A72 samples.
In the WA20 samples, the Marinicella (5.36%), Paraglaciecola (4.83%), Rhodopirellula (3.86%), and Woeseia (1.78%) genera prevailed. In the WA43 samples, the most abundant genera were Woeseia (5.28%), Unidentified_Gammaproteobacteria (1.77%), and Vibrio (1.55%). In the WA72 samples, Woeseia (5.76%), Unidentified_Gammaproteobacteria (5.09%), and Magnetospira (1.22%) were the dominant genera. The results indicate obvious differences in bacterial community structures at the genus level between the samples obtained from different treatments on the corrugated plates in the A. japonicus culture tank.
Response of the Predominant Taxa to Seawater Acidification and Warming
In this study, Rhodobacteraceae and Flavobacteriaceae were the most abundant taxa in the samples obtained from the CK group. We analyzed the variation in the relative abundance of Rhodobacteraceae and Flavobacteriaceae in all the samples during different periods to assess the effects of seawater acidification and warming on the dominant taxa on the culturing plate of post-settlement sea cucumbers. The relative abundance of Rhodobacteraceae and Flavobacteriaceae decreased obviously in the middle and later periods (43 and 72 days) compared to the early period (20 days) in the three other groups (Figure 4). More interestingly, the seawater acidification (A group) significantly increased the relative abundance of Rhodobacteraceae and Flavobacteriaceae in the early period. Meanwhile, the combination of acidification and warming significantly reduced the relative abundance of Rhodobacteraceae in the early and middle periods (20 and 43 days).
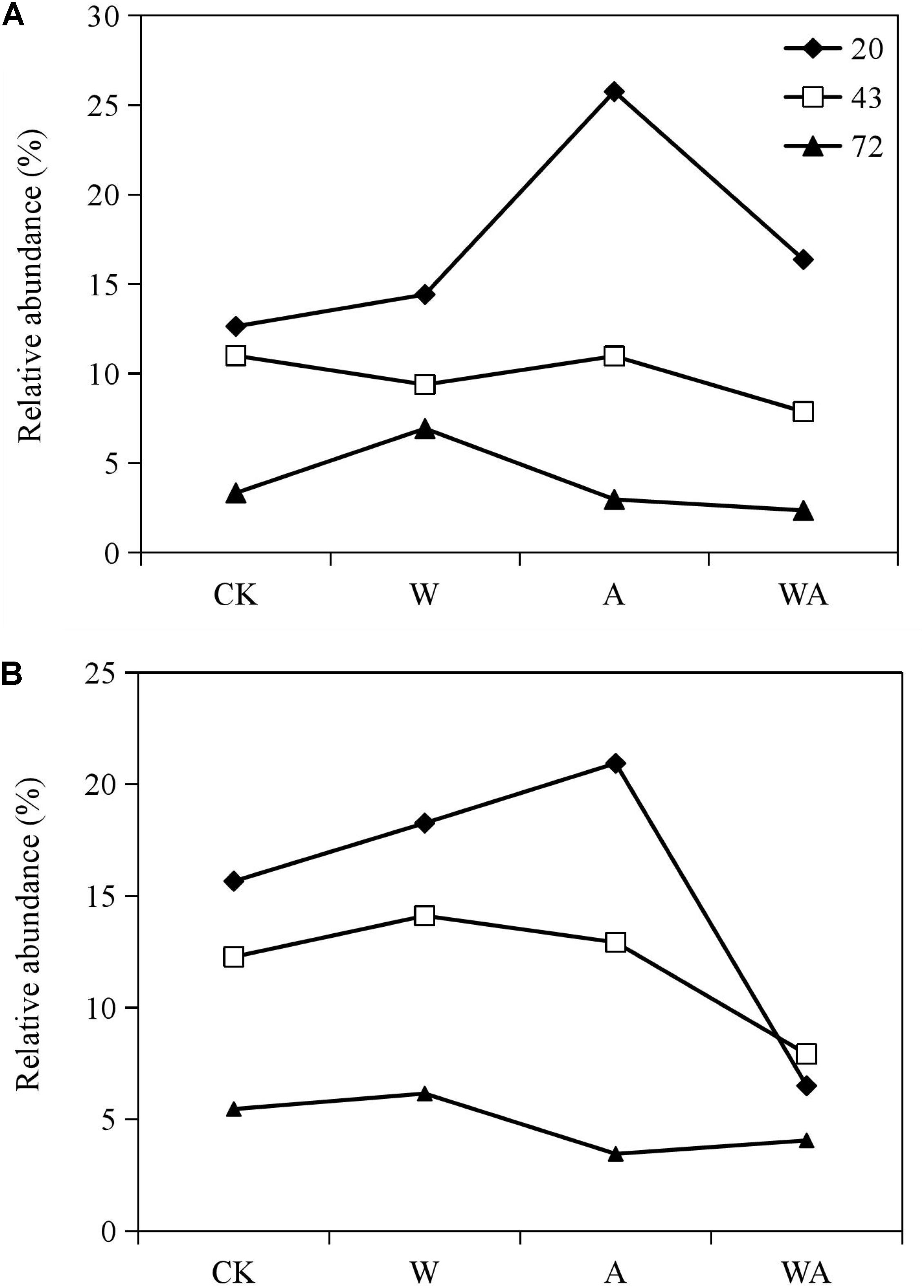
Figure 4. Relative abundance of the predominant families Flavobacteriaceae (A) and Rhodobacteraceae (B) in samples from different groups in different periods. CK, control; W, warming treatment; A, acidification treatment; WA, acidification and warming treatment. 20, 43, and 72: samples were collected at 20, 43, and 72 days.
Seawater acidification or warming obviously affected the bacterial diversity and composition on the corrugated plates of post-settlement A. japonicus in the early period. Especially, acidification significantly changed the abundance of the predominant taxa in the 20-day period. Then, the bacterial diversity and community structures reverted to the level of the control group.
Discussion
Numerous studies on the response of marine microbiota to seawater acidification and/or warming have been conducted and have received increased interest because of the vital role of microbiota in marine ecosystems (Currie et al., 2017; Smale et al., 2017; Mensch et al., 2020). Environmental/Sediment bacteria could be an important dietary component of sea cucumbers (Gao et al., 2014b). For instance, the sea cucumber Holothuria tubulosa has a high assimilation efficiency for bacteria, averaging approximately 30% (Amon and Herndl, 1991). Thus, microorganisms in the cultured environment can be ingested by A. japonicus and might contribute to the intestinal microbial composition and modulation of A. japonicus during the early developmental stage. Thus, we must assess the effects of seawater acidification and warming on the bacterial community on the corrugated plates settled by the larval development of sea cucumber A. japonicus. The results suggest that the bacterial diversity and community on the corrugated plates of post-settlement A. japonicus were affected in the early period and could then acclimatize to seawater acidification and warming.
The analysis of the bacterial community on the corrugated plates in all the groups revealed significant differences between the different periods of incubation. Wang et al. (2021) obtained similar results in response to high temperature noting the obvious evolution of the bacterial community in the water of the A. japonicus cultured pond in summer. The bacterial community composition on Fucus vesiculosus and in the surrounding seawater are affected by warming but vary in time (Mensch et al., 2020). Our findings and those of a previous study indicate temporal changes in bacterial communities in the aquaculture system response to seawater acidification and warming (Figure 1). The diversity and structure of the bacterial communities also varied in the W and A groups compared to CK group in the same period, meaning that seawater acidification and warming affected the microbial communities on the plates in the culture tanks.
A previous study indicates that many marine organisms are likely to be susceptible to ocean acidification and thereby likely to change their contribution to the ecosystem (Hancock et al., 2020). In the present study, seawater acidification significantly reduced the Shannon index and Chao1 richness of bacteria in the early period (day 20) and then increased them in the later period (day 72). Conversely, warming treatment increased the bacterial α-diversity in the early period, whereas the combined treatment showed a non-additive effect throughout the experiment.
Our data show small but significant differences in bacterial community composition under increased CO2 or increased temperature treatments (W and A groups) after 20 days of incubation, with increases to the relative abundance of predominant taxa (Rhodobacteraceae, Flavobacteriaceae, Sphingomonadaceae, and Vibrionaceae) and decreases to some taxa (such as Woeseiaceae, Alteromonadaceae, and Unidentified _Gammaproteobacteria) (Figure 2 and Supplementary Figure 4). Supporting our results, a study shows the positive effects of elevated temperature on the abundance of Rhodobacteraceae, Flavobacteriaceae, and Vibrionaceae (Zhang et al., 2019c). Some previous studies that assessed the effects of increased CO2 and temperature on marine microbial community composition show different responses, with some suggesting no significant differences in community abundance, structure, or composition (Kerfahi et al., 2014; Oliver et al., 2015). Other findings show that marine benthic microorganisms are susceptible to changes in ocean CO2 concentration and seawater temperature (Currie et al., 2017). A few studies revealed that the bacterial community composition is strongly influenced by temperature but not acidification (Bergen et al., 2016; Mensch et al., 2020). Other incubation experiments with marine sediments and biofilms resulted either in rapid responses of microbial community compositions and activities to seawater acidification (Witt et al., 2011; Braeckman et al., 2014; Tait et al., 2014) or in minor effects of acidification on microbial communities and activity (Tait et al., 2013; Gazeau et al., 2014). Moreover, different microbial groups in marine sediments have different pH optima, and the impact of elevated CO2 on their function may be dependent on their relative abundances (Braeckman et al., 2014; Tait et al., 2014). These findings suggest that the microbial response to seawater acidification and warming is species and factor specific.
At the genus level, the bacterial community compositions varied obviously in the W and A groups in the early period (day 20), indicating that the marine microorganisms in the A. japonicus culture tank were susceptible to changes in seawater acidification and increased temperature in the early period, which may ultimately have an impact on the gut microbiota of A. japonicus. Meanwhile, microbes can rapidly acclimatize to changing environmental conditions (Hicks, 2017). Thus, the compositions tended to recover in the W and A groups in the later period. Our results are consistent with previous findings, which reveal that the microbiota could enhance its resistance to increased temperature by changing its community structure and functions, thereby maintaining a microecological balance in the A. japonicus culture pond ecosystem (Wang et al., 2021). The benthic microbial communities in sediments are likely well adapted to low pH (Braeckman et al., 2014). Thus, the microbial communities in aquaculture systems may be affected in the early period and could then adapt to increased CO2 and temperature.
Increasing water acidity and seawater temperatures is likely to impose considerable pressure on microbial communities (Currie et al., 2017). The combined treatment (WA group) showed a non-additive effect on bacterial diversity and community structures throughout the experiment. However, understanding the impacts on microbial communities is the key to predicting long-term changes in aquaculture systems.
Another interesting aspect is that this study saw a clear response in Rhodobacteraceae and Flavobacteriaceae by day 20, that is, their relative abundance in the acidification group increased significantly and tended to recover in the middle and later periods (days 43 and 72). The result suggests that microbiota could increase the abundance of predominant taxa to adapt to increased CO2 concentration and reconstruct a stable community structure. Furthermore, a previous study found that Rhodobacterales retaining polyhydroxybutyrate (PHB) metabolism genes may promote the growth of A. japonicus (Yamazaki et al., 2016). In addition, we previously reported that Rhodobacteraceae and Flavobacteriaceae may function as keystone taxa in the intestinal microbial community of A. japonicus during intestine regrowth (Zhang et al., 2019a). Rhodobacteraceae are related to PHB production and Flavobacteriaceae to carbohydrate production, which are beneficial to the regrowth intestine of A. japonicus (Zhang et al., 2020). Therefore, changes in the abundance of these microorganisms may impact intestinal microbiota and the growth of A. japonicus.
Our previous study under the same conditions revealed the effects of seawater acidification and warming on growth performance, and body pigmentation in the key stage of post-settlement A. japonicus (Song et al., 2020). Song et al. (2020) found that a pH decrease of 0.23 units (1,000 ppm) delays growth and slows pigmentation from “white dot” to “black dot” in post-settlement sea cucumber A. japonicus. However, a 2°C temperature increase accelerates the growth and pigmentation of juveniles, potentially neutralizing the negative effects of pH decrease. In the current study, the effects of seawater acidification and warming on the microbial community during the development from “white dot” to “black dot” indicate that the evolution of microbiota on the corrugated plates in which juvenile sea cucumbers are sheltered could have an indirect influence on the juvenile development (e.g., growth and pigmentation) by micro-environment, but the direct influence remains unclear. Additional experiments should be conducted to further assess the intestinal microbiota response to seawater acidification and warming and to further study the relationship between environment and gut microbiota; then, we could infer their effects on host animals.
The results in this study suggest that microbiota can increase the abundance of predominant taxa to adapt to increased CO2 concentration and temperature and reconstruct a stable community structure on the corrugated plates in the A. japonicus culture tanks. Furthermore, the adaptation of microbiota in the aquaculture environment to seawater pH decrease and temperature increase in our current study could imply a stable trophic relationship between the sediment microbe community and sea cucumbers in the natural ecosystem under the end-of-21st century scenario of OAW. This finding illustrates that OAW may not influence the food supply to sea cucumber because the microbe in the sediment plays an important role in the food chains of the sea-cucumber-dependent ocean (Gao et al., 2014b).
In conclusion, this study highlights a significant effect of seawater acidification and warming on the bacterial diversity and community composition in the early period. The diversity and composition of the microbiota tended to recover in the middle and later periods, suggesting that their great adaptability to increased CO2 concentration and temperature in the aquaculture systems. The results reveal that the response of predominant taxa to seawater warming was less pronounced, with the most noticeable response seen in the acidification treatment. No interaction effect of both factors was observed in the combined group. There will be considerable independent effects of seawater acidification and warming on microbial communities in the aquaculture system. To our knowledge, the information on adaption and acclimation in microbial communities in aquaculture systems is very limited, requiring emphasis on long-term responses.
Data Availability Statement
The datasets presented in this study can be found in online repositories. The names of the repository/repositories and accession number(s) can be found below: NCBI (accession: PRJNA770452).
Author Contributions
HZ: data curation, formal analysis, and writing – original draft. MS: investigation, data curation, formal analysis, and writing – review and editing. LW: methodology and supervision. AZ and XYa: supervision and investigation. YL: methodology. XYu: conceptualization, funding acquisition, methodology, and writing – review and editing. All authors provided critical feedback and helped to conduct the research, analysis and manuscript.
Funding
This research was supported by the National Natural Science Foundation of China (41676164).
Conflict of Interest
The authors declare that the research was conducted in the absence of any commercial or financial relationships that could be construed as a potential conflict of interest.
Publisher’s Note
All claims expressed in this article are solely those of the authors and do not necessarily represent those of their affiliated organizations, or those of the publisher, the editors and the reviewers. Any product that may be evaluated in this article, or claim that may be made by its manufacturer, is not guaranteed or endorsed by the publisher.
Supplementary Material
The Supplementary Material for this article can be found online at: https://www.frontiersin.org/articles/10.3389/fmars.2021.802023/full#supplementary-material
Footnotes
References
Amon, R., and Herndl, G. (1991). Deposit feeding and sediment: II. Decomposition of fecal pellets of Holothuria tubulosa (Holothurioida, Echinodermata). Mar. Ecol. 12, 175–184. doi: 10.1111/j.1439-0485.1991.tb00251.x
Bergen, B., Endres, S., Engel, A., Zark, M., Dittmar, T., Sommer, U., et al. (2016). Acidification and warming affect prominent bacteria in two seasonal phytoplankton bloom mesocosms. Environ. Microbiol. 18, 4579–4595. doi: 10.1111/1462-2920
Birte, M., Neulinger, S. C., Angelika, G., Andreas, P., Sven, K., Fischer, M. A., et al. (2016). Restructuring of epibacterial communities on fucus vesiculosus forma mytili in response to elevated pCO2 and increased temperature levels. Front. Microbiol. 7:434. doi: 10.3389/fmicb.2016.00434
Blancheton, J. P., Attramadal, K., Michaud, L., D’Orbcastel, E., and Vadstein, O. (2013). Insight into bacterial population in aquaculture systems and its implication. Aquacult. Eng. 53, 30–39. doi: 10.1016/j.aquaeng.2012.11.009
Braeckman, U., Colen, C. V., Guilini, K., Gansbeke, D. V., and Vopel, K. (2014). Empirical evidence reveals seasonally dependent reduction in nitrification in coastal sediments subjected to near future ocean acidification. PLoS One 9:e108153. doi: 10.1371/journal.pone.0108153
Caporaso, J. G., Kuczynski, J., Stombaugh, J., Bittinger, K., Bushman, F. D., Costello, E. K., et al. (2010). QIIME allows analysis of high-throughput community sequencing data. Nat. Methods 7, 335–336. doi: 10.1038/nmeth.f.303
Currie, A. R., Karen, T., Helen, P., Beatriz, F. M., Natalie, H., Mark, O. A., et al. (2017). Marine microbial gene abundance and community composition in response to ocean acidification and elevated temperature in two contrasting coastal marine sediments. Front. Microbiol. 8:1599. doi: 10.3389/fmicb.2017.01599
Gao, F., Li, F., Tan, J., Yan, J., and Sun, H. (2014a). Bacterial community composition in the gut content and ambient sediment of sea cucumber Apostichopus japonicus revealed by 16S rRNA gene pyrosequencing. PLoS One 9:e100092. doi: 10.1371/journal.pone.0100092
Gao, F., Tan, J., Sun, H., and Yan, J. (2014b). Bacterial diversity of gut content in sea cucumber (Apostichopus japonicus) and its habitat surface sediment. J. Ocean U. China 13, 303–310. doi: 10.1007/s11802-014-2078-7
Gazeau, F., Rijswijk, P. V., Pozzato, L., and Middelburg, J. J. (2014). Impacts of ocean acidification on sediment processes in shallow waters of the arctic ocean. PLoS One 9:e94068. doi: 10.1371/journal.pone.0094068
Hancock, A. M., King, C. K., Stark, J. S., Mcminn, A., and Davidson, A. T. (2020). Effects of ocean acidification on Antarctic marine organisms: A meta-analysis. Ecol. Evol. 10, 4495–4514. doi: 10.1002/ece3.6205
Hicks, N. (2017). Oxygen dynamics in shelf seas sediments incorporating seasonal variability. Biogeochemistry 135, 35–47. doi: 10.1007/s10533-017-0326-9
IPCC (2021). Climate Change 2021: the physical science basis. Contribution of working group I to the sixth assessment report of the intergovernmental panel on climate change. Cambridge, MA: Cambridge University Press.
Jian, S., Zhang, J., Zhang, H. H., and Yang, G. P. (2019). Effects of ocean acidification and short-term light/temperature stress on biogenic dimethylated sulfur compounds cycling in the Changjiang River Estuary. Environ. Chem. 16, 197–211. doi: 10.1071/EN18186
Josiane, M., Maurice, L., Martine, L., Michael, S., Jean-Éric, T., Philippe, T., et al. (2016). Impact of ocean acidification on phytoplankton assemblage, growth, and DMS production following Fe-dust additions in the NE Pacific high-nutrient, low-chlorophyll waters. Biogeosciences 13, 1677–1692. doi: 10.5194/bg-13-1677-2016
Kerfahi, D., Hall-Spencer, J. M., Tripathi, B. M., Milazzo, M., and Adams, J. M. (2014). Shallow water marine sediment bacterial community shifts along a natural CO2 gradient in the Mediterranean Sea Off Vulcano. Italy. Microb. Ecol. 67, 819–828. doi: 10.1007/s00248-014-0368-7
Kerfahi, D., Harvey, B. P., Agostini, S., Kon, K., and Hall-Spencer, J. M. (2020). Responses of intertidal bacterial biofilm communities to increasing pCO2. Mar. Biotechnol. 22, 727–738. doi: 10.1007/s10126-020-09958-3
Krause, E., Wichels, A., Giménez, L., Lunau, M., Schilhabel, M. B., Gerdts, G., et al. (2012). Small changes in pH have direct effects on marine bacterial community composition: A microcosm approach. PLoS One 7:e47035. doi: 10.1371/journal.pone.0047035
Lenz, B., Fogarty, N. D., Figueiredo, J., and Lenz, B. (2019). Effects of ocean warming and acidification on fertilization success and early larval development in the green sea urchin Lytechinus variegatus. Mar. Pollut. Bull. 141, 70–78. doi: 10.1016/jmarpolbul.2019.02.018
Lewis, E., Wallace, D., and Allison, L. J. (1998). Program developed for CO2 system calculations. Oak Ridge, TN: Carbon Dioxide Information Analysis Center, Oak Ridge National Laboratory, U.S. Department of Energy.
Li, J., Liao, M. J., Li, B., Wang, Y. G., Rong, X. J., Zhang, Z., et al. (2019). The characteristics of culturable bacterial microflora in the gastrointestinal tract of cultured sea cucumber (Apostichopus japonicus) during the early developmental stage and its relationship with environmental flora. Prog. Fish. Sci. 40, 122–131.
Lidbury, I., Johnson, V., Hall-Spencer, J. M., Munn, C. B., and Cunliffe, M. (2012). Community-level response of coastal microbial biofilms to ocean acidification in a natural carbon dioxide vent ecosystem. Mar. Pollut. Bull. 64, 1063–1066. doi: 10.1016/j.marpolbul.2012.02.011
Mensch, B., Neulinger, S. C., Künzel, S., Wahl, M., and Schmitz, R. A. (2020). Warming, but not acidification, restructures epibacterial communities of the baltic macroalga Fucus vesiculosus with seasonal variability. Front. Microbiol. 11:1471. doi: 10.3389/fmicb.2020.01471
Oliver, A. E., Newbold, L. K., Whiteley, A. S., and Van, D. (2015). Marine bacterial communities are resistant to elevated carbon dioxide levels. Environ.Microbiol. Rep. 6, 574–582. doi: 10.1111/1758-2229.12159
Orr, J., Fabry, V., Aumont, O., Bopp, L., Doney, S., Feely, R., et al. (2005). Anthropogenic ocean acidification over the twenty-first century and its impact on calcifying organisms. Nature 437, 681–686. doi: 10.1038/nature04095
Qiu, T. (2013). Research and application of key technology principle of sea cucumber Apostichopus japonicas ecological offspring breeding (PhD thesis). Shandong Sheng: Institute of Oceanology, Chinese Academy of Sciences.
Rogelj, J., Huppmann, D., Krey, V., Riahi, K., Clarke, L., Gidden, M., et al. (2019). A new scenario logic for the Paris Agreement long-term temperature goal. Nature 573, 357–363. doi: 10.1038/s41586-019-1541-4
Smale, D. A., Taylor, J. D., Coombs, S. H., Moore, G., and Cunliffe, M. (2017). Community responses to seawater warming are conserved across diverse biological groupings and taxonomic resolutions. P. Roy. Soc. B: Biol. Sci. 284:20170534. doi: 10.1098/rspb.2017.0534
Song, M., Yang, X., Zhang, A., Liu, Y., Yang, D., and Yuan, X. (2020). Effects of seawater acidification and warming on growth and pigmentation in juvenile sea cucumber Apostichopus japonicas. Chinese J. Ecol. 39, 1609–1616. doi: 10.13292/j.1000-4890.202005.033
Sunday, J. M., Fabricius, K. E., Kroeker, K. J., Anderson, K. M., Brown, N. E., Barry, J. P., et al. (2016). Ocean acidification can mediate biodiversity shifts by changing biogenic habitat. Nat. Clim. Change 7, 81–85. doi: 10.1038/nclimate3161
Tait, K., Laverock, B., Shaw, J., Somerfield, P. J., and Widdicombe, S. (2013). Minor impact of ocean acidification to the composition of the active microbial community in an Arctic sediment. Environ. Microbiol. Rep. 5, 851–860. doi: 10.1111/1758-2229.12087
Tait, K., Laverock, B., and Widdicombe, S. (2014). Response of an arctic sediment nitrogen cycling community to increased CO2. Estuar. Coast. 37, 724–735. doi: 10.1007/s12237-013-9709-x
Wang, L., Wei, C., Chang, Y., and Ding, J. (2021). Response of bacterial community in sea cucumber Apostichopus japonicus intestine, surrounding water and sediment subjected to high-temperature stress. Aquaculture 535:736353. doi: 10.1016/jaquaculture.2021.736353
Witt, V., Wild, C., Anthony, K., Diaz-Pulido, G., and Uthicke, S. (2011). Effects of ocean acidification on microbial community composition of, and oxygen fluxes through, biofilms from the Great Barrier Reef. Environ. Microbiol. 13, 2976–2989. doi: 10.1111/j.1462-2920.2011.02571.x
Yamazaki, Y., Meirelles, P. M., Mino, S., Suda, W., Oshima, K., Hattori, M., et al. (2016). Individual Apostichopus japonicus fecal microbiome reveals a link with polyhydroxybutyrate producers in host growth gaps. Sci. Rep. 6, 1–10. doi: 10.1038/srep21631
Yang, H., Hamel, J. F., and Mercier, A. (2015). The sea cucumber Apostichopus japonicus: History, biology and aquaculture. Developments in Aquaculture and Fisheries Science 39. Boston: Academic Press.
Yuan, X., Shao, S., Dupont, S., Meng, L., Liu, Y., and Wang, L. (2015). Impact of CO2-driven acidification on the development of the sea cucumber Apostichopus japonicus (Selenka) (Echinodermata: Holothuroidea). Mar. Pollut. Bull. 95, 195–199. doi: 10.1016/j.marpolbul.2015.04.021
Yuan, X., Shao, S., Yang, X., Yang, D., Xu, Q., Zong, H., et al. (2016). Bioenergetic trade-offs in the sea cucumber Apostichopus japonicus (Echinodermata: Holothuroidea) in response to CO2-driven ocean acidification. Environ.Sci.Pollut. Res. 23, 8453–8461. doi: 10.1007/s11356-016-6071-0
Zhang, H., Wang, Q., Zhao, J., Liu, S., Zhang, L., Zhao, Y., et al. (2020). Quantitative microbiome profiling links microbial community variation to the intestine regeneration rate of the sea cucumber Apostichopus japonicus. Genomics 112, 5012–5020. doi: 10.22541/au.159248860.02406768
Zhang, Q., and Liu, Y. (1998). Techniques for culturing sea cucumbers and sea urchins. Qingdao: Qingdao Ocean University press
Zhang, Z., Zhang, W., Hu, Z., Li, C., Shao, Y., Zhao, X., et al. (2019c). Environmental factors promote pathogen-induced skin ulceration syndrome outbreak by readjusting the hindgut microbiome of Apostichopus japonicus. Aquaculture 507, 155–163. doi: 10.1016/j.aquaculture.2019.03.054
Zhang, H., Wang, Q., Liu, S., Huo, D., Zhao, J., Zhang, L., et al. (2019a). Genomic and metagenomic insights into the microbial community in the regenerating intestine of the sea cucumber Apostichopus japonicus. Front. Microbiol. 10:1165. doi: 10.3389/fmicb.2019.01165
Keywords: climate change, microbial community, Apostichopus japonicus, post-settlement, aquaculture system
Citation: Zhang H, Song M, Wang L, Zhang A, Yang X, Liu Y and Yuan X (2021) Response of Microbial Communities on Culturing Plates of Post-settlement Sea Cucumbers to Seawater Acidification and Warming. Front. Mar. Sci. 8:802023. doi: 10.3389/fmars.2021.802023
Received: 26 October 2021; Accepted: 12 November 2021;
Published: 01 December 2021.
Edited by:
Jinghui Fang, Yellow Sea Fisheries Research Institute, Chinese Academy of Fishery Sciences (CAFS), ChinaReviewed by:
Chuanxin Qin, South China Sea Fisheries Research Institute, Chinese Academy of Fishery Sciences (CAFS), ChinaMuyan Chen, Ocean University of China, China
Copyright © 2021 Zhang, Song, Wang, Zhang, Yang, Liu and Yuan. This is an open-access article distributed under the terms of the Creative Commons Attribution License (CC BY). The use, distribution or reproduction in other forums is permitted, provided the original author(s) and the copyright owner(s) are credited and that the original publication in this journal is cited, in accordance with accepted academic practice. No use, distribution or reproduction is permitted which does not comply with these terms.
*Correspondence: Xiutang Yuan, eHR5dWFuQHlpYy5hYy5jbg==