- 1MARE - Centro de Ciências do Mar e do Ambiente, Faculdade de Ciências, Universidade de Lisboa, Campo Grande, Portugal
- 2IPMA - Instituto Português do Mar e da Atmosfera, Olhão, Portugal
- 3CCMAR - Centre of Marine Sciences, Universidade do Algarve, Faro, Portugal
- 4Departamento de Biologia Vegetal, Faculdade Ciências da Universidade de Lisboa, Campo Grande, Portugal
- 5Departamento de Biologia Animal, Faculdade Ciências da Universidade de Lisboa, Campo Grande, Portugal
Cockles are amongst the most exploited bivalve species in Portugal, playing an important ecological and socioeconomic role in coastal ecosystems. Two sympatric species of cockles, Cerastoderma edule (Linnaeus, 1758) and Cerastoderma glaucum (Bruguière, 1789–1792) may co-occur in estuaries and coastal lagoons in mixed populations along the European Atlantic coast, namely in Portugal, France and the United Kingdom. The increasing importance of shellfish harvesting in Portugal requires a good knowledge of cockle stocks and temporal variability in stock levels to better inform sustainable management practices. Therefore, this study aimed at assessing spatial and temporal variations in cockle populations in two Portuguese estuarine systems where the species are exploited at low levels. Sampling was carried out using a clam dredge, covering the entire potential area of occurrence of cockles in the Tagus and Sado estuaries at around the same time of the year in each of the three sampling years (2015, 2018, and 2019). The abundance, spatial distribution and population structure of cockles were examined at each system. Moreover, several water and sediment parameters were measured to understand the influence of environmental conditions on the spatial distribution and abundance of cockles. The results obtained showed that cockles occur mostly in the intermediate areas of both estuarine systems and are more abundant in the Tagus estuary. Depth, average sediment grain size and the species Ruditapes philippinarum were the factors that better explained the probability of species occurrence. The population structure analysis indicated that natural mortality is constraining the cockle communities given the low abundance of adult individuals with marketable size in both estuaries. This study highlights the need for appropriate management measures to ensure the sustainability of these bivalve population stocks that have significant socioeconomic importance for local populations.
Introduction
Estuaries are considered one of the most productive ecosystems in the world due to their biological, physical and chemical characteristics, providing food, shelter and nursery areas for several species of high economic interest (Day et al., 1988; Crespo et al., 2010; Barbier et al., 2011).
Bivalves are an essential component of these estuarine ecosystems, playing an important ecological and socioeconomic role (Ysebaert et al., 2003; Callaway et al., 2013). They are considered keystone species, acting as ecosystem engineers creating, modifying and maintaining habitats for other species (Vaughn and Hoellein, 2018). Additionally, they are a key element of estuarine food webs due to their suspension-feeding activity and for being a major food source for wading birds, fish, and crustaceans. Furthermore, by being part of human diets, they support locally important commercial fisheries. For these reasons, the identification of factors that influence cockle populations distribution and abundance is of the utmost importance (Magalhães et al., 2018).
Among bivalves, cockles are one of the most abundant and exploited species in the estuaries of Europe (Magalhães et al., 2018). In Portugal, they are an exploited living resource with particular important socio-economic relevance, that is harvested and commercialized from the North to the South (Oliveira et al., 2013; Magalhães et al., 2018). Furthermore, cockles play a major role in ecosystem function, being responsible for several ecosystem services such as carbon storage, biodiversity support, habitat modifying and food source for people (Carss et al., 2020).
In Europe, there are two species of cockle, the common cockle Cerastoderma edule (Linnaeus, 1758) and the lagoon cockle Cerastoderma glaucum (Bruguière, 1789–1792). The common cockle is most likely to be found in coastal lagoons and estuaries along the Atlantic coast, between the Barents Sea and Mauritania (Freitas et al., 2014), while the lagoon cockle tends to occur in non-tidal areas such as lagoons and marshes, from the Baltic to the Black Sea (Malham et al., 2012). In Portugal, both species are usually found in estuarine and lagoon systems, where they can co-exist and form sympatric populations (Machado and Costa, 1994). Its differentiation is particularly difficult given the morphological similarities and the considerable variability inherent in each species (Machado and Costa, 1994; Mariani et al., 2002; Freire et al., 2011). Since there is no scientific agreement or studies that support which of the species occurs in the Tagus and Sado estuaries, or whether both co-exist, this study focuses on the genus Cerastoderma spp., assuming it can include specimens of both species.
Cockle species reach maturity between 15 and 20 mm in shell length, living up to 9 years in some habitats but more commonly between 2 and 4 years. They live buried at a maximum depth of 5 cm from the surface, in sandy, muddy sandy, mud or muddy gravel sediments of the intertidal and subtidal zones and are generally found in sites with salinities of 15–35 psu but can tolerate salinities as low as 10 (Tyler-Walters, 2007).
Cockle population dynamics are controlled by both biotic factors, such as predation, bioturbation, parasitism, food availability, and abiotic factors such as temperature, immersion time, water velocity and sediment dynamics (Ramón, 2003; Gam et al., 2010). These factors can affect the physiological processes of cockles in juvenile or adult stages. Therefore, cockle populations suffer great temporal fluctuations in abundance and biomass due to the variations in production, growth, and mortality. The main cause of this variability is the success or failure of recruitment (Ramón, 2003).
Despite being a species that supports commercial fisheries and that is of conservation interest, mortality episodes and decline of their populations have been reported over the years, with diseases, climatic events and overfishing being pointed out as some of the main factors (Malham et al., 2012; Callaway et al., 2013; Burdon et al., 2014) and, as such, it is extremely important to monitor these populations.
The assessment of the abundance, distribution and populational structure of a given species is a requirement for the assessment of stocks. The analysis of length-frequency distributions is one of the methods used to study the population structure and may give indications about the success of the recruitment process (Iglesias and Navarro, 1990; Ramón, 2003). Given that cockles represent an important economic resource subject to multiple anthropogenic pressures, the present work assumes an important contribution so that in the future, stocks of these populations can be managed more sustainably, with adapted management policies.
Bivalves represent a significant portion of the world fishing industry. In 2018, cockle production from fisheries and aquaculture was almost 19,613 t in Europe, with 2,077 t of this from aquaculture and 17,536 t from fisheries landings (according to FAO database). In Portugal, the exploitation of bivalves is also an activity of great socioeconomic importance. Several species are harvested in Portugal, including clams, cockles, and oysters but cockles accounted for most landings in the past 20 years. In 2018, cockles accounted for ∼5,330 t of the ∼9,609 t of bivalve catches (according to DGRM database). However, in 2019, this value decreased by half, with only ∼2,285 t of cockle species being caught among the total ∼7,134 t of bivalve landings, a situation enhanced by the periods of capture prohibitions of this species due to the occurrence of microbiological contamination (INE, 2020).
The main objective of the present study was assessing spatial and temporal variations in cockle populations in two Portuguese estuarine systems with low directed fishing pressure. The specific aims were: (i) to analyze spatial and temporal variability in the distribution and abundance of cockle populations; (ii) to assess the role of environmental variables and other bivalve species in the observed spatial and temporal variability in cockle populations; and (iii) to determine cockle populations structure and its relationship with fisheries.
Materials and Methods
Study Area
This study was conducted at the Tagus and Sado estuaries, the two largest estuaries in Portugal.
The Tagus estuary, with an area of 320 km2, a mean depth of 5 m and a mean river flow of 300 m3s–1 (Gaudêncio and Cabral, 2007), is a mesotidal well-mixed estuary with irregular river discharge both seasonally and interannually (Cabral et al., 2001; Bettencourt et al., 2003). It is part of the Tagus catchment which covers a total area of about 80,629 km2, of which 24,800 km2 are in Portugal (Fernandes et al., 2020). Salinity is strongly influenced by tidal and seasonal variation (Chainho et al., 2008; Cabral et al., 2020) and tidal range varies from 1 m in neap tides to 4 m in spring tides (Vale and Sundby, 1987; Salgado et al., 2007). Water temperature ranges from 8 to 26°C (Cabral et al., 2001). This estuary is characterized by extensive tidal flats and salt marshes (Dias et al., 2013).
The Sado estuary, with an area of 180 km2 (Lillebø et al., 2011), a mean depth of 8 m and a mean river flow of 40 m3s–1 (França et al., 2009), is a mesotidal well-mixed estuary with irregular river discharge. It is part of Sado catchment which has an area of approximately 7,692 km2. Tidal currents dominate the estuarine dynamics given the limited freshwater discharge of the Sado river (Freitas et al., 2008). Consequently, the salinity remains close to normal marine water in most of the estuary, and is highly influenced by tidal currents and seasonal variations (ICNF, 2007; Biguino et al., 2021). This estuary has an average tidal range of 2.7 m, varying between 1.3 m at neap tides to 3.5 m at spring tides (Freitas et al., 2008). It comprises a wide bay characterized by two channels, the Northern channel with weaker residual currents and a highly dynamic Southern channel separated from the North channel by sand banks (Caeiro et al., 2005; Biguino et al., 2021). This estuary is also characterized by large areas of salt marshes and intertidal flats, and sandy sediments of marine origin, in the area closest to the mouth. Water temperature ranges from 10 to 26°C (Cabeçadas et al., 1999). These two estuaries were chosen because we wanted to understand the status of cockle populations in systems where cockle exploitation is low.
Sampling Surveys
Samples were collected in three different years in the Tagus estuary (May 2015, May 2018, and May 2019) and in the Sado estuary (July 2015, May 2018, and June 2019). Samples were collected at 48 and 35 sampling stations randomly selected along the Tagus and Sado estuarine gradients, respectively, to cover all the potential cockle distribution area (Figure 1).
Samples were collected at a maximum sediment depth of 12 cm, using a clam dredge with a mesh size of 3 cm (Supplementary Table 1) deployed from a professional fishing vessel that was towed once for 30 s at a mean speed of 1.5 knots in every sampling station (Supplementary Figure 1). Despite the mesh size was 3 cm, the clam dredge sampled smaller sized cockles, but there may have been some standardized undersampling of smaller sized classes.
Samples were sorted onboard by visual selection in a sorting tray and only living organisms were collected and placed in properly labeled bags, with the indication of the respective sampling station. Although all living organisms were collected only bivalves were used in further analyses.
Several environmental parameters were measured at each sampling station using an YSI multiparametric sonde, namely depth (m), water temperature (°C), salinity and dissolved oxygen (%). Sediment samples were also collected at each sampling station with a van Veen grab (0.05 m2) to determine the sediment grain size and the total organic matter content (%).
Laboratory Procedures
Bivalve molluscs were identified to the lowest possible taxonomic level and counted, weighed individually and shell length measured.
Sediment grain size was determined by separating sedimentary fractions through a column of four “AFNOR” sieves (0.063, 0.250, 0.500, and 2.000 mm), after drying at 60°C for 24 h. Then a mean grain size indicator, phi, was calculated according to Folk and Ward (1957) using GRADISTAT v8.
The organic matter content of the sediment was determined by the difference between the weight of a dry sample at 60°C, in an oven after 24 h, and the weight of the same sample after combustion in a furnace at 550°C, for 4 h (Luczak et al., 1997).
Data Analysis
For a better understanding of the distribution of cockle populations throughout the study area, two maps were produced using ArcGIS software, representing their distribution and abundance at each sampling station for all the three years where sampling took place in each estuary.
The frequency of occurrence of cockle species, that is the number of sampling stations they occur at divided by all sampling stations was estimated for each estuary. To test if there were significant differences in the frequency of occurrence of cockles between years in each estuary, a Pearson’s chi-square test of independence (significance level p > 0.05) was performed.
Differences in the abundance of cockle populations among estuaries and years were tested using a Permutational Multivariate Analysis of Variance (PERMANOVA) with a two-way factorial design: Year (fixed, 3 levels: 2015, 2018, and 2019) nested in Estuary (fixed, 2 levels: Tagus and Sado estuary). Data were square root transformed, and afterward a Bray-Curtis similarity matrix of cockle abundances was calculated.
To determine which environmental factors and which other bivalve species better explain cockle distribution and abundance, a Zero-inflated Poisson regression model was developed due to an excess of zeros of the data. The zero-inflated Poisson regression model is composed of two parts: a Poisson count model and the logit model for the excess zeros. A Poisson model is generated to explain cockle abundance and then a logit model is generated for zeros, i.e., to explain the probability of the species not being present.
The explanatory environmental factors were salinity, depth, water temperature, dissolved oxygen, sediment organic matter content and average sediment grain size (phi), and the categorical variable Estuary (Tagus and Sado). The bivalve species that accounted for 80–90% of the total abundance were selected to be part of the model as well, namely Ruditapes philippinarum, Scrobicularia plana, Ostrea stentina, Magallana angulata, and Solen Marginatus. Collinearity between the environmental factors was investigated to avoid the inclusion of redundant variables in the model. A correlation matrix with Pearson’s correlation coefficients and the respective p-value was performed (Zuur et al., 2009) using the “hetcor” function of the “polycor” statistical package (Fox, 2016). Correlations higher than 0.8 were considered significant, according to Snelder and Lamouroux (2010). To select the best model the AIC criterion (Akaike Information Criteria) was used (Akaike, 1997), which estimates the relative information lost by a given model, so the smaller its value, the more suitable the model.
Differences in the shell lengths of cockle populations among estuaries and years were tested using a PERMANOVA with a two-way factorial design: Year (fixed, 3 levels: 2015, 2018, and 2019) nested in Estuary (fixed, 2 levels: Tagus and Sado estuary). Data were square root transformed, and afterward a Bray-Curtis similarity matrix of cockle lengths was calculated. Significant differences were further analyzed using a posteriori pair-wise comparison.
To assess cockle population structure in each year and at each estuary, a graphical analysis of shell length-frequency distribution was performed. Dimensional classes were defined with an interval of 1 mm and specimens below and above the minimum legal size for catching (25 mm) were represented. Pearson’s chi-square test of independence, the Zero-Inflated Poisson regression model and the graphical analysis of shell length-frequency distribution routines were conducted using RStudio Team (2020). PERMANOVA analysis were conducted using software PRIMER v.6 with add on PERMANOVA (Anderson, 2001; Clarke and Gorley, 2006; Anderson et al., 2008).
Results
Temporal and Spatial Distribution
A total of 78,176 bivalve specimens were collected in all sampling stations, 57,077 in the Tagus estuary and 21,099 in the Sado estuary, during the 3 years of the study. Cockles (Cerastoderma spp.), peppery furrow (Scrobicularia plana), Manila clam (Ruditapes philippinarum) and the dwarf oyster (Ostrea stentina), were the most abundant species in the Tagus estuary over the 3 years. In the Sado estuary, there was a large abundance of the dwarf oyster but cockles, the Manila clam, the Portuguese oyster (Magallana angulata), the razor clam (Solen marginatus) and the peppery furrow were also well represented (Figure 2).
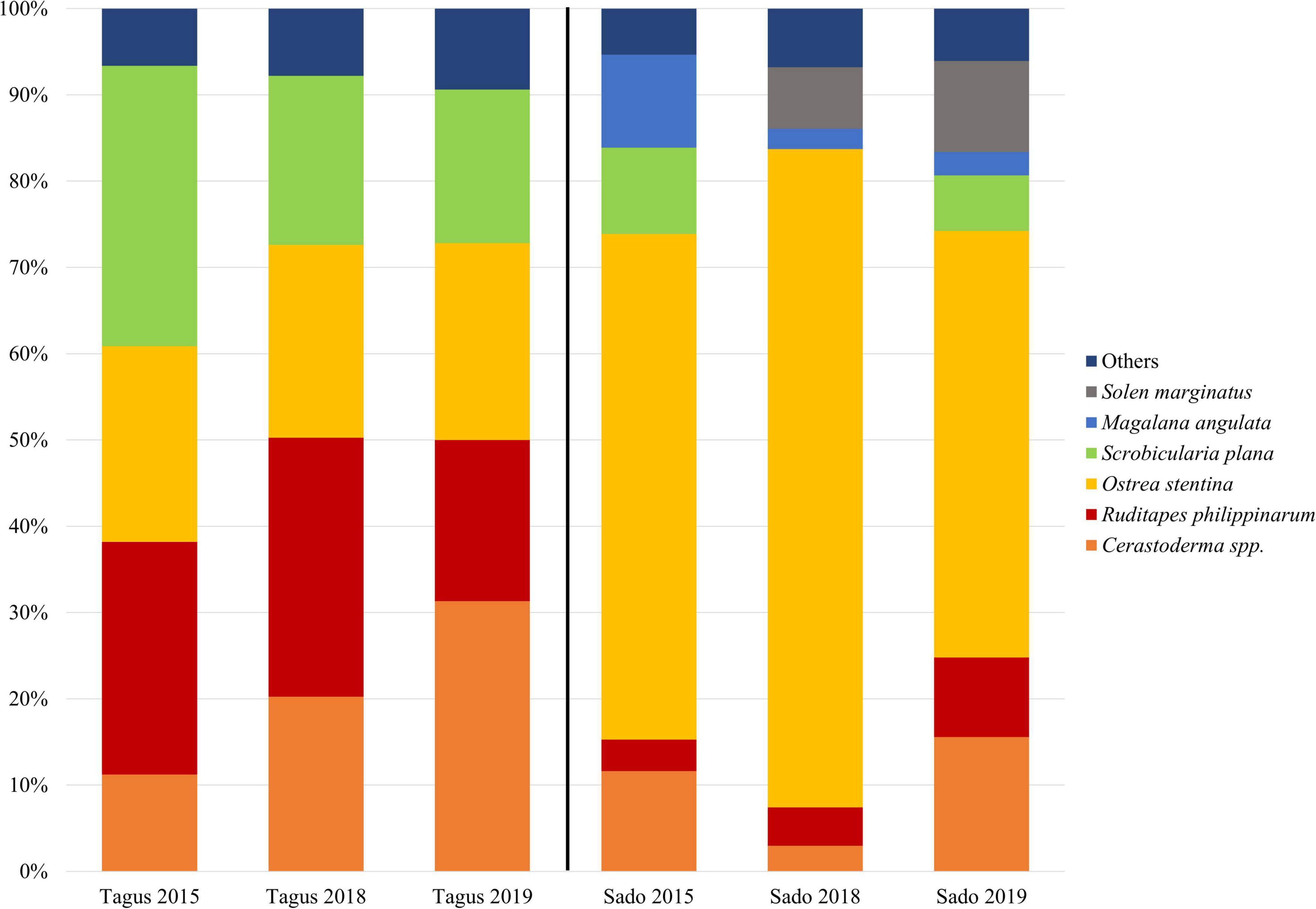
Figure 2. Relative abundance of the most representative bivalve species in the sampling campaigns conducted in the Tagus and Sado estuaries, in 2015, 2018, and 2019.
In the Tagus estuary, cockles were mostly found in the upstream and intermediate areas of the estuary and in the bays. However, cockles occur in greater abundance and more frequently in the upstream and bay areas over the 3 years. In the Sado estuary, the same pattern is observed. Cockle distribution was wider, occurring almost in the whole extent of the estuary. However, they occur in greater abundance in the upstream and bay area of the estuary (Figure 3).
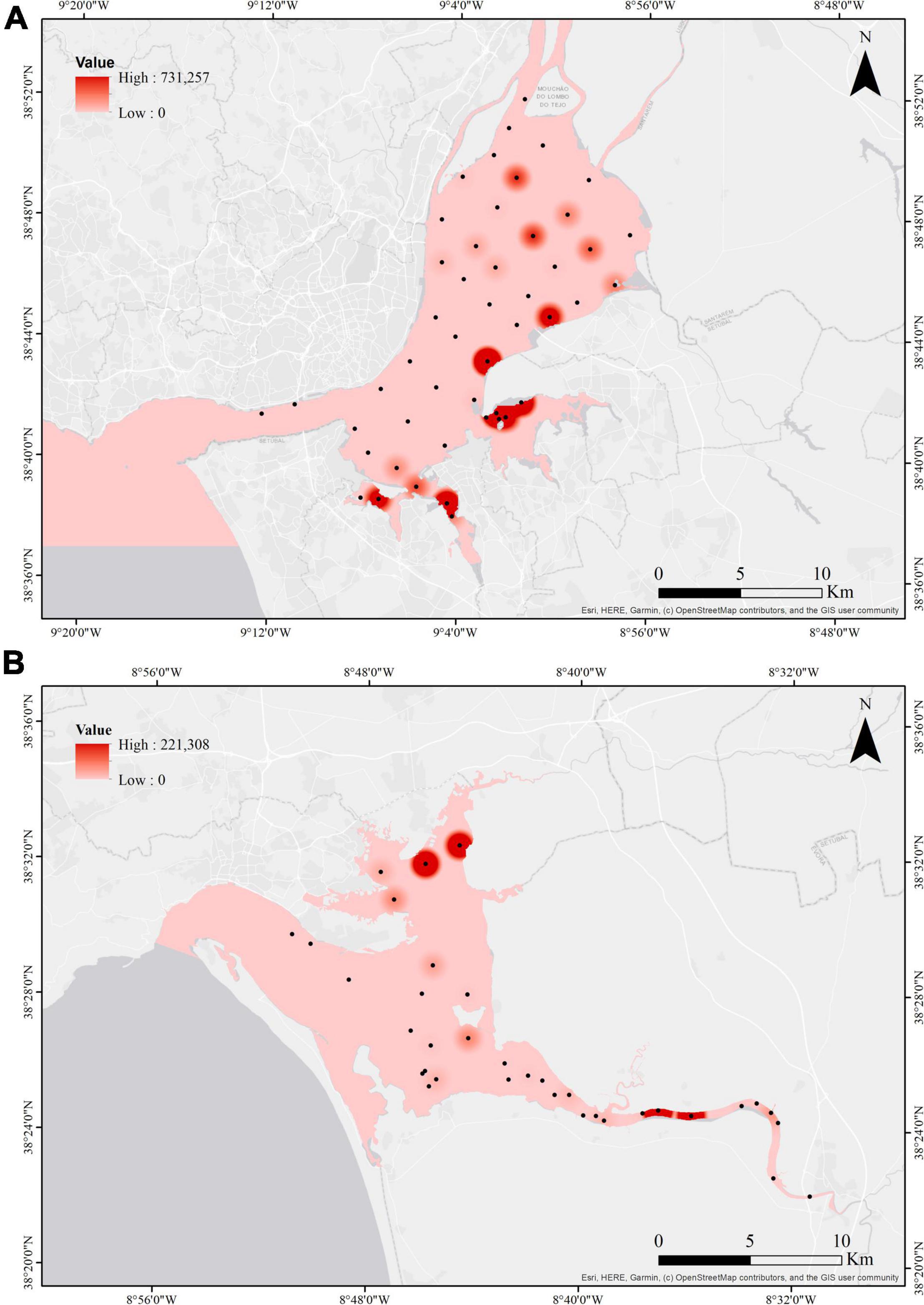
Figure 3. Illustrative map of cockle distribution and mean cockle abundance over the three years (2015, 2018, and 2019) at each sampling station in the Tagus (A) and Sado estuaries (B).
Additionally, it can be noted that in both estuaries cockles generally occur at the same sampling sites as the species Ruditapes philippinarum. In the Tagus estuary cockles are less abundant or do not occur at the sampling sites where Ostrea stentina and Scrobicularia plana are present. In the Sado estuary cockles are less abundant or do not occur at the sampling sites where Ostrea stentina, Magallana angulata, Scrobicularia plana, and Solen marginatus are present (Supplementary Tables 2, 3).
For the Tagus estuary during 2015, 2018, and 2019, cockle specimens accounted for 11%, 20%, and 31% of the total individuals caught, respectively. For the Sado estuary, during the years 2015, 2018, and 2019, cockle specimens represented 11%, 2%, and 15%, of the total number of individuals caught, respectively.
The frequency of occurrence of cockles was independent of the year, as determined by the results of the Pearson’s chi-square test of independence for Tagus estuary (p > 0,05) and for Sado estuary (p > 0,05), suggesting that the number of stations where they occurred over years has not changed significantly.
The PERMANOVA analysis indicates that significant differences in cockle abundance were found between estuaries, with higher abundances in the Tagus estuary, but not between years (Table 1). The correlation matrix between the factors selected as likely to integrate the explanatory model of cockle spatial distribution as a function of environmental factors demonstrated that there was no highly significant correlation (r > 0.80) between them, so all factors were kept in the initial model.
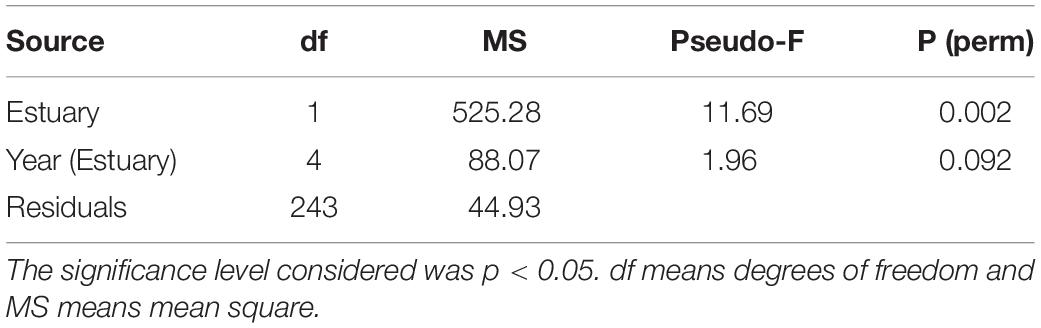
Table 1. Permutational Multivariate Analysis of Variance (PERMANOVA) analysis results on cockle abundance in the Tagus and Sado estuaries, in 2015, 2018, and 2019.
The Poisson count model revealed that cockle abundance is influenced by all the variables included in the initial model: depth, temperature, dissolved oxygen, salinity, sediment organic matter content, granulometry, average sediment grain size (phi), the categorical variable Estuary (Tagus and Sado) and the species Ruditapes philippinarum, Scrobicularia plana, Ostrea stentina, Magallana angulata, and Solen marginatus. Whereas the logit model included only three variables: depth, average sediment grain size (phi) and the species Ruditapes philippinarum (Table 2).
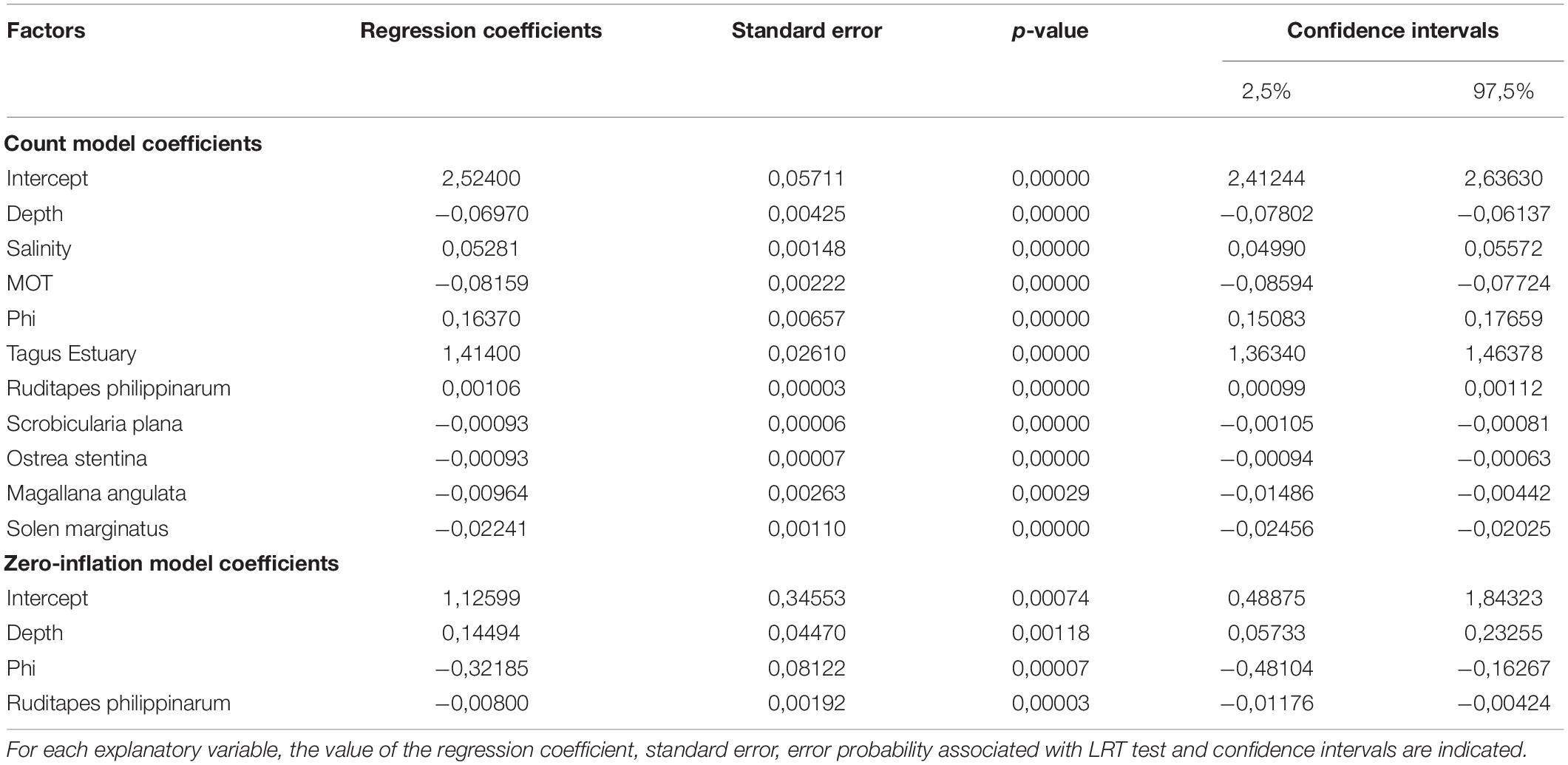
Table 2. Characterization of the explanatory environmental factors selected as integrating the final model explaining cockle distribution in the Tagus and Sado estuaries.
The Poisson count model indicated that there is a higher abundance of cockles when depth, temperature and sediment organic matter content is lower, when dissolved oxygen, salinity, average sediment grain size (phi) is higher and when it is the Tagus estuary. Regarding the other bivalve species, the model indicated that there is a higher cockle abundance in sites with higher abundance of the species Ruditapes philippinarum and lower abundance of the species Scrobicularia plana, Ostrea stentina, Magallana angulata, and Solen marginatus.
The logit model indicated a higher probability of cockles being absent in deeper areas of the estuaries, with lower phi values (i.e., when the sediment has a greater grain size) and in areas of the estuaries with lower abundance of Ruditapes philippinarum.
Population Structure and Cockle Fisheries
The PERMANOVA analysis showed that no significant differences in cockle shell lengths were found between estuaries. However, there were significant differences between the years for the Tagus estuary (Table 3), namely between the years of 2015 and 2018, and 2018 and 2019, with larger specimens collected in 2019.
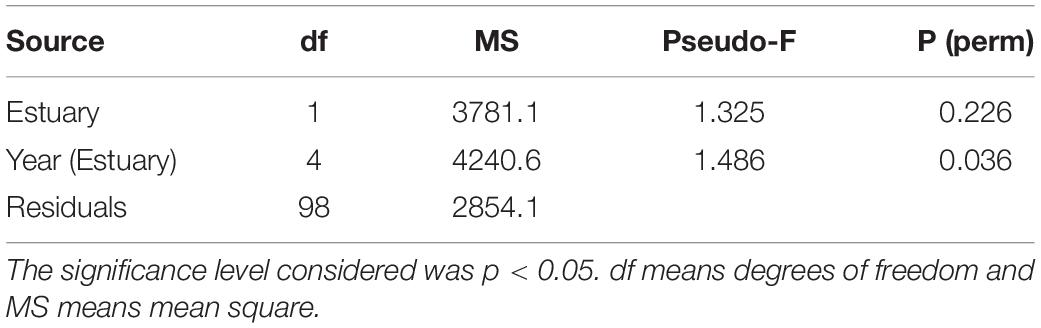
Table 3. Results of the PERMANOVA analysis comparing cockle shell lengths between years (fixed, 3 levels: 2015, 2018, and 2019) nested in estuaries (fixed, 2 levels: Tagus and Sado).
At the sampling sites located in the bays of both estuaries there are usually individuals with larger size, however at the sampling stations in the upper and intermediate zone of the estuaries cockle shell average length is quite variable over the years, so it’s not possible to observe a clear pattern (Supplementary Tables 4, 5). The cockle shell length distribution was determined in all years for both estuaries (Figure 4). For the Tagus estuary, shell length of captured individuals ranged from 9 mm to 33 mm and higher abundance was registered for lengths between 19 mm and 20 mm. For Sado estuary shell sizes varied between 11 mm and 39 mm with higher abundance between 20 mm and 23 mm. Most of the individuals caught (around 90% in both estuaries) were below the minimum catch size.
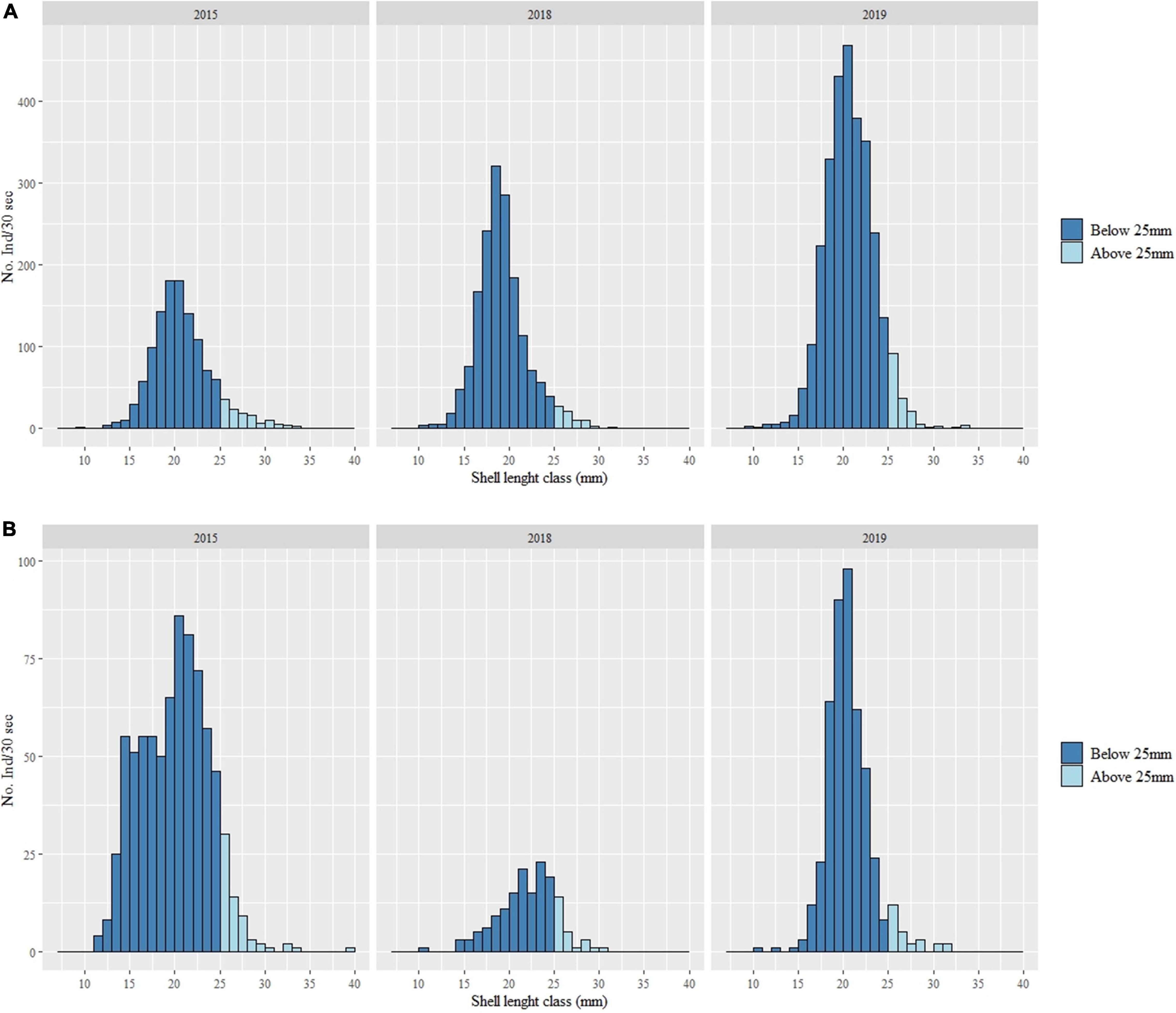
Figure 4. Shell length-frequency distribution of Cerastoderma spp. in the Tagus estuary (A) and Sado estuary (B), in the years 2015, 2018, and 2019. The dark blue bars represent the shell length classes below the minimum catch size (25 mm).
Discussion
Bivalve molluscs are an essential component of estuarine systems, namely in the Tagus and Sado estuaries. The Manila clam (Ruditapes philippinarum), a non-indigenous species, was one of the most abundant species in the Tagus and Sado estuaries. This species was first recorded in 2000 and 2010 in the Tagus and Sado estuaries, respectively, and its abundance has increased since then (Cabral et al., 2020). The dwarf oyster (Ostrea stentina) was the most abundant species in the Sado estuary over the years, being found mainly in the middle area of the system in stations with subtidal predominance, which was also observed by Lapégue et al. (2006) and Portela (2016). It is a species that inhabits the subtidal areas of estuaries and can be found mainly on rocky substrates or attached to other bivalve shells. This feature was supported by the present study as this oyster species was found mostly attached to shells of the Portuguese oyster (Magallana angulata), another oyster species present within this estuary.
Several physical and biological factors influence cockle distribution and abundance along the estuarine gradient. The factors that seem to have the most relevance in explaining cockle abundance (count model) were depth, temperature, dissolved oxygen, salinity, sediment organic matter content, average grain size (phi), Tagus estuary, Ruditapes philippinarum, Scrobicularia plana, Ostrea stentina, Magallana angulata, and Solen marginatus. On the other hand, the factors that seem to have the most relevance in explaining the probability of cockle absence (logit model) in both estuaries were depth, granulometry and the species Ruditapes philippinarum. Additionally, the analysis revealed that these species are more likely to occur in the Tagus estuary rather than in the Sado estuary.
Depth was one of the environmental factors identified as being relevant to explain the cockle spatial distribution and abundance in this study, being positively correlated with the probability of cockles absence, suggesting that this species doesn’t occur at deeper depths but rather in shallow depths and is negatively correlated with cockles abundance, which suggests that there is a higher abundance in shallow depths. This is similar to what was observed by Ysebaert et al. (2002) when developing predictive models of the response of several species of benthic macroinvertebrates (including the species Cerastoderma edule) as a function of environmental factors, who found that the probability of cockle occurrence increases with decreasing depth. In fact, cockle species show a higher growth rate when the depth is shallower, and are often found in shallow intertidal and subtidal estuarine areas (Burdon et al., 2014). Kater et al. (2006) also found that in the Scheldt estuary, one of the most determining environmental factors affecting cockle distribution was the emersion time, a measure inversely equated to depth, which means that the probability of cockles occurring rises with increasing emersion time.
Cockles are often found in substrates composed of sandy to muddy sediments (Soissons et al., 2019), in areas with low current velocities that promote juvenile settlement (Coosen et al., 1994; Burdon et al., 2014). In the explanatory model of cockle spatial distribution and abundance as a function of environmental factors and other bivalve species developed in the present study, cockle absence was found to be negatively correlated with mean sediment grain size (phi), i.e., when the value of phi is lower (more coarser sediment), the more likely the species is not present. In contrast, cockle abundance is positively correlated with mean sediment grain size (phi), which suggests that its abundance is higher in sandy-muddy sediments. Ysebaert et al. (2002) also found, in the models they developed, that the probability of cockles occurring is higher in sediments between 100 and 150 μm, which correspond to fine to very fine sand sediments.
Sandy sediments generally have lower organic matter and according to the model, cockles tend to occur in sites with lower sediment organic matter content. These results were also observed previously by Ysebaert et al. (2002) in the Schelde estuary.
Oxygen depletion can potentially cause mass mortalities in cockle populations (Burdon et al., 2014). In this study it is shown that dissolved oxygen is positively correlated with cockle abundance. Therefore, the higher the dissolved oxygen content in the water the more abundant the cockles will be. Temperature is negatively correlated with cockle abundance. There is a greater cockle abundance in sites with lower temperatures (in the temperature range measured in this study, 14–27°C). Cockles are able to tolerate a wide range of temperatures, however their survival is mainly affected by high temperatures (Malham et al., 2012; Verdelhos et al., 2015).
Cockle abundance is also influenced by salinity, being higher in sampling sites with higher salinity. Several studies have shown that cockle distribution is limited by salinities below 15 psu (Kater et al., 2006; Malham et al., 2012; Peteiro et al., 2018), which was also the case in this study, where only occasional presence in stations with salinity below this value was observed. Ysebaert et al. (2002) modeled the Western Scheldt cockle population and predicted cockles would thrive at salinities between 10‰ and 30‰, with the optimum at 25‰ (Kater et al., 2006).
Although cockle populations were found in both estuaries under study, Zero-inflated Poisson regression model analysis showed that cockle abundance is higher at Tagus estuary. This might be explained by the fact that this system has a larger area than the Sado estuary consisting of fine sand and mud sediments, conditions in which greater cockle abundance seems to occur (Soissons et al., 2019). Additionally, cockles were mostly found in the sheltered bays where hydrodynamic stress is usually lower, which may favor recruitment and larval settlement in these areas, and therefore a greater number of cockles (de Fouw et al., 2020).
Cockle distribution and abundance is also influenced by other bivalve species presence and abundance. Cockle abundance is positively correlated with manila clam (Ruditapes philippinarum) abundance, which suggests that its abundance is higher when it occurs where this species is also present and more abundant. Dias et al. (2019) demonstrated in their study in the Tagus estuary that cockles relied on the same food sources as R. philippinarum and that the trophic niche of R. philippinarum overlapped with Cerastoderma glaucum by 23%.
Conversely, cockle abundance is negatively correlated with the abundance of S, plana, O. stentina, M. angulata, and S. marginatus which suggests that the lowest abundances or absence of cockles are found in sites with higher abundances of these species.
Previous studies have shown that oysters are more common in habitats that are not suitable for cockles (Kater et al., 2006) which is in line with the results of this study. S. plana occurs in sites with higher amount of organic matter and more muddy sediments, while cockles occur mainly in sites with lower amount of organic matter and muddy-sandy sediments. S. marginatus occurs mainly in coarser sediments where cockles are generally not present. In general, the populations’ size and structure in both estuaries was dominated by commercially undersized cockles (minimum catch size = 25 mm), which reveals adequate recruitment in these two estuaries. However, there is a low proportion of individuals above the minimum catch size that can be harvested. This may be due to natural mortality events and/or the continuous removal of commercially sized cockles by intensive harvesting that may lead to resource overexploitation. However, the latter is less likely to have occurred because despite being one of the most abundant species in both estuaries (Cabral et al., 2020), cockle landings are low compared with other species, suggesting that professional fishing of this resource is not so relevant in these estuarine systems. Conversely, landings for the Ria de Aveiro and Ria Formosa are high compared to other species, which suggests that professional fishing for this resource might be more relevant in these systems. However, although professional fishing does not seem to have as much relevance in the Tagus and Sado estuary, it should be taken into account that an undetermined amount of cockle trade is made through a clandestine market, in which the catches are not officially declared at the fishing ports. Additionally, there is also an undetermined number of recreational harvesters, who exploit the most accessible cockle beds, and generally do not meet the criteria of minimum catch size and closed seasons, thus undermining the sustainability of the exploitation of this resource. Monitoring of these populations in these systems is therefore crucial.
The size of cockle populations varies significantly, mainly as a consequence of annual variability in reproductive success (Beukema and Cadée, 1996). In other places where cockles are exploited as a resource, populations have been declining. In Ria de Arousa, in Spain, this decrease seems to be mainly related to a disease caused by a parasite from the genus Marteilia (Villalba et al., 2014). On the other hand, in the Wadden Sea, this decrease seems to be mainly a result of low recruitment, which can be explained by an increase in predation on the species under study (Beukema and Dekker, 2005). In Portugal, in the Mondego estuary, overexploitation seems to have been the cause of the declining stocks (Crespo et al., 2010). The increase of the Manila clam populations can also be a threat to cockle populations. Manila clam is currently one of the dominant species at Tagus estuary (Cabral et al., 2020) and although it still has a relatively low occurrence in the Sado estuary, the spatial overlap with the cockle distribution area is high. A recent study conducted in the Tagus estuary using stable isotopes indicated that there is 23% overlap between the trophic niches of the Manilla clam and the Lagoon cockle, highlighting the high potential for trophic competition between these sympatric species (Dias et al., 2019; Soissons et al., 2019). Considering the challenges indicated above there would appear to be several causes that could trigger a decrease in cockle populations in both systems.
Cockles are known to have high temporal and spatial variability due to biotic and abiotic factors (Beukema et al., 2010; Peteiro et al., 2018). Additionally, global change is occurring, due to climate change and anthropogenic activities with consequent changes in environmental parameters, which reinforces the need for cockle population dynamics and their health to be monitored and used to guide appropriate and effective management measures to ensure future sustainable fisheries.
Data Availability Statement
The raw data supporting the conclusions of this article will be made available by the authors, without undue reservation.
Author Contributions
CS wrote the original draft of the manuscript. CS, JC, and PC conceptualized the study. CS, FC, JR, PC, AS, and SC conducted the data collection. CS, FC, GS, JM, PC, AS, SC, and TG conducted the laboratory procedures. CS and JM performed the statistical analysis. AB, JC, MG, and PC assisted in funding acquisition. All authors reviewed the manuscript, contributed to the article and approved the submitted version.
Funding
This publication was financed by Portuguese national funds through FCT–Fundação IP under project reference UIDB/04292/2020, and by the European Union’s Horizon 2020 Research and Innovation Programme under grant agreement N810139: Project Portugal Twinning for Innovation and Excellence in Marine Science and Earth Observation–PORTWIMS. This study had the funding support from the following projects: NIPOGES (MAR-01.03.02-FEAMP-0013); AQUASADO (MAR-02.01.01-FEAMP-0051); COCKLES (652 EAPA_458/2016); COASTNET (PINFRA/22128/2016). FCT, I.P. provided support via a researcher contract attributed to PC and Ph.D. grants attributed to FC (2020.06325. BD), SC (SFRH/BD/145746/2019) and TG (2020.05001. BD). AB and PC were funded by the Fundação para a Ciência e a Tecnologia (FCT), through the Scientific Employment Stimulus Programme (CEECIND/0095/2017 and 2020.01797.CEECIND).
Conflict of Interest
The authors declare that the research was conducted in the absence of any commercial or financial relationships that could be construed as a potential conflict of interest.
Publisher’s Note
All claims expressed in this article are solely those of the authors and do not necessarily represent those of their affiliated organizations, or those of the publisher, the editors and the reviewers. Any product that may be evaluated in this article, or claim that may be made by its manufacturer, is not guaranteed or endorsed by the publisher.
Acknowledgments
A special thanks to “Direção Geral de Recursos Naturais, Segurança e Recursos Marítimos (DGRM)” and to “Instituto da Conservação da Natureza e das Florestas (ICNF)” for their support during diferente stages of this study. We are also thankful to António Fernandes, Carla Mirra, Miguel Letra, Erica Sá, and Teresa Portela, who assisted us during field work.
Supplementary Material
The Supplementary Material for this article can be found online at: https://www.frontiersin.org/articles/10.3389/fmars.2022.699622/full#supplementary-material
References
Akaike, H. (1997). Information Theory and an Extension of the Maximum Likelihood Principle. Berlin: Springer.
Anderson, M. J. (2001). A new method for non-parametric multivariate analysis of variance. Austral Ecol. 26, 32–46. doi: 10.1046/j.1442-9993.2001.01070.x
Anderson, M., Gorley, R. N., and Clarke, K. R. (2008). PERMANOVA + for PRIMER: Guide to Software and Statistical Methods. Plymouth: PRIMER-E.
Barbier, E. B., Hacker, S. D., Kennedy, C., Koch, E. W., Stier, A. C., and Silliman, B. R. (2011). The value of estuarine and coastal ecosystem services. Ecol. Monogr. 81, 169–193. doi: 10.1890/10-1510.1
Bettencourt, A., Bricker, S. B., Ferreira, J. G., Franco, A., Marques, J. C., Melo, J. J., et al. (2003). Typology and Reference Conditions for Portuguese Transitional and Coastal Waters. Lisbon: Development of guidelines for the application of the European Union Water Framework Directive.
Beukema, J. J., and Cadée, G. C. (1996). Consequences of the sudden removal of nearly all mussels and cockles from the Dutch Wadden Sea. Mar. Ecol. 17, 279–289. doi: 10.1111/j.1439-0485.1996.tb00508.x
Beukema, J. J., and Dekker, R. (2005). Decline of recruitment success in cockles and other bivalves in the Wadden Sea: possible role of climate change, predation on postlarvae and fisheries. Mar. Ecol. Prog. Ser. 287, 149–167. doi: 10.3354/meps287149
Beukema, J. J., Dekker, R., and Philippart, C. J. M. (2010). Long-term variability in bivalve recruitment, mortality, andgrowth and their contribution to fluctuations in food stocksof shellfish-eating birds. Mar. Ecol. Prog. Ser. 414, 117–130. doi: 10.3354/meps08706
Biguino, B., Sousa, F., and Brito, A. C. (2021). Variability of currents and water column structure in a temperate estuarine system (Sado estuary. Portugal). Water (Switzerland) 13, 1–13. doi: 10.3390/w13020187
Bruguière, J. G. (1789–1792). Encyclopédie Méthodique ou par Ordre de Matières. Histoire Naturelle des vers, Volume 1. Paris: Pancoucke. Pp. i–xviii, 1–344 [Livraison 32, June 1789]; 345–757 [Livraison 48, 13 Feb. 1792] [Dates after Evenhuis, 2003, Zootaxa, 166: 37; Zootaxa, 207].
Burdon, D., Callaway, R., Elliott, M., Smith, T., and Wither, A. (2014). Mass mortalities in bivalve populations: a review of the edible cockle Cerastoderma edule (L.). Estuar. Coast. Shelf Sci. 150, 271–280. doi: 10.1016/j.ecss.2014.04.011
Cabeçadas, G., Nogueira, M., and Brogueira, M. J. (1999). Nutrient dynamics and productivity in three european estuaries. Mar. Pollut. Bull. 38, 1092–1096. doi: 10.1016/S0025-326X(99)00111-113
Cabral, H. N., Costa, M. J., and Salgado, J. P. (2001). Does the Tagus estuary fish community reflect environmental changes? Clim. Res. 18, 119–126. doi: 10.3354/cr018119
Cabral, S., Carvalho, F., Gaspar, M., Ramajal, J., Sá, E., Santos, C., et al. (2020). Non-indigenous species in soft-sediments: are some estuaries more invaded than others? Ecol. Indic. 110:105640. doi: 10.1016/j.ecolind.2019.105640
Caeiro, S., Costa, M. H., Ramos, T. B., Fernandes, F., Silveira, N., Coimbra, A., et al. (2005). Assessing heavy metal contamination in Sado Estuary sediment: an index analysis approach. Ecol. Indic. 5, 151–169. doi: 10.1016/j.ecolind.2005.02.001
Callaway, R., Burdon, D., Deasey, A., Mazik, K., and Elliott, M. (2013). The riddle of the sands: how population dynamics explains causes of high bivalve mortality. J. Appl. Ecol. 50, 1050–1059. doi: 10.1111/1365-2664.12114
Carss, D. N., Brito, A. C., Chainho, P., Ciutat, A., de Montaudouin, X., Fernández Otero, R. M., et al. (2020). Ecosystem services provided by a non-cultured shellfish species: the common cockle Cerastoderma edule. Mar. Environ. Res. 158:104931. doi: 10.1016/j.marenvres.2020.104931
Chainho, P., Chaves, M. L., Costa, J. L., Costa, M. J., and Dauer, D. M. (2008). Use of multimetric indices to classify estuaries with different hydromorphological characteristics and different levels of human pressure. Mar. Pollut. Bull. 56, 1128–1137. doi: 10.1016/j.marpolbul.2008.03.018
Clarke, K. R., and Gorley, R. N. (2006). Getting Started with PRIMER v6: User Manual/Tutorial. Plymouth: PRIMER-E.
Coosen, J., Twisk, F., van der Tol, M. W. M., Lambeck, R. H. D., van Stralen, M. R., and Meire, P. M. (1994). Variability in stock assessment of cockles (Cerastoderma edule L.) in the Oosterschelde (in 1980-1990), in relation to environmental factors. Hydrobiologia 28, 381–395. doi: 10.1007/BF00024643
Crespo, D., Verdelhos, T., Dolbeth, M., and Pardal, M. Â (2010). Effects of the over harvesting on an edible cockle (Cerastoderma edule Linaeus, 1758) population on a southern european estuary. Fresenius Environ. Bull. 19, 2801–2811.
Day, J. W., Hall, C. A. S., Kemp, W. M., and Yanez-Arancibia, A. (1988). Estuarine Ecology, 1st Edn. New York, NY: John Wiley and Sons.
de Fouw, J., van der Zee, E. M., van Gils, J. A., Eriksson, B. K., Weerman, E. J., Donadi, S., et al. (2020). The interactive role of predation, competition and habitat conditions in structuring an intertidal bivalve population. J. Exp. Mar. Bio. Ecol. 523:151267. doi: 10.1016/j.jembe.2019.151267
Dias, E., Chainho, P., Barrocas-Dias, C., and Adão, H. (2019). Food sources of the non-indigenous bivalve Ruditapes philippinarum (Adams and Reeve, 1850) and trophic niche overlap with native species. Aquat. Invasions 14, 638–655. doi: 10.3391/ai.2019.14.4.05
Dias, J. M., Valentim, J. M., and Sousa, M. C. (2013). A numerical study of local variations in tidal regime of Tagus estuary, Portugal. PLoS One 8:e80450. doi: 10.1371/journal.pone.0080450
Fernandes, M. R., Aguiar, F. C., Martins, M. J., Rivaes, R., and Ferreira, M. T. (2020). Long-term human-generated alterations of Tagus River: effects of hydrological regulation and land-use changes in distinct river zones. Catena 188:104466. doi: 10.1016/j.catena.2020.104466
Folk, R. L., and Ward, W. C. (1957). Brazos River bar [Texas]; a study in the significance of grain size parameters. J. Sediment. Res. 27, 3–26. doi: 10.1306/74D70646-2B21-11D7-8648000102C1865D
Fox, J. (2016). Polychoric and Polyserial Correlations. Available online at: https://r-forge.r-project.org/projects/polycor/ (accessed October 18, 2020).
França, S., Costa, M. J., and Cabral, H. N. (2009). Assessing habitat specific fish assemblages in estuaries along the Portuguese coast. Estuar. Coast. Shelf Sci. 83, 1–12. doi: 10.1016/j.ecss.2009.03.013
Freire, R., Arias, A., Méndez, J., and Insua, A. (2011). Identification of European commercial cockles (Cerastoderma edule and C. glaucum) by species-specific PCR amplification of the ribosomal DNA ITS region. Eur. Food Res. Technol. 232, 83–86. doi: 10.1007/s00217-010-1369-1365
Freitas, M. C., Andrade, C., Cruces, A., Munhá, J., Sousa, M. J., Moreira, S., et al. (2008). Anthropogenic influence in the Sado estuary (Portugal): a geochemical approach. J. Iber. Geol. 34, 271–286. doi: 10.5209/JIGE.33892
Freitas, R., Martins, R., Campino, B., Figueira, E., Soares, A. M. V. M., and Montaudouin, X. (2014). Trematode communities in cockles (Cerastoderma edule) of the Ria de Aveiro (Portugal): influence of inorganic contamination. Mar. Pollut. Bull. 82, 117–126. doi: 10.1016/j.marpolbul.2014.03.012
Gam, M., de Montaudouin, X., and Bazairi, H. (2010). Population dynamics and secondary production of the cockle Cerastoderma edule: a comparison between Merja Zerga (Moroccan Atlantic Coast) and Arcachon Bay (French Atlantic Coast). J. Sea Res. 63, 191–201. doi: 10.1016/j.seares.2010.01.003
Gaudêncio, M. J., and Cabral, H. N. (2007). Trophic structure of macrobenthos in the Tagus estuary and adjacent coastal shelf. Hydrobiologia 587, 241–251. doi: 10.1007/s10750-007-0686-686
ICNF (2007). Plano de Ordenamento e Gestão Para a Reserva Natural do Estuário do Sado. Alpharetta, GA: ICNF.
Iglesias, J. I. P., and Navarro, E. (1990). Shell growth of the cockle Cerastoderma edule in the mundaca estuary (North Spain). J. Molluscan Stud. 56, 229–238. doi: 10.1093/mollus/56.2.229
Kater, B. J., Geurts Van, Kessel, A. J. M., and Baars, J. J. M. D. (2006). Distribution of cockles cerastoderma edule in the eastern scheldt: habitat mapping with abiotic variables. Mar. Ecol. Prog. Ser. 318, 221–227. doi: 10.3354/meps318221
Lapégue, S., Ben Salah, I., Batista, F. M., Heurtebise, S., Neifar, L., and Boudry, P. (2006). Phylogeographic study of the dwarf oyster, Ostreola stentina, from Morocco, Portugal and Tunisia: evidence of a geographic disjunction with the closely related taxa, Ostrea aupouria and Ostreola equestris. Mar. Biol. 150, 103–110. doi: 10.1007/s00227-006-0333-1
Lillebø, A. I., Coelho, P. J., Pato, P., Válega, M., Margalho, R., Reis, M., et al. (2011). Assessment of mercury in water, sediments and biota of a southern European estuary (Sado Estuary, Portugal). Water. Air. Soil Pollut. 214, 667–680. doi: 10.1007/s11270-010-0457-452
Linnaeus, C. (1758). Systema Naturae per Regna tria Naturae, Secundum Classes, Ordines, Genera, Species, cum Characteribus, Differentiis, Synonymis, Locis, 10th revised Edn. Vol. 1. Holmiae: Laurentius Salvius, 824.
Luczak, C., Janquin, M. A., and Kupka, A. (1997). Simple standard procedure for the routine determination of organic matter in marine sediment. Hydrobiologia 345, 87–94. doi: 10.1023/A:1002902626798
Machado, M. M., and Costa, A. M. (1994). Enzymatic and morphological criteria for distinguishing between Cardium edule and C. glaucum of the Portuguese coast. Mar. Biol. 120, 535–544. doi: 10.1007/BF00350073
Magalhães, L., Correia, S., de Montaudouin, X., and Freitas, R. (2018). Spatio-temporal variation of trematode parasites community in Cerastoderma edule cockles from Ria de Aveiro (Portugal). Environ. Res. 164, 114–123. doi: 10.1016/j.envres.2018.02.018
Malham, S. K., Hutchinson, T. H., and Longshaw, M. (2012). A review of the biology of European cockles (Cerastoderma spp.). J. Mar. Biol. Assoc. United Kingdom 92, 1563–1577. doi: 10.1017/S0025315412000355
Mariani, S., Piccari, F., and De Matthaeis, E. (2002). Shell morphology in Cerastoderma spp. (Bivalvia: Cardiidae) and its significance for adaptation to tidal and non-tidal coastal habitats. J. Mar. Biol. Assoc. United Kingdom 82, 483–490. doi: 10.1017/S0025315402005751
Oliveira, J., Castilho, F., Cunha, Â, Pereira, M. J., Oliveira, J., and Castilho, F. (2013). Bivalve harvesting and production in portugal: an overview. J. Shellfish Res. 32, 911–924. doi: 10.2983/035.032.0334
Peteiro, L. G., Woodin, S. A., Wethey, D. S., Costas-Costas, D., Martínez-Casal, A., Olabarria, C., et al. (2018). Responses to salinity stress in bivalves: evidence of ontogenetic changes in energetic physiology on Cerastoderma edule. Sci. Rep. 8:8329. doi: 10.1038/s41598-018-26706-26709
Portela, T. (2016). Distribuição e Recrutamento da Ostra-portuguesa, Crassostrea angulata (Lamarck, 1819), no Estuário do Sado. Disseration Mestrado, Lisboa: Faculdade de Ciências da Universidade de Lisboa.
Ramón, M. (2003). Population dynamics and secondary production of the cockle Cerastoderma edule (L.) in a backbarrier tidal flat of the Wadden Sea. Sci. Mar. 67, 429–443. doi: 10.3989/scimar.2003.67n4429
Salgado, J. P., Cabral, H. N., and Costa, M. J. (2007). Spatial and temporal distribution patterns of the macrozoobenthos assemblage in the salt marshes of Tejo estuary (Portugal). Hydrobiologia 587, 225–239. doi: 10.1007/s10750-007-0685-687
Snelder, T. H., and Lamouroux, N. (2010). Co-variation of fish assemblages, flow regimes and other habitat factors in French rivers. Freshwater Biol. 55, 881–892. doi: 10.1111/j.1365-2427.2009.02320.x
Soissons, L. M., Gomes, da Conceiçâo, T., Bastiaan, J., van Dalen, J., Ysebaert, T., et al. (2019). Sandification vs. muddification of tidal flats by benthic organisms: a flume study. Estuar. Coast. Shelf Sci. 228:106355. doi: 10.1016/j.ecss.2019.106355
Tyler-Walters, H. (2007). “Cerastoderma edule common cockle,” in Marine Life Information Network: Biology and Sensitivity Key Information Reviews, eds H. Tyler-Walters and K. Hiscock (Plymouth: Marine Biological Association of the United Kingdom).
Vale, C., and Sundby, B. (1987). Suspended sediment fluctuations in the Tagus estuary on semi-diurnal and fortnightly time scales. Estuar. Coast. Shelf Sci. 25, 495–508. doi: 10.1016/0272-7714(87)90110-90117
Vaughn, C. C., and Hoellein, T. J. (2018). Bivalve impacts in freshwater and marine ecosystems. Annu. Rev. Ecol. Evol. Syst. 49, 183–208. doi: 10.1146/annurev-ecolsys-110617-162703
Verdelhos, T., Marques, J. C., and Anastácio, P. (2015). Behavioral and mortality responses of the bivalves Scrobicularia plana and Cerastoderma edule to temperature, as indicator of climate change’s potential impacts. Ecol. Indic. 58, 95–103. doi: 10.1016/j.ecolind.2015.05.042
Villalba, A., Iglesias, D., Ramilo, A., Darriba, S., Parada, J. M., No, E., et al. (2014). Cockle cerastoderma edule fishery collapse in the Ría de Arousa (Galicia, NW Spain) associated with the protistan parasite marteilia cochilli. Dis. Aquat. Organ. 109, 55–80. doi: 10.3354/dao02723
Ysebaert, T., Herman, P. M. J., Meire, P., Craeymeersch, J., Verbeek, H., and Heip, C. H. R. (2003). Large-scale spatial patterns in estuaries: Estuarine macrobenthic communities in the Schelde estuary, NW Europe. Estuar. Coast. Shelf Sci. 57, 335–355. doi: 10.1016/S0272-7714(02)00359-351
Ysebaert, T., Meire, P., Herman, P. M. J., and Verbeek, H. (2002). Macrobenthic species response surfaces along estuarine gradients: prediction by logistic regression. Mar. Ecol. Prog. Ser. 225, 79–95. doi: 10.3354/meps225079
Keywords: spatial distribution, environmental factors, population structure, Tagus estuary, Sado estuary, Portugal
Citation: Santos C, Cabral S, Carvalho F, Sousa A, Goulding T, Ramajal J, Medeiros JP, Silva G, Angélico MM, Gaspar MB, Brito AC, Costa JL and Chainho P (2022) Spatial and Temporal Variations of Cockle (Cerastoderma spp.) Populations in Two Portuguese Estuarine Systems With Low Directed Fishing Pressure. Front. Mar. Sci. 9:699622. doi: 10.3389/fmars.2022.699622
Received: 23 April 2021; Accepted: 15 February 2022;
Published: 30 March 2022.
Edited by:
Irene Martins, University of Porto, PortugalReviewed by:
Jenny R. Hillman, The University of Auckland, New ZealandRuth Callaway, Swansea University, United Kingdom
Copyright © 2022 Santos, Cabral, Carvalho, Sousa, Goulding, Ramajal, Medeiros, Silva, Angélico, Gaspar, Brito, Costa and Chainho. This is an open-access article distributed under the terms of the Creative Commons Attribution License (CC BY). The use, distribution or reproduction in other forums is permitted, provided the original author(s) and the copyright owner(s) are credited and that the original publication in this journal is cited, in accordance with accepted academic practice. No use, distribution or reproduction is permitted which does not comply with these terms.
*Correspondence: Cátia Santos, Y3NzYW50b3NAZmMudWwucHQ=