- 1Shandong Key Laboratory of Eco-Environmental Science for the Yellow River Delta, Binzhou University, Binzhou, China
- 2Wetland Ecosystem Research Station of Hangzhou Bay, Research Institute of Subtropical Forestry, Chinese Academy of Forestry, Hangzhou, China
- 3State Key Laboratory of Estuarine and Coastal Research, East China Normal University, Shanghai, China
As a common reclamation practice in global estuaries and coasts, conversion of natural wetlands to aquaculture ponds for food demand had significant effects on nitrogen (N) cycling processes. However, the response of sedimemt N fixation process on this conversion remains poorly understood. Here, slury incubation experiment combined with 15N isotope tracing method and molecular analysis technique were used to investigate the influences of conversion of natural marshland to shrimp ponds on sediemt N fixation process. The results showed that conversion of natural marshland to shrimp ponds significantly promoted sediment N fixation rate. The increasing of sediment N fixation rate may be mainly attributed to the change of sediment EC, TOC and Fe2+/Fe3+ rather than the change of N fixation gene abundance. In addition, there was no obvious difference in sediment N fixation rate between 5-year-old shrimp ponds and 18-year-old shrimp ponds, which may be ascribed to the increasing inhibiting effect of inorganic N concentration with reclamation time. Overall, our findings highlighted that conversion of natural wetlands to aquaculture ponds can affect the N budgets in estuarine and coastal regions by altering the sediment N fixation process.
Introduction
Coastal wetlands provide a variety of importance ecosystem services, including natural coastal protection, contaminant purification, bird habitat and nutrient cycle (Schuerch et al., 2018). Over the past few decades, reactive nitrogen (N) loadings in estuarine and coastal zones have increased significantly, causing a series of eco-environmental problems, such as eutrophication, algal bloom and hypoxia (Deegan et al., 2012; Damashek and Francis, 2018). Anthropogenic input of reactive N is regarded as the major reason for the overloaded N in this ecosystem (Capone et al., 2005; Fulweiler et al., 2015; Lin and Lin, 2022). However, microbial N fixation, the reduction of atmospheric dinitrogen gas (N2) to available ammonia, may also be an important and internal source of reactive N in estuarine and coastal environments (Gardner et al., 2006). Thus, increasing attention to microbial N fixation process in estuarine and coastal ecosystems has been raised in recent years (Fulweiler et al., 2013; Lin et al., 2017; Hou et al., 2018).
Numerous studies have examined the spatio-temporal variability of the N fixation process across the estuarine and coastal ecosystems, and noted that the process rate is affected by temperature, pH, salinity, sulfide, carbon and N substrate availability to a certain extent (Fulweiler et al., 2013; Wang et al., 2018a; Li et al., 2021). For instance, Wang et al. (2018a) found that N fixation rates in the East China Sea were significantly related to temperature, salinity, sulfide, iron and C/N. While in the intertidal wetlands of Yangtze Estuary, the N fixation process was closely associated with salinity, pH, organic carbon and NH4+ (Hou et al., 2018). Saltmarsh plants can alter sediment physicochemical properties and further affect the N fixation process (Huang et al., 2016). In addition, a recent study reported that increased overlying NO3– contents can reduce microbial N fixation rates at different organic carbon concentrations (Li et al., 2021). Sediment microbial communities could also control the N fixation, with the abundance of nifH gene being indicative of N fixation activities in estuarine and coastal wetlands (Huang et al., 2016). Previous study found that the nifH gene abundance had similar spatial variation trend with the N fixation rate in the Yangtze Estuary, and a positive relationship was also observed for these two parameters (Hou et al., 2018). However, Wang et al. (2018a) reported that sediment physicochemical variables rather than functional microbes mediated the N fixation process rate in the East China Sea. Although a lot studies have been conducted to investigate N fixation process, the influencing factors and mechanisms controlling N fixation process in coastal wetlands remain largely uncertain due to the complexity of coupled biotic and abiotic conditions.
Reclamation of estuarine and coastal wetlands for agriculture is a common practice around the world and has resulted in a significant deterioration of wetland ecosystem functions (Sun et al., 2015; Murray et al., 2019). The aquaculture pond is one of the most common types of agricultural reclamation in estuarine and coastal wetlands, which can profoundly affect sediment element cycles due to the changed hydrology and management modes (Yang et al., 2017b). Generally, intensive aquaculture is maintained based on the daily addition of feeds, and the majority of feeds are accumulated in the sediments because only a small proportion of feeds is utilized by cultured animals (Chen et al., 2016). Therefore, aquaculture reclamation could dramatically increase sediment carbon and N contents (Murphy et al., 2016; Gao et al., 2018). In addition, remarkable changes of greenhouse gases emission were observed when natural saltmarsh conversion to aquaculture ponds (Yang et al., 2017a and Yang et al., 2019). Recently, some studies have explored the impacts of aquaculture on sediment nitrification, denitrification, anammox and dissimilatory nitrate reduction to ammonium (DNRA), and they noted that aquaculture can affect N budgets of aquatic ecosystems through changing microbial N transformation processes (Murphy et al., 2016; Gao et al., 2019b). In fact, sediment N fixation is also an important N source in estuarine and coastal ecosystems, but it is not clear how the N fixation process change after natural saltmarsh conversion to aquaculture ponds. Considering that China has the largest aquaculture industry in the world with a total area of 2.6 × 106 ha (Chen et al., 2016), we selected a typical shrimp aquaculture reclamation areas in the Min River estuary of southeastern China as study areas to reveal the influences of aquaculture reclamation on sediment N fixation process. The specific aims of this study were (1) to explore the changes in rate and associated functional gene abundance of sediment N fixation process after coastal saltmarsh conversion to shrimp aquaculture ponds, (2) to reveal the main environmental factors governing the sediment N fixation process, and (3) to assess the environmental implication changes of sediment N fixation process after the conversion of coastal saltmarsh to shrimp ponds.
Materials and methods
Study area and sample collection
The study was conducted in Shanyutan wetland (22°00′36″–26°03′42″N, 119°34′12″–119°41′40″E, Figure 1), the brackish-water tidal wetland in the Minjiang River estuary in Fujian Province, Southeast China (Tong et al., 2012). The study area is characterized by a subtropical monsoonal climate with hot and humid in summer and dry and cold in winter (Wang et al., 2018b). The mean annual temperature and precipitation were 19.6°C and 1350 mm, respectively (Tong et al., 2010). Shanyutan wetland is subjected to the formal semi-diurnal tide, with a tide range of 2.5 – 6 m (Zhang et al., 2015). The study area has a high diversity of vegetation species, with native Phragmite australis, Scirpus triqueter and Cyperus malaccensis and exotic invasive Spartina alterniflora being the dominant vegetation species (Zhang et al., 2011).
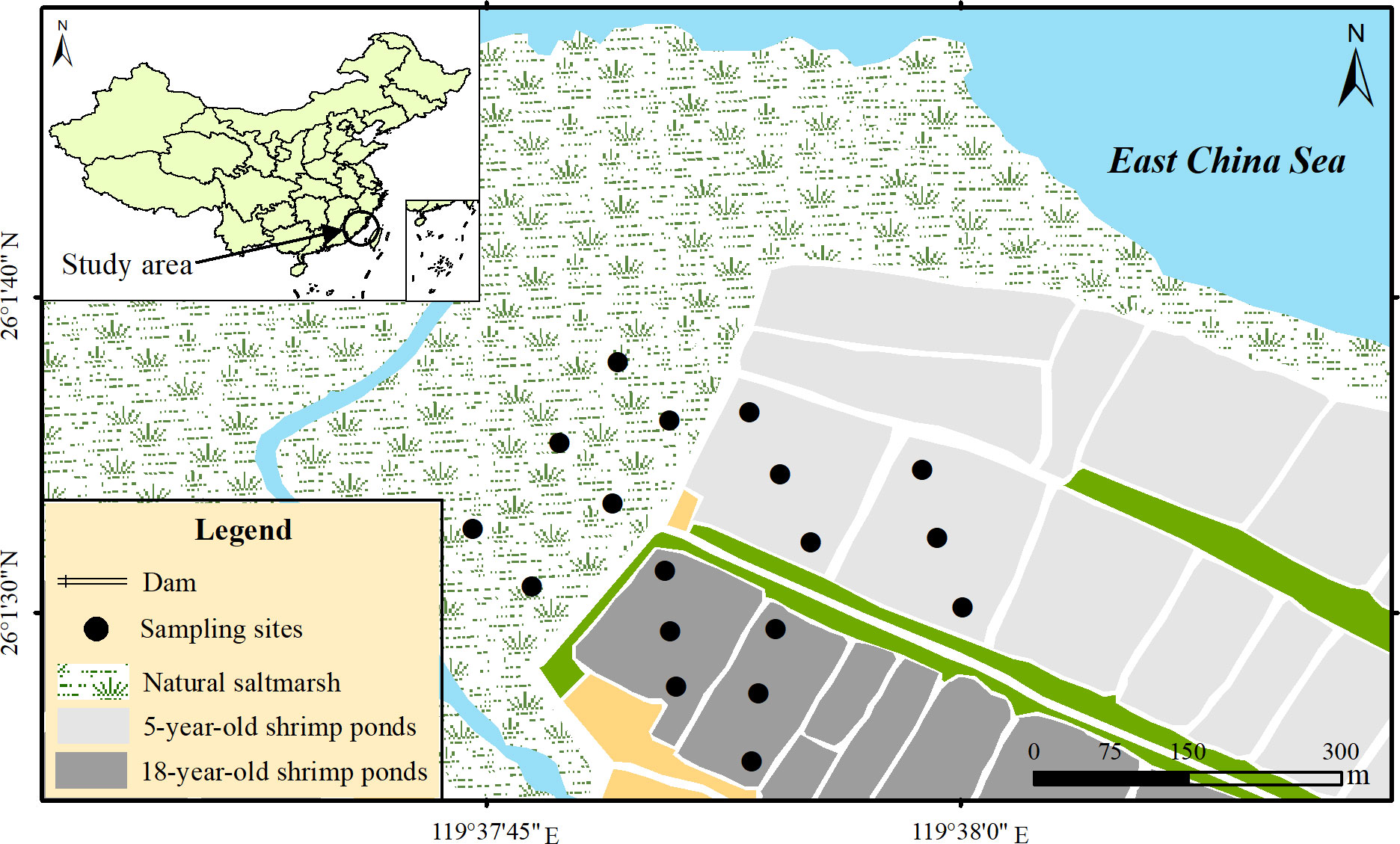
Figure 1 Study area and sampling sites (Figure 1 is quoted from our previous research (Gao et al., 2019b).
In recent years, large-scale conversion of natural wetland was performed in Shanyutan, and almost all of the natural wetland was converted to aquaculture ponds to increase seafood demand (Yang et al., 2017a). Shrimp pond is one of the most important aquaculture ponds (Yang et al., 2019). The shrimp culture period usually starts in June and ends in November, with only harvest once a year (Yang et al., 2017a). Before breeding, saltwater from the adjacent estuary was injected into the shrimp pond using a submerged pump at once to retain the mean water depth of 1.4 m during the shrimp culture period (Yang et al., 2019). After harvest, the pond water was discharged into the adjacent estuary. During the shrimp culture period, feeding and aeration were operated twice and four times per day, respectively.
According to reclamation time, the shrimp ponds in Shanyutan could be mainly divided into two categories: shrimp ponds reclaimed in 1998 and 2011. To assess the impact of natural wetland conversion to shrimp culture pond on sediment N fixation process, sediment cores (5 cm deep) were retrieved twice in July and November 2016 in three typical sampling sites: natural saltmarsh, 5-year-old shrimp ponds (ponds reclaimed in 2011), and 18-year-old shrimp ponds (ponds reclaimed in 1998), each of which had six replicate locations. In this study, the sediment core was retrieved using an acrylic tube (7.0 cm diameter and 20 cm deep), which was installed into a surface-operated coring device equipped with a core cylinder and a one-way check valve (Gao et al., 2019b). After collection, the sediment cores were preserved in sterile bags and transported to the laboratory immediately. In the laboratory, sediment sample was mixed homogeneously and divided into two parts. One fresh part was used to determine the sediment N fixation rate and parameters. The other part was preserved at -80°C for analyses of molecular microbiology. In addition, 5 L overlying water was taken from each location for the determination of the sediment N fixation rate.
Analysis of sediment parameters
The in situ pH and electrical conductivity (EC) of sediment were measured using IQ150 pH meter (IQ Scientific Instruments, USA) and 2265FS EC meter (Spectrum Technologies Inc., USA), respectively. The water content was determined based on the weight loss of fresh sediment through oven drying (Hou et al., 2018). The TOC and TN of sediment were analyzed using Vario EL elemental analyzer (Elementar, Germany) as described by Gao et al. (2019a). Sediment NH4+ and NOx– were extracted using 1 M KCl and then measured by a continuous flow analyzer (Skalar Analytical SAN++, Netherlands) (Hou et al., 2015). The Fe2+ and Fe3+ of sediment were extracted with 0.5 M HCl and then analyzed using the phenanthroline method (Lovley and Phillips, 1987).
Determination of sediment potential N fixation rate
In this study, sediment potential N fixation rates (NFR) was determined by using slurry incubation experiment in combination with 15N isotope tracing technique which was improved on the basis of the previous method described by Hou et al. (2018). Briefly, the slurry was prepared by mixing the overlying water and sediment at a ratio of 7:1. Then, the homogeneous slurry was purged with helium (He) for 30 min to remove background N2 and transferred into 12 mL gas-tight vials (Exetainers, Labco, UK) in He-filled glove box. All vials were divided into two parts. One part was immediately injected with 0.2 mL saturated ZnCl2 solution to inhibit microbial activity and designated as initial sample. The other part was injected with 0.5 mL 99 atom% 15N-N2 (Campro Scientific, Germany) and incubated in dark at near in situ temperature for about 24 h. These vials were preserved by adding 0.2 mL ZnCl2 solution and designated as final samples. Then, all vials were purged with He for 30 min to remove N2 and injected with hypobromite iodine solution to oxidize 15N-labeled products generated by N fixation into N2. The concentrations of 29N2 and 30N2 in all samples were determined by membrane inlet mass spectrometry (HPR-40, Hiden Analytical, UK). The differences of 15N-labeled N2 concentrations between final and initial samples were used to calculate NFR. This method is especially suitable for relative comparisons of different N transformation rates (Aoki and McGlathery, 2017). Nevertheless, because slurry mixing removed sediment biogeochemical gradients, the method may not reflect the actual N fixation rate in situ environment (Behrendt et al., 2013).
Sediment DNA extraction and quantitative polymerase chain reaction
We quantified the nifH encoding the key enzyme for N fixation to explore the potential impact of diazotrophs on N fixation process (Hou et al., 2018). According to the manufacturer’s instruction, the sediment DNA was extracted with Powersoil™ DNA Isolation Kits (MO BIO, USA). The extracted sediment DNA was stored at -80°C before subsequent analysis. The nifH gene fragment was amplified using nifHfw (5’-GGH AAR GGH GGH ATH GGN AAR TC-3’) and nifHrv (5’-GGC ATN GCR AAN CCV CCR CAN AC-3’) primers (Wang et al., 2018a). Then, the abundance of nifH was quantified by q-PCR using an ABI 7500 Sequence Detection System (Applied Biosystems, Canada) and SYBR green method. The detailed information of q-PCR protocol was shown in Table S1. The relative abundance of nifH gene in sediment was calculated by the standard curve which was constructed by diluting a known amount of plasmid DNA including nifH fragment into six gradients (from 2.50 × 104 to 2.50 × 109 copies μL-1). The amplification efficiency was above 96% in this study, and the correlation coefficient was higher than 0.99.
Statistical analysis
The data were checked for normal distribution and homogeneity of variance and transformed if necessary before statistical analysis (Täumer et al., 2020). Pearson’s correlation analysis (SPSS 22.0) and Redundancy analysis (RDA) (Canoco 5) were performed to reveal relationships between sediment parameters, nifH abundance, and N fixation rate. Path analysis (AMOS 22.0) was used to futher explore the effects of shrimp aquaculture reclamation on N fixation process. Before path analysis, a conceptual model was constructed based on existing literature and ecological principles (Liu et al., 2018). Then, the model was improved in a stepwise manner. In present study, column and box charts were drawn using Origin 2021.
Results
Sediment physicochemical parameters
Measured sediment physicochemical parameters were shown in Figure 2 (This data was quoted from our previous studies (Gao et al., 2019b)). The water content of sediment was generally higher in 18-year-old ponds than in 5-year-old ponds and natural saltmarsh, but no obvious difference was observed between the latter two. Sediment pH ranged from 6.28 to 7.11 and 6.16 to 6.98 in summer and winter, respectively, with no significant difference among the natural saltmarsh, 5-year-old ponds and 18-year-old ponds. Summer EC in natural saltmarsh and 5-year-old ponds were significantly higher than that of 18-year-old ponds, while in winter, sediment EC was higher in natural saltmarsh than in 5-year-old ponds and 18-year-old ponds. Sediment TOC content varied from 16.03 to 33.24 g kg-1 and 16.01 to 35.40 g kg-1 in summer and winter, respectively, and the content was generally higher in shrimp ponds (5-year-old ponds and 18-year-old ponds) than in natural saltmarsh. The NH4+ and NOx– concentrations of sediment ranged from 15.78 to 98.31 mg kg-1 and 1.39 to 3.45 mg kg-1, respectively, and their changes patterns were similar to TOC. There was no significant difference in TN content between the natural saltmarsh and shrimp ponds in summer, but the TN content in 18-year-old ponds was higher than that of the natural saltmarsh in winter. Sediment Fe2+/Fe3+ in shrimp ponds (1.44 – 2.52) was generally higher than that of natural saltmarsh (0.64 – 0.91), although some difference was not significant.
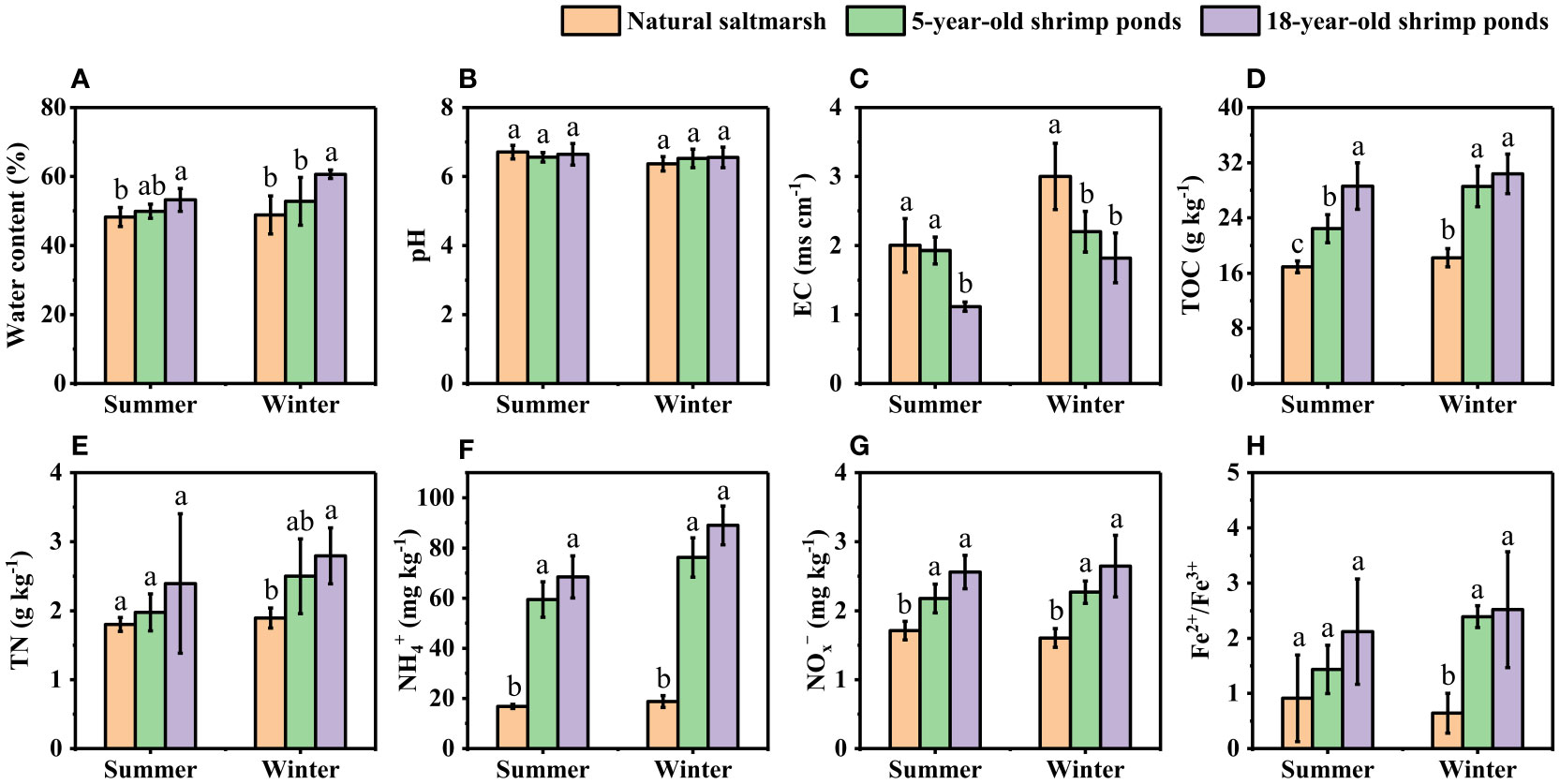
Figure 2 Sediment physicochemical properties of natural saltmarsh and shrimp ponds. Different lowercase letters indicate significant differences (p < 0.05) among natural saltmarsh and shrimp ponds in the same season. (A) Water content, (B) pH, (C) EC, (D) TOC, (E) TN, (F) NH4+, (G) NOx-, (H) Fe2+/Fe3+.
Sediment potential N fixation rates and nifH gene abundance
Sediment potential N fixation rates ranged from 6.16 to 10.31 nmol g-1 h-1 and from 3.71 to 7.84 nmol g-1 h-1 in summer and winter, respectively, with higher N fixation rates in summer (Figure 3). Compared with natural saltmarsh, shrimp ponds had higher N fixation rates in both summer and winter (Figure 3). However, There was no obvious difference in N fixation rates between 5-year-old and 18-year-old shrimp ponds (Figures 3, 5). Sediment nifH gene abundance varied from 1.26 × 108 to 1.26 × 108 copies g-1 and 7.33× 107 to 1.04 × 108 copies g-1 in summer and winter, respectively, with no significant difference among the natural saltmarsh, 5-year-old and 18-year-old shrimp ponds (Figure 4). In addition, the mean abundance of nifH gene was also higher in summer than that in winter (Figure 4).
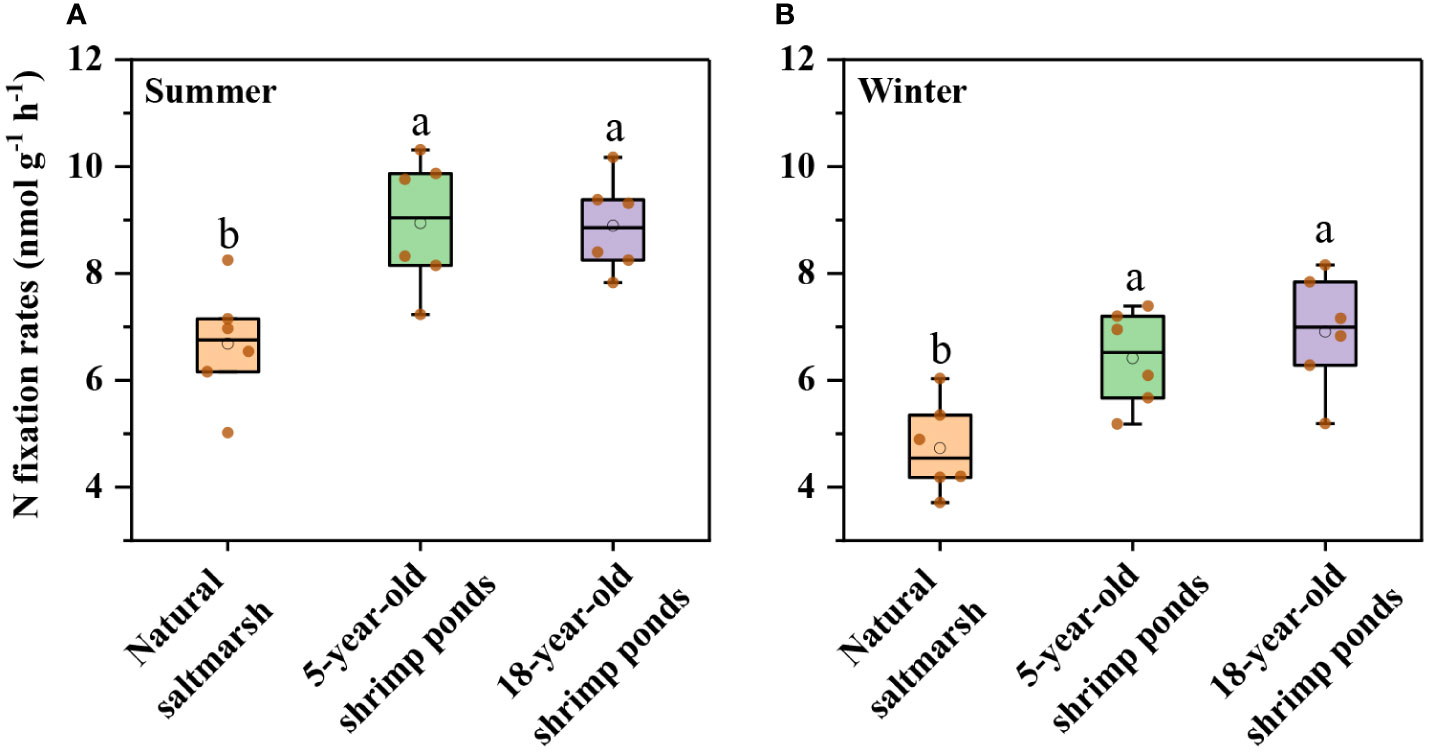
Figure 3 Sediment N fixations rates of natural saltmarsh and shrimp ponds in (A) Summer and (B) Winter. Different lowercase letters indicate significant differences (p < 0.05) among natural saltmarsh and shrimp ponds in the same season.
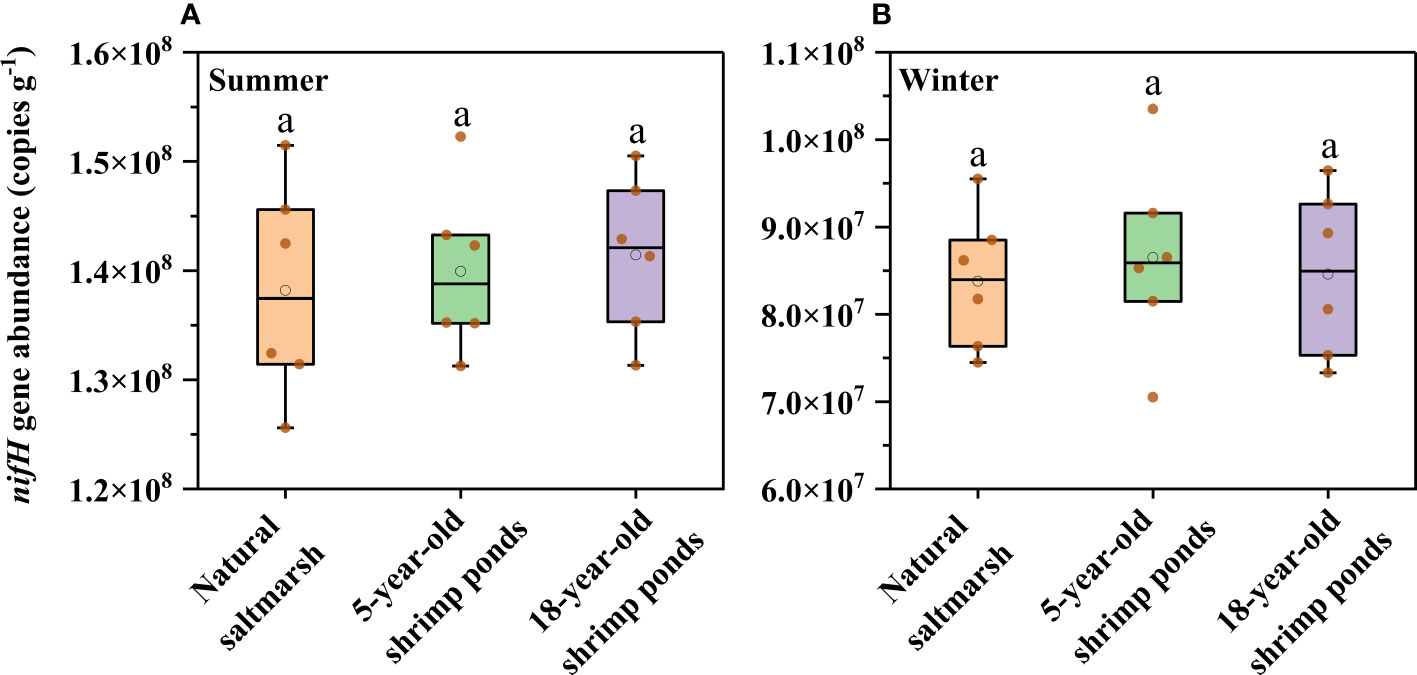
Figure 4 Sediment nifH gene abundance of natural saltmarsh and shrimp ponds in (A) Summer and (B) Winter. Different lowercase letters indicate significant differences (p < 0.05) among natural saltmarsh and shrimp ponds in the same season.
Influences of sediment parameters on N fixation rates
Potential N fixation rate was significantly related to sediment EC, TOC, NH4+, NOx− and Fe2+/Fe3+ in summer and winter, respectively, and only significantly related to TN in winter (Table 1). For bulked two-season data, the N fixation rate was significantly associated with sediment EC, NH4+, NOx− and nifH gene (Table 1). In addition, results from the RDA of sediment physicochemical parameters, nifH gene abundance and N fixation rate showed that sediment samples in natural saltmarsh were clearly separated from those in shrimp ponds along Axis 1 (Figure 5). The sum of Axis 1 and Axis 2 accounted for 60.4% and 55.3% of the variation in sediment parameters measured in summer and winter, respectively (Figure 5). Sediment N fixation rate, EC, TOC, NH4+, NO3– and Fe2+/Fe3+ contributed significantly to the variation of Axis 1, while nifH gene abundance was minor importance in the variation of Axis 1 (Figure 5). Predicted path model further showed that reclamation time can influence N fixation rate by affecting sediment EC, NH4+ and nifH gene abundance (Figure 6).
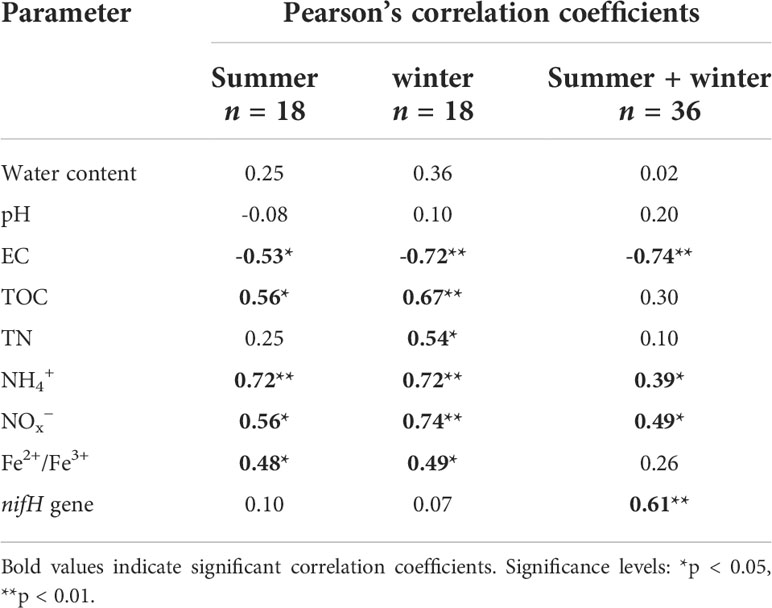
Table 1 Pearson’s correlation coefficients for relations between sediment parameters and N fixation rates.
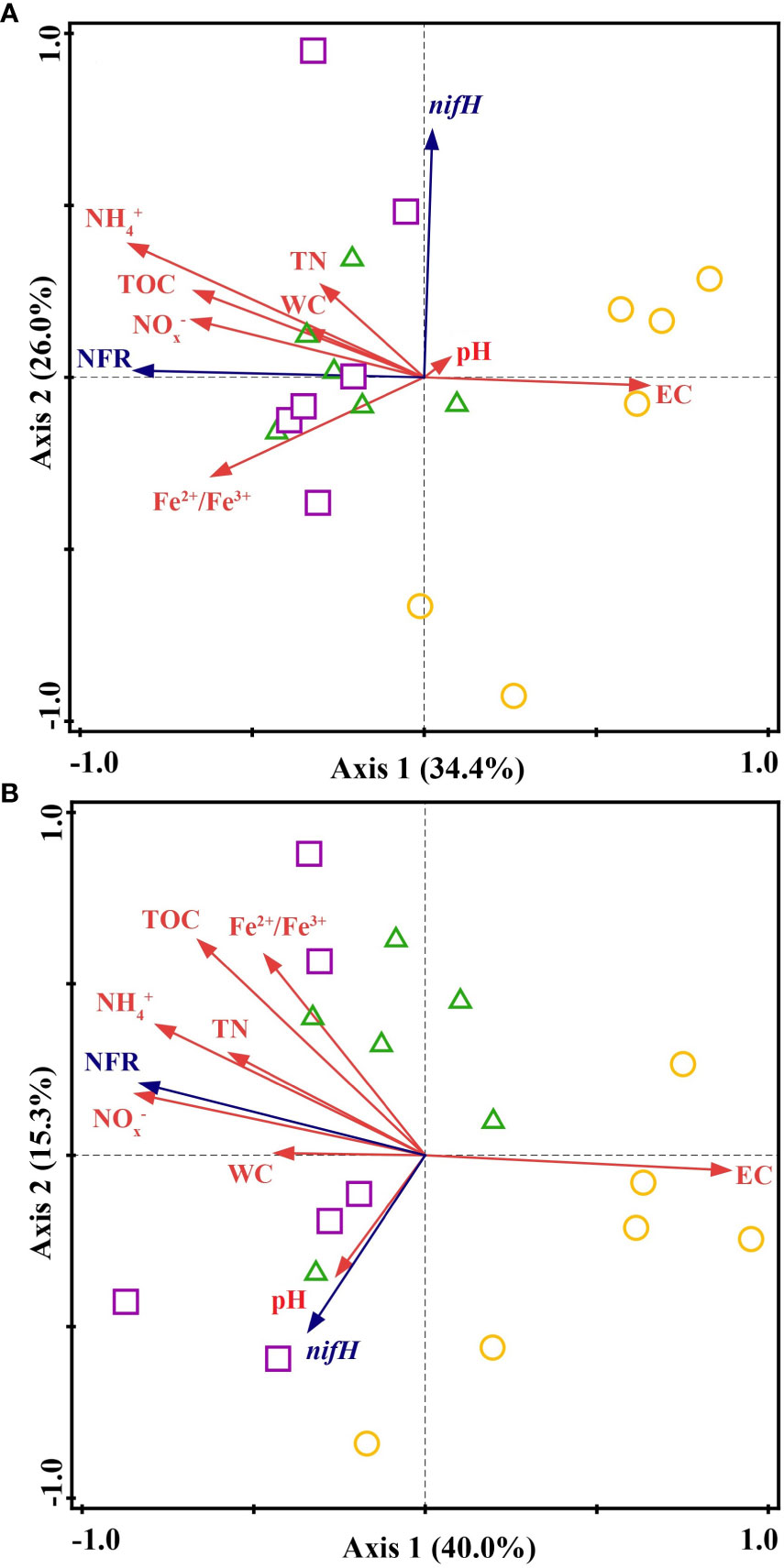
Figure 5 The redundancy analysis (RDA) of N fixation rates (NFR) and nifH gene abundance with sediment parameters. The yellow circles, green triangles and purple squares represent natural saltmarsh, 5-year-old shrimp ponds and 18-year-old shrimp ponds in (A) summer and (B) winter, respectively.
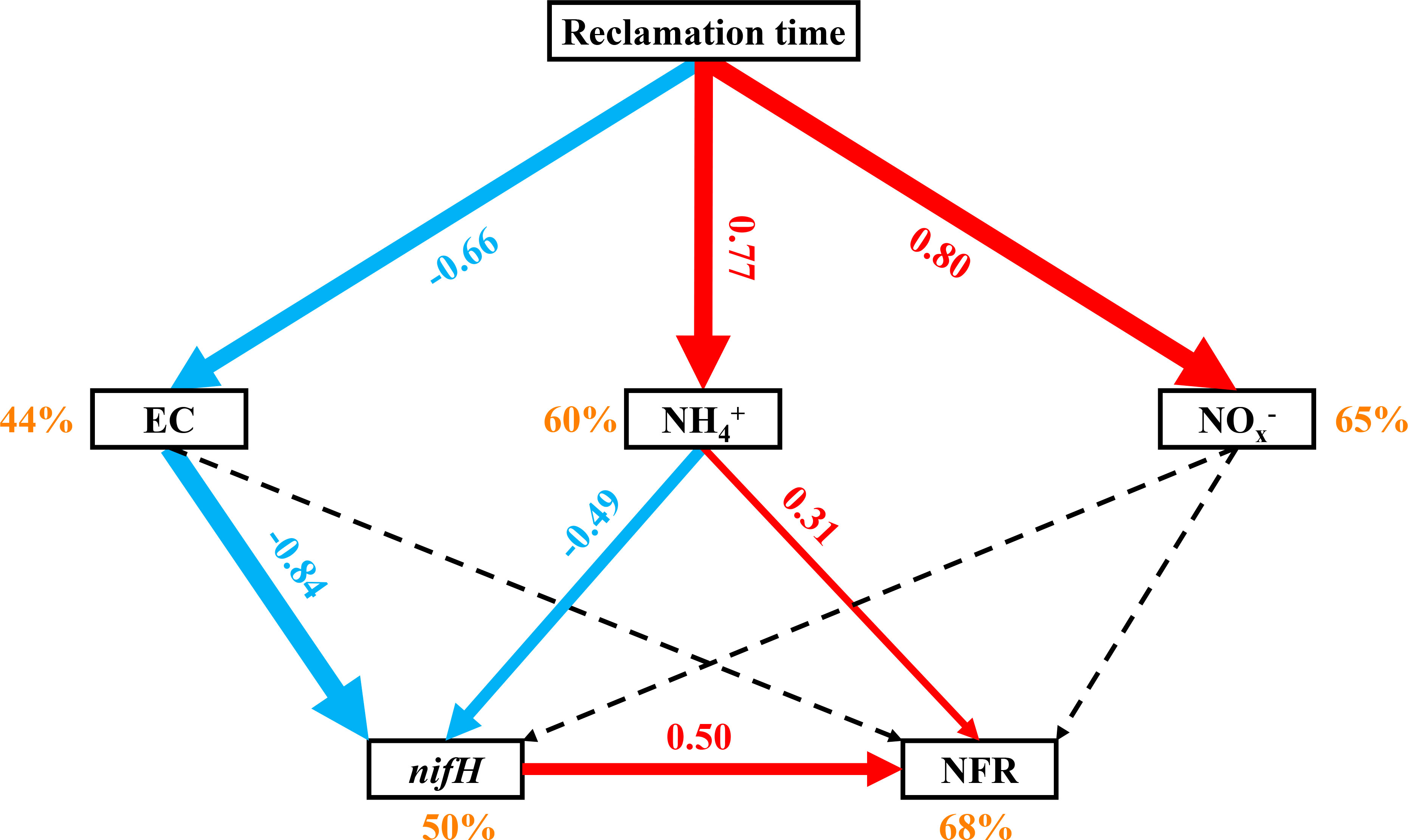
Figure 6 Predicted path models relating reclamation time to N fixation rates (NFR). Solid red lines indicate significant positive effects, solid blue lines indicate significantly negative effects, and black dashed lines indicate insignificant effects. Numbers adjacent to the lines are standardized path coefficients, also shown by the width of the lines. Percentages adjacent to the variables indicate their explained variance.
Discussion
Previous studies suggested that the conversion of natural saltmarsh to aquaculture ponds has a significant influence on nutrient element biogeochemical cycle (Murphy et al., 2016; Gao et al., 2019b). In present study, the sediment potential N fixation rates in shrimp ponds were significantly higher than those in natural saltmarsh (Figure 3), which verified previous studies (Gao et al., 2019b). RDA further indicated that the sediment potential N fixation rates in shrimp ponds were clearly separated from those in natural saltmarsh (Figure 5). This phenomenon could be explained by the changes of sediment physicochemical parameters.
Generally, low salinity was in favor of the metabolism of diazotrophic and N fixation process (Tel-or, 1980; Severin et al., 2012). Previous study shown that shrimp ponds water extracted from adjacent estuary could decrease sediment salinity of original natural saltmarsh (Yang et al., 2017b). In this study, the sediment EC of shrimp ponds were lower than those of natural saltmarsh, which was consistent with previous study (Figure 2C). Thus, decreased sediment EC provided a favorable environment for the N fixation process, which was supported by the significant negative correlation between sediment EC and N fixation rate (Table 1). TOC is another important factor in controlling N fixation process due to its supplement of energy for heterotrophic organisms (Gier et al., 2016; Moseman-Valtierra et al., 2016; Wang et al., 2018a). In present study, sediment TOC content increased significantly after the conversion of natural saltmarsh to shrimp ponds (Figure 2D), which could explain the increasing of the sediment potential N fixation rates. The significant positive correlatin between TOC content and N fixation rate further confirmed this explanation (Table 1). It has been noted that in addition to the quantity of organic matter, the quality of organic matter may play a more important role in mediating the N fixation (Newell et al., 2016). However, we did not analyze the components of organic carbon and future studies will be conducted to explore the impact of different organic carbon fractions on N fixation process. Futhermore, we observed sediment Fe2+/Fe3+ ratios in shrimp ponds were generally higher than those in natural saltmarsh (Figure 2H), indicating that shrimp ponds can stimulate the reduction of Fe3+ to Fe2+ (Gao et al., 2019b). The significant positive correlation of Fe2+/Fe3+ ratio with N fixation rate was observed in present study (Table 1 and Figure 5). Previous studies have noted that iron-reducing bacteria may be also responsible for the N fixation process (Bertics et al., 2010; Gier et al., 2017). Thus, we hypothesize that the reduction of Fe3+ to Fe2+ can indirectly promote the process rates of N fixation.
In addition to environmental parameters, many works have noted that the N fixation process was influenced largely by relevant microorganisms, and nifH gene can be regarded as a critical indicator of N fixation process (Huang et al., 2016; Damashek and Francis, 2018; Hou et al., 2018). Despite the nifH gene abundance in shrimp ponds was higher than that in natural saltmarsh, there was no obvious correlation between nifH gene abundance and sediment potential N fixation rate (Figure 4 and Figure 5), which indicated that compared with the change of sediment physicochemical factors, the change of nifH gene abundance may not be the main factor affecting the N fixation during the conversion of natural saltmarsh to shrimp ponds. In addition to functional gene abundance, the expression of functional gene and the structure and composition of microbial community may have significant impacts on microbial activitiy (Gao et al., 2017). Therefore, the role of microbe on N fixation should be further explored.
Compare with 5-year-old shrimp ponds, 18-year-old shrimp ponds had higher TOC contents and Fe2+/Fe3+ ratios and lower sediment EC (Figure 2), which was expected to cause a higher N fixation rates in 18-year-old shrimp ponds than those in 5-year-old shrimp ponds. Unexpectedly, there was no significant difference in sediment potential N fixation rate between shrimp ponds with different reclamation time (Figure 3 and Figure 5). This phenomenon might be attributed to the inhibiting effect of inorganic N (Capone et al., 2005). Many previous studies found that high concentration of inorganic N, especially NH4+, may inhibit N fixation (Bertics et al., 2010; Moseman-Valtierra et al., 2016). Sediment NH4+ and NOx− in 18-year-old shrimp ponds were generally higher than those in 5-year-old shrimp ponds, which may be the important reason for this unexpected phenomenon. Predicted path model futher indicated that the increasing of reclamation time could promote the concentraions of NH4+, which could inhibit N fixation process (Figure 6).
It’s noteworthy that natural saltmarsh and shrimp ponds had same seasonal dynamics of N fixation process with high N fixation rates in summer and low N fixation rates in winter (Figure 3). This phenomenon might be attributed to the temperature change. Previous studies have documented that temperature rising could directly promote N fixation rate in estuarine and coastal ecosystems (Hou et al., 2018). The temperature of the Min River Estuary was higher in July (31.2 – 33.9°C) than that in November (19.6 − 21.1°C) (Gao et al., 2019b), which caused that both natural saltmarsh and shrimp ponds in Min River Estuary had higher N fixation rates in summer than in winter. In addition to direct effect, temperature may regulate the N fixation process through its impact on relevant microorganisms (Huang et al., 2016; Damashek and Francis, 2018). Our result showed that nifH gene abundance in summer was significantly higher than that in winter, implying the functional gene may be an important factor in controlling the seasonal dynamics of N fixation process. For bulked two-season data, the N fixation rate were significantly associated with the nifH gene abundance, which also supported the aforesaid opinion (Table 1).
In last few decades, anthropogenic reactive N has continually increased due to intensive industrial and agricultural activities (Davidson, 2009). Since about 20–30% of reactive N has been delivered into estuarine and coastal ecosystems, the N transformation is important for the N balance in such ecosystems (Plummer et al., 2015; Huang et al., 2021). Reclamation is one of the main anthropogenic disturbances in coastal wetlands that could change the intrinsic N balance. Based on the measured N fixation rates and sediment densities, we estimated that the amounts of N fixation in natural marshland, 5-year-old and 18-year-old shrimp ponds were about 50.7, 68.3 and 70.2 g N m-2 yr.-1, respectively. Previous study showed that the amounts of N loss in natural marshland, 5-year-old and 18-year-old shrimp ponds were about 88.9, 116.6 and 130.6 g N m-2 yr.-1, respectively (Gao et al., 2019b). By comparing the amounts of N fixation and N loss, we found that natural marshland, 5-year-old and 18-year-old shrimp ponds had same ratio of N fixation to N loss (about 55%), which suggested that although conversion of natural saltmarsh to shrimp ponds simultaneously promoted N fixation and loss, it had no obvious effects on the whole N cycling. Thus, when we evaluate the effects of reclamation on the N budgets in estuarine and coastal wetlands, comprehensive N cycling processes should be taken into full consideration.
Conclusion
This study elucidated the impacts of conversion of natural marshland to shrimp ponds on sediment N fixation process in estuarine and coastal areas. This conversion significantly promoted the process rate of sediment N fixation. However, different reclamation time of shrimp ponds had no significant effects on the sediment N fixation process. The variation of sediment EC, TOC, Fe2+/Fe3+ and inorganic N driven by this conversion were key factors to control sediment N fixation. Futher, the conversion of natural marshland to shrimp ponds simultaneously facilitated sediment N fixation and N loss, highlighting the significance of considering comprehensive N cycling processes when evaluating the environmental implications of aquaculture reclamation on the N budgets in estuarine and coastal wetlands.
Data availability statement
The original contributions presented in the study are included in the article/Supplementary Material. Further inquiries can be directed to the corresponding author.
Author contributions
CL: Investigation, Formal analysis, Writing-original draft, Writing-review and editing. NL: Writing-review and editing. XS: Conceptualization, Funding acquisition, Methodology, Formal analysis, Writing-review and editing, Supervision. DG: Conceptualization, Funding acquisition, Project administration. JX, QC and DZ: Investigation. All authors contributed to the article and approved the submitted version.
Funding
This work was supported by the Natural Science Foundation of China (grant numbers: 31870597, 41901118 and 42101111). It was also funded by the Special fund for cooperation of Zhejiang Province and Chinese academy of forestry (2021SY03); the Youth Innovation Support Program of Shandong Universities [grant number 2021KJ081]; the Shandong Provincial Natural Science Foundation (grant number ZR2021QD101); grants from China Postdoctoral Science Foundation (2021M691020); Open Fund of Key Laboratory of Geographic Information Science (Ministry of Education), East China Normal University [grant number KLGIS2021A03]; the Fundamental Research Funds for the Central Universities; and the PhD research startup foundation of Binzhou University [grant numbers 2021Y14 and 2021Y36].
Acknowledgments
Thanks are given to the editor and anonymous reviewers for valuable comments on this manuscript.
Conflict of interest
The authors declare that the research was conducted in the absence of any commercial or financial relationships that could be construed as a potential conflict of interest.
Publisher’s note
All claims expressed in this article are solely those of the authors and do not necessarily represent those of their affiliated organizations, or those of the publisher, the editors and the reviewers. Any product that may be evaluated in this article, or claim that may be made by its manufacturer, is not guaranteed or endorsed by the publisher.
Supplementary material
The Supplementary Material for this article can be found online at: https://www.frontiersin.org/articles/10.3389/fmars.2022.1034145/full#supplementary-material
References
Aoki L. R., McGlathery K. J. (2017). Push-pull incubation method reveals the importance of denitrification and dissimilatory nitrate reduction to ammonium in seagrass root zone. Limnol. Oceanogr-Meth. 15, 766–781. doi: 10.1002/lom3.10197
Behrendt A., de Beer D., Stief P. (2013). Vertical activity distribution of dissimilatory nitrate reduction in coastal marine sediments. Biogeosciences. 10, 7509–7523. doi: 10.5194/bgd-10-8065-2013
Bertics V. J., Sohm J. A., Treude T., Chow C. E. T., Capone D. G., Fuhrman J. A., et al. (2010). Burrowing deeper into benthic nitrogen cycling: the impact of bioturbation on nitrogen fixation coupled to sulfate reduction. Mar. Ecol. Prog. Ser. 409, 1–15. doi: 10.3354/meps08639
Capone D. G., Burns J. A., Montoya J. P., Subramaniam A., Mahaffey C., Gunderson T., et al. (2005). Nitrogen fixation by trichodesmium spp.: An important source of new nitrogen to the tropical and subtropical north Atlantic ocean. Global. Biogeochem. Cy. 19, GB2024. doi: 10.1029/2004gb002331
Chen Y., Dong S., Wang F., Gao Q., Tian X. (2016). Carbon dioxide and methane fluxes from feeding and no-feeding mariculture ponds. Environ. pollut. 212, 489–497. doi: 10.1016/j.envpol.2016.02.039
Damashek J., Francis C. A. (2018). Microbial nitrogen cycling in estuaries: From genes to ecosystem processes. Estuar. Coast. 41, 626–660. doi: 10.1007/s12237-017-0306-2
Davidson E. A. (2009). The contribution of manure and fertilizer nitrogen to atmospheric nitrous oxide since 1860. Nat. Geosci. 2, 659–662. doi: 10.1038/ngeo608
Deegan L. A., Johnson D. S., Warren R. S., Peterson B. J., Fleeger J. W., Fagherazzi S., et al. (2012). Coastal eutrophication as a driver of salt marsh loss. Nature. 490, 388–392. doi: 10.1038/nature11533
Fulweiler R. W., Brown S. M., Nixon S. W., Jenkins B. D. (2013). Evidence and a conceptual model for the co-occurrence of nitrogen fixation and denitrification in heterotrophic marine sediments. Mar. Ecol. Prog. Ser. 482, 57–68. doi: 10.3354/meps10240
Fulweiler R. W., Heiss E. M., Rogener M. K., Newell S. E., LeCleir G. R., Kortebein S. M., et al. (2015). Examining the impact of acetylene on n-fixation and the active sediment microbial community. Front. Microbiol. 6. doi: 10.3389/fmicb.2015.00418
Gao D. Z., Chen G. X., Li X. F., Lin X. B., Zeng C. S. (2018). Reclamation culture alters sediment phosphorus speciation and ecological risk in coastal zone of southeastern China. CLEAN-Soil. Air. Water. 46, 1700495. doi: 10.1002/clen.201700495
Gao D. Z., Hou L. J., Li X. F., Liu M., Zheng Y. L., Yin G. Y., et al. (2019a). Exotic Spartina alterniflora invasion alters soil nitrous oxide emission dynamics in a coastal wetland of China. Plant Soil. 442, 233–246. doi: 10.1007/s11104-019-04179-7
Gao D. Z., Li X. F., Lin X. B., Wu D. M., Jin B. S., Huang Y. P., et al. (2017). Soil dissimilatory nitrate reduction processes in the spartina alterniflora invasion chronosequences of a coastal wetland of southeastern China: Dynamics and environmental implications. Plant Soil. 421, 383–399. doi: 10.1007/s11104-017-3464-x
Gao D. Z., Liu M., Hou L. J., Lai D. Y. F., Wang W. Q., Li X. F., et al. (2019b). Effects of shrimp-aquaculture reclamation on sediment nitrate dissimilatory reduction processes in a coastal wetland of southeastern China. Environ. pollut. 255, 113219. doi: 10.1016/j.envpol.2019.113219
Gardner W. S., McCarthy M. J., An S., Sobolev D., Sell K. S., Brock D. (2006). Nitrogen fixation and dissimilatory nitrate reduction to ammonium (DNRA) support nitrogen dynamics in Texas estuaries. Limnol. Oceanogr. 51, 558–568. doi: 10.4319/lo.2006.51.1_part_2.0558
Gier J., Löscher C. R., Dale A. W., Sommer S., Lomnitz U., Treude T. (2017). Benthic dinitrogen fixation traversing the oxygen minimum zone off Mauritania (NW Africa). Front. Mar. Sci. 4. doi: 10.3389/fmars.2017.00390
Gier J., Sommer S., Löscher C. R., Dale A. W., Schmitz R. A., Treude T. (2016). Nitrogen fixation in sediments along a depth transect through the Peruvian oxygen minimum zone. Biogeosciences. 13, 4065–4080. doi: 10.5194/bg-13-4065-2016
Hou L. J., Wang R., Yin G. Y., Liu M., Zheng Y. L. (2018). Nitrogen fixation in the intertidal sediments of the Yangtze estuary: Occurrence and environmental implications. J. Geophys. Res-Biogeo. 123, 936–944. doi: 10.1002/2018jg004418
Hou L. J., Zheng Y. L., Liu M., Li X. F., Lin X. B., Yin G. Y., et al. (2015). Anaerobic ammonium oxidation and its contribution to nitrogen removal in china’s coastal wetlands. Sci. Rep-UK. 5, 15621. doi: 10.1038/srep15621
Huang F. J., Lin X. B., Hu W. F., Zeng F., He L., Yin K. D. (2021). Nitrogen cycling processes in sediments of the pearl river estuary: Spatial variations, controlling factors, and environmental implications. Catena. 206, 105545. doi: 10.1016/j.catena.2021.105545
Huang J. X., Xu X., Wang M., Nie M., Qiu S. Y., Wang Q., et al. (2016). Responses of soil nitrogen fixation to Spartina alterniflora invasion and nitrogen addition in a Chinese salt marsh. Sci. Rep-UK. 6, 20384. doi: 10.1038/srep20384
Lin X. B., Li X. F., Gao D. Z., Liu M., Cheng L. (2017). Ammonium production and removal in the sediments of shanghai river networks: Spatiotemporal variations, controlling factors, and environmental implications. J. Geophys. Res-Biogeo. 122, 2461–2478. doi: 10.1002/2017jg003769
Lin G. M., Lin X. B. (2022). Bait input altered microbial community structure and increased greenhouse gases production in coastal wetland sediment. Water. Res. 218, 118520. doi: 10.1016/j.watres.2022.118520
Li S., Twilley R. R., Hou A. X. (2021). Heterotrophic nitrogen fixation in response to nitrate loading and sediment organic matter in an emerging coastal deltaic floodplain within the Mississippi river delta plain. Limnol. Oceanogr. 66, 1961–1978. doi: 10.1002/lno.11737
Liu W. Z., Yao L., Jiang X. L., Guo L. D., Cheng X. L., Liu G. H. (2018). Sediment denitrification in Yangtze lakes is mainly influenced by environmental conditions but not biological communities. Sci. Total. Environ. 616–617, 978–987. doi: 10.1016/j.scitotenv.2017.10.221
Lovley D. R., Phillips E. J. P. (1987). Rapid assay for microbially reducible ferric iron in aquatic sediments. Appl. Microbiol. Biot. 53, 1536–1540. doi: 10.1128/aem.53.7.1536-1540.1987
Moseman-Valtierra S., Levin L. A., Martin R. M. (2016). Anthropogenic impacts on nitrogen fixation rates between restored and natural Mediterranean salt marshes. Mar. Ecol. 37, 370–379. doi: 10.1111/maec.12289
Murphy A. E., Anderson I. C., Smyth A. R., Song B., Luckenbach M. W. (2016). Microbial nitrogen processing in hard clam (Mercenaria mercenaria) aquaculture sediments: The relative importance of denitrification and dissimilatory nitrate reduction to ammonium (DNRA). Limnol. Oceanogr. 61, 1589–1604. doi: 10.1002/lno.10305
Murray N. J., Phinn S. R., DeWitt M., Ferrari R., Johnston R., Lyons M. B., et al. (2019). The global distribution and trajectory of tidal flats. Nature. 565, 222–225. doi: 10.1038/s41586-018-0805-8
Newell S. E., McCarthy M. J., Gardner W. S., Fulweiler R. W. (2016). Sediment nitrogen fixation: A call for re-evaluating coastal n budgets. Estuar. Coast. 39, 1626–1638. doi: 10.1007/s12237-016-0116-y
Plummer P., Tobias C., Cady D. (2015). Nitrogen reduction pathways in estuarine sediments: Influences of organic carbon and sulfide. J. Geophys. Res-Biogeo. 120, 1958–1972. doi: 10.1002/2015jg003057
Schuerch M., Spencer T., Temmerman S., Kirwan M. L., Wolff C., Lincke D. (2018). Future response of global coastal wetlands to sea-level rise. Nature. 561, 231–234. doi: 10.1038/s41586-018-0476-5
Severin I., Confurius-Guns V., Stal L. J. (2012). Effect of salinity on nitrogenase activity and composition of the active diazotrophic community in intertidal microbial mats. Arch. Microbiol. 194, 483–491. doi: 10.1007/s00203-011-0787-5
Sun Z. G., Sun W. G., Tong C., Zeng C. S., Yu X., Mou X. J. (2015). China’s coastal wetlands: Conservation history, implementation efforts, existing issues and strategies for future improvement. Environ. Int. 79, 25–41. doi: 10.1016/j.envint.2015.02.017
Täumer J., Kolb S., Boeddinghaus R. S., Wang H. T., Schöning I., Schrumpf M., et al. (2020). Divergent drivers of the microbial methane sink in temperate forest and grassland soils. Global. Change. Biol. 27, 929–940. doi: 10.1111/gcb.15430
Tel-Or E. (1980). Response of N2-fixing cyanobacteria to salt. Appl. Environ. Microb. 40, 689–693. doi: 10.1128/aem.40.4.689-693.1980
Tong C., Wang W. Q., Huang J. F., Gauci V., Zhang L. H., Zeng C. S. (2012). Invasive alien plants increase CH4 emissions from a subtropical tidal estuarine wetland. Biogeochemistry. 111, 677–693. doi: 10.1007/s10533-012-9712-5
Tong C., Wang W. Q., Zeng C. S., Marrs R. (2010). Methane emissions from a tidal marsh in the Min river estuary, southeast China. J. Environ. Sci. Heal. A. 45, 506–516. doi: 10.1080/10934520903542261
Wang W. M., Hu M. J., Ren H. C., Li J. B., Tong C., Musenze R. S. (2018b). Seasonal variations of nitrous oxide fluxes and soil denitrification rates in subtropical freshwater and brackish tidal marshes of the Min river estuary. Sci. Total. Environ. 616–617, 1404–1413. doi: 10.1016/j.scitotenv.2017.10.175
Wang R., Li X. F., Hou L. J., Liu M., Zheng Y. L., Yin G. Y., et al. (2018a). Nitrogen fixation in surface sediments of the East China Sea: Occurrence and environmental implications. Mar. pollut. Bull. 137, 542–548. doi: 10.1016/j.marpolbul.2018.10.063
Yang P., Bastviken D., Lai D. Y. F., Jin B. S., Mou X. J., Tong C., et al. (2017a). Effects of coastal marsh conversion to shrimp aquaculture ponds on CH4 and N2O emissions. Estuar. Coast. Shelf. S. 199, 125–131. doi: 10.1016/j.ecss.2017.09.023
Yang P., Lai D. Y., Jin B. S., Bastviken D., Tan L. S., Tong C. (2017b). Dynamics of dissolved nutrients in the aquaculture shrimp ponds of the Min river estuary, China: Concentrations, fluxes and environmental loads. Sci. Total. Environ. 603, 256–267. doi: 10.1016/j.scitotenv.2017.06.074
Yang P., Zhang Y., Yang H., Zhang Y. F., Xu J., Tan L. S., et al. (2019). Large Fine-scale spatiotemporal variations of CH4 diffusive fluxes from shrimp aquaculture ponds affected by organic matter supply and aeration in southeast China. Biogeosciences. 124, 1290–1307. doi: 10.1029/2019jg005025
Zhang W. L., Zeng C. S., Tong C., Zhai S. J., Lin X., Gao D. Z. (2015). Spatial distribution of phosphorus speciation in marsh sediments along a hydrologic gradient in a subtropical estuarine wetland, China. Estuar. Coast. Shelf. S. 154, 30–38. doi: 10.1016/j.ecss.2014.12.023
Keywords: N fixation, N cycling, agricultural reclamation, shrimp aquaculture, coastal wetland, environmental implication
Citation: Liu C, Li N, Shao X, Gao D, Xia J, Cui Q and Zhang D (2022) Effects of coastal marsh conversion to shrimp aquaculture ponds on sediment nitrogen fixation. Front. Mar. Sci. 9:1034145. doi: 10.3389/fmars.2022.1034145
Received: 01 September 2022; Accepted: 14 September 2022;
Published: 28 September 2022.
Edited by:
Xianbiao Lin, Ocean University of China, ChinaCopyright © 2022 Liu, Li, Shao, Gao, Xia, Cui and Zhang. This is an open-access article distributed under the terms of the Creative Commons Attribution License (CC BY). The use, distribution or reproduction in other forums is permitted, provided the original author(s) and the copyright owner(s) are credited and that the original publication in this journal is cited, in accordance with accepted academic practice. No use, distribution or reproduction is permitted which does not comply with these terms.
*Correspondence: Xuexin Shao, c2hhb3h1ZXhpbkAxMjYuY29t