- 1School of Marine and Environmental Programs, University of New England, Biddeford, ME, United States
- 2Department of Marine Biology, Texas A&M University at Galveston, Galveston, TX, United States
- 3Department of Biological Oceanography, Center for Scientific Research and Higher Education of Ensenada, Ensenada, Mexico
- 4Jackson School of Geosciences, University of Texas at Austin, Austin, TX, United States
The conservation and management of highly migratory sharks relies on understanding age-related movements and nursery habitat utilization. We reconstructed the habitat use and migratory history of young White Sharks (Carcharodon carcharias), a highly protected species, by utilizing natural chemical tracers (element:Ca ratios and stable isotope analysis, SIA) in vertebral cartilage growth bands. Two nursery areas in the northeastern Pacific are known, but migration patterns of immature White Sharks within the Gulf of California (GC) and natal philopatry are poorly understood. Vertebrae from coastal Mexican artisanal fisheries off central Baja California in the Pacific (12 neonates and juveniles; 139-280 cm total length) and the GC (3 subadults; 289-355 cm TL) were analyzed to characterize (1) trophic histories from collagen δ13C and δ15N values, and (2) in utero patterns and post-birth environmental histories from element:Ca time-series. Mean δ15N values from vertebral edges of GC sharks, representing the most recent feeding, was +5‰ higher than in the Pacific, reflecting the intense denitrification that permeates the regional food web and supporting SIA as tracers of migration between regions. A subadult from the GC likely resided within the system throughout its life, and two subadults migrated into the GC. Most neonate and juvenile sharks caught in the Pacific had SIA that did not overlap with those of the GC, but a single subadult likely migrated to the GC. Element:Ca ratios displayed ontogenetic trends, with Li:Ca, Zn:Ca, and Ba:Ca significantly higher before the birth mark in sharks captured in the GC. Edge values were significantly higher in Zn:Ca and Ba:Ca in the GC compared to the Pacific, suggesting elemental ratios may serve as tracers of migration between regions. Subadult sharks collected from GC displayed elevated maternal Zn:Ca and Ba:Ca, suggesting mothers may have resided in the GC for an extensive period pre-birth. Some White Sharks may reside within the GC from birth until at least the subadult stage (ca. 3 m TL), and there may be an unidentified nursery. Chemical tracers, coupled with genomic and tagging studies, should improve understanding of the importance of the GC to White Shark populations in the northeast Pacific.
1. Introduction
Migration patterns and habitat use of White Sharks (Carcharodon carcharias) in the eastern North Pacific are relatively well understood. Immature White Sharks use nearshore habitats as nurseries (Weng et al., 2007; Anderson et al., 2021). Two nursery areas have been confirmed for this species; the Southern California Bight in the United States and Vizcaino Bay, which is off the central Baja California Peninsula, Mexico (Lowe et al., 2012; Oñate-González et al., 2017; Anderson et al., 2021). Young sharks migrate from southern Californian to Mexican waters during late summer and fall, when water temperature decreases (Weng et al., 2007; Weng et al., 2012; White et al., 2019) and some individuals may enter the Gulf of California (GC) (Weng et al., 2012). Immature sharks find high food availability and suitable environmental conditions off the western coast of the Baja California peninsula year-round (White et al., 2019; Anderson et al., 2021; García-Rodríguez et al., 2021).
Younger sharks (< 2 m total length; TL) feed mostly on demersal species, like small elasmobranchs and bony fishes (Tricas and McCosker, 1984; Santana-Morales et al., 2012; García-Rodríguez et al., 2021). Larger juveniles and sub-adults (2-3.5 m TL) switch their diets to larger bony fishes and marine mammals (Carlisle et al., 2012; Hussey et al., 2012). Concurrent with the switch in diet, White Sharks move from coastal areas to adult and subadult aggregation sites around the islands off central California and Guadalupe Island (Klimley et al., 1992; Domeier and Nasby-Lucas, 2007; Jorgensen et al., 2010),. White Sharks leave those aggregation sites seasonally, migrating to offshore feeding areas in the Pacific Ocean and eventually returning to the aggregation regions, with some sharks entering the GC (Jorgensen et al., 2010; Domeier and Nasby-Lucas, 2013). Although the species is protected in the US and Mexico, incidental catches of White Sharks are documented in the Pacific off southern California, the Baja California Peninsula and in the GC (Lowe et al., 2012, Santana-Morales et al., 2012, García-Rodríguez and Sosa-Nishizaki, 2020; Madigan et al., 2021). Catches of White Sharks within the GC are considered rare, and more detailed knowledge about their habitat use is needed to inform conservation efforts (Galván-Magaña et al., 2010, Malpica-Cruz et al., 2013). Specifically, the migration of immature White Sharks from nursery habitats and oceanic areas in the Pacific to the GC is poorly understood, and whether some sharks are born in the GC and become permanent residents is unknown.
Stable isotope analyses (SIA) of fish tissues are powerful intrinsic tracers that have contributed to the ecological understanding of highly migratory and protected shark species (Estrada et al., 2003; MacNeil et al., 2005; Hussey et al., 2012; Bird et al., 2018). Chronometric structures such as the mineralized vertebral cartilage of several shark species have been used as chemical archives to characterize life-history traits (Hussey et al., 2012), infer ontogenetic changes in habitat and feeding habits (Estupiñán-Montaño et al., 2019; Estupiñán-Montaño et al., 2021a), reconstruct environmental histories (Carlisle et al., 2015), and track movements between oceanic and coastal marine ecosystems (Carlisle et al., 2015; Estupiñán-Montaño et al., 2021b). The δ13C and δ15N values of subsamples taken from the recently deposited vertebral edge of sharks reflects local foraging, and in the case of young sharks, the material laid down after the birth mark indicates a mixture of both maternal feeding from eggs within the womb and maternal supply (e.g. uterine milk, yolk sac placenta) in late-term embryos (Estupiñán-Montaño et al., 2019; Tomita et al., 2022), as well as foods consumed in their nursery habitats as neonates (Estrada et al., 2006; Shen et al., 2021). In older sharks, determination of isotopic values of vertebrae subsamples taken longitudinally along the axis of outward radial growth allows changes in feeding habits and foraging regions to be characterized throughout ontogeny (Estrada et al., 2006; Kim et al., 2012; Estupiñán-Montaño et al., 2019; Estupiñán-Montaño et al., 2021b).
The SIA of vertebral tissue provides insight into the ecology of White Sharks throughout life. SIA of subsamples extracted from the corpus calcareum of vertebrae were used to identify an increase in the trophic position of sharks with size (Estrada et al., 2006). Kerr et al. (2006) analyzed vertebrae samples of White Sharks sampled in the northeastern Pacific off California, and inferred movement into offshore and deeper waters with age. These studies indicate that large White Sharks feed at high trophic levels, consistent with their known consumption of marine mammals in the region (Estrada et al., 2006; Carlisle et al., 2012; Hussey et al., 2012). Based on the SIA of subadult and adult White Shark vertebrae collected between 1957 and 2000 off the California coast, Kim et al. (2012) identified a variety of feeding patterns and a high degree of specialization among individuals, which they attributed to individual prey preferences or availability, and changes in foraging locations during ontogeny. However, some of this variability could be confounded by ecosystem-level changes associated with decadal or interannual-variations, which are known to influence the baseline isotope ratios of the California Current System in the northeast Pacific (Décima et al., 2013; Ruiz-Cooley et al., 2017), and the onshore-offshore migration patterns documented by Kerr et al. (2006) were not considered.
Trace elements deposited in mineralized vertebral cartilage provide additional life history information as environmental tracers (McMillan et al., 2017; Livernois et al., 2021). Controlled laboratory experiments with model elasmobranch species have demonstrated that temperature and dissolved water chemistry affect uptake of barium (Ba:Ca) in round stingrays Urobatis halleri (Smith et al., 2013) and temperature influences manganese (Mn:Ca), strontium (Sr:Ca), and Ba:Ca uptake in Port Jackson sharks (Heterodontus portusjacksoni) (Pistevos et al., 2019). No controlled lab studies to date have examined the influence of diet on elasmobranch element incorporation. Investigations of White Shark vertebrae elemental chemistry have suggested that general decreases in Sr:Ca with growth may represent ontogenetic movements into colder water temperatures with age (Christiansen, 2011). Oscillations in U:Ca and Ba:Ca were hypothesized to reflect feeding and upwelling histories, respectively (Christiansen, 2011). The chemistry of maternal vertebral cartilage might represent movements of pregnant White Sharks, as documented in north Atlantic White Sharks (Hamady et al., 2014). Few studies have combined both SIA and element:Ca ratios of individual White Sharks (see Hamady et al., 2014), and none have combined these tracers in Pacific White Shark vertebrae samples.
In the northeastern Pacific Ocean, White Sharks are found in the southern California Current System (CCS), as well as within the GC. These two oceanographic regions have different water masses (Lavín and Marinone, 2003; Portela et al., 2016). The CCS flows southward along the western coast of North America, and the alongshore wind field leads to seasonal upwelling that supports a highly productive ecosystem (Checkley and Barth, 2009). The GC is characterized by seasonal upwelling as well as high stratification during the summer months. Upper water mass layers of the GC are strongly influenced by seasonally induced wind-driven circulation and heat exchange, as well as interaction with the Pacific Ocean, with CCS waters sometimes reaching its mouth during the spring upwelling season (see review by Lavín and Marinone, 2003). An important feature of the tropical eastern Pacific is the presence of a broad oxygen minimum zone (OMZ), which leads to strong denitrification and high δ15N values of dissolved inorganic nitrogen (Altabet et al., 1999; Sigman et al., 2005). This produces an enriched 15N baseline within the GC, with consumers feeding within the system having higher tissue δ15N values that those from the Pacific, including off Baja California (e.g., Aurioles-Gamboa et al., 2013; Whitehead et al., 2020; García-Rodríguez et al., 2021). If chemical and isotopic differences in regional water masses and associated biogeochemical processes are recorded in White Shark vertebrae during growth, it may be practical to differentiate individuals that have resided either in the northeastern Pacific or GC, or that migrated between these areas.
For this study, we used SIA and element:Ca ratio analysis of vertebral subsamples of White Sharks caught in the Pacific off Baja California and within the GC to (1) evaluate whether the chemical composition of vertebral edges differ between the two regions, thereby serving as a tracer of habitat use, and (2) evaluate the chemical composition of vertebrae throughout ontogeny to infer residence or migration between the Pacific and GC. Concomitant analysis of these two complementary intrinsic tracers from the same individuals allowed us to test for consistency in the observed ontogenetic changes and inferred habitat use patterns.
2. Materials and methods
2.1. Field sampling
Vertebrae from the dorso-anterior region of 15 White Sharks were collected opportunistically (2010-2019) from artisanal fishing camps along the northwestern coast of Mexico. Vertebrae were stored in labeled bags over ice, and frozen at -20 °C at the Fisheries Ecology Laboratory at CICESE. Five of the six localities where the sharks were caught are on the Pacific coast of Baja California and one is within the GC (El Barril; Figure 1). Total length was recorded (TL, cm) for most individuals. In five of the White Sharks sampled, TL measurements were unavailable (Table 1). Therefore, TL was estimated using a linear regression calculated from the vertebral diameter against the TL of the remaining ten White Sharks measured in the field. The equation was VD = 0.22*TL -16.49 (r2 = 0.954), where VD is the vertebral diameter (mm), and TL is the total length (cm). Age of each specimen was back-calculated based on the Von Bertalanffy growth model parameters estimated by Cailliet et al. (1985) for the northeastern Pacific White Shark population.
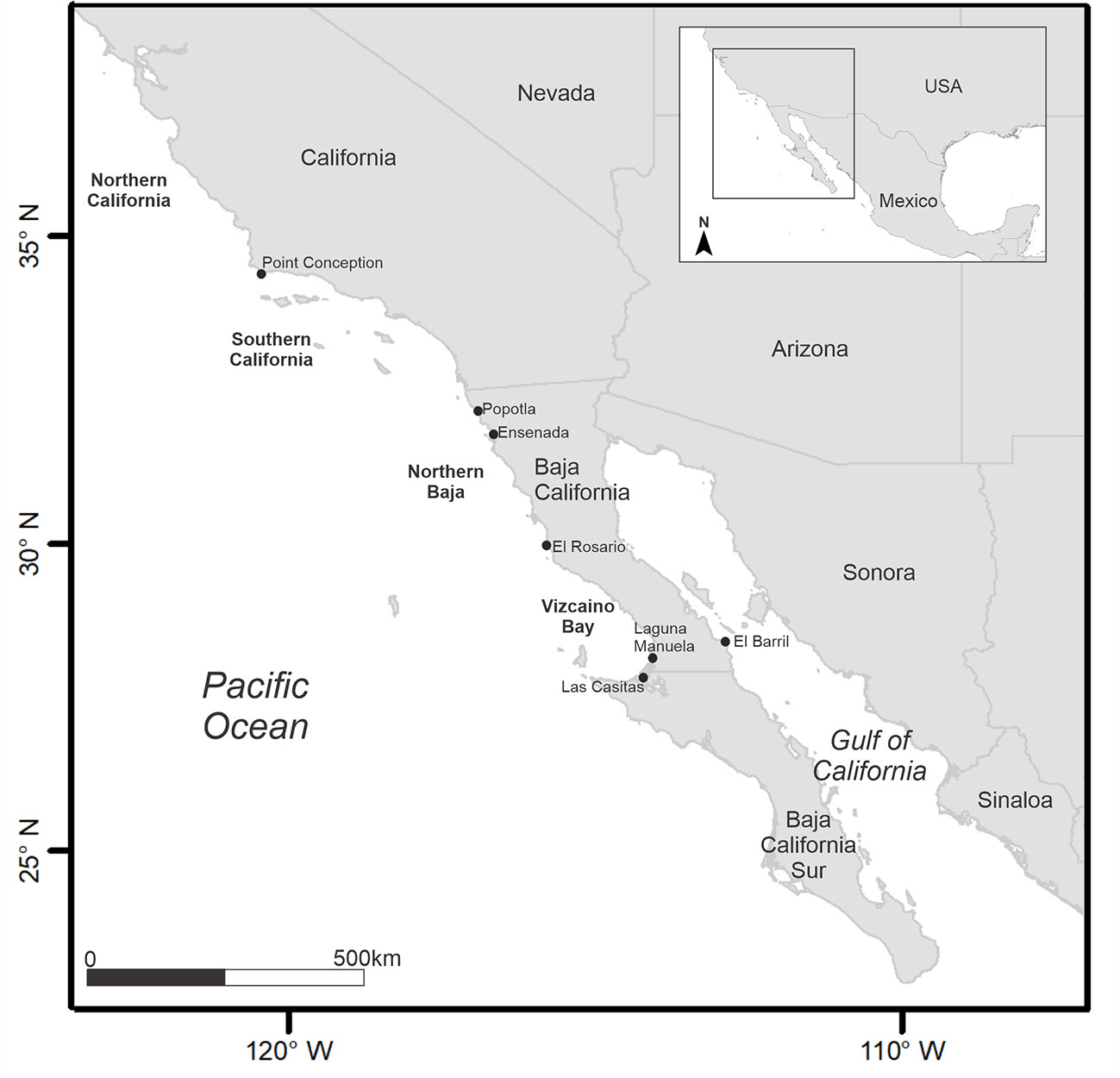
Figure 1 Location of artisanal fishing camps on the coasts of the Baja California peninsula where White Sharks were sampled following their incidental capture.
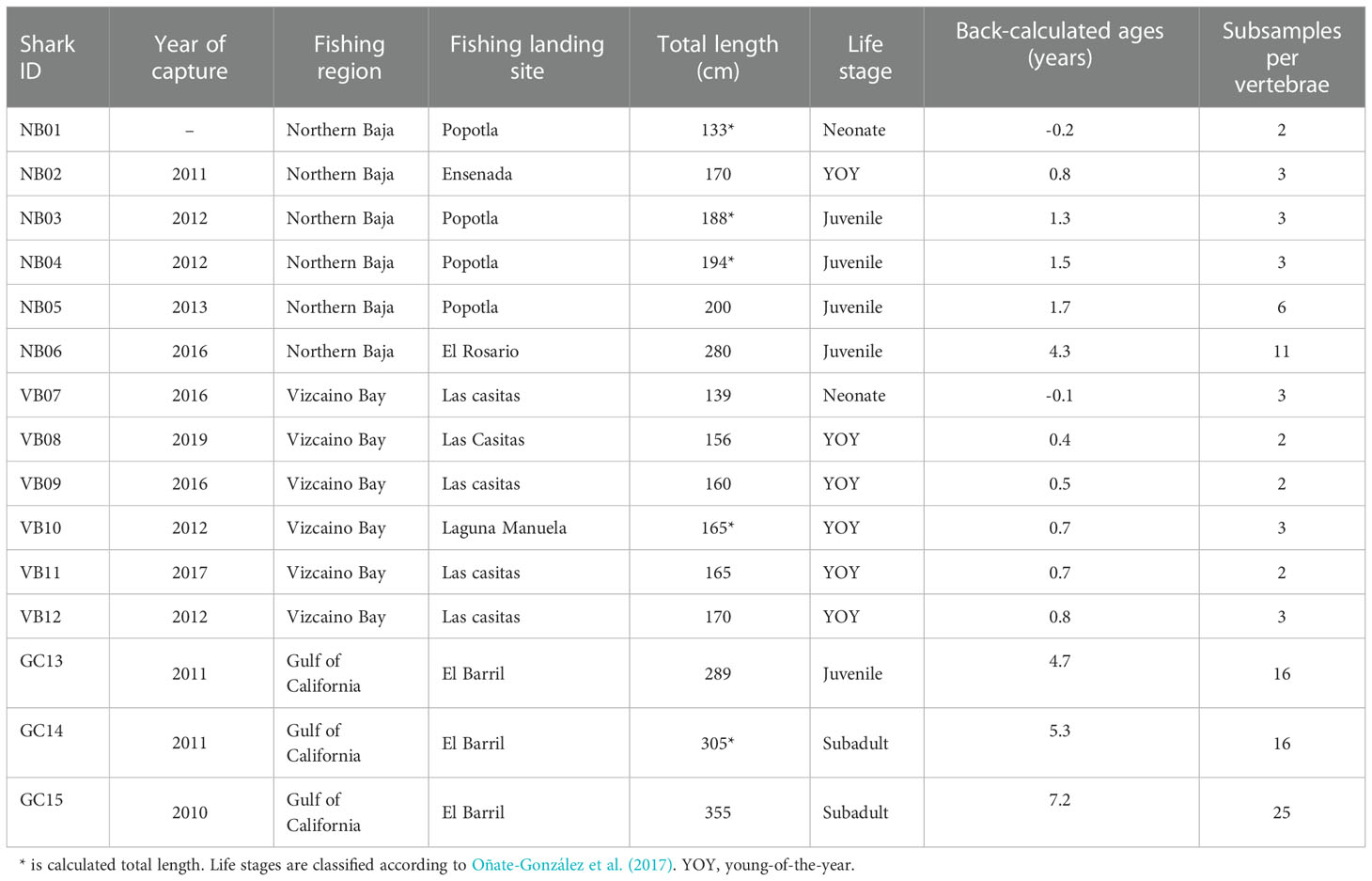
Table 1 Summary of capture location, year and total length of white sharks collected off the Pacific coast of Baja California peninsula (NB, Northern Baja; VB, Vizcaino Bay) and the Gulf of California (GC).
2.2. Stable isotope analysis
One vertebrae from each White Shark was cleaned by removing the neural arch and connective tissue, and dried at room temperature. Vertebrae were cross-sectioned using a slow-speed diamond saw (Buehler, ISOMET™) along a central longitudinal plane to obtain a 1-cm thick section. Each section was mounted on a glass slide using thermoplastic adhesive (Crystalbond). Subsamples of vertebral collagen were sequentially extracted along the corpus calcareum, beginning before the birth mark and continuing to the marginal edge, using a high precision micromill (ESI New Wave Micromill) at CICESE. Each sample consisted of powder (~1200 µg) recovered from a 500x500x700 μm (LxWxD) volume. Sample midpoint distances relative to the marginal edge are used to plot C and N isotopic compositions through life and provide datums for correlation with corresponding element time-series. (Figure 2A). Because vertebral growth rates slow with age, our usage of standard sample volumes means that samples approaching marginal edges of largest individuals will integrate more time.
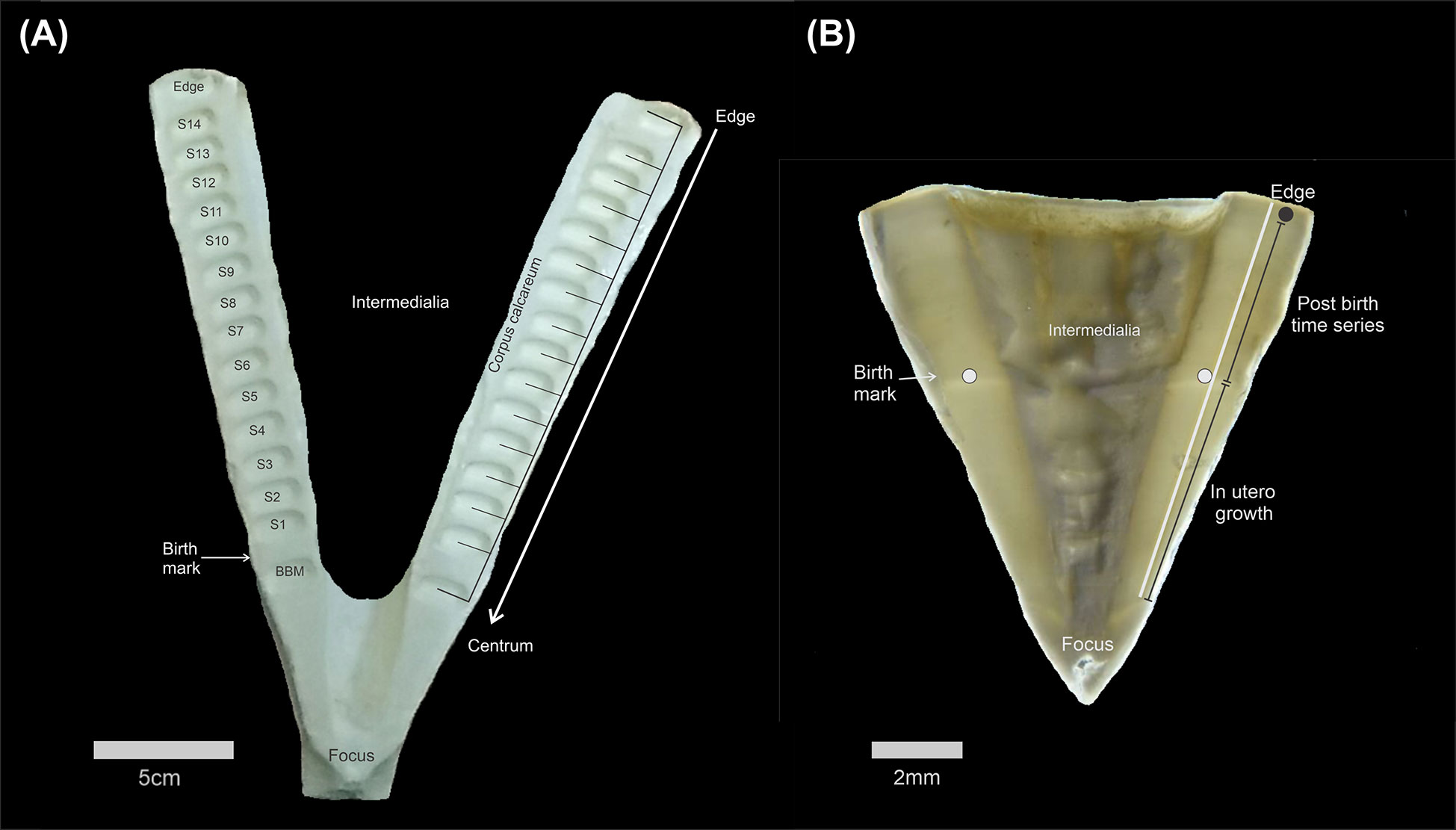
Figure 2 Transversal section of a vertebral centrum from White Shark vertebrae illustrating (A) the location of milled subsamples with a dark arrow indicating the middle of each raster used for isotopic subsampling; (B) the location of laser ablation trace (white line) along the corpus calcareum.
Carbon and nitrogen isotope compositions of vertebral collagen were analyzed using a PDZ Europa ANCA-GSL elemental analyzer interfaced to a PDZ Europa 20-20 isotope ratio mass spectrometer (IRMS; Sercon Ltd., Cheshire, UK) at the Stable Isotope Facility of the University of California at Davis, USA. Accuracy of internal laboratory standards (bovine liver, glutamic acid) was within 0.07 ‰ for both elements, with a precision of ~0.1‰ or lower (SD = 0.11 ‰). The C:N ratio was estimated to determine whether the lipid content in tissues have an influence on δ13C values; pure protein would be expected to have a C:N close 3, and hence this value was used an a reference for the absence of lipids (Hussey et al., 2012). Note that cartilage is not expected to contain lipids (Hussey et al., 2012). Isotope ratios are reported in delta (δ) notation relative to Vienna Pee Dee Belemnite for δ13C and atmospheric N2 for δ15N as international standards:
where R is the abundance of the heavy to light isotope ratio for the sample or standard.
2.3. Elemental ratios
Elemental concentrations through radial growth in White Shark vertebra (N=14, one of the smallest samples excluded) were measured by LA-ICP-MS in two consecutive daily sessions (January 24-25, 2018) at the University of Texas at Austin Department of Geosciences, using an ESI NWR193 excimer laser ablation system (193nm, 4ns pulse width) coupled to an Agilent 7500ce ICP-MS. The LA-ICP-MS system is equipped with a large format, two-volume, sample cell with fast washout (<1s) that accommodated all samples and standards in a single cell loading. The system was optimized daily for sensitivity across the AMU mass range and low oxide production (ThO/Th: 0.39 ± 0.08%) by tuning on a standard (NIST 612), and these parameters checked from trial transects on representative specimens. Following pre-ablation (125µm spot, 50µm/s scan rate, 2.2 J/cm2 fluence) to remove shallow surface contaminants, transects running from the corpus calcareum focus to the vertebral edge were performed on each specimen using a 25x100µm rectangular aperture-with the short axis maintained parallel to the growth axis, 5µm/s scan rate, 3.36 ± 0.04 J/cm2 energy density (fluence), 20 Hz repetition rate, and carrier gas flows of 0.8 L/min for Ar and He. Due to the large size of the vertebrae, several nodes were placed along the lines to ensure that the sample surface remained in focus throughout transects (Figure 2B). Baseline intensities before each standard and sample analysis were determined from 30 s gas blank measurements, made while the laser was off and all masses were scanned by the quadrupole. The quadrupole time-resolved method measured 13 masses with integration times of 10ms (24-25Mg, 43Ca, 55Mn, 88Sr), 20ms (7Li, 63-65Cu,66-68Zn, 111Cd, 138Ba, 208Pb), and 25ms (137Ba). The quadrupole duty cycle of 0.2422s corresponds to 88.8% measurement time, with corresponding linear sampling rates of 1.211µm, equivalent to 20.6 measurements within the footprint of the 25µm-tall aperture. Measured intensities were converted to elemental concentrations (ppm) with iolite software (Paton et al., 2011) and using 43Ca as the internal standard and a Ca index concentration value of 35 wt% for vertebra unknowns (McMillan et al., 2017). USGS MAPS-4 (synthetic bone) was used as the primary calibration standard. USGS MACS-3 (synthetic aragonite) and NIST 612 were used as secondary reference standards. Standards were analyzed hourly in triplicate for 60s. Over the two days, the grand average of analyte recoveries was within 4% and 7% of reference values (http://georem.mpch-mainz.gwdg.de) for MACS-3 (n=54) and NIST 612 (n=60), respectively. The derived elemental time-series were smoothed by consecutive moving median and average filters using a 7-point boxcar width (8.477 µm equivalent distance), resulting in smooth, locally weighted, signals that are free from high-frequency outliers. Signals were converted to distance (µm) based on the scan rate and duty cycle and then concentration data was expressed as molar ratios to calcium. Because signals for elements measured with multiple isotopes were virtually identical, we use the higher abundance isotope (24Mg, 63Cu, 66Zn, 138Ba) for plotting and statistical analysis. Outliers were still present after the 7-point smoothing, especially near the vertebrae edge, where the mineralized corpus calcareum intersects with non-mineralized cartilage. A decrease in 43Ca and increase in 63Cu were therefore used to approximate the shift in vertebrae material near the edges. The final 500 µm of element data were plotted to examine the edge spikes in detail and data was trimmed to ensure only the corpus calcareum element data was used for regional comparisons (see below).
2.4. Data analysis
Although both SIA and elemental:Ca ratios represent a longitudinal transect along each individual’s life, the temporal scale reflected by each set of measurements differs. The δ13C and δ15N values were plotted longitudinally by position along vertebrae and binned into three ontogenetic subgroups: (1) before the birthmark (BM), (2) after the BM, and (3) along the corpus calcareum and edge (E). In addition, isotope ratios were plotted as a function of estimated TL by back-calculating the size corresponding to the position of each subsample, This assumes a proportional relationship between shark TL and vertebral diameter that is supported by the linear relationship reported above.
Two sample t-tests with unequal variance were performed to test for differences in the isotopic composition of edges of sharks caught in the Pacific off Northern Baja, in Vizcaino Bay and the GC.
For statistical comparison of elemental ratios with SIA data, average element:Ca ratios were calculated for the entire maternal region (distances of 5,000 to 6,500 μm from the vertebrae focus to the BM), which corresponds to vertebral development during gestation before birth, and for most recent vertebrae development within the final ~500 microns of the marginal edge. which represent the most recently formed material. Due to small sample size, a nonparametric Kruskal-Wallis test with Dunn’s multiple comparison was performed to compare median elemental ratio differences of maternal and vertebrae edges among capture regions.
Due to the highly variable size of vertebrae, (i.e., smaller sharks with mostly maternal cartilage, larger sharks spanning many years post birth), element:Ca ratios were visualized in several ways. All vertebrae were color coded by region of collection. Digital images of each vertebrae were used to measure the distance of the beginning of the laser path from the vertebrae focus to the birthmark, and from the birthmark to the vertebrae edge, using ImageJ. The measured distances were then used to parse the data in maternal time-series profiles, neonate to juveniles, and juvenile/subadult. Separating the high-resolution element:Ca ratio data on different distance scales was required to observe underlying patterns.
3. Results
White Shark sizes ranged from 133 to 355 cm TL, which corresponds to neonates to sub-adults from zero to seven years old (Table 1). The smallest sharks were caught in the Pacific coast off Baja California, and the largest in the GC. To aid interpretation of stable isotope ratios and elemental ratios relative to life stages, White Sharks were classified by size following Oñate-González et al. (2017) into four classes: newborn or neonate (102-150 cm TL), young of the year (YOY, 150-175 cm TL), juveniles (175-300 cm TL), and subadults (>300 cm TL). No adults (>360 cm TL for males and >480 cm TL for females) were sampled.
3.1. Stable isotope analysis
Except for one subsample, all C:N ratios were lower than 3.2 (3.0 avg), indicating no effect of lipids on results and a sample pretreatment for decalcification was therefore not necessary (Supplementary Table 1). Vertebrae δ13C and δ15N values showed a broad range, with -11.3 to -16.5‰ for carbon and 15.4 to 21.2‰ for nitrogen (Supplementary Table 1). Averages and standard deviations calculated as a function of location along the corpus calcareum and pooling subsamples from all sharks, demonstrate a positive relationship between carbon and nitrogen isotope ratios (Figure 3). Material deposited before and after the birth mark (BM) are coded as position 1 and 2, respectively. For the smallest sharks, edge values were deposited at or near the BM (position 2 and/or 3 in graph). A broad range of values was observed in subsamples corresponding to both before (position 1) and after BM (positions 2+). Vertebrae margin edge samples had a lower range of isotopic values, 3.5‰ for δ13C and 5.5‰ for δ15N.
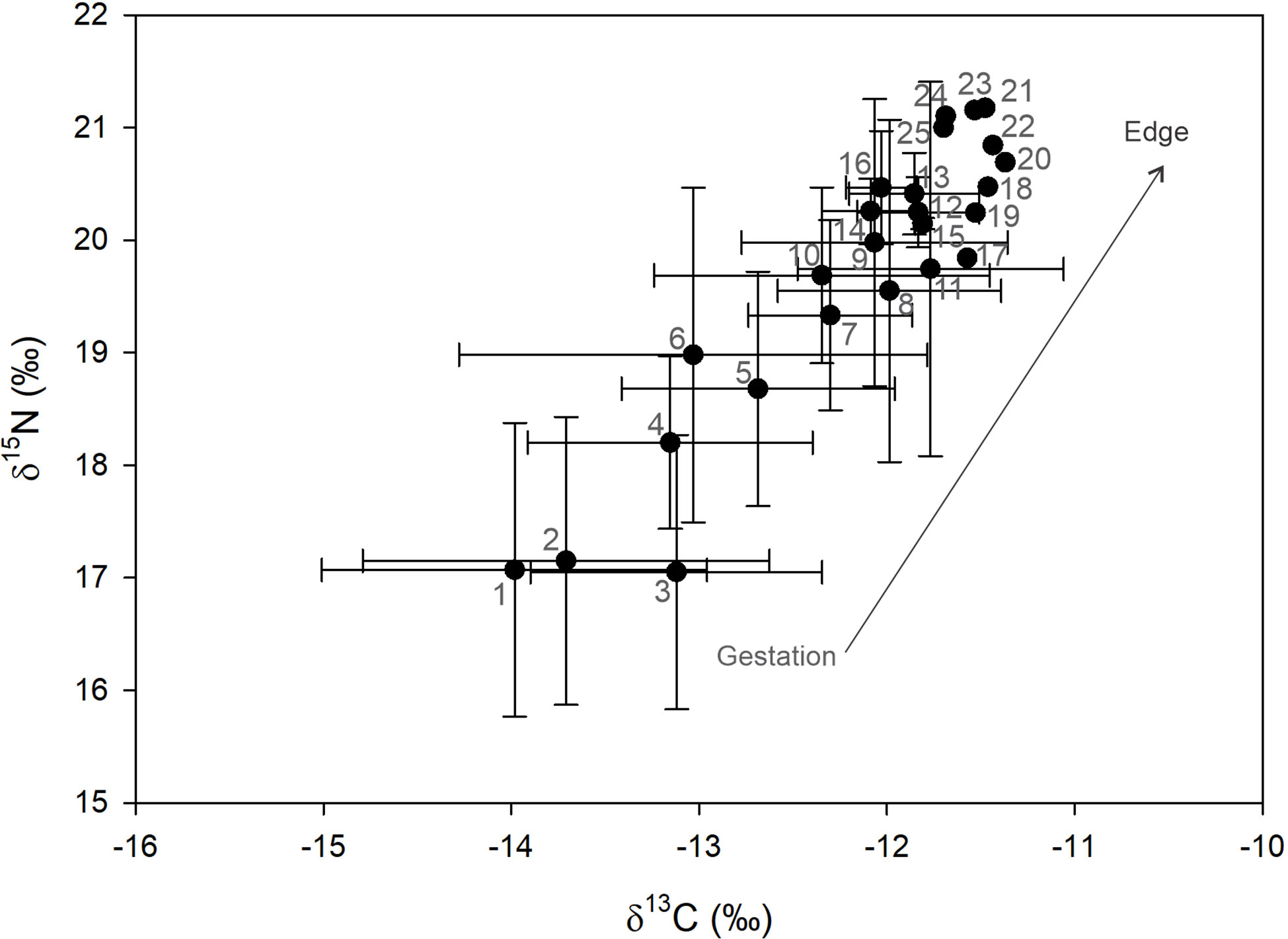
Figure 3 Mean ± SD of carbon and nitrogen isotope ratios for White Shark vertebrae sampled sequentially from before the birth mark (gestation period; number 1 on graph) to the subsample of the edge from the largest shark from which 25 subsamples were obtained (number 25 on graph). Since raster size was similar, sequential subsamples should correspond roughly to the same shark size.
Longitudinal isotopic data for individual sharks indicated two distinct groups: those captured in the GC that included the largest sharks (GC13, GC14 and GC15), and those caught in the Pacific coast off Baja California (Figure 4). Sharks caught in the GC had higher δ13C (ca. -12‰) and δ15N (ca. 20-21‰) values in subsamples from close to the vertebral edge (Figures 4A, C). Shark GC15 had similar C and N isotope ratios throughout its ontogeny, and a different isotopic composition than Pacific coast sharks even in early life (Figures 4B, D). In contrast, early life White Sharks GC13 and GC14 had similar isotopic ratios to the edges of sharks caught in the Pacific coast off Baja California, suggesting they may have been born there and later migrated into the GC. White Shark NB06 caught in El Rosario (Pacific coast off Baja California) also had a similar isotopic ratio early in life to that of subadults caught in the GC, suggesting it may have been reared in the GC and subsequently migrated to the Pacific.
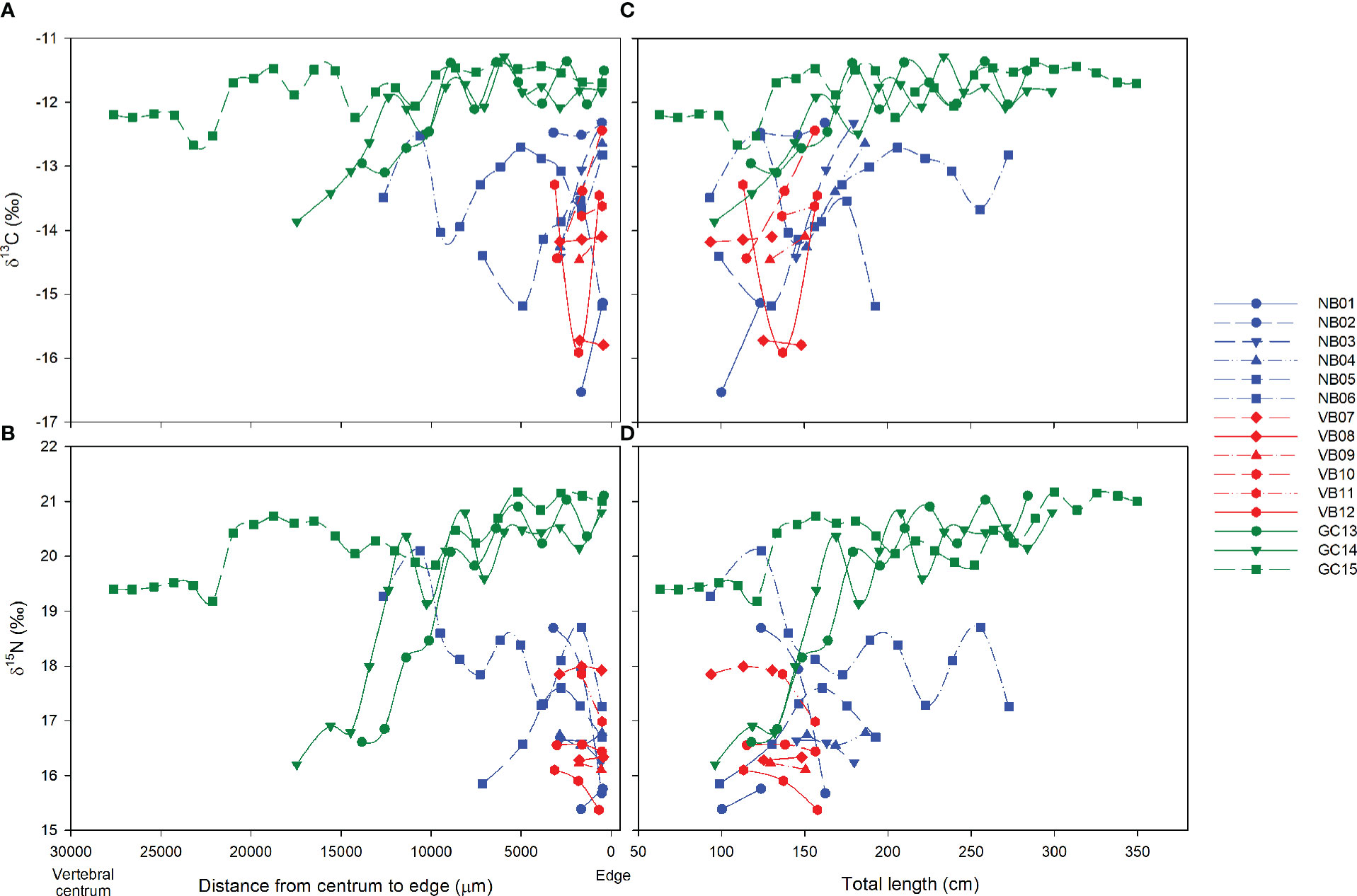
Figure 4 Longitudinal δ13C (A) and δ15N (B) values from before the birth mark (growth in the uterus) to the edge. Panels (C) and (D) present isotope ratios as a function if estimated total length. Zero indicates the edge. NB, Northern Baja; VB, Vizcaino Bay; GC, Gulf of California.
3.2. Vertebrae edge stable isotope analysis
Comparison of mean ± SD of C and N isotopes by capture region (from edge subsamples) shows a wide range of isotope ratios (Figure 5). However, sharks caught in the GC had higher δ15N values (by 4-5 ‰) and δ13C values (by 2 ‰) than those captured from the Pacific coast off Baja California, Differences were highly significant for carbon and nitrogen isotope ratios (p = 0.004 and p<0.001, respectively).
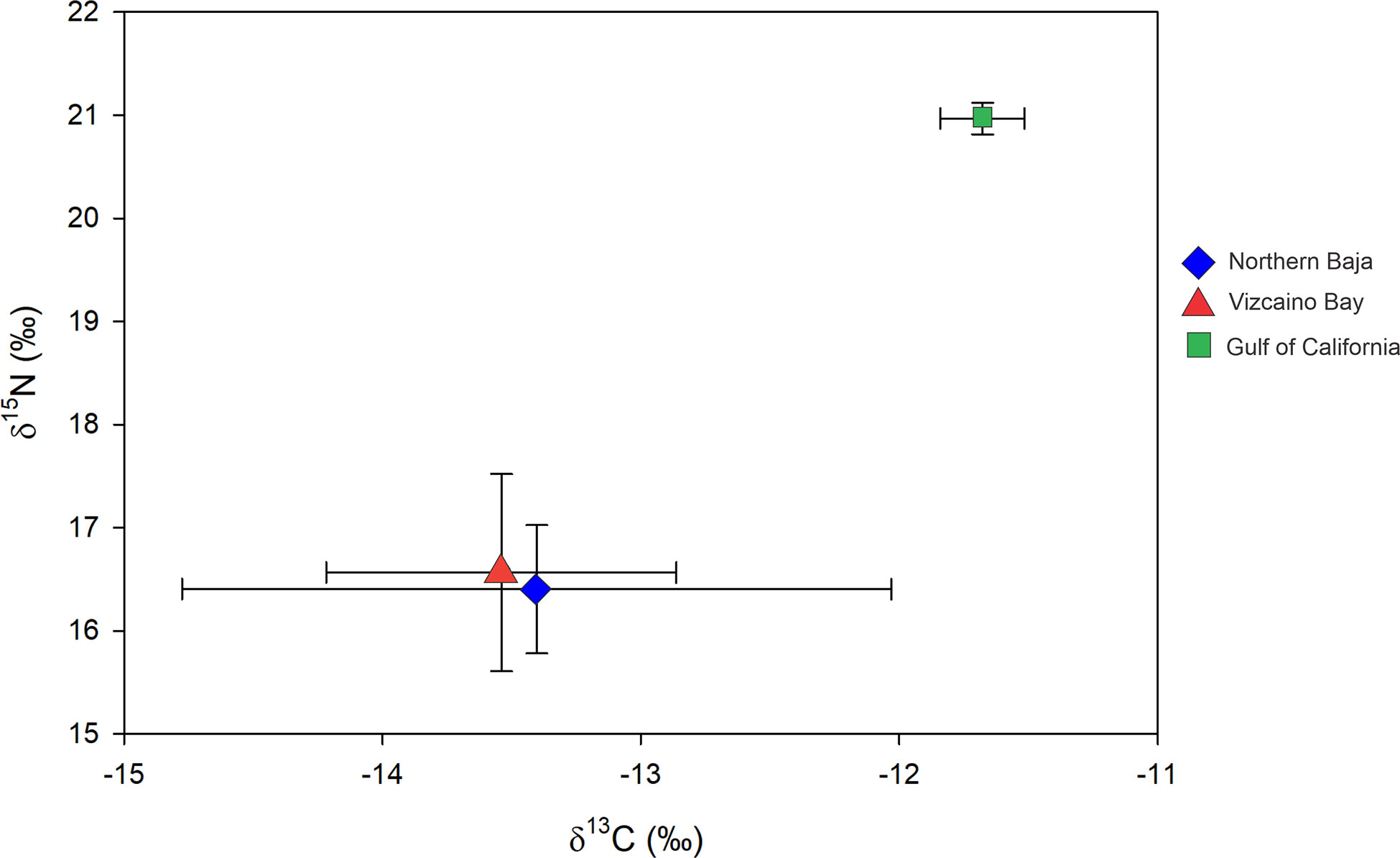
Figure 5 Mean ± SD isotope ratios of edge subsamples of White Shark vertebrae collected in Northern Baja, Vizcaino Bay and Gulf of California.
3.3. Element:Ca ratios
Maternal element:Ca ratios were generally stable and consistent for Li:Ca and Sr:Ca, with sharks from all regions exhibiting overlapping values (Figure 6). Mg:Ca was consistent from the focus until just prior to the BM and then increased. Mn:Ca, Zn:Ca, and Ba:Ca also varied just before the BM. White Sharks collected from GC tended to have higher Ba:Ca and Zn:Ca, compared to those from northern Baja and Vizcaino Bay (Figure 6). Mn:Ca and Zn:Ca were generally elevated near the vertebral focus, and then gradually decreased towards the BM.
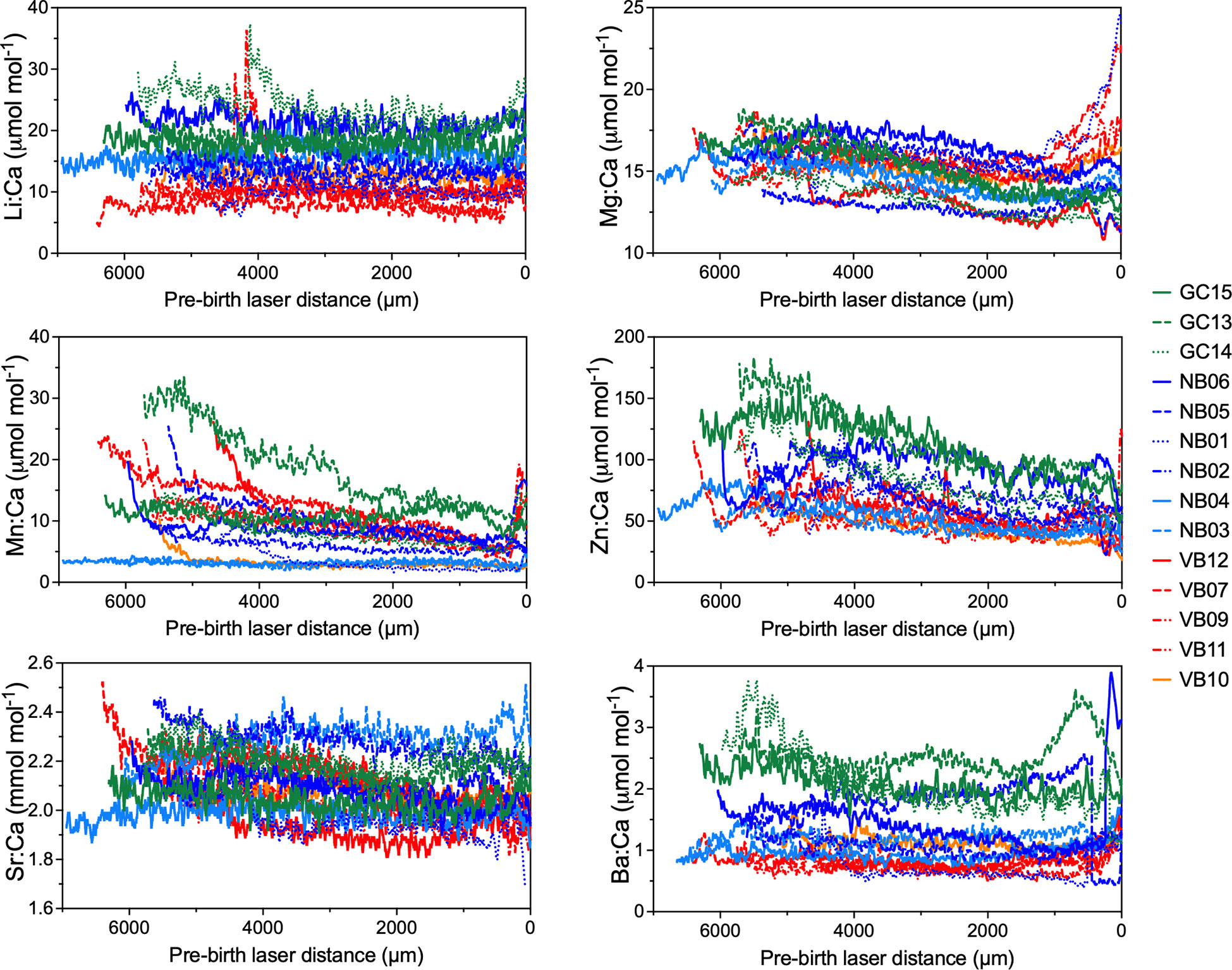
Figure 6 Elemental ratio profiles of maternal vertebrae regions. Laser ablation started near the focus of the corpus calcareum and proceeded to the birth band (distance = 0). Sharks are color coded by region of sample collection (see Table 1 for sample metadata), with color tones and dashed lines representing individuals.
Post-birth mark (BM) element:Ca ratios were highly variable in juvenile White Sharks from northern Baja, Vizcaino Bay, and the GC (Figure 7). Most element:Ca values displayed oscillatory patterns with sharks from different regions overlapping except GC15 and GC13, which had higher and longer duration Mn:Ca peaks. All sharks showed an increase of Mg:Ca near the vertebrae edge. In GC subadult White Shark vertebrae, Li:Ca and Sr:Ca showed a gradual decrease, and high degree of overlap with northern Baja specimens (Figures 8A, B). Similar to the juveniles, subadult vertebral Mg:Ca increased rapidly near the vertebrae edge and Mn:Ca oscillated with higher maximum peaks in sharks from the GC (Figure 8A). White Sharks collected from GC also exhibited highly oscillatory Zn:Ca and Ba:Ca that were higher than other capture localities (Figure 8B). A large shark collected in the Pacific coast off Baja California (NB06) had notably lower Ba:Ca and Mn:Ca compared to other GC sharks (Figures 8A, B).
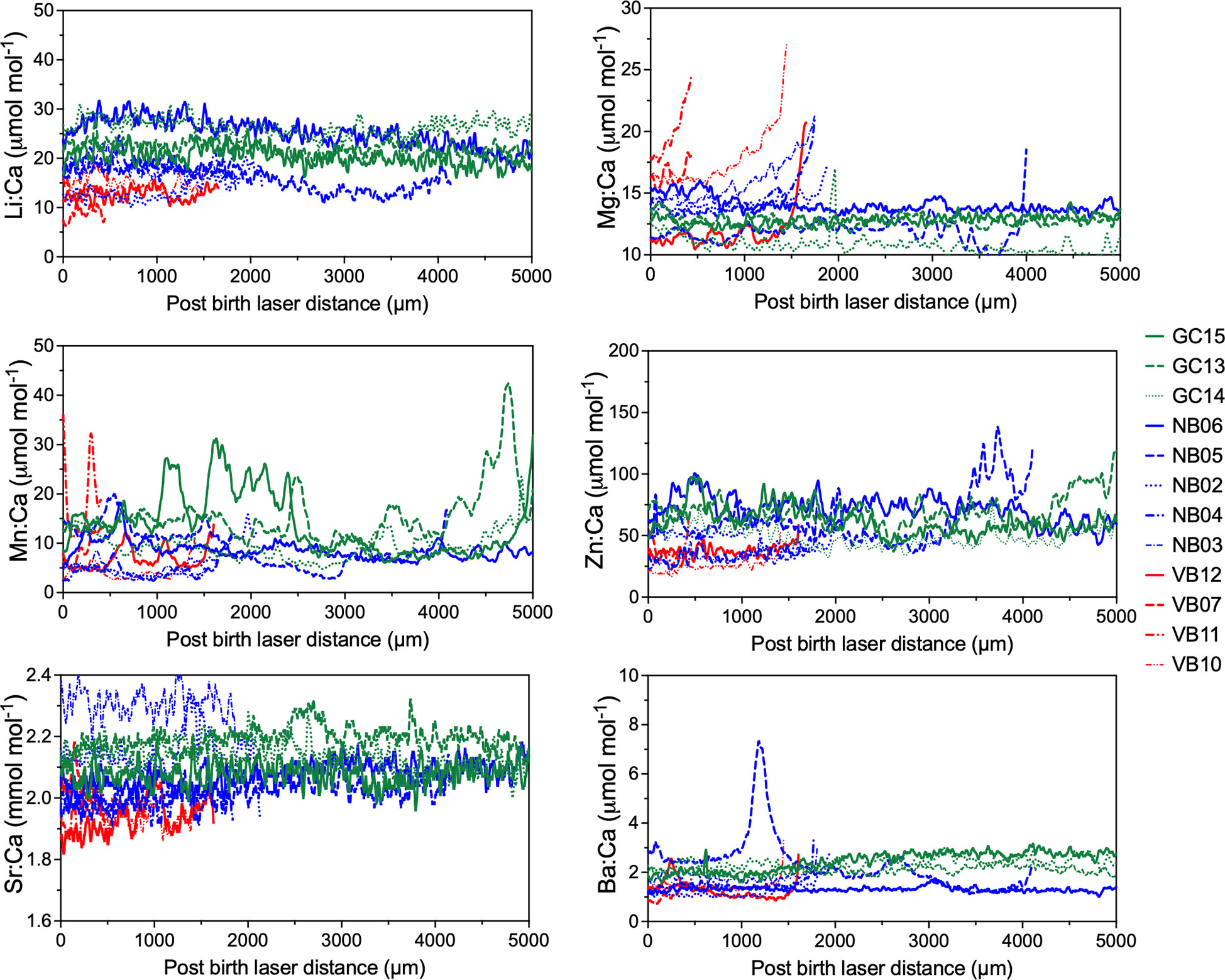
Figure 7 Post-birth elemental ratio profiles of juvenile (see Table 1) White Shark vertebrae. Distance zero indicates the birth band with distances (up to 5000 microns) varying depending on shark size. Blue lines represent sharks caught off northern Baja California while red indicates samples collected in Vizcaino Bay and green profiles are sharks collected from Gulf of California.
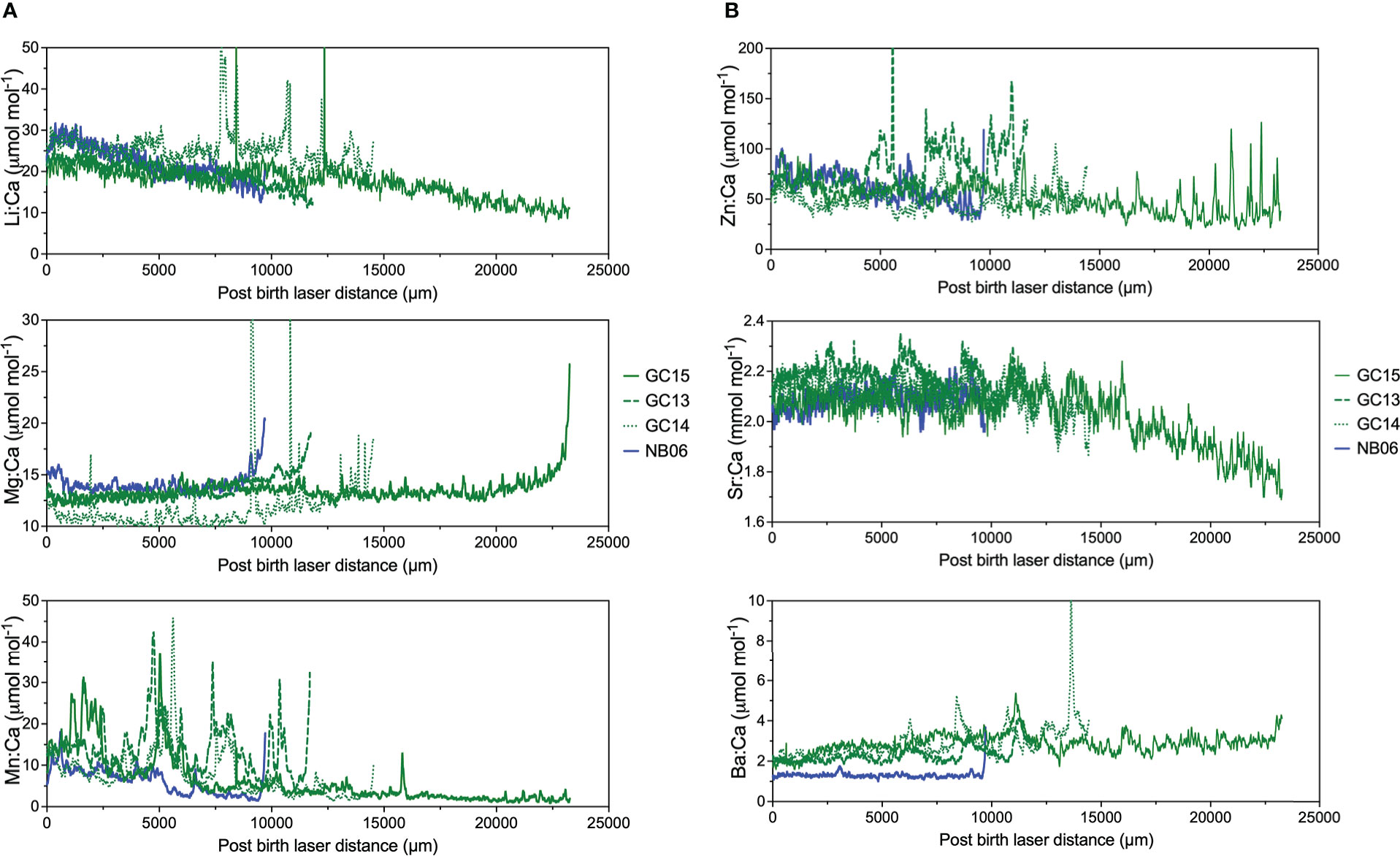
Figure 8 (A) Elemental ratio profiles (Li:Ca, Mg:Ca, Mn:Ca) of sub-adult White Shark vertebrae (see Table 1). Green color indicates samples from the Gulf of California, blue indicates northern Baja. (B) Element profiles (Zn:Ca, Sr:Ca, Ba:Ca) of sub-adult White Shark vertebrae (see Table 1). Shark NB06, collected in the northern Baja, was included for comparative purposes since stable isotope analysis indicated it migrated from the GC to the Pacific.
3.4. Maternal and edge element: Ca ratio comparison
Average element:Ca ratios for vertebral growth during gestation differed among all capture localities except northern Baja for Li:Ca, Zn:Ca, and Ba:Ca, with significantly higher values for sharks caught in the GC compared to Vizcaino Bay (Figure 9). Maternal Mg:Ca, Mn:Ca, Sr:Ca were generally comparable among White Sharks collected in the three regions. Average edge values, representing recent life prior to capture, were significantly higher in Zn:Ca and Ba:Ca in GC captures compared to Vizcaino Bay (Figure 10), whereas Li:Ca, Mg:Ca, Mn:Ca, and Sr:Ca did not differ among sampling regions.
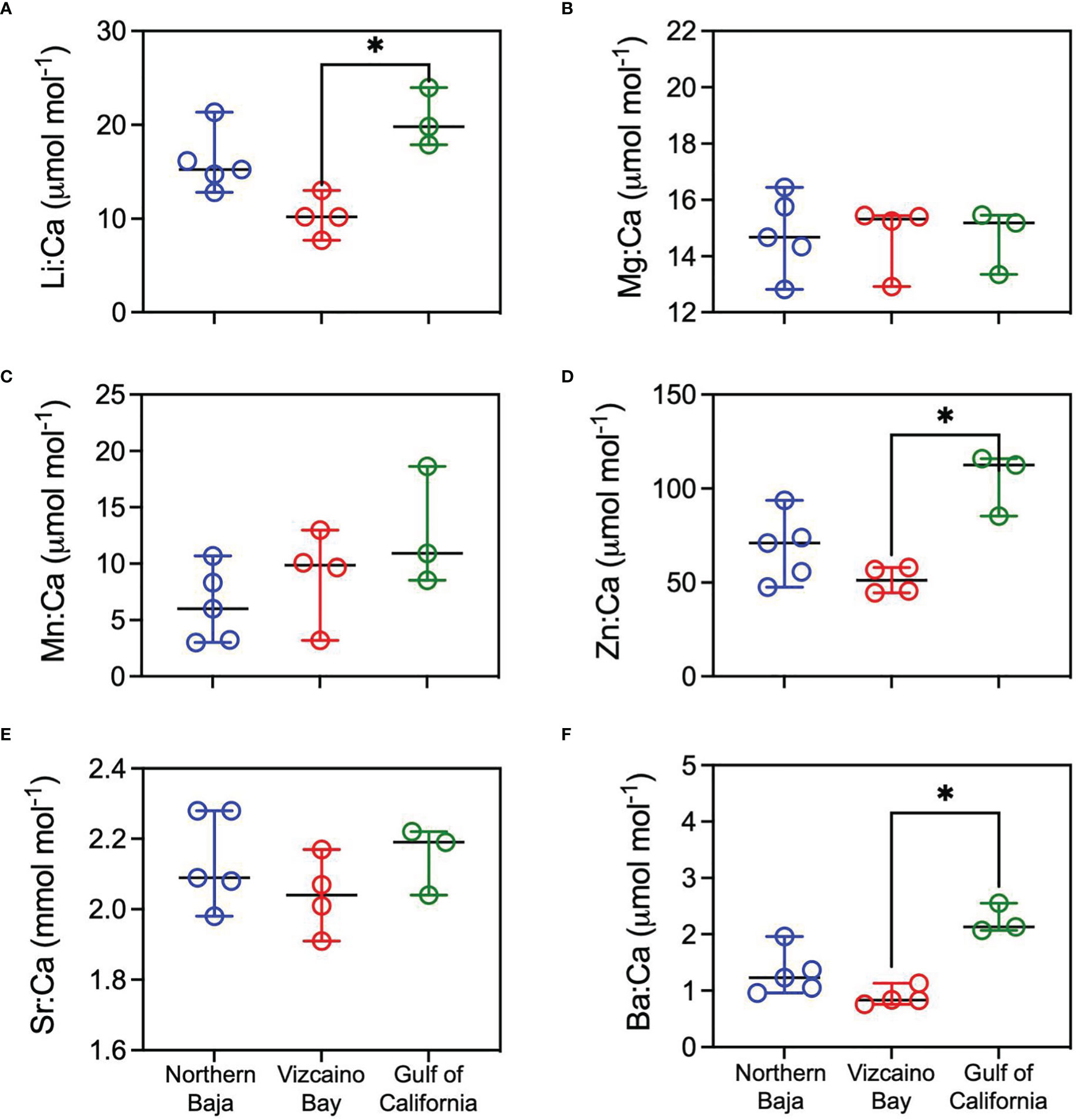
Figure 9 Scatter dot plots (line=median, error bars=95% confidence intervals) of average elemental ratio concentration of maternal vertebrae regions (pre-birth band). Sharks are grouped by region of sample collection: Northern Baja=blue, Vizcaino Bay=red; Gulf of California=green. Nonparametric Kruskal-Wallis tests compared median values among regions, with an asterisk indicating significant differences (p < 0.05) based on Dunn’s multiple comparisons test. (A) Li:Ca, (B) Mg:Ca, (C) Mn:Ca, (D) Zn:Ca, (E) Sr:Ca, (F) Ba: Ca.
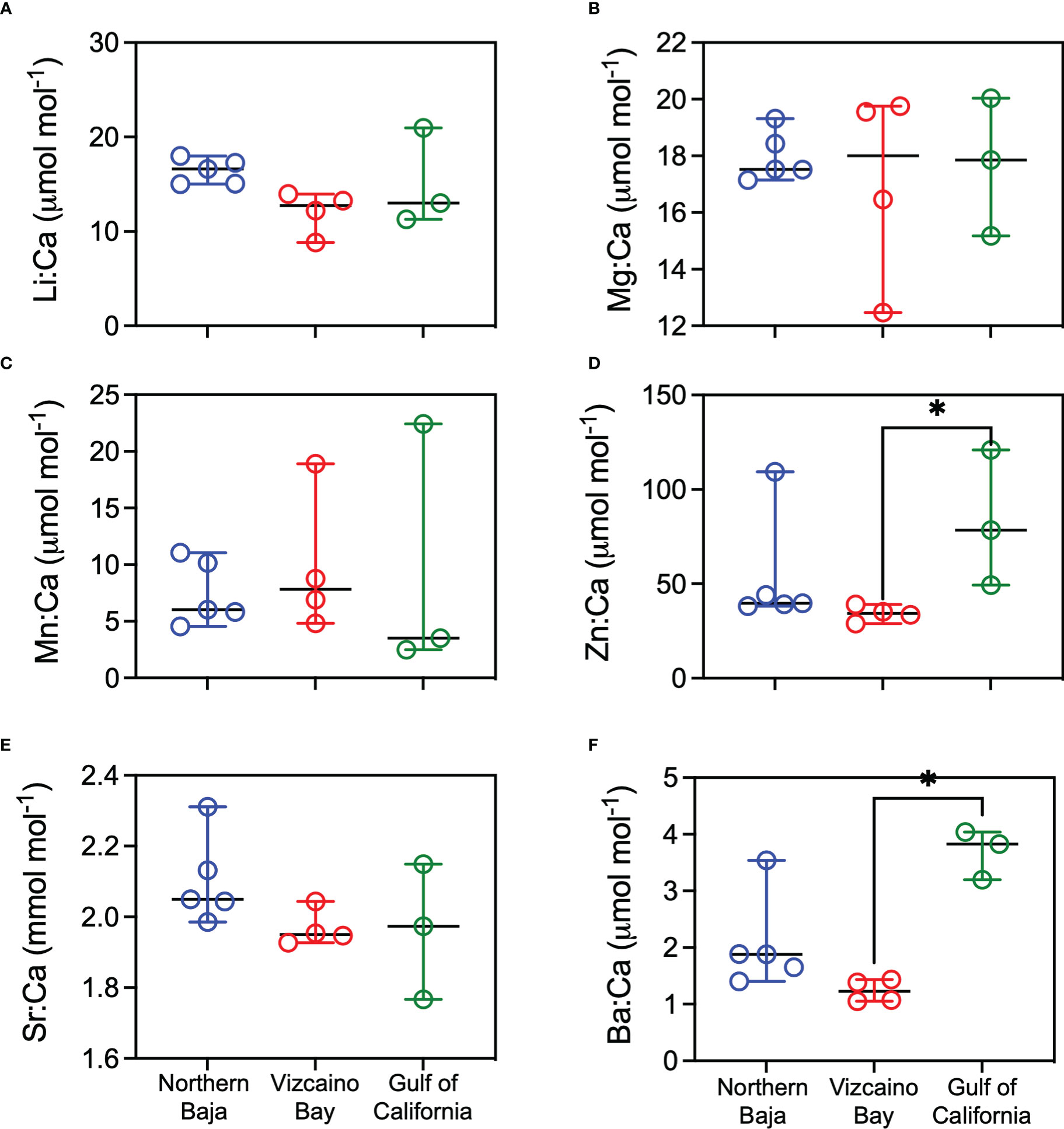
Figure 10 Scatter dot plots (line=median, error bars=95% confidence intervals) of average elemental ratio concentration of vertebrae edges (final 500 microns). Sharks are grouped by region of sample collection: Northern Baja=blue, Vizcaino Bay=red; Gulf of California=green. Nonparametric Kruskal-Wallis tests compared median values among regions, with an asterisk indicating significant differences (p < 0.05) with Dunn’s multiple comparisons test. (A) Li:Ca, (B) Mg:Ca, (C) Mn:Ca, (D) Zn:Ca, (E) Sr:Ca, (F) Ba: Ca.
4. Discussion
The consistent increase in δ13C and δ15N values of subsamples collected from before the BM to the edge is broadly consistent with feeding at higher trophic levels during ontogeny, as has been documented for White Sharks based on gut content analysis, behavioral observations of feeding and previous δ13C and δ15N analyses of soft tissues (Tricas and McCosker, 1984; Estrada et al., 2006). However, in our study this pattern is likely confounded by regional differences in isotopic baselines and hence the isotopic composition of the prey on which individuals fed throughout their lives. Hussey et al. (2010) reported trophic discrimination factors for vertebral tissues of 1.5 and 3.8 ‰ for carbon and nitrogen, respectively, based on semi-controlled feeding experiments on large sharks. Off central Baja California, small White Sharks (200 cm TL) feed mostly on demersal fishes, with a limited contribution of pelagic fishes (Tamburin et al., 2020, García-Rodríguez et al., 2021). However, the largest shark caught in the Pacific and those caught in the GC should feed on marine mammals based on their size and known ontogenetic diet shifts (Tricas and McCosker, 1984). Although the three sharks caught in the GC are larger than most of the sharks caught off the west coast of Baja California (289 to 355 cm TL), the largest one (NB06, 280 cm TL) had an edge δ15N value of 17‰, which is about 4-5‰ lower than the mean for edge subsamples of GC sharks. In the absence of differences in the isotopic composition of the isotopic baseline, these subadults would have similar isotope ratios, which is not the case.
The enrichment in 15N observed in the vertebral edges of sharks caught in the GC likely reflects well-documented intense denitrification associated with the minimum oxygen zone of the tropical eastern Pacific, which extends to the GC and leads to high nitrate values that propagate throughout the food web (Altabet et al., 1999; Voss et al., 2001; Sigman et al., 2005; Aurioles-Gamboa et al., 2013). Although the sharks analyzed in this study were caught over the course of nine years and decadal-scale or interannual variations in the isotopic baseline are possible (e.g. Lorrain et al., 2020), intense denitrification in the tropical and subtropical eastern Pacific spans millennia and persists even during El Niño/La Niña Events (Thunell and Kepple, 2004; Yang et al., 2017). In addition, examination of C and N isotope ratios of young sharks sampled off central Baja California did not show marked differences between 2013 and 2017 (García-Rodríguez et al., 2021). Multiple studies over time have shown the GC’s food web to be enriched in 13C and 15N relative to the Pacific (e.g. Porras-Peters et al., 2008; Ruiz-Cooley et al., 2011; Aurioles-Gamboa et al., 2013; Richert et al., 2015; Ordiano-Flores et al., 2021), including studies that have analyzed the same tissue type from the same species in both regions (Elorriaga-Verplancken and García-Aguilar, 2018; Rosas-Hernández et al., 2018; Busquets-Vass et al., 2021). Fur from adult female California sea lions (Zalophus californianus) sampled in the Pacific off the central Baja California Peninsula had lower δ15N values than those from the southwestern GC (17.8 ± 0.2‰ vs 20.7 ± 1.1‰, Elorriaga-Verplancken and García-Aguilar, 2018). Similarly, vibrissa (whiskers) of California sea lions samples in the central GC and in southern Baja’s Pacific waters indicated δ15N values that were ca. 1‰ higher in the GC (Rosas-Hernández et al., 2018). In this case, the limited difference in nitrogen isotopic composition is likely due to the influence of denitrified waters off the southern extent of the Baja California Peninsula (Altabet et al., 1999). Skin tissue of filter-feeding blue whales (Balaenoptera musculus) was also significantly higher in the GC compared to the Pacific (14.8 ± 0.1‰ vs. 13.3 ± 0.1‰; Busquets-Vass et al., 2021). Therefore, the δ15N values reported in these studies are consistent with the well-documented enrichment in 15N within the GC, which is consistent among years.
Based on the premise that consumers in the GC consistently have higher tissue δ13C and δ15N values, longitudinal analysis of the largest shark sampled (GC15: a 355 mm TL subadult) indicates that it was a lifelong resident in the GC. This implies that there may be unrecognized White Shark birthing and nursery areas in the GC that could add to known northeastern Pacific nursery habitats in the Southern California Bight and Vizcaino Bay (Domeier et al., 2012, Santana-Morales et al., 2012; Oñate-González et al., 2017). Based on δ13C and δ15N values from the BM and later life, two subadult sharks caught in the GC (GC13 and 14) were likely born in the Pacific coast of Baja California and then migrated to the GC until capture. Since we only had access to samples from three individuals caught in the GC, it is difficult to extrapolate our findings to the population level. Our results nevertheless indicate that some White Sharks are born in the GC, and that some proportion of young juveniles migrate from the Pacific into the Gulf. Little is known about these specific migration patterns, however, and future studies are warranted.
Given that the isotopic composition of edge subsamples reflects an individual’s most recent feeding history, the distinct vertebral δ13C and δ15N values of sharks captured in the Pacific coast off Baja California and the GC supports our proposition that migration of individual sharks between the Pacific and Baja California is traceable. For example, based on the size of the vertebrae when the isotopic composition shifted, at around 10,000 µm in GC13 and 12,000 µm in GC14 (Figure 4), we estimate that migration into the GC occurred at a TL of around 164 cm and 157 cm, respectively, which corresponds to the YOY stage (Oñate-González et al., 2017). Since the BM isotopic compositions suggest that these sharks fed in the Pacific coast off Baja California during early life, we infer that they migrated into the GC as YOY, where they remained until capture. Consistent with the possibility, Weng et al. (2012) reported that a YOY White Shark held in captivity for half a year, tagged and released off Monterey, California migrated southward along the coast, entered the GC, and continued migrating to the central Gulf.
With one exception (NB06), δ13C and δ15N values of all sharks (11 individuals) caught in the Pacific coast off Baja California were lower than sharks caught in the GC. Since all these sharks were neonates and juveniles (133-180 cm TL), this is consistent with their birth and residency in the Pacific coast. However, their small size at capture does not preclude the possibility that larger sharks migrate from the Pacific coast of Baja California to the GC, as was the case for NB06 (280 cm TL), which likely migrated to the GC during the juvenile stage. The broad range of δ13C and δ15N values in edge subsamples of sharks caught in the Pacific coast of Baja California indicates that feeding could have occurred in different areas, including either nursery ground as well as the migratory corridor between them described by Weng et al., (2007; 2012), White et al. (2019), and García-Rodríguez et al. (2021).
Some recent studies have documented that the late-term White Shark embryos feed on uterine milk (Sato et al., 2016; Tomita et al., 2022). Given the extensive seasonal migration from oceanic to coastal habitats by pregnant females to give birth (Domeier and Nasby-Lucas, 2013) and the low consumption rate of female sharks in oceanic areas (Carlisle et al., 2012), the isotopic composition of the uterine milk could reflect different feeding areas in northeastern Pacific Ocean. Since neonates are known to start feeding immediately after birth (Logan et al., 2018; García-Rodríguez et al., 2021), the small variation in δ13C and δ15N values before and after BM (less than 1‰) among individuals for our study, suggests that the isotopic composition of neonate vertebrae may mark a gradual transition from intrauterine milk to external coastal prey. This raises the possibility that nursery areas may be distinguished based on the isotopic composition of a vertebrae’s edge of neonates and young of the year who are still feeding in the nursery area. In contrast, the isotopic composition of the vertebral tissue laid down after the BM in oceanic habitat-dwelling sharks that undergo intrauterine placental feeding, such as the oceanic White Tip Shark (Carcharhinus longimanus) and the Blue Shark (Prionace glauca), appears to solely follow the maternal contribution and can thus be used to infer feeding region, rather than nursery areas of the young (Estupiñán-Montaño et al., 2019; Shen et al., 2021).
To assess latitudinal gradients in the isotopic composition of White Shark vertebrae at catch locations throughout their distribution in the northeastern Pacific, we compared our data with Kerr et al. (2006) and Kim et al. (2012), who analyzed historical samples from White Sharks caught off California. Sharks in these two studies were older and larger (9-20 years old in Kim et al., 2012 with sizes not reported, and 239 to 463 cm TL in Kerr et al., 2006) than sharks analyzed in this study (0-7 years) and were likely feeding at a higher trophic level. Mean δ13C vertebral edge values of sharks caught north and south of Point Conception, California, are -12.6 ‰ and -13.7 ‰, respectively, which is similar to mean values for sharks in our study from the Pacific coast off Baja California (ca. -13.5‰) but distinct from those from the GC (ca. -11.8‰). Mean δ15N values of 18.6‰ and 18.3‰ for sharks caught north and south of Point Conception are higher than sharks collected in this study off northern and central Baja California (Figure 5). In our study, δ15N values from vertebral edges of sharks caught in the GC are even higher (ca. 21‰). The nitrogen isotope composition of vertebra for the White Shark that migrates from the GC to the Pacific coast off Baja California or vice-versa should therefore be distinguishable, suggesting that this intrinsic tracer could be useful in future migration studies. We note that samples in Kerr et al. (2006) and Kim et al. (2012) were pretreated with HCl and EDTA, respectively, to remove the mineralized carbon fraction. EDTA treatment leads to differences of about 0.15 to 0.5‰ in δ13C values and about ca. 0.4‰ in δ15N values and depends on the shark species (Christiansen, 2011). The potential impact of EDTA on δ15N values is smaller than the difference between edge δ15N values for sharks captured from the Pacific coast off Baja California and GC. In our study, any pretreatment was omitted to allow for stable isotope and elemental ratio measurements on the same vertebra.
Element:Ca ratio profiles in White Shark vertebrae offered similar inferences about habitat use and movement between the GC and Pacific coast of Baja California for some individuals. However, our current understanding of the influence of external environmental factors (i.e. temperature) and internal biological processes like digestion on vertebral cartilage mineralization, limits the precise interpretation of element patterns. Zinc is an essential element for biological processes including cell signaling and protein regulation (Vallee, 1983) and vertebral Zn:Ca in elasmobranchs was related to somatic growth rates in controlled lab experiments (Smith et al., 2013). Barium is a non-essential element in elasmobranchs and likely reflects environmental concentrations, such as the degree of upwelling (Mohan et al., 2018) or decreased temperatures (Smith et al., 2013) and upwelled waters are typically colder and nutrient rich. Vertebral edge element values most representative of the capture habitat suggest the GC was enriched in Ba:Ca and Zn:Ca compared to Pacific coast nurseries. Larger subadults (GC13, GC14 and GC15) with higher Ba:Ca, Zn:Ca and Mn:Ca throughout life compared to younger sharks caught in the Pacific coast off Baja California, suggest longer term residence in the GC, which is consistent with our interpretation of the stable isotope ratios for these individuals. Shark GC13 showed an increase in Zn:Ca at about 4500 µm that was not observed in GC14; however, Mn:Ca patterns displayed the widest peaks of variation, potentially indicating residence in the different areas of the GC. Dissolved Mn2+ concentrations could reflect redox conditions related to the OMZ in the GC (Delgadillo-Hinojosa et al., 2006), as low oxygen promotes solubility of Mn2+ from particulate Mn oxides. The Pacific-collected shark NB06, displayed elevated Zn:Ca and Mn:Ca in earlier life, but those concentrations decreased as it moved from the GC to the Pacific coast off Baja California. This movement pattern is consistent with the nitrogen stable isotope data described above.
Examination of maternal regions of White Shark vertebrae suggest that mothers of three subadults collected in the GC (GC13, GC14 and GC15) could have been in the GC for the duration of pregnancy, since both Zn:Ca and Ba:Ca were elevated in those sharks. Two sharks collected in the Pacific coast off Baja California, (NB06 and NB05), with elevated maternal Zn:Ca and Ba:Ca may also indicate the mother spent time in the GC before migrating out to the Pacific coast to give birth. If so, some pregnant females may explore the GC and then leave to give birth in the Pacific coast, while others reside entirely within the GC during fetal development. Tagging of pregnant females may provide further information about migration during gestations since the mechanisms controlling Zn:Ca and Ba:Ca levels in White Shark vertebrae will be difficult to study under controlled laboratory conditions.
Overall, Mg:Ca, Mn:Ca, and Zn:Ca tended to decrease through gestation which may relate to a switch from yolk to uterine milk nourishment during embryonic development (Sato et al., 2016; Tomita et al., 2022) as described above for SIA. Previous studies have suggested that maternal Sr:Ba and Pb:Ca might be indicators of offshore to nearshore movements in pregnant Scalloped Hammerhead Sharks (Coiraton and Amezcua, 2020), but our study on younger sharks evaluates migration over more regional scales, making comparisons difficult. Maternal offloading of both essential (Mn, Zn) and nonessential (Ba) elements between mother and offspring muscle and liver tissue has been reported in other shark species (de Souza-Araujo et al., 2020).
There was a mismatch between SIA results and elemental ratios for GC13 and GC14. The higher Zn:Ca and Ba:Ca values for these specimens suggests they spent more time residing within the GC prior to capture, whereas SIA results suggested recent movements beyond GC. Given the lack of understanding of the mechanisms that drive element variation in elasmobranch cartilage, and that ages for these sharks varied by 4-6 years, it is possible that temporal variability in water chemistry or food compositions could be responsible for the mismatch. Temporal variability in elemental baselines is a factor that can confound spatial interpretations of chemical tracers (Gillanders, 2002).
5. Conclusions
Our results demonstrate that the SIA and element:Ca ratios of Pacific White Shark vertebrae can be useful for determining natal origins and regional migrations with respect to Pacific and GC nursery and capture areas. Despite differences in the integration period of isotope subsamples and longitudinal traces of element:Ca ratios, our results are consistent with the proposition made by Domeier and Nasby-Lucas (2013) that the GC might be an alternative nursery area in the northeastern Pacific. Indeed, satellite tagging studies have shown that some adult female White Sharks move into the GC during the parturition period of their reproductive cycle (Domeier and Nasby-Lucas, 2013).
The GC is a highly productive ecosystem that can provide White Sharks with abundant demersal and pelagic prey throughout ontogeny (Lluch-Cota et al., 2007), and possibly support a local White Shark population. However, some of their prey are targeted by local artisanal and industrial fisheries, which explains by-catch of White Sharks by local fisheries inside the GC (Galván-Magaña et al., 2010; Madigan et al., 2021; Malpica-Cruz et al., 2021). Further, thirteen California sea lion (Zalophus californianus) colonies are found in the GC mainly in the central and northern reaches which might serve as an important food source for adult White Sharks (Masper et al., 2019; Pelayo-González et al., 2021).
Future research efforts using vertebrae composition, genomic and tagging studies, in concert with local fishermen, may help determine if the GC serves as a nursery habitat for young White Sharks and supports a local population of lifetime residents as suggested by the chemical composition of GC15. Verification of a local White Shark population within the GC would indicate that the regional northeastern Pacific population has a more complex population structure than previously thought (Oñate-González et al., 2017) and present important considerations for conservation efforts. Although the White Shark is a protected species by Mexican regulations, a true commitment to working collaboratively with fishers and stakeholders in addition to further enforcement efforts inside the GC are needed to assure its conservation (Malpica-Cruz et al., 2021).
Data availability statement
The original contributions presented in the study are included in the article/Supplementary Material. Further inquiries can be directed to the corresponding author.
Ethics statement
The sampling of sharks was done opportunistically in collaboration with regional small-scale fisheries that capture White Sharks incidentally.
Author contributions
SH, OS-N, and RW designed and financed the study. AERC performed C and N sampling, JM and NM conducted elemental analyses. All authors contributed to the data analysis, interpretation, and writing. All authors contributed to the article and approved the submitted version.
Funding
Funding was provided by Texas A&M University-CONACYT research grant 2016-026 awarded to SH and RW.
Acknowledgments
We thank members of the Fisheries Ecology Laboratory and fishermen from Baja California for collecting the White Shark vertebrae, and Carmen Rodríguez Medrano for processing vertebrae cuttings. All Mexico samples were collected under permits issued by the Wildlife Direction from the Council of Environment and Natural Resources. Permits SGPA/DGVS/03661/11, SGPA/DGVS/04180/13, SGPA/DGVS/06243/14, SGPA/DGVS/06777/15, SGPA/DGVS/06294/16, SGPA/DGVS/07290/17, SGPA/DGVS/00131/19. Samples were exported under CITES Permit MX104208.
Conflict of interest
The authors declare that the research was conducted in the absence of any commercial or financial relationships that could be construed as a potential conflict of interest.
Publisher’s note
All claims expressed in this article are solely those of the authors and do not necessarily represent those of their affiliated organizations, or those of the publisher, the editors and the reviewers. Any product that may be evaluated in this article, or claim that may be made by its manufacturer, is not guaranteed or endorsed by the publisher.
Supplementary material
The Supplementary Material for this article can be found online at: https://www.frontiersin.org/articles/10.3389/fmars.2022.1082219/full#supplementary-material
References
Altabet M. A., Pilskaln C., Thunell R., Pride C., Sigman D., Chavez F., et al. (1999). The nitrogen isotope biogeochemistry of sinking particles from the margin of the Eastern North Pacific. Deep Sea Res. Part I Oceanogr. Res. Pap. 46 (4), 655–679. doi: 10.1016/s0967-0637(98)00084-3
Anderson J. M., Burns E. S., Meese E. N., Farrugia T. J., Stirling B. S., White C. F., et al. (2021). Interannual nearshore habitat use of young of the year White Sharks off southern California. Front. Mar. Sci. 8. doi: 10.3389/fmars.2021.645142
Aurioles-Gamboa D., Rodríguez-Pérez M. Y., Sánchez-Velasco L., Lavín M. F. (2013). Habitat, trophic level, and residence of marine mammals in the Gulf of California assessed by stable isotope analysis. Mar. Ecol. Prog. Ser. 488, 275–290. doi: 10.3354/meps10369
Bird C. S., Verissimo A., Magozzi S., Abrantes K. G., Aguilar A., Al-Reasi H., et al. (2018). A global perspective on the trophic geography of sharks. Nat. Ecol. Evol. 2 (2), 299–305. doi: 10.1038/s41559-017-0432-z
Busquets-Vass G., Newsome S. D., Pardo M. A., Calambokidis J., Aguiniga-Garcia S., Paez-Rosas D., et al. (2021). Isotope-based inferences of the seasonal foraging and migratory strategies of blue whales in the eastern Pacific Ocean. Mar. Environ. Res. 163, 105201. doi: 10.1016/j.marenvres.2020.105201
Cailliet G. M., Natanson L. J., Welden B. A., Ebert D. A. (1985). Preliminary studies on the age and growth of the White Shark, Carcharodon carcharias, using vertebral bands. Mem. South. Calif. Acad. Sci. 9 (4), 49–60.
Carlisle A. B., Kim S. L., Semmens B. X., Madigan D. J., Jorgensen S. J., Perle C. R., et al. (2012). Using stable isotope analysis to understand the migration and trophic ecology of northeastern Pacific White Sharks (Carcharodon carcharias). PloS One 7 (2), e30492. doi: 10.1371/journal.pone.0030492
Carlisle A. B., Litvin S. Y., Hazen E. L., Madigan D. J., Goldman K. J., Lea R. N., et al. (2015). Reconstructing habitat use by juvenile Salmon Sharks links upwelling to strandings in the California current. Mar. Ecol. Prog. Ser. 525, 217–228. doi: 10.3354/meps11183
Checkley D. M., Barth J. A. (2009). Patterns and processes in the California current system. Prog. Oceanogr. 83, 49–64. doi: 10.1016/j.pocean.2009.07.028
Christiansen H. (2011). Developing and applying elemental composition of shark vertebrae as a tool for quantifying life history characteristics over ontogeny. Electronic theses and dissertations (University of Windsor). Available at: https://scholar.uwindsor.ca/etd/5585.
Coiraton C., Amezcua F. (2020). In utero elemental tags in vertebrae of the Scalloped Hammerhead Shark Sphyrna lewini reveal migration patterns of pregnant females. Sci. Rep. 10, 1–13. doi: 10.1038/s41598-020-58735-8
Décima M., Landry M. R., Popp B. N. (2013). Environmental perturbation effects on baseline δ15N values and zooplankton trophic flexibility in the southern California current ecosystem. Limnol. Oceanogr. 58 (2), 624–634. doi: 10.4319/lo.2013.58.2.0624624
Delgadillo-Hinojosa F., Segovia-Zavala J. A., Huerta-Díaz M. A., Atilano-Silva H. (2006). Influence of geochemical and physical processes on the vertical distribution of manganese in Gulf of California waters. Deep Sea Res. Part I Oceanogr. Res. Pap. 53 (8), 1301–1319. doi: 10.1016/j.dsr.2006.06.002
de Souza-Araujo J., Andrades R., de Oliveira Lima M., Hussey N. E., Giarrizzo T. (2020). Maternal and embryonic trace element concentrations and stable isotope fractionation in the Smalleye Smooth-hound (Mustelus higmani). Chemosphere 257, 127183. doi: 10.1016/j.chemosphere.2020.127183
Domeier M. L., Nasby-Lucas N. (2007). Annual re-sightings of photographically identified White Sharks (Carcharodon carcharias) at an eastern Pacific aggregation site (Guadalupe island, Mexico). Mar. Biol. 150, 977–984. doi: 10.1007/s00227-006-0380-7
Domeier M. L. (2012). Two-year migration of adult female White Sharks (Carcharodon carcharias) reveals widely separated nursery areas and conservation concerns. Global perspectives on the biology and life history of the white shark. (CRC Press Taylor & Francis Group) 199–224.
Domeier M. L., Nasby-Lucas N. (2013). Two-year migration of adult female White Sharks (Carcharodon carcharias) reveals widely separated nursery areas and conservation concerns. Anim. Biotelemetry 1, 2. doi: 10.1186/2050-3385-1-2
Elorriaga-Verplancken F. R., García-Aguilar M. C. (2018). Interannual, (2002-2016) variation in the natality rate of the northern elephant seal (Mirounga angustirostris) at the San Benito colony, Baja California, Mexico. Mar. Mamm. Sci. 34, 823–828. doi: 10.1111/mms.12486
Estrada J., Rice A., Lutcavage M., Skomal G. (2003). Predicting trophic position in sharks of the north-west Atlantic ocean using stable isotope analysis. J. Mar. Biolog. Assoc. 83 (6), 1347–1350. doi: 10.1017/S0025315403008798
Estrada J. A., Rice A. N., Natanson L. J., Skomal G. B. (2006). Use of isotopic analysis of vertebrae in reconstructing ontogenetic feeding ecology in White Sharks. Ecology 87 (4), 829–834. doi: 10.1890/0012-9658(2006)87[829:UOIAOV]2.0.CO;2
Estupiñán-Montaño C., Galván-Magaña F., Elorriaga-Verplancken F., Zetina-Rejón M. J., Sánchez-González A., Polo-Silva C. J., et al. (2021a). Ontogenetic feeding ecology of the scalloped hammerhead shark Sphyrna lewini in the Colombian Eastern tropical Pacific. Mar. Ecol. Prog. Ser. 663, 127–143. doi: 10.3354/meps13639
Estupiñán-Montaño C., Galván-Magaña F., Sánchez-González A., Elorriaga-Verplancken F. R., Delgado-Huertas A., Páez-Rosas D. (2019). Dietary ontogeny of the Blue Shark, Prionace glauca, based on the analysis of δ13C and δ15N in vertebrae. Mar. Biol. 166 (8), 1–13. doi: 10.1007/s00227-019-3550-0
Estupiñán-Montaño C., Tamburin E., Delgado-Huertas A. (2021b). Stable isotope evidence for movements of Hammerhead Sharks Sphyrna lewini, connecting two natural protected areas in the Colombian Pacific. Mar. Biodivers 51, 1–8. doi: 10.1007/s12526-021-01215-7
Galván-Magaña F., Hoyos-Padilla E. M., Navarro-Serment C. J., Márquez-Farías F. (2010). Records of White Shark, Carcharodon carcharias, in the Gulf of California, Mexico. Mar. Biodivers. Rec. 3, 1–6. doi: 10.1017/S1755267210000977
García-Rodríguez E., Herzka S. Z., Sosa-Nishizaki O., Lowe C. G., O’Sullivan J. B. (2021). Stable isotope analysis of juvenile White Sharks inside a nursery area reveals foraging in demersal-inshore habitats and trophic overlap with sympatric sharks. Front. Mar. Sci. 8. doi: 10.3389/fmars.2021.687738
García-Rodríguez E., Sosa-Nishizaki O. (2020). Artisanal fishing activities and their documented interactions with juvenile White Shark insider a nursery area. Aquatic Conserv. Mar. Freshw. Ecosyst. 30, 903–914. doi: 10.1002/aqc.3300
Gillanders B. M. (2002). Temporal and spatial variability in elemental composition of otoliths: Implications for determining stock identity and connectivity of populations. Can. J. Fish. Aquat. Sci. 59, 669–679. doi: 10.1139/f02-040
Hamady L. L. (2014). Age, movements, and feeding ecology of northwest Atlantic White Sharks estimated from ecogeochemical profiles in vertebrae. Massachusetts Institute of Technology (MIT-WHOI Joint Program in Oceanographic/Applied Ocean Science and Engineering). Available at: https://core.ac.uk/download/pdf/222883763.pdf.
Hussey N. E., Brush J., McCarthy I. D., Fisk A. T. (2010). δ15N and δ13C diet– tissue discrimination factors for large sharks under semi-controlled conditions. Comp. Biochem. Physiol. A 155, 445–453. doi: 10.1016/j.cbpa.2009.09.023
Hussey N. E., MacNeil M. A., Olin J. A., McMeans B. C., Kinney M. J., Chapman D. D., et al. (2012). Stable isotopes and elasmobranchs: tissue types, methods, applications and assumptions. J. Fish Biol. 80, 1449–1484. doi: 10.1111/j.1095-8649.2012.03251.x
Hussey N., McCann H., Cliff G., Dudley S., Wintner S., Fisk A. (2012). “Size-based analysis of diet and trophic position of the White Shark, Carcharodon carcharias, in south African waters,” in Global perspectives on the biology and life history of the White Shark. Ed. Domeier M. L. (Boca Raton: CRC Press), 27–50. doi: 10.1201/b11532-5
Jorgensen S. J., Reeb C. A., Chapple T. K., Anderson S., Perle C., Van Sommeran S. R., et al. (2010). Philopatry and migration of Pacific White Sharks. Proc. R. Soc B Biol. Sci. 277, 679–688. doi: 10.1098/rspb.2009.1155
Kerr L. A., Andrews A. H., Cailliet G. M., Brown T. A., Coale K. H. (2006). “Investigations of Δ14C, δ13C, and δ15N in vertebrae of White Shark (Carcharodon carcharias) from the eastern North Pacific Ocean,” in Special issue: Age and growth of chondrichthyan fishes: New methods, techniques and analysis (Dordrecht: Springer), 337–353. doi: 10.1007/978-1-4020-5570-6_14
Kim S. L., Tinker M. T., Estes J. A., Koch P. L. (2012). Ontogenetic and among-individual variation in foraging strategies of northeast Pacific White Sharks based on stable isotope analysis. PLoS One 7 (9), e45068. doi: 10.1371/journal.pone.0045068
Klimley A. P., Anderson S. D., Pyle P., Henderson R. P. (1992). Spatiotemporal patterns of White Shark (Carcharodon carcharias) predation at the South Farallon Islands, California. Copeia 1992, 680–690. doi: 10.2307/1446143
Lavín M. F., Marinone S. G. (2003). “An overview of the physical oceanography of the Gulf of California,” in Nonlinear processes in geophysical fluid dynamics. Eds. Velasco Fuentes O. U., Sheinbaum J., Ochoa J. (Dordrecht: Springer). doi: 10.1007/978-94-010-0074-1_11
Livernois M. C., Mohan J. A., TinHan T. C., Richards T. M., Falterman B. J., Miller N. R., et al. (2021). Ontogenetic patterns of elemental tracers in the vertebrae cartilage of coastal and oceanic sharks. Front. Mar. Sci. 8. doi: 10.3389/fmars.2021.704134
Lluch-Cota S. E., Aragón-Noriega E. A., Arreguín-Sánchez F., Aurioles-Gamboa D., Bautista-Romero J. J., Brusca R. C., et al. (2007). The Gulf of California: Review of ecosystem status and sustainability challenges. Prog. Oceanogr. 73, 1–26. doi: 10.1016/j.pocean.2007.01.013
Logan R. K., White C. F., Winkler C., Jorgensen S. J., O’Sullivan J. B., Lowe C. G., et al. (2018). An evaluation of body condition and morphometric relationships within southern California juvenile White Sharks Carcharodon carcharias. J. Fish Biol. 93, 842–849. doi: 10.1111/jfb.13785
Lorrain A., Pethybridge H., Cassar N., Receveur A., Allain V., Bodin N., et al. (2020). Trends in tuna carbon isotopes suggest global changes in pelagic phytoplankton communities. Glob Chang Biol. 26 (2), 458–470. doi: 10.1111/gcb.14858
Lowe C. G., Blasius M. E., Jarvis E. T., Mason T. J., Goodmanlowe G. D., O’Sullivan J. B. (2012). “Historic fisheries interactions with White Sharks in the Southern California Bight,” in Global perspectives on the biology and life history of the White Shark. Ed. Domeier M. L. (USA: CRC Press), 169–186.
MacNeil M. A., Skomal G. B., Fisk A. T. (2005). Stable isotopes from multiple tissues reveal diet switching in sharks. Mar. Ecol. Prog. Ser. 302, 199–206. doi: 10.1111/fog.12034
Madigan D. J., Arnoldi N. S., Hussey N. E., Carlisle A. B. (2021). An illicit artisanal fishery for North Pacific White Sharks indicates frequent occurrence and high mortality in the Gulf of California. Conserv. Lett. 14, 1–13. doi: 10.1111/conl.12796
Malpica-Cruz L., Abadía-Cardoso A., Aquino-Baleytó M., Beas-Luna R., Becerril-García E. E., Castillo-Géniz J. L., et al. (2021). Empowering fishers for great White Shark stewardship: Reply to madigan et al. 2021. Conserv. Lett. 14 (4), 1–3. doi: 10.1111/conl.12828
Malpica‐Cruz L., Herzka S. Z., Sosa‐Nishizaki O., Escobedo‐Olvera M. A. (2013). Tissue‐specific stable isotope ratios of shortfin mako (Isurus oxyrinchus) and white (Carcharodon carcharias) sharks as indicators of size‐based differences in foraging habitat and trophic level. Fisheries Oceanography. 22 (6),429–445. doi: 10.1111/fog.12034
Masper A., Gallo-Reynoso-Reynoso J. P., Cisneros-Mata M.Á., García-Hernández J. (2019). Review of California sea lion (Zalophus californianus) abundance, and population dynamics in the Gulf of California. Rev. Biol. Trop. 67, 833–849. doi: 10.15517/rbt.v67i4.35965
McMillan M. N., Izzo C., Wade B., Gillanders B. M. (2017). Elements and elasmobranchs: Hypotheses, assumptions and limitations of elemental analysis. J. Fish Biol. 90, 559–594. doi: 10.1111/jfb.13189
Mohan J. A., Miller N. R., Herzka S. Z., Sosa-Nishizaki O., Dewar H., Kinney M., et al. (2018). Elements of time and place: manganese and barium in shark vertebrae reflect age and upwelling histories. Proc. R. Soc. B 285, 20181760. doi: 10.1098/rspb.2018.1760
Oñate-González E. C., Sosa-Nishizaki O., Herzka S. Z., Lowe C. G., Lyons K., Santana-Morales O., et al. (2017). Importance of Bahia Sebastian Vizcaino as a nursery area for White Sharks (Carcharodon carcharias) in the northeastern Pacific: A fishery dependent analysis. Fish. Res. 188, 125–137. doi: 10.1016/j.fishres.2016.12.014
Ordiano-Flores A., Galván-Magana F., Sánchez-González A., Soto-Jiménez M. F., Paez-Osuna F. (2021). Mercury, selenium, and stable carbon and nitrogen isotopes in the Striped Marlin Kajikia audax and Blue Marlin Makaira nigricans food web from the Gulf of California. Mar. pollut. Bull. 170, 112657. doi: 10.1016/j.marpolbul.2021.112657
Paton C., Hellstrom J., Paul B., Woodhead J., Hergt J. (2011). Iolite: Freeware for the visualization and processing of mass spectrometric data. J. Anal. At. Spectrom. 26, 2508–2518. doi: 10.1039/C1JA10172B
Pelayo-González L., González-Rodríguez E., Ramos-Rodríguez A., Hernández-Camacho C. J. (2021). California Sea lion population decline at the southern limit of its distribution during warm regimes in the Pacific Ocean. Reg. Stud. Mar. Sci. 48, 102040. doi: 10.1016/j.rsma.2021.102040
Pistevos J. C. A., Reis-Santos P., Izzo C., Gillanders B. M. (2019). Element composition of shark vertebrae shows promise as a natural tag. Mar. Freshw. Res. 70, 1722–1733. doi: 10.1071/MF18423
Porras-Peters H., Aurioles-Gamboa D., Cruz-Escalona V. H., Koch P. L. (2008). Trophic level and overlap of sea lions (Zalophus californianus) in the Gulf of California, Mexico. Mar. Mamm. Sci. 24 (3), 554–576. doi: 10.1111/j.1748-7692.2008.00197.x
Portela E., Beier E., Barton E. D., Castro R., Godínez V., Palacios-Hernández E., et al. (2016). Water masses and circulation in the tropical Pacific off central Mexico and surrounding areas. J. Phys. Oceanogr. 46, 3069–3081. doi: 10.1175/jpo-d-16-0068.1
Richert J. E., Galván-Magaña F., Klimley A. P. (2015). Interpreting nitrogen stable isotopes in the study of migratory fishes in marine ecosystems. Mar. Biol. 162, 1099–1110. doi: 10.1007/s00227-015-2652-6
Rosas-Hernández M. P., Aurioles-Gamboa D., Hernández-Camacho C. J. (2018). Differences in the isotopic niche and trophic position of female California sea lions (Zalophus californianus) in distinct oceanographic conditions. Aquat. Mamm. 44, 374–388. doi: 10.1578/am.44.4.2018.374
Ruiz-Cooley R. I., Engelhaupt D. T., Ortega-Ortiz J. G. (2011). Contrasting C and N isotope ratios from sperm whale skin and squid between the Gulf of Mexico and Gulf of California: effect of habitat. Mar. Biol. 159 (1), 151–164. doi: 10.1007/s00227-011-1795-3
Ruiz-Cooley R. I., Gerrodette T., Fiedler P. C., Chivers S. J., Danil K., Ballance L. T. (2017). Temporal variation in pelagic food chain length in response to environmental change. Sci. Adv. 3, 1–9. doi: 10.1126/sciadv.1701140
Santana-Morales O., Sosa-Nishizaki O., Escobedo-Olvera M., Onate-Gonzalez E., O’Sullivan J., Cartamil D. (2012). “Incidental catch and ecological observations of juvenile White Sharks, Carcharadon carcharias, in western Baja California, mexico. conservation implications,” in Global perspectives on the biology and life history of the White Shark. Ed. Domeier M. L. (USA: CRC Press), 187–198.
Sato K., Nakamura M., Tomita T., Toda M., Miyamoto K., Nozu R. (2016). How great White Sharks nourish their embryos to a large size: Evidence of lipid histotrophy in lamnoid shark reproduction. Biol. Open 5, 1211–1215. doi: 10.1242/bio.017939
Shen Y., Gong Y., Wu F., Li Y. (2021). Retrospective stable isotopes of vertebrae reveal sexual ontogenetic patterns and trophic ecology in oceanic Whitetip Shark, Carcharhinus longimanus. Ecol. Evol. 12, e8452. doi: 10.1002/ece3.8452
Sigman D. M., Granger J., DiFiore P. J., Lehmann M. M., Ho R., Cane G., et al. (2005). Coupled nitrogen and oxygen isotope measurements of nitrate along the eastern North Pacific margin. Global Biogeochem. Cycles 19 (4), 1–14. doi: 10.1029/2005gb002458
Smith W. D., Miller J. A., Heppell S. S. (2013). Elemental markers in elasmobranchs: Effects of environmental history and growth on vertebral chemistry. PloS One 8, 1–19. doi: 10.1371/journal.pone.0062423
Tamburin E., Elorriaga-Verplancken F. R., Estupiñan-Montaño C., Madigan D. J., Sánchez-González A., Padilla M. H., et al (2020). New insights into the trophic ecology of young white sharks (Carcharodon carcharias) in waters off the Baja California Peninsula, Mexico. Marine Biology. 16 (5),1–14. doi: 10.1007/s00227-020-3660-8
Thunell R. C., Kepple A. B. (2004). Glacial-Holocene δ15N record from the Gulf of tehuantepec, Mexico: Implications for denitrification in the eastern equatorial Pacific and changes in atmospheric N2O. Global Biogeochemical Cycles 18 (1), 1–12. doi: 10.1029/2002gb002028
Tomita T., Nakamura M., Nozu R., Ogawa N., Toda M., Sato K. (2022). Mode of uterine milk secretion in the White Shark. Anat. Rec. 305, 1724–1731 doi: 10.1002/ar.24860
Tricas T. C., McCosker J. E. (1984). Predatory behavior of the White Shark (Carcharodon carcharias), with notes on its biology. Proc. Calif. Acad. Sci. 43, 221–238.
Vallee B. L. (1983). “Zinc in biology and biochemistry,” in TG sprio, zinc enzymes, metal ions in biology, vol. Vol 5. (New York: John Wiley & Sons).
Voss M., Dippner J. W., Montoya J. P. (2001). Nitrogen isotope patterns in the oxygen-deficient waters of the eastern tropical North Pacific Ocean. Deep Sea Res. Part I Oceanogr. Res. Pap. 48, 1905–1921. doi: 10.1016/s0967-0637(00)00110-2
Weng K. C., O’Sullivan J. B., Lowe C. G., Winkler C. E., Dewar H., Block B. A. (2007). Movements, behavior and habitat preferences of juvenile White Sharks Carcharodon carcharias in the eastern Pacific. Mar. Ecol. Prog. Ser. 338, 211–224. doi: 10.3354/meps338211
Weng K. C., Sullivan J. B. O., Lowe C. G., Winkler C. E., Blasius M. E., Locke-Smith K. A., et al. (2012). “Back to the wild release of juvenile White Sharks from the Monterey bay aquarium,” in Global perspectives on the biology and life history of the White Shark. Ed. Domeier M. L. (USA: CRC Press) 419–446.
Whitehead D. A., Murillo-Cisneros D., Elorriaga-Verplancken F. R., Hacohen-Domene A., de la Parra R., Gonzalez-Armas R., et al. (2020). Stable isotope assessment of whale sharks across two ocean basins: Gulf of California and the Mexican Caribbean. J. Exp. Mar. Biol. Ecol 527, 151359. doi: 10.1016/j.jembe.2020.151359
White C. F., Lyons K., Jorgensen S. J., O’Sullivan J., Winkler C., Weng K. C., et al. (2019). Quantifying habitat selection and variability in habitat suitability for juvenile White Sharks. PloS One 14, 1–20. doi: 10.1371/journal.pone.0214642
Keywords: White Shark (Carcharodon carcharias), stable isotope, trace elements, vertebrae, migration, Pacific Ocean (Northeast), Gulf of California
Citation: Mohan JA, Romo-Curiel AE, Herzka SZ, Wells RJD, Miller NR, Sosa-Nishizaki O and García-Rodríguez E (2023) Inferring habitat use of the Pacific White Shark using vertebral chemistry. Front. Mar. Sci. 9:1082219. doi: 10.3389/fmars.2022.1082219
Received: 27 October 2022; Accepted: 31 December 2022;
Published: 19 January 2023.
Edited by:
Carlos Polo, Universidad de Bogotá Jorge Tadeo Lozano, ColombiaReviewed by:
Arturo Tripp, Instituto Politécnico Nacional (IPN), MexicoJohn Logan, Massachusetts Division of Marine Fisheries, United States
Copyright © 2023 Mohan, Romo-Curiel, Herzka, Wells, Miller, Sosa-Nishizaki and García-Rodríguez. This is an open-access article distributed under the terms of the Creative Commons Attribution License (CC BY). The use, distribution or reproduction in other forums is permitted, provided the original author(s) and the copyright owner(s) are credited and that the original publication in this journal is cited, in accordance with accepted academic practice. No use, distribution or reproduction is permitted which does not comply with these terms.
*Correspondence: Sharon Z. Herzka, c2hlcnprYUBjaWNlc2UubXg=
†These authors have contributed equally to this work