Water quality negatively impacts coral occurrence in eutrophic nearshore environments of the Florida Keys
- Department of Biology, University of Miami, Miami, FL, United States
Introduction: Coral species are negatively impacted by anthropogenic stressors worldwide. Nearshore coastal ecosystems provide an excellent study system for the investigation of the impacts of local land-based stressors such as nutrients on coral assemblages due to their proximity to land based sources of pollution. The nearshore environs of the Florida Keys are an intensively developed and intensively managed system; Florida Keys waters have been characterized by chronic eutrophication since 1999. Benthic communities are known to change or “shift” under chronic eutrophication.
Objectives: This research examines the patterns of occurrence in stony corals correlating to detailed nearshore water quality measurements. The questions addressed are a) What are the coral assemblages within 500 meters of the shoreline, and which species are resilient to these environs? b) How does water quality impact coral occurrence?
Methods: This study examined the immediate nearshore (0-500m) areas of 13 sites using a randomized blocked experimental design for both water quality and benthic sampling. Water quality parameters measured included chlorophyll-a, nitrates + nitrites (NOx), total nitrogen, total Kjeldahl nitrogen, total phosphorus, Salinity, pH, temperature, and dissolved oxygen. Benthic surveys documented stony coral occurrence using belt transects with point intercept measurements.
Results: Stony coral species occurrence was limited at most of the study sites. Siderastrea radians and Porites divaricata were the two common species that appear to have a higher tolerance to eutrophic conditions under these shallow water conditions. Of the 13 study sites, 2 sites had no coral species occurring; this analysis was based on the comparison between sites with and without stony coral species. Water column Chlorophyll-a, pH, dissolved oxygen saturation, temperature, NOx, N:P ratio, total nitrogen and total phosphorus were found to be significantly different between the sites with and without corals. Further multivariate analysis showed a clear clustering of the two groups.
Conclusion: The low overall diversity and the higher occurrences of stress tolerant species provide evidence of the effects of coastal eutrophication on the coastal Florida Keys ecosystem. Our data suggest that more conservative water quality thresholds should be posed to improve nearshore biodiversity and ecological functioning of coastal systems.
1 Introduction
Worldwide, reef-building corals are experiencing an unprecedented decline due to human-induced stressors (Putnam et al., 2017; Good and Bahr, 2021). Corals are impacted by both global and local stressors, while in many instances, multiple stressors work synergistically to deteriorate coral survival and health (Ban et al., 2014; Ellis et al., 2019). Coral reef decline is often linked to the effect of localized stressors such as pollution, overfishing and coastal development (Zaneveld et al., 2016). Water quality has been recognized as one of the most important anthropogenic factors that affects coral health and survival (De’ath and Fabricius, 2010; Browne et al., 2015), critical indicators of coral health include coral biodiversity (De’ath and Fabricius, 2010) and species assemblage composition (Uthicke et al., 2010). Coral assemblages in nearshore habitats may play an important role in supporting and replenishing coral reefs through increasing their genetic diversity. Nearshore corals are exposed to greater environmental variability within these shallow habitats and might thus benefit coral resilience and influence long-term metapopulation persistence (Semon, 2007)
The Florida Keys (Florida, USA) is an intensely used and intensively managed system associated with the larger Everglades ecosystem of South Florida, and the Comprehensive Everglades Restoration Plan (CERP), the largest wetland restoration project in the world (McIvor et al., 1994; Lott, 1996, Office of National Marine Sanctuaries, 2011). Moreover, anthropogenic pressures such as coastal development, and tourism have had and continue to have an immense impact on the nearshore water quality of the area. This, in combination with a history of leaching from cesspits since 1940’s until advanced wastewater treatment installation in 2017, has contributed to poor water quality with the accumulation of nutrients in the nearshore environs (Lapointe et al., 1990; Paul et al., 1997; Montenero et al., 2020). A central component of Florida Keys coastal development is the presence of over 500 dredged residential canals and marinas; these exacerbate the accumulation of nutrients through a variety of processes (Montenero et al., 2020).
Water quality was first identified as a central challenge to Florida Keys marine resources by the Environmental Protection Agency (EPA) in 1999, and by 2017, advanced wastewater treatment had been implemented throughout the Florida Keys to reduce nutrient loading to the nearshore waters. Monroe County addressed this challenge of canal remediation within selected residential canals through demonstration projects designed to address nutrient loading. Elevated nutrient concentrations in the water column have been linked to the alteration and loss of diversity in nearshore marine communities (Lapointe et al., 1994; Fourqurean et al., 1995; Ferdie and Fourqurean, 2004; López et al., 2019). Nutrient pollution was cited as a major contributor to coral reef decline (NOAA,1988); sewage leaching into coastal waters was one of the factors that led to the formation of the Florida Keys National Marine Sanctuary (FKNMS) Act in 1990 which mandated a multi-agency Water Quality Protection Program (WQPP) (NOAA,1996). The WQPP program recognized that both local (within the FKNMS) and regional (i.e., on the South Florida mainland; wider Caribbean) sources of nutrient enrichment, must be addressed in coral reef management.
The effects of eutrophication on benthos in the Florida Keys have been well documented for seagrass communities. Seagrass exposed to eutrophic conditions experience excessive epiphyte growth (Frankovich and Fourqurean, 1997); seagrass communities can be displaced by competitive fast-growing benthic algal species (Armitage et al., 2005). Hard corals are affected by nutrients (Koop et al., 2001; Adam et al., 2021), though there is limited experimental evidence for the mechanisms by which elevated nutrient availability leads to coral decline (Szmant, 2002; Lesser, 2021). The creation of the FKNMS has led to long-term research initiatives to address the threats facing coral reefs (Lirman et al., 2011; Vega Thurber et al., 2014; Enochs et al., 2015; Neely et al., 2021). Less attention has been given to nearshore patch reefs and hardbottom communities that fall outside the FKNMS jurisdiction and are managed by Monroe County and State of Florida agencies. Nearshore communities have greater exposure for land-based sources of pollutants (Lapointe et al., 2004; Lapointe et al., 2019). This study is focused on the occurrence of stony corals in the immediate nearshore environs (i.e., adjacent to the shoreline up to 500m offshore) and the effects of local stressors on these communities.
Nutrient pollution has negative effects on coral ecology, from coral demography (Duprey et al., 2016) to specific physiological responses and promoting diseases at the population and species level (Browne et al., 2015). The symbiotic relationship of Scleractinian coral species with dinoflagellate algae (zooxanthellae) hosted in the tissue of the coral (Muscatine, 1990) is easily disrupted with water quality changes. Previous experiments have shown that elevated nutrient availability might negatively impact coral functions such as reproduction and calcification (Stambler et al., 1991; Loya et al., 2004; Dunn et al., 2012). Conversely, other studies have found a positive relationship between nutrients and coral functions such as growth rates (Koop et al., 2001; Bongiorni et al., 2003; Dunn et al., 2012). Nevertheless, it has been suggested that values in both the extremely low and extreme high levels of the nutrient concentrations on coral reefs are not optimal for coral health.
Therefore, levels beyond certain thresholds might be detrimental for coral health and occurrence, although these thresholds can be species-specific (D’Angelo and Wiedenmann, 2014). Past research suggested that concentrations of chlorophyll-a above 0.2 mg m−3, especially under chronic eutrophic stress can be unsustainable for coral communities at Great Barrier Reef (Bell et al., 2014). Further, stony coral percent cover in the Florida Keys (Looe Key, Lower Keys), have been negatively affected by anthropogenic nutrient inputs, over the past 30 years with Dissolved Inorganic Nitrogen and chlorophyll-a concentrations being highly correlated with coral degradation (Lapointe et al., 2019). Particularly, since the early 1990s chlorophyll-a concentrations have peaked at approximately 0.6 mg/l and have averaged 0.25 mg/l since 2000. For reference, the respective baseline value in the 1980s was 0.14 mg/l and thus an almost two-fold change has been observed (Lapointe et al., 2019).
This study examines a fine-scale water quality dataset collected over two years in nearshore waters linked to the occurrence of corals in the nearshore environs of the Florida Keys. The study elucidates the species of corals remaining in eutrophic areas throughout the Keys. The research addressed two questions: a) What coral species currently occur in the very nearshore environs in the Florida Keys? and b) Are there significant differences in the nearshore water quality between areas where corals are present and areas where corals are absent? Our initial assumption, based on benthic habitat maps created in the Florida Keys, is that corals should occur throughout the nearshore environs as suitable habitats are available, including seagrass beds, hard bottom, and patch reefs. We wish to understand the severity and extent of nearshore eutrophication, and how water quality may have impacted nearshore coral occurrence.
2 Materials and methods
2.1 Study areas and sampling methods
Thirteen study coastal sites were selected throughout the Florida Keys (Upper, Middle and Lower) on both the bay and ocean sides of the islands. The nearshore environs that extended from the shoreline up to 500m offshore were investigated for all sites. These sites were adjacent to both developed and undeveloped shorelines (Figure 1A). Water Quality and benthic sampling started in January 2019 and continued through May 2021.
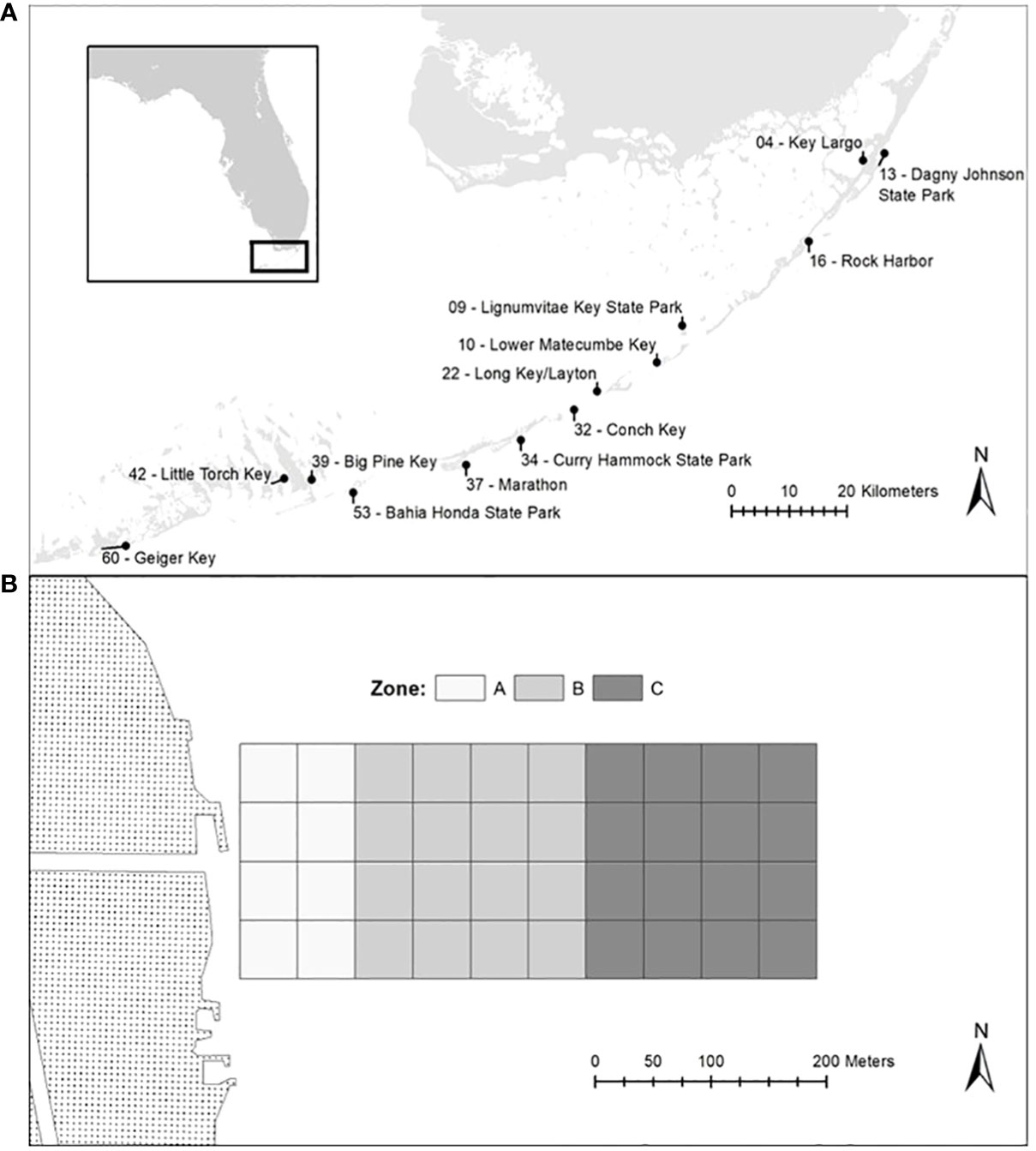
Figure 1 Map of the Florida Keys depicting the locations of all 13 study sites (A) and map showing the blocked experimental design (B).
This research is part of a larger project that examined the impact of water quality on nearshore diversity of the Florida Keys. The research design employed a stratified random block within a 40-block grid (200 m * 500 m grid subdivided into 50 x 50 m survey blocks). The blocks were divided in three different zones in which 0 to 100m correspond to Zone A; 100 to 300m correspond to Zone B; 300 to 500m correspond toZone C (Figure 1B; see Patus et al., 2022).
Benthic surveys were carried out throughout the sampling period, and a point intercept method was used along a 25-meter transect in randomly selected blocks. Each survey consisted of six replicates of a 0.5m * 0.5m quadrat deployed randomly along the transect line within each surveyed block. Quadrats were divided into a 16-point grid, and benthic epifauna and submerged aquatic vegetation species were recorded below each of these 16 points. Species occurrences were recorded at the functional group level (i.e., algae, seagrass, hard corals, soft corals, sponges, and other invertebrates) and at the species level. This research reports on the stony coral (Scleractinia) and fire coral (Hydrozoa; Milleporidae) occurrence across these three zones as related to 8 quarters of water quality measurements.
For the water quality measurements, the water column was sampled for nine water quality parameters over eight quarters (two years), resulting in a complete dataset for all 13 sites and all quarters; except the data corresponding to the first quarter of sampling at Long Key (22) site. Water quality measurements followed FL-DEP guidelines and all data is archived in the Florida Watershed Information Network (FL-WIN). Surface measurements of dissolved oxygen saturation (DO), pH, salinity, and temperature were recorded by a Yellow Springs Instruments ProQuatro multiparameter meter equipped with conductivity, pH, and DO sensors. Surface water grabs were collected, processed, and preserved and sent to a NELAP-accredited laboratory for determination of chlorophyll-a content, total phosphorous (TP), total nitrogen (TN), total Kjeldahl nitrogen (TKN), and nitrate + nitrite (NOx) concentration. The molar nitrogen-phosphorous ratio (N:P) was calculated by replacement of zero TP concentrations with the minimum nonzero value found within the TP dataset.
A large water quality dataset was developed in a two-year project studying nearshore Florida Keys ecology. Details of the water quality sampling and results are given in Patus et al., 2022. Each of the water quality parameters was subjected to interpolation by empirical Bayesian kriging (EBK) by quarter, within sites using ArcMap 10.5. Following the generation of these interpolated surfaces, the zonal statistics tool was used to extract the mean interpolated parameter value within each of the 40 blocks in each study area to be used for further statistical analysis. Quarterly data were condensed into a single value for each water quality parameter at each block in the sample area by calculating the bootstrapped (x 10,000) geometric mean of each indicator’s quarterly dataset within each block, resulting in a mean value for each parameter related to the centroid of every block in the sample areas. Due to the occurrence of zero values in the dataset, a modified formula for the calculation of geometric means that accounts for the presence of non-positive values (Habib, 2012) was used.
The data set compiled for this research only included the corresponding blocks that were surveyed for benthic community transects and the corresponding water quality dataset.
2.2 Summary statistics and statistical analysis
2.2.1 Coral occurrence description
To visualize coral species richness, a map with all the study sites and their corresponding species richness was constructed using ArcMap 10.5. The total occurrence of each species across all sites (measured in number of blocks in which each species was recorded) was also reported to allow for identification of the most abundant coral species occurring in the nearshore areas of the Florida Keys. A heatmap was designed to visualize the relative percent occurrence of each coral species within each study site, using the ggplot2 R package (Wickham, 2016). Due to the variation in sampling effort across sites, the coral occurrence values were normalized by site, as the quotient of the number of coral occurrences divided by the total number of points surveyed to allow for comparisons between sites (Supplementary Table 1). The small discrepancy between the overall normalized coral occurrences within each site (Supplementary Table 1) and the relative percent occurrence for each coral species within each site (Figure 2), are due to the fact that the former values were rounded to be used for the heatmap construction and that species with lower than 0.1% occurrence were excluded from the heatmap. Additional graphs depicting the species richness, Shannon’s diversity, and Pielou’s evenness per site were added as a supplemental figure to allow for diversity comparisons among Coral Present sites (Supplementary Figure 1).
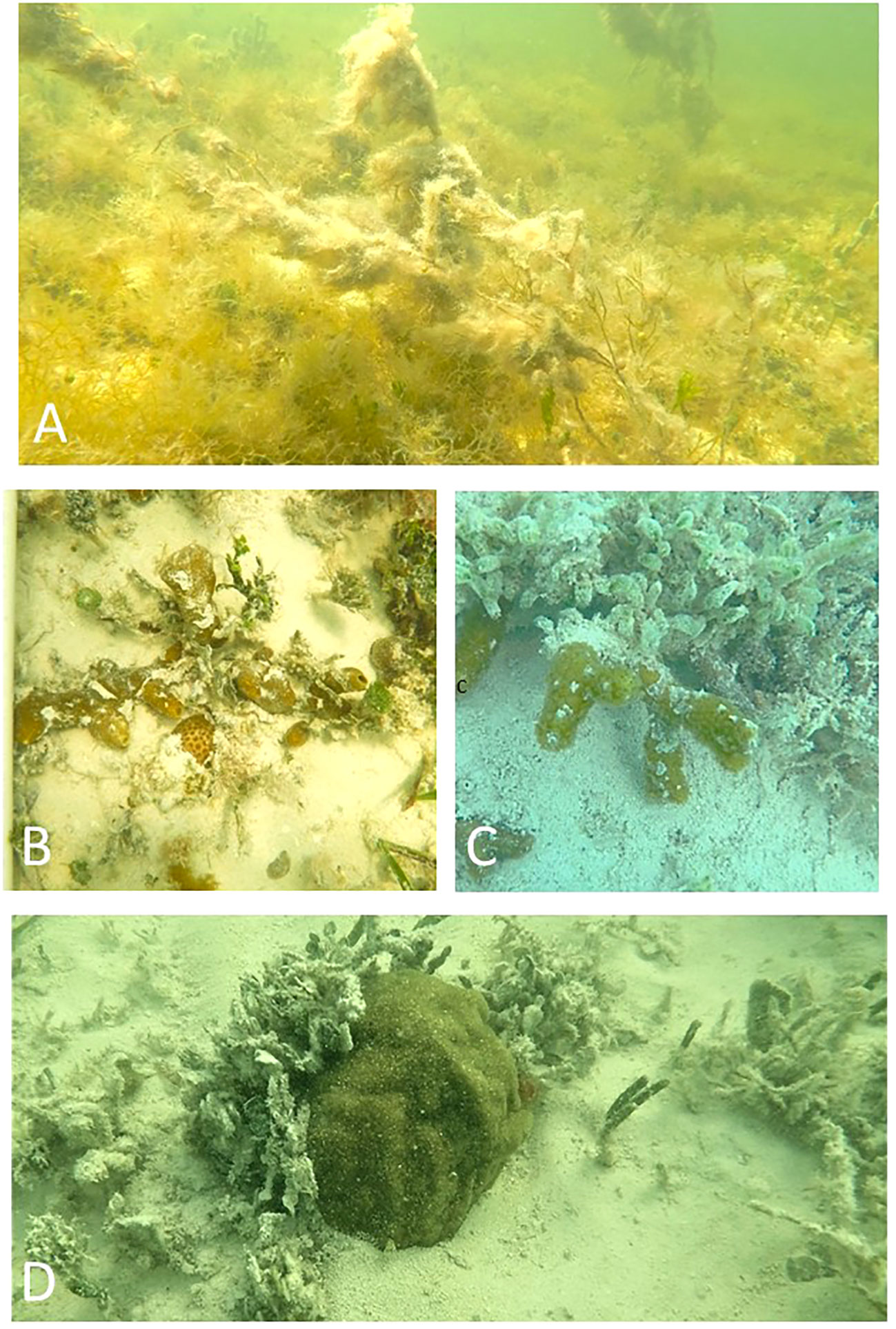
Figure 2 (A) Eutrophic conditions in nearshore benthic communities have created environmental conditions that are difficult for stony corals to recruit, survive and reproduce. (B) S. radians colonies in a hard bottom community off Lignum Vitae Key (C) P. divaricata colony in hardbottom community off Bahia Honda, and (D) P. astreoides off Geiger Key.
2.2.2 Water quality and coral occurrence
To explore the relationship between water quality and coral occurrence, two groups were formed. Sites 34 (Curry Hammock State Park) and 39 (Big Pine Key) comprised the “Coral Absent” group, since no coral species were recorded within these sites. All other sites, where there was at least one species recorded, comprised the “Coral Present” group. To test for statistically significant differences in water quality between Coral Present and Coral Absent groups, the mean water quality differences of the two groups (i.e., Coral Present - Coral Absent) were bootstrapped (R=1000, resample with replacement) for each parameter, and frequency histograms were created for each parameter. The corresponding mean values and 0.90 confidence intervals of the bootstrapped mean differences were used to test for significance between the groups. The simpleboot R package (Peng, 2019) was used to conduct the bootstrap and the ggplot2 R package (Wickham, 2016) to construct the histograms.
A Principal Component Analysis (PCA) was performed to further investigate the water quality and nutrient parameters that contribute the most to the differences shown between the two groups. Only the water quality parameters that presented significant differences between the two groups were included in this analysis. To reduce the noise in the graph, blocks were grouped by zones within each site and the mean within the zone for each parameter was used as input for the PCA. An Analysis of Similarities (ANOSIM) was conducted on this data set using Bray-Curtis dissimilarity distance metric and based on coral presence/absence grouping using the vegan R package (Oksanen J. et al., 2020). The contribution of each water quality variable to each of the dimensions of the PCA, was shown using a correlation plot, constructed using the corrplot R package (Wei and Simko, 2021). The ggplot R package (Wickham, 2016) and the ggpubr R package (Kassambara, 2020) were used to construct the PCA plot. All statistical analysis was conducted in R (R Core Team, 2020) using R Studio (RStudio Team, 2020).
3 Results
3.1 Coral occurrence and diversity
Several conspicuous coral species of the nearshore benthos in the Florida Keys are shown in Figure 3. Coral species richness is shown on a map of study sites with the corresponding number of stony coral species occurring. Two sites had no corals, 11 sites had from 8 to 2 coral species occurring. Two sites in the Lower Keys, Geiger Key and Bahia Honda State Park, had the highest number of coral species present, with 8 and 7 different species recorded respectively. Two sites in the Middle Keys, Marathon and Lignumvitae Key State Park, had 6 coral species occurring. Three sites in the Upper Keys presented the lowest coral species occurrence with 2 to 4 species occurring (Figure 4).
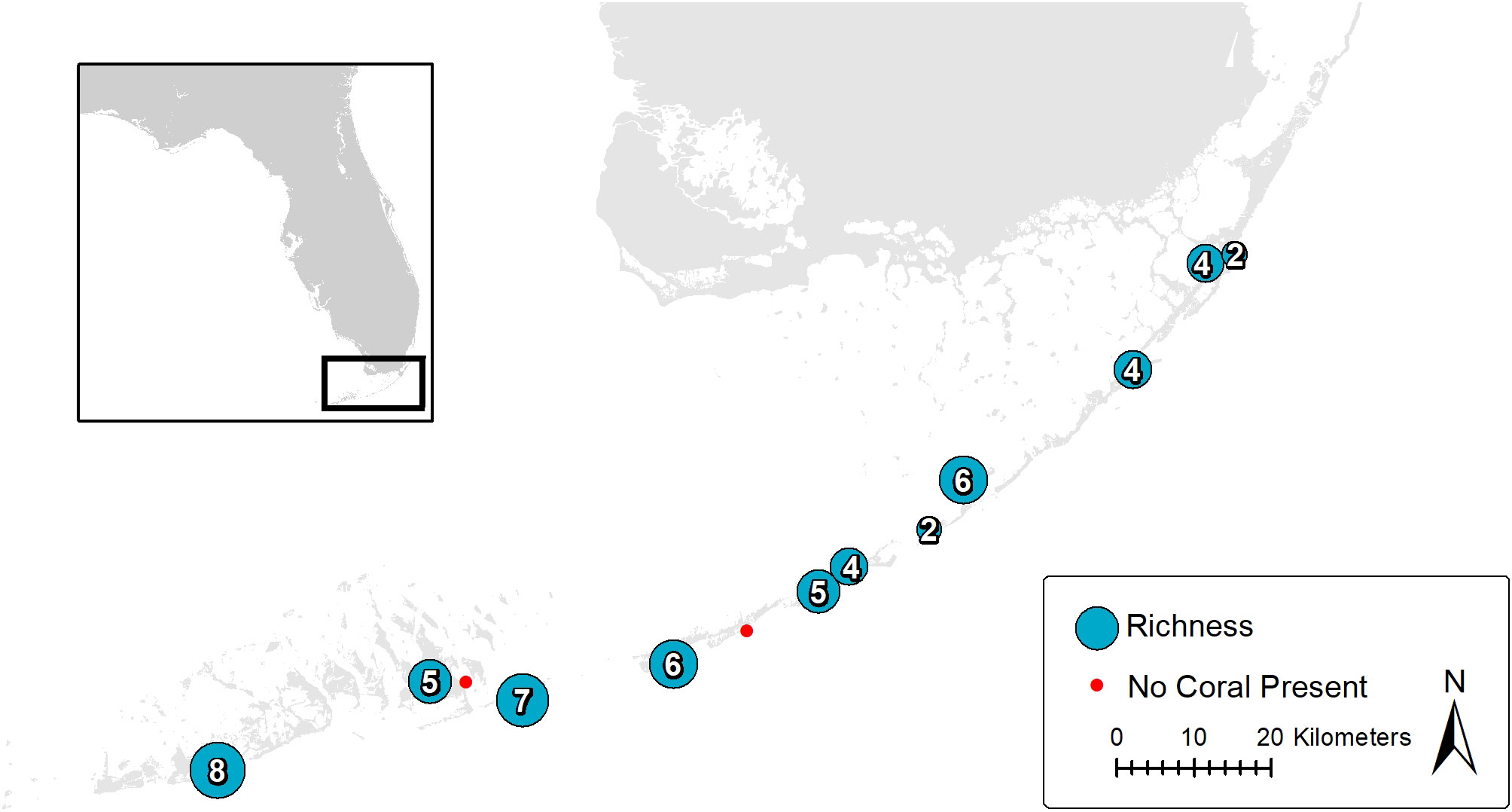
Figure 3 Map of the study area in the Florida Keys. Each circle represents the coral species richness within the corresponding site. The two red dots represent study sites within which no coral species were recorded.
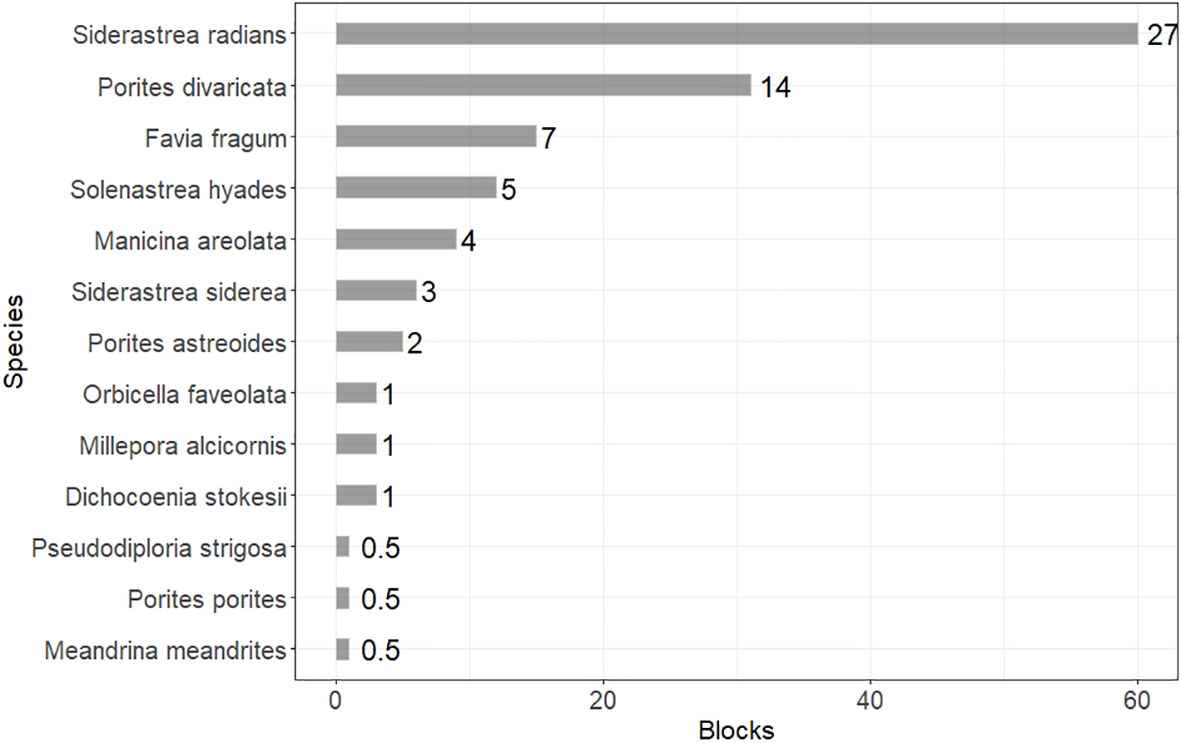
Figure 4 Bar plot of coral species occurrence. Occurrence is measured in overall number of blocks that each species was recorded, while the aggregated percent occurrence (i.e., number of blocks where the species was recorded to total number of blocks surveyed) is shown next to each bar.
Small weedy coral species characterized the nearshore areas of the Florida Keys, and the most common coral species were Siderastrea radians and Porites divaricata with occurrences within 60 and 31 blocks respectively. The aggregated percent occurrence (i.e., number of blocks where the species was recorded to total number of blocks surveyed) was 27% and 14%. Favia fragum was present at 15 blocks with 7% aggregated percent occurrence and Solenastrea hyades was recorded within 12 blocks and 5% percent occurrence. Manicina areolata was present within 9 blocks overall (4% aggregated percent occurrence), S. siderea in 6 blocks (3%), P. astreoides in 5 blocks (2%). Dichocoenia stokesii, Millepora alcicornis, and Orbicella faveolata were recorded in 3 blocks (1%), while Meandrina meandrites, P. porites, and Pseudodiploria strigosa were the least common species and occurred within only one block (<1%) (Figure 2).
The within sites percent occurrence followed a similar pattern as the within sites species richness, with two sites in the Lower Keys, Bahia Honda, and Geiger Key, and Lignumvitae Key in the Middle Keys presenting the highest total percent occurrences, with 7%, 5.7%, and 4.2% respectively (Figure 5, Supplementary Table 1). Seven different coral species were recorded at Bahia Honda. S. radians and P. divaricata presented the highest percent occurrences within this site with 2.8% and 2.5% occurrence respectively. O. faveolata and F. fragum presented 0.6% occurrence, while S. siderea(0.2%), P. asteoides(0.2%), and M. alcicornis (0.1%) occurred in lower percentages (Figure 5, Supplementary Table 1) Within Geiger Key, S.radians presented the highest percent occurrence (4.4%), followed by P. divaricata(0.6%). F. fragum (0.3%), D. stokesii, P. astreoides, P.strigosa, and M. areolata were present in lower percent occurrences (0.1%). S. radians also occurred the most within Lignumvitae Key, with a 3.7% occurrence. P. divaricata (0.2%), S. hyades (0.2%), and S. siderea (0.1%) were present in lower percent occurrences, while P. porites and F.fragum were also part of the coral assemblage of this site yet their occurrences were lower than 0.1% and thus are not depicted in Figure 5.
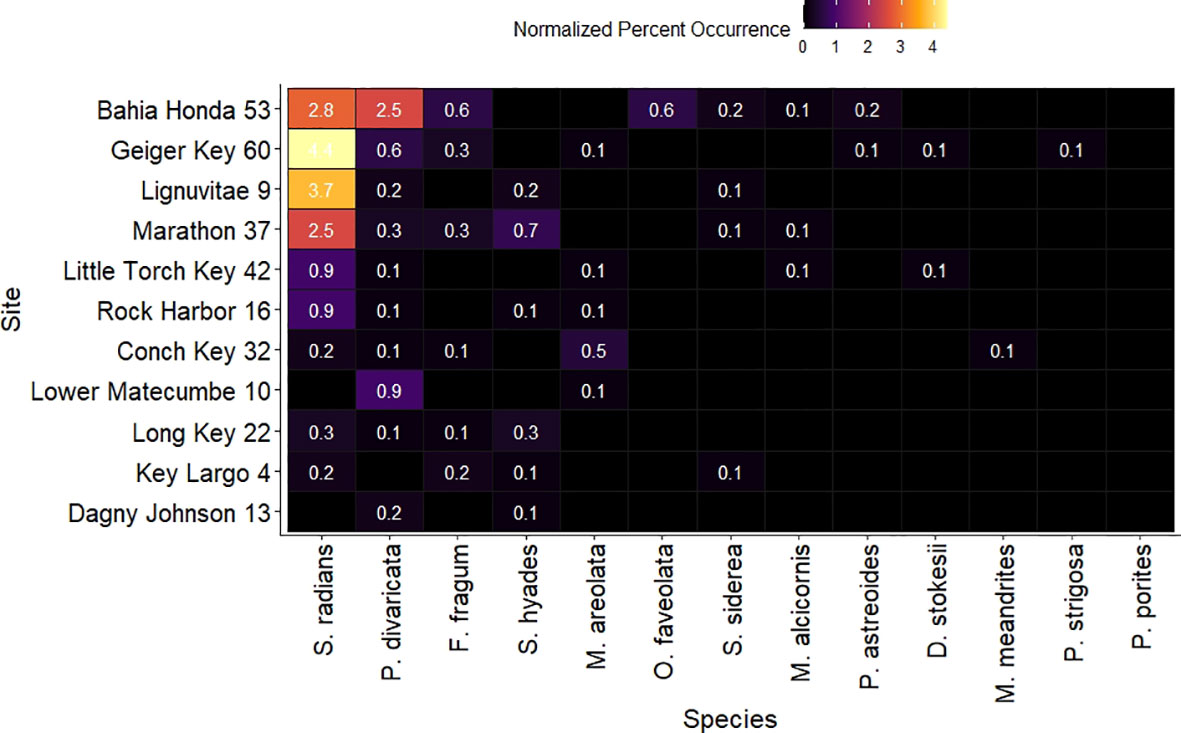
Figure 5 Heatmap depicting hard coral normalized percent occurrence for all species present within each study site. Percent occurrences lower than 0.1% for species within certain sites were excluded from the graph. Site names are shown on the y axis and species names on the x axis. Both sites and species are ordered from highest to lowest mean percent occurrence.
All sites in the Middle Keys presented an intermediate percent coral occurrence. At Marathon, the overall percent coral occurrence was 4%, from which 2.5% corresponded to S. radians occurrence, 0.7% to S. hyades, 0.3% to P. divaricata and F. fragum, and 0.1% to S. siderea and M. alcicornis (Figure 5, Supplementary Table 1). Rock harbor had 1.3% total coral occurrence, 0.9% of which was represented by S. radians, and 0.1% by each of the species S. hyades, P. divaricata, and M. areolata. The total percent occurrence for Little Torch Key was 1.2%. From this, 0.9% was S. radians, and P. divaricata, M. alcicornis, M. areolata, and D. stokesii, each accounted for 0.1% occurrence. P. divaricata was the most common species within Lower Matecumbe site with 0.9% occurrence, while M. areolata was the only other species found within this site with o 0.1% occurrence, accounting together for an overall 1% coral occurrence within this site. Conch Key presented a total coral occurrence of 0.9%, and M.areolata accounted for 0.5%, S. radians for 0.2%, while P. divaricata, F. fragum and M. meandrites accounted for 0.1% each. At Long Key, the total coral occurrence was 0.8%. S. radians and S. hyades occurred at 0.3% each of the total point surveyed, while P. divaricata and F. fragum were also present with 0.1% occurrence.
The Upper Keys presented the lowest coral percent occurrence. At Key Largo and Dagny Johnson, the total percent occurrences were 0.6% and 0.2% respectively. At Key Largo, F. fragum and S. radians accounted for 0.2% each. In addition, S. hyades and S.siderea accounted for 0.1% each. Dagny Johnson presented the lowest percent occurrence compared to all other sites with 0.3% total coral occurrence. P. divaricata accounted for 0.2% and S. hyades for 0.1%. (Figure 5, Supplementary Table 1).
A summary figure depicted species diversity values for each site. Bahia Honda presented the highest Shannon’s diversity index, species richness, and Pielou’s evenness index. At Geiger Key although the number of species was the highest, Shannon’s diversity was relatively low compared to all other sites, and evenness was the lowest of all sites. Dagny Johnson and Lower Matecumbe were the two sites with the lowest number of species and lowest Shannon’s diversity. Dagny Johnson presented low evenness yet Lower Matecumbe had a relatively high evenness (Supplementary Figure 1). A summary table is presented to allow for a numeric comparison between Coral Absent and Coral Present groups for all water quality parameters. This table gives the means and standard deviations by group for each water quality parameter (Table 1).
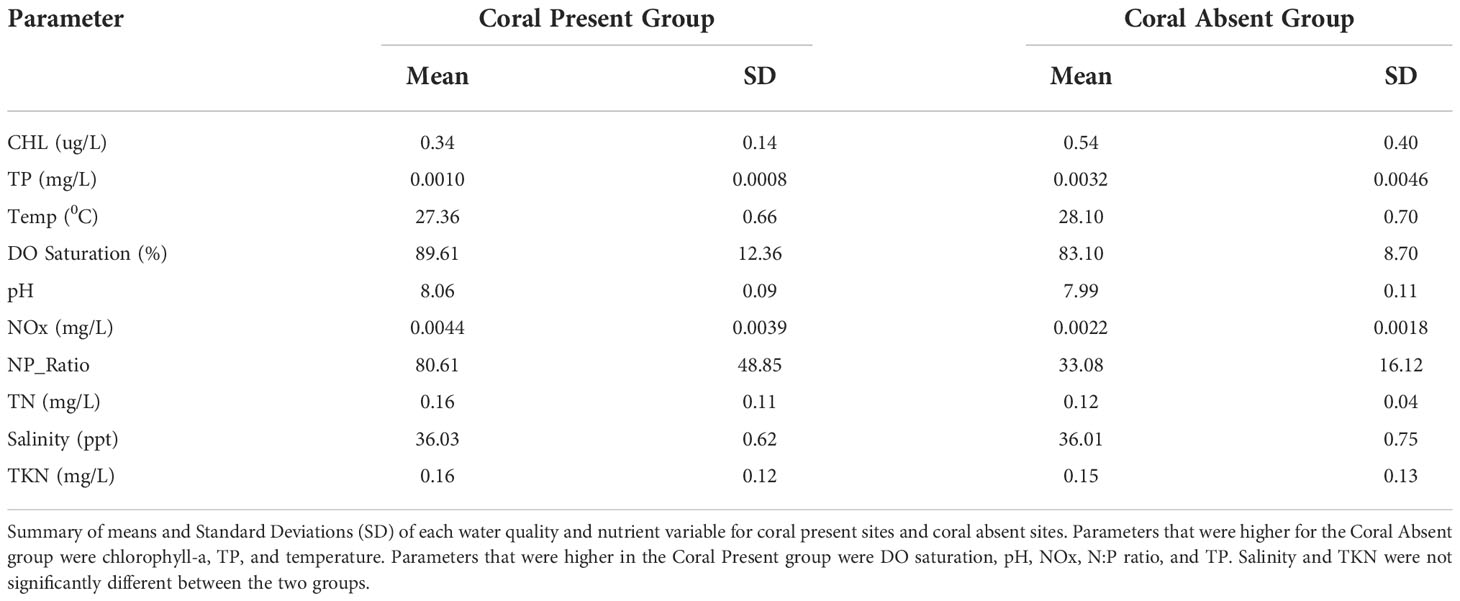
Table 1 Summary of means and Standard Deviations (SD) of each water quality and nutrient variable for coral present sites and coral absent sites.
3.2 Water quality and coral occurrence
Chlorophyll-a, TP, and temperature were significantly higher for the Coral Absent group (The 90% confidence intervals around the mean differences between groups included only values < 0 when subtracting Coral Present from Coral Absent values). The mean chlorophyll-a concentration for the Coral Absent group was 0.54 ug/L and 0.34 ug/L for the Coral Present group (Figure 6A, Table 1).TP average value for the Coral Absent group was 0.0032mg/L while for the Coral Present group the corresponding value was 0.0010mg/L (Figure 6B, Table 1). Temperature mean value for the Coral Present sites was 27.36 °C, and 28.10 °C for the Coral Absent sites (Figure 6C, Table 1).
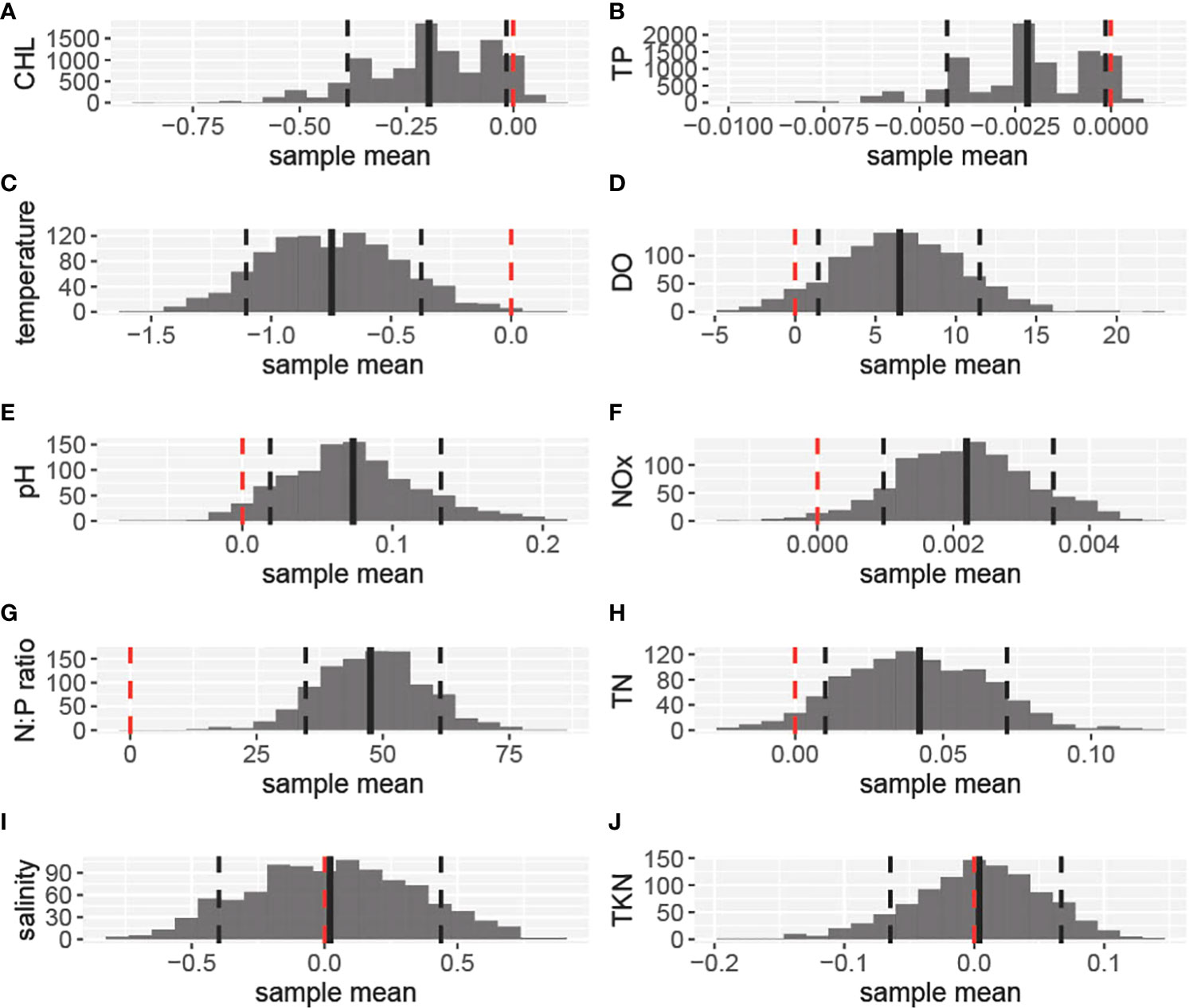
Figure 6 Histograms depicting the frequency of bootstrapped mean differences for each of the water quality and nutrient parameters: (A). depicting chlorophyll-a; (B). Total Phosphorus (TP); (C). temperature; (D). Dissolved Oxygen (DO); (E). pH units; (F). Nitrates and Nitrates (NOx); (G). N:P ratio; (H). Total Nitrogen (TN); (I). Salinity in ppt; and (J). Total Kjeldahl Nitrogen (TKN). The differences were calculated by subtracting the coral absence group values from the coral presence group values (i.e., coral presence- coral absence). For each parameter, the mean value of the differences is depicted with a vertical solid black line, while the confidence intervals (0.10, 0.90) are depicted with dashed lines. The red lines correspond to the zero value and are used for significance reference.
DO, pH, NOx, N:P ratio, and TN were significantly lower for sites within the Coral Absent group (the 90% confidence intervals around the mean differences between groups included only values > 0 when subtracting Coral Present from Coral Absent values). DO was lower on average within the Coral Absent sites (83.10%), compared to the Coral Present sites (89.61%) (Figure 6D, Table 1). The Coral Present group had a mean pH value of 8.07, and the corresponding value for the Coral Absent group was 7.99 (Figure 6E, Table 1). The mean NOx value was higher for the Coral Present sites (0.0044 mg/L) and lower for the Coral Absent sites (0.0022 mg/L) (Figure 6F, Table 1). The mean TN value within the Coral Present group was 0.16 mg/L and within the Coral Absent group was 0.12 mg/L mean value (Figure 5H, Table 1). As expected, the same was true for N:P ratio for which Coral Present sites had a value of 80.61, while Coral Absent sites presented a mean value of 33.08 (Figure 6G, Table 1).
The parameters that did not show significant differences between the two groups (the 90% confidence intervals around the mean differences between groups included only values = 0 when subtracting Coral Present from Coral Absent values) were salinity, and TKN. The mean salinity value for the Coral Present group was 36.03 ppt, and 36.01 ppt for the Coral Absent group (Figure 6I, Table 1). The TKN mean value was 0.15 mg/L for the Coral Absent group and 0.16 mg/L for the Coral Present group (Figure 6J, Table 1).
The significance of the overall model of the PCA (ANOSIM, R2 = 0.26, p=0.05), showed a clear separation of the overall water quality characteristics between the two groups as shown in the PCA biplot (Figure 7). Chlorophyll-a, pH, TP, followed by DO are strongly correlated with the first axis of the PCA. N:P ratio and TN were the parameters that were most strongly correlated with the second axis, followed TN(Supplementary Figure 2). The Coral Absent group is located in the lower left and lower right quadrants of the PCA. The variables (arrows) pH, and DO, were positively correlated with each other, while they are negatively correlated to chlorophyll-a, T, and TP. N:P, TN, and NOx are positively correlated to each and explain most of the variance within the Coral Present group. The variable T seemed to contribute the least to the overall variation of the samples as shown by the length of the corresponding arrow on the graph (Figure 7).
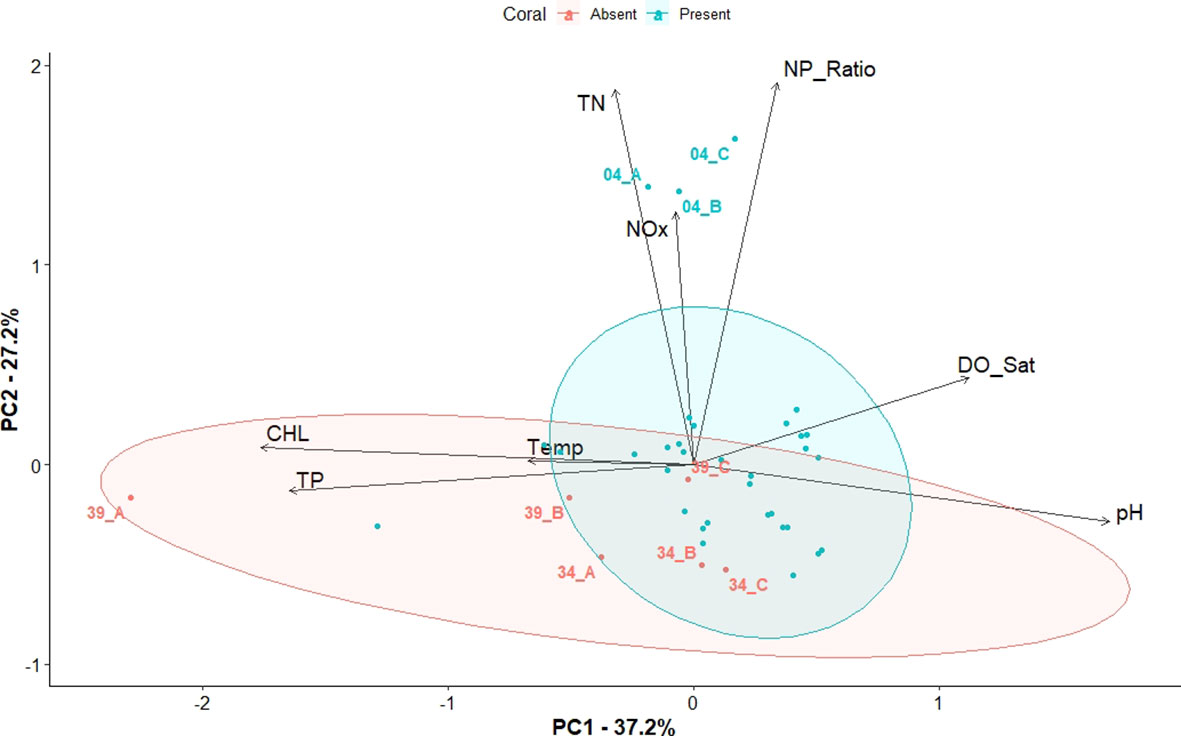
Figure 7 Principal Component Analysis (PCA) biplot representing both PC of sites (depicted as dots) and water quality parameters (depicted as arrows). The length of the arrows represents the level of influence they have on the corresponding PC. Coral present sites are shown in blue color, while coral absent sites are shown in red color. Concentration ellipses are drawn around the 0.90 confidence intervals.
4 Discussion
The assumption entering in this study is that certain stony corals were and should be present in Florida Keys nearshore benthic communities. More specifically, 20 stony coral species were identified cumulatively during the years 1989-1996 within only one nearshore hardbottom site (Sullivan, 1993; Thanopoulou, 2022a). The coral assemblages in the nearshore Florida Keys today present an unprecedented loss of species diversity. This loss seems to be exacerbated by the chronic eutrophic conditions in these nearshore environs as confirmed by the present and previous studies, to the point where corals were completely absent from sites with more extreme eutrophic conditions. These results suggest that hard corals have water quality thresholds, beyond which corals may not persist in ecologically meaningful populations. The research addressed two questions: a) What is the stony coral species assemblage in the nearshore environs in the Florida Keys? and b) Are water quality parameters significantly different between sites characterized by coral presence and sites that are completely devoid of corals? The results showed very few species of corals in the nearshore environs, and a strong correlation between coral occurrence and eutrophic conditions.
4.1 Loss of Coral Species in Nearshore environs
The nearshore coral assemblages in the Florida Keys are now characterized by low species richness and low overall occurrence, with only a few sites and few species deviating from this pattern. This research illustrates the paucity of coral species in nearshore environs of the Florida Keys, a finding that was inconsistent with past assessments. Surveys of Florida Keys nearshore benthic communities from 1989 to 1993 reported Solenastrea bournoni and Occulina diffusa accounted for over 90% of the total hard coral cover (Chiappone and Sullivan, 1994). However, neither species was recorded in the present study. Moreover, up to 12 hard coral species were reported within only one study site (Chiappone and Sullivan, 1994) whereas in the present study, the highest species richness within a single site was 8 species, with an overall Keys-wide richness of 13 species. Nonetheless, the hard coral species assemblage described in 1994 was similar to the species assemblage described in this study, except for the two aforementioned species. Loss of stony coral species is a widespread phenomenon across the Florida Keys, with the Florida Reef Tract and the nearshore patch reefs presenting a striking decline in species richness and live coral tissue cover over the past years (Montenero et al., 2020).
Regarding the diversity indexes, the two sites with the highest species richness (i.e., Bahia Honda and Geiger Key) presented a different pattern in terms of Shannon’s diversity and evenness. The low diversity and the even lower evenness at Geiger Key suggest that there was dominance of certain coral species, which was not true for Bahia Honda. As suggested by Figure 5, S. radians was the dominant species within Geiger Key and is presumably the species that drove evenness so low. A similar trend is observed within the two sites with the lowest species richness: Lower Matecumbe and Dagny Johnson, where the first presented high evenness relative to the second. Again, one of the most common species across our study sites (P. divaricata) was the driver of this divergence between these two sites. These inconsistencies in species diversity and evenness between sites characterized by the same species richness suggest that S. radians and P. divaricata often occur in unbalanced abundances compared to other coral species within the nearshore areas of the Florida Keys. The species S. radians and P. divaricata were the most common within these nearshore environs (Figures 2 and Figure 5). This finding is in agreement with previous studies that have described these two species as common in the nearshore hard-bottom communities (Chiappone and Sullivan, 1994; Fletcher et al., 2013). Moreover, S. radians is a species well known for its resilience in environments with high sedimentation and environmental fluctuations such as salinity abnormalities (Lirman and Manzello, 2009). Despite this naturally occurring stress tolerance due to often exposure to environmental extremes, S. radians’ resilience can be significantly reduced leading to increased mortality under chronic stressors (Lirman and Manzello, 2009). Further, the stress tolerance of S. radians and P. divaricata to temperature and salinity extremes did not make them immune to other stressors such as ocean acidification which was shown to heavily impact S. radians’ calcification rates (Okazaki et al., 2013) and the survival of P. divaricata under extreme acidic pH conditions (Crook et al., 2012). Besides, the health and reproductive output of these populations seems to be impaired. S. radians is a small gonochoric encrusting species whose larvae release is not based on lunar cycles (Szmant, 1986), therefore a continuous presence of recruits would be expected within a healthy population in an ideal environment. A past study suggested that healthy coral populations (including S. radians populations) produced higher number of larvae per unit cover when compared to declining populations (Hartmann et al., 2018). Although not directly measured in this study, the presence of coral recruits of this species was scarce (only observed at Lignumvitae Key). These two findings combined, suggest that even in species that seemingly persist within these environments, unobserved sublethal effects might undermine their long-term viability. Sublethal effects such as stony coral disease leading to loss of coral populations have been previously linked to poor water quality and heat stress (Precht et al., 2016; Lapointe et al., 2019).
An additional factor that may have played a role in the low observed hard coral occurrence of this study is the lack of appropriate substrate. The nearshore benthic communities in the Florida Keys are comprised largely by seagrass beds as well as hard bottom communities (Lirman et al., 2019). In fact, seagrass was the dominant benthic community type within our study sites and occurred within over half of the total area surveyed (Thanopoulou, 2022b). Therefore, the dominance of seagrass meadows versus hardbottom communities that are considered as a more appropriate substrate for corals may have played a role in the low overall coral occurrence within our study sites. However, the presence of certain Scleractinia coral families such as Poritidae and Siderastreidae has been previously associated with seagrass communities (Lohr et al., 2017) and this pattern was also observed within the present study, where the species P. divaricata was common within seagrass communities (Thanopoulou, 2022b). Additionally, hard-bottom communities were also common within our study sites and thus it may not be inferred that the lack of appropriate substrate was the sole reason for the low coral occurrence. This variability in naturally occurring habitats was also true for the Coral Absent sites, where four different types of communities were recorded: hardbottom, seagrass beds, algal beds, and infauna/soft sediment communities (Thanopoulou, 2022b) making it unlikely for the complete lack of corals to be attributed to the absence of coral settlement appropriate habitat.
4.2 Water quality and chronic eutrophic conditions at Coral Absent sites
The significant differences observed between Coral Absent and Coral Present sites for numerous nutrient and water quality parameters support the idea that water quality plays an important role in corals’ occurrence. The Coral Absent sites were characterized by higher chlorophyll-a, TP, and temperature, more acidic pH, lower DO, NOx, TN, and N:P ratio (Figure 6).
The high concentrations of chlorophyll-a in the water column throughout the 2-year study, suggest that the nearshore areas of the Florida Keys are suffering from chronic eutrophication. High chlorophyll-a concentrations have been previously linked with coral reef decline (Bell et al., 2014; Seemann et al., 2014; Guo et al., 2019). The negative effects of eutrophication on coral reefs (Bell, 1992) include reduced biodiversity (Duprey et al., 2016) and increased coral-algae competition by favoring the increase of benthic algae (Koop et al., 2001). The mean values of both groups (Coral Present and Coral Absent) for chlorophyll-a were above the limit set by the Florida DEP (0.2-0.3 ug/L), suggesting that the nearshore areas are highly eutrophic. Furthermore, previous studies in the Florida Keys and elsewhere have suggested that for chlorophyll-a concentrations above 0.2 ug/L that persist through time, impacts on coral communities may be detrimental (Bell et al., 2014; Lapointe et al., 2019). Even so, some coral species seem to have been adapted within these highly eutrophic environments (Figure 4). However, the higher chlorophyll-a content within the Coral Absent sites may indicate that even coral species seemingly adapted within these environments may present specific chlorophyll-a thresholds beyond which their survival is threatened.
Temperature has been linked to severe effects on corals (Hoegh-Guldberg, 1999) and was higher within the Coral Absent sites. Temperature anomalies and especially higher than normal temperatures have been shown to affect the coral holobiont both directly, for example by causing bleaching (Brown, 1997; McWilliams et al., 2005) and indirectly by increasing coral susceptibility to pathogens (Rosenberg and Ben-Haim, 2002). Moreover, temperature has been suggested as one of the most influencing stressors when it comes to synergistic stressor effects on coral reefs (Ban et al., 2014). In the present study, although the mean value for either of the groups was not extremely high, temporal variation in temperature was not captured in the mean values and thus temperature extremes were not represented by the analysis. Furthermore, these shallow areas might be disproportionately affected by temperature extremes when compared to coral reefs in deeper environments. The increased turbidity in the water with higher Chlorophyll-a concentrations can add to the heat absorption and higher temperatures in very nearshore areas. This interconnection between temperature and water turbidity was a decisive factor for the inclusion of a physical parameter such as temperature in an analysis mostly composed by anthropogenic eutrophication stressors.
DO plays an important role in coral occurrence. The lower saturation of DO within the Coral Absent compared to Coral Present sites illustrates the necessity of higher dissolved oxygen concentrations for corals to occur. Hypoxia is yet another factor that is increasingly threatening coral reefs, and a few recent studies have tried to raise awareness concerning this matter (Nelson and Altieri, 2019). Oxygen affects various aspects of coral physiology such as photosynthesis (Finelli et al., 2006), calcification (Colombo-Pallotta et al., 2010), and respiration (Shick, 1990). Both hypoxia and hyperoxia may act as stressors on coral reefs and their direct and indirect effects have been effectively summarized in a recent study (Nelson and Altieri, 2019). Hypoxia tolerance among different taxa and different species naturally varies and therefore a single threshold is impossible to implement, considering this variation. A past study showed that hypoxia may vary vastly between taxa, with certain species being impacted by DO as low as 0% while others present a hypoxia threshold for certain functions as high as 75% saturation (Gray et al., 2002). Moreover, duration, frequency, and severity of hypoxic conditions should be considered when imposing dissolved oxygen thresholds. The DEP limit seems to be reflecting this, since it is stated that the 30-day average DO% saturation should not be below 56% more than once per year (DEP, 2016). Although this limit was not exceeded even within the Coral Absent group, it should be mentioned that often hypoxia thresholds fail to consider sublethal effects and might therefore not be appropriate for the survival and health of all benthic organisms.
Various studies have experimentally confirmed the negative influence of low pH on coral growth (Venn et al., 2013) and reproductive success (Albright and Langdon, 2011). Additional studies have suggested that ocean acidification may work in tandem with other stressors such as global warming and high nutrient concentrations to exacerbate coral health by causing bleaching and increasing bioerosion rates (Anthony et al., 2008; DeCarlo et al., 2015). These findings support the idea that pH plays an important role in coral occurrence in the present study, since the Coral Absent sites presented significantly lower pH (more acidic) when compared to the Coral Present sites (Figure 6E). As shown in Table 1, the difference is only in the range of <0.1 units, however, calcifying organisms such as corals are highly susceptible to such small changes. Nevertheless, this ecologically significant sensitivity does not seem to be reflected in the pH limits set by the Florida DEP. The lower DEP limit is set to pH=6.5 (DEP, 2016) and is unreasonably acidic for calcifying organisms to survive.
Nutrient enrichment is the driving factor behind eutrophication and nitrogen and phosphorus are two of the elements that studies have focused on when discussing the effects of nutrient enrichment on coral reefs. High nitrogen concentrations have been found to negatively impact coral physiology by reducing their heat tolerance and calcification rates. Moreover, nitrogen enrichment has been found to cause various indirect effects such as increased coral disease vulnerability via increases stimulation of viruses (Thurber et al., 2017) and pathogenic bacteria (Barott and Rohwer, 2012). Predominantly, skewed stoichiometries of inorganic nitrogen and phosphorus have been shown to impact zooxanthellate coral species (Wiedenmann et al., 2013; D’Angelo and Wiedenmann, 2014; Rosset et al., 2017). A recent study that investigated the impact of nitrogen and phosphorus enrichment and skewed N:P stoichiometry on coral skeletal growth, suggested that under nutrient limited (i.e., either N or P is in low concentrations) conditions, skeletal growth was limited (Buckingham et al., 2022). Nevertheless, the authors pointed out that sensitivity of coral responses to nutrient changes is taxonomy specific and that N:P environmental ratio should be considered when drawing conclusions.
In the present study, the Coral Absent sites had an overall lower TN and NOx concentration (nitrites and nitrates), as well as lower N:P ratios than the Coral Present sites, while at the same time the TP concentrations were higher. Even though the Coral Present sites had higher NOx concentrations and N:P ratios when compared to the Coral Absent sites, both groups seem to have a highly skewed N:P stoichiometry. Although the higher N values within the Coral Present sites are opposite to what we would have anticipated, the fact that both groups deviated markedly from the expected 16:1 N:P Redfield Ratio (Redfield, 1958) suggests that the nearshore environs of the Florida Keys are suffering from nutrient enrichment that has possibly shaped the corresponding coral assemblages. However, it is worth noting that as shown in the PCA, the high nitrogen values within the Coral Present group were strongly related to the site 04- Key Largo (Figure 7) which was one of the sites that presented the lowest overall percent occurrence of corals (Figure 5). Moreover, the low concentrations of TP within both groups are driving the N:P ratio even higher, adding to the negative effects of skewed stoichiometry on coral health. In fact, the N:P ratio presented a fivefold deviation from the Redfield ratio for the Coral Present sites and a little over twofold increase for the Coral Absent sites. In summary, our results show a positive correlation of Coral Absent sites with high TP, but all nitrogen related parameters did not correlate as direct drivers of coral loss. However, the highly skewed N:P stoichiometry at all sites suggests that although nitrogen was not directly correlated with coral loss, it may act a secondary stressor to stony corals throughout these nearshore areas. Nevertheless, it should be mentioned here that most studies have been focused on the sublethal effects of nutrification and have taken place in controlled experimental settings. The analysis in the present study has focused on the differences between Coral Present and Coral Absent sites and did not consider any coral physiology responses. Therefore, additional ecosystem processes and/or environmental parameters not taken into consideration in this analysis might be playing a role since this was an in-situ study with no nutrient concentration manipulation.
Although salinity is often a factor considered as a stressor for coral survival (Kuanui et al., 2015) and coral physiology and health (Hoegh-Guldberg and Smith, 1989; Dias et al., 2019), there was no direct indication in the present study that this parameter might have affected coral presence. This might be explained by the fact that while nutrients may enter the water column through point sources of pollution, salinity is influenced at a more regional scale. Therefore, highly localized salinity conditions were not expected to be observed. The same was true for TKN which represents the amount of organic and ammonia forms of N, for which no differences between the two groups were observed. However, sampling frequency in this study may have missed the possible effects of extreme rainfall events that might have caused large salinity fluctuations within these nearshore environments.
The idea that specific water quality and nutrients are strongly influencing coral survival within the study sites, was further supported by the findings of the PCA (Figure 7). The clustering of the sites corresponding to the Coral Absent and Coral Present groups reflects the differences of water quality and nutrient conditions observed between groups. Most of the parameters previously found to have significant differences between the groups are shown to be driving the presence/absence of corals. More specifically, Coral Absent sites are characterized by higher chlorophyll-a, higher TP, higher T, lower DO, and more acidic pH. However, the PCA suggested that N was not one of the nutrients that influenced coral presence/absence, yet it explained some of the overall model variance most notably due to the high N values within the Coral Present site 4 (Key Largo) (Figure 7).
Overall, quarterly sampling may have missed a large amount of variance of water quality parameters as well as large and rapid fluctuations such as these caused by extreme weather events. Thus, the data may have been kept within a narrow and less representative of reality range of values which would not be expected, in-and-of themselves, to affect coral survival.
4.3 Conclusions and implications for coastal management
Water quality, including physical characteristics and nutrient concentrations play a crucial role in coral ecology in nearshore benthic environs of the Florida Keys. Our results suggest that the role of pH, DO, chlorophyll-a, and TP as water quality parameters that shape coral assemblages to the point of determining coral presence or absence. Annual mean values of pH ≤ 7.99, DO ≤ 83.10%, Chlorophyll-a ≥ 0.54 ug/L, and TP ≥ 0.0032 mg/L are detrimental for coral survival. These mean parameter value thresholds can be used as a baseline for future ecologically meaningful management and conservation efforts. This study suggests that if eutrophication within these nearshore communities persists, exacerbated water quality impacts will result in a decrease in hard coral occurrence, with a potential consequence of the complete disappearance of functional coral populations.
Future studies examining the impact of eutrophication on coral assemblages in the nearshore areas should also focus on the sublethal effects on corals. Furthermore, additional water quality parameters known to be affecting coral survival such as turbidity should also be investigated. This study highlights the need to reassess the way in which water quality regulations are defined in terms of ecological impacts, especially phase shifts in coastal systems. Ecosystem health in general and more specifically coral health should be considered when mitigating water quality in management and conservation planning. Nutrients and the abiotic parameters measured in this study are only one aspect of the overall water quality in the nearshore areas of the Florida Keys. Other pollutants have been shown to affect marine organisms and especially corals (Nalley et al., 2021) and were not considered in this study. Contaminants and pathogens are also major components of water quality and may play an important role in coral occurrence. We suggest that water quality thresholds linked to ecological metrics should be established to account for the synergistic effects of stressors (such as climate change -induced thermal stress) acting upon benthic communities. Stony corals may be a critical component of benthic biological diversity that can be monitored to measure success of water quality mitigation measures.
Data Availability Statement
All water quality data is archived with the State of Florida Watershed Information Network (WIN), Florida Department of Environmental Protection, Division of Environmental Assessment & Restoration - WSP, 2600 Blair Stone Road (MS 3526) Tallahassee, FL 32399-2400. The corresponding electronic data repository can be found here: https://floridadep.gov/dear/watershed-services-program/content/winstoret. The raw coral assemblage data are included in the article/Supplemental Material. Further inquiries may be directed to the corresponding author..
Author contributions
ZT participated in data collection, conducted the statistical analysis, prepared the figures, and led the writing. JP participated in data collection, helped with figure preparation, and assisted with the writing. KS conceptualized the project, participated in data collection and assisted with the writing. All authors contributed to the article and approved the submitted version.
Funding
This research was sponsored by a contract to the University of Miami (K. Sealey, P.I.) for performing two projects: 1) water quality monitoring services for the update to the Florida Keys Reasonable Assurance Document (FRAD) and 2) Florida Keys Residential canal water quality studies as requested by the Monroe County Board of County Commissioners (Agreement MN008).
Acknowledgments
We would like to acknowledge Caitlin Camarena, Natalie Bryce, Ellery Lennon, John MacDougall and Ashley Goncalves for assisting with the field work and the data collection. We would also like to thank Captain Chad Bohn.
Conflict of interest
The authors declare that the research was conducted in the absence of any commercial or financial relationships that could be construed as a potential conflict of interest.
Publisher’s note
All claims expressed in this article are solely those of the authors and do not necessarily represent those of their affiliated organizations, or those of the publisher, the editors and the reviewers. Any product that may be evaluated in this article, or claim that may be made by its manufacturer, is not guaranteed or endorsed by the publisher.
Supplementary material
The Supplementary Material for this article can be found online at: https://www.frontiersin.org/articles/10.3389/fmars.2022.1005036/full#supplementary-material
Supplementary Figure 1 | Diversity plots depicting Shannon’s diversity indexes (A), species richness (B), and Pielou’s evenness (C) for each Coral Present study site. Sites are presented in descending order for species richnes (B) and each site is represented with a different dot color.
Supplementary Figure 2 | Correlation plot of contributing variables and dimensions of the principal components to highlight the most contributing variables for each dimension.
References
Adam T. C., Burkepile D. E., Holbrook S. J., Carpenter R. C., Claudet J., Loiseau C., et al. (2021). Landscape-scale patterns of nutrient enrichment in a coral reef ecosystem: implications for coral to algae phase shifts. Ecol. Appl. 31 (1), e2227. doi: 10.1002/eap.2227
Albright R., Langdon C. (2011). Ocean acidification impacts multiple early life history processes of the Caribbean coral porites astreoides. Global Change Biol. 17 (7), 2478–2487. doi: 10.1111/j.1365-2486.2011.02404.x
Anthony K. R., Kline D. I., Diaz-Pulido G., Dove S., Hoegh-Guldberg O. (2008). Ocean acidification causes bleaching and productivity loss in coral reef builders. Proc. Natl. Acad. Sci. 105 (45), 17442–17446. doi: 10.1073/pnas.0804478105
Armitage A. R., Frankovich T. A., Heck K. L., Fourqurean J. W. (2005). Experimental nutrient enrichment causes complex changes in seagrass, microalgae, and macroalgae community structure in Florida bay. Estuaries 28 (3), 422–434. doi: 10.1007/BF02693924
Ban S. S., Graham N. A., Connolly S. R. (2014). Evidence for multiple stressor interactions and effects on coral reefs. Global Change Biol. 20 (3), 681–697. doi: 10.1111/gcb.12453
Barott K. L., Rohwer F. L. (2012). Unseen players shape benthic competition on coral reefs. Trends Microbiol. 20 (12), 621–628. doi: 10.1016/j.tim.2012.08.004
Bell P. (1992). Eutrophication and coral reefs–some examples in the great barrier reef lagoon. Water Res. 26 (5), 553–568. doi: 10.1016/0043-1354(92)90228-V
Bell P. R., Elmetri I., Lapointe B. E. (2014). Evidence of large-scale chronic eutrophication in the great barrier reef: quantification of chlorophyll a thresholds for sustaining coral reef communities. Ambio 43 (3), 361–376. doi: 10.1007/s13280-013-0443-1
Bongiorni L., Shafir S., Angel D., Rinkevich B. (2003). Survival, growth and gonad development of two hermatypic corals subjected to in situ fish-farm nutrient enrichment. Mar. Ecol. Prog. Ser. 253, 137–144. doi: 10.3354/meps253137
Brown B. (1997). Coral bleaching: causes and consequences. Coral Reefs 16 (1), S129–S138. doi: 10.1007/s003380050249
Browne N. K., Tay J. K. L., Low J., Larson O., Todd P. A. (2015). Fluctuations in coral health of four common inshore reef corals in response to seasonal and anthropogenic changes in water quality. Mar. Environ. Res. 105, 39–52. doi: 10.1016/j.marenvres.2015.02.002
Buckingham M., D’Angelo C., Chalk T., Foster G., Johnson K., Connelly Z., et al. (2022). Impact of nitrogen (N) and phosphorus (P) enrichment and skewed n: P stoichiometry on the skeletal formation and microstructure of symbiotic reef corals. Coral Reefs 20, 1–13. doi: 10.1007/s00338-022-02223-0
Chiappone M., Sullivan K. (1994). Ecological structure and dynamics of nearshore hard-bottom communities in the Florida keys. Bull. Mar. Sci. 54 (3), 747–756.
Colombo-Pallotta M., Rodríguez-Román A., Iglesias-Prieto R. (2010). Calcification in bleached and unbleached montastraea faveolata: evaluating the role of oxygen and glycerol. Coral Reefs 29 (4), 899–907. doi: 10.1007/s00338-010-0638-x
Crook E. D., Potts D., Rebolledo-Vieyra M., Hernandez L., Paytan A. (2012). Calcifying coral abundance near low-pH springs: implications for future ocean acidification. Coral Reefs 31 (1), 239–245. doi: 10.1007/s00338-011-0839-y
D’Angelo C., Wiedenmann J. (2014). Impacts of nutrient enrichment on coral reefs: new perspectives and implications for coastal management and reef survival. Curr. Opin. Environ. Sustainability 7, 82–93. doi: 10.1016/j.cosust.2013.11.029
De’ath G., Fabricius K. (2010). Water quality as a regional driver of coral biodiversity and macroalgae on the great barrier reef. Ecol. Appl. 20 (3), 840–850. doi: 10.1890/08-2023.1
DeCarlo T. M., Cohen A. L., Barkley H. C., Cobban Q., Young C., Shamberger K. E., et al. (2015). Coral macrobioerosion is accelerated by ocean acidification and nutrients. Geology 43 (1), 7–10. doi: 10.1130/G36147.1
Department of Environmental Protection (2016)Surface water quality standards, chapter 62-302. In: . Available at: https://www.flrules.org/gateway/ChapterHome.asp?Chapter=62-302 (Accessed May 15, 2022).
Dias M., Madeira C., Jogee N., Ferreira A., Gouveia R., Cabral H., et al. (2019). Oxidative stress on scleractinian coral fragments following exposure to high temperature and low salinity. Ecol. Indic. 107, 105586. doi: 10.1016/j.ecolind.2019.105586
Dunn J. G., Sammarco P. W., LaFleur G. (2012). Effects of phosphate on growth and skeletal density in the scleractinian coral acropora muricata: A controlled experimental approach. J. Exp. Mar. Biol. Ecol. 411, 34–44. doi: 10.1016/j.jembe.2011.10.013
Duprey N. N., Yasuhara M., Baker D. M. (2016). Reefs of tomorrow: eutrophication reduces coral biodiversity in an urbanized seascape. Global Change Biol. 22 (11), 3550–3565. doi: 10.1111/gcb.13432
Ellis J. I., Jamil T., Anlauf H., Coker D. J., Curdia J., Hewitt J., et al. (2019). Multiple stressor effects on coral reef ecosystems. Global Change Biol. 25 (12), 4131–4146. doi: 10.1111/gcb.14819
Enochs I. C., Manzello D. P., Carlton R. D., Graham D. M., Ruzicka R., Colella M. A. (2015). Ocean acidification enhances the bioerosion of a common coral reef sponge: implications for the persistence of the Florida reef tract. Bull. Mar. Sci. 91, 271–290. doi: 10.5343/bms.2014.1045
Ferdie M., Fourqurean J. W. (2004). Responses of seagrass communities to fertilization along a gradient of relative availability of nitrogen and phosphorus in a carbonate environment. Limnol Oceanogr 49 (6), 2082–2094. doi: 10.4319/lo.2004.49.6.2082
Finelli C. M., Helmuth B. S., Pentcheff N. D., Wethey D. S. (2006). Water flow influences oxygen transport and photosynthetic efficiency in corals. Coral Reefs 25 (1), 47–57. doi: 10.1007/s00338-005-0055-8
Fletcher P. J., Nuttle W. K., Ault J. S., Browder J., Riegl B. M., Gillam D. S., et al. (2013) Integrated conceptual ecosystem model development for the southeast Florida coastal marine ecosystem: MARine estuarine goal setting (MARES) for south Florida. Available at: https://repository.library.noaa.gov/view/noaa/9408 (Accessed April 18, 2022). NOAA technical memorandum OAR AOML 103; NOAA technical memorandum NOS NCCOS; 163.
Fourqurean J. W., Powell G. V., Kenworthy W. J., Zieman J. C. (1995). The effects of long-term manipulation of nutrient supply on competition between the seagrasses thalassia testudinum and halodule wrightii in Florida bay. Oikos, 72, 349–358. doi: 10.2307/3546120
Frankovich T. A., Fourqurean J. W. (1997). Seagrass epiphyte loads along a nutrient availability gradient, Florida bay, USA. Mar. Ecol. Prog. Ser. 159, 37–50. doi: 10.3354/meps159037
Good A. M., Bahr K. D. (2021). The coral conservation crisis: interacting local and global stressors reduce reef resiliency and create challenges for conservation solutions. SN Appl. Sci. 3 (3), 312. doi: 10.1007/s42452-021-04319-8
Gray J. S., Wu R. S., Or Y. Y. (2002). Effects of hypoxia and organic enrichment on the coastal marine environment. Mar. Ecol. Prog. Ser. 238, 249–279. doi: 10.3354/meps238249
Guo J., Yu K., Wang Y., Zhang R., Huang X., Qin Z. (2019). Potential impacts of anthropogenic nutrient enrichment on coral reefs in the south China Sea: Evidence from nutrient and chlorophyll a levels in seawater. Environ. Sci: Processes Impacts 21 (10), 1745–1753. doi: 10.1039/C9EM00331B
Habib E. A. (2012). Geometric mean for negative and zero values. Int. J. Res. Rev. Appl. Sci. 11 (3), 419–432.
Hartmann A. C., Marhaver K. L., Vermeij M. J. (2018). Corals in healthy populations produce more larvae per unit cover. Conserv. Lett. 11 (3), e12410. doi: 10.1111/conl.12410
Hoegh-Guldberg O. (1999). Climate change, coral bleaching and the future of the world’s coral reefs. Mar. Freshw. Res. 50 (8), 839–866. doi: 10.1071/MF99078
Hoegh-Guldberg O., Smith G. J. (1989). The effect of sudden changes in temperature, light and salinity on the population density and export of zooxanthellae from the reef corals stylophora pistillata esper and seriatopora hystrix Dana. J. Exp. Mar. Biol. Ecol. 129 (3), 279–303. doi: 10.1016/0022-0981(89)90109-3
Kassambara A. (2020). “Ggpubr: ‘ggplot2’ based publication ready plots,” in R package version 0.4.0. Available at: https://CRAN.R-project.org/package=ggpubr.
Koop K., Booth D., Broadbent A., Brodie J., Bucher D., Capone D., et al. (2001). ENCORE: the effect of nutrient enrichment on coral reefs. synthesis of results and conclusions. Mar. pollut. Bull. 42 (2), 91–120. doi: 10.1016/S0025-326X(00)00181-8
Kuanui P., Chavanich S., Viyakarn V., Omori M., Lin C. (2015). Effects of temperature and salinity on survival rate of cultured corals and photosynthetic efficiency of zooxanthellae in coral tissues. Ocean Sci. J. 50, 263–268. doi: 10.1007/s12601-015-0023-3
Lapointe B. E., Barile P. J., Matzie W. R. (2004). Anthropogenic nutrient enrichment of seagrass and coral reef communities in the lower Florida keys: discrimination of local versus regional nitrogen sources. J. Exp. Mar. Biol. Ecol. 308 (1), 23–58. doi: 10.1016/j.jembe.2004.01.019
Lapointe B. E., Brewton R. A., Herren L. W., Porter J. W., Hu C. (2019). Nitrogen enrichment, altered stoichiometry, and coral reef decline at looe key, Florida keys, USA: a 3-decade study. Mar. Biol. 166, 1–31. doi: 10.1007/s00227-019-3538-9
Lapointe B. E., O’Connell J. D., Garrett G. S. (1990). Nutrient couplings between on-site sewage disposal systems, groundwaters, and nearshore surface waters of the Florida keys. Biogeochemistry 10 (3), 289–307. doi: 10.1007/BF00003149
Lapointe B. E., Tomasko D. A., Matzie W. R. (1994). Eutrophication and trophic state classification of seagrass communities in the Florida keys. Bull. Mar. Sci. 54 (3), 696–717.
Lesser M. P. (2021). Eutrophication on coral reefs: What is the evidence for phase shifts, nutrient limitation and coral bleaching. BioScience 71 (12), 1216–1233. doi: 10.1093/biosci/biab101
Lirman D., Ault J. S., Fourqurean J. W., Lorenz J. J. (2019). “The coastal marine ecosystem of south Florida, united states,” in World seas: an environmental evaluation, 2nd edition, editor C. Sheppard. (London, UK: Elsevier), 427–444. doi: 10.1016/B978-0-12-805068-2.00021-8
Lirman D., Manzello D. (2009). Patterns of resistance and resilience of the stress-tolerant coral siderastrea radians (Pallas) to sub-optimal salinity and sediment burial. J. Exp. Mar. Biol. Ecol. 369 (1), 72–77. doi: 10.1016/j.jembe.2008.10.024
Lirman D., Schopmeyer S., Manzello D., Gramer L. J., Precht W. F., Muller-Karger F., et al. (2011). Severe 2010 cold-water event caused unprecedented mortality to corals of the Florida reef tract and reversed previous survivorship patterns. PloS One 6 (8), e23047. doi: 10.1371/journal.pone.0023047
Lohr K. E., Smith D. J., Suggett D. J., Nitschke M. R., Dumbrell A. J., Woodcock S.and Camp E. F. (2017). Coral community structure and recruitment in seagrass meadows. Front. Mar. Sci. 4, 388. doi: 10.3389/fmars.2017.00388
López I. G. M., van Den Akker M., Walk L., van Katwijk M. M., van der Heide T., van Tussenbroek B. I. (2019). Nutrient availability induces community shifts in seagrass meadows grazed by turtles. PeerJ 7, e7570. doi: 10.7717/peerj.7570
Lott C., Dye R., Sullivan K. (1996). Historical overview of development and natural history of the Florida Keys. In Site Characterization for the Florida Keys, National Marine Sanctuary and Environs. vol. 3. (Zenda, Wisconsin: The Nature Conservancy, The Preserver), pp. 5-49.
Loya Y., Lubinevsky H., Rosenfeld M., Kramarsky-Winter E. (2004). Nutrient enrichment caused by in situ fish farms at eilat, red Sea is detrimental to coral reproduction. Mar. pollut. Bull. 49 (4), 344–353. doi: 10.1016/j.marpolbul.2004.06.011
McIvor C. C., Ley J. A., Bjork R. D. (1994). “Changes in freshwater inflow from the Everglades to Florida bay including effects on biota and biotic processes: a review,” in Everglades: The ecosystem its restoration, (Boca Raton, FL, USA: St. Lucie Press) 117–146.
McWilliams J. P., Côté I. M., Gill J. A., Sutherland W. J., Watkinson A. R. (2005). Accelerating impacts of temperature-induced coral bleaching in the Caribbean. Ecology 86, 2055–2060. doi: 10.1890/04-1657
Montenero K. A., Kelble C. R., Spooner E. (2020)Florida Keys integrated ecosystem assessment ecosystem status report (Accessed June 10, 2022).
Muscatine L. (1990). The role of symbiotic algae in carbon and energy flux in reef corals. Ecosyst. World. Coral Reefs. 25, 75–87.
Nalley E. M., Tuttle L. J., Barkman A. L., Conklin E. E., Wulstein D. M., Richmond R. H., et al. (2021). Water quality thresholds for coastal contaminant impacts on corals: A systematic review and meta-analysis. Sci. Total Environ. 794, 148632. doi: 10.1016/j.scitotenv.2021.148632
National Oceanic and Atmospheric Administration (1996). Results of a workshop on coral reef research and management in the Florida Keys: a blueprint for action. volume 88 (Key Largo, Florida: National Undersea Research Program), 1–25.
Neely K. L., Lewis C. L., Lunz K. S., Kabay L. (2021). Rapid population decline of the pillar coral dendrogyra cylindrus along the Florida reef tract. Front. Mar. Sci. 8, 434. doi: 10.3389/fmars.2021.656515
Nelson H. R., Altieri A. H. (2019). Oxygen: the universal currency on coral reefs. Coral Reefs 38, 177–198. doi: 10.1007/s00338-019-01765-0
Office of National Marine Sanctuaries. (2011). Florida Keys National Marine Sanctuary Condition Report 2011. U.S. Department of Commerce, National Oceanic and Atmospheric Administration, Office of National Marine Sanctuaries, (Silver Spring, MD). 105 pp.
Okazaki R. R., Swart P. K., Langdon C. (2013). Stress-tolerant corals of Florida bay are vulnerable to ocean acidification. Coral Reefs 32 (3), 671–683. doi: 10.1007/s00338-013-1015-3
Oksanen J., Guillaume Blanchet F., Friendly M., Kindt R., Legendre P., McGlinn D., et al. (2020). “Vegan: Community ecology package,” in R package version 2, 5–7. Available at: https://CRAN.R-project.org/package=vegan.
Patus J. A. (2022). Large dataset analysis to identify anthropogenic shifts in ecosystem function for the nearshore environs of small carbonate islands. Doctoral dissertation, (University of Miami, Miami: ProQuest Dissertations Publishing).
Paul J. H., Rose J. B., Jiang S. C., Zhou X., Cochran P., Kellogg C., et al. (1997). Evidence for groundwater and surface marine water contamination by waste disposal wells in the Florida keys. Water Res. 31 (6), 1448–1454. doi: 10.1016/S0043-1354(96)00374-0
Peng R. D. (2019). “Simpleboot: Simple bootstrap routines,” in R package version 1, 1–7. Available at: https://CRAN.R-project.org/package=simpleboot.
Precht W. F., Gintert B. E., Robbart M. L., Fura R.and Van Woesik R. (2016). Unprecedented disease-related coral mortality in southeastern Florida. Sci. Rep. (Nature Publisher Group) 6, 31374. doi: 10.1038/srep31374
Putnam H. M., Barott K. L., Ainsworth T. D., Gates R. D. (2017). The vulnerability and resilience of reef-building corals. Curr. Biol. 27 (11), R528–R540. doi: 10.1016/j.cub.2017.04.047
R Core Team (2020). R: A language and environment for statistical computing (Vienna, Austria: R Foundation for Statistical Computing). Available at: https://www.R-proj.
Redfield A. C. (1958). The biological control of chemical factors in the environment. Am. Scientist 46 (3), 230A–2221.
Rosenberg E., Ben-Haim Y. (2002). Microbial diseases of corals and global warming. Environ. Microbiol. 4 (6), 318–326. doi: 10.1046/j.1462-2920.2002.00302.x
Rosset S., Wiedenmann J., Reed A. J., D’Angelo C. (2017). Phosphate deficiency promotes coral bleaching and is reflected by the ultrastructure of symbiotic dinoflagellates. Mar. pollut. Bull. 118 (1-2), 180–187. doi: 10.1016/j.marpolbul.2017.02.044
RStudio Team (2020). RStudio: Integrated development environment for r (Boston, MA: RStudio, PBC). Available at: http://www.rstudio.com/.
Seemann J., González C. T., Carballo-Bolaños R., Berry K., Heiss G. A., Struck U., et al. (2014). Assessing the ecological effects of human impacts on coral reefs in bocas del toro, Panama. Environ. Monit. Assess. 186 (3), 1747–1763. doi: 10.1007/s10661-013-3490-y
Semon K. L. (2007). Life history strategies and population dynamics of stony corals (Cnidaria: Scleractinia) in marginal habitats of the bahama banks: Implications for long-term survival and persistence of coral communities (Miami: University of Miami).
Shick J. M. (1990). Diffusion limitation and hyperoxic enhancement of oxygen consumption in zooxanthellate sea anemones, zoanthids, and corals. Biol. Bull. 179 (1), 148–158. doi: 10.2307/1541749
Stambler N., Popper N., Dubinsky Z. V. Y., Stimson J. (1991). Effects of nutrient enrichment and water motion on the coral pocillopora damicornis. Pacific Sci. 45 (3), 299–307.
Sullivan K., Chiappone M. (1993). Hierarchical methods and sampling design for conservation monitoring of tropical marine hard bottom communities. Aquatic Conservation: Marine and Freshwater Ecosystems, 3(3), 169–187.
Szmant A. M. (1986). Reproductive ecology of Caribbean reef corals. Coral Reefs 5 (1), 43–53. doi: 10.1007/BF00302170
Szmant A. M. (2002). Nutrient enrichment on coral reefs: Is it a major cause of coral reef decline? Estuaries 25 (4), 743–766. doi: 10.1007/BF02804903
Thanopoulou Z. (2022a). Loss of benthic invertebrate diversity suggests irreversible changes in nearshore communities in the Florida Keys. (Ph.D.), University of Miami, (Chapter 4), Miami.
Thanopoulou Z. (2022b). Patterns of benthic communities in nearshore areas: Evidence of phase shifts and loss of epifauna diversity. (Ph.D.), University of Miami, (Chapter 2), Miami.
Thurber R. V., Payet J. P., Thurber A. R., Correa A. M. (2017). Virus–host interactions and their roles in coral reef health and disease. Nat. Rev. Microbiol. 15 (4), 205–216. doi: 10.1038/nrmicro.2016.176
Uthicke S., Thompson A., Schaffelke B. (2010). Effectiveness of benthic foraminiferal and coral assemblages as water quality indicators on inshore reefs of the great barrier reef, Australia. Coral Reefs 29 (1), 209–225. doi: 10.1007/s00338-009-0574-9
Vega Thurber R. L., Burkepile D. E., Fuchs C., Shantz A. A., McMinds R., Zaneveld J. R. (2014). Chronic nutrient enrichment increases prevalence and severity of coral disease and bleaching. Global Change Biol. 20 (2), 544–554. doi: 10.1111/gcb.12450
Venn A. A., Tambutté E., Holcomb M., Laurent J., Allemand D., Tambutté S. (2013). Impact of seawater acidification on pH at the tissue–skeleton interface and calcification in reef corals. Proc. Natl. Acad. Sci. 110 (5), 1634–1639. doi: 10.1073/pnas.1216153110
Wei T., Simko V. (2021). R package ‘corrplot’: Visualization of a Correlation Matrix. (Version 0.92). Available at: https://github.com/taiyun/corrplot
Wickham H. (2016). ggplot2: Elegant graphics for data analysis (New York: Springer-Verlag). doi: 10.1007/978-3-319-24277-4
Wiedenmann J., D’Angelo C., Smith E. G., Hunt A. N., Legiret F.-E., Postle A. D., et al. (2013). Nutrient enrichment can increase the susceptibility of reef corals to bleaching. Nat. Climate Change 3, 160–164. doi: 10.1038/nclimate1661
Keywords: stony corals, nutrients, eutrophication, nearshore, coastal management, Florida Keys
Citation: Thanopoulou Z, Patus J and Sealey KS (2022) Water quality negatively impacts coral occurrence in eutrophic nearshore environments of the Florida Keys. Front. Mar. Sci. 9:1005036. doi: 10.3389/fmars.2022.1005036
Received: 27 July 2022; Accepted: 31 October 2022;
Published: 21 November 2022.
Edited by:
Christopher R. Kelble, Atlantic Oceanographic and Meteorological Laboratory (NOAA), United StatesReviewed by:
Robert H. Richmond, University of Hawaii at Manoa, United StatesJames W. Porter, University of Georgia, United States
Copyright © 2022 Thanopoulou, Patus and Sealey. This is an open-access article distributed under the terms of the Creative Commons Attribution License (CC BY). The use, distribution or reproduction in other forums is permitted, provided the original author(s) and the copyright owner(s) are credited and that the original publication in this journal is cited, in accordance with accepted academic practice. No use, distribution or reproduction is permitted which does not comply with these terms.
*Correspondence: Zoi Thanopoulou, zxt89@miami.edu