- 1CAS Key Laboratory of Marine Ecology and Environmental Sciences, Institute of Oceanology, Chinese Academy of Sciences, Qingdao, China
- 2Laboratory for Marine Ecology and Environmental Science, Qingdao National Laboratory for Marine Science and Technology, Qingdao, China
- 3University of Chinese Academy of Sciences, Beijing, China
- 4Shandong Hydrobios Resources Conservation and Management Center, Yantai, China
- 5Center for Ocean Mega-Science, Chinese Academy of Sciences, Qingdao, China
Estuaries, where fresh and salty water converge, provide abundant nutrients for ichthyoplankton. Ichthyoplankton, including fish eggs, larvae, and juveniles, are important fishery recruitment resources. The Yangtze Estuary and its adjacent waters comprise a typical large-scale estuary and supply many important fish spawning, feeding, and breeding areas. In this study, 1,291 ichthyoplankton individuals were collected in the Yangtze Estuary in spring, from 2013 to 2020. The aims of the study were to provide detailed information on characteristics of the ichthyoplankton assemblage, explore interannual variation, and evaluate the effects of environmental variables on the temporal variation in assemblage structure. Twenty-six species in seventeen families were identified. The dominant species were Coilia mystus, Chelidonichthys spinosus, Engraulis japonicus, Hypoatherina valenciennei, Larimichthys polyactis, Salanx ariakensis, Stolephorus commersonnii, and Trachidermus fasciatus. The ichthyoplankton assemblage changed significantly over time, and Chelidonichthys spinosus became one of the dominant species. Canonical correspondence analysis showed that temperature and chlorophyll a were the key factors affecting the assemblage structure in the Yangtze Estuary in spring.
Introduction
Estuaries are semi-closed coastal water bodies that are freely connected to the open sea, in which the seawater is diluted by fresh water produced by land drainage (Day, 1981; Potter et al., 1990). The interactions of physical, chemical, biological, and geological processes lead to complex change processes and a sensitive and unique estuarine environment that differs from adjacent fresh water and marine environments (Luo, 1994; Shan et al., 2004). Estuaries are considered to be among the most productive and nutrient-rich ecosystems on earth, and provide important habitat for a variety of species (Hodgson et al., 2020). Many species complete some or all of their life histories in estuaries, and these areas are particularly important foraging and breeding habitats for many fish species (Sheaves et al., 2015; Xian et al., 2016; Lefcheck et al., 2019). As a result of their unique geographical location, estuaries have been sites of human development for thousands of years (Limburg, 1999; Lotze, 2010). Currently, about 40% of the world’s population lives in coastal areas (Barragán and de Andrés, 2015). More than 90% of economical species, including fish, have been depleted, and biomass is lower than 50% of its historical abundance in some estuaries. The main drivers of this change are resource overexploitation and habitat loss (Lotze et al., 2006).
Ichthyoplankton, including eggs, larvae, and juveniles, are significant stages during the growth and development of fish (Butler et al., 2003; Shan et al., 2004) and are the basis for the sustainable utilization of fishery resources (Miller and Kendall, 2009; Zhang et al., 2015). Although these planktonic stages can be very short, they are the most vulnerable in a fish’s life history (Shao et al., 2001; Jiang et al., 2006) and can be strongly affected by environmental variability. The abundance and survival of ichthyoplankton are indicators of available biomass and interannual dynamics of fish populations in the future (Butler et al., 2003; Song et al., 2019). In addition, ichthyoplankton play a significant role in energy transfer in estuarine ecosystems (Wan and Sun, 2006), consuming and converting bioenergy as a key link in aquatic food webs (Wan and Jiang, 2000).
Ichthyoplankton assemblages, species composition, and distribution are affected by the interactions of a range of biotic and abiotic processes (Zhang et al., 2015). Biological factors include the location, timing, and modes of spawning; larval life history and behavior; rates of predation; and feeding (Azeiteiro et al., 2006). Physical factors include salinity (Wang and Zhang, 1998; Wooldridge, 1999; Campfield and Houde, 2011); temperature (Neira and Potter, 1992; Primo et al., 2011; Wang et al., 2017); turbidity (Harris and Cyrus, 1995); dissolved oxygen (Rakocinski et al., 1996); depth (Zhang et al., 2015); light (Shoji et al., 2006); and hydrographic events such as river flow (Taylor, 1982), tides (Chen et al., 1997), currents (Wu, 1989), upwelling (Ramos et al., 2006; Valencia et al., 2019), and winds (Tzeng et al., 2002). Studies of ichthyoplankton provide a foundation for understanding trends in fishery resources, determining spawning sites and cycles, and clarifying fishing mechanisms; they can also be used to monitor ecosystem health.
The Yangtze Estuary is the link between the Yangtze River and the East China Sea. The estuary is influenced by fresh water from the Yangtze River, as well as the Yellow Sea cold water mass and the Taiwan warm current. The estuary is generally considered to be important nursery habitat used by many fishes to reproduce, forage, and grow (Luo and Shen, 1994). Although researchers have previously investigated ichthyoplankton composition and distribution in the Yangtze Estuary and the relationships between ichthyoplankton assemblages and environmental factors, the duration of these studies was short (Yang et al., 1990; Zhu et al., 2002; Jiang et al., 2006; Liu et al., 2008; Zhang et al., 2019) or discontinuous (Zhang et al., 2015, 2016). A clear understanding of how the physical and chemical characteristics of the Yangtze Estuary affect ichthyoplankton assemblages is still lacking.
The present study was based on consecutive survey data from spring 2013 to 2020. The aims of the study were to provide detailed information on the characteristics of the ichthyoplankton assemblage in the Yangtze Estuary in spring, explore interannual variability in ichthyoplankton assemblages, and evaluate the effects of environmental variables on temporal variations in assemblage structure.
Materials and Methods
Data Source
Forty stations were established in the Yangtze Estuary (30°45′-32°00′ N, 121°00′-123°20′ E), where the biological and oceanographic data were collected in eight springs (05/2013, 05/2014, 05/2015, 05/2016, 05/2017, 05/2018, 05/2019, 05/2020) (Figure 1).
In total, 320 ichthyoplankton samples were collected by surface tows using a plankton net (0.8 m mouth diameter, 2.8 m length, 0.505 mm mesh size) equipped with a flow meter. At each station, the net was towed against the tidal flow for 10 min at a depth of 0.5 m from the surface, with a towing speed of approximately 2–3 knots. After completion of each tow, the nets were cleaned and the samples were fixed and preserved in 5% buffered formaldehyde–seawater solution.
The geographical locations of sampling stations were determined using GPS (Magellan 315) (Zhang et al., 2016). A conductivity, temperature, and depth device (CTD) was used to measure depth (D), salinity (S), and temperature (T). Other environmental indicators, including dissolved oxygen (DO), pH, total phosphorus (TP), total nitrogen (TN), suspended particulate matter (SPM), chemical oxygen demand (COD), and chlorophyll a (Chla), were determined in the study according to GB/T 12763-2007 specifications for oceanographic surveys (Standardization Administration of China (SAC), 2007).
Data Analysis
Ichthyoplankton were identified in the laboratory to the lowest possible taxonomic level according to their morphological characteristics. Numerical density for each species was standardized to catch per unit effort (CPUE) as abundance per tow every 10 min.
The dominant species were determined using the index of relative importance (IRI) (Zhu et al., 2002), calculated as
where N 100% and F 100% are the relative abundance and frequency of occurrence, respectively. The IRI of the dominant species should be greater than 100.
To determine the significance of interannual trends in assemblage structure from 2013 to 2020, non-parametric ANOSIM analysis was performed based on a Bray–Curtis similarity matrix calculated using log(x + 1) transformed data (Clarke and Warwick, 2001). R-statistic values of paired comparisons provided by ANOSIM were used to determine differences between groups. R values close to 1 indicate that the differences between groups are greater than those within groups, and R values close to 0 indicate little difference between groups.
The ichthyoplankton assemblage structure and its relationship with environmental factors was analyzed by canonical correspondence analysis (CCA) (CANOCO Software, version 5.0). Only species that occurred in >0.5% of the catches were included in the analysis. Fourteen species found between 2013 and 2020 were included in the analyses. The species abundance data were lg(x + 1) transformed to reduce the dominance effects of some species (Clarke and Warwick, 2001; Zhang et al., 2015). These 14 species and 10 environmental factors were used in the CCA.
Results
Ichthyoplankton Composition
In total, 1,291 ichthyoplankton individuals, belonging to 26 species in 17 families, were captured in the Yangtze Estuary in spring 2013–2020. The scientific names, family, ecological guilds, and IRI values for the assemblages are shown in Table 1. The dominant species were Coilia mystus, Chelidonichthys spinosus, Engraulis japonicus, Hypoatherina valenciennei, Larimichthys polyactis, Salanx ariakensis, Stolephorus commersonnii, and Trachidermus fasciatus. Most of the species with low abundance were collected occasionally.
Interannual Variation of Assemblage Structure
The assemblage structure changed significantly in the Yangtze Estuary according to the IRI values for spring 2013–2020 (Table 2). Although Engraulis japonicus was always the dominant species in the Yangtze Estuary and adjacent waters, the composition of the other dominant species changed significantly, highlighted by the shift in the relative abundances of Coilia mystus and Chelidonichthys spinosus. The assemblage composition based on presence–absence methods varied among years and the average dissimilarity among years was very high (Table 3).
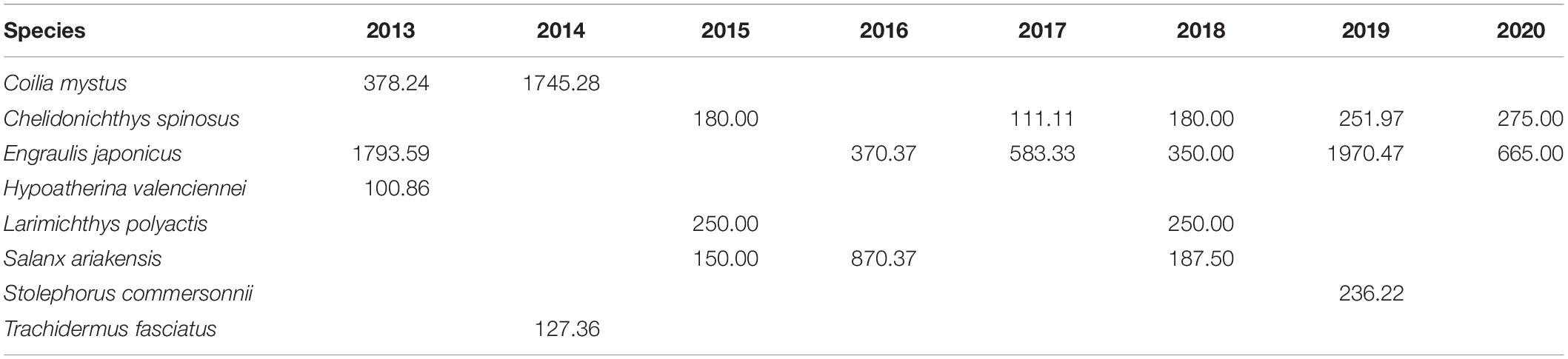
Table 2. Dominant species determined by index of relative importance (IRI) in the Yangtze Estuary in spring 2013–2020.
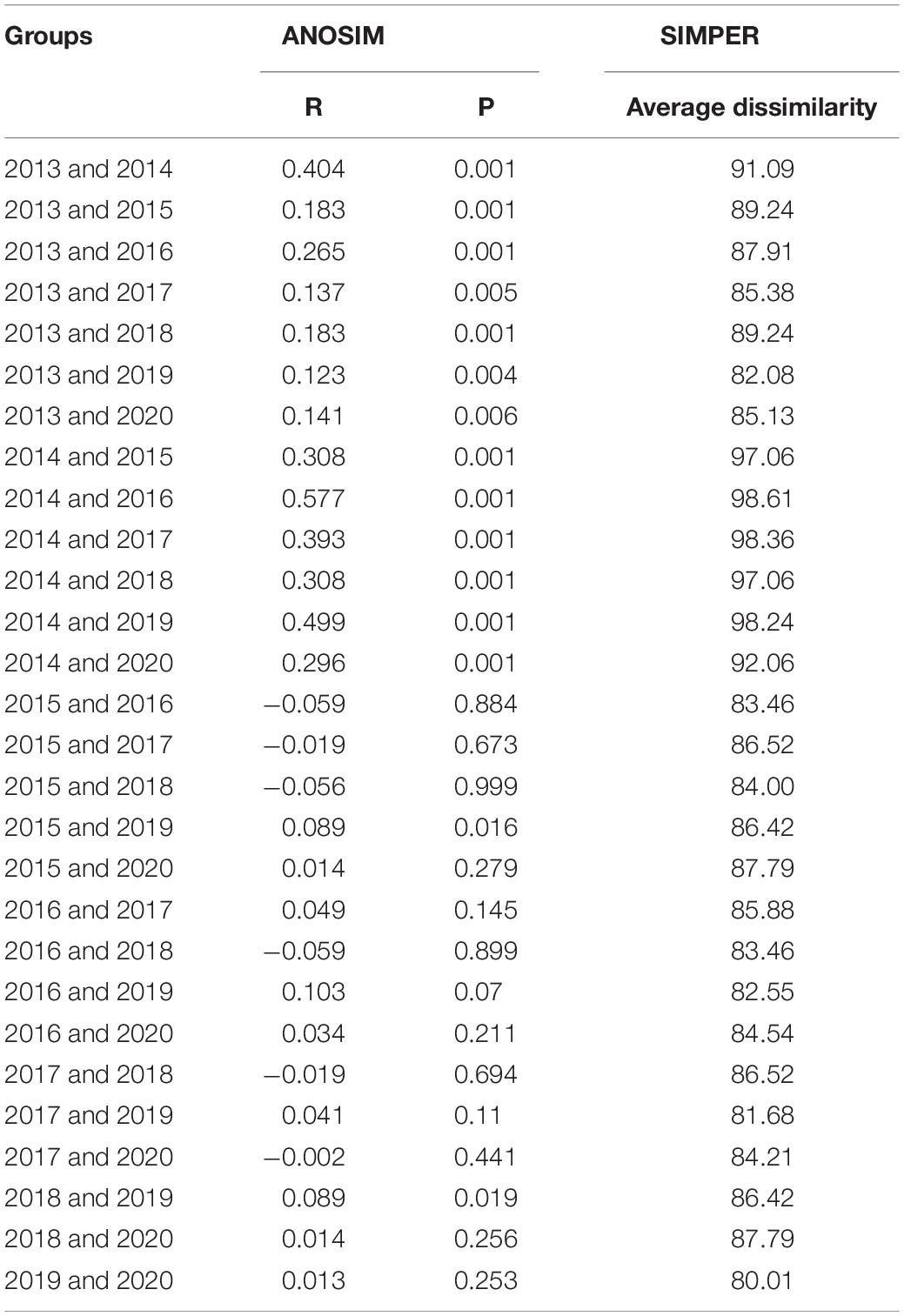
Table 3. Inter-annual comparison of the assemblage structure according to one-way ANOSIM (R value and significance level) and SIMPER analysis.
Ichthyoplankton Assemblages and Environmental Variables
The CCA results are shown in Table 4. Eigenvalues (0–1) indicate the importance of the CCA axes. The sum of all canonical eigenvalues and unconstrained eigenvalues were 1.338 and 7.729, respectively. The former only accounted for 17.31% of the latter, indicating the restrictive effect of building environmental relationships into the CCA model. The eigenvalues of the first four axes were 0.362 (CCA 1), 0.334 (CCA 2), 0.233 (CCA 3), and 0.167 (CCA 4) in the present study. The eigenvalues of the first two axes were moderately high, with correlation coefficients of 0.716 and 0.646, whereas those of the latter two were relatively low (<0.3). Consequently, the results for the first two axes were plotted (Figure 2), which explained 9.01 and 64.62% of the cumulative percentage variance of species and species–environment, respectively (Table 4).
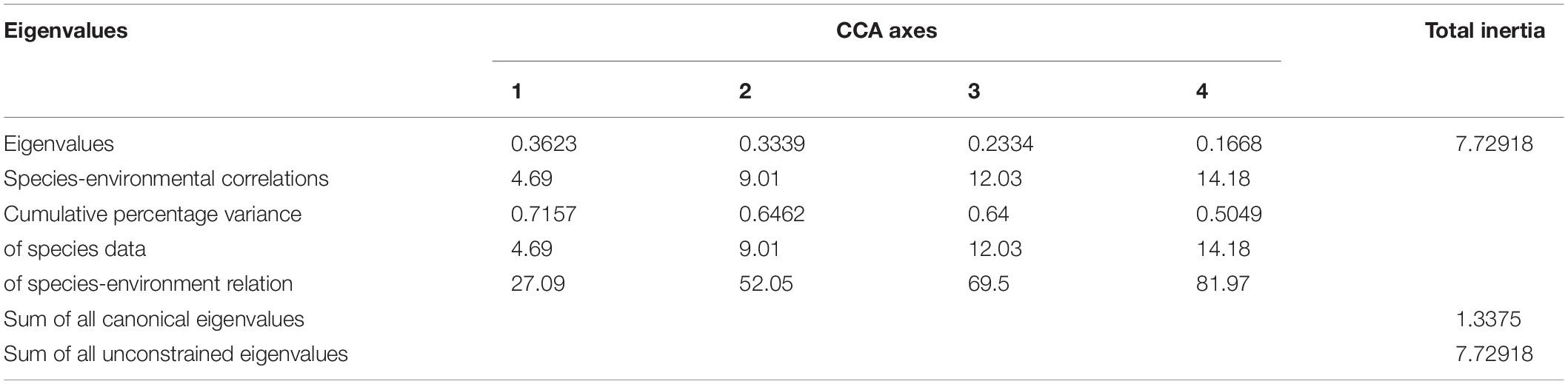
Table 4. Results of canonical correspondence analysis (CCA) relating ichthyoplankton abundance data to environmental factors in the Yangtze Estuary in spring 2013–2020.
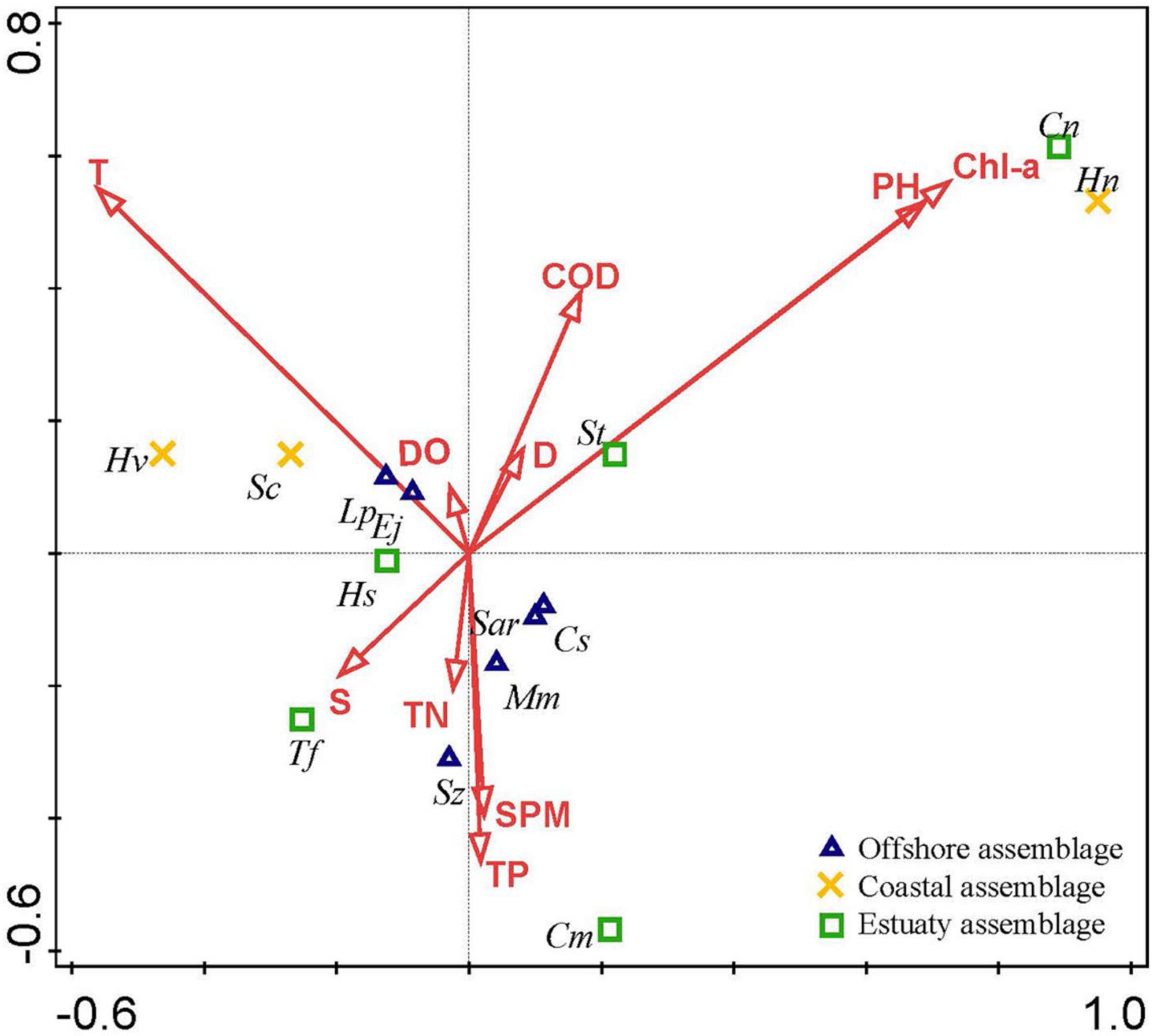
Figure 2. Canonical correspondence analysis (CCA) biplot of ichthyoplankton species in the Yangtze Estuary in spring 2013–2020. The black font indicated the abbreviate name of the species as shown in Table 1.
On the basis of the Monte Carlo tests of F-ratios (P < 0.05), T and Chla were the most significant environmental variables affecting the ichthyoplankton assemblages (Table 5). In addition to these variables, the other eight environmental variables were evaluated by their inter-set correlations. There are two types of scores in this plot. (1) Environmental variable arrows, where each arrow points in the direction in which the value of the variable increases most sharply. The length of the arrow represents the strength of the environmental variable on the axis. The angle between arrows represents the correlation between the variables. More accurately, the approximate correlation between two environmental variables can be found by projecting the arrow of one variable onto an imaginary line running along the arrow direction of the other. (2) Species symbols: the distance between symbols is similar to the difference in the distribution of the relative abundance of these species in the sample, measured by chi-square distance. Adjacent points correspond to species that often occur at the same time. The species symbol can be projected vertically onto the line above the arrow of a specific environmental variable. These predictions can be used to approximate the optimal value of an environmental variable for a single species. The projection points for the species are arranged in the order of increasing value of the predictive variable. According to these rules, some environmental variables were inferred to be important correlates of one or both of the first two CCA axes. pH, Chla, S, and T were highly correlated with the first axis (CCA 1), while TN, SPM, TP, D, DO, and COD were strongly correlated with the second axis (CCA2). Although there was no obvious correlation between temperature and chlorophyll a, they both affected the interannual variation in ichthyoplankton (Figure 2).
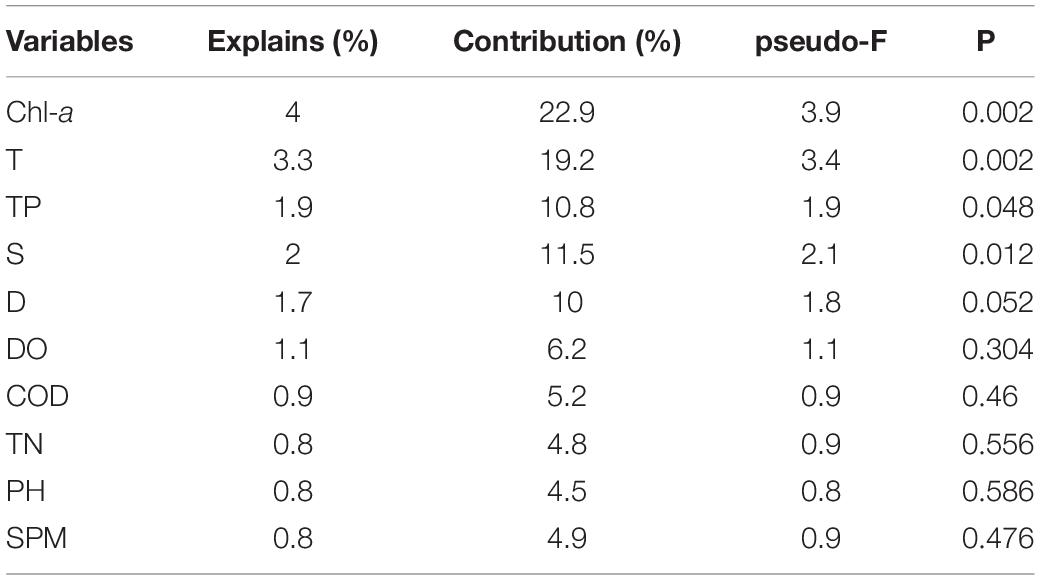
Table 5. Conditional effects and correlations of environmental variables with the canonical correspondence analysis (CCA) axes.
Ichthyoplankton were divided into three species groups according to their ecological guilds (Table 1 and Figure 2). Offshore assemblage: six species (Chelidonichthys spinosus, Sardinella zunasi, Minous monodactylus, Salanx ariakensis, Larimichthys polyactis, and Engraulis japonicus) distributed close to CCA axis 2 and mainly correlated with T. All species, except for Sardinella zunasi and Minous monodactylus, were dominant. Coastal assemblage: three species (Hypoatherina valenciennei, Stolephorus commersonnii, and Harpadon nehereus) distributed in brackish waters. Hypoatherina valenciennei and Stolephorus commersonnii were dominant species. Estuarine assemblage: five species (Hyporhamphus sajori, Setipinna tenuifilis, Trachidermus fasciatus, Coilia mystus, and Coilia nasus) with dispersed distribution and mainly correlated with S, pH, S, and Chla. Trachidermus fasciatus and Coilia mystus were dominant species.
Discussion
Variation on Ichthyoplankton Composition
The ichthyoplankton assemblage in the Yangtze Estuary is changing, as reflected in the reduction in species number and shifts in species composition. In the 1980s, 53 families and 94 species of ichthyoplankton were recorded in the Yangtze Estuary (Yang et al., 1990). By 2000–2003, the number of species collected had decreased sharply, with only 30 families and 45 species (Jiang et al., 2006). From 2012 to 2014, the number of ichthyoplankton species decreased again, with only 38 species in 18 families (Kindong et al., 2020). In this study (2013–2020), 17 families and 26 species of ichthyoplankton were collected, which is only 27.66% of the diversity observed in the 1980s. These results show that the ichthyoplankton species composition in the Yangtze Estuary has changed significantly in the last 35 years.
The abundance of ichthyoplankton in estuaries is determined by the environmental characteristics and nutritional status of the estuarine ecosystem. The community structure of ichthyoplankton in the estuary was composed of a few high abundance species and a large number of rare species, which is a common feature of estuaries (Gaughan et al., 1990; Harrison and Whitfield, 1990; Drake and Arias, 1991). For example, ichthyoplankton communities in warm water estuaries were found to be dominated by Gobiidae, which live in estuaries, and Clupeidae and Engraulidae, which lay eggs seasonally in estuaries (Talbot and Able, 1984; Drake and Arias, 1991). Our results are in accordance with those of previous studies. The eight dominant species accounted for 94.58% of all ichthyoplankton sampled. Interestingly, Engraulis japonicus was the dominant species in the Yangtze Estuary from 2013 to 2020 in our study, accounting for 44.15% of the total catch, which is consistent with previous reports in which this species represented 67.91% of the total catch (Shen et al., 2011; Zhang et al., 2015). In addition, Chelidonichthys spinosus gradually became one of the dominant species after 2015, although it accounted for only 4.96% of the total catch. As far as we know, this finding is the first report of this shift.
Interannual Variability of Assemblage Structure
Estuaries have complex and variable physical, chemical, and hydrological conditions, with environmental factors that change dramatically in time and space (Whitfield, 1994; Harris and Cyrus, 1995; Hettler and Hare, 1998). Ichthyoplankton are very sensitive to environmental conditions (Shao et al., 2001). Thus, ichthyoplankton assemblages usually show high interannual variability in abundance and composition. By comparing the shifts in dominant species between this study (2013–2020) and previous ones (1999, 2001, 2004, and 2007), it was found that the dominant species of ichthyoplankton had decreased significantly, and the species composition of dominant species had changed (Figure 3). During the study period, there were obvious changes in the structure of the ichthyoplankton community in the Yangtze Estuary, indicating that environmental variables have a significant effect on interannual changes in ichthyoplankton community structure. However, there were no significant differences in the structure of the ichthyoplankton community in the spring in 1999, 2001, 2004, and 2007 (Zhang et al., 2015). Distribution patterns of ichthyoplankton are closely related to the reproductive strategy and life history of the adult populations, which are often associated with oceanography and meteorology (Hernández-Miranda et al., 2003). Therefore, the ability of juvenile fish to survive in the pelagic environment and migrate to suitable adult habitats may depend on their ability to regulate their diffusion or migration (Muhling et al., 2007).
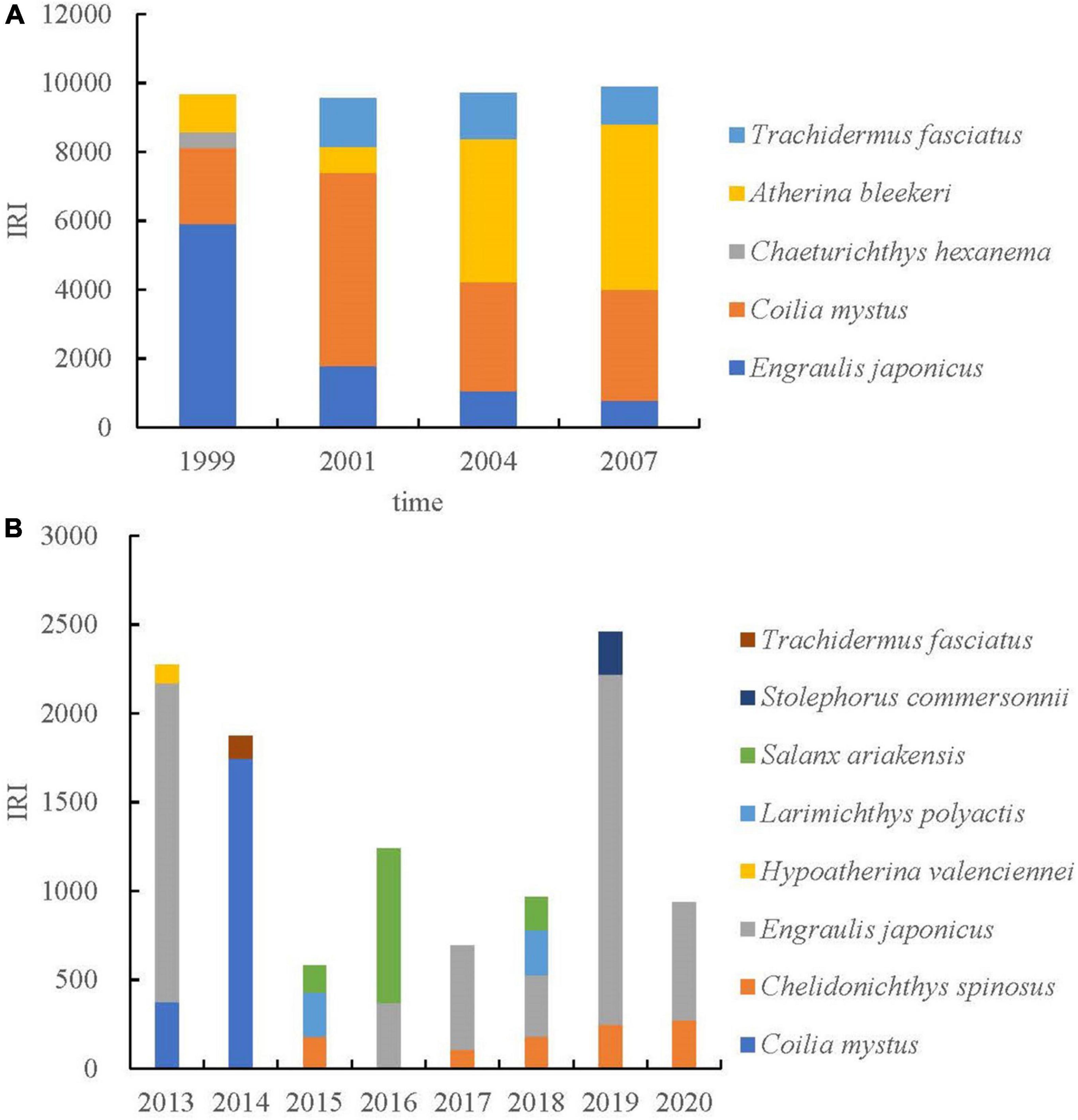
Figure 3. Variations on ichthyoplankton dominance (IRI) and species composition in the Yangtze Estuary in spring. Panel (A) represented published data (Zhang et al., 2015); panel (B) represented the data of this study. Because the original abundance data were not obtained in the literature, the dominance data (IRI) were used for comparison.
Stochastic climatic events (cyclones) can alter the community composition of ichthyoplankton in estuaries by bringing intense rainfall, which significantly changes the distribution and abundance of estuary residents and marine migratory species (Martin et al., 1992). In addition, long-term climate trends, such as climate change and El Niño events, can also drive changes in community structure (Franco-Gordoa et al., 2004; Acha et al., 2012). Climate change is altering the temperature, salinity, dissolved oxygen, and other estuarine conditions, resulting in changes to the temporal and spatial distribution of ichthyoplankton (Sloterdijk et al., 2017). Increasing temperatures could lead to earlier fish spawning (Thaxton et al., 2020), extension of the spawning time (Primo et al., 2011; Acha et al., 2012), shortening of egg hatching time (Hassell et al., 2008), early gonadal maturation (Thaxton et al., 2020), and northward shift of spawning areas (Auth et al., 2018; Zhang et al., 2019), resulting in changes in ichthyoplankton community structure.
In recent years, the modernization of fishing gear and improvements in fishing technology have led to increases in fishing intensity, which now far exceeds the capacity of resource replenishment. Some important economic species have become rare, and even endangered (Zhang et al., 2009). In the Yangtze Estuary, overfishing of important economic species, such as Trichiurus japonicus and Larimichthys polyactis, has led to fish miniaturization, early maturity, and juvenile structure (Shan et al., 2004). The main reason for the miniaturization of fish communities is the uncontrolled overuse of marine resources in coastal waters (Zhu et al., 2002). Overfishing is the main reason for the significant reductions in fishery resources (Song et al., 2019). Under the joint influences of overfishing and environmental change, the population and community structure of fish resources in estuarine ecosystems have changed significantly (Bian et al., 2010), leading to loss of ecosystem resilience and integrity (Ryder et al., 1981).
Ichthyoplankton Assemblage Structure and Its Influencing Factors
The ichthyoplankton community in the Yangtze Estuary was divided into three groups: estuarine, coastal, and offshore species (Zhang et al., 2015). It is generally believed that the distribution patterns of ichthyoplankton communities is affected by both biological and environmental factors (Zhu et al., 2002). In this study, CCA was used to determine the correlations between ichthyoplankton abundance (time–abundance data) and environmental variables (time–environment data). In general, T and Chla were the most significant factors affecting community structure. The other factors, such as pH, D, COD, DO, TN, and SPM, had no significant effect on ichthyoplankton assemblage structure.
Temperature has a significant and direct effect on fish reproduction, physiology, growth, and behavior (Sabates et al., 2006; Santos and Severi, 2019), which is very important for the abundance and spatial distribution of ichthyoplankton (Bruno et al., 2014; Chermahini et al., 2021). Estuaries are generally shallow, with large temperature changes, and diurnal and seasonal changes, therefore estuarine species adapted to a wide temperature range often dominate (Yang et al., 2006). In temperate and subtropical estuaries, the peak number of species usually occurs in the spring and summer because of the annual changes in temperature (Neira and Potter, 1992; Primo et al., 2011; Wang et al., 2017). Therefore, during the growth and developmental stages of larvae and juveniles, temperature could have an important influence on growth, density, and reproduction (Ramos et al., 2006). Here, interannual changes in the abundance of Engraulis japonicus and Larimichthys polyactis were closely correlated with increasing temperature, and interannual changes in Salanx ariakensis and Chelidonichthys spinosus abundance were closely associated with decreasing temperatures.
Chla is an important environmental variable that has a significant influence on ichthyoplankton community structure, and was positively correlated with pH and negatively correlated with S. Chla is a relatively accurate reflection of phytoplankton standing stock; the larger the phytoplankton biomass, the higher the primary productivity (Whitfield, 1999). Chla is closely associated with fresh water nutrient levels entering the estuary. When nutrient levels are high, the Chla level in the estuary is also high, and food resources are rich, which is necessary for the development and growth of larvae and juveniles. Abundant food resources also improved the survival rate and successful foraging rate (Zhang et al., 2015). In this study, three estuarine species (Trachidermus fasciatus, Coilia nasus, and Setipinna tenuifilis) were closely associated with the content of Chla (Figure 2). Zhang et al. (2016) also reported that Chla was closely related to the distribution of three freshwater species.
In addition to T and Chla, other environmental variables also affected the community structure of ichthyoplankton. TP and TN represent nutritional status, S, DO, COD, SPM, and pH represent water quality. Salinity is a key abiotic factor determining the structure of the ichthyoplankton community in estuaries (Wooldridge, 1999; Campfield and Houde, 2011). A decrease in salinity level can lead to peaks in fish eggs and larvae, and low salinity coastal systems have a significant influence on the hatching and development of fish eggs and larvae (Jiang et al., 2006). SPM is one of the most important factors affecting the density of estuarine species (Harris and Cyrus, 1995). Rakocinski et al. (1996) used canonical correlation analysis to analyze the changes in ichthyoplankton community structure in the Mississippi River and found that dissolved oxygen had a strong influence, as did temperature and salinity.
The variation in species distribution explained by the first four axes of the CCA was only 14.18%, which indicates that other factors are affecting the ichthyoplankton assemblage structure in the Yangtze Estuary. Biological and human factors were not included in this study and the influence of these variables should be investigated in the Yangtze Estuary.
Conclusion
In this study, 1,291 ichthyoplankton individuals were collected in the Yangtze Estuary from 2013 to 2020, and identified as 26 species in 17 families. The dominant species were Coilia mystus, Chelidonichthys spinosus, Engraulis japonicus, Hypoatherina valenciennei, Larimichthys polyactis, Salanx ariakensis, Stolephorus commersonnii, and Trachidermus fasciatus. The interannual ichthyoplankton assemblage has changed significantly, reflected in the replacement of some dominant species and shifts in species composition. In particular, Chelidonichthys spinosus became a dominant species. Temperature and chlorophyll a were the key factors affecting the ichthyoplankton assemblage in the Yangtze Estuary in spring. Temperature and chlorophyll a were closely related to the abundance of offshore and estuarine species assemblages, respectively.
Data Availability Statement
The original contributions presented in the study are included in the article/supplementary material, further inquiries can be directed to the corresponding authors.
Ethics Statement
Ethical review and approval was not required for the animal study because this study did not involve animal ethics.
Author Contributions
YW analyzed the data and completed the first draft. HZ and WX provided guidance on the structure of the manuscript. ZC and SL provided the suggestions on this manuscript. YW, CL, and HZ modified the manuscript. All authors are contributed to revise the manuscript.
Funding
The present work was supported by the National Natural Science Foundation of China (Nos. 41976094 and 31872568), the Key Deployment Project of Center for Ocean Mega-Science, Chinese Academy of Sciences (COMS2019Q14), and the Youth Innovation Promotion Association CAS (No. 2020211).
Conflict of Interest
The authors declare that the research was conducted in the absence of any commercial or financial relationships that could be construed as a potential conflict of interest.
Publisher’s Note
All claims expressed in this article are solely those of the authors and do not necessarily represent those of their affiliated organizations, or those of the publisher, the editors and the reviewers. Any product that may be evaluated in this article, or claim that may be made by its manufacturer, is not guaranteed or endorsed by the publisher.
Acknowledgments
We are grateful to the editors and reviewers for their constructive feedback and concerning on our work.
References
Acha, E. M., Simionato, C. G., Carozza, C., and Mianzan, H. (2012). Climate-induced year-class fluctuations of whitemouth croaker Micropogonias furnieri (Pisces, Sciaenidae) in the Rio de la Plata estuary, Argentina-Uruguay. Fish. Oceanogr. 21, 58–77. doi: 10.1111/j.1365-2419.2011.00609.x
Auth, T. D., Daly, E. A., Brodeur, R. D., and Fisher, J. L. (2018). Phenological and distributional shifts in ichthyoplankton associated with recent warming in the northeast Pacific Ocean. Glob. Chang. Biol. 24, 259–272. doi: 10.1111/gcb.13872
Azeiteiro, U. M., Bacelar-Nicolau, L., Resende, P., Goncalves, F., and Pereira, M. J. (2006). Larval fish distribution in shallow coastal waters off North Western Iberia (NE Atlantic). Estuar. Coast. Shelf Sci. 69, 554–566. doi: 10.1016/j.ecss.2006.05.023
Barragán, J. M., and de Andrés, M. (2015). Analysis and trends of the world’s coastal cities and agglomerations. Ocean Coast. Manage. 114, 11–20. doi: 10.1016/j.ocecoaman.2015.06.004
Bian, X. D., Zhang, X. M., Gao, T. X., Wan, R. J., and Zhang, P. D. (2010). Category composition and distributional patterns of ichthyoplankton in the Yellow River estuary during spring and summer 2007. J. Fish. Sci. China 17, 815–827.
Bruno, D. O., Delpiani, S. M., Cousseau, M. B., de Astarloa, J. M. D., Blasina, G. E., Mabragana, E., et al. (2014). Ocean-estuarine connection for ichthyoplankton through the inlet channel of a temperate choked coastal lagoon (Argentina). Mar. Freshw. Res. 65, 1116–1130. doi: 10.1071/Mf13128
Butler, J. L., Jacobson, L. D., Barnes, J. T., and Moser, H. G. (2003). Biology and population dynamics of cowcod (Sebastes levis) in the southern California Bight. Fish. Bull. 101, 260–280.
Campfield, P. A., and Houde, E. D. (2011). Ichthyoplankton community structure and comparative trophodynamics in an estuarine transition zone. Fish. Bull. 109, 1–19.
Chen, Y. H., Shaw, P. T., and Wolcott, T. G. (1997). Enhancing estuarine retention of planktonic larvae by tidal currents. Estuar. Coast. Shelf Sci. 45, 525–533.
Chermahini, M. A., Shabani, A., Naddafi, R., Ghorbani, R., Rabbaniha, M., and Noorinejad, M. (2021). Diversity, distribution, and abundance patterns of ichthyoplankton assemblages in some inlets of the northern Persian Gulf. J. Sea Res. 167:101981. doi: 10.1016/j.seares.2020.101981
Clarke, K. R., and Warwick, R. M. (2001). Changes in marine communities: an approach to statistical analysis and interpretation. Mt. Sinai J. Med. 40, 689–692.
Day, J. (1981). “The nature, origin and classification of estuaries,” in Estuarine Ecology with Particular Reference to Southern Africa, ed. J. H. Day (Rotterdam: CRC Press), 1–6.
Drake, P., and Arias, A. M. (1991). Composition and seasonal fluctuations of the ichthyoplankton community in a shallow tidal channel of cadiz bay (Sw Spain). J. Fish Biol. 39, 245–263. doi: 10.1111/j.1095-8649.1991.tb04360.x
Franco-Gordoa, C., Godínez-Domíngueza, E., Filonovc, A. E., Tereshchenkoc, I. E., and Freireb, J. (2004). Plankton biomass and larval fish abundance prior to and during the El Niño period of 1997–1998 along the central Pacific coast of México. Prog. Oceanogr. 63, 99–123.
Gaughan, D. J., Neira, F. J., Beckley, L. E., and Potter, I. C. (1990). Composition, seasonality and distribution of the ichthyoplankton in the lower swan estuary, South-Western Australia. Aust. J. Mar. Freshw. Res. 41, 529–543. doi: 10.1071/mf9900529
Harris, S. A., and Cyrus, D. P. (1995). Occurrence of fish larvae in the St Lucia Estuary, Kwazulu-Natal, South Africa. Afr. J. Marine Sci. 16, 333–350. doi: 10.2989/025776195784156601
Harrison, T. D., and Whitfield, A. K. (1990). Composition, distribution and abundance of ichthyoplankton in the sundays river estuary. Afr. J. Zool. 25, 161–168. doi: 10.1080/02541858.1990.11448206
Hassell, K. L., Coutin, P. C., and Nugegoda, D. (2008). Hypoxia, low salinity and lowered temperature reduce embryo survival and hatch rates in black bream Acanthopagrus butcheri (Munro, 1949). J. Fish Biol. 72, 1623–1636. doi: 10.1111/j.1095-8649.2008.01829.x
Hernández-Miranda, E., Palma, A. T., and Ojeda, F. P. (2003). Larval fish assemblages in nearshore coastal waters off central Chile: temporal and spatial patterns. Estuar. Coast. Shelf Sci. 56, 1075–1092. doi: 10.1016/s0272-7714(02)00308-6
Hettler, W. F. Jr., and Hare, J. A. (1998). Abundance and size of larval fishes outside the entrance to Beaufort inlet, North Carolina. Estuaries 21, 476–499. doi: 10.2307/1352846
Hodgson, E. E., Wilson, S. M., and Moore, J. W. (2020). Changing estuaries and impacts on juvenile salmon: a systematic review. Glob. Chang. Biol. 26, 1986–2001. doi: 10.1111/gcb.14997
Jiang, M., Shen, X., and Chen, L. (2006). Relationship between with abundance distribution of fish eggs, larvae and environmental factors in the Changjiang Estuary and vicinity waters in spring. Mar. Environ. Sci. 25, 37–39.
Kindong, R., Wu, J., Gao, C., Dai, L., Tian, S., Dai, X., et al. (2020). Seasonal changes in fish diversity, density, biomass, and assemblage alongside environmental variables in the Yangtze River Estuary. Environ. Sci. Pollut. Res. 27, 25461–25474. doi: 10.1007/s11356-020-08674-8
Lefcheck, J. S., Hughes, B. B., Johnson, A. J., Pfirrmann, B. W., Rasher, D. B., Smyth, A. R., et al. (2019).). Are coastal habitats important nurseries? A meta-analysis. Conserv. Lett. 12:e12645. doi: 10.1371/journal.pone.0114715
Limburg, K. E. (1999). Estuaries, ecology, and economic decisions: an example of perceptual barriers and challenges to understanding. Ecol. Econ. 30, 185–188. doi: 10.1016/s0921-8009(99)00045-2
Liu, S. D., Xian, W. W., and Liu, D. (2008). Characters of ichthyoplankton assemblages in Yangtze Estuary and adjacent waters in spring. Chin. J. Appl. Ecol. 19, 2284–2292. doi: 10.13287/j.1001-9332.2008.0374
Lotze, H. K. (2010). “Historical reconstruction of human-induced changes in US estuaries,” in Oceanography and Marine Biology: An Annual Review, eds R. N. Gibson, R. J. A. Atkinson, and J. D. M. Gordon (Boca Raton, FL: CRC Press), 267–338. doi: 10.1201/ebk1439821169-5
Lotze, H. K., Lenihan, H. S., Bourque, B. J., Bradbury, R. H., Cooke, R. G., Jackson, J. B. C., et al. (2006). Depletion, degradation, and recovery potential of estuaries and coastal seas. J. Sci. 312, 1806–1809. doi: 10.1126/science.1128035
Luo, B. Z. (1994). Three Gorges Project and Estuarine Ecological Environment. Beijing: Science Press.
Luo, B. Z., and Shen, H. T. (1994). The Three Gorges Project and the Ecological Environment of Yangtze Estuary. Beijing: Science Press.
Martin, T. J., Cyrus, D. P., and Forbes, A. T. (1992). Episodic events - the effects of cyclonic flushing on the ichthyoplankton of St-Lucia estuary on the Southeast Coast of Africa. Neth. J. Sea Res. 30, 273–278. doi: 10.1016/0077-7579(92)90065-M
Miller, B. S., and Kendall, A. W. (2009). Early Life History of Marine Fishes. Berkeley, CA: University of California Press.
Muhling, B. A., Beckley, L. E., and Olivar, M. P. (2007). Ichthyoplankton assemblage structure in two meso-scale Leeuwin current eddies, eastern Indian Ocean. Deep Sea Res. Part II 54, 1113–1128. doi: 10.1016/j.dsr2.2006.05.045
Neira, F. J., and Potter, I. C. (1992). Movement of larval fishes through the entrance channel of a seasonally open estuary in Western Australia. Estuar. Coast. Shelf Sci. 35, 213–224. doi: 10.1016/S0272-7714(05)80114-3
Potter, I. C., Beckley, L. E., Whitfield, A. K., and Lenanton, R. C. (1990). Comparisons between the roles played by estuaries in the life cycles of fishes in temperate Western Australia and Southern Africa. Environ. Biol. Fishes 28, 143–178. doi: 10.1007/978-94-009-2065-1_9
Primo, A. L., Azeiteiro, U. M., Marques, S. C., and Pardal, M. A. (2011). Impact of climate variability on ichthyoplankton communities: an example of a small temperate estuary. Estuar. Coast. Shelf Sci. 91, 484–491. doi: 10.1016/j.ecss.2010.11.009
Rakocinski, C. F., LyczkowskiShultz, J., and Richardson, S. L. (1996). Ichthyoplankton assemblage structure in Mississippi sound as revealed by canonical correspondence analysis. Estuar. Coast. Shelf Sci. 43, 237–257. doi: 10.1006/ecss.1996.0067
Ramos, S., Cowen, R. K., Paris, C., Re, P., and Bordalo, A. A. (2006). Environmental forcing and larval fish assemblage dynamics in the Lima River estuary (Northwest Portugal). J. Plankton Res. 28, 275–286. doi: 10.1093/plankt/fbi104
Ryder, R. A., Kerr, S. R., Taylor, W. W., and Larkin, P. A. (1981). Community consequences of fish stock diversity. Can. J. Fish. Aquat. Sci. 38, 1856–1866. doi: 10.1139/f81-231
Sabates, A., Martin, P., Loret, J., and Raya, V. (2006). Sea warming and fish distribution: the case of the small pelagic fish, Sardinella aurita, in the western Mediterranean. Glob. Chang. Biol. 12, 2209–2219. doi: 10.1111/j.1365-2486.2006.01246.x
Santos, R. V. S., and Severi, W. (2019). Dynamics of early life-history stages of fish along an estuarine gradient. Fish. Oceanogr. 28, 402–418. doi: 10.1111/fog.12420
Shan, X. J., Xian, W. W., and Wu, Y. F. (2004). Progress of studies on ichthyoplankton ecology of Changjiang river estuary. J. Oceanol. Limnol. 0, 87–93.
Shao, G., Yang, R., and Chen, K. (2001). An Identification Guide of Marine Fish Eggs From Taiwan. Taibei: Academia Sinica.
Sheaves, M., Baker, R., Nagelkerken, I., and Connolly, R. M. (2015). True value of estuarine and coastal nurseries for fish: incorporating complexity and dynamics. Estuar. Coast. 38, 401–414. doi: 10.1007/s12237-014-9846-x
Shen, X., Shi, Y., Chao, M., Huang, H., and Tian, F. (2011). Fish community structure of the Yangtze River Estuary in summer and autumn. J. Fish. China 35, 700–710.
Shoji, J., Ohta, T., and Tanaka, M. (2006). Effects of river flow on larval growth and survival of Japanese seaperch Lateolabrax japonicus (Pisces) in the Chikugo River estuary, upper Ariake Bay. J. Fish Biol. 69, 1662–1674. doi: 10.1111/j.1095-8649.2006.01235.x
Sloterdijk, H., Brehmer, P., Sadio, O., Muller, H., Doring, J., and Ekau, W. (2017). Composition and structure of the larval fish community related to environmental parameters in a tropical estuary impacted by climate change. Estuar. Coast. Shelf Sci. 197, 10–26. doi: 10.1016/j.ecss.2017.08.003
Song, Y. F., Zhang, L. J., and Luo, X. X. (2019). Spatiotemporal distribution of fish eggs and larvae in the Huanghe (Yellow) River estuary, China in 2005-2016. J. Oceanol. Limnol. 37, 1625–1637. doi: 10.1007/s00343-019-8167-0
Standardization Administration of China (SAC) (2007). Specifications for Oceanographic Survey, Part 4: Survey of Chemical Parameters in sea Water (GB/T 12763-2007). Beijing: SAC.
Talbot, C. W., and Able, K. W. (1984). Composition and distribution of larval fishes in New Jersey high marshes. Estuaries 7, 434–443. doi: 10.2307/1351624
Taylor, R. H. (1982). “The St. Lucia estuary: the aquatic environment: physical and chemical characteristics,” in St. Lucia Research Review, Proceedings of the Natal Parks Board 1992, ed. R. H. Taylor (South Africa), 42–56.
Thaxton, W. C., Taylor, J. C., and Asch, R. G. (2020). Climate-associated trends and variability in ichthyoplankton phenology from the longest continuous larval fish time series on the east coast of the United States. Mar. Ecol. Prog. Ser. 650, 269–287. doi: 10.3354/meps13404
Tzeng, W. N., Wang, Y. T., and Chang, C. W. (2002). Spatial and temporal variations of the estuarine larval fish community on the west coast of Taiwan. Mar. Freshw. Res. 53, 419–430. doi: 10.1071/mf01136
Valencia, B., Giraldo, A., Rivera-Gomez, M., Izquierdo-Pena, V., and Cuellar-Chacon, A. (2019). Effects of seasonal upwelling on hydrography and mesozooplankton communities in a Pacific tropical cove off Colombia. Rev. Biol. Trop. 67, 945–962.
Wan, R., and Jiang, Y. (2000). The species and biological characteristics of the eggs and larvae of osteichthyes in the Bohai and Yellow Sea. J. Shanghai Fish. Univ. 9, 290–297.
Wan, R., and Sun, S. (2006). The category composition and abundance of ichthyoplankton in the ecosystem of the Yellow Sea and the East China Sea. J. Acta. Zool. Sin. 52, 28–44.
Wang, H. T., and Zhang, P. J. (1998). Effects of environmental conditions on fertilized eggs and early larva of marine fishes. Mar. Sci. 4, 50–52.
Wang, X. G., Sun, D., and Lin, S. Q. (2017). The ecological study of fish larvae in the Yangtze River Estuary and adjacent waters. J. Shanghai Fish. Univ. 26, 733–742.
Whitfield, A. K. (1994). Abundance of larval and juvenile marine fishes in the lower reaches of 3 Southern African estuaries with differing fresh-water inputs. Mar. Ecol. Prog. Ser. 105, 257–267. doi: 10.3354/meps105257
Whitfield, A. K. (1999). Ichthyofaunal assemblages in estuaries: a South African case study. Rev. Fish Biol. Fish. 9, 151–186.
Wooldridge, T. (1999). Estuarine Zooplankton Community Structure and Dynamics. Cambridge: Cambridge University Press.
Wu, G. Z. (1989). The ecological characteristics of distribution of eggs, larvae and juveniles of the Engraulis japonicus (temminck & schlegel) and Anchoviella commersonii in the Changjiang River Estuary. J. Oceanol. Limnol. 3, 217–229.
Xian, W. W., Zhang, H., and Liu, S. D. (2016). Research advance in estuarine ichthyoplankton ecology. Stud. Mar. Sin. 2016, 167–180.
Yang, D., Wu, G., and Sun, J. (1990). The investigation ok pelagic eggs, larvae and juveniles of fishes at the mouth of the Changjiang river and adjacent areas. J. Oceanol. Limnol. 4, 346–355.
Yang, Y. F., Wang, Q., Chen, J. F., and Pang, S. X. (2006). Research advance in estuarine zooplankton ecology. Acta Ecol. Sin. 26, 576–585.
Zhang, H., Xian, W., and Liu, S. (2015). Ichthyoplankton assemblage structure of springs in the Yangtze Estuary revealed by biological and environmental visions. PeerJ 3:e1186. doi: 10.7717/peerj.1186
Zhang, H., Xian, W., and Liu, S. (2016). Autumn ichthyoplankton assemblage in the Yangtze Estuary shaped by environmental factors. PeerJ 4:e1922. doi: 10.7717/peerj.1922
Zhang, H., Xian, W., and Liu, S. (2019). Seasonal variations of the ichthyoplankton assemblage in the Yangtze Estuary and its relationship with environmental factors. PeerJ 7:e6482. doi: 10.7717/peerj.6482
Zhang, H., Zhu, G., and Lu, J. (2009). Fish species composition and diversity of Yangtze River estuarine wetlands. Biodiversity Sci. 17, 76–81. doi: 10.3724/sp.j.1003.2009.08140
Keywords: fish eggs, larvae and juveniles, environmental factors, assemblage structure, interannual variability
Citation: Wang Y, Liang C, Chen Z, Liu S, Zhang H and Xian W (2021) Spring Ichthyoplankton Assemblage Structure in the Yangtze Estuary Under Environmental Factors. Front. Mar. Sci. 8:806096. doi: 10.3389/fmars.2021.806096
Received: 31 October 2021; Accepted: 29 November 2021;
Published: 16 December 2021.
Edited by:
Liping Liu, Shanghai Ocean University, ChinaReviewed by:
Sher Khan Panhwar, University of Karachi, PakistanMing Liu, Morgan State University, United States
Copyright © 2021 Wang, Liang, Chen, Liu, Zhang and Xian. This is an open-access article distributed under the terms of the Creative Commons Attribution License (CC BY). The use, distribution or reproduction in other forums is permitted, provided the original author(s) and the copyright owner(s) are credited and that the original publication in this journal is cited, in accordance with accepted academic practice. No use, distribution or reproduction is permitted which does not comply with these terms.
*Correspondence: Hui Zhang, emhhbmdodWlAcWRpby5hYy5jbg==; Weiwei Xian, d3d4aWFuQHFkaW8uYWMuY24=
†Lead corresponding author