- 1Center for Marine and Environmental Research (CIMA), University of Algarve, Faro, Portugal
- 2Facultad de Ciencias del Mar y Ambientales, University of Cádiz, Cádiz, Spain
- 3Faculdade de Ciências e Tecnologia (FCT), University of Algarve, Faro, Portugal
- 4Belthorn, Broadway, United Kingdom
- 5Sagremarisco Lda., Vila do Bispo, Portugal
Changes in the composition of dinoflagellates from 1994 to 2001 at a station influenced by wind-induced seasonal upwelling off SW Portugal were analyzed in relation to oceanography. 194 taxa of dinoflagellates were detected, the most frequent belonged to the genera Tripos, Protoperidinium, Dinophysis, Diplopsalopsis, Prorocentrum and Lingulodinium. The composition of dinoflagellate communities followed a seasonal pattern, in association with oceanographic forcing and change of upwelling conditions. Harmful species such as Dinophysis acuminata, D. acuta, D. caudata, Gonyaulax spp. and Lingulodinium polyedra were found to develop during the upwelling season, typically comprising summer and early autumn in the West Iberian upwelling system, and also occasionally in the conditions following upwelling events in other seasons.
Introduction
Dinoflagellates are a common group of the phytoplankton in all aquatic habitats (Hackett et al., 2004). Under marine conditions, they make a substantial contribution to the primary production of the oceans (Taylor et al., 2008), but they also produce several classes of potent biotoxins. Indeed, Cembella (2003) suggests that 75–80% of potentially toxic phytoplankton are dinoflagellates. They have diverse feeding strategies, ecological interactions, and often complex life-cycles (Smayda and Reynolds, 2003). Members of the group have adapted to exploit a wide range of ecological conditions and types of water masses, sometimes forming high density blooms (Smayda and Reynolds, 2001).
Although dinoflagellates have considerable ecological importance for their contribution to marine primary production, they also have economic consequences from the harmful effects that can take place both during dense blooms (HAB), but also at very low densities through bioaccumulation of toxins, for example, in the tissues of filter feeding mollusks (Shumway et al., 2018). Dinoflagellates are an important issue for marine aquaculture and tourism, causing risk of food poisoning, skin irritations and fish kills. The conditions for dinoflagellate-related HAB can be diverse (e.g., oceanography, meteorological forcing, phytoplankton succession, natural eutrophication, sediment disturbance, etc.) and not only connected with anthropogenic eutrophication or other ecosystem disturbance (Smayda, 2008; Kudela et al., 2010; Gowen et al., 2012).
Studies of oceanographic conditions, life cycles and ecological interactions of harmful dinoflagellate species have identified that conditions for HAB development often have complex multi-factor characteristics, and therefore remain unpredictable with many specific regional and local aspects (Smayda, 1997, 2008). In view of this, for the tasks such as HAB monitoring and prevention, seafood quality assurance, management of aquaculture, it is important to improve knowledge of HAB species ecophysiology at the scale of regional and local coastal systems, including their geographical distribution, population dynamics, and patterns of occurrence in relation to oceanographic and other environmental factors.
The dinoflagellate species assemblages of different ecophysiological life forms develop depending on the habitat conditions. Up to nine characteristic communities of species are mainly defined by the gradient of nutrient availability, water column mixing-stratification, and coastal—offshore conditions (Smayda and Reynolds, 2001). The upwelling systems, such as West Iberian where this study site was located, represent dynamic and complex marine habitat with wide range of oceanographic conditions, and corresponding ecologically and morphologically diverse dinoflagellate species (Smayda and Trainer, 2010).
The study area (Figure 1) was located in the vicinity of the Cape Saint Vincent (CSV) at the South-Western point of the Iberian Peninsula, and belongs to the West Iberian upwelling system. Northerly winds are prevailing in spring and summer (Fiúza et al., 1982; Loureiro et al., 2011), causing upwelling regime along the West coast of the Iberian peninsula (Moita et al., 2003). Under such conditions, upwelled water may propagate further east around the CSV, and may form structures such as upwelling filaments (Cravo et al., 2010) or a jet current separated from the coast (Relvas and Barton, 2005; Relvas et al., 2007), which spread the influence of the upwelling further along the southern coast of Portugal. Additionally, the coast of Southern Portugal lies sub-latitudinally, so that offshore Ekman transport can be also induced by the westerly winds. The study area may also be affected by the warm coastal counter-current from the Gulf of Cadiz (Relvas and Barton, 2005; Relvas et al., 2007). The onshore and offshore components of the near-shelf circulation along the southern coast are subject to fluctuations (Sánchez et al., 2006; Garel et al., 2016) mainly driven by wind stress. Due to this complex nature of oceanographic conditions in the region, the study area is characterized by the changes between the dominant upwelling conditions during the warm part of the year and upwelling relaxation in winter months. The existence of the along-shore counter-currents creates the possibility for the phytoplankton transport between enclosed coastal habitats (lagoons, estuaries and embayments, e.g., Ria Formosa lagoon) and the open sea along the southern coast of Portugal.
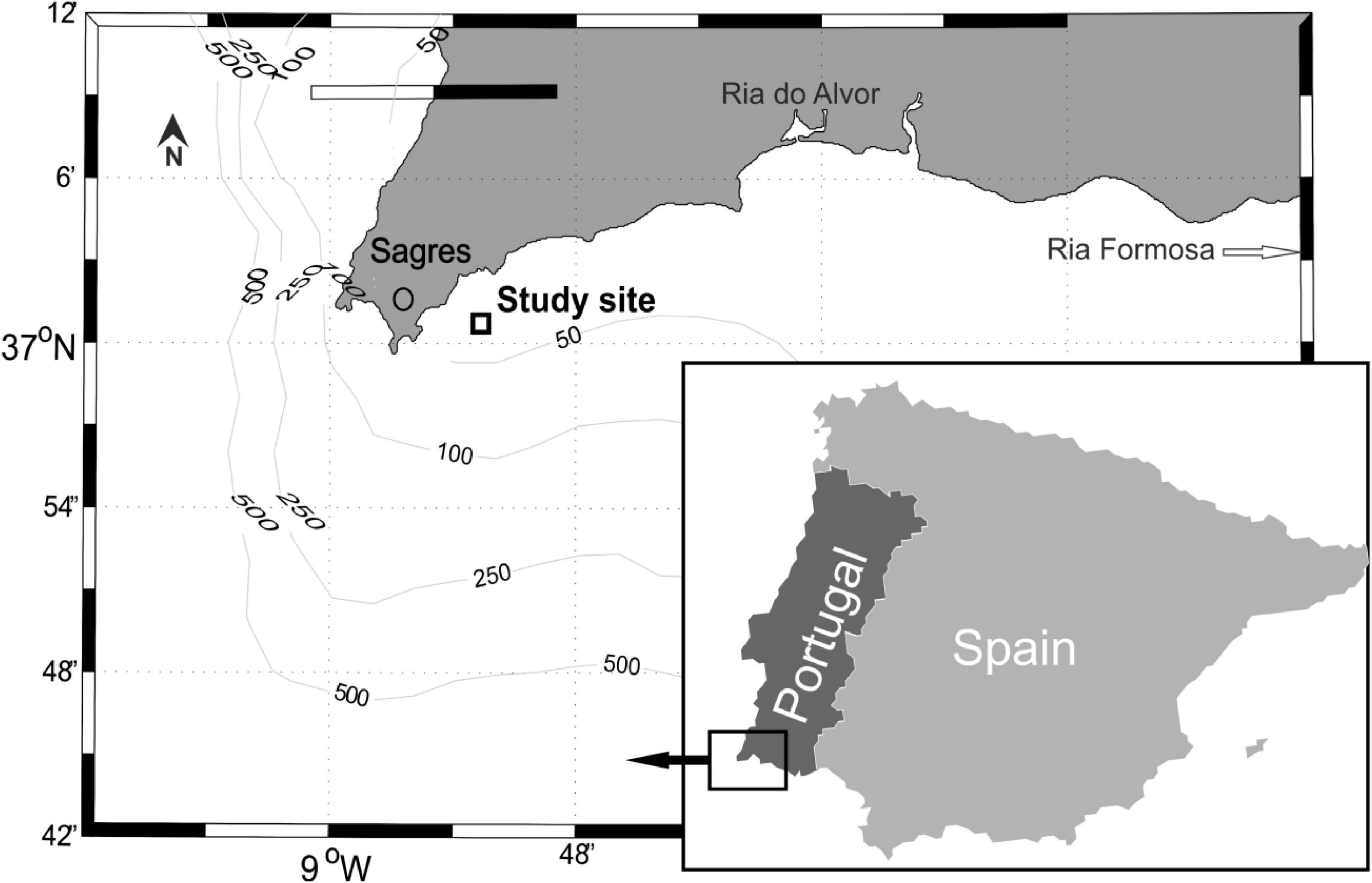
Figure 1. Study site location shown on the higher scale map off Sagres in the vicinity of Cape Saint Vincent, South-West Portugal. Aquaculture areas Ria do Alvor and Ria Formosa are shown to the east of the main study site.
In the study area, dominance of diatoms is typical for seasonal spring-summer blooms related to upwelling and the elongation of the photoperiod, while assemblages dominated by dinoflagellates develop during upwelling relaxation in coastal areas (Moita et al., 2003; Oliveira et al., 2009; Silva et al., 2009). The occurrence of toxic dinoflagellates (e.g., Gymnodinium catenatum) has been linked to late stages of upwelling events (Moita et al., 2003) in the region. The study of the cysts of dinoflagellates Gymnodinium catenatum and Lingulodinium polyedra in the coastal sediments (Amorim et al., 2002) suggests regular development of these toxic species along the S and SW coasts of Portugal that are affected by upwelling, including the Sagres area. Recent studies at Sagres (Loureiro et al., 2005, 2011; Goela et al., 2014, 2015; Danchenko et al., 2019; Santos et al., 2020) reported phytoplankton blooms dominated by diatoms and demonstrated a frequent presence of harmful phytoplankton species, including dinoflagellates of genera Dinophysis, Prorocentrum, Lingulodinium, Gymnodinium, Karlodinium, Azadinium and their relation with seasonal upwelling.
The focus in this paper is on the dinoflagellate assemblages in the upwelling region, and their response to oceanographic conditions. This study represents an 8-year long survey of mainly armored (thecate) dinoflagellates, conducted at the coastal site, influenced by pronounced seasonal upwelling, located in the West-Iberian upwelling system in the North-East Atlantic, at the South-West coast of Portugal. The aim of this research was to examine the relation between seasonally changing oceanographic conditions and composition of dinoflagellate community, including the appearance of harmful species. The objectives were: (1) to reveal the diversity of dinoflagellates over the 8-year observation period, (2) to examine the relation between oceanographic conditions and changes in the dinoflagellates community, (3) to identify the conditions under which communities containing harmful species are likely to occur in the study area.
The developing aquaculture sector in the study area is affected by frequent closures (Anonymous, 2013), following the detection of harmful algal species and toxins by authorities. DSP and PSP risk caused by dinoflagellates Dinophysis, Prorocentrum, Gymnodinium catenatum, Alexandrium and Lingulodinium polyedra has been reported (Loureiro et al., 2011; Anonymous, 2013) from Sagres area, alongside with ASP risk by diatom genus Pseudo-nitzschia. The outcome of the study may provide information for improvements to bivalve aquaculture management in the area, accounting for upwelling conditions, season and likelihood of HAB, and to contribute to water quality monitoring.
Materials and Methods
Study Area and Sampling
The Sagres peninsula and Cape Saint Vincent are located in the extreme south-west of the Iberian peninsula (Figure 1). The coastal area has minimal input of terrestrial nutrients due to a low human population of circa 7,000 inhabitants (Loureiro et al., 2011), geographic position and an absence of significant terrestrial fluvial input. Insignificance of the terrestrial sources suggests the main source of nutrient enrichment of the coastal waters in the study area is wind-driven upwelling from nutrient-rich deep waters. The sampling station was located approximately 5 km east from Cape Saint Vincent, and 1 km south of Sagres port (37°00′39″ N, 8°53′58″ W), near the offshore long-line installations for bivalve aquaculture (Figure 1), and has a depth of 25 m. In total, 266 samples were obtained, over the 8-year period starting from 27th January 1994 until 16th October 2001. Periodicity of the sampling was not regular, if sea conditions permitted, usually bi-weekly, with a 45 μm mesh size plankton net to target dinoflagellates of medium and large size; the net was towed horizontally for 5 min in the surface water layer at 1 m depth; sampling took place during late mornings at 11–12 a.m. to minimize the effect of diurnal vertical migration. The filtration method was chosen to focus on the collection of armored dinoflagellates that were usually present in low densities and to increase the probability of detecting rare species, simultaneously some unarmored species larger than the mesh size (45 μm) were also collected. Samples were fixed with 1% formaldehyde and stored refrigerated (+8°C) before analysis. Microscopic analysis was performed using 100× to 400× magnifications. Approximately one hundred (on average 106, min. 78, max. 185) random cells were identified to species level from each sample. Taxa identification was performed using Dodge (1982) and Steidinger and Tangen (1996) as sources of taxonomic information. Therefore, the dinoflagellate species abundance data was proportional, reflecting relative abundances of dinoflagellate species compared to the total number of the individuals identified in each sample, which was in most cases close to one hundred cells per sample.
Sea Surface Temperature, Wind and Upwelling Index
Upwelling conditions were characterized based on upwelling indices derived from wind stress and sea surface temperature (SST) to study the influence of upwelling on the phytoplankton community.
Sea surface temperature (SST) time series were obtained from the NOAA “SST Daily Optimum Interpolation (OI), AVHRR Only, Version 2.1, Final, Global, 0.25°, 1981-present” product (Reynolds et al., 2007), for the data grid square containing sampling station, with coordinates range N 36.75° to N 37.00°, W 8.75° to W 9.00°. Wind speed and direction components were obtained from the “Blended Daily Averaged 0.25-degree Sea Surface Winds product” (at 10 m level), made available by the National Oceanic and Atmospheric Administration (NOAA) and National Climatic Data Center (Zhang et al., 2006), from the grid square centered at N 37.00°, W 9.00°. The wind conditions at that point were considered representative of the local upwelling, although the study area belongs to the broader upwelling system, influenced by winds along the coastal stretch of Western Iberia. It was previously shown (Gonzalez-Nuevo et al., 2014) that upwelling indices based on local data were a good approximation for regional conditions for the NW Iberian upwelling system.
Wind stress upwelling index (UI) was based on Ekman transport (Qx,y) of surface water calculated following Bakun, 1973 and Cropper et al. (2014), as
where τx,y is the wind stress, u and v are the wind speeds in longitudinal and meridional directions respectively, w is the module of wind speed, ρa is the air density (1.22 kg m–3), Cd is the dimensionless drag coefficient (1.14 × 103) (Large and Pond, 1982), f is the Coriolis parameter (8.78 × 10–5 s–1 for the study area) and ρw is the density of sea water (1,025 kg m–3).
The Ekman transport latitudinal (Qx) and longitudinal (Qy) components were used to represent the upwelling indices for the western and southern coasts, because the coastline directions in the study area were roughly parallel to the meridian and the equator. Negative values of Qx and Qy indicated upwelling favorable conditions along the western and southern coasts in the study area, respectively. The latitudinal upwelling component of the western coast (Qx) is more important in Sagres area (Krug et al., 2017), as its influence spreads around the CSV. Cumulative upwelling index (CUI) was used to represent summation of the daily upwelling indices (Qx) from January 1st to the end of every year, and contributed to the estimation of the length of upwelling season and general upwelling intensity through the years [as in Díaz et al. (2016)].
Surface Chl a was used as a proxy of total phytoplankton independent of the dinoflagellates abundance, based on the results of previous studies on phytoplankton pigments in the same area (Goela et al., 2014). SeaWiFS Chl a product was available for the dates from 15th September 1997 until the end of the study period in 2001. Data on Chl a was obtained via the NOAA Environmental Research Division Data Access Program (ERDDAP) via web-site http://upwell.pfeg.noaa.gov/erddap/index.html.
Data Analysis
Statistica 6.0 was used for correlation analysis and basic statistical tests. Principal component analysis (PCA) was used as an exploration method to reveal the structure of species composition. The set of species used for this analysis was selected by their abundance across samples, in order to focus on bloom forming species. Usually abundant species tended to be highly frequent as well, so this approach did not distort the representation of common species. As PCA is sensitive to values scale, abundant species would account for greater explanation of the variance. The data was normalized (across samples) and standardized (by subtracting the mean and dividing by standard deviation) before analysis to account for both the higher and the lesser abundant species. PRIMER-E version 6.1.6 (Clarke and Warwick, 2001) was used for calculations of PCA and Similarity Percentages of species contributions (SIMPER) analysis and data transformations.
Results
Oceanographic Conditions
Interannual Variability of Upwelling
Upwelling favorable winds were predominant in the study area during most months of the year, except December (Figure 2). Multiannual mean upwelling index for the study period 1994–2001 was 296 m3 km–1 s–1 for zonal Ekman transport (Qx) and 180 m3 km–1 s–1 for meridional transport (Qy). For a longer period 1989–2008 it was, respectively, 356 m3 km–1 s–1 (Qx) and 152 m3 km–1 s–1 (Qy), these were considered as average multiannual conditions to assess seasonality. Upwelling season for the purpose of this study was defined as a period during the warm part of the year during which monthly mean upwelling indices (Qx, Qy) were above the 20-year average 1989–2008. The main upwelling season (MUS) using such criterion lasted April to September (Figure 2B). During the warm half of the year the oceanography was shaped by a succession of upwelling events, associated with N and W winds, interrupted by periods of relaxation. The peak upwelling season was observed during meteorological summer (June to August), with a monthly mean Qx in excess of 500 m3 km–1 s–1. Standard errors of the mean (SE) were relatively smaller during summer months, compared to higher SE observed in April, May, and September. This can be interpreted as more stable upwelling favorable conditions during the peak summer months (June to August), and highly variable conditions in the beginning and before the end of the upwelling season (April, May, and September). February and March were the months with high Qx (331 and 294 m3 km–1 s–1) outside of the main upwelling season, because of the favorable high zonal upwelling transport Qx (indicated by high SE) and on average slightly unfavorable meridional transport Qy (Figure 2B). Such spring upwelling characteristics may have contributed to spring phytoplankton blooms, together with seasonal SST growth and photoperiod. Overall, the main upwelling season from April to September was characterized by both high zonal and meridional transport, with especially high values of zonal transport Qx during peak upwelling season June to August, and lower deviations of these variables, indicating relative stability of wind regime, compared to spring and winter.
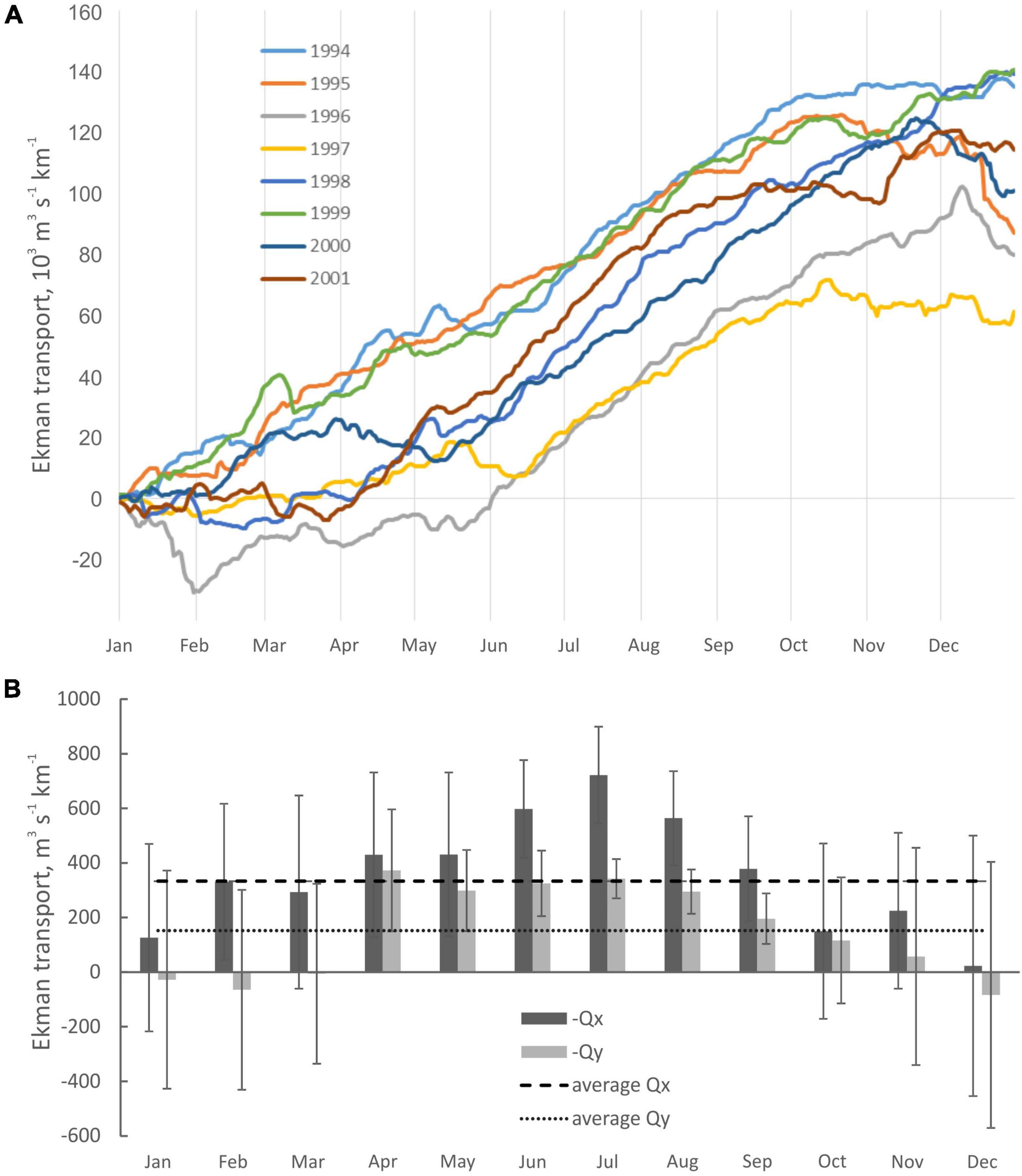
Figure 2. (A) Cumulative annual upwelling index (CUI, Qx) (m3s–1km–1), 1994–2001; (B) Monthly average Ekman transport along the western coast (Qx), and southern coasts (Qy) calculated from the wind stress during 1989–2009 (lower panel). Values converted to positive, bars = SE. Above average positive values of Qx and Qy indicate significant upwelling-favorable conditions (monthly means 1989–2009: Qx = 356, Qy = 152 m3s–1km–1).
Daily time-series of Ekman transport and SST (Figure 3) demonstrated that the study area was usually under upwelling conditions during the warm part of the year, mainly related to the northerly wind along the western coast. The main upwelling periods are denoted by shaded areas in Figure 3. The actual main upwelling period length and beginning and ending dates varied between years, and in several cases notable upwelling events were identified outside of the main upwelling season. Cumulative upwelling index (CUI, Figure 2A) demonstrated interannual differences in the start of main seasonal upwelling, as well as total annual upwelling intensity. The years with high CUI were 1994, 1998 and 1999, while 1996 and 1997 were the years with low total upwelling, with 1996 characterized by weak spring upwelling and late onset of the main season.
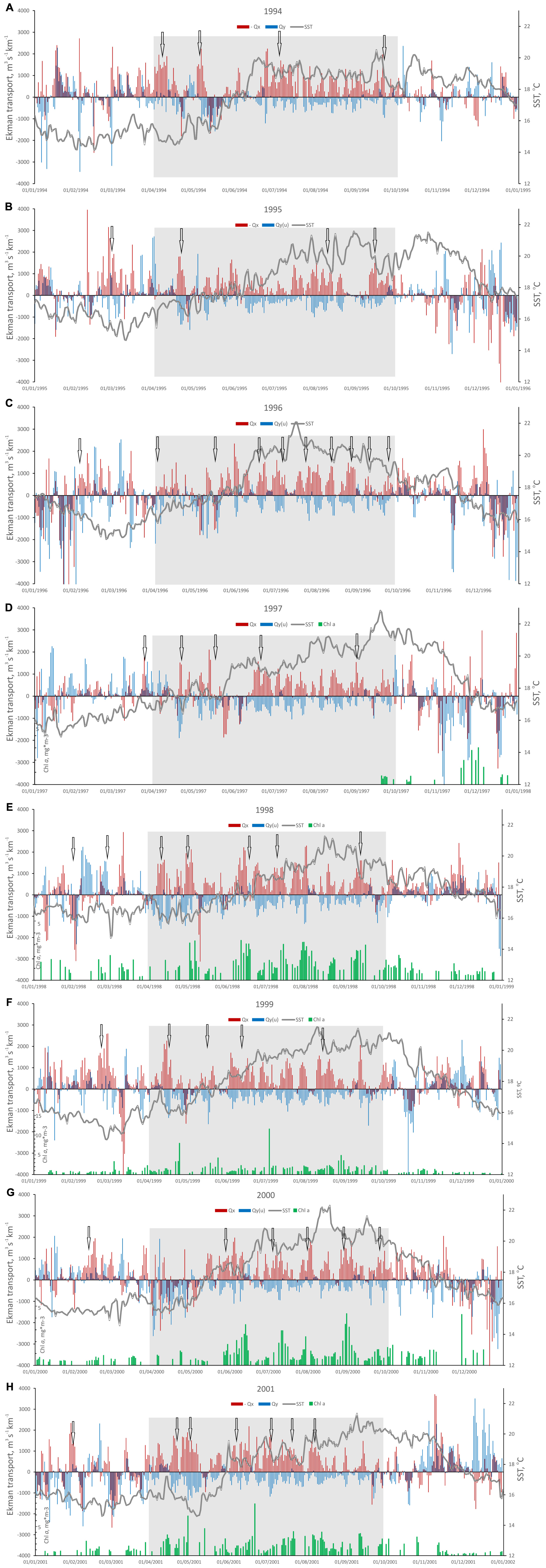
Figure 3. Daily variation of eastward (-Qx) and northward (Qy) Ekman transport, Optimal Interpolation SST (NOAA) and Chl a (SeaWiFS) from 1994 to 2001: (A) 1994, (B) 1995, (C) 1996, (D) 1997, (E) 1998, (F) 1999, (G) 2000, and (H) 2001. Qx and Qy represent zonal and meridional upwelling indices along the western and southern coasts, respectively; Chl a SeaWiFS has data gaps. Shaded gray areas indicate main upwelling periods, arrows point at pronounced upwelling events.
The following actual upwelling seasons were identified for each year, and considered for further analysis. During 1994, spring upwelling event was observed between 20th March and 15th April, then after the change of conditions upwelling regime established again around 20th of June and continued until the beginning of October (Figure 3A). In 1995 pronounced spring upwelling started early, around 20th of February and lasted until 20th of March, the summer upwelling season started in the mid-April and continued until the end of September, although with noticeable relaxation in the beginning of that month (Figure 3B). 1996 was characterized by more typical seasonality, with no significant upwelling events in spring, and summer upwelling period established from 20th of May and lasted until approximately 10th of October (Figure 3C). In 1997 the summer upwelling conditions started around 15th June and continued until 15th of October (Figure 3D). In 1998 the upwelling season started in the beginning of April and lasted until the middle of October (Figure 3E). During 1999 the spring upwelling events were observed in February—early March and in mid-April, later on the main upwelling season lasted from the beginning of June until around 10th of October (Figure 3F). Similarly, in 2000 there was a short upwelling event in February, and the summer upwelling period extended from 15th of May until October (Figure 3G). During 2001 the upwelling season started in spring (Figure 3H), from April and finished around 15th of September, although upwelling favorable transport was weak during August.
Four meteorological seasons in the study area had the following characteristics: (1) winter, December—February, with S and SE winds and low SST, upwelling events in February related to N winds; (2) spring, March—May, with seasonal increase in SST and photoperiod, and start of the annual main upwelling season; (3) summer, June—August with high SST, with SST decreases related to both N and W winds; (4) autumn, September—November, marked by seasonal decrease in SST and relaxation of upwelling conditions. The main upwelling season in terms of meteorological seasons comprised late spring, summer and early autumn (April to September).
Dinoflagellate Species Richness
The total species richness of dinoflagellates during the 8-year study period comprised 194 species, across the 266 samples examined. Taxa names with the respective short codes and frequency of occurrence are listed in the Supplementary Appendix 1. Sixty most common species occurred in not less than 26 samples (10% frequency) and 41 species in not less than 53 samples (20% occurrence frequency) out of the total 266 samples examined (Table 1). The most frequently identified species belonged to genera Tripos, Protoperidinium, Dinophysis, Diplopsalopsis, and Prorocentrum. On average 25.5 species per sample were identified, the average Shannon community diversity index was 2.53 and ranged from 0.34 to 3.40. Only 107 species occurred in quantity of more than 10 individuals and 62 species of more than 50 individuals throughout the study period, indicating that the sampling method was effective for detecting rare species.
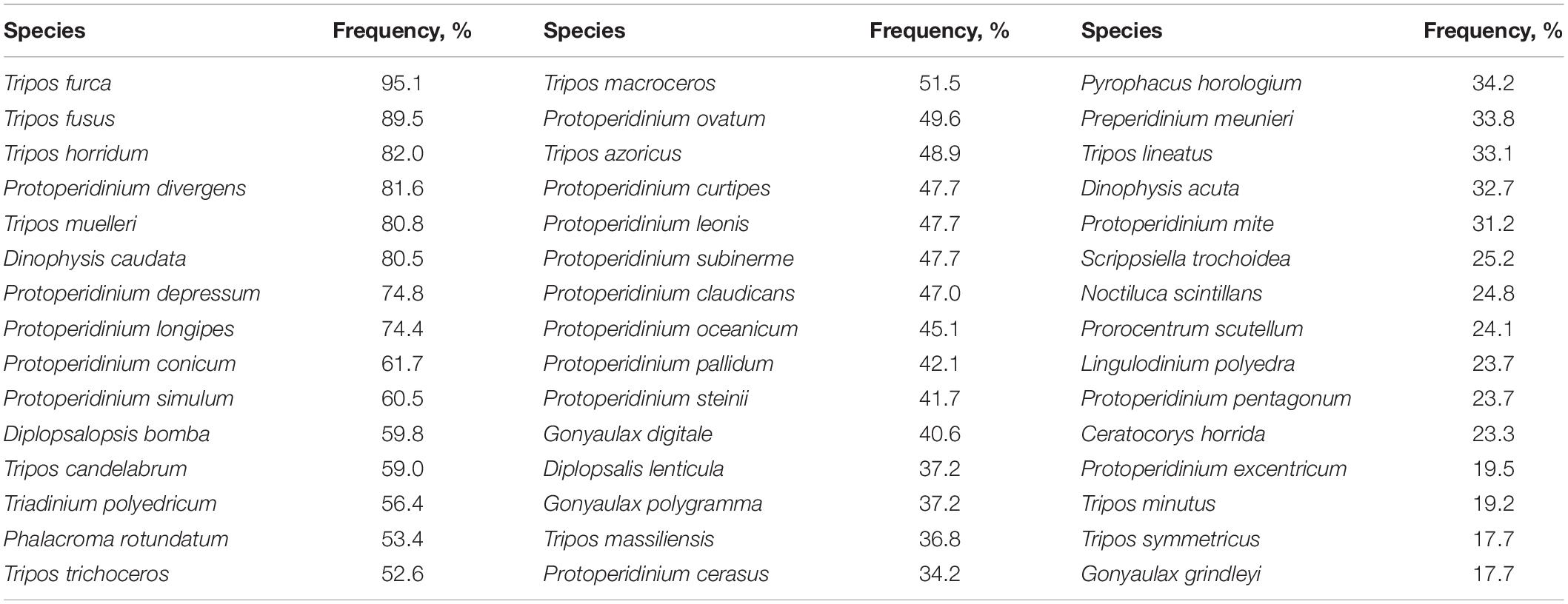
Table 1. Frequencies of occurrence of the most common species of dinoflagellates (samples N = 266; taxa authority listed in Supplementary Appendix 1).
The most frequently occurring potential HAB species by order of occurrence were Dinophysis caudata, Phalacroma rotundatum, Gonyaulax digitale, G. polygramma, Dinophysis acuta, Lingulodinium polyerda, Gonyaulax grindleyi, G. spinifera, Alexandrium affine, D. acuminata, D. ovum, Prorocentrum gracile, and P. micans.
Harmful Algal Bloom Genera Occurrence
Dinophysis and Phalacroma
The DSP-producing Dinophysis was the most common HAB genus, represented by 21 species, and was consistently present in almost all samples (n = 248, or 93.2% of samples), but exhibited highly variable occurrence seasonally and annually. The most common species detected with more than 5% frequency were: Dinophysis caudata (n = 214, 80.5% of samples), D. acuta (n = 87 and 32.7%), D. fortii (n = 45 and 16.9%), D. acuminata (n = 31 and 11.7%), D. ovum (n = 23 and 8.7%), D. hastata (n = 19 and 7.1%), D. parva (n = 19 and 7.1%), and D. porosa (n = 15 and 5.6%). Taxonomically related Phalacroma was frequently present (P. rotundatum, n = 142 or 53.4% of samples), P. rapa (n = 16 and 6). Periods of significant (more than approximately 10% of community) Dinophysis contribution were the following: (1) June to September 1994 (peaking at 36.7% in July 1994, D. caudata and D. acuta, coincident with Phalacroma rotundatum); (2) Late March and April, then June to early August 1995, then again from September until December 1995 (with peak of 66% of community on 17th November 1995, consisting of D. caudata, D. acuta and P. rotundatum). Then significant presence of between 5 and 17% continued into January to June 1996, peaking on 29th May 1996 when the bloom of Dinophysis caudata contributed 73.6% of the dinoflagellate community. After the continuous bloom period during 1995 and 1996, Dinophysis had very low relative numbers in summer and autumn 1996; (3) The next period of elevated numbers was observed from June to October 1997, with the maximum of 62% on 11th September and consistently around 50% contribution during late September to early October 1997 (dominated by D. caudata with the presence of D. acuminata, D. acuta, D. fortii and D. tripos). There existed stable upwelling conditions from June to October, preceded by low UI in winter 1996–1997, with a pause in upwelling indicated by negative slope of the CUI in May and first half of June, and a low annual CUI; (4) During 1998, a short increase of D. acuminata and D. caudata was observed in late February to early March (up to 20% of community), and on 28th April (16.8% of D. caudata). The long summer-autumn period of Dinophysis proliferation lasted from late June until end of September 1998, when on 20th August the maxima of 62.5% in community was observed, contributed mostly by D. acuta, while D. caudata was present through autumn until January 1999; (5) During the summer 1999, from May to mid-July with the most significant contribution reaching 20–26.4%, an autumnal small peak was observed between 30th October and 12th November (up to 9%); (6) Late February and March 2000 (up to 15.3%), then long period from July 2000 until January 2001, when the most significant proportions (typically higher than 20%) were observed during September to November, with the maximum of 72.8% on 15th October, and unusually high values for the cold season on 21st November (45%) and 22nd of January (35.4%); (7) During summer and autumn 2001, Dinophysis development was observed from late August until the end of sampling in October, with the highest recorded share in community (59.8%) on 15th September.
Prorocentrum
A potential HAB genus, with several species producing DSP toxins, was represented by 9 species, and found in 126 samples (45.9% of total). The most frequent species were P. scutellum (24.1%), P. triestinum (10.9%), P. micans (7.9%), P. gracile (7.9%) and P. rostratum (6.8%). The periods with significant Prorocentrum contribution to community were as follows: (1) October 1994 to early January 1995, with the highest share of 38.2% of total dinoflagellates, comprised mainly by P. rostratum and P. scutellum, with the presence of P. balticum and P. compressum; (2) July to August 1995, marked by the development of P. triestinum (up to 48.2% on 9th August) and P. micans, peaked on 23th August at 53.9% contribution of these two species combined; (3) July 1998 when P. scutellum reached 17.5% share; (4) The highest level of Prorocentrum contribution was reached from 27th August to 17th September 1999, when P. triestinum comprised between 35% and up to 75% (30th August) of total community; (5) From August to October 2001 the genus was present at up to 7.7% share with P. gracile being common.
Gonyaulax
This HAB genus was represented by 14 species, of which three species were the most common: Gonyaulax digitalis (40.6% samples), G. polygramma (37% samples) and G. spinifera (16.5% samples). This genus was generally not dominant amongst the dinoflagellates with less than 5% contribution to community at most sampling dates. However, it demonstrated somewhat elevated contribution during some periods, such as: (1) October 1994 to February 1995, when G. polygramma and G. fragilis contributed between 2 and 7%; (2) July to September 1995, when Gonyaulax contribution occasionally reached between 22 and 31% of community, possibly because of pronounced upwelling-relaxation changes during that time; (3) April and July to September 1997 (G. spinifera, up to 49% on 16th September); (4) The highest dominance of Gonyaulax occurred in September—October 1999, when G. spinifera and G. digitale constituted together from 55 to 89% of community; (5) on the 7th September 2001, when G. digitalis reached 13.6% of community.
Lingulodinium polyedra (F. Stein) J. D. Dodge
This yessotoxin producer was absent during 1994 and 1995, and first found in February 1996, then continuously recorded from August to November 1996. The highest contribution of L. polyedra was observed when it dominated the dinoflagellate community during October 1996, reaching numbers from 31 (9th October) up to 57 cells per sample (15th and 21st October 1996); although absolute densities were not obtained by the sampling method, such numbers indicated that it may have formed a bloom on these dates. This species continued to occur in samples during 1997, with notable presence in periods from March to April (up to 9 cells per sample on 23rd April), and June to September (8 cells per sample 28th July). During 1998 L. polyedra appeared sporadically from April to November, and only reached significant numbers of 32 cells per sample once on 24th June. Throughout 1999 to April 2000 there were almost no records, but then it appeared consistently in summer 2000 from 16th May to 8th September, reaching 25 cells per sample on 4th August. The next period of continuous presence was from June to September 2001, with highest count of 15 cells per sample observed on 18th August. Generally, L. polyedra exhibited irregular inter-annual appearance, and tended to occur during the warm half of the year, usually April to October, and probably formed a continuous bloom in October 1996, with shorter events detected in June 1998 and August 2000. The strong northerly winds blowing during October 1996 formed upwelling conditions at the western coast; when the winds changed to westerly, they created conditions for water to move East around the Cape San Vincent, also contributing to increase of upwelling index at the South coast. These conditions may have contributed to Lingulodinium development and persistence in the plankton during a prolonged time.
The relative abundance of potentially HAB genera was different between sampling dates with upwelling and non-upwelling conditions (Figure 4). Totals of Dinophysis, Phalacroma, Gonyaulax and Prorocentrum had higher proportions in the communities during upwelling seasons and events. The Mann–Whitney–Wilcoxon U-tests (Table 2.) demonstrated significant at p < 0.05 differences between medians of relative abundances of Dinophysis, Phalacroma and L. polyedra during upwelling against non-upwelling sampling dates.
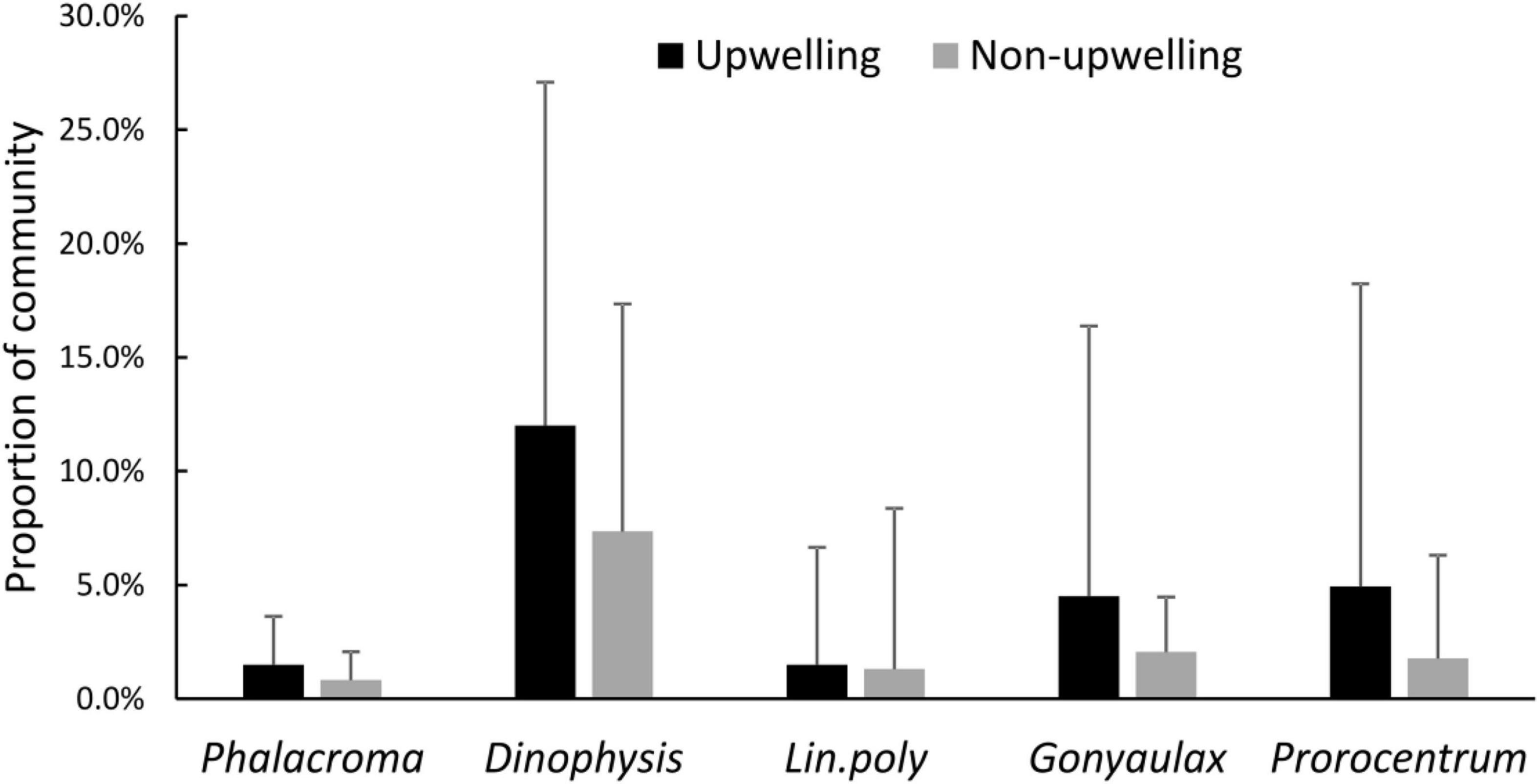
Figure 4. Potentially HAB dinoflagellate genera relative abundance, means for upwelling and non-upwelling periods; bars: standard error.
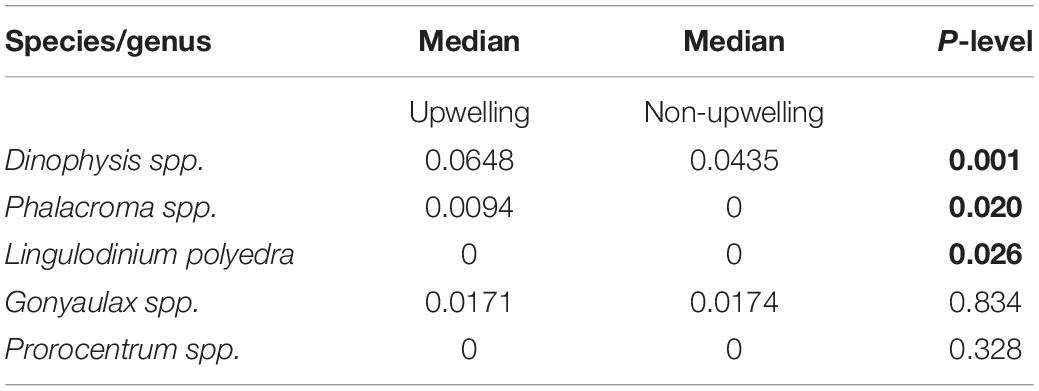
Table 2. Summary of the Mann–Whitney U-test results of the potential HAB species contributions between samples of upwelling and non-upwelling conditions; significant results at p < 0.05 are highlighted in bold, N = 143 (upwelling) and N = 123 (non-upwelling).
Multivariate Species Assemblage Analysis
Principal component analysis (PCA) was chosen to study community structure, as it was considered to be the appropriate method for the analysis of proportional datasets, i.e., lacking density values and describing community in terms of relative proportions of species. The PCA analysis was performed on standardized and log transformed abundances of the 50 most frequent species (Figure 5), to reduce the influence of the most abundant species and reveal the variability associated with relatively rare species.
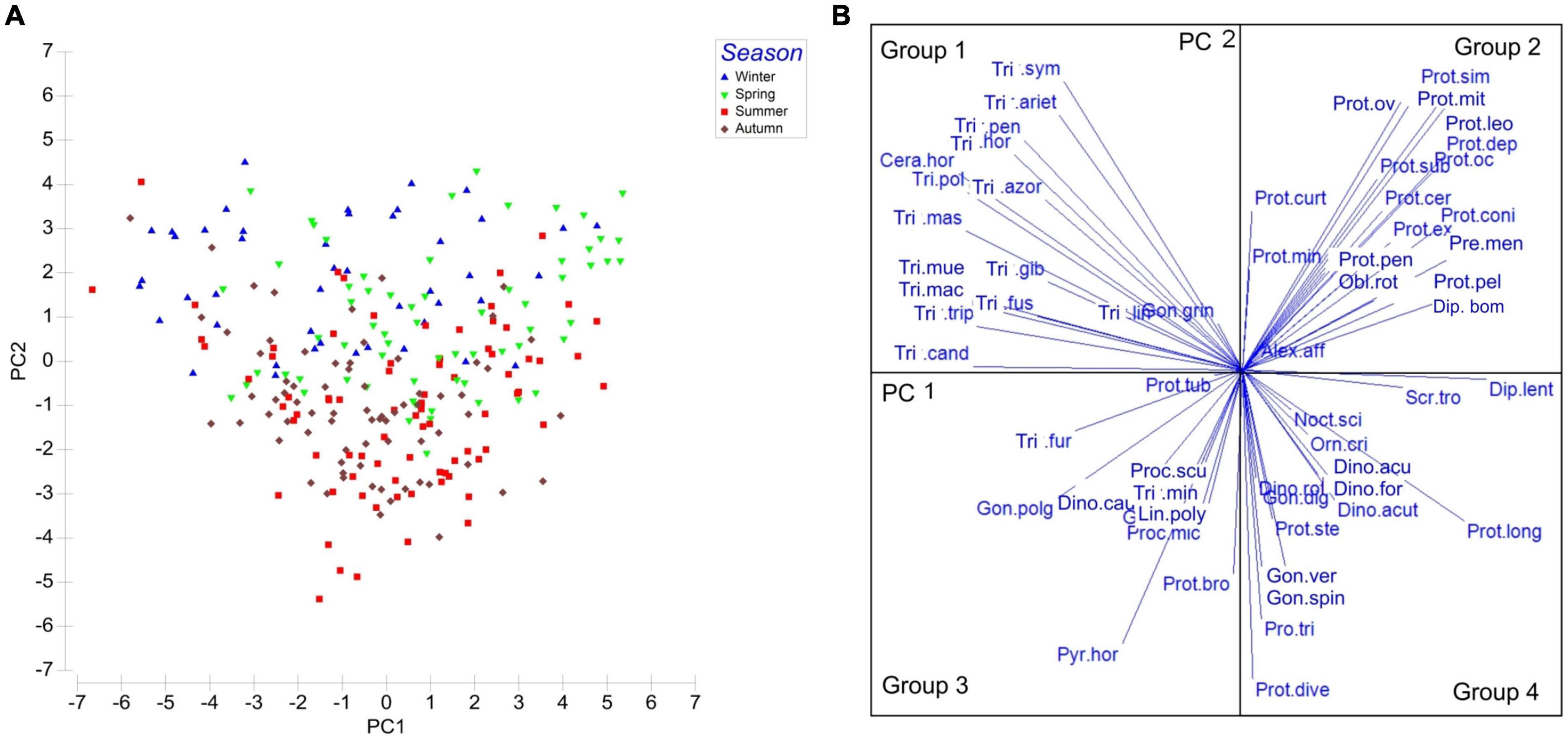
Figure 5. PCA distribution of samples in the space defined by the principal components performed on normalized and standardized abundance of the 50 most abundant dinoflagellate species: (A); PC1 plus PC2 account for 16.2%, cumulatively with PC3 for 20.7% of variance: (B) Vectors of species variables.
Sampling dates were separated by the seasonal factor, summer and autumn samples were distributed mainly below the center of diagram (negative PC2 scores). Winter and spring samples were associated with positive scores on PC2, but were widely scattered along the PC1, probably because of the influence of the most frequent species. Group 1 (as in Table 3), consisting mostly of Tripos spp., was clearly associated with sampling dates from November through April-May. Group 2 (Protoperidinium spp.) was distinctly separated and associated with spring (February to May) sampling dates. Vectors of species belonging to Groups 3 and 4 were more weakly separated, but linked with summer and autumn sampling dates. These two groups contained most of the HAB species typical of the area, belonging to genera Dinophysis, Prorocentrum, Gonyaulax, and Lingulodinium polyedra.
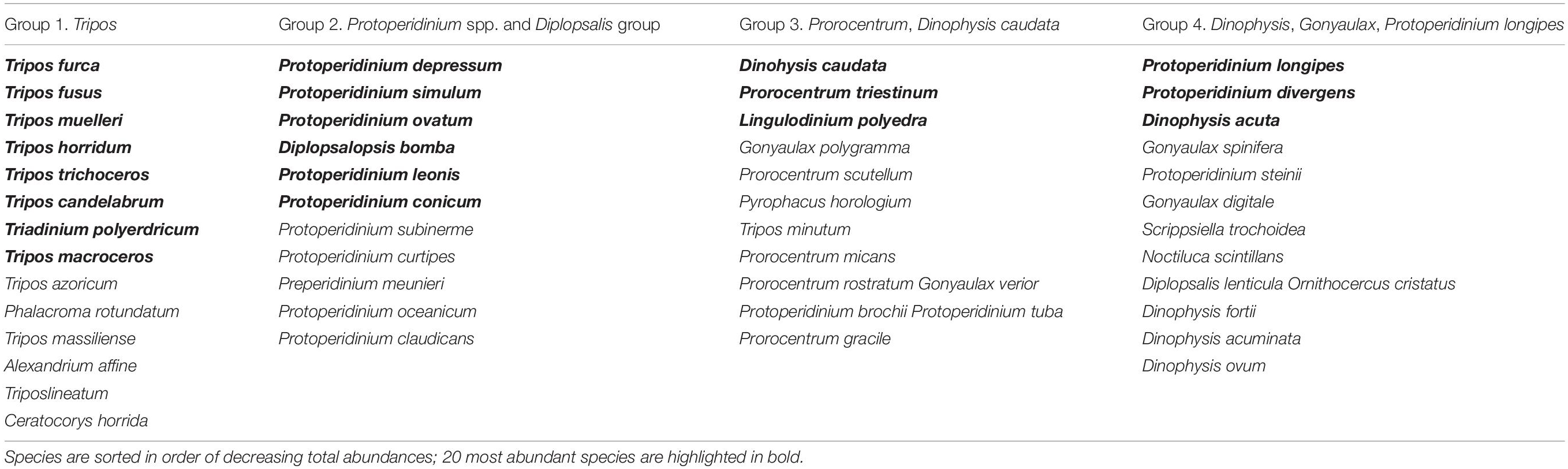
Table 3. Dinoflagellate assemblages grouping abundant species revealed by principal components analysis (PCA) applied to standardized fourth-root transformed data; PC1 plus PC2 account for 16.6% of taxonomic variability.
Taking into consideration the low total variance explained by the first three PCs (20.76%); a consequence of a large number of variables (species), reflecting the complexity of community. Therefore, the results for the PCA are treated as descriptive, but four types of dinoflagellate assemblages were separated by the combined effect of the variances associated with the first two principal components (Figure 5).
Seasonal Patterns of Occurrence
In order to further reveal seasonal differences, PCA analysis was applied to species proportions averaged by season of each year. The resulting plot demonstrated seasonal differences in the composition of dinoflagellate community (Figure 6). Winter and spring communities were distinctly separated from summer and autumn, the latter two being more similar. Comparison of the species variables vectors with data points in the principal components space demonstrated that species groups responsible for seasonal differences were similar to groups identified in the previous PCA analysis done on the non-averaged data. Winter communities were mainly characterized by the Tripos group (T. furca, fusus, horridum) and others, Triadinium polyedra, and the Protoperidinium group (P. leonis, mite). In spring, the more numerous of the Protoperidinium group (P. longipes, P. depressum) and others were highly represented, together with the Diplopsalis group (incl. Preperidinium meunieri) and the constantly present Tripos genus. Summer and autumn communities showed less distinct separation, with main contribution from the presence of Protoperidinium divergens, Dinophysis caudata, Lingulodinium polyedra and Prorocentrum triestinum. Summer and autumn were more likely to contain HAB species.
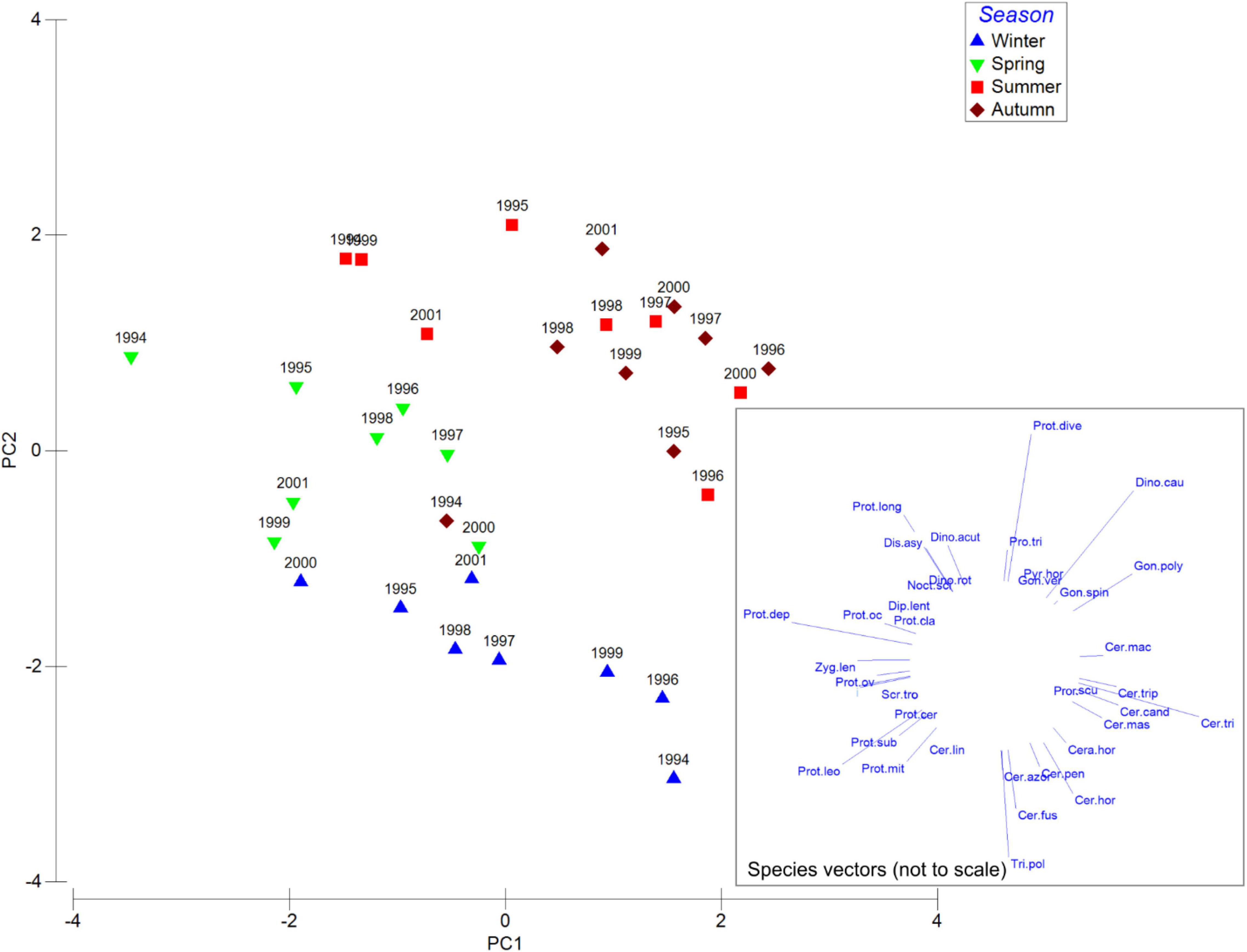
Figure 6. PCA analysis of seasonally averaged standardized abundances of the 50 most common dinoflagellates species. PC1 and PC2 explain 14.6% and 12.2% variability respectively. Species codes are listed in the Supplementary Appendix 1.
Similarity percentages analysis (SIMPER) was further used to determine the contributions of individual species to the similarities between samples within different seasonal groups (Table 4), based on the Bray-Curtis similarities of the resemblance matrix. In each case, the average similarity between samples belonging to seasonal groups was in the range from 42 to 46%, with 12–16 most significant species responsible for 75% of total similarity. Generally, the most frequent and relatively abundant species contributed the most to similarity in all seasonal groups. In winter, Tripos furca, fusus, horridum and muelleri, Dinophysis caudata and several Protoperidinium spp. were the main contributing species. In spring samples, similarity was driven by the same Tripos species with a higher contribution of Protoperidinium depressum, longipes, divergens, and ovatum. In summer, Dinophysis caudata contributed to similarities, together with the same frequent Tripos and Protoperidinium species. Autumn samples were characterized by decrease of contribution of Protoperidinium, with Tripos furca, T. fusus, T. muelleri and Dinophysis caudata being the most important contributors. In general, similarities between communities of the same season revealed by SIMPER were attributed to the most common and abundant species, but not specifically to HAB species. The exception was Dinophysis caudata, which was the most common harmful species that contributed to seasonal dissimilarity.
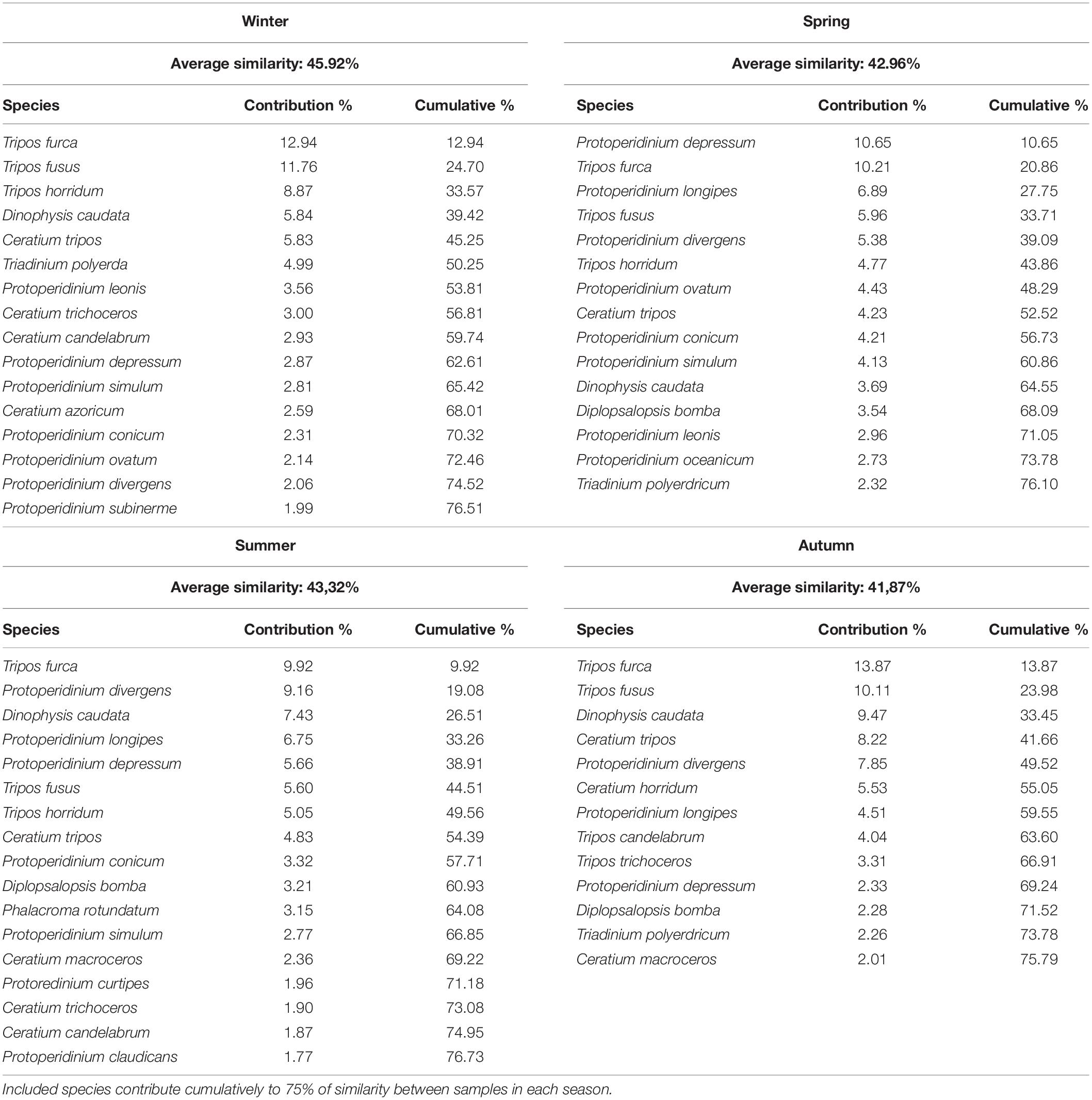
Table 4. Contribution of species to the similarities between seasonally grouped samples obtained by SIMPER analysis applied to standardized log transformed data for species proportions.
Discussion
Dinoflagellate Groups
The species assemblages described in this study were defined as the result of observations of coincident occurrence, and the study did not examine any inter-species interactions; therefore, we did not consider here the existence or influence of such interactions on the community composition. The data collected was limited only to larger dinoflagellates and therefore, other primary producers, such as other phytoflagellate classes and diatoms, were not included. Considering this, the composition of the dinoflagellate groups is treated here as a contingent abundance of species, that are promoted by favorable oceanographic conditions. Predation and other ecological interactions could not be assessed because of the limitations with the method.
Taxonomically closely related species, i.e., belonging to the same genera tended to co-occur together, as revealed by multivariate analysis. This likely reflects similarity in their ecological requirements. This relation in occurrence was especially pronounced in common genera such as Tripos, Dinophysis and Protoperidinium. A high similarity was observed between Tripos furca, T. fusus, T. horridum and T. muelleri that occurred in association with Protoperidinium longipes, P. divergens and Dinophysis caudata, meaning that such frequent species tended to have similar occurrence and abundance patterns, therefore, were favored by the same environmental conditions.
Observed grouping of the taxa in this study was similar to dinoflagellate life-form types delimited by Smayda and Reynolds (2001, 2003). In the present study, the “Tripos” community (Group 1) seemed to be associated with winter and spring samples, so outside of the upwelling period, but in some cases also autumn (Figure 5). We found Group 1 of this research (“Tripos”) to be comparable with Type III by Smayda and Reynolds (2003). This dinoflagellate type was described by the same authors to bloom during summer and autumn stratification in coastal waters, but also favored by elevated nutrients concentrations, which was partly opposite to our observations. This could be explained by the prevalence of upwelling conditions during summer, while less active upwelling combined with relatively high SST during Mediterranean winter were possibly favorable for the “Tripos” group of species.
Group 2 was very distinct and contained almost exclusively Protoperidinium and taxonomically related genera Diplopsalopsis, Oblea, Preperidinium with Protoperidinium depressum, P. simulum, P. ovatum, P. leonis, P. conicum and Diplopsalopsis bomba occurring as the most abundant species. It was usually associated with spring and summer sampling dates but occasionally found in autumn. It can be compared with Type II (nutrient enriched warm conditions) in Smayda and Reynolds (2003). Data obtained at Sagres area during 2010–2012 (Goela et al., 2014) suggests that high Protoperidinium and Diplopsalis-type abundance was associated with spring-summer blooms characterized by high biomass chain forming diatom development (Guinardia spp., Chaetoceros spp.) and was related with upwelling conditions favoring development of diatoms (nutrient enrichment, deep mixed layer, turbulence).
Group 3 contained such HAB species as Dinophysis caudata and Lingulodinium polyedra together with Prorocentrum micans and P. scutellum. These species can be compared with Type II assemblage described by Smayda and Reynolds (2001, 2003) that are formed during summers in conditions of rather elevated nutrient levels and local stratification. In this study, Group 3 was clearly associated with the end of summer upwelling season and early autumn (July—November), and so corresponded with Type II habitat conditions. Type V (Upwelling relaxation taxa) also can be compared to Group 3 (incl. by the presence of L. polyedra). Therefore, Group 3 species combined features described for Types II and V assemblages and could be associated with the conditions of establishing stratification at the end of the upwelling season during summer and autumn.
Group 4 was the most taxonomically diverse and contained Protoperidinium longipes and Dinophysis acuta, as well as other Dinophysis species (D. fortii, D. acuminata, D. ovum), Gonyaulax spinifera, Scrippsiella trochoidea, Pyrophacus sp., Torodinium sp., Ornithocercus sp. and Noctiluca scintillans. It was typically associated with spring and summer samples and by taxa composition possessing the traits of Type VII (Dinophysoids) and VIII (Tropical taxa) described by Smayda and Reynolds. These types represent habitat range from small scale upwelling currents to oligotrophic stratified and usually offshore conditions.
Type I (Gymnodiniods) by Smayda and Reynolds (2001) could not be compared with the results of this study because only larger armored dinoflagellates were collected. However, in the data obtained at the same location during 2010–2016 (Goela et al., 2014; Danchenko et al., 2019) Gymnodinioid species were frequently present during summer upwelling season, often concurrently with diatom blooms.
Seasonal Patterns of Dinoflagellate Assemblages
The present study does not allow direct comparison of dinoflagellate community development with that of the other phytoplankton groups (diatoms, flagellates, coccolithophorids). Described patterns of seasonal blooms of diatoms, nanoflagellates and cyanobacteria (Loureiro et al., 2005, 2011; Goela et al., 2014) in the same area indicated that late autumn and winter (November to February) are the seasons with generally low abundance of phytoplankton, dominated by nanoflagellates. The corresponding winter dinoflagellate community in this study contained species of the “Tripos” Group 1 and some rare species in low proportions. Spring and early summer at study site were characterized (Loureiro et al., 2005, 2011; Goela et al., 2014, 2015; Danchenko et al., 2019) by the proliferation of blooms, mainly composed of diatoms belonging to genera Chaetoceros, Guinardia, Pseudo-nitzschia and marked by an increase in Chl a. These spring blooms could be initiated by the upwelling-driven increase in nutrients, increasing photoperiod and SST (Loureiro et al., 2005, 2011; Goela et al., 2014). In the current study, the dinoflagellate community that developed during spring was characterized by Group 2 (“Protoperidinium”). June and August were normally the periods of active upwelling associated with N and W winds, which decreased by the end of September—October (Haynes et al., 1993; Goela et al., 2016). Diatom blooms are known to decline in such conditions and replaced by community dominated by nanoflagellates and by warm oligotrophic water taxa of dinoflagellates that we described as Group 4 (Dinophysis acuta, D. fortii, D. acuminata, D. ovum and Gonyaulax spp.). Groups 3 and 4 contained a majority of potentially HAB species. Therefore, upwelling relaxation in summer and autumn is the season when conditions for harmful blooms of dinoflagellates can be expected in the study area.
It was revealed by both PCA and SIMPER analyses that the most common and frequently occurring species made the main contribution to statistical measures of similarities between samples of the same season and similar oceanographic conditions, as well as to dissimilarities between different seasons. Harmful species, except for Dinophysis spp., were not found to be among the most abundant and frequently occurring (Table 5). Other factors affecting communities (such as ecological, competition, life-cycle and others) were not examined in this study, but possibly contributed to the high temporal variability observed.
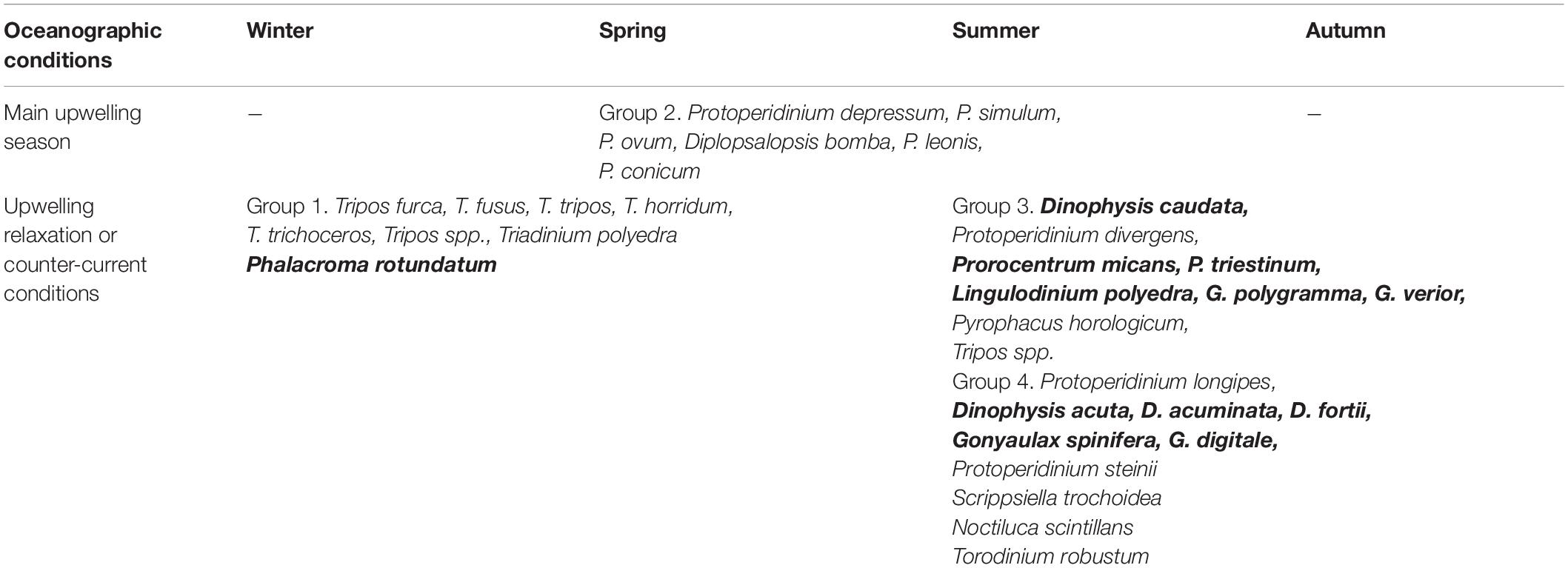
Table 5. Dinoflagellate groups with dominant species and the seasonality of their appearance; potentially harmful species highlighted in bold.
Implications for Aquaculture
One of the objectives was to provide information on harmful algae monitoring in the interests of shellfish aquaculture activities. The observed co-occurrence between HAB-containing types of assemblages with those of the more common species suggest the requirement for similar ecological conditions. The communities with harmful species had a general tendency to develop during the relaxation conditions of late summer-autumn, that followed a long summer upwelling season. There were nevertheless cases of HAB containing assemblages in winter (e.g., Dinophysis spp. in spring 1995, 1996 and notably from summer 1997 through winter until spring 1998, that corresponded with DSP event; L. polyedra present since March to August 1997). Dinophysis species, especially D. acuminata were often detected in low numbers throughout the annual cycle, allowing them to increase in any season under favorable conditions.
In the West Iberian region (Galicia, West Portugal), the main source of dinoflagellate-related HABs are Dinophysis species (Escalera et al., 2010; Díaz et al., 2016; Moita et al., 2016). Early onset of upwelling and upwelling events during spring were reported to promote D. acuminata blooms in Galician Rias (Díaz et al., 2013). Hot summers with positive SST anomalies, with weak upwelling conditions were found to be associated with the early decline of population of D. acuminata and its exchange to Dinophysis acuta (Díaz et al., 2016, 2019). During this study, the most pronounced Dinophysis contribution was observed starting summer 1997, and lasted through the winter, and during February to September 1998 coincided with DSP outbreak in the Algarve, reported by Vale et al., 1999. Dinophysis bloom in February-March 1998 was represented mostly by D. acuminata and to lesser degree by D. caudata, later in summer D. acuta appeared and reached high numbers (together with D. caudata) in July to October 1998. Such pattern is similar with reports from N Iberia by Díaz et al. (2013). D. acuta was also significantly represented in other years, e.g., 1994. Study sampling site at Sagres was located to the West from Ria de Alvor and Ria Formosa, where the DSP health event was observed, therefore a direct comparison is not feasible. But nevertheless, the coincidence in time and lengths with the recorded outbreak suggested that similar conditions for Dinophysis blooms existed along the South Portugal coast. Coastal counter-currents directed East to West were found to be independent of the local wind forcing, defined by regional barometric features and frequent (Sánchez et al., 2006; Garel et al., 2016) along the S Algarve coastline. These warmer counter-currents may have contributed to warmer and more stratified conditions at the shelf, contributing to the DSP event in 1998 (Vale et al., 1999), and the increase in Lingulodinium polyedra observed in some years. The influence of Gulf of Cadiz counter-current is likely to be more pronounced in the Eastern Algarve coast, compared to the sampling site at Sagres near Cape San Vincent.
The regular shellfish quality control required by law is implemented by IPMA (Instituto Português do Mar e da Atmosfera) since 1986, and includes sampling of phytoplankton and commercial bivalves (Vale et al., 2008; Anonymous, 2013). The reports were made public since 2014, and so do not cover the period of this study. The suggestion resulting from this study would be to manage the timing of collection of shellfish so that it does not coincide with upwelling relaxation in summer-autumn, which can be assessed from the published meteorological data. In the Sagres and Cape Saint Vincent area this means relaxation of prevailing N and NW winds and change to winds of other directions during late summer and autumn. Adjustment of harvesting based on the knowledge of weather and oceanographic conditions may assist the bivalve aquaculture stakeholders to reduce the probability of toxicity in shellfish and compliance with safety requirements.
Harmful Algal Bloom Species and Climate Trends
Long term studies of trends in climatological wind patterns (Leitão et al., 2018) indicated that the annual Northerly wind component has tendency to increase along the coasts of Iberia, including South and South-West coasts over the recent decades. At the same time, no changes of wind trends were found for summer, and decrease of N winds was observed for September. With regards to upwelling in the study area, such trends point to possible future weakening of upwelling in the late summer-early autumn, consistent with climatic SST increase observed (Baptista et al., 2018). As some HAB dinoflagellates (Dinophysis, Lingulodinium) were observed in this study to be favored by weaker upwelling and warmer water in late summer and autumn, such climatic trends may increase the likelihood of their development. At the same time, upwelling conditions along the West coast of Iberia, including the study area, demonstrated a tendency to increase during colder half of the year, i.e., in period when water column is supposedly more mixed due to wind conditions. This may possibly promote more intensive phytoplankton blooms in spring related with upwelling events outsides of the main upwelling season. Such blooms are initially dominated by diatoms and flagellates in the study area (Loureiro et al., 2005; Goela et al., 2014), but at the later stages of succession dinoflagellates may be promoted, including HAB species e.g., as observed in spring 2016, when diatom bloom was followed by the appearance of HAB dinoflagellates (Danchenko et al., 2019).
Pronounced upwelling events in spring were observed repeatedly in this study, sometimes as early as February (e.g., in 1995, 1999 and 2000). Consecutively, the species of dinoflagellates that occurred after such short upwelling pulse has diminished (e.g., Dinophysis spp.) often resembled the community characteristic of the autumn succession in the end of upwelling season. Therefore, upwelling events during cold part of the year showed the potential to favor HAB species, which usually appear later during summer, and such prolonged presence of potential HAB organisms may pose a problem for aquaculture, as monitoring programs may require more frequent and longer closures of bivalve mollusk collection.
Conclusion
1. The armored dinoflagellate communities in the region were taxonomically very diverse, with 194 species detected at one study site during 8 years of monitoring.
2. Conditions that favored development of assemblages containing potentially HAB dinoflagellate taxa were usually observed during summer and early autumn, i.e., during the seasonal main upwelling period, but also occasionally in spring or winter, in favorable conditions.
3. Climatic trends in the region, such as weakening of summer upwelling and increase in frequency of upwelling events during colder seasons have potential to alter the normal patterns of HAB species occurrence.
4. Knowledge about the response of the dinoflagellate communities to the oceanographic conditions change could be employed to improve the bivalve aquaculture monitoring and adjust harvest timing for timing of collection in order to reduce the risk of toxicity and economic losses due to safety measures.
Data Availability Statement
The raw data on dinoflagellate composition is made available as the Supplementary Appendix 2. Oceanographic data can be accessed from NOAA Environmental Research Division Data Access Program (ERDDAP).
Author Contributions
SD analyzed the data and wrote the manuscript. JD performed taxonomic identifications of phytoplankton. JI conceptualized the study, performed field work, and wrote the manuscript. AN provided resources, supervision, and corrected the manuscript. All authors contributed to the article and approved the submitted version.
Funding
SD was funded by the European Union’s Erasmus Mundus MACOMA program; AN was funded by the Chinese Academy of Sciences (CAS-YIC) scholarship and an SKLECECNU project 111 scholarship; JD was supported by Royal Holloway, University of London. We would like to acknowledge the financial support of the Portuguese Foundation of Science and Technology (FCT) to CIMA through UIDP/00350/2020. JI thanks the EU Horizon Research and Innovation Program under grant agreement No 77330 (GAIN-Green Aquaculture Intensification in Europe) for the funding of this article.
Conflict of Interest
JI was employed by the company Sagremarisco Lda.
The remaining authors declare that the research was conducted in the absence of any commercial or financial relationships that could be construed as a potential conflict of interest.
Publisher’s Note
All claims expressed in this article are solely those of the authors and do not necessarily represent those of their affiliated organizations, or those of the publisher, the editors and the reviewers. Any product that may be evaluated in this article, or claim that may be made by its manufacturer, is not guaranteed or endorsed by the publisher.
Acknowledgments
We would like to acknowledge the support and contribution of Future Earth Coasts (FEC), Integrated Marine Biosphere Research (IMBeR), Future Earth Oceans Knowledge-Action Network, and Scientific Committee on Oceanic Research (SCOR). We sincerely thank the reviewers, whose valuable comments significantly improved this article.
Supplementary Material
The Supplementary Material for this article can be found online at: https://www.frontiersin.org/articles/10.3389/fmars.2022.591759/full#supplementary-material
References
Amorim, A., Moita, M., and Oliveira, P. (2002). “Harmful Algae 2002,” in Florida Fish and Wildlife Conservation Commission, Florida Institute of Oceanography, and Intergovernmental Oceanographic Commission of UNESCO, eds K. A. Steidinger, J. H. Landsberg, C. R. Tomas, and G. A. Vargo (Florida, USA: St. Petersburg), 89–91.
Anonymous (2013). Plano de Açao Sistema Nacional de Monitorização de Moluscos Bivalves. Novembro 2013. Lisboa : Instituto Português do Mar e de Atmosfera, I.P.
Bakun, A. (1973). Coastal Upwelling Indices, West Coast of North America. Technical Report NMFS SSRF-671. Seattle, WA: NOAA.
Baptista, V., Silva, P. L., Relvas, P., Teodósio, M. A., and Leitão, F. (2018). Sea surface temperature variability along the Portuguese coast since 1950. Int. J. Climatol. 38, 1145–1160. doi: 10.1002/joc.5231
Cembella, A. D. (2003). Chemical ecology of eukaryotic microalgae in marine ecosystems. Phycologia 42, 420–447.
Clarke, K. R., and Warwick, R. M. (2001). A further biodiversity index applicable to species lists: Variation in taxonomic distinctness. Mar. Ecol. Prog. Ser. 216, 265–278. doi: 10.3354/meps216265
Cravo, A., Relvas, P., Cardeira, S., Rita, F., Madureira, M., and Sánchez, R. (2010). An upwelling filament off southwest Iberia: Effect on the chlorophyll a and nutrient export. Cont. Shelf Res. 30, 1601–1613. doi: 10.1016/j.csr.2010.06.007
Cropper, T. E., Hanna, E., and Bigg, G. R. (2014). Spatial and temporal seasonal trends in coastal upwelling off Northwest Africa, 1981-2012. Deep. Res. Part I Oceanogr. Res. Pap. 86, 94–111. doi: 10.1016/j.dsr.2014.01.007
Danchenko, S., Fragoso, B., Guillebault, D., Icely, J., Berzano, M., and Newton, A. (2019). Harmful phytoplankton diversity and dynamics in an upwelling region (Sagres, SW Portugal) revealed by ribosomal RNA microarray combined with microscopy. Harmful Algae 82, 52–71. doi: 10.1016/j.hal.2018.12.002
Díaz, P. A., Reguera, B., Moita, T., Bravo, I., Ruiz-Villarreal, M., and Fraga, S. (2019). Mesoscale dynamics and niche segregation of two Dinophysis species in Galician-Portuguese coastal waters. Toxins 11:37. doi: 10.3390/toxins11010037
Díaz, P. A., Reguera, B., Ruiz-Villarreal, M., Pazos, Y., Velo-Suárez, L., Berger, H., et al. (2013). Climate variability and oceanographic settings associated with interannual variability in the initiation of Dinophysis acuminata blooms. Mar. Drugs 11, 2964–2981. doi: 10.3390/md11082964
Díaz, P. A., Ruiz-Villarreal, M., Pazos, Y., Moita, T., and Reguera, B. (2016). Climate variability and Dinophysis acuta blooms in an upwelling system. Harmful Algae 53, 145–159. doi: 10.1016/j.hal.2015.11.007
Escalera, L., Reguera, B., Moita, T., Pazos, Y., Cerejo, M., Cabanas, J. M., et al. (2010). Bloom dynamics of Dinophysis acuta in an upwelling system: In situ growth versus transport. Harmful Algae 9, 312–322. doi: 10.1016/j.hal.2009.12.002
Fiúza, A. F. D., de Macedo, M. E., and Guerreiro, M. R. (1982). Climatological space and time variation of the Portuguese coastal upwelling. Oceanol. Acta 5, 31–40.
Garel, E., Laiz, I., Drago, T., and Relvas, P. (2016). Characterisation of coastal counter-currents on the inner shelf of the Gulf of Cadiz. J. Mar. Syst. 155, 19–34. doi: 10.1016/j.jmarsys.2015.11.001
Goela, P. C., Cordeiro, C., Danchenko, S., Icely, J., Cristina, S., and Newton, A. (2016). Time series analysis of data for sea surface temperature and upwelling components from the southwest coast of Portugal. J. Mar. Syst. 163, 12–22. doi: 10.1016/j.jmarsys.2016.06.002
Goela, P. C., Danchenko, S., Icely, J. D., Lubian, L. M., Cristina, S., and Newton, A. (2014). Using CHEMTAX to evaluate seasonal and interannual dynamics of the phytoplankton community off the South-west coast of Portugal. Estuar. Coast. Shelf Sci. 151, 112–123. doi: 10.1016/j.ecss.2014.10.001
Goela, P. C. P. C., Icely, J., Cristina, S. S., Danchenko, S., DelValls, T. A., Newton, A., et al. (2015). Using bio-optical parameters as a tool for detecting changes in the phytoplankton community (SW Portugal). Estuar. Coast. Shelf Sci. 167, 125–137. doi: 10.1016/j.ecss.2015.07.037
Gonzalez-Nuevo, G., Gago, J., and Cabanas, J. M. (2014). Upwelling index: a powerful tool for marine research in the NW Iberian upwelling system. J. Oper. Oceanogr. 7, 47–57. doi: 10.1080/1755876x.2014.11020152
Gowen, R. J., Tett, P., and Smayda, T. J. (2012). Phytoplankton and the balance of nature: An opinion. Estuar. Coast. Shelf Sci. 113, 317–323. doi: 10.1016/j.ecss.2012.08.009
Hackett, J. D., Anderson, D. M., Erdner, D. L., and Bhattacharya, D. (2004). Dinoflagellates: a remarkable evolutionary experiment. Am. J. Bot. 91, 1523–1534. doi: 10.3732/ajb.91.10.1523
Haynes, R., Barton, E. D., and Pilling, I. (1993). Development, persistence, and variability of upwelling filaments off the Atlantic coast of the Iberian Peninsula. J. Geophys. Res. 98:22681. doi: 10.1029/93JC02016
Krug, L. A., Platt, T., Sathyendranath, S., and Barbosa, A. B. (2017). Unravelling region-specific environmental drivers of phytoplankton across a complex marine domain (off SW Iberia). Remote Sens. Environ. 203, 162–184. doi: 10.1016/j.rse.2017.05.029
Kudela, R. M. M., Seeyave, S., and Cochlan, W. P. P. (2010). The role of nutrients in regulation and promotion of harmful algal blooms in upwelling systems. Prog. Oceanogr. 85, 122–135. doi: 10.1016/j.pocean.2010.02.008
Large, W. G., and Pond, S. (1982). Sensible and latent heat flux measurements over the ocean. J. Phys. Oceanogr. 12, 464–482. doi: 10.1175/1520-04851982012
Leitão, F., Relvas, P., Cánovas, F., Baptista, V., and Teodósio, A. (2018). Northerly wind trends along the Portuguese marine coast since 1950. Theor. Appl. Climatol. 137, 1–19. doi: 10.1007/s00704-018-2466-9
Loureiro, S., Newton, A., and Icely, J. D. (2005). Microplankton composition, production and upwelling dynamics in Sagres (SW Portugal) during the summer of 2001. Sci. Mar. 69, 323–341. doi: 10.3989/scimar.2005.69n3323
Loureiro, S., Reñé, A., Garcés, E., Camp, J., and Vaqué, D. (2011). Harmful algal blooms (HABs), dissolved organic matter (DOM), and planktonic microbial community dynamics at a near-shore and a harbour station influenced by upwelling (SW Iberian Peninsula). J. Sea Res. 65, 401–413. doi: 10.1016/j.seares.2011.03.004
Moita, M. T., Oliveira, P. B., Mendes, J. C., and Palma, A. S. (2003). Distribution of chlorophyll a and Gymnodinium catenatum associated with coastal upwelling plumes off central Portugal. Acta Oecol. 24, S125–S132. doi: 10.1016/S1146-609X(03)00011-0
Moita, M. T., Pazos, Y., Rocha, C., Nolasco, R., and Oliveira, P. B. (2016). Toward predicting Dinophysis blooms off NW Iberia: A decade of events. Harmful Algae 53, 17–32. doi: 10.1016/j.hal.2015.12.002
Oliveira, P. B., Moita, T., Silva, A., Monteiro, I. T., and Sofia Palma, A. (2009). Summer diatom and dinoflagellate blooms in Lisbon Bay from 2002 to 2005: Pre-conditions inferred from wind and satellite data. Prog. Oceanogr. 83, 270–277. doi: 10.1016/j.pocean.2009.07.030
Relvas, P., and Barton, E. D. (2005). A separated jet and coastal counterflow during upwelling relaxation off Cape São Vicente (Iberian Peninsula). Cont. Shelf Res. 25, 29–49. doi: 10.1016/j.csr.2004.09.006
Relvas, P., Barton, E. D., Dubert, J., Oliveira, P. B., Peliz, Á, da Silva, J. C. B., et al. (2007). Physical oceanography of the western Iberia ecosystem: Latest views and challenges. Prog. Oceanogr. 74, 149–173. doi: 10.1016/j.pocean.2007.04.021
Reynolds, R. W., Smith, T. M., Liu, C., Chelton, D. B., Casey, K. S., and Schlax, M. G. (2007). Daily high-resolution-blended analyses for sea surface temperature. J. Clim. 20, 5473–5496. doi: 10.1175/2007JCLI1824.1
Sánchez, R. F., Mason, E., Relvas, P., da Silva, A. J., and Peliz, Á (2006). On the inner-shelf circulation in the northern Gulf of Cádiz, southern Portuguese shelf. Deep. Res. Part II Top. Stud. Oceanogr. 53, 1198–1218. doi: 10.1016/j.dsr2.2006.04.002
Santos, M., Moita, M. T., Oliveira, P. B., and Amorim, A. (2020). Phytoplankton communities in two wide-open bays in the Iberian upwelling system. J. Sea Res. 167:101982. doi: 10.1016/j.seares.2020.101982
Shumway, S. M., Burkholder, J., and Morton, S. (2018). Harmful Algal Blooms: A Compendium Desk Reference. New Jersey, NJ: Wiley.
Silva, A., Palma, S., Oliveira, P. B., and Moita, M. T. (2009). Composition and interannual variability of phytoplankton in a coastal upwelling region (Lisbon Bay, Portugal). J. Sea Res. 62, 238–249. doi: 10.1016/j.seares.2009.05.001
Smayda, T. J. (1997). Harmful algal blooms: Their ecophysiology and general relevance to phytoplankton blooms in the sea. Limnol. Oceanogr. 42, 1137–1153. doi: 10.4319/lo.1997.42.5_part_2.1137
Smayda, T. J. (2008). Complexity in the eutrophication-harmful algal bloom relationship, with comment on the importance of grazing. Harmful Algae 8, 140–151. doi: 10.1016/j.hal.2008.08.018
Smayda, T. J., and Reynolds, C. S. (2001). Community assembly in marine phytoplankton: Application of recent models to harmful dinoflagellate blooms. J. Plankton Res. 23, 447–461. doi: 10.1093/plankt/23.5.447
Smayda, T. J., and Reynolds, C. S. (2003). Strategies of marine dinoflagellate survival and some rules of assembly. J. Sea Res. 49, 95–106. doi: 10.1016/S1385-1101(02)00219-8
Smayda, T. J., and Trainer, V. L. (2010). Dinoflagellate blooms in upwelling systems: Seeding, variability, and contrasts with diatom bloom behaviour. Prog. Oceanogr. 85, 92–107. doi: 10.1016/j.pocean.2010.02.006
Steidinger, K. A., and Tangen, K. (1996). “Dinoflagellates,” in Identifying Marine Phytoplankton, ed. C. R. Tomas (San Diego: Academic Press), 387–584.
Taylor, F. J. R., Hoppenrath, M., and Saldarriaga, J. F. (2008). Dinoflagellate diversity and distribution. Biodiv. Cons. 17, 407–418. doi: 10.1007/s10531-007-9258-3
Vale, P., Antónia, M., and Sampayo, M. (1999). Esters of okadaic acid and dinophysistoxin-2 in Portuguese bivalves related to human poisonings. Toxicon 37, 1109–1121. doi: 10.1016/S0041-0101(98)00247-5
Vale, P., Botelho, M. J., Rodrigues, S. M., Gomes, S. S., and Sampayo, M. A. D. (2008). Two decades of marine biotoxin monitoring in bivalves from Portugal (1986-2006): A review of exposure assessment. Harmful Algae 7, 11–25. doi: 10.1016/j.hal.2007.05.002
Keywords: dinoflagellate, upwelling systems, phytoplankton, harmful algal bloom (HAB), aquaculture management
Citation: Danchenko S, Dodge J, Icely J and Newton A (2022) Dinoflagellate Assemblages in the West Iberian Upwelling Region (Sagres, Portugal) During 1994–2001. Front. Mar. Sci. 9:591759. doi: 10.3389/fmars.2022.591759
Received: 05 August 2020; Accepted: 03 February 2022;
Published: 25 March 2022.
Edited by:
Bernardo Antonio Perez Da Gama, Fluminense Federal University, BrazilReviewed by:
Peter Allan Thompson, Commonwealth Scientific and Industrial Research Organization (CSIRO), AustraliaManuel Ruiz Villarreal, Centro Oceanográfico da Coruña, Instituto Español de Oceanografía (CSIC), Spain
Copyright © 2022 Danchenko, Dodge, Icely and Newton. This is an open-access article distributed under the terms of the Creative Commons Attribution License (CC BY). The use, distribution or reproduction in other forums is permitted, provided the original author(s) and the copyright owner(s) are credited and that the original publication in this journal is cited, in accordance with accepted academic practice. No use, distribution or reproduction is permitted which does not comply with these terms.
*Correspondence: Sergei Danchenko, sergei.danchenko.bio@gmail.com