- 1Japan Agency for Marine-Earth Science and Technology (JAMSTEC), Yokosuka, Japan
- 2Department of Biology, Nordcee and Danish Center for Hadal Research, University of Southern Denmark, Odense, Denmark
- 3Graduate School of Science and Technology for Innovation, Yamaguchi University, Yamaguchi, Japan
- 4Faculty of Advanced Science and Technology, Kumamoto University, Kumamoto, Japan
- 5RZSS WildGenes, Royal Zoological Society of Scotland, Edinburgh, United Kingdom
Extreme storms, such as tropical cyclones, are responsible for a significant portion of the plastic debris transported from land to sea yet little is known about the storm response of microplastics and other debris in offshore and open waters. To investigate this, we conducted floating plastic surveys in the center of Sagami Bay, Japan approximately 30 km from the coastline, before and after the passage of a typhoon. The concentrations (number of particles/km2) of micro- and mesoplastics were two orders of magnitude higher 1-day after the typhoon than the values recorded pre-typhoon and the mass (g/km2) of plastic particles (sum of micro- and mesoplastics) increased 1,300 times immediately after the storm. However, the remarkably high abundance of micro- and mesoplastics found at 1-day after the typhoon returned to the pre-typhoon levels in just 2 days. Model simulations also suggested that during an extreme storm a significant amount of micro- and mesoplastics can be rapidly swept away from coastal to open waters over a short period of time. To better estimate the annual load of plastics from land to sea it is important to consider the increase in leakages of plastic debris into the ocean associated with extreme storm events.
Introduction
Each year, approximately 20 million metric tons of land-based plastic debris makes its way into aquatic systems, including oceans and freshwater networks, and this figure is only likely to rise (Borrelle et al., 2020). Once they end up in the sea, plastic debris eventually accumulates in the marine environment as most plastics are believed to take several hundred years, or even longer, to break down completely (Derraik, 2002; Turner et al., 2020).
One of the main sources of plastic debris that ends up in the sea is mismanaged plastic waste that is leaked directly from shorelines or indirectly through riverine waterways (Lebreton et al., 2017), urban runoff (Weideman et al., 2020), sewage discharge (Browne et al., 2011) and wind dispersal (Schmidt et al., 2017). Particularly, flood events caused by heavy rainfall enhance the movement of plastic debris of land-based origin into the marine environments from multiple source points (Moore et al., 2002; Axelsson and van Sebille, 2017; Kataoka et al., 2019; Nihei et al., 2020). Therefore, extreme weather events that cause strong winds, heavy rainfall, and associated flooding can greatly enhance the leakage of plastic and other debris into the sea (Veerasingam et al., 2016; Gündoğdu et al., 2018; Hitchcock, 2020; Ockelford et al., 2020).
Tropical cyclones, including typhoons and hurricanes, are the most extreme episodic weather events occurring at low- and mid-latitudes. They bring heavy precipitation resulting in increased river flow and terrestrial runoff (Tsuchiya et al., 2015), and when coupled with the powerful winds they generate tropical cyclones can be a significant event that enhances the loading of plastic debris from land to the marine environment (Pelamatti et al., 2019; Wang et al., 2019; Hitchcock, 2020; Lo et al., 2020; Garcés-Ordóñez et al., 2021). For example, a single typhoon can contribute to more than 80% of the annual transport of microplastics that flow in the Edo River in Japan (Kudo et al., 2018). As such, an important focus of recent plastic research has been to establish the role of extreme storm events on plastic pollution and to determine how plastic debris varies in space and time after such events (Wang et al., 2019). Studies of past tropical cyclones have suggested that global warming may lead to increasing intensities of these episodic events (Kunkel et al., 2013; Tu and Chou, 2013) and therefore the impacts of tropical cyclones on the transport of marine debris will also be augmented. Understanding the leakage patterns of marine plastic debris by tropical cyclones and other storm events is essential for determining strategies to mitigate further pollution, especially in regions where there are frequent typhoon or hurricane events.
At present, most studies investigating how microplastics and other debris respond to storm disturbances are based on observing succession patterns by revisiting rivers (Hitchcock, 2020), beaches (Lo et al., 2020), shores (Zhao et al., 2015) or nearshore waters (<10 km) (Gündoğdu et al., 2018; Chen et al., 2019) after storm events. Although these studies provide valuable information on the storm response of microplastics and other debris in rivers, coasts and shallow waters, studies investigating the responses offshore or in open waters are scarce. Debris flowing into the coastal waters through rivers and shores via storm events will experience rougher sea conditions, such as higher waves and rapid currents, which may cause; an increase in transportation of debris out to the open waters, debris to be re-deposited into coastlines, or debris to sink to the sea bottom (Kukulka et al., 2012). As a result, the abundance of debris flowing into coastal waters may vary significantly over time after extreme storms. Yet, the true extent of plastic debris that is flowing into coastal waters and offshore through extreme weather events remains unclear.
In September 2019, Typhoon Faxai, equivalent to a Category 4 typhoon made landfall near Tokyo, Japan (Suzuki et al., 2020; Tamura et al., 2021). The destructive winds and storm surged with a maximum wind gust speed of 210 km h–1 which caused landslides, damage to buildings and homes, knocking out power and uprooting trees across central Japan, resulting in 25 billion USD economic loss (Eckstein et al., 2021). However, this typhoon event provided unique research opportunities for estimating the influence of extreme storm events on plastic debris in the marine environment.
Here we present the study of floating micro- and mesoplastics debris in the center of Sagami Bay, some 30 km away from the Japanese coastline, at 3-days before, 1-day after and 3-days the typhoon Faxai’s passage. Specifically, our study addressed two key topics regarding typhoon derived debris through observations and model simulations: (1) how much does the concentration of floating plastic debris offshore change before and after a typhoon passage? and (2) where does the debris that is carried from the land to coastal waters by the typhoon go? This will significantly contribute to our understanding of the fate of plastic debris after an extreme storm.
Materials and Methods
Typhoon Faxai
Faxai (T1915) occurred east of the International Dateline presenting as a tropical depression on 2nd September 2019 before being upgraded to a typhoon on 5th September near Minami-Torishima Island as it moved across the Pacific Ocean (Japan Meteorological Agency, 2020; Figure 1A). The lowest central pressure of Faxai was 955 hPa with maximum sustained winds of 157 km h–1. Shortly before 03:00 JST (Japan Standard time, + 9 GMT) on 9th September 2019, Faxai passed through Sagami Bay in central Japan with the largest diameter of storm wind determined to be 180 km (Figure 1B). The storm caused heavy precipitation with a maximum of 109 mm of rain in 1 h, resulting in urban flooding in Tokyo metropolitan area (Tokyo Regional Headquarters, 2019). This was one of the strongest typhoons to ever hit the region on record (Tamura et al., 2021). After Faxai passed through the Tokyo area, it was downgraded to an extra-tropical cyclone on 10th September 2019 off the east coast of Japan.
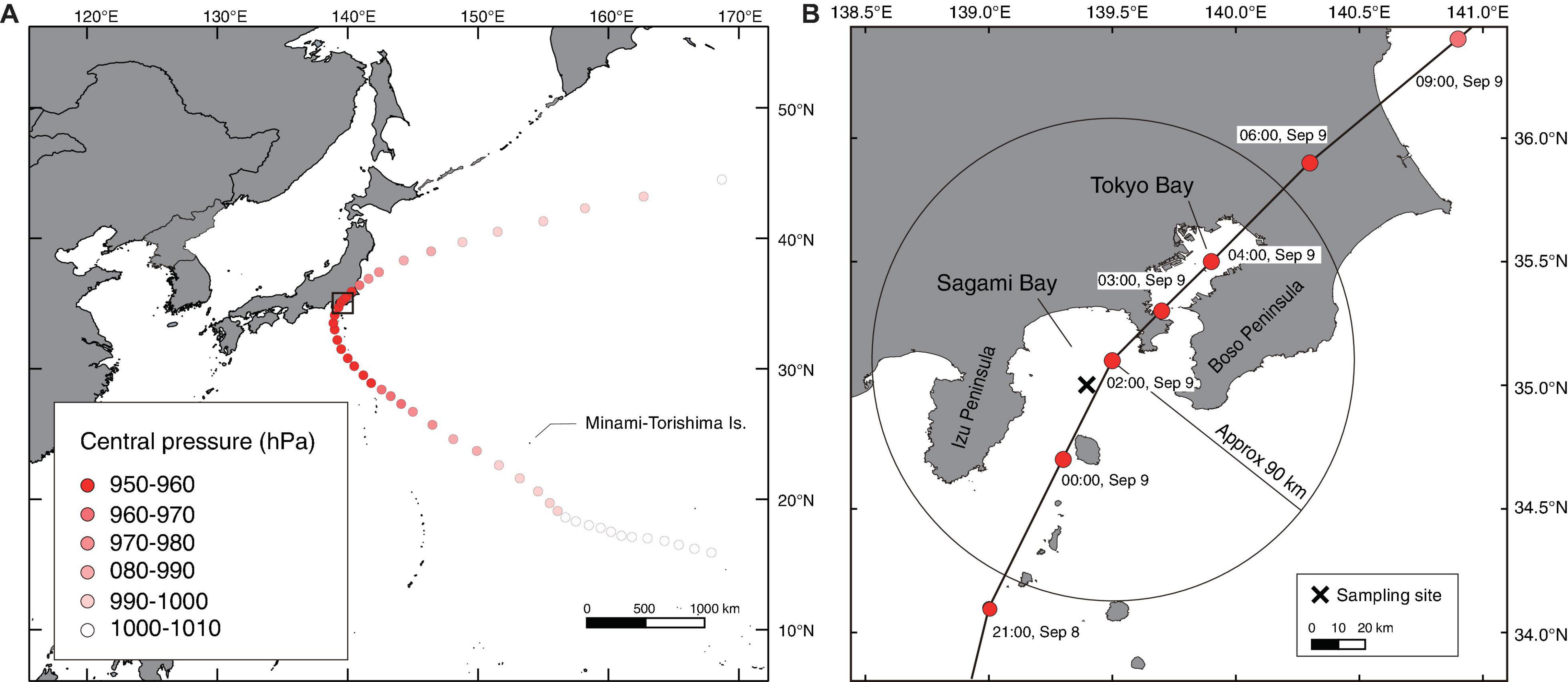
Figure 1. Track of typhoon Faxai off (A) the east coast of Japan and (B) over Sagami Bay. The colors of circles on (A,B) donate the central pressure (hPa) of Faxai. Large circle on (B) represents the area of the storm with the wind speed of > 25 m/s (02:00 September 9th). Cross on (B) donates sampling site.
Plastic Sampling
Sampling was conducted at a location in the center of Sagami Bay (35°1.25′N; 139°22.65′E) over a water depth of 1,400 m (Figure 1B). This site was located near the center of the anticyclonic eddy of the bay (Iwata and Matsuyama, 1989) and thus an accumulation of debris was expected. We conducted sampling 3-days before Faxai reached the bay (September 7th), and revisited the same survey site at 1-day after (September 10th) and 3-days after (September 12th) the typhoon passed during a cruise on the R/V Yokosuka (YK19-11).
Floating micro- and mesoplastic particles were collected during the day (6:00–13:00) using a neuston net (mesh size, 333-μm; mouth opening size, 1.0 m width and 0.75 m height) equipped with a pre-calibrated flow-meter (Rigo). Prior to towing, the net without cod-end was rinsed from the outside with running seawater in order to remove any contaminants in the net. The neuston net was deployed 4 m from the ship’s starboard side using a crane and dragged for 20 min at a speed of 1–2 knots in the upper 0.4 m of the sea surface, where samples were collected in the cod-end of the net (Michida et al., 2019). Triplicate samples were collected at each sampling period by repeating the net towing. Once onboard, the net was thoroughly rinsed from the outside of net with running seawater to concentrate all particles into the cod-end. Samples were then transferred to 450 ml glass jars, fixed with 5% formalin and stored for laboratory analysis. The formalin was filtered before usage applying a 30 μm stainless mesh screen to avoid external contamination.
In addition to the neuston net sampling, floating macroplastic debris was visually counted 3-days before and 1-day after the storm. No visual observation was conducted 3-days after the storm. Three to four researchers were stationed on both the port and starboard side of the ship to observe floating debris for 70–90 min while the ship was running at a speed of 1 knot. All debris > 25 mm within 4 m from the side of the ship was visually counted. The lids of standard plastic bottles (ca. 28 mm in diameter), which were often observed during the observation, were used as a nominal calibration measure during the enumeration of debris > 25 mm.
Laboratory Analysis
Plastic-fiber free lab coats were worn in the laboratory and work surfaces were precleaned and dust-free prior to working. Sample processing was conducted in a laminar flow cabinet where possible. The neuston net samples were purified using 30% H2O2 at room temperature for 7 days (Hurley et al., 2018) and then visually inspected using a dissecting stereo microscope (Olympus, SZX16). All potential plastic particles were sorted out by hand using forceps and transferred to glass multiwell plates with glass lids. Particles were then dried at room temperature for 3 days, photographed with a connected camera (Olympus, DP74) to the microscope, and categorized according to their shape: fragments (mixture of hard fragments and films), line, foam, and pellet (Michida et al., 2019). Length and width of each particle were measured using an image software (Olympus, cellSens Dimension 2.1). Textile microfibers were excluded from the analysis due to the large mesh size of the net and to minimize the inclusion of external contamination. For the samples collected at the 1-day after the storm with many particles, large (>5 mm) or prominent plastic particles were sorted first where possible, then the remaining sample was split (1/8) using a stainless-steel splitter. All potential plastic particles, except for foamed particles, were then sorted from the split samples. As foamed particles were numerous in the split samples these samples were split further (1/8) and all foamed particles were sorted.
Polymer types for each of the sorted plastic-like particles were identified using Fourier Transform Infrared spectroscopy (FT-IR) with a single reflection diamond Attenuated Total Reflection (ATR) (Nicolet iS5, Thermo Fisher Scientific). The samples were positioned onto the ATR crystal and then compressed using the instrument clamp to achieve optical contact, allowing at 4 cm–1 spectral resolution by co-adding 16 scans per sample, and a background in air was collected every 60 min during measurement. Each collected spectrum was compared to multiple spectral database libraries containing both synthetic polymers and non-synthetic materials (Omnic 9, Thermo Fisher Scientific). A hit quality > 60% was used as the threshold for polymer types (Galgani et al., 2013). In total, 1,821 particles were identified as plastic polymers with FT-IR in this study.
Particles identified as plastic were pooled by each net tow sample and transferred to a pre-weighed glass vial, then the weights of the plastic particles were measured. The plastic particles collected from the neuston nets were characterized into three size-categories: microplastic (<5 mm), mesoplastic (5–25 mm), and macroplastic (>25 mm) based on the lengths of each particle (GESAMP, 2019). After the counting of individual plastic particles in each sample, densities (number of particles per square kilometer) were calculated from the towed distance measured by the flow-meter and the frequency of sample split.
Wooden and plant debris from the neuston net samples were sorted out using forceps prior to the plastic analysis and pooled by net tow sample. These pooled samples were dried for 24 h at 60°C and then weighed. In addition, prominent insects were also counted and sorted out, and identified to family level wherever possible.
Particle Tracking Simulations After Storm
We conducted particle tracking simulations at the surface of Sagami Bay before and after the typhoon passage using the surface current reproduced from a tide-resolution general circulation model with the horizontal resolution of 1/36 (JCOPE-T DA) (Miyazawa et al., 2021). See Supplementary Material for the details of the model configuration. We aligned 100 model particles on the coast of Sagami Bay 2, 7, 9, and 12 h after the storm, and 1 week before the storm to see how many particles could be flushed out of the Sagami Bay across different time scales. We defined the extent of the bay as the inner side of the line connecting the edge of Boso Peninsula and the edge of Izu Peninsula (Figure 1B), and the particles which moved out of this line were considered to have been transported out of the bay. Finally, we compared the relative abundance of residual particles in the bay between the different time set ups.
Statistical Analysis
The difference in the density, mass, and length of microplastics and mesoplastics between different sampling periods (3-days before, and 1- and 3-days after the storm passage) was determined using a one-way ANOVA and differences among means were then analyzed using Tukey-Kramer multiple comparison tests. A difference at p < 0.05 was considered significant.
Results
Changes in the Density and Mass of Plastic and Other Debris
At 3-days before the typhoon passed (September 7th), the densities of micro- and mesoplastics were on average (± SD) 101,762 ± 13,213 pieces km–2 and 11,340 ± 7,198 pieces km–2, respectively (Figures 2A,B). At 1-day after the typhoon passage (September 10th), the concentrations of micro- and mesoplastics significantly increased by two orders of magnitude (p < 0.01 for both micro- and mesoplastics, Tukey-Kramer), being 226 times and 274 times greater than those at the pre-typhoon period, respectively. Mean concentration of micro- and mesoplastics 1-day after the typhoon passage reached 23,032,769 ± 8,372,347 pieces km–2 and 3,104,930 ± 1,847,652 pieces km–2, respectively. At 3-days after the typhoon passage, the concentration of micro- and mesoplastics significantly (p < 0.05 for both micro- and mesoplastics, Tukey-Kramer) dropped to concentrations similar to pre-typhoon levels (microplastic, 77,698 ± 10,863 pieces km–2; mesoplastics, 12,143 ± 10,244 pieces km–2). There were no significant differences in the density of microplastics (p > 0.05) and mesoplastics (p > 0.05) between the samples taken pre-typhoon and 3-days after the typhoon passed.
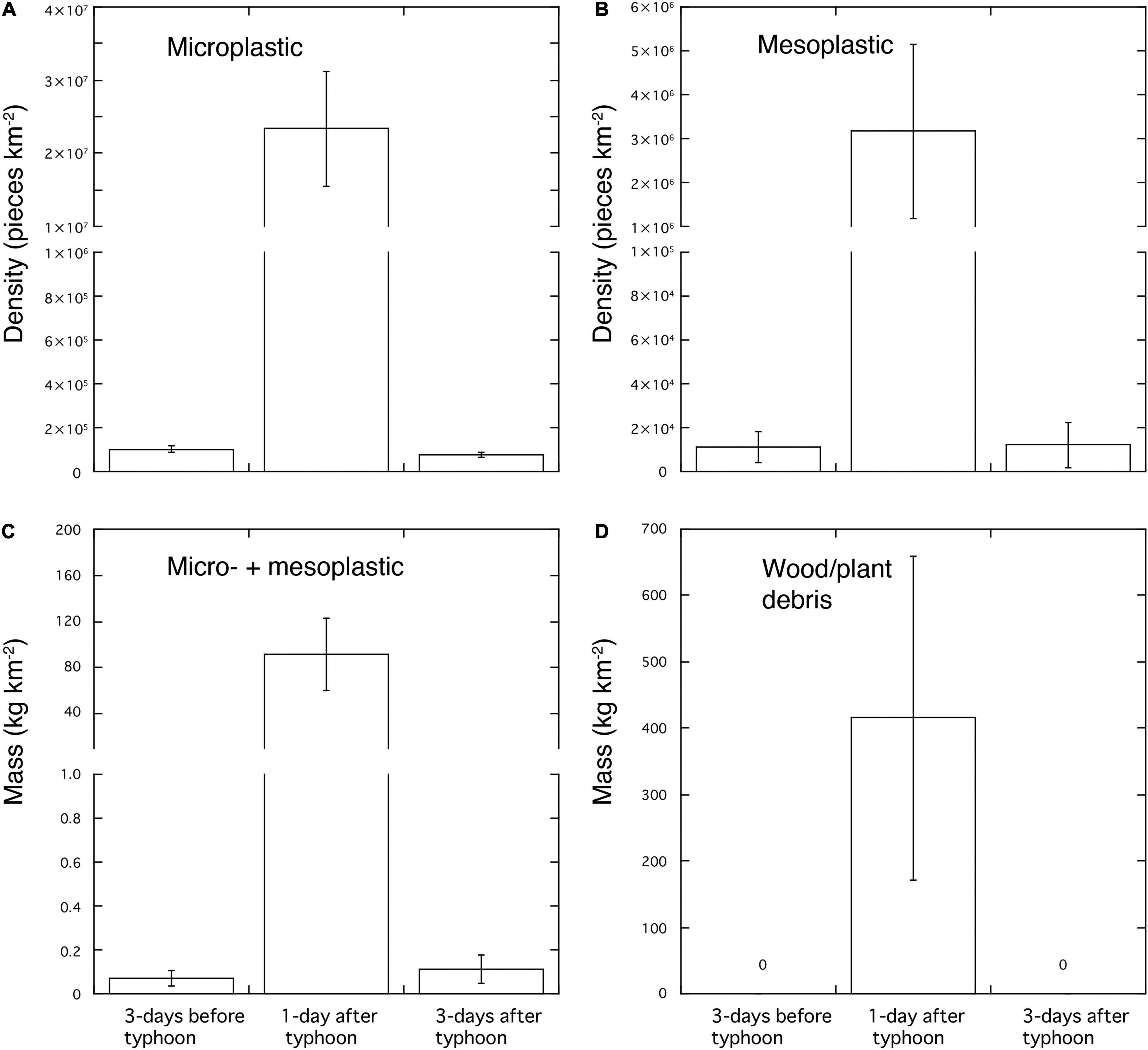
Figure 2. Changes in the density and mass of plastic and other debris 3-days before, and 1- and 3-days after typhoon passage: density (pieces km–2) of (A) microplastic and (B) mesoplastics, and mass (kg km–2) of (C) micro− + mesoplastics and (D) wood and plant debris. Error bars indicate standard deviation of mean.
The mass (g km–2) of plastic particles collected by the net (sum of micro- and mesoplastics) showed the same trend as the density data (Figure 2C). The mass was on average 0.070 ± 0.035 kg km–2 before the typhoon passage, which significantly (p < 0.01, Tueky-Kramer) increased 1,309 times immediately after the storm to 91.6 ± 30.9 kg km–2. Then, it significantly (p < 0.01, Tueky-Kramer) decreased to the pre-typhoon level at 3-days after the storm (0.11 ± 0.062 kg km–2). There was no significant difference in the mass between pre- and 3-days post typhoon (p > 0.05, Tueky-Kramer).
In addition to plastic debris, a markedly large amount of fine wooden debris and plant debris was collected from the net 1-day after typhoon, reaching 415.3 ± 243.8 kg km–2 (Figure 2D). Wood and other plant debris were not collected before the typhoon or 3-days after the typhoon. Some insects, including wingless insects such as ants (Formicidae), were also only collected at 1-day after the storm (Supplementary Figure 1).
Large plastic debris was also prominent on the sea-surface 1-day after the storm. At 3-days before the typhoon passed, the density of visually counted macroplastic (> 25 mm) ranged from 1,824 to 2,183 pieces km–2 with a mean of 2004 pieces km–2 (Supplementary Figure 2A). At 1-day after the typhoon passed the mean density was 5.2 times greater than the pre-typhoon period, ranging from 10,092 to 10,698 pieces km–2 with a mean of 10,392 pieces km–2. The mean density of visually counted macroplastic was lower than that obtained from the net sampling at 1-day after the storm (29,269 ± 26,713 pieces km–2), though no macroplastics were collected by the net 3-days before or 3-days after the typhoon (Supplementary Figure 2B).
Changes in the Polymer Type, Shape and Size of Micro- and Mesoplastics
Polyethylene (PE) and polypropylene (PP) were dominant in microplastics and mesoplastics before the typhoon passed (PE 59.7 ± 10.3% and PP 30.2 ± 6.3% in microplastics; PE 80.0 ± 20.0% and PP 13.3 ± 11.5% in mesoplastics) (Figures 3A,B). At 1-day after the typhoon, microplastics consisted mostly of polystyrene (PS) (76.5 ± 14.1%) while mesoplastics were comprised mainly of PE (38.4 ± 24.9%) and PS (37.2 ± 18.3%). At 3-days after the storm, PS was still the highest proportion of polymer seen in microplastics (63.4 ± 3.3%) while mesoplastics were comprised mainly of PE (58.3 ± 52.0%) and PS (33.3 ± 57.7%).
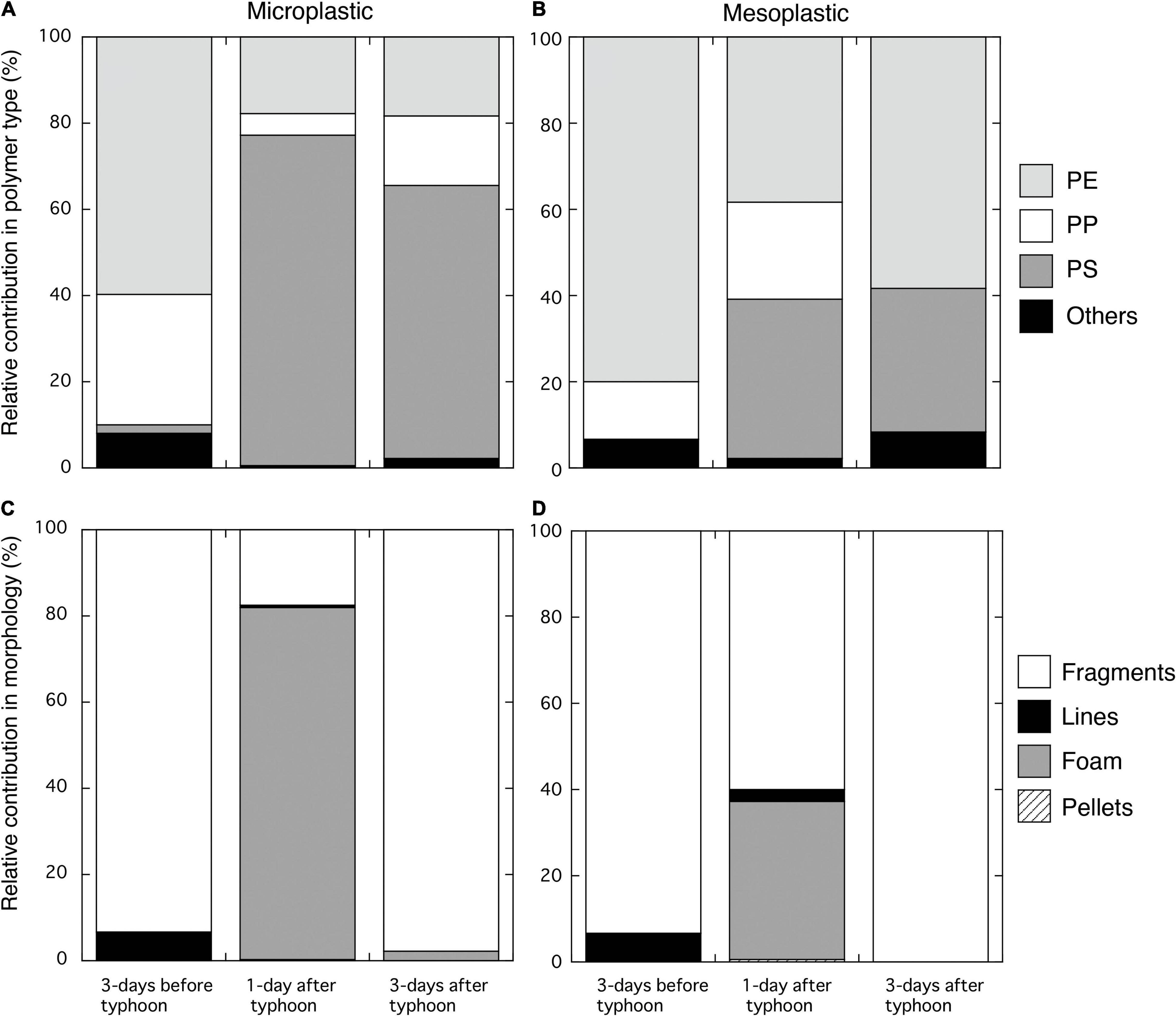
Figure 3. Changes in the composition of (A,B) polymer type and (C,D) shape of microplastics and mesoplastics 1-day before and 1- and 3-days after the typhoon passage. PE, polyethylene; PP, polypropylene; PS, polystyrene. Fragments on (C,D) include hard fragments and films.
The morphological shapes of microplastics and mesoplastics collected before the typhoon passed were predominantly fragments including hard fragments and films (93.4 ± 5.9% in microplastics; 80.0 ± 20.0% in mesoplastics) followed by lines (6.6 ± 5.9% in microplastics; 13.3 ± 11.5% in mesoplastics) (Figures 3C,D). At 1-day after the typhoon, foamed particles, namely particles of expanded PS (EPS), were dominant in microplastics (81.7 ± 9.9%). Foamed particles were also an important component in mesoplastics (37.1 ± 17.9%) 1 day after the storm but the most dominant shapes seen were fragments (59.8 ± 15.1%). At 3-days after the storm, both microplastics and mesoplastics consisted mostly or entirely of fragments (97.8 ± 3.8 and 100.0 ± 0.0%, respectively).
Boxplots of particle length (μm) of microplastics and mesoplastics before and after the typhoon showed a presence of larger particles in microplastics 1-day after the storm (Figure 4). The mean size of microplastic particles at 1-day after the storm was significantly higher (p < 0.01, Tueky-Kramer) than before and 3-days after the storm, yet no significant difference was observed between before and 3-days after the storm (Figure 4A). For mesoplastics, no significant difference was found in particle size across the study periods (p > 0.05, Tueky-Kramer) (Figure 4B).
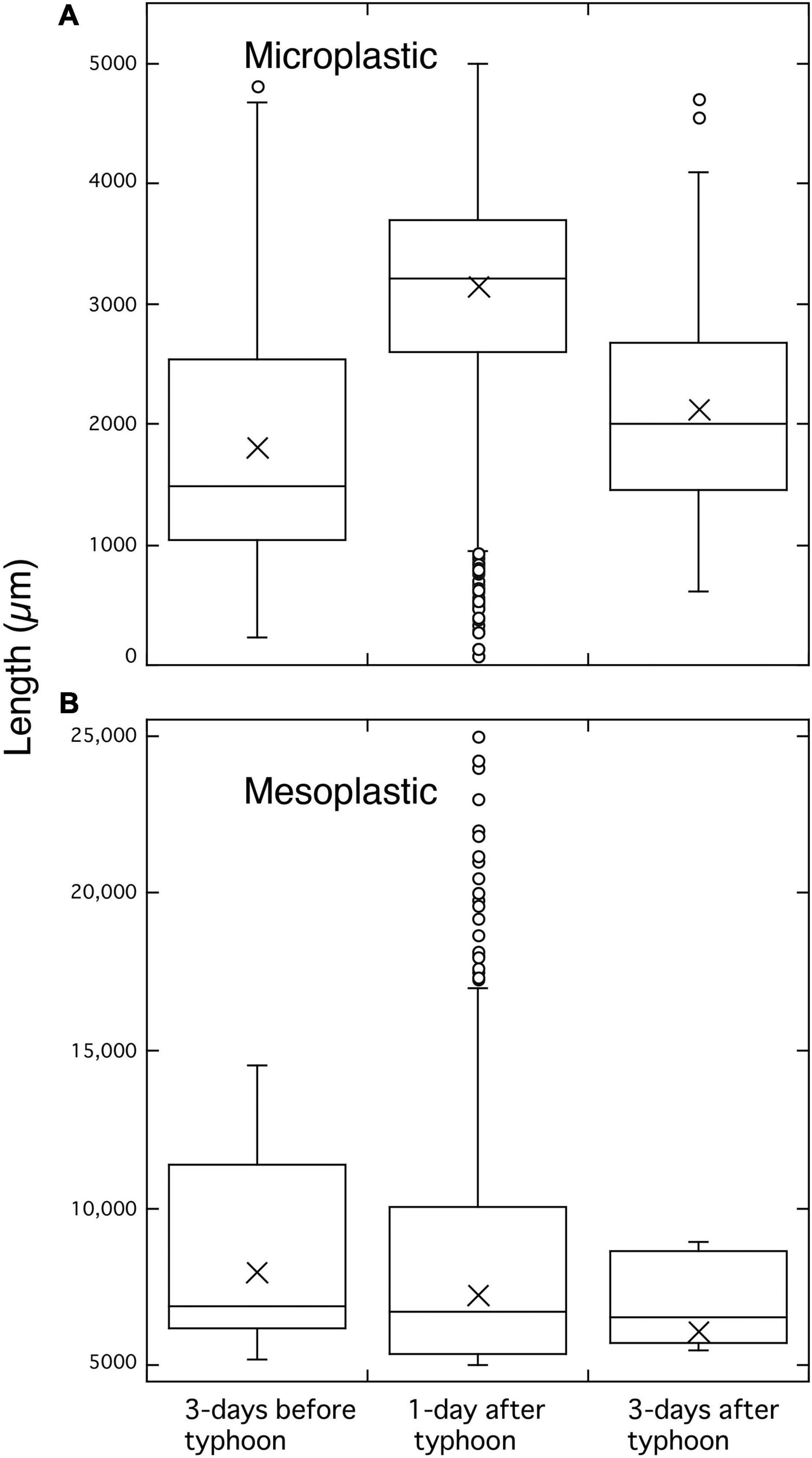
Figure 4. Changes in the length (μm) of (A) microplastics and (B) mesoplastics 1-day before and 1-day and 3-days after typhoon passage. The line in the middle of the boxes represents the median values; the tops and bottoms of the boxes denote the 75 and 25% quartiles, respectively, and the top and bottom of the errors show the maximum and minimum value, excluding outliers (circles). Crosses denote the mean data.
Particle-Tracking Simulations
Particle model analysis showed that plastic particles entering the bay immediately after the passage of the typhoon were highly likely to be transported out of the bay to the open ocean. In contrast, plastic particles that entered the bay before the typhoon, or more than 12 h after the passage of the typhoon, were less likely to be transported from the bay to the open ocean (Figure 5, see also movies in Supplementary Material). Most particles released from the coastline at 2 and 7 h after the typhoon passed (i.e., 04:00 and 09:00 September 9th) remained in the bay for the first 24 h (>90%), but only < 50% (45.7–49.3%) continued to persist in the bay after 120 h due to leakage into the open waters (Figure 5). Similarly, particles released 9 h after the typhoon passage (11:00 September 9th) showed persistence of ca. 60% in the bay after 120 h. In contrast, particles released 12 h after the typhoon, or 1 week before the typhoon, showed relatively higher persistence in the bay with 78–82% still residing after 120 h.
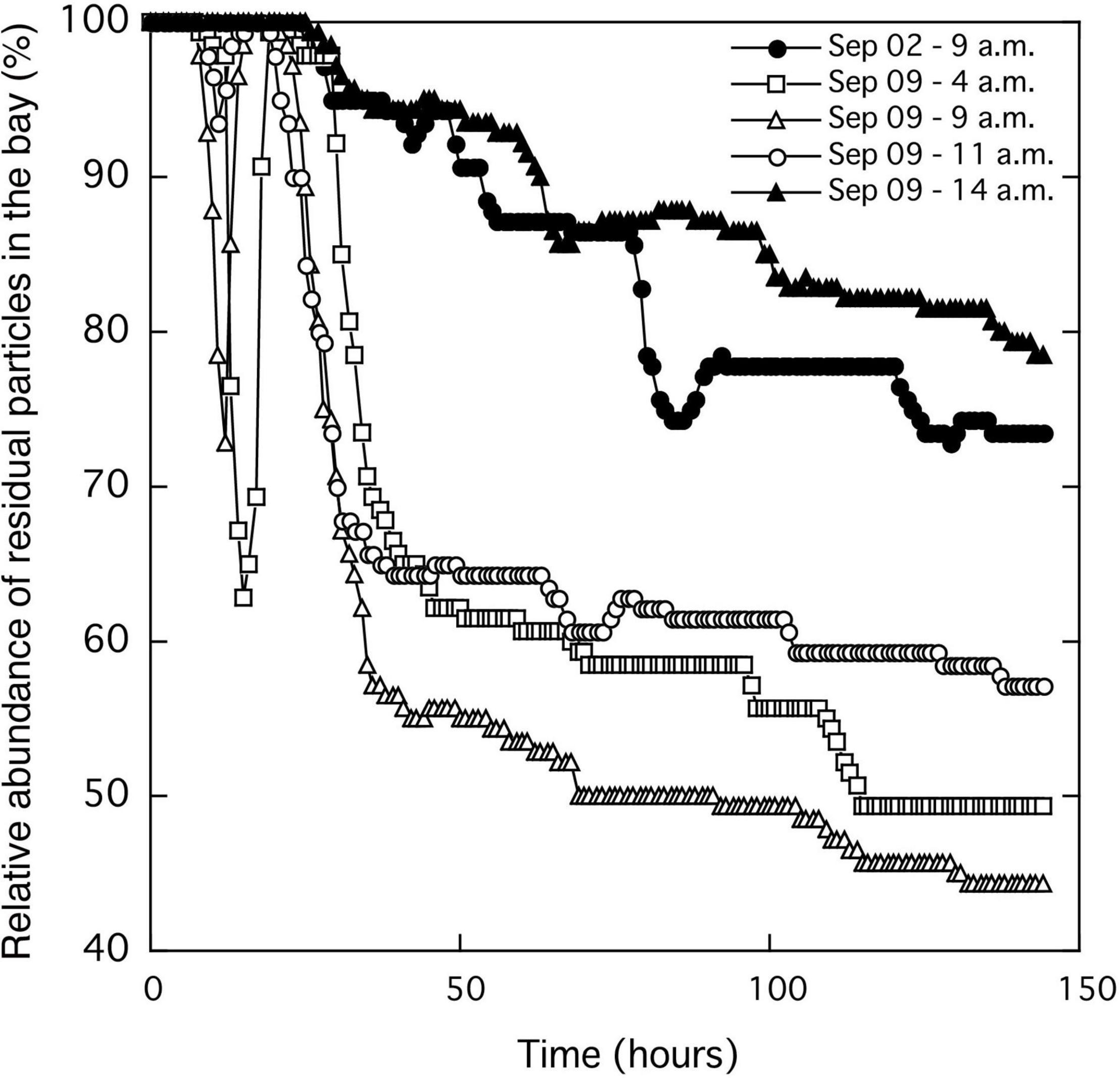
Figure 5. Relative abundance (%) of residual particles in Sagami Bay after releasing particles from the shoreline at different time intervals based on the typhoon passage: 1 week before (09:00 September 2nd), 2 h after (04:00 September 9th), 7 h after (09:00 September 9th), 9 h after (11:00 September 9th) and 12 h after (14:00 September 9th).
Discussion
Here we provide evidence that extreme weather events are one of the major driving forces responsible for transporting large amounts of plastic debris from the land to the sea. Although earlier studies have documented debris succession patterns before and after storm events in rivers, coasts, and shallow nearshore waters, our results highlight the degree of debris found offshore far from the coast (> 30 km) after an extreme storm event.
Flood events caused by heavy precipitation enhance the movement of plastic debris of terrestrial origin into the marine environments from multiple points, of which rivers are considered one of the major pathways (Kudo et al., 2018; Nihei et al., 2020). One typical debris that is abundant in rivers during heavy rainfalls is wooden debris (Doong et al., 2011). Considering the large amount of wooden debris found in this study, as well as the presence of wingless insects (ants), the plastic debris collected 1-day after the storm may be of river-based origin. This hypothesis is further supported by satellite image data taken at 1-day after the storm by Sentinel-2 showing muddy water flowing from the coastlines to the center of Sagami Bay which are occurrences of river runoff (Sentinel, 2019). In addition, the surface chlorophyll data provided by the satellite Shikisai (GCOM-C) showed that the bay at 1-day after the storm the bay experienced very little influx of oceanic seawater that could be a source of microplastics (Japan Aerospace Exploration Agency, 2019). Microplastics on the seafloor can be resuspended after storms that cause sufficient turbulence in the water column (Lattin et al., 2004). However, the study site has a total water depth of 1,400 m making resuspension of plastic debris from sediments at this depth is unlikely. As such, we can infer that the majority of the debris observed in the center of Sagami Bay after the typhoon most likely originated from river sources.
Although comparatively fewer studies exist on plastic debris before and after tropical cyclones, it is apparent that extreme storms are responsible for a significant portion of the plastic debris transported from land to aquatic ecosystems. Previous investigations examining plastic debris before and after tropical cyclones, and other storm events have revealed that an increase in the abundance of microplastics and macroplastics is typically observed after storms (Table 1). For example, measurements of macroplastic debris densities in Hong Kong beaches during typhoon Mangkhut in 2018 showed a 12-fold increase compared with measurements taken before the typhoon passage (Lo et al., 2020). Similarly, typhoon Meranti in 2016 caused a 10 times increase in floating macroplastics in Xiamen Bay, China (Chen et al., 2019). Our results show that macroplastics (> 50 mm) yielded a 5-fold increase in abundance immediately after the storm, which is comparable to previous studies.
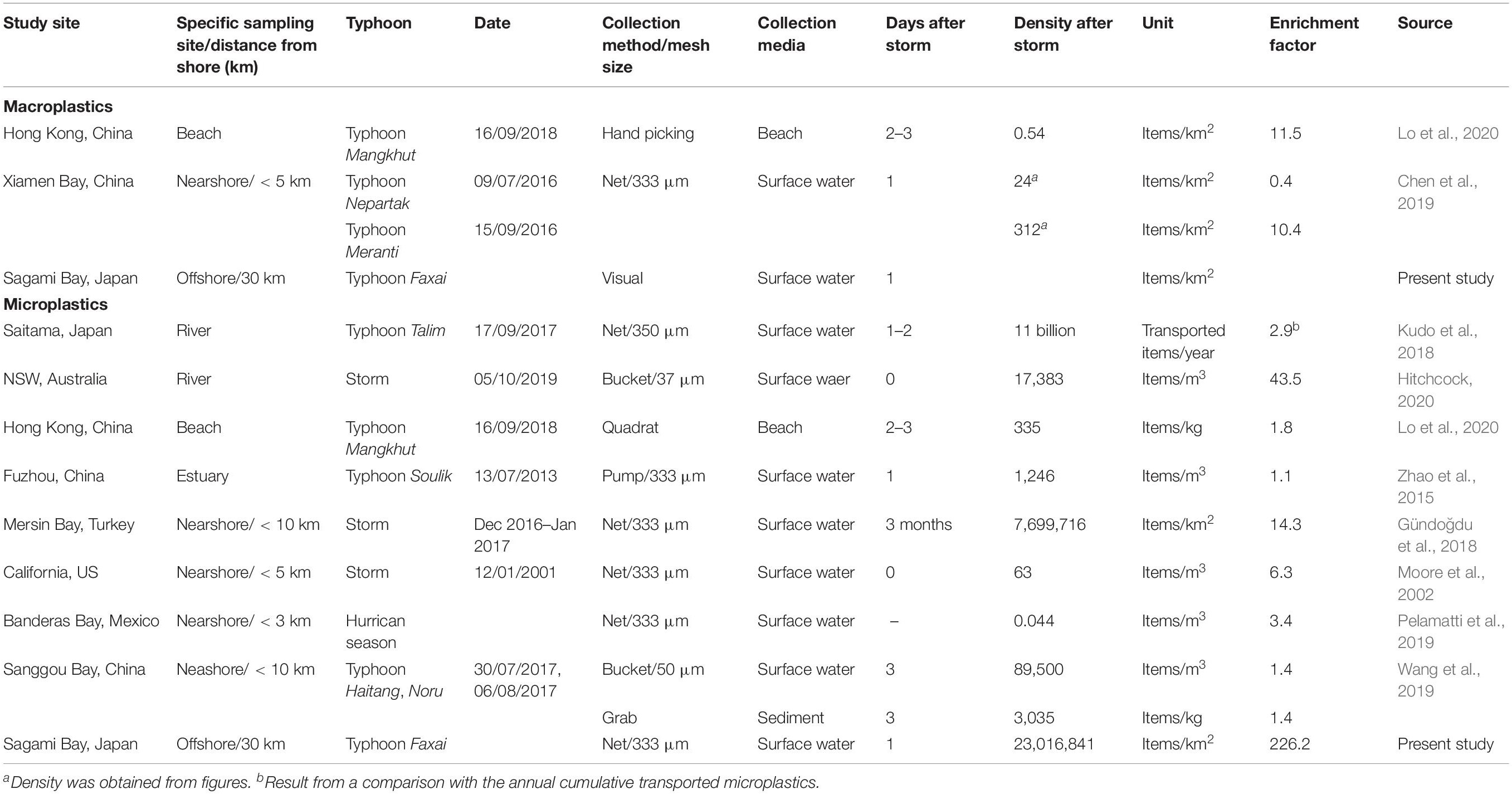
Table 1. Comparative summary of the enrichment factors of macro- and microplastics in several bodies of water after storm events.
In studies of microplastics, previous investigations revealed that storm events caused 1.1–43.5-fold increase in the density of microplastics from rivers, beaches, estuary and nearshore waters (Table 1). However, in our study, microplastics (<5 mm) from offshore sites showed a 280-fold increase in density, which is the highest recorded value to date. Unlike microplastics found in rivers and shores, where microplastics continue to be transited from the land to the sea, the offshore study site is located near the center of an eddy in the bay and, as such, microplastics are likely to be accumulated. This propensity for accumulation at the offshore site compared to rivers and shores may explain the higher enrichment factor in microplastic density seen there. It is interesting to note that the study of the beaches in Hong Kong (Lo et al., 2020) showed a higher enrichment factor in the density of macroplastics than that of microplastics (11.5 vs. 1.8, respectively), which contradicts our offshore results that showed a higher enrichment factor for microplastics. This suggests that smaller plastic particles may be more likely to be transported offshore by storm events. Previous studies showed that microplastics have a much shorter residence time in shore waters than macroplastics so microplastics may be more easily transported to offshore waters via backwash waves (Hinata et al., 2017). This may also explain the higher microplastics enrichment factor seen for our offshore site. Additionally, the breakage of fragile debris by rougher seas could also contribute to the increased number of small plastic particles recorded.
The most significant contributor to the increase in densities of micro- and macroplastics after the typhoon passed was polystyrene foam or expanded PS (EPS) particles. Polystyrene foams are extensively used as disposable take-way food boxes, heat-insulated containers and buoys, where polystyrene foam litter has been widely reported in the Asian-Pacific region (Yamashita and Tanimura, 2007; Kim et al., 2015; Fok et al., 2017). Most EPS particles found in this study were 2–3 mm in length, which largely contributed to the size shift of microplastics toward larger particles. Although the source of the EPS particles is unclear in our study, one possible source could be insulated containers such as the type used for transporting seafood products amongst other things as many EPS insulated containers are made of ca. 1–3 mm diameter of spherical EPS beads bonded together (Subhan, 2006). EPS made containers are fragile and are easily fragmented by external energy releasing small EPS particles (Majanga et al., 2015; Song et al., 2020). High physical energy associated with strong winds and rough ocean waves during typhoons could encourage the breakdown of exposed EPS containers into parts which could be further fragmented into smaller pieces that drift into offshore waters (Lee et al., 2013). As such, it is important to devise measures to prevent EPS containers from leaking into the environment in order to reduce microplastic leakage into the ocean.
The remarkably high abundance of micro- and mesoplastics found 1-day after the typhoon (91 kg/km2) returned to the pre-typhoon level in just 2 days (0.1 kg/km2). Essentially, ca. 90 kg/km2 of plastic particles moved on from the survey site within a few days and it is not immediately clear where they have relocated to. It is unlikely that most plastic particles sank in just 2 days given that the predominant polymers were PP, PE and EPS, which have lower specific gravity than seawater. In fact, it would normally take several months to several years for buoyant plastic debris to move from the surface to the deeper water via events such as biofouling (Isobe et al., 2019; Nakajima et al., 2021). As such, the plastic particles may have been pushed back to the shore or swept away from the bay over a relatively short time scale. Our model shows that particles remain in the bay for a relatively longer period when they are not under the influence of a storm (i.e., 1 week before and 12 h after typhoon passage) probably due to the anticyclonic eddy in the Sagami Bay (Iwata and Matsuyama, 1989). On the contrary, half of the particles that moved into the bay right after the typhoon passed were shown to have been swept away from the bay in a few days probably due to the higher outflow from Tokyo Bay, next to Sagami Bay. The higher outflow from the mouth of Tokyo Bay, resulting from the typhoon which rotated counterclockwise and increased water volume in the bay due to increased precipitation (Okada et al., 2011), could have been responsible for the higher leakage of particles from the mouth of the Sagami Bay to the open waters. These results suggest that a significant amount of micro- and mesoplastics can be swept away rapidly from coastal waters to open waters by extreme storms. As such, it is important to consider the enhanced leakages of plastic debris into the ocean associated with extreme storm events to better estimate the annual plastic load from land to sea. This will be further emphasized in low- and mid-latitudes regions with frequent occurrences of tropical cyclones including typhoons and hurricanes. Notably East and Southeast Asia, the regions with frequent typhoons, account for approximately half of the plastic that ends up in the ocean (Jambeck et al., 2015), which could be upwardly revised considering the effect of typhoons. As the intensity of storm events is expected to increase with a changing climate (Knutson et al., 2010; Kossin et al., 2020), this study highlights the significance of the understanding the leakage patterns of marine plastic debris caused by extreme weather events. This will allow for the development of better strategies to mitigate plastic pollution, especially in regions where there are frequent typhoon or hurricane events.
It is important to note that while the effect of only a single typhoon was analyzed in this study, we believe that this adequately covers the significance of the debris leakage from land to sea. This is evidenced by the highest enrichment factor being recorded as a result of the typhoon Faxai. It should also be noted that, unlike rivers or coasts which are relatively easily accessible when typhoons occur, it is difficult to immediately investigate the changes in debris abundances at such an offshore site, some 30 km away from the coastline.
Conclusion
We provide insights into the fate of floating plastic debris transported offshore when a typhoon passed over Sagami Bay, Japan. Our investigation confirms the view that a tremendous amount of waste can be transported from the land to sea by an extreme storm event. This can result in two or three orders of magnitude higher abundance of micro- and macroplastics being recorded immediately after the typhoon compared to the values recorded pre-typhoon. However, the remarkably high abundance of micro- and mesoplastics found 1-day after the typhoon returned to the pre-typhoon levels in just 2 days, because the majority of the debris was swept away from the bay to the open ocean over a short period of time. The annual load of plastic debris being transported from land to sea will be underestimated if episodic storm events are not fully considered. In order to halt the leakage of plastic debris from land to sea, it is important to take measures that consider unexpected natural disasters such as typhoons.
Data Availability Statement
The original contributions presented in the study are included in the article/Supplementary Material, further inquiries can be directed to the corresponding author/s.
Author Contributions
RN: conceptualization, methodology, formal analysis, investigation, writing—original draft, and visualization. TM: methodology, formal analysis, and visualization. TK, NI, YN, TI, KO, TYo, YM, KK, MS, TYa, and HR: investigation and writing—review and editing. MT and KF: supervision and writing—review and editing. AY: conceptualization, methodology, investigation, and writing—review and editing. All authors contributed to the article and approved the submitted version.
Funding
This study was partially funded by the New Energy and Industrial Technology Development Organization (NEDO) project (JPNP18016) to RN, NI, YN and JSPS KAKENHI grant (19H04262) awarded to RN.
Conflict of Interest
The authors declare that the research was conducted in the absence of any commercial or financial relationships that could be construed as a potential conflict of interest.
Publisher’s Note
All claims expressed in this article are solely those of the authors and do not necessarily represent those of their affiliated organizations, or those of the publisher, the editors and the reviewers. Any product that may be evaluated in this article, or claim that may be made by its manufacturer, is not guaranteed or endorsed by the publisher.
Acknowledgments
We thank the captain and the crew of R/V Yokosuka for their efforts to make the sampling possible, and Tomoko Morotomi, Rie Matsui and Nozomi Noha for their help in sample analysis. We also thank M. Morioka, Nippon Marine Enterprises, Ltd. for her technical help during the cruise.
Supplementary Material
The Supplementary Material for this article can be found online at: https://www.frontiersin.org/articles/10.3389/fmars.2021.806952/full#supplementary-material
References
Axelsson, C., and van Sebille, E. (2017). Prevention through policy: urban macroplastic leakages to the marine environment during extreme rainfall events. Mar. Pollut. Bull. 124, 211–227. doi: 10.1016/j.marpolbul.2017.07.024
Borrelle, S. B., Ringma, J., Law, K. L., Monnahan, C. C., Lebreton, L., McGivern, A., et al. (2020). Predicted growth in plastic waste exceeds efforts to mitigate plastic pollution. Science 369, 1515–1518. doi: 10.1126/science.aba3656
Browne, M. A., Crump, P., Niven, S. J., Teuten, E., Tonkin, A., Galloway, T., et al. (2011). Accumulation of microplastic on shorelines woldwide: sources and sinks. Environ. Sci. Technol. 45, 9175–9179. doi: 10.1021/es201811s
Chen, H., Wang, S., Guo, H., Lin, H., Zhang, Y., Long, Z., et al. (2019). Study of marine debris around a tourist city in East China: implication for waste management. Sci. Total Environ. 676, 278–289. doi: 10.1016/j.scitotenv.2019.04.335
Derraik, J. G. B. (2002). The pollution of the marine environment by plastic debris: a review. Mar. Pollut. Bull. 44, 842–852. doi: 10.1016/s0025-326x(02)00220-5
Doong, D.-J., Chuang, H.-C., Shieh, C.-L., and Hu, J.-H. (2011). Quantity, distribution, and impacts of coastal driftwood triggered by a typhoon. Mar. Pollut. Bull. 62, 1446–1454. doi: 10.1016/j.marpolbul.2011.04.021
Eckstein, D., Künzel, V., and Schäfer, L. (2021). Global Climate Risk Index 2021. Who Suffers Most from Extreme Weather Events? Weather-Related Loss Events in 2019 and 2000-2019. Berlin: Germanwatch e.V.
Fok, L., Cheung, P. K., Tang, G., and Li, W. C. (2017). Size distribution of stranded small plastic debris on the coast of Guangdong. South China. Environ. Pollut. 220, 407–412. doi: 10.1016/j.envpol.2016.09.079
Galgani, F., Hanke, G., Werner, S., Oosterbaan, L., Nilsson, P., Fleet, D., et al. (2013). Guidance on Monitoring of Marine Litter in European Seas. Luxembourg: Publications Office of the European Union, doi: 10.2788/99475
Garcés-Ordóñez, O., Saldarriaga-Vélez, J. F., and Espinosa-Díaz, L. F. (2021). Marine litter pollution in mangrove forests from Providencia and Santa Catalina islands, after Hurricane IOTA path in the Colombian Caribbean. Mar. Pollut. Bull. 168:112471. doi: 10.1016/j.marpolbul.2021.112471
GESAMP (2019). Guidelines for the Monitoring and Assessment of Plastic Litter in the Ocean-GESAMP. Reports and Studies No. 99. Nairobi: United Nations Environment Programme, 130.
Gündoğdu, S., Çevik, C., Ayat, B., Aydoğan, B., and Karaca, S. (2018). How microplastics quantities increase with flood events? An example from Mersin Bay NE Levantine coast of Turkey. Environ. Pollut. 239, 342–350. doi: 10.1016/j.envpol.2018.04.042
Hinata, H., Mori, K., Ohno, K., Miyao, Y., and Kataoka, T. (2017). An estimation of the average residence times and onshore-offshore diffusivities of beached microplastics based on the population decay of tagged meso-and macrolitter. Mar. Pollut. Bull. 122, 17–26. doi: 10.1016/j.marpolbul.2017.05.012
Hitchcock, J. N. (2020). Storm events as key moments of microplastic contamination in aquatic ecosystems. Sci. Total Environ. 734:139436. doi: 10.1016/j.scitotenv.2020.139436
Hurley, R. R., Lusher, A. L., Olsen, M., and Nizzetto, L. (2018). Validation of a method for extracting microplastics from complex, organic-rich, environmental matrices. Environ. Sci. Technol. 52, 7409–7417. doi: 10.1021/acs.est.8b01517
Isobe, A., Iwasaki, S., Uchida, K., and Tokai, T. (2019). Abundance of non-conservative microplastics in the upper ocean from 1957 to 2066. Nat. Commun. 10:417. doi: 10.1038/s41467-019-08316-9
Iwata, S., and Matsuyama, M. (1989). Surface circulation in Sagami bay: the response to variations of the Kuroshio axis. J. Oceanogr. 45, 310–320.
Jambeck, J. R., Geyer, R., Wilcox, C., Siegler, T. R., Perryman, M., Andrady, A., et al. (2015). Plastic waste inputs from land into the ocean. Science 347, 768–771. doi: 10.1126/science.1260352
Japan Aerospace Exploration Agency (2019). Shikisai GCOM-C Surface Chlorophyll. Available online at: https://www.eorc.jaxa.jp/JASMES/SGLI_NRT/data/CHLA/2019/09/10/GC1SG1_201909100120004900_L2MG_IWPRQ_2000_CHLA_11.png (accessed November 27, 2021).
Japan Meteorological Agency (2020). Annual Report on the Activities of the RSMC Tokyo-Typhoon Center 2019. Available online at: https://www.jma.go.jp/jma/jma-eng/jma-center/rsmc-hp-pub-eg/AnnualReport/2019/Text/Text2019.pdf (accessed November 27, 2021).
Kataoka, T., Nihei, Y., Kudou, K., and Hinata, H. (2019). Assessment of the sources and inflow processes of microplastics in the river environments of Japan. Environ. Pollut. 244, 958–965. doi: 10.1016/j.envpol.2018.10.111
Kim, I.-S., Chae, D.-H., Kim, S.-K., Choi, S., and Woo, S.-B. (2015). Factors influencing the spatial variation of microplastics on high-tidal coastal beaches in Korea. Arch. Environ. Contam. Toxicol. 69, 299–309. doi: 10.1007/s00244-015-0155-6
Knutson, T. R., McBride, J. L., Chan, J., Emanuel, K., Holland, G., Landsea, C., et al. (2010). Tropical cyclones and climate change. Nat. Geosci. 3, 157–163.
Kossin, J. P., Knapp, K. R., Olander, T. L., and Velden, C. S. (2020). Global increase in major tropical cyclone exceedance probability over the past four decades. Proc. Natl. Acad. Sci. U.S.A. 117, 11975–11980.
Kudo, K., Kataoka, T., Nihei, Y., and Kitaura, F. (2018). Estimation of temporal variations and annual flux of microplastics in rivers under-and high-flow conditions. J. Jpn. Soc. Civ. Eng. Ser. B1 (Hydraul. Eng.). 74, 529–534.
Kukulka, T., Proskurowski, G., Morét-Ferguson, S., Meyer, D. W., and Law, K. L. (2012). The effect of wind mixing on the vertical distribution of buoyant plastic debris. Geophys. Res. Lett. 39:L07601.
Kunkel, K. E., Karl, T. R., Brooks, H., Kossin, J., Lawrimore, J. H., Arndt, D., et al. (2013). Monitoring and understanding trends in extreme storms: state of knowledge. Bull. Am. Meteorol. Soc. 94, 499–514.
Lattin, G. L., Moore, C. J., Zellers, A. F., Moore, S. L., and Weisberg, S. B. (2004). A comparison of neustonic plastic and zooplankton at different depths near the southern California shore. Mar. Pollut. Bull. 49, 291–294. doi: 10.1016/j.marpolbul.2004.01.020
Lebreton, L. C. M., Van der Zwet, J., Damsteeg, J.-W., Slat, B., Andrady, A., and Reisser, J. (2017). River plastic emissions to the world’s oceans. Nat. Commun. 8:15611.
Lee, J., Hong, S., Song, Y. K., Hong, S. H., Jang, Y. C., Jang, M., et al. (2013). Relationships among the abundances of plastic debris in different size classes on beaches in South Korea. Mar. Pollut. Bull. 77, 349–354. doi: 10.1016/j.marpolbul.2013.08.013
Lo, H.-S., Lee, Y.-K., Po, B. H.-K., Wong, L.-C., Xu, X., Wong, C.-F., et al. (2020). Impacts of Typhoon Mangkhut in 2018 on the deposition of marine debris and microplastics on beaches in Hong Kong. Sci. Total Environ. 716:137172. doi: 10.1016/j.scitotenv.2020.137172
Majanga, B. D., Fujieda, S., Nishi, R., and Hosotani, K. (2015). Marine debris distribution, variation and pattern/seasonal changes along the coast and on sea surface of the Kagoshima Bay. J. Jpn. Soc. Civ. Eng. Ser. B3 (Ocean Eng.). 71, I_503–I_508.
Michida, Y., Chavanich, S., Cabañas, A. C., Hagmann, P., Hinata, H., Isobe, A., et al. (2019). Guidelines for Harmonizing Ocean Surface Microplastic Monitoring Methods. Tokyo: Ministry of the Environment Japan.
Miyazawa, Y., Varlamov, S. M., Miyama, T., Kurihara, Y., Murakami, H., and Kachi, M. (2021). A nowcast/forecast system for Japan’s coasts using daily assimilation of remote sensing and in situ data. Remote Sens. 13:2431. doi: 10.3390/rs13132431
Moore, C. J., Moore, S. L., Weisberg, S. B., Lattin, G. L., and Zellers, A. F. (2002). A comparison of neustonic plastic and zooplankton abundance in southern California’s coastal waters. Mar. Pollut. Bull. 44, 1035–1038. doi: 10.1016/s0025-326x(02)00150-9
Nakajima, R., Tsuchiya, M., Yabuki, A., Masuda, S., Kitahashi, T., Nagano, Y., et al. (2021). Massive occurrence of benthic plastic debris at the abyssal seafloor beneath the Kuroshio Extension, the North West Pacific. Mar. Pollut. Bull. 166:112188. doi: 10.1016/j.marpolbul.2021.112188
Nihei, Y., Yoshida, T., Kataoka, T., and Ogata, R. (2020). High-resolution mapping of Japanese microplastic and macroplastic emissions from the land into the sea. Water 12:951.
Ockelford, A., Cundy, A., and Ebdon, J. E. (2020). Storm response of fluvial sedimentary microplastics. Sci. Rep. 10:1865. doi: 10.1038/s41598-020-58765-2
Okada, T., Nakayama, K., Takao, T., and Furukawa, K. (2011). Influence of freshwater input and bay reclamation on long-term changes in seawater residence times in Tokyo Bay, Japan. Hydrol. Process. 25, 2694–2702. doi: 10.1002/hyp.8010
Pelamatti, T., Fonseca-Ponce, I. A., Rios-Mendoza, L. M., Stewart, J. D., Marín-Enríquez, E., Marmolejo-Rodriguez, A. J., et al. (2019). Seasonal variation in the abundance of marine plastic debris in Banderas Bay, Mexico. Mar. Pollut. Bull. 145, 604–610. doi: 10.1016/j.marpolbul.2019.06.062
Schmidt, C., Krauth, T., and Wagner, S. (2017). Export of plastic debris by rivers into the sea. Environ. Sci. Technol. 51, 12246–12253. doi: 10.1021/acs.est.7b02368
Sentinel (2019). Sentinel-1 L2C True Color. Available online at: https://apps.sentinel-hub.com/eo-browser/?zoom=10&lat=35.10755&lng=139.34647&themeId=DEFAULT-THEME&visualizationUrl=https%3A%2F%2Fservices.sentinel-hub.com%2Fogc%2Fwms%2F42924c6c-257a-4d04-9b8e-36387513a99c&datasetId=S2L1C&fromTime=2019-09-10T00%3A00%3A00 (accessed November 27, 2021).
Song, Y. K., Hong, S. H., Eo, S., Han, G. M., and Shim, W. J. (2020). Rapid production of micro-and nanoplastics by fragmentation of expanded polystyrene exposed to sunlight. Environ. Sci. Technol. 54, 11191–11200. doi: 10.1021/acs.est.0c02288
Subhan, T. F. L. (2006). Lightweight high strength concrete with expanded polystyrene beads. Mektek 8, 9–15.
Suzuki, T., Tajima, Y., Watanabe, M., Tsuruta, N., Takagi, H., Takabatake, T., et al. (2020). Post-event survey of locally concentrated disaster due to 2019 Typhoon Faxai along the western shore of Tokyo Bay, Japan. Coast. Eng. J. 62, 146–158. doi: 10.1080/21664250.2020.1738620
Tamura, H., Kawaguchi, K., Iwamoto, T., and Fujiki, T. (2021). Coastal destruction and unusual wave spectra induced by Typhoon Faxai in 2019. Coast. Eng. J. 63, 92–105. doi: 10.1080/21664250.2021.1877944
Tokyo Regional Headquarters. (2019). Preliminary Weather Report of Typhoon Faxai 2019. Tokyo: Tokyo Regional Headquarters.
Tsuchiya, K., Kuwahara, V. S., Hamasaki, K., Tada, Y., Ichikawa, T., Yoshiki, T., et al. (2015). Typhoon-induced response of phytoplankton and bacteria in temperate coastal waters. Estuar. Coast. Shelf Sci. 167, 458–465. doi: 10.1016/j.ecss.2015.10.026
Tu, J.-Y., and Chou, C. (2013). Changes in precipitation frequency and intensity in the vicinity of Taiwan: typhoon versus non-typhoon events. Environ. Res. Lett. 8:14023.
Turner, A., Arnold, R., and Williams, T. (2020). Weathering and persistence of plastic in the marine environment: lessons from LEGO. Environ. Pollut. 262:114299. doi: 10.1016/j.envpol.2020.114299
Veerasingam, S., Saha, M., Suneel, V., Vethamony, P., Rodrigues, A. C., Bhattacharyya, S., et al. (2016). Characteristics, seasonal distribution and surface degradation features of microplastic pellets along the Goa coast, India. Chemosphere 159, 496–505. doi: 10.1016/j.chemosphere.2016.06.056
Wang, J., Lu, L., Wang, M., Jiang, T., Liu, X., and Ru, S. (2019). Typhoons increase the abundance of microplastics in the marine environment and cultured organisms: a case study in Sanggou Bay, China. Sci. Total Environ. 667, 1–8. doi: 10.1016/j.scitotenv.2019.02.367
Weideman, E. A., Perold, V., Arnold, G., and Ryan, P. G. (2020). Quantifying changes in litter loads in urban stormwater run-off from Cape Town, South Africa, over the last two decades. Sci. Total Environ. 724:138310. doi: 10.1016/j.scitotenv.2020.138310
Yamashita, R., and Tanimura, A. (2007). Floating plastic in the Kuroshio current area, western North Pacific Ocean. Mar. Pollut. Bull. 4, 485–488. doi: 10.1016/j.marpolbul.2006.11.012
Keywords: hurricanes, macroplastics, mesoplastics, microplastics, Sagami Bay, tropical cyclones, typhoon
Citation: Nakajima R, Miyama T, Kitahashi T, Isobe N, Nagano Y, Ikuta T, Oguri K, Tsuchiya M, Yoshida T, Aoki K, Maeda Y, Kawamura K, Suzukawa M, Yamauchi T, Ritchie H, Fujikura K and Yabuki A (2022) Plastic After an Extreme Storm: The Typhoon-Induced Response of Micro- and Mesoplastics in Coastal Waters. Front. Mar. Sci. 8:806952. doi: 10.3389/fmars.2021.806952
Received: 01 November 2021; Accepted: 01 December 2021;
Published: 13 January 2022.
Edited by:
Xiaoshan Zhu, Tsinghua University, ChinaReviewed by:
Atsuhiko Isobe, Kyushu University, JapanMuhammad Reza Cordova, Indonesian Institute of Sciences, Indonesia
Copyright © 2022 Nakajima, Miyama, Kitahashi, Isobe, Nagano, Ikuta, Oguri, Tsuchiya, Yoshida, Aoki, Maeda, Kawamura, Suzukawa, Yamauchi, Ritchie, Fujikura and Yabuki. This is an open-access article distributed under the terms of the Creative Commons Attribution License (CC BY). The use, distribution or reproduction in other forums is permitted, provided the original author(s) and the copyright owner(s) are credited and that the original publication in this journal is cited, in accordance with accepted academic practice. No use, distribution or reproduction is permitted which does not comply with these terms.
*Correspondence: Ryota Nakajima, bmFrYWppbWFyQGphbXN0ZWMuZ28uanA=