- 1State Key Laboratory of Freshwater Ecology and Biotechnology, Institute of Hydrobiology, Chinese Academy of Sciences, Wuhan, China
- 2College of Life Sciences, China West Normal University, National Freshwater Fisheries Engineering Technology Research Center (Wuhan) Southwest Branch, Nanchong, China
- 3College of Advanced Agricultural Sciences, University of Chinese Academy of Sciences, Beijing, China
- 4Chinese Sturgeon Research Institute, China Three Gorges Corporation, Yichang, China
- 5Hubei Key Laboratory of the Three Gorges Project for Conservation of Fishes, Yichang, China
- 6Key Laboratory of Freshwater Biodiversity Conservation and Utilization, Ministry of Agriculture/Yangtze River Fisheries Research Institute, Chinese Academy of Fisheries Science, Wuhan, China
- 7Fish Ecology and Conservation Physiology Laboratory, Department of Biology and Institute of Environmental and Interdisciplinary Science, Carleton University, Ottawa, ON, Canada
Differences in individual personality are common amongst animals, which can play an ecological and evolutionary role given links to fitness. Personality affects animal life processes and outputs (e.g., behavior, life history, growth, survival, reproduction), and has become a common theme in animal behavioral ecology research. In the present study, we used Siberian Sturgeon to explore how personality traits of boldness and shyness are related to swimming performance, post exercise recovery and phenotypic morphology. Firstly, our results indicated that the Siberian sturgeon juveniles of shyness were better swimmers, validating evolutionary biology trade-off theory. The critical swimming speed (Ucrit) of the shy groups was higher than that of the bold groups. Secondly, the shy groups were more resilient after exercise fatigue. The swimming fatigue recovery ability, the glucose and lactic acid concentration recovery ability of shy groups were greater than that of bold groups. Thirdly, the shy groups were more streamlined. Compared with bold groups, shy groups had smaller caudate stalk lengths, caudate stalk heights, superior caudal lobes, and inferior caudal lobes. In general, we demonstrated that shy Siberian sturgeon had better swimming performance from physiology and morphology. These research results further enrich the theoretical viewpoints of fish behavior biology, more importantly, which provided a good example for studying the relationship between sturgeon’s “personality” and swimming performance.
Introduction
Animal personality has been broadly defined as interindividual differences in behavior that remain consistent over time and contexts (Slater, 1981; Smith, 1982; Caro and Bateson, 1986; Clark and Ehlinger, 1987; Réale et al., 2007; Roche et al., 2016). Research on animal personality has covered diverse taxa including mammals, birds, reptiles, amphibians, fish, arthropods and mollusks (Gosling, 2001). Personality affects animal life processes and fitness, and has become the basis for much research in behavioral ecology (Réale et al., 2007). One of the most commonly focused dimensions of personality is the shyness-boldness continuum. The terms shy and bold refer to the propensity of an individual to take risks, especially in novel environments (Brown et al., 2007). Shy individuals react to novelty by retreating, reducing activity levels, and becoming more vigilant. In contrast, bold individuals are more likely to approach novel objects and increase activity levels and exploratory behavior (Brown et al., 2007). In the case of fish, personality influences various behaviors (including feeding, reproduction, migration, etc.) and is related to physiological processes (e.g., metabolism, immunity), cognitive abilities, morphology, and thus to growth and survival (Zhang and Wang, 2021).
The study of swimming performance in fish species has a long history, classified as steady or unsteady swimming (Webb, 1984; Langerhans, 2009b; Domenici, 2010). The trade-off between the two primary swimming modes, steady and unsteady swimming, is presumed to exist in fish species employing relatively coupled locomotor systems (i.e. the same morphological structures are used for propulsion during both steady and unsteady swimming) (Webb, 1978; Webb, 1984). The trade-off is thought to be of general evolutionary importance in fish (Lighthill, 1969; Webb, 1982; Lighthill, 2006; Langerhans, 2007; Langerhans, 2008). From an ecological and evolutionary perspective, the trade-off can occur when an increase in fitness due to a change in one trait is opposed by a decrease in fitness due to a concomitant change in another characteristic (Stephens and Krebs, 1986; Roff, 1992; Reznick et al., 2000). For example, the northern pike (Esox lucius) has much higher swimming velocities during acceleration tests but is a poor endurance swimmer (Harper and Blake, 1990). Fitness trade-off is an ecological mechanism that produces and maintains individual behavioral differences within a population (Kern et al., 2016). Personality may be one of the reasons for differences in individual swimming performance within a population. Shy zebrafish (Danio rerio) often avoid predators (‘flight’) and exhibit higher steady-swimming performance and, thus, greater energetic efficiency during routine activities. In contrast, bold zebrafish usually adopt a defensive strategy against predators (‘fight’), showing higher fast-start swimming performance, achieving greater access to food resources and higher foraging efficiency; meanwhile, the risk of predation is higher (Kern et al., 2016). The cost-benefit trade-offs associated with energy expenditure and personality differences may be one of the most critical ecological mechanisms for the generation and coexistence of different swimming performances in fish (Stamps, 2007).
Significant variations in swimming performance exist among fishes, and morphology is considered the primary determinant of this diversity of swimming performance (Webb, 1984). Morphological characteristics associated with swimming can affect the performance of critical activities such as foraging, predator evasion and migration (Bruckerhoff and Magoulick, 2017). Morphology differences in body and fin shape are thought to be strong selective pressures acting upon swimming phenotypes (Webb, 1984; Blake, 2010). For instance, Australian smelt (Retropinna semoni) from river populations tended to have dorso-ventrally deeper bodies and larger heads compared to reservoir conspecifics, with a narrower, fusiform body shape and smaller head (Svozil et al., 2020). Morphological variation associated with different personality types may also be an essential manifestation of variation in fish swimming performance. Studies on zebrafish across numerous generations have found that bold, exploratory, and risk-taking individuals tend to have a more slender body and more significant caudal regions, with high fast-start swimming performance and low, stable swimming performance, which can improve survival rates in predatory encounters (Walker et al., 2005; Langerhans, 2009a). In some cases, certain personalities only enhance swimming performance when combined with a specific morphological characteristic (Roff and Fairbairn, 2012). The association between the morphology and swimming performance of fish with different personality traits requires further research.
Physiological variation associated with different personality types may also be an essential manifestation of variation in fish swimming performance (Careau et al., 2008; Biro and Stamps, 2010; Reale et al., 2010). Physiological specialization is considered one of the main determinants of diversity in locomotor capacities (Reidy et al., 2000). The metabolic rate of risk-taking carp (Cyprinus carpio) is significantly higher than that of risk-avoiding individuals (Huntingford et al., 2010). Moreover, cortisol receptor gene expression, plasma glucose and lactate levels are considerably lower in risk-taking carp than in risk-avoiding conspecifics (Huntingford et al., 2010). Bold mulloway (Argyosomus japonicus) exhibits lower cortisol levels than shy individuals (Raoult et al., 2012). During uniformly accelerated swimming to fatigue of White bream (Parabramis pekinensis), plasma glucose concentration decreased while lactic acid concentration increased (Wang, 2015). Yet, these studies focus on a single relationship between personality and physiology or swimming performance. Research on how fitness regulates the relationship between physiological performance and swimming performance of fish with different personalities is notably lacking.
Siberian sturgeon (Acipenser baerii), belonging to the class Osteichthyes, Actinopterygii, Chondrostei, Acipenseriformes, is the earliest specific trans-estuarine fish group. The species achieves a large body size, has a long life span, and has extremely important scientific value in studying evolutionary biology (Birstein et al., 1997). Here, we tested some theoretical concepts in evolutionary biology, 1) Are there personality differences between individuals of Siberian sturgeon? 2) What are the effects of personality differences on swimming performance? 3) What are the effects of personality differences on morphology and physiological performance, which correlates with swimming performance? Findings from this work will further enrich the theoretical knowledge pertaining to sturgeon evolutionary biology and may help inform conservation efforts for endangered sturgeon species in artificial propagation programs.
Materials and methods
Source of fish and rearing condition
One hundred juvenile Siberian sturgeon (mean total length ± SD =15.48 ± 1.80 cm and mean body weight ± SD = 13.13 ± 3.82 g) were collected from Wanming Fishery hatchery farm in Jingmen City, Hubei Province, China. Sturgeons are transported and housed in a recirculating aquaculture system (the following abbreviation is RAS; Yuya Technology (Shanghai) Co., LTD) at the laboratory of Institute of Hydrobiology, Chinese Academy of Sciences. Water temperature was maintained at 23.0 ± 0.5°C; dissolved oxygen was greater than 6 mg/L; pH was maintained at 7~8; And 12 h:12 h light: dark regime were based on natural lighting (lights on at 0700 hours). The Siberian sturgeons were fed on 3% of body weight commercial food twice at 8:00 am and 6:00 pm per day. Healthy fish were randomly selected for experimentation with food withheld for 48h before each experiment.
Experimental overview
To control for acclimatization effects, experiments started a month after laboratory housing. We started with the personality test and subjected fish to a classic “boldness” assay (Kern et al., 2016). We then assigned individuals to the boldness and shyness group and returned them to the RAS. After 2 rest days, we conducted the swimming performance test. A total of 30 bold individuals and 30 shy individuals were tested. Once the swimming performance test was completed, the individual was anaesthetized with MS-222 (0.033 g/L), and blood was sampled for physiological status analysis. Body weight was measured, and pictures of the body were taken (Nikon D810) for morphology analysis. To control the potential circadian rhythm affect, all the tests were conducted from 8:00 am to 12:00 am.
Personality test
A dark/light maze that was divided by a baffle into one shaded (“dark”) arm (50 × cm) with a lid on top and one exposed (“light”) arm (50 × cm) was used to assess “boldness” (Figure 1). The black cloth around the maze minimized external visual disturbance. The behavior of the test fish was recorded with a web camera (BASLER, acA1920-155uc, made in Germany, 25 frames/s) placed about 1.5 m above the maze. For each personality experiment, fish were selected randomly and transferred into a black container (10L) positioned adjacent to the test device. The container was filled with water with the same properties as the RAS. Fish were gently transferred into the shaded arms with the baffle “closed”. After a two-minute acclimation period, the baffle was removed, and the exploratory behavior of the tested fish was recorded with cameras. A total of eight minutes were recorded, after which individuals were returned to a separate glass holding tank for further experiments.
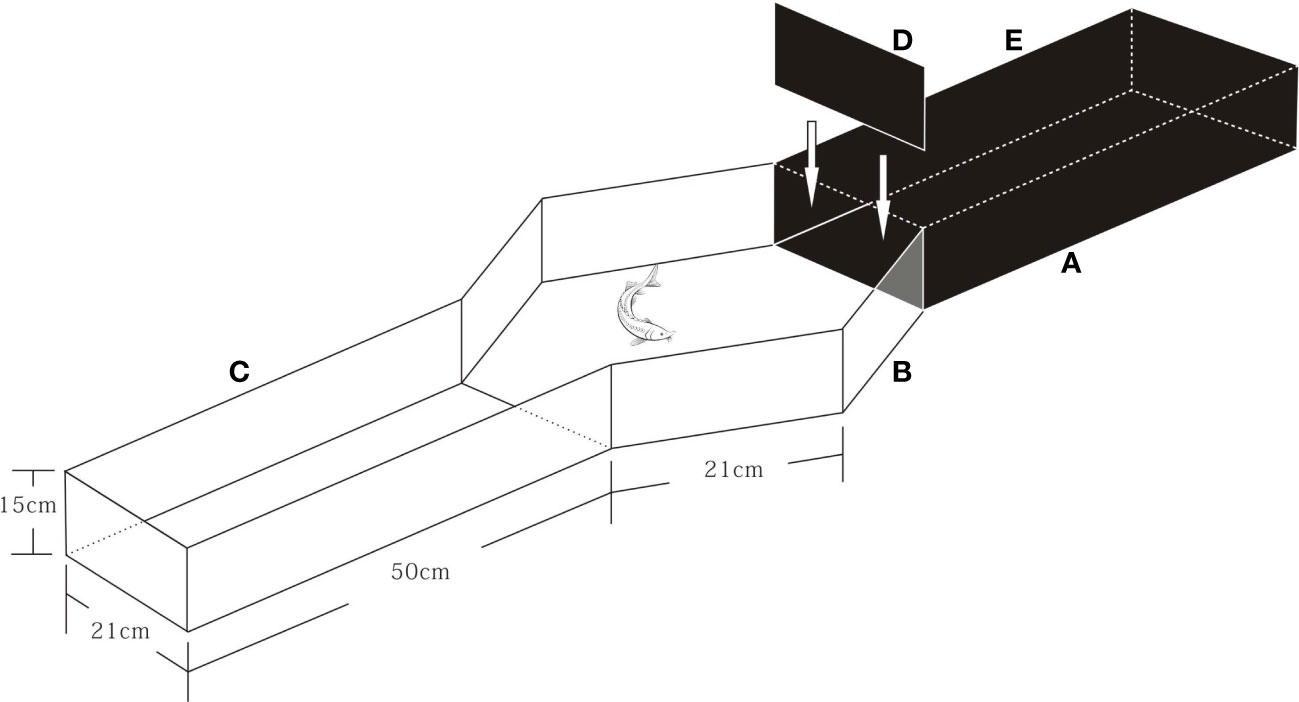
Figure 1 Experimental apparatus for testing fish personality. The device was divided into 3 parts: (A) is a cuboid dark area (length×width×height = 50cm×21cm×15cm), equipped with a black opaque lid (E) and an opaque baffle (D); (B) is a hexagon lit area (side length = 21cm); (C) is a lit area with the same shape as A.
Time spent in the dark arms was an indication of “boldness”. Bold individuals were defined as individuals that spent more than four minutes in the exposed arms as per (Brown et al., 2005; Maximino et al., 2010), and vice versa. However, if the fish still had not emerged after eight minutes, the trial was terminated, and the individual was allocated a ceiling value of 480 s (Brown et al., 2005; Maximino et al., 2010). In addition, the animal behavior analysis system (Noldus, Ethovision XT10.0, The Netherlands) was also used to analyze the cumulative exploration time of experimental fish in the exposed arms. The water within the maze was replaced completely between individuals for each experiment. Each individual was tested no more than three times, with each retest occurring three days apart to assess short-term repeatability. The fish with the same results in two continuous tests was defined as the bold individual or shy individual. Otherwise, the experimental fish will be discarded, and the selection will be made again.
Swimming performance test
We conducted the swimming performance test following the personality test. A self-manufactured swimming tunnel combined with a web camera (fixed 1.5 m above the swimming device; BASLER, acA1920-155uc, made in Germany, 25 frames/s) was used to assess swimming performance (Figure 2). The LS300-type portable flow rate measuring instrument was used to calibrate the flow rate, and a linear relationship was established between the flow rate (Y) and the adjustment frequency (X): Y= 0.2517X + 0.6231, R=0.9975 (Figure 3).
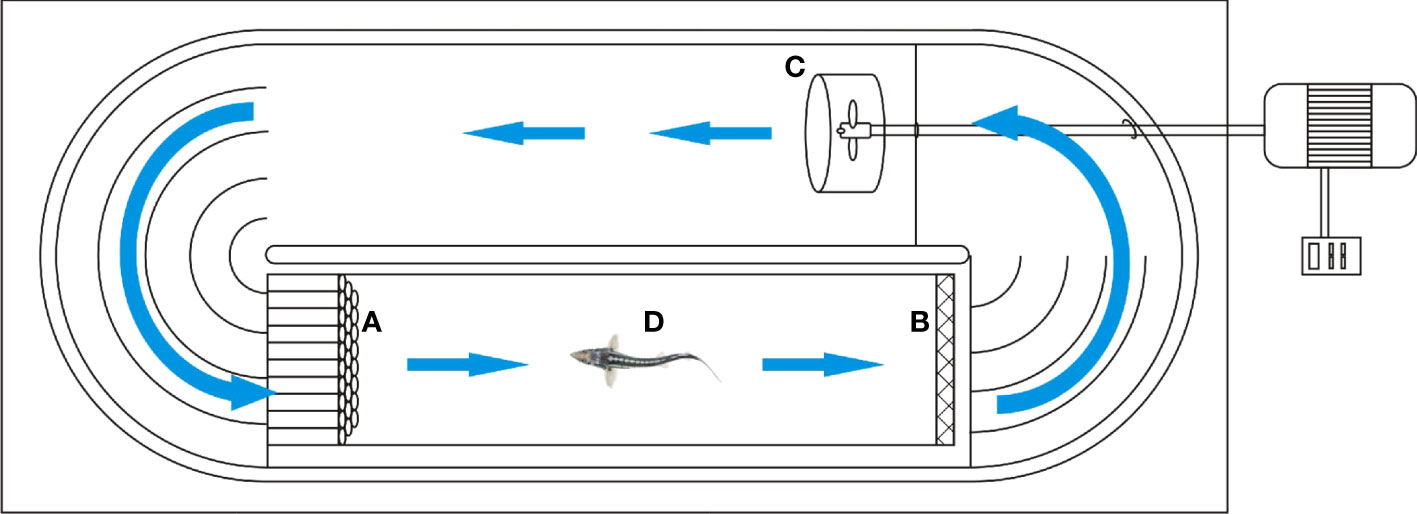
Figure 2 Experimental apparatus for testing swimming performance. (A) is the rectifier; (B) is the wire mesh; (C) is the propeller; (D) is the swimming test area (50cm×10cm×10cm); The frequency converter controls the motor, and the motor controls the three-blade propeller to create water flow, and the water circulates in the oval swimming tank, and the direction of the blue arrow is the direction of the water flow.
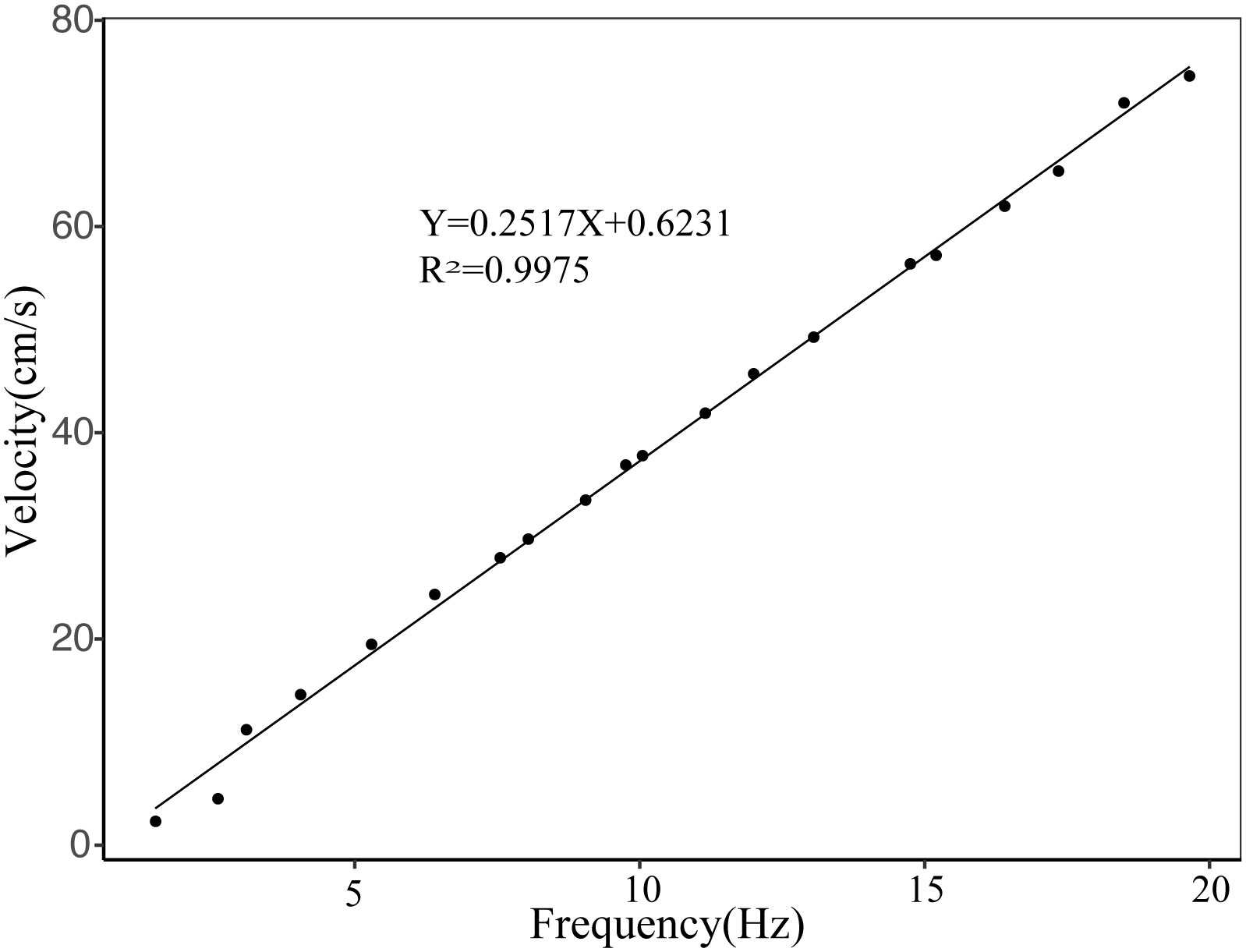
Figure 3 The relationship between frequency and velocity. Control the speed of water flow by adjusting the frequency of the inverter of the device of swimming performance test.
Stepped velocity tests were carried out to measure the critical swimming speed of each fish (Ucrit) (Hammer, 1995). The test fish was measured for body length and introduced to the tunnel to acclimate at 0.5 BL/s for 2 h. Then the water velocity was increased by 1 BL/s every 20 min until the fish became fatigued (Shi et al., 2012; Downie and Kieffer, 2017). Fatigue was defined as the point at which the fish could no longer swim away from the steel wire mesh positioned downstream of the swimming area (> 20 s) (Lee et al., 2003). The water velocity and swimming fatigue time (Endurance: defined as the time it took for the fish to stop swimming and fall back to the downstream wire mesh) were recorded. The flow speed was then decreased to 0.5 BL/s for a 60 min recovery test, and the recovery time was defined as the time the fish removed itself from the steel mesh and began swimming once again. Then a second stepped velocity test was undertaken (the test method was the same as the first time). In each trial, the water in the tunnel was the same as that of the RAS described above. The water was kept aerated when no fish were tested. Videos were playback by Quick Time Player at 0.5 times the speed for the tail beat frequency analysis.
Ucrit were calculated according to equation (1) (Brett, 1964):
where U (cm/s) is the highest velocity at which fish swam for the full-time interval ΔU, m/s) is the speed step, t (min) is the time to fatigue during the last velocity step, and Δt (min) is the time step (20 min). The body cross-sectional area of the test fish in this study did not exceed 10% of the cross-sectional area of the swimming area, minimizing the need for a Ucrit correction (Jain, 2003; Wang, 2009).
The recovery ratio (R) of Ucrit (cm/s) was a measure of a fish’s ability to recover from fatigue. A lower R-value indicates incomplete recovery and a larger effect of fatigue on subsequent swimming performance. The value of R was calculated using equation (2) (Farrell et al., 1998):
where Ucrit1 and Ucrit2 indicated the Ucrit of the first stepped velocity test and second stepped velocity test, respectively.
One minute per five minutes videos was selected for swimming states analysis, and the average value for each flow velocity gradient in the test was calculated. The swimming states of fish were classified into four types according to swimming speed: countercurrent forward: V=M+N, countercurrent stationery: V=M, Float downstream: V=M-N, and countercurrent backward: V=N-M. The proportion of each swimming state (P) was also calculated (Iwata, 1995). The value of P (%) was calculated using equation (3):
Where V is the actual swimming speed, M is the flow velocity, and N is the instantaneous swimming speed of the experimental fish measured by the Ethovision XT10.0. t1 is the duration of a specific swimming state (s), and T is the total observation time (s).
The tail beat frequency (TBF) is the complete tail-beating process of the experimental fish while swimming (Ohlberger et al., 2007). Every five minutes, a 20 s period of the video was selected from the whole video to calculate TBF. For each velocity, the value of TBF (times/s) was calculated using equation (4):
TBT is the total number of tail beats of each fish during the period of observation, and t is the total time of observation.
Physiological status test
After the swimming performance test was completed, the fish was anaesthetized with MS-222 (33 mg/L), and blood was sampled. Body weight was measured, and pictures of the body were taken (Nikon D810) for morphology analysis. Then returned, the fish to a holding tank (70×50×20 cm) for recovery. Blood was sampled three times at 0 h, 3 h and 24 h, respectively, to understand the recovery pattern of these blood physiology parameters following swimming. In addition, the blood of eight untreated fishes was sampled and analyzed to rule out the effect of MS-222. The blood samples were stored in a refrigerator (4°C) for 2 hours prior to centrifugation (4000 r/min, 20 min), while the centrifugal supernatant was stored separately at -20°C. The supernatant sample was bathed in 4°C water to prevent sample distortion before being tested. Physiological indicators were measured using kits developed by the Nanjing Jiancheng Institute of Biological Engineering and a 721 UV spectrophotometer (Svalheim et al., 2017; Yuan et al., 2018). Glucose, lactic acid and total protein were analyzed to characterize energy consumption in this study, which was determined by the glucose oxidase, colorimetric and biuret methods. All tests were carried out in strict accordance with the kit’s instructions (http://www.njjcbio.com).
Morphological characteristics measurement
Morphological characteristics were analyzed by TPS series software (http://life.bio.sunysb.edu/morph). Twenty-one landmark points were selected to obtain the corresponding x, y coordinate values (2D) for geometric morphometry by tpsDig2 (Figure 4) (Bookstein, 1997). The validity of the landmark points was tested by tpsSmall. Procrustes analysis was used to superimpose the landmark points of all samples by tpsRelw, and the landmark points of each sample were translated, centered, rotated and zoomed. The centroid distance and mean shape were calculated, and the partial warp and relative warp principal component analyses (RWA) were performed. Lastly, the relative warp scores (RW) were used for subsequent analysis (Rohlf, 1990; Rohlf, 1993). The relative warp (RW) was mapped on the thin plate spline to visualize the results (Bookstein, 1989), while a grid deformation map of all samples was constructed using tpsRegr to analyze and compare morphological differences.
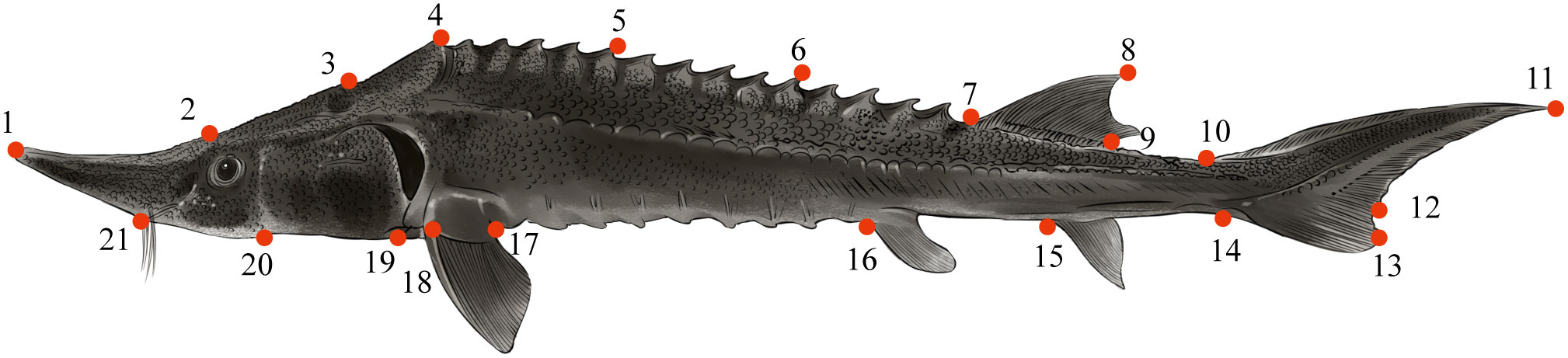
Figure 4 The 21 landmarks used for examination of lateral body shape (boldness depicted). 1. Snout tip; 2. Upper snout end; 3. Upper head end; 4. Dorsal origin; 5-6. Dorsal rhomboid primitive spikes; 7. Origin of dorsal fin base; 8. Highest point of dorsal fin; 9. Base end of dorsal fin; 10. Dorsal origin of caudal fin; 11. Upper lobe terminal of caudal fin; 12. Deepest part of the caudal fin; 13. Lower lobe terminal caudal fin; 14. Ventral origin of caudal fin; 15. Origin of anal fin; 16. Origin of pelvic fin; 17. End of pectoral fin; 18. Origin of pectoral fin; 19. Lower head end; 20. Base of operculum; 21. Lower snout end.
Data analysis
T-tests were used to compare the initial differences in body length and weight between bold and shy groups. Two-way repeated measures ANOVA in a general linear model (GLM) was used to assess for differences in swimming performance an physiological indicators between different personality types (shy and bold) over time. The subject’s internal factors included personality (two levels: “bold” and “shy”) and test (two levels of test: the first and the second stepped velocity test, which formed 4 treatment groups; physiological test at three levels: 0 h, 3 h, 24 h, a total of 6 treatment groups). The dependent variables were swimming performance which included critical swimming speed, fatigue time, fatigue recovery time, and physiological index. For each treatment group, all response variables were evaluated for normality and equal variance using the Shapiro-Wilkins normality test as well as density plots. If the data was not normally distributed, it was converted logarithmically. Before assessing whether there was an interaction between personality and testing time, it was necessary to evaluate whether the interaction conforms to Mauchly’s Test of Sphericity. When the conditions of the spherical hypothesis were violated (p< 0.05), an epsilon(ϵ) correction was required, and for epsilon(ϵ)< 0.75, the Greenhouse-Geisser method was used for correction, while for epsilon(ϵ) > 0.75, the Huynh-Feldt method was used. When the condition of the spherical hypothesis was satisfied, the covariance matrix of the dependent variable for interaction term personality*time was equal (p > 0.05). Then, we analyzed whether the effect of the interaction on the dependent variables was statistically significant. If the interactions were significant, differences in the swimming performance and physiological index concentrations between the bold and shy groups were compared at different time levels to test the separate effects of the treatment group and to examine the impact of time alone by comparing differences in time factors between the two personality groups. When only two groups were compared, there was no need to test the spherical hypothesis. Otherwise, the main effects of personality and time were analyzed. If the main effect of a factor with more than two intra-level groups was significant, a pairwise comparison was required. In addition, a one-way analysis of variance (ANOVA) was used to compare differences in the physiological index concentrations at different time levels between the bold and shy groups and the control group. Differences in the time ratio of swimming state and the tail beat frequency across personality groups were tested by t-test. Hotelling T2 method of multivariate analysis of variance (MANOVA) in a general linear model (GLM) was used to examine and compare differences in relative distortion scores of fish with either personality group. All data analysis and plots were performed in R Studio Version 1.3.1, and significance was considered at an α level of 0.05.
Results
Personality identification
Consistent interindividual variation in fish’s tendency to explore the exposed arms was founded. According to the proportion of the cumulative time spent exploring the bright area, 60 individuals could be clearly identified as a personality group, which included 30 bold individuals and 30 shy individuals.
Swimming performance of different personalities of the Siberian sturgeon
There were no differences in body length and weight between the bold and shy groups. For the swimming performance experiment, the interaction of personality and time significantly affected Ucrit (F1,23 = 9.16, p< 0.001). The single effects of personality indicated that the Ucrit of shy groups was significantly higher than that of bold groups (F1,23 = 18.51, p< 0.05). The Ucrit of the shy groups increased in the second time test (F1,23 = 1.12, p > 0.05), while that of the bold groups decreased significantly (F1,32 = 8.87, p< 0.05) (Table 1; Figures 5A, B). In addition, the recovery rate of the shy groups (R=104%) was higher than (p > 0.05) for the bold groups (R=95%). The interaction between personality and time had no significant effect on the fatigue recovery time of test fish (F1,22 = 1.59, p > 0.05), and the main effects of personality and time had no significant impact on the fatigue recovery time of test fish (p > 0.05) (Table 1; Figure 5C).
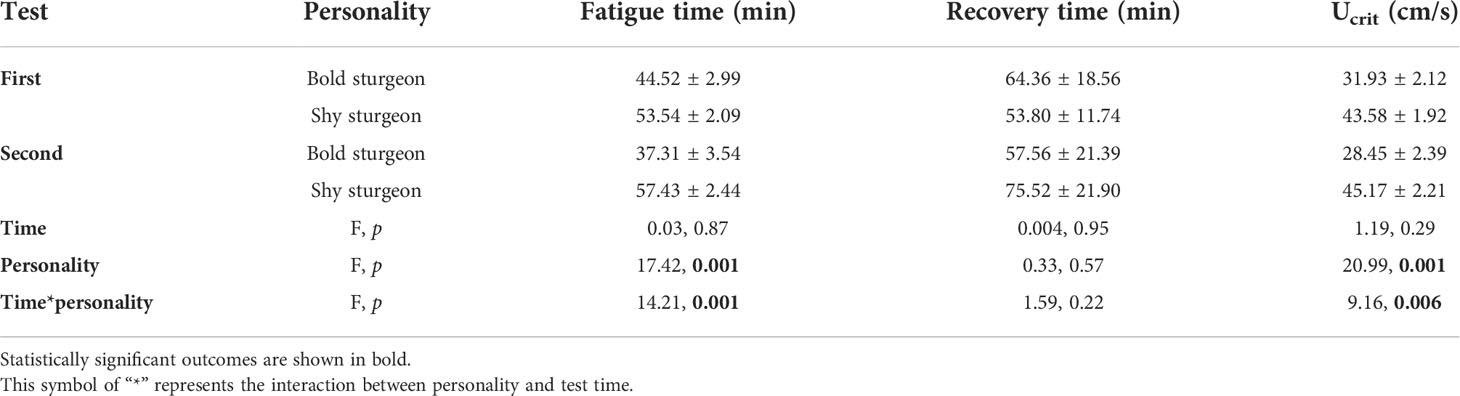
Table 1 Summary statistics for swimming performance parameters and the results of general linear models (GLM) examining variation in swimming performance parameters between bold and shy Siberian sturgeon.
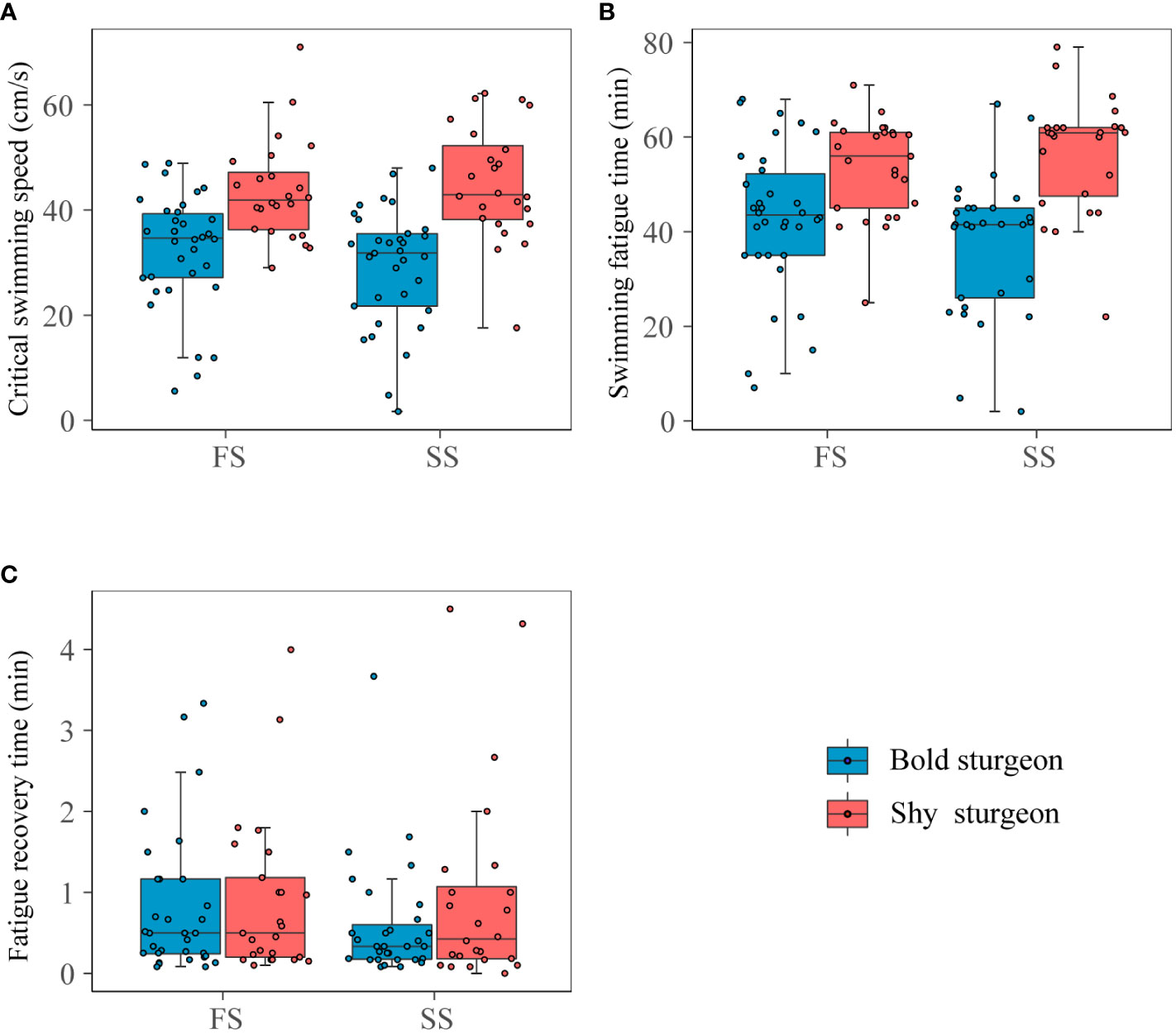
Figure 5 Swimming performance of different personalities. Blue and red represented bold sturgeon and shy sturgeon respectively; FS and SS represented the first and second swimming performance test respectively. (A) was the critical swimming speed, (B) was the swimming fatigue time (endurance), and (C) was the fatigue recovery time.
The proportion of time of swimming states and tail beat frequency of fish under different flow velocities indicated that bold groups stay in a state of countercurrent forward swimming for a longer period than shy groups (p< 0.05) but spend less time in countercurrent stationery and float downstream swimming states than shy groups (p< 0.05) (Table 2; Figure 6A). Moreover, the tail beat frequency of fish in the shy group was significantly higher than that of fish in the bold group as the flow velocity increased (p< 0.05) (Table 2; Figure 6B).
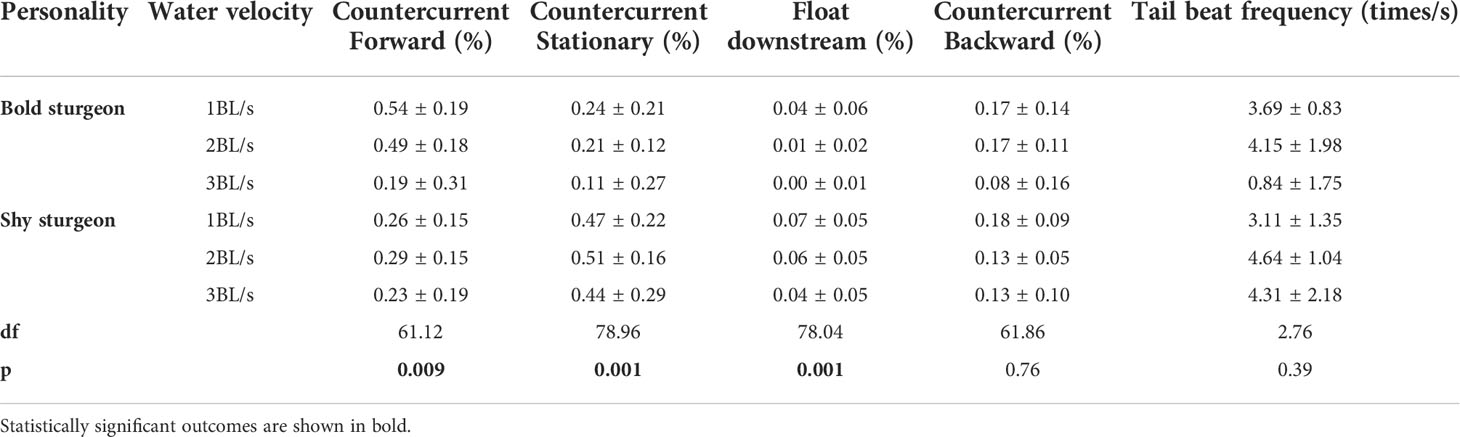
Table 2 Changes in the proportion of time spent in swimming state with individual differences and the tail beat frequency.
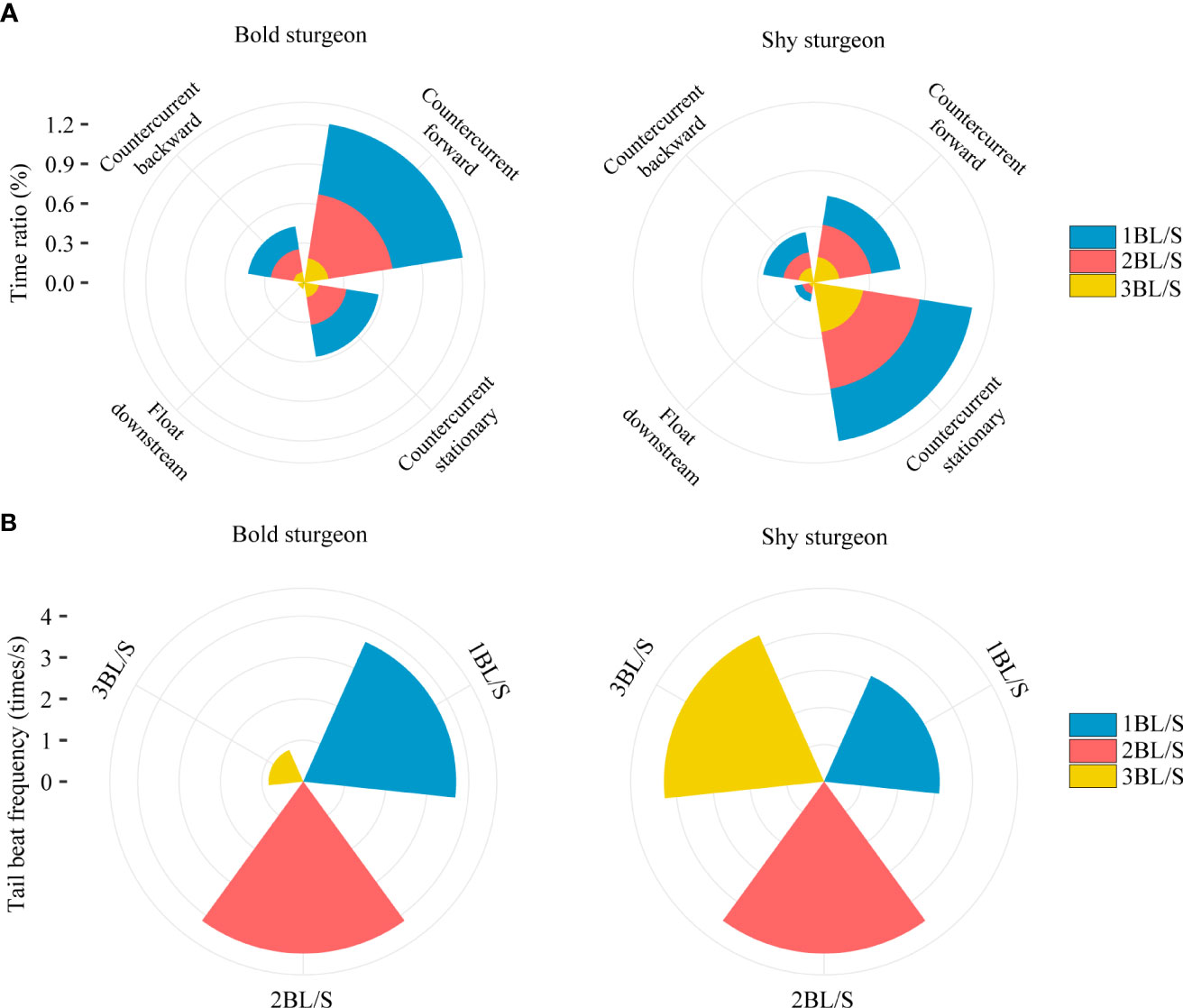
Figure 6 Changes in the proportion of time spent in swimming state and the tail beat frequency with different personalities. Blue, red and yellow represented 1BL/s flow rate, 2BL/s flow rate and 3BL/s flow rate, respectively. (A) showed the time proportion of swimming state of sturgeon with two personalities at different flow velocity. (B) showed the tail beat frequency of two personalities at different flow velocity.
Physiological characteristics of different personalities of the Siberian sturgeon
The personality and time interaction had a statistically significant effect on plasma glucose and lactate concentration (p< 0.05). The separate effect of personality at each time level showed that the glucose and lactate concentrations of shy groups were higher than that of bold groups (p< 0.05). The separate effect of time showed that the plasma glucose and lactate acid concentrations in the bold and shy groups changed significantly over time (p< 0.05). The results of the one-way analysis of variance revealed that at the 24th hour of recovery, the plasma glucose concentration of the shy groups was significantly higher than that of the control group (F1, 24 = 14.38, p< 0.05), indicating that the plasma glucose concentration of the shy groups rose faster than that of the bold groups. At the beginning of the recovery (i.e., at0 h), the plasma lactate concentration of fish in the shy group was significantly higher than the control groups (F1, 24 = 11.05, p< 0.05); at 24 h, there was no significant difference compared to the control group, indicating that the plasma lactate recovery ability of shy groups was greater than that of bold groups (Table 3; Figures 7A, B).
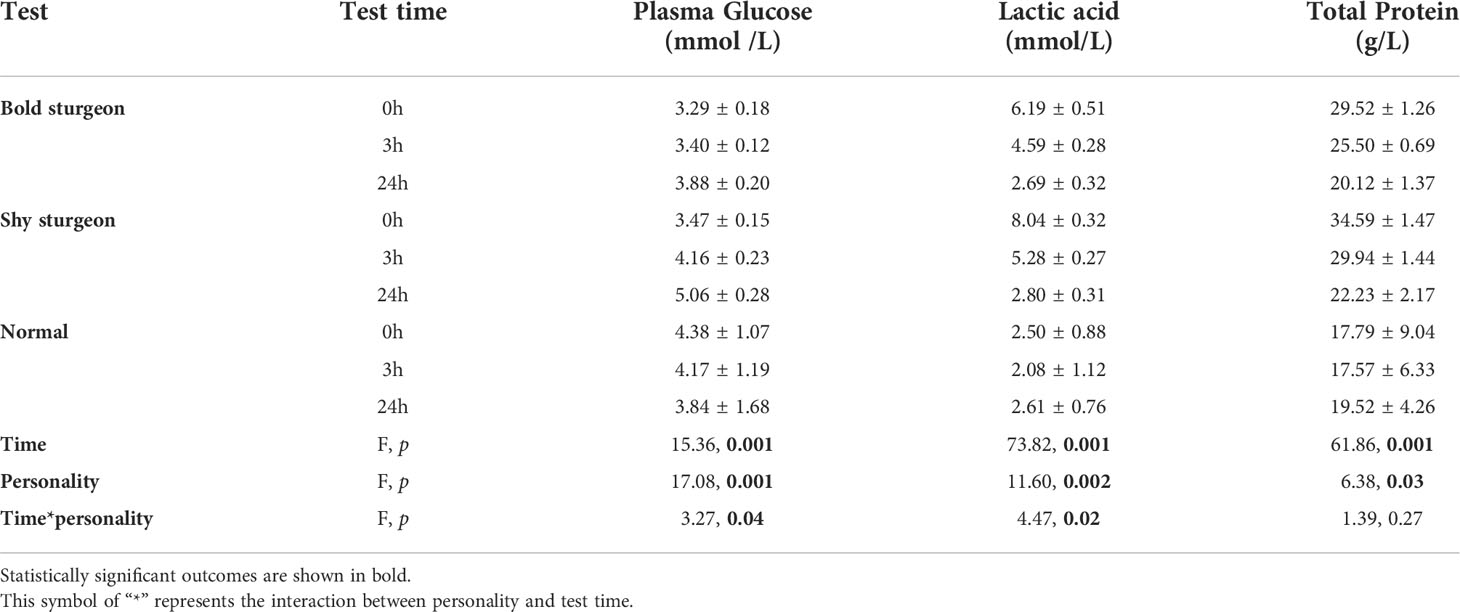
Table 3 Blood of plasma glucose, lactic acid and total protein levels (Mean±SD) in individual differences test fish at each test stage, and the results of general linear models (GLM) examining variation in different physiological indicators between bold and shy test Siberian sturgeon.
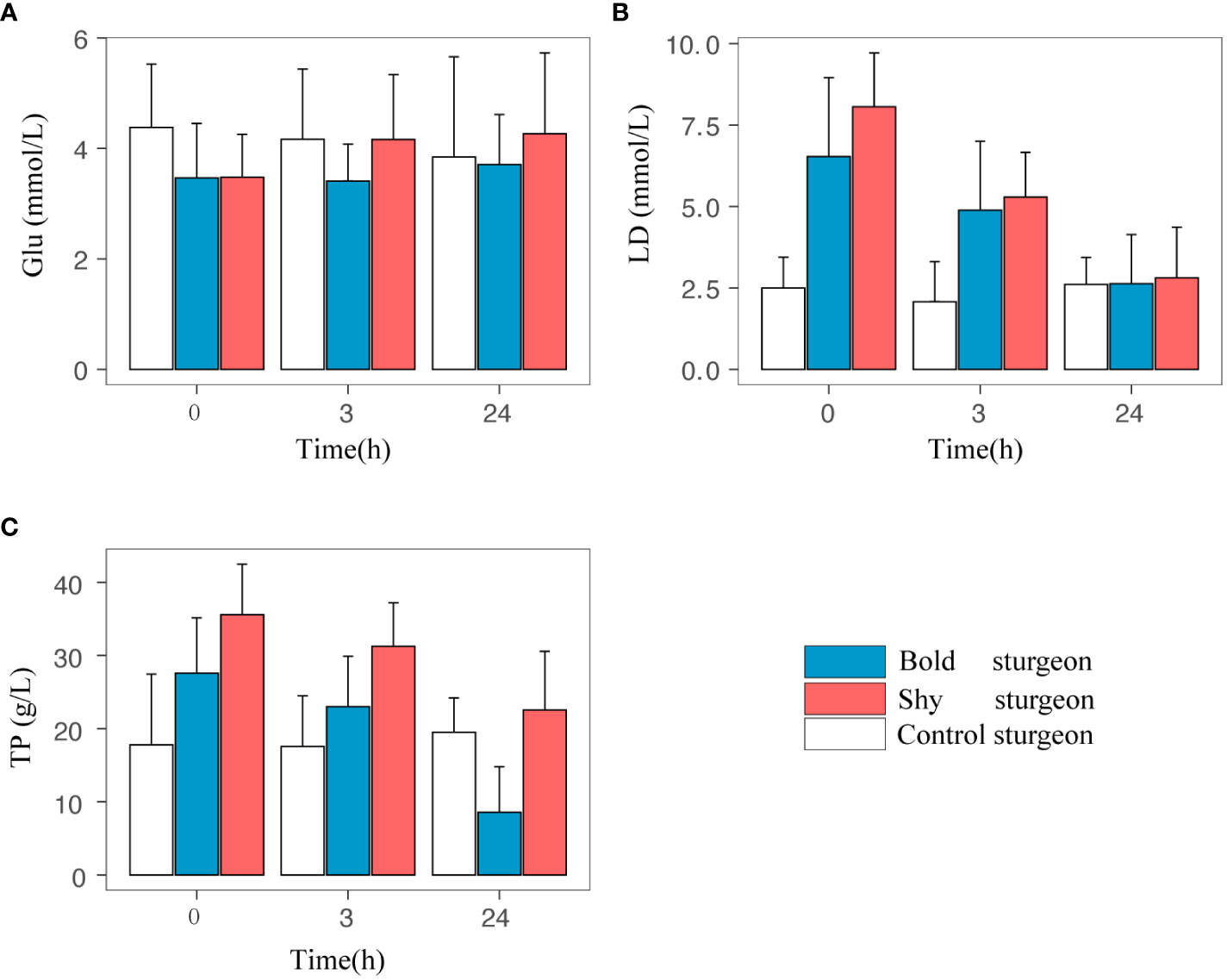
Figure 7 Physiological changes in different personalities. 0h, 3h and 24h are the fatigue recovery time of the test Siberian sturgeon; Glu, LD, TP are the concentration of glucose, lactic acid and total protein in the blood respectively.
For total protein concentrations in plasma, the interaction between personality and time was not statistically significant (F1.19, 10.68 = 1.390, p > 0.05). The main effect of personality on total protein concentration was statistically significant, with the mean total protein concentration in the shy group being higher than that of the bold group (F1, 9 = 6.38, p< 0.05). For the main effect of time, the influence of time on total protein concentration was statistically significant (F2, 18 = 61.86, p< 0.001). The results of the one-way analysis of variance showed that at 0 h, the total protein concentration of the bold group and the shy group was significantly higher than that of the control group (p< 0.05); at 24 h, there was no significant difference between the total protein concentration of the two groups and the control group. Overall, the total protein concentration of fish declined over time after the swimming performance test, and it was always higher in the shy group than that of the bold group (Table 3; Figure 7C).
The effect of personality on morphological characteristics of the Siberian sturgeon
In the geometric analysis based on landmark points, the regression coefficients were all greater than 0.99, emphasizing the validity of the landmark points selected. The relative warp principal component analysis extracted a total of 24 principal components, of which the eigenvalues of the first three principal components were 0.331, 0.123, and 0.108, and the total contribution rate was 66.89%, 9.22%, and 7.20%. A total of 83.31% of the intraspecific morphological differences were explained (Table 4). The contribution rate of 21 landmark points in the relative distortion principal component analysis showed that landmark points 12 (deepest part of the caudal fin) and 13 (lower lobe terminal caudal fin) have the largest contribution rate, followed by landmark points 9 (base end of the dorsal fin), 10 (dorsal origin of caudal fin), 14 (ventral origin of caudal fin), 18 (origin of pectoral fin), and 19 (lower head end). The total contribution rate of these seven traits was 86.12%. There were significant differences in the caudal fin, caudal stalk, pectoral fin, and head of fishes with different personalities. The Hotelling T2 test showed that the bold and shy groups had significant differences in the former three relative distortions (F3,21 = 0.914, p< 0.05) and that the two personalities of sturgeons had significant differences in RW1 (p< 0.05). When the first relative warp was mapped on the thin plate spline, we could visualize its shape (Figure 8). The caudate stalk length, upper caudal lobe and the lower caudal lobe of the bold groups were significantly larger than those of the shy groups, while the head height, snout thickness, snout length, body height, and body length of the bold groups were significantly smaller than those of the shy groups.

Table 4 Eigenvalues and contribution rates of the first 5 principal components of relative warps scores of different morphological features of the test Siberian sturgeon.
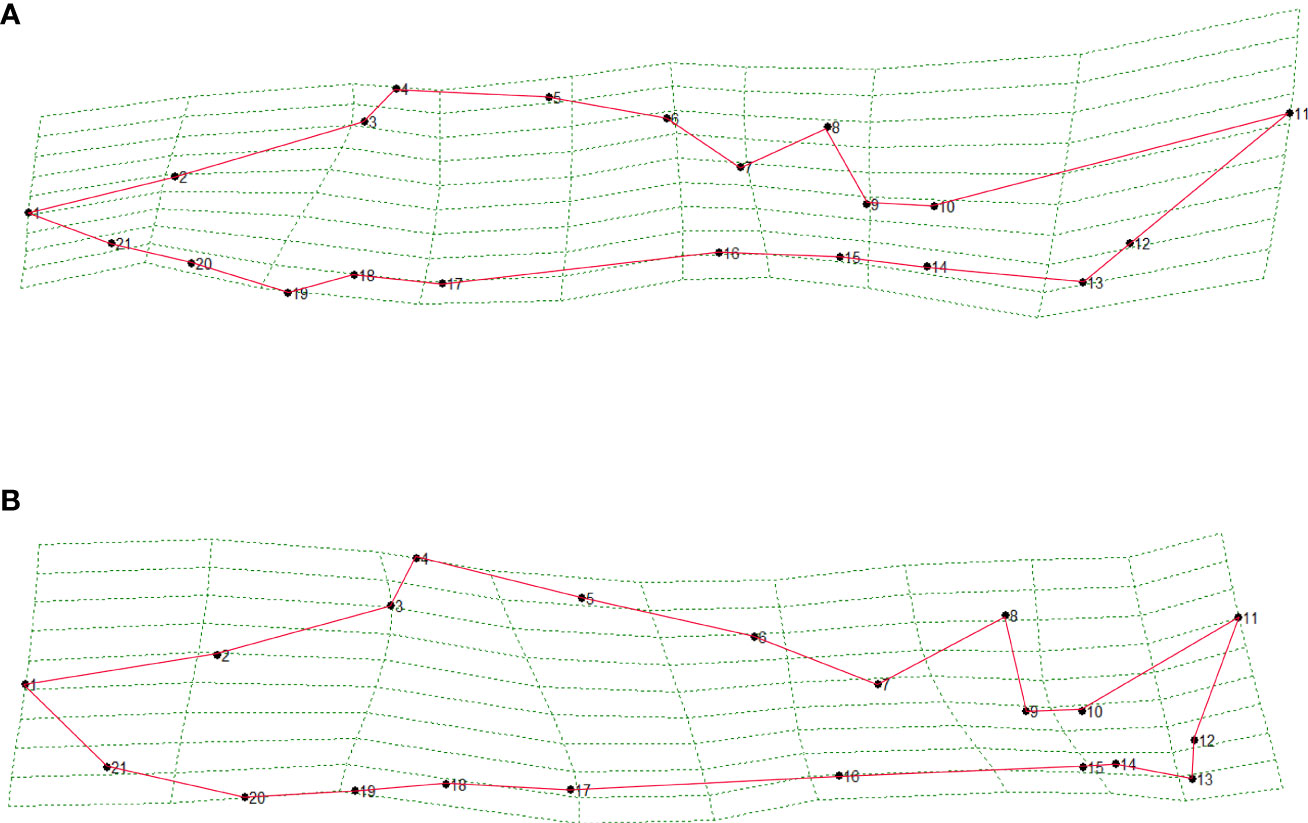
Figure 8 Grid deformation and variation visualization of morphological features of bold (A) and shy (B) test Siberian sturgeon.
Discussion
Our research showed that there were indeed individuals with different personalities in the Siberian sturgeon population. Individuals with different personalities have evolved not only a series of behavioral differences but also divergences in physiological performance and morphology. First, we found that shy groups had a significantly higher Ucrit than bold groups. Other studies have shown that personality can affect the swimming performance of fish. For instance, in Trinidadian killifish (Rivulus hartii), individuals from high-predation sites were faster sprinters but had reduced Ucrit (Oufiero et al., 2011). Shy wild-type zebrafish (Danio rerio) were slower in movement and exhibited higher stable swimming performance, while bold fish were more agile and exhibited higher quick-start swimming performance (Kern et al., 2016). Fish with different personalities have evolved different swimming strategies depending on their prey and habitat, often at the expense of another form of locomotion (Webb, 1978; Webb, 1984). This phenomenon is manifested as a trade-off between stable swimming performance (aerobic swimming) and unstable swimming performance (anaerobic swimming), and its ecological mechanism reflects a trade-off between energy gain and cost (Webb, 1984; Langerhans, 2007; Langerhans, 2008). In addition, we also found that the proportion of time that bold groups swam countercurrent forward during the experiment was higher than that of shy groups, while the proportion of time that they were countercurrent stationery and ‘float downstream’ was lower than that of shy groups. This may be related to the fact that bold animals are highly exploratory and aggressive, tend to engage in active avoidance, or cope with stressful stimuli through a “fight or flight” response. Conversely, shy animals typically undertake a passive “freeze and hide” response (Benhaïm et al., 2016). The higher tail beat frequency of the shy group with higher flow rates may be related to swimming fatigue in the bold group before reaching the Ucrit of the shy group.
Our research revealed that the morphology of fish differed based on their personality type (shy vs. bold). Shy groups had smaller caudate stalk lengths, caudate stalk heights, superior caudal lobes, and inferior caudal lobes compared with bold groups, while the head height, body height, snout thickness, snout length, and body length were larger in bold fish. Other studies have shown that strong selective pressure affects the morphology of fish with different personalities and that morphology further affects swimming performance. Western mosquito fish (Gambusia affinis) from high predation risk areas have smaller heads and larger tails and have a stronger ability to start swimming quickly compared to those from low predation risk areas that have larger heads, smaller tails, and more streamlined, stable swimming (Langerhans, 2009). It has long been suggested that the divergences in swimming performance occurred, at least partially, via morphological change, as morphology has a significant impact on swimming transport costs (Peres-Neto and Magnan, 2004; Langerhans, 2008; Langerhans, 2009a). Changes in body shape would affect fish hydrodynamics and, ultimately drag coefficients and Ucrit (Ostenfeld, 1998; Lee et al., 2003). Morphological variations that are poorly suited to produce maximum thrust and minimum drag in GH-transgenic C. carpio may be responsible for their lower swimming performance compared with non-transgenic controls (Li et al., 2009). The morphology of the fins of juvenile Atlantic salmon (Salmon salar) living in slow- and fast-flowing environments differs significantly (Pa´ez et al., 2008). In this study, we believe that the larger head and smaller caudal of shy groups made the overall body shape more streamlined, which may minimize recoil energy loss and enhance stable swimming performance (Webb, 1984).
In addition to morphological performance, we also found that the physiological performance of fish with different personalities was different. The ability to recover following swimming fatigue was greater in shy vs. bold groups following the two swimming performance tests. With respect to physiology, the blood glucose concentration of shy fish increased faster than that of bold groups, and the ability to recover from elevated blood lactate levels scavenging was greater in shy fish. Further, their total protein concentrations were consistently higher than that of bold fish. These findings suggest that personality affects the physiological performance of fish, which in turn affects the swimming performance of fish. Prolonged exercise training on the swimming performance of juvenile common carp (Cyprinus carpio) revealed that trained fish exhibited higher lactate clearance and significantly increased aerobic swimming performance (He et al., 2013). Studies have shown that strenuous exercise led to accumulations of total protein in blood serum, and the increased levels of total protein resulted primarily from lactate accumulation due to anaerobic metabolism in white muscle tissue (Genz et al., 2013). Previous studies have shown that physiological traits represent different adaptive strategies to respond to environmental challenges (Kristiansen and Fern, 2007). Various degrees of physiological resilience may be influenced mainly by genetics, environment, and the interaction between genetic and environmental factors (Julia et al., 2011; Careau and Garland, 2012). In this study, individual differences were measured in the absence of social interaction and with equal and predominantly constant environmental conditions, suggesting that the stronger physiological resilience of shy individuals may be influenced primarily by a genetic basis. In addition, we believe that due to the sufficient energy accumulation caused by the passive “freeze and hide” response of shy groups, high-level energy reserves could be prioritized for physiological recovery (Magnhagen and Borcherding, 2008). Previous studies have shown that individual differences in personality type and energy utilization efficiency affect individual fitness (Biro and Stamps, 2008; Biro and Dingemanse, 2009). For instance, it is possible that bolder katydid spend more time gathering information about all the available options but owing to the limited time and attention, this leads to lower biomass consumed (i.e. lower foraging efficiency) when compared to shy katydids (Tan et al., 2018). We speculate that the bold groups in this study may have spent more time gathering information about potential food choices within the environment and resulting in less time for food (Tan et al., 2018). This condition allowed shy groups to benefit by having a higher food intake, maximizing their growth (Tan et al., 2018). In addition, because proactive individuals are usually aggressive, dominant, and bold, they are more likely to engage in energetically costly behavior, which leads to higher daily energy expenditure (DEE) and resting standard metabolism (Stamps, 2007). Therefore, it was possible that because the metabolic intensity of bold groups was higher than that of shy groups, the physiological indicators of bold groups were always lower than those of shy groups in the process of fatigue recovery (Huntingford et al., 2010).
Conclusion
In the present study, different personality types affected the swimming performance of Siberian sturgeon. In general, we demonstrated that shy Siberian sturgeon had better swimming performance from physiology and morphology. Morphology and physiology also differed across personality types, which may have contributed to the observed differences in swimming performance. Our research results further validate the theoretical viewpoints pertaining to the energetic trade-offs associated with an animal’s life-history strategy. A lower Ucrit and slower physiological recovery rate may place bold groups at a disadvantage when competing with shy wild-type groups in their natural habitat, though further research is needed to assess this hypothesis. Our results also provide further knowledge on the links between personality and swimming performance in sturgeon and theoretical support for improving the conservation of sturgeon. For example, sturgeon could be selectively cultivated with different risk coping styles so that they evolve not only a range of behavioral differences but also differences in morphological performance and physiological performance, and selectively release sturgeon with different personality traits for the actual conditions of the natural environment. Future research could investigate other aspects of personality and how these relate to physiology, morphology, and swimming performance in sturgeon.
Data availability statement
The original contributions presented in the study are included in the article/supplementary material. Further inquiries can be directed to the corresponding authors.
Ethics statement
This study was reviewed and approved by Research Ethics Committee of Institute of Hydrology, CAS.
Author contributions
LX (Co-first authors): Conceptualization, Methodology, Software, Investigation, Formal Analysis, Writing-Original Draft. XM(Co-first authors): Conceptualization, Methodology, Software, Investigation, Writing-Original Draft. YD: Resources, Supervision. YZ: Writing-Review and Editing. WJ: Resources, Supervision. HD: Resources. WT: Writing-Review. SC: Writing-Review. JB (Co-corresponding authors): Conceptualization, Writing-Review and Editing. MD (Co-corresponding authors):Conceptualization, Funding Acquisition, Writing-Review and Editing. All authors contributed to the article and approved the submitted version.
Funding
This work was financially supported by the National Natural Science Foundation of China (grant number 32172955), the Hubei Key Laboratory of Three Gorges Project for Conservation of Fishes (grant number 201902), the National Natural Science Foundation of China (grant number 32202914), the Open Innovation Fund of Changjiang Survey Planning Design and ResearchCo., Ltd (grant number CX2021K03), the National Key R & D Program of China (grant number 2018YFD 0900605), the Natural Science Foundation of Hubei Province (grant number 2020CFB832), and the Scientific Instrument Developing Project of the Chinese Academy of Sciences (grant number YJKYYQ20190055). The funders were not involved in the study design, collection, analysis, interpretation of data, the writing of this article, or the decision to submit it for publication.
Conflict of interest
Authors YD and WJ were employed by the Chinese Sturgeon Research Institute, China Three Gorges Corporation.
The remaining authors declare that the research was conducted in the absence of any commercial or financial relationships that could be construed as a potential conflict of interest.
Publisher’s note
All claims expressed in this article are solely those of the authors and do not necessarily represent those of their affiliated organizations, or those of the publisher, the editors and the reviewers. Any product that may be evaluated in this article, or claim that may be made by its manufacturer, is not guaranteed or endorsed by the publisher.
Supplementary material
The Supplementary Material for this article can be found online at: https://www.frontiersin.org/articles/10.3389/fmars.2022.1040225/full#supplementary-material
References
Benhaïm M. D., Ferrari S., Chatain B., Bégout M. (2016). The shy prefer familiar congeners. Behav. Processes. 126, 113–120. doi: 10.1016/j.beproc.2016.03.008
Biro P. A., Dingemanse N. J. (2009). Sampling bias resulting from animal personality. Trends Ecol. Evol. 24 (2), 66–67. doi: 10.1016/j.tree.2008.11.001
Biro P. A., Stamps J. A. (2008). Are animal personality traits linked to life-history. productivity? Trends Ecol. Evol. 23 (7), 361–368. doi: 10.1016/j.tree.2008.04.003
Biro P. A., Stamps J. A. (2010). Do consistent individual differences in metabolic rate. promote consistent individual differences in behavior? Trends Ecol. Evol. 25 (11), 653–659. doi: 10.1016/j.tree.2010.08.003
Birstein V. J., Bemis W. E., Waldman J. R. (1997). The threatened status of acipenseriform. species: a summary. Environ. Biol. Fishes. 48, 427–435. doi: 10.1007/0-306-46854-9_33
Blake R. W. (2010). Fish functional design and swimming performance. J. Fish Biol. 65 (5), 1193–1222. doi: 10.1111/j.0022-1112.2004.00568.x
Bookstein F. L. (1989). Principal Warps: thin-plate splines and the decomposition of deformation. IEEE Transactions on Pattern Analysis and Machine Intelligence, 6(11), 567–585. doi: 10.1109/34.24792
Bookstein F. (1997). Landmark methods for forms without landmarks: morphometrics of group differences in outline shape. Med. Image Anal. 1, 225–243. doi: 10.1109/MMBIA.1996.534080
Brett J. R. (1964). The respiratory metabolism and swimming performance of young. sockeye salmon. J.Fish.Res.Bd.Can 21 (5), 1183–1226. doi: 10.1139/f64-103
Brown C., Jones F., Braithwaite V. (2005). In situ examination of boldness–shyness traits in the tropical poeciliid, brachyraphis episcopi. Anim. Behav. 70 (Pt5), 1003–1009. doi: 10.1016/j.anbehav.2004.12.022
Brown C., Jones F., Braithwaite V. A. (2007). Correlation between boldness and body mass in natural populations of the poeciliid brachyrhaphis episcopi. J. Fish Biol. 71 (6), 1590–1601. doi: 10.1111/j.1095-8649.2007.01627.x
Bruckerhoff L. A., Magoulick D. D. (2017). Hydrologic regimes as potential drivers of morphologic divergence in fish. Evol. Ecol. 31 (4), 517–531. doi: 10.1007/s10682-017-9897-0
Careau V., Garland T. (2012). Performance, personality, and energetics: correlation, causation, and mechanism. Physiol. Biochem. Zool 85 (6), 543–571. doi: 10.1086/666970
Careau V., Thomas D., Humphries M. M., D Réale (2008). Energy metabolism and. animal personality. Oikos 117 (5), 641–653. doi: 10.1111/j.0030-1299.2008.16513.x
Caro T. M., Bateson P. (1986). Organization and ontogeny of alternative tactics. Anim. Behav. 34 (5), 1483–1499. doi: 10.1016/S0003-3472(86)80219-6
Clark A. B., Ehlinger T. J. (1987). Pattern and adaptation in individual behavioral differences. Perspect. Ethology, 7, 1–47. doi: 10.1007/978-1-4613-1815-6_1
Domenici P. (2010). “Escape responses in fish: kinematics, performance and behavior,” in Fish locomotion: an eco-ethological perspective. Eds. Domenici P., Kapoor B. G.. Florida, US. 123–170.
Downie A. T., Kieffer J. D. (2017). Swimming performance in juvenile shortnose sturgeon (Acipenser brevirostrum): the influence of time interval and velocity increments on critical swimming tests. Conserv. Physiol. 5 (1), 1–12. doi: 10.1093/conphys/cox038
Farrell A. P., Gamperl A. K., Birtwell I. K. (1998). Prolonged swimming, recovery and repeat swimming performance of mature sockeye salmon oncorhynchus nerka exposed to moderate hypoxia and pentachlorophenol. J. Exp. Biol. 201 (Pt14), 2183–2193. doi: 10.1242/jeb.201.14.2183
Genz J., Jyde M. B., Svendsen J. C., Steffensen J. F., Ramlov H. (2013). Excess post-hypoxic oxygen consumption is independent from lactate accumulation in two cyprinid fishes. Comp. Biochem. Phys. A 165 (1), 54–60. doi: 10.1016/j.cbpa.2013.02.002
Gosling S. D. (2001). From mice to men: what can we learn about personality from animal research? Psychol. Bull. 127 (1), 45–86. doi: 10.1037/0033-2909.127.1.45
Hammer C. (1995). Fatigue and exercise tests with fish. Comp. Biochem. Physiol. A-Mol. Integr. Physiol. 112 (1), 1–20. doi: 10.1016/0300-9629(95)00060-K
Harper D. G., Blake R. W. (1990). Fast-start performance of rainow trout salmo gairdneri and northern pike esox lucius. J. Exp. Biol. 150, 321–342.
He W., Xia W., Cao Z. D., Fu S. J. (2013). The effect of prolonged exercise training on swimming performance and the underlying biochemical mechanisms in juvenile common carp (Cyprinus carpio). Comp. Biochem. Physiol. Part A Mol. Integr. Physiol. 166 (2), 308–315. doi: 10.1016/j.cbpa.2013.07.003
Huntingford F. A., Andrew G., Mackenzie S., Morera D., Coyle S. M., Pilarczyk M., et al. (2010). Coping strategies in a strongly schooling fish, the. common carp cyprinus carpio. J. Fish Biol. 76 (7), 1576–1591. doi: 10.1111/j.1095-8649.2010.02582.x
Iwata M. (1995). Downstream migratory behavior of salmonids and its relationship with cortisol and thyroid hormones: A review. Aquaculture 135 (1-3), 131–139. doi: 10.1016/0044-8486(95)01000-9
Jain K. E. (2003). Influence of seasonal temperature on the repeat swimming performance of rainbow trout oncorhynchus mykiss. J. Exp. Biol. 206 (20), 3569–3579. doi: 10.1242/jeb.00588
Mas-Munoz J., Komen H., Schneider O., Visch S. W., Schrama J. W. (2011). Feeding behaviour, swimming activity and boldness explain variation in feed intake and growth of sole (Solea solea) reared in captivity. PloS One 6 (6), e21393. doi: 10.1371/journal.pone.0021393
Kern E., Robinson D., Gass E., Godwin J., Langerhans R. B. (2016). Correlated evolution of personality, morphology and performance. Anim. Behav. 117, 79–86. doi: 10.1016/j.anbehav.2016.04.007
Kristiansen T. S., Fern A. (2007). Individual behaviour and growth of halibut ( hippoglossus l.) fed sinking and floating feed: Evidence of different coping styles. Appl. Anim. Behav. Sci. 104 (3-4), 236–250. doi: 10.1016/j.applanim.2006.09.007
Langerhans R. B. (2009). Trade-off between steady and unsteady swimming underlies predator-driven divergence in gambusia affinis. J. Evol. Biol. 22 (5), 1057–1075. doi: 10.1111/j.1420-9101.2009.01716.x
Langerhans R. B. (2007). Evolutionary consequences of predation: avoidance, escape, reproduction, and diversification. Springer Berlin Heidelberg, 177–220. doi: 10.1007/978-3-540-46046-6_10
Langerhans R. B. (2008). Predictability of phenotypic differentiation across flow regimes in fishes. Integr. Comp. Biol. 48, 750–768. doi: 10.1093/icb/icn092
Langerhans R. B. (2009a). Morphology, performance, fitness: functional insight into a. post-pleistocene radiation of mosquitofish. Biol. Lett. 5 (4), 488–491. doi: 10.1098/rsbl.2009.0179
Langerhans R. B. (2009b). Trade-off between steady and unsteady swimming underlies predator-driven divergence in gambusia affinis. J. Evolutionary Biol. 22 (5), 1057–1075. doi: 10.1111/j.1420-9101.2009.01716.x
Lee C. G., Devlin R. H., Farrell A. P. (2003). Swimming performance, oxygen consumption and excess post-exercise oxygen consumption in adult transgenic and ocean-ranched coho salmon. J. Fish Biol. 62, 753–766. doi: 10.1046/j.1095-8649.2003.00057.x
Lee C. G., Farrell A. P., Lotto A., Hinch S. G., Healey M. C. (2003). Excess post-exercise oxygen consumption. in adult sockeye(Oncorhynchus nerka)and coho(O.kisutch)salmon following critical speed swimming. J. Exp. Biol. 206, 3253–3260. doi: 10.1242/jeb.00548
Lighthill M. J. (1969). Hydromechanics of aquatic animal propulsion. Annu. Rev. Fluid Mech. 1 (1), 413–446. doi: 10.1146/annurev.fl.01.010169.002213
Lighthill M. J. (2006). Aquatic animal propulsion of high hydromechanical efficiency. J. Fluid Mech. 44 (02), 265–301. doi: 10.1017/S0022112070001830
Li D., Hu W., Wang Y., Zhu Z., Fu C. (2009). Reduced swimming abilities in fast-growing transgenic common carp cyprinus carpio associated with their morphological variations. J. Fish Biol. 74 (1), 186–197. doi: 10.1111/j.1095-8649.2008.02128.x
Magnhagen C., Borcherding J. (2008). Risk-taking behaviour in foraging perch: does predation pressure influence age-specific boldness? Anim. Behav. 75 (2), 509–517. doi: 10.1016/j.anbehav.2007.06.007
Maximino C., Thiago M., Dias C., Gouveia A., Morato S. (2010). Scototaxis as anxiety-like behavior in fish. Nat. Protoc. 5 (2), 209. doi: 10.1038/nprot.2009.225
Ohlberger J., Staaks G., Hlker F. (2007). Estimating the active metabolic rate (AMR) in fish based on tail beat frequency (TBF) and body mass. J. Exp. Zool. Part A. 307 (5), 296–300. doi: 10.1002/jez.384
Ostenfeld T. (1998). Transgenesis changes body and head shape in pacific salmon. J. Fish Biol. 52, 850–854. doi: 10.1111/j.1095-8649.1998.tb00825.x
Oufiero C. E., Walsh M. R., Reznick D. N., Garland T. (2011). Swimming performancetrade-offsfs across a gradient in community composition in trinidadian killifish (Rivulus hartii). Ecology 92, 170–179. doi: 10.1890/09-1912.1
Pa´ez D. J., Hedger R., Bernatchez L., Dodson J. J. (2008). The morphological plastic. response to water current velocity varies with age and sexual state in juvenile Atlantic salmon, salmo salar. Freshw. Biol. . 53, 1544–1554. doi: 10.1111/j.1365-2427.2008.01989.x
Peres-Neto P. R., Magnan P. (2004). The influence of swimming demand on phenotypic plasticity and morphological integration: a comparison of two polymorphic charr species. Oecologia 140, 36–45. doi: 10.1007/s00442-004-1562-y
Raoult V., Brown C., Zuberi A., Williamson J. E. (2012). Blood cortisol concentrations predict boldness in juvenile mulloway (Argyosomus japonicus). J. Ethol. 30 (2), 225–232. doi: 10.1007/s10164-011-0314-9
Reale D., Garant D., Humphries M. M., Bergeron P., Careau V., Montiglio P. O. (2010). Personality and the emergence of the pace-of-life syndrome concept at the population level. Philos. Trans. R. Soc Lond. Ser. A-Math. Phys. Eng. Sci. 365 (1560), 4051–4063. doi: 10.1098/rstb.2010.0208
Réale D., Reader S. M., Sol D., McDougall P. T., Dingemanse N. J. (2007). Integrating animal temperament within ecology and evolution. Biol. Rev. 82, 291–318. doi: 10.1111/j.1469-185X.2007.00010.x
Reidy S. P., Kerr S. R., Nelson J. A. (2000). Aerobic and anaerobic swimming performance of individual Atlantic cod. J. Exp. Biol. 203 (Pt 2), 347–357. doi: 10.1242/jeb.203.2.347
Reznick D., Nunney L., Tessier A. (2000). Big houses, big cars, superfleas and the costs of reproduction. Trends Ecol. Evol. 15, 421–425. doi: 10.1016/S0169-5347(00)01941-8
Roche D. G., Careau V., Binning S. A. (2016). Demystifying animal 'personality' (or not): why individual variation matters to experimental biologists. J. Exp. Biol. 219, 3832–3843. doi: 10.1242/jeb.146712
Roff D. A. (1992). The evolution of life histories : theory and analysis (New York: Chapman and Hall). doi: 10.1002/9781444304312.ch2
Roff D. A., Fairbairn D. J. (2012). A test of the hypothesis that correlational selection generates genetic correlations. Evolution 66 (9), 2953–2960. doi: 10.1111/j.1558-5646.2012.01656.x
Rohlf F. J. (1990). Fitting curves to outlines (The University of Michigan Museum of Zoology: Michigan Morphometrics Workshop).
Rohlf F. J. (1993). Relative warp analysis and an example of its application to mosquito wings. Contribution to Morphometrics. 8, 131–159.
Shi X. T., Chen Q. W., Liu D. F., Huang Y, Zhuang P, Chen Z. Q, et al. (2012). Critical swimming speed of juvenile mullet. J. Hydrobiology. 36 (01), 133–136. doi: 10.3724/SP.J.1035.2012.00133
Slater P. (1981). Individual differences in animal behavior. SpringerPlus, 35–39. doi: 10.1007/978-1-4615-7575-7_2
Smith J. M. (1982). Evolution and the theory of games: Frontmatter. Cambridge, UK: Cambridge University Press, 1–4 doi: 10.1017/CBO9780511806292
Stamps J. A. (2007). Growth-mortalittrade-offsfs and "personality traits" in animals. Ecol. Lett. 10 (5), 355–363. doi: 10.1111/j.1461-0248.2007.01034.x
Svalheim R. A., Karlsson-Drangsholt A., Olsen S. H., Johnsen H. K., Aas-Hansen O. (2017). Effects of exhaustive swimming and subsequent recuperation on flesh quality in unstressed Atlantic cod (Gadus morhua). Fish Res. 193, 158–163. doi: 10.1016/j.fishres.2017.04.008
Svozil D. P., Baumgartner L. J., Fulton C. J., Kopf R. K., Watts R. J. (2020). Morphological predictors of swimming speed performance in river and reservoir populations of Australian smelt retropinna semoni. J. Fish Biol. 97, 1632–1643. doi: 10.1111/jfb.14494
Tan M. K., Chang C. C., Hugh T. W. (2018). Shy herbivores forage more. efficiently than bold ones regardless of information-processing overload. Behav. Processes. 149 (1), 52–58. doi: 10.1016/j.beproc.2018.02.003
Walker J. A., Ghalambor C. K., Griset O. L., et al. (2005). Do faster starts increase the probability of evading predators? Funct. Ecol. 19 (5), 808–815. doi: 10.1111/j.1365-2435.2005.01033.x
Wang P. Y. X. (2009). Effect of temperature on swimming performance in juvenile southern catfish (Silurus meridionalis). Comp. Biochem. Phys. A 153 (2), 125–130. doi: 10.1016/j.cbpa.2009.01.013
Wang J. W. (2015). Effects of hypoxia on critical swimming and uniform accelerated swimming ability of juvenile parabramis bream and its biochemical mechanism. Chongqing Normal University. 11-15.
Webb P. W. (1978). Sprint performance and body form in seven species of teleost. J. Exp. Biol. 74, 211–226. doi: 10.1242/jeb.74.1.211
Webb P. W. (1982). Locomotor patterns in the evolution of actinopterygian fishes. Am. Zool. 22 (2), 329–342. doi: 10.1093/icb/22.2.329
Webb P. W. (1984). Body form, locomotion and foraging in aquatic vertebrates. Am. Zool. 24, 107–120. doi: 10.1093/icb/24.1.107
Yuan X., Huang Y. P., Cai L., Johnson D., Tu Z. Y., Zhou Y. H. (2018). Physiological responses to swimming fatigue in juvenile largemouth bronze gudgeon coreius guichenoti. J. Fish Biol. 92(4), 1192–1197. doi: 10.1007/s10750-019-04049-4
Keywords: individual differences, Siberian sturgeon (Acipenser baerii), swimming performance trade-off, morphology, physiology
Citation: Xiang L, Mi X, Dang Y, Zeng Y, Jiang W, Du H, Twardek WM, Cooke SJ, Bao J and Duan M (2022) Shyer fish are superior swimmers in Siberian sturgeon (Acipenser baerii). Front. Mar. Sci. 9:1040225. doi: 10.3389/fmars.2022.1040225
Received: 09 September 2022; Accepted: 28 September 2022;
Published: 18 October 2022.
Edited by:
Ce Shi, Ningbo University, ChinaCopyright © 2022 Xiang, Mi, Dang, Zeng, Jiang, Du, Twardek, Cooke, Bao and Duan. This is an open-access article distributed under the terms of the Creative Commons Attribution License (CC BY). The use, distribution or reproduction in other forums is permitted, provided the original author(s) and the copyright owner(s) are credited and that the original publication in this journal is cited, in accordance with accepted academic practice. No use, distribution or reproduction is permitted which does not comply with these terms.
*Correspondence: Ming Duan, ZHVhbm1pbmdAaWhiLmFjLmNu; Jianghui Bao, YmFvamlhbmdodWlAaWhiLmFjLmNu
†These authors share first authorship