- 1Institute of Marine and Antarctic Studies University of Tasmania, Battery Point, TAS, Australia
- 2Integrated Marine Observing System (IMOS) Animal Tagging, Sydney Institute of Marine Science, Mosman, NSW, Australia
- 3School of Natural Sciences, Macquarie University, North Ryde, NSW, Australia
- 4d’Etudes Biologiques de Chizé, Centre National de la Recherche Scientifique (CNRS), Villiers en Bois, France
- 5Australian Antarctic Division, Channel Highway, Kingston, TAS, Australia
Indirect ecological interactions such as competition for resources between fisheries and marine predators have often been proposed but can be difficult to demonstrate empirically. The Kerguelen Plateau in the Southern Indian Ocean supports fisheries for both Patagonian toothfish and mackerel icefish and is also an important foraging ground for several avian and mammalian predators, including the southern elephant seal. We quantified the spatio-temporal use of the plateau by southern elephant seals and found that males and females spent 30% of their time on the plateau within the commonly used fishing grounds, indicating the possibility of competition for resources there. We then contrasted the seals’ use of two habitat types, the benthos (where interactions with the long-line fisheries are most likely) and the epi-pelagic zone. The likelihood of feeding on the benthos declined as ocean depth increased and was also less likely at night. Males were also more likely to feed on the benthos than females. The sub-adult male seals consumed an estimated 6,814 – 14,848 tons of high energy content prey (including toothfish) and females 7,085 – 18,037 tons from the plateau during the post-molt winter months. For males this represented 79.6 - 173.4% of the mean annual catch by the Kerguelen fishery compared to 82.8 - 210.7% for adult females. When considering the seals consumption of fish from the benthos within the fishing grounds these estimates decreased to 3.6 - 15.1% of the fishery’s total annual catch for females and 7.8 - 19.1% for males. While this further indicates the possibility of indirect ecological interactions (with the fishery taking more fish than the seals), the lack of detailed diet information for the seals precludes us from establishing the degree or nature of the possible interactions because the importance of toothfish and icefish in the diet of the seals is unknown. However, the unique life history and highly polygynous nature of this species, and the lack of evidence of a measurable effect on either the seal’s population growth rates or the catch per unit of the fishery, suggest that any indirect ecological interactions are not of sufficient magnitude to affect either the seal population or the fishery.
Introduction
Interactions between marine predators and fishing activities are important issues for many fisheries. Interactions can be direct, such as by-catch and depredation, or indirect ecological interactions such as competition for the same resources (Jog et al., 2022). There has been considerable focus on direct interactions, both the economic costs of interference and depredation on fisheries (Werner et al., 2015; Janc et al., 2018; Tulloch et al., 2019) and quantifying how fisheries directly affect predator’s vital rates through increased mortality resulting from by-catch or entanglement (Robertson et al., 2014; Nelms et al., 2021). However, while the possibility of indirect effects have long been recognized, they remain complex and poorly understood with relatively little directly known about competitive interactions (Nelms et al., 2021).
The Kerguelen Plateau supports two large long-line fisheries for Patagonian toothfish (Dissostichus eleginoides, henceforth toothfish) and a smaller mackerel icefish (Champsocephalus gunnari) trawl fishery. Toothfish have a large geographic range with individuals found from the slope of the Antarctic continent to the cold deep waters off the Ecuadorian coast (Reid, 2018). Most individuals, however, are found around the Sub-Antarctic islands, banks and seamounts, and the South American continental slope within the Southern Ocean (Peron et al., 2016; Reid, 2019). Inhabiting the largest depth range of any teleost species (10 - 2500 m), toothfish are believed to migrate from shallow to deeper waters as they increase in size and age (Peron et al., 2016; Duhamel et al., 2019; Farmer et al., 2019). The toothfish fishery in CCAMLR Area 58.2.1 (France) began in 1992 and has taken an average reported catch of 4814 ± 1351 tons annually since then (Duhamel et al., 2019). Fishing for toothfish in Area 58.2.2 (Australia) began in 1997 and supports an average reported catch of 2813 ± 483 tons. The toothfish fishery currently uses longlines in the deep-sea (500-2000m), but initially was predominantly trawl based, only replaced by longlining in the early 2000s. In the early period of the fishery there was also a substantial illegal and unreported fishing (IUU) on the plateau, with an estimated 13,211 tons taken in 1997, but this declined rapidly with none estimated since 2011.
Mackerel icefish are limited to depths shallower than 500 m associated with sub-Antarctic islands and continental shelves in the Atlantic and Indian sectors of the Southern Ocean between the Polar Front and the Southern Boundary of the Antarctic Circumpolar Current (Maschette and Welsford, 2019). Mackerel icefish were overexploited in the 1970s by a fleet of trawlers fishing simultaneously and the stock was heavily depleted by the late 1970s (Duhamel and Agnew, 1990). Fisheries on the Kerguelen Plateau are now subject to CCAMLR regulation as well as two national Economic Exclusion Zones (EEZs) (France and Australia) (Figure 1). Whilst both EEZs have catch limits for Mackerel Icefish, only the Heard Island fishery (CCAMLR Statistical area 58.5.2) is regularly fished, taking an average 503.76 ± 76.1 tons annually since 1997. This fishery which is managed very precautionarilly, appears to be operating sustainably due to both the steadily increasing biomass estimates and the consistent level of effort needed to fill fishing quotas (Subramaniam et al., 2020).
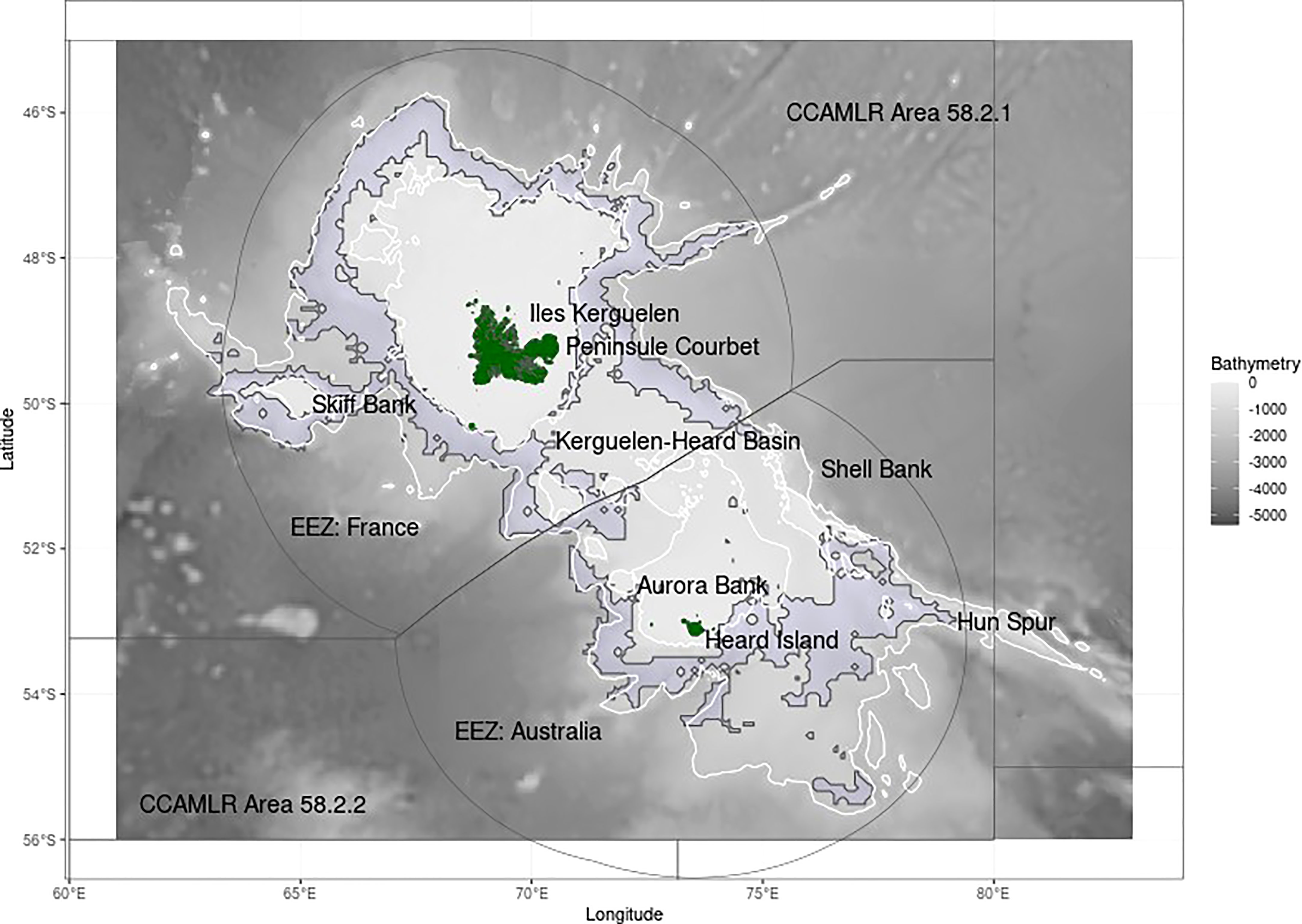
Figure 1 Map of the Kerguelen Plateau, indicating the approximate position of the fishing grounds (blue shaded area after Peron et al. (2016)), and the major geographic features. Also shown are the; -2000m and -500m bathymetric contours (GEBCO 19), the boundaries of CCAMLR managements areas and the 200nm French and Australian Economic Exclusion Zones (EEZs).
There have been a number of documented direct interactions between the fishery and marine mammals and birds in the region including albatross, sperm whales (Physeter macrocephalus), killer whales (Orcinus orca) and southern elephant seals (Mirounga leonina)(van den Hoff et al., 2017; Janc et al., 2018; Corbeau et al., 2021a; Corbeau et al., 2021b; Amelot et al., 2022). However, little is known about the nature and magnitude of the indirect ecological interactions with the major fish predators of the region, such as southern elephant seals.
Southern elephant seals have a circumpolar distribution and predominantly breed on sub-Antarctic islands with four distinct populations being recognized (Slade et al., 1998;Mcmahon et al., 2005a; Corrigan et al., 2016). All of the southern elephant seal populations have undergone considerable changes in the last four decades. Some populations are exhibiting long-term and constant declines, with the Macquarie Island stock declining at a rate of 0.5% per annum (Hindell et al., 2016). In contrast the South Georgia stock has remained stable, while the Kerguelen stock exhibited initial declines followed by a period of stability (Authier et al., 2011) and is now increasing at 2.7% per annum Laborie et al., submitted. Southern elephant seals constitute one of the largest predator biomasses on the Kerguelen Plateau (Subramaniam et al., 2020), and they are known to feed on bentho-pelagic prey, although details of their diet are unclear because seals are sampled while they are on land, which can be a considerable distance and time from their primary foraging grounds (Cherel et al., 2008; Banks et al., 2014). Nonetheless, studies of elephant seal diving behaviour while on the Plateau indicate that a large proportion of their time is spent feeding on the benthos, and that this behaviour is more common in young males than adult females (Hindell et al., 2021). Consequently, there is the potential for elephant seals to interact with the fishery directly and indirectly. For example, there are a small number of direct interactions between southern elephant seals (SES) and the Australian fishery, with 2-3 males being entangled each year (Van Den Hoff et al., 2017).
Identifying indirect ecological interactions between marine predators and fisheries has been difficult and controversial (Trites et al., 1997; Guénette et al., 2006). Seals have been proposed to compete with humans for fish resources in several fisheries, such as the north Atlantic cod and the South African pelagic and demersal fisheries (Yodzis, 2002; Pichegru et al., 2009) While there is ecological modelling that indicates it is possible, albeit complex (Yodzis, 2002; Pichegru et al., 2009; Doll and Jacquemin, 2019). To quantitively demonstrate these interactions requires several lines of evidence (Harwood and Croxall, 1988; Harwood and Stokes, 2003). First, there must be spatial and temporal overlap between the wild predators and the fishery. It must then be demonstrated that the predators are consuming enough of the resource to be a potential competitor for the fishery (or visa-versa). Finally, the predators and the fisheries must be shown to be using the same resource, i.e. the same size and age classes. The inherent difficulties in collecting all three lines of evidence on free ranging marine predators means that this has never been unambiguously demonstrated. A recent review (Jog et al., 2022) delves into the detail of the interactions between marine mammals and the fisheries across the globe, concluding that managing and understanding completely the interactions remains elusive.
We assess the potential for indirect ecological interactions between elephant seals and the Kerguelen Plateau fisheries, defined as one where the seals share a resource with the fishery leading to the potential for competition between the two. This study used a large body of elephant seal tracking data collected over 15 years by the Centre National de la Recherche Scientifique (France) and the Integrated Marine Observing System (Australia) to quantify the degree of spatial overlap between the seals and the fisheries, and then estimate seal consumption of prey within the area used by the fishery satisfying the three key elements to quantify the ecological interactions between seals and the fishery. Specifically, we:
1. Quantify the use of the Kerguelen Plateau by southern elephant seals during the autumn and winter months
2. Describe foraging behaviour in terms of benthic and pelagic diving. Feeding on the benthos is where seals are most likely to interact with the fishery
3. Quantify the degree of overlap between the footprint of the fishery and seal distributions in general and benthic foraging in particular
4. Estimate elephant seal prey consumption rates within and outside the fishing footprint and compare this to the total catch of fisheries to assess the potential for ecological interactions.
Methods
Methodological overview
The overarching objective was to examine the potential for indirect ecological interactions between seals and the Kerguelen Plateau fishery within the footprint of that fishery. The study has three sequential steps: (1) quantifying the spatial use of the Kerguelen Plateau and the associated fishing grounds by elephant seals during their post molt period at sea, (2) developing a spatial representation of seal foraging types (i.e. benthic vs. pelagic) and (3) combining steps 1 and 2 with diet and energetics information to derive spatially explicit estimates of prey consumption for the plateau as a whole and for within the fishing grounds. Each step has varying levels of uncertainty which must be recognized for proper interpretation of the results. We regard step 1 as having the least uncertainty because it is based on well understood satellite tracking technology and statistical modelling, although tracking data are not available for all colonies on the plateau. We regard step 2 as having intermediate uncertainty because it relies on a machine learning algorithm which has a 20% error rate when assigning dive types (see Supplementary Material 1). We rate step 3 as having the most uncertainty as it relies on parameters which are not well known for elephant seals, primarily field metabolic rate and diet composition (see below for more details). Our final prey consumption estimates should therefore be regarded as conservative estimates as we use minimum values for metabolic rate and seal body mass.
Study area
We used GEBCO 19 (GEBCO Compilation Group 2020; 15 arc-second grid resolution) bathymetry to define the Kerguelen Plateau as the region less than -2000 m in depth, and north of -56°S. We used Peron et al. (2016) to identify the principal fishing grounds on the plateau, which were broadly on the slope between -500 and -1500 m. Although the French fishery tends to use the same regions each year, the Australian fishing fleet is more variable in its fishing areas, and has recently expanded to use more of the southern plateau (Farmer et al., 2019).
Tag deployments and diagnostics
We attached conductivity temperature depth satellite relayed data loggers (CTD-SRDLs; Sea Mammal Research Unit, University of St Andrews, UK) to 344 post-molt female and sub-adult male southern elephant seals on the Péninsule Courbet on the east coast of Iles Kerguelen (Figure 1). All deployments were made on post-molt seals that feed at sea between February and November (Hindell et al., 2021). The deployments were done each year between 2004 and 2022 (except in 2006). The capture, handling, sedation and attachment procedures are described in full elsewhere (Mcmahon et al., 2000; Field et al., 2002; Mcmahon et al., 2008; Field et al., 2012). The CTD-SRDLs record dive depth data summarized using a 6-point broken-stick algorithm (Photopoulou et al., 2015) that are transmitted through the ARGOS satellite system (Argos, 2016). The at-sea Doppler-derived locations provided by Argos were filtered using a state-space model in the R package foieGras (Jonsen et al., 2020; Jonsen and Patterson, 2020) after removing locations before the start and end of the foraging trip and also removing seals with fewer than five days of data. We used a timestep of 6 hours in a random walk model with a maximum rate of travel of 4 m/sec. Each resulting track also underwent a visual quality control step and seals with poor model performance (e.g. loops and spikes due to implausible interpolation through data gaps) were removed leaving 338 post-molt seals (182 males and 156 females) for the subsequent analysis.
Step 1. Spatial use of the Kerguelen plateau by southern elephant seals (low uncertainty)
We focused on the post-molt trips of adult females and sub-adult males as these were age and sex classes for which we had sufficient data to reliably characterize their use of the Kerguelen Plateau. The analysis was raster-based, using a grid of 5 x 5 km cells (Harcourt et al., 2021). This grid size was slightly coarser than the location uncertainty of elephant seal positions after state-space modelling (Jonsen et al., 2020). For the same reason we also included a 5 km buffer around the coast to exclude possible locations on land. We then produced sex-specific: (i) stacks of rasters representing the time that each individual seal spent in each 5x5 km cell on the plateau. This was done for the entire trip, including the time seals spent off the plateau to avoid spurious interpolations over long absences, and we then cropped this to just the plateau. (ii) A single raster per sex of the overall mean time spent in each 5 x 5 km cell. (iii) A single raster for each sex of the number of seals that used each cell (density). The product of the density and mean time spent rasters provided the Utilization Distribution of the sample of seals (UDs) expressed as the number of seal days per cell. Finally, we calculated the 50 and 90 percent contours of the UDs.
Representativeness
We estimated the representativeness of the spatial use of the plateau by our sample of seals for each sex compared to the theoretical area used by the entire population of seals after Hindell et al. (2003). This used a bootstrap approach that estimated the total area occupied by an increasing number of seals in the sample. A single seal was drawn at random from the total pool of seals and the area occupied by that seal calculated. A second seal was drawn from the remaining seals and the total area occupied by the two seals calculated (i.e. sampling without replacement). This was repeated until all the seals contributed to the area estimate. This process was repeated 50 times to provide an estimate of the variation around the relationship between sample size and area used. We then fit a Michaelis-Menten form of a power curve to these data to examine if an asymptote was reached (i.e. whether increasing the sample of seals did or did not substantially increase the area of the plateau use by the seals).
Step 2. Spatial representation of seal foraging types (medium uncertainty)
We used the summarized 6-point dive profiles transmitted by the CTD-SLDRs to identify two types of foraging behaviour, benthic (on the ocean floor) and pelagic (in the water column). A simple automated quality control of the dive data was performed by removing dives deeper than 2000m and using a quantile regression to identify the lower edge in the dive depth/duration scatter plot, excluding the 1% of dives falling below the edge. These were dives that required an unrealistic rate of vertical travel to reach the maximum depth, most likely as a result of erroneous depth records. A location was then estimated for each remaining dive, using the state-space model (foieGras package) based on the time of each dive.
Assigning dive types
We developed a boosted regression tree (BRT) machine learning algorithm to assign dive type based on the 6-point dive profile data supplied by the tags (see Supplementary Material 1 for full details). The model was trained on dives from a subset of 14 seals for which high temporal resolution dive data (every 4 s) were available in addition to the low-resolution dive data, using dives visually classified as either benthic or non-benthic independently by two observers (MAH and CRM). Benthic dives were identified primarily by their characteristic flat bottoms and lack of diurnal variation in depth. Seventeen variables (Supplementary Material 1) were derived from the 6-point dive data for all of those visually identified dives where both observers agreed. These variables were all independent of bathymetry to ensure that classifications were not influenced by estimates of ocean depth which are often unreliable in the Southern Ocean (Padman et al., 2010; Ribeiro et al., 2021). We used this model to assign dive types to our data set of 332,244 seal dives made on the Kerguelen Plateau. Finally, we added information on sex, time of day (day, night or twilight), dive depth (m), dive duration (s) and surface time (s), all of which were used to spatially estimate the time the seals spent feeding benthically - see below).
Spatial distribution of dive types
Most tags did not transmit for the full deployment (Figure 2A), so the UDs were re-scaled to provide an estimate of the total time spent per cell over the full winter period. This required an estimate of the total duration of full post-molt trips. These were calculated from the tracking data, using only seals with full trips (i.e. seals that returned to within 5 km of the Kerguelen coastline at the end of the winter). For males, we also excluded trips that finished before September as these could represent the start of mid-year haul-outs (Hindell and Burton, 1988) rather than the end of the overall winter post-molt trip. We then adjusted the individual time spent rasters to account for the time after the tags failed, using the average trip duration for females and males. Using this we re-scaled each UD to represent the number of seal days over the entire post-molt period, assuming that each seal was at sea for the average time, and that its use of the plateau did not change after the tag stopped transmitting.
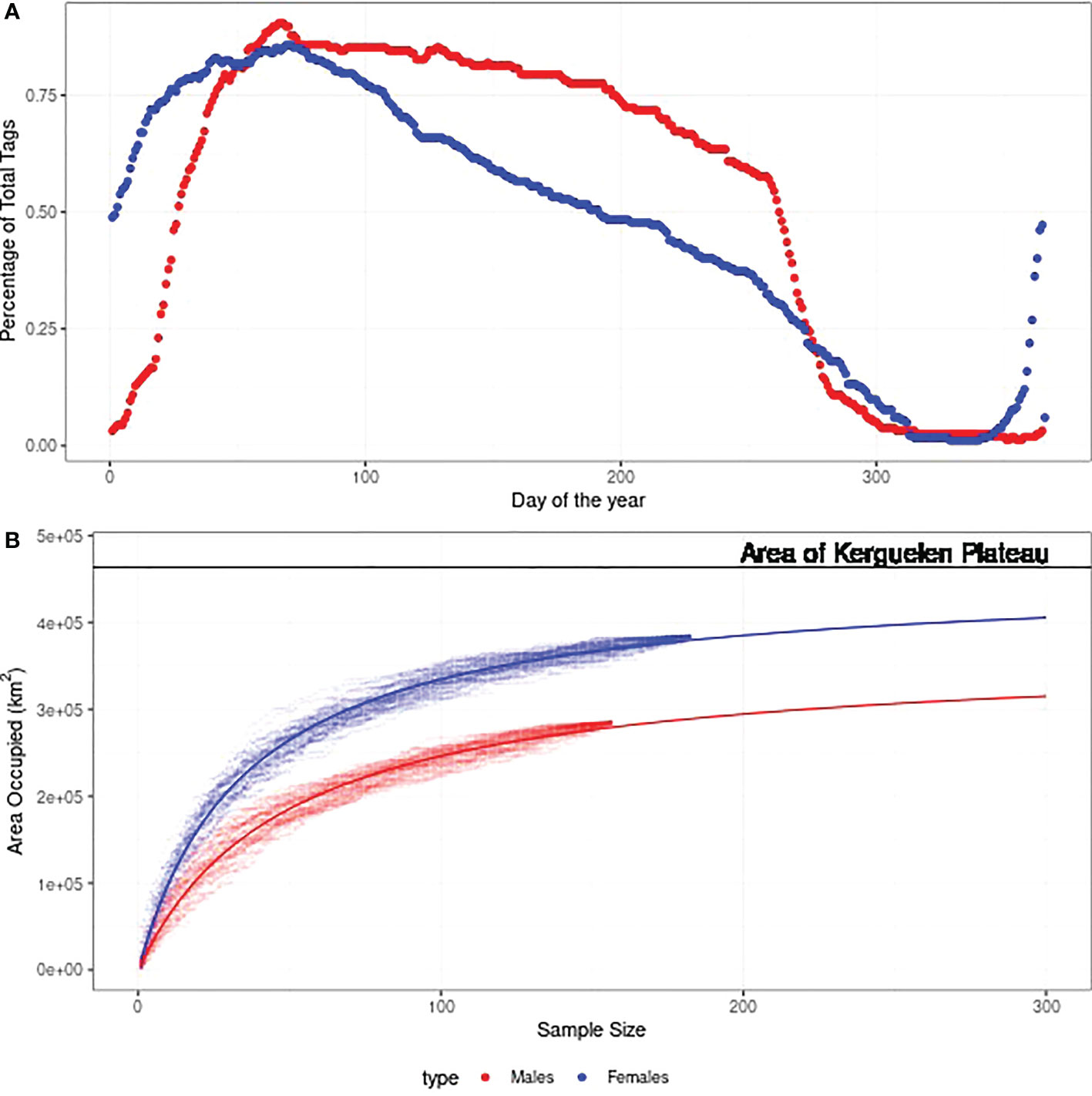
Figure 2 Basic diagnostics of the 338 CTD SLDR tags deployed on the sample of southern elephant seals at Iles Kerguelen. (A) Tag performance in terms of transmission duration, showing the percentage of the total number of tags deployed transmitting each day of the year. (B) Saturation curves to demonstrate how representative the sample of 182 males and 156 female seals were of the overall, unsampled population. The dots represent the total area occupied by increasing samples of seals randomized 50 times. A power curve was fitted to these data using a Michaelis-Menten formulation to demonstrate the theoretical asymptotic area. Also shown as a horizontal black line the total area of the Kerguelen Plateau, 462,975 km2 (defined as shallower than -2000m and excluding >0m).
We then used these re-scaled UD datasets, combined with the dive type datasets, to make rasters of the time spent making benthic dives per cell. Raster stacks of the percentage of total time in a cell spent on benthic dives were multiplied by the overall time spent to estimate the total number of days making benthic dives. These rasters were used in the subsequent overlap analysis and in our prey consumption estimates.
Step 3. Spatially explicit estimates of population level prey consumption
Our estimates of population level prey consumption required; (i) the estimated energy expenditure (field metabolic rate) of individual seals; (ii) estimates of the diet composition; (iii) estimates of the energy content of prey and (iv) estimates of the number of seals in each sex and age class. These data were combined with the individual UDs (total seal days over the winter per 5x5 km pixel) and information on body size to provide estimates of total prey consumed in each pixel over the winter months by the full population of seals in each age and sex class.
Energy expenditure
An initial requirement when estimating the amount of prey consumed by an individual is some knowledge of its metabolic rates, and here we used the best empirically derived estimate of field metabolic rate of 106.5 kJ kg-1 day-1 for northern elephant seals (Maresh et al., 2014).
Diet
Southern elephant seal diets, despite much research (Green and Burton, 1993; Slip, 1995; Bradshaw et al., 2004; Cherel et al., 2008; Authier et al., 2012; Banks et al., 2014; Walters et al., 2014), remain difficult to describe in detail. Male elephant seals on the Kerguelen Plateau use benthic habitats more than females (Hindell et al., 2021), and this is likely to be reflected in their diets. However, despite multiple sampling methods (stomach lavage, isotope ratios and fatty acid analyses), no clear consensus exists of the specific diets of elephant seals of either sex. For example, stomach content analyses show the presence of both squid and fish (including D. eleginoides and C. gunneri) in the diet (Green and Burton, 1993; Slip, 1995), while stable isotopes suggest a preponderance of myctophids (Electrona spp.)in the diet of females (Cherel et al., 2008).
Energy content of prey
We used published information to estimate the mean energy content (Scientific Committee on Antarctic Research (2021) Southern Ocean Diet and Energetics Database. doi:10.5281/zenodo.5708934) of the species most likely eaten by elephant seals on the Plateau (Croxall and Prince, 1982; Schaafsma et al., 2018). Energy content of potential prey vary considerably, with Ice fish and most notothenids, having relatively low energy density similar to that of some squids, while toothfish and myctophids have relatively high energy contents (Schaafsma et al., 2018). To account for the uncertainty in diet composition, and variability in prey energy content, we have taken a broad approach in our analysis by using three diet composition scenarios for both sexes: (i) 75% high energy prey (toothfish, myctophids and some squid; mean energy content of 9.100 kJ kg-1 wet weight) and 25%to low energy prey diet (some squid and icefish; mean energy content of 3.470 kJ kg-1 wet weight), (ii) a diet of equal amounts of high energy prey (50%) and low energy prey (50%) and (iii) a 25% high energy prey and 75% low energy prey diet, to estimate prey consumption on the Kerguelen Plateau. We finally incorporated an estimate of the prey energy assimilation rates. We used an assimilation rate of 90% which was similar to observed ranges, 91% - 94%, and rates observed in other seals (Prime and Hammond, 1987; Fisher et al., 1989; Krockenberger and Bryden, 1993) but higher than that (75%) used by Hindell et al. (2003).
Population estimates
Life tables are an effective way of summarizing birth and death rates for seals at different stages of their lives, and of allocating the number of individuals to specific age classes (Caughley, 1977). There is no life table available for elephant seals from Iles Kerguelen, so we constructed one extending to age 15 years as in Boyd et al. (1994)) for males and for female elephant seals to age 23-years from previously published age-specific survival estimates from Macquarie Island (Mcmahon et al., 2005b; Goedegebuure et al., 2018), South Georgia and Marion Island (Supplementary Material 2). The life tables summarized the annual survivorship (lx) of seals and provided an estimate of the age-specific standing proportion of seals in the total population of 340 000 seals at Iles Kerguelen Laborie et al., submitted. We used these proportions of seals in each age class in our estimates of age-specific and mass-specific prey consumption rates.
Prey consumption model
We used a bootstrap approach to estimate total prey consumption for each age and sex class for which we had tracking data. We; (i) randomly selected an individual UD from our total sample of individual UDs; (ii) randomly selected a body mass from the sample of body masses for that age and sex class; (iii) used the BMR equation to estimate daily energy requirement for that seal in each pixel (based on the seal’s mass and the number of days spent in the pixel over the post-molt period); (iv) corrected this by the energy assimilation rate to give total energy consumption per pixel; (v) estimated how much of this total energy came from each prey type (high or low energy content) (total energy consumed * proportion of prey type in the diet); (vi) estimated the mass of each prey type by dividing this by the energy content of each prey type; (vii) combined the mass consumed of each prey type to give total prey consumption (kg) for that seal in each pixel; (viii) the values from each pixel were combined to give the overall prey consumption and the fish only consumption for that seal for the time that it spent (i) on the entire plateau and (ii) within the fishing grounds. This was repeated 1000 times for each age and sex class and for each of the three diet compositions to allow a range of possible prey consumptions to be estimated. A key assumption of this approach is that our sample of seals is a reasonable representation of the entire population, which is supported by the representativeness analysis.
For each age and sex class we derived four groups of prey consumption estimates: (i) for all foraging on the entire Kerguelen Plateau; (ii) for all foraging within the fishing grounds; (iii) for foraging on the benthos for the entire Kerguelen Plateau; and (iv) for foraging on the benthos within the fishing grounds.
Results
Tag diagnostics
Overall, the tags transmitted for an average of 183.5± 85.3 days (mean ± SD, maximum=351.5 days). Tags on females transmitted for an average of 193.8 ± 77.4 days, which was 70.1% of the mean duration of a full post-molt trip for an adult female (274.3 ± 35.11 days, n=25 seals). Tags on males transmitted for 174.6 ± 91.8 days, or 61.0% of the mean duration of a full post-molt trip for a sub-adult male (286.1± 18.5 days, n=12 seals). Between day 68 and 250 (day 250 is the approximate start date of the female breeding season), the proportion of male tags transmitting decreased from 82% to 35% (overall decrease of 47%), whereas over the same period the proportion of female tags transmitting dropped from 88% to 61% (overall decrease of 27%) (Figure 2A).
The representativeness analysis approached an asymptote indicating that our sample of seals utilized most of the space that the full population of seals from the sampled colonies might be expected to use (Figure 2B). Increasing the sample of seals tracked initially resulted in a rapid increase in the total area occupied, but with a much less rapid increase beyond 100 seals tracked. Our final sample of 182 males occupied 379,266km2. Doubling the sample to 364 seals would have increased this to 413,056 km2, only an 8.9% change. The females, with a slightly smaller sample, were further from an asymptote, but nonetheless our sample of 156 female seals occupied 279,038 km2 and doubling the number of seals to 312 would increase this to 316,742km2, a change of only 13.5%. Thus, while it is unlikely that the seals used every part of the plateau with the area used by a theoretical sample of 300 seals not reaching the total area of the plateau (Figure 2B), we can nevertheless be confident that our sample seals provide a reasonable indication of the total area of the Kerguelen Plateau that is used by adult female and sub-adult male elephant seals from the Péninsule Courbet.
General distribution
Our sample of seals dispersed widely throughout the Southern Ocean, ranging from -9.94° to 149.70° West and -36.95° to -70.04° South. Overall, 24.1% of all 6 hourly at-sea locations from the State Space Models (n=243,588) were on the Kerguelen Plateau. Each individual seal spent some time on the Kerguelen Plateau, although many simply transited across it as they moved to more distant foraging grounds (Figures 3A, C). We defined a seal as a plateau specialist if more than 50% of its total locations were on the plateau. For post-molt females, only 10.5% were plateau specialists, compared to 33.1% of post-molt males. Seals of both sexes from the Péninsule Courbet had most of their plateau locations east of 70° E. The region north and west of lles Kerguelen was rarely used, especially the shallow waters less than 500m in depth. The regions of the plateau with highest use (represented as the number of seal days per 5x5 km pixel) were in the relatively deep water (-500 to -2000m) of the Kerguelen-Heard Basin, and to a lesser extent the Shell Bank and Williams Ridge (Figures 3B, D).
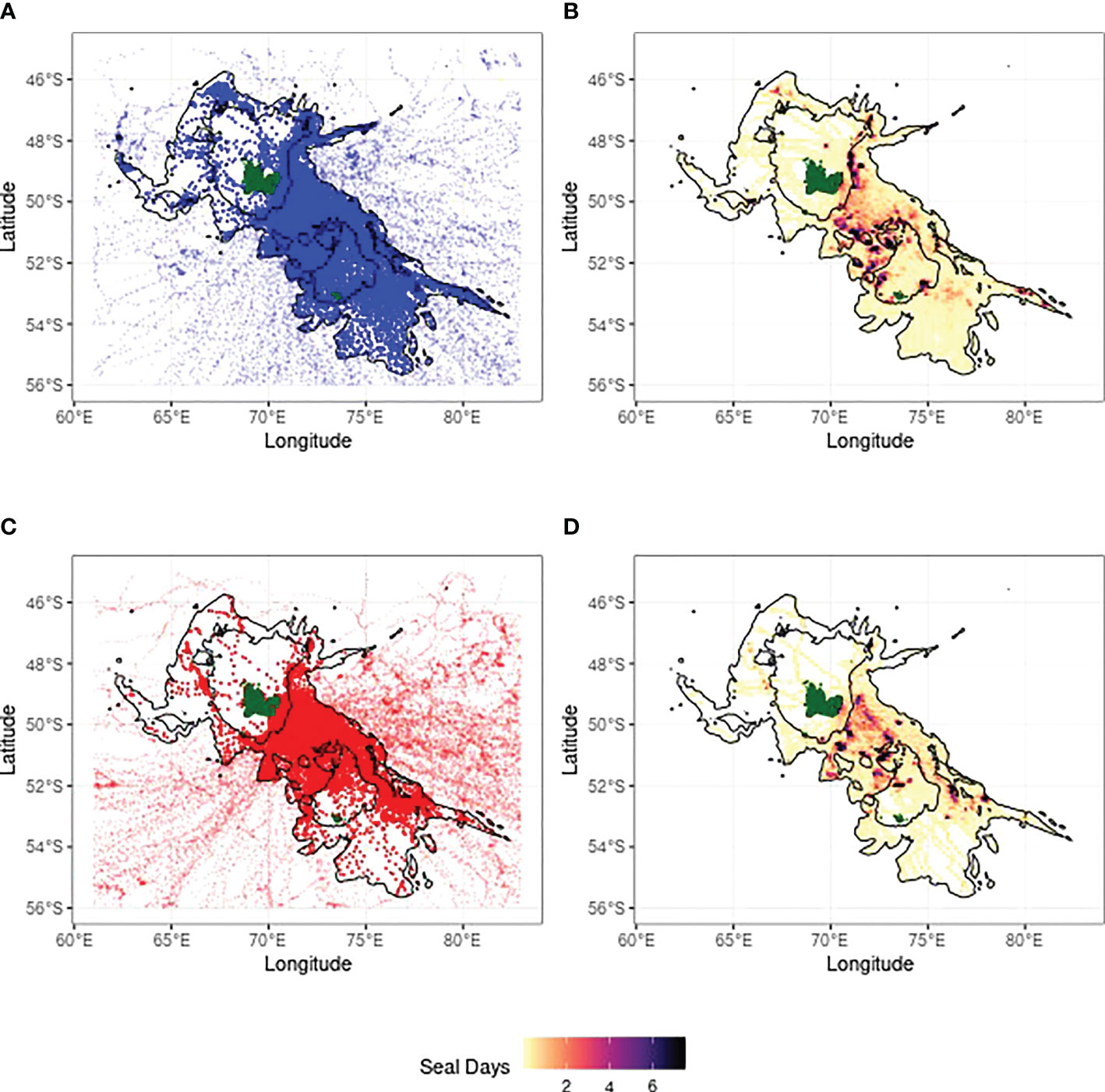
Figure 3 Distribution of male (blue, n=182) and female (red, n=156) post-molt elephant seals on the Kerguelen Plateau. (A, C) show those 6 hourly, state-space model-estimated locations that occurred within the overall study domain. (B, D) depict the utilization distribution, presented as seal days per 5x5 km cell, restricted to waters shallower than -2000m. The contours represent the -500 and -2000 m bathymetry.
Differences between male and female utilization distributions
Broadly speaking, the sub-adult males and adult females used similar areas of the Kerguelen Plateau during their post-molt trips to sea. The 90% utilization distribution of the sample of seals (UDs) of both sexes were concentrated in the Kerguelen-Heard Basin (Figure 3), and in the deeper waters to its south. Males used the area south of the 500m contour to the south of Heard Island more than the females, and females used the deep water to the west of Shell Bank area more than males. The sexes had considerable overlap in their 90% UDs (Figure 4): the region common to both sexes occupied 58% of the total male 90% UDs, compared to 71% of the female 90% UDs.
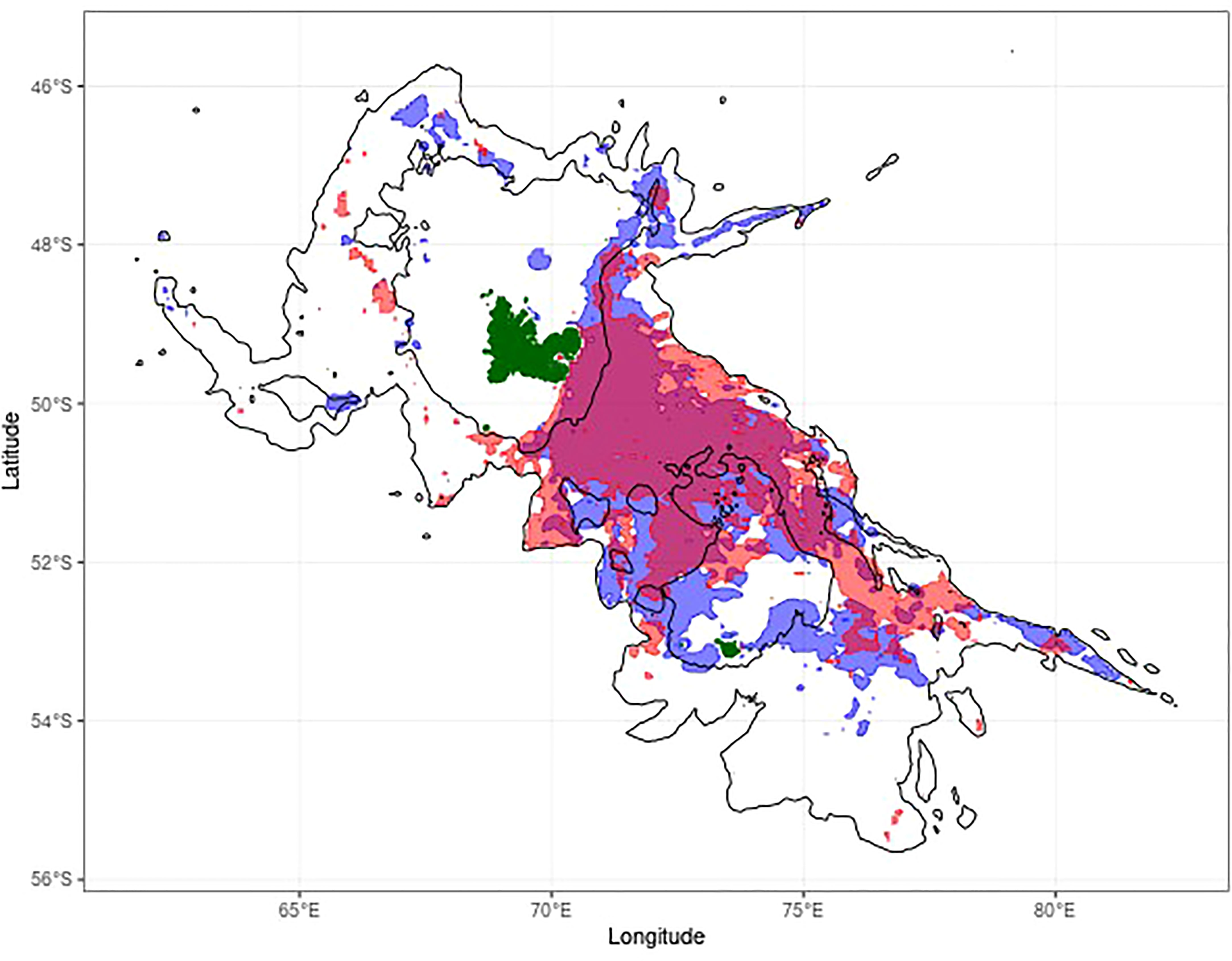
Figure 4 Comparison of 90% utilization distributions of post-molt males (blue) and female (red) elephant seals tagged at Iles Kerguelen. The contours represent the -500 and -2000 m bathymetry.
Diving behaviour: Benthic and pelagic dives
The boosted regression tree performed well when classifying dives as either benthic or pelagic (Supplementary Material 1). The final BRT model (based on all 15 seals with high resolution data) had a training data correlation of 0.943 (cross-validation correlation = 0.853, s.e. = 0.006), and a training data AUC score of 0.995 (cross-validation AUC score = 0.968, s.e. = 0.002). The most influential variable was ROC3 (the rate of vertical displacement in segment 3 of the profile) with a relative contribution of 27.8% (Supplementary Material 1), followed by ROC2 (17.0%), and pdep (the depth relative to the maximum dive depth that day) (10.1%). All other variables contributed less than 10%. Lower rates of depth change within a dive segment were associated with benthic dives which also spent longer within 20% of the maximum daily depth. The external 5-fold cross validation correctly identified benthic dives 81% of the time and pelagic dives 82% of the time (Supplementary Material 1).
Using the BRT model to assign dive types across the full data set of 332,244 dives made on the Kerguelen Plateau, resulted in 35.0 ± 16.2% of the dives being assigned as benthic (Table 1). This proportion varied between the sexes: females made more pelagic dives than males (70.7 vs. 60.1%), and therefore fewer benthic dives than males (29.3 vs. 39.9%) (Table 1).
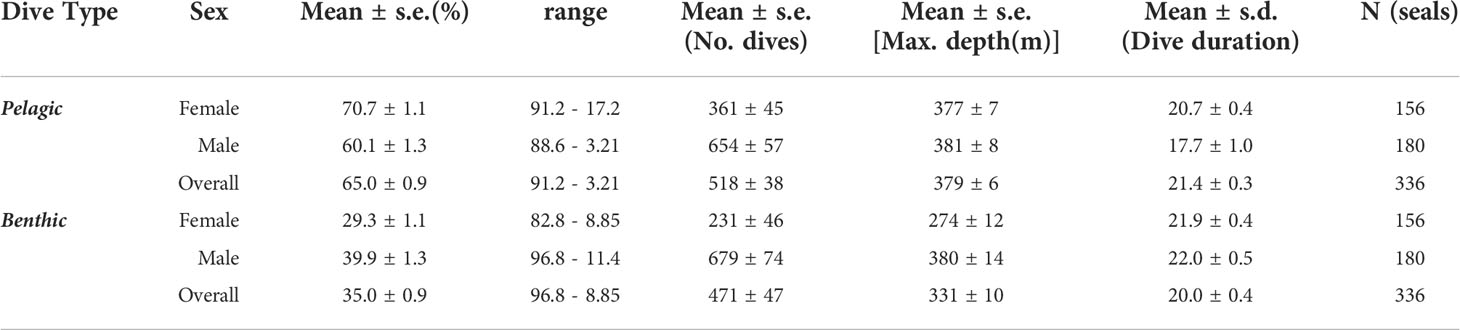
Table 1 Summary statistics of two types of foraging behaviour (benthic and pelagic) performed by adult female and sub-adult males on the Kerguelen Plateau.
The probability of making a benthic dive on the Kerguelen Plateau was influenced by bathymetry, sex and time of day. The best GLMM, including individual seal as a random term, exploring these effects include all three terms and their interactions and had a conditional R2 (Nakagawa et al., 2013) of 0.21 (Supplementary Material 3). Although having a relatively low R-squared, this model still performs better that any of the alternatives, indicating that each of its terms are having an effect on the likelihood of making a benthic dive. Overall, the probability of a benthic dive decreased with increasing ocean depth, and males were more likely to make benthic dives than females (Figure 5). Time of day was another strong effect, with benthic dives being more likely during the day. This relationship also differed between the sexes, with females much less likely to make benthic dives than males at night.
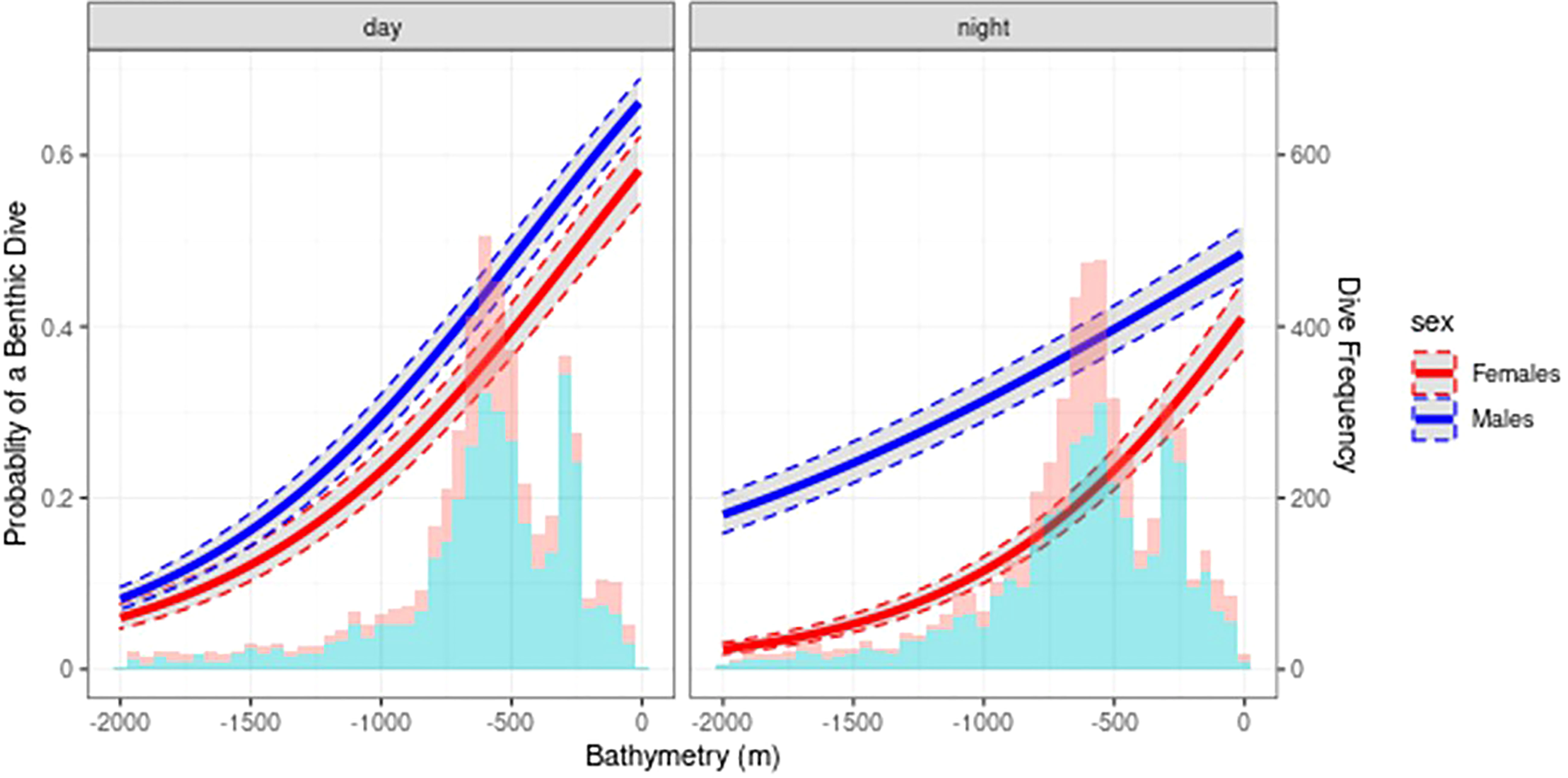
Figure 5 The probability of an elephant seal performing a benthic dive on the Kerguelen Plateau during their post-molt time at sea, with respect to bathymetry, time of day and sex. The solid lines represent the predicted values from a GLMM (dive Type~sex*bathymetry*time of day, with seal ID as a random term), and the dotted lines the 95% confidence limit of those predictions. The histograms in the background indicate the frequency distribution of bathymetric values used in the analysis.
The distribution of benthic foraging broadly reflected the overall distribution of the seals (Figure 3B, 6A, C). For females, benthic foraging was concentrated on the Heard-Kerguelen Basin. For males benthic foraging was a little more widespread, occurring in the Heard-Kerguelen Basin, but also occurring in the relatively shallow (> -500 m) surrounding Iles Kerguelen, and in deeper waters at the southern edge of the plateau. The highest concentration of benthic foraging for males was associated within the -500m bathymetric contour to the south of Iles Kerguelen.
Spatial overlap of elephant seals and fishing grounds on the Kerguelen plateau
Overall, most of the seals spent some time in the fishing grounds described in Peron et al. (2016), with only one male never visiting them. The use of fishing grounds was similar between the sexes: females spent on average 31.5 ± 18.7% of their time on the plateau within the fishing grounds, compared to males which spent 29.9 ± 20.2% of their time on the plateau there (Figures 6B, D). Not all seals exhibited benthic foraging behaviour within the fishing grounds, with eight females and seven males making no benthic dives there. Only 25.1 ± 22.3% of all females benthic foraging time occurred in the fishing grounds similar to 24.5 ± 24.0% of male benthic foraging time.
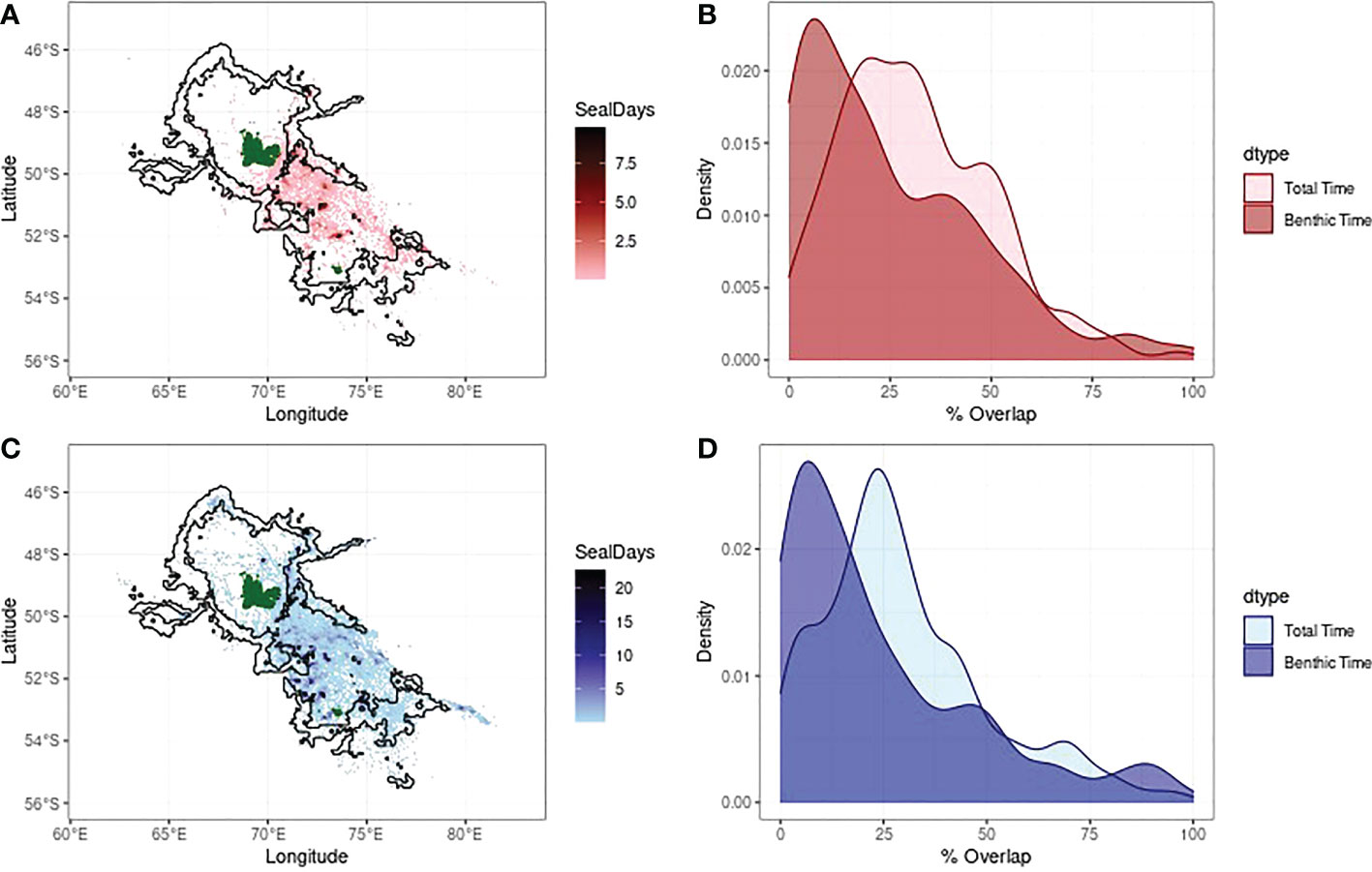
Figure 6 Degree of overlap between female and male elephant seals and commercial fishing on the Kerguelen Plateau. (A) Females: the number of seal days spent on benthic diving on the Kerguelen Plateau. Black contours indicate the commercial fishing grounds described in Peron et al., 2016). (B) Females: The frequency distribution (expressed as a kernel density) of the percentage of total time that individual seals spent in the commercial fishing grounds (% overlap), presented for both the total time diving and for benthic dives alone. (C, D) show the same information for males.
Estimates of total prey and fish consumption
A summary of elephant seal prey consumption estimates are presented in Supplementary Materials 4, 5, showing the maximum and minimum estimates for each sex based on the bootstrap analysis incorporating individual tracks, body size and a range of dietary energy compositions. Sub-adult males consumed comparable levels of total prey (17,321 – 31,108 tons) to adult females (19,493 – 34,899 tons) despite their greater use of the plateau, which is offset by the greater number of adult females in the population.
When just the high energy content prey component of the diet is considered (which includes toothfish), this reduces to 6,814 – 14,848 and 7,085 – 18,037 tons for males and females respectively (Figure 7). Over the same period of time the Kerguelen Plateau fishery, combining all target species and both reported and estimated IUU, has taken on average 8561 ± 334 tons per year (based on 17 years of catch data from 2004 to 2021) for CCAMLR Areas 85.2.1 and 85.2.2 (CCAMLR Secretariat, 2022b; CCAMLR Secretariat, 2022a). The high energy content prey consumption on the entire plateau for the males was 79.6 - 173.4% of the mean annual total catch (i.e. 2.0 to 3.6 times greater) by the Kerguelen fishery (CCAMLR Secretariat, 2022b; CCAMLR Secretariat, 2022a), compared to 82.8 - 210.7% for adult females. However, when considering high energy content prey only consumption within the fishing grounds these estimates decreased to 23.2 - 55.8% of the fishery’s total annual catch for males and 22.8 - 68.4% for females (Supplementary Materials 4).
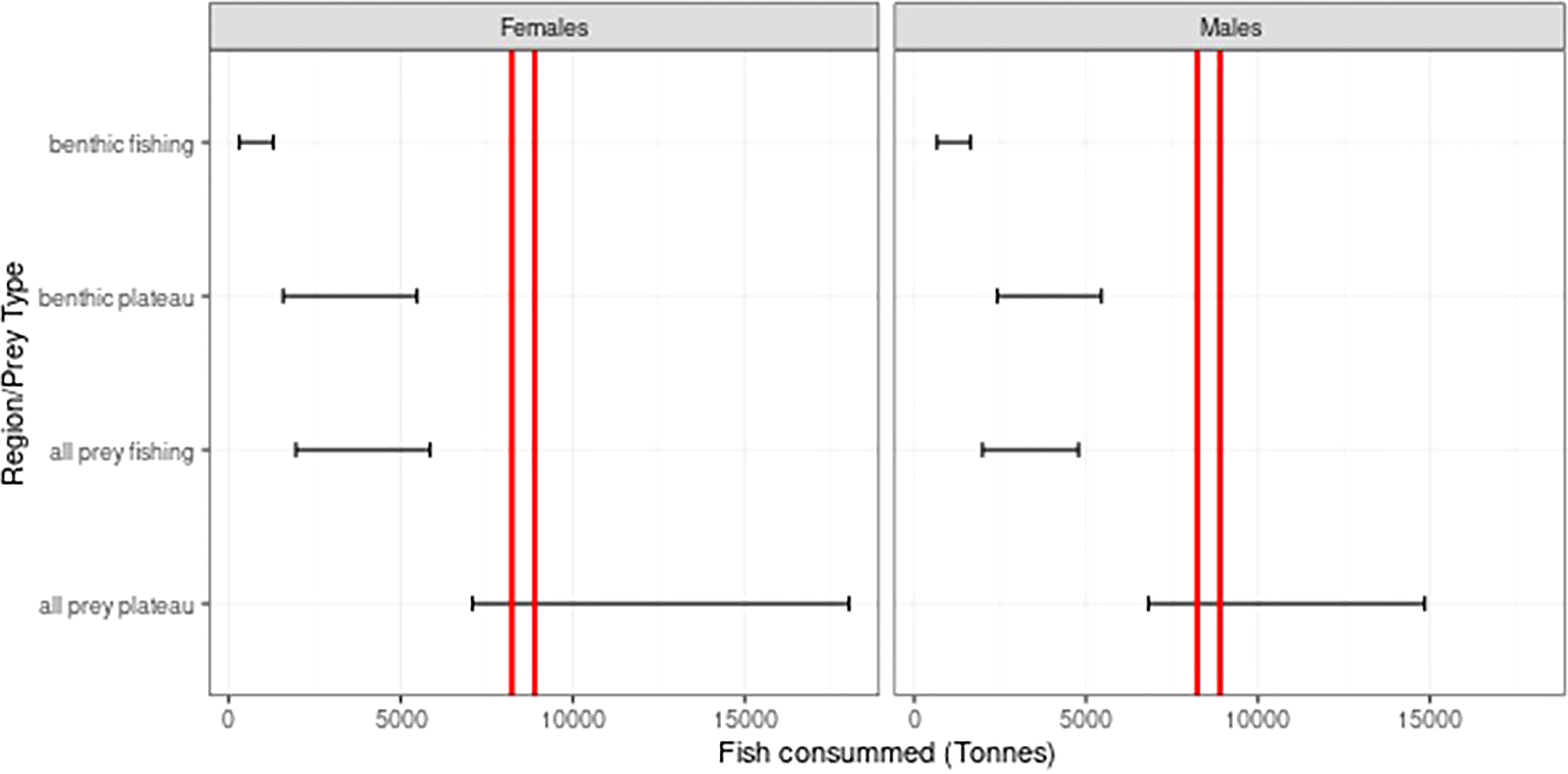
Figure 7 Estimates of fish (high energy content prey) consumption (tons) by southern elephant seals on the Kerguelen Plateau during their post-molt time at sea. Consumption estimates are separated regionally and by foraging the habitat identified from the seals dive behaviour (all benthos and water column plus benthos): Benthic prey within the fishing grounds (Peron et al., 2016) (Benthic Fishing), benthic prey for the entire Plateau (Benthic Plateau), All prey types (benthic and epi-pelagic) within the fishing grounds (All Prey Fishing) and All prey types for the entire Plateau (All Prey Plateau) Estimates are reported for adult females and three age classes of sub-adult males combined. Seal consumptions are presented as the maximum and minimum estimates for that sex/age class based on bootstrap analysis incorporating individual tracks, body size and a range of diet mixes (25% high energy content prey:75% low energy content prey, 50% high energy content prey:50% low energy content prey and 75% high energy content prey:25% low energy content prey) which are presented in more detail in Supplementary Materials 4, 5). The vertical red lines represent the upper and lower 95% CI of reported total catch rates (icefish, toothfish and IUU) on the plateau (8561 ± 334) tons) based on 17 years of catch data from 2004 to 2021 for CCAMLR Areas 85.2.1 and 85.2.2 (CCAMLR Secretariat, 2022b; CCAMLR Secretariat, 2022a).
Post-molt adult males consume between 5815 – 11690 tons of prey from the benthos on the Kerguelen plateau each year, compared to between 5222 – 9005 tons for females. Of this, males take between 1636 – 3531 tons from benthos on the fishing grounds, with 1093 – 1985 tons for the females, i.e. 7.8 - 19.1% of the mean annual high energy prey catch for males and 3.6 - 15.1% for females.
Discussion
Of the three necessary lines of evidence to demonstrate indirect ecological interactions between elephant seals and the Kerguelen fishery we could: (i) confidently assess the degree of spatial overlap between the predators and the fishery, (ii) broadly estimate seal prey consumption in the fishing grounds and compare these to the take by the fishery, but we were unable to demonstrate convincingly (iii) that the seals and the fishery were targeting the same species due to the lack of detailed seal diet information. However, combining the unique life history and highly polygynous nature of this species (see below), and given there is no evidence of a measurable effect on either the seal’s population growth rates (in fact the population is increasing), or changes in the catch per unit effort of the fishery, there is enough information to infer that indirect ecological interactions are not of sufficient magnitude to affect either the seal population or the fishery.
The Kerguelen Plateau is an important foraging habitat for southern elephant seals, particularly for sub-adult males, 1/3 of which spend most of their time there. Adult females also use the plateau but to a lesser extent, with the majority of the individuals using deep oceanic waters (Hindell et al., 2021). When on the plateau, the seals have the potential for direct and indirect (ecological) interactions with the Kerguelen fishery (Van Den Hoff et al., 2017; Subramaniam et al., 2020), because they spend 30% of that time on the main fishing grounds where they take prey from both benthic and epi-pelagic habitats. This goes some way to indicating the potential for ecological interactions within the fishing grounds, where the commercial catches are 2-3 times the estimated weight of prey taken by the seals. At least some of these prey are likely to be commercially harvested species (Green and Burton, 1993; Slip, 1995) caught on the benthos. The extent of the interactions depends on the age and sex class of the seals, their foraging behaviour and diet.
Our sample of 344 seals is sufficiently large to provide a reliable description of how elephant seals from the Péninsule Courbet use the Kerguelen Plateau. However, uncertainty remains about the at-sea distribution for elephant seals from colonies on the island which were not studied, and which constitute about 33% of the Kerguelen population Laborie et al., submitted. Our sample also doesn’t include seals from nearby Heard Island with a population of 61 933 in the 1990s (Slip and Burton, 1999), which is 28% of the total Kerguelen Plateau elephant seal population. Our estimates of spatial overlap and prey consumption rely on the assumption that these other colonies have similar patterns of habitat use, but there are few data with which to test this. One study of post-breeding female seals from the north of the island indicated that they used the area to the northwest, the region least used by our sample of seals (Godard et al., 2020). However, post-breeding seals have distinctly different foraging ranges to post-molt seals (Hindell et al., 2021) dispersing over much shorter distances, so it is difficult to know if this difference persists over the winter. The northwest region of the plateau that is least used by the seals is an area of active fishing by the French fleet, but there are no reported instances of elephant seal by-catch there (Van Den Hoff et al., 2017), which supports our observation that although some seals use this area their density is likely to be low and it is unlikely that there would be appreciable levels seal-fishery interactions.
Our descriptions of spatial use, foraging behaviour and prey consumption only relate to those components of the elephant seal population for which there are good tracking data; adult females and sub-adult males. Therefore, our estimates of total seal days and prey consumption in the fishing grounds are minimum estimates. The two major groups missing from our analyses are juveniles (sub-yearling, one- and two-year old seals) and adult males (6- to 15-year-old seals). Juvenile seals constitute approximately 51% of the total population, and adult males 24% i.e. 75% of the total population (Supplementary Material 1). However, the lack of data on the diet and at-sea distribution of these components of the population makes it impossible to assess their prey consumption on the plateau.
Our study is characterized by having increasing levels of uncertainty associated with each of the lines of evidence for ecological interactions. Our estimates of prey consumption rely on knowing the at-sea energy requirements of the seals, and this has been a considerable challenge for most species of marine mammal (Ponganis et al., 1990), where long periods of time away from land preclude the use of techniques such as isotope turnover to estimate this. Further, deep diving marine mammals such as elephant seals likely employ some degree of hypo-metabolism while diving (part of the dive response (Kooyman, 1976; Kooyman et al., 1983; Kooyman et al., 1992), whereby metabolic activity in some tissues is reduced to conserve oxygen and prolong dive duration (Hindell et al., 1991). Therefore, relying on simple allometric relationships of body size and metabolism based on other species are likely to overestimate metabolic rates. Indeed, the field metabolic rate of 106.5 kJ kg-1 day-1 for northern elephant seals (Maresh et al., 2014) derived from a translocation experiment (Andrews et al., 1997; Andrews et al., 2000) is close to the resting metabolic rate for an animal of equivalent size (Maresh et al., 2014), consistent with hypo-metabolism while diving. A second and likely more serious source of uncertainty is the diet of the seals. Again, the long periods at sea make it difficult to quantitatively determine the degree to which the seals are eating commercial target species (potential for direct competition) or not (more complex food-web interactions). The diet information for elephant seals on the Kerguelen Plateau are either derived from small samples of stomach contents from animals ashore at Heard Is (Green and Burton, 1993; Slip, 1995), which are typically highly digested and likely under-represent some species (Deagle and Tollit, 2007), or based on stable isotope ratios (Cherel et al., 2008) fixed in tissues such as blood and whiskers, which have indeterminate timelines and coarse taxonomic resolution.
Despite these uncertainties, our study suggests that there is potential for competition within the fishing grounds, but less so on the plateau overall. However, the nature of the interaction in the fishing ground is unclear and is likely to remain so until a more comprehensive understanding of elephant seal diet is available. Nonetheless, it is likely that toothfish are a minor component of their diet in the fishing grounds because toothfish are not a commonly reported prey item overall (Slip, 1995) and the bottom waters deeper than 500 m tend to be home to larger and older fish (Farmer et al., 2019), which may be too hard for the seals to catch (but similar sized Weddell seals, Leptonychotes weddellii, do capture some large fish Harcourt et al. (2021)). Ice fish are also patchily distributed throughout the fishing grounds and are not targeted in the French EEZ). Combined with the decreasing likelihood of using benthic habitats in deeper waters, the seals may be getting most of their prey in the fishing grounds from the epipelagic zone targeting myctophids and squid (Cherel et al., 2008). Benthic prey such as skates and nototheniids are also rarely reported in the seal diets and along with the low incidences of their presence in the fishery as bycatch suggests that this is not a point of conflict. Rather than competing for prey, the interactions between the seals and the fishery if they occur may be more complex with multiple intermediate steps involving other potential competitors (Yodzis, 2001).
In conclusion, we found little to suggest that the level of competition between the Kerguelen fisheries and the elephant seal populations is sufficient to pose a substantial threat to those populations There are three lines of evidence for this: (1) The population of elephant seals at Iles Kerguelen has been increasing by 2.7% per annum over the last two decades Laborie et al., submitted. (2) Females feed primarily in oceanic waters and only briefly transit across the fishing grounds in January/February and again September/October on the way to and from their distant foraging grounds. Consequently, the female seals are unlikely to be relying on these plateau areas to fuel their reproductive efforts. Individual females also have few opportunities for competition or direct negative interactions such as being caught on the fishing lines given the short periods they spend in proximity to the fisheries. (3) Some sub-adult males do spend longer periods feeding on the fishing grounds and therefore have increased risks of competition and likelihood of being caught on fishing lines evidenced by male seals entangled in fishing lines (Van Den Hoff et al., 2017). Nonetheless, it is unlikely that these interactions affect population growth rates because sub-adults do not play an active role in breeding. Also, only a small subset of these animals will become adult and even fewer (4% of pups born) will become beachmasters and breed and so most young males don’t contribute to population growth. Given only a small number of males hold harems some mortality due to fisheries interactions in this age group is unlikely to have any appreciable effects at the population level as many of these young males will succumb to natural mortality (Mccann, 1980; Mccann, 1985) and the polygynous mating system means that only a relatively small number of males are required for breeding.
Data availability statement
The datasets presented in this study can be found in online repositories. The names of the repository/repositories and accession number(s) can be found below: The Australian Ocean Data Network (AODN), https://portal.aodn.org.au/.
Ethics statement
The animal study was reviewed and approved by University of Tasmania Animal Ethics Committee (A12141, A14523) The Comité d’éthique Anses/ENVA/UPEC (no. APAFiS: 21375) Macquarie University Ethics Committee ARA 2014_057.
Author contributions
Fieldwork was coordinated by CG and CM. Analysis was conducted by MH, CM, and BR and the manuscript was written by all the authors. All authors contributed to the article and approved the submitted version.
Funding
This research was funded by the Australian Research Council Discovery Project DP180101667. The fieldwork to deploy the instruments on elephant seals was financially and logistically supported by the Institut Paul Emile Victor (IPEV, France) under the Antarctic research program 109 (H. Weimerskirch), 1201 (C. Gilbert and CG) and 1182 (J.B. Charrassin) in collaboration with the Integrated Marine Observing System (IMOS, Australia; https://imos.org.au/) and the Program Terre-Océan-Surface Continentale-Atmosphère from the Centre National d’Etudes Spatiales (TOSCA-CNES, France). IMOS is a national collaborative research infrastructure, supported by the Australian Government. It is operated by a consortium of institutions with the University of Tasmania as lead agent.
Conflict of interest
The authors declare that the research was conducted in the absence of any commercial or financial relationships that could be construed as a potential conflict of interest.
Publisher’s note
All claims expressed in this article are solely those of the authors and do not necessarily represent those of their affiliated organizations, or those of the publisher, the editors and the reviewers. Any product that may be evaluated in this article, or claim that may be made by its manufacturer, is not guaranteed or endorsed by the publisher.
Supplementary material
The Supplementary Material for this article can be found online at: https://www.frontiersin.org/articles/10.3389/fmars.2022.1006120/full#supplementary-material
References
Amelot M., Plard F., Guinet C., Arnould J. P. Y., Gasco N., Tixier P. (2022). Increasing numbers of killer whale individuals use fisheries as feeding opportunities within subantarctic populations. Biol. Lett. 18, 20210328. doi: 10.1098/rsbl.2021.0328
Andrews R. D., Costa D. P., Le Boeuf B. J., Jones D. R. (2000). Breathing frequencies of northern elephant seals at sea and on land revealed by heart rate spectral analysis. Respiration Physiol. 123, 71–85. doi: 10.1016/S0034-5687(00)00168-7
Andrews R. D., Jones D. R., Williams J. D., Thorson P. H., Oliver G. W., Costa D. P., et al. (1997). Heart rates of northern elephant seals diving at sea and resting on the beach. J. Exp. Biol. 200, 2083–2095. doi: 10.1242/jeb.200.15.2083
Argos (2016). Argos User’s manual: Worldwide tracking and environmental monitoring by satellite (Toulouse, France: CLS).
Authier M., Delord K., Guinet C. (2011). Population trends of female elephant seals breeding on the Courbet Peninsula, Iles Kerguelen. Polar Biol. 34, 319–328. doi: 10.1007/s00300-010-0881-1
Authier M., Dragon A.-C., Richard P., Cherel Y., Guinet C. (2012). O’ mother where wert thou? maternal strategies in the southern elephant seal: a stable isotope investigation. Proc. R. Soc. B-Biological Sci 2681–2690. doi: 10.1098/rspb.2012.01991471-2954
Banks J. C., Lea M.-A., Mcmahon C. R., Hindell M. A. (2014). Combining bio-logging and fatty acid signature analysis indicates spatio-temporal variation in the diet of the southern elephant seal, Mirounga leonina. J. Exp. Mar. Biol. Ecol. 450, 79–90. doi: 10.1016/j.jembe.2013.10.024
Boyd I. L., Arnbom T., Fedak M. A. (1994). “Biomass and energy consumption of the South Georgia population of southern elephant seals,” in Elephant seals: population ecology, behaviour, and physiology. Eds. Boeuf B. J. Le, Laws R. M. (Berkeley: University of California Press), 98–117.
Bradshaw C. J. A., Hindell M. A., Sumner M. D., Michael K. J. (2004). Loyalty pays: potential life history consequences of fidelity to marine foraging regions by southern elephant seals. Anim. Behav. 68, 1349–1360. doi: 10.1016/j.anbehav.2003.12.013
Ccamlr Secretariat (2022a). Fishery report 2021: Dissostichus eleginoides at Heard Island (Division 58.5.2) (Hobart: CCAMLR).
Ccamlr Secretariat (2022b). “Fishery report 2021: Dissostichus eleginoides at kerguelen islands French EEZ (Division 58.5.1),” (Hobart: CCAMLR).
Cherel Y., Ducatez S., Fontaine C., Richard P., Guinet C. (2008). Stable isotopes reveal the trophic position and mesopelagic fish diet of female southern elephant seals breeding on the Kerguelen islands. Mar. Ecology-Progress Ser. 370, 239–247. doi: 10.3354/meps07673
Corbeau A., Collet J., Orgeret F., Pistorius P., Weimerskirch H. (2021a). Fine-scale interactions between boats and large albatrosses indicate variable susceptibility to bycatch risk according to species and populations. Anim. Conserv. 24, 689–699. doi: 10.1111/acv.12676
Corbeau A., Collet J., Pajot A., Joo R., Thellier T., Weimerskirch H. (2021b). Differences in foraging habitat result in contrasting fisheries interactions in two albatross populations. Mar. Ecol. Prog. Ser. 663, 197–208. doi: 10.3354/meps13648
Corrigan L. J., Fabiani A., Chauke L. F., Mcmahon C. R., De Bruyn M., Bester M. N., et al. (2016). Population differentiation in the context of Holocene climate change for a migratory marine species, the southern elephant seal. J. Evol Biol. 29, 1667–1679. doi: 10.1111/jeb.12870
Croxall J. P., Prince P. A. (1982). Calorific content of squid (Mollusca: Cephalopoda). Br. Antarctic Survey Bull. 55, 27–31.
Deagle B. E., Tollit D. J. (2007). Quantitative analysis of prey DNA in pinniped faeces: potential to estimate diet composition? Conserv. Genet. 8, 743–747. doi: 10.1007/s10592-006-9197-7
Doll J. C., Jacquemin S. J. (2019). Bayesian Model selection in fisheries management and ecology. J. Fish Wildlife Manage. 10, 691–707. doi: 10.3996/042019-JFWM-024
Duhamel G., Agnew D. (1990). A re-analysis of the Kerguelen Shelf and skiff bank stocks of champsocephalus gunnari In: Welsford D., Dell J., Duhamel G. (Eds). The Kerguelen Plateau: marine ecosystem and fisheries. Proceedings of the Second Symposium. Australian Antarctic Division, Kingston, Tasmania, Australia.
Duhamel G., Péron C., Sinègre R., Chazeau C., Gasco N., Hautecœur M., et al. (2019). “Important readjustments in the biomass and distribution of groundfish species in the northern part of the Kerguelen Plateau and skiff bank,” in Second Kerguelen Plateau Symposium: marine ecosystem and fisheries, Vol. 135–184.
Elith J., Leathwick J. R., Hastie T. (2008). A working guide to boosted regression trees. J. Anim. Ecol. 77, 802–813. doi: 10.1111/j.1365-2656.2008.01390.x
Farmer B., Nowara G. B., Barnes T. C., Burch P., Woodcock E., Ziegler P., et al. (2019). “Modelling the spatial distribution of Patagonian toothfish (Dissostichus eleginoides) by length and age around heard island and McDonald islands on the kerguelen plateau,” in The Kerguelen Plateau: marine ecosystem and fisheries. proceedings of the second symposium. Eds. Welsford D. C., Dell J., Duhamel G. (Kingston, Tasmania: Australian Antarctic Division), 219–235.
Field I. C., Bradshaw C. J. A., Mcmahon C. R., Harrington J., Burton H. R. (2002). Effects of age, size and condition of elephant seals (Mirounga leonina) on their intravenous anaesthesia with tiletamine and zolazepam. Veterinary Rec. 151, 235–240. doi: 10.1136/vr.151.8.235
Field I. C., Harcourt R. G., Boehme L., De Bruyn P. J. N., Charrassin J. B., Mcmahon C. R., et al. (2012). Refining instrument attachment on phocid seals. Mar. Mammal Sci. 28, E325–E332. doi: 10.1111/j.1748-7692.2011.00519.x
Fisher K. I., Stewart R. E. A., Kastelein R. A. (1989). “Apparent digestive efficiency in the walrus, odobenus rosmarius,” in Eighth biennial conference on the biology of marine mammals (Pacific Grove, California: Society for Marine Mammalogy).
Godard M., Manté C., Guinet C., Picard B., Nerini D. (2020). Diving behavior of Mirounga leonina: A functional data analysis approach. Front. Mar. Sci. 7. doi: 10.3389/fmars.2020.00595
Goedegebuure M., Melbourne-Thomas J., Corney S. P., Mcmahon C. R., Hindell M. A. (2018). Modelling southern elephant seals Mirounga leonina using an individual-based model coupled with a dynamic energy budget. PloS One 13, e0194950. doi: 10.1371/journal.pone.0194950
Green K., Burton H. R. (1993). Comparison of the stomach contents of the southern elephant seal, Mirounga leonina, at Macquarie and Heard Islands. Mar. Mammal Sci. 9, 10–22. doi: 10.1111/j.1748-7692.1993.tb00422.x
Guénette S., Heymans S. J. J., Christensen V., Trites A. W. (2006). Ecosystem models show combined effects of fishing, predation, competition, and ocean productivity on steller sea lions (Eumetopias jubatus) in Alaska. Can. J. Fish Aquat. Sci. 63, 2495–2517. doi: 10.1139/f06-136
Harcourt R., Hindell M. A., Mcmahon C. R., Goetz K. T., Charrassin J.-B., Heerah K., et al. (2021). Regional variation in winter foraging strategies by Weddell seals in Eastern Antarctica and the Ross Sea. Front. Mar. Sci. doi: 10.3389/fmars.2021.720335
Harwood J., Croxall J. P. (1988). The assessment of competition between seals and commercial fisheries in the North Sea and the Antarctic1. Mar. Mammal Sci. 4, 13–33. doi: 10.1111/j.1748-7692.1988.tb00179.x
Harwood J., Stokes K. (2003). Coping with uncertainty in ecological advice: lessons from fisheries. Trends Ecol. Evol. 18, 617–622. doi: 10.1016/j.tree.2003.08.001
Hindell M. A., Bradshaw C. J. A., Sumner M. D., Michael K. J., Burton H. R. (2003). Dispersal of female southern elephant seals and their prey consumption during the austral summer: relevance to management and oceanographic zones. J. Appl. Ecol. 40, 703–715. doi: 10.1046/j.1365-2664.2003.00832.x
Hindell M. A., Burton H. R. (1988). Seasonal haul-out patterns of the southern elephant seal (Mirounga leonina) at Macquarie Island. J. Mammal 69, 81–88. doi: 10.2307/1381750
Hindell M. A., Mcmahon C. R., Bester M. N., Boehme L., Costa D., Fedak M. A., et al. (2016). Circumpolar habitat use in the southern elephant seal: implications for foraging success and population trajectories. Ecosphere 7, e01213. doi: 10.1002/ecs2.1213
Hindell M. A., Mcmahon C. R., Harcourt R., Arce F., Guinet C., Jonsen I. (2021). Inter- and intra-sex habitat partitioning in the highly dimorphic southern elephant seal. Ecol. Evol 1620–1633. doi: 10.1002/ece1003.7147
Hindell M. A., Slip D. J., Burton H. R. (1991). The diving behaviour of adult male and female southern elephant seals, Mirounga leonina (Pinnipedia, phocidae). Aust. J. Zool 39, 595–619. doi: 10.1071/ZO9910595
Janc A., Richard G., Guinet C., Arnould J. P. Y., Villanueva M. C., Duhamel G., et al. (2018). How do fishing practices influence sperm whale (Physeter macrocephalus) depredation on demersal longline fisheries? Fish Res. 206, 14–26.
Jog K., Sutaria D., Diedrich A., Grech A., Marsh H. (2022). Marine mammal interactions with fisheries: Review of research and management trends across commercial and small-scale fisheries. Front. Mar. Sci. 9. doi: 10.3389/fmars.2022.758013
Jonsen I. D., Patterson T. A. (2020). “foieGras: Fit continuous-time state-space and latent variable models for filtering Argos satellite (and other) telemetry data and estimating movement behaviour,” in R package version 0.6.0 (CRAN).
Jonsen I., Patterson T. A., Doherty P. D., Godley B. J., Grecian W. J., Guinet C., et al. (2020). A continuous-time state-space model for rapid quality-control of Argos locations from animal-borne tags. Movement Ecol. 8, Article 31, 1–13. doi: 10.1186/s40462-020-00217-7
Kooyman G. L., Castellini M. A., Davis R. W., Maue R. A. (1983). Aerobic diving limits of immature Weddell seals. J. Comp. Physiol. 151, 171–174. doi: 10.1007/BF00689915
Kooyman G. L., Cherel Y., Lemaho Y., Croxall J. P., Thorson P. H., Ridoux V. (1992). Diving behavior and energetics during foraging cycles in king penguins. Ecol. Monogr. 62, 143–163. doi: 10.2307/2937173
Krockenberger M. B., Bryden M. M. (1993). Energy intake of immature southern elephant seals, Mirounga leonina (Carnivora: Phocidae). Aust. J. Zool 41, 589–597. doi: 10.1071/ZO9930589
Maresh J. L., Simmons S. E., Crocker D. E., Mcdonald B. I., Williams T. M., Costa D. P. (2014). Free-swimming northern elephant seals have low field metabolic rates that are sensitive to an increased cost of transport. J. Exp. Biol. 217, 1485–1495. doi: 10.1242/jeb.094201
Maschette D., Welsford D. (2019). Population dynamics and life-history plasticity of mackerel icefish (Champsocephalus gunnari) within the vicinity of Heard Island and the McDonald Islands. In: Welsford D., Dell J., Duhamel G. (Eds). The Kerguelen Plateau: marine ecosystem and fisheries. Proceedings of the Second Symposium. Kingston, Tasmania, Australia: Australian Antarctic Division
Mccann T. S. (1980). Population structure and social organization of southern elephant seals Mirounga leonina (L). Biol. J. Linn. Soc. 14, 133–150. doi: 10.1111/j.1095-8312.1980.tb00102.x
Mccann T. S. (1985). “Size, status and demography of southern elephant seal (Mirounga leonina) populations,” in Sea Mammals in south latitudes: Proceedings of a symposium of the 52nd ANZAAS congress. Eds. Ling J. K., Bryden M. M. (Adelaide: South Australian Museum), 1–17.
Mcmahon C. R., Bester M. N., Burton H. R., Hindell M. A., Bradshaw C. J. A. (2005a). Population status, trends and a re-examination of the hypotheses explaining the recent declines of the southern elephant seal Mirounga leonina. Mammal Rev. 35, 82–100. doi: 10.1111/j.1365-2907.2005.00055.x
Mcmahon C. R., Burton H. R., Mclean S., Slip D., Bester M. N. (2000). Field immobilisation of southern elephant seals with intravenous tiletamine and zolazepam. Veterinary Rec. 146, 251–254. doi: 10.1136/vr.146.9.251
Mcmahon C. R., Field I. C., Bradshaw C. J. A., White G. C., Hindell M. A. (2008). Tracking and data-logging devices attached to elephant seals do not affect individual mass gain or survival. J. Exp. Mar. Biol. Ecol. 360, 71–77. doi: 10.1016/j.jembe.2008.03.012
Mcmahon C. R., Hindell M. A., Burton H. R., Bester M. N. (2005b). Comparison of southern elephant seal populations, and observations of a population on a demographic knife-edge. Mar. Ecol. Prog. Ser. 288, 273–283. doi: 10.3354/meps288273
Nakagawa S., Schielzeth H., O'hara R. B. (2013). A general and simple method for obtainingR2from generalized linear mixed-effects models. Methods Ecol. Evol. 4, 133–142. doi: 10.1111/j.2041-210x.2012.00261.x
Nelms S. E., Alfaro-Shigueto J., Arnould J. P. Y., Avila I. C., Bengtson Nash S., Campbell E., et al. (2021). Marine mammal conservation: over the horizon. Endangered Species Res. 44, 291–325. doi: 10.3354/esr01115
Padman L., Costa D. P., Bolmer S. T., Goebel M. E., Huckstadt L. A., Jenkins A., et al. (2010). Seals map bathymetry of the Antarctic continental shelf. Geophysical Res. Lett. 37, L21601. doi: 10.1029/2010GL044921
Peron C., Welsford D. C., Ziegler P., Lamb T. D., Gasco N., Chazeau C., et al. (2016). Modelling spatial distribution of Patagonian toothfish through life-stages and sex and its implications for the fishery on the Kerguelen Plateau. Prog. Oceanogr 141, 81–95. doi: 10.1016/j.pocean.2015.12.003
Photopoulou T., Lovell P., Fedak M. A., Thomas L., Matthiopoulos J. (2015). Efficient abstracting of dive profiles using a broken-stick model. Methods Ecol. Evol. 6, 278–288. doi: 10.1111/2041-210X.12328
Pichegru L., Ryan P. G., Le Bohec C., van der Lingen C. D., Navarro R., Petersen S., et al. (2009). Overlap between vulnerable top predators and fisheries in the Benguela upwelling system: implications for marine protected areas. Mar. Ecol. Prog. Ser. 391, 199–208. doi: 10.3354/meps08283
Ponganis P. J., Kooyman G. L., Zornow M. H., Castellini M. A., Croll D. A. (1990). Cardiac-output and stroke volume in swimming harbor seals. J. Comp. Physiol. B-Biochemical Systemic Environ. Physiol. 160, 473–482.
Prime J. H., Hammond P. S. (1987). “Quantitative assessment of grey seal diet from faecal analysis,” in Approaches to marine mammal energetics. Eds. Huntley A. C., Costa D. P., Worthy G., Castellini M. A. (Kansas: Society for Marine Mammalogy Special Publication No 1, Allen Press), 165–188.
Reid K. (2018). “Climate change impacts, vulnerabilities and adaptations: Southern ocean marine fisheries, in , eds. 2018. . FAO fisheries and aquaculture technical paper no. 627. Rome, FAO. 628 pp,” in Impacts of climate change on fisheries and aquaculture: synthesis of current knowledge, adaptation and mitigation options. Eds. Barange M., Bahri T., Beveridge M. C. M., Cochrane K. L., Funge-Smith S., Poulain F. (Rome: FAO), 363–374.
Reid K. (2019). Climate change impacts, vulnerabilities and adaptations: Southern ocean marine fisheries. Impacts of climate change on fisheries and aquaculture, 363
Ribeiro N., Herraiz-Borreguero L., Rintoul S. R., Mcmahon C. R., Hindell M., Harcourt R., et al. (2021). Warm modified circumpolar deep water intrusions drive ice shelf melt and inhibit dense shelf water formation in Vincennes bay, East Antarctica. J. Geophysical Res: Oceans 126.
Robertson G., Moreno C., Arata J. A., Candy S. G., Lawton K., Valencia J., et al. (2014). Black-browed albatross numbers in Chile increase in response to reduced mortality in fisheries. Biol. Conserv. 169, 319–333. doi: 10.1016/j.biocon.2013.12.002
Schaafsma F. L., Cherel Y., Flores H., Van Franeker J. A., Lea M. A., Raymond B., et al. (2018). Review: the energetic value of zooplankton and nekton species of the Southern Ocean. Mar. Biol. 165, 129. doi: 10.1007/s00227-018-3386-z
Scientific Committee on Antarctic Research. (2021). SCAR Southern Ocean Diet and Energetics Database (2021-11-18) [Data set]. Zenodo. doi: 10.5281/zenodo.5708934
Slade R. W., Moritz C., Hoelzel A. R., Burton H. R. (1998). Molecular population genetics of the southern elephant seal Mirounga leonina. Genetics 149, 1945–1957. doi: 10.1093/genetics/149.4.1945
Slip D. J. (1995). The diet of southern elephant seals (Mirounga leonina) at heard island. Can. J. Zoology-Revue Can. Zoologie 73, 1519–1528. doi: 10.1139/z95-180
Slip D. J., Burton H. R. (1999). Population status and seasonal haulout patterns of the southern elephant seal (Mirounga leonina) at Heard Island. Antarctic Sci. 11, 38–47. doi: 10.1017/S0954102099000061
Subramaniam R. C., Melbourne-Thomas J., Corney S. P., Alexander K., Péron C., Ziegler P., et al. (2020). Time-dynamic food web modeling to explore environmental drivers of ecosystem change on the Kerguelen Plateau. Front. Mar. Sci. 7. doi: 10.3389/fmars.2020.00641
Trites A. W., Christensen V., Pauly D. (1997). Competition between fisheries and marine mammals for prey and primary production in the pacific ocean. J. Northwestern Atl Fish Sci. 22, 173–187. doi: 10.2960/J.v22.a14
Tulloch V., Pirotta V., Grech A., Crocetti S., Double M., How J., et al. (2019). Long-term trends and a risk analysis of cetacean entanglements and bycatch in fisheries gear in Australian waters. Biodivers Conserv. 29, 251–282. doi: 10.1007/s10531-019-01881-x
Van Den Hoff J., Kilpatrick R., Welsford D. (2017). Southern elephant seals (Mirounga leonina linn.) depredate toothfish longlines in the midnight zone. PloS One 12, e0172396.
Walters A., Lea M. A., Van Den Hoff J., Field I. C., Virtue P., Sokolov S., et al. (2014). Spatially explicit estimates of prey consumption reveal a new krill predator in the Southern Ocean. PloS One 9. doi: 10.1371/journal.pone.0086452
Werner T. B., Northridge S., Press K. M., Young N. (2015). Mitigating bycatch and depredation of marine mammals in longline fisheries. ICES J. Mar. Sci. 72, 1576–1586. doi: 10.1093/icesjms/fsv092
Yodzis P. (2001). Must top predators be culled for the sake of fisheries? Trends Ecol. Evol. 16, 78–84.
Keywords: Kerguelen Plateau, southern elephant seal, fisheries interactions, Patagonian toothfish, mackerel icefish
Citation: Hindell MA, McMahon CR, Guinet C, Harcourt R, Jonsen ID, Raymond B and Maschette D (2022) Assessing the potential for resource competition between the Kerguelen Plateau fisheries and southern elephant seals. Front. Mar. Sci. 9:1006120. doi: 10.3389/fmars.2022.1006120
Received: 29 July 2022; Accepted: 28 October 2022;
Published: 18 November 2022.
Edited by:
Randall William Davis, Texas A&M University at Galveston, United StatesReviewed by:
Luis Cardona, University of Barcelona, SpainLeslie Cornick, University of Washington Bothell, United States
Richard Kindong, Shanghai Ocean University, China
Copyright © 2022 Hindell, McMahon, Guinet, Harcourt, Jonsen, Raymond and Maschette. This is an open-access article distributed under the terms of the Creative Commons Attribution License (CC BY). The use, distribution or reproduction in other forums is permitted, provided the original author(s) and the copyright owner(s) are credited and that the original publication in this journal is cited, in accordance with accepted academic practice. No use, distribution or reproduction is permitted which does not comply with these terms.
*Correspondence: Mark Andrew Hindell, bWFyay5oaW5kZWxsQHV0YXMuZWR1LmF1
†These authors have contributed equally to this work and share first authorship